
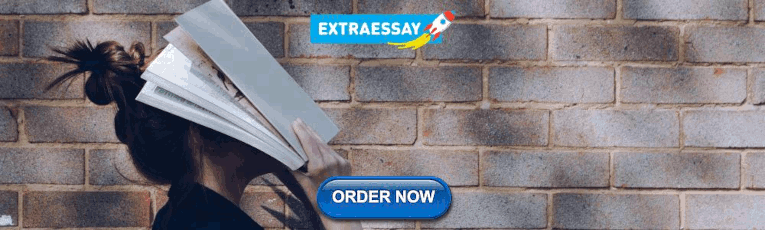
200+ Unique And Interesting Biology Research Topics For Students In 2023
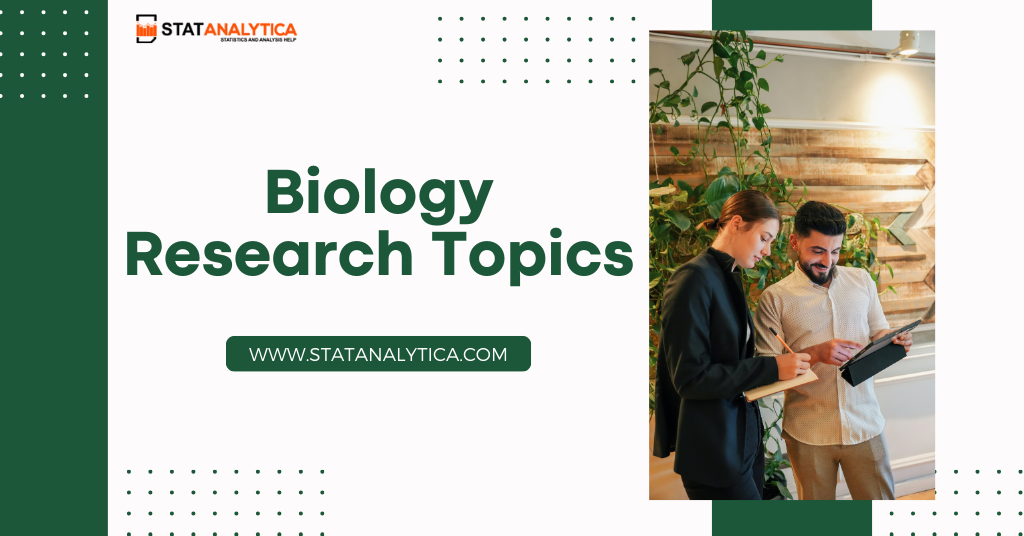
Are you curious about the fascinating world of biology and its many research possibilities? Well, you are in the right place! In this blog, we will explore biology research topics, exploring what biology is, what constitutes a good research topic, and how to go about selecting the perfect one for your academic journey.
So, what exactly is biology? Biology is the study of living organisms and their interactions with the environment. It includes everything from the tiniest cells to the largest ecosystems, making it a diverse and exciting field of study.
Stay tuned to learn more about biology research topics as we present over 200 intriguing research ideas for students, emphasizing the importance of selecting the right one. In addition, we will also share resources to make your quest for the perfect topic a breeze. Let’s embark on this scientific journey together!
If you are having trouble with any kind of assignment or task, do not worry—we can give you the best microbiology assignment help at a value price. Additionally, you may look at nursing project ideas .
What Is Biology?
Table of Contents
Biology is the study of living things, like animals, plants, and even tiny organisms too small to see. It helps us understand how these living things work and how they interact with each other and their environment. Biologists, or scientists who study biology, explore topics like how animals breathe, how plants grow, and how our bodies function. By learning about biology, we can better care for the Earth and all its living creatures.
What Is A Good Biology Research Topic?
A good biology research topic is a question or problem in the field of biology that scientists want to investigate and learn more about. It should be interesting and important, like studying how a new medicine can treat a disease or how animals adapt to changing environments. The topic should also be specific and clear, so researchers can focus on finding answers. Additionally, it’s helpful if the topic hasn’t been studied extensively before, so the research can contribute new knowledge to the field of biology and help us better understand the natural world.
Tips For Choosing A Biology Research Topics
Here are some tips for choosing a biology research topics:
1. Choose What Interests You
When picking a biology research topic, go for something that you personally find fascinating and enjoyable. When you’re genuinely curious about it, you’ll be more motivated to study and learn.
2. Select a Significant Topic
Look for a subject in biology that has real-world importance. Think about whether your research can address practical issues, like finding cures for diseases or understanding environmental problems. Research that can make a positive impact is usually a good choice.
3. Check If It’s Doable
Consider if you have the necessary tools and time to carry out your research. It’s essential to pick a topic that you can actually study with the resources available to you.
4. Add Your Unique Perspective
Try to find a fresh or different angle for your research. While you can build upon existing knowledge, bringing something new or unique to the table can make your research more exciting and valuable.
5. Seek Guidance
Don’t hesitate to ask for advice from your teachers or experienced researchers. They can provide you with valuable insights and help you make a smart decision when choosing your research topic in biology.
Biology Research Topics For College Students
1. Investigating the role of genetic mutations in cancer development.
2. Analyzing the impact of climate changes on wildlife populations.
3. Studying the ecology of invasive species in urban environments.
4. Investigating the microbiome of the human gut and its relationship to health.
5. Analyzing the genetic diversity of endangered species for conservation.
6. Studying the evolution of antibiotic resistance in bacteria.
7. Investigating the ecological consequences of deforestation.
8. Analyzing the behavior and communication of social insects like ants and bees.
9. Studying the physiology of extreme environments, such as deep-sea hydrothermal vents.
10. Investigating the molecular mechanisms of cell division and mitosis.
Plant Biology Research Topics For College Students
11. Studying the impact of different fertilizers on crop yields and soil health.
12. Analyzing the genetics of plant resistance to pests and diseases.
13. Investigating the role of plant hormones in growth and development.
14. Studying the adaptation of plants to drought conditions.
15. Analyzing the ecological interactions between plants and pollinators.
16. Investigating the use of biotechnology to enhance crop traits.
17. Studying the genetics of plant breeding for improved varieties.
18. Analyzing the physiology of photosynthesis and carbon fixation in plants.
19. Investigating the effects of soil microbiota on plant health.
20. Studying the evolution of plant species in response to changing environments.
Biotechnology Research Topics For College Students
21. Investigating the use of CRISPR-Cas9 technology for genome editing.
22. Analyzing the production of biofuels from microorganisms.
23. Studying the application of biotechnology in medicine, such as gene therapy.
24. Investigating the use of bioplastics as a sustainable alternative to conventional plastics.
25. Analyzing the role of biotechnology in food production, including GMOs.
26. Studying the development of biopharmaceuticals and monoclonal antibodies.
27. Investigating the use of bioremediation to clean up polluted environments.
28. Studying the potential of synthetic biology for creating novel organisms.
29. Analyzing the ethical and social implications of biotechnological advancements.
30. Investigating the use of biotechnology in forensic science, such as DNA analysis.
Molecular Biology Research Topics For Undergraduates
31. Studying the structure and function of DNA and RNA molecules.
32. Analyzing the regulation of gene expression in eukaryotic cells.
33. Investigating the mechanisms of DNA replication and repair.
34. Studying the role of non-coding RNAs in gene regulation.
35. Analyzing the molecular basis of genetic diseases like cystic fibrosis.
36. Investigating the epigenetic modifications that control gene activity.
37. Studying the molecular mechanisms of protein folding and misfolding.
38. Analyzing the molecular pathways involved in cancer progression.
39. Investigating the molecular basis of neurodegenerative diseases.
40. Studying the use of molecular markers in genetic diversity analysis.
Life Science Research Topics For High School Students
41. Investigating the effects of different diets on human health.
42. Analyzing the impact of exercise on cardiovascular fitness.
43. Studying the genetics of inherited traits and diseases.
44. Investigating the ecological interactions in a local ecosystem.
45. Analyzing the diversity of microorganisms in soil or water samples.
46. Studying the anatomy and physiology of a specific organ or system.
47. Investigating the life cycle of a local plant or animal species.
48. Studying the effects of environmental pollutants on aquatic organisms.
49. Analyzing the behavior of a specific animal species in its habitat.
50. Investigating the process of photosynthesis in plants.
Biology Research Topics For Grade 12
51. Investigating the genetic basis of a specific inherited disorder.
52. Analyzing the impact of climate change on a local ecosystem.
53.Studying the biodiversity of a particular rainforest region.
54. Investigating the physiological adaptations of animals to extreme temperatures.
55. Analyzing the effects of pollution on aquatic ecosystems.
56. Studying the life history and conservation status of an endangered species.
57. Investigating the molecular mechanisms of a specific disease.
58. Studying the ecological interactions within a coral reef ecosystem.
59. Analyzing the genetics of plant hybridization and speciation.
60. Investigating the behavior and communication of a particular bird species.
Marine Biology Research Topics
61. Studying the impact of ocean acidification on coral reefs.
62. Analyzing the migration patterns of marine mammals.
63. Investigating the physiology of deep-sea creatures under high pressure.
64. Studying the ecology of phytoplankton and their role in the marine food web.
65. Analyzing the behavior of different species of sharks.
66. Investigating the conservation of sea turtle populations.
67. Studying the biodiversity of deep-sea hydrothermal vent communities.
68. Analyzing the effects of overfishing on marine ecosystems.
69. Investigating the adaptation of marine organisms to extreme cold in polar regions.
70. Studying the bioluminescence and communication in marine organisms.
AP Biology Research Topics
71. Investigating the role of specific enzymes in cellular metabolism.
72. Analyzing the genetic variation within a population.
73. Studying the mechanisms of hormonal regulation in animals.
74. Investigating the principles of Mendelian genetics through trait analysis.
75. Analyzing the ecological succession in a local ecosystem.
76. Studying the physiology of the human circulatory system.
77. Investigating the molecular biology of a specific virus.
78. Studying the principles of natural selection through evolutionary simulations.
79. Analyzing the genetic diversity of a plant species in different habitats.
80. Investigating the effects of different environmental factors on plant growth.
Cell Biology Research Topics
81. Investigating the role of mitochondria in cellular energy production.
82. Analyzing the mechanisms of cell division and mitosis.
83. Studying the function of cell membrane proteins in signal transduction.
84. Investigating the cellular processes involved in apoptosis (cell death).
85. Analyzing the role of endoplasmic reticulum in protein synthesis and folding.
86. Studying the dynamics of the cytoskeleton and cell motility.
87. Investigating the regulation of cell cycle checkpoints.
88. Analyzing the structure and function of cellular organelles.
89. Studying the molecular mechanisms of DNA replication and repair.
90. Investigating the impact of cellular stress on cell health and function.
Human Biology Research Topics
91. Analyzing the genetic basis of inherited diseases in humans.
92. Investigating the physiological responses to exercise and physical activity.
93. Studying the hormonal regulation of the human reproductive system.
94. Analyzing the impact of nutrition on human health and metabolism.
95. Investigating the role of the immune system in disease prevention.
96. Studying the genetics of human evolution and migration.
97. Analyzing the neural mechanisms underlying human cognition and behavior.
98. Investigating the molecular basis of aging and age-related diseases.
99. Studying the impact of environmental toxins on human health.
100. Analyzing the genetics of organ transplantation and tissue compatibility.
Molecular Biology Research Topics
101. Investigating the role of microRNAs in gene regulation.
102. Analyzing the molecular basis of genetic disorders like cystic fibrosis.
103. Studying the epigenetic modifications that control gene expression.
104. Investigating the molecular mechanisms of RNA splicing.
105. Analyzing the role of telomeres in cellular aging.
106. Studying the molecular pathways involved in cancer metastasis.
107. Investigating the molecular basis of neurodegenerative diseases.
108. Studying the molecular interactions in protein-protein networks.
109. Analyzing the molecular mechanisms of DNA damage and repair.
110. Investigating the use of CRISPR-Cas9 for genome editing.
Animal Biology Research Topics
111. Studying the behavior and communication of social insects like ants.
112. Analyzing the physiology of hibernation in mammals.
113. Investigating the ecological interactions in a predator-prey relationship.
114. Studying the adaptations of animals to extreme environments.
115. Analyzing the genetics of inherited traits in animal populations.
116. Investigating the impact of climate change on animal migration patterns.
117. Studying the diversity of marine life in coral reef ecosystems.
118. Analyzing the physiology of flight in birds and bats.
119. Investigating the molecular basis of animal coloration and camouflage.
120. Studying the behavior and conservation of endangered species.
- Neuroscience Research Topics
- Mental Health Research Topics
Plant Biology Research Topics
121. Investigating the role of plant hormones in growth and development.
122. Analyzing the genetics of plant resistance to pests and diseases.
123. Climate change and plant phenology are being examined.
124. Investigating the ecology of mycorrhizal fungi and their symbiosis with plants.
125. Investigating plant photosynthesis and carbon fixing.
126. Molecular analysis of plant stress responses.
127. Investigating the adaptation of plants to drought conditions.
128. Studying the role of plants in phytoremediation of polluted environments.
129. Analyzing the genetics of plant hybridization and speciation.
130. Investigating the molecular basis of plant-microbe interactions.
Environmental Biology Research Topics
131. Analyzing the effects of pollution on aquatic ecosystems.
132. Investigating the biodiversity of a particular ecosystem.
133. Studying the ecological consequences of deforestation.
134. Analyzing the impact of climate change on wildlife populations.
135. Investigating the use of bioremediation to clean up polluted sites.
136. Studying the environmental factors influencing species distribution.
137. Analyzing the effects of habitat fragmentation on wildlife.
138. Investigating the ecology of invasive species in new environments.
139. Studying the conservation of endangered species and habitats.
140. Analyzing the interactions between humans and urban ecosystems.
Chemical Biology Research Topics
141. Investigating the design and synthesis of new drug compounds.
142. Analyzing the molecular mechanisms of enzyme catalysis.
143.Studying the role of small molecules in cellular signaling pathways.
144. Investigating the development of chemical probes for biological research.
145. Studying the chemistry of protein-ligand interactions.
146. Analyzing the use of chemical biology in cancer therapy.
147. Investigating the synthesis of bioactive natural products.
148. Studying the role of chemical compounds in microbial interactions.
149. Analyzing the chemistry of DNA-protein interactions.
150. Investigating the chemical basis of drug resistance in pathogens.
Medical Biology Research Topics
151. Investigating the genetic basis of specific diseases like diabetes.
152. Analyzing the mechanisms of drug resistance in bacteria.
153. Studying the molecular mechanisms of autoimmune diseases.
154. Investigating the development of personalized medicine approaches.
155. Studying the role of inflammation in chronic diseases.
156. Analyzing the genetics of rare diseases and genetic syndromes.
157. Investigating the molecular basis of viral infections and vaccines.
158. Studying the mechanisms of organ transplantation and rejection.
159. Analyzing the molecular diagnostics of cancer.
160. Investigating the biology of stem cells and regenerative medicine.
Evolutionary Biology Research Topics
161. Studying the evolution of human ancestors and early hominids.
162. The genetic variety of species and between species is being looked at.
163. Investigating the role of sexual selection in animal evolution.
164. Studying the co-evolutionary relationships between parasites and hosts.
165. Analyzing the evolutionary adaptations of extremophiles.
166. Investigating the evolution of developmental processes (evo-devo).
167. Studying the biogeography and distribution of species.
168. Analyzing the evolution of mimicry in animals and plants.
169. Investigating the genetics of speciation and hybridization.
170. Studying the evolutionary history of domesticated plants and animals.
Cellular Biology Research Topics
171. Investigating the role of autophagy in cellular homeostasis.
172. Analyzing the mechanisms of cellular transport and trafficking.
173. Studying the regulation of cell adhesion & migration.
174. Investigating the cellular responses to DNA damage.
175. Analyzing the dynamics of cellular membrane structures.
176. Studying the role of cellular organelles in lipid metabolism.
177. Investigating the molecular mechanisms of cell-cell communication.
178. Studying the physiology of cellular respiration and energy production.
179. Analyzing the cellular mechanisms of viral entry and replication.
180. Investigating the role of cellular senescence in aging and disease.
Good Biology Research Topics Related To Brain Injuries
181. Analyzing the molecular mechanisms of traumatic brain injury.
182. Investigating the role of neuroinflammation in brain injury recovery.
183. Studying the impact of concussions on long-term brain health.
184. Analyzing the use of neuroimaging in diagnosing brain injuries.
185. Investigating the development of neuroprotective therapies.
186. Studying the genetics of susceptibility to brain injuries.
187. Analyzing the cognitive and behavioral effects of brain trauma.
188. Investigating the role of rehabilitation in brain injury recovery.
189. Studying the cellular and molecular changes in axonal injury.
190. Looking into how stem cell therapy might be used to help brain injuries.
Biology Quantitative Research Topics
191. Investigating the mathematical modeling of population dynamics.
192. Analyzing the statistical methods for biodiversity assessment.
193. Studying the use of bioinformatics in genomics research.
194. Investigating the quantitative analysis of gene expression data.
195. Studying the mathematical modeling of enzyme kinetics.
196. Analyzing the statistical approaches for epidemiological studies.
197. Investigating the use of computational tools in phylogenetics.
198. Studying the mathematical modeling of ecological systems.
199. Analyzing the quantitative analysis of protein-protein interactions.
200. Investigating the statistical methods for analyzing genetic variation.
Importance Of Choosing The Right Biology Research Topics
Here are some importance of choosing the right biology research topics:
1. Relevance to Your Interests and Goals
Choosing the right biology research topic is important because it should align with your interests and goals. Studying something you’re passionate about keeps you motivated and dedicated to your research.
2. Contribution to Scientific Knowledge
Your research should contribute something valuable to the world of science. Picking the right topic means you have the chance to discover something new or solve a problem, advancing our understanding of the natural world.
3. Availability of Resources
Consider the resources you have or can access. If you pick a topic that demands resources you don’t have, your research may hit a dead end. Choosing wisely means you can work efficiently.
4. Feasibility and Manageability
A good research topic should be manageable within your time frame and capabilities. If it’s too broad or complex, you might get overwhelmed. Picking the right topic ensures your research is doable.
5. Real-World Impact
Think about how your research might benefit the real world. Biology often has implications for health, the environment, or society. Choosing a topic with practical applications can make your work meaningful and potentially change lives.
Resources For Finding Biology Research Topics
There are numerous resources for finding biology research topics:
1. Online Databases
Look on websites like PubMed and Google Scholar. They have lots of biology articles. Type words about what you like to find topics.
2. Academic Journals
Check biology magazines. They talk about new research. You can find ideas and see what’s important.
3. University Websites
Colleges show what their teachers study. Find teachers who like what you like. Ask them about ideas for your own study.
4. Science News and Magazines
Read science news. They tell you about new things in biology. It helps you think of research ideas.
5. Join Biology Forums and Communities
Talk to other people who like biology online. You can ask for ideas and find friends to help you. Use websites like ResearchGate and Reddit for this.
Conclusion
Biology Research Topics offer exciting opportunities for exploration and learning. We’ve explained what biology is and stressed the importance of picking a good research topic. Our tips and extensive list of over 200 biology research topics provide valuable guidance for students.
Selecting the right topic is more than just getting good grades; it’s about making meaningful contributions to our understanding of life. We’ve also shared resources to help you discover even more topics. So, embrace the world of biology research, embark on a journey of discovery, and be part of the ongoing effort to unravel the mysteries of the natural world.
Related Posts
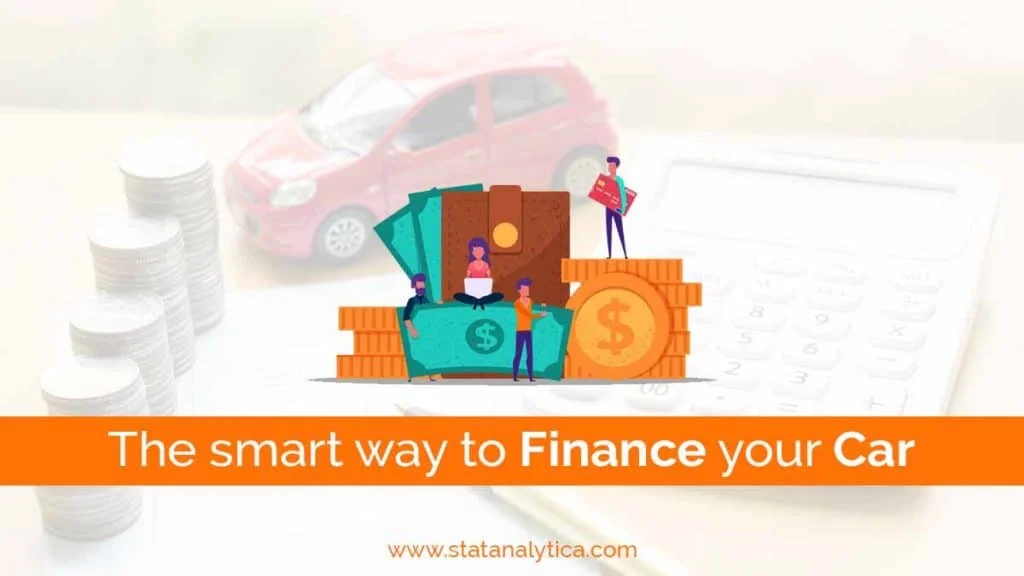
Step by Step Guide on The Best Way to Finance Car
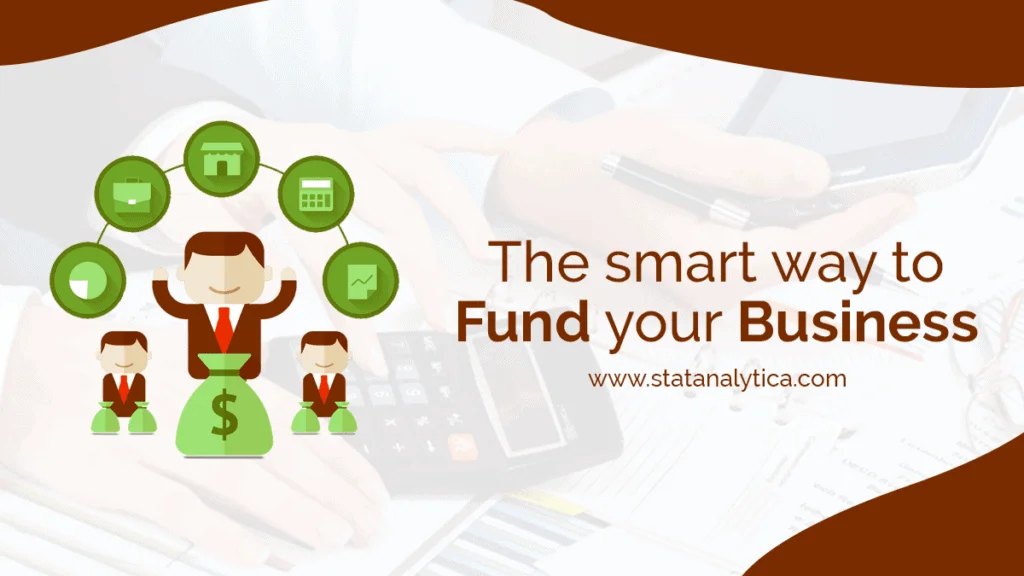
The Best Way on How to Get Fund For Business to Grow it Efficiently
- How it works
Published by Robert Bruce at August 29th, 2023 , Revised On September 5, 2023
Biology Research Topics
Are you in need of captivating and achievable research topics within the field of biology? Your quest for the best biology topics ends right here as this article furnishes you with 100 distinctive and original concepts for biology research, laying the groundwork for your research endeavor.
Table of Contents
Our proficient researchers have thoughtfully curated these biology research themes, considering the substantial body of literature accessible and the prevailing gaps in research.
Should none of these topics elicit enthusiasm, our specialists are equally capable of proposing tailor-made research ideas in biology, finely tuned to cater to your requirements.
Thus, without further delay, we present our compilation of biology research topics crafted to accommodate students and researchers.
Research Topics in Marine Biology
- Impact of climate change on coral reef ecosystems.
- Biodiversity and adaptation of deep-sea organisms.
- Effects of pollution on marine life and ecosystems.
- Role of marine protected areas in conserving biodiversity.
- Microplastics in marine environments: sources, impacts, and mitigation.
Biological Anthropology Research Topics
- Evolutionary implications of early human migration patterns.
- Genetic and environmental factors influencing human height variation.
- Cultural evolution and its impact on human societies.
- Paleoanthropological insights into human dietary adaptations.
- Genetic diversity and population history of indigenous communities.
Biological Psychology Research Topics
- Neurobiological basis of addiction and its treatment.
- Impact of stress on brain structure and function.
- Genetic and environmental influences on mental health disorders.
- Neural mechanisms underlying emotions and emotional regulation.
- Role of the gut-brain axis in psychological well-being.
Cancer Biology Research Topics
- Targeted therapies in precision cancer medicine.
- Tumor microenvironment and its influence on cancer progression.
- Epigenetic modifications in cancer development and therapy.
- Immune checkpoint inhibitors and their role in cancer immunotherapy.
- Early detection and diagnosis strategies for various types of cancer.
Also read: Cancer research topics
Cell Biology Research Topics
- Mechanisms of autophagy and its implications in health and disease.
- Intracellular transport and organelle dynamics in cell function.
- Role of cell signaling pathways in cellular response to external stimuli.
- Cell cycle regulation and its relevance to cancer development.
- Cellular mechanisms of apoptosis and programmed cell death.
Developmental Biology Research Topics
- Genetic and molecular basis of limb development in vertebrates.
- Evolution of embryonic development and its impact on morphological diversity.
- Stem cell therapy and regenerative medicine approaches.
- Mechanisms of organogenesis and tissue regeneration in animals.
- Role of non-coding RNAs in developmental processes.
Also read: Education research topics
Human Biology Research Topics
- Genetic factors influencing susceptibility to infectious diseases.
- Human microbiome and its impact on health and disease.
- Genetic basis of rare and common human diseases.
- Genetic and environmental factors contributing to aging.
- Impact of lifestyle and diet on human health and longevity.
Molecular Biology Research Topics
- CRISPR-Cas gene editing technology and its applications.
- Non-coding RNAs as regulators of gene expression.
- Role of epigenetics in gene regulation and disease.
- Mechanisms of DNA repair and genome stability.
- Molecular basis of cellular metabolism and energy production.
Research Topics in Biology for Undergraduates
- 41. Investigating the effects of pollutants on local plant species.
- Microbial diversity and ecosystem functioning in a specific habitat.
- Understanding the genetics of antibiotic resistance in bacteria.
- Impact of urbanization on bird populations and biodiversity.
- Investigating the role of pheromones in insect communication.
Synthetic Biology Research Topics
- Design and construction of synthetic biological circuits.
- Synthetic biology applications in biofuel production.
- Ethical considerations in synthetic biology research and applications.
- Synthetic biology approaches to engineering novel enzymes.
- Creating synthetic organisms with modified functions and capabilities.
Animal Biology Research Topics
- Evolution of mating behaviors in animal species.
- Genetic basis of color variation in butterfly wings.
- Impact of habitat fragmentation on amphibian populations.
- Behavior and communication in social insect colonies.
- Adaptations of marine mammals to aquatic environments.
Also read: Nursing research topics
Best Biology Research Topics
- Unraveling the mysteries of circadian rhythms in organisms.
- Investigating the ecological significance of cryptic coloration.
- Evolution of venomous animals and their prey.
- The role of endosymbiosis in the evolution of eukaryotic cells.
- Exploring the potential of extremophiles in biotechnology.
Biological Psychology Research Paper Topics
- Neurobiological mechanisms underlying memory formation.
- Impact of sleep disorders on cognitive function and mental health.
- Biological basis of personality traits and behavior.
- Neural correlates of emotions and emotional disorders.
- Role of neuroplasticity in brain recovery after injury.
Biological Science Research Topics:
- Role of gut microbiota in immune system development.
- Molecular mechanisms of gene regulation during development.
- Impact of climate change on insect population dynamics.
- Genetic basis of neurodegenerative diseases like Alzheimer’s.
- Evolutionary relationships among vertebrate species based on DNA analysis.
Biology Education Research Topics
- Effectiveness of inquiry-based learning in biology classrooms.
- Assessing the impact of virtual labs on student understanding of biology concepts.
- Gender disparities in science education and strategies for closing the gap.
- Role of outdoor education in enhancing students’ ecological awareness.
- Integrating technology in biology education: challenges and opportunities.
Biology-Related Research Topics
- The intersection of ecology and economics in conservation planning.
- Molecular basis of antibiotic resistance in pathogenic bacteria.
- Implications of genetic modification of crops for food security.
- Evolutionary perspectives on cooperation and altruism in animal behavior.
- Environmental impacts of genetically modified organisms (GMOs).
Biology Research Proposal Topics
- Investigating the role of microRNAs in cancer progression.
- Exploring the effects of pollution on aquatic biodiversity.
- Developing a gene therapy approach for a genetic disorder.
- Assessing the potential of natural compounds as anti-inflammatory agents.
- Studying the molecular basis of cellular senescence and aging.
Biology Research Topic Ideas
- Role of pheromones in insect mate selection and behavior.
- Investigating the molecular basis of neurodevelopmental disorders.
- Impact of climate change on plant-pollinator interactions.
- Genetic diversity and conservation of endangered species.
- Evolutionary patterns in mimicry and camouflage in organisms.
Biology Research Topics for Undergraduates
- Effects of different fertilizers on plant growth and soil health.
- Investigating the biodiversity of a local freshwater ecosystem.
- Evolutionary origins of a specific animal adaptation.
- Genetic diversity and disease susceptibility in human populations.
- Role of specific genes in regulating the immune response.
Cell and Molecular Biology Research Topics
- Molecular mechanisms of DNA replication and repair.
- Role of microRNAs in post-transcriptional gene regulation.
- Investigating the cell cycle and its control mechanisms.
- Molecular basis of mitochondrial diseases and therapies.
- Cellular responses to oxidative stress and their implications in ageing.
These topics cover a broad range of subjects within biology, offering plenty of options for research projects. Remember that you can further refine these topics based on your specific interests and research goals.
Frequently Asked Questions
What are some good research topics in biology?
A good research topic in biology will address a specific problem in any of the several areas of biology, such as marine biology, molecular biology, cellular biology, animal biology, or cancer biology.
A topic that enables you to investigate a problem in any area of biology will help you make a meaningful contribution.
How to choose a research topic in biology?
Choosing a research topic in biology is simple.
Follow the steps:
- Generate potential topics.
- Consider your areas of knowledge and personal passions.
- Conduct a thorough review of existing literature.
- Evaluate the practicality and viability.
- Narrow down and refine your research query.
- Remain receptive to new ideas and suggestions.
Who Are We?
For several years, Research Prospect has been offering students around the globe complimentary research topic suggestions. We aim to assist students in choosing a research topic that is both suitable and feasible for their project, leading to the attainment of their desired grades. Explore how our services, including research proposal writing , dissertation outline creation, and comprehensive thesis writing , can contribute to your college’s success.
You May Also Like
A preliminary literature review is an initial exploration of existing research on a topic, setting the foundation for in-depth study.
Learn how to write a finance thesis and more than 30 finance thesis topics to choose from. Start your research with the help of our guide.
Want to score band 9 on your IELTS essay? Here are some tips and IELTS sample essays to help you get the score you want.
Ready to place an order?
USEFUL LINKS
Learning resources, company details.
- How It Works
Automated page speed optimizations for fast site performance
Quantitative Biology Research Topics Ideas [MS-PhD]
Research Area/ Research Interest: Quantitative Biology
Research Paper Topics for Masters and Ph.D. Thesis and publication
1. The impact of integrated STEM modeling on elementary preservice teachers’ self‐efficacy for integrated STEM instruction: A co‐teaching approach
2. Desynchronization of jammed oscillators by avalanches 3. Ten simple rules for tackling your first mathematical models: A guide for graduate students by graduate students 4. Novel quantitative structure–activity relationship model to predict activities of natural products against COVID‐19 5. Quantitative genetics of extreme insular dwarfing: The case of red deer on Jersey 6. On the Edge-version of Topological Indices of Titanium Dioxide Nanotube and Nanosheet 7. Temporal Systematics 8. Quantitative determination of tip undercooling of faceted sea ice with in situ experiments 9. The genetic architecture of behavioral traits in a spider 10. A bioturbator, a holobiont, and a vector: The multifaceted role of Chironomus plumosus in shaping N‐cycling 11. A new efficient medical image cipher based on hybrid chaotic map and DNA code
12. Student Perceptions of the Online Introductory Biology Laboratory Curriculum Using a Framework of Vision and Change and its Core Competencies 13. Gender Differences in Graduate Employability: Why They Matter for Math Teacher Education 14. Adding CURE to Traditional Labs: Hands-On Microplastics Research in Freshwater Systems Conducted by First-Year Biology Students 15. Impact of ground cover vegetation types on the diversity and similarity of spider assemblage at two adjacent sites 16. Biozobots: An Investigation into Using Robotics in an Introductory Biology Course. 17. Chemistry and Grit: The Impact of Prerequisites vs Perseverance on Academic Success in Biology 18. Can a self‐expanding pediatric stent expand with an artery? Relationship of stent design to vascular biology 19. Activation function dependence of the storage capacity of treelike neural networks 20. System for quantitative evaluation of DAB&H-stained breast cancer biopsy digital images (CHISEL) 21. Rhodococcus comparative genomics reveals a phylogenomic-dependent non-ribosomal peptide synthetase distribution: insights into biosynthetic gene …
22. Math counts: major and gender differences in college mathematics coursework 23. Importance of assessing spatial ability in intellectually talented young adolescents: A 20-year longitudinal study. 24. Quantitative studies on gonad cycle, fecundity, reproductive output and recruitment in a Baltic Mytilus edulis population 25. A maximum entropy approach to species distribution modeling 26. Encouraging Competence in Basic Mathematics in Hydrology using The Math You Need 27. Robust and efficient parameter estimation in dynamic models of biological systems 28. Women’s selection of quantitative undergraduate fields of study: Direct and indirect influences 29. On the mechanism and biology of cytochrome oxidase inhibition by nitric oxide 30. Modeling biomolecular networks in cells: structures and dynamics 31. Making a difference for minorities: Evaluation of an educational enrichment program
32. Prescribed active learning increases performance in introductory biology 33. The power of a good idea: Quantitative modeling of the spread of ideas from epidemiological models 34. Quantitative synthesis of context dependency in ant–plant protection mutualisms 35. Gender differences in abilities and preferences among the gifted: Implications for the math-science pipeline 36. Continuing motivation beyond the high school classroom 37. Mathematical modeling of cancer: the future of prognosis and treatment 38. Math Workout for the GMAT 39. Topology and life: in search of general mathematical principles in biology and sociology 40. Math Book: Bibliography 41. Stereotype threat and women’s math performance
42. Mathematical modeling in systems biology: an introduction 43. UNDERGRADUATE SCIENCE AND MATH SYMPOSIUM 44. Antibodies exhibit multiple paratope states influencing VH–VL domain orientations 45. Overcoming math anxiety 46. Global systems biology, personalized medicine and molecular epidemiology 47. Physical biology of the cell 48. An investigation of the impact of science course sequencing on student performance in high school science and math 49. When seeing isn’t believing: How math can guide our interpretation of measurements and experiments 50. A quantitative approach to the study of cell shapes and interactions during early chordate embryogenesis 51. Explaining the gender gap in math test scores: The role of competition
52. Early experiences and integration in the persistence of first‐generation college students in STEM and non‐STEM majors 53. Statistical issues with microarrays: processing and analysis 54. Exploring and understanding Maryland’s math and science teachers’ perspectives on NCLB and increase testing: Employing a phenomenological inquiry approach 55. Quantitative and logic modelling of molecular and gene networks 56. TEASIng apart alien species risk assessments: a framework for best practices 57. Power of variance component linkage analysis to detect quantitative trait loci 58. Extensive quantitative remodeling of the proteome between normal colon tissue and adenocarcinoma 59. Using math in physics–2. Estimation 60. Quantitative genetic properties of four measures of deformity in yellowtail kingfish Seriola lalandi Valenciennes, 1833 61. Simultaneous mapping of filamentous actin flow and turnover in migrating cells by quantitative fluorescent speckle microscopy
62. From plant traits to plant communities: a statistical mechanistic approach to biodiversity 63. Beneath the numbers: A review of gender disparities in undergraduate education across science, technology, engineering, and math disciplines 64. Math self-efficacy and the likelihood of pursuing a STEM-based career: a gender-based analysis 65. The function of dendritic spines: a review of theoretical issues 66. Noninvasive functional optical spectroscopy of human breast tissue 67. A Gateway to Opportunities 68. Regulation of gene expression by small non‐coding RNAs: a quantitative view 69. A Letter from the Guest Editors 70. Robustness analysis and tuning of synthetic gene networks 71. Animal groups in three dimensions: how species aggregate
72. Multiscale modeling in biology: New insights into cancer illustrate how mathematical tools are enhancing the understanding of life from the smallest scale to the … 73. Active learning narrows achievement gaps for underrepresented students in undergraduate science, technology, engineering, and math 74. Scaling metagenome sequence assembly with probabilistic de Bruijn graphs 75. Advanced placement math and science courses: Influential factors and predictors for success in college STEM majors 76. Suppression of mutations in the alkaline phosphatase structural cistron of E. coli 77. Feature point tracking and trajectory analysis for video imaging in cell biology 78. Quantitative localized 1H MR spectroscopy for clinical use 79. Simplified equation to extract diffusion coefficients from confocal FRAP data 80. Chlorophylls and carotenoids: Measurement and characterization by UV‐VIS spectroscopy 81. Controlled vocabularies and semantics in systems biology
82. A method for detecting modules in quantitative bipartite networks 83. Deep learning automates the quantitative analysis of individual cells in live-cell imaging experiments 84. Measuring Attitude Towards Chemistry, Biology, and Math at a Hispanic-Serving Institution 85. Quantitative analysis of culture using millions of digitized books 86. A quantitative genetic model for growth, shape, reaction norms, and other infinite-dimensional characters 87. Evolving a lingua franca and associated software infrastructure for computational systems biology: the Systems Biology Markup Language (SBML) project 88. Anniversary Paper: History and status of CAD and quantitative image analysis: The role of Medical Physics and AAPM 89. Receptors: models for binding, trafficking, and signaling 90. A quantitative measure of migratory connectivity 91. Admission test analysis of pre-service math-science teacher based on its related factors
92. Systems biology and the virtual physiological human 93. Non-graduate teacher recruitment and retention: some factors affecting teacher effectiveness in Tanzania 94. On the changing concept of evolutionary population stability as a reflection of a changing point of view in the quantitative theory of evolution 95. The MicroAnalysis Toolkit: X‐ray fluorescence image processing software 96. Risk analysis of complex and uncertain systems 97. The evolution of protein structures 98. Phenotypic diversity and ecosystem functioning in changing environments: a theoretical framework 99. Modeling transcriptional control in gene networks—methods, recent results, and future directions 100. Commentary: Carcinogenesis as Darwinian evolution? Do the math! 101. A quantitative trait locus associated with cognitive ability in children
102. Bayesian methods in bioinformatics and computational systems biology 103. Integrative mathematical oncology 104. Contributed Session 04: Modelling and Math Biology 105. Connecting mathematics and science: A learning community that helps math-phobic students 106. A quantitative map of the circuit of cat primary visual cortex 107. Homework and students’ achievement in math and science: A 30-year meta-analysis, 1986–2015 108. Math literacy in American schools and society: the accessibility and promotion of math education 109. The case for an error minimizing standard genetic code 110. Neutral additive genetic variance in a metapopulation 111. Supporting girls’ and boys’ engagement in math and science learning: A mixed methods study
112. Local graph alignment and motif search in biological networks 113. FragSeq: transcriptome-wide RNA structure probing using high-throughput sequencing 114. Metabolic network reconstruction of Chlamydomonas offers insight into light‐driven algal metabolism 115. MATH COUNTS Computing for learning math and other disciplines 116. Fractal physiology for physicists: Lévy statistics 117. Minimum information requested in the annotation of biochemical models (MIRIAM) 118. The golden age of theoretical ecology: 1923–1940 119. Mechanics of DNA packaging in viruses 120. Machine learning methods for quantitative radiomic biomarkers 121. Strategies for the physiome project
122. Quantitative measurement of microvascular permeability in human brain tumors achieved using dynamic contrast-enhanced MR imaging: correlation with histologic … 123. Stochastic evolutionary differential games toward a systems theory of behavioral social dynamics 124. The teaching practices inventory: A new tool for characterizing college and university teaching in mathematics and science 125. Randomization, bootstrap and Monte Carlo methods in biology 126. Exploring the inhibitory effect of membrane tension on cell polarization 127. Measuring individual‐level resource specialization 128. Math matters 129. Wavelets: a mathematical tool for signal analysis 130. Classification of biological networks by their qualitative dynamics 131. A perspective on systems analysis in crop production and insect pest management
132. Asymptotic behavior of Markov systems 133. NSF: New Study on Science, Math Teachers in High Schools Focuses on Their Education, Assignment 134. Resolution enhancement techniques in microscopy 135. Predictors of high academic achievement in mathematics and science by mathematically talented students: A longitudinal study. 136. Using qualitative methods to develop a survey measure of math and science engagement 137. The effects of self-efficacy beliefs and metacognition on academic performance: A mixed method study 138. A specific complement-fixing antigen present in SV40 tumor and transformed cells 139. “But I’m not good at math”: The changing salience of mathematical self-concept in shaping women’s and men’s STEM aspirations 140. Gender and course selection in upper secondary education: Effects of academic self-concept and intrinsic value 141. Sex, Math and Scientific Achievement: Why do men dominate the fields of science, engineering and mathematics?
142. Optimality principles in biology 143. Evaluation of an iterative reconstruction method for quantitative elastography 144. Time without end: Physics and biology in an open universe 145. Quantifying absolute protein synthesis rates reveals principles underlying allocation of cellular resources 146. Div. of Applied Math Div. of Biology and Dept. of Mathematics Brown University Medicine Southern Methodist Univ. 147. Anatomy of the normal left atrial appendage: a quantitative study of age-related changes in 500 autopsy hearts: implications for echocardiographic examination 148. Numerical simulation of blowup solutions A white paper submitted to the 2017 DOE ASCR Applied Math Meeting addressing Question 2 (a) 149. Middle school students’ attitudes toward pursuing careers in science, technology, engineering, and math 150. Matrices and graphs: stability problems in mathematical ecology 151. Identification of nucleocytoplasmic cycling as a remote sensor in cellular signaling by databased modeling
152. The relationship among student achievement scores on the math and science end-of-course-tests and scores on the high school graduation test 153. Clinical quantitative susceptibility mapping (QSM): Biometal imaging and its emerging roles in patient care 154. Numeracy: The new literacy for a data-drenched society 155. The role of Socratic questioning in thinking, teaching, and learning 156. Quantitative descriptions of development 157. Evaluation of Teacher Professional Development Mix it Up: Correlated Science and Math Instructional Model 158. Enhancing Math and Science Education with Graphing Technology: Integrating Technology into Preservice Methods Courses 159. Population biology, evolution, and infectious disease: convergence and synthesis 160. Numbers and Math are Nice, but… 161. Finding cycles in synchronous Boolean networks with applications to biochemical systems
162. Ergodic theorems in demography 163. The effectiveness of games for educational purposes: A review of recent research 164. The effects of spontaneous mutation on quantitative traits. I. Variances and covariances of life history traits. 165. Quantitative metrics of net proliferation and invasion link biological aggressiveness assessed by MRI with hypoxia assessed by FMISO-PET in newly diagnosed … 166. Statistical significance of variables driving systematic variation in high-dimensional data 167. A simple model for phase locking of biological oscillators 168. Math and science attitudes and achievement at the intersection of gender and ethnicity 169. Digital literacy skills of math students through e-learning in COVID-19 era: a case study in Universitas Riau 170. How does clickthrough data reflect retrieval quality? 171. Understanding systems-level properties: timely stories from the study of clocks
172. Some new directions in control theory inspired by systems biology 173. Integrating Math & Computer Skills in the Biology Classroom: An Example using Spreadsheet Simulations to Teach Fundamental Sampling Concepts 174. Biophysics for beginners: a journey through the cell nucleus 175. Quantitative analysis of cell-free Epstein-Barr virus DNA in plasma of patients with nasopharyngeal carcinoma 176. Rarefaction, relative abundance, and diversity of avian communities 177. PESTO: parameter estimation toolbox 178. Virtual Cell modelling and simulation software environment 179. Mathematical modeling of gene expression: a guide for the perplexed biologist 180. Selection and phenotypic plasticity in evolutionary biology and animal breeding 181. Critical exponents for a nonlinear reaction-diffusion system with fractional derivatives
182. Molecular driving forces: statistical thermodynamics in biology, chemistry, physics, and nanoscience 183. NetMHCpan, a Method for Quantitative Predictions of Peptide Binding to Any HLA-A and -B Locus Protein of Known Sequence 184. When do gender stereotypes impair math performance? A study of stereotype threat among Ugandan adolescents 185. Microfluidics for mammalian cell chemotaxis 186. Improving the phenotype predictions of a yeast genome‐scale metabolic model by incorporating enzymatic constraints 187. Quantitative fatty acid signature analysis: a new method of estimating predator diets 188. Sequence‐specific determination of protein and peptide concentrations by absorbance at 205 nm 189. Culturally Based Math Education as a Way to Improve Alaska Native Students’ Math Performance. 190. Mathematical models for infectious disease statistics 191. Structural and immunohistological modifications in olfactory bulb of the staggerer mutant mouse
192. The engineering of gene regulatory networks 193. Math and Leaves 194. Traveling the road to success: A discourse on persistence throughout the science pipeline with African American students at a predominantly white institution 195. Noise in biology 196. Update on brain tumor imaging: from anatomy to physiology 197. Molecular systems biology and control 198. Sex differences in visual-spatial ability: The role of performance factors 199. The quantitative limitations of exponential curve fitting 200. Teaching introductory STEM with the Marble Game 201. FEATURE ARTICLE Integrating Math & Computer Skills in the Biology Classroom: An Example Using Spreadsheet Simulations to Teach Fundamental Sampling …
202. Emerging trends in peer review—a survey 203. Possible global minimum lattice configurations for Thomson’s problem of charges on a sphere 204. Dynamic modelling and analysis of biochemical networks: mechanism-based models and model-based experiments 205. The approximate number system and its relation to early math achievement: Evidence from the preschool years 206. Identification and fine mapping of quantitative trait loci for seed vigor in germination and seedling establishment in rice 207. Student-driven research at the mathematical and theoretical biology institute 208. The relationships among high school STEM learning experiences and students’ intent to declare and declaration of a STEM major in college 209. Quantitative clinical radiobiology of early and late lung reactions 210. The math gender gap: The role of culture 211. How to quantify the temporal storage effect using simulations instead of math
212. Dynamic energy budget theory restores coherence in biology 213. Implicit theories of intelligence and academic achievement: A meta-analytic review 214. Metapopulation theory for fragmented landscapes 215. A critical assessment of Levins’s The strategy of model building in population biology (1966) 216. Metabolomics and systems biology: making sense of the soup 217. Emotional presence in a relationship of inquiry: The case of one-to-one online math coaching. 218. Effects of molecular memory and bursting on fluctuations in gene expression 219. Math, magnets, and medicine: Enabling personalized oncology 220. Bacterial growth laws and their applications 221. Emergent cooperation in microbial metabolism
222. Minimizing math anxiety: Help for the sciences 223. BioModels database: a repository of mathematical models of biological processes 224. Quantitative human physiology: an introduction 225. Mathematical and computational challenges in population biology and ecosystems science 226. Serine synthesis through PHGDH coordinates nucleotide levels by maintaining central carbon metabolism 227. A methodology for performing global uncertainty and sensitivity analysis in systems biology 228. Modeling approaches for qualitative and semi-quantitative analysis of cellular signaling networks 229. Qualitative and quantitative risk assessment 230. The relationship between geodiversity and habitat richness in Šumava. National Park and Křivoklátsko Pla (Czech Republic): a quantitative analysis approach 231. Advancing the cancer genome atlas glioma MRI collections with expert segmentation labels and radiomic features
232. The application of statistical physics to evolutionary biology 233. Connecting math achievement to parental influences 234. Quantitative drug design: a critical introduction 235. Student characteristics, pre-college, college, and environmental factors as predictors of majoring in and earning a STEM degree: An analysis of students attending a … 236. An evolutionary model of carcinogenesis 237. Structural imprints in vivo decode RNA regulatory mechanisms 238. On cellular automaton approaches to modeling biological cells 239. Alleviating math anxiety in students 240. Quantitative analysis of a molecular model of mitotic control in fission yeast 241. Low Mutant Allele Tumor Heterogeneity (MATH) is Associated with Activation of the Immune Response and Improved Survival of Breast Cancer Patients
242. Quantitative phase imaging in biomedicine 243. PLABQTL: a program for composite interval mapping of QTL 244. Using Whole-Genome Sequence Data to Predict Quantitative Trait Phenotypes in Drosophila melanogaster 245. TRANSDISCIPLINARY PREPARATION OF PRESERVICE SECONDARY MATH AND SCIENCE TEACHERS 246. Reverse engineering and identification in systems biology: strategies, perspectives and challenges 247. Boolean modeling in systems biology: an overview of methodology and applications 248. Gypsy moth invasion in North America: a quantitative analysis 249. Functional mapping of quantitative trait loci underlying the character process: a theoretical framework 250. Math, the Genetic Code and Living Organisms 251. A quantitative characterization of the yeast heterotrimeric G protein cycle
252. A review of state-of-the-art stereology for better quantitative 3D morphology in cardiac research 253. Network ‘small-world-ness’: a quantitative method for determining canonical network equivalence 254. Quantitative analysis of fiddler crab flock movement: evidence for ‘selfish herd’behaviour 255. Individuals’ math and science motivation and their subsequent STEM choices and achievement in high school and college: A longitudinal study of gender and college … 256. An invitation to biomathematics 257. Complex networks and simple models in biology 258. Patterns of phase compromise in biological cycles 259. A QSPR study of hydrophobicity of phenols and 2-(aryloxy-α-acetyl)-phenoxathiin derivatives using the topological index ZEP
260. Quantitative kinetic analysis of the bacteriophage λ genetic network 261. Microfluidics-based systems biology 262. Quantitative phase imaging for medical diagnosis 263. A beautiful math: John Nash, game theory, and the modern quest for a code of nature 264. Probabilistic Boolean networks: the modeling and control of gene regulatory networks 265. Bayesian integrated testing strategy (ITS) for skin sensitization potency assessment: a decision support system for quantitative weight of evidence and adaptive testing … 266. Instructional systems of practice: A multidimensional analysis of math and science undergraduate course planning and classroom teaching 267. Quantitative analysis of complex glioma cell migration on electrospun polycaprolactone using time-lapse microscopy 268. Of mice and math: A systems biology model for Alzheimer’s disease 269. Intuitive conceptions of probability and the development of basic math skills
270. Chinese parents’ effect on children’s math and science achievements in schools with different SES 271. Carbon input by roots into the soil: quantification of rhizodeposition from root to ecosystem scale 272. Enhanced brain connectivity in math-gifted adolescents: An fMRI study using mental rotation 273. Reflections on math reforms in the US: A cross-national perspective 274. “It’s Wrong to Exclude Girls From Something They Love.” Adolescents’ Attitudes About Sexism in Science, Technology, Engineering, and Math 275. Adding new dimensions to case study evaluations: The case of evaluating comprehensive reforms 276. Math I Benchmark Testing: Friend or Foe? 277. The genetics of human populations 278. Clinical utility of Stewart’s method in diagnosis and management of acid-base disorders 279. Mechanism of action of colicines
280. A novel multiple subdivision-based algorithm for quantitative assessment of retinal vascular tortuosity 281. The influence of quantitative and qualitative aspects of habitat complexity in tropical sea-grass meadows 282. Georgia’s higher education initiatives focus on graduation: All our initiatives are working in one direction; they are focused on increasing graduation numbers 283. Implications for teacher supply and methodological lessons for research 284. Quantitative Fourier analysis of approximation techniques. I. Interpolators and projectors 285. TPT NOTES: Interdepartmental Team Teaching—A Physics-Math Course 286. A cell-centered approach to developmental biology 287. Application of regression techniques to studies of relative growth in crustaceans 288. The relationship between the volume of antimicrobial consumption in human communities and the frequency of resistance 289. Short technical report processing of gene expression data generated by quantitative real-time RT-PCR
290. The math myth: And other STEM delusions 291. The origin of universal scaling laws in biology 292. Not just math and English: Courses that pose obstacles to community college completion 293. Modeling and simulation of molecular biology systems using petri nets: modeling goals of various approaches 294. Moment-closure approximations for mass-action models 295. Gender Issues and the Math/Science Curricula: Effects on Females. 296. Computational improvements reveal great bacterial diversity and high metal toxicity in soil 297. Using advance organizers to enhance students’ motivation in learning biology 298. Math j Bio 299. SPECIAL ACADEMIC OFFERINGS
300. Quantitative proteomic analysis reveals induction of premature senescence in human umbilical vein endothelial cells exposed to chronic low‐dose rate gamma … 301. Estimating quantitative genetic parameters in wild populations: a comparison of pedigree and genomic approaches 302. Computer applications to biochemical kinetics 303. CellProfiler 3.0: Next-generation image processing for biology 304. New quantitative descriptors of amino acids based on multidimensional scaling of a large number of physical–chemical properties 305. Pervasive robustness in biological systems 306. The mediating role of number-to-magnitude mapping precision in the relationship between approximate number sense and math achievement depends on the … 307. Mathematics of cell motility: have we got its number? 308. Math 132 Calculus Lab Manual 309. Using a neural network to identify secondary RNA structures quantified by graphical invariants
310. Evolution of phenotypic plasticity in colonizing species 311. Prospective and retrospective perturbation analyses: their roles in conservation biology 312. Mechanical behavior in living cells consistent with the tensegrity model 313. When approximate number acuity predicts math performance: The moderating role of math anxiety 314. Evaluating the Relationships Between Science Class Success and Math Placement in High School 315. INTRODUCING BUSINESS EDUCATION IN EARLY MIDDLE SCHOOL–MATH PERSPECTIVE 316. Structural and practical identifiability analysis of partially observed dynamical models by exploiting the profile likelihood 317. A practitioner’s perspective of the role of quantitative structure-activity analysis in medicinal chemistry 318. Addressing the “math and science deficit” in Muslim learning 319. Do faculty serve as role models? The impact of instructor gender on female students
320. Mechanism of topology simplification by type II DNA topoisomerases 321. Quantitative studies of the structure of proteins in solution by Fourier-transform infrared spectroscopy 322. MATH/BTB CRL3 receptors target the homeodomain-leucine zipper ATHB6 to modulate abscisic acid signaling 323. Quantification of skeletal muscle mitochondrial function by 31P magnetic resonance spectroscopy techniques: a quantitative review 324. Delivery of Alkaline Phosphatase Promotes Periodontal Regeneration in Mice 325. Efficiency of DNA replication in the polymerase chain reaction 326. PTH and vitamin D repress DMP1 in cementoblasts 327. Stochastic modelling for systems biology 328. The strength of indirect selection on female mating preferences 329. Does anxiety in science classrooms impair math and science motivation?-Gender differences beyond the mean level
330. Algebraic statistics for computational biology 331. Math| Bio 332. Mathematical modeling of endocytic actin patch kinetics in fission yeast: disassembly requires release of actin filament fragments 333. Modeling a synthetic multicellular clock: repressilators coupled by quorum sensing 334. Quantitative cell-free DNA, KRAS, and BRAF mutations in plasma from patients with metastatic colorectal cancer during treatment with cetuximab and irinotecan 335. Acceleration across California: Shorter pathways in developmental English and math 336. Teacher education that works: Preparing secondary-level math and science teachers for success with English language learners through content-based instruction 337. Invited review: Integrating quantitative findings from multiple studies using mixed model methodology 338. Sampling bias is a challenge for quantifying specialization and network structure: lessons from a quantitative niche model 339. Forecasting extinction risk of ectotherms under climate warming: an evolutionary perspective
340. Platform for Math Learning with Audio-Tactile Graphics for Visually Impaired Students 341. Boys+ girls+ math 342. On growth and form 343. Evidence for complex, collective dynamics and emergent, distributed computation in plants 344. Why Our Kids Don’t Get Math 345. The First Summer Undergraduate Research Program at Clayton State University 346. Conformation gating as a mechanism for enzyme specificity 347. Exploring the Relationship Between Math Anxiety, Working Memory, and Experiences 348. Variation and selection of quantitative traits in plant pathogens 349. Estimating hydration changes upon biomolecular reactions from osmotic stress, high pressure, and preferential hydration experiments
350. Stochastic processes in cell biology 351. Excitation dynamics: insights from simplified membrane models 352. Domestication quantitative trait loci in Triticum dicoccoides, the progenitor of wheat 353. A simple regression method for mapping quantitative trait loci in line crosses using flanking markers 354. The effect of SUV discretization in quantitative FDG-PET Radiomics: the need for standardized methodology in tumor texture analysis 355. The effects of math and math-related courses in high school 356. Multi-scale modelling and simulation in systems biology 357. Vesicle pools and Ca2+ microdomains: new tools for understanding their roles in neurotransmitter release. 358. Quantitative modelling of DNA damage using Monte Carlo track structure method 359. A study of persistence of undergraduate women majoring in engineering and math
360. Patterns of quantitative genetic variation in multiple dimensions 361. College-readiness in math: A conceptual analysis of the literature 362. Association between individual differences in non-symbolic number acuity and math performance: A meta-analysis 363. Free fitness that always increases in evolution 364. A decision analytic model to guide early‐stage government regulatory action: Applications for synthetic biology 365. Up hill, down dale: quantitative genetics of curvaceous traits 366. Comparison of microfluidic digital PCR and conventional quantitative PCR for measuring copy number variation 367. Science education as conceptual change 368. Teachers’ emotions and emotion regulation strategies: Self-and students’ perceptions 369. ConfChem conference on mathematics in undergraduate chemistry instruction: MUST-know pilot study—math preparation study from Texas
370. Quantitative image analysis of cellular heterogeneity in breast tumors complements genomic profiling 371. Conductivity tensor mapping of the human brain using diffusion tensor MRI 372. The mutualism–parasitism continuum in ectomycorrhizas: a quantitative assessment using meta‐analysis 373. Hands-on biology: A museum-school-university partnership for enhancing students’ interest and learning in science 374. Natural selection and quantitative genetics of life‐history traits in western women: A twin study 375. Negotiating the “White male math myth”: African American male students and success in school mathematics 376. Quantitative analysis of dose-effect relationships: the combined effects of multiple drugs or enzyme inhibitors 377. Sex differences in college major 378. Modeling theory for math and science education 379. Math Hits the Sweet Spot
380. Analysis of Mixtures of Radioactive Isotopes by γ-Ray Measurements. Application of the Method to , , and 381. A comparative study for the quantitative determination of soluble solids content, pH and firmness of pears by Vis/NIR spectroscopy 382. A workflow to process 3D+ time microscopy images of developing organisms and reconstruct their cell lineage 383. Parent–offspring conflict and co-adaptation: behavioural ecology meets quantitative genetics 384. Probabilistic models in cluster analysis 385. Measuring Community College Math Students Self-Report of Learning Engagement when Interactive Whiteboards Are Used in Classroom Teaching 386. Exploring gender differences across elementary, middle, and high school students’ science and math attitudes and interest 387. Mapping of quantitative trait loci for oil content in cottonseed kernel 388. Simple thinking using complex math vs. complex thinking using simple math—A study using model eliciting activities to compare students’ abilities in standardized … 389. How Project-Based Learning promotes 7th grade students’ motivation and attitudes towards studying biology
390. 2 H2 391. 3 H3 Why are computational neuroscience and systems biology so separate? 392. Androgen Regulation of the Pem Homeodomain Gene in Mice and Rat Sertoli and Epididymal Cells 393. Calculation of absolute protein–ligand binding free energy from computer simulations 394. Estimating the genetic architecture of quantitative traits 395. Increased course structure improves performance in introductory biology 396. Critical thinking in higher education: The influence of teaching styles and peer collaboration on science and math learning 397. Lung morphometry: a new generation of tools and experiments for organ, tissue, cell, and molecular biology 398. Olfactory search at high Reynolds number 399. Widespread evidence for incipient ecological speciation: a meta‐analysis of isolation‐by‐ecology 400. Quantification of the hydrophobic interaction by simulations of the aggregation of small hydrophobic solutes in water
401. A theory of how the brain might work 402. Phenotypic switching in gene regulatory networks 403. Effects of straw carbon input on carbon dynamics in agricultural soils: a meta‐analysis 404. Getting under the hood: How and for whom does increasing course structure work? 405. What Fundamental Questions in Cancer Would Most Benefit from Math, Engineering, and AI? 406. Fractional-order delayed predator–prey systems with Holling type-II functional response 407. Neuromodulation and cortical function: modeling the physiological basis of behavior 408. Quantitative analysis of protein-RNA interactions by gel mobility shift 409. Biological physics 410. Just the facts? Introductory undergraduate biology courses focus on low-level cognitive skills
411. Fractal analyses of animal movement: a critique 412. Complex adaptive systems: exploring the known, the unknown and the unknowable 413. Dynamics of cancer progression 414. Using math in physics: Overview 415. Quantitative structure–property relationship modeling of diverse materials properties 416. 23. Case Study: Math and Computer Science Academic Support Services at Bethel University 417. Retention through Community Building: Secondary Science and Math Noyce Scholars’ Use of a Chat Room 418. Building Teachers’ Capacity to Integrate Science and Math Content: Implications for Professional Development and Learning 419. Information Theory in Biology after 18 Years: Information theory must be modified for the description of living things. 420. Mechanics of fibroblast locomotion: quantitative analysis of forces and motions at the leading lamellas of fibroblasts.
421. Why are some STEM fields more gender balanced than others? 422. Quantitative genetics of geometric shape in the mouse mandible 423. An active-learning lesson that targets student understanding of population growth in ecology 424. Math envy 425. Determination of species composition in the Enallagma damselfly assemblages of permanent lakes 426. Measuring lymphocyte proliferation, survival and differentiation using CFSE time-series data 427. Quantitative analysis of multiparametric prostate MR images: differentiation between prostate cancer and normal tissue and correlation with Gleason score—a … 428. MATH 111A (Fall Quarter 2011). Introduction to Mathematical Modelling 429. Understanding and using quantitative genetic variation 430. Systems biology of stem cell fate and cellular reprogramming
431. Recent advances in parameteridentification techniques for ode 432. Bacteriophage-induced mutation in Escherichia coli 433. the nature of both processes and have been ap-practical use of such formulæ in actuarial math 434. The effect of vaccines on backward bifurcation in a fractional order HIV model 435. Quantifying sustainability: Resilience, efficiency and the return of information theory 436. Quantitative analysis of a singularly perturbed shape optimization problem in a polygon 437. Math preparation of undergraduates in general chemistry, a gatekeeper course required for biophysicists 438. Proving Darwin: making biology mathematical 439. What is the total number of protein molecules per cell volume? A call to rethink some published values 440. What dysarthrias can tell us about the neural control of speech 441. Optical imaging techniques in cell biology 442. Men and women at promise for scientific excellence: Similarity not dissimilarity 443. Quantitative general theory for periodic breathing in chronic heart failure and its clinical implications 444. RInSp: an r package for the analysis of individual specialization in resource use 445. Helically chiral polymers: A class of ligands for asymmetric catalysis 446. Why do populations cycle? A synthesis of statistical and mechanistic modeling approaches 447. Integration of science, technology, engineering, and math into a food and nutrition curriculum in Utah 448. Gender, mathematics, and science 449. Signaling text-picture relations in multimedia learning: A comprehensive meta-analysis
450. Identity, critical agency, and engineering: An affective model for predicting engineering as a career choice 451. Using Primary Sources in Math and Science–An Examination of How Engaging, Challenging, and Effective Teachers Rate Lesson Plans 452. Impact of Physics First on PSAE achievement in science and math 453. MR1839210 (2002f: 92031) 92D40 (92D25) 454. Math for Soil Scientists, Mark S. Coyne, James A. Thompson, Thomson Delmar Learning, Clifton Park, NY (2006), US $26.95, 285 pp., ISBN: 0-7668-4268 … 455. Glutamine‐driven oxidative phosphorylation is a major ATP source in transformed mammalian cells in both normoxia and hypoxia 456. An algorithmic framework for predicting side effects of drugs 457. The theoretical population genetics of autopolyploidy 458. Dimensional comparison theory: Paradoxical relations between self-beliefs and achievements in multiple domains 459. The MATH-BTB BPM3 and BPM5 subunits of Cullin3-RING E3 ubiquitin ligases target PP2CA and other clade A PP2Cs for degradation
460. CONSeQuence: prediction of reference peptides for absolute quantitative proteomics using consensus machine learning approaches 461. Coexistence of plant species with similar niches 462. Math self-efficacy and STEM intentions: A person-centered approach 463. Partial metrics, valuations, and domain theory 464. Understanding sensorimotor feedback through optimal control 465. Science Blogs 466. Mathematical population biology 467. Ecological‐niche factor analysis: how to compute habitat‐suitability maps without absence data? 468. Three basic epidemiological models 469. Women in academic science: A changing landscape
470. The science of sex differences in science and mathematics 471. Keystone species and food webs 472. Heritability and tissue specificity of expression quantitative trait loci 473. Observation of Kuznetsov-Ma soliton dynamics in optical fibre 474. Math in Physics 475. TEEMSS2: technology enhanced elementary math and science-year 1 report 476. Multi-laboratory assessment of reproducibility, qualitative and quantitative performance of SWATH-mass spectrometry 477. The phenomenology of niche evolution via quantitative traits in a ‘black-hole’sink 478. Feedback control in systems biology 479. Thinking and acting like a scientist: Investigating the outcomes of introductory science and math courses
480. Assessment of the impact of case studies on student learning gains in an introductory biology course 481. Pharmacometrics: a multidisciplinary field to facilitate critical thinking in drug development and translational research settings 482. Cell lineage reconstruction of early zebrafish embryos using label-free nonlinear microscopy 483. Understanding the home math environment and its role in predicting parent report of children’s math skills 484. Towards quantitative 3D imaging of the osteocyte lacuno-canalicular network 485. Math Placement Testing for College-Bound Students 486. Math teachers attitudes towards photo math application in solving mathematical problem using mobile camera 487. Quantitative genetic analysis of multivariate evolution, applied to brain: body size allometry 488. Ecological niche modeling and differentiation of populations of* Triatoma brasiliensis* Neiva, 1911, the most important Chagas disease vector in northeastern Brazil … 489. Computational complexity of inferring phylogenies from dissimilarity matrices
490. Fundamental issues in systems biology 491. Infusion of collaborative inquiry throughout a biology curriculum increases student learning: a four-year study of “Teams and Streams” 492. Fast semi-analytical model-based acoustic inversion for quantitative optoacoustic tomography 493. Domains in chromatin structure 494. Increasing achievement and higher-education representation of under-represented groups in science, technology, engineering, and mathematics fields: A review of … 495. Equation-free multiscale computation: Algorithms and applications 496. Estimation of quantitative genetic parameters 497. Math PhD careers: New opportunities emerging amidst crisis 498. A review of cell-based computational modeling in cancer biology 499. Genome-wide probing of RNA structure reveals active unfolding of mRNA structures in vivo
500. The structure of sodium thymonucleate fibres. II. The cylindrically symmetrical Patterson function 501. Matrix Metalloproteinase-9 Expression in Alveolar Extraction Sockets of Zoledronic Acid–Treated Rats 502. The Mathematics of Sex: How Biology and Society Conspire to Limit Talented Women and Girls 503. Integrating quantitative and qualitative methods in research 504. Extinction risk depends strongly on factors contributing to stochasticity 505. eQED: an efficient method for interpreting eQTL associations using protein networks 506. Computational analysis of the synergy among multiple interacting genes 507. Importance of math prerequisites for performance in introductory physics 508. Cognition math based on factor space 509. Thermal adaptation: a theoretical and empirical synthesis
510. Programming an in vitro DNA oscillator using a molecular networking strategy 511. Exogenous and endogenous components in circadian rhythms 512. Number-specific and general cognitive markers of preschoolers’ math ability profiles 513. Research methods in anthropology: Qualitative and quantitative approaches 514. Transcriptome meets metabolome: hierarchical and metabolic regulation of the glycolytic pathway 515. Data2Dynamics: a modeling environment tailored to parameter estimation in dynamical systems 516. Advanced statistical matrices for texture characterization: application to cell classification 517. Automated descriptor selection for quantitative structure-activity relationships using generalized simulated annealing 518. Blogging about Science and Math: Elementary Students’ Learning Explorations 519. Great scientist≠ good at math
520. Predictors of Success in High School Advanced Math and Science Coursework 521. Visual rating of age-related white matter changes on magnetic resonance imaging: scale comparison, interrater agreement, and correlations with quantitative … 522. Application of Bloom’s Taxonomy Debunks the MCAT Myth” 523. Genome-wide discovery of loci influencing chemotherapy cytotoxicity 524. A quantitative model of human DNA base excision repair. I. Mechanistic insights 525. Enhancing Collaboration among Math and Career and Technical Education Teachers: Is Technology the Answer?. 526. Neutral theory of quantitative genetic variance in an island model with local extinction and colonization 527. The search for patterns in the balance of nature: advances and retreats 528. Girls Are… Boys Are… Myths, Stereotypes, and Gender Differences. Math and Science for the Coed Classroom. 529. Increasing Problem Solving Ability and Motivation Learning Through Grand of Math Teacher Comments on Results Homework (PR)
530. Systems biology approaches identify ATF3 as a negative regulator of Toll-like receptor 4 531. Fungal associations of roots of dominant and sub-dominant plants in high-alpine vegetation systems with special reference to mycorrhiza 532. Drawing-to-learn: a framework for using drawings to promote model-based reasoning in biology 533. The role of photodynamic therapy (PDT) physics 534. A combinatorial partitioning method to identify multilocus genotypic partitions that predict quantitative trait variation 535. Division of Science and Math Curricular Changes 00/03/2010 536. Professional development for K–12 math and science teachers: What do we really know? 537. Time-resolved analysis and visualization of dynamic processes in living cells 538. MATH 170-02 Calculus I 539. Biased random walk models for chemotaxis and related diffusion approximations
540. Quantitative structure‐activity relationships for toxicity of phenols using regression analysis and computational neural networks 541. Importance of undergraduate research for minority persistence and achievement in biology 542. The role of qualitative research in science education 543. Distributions of tree comparison metrics—some new results 544. Automated analysis of high‐content microscopy data with deep learning 545. The Impact of an Online, Mastery, and Project-Based Developmental Math Curriculum on Student Achievement and Attitude 546. Comparative Genomics Reveals Adaptation by Alteromonas sp. SN2 to Marine Tidal-Flat Conditions: Cold Tolerance and Aromatic Hydrocarbon Metabolism 547. How Knowledge-in-Pieces Informs Research in Math-Bio Education 548. Structural model analysis of multiple quantitative traits 549. Gender differences in large-scale math assessments: PISA trend 2000 and 2003
550. Cell-size control and homeostasis in bacteria 551. Quantitative evaluation and modeling of two-dimensional neovascular network complexity: the surface fractal dimension 552. The Metabolism of and Its Daughters and by Rats 553. On entropy-based molecular descriptors: Statistical analysis of real and synthetic chemical structures 554. Quantitative analysis of the development of cellular slime molds 555. A School-University Math and Science P-16 Partnership: Lessons Learned in Promoting College and Career Readiness. 556. Modeling in systems biology: the Petri net approach 557. STUDENTS’ABILITY IN TRANSLATING MATHEMATIC TEXT OF FIFTH SEMESTER STUDENTS IN MATH EDUCATION PROGRAM STKIP YPM BANGKO 558. Response of vegetation phenology to urbanization in the conterminous United States 559. Live-cell mass profiling: an emerging approach in quantitative biophysics
560. Theories of Learning in Math and Science Educational Software 561. Interval mapping of viability loci causing heterosis in Arabidopsis. 562. Small–angle neutron scattering (sans) and wide–angle x–ray diffraction (WAXD) comparative study on malignant and benign human cancer cells and tissues … 563. The fibroblast-populated collagen microsphere assay of cell traction force—Part 2: Measurement of the cell traction parameter 564. Delay differential equations and applications to biology 565. Experimental design for parameter estimation of gene regulatory networks 566. The gender gap in STEM fields: The impact of the gender stereotype of math and science on secondary students’ career aspirations 567. A model of excitation and adaptation in bacterial chemotaxis 568. Ohio State nav bar 569. Learning and teaching early math: The learning trajectories approach
570. Motivational pathways to STEM career choices: Using expectancy–value perspective to understand individual and gender differences in STEM fields 571. Computer simulation of glioma growth and morphology 572. So you want to stop devouring ecosystems? Do the math! 573. The phenomenon of biodiversity in conservation biology 574. Quantitative fisheries stock assessment: choice, dynamics and uncertainty 575. Wedded bliss: Lessons learned from uniting math placement with math pathways 576. Vancomycin-resistant enterococci in intensive-care hospital settings: transmission dynamics, persistence, and the impact of infection control programs 577. Experimental studies and quantitative modeling of Turing patterns in the (chlorine dioxide, iodine, malonic acid) reaction 578. The Eigenfactor MetricsTM: A network approach to assessing scholarly journals 579. MicroRNA binding sites in the coding region of mRNAs: Extending the repertoire of post‐transcriptional gene regulation
580. THE JARIMATIKA METHOD ON MATH LEARNING: HOW TO IMPROVE MATH SKIL 581. On the distribution of the mean and variance of a quantitative trait under mutation-selection-drift balance. 582. Using math in physics: 5. Functional dependence 583. Bridging generality and specificity: The amusement park theoretical (APT) model of creativity 584. Differential signaling via the same axon of neocortical pyramidal neurons 585. Fourier analysis of cell motility: correlation of motility with metastatic potential 586. Sensors and sensing in biology and engineering 587. Bioinformatics 588. The Effectiveness of Discovery and Inquiry Learning Strategies in Improving Students’ Early Math Skills 589. Closing the gap: inverting the genetics curriculum to ensure an informed public
590. How to infer gene networks from expression profiles 591. Enhancing the validity and usefulness of large-scale educational assessments: II. NELS: 88 science achievement 592. A data integration methodology for systems biology 593. Responding to the Crisis in Math and Science Teaching: Four Initiatives. Final Report. 594. Mechanisms of calcium oscillations and waves: a quantitative analysis 595. Quantitative genetic models for the coevolution of character displacement 596. Better math for better brain images 597. Biology by numbers: mathematical modelling in developmental biology 598. Math goes to the movies 599. Periodicity in epidemiological models
600. Math or science? Using longitudinal expectations data to examine the process of choosing a college major 601. Math science partnership (MSP) program: title II, part B-Rockdale County MSP grant 602. Mammographic Density Measured with Quantitative Computer-aided Method: Comparison with Radiologists’ Estimates and BI-RADS Categories1 603. The rise of computational biology 604. Functional mapping—how to map and study the genetic architecture of dynamic complex traits 605. DEPARTMENT OF MATH, SCIENCE, & TECHNOLOGY 606. Making sense of life 607. Quantitative review finds no evidence of cognitive effects in healthy populations from single-session transcranial direct current stimulation (tDCS) 608. It’s not maths; it’s science: exploring thinking dispositions, learning thresholds and mindfulness in science learning 609. Quantitative molecular magnetic resonance imaging of tumor angiogenesis using cNGR-labeled paramagnetic quantum dots
610. When physics takes over: BAR proteins and membrane curvature 611. Evolutionary quantitative genetics: how little do we know? 612. Computational systems biology: From molecular mechanisms to disease 613. Evolutionary population genetics of promoters: predicting binding sites and functional phylogenies 614. … of androgen, estrogen (ERα and ERβ), and progesterone receptor expression in human prostate cancer by real-time quantitative reverse transcription-polymerase … 615. SELECTING SUCCESSFUL GRADUATE STUDENTS: IN‐DEPTH INTERVIEWS WITH GRE® USERS 616. Globin mRNA sequences: analysis of base pairing and evolutionary implications 617. Work preferences, life values, and personal views of top math/science graduate students and the profoundly gifted: Developmental changes and gender differences … 618. Quantitative ecology: spatial and temporal scaling 619. From graphs to spatial graphs
620. Gender gaps in achievement and participation in multiple introductory biology classrooms 621. Topological models in biology 622. Molecular circuits for dynamic noise filtering 623. Teacher and school characteristics and their influence on curriculum implementation 624. Math and Chemistry connections 625. Opening the Math Gate: Creating a Math Learning Center to Teach Service Mathematics and Statistics Classes 626. Grazing intensity significantly affects belowground carbon and nitrogen cycling in grassland ecosystems: A meta‐analysis 627. Computational approaches to cellular rhythms 628. Enhancing diversity in science: is teaching science process skills the answer? 629. Math anxiety—contributing school and individual level factors
630. Escaping free-energy minima 631. Teaching and curricular practices contributing to success in gateway courses for freshman and sophomore students in math, science, engineering, and technology … 632. Towards a quantitative understanding of the within-host dynamics of influenza A infections 633. Some mechanistically derived population models 634. Morphometrics 635. Detecting genetic drift versus selection in human evolution 636. Educational research: Quantitative, qualitative, and mixed approaches 637. Status, testosterone, and human intellectual performance: Stereotype threat as status concern 638. The minimal area problem in invertebrate communities of Mediterranean rocky substrata 639. AVID: A global alignment program
640. Social research methods: Qualitative and quantitative approaches 641. Effective intercellular communication distances are determined by the relative time constants for cyto/chemokine secretion and diffusion 642. Quantitative genetics and the evolution of reaction norms 643. Evolutionary theory predicts late-life mortality plateaus 644. Beyond academic math: The role of applied STEM course taking in high school 645. Cancer systems biology: a peek into the future of patient care? 646. Sequential sampling models in cognitive neuroscience: Advantages, applications, and extensions 647. Modelling the molecular mechanisms of synaptic plasticity using systems biology approaches 648. Model selection in systems and synthetic biology 649. Stochastic simulation in systems biology
650. Equations for tracer experiments 651. Germline-specific MATH-BTB substrate adaptor MAB1 regulates spindle length and nuclei identity in maize 652. Effect of electrolyte concentration on the stern layer thickness at a charged interface 653. The biology hidden inside residual within‐individual phenotypic variation 654. Mathematical models in cell biology and cancer chemotherapy 655. Using math in physics–3. Anchor equations 656. Predictive oncology: a review of multidisciplinary, multiscale in silico modeling linking phenotype, morphology and growth 657. Petri nets for systems and synthetic biology 658. The reciprocal interaction of angiosperm evolution and tetrapod herbivory 659. Reflections on math reforms in the US
660. Project Pathways: Opening Routes to Math & Science Success for All Students 661. Spatiotemporal patterns in ecology and epidemiology: theory, models, and simulation 662. Stereotype threat leads to reduction in number of math problems women attempt. 663. Influence of the Reflex Math Fact Fluency Program on Math Scores 664. MR2449595 (2010j: 37018) 37B10 665. The Princeton guide to ecology 666. MCA has more to say 667. The evolution of genetic architecture 668. Cognitive appraisals, achievement emotions, and students’ math achievement: A longitudinal analysis. 669. Scalable parameter estimation for genome-scale biochemical reaction networks
670. Quantifying ubiquitin signaling 671. North Carolina Math/Science/Special Education (MSSE) $1,800 Teacher Bonus Program: An Initial Evaluation. Policy Matters. 672. The free-energy cost of accurate biochemical oscillations 673. Correlated random walks in heterogeneous landscapes: Derivation, homogenization, and invasion fronts 674. Graduate Students’ Conceptions Of The Tenure-Track Hiring Process In Science, Technology, Engineering, And Math Fields. 675. Seasonal carbon dynamics and water fluxes in an a mazon rainforest 676. KOHLEN-BECKENS. By OH SCHINDEWOLF. Akad. Wiss. Liter. Mainz (Abhandl. Math.-naturw. Kl.). Jahrg. 1952, Nr. 4, pp. 143-227, with 29 figs, and 2 plates. M … 677. Approaching math increases math= me and math= pleasant 678. Evolvability and hierarchy in rewired bacterial gene networks 679. Spectral measures of bipartivity in complex networks
680. Human nature in nineteenth-century British novels: Doing the math 681. Stochastic processes in the neurosciences 682. Speeds of invasion in a model with strong or weak Allee effects 683. The internal/external frame of reference (I/E) model: Extension to five school subjects and invariance across German secondary school ability tracks 684. Different time courses for visual perception and action priming 685. The school for science and math at Vanderbilt: An innovative research-based program for high school students 686. Quantitative assessment of regional cerebral blood flows by perfusion CT studies at low injection rates: a critical review of the underlying theoretical models 687. To walk or to fly? How birds choose among foraging modes 688. Visual literacy in higher education 689. Language-selective and domain-general regions lie side by side within Broca’s area
690. Neurobiological underpinnings of math and reading learning disabilities 691. What are grades made of? 692. Reproducing kernel Hilbert spaces regression methods for genomic assisted prediction of quantitative traits 693. The Remedy That’s Killing: CUNY, LaGuardia, and The Fight for Better Math Policy 694. Mapping quantitative trait loci in complex pedigrees: a two-step variance component approach 695. Modular cell biology: retroactivity and insulation 696. Assessing habitat-suitability models with a virtual species 697. De novo identification and biophysical characterization of transcription-factor binding sites with microfluidic affinity analysis 698. Effects of a Humorous Collaborative and Competitive Whiteboard Game on Math Anxiety and Proficiency in African-American College Students 699. Salivary proteomics in bisphosphonate‐related osteonecrosis of the jaw
700. Novel cell segmentation and online SVM for cell cycle phase identification in automated microscopy 701. Intersectionality in quantitative psychological research: II. Methods and techniques 702. The role of the airline transportation network in the prediction and predictability of global epidemics 703. Online testing integrity in a general education math course: A correlational study 704. The Fractal Organization of the Nervous System 705. A general model for the origin of allometric scaling laws in biology 706. Quantitative genetics of continuous reaction norms: thermal sensitivity of caterpillar growth rates 707. Interaction of EGF receptor and grb2 in living cells visualized by fluorescence resonance energy transfer (FRET) microscopy 708. Period three implies chaos 709. Body masses, functional responses and predator–prey stability
710. Evolution of acquired resistance to anti-cancer therapy 711. Induced active transport of ions in mitochondria. 712. The effects of the language of instruction in the science and math achievement of Lebanese students.(c2014) 713. Thermodynamics of stoichiometric biochemical networks in living systems far from equilibrium 714. The Right Math at the Right Time for Michigan:“Coalition of the Willing” Strengthens Math Pathways in 715. Participation in an all-female algebra I class: Effects on high school math and science course selection 716. Image-based quantitative analysis of gold immunochromatographic strip via cellular neural network approach 717. Advances in real-time multispectral optoacoustic imaging and its applications 718. IS GAISE EVIDENT? COLLEGE STUDENTS’PERCEPTIONS OF STATISTICS CLASSES AS” ALMOST NOT MATH”. 719. The 2.1-D sketch
720. A consumer–resource approach to the density‐dependent population dynamics of mutualism 721. Recent Bayesian stable‐isotope mixing models are highly sensitive to variation in discrimination factors 722. Stochastic formulation of ecological models and their applications 723. Perspectives of students with intellectual disabilities about their experiences with paraprofessional support 724. Quantitative comparisons of image registration techniques based on high-resolution MRI of the brain 725. Fluorescence correlation spectroscopy: diagnostics for sparse molecules 726. The dynamic range of the human metabolome revealed by challenges 727. AN EMPIRICAL APPROACH TO STUDY THE STABILITY OF GENERALIZED LOGISTIC MAP IN SUPERIOR ORBIT 728. Learning and teaching with Moodle-based E-learning environments, combining learning skills and content in the fields of Math and Science & Technology 729. A survey of models for tumor-immune system dynamics
730. COURSE ENROLLMENT AS SELF-REGULATORY BEHAVIOR://HO) TAKES OPTIONAL HIGH SCHOOL MATH COURSES? 731. Robust perfect adaptation in bacterial chemotaxis through integral feedback control 732. A statistical model of criminal behavior 733. Electron microscopic examination of subcellular fractions: II. Quantitative analysis of the mitochondrial population isolated from rat liver 734. Pleiotropic models of quantitative variation. 735. A multi-stage model for quantitative PCR 736. T1-weighted DCE imaging concepts: modelling, acquisition and analysis 737. Circumpolar assessment of permafrost C quality and its vulnerability over time using long‐term incubation data 738. The Multidisciplfnmy Impact of Math and Computer Science Is Reflected fn the 100 Most-Cited Articles fn CompuMath Citation Index, 1976-1980 739. Geometric morphometrics
740. Reconstructing folding energy landscapes by single-molecule force spectroscopy 741. Math wars: A guide for parents and teachers 742. A QUANTITATIVE GENETIC ANALYSIS OF THERMAL SENSITIVITY IN THE LOCOMOTOR PERFORMANCE CURVE OF APHIDIUS ERVI 743. Increased preclass preparation underlies student outcome improvement in the flipped classroom 744. Analysis of the transcriptional activation and post‐transcriptional regulation of transient receptor potential mucolipin‐2 gene (561.5) 745. Advances in quantitative electroencephalogram analysis methods 746. Active gel physics 747. Population genetics, molecular evolution, and the neutral theory: selected papers
Research Topics Biology
Related posts:.
- Mathematical Biology Research Topics Ideas [MS PhD]
- information visualization Research Topics Ideas [MS PhD]
- Molecular Computing Research Topics Ideas [MS PhD]
- Software Security Research Topics Ideas [MS PhD]
- Stochastic Networks Research Topics Ideas [MS PhD]
- VLSI Research Topics Ideas [MS PhD]
You must be logged in to post a comment.
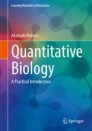
Quantitative Biology pp 1–10 Cite as
Introduction to Quantitative Biology
- Akatsuki Kimura 2
- First Online: 04 January 2022
1223 Accesses
Part of the book series: Learning Materials in Biosciences ((LMB))
In this introductory chapter, we begin by discussing what quantitative biology is. Although the term is used quite often these days, it is difficult to define. In fact, there seems to be no clear-cut and universal definition. We will review historical background leading to interest in the field of quantitative biology. We next discuss why a student or scientist new to the field may want to study quantitative biology using this book. Intended readers of the book are biology students and scientists who are not familiar with quantitative approaches, such as statistical analyses and computer modeling. We hope to convince readers that quantitative ways of thinking and skills are inevitable in modern biology. We will focus on the construction of quantitative models and analyses using computational modeling as a basic skill in quantitative biology. At the end of the chapter, we will discuss the importance of quantitative modeling in quantitative biology and the factors that contribute to a good model.
- Quantitative biology
- Systems biology
This is a preview of subscription content, log in via an institution .
Buying options
- Available as PDF
- Read on any device
- Instant download
- Own it forever
- Available as EPUB and PDF
- Compact, lightweight edition
- Dispatched in 3 to 5 business days
- Free shipping worldwide - see info
Tax calculation will be finalised at checkout
Purchases are for personal use only
Einstein A. The ultimate quotable Einstein. Princeton University Press; 2010.
Google Scholar
Feynman RP. The character of physical law. London: Penguin; 2007.
Hey AJG. The fourth paradigm. Microsoft Research; 2009.
Hlavacek WS, Edwards JS, Jiang Y, Nemenman I, Wall ME, Faeder JR. Editorial: selected papers from the first q-bio conference on cellular information processing. IET Syst Biol. 2008;2:203–5.
Article Google Scholar
Jesseph D. Ratios, quotients, and the language of nature. The language of nature. University of Minnesota Press; 2016. p. 160–77.
Kimura K, Mamane A, Sasaki T, et al. Endoplasmic-reticulum-mediated microtubule alignment governs cytoplasmic streaming. Nat Cell Biol. 2017;19:399–406.
Article CAS Google Scholar
Kitano H. Perspectives on systems biology. New Gen Comput. 2000;18:199–216.
Lewis M. Moneyball: the art of winning an unfair game. W W Norton & Co Inc; 2003.
Mirny LA. The fractal globule as a model of chromatin architecture in the cell. Chromosome Res. 2011;19:37–51.
Article CAS PubMed Central Google Scholar
Phillips R, Kondev J, Theriot J, Orme N. Physical biology of the cell. Garland Pub; 2013.
Weatherall JO. The physics of wall street: a brief history of predicting the unpredictable. Houghton Mifflin Harcourt; 2013.
Further Reading
Milo R, Phillips R. Cell biology by the numbers. Garland Science; 2015.
Book Google Scholar
Download references
Author information
Authors and affiliations.
National Institute of Genetics, and The Graduate University for Advanced Studies (SOKENDAI), Mishima, Japan
Akatsuki Kimura
You can also search for this author in PubMed Google Scholar
Rights and permissions
Reprints and permissions
Copyright information
© 2022 Springer Nature Singapore Pte Ltd.
About this chapter
Cite this chapter.
Kimura, A. (2022). Introduction to Quantitative Biology. In: Quantitative Biology. Learning Materials in Biosciences. Springer, Singapore. https://doi.org/10.1007/978-981-16-5018-5_1
Download citation
DOI : https://doi.org/10.1007/978-981-16-5018-5_1
Published : 04 January 2022
Publisher Name : Springer, Singapore
Print ISBN : 978-981-16-5017-8
Online ISBN : 978-981-16-5018-5
eBook Packages : Biomedical and Life Sciences Biomedical and Life Sciences (R0)
Share this chapter
Anyone you share the following link with will be able to read this content:
Sorry, a shareable link is not currently available for this article.
Provided by the Springer Nature SharedIt content-sharing initiative
- Publish with us
Policies and ethics
- Find a journal
- Track your research
- USC Libraries
- Research Guides
- *Biological and Life Sciences
Quantitative Biology
*biological and life sciences: quantitative biology.
- Human Biology
- Computational Biology and Bioinformatics
- Biotechnology
- Molecular Genetics and Biochemistry
- Neuroscience
- Human Development and Aging
- Health Promotion and Disease Prevention
- Human Movement Sciences
- Natural Science
- Marine Biology
- Environmental Science
These databases will help you with specific research in quantative biology:
- arXiv Started in August 1991, arXiv.org (formerly xxx.lanl.gov) is a highly-automated electronic archive and distribution server for research articles in the areas of physics, mathematics, computer science, nonlinear sciences, quantitative biology and statistics. arXiv is maintained and operated by the Cornell University Library.
- Cold Spring Harbor Laboratory Press This resource includes access to all six of their journals; their Protocols; and the Cold Spring Harbor Symposia on Quantitative Biology. This is especially strong for molecular biology topics.
- Colloquium Digital Library of Life Sciences The Colloquium Digital Library is an original collection of PDF e-books for researchers, instructors, and advanced-level students in the biomedical life sciences. Colloquium Lectures are organized by subject area, or Series including Biotechnology; Building Blocks of the Cell: Cell Structure & Function; Cell Biology of Medicine; The Developing Brain; Developmental Biology; The Genetic Basis of Human Disease; Genomic and Molecular Medicine; Integrated Systems Physiology: From Molecule to Function to Disease; Neurobiology of Alzheimer's Disease; Neuroglia in Biology and Medicine: From Physiology to Disease; Neuropeptides; Protein Activation and Cancer; Stem Cell Biology; and Quantitative Cell Biology.
- Scopus Scopus is an expertly curated database that focuses on life sciences, physical sciences, and health sciences research. Find the latest research in the field.
Here are recent ebooks that can get you started with quantative biology:
- Quantitative Biology: Dynamics of Living Systems by Akatsuki Kimura ; Jason Edward Shoemaker ; Rinshi S. Kasai ; Ziya Kalay ; Hiroaki Takagi ; Douglas B. Murray ; Chun - Biu Li ; Akira Funahashi ; Tetsuya J. Kobayashi ; Viji M. Draviam ; Noriko Hiroi ; Naoki A. Irie ISBN: 9782889452132 Publication Date: 2017 With the emergence of Systems Biology, there is a greater realization that the whole behavior of a living system may not be simply described as the sum of its elements. To represent a living system using mathematical principles, practical quantities with units are required. Quantities are not only the bridge between mathematical description and biological observations; they often stand as essential elements similar to genome information in genetics. This important realization has greatly rejuvenated research in the area of Quantitative Biology. Because of the increased need for precise quantification, a new era of technological development has opened. For example, spatio-temporal high-resolution imaging enables us to track single molecule behavior in vivo. Clever artificial control of experimental conditions and molecular structures has expanded the variety of quantities that can be directly measured. In addition, improved computational power and novel algorithms for analyzing theoretical models have made it possible to investigate complex biological phenomena. This research topic is organized on two aspects of technological advances which are the backbone of Quantitative Biology: (i) visualization of biomolecules, their dynamics and function, and (ii) generic technologies of model optimization and numeric integration. We have also included articles highlighting the need for new quantitative approaches to solve some of the long-standing cell biology questions.
Here are some of the academic journals that USC Libraries subscribes to that are related to quantative biology:
- Cold Spring Harbor Symposia on Quantitative Biology Since 1933, major discoveries in biology--such as the structure of DNA, the genetic code, the polymerase chain reaction (PCR), and RNA interference (RNAi)--have been presented and debated at the Symposium held every summer at Cold Spring Harbor Laboratory in New York. Each Symposium focuses on a different and timely area of biological research and is attended by the leading figures in the field. The speakers are handpicked luminaries and rising stars who also publish a detailed discussion of the work they present in the annual Cold Spring Harbor Symposia on Quantitative Biology volume.
- << Previous: Human Biology
- Next: Computational Biology and Bioinformatics >>
- Last Updated: Dec 12, 2023 1:16 PM
- URL: https://libguides.usc.edu/biology
Thank you for visiting nature.com. You are using a browser version with limited support for CSS. To obtain the best experience, we recommend you use a more up to date browser (or turn off compatibility mode in Internet Explorer). In the meantime, to ensure continued support, we are displaying the site without styles and JavaScript.
- View all journals
- Explore content
- About the journal
- Publish with us
- Sign up for alerts
- Published: December 2012
Towards quantitative cell biology
- Prisca Liberali 1 &
- Lucas Pelkmans 1
Nature Cell Biology volume 14 , page 1233 ( 2012 ) Cite this article
5241 Accesses
8 Citations
8 Altmetric
Metrics details
- Cell biology
- Systems biology
Cell biologists must decide whether to embrace the maturing field of systems biology. We argue that a fusion of the two is urgently needed to strengthen both fields.
As researchers working in both cell biology and systems biology, and making attempts to connect the two fields, we realise that the majority of cell biologists are not familiar with the merits of systems biology. Instead, they often seem more familiar with its pitfalls. This is unfortunate, as cell biologists are in a prime position to harness the power of the technologies that systems biology has brought forth. We do not merely suggest the application of these new technologies to classical cell biological questions, but rather that the fundamental approaches of systems biology, which are unbiased, large-scale, quantitative and multivariate, are integrated into the core of molecular cell biology in the future. This should be seen as a complementary and much-needed extension of traditional approaches in cell biology.
Systems biology has produced staggering amounts of complex data. Although the fields of functional genomics, proteomics and metabolomics are mining and integrating these data, molecular cell biology lags behind. Most importantly, in the last few years, systems biology has embraced the single-cell revolution, applying functional genomics, proteomics and metabolomics at the single-cell level — thereby allowing the analysis of collectives of molecules and the structures they form simultaneously within single cells. Ironically, the single cell has traditionally been the domain of cell biology, but the experiments were not large-scale, quantitative and multivariate. As a consequence, classical approaches in molecular cell biology can be particularly sensitive to experimental bias. Most experiments study only a small fraction or an average of the possible states of a cell biological process. This occurs in cell lysates, homogenized mixes of cellular extracts from millions of cells, representing an average of a complex mixture of states, and also in microscopy, where often only a small number of single cells or subcellular structures are analysed. To reduce such bias, an experiment must be large-scale in the sense that it consists of a large number of samplings. Although this can occasionally be achieved manually, it is clear that this is an ideal task for computers. This not only allows an individual to easily obtain large numbers of samplings, but it also decreases bias. This requires methods that yield results in formalized and quantitative formats (since these are machine-readable) for many molecular, morphological and spatial properties of subcellular structures, single cells and cell populations. With such multivariate measurements, correlation analysis and causal inference can be applied to learn the functional molecular causality underlying cellular activities across different scales. Embracing these quantitative approaches will allow cell biologists to provide detailed information about their own experiments in a more formalized and structured manner. This can provide a much-needed extension of functional annotation and interaction databases such as DAVID and STRING, or manually curated databases such as KEGG pathways, as these lack accurate information about molecular machines and processes involved in subcellular compartmentalization, membrane trafficking and cytoskeletal regulation. This is necessary, as it is clear that a cornerstone of future scientific endeavours will be to devise strategies to obtain useful information from such data sets.
These quantitative and unbiased approaches hold many benefits for cell biologists. It may allow them to identify particular properties of single cells or subcellular regions that enrich for an otherwise rare phenotype, allowing the design of new experiments that favour the appearance of that specific phenotype. Most importantly, many fundamental properties of a system will only become visible when the spectrum of cellular states is sufficiently sampled. This is, for instance, illustrated in the analysis of quantile assembly of caveolae, heterogeneous dynamics of clathrin-coated pits, or patterns of cell-to-cell variability in virus infection. Also, it is highly relevant for the interpretation of perturbations. Although each single cell may display a normal level of activity corresponding to its state, altered proportions of cellular states in a population (for example, dense and small cells versus sparse and large cells) may lead to the incorrect conclusion that the activity itself is perturbed. Such an indirect perturbation is fundamentally different from a direct perturbation of the cellular activity. Similarly, perturbing a key factor in early endosome function may affect a cellular activity by causing changes in plasma membrane lipid composition on which the cellular activity depends, not because the cellular activity is directly controlled by endocytosis. Although further experiments must always be performed to test for such indirect effects, the reality is that confounding factors may often not be apparent, and thus remain untested, when an unbiased multivariate approach is not used.
By far the most important reason for building these approaches into the core of cell biology is that it allows the field to adopt the language and scientific rigour of the exact sciences, without sacrificing the characteristics that distinguish it from biophysics and bioinformatics. This transformation is necessary for cell biology to maintain its important central position in the rapidly evolving molecular life sciences. Implementing image analysis algorithms, applying multivariate statistics and deriving data-driven models must become second nature for a cell biologist.
Author information
Authors and affiliations.
Prisca Liberali and Lucas Pelkmans are at the Institute of Molecular Life Sciences, University of Zurich, Winterthurerstrasse 190, CH-8057 Zurich, Switzerland,
Prisca Liberali & Lucas Pelkmans
You can also search for this author in PubMed Google Scholar
Corresponding author
Correspondence to Lucas Pelkmans .
Rights and permissions
Reprints and permissions
About this article
Cite this article.
Liberali, P., Pelkmans, L. Towards quantitative cell biology. Nat Cell Biol 14 , 1233 (2012). https://doi.org/10.1038/ncb2648
Download citation
Issue Date : December 2012
DOI : https://doi.org/10.1038/ncb2648
Share this article
Anyone you share the following link with will be able to read this content:
Sorry, a shareable link is not currently available for this article.
Provided by the Springer Nature SharedIt content-sharing initiative
This article is cited by
Quantitative techniques for imaging cells and tissues.
- Christopher S. von Bartheld
- Fred S. Wouters
Cell and Tissue Research (2015)
Finding the shape-shifter genes
- Michael F. Olson
Nature Cell Biology (2013)
Quick links
- Explore articles by subject
- Guide to authors
- Editorial policies
Sign up for the Nature Briefing newsletter — what matters in science, free to your inbox daily.

49 Most Interesting Biology Research Topics
August 21, 2023
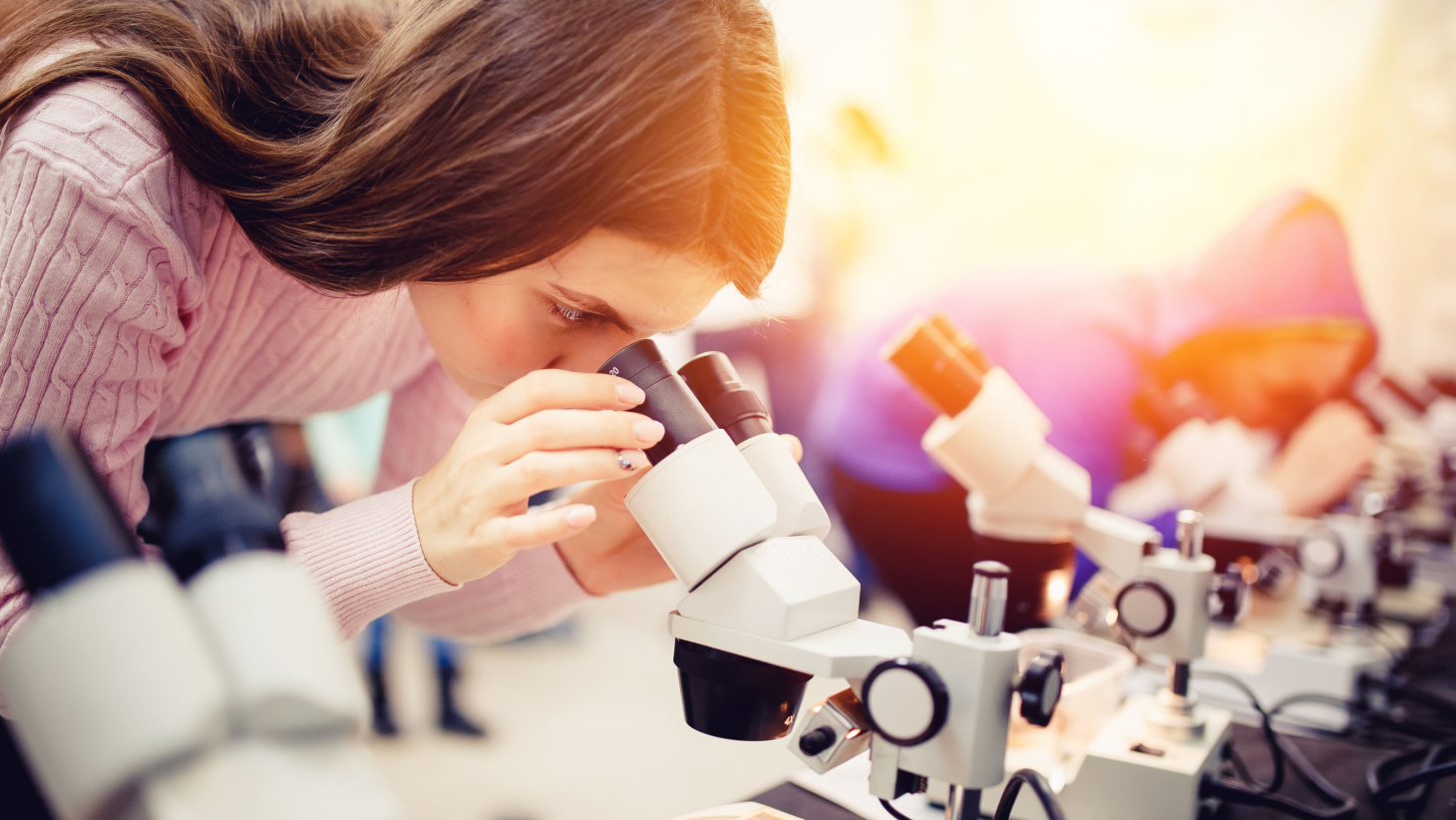
In need of the perfect biology research topics—ideas that can both showcase your intellect and fuel your academic success? Lost in the boundless landscape of possible biology topics to research? And afraid you’ll never get a chance to begin writing your paper, let alone finish writing? Whether you’re a budding biologist hoping for a challenge or a novice seeking easy biology research topics to wade into, this blog offers curated and comprehensible options.
And if you’re a high school or transfer student looking for opportunities to immerse yourself in biology, consider learning more about research opportunities for high school students , top summer programs for high school students , best colleges for studying biomedical engineering , and best colleges for studying biology .
What is biology?
Well, biology explores the web of life that envelops our planet, from the teeny-tiny microbes to the big complex ecosystems. Biology investigates the molecular processes that define existence, deciphers the interplay of genes, and examines all the dynamic ways organisms interact with their environments. And through biology, you can gain not only knowledge, but a deeper appreciation for the interconnectedness of all living things. Pretty cool!
There are lots and lots of sub-disciplines within biology, branching out in all directions. Throughout this list, we won’t follow all of those branches, but we will follow many. And while none of these branches are truly simple or easy, some might be easier than others. Now we’ll take a look at a few various biology research topics and example questions that could pique your curiosity.
Climate change and ecosystems
The first of our potentially easy biology research topics: climate change and ecosystems. Investigate how ecosystems respond and adapt to the changing climate. And learn about shifts in species distributions , phenology , and ecological interactions .
1) How are different ecosystems responding to temperature changes and altered precipitation patterns?2) What are the implications of shifts in species distributions for ecosystem stability and functioning?
2) Or how does phenology change in response to climate shifts? And how do those changes impact species interactions?
3) Which underlying genetic and physiological mechanisms enable certain species to adapt to changing climate conditions?
4) And how do changing climate conditions affect species’ abilities to interact and form mutualistic relationships within ecosystems?
Microbiome and human health
Intrigued by the relationship between the gut and the rest of the body? Study the complex microbiome . You could learn how gut microbes influence digestion, immunity, and even mental health.
5) How do specific gut microbial communities impact nutrient absorption?
6) What are the connections between the gut microbiome, immune system development, and susceptibility to autoimmune diseases?
7) What ethical considerations need to be addressed when developing personalized microbiome-based therapies? And how can these therapies be safely and equitably integrated into clinical practice?
8) Or how do variations in the gut microbiome contribute to mental health conditions such as anxiety and depression?
9) How do changes in diet and lifestyle affect the composition and function of the gut microbiome? And what are the subsequent health implications?
Urban biodiversity conservation
Next, here’s another one of the potentially easy biology research topics. Examine the challenges and strategies for conserving biodiversity in urban environments. Consider the impact of urbanization on native species and ecosystem services. Then investigate the decline of pollinators and its implications for food security or ecosystem health.
10) How does urbanization influence the abundance and diversity of native plant and animal species in cities?
11) Or what are effective strategies for creating and maintaining green spaces that support urban biodiversity and ecosystem services?
12) How do different urban design and planning approaches impact the distribution of wildlife species and their interactions?
13) What are the best practices for engaging urban communities in biodiversity conservation efforts?
14) And how can urban agriculture and rooftop gardens contribute to urban biodiversity conservation while also addressing food security challenges?
Bioengineering
Are you a problem solver at heart? Then try approaching the intersection of engineering, biology, and medicine. Delve into the field of synthetic biology , where researchers engineer biological systems to create novel organisms with useful applications.
15) How can synthetic biology be harnessed to develop new, sustainable sources of biofuels from engineered microorganisms?
16) And what ethical considerations arise when creating genetically modified organisms for bioremediation purposes?
17) Can synthetic biology techniques be used to design plants that are more efficient at withdrawing carbon dioxide from the atmosphere?
18) How can bioengineering create organisms capable of producing valuable pharmaceutical compounds in a controlled and sustainable manner?
19) But what are the potential risks and benefits of using engineered organisms for large-scale environmental cleanup projects?
Neurobiology
Interested in learning more about what makes creatures tick? Then this might be one of your favorite biology topics to research. Explore the neural mechanisms that underlie complex behaviors in animals and humans. Shed light on topics like decision-making, social interactions, and addiction. And investigate how brain plasticity and neurogenesis help the brain adapt to learning, injury, and aging.
20) How does the brain’s reward circuitry influence decision-making processes in situations involving risk and reward?
21) What neural mechanisms underlie empathy and social interactions in both humans and animals?
22) Or how do changes in neural plasticity contribute to age-related cognitive decline and neurodegenerative diseases?
23) Can insights from neurobiology inform the development of more effective treatments for addiction and substance abuse?
24) What are the neural correlates of learning and memory? And how can our understanding of these processes be applied to educational strategies?
Plant epigenomics
While this might not be one of the easy biology research topics, it will appeal to plant enthusiasts. Explore how epigenetic modifications in plants affect their ability to respond and adapt to changing environmental conditions.
25) How do epigenetic modifications influence the expression of stress-related genes in plants exposed to temperature fluctuations?
26) Or what role do epigenetic changes play in plants’ abilities to acclimate to changing levels of air pollution?
27) Can certain epigenetic modifications be used as indicators of a plant’s adaptability to new environments?
28) How do epigenetic modifications contribute to the transgenerational inheritance of traits related to stress resistance?
29) And can targeted manipulation of epigenetic marks enhance crop plants’ ability to withstand changing environmental conditions?
Conservation genomics
Motivated to save the planet? Conservation genomics stands at the forefront of modern biology, merging the power of genetics with the urgent need to protect Earth’s biodiversity. Study genetic diversity, population dynamics, and how endangered species adapt in response to environmental changes.
30) How does genetic diversity within endangered species influence their ability to adapt to changing environmental conditions?
31) What genetic factors contribute to the susceptibility of certain populations to diseases, and how can this knowledge inform conservation strategies?
32) How can genomic data be used to inform captive breeding and reintroduction programs for endangered species?
33) And what are the genomic signatures of adaptation in response to human-induced environmental changes, such as habitat fragmentation and pollution?
34) Or how can genomics help identify “hotspots” of biodiversity that are particularly important for conservation efforts?
Zoonotic disease transmission
And here’s one of the biology research topics that’s been on all our minds in recent years. Investigate the factors contributing to the transmission of zoonotic diseases , like COVID-19. Then posit strategies for prevention and early detection.
35) What are the ecological and genetic factors that facilitate the spillover of zoonotic pathogens from animals to humans?
36) Or how do changes in land use, deforestation, and urbanization impact the risk of zoonotic disease emergence?
37) Can early detection and surveillance systems be developed to predict and mitigate the spread of zoonotic diseases?
38) How do social and cultural factors influence human behaviors that contribute to zoonotic disease transmission?
39) And can strategies be implemented to improve global pandemic preparedness?
Bioinformatics
Are you a data fanatic? Bioinformatics involves developing computational tools and techniques to analyze and interpret large biological datasets. This enables advancements in genomics, proteomics, and systems biology. So delve into the world of bioinformatics to learn how large-scale genomic and molecular data are revolutionizing biological research.
40) How can machine learning algorithms predict the function of genes based on their DNA sequences?
41) And what computational methods can identify potential drug targets by analyzing protein-protein interactions in large biological datasets?
42) Can bioinformatics tools be used to identify potential disease-causing mutations in human genomes and guide personalized medicine approaches?
43) What are the challenges and opportunities in analyzing “omics” data (genomics, proteomics, transcriptomics) to uncover novel biological insights?
44) Or how can bioinformatics contribute to our understanding of microbial diversity, evolution, and interactions within ecosystems?
Regenerative medicine
While definitely not one of the easy biology research topics, regenerative medicine will appeal to those interested in healthcare. Research innovative approaches to stimulate tissue and organ regeneration, using stem cells, tissue engineering, and biotechnology. And while you’re at it, discover the next potential medical breakthrough.
45) How can stem cells be directed to differentiate into specific cell types for tissue regeneration, and what factors influence this process?
46) Or what are the potential applications of 3D bioprinting in creating functional tissues and organs for transplantation?
47) How can bioengineered scaffolds enhance tissue regeneration and integration with host tissues?
48) What are the ethical considerations surrounding the use of stem cells and regenerative therapies in medical treatments?
49) And can regenerative medicine approaches be used to treat neurodegenerative disorders and restore brain function?
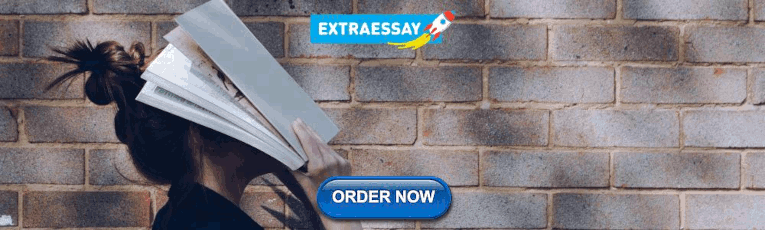
Biology Research Topics – Final thoughts
So as you take your next steps, try not to feel overwhelmed. And instead, appreciate the vast realm of possibilities that biology research topics offer. Because the array of biology topics to research is as diverse as the ecosystems it seeks to understand. And no matter if you’re only looking for easy biology research topics, or you’re itching to unravel the mysteries of plant-microbe interactions, your exploration will continue to deepen what we know of the world around us.
- High School Success

Mariya holds a BFA in Creative Writing from the Pratt Institute and is currently pursuing an MFA in writing at the University of California Davis. Mariya serves as a teaching assistant in the English department at UC Davis. She previously served as an associate editor at Carve Magazine for two years, where she managed 60 fiction writers. She is the winner of the 2015 Stony Brook Fiction Prize, and her short stories have been published in Mid-American Review , Cutbank , Sonora Review , New Orleans Review , and The Collagist , among other magazines.
- 2-Year Colleges
- Application Strategies
- Big Picture
- Career & Personality Assessment
- College Essay
- College Search/Knowledge
- College Success
- Costs & Financial Aid
- Dental School Admissions
- Extracurricular Activities
- Graduate School Admissions
- High Schools
- Law School Admissions
- Medical School Admissions
- Navigating the Admissions Process
- Online Learning
- Private High School Spotlight
- Summer Program Spotlight
- Summer Programs
- Test Prep Provider Spotlight

“Innovative and invaluable…use this book as your college lifeline.”
— Lynn O'Shaughnessy
Nationally Recognized College Expert
College Planning in Your Inbox
Join our information-packed monthly newsletter.
Sign Up Now
- How It Works
212 Unique Biology Research Topics For Students And Researchers
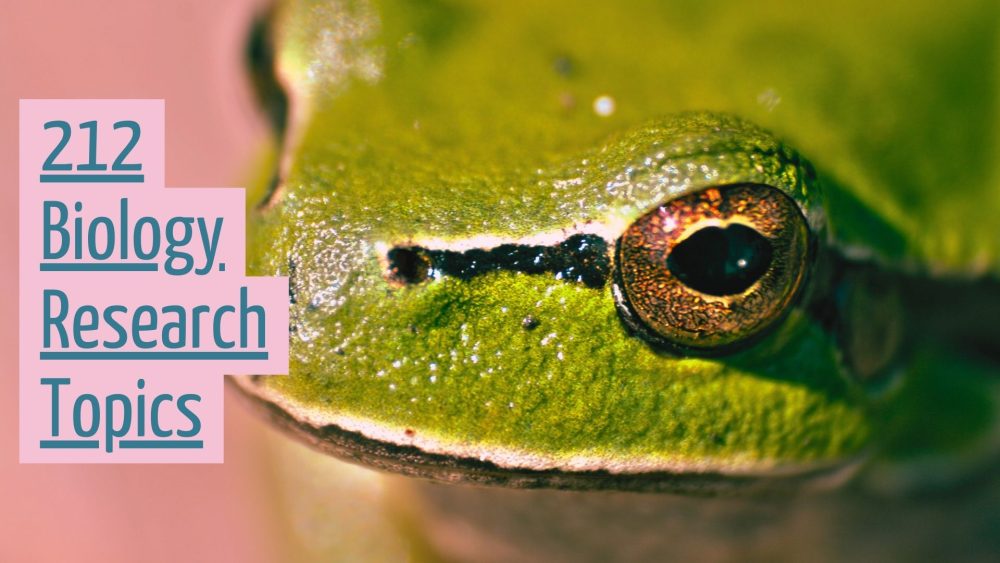
Every student studying something related to biology — botany, marine, animal, medicine, molecular or physical biology, is in an interesting field. It’s a subject that explores how animate and inanimate objects relate to themselves. The field unveils the past, the present, and what lies in the future of the relationship between the living and nonliving things.
This is precisely why you need custom and quality biology topics for your college and university essay or project. It’ll make it easy to brainstorm, research, and get to writing straight away. Before the deep dive, what is biology?
What Is Biology?
Everyone knows it’s the scientific study of life, but beyond that, biology facilitates the comprehension of living and nonliving things. It’s a branch that explores their anatomy, behavior, distribution, morphology, and physiology.
For example, it understands how genes are classified and constituted into generations. It encompasses various branches, including botany, medicine, genetics, ecology, marine biology, zoology, and molecular biology.
Here are what some of these mean:
Botany: This study of plants examines their structure, physiology, ecology, economic importance, and distribution, among others. It also deals with their biochemical processes, properties, and social interactions between plants. It extends to how plants are vital for human life, survival, and growth and how they play a significant role in stabilizing environmental health. Zoology: Zoology studies animal behavior, brain, structure, physiology, class, and distribution. It’s the general study of the lives of both living and extinct animals. It explains animal classification, the animal kingdom, evolution, habitat, embryology, and life span. Physiology: Physiology deals with the daily functions of the human body: How it works and the factors that make it work. It examines molecular behavior, the chemistry and physics behind locomotion, and how the cells in the living organisms’ body function. It helps understand how humans and animals get sick and what can be done to alleviate pain. Microbiology: Dealing with microorganisms, it examined how viruses, algae, fungi, bacteria, protozoa, and slime molds become parts of human life. They’re regarded as microbes, which play substantial roles in the human biochemical processes, including climate change, biodegradation, biodeterioration, food spoilage, biotech, and epidemiology. Marine Biology: This is the scientific study of organs in the sea. It understands their family classification, how they survive, and what makes wild marine animals different from domesticated and consumable ones. It also explores their interaction with the environment through several processes. The marine biologist studies marines in their natural environment, collects data on their characteristics, human impact on their living, and how they relate with themselves.
Now that you know all these, here are some custom biology topics to research for your university or college essay and paper.
Controversial Biology Topics
There are many controversial subjects in every field, and biology isn’t exempt from controversy. If you’d like to create an original essay through diverse opinions, here are biology topics for you:
- What are your thoughts on the post-Roe V Wade world?
- How can the post-Roe V Wade policy affect developing countries looking up to America for their laws?
- Abortion and feminism: discuss
- Does saving life justify cloning?
- Explain the principle of abortion in medical practice
- The effects of cloning in medicine
- How does genetics contribute to obesity?
- Explain why a parent could have Hepatitis B virus and only one of five offspring have the virus
- Is homosexuality really in the gene?
- How does depression correlate with genetics?
- Additives and how they affect the genes
- Examine how genetic mutations work
- Discuss the grounds that you could prove for legalizing human cloning
- Which is more immoral: Human or animal cloning?
- How is nanotechnology different from biotechnology?
- Discuss the manifestation of nanotechnology in science
- Explain three instances where public opinion has held back scientific inventions
- How does transgenic crop work?
- Would you say genetically modified food is safe for consumption?
- Explain why sexual abuse leads to trauma.
Biology Research Paper Topics
You’d need to write an extensive paper on biology one day. This could be when you’re in your final year in college or the university or submitting to a competition. You’d need Biology topics to research for brainstorming, and here are 30 of them:
- Stem cells and tissue formation processes
- Why are there different congenital disabilities?
- Mixtures in anticancer drugs?
- What are the complexities of existing HIV drugs?
- What is the contribution of chemotherapy to cancer?
- Examine the chemotherapy process and why it doesn’t work for some patients.
- Explain the origin of developmental diseases
- How do germs affect the cells?
- What are the consequences of the sun on the white person’s and black person’s skin?
- Why are some diseases treatable through drugs while some are not?
- Scientific lessons learned from COVID-19 and ideas to tackle the next virus
- If animals are carriers of the virus, what should be done to them?
- Examine five animals in extinction and what led to it
- Discuss the subject of endangered species and why people should care
- Is a plant-based diet sustainable for human health?
- Account for the consequence of living on Mars on human health
- Discuss the inconveniences involved in space travel
- How does space flight contribute to environmental disasters
- Discuss the emergence of leukemia
- Explain how the immune systems in humans work
- Evaluate the factors that weaken the immunological system
- What would you consider the deadliest virus?
- Autoimmune: what is it, origin and consequences
- Immune disorder: origin and how it affects the body
- Does stress affect the ability to have sex?
- Contribution of vaccine to eradicating disease: Discuss
- What are the complexities in taking the Hepatitis B vaccine while being positive?
- Allergies: why do humans have them?
- DNA modification: how does it work?
- Explain the misconceptions about the COVID-19 vaccines.
Interesting Biology Topics
Biology doesn’t have to be boring. Different aspects of biology could be fun to explore, especially if you’ve had a flair for the study since your elementary school classes.
You can either write an essay or paper with the following interesting biology research topics:
- Human emotions and conflicts with their intellectual intelligence
- Emotions: Its influence on art and music and how the perception of art influences the world
- The consequences of marijuana and alcohol on teenagers
- Compare and contrast how alcohol affects teenagers and adults
- Discuss the contributions of neuroscience to the subject of emotional pain
- Explain how the brain process speech
- Discuss the factors that cause autism
- Explain what is meant when people say humans are animals
- Why do scientists say humans are pessimists?
- Factors contributing to the dopamine levels human experience
- How does isolation affect the human brain?
- What factors contribute to instinctive responses?
- Noise pollution: how it affects living organisms
- Fire ecology: The contributions of plants to fire outbreak
- Explain the science behind how hot temperature, soil, and dry grass start a fire
- Microbes: what do you understand by bioremediation?
- Explain urban ecology and the challenges it pokes to solve
- Discuss how excessive internet usage affects the human memory
- Evaluate how conservation biology contributes to the extinction prevention efforts
- Discuss the role of satellites and drones in understanding the natural world
- Why do we need space travel and studies?
- Explain the limitations of limnology studies
- What are infectious-disease-causing agents all about?
- Discuss what epigenetics studies encompass
- Why is cancer research essential to the world?
- Discuss climate change: Governments are not interested, and there is no alternative
- How is behavioral science studies a core part of the understanding of the world?
- Discuss the issues with genetic engineering and why it’s a challenge
- Evaluate the strengths and weaknesses in the arguments for a plant-based diet
- Create a survey amongst students of biology asking why they chose to study the course.
Biology Research Topics For College Students
If you find any of the above beyond your intellectual and Research capacity, here are some topics you can handle. You can use these for your essays, projects, quizzes, or competitions.
These custom yet popular biology research topics will examine famous personalities and other discourse in biology:
- Effects of the human hormone on the mind
- Why do men get erect even when they’re absentminded?
- How does women’s arousal work?
- How can melatonin be valuable for therapy?
- Risky behavior: Hormones responsible for the risk
- Stem and cloning: what is the latest research on the subject?
- Hormones: changes in pregnancy
- Why do pregnant women have an appetite for random and remote things?
- The role of physical activities in hormone development
- Examine the benefits and threats of transgenic crops
- The fight against COVID-19: assess current successes
- The fight against smallpox: assess current successes
- The fight against HIV: history, trends, and present research
- Discuss the future of prosthetic appliances
- Examine the research and the future of mind-controlled limbs
- What does cosmetic surgery mean, and why is it needed?
- Analyze the meaning and process of vascular surgery
- Discuss the debate around changes in genital organs for males and females in transgender bodies
- How do donors and organ transplants work?
- Account for the work of Dr. Malcom E Miller
- Discuss the contribution of Charles Darwin to human evolution
- Explain the trends in biomedicine
- Discuss the functions of x-rays in botany
- Assess the most efficient systems for wildlife preservation
- Examine how poverty contributes to climate hazards
- Discuss the process involved in plant metabolism
- The transformation of energy into a living thing: discuss
- Prevention for sexually transmitted disease: What are the misconceptions?
- Analyze how the human body reacts to poison
- Russian Poisoning: What are the lessons scientists must learn?
- COVID-19: Discuss the efforts by two or three governments to prevent the spread
- Discuss the contributions of Pfizer during the pandemic.
Marine Biology Research Topics
This subject explains orgasms in the sea, how they survive, and their interaction with their environment. If you have a flair for this field, the following Biology research topics may interest you:
- Discuss what quantitative ecology through modeling means
- Smallest diatoms and marine logistics: discuss
- How is the shark studied?
- Acidification of seas: Causes and consequences
- Discuss the concept of the immortality of Jellyfishes
- Discuss the differences between seawater and freshwater in marine study
- Account for some of the oldest marine species
- Discuss the evolution of the deep sea
- Explain whales’ communication techniques
- What does plankton ecology encompass?
- The importance of coral reefs to seawater
- Challenges that encompass geological oceanography
- How tourism affects natural animal habitat
- Discuss some instances of the domestication of wild marine animals
- Coastal zone: pros and cons of living in such areas
- How do sharks perceive enemies?
- Analyze why some animals can live in water but can’t live on land
- Explain how plants survive in the sea
- Compare and contrast the different two species of animals in the water
- How can marine energy be generated, stored, and used?
Molecular Biology Research Topics
Focusing on the construct of cells and analysis of their composition, it understands the alteration and maintenance of cellular processes. If you’d like to focus on molecular biology, here are 15 good biology research topics for you:
- Ethical considerations in molecular genetics
- Discuss the structure and component of the gene
- Examine the restrictions in DNA
- What are the peculiarities in modern nucleic acid analysis
- What goes into the Pharmaceutical production of drugs
- Evaluate the building blocks of life
- Discuss the systems of RNA translation to protein
- PCR: How DNA is tested and analyzed
- Why is prion disease so dangerous?
- Compare and contrast recessive genes vs. dominant genes
- Can there be damage to the human DNA, and can it be repaired?
- Constraints in the research of microarray data analysis
- Protein purification: How it evolves
- Objectives of nucleic acid
- Explain the structure of a prion.
Biology Research Topics For High School
Your teachers and professors will be awed if you create impeccable essays for your next report. You need to secure the best grades as you move closer to graduation, and brainstorming any of these popular biology research topics will help:
- Identify the most endangered species
- The challenges to animal extinction
- What are the things everyone should know about sea life?
- Discuss the history of genetics
- Explain the biological theory of Charles Darwin
- How did the lockdown affect social interaction?
- Why do some people refuse the vaccine?
- Origin of genetics
- What is animal hunting, and why is it fashionable
- Explain the evolution of a virus
- Role of lockdown in preventing deaths and illnesses
- Invasive species: What does it mean?
- Endangered animals: How do they survive in the face of their hazards?
- Lockdown and their role in reducing coronavirus transmission
- Vaccine distribution: Ideas for global distribution
- Why can viruses become less virulent?
- Discuss the evolution of the world
- Explain the evolution of the planet
- Explain what Elon Musk means when he says life on Mars is possible
- What does herd immunity mean?
- Flu: why is there a low incidence in 2020?
- Relationship between archaeology and biology
- Antiviral drug: What it means
- Factors leading to the evolution of humans
- Give instances of what natural selection means
- What is considered the dead branches of evolution
- Whale hunting: What it means and the present trends
- Who is Stephen Jay, and what is his role in paleontology?
- Origin of diseases: why must humans fall sick?
- Why are humans called higher animals?
Human Biology Research Topics
Human biology understands humans and their relationship between themselves and their environment. It also studies how the body works and the impediments to health. Here are some easy biology research topics to explore on the subject:
- How do gut bacteria affect the brain?
- What are the ethical concerns around organ transplants?
- The consequence of alcohol on the liver
- The consequences of extreme salt on the human body
- Why do humans need to deworm regularly?
- The relationship between obesity and genetics
- Genetically modified foods: Why are they needed?
- How sun exposure affects human skin
- Latest trends: Depression is hereditary
- Influence of music on the human brain
- What are the stages of lung cancer
- Forensic DNA: latest trends
- How visual consumptions affect how humans think
- What is the process that leads to pregnancy?
- Explain the role of nanotechnology in HIV research
- Discuss any experiment with stem cells you know about
- Explain how humans consume food
- Discuss the process of metabolism as well as its criticality to human health
- Explore the consistent challenges technology poses to human health
- Explain the process of body decay to a skeleton.
Cell Biology Research Topics
There are many evolutionary biology research paper topics formed not by the nomenclature but for what they stand for. Cell biology is one of the most complex branches of the field.
It examines minor units and the living organisms that make them up. The focus is on the relationship between the cytoplasm, membrane, and parts of the cell. Here are some topics to explore for your scientific dissertation writing :
- How does chromatin engage in the alterations of gene expression?
- What are the usual cell infections, and why does the body have immunity defections?
- Identify and account for the heritage of Robert Brown in his core career focus
- Explain the structure of the animal cell and why It’s what it is
- Identify the cells in the human body as well as their functions
- Explain a scenario and justify the context of animals photosynthesizing like plants
- Why do bacteria invade the body, and how do they do it?
- Why are mitochondria considered the powerhouse of the cell
- Use the molecular analysis tool to explain multicellular organisms
- Examine how the White blood cells fight disease
- What do you understand about the role of cell biology in the treatment of Alzheimer’s Disease
- What are the latest research methods in cell biology?
- Identify the characteristics of viruses and why they threaten human existence.
- Discuss the differences between DNA and RNA
- What part of the body is responsible for human functionality for as long as the individual wants?
Get Biology Research Help As Soon As Possible
Creating the best essays or papers is easier now that you have custom biology research topics. However, you may still need support writing your paper beyond these topic ideas. After all, the first stage of writing like experts is brainstorming ideas and researching which is most feasible to write about.
If you truly want to wow your professor or teacher but can’t afford to dedicate all the required time, here’s an alternative. You can hire writing helpers online for quality papers at a cheap price, and we can help with that. We are a team of writers with many years of writing experience for students in Europe and North America. You can even buy thesis online with us, as well as editing services.
Each paper is assigned to writers with expertise in a specific field. This enables them to provide in-depth analysis as your assignment requires. We’re based online, which means you won’t have issues with accessibility and availability. Just tell us what you need, and we will get it done.
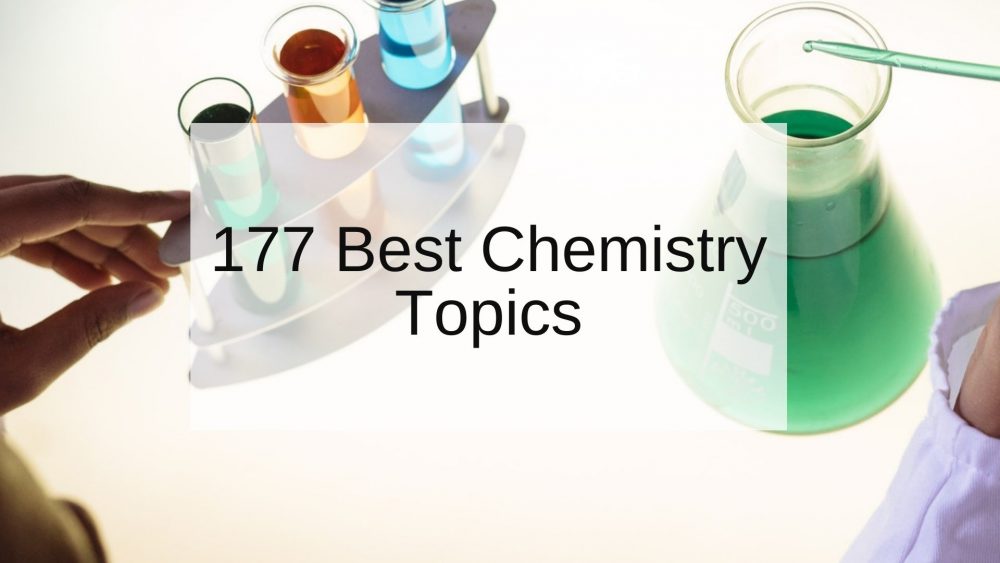
Leave a Reply Cancel reply
Your email address will not be published. Required fields are marked *
Comment * Error message
Name * Error message
Email * Error message
Save my name, email, and website in this browser for the next time I comment.
As Putin continues killing civilians, bombing kindergartens, and threatening WWIII, Ukraine fights for the world's peaceful future.
Ukraine Live Updates
Custom Essay, Term Paper & Research paper writing services
- testimonials
Toll Free: +1 (888) 354-4744
Email: [email protected]
Writing custom essays & research papers since 2008
Top 100 biology research topics for high school and college.
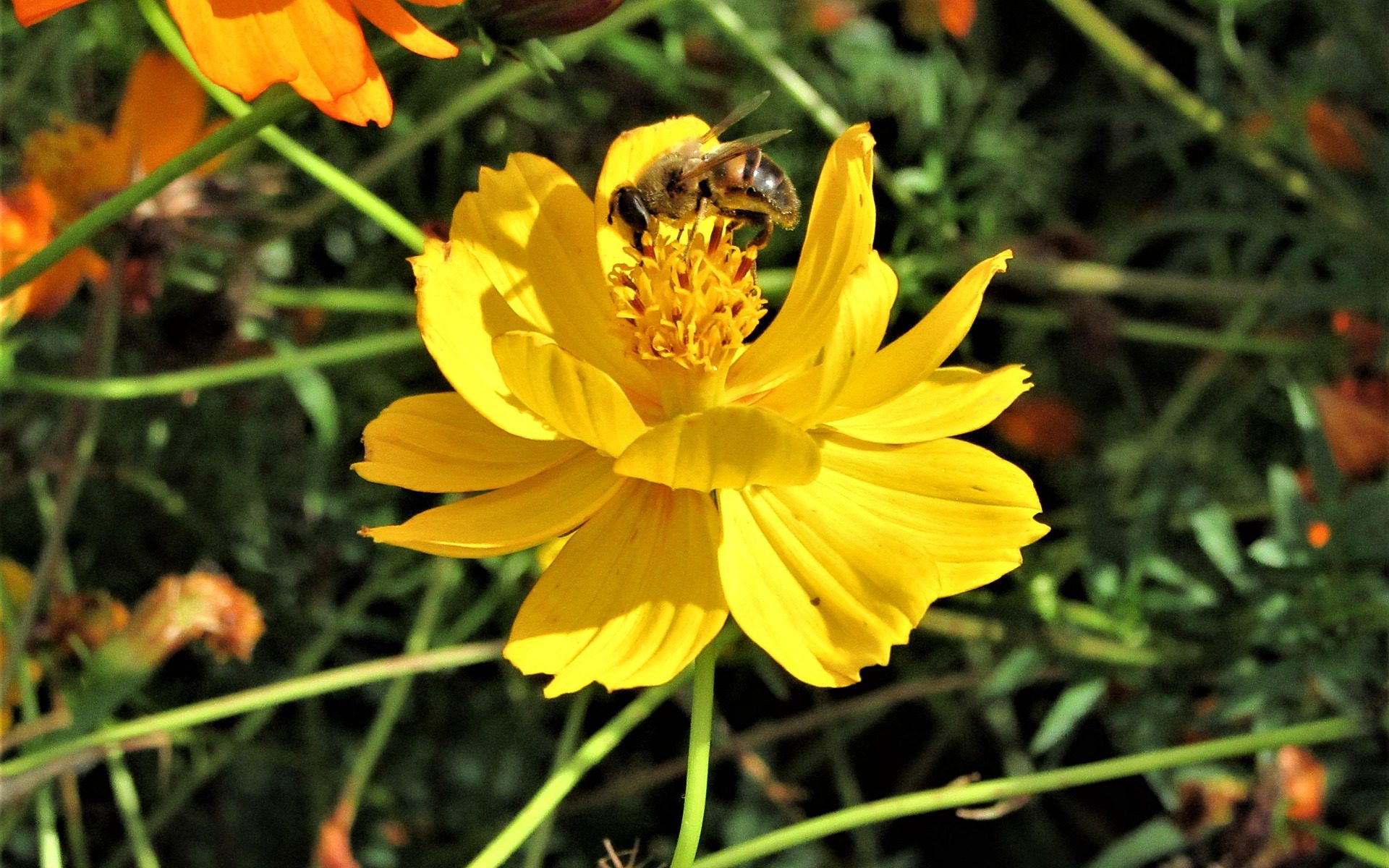
Writing a biology essay may not sound like a very difficult thing to do. In fact, most students really like this subject. The problem is not that you can’t write a good paper on a topic in biology. The problem is with finding excellent biology research topics. Now, you may be wondering why you would want to invest so much time into finding great biology research paper topics. After all, what you write in the essay matters more than the topic, right? Wrong! We are here to tell you that professors really appreciate interesting and unique topics.
And it makes a lot of sense, if you think about it. If you simply pick one of the most popular biology research topics, you will never be able to pique the interest of your teacher. He has read dozens, if not hundreds, or papers on that exact same topic. What you want to do is come up with interesting biology research topics. You want to find topics that none of your classmates are thinking of writing an academic paper about. You will shortly see why this is important. And we will also give you 100 biology topics for research projects that you can use for free – right now!
Biology Research Paper Topics Really Are Important!
It doesn’t matter what area of biology you need to write about. This information applies to everything from zoology and botany to anatomy. The reality is that your professor will really appreciate good topics. And you can rest assured that he or she knows how to spot them. The moment the professor starts to read your paper, he or she will immediately realize that you really did your best to find an excellent topic. And if you write a good introduction paragraph (which contains a captivating thesis statement as well), you are in the best position to earn bonus points.
You may not be aware of it, but teachers are willing to treat great papers with more leniency. This means that you will not get penalized for minor mistakes if you come up with a great topic. In other words, you will get a better grade on your papers if you manage to come up with good research topics for biology. This is a fact and it is based on thousands of pieces of feedback from our readers.
How Do You Choose Good Biology Research Topics?
Choosing research topics for biology can be a daunting task. Frankly, the research paper topics biology students are looking for are not easy to come by. The first thing you want to avoid is going to the first website that pops up in Google and getting your ideas from there. Most of your peers will do the same. Also, avoid topics that are extremely simple. You will simply not have enough ideas to write about. Of course, you should avoid overly complex topics because finding information about them may be extremely difficult.
The best way to find a good topic, in our opinion, is to get in touch with an academic writing company. You will get access to a professional writer who knows exactly what professors are looking for. A writer will quickly give you an amazing research topic in biology.
Eloquent Examples of Popular Biology Research Topics
To make things as simple as possible for you, we’ve put together a list of biology research project ideas. You will find 100 topics on various subjects below. Of course, you can use any of our topics for free. However, keep in mind that even though we are doing our best to maintain this list fresh, other students will find it as well. If you need new topics for your next biology essay, we recommend you to get in touch with us. We monitor our email address, so we can help you right away. Also, you can buy a research paper from our service.
Biology Research Topics for High School
Are you looking for biology research topics for high school? These are relatively simple when compared to college-level topics. Here are a couple of topic ideas that high school students will surely appreciate:
- Identifying Three Dead Branches of Evolution.
- What Is Sleep?
- How Does Physical Exercise Affect the Metabolism?
- A Behavioral Study of Birds.
- How Does Music Affect Your Brain?
- Climate Change and Biodiversity.
- Are Bees Really Becoming Extinct?
- Rainforest Extinction Is Dangerous.
- The Benefits of Organic Farming.
- Can the Brain Repair Itself?
- The Effect of Bacteria on Depression.
- How Do Sea Animals Camouflage?
Research Topics in Biology for Undergraduates
Research topics in biology for undergraduates are more complex than high school or college topics. Our researchers did their best to find topics that are relatively complex. However, each one of the following topics has plenty of information about it online:
- What Is the Mechanism of Metastasis in Cancer Patients?
- How Do Tumor Suppressor Genes Appear?
- How Can We Destroy Cancer Cells Without Damaging Other Cells?
- The Benefits of Gene Therapy.
- Analyzing the Huntington’s Disease (the HTT Gene).
- How Does the down Syndrome (Trisomy of 21st Chromosome) Appear?
- Analyzing the Brain Activity During an Epileptic Seizure.
- How Are Our Memories Formed and Preserved?
- The Effect of Probiotics on Infections.
- Analyzing Primate Language.
- Analyzing Primate Cognitive Functions.
- The Link Between Darwin’s Theory and Biology.
Biology Research Topics for College Students
Biology research topics for college students are of moderate difficulty. They are easier than undergrad topics and more complex than high school topics. While compiling this list, we made sure you have more than enough information online to write the paper quickly:
- Using DNA Technology in the Field of Medical Genetics.
- The Effect of Drinking on Embryonic Development.
- How Are Genes Mapped and Cloned?
- Explain What Genetic Polymorphism Is.
- What Is a Hereditary Disease?
- The Effect of Drugs on Embryonic Development.
- Describing Oligogenic Diseases (like Hirschsprung Disease)
- What Is the Mendelian Inheritance?
- How Transcriptomics and Proteomics Changed Modern Medicine.
- The Risk Factors of Infertility Explained.
- How Does Aging Effect Infertility?
- What Do Ash Elements Do in a Plant?
- Explaining the Pigments in a Plant Cell.
- How Is Photosynthesis Done?
- The Role of Fats in Plant Cells.
- The Effect of Smoking on Embryonic Development.
Cell Biology Research Topics
Some of the best biology topics are cell biology research topics. The scientific community is constantly making progress in this area, so there is always something new to write about. Here are some of the best examples:
- What Is Regenerative Medicine?
- A Closer Look at Tissue Engineering.
- Discuss the Future of Regenerative Medicine.
- Analyzing Therapeutic Cloning.
- The Pros and Cons of Creating Artificial Organs.
- How Do Cell Age?
- Can We Reverse Cell Aging?
- Advances in Cell Therapy.
- What Is Cell Adhesion?
- Explaining Cell Division.
- What Is Cellular Metabolism?
- Describe Active and Passive Transport in Cells.
- What Are Cell Plastids?
Evolutionary Biology Research Paper Topics
If you want something more complex, you can try your hand at writing on evolutionary biology research paper topics. As with all our topics, you will be able to find a lot of ideas and information online. Here are our picks:
- Where Did Plants Come From? (The Evolutionary Theory)
- Explaining the Host-parasite Coevolution.
- How Did Parasites Evolve over Time?
- What Is Natural Selection and How Does It Work?
- Explain Sexual Selection.
- Explain Sexual Conflict.
- How Did Our Immune Systems Evolve?
- How Do New Species Appear in the Wild?
- The Evolution of Cell Respiration.
- What Is the Hippo Pathway? (Developmental Biology)
Various Topics
Antibiotics resistance, agriculture and cloning are hot subjects nowadays. Your professor will surely be interested to learn more about biology research topics. Here is a mix of topic ideas from our established community of academic writers:
- The Problem of Using Antibiotics on Large Scale.
- Examining the Effects of Salt on Plants.
- What Is DNA Technology?
- The Effects of GMOs on the Human Body.
- How Is the Quality of Antibiotics Controlled?
- How Are GMO Food Crops Created?
- The Effect of Veterinary Antibiotics on Humans.
- The Allergic Reactions to Specific Antibiotics.
- A Look at How Penicillin Works in the Human Body.
- How Are Antibiotics Obtained?
- What Are Natural Biochemicals with Pest-repellent Properties?
- The 3 Most Toxic Effects of Antibiotics
- How the Human Body Develops Resistance to Antibiotics.
- The Impact of Biology on the Us Agriculture.
- What Is the Green Revolution?
- Analyzing the Minerals in the Plant Cell.
- Analyzing Muscle Development and Regeneration
- The Uses of Cancer Stem Cells.
Marine Biology Research Topics
There is a lot of talk about global warming, about microplastics in our oceans, and about endangered marine species. This means that marine biology research topics are a very hot topic today. Here are some of our best ideas:
- Can GMO Organisms Break down Oil after Maritime Accidents?
- Pollution-absorbing Bio-films.
- Microbes That Can Absorb Toxic Compounds in the Water.
- Can We Really Use Bioluminescence?
- How Is Bio-diesel Created?
- Analyzing the Coral Reef Biology.
- Why Is the Lobster Population Dwindling?
- The Effect of Mass Fishing on the World’s Oceans.
- Global Warming and Its Effect on Marine Microorganisms.
Molecular Biology Research Topics
Writing about molecular biology research topics is not easy. However, it’s a foolproof way to get a top grade. Your professor will really appreciate your willingness to write an essay about a complex topic. Just make sure you know what you are talking about. Below you can find some of the best topics:
- How Is Insulin Produced?
- How Is the Growth Hormone Produced?
- Analyzing the Repropagation of Translation.
- What Is DNA-telomerase?
- The Process of Sequencing Nucleotides in DNA.
- What Is Telomerase?
- The Link Between Telomerase and Cancer.
- The Link Between Telomerase and Aging.
- How Does DNA Forensics Work?
- Describe the Process of Protein Metabolism.
There is no such thing as easy biology research topics. When the topic is too simple, you end up getting penalized. You can’t write 500 words about it without straying away from the subject. Also, no matter how interesting the topic may be, you should make sure that the essay is written perfectly. This means that not even interesting biology research topics can save you from a bad grade if you fail to follow all applicable academic writing standards.
Find it hard to cope with your college paper? Great news! Use promo “ mypaper20 ” and enjoy 20% discount on a biology writing assignment from our profs!
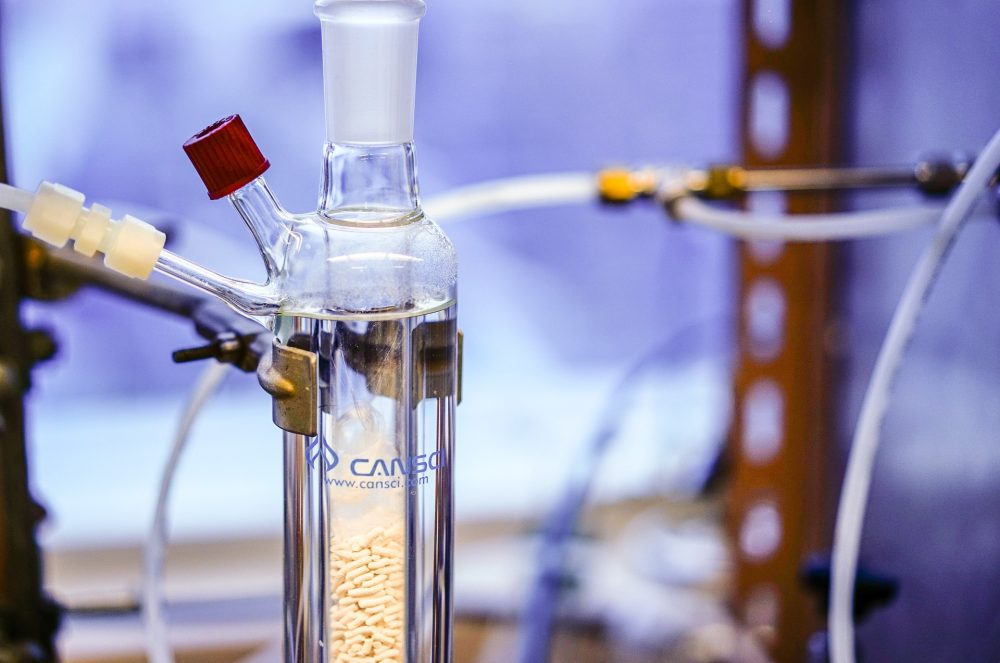
- Write my thesis
- Thesis writers
- Buy thesis papers
- Bachelor thesis
- Master's thesis
- Thesis editing services
- Thesis proofreading services
- Buy a thesis online
- Write my dissertation
- Dissertation proposal help
- Pay for dissertation
- Custom dissertation
- Dissertation help online
- Buy dissertation online
- Cheap dissertation
- Dissertation editing services
- Write my research paper
- Buy research paper online
- Pay for research paper
- Research paper help
- Order research paper
- Custom research paper
- Cheap research paper
- Research papers for sale
- Thesis subjects
- How It Works
100+ Quantitative Research Topics For Students
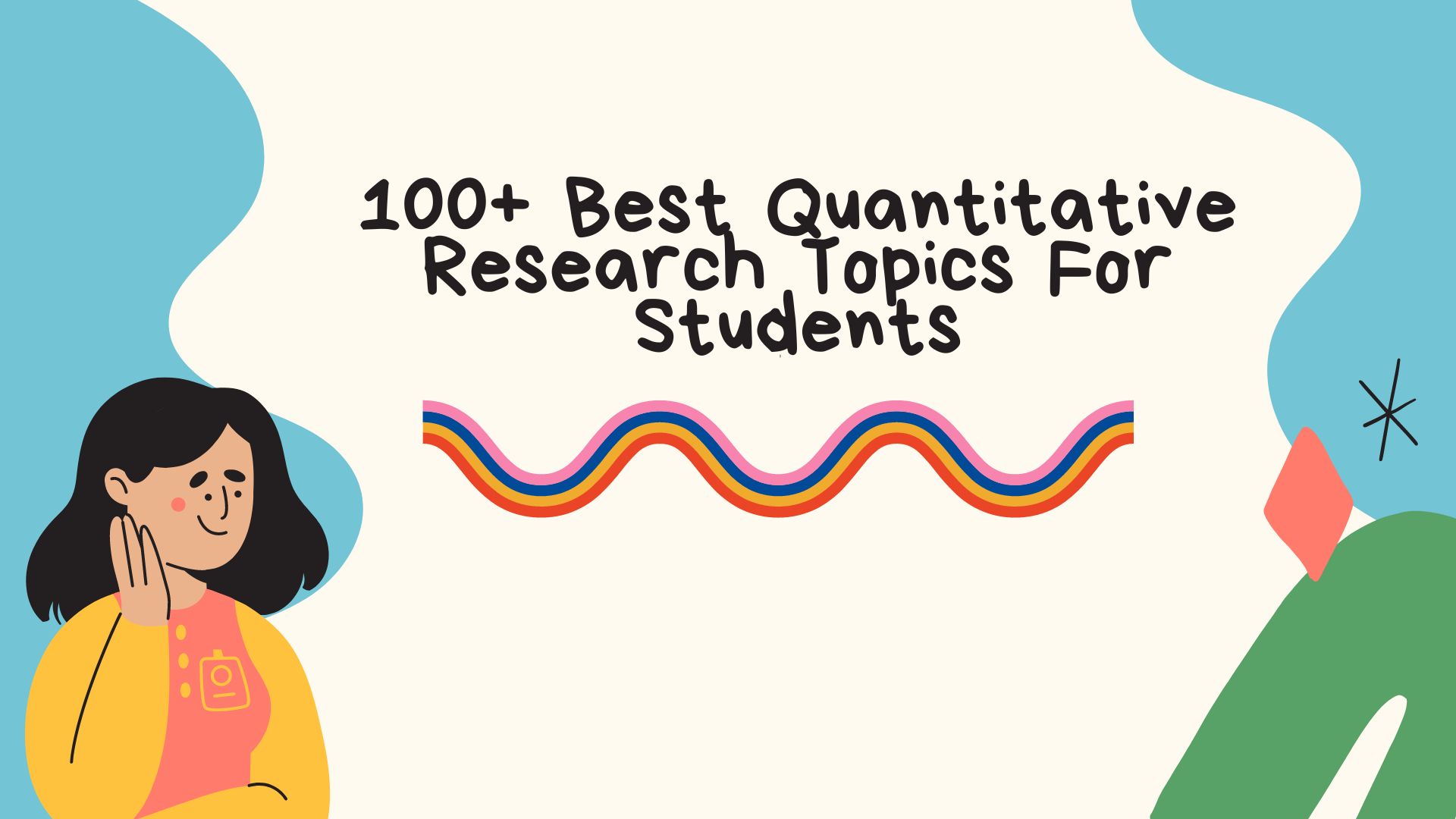
Quantitative research is a research strategy focusing on quantified data collection and analysis processes. This research strategy emphasizes testing theories on various subjects. It also includes collecting and analyzing non-numerical data.
Quantitative research is a common approach in the natural and social sciences , like marketing, business, sociology, chemistry, biology, economics, and psychology. So, if you are fond of statistics and figures, a quantitative research title would be an excellent option for your research proposal or project.
How to Get a Title of Quantitative Research
How to make quantitative research title, what is the best title for quantitative research, amazing quantitative research topics for students, creative quantitative research topics, perfect quantitative research title examples, unique quantitative research titles, outstanding quantitative research title examples for students, creative example title of quantitative research samples, outstanding quantitative research problems examples, fantastic quantitative research topic examples, the best quantitative research topics, grade 12 quantitative research title for students, list of quantitative research titles for high school, easy quantitative research topics for students, trending topics for quantitative research, quantitative research proposal topics, samples of quantitative research titles, research title about business quantitative.
Finding a great title is the key to writing a great quantitative research proposal or paper. A title for quantitative research prepares you for success, failure, or mediocre grades. This post features examples of quantitative research titles for all students.
Putting together a research title and quantitative research design is not as easy as some students assume. So, an example topic of quantitative research can help you craft your own. However, even with the examples, you may need some guidelines for personalizing your research project or proposal topics.
So, here are some tips for getting a title for quantitative research:
- Consider your area of studies
- Look out for relevant subjects in the area
- Expert advice may come in handy
- Check out some sample quantitative research titles
Making a quantitative research title is easy if you know the qualities of a good title in quantitative research. Reading about how to make a quantitative research title may not help as much as looking at some samples. Looking at a quantitative research example title will give you an idea of where to start.
However, let’s look at some tips for how to make a quantitative research title:
- The title should seem interesting to readers
- Ensure that the title represents the content of the research paper
- Reflect on the tone of the writing in the title
- The title should contain important keywords in your chosen subject to help readers find your paper
- The title should not be too lengthy
- It should be grammatically correct and creative
- It must generate curiosity
An excellent quantitative title should be clear, which implies that it should effectively explain the paper and what readers can expect. A research title for quantitative research is the gateway to your article or proposal. So, it should be well thought out. Additionally, it should give you room for extensive topic research.
A sample of quantitative research titles will give you an idea of what a good title for quantitative research looks like. Here are some examples:
- What is the correlation between inflation rates and unemployment rates?
- Has climate adaptation influenced the mitigation of funds allocation?
- Job satisfaction and employee turnover: What is the link?
- A look at the relationship between poor households and the development of entrepreneurship skills
- Urbanization and economic growth: What is the link between these elements?
- Does education achievement influence people’s economic status?
- What is the impact of solar electricity on the wholesale energy market?
- Debt accumulation and retirement: What is the relationship between these concepts?
- Can people with psychiatric disorders develop independent living skills?
- Children’s nutrition and its impact on cognitive development
Quantitative research applies to various subjects in the natural and social sciences. Therefore, depending on your intended subject, you have numerous options. Below are some good quantitative research topics for students:
- The difference between the colorific intake of men and women in your country
- Top strategies used to measure customer satisfaction and how they work
- Black Friday sales: are they profitable?
- The correlation between estimated target market and practical competitive risk assignment
- Are smartphones making us brighter or dumber?
- Nuclear families Vs. Joint families: Is there a difference?
- What will society look like in the absence of organized religion?
- A comparison between carbohydrate weight loss benefits and high carbohydrate diets?
- How does emotional stability influence your overall well-being?
- The extent of the impact of technology in the communications sector
Creativity is the key to creating a good research topic in quantitative research. Find a good quantitative research topic below:
- How much exercise is good for lasting physical well-being?
- A comparison of the nutritional therapy uses and contemporary medical approaches
- Does sugar intake have a direct impact on diabetes diagnosis?
- Education attainment: Does it influence crime rates in society?
- Is there an actual link between obesity and cancer rates?
- Do kids with siblings have better social skills than those without?
- Computer games and their impact on the young generation
- Has social media marketing taken over conventional marketing strategies?
- The impact of technology development on human relationships and communication
- What is the link between drug addiction and age?
Need more quantitative research title examples to inspire you? Here are some quantitative research title examples to look at:
- Habitation fragmentation and biodiversity loss: What is the link?
- Radiation has affected biodiversity: Assessing its effects
- An assessment of the impact of the CORONA virus on global population growth
- Is the pandemic truly over, or have human bodies built resistance against the virus?
- The ozone hole and its impact on the environment
- The greenhouse gas effect: What is it and how has it impacted the atmosphere
- GMO crops: are they good or bad for your health?
- Is there a direct link between education quality and job attainment?
- How have education systems changed from traditional to modern times?
- The good and bad impacts of technology on education qualities
Your examiner will give you excellent grades if you come up with a unique title and outstanding content. Here are some quantitative research examples titles.
- Online classes: are they helpful or not?
- What changes has the global CORONA pandemic had on the population growth curve?
- Daily habits influenced by the global pandemic
- An analysis of the impact of culture on people’s personalities
- How has feminism influenced the education system’s approach to the girl child’s education?
- Academic competition: what are its benefits and downsides for students?
- Is there a link between education and student integrity?
- An analysis of how the education sector can influence a country’s economy
- An overview of the link between crime rates and concern for crime
- Is there a link between education and obesity?
Research title example quantitative topics when well-thought guarantees a paper that is a good read. Look at the examples below to get started.
- What are the impacts of online games on students?
- Sex education in schools: how important is it?
- Should schools be teaching about safe sex in their sex education classes?
- The correlation between extreme parent interference on student academic performance
- Is there a real link between academic marks and intelligence?
- Teacher feedback: How necessary is it, and how does it help students?
- An analysis of modern education systems and their impact on student performance
- An overview of the link between academic performance/marks and intelligence
- Are grading systems helpful or harmful to students?
- What was the impact of the pandemic on students?
Irrespective of the course you take, here are some titles that can fit diverse subjects pretty well. Here are some creative quantitative research title ideas:
- A look at the pre-corona and post-corona economy
- How are conventional retail businesses fairing against eCommerce sites like Amazon and Shopify?
- An evaluation of mortality rates of heart attacks
- Effective treatments for cardiovascular issues and their prevention
- A comparison of the effectiveness of home care and nursing home care
- Strategies for managing effective dissemination of information to modern students
- How does educational discrimination influence students’ futures?
- The impacts of unfavorable classroom environment and bullying on students and teachers
- An overview of the implementation of STEM education to K-12 students
- How effective is digital learning?
If your paper addresses a problem, you must present facts that solve the question or tell more about the question. Here are examples of quantitative research titles that will inspire you.
- An elaborate study of the influence of telemedicine in healthcare practices
- How has scientific innovation influenced the defense or military system?
- The link between technology and people’s mental health
- Has social media helped create awareness or worsened people’s mental health?
- How do engineers promote green technology?
- How can engineers raise sustainability in building and structural infrastructures?
- An analysis of how decision-making is dependent on someone’s sub-conscious
- A comprehensive study of ADHD and its impact on students’ capabilities
- The impact of racism on people’s mental health and overall wellbeing
- How has the current surge in social activism helped shape people’s relationships?
Are you looking for an example of a quantitative research title? These ten examples below will get you started.
- The prevalence of nonverbal communication in social control and people’s interactions
- The impacts of stress on people’s behavior in society
- A study of the connection between capital structures and corporate strategies
- How do changes in credit ratings impact equality returns?
- A quantitative analysis of the effect of bond rating changes on stock prices
- The impact of semantics on web technology
- An analysis of persuasion, propaganda, and marketing impact on individuals
- The dominant-firm model: what is it, and how does it apply to your country’s retail sector?
- The role of income inequality in economy growth
- An examination of juvenile delinquents’ treatment in your country
Excellent Topics For Quantitative Research
Here are some titles for quantitative research you should consider:
- Does studying mathematics help implement data safety for businesses
- How are art-related subjects interdependent with mathematics?
- How do eco-friendly practices in the hospitality industry influence tourism rates?
- A deep insight into how people view eco-tourisms
- Religion vs. hospitality: Details on their correlation
- Has your country’s tourist sector revived after the pandemic?
- How effective is non-verbal communication in conveying emotions?
- Are there similarities between the English and French vocabulary?
- How do politicians use persuasive language in political speeches?
- The correlation between popular culture and translation
Here are some quantitative research titles examples for your consideration:
- How do world leaders use language to change the emotional climate in their nations?
- Extensive research on how linguistics cultivate political buzzwords
- The impact of globalization on the global tourism sector
- An analysis of the effects of the pandemic on the worldwide hospitality sector
- The influence of social media platforms on people’s choice of tourism destinations
- Educational tourism: What is it and what you should know about it
- Why do college students experience math anxiety?
- Is math anxiety a phenomenon?
- A guide on effective ways to fight cultural bias in modern society
- Creative ways to solve the overpopulation issue
An example of quantitative research topics for 12 th -grade students will come in handy if you want to score a good grade. Here are some of the best ones:
- The link between global warming and climate change
- What is the greenhouse gas impact on biodiversity and the atmosphere
- Has the internet successfully influenced literacy rates in society
- The value and downsides of competition for students
- A comparison of the education system in first-world and third-world countries
- The impact of alcohol addiction on the younger generation
- How has social media influenced human relationships?
- Has education helped boost feminism among men and women?
- Are computers in classrooms beneficial or detrimental to students?
- How has social media improved bullying rates among teenagers?
High school students can apply research titles on social issues or other elements, depending on the subject. Let’s look at some quantitative topics for students:
- What is the right age to introduce sex education for students
- Can extreme punishment help reduce alcohol consumption among teenagers?
- Should the government increase the age of sexual consent?
- The link between globalization and the local economy collapses
- How are global companies influencing local economies?
There are numerous possible quantitative research topics you can write about. Here are some great quantitative research topics examples:
- The correlation between video games and crime rates
- Do college studies impact future job satisfaction?
- What can the education sector do to encourage more college enrollment?
- The impact of education on self-esteem
- The relationship between income and occupation
You can find inspiration for your research topic from trending affairs on social media or in the news. Such topics will make your research enticing. Find a trending topic for quantitative research example from the list below:
- How the country’s economy is fairing after the pandemic
- An analysis of the riots by women in Iran and what the women gain to achieve
- Is the current US government living up to the voter’s expectations?
- How is the war in Ukraine affecting the global economy?
- Can social media riots affect political decisions?
A proposal is a paper you write proposing the subject you would like to cover for your research and the research techniques you will apply. If the proposal is approved, it turns to your research topic. Here are some quantitative titles you should consider for your research proposal:
- Military support and economic development: What is the impact in developing nations?
- How does gun ownership influence crime rates in developed countries?
- How can the US government reduce gun violence without influencing people’s rights?
- What is the link between school prestige and academic standards?
- Is there a scientific link between abortion and the definition of viability?
You can never have too many sample titles. The samples allow you to find a unique title you’re your research or proposal. Find a sample quantitative research title here:
- Does weight loss indicate good or poor health?
- Should schools do away with grading systems?
- The impact of culture on student interactions and personalities
- How can parents successfully protect their kids from the dangers of the internet?
- Is the US education system better or worse than Europe’s?
If you’re a business major, then you must choose a research title quantitative about business. Let’s look at some research title examples quantitative in business:
- Creating shareholder value in business: How important is it?
- The changes in credit ratings and their impact on equity returns
- The importance of data privacy laws in business operations
- How do businesses benefit from e-waste and carbon footprint reduction?
- Organizational culture in business: what is its importance?
We Are A Call Away
Interesting, creative, unique, and easy quantitative research topics allow you to explain your paper and make research easy. Therefore, you should not take choosing a research paper or proposal topic lightly. With your topic ready, reach out to us today for excellent research paper writing services .
Leave a Reply Cancel reply
- Privacy Policy
Buy Me a Coffee

Home » 350+ Biology Research Topics
350+ Biology Research Topics

Biology is a vast field of study that explores the diverse aspects of life, from the smallest organisms to the complex ecosystems they inhabit. With new discoveries being made every day, the field of biology is constantly evolving and expanding. As a result, there are numerous research topics within biology that can capture the imagination of students, researchers , and professionals alike. Whether you’re interested in genetics, ecology, microbiology, or any other subfield of biology, there is no shortage of fascinating topics to explore. In this post, we will discuss some of the most compelling biology research topics that you can delve into.
Biology Research Topics
Biology Research Topics are as follows:
- The role of gut microbiota in human health and disease.
- The effects of climate change on animal behavior and physiology.
- The molecular mechanisms of cancer development and progression.
- The evolutionary origins of human language.
- The impact of pesticides on insect populations and ecosystems.
- The genetic basis of aging and longevity.
- The ecological importance of microbial communities in soil.
- The physiology and behavior of marine mammals.
- The molecular mechanisms of viral infections.
- The evolutionary history of flowering plants.
- The ecological impacts of invasive species.
- The role of epigenetics in gene regulation and disease.
- The evolution of social behavior in animals.
- The physiology and ecology of birdsong.
- The impact of antibiotics on gut microbiota and human health.
- The role of the microbiome in psychiatric disorders.
- The evolutionary history of human migrations.
- The ecological and physiological effects of light pollution on animals.
- The mechanisms of cell division and differentiation.
- The ecological impacts of deforestation.
- The molecular mechanisms of drug addiction.
- The genetic basis of plant resistance to pests and diseases.
- The evolutionary history of human diet and nutrition.
- The molecular mechanisms of neurodegenerative diseases.
- The ecology and evolution of sexual selection.
- The physiological and behavioral effects of air pollution on animals.
- The role of epigenetics in plant development and stress response.
- The evolutionary history of animal domestication.
- The molecular mechanisms of genetic diseases.
- The ecological impacts of climate change on plants.
- The evolutionary history of human mating systems.
- The physiological and behavioral effects of noise pollution on animals.
- The genetic basis of intelligence and cognitive abilities.
- The ecological and physiological effects of ocean acidification on marine organisms.
- The molecular mechanisms of immune system function and dysfunction.
- The evolutionary history of human social structures.
- The ecological impacts of plastic pollution on marine ecosystems.
- The genetic basis of animal migration.
- The physiological and behavioral effects of light and dark cycles on animals.
- The ecological and evolutionary dynamics of symbiosis.
- The molecular mechanisms of gene regulation and expression.
- The evolutionary history of human disease resistance.
- The ecological impacts of overfishing on marine ecosystems.
- The genetic basis of animal communication.
- The physiological and behavioral effects of temperature changes on animals.
- The ecological and evolutionary dynamics of parasitism.
- The molecular mechanisms of circadian rhythms.
- The evolutionary history of human social cognition.
- The ecological impacts of urbanization on wildlife.
- The genetic basis of antibiotic resistance in bacteria.
- The impact of climate change on insect population dynamics.
- The role of the microbiome in the development of autoimmune diseases.
- The genetic basis of complex human diseases such as diabetes and heart disease.
- The evolution of plant secondary metabolites and their ecological functions.
- The effects of anthropogenic noise on animal communication and behavior.
- The molecular mechanisms of protein synthesis and folding.
- The role of RNA in gene expression and regulation.
- The ecology and evolution of microbial symbioses in plants.
- The physiological and behavioral effects of air temperature changes on animals.
- The genetic basis of crop domestication and improvement.
- The evolution of reproductive strategies in animals.
- The impacts of plastic pollution on terrestrial ecosystems.
- The molecular mechanisms of stem cell differentiation and regeneration.
- The ecological dynamics of predator-prey interactions.
- The role of gut microbiota in the regulation of host metabolism.
- The genetic basis of host-pathogen coevolution.
- The evolution of social cognition and cooperation in animals.
- The ecological and physiological effects of wildfires on ecosystems.
- The molecular mechanisms of transcriptional regulation in eukaryotic cells.
- The role of microorganisms in soil nutrient cycling and ecosystem functioning.
- The genetic basis of plant-pathogen interactions.
- The ecology and evolution of microbial communities in the ocean.
- The physiological and behavioral effects of water pollution on aquatic organisms.
- The molecular mechanisms of protein degradation and turnover.
- The impact of urbanization on pollinator populations and plant-pollinator interactions.
- The genetic basis of insecticide resistance in pests.
- The evolution of animal cognition and perception.
- The ecological and evolutionary dynamics of host-parasite interactions.
- The role of epigenetic modifications in plant adaptation to environmental stress.
- The physiological and behavioral effects of endocrine disruptors on animals.
- The molecular mechanisms of DNA replication and repair.
- The impact of ocean warming on coral reef ecosystems.
- The genetic basis of animal personality traits.
- The ecology and evolution of microbial symbioses in animals.
- The physiological and behavioral effects of light quality on plants.
- The molecular mechanisms of RNA editing and splicing.
- The role of microbial communities in plant-pathogen interactions.
- The ecological and evolutionary dynamics of seed dispersal.
- The genetic basis of animal coloration and pattern.
- The impact of climate change on plant phenology and productivity.
- The molecular mechanisms of signal transduction in cells.
- The role of microbial communities in the human gut-brain axis.
- The ecology and evolution of animal migrations.
- The physiological and behavioral effects of chemical pollution on animals.
- The genetic basis of animal development and morphogenesis.
- The evolution of animal social behavior and communication.
- The ecological dynamics of plant-pollinator networks.
- The molecular mechanisms of intracellular trafficking and transport.
- The role of microbial communities in the degradation of pollutants.
- The ecological and evolutionary dynamics of species interactions in ecological communities.
- The role of epigenetics in cancer development and progression.
- The molecular basis of antibiotic resistance in bacteria.
- The impact of climate change on biodiversity and ecosystem functioning.
- The genetic basis of aging and age-related diseases.
- The evolution of social organization in primates.
- The ecological dynamics of plant-fungal interactions.
- The role of microbiota in immune system development and function.
- The molecular mechanisms of DNA damage and repair.
- The physiological and behavioral effects of climate change on marine organisms.
- The genetic basis of human variation and diversity.
- The evolution of sexual selection and mate choice in animals.
- The ecological and evolutionary dynamics of species invasions.
- The role of microbiota in brain function and behavior.
- The molecular mechanisms of immune system activation and regulation.
- The physiological and behavioral effects of pollution on wildlife.
- The genetic basis of behavioral disorders and mental illness.
- The evolution of plant-pollinator mutualisms.
- The ecological dynamics of predator-prey coevolution.
- The role of microbiota in metabolic diseases such as obesity and diabetes.
- The molecular mechanisms of protein-protein interactions and signaling.
- The genetic basis of complex traits such as intelligence and personality.
- The evolution of animal communication and language.
- The ecological and evolutionary dynamics of mutualistic interactions in ecological communities.
- The role of microbiota in the development and maintenance of gut homeostasis.
- The molecular mechanisms of neurotransmitter synthesis and release.
- The physiological and behavioral effects of artificial light at night on wildlife.
- The genetic basis of developmental disorders such as autism and ADHD.
- The evolution of host-parasite coevolution and adaptation.
- The ecological dynamics of plant-herbivore interactions.
- The role of microbiota in the regulation of metabolism and energy balance.
- The molecular mechanisms of membrane transport and signaling.
- The physiological and behavioral effects of habitat fragmentation on wildlife.
- The genetic basis of circadian rhythms and sleep disorders.
- The evolution of animal cognition and decision-making.
- The ecological and evolutionary dynamics of trophic cascades.
- The role of microbiota in the development and function of the respiratory system.
- The molecular mechanisms of epigenetic inheritance.
- The physiological and behavioral effects of endocrine disruptors on wildlife.
- The genetic basis of developmental plasticity and adaptation.
- The evolution of animal social learning and culture.
- The ecological dynamics of predator-prey interactions in aquatic systems.
- The role of microbiota in the regulation of host immunity and inflammation.
- The molecular mechanisms of RNA interference and gene silencing.
- The physiological and behavioral effects of climate change on migratory animals.
- The genetic basis of drug addiction and substance abuse disorders.
- The evolution of animal cooperation and conflict resolution.
- The ecological and evolutionary dynamics of niche construction.
- The role of microbiota in the regulation of host-microbe interactions.
- The molecular mechanisms of gene regulation by non-coding RNAs.
- The role of epigenetics in gene expression and regulation.
- The molecular mechanisms of DNA damage response and repair.
- The impact of environmental toxins on human health.
- The evolutionary origins of viruses and their impact on hosts.
- The genetics of aging and age-related diseases.
- The impact of ocean acidification on marine organisms.
- The molecular basis of cancer development and progression.
- The genetic basis of behavior in animals.
- The impact of environmental stressors on plant growth and productivity.
- The evolution of sex determination and sexual selection.
- The role of the immune system in host-microbe interactions.
- The molecular mechanisms of circadian rhythms and sleep.
- The impact of air pollution on respiratory health.
- The genetic basis of speciation and hybridization.
- The role of neurotransmitters in brain function and behavior.
- The ecological dynamics of microbial communities in soil.
- The impact of climate change on biodiversity and ecosystem services.
- The molecular mechanisms of viral entry, replication, and release.
- The genetics of plant domestication and diversification.
- The role of mitochondrial DNA in aging and disease.
- The impact of deforestation on ecosystem functioning.
- The molecular basis of drug addiction and treatment.
- The genetic basis of adaptation and evolution in response to environmental change.
- The role of gut-brain signaling in behavior and disease.
- The impact of noise pollution on wildlife populations.
- The genetic basis of plant morphology and development.
- The role of the microbiome in disease susceptibility and resistance.
- The ecological dynamics of plant-insect interactions.
- The impact of agricultural practices on soil health and biodiversity.
- The molecular mechanisms of gene regulation in development and disease.
- The genetic basis of complex traits in humans and animals.
- The role of cytokines in immune response and inflammation.
- The ecological dynamics of microbial communities in aquatic ecosystems.
- The impact of plastic waste on marine ecosystems.
- The molecular mechanisms of genome stability and repair.
- The genetics of rare and common genetic diseases.
- The role of the endocannabinoid system in health and disease.
- The ecological dynamics of competition and cooperation in populations.
- The impact of light pollution on wildlife behavior and ecology.
- The genetic basis of animal migration and navigation.
- The role of the microbiome in host metabolism and energy balance.
- The impact of climate change on agricultural productivity and food security.
- The molecular mechanisms of epigenetic inheritance and transmission.
- The genetics of human brain development and disorders.
- The role of pheromones in animal communication and behavior.
- The ecological dynamics of host-microbe-pathogen interactions.
- The effect of diet and nutrition on gut microbiome diversity and composition.
- The ecology and evolution of microbial interactions in the soil.
- The role of epigenetic modifications in cancer development and progression.
- The impact of climate change on marine biodiversity and ecosystem functioning.
- The molecular mechanisms of mitochondrial respiration and ATP synthesis.
- The role of non-coding RNAs in gene regulation and disease.
- The evolution and diversification of flowering plants.
- The effects of artificial light at night on animal behavior and physiology.
- The genetic basis of adaptation to extreme environments.
- The ecology and evolution of plant-microbe interactions.
- The physiological and behavioral effects of noise pollution on wildlife.
- The molecular mechanisms of DNA methylation and histone modification.
- The role of microbial communities in the cycling of nutrients in aquatic ecosystems.
- The evolution of animal color vision and perception.
- The ecological and evolutionary dynamics of mutualistic interactions.
- The impact of deforestation on soil fertility and carbon storage.
- The molecular mechanisms of viral replication and pathogenesis.
- The role of microorganisms in the biodegradation of plastics.
- The ecology and evolution of microbial communities in the human gut.
- The physiological and behavioral effects of climate change on birds.
- The impact of invasive species on native ecosystems.
- The genetic basis of developmental disorders and intellectual disabilities.
- The evolution of animal behavior and communication in response to anthropogenic change.
- The ecological dynamics of soil carbon sequestration and storage.
- The role of microbial communities in the decomposition of organic matter.
- The physiological and behavioral effects of air pollution on plants.
- The molecular mechanisms of cellular differentiation and tissue development.
- The ecology and evolution of plant-animal interactions.
- The genetic basis of resistance to herbicides and pesticides in crops.
- The impact of urbanization on bird diversity and distribution.
- The role of microorganisms in the cycling of carbon and nitrogen in soil.
- The ecological and evolutionary dynamics of invasive species interactions.
- The physiological and behavioral effects of climate change on reptiles and amphibians.
- The role of microbial communities in the degradation of petroleum hydrocarbons.
- The genetic basis of plant development and growth.
- The evolution of animal migration and dispersal.
- The impact of land use change on freshwater biodiversity.
- The molecular mechanisms of membrane transport and ion channels.
- The role of microorganisms in the cycling of sulfur and phosphorus in soil.
- The physiological and behavioral effects of ocean acidification on marine organisms.
- The genetic basis of behavior and personality traits in humans.
- The evolution of plant reproductive strategies and pollination systems.
- The ecological and evolutionary dynamics of predator-prey coevolution.
- The impact of environmental stressors on gene expression and epigenetics.
- The evolution of sexual reproduction and mating systems in plants.
- The role of microorganisms in bioremediation of contaminated sites.
- The physiological and behavioral effects of climate change on fish.
- The molecular mechanisms of chromatin remodeling and gene regulation.
- The genetic basis of adaptation to high altitude environments.
- The ecology and evolution of plant-insect interactions.
- The impact of pesticide use on insect biodiversity and ecosystem functioning.
- The role of microorganisms in nitrogen fixation and cycling.
- The genetic basis of neurodegenerative diseases and cognitive decline.
- The evolution of social behavior and cooperation in animals.
- The ecological and evolutionary dynamics of plant invasions.
- The physiological and behavioral effects of noise pollution on humans.
- The molecular mechanisms of RNA splicing and alternative splicing.
- The role of microorganisms in biogeochemical cycling of trace elements.
- The genetic basis of adaptation to extreme temperatures.
- The ecology and evolution of microbial communities in soil and water.
- The impact of climate change on insect phenology and distribution.
- The molecular mechanisms of protein folding and misfolding.
- The role of microorganisms in biodegradation of environmental pollutants.
- The evolution of animal cognition and intelligence.
- The ecological and evolutionary dynamics of predator-prey interactions.
- The impact of anthropogenic noise on marine mammals.
- The role of microorganisms in biofilm formation and quorum sensing.
- The genetic basis of speciation and hybridization in plants.
- The evolution of parental care and offspring development in animals.
- The ecological and evolutionary dynamics of food web interactions.
- The physiological and behavioral effects of air pollution on human health.
- The molecular mechanisms of transcriptional regulation and gene expression.
- The role of microorganisms in plant growth promotion and disease suppression.
- The genetic basis of adaptation to drought stress in crops.
- The ecology and evolution of microbial interactions in the ocean.
- The impact of land use change on soil erosion and nutrient cycling.
- The molecular mechanisms of autophagy and programmed cell death.
- The role of microorganisms in biodegradation of pharmaceuticals.
- The genetic basis of immune system variation and disease susceptibility.
- The evolution of animal social networks and communication systems.
- The ecological and evolutionary dynamics of biodiversity loss.
- The physiological and behavioral effects of light pollution on nocturnal animals.
- The molecular mechanisms of DNA repair and genome stability.
- The role of microorganisms in the production of biofuels and bioplastics.
- The genetic basis of adaptation to salinity stress in plants.
- The ecology and evolution of microbial symbioses with plants and animals.
- The impact of climate change on plant-pollinator interactions.
- The molecular mechanisms of cellular senescence and aging.
- The role of microorganisms in biodegradation of synthetic organic compounds.
- The genetic basis of variation in complex traits in humans.
- The evolution of animal social behavior and cultural transmission
- The genetic basis of cancer development and progression.
- The role of microorganisms in the gut microbiome and human health.
- The genetic basis of phenotypic plasticity and adaptation in plants.
- The evolution of animal migration and navigation.
- The ecological and evolutionary dynamics of community assembly.
- The physiological and behavioral effects of light and dark cycles on circadian rhythms.
- The molecular mechanisms of protein synthesis and degradation.
- The role of microorganisms in nitrogen and carbon cycling in aquatic ecosystems.
- The genetic basis of sex determination and differentiation in animals.
- The ecology and evolution of predator-prey coevolution.
- The impact of anthropogenic activities on marine biodiversity and ecosystems.
- The role of microorganisms in bioleaching and biomining of metals.
- The genetic basis of inherited disorders and genetic diseases.
- The evolution of animal social behavior and communication systems.
- The ecological and evolutionary dynamics of competition and coexistence.
- The physiological and behavioral effects of endocrine disruptors on human health.
- The molecular mechanisms of cell division and mitosis.
- The role of microorganisms in biodegradation of plastics and synthetic materials.
- The genetic basis of epigenetic inheritance and regulation.
- The ecology and evolution of mutualistic symbioses in plants and animals.
- The impact of habitat fragmentation on species diversity and ecosystem functioning.
- The role of microorganisms in bioremediation of oil spills.
- The genetic basis of drug resistance in pathogens and cancer cells.
- The evolution of animal personality and individual variation.
- The ecological and evolutionary dynamics of biotic interactions in freshwater ecosystems.
- The physiological and behavioral effects of artificial sweeteners on human health.
- The molecular mechanisms of intracellular trafficking and secretion.
- The role of microorganisms in biocontrol of plant pathogens and pests.
- The genetic basis of hybridization and introgression in animals and plants.
- The ecology and evolution of plant-pollinator mutualisms.
- The impact of climate change on marine ecosystems and fisheries.
- The molecular mechanisms of genome editing and gene therapy.
- The role of microorganisms in biogas production and carbon capture.
- The genetic basis of developmental disorders and birth defects.
- The evolution of animal coloration and camouflage.
- The ecological and evolutionary dynamics of invasive species.
- The physiological and behavioral effects of air pollution on wildlife.
- The molecular mechanisms of signal transduction and cell signaling.
- The role of microorganisms in biodegradation of pharmaceuticals and personal care products.
- The genetic basis of reproductive isolation and speciation.
- The ecology and evolution of microbial interactions with plants and insects.
- The impact of climate change on bird migration and breeding patterns.
- The molecular mechanisms of protein-protein interactions and protein complexes.
- The role of microorganisms in bioremediation of heavy metals.
- The evolution of animal cognition and learning.
- The ecological and evolutionary dynamics of biodiversity hotspots.
- The impact of ocean acidification on marine ecosystems.
- The genetics of complex diseases and personalized medicine.
- The evolution of plant defense mechanisms against herbivores.
- The role of microorganisms in soil carbon sequestration.
- The physiological and behavioral effects of light on plant growth and development.
- The molecular mechanisms of cancer metastasis and invasion.
- The ecology and evolution of microbial communities in the human body.
- The impact of climate change on migratory bird populations.
About the author
Muhammad Hassan
Researcher, Academic Writer, Web developer
You may also like

500+ Quantitative Research Titles and Topics

500+ Criminal Justice Research Topics

500+ Google Scholar Research Topics

300+ AP Research Topic Ideas

300+ American History Research Paper Topics

1000+ Sociology Research Topics
- Frontiers in Systems Biology
- Translational Systems Biology and In Silico Trials
- Research Topics
Quantitative Systems Pharmacology meets Systems Biology
Total Downloads
Total Views and Downloads
About this Research Topic
Quantitative Systems Pharmacology (QSP) has emerged as the convolution of four distinct areas: a) systems biology, which focuses on modeling the dynamics of molecular and cellular mechanisms and networks; b) systems pharmacology, which aims at incorporating links between therapeutic interventions and ...
Keywords : Quantitative Systems Pharmacology, systems pharmacology, systems physiology, data science, in silico models, best practices
Important Note : All contributions to this Research Topic must be within the scope of the section and journal to which they are submitted, as defined in their mission statements. Frontiers reserves the right to guide an out-of-scope manuscript to a more suitable section or journal at any stage of peer review.
Topic Editors
Topic coordinators, recent articles, submission deadlines.
Submission closed.
Participating Journals
Total views.
- Demographics
No records found
total views article views downloads topic views
Top countries
Top referring sites, about frontiers research topics.
With their unique mixes of varied contributions from Original Research to Review Articles, Research Topics unify the most influential researchers, the latest key findings and historical advances in a hot research area! Find out more on how to host your own Frontiers Research Topic or contribute to one as an author.
- Search This Site All UCSD Sites Faculty/Staff Search Term
- School Factsheet
- Faculty Honors
- Dean's Leadership Council
- Research Topics
- Academic Departments
- Initiatives and Units
- Facilities and Resources
- Undergraduate
- Student Success
- Contiguous BS/MS Program
- Concurrent Enrollment
- Co-Op Program
- Message from the Director
- Who are we?
- Faculty Expectations
- Get Involved
- Accountability
- Postdoctoral
- Instructional
- Professional Researcher
- Instructional Assistants
- Summer Session
- Alumni Spotlights
- Ways to Give
- Eureka! Scholars Program
- Units & Resources
- Directories

Biological Literature: Quantitative vs Qualitative Research
- The Scientific Method Explained
- Parts of a Scientific Article
- How to Read Scientific Articles
- Research vs Review Articles
- Quantitative vs Qualitative Research
- How do I find a Quantitative article?
- Find Books/eBooks
- Linking Google Scholar to Gee Library Resources
- Related Websites
- Evaluating Resources
- Documenting Sources (Citations)
- Avoiding Plagiarism
- Choosing Your Topic This link opens in a new window
- Creating a Literature Review
- Related Guides
- Library Session Materials
Differences in a Nutshell
In the world of research, there are two general approaches to gathering and reporting information: qualitative and quantitative approaches. Qualitative research generates non-numerical data while quantitative research generates numerical data or information that can be converted into numbers.
Comparison of the Characteristics of Qualitative & Quantitative Articles

Page Attribution
UTA Libraries. Biological Literature: Q uantitative and Qualitative Research . October 3, 2022, 2:28 p.m. CST. URL: https://libguides.uta.edu/quantitative_and_qualitative_research/differences
How to determine which type of article you may have found
1. Does it have Method and Results sections?
EXAMPLE ABSTRACT
a) If not, the article is secondary research (typically a review) .
b) If it does have Method and Results sections, continue to step 2.
2. In the Method section , does it talk about a literature search strategy?
a) If you answered yes, i t is a systematic review or meta-analysis .
b) If you answered no, and that section describes a research study (research participants, an intervention, etc.), it is original research . Continue to step 3 to determine its type.
STEP 3: Now, let's determine what type of original research this is.
3a. In the Method section, is the study described as an interview, observation, or questionnaire? Does the study involve looking into self-reported beliefs, thoughts, etc. ?
a ) If you answered yes, it’s a qualitative study .
b) If you answered no, see 3b.
3b. In the Method section, does the study describe the use of already-existing data (for example, reviewing patient admissions from the past 3 months)?
a ) If you answered yes, it’s a retrospective study .
b) If you answered no, see 3c.
3c. In the Method or Results sections/areas , does it mention using quantitative analyses or statistical tests ( e.g. , ANOVA, t-test, p values)?
EXAMPLE ARTICLE EXCERPT
a ) If you answered yes, it’s a quantitative study .
b) If you answered no, it’s qualitative.
BONUS. Are 3a AND 3c both true? In other words, does the study use BOTH quantitative and qualitative methods?
If so, it's a mixed methods study.
- << Previous: Research vs Review Articles
- Next: How do I find a Quantitative article? >>
- Last Updated: Oct 3, 2022 2:30 PM
- URL: https://libguides.mssu.edu/c.php?g=865170
This site is maintained by the librarians of George A. Spiva Library . If you have a question or comment about the Library's LibGuides, please contact the site administrator .

An official website of the United States government
The .gov means it’s official. Federal government websites often end in .gov or .mil. Before sharing sensitive information, make sure you’re on a federal government site.
The site is secure. The https:// ensures that you are connecting to the official website and that any information you provide is encrypted and transmitted securely.
- Publications
- Account settings
Preview improvements coming to the PMC website in October 2024. Learn More or Try it out now .
- Advanced Search
- Journal List
- Quant Plant Biol
- PMC10095877

What is quantitative plant biology?
Daphné autran.
1 DIADE, University of Montpellier, IRD, CIRAD, Montpellier, France
George W. Bassel
2 School of Life Sciences, University of Warwick, Coventry, United Kingdom
Eunyoung Chae
3 Department of Biological Sciences, National University of Singapore, Singapore, Singapore
Daphne Ezer
4 The Alan Turing Institute, London, United Kingdom
5 Department of Statistics, University of Warwick, Coventry, United Kingdom
6 Department of Biology, University of York, York, United Kingdom
Ali Ferjani
7 Department of Biology, Tokyo Gakugei University, Tokyo, Japan
Christian Fleck
8 Freiburg Center for Data Analysis and Modeling (FDM), University of Freiburg, Breisgau, Germany
Olivier Hamant
9 Laboratoire de Reproduction et Développement des Plantes, École normale supérieure (ENS) de Lyon, Université Claude Bernard Lyon (UCBL), Lyon, France
10 Institut national de recherche pour l’agriculture, l’alimentation et l’environnement (INRAE), CNRS, Université de Lyon, Lyon, France
Félix P. Hartmann
11 Université Clermont-Auvergne, INRAE, PIAF, Clermont-Ferrand, France
Yuling Jiao
12 State Key Laboratory of Plant Genomics and National Center for Plant Gene Research (Beijing), Institute of Genetics and Developmental Biology, The Innovative Academy of Seed Design, Chinese Academy of Sciences, Beijing, China
13 University of Chinese Academy of Sciences, Beijing, China
Iain G. Johnston
14 Department of Mathematics, University of Bergen, Bergen, Norway
Dorota Kwiatkowska
15 Institute of Biology, Biotechnology and Environment Protection, Faculty of Natural Sciences, University of Silesia in Katowice, Katowice, Poland
Boon L. Lim
16 School of Biological Sciences, University of Hong Kong, Hong Kong, China
Ari Pekka Mahönen
17 Institute of Biotechnology, HiLIFE, University of Helsinki, Helsinki, Finland
18 Organismal and Evolutionary Biology Research Programme, Faculty of Biological and Environmental Sciences, University of Helsinki, Helsinki, Finland
19 Viikki Plant Science Centre, University of Helsinki, Helsinki, Finland
Richard J. Morris
20 Computational and Systems Biology, John Innes Centre, Norwich, United Kingdom
Bela M. Mulder
21 Department of Living Matter, Institute AMOLF, Amsterdam, The Netherlands
Naomi Nakayama
22 Department of Bioengineering, Imperial College London, London, United Kingdom
Ross Sozzani
23 Department of Plant and Microbial Biology, North Carolina State University, Raleigh, North Carolina USA
Lucia C. Strader
24 Department of Biology, Duke University, Durham, North Carolina, USA
25 NSF Science and Technology Center for Engineering Mechanobiology, Department of Biology, Washington University in St. Louis, St. Louis, Missouri USA
Kirsten ten Tusscher
26 Theoretical Biology, Department of Biology, Utrecht University, Utrecht, The Netherlands
Minako Ueda
27 Graduate School of Life Sciences, Tohoku University, Sendai, Japan
Sebastian Wolf
28 Centre for Organismal Studies (COS) Heidelberg, Heidelberg University, Heidelberg, Germany
Associated Data
Data availability is not applicable to this article as no new data were created or analysed in this study.
Peer Review Summary
Quantitative plant biology is an interdisciplinary field that builds on a long history of biomathematics and biophysics. Today, thanks to high spatiotemporal resolution tools and computational modelling, it sets a new standard in plant science. Acquired data, whether molecular, geometric or mechanical, are quantified, statistically assessed and integrated at multiple scales and across fields. They feed testable predictions that, in turn, guide further experimental tests. Quantitative features such as variability, noise, robustness, delays or feedback loops are included to account for the inner dynamics of plants and their interactions with the environment. Here, we present the main features of this ongoing revolution, through new questions around signalling networks, tissue topology, shape plasticity, biomechanics, bioenergetics, ecology and engineering. In the end, quantitative plant biology allows us to question and better understand our interactions with plants. In turn, this field opens the door to transdisciplinary projects with the society, notably through citizen science.
1. Introduction
Strictly speaking, taking quantitative biology approach means that we use numbers, and typically also mathematics, to describe biological processes ( Figure 1 ). However, this is not merely a nice-to-have extra or a technological increment; it actually revolutionises knowledge production. Quantitation leads to identifying and modelling dependencies between different measurements, which is the way to form new hypotheses. Statistical approaches measure the strength of such dependencies. Modelling then allows a preliminary test of the hypothesis in silico, with predictions tested again in experiments with quantitative results, while all along probability theory is used to make sure that we draw sound inferences, and account for noise and robustness. This is what a quantitative approach in the multiple senses used in this review means—an iterative approach of measurement, statistical analyses, hypothesis testing in silico, in vitro and in planta, and back to the next cycle with the gained knowledge we have just obtained.

A quantitative revolution in plant science. Whereas molecular insights in plant biology could simply provide a molecular catalogue of plant ontology, the integration of mathematics and computational modelling has instead helped to identify new questions with the aim to unravel the principles of plant life. Hypotheses are formalised and tested in computational models, and results from simulations fuel further experimental analysis. Assessment of the validity of the results, from molecules to ecosystems, involves statistical validation and further quantitative exploration. Background image ( Primula officinalis ) taken from Atlas des plantes de France, A. Masclef, Paul Klincksieck Ed., Paris, 1890. Quantitative examples extracted from Verna et al., 2019 eLife; Chakrabortty et al., 2018 Curr. Biol.; Bastien et al., 2013 PNAS; Brestovitsky et al., 2019 Plant Direct; Zhao et al., 2020 Curr. Biol.; Woolfenden et al., 2017 Plant J; Cummins, 2018 Nature; Allard, 2010 Mol. Biol. Cell. and Fache et al., 2010 Plant Cell.
Furthermore, such an interdisciplinary approach also fuels creativity and triggers new questions for two reasons: (a) being able to formalise questions within a defined mathematical framework also means that hypotheses become truly testable and interoperable. This is key to understand plants as multiscale (both in space and in time) systems, (b) because interdisciplinary settings imply that not everybody is an expert in all techniques, the focus remains on the question at hand, and not the techniques and their development. This means that quantitative biology is one of the best ways to open new avenues of research, and identify new questions ( Figure 2 ). This is what we are attempting to elaborate on in this review.

Examples of research topics at the crossroad between plant biology, physics and maths. Quantitative plant biology explores these topics, and the common mathematical framework allows their integration, across spatial and temporal scales. See main text for details.
2. Signalling networks
Signalling networks primarily act to process and integrate information perceived through a multitude of receptor systems, and relay this information to cellular effectors, which, in turn, enact a response tailored to the conditions. This definition implies that information is encoded and decoded, and thus can be formalised. This is a thriving field of study in computational modelling (Long et al., 2008 ). In terms of identifying complex relationships between inputs and outputs, machine learning approaches are becoming increasingly popular. Furthermore, exciting developments in technologies coupled with statistical techniques now allow for the inference signalling networks from large genomic datasets which are becoming readily available (Carré et al., 2017 ).
Most studies on signalling networks have emphasised the identification of the molecular components critical for signal transduction pathways in a mostly binary manner (‘on’ vs. ‘off’), often considering a reduced set of actors (minimally a receptor and its ligand), under controlled-lab growth conditions. To expand our knowledge beyond the description of pathway architecture and understand how integrated signalling networks behave in varying conditions, quantitative biology approaches are required. For example, how can signals be discriminated from each other when they simultaneously occur? How can priorities be established and an integrated response achieved when a cell is challenged with multiple inputs, beyond the so-called ‘hormonal cross-talk’? How are thresholding and noise filtering mechanisms molecularly encoded to prevent spurious pathway activation? Answering these questions requires accepting a number of technical challenges, for example, concerning the quantification of signalling molecules and graded responses.
A major achievement in plant signalling has been the identification of the core components of ‘receptor–ligand’ pairs for many signal transduction pathways, through the isolation of mutants that have modified responses to a particular signal, that either fail to respond, or respond constitutively even in the absence of that signal. Genetics further uncovered the hierarchy and interactions among transduction pathway components and showed how complex the networks involved in the integration of signalling inputs and outputs are. This includes feedback mechanisms, where signalling sometimes provides unexpected compensatory responses. For instance, a dwarf cellulose synthase mutant is partially rescued when it bears another mutation in the wall integrity pathway: the plant becomes unable to detect the damages in its cell wall and thus does not trigger growth arrest mechanisms (Hématy et al., 2007 ).
Compared to other systems, the contribution of signalling dynamics, that is, the duration, frequency and amplitude of a signal for downstream responses, has been somewhat neglected in plants (Purvis & Lahav, 2013 ). For example, in mammalian cells, transient activation of extracellular signal-regulated kinase (ERK) through epidermal growth factor can result in cell proliferation, whereas sustained activation by nerve growth factor can lead to cell differentiation (Avraham & Yarden, 2011 ). It has been predicted and experimentally validated that modulation of feedback strength in a single inhibitory loop from ERK to one of its upstream kinases (RAF) can result in a variety of stable output states ranging from a sustained monotone response to a transient adapted output, to oscillation, or to bi-stable, switch-like responses (Kholodenko et al., 2010 ). In plants, our understanding of this temporal dimension of information encoding is far less developed and thus opens many avenues for pioneering studies in quantitative plant biology.
Despite increased efforts, the availability of quantitative data on signalling events remains the major constraint for approaches aiming at a deeper understanding and modelling of signalling networks. An ever-expanding set of biosensors, which allow for the in vivo visualisation and quantification of signalling molecules with cellular or even subcellular resolution, are among the most promising remedies for this lingering ailment. In fact, very recently, biosensor-based approaches have brought breakthroughs to the field of plant signalling. For example, a study by Toyota and colleagues has elegantly elucidated ‘Rapid, long-distance signalling in plants’ showing that when injured on one leaf by a nibbling insect, a plant can alert its other leaves to begin anticipatory defence responses (Toyota et al., 2018 ). This development went hand in hand with quantitative approaches and mathematical modelling that led to the proposal of a propagation mechanism (Evans et al., 2016 ). In addition, emerging systems biology tools may provide new ways to perturb signalling network components in a spatially and temporally controlled manner to illustrate network behaviour.
Finally, beyond the computational models of networks, validation with molecular genetics and biosensors, quantitative approaches on signalling also need to deal with numerous players, their redundancy, synergy and antagonism. Crucial gene activities are often shared by several redundant homologs, and thus single mutations in such genes cause only partial defects. It is also challenging to identify the primary defect from all the secondary defects. These difficulties can be solved by extensive phenotype quantification. For example, the roles of various miRNAs, which were identified by small RNA sequencing of Arabidopsis embryos, were clarified based on their mutant phenotypes on each embryonic tissue and developmental stage (Plotnikova et al., 2019 ). Such quantification can be further combined with CRISPR/Cas9-based genome editing techniques that enable tissue-specific and conditional gene manipulation of target gene (Decaestecker et al., 2019 ; Wang et al., 2020 ). With the help of such systems, one can knockout genes in specific cell types at will, thus enabling the identification of the distinct roles of the identified genes.
3. Noise and robustness
Another layer of complexity is brought about by a prevalent factor in biology: noise. Stochastic, or random, effects pervade biology across scales (Lestas et al., 2010 ; Tsimring, 2014 ), from cells where molecules are constantly buffeted by thermal noise, to the robust formation of organs by collections of cells, to the environmental fluctuations experienced by crops in the field. Noise also invades our efforts to measure the biological world, leading to technical variation and requiring transparent quantitative methods for responsible analysis. Unavoidable, multiscale noise in biology provides both challenges and opportunities for plants, and a large and growing body of work seeks to elucidate how plants attempt to filter out, or exploit, randomness. We cannot hope here to give a comprehensive survey of the many ways stochastic influences shape plant biology, but hope that a few classic examples across scales will illustrate the ubiquity, and importance, of stochasticity in plant biology (Abley et al., 2016 ).
At the most fundamental level, stochasticity in the form of spontaneous mutations and other events underlies all plant evolution (e.g., Rose et al., 2002 ) studying the interplay of stochastic variation and fitness in thistle populations. With the view of neutral theory of molecular evolution, stochastic events continually shape plant population structure (e.g., Menges, 2014 , modelling the impact of stochastic extinction events on plant populations).
Within plants, cellular noise impacts vital processes across scales including the circadian clock (Guerriero et al., 2012 ), gene expression (Araújo et al., 2017 ; Wang et al., 2004 ), internal signalling (Trewavas, 2012 ), tropisms (Meroz & Bastien, 2014 ), patterning (Meyer et al., 2017 ) organ shape plasticity (Hong et al., 2018 ) and seed germination (see below). The cytoskeletal polymer networks formed by actin and microtubules are prime examples of why quantitative approaches are inevitable: they are far-out of equilibrium, stochastic, interacting many-particle systems with a high number of spatial degrees of freedom. Emergent properties from such behaviour include the formation of parallel arrays or cell division plane orientations. As such, they are at the cutting edge of statistical physics, a vibrant field of highly quantitative research in its own right (Deinum & Mulder, 2013 ; Wasteneys & Ambrose, 2009 ).
Stochastic modelling can provide a powerful framework to understand the interactions of plants with their environments (e.g., Katul et al., 2007 ). Elegant modelling work coupling mechanical and stochastic influences has been used to describe whole-plant development (e.g., Costes et al., 2008 ; for apple trees). The explosion of multiomics technology has allowed genetic features shaping noise levels in transcripts and metabolites to be discovered (Jimenez-Gomez et al., 2011 ).
Some of these stochastic influences constitute challenges for plants—for example, cellular noise in signalling pathways means that plants need to invest extra resources in maintaining the fidelity of signals. However, noise can also be beneficial, providing a useful source of variability in plants (Muller et al., 2019 ). Bet-hedging in seeds provides a compelling example of plants exploiting noise: a generation of seeds that germinate at different times will be more robust to unpredictable environmental change than one that germinates synchronously. Johnston and Bassel ( 2018 ) identified a network motif encoding a variability enabling bet-hedging in seeds. In this system, noisy positive feedback onto both ABA synthesis and ABA degradation can result in significantly varying final hormone levels. Noise can even help signals to become detectable, because noise on top of a weak signal can make the signal detectable, a phenomenon called ‘stochastic resonance’ (Rué et al., 2012 ).
Variability in environmental inputs is also leveraged by dormant seeds. Topham et al. ( 2017 ) identified a system whereby seeds make preferential use of fluctuating ambient and low temperatures over constant low temperature to break their dormancy. This mechanism indicates that the perception of low temperature in seeds is not a matter of the linear accumulation of cold, but rather complex processing of these fluctuating inputs. The adaptive significance of this may relate to daily temperature fluctuations being greater in the spring and autumn, with these variable signals acting as indicators of the changing seasons.
The relationship between the variability in single cells and their collective contribution towards robust organ shape and size has also been investigated. It is proposed that noise across molecular and cellular scales can be amplified to prime organogenesis (Uyttewaal et al., 2012 ) or filtered in order to ultimately result in the formation of organs having consistent morphologies (Hervieux et al., 2017 ).
The study of these vital influences is quantitative in essence. Statistical measures to quantify variability and heterogeneity across scales have been developed. The characterisation of noise requires these quantitative measures (for example, a measure of dispersion like the coefficient of variation or Fano factor; Tsimring, 2014 ). Large numbers of quantitative observations are required for accuracy in these measurements, and to distinguish mechanistic hypotheses when noise is involved. It is only through suitably coupled quantitative models, experiments and statistical methods that we can hope to unravel the sources and effects of stochasticity in plant biology, and the mechanisms by which plants deal with, and exploit, the resulting variability. There is much at stake since robust agrosystems increasingly build on genetic heterogeneity (agroforestry, agroecology and mixed varieties) while facing increasing environmental fluctuations. The future of food security will involve careful assessment of uncertainties and better ways to build on the added values of stochasticity.
Another issue related to noise exists in concert—perhaps less biologically exciting but equally, if not more, societally important. Statistical misunderstandings of the role noise plays in experiments has led to most published research findings being wrong, at least in some scientific fields (Ioannidis, 2005 ). To combat this, we need both to embrace rigorous (but not necessarily complicated) statistical methods and a shift in quantitative philosophy, where noise and uncertainty are more transparently and openly addressed and critically analysed. The p < .05 paradigm, and accompanying focus on statistical rather than scientific significance, has been much criticised (Ziliak & McCloskey, 2008 ). Even within this paradigm, statistical tests are frequently misused. A common example is using a t -test for integers or non-negative entities when the standard deviation (not the standard error!) is of similar magnitude to the mean. Clearly, such a sample cannot be normally distributed, and other tests (like Mann–Whitney) should be favoured (Saxon, 2015 ). The series of articles in Saxon ( 2015 ) highlights several other statistical misuses that confound scientific progress. As the transition towards more quantitative and data-rich plant biology continues, we urge researchers to adapt their statistical approaches to ensure this exciting world is understood as accurately as possible.
4. Tissue topology as an instructive cue
Signalling and noise occur in cells that reside in tissues. An individual cell’s function is thus highly biased by that of its neighbours. Beyond the molecular aspects, plant biology thus embeds another strong quantitative component: topology.
Cell-to-cell communication is central to plants. Coordination between cells is needed to orchestrate growth and development, as well as responses to their environment. Plant cells can transmit information using chemical, mechanical and electrical signals. Chemical signals typically move between cells through a secreted peptide sensed by a transmembrane receptor kinase, through symplastic channels called ‘plasmodesmata’ or via efflux and influx transporters. Mechanical signals are likely to be transduced via the connecting cell wall surfaces, although the precise mechanisms for this remain to be elucidated. Electrical signals could be transferred through membrane voltage through plasmodesmata or the transport of ions. All these mechanisms are dependent on either symplastic connectivity or common surface areas between cells. Topology is thus an essential part of plant biology.
As cells divide, the daughter cells may lose connections to some of the previously neighbouring cells. Growth can cause movement or deformation of cells, thus leading to changes in the contact surface area with neighbouring cells and potentially new connections. One would therefore expect that growth and development would lead to changes in communication between cells. How could plant cells then know, in this ever-changing environment, how to act and whether they should divide or differentiate (Jackson et al., 2019 )? How is this dynamic cell-to-cell communication achieved and coordinated (Bassel, 2018 )?
One possibility is spatial restriction acting to modulate the expression of key proteins, whose flux in concentration gradients create unique microenvironments. The microenvironment of a cell is established by a combination of signals within the original cell, known as cell autonomous signalling, along with external signals known as non cell autonomous signals. These signals originate from neighbouring cells, such as phytohormones or mobile proteins, executing non cell autonomous functions in plants. The importance of regulatory mobile proteins in establishing a unique microenvironment has been shown to be central for the proper cellular organisation of the root stem cell niche, and thus cell-to-cell communication in this region. For example, non cell autonomous signalling of SHORTROOT (SHR) and its binding partner SCARECROW (SCR) guide the timing of cell division and determine cell fate of quiescent centre (QC) cells and cortex-endodermis initial (CEI) cells in the Arabidopsis root stem cell niche (Cruz-Ramírez et al., 2012 ).
Studying the communication between cells is critical both to address complex problems at the whole-organism level, and to understand systemwide cellular behaviours. To accommodate the study of cell-to-cell communication, several exciting approaches have been used. Using scanning fluorescence correlation spectroscopy, one can quantify protein characteristics within a specific cell type, such as complex stoichiometry, as well as molecular dynamics between different cells, such as protein movement (Clark et al., 2016 ). SHR and SCR expression activity differs in QC and CEI cells, as they form complexes in each cell type with different stoichiometric proportions. Methods like raster image correlation spectroscopy, pair correlation function and number and brightness allow mobile transcription factor motility and expression levels to be analysed, thus quantifying how they contribute to developmental processes. Computational modelling can then be used to predict cellular behaviour, such as cell division or differentiation timing, as well as expression dynamics of key regulatory proteins in specific QC and CEI cells. The latter can be accomplished through mathematical methods such as ordinary differential equations, which can take into consideration the number and concentration of stoichiometric complexes of SHR and SCR in each cell type, the difference in SHR and SCR expression between cell types QC and CEI and relevant upstream regulatory elements (Clark et al., 2020 ).
Mathematical modelling also has a key role to play in developing and testing hypotheses on communication through plasmodesmata. Our understanding of how this process works is still relatively poor, but two mathematical models have recently offered new insights into how this may work. Deinum et al. ( 2019 ) developed a model of diffusion from cell to cell through plasmodesmata. The authors built a detailed multilevel model based on realistic plasmodesmatal geometries and investigated the impact of different geometrical parameters and plasmodesmatal distributions. This model allows for wall permeabilities, as a function of geometrical parameters, to be inferred from experimental data. Park et al. ( 2019 ) modelled cell-to-cell communication via plasmodesmatal flux as a function of turgor pressure. They hypothesised a plasmodesmata closing mechanism based on mechanosensing. This model offers an explanation for rapid closing for which the alternate model of callose deposition seems too slow. Further work, both theoretical and experimental, is needed to elucidate the mechanisms underpinning the functioning of these important communication channels.
An interdisciplinary approach is required for another key aspect of this topic: ‘decoding’ the information involved in cell communication. Increasingly, detailed experiments and bioinformatics are revealing the mechanisms of production, and dynamics, of different signals (molecular and biophysical). But how such complex signals are used by the plant to convey information is an open question. What processing converts an intracellular combination of hormone concentrations into an actionable signal, for example? Over what length and timescales can signals of different physical forms be sent and received through the plant? How is the fidelity of such signals retained in the face of inevitable noise (Lestas et al., 2010 )? Quantitative answers to these questions have tremendous potential for basic biology and agronomical resilience, but will require a cross-disciplinary approach using information theory, modelling and statistics to harness exciting new data.
5. Morphometric atlas for morphogenesis
Scaling up from tissue topology, another quantitative aspect of plant biology is shape, which can be complex in case of many organs, and morphogenesis, that is, shape changes in time or its maintenance despite organ growth. At the tissue scale, quantitative plant biology can take the form of growth kinematics, which is growth description of high spatiotemporal resolution that is necessary to understand plant growth dynamics, that is, how the plants develop (Silk, 1984 ). The most influential proponents of quantitative studies of plant growth and development were Ralph O. Erickson (1914–2006) and his students or followers, the late Zygmunt Hejnowicz (1929–2016) and Paul B. Green (1931–1998), as well as Wendy K. Silk (Meicenheimer & Silk, 2006 ). These scientists have used quantitative and interdisciplinary approaches despite the average biologists’ prejudice against math and statistics at that time (Erickson, 1988 ). In his review devoted to modelling of plant growth, Erickson ( 1976 ) summed up their approach writing that ‘in any attempt at modelling it should be possible to relate the experimental data to the differential equations which represent the process being modelled’. Experimental data thus must have to be extensive in terms of precision and robust, through large sample size. In such endeavours, the required quantitative analysis is often complemented by modelling.
Kinematics of plant growth has been studied from two biophysical perspectives: fluid dynamics (Silk, 1984 ) and solid body mechanics (Hejnowicz & Romberger, 1984 ). The first perspective is based on the analogy between plant growth and fluid flow: individual cells ‘flow’ through a growing plant organ that maintains an almost steady shape. The second perspective focuses on the continuous character of the symplastic growth, typical for plant tissues, which is cell growth coordinated at tissue and organ levels. The symplastic growth of plant organs, which is often also anisotropic, is the irreversible tissue deformation that observes the continuum condition of solid body mechanics. Both perspectives, fluid dynamics and solid body mechanics, put forward the tensorial nature of plant organ growth, which implies elaborate quantifications and modelling (Hejnowicz & Romberger, 1984 ; Silk, 1984 ).
Empirical studies of plant growth and development as well as its complex regulation are technically challenging, because growth is often unsteady and inhomogeneous. Therefore, critical for the progress of our understanding of plant morphogenesis are techniques enabling the acquisition of high spatiotemporal resolution, high quality and well-quantified imaging data and its subsequent analysis. Recently, various techniques were developed to support live imaging, such as autotracking of moving samples and in vitro tissue cultivation under microscopes. These techniques were further combined with minimally invasive microscopy, including light sheet and two-photon excitation systems, and enabled long-term time-lapse imaging to visualise the four-dimensional dynamics during pattern formation. For example, the combination of Arabidopsis ovule cultivation and two-photon excitation microscopy revealed the 4D atlas of cell lineages during embryo patterning (Gooh et al., 2015 ). Furthermore, high-resolution live imaging enabled to monitor the intracellular behaviour of developing cells, such as cytoskeletal rearrangement during lateral root initiation and vacuolar shape change during zygote polarisation (Kimata et al., 2019 ; Vilches Barro et al., 2019 ).
The advanced imaging technologies provide a vast amount of spatiotemporal data, and this can mask important information. Therefore, image quantification is essential to extract key properties from the 4D big data. For example, cell volume and division orientation were quantified at various stages of embryogenesis, and these values were utilised for simulation modelling, which revealed the importance of geometric cell division rules and its modification by plant hormone auxin in embryo patterning (Yoshida et al., 2014 ; Moukhtar et al. , 2019 ). Thus, the combination of 4D imaging and detailed quantification can provide a powerful tool to identify fundamental rules underlying plant morphogenesis. As shown next, such morphometric analyses are now integrated with gene networks and cell identities in comprehensive 4D atlases to identify patterning rules.
6. Patterning spatial and temporal information
It has long been recognised that plants exploit algorithm-like patterns in their development (Prusinkiewicz & Hanan, 1989 ). We are only now beginning to uncover the complex mechanisms based on long-range transport and sensing of small metabolites, such as the ubiquitous auxin, in some sense the charge-carrier of the analogue ‘electronics’ steering plant development.
To integrate 4D imaging-based quantitative information of dynamic transcriptional or signalling networks, developmental atlases are arising as functional tools, complementary to computational modelling (Refahi et al., 2021 ). For instance, this coupling of approaches, allowing the precise spatial registration of auxin maxima and signalling in the shoot apical meristem, uncovered a novel mechanistic framework to explain phyllotaxis (Galvan-Ampudia et al., 2020 ).
Other examples include the formation of trichomes, the division profile of the stomatal lineage, the emergence of lateral roots and the positioning of root hairs. Such patterning processes often involve local signalling modules, with positive and negative feedback loops. Interestingly, while many of these networks build on biochemical interactions, as, for example, in reaction-diffusion Turing-like patterns, there is increasing interests in more holistic models that also include mechanics. For instance, lateral root emergence from the pericycle, and through cortical tissues, involves adjacent cells and their mechanical accommodation to such an invasion (Ditengou et al., 2008 ; Lucas et al., 2013 ; Vermeer et al., 2014 ). Similarly, the stomatal lineage patterning involves a tight control of polarity, which also involves large-scale patterns of tissue tension across the leaf (Bringmann & Bergmann, 2017 ). Finally, some of the feedbacks involved in patterning can be geometrical. For instance, it has been proposed that the positioning and size of the WUS-expressing domain at the shoot apical meristem depends on cytokinins diffusing from the epidermis, and thus scales with meristem shape (Gruel et al., 2016 ).
Importantly, integrated quantitative approaches also allow us to address how stereotypical patterns and organ shapes are. Defining stereotypes, in turn, paves the way to quantify the variability of morphogenesis, which is likely a crucial component per se of development (Waddington, 1942 ). In plants, mechanisms controlling specifically plasticity and robustness of development are starting to be explored (Hong et al., 2018 ), and will shed new lights on plant growth and form.
7. Shape plasticity
Whereas many animals can developmentally rest on their laurels after reaching maturity, plant morphogenesis is by definition an unfinished project. As such, postembryonic development is central to plant developmental biology. Organogenesis can be plastic and nonuniform, and organ shapes can respond to environmental cues. While in animals, symmetry, repeatability and scalability are key due to the importance of motion for animal survival, in plants instead plastic development in response to environmental conditions is central to plant fitness. It is this plasticity that enables plants, for instance, to generate new lateral roots where nutrients or water is found, yet save valuable resources by not investing in growth elsewhere.
Heterogeneity in growth, ranging from organ shape deformations to tropisms, are key to plant adaption. Organ or architecture plasticity is observed not only across plant evolution between species, but also within single individuals during development, or in response to changing environments. Such plasticity can also be predictable, meaning that quantitative approaches can measure, describe and help to understand its features. Toward this aim, robust shape descriptors have been developed. For instance, leaf shape plasticity has been assessed quantitatively in grapevine, using more than 5,500 leaves representing 270 vines from more than 11 species, to understand environmental impact (Chitwood & Sinha, 2016 ). Similarly, at the cellular level, careful cell shape description in 3D identified geometric cues predicting specific formative asymmetric division, shifting organism growth from 2D to 3D in a basal plant, the moss Physcomistrella patens (Tang et al., 2020 ).
Cell growth orientation and anisotropy, defining final cell shape, clearly determine asymmetric cell divisions, but also symmetric cell divisions in proliferative tissues, as cell division plane depends on geometrical and mechanical rules (von Wangenheim et al., 2016 ; Willis et al., 2016 ; Yoshida et al., 2014 ). As such, cell shape defines cell division patterns, which are key in various aspect of plant morphogenesis, such as stomata, root development or early embryogenesis in Arabidopsis. However, mutants displaying random cell division patterns, like tonneau , are still able to pattern normal fates territories (Traas et al., 1995 ), and embryo patterning occurs in monocots despite highly variable cell division patterns (Zhao et al., 2017 ). These examples suggest that supracellular mechanisms operate to control morphogenesis, as predicted by the organismal theory (Kaplan, 1992 ).
Shape robustness entails a control of organ shape plasticity to ensure reproducible final shapes while facing external and internal perturbations. This implies the coordination of heterogeneous cellular behaviours. This coordination involves various mechanisms ranging from mechanical stress to mobile morphogens controlled by cell-to-cell communications (e.g., Hervieux et al., 2017 ). Importantly, local cellular growth variability can be as well used by the plant to buffer shape variations at the organ or tissue level, as shown, for instance, during Arabidopsis sepal development (Hong et al., 2016 ), or leaf primordia initiation at the shoot apical meristem (Uyttewaal et al., 2012 ), with a primary role of the epidermis (Malivert et al., 2018 ; Zhou et al., 2020 ). Finally, shape definition during plant development is a multiscale and integrated process, which is plastic by nature. Indeed, the gradual production of new cells during iterative development imposes continuously new geometric, topological and mechanical constraints, which, in turn, canalise individual cells growth and shapes.
Plastic cellular patterns can rely on local fluctuations in gene expression, establishing thresholds determining cell fate trajectories. Indeed, the coupling of variations in gene expression and in cell fate has been observed for individual genes, such as ATML1 in leaf and sepal epidermal cells. In epidermal cells arrested in G2 phase of the cell cycle, when ATML1 level exceeds a certain threshold, endoreduplication starts, allowing differential cell growth (Meyer et al., 2017 ). Recent years have seen the rise of single cell transcriptome techniques. To obtain separate cells for single cell analysis, cell walls need to be removed (i.e., generation of protoplasts) quickly from plant tissues, whose effect on gene expression remains to be comprehensively assayed. Protoplasts are easy to extract from the root meristem of Arabidopsis, and therefore several papers describing the single cell transcriptome of that tissue have recently been published. These pioneering studies confirmed the identities of different cell types in the root meristem, but most interestingly, with the help of bioinformatics, they also revealed cell differentiation trajectories (Efroni et al., 2016 ). Differentiation of the stomatal lineage have also been addressed recently (Lee et al., 2019 ), showing that more and more plant tissues can be amenable to single cell transcriptomic approaches. Interestingly, differentiation trajectories can be mapped on pseudotime curves, by coupling single cell techniques to careful tissue staging, as recently shown for male germline precursors in maize (Nelms & Walbot, 2019 ). Through spatial transcriptomics (Duncan et al., 2016 ; Giacomello et al., 2017 ), we will obtain information on transcriptional programs during morphogenesis at a temporal and spatial resolution we thought impossible just a while ago. Such high-resolution transcriptome technology would allow us to understand how individual cells dynamically determine their cell fates in response to diverse inputs, such as nutrient amount mechanical stress and the dynamics of neighbouring cells, to actualise plastic plant development.
8. Mechanics behind growth and motion
A quantitative approach is also indispensable in the field of plant biomechanics. Cell growth is a classical and enlightening example of how quantitative descriptions and mechanical reasoning can shed light on a key biological process. It has been quantitatively studied since the 19th century, with experiments on osmosis by Wilhelm Pfeffer (1845–1920). Many control factors are involved (temperature, hormones, osmole concentration, oxygen etc.). Although turgor pressure is understood to be the force behind cell growth, its action appears contradictory: On the one hand, turgor pushes on the cell wall, promoting growth; on the other hand, it raises the water potential, inhibiting water entry into the cell. By modelling the cell wall as a viscoplastic material, Lockhart ( 1965 ) was able to combine these two opposite tendencies into a single equation, from which turgor is absent. In Lockhart’s equation, the relative growth rate becomes nonzero when the difference in osmotic pressure Δπ rises above a yield pressure P Y :

where φ is the extensibility of the cell wall and L is its relative hydraulic conductance. As argued by Green ( 1996 ), these two physical parameters enter the equation in a nonlinear way, which could not have been deduced from co-variation studies. Biophysical modelling, supported by quantitative data, was therefore a necessary step. Ortega ( 1985 ) added an elastic component to the equation, accounting for reversible changes in cell volume, which can be significant, for instance, in diurnal variations in tree stem diameter.
Another biophysical approach to cell growth is based on the principle of minimum energy (Hejnowicz, 2011 ). However, neither this nor the Lockhart–Ortega equation directly accounts for the effects of temperature, hormones or other factors. Actually, these factors act on the extensibility of the cell wall. Investigating the corresponding relationships requires more detailed models of the chemo-rheological processes occurring in the cell wall (e.g., Ali & Traas, 2016 ), assessed against quantitative data (Proseus et al., 2000 ).
Upscaling mechanical properties from a single cell to an organ(ism) is nontrivial. The whole structure has to be closely considered. Because cell walls are stiffer than protoplasts and are under tensile stress generated by turgid protoplasts, the apoplast is the load bearing and load transmitting part of the organ. The green organ can thus be regarded as made of a pressurised cellular solid, where the contribution of various organ tissues into its mechanical properties/stiffness depends on tissue distribution and structure. One of the first plant morphologists who studied biophysical aspects of plant structure and development was Hofmeister, who also noted that cell walls are under tension. His ‘ability to combine exceptional observational detail with an emphasis on experimental methodology’ (Kaplan & Cooke, 1996 ) was an early manifestation of quantitative plant biology. The structure of plant organs was studied from a mechanical perspective and looked at as a supportive system also by other pioneers of plant anatomy, such as Sachs and Simon Schwendener (1829–1919), although later these aspects of plant structure were generally neglected for decades (Romberger & Hejnowicz, 1993 ).
Mechanics also explains plant movements, such as the operation of contractile roots, the catapult-like action of fern sporangia (Noblin et al., 2012 ) or the closing of the Venus flytrap (Forterre et al., 2005 ). Some of these movements, including nastic ones, have important morphogenetic implications, for instance, to explain how leaves flatten as they grow (Derr et al., 2018 ). All these phenomena in living organisms have to be studied observing empirical rigors of physics, and thus contribute to quantitative plant biology.
As for biochemistry, mechanics is not only an output of the gene network, but also an input. Growth and geometry changes cause mechanical tension and stress during morphogenesis, and this, in turn, feeds back to tissue patterning (Heisler et al., 2010 ; Nakayama et al., 2012 ). A growing number of studies report that both short- and long-distance signalling between plant cells is accompanied by tension/stress sensing mechanisms enabling correct morphogenetic processes (Trinh et al., 2021 ). Because forces are invisible in essence, and as these regulatory mechanisms take place in three dimensions and at different timescales, computational modelling has become a vital tool to understand patterning processes ranging from the tissue, organ to whole plant scale.
Because plants develop ‘in-place’, they might be more geometry-aware than many other organisms. This is apparent even at the level of individual plant cells, which like plants themselves largely develop ‘in-place’. The unique properties of the semirigid plant cell wall, means that plant cells need to maintain and hence be able to sense their geometry often at a size scale of tens of microns. It is remarkable that Paul Green in a landmark paper (Green, 1962 ; Green & King, 1966 ) basically predicted the existence of cellulose microfibril reorientation by mechanical stress on the basis of his observations of the plant cell wall, even before microtubules were discovered (Ledbetter & Porter, 1963 ). Microtubules were later on found to guide the synthesis and orientation of cellulose microfibrils, which determine cell growth orientation, through live imaging (Paredez et al., 2006 ).
Not only the role of mechanical stress in development is well established (Green, 1999 ; Hamant et al., 2008 ; Lynch & Lintilhac, 1997 ), but it is also widely accepted that mechanics is at the basis of the supportive functional system of plant organs (Romberger et al., 1993). Noteworthy, plant organs are often prestressed constructions. Namely, an important role in this system is played by tissue stresses (Hejnowicz & Sievers, 1996; Kutschera, 1989 ), that is, the tensegrity at the organ level. The structural tissue stresses, an indirect result of the turgor pressure, exist in plant organs that are composed of turgid tissues that differ in cell size as well as thickness and mechanical properties of the cell walls.
Due to their size and perennity, trees are of special interest for plant biomechanics. As slender structures, they lend themselves well to engineering approaches like beam theory (Niklas, 1992 ). Prompted by the pioneering works of Schwendener (Schwendener, 1874 ), biomechanicians started to investigate the quantitative constraints put on tree growth by their own weight (Greenhill, 1881 ) or external loads like wind (Metzger, 1893 ). Trees greatly differ from engineered structures as they change their mass and size by large factors during their lifetime and experience a wide range of mechanical loads. In addition, they explore their environment and adapt to it (see, e.g., Alonso-Serra et al., 2020 ; Eloy et al., 2017 ). Defining integrative biomechanical traits makes it possible to quantify how well a tree is adapted to its environment and to infer which ecological strategy it follows (Fournier et al., 2013 ). This also illustrates how physics, engineering and plant ecology can work hand in hand.
9. Bioenergetics
As is the case for all living organisms, plant growth, development and physiology involve continuous dynamic energy conversion, abiding the laws of thermodynamics. Photosynthesis is the most important energy-harvesting process on Earth. By driving the flow of electrons extracted from water molecules through several highly organised photosynthetic complexes, sun energy is momentarily converted into two chemical energy currencies of the cells, ATP and NADPH. At the same time, it drives the assimilation of carbon, nitrogen and sulphur. The chemical energy generated from photosystems then fuels many anabolic metabolisms that promote the mechanisms behind plant growth described above.
As shown for other themes, cell bioenergetics is examined by quantitative approaches, such as absorption spectrometry, chlorophyll fluorescence, irradiance measurement, optical microscopy, gas analysis, electrodes or isotopic labelling. These methods allow the estimation of photosynthetic parameters, carbon assimilation rate, electron flows, metabolic fluxes, stomatal conductance or respiration rate (Fernandez-Jaramillo et al., 2012 ).
The acquired data are integrated into metabolic networks and flux-balance analysis models to describe energy metabolism in photosynthetic organisms (Cheung et al., 2014 ; Rügen et al., 2015 ). One example is the Farquhar, von Caemmerer and Berry model for predicting net CO 2 uptake (A) in C3 plants by linking A to the carboxylation rate of ribulose 1,5-bisphosphate (RuBP), oxygenation rate of RuBP and mitochondrial respiration in the light (Farquhar et al., 1980 ). This model, which has been cited more than 7,500 times since its publication 40 years ago, has been applied to a wide range of studies, from investigating C3 bioenergetics to predicting photosynthetic fluxes of ecosystems on a global scale.
In fact, the importance of modelling and quantitative studies of photosynthesis goes beyond the cellular, tissue or organismal levels. Field and global measurements of solar-induced vegetation fluorescence by recent remote sensing technologies using airborne sensors or satellite systems allow scientists to monitor the temporal and seasonal changes of vegetation and the fluxes of carbon, water and energy on a regional or a global scale, and to study the interaction between vegetation, primary productivity, environmental stresses and climate changes (Wu et al., 2016 ). Coupled with data gathered in the field, this opens many opportunities to bridge scales and revisit the essential role of plant productivity for our civilisation and ecosystem.
10. Integrating fluctuating and diverse abiotic environmental factors
Within a single day, plants may experience all kinds of challenges, including fluctuating light intensity and/or quality, temperature changes, wind, rain or snow. Each of such fluctuations in the surrounding environment factors may represent a threat to plants, unless dealt with properly.
The information contained in abiotic signals needs to be processed and integrated in order to arrive to developmental decisions. To elucidate this process, a quantitative systems approach is required, because the intricate interplay between the several parts of the signalling networks often escapes intuitive reasoning (Boer et al., 2020 ). There are numerous quantitative challenges related to: (a) learning the structure of environmental signal integration networks, (b) understanding the dynamic properties of these networks and (c) designing genetic changes to the network that would cause the plant to respond to environmental parameters in a specific way.
One of the most thoroughly studied abiotic response systems in plants is light and temperature signal integration. Plants sense the quantity and quality of light, for which a variety of photosensors are used ranging from the UV to the red part of the spectrum (Möglich et al., 2010 ; Paik & Huq, 2019 ). For instance, measuring the duration of the day by sensing dawn and dusk is important for the entrainment of the circadian clock (Seaton et al., 2018 ; Wenden et al., 2011 ). But also other responses, such as germination, de-etiolation, regulation of flowering and responses to canopy shade, are regulated by light sensing networks (Chen et al., 2004 ; Galvão & Fankhauser, 2015 ; Kami et al., 2010 ; Paik & Huq, 2019 ). Plants respond differently to different spectral distributions of the incident light, which is achieved not by the light sensors alone, but by an interplay between the sensors and their interacting factors, that is, by the light sensing network (Galvão & Fankhauser, 2015 ; Klose et al., 2015 ; Paik & Huq, 2019 ; Rausenberger et al., 2010 ). Due to this, the plant’s response to environmental light is a system property and cannot be understood by analysing the properties of the photoreceptors alone, besides simple cases.
A prominent example of this is phytochrome A (phyA) in Arabidopsis thaliana. The spectral response of phyA depends on the light intensity; for low intensities, phyA responds to red (660 nm) light, whereas for high intensities, it responds to far-red light (720 nm; Franklin et al., 2007 ). This intensity-dependent change of the spectral response without a change of the physical properties of phyA can only be understood, if one analyses the signalling network (Possart et al., 2014 ; Rausenberger et al., 2011 ; Schäfer, 1975 ).
The understanding of the light signalling networks and their different responses to the light spectrum is very challenging and requires joining the forces of experiments and mathematical modelling. This is even more true if one aims to unravel the integration and processing of light and temperature signals in plants. Plants are sensitive to temperature changes, and many aspects of plant growth and development respond to temperature, for example, hypocotyl elongation or flowering (Wigge, 2013 ). However, both processes are also sensitive to light, and therefore the temperature and the light signals need to be taken into account together.
In principle, temperature and light could be sensed by two separate networks, and the integration could be achieved through further downstream elements. Nevertheless, it has become clear that in Arabidopsis thaliana , temperature and light sensing and signal integration are done by the same network (Franklin, 2009 ; Jung et al., 2016 ; Legris et al., 2016 ; Seaton et al., 2018 ). Again, without mathematical analysis of the data and knowledge integration into dynamic mathematical models, progress in our conceptual understanding of how plants analyse ambient temperature and light conditions in a coordinated manner would be very difficult. The mathematical models allow for studying the effect of the different parts of the network, their interplay and role in the signal processing.
While dynamical systems approaches have been useful for understanding the interplay between temperature and light signal integration, many of the environmental signal integration networks in plants have been primarily interrogated with ‘Big Data’-based approaches that also provide insight into the structure and behaviours of signal integration networks. One research aim has been to infer the structure of large biological networks used in signal integration using transcriptomics data and either experimental or computational predictions of transcription factor binding (Brooks et al., 2019 ; Ezer et al., 2017 ; Gamboa-Tuz et al., 2018 ; Greenham et al., 2017 ; Walker et al., 2017 ). The resulting networks can be too complicated for us to easily comprehend how plants integrate environmental signals, and so there is an ongoing effort to identify submodules that perform specific environmental sensing and integration roles (Polanski et al., 2014 ).
When environmental signal integration networks are too complicated to model with dynamical systems, an alternative approach to understanding their behaviours is to directly predict phenotypic traits of interest from environmental input variables. Here, the aim is not to learn conceptually or model the precise network that plants use intrinsically to integrate signals, but rather to find a model that makes accurate predictions. These kinds of phenotypic forecasts have been especially useful in agricultural applications, where the primary aim of the researcher is to predict agriculturally relevant traits, given a certain set of abiotic environmental parameters. Innovations in this area come from deep learning techniques that integrate networks of sensor and satellite data (Aruul Mozhi Varman et al., 2017 ; Wang et al., 2018 ; Wolanin et al., 2020 ) and statistical advances that integrate more time-series environmental data into these models, taking into account that a plants’ response to an environmental stimulus is time-of-day and season-dependent (Brestovitsky & Ezer, 2019 ; Kocian et al., 2020 ; Newlands et al., 2014 ).
There are a number of open challenges related to the study of how abiotic factors are integrated by plants, which merit quantitative investigation. For instance, there is a limit to how many combinations of environmental parameters can be perturbed in an experiment, so there is a need for new tools to help scientists iteratively design experiments (Ezer & Keir, 2019 ). These would help them choose growth conditions that would help them learn as much as possible about how plants integrate environmental signals, while adhering to time and budget constraints. A second major challenge is to efficiently reverse-engineer specific responses to abiotic stimuli, so we can efficiently engineer crops that are sustainable in the light of climate change.
11. Ecosystem complexity: interactions with pathogens and microbiome
Plant phenotype goes beyond their individual body: to understand their biology, one needs to account for the myriad of living organisms with which they interact. This represents a large field of research, notably because of the threat posed by pathogens on plant development. Needless to say, quantitative thinking is also at the heart of such biotic interactions.
The research field of plant immunity is deeply rooted in crop sciences with phytopathology and breeding for disease resistance traits. The gene-for-gene hypothesis by Flor established the field under the concept of qualitative disease resistance: the presence or absence of a matching pair of host resistance gene and pathogenic avirulent factor determines the qualitative traits, resistance or susceptibility (Flor, 1942 ). This simple assumption has greatly contributed to the development of the plant immunity research field, resulting in a growing list of important immune receptors both in model and crop plant species and the deployment of such robust resistance traits in the crop fields.
Despite its initial contributions, the simple qualitative concept has been challenged with quantitative counterarguments. A resistance trait based on the one-on-one relationship would be predicted to break easily if fast-evolving pathogens come up with a strategy to overcome the resistance. However, most natural populations withstand stochastic pathogenic loads, reflecting their complex immune systems that would consist of resistance genes serving to recognise more than one pathogenic avirulent factor.
As the research field matures, evidence for the quantitative nature of disease resistance and plant immunity is accumulating. An obvious numbers game comes from a genomic view of plant immunity. Numerous genome sequencing datasets point out that the list of immune receptors on our hands, mostly discovered under the gene-for-gene concept, is only a tiny fraction of what a given plant species could carry. For example, out of approximately 160 genes belonging to NLR-type (formerly known as NBS-LRR) resistance genes found in Arabidopsis thaliana , only less than two dozen have been functionally assigned to resistance. The fraction of knowns in other species is far less than those found in the most well-studied model species (Kourelis et al., 2020 ). What are the functions of the rest of these unannotated immune genes present in the plant genome? Are they ‘reservoir’ immune genes that would ensure the plant populations to be ready for stochastic pathogenic pressures? In this sense, does the immune complexity revealed from the functional genomics of plant immunity research reflect the phenotypic plasticity wired in their immune network? How much is the contribution of cryptic genetic variation accumulated in the system to overall plant fitness? Can the complexity be utilised to develop durable resistance in the field?
The current research focus is shifting towards a systematic and quantitative approach to understand the robustness of the plant immune system. Recent molecular findings point to cooperative modules of immune receptors and a diffuse relationship between receptor and ligands in the plant immune system (Cesari et al., 2014 ; Karasov et al., 2014 ; Wang et al., 2015 ; Williams et al., 2014 ). These findings suggest that we should adopt a network view on plant immunity to better understand innate complexity in the plant immune system (Adachi et al., 2019 ).
Copious omics-driven large-scale research has revealed that despite an obvious expansion in the peripheral nodes of the network which accommodates diverse recognition modes, plants have evolved a core machinery that activates rather conserved immune responses (Hillmer et al., 2017 ; Mukhtar et al., 2011 ; Tsuda & Katagiri, 2010 ; Wessling et al., 2014 ). Characteristic plant immune responses often culminate in immunological cell death events that would dispose of the infected local areas, while the activation of immune responses in return sacrifice growth in general. The two topics, the canalisation of diverse recognition events to canonical immune responses and the trade-off between immunity and growth, are the major areas that could benefit from the quantitative approaches already implemented in other areas of biology, particularly in plant development and signalling.
If one can visualise characteristic immune responses, for example by defining and utilising immune responsive elements, detailed image analysis in a spatiotemporal manner would certainly uncover the hidden rules of immune signalling and cell death execution. Such details in immune responses in plants are expected to provide a way to modulate the responses to quantitatively pinpoint how much either acute or residual immune responses affect plant performance during the course of development. As local cell death could disturb the developmental system drastically, rerouting of developmental signalling in a quantitative matter would strengthen our understanding of phenotypic plasticity of plants under external stress.
Another important research area of plant immunity lies in its connection to field sciences. Microbial ecology is an inseparable research area from that of plant immunity. With recent advances in microbiome research platforms, the relative importance of the host-microbe interaction starts to be unveiled in its contribution to plant performance (Chen et al., 2020 ; Hacquard et al., 2017 ). Such efforts widen the past focus on plant–pathogen interactions to embrace different kinds of plant–microbe interactions including symbiosis. Definitely, the expansion of the plant immunity field to embrace the multitude of interactions between plants and microbes as well as between plant immune components will require sophisticated quantitative tools for analysis to build up a comprehensive view of the plant immune system.
12. Back to society: plants and humans
Engineering technology and approaches have always been a weighty facilitator of research and innovation in life sciences, including plant sciences. In turn, many areas of plant sciences are identified as the next frontiers of bioengineering, particularly in light of working towards mitigating climate disasters and realising a sustainable future (Wintle et al., 2017 ). The interface between biology and engineering can be classified into three types: engineering for biology, with biology and inspired by biology.
First, engineering can help biology with quantitative tools. It could be a totally new method, or more often a new improved version of an available technology. For example, how fast and cost-effectively we can sequence DNA has kept transforming the size of the datasets we can afford to produce, and hence the depth and breadth of information we can extract from them. Plant genomes tend towards the large end of the spectrum compared to ones from the other kingdoms (e.g., Nystedt et al., 2013 ). The phenotypic variation is high even within a species, which has prompted genomic sequencing of a collection of accessions (e.g., Alonso-Blanco et al., 2016 ). New bioimaging technology has revealed more and more about plant structures, ever since Robert Hooke saw ‘cells’ in oak cork. In the past 20 years, plant science has been leading the emerging field of morphodynamics. Community efforts to capture growth and development in 4D have kept improving the capacity in data acquisition, processing, extraction and analysis (e.g., Barbier de Reuille et al., 2015 ; Wolny et al., 2020 ). Phenotypic platforms have been developed, driving innovative solutions to capture morphodynamics in situ, generating a wealth of quantitative data. Even root development that occurs underground and is usually optically inaccessible has been visualised, with such technologies as transparent soil and CT scan protocols (Bao et al., 2014 ; Rellán-Álvarez et al., 2015 ). Satellite data, together with machine learning techniques, are increasingly used to monitor ecosystem evolution at continent scale (Newman & Furbank, 2021 ).
Bioengineering technology is not limited to hardware or data acquisition. Breakthroughs in in silico platforms for data processing, analysis and sharing underscore rapid progress in quantitative experimentation, lately including AI and machine learning. These are only few examples; engineering is constantly rewriting what is possible to find in the chemistry and physics of living organisms, and it has been driving biology towards quantitative data collection.
Second, engineering with biology involves a much deeper connection. Notably, through domestication, this corresponds to a form of co-evolution between plants and humans. Since the dawn of civilisation, people have indeed engineered food, drugs and other health remedies, materials and energy sources with living organisms, in the forms of agriculture, horticulture, forestry, fermentation and so on. Genetic engineering deepened the level of orientation towards engineering in that it is design-led.
In the past 20 years, the new field of ‘synthetic biology’ has been expanding rapidly in microbial classes first but later in multicellular systems including plants. Synthetic biology is sometimes called ‘engineering biology’, for its commitment to the engineering principles in design-led problem solving, such as simplicity, universality, efficiency, consistency and predictability. Because of its streamlined and user-considered designs, synthetic biology enables complex genetic engineering; small and well-characterised standard parts of promoters, terminators, functional coding sequences and logic gates all promote efficient cloning of many-part, multigene constructs. Simpler and faster genetic construction calls for large-scale and efficient, likely automated, characterisation platforms. Tools are constantly expanded (while each of them simplified); new technology, should it be CRISPR or directed evolution, is adopted quickly and transformed into usable and sharable parts. Predictability and reproducibility are overarching goals in synthetic biology, and thus predictive modelling plays a crucial role. Synthetic biology builds upon systems biology, or systems biology prompted synthetic biology; synthetic biology could be viewed as an experimental platform for systems biology, and systems biology as a theoretical platform for synthetic biology.
The two most major industrial applications of plant sciences—metabolic engineering and molecular breeding—entail complex genetic engineering to tinker with biosynthetic or developmental pathways that are controlled by multiple genes that often cross-regulate among themselves. As such, synthetic biology technology has already been employed to the pioneering crop engineering projects such as the GM omega-3 project, C4 Rice Project and RIPE (Realizing Increased Photosynthesis Enhancement) Project (Napier et al., 2014 ; Parry et al., 2013 ; Wang et al., 2016 ). Step changes in biotechnology and agriculture are anticipated, as more countries approve gene-edited organisms in their policy and regulation.
Development of universal standards is a key objective of the synthetic biology field, and plant synthetic biologists, in particular, have been leading the efforts to enhance community sharing. The unified design overhangs for standard parts to make DNA assembly compatible named PhytoBricks, and the simple and open material transfer agreement called ‘Open MTA’ were both proposed and developed by plant scientists first and then adopted by wider circles of synthetic biologists (e.g., the student genetic engineering competition iGEM and the largest DNA repository Addgene; Patron et al., 2015 ; Kahl et al., 2018 ).
Finally, biology can also inspire engineering. Among all the living organisms great and small that together exhibit a fantastic variety of functional and structural features, plants have been the primary source of inspiration for engineering. This may be due to their sessile nature. The plant mode of living is not as dependent on rapid and constant movement, and thus their structures are closer to engineered constructions. Many functional structures are made of no-longer living components and feasible to emulate via engineering. For example, the functional appendages that aid seed flight in winged or haired diaspores (such as of maples and dandelions) are made solely of the cell wall remnants of no-longer living cells. The intricate surface textures that confer differential functionalities, such as structural colour and hydrophobicity, are patterned with wax. In fact, the representative examples of biomimetic innovations, such as the burr-mimicking VELCRO and self-cleaning materials that resemble the lotus leaf surface, were inspired by plant structures (Koch et al., 2009 ). This trend continues in robotics, and plant tropisms motivated a large interdisciplinary consortium effort to create self-growing robots, which also embody sensors and modify their growth in response to physical cues from the environment (Fiorello et al., 2020 ).
Engineered mimics often highlight what we do not as yet know about the living structures that they emulate. Therefore, biomimetic engineering, as well as engineering with biology, can be used as learning via building . This is why in bioengineering, Richard Feynman’s famous words—‘What I cannot create, I do not understand.’—are so often quoted. Reconstruction and recapitulation are a highly effective process of redefining questions. The engineering investigative framework dictates the circular iteration of design–build–test stages. They are reminiscent of the modern scientific framework in which a question is defined as hypothesis that is tested via experimentation. The engineering cycle is indeed a variation of the general framework in research and project development of any kinds. However, there is a benefit to explicitly casting this concept onto applied and basic research in natural sciences, because of the emphasis on its interactive nature. Research typically does not end with the ‘test’ stage with a clear and definitive conclusion. Any project or scientific endeavour is not a linear process; rather, it is iterations of inquisition. There is no failure of a project; any sound scientific experimentation only brings us closer to new insights and findings about plants and how to apply such gained knowledge better.
13. Conclusion: a proposition
At the end, this overview of quantitative plant biology in the 21st century is an invitation to explore new paradigm changes in fields including high-resolution cell dynamics and computational reconstruction of gene networks, or plant and environment interactions through multiscale metamodels. This synthesis must also include yet another important player: the citizen. With the rise of participative science, notably through education and digital technologies, it appears that data acquisition, analysis and interpretation is more and more open to plant amateurs. This is facilitated by fair science and open access to data. By sharing lab and citizen data, new questions arise. How to deal with heterogeneous measurements, from people with different expertise? How to compare results obtained in controlled conditions but in small numbers and results obtained in the field in large numbers? Even more interestingly, situated knowledge, for instance, relating to agronomical practices, are now fuelling basic research in the labs. This notably includes the synergistic properties of varietal mixtures, an emerging field of study in genetics and ecophysiology. It seems therefore that quantitative plant biology is taking a turn from inter- to transdisciplinary research.
Building on this fertile field and these exciting developments, we proposed the founding of a new journal, Quantitative Plant Biology , that also includes a new transdisciplinary field of research, involving scientists and nonscientists. This will likely require new ways to coordinate between disciplines and community resources, for instance, when considering stock centres [special code repositories, coordination on file specifications (like SBML did for other areas of biology), and stock centres of synthetic biology parts, databases of images and shared software for image storage and analysis] or new standards in article format and reviewing (e.g., running scripts and models in published notebooks with the article, access to code and data). This will also require the formation of the next generation of inter- and transdisciplinarians. Several summer schools and workshops are supporting these efforts. We hope this open access journal, affiliated to both a public research institution like the John Innes Centre and a nonprofit publisher (Cambridge University Press), is the ideal forum for these stimulating endeavours.
Acknowledgement
We are thankful to our colleagues and the larger community for fruitful discussions on this topic.
Financial support
This work received no specific grant from any funding agency, commercial or not-for-profit sectors.
Conflict of interest
The authors declare that there is no conflict of interest.
Authorship contributions
All authors contributed to specific sections of the article, and all reviewed the entire manuscript.
Data availability statement
Ethical standards.
All the authors comply with the journal’s publishing ethics.
- Abley, K. , Locke, J. C. W. , & Leyser, H. M. O. (2016). Developmental mechanisms underlying variable, invariant and plastic phenotypes . Annals of Botany , 117 , 733–748. [ PMC free article ] [ PubMed ] [ Google Scholar ]
- Adachi, H. , Derevnina, L. , & Kamoun, S. (2019). NLR singletons, pairs, and networks: Evolution, assembly, and regulation of the intracellular immunoreceptor circuitry of plants . Current Opinion in Plant Biology , 50 , 121–131. [ PubMed ] [ Google Scholar ]
- Allard, Jun F. , Wasteneys, Geoffrey O. , & Cytrynbaum, Eric N. (2010). Mechanisms of self-organization of cortical microtubules in plants revealed by computational simulations. Mol Biol Cell 21 ( 2 ):278–86. doi: 10.1091/mbc.e09-07-0579. [ PMC free article ] [ PubMed ] [ Google Scholar ]
- Ali, O. , & Traas, J. (2016). Force-driven polymerization and turgor-Induced Wall expansion . Trends in Plant Science , 21 , 398–409. [ PubMed ] [ Google Scholar ]
- Alonso-Blanco, C. , Andrade, J. , Becker, C. , Bemm, F. , Bergelson, J. , Borgwardt, K. M. , Cao, J. , Chae, E. , Dezwaan, T. M. , Ding, W. , Ecker, J. R. , Exposito-Alonso, M. , Farlow, A. , Fitz, J. , Gan, X. , Grimm, D. G. , Hancock, A. M. , Henz, S. R. , Holm, S. , & Zhou, X. (2016). 1,135 genomes reveal the global pattern of polymorphism in Arabidopsis thaliana . Cell , 166 , 481–491. [ PMC free article ] [ PubMed ] [ Google Scholar ]
- Alonso-Serra, J. , Shi, X. , Peaucelle, A. , Rastas, P. , Bourdon, M. , Immanen, J. , Takahashi, J. , Koivula, H. , Eswaran, G. , Muranen, S. , Help, H. , Smolander, O. P. , Su, C. , Safronov, O. , Gerber, L. , Salojärvi, J. , Hagqvist, R. , Mähönen, A. P. , Helariutta, Y. , & Nieminen, K. (2020). ELIMÄKI locus is required for vertical proprioceptive response in birch trees . Current Biology: CB , 30 , 589–599.e5. [ PubMed ] [ Google Scholar ]
- Araújo, I. S. , Pietsch, J. M. , Keizer, E. M. , Greese, B. , Balkunde, R. , Fleck, C. , & Hülskamp, M. (2017). Stochastic gene expression in Arabidopsis thaliana . Nature Communications , 8 , 2132. [ PMC free article ] [ PubMed ] [ Google Scholar ]
- Aruul Mozhi Varman, S. , Baskaran, A. R. , Aravindh, S. , & Prabhu, E. (2017). Deep learning and IoT for smart agriculture using WSN. In 2017 IEEE International Conference on Computational Intelligence and Computing Research (ICCIC) (pp. 1–6).
- Avraham, R. , & Yarden, Y. (2011). Feedback regulation of EGFR signalling: Decision making by early and delayed loops . Nature Reviews. Molecular Cell Biology , 12 , 104–117. [ PubMed ] [ Google Scholar ]
- Bao, Y. , Aggarwal, P. , Robbins, N. E. , Sturrock, C. J. , Thompson, M. C. , Tan, H. Q. , Tham, C. , Duan, L. , Rodriguez, P. L. , Vernoux, T. , Mooney, S. J. , Bennett, M. J. , & Dinneny, J. R. (2014). Plant roots use a patterning mechanism to position lateral root branches toward available water . Proceedings of the National Academy of Sciences , 111 , 9319–9324. [ PMC free article ] [ PubMed ] [ Google Scholar ]
- Barbier de Reuille, P. , Routier-Kierzkowska, A.-L. , Kierzkowski, D. , Bassel, G. W. , Schüpbach, T. , Tauriello, G. , Bajpai, N. , Strauss, S. , Weber, A. , Kiss, A. , Burian, A. , Hofhuis, H. , Sapala, A. , Lipowczan, M. , Heimlicher, M. B. , Robinson, S. , Bayer, E. M. , Basler, K. , Koumoutsakos, P. , & Smith, R. S. (2015). MorphoGraphX: A platform for quantifying morphogenesis in 4D . eLife , 4 , 05864. [ PMC free article ] [ PubMed ] [ Google Scholar ]
- Bassel, G. W. (2018). Information processing and distributed computation in plant organs . Trends in Plant Science , 23 , 994–1005. [ PubMed ] [ Google Scholar ]
- Bastien, R. , Bohr, T. , Moulia, B. , & Douady, S. (2013). Unifying model of shoot gravitropism reveals proprioception as a central feature of posture control in plants . Proc Natl Acad Sci U S A , 110 , 755–60. 10.1073/pnas.1214301109. [ PMC free article ] [ PubMed ] [ CrossRef ] [ Google Scholar ]
- Boer, M. D. , Santos Teixeira, J. , & Ten Tusscher, K. H. (2020). Modeling of root nitrate responses suggests preferential foraging arises from the integration of demand, supply and local presence signals . Frontiers in Plant Science , 11 , 708. [ PMC free article ] [ PubMed ] [ Google Scholar ]
- Brestovitsky, A. , & Ezer, D. (2019). A mass participatory experiment provides a rich temporal profile of temperature response in spring onions . Plant Direct , 3 , e00126. doi: 10.1002/pld3.126. [ PMC free article ] [ PubMed ] [ CrossRef ] [ Google Scholar ]
- Bringmann, M. , & Bergmann, D. C. (2017). Tissue-wide mechanical forces influence the polarity of stomatal stem cells in Arabidopsis . Current Biology: CB , 27 , 877–883. 10.1016/j.cub.2017.01.059. [ PubMed ] [ CrossRef ] [ Google Scholar ]
- Brooks, M. D. , Cirrone, J. , Pasquino, A. V. , Alvarez, J. M. , Swift, J. , Mittal, S. , Juang, C.-L. , Varala, K. , Gutiérrez, R. A. , Krouk, G. , Shasha, D. , & Coruzzi, G. M. (2019). Network walking charts transcriptional dynamics of nitrogen signaling by integrating validated and predicted genome-wide interactions . Nature Communications , 10 , 1569. [ PMC free article ] [ PubMed ] [ Google Scholar ]
- Carré, C. , Mas, A. , & Krouk, G. (2017). Reverse engineering highlights potential principles of large gene regulatory network design and learning . NPJ Systems Biology and Applications , 3 , 17. [ PMC free article ] [ PubMed ] [ Google Scholar ]
- Cesari, S. , Kanzaki, H. , Fujiwara, T. , Bernoux, M. , Chalvon, V. , Kawano, Y. , Shimamoto, K. , Dodds, P. , Terauchi, R. , & Kroj, T. (2014). The NB-LRR proteins RGA4 andRGA5 interact functionally and physically to confer disease resistance . EMBO J , 33 , 1941–1959. [ PMC free article ] [ PubMed ] [ Google Scholar ]
- Chakrabortty, B. , Willemsen, V. , Zeeuw, T. , Liao, C. , Weijers, D. , Mulder, B. , & Scheres, B. (2018). A Plausible Microtubule-Based Mechanism for Cell Division Orientation in Plant Embryogenesis . Curr Biol , 28 , 3031–3043.e2. 10.1016/j.cub.2018.07.025. [ PubMed ] [ CrossRef ] [ Google Scholar ]
- Chen, M. , Chory, J. , & Fankhauser, C. (2004). Light signal transduction in higher plants . Annual Review of Genetics , 38 , 87–117. 10.1146/annurev.genet.38.072902.092259. [ PubMed ] [ CrossRef ] [ Google Scholar ]
- Chen, T. , Nomura, K. , Wang, X. , Sohrabi, R. , Xu, J. , Yao, L. , Paasch, B. C. , Ma, L. , Kremer, J. , & Chen g, Y. , et al. (2020). A plant genetic network for preventing dysbiosis in the phyllosphere . Nature , 580 , 653–657. [ PMC free article ] [ PubMed ] [ Google Scholar ]
- Cheung, C. Y. M. , Poolman, M. G. , Fell, D. A. , Ratcliffe, R. G. , & Sweetlove, L. J. (2014). A diel flux balance model captures interactions between light and dark metabolism during day-night cycles in C3 and Crassulacean acid metabolism leaves . Plant Physiology , 165 , 917–929. [ PMC free article ] [ PubMed ] [ Google Scholar ]
- Chitwood, D. H. , & Sinha, N. R. (2016). Evolutionary and environmental forces sculpting leaf development . Current Biology: CB , 26 , R297–R306. [ PubMed ] [ Google Scholar ]
- Clark, N. M. , Fisher, A. P. , Berckmans, B. , den Broeck, L. V. , Nelson, E. C. , Nguyen, T. T. , Bustillo-Avendaño, E. , Zebell, S. G. , Moreno-Risueno, M. A. , Simon, R. , Gallagher, K. L. , & Sozzani, R. (2020). Protein complex stoichiometry and expression dynamics of transcription factors modulate stem cell division . Proceedings of the National Academy of Sciences , 117 , 15332–15342. [ PMC free article ] [ PubMed ] [ Google Scholar ]
- Clark, N. M. , Hinde, E. , Winter, C. M. , Fisher, A. P. , Crosti, G. , Blilou, I. , Gratton, E. , Benfey, P. N. , & Sozzani, R. (2016). Tracking transcription factor mobility and interaction in Arabidopsis roots with fluorescence correlation spectroscopy . eLife , 5 , e14770. [ PMC free article ] [ PubMed ] [ Google Scholar ]
- Costes, E. , Smith, C. , Renton, M. , Guédon, Y. , Prusinkiewicz, P. , & Godin, C. (2008). MAppleT: Simulation of apple tree development using mixed stochastic and biomechanical models . Functional Plant Biology , 35 , 936. [ PubMed ] [ Google Scholar ]
- Cruz-Ramírez, A. , Díaz-Triviño, S. , Blilou, I. , Grieneisen, V. A. , Sozzani, R. , Zamioudis, C. , Miskolczi, P. , Nieuwland, J. , Benjamins, R. , Dhonukshe, P. , Caballero-Pérez, J. , Horvath, B. , Long, Y. , Mähönen, A. P. , Zhang, H. , Xu, J. , JAH, M. , Benfey, P. N. , Bako, L. , & Scheres, B. (2012). A bistable circuit involving SCARECROW-RETINOBLASTOMA integrates cues to inform asymmetric stem cell division . Cell , 150 , 1002–1015. [ PMC free article ] [ PubMed ] [ Google Scholar ]
- Cummins, C. , Seale, M. , Macente, A. , Certini, D. , Mastropaolo, E. , Maria Viola, I. , & Nakayama, N. (2018). A separated vortex ring underlies the flight of the dandelion . Nature , 562 , 414–418. 10.1038/s41586-018-0604-2. [ PubMed ] [ CrossRef ] [ Google Scholar ]
- Decaestecker, W. , Buono, R. A. , Pfeiffer, M. L. , Vangheluwe, N. , Jourquin, J. , Karimi, M. , Van Isterdael, G. , Beeckman, T. , Nowack, M. K. , & Jacobs, T. B. (2019). CRISPR-TSKO: A technique for efficient mutagenesis in specific cell types, tissues, or organs in Arabidopsis . The Plant Cell , 31 , 2868–2887. [ PMC free article ] [ PubMed ] [ Google Scholar ]
- Deinum, E. E. , & Mulder, B. M. (2013). Modelling the role of microtubules in plant cell morphology . Current Opinion in Plant Biology , 16 , 688–692. [ PubMed ] [ Google Scholar ]
- Deinum, E. E. , Mulder, B. M. , & Benitez-Alfonso, Y. (2019). From plasmodesma geometry to effective symplasmic permeability through biophysical modelling . eLife , 8 , e49000. [ PMC free article ] [ PubMed ] [ Google Scholar ]
- Derr, J. , Bastien, R. , Couturier, É. , & Douady, S. (2018). Fluttering of growing leaves as a way to reach flatness: Experimental evidence on Persea americana . Journal of the Royal Society Interface , 15 , 20170595. [ PMC free article ] [ PubMed ] [ Google Scholar ]
- Ditengou, F. A. , Teale, W. D. , Kochersperger, P. , Flittner, K. A. , Kneuper, I. , van der Graaff, E. , Nziengui, H. , Pinosa, F. , Li, X. , Nitschke, R. , Laux, T. , & Palme, K. (2008). Mechanical induction of lateral root initiation in Arabidopsis thaliana . Proceedings of the National Academy of Sciences of the United States of America , 105 , 18818–18823. [ PMC free article ] [ PubMed ] [ Google Scholar ]
- Duncan, S. , Olsson, T. S. G. , Hartley, M. , Dean, C. , & Rosa, S. (2016). A method for detecting single mRNA molecules in Arabidopsis thaliana . Plant Methods , 12 , 13. [ PMC free article ] [ PubMed ] [ Google Scholar ]
- Efroni, I. , Mello, A. , Nawy, T. , Ip, P.-L. , Rahni, R. , DelRose, N. , Powers, A. , Satija, R. , & Birnbaum, K. D. (2016). Root regeneration triggers an embryo-like sequence guided by hormonal interactions . Cell , 165 , 1721–1733. [ PMC free article ] [ PubMed ] [ Google Scholar ]
- Eloy, C. , Fournier, M. , Lacointe, A. , & Moulia, B. (2017). Wind loads and competition for light sculpt trees into self-similar structures . Nature Communications , 8 , 1014. [ PMC free article ] [ PubMed ] [ Google Scholar ]
- Erickson, R. O. (1976). Modeling of plant growth . Annual Review of Plant Physiology , 27 407–34. [ Google Scholar ]
- Erickson, R. O. (1988). Growth and development of a botanist . Annual Review of Plant Physiology and Plant Molecular Biology , 39 , 1–22. [ Google Scholar ]
- Evans, M. J. , Choi, W.-G. , Gilroy, S. , & Morris, R. J. (2016). A ROS-assisted calcium wave dependent on the AtRBOHD NADPH oxidase and TPC1 Cation Channel propagates the systemic response to Salt stress . Plant Physiology , 171 , 1771–1784. [ PMC free article ] [ PubMed ] [ Google Scholar ]
- Ezer, D. , & Keir, J. (2019). NITPicker: Selecting time points for follow-up experiments . BMC Bioinformatics , 20 , 166. [ PMC free article ] [ PubMed ] [ Google Scholar ]
- Ezer, D. , Shepherd, S. J. K. , Brestovitsky, A. , Dickinson, P. , Cortijo, S. , Charoensawan, V. , Box, M. S. , Biswas, S. , Jaeger, K. E. , & Wigge, P. A. (2017). The G-Box transcriptional regulatory code in Arabidopsis . Plant Physiology , 175 , 628–640. [ PMC free article ] [ PubMed ] [ Google Scholar ]
- Fache, V. , Gaillard, J. , Van Damme, D. , Geelen, D. , Neumann, E. , Stoppin-Mellet, V. , & Vantard, M. (2010). Arabidopsis kinetochore fiber-associated MAP65-4 cross-links microtubules and promotes microtubule bundle elongation . Plant Cell , 22 , 3804–15. 10.1105/tpc.110.080606. [ PMC free article ] [ PubMed ] [ CrossRef ] [ Google Scholar ]
- Farquhar, G. D. , von Caemmerer, S. , & Berry, J. A. (1980). A biochemical model of photosynthetic CO 2 assimilation in leaves of C3 species . Planta , 149 , 78–90. [ PubMed ] [ Google Scholar ]
- Fernandez-Jaramillo, A. A. , Duarte-Galvan, C. , Contreras-Medina, L. M. , Torres-Pacheco, I. , de J Romero-Troncoso, R. , Guevara-Gonzalez, R. G. , & Millan-Almaraz, J. R. (2012). Instrumentation in developing chlorophyll fluorescence biosensing: A review . Sensors , 12 , 11853–11869. [ PMC free article ] [ PubMed ] [ Google Scholar ]
- Fiorello, I. , Del Dottore, E. , Tramacere, F. , & Mazzolai, B. (2020). Taking inspiration from climbing plants: Methodologies and benchmarks—A review . Bioinspiration & Biomimetics , 15 , 031001. [ PubMed ] [ Google Scholar ]
- Flor, H. H. (1942). Inheritance of pathogenicity in Melampsora lini . Phytopathology , 32 ,653–669. [ Google Scholar ]
- Forterre, Y. , Skotheim, J. M. , Dumais, J. , & Mahadevan, L. (2005). How the Venus flytrap snaps . Nature , 433 , 421–425. [ PubMed ] [ Google Scholar ]
- Fournier, M. , Dlouhá, J. , Jaouen, G. , & Almeras, T. (2013). Integrative biomechanics for tree ecology: Beyond wood density and strength . Journal of Experimental Botany , 64 , 4793–4815. [ PubMed ] [ Google Scholar ]
- Franklin, K. A. (2009). Light and temperature signal crosstalk in plant development . Current Opinion in Plant Biology , 12 , 63–68. [ PubMed ] [ Google Scholar ]
- Franklin, K. A. , Allen, T. , & Whitelam, G. C. (2007). Phytochrome A is an irradiance-dependent red light sensor . The Plant Journal , 50 , 108–117. [ PubMed ] [ Google Scholar ]
- Galvan-Ampudia, C. S. , Cerutti, G. , Legrand, J. , Brunoud, G. , Martin-Arevalillo, R. , Azais, R. , Bayle, V. , Moussu, S. , Wenzl, C. , Jaillais, Y. , Lohmann, J. U. , Godin, C. , & Vernoux, T. (2020). Temporal integration of auxin information for the regulation of patterning . eLife , 9 , e55832. 10.7554/eLife.55832. [ PMC free article ] [ PubMed ] [ CrossRef ] [ Google Scholar ]
- Galvão, V. C. , & Fankhauser, C. (2015). Sensing the light environment in plants: Photoreceptors and early signaling steps . Current Opinion in Neurobiology , 34 , 46–53. [ PubMed ] [ Google Scholar ]
- Gamboa-Tuz, S. D. , Pereira-Santana, A. , Zamora-Briseño, J. A. , Castano, E. , Espadas-Gil, F. , Ayala-Sumuano, J. T. , Keb-Llanes, M. A. , Sanchez-Teyer, F. , & Rodríguez-Zapata, L. C. (2018). Transcriptomics and co-expression networks reveal tissue-specific responses and regulatory hubs under mild and severe drought in papaya (Carica papaya L.) . Scientific Reports , 8 , 14539. [ PMC free article ] [ PubMed ] [ Google Scholar ]
- Giacomello, S. , Salmén, F. , Terebieniec, B. K. , Vickovic, S. , Navarro, J. F. , Alexeyenko, A. , Reimegård, J. , LS, M. K. , Mannapperuma, C. , Bulone, V. , Ståhl, P. L. , Sundström, J. F. , Street, N. R. , & Lundeberg, J. (2017). Spatially resolved transcriptome profiling in model plant species . Nature Plants , 3 , 17061. [ PubMed ] [ Google Scholar ]
- Gooh, K. , Ueda, M. , Aruga, K. , Park, J. , Arata, H. , Higashiyama, T. , & Kurihara, D. (2015). Live-cell imaging and optical manipulation of Arabidopsis early embryogenesis . Developmental Cell , 34 , 242–251. [ PubMed ] [ Google Scholar ]
- Green, P. , & King, A. (1966). A mechanism for the origin of specifically oriented textures in development with special reference to Nitella wall texture . Australian Journal of Biological Sciences , 19 , 421–437. [ Google Scholar ]
- Green, P. B. (1962). Mechanism for plant cellular morphogenesis . Science , 138 , 1404–1405. [ PubMed ] [ Google Scholar ]
- Green, P. B. (1996). Transductions to generate plant form and pattern: an essay on cause and effect . Annals of Botany , 78 ,9–281. [ PubMed ] [ Google Scholar ]
- Green, P. B. (1999). Expression of pattern in plants: Combining molecular and calculus-based biophysical paradigms . American Journal of Botany , 86 , 1059–1076. [ PubMed ] [ Google Scholar ]
- Greenham, K. , Guadagno, C. R. , Gehan, M. A. , Mockler, T. C. , Weinig, C. , Ewers, B. E. , & McClung, C. R. (2017). Temporal network analysis identifies early physiological and transcriptomic indicators of mild drought in Brassica rapa . eLife , 6 , e29655. [ PMC free article ] [ PubMed ] [ Google Scholar ]
- Greenhill, A.-G. (1881). Determination of the greatest height consistent with stability that a vertical pole or mast can be made, and the greatest height to which a tree of given proportions can grow . Proceedings of the Cambridge Philosophical Society , 4 , 65–73. [ Google Scholar ]
- Gruel, J. , Landrein, B. , Tarr, P. , Schuster, C. , Refahi, Y. , Sampathkumar, A. , Hamant, O. , Meyerowitz, E. M. , & Jönsson, H. (2016). An epidermis-driven mechanism positions and scales stem cell niches in plants . Science Advances , 2 , e1500989. [ PMC free article ] [ PubMed ] [ Google Scholar ]
- Guerriero, M. L. , Pokhilko, A. , Fernández, A. P. , Halliday, K. J. , Millar, A. J. , & Hillston, J. (2012). Stochastic properties of the plant circadian clock . Journal of the Royal Society Interface , 9 , 744–756. [ PMC free article ] [ PubMed ] [ Google Scholar ]
- Hacquard, S. , Spaepen, S. , Garrido-Oter, R. , & Schulze-Lefert, P. (2017). Interplay Between Innate Immunity and the Plant Microbiota . Annu Rev Phytopathol , 55 , 565–589. [ PubMed ] [ Google Scholar ]
- Hamant, O. , Heisler, M. G. , Jonsson, H. , Krupinski, P. , Uyttewaal, M. , Bokov, P. , Corson, F. , Sahlin, P. , Boudaoud, A. , Meyerowitz, E. M. , Couder, Y. , & Traas, J. (2008). Developmental patterning by mechanical signals in Arabidopsis . Science , 322 , 1650–1655. [ PubMed ] [ Google Scholar ]
- Heisler, M. G. , Hamant, O. , Krupinski, P. , Uyttewaal, M. , Ohno, C. , Jönsson, H. , Traas, J. , & Meyerowitz, E. M. (2010). Alignment between PIN1 polarity and microtubule orientation in the shoot apical meristem reveals a tight coupling between morphogenesis and auxin transport . PLoS Biology , 8 , e1000516. 10.1371/journal.pbio.1000516. [ PMC free article ] [ PubMed ] [ CrossRef ] [ Google Scholar ]
- Hejnowicz, Z. , & Romberger, J. A. (1984). Growth tensor of plant organs . Journal of Theoretical Biology , 110 , 93–114. [ Google Scholar ]
- Hejnowicz, Z. , & Sievers, A. (1996). Tissue stresses in organs of herbaceous plants. III. Elastic properties of the tissues of sunflower hypocotyl and origin of tissue stresses . Journal of Experimental Botany , 47 , 519–528. [ Google Scholar ]
- Hejnowicz, Z. (2011). Plants as mechano-osmotic transducers. In, Mechanical integration of plant cells and plants P. Wojtaszek (ed.) (pp. 241–267). Springer. [ Google Scholar ]
- Hématy, K. , Sado, P. E. , Van Tuinen, A. , Rochange, S. , Desnos, T. , Balzergue, S. , Pelletier, S. , Renou, J.-P. , & Höfte, H. (2007). A receptor-like kinase mediates the response of Arabidopsis cells to the inhibition of cellulose synthesis . Current Biology: CB , 17 , 922–931. [ PubMed ] [ Google Scholar ]
- Hervieux, N. , Tsugawa, S. , Fruleux, A. , Dumond, M. , Routier-Kierzkowska, A. L. , Komatsuzaki, T. , Boudaoud, A. , Larkin, J. C. , Smith, R. S. , Li, C. B. , & Hamant, O. (2017). Mechanical shielding of rapidly growing cells buffers growth heterogeneity and contributes to organ shape reproducibility . Current Biology: CB , 27 , 3468–3479.e4. [ PubMed ] [ Google Scholar ]
- Hillmer, R. A. , Tsuda, K. , Rallapalli, G. , Asai, S. , Truman, W. , Papke, M. D. , Sakakibara, H. , Jones, J. D. G. , Myers, C. L. , & Katagiri, F. (2017). The highly buffered Arabidopsis immune signaling network conceals the functions of its components . PLoS Genet , 13 , e1006639. [ PMC free article ] [ PubMed ] [ Google Scholar ]
- Hong, L. , Dumond, M. , Tsugawa, S. , Sapala, A. , Routier-Kierzkowska, A.-L. , Zhou, Y. , Chen, C. , Kiss, A. , Zhu, M. , Hamant, O. , Smith, R. S. , Komatsuzaki, T. , Li, C.-B. , Boudaoud, A. , & Roeder, A. H. K. (2016). Variable cell growth yields reproducible OrganDevelopment through spatiotemporal averaging . Developmental Cell , 38 , 15–32. [ PubMed ] [ Google Scholar ]
- Hong, L. , Dumond, M. , Zhu, M. , Tsugawa, S. , Li, C.-B. , Boudaoud, A. , Hamant, O. , & Roeder, A. H. K. (2018). Heterogeneity and robustness in plant morphogenesis: From cells to organs . Annual Review of Plant Biology , 69 , 469–495. [ PubMed ] [ Google Scholar ]
- Ioannidis, J. P. A. (2005). Why most published research findings are false . PLoS Medicine , 2 , e124. [ PMC free article ] [ PubMed ] [ Google Scholar ]
- Jackson, M. D. B. , Duran-Nebreda, S. , Kierzkowski, D. , Strauss, S. , Xu, H. , Landrein, B. , Hamant, O. , Smith, R. S. , Johnston, I. G. , & Bassel, G. W. (2019). Global topological order emerges through local mechanical control of cell divisions in the Arabidopsis shoot apical meristem . Cell Systems , 8 , 53–65.e3. [ PMC free article ] [ PubMed ] [ Google Scholar ]
- Jimenez-Gomez, J. M. , Corwin, J. A. , Joseph, B. , Maloof, J. N. , & Kliebenstein, D. J. (2011). Genomic analysis of QTLs and genes altering natural variation in stochastic noise . PLoS Genetics , 7 , e1002295. [ PMC free article ] [ PubMed ] [ Google Scholar ]
- Johnston, I. G. , & Bassel, G. W. (2018). Identification of a bet-hedging network motif generating noise in hormone concentrations and germination propensity in Arabidopsis . Journal of the Royal Society Interface , 15 , 20180042. [ PMC free article ] [ PubMed ] [ Google Scholar ]
- Jung, J.-H. , Domijan, M. , Klose, C. , Biswas, S. , Ezer, D. , Gao, M. , Khattak, A. K. , Box, M. S. , Charoensawan, V. , Cortijo, S. , Kumar, M. , Grant, A. , JCW, L. , Schäfer, E. , Jaeger, K. E. , & Wigge, P. A. (2016). Phytochromes function as thermosensors in Arabidopsis . Science , 354 , 886–889. [ PubMed ] [ Google Scholar ]
- Kahl, L. , Molloy, J. , Patron, N. , Matthewman, C. , Haseloff, J. , Grewal, D. , Johnson, R. , & Endy, D. (2018). Opening options for material transfer . Nature Biotechnology , 36 , 923–927. [ PMC free article ] [ PubMed ] [ Google Scholar ]
- Kami, C. , Lorrain, S. , Hornitschek, P. , & Fankhauser, C. (2010). Light-regulated plant growth and development . Current Topics in Developmental Biology , 91 , 29–66. [ PubMed ] [ Google Scholar ]
- Kaplan, D. (1992). The Relationship of Cells to Organisms in Plants: Problem and Implications of an Organismal Perspective . International Journal of Plant Sciences , 153 , 28–37. [ Google Scholar ]
- Kaplan, D. R. , & Cooke, T. J. (1996). The genius of Wilhelm Hofmeister: The origin of causal-analytical research in plant development . American Journal of Botany , 83 , 1647–1660. [ Google Scholar ]
- Karasov, T. L. , Kniskern, J. M. , Gao, L. , DeYoung, B. J. , Ding, J. , Dubiella, U. , Lastra, R. O. , Nallu, S. , Roux, F. , & Innes, R. W. , et al. (2014). The long-term maintenance of a resistance polymorphism through diffuse interactions . Nature , 512 ,36–440. [ PMC free article ] [ PubMed ] [ Google Scholar ]
- Katul, G. , Porporato, A. , & Oren, R. (2007). Stochastic dynamics of plant–water interactions . Annual Review of Ecology, Evolution, and Systematics , 38 , 767–791. [ Google Scholar ]
- Kholodenko, B. N. , Hancock, J. F. , & Kolch, W. (2010). Signalling ballet in space and time . Nature Reviews Molecular Cell Biology , 11 , 414–426. [ PMC free article ] [ PubMed ] [ Google Scholar ]
- Kimata, Y. , Kato, T. , Higaki, T. , Kurihara, D. , Yamada, T. , Segami, S. , Morita, M. T. , Maeshima, M. , Hasezawa, S. , Higashiyama, T. , Tasaka, M. , & Ueda, M. (2019). Polar vacuolar distribution is essential for accurate asymmetric division of Arabidopsis zygotes . Proceedings of the National Academy of Sciences , 116 , 2338–2343. [ PMC free article ] [ PubMed ] [ Google Scholar ]
- Klose, C. , Venezia, F. , Hussong, A. , Kircher, S. , Schäfer, E. , & Fleck, C. (2015). Systematic analysis of how phytochrome B dimerization determines its specificity . Nature Plants , 1 , 15090. [ PubMed ] [ Google Scholar ]
- Koch, K. , Bhushan, B. , & Barthlott, W. (2009). Multifunctional surface structures of plants: An inspiration for biomimetics . Progress in Materials Science , 54 , 137–178. [ Google Scholar ]
- Kocian, A. , Carmassi, G. , Cela, F. , Incrocci, L. , Milazzo, P. , & Chessa, S. (2020). Bayesian sigmoid-type time series forecasting with missing data for greenhouse crops . Sensors , 20 , 3246. [ PMC free article ] [ PubMed ] [ Google Scholar ]
- Kourelis, J. , Sakai, T. , Adachi, H. , & Kamoun, S. (2020). RefPlantNLR: A comprehensive collection of experimentally validated plant NLRs. Plant Biology . Preprint. Retrieved from http://biorxiv.org/lookup/doi/10.1101/2020.07.08.193961 . [ PMC free article ] [ PubMed ]
- Kutschera, U. (1989). Tissue stresses in growing plant organs . Physiologia Plantarum , 77 , 157–163. [ Google Scholar ]
- Ledbetter, M. C. , & Porter, K. R. (1963). A ‘microtubule’ in plant cell fine structure . The Journal of Cell Biology , 19 , 239–250. [ PMC free article ] [ PubMed ] [ Google Scholar ]
- Lee, L. R. , Wengier, D. L. , & Bergmann, D. C. (2019). Cell-type-specific transcriptome and histone modification dynamics during cellular reprogramming in the Arabidopsis stomatal lineage . Proceedings of the National Academy of Sciences of the United States of America , 116 , 21914–21924. [ PMC free article ] [ PubMed ] [ Google Scholar ]
- Legris, M. , Klose, C. , Burgie, E. S. , Rojas, C. C. , Neme, M. , Hiltbrunner, A. , Wigge, P. A. , Schäfer, E. , Vierstra, R. D. , & Casal, J. J. (2016). Phytochrome B integrates light and temperature signals in Arabidopsis . Science , 354 , 897–900. [ PubMed ] [ Google Scholar ]
- Lestas, I. , Vinnicombe, G. , & Paulsson, J. (2010). Fundamental limits on the suppression of molecular fluctuations . Nature , 467 , 174–178. [ PMC free article ] [ PubMed ] [ Google Scholar ]
- Lockhart, J. A. (1965). An analysis of irreversible plant cell elongation . Journal of Theoretical Biology , 8 , 264–275. [ PubMed ] [ Google Scholar ]
- Long, T. A. , Brady, S. M. , & Benfey, P. N. (2008). Systems approaches to identifying gene regulatory networks in plants . Annual Review of Cell and Developmental Biology , 24 , 81–103. [ PMC free article ] [ PubMed ] [ Google Scholar ]
- Lucas, M. , Kenobi, K. , von Wangenheim, D. , Voβ, U. , Swarup, K. , De Smet, I. , Van Damme, D. , Lawrence, T. , Péret, B. , Moscardi, E. , Barbeau, D. , Godin, C. , Salt, D. , Guyomarc’h, S. , EHK, S. , Maizel, A. , Laplaze, L. , & Bennett, M. J. (2013). Lateral root morphogenesis is dependent on the mechanical properties of the overlaying tissues . Proceedings of the National Academy of Sciences , 110 , 5229–5234. [ PMC free article ] [ PubMed ] [ Google Scholar ]
- Lynch, T. M. , & Lintilhac, P. M. (1997). Mechanical signals in plant development: A new method for single cell studies . Developmental Biology , 181 , 246–256. [ PubMed ] [ Google Scholar ]
- Meicenheimer, R. , & Silk, W. K. (2006). In Memoriam: Ralph Erickson 1914 - 2006 . Plant Science Bulletin , 52 , 88–91. [ Google Scholar ]
- Malivert, A. , Hamant, O. , & Ingram, G. (2018). The contribution of mechanosensing to epidermal cell fate specification . Current Opinion in Genetics & Development , 51 , 52–58. [ PubMed ] [ Google Scholar ]
- Menges, E.S. (1992). Stochastic modeling of extinction in plant populations . In Conservation biology 253–275. Springer, Boston, MA. [ Google Scholar ]
- Meroz, Y. , & Bastien, R. (2014). Stochastic processes in gravitropism . Frontiers in Plant Science , 5 , 674. [ PMC free article ] [ PubMed ] [ Google Scholar ]
- Metzger, C. (1893). Der wind als maßgebender Faktor für das Wachsthum der Bäume . Mündener Forstliche Hefte , 5 , 35–86. [ Google Scholar ]
- Meyer, H. M. , Teles, J. , Formosa-Jordan, P. , Refahi, Y. , San-Bento, R. , Ingram, G. , Jönsson, H. , JCW, L. , & Roeder, A. H. K. (2017). Fluctuations of the transcription factor ATML1 generate the pattern of giant cells in the Arabidopsis sepal . eLife , 6 , e19131. 10.7554/eLife.19131. [ PMC free article ] [ PubMed ] [ CrossRef ] [ Google Scholar ]
- Möglich, A. , Yang, X. , Ayers, R. A. , & Moffat, K. (2010). Structure and function of plant photoreceptors . Annual Review of Plant Biology , 61 , 21–47. [ PubMed ] [ Google Scholar ]
- Moukhtar, J. , Trubuil, A. , Belcram, K. , Legland, D. , Khadir, Z. , Urbain, A. , Palauqui, J. , & Andrey, P. (2019). Cell geometry determines symmetric and asymmetric division plane selection in Arabidopsis early embryos. PLoS Comput Biol , 15 , e1006771. 10.1371/journal.pcbi.1006771. [ PMC free article ] [ PubMed ] [ CrossRef ] [ Google Scholar ]
- Mukhtar, M. S. , Carvunis, A. R. , Dreze, M. , Epple, P. , Steinbrenner, J. , Moore, J. , Tasan, M. , Galli, M. , Hao, T. , & Nishimura, M.T. , et al. (2011). Independently evolved virulence effectors converge onto hubs in a plant immune system network . Science , 333 , 596–601. [ PMC free article ] [ PubMed ] [ Google Scholar ]
- Muller, B. , Guédon, Y. , Passot, S. , Lobet, G. , Nacry, P. , Pagès, L. , Wissuwa, M. , & Draye, X. (2019). Lateral roots: Random diversity in adversity . Trends in Plant Science , 24 , 810–825. [ PubMed ] [ Google Scholar ]
- Nakayama, N. , Smith, R. S. , Mandel, T. , Robinson, S. , Kimura, S. , Boudaoud, A. , & Kuhlemeier, C. (2012). Mechanical regulation of auxin-mediated growth . Current Biology: CB , 22 , 1468–1476. [ PubMed ] [ Google Scholar ]
- Napier, J. A. , Haslam, R. P. , Beaudoin, F. , & Cahoon, E. B. (2014). Understanding and manipulating plant lipid composition: Metabolic engineering leads the way . Current Opinion in Plant Biology , 19 , 68–75. [ PMC free article ] [ PubMed ] [ Google Scholar ]
- Nelms, B. , & Walbot, V. (2019). Defining the developmental program leading to meiosis in maize . Science , 364 , 52–56. [ PubMed ] [ Google Scholar ]
- Newlands, N. K. , Zamar, D. S. , Kouadio, L. A. , Zhang, Y. , Chipanshi, A. , Potgieter, A. , Toure, S. , & Hill, H. S. J. (2014). An integrated, probabilistic model for improved seasonal forecasting of agricultural crop yield under environmental uncertainty . Frontiers in Environmental Science , 2 . 10.3389/fenvs.2014.00017. [ CrossRef ] [ Google Scholar ]
- Newman, S. J. , & Furbank, R. T. (2021). Explainable machine learning models of major crop traits from satellite-monitored continent-wide field trial data. Plant Biology . Preprint. Retrieved from http://biorxiv.org/lookup/doi/10.1101/2021.03.08.434495 . [ PubMed ]
- Niklas, K. J. (1992). Plant biomechanics: An engineering approach to plant form and function . University of Chicago Press. [ Google Scholar ]
- Noblin, X. , Rojas, N. O. , Westbrook, J. , Llorens, C. , Argentina, M. , & Dumais, J. (2012). The fern sporangium: A unique catapult . Science , 335 , 1322. [ PubMed ] [ Google Scholar ]
- Nystedt, B. , Street, N. R. , Wetterbom, A. , Zuccolo, A. , Lin, Y.-C. , Scofield, D. G. , Vezzi, F. , Delhomme, N. , Giacomello, S. , Alexeyenko, A. , Vicedomini, R. , Sahlin, K. , Sherwood, E. , Elfstrand, M. , Gramzow, L. , Holmberg, K. , Hällman, J. , Keech, O. , Klasson, L. , & Jansson, S. (2013). The Norway spruce genome sequence and conifer genome evolution . Nature , 497 , 579–584. [ PubMed ] [ Google Scholar ]
- Ortega, J. K. E. (1985). Augmented growth equation for cell wall expansion . Plant Physiology , 79 , 318–320. [ PMC free article ] [ PubMed ] [ Google Scholar ]
- Paik, I. , & Huq, E. (2019). Plant photoreceptors: Multi-functional sensory proteins and their signaling networks . Seminars in Cell & Developmental Biology , 92 , 114–121. [ PMC free article ] [ PubMed ] [ Google Scholar ]
- Paredez, A. R. , Somerville, C. R. , & Ehrhardt, D. W. (2006). Visualization of cellulose synthase demonstrates functional association with microtubules . Science , 312 , 1491–1495. [ PubMed ] [ Google Scholar ]
- Park, K. , Knoblauch, J. , Oparka, K. , & Jensen, K. H. (2019). Controlling intercellular flow through mechanosensitive plasmodesmata nanopores . Nature Communications , 10 , 3564. [ PMC free article ] [ PubMed ] [ Google Scholar ]
- Parry, M. A. J. , Andralojc, P. J. , Scales, J. C. , Salvucci, M. E. , Carmo-Silva, A. E. , Alonso, H. , & Whitney, S. M. (2013). Rubisco activity and regulation as targets for crop improvement . Journal of Experimental Botany , 64 , 717–730. [ PubMed ] [ Google Scholar ]
- Patron, N. J. , Orzaez, D. , Marillonnet, S. , Warzecha, H. , Matthewman, C. , Youles, M. , Raitskin, O. , Leveau, A. , Farré, G. , Rogers, C. , Smith, A. , Hibberd, J. , AAR, W. , Locke, J. , Schornack, S. , Ajioka, J. , Baulcombe, D. C. , Zipfel, C. , Kamoun, S. , & Haseloff, J. (2015). Standards for plant synthetic biology: A common syntax for exchange of DNA parts . New Phytologist , 208 , 13–19. [ PubMed ] [ Google Scholar ]
- Plotnikova, A. , Kellner, M. J. , Schon, M. A. , Mosiolek, M. , & Nodine, M. D. (2019). MicroRNA dynamics and functions during Arabidopsis embryogenesis . The Plant Cell , 31 , 2929–2946. [ PMC free article ] [ PubMed ] [ Google Scholar ]
- Polanski, K. , Rhodes, J. , Hill, C. , Zhang, P. , Jenkins, D. J. , Kiddle, S. J. , Jironkin, A. , Beynon, J. , Buchanan-Wollaston, V. , Ott, S. , & Denby, K. J. (2014). Wigwams: Identifying gene modules co-regulated across multiple biological conditions . Bioinformatics , 30 , 962–970. [ PMC free article ] [ PubMed ] [ Google Scholar ]
- Possart, A. , Fleck, C. , & Hiltbrunner, A. (2014). Shedding (far-red) light on phytochrome mechanisms and responses in land plants . Plant Science , 217–218, 36–46. [ PubMed ] [ Google Scholar ]
- Proseus, T. E. , Zhu, G. , & Boyer, J. S. (2000). Turgor, temperature and the growth of plant cells: Using Chara corallina as a model system . Journal of Experimental Botany , 51 , 1481–1494. [ PubMed ] [ Google Scholar ]
- Prusinkiewicz, P. , & Hanan, J. (1989). Lindenmayer systems, fractals, and plants . Springer. [ Google Scholar ]
- Purvis, J. E. , & Lahav, G. (2013). Encoding and decoding cellular information through signaling dynamics . Cell , 152 , 945–956. [ PMC free article ] [ PubMed ] [ Google Scholar ]
- Rausenberger, J. , Hussong, A. , Kircher, S. , Kirchenbauer, D. , Timmer, J. , Nagy, F. , Schäfer, E. , & Fleck, C. (2010). An integrative model for phytochrome B mediated photomorphogenesis: From protein dynamics to physiology . PLoS One , 5 , e10721. [ PMC free article ] [ PubMed ] [ Google Scholar ]
- Rausenberger, J. , Tscheuschler, A. , Nordmeier, W. , Wüst, F. , Timmer, J. , Schäfer, E. , Fleck, C. , & Hiltbrunner, A. (2011). Photoconversion and nuclear trafficking cycles determine phytochrome A’s response profile to far-red light . Cell , 146 , 813–825. [ PubMed ] [ Google Scholar ]
- Refahi, Y. , Zardilis, A. , Michelin, G. , Wightman, R. , Leggio, B. , Legrand, J. , Faure, E. , Vachez, L. , Armezzani, A. , Risson, A.-E. , Zhao, F. , Das, P. , Prunet, N. , Meyerowitz, E. M. , Godin, C. , Malandain, G. , Jönsson, H. , & Traas, J. (2021). A multiscale analysis of early flower development in Arabidopsis provides an integrated view of molecular regulation and growth control . Developmental Cell , 56 , 540–556.e8. [ PMC free article ] [ PubMed ] [ Google Scholar ]
- Rellán-Álvarez, R. , Lobet, G. , Lindner, H. , Pradier, P.-L. , Sebastian, J. , Yee, M.-C. , Geng, Y. , Trontin, C. , LaRue, T. , Schrager-Lavelle, A. , Haney, C. H. , Nieu, R. , Maloof, J. , Vogel, J. P. , & Dinneny, J. R. (2015). GLO-roots: An imaging platform enabling multidimensional characterization of soil-grown root systems . eLife , 4 , e07597. [ PMC free article ] [ PubMed ] [ Google Scholar ]
- Romberger, J. A. , & Hejnowicz, Z. (1993). Plant structure: Function and development . Springer. [ Google Scholar ]
- Romberger, J. A. , Hejnowicz, Z. , & Hill, J. F. (1993). Plant structure: function and development . Springer Verlag , Berlin. [ Google Scholar ]
- Rose, K. E. , Rees, M. , & Grubb, P. J. (2002). Evolution in the real world: Stochastic variation and the determinants of fitness in CARLINA vulgaris . Evolution , 56 , 1416–1430. [ PubMed ] [ Google Scholar ]
- Rué, P. , Domedel-Puig, N. , Garcia-Ojalvo, J. , & Pons, A. J. (2012). Integration of cellular signals in chattering environments . Progress in Biophysics and Molecular Biology , 110 , 106–112. [ PubMed ] [ Google Scholar ]
- Rügen, M. , Bockmayr, A. , & Steuer, R. (2015). Elucidating temporal resource allocation and diurnal dynamics in phototrophic metabolism using conditional FBA . Scientific Reports , 5 , 15247. [ PMC free article ] [ PubMed ] [ Google Scholar ]
- Saxon, E. (2015). Beyond bar charts . BMC Biology , 13 , 60. [ PMC free article ] [ PubMed ] [ Google Scholar ]
- Schäfer, E. (1975). A new approach to explain the ‘high irradiance responses’ of photomorphogenesis on the basis of phytochrome . Journal of Mathematical Biology , 2 , 41–56. [ Google Scholar ]
- Schwendener, S. (1874). Das mechanische Princip in anatomischen Bau der Monocotylen: Mit vergleichenden Ausblicken auf übrigen Pflanzenklassen . Engelmann. [ Google Scholar ]
- Seaton, D. D. , Toledo-Ortiz, G. , Ganpudi, A. , Kubota, A. , Imaizumi, T. , & Halliday, K. J. (2018). Dawn and photoperiod sensing by phytochrome A . PNAS , 115 , 10523–10528. [ PMC free article ] [ PubMed ] [ Google Scholar ]
- Silk, W. K. (1984). Quantitative descriptions of development . Annual Review of Plant Physiology , 35 , 479–518. [ Google Scholar ]
- Tang, H. , Duijts, K. , Bezanilla, M. , Scheres, B. , Vermeer, J. E. M. , & Willemsen, V. (2020). Geometric cues forecast the switch from two- to three-dimensional growth in Physcomitrella patens . New Phytologist , 225 , 1945–1955. [ PMC free article ] [ PubMed ] [ Google Scholar ]
- Topham, A. T. , Taylor, R. E. , Yan, D. , Nambara, E. , Johnston, I. G. , & Bassel, G. W. (2017). Temperature variability is integrated by a spatially embedded decision-making center to break dormancy in Arabidopsis seeds . Proceedings of the National Academy of Sciences , 114 , 6629–6634. [ PMC free article ] [ PubMed ] [ Google Scholar ]
- Toyota, M. , Spencer, D. , Sawai-Toyota, S. , & Gilroy, S. (2018). Glutamate triggers long-distance, calcium-based plant defense signaling . Science , 361 , 1112–1115. [ PubMed ] [ Google Scholar ]
- Traas, J. , Bellini, C. , Nacry, P. , Kronenberger, J. , Bouchez, D. , & Caboche, M. (1995). Normal differentiation patterns in plants lacking microtubular preprophase bands . Nature , 375 , 676–677. [ Google Scholar ]
- Trewavas, A. (2012). Information, noise and communication: thresholds as controlling elements in development. In Biocommunication of plants , 11–35. Springer, Berlin, Heidelberg. [ Google Scholar ]
- Trinh, D.-C. , Alonso-Serra, J. , Asaoka, M. , Colin, L. , Cortes, M. , Malivert, A. , Takatani, S. , Zhao, F. , Traas, J. , Trehin, C. , & Hamant, O. (2021). How mechanical forces shape plant organs . Current Biology: CB , 31 , R143–R159. [ PubMed ] [ Google Scholar ]
- Tsimring, L. S. (2014). Noise in biology . Reports on Progress in Physics. Physical Society (Great Britain) , 77 , 026601. [ PMC free article ] [ PubMed ] [ Google Scholar ]
- Tsuda, K. , & Katagiri, F. (2010). Comparing signaling mechanisms engaged in pattern-triggered and effector-triggered immunity . Curr Opin Plant Biol , 13 , 459–465. [ PubMed ] [ Google Scholar ]
- Uyttewaal, M. , Burian, A. , Alim, K. , Landrein, B. , Borowska-Wykręt, D. , Dedieu, A. , Peaucelle, A. , Ludynia, M. , Traas, J. , Boudaoud, A. , Kwiatkowska, D. , & Hamant, O. (2012). Mechanical stress acts via katanin to amplify differences in growth rate between adjacent cells in Arabidopsis . Cell , 149 , 439–451. [ PubMed ] [ Google Scholar ]
- Vermeer, J. E. M. , von Wangenheim, D. , Barberon, M. , Lee, Y. , EHK, S. , Maizel, A. , & Geldner, N. (2014). A spatial accommodation by neighboring cells is required for organ initiation in Arabidopsis . Science , 343 , 178–183. [ PubMed ] [ Google Scholar ]
- Verna, Carla. , Sree, Janani Ravichandran , Sawchuk, Megan G. , Nguyen, Manh Linh & Enrico, Scarpella , (2019). Coordination of tissue cell polarity by auxin transport and signaling Elife, 8, e51061. 10.7554/eLife.51061. [ PMC free article ] [ PubMed ] [ CrossRef ]
- Vilches Barro, A. , Stöckle, D. , Thellmann, M. , Ruiz-Duarte, P. , Bald, L. , Louveaux, M. , von Born, P. , Denninger, P. , Goh, T. , Fukaki, H. , Vermeer, J. E. M. , & Maizel, A. (2019). Cytoskeleton dynamics are necessary for early events of lateral root initiation in Arabidopsis . Current Biology: CB , 29 , 2443–2454.e5. [ PubMed ] [ Google Scholar ]
- von Wangenheim, D. , Fangerau, J. , Schmitz, A. , Smith, R. S. , Leitte, H. , EHK, S. , & Maizel, A. (2016). Rules and self-organizing properties of post-embryonic plant organ cell division patterns . Current Biology: CB , 26 , 439–449. [ PubMed ] [ Google Scholar ]
- Waddington, C. H. (1942). Canalization of development and the inheritance of acquired characters . Nature , 150 , 563–565. [ Google Scholar ]
- Walker, L. , Boddington, C. , Jenkins, D. , Wang, Y. , Grønlund, J. T. , Hulsmans, J. , Kumar, S. , Patel, D. , Moore, J. D. , Carter, A. , Samavedam, S. , Bonomo, G. , Hersh, D. S. , Coruzzi, G. M. , Burroughs, N. J. , & Gifford, M. L. (2017). Changes in gene expression in space and time orchestrate environmentally mediated shaping of root architecture . The Plant Cell , 29 , 2393–2412. [ PMC free article ] [ PubMed ] [ Google Scholar ]
- Wang, A. X. , Tran, C. , Desai, N. , Lobell, D. , & Ermon, S. (2018). Deep transfer learning for crop yield prediction with remote sensing data. In, Proceedings of the 1st ACM SIGCAS Conference on Computing and Sustainable Societies (pp. 1–5). Association for Computing Machinery. [ Google Scholar ]
- Wang, J. , Tian, L. , Madlung, A. , Lee, H.-S. , Chen, M. , Lee, J. J. , Watson, B. , Kagochi, T. , Comai, L. , & Chen, Z. J. (2004). Stochastic and epigenetic changes of gene expression in Arabidopsis polyploids . Genetics , 167 , 1961–1973. [ PMC free article ] [ PubMed ] [ Google Scholar ]
- Wang, G. , Roux, B. , Feng, F. , Guy, E. , Li, L. , Li, N. , Zhang, X. , Lautier, M. , Jardinaud, M. F. , & Chabannes, M. , et al. (2015). The Decoy Substrate of a PathogenEffector and a Pseudokinase Specify Pathogen-Induced Modified-Self Recognition and Immunity in Plants . Cell Host Microbe , 18 , 285–295. [ PubMed ] [ Google Scholar ]
- Wang, P. , Vlad, D. , & Langdale, J. A. (2016). Finding the genes to build C4 rice . Current Opinion in Plant Biology , 31 , 44–50. [ PubMed ] [ Google Scholar ]
- Wang, X. , Ye, L. , Lyu, M. , Ursache, R. , Löytynoja, A. , & Mähönen, A. P. (2020). An inducible genome editing system for plants . Nature Plants , 6 , 766–772. [ PMC free article ] [ PubMed ] [ Google Scholar ]
- Wessling, R. , Epple, P. , Altmann, S. , He, Y. , Yang, L. , Henz, S. R. , McDonald, N. , Wiley, K. , Bader, K. C. , & Glasser, C. , et al. (2014). Convergent targeting of acommon host protein-network by pathogen effectors from three kingdoms of life . Cell Host Microb ,e 16, 364–375. [ PMC free article ] [ PubMed ] [ Google Scholar ]
- Wasteneys, G. O. , & Ambrose, J. C. (2009). Spatial organization of plant cortical microtubules: Close encounters of the 2D kind . Trends in Cell Biology , 19 . 10.1016/j.tcb.2008.11.004. [ PubMed ] [ CrossRef ] [ Google Scholar ]
- Wenden, B. , Kozma-Bognár, L. , Edwards, K. D. , Hall, A. J. W. , Locke, J. C. W. , & Millar, A. J. (2011). Light inputs shape the Arabidopsis circadian system . The Plant Journal: For Cell and Molecular Biology , 66 , 480–491. 10.1111/j.1365-313X.2011.04505.x. [ PubMed ] [ CrossRef ] [ Google Scholar ]
- Wigge, P. A. (2013). Ambient temperature signalling in plants . Current Opinion in Plant Biology , 16 , 661–666. [ PubMed ] [ Google Scholar ]
- Willis, L. , Refahi, Y. , Wightman, R. , Landrein, B. , Teles, J. , Huang, K. C. , Meyerowitz, E. M. , & Jönsson, H. (2016). Cell size and growth regulation in the Arabidopsis thaliana apical stem cell niche . Proceedings of the National Academy of Sciences of the United States of America , 113 , E8238–E8246. [ PMC free article ] [ PubMed ] [ Google Scholar ]
- Williams, S.J. , Sohn, K.H. , Wan, L. , Bernoux, M. , Sarris, P.F. , Segonzac, C. , Ve, T. , Ma, Y. , Saucet, S.B. , & Ericsson, D.J. , et al. (2014). Structural basis for assembly and function of a heterodimeric plant immune receptor . Science , 344 , 299–303. [ PubMed ] [ Google Scholar ]
- Wintle, B. C. , Boehm, C. R. , Rhodes, C. , Molloy, J. C. , Millett, P. , Adam, L. , Breitling, R. , Carlson, R. , Casagrande, R. , Dando, M. , Doubleday, R. , Drexler, E. , Edwards, B. , Ellis, T. , Evans, N. G. , Hammond, R. , Haseloff, J. , Kahl, L. , Kuiken, T. , & Sutherland, W. J. (2017). A transatlantic perspective on 20 emerging issues in biological engineering . eLife , 6 , e30247. [ PMC free article ] [ PubMed ] [ Google Scholar ]
- Wolanin, A. , Mateo-García, G. , Camps-Valls, G. , Gómez-Chova, L. , Meroni, M. , Duveiller, G. , Liangzhi, Y. , & Guanter, L. (2020). Estimating and understanding crop yields with explainable deep learning in the Indian Wheat Belt . Environmental Research Letters , 15 , 024019. [ Google Scholar ]
- Wolny, A. , Cerrone, L. , Vijayan, A. , Tofanelli, R. , Barro, A. V. , Louveaux, M. , Wenzl, C. , Strauss, S. , Wilson-Sánchez, D. , Lymbouridou, R. , Steigleder, S. , Pape, C. , Bailoni, A. , Duran-Nebreda, S. , Bassel, G. W. , Lohmann, J. U. , Tsiantis, M. , Hamprecht, F. A. , Schneitz, K. , Maizel, A. , et al. (2020). Accurate and versatile 3D segmentation of plant tissues at cellular resolution. Elife . 29 ; 9 :e57613. [ PMC free article ] [ PubMed ] [ Google Scholar ]
- Woolfenden, H.C. , Bourdais, G. , Kopischke, M. , Miedes, E. , Molina, A. , Robatzek, S. , & Morris, R. J. (2017). A computational approach for inferring the cell wall properties that govern guard cell dynamics. Plant J , 92 , 5–18. 10.1111/tpj.13640. [ PMC free article ] [ PubMed ] [ CrossRef ] [ Google Scholar ]
- Wu, J. , Albert, L. P. , Lopes, A. P. , Restrepo-Coupe, N. , Hayek, M. , Wiedemann, K. T. , Guan, K. , Stark, S. C. , Christoffersen, B. , Prohaska, N. , Tavares, J. V. , Marostica, S. , Kobayashi, H. , Ferreira, M. L. , Campos, K. S. , da Silva, R. , Brando, P. M. , Dye, D. G. , Huxman, T. E. , & Saleska, S. R. (2016). Leaf development and demography explain photosynthetic seasonality in Amazon evergreen forests . Science , 351 , 972–976. [ PubMed ] [ Google Scholar ]
- Yoshida, S. , Barbier de Reuille, P. , Lane, B. , Bassel, G. W. , Prusinkiewicz, P. , Smith, R. S. , & Weijers, D. (2014). Genetic control of plant development by overriding a geometric division rule . Developmental Cell , 29 , 75–87. [ PubMed ] [ Google Scholar ]
- Zhao, P. , Begcy, K. , Dresselhaus, T. , & Sun, M.-X. (2017). Does early embryogenesis in eudicots and monocots involve the same mechanism and molecular players? Plant Physiology , 173 , 130–142. [ PMC free article ] [ PubMed ] [ Google Scholar ]
- Zhao, F. , Du, F. , Oliveri, H. , Zhou, L. , Ali, O. , Chen, W. , Feng, S. , Wang, Q. , Lü, S. , Long, M. , Schneider, R. , Sampathkumar, A. , Godin, C. , Traas, J. , & Jiao, Y. (2020). Microtubule-Mediated Wall Anisotropy Contributes to Leaf Blade Flattening . Curr Biol , 30 , 3972–3985.e6. 10.1016/j.cub.2020.07.076. [ PMC free article ] [ PubMed ] [ CrossRef ] [ Google Scholar ]
- Zhou, L. , Du, F. , Feng, S. , Hu, J. , Lü, S. , Long, M. , & Jiao, Y. (2020). Epidermal restriction confers robustness to organ shapes . Journal of Integrative Plant Biology , 62 , 1853–1867. [ PubMed ] [ Google Scholar ]
- Ziliak, S. , & McCloskey, D.N. (2008). The cult of statistical significance: How the standard error costs us jobs, justice, and lives. University of Michigan Press .
Author comment: What is quantitative plant biology? — R0/PR1
RDP, ENS de Lyon, France
Dear Enrico,
Here is our opinion piece on the definition of Quantitative Plant Biology. Although most of the editorial board is involved, we still need to have external reviewers for this. Reviewers may simply give their opinion on areas we should have covered or important things we have missed or shortening suggestions (it’s quite long!).
Best wishes,
Review: What is quantitative plant biology? — R0/PR2
Elliot meyerowitz.
Biology and Biological Engineering, California Institute of Technology, United States
Comments to Author : This is a comprehensive review covering the remarkable array of research areas in plant biology that have and can in the future benefit from a quantitative approach. As such it is an invitation for paper submissions to the new journal for which it is written, and makes clear that quantitative plant biology covers a great diversity of research areas and approaches, papers from all of which are apparently invited.
The review also makes clear that what is meant by quantitative in this context is diverse, less by explicit discussion than by using the term “quantitative” to describe approaches to research to mean a multiplicity of different things. One is measurements of outputs such as gene expression levels, organ and cell shapes, growth rates, mutation and allele frequency changes, and much more. Another meaning given to quantitative in the review is the use of mathematical approaches to hypothesis testing, such as construction of differential equation models to simulate experimental outputs based on varied parameter changes. Yet another meaning is statistical evaluation of measurement variability, stochastic outcomes, and experimental significance. It is a fact that all of these are quantitative, or at least mathematical, as they involve numbers or equations – but they are very different things. All are apparently invited to the new journal, but perhaps it would be worth being explicit, early in the article, about what is meant by “quantitative approaches” to better organize the review, and to increase clarity about what exactly is meant by quantitative plant biology.
In this context, perhaps the authors could add a box, that lists some of the many possibilities discussed (and not discussed), so as not to interrupt the flow of text? In my mind I see a matrix with organization level (molecular, cellular, tissue, organismal, ecological) on one axis, and valid quantitative approaches (measurements of gene expression, protein levels and activities, shape, growth, image segmentation, mechanical properties, environmental inputs such as light intensity and spectrum, temperature, nutrition, pathogens…); modeling (differential equation, Boolean, Cellular Potts, omics, network analysis…); statistical analysis (of noise, variation, significance…) with possible examples or citations in each square, but I’m not sure this would be the best way to show the broad range of areas under consideration (and it may not be a finite exercise) – what, if anything, to do is up to the authors.
Another thing that could be added to the review is a bit more about why quantitative approaches are important or useful. The authors say that a quantitative approach “allows us to question and better understand our interactions with plants” and that a quantitative approach “serves as a framework to understand plant complexity and provides a systemic view of plant life.” I think they could go farther than this, and point out that quantitation leads to looking at and modeling correlations between different measurements, which is the way to form new hypotheses. Statistical approaches measure the strength of the correlations. Modeling then allows a preliminary test of the hypothesis in silico, with predictions tested again in experiments with quantitative results. While all along a statistical analysis assesses likelihood that the tests are meaningful, and allows measurements of noise and robustness. This is what a quantitative approach in the multiple senses used in this review means – an iterative approach of measurement, correlation, hypothesis, testing in silico and in vivo, back to the start. This approach applies at all levels of organization, from atomic to ecosystem.
A review should end with a bold proposal. This one does – the founding of a new journal, and a new transdisciplinary field of research, involving scientists and non-scientists. I think it may be worth expounding a bit more on this so as to emphasize what is being proposed, and to use the opportunity to address this new field to say even more about what types of explicit coordination between disciplines will lead to this new field, and what community resources will be necessary for success. Genomics needs stock centers, for example: does this new field need special code repositories, coordination on file specifications (like SBML did for other areas of biology), stock centers of synthetic biology parts, databases of images, shared software for image storage and analysis? Are new standards or types of paper review needed [and will we finally be able to see papers where the main text is the modeling, and the experiments only in the supplementary material?].
The proposal for this new field could go farther than a journal, to a recommendation (for scientists as well as their funding agencies) for a coordinated approach that will create the revolution in plant science that is necessary not only to understand plants, but to understand the principles of living things by comparison of plants to other kingdoms, and to use plants to ameliorate the environment and the human condition.
A few specific comments: first, a request for some light editing to align the text with English grammatical rules such as agreement of plurals and such – always an issue for an article with many authors of many different native languages, and something that perhaps the journal can provide.
In the abstract and again at the bottom of page 11, the term “systemic” is used, and I do not know what is meant by it in either context.
In the middle of page 6, I don’t see what is “counterintuitive” about losing a cellular response pathway when the signaling mechanism is abrogated.
At the top of page 11 it is stated that chemical signals between plant cells “typically move between cells through symplastic channels called plasmodesmata or via efflux
and influx transporters.” The typical signal is in fact a secreted peptide sensed by a transmembrane receptor kinase (of which there are many more examples known than of plasmodesmatal signals or those that require transporters). The peptide mode should be mentioned here with the others (it already is discussed earlier in the article).
At the bottom of page 15 the standard botanist’s description of animal development being complete at maturity really isn’t true for many animals, including for example tape worms, which constantly create new segments behind the scolex, just like a shoot meristem; or some annelids, which generate new segments throughout their lives from a posterior pygidium, just like a root meristem. There are even two species of worms (Ramisyllus multicaudata and Syllis ramosa) that branch like plants, from lateral buds. They reproduce from stolons at the end of the branches. The statement in the review can be fixed easily enough by just writing “many animals.” And I’ve had a bit of fun describing some of the exceptions!
At the top of page 26 there is a note to complete a sentence – “see sections of
XX/link to the other sites” – that needs to be dealt with.
The second line of page 30 has the phrase “fait science” – what does this mean?
Review: What is quantitative plant biology? — R0/PR3
Comments to Author : This is an excellent review. I have made some suggestions directly in the PDF. What i could recommend is perhaps a paragraph on education required to get more scientists/students into quantitative biology. One reason why quantitative biology has not picked up is current education often does encourage courses that incorporate this aspect. Something akin to phage biology course in Cold spring harbor I think which introduced biology to a lot of physicists and laid a ground work for molecular biology would be useful and commented on?
Recommendation: What is quantitative plant biology? — R0/PR4
Comments to Author : Dear Dr. Hamant,
Thank you for submitting your manuscript to Quantitative Plant Biology. I read with great interest your work and the reviewers’ comments. Reviewer 2 had very few and very minor editorial suggestions; I leave to you whether they should be addressed. By contrast, Reviewer 1 offers very valuable insights on how to improve significantly the manuscript; I believe addressing those comments will greatly advance its impact. I would therefore like to ask you to take those suggestions into full consideration while preparing a revised manuscript.
At submission, please upload in addition to the revised manuscript:
(1) A point-by-point response to the reviewers’ comments; please respond to all comments: if you disagree with some of them, please explain why that is so, instead of ignoring them.
(2) A version of your manuscript in which the changes made are clearly visible (e.g., a PDF of a DOC(X) file in which the changes made had been tracked with the "Track Changes" option).
I look forward to receiving your revised manuscript soon.
Sincerely yours,
Dr. Enrico Scarpella
Associate Editor
Quantitative Plant Biology
Decision: What is quantitative plant biology? — R0/PR5
No accompanying comment.
Author comment: What is quantitative plant biology? — R1/PR6
Review: what is quantitative plant biology — r1/pr7.
Comments to Author : All of my earlier questions have been answered, and suggestions considered (and mostly taken). Two examples of the word "systemic" have survived from the previous version (Abstract line 2, and near the bottom of page 9. In both cases I think what is meant is "system-wide," but I am not entirely certain. I like the review very much - it is comprehensive (even encyclopedic) and serves as a broad-based introduction to the newly enlarged field, and the new journal. It is time to publish it!
Recommendation: What is quantitative plant biology? — R1/PR8
Comments to Author : Dear Dr. Hamant,
After reading your revised manuscript and your response to Reviewer 1’s comments, I am pleased to accept your manuscript for publication in Quantitative Plant Biology. My only suggestion would be that, at the proof editing stage, of clarifying the two instances of the unclear use of the word "systemic" Reviewer 1 refers to. Other than that, congratulations!
Yours sincerely,
Dr. Enrico Scarpella
Associate Editor
Decision: What is quantitative plant biology? — R1/PR9
Author comment: what is quantitative plant biology — r2/pr10.
This is a unique review on quantitative plant biology, much longer than the regular format in the journal. It should play its integrative role for this community, and may even be viewed as a written conference report on the past two decade work in this emerging field.
Recommendation: What is quantitative plant biology? — R2/PR11
Decision: what is quantitative plant biology — r2/pr12.
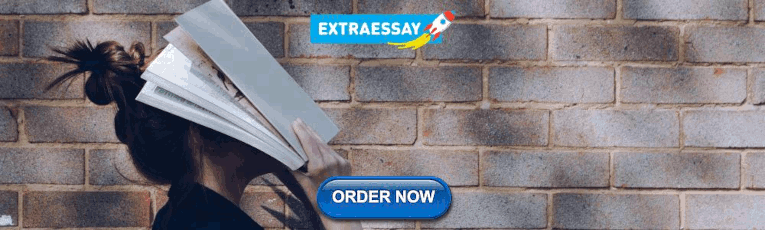
IMAGES
VIDEO
COMMENTS
Molecular Biology Research Topics For Undergraduates. 31. Studying the structure and function of DNA and RNA molecules. 32. Analyzing the regulation of gene expression in eukaryotic cells. 33. Investigating the mechanisms of DNA replication and repair. 34. Studying the role of non-coding RNAs in gene regulation.
Research Topics in Biology for Undergraduates. 41. Investigating the effects of pollutants on local plant species. Microbial diversity and ecosystem functioning in a specific habitat. Understanding the genetics of antibiotic resistance in bacteria. Impact of urbanization on bird populations and biodiversity. Investigating the role of pheromones ...
A List of Researchable Topics for Biology. A list of researchable topics for biology students starts with several interesting biological topics concerning sociological perspective and ethical issues. The most debatable subjects are abortion, human cloning, genetic researches and the new ethics that should be created to resolve these issues.
Research Area/ Research Interest: Quantitative Biology. Research Paper Topics for Masters and Ph.D. Thesis and publication. 1. The impact of integrated STEM modeling on elementary preservice teachers' self‐efficacy for integrated STEM instruction: A co‐teaching approach. 2.
Quantitative biology is a trending area in modern biology. It emphasizes quantitative approaches, such as data quantification, calculation, or quantitative modeling. Because biology, of course, is a branch of the natural sciences, it is hard to imagine a non-quantitative form of biology.
Some biology topics are easier for non-biologists to get more excited about than others, though. Whether you're looking for a research topic for a college paper or an area to specialize in if you're majoring in biology, here are some of the most interesting things going on in the biology world right now. 1. CRISPR and Genetic Engineering
About the Journal. Quantitative Biology (QB) is an open-access journal published quarterly with no page charges. Contributions may include cutting-edge research, reviews, methods, software, protocol and tutorial, science news, commentaries, and perspectives. All submitted manuscripts will be peer-reviewed before being considered for acceptance ...
Abstract. Quantitative methods and approaches have been playing an increasingly important role in cell biology in recent years. They involve making accurate measurements to test a predefined hypothesis in order to compare experimental data with predictions generated by theoretical models, an approach that has benefited physicists for decades.
This research topic is organized on two aspects of technological advances which are the backbone of Quantitative Biology: (i) visualization of biomolecules, their dynamics and function, and (ii) generic technologies of model optimization and numeric integration.
Quantitative Biology is a new approach to understand how biological systems are controlled and this has been backboned by recent advances in measuring, mathematical modeling and conditionally perturbing the dynamics of physiological processes. In this Research Topic, we focus on the following points: *Theory, imaging and analyses for exploring the mesoscopic world *Power of engineering in new ...
Towards quantitative cell biology. Cell biologists must decide whether to embrace the maturing field of systems biology. We argue that a fusion of the two is urgently needed to strengthen both ...
She is the winner of the 2015 Stony Brook Fiction Prize, and her short stories have been published in Mid-American Review, Cutbank, Sonora Review, New Orleans Review, and The Collagist, among other magazines. The 49 most interest biology research topics are explored. We also offer links to variety of resources for research topics in biology.
Quantitative plant biology is an interdisciplinary field that builds on a long history of biomathematics and biophysics. Today, thanks to high spatiotemporal resolution tools and computational modelling, it sets a new standard in plant science. ... Examples of research topics at the crossroad between plant biology, physics and maths ...
Quantitative research involves collecting and analyzing numerical data to identify patterns, trends, and relationships among variables. This method is widely used in social sciences, psychology, economics, and other fields where researchers aim to understand human behavior and phenomena through statistical analysis. If you are looking for a quantitative research topic, there are numerous areas ...
You can either write an essay or paper with the following interesting biology research topics: Human emotions and conflicts with their intellectual intelligence. Emotions: Its influence on art and music and how the perception of art influences the world. The consequences of marijuana and alcohol on teenagers.
To make things as simple as possible for you, we've put together a list of biology research project ideas. You will find 100 topics on various subjects below. Of course, you can use any of our topics for free. However, keep in mind that even though we are doing our best to maintain this list fresh, other students will find it as well.
An example of quantitative research topics for 12 th -grade students will come in handy if you want to score a good grade. Here are some of the best ones: The link between global warming and climate change. What is the greenhouse gas impact on biodiversity and the atmosphere.
Biology is a vast field of study that explores the diverse aspects of life, from the smallest organisms to the complex ecosystems they inhabit. With new discoveries being made every day, the field of biology is constantly evolving and expanding. As a result, there are numerous research topics within biology that can capture the imagination of students, researchers, and professionals alike.
Quantitative research is the opposite of qualitative research, which involves collecting and analyzing non-numerical data (e.g., text, video, or audio). Quantitative research is widely used in the natural and social sciences: biology, chemistry, psychology, economics, sociology, marketing, etc. Quantitative research question examples
Quantitative Systems Pharmacology (QSP) has emerged as the convolution of four distinct areas: a) systems biology, which focuses on modeling the dynamics of molecular and cellular mechanisms and networks;b) systems pharmacology, which aims at incorporating links between therapeutic interventions and drug mechanisms; c) systems physiology, which describes disease mechanisms driving the dynamics ...
Major research topics in the School of Biological Sciences
In the world of research, there are two general approaches to gathering and reporting information: qualitative and quantitative approaches. Qualitative research generates non-numerical data while quantitative research generates numerical data or information that can be converted into numbers.
Quantitative plant biology is an interdisciplinary field that builds on a long history of biomathematics and biophysics. Today, thanks to high spatiotemporal resolution tools and computational modelling, it sets a new standard in plant science. ... Examples of research topics at the crossroad between plant biology, physics and maths ...