Centers & Institutes
The following research centers and institutes are under the organizational structure of the Office of the Vice President for Research. They are non-degree granting and multidisciplinary in scope.
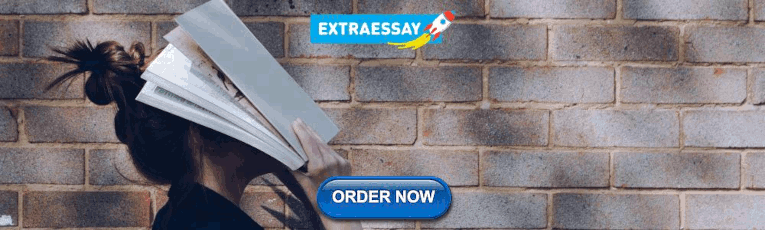
Center for Applied Energy Research (CAER)
Staffed by more than 100 chemists, geologists and engineers, CAER is internationally recognized for research in fields including algae for CO2 clean up, ash management, automotive catalysis, biodiesel, biomass, carbon materials (including nanotube synthesis and applications), catalysis (including Fischer-Tropsch), coal cleaning, concrete and cement (low energy), emissions control in utilities, energy policy, fuels research, hydrogen, materials characterization, petroleum processing and plant optimization. The center is funded by state, industry and federal agencies.
Center for Clinical and Translational Science (CCTS)
The CCTS brings together clinicians, researchers, and communities to accelerate the translation of basic science discoveries to tangible improvements in health. With support from a Clinical and Translational Science Award from NIH, the CCTS fosters innovative team science across multiple campuses and states. The center provides infrastructure, funding and research support services, and trains the next generation of clinical and translational researchers. The CCTS focuses on addressing chronic health disparities in rural and underserved populations throughout the Central Appalachian region.
Center for Computational Sciences
The center works to enhance the success of UK researchers and collaborators whose work will benefit from research computing solutions. Its goals are to increase publications and research funding linked to research computing and increase access to specialized computational resources (hardware, software applications, computational methodologies, collaborative relationships, and people/expertise). Major research projects, funded by NSF, include ParaChem and the Kentucky Virtual Observatory and Ecological Informatics System.
Center for Health Services Research (CHSR)
The Center for Health Services Research (CHSR) serves as a focal point for health services research across the University of Kentucky campus. By bringing together faculty, staff, and students across disciplines and colleges, we facilitate research on effective ways to organize, finance, and deliver high-quality health care.
Center for Research on Violence Against Women (CRVAW)
Center for structural biology.
The center provides services and equipment for protein production and characterization to the UK research community. This includes equipment for bacterial, yeast, insect and mammalian cell culture, devices for cell-cracking and automated chromatography systems for protein and peptide purification, analytical centrifugation, spectroscopic instrumentation (circular dichroism, dynamic light scattering, fluorometers), a TIRF illuminated microscope for single molecule and cellular fluorescence experiments and state-of-the-art instruments for protein crystal growth and collection of X-ray data.
Center for the Environment
The UK Center for Environment is a multidisciplinary research center and institution-wide educational unit. The center recognizes that the environmental challenges faced by the Commonwealth, our nation and the world are complex and require multidisciplinary collaboration from across academic, government, and private sectors. As a land-grant institution, UK was built on the premise that the environment is integral to civilization and critical in our three-fold mission of teaching, research, and community partnerships.
Center of Membrane Sciences
The center fosters research on biological and synthetic membranes and their interface, provides scientific and technological leadership and facilities to develop basic research and to promote partnerships fundamental to knowledge and technology transfer. State-of-the-art equipment for membrane research includes hollow-fiber spinning and continuous sheet casting facilities, high-field NMR, ESR, and fluorescence spectrometers, gamma camera, neutron sources, ATR-FTIR, TRIM, micropipette aspiration, adhesion measurements, fluorescence microscopes, computer-controlled HPLCs, pore-volume analyzers, computer-controlled fermenter, polymer dielectric relaxation and dynamics equipment.
Human Development Institute (HDI)
With federal and state funding, HDI focuses on interdisciplinary education, research and development, information sharing, advocacy and outreach. HDI conducts research, development, and model demonstration efforts to continually improve state-of-the-art best practices. HDI staff provide outreach services and consultation to agency staff to identify and resolve the challenges involved in promoting and achieving independence, productivity and inclusion of persons with disabilities throughout life.
Institute for Biomedical Informatics (IBI)
The Institute for Biomedical Informatics (IBI) facilitates data-intensive, multidisciplinary team science to improve the health of patients and populations, in Kentucky and beyond. IBI provides services through the Center for Clinical and Translational Science's Biomedical Informatics Core.
Kentucky Geological Survey (KGS)
As the official geologic research organization for the Commonwealth of Kentucky, KGS is focused on research in earth resources and processes, public service, and the dissemination of data, knowledge, and experience to stakeholders in industry, government, educational institutions, and the general public. With 48 full-time staff, KGS is increasing knowledge and understanding of the mineral, energy and water resources, geologic hazards, and geology of Kentucky for the benefit of the Commonwealth of Kentucky and the nation.
Kentucky Water Research Institute (KWRI)
The institute focuses on water resources and water-related environmental issues important to the state of Kentucky. In keeping with its statewide mission, the KWRI works to foster research collaborations between faculty and research staff at UK, as well as faculty and research staff affiliated with the various regional universities across the state. With a mission that encompasses research, education, training and technology transfer, the institute works with federal and state agencies.
Markey Cancer Center
In July 2013, Markey was designated by the National Cancer Institute (NCI) to receive research funding and many other opportunities available only to the nation’s best cancer centers. Markey is organized into four thematic research programs (Cancer Cell Biology and Signaling; Cancer Prevention and Control; Drug Discovery, Delivery and Translational Therapeutics; and Redox Injury and Repair) and has five established shared resource facilities (Biospecimen and Tissue Procurement; Biostatistics and Bioinformatics; Cancer Research Informatics; Flow Cytometry and Cell Sorting; and Free Radical Biology in Cancer).
Tracy Farmer Institute for Sustainability and the Environment (ISE)
The institute’s mission is to enhance the health and well being of Kentuckians and the global community by improving built, managed and natural environments. This involves promoting sustainable development, sound energy policies, and effective environmental stewardship through an integrated transdisciplinary program of fundamental and applied research in the physical, life, economic, and social sciences through education, as well as public service and stakeholder engagement. The research agenda of the institute is supported, developed and implemented by a group of 150 faculty representing 14 colleges.
Other Research Centers & Institutes
- Agricultural Experiment Station
- Advanced Science and Technology Commercialization Center (ASTeCC)
- African American and Africana Studies (AAAS)
- Appalachian Center & Appalachian Studies
- Area Health Education Centers (AHEC)
- Barnstable Brown Kentucky Diabetes and Obesity Center
- Center for Advanced Training & Simulation (CATS)
- Center for Advanced Translational Stroke Science (CATSS)
- Center for Aluminum Technology (CAT)
- Center for Appalachian Research in Environmental Sciences (UK-CARES)
- Center for Business and Economic Research (CBER)
- Center for Cancer and Metabolism (COBRE)
- Center for Microelectrode Technology (CenMeT)
- Center for Molecular Medicine (COBRE)
- Center for Muscle Biology
- Center for Nanoscale Science and Engineering (CeNSE)
- Center for Obesity and Cardiovascular Disease (COCVD) COBRE
- Center for Oral Health Research
- Center for Innovation in Population Health
- Center for Poverty Research (UKCPR)
- Center for Rural Development
- Center for Visualization and Virtual Environments (CVVE)
- Center of Biomedical Research Excellence (COBRE) in Women's Health
- Center of Excellence in Rural Health
- Center on Drug and Alcohol Research (CDAR)
- Center on Trauma and Children (CTAC)
- Community and Economic Development Initiative of Kentucky (CEDIK)
- Donald T. Frazier Science Outreach Center
- Gill Heart Institute
- Gluck Equine Research Center
- Graduate Center for Biomedical Engineering
- Graduate Center for Nutritional Sciences
- Graduate Center for Toxicology
- Institute for Biomedical Informatics
- Institute for Educational Research
- Institute for Rural Journalism and Community Issues
- Institute for Sustainable Manufacturing
- Institute of Research for Technology Development (IR4TD)
- John Jacob Niles Center for American Music
- Kentucky Center for School Safety
- Kentucky Center for Smoke-free Policy
- Kentucky Injury Prevention and Research Center (KIPRC)
- Kentucky Neuroscience Institute (KNI)
- Kentucky Research Data Center
- Kentucky Tobacco Research and Development Center (KTRDC)
- Kentucky Transportation Center
- LINKS Center for Social Network Analysis
- Martin School of Public Policy and Administration
- Morris K. Udall Parkinson's Disease Research Center of Excellence
- Saha Cardiovascular Research Center
- Sanders-Brown Center on Aging
- Southeast Center for Agricultural Health & Injury Prevention
- Spinal Cord & Brain Injury Research Center
- Sports Medicine Research Institute (SMRI)
- Superfund Research Center (SRC)
- Training Resource Center (Social Work)
- Ultrasound Core (Saha Cardiovascular Research Center)
- Veterinary Diagnostic Laboratory (VDL)
- Vibro-Acoustics Consortium
Alzheimer's Disease Research Center
An integral part of Sanders-Brown Center on Aging (SBCoA) is the National Institute on Aging-funded University of Kentucky Alzheimer's Disease Research Center (UK-ADRC). Over the past 35 years, the UK-ADRC has developed a vigorous program in the clinical, neuropathological, educational, and research aspects of Alzheimer's disease that serves as a critical resource for the university, community, state, and nation.
September 2022 — A team of researchers at the University of Kentucky’s Sanders-Brown Center on Aging (SBCoA) is awarded a $20.5 million grant from the National Institute on Aging (NIA) of the National Institutes of Health (NIH) to continue and further research and clinical initiatives geared toward beating Alzheimer’s disease and other forms of dementia. Currently, only 33 designated Alzheimer's Disease Research Centers exist in the U.S. In 1985, SBCoA was among the first 10 ADCs funded by the NIH and has been continuously funded since the designation was launched.
The University of Kentucky Alzheimer’s Disease Research Center (UK-ADRC) is a NIH-funded (P30 AG072946) mature and experienced ADRC. The UK-ADRC is housed in the Sanders-Brown Center on Aging (SBCoA), a Kentucky Center of Excellence that facilitates clinical and basic research in healthy brain aging and neurodegenerative disorders.
Our principal mission is to serve as the focal point for all Alzheimer’s disease-related activities at UK and the Commonwealth of Kentucky, by providing an environment and core resources that catalyze innovative research, outreach, education, and clinical programs.
Over the past 35 years, we have developed a vigorous program in the clinical, neuropathological, educational, and research aspects of Alzheimer’s disease that serves as a critical resource for the university, community, state, and nation.
Two of the historically outstanding facets of the UK-ADRC are:
Autopsy Program
A strong autopsy program providing clinical-neuropathological correlation and short postmortem interval (PMI) research material.
Data / Subjects
A unique, continuously replenished group of ~500 cognitively intact subjects followed longitudinally, together with the initially normal who transition to MCI or Alzheimer’s disease, and all committed to brain donation upon death.
These signature resources have contributed to our becoming one of the premier center’s defining pathogenic mechanisms underlying the transitions from normal cognitive aging to Alzheimer’s disease.
The UK-ADRC focus is on two interrelated themes: transitions and translation. Our overall emphasis is to more effectively bridge the gap between basic research and clinical studies by facilitating translational efforts. We will also carefully characterize transitions across the spectrum of cognitive impairment (normal/preclinical Alzheimer’s disease/ MCI/ dementia), with focus on definition of early disease.
The ADRC provides an infrastructure and environment that focus on these integrated themes and advance multidisciplinary, innovative Alzheimer’s disease research through the pursuit of six overall aims.
Provide strong leadership and an administrative structure that support and facilitate basic and clinical research to understand the early transitions to cognitive decline and identify potential intervention strategies that promote cohesiveness and dynamic interactions among the cores to create new opportunities for innovative Alzheimer’s disease research at UK and beyond, and support NIA major initiatives.
Support a clinical core that maintains a central longitudinal cohort of 500 cognitively intact subjects and 300 cognitively impaired subjects with a major focus on early cognitive transitions from normal and from mild cognitive impairment to facilitate research projects, and use this infrastructure to support an integrated translational clinical trials team to evaluate potential new therapeutic interventions.
Maintain and expand the successful Minority Gateway Satellite Clinic to recruit African Americans, with overall goals that emphasize clinical evaluation and longitudinal follow-up, education of the community about Alzheimer’s disease, and increased participation in research.
Data Management and Statistical Core
Continue the data management and statistical core (DMSC) that maintains an integrated centralized database, provides expertise on experimental design and analysis, and interfaces with other ADRCs and with NACC. The BDMC will contribute innovative statistical expertise to characterize clinical transitions.
Neuropathology
Maintain a neuropathology core that performs state-of-the-art neuropathological services for the UK-ADRC clinical cohort and maintains a tissue bank from well-characterized, short PMI autopsy subjects from across the cognitive spectrum, as well as serum and CSF from living subjects to support research.
Outreach, Recruitment, and Education Core
Continue an education and information transfer core (EITC) to support recruitment and retention activities, increase community awareness of Alzheimer’s disease, and support educational initiatives.
- Set your location
- Change your location
- to see results near you
- Set Your Location
- Clear Location
- Set my preferred location
- Change my preferred location
- to show options closest to me
- Set Your location
Suggested Searches
- Online Bill Pay
- Weight Control
- Maternity Tours
- Nursing Jobs
Research at Baptist Health Lexington
A leader in medical research for over 30 years.
Medical research is an essential tool for evaluating the most effective therapies and diagnostic methods. At Baptist Health Lexington, we’ve been conducting medical research for over 30 years. Our clinical trials have played a significant role in the development of new treatments for diseases. Our studies allow patients to remain in their community and continue to see their doctors and other medical providers while taking advantage of new drugs and therapies tested on a national level. Patients enrolling in research at Baptist Health Lexington participate in treatments at the forefront of care.
Clinical Expertise Is Our Core Strength
Our medical research team is part of the Baptist Health Research program which is located at Baptist Health Lexington hospital. The Lexington team maintains a professional staff comprised of dedicated research management , clinical research coordinators , data specialists, and are supported by the Baptist Health Office for Research Administration. The administrative office includes research nurses, regulatory specialists, finance and business staff, and an internal compliance specialist . Most of our staff have been with the Clinical Research Center for multiple years, leading to more than nine decades of collective research experience.
Research Highlights
Below are highlights of some of the research currently being conducted at Baptist Health Lexington:
- ONYX Study. This study is focused on investigational treatment and routine care for patients with previously untreated extensive-stage, small-cell lung cancer. Principal investigator Firas Badin, MD, a hematologist and oncologist, has been recognized as the top enroller for phase 1b/2. Dr. Badin presented preliminary data from the study at the 2015 World Lung Conference in Denver, Colorado.
- LEADLESS II Study. LEADLESS II is designed to evaluate the safety and effectiveness of the Nanostim Leadless Cardiac Pacemaker System in patients indicated for a VVI(R) pacemaker. The cardiac-pacemaker system has potential to eliminate leads, pockets, and connectors required by conventional pacemakers and to reduce associated complications. Baptist Health Lexington is only location in Kentucky to offer this cutting-edge technology.
- FIX HF Trial. This trial’s purpose is to evaluate the Optimizer System, a new technology for managing the symptoms of patients with moderate to severe heart failure. Use of this device in Kentucky is limited to individuals participating in the clinical trial at Baptist Health Lexington.
- Publication and presentation. The medical research staff at Baptist Health Lexington shares the findings of its clinical studies with the world. One example is John Barton, MD, an obstetrician-gynecologist and leader in the field of maternal fetal medicine. He has presented his research in numerous professional settings, including the 10th Congress of Obstetric Critical Care in Shanghai, China, during 2015.
Looking for Cures in All the Right Places
Since its establishment in 1999, the Clinical Research Center has conducted several hundred trials across a wide range of therapeutic areas, including but not limited to:
- Oncology research
- Hematology research
- Bariatric research
- Cardiac research
- Electrophysiology (EP) research
- Obstetrics research
- Neonatal research
- Stroke research
- Neuro-interventional research
- Pulmonary Medicine research
- Orthopedic research
In addition, we offer trial-matching services for research being conducted at other facilities, both inside and outside the Baptist Health network. Our goal is to offer patients the widest possible range of options for advanced clinical treatment.
Areas of Emphasis
Baptist Health Lexington offers both observational trials focusing on prevention, diagnosis, and population health with respect to specific medical conditions, as well as treatment trials for investigating the efficacy and safety of new drugs and medical devices. We are currently undertaking a range of commercially and federally sponsored trials in the areas of orthopedic surgery, neurology, cardiology, electrophysiology, neonatal care, pulmonary medicine, stroke, neuro-intervention, obstetrics, and hematology/oncology, with a particular emphasis on oncology, electrophysiology, obstetrics, and neuro-intervention.
Program Highlights
The research program at Baptist Health Lexington is nationally recognized for its excellence:
- More than 40 physicians currently serve as investigators or co-investigators on research projects.
- The program’s medical personnel are leaders in their fields. Many serve on national scientific advisory boards.
- A majority of the program’s support staff have been certified as Clinical Research Professionals through the Society of Clinical Research Administrators (SOCRA) or the Association of Clinical Research Professionals (ACRP).
- Baptist Health Lexington is affiliated with the National Cancer Institute’s adult oncology treatment group, NRG Oncology.
- Baptist Health Lexington collaborates with the Guardian Research Network , a consortium of community health systems that expand patient access to leading treatments and therapies.
How to Contact Us
For general questions about the research program at Baptist Health Lexington, Richmond, or Corbin, please contact the hospital’s research site managers:
Franklin Echevarria Research Site Manager Phone: 859.260.3197 Email: [email protected]
Karli Herald Research Site Manager Phone: 859.260.6362 Email: [email protected]
If you are currently participating in a trial and have a question, please contact your study coordinator, your physician, or the physician overseeing your study. Contact information was included with the consent documents you signed when you enrolled in the trial.
Where We’re Located
Baptist Health Lexington Clinical Research Center 1740 Nicholasville Road Lexington, KY 40503
Micheal F. Stephens
Associate Vice President, Research
Baptist Office of Research Administration
Phone: 859-260-6456
Email: [email protected]
Lexington, Kentucky
- Actively Recruiting
Center Director – Sharon Walsh, Ph.D.
The Center on Drug and Alcohol Research (CDAR) began in 1990. CDAR conducts research into the biological, psychological, sociopolitical, and clinical aspects of substance abuse and related behavior. The Center conducts federally funded and state-funded research on substance abuse and related problems areas such as intimate violence, criminal justice settings and characteristics of rural substance use. Our multidisciplinary center also provides consultation services to public agencies and state and local government. The Center has a history of providing training on evidence-based treatment, intervention and prevention approaches, as well as providing service to the community.
Faculty and Center Associates
Meet the Faculty and Associates involved with the research conducted in the center.
Faculty »
Behavioral Health Outcome Studies
Explore the research projects centered on behavioral health conducted by the center.
BHOS »
Participate in Current Research Studies
You may be eligible to participate in current research studies.
Current Research Studies »
- All Services A-Z
- Markey Cancer Center
- Gill Heart & Vascular Institute
- Barnstable Brown Diabetes Center
- Kentucky Children's Hospital
- Kentucky Neuroscience Institute
- Orthopaedic Surgery & Sports Medicine
- Digestive Health
- Primary Care
- Emergency Department
- Appointments
- Tests & Exams
- Find a Doctor
- Schedule an Appointment
- For Patients
- Amenities & Resources A-Z
- Appointment request online form
- Appointment reminders
- Patient Notice of Privacy Practices
- Become a Patient
- Clinical Trials
- Don and Cathy Jacobs Health Education Center
- Interpreter Services
- In-Network Insurance Plans
- Visit MyChart
- Maps & Directions
- Pastoral Care
- Office of Patient Experience
- Pharmacy Locations
- About Advance Directives
- Privacy Policy
- Notice of Nondiscrimination for UK Health Programs & Activities
- Subscribe to HealthMatters
- Wellness & Community
- For Visitors
- Food & Lodging
- Pay your bill
- Patient's Condition & Phone Number
- Tobacco Free Policy
- Authorizations & agreements
- Autorizaciones y Convenios
- Mail & eGreetings
- Thank a Great Provider
- Refer a Patient
- Request a Login
- Corporate Compliance
- Information Technology
- Manage Your Profile
- Brand Strategy
- Employee Health
- Enterprise Learning
- UK Human Resources
- Department Phone Directory
- Quality & Safety
- Cancer Clinical Trials
- Emergency Transport
- Physician Liaison Program
- UK•MDs Medical Contact Center
- Mission/Vision
- Employment at Eastern State Hospital
- Nurse Residency Program
- Continuing Nursing Education
- Nursing Faculty Orientation
- Nursing Staff Development
- Registered Nurse (RN) Internships
- Nursing Scholarships
- Student Nurse Academic Practicum (SNAP)
- Tuition Reimbursement
- Acute Care Nursing
- Behavioral Health Nurse Services
- Central Monitoring System
- Critical Care Nursing
- Emergency Services
- Maternal Care Area Nursing
- Markey Cancer Center Nursing
- Nursing at Kentucky Children's Hospital
- Per Diem Nursing Pool
- Perioperative Nursing Services
- Rapid Response Nursing Teams
- Nursing Special Services
- Ambulatory Care Nursing Services
- Advanced Practice
- Care Delivery Model
- Certifications
- Career Center
- Clinical Nurse Expert
- Community Outreach
- Professional Advancement
- Professional Practice Model
- Service Line Triads
UK HealthCare Research
- Genomics Core Laboratory
- Sanders-Brown Center on Aging
- Cancer Researcher Directory
- Making a Difference
- Awards & Recognitions
- Strategic Plan
- Visual Arts
- Performing Arts
- Learn about volunteering
- Office of Observation and Learning Experience
- Diversity, Equity & Inclusion
- Healthcare Jumpstart Program
- Application Process
- How to become a RD
- Accreditation
- Tour facility
- Patient Stories
- Request an Appointment
- Medical Records
- Daisy Award Nomination Form
- Community Commitment
- Cooperative Extension Service
- Community Resources
- Office of Opioid Safety
- Safe Kids Fayette County
- UKHC Digital Platforms
- Learn about MyChart
- Log in to MyChart
- Request a MyChart account
- Proxy accounts
Appointment
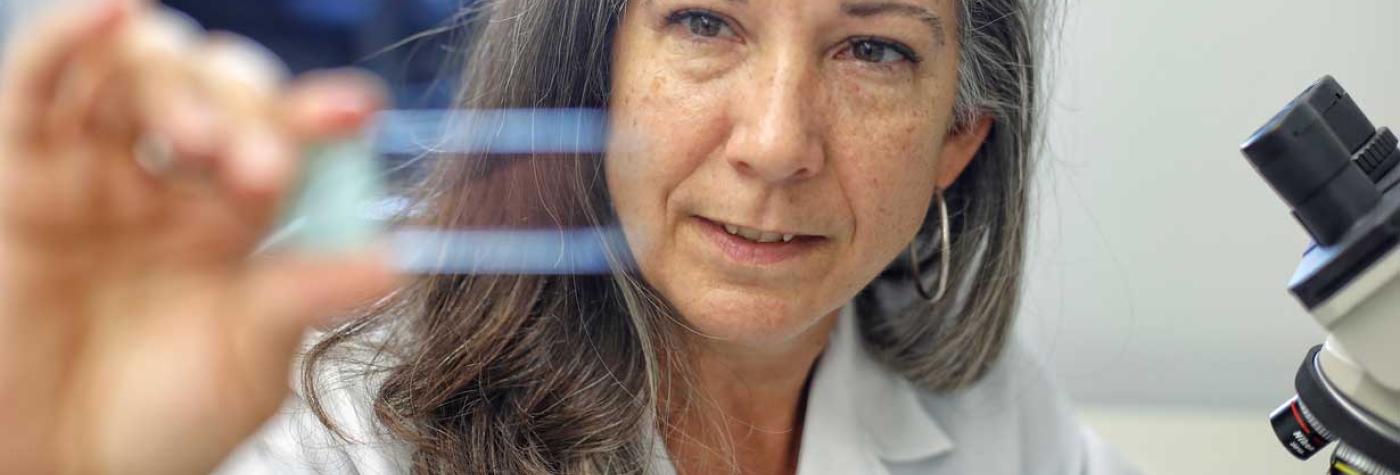
Our Research Leads to Breakthroughs in Treatment
Guidance for research participants The University of Kentucky is pausing select human subject research studies. The health and safety of our community is of paramount importance during the COVID-19 emergency. Read the full statement »
UK HealthCare is part of an academic medical center that has a thriving research community.
Medical advances are frequently made in a wide variety of health care disciplines. By participating in a research-based clinical trial, you can play a more active role in your health care and gain access to new research treatments before they are widely available.
You can also help others by contributing to medical research. Ask your physician if there are any research studies being conducted at UK that may benefit you. To help you decide, the researchers involved will explain the details of the study.
- Research at Markey Cancer Center
- Research at Kentucky Neuroscience Institute
Treating Cancer at the Molecular Level
The UK Markey Cancer Center, Kentucky’s only NCI-designated comprehensive cancer center, is proud to be on the leading edge of cancer research and treatment. Our Molecular Tumor Board, the first of its kind in the Commonwealth, uses precision medicine to analyze the genetic makeup of a patient’s cancer and then enlists a team of experts to determine the best treatment for that unique cancer – and that patient. It’s just one way we’re harnessing the power of advanced medicine to tackle the very real challenges cancer poses for our patients and our state.
- All Programs

I'm searching for
College of Public Health
- Health Services Research (Graduate)
- Home /
- Registrar /
- Programs /
- Financial Aid
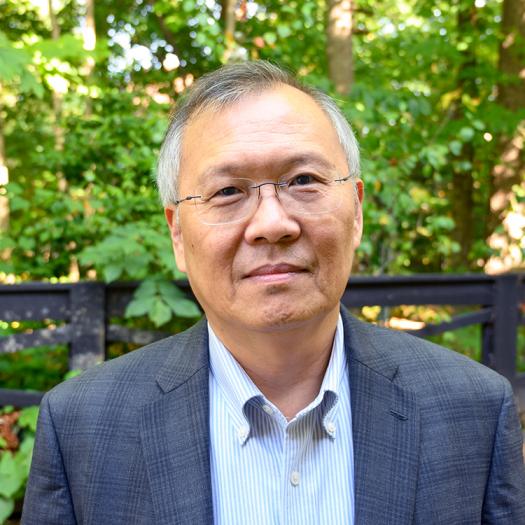
Min-Woong Sohn
Director of Graduate Studies
111 Washington Ave, Suite 101
Lexington, KY
859-257-5678
Other programs from the College of Public Health:
Gerontology - graduate, epidemiology and biostatistics phd.
The PhD program in Health Services Research at the University of Kentucky College of Public Health prepares professionals for a career in conducting data-driven health services research. This unique program strongly emphasizes applied health services research skills, including study design, data management, statistics and other quantitative methods. There are opportunities to organize coursework based on interests such as health economics or health outcomes.
Transform our healthcare system
Graduates will be prepared to address the practical challenges of conducting health services research in the multidisciplinary research environments of academia, government, consulting and industry. The mentored research program will prepare independent researchers skilled at designing and conducting health services research, leveraging a variety of study designs, using primary data collection approaches, and navigating primary and secondary databases to inform healthcare delivery and health policy.
- Understand critical health services research issues, including access, quality, and efficiency.
- Apply theoretical knowledge and conceptual models in support of health services research.
- Describe key issues and methods in a concentrated substantive area, such as health economics or health outcomes.
Application Requirements
Application Checklist
Applicants must meet the Graduate School admission requirements .
Application Deadlines:
- Final application deadline for all applicants: February 1
About BREATHE
BREATHE (Bridging Research Efforts and Advocacy Toward Healthy Environments), located at 2265 Harrodsburg Road in Lexington, Kentucky, is a multi-disciplinary research, outreach, and practice collaborative of the University of Kentucky (UK) College of Nursing, with partners internal and external to UK. BREATHE’S Vision is that all people will have access to clean air and live in healthy environments. BREATHE’S Mission is to promote lung health and healthy environments with at risk populations to achieve health equity through a) research; b) community outreach and empowerment; c) advocacy and policy development; and d) access to health services.
BREATHE, formerly known as the Clean Indoor Air Partnership, was recognized as one of UK’s Commonwealth Collaboratives, and it received the Exemplary Project Award for community engagement in 2011 from the Association of Public Land-Grant Universities (APLU). BREATHE has administrative and biostatistics cores, as well as an air quality monitoring laboratory. BREATHE consists of a large team of faculty researchers and clinicians, staff from a variety of disciplines, and students from both undergraduate and graduate programs. BREATHE provides facilitation for the UK Markey Cancer Center’s Comprehensive Lung Cancer Translational Research Group.
BREATHE is directed by Dr. Ellen J. Hahn, PhD, RN, FAAN with critical expert guidance provided by the BREATHE Advisory Board and over 80 organizational community partners. BREATHE is a clearinghouse for information, research and analysis, and policy development, as well as community education and outreach related to smoke- and tobacco- free policies, tobacco health disparities, electronic smoking devices, tobacco prevention and treatment, and radon reduction. BREATHE has had success working with both rural and urban communities and in economically diverse localities. BREATHE is comprised of four divisions: Tobacco Policy, Tobacco Prevention & Treatment, Radon Policy, and Special Populations. The Administration and Data Management & Outcomes units support faculty, staff, and students. See the BREATHE organizational chart here .
The Tobacco Policy Division includes the Kentucky Center for Smoke-free Policy (KCSP) and Go Tobacco-free . KCSP is a dissemination and implementation research center that assists communities with the development and implementation of smoke-free policy including protections from the use and distribution of emerging tobacco products. KCSP was created in December 2004 with seed money from The Robert Wood Johnson Foundation’s Tobacco Policy Change Program after launching a successful smoke-free campaign in Lexington, Kentucky. Go Tobacco-free is a valuable resource for campuses with tobacco-free policies or for those who want to adopt a tobacco-free policy. Go Tobacco-free provides technical assistance via email, conference calls, webinars, Skype and/or in person using an evidence-based policy implementation approach with a focus on compliance and evaluation. Go Tobacco-free is a collaborative network for individuals doing similar work, recognizing the importance of sharing lessons learned with others advocating for or implementing tobacco-free campus policies. Go Tobacco-free disseminates evidence-based resources including a monthly Campus Spotlight highlighting successful tobacco-free policies. Go Tobacco-free partners with organizations and campuses to conduct research and develop evidence-based tobacco-free policy practices.
The Tobacco Prevention & Treatment Division (TPT) develops and enhances tobacco screening and personalized treatment services in healthcare and community settings through prevention, treatment, education, and research. The TPT team offers a variety of trainings for health providers and professionals, including an intensive tobacco treatment specialist (TTS) training program . These trainings are intended to enhance engagement in tobacco dependence treatment and education with a focus on disparately affected populations.
The Radon Policy Division, developed in 2006 and funded in part by the Kentucky State Radon Program, houses an evidence-based community outreach and research center to raise awareness about the dangers of radon exposure and prompt action to reduce radon risks using evidence-based practices. Radon research focuses on reducing the synergistic risks of radon and tobacco smoke exposure. The two major programs within the Radon Policy Division are Provider Education and Geological Mapping. The Provider Education Program offers free continuing education credits for healthcare and public health professionals as well as materials to share with patients, providers, and community members. The Geological Mapping Program partners with the Kentucky Geological Survey, also located at the University of Kentucky, to evaluate radon potential using observed radon values and rock formation type and to create county-specific maps and information. The Radon Policy Division’s faculty and staff serve on the American Association of Radiation Scientists and Technologists (AARST) Foundation Board and the Kentucky Governor’s Radon Advisory Committee.
The Special Populations Division aims to reduce tobacco-related health disparities. Affiliated faculty are experts in tobacco use and tailored treatment approaches for vulnerable populations including women and children, youth and young adults, individuals with mental illness or substance use disorders, and members of the LGBT community. BREATHE faculty serve on the Substance Abuse and Mental Health Administration (SAMHSA) State Tobacco Policy Academy on Tobacco Control and Behavioral Health, a statewide collaborative process to address tobacco use among those with a behavioral health disorder. BREATHE faculty also serve on the advisory board for LGBT Healthlink, a CDC funded cancer and tobacco disparity network, which is a community driven group of advocates and professionals working to reduce tobacco use and cancer.
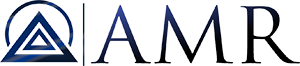
Our Locations
Strategically positioned to serve a diverse population., our vast experience and excellence in clinical research allow us to custom design a plan that works best for you in any of our 32 locations..
“Especially when I have large studies that need large numbers of subjects enrolled quickly and with quality and good investigator oversight, I always involve AMR sites.”
“AMR sites were pivotal to the success of our seasonal vaccine program. All AMR sites met and exceeded enrollment expectations. The variety of experience and expertise among AMR sites has helped us enroll a broad therapeutic range of clinical trials.”
“I’ve been doing this job for 18 years and have met with literally thousands of customers. After all that, today was the single best customer visit I’ve ever had, I had high expectations going into the meeting, but you blew my expectations away. The combination of your incredible capacity and performance in clinical trials combined with your hospitality made this the absolute perfect day.”
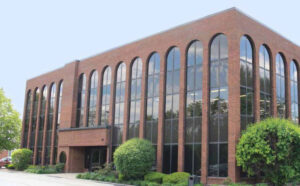
Chicago, IL
Amr chicago brings together physicians in multiple specialties who offer clinical trial services to the highest standards of good clinical practice..
AMR Chicago was formerly known as Affinity Health.
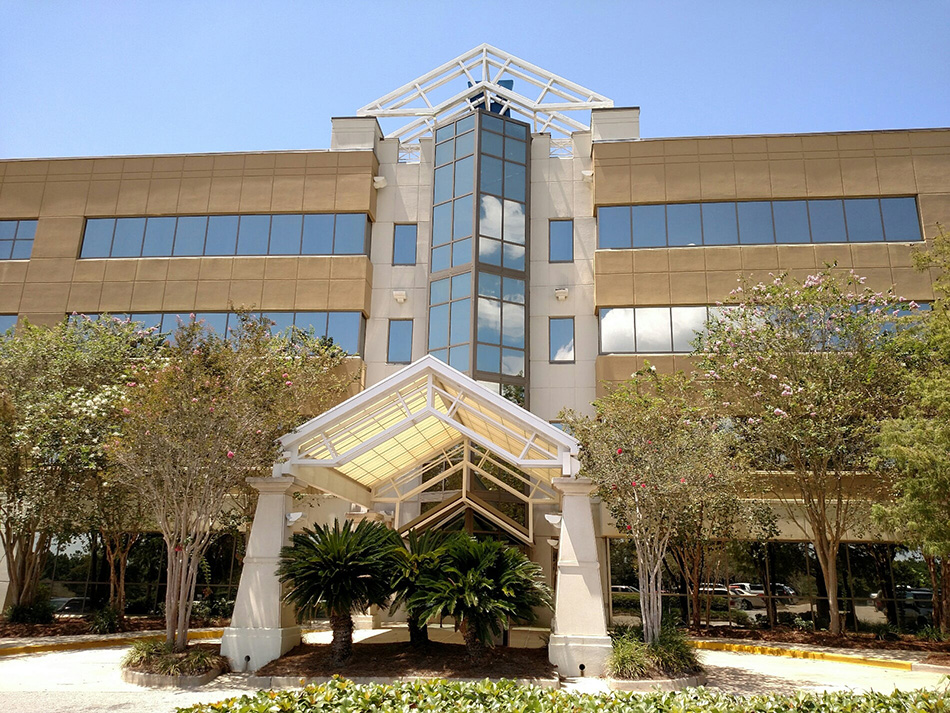
AMR Daphne is Located in a professional medical office building attached to the Sleep & Cardiovascular health practice run by Dr. Ledet.
Founded in 2022 27+ clinical trials Phase I, II, III, and IV
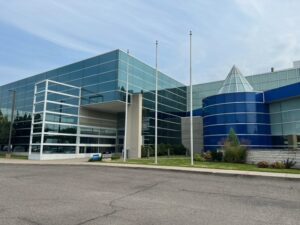
Dearborn, MI
Amr dearborn is our newest clinical research center conveniently located in dearborn, michigan that will primarily focus on cardiology clinical trials as well as hypercholesterolemia, obesity, and vaccines..
Founded in 2023 Phase I, II, III, and IV Arabic Also Spoken
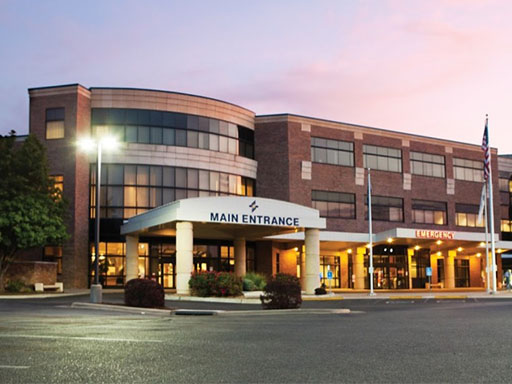
El Dorado, KS
Amr el dorado is located in the susan b. allen memorial hospital..
Founded in 1991 1,400+ clinical trials Phase I, II, III, and IV
AMR El Dorado was formerly known as Heartland Research Associates.
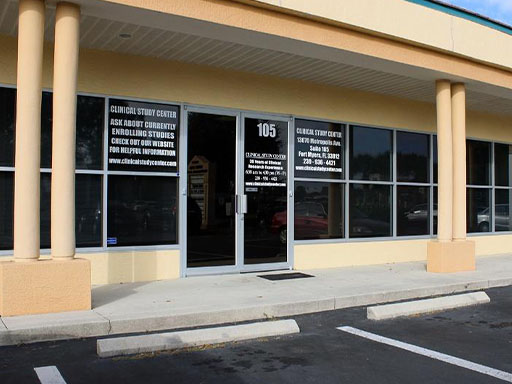
Fort Myers, FL
Amr fort myers is across the street from the newest hospital in fort myers..
Founded in 1978 700+ clinical trials Phase I, II, III, and IV
AMR Fort Myers was formerly known as Clinical Physiology Associates.
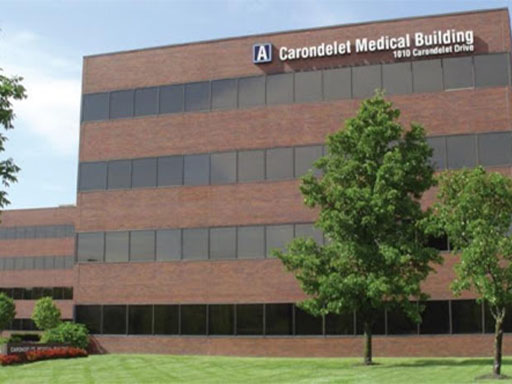
Kansas City, MO
Amr kansas city has conducted more trials since 1986 and owns a database of more than 33,000 volunteers..
Founded in 1986 800+ clinical trials Phase I, II, III, and IV
AMR Kansas City was formerly known as The Center for Pharmaceutical Research.
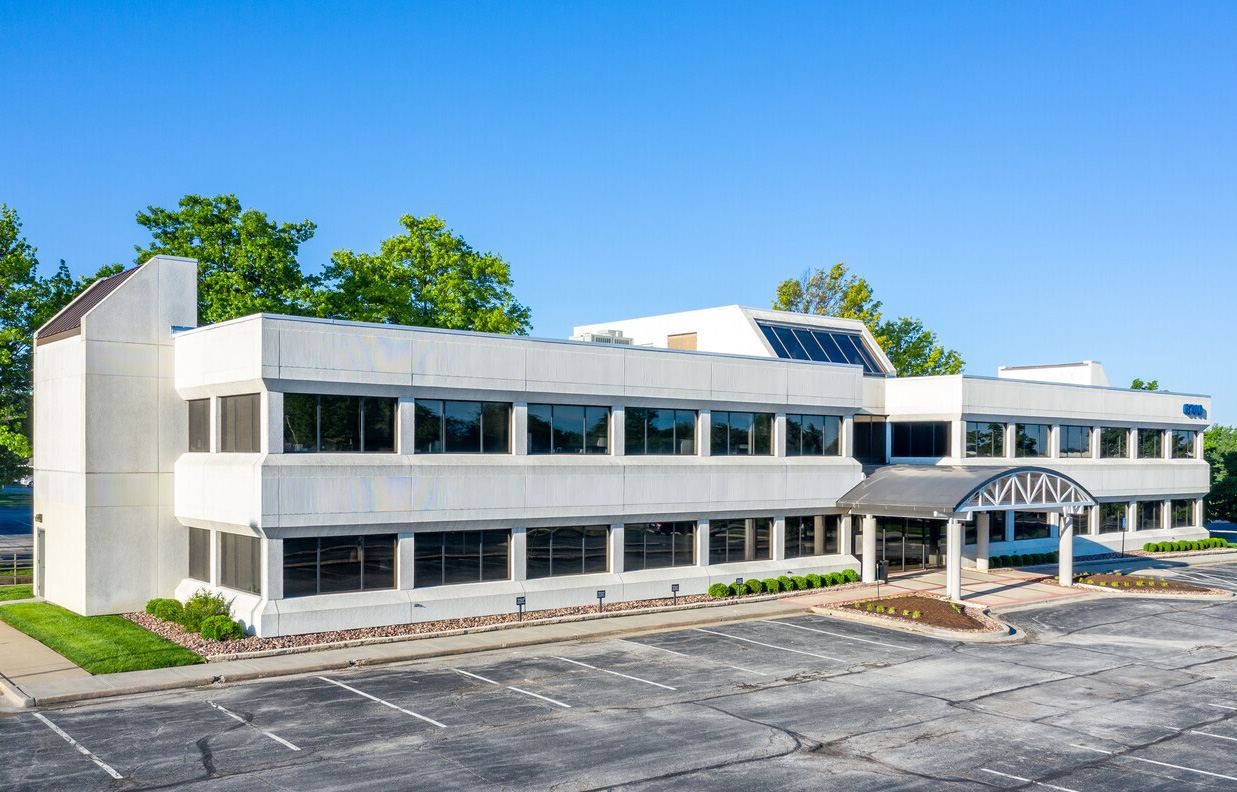
Kansas City Oncology, KS
Amr kansas city oncology.
75 clinical trials
5 Oncologists and 10 oncology nurse practitioners
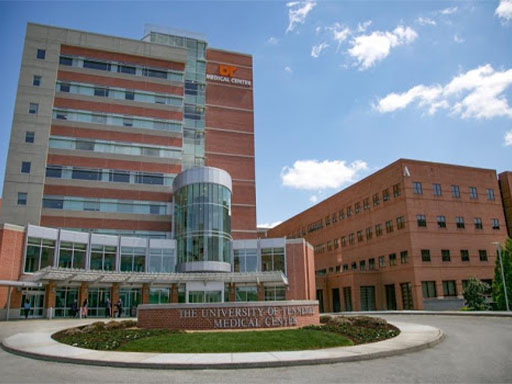
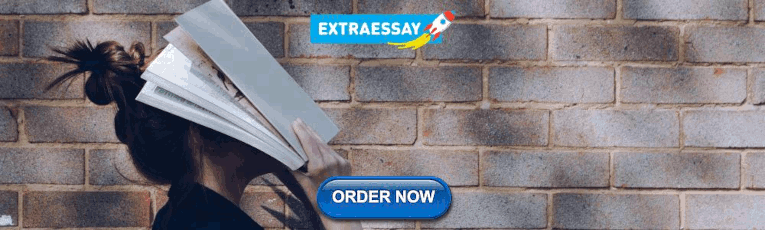
Knoxville, TN
Amr knoxville is located in an academic medical center with state-of-the-art capabilities..
Founded in 1985 1,900+ clinical trials Phase I, II, III, and IV
AMR Knoxville was formerly known as Volunteer Research Group/NOCCR-Knoxville.
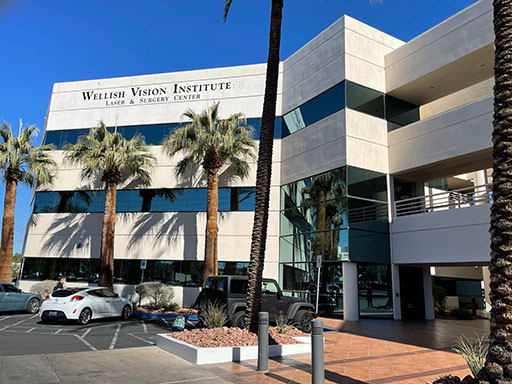
Las Vegas, NV
Amr las vegas is located on a medical campus across the street from desert springs hospital..
Founded in 2003 250+ clinical trials Phase I, II, III, and IV
AMR Las Vegas was formerly known as Clinical Research Consortium in Las Vegas.
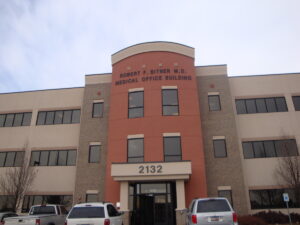
Founded in 2000 670+ clinical trials Phase I, II, III, and IV
AMR Layton was formerly known as Pharmaceutical Research Organization.
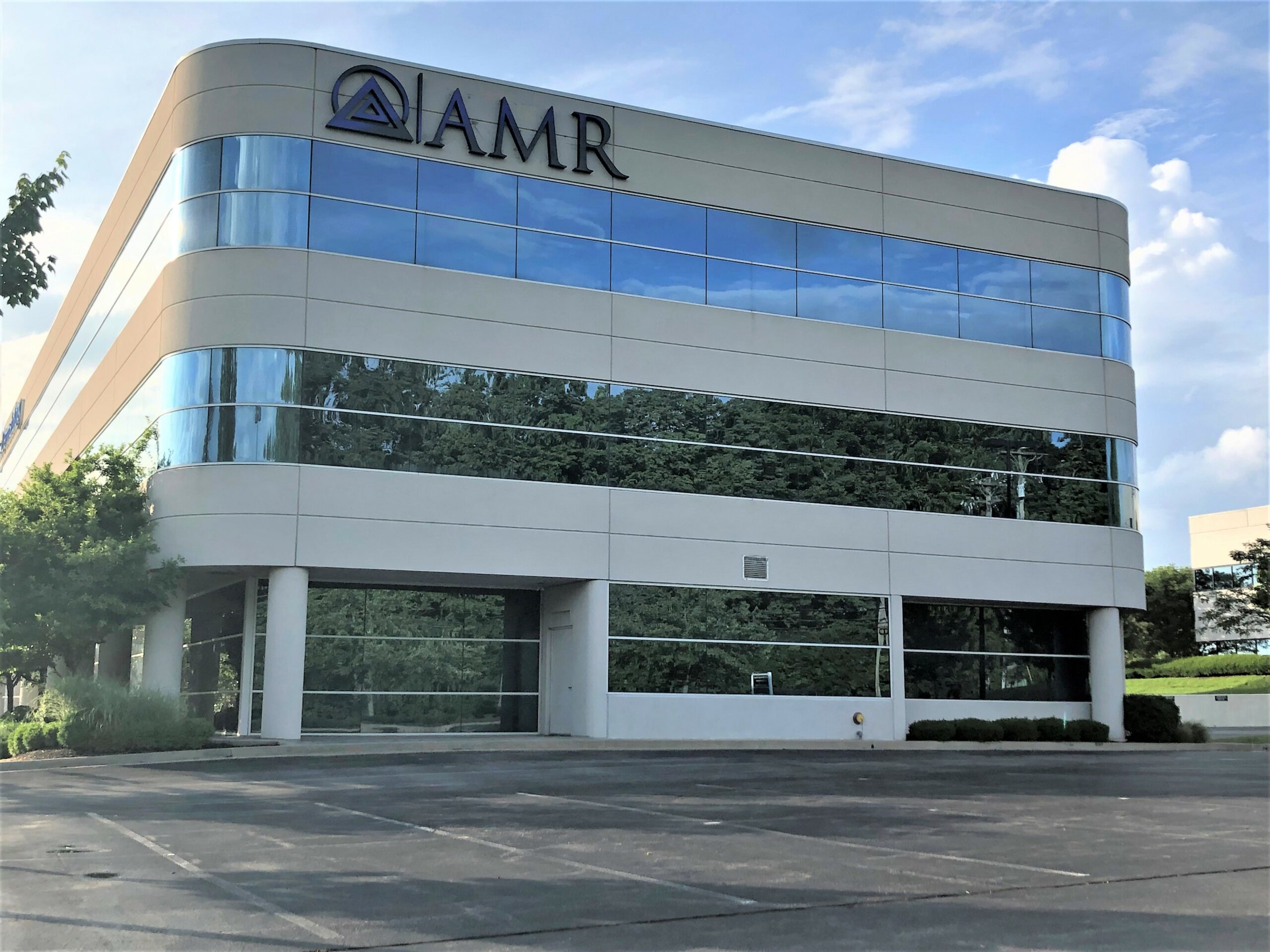
Lexington, KY
Amr lexington was kentucky’s first independent clinical drug research facility..
Founded in 1991 600+ clinical trials Phase I, II, III, and IV
AMR Lexington was formerly known as Central Kentucky Research Associates.
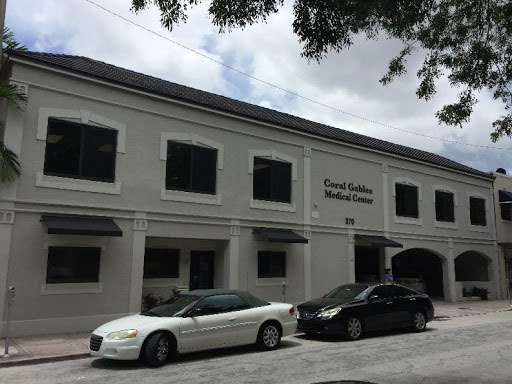
AMR Miami in Coral Gables, Florida has recently invested in a PBMC lab, which became functional in May 2018.
Founded in 1982 813+ clinical trials Phase I, II, III, and IV
AMR Miami was formerly known as Clinical Research of South Florida.
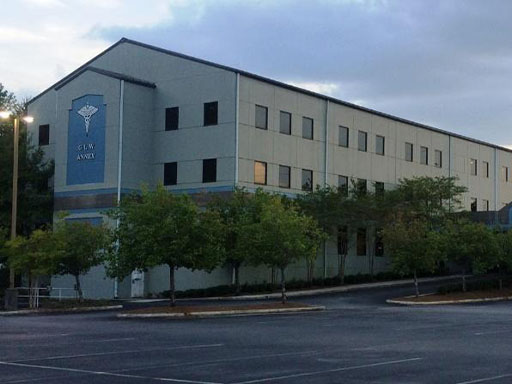
AMR Mobile was the first free-standing multispecialty research site in the state of Alabama.
Founded in 1987 850+ clinical trials Phase I, II, III, and IV
AMR Mobile was formerly known as Coastal Clinical Research.
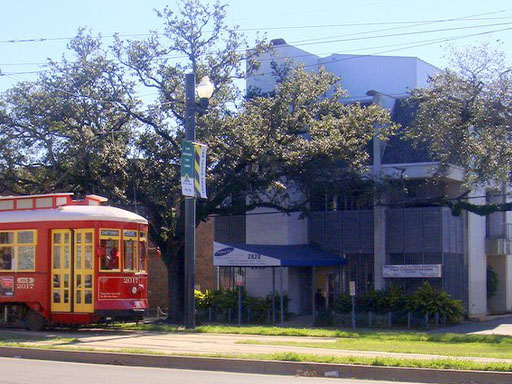
New Orleans, LA
Amr new orleans is a dedicated research clinic located in the mid-city area on canal street..
Founded in 1985 900+ clinical trials Phase I, II, III, and IV
AMR New Orleans was formerly known as NOCCR.
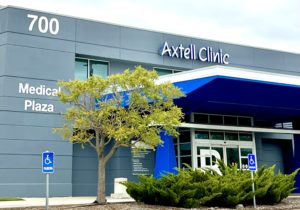
AMR Newton is connected to the Axtell Clinic, which has provided care for over 100 years.
AMR Newton was formerly known as Heartland Research Associates.
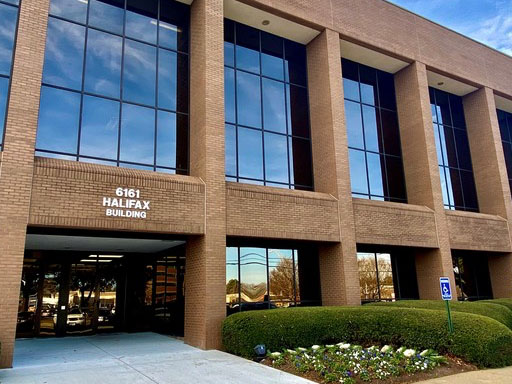
Norfolk, VA
Amr norfolk is located on the campus of eastern virginia medical complex..
Founded in 1983 700+ clinical trials Phase I
AMR Norfolk was formerly known as Clinical Research Associates of Tidewater.
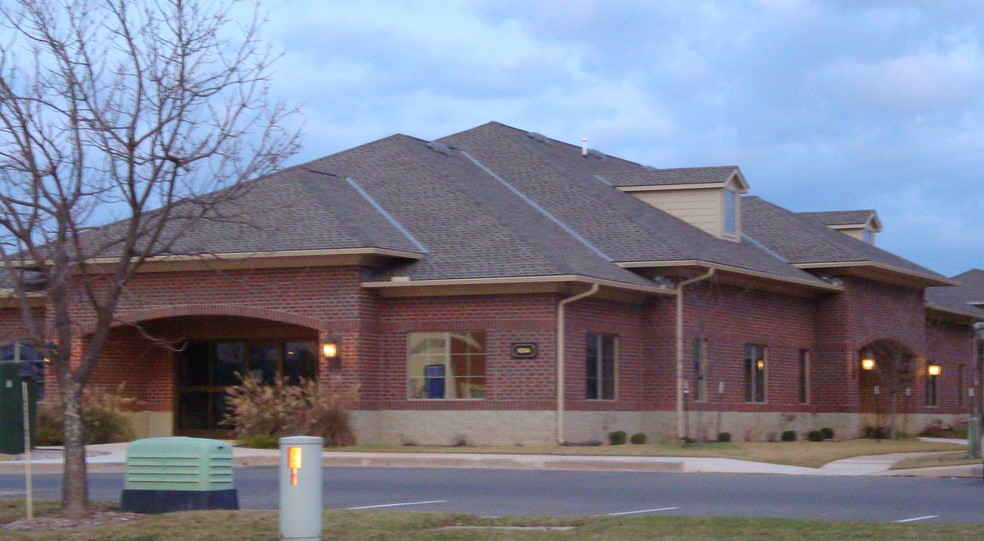
AMR Norman is located in the heart of the Midwest – just 30 minutes from Oklahoma City in Norman, Oklahoma.
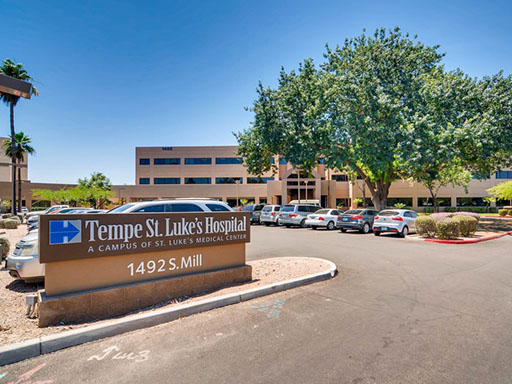
Phoenix, AZ
Amr phoenix is located in the medical office building on the campus of tempe st. luke’s hospital..
Founded in 2011 250+ clinical trials Phase I, II, III, and IV
AMR Phoenix was formerly known as Clinical Research Consortium.
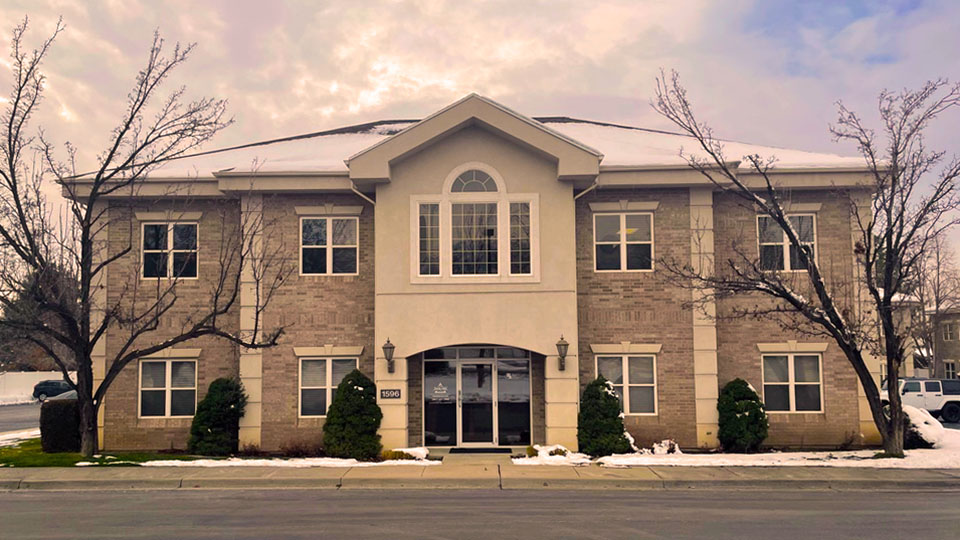
Salt Lake City, UT
AMR Utah was formerly known as Pharmaceutical Research Organization.
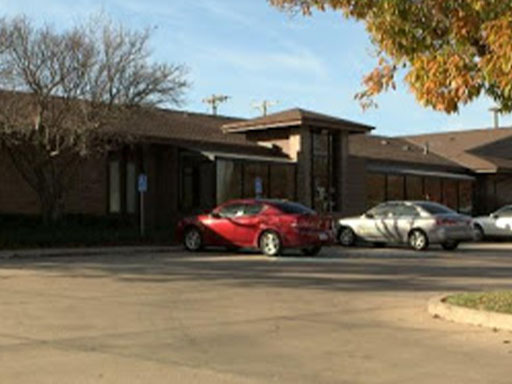
Wichita - East, KS
Amr wichita – east is within family medicine east, a private practice clinic..
AMR Wichita-East was formerly known as Heartland Research Associates.
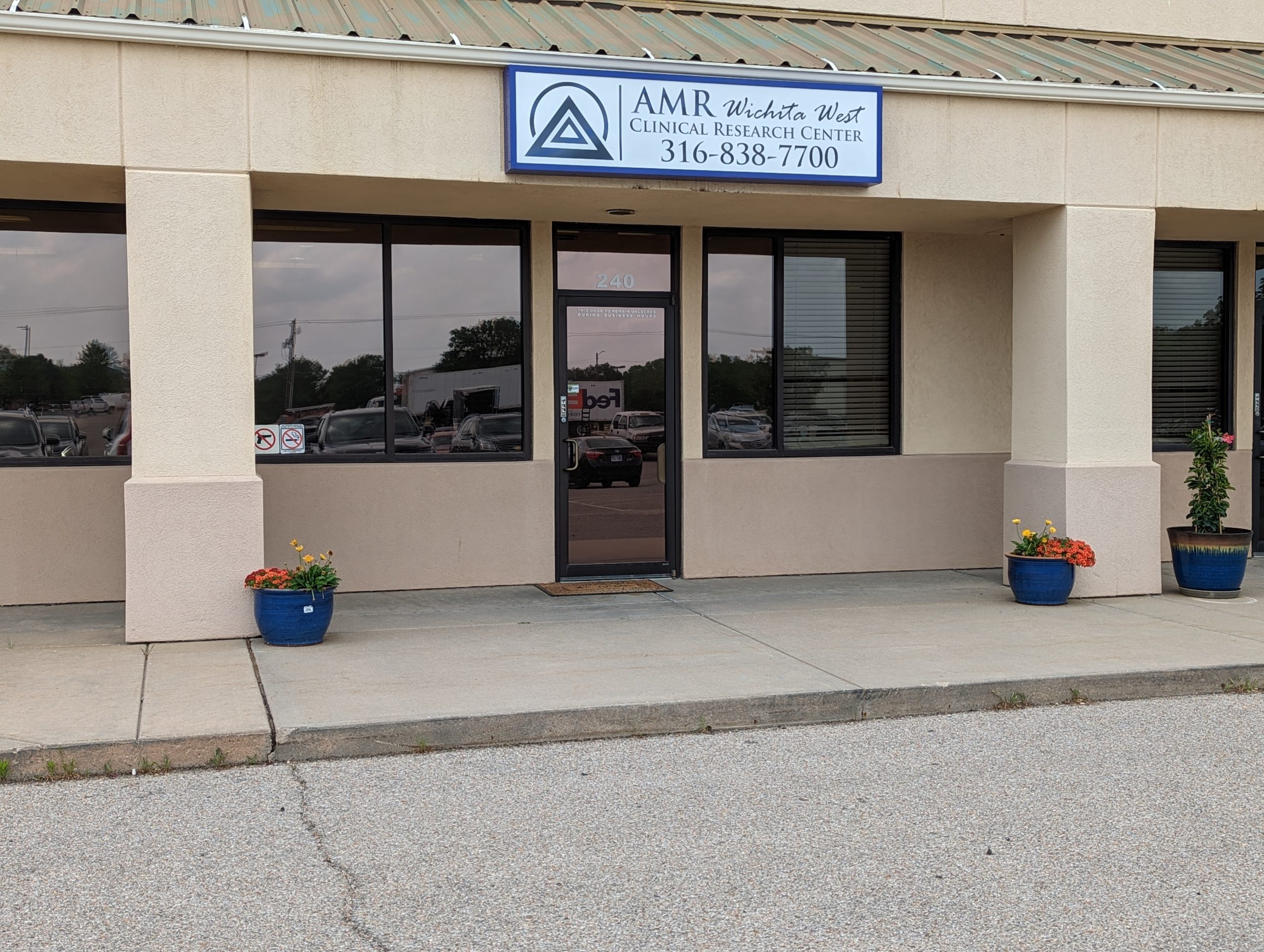
Wichita - West, KS
Amr wichita.
AMR Wichita-West was formerly known as Heartland Research Associates.
Find Your Nearest AMR Center
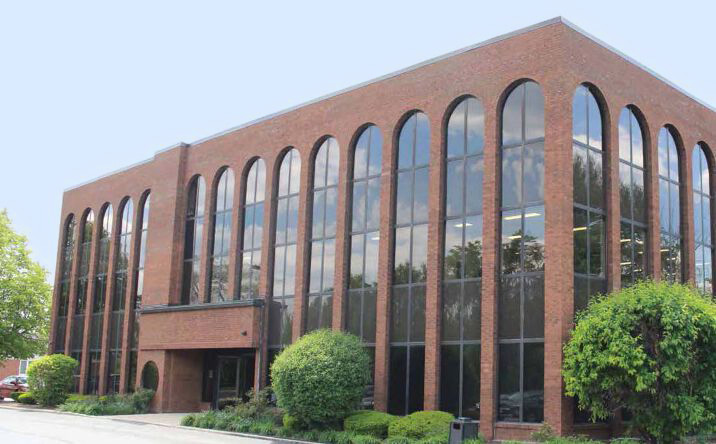
AMR Chicago aims to deliver quality healthcare with a patient-centered approach. We provide variety of medical services & clinical research trials with compassion, while ensuring exceptional patient care and safety.
2001 Midwest Road, Suite 305 Oak Brook, IL 60523
630-491-1900 [email protected]
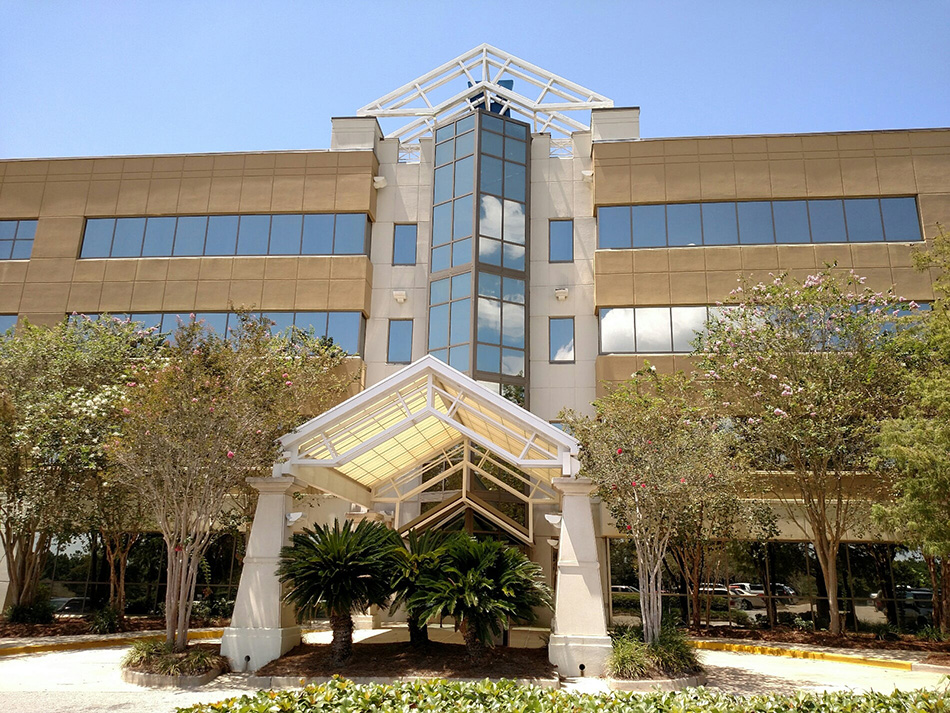
AMR Daphne is Located in a professional medical office building attached to the Sleep & Cardiovascular health practice run by Dr. Ledet, who also serves as Medical Director at Southeast Regional Center for Sleep/Wake Disorders since its inception in the early 1990s. His focus of healthcare is on sleep and cholesterol disorders to prevent and manage cardiovascular disease, which includes caring for risk factors such as insulin resistance. Our full-time research team includes clinical research coordinators, back-up coordinators, patient recruiters, lab coordinator, data entry coordinator, regulatory specialist, and administrative staff. We provide our community with innovative treatments and the highest quality of care throughout each clinical research trial.
7101 Hwy 90, Suite 102 Daphne, AL 36526
251-901-2111 [email protected]
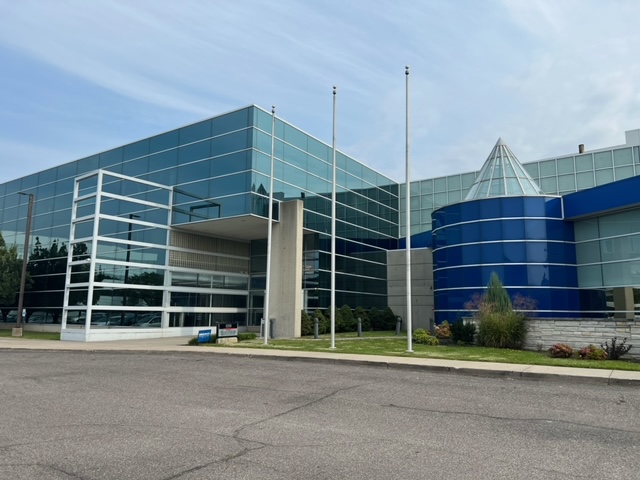
AMR currently has two investigators to conduct clinical research trials in the Dearborn community, Dr. Elias H. Kassab and Dr. Ashok K. Kondur. Dr. Kassab serves as Principal Investigator. Dr. Kassab completed his graduate training and fellowship in cardiology throughout Michigan after completing his education at Universite Libre de Bruxelles, Brussels, Belgium in 1982. In addition to clinical research, his experience includes Cardiology, Endovascular and Vascular Management. Dr. Kondur is the Sub Investigator for the site. Dr. Kondur is board-certified in PET imaging, Nuclear Medicine, Vascular Medicine and Coronary, Peripheral, Structural and Endovascular Interventions. Both Dr. Kondur and Dr. Kassab will focus primarily on Cardiology clinical trials as well as conducting trials focused on Hypercholesterolemia, Obesity, and Vaccines.
5250 Auto Club Drive, Suite 310B Dearborn, Michigan 48126
251-901-2111 [email protected]
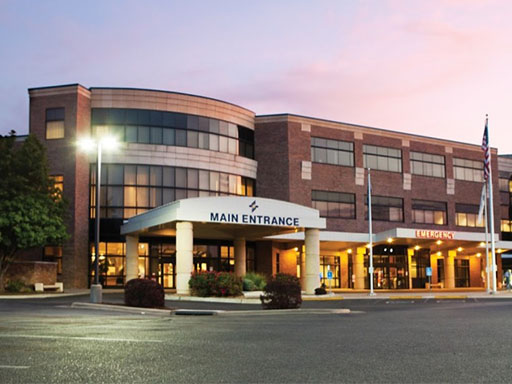
AMR Heartland Research Associates – El Dorado, Kansas (previously located in Augusta, Kansas) is located in the Susan B. Allen Memorial Hospital. It has conducted more than 75 Phase I–IV pharmaceutical and device trials since opening in 2010. Three family practice clinics in the Augusta / El Dorado and neighboring communities are owned by the center’s research investigator. Access to these subjects makes enrollment efforts highly successful.
- General Manager: Jill Hiebert
- Managing Principal Investigators: Thomas Klein, MD, FAAFP, CPI; Terry Klein, MD, DABFM, CPA; Tracy Klein, MD, DABFM, CPI, Terry Poling, MD, DABFM, FAAFP, CPI
- Founded in 1991
- Conducted 1,400+ clinical trials
- Phase I, II, III, and IV trials
- Limited overnight stay capabilities (expanded phase I capabilities coming soon)
Specialties:
- Infant Vaccines
- Adult Vaccines
- Maternal Health
700 W. Central, Suite 101 El Dorado, KS 67042 316-600-5127
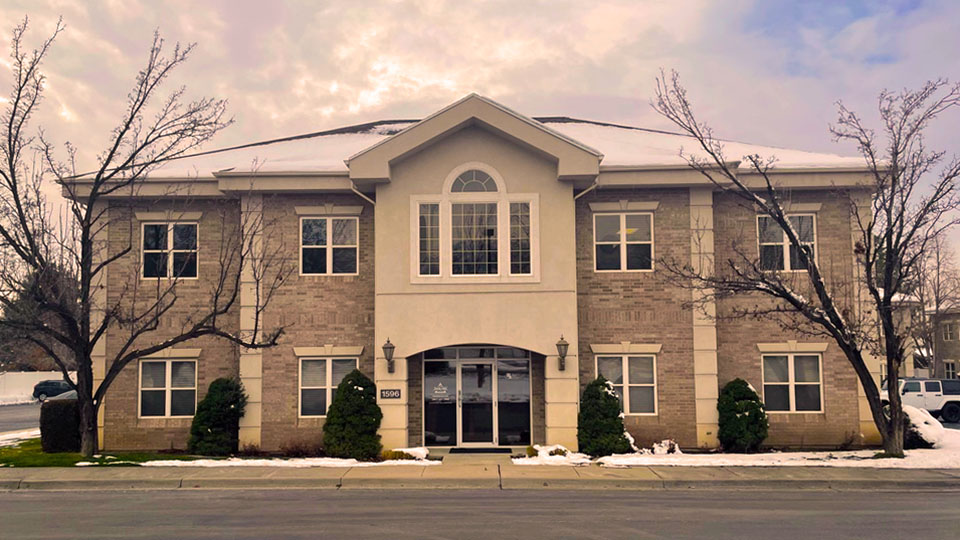
Pharmaceutical Research Organization, now AMR Utah, conducts and manages the day-to-day clinical research trial at the investigative site level under the direction of the principal investigator. While we manage a wide-range of trial types, AMR Utah has expertise and a focus in pediatric vaccines.
- Site Manager: Shelly Searle
- Business Development & Community Relations: Luke Johnson
- Founded in 2000
- Conducted 670+ clinical trials
- Mainly Phase II, III, and IV clinical research protocols
- Dermatology
- Family Practice
- Gastroenterology
- Internal Medicine
- Obstetrics & Gynecology
- Ophthalmology
- Pediatric Dentistry
1492 West Antelope Drive, Suite 209 Layton, Utah 84041 (801) 294-9911
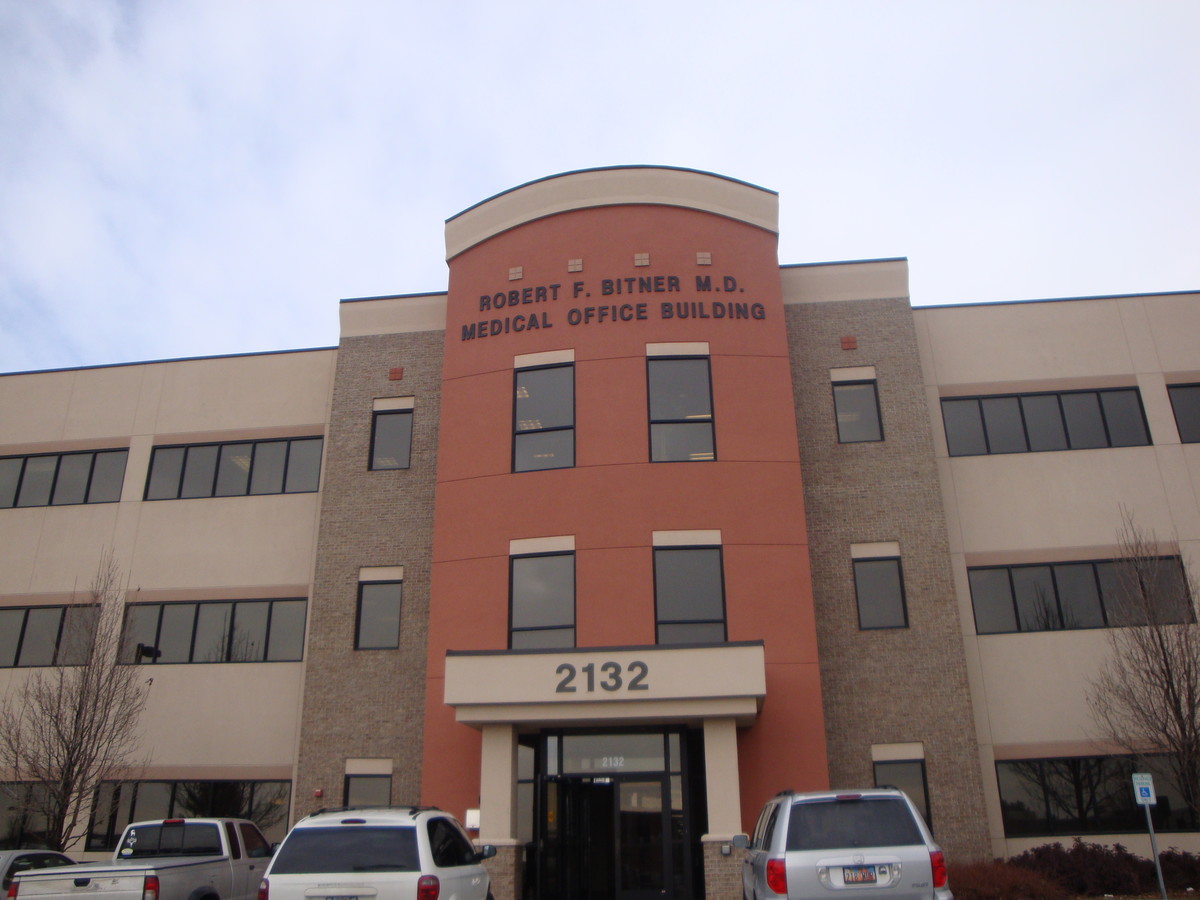
Pharmaceutical Research Organization, now AMR Layton, conducts and manages the day-to-day clinical research trial at the investigative site level under the direction of the principal investigator. While we manage a wide-range of trial types, AMR Layton has expertise and a focus in pediatric vaccines.
- Site Manager: Curtis Thomas
- Business Developmen: Luke Johnson
2132 N 1700 W, Suite 200 Layton, UT 84041 (801) 679-4153
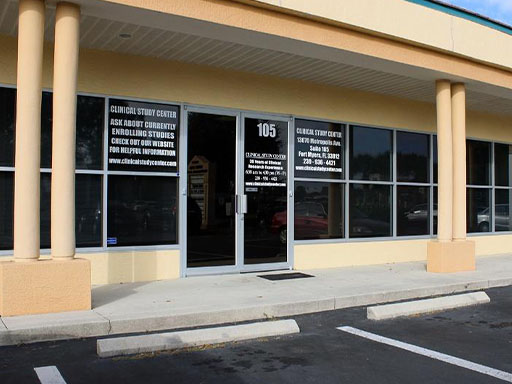
AMR Fort Myers (aka Clinical Physiology Associates and the Clinical Study Center), Fort Myers, Florida has conducted more than 800 Phase I–IV clinical research trials since 1978. The center is located across the street from the newest hospital in Fort Myers, which recently completed a $350 million expansion. Led by principal investigator, Dr. Pedro Ylisisatigui, AMR Fort Myers has developed research relationships with local doctors focusing on internal medicine, women’s health, dermatology, endocrinology and pain management. The Ft. Myers location has a long-standing research relationship with Premier Women’s Care, the largest OB/GYN group in the area and has completed more than 130 women’s health studies in the past 15 years. The center has two beds for overnight studies.
- General Manager: Ken Aschom
- Founded in 1978
- Conducted 800+ clinical trials
- Limited overnight stay capabilities
- Women’s and Men’s Health
- Musculoskeletal
- Endocrinology
- Pain Management
13670 Metropolis Ave. Suite 105 Fort Myers, FL 33912 239-936-4421 239-936-2865 fax
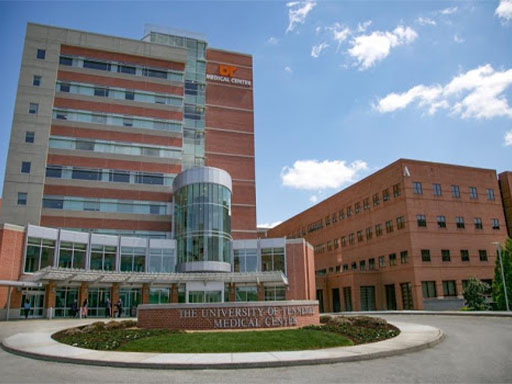
AMR Volunteer Research Group for Clinical Research(Knoxville, TN) and AMR New Orleans Center for Clinical Research(New Orleans, LA) operate as three distinct units;
- 60-bed inpatient PK unit in Knoxville at the University of Tennessee Medical Center
- an outpatient unit in Knoxville
- the original facility, which is now an outpatient unit in New Orleans
These centers have conducted more than 1,900 Phase I–IV trials since opening in 1991. The inpatient unit employs 60 full-time staff and conducts a variety of trials including NHV, SAD/MAD, FIH, special population trials including renal, hepatic, diabetic, obese and elderly among others, as well as hybrid studies starting in HNV and bridging into patient populations such as Alzheimer’s disease, Parkinson’s disease, COPD, ALS as well as cancer subjects. It also conducts inpatient vaccine and smoking-related trials. The site focuses on complex studies which lend themselves to being performed in a level-one trauma facility with 24/7 rapid-response code team coverage. Located in an academic medical center, the site has relationships with more than 800 physicians, unique state-of-the-art imaging, diagnostic and laboratory capabilities.
The outpatient units in Knoxville and New Orleans function as Phases II-IV research sites conducting efficacy trials from vaccine, obesity, migraine, diabetes and hypertension to women’s and men’s health, COPD and generally all internal medicine-related indications. The New Orleans location has been in operation since the early 1980s and was a base of operation until Hurricane Katrina forced relocation in 2005. The Knoxville location has been in operation since 1998.
Staff has an average longevity of 15 years. The site has an exemplary track record of compliancy, having successfully passed the last five FDA inspections in which NOCCR was found to be in full compliance with FDA rules and regulations.
- General Manager: Nyda Brook
- Founded in 1985
- Conducted 1,900+ clinical trials
- Cardiovascular
- Neuropsychology
- Complex Inpatient Trials
- Sleep Disorders
- Elderly PK and Healthy Phase I
- Tobacco Related
1924 Alcoa Hwy 4th & 5th Floors North Tower Knoxville, TN 37920
801 North Weisgarber Rd. Suite 100 Knoxville, TN 37909 865-305-9100 865-305-8381 fax
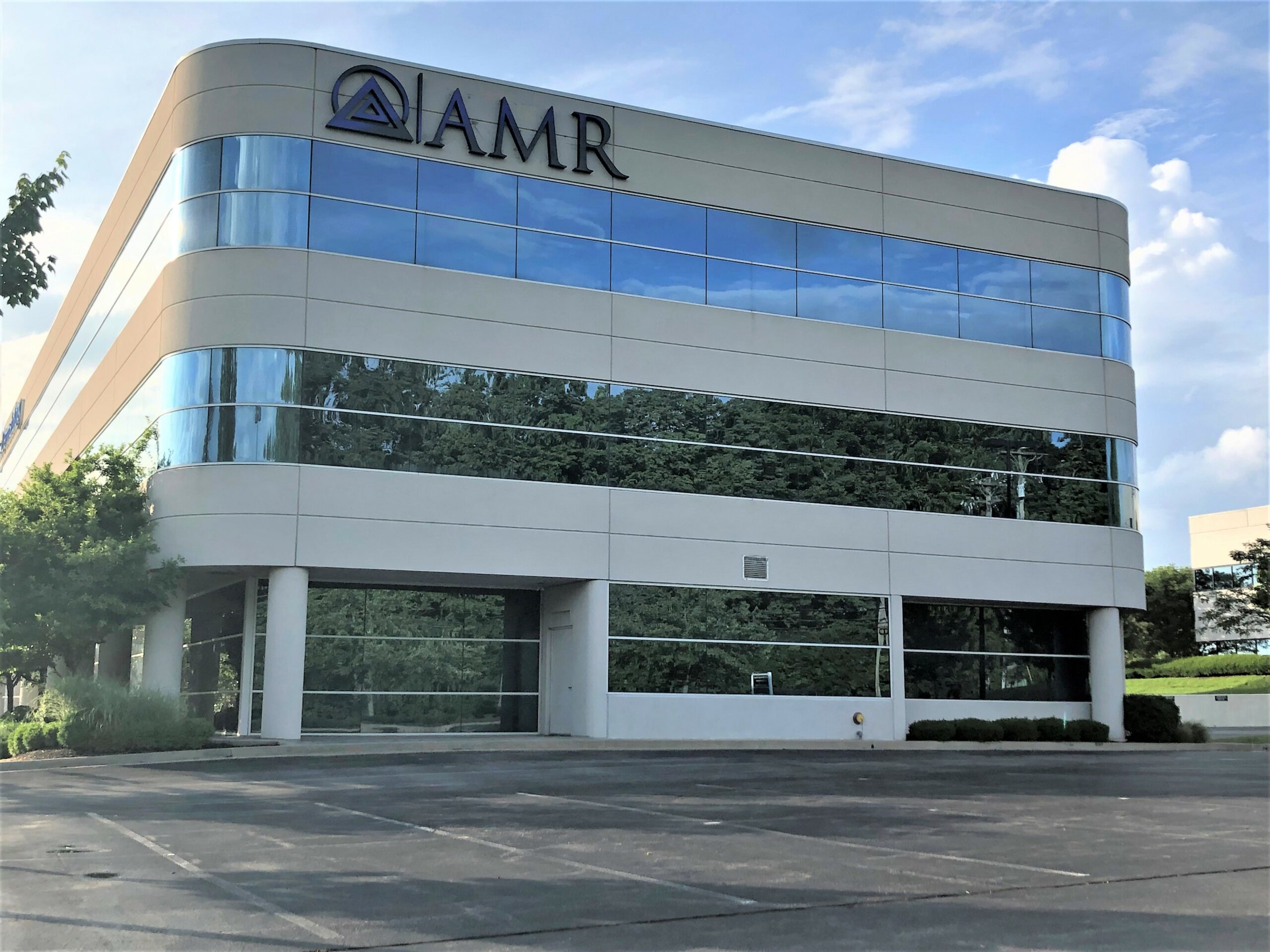
AMR – Lexington, Central Kentucky Research Associates, in Lexington, Kentucky has conducted 608 Phase I–IV trials since opening in 1991. This was Kentucky’s first independent clinical drug research facility and is housed in a 17,000 square foot facility that contains both an outpatient Phase II-IV clinic and an inpatient 23-bed Phase I and overnight unit.
AMR Lexington is fully staffed and has educated coordinator staff who are supported by a strong team of professionals who assist with regulatory, operations, data management, and quality assurance. The location also has trained PRN employees who can be called upon to assist in the conduct of overnight and Phase I trials. Staff at the Lexington location have 75-plus years of combined clinical research experience.
- General Manager: Ginger Switzer
- Conducted >600 clinical trials
- Full service phase I unit with 25 beds
- Tobacco and Vape
- Hypertension
3475 Richmond Rd. 3rd Floor Lexington, KY 40509 859-264-8999
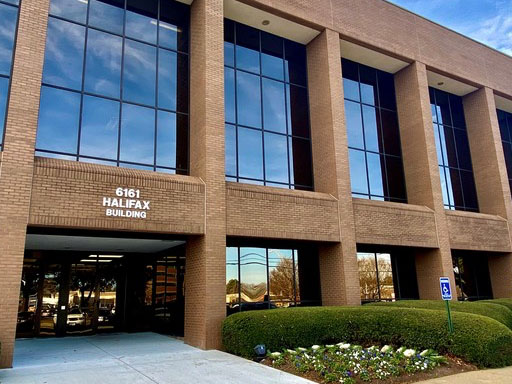
AMR Clinical Research Associates of Tidewater/(CRAT) in Norfolk, Virginia was founded in 1983 and has conducted 700 Phase I–IV trials. The center is conveniently located on the campus of Eastern Virginia Medical Complex, home to the area’s only Level One Trauma Center; Children’s Hospital of the King’s Daughters and Eastern Virginia Medical School. The center has four beds, which allows for the conduct of overnight studies. The Norfolk location is currently investing in a PBMC laboratory, which opened in 2018.
- Founded in 1983
- Conducted more than 600 clinical trials
- Primary specialties include: vaccines, women’s health, men’s health, osteoarthritis, smoking/smoking cessation, obesity, neurology (DPN, PHN, migraines) and dermatology
- Women’s & men’s health
- Tobacco/smoking cessation
6161 Kempsville Circle Suite 225 Norfolk, VA 23502 757-627-7446 757-624-1121 fax
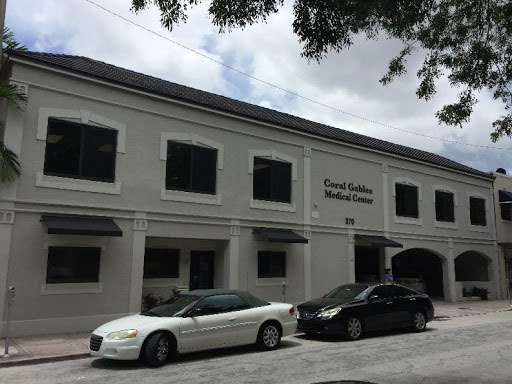
Founded in Coral Gables, Florida in 1982, AMR Miami conducted 842 Phase I-IV clinical trials in a wide range of therapeutic areas. This facility has two beds for overnight studies and has access to a 16-bed fully staffed Phase I center. In addition, AMR Miami has access to two PBMC labs which are actively in use. AMR Miami has strong ties with the local community and its diverse population. This site also maintains a strategic partnership with PrimeHealth Physicians, a 40-provider primary care practice in the Miami/Coral Gables area; a key partner for the sourcing of trial participants.
- Healthy Blood Draw
- Rheumatology/Musculoskeletal
370 Minorca Ave Coral Gables, Florida 33134 305-445-5637 [email protected]
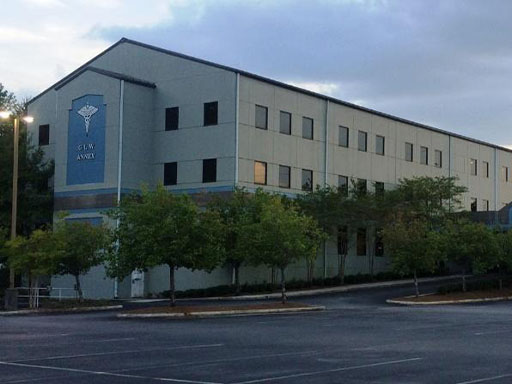
Founded in 1987, AMR Coastal Clinical Research was the first free-standing multispecialty research site in the state of Alabama. To date, the Mobile location has conducted more than 851 biotech and pharmaceutical-sponsored clinical research trials in Phases I-IV.
The Mobile location features a unique mixture of seasoned Investigators with different board-certified specialties that has resulted in stability and steady growth during their 30+ years in business. Mobile’s database includes subjects from the local community, surrounding areas, and parts of Mississippi, Florida and Louisiana. The Mobile location is also on the grounds of Springhill Medical Center in Mobile, AL, with access to all of their diagnostic and medical equipment. The site has an overnight capacity of 8 beds.
This location is the largest dedicated research site in Mobile with competition insignificant to new business and the growth of its database. The Investigator’s reputable private practices are separate from CCR and provide another avenue for recruiting quality subjects.
- Orthopedics
- Smoking Cessation
100 Memorial Hospital Drive Annex Building, Suite 3-B Mobile, AL 36608 251-414-1984 [email protected]
New Orleans
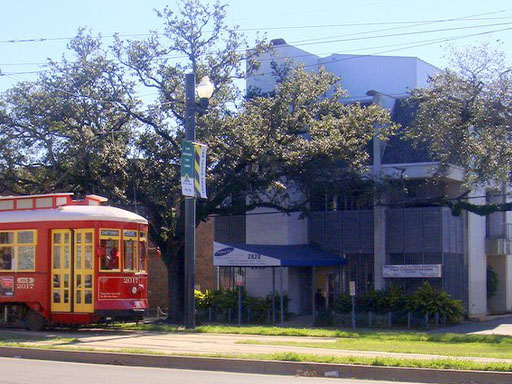
NOCCR-New Orleans was established in 1985 and has conducted over 900 trials in Phase I-IV. NOCCR is a dedicated research clinic located in the Mid-City area on Canal Street. The unit has extensive experience in tobacco trials, women’s and men’s health, vaccines, diabetes, hypertension as well as other indications. Being located close to Tulane, Ochsner and University Medical Centers allows NOCCR to have remote access to DEXA, Interventional Radiology, EEGs, Treadmills, etc. The unit has a catchment area of approximately 1.3 million people and maintain a subject database of over 45,000 subjects.
- Healthy volunteer
- Rheumatology / musculoskeletal
- Internal medicine
- Smoking cessation
2820 Canal Street New Orleans, LA 70119 504-821-8158 [email protected]
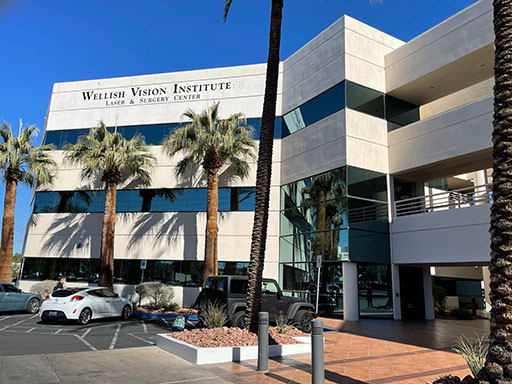
AMR Las Vegas, formerly Clinical Research Consortium, was founded in 2003 and has conducted 250+ Phase I-IV trials. The Las Vegas, NV location has 8 overnight beds and has recently doubled the size of the recruitment staff to six employees. Located across the street from Desert Springs Hospital, AMR Las Vegas has strong ties with the local community and its diverse population. The center has an active daily pre-screening clinic to pre-identify potential subjects for various studies that are ongoing and upcoming.
- Founded in 2003
- Conducted >250 clinical trials
- Full-service phase 1 unit with 8 beds
- Primary specialties include: vaccines, respiratory/pulmonary, musculoskeletal, immunology, genitourinary, endocrine/metabolic, chronic pain, devices.
- Tobacco/Smoking Cessation
- Healthy Volunteer
2110 E Flamingo Rd Suite 308 Las Vegas, NV 89119 To reach the receptionist, please call: 702-597-9825 If you are interested in participating in a study, please call: 702-545-6840
702-597-1596 fax
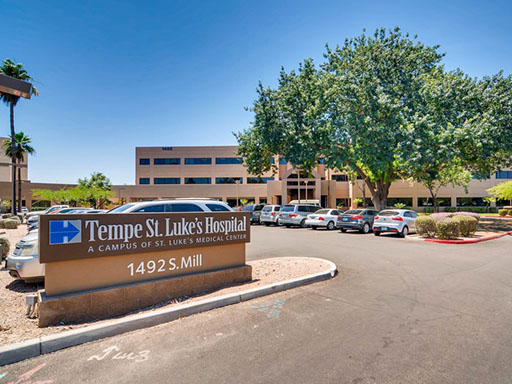
AMR Phoenix, formerly Clinical Research Consortium, is a dedicated multispecialty clinical research center that has been in operation since 2011 and has conducted 250+ Phase I-IV Clinical Trials. Dr. Corey Anderson, Principal Investigator, has over 18 years of clinical trials experience across an ever-expanding array of therapeutic areas and indications. AMR Phoenix is conveniently located in the medical office building on the campus of Tempe St. Luke’s Hospital and has 8 overnight beds. The center has an active daily pre-screening clinic to pre-identify potential subjects for various studies that are ongoing and upcoming.
- Founded in 2011
- Primary specialties include: vaccines, respiratory/pulmonary, musculoskeletal, immunology, genitourinary, endocrine/metabolic, chronic pain, device, dermatology.
1492 S. Mill Ave. Suite 312 Tempe, AZ 85281 602-759-7559
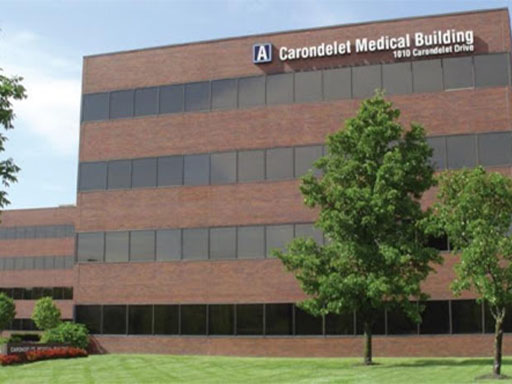
AMR’s Kansas City, Missouri location, the Center for Pharmaceutical Research, has conducted more than 730 Phase I–IV trials since 1986 and owns a database of more than 29,000 volunteers. Staff includes more than 33 full-time employees with 274-plus years of combined research experience. The Kansas City center is located on the Joint Commission-accredited St. Joseph Medical Center campus and works with a major imaging center to include access to a 3.0 Tesla MRI.
Since 2005, the Kansas City team has conducted more than 90 vaccine trials, including 12 Phase I vaccine studies and numerous complex vaccine trials including PBMC samples, with Institutional Biosafety Committees, government contracts, complex vaccine compounding, and Biosafety Level -2 certification. Kansas City has successfully passed four FDA audits, not for cause, with no findings or 483s. In addition, this ocation has recently finalized a relationship that will allow access to 32 hospital beds for overnight studies.
- General Manager: Barbara Bradshaw
- Founded in 1986
- Conducted >730 clinical trials
1010 Carondelet Drive Suite 426 Kansas City, MO 64114 816-943-0770 [email protected]
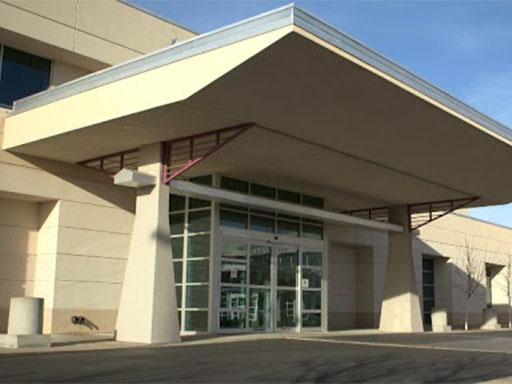
AMR – Newton has been conducting high-quality clinical research since 2003 as Heartland Research Associates; including more than 375 Phase I–IV pharmaceutical and device trials. Over the years, they have completed two not-for-cause FDA audits of which neither resulted in any findings or any FDA Form 483s being issued.
The Newton location is connected to Axtell Clinic, a large, five-provider owned, family practice medical facility, which has been providing quality medical care to Newton, Kansas and the surrounding communities for more than 100 years. This creates an excellent partnership between research and practicing medicine in which the subjects have an established history of trust with the center’s investigators. This location employs 22 staff and has six dedicated research exam rooms and 20 overflow exam rooms available.
- General Manager: Shannon Thomas
- Managing Principal Investigators: Richard M. Glover II,MD; Stacy B. Slechta, DO; William R. Beck, MD; Robyn D. Hartvickson, MD; Troy A. Holdeman, MD
- Conducted >1,400 clinical trials
- Adult vaccines
- Infant vaccines and maternal health
- Women’s health
700 Medical Center Drive Suite 110 Newton, KS 67114 316-804-7301
Wichita – East
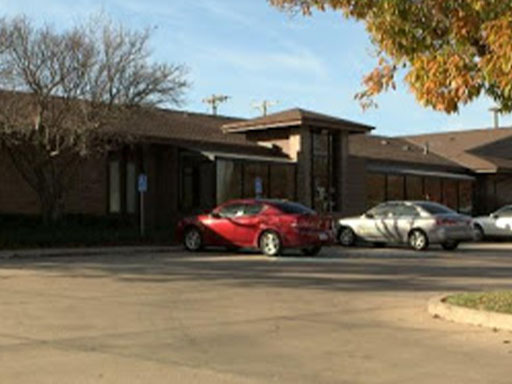
AMR Heartland Research Associates, Wichita – East has been a leader in clinical research studies since 1991, conducting more than 730 Phase I–IV pharmaceutical and device trials. The center has completed nine FDA audits, not-for cause, of which none resulted in any findings or 483s. The group is located in the well-established Wichita, Kansas area, with its research operation constructed within Family Medicine East, a private practice clinic, which is also owned and operated by primary investigators. The strong and trusting physician/patient relationships aid in successful recruitment, compliance and retention. This location employs 36 staff, has 6 dedicated research exam rooms, 30 overflow exam rooms, and two beds for overnight studies.
- Primary specialties include: vaccines, women’s health, diabetes, osteoarthritis, obesity, dermatology, and gastroenterology
Speciaties:
- Plastic surgery
- Rheumatology
- Alzheimer’s disease
- Intra-articular injections
- Metabolic disorders
1709 S. Rock Road Wichita, KS 67207 316-689-6635
Wichita – West
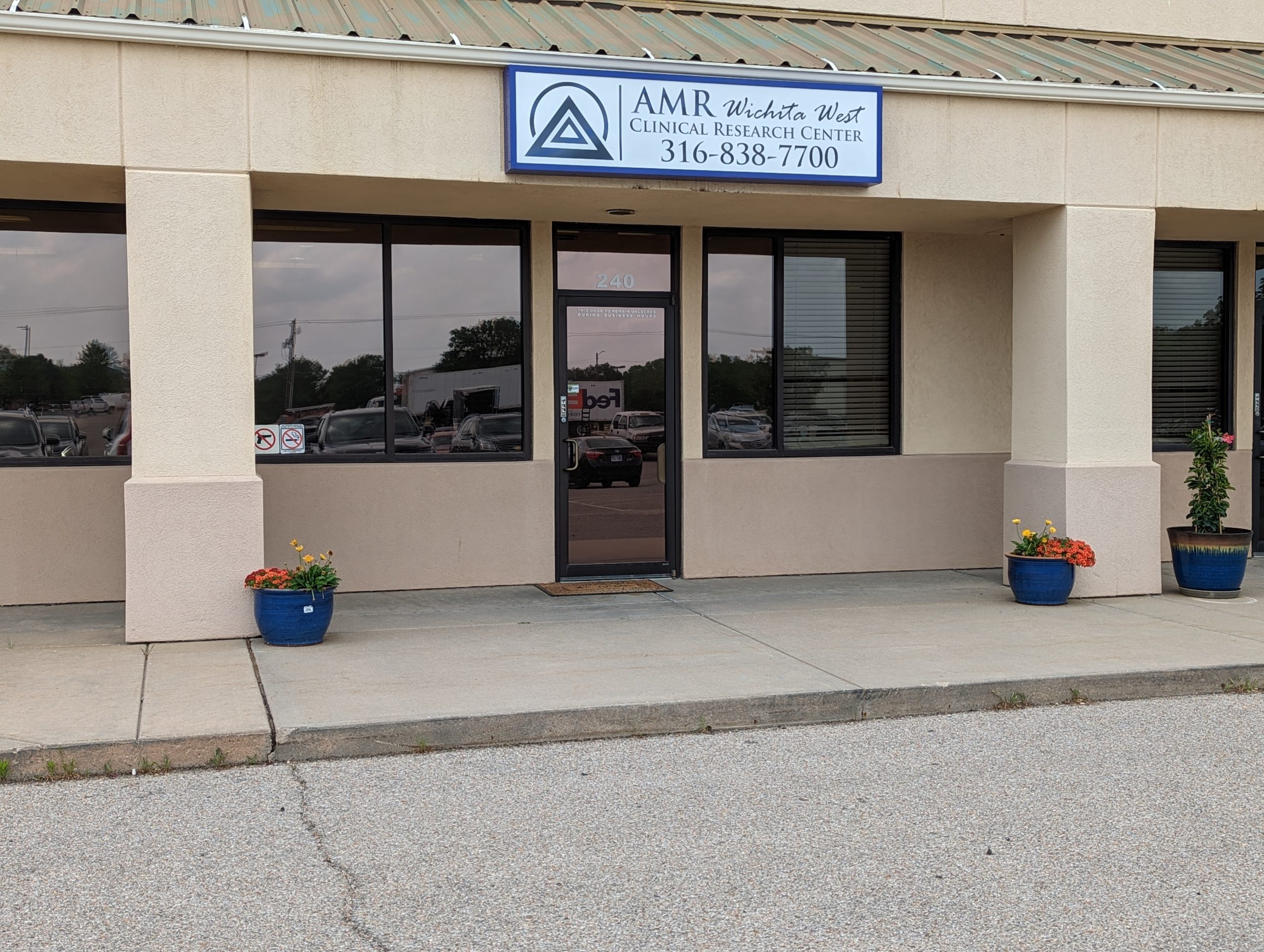
AMR Heartland Research Associates Wichita – West was established in 2001 and has proudly conducted more than 215 Phase I–IV pharmaceutical and device trials. Recently, the site completed a not-for-cause FDA audit, which did not result in any findings and no FDA Form 483s were issued. This large database of established patients, who trust in the center’s investigators, makes for quality subjects with verifiable medical histories. This location employs 11 staff, has five dedicated research exam rooms, and 20 overflow exam rooms available.
- General Manager: Shannon Thomas
- Managing Principal Investigators: Richard Egelhof, MD; William Simon, DO; Paula Worley, MD
- Women’s health
- Alzheimer’s
2260 N. Ridge Rd, Suite 240 Wichita, KS 67205 316-838-7700
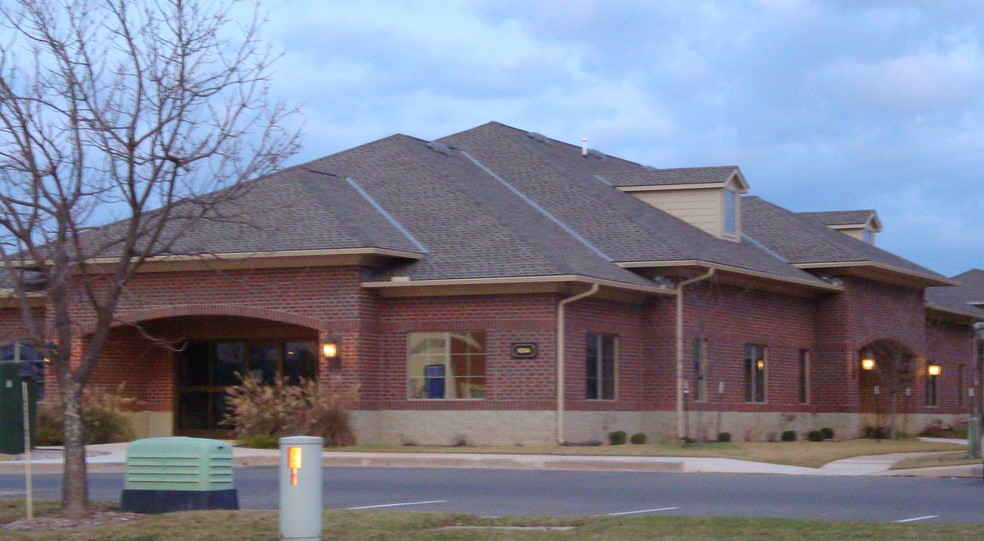
AMR Norman is located in the heart of the Midwest – just minutes from Oklahoma City in Norman, Oklahoma. Previously Intend Research, this research center joined AMR officially in mid 2022. Lisa Connery, MD, has provided her medical experience as the site’s Principal Investigator since 2016. AMR Norman has experience with anemia, chronic lower back pain, constipation, diabetes (Type I and Type II), fibromyalgia, hypertension and more. Our full-time research team includes clinical research coordinators, back-up coordinators, patient recruiters, lab coordinator, data entry coordinator, regulatory specialist, and administrative staff. We provide our community with innovative treatments and the highest quality of care throughout each clinical research trial.
1016 24th Ave., NW Suite 100 Norman, OK 73069 405-701-8999
Kansas City Oncology
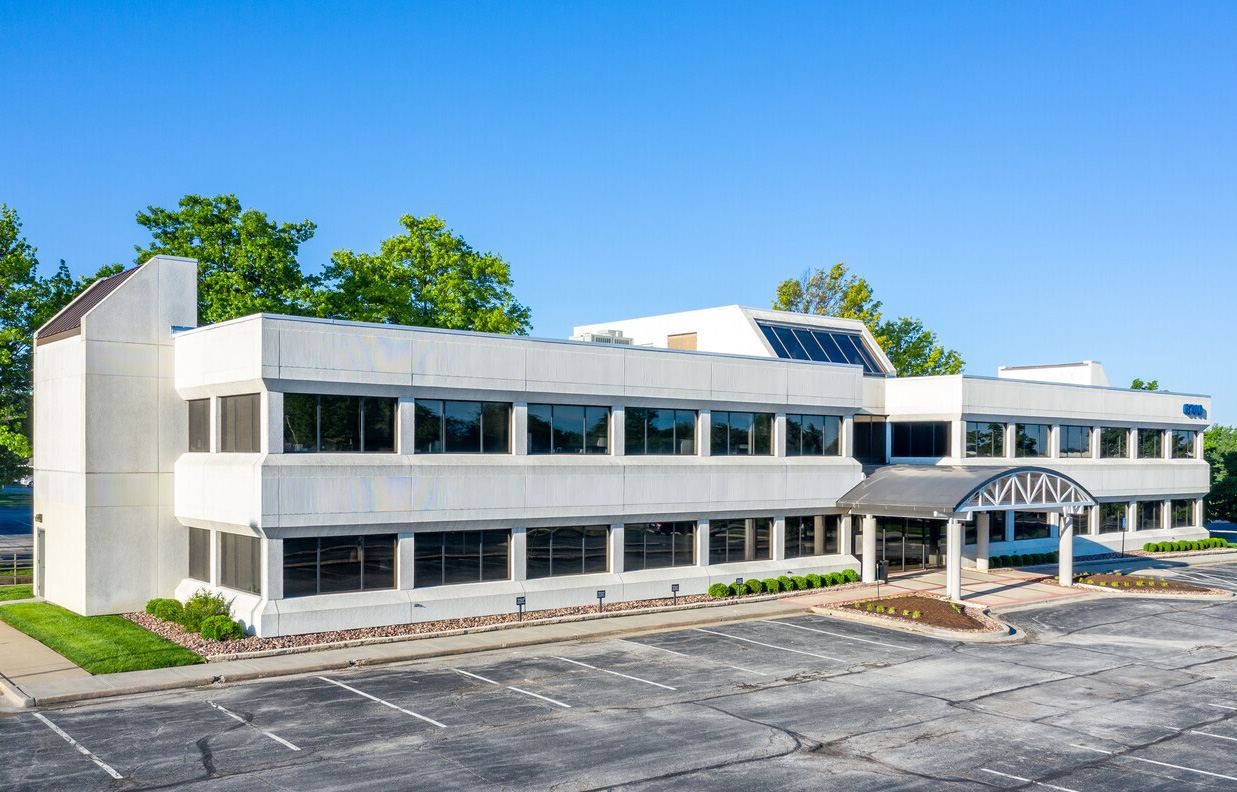
AMR Kansas City Oncology has conducted 75 clinical trials. Recent experience includes Breast Cancer, Cancer Associated Pain, Carcinoma of unknown primary site, Colon Cancer, Esophageal Cancer, Hepatocellular Carcinoma (HCC), Immune Thrombocytopenia (ITP), Leukemia, Lung Cancer, Lymphoma, Melanoma, Myelodysplastic syndromes, Myeloma, Neuroendocrine Cancer, Prostate Cancer, Solid Tumors, Squamous Cell Carcinoma, and Pancreatic Cancer.
6740 Antioch Rd #101 Merriam, KS 66204 913-386-7556
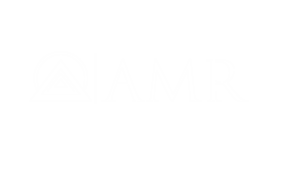
801 North Weisgarber Rd, Suite 100 Knoxville, TN 37909 (615) 591-0211 [email protected]
Join Our Mailing List
- Name First Last
- Phone This field is for validation purposes and should be left unchanged.
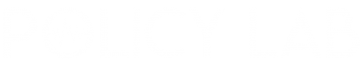
- Diversity in Clinical Research
- Clinical Research for Older Populations
- Clinical Research for Compensation
- Clinical Trial Information for Parents
- What Is A Clinical Trial?
Kentucky Paid Clinical Trials
Match to clinical trials, popular cities, search clinical trials in kentucky by condition.
This content is for informational and educational purposes only. It is not intended to provide medical advice or to take the place of such advice or treatment from a personal physician. All readers/viewers of this content are advised to consult their doctors or qualified health professionals regarding specific health questions. Policylab.us does not take responsibility for possible health consequences of any person or persons reading or following the information in this educational content. All viewers of this content, especially those taking prescription or over-the-counter medications, should consult their physicians before beginning any nutrition, supplement or lifestyle program
Subscribe To Our Newsletter | Make a Donation
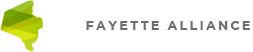
We fund research to provide objective data to support our advocacy work and inform decisions around land-use policy in Lexington-Fayette County.
It’s not “if” we grow, it’s “how.” Fayette Alliance focuses on “how” we grow by working with experts to conduct research on the complex issues facing our community’s development.
By basing our positions on objective research and studies, Fayette Alliance advocates for intentional growth strategies that leverage, not threaten, the things that make Lexington a great place to live, work, and play.
By compiling facts related to our land-use and economic development , Fayette Alliance keeps you and our decision-makers better informed.
By advocating for data-driven policies and laws, Fayette Alliance promotes real change.
By sponsoring research related to local land-use and economic development, we encourage data-driven policies and solutions for our citizens.
2022 Breeders’ Cup Economic Impact Study
View the Economic Impact Study
Lexington Grow Smart Study, Lord Aeck Sargent, 2021
See the Grow Smart Study
Fayette County Housing Demand Study, Urban Partners, 2017 See Fayette County Housing Demand Study
Agriculture Impact Study, Community and Economic Development Initiative of Kentucky College of Agriculture, Food, and Environment University of Kentucky, 2017 See Agriculture Impact Study
Cost of Community Services Study, Community and Economic Development Initiative of Kentucky College of Agriculture, Food, and Environment University of Kentucky, 2017 See Cost of Community Services Study
Underutilized Properties Study, Lord Aeck Sargent, 2017 See Underutilized Property Study
Rural Trails Plan, Part of Grow Smart 2017, Lord Aeck Sargent See Rural Trails
Lexington Quality of Life Survey, The Matrix Group, 2013 See Lexington Quality of Life Study
Kentucky Equine Survey, Kentucky field office of the National Agricultural Statistics Service, 2012 See Kentucky Equine Survey
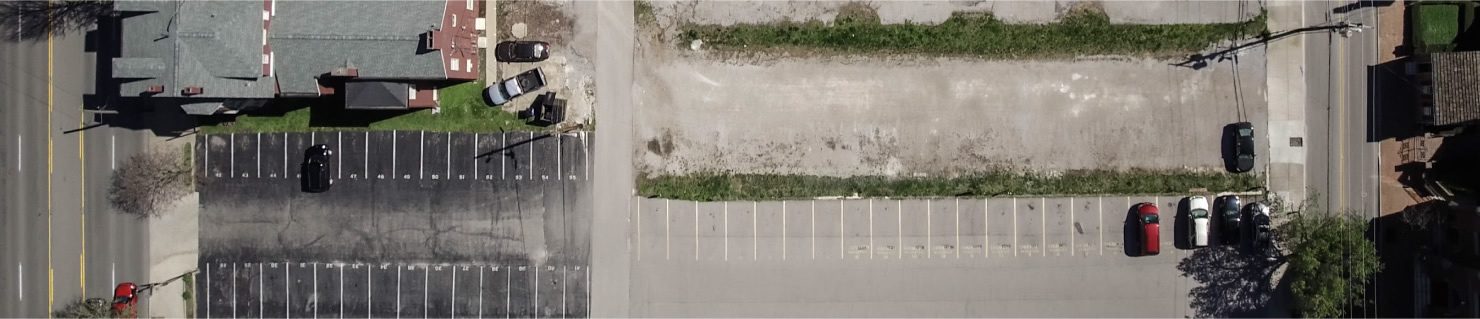
169 N. Limestone, Suite 1B Lexington, KY 40507 (859) 281-1202
Facebook Twitter Instagram
- Annual Reports
- Name This field is for validation purposes and should be left unchanged.
Receive our monthly newsletter with curated land-use content related to Fayette County. In addition, we occasionally send advocacy alerts, event notifications, and breaking news.
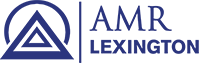
Bringing better medicine to life.
Participate in studies, with your help, we can help advance medical knowledge and improve the health and well-being of countless others., 〉 available studies, 〉 frequently asked questions, 〉 volunteer form.
Our goal is to assist the pharmaceutical and medical device industries develop new and effective products to treat a multitude of medical conditions and illnesses. We perform this important work through the administration of clinical trials.
Please consider joining us in this effort. Our experienced and professional clinical research staff are highly qualified and fully committed to engaging you and your primary care physician as informed partners in everything we do.
Available Studies
See if you pre-qualify for any of the research studies we are now conducting in your area by following one of the links below., asthma clinical research study.
AMR Lexington is accepting volunteers for an investigational Asthma clinical research study.
Qualified participants must:
· be 18 to 55 years of age · have moderate to severe uncontrolled asthma · have documented diagnosis of asthma 12 months prior to screening visit · meet other study criteria
Qualified participants will receive at no cost:
· all study-related medical exams · lab tests · investigational medication or placebo · study-related care overseen by a licensed Physician
Compensation up to $1,240.00 is available for time and travel. Insurance is not required.
Healthy Volunteers Vaccine Studies
Gout treatment 18 to 85.
AMR Lexington is seeking volunteers for investigational gout clinical research studies.
· be ages 18 to 85 · meet other study criteria
· all study-related medical examinations and lab work · the investigational medication
Compensation is available for time and travel. Insurance is not required. Contact AMR Lexington at 888-259-1231 to learn more today!
Investigational Gout Study
An attack of gout can occur suddenly, often waking sufferers up in the middle of the night with the sensation that one’s big toe is on fire. The affected joint is hot, swollen and tender to the touch. While Hyperuricemia and gout – a form of arthritis – can occur in any joint, it is primarily diagnosed in patients’ feet and toes.
That is why AMR Lexington is conducting a clinical research study of an investigational medication for Hyperuricemia and gout.
· be otherwise healthy adults ages 18 to 85 · have Hyperuricemia and a history or presence of gout · meet other study criteria
· all study-related medical exams and lab work · investigational medication
Compensation up to $1,624 is available for time and travel. Health insurance is not required. Contact AMR Lexington today at 888-259-1231 to see if you qualify.
Investigational Cardiovascular Disease Clinical Study
Cardiovascular disease is risky business! That’s why AMR Lexington is conducting a clinical trial of an investigational medication to potentially reduce major adverse cardiovascular events in those with a previous history of adverse cardiovascular events and in those who are at high cardiovascular risk.
· be ages 18 and older with a history of a major cardiovascular event Or · be ages 50 and older who are at high risk for having their first major cardiovascular event · meet other study criteria
Qualified participants will receive:
· all study-related care and the investigational medication or placebo at no cost · compensation up to $2,550.00 for time and travel
Learn more by calling AMR Lexington at 888-259-1231 today!
High Cholesterol Treatment 18+
AMR is currently accepting volunteers to participate in a high cholesterol clinical research study. Qualified participants are those ages 18 and older who meet other study criteria. Qualified participants will receive all study-related care and the investigational treatment or placebo at no cost. Compensation may be available up to $1,350.00 for study-related time and travel.
Investigational Medication for Diabetic Peripheral Neuropathic Pain
Diabetic Peripheral Neuropathic Pain: the most common type of diabetic neuropathy that affects the feet and legs first. It significantly impacts the quality of life in walking ability, sleep, work, and general activity. Currently, there are only four medications approved by the FDA for DPNP and clinical guidelines recommend pain relief through antidepressants, opioids, and topical medications. AMR Lexington is evaluating an investigational medication in adult participants with Diabetic Peripheral Neuropathic Pain to potentially lessen pain intensity.
· be 18 and older · have Type 1 Diabetes or Type 2 Diabetes diagnosed at least six months prior · be on a stable diabetes treatment · be willing to have a metformin dose modification · meet other study criteria
· all study-related care and health monitoring · the investigational medication or placebo
Compensation is available up to $1,533.00 for time and travel. To learn more, call AMR Lexington at 888-259-1231 today.
Investigational RSV/hMPV Vaccine Clinical Study
Join a clinical study of an investigational RSV/hMPV mRNA vaccine. AMR is conducting an investigational Respiratory Syncytial Virus and human MetaPneumo Virus mRNA vaccine clinical trial and is seeking healthy seniors to participate.
· be 60 and older · be considered healthy · meet other study criteria
Receive study-related care and the investigational vaccine or placebo at no cost. Compensation is available up to $2,010.00 for time and travel. Learn more by calling AMR at 888-259-1231 today.
Clinical Study of an Investigational Weight Loss Medication for Type 2 Diabetics
AMR Lexington is conducting a study to test an investigational weight loss medication for overweight or obese Type 2 Diabetics.
· be ages 18 and older · be on stable treatment or diet and exercise for Type 2 Diabetes · have a BMI of 27 or greater · meet other study criteria
· all study-related care · the investigational study drug or placebo
Compensation for time and travel may be provided. Learn more today! Call AMR Lexington at 888-259-1231.
Investigational Weight Loss Medication Clinical Study
AMR Lexington is currently studying an investigational medication to potentially help people lose weight who are living with obesity.
· be ages 18 and older · have a BMI of 30 or more; or · have a BMI of 27 or more with at least one weight-related complication such as hypertension, dyslipidemia, obstructive sleep apnea, cardiovascular disease, or non-alcoholic fatty liver · not have Diabetes · meet other study criteria
Compensation for time and travel may be available. To learn more, call AMR at 888-259-1231 today!
Research Study of Investigational Heated Tobacco Products
Frequently asked questions, how are clinical trials conducted.
In Phase I trials, researchers test an experimental drug or treatment in a small group of people (20-80) for the first time to evaluate its safety, determine a safe dosage range, and identify side effects.
In Phase II trials, the experimental study drug or treatment is given to a larger group of people (100-300) to see if it is effective and to further evaluate its safety.
What is a protocol?
A protocol is a study plan on which all clinical trials are based. The plan is carefully designed to safeguard the health of the participants as well as answer specific research questions. A protocol describes what types of people may participate in the trial; the schedule of tests, procedures, medications, and dosages; and the length of the study. While in a clinical trial, participants following a protocol are seen regularly by the research staff to monitor their health and to determine the safety and effectiveness of their treatment.
Who sponsors clinical trials?
How is the safety of the participant protected, what are the benefits and risks of participating in a clinical trial.
Clinical trials that are well-designed and well-executed are the best approach for eligible participants to:
Play an active role in their own health care. Gain access to new research treatments before they are widely available. Obtain expert medical care at leading healthcare facilities during the trial. Help others by contributing to medical research. Risks
There are risks to clinical trials.
What is informed consent?
What happens during a clinical trial, who can participate in a clinical trial.
All clinical trials have guidelines about who can participate. Using inclusion/exclusion criteria is an important principle of medical research that helps to produce reliable results. The factors that allow someone to participate in a clinical trial are called “inclusion criteria” and those that disallow someone from participating are called “exclusion criteria”. These criteria are based on such factors as age, gender, the type and stage of a disease, previous treatment history, and other medical conditions. Before joining a clinical trial, a participant must qualify for the study. Some research studies seek participants with illnesses or conditions to be studied in the clinical trial, while others need healthy participants. It is important to note that inclusion and exclusion criteria are not used to reject people personally. Instead, the criteria are used to identify appropriate participants and keep them safe. The criteria help ensure that researchers will be able to answer the questions they plan to study
Why participate in a clinical trial?
Participants in clinical trials can play a more active role in their own health care, gain access to new research treatments before they are widely available, and help others by contributing to medical research.
What is a clinical trial?
Although there are many definitions of clinical trials, they are generally considered to be biomedical or health-related research studies in human beings that follow a predefined protocol. ClinicalTrials.gov includes both interventional and observational types of studies. Interventional studies are those in which the research subjects are assigned by the investigator to a treatment or other intervention, and their outcomes are measured. Observational studies are those in which individuals are observed and their outcomes are measured by the investigators.
Volunteer Form
By volunteering for clinical studies, you authorize AMR to store your personal information on file. In accordance with HIPAA regulations, AMR will never share your information with anyone.
Respiratory Syncytial Virus
It is estimated that more than 177,000 older adults are hospitalized and 14,000 of them die in the us due to rsv infections per year. in 2018, more elder americans died from rsv infection than the common flu., to learn more, call our enrollment line:, refer-a-friend, earn extra cash* by recommending a friend to us and receive up to $100 for each friend you refer.
Read Important Instructions
* Some restrictions apply. Not all studies are eligible for the Refer-A-Friend program. Ask an AMR representative for details.
Fill out the Refer-A-Friend form. You can download the form here or pick one up at one of our offices. The referrer (you) must fill out the form.
→ Download Referral Form
Your friend or family member brings the completed referral form to CRC on their first visit to our office and gives it to a staff member upon checking in.
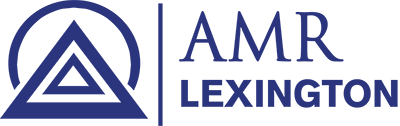
Join Our Mailing List
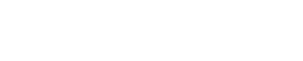
- Buy Lexington Monopoly
- Current Issue
- Health Kentucky
- Advertise in The Lane Report
- Ad Dimensions
- Construction
- Economic Development
- Hospitality
- State Government
- National Government
- Manufacturing
- Real Estate
- Transportation
- Philanthropy
- Wealth Management
- Workforce Development
- Big Moves Submissions
- Executive Profile Submission
- Sponsored E Blast Submission
- Seeking Employment
- Submit Your Upcoming Event
- Lane Report
- General Questions
- Writer’s Guidelines
- Privacy Policy
- Google Plus
Markey research uncovers disruption pathway for breast cancer treatment
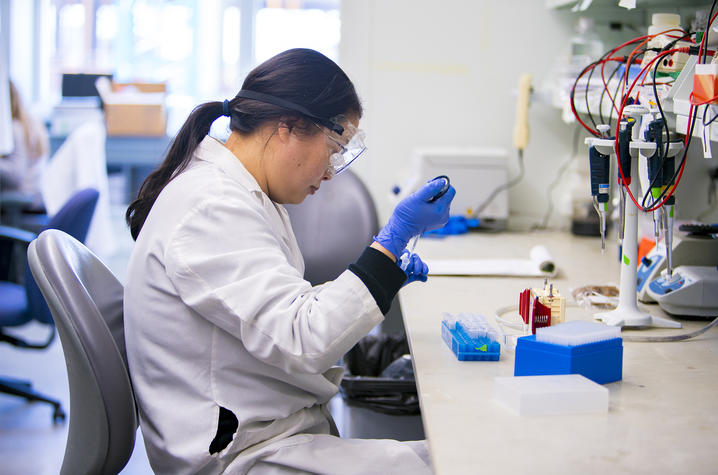
LEXINGTON, Ky. — A recent University of Kentucky Markey Cancer Center study published in PNAS uncovers a critical pathway involved in immune evasion by breast cancer cells that addresses a crucial gap in the understanding of how breast cancer fosters immune evasion and offers a new potential target for cancer therapies.
The study is led by UK Markey Cancer Center researcher Yadi Wu, Ph.D.
The research suggests a chain reaction between three different proteins – CDK9, RNF20 and LSD1 – helps breast cancer cells evade the immune system. By disrupting this chain, researchers made the cells more susceptible to immunotherapy treatment.
“The results suggest targeting this pathway in the development of cancer therapies ,” said Wu, an associate professor in the UK College of Medicine department of Pharmacology and Nutritional Sciences . “This new knowledge could potentially lead to more effective breast cancer treatments for patients.”
Cancer cells can avoid immune detection by manipulating genetic signals. One such way is through LSD1, a protein involved in regulating gene expression. High levels of LSD1 are found in many cancers and are linked to worse outcomes for patients. Studies show that blocking LSD1 can help immune cells fight cancer more effectively.
The lab study on breast cancer cells revealed that CDK9, another protein involved in regulating gene expression, plays a crucial role in stabilizing LSD1 and therefore causing immune suppression. This stabilization is achieved through a process involving the third protein, RNF20.
When researchers disrupted this interplay by removing RNF20, breast cancer cells became sensitized to a type of immunotherapy treatment called anti-PD-1.
“This discovery is a significant step forward in our understanding of how cancer cells evade the immune system,” said Wu. “Our findings highlight the potential importance of targeting the CDK9-RNF20-LSD1 pathway in the development of new cancer therapies, specifically immunotherapies.”
— By Elizabeth Chapin
You may also like
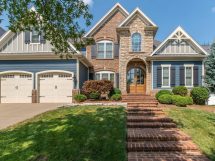
Kentucky realtors reacting to NAR commission settlement
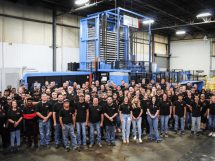
Store checkout fixture maker Pan-Oston investing $7M in expansion
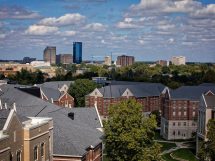
- Construction projects to begin on UK’s south campus
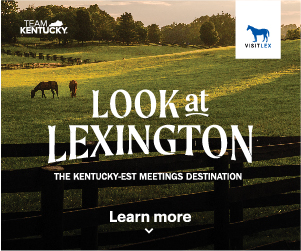
Popular Stories
Diageo na expands don’t trash glass recycling to kentucky.
- Ky-made Camry, Hybrid Camry named Best Family Midsize
March mindfulness: Staying calm amidst the ‘Madness’
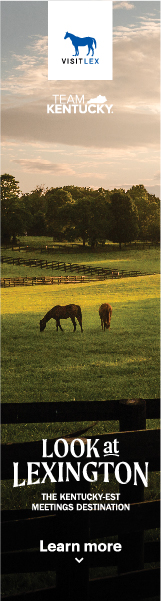
The Lane Report
- March mindfulness: Staying calm amidst the ‘Madness’
- Diageo NA expands Don’t Trash Glass recycling to Kentucky
- More flights, bigger aircraft for Derby 150 at Louisville
- Holley Performance to engage 500,000 enthusiasts at 2024 events
- Big Dig returns in NKy for skilled construction workers of tomorrow
- Event connects minority business with procurement network
- More smoking cessation needed in cancer care, study finds
- UK folklorist explains 1500-year-old history of St. Patrick’s Day
- Campus News
- Student News
- UK HealthCare
- UK Happenings
- Arts & Culture
- Professional News
Register for the UK Women Innovators Network Conference: ‘Empowering Women’
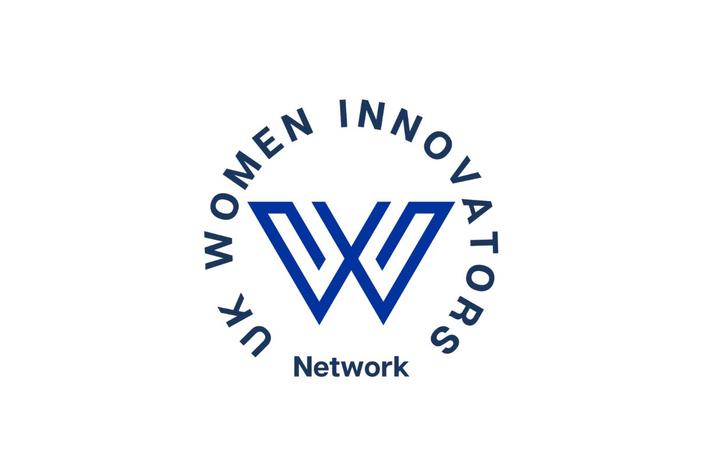
LEXINGTON, Ky. (March 18, 2024) — The University of Kentucky Office of Technology Commercialization (OTC) is hosting its annual UK Women Innovators Network (UKWIN) conference next week.
This year’s conference focuses on the theme "Empowering Women: Leveraging Tech Transfer & Entrepreneurial Resources for Innovation Commercialization.” The event will take place 3-5 p.m. Tuesday, March 19, in Ballroom 212A of the Gatton Student Center.
The UKWIN program has a mission to increase the number of UK women who participate in innovation. This conference offers opportunities for UK and Lexington women to connect, network and learn from one another.
“Empowering women in innovation is not just about breaking barriers, it’s about reshaping the future. I hope that people will join us in celebrating the strength, creativity and leadership of women driving change in the world of innovation,” said OTC Director Taunya Phillips.
The keynote speaker is Almesha Campbell, Ph.D., the assistant vice president for research and economic development at Jackson State University (JSU) and past chair of AUTM, “the non-profit leader in efforts to educate, promote and inspire professionals to support the development of academic research.”
Campbell designs and manages programs around innovation, technology transfer and commercialization to broaden the participation of underrepresented minorities in these areas. She also co-led the development of the JSU Center for Innovation and Entrepreneurship and created the JSU Innovation Fellows Program.
Kendra Stenzel, Ph.D., OTC’s commercialization manager for medical devices, will serve as the panelist moderator for the event.
The panel includes Janine Barnett, perinatal research assistant director in the College of Nursing; April Fernando, Ph.D., associate director of the Center for Innovation in Population Health in the College of Public Health (CPH); Michelle Fernando, CPH operations director; and Angela Gutierrez Echeverri, Ph.D., UK Superfund Research Center (SRC) liaison and a postdoctoral trainee.
Anyone interested in attending the event can register online here .
About OTC The Office of Technology Commercialization (OTC) mission is to advance innovation that makes a difference. OTC supports the university’s strategic plan by committing to help build Kentucky’s innovation ecosystem and collaborate with industry partners worldwide; work cooperatively with innovators to strategically assess, protect, and license early-stage technologies; and to cultivate entrepreneurship and co-create new technology startups.
As the state’s flagship, land-grant institution, the University of Kentucky exists to advance the Commonwealth. We do that by preparing the next generation of leaders — placing students at the heart of everything we do — and transforming the lives of Kentuckians through education, research and creative work, service and health care. We pride ourselves on being a catalyst for breakthroughs and a force for healing, a place where ingenuity unfolds. It's all made possible by our people — visionaries, disruptors and pioneers — who make up 200 academic programs, a $476.5 million research and development enterprise and a world-class medical center, all on one campus.
In 2022, UK was ranked by Forbes as one of the “Best Employers for New Grads” and named a “Diversity Champion” by INSIGHT into Diversity, a testament to our commitment to advance Kentucky and create a community of belonging for everyone. While our mission looks different in many ways than it did in 1865, the vision of service to our Commonwealth and the world remains the same. We are the University for Kentucky.
Latest Stories
Heather bush named permanent dean of uk college of public health, behind the blue: heart surgeon tessa london-bounds discusses study on access, equity for breastfeeding surgeons, uk student, ffa officer represents kentucky for international leadership experience in australia, projects to begin on uk’s south campus, march mindfulness: staying calm amidst the 'madness'.
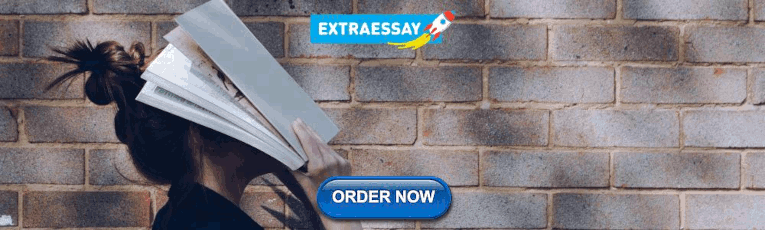
IMAGES
VIDEO
COMMENTS
Learn more about participating in research studies! ... The program allows students from underrepresented backgrounds and communities to get a jumpstart in health equity research early in their college experience. ... Lexington, Kentucky 40506; 859.323.2615; Social Media. Feedback.
Central Kentucky Research Associates is now AMR Lexington. ... principal investigator. We handle all regulatory correspondence, contracts and budgets, plus training and placement of study-dedicated research coordinators. We recruit participants, gather data, provide complete documentation and follow study participants. ... 3475 Richmond Rd ...
Research and development at UK has a direct economic impact on Kentucky. The $476.5 million UK spent on R&D in FY22 generated $873 million in economic activity across Kentucky. ... The Campbell House, 1375 S Broadway, Lexington, KY 40504. 1:00pm - 7:00pm. Mar. 19. 2024 UK WOMEN INNOVATORS NETWORK EVENT. Ballroom 212A, Gatton Student Center. 3 ...
Match to Clinical Trials. There are currently 547 clinical trials in Lexington, Kentucky looking for participants to engage in research studies. Trials are conducted at various facilities, including University of Kentucky, University of Kentucky/Markey Cancer Center, Saint Joseph Hospital East and Baptist Health Lexington.
We have a variety of studies for individuals with and without memory loss. To discuss how you can participate please call us at (859) 323-5550. Alternatively, you can give us some basic information by completing our online research interest form and we will get back in touch with you soon. Click here to access the research interest form.
Explore current research participation opportunities at the University of Kentucky. If you find a study that interests you, you can contact the research team directly or submit your contact information on the study page and they'll get in touch with more info. ... Lexington, Kentucky 40506; 859.323.2615; Social Media. Feedback. University of ...
For questions about research studies, visit any of our Participant Recruitment Services webpages. For questions about your rights as a participant, contact the Office of Research Integrity at 859-257-9428 or 1-866-400-9428. Clinical Trials Help UK Serve, Heal Kentuckians. Watch on.
If you have questions about participating in research or current studies at UK, please contact us at [email protected] or call 859-218-6822 or 859-218-6765.
The research agenda of the institute is supported, developed and implemented by a group of 150 faculty representing 14 colleges. 311 Main Building. University of Kentucky. Lexington, KY 40506-0032.
At Markey, we have several clinical trials and research studies open at any one time. In fact, through our Research Network, we offer patients access to clinical trials in multiple locations in Kentucky and West Virginia, serving the people of Kentucky and Appalachia close to home. Markey has more open clinical trials than any other cancer ...
The University of Kentucky Alzheimer's Disease Research Center (UK-ADRC) is a NIH-funded (P30 AG072946) mature and experienced ADRC. The UK-ADRC is housed in the Sanders-Brown Center on Aging (SBCoA), a Kentucky Center of Excellence that facilitates clinical and basic research in healthy brain aging and neurodegenerative disorders.
Baptist Health Lexington Clinical Research Center 1740 Nicholasville Road Lexington, KY 40503. Micheal F. Stephens. Associate Vice President, Research. Baptist Office of Research Administration. Phone: 859-260-6456. Email: [email protected]
Students will receive a foundation in key, introductory elements of research and policy studies through the Core Courses along with additional specialization into various areas of concentration, including quantitative methods, evaluation, or research design, policy studies. ... Lexington KY 40506. Other programs from the College of Education ...
300 N. Washington St., Suite 200 Falls Church, VA 22046, USA Phone: 617.948.5100 Toll Free: 866.219.3440 . Cookie Settings. About Us; Contact Us; Disclaimer
Formerly Central Kentucky Research Associates. AMR Lexington was originally founded in 1991 as Central Kentucky Research Associates (CKRA) by Deborah Dyer, RN, BSN, and the late Jacqueline Smith, RN, BSN. CKRA was Kentucky's first independent clinical drug research facility and one of only a few such facilities in the nation that was owned ...
The Center on Drug and Alcohol Research (CDAR) began in 1990. CDAR conducts research into the biological, psychological, sociopolitical, and clinical aspects of substance abuse and related behavior. The Center conducts federally funded and state-funded research on substance abuse and related problems areas such as intimate violence, criminal ...
Guidance for research participants. The University of Kentucky is pausing select human subject research studies. The health and safety of our community is of paramount importance during the COVID-19 emergency. Read the full statement » UK HealthCare is part of an academic medical center that has a thriving research community.
Open Trials AMR locations nationwide are conducting research studies. Please scroll down to see our current opportunities. Find a clinical study near you. 1-888-259-1231. Skip to content (615 ... Lexington, Kentucky: 859-264-8999 [email protected]. Mobile, Alabama: 251-414-1984 [email protected].
The mentored research program will prepare independent researchers skilled at designing and conducting health services research, leveraging a variety of study designs, using primary data collection approaches, and navigating primary and secondary databases to inform healthcare delivery and health policy. ... Lexington, KY. 859-257-5678. Other ...
BREATHE (Bridging Research Efforts and Advocacy Toward Healthy Environments), located at 2265 Harrodsburg Road in Lexington, Kentucky, is a multi-disciplinary research, outreach, and practice collaborative of the University of Kentucky (UK) College of Nursing, with partners internal and external to UK.BREATHE'S Vision is that all people will have access to clean air and live in healthy ...
AMR - Lexington, Central Kentucky Research Associates, in Lexington, Kentucky has conducted 608 Phase I-IV trials since opening in 1991. ... East has been a leader in clinical research studies since 1991, conducting more than 730 Phase I-IV pharmaceutical and device trials. The center has completed nine FDA audits, not-for cause, of which ...
Kentucky is currently home to 1093 active clinical trials, seeking participants for engagement in research studies. These trials take place at a variety of cities in the state, including Louisville, Lexington, Owensboro and Paducah.Whether you're a healthy volunteer interested in paid medical research or someone seeking trials related to a specific condition, the state offers a diverse array ...
By sponsoring research related to local land-use and economic development, we encourage data-driven policies and solutions for our citizens. 2022 Breeders' Cup Economic Impact Study. View the Economic Impact Study. Lexington Grow Smart Study, Lord Aeck Sargent, 2021. See the Grow Smart Study . Fayette County Housing Demand Study, Urban ...
That is why AMR Lexington is conducting a clinical research study of an investigational medication for Hyperuricemia and gout. Compensation up to $1,624 is available for time and travel. Health insurance is not required. Contact AMR Lexington today at 888-259-1231 to see if you qualify.
The study is led by UK Markey Cancer Center researcher Yadi Wu, Ph.D. The research suggests a chain reaction between three different proteins - CDK9, RNF20 and LSD1 - helps breast cancer cells ...
LEXINGTON, Ky. (March 18, 2024) — The University of Kentucky Office of Technology Commercialization (OTC) is hosting its annual UK Women Innovators Network (UKWIN) conference next week. This year's conference focuses on the theme "Empowering Women: Leveraging Tech Transfer & Entrepreneurial Resources for Innovation Commercialization."