- Earth and Environment
- Literature and the Arts
- Philosophy and Religion
- Plants and Animals
- Science and Technology
- Social Sciences and the Law
- Sports and Everyday Life
- Additional References

- Biology and Genetics
- Cell Biology
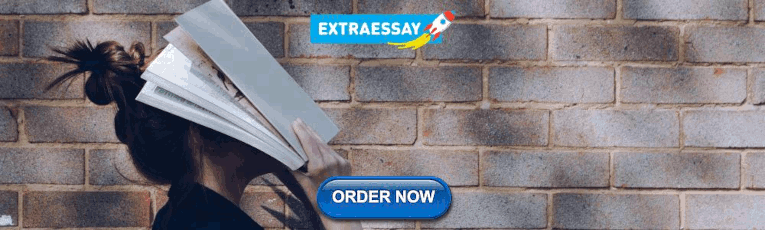
Signal Hypothesis
Signal hypothesis.
The signal hypothesis was proposed to explain how proteins that were destined for export from bacteria or for targeting to certain regions within eucaryotic microorganisms (e.g., yeast ) achieved their target. The hypothesis was proposed in the 1970s by G ü nter Blobel, who was then as now a molecular biologist at the Rockefeller University in New York . Blobel's work received the 1999 Nobel Prize in medicine or physiology.
The signal hypothesis proposes that proteins destined for secretion, which involves the movement of the protein across a biological membrane, are originally manufactured with an initial sequence of amino acids that may or may not present in the mature protein.
Work by Blobel and others over two decades established the validity of the proposal. The so-called signal sequence is now known to be only some 20 amino acids in length. The arrangement of amino acids in the signal sequence is not random. Rather, the beginning of the sequence, along with a few amino acid residues in the center of the sequence, is comprised of amino acids that are hydrophilic ("water-loving"). Sandwiched between these regions is a central portion that is made up of amino acids that are hydrophobic ("water-hating").
The hydrophilic beginning of the signal sequence, which emerges first as the protein is made, associates with the inner hydrophilic surface of the membrane. As the hydrophilic region of the protein merges, it burrows into the core of the membrane bilayer. The short hydrophilic stretch within the signal sequence anchors in the hydrophilic region on the opposite side of the membrane. Thus, the sequence provides an anchor for the continued extrusion of the emerging protein. In some proteins, the signal sequence can be enzymatically clipped off the remainder of the protein. Proteins of Gramnegative bacteria that are exported from the inside of the cell to the periplasmic space between the inner and outer membranes are examples of such processed proteins. Alternatively, the protein may remain anchored to the membrane via the embedded signal sequence.
The signal hypothesis has been demonstrated in plant cells, animal cells, single-celled eukaryotes (e.g., yeast), and in bacteria. The malfunction of the signal mechanism can be detrimental in all these systems. In contrast, the use of signal sequences has proven beneficial for the export of bio-engineered drugs from bacteria.
See also Bacterial membranes and cell wall; Prokaryotic membrane transport
Cite this article Pick a style below, and copy the text for your bibliography.
" Signal Hypothesis . " World of Microbiology and Immunology . . Encyclopedia.com. 18 Mar. 2024 < https://www.encyclopedia.com > .
"Signal Hypothesis ." World of Microbiology and Immunology . . Encyclopedia.com. (March 18, 2024). https://www.encyclopedia.com/science/encyclopedias-almanacs-transcripts-and-maps/signal-hypothesis
"Signal Hypothesis ." World of Microbiology and Immunology . . Retrieved March 18, 2024 from Encyclopedia.com: https://www.encyclopedia.com/science/encyclopedias-almanacs-transcripts-and-maps/signal-hypothesis
Citation styles
Encyclopedia.com gives you the ability to cite reference entries and articles according to common styles from the Modern Language Association (MLA), The Chicago Manual of Style, and the American Psychological Association (APA).
Within the “Cite this article” tool, pick a style to see how all available information looks when formatted according to that style. Then, copy and paste the text into your bibliography or works cited list.
Because each style has its own formatting nuances that evolve over time and not all information is available for every reference entry or article, Encyclopedia.com cannot guarantee each citation it generates. Therefore, it’s best to use Encyclopedia.com citations as a starting point before checking the style against your school or publication’s requirements and the most-recent information available at these sites:
Modern Language Association
http://www.mla.org/style
The Chicago Manual of Style
http://www.chicagomanualofstyle.org/tools_citationguide.html
American Psychological Association
http://apastyle.apa.org/
- Most online reference entries and articles do not have page numbers. Therefore, that information is unavailable for most Encyclopedia.com content. However, the date of retrieval is often important. Refer to each style’s convention regarding the best way to format page numbers and retrieval dates.
- In addition to the MLA, Chicago, and APA styles, your school, university, publication, or institution may have its own requirements for citations. Therefore, be sure to refer to those guidelines when editing your bibliography or works cited list.
signal hypothesis
" signal hypothesis . " A Dictionary of Biology . . Encyclopedia.com. 18 Mar. 2024 < https://www.encyclopedia.com > .
"signal hypothesis ." A Dictionary of Biology . . Encyclopedia.com. (March 18, 2024). https://www.encyclopedia.com/science/dictionaries-thesauruses-pictures-and-press-releases/signal-hypothesis
"signal hypothesis ." A Dictionary of Biology . . Retrieved March 18, 2024 from Encyclopedia.com: https://www.encyclopedia.com/science/dictionaries-thesauruses-pictures-and-press-releases/signal-hypothesis
More From encyclopedia.com
About this article, you might also like.
- nucleoproteins
- Membrane Proteins
- oxyntic cells
- Cell, Eukaryotic
- RECEPTOR: NMDA (N-METHYL D-ASPARTIC ACID)
- Protein Export
- oxyntic cell
NEARBY TERMS
C2006/F2402 '07 OUTLINE OF LECTURE #7
(c) 2007 Dr. Deborah Mowshowitz, Columbia University, New York, NY . Last update 02/06/2007 07:26 PM
Handouts: 6B -- Signal Hypothesis -- Co-translational Import ; 7A = How proteins insert into ER membrane 7B = Golgi & Lysosomes; not on web.
For a nice video (& explanation) of a pulse chase experiment, try this link: http://www.sumanasinc.com/webcontent/anisamples/majorsbiology/pulsechase/pulsechase.html
I. ER -- How does Co-translational Import Work?
A. Signal hypothesis -- How ribosomes get to the ER & Protein enters ER -- Handout 6B . Steps below refer to handout. See Becker fig. 22-16 (20-16) or Purves 12.15
1. What is the Signal Hypothesis? Ribosome unattached to ER starts making protein. (step 1) If nascent (growing, incomplete) peptide has a "signal peptide," then ribosome plus growing chain will attach to ER membrane, and growing chain will enter ER as it grows. 2. How does ribosome get to the ER? a. Signal peptide (SP) = section of growing peptide (usually on amino end) binds SRP. (step 2) b. SRP = s ignal r ecognition p article Role: temporarily blocks translation; ferries nascent protein to ER. Structure: SRP contains proteins + RNA = Large particle containing both ribonucleic acid & protein like a ribosome or spliceosome . Called an 'RNP' for r ibo n ucleoprotein p article. c. SRP receptor on ER = docking protein. Binds SRP. (step 3). Translocon (gated pore or channel through membrane) is still closed.
3. How does growing chain enter the ER?
a . Ribosome/translocon complex formation occurs. (step 4) SRP is released, recycles -- GTP split Ribosome binds to pore/translocon Translocon opens & peptide enters (as loop). Ribosome resumes translation. b. Translation (and movement through translocon) continues (step 5) Handout shows how a soluble protein ends up inside the lumen of the ER. How a transmembrane protein -- single pass or multipass -- ends up inserted in the ER membrane is explained below. c. Translation (and translocation) are completed, translocon closes. (step 6) e. Signal Peptidase (usually) cuts off signal peptide at arrow. (step 7) Protein is released into lumen of ER Ribosome and mRNA are released
Now try problem 3-4, especially part D. (If some of the parts are not obvious, wait until later.)
B. How do proteins cross or enter the ER membrane? (See handout 7A, bottom of 6B , and/or fig. 22-17 (20-17) of Becker)
1 . How proteins enter/pass through the membrane -- important points a. ER membrane contains gated pore (also called channel or translocon) for the growing chain. Note on terminology: The term "translocon" is used in (at least) 2 different ways. Sometimes used to mean only the channel itself, and sometimes it is used to mean the whole complex of proteins required for translocation of proteins across the membrane -- the channel, SRP receptor, etc. It should be clear from context which usage is meant at any one time.
b. SP probably forms loop not arrow. Loop enters channel (translocon) in membrane. SP loop is probably what opens (gates) the channel on the cytoplasmic side. c. Growing protein chain passes through channel as chain is synthesized. (Co-translational import.) d. Where will protein end up? Protein can go all the way through the membrane and end up as a soluble protein in the lumen (as in example above) or protein can go part way through and end up as a transmembrane protein (see below). e. How do transmembrane proteins get anchored in the membrane? A hydrophobic sequence may trigger opening of the pore sideways, so protein slides out of pore, laterally, into lipid bilayer. These hydrophobic sequences are called 'stop-transfer' sequences and/or 'anchor' sequences.
2 . Types of Proteins that can result (see handout 7A)
a. Soluble protein in lumen . Happens if protein passes all the way through the membrane and SP (on amino end) is removed, as above. b. Integral membrane protein anchored in membrane by SP with no cytoplasmic domain. This happens if SP is on the amino end and is not removed. c. Single Pass transmembrane protein -- get one of 2 possibilities: (1). Type 1: Amino end is on lumen side of membrane (on E side); Carboxyl end is in cytoplasm (on P side of membrane) One way this could happen: If SP is on amino end, and SP removed, and there is a hydrophobic sequence (acting as a stop-transfer sequence) in the middle of the peptide. (2). Type 2: Carboxyl end is on lumen side of membrane (on E side); Amino end is in cytoplasm (on P side) One way this could happen: If SP is in the middle, not on amino end. SP in this case is not removed -- it becomes the transmembrane domain of the protein. (SP doubles as stop-transfer sequence.)
d. Multipass transmembrane protein. (Requires one SP and several hydrophobic (start/stop) sequences.
(1). Hydrophobic sequences can stop the process (of moving through pore) and anchor protein in membrane, as explained above. (2). Hydrophobic sequence s in the middle of the peptide can restart looping → multipass protein. These are usually called "start-transfer" sequences. (3). 'Start-transfer' and 'stop-transfer' sequences are probably equivalent. Role depends on where in protein they occur.
(4). Every SP is also a "start transfer sequence" but not every start/stop sequence is necessarily an SP. To act as an SP, a sequence must bind to the SRP. If a hydrophobic sequence does not bind the SRP, it will not direct ribosomes to the ER.
e. Lipid Anchored Proteins: Proteins to be anchored to lipids on the outside of the plasma membrane are generally made as follows: Protein is made on RER and inserted into the ER membrane. After the protein reaches the plasma membrane, the extracellular domain is detached from the rest of the protein and attached to lipid. (Proteins to be anchored to the plasma membrane on the inside are made on cytoplasmic ribosomes.) See Becker if you are curious about the details.
Now finish problem 3-1 & try 3-2, A -C. To test yourself on structure/insertion of integral membrane proteins, try problem 3-3.
II. What Else Happens in/on the ER?
A . What happens inside ER
1. Terminal SP usually removed by signal peptidase (enzyme) inside ER. 2. Folding of protein -- requires chaperones. a. Chaperones (also called chaperonins) -- proteins needed to assist in protein folding. Chaperones are used every time a protein remains unfolded or becomes unfolded to cross a membrane (or refolds on the other side). Two or more types of chaperones are involved in protein folding-- different ones are found in different parts of the cell. b. Chaperones are of two major types (families) -- HSP 60 (forms barrel) or HSP 70 (binds to hydrophobic regions). Differ in molecular weight (60 K vs 70 K) and mode of action. (See an advanced text if you are curious about the mechanisms.) c. The major chaperone inside the ER is a member of the HSP 70 family, also called "BiP" d. Often called HSP = heat shock proteins, because these proteins are made in large amounts after exposure to high temperatures. (That's how they were first discovered.)
3. Enzymatic Modifications. The appropriate enzymes inside the ER catalyze the following:
a. Making of S-S bonds . In eukaryotes, all S-S bonds are formed in proteins inside the ER. Proteins made in the cytoplasm do not have S-S bonds. Cytoplasmic proteins do contain cysteines and have free SH groups. b. Start of N-glycosylation (oligosaccacharides are added to the N of the amide of asparagine side chains -- called N glycosylation.) See Becker fig. 12-7 if you are curious about the biochemical details.
4. Some proteins stay in ER (in lumen or membrane); most move on to Golgi.
5. What happens to proteins in ER that do not fold properly? See Becker p. 755-757 (733-734).
a. Transport to cytosol -- Unfolded proteins are transported back to the cytosol (through the translocon -- mechanism unknown). b. Ubiquitin addition -- in cytosol, proteins to be degraded are marked for destruction by addition of a multiple molecules of a small protein called ubiquitin to side chains of lysine. (See Becker or advanced texts if you are interested in the enzymatic details.) c. Role of Proteasome = a large protein complex in cytosol that degrades ubiquitinylated proteins to fragments, at expense of ATP. Major site of degradation of intracellular proteins. (Proteins from outside are generally degraded in lysosomes.) d. What goes to the proteasome? Proteins that are misfolded, damaged, or have served their function. A major proportion of all proteins made in cell do not fold properly and are degraded. Destruction of many proteins is regulated -- level of protein activity can be controlled by protein degradation as well as by rate of synthesis, feed back of activity, modification, etc. More details and/or examples to follow. e. 2 004 Nobel Prize for Chemistry was awarded to Aaron Ciechanover , Avram Hershko and Irwin Rose for the discovery of the ubiquitin/proteasome system.
B. What happens on outside of ER (besides protein synth esis )
1. Lipid synthesis -- a. Insertion: Lipids made and inserted on cytoplasmic side of membrane by enzymes attached to/in membrane. b. Flipping: Enzymes ('flippases') required to move amphipathic lipids from one leaflet (P side) of membrane to other leaflet (E side). c. Transport: Lipids can reach parts of cell not connected to ER through vesicles and/or transport (exchange) prot eins.
2. Some detoxifications and other reactions are catalyzed by proteins on the cyto side of ER. See text for details if interested.
To review the structure and function of the ER, try problem 3-4.
III. Golgi Complex -- Structure & Function
A. How things get there -- from ER in coated vesicles ( coat made of protein called coatomer or COP instead of clathrin). For animation of how materials pass from ER to and through Golgi click here.
B. Structure & Overall Traffic Flow - S ee Purves, fig 4.12 or Becker fig. 12-4 & 12-8, and handout 7B.
1. Two sides of stack a. cis /forming face (side closest to nucleus & ER) b. trans /maturing face (away from nucleus)
2. Three basic parts or compartments in a stack
a. CGN (cis-Golgi network) or cis Golgi b. medial cisternae (sacs) -- part in between 'cis' and 'trans' Golgi c. TGN (trans-Golgi network) or trans Golgi
3 . Different marker enzymes/functions found in different parts. (See Becker figs 12-5 & 12-6) Enzymes unique to any one cell organelle or compartment are called 'marker enzymes' = their presence is a 'marker' for the presence of that compartment or organelle.
4. Sacs in stack connected by vesicle traffic -- not completely clear which way transport vesicles go or what they carry. It is clear that newly made protein and lipid passes through the Golgi from the cis face to the trans face, and that vesicles from one stack can fuse with another in vitro . Models for transport through the Golgi are discussed in more detail later.
C. Function -- what reactions take place inside Golgi?
1. Finish N glycosylation -- oligosacch. that was added to glycoproteins in ER is modified. Oligosacch. is attached to "N" of amide side chains of asparagines (asn's). 2. Do O glycosylation of glycoproteins. Sugars are added to "O" of the hydroxyl of the side chain of ser & thr . 3. Assemble sugars of proteoglycans (linear chains of repeating sequence = GAGs) 4. Concentrate, sort proteins . This occurs at trans face (TGN). Different areas of Golgi have receptors that trap proteins going to different destinations.
To review how proteins are directed to the right place and modified in the ER and Golgi, try problem 3-2.
IV. T ransport Through the Golgi -- Are cisternae stationary or do they progress? A. Background
1. Transport vesicles can bud off one sac (cisterna) of the Golgi and fuse with another. However it is not completely clear which way the transport vesicles go or what they carry. a. Direction of vesicle traffic: is it cis to trans = forward = anterograde, or vice versa = retrograde? (Older models assumed it was forward, but current evidence indicates it is both -- some vesicles go in one direction and some the other, as shown in Becker fig. 12-8.) b. What do the vesicles carry? 1. Cargo proteins -- Newly made proteins from the ER, and/or 2. Modification Enzymes -- Used in the Golgi to modify the newly made cargo proteins
2. Net Direction of Transport. It is clear that newly made protein and lipid pass through the Golgi from the cis face to the trans face, as shown on this animation.
3. Vesicles from one stack can fuse with cisternae of another stack in vitro . (See problem 3-10.)
B. Models
1. "Vesicle Transport Model" or Stationary Cisternae Model a. Transport vesicles move primarily forward (anterograde) -- towards trans face. For an animation of this process, see here. b. Vesicles carry cargo -- vesicles carry newly made proteins from one part of Golgi to next part for additional modifications. c. Sacs of Golgi (& their characteristic enzymes) stay put -- newly made proteins (cargo) pass from sac to sac by means of vesicles. d. Enzyme composition of each sac stays the same . Each part stays in the same place and holds on to its characteristic ('marker') enzymes. It is the substrates of the enzymes (the newly made cargo proteins) that pass through, carried by the vesicles from sac to sac.
2. "Cisternal Maturation Model" = a modified Progression Model
a. Transport vesicles move primarily retrograde -- towards cis face. b. Vesicles carry enzymes to modify and sort proteins. They do not carry the newly made proteins from the ER. c. Sacs of Golgi move , carrying newly made proteins & lipids inside. New sacs are constantly formed at the cis face from material transported from the ER. Old sacs are lost from the trans face as they age. d. Enzyme composition of each sac changes with time. The enzyme composition of each individual sac is constantly changing as it ages and passes from cis to trans face. However the characteristic enzymes found in the sacs at each position of the Golgi (cis, medial & trans) remain the same, because the enzymes are "passed back." The vesicles retrieve the enzymes of "older" sacs (closer to the trans face) and carry them back to newer sacs (closer to the cis face).
3. Connection Model -- another possibility is that the Golgi sacs are actually connected (although they seem to be separate), and that proteins move back and forth from one sac to the next, although net bulk flow of newly made proteins is from cis to trans . There isn't much evidence or enthusiasm for this model, but some recent data using genetically modified protein tagged with GFP has been interpreted in support of this model.
4. What really happens? The models above are not mutually exclusive, so a hybrid model is possible. Only new experiments generating new data will settle the question of how material actually moves. Not all materials may move through the Golgi in the same way. For a review of the models and the data as of 1998 (the 100th anniversary of the discovery of the Golgi) see Coming to Grips with the Golgi .
To review traffic through the Golgi, try problem 3-10.
V. Lysosomes -- an example of sorting at end of the Golgi
A. How proteins get to lysosomes (See Becker fig. 12-9) -- Normal Pathway (steps refer to numbers on handout 7B)
1. How do hydrolases reach the Golgi? a. Enzyme synthesis: Hydrolases are made on ribosomes bound to ER. Enzymes enter ER co-translationally (as they are made). b. Transport to Golgi: Hydrolases are transported in vesicles to Golgi. Steps 1 & 2 .
2 . How are hydrolases identified & tagged for transport to lysosomes?
a. Localization Signal -- Most hydrolases destined for lysosomes have a special sequence/patch. b. Reading the LS -- Enzyme(s) recognize the sequence/patch and add Mannose-6-P (N-glycosylation starts in ER by addition of standard oligosaccharide. Modification of standard sugars to M6P occurs in Golgi -- this modification only happens to proteins with the proper amino acid sequence = soluble hydrolases bound for lysosomes) Step 3.
3. Role of M6P Receptor -- how is the tag recognized?
a. Binding the M6P -- Receptor in special part of trans Golgi binds proteins with M6P. Step 4. b. Sorting in trans Golgi -- Proteins with M6P and their receptors accumulate in coated pits ( step 5 ) and bud off ( step 6 ) and go to a sorting vesicle/endosome ( step 7 ).
4. Sorting after the Golgi
a. Sorting vesicle/Endosome sorts multiple types of receptors and hydrolases. Same as what happens during RME when receptors and ligands are sorted. ( step 7 ) b. Recycling: M6P Receptors recycle back to Golgi ( steps 8A & 10) ; vesicles with hydrolases add to old lysosome or form new one ( steps 8B & 9 ). Note that 8A & 8B are equivalent to the same numbers on the handout of RME. 8B goes to the lysosome and 8A recycles back to the membrane from which it came. c. SNAREs. How do various vesicles fuse when they reach the proper target? There are matching transmembrane proteins (SNAREs) on the target membrane and the vesicle membrane. The cytoplasmic domains of the proteins are complementary, and pair up with each other. See SNARE hypothesis in Becker, p. 348-349 (352-353), if you are interested in more details.
B. Lysosomal diseases -- next time -- what if hydrolases are not made and/or do not reach the lysosome?
Next Time: Wrap up of lysosomal diseases -- Can you treat any lysosomal storage diseases? How do enzymes get to peroxisomes, nuclei and mitochondria?
- Subscriber Services
- For Authors
- Publications
- Archaeology
- Art & Architecture
- Bilingual dictionaries
- Classical studies
- Encyclopedias
- English Dictionaries and Thesauri
- Language reference
- Linguistics
- Media studies
- Medicine and health
- Names studies
- Performing arts
- Science and technology
- Social sciences
- Society and culture
- Overview Pages
- Subject Reference
- English Dictionaries
- Bilingual Dictionaries
Recently viewed (0)
- Save Search
- Share This Facebook LinkedIn Twitter
Related Content
Related overviews.
agency relationship
asymmetric information
agency problem
'signalling hypothesis' can also refer to...
signal hypothesis
More Like This
Show all results sharing these subjects:
- Business and Management
signalling hypothesis
Quick reference.
The idea that many actions taken by economic agents are motivated chiefly by the wish to send a positive ‘signal’ to other agents, rather than by their ostensible purpose. This can be a means of overcoming the problem of asymmetric information between transactors. For example, a business may choose to spend an ostentatious amount of money on advertising a new product – not because this is the most cost-effective form of publicity, but because a large outlay by the company signals its faith in the product to investors, distributors, and other stakeholders. Similarly, a generous dividend policy can be a means by which management signals its positive view of the firm's position and future prospects to the financial markets. In this case, such signalling is a means of overcoming the mismatch between the information available to managers and that available to shareholders. See also agency relationship.
From: signalling hypothesis in A Dictionary of Business and Management »
Subjects: Social sciences — Business and Management
Related content in Oxford Reference
Reference entries.
View all related items in Oxford Reference »
Search for: 'signalling hypothesis' in Oxford Reference »
- Oxford University Press
PRINTED FROM OXFORD REFERENCE (www.oxfordreference.com). (c) Copyright Oxford University Press, 2023. All Rights Reserved. Under the terms of the licence agreement, an individual user may print out a PDF of a single entry from a reference work in OR for personal use (for details see Privacy Policy and Legal Notice ).
date: 23 March 2024
- Cookie Policy
- Privacy Policy
- Legal Notice
- Accessibility
- [66.249.64.20|162.248.224.4]
- 162.248.224.4
Character limit 500 /500
Advertisement
Lost in translation : the signal hypothesis
- Standard View
- Article contents
- Figures & tables
- Supplementary Data
- Peer Review
- Open the PDF for in another window
- Get Permissions
- Cite Icon Cite
- Search Site
Mitch Leslie; Lost in translation : the signal hypothesis . J Cell Biol 1 August 2005; 170 (3): 338. doi: https://doi.org/10.1083/jcb1703fta1
Download citation file:
- Ris (Zotero)
- Reference Manager
It was cell biology's version of the ship in the bottle. How do proteins a cell intends to secrete end up in the endoplasmic reticulum? Winkling out the details of the translocation mechanism that spirits these proteins into the ER required more than 20 years and earned Günter Blobel of Rockefeller University the 1999 Nobel Prize in Physiology or Medicine.
Another Rockefeller laureate, George Palade, had demonstrated that ribosomes free in the cytoplasm manufactured nonsecreted proteins, whereas ribosomes stuck to the ER made proteins for export. Cell biologists searched in vain for distinctions between free and attached ribosomes that might explain their contrasting behavior. A new assistant professor at Rockefeller and Palade's protege, Blobel suspected that the difference must lie in the proteins themselves. He and colleague David Sabatini conjectured that secretory proteins might carry a short segment near the NH 2 terminus (Blobel and Sabatini, 1971). Once this sequence protruded from the ribosome during translation, a “binding factor” would hook onto the protein and guide it and the ribosome to the ER membrane. Continued translation would then thread the elongating protein into the ER's interior. “It was a beautiful idea,” says Blobel. It was also, he admits, “pure speculation.”

The signal hypothesis in 1975, with the signal peptide as a dotted line.
Unaware of Blobel and Sabatini's hypothesis, Cesar Milstein of Cambridge University proposed a similar idea based on his team's cell-free system. It also pumped out an overweight light chain, but when the researchers checked the output of microsomes (ER fragments), they found only the normal-sized protein (Milstein et al., 1972). Milstein speculated that the extra amino acids help direct the growing protein to the ER.
Despite this suggestive data, detractors argued that the protein's extra heft was an artifact of in vitro translation or isolation errors, Blobel recalls. To answer their complaints, he crafted a protein-synthesizing system with help from post-doc Bernhard Dobberstein (now at the University of Heidelberg). Using detergent, they dislodged ribosomes from rough microsomes, and then slipped the particles—which carried unfinished light chains—into a solution that allowed protein making to resume. Because the researchers also added a compound that blocks new translation, the ribosomes could only complete chains they had started.
At first, only the smaller, processed chain appeared (Blobel and Dobberstein, 1975a). These proteins came from ribosomes that were well into translation when they parted from microsomes, the researchers concluded, and the chains they held had already undergone pruning to remove the signal sequence. After a few minutes, however, the synthesis mixture started producing longer chains as well. The bulkier proteins emerged from ribosomes that had just started translating when isolated from microsomes. At the time, they bore stubby chains that hadn't yet shed their signal sequence. When translation restarted, these short chains didn't lose the sequence—evidence that the processing enzyme that removes the signal is part of the ER membrane.
In another key experiment, Blobel and Dobberstein let rough microsomes—which carry ribosomes and some associated mRNA—produce proteins. The scientists detected only the shorter version. Adding the protein-dissolving enzymes trypsin and chymotrypsin (which rarely enter the microsomes) did not digest most of the chains, confirming that the trimmed protein ends up tucked away within the microsomes, as the signal hypothesis predicted.

Ribosomes severed from microsomes make first a smaller, processed protein (left) and later a longer form with signal sequence intact (upper band on right).
Blobel, G., and B. Dobberstein. 1975 a. J. Cell Biol. 67 : 835 –851.
Blobel, G., and B. Dobberstein. 1975 b. J. Cell Biol. 67 : 852 –862.
Blobel, G., and D.D. Sabatini. 1971. In Biomembranes. L.A. Manson, ed. 2:193–195.
Milstein, C., et al. 1972 . Nat. New Biol. 239 : 117 –120.
Schechter, I. 1973 . Proc. Natl. Acad. Sci. USA. 70 : 2256 –2260.
Swan, D., et al. 1972 . Proc. Natl. Acad. Sci. USA. 69 : 1967 –1971.
Tonegawa, S., and I. Baldi. 1973 . Biochem. Biophys. Res. Commun. 51 : 81 –87.
Data & Figures
- Previous Article
- Next Article
Supplements
Related & metrics, suggested content, email alerts, affiliations.
- Newest Articles
- Current Issue
- Email Alerts
- Submit a Manuscript
- Instructions for Authors
- For Librarians
- Editors & Staff
- Policies & Permissions
- Accessibility Statement
- Privacy Policy
- Online ISSN 1540-8140
- Print ISSN 0021-9525
This Feature Is Available To Subscribers Only
Sign In or Create an Account

An official website of the United States government
The .gov means it’s official. Federal government websites often end in .gov or .mil. Before sharing sensitive information, make sure you’re on a federal government site.
The site is secure. The https:// ensures that you are connecting to the official website and that any information you provide is encrypted and transmitted securely.
- Publications
- Account settings
Preview improvements coming to the PMC website in October 2024. Learn More or Try it out now .
- Advanced Search
- Journal List
- HHS Author Manuscripts

GENETIC ANALYSIS OF PROTEIN TRANSLOCATION
Thomas j. silhavy.
a Department of Molecular Biology, Princeton University, Princeton, New Jersey, USA
Angela M. Mitchell
Cells in all domains of life must translocate newly synthesized proteins both across membranes and into membranes. In eukaryotes, proteins are translocated into the lumen of the ER or the ER membrane. In prokaryotes, proteins are translocated into the cytoplasmic membrane or through the membrane into the periplasm for Gram-negative bacteria or the extracellular space for Gram-positive bacteria. Much of what we know about protein translocation was learned through genetic selections and screens utilizing lacZ gene fusions in Escherichia coli . This review covers the basic principles of protein translocation and how they were discovered and developed. In particular, we discuss how lacZ gene fusions and the phenotypes conferred were exploited to identify the genes involved in protein translocation and provide insights into their mechanisms of action. These approaches, which allowed the elucidation of processes that are conserved throughout the domains of life, illustrate the powerof seemingly simple experiments.
The term outer membrane (OM), as it is currently applied to Gram-negative bacteria, first appeared in the literature in 1964 [ 1 ]. The discovery that these bacteria have two membranes led to the recognition of a distinct, aqueous cellular compartment between the two membranes called the periplasm, a term credited to Peter Mitchell [ 2 ]. The peptidoglycan cell wall is a distinct structure located in the periplasm. By the end of the decade, the anatomy of the Gram-negative cell envelope had finally been clarified [ 3 ].
Fractionation methods were developed to separate the two membranes and to isolate the proteins present in the periplasm [ 4 – 7 ]. These showed that the OM, which was protein rich, contained a small number of very abundant proteins. In contrast, the inner membrane (IM) contained the enzymes, such as NADH dehydrogenase, that are typically found in the cellular organelles of eukaryotic cells. The periplasm was shown to contain degradative enzymes such as alkaline phosphatase (PhoA) and soluble sugar- or amino acid-binding proteins that functioned as components of the transport systems for these molecules [ 8 ]. Clearly each different cellular compartment had a distinct set of proteins. How were these proteins translocated from their site of synthesis in the cytoplasm to their final destination and how did the cell know the location to which they should be transported? It is the first question that this article is concerned with. In particular, it is concerned with the genetic approaches that were used to identify the cellular components required for this essential, protein translocation process.
In a similar time frame, work on protein translocation in eukaryotic cells was focused on the endoplasmic reticulum. Palade had shown that proteins destined for secretion are made on ribosomes attached to the endoplasmic reticulum (ER) and this provided strong evidence that secreted proteins were translocated into the ER lumen as they were being translated [ 9 ]. Blobel and Sabatini (1971) suggested that the signal that directed ribosomes making secreted proteins to the ER was located at the amino terminus of these proteins [ 10 ]. Milstein et al. (1972) showed that the antibody light chain was made initially in precursor form [ 11 ]. The signal hypothesis was born when Blobel and Dobberstein (1975) developed an in vitro system and a protease protection assay to show that the precursor form of this protein is processed during the translocation reaction [ 12 , 13 ]. Inouye and Beckwith (1977) showed that PhoA from Escherichia coli is made in vitro in precursor form providing evidence that the process of protein translocation was conserved in all of the domains of life [ 14 ]. Accordingly, it was clear that bacterial genetics could be used to study this process.
Unfortunately, there was no obvious way to approach the protein translocation problem genetically. To illustrate the problem, consider the lamB gene, which specifies an OM protein that functions as the receptor for bacteriophage λ and as maltoporin [ 15 – 17 ]. Cells lacking LamB can grow on maltose, because the disaccharide is small enough to cross the OM through the general porins OmpF and OmpC [ 18 ]. However, transport of maltose polymers (dextrins) requires LamB absolutely [ 19 ]. The lamB gene thus provides a direct selection for the loss of the protein (resistance to phage λ, λ R ) and a direct selection for restoration of the protein in the OM (growth on dextrins as sole carbon source, Dex + ). Despite these very useful phenotypes, classical genetic approaches failed to find lamB mutations that block translocation of LamB. The basic problem here is that mutations that prevent targeting of LamB to the OM will confer the same phenotype as mutations that prevent synthesis of LamB (Dex − , λ R ), and the latter are far more frequent than the former. Mutations that alter the signal sequence of LamB do confer λ R , but when they were discovered and mapped, it became clear that they lie earlier in the gene than any of the hundreds of lamB mutations known at that time [ 20 ]. New approaches were required to overcome the failure of more classical methods.
A BRIEF SUMMARY OF THE TRANSLOCATION PROCESS
To facilitate discussion of the approaches developed, a brief summary of what is currently know about the translocation process is warranted. Protein translocation from the cytoplasm in bacteria like E. coli is catalyzed by a heterotrimeric complex of IM proteins, SecYEG, and it is driven by the ATPase SecA and the proton motif force (for review see [ 21 ]). SecYE and SecA are sufficient for protein translocation in vitro [ 22 ]. SecG is not essential, but it does increase translocation in vitro in in some strains at low growth temperatures [ 23 ]. In the cell, seven different membrane proteins, SecYEG-SecDF-YajC-YidC, make up what is called the holo-translocon [ 24 ]. SecDF couple the proton motive force to protein translocation [ 25 ]. YidC functions in the insertion of IM proteins [ 26 ]. The function of YajC is not known, but it is not required for cell viability or protein translocation [ 27 ].
Two different pathways target protein to the translocon ( Fig. 1 ). Many proteins like LamB and MalE are secreted post-translationally and are kept in a secretion-competent conformation by the cytoplasmic chaperone SecB [ 28 ]. In contrast, many proteins destined for the IM like MalF are targeted to the translocon by Signal Recognition Particle (SRP) and are translocated cotranslationally [ 29 ]. Nearly all of the genes mentioned in this brief summary were discovered using the approaches described below.

The SecYEG complex is responsible for the translocation of unfolded proteins through the IM [ 22 ]. Two recognition pathways allow the either the translocation of the protein into the periplasm or the insertion of the protein into the IM. Post-translationally secreted polypeptides are recognized by SecB after translation and SecB maintains their unfolded state [ 28 ] while bringing them to the SecYEG complex through an interaction with SecA [ 97 ]. The polypeptide is then translocated through SecYEG. After secretion, the signal sequence is cleaved by signal peptidase 1 (SPase) releasing the polypeptide into the periplasm [ 98 ] where it can fold or can be protected by chaperones until OM insertion. Lipoproteins are lipidated following translocation and their signal sequences are cleaved by signal peptidase II [ 99 , 100 ]. In contrast, the highly hydrophobic signal sequence of co-translationally secreted proteins is recognized by SRP and the translating ribosome is brought to Sec through an interaction between SRP and FtsY, the SRP receptor [ 29 , 88 ]. Sec inserts the transmembrane helices of the IM proteins into the membrane as the protein is translated. Accessory Sec proteins are omitted from the diagram for clarity.
GENE FUSIONS
The first hybrid gene was made by a spontaneous deletion that fused the T4 rIIA gene to the rIIB gene. This gene fusion was isolated by Benzer and Champe (1962) and exploited by them to show that nonsense mutations are chain terminating [ 30 ]. It was also used by Crick et al. (1961) to provide evidence that frameshift mutations alter the reading frame and that there is a fixed starting point for translation [ 31 ]. Jacob et al. (1965) identified a deletion that fused the intact lacY gene to the purA promoter [ 32 ] and Beckwith and colleagues (1966) learned more about operon structure from the study of various fusions that placed the lac genes under the control of the trp promoter [ 33 ]. However, the methods used to generate these useful tools were not easy to generalize.
Malcolm Casadaban appreciated the power of gene fusions and using nothing but toothpicks and logic, he developed a method that allowed one to fuse LacZ to any non-essential gene in E. coli [ 34 ]. At the time, Casadaban was a graduate student in Jon Beckwith’s lab and Beckwith envisioned using this technology to study the protein translocation problem. From today’s vantage point, it is hard to appreciate the intellectual leap this required. Beckwith saw LacZ as what would now be called an epitope tag, albeit a very large one, and he felt this tag could be used to follow amino-terminal fragments of cell envelope proteins on their journey through the cell envelope. Nowadays this logic is so commonly used it is hard to find a cell biology paper in either prokaryotes or eukaryotes that does not use it.
The maltose transport system in E. coli is specified by five genes located in two divergently transcribed operons [ 35 ] ( Fig. 2a ), and it seemed a useful system for studying protein targeting because there was at least one maltose transport protein located in each cellular compartment. As noted above, LamB is located in the OM ( Fig. 3 ) [ 15 , 16 ]. Maltose binding protein is located in the periplasm. It accepts substrates that diffuse through LamB and delivers them to a paradigmatic ABC (ATP-Binding Cassette) transporter located in the IM [ 36 ]. This transporter is composed of two IM proteins, MalF and MalG, and an associated, cytoplasmic ATPase, MalK [ 37 – 39 ].

(a) The genes responsible for maltose import are found in divergent operons [ 35 ]. The transcription of these operons is activated by maltose bound MalT [ 101 ]. While one operon contains malE, malF , and malG , the other contains malK and lamB . (b) In the lamB-lacZ fusion, lacZ, lacY , and λ DNA is inserted into the genome in the lamB gene. This fuses the 5’ end of the lamB gene to the lacZ gene [ 42 ]. (c) In the malE-lacZ fusion, the insertion is located in the malE gene. This fuses the 5’ end of the malE gene to the lacZ gene [ 43 ].

Although maltose can cross the OM through general porins, large maltodextrins (maltose oligomers) require LamB, the OM maltoporin, to enter the periplasm [ 19 ]. After entering the periplasm, maltose and maltodextrins are bound by the periplasmic MalE protein, which brings them to the IM import pathway [ 36 ]. Binding of MalE and maltose to the periplasmic face of MalFG causes ATP hydrolysis by MalK and import of the maltose or maltodextrin to the cytoplasm [ 102 ]. MalP and MalQ release glucose monomers from the maltodextrins to be used in glycolysis [ 103 , 104 ].
The value of gene fusion technology for the analysis of protein targeting was first demonstrated with malF-lacZ fusions [ 40 ]. The amino-terminal fragment of MalF present in the hybrid proteins described was able to direct β-galactosidase to the IM. Since these fusion strains required LacY for growth on lactose, it was concluded that LacZ sequences were located in the cytoplasm. We now know that in one of these fusions, LacZ is fused to MalF at a point between transmembrane helices 6 and 7, which would position LacZ in the cytoplasm verifying this prediction [ 41 ]. The significance of this is discussed in more detail below.
Gene fusions that attach sequences of non-cytoplasmic proteins to β-galactosidase can confer one or two distinctive phenotypes that are closely associated with protein targeting ( Fig. 2bc ). Accordingly, these phenotypes could be exploited to obtain mutations that were targeting-defective. Information gained from the analysis of these fusions and the mutations obtained has provided key insights into both the signals and the cellular machinery responsible for protein targeting.
SECRETION-RELATED PHENOTYPES OF LACZ FUSION STRAINS
Perhaps the most striking phenotype exhibited by lamB-lacZ or malE-lacZ strains is a pronounced sensitivity to inducer, i.e. induction, which causes high level expression of these hybrid genes is lethal [ 42 , 43 ]. A second useful phenotype concerns the LacZ activity exhibited by these strains. Both fusion strains are Lac + , i.e. they will grow on lactose minimal media. However, the LacZ activity exhibited by the fusion strains is much lower than expected [ 44 ]. Before recounting how these phenotypes were exploited in the study of protein translocation, in the interest of clarity, a description of what we now know about the molecular basis for these phenotypes is provided.
It has long been clear that induction of lamB-lacZ or malE-lacZ fusions causes a “jamming” of the secretion machinery ( Fig. 4 ). Signal peptidase is located in the periplasm, so if a protein cannot be translocated, the signal cannot be removed. The lack of translocator activity in the dying fusion strains was evidence by the accumulation of the precursor form of other periplasmic and outer membrane proteins [ 45 ]. The cell is trying to translocate these hybrid proteins in a posttranslational manner. In its native, cytoplasmic environment, LacZ is folding and the translocator cannot deal effectively with folded proteins. When uninduced, the translocator has enough time to handle the low levels of hybrid protein produced ( Fig. 4a ). Under these conditions, a significant fraction of the hybrid protein is translocated into the periplasm where it is inactivated (see below). The low level of LacZ activity observed under noninducing conditions is due to a fraction of the hybrid protein that is not translocated and remains in the cytoplasm. The translocators cannot deal effectively with the large amounts of hybrid protein that are made under inducing conditions and this leads to cell death ( Fig. 4b ).

(a) Without induction, the mal operons are transcribed at a low level. The low levels of hybrid protein produced allows adequate time for the LamB-LacZ fusion to be secreted despite the propensity for LacZ to fold in the cytoplasm [ 42 ]. In the periplasm, aberrant disulfide bonds prevent LacZ activity [ 48 ]. The secretion of MalE is not affected. (b) When maltose is present, the transcription of the mal operon is activated by maltose bound MalT [ 101 ]. The protein secretion machinery can no longer keep up with the increased levels of LamB-LacZ and LacZ begins to fold in the cytoplasm. The folded LacZ domain clogs the Sec translocation machinery, which is eventually degraded causing cell death [ 46 , 42 ]. The clogging and degradation of Sec prevents the translocation of MalE.
Why translocator jamming leads to cell death is not clear. Induction of the lamB-lacZ fusion leads to the degradation of the jammed translocator by the protease FtsH [ 46 ]. However, this does not happen with malE-lacZ fusion strains; here death seems dependent on reactive oxygen species [ 47 ]. In the malE-lacZ fusion strain, the malE gene is disrupted and the expression of malF and malG is prevented by polarity ( Fig. 2c ). Accordingly, to see induction, malEFG must be provided in trans. The lamB gene is not required for growth on maltose so haploid lamB-lacZ fusion strains can be induced. If this strain is made diploid, the extra copies of the divergent promoters that control expression of the malEFG and the malK lamB genes titrate the positive activator MalT and reduce expression and the toxicity of the lamB-lacZ hybrid gene [ 48 ]. It would not be surprising if lethal jamming of the diploid lamB-lacZ fusion would phenocopy lethal jamming of the diploid malE-LacZ fusion.
Manoil and Beckwith devised a genetic approach to analyze the topology of IM proteins using the periplasmic enzyme PhoA [ 49 ]. This protein contains disulfide bonds and their formation is catalyzed by the periplasmic enzyme DsbA [ 50 ]. Consequently, even though the precursor form of PhoA is active in vitro [ 14 ], in vivo PhoA is only active in the periplasm. So if PhoA is fused to a periplasmic loop of an IM protein it will exhibit high activity. If fused to an internal loop it will exhibit very low activity.
LacZ behaves in the opposite fashion from PhoA [ 41 ]. If fused to a periplasmic loop it is inactive. Active LacZ requires fusion to an internal loop. Recall that IM proteins like MalF are targeted to the translocon by SRP and are translocated cotranslationally. Accordingly, LacZ sequences never exist in the cytoplasm and therefore, jamming does not occur. Indeed, if the signal sequence of LamB is made more hydrophobic so that it becomes SRP dependent then the altered LamB-LacZ hybrid protein is directed efficiently to the periplasm [ 51 ]. LacZ has a number of cysteine residues but no disulfide bonds. In the periplasm, DsbA oxidizes LacZ and forms disulfide-bonded aggregates. With LamB-LacZ these periplasmic aggregates accumulate to toxic levels. With MalF-LacZ expression levels are much lower and toxicity is not seen unless the hybrid protein is purposely overproduced. With either fusion, removal of DsbA allows LacZ to fold normally into a non-toxic, active enzyme [ 48 ].
SIGNAL SEQUENCE MUTATIONS
The inducer-sensitive (Mal s ) phenotype exhibited by both the lamB-lacZ and malE-lacZ fusion strains is clearly related to the cell’s attempted translocation of these hybrid proteins from the cytoplasm. The most common survivors of a selection for resistance to inducer, which in these cases is a selection for growth on maltose (Mal + ), are simply mutants that for one reason or another fail to make the toxic hybrid protein. As noted above, the problem with classical approaches for targeting defective mutations was always that the phenotype of mutants that lack the protein and mutants that target the protein incorrectly are identical. However, gene fusion provides a simple way to distinguish these two classes of mutation. Mutants that don’t make the hybrid protein will be LacZ − , mutants that make the hybrid protein, but fail to target it from the cytoplasm will be LacZ + . Using this logic, mutations that alter the signal sequence of LamB and MalE were identified [ 20 , 43 , 52 , 53 ]. Generally speaking, these mutations either reduced the size or introduced charge into the hydrophobic core of the signal sequence. When these signal sequence mutations were recombined back into the wild-type lamB or malE gene, they blocked translocation and caused the precursor form of the protein to accumulate in the cytoplasm. Thus, they provided the first direct evidence for Gunther Blobel’s signal hypothesis [ 12 , 13 ].
MUTATIONS THAT ALTER THE TRANSLOCATION MACHINERY
Two general approaches were taken initially to identify the genes that specify components of the translocation machinery. One exploited the signal sequence mutations that were obtained using gene fusions as described above. Basically, this approach sought dominant, gain-of-function mutations that enabled translocation of an internalized protein. The mutations obtained using this approach were termed prl (PRotein Localization). The other approach exploited the low level of LacZ activity exhibited by the LacZ fusion strains, in particular the malE-lacZ fusion, to identify recessive, loss-of-function mutations that internalized a translocated protein, increasing LacZ activity. The mutations isolated using this approach were termed sec (SECretion). Satisfyingly, these two distinct approaches led to the identification of the same gene multiple times, providing strong evidence that these gene products functioned directly in protein translocation. Today, these genes are still known by both the prl and the sec mnemonic. In what follows, we will often refer to a gene using both names. When doing so, the name that appears first reflects the mutation in question. For example, prlA ( secY ) mutations are dominant suppressors of signal sequence mutations and secY ( prlA ) mutations are recessive loss of function mutations that compromise the protein translocation process.
Suppressors of signal sequence mutations
The signal sequence mutations likely block protein translocation because they prevent recognition of the mutant precursor protein by the translocation machinery. If this was true, then genetic logic suggests that it may be possible to identify mutations that alter the translocation machinery and restore recognition of the mutant signal. This approach, termed interactive suppressors, conformational suppressors, or allele-specific suppressors, was first used in prokaryotes by Jarvik and Botstein in their study of phage P22 proteins [ 54 ]. It is difficult to prove direct interaction genetically and the best that can be done is to demonstrate strict allele specificity. You can imagine a mutant key that won’t fit a mutant lock, but a “suppressor” lock mutation that fits one mutant key should not fit any other mutant key. There are many examples where this approach has been applied successfully. For example, this technique was used to show that λ cl activates transcription by direct interaction with the σ subunit of RNA polymerase [ 55 ] and the direct interaction between the amino acids affected by these two mutations in the wild-type proteins has been confirmed by structural analysis [ 56 ].
The lamB signal sequence mutations were especially useful for this type of suppressor analysis for the following reason. Suppressors can be selected by demanding growth on maltodextrins. It turns out that there are mutations that allow growth on maltodextrins (Dex + ) by bypassing the requirement for LamB altogether. Such mutations can increase expression of a normally cryptic porin [ 57 ], increase the pore size of a typical porin like OmpC [ 58 ], or they can disrupt the OM permeability barrier [ 59 ]. However, a suppressor that restores translocation of a mutant LamB protein with a defective signal sequence will not only be Dex + but it will also become sensitive to phage λ, whereas the bypass suppressors mentioned above will remain phage resistant.
Suppressors of MalE signal sequence mutations can be obtained by demanding growth on maltose. Mutations that bypass the maltose transport system are known [ 60 ], so restoration of MalE translocation must be confirmed biochemically.
The first suppressors of signal sequence mutations (lamB) identified mapped to a gene in the largest ribosomal gene cluster in E. coli and were termed prlA [ 61 ]. Subsequent work showed that the suppressor mutations mapped to a previously unidentified gene at the end of this operon [ 62 ]. Following localized mutagenesis, Ito et al. identified a temperature-sensitive lethal mutation that mapped to this region [ 63 ]. When shifted to the non-permissive temperature, pulse-chase experiments show that this mutant, like the other sec mutants described below, accumulated the precursor form of MalE, demonstrating a block to translocation. The Nomura lab had identified an open reading frame at the end of this operon and called it Y [ 64 ]. Accordingly, Ito et al. named the gene secY [ 63 ].
The first gene identified using the LacZ fusion approach described in the next section was secA [ 44 ]. Suppressors of signal sequence mutations that mapped to secA , termed prlD mutations, were identified by Fikes and Bassford [ 65 ]. A more extensive hunt for suppressors of lamB signal sequence mutations revealed additional prlA and prlD suppressors but also suppressors in a new gene termed prlG [ 66 ]. The paper describing this work appeared back-to-back with a paper describing a new sec gene, secE that was obtained as described below [ 67 ]. By screening PCR mutagenized secG libraries, suppressors of MalE signal sequence mutations have also been obtained and these are termed prlH [ 68 ].
It is clear that none of the prl suppressors restores recognition of the mutant signal sequence used for their selection. These suppressors do not exhibit allele-specificity. Indeed all of the prl mutations except for prlD will suppress a complete signal sequence deletion [ 69 , 70 , 68 ]. As noted below, the prlD mutations act by a distinct mechanism. The prlAGH suppressors are really bypass suppressors; they bypass the requirement for a signal sequence [ 71 ].
Besides identifying genes, the prl mutations also provided insight into mechanism. Studies with double mutants revealed several pairs of prlA and prlG mutations that exhibited allele-specific synthetic lethality. Moreover, these pairs of alleles were also topologically specific, i.e. a mutation in a transmembrane helix of one protein was synthetically lethal with a mutation in the other protein that was also in a transmembrane helix. This suggested that these mutations identified domains of interaction between the two proteins. Lethality occurred because together these mutations prevented an essential interaction between the two proteins [ 72 ].
One of the synthetically lethal prlA and prlG mutant pairs alters an amino acid in an external loop of both proteins. In order to demonstrate that these amino acids were in close proximity in vivo , the amino acids were changed to cysteine. Expression of the cysteine mutant pair resulted in a DsbA-dependent lethal phenotype. The lethality is dominant to either wild-type secY ( prlA ) or secE ( prlG ) provided in trans and protein translocation is largely unperturbed in the dying cells demonstrating that it is the disulfide-bonded complex itself that is causing toxicity [ 73 ].
The predictions about interactive domains from the synthetic lethal phenotypes were confirmed when the structure of the SecYEG complex was determined with one glaring exception [ 74 ]. The residues that could be crosslinked by disulfide bond in vivo were more than 20A apart. The structure determined is the “closed” form of the complex and, in this structure, the external loop where the cysteine residue in SecY introduced by the prlA mutation is located is folded back into the channel forming a plug ( Fig. 5 ). The disulfide bonded complex explained how the plug moves to open the channel during the translocation reaction. In fact, the disulfide bonded complex is locked open explaining its dominant lethality.

In the closed, inactive conformation of SecYEG, transmembrane helix (TM) 2 and TM7 are located close together and the protein translocation channel is blocked by the plug. SecA binds to the open conformation of SecYEG through interactions with the C4/C5 loop and SecE. TM2 and TM7 separate and the plug is moved out of the protein translocation channel, allowing translocation of the secreted protein [ 74 ]. The yellow line in the diagram of the open conformation indicates synthetic lethal cross-linking formed by cysteine mutations in SecY and SecE [ 73 ]. Adapted from Corey, et al. 2016 [ 105 ].
The closed and open forms of the SecYEG complex exist in equilibrium and signal sequence recognition pushes the equilibrium towards the open form [ 74 ]. The prl mutations either stabilize the open form or destabilize the closed form thus reducing the requirement for a signal sequence [ 75 ]. Nonetheless, to be translocated, a protein must be delivered to the translocator. This happens because these precursors lacking a signal sequence are still recognized by SecB and SecB delivers them to SecA and thus to SecYEG. Suppression of signal sequence deletions requires SecB absolutely [ 69 , 70 ].
The secA ( prlD ) mutations work by a different mechanism(s). Most of the prlD alleles also confer resistance to azide [ 76 ]. In fact, the first suppressor of signal sequence mutations was actually isolated by Joshua Lederberg [ 77 ]. SecA is an ATPase and it is likely that the prlD alleles affect the nucleotide binding and hydrolysis cycles [ 78 ].
Mutations that increase the LacZ activity gene fusion strains
As noted above, lamB-lacZ and malE-lacZ fusion strains exhibit two unusual phenotypes. One of these is inducer sensitivity and this was exploited as described above to identify signal sequence mutations. The other phenotype is the low level of LacZ activity. Inducer sensitivity is caused by translocator jamming [ 46 ]; low LacZ activity is caused by the targeting of LacZ to the periplasm [ 48 ] ( Fig. 4 ).
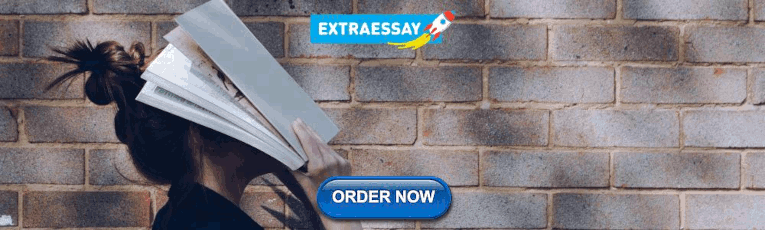
malE-lacZ phoA-lacZ and lamB-lacZ fusions .
Oliver and Beckwith noted that mutations that alter the malE signal sequence in the malE-lacZ fusion increase the uninduced LacZ activity dramatically [ 44 ]. They reasoned therefore, that mutations that decrease the activity of the protein translocation machinery should also increase the LacZ activity of MalE-LacZ because a higher percentage of the hybrid protein would remain in the cytoplasm where LacZ is fully active. Using lactose tetrazolium agar, they searched for mutant Lac + papillae growing out of a lawn of the malE-lacZ fusion strain, which appears Lac − on this media. Assuming that the genes they sought were essential, they screened for mutations that compromise the translocation machinery at low temperatures (30°) but kill the cell at high growth temperatures (TS lethal at 42°). In this way they could avoid signal sequence mutations and mutations that simply increase expression of the hybrid protein. They found a new gene, which they named secA [ 79 ]. At the non-permissive temperature, the precursor forms of periplasmic and OM proteins could be detected in pulse-chase experiments.
Kumamoto and Beckwith performed an extensive hunt for Lac + derivatives of the malE-lacZ fusion strain and found an additional gene that they termed secB [ 80 ]. Unlike secA, secB is not essential, but they showed that strains lacking this protein show pleiotropic defects in protein translocation. Similar Lac + selections with phoA-lacZ and lamB-lacZ fusions yield mutations at another locus termed secD [ 81 ]. Subsequent analysis showed that the secD gene is in an operon with another gene termed secF . Both of these proteins are membrane proteins and strains lacking either are very cold sensitive.
It is remarkable that the Lac + selections with the malE-lacZ and the phoA-lacZ and the lamB-lacZ fusion strains found different, non-overlapping genes. Also, remarkable is the fact that mutations in secY ( prlA ) did not answer any of them. As noted above, the secY mutations were found following localized mutagenesis of the prlA region of the chromosome. Why this is so has never been clarified.
secA-lacZ fusions.
Oliver and Beckwith discovered that SecA production is increased up to 10-fold when protein translocation is compromised [ 82 ]. We now know that the secA gene is located in an operon downstream of a gene called secM . This gene specifies a periplasmic protein that is rapidly degraded; its only real function is in regulation. If protein translocation is compromised, a sequence near the carboxy terminus of SecM gets stuck in the ribosome exit tunnel, and this changes the conformation of the downstream mRNA exposing the Shine-Dalgarno sequence of secA . If the translocation machinery is not compromised and is working properly it actually pulls SecM out of the ribosome, the mRNA structure is not altered and SecA synthesis is kept at a low level (for review see [ 83 ]).
Riggs et al. exploited this novel translational regulation of secA to design a new screen for mutations that alter the protein translocation machinery [ 84 ]. Starting with a strain that carried a secA-lacZ fusion in trans to secA + , they looked for mutations that increased LacZ production. Mutations in secY ( prlA ), secA ( prlD ), secDF [ 84 ] and secE ( prlG ) [ 67 ] answered this screen. It is not surprising that secB and secG mutations were not obtained because it was known that these mutations do not increase SecA production. The secA-lacZ screen proved ultimately to be the most generally useful screen for mutants with protein translocation defects.
Many of the sec alleles confer cold-sensitive growth defects. The first secE alleles were cold sensitive, but they did not alter the protein sequence. Rather, they reduced synthesis by reducing translation [ 85 ]. This likely reflects the fact that protein translocation is an inherently cold-sensitive process.
malF-lacZ fusions.
In eukaryotic cells, Signal Recognition Particle (SRP) recognizes signal sequences as they emerge from the ribosome and delivers the ribosome and the nascent chain to the endoplasmic reticulum where translation resumes and the protein is simultaneously translocated from the cytoplasm [ 86 ]. DNA sequence analysis revealed that the E. coli chromosome contains homologues of the protein subunit that binds signal sequences, a protein called Ffh (Fifty-Four Homologue) [ 87 – 89 ], as well as a homologue of 7S RNA, an RNA called Ffs (Four point Five S RNA) [ 90 , 91 ]. Ffh [ 92 ] and Ffs are essential, but that might reflect a role in translation for Ffs [ 93 , 94 ]. Using depletion strains some defects in protein translocation could be detected, but the observed defects were clearly not as severe as the defect observed in the sec mutants [ 90 – 92 ]. Moreover, extensive genetic and biochemical analysis had failed to identify a role for the diminutive prokaryotic SRP. We now know that this is because the genetics and biochemistry had been done using periplasmic and outer membrane proteins as model systems, but as noted above, in bacteria, SRP is primarily used for inner membrane proteins [ 29 ].
Using the logic described above with malE-lacZ fusion strains, the Beckwith lab had designed a selection using a malF-lacZ fusion for mutants defective in the assembly of inner membrane proteins. The malF-lacZ fusion employed exhibited low LacZ activity because LacZ was fused to a periplasmic loop. However, Bardwell et al. discovered that mutations that increased LacZ activity only yielded mutations that disrupted disulfide bond formation [ 50 ]. We now know that this is because the malF-lacZ fusion directed LacZ to the periplasm where DsbA inactivated the enzyme by forming disulfide-bonded aggregates. In the absence of DsbA, LacZ folds normally into an active enzyme [ 48 ]. Nearly a decade later, in 2000, Tian et al. modified the malF-lacZ selection to get the mutations that were originally sought [ 95 ]. Basically, instead of selecting mutants with strongly increased LacZ activity, they isolated mutants that only partially restored LacZ activity. These mutants had defects in SRP.
CONCLUSIONS
LacZ gene fusions enabled the power of bacteria genetics to be applied to the study of protein translocation and this stimulated both progress and interest in the new field of bacterial cell biology. It was an exciting time in part because of the realization that eukaryotic and prokaryotic researchers had much to learn from each other. We’d like to close with a sentence from the announcement for Blobel’s Nobel Prize: The signal hypothesis describes a process that “is a universal one: it operates similarly in plant, yeast and animal cells, including those of humans” [ 96 ]. Actually, it is even more universal than that!
Acknowledgments
Funding Funding was provided by National Institute of General Medical Sciences (Grant No. R35GM118024).
Thank you for visiting nature.com. You are using a browser version with limited support for CSS. To obtain the best experience, we recommend you use a more up to date browser (or turn off compatibility mode in Internet Explorer). In the meantime, to ensure continued support, we are displaying the site without styles and JavaScript.
- View all journals
- Explore content
- About the journal
- Publish with us
- Sign up for alerts
- Published: November 1999
A Nobel Prize for cell biology
Nature Cell Biology volume 1 , page E169 ( 1999 ) Cite this article
15k Accesses
4 Citations
10 Altmetric
Metrics details
This year’s Nobel Prize in Physiology or Medicine has been awarded to Günter Blobel, of the Rockefeller University, for ‘‘the discovery that proteins have intrinsic signals that govern their transport and localisation in the cell’’. Angus Lamond of the University of Dundee says that the prize is “a landmark in its recognition of the fundamental importance of basic research in molecular cell biology for modern medicine and the fight against disease”.
The idea that proteins contain targeting zip codes originated in 1971, when Blobel and his colleague David Sabatini postulated that the information needed to direct a nascent peptide to the membrane of the endoplasmic reticulum is contained within the peptide itself. A year later, César Milstein and colleagues provided experimental evidence for a transient signal sequence at the amino-terminal end of a secretory protein. Building on this important discovery and work from his own laboratory, Blobel, together with Bernhard Dobberstein, formulated the ‘signal hypothesis’. In a 1975 paper — which has since become a citation classic — they predicted that newly synthesized proteins have a built-in signal which directs them to the endoplasmic reticulum and through a channel in the membrane. This principle has turned out to be a common mechanism, operating in a variety of intracellular pathways in all organisms, from bacteria to yeast to man. According to Peter Walter of the University of San Francisco, ‘‘Günter’s accomplishment is not defined by a single paper or even a set of papers, but rather is the fruit of his casting a wide net over a fundamentally important problem in biology. Many firsts came out of his lab, but it’s the sum of the individual pieces that adds up to the big picture’’.
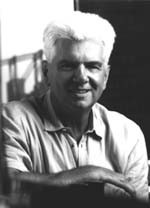
As well as his proposal of the signal hypothesis, it is Blobel’s imaginative approach towards the in vitro reconstitution of protein transport that has revolutionized the field of cell biology. ‘‘The early realisation that many, perhaps most, reactions that occur in the cell could ultimately be recapitulated in vitro ,’’ says Hidde Ploegh of Harvard University, ‘‘is in no small measure due to Blobel’s work in this area’’. According to Randy Schekman of the University of California, Berkeley, it was the first time that ‘‘a cell-free system was developed to dissect the process of intracellular transport using the tools of the enzymologist. Before this landmark event, the field had relied essentially exclusively on morphology and cell fractionation, techniques that by themselves had little prospect of elucidating the machinery required to move proteins from one place to another in the cell’’. Blobel’s in vitro experimental system inspired other cell biologists and, according to Graham Warren of Yale University, it ‘‘made people believe that any cellular function, no matter how complicated, can be mimicked in the test tube’’.
Founder of a distinguished scientific lineage
Like any high-profile award, this Nobel Prize has sparked some controversy and speculation. It is, however, undeniable that Blobel has played a pivotal part in training an impressive group of cell biologists, including Bernhard Dobberstein, Peter Walter, Reid Gilmore, Larry Gerace, Vishwanath Lingappa, Gerry Waters, David Anderson, Sandy Simon and Mary Moore, to name just a few. These scientists are now shaping the field of molecular cell biology. Blobel himself was trained by the 1974 Nobel laureate George Palade, who fondly remembers him as a ‘‘young, impetuous and highly promising postdoctoral fellow’’. Palade, who is well known for his dedication to his students and postdoctoral fellows, no doubt provided an excellent role model in this regard. Waters, who was a graduate student with Blobel, says that he ‘‘was always available to discuss science, and was an extraordinarily supportive and enthusiastic graduate advisor’’. Lingappa ‘‘is, and always has been, immensely proud to be one of his scientific offsprings’’. It seems that Blobel’s extraordinary track record as a mentor reflects his ability to bring out the best in people by making them believe in themselves. Perhaps it is this, and not the Nobel Prize, that is his greatest achievement.
Rights and permissions
Reprints and permissions
About this article
Cite this article.
A Nobel Prize for cell biology. Nat Cell Biol 1 , E169 (1999). https://doi.org/10.1038/15602
Download citation
Issue Date : November 1999
DOI : https://doi.org/10.1038/15602
Share this article
Anyone you share the following link with will be able to read this content:
Sorry, a shareable link is not currently available for this article.
Provided by the Springer Nature SharedIt content-sharing initiative
This article is cited by
Blobel and sabatini’s “beautiful idea”: visual representations of the conception and refinement of the signal hypothesis.
- Michelle Lynne LaBonte
Journal of the History of Biology (2017)
Controlling Protein Compartmentalization to Overcome Disease
- James R. Davis
- Mudit Kakar
- Carol S. Lim
Pharmaceutical Research (2006)
Quick links
- Explore articles by subject
- Guide to authors
- Editorial policies
Sign up for the Nature Briefing newsletter — what matters in science, free to your inbox daily.

Digital Commons @ RU
- < Previous
Home > MARKUS_LIBRARY > Exhibits > BLOBEL-MOLECULAR-BIOLOGY > 28
Günter Blobel: Pioneer of Molecular Cell Biology
Signal hyposesis. Illustration
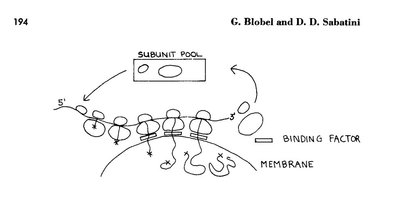
Creation Date
Description.
The signal hypothesis for secretory proteins formulated by Günter Blobel and David Sabatini.
Blobel and Sabatini hypothesized in 1971 that a nascent peptide, bearing a terminal ‘signal’, interacts with a binding factor attached to the endoplasmic reticulum (ER) membrane, allowing docking of the ribosome and its associated mRNA. Upon passage of the newly synthesized protein across the ER membrane, the ribosome is able to dissociate from the site of synthesis and re-enter the cytoplasmic pool, ready for another round of synthesis. From Blobel, G. and Sabatini, D. (1971) in Biomembranes , pp.193-195, Plenum Press, New York
Since October 19, 2018
David Sabatini, Günter Blobel, signal hypothesis
Image Location
Advanced Search
- Notify me via email or RSS
- Collections
- Disciplines
Author Corner
- The Rockefeller University
- The Rita and Frits Markus Library
Gallery Locations
- View gallery on map
- View gallery in Google Earth
Home | About | FAQ | My Account | Accessibility Statement
Privacy Copyright
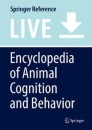
Encyclopedia of Animal Cognition and Behavior pp 1–3 Cite as
Handicap Hypothesis
- James P Higham 3
- Living reference work entry
- First Online: 12 July 2018
52 Accesses
Costly signaling ; Handicap principle
The hypothesis that in order to be honest, animal signals must be costly.
The Handicap Hypothesis
The handicap hypothesis is an idea proposed in 1975 by Amotz Zahavi as a mechanism to explain how honesty is maintained in animal communication. The hypothesis proposes that in order to be honest, signals must have costs that are borne by signalers. Under this hypothesis, only high-quality individuals can afford to bear the costs of a high-quality signal, leading to honesty in communication. The hypothesis was critiqued by a number of authors through the 1970s and 1980s, including one of the preeminent theoretical evolutionary biologists of the era, John Maynard Smith. The handicap hypothesis remained largely discredited within the field until a game theoretic model was produced by Alan Grafen in 1990. In this model, a separating equilibrium was found in which the optimal strategy for signalers of different quality was to give a...
This is a preview of subscription content, log in via an institution .
Biernaskie, J. M., Grafen, A., & Perry, J. C. (2014). The evolution of index signals to avoid the cost of dishonesty. Proceedings of the Royal Society of London B, 281 , 20140876.
Article Google Scholar
Grafen, A. (1990). Biological signals as handicaps. Journal of Theoretical Biology, 144 , 517–546.
Madden, J. R. (2002). Bower decorations attract females but provoke other male spotted bowerbirds: Bower owners resolve this trade-off. Proceedings of the Royal Society of London B, 269 , 1347–1351.
Spence, M. (1973). Job market signaling. Quarterly Journal of Economics, 87 , 355–374.
Zahavi, A. (1975). Mate selection–a selection for a handicap. Journal of Theoretical Biology, 53 , 205–214.
Download references
Author information
Authors and affiliations.
New York University, New York, NY, USA
James P Higham
You can also search for this author in PubMed Google Scholar
Corresponding author
Correspondence to James P Higham .
Editor information
Editors and affiliations.
Oakland University , Rochester, MI, USA
Jennifer Vonk
Department of Psychology, Oakland University Department of Psychology, Rochester, MI, USA
Todd Shackelford
Section Editor information
University of Cambridge, Cambridge, UK
Constance Dubuc
Rights and permissions
Reprints and permissions
Copyright information
© 2018 Springer International Publishing AG, part of Springer Nature
About this entry
Cite this entry.
Higham, J.P. (2018). Handicap Hypothesis. In: Vonk, J., Shackelford, T. (eds) Encyclopedia of Animal Cognition and Behavior. Springer, Cham. https://doi.org/10.1007/978-3-319-47829-6_353-1
Download citation
DOI : https://doi.org/10.1007/978-3-319-47829-6_353-1
Received : 05 September 2017
Accepted : 24 June 2018
Published : 12 July 2018
Publisher Name : Springer, Cham
Print ISBN : 978-3-319-47829-6
Online ISBN : 978-3-319-47829-6
eBook Packages : Springer Reference Behavioral Science and Psychology Reference Module Humanities and Social Sciences Reference Module Business, Economics and Social Sciences
- Publish with us
Policies and ethics
- Find a journal
- Track your research
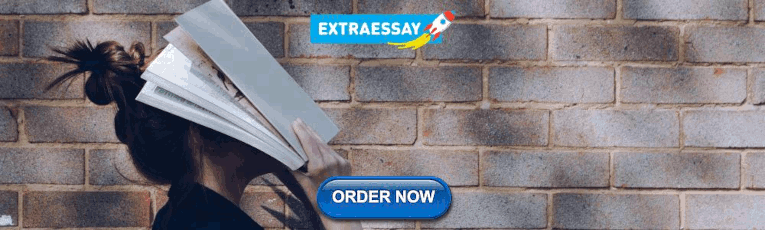
IMAGES
VIDEO
COMMENTS
signal hypothesis A hypothesis to explain how ribosomes become attached to membranes within cells in order to deliver the appropriate proteins to cell organelles, such as mitochondria and chloroplasts, or transport proteins outside the cell membrane.It proposes that the leading end of the nascent polypeptide chain consists of a signal peptide.This sticks out from the ribosome and is recognized ...
The signal hypothesis was originally proposed by Günter Blobel and David Sabatini in 1971, and demonstrated by Blobel and colleagues in 1975. The signal hypothesis showed that cytoplasmically synthesised proteins targeted to the ER use a signal sequence to direct them to the ER membrane. A signal sequence is a short peptide that is part of the ...
The signal hypothesis also laid the foundations of modern biotechnology. Proteins such as human insulin and human growth hormone could be tagged for mass production in bacteria, secreted and ...
In 1971, Günter Blobel and David Sabatini formulated the signal hypothesis, which proposed that proteins contain signal sequences that target them for secretion. Over the past 40 years this ...
c. Translation (and translocation) are completed, translocon closes. (step 6) e. Signal Peptidase (usually) cuts off signal peptide at arrow. (step 7) Protein is released into lumen of ER. Ribosome and mRNA are released. Now try problem 3-4, especially part D. (If some of the parts are not obvious, wait until later.) B.
Search for: 'signalling hypothesis' in Oxford Reference ». The idea that many actions taken by economic agents are motivated chiefly by the wish to send a positive 'signal' to other agents, rather than by their ostensible purpose. This can be a means of overcoming the problem of asymmetric information between transactors.
Günter Blobel won a Nobel Prize for his "signaling hypothesis" explaining how signal peptides target secretory proteins to a translocase channel embedded in the membrane of the endoplasmic reticulum ( 2 ). Once the proteins have passed through this channel into the lumen of the ER, the signal peptide is removed by proteases and the mature ...
The signal hypothesis proposes that the N-terminus of a secreted protein has a signal sequence whose presence marks it for membrane insertion. Once the protein chain is well inserted into the membrane, the signal sequence is cleaved off by a protease within the membrane and the protein can then enter or even pass through the membrane.
The signal hypothesis, which describes how secretory and membrane proteins are targeted to the endoplasmic reticulum, was proposed in 1971 by Günter Blobel and David Sabatini and demonstrated by Blobel and Bernhard Dobberstein in 1975. Subsequent research identified the key components of the membrane insertion and translocation machinery ...
The signal hypothesis was found to be applicable to protein translocation in all eukaryote and prokaryotes and the concept of signal-mediated targeting of proteins was expanded into a generalised hypothesis of protein topogenesis by Gunter Blobel in 1980. Abstract The signal hypothesis, which describes how secretory and membrane proteins are targeted to the endoplasmic reticulum, was proposed ...
The signal hypothesis in 1975, with the signal peptide as a dotted line. BLOBEL. system concocted by Philip Leder and colleagues (Swan et al., 1972) churned out an antibody light chain that was 6 to 8 amino acids longer than the normal secreted version in the body. Tonegawa and Baldi (1973) and Schechter (1973) obtained similar results. ...
The signal hypothesis, first formulated in 1971 by Günter Blobel and David Sabatini and developed by Blobel and Bernhard Dobberstein in 1975, showed how proteins that are destined for export from ...
The signal hypothesis, which describes how secretory and membrane proteins are targeted to the endoplasmic reticulum, was proposed in 1971 by Günter Blobel and David Sabatini and demonstrated by ...
"The signal hypothesis". Proteins which are to be exported out of the cell are synthesized by ribosomes, associated with the endoplasmic reticulum. The genetic information from DNA is transferred via messenger RNA (mRNA). This information determines how the amino acids build up the proteins. First, a signal peptide is formed as a part of ...
The signal can be the call itself, the intensity of a call, its variation style, its repetition rate, and so on. Various hypotheses seek to explain why females would select for one call over the other. The sensory exploitation hypothesis proposes that pre-existing preferences in female receivers can drive the evolution of signal innovation ...
The signal hypothesis was born when Blobel and Dobberstein (1975) developed an in vitro system and a protease protection assay to show that the precursor form of this protein is processed during the translocation reaction [12,13]. Inouye and Beckwith (1977) ...
Signal hypothesis was proposed in the early 1971 by Gunter Blobel and David Sabatini. Their work received Nobel prize in Physiology or Medicine in 1999. Signal hypothesis describes how secretory and membrane proteins are targeted to E.R . Although the process of protein synthesis takes place in the cytosol through the interaction of ribosomes ...
A major contribution to this end has been the confirmation of the signal hypothesis proposed in the early 1970s by Blobel and Sabatini. According to this hypothesis (Fig. 15-19), proteins that are to be either (a) secreted from the cell, (b) dispatched to lysosomes, or (c) incorporated into the plasma membrane or membranes of the endoplasmic ...
Hypothesis Testing INTRODUCTION The topic of hypothesis testing arises in many contexts in signal processing and communications, as well as in medicine, statistics and other settings in which a ... produces a signal at the channel output that we represent by s(t) = p(t) ∗ h(t). Figure 13.1 thus reduces to the overall system shown in Figure 13.2.
As well as his proposal of the signal hypothesis, it is Blobel's imaginative approach towards the in vitro reconstitution of protein transport that has revolutionized the field of cell biology ...
The signal hypothesis for secretory proteins formulated by Günter Blobel and David Sabatini. Blobel and Sabatini hypothesized in 1971 that a nascent peptide, bearing a terminal 'signal', interacts with a binding factor attached to the endoplasmic reticulum (ER) membrane, allowing docking of the ribosome and its associated mRNA. Upon passage of the newly synthesized protein across the ER ...
It originated in radar technology and now applies to psychology. The theory investigates how strong a signal must be for detection. It considers two variables: D Prime (signal strength) and C (strategy). Strategies can be conservative (saying "no" unless certain) or liberal (often saying "yes"). Created by Ronald Sahyouni.
The Handicap Hypothesis. The handicap hypothesis is an idea proposed in 1975 by Amotz Zahavi as a mechanism to explain how honesty is maintained in animal communication. The hypothesis proposes that in order to be honest, signals must have costs that are borne by signalers. Under this hypothesis, only high-quality individuals can afford to bear ...