A comprehensive review of water quality indices (WQIs): history, models, attempts and perspectives
- Review paper
- Published: 11 March 2023
- Volume 22 , pages 349–395, ( 2023 )
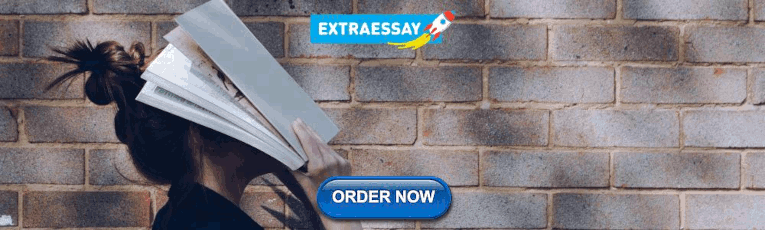
Cite this article
- Sandra Chidiac ORCID: orcid.org/0000-0002-1822-119X 1 ,
- Paula El Najjar 1 , 2 ,
- Naim Ouaini 1 ,
- Youssef El Rayess 1 &
- Desiree El Azzi 1 , 3
16k Accesses
20 Citations
1 Altmetric
Explore all metrics
Water quality index (WQI) is one of the most used tools to describe water quality. It is based on physical, chemical, and biological factors that are combined into a single value that ranges from 0 to 100 and involves 4 processes: (1) parameter selection, (2) transformation of the raw data into common scale, (3) providing weights and (4) aggregation of sub-index values. The background of WQI is presented in this review study. the stages of development, the progression of the field of study, the various WQIs, the benefits and drawbacks of each approach, and the most recent attempts at WQI studies. In order to grow and elaborate the index in several ways, WQIs should be linked to scientific breakthroughs (example: ecologically). Consequently, a sophisticated WQI that takes into account statistical methods, interactions between parameters, and scientific and technological improvement should be created in order to be used in future investigations.
Similar content being viewed by others
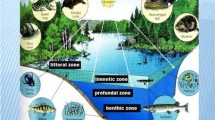
Water quality assessment of lake water: a review
Rachna Bhateria & Disha Jain
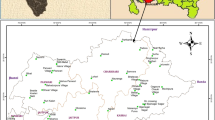
Groundwater quality assessment using water quality index (WQI) under GIS framework
Arjun Ram, S. K. Tiwari, … Y. V. Singh
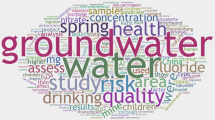
Drinking Water Quality and Public Health
Peiyue Li & Jianhua Wu
Avoid common mistakes on your manuscript.
1 Introduction
Water is the vital natural resource with social and economic values for human beings (Kumar 2018 ). Without water, existence of man would be threatened (Zhang 2017 ). The most important drinking sources in the world are surface water and groundwater (Paun et al. 2016 ).
Currently, more than 1.1 billion people do not have access to clean drinking water and it is estimated that nearly two-thirds of all nations will experience water stress by the year 2025 (Kumar 2018 ).
With the extensive social and economic growth, such as human factors, climate and hydrology may lead to accumulation of pollutants in the surface water that may result in gradual change of the water source quality (Shan 2011 ).
The optimal quantity and acceptable quality of water is one of the essential needs to survive as mentioned earlier, but the maintenance of an acceptable quality of water is a challenge in the sector of water resources management (Mukate et al. 2019 ). Accordingly, the water quality of water bodies can be tested through changes in physical, chemical and biological characteristics related to anthropogenic or natural phenomena (Britto et al. 2018 ).
Therefore, water quality of any specific water body can be tested using physical, chemical and biological parameters also called variables, by collecting samples and obtaining data at specific locations (Britto et al. 2018 ; Tyagi et al. 2013 ).
To that end, the suitability of water sources for human consumption has been described in terms of Water Quality Index (WQI), which is one of the most effective ways to describe the quality of water, by reducing the bulk of information into a single value ranging between 0 and 100 (Tyagi et al. 2013 ).
Hence, the objective of the study is to review the WQI concept by listing some of the important water quality indices used worldwide for water quality assessment, listing the advantages and disadvantages of the selected indices and finally reviewing some water quality studies worldwide.
2 Water quality index
2.1 history of water quality concept.
In the last decade of the twentieth century, many organizations involved in water control, used the water quality indices for water quality assessment (Paun et al. 2016 ). In the 1960’s, the water quality indices was introduced to assess the water quality in rivers (Hamlat et al. 2017 ).
Horton ( 1965 ), initially developed a system for rating water quality through index numbers, offering a tool for water pollution abatement, since the terms “water quality” and “pollution” are related. The first step to develop an index is to select a list of 10 variables for the index’s construction, which are: sewage treatment, dissolved oxygen (DO), pH, coliforms, electroconductivity (EC), carbon chloroform extract (CCE), alkalinity, chloride, temperature and obvious pollution. The next step is to assign a scale value between zero and 100 for each variable depending on the quality or concentration. The last step, is to designate to each variable is a relative weighting factor to show their importance and influence on the quality index (the higher the assigned weight, the more impact it has on the water quality index, consequently it is more important) (Horton 1965 ).
Later on, Brown et al. ( 1970 ) established a new water quality index (WQI) with nine variables: DO, coliforms, pH, temperature, biochemical oxygen demand (BOD), total phosphate, nitrate concentrations, turbidity and solid content based on a basic arithmetic weighting using arithmetic mean to calculate the rating of each variable. These rates are then converted not temporary weights. Finally, each temporary weight is divided by the sum of all the temporary weights in order to get the final weight of each variable (Kachroud et al. 2019a ; Shah and Joshi 2017 ). In 1973, Brown et al., considered that a geometric aggregation (a way to aggregate variables, and being more sensitive when a variable exceeds the norm) is better than an arithmetic one. The National Sanitation Foundation (NSF) supported this effort (Kachroud et al. 2019a ; Shah and Joshi 2017 ).
Steinhart et al. ( 1982 ) developed a novel environmental quality index (EQI) for the Great Lakes ecosystem in North America. Nine variables were selected for this index: biological, physical, chemical and toxic. These variables were: specific conductance or electroconductivity, chloride, total phosphorus, fecal Coliforms, chlorophyll a , suspended solids, obvious pollution (aesthetic state), toxic inorganic contaminants, and toxic organic contaminants. Raw data were converted to subindex and each subindex was multiplied by a weighting factor (a value of 0.1 for chemical, physical and biological factors but 0.15 for toxic substances). The final score ranged between 0 (poor quality) and 100 (best quality) (Lumb et al. 2011a ; Tirkey et al. 2015 ).
Dinius ( 1987 ), developed a WQI based on multiplicative aggregation having a scale expressed with values as percentage, where 100% expressed a perfect water quality (Shah and Joshi 2017 ).
In the mid 90’s, a new WQI was introduced to Canada by the province of British Columbia, and used as an increasing index to evaluate water quality (Lumb et al. 2011b ; Shah and Joshi 2017 ). A while after, the Water Quality Guidelines Task Group of the Canadian Council of Ministers of the Environment (CCME) modified the original British Columbia Water Quality Index (BCWQI) and endorsed it as the CCME WQI in 2001(Bharti and Katyal 2011 ; Lumb et al. 2011b ).
In 1996, the Watershed Enhancement Program (WEPWQI) was established in Dayton Ohio, including water quality variables, flow measurements and water clarity or turbidity. Taking into consideration pesticide and Polycyclic Aromatic Hydrocarbon (PAH) contamination, is what distinguished this index from the NSFWQI (Kachroud et al. 2019a , b ).
Liou et al. (2003) established a WQI in Taiwan on the Keya River. The index employed thirteen variables: Fecal coliforms, DO, ammonia nitrogen, BOD, suspended solids, turbidity, temperature, pH, toxicity, cadmium (Cd), lead (Pb), copper (Cu) and zinc (Zn). These variables were downsized to nine based on environmental and health significance: Fecal coliforms, DO, ammonia nitrogen, BOD, suspended solids, turbidity, temperature, pH and toxicity. Each variable was converted into an actual value ranging on a scale from 0 to 100 (worst to highest). This index is based on the geometric means (an aggregation function that could eliminate the ambiguous caused from smaller weightings) of the standardized values (Akhtar et al. 2021 ; Liou et al. 2004 ; Uddin et al. 2021 ).
Said et al. ( 2004 ) implemented a new WQI using the logarithmic aggregation applied in streams waterbodies in Florida (USA), based on only 5 variables: DO, total phosphate, turbidity, fecal coliforms and specific conductance. The main idea was to decrease the number of variables and change the aggregation method using the logarithmic aggregation (this function does not require any sub-indices and any standardization of the variables). This index ranged from 0 to 3, the latter being the ideal value (Akhtar et al. 2021 ; Kachroud et al. 2019a , b ; Said et al. 2004 ; Uddin et al. 2021 ).
The Malaysian WQI (MWQI) was carried out in 2007, including six variables: DO, BOD, Chemical Oxygen Demand (COD), Ammonia Nitrogen, suspended solids and pH. For each variable, a curve was established to transform the actual value of the variable into a non-dimensional sub-index value.
The next step is to determine the weighting of the variables by considering the experts panel opinions. The final score is determined using the additive aggregation formula (where sub-indices values and their weightings are summed), extending from 0 (polluted) to 100 (clean) (Uddin et al. 2021 ).
The Hanh and Almeida indices were established respectively in 2010 on surface water in Vietnam and 2012 on the Potrero de los Funes in Argentina, based on 8 (color, suspended solids, DO, BOD, COD, chloride, total coliforms and orthophosphate) and 10 (color, pH, COD, fecal coliforms, total coliforms, total phosphate, nitrates, detergent, enterococci and Escherichia coli .) water quality variables. Both indices were based on rating curve- based sum-indexing system (Uddin et al. 2021 ).
The most recent developed WQI model in the literature was carried out in 2017. This index tried to reduce uncertainty present in other water quality indices. The West Java Water Quality Index (WJWQI) applied in the Java Sea in Indonesia was based on thirteen crucial water quality variables: temperature, suspended solids, COD, DO, nitrite, total phosphate, detergent, phenol, chloride, Zn, Pb, mercury (Hg) and fecal coliforms. Using two screening steps (based on statistical assessment), parameter (variable) redundancy was determined to only 9: temperature, suspended solids, COD, DO, nitrite, total phosphate, detergent, phenol and chloride. Sub-indices were obtained for those nine variables and weights were allocated based on expert opinions, using the same multiplicative aggregation as the NSFWQI. The WJWQI suggested 5 quality classes ranging from poor (5–25) to excellent (90–100) (Uddin et al. 2021 ).
2.2 Phases of WQI development
Mainly, WQI concept is based on many factors as displayed in Fig. 1 and described in the following steps:
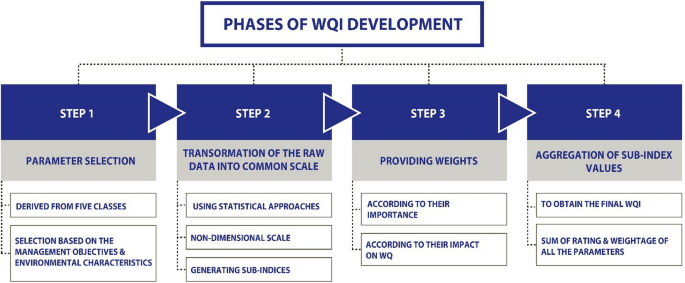
Phases of WQI development
Parameter selection for measurement of water quality (Shah and Joshi 2017 ):
The selection is carried out based on the management objectives and the environmental characteristics of the research area (Yan et al. 2015 ). Many variables are recommended, since they have a considerable impact on water quality and derive from 5 classes namely, oxygen level, eutrophication, health aspects, physical characteristics and dissolved substances (Tyagi et al. 2013 ).
Transformation of the raw data parameter into a common scale (Paun et al. 2016 ):
Different statistical approach can be used for transformation, all parameters are transformed from raw data that have different dimensions and units (ppm, saturation, percentage etc.) into a common scale, a non-dimensional scale and sub-indices are generated (Poonam et al. 2013 ; Tirkey et al. 2015 ).
Providing weights to the parameters (Tripathi and Singal 2019 ):
Weights are assigned to each parameter according to their importance and their impact on water quality, expert opinion is needed to assign weights (Tirkey et al. 2015 ). Weightage depends on the permissible limits assigned by International and National agencies in water drinking (Shah and Joshi 2017 ).
Aggregation of sub-index values to obtain the final WQI:
WQI is the sum of rating and weightage of all the parameters (Tripathi and Singal 2019 ).
It is important to note that in some indices, statistical approaches are commonly used such as factor analysis (FA), principal component analysis (PCA), discriminant analysis (DA) and cluster analysis (CA). Using these statistical approaches improves accuracy of the index and reduce subjective assumptions (Tirkey et al. 2015 ).
2.3 Evolution of WQI research
2.3.1 per year.
According to Scopus ( 2022 ), the yearly evolution of WQI's research is illustrated in Fig. 2 (from 1978 till 2022).
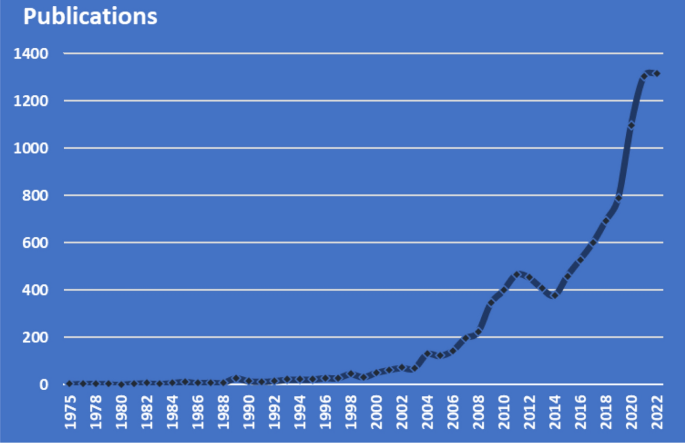
Evolution of WQI research per year (Scopus 2022 )
Overall, it is clear that the number of research has grown over time, especially in the most recent years. The number of studies remained shy between 1975 and 1988 (ranging from 1 to 13 research). In 1998, the number improved to 46 studies and increased gradually to 466 publications in 2011.The WQI's studies have grown significantly over the past decade, demonstrating that the WQI has become a significant research topic with the goal of reaching its maximum in 2022 (1316 studies) (Scopus, 2022 ).
2.3.2 Per country
In Fig. 3 , the development of WQI research is depicted visually per country from 1975 to 2022.
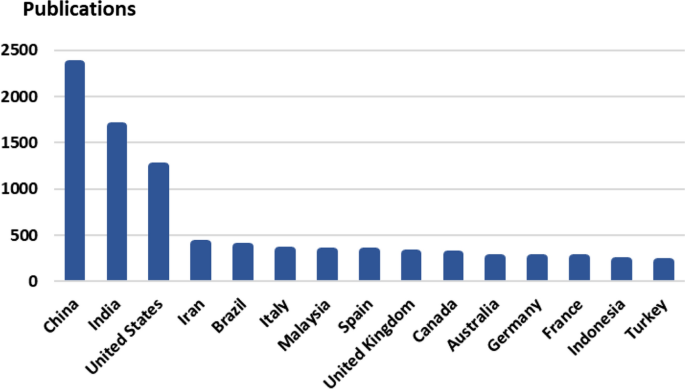
Evolution of WQI research per country (Scopus 2022 )
According to Scopus ( 2022 ), the top three countries were China, India and the United States, with 2356, 1678 and 1241 studies, respectively. Iran, Brazil, and Italy occupy the fourth, fifth, and sixth spots, respectively (409, 375 and 336 study). Malaysia and Spain have approximately the same number of studies, respectively 321 and 320 study. The studies in the remaining countries decrease gradually from 303 document in Spain to 210 documents in Turkey. This demonstrates that developing nations, like India, place a high value on the development of water quality protection even though they lack strong economic power, cutting-edge technology, and a top-notch scientific research team. This is because water quality is crucial to the long-term social and economic development of those nations (Zhang 2019 ).
2.4 Different methods for WQI determination
Water quality indices are tools to determine water quality. Those indices demand basic concepts and knowledge about water issues (Singh et al. 2013 ). There are many water quality indices such as the: National Sanitation Foundation Water Quality Index (NSFWQI), Canadian Council of Ministers of Environment Water Quality Index (CCMEWQI), Oregon Water Quality Index (OWQI), and Weight Arithmetic Water Quality Index (WAWQI) (Paun et al. 2016 ).
These water quality indices are applied in particular areas, based on many parameters compared to specific regional standards. Moreover, they are used to illustrate annual cycles, spatio-temporal variations and trends in water quality (Paun et al. 2016 ). That is to say that, these indices reflect the rank of water quality in lakes, streams, rivers, and reservoirs (Kizar 2018 ).
Accordingly, in this section a general review of available worldwide used indices is presented.
2.4.1 National sanitation foundation (NSFWQI)
The NSFWQI was developed in 1970 by the National Sanitation Foundation (NSF) of the United States (Hamlat et al. 2017 ; Samadi et al. 2015 ). This WQI has been widely field tested and is used to calculate and evaluate the WQI of many water bodies (Hamlat et al. 2017 ). However, this index belongs to the public indices group. It represents a general water quality and does not take into account the water’s use capacities, furthermore, it ignores all types of water consumption in the evaluation process (Bharti and Katyal 2011 ; Ewaid 2017 ).
The NSFWQI has been widely applied and accepted in Asian, African and European countries (Singh et al. 2013 ), and is based on the analysis of nine variables or parameters, such as, BOD, DO, Nitrate (NO 3 ), Total Phosphate (PO 4 ), Temperature, Turbidity, Total Solids(TS), pH, and Fecal Coliforms (FC).
Some of the index parameters have different importance, therefore, a weighted mean for each parameter is assigned, based on expert opinion which have grounded their opinions on the environmental significance, the recommended principles and uses of water body and the sum of these weights is equal to 1 (Table 1 ) (Ewaid 2017 ; Uddin et al. 2021 ).
Due to environmental issues, the NSFWQI has changed overtime. The TS parameter was substituted by the Total Dissolved Solids (TDS) or Total Suspended Solids (TSS), the Total Phosphate by orthophosphate, and the FC by E. coli (Oliveira et al. 2019 ).
The mathematical expression of the NSFWQI is given by the following Eq. ( 1 ) (Tyagi et al. 2013 ):
where, Qi is the sub-index for ith water quality parameter. Wi is the weight associated with ith water quality parameter. n is the number of water quality parameters.
This method ranges from 0 to 100, where 100 represents perfect water quality conditions, while zero indicates water that is not suitable for the use and needs further treatment (Samadi et al. 2015 ).
The ratings are defined in the following Table 2 .
In 1972, the Dinius index (DWQI) happened to be the second modified version of the NSF (USA). Expended in 1987 using the Delphi method, the DWQI included twelve parameters (with their assigned weights): Temperature (0.077), color (0.063), pH (0.077), DO (0.109), BOD (0.097), EC (0.079), alkalinity (0.063), chloride (0.074), coliform count (0.090), E. coli (0.116). total hardness (0.065) and nitrate (0.090). Without any conversion process, the DWQI used the measured variable concentrations directly as the sub-index values (Kachroud et al. 2019b ; Uddin et al. 2021 ).
Sukmawati and Rusni assessed in 2018 the water quality in Beratan lake (Bali), choosing five representative stations for water sampling representing each side of the lake, using the NSFWQI. NSFWQI’s nine parameters mentioned above were measured in each station. The findings indicated that the NSFWQI for the Beratan lake was seventy-eight suggesting a good water quality. Despite this, both pH and FC were below the required score (Sukmawati and Rusni 2019 ).
The NSFWQI indicated a good water quality while having an inadequate value for fecal coliforms and pH. For that reason, WQIs must be adapted and developed so that any minor change in the value of any parameter affects the total value of the water quality index.
A study conducted by Zhan et al. ( 2021 ) , concerning the monitoring of water quality and examining WQI trends of raw water in Macao (China) was established from 2002 to 2019 adopting the NSFWQI. NSFWQI's initial model included nine parameters (DO, FC, pH, BOD, temperature, total phosphates, and nitrates), each parameter was given a weight and the parameters used had a significant impact on the WQI calculation outcomes. Two sets of possible parameters were investigated in this study in order to determine the impact of various parameters. The first option was to keep the original 9-parameter model, however, in the second scenario, up to twenty-one parameters were chosen, selected by Principal Component Analysis (PCA).
The latter statistical method was used to learn more about the primary elements that contributed to water quality variations, and to calculate the impact of each attribute on the quality of raw water. Based on the PCA results, the 21-parameter model was chosen. The results showed that the quality of raw water in Macao has been relatively stable in the period of interest and appeared an upward trend overall. Furthermore, the outcome of environmental elements, such as natural events, the region's hydrology and meteorology, can have a significant impact on water quality. On the other hand, Macao's raw water quality met China's Class III water quality requirements and the raw water pollution was relatively low. Consequently, human activities didn’t have a significant impact on water quality due to effective treatment and protection measures (Zhan et al. 2021 ).
Tampo et al. ( 2022 ) undertook a recent study in Adjougba (Togo), in the valley of Zio River. Water samples were collected from the surface water (SW), ground water (GW) and treated wastewater (TWW), intending to compare the water quality of these resources for irrigation and domestic use.
Hence, WQIs, water suitability indicators for irrigation purposes (WSI-IPs) and raw water quality parameters were compared using statistical analysis (factor analysis and Spearman’s correlation).
Moreover, the results proposed that he water resources are suitable for irrigation and domestic use: TWW suitable for irrigation use, GW suitable for domestic use and SW suitable for irrigation use.
The NSFWQI and overall index of pollution (OPI) parameters were tested, and the results demonstrated that the sodium absorption ratio, EC, residual sodium carbonate, Chloride and FC are the most effective parameters for determining if water is suitable for irrigation.
On the other hand, EC, DO, pH, turbidity, COD, hardness, FC, nitrates, national sanitation foundation's water quality index (NSFWQI), and overall index of pollution (OPI) are the most reliable in the detection of water suitability for domestic use (Tampo et al. 2022 ).
Following these studies, it is worth examining the NSFWQI. This index can be used with other WQI models in studies on rivers, lakes etc., since one index can show different results than another index, in view of the fact that some indices might be affected by other variations such as seasonal variation.
Additionally, the NSFWQI should be developed and adapted to each river, so that any change in any value will affect the entire water quality. It is unhelpful to have a good water quality yet a low score of a parameter that can affect human health (case of FC).
2.4.2 Canadian council of ministers of the environment water quality index (CCMEWQI)
The Canadian Water Quality Index adopted the conceptual model of the British Colombia Water Quality Index (BCWQI), based on relative sub-indices (Kizar 2018 ).
The CCMEWQI provides a water quality assessment for the suitability of water bodies, to support aquatic life in specific monitoring sites in Canada (Paun et al. 2016 ). In addition, this index gives information about the water quality for both management and the public. It can furthermore be applied in many water agencies in various countries with slight modification (Tyagi et al. 2013 ).
The CCMEWQI method simplifies the complex and technical data. It tests the multi-variable water quality data and compares the data to benchmarks determined by the user (Tirkey et al. 2015 ). The sampling protocol requires at least four parameters sampled at least four times but does not indicate which ones should be used; the user must decide ( Uddin et al. 2021 ). Yet, the parameters may vary from one station to another (Tyagi et al. 2013 ).
After the water body, the objective and the period of time have been defined the three factors of the CWQI are calculated (Baghapour et al. 2013 ; Canadian Council of Ministers of the Environment 1999 ):
The scope (F1) represents the percentage of variables that failed to meet the objective (above or below the acceptable range of the selected parameter) at least once (failed variables), relative to the total number of variables.
The frequency (F2) represents the percentage of tests which do not meet the objectives (above or below the acceptable range of the selected parameter) (failed tests).
The amplitude represents the amount by which failed tests values did not meet their objectives (above or below the acceptable range of the selected parameter). It is calculated in three steps.
The excursion is termed each time the number of an individual parameter is further than (when the objective is a minimum, less than) the objective and is calculated by two Eqs. ( 4 , 5 ) referring to two cases. In case the test value must not exceed the objective:
For the cases in which the test value must not fall below the objective:
The normalized sum of excursions, or nse , is calculated by summing the excursions of individual tests from their objectives and diving by the total number of tests (both meetings and not meeting their objectives):
F3 is then calculated an asymptotic function that scales the normalized sum of the excursions from objectives (nse) to yield a range between 0 and 100:
Finally, the CMEWQI can be obtained from the following equation, where the index changes in direct proportion to changes in all three factors.
where 1.732 is a scaling factor and normalizes the resultant values to a range between 0 and 100, where 0 refers to the worst quality and one hundred represents the best water quality.
Once the CCME WQI value has been determined, water quality in ranked as shown in Table 3
Ramírez-Morales et al. ( 2021 ) investigated in their study the measuring of pesticides and water quality indices in three agriculturally impacted micro catchments in Costa Rica between 2012 and 2014. Surface water and sediment samples were obtained during the monitoring experiment.
The specifications of the water included: Pesticides, temperature, DO, oxygen saturation, BOD, TP, NO3, sulfate, ammonium, COD, conductivity, pH and TSS.
Sediment parameters included forty-two pesticides with different families including carbamate, triazine, organophosphate, phthalimide, pyrethroid, uracil, benzimidazole, substituted urea, organochlorine, imidazole, oxadiazole, diphenyl ether and bridged diphenyl.
WQIs are effective tools since they combine information from several variables into a broad picture of the water body's state. Two WQIs were calculated using the physicochemical parameters: The Canadian Council of Ministers of the Environment (CCME) WQI and the National Sanitation Foundation (NSF) WQI.
These were chosen since they are both extensively used and use different criteria to determine water quality: The NSF WQI has fixed parameters, weights, and threshold values, whereas the CCME has parameters and threshold values that are customizable.
The assessment of water quality using physico-chemical characteristics and the WQI revealed that the CCME WQI and the NSF WQI have distinct criteria. CCME WQI categorized sampling point as marginal/bad quality, while most sampling locations were categorized as good quality in the NSF WQI. Seemingly, the water quality classifications appeared to be affected by seasonal variations: during the wet season, the majority of the CCME WQI values deteriorated, implying that precipitation and runoff introduced debris into the riverbed. Thus, it’s crucial to compare WQIs because they use various factors, criteria, and threshold values, which might lead to different outcomes (Ramírez-Morales et al. 2021 ).
Yotova et al. ( 2021 ) directed an analysis on the Mesta River located between Greece and Bulgaria. The Bulgarian section of the Mesta River basin, which is under the supervision of the West-Aegean Region Basin Directorate, was being researched. The goal was to evaluate the surface water quality of ten points of the river using a novel approach that combines composite WQI developed by the CCME and Self organizing map (SOM) on the required monitoring data that include: DO, pH, EC, ammonium, nitrite, nitrate, total phosphate, BOD and TSS.
The use of WQI factors in SOM calculations allows for the identification of specific WQI profiles for various object groups and identifying groupings of river basin which have similar sampling conditions. The use of both could reveal and estimate the origin and magnitude of anthropogenic pressure. In addition, it might be determined that untreated residential wastewaters are to blame for deviations from high quality requirements in the Mesta River catchment.
Interestingly, this study reveals that WQI appear more accurate and specific when combined with a statistical test such as the SOM (Yotova et al. 2021 ).
2.4.3 Oregon water quality index (OWQI)
The Oregon Water Quality Index is a single number that creates a score to evaluate the water quality of Oregon’s stream and apply this method in other geographical region (Hamlat et al. 2017 ; Singh et al. 2013 ). The OWQI was widely accepted and applied in Oregon (USA) and Idaho (USA) (Sutadian et al. 2016 ).
Additionally, the OWQI is a variant of the NSFWQI, and is used to assess water quality for swimming and fishing, it is also used to manage major streams (Lumb et al. 2011b ). Since the introduction of the OWQI in 1970, the science of water quality has improved noticeably, and since 1978, index developers have benefited from increasing understanding of stream functionality (Bharti and Katyal 2011 ). The Oregon index belongs to the specific consumption indices group. It is a water classification based on the kind of consumption and application such as drinking, industrial, etc. (Shah and Joshi 2017 ).
The original OWQI dropped off in 1983, due to excessive resources required for calculating and reporting results. However, improvement in software and computer hardware availability, in addition to the desire for an accessible water quality information, renewed interest in the index (Cude 2001 ).
Simplicity, availability of required quality parameters, and the determination of sub-indexes by curve or analytical relations are some advantages of this approach (Darvishi et al. 2016a ). The process combines eight variables including temperature, dissolved oxygen (percent saturation and concentration), biochemical oxygen demand (BOD), pH, total solids, ammonia and nitrate nitrogen, total phosphorous and bacteria (Brown 2019 ). Equal weight parameters were used for this index and has the same effect on the final factor (Darvishi et al. 2016a ; Sutadian et al. 2016 ).
The Oregon index is calculated by the following Eq. 9 (Darvishi et al. 2016a ):
where,n is the number of parameters (n = 8) SI i is the value of parameter i.
Furthermore, the OWQI scores range from 10 for the worse case to 100 as the ideal water quality illustrated in the following Table 4 (Brown 2019 ).
Kareem et al. ( 2021 ) using three water quality indices, attempted to analyze the Euphrates River (Iraq) water quality for irrigation purposes in three different stations: WAWQI, CCMEWQI AND OWQI.
For fifteen parameters, the annual average value was calculated, which included: pH, BOD, Turbidity, orthophosphate, Total Hardness, Sulphate, Nitrate, Alkalinity, Potassium Sodium, Magnesium, Chloride, DO, Calcium and TDS.
The OWQI showed that the river is “very poor”, and since the sub-index of the OWQI does not rely on standard-parameter compliance, there are no differences between the two inclusion and exclusion scenarios, which is not the case in both WAWQI and CCMEWQI (Kareem et al. 2021 ).
Similarly, the OWQI showed a very bad quality category, and it is unfit for human consumption, compared to the NSFWQI and Wilcox indices who both showed a better quality of water in Darvishi et al., study conducted on the Talar River (Iran) (Darvishi et al. 2016b ).
2.4.4 Weighted arithmetic water quality index (WAWQI)
The weighted arithmetic index is used to calculate the treated water quality index, in other terms, this method classifies the water quality according to the degree of purity by using the most commonly measured water quality variables (Kizar 2018 ; Paun et al. 2016 ).This procedure has been widely used by scientists (Singh et al. 2013 ).
Three steps are essential in order to calculate the WAWQI:
Further quality rating or sub-index was calculated using the following equation (Jena et al. 2013 ):
Qn is the quality rating for the nth water quality parameter.
Vn is the observed value of the nth parameter at a given sampling station.
Vo is the ideal value of the nth parameter in a pure water.
Sn is the standard permissible value of the nth parameter.
The quality rating or sub index corresponding to nth parameter is a number reflecting the relative value of this parameter in polluted water with respect to its permissible standard value (Yogendra & Puttaiah 2008 ).
The unit weight was calculated by a value inversely proportional to the recommended standard values (Sn) of the corresponding parameters (Jena et al. 2013 ):
Wn is the unit weight for the nth parameter.
K is the constant of proportionality.
Sn is the standard value of the nth parameter.
The overall WQI is the aggregation of the quality rating (Qn) and the unit weight (Wn) linearly (Jena et al. 2013 ):
After calculating the WQI, the measurement scale classifies the water quality from “unsuitable water” to “excellent water quality” as given in the following Table 5 .
Sarwar et al. ( 2020 ) carried out a study in Chaugachcha and Manirampur Upazila of Jashore District (Bangladesh). The goal of this study was to determine the quality of groundwater and its appropriateness for drinking, using the WAWQI including nine parameters: turbidity, EC, pH, TDS, nitrate, ammonium, sodium, potassium and iron. Many samplings point was taken from Chaugachcha and Manirampur, and WQI differences were indicated (ranging from very poor to excellent). These variations in WQI were very certainly attributable to variances in geographical location. Another possibility could be variations in the parent materials from which the soil was created, which should be confirmed using experimental data. It is worth mentioning that every selected parameter was taken into consideration during calculation. Similarly, the water quality differed in Manirampur due to the elements contained in the water samples that had a big impact on the water quality (Sarwar et al. 2020 ).
In 2021, García-Ávila et al. undertook a comparative study between the CCMEWQI and WAWQI for the purpose of determining the water quality in the city of Azogues (Ecuador). Twelve parameters were analyzed: pH, turbidity, color, total dissolved solids, electrical conductivity, total hardness, alkalinity, nitrates, phosphates, sulfates, chlorides and residual chlorine over 6 months. The average WAWQI value was calculated suggesting that 16.67% of the distribution system was of 'excellent' quality and 83.33% was of 'good' quality, while the CCMEWQI indicated that 100% of the system was of ‘excellent’ quality.
This difference designated that the parameters having a low maximum allowable concentration have an impact on WAWQI and that WAWQI is a valuable tool to determine the quality of drinking water and have a better understanding of it (García-Ávila et al. 2022a , b ).
2.4.5 Additional water quality indices
The earliest WQI was based on a mathematical function that sums up all sub-indices, as detailed in the 2.1. History of water quality concept section (Aljanabi et al. 2021 ). The Dinius index (1972), the OWQI (1980), and the West Java index (2017) were later modified from the Horton index, which served as a paradigm for later WQI development (Banda and Kumarasamy 2020 ).
Based on eleven physical, chemical, organic, and microbiological factors, the Scottish Research Development Department (SRDDWQI) created in 1976 was based on the NSFWQI and Delphi methods used in Iran, Romania, and Portugal. Modified into the Bascaron index (1979) in Spain, which was based on 26 parameters that were unevenly weighted with a subjective representation that allowed an overestimation of the contamination level. The House index (1989) in the UK valued the parameters directly as sub-indices. The altered version was adopted as Croatia's Dalmatian index in 1999.
The Ross WQI (1977) was created in the USA using only 4 parameters and did not develop into any further indices.
In 1982, the Dalmatian and House WQI were used to create the Environmental Quality Index, which is detailed in Sect. 2.1 . This index continues to be difficult to understand and less powerful than other indices (Lumb et al. 2011a ; Uddin et al. 2021 ).
The Smith index (1990), is based on 7 factors and the Delphi technique in New Zealand, attempts to eliminate eclipsing difficulties and does not apply any weighting, raising concerns about the index's accuracy (Aljanabi et al. 2021 ; Banda and Kumarasamy 2020 ; Uddin et al. 2021 ).
The Dojildo index (1994) was based on 26 flexible, unweighted parameters and does not represent the water's total quality.
With the absence of essential parameters, the eclipse problem is a type of fixed-parameter selection. The Liou index (2004) was established in Taiwan to evaluate the Keya River based on 6 water characteristics that were immediately used into sub-index values. Additionally, because of the aggregation function, uncertainty is unrelated to the lowest sub-index ranking (Banda and Kumarasamy 2020 ; Uddin et al. 2021 ).
Said index (2004) assessed water quality using only 4 parameters, which is thought to be a deficient number for accuracy and a comprehensive picture of the water quality. Furthermore, a fixed parameter system prevents the addition of any new parameters.
Later, the Hanh index (2010), which used hybrid aggregation methods and gave an ambiguous final result, was developed from the Said index.
In addition to eliminating hazardous and biological indicators, the Malaysia River WQI (MRWQI developed in the 2.1 section) (2007) was an unfair and closed system that was relied on an expert's judgment, which is seen as being subjective and may produce ambiguous findings (Banda and Kumarasamy 2020 ; Uddin et al. 2021 ).
Table illustrated the main data of the studies published during 2020–2022 on water quality assessments and their major findings:
2.5 Advantages and disadvantages of the selected water quality indices
A comparison of the selected indices is done by listing the advantages and disadvantages of every index listed in the Table 7 below.
2.6 New attempts of WQI studies
Many studies were conducted to test the water quality of rivers, dams, groundwater, etc. using multiple water quality indices throughout the years. Various studies have been portrayed here in.
Massoud ( 2012 ) observed during a 5-year monitoring period, in order to classify the spatial and temporal variability and classify the water quality along a recreational section of the Damour river using a weighted WQI from nine physicochemical parameters measured during dry season. The WWQI scale ranged between “very bad” if the WQI falls in the range 0–25, to “excellent” if it falls in the range 91–100. The results revealed that the water quality of the Damour river if generally affected by the activities taking place along the watershed. The best quality was found in the upper sites and the worst at the estuary, due to recreational activities. If the Damour river is to be utilized it will require treatment prior any utilization (Massoud 2012 ).
Rubio-Arias et al. ( 2012 ) conducted a study in the Luis L. Leon dam located in Mexico. Monthly samples were collected at 10 random points of the dam at different depths, a total of 220 samples were collected and analyzed. Eleven parameters were considered for the WQI calculation, and WQI was calculated using the Weighted WQI equation and could be classified according to the following ranges: < 2.3 poor; from 2.3 to 2.8 good; and > 2.8 excellent. Rubio-Arias et al., remarked that the water could be categorized as good during the entire year. Nonetheless, some water points could be classified as poor due to some anthropogenic activities such as intensive farming, agricultural practices, dynamic urban growth, etc. This study confirms that water quality declined after the rainy season (Rubio-Arias et al. 2012 ).
In the same way, Haydar et al. ( 2014 ) evaluated the physical, chemical and microbiological characteristics of water in the upper and lower Litani basin, as well as in the lake of Qaraaoun. The samples were collected during the seasons of 2011–2012 from the determined sites and analyzed by PCA and the statistical computations of the physico-chemical parameters to extract correlation between variables. Thus, the statistical computations of the physico-chemical parameters showed a correlation between some parameters such as TDS, EC, Ammonium, Nitrate, Potassium and Phosphate. Different seasons revealed the presence of either mineral or anthropogenic or both sources of pollution caused by human interference from municipal wastewater and agricultural purposes discharged into the river. In addition, temporal effects were associated with seasonal variations of river flow, which caused the dilution if pollutants and, hence, variations in water quality (Haydar et al. 2014 ).
Another study conducted by Chaurasia et al., ( 2018 ), proposed a groundwater quality assessment in India using the WAWQI. Twenty-two parameters were taken into consideration for this assessment, however, only eight important parameters were chosen to calculate the WQI. The rating of water quality shows that the ground water in 20% of the study area is not suitable for drinking purpose and pollution load is comparatively high during rainy and summer seasons. Additionally, the study suggests that priority should be given to water quality monitoring and its management to protect the groundwater resource from contamination as well as provide technology to make the groundwater fit for domestic and drinking (Chaurasia et al. 2018 ).
Daou et al. ( 2018 ) evaluated the water quality of four major Lebanese rivers located in the four corners of Lebanon: Damour, Ibrahim, Kadisha and Orontes during the four seasons of the year 2010–2011. The assessment was done through the monitoring of a wide range of physical, chemical and microbiological parameters, these parameters were screened using PCA. PCA was able to discriminate each of the four rivers according to a different trophic state. The Ibrahim River polluted by mineral discharge from marble industries in its surroundings, as well as anthropogenic pollutants, and the Kadisha river polluted by anthropogenic wastes seemed to have the worst water quality. This large-scale evaluation of these four Lebanese rivers can serve as a water mass reference model (Daou et al. 2018 ).
Moreover, some studies compared many WQI methods. Kizar ( 2018 ), carried out a study on Shatt Al-Kufa in Iraq, nine locations and twelve parameters were selected. The water quality was calculated using two methods, the WAWQI and CWQI. The results revealed the same ranking of the river for both methods, in both methods the index decreased in winter and improved in other seasons (Kizar 2018 ).
On the other hand, Zotou et al. ( 2018 ), undertook a research on the Polyphytos Reservoir in Greece, taking into consideration thirteen water parameters and applying 5 WQIs: Prati’s Index of Pollution (developed in 1971, based on thirteen parameter and mathematical functions to convert the pollution concentration into new units. The results of PI classified water quality into medium classes (Gupta and Gupta 2021 ). Bhargava’s WQI (established in 1983, the BWQI categorize the parameters according to their type: bacterial indicators, heavy metals and toxins, physical parameters and organic and inorganic substances. The BWQI tends to classify the water quality into higher quality classes, which is the case in the mentioned study (Gupta and Gupta 2021 ). Oregon WQI, Dinius second index, Weighted Arithmetic WQI, in addition to the NSF and CCMEWQI. The results showed that Bhargava and NSF indices tend to classify the reservoir into superior quality classes, Prati’s and Dinius indices fall mainly into the middle classes of the quality ranking, while CCME and Oregon could be considered as “stricter” since they give results which range steadily between the lower quality classes (Zotou et al. 2018 ).
In their study, Ugochukwu et al. ( 2019 ) investigated the effects of acid mine drainage, waste discharge into the Ekulu River in Nigeria and other anthropogenic activities on the water quality of the river. The study was performed between two seasons, the rainy and dry season. Samples were collected in both seasons, furthermore, the physic-chemistry parameters and the heavy metals were analyzed. WQI procedure was estimated by assigning weights and relative weights to the parameters, ranking from “excellent water” (< 50) to “unsuitable for drinking” (> 300). The results showed the presence of heavy metals such as lead and cadmium deriving from acid mine drainage. In addition, the water quality index for all the locations in both seasons showed that the water ranked from “very poor” to “unsuitable for drinking”, therefore the water should be treated before any consumption, and that enough information to guide new implementations for river protection and public health was provided (Ugochukwu et al. 2019 ).
The latest study in Lebanon related to WQI was carried out by El Najjar et al. ( 2019 ), the purpose of the study was to evaluate the water quality of the Ibrahim River, one of the main Lebanese rivers. The samples were collected during fifteen months, and a total of twenty-eight physico-chemical and microbiological parameters were tested. The parameters were reduced to nine using the Principal Component Analysis (PCA) and Pearson Correlation. The Ibrahim WQI (IWQI) was finally calculated using these nine parameters and ranged between 0 and 25 referring to a “very bad” water quality, and between 91 and 100 referring to an “excellent” water quality. The IWQI showed a seasonal variation, with a medium quality during low -water periods and a good one during high-water periods (El Najjar et al. 2019 ).
3 Conclusion
WQI is a simple tool that gives a single value to water quality taking into consideration a specific number of physical, chemical, and biological parameters also called variables in order to represent water quality in an easy and understandable way. Water quality indices are used to assess water quality of different water bodies, and different sources. Each index is used according to the purpose of the assessment. The study reviewed the most important indices used in water quality, their mathematical forms and composition along with their advantages and disadvantages. These indices utilize parameters and are carried out by experts and government agencies globally. Nevertheless, there is no index so far that can be universally applied by water agencies, users and administrators from different countries, despite the efforts of researchers around the world (Paun et al. 2016 ). The study also reviewed some attempts on different water bodies utilizing different water quality indices, and the main studies performed in Lebanon on Lebanese rivers in order to determine the quality of the rivers (Table 6 ).
As mentioned in the article (Table 7 ); WQIs may undergo some limitations. Some indices could be biased, others are not specific, and they may not get affected by the value of an important parameter. Therefore, there is no interaction between the parameters.
Moreover, many studies exhibited a combination between WQIs and statistical techniques and analysis (such as the PCA, Pearson’s correlation etc.). with a view to obtain the relation between the parameters and which parameter might affect the water quality.
In other research, authors compared many WQIs to check the difference of water quality according to each index. Each index can provide different values depending on the sensitivity of the parameter. For that reason, WQIs should be connected to scientific advancements to develop and elaborate the index in many ways (example: ecologically). Therefore, an advanced WQI should be developed including first statistical techniques, such as Pearson correlation and multivariate statistical approach mainly Principal Component Analysis (PCA) and Cluster Analysis (CA), in order to determine secondly the interactions and correlations between the parameters such as TDS and EC, TDS and total alkalinity, total alkalinity and chloride, temperature and bacteriological parameters, consequently, a single parameter could be selected as representative of others. Finally, scientific and technological advancement for future studies such as GIS techniques, fuzzy logic technology to assess and enhance the water quality indices and cellphone-based sensors for water quality monitoring should be used.
Akhtar N, Ishak MIS, Ahmad MI, Umar K, Md Yusuff MS, Anees MT, Qadir A, Ali Almanasir YK (2021) Modification of the Water Quality Index (WQI) process for simple calculation using the Multi-Criteria Decision-Making (MCDM) Method: a review. Water 13:905. https://doi.org/10.3390/w13070905
Article CAS Google Scholar
Alexakis DE (2020) Meta-evaluation of water quality indices application into groundwater resources. Water 12:1890. https://doi.org/10.3390/w12071890
Article Google Scholar
Aljanabi ZZ, Jawad Al-Obaidy AHM, Hassan FM (2021) A brief review of water quality indices and their applications. IOP Conf Ser: Earth Environ Sci 779:012088. https://doi.org/10.1088/1755-1315/779/1/012088
Al-Kareem SA, ALKzwini RS (2022) Statistical analysis for water quality index for Shatt-Al-Hilla river in Babel city. Water Pract Technol 17:567–586. https://doi.org/10.2166/wpt.2022.004
Baghapour MA, Nasseri S, Djahed B (2013) Evaluation of Shiraz wastewater treatment plant effluent quality for agricultural irrigation by Canadian Water Quality Index (CWQI). Iran J Environ Health Sci Eng 10:27. https://doi.org/10.1186/1735-2746-10-27
Banda T, Kumarasamy M (2020) Development of a universal water quality index (UWQI) for South African River Catchments. Water 12:1534. https://doi.org/10.3390/w12061534
Betis H, St-Hilaire A, Fortin C, Duchesne S (2020) Development of a water quality index for watercourses downstream of harvested peatlands. Water Qual Res J 55:119–131. https://doi.org/10.2166/wqrj.2020.007
Bharti N, Katyal D (2011) Water quality indices used for surface water vulnerability assessment. Int J Environ Sci 2:154–173
CAS Google Scholar
Britto FB, do Vasco AN, Aguiar Netto ADO, Garcia CAB, Moraes GFO, Silva MGD (2018) Surface water quality assessment of the main tributaries in the lower São Francisco River, Sergipe. RBRH 23:6–23. https://doi.org/10.1590/2318-0331.231820170061
Brown D (2019) Oregon Water Quality Index: background, analysis and usage. State of Oregon Department of Environmental Quality, Laboratory and Environmental Assessment Program
Brown RM, McClelland NI, Deininger RA, Tozer RG (1970) A water quality index-do we dare. Water Sew Work 117:339–343
Calmuc M, Calmuc V, Arseni M, Topa C, Timofti M, Georgescu LP, Iticescu C (2020) A comparative approach to a series of physico-chemical quality indices used in assessing water quality in the lower Danube. Water 12:3239. https://doi.org/10.3390/w12113239
Canadian Council of Ministers of the Environment 2001 (1999) Canadian water quality guidelines for the protection of aquatic life: CCME Water Quality Index 1.0, Technical Report. Canadian environmental quality guidelines, Canadian Council of Ministers of the Environment, Winnipeg
Chaurasia AK, Pandey HK, Tiwari SK, Prakash R, Pandey P, Ram A (2018) Groundwater quality assessment using Water Quality Index (WQI) in parts of Varanasi District, Uttar Pradesh, India. J Geol Soc India 92:76–82. https://doi.org/10.1007/s12594-018-0955-1
Chen L, Tian Z, Zou K (2020) Water quality evaluation based on the water quality index method in Honghu Lake: one of the largest shallow lakes in the Yangtze River Economic Zone. Water Supp 20:2145–2155. https://doi.org/10.2166/ws.2020.111
Choi B, Choi SS (2021) Integrated hydraulic modelling, water quality modelling and habitat assessment for sustainable water management: a case study of the Anyang-Cheon stream. Korea Sustain 13:4330. https://doi.org/10.3390/su13084330
Choque-Quispe D, Froehner S, Palomino-Rincón H, Peralta-Guevara DE, Barboza-Palomino GI, Kari-Ferro A, Zamalloa-Puma LM, Mojo-Quisani A, Barboza-Palomino EE, Zamalloa-Puma MM, Martínez-Huamán EL, Calla-Florez M, Aronés-Medina EG, Solano-Reynoso AM, Choque-Quispe Y (2022) Proposal of a water-quality index for high Andean Basins: application to the Chumbao River, Andahuaylas. Peru Water 14:654. https://doi.org/10.3390/w14040654
Cong Thuan N (2022) Assessment of surface water quality in the Hau Giang province using geographical information system and statistical Aaproaches. J Ecol Eng 23:265–276. https://doi.org/10.12911/22998993/151927
Cristable RM, Nurdin E, Wardhana W (2020) Water quality analysis of Saluran Tarum Barat, West Java, based on National Sanitation Foundation-Water Quality Index (NSF-WQI). IOP Conf Ser: Earth Environ Sci 481:012068. https://doi.org/10.1088/1755-1315/481/1/012068
Cude CG (2001) Oregon Water Quality Index a tool for evaluating water quality management effectiveness. J Am Water Resour as 37:125–137. https://doi.org/10.1111/j.1752-1688.2001.tb05480.x
Da Silveira VR, Kunst Valentini MH, dos Santos GB, Nadaleti WC, Vieira BM (2021) Assessment of the water quality of the Mirim Lagoon and the São Gonçalo channel through qualitative indices and statistical methods. Water Air Soil Poll 232:217. https://doi.org/10.1007/s11270-021-05160-w
Daou C, Salloum M, Legube B, Kassouf A, Ouaini N (2018) Characterization of spatial and temporal patterns in surface water quality: a case study of four major Lebanese rivers. Environ Monit Assess 190:485. https://doi.org/10.1007/s10661-018-6843-8
Darvishi G, Kootenaei FG, Ramezani M, Lotfi E, Asgharnia H (2016a) Comparative investigation of river water quality by OWQI, NSFWQI and Wilcox indexes (Case study: The Talar River – IRAN). Arch Environ Prot 42:41–48. https://doi.org/10.1515/aep-2016-0005
Darvishi G, Kootenaei FG, Ramezani M, Lotfi E, Asgharnia H (2016b) Comparative investigation of river water quality by OWQI, NSFWQI and Wilcox indexes (Case study: The Talar River – IRAN). Arch Environ Prot. https://doi.org/10.1515/aep-2016-0005
De Oliveira MD, de Rezende OLT, de Fonseca JFR, Libânio M (2019) Evaluating the surface water quality index fuzzy and its influence on water treatment. J Water Process Eng 32:100890. https://doi.org/10.1016/j.jwpe.2019.100890
Deep A, Gupta V, Bisht L, Kumar R (2020) Application of WQI for water quality assessment of high-altitude snow-fed sacred Lake Hemkund. Garhwal Himal Sustain Water Resour Manag 6:89. https://doi.org/10.1007/s40899-020-00449-w
Deng L, Shahab A, Xiao H, Li J, Rad S, Jiang J, GuoYu Jiang P, Huang H, Li X, Ahmad B, Siddique J (2021) Spatial and temporal variation of dissolved heavy metals in the Lijiang River, China: implication of rainstorm on drinking water quality. Environ Sci Pollut R 28:68475–68486. https://doi.org/10.1007/s11356-021-15383-3
Dinius SH (1987) Design of an index of water quality. Water Resour Bull 23:833–843
Doderovic M, Mijanovic I, Buric D, Milenkovic M (2020) Assessment of the water quality in the Moraca River basin (Montenegro) using water quality index. Glas Srp Geogr Drus 100:67–81. https://doi.org/10.2298/GSGD2002067D
El Najjar P, Kassouf A, Probst A, Probst JL, Ouaini N, Daou C, El Azzi D (2019) High-frequency monitoring of surface water quality at the outlet of the Ibrahim River (Lebanon): a multivariate assessment. Ecol Indic 104:13–23. https://doi.org/10.1016/j.ecolind.2019.04.061
En-nkhili H, Najy M, Etebaai I, Talbi FZ, El Kharrim K, Belghyti D (2020) Application of water quality index for the assessment of Boudaroua lake in the Moroccan pre-rif. Conference GEOIT4W-2020: 1–5. https://doi.org/10.1145/3399205.3399248
Ewaid SH (2017) Water quality evaluation of Al-Gharraf river by two water quality indices. Appl Water Sci 7:3759–3765. https://doi.org/10.1007/s13201-016-0523-z
Fadel A, Kanj M, Slim K (2021) Water quality index variations in a Mediterranean reservoir: a multivariate statistical analysis relating it to different variables over 8 years. Environ Earth Sci 80:65. https://doi.org/10.1007/s12665-020-09364-x
Fraga MDS, da Silva DD, Reis GB, Guedes HAS, Elesbon AAA (2021) Temporal and spatial trend analysis of surface water quality in the Doce River basin, Minas Gerais, Brazil. Environ Dev Sustain 23:12124–12150. https://doi.org/10.1007/s10668-020-01160-8
Frîncu RM (2021) Long-term trends in water quality indices in the lower Danube and tributaries in Romania (1996–2017). Int J Environ Res Pub He 18:1665. https://doi.org/10.3390/ijerph18041665
Fu D, Chen S, Chen Y, Yi Z (2022) Development of modified integrated water quality index to assess the surface water quality: a case study of Tuo River. China Environ Monit Assess 194:333. https://doi.org/10.1007/s10661-022-09998-3
Galarza E, Cabrera M, Espinosa R, Espitia E, Moulatlet GM, Capparelli MV (2021) Assessing the quality of Amazon aquatic ecosystems with multiple lines of evidence: the case of the Northeast Andean foothills of Ecuador. B Environ Contam Tox 107:52–61. https://doi.org/10.1007/s00128-020-03089-0
Gamvroula DE, Alexakis DE (2022) Evaluating the performance of water quality indices: application in surface water of lake union, Washington State-USA. Hydrology 9:116. https://doi.org/10.3390/hydrology9070116
García-Ávila F, Jiménez-Ordóñez M, Torres-Sánchez J, Iglesias-Abad S, Cabello Torres R, Zhindón-Arévalo C (2022a) Evaluation of the impact of anthropogenic activities on surface water quality using a water quality index and environmental assessment. J Water Land Dev 53:58–67. https://doi.org/10.24425/JWLD.2022.140780
García-Ávila F, Zhindón-Arévalo C, Valdiviezo-Gonzales L, Cadme-Galabay M, Gutiérrez-Ortega H, del Pino LF (2022b) A comparative study of water quality using two quality indices and a risk index in a drinking water distribution network. Environ Technol Rev 11:49–61. https://doi.org/10.1080/21622515.2021.2013955
Ghani J, Ullah Z, Nawab J, Iqbal J, Waqas M, Ali A, Almutairi MH, Peluso I, Mohamed HRH, Shah M (2022) Hydrogeochemical characterization, and suitability assessment of drinking groundwater: application of geostatistical approach and geographic information system. Front Environ Sci 10:874464. https://doi.org/10.3389/fenvs.2022.874464
Giao NT, Nhien HTH, Anh PK, Van Ni D (2021) Classification of water quality in low-lying area in Vietnamese Mekong delta using set pair analysis method and Vietnamese water quality index. Environ Monit Assess 193:319. https://doi.org/10.1007/s10661-021-09102-1
Gomes FDG (2020) Climatic seasonality and water quality in watersheds: a study case in Limoeiro River watershed in the western region of São Paulo State, Brazil. Environ Sci Pollut Res 27:30034–30049. https://doi.org/10.1007/s11356-020-09180-7
Gruss L, Wiatkowski M, Pulikowski K, Kłos A (2021) Determination of changes in the quality of surface water in the river reservoir system. Sustainability 13:3457. https://doi.org/10.3390/su13063457
Gupta S, Gupta SK (2021) A critical review on water quality index tool: genesis, evolution and future directions. Ecol Inform 63:101299. https://doi.org/10.1016/j.ecoinf.2021.101299
Hachi T, Hachi M, Essabiri H, Boumalkha O, Doubi M, Khaffou M, Abba EH (2022) Statistical assessment of the water quality using water quality index and organic pollution index—Case study, Oued Tighza, Morocco. Mor J Chem 10:500–508. https://doi.org/10.48317/IMIST.PRSM/MORJCHEM-V10I3.33139
Hamlat A, Guidoum A, Koulala I (2017) Status and trends of water quality in the Tafna catchment: a comparative study using water quality indices. J Water Reuse Desal 7:228–245. https://doi.org/10.2166/wrd.2016.155
Haydar CM, Nehme N, Awad S, Koubaissy B, Fakih M, Yaacoub A, Toufaily J, Villeras F, Hamieh T (2014) Water quality of the upper Litani river Basin, Lebanon. Physcs Proc 55:279–284. https://doi.org/10.1016/j.phpro.2014.07.040
Horton RK (1965) An index-number system for rating water quality. J Water Pollut Con F 37:292–315
Google Scholar
Hu L, Chen L, Li Q, Zou K, Li J, Ye H (2022) Water quality analysis using the CCME-WQI method with time series analysis in a water supply reservoir. Water Supply 22:6281–6295. https://doi.org/10.2166/ws.2022.245
Jena V, Dixit S, Gupta S (2013) Assessment of water quality index of industrial area surface water samples. Int J Chemtech Res 5:278–283
Kachroud M, Trolard F, Kefi M, Jebari S, Bourrié G (2019a) Water quality indices: challenges and application limits in the literature. Water 11:361. https://doi.org/10.3390/w11020361
Kachroud M, Trolard F, Kefi M, Jebari S, Bourrié G (2019b) Water quality indices: challenges and application limits in the literature. Water. https://doi.org/10.3390/w11020361
Kareem SL, Jaber WS, Al-Maliki LA, Al-husseiny RA, Al-Mamoori SK, Alansari N (2021) Water quality assessment and phosphorus effect using water quality indices: Euphrates river- Iraq as a case study. Groundw Sustain Dev 14:100630. https://doi.org/10.1016/j.gsd.2021.100630
Khan I (2022) Hydrogeochemical and health risk assessment in and around a Ramsar-designated wetland, the Ganges River Basin, India: implications for natural and human interactions. Environ Monit and Asses 194:1–24. https://doi.org/10.1007/s10661-022-10154-0
Khan R, Saxena A, Shukla S, Sekar S, Goel P (2021) Effect of COVID-19 lockdown on the water quality index of river Gomti, India, with potential hazard of faecal-oral transmission. Environ Sci Pollut R 28:33021–33029. https://doi.org/10.1007/s11356-021-13096-1
Kizar FM (2018) A comparison between weighted arithmetic and Canadian methods for a drinking water quality index at selected locations in shatt al-kufa. IOP Conf Ser: Mater Sci Eng 433:012026. https://doi.org/10.1088/1757-899X/433/1/012026
Kothari V, Vij S, Sharma S, Gupta N (2021) Correlation of various water quality parameters and water quality index of districts of Uttarakhand. Environ Sustain Indic 9:100093. https://doi.org/10.1016/j.indic.2020.100093
Kulisz M, Kujawska J (2021) Application of artificial neural network (ANN) for water quality index (WQI) prediction for the river Warta. Poland. J Phys Conf Ser 2130:012028. https://doi.org/10.1088/1742-6596/2130/1/012028
Kumar A, Bojjagani S, Maurya A, Kisku GC (2022) Spatial distribution of physicochemical-bacteriological parametric quality and water quality index of Gomti river, India. Environ Monit Assess 194:159. https://doi.org/10.1007/s10661-022-09814-y
Kumar P (2018) Simulation of Gomti River (Lucknow City, India) future water quality under different mitigation strategies. Heliyon 4:e01074. https://doi.org/10.1016/j.heliyon.2018.e01074
Kunst Valentini MH, dos Santos GB, Duarte VH, Franz HS, Guedes HAS, Romani RF, Vieira BM (2021) Analysis of the influence of water quality parameters in the final WQI result through statistical correlation methods: Mirim lagoon, RS, Brazil, case study. Water Air Soil Pollut 232:363. https://doi.org/10.1007/s11270-021-05321-x
Lencha SM, Tränckner J, Dananto M (2021) Assessing the water quality of lake Hawassa Ethiopia—trophic state and suitability for anthropogenic uses—applying common water quality indices. Int J Environ Res Pub He 18:8904. https://doi.org/10.3390/ijerph18178904
Liou SM, Lo SL, Wang SH (2004) A generalized water quality index for Taiwan. Environ Monit Assess 96:35–52. https://doi.org/10.1023/B:EMAS.0000031715.83752.a1
Losa MS, González ARM, Hurtado DC (2022) Assessment of water quality with emphasis on trophic status in bathing areas from the central-southern coast of Cuba. Ocean Coast Res 70:e22019. https://doi.org/10.1590/2675-2824070.21096msl
Lumb A, Sharma TC, Bibeault JF (2011) A review of genesis and evolution of Water Quality Index (WQI) and some future directions. Water Qual Expos Hea. https://doi.org/10.1007/s12403-011-0040-0
Lumb LA, Sharma TC, Bibeault JF (2011) A review of genesis and evolution of Water Quality Index (WQI) and some future directions. Water Qual Expos Hea 3:11–24. https://doi.org/10.1007/s12403-011-0040-0
Luo P, Xu C, Kang S, Huo A, Lyu J, Zhou M, Nover D (2021) Heavy metals in water and surface sediments of the Fenghe river basin, China: assessment and source analysis. Water Sci Technol 84:3072–3090. https://doi.org/10.2166/wst.2021.335
Maity S, Maiti R, Senapati T (2022) Evaluation of spatio-temporal variation of water quality and source identification of conducive parameters in Damodar River, India. Environ Monit Assess 194:1–23. https://doi.org/10.1007/s10661-022-09955-0
Makubura R, Meddage DPP, Azamathulla H, Pandey M, Rathnayake U (2022) A simplified mathematical formulation for water quality index (WQI): a case study in the Kelani River Basin. Sri Lanka Fluids 7:147. https://doi.org/10.3390/fluids7050147
Massoud MA (2012) Assessment of water quality along a recreational section of the Damour River in Lebanon using the water quality index. Environ Monit Assess 184:4151–4160. https://doi.org/10.1007/s10661-011-2251-z
Hamdi KM, Lihan S, Hamdan N, Tay MG (2022) Water quality assessment and the prevalence of antibiotic- resistant bacteria from a recreational river in Kuching, Sarawak, Malaysia. J Sustain Sci Manag 17:37–59. https://doi.org/10.46754/jssm.2022.05.004
Moskovchenko DV, Babushkin AG, Yurtaev AA (2020) The impact of the Russian oil industry on surface water quality (a case study of the Agan River catchment, West Siberia). Environ Earth Sci 79:1–21. https://doi.org/10.1007/s12665-020-09097-x
Mukate S, Wagh V, Panaskar D, Jacobs JA, Sawant A (2019) Development of new integrated water quality index (IWQI) model to evaluate the drinking suitability of water. Ecol Indic 101:348–354. https://doi.org/10.1016/j.ecolind.2019.01.034
Muniz DHF, Malaquias JV, Lima JE, Oliveira-Filho EC (2020) Proposal of an irrigation water quality index (IWQI) for regional use in the Federal District, Brazil. Environ Monit Assess 192:1–15. https://doi.org/10.1007/s10661-020-08573-y
Murillo-Delgado JO, Jimenez-Torres HD, Alvarez-Bobadilla JI, Gutierrez-Ortega JA, Camacho JB, Valle PFZ, Barcelo-Quintal ID, Delgado ER, Gomez-Salazar S (2021) Chemical speciation of selected toxic metals and multivariate statistical techniques used to assess water quality of tropical Mexican Lake Chapala. Environ Monit Assess 193:1–25. https://doi.org/10.1007/s10661-021-09185-w
Muvundja FA, Walumona JR, Dusabe MC, Alunga GL, Kankonda AB, Albrecht C, Eisenberg J, Wüest A (2022) The land–water–energy nexus of Ruzizi River Dams (Lake Kivu outflow, African Great Lakes Region): status, challenges, and perspectives. Front Environ Sci 10:892591. https://doi.org/10.3389/fenvs.2022.892591
Nair HC, Joseph A, Padmakumari Gopinathan V (2020) Hydrochemistry of tropical springs using multivariate statistical analysis in Ithikkara and Kallada river basins, Kerala, India. Sustain Water Resour Manag 6:1–21. https://doi.org/10.1007/s40899-020-00363-1
Najah A, Teo FY, Chow MF, Huang YF, Latif SD, Abdullah S, Ismail M, El-Shafie A (2021) Surface water quality status and prediction during movement control operation order under COVID-19 pandemic: case studies in Malaysia. Int J Environ Sci Te 18:1009–1018. https://doi.org/10.1007/s13762-021-03139-y
Nong X, Shao D, Zhong H, Liang J (2020) Evaluation of water quality in the South-to-North Water diversion project of China using the water quality index (WQI) method. Water Res 178:115781. https://doi.org/10.1016/j.watres.2020.115781
Ortega-Samaniego QM, Romero I, Paches M, Dominici A, Fraíz A (2021) Assessment of physicochemical and bacteriological parameters in the surface water of the Juan Diaz River, Panama. WIT Trans Ecol Environ 251:95–104. https://doi.org/10.2495/WS210101
Othman F, Alaaeldin ME, Seyam M, Ahmed AN, Teo FY, Fai CM, Afan HA, Sherif M, Sefelnasr A, El-Shafie A (2020) Efficient River water quality index prediction considering minimal number of inputs variables. Eng Appl Comp Fluid Mech 14:751–763. https://doi.org/10.1080/19942060.2020.1760942
Panneerselvam B, Muniraj K, Duraisamy K, Pande C, Karuppannan S, Thomas M (2022) An integrated approach to explore the suitability of nitrate-contaminated groundwater for drinking purposes in a semiarid region of India. Environ Geochem Hlth 10:1–7. https://doi.org/10.1007/s10653-022-01237-5
Parween S, Siddique NA, Mahammad Diganta MT, Olbert AI, Uddin MG (2022) Assessment of urban river water quality using modified NSF water quality index model at Siliguri city, West Bengal, India. Environ Sustain Indic 16:100202. https://doi.org/10.1016/j.indic.2022.100202
Paun I, Cruceru L, Chiriac FL, Niculescu M, Vasile GG, Marin NM (2016) Water quality indices—methods for evaluating the quality of drinking water. In: Proceedings of the 19th INCD ECOIND International Symposium—SIMI 2016, “The Environment and the Industry”, Bucharest, Romania, 13–14 October 2016: 395–402. https://doi.org/10.21698/simi.2016.0055
Peluso J (2021) Comprehensive assessment of water quality through different approaches: physicochemical and ecotoxicological parameters. Sci Total Environ 800:149510. https://doi.org/10.1016/j.scitotenv.2021.149510
Peng H (2022) Hydrochemical characteristics and health risk assessment of groundwater in karst areas of southwest China: a case study of Bama, Guangxi. J Clean Prod 341:130872. https://doi.org/10.1016/j.jclepro.2022.130872
Phadatare SS, Gawande S (2016) Review paper on development of water quality index. Int Res J Eng Technol 5:765–767. https://doi.org/10.17577/IJERTV5IS050993
Poonam T, Tanushree B, Sukalyan C (2013) Water quality indices- important tools for water quality assessment: a review. Int J Adv Chem 1:15–28. https://doi.org/10.5121/ijac.2015.1102
Qu X, Chen Y, Liu H, Xia W, Lu Y, Gang DD, Lin LS (2020) A holistic assessment of water quality condition and spatiotemporal patterns in impounded lakes along the eastern route of China’s South-to-North water diversion project. Water Res 185:116275. https://doi.org/10.1016/j.watres.2020.116275
Radeva K, Seymenov K (2021) Surface water pollution with nutrient components, trace metals and metalloidsin agricultural and mining-affected river catchments: a case study for three tributaries of the Maritsa River, Southern Bulgaria. Geogr Pannonica 25:214–225. https://doi.org/10.5937/gp25-30811
Ramírez-Morales D, Pérez-Villanueva ME, Chin-Pampillo JS, Aguilar-Mora P, Arias-Mora V, Masís-Mora M (2021) Pesticide occurrence and water quality assessment from an agriculturally influenced Latin-American tropical region. Chemosphere 262:127851. https://doi.org/10.1016/j.chemosphere.2020.127851
Ristanto D, Ambariyanto A, Yulianto B (2021) Water quality assessment based on national sanitations foundation water quality index during rainy season in Sibelis and Kemiri estuaries Tegal City. IOP Conf Ser: Earth and Environ Sci 750:012013. https://doi.org/10.1088/1755-1315/750/1/012013
Rizani S, Feka F, Fetoshi O, Durmishi B, Shala S, Çadraku H, Bytyçi P (2022) Application of water quality index for the assessment the water quality in River Lepenci. Ecol Eng Environ Tech 23:189–201. https://doi.org/10.12912/27197050/150297
Roozbahani MM, Boldaji MN (2013) Water quality assessment of Karoun river using WQI. Int Res J Appl Basic Sci 5:628–632
Roșca OM (2020) Impact of anthropogenic activities on water quality parameters of glacial lakes from Rodnei mountains, Romania. Environ Res 182:109136. https://doi.org/10.1016/j.envres.2020.109136
Rubio-Arias H, Contreras-Caraveo M, Quintana RM, Saucedo-Teran RA, Pinales-Munguia A (2012) An overall water quality index (WQI) for a man-made aquatic reservoir in Mexico. Int J Env Res Pub He 9:1687–1698. https://doi.org/10.3390/ijerph9051687
Said A, Stevens DK, Sehlke G (2004) An innovative index for evaluating water quality in streams. Environ Manage 34:406–414. https://doi.org/10.1007/s00267-004-0210-y
Samadi MT, Sadeghi S, Rahmani A, Saghi MH (2015) Survey of water quality in Moradbeik river basis on WQI index by GIS. Environ Eng Manag J 2:7–11
Sarwar S, Ahmmed I, Mustari S, Shaibur MR (2020) Use of Weighted Arithmetic Water Quality Index (WAWQI) to determine the suitability of groundwater of Chaugachcha and Manirampur Upazila, Jashore, Bangladesh. Environ Biolog Res 2:22–30
Scopus (2022) Analyze search results Retrieved February 22, 2023, from https://www.scopus.com/term/analyzer.uri?sid=8eeff2944308f3417393fe6b0de5b7e1&origin=resultslist&src=s&s=TITLE-ABS-KEY%28water+quality+index%29&sort=cp-f&sdt=b&sot=b&sl=34&count=38419&analyzeResults=Analyze+results&txGid=68cf75652b70f07c51075648639736f3
Shah KA, Joshi GS (2017) Evaluation of water quality index for River Sabarmati, Gujarat. India Appl Water Sci 7:1349–1358. https://doi.org/10.1007/s13201-015-0318-7
Shan W (2011) Discussion on parameter choice for managing water quality of the drinking water source. Procedia Environ Sci 11:1465–1468. https://doi.org/10.1016/j.proenv.2011.12.220
Singh PK, Tiwari AK, Panigary BP, Mahato K (2013) Water quality indices used for water resources vulnerability assessment using GIS technique: a review. Int J Earth Sci Eng 6:1594–1600
Sofi MS, Hamid A, Bhat SU, Rashid I, Kuniyal JC (2022) Impact evaluation of the run-of-river hydropower projects on the water quality dynamics of the Sindh River in the Northwestern Himalayas. Environ Monit Assess 194:626. https://doi.org/10.1007/s10661-022-10303-5
Steinhart CE, Shcierow LJ, Sonzogni WC (1982) Environmental quality index for the great lakes. Water Resour Bull 18:1025–1031
Stričević L, Pavlović M, Filipović I, Radivojević A, Martić Bursać N, Gocić M (2021) Statistical analysis of water quality parameters in the basin of the Nišava River (Serbia) in the period 2009–2018. Geografie 126:55–73. https://doi.org/10.37040/geografie2021126010055
Sudhakaran S, Mahadevan H, Arun V, Krishnakumar AP, Krishnan KA (2020) A multivariate statistical approach in assessing the quality of potable and irrigation water environs of the Netravati River basin (India). Groundw Sustain Dev 11:100462. https://doi.org/10.1016/j.gsd.2020.100462
Sukmawati NMH, Rusni NW (2019) Assessment of Water Quality Index of Beratan lake using NSF WQI indicator. Warmadewa Med J 4:39–43
Sutadian AD, Muttil N, Yilmaz AG, Perera BJC (2016) Development of river water quality indice- A review. Environ Monit Assess 188:58. https://doi.org/10.1007/s10661-015-5050-0
Taloor AK, Pir RA, Adimalla N, Ali S, Manhas DS, Roy S, Singh AK (2020) Spring water quality and discharge assessment in the Basantar watershed of Jammu Himalaya using geographic information system (GIS) and water quality Index (WQI). G Groundw Sustain Dev 10:100364. https://doi.org/10.1016/j.gsd.2020.100364
Tampo L, Alfa-Sika Mande SL, Adekanmbi AO, Boguido G, Akpataku KV, Ayah M, Tchakala I, Gnazou MDT, Bawa LM, Djaneye-Boundjou G, Alhassan EH (2022) Treated wastewater suitability for reuse in comparison to groundwater and surface water in a peri-urban area: Implications for water quality management. Sci Total Environ 815:152780. https://doi.org/10.1016/j.scitotenv.2021.152780
Teodorof L, Ene A, Burada A, Despina C, Seceleanu-Odor D, Trifanov C, Ibram O, Bratfanof E, Tudor MI, Tudor M, Cernisencu I, Georgescu LP, Iticescu C (2021) Integrated assessment of surface water quality in Danube River Chilia branch. Appl Sci 11:9172. https://doi.org/10.3390/app11199172
Tirkey P, Bhattacharya T, Chakraborty S (2015) Water quality indices-important tools for water quality assessment: a review. Int J Adv Chem 1:15–28
Tripathi M, Singal SK (2019) Allocation of weights using factor analysis for development of a novel water quality index. Ecotox Environ Safe 183:109510. https://doi.org/10.1016/j.ecoenv.2019.109510
Tyagi S, Sharma B, Singh P, Dobhal R (2013) Water quality assessment in terms of water quality index. Am J Water Resour 1:34–38. https://doi.org/10.12691/ajwr-1-3-3
Uddin MG, Nash S, Rahman A, Olbert AI (2022) A comprehensive method for improvement of water quality index (WQI) models for coastal water quality assessment. Water Res 219:118532. https://doi.org/10.1016/j.watres.2022.118532
Uddin MG, Nash S, Olbert AI (2021) A review of water quality index models and their use for assessing surface water quality. Ecol Indic 122:107218. https://doi.org/10.1016/j.ecolind.2020.107218
Udeshani WAC, Dissanayake HMKP, Gunatilake SK, Chandrajith R (2020) Assessment of groundwater quality using water quality index (WQI): a case study of a hard rock terrain in Sri Lanka. Groundw Sustain Dev 11:100421. https://doi.org/10.1016/j.gsd.2020.100421
Ugochukwu U, Onuora O, Onuarah A (2019) Water quality evaluation of Ekulu river using water quality index (WQI). J Environ Stud 4:4. https://doi.org/10.13188/2471-4879.1000027
Uning R, Suratman S, Bedurus EA, Nasir FAM, Hock Seng T, Latif MT, Mostapa R (2021) The water quality and nutrients status in the Dungun River Basin, Terengganu. Am Soc Microbiol Sci J 16:1–14. https://doi.org/10.32802/asmscj.2021.837
Vaiphei SP, Kurakalva RM (2021) Hydrochemical characteristics and nitrate health risk assessment of groundwater through seasonal variations from an intensive agricultural region of upper Krishna River basin, Telangana. India. Ecotox Environ Safe 213:112073. https://doi.org/10.1016/j.ecoenv.2021.112073
Valentini M, dos Santos GB, Muller Vieira B (2021) Multiple linear regression analysis (MLR) applied for modeling a new WQI equation for monitoring the water quality of Mirim Lagoon, in the state of Rio Grande do Sul—Brazil. SN Appl Sci 3:70. https://doi.org/10.1007/s42452-020-04005-1
Varol S, Davraz A, Şener Ş, Şener E, Aksever F, Kırkan B, Tokgözlü A (2021) Assessment of groundwater quality and usability of Salda Lake Basin (Burdur/Turkey) and health risk related to arsenic pollution. J Environ Health Sci 19:681–706. https://doi.org/10.1007/s40201-021-00638-5
Vasistha P (2020) Assessment of spatio-temporal variations in lake water body using indexing method. Environ Sci Pollut R 27:41856–41875
Wang Q, Li Z, Xu Y, Li R, Zhang M (2022) Analysis of spatio-temporal variations of river water quality and construction of a novel cost-effective assessment model: a case study in Hong Kong. Environ Sci Pollut R 29:28241–28255. https://doi.org/10.1007/s11356-021-17885-6
Wong YJ, Shimizu Y, He K, Nik Sulaiman NM (2020) Comparison among different ASEAN water quality indices for the assessment of the spatial variation of surface water quality in the Selangor River basin. Malaysia Environ Monit Assess 192:644. https://doi.org/10.1007/s10661-020-08543-4
Xiao L, Zhang Q, Niu C, Wang H (2020) Spatiotemporal patterns in river water quality and pollution source apportionment in the Arid Beichuan River Basin of Northwestern China using positive matrix factorization receptor modeling techniques. Int J Env Res Pub He 17:5015. https://doi.org/10.3390/ijerph17145015
Xiong F, Chen Y, Zhang S, Xu Y, Lu Y, Qu X, Gao W, Wu X, Xin W, Gang DD, Lin LS (2022) Land use, hydrology, and climate influence water quality of China’s largest river. J Environ Manage 318:115581. https://doi.org/10.1016/j.jenvman.2022.115581
Yan F, Liu L, You Z, Zhang Y, Chen M, Xing X (2015) A dynamic water quality index model based on functional data analysis. Ecol Indic 57:249–258. https://doi.org/10.1016/j.ecolind.2015.05.005
Yang Z, Bai J, Zhang W (2021) Mapping and assessment of wetland conditions by using remote sensing images and POI data. Ecol Indic 127:107485. https://doi.org/10.1016/j.ecolind.2021.107485
Yılmaz E, Koç C, Gerasimov I (2020) A study on the evaluation of the water quality status for the Büyük Menderes River, Turkey. Sustain Water Resour Manag 6:100. https://doi.org/10.1007/s40899-020-00456-x
Yogendra K, Puttaiah ET (2008) Determination of water quality index and suitability of an urban waterbody in Shimoga Town, Karnataka. Proceedings of Taal 2007: The 12th world lake conference 342: 346
Yotova G, Varbanov M, Tcherkezova E, Tsakovski S (2021) Water quality assessment of a river catchment by the composite water quality index and self-organizing maps. Ecol Indic 120:106872. https://doi.org/10.1016/j.ecolind.2020.106872
Yuan H, Yang S, Wang B (2022) Hydrochemistry characteristics of groundwater with the influence of spatial variability and water flow in Hetao Irrigation District, China. Environ Sci Pollut R 20:1–5. https://doi.org/10.1007/s11356-022-20685-1
Zakir HM, Sharmin S, Akter A, Rahman MS (2020) Assessment of health risk of heavy metals and water quality indices for irrigation and drinking suitability of waters: a case study of Jamalpur Sadar area, Bangladesh. Environ Adv 2:100005. https://doi.org/10.1016/j.envadv.2020.100005
Zhan S, Zhou B, Li Z, Li Z, Zhang P (2021) Evaluation of source water quality and the influencing factors: a case study of Macao. Phys Chem Earth Parts a/b/c 123:103006. https://doi.org/10.1016/j.pce.2021.103006
Zhang L (2017) Different methods for the evaluation of surface water quality: the case of the Liao River, Liaoning Province, China. Int Rev Spat Plan Sustain Dev 5:4–18. https://doi.org/10.14246/irspsd.5.4_4
Zhang L (2019) Big data, knowledge mapping for sustainable development: a water quality index case study. Emerg Sci J 3:249–254. https://doi.org/10.28991/esj-2019-01187
Zhang ZM, Zhang F, Du JL, Chen DC (2022) Surface water quality assessment and contamination source identification using multivariate statistical techniques: a case study of the Nanxi River in the Taihu Watershed, China. Water 14:778. https://doi.org/10.3390/w14050778
Zhu X, Wang L, Zhang X, He M, Wang D, Ren Y, Yao H, Net Victoria Ngegla J, Pan H (2022) Effects of different types of anthropogenic disturbances and natural wetlands on water quality and microbial communities in a typical black-odor river. Ecol Indic 136:108613. https://doi.org/10.1016/j.ecolind.2022.108613
Zotou I, Tsihrintzis VA, Gikas GD (2018) Comparative assessment of various water quality indices (WQIs) in Polyphytos reservoir-Aliakmon River. Greece Proc 2:611. https://doi.org/10.3390/proceedings2110611
Download references
Author information
Authors and affiliations.
Department of Agricultural and Food Engineering, School of Engineering, Holy Spirit University of Kaslik, P.O.Box 446, Jounieh, Lebanon
Sandra Chidiac, Paula El Najjar, Naim Ouaini, Youssef El Rayess & Desiree El Azzi
FMPS HOLDING BIOTECKNO s.a.l. Research & Quality Solutions, Naccash, P.O. Box 60 247, Beirut, Lebanon
Paula El Najjar
Syngenta, Environmental Safety, Avenue des Près, 78286, Guyancourt, France
Desiree El Azzi
You can also search for this author in PubMed Google Scholar
Corresponding authors
Correspondence to Sandra Chidiac or Desiree El Azzi .
Ethics declarations
Conflict of interest.
All authors declare that they have no conflict of interest.
Additional information
Publisher's note.
Springer Nature remains neutral with regard to jurisdictional claims in published maps and institutional affiliations.
Rights and permissions
Springer Nature or its licensor (e.g. a society or other partner) holds exclusive rights to this article under a publishing agreement with the author(s) or other rightsholder(s); author self-archiving of the accepted manuscript version of this article is solely governed by the terms of such publishing agreement and applicable law.
Reprints and permissions
About this article
Chidiac, S., El Najjar, P., Ouaini, N. et al. A comprehensive review of water quality indices (WQIs): history, models, attempts and perspectives. Rev Environ Sci Biotechnol 22 , 349–395 (2023). https://doi.org/10.1007/s11157-023-09650-7
Download citation
Received : 07 December 2022
Accepted : 23 February 2023
Published : 11 March 2023
Issue Date : June 2023
DOI : https://doi.org/10.1007/s11157-023-09650-7
Share this article
Anyone you share the following link with will be able to read this content:
Sorry, a shareable link is not currently available for this article.
Provided by the Springer Nature SharedIt content-sharing initiative
- Water quality index (WQI)
- Water quality parameters
- Surface water
- Ground water
- Find a journal
- Publish with us
- Track your research
Click through the PLOS taxonomy to find articles in your field.
For more information about PLOS Subject Areas, click here .
Loading metrics
Open Access
Peer-reviewed
Research Article
Microplastic contamination of drinking water: A systematic review
Roles Conceptualization, Data curation, Formal analysis, Funding acquisition, Investigation, Methodology, Visualization, Writing – original draft, Writing – review & editing
* E-mail: [email protected]
Affiliation Hull York Medical School, University of Hull, Hull, United Kingdom

Roles Project administration, Supervision, Validation, Writing – review & editing
Roles Funding acquisition, Project administration, Supervision, Writing – review & editing
Affiliation Department of Biological and Marine Sciences, University of Hull, Hull, United Kingdom
- Evangelos Danopoulos,
- Maureen Twiddy,
- Jeanette M. Rotchell
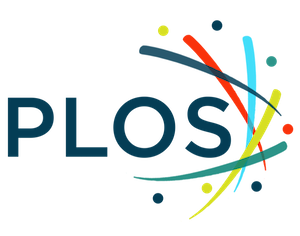
- Published: July 31, 2020
- https://doi.org/10.1371/journal.pone.0236838
- Reader Comments
Microplastics (MPs) are omnipresent in the environment, including the human food chain; a likely important contributor to human exposure is drinking water.
To undertake a systematic review of MP contamination of drinking water and estimate quantitative exposures.
The protocol for the systematic review employed has been published in PROSPERO (PROSPERO 2019, Registration number: CRD42019145290). MEDLINE, EMBASE and Web of Science were searched from launch to the 3rd of June 2020, selecting studies that used procedural blank samples and a validated method for particle composition analysis. Studies were reviewed within a narrative analysis. A bespoke risk of bias (RoB) assessment tool was used.
12 studies were included in the review: six of tap water (TW) and six of bottled water (BW). Meta-analysis was not appropriate due to high statistical heterogeneity (I 2 >95%). Seven studies were rated low RoB and all confirmed MP contamination of drinking water. The most common polymers identified in samples were polyethylene terephthalate (PET) and polypropylene (PP), Methodological variability was observed throughout the experimental protocols. For example, the minimum size of particles extracted and analysed, which varied from 1 to 100 μm, was seen to be critical in the data reported. The maximum reported MP contamination was 628 MPs/L for TW and 4889 MPs/L for BW, detected in European samples. Based on typical consumption data, this may be extrapolated to a maximum yearly human adult uptake of 458,000 MPs for TW and 3,569,000 MPs for BW.
Conclusions
This is the first systematic review that appraises the quality of existing evidence on MP contamination of drinking water and estimates human exposures. The precautionary principle should be adopted to address concerns on possible human health effects from consumption of MPs. Future research should aim to standardise experimental protocols to aid comparison and elevate quality.
Citation: Danopoulos E, Twiddy M, Rotchell JM (2020) Microplastic contamination of drinking water: A systematic review. PLoS ONE 15(7): e0236838. https://doi.org/10.1371/journal.pone.0236838
Editor: Amitava Mukherjee, VIT University, INDIA
Received: May 1, 2020; Accepted: July 14, 2020; Published: July 31, 2020
Copyright: © 2020 Danopoulos et al. This is an open access article distributed under the terms of the Creative Commons Attribution License , which permits unrestricted use, distribution, and reproduction in any medium, provided the original author and source are credited.
Data Availability: All relevant data are within the manuscript and its Supporting Information files.
Funding: This research is supported by a PhD scholarship to ED within the “Health Inequalities and emerging environmental contaminants – Places and People” cluster funded by the University of Hull. The funder had no role in study design, data collection and analysis, decision to publish, or preparation of the manuscript.
Competing interests: The authors have declared that no competing interests exist.
Introduction
Microplastics (MPs) are particles of predominantly synthetic polymeric composition in the micro scale [ 1 , 2 ], and while a consensus on size range has not been reached, the typical range is between 1 μm and 5 mm. MPs have been identified in all aquatic environments: marine [ 3 – 7 ] and freshwater (lakes, rivers, reservoirs, groundwater) [ 8 – 15 ], but research has so far concentrated more on marine environments. MP contamination of aquatic environments is expected to rise, hand-in-hand with the continuous rise in plastic production, use and waste [ 16 – 20 ]. MPs have also entered the food web, thus becoming an emerging food safety issue and risk [ 21 – 25 ]. Emerging risk in terms of food safety is defined as a risk posed by possible significant exposures to a recently identified (emerging) hazard [ 26 , 27 ].
Human exposure pathways include ingestion and inhalation and the presence of MPs in human stool samples has recently been verified [ 28 ]. Drinking water is considered as one possible medium for the introduction of MPs into the human body [ 24 ]. There is a growing interest around the prevalence of MPs in drinking water underpinned by recent research but a systematic review of available evidence is lacking [ 29 – 35 ]. None of the existing reviews have used the methodology [ 36 ] on which systematic reviews are based. Systematic reviews synthesize the findings quantitatively and qualitatively in a standardised way, avoiding the introduction of bias. Although human health effects are still under examination, lessons from toxicology inform us that the effects will be dose dependent [ 37 – 39 ]. Determining exposure levels is key in formulating a risk assessment framework for this emerging environmental contaminant. Health effects will be caused by: their physical attributes, the chemical properties of the polymers, the plasticisers, or other chemicals added in the manufacturing process, and the chemicals they can absorb in nature as well as the microbes that can grow on their surface [ 40 – 42 ].
This review focuses on water intended for human consumption, including tap water (TW) that is available to consumers via water treatment plants (WTP) and bottled water (BW). BW is further divided into table, spring and natural mineral water. Specific regulations govern their categorization according to their source and the processes that they are allowed to undergo before being bottled [e.g. 43 – 45 ]. Both natural mineral and spring water come from underground water sources, in principle, protected from pollution and are bottled in situ . In contrast, bottled table water can come from any source, including municipal mains (tap water), as long as it conforms to water safety specifications [ 43 ]. Water from different categories will vary in quality depending on the initial water quality, and the processes they are subjected to ensure food safety, transportation and packaging.
The aim of this review was to identify all available research on MP contamination of drinking waters and assess their quality to determine the state of the evidence and consequently, attempt quantification of human exposures in the prism of an emerging food safety issue. We also aim to compare water of different origins (TW and BW) in terms of MP contamination load. Further, we address the methodological issues in the field of environmental MPs research regarding study design, execution and reporting.
This review follows a protocol published in PROSPERO (PROSPERO 2019, Registration number: CRD42019145290) available from: https://www.crd.york.ac.uk/prospero/display_record.php?ID=CRD42019145290 and in the S1 Protocol (available in the Supporting Information). The protocol was developed according to the guidelines set by the Preferred Reporting Items for Systematic Reviews and Meta-Analyses protocols (PRISMA-P) [ 46 , 47 ]. The protocol was designed to include available research on all food categories which were determined by a preceding scoping review. In brief, only descriptive and analytic observational study designs (and not experimental) were included [ 48 ]. No time limit on publication date was set and databases were searched from launch date to 10 th July 2019. The searches were repeated on the 3rd of June 2020 to include the most recently published papers. Only studies that reported on ‘water intended for human consumption’ as defined by Directive 2009/54/EC [ 44 ] and Regulation (EC) No 178 [ 49 ] were included. Eligible studies must have used one (or more) of the four currently validated processes for the identification of microparticle composition: Fourier-transform infrared spectroscopy (FTIR), Raman spectroscopy (RM), pyrolysis gas chromatography/ mass spectrometry (Pyr-GC-MS) and scanning electron microscopy plus energy-dispersive X-ray spectroscopy (SEM/EDS). The use of procedural blank samples was also mandatory. Articles that were not published in the English language were excluded.
Information sources were MEDLINE (OVID interface, 1946 onwards), EMBASE (OVID interface, 1974 onwards) and the Web of Science core collection (Web of Science, 1900 onwards). The search strategy was developed for MEDLINE and EMBASE (OVID interface) using free text and MeSH, for all food categories. Search terms included: microplastic, nanoplastic, food contamination, water contamination (full search strategy can be found in S1 Table ). Study selection was executed using a two-level screening by two independent reviewers against the inclusion/exclusion criteria. Any discrepancies were resolved by a third-party arbitrator. Inter-rater agreement level for the first level screening was 90%, Cohen’s k: 0.34, and for the second level: 100%, Cohen’s k: 1 [ 50 ]. A form previously developed and verified for a scoping review was used for data extraction.
The quality of the studies was assessed with the use of a bespoke risk of bias (RoB) assessment tool, which was developed because the existing tools were not suitable for the scope of the review [ 51 ]. Assessment tool development was based on guidelines set by the Centre for Reviews and Dissemination [ 52 ], the STROBE Statement checklist [ 53 ], the Agency for Healthcare Research and Quality of the U.S. Department of Health and Human Services [ 54 ], the Environmental-Risk of Bias Tool [ 55 ] regarding evidence in environmental science and the Cochrane Collaboration’s tool for assessing RoB [ 56 ]. The RoB tool, is a checklist ( S2 Table ), that prompts questions across four domains: study design, sampling, analysis and reporting, leading to an overall assessment with justification for each entry [ 57 ]. There were three ratings: high risk, low risk or unclear RoB and the results were used to assess study quality and overall certainty of evidence.
The primary outcome of interest was MP content in the sample expressed in a quantitative measure in any available units of measurements. Further information of interest included the methodological specifications of the experimental protocols. The studies were reviewed in a narrative analysis according to the guidelines set down by the Centre for Reviews and Dissemination [ 52 ] and the results were reported according to the Preferred Reporting Items for Systematic Reviews and Meta-Analyses (PRISMA) Statement [ 58 , 59 ] ( S1 PRISMA Checklist ).
Study selection
2467 citations were identified by the search strategy, after duplicates were removed, and 2307 citations were dismissed in the first-level screening based on their title and abstract ( Fig 1 ). During the second-level screening, the full papers were scrutinized, and 112 studies were removed with reasons ( S1 Appendix ) and seven were included. When the searches were re-run, five more studies were included after the first and second level screening ( Fig 1 ), resulting in 12 studies [ 60 – 71 ] finally included in this systematic review.
- PPT PowerPoint slide
- PNG larger image
- TIFF original image
The flow chart presents the results and screening process of the original searches and the rerun of the searches.
https://doi.org/10.1371/journal.pone.0236838.g001
Study characteristics
All the studies included analysed water readily available for human consumption. The study characteristics are presented in S3 Table . Six studies used samples of BW (table and mineral) and six studies used TW. The overall sample size for BW was n = 91 brands (n = 435 bottles) and for the TW, n = 155 samples. All of the studies used different techniques to extract particles from their samples. One study used FTIR [ 61 ], three studies used m-FTIR [ 62 , 67 , 70 ], one study used RM [ 68 ], four used m-RM [ 63 , 65 , 66 , 69 ], one both FTIR and RM [ 60 ], one used both m-FTIR and m-RM [ 64 ] and one SEM-EDX [ 71 ] to identify the composition of the extracted particles. Ten of the studies reported the results by MP particles per volume, one provides only the range of MP content and one the frequency of occurrence.
Risk of bias within studies
RoB was assessed in a systematic way using the RoB tool created for this review. The results of the assessment are illustrated in Figs 2 and 3 . Two studies were assessed as of high RoB [ 69 , 71 ] and three of unclear RoB [ 66 – 68 ]. The RoB assessment is used in the analysis part of the review.
The figure shows the rating for the four domains and the overall rating for each study. Red (-) indicates high RoB, green (+) indicates low RoB and yellow (?) indicates unclear RoB (Unclear RoB is given to a study when substantial information to make an informed assessment have not been reported).
https://doi.org/10.1371/journal.pone.0236838.g002
https://doi.org/10.1371/journal.pone.0236838.g003
Results of MPs contamination
The results are presented in Table 1 as two categories of TW and BW. The results from Mintenig et al. [ 62 ] were converted from MPs/m 3 to MPs/L content for ease of comparison to the remaining studies. Mason et al. [ 61 ] divided the results in two sections: one including particles ≥100 μm that were verified as MPs through FTIR spectral analysis and particles <100 μm that were only tagged using Nile Red solution to dye them. In line with our eligibility criteria, only the results of the FTIR verified particles will be included in this review. Visual observation for the identification of MP particles can lead to under or overestimations [ 31 ]. The use of instruments which identify the chemical composition in a standardized way based on a physical or electronic output (spectra, pyrograms etc.) exclude the introduction of human error and enable reproducibility and transparency of the results.
https://doi.org/10.1371/journal.pone.0236838.t001
Regarding studies other than BW, when results were presented for both untreated and treated water, only the latter are presented.
Six studies [ 62 , 64 , 66 – 68 , 70 ] sampled and analysed TW that was readily available to consumers via a public service. The percentage of samples containing MPs across the studies ranged from 24% to 100% and the MPs content from 0–1247 MPs/L. The most common shapes identified were fragments and second most common was fibres. A key difference between the samples is that Pivokonsky et al. [ 64 ] used water coming from surface waters (reservoirs), which are open aquatic systems exposed to contamination, while Mintenig et al. [ 62 ] used water from underground and therefore protected sources. Shruti et al. [ 66 ] used water from a variety of sources but the majority came from local aquifers. Strand et al. [ 67 ], Tong et al. [ 68 ] and Zhang et al. [ 70 ] did not provide information on the origin of the water. It is reasonable to assume that water quality before it entered the WTP would vary and directly affect the quality of the water after processing [ 8 ].
Four of the studies [ 64 , 66 , 68 , 70 ] provided the necessary data to attempt a meta-analysis. In order to test whether the results were appropriate for meta-analysis, the statistical heterogeneity was measured using a Higgins I 2 test [ 72 ], calculated using R (version 3.6.0) [ 73 ], executing all analysis via RStudio, (version 1.2.1335) [ 74 ], and using the additional packages meta (version 4.9–7) [ 75 ], metaphor (version 2.1–0) [ 76 ], dmetar [ 77 ], robvis [ 78 ] and ggplot2 [ 79 ]. A random-effects model was fitted [ 80 , 81 ] and heterogeneity was found to be high, I 2 = 99.8% (see forest plot in S1 Fig ). In order to detect the origin of heterogeneity, a series of random-effects models were fitted excluding two studies [ 64 , 68 ] that were identified as statistical outliers. The exclusion of the studies did not improve heterogeneity which remained high (100%). Therefore, the data were found to be inappropriate for meta-analysis. Heterogeneity is either caused by clinical (sample) or methodological variability [ 36 , 82 ] and is further discussed in the narrative analysis section.
Sample treatment/particle extraction.
The experimental protocol for the extraction of particles differed between the six studies in terms of sample collection, treatment and filtering. Mintenig et al. [ 62 ] filtered the water directly at the sampling sites using stainless steel filter cartridges (3 μm) and then further treated the residue on the filters at the lab. A solution of hydrochloric acid was used to dissolve inorganic material, such as calcium carbonate and iron precipitates, followed by a second filtering through another 3 μm stainless steel filter. The residue was treated again using hydrogen peroxide before the third and final filtration on 0.2 μm aluminium oxide filters. An additional density separation step was used for the raw water samples, employing a zinc chloride solution to remove further iron oxide particles. Strand et al. [ 67 ] also filtered the samples at the sampling sites but using a stainless-steel filter with absolute filtering ability of 11–12 μm. The sample was then treated using a solution of acetic acid. For the collection of the particles used for the spectral analysis, a backwashing procedure with detergent solution was used, this was pre-filtered water and then ethanol under vacuum suction on an Anodisc filter (0.2 μm). Four studies [ 64 , 66 , 68 , 70 ] collected the samples in bottles and then transported them to the lab for processing. Pivokonsky et al. [ 64 ] used wet peroxide oxidation and heat treatment at 75°C for digestion, followed by a double filtration through 5 μm and then 0.2 μm membrane filters (PTFE). Tong et al. [ 68 ] used hydrochloric acid for digestion followed by filtering through 0.2 μm aluminium oxide filters. In contrast, Shruti et al. [ 66 ] and Zhang et al. [ 70 ] did not treat the samples prior to filtering, using 0.22 μm and 0.45 μm pore size filters respectively.
The difference in the pore size of the filters used in the different stages reflects the sizes of the particles extracted which were subsequently further analysed for composition identification, and has thus directly affected the measured MP content. On the other hand, the use of a digestion step to dissolve particulate matter is employed only by some of the studies to extract water impurities and optimize the filtration process.
Spectral analysis.
Differences in the methodology of the studies were identified while important information such as the number of extracted particles and the number of particles that were analysed for composition were not reported ( Table 2 ). Three studies used FTIR for spectral analysis, while Pivokonsky et al. [ 64 ] also used RM for the smaller size range of 1–10 μm. One study used m-FTIR, one RM and one m-RM. A key difference between them is the technical limitation of the instrument regarding the minimum particle size detected. FTIR and RM technical specifications are in the range of 40 μm and 10 μm, respectively. When these methods are used in conjunction with microscopes, it becomes possible to analyse particles down to the size of 10 μm (m-FTIR) and 1 μm (m-RM) [ 31 , 83 – 86 ]. Mintenig et al. [ 62 ] and Zhang et al. [ 70 ] analysed 100% of the filters’ surface, Pivokonsky et al. [ 64 ] about 25% of the sample and Strand et al. [ 67 ] 10% of the filter but coming from only three out of the 17 sampling sites/samples. Shruti et al. [ 66 ] and Tong et al. [ 68 ] did not report the amount of the sample analysed.
https://doi.org/10.1371/journal.pone.0236838.t002
None of the studies reported the final number of particles analysed and only Strand et al. [ 67 ] reported the success rate of conclusive identification (44%) and the proportion that was identified as MPs (3%). Only the two studies by Pivokonsky et al. [ 64 ] and Zhang et al. [ 70 ] reported the similarity index for the spectral analysis, 80% and 70%, respectively. Although scientific guidance on the particles that need to be analysed does not exist, it is reasonable to assume that larger proportions would lead to more robust results. Mintenig et al. [ 62 ] did not analyse the fibres at all. Although a larger number of fibres were discovered compared with ‘particles’ in the samples, spectral analysis was not utilised because the fibre presence was attributed to their presence as post-sampling contamination. Fibres are a high proportion of MPs and their complete exclusion from the results might have resulted in an underestimation of MP content.
Particle size.
The key difference in the studies’ protocol is the size of the particles identified and verified via spectral analysis and is directly connected to the extraction process and the composition identification process used. Shruti et al. [ 66 ] only analysed particles >500 μm, Mintenig et al. [ 62 ] ≥20 μm, Strand et al. [ 67 ] and Zhang et al. [ 70 ] ≥10 μm, Pivokonsky et al. [ 64 ] ≥1 μm, while Tong et al. [ 68 ] did not report the minimum size. The study by Pivokonsky et al. [ 64 ] reported the highest MP content ranging from 338 ±76 to 628 ±28 MPs/L and stated that 25–60% of the MPs were in the range of 1–5 μm and 30–50% in the range of 5–10 μm. Tong et al. [ 68 ] reported content in the same magnitude of 440 ±275 MPs/L, and state that MPs <50 μm were significantly dominant. It must be noted that Tong et al. [ 68 ] used only Nile Red dying and visual identification for the determination of particle size in a reported range of 3–4453 μm. The results from these two studies present a noteworthy difference. When the MPs’ size range is taken into consideration it becomes clear that this variance could be attributed to the fact that the other four studies were not able to detect that same range of sizes ( Fig 4 ). In addition, it should be noted that although Strand et al. [ 67 ] state that particles were measured down to 10 μm, the majority of the results were based on particles ≥100 μm. The inverse relationship between the size of MPs and their abundance is further supported by the findings of Shruti et al. [ 66 ] who reported that 75% of the particles were in the range of 100 μm– 1 mm, Zhang et al. [ 70 ] who reported that 46% were in the range of 500 μm -1 mm and Mintenig et al. [ 62 ] who found that all particles were in the range of 50–150 μm.
MP content (MPs/L) is illustrated in the left-hand side y axis in log 10 scale. BW: diagonal stripes, TW: chequerboard, Minimum particle size included in each study is illustrated in the right-hand side y axis. Studies by Tong et al. [ 68 ], Wiesheu et al. [ 69 ] and Zuccarello et al. [ 71 ] were not included because they were rated as of high RoB.
https://doi.org/10.1371/journal.pone.0236838.g004
Bottled water
Six studies samples BW ( Table 3 ). Kankanige and Babel [ 60 ] sampled spring and TW, Mason et al. [ 61 ] sampled table and mineral water and the rest of the studies sampled only mineral water. Three different container materials were selected: plastic (single-use and reusable), glass and carton. MPs content ranged from 0 to 1.1 X 10 8 MPs/L across all containers. The percentage of samples containing MPs ranged from 92% to 100%. Fragments and films were the most commonly identified shape.
https://doi.org/10.1371/journal.pone.0236838.t003
Meta-analysis was attempted using the results from four of the studies [ 51 , 60 , 65 , 71 ] which provided the necessary data. Statistical heterogeneity as measured by Higgins I 2 test [ 72 ] in a random-effects model was found to be high, I 2 = 99%, even when the high RoB study by Zuccarello et al. [ 71 ] was excluded ( S2 and S3 Figs). Examining the four different types of containers separately in a mixed-effects subgroup analysis [ 80 , 81 ], statistical heterogeneity within the groups still remained high I 2 <84% ( S4 Fig ). The pooled effect estimate was accompanied by a 95% confidence interval which included negative values for all categories, further showing that meta-analysis was not appropriate. The results of the analysis showed that pooling of the data was not appropriate. The origin of heterogeneity is addressed in the narrative analysis.
Four studies [ 60 , 61 , 65 , 69 ] did not use a digestion process. Mason et al. [ 61 ] used glass-fibre filters (1.5 μm pore size), Schymanski et al. [ 65 ] used gold-coated poly-carbonate filters (3.0 μm pore size) while both studies by Kankanige and Babel [ 60 ] and Wiesheu et al. [ 69 ] used cellulose nitrate filters (0.45 μm pore size). Oßmann et al. [ 63 ] implemented a digestion process using an ethylene diamine tetra-acetic acid tetrasodium salt (EDTA) solution then followed by a density separation (flotation) step via a detergent solution of sodium dodecyl sulphate (SDS) and filtration through aluminium-coated polycarbonate membrane filters (0.4 μm pore size). Zuccarello et al. [ 71 ] did not employ a digestion nor a filtration process, opting for a newly developed method to target MPs <10 μm, which differs significantly from previous studies and cannot thus be directly compared to the rest of the studies. The alternative approach used nitric acid and a high temperature incubation (60° C for 24 hours) for mineralization of the samples to remove carbon-based particles. This was followed by vortexing, centrifugation, addition of dichloromethane, resuspension using acetonitrile and drying. The sample was then deposited on an aluminium and copper alloy stub to be coated with gold before SEM-EDX analysis [ 87 ]. The methods used by this study have already been highlighted [ 88 ] under the reporting and verification sections of the analytical methods which was partially addressed by a corrigendum of the authors [ 87 ]. The scientific base of the process employed is a publication that is not available in English [ 89 ] and therefore cannot be assessed, as well as a second publication [ 90 ] concerning MPs extraction method from the gastrointestinal tract of fish. The latter describes a different method (two-step digestion process using sodium hydroxide and nitric acid, followed by filtration, density separation and verification by visual identification alone, that subsequently targets MPs of a completely different size of >100 μm).
Schymanski et al. [ 65 ] examined the largest number of particles in RM spectral analysis, analysing 100% of the particles or a maximum of 1000 (in the 5–10 μm size fraction) on each of the filters, corresponding to each of the 38 samples ( Table 3 ). The verified MP particles ranged from 0.03 to 10.7% of the analysed particles, using a ≥ 70% spectral similarity index. Kankanige and Babel [ 60 ] analysed 100% of the extracted particles (>50 μm), using FTIR and a 60% spectral similarity index, verifying 45.8% of them as MPs. RM analysis was used for particles of the lower range of 1–10 μm but these findings are not reported in the details of the analysis. Mason et al. [ 61 ] also used FTIR but only for particles ≥100 μm and examined around 1000 particles which was almost 50% of the particles extracted, using a ≥ 70% similarity index verifying 40% of the particles as MPs. Oßmann et al. [ 63 ] on the other hand, did not provide information on the number of extracted particles, reporting the analysis of 4.4% of the surface of each filter using RM, but not reporting how many were finally verified as MPs. Oßmann et al. [ 63 ] did not use an automated software option in which spectral similarity is calculated automatically but a mix of semi-automated methods. In this sense, a standardized spectral similarity index was not utilised, which might have introduced experimental error into this protocol. Wiesheu et al. [ 69 ] only analysed the one fibre extracted from the samples isolated, not providing further details on the methods employed.
Zuccarello et al. [ 71 , 87 ] used SEM-EDX for the identification of MPs. No digestion or filtration process for the extraction of the mineral water impurities was employed. The authors suggest that the mineralization process extracts all carbon-containing particles that are not plastic. This removal needs to be done with near unit efficiency due to the fact that typical concentrations of carbonates in mineral water exceed, by many orders of magnitude, the reported MP concentrations in BW samples in other studies. The specificity of this method has not been proven as mentioned in the previous section. The aim of the method was to quantify the number of MPs per volume in the size range of 0.5–10 μm and a further objective was to calculate the mass of MPs per volume, using the density of the plastic bottles containing the water. The reported validation of the process used is weak in that the mass of MPs per volume was measured in three samples spiked with MPs (whose size was not reported), and then a calculation of MPs per volume was conducted, which is the opposite way round to the calculation made with the unknown samples and may introduce systematic error.
Mason et al. [ 61 ] used FTIR only for particles ≥100 μm but reported that 95% of particles were between 6.5 and 100 μm. The MP content for all sizes was 325 MPs/L, whereas for particles ≥100 μm it was only 10.4 MPs/L. In addition, it was not clear what maximum size cut-off was employed. Kankanige and Babel [ 60 ] used FTIR for particles ≥50 μm but extrapolated the findings to the smaller size range 6.5–50 μm, reporting MPs contents of 140 ±19 MPs/L for plastic bottles and 52 ±4 MPs/L for glass bottles. The size range of 6.5–20 μm was identified as the most dominant. Schymanski et al. [ 65 ] extracted and analysed particles including even smaller sizes of ≥5 μm and reported that 80% of the verified MPs were in the range of 5 and 20 μm, with MP contents of 14 ±14 MPs/L for single use plastic bottles, 118 ± 88 MPs/L for reusable plastic bottles, 11 ± 8 MPs/L for carton and 50 ± 52 MPs/L for glass bottles. Oßmann et al. [ 63 ] decreased the size of the included particles to ≥1 μm reporting much higher MP contents of 2649 ± 2857 MPs/L for single use PET bottles, 4889 ± 5432 MPs/L for reusable PET bottles and 6292 ± 10521 MPs/L for glass bottles. The same authors also highlight that more than 95% of MPs were smaller than 5 μm and 50% smaller than 1.5 μm. Zuccarello et al. [ 71 ] focused on the 0.5–10 μm size range, reporting high concentrations of 5.42 ± 1.95 X 10 7 MPs/L. Although the size range of the identified MPs (1.28–4.2 μm) is similar to the Oßmann et al. [ 63 ] study (>1 μm), the results differ by a factor of 11000, further highlighting the possible quality issues of the study. The results of the Wiesheu et al. [ 69 ] study on MPs content were inconclusive. As can be seen in Fig 4 , as the size of the identified particles decreases, the MP content increases significantly.
Twelve studies were systematically reviewed, which collectively analysed more than 40000 L of TW and 435 bottles of BW (table and mineral water). It would not be reasonable to collate the evidence from the twelve studies included in this systematic review due to key differences that were identified in the experimental protocols and high sample heterogeneity. In addition, the lack of key information (e.g. SE, SD) and high statistical heterogeneity hinder the execution of meta-analysis in an attempt to quantify MP content. RoB was found to be low in the majority the studies. Two studies were rated as of high RoB and therefore the results of these are excluded. The study by Zuccarello et al. [ 71 ] was rated high RoB in the two domains of sampling and reporting, while the study by Wiesheu et al. [ 69 ] was rated high RoB in the domains of analysis and reporting.
All studies reported some level of MP contamination. Samples positive for contamination ranged from 24–100% in TW and 92–100% for BW. Comparing the results between the different water origins, specifically between the two studies [ 63 , 64 ] that targeted similar MP sizes of minimum 1 μm, MP content was higher in BW (plastic and glass bottles) than TW ( Fig 4 ). Therefore, current evidence suggests that there are higher rates of MP contamination in BW compared with TW, both in terms of frequency and quantity. Regarding the primary origin of BW, Mason et al. [ 61 ] analysed table and mineral BW and Kankanige and Babel [ 60 ] tap and spring BW, but did not report a comparison between the different water origins which could shed some light on the possible differences.
The methodology used in the studies varied in both sampling and analysis. Standardization of the experimental protocols is key in order to increase confidence in the quality of the studies and certainty of the evidence. The first step in obtaining comparable and trustworthy results is the use of a verified composition identification process, which was employed by all of the studies included in this review. Not using such a process has been proven to lead to gross under- or over-estimations [ 31 , 91 , 92 ]. Even with all the studies using either FTIR, RM or SEM-EDX, there were still differences in the spectral similarity index, the number and proportion of the particles analysed, and the spectral library used. Furthermore, poor reporting hindered the assessment of the experimental protocols’ effectiveness; only one study [ 60 ] reported how many particles were retrieved from the extraction process and only four [ 60 , 61 , 65 , 69 ] reported how many particles were analysed for composition identification.
The most significant difference in the methods is the size of the particles that were extracted from the samples and analysed for composition identification. Studies using FTIR and RM were able to analyse particles down to 1 μm which significantly influenced the results. The degradation of MPs in the marine environment and the exponential increase of the number as the size decreases has been experimentally and mathematically explored [ 93 – 95 ]. This would suggest that the same fragmentation pattern may also apply to other aquatic environments as well.
On the other hand, only seven [ 62 – 64 , 66 , 68 – 71 ] of the twelve studies reported the upper limit of the range in MP size. The importance of defining and reporting the size range of the identified MPs has a double significance as follows. As a methodology parameter it is connected to the quantified MP content results. As a food contamination parameter, it is indicative of the potential health effects. MPs <1.5 μm are characterized as more dangerous since they are, in theory, capable of crossing the gut epithelium, further progressing into the human body and thus possibly causing an adverse health effect [ 23 ].
Differences in sample size were striking, ranging from 36 to 32000 L (per study) for TW and 3 to (>)130 L for BW. At the moment, methodological consensus concerning sample size does not exist. Koelmans et al. [ 30 ], in a recent review, proposed a minimum of 1000 L for TW and 500 L for BW. In the first instance, sample size is dictated by the objectives and design of the study which in many cases are a function of the available resources [ 96 , 97 ]. Sample size should be directly connected to the contaminant under examination. The volume of the samples as well as the sampling frequency can only be set when there is enough evidence to support what a meaningful MP content is. Meaningful being expressed in terms of food safety linked to human health and what is considered to be ‘wholesome and clean’ water intended for human consumption, which is the requirement of relevant European regulations and universal standards [ 43 , 49 , 98 ]. At the moment, there is not enough evidence to formulate an informed guideline for sampling sizes, nevertheless scientific experience points to larger sample sizes being more robust and reliable [ 99 ].
Another area of importance is quality assurance of sampling and sample handling to avoid cross contamination via airborne MPs. This issue was addressed by our RoB assessment tool in the sampling domain. In addition, only studies that employed blank procedural samples to account for this type of experimental error were included [ 100 , 101 ]. The lack of detailed information on the results and the significance of procedural blank samples downgraded the quality of the study as assessed by the RoB assessment tool. The bespoke RoB tool used did not employ scales to rank the studies as done by other reviews in the field [ 30 ] but is a domain-based evaluation according to the guidance of leading methodology regarding systematic reviews [ 36 ]. The use of scales in RoB assessment is explicitly discouraged as research experience has shown that they can be unreliable [ 57 ].
Seven studies used samples from Europe (3 TW, 4 BW), three from Asia (2 TW, 1 BW), one from North America (TW), and one from multiple continents (BW) ( S3 Table ). The highest MPs content are reported in Europe for both TW and BW. Regarding TW, the highest reported MPs content for Europe and Asia were in the same magnitude but almost 25 times higher than those reported for the samples from North America. In BW, the maximum reported MPs content in Europe was 35 times higher than that reported in Asia. However, it is not clear if this is due to the number of existing studies and the varying methodology employed, or the geographical location. Recent research has shown that MP contamination of the environment is directly linked to waste management, which is compromised in developing countries [ 102 , 103 ]. In this sense, it would be reasonable to expect higher MPs contamination of potable water in these countries, where further research is needed. In terms of polymeric composition, PET and PP were the most prevalent polymers identified in BW. The differences between the polymeric composition in the various BW studies can be attributed to the different origin of the water, processing, the material used for packaging but also to the different particle sizes the studies extracted and analysed since degradation rates between polymers vary [ 2 , 104 ]. In TW, polymeric composition varied with PET and PP present along with polyester, PTT and rayon. This may possibly due to the wide geographical and environmental origin of the water samples. Rayon is a man-made but not synthetic fibre and is not included in most MP research. It should be noted that the most produced and used polymers for the last 15 years have been PE and PP, whose prevalence would be anticipated to be the highest in terms of environmental contamination although geographical variation is expected [ 17 – 20 ]. Fragments and fibres were the prevalent MP shape in both categories, highlighting an agreement in the findings across all studies. Polymeric composition and shape characteristics can be used as guides to the origin of MPs as well as to focus future toxicological research.
A recent review by Koelmans et al. [ 30 ] has recently addressed the issue of MPs contamination of drinking water. Koelmans et al. [ 30 ] focused not only on drinking water but also on freshwater MP contamination and experimental methodology and did not attempt quantitative collation of the evidence. The study assessed the quality of the studies using a bespoke rating system, focusing on different aspects of experimental design and execution using a scoring system. The use of scoring scales in quality assessment is explicitly discouraged by the Cochrane Collaboration, which is the leading body of systematic reviews, as research experience has shown that they can be unreliable due to the lack of justification for the ratings [ 36 , 51 , 57 , 105 ]. The World Health Organization (WHO) delivered a report [ 24 ] based on a commissioned systematic review by Koelmans et al. [ 30 ], yet the authors make no claim that it is systematic, nor is there a description of the relevant review methods utilised, such as the existence of a published protocol.
Human MPs exposure via drinking water
Water intake varies in adults depending on gender, climate, diet and physical activity. The WHO guideline value for water daily consumption is 2 L for adults (with a default body weight of 60 kg), 1 L for children (default body weight of 10 kg) and 0.75 L for infants (default body weight of 5 kg) [ 98 ]. Maximum daily human exposures were calculated by using the highest MP content evidence that have been rated of low and unclear RoB for the three continents, and the WHO values for daily water consumption and use [ 98 ]. The highest daily possible exposures were calculated in Europe at 1260 MPs for TW and 9800 MPs for BW ( Table 4 ). These exposures are significant underestimations since they assume that all populations have access to treated drinking water which is not the case. These high exposure levels are driven more by the amount of water we consume and less the absolute MP content of water compared to other food categories.
https://doi.org/10.1371/journal.pone.0236838.t004
After the ingestion of MPs, particles <1.5 μm could pass the gut barrier and translocate to other organs. Paradigms from studies on plastic material that have been used for orthopaedic replacement prosthetics have proved translocation of plastic particles to organs such as the liver, spleen and lymph nodes [ 106 – 109 ]. The effects of MPs will depend on their size, polymeric composition, additives (plasticisers), the chemicals that they might have absorbed from the environment, their chemical state and where they are located in the human body [ 41 , 110 ].
An additional possible exposure pathway that has not yet been investigated may occur from the use of MP contaminated water for incorporation into food. According to WHO estimations, 7.5 L of water per capita per day [ 98 ] is used by most people in most situations around the world for hydration and incorporation into food. This is a complex issue since it is not clear to what extent MPs in the water would be taken up into the foodstuffs. This would depend on how the food is prepared and have geographic, cultural variation. Nevertheless, further research into this issue is clearly warranted as it is another potential pathway for MPs in water to enter the human body.
Strengths and limitations
To our knowledge this is the first systematic review focusing on MP contamination of water intended for human consumption. The review was based on a protocol which was created beforehand, outlining the methodology used throughout. The protocol ensures that bias is not introduced. In addition, the quality of studies was assessed using a systematic RoB tool tailored to the needs of the review, addressing every stage of design, execution and reporting of research. The review was limited to a narrative analysis and did not include a meta-analysis due to high sample, experimental and statistical heterogeneity as well as poor reporting in a fraction of the studies. The majority of the studies were assessed to be low RoB.
Research methodology in the field of MPs environmental contamination has advanced in recent years, especially with the use of FTIR and RM validation of particle characteristics, but is still lacking in quality and robustness. The systematic review identified specific areas where further development and standardization is needed:
- Sampling methodology: sampling size, location, frequency, instruments, quality assurance, procedural blanks, replicate samples.
- Registry of all relevant sample characteristics when available: brand, geographical and environmental origin, volumes, production dates, information on water treatment and additives.
- Particle extraction process specifications: sample volumes, chemicals used for digestion and density separation, type and pore size of filters.
- ○. Use of one of the currently validated methods: FTIR, RM, SEM, Pyr-GC-MS and SEM/EDS.
- ○. Proportion of extracted particles for analysis.
- ○. Spectral similarity index and which spectral libraries are used (bespoke or commercially available).
- Post-sampling handling: measures to protect cross-contamination and use of procedural blank samples in all experimental aspects to ensure effectiveness and account for experimental errors.
- Detailed reporting of all aspects of research including design, execution and statistical analysis.
In terms of future research there is a clear need for research on MP contamination of drinking water in countries beyond Europe where there is less data. Comparison between table water, natural mineral and spring waters to detect differences is another area that has not been explored. The additional exposure pathway via the use of MP contaminated water for incorporation into food also merits further research.
As this review shows, there are still relatively few studies examining MP contamination in drinking water, and levels vary significantly. The presence of MP in human stool samples has recently been verified [ 28 ], although the effects on human health are still under examination [ 28 , 41 , 111 – 113 ]. Given the amount of water humans drink and its use for incorporation into food, a clearer understanding of the levels of MP present in drinking water is needed, in order to better assess the risks that MPs in water present. Quantification of MPs human exposures is an integral part of the exposure assessment in the wider frame of a risk assessment to determine the likelihood of MPs having adverse human health effects [ 114 , 115 ].
Our findings support the omnipresent MPs contamination of drinking water. Current food and drinking water safety regulation and standards around the world [ 49 , 116 , 117 ] adopt the precautionary principle [ 118 , 119 ] on food safety risk management. The principle dictates that in the face of scientific uncertainty concerning possible harmful effects, after an initial assessment of available evidence has been completed and a comprehensive risk assessment is anticipated, risk management measures must be adopted in order to ensure the protection of health. The weight of the current evidence suggests that the time may have come to implement protective measures against the ingestion of MPs.
Supporting information
S1 checklist. prisma checklist..
https://doi.org/10.1371/journal.pone.0236838.s001
S1 Protocol. Systematic review protocol.
Published in the International prospective register of systematic reviews (PROSPERO)
https://doi.org/10.1371/journal.pone.0236838.s002
S1 Appendix. Exclusion reasons during the second level screening.
https://doi.org/10.1371/journal.pone.0236838.s003
S1 Table. Search strategy for MEDLINE (OVID).
https://doi.org/10.1371/journal.pone.0236838.s004
S2 Table. Risk of bias (RoB) assessment tool template.
https://doi.org/10.1371/journal.pone.0236838.s005
S3 Table. Study characteristics.
https://doi.org/10.1371/journal.pone.0236838.s006
S1 Fig. Forest plot of TW studies random-effects model analysis.
The x axis represents the standardized mean difference (SMD) expressed in MPs/L. The vertical line is the line of null effect where MP content is 0. The grey boxes represent the pooled effect estimate and the lines the CI 95%. The size of the boxes is proportional to the study weight. The diamond is the combined point estimate and CI for each of the subgroups.
https://doi.org/10.1371/journal.pone.0236838.s007
S2 Fig. Forest plot of BW studies random-effects model analysis.
The x axis represents the standardized mean difference (SMD) expressed in MPs/L. The vertical line is the line of null effect where MP content is 0. The grey boxes represent the pooled effect estimate and the lines the CI 95%. The size of the boxes is proportional to the study weight.
https://doi.org/10.1371/journal.pone.0236838.s008
S3 Fig. Forest plot of BW studies random-effects model analysis excluding the high RoB study.
https://doi.org/10.1371/journal.pone.0236838.s009
S4 Fig. Forest plot of sub-group analysis of BW studies.
Mixed-effects (plural model) analysis. The x axis represents the standardized mean difference (SMD) expressed in MPs/ L. The vertical line is the line of null effect where MP content is 0. The grey boxes represent the pooled effect estimate and the lines the CI 95%. The size of the boxes is proportional to the study weight. The diamonds are the combined point estimates and CI for each of the subgroups. The red square is the overall pooled effect for all subgroups.
https://doi.org/10.1371/journal.pone.0236838.s010
- View Article
- PubMed/NCBI
- Google Scholar
- 17. Plastics Europe. The Compelling Facts About Plastics. An analysis of plastics production, demand and recovery for 2006 in Europe. Plastics Europe, 2008.
- 18. Plastics Europe. Plastics: the Facts 2017: An analysis of European plastics production, demand and waste data. 2017.
- 19. Plastics Europe. Plastics–the Facts 2018; An analysis of European plastics production, demand and waste data. 2018.
- 20. Plastics Europe. Plastics–the Facts 2019; An analysis of European plastics production, demand and waste data. Plastics Europe, 2019.
- 24. WHO. Microplastics in drinking-water. Geneva: World Health Organization, 2019 Report No.: 9241516194.
- 27. EFSA. Definition and description of “emerging risks” within the EFSA's mandate. (adopted by the Scientific Committee on 10 July 2007). Parma: European Food Safety Authority, 2007.
- 36. Higgins JPT, Thomas J, Chandler J, Cumpston M, Li T, Page MJ, et al. Cochrane Handbook for Systematic Reviews of Interventions. Version 6.0 (updated July 2019). Cochrane.: Cochrane; 2019. Available from: www.training.cochrane.org/handbook .
- 37. FAO, WHO. Principles and methods for the risk assessment of chemicals in food: Environmental Health Criteria 240. Stuttgart, Germany: Food and Agriculture Organization of the United Nations and the World Health Organization, 2009.
- 38. IPCS. World Health Organization human health risk assessment toolkit: chemical hazards. Geneva: World Health Organization, 2010.
- 39. WHO. International Programme on Chemical Safety (IPCS): Principles for the assessment of risks to human health from exposure to chemicals. Geneva: World Health Organization: 1999.
- 43. Council directive 98/83/EC. On the quality of water intended for human consumption. European Council. 1998.
- 44. Directive 2009/54/EC. On the exploitation and marketing of natural mineral waters. European parliament and European Council. 2009.
- 45. The Natural Mineral Water Spring Water and Bottled Drinking Water (England) (Amendment) Regulations. United Kingdom. 2018;SI 2018:352.
- 48. Centre for Evidence-Based Medicine [Internet]. Study Designs 2019 [16–10–2019]. Available from: https://www.cebm.net/2014/04/study-designs/ .
- 49. Regulation (EC) No 178. Laying down the general principles and requirements of food law, establishing the European Food Safety Authority and laying down procedures in matters of food safety. European Parliament and Council. 2002.
- 52. Centre for Reviews and Dissemination (CRD). Systematic reviews: CRD's guidance for undertaking reviews in health care. York, United Kingdom: Centre for Reviews and Dissemination, University of York; 2009.
- 54. West S, King V, Carey T, Lohr K, McKoy N, Sutton S, et al. Systems to rate the strength of scientific evidence: summary. Rockville, MD, USA: Agency for Healthcare Research and Quality (US), 2002.
- 57. Higgins JPT, Green SP, Cochrane C. Cochrane handbook for systematic reviews of interventions. Chichester, United KIngdom: John Wiley & Sons; 2011.
- 67. Strand J, Feld L, Murphy F, Mackevica A, Hartmann NB. Analysis of microplastic particles in Danish drinking water. DCE-Danish Centre for Environment and Energy, 2018 877156358X.
- 73. R Core Team. R: A language and environment for statistical computing. Vienna, Austria: R Foundation for Statistical Computing; 2019.
- 74. RStudio Team. RStudio: Integrated Development for R. RStudio. Boston, MA: Inc.; 2018.
- 77. Harrer M, Cuijpers P, Furukawa T, Ebert D-D. dmetar: Companion R Package For The Guide 'Doing Meta-Analysis in R'. 2019.
- 78. McGuinness L, Kothe E. robvis: Visualize the Results of Risk-of-Bias (ROB) Assessments. 2019.
- 79. Hadley W. ggplot2: Elegant Graphics for Data Analysis. New York: Springer-Verlag.; 2016.
- 80. Chen DG, Peace KE. Applied meta-analysis with R. Peace KEa, editor. Boca Raton, Florida, USA: CRC Press; 2013.
- 84. Bergmann M. Marine Anthropogenic Litter. Gutow L, Klages M, editors. Cham: Springer; 2015.
- 96. EPA. Guidance for Data Quality Assessment. Practical Methods for Data Analysis. United States Environmental Protection Agency, 2000 Contract No.: EPA/600/R-96/084.
- 97. EPA. Guidance on choosing a sampling design for environmental data collection, for Use in Developing a Quality Assurance Project Plan. United States Environmental Protection Agency, 2002 Contract No.: EPA QA/G-5S.
- 98. WHO. Guidelines for drinking-water quality, fourth edition incorporating the first addendum. Geneva: World Health Organization, 2017.
- 99. Zhang C. Fundamentals of environmental sampling and analysis. Hoboken, N.J., USA: Wiley; 2007.
- 105. Whiting P, Savović J, Higgins JPT, Caldwell DM, Reeves BC, Shea B, et al. ROBIS: Tool to assess risk of bias in systematic reviews; Guidance on how to use ROBIS2016 01-03-2019. Available from: https://vle.york.ac.uk/bbcswebdav/pid-2989084-dt-content-rid-6036201_2/courses/Y2016-002677/robisguidancedocument.pdf .
- 109. GESAMP. Sources, fate and effects of microplastics in the marine environment: a global assessment. London, UK: The Joint Group of Experts on Scientific Aspects of Marine Environmental Protection, Working Group 40, 2015.
- 110. GESAMP. Sources, fate and effects of microplastics in the marine environment: part two of a global assessment. London, UK: The Joint Group of Experts on Scientific Aspects of Marine Environmental Protection, Working Group 40, 2016.
- 114. SAM. Environmental and Health Risks of Microplastic Pollution; Scientific Advice Mechanism. Group of Chief Scientific Advisors. Luxembourg: European Commission, 2019.
- 115. SAPEA. A Scientific Perspective on Microplastics in Nature and Society. Berlin: Science Advice for Policy by European Academies, 2019.
- 116. ISO. ISO 22000:2018. Food safety management systems—Requirements for any organization in the food chain. 2018 [13–3–2020]. Available from: https://www.iso.org/standard/65464.html .
- 117. Wallace C. HACCP a food industry briefing. Second edition. ed. Chichester, West Sussex, United Kingdom: Wiley-Blackwell; 2015.
- 119. Jackson W, Steingraber S. Protecting public health and the environment: implementing the precautionary principle. Washington DC, USA: Island Press; 1999.
Thank you for visiting nature.com. You are using a browser version with limited support for CSS. To obtain the best experience, we recommend you use a more up to date browser (or turn off compatibility mode in Internet Explorer). In the meantime, to ensure continued support, we are displaying the site without styles and JavaScript.
- View all journals
- My Account Login
- Explore content
- About the journal
- Publish with us
- Sign up for alerts
- Open access
- Published: 11 April 2022
Water quality assessment and evaluation of human health risk of drinking water from source to point of use at Thulamela municipality, Limpopo Province
- N. Luvhimbi 1 ,
- T. G. Tshitangano 1 ,
- J. T. Mabunda 1 ,
- F. C. Olaniyi 1 &
- J. N. Edokpayi 2
Scientific Reports volume 12 , Article number: 6059 ( 2022 ) Cite this article
19k Accesses
19 Citations
1 Altmetric
Metrics details
- Environmental sciences
- Risk factors
Water quality has been linked to health outcomes across the world. This study evaluated the physico-chemical and bacteriological quality of drinking water supplied by the municipality from source to the point of use at Thulamela municipality, Limpopo Province, South Africa; assessed the community practices regarding collection and storage of water and determined the human health risks associated with consumption of the water. Assessment of water quality was carried out on 114 samples. Questionnaires were used to determine the community’s practices of water transportation from source to the point-of-use and storage activities. Many of the households reported constant water supply interruptions and the majority (92.2%) do not treat their water before use. While E. coli and total coliform were not detected in the water samples at source (dam), most of the samples from the street taps and at the point of use (household storage containers) were found to be contaminated with high levels of E. coli and total coliform. The levels of E. coli and total coliform detected during the wet season were higher than the levels detected during the dry season. Trace metals’ levels in the drinking water samples were within permissible range of both the South African National Standards and World Health Organisation. The calculated non-carcinogenic effects using hazard quotient toxicity potential and cumulative hazard index of drinking water through ingestion and dermal pathways were less than unity, implying that consumption of the water could pose no significant non-carcinogenic health risk. Intermittent interruption in municipal water supply and certain water transportation and storage practices by community members increase the risk of water contamination. We recommend a more consistent supply of treated municipal water in Limpopo province and training of residents on hygienic practices of transportation and storage of drinking water from the source to the point of use.
Similar content being viewed by others
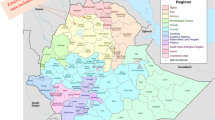
Access to basic drinking water services, safe water storage, and household water treatment practice in rural communities of northwest Ethiopia
Zemichael Gizaw, Mulat Gebrehiwot, … Adane Nigusie
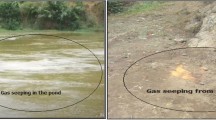
Ecological and health risk assessment of trace metals in water collected from Haripur gas blowout area of Bangladesh
M. Farhad Howladar, Md. Numan Hossain, … Debjani Das
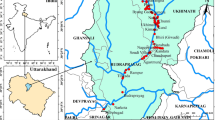
A systematic study on occurrence, risk estimation and health implications of heavy metals in potable water from different sources of Garhwal Himalaya, India
Mukesh Prasad, R. S. Aswal, … R. C. Ramola
Introduction
Water is among the major essential resources for the sustenance of humans, agriculture and industry. Social and economic progress are based and sustained upon this pre-eminent resource 1 . Availability and easy access to safe and quality water is a fundamental human right 2 and availability of clean water and sanitation for all has been listed as one of the goals to be achieved by the year 2030 for sustainable development by the United Nations General Assembly (UNGA) 3 .
The physical, chemical, biological and aesthetic properties of water are the parameters used to describe its quality and determine its capability for a variety of uses including the protection of human health and the aquatic ecosystem. Most of these properties are influenced by constituents that are either dissolved or suspended in water and water quality can be influenced by both natural processes and human activities 4 , 5 . The capacity of a population to safeguard sustainable access to adequate quantities and acceptable quality of water for sustaining livelihoods of human well-being and socioeconomic growth; as well as ensuring protection against pollution and water related disasters; and for conserving ecosystems in a climate of peace and political balance is regarded to as water security 6 .
Although the world’s multitudes have access to water, in numerous places, the available water is seldom safe for human drinking and not obtainable in sufficient quantities to meet basic health needs 7 . The World Health Organization (WHO) estimated that about 1.1 billion people globally drink unsafe water and most diarrheal diseases in the world (88%) is attributed to unsafe water, poor sanitation and unhygienic practices. In addition, the water supply sector is facing enormous challenges due to climate change, global warming and urbanization. Insufficient quantity and poor quality of water have serious impact on sustainable development, especially in developing countries 8 .
The quality of water supplied by the municipality is to be measured against the national standards for drinking water developed by the federal governments and other relevant bodies 9 . These standards considered some attributes to be of primary importance to the quality of drinking water, while others are considered to be of secondary importance. Generally, the guidelines for drinking water quality recommend that faecal indicator bacteria (FIB), especially Escherichia coli ( E. coli ) or thermo tolerant coliform (TTC), should not be found in any 100 mL of drinking water sample 8 .
Despite the availability of these standards and guidelines, numerous WHO and United Nations International Children Emergency Fund (UNICEF) reports have documented faecal contamination of drinking water sources, including enhanced sources of drinking water like the pipe water, especially in low-income countries 10 . Water-related diseases remain the primary cause of a high mortality rate for children under the age of five years worldwide. These problems are specifically seen in rural areas of developing countries. In addition, emerging contaminants and disinfection by-products have been associated with chronic health problems for people in both developed and developing countries 11 . Efforts by governmental and non-governmental organizations to ensure water security and safety in recent years have failed in many areas due to a lack of sustainability of water supply infrastructures 12 .
Water quality, especially regarding the microbiological content, can be compromised during collection, transport, and home storage. Possible sources of drinking water contamination are open field defecation, animal wastes, economic activities (agricultural, industrial and businesses), wastes from residential areas as well as flooding. Any water source, especially is vulnerable to such contamination 13 . Thus, access to a safe source alone does not ensure the quality of water that is consumed, and a good water source alone does not automatically translate to full health benefits in the absence of improved water storage and sanitation 14 . In developing countries, it has been observed that drinking-water frequently becomes re-contaminated following its collection and during storage in homes 15 .
Previous studies in developing countries have identified a progressive contamination of drinking water samples with E. coli and total coliforms from source to the point of use in the households, especially as a result of using dirty containers for collection and storage processes 16 , 17 , 18 . Also, the type of water treatment method employed at household levels, the type of container used to store drinking water, the number of days of water storage, inadequate knowledge and a lack of personal and domestic hygiene have all been linked with levels of water contamination in households 19 , 20 .
In South Africa, many communities have access to treated water supplied by the government. However, the water is more likely to be piped into individual households in the urban than rural areas. In many rural communities, the water is provided through the street taps and residents have to collect from those taps and transport the water to their households. Also, water supply interruptions are frequently experienced in rural communities, hence, the need for long-term water storage. A previous study of water quality in South Africa reported better quality of water at source than the water samples obtained from the household storage containers, showing that water could be contaminated in the process of transporting it from source to the point of use 21 .
This study was conducted in a rural community at Thulamela Municipality, Limpopo province, South Africa, to describe the community’s drinking water handling practices from source to the point of use in the households and evaluate the quality of the water from source (the reservoir), main distribution systems (street taps), yard connections (household taps) and at the point of use (household storage containers). Water quality assessment was done by assessing the microbial contamination and trace metal concentrations, and the possible health risks due to exposure of humans to the harmful pathogens and trace metals in the drinking water were determined.
The study was conducted at Lufule village in Thulamela municipality, Limpopo Province, South Africa. The municipality is situated in the eastern subtropical region of the province. The province is generally hot and humid and it receives much of its rainfall during summer (October–March) 22 . Lufule village is made up of 386 households and a total population of 1, 617 residents 23 . The study area includes Nandoni Dam (main reservoir) which acquires its raw water from Luvuvhu river that flows through Mutoti and Ha-Budeli villages just a few kilometers away from Thohoyandou town. Nandoni dam is where purification process takes place to ensure that the water meets the standards set for drinking water. This dam is the main source of water around the municipality, and it is the one which supplies water to selected areas around the dam, including Lufule village. Water samples for analysis were collected from the dam (D), street taps (ST), household taps (HT) and household storage containers (HSC) (Fig. 1 ).
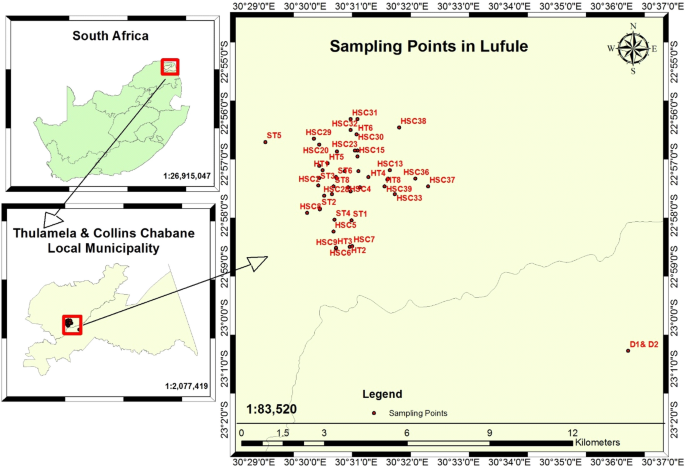
Map of the study area showing water samples’ collection areas.
Research design
This study adopted a quantitative design comprising of field survey and water analysis.
Field survey
The survey was done to identify the selected households and their shared source of drinking water (street taps). The village was divided into 10 quadrants for sampling purposes. From each quadrant, 6 households were randomly selected where questionnaires were distributed and household water samples were also collected for analysis.
Quantitative data collection
A structured interviewer-administered questionnaire was employed for data collection in the selected households. The population of Lufule village residents aged 15–69 years is 1, 026 (Census, 2011). About 10% of the adult population (~ 103) was selected to complete the questionnaires to represent the entire population. However, a total of 120 questionnaires were distributed, to take care of those which might be lacking vital information and therefore would not qualify to be analysed. Adults between the ages of 18 and 69 years were randomly selected to complete the questionnaire which includes questions concerning demographic and socio-economic statuses of the respondents, water use practices, sanitation, hygiene practices as well as perception of water quality and health. The face validity of the instrument was ensured by experts in the Department of Public Health, University of Venda, who reviewed questionnaire and confirmed that the items measure the concepts of interest relevant to the study 24 . Respondents were given time to go through the questionnaire and the researcher was present to clear any misunderstanding that may arise.
Water sampling
Permission to collect water samples from the reservoir tank at the Nandoni water treatment plant and households was obtained from the plant manager and the households’ heads respectively. Two sampling sites were identified at the dam, from where a water sample each was collected during the dry and the wet season. Similarly, 8 sampling sites were identified from the street and household taps, while 60 sampling sites were targeted for the household storage containers. However, only 39 household sites were accessible for sample collection, due to unavailability of the residents at the times of the researcher’s visit. Thus, water samples were collected from a total of 57 sites. Samples were collected from each of the sites during the dry (12th–20th April, 2019) and wet seasons (9th–12th December, 2019) between the hours of 08h00 and 14h30. A total of 114 samples were collected during the sampling period: 4 from the reservoir, 16 from street taps, 16 from household taps and 78 from households’ storage systems. Water samples were collected in 500 mL sterile polyethylene bottles. After collection, the containers were transported to the laboratory on ice in a cooler box. Each of the samples was tested for physico-chemical parameters, microbial parameters and trace metals’ concentration.
Physicochemical parameters’ analysis
Onsite analysis of temperature, pH, Electrical conductivity (EC) and Total Dissolved Solids (TDS) were performed immediately after sampling using a multimeter (model HI “HANNA” instruments), following the standards protocols and methods of American Public Health Association (APHA) 25 . The instrument was calibrated in accordance with the manufacturer’s guideline before taking the measurements. The value of each sample was taken after submerging the probe in the water and held for a couple of minutes to achieve a reliable reading. After measurement of each sample, the probe was rinsed with de-ionized water to avoid cross contamination among different samples.
ICP-OES and ICP-MS analyses of major and trace elements
An inductively coupled plasma optical emission spectrophotometer (ICP-OES) was used to analyse the major metals (Calcium (Ca), Sodium (Na), Potassium (K) and Magnesium (Mg)) in the water samples while inductively coupled plasma mass spectrophotometer (ICP-MS) was used to analyze the trace metals. The instrument was standardized with a multi-element calibration standard IV for ICP for Copper (Cu), Manganese (Mn), Iron (Fe), Chromium (Cr), Cadmium (Cd), Arsenic (As), Nickel (Ni), Zinc (Zn), Lead (Pb) and Cobalt (Co) and analytical precision was checked by frequently analysing the standards as well as blanks. ICP multi Standard solution of 1000 ppm for K, Ca, Mg and Na was prepared with NH 4 OAC for analysis to verify the accuracy of the calibration of the instrument and quantification of selected metals before sample analysis, as well as throughout the analysis to monitor drift.
Microbiological water quality analysis
Analysis of microbial parameters was conducted within 6 h of collection as recommended by APHA 25 . Viable Total coliform and E. coli were quantified in each sample using the IDEXX technique approved by the United States Environmental Protection Agency (USEPA). Colilert media was added to 100 mL sample and mixed until dissolved completely. The solution was poured into an IDEXX Quanti-Tray/2000 and sealed using the Quanti-Tray sealer 26 . The samples were incubated at 35 °C for 24 h. Trays were scanned using a fluorescent UV lamp to count fluorescent wells positive for E. coli concentration and counted with the most probable number (MPN) table provided by the manufacturer 27 .
Health risk assessment
Risk assessment have been estimated for ingestion and dermal pathways. Exposure pathway to water for ingestion and dermal routes are calculated using Eqs. ( 1 ) and ( 2 ) below:
where Exp ing : exposure dose through ingestion of water (mg/kg/day); BW: average body weight (70 kg for adults; 15 kg for children); Exp derm : exposure dose through dermal absorption (mg/kg/day); C water : average concentration of the estimated metals in water (μg/L); IR: ingestion rate in this study (2.0 L/day for adults; 1.0 L/day for children); ED: exposure duration (70 years for adults; and 6 years for children);AT: averaging time (25,550 days for an adult; 2190 days for a child); EF: exposure frequency (365 days/year) SA: exposed skin area (18.000 cm 2 for adults; 6600 cm 2 for children); K p : dermal permeability coefficient in water, (cm/h), 0.001 for Cu, Mn, Fe and Cd, while 0.0006 for Zn; 0.002 for Cr and 0.004 for Pb; ET: exposure time (0.58 h/ day for adults; 1 h/day for children) and CF: unit conversion factor (0.001 L/cm 3 ) 28 .
The hazard quotient (HQ) of non-carcinogenic risk by ingestion pathway can be determined by Eq. ( 3 )
where RfD ing is ingestion toxicity reference dose (mg/kg/day). An HQ under 1 is assumed to be safe and taken as significant non-carcinogenic, but HQ value above 1 may indicate a major potential health concern associated with over-exposure of humans to the contaminants 28 .
The total non-carcinogenic risk is represented by hazard index (HI). HI < 1 means the non-carcinogenic risk is acceptable, while HI > 1 indicates the risk is beyond the acceptable level 29 . The HI of a given pollutant through multiple pathways can be calculated by summing the hazard quotients by Eq. ( 4 ) below.
Carcinogenic risks for ingestion pathway is calculated by Eq. ( 5 ). For the selected metals in the study, carcinogenic risk (CR ing ) can be defined as the probability that an individual will develop cancer during his lifetime due to exposure under specific scenarios 30 .
where CRing is carcinogenic risk via ingestion route and SF ing is the carcinogenic slope factor.
Data analysis
Data obtained from the survey were analysed using Microsoft Excel and presented as descriptive statistics in the form of tables and graphs. The experimental data obtained was compared with the South African National Standards (SANS) 31 and Department of Water Affairs and Forestry (DWAF) 32 guidelines for domestic water use.
Ethics approval and consent to participate
The ethical clearance for this study was granted by the University of Venda Health, Safety and Research Ethics’ Committee (SHS/19/PH/14/1104). Permission to conduct the study was obtained from the Department of Water affairs, Limpopo province, Vhembe district Municipality and the selected households. Respondents were duly informed about the study and informed consent was obtained from all of them. The basic ethical principles of voluntary participation, informed consent, anonymity and confidentiality of respondents were duly complied with during data collection, analysis and reporting.
Consent for publication
Not applicable.
Socio-demographic characteristics of respondents
A total of 120 questionnaires were distributed but only 115 were completed, making a good response rate of 95%. The socio-demographic characteristics of the respondents are presented in Table 1 .
Household water supply
Many households (68.7%) had their primary water source from the municipality piped into their yards, but only 5.2% have the water flowing within their houses. The others have to fetch water at their neighbours’ yards or use the public taps on the streets. When the primary water supply is interrupted (i.e. when there is no water flowing through the pipes within the houses, yards or the public taps due to water rationing activities by the municipality, leakage of water distribution pipes, vandalization of pipes during road maintenance, etc.), the interruption usually lasts between a week or two, during which the respondents resort to other alternative sources. A return trip to the secondary source of water usually takes between 10 and 30 min for more than half of the respondents (53.0%) (Table 2 ).
Water storage and treatment practices at the household
Household water was most frequently stored in plastic buckets (n = 78, 67.8%), but ceramic vessels, metal buckets and other containers are also used for water storage (Fig. 2 ). Most households reported that their drinking water containers were covered (n = 111, 96.5%). More than half (53.9%) of the respondents used cups with handles to collect water from the storage containers whereas 37.4% used cups with no handles. Only 7.8% households reported that they treat their water before use mainly by boiling. Approximately 82.6% of respondent are of the opinion that one cannot get sick from drinking water and only 17.4% knew the risks that come with untreated water, and cited diarrhoea, schistosomiasis, cholera, fever, vomiting, ear infections, malnutrition, rash, flu and malaria as specific illnesses associated with water. Despite these perceptions, the majority (76.5%) were satisfied with their current water source. The few (23.5%) who were not satisfied cited poor quality, uncleanness, cloudiness, bad odour and taste in the water as reasons for their dissatisfaction (Table 3 ).
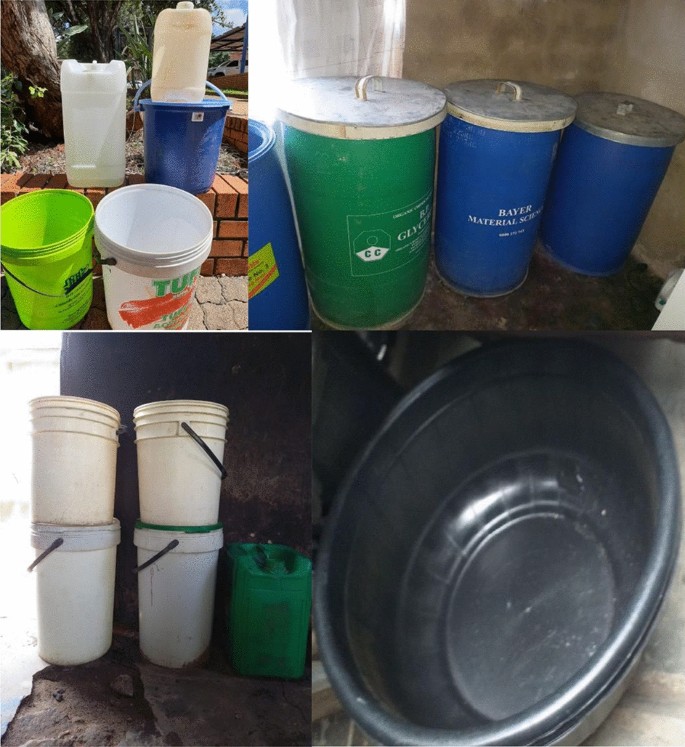
Examples of household water storage containers, some with lids and others without lids (photo from fieldwork).
Sanitation practices at the household level
More than half of the respondents (67%) use pit toilets, whereas only 26.1% use the flush to septic tank system, most of the toilets (93.9%) have a concrete floor. About 76.5% of households do not have designated place to wash their hands, however, all respondents indicated that they always wash their hands with soap or any of its other alternatives before preparing meals and after using the toilet (Table 4 ).
Water samples analysis
The water samples analyses comprise of microbial analysis, physico-chemical analysis and trace metals' parameters.
Microbial analysis
The samples from the reservoir during dry and wet season had 0 MPN/100 mL of total coliform and E. coli and were within the recommended limits of WHO and SANS for drinking water. During the wet season, seven out of the eight water samples collected from the street taps were contaminated with total coliform, while four of the samples taken from the same source were contaminated with total coliform during the dry season. Water samples from street taps 3 and 7 (ST 3 and ST7) were contaminated with total coliform during both seasons, however, the total coliform counts during the wet season were more than the counts during the dry season. None of the samples was contaminated with E. coli during the dry season, however, 2 samples from the street taps (ST3 & ST6) were found to be contaminated with E. coli during the wet season. Samples from household taps showed a similar trend with the street taps—with all samples being contaminated with total coliform during the wet season. Though 7 of the 8 samples taken from the household taps were contaminated with total coliform during the dry season, the samples from the same sources showed a higher level of total coliform in the wet season, with almost all the samples showing contamination at maximum detection levels of more than 2000 MPN/100 mL, except one sample (HT8) which showed a higher level of contamination with total coliform during the dry compared with the wet season. Only one sample (HT4) was found to be contaminated with E. coli during both dry and wet season. This shows that total coliform contamination levels are higher during the wet season than the dry season (Table 5 ).
Water samples from household storage containers (HSC) showed a higher level of total coliform during the wet season than the dry season and more samples were contaminated with E. coli during the wet season also (Table 6 ). A higher level of contamination was recorded for the HSCs compared to the street and household taps.
Physico-chemical analysis
In the reservoir samples, the pH value ranged from 8.37 to 8.45, EC ranged between 183 and 259 µS/cm whereas TDS varied between 118 and 168 mg/L. Similarly, in the street tap samples, pH value ranged from 7.28 and 9.33, EC ranged between 26 and 867 µS/cm whereas TDS varied between 16 and 562 mg/L (Fig. 3 ).
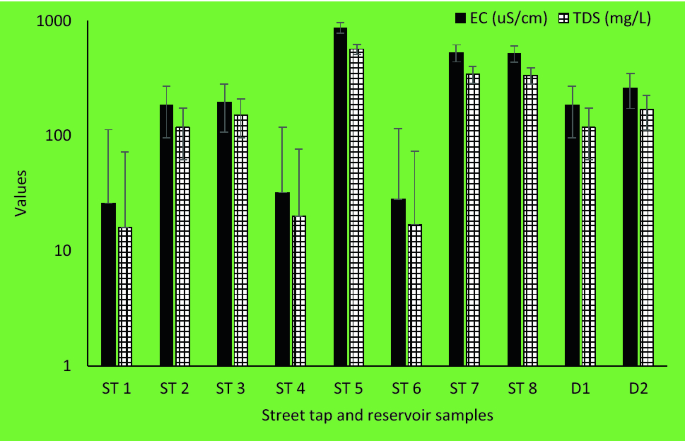
EC and TDS levels for the street taps and reservoir samples.
In the household taps, pH value ranged from 7.70–9.98, EC range between 28–895 µS/cm and TDS varied between 18 and 572 mg/L (Fig. 4 ).
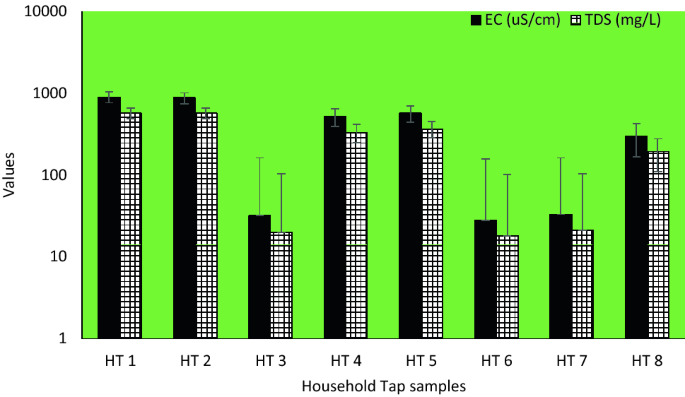
EC and TDS levels for household taps.
In household storage container samples, the pH value ranges from 7.67–9.77, EC ranged between 19–903 µS/cm and TDS values ranged from 12–1148 mg/L (Fig. 5 ).
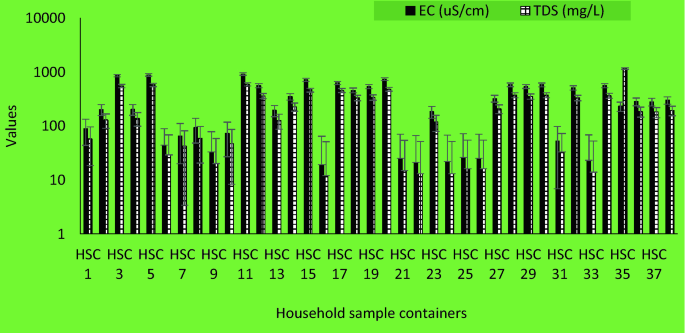
EC and TDS levels for household storage container samples.
Analysis of cations and trace metals in water
To detect the cations’ and trace metals’ concentrations in the water samples, representative samples from each of the sources were selected for analysis. The concentration of Calcium ranged between 2.14 and 31.65 mg/L, Potassium concentration ranged from 0.14 to 1.85 mg/L, Magnesium concentration varied from 1.32 to 16.59 mg/L, Sodium ranged from 0.18 to 12.96 mg/L (Table 7 ).
Trace metals’ analysis
The minimum and maximum concentrations of trace metals (Al, Mn, Fe, Co, Ni, Cu, Zn, As and Pb) present in water samples from selected street taps, household taps and household storage containers are presented in Table 8 .
Hazard quotient (HQ) and carcinogenic risk assessment
Table 9 presents the exposure dosage and hazard quotient (HQ) for ingestion and dermal pathway for metals. The HQ ing and HQ derm for all analyzed trace metals in both children and adults were less than one unit, indicating that there are no potential non-carcinogenic health risks associated with consumption of the water. Table 10 presents the total Hazard Quotient and Health risk index (HI) for trace metals in the water samples, showing that residents of the study area are not susceptible to non-cancer risks due to exposure to trace metals in drinking water. Table 11 presents the cancer risk associated with the levels of Ni, As and Pb in the drinking water samples. The table shows that only the maximum levels of lead had the highest chance of cancer risks for both adults and children.
This study provides information about the quality of drinking water in a selected rural community of Thulamela municipality of Limpopo province, South Africa, taking into consideration the physicochemical, microbiological and trace metals’ parameters of the treated water supplied to the village by the government, through the municipality. Many participants in the study have their primary source of water piped into their yards, while very few have water in their houses. This implies that getting water for household use would involve collecting the water from the yard and then into the storage containers. Those who do not have the taps in their yards have to collect water from the neighbours’ yards or the street taps. This observation is not restricted to the study area, as a similar situation has been observed in other rural communities of Limpopo Province 21 . This need to pass water through multiple containers before the point of use increases the risk of contamination.
Residents of the study area, just like residents of other settlements in Thulamela Municipality 21 , store their drinking water in plastic buckets, ceramic vessels, jerry cans and other containers. Almost all the respondents (96.5%) claim that their water storage vessels are covered and that their drinking water usually stays for less than a week in the storage containers (87.8%). Covering of water storage containers reduces the risk of water contamination from dust or other airborne particles. However, intermittent interruption of municipal water supply lasting for a week or more in the study area and the consequent use of alternative sources of water predispose the residents to various health risks as intermittent interruption in water supply has been linked to higher chances of contamination in the distribution systems, compared with continuous supply; in addition, the alternative sources of water may not be of a good quality as the treated municipal water 33 , 34 , yet, more than half of the respondents in this study (53%) use water directly from source without any form of treatment. This is because many residents in rural communities of Limpopo province believe that the water they drink is of good quality and thus do not need any further treatment 21 . The few who treat their water before drinking mostly use the boiling method. While boiling and other home-based interventions like solar disinfection of water have been reported to improve the quality of drinking water; drinking vessels, like cups, have also been implicated in water re-contamination of treated water at the point of use 16 and most respondents (91.3%) in this study admittedly use cups to collect water from the storage containers. The risk of contamination is even increased when cups without handles are used, where there is a higher chance that the water collector would touch the water in the container with his/her fingers. The Centres for Disease Control and Prevention (CDC) recommends that containers for drinking water should be fitted with a small opening with a cover or a spigot, through which water can be collected while the container remains closed, without dipping any potentially contaminated object into the container 35 . However, it is noteworthy that all the respondents claim to always wash their hands with soap (or its equivalents) and water after using the toilets, a constant practice of hand washing after using the toilet has been associated with a reduced risk of water contamination with E. coli 19 .
Treated water from the dam tested negative for both total coliform and E. coli hence complied with regulatory standards of SANS 31 and WHO 8 . The results could probably be due to the use of chlorine as a disinfectant in the treatment plant. Using disinfectants, pathogenic bacteria from the water can be killed and water made safe for the user. Similar studies have also reported that treated water in urban water treatment plants contains no total coliforms and E. coli 36 . In contrast, treated water sources in rural areas have been reported to have considerable levels of total coliform and E. coli 37 . The reason alluded to this include lack of disinfectant, no residual chlorine in the treated water, high prevalence of open defecation and unhygienic practices in proximity to water sources 38 .
From the water samples collected from the street taps, 62.5% were found to be contaminated with total coliform during the dry season, while the percentage rose to 87.5% during the wet season. The street tap which is about 13 km from the reservoir recorded high levels of total coliform ranging from 1.0 -2000 MPN/100 mL with most of the sites exceeding the WHO guidelines of 10 MPN/100 mL 8 . In both seasons, all the samples tested negative for E. coli , this complies with the WHO guideline of 0 MPN/100 mL. While the water leaving the treatment plant met bacteriological standards, the detection of coliform bacteria in the distribution lines suggest that the water is contaminated in the distribution networks. This could be due to the adherence of bacteria onto biofilms or accidental point source contamination by broken pipes, installation and repair works 39 . Furthermore, the water samples from households’ storage containers were contaminated by total coliform (73% and 85%) and E. coli (10.4% and 13.2%) during the dry and wet season, respectively. Microbiological contamination of household water stored in containers could be due to unhygienic practices occurring between the collection point and the point-of-use 40 , 41 .
Generally, higher levels of contamination were recorded in the wet season than in the dry season. The wet season in Thulamela Municipality is often characterized with increased temperature which could lead to favourable condition for microbial growth. Also, the treatment plant usually makes use of the same amount of chlorine for water purification during both seasons, even though influent water would be of a higher turbidity during the wet season, hence reducing the levels of residual chlorine 42 .
The pH of the analyzed samples from the study area ranged from 7.15 to 9.92. Most of the samples were within the values recommended by SANS (5 to 9.7) and comparable to results from previous similar studies 31 , 43 . Also, the electrical conductivity of all water samples from this study ranged from 28 µS/cm to 903 µS/cm which complied with the recommended value of SANS: < 1700 µS/cm 31 . The presence of dissolved solids such as calcium, chloride, and magnesium in water samples is responsible for its electrical conductivity 44 .
Total dissolved solids are the inorganic salts and small amounts of organic substance, which are present as solution in water 45 . Water has the ability to dissolve a wide range of inorganic and some organic minerals or salts such as potassium, calcium, sodium, bicarbonates, chlorides, magnesium, sulphates, etc. These minerals produced unwanted taste and colour in water 46 . A high TDS value indicates that water is highly mineralised. The recommended TDS value set for drinking water quality is ≤ 1200 mg/L 31 . In this study, the TDS values ranged from 18 mg/L to 572 mg/L. Hence, the TDS of all the household’s storage samples complied with the guidelines and consistent with previous studies 47 .
The analysis of magnesium (1.32 to 16.59 mg/L) and calcium (2.14 to 31.65 mg/L) concentrations showed that they were within the permissible range recommended for drinking water by SANS 31 and WHO 8 . All living organisms depend on magnesium in all types of cells, body tissues and organs for variety of functions while calcium is very important for human cell physiology and bones. Similar studies in Ethiopia and Turkey also showed acceptable levels of these metals in drinking water 46 , 48 . Likewise, the levels of potassium (0.14 to 1.85 mg/L) and sodium (0.18 to 12.96 mg/L) were within the permissible limit of WHO and SANS and may not cause health related problems. Sodium is essential in humans for the regulation of body fluid and electrolytes, and for proper functioning of the nerves and muscles, however, excessive sodium in the body can increase the risk of developing a high blood pressure, cardiovascular diseases and kidney damage 49 , 50 . Potassium is very important for protein synthesis and carbohydrate metabolism, thus, it is very important for normal growth and body building in humans, but, excessive quantity of potassium in the body (hyperkalemia) is characterized with irritability, decreased urine production and cardiac arrest 51 .
Metals like copper (Cu), cobalt (Co) and zinc (Zn) are essential requirements for normal body growth and functions of living organisms, however, in high concentrations, they are considered highly toxic for human and aquatic life 42 . Elevated trace metal(loids) concentrations could deteriorate water quality and pose significant health risks to the public due to their toxicity, persistence, and bio accumulative nature 52 . In this study, the concentrations of Manganese, Cobalt, Nickel and Copper all complied with the recommended concentration by SANS for domestic water use.
Aluminum concentration in the drinking water samples ranged from 1.25—13.46 µg/L. All analysed samples complied with the recommended concentration of ≤ 300 µg/L for domestic water use 31 . The recorded levels of Al in water from this study should not pose any health risk. At a high concentration, aluminium affects the nervous system, and it is linked to several diseases, such as Parkinson’s and Alzheimer’s diseases 53 . Iron (Fe) is an essential element for human health, required for the production of protein haemoglobin, which carries oxygen from our lungs to the other parts of the body. Insufficient or excess levels of iron can have negative effect on body functions 54 . The recommended concentration of iron in drinking water is ≤ 2000 µg/L 31 . In this study, the concentration of iron in the samples ranged from 0.96 to 73.53 µg/L. Similar results were reported by Jamshaid et al. in Khyber Pakhtunkhwa province 55 . A high concentration of Fe in water can give water a metallic taste, even though it is still safe to drink 56 .
The levels of Pb, As and Zn were in the range of 0.02–0.57 µg/L, 0.02–0.17 µg/L, and 2.54–194.96 µg/L, respectively whereas Cr was not detected in the samples collected. The levels recorded complied with the SANS 31 and WHO 8 guidelines for drinking water. Similar results were reported by Mohod and Dhote 57 . Lead is not desirable in drinking water because it is carcinogenic and can cause growth impairment in children 41 . Inorganic arsenic is a confirmed carcinogen and is the most significant chemical contaminant in drinking-water globally 44 . Zinc deficiency can cause loss of appetite, decreased sense of taste and smell, slow wound healing and skin sores 58 . Cr is desirable at low concentration but can be harmful if present in elevated levels.
The hazard quotient (HQ) takes into consideration the oral toxicity reference dose for a trace metal that humans can be exposed to 59 . Health related risk associated with the exposure through ingestion depends on the weight, age and volume of water consumed by an individual. HQ ing and HQ derm for all analyzed trace metals in both children and adults were less than one unit (Table 9 ), indicating that there are no potential non-carcinogenic health risks associated with the consumption of the water from the study area either by children or adults. The calculated average cumulative health risk index (HI) for children and adult was 3.88E-02 and 1.78E-02, respectively. HQ across metals serve as a conservative assessment tool to estimate high-end risk rather than low end-risk in order to protect the public. This served as a screen value to determine whether there is major significant health risk 60 . The results in this study signifies that the population of the investigated area are not susceptible to non-cancer risks due to exposure to trace metals in drinking water. Similar observation has been reported by Bamuwamye et al. after investigating human health risk assessment of trace metals in Kampala (Uganda) drinking water 61 . It should be noted that the hazard index values for children were higher than that of adult, suggesting that children were more susceptible to non-carcinogenic risk from the trace metals.
Drinking water with trace metals such as Pb, As, Cr and Cd could potentially enhance the risk of cancer in human beings 62 , 63 . Long term exposure to low amounts of toxic metals might, consequently, result in many types of cancers. Using As, Ni and Pb carcinogens, the total exposure risks of the residents in Table 11 . For trace metals, an acceptable carcinogenic risk value of less than 1 × 10 −6 is considered as insignificant and the cancer risk can be neglected; while an acceptable carcinogenic risk value of above 1 × 10 –4 is considered as harmful and the cancer risk is worrisome. Amongst the studied trace metals, only the maximum levels of lead for both adults and children had the highest chance of cancer risks (1.93E−03 and 4.46E−03) while Arsenic and Nickel have no chance of cancer risk with values of 3.34E−06; 7.72E−06 and 2.24E−05; 5.18E−05, in both adults and children respectively. The only cancer risk to residents of the studied area could be from the cumulative ingestion of lead in their drinking water. The levels of Pb recorded in this study complied to the SANS guideline value for safe drinking water. While the levels of Pb from the dam and the street pipes were relatively low, higher levels where recorded at household taps and storage containers and this may be due to the kind of storage containers and pipes used in those households. Generally, the water supply is of low Pb levels which should not pose any health risk to the consumers. However, the residents in rural areas should be properly educated on the kind of materials to be used for safe storage of water which should not pose an additional health burden. The likelihood of cancer risk was only associated with the consumption of the highest levels of Pb reported for a life time for adults (set at 70 years) and 6 years for children. Consistent consumption of water from the same source throughout an adult’s lifetime is unlikely as residents in those communities may change their locations at some points, hence reducing the possible risk associated with consistent exposure to the same levels of Pb.
Conclusions
The study shows that as distance increases from the treatment reservoir to distribution points, the cross-contamination rate also increases, therefore, good hygienic practices is required while transporting, storing and using water. Unhygienic handling practices at any point between collection and use contribute to the deterioration of drinking water quality.
The physicochemical, bacteriological quality and trace metals’ concentration of water samples from treated source, street taps and household storage containers were majorly within the permissible range of both WHO and SANS drinking water standards. HQ for both children and adults were less than unity, showing that the drinking water poses less significance health threat to both children and adults. Amongst the studied trace metals, only the maximum level of lead for both adults and children has the highest chance of cancer risks.
We recommend that appropriate measures should be taken to maintain residual free chlorine at the distribution points, supply of municipal treated water should be more consistent in all the rural communities of Thulamela municipality, Limpopo province and residents should be trained on hygienic practices of transportation and storage of drinking water from the source to the point of use.
Data availability
The datasets used and analysed during the current study are available from the first author on reasonable request.
Abbreviations
American Public Health Association
Centres for Disease Control and Prevention
Department of Water Affairs and Forestry
Electrical conductivity
Health risk index
Hazard quotient
Household storage containers
Household taps
Inductively coupled plasma mass spectrophotometer
Inductively coupled plasma optical emission spectrophotometer
Most probable number
South African National Standards
Street taps
Total Dissolved Solids
United Nations General Assembly
United Nations International Children Emergency Fund
United States Environmental Protection Agency
World Health Organization
Taiwo, A.M., Olujimi, O.O., Bamgbose, O. & Arowolo, T.A. Surface water quality monitoring in Nigeria: Situational analysis and future management strategy. In Water Quality Monitoring and Assessment (ed. Voudouris, K) 301–320 (IntechOpen, 2012).
Corcoran, E., et al. Sick water? The central role of wastewater management in sustainable development: A rapid response assessment. United Nations Enviromental Programme UN-HABITAT, GRID-Arendal. https://wedocs.unep.org/20.500.11822/9156 (2010).
United Nations, The 2030 Agenda and the Sustainable Development Goals: An opportunity for Latin America and the Caribbean (LC/G.2681-P/Rev.3), Santiago (2018).
Hubert, E. & Wolkersdorfer, C. Establishing a conversion factor between electrical conductivity and total dissolved solids in South African mine waters. Water S.A. 41 , 490–500 (2015).
Article CAS Google Scholar
Department of Water Affairs (DWA). Groundwater Strategy. Department of Water Affairs: Pretoria, South Africa. 64 (2010).
Lu, Y., Nakicenovic, N., Visbeck, M. & Stevance, A. S. Policy: Five priorities for the UN sustainable development goals. Nature 520 , 432–433 (2015).
Article ADS PubMed Google Scholar
Shaheed, A., Orgil, J., Montgomery, M. A., Jeuland, M. A. & Brown, J. Why, “improved” water sources are not always safe. Bull. World Health Organ. 92 , 283–289 (2014).
Article PubMed PubMed Central Google Scholar
WHO. Guidelines for Drinking Water Quality 4th Edn (World Health Organization, Geneva, Switzerland, 2011). http://apps.who.int/iris/bitstream/10665/44584/1/9789241548151_eng.pdf .
Patil, P. N., Sawant, D. V. & Deshmukh, R. N. Physico-chemical parameters for testing of water—a review. Int. J. Environ. Sci. 3 , 1194–1207 (2012).
CAS Google Scholar
Bain, R. et al. Fecal contamination of drinking-water in low-and middle-income countries: A systematic review and meta-analysis. PLoS Med. 11 , e1001644 (2014).
Younos, T. & Grady, C.A. Potable water, emerging global problems and solutions. In The Handbook of Environmental Chemistry 30 (2014).
Tigabu, A. D., Nicholson, C. F., Collick, A. S. & Steenhuis, T. S. Determinants of household participation in the management of rural water supply systems: A case from Ethiopia. Water Policy. 15 , 985–1000 (2013).
Article Google Scholar
Oljira, G. Investigation of drinking water quality from source to point of distribution: The case of Gimbi Town, in Oromia Regional State of Ethiopia (2015).
Clasen, T., Haller, L., Walker, D., Bartram, J. & Cairncross, S. Cost-effectiveness of water quality interventions for preventing diarrhoeal disease in developing countries. J. Water Health 5 , 599–608 (2007).
Article PubMed Google Scholar
Too, J. K., Sang, W. K., Ng’ang’a, Z. & Ngayo, M. O. Fecal contamination of drinking water in Kericho District, Western Kenya: Role of source and household water handling and hygiene practices. J. Water Health 14 , 662–671 (2016).
Rufener, S., Mausezahl, D., Mosler, H. & Weingartner, R. Quality of drinking-water at source and point-of-consumption—drinking cup as a high potential recontamination risk: A field Study in Bolivia. J. Health Popul. Nutri. 28 , 34–41 (2010).
Google Scholar
Nsubuga, F. N. W., Namutebi, E. N. & Nsubuga-ssenfuma, M. Water resources of Uganda: An assessment and review. Water Resour. Prot. 6 , 1297–1315 (2014).
Rawway, M., Kamel, M. S. & Abdul-raouf, U. M. Microbial and physico-chemical assessment of water quality of the river Nile at Assiut Governorate (Upper Egypt). J. Ecol. Health Environ. 4 , 7–14 (2016).
Agensi, A., Tibyangye, J., Tamale, A., Agwu, E. & Amongi C. Contamination potentials of household water handling and storage practices in Kirundo Subcounty, Kisoro District, Uganda. J. Environ. Public Health. Article ID 7932193, 8 pages (2019).
Mahmud, Z. H. et al. Occurrence of Escherichia coli and faecal coliforms in drinking water at source and household point-of-use in Rohingya camps, Bangladesh. Gut Pathog. 11 , 52. https://doi.org/10.1186/s13099-019-0333-6 (2019).
Edokpayi, J. N. et al. Challenges to sustainable safe drinking water: A case study of water quality and use across seasons in rural communities in Limpopo Province, South Africa. Water 10 , 159 (2018).
Article PubMed PubMed Central CAS Google Scholar
Musyoki, A., Thifhulufhelwi, R. & Murungweni, F. M. The impact of and responses to flooding in Thulamela Municipality, Limpopo Province, South Africa, Jàmbá. J. Disaster Risk Stud. 8 , 1–10 (2016).
Census 2011. Main Place: Lufule. Accessed from Census 2011: Main Place: Lufule (adrianfrith.com) on 30/01/2022.
Bolarinwa, O. A. Principles and methods of validity and reliability testing of questionnaires used in social and health science researches. Niger. Postgrad. Med. J. 22 , 195–201 (2015).
Association, A. P. H. Standard Methods for the Examination of Water and Waste Water 16th edn. (American Public Health Association, Washington, 1992).
Bernardes, C., Bernardes, R., Zimmer, C. & Dorea, C. C. A simple off-grid incubator for microbiological water quality analysis. Water 12 , 240 (2020).
Rich CR, Sellers JM, Taylor HB, IDEXX Laboratories Inc. Chemical reagent test slide. U.S. Patent Application 29/218,589. (2006).
Naveedullah, et al. Concentrations and human health risk assessment of selected heavy metals in surface water of siling reservoir watershed in Zhejiang Province, China. Pol. J. Environ. Stud. 23 , 801–811 (2014).
Wu, J. & Sun, Z. Evaluation of shallow groundwater contamination and associated human health risk in an alluvial plain impacted by agricultural and industrial activities, mid-west China. Expos. Health. 8 , 311–329 (2016).
Wu, L., Zhang, X. & Ju, H. Amperometric glucose sensor based on catalytic reduction of dissolved oxygen at soluble carbon nanofiber. Biosens. Bioelectron. 23 , 479–484 (2007).
Article PubMed CAS Google Scholar
South African National Standard (SANS). 241-1: Drinking Water, Part 1: Microbiological, Physical, Aesthetic and Chemical Determinants. 241-2: 2015 Drinking Water, Part 2: Application of SANS 241-1 (2015).
Department of Water Affairs and Forestry (DWAF). South African Water Quality Guidelines (second edition). Volume 1: Domestic Use, (1996).
Drake, M.J. & Stimpfl, M. Water matters. In Lunar and Planetary Science Conference , Vol. 38, 1179 (2007).
Kumpel, E. & Nelson, K. L. Intermittent water supply: prevalence, practice, and microbial water quality. Environ. Sci. Technol. 50 , 542–553 (2016).
Article ADS CAS PubMed Google Scholar
Centers for Disease Control and Prevention. The safe water system: Safe storage of drinking water. Accessed from CDC Fact Sheet on 30/01/2022 (2012).
Hashmi, I., Farooq, S. & Qaiser, S. Chlorination and water quality monitoring within a public drinking water supply in Rawalpindi Cantt (Westridge and Tench) area, Pakistan. Environ. Monit. Assess. 158 , 393–403 (2009).
Article CAS PubMed Google Scholar
Onyango, A. E., Okoth, M. W., Kunyanga, C. N. & Aliwa, B. O. Microbiological quality and contamination level of water sources in Isiolo country in Kenya. J. Environ. Public Health . 2018 , 2139867 (2018).
Gwimbi, P., George, M. & Ramphalile, M. Bacterial contamination of drinking water sources in rural villages of Mohale Basin, Lesotho: Exposures through neighbourhood sanitation and hygiene practices. Environ. Health Prev. Med. 24 , 33 (2019).
Karikari, A. Y. & Ampofo, J. A. Chlorine treatment effectiveness and physico-chemical and bacteriological characteristics of treated water supplies in distribution networks of Accra-Tema Metropolis, Ghana. Appl. Water Sci. 3 , 535–543 (2013).
Article ADS CAS Google Scholar
Thompson, T., Sobsey, M. & Bartram, J. Providing clean water, keeping water clean: An integrated approach. Int. J. Environ. Health Res. 13 , S89–S94 (2003).
Cronin, A. A., Breslin, N., Gibson, J. & Pedley, S. Monitoring source and domestic water quality in parallel with sanitary risk identification in Northern Mozambique to Prioritise protection interventions. J. Water Health 4 , 333–345 (2006).
Edokpayi, J. N., Enitan, A. M., Mutileni, N. & Odiyo, J. O. Evaluation of water quality and human risk assessment due to heavy metals in groundwater around Muledane area of Vhembe District, Limpopo Province, South Africa. Chem. Cent. J. 12 , 2 (2018).
Article CAS PubMed PubMed Central Google Scholar
Edimeh, P. O., Eneji, I. S., Oketunde, O. F. & Sha’Ato, R. Physico-chemical parameters and some heavy metals content of Rivers Inachalo and Niger in Idah, Kogi State. J. Chem. Soc. Nigeria 36 , 95–101 (2011).
Rahmanian, N., et al . Analysis of physiochemical parameters to evaluate the drinking water quality in the State of Perak, Malaysia. J. Chem. 1–10. Article ID 716125 (2015).
WHO/FAO. Diet, Nutrition, and the Prevention of Chronic Diseases (World Health Organisation, Geneva, 2003).
Meride, Y. & Ayenew, B. Drinking water quality assessment and its effects on resident’s health in Wondo genet campus, Ethiopia. Environ. Syst. Res. 5 , 1 (2016).
Mapoma, H. W. & Xie, X. Basement and alluvial aquifers of Malawi: An overview of groundwater quality and policies. Afr. J. Environ. Sci. Technol. 8 , 190–202 (2014).
Soylak, M., Aydin, F., Saracoglu, S., Elci, L. & Dogan, M. Chemical analysis of drinking water samples from Yozgat. Turkey Pol. J. Environ. Stud. 11 , 151–156 (2002).
Munteanu, C. & Iliuta, A. The role of sodium in the body. Balneo Res. J. 2 , 70–74 (2011).
Strazzullo, P. & Leclercq, C. Sodium. Adv. Nutr. 5 , 188–190 (2014).
Pohl, H. R., Wheeler, J. S. & Murray, H. E. Sodium and potassium in health and disease. Met. Ions Life Sci. 13 , 29–47 (2013).
Muhammad, S., Shah, M. T. & Khan, S. Health risk assessment of heavy metals and their source apportionment in drinking water of Kohistan region, northern Pakistan. Microchem. J. 98 , 334–343 (2011).
Inan-Eroglu, E. & Ayaz, A. Is aluminum exposure a risk factor for neurological disorders?. J. Res. Med. Sci. 23 , 51 (2018).
Milman, N. Prepartum anaemia: Prevention and treatment. Ann. Hematol. 87 , 949–959 (2008).
Jamshaid, M., Khan, A. A., Ahmed, K. & Saleem, M. Heavy metal in drinking water its effect on human health and its treatment techniques—a review. Int. J. Biosci. 12 , 223–240 (2018).
Tagliabue, A., Aumont, O. & Bopp, L. The impact of different external sources of iron on the global carbon cycle. Geophys. Res. Lett. 41 , 920–926 (2014).
Mohod, C. V. & Dhote, J. Review of heavy metals in drinking water and their effect on human health. Int. J. Innov. Res. Technol. Sci. Eng. 2 , 2992–2996 (2013).
Bhowmik, D., Chiranjib, K. P. & Kumar, S. A potential medicinal importance of zinc in human health and chronic. Int. J. Pharm. 1 , 05–11 (2010).
Mahmud, M. A. et al. Low temperature processed ZnO thin film as electron transport layer for efficient perovskite solar cells. Sol. Energy Mater. Sol. Cells. 159 , 251–264 (2017).
Rajan, S. & Ishak, N. S. Estimation of target hazard quotients and potential health risks for metals by consumption of shrimp ( Litopenaeus vannamei ) in Selangor, Malaysia. Sains Malays. 46 , 1825–1830 (2017).
Bamuwamye, M. et al. Human health risk assessment of heavy metals in Kampala (Uganda) drinking water. J. Food Res. 6 , 6–16 (2017).
Saleh, H. N. et al. Carcinogenic and non-carcinogenic risk assessment of heavy metals in groundwater wells in Neyshabur Plain, Iran. Biol. Trace Elem. Res. 190 , 251–261 (2019).
Tani, F. H. & Barrington, S. Zinc and copper uptake by plants under two transpiration rates Part II Buckwheat ( Fagopyrum esculentum L.). Environ. Pollut. 138 , 548–558 (2005).
Download references
Acknowledgements
The authors wish to thank the University of Venda Health, Safety and Research Ethics’ Committee, the Department of Water affairs, Limpopo province and Vhembe district Municipality for granting the permission to conduct this study. We also thank all the respondents from the selected households in Lufule community.
The study was funded by the Research and Publication Committee of the University of Venda (Grant number: SHS/19/PH/14/1104).
Author information
Authors and affiliations.
Department of Public Health, School of Health Sciences, University of Venda, Thohoyandou, 0950, South Africa
N. Luvhimbi, T. G. Tshitangano, J. T. Mabunda & F. C. Olaniyi
Department of Hydrology and Water Resources, School of Environmental Sciences, University of Venda, Thohoyandou, 0950, South Africa
J. N. Edokpayi
You can also search for this author in PubMed Google Scholar
Contributions
L.N. and J.N.E. conceptualized the study, L.N. collected and analysed the data, T.G.T., J.T. M., and J.N.E. supervised the data collection and analysis. F.C.O. drafted the original manuscript, J.N.E. reviewed and edited the original manuscript. All authors approved the final manuscript.
Corresponding author
Correspondence to F. C. Olaniyi .
Ethics declarations
Competing interests.
The authors declare no competing interests.
Additional information
Publisher's note.
Springer Nature remains neutral with regard to jurisdictional claims in published maps and institutional affiliations.
Rights and permissions
Open Access This article is licensed under a Creative Commons Attribution 4.0 International License, which permits use, sharing, adaptation, distribution and reproduction in any medium or format, as long as you give appropriate credit to the original author(s) and the source, provide a link to the Creative Commons licence, and indicate if changes were made. The images or other third party material in this article are included in the article's Creative Commons licence, unless indicated otherwise in a credit line to the material. If material is not included in the article's Creative Commons licence and your intended use is not permitted by statutory regulation or exceeds the permitted use, you will need to obtain permission directly from the copyright holder. To view a copy of this licence, visit http://creativecommons.org/licenses/by/4.0/ .
Reprints and permissions
About this article
Cite this article.
Luvhimbi, N., Tshitangano, T.G., Mabunda, J.T. et al. Water quality assessment and evaluation of human health risk of drinking water from source to point of use at Thulamela municipality, Limpopo Province. Sci Rep 12 , 6059 (2022). https://doi.org/10.1038/s41598-022-10092-4
Download citation
Received : 02 December 2021
Accepted : 30 March 2022
Published : 11 April 2022
DOI : https://doi.org/10.1038/s41598-022-10092-4
Share this article
Anyone you share the following link with will be able to read this content:
Sorry, a shareable link is not currently available for this article.
Provided by the Springer Nature SharedIt content-sharing initiative
This article is cited by
Characterization of natural zeolite and determination of its ion-exchange potential for selected metal ions in water.
- G. M. Wangi
- P. W. Olupot
- R. Kulabako
Environmental Processes (2023)
Relations between personal exposure to elevated concentrations of arsenic in water and soil and blood arsenic levels amongst people living in rural areas in Limpopo, South Africa
- Thandi Kapwata
- Caradee Y. Wright
- Angela Mathee
Environmental Science and Pollution Research (2023)
By submitting a comment you agree to abide by our Terms and Community Guidelines . If you find something abusive or that does not comply with our terms or guidelines please flag it as inappropriate.
Quick links
- Explore articles by subject
- Guide to authors
- Editorial policies
Sign up for the Nature Briefing newsletter — what matters in science, free to your inbox daily.

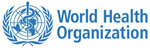
- Previous Article
- Next Article
INTRODUCTION
Conclusions, data availability statement, conflict of interest, a bibliometric analysis of global research on drinking water and health in low- and lower-middle-income countries.

- Article contents
- Figures & tables
- Supplementary Data
- Open the PDF for in another window
- Guest Access
- Cite Icon Cite
- Permissions
- Search Site
Alexa Bennett , Jeffrey Demaine , Caetano Dorea , Alexandra Cassivi; A bibliometric analysis of global research on drinking water and health in low- and lower-middle-income countries. J Water Health 1 March 2023; 21 (3): 417–438. doi: https://doi.org/10.2166/wh.2023.293
Download citation file:
- Ris (Zotero)
- Reference Manager
Heightened interest in drinking water research in recent decades has been aimed at narrowing the knowledge gaps surrounding water and health in a global pursuit to provide safely managed drinking water services to populations who continue to lack access. This study used bibliometrics and network analysis to produce a global overview of publications and groups that have contributed to research on drinking water and health in low- and lower-middle-income countries (LLMICs). The United States and the United Kingdom, which have historically dominated the field based on the production and impact of scientific literature, remain at the center of international collaborative research partnerships with emerging countries. However, in recent years, the volume of publications produced by India has surpassed that of the United States while Bangladesh is ranked third for the strongest international collaborations. Iran and Pakistan are also emerging as major producers of research, yet publications out of these countries and India remain disproportionately restricted behind paywalls. Contamination, diarrheal disease, and water resources are the themes that characterize the majority of research on water and health. These findings may be used to accelerate equitable, inclusive research in the realm of water and health, thereby enabling gaps in global drinking water inequalities to be filled.
A global overview of published literature on water and health in low and lower-middle-income countries using bibliometric data
Critical insights into research gaps pertaining to the literature on water and health
May inform future research priorities, facilitate collaboration, and identify where national research systems must be strengthened
Graphical Abstract

Access to safe drinking water is a fundamental human right and has been acknowledged as such by the United Nations General Assembly through the human right to water ( United Nations General Assembly 2010 ). The provision of safe, affordable, and reliable water is essential to human health and is indispensable to sustainable development. Nonetheless, in 2021, two billion people around the globe continue to lack access to safely managed drinking water services, which is defined as an improved water source that is located on premise, available when needed, and free from contamination ( UN Water 2021 ). Most people who lack access to safe drinking water are situated in low- and lower-middle-income countries (LLMICs), which are consequently the regions disproportionately affected by the health burdens associated with the inadequate provision of water ( WHO & UNICEF 2021 ). Some of these conditions include infectious diseases caused by waterborne pathogens, neglected tropical diseases, and adverse health outcomes such as stunting, wasting, and being underweight ( Deshpande et al. 2020 ). It is estimated that universal access to drinking water, together with guaranteeing sanitation and hygiene services, would prevent 1.6 million deaths per year, accounting for 2.8% of global mortalities ( Prüss-Ustün et al. 2019 ).
Lack of access to water also affects human health and development through several secondary mechanisms, making targeted health interventions complex. The inadequate provision of safe and reliable water is associated with food insecurity ( Brewis et al. 2020 ) and reduced hygiene practices ( Staddon et al. 2020 ), which can in turn lead to heightened rates of malnutrition and infectious diseases, respectively. Moreover, the ramifications of drinking water insecurity extend beyond the development of biological conditions and also involve potential consequences for mental health and well-being, including increased levels of emotional/perceived stress, depression, and anxiety ( Wutich et al. 2020 ). As women and girls are primarily responsible for household water management in LLMICs, they bear the brunt of these mental health burdens ( Hadley & Wutich 2009 ) and often experience heightened vulnerability related to water insecurity during periods of menstruation, pregnancy, and personal or household illness ( Stevenson et al. 2012 ; Collins et al. 2019 ; Adams et al. 2021 ). Moreover, evidence suggests that the provision of on-site access to water is associated with an increase in educational and economic opportunities, with these benefits skewing heavily toward women ( WaterAid 2021 ). Thus, obtaining universal access to drinking water is not only a prerequisite for health but is also necessary for achieving progress toward eliminating hunger, advancing women's empowerment, and enabling greater opportunities for economic development.
In recent decades, heightened interest in drinking water research that has been maintained by supportive funding sources has narrowed the gap in knowledge surrounding water and health. The volume of publications in the field has expanded as researchers investigate diverse perspectives on drinking water, such as those related to quality, availability, and accessibility and a multitude of themes, including major water-related diseases, contaminants of emerging concern, water and gender equality, and water governance. Yet it remains unclear exactly which interests dominate the field of drinking water research in LLMICs. Further, the globalization of research on global health topics has accelerated in recent decades, pointing to shifting collaborative efforts that have become increasingly diverse both geographically and across socioeconomic parameters ( Addo-Atuah et al. 2020 ). However, research outputs from High-Income Countries (HICs) continue to dominate several fields of global health research with less representation from LLMICs where the disease burden is disproportionately higher ( Ghani et al. 2021 ). The distribution of global research on drinking water and health in LLMICs needs to be better understood to identify whether these gaps exist with regard to drinking water research.
It is relevant to explore emerging trends in scientific activity to understand where research has been undertaken and by whom. Past bibliometric studies have examined specific topics on drinking water ( Hu et al. 2010 ; Abejon & Garea 2015 ; Tang et al. 2020 ; Ekundayo et al. 2021 ) and concentrated on research out of specific geographical regions ( Wambu & Ho 2016 ), or narrow time periods ( Fu et al. 2013 ). Recent studies have also emerged to assess research on Sustainable Development Goal (SDG) 6 ( Basu et al. 2021 ; Dibbern et al. 2022 ; Roy et al. 2022 ), which include a wide range of water, sanitation, and hygiene-related priorities and are again restricted to recent years. A comprehensive analysis examining research on drinking water and health that highlights changing trends over time and analyses data from the perspective of country-level income has yet to be conducted. Hence, the current study aims to assess research trends on water and health in LLMICs from 1980, focusing on progress made in closing research disparities over time. It aims to explore possible pathways forward in the global pursuit toward a more equitable scientific community, such as through initiatives that promote Open Access (OA) content. A comprehensive overview of the field may be used to inform future research priorities and decision-making processes, facilitate improved collaboration on research outputs, and identify where national research systems must be strengthened. In doing so, the following questions would be addressed: What are the overall volume, type, and growth rate of all published documents on drinking water and health in LLMICs? What countries, institutions, journals, or funding agencies have had the greatest influence on research related to drinking water and health in LLMICs? What relationships are present between influential groups? What percentage of global research on drinking water and health in LLMICs is universally accessible (i.e. OA)? What are the most frequently studied topics on drinking water and health in LLMICs?
A bibliometric analysis was conducted to produce an overview of publications and groups that have contributed to research on drinking water and health in LLMICs since 1980. The Web of Science Core Collection (WOSCC), a leading bibliographic source ( Mongeon & Paul-Hus 2016 ), was used to extract data sets. The final search was performed on 28 June 2022.
Search strategy
Search strategy used to retrieve relevant records from WOSCC (TS = topic)
LLMICs were determined based on the ‘list of ODA recipients effective for reporting on 2022 and 2023 flows,’ published by the Organisation for Economic Co-operation and Development (OECD) ( DAC 2021 ). For inclusion in this study, only research on low-income countries (LICs) and lower-middle-income countries (LMICs) was searched, which are defined as nations with GNI per capita below $1,045 and between $1,046 and $4,095, respectively ( Hamadeh et al. 2021 ). Literature on upper-middle-income countries (UMICs) and HICs was not explicitly searched as a part of this study due to the considerably higher rates of drinking water access in these regions. To retrieve relevant records, synonyms for LLMICs included now outdated terms such as ‘third world,’ as well as imprecise terms such as ‘global south’ ( Khan et al. 2022 ; Lencucha & Neupane 2022 ). The use of this vocabulary, however, is not endorsed and henceforth will refer to LLMICs as a generic term. In recognizing the role that language plays in perpetuating or minimizing the marginalization of certain groups, this term was chosen for its comparatively objective connotation in this study and elsewhere. However, it should be acknowledged that any attempt to classify groups of people based on indicators, like national income status, will present inherent shortcomings, as reflected by the drastic income inequalities that persist within nations.
A subset of the search queries was reviewed to ensure the search strategy produced results that fit within the scope of this study. A total of 7,899 publications were retrieved from WOSCC and included in the analysis.
Analysis and science mapping
Analysis of the structure and dynamics of the research field was conducted using the functionality offered by Clarivate's InCites tool, in conjunction with the Bibliometrix R package. The leading institutions, countries, authors, and journals were identified using publication-related metrics (indicating productivity) and citation-related metrics (indicating research impact and academic influence). To minimize the likelihood that results were skewed by outliers, thresholds to limit the search were established. For example, during impact analysis (i.e. examining Category Normalized Citation Impact (CNCI)), publications were limited to those with up to 25 authors and from institutions/countries with a minimum of 25 publications that contributed to the field. This is done to ensure that publications with an abnormally high number of co-authors, as well as institutions/countries with minimal publications, do not have unduly influence on interpretation. To explore recent trends, the time period was adjusted to the past five complete years (2016–2021).
By examining the records retrieved, the author affiliations and citations that link documents were leveraged to identify relationships among researchers, institutions, and countries. Network analysis was performed to draw attention to the relative importance of research groups to the field and the strength of relational ties between institutions/countries was measured. The software tool VOSviewer 1.6.18 was used to generate, visualize, and analyze these bibliometric networks.
Bibliographic coupling was utilized to explore the topical research similarities at the institutional- and country-levels ( Kessler, 1963 ). Additionally, co-authorship analysis was utilized to illustrate regional contributions to the field and inter-country collaboration patterns. Mapping such collaborations may be particularly relevant for highlighting countries or regions that typically partner to produce research outputs, which may have the potential to spark new collaborative networks that integrate underrepresented regions.

Annual growth of scientific production on drinking water and health in LLMICs (1980–2021).
Key players
Institutions.

Top 10 institutions of published research on drinking water and health in LLMICs by publication volume (1980–2022).
Top 12 organizations having published more than 100 articles from 1980 to 2022, ranked by CNCI
Note: Child/parent organizations are unified.
Countries/regions

Leading countries of published research on drinking water and health in LLMICs by publication volume (1980–2022): (a) Percent; (b) Trend.
Top 10 countries producing published research on drinking water and health in LLMICs (past 5 years)
Authors and publications
Since 1980, 9 of the top 10 most influential authors based on the H-index are affiliated with UMICs/HICs. On average, LLMIC-affiliated publications have an Impact Relative to World indicator of 0.84, in contrast to 1.23 from UMICs/HICs. This indicator is used to determine the relative research performance where the world average is equal to one.
For each article, InCites associates the Journal Impact Factor (JIF) of the journal in which it was published. As the ratio of incoming citations to outgoing references, the JIF provides an index of the relative importance of a journal in the field. A journal with a high JIF is widely read and contains articles that have attracted more citations than they themselves cite. While this journal-level metric does not confer any level of significance to a given article, it serves as a marker of the general quality of research found in that journal, as editors of a high JIF journal can be very selective of which manuscripts they accept. Results show that 47% of publications generated by researchers affiliated with UMICs/HICs are published in journals that have a JIF in the top quartile (i.e. attract more citations than 75% of all journals in the field), compared to 35% of publications produced by LLMIC-affiliated researchers. This indicates that as a percentage of the total published literature, documents produced by LLMIC-affiliated researchers are less likely to be published in highly cited journals.
The most relevant sources were Science of the Total Environment (236 articles), Environmental Monitoring and Assessment (172 articles), Environmental Science and Pollution Research (172 articles), International Journal of Environmental Research and Public Health (154 articles), American Journal of Tropical Medicine and Hygiene (146 articles), Journal of Water and Health (140 articles), Environmental Geochemistry and Health (133 articles), PLOS One (130 articles), Journal of Water Sanitation and Hygiene for Development (120 articles), and Environmental Earth Sciences (119 articles).
Major global networks
International collaboration.

Co-authorships between countries. (Maximum of 25 countries per document, minimum of 100 publications per country. Minimum 25 co-authorships between countries).
As a property of each node in the network, the sum of a country's collaborations is given as total link strength . The United States has the most collaborations (1,486 total link strength), followed by England (815), Bangladesh (745), and India (594), indicating that these countries have the strongest collaborative research ties. England had a total of 57 country links, followed by India (55), the United States (54), and Germany (49), which represent the countries with the most diverse range of collaborative ties. The strongest bi-lateral connections are observed between the United States and the following countries: Bangladesh (with link strength of 295), England (198), India (150), and Kenya (104). This was followed by connections between Bangladesh and Japan (94 link strengths) and between Pakistan and China (93).
Thematic clusters

Network visualization of bibliographic coupling of countries (minimum link strength of 1,000). Please refer to the online version of this paper to see this figure in colour: http://dx.doi.org/10.2166/wh.2023.293 .

Topical map of bibliographic coupling of institutions (minimum link strength of 1,000). Please refer to the online version of this paper to see this figure in colour: http://dx.doi.org/10.2166/wh.2023.293 .
Research accessibility
Definitions of OA journal types

Percentage of publications that are OA by country (minimum 100 publications, 2017–2021).

Trend indicating the per cent of OA documents on drinking water and health in LLMICs, by country (1980–2022).
Research priorities

Trend data of top five meso-level research areas of published research on drinking water and health in LLMICs.
In the past 5 years (2016–2021), the publications targeting the SDGs have primarily focused on SDG 3: good health and well-being (3,311 documents and 38,305 times cited), SDG 6: clean water and sanitation (2,171 documents and 27,089 times cited), and SDG 14: life below water (1,555 documents and 18,996 times cited).
Leading global funders of published research on drinking water and health in LLMICs
Of the global research on drinking water and health in LLMICs published since 1980, the United States and the United Kingdom have dominated the field based on the production and impact of scientific literature. India, Iran, Bangladesh, and Pakistan are emerging as key players, yet, except for Bangladesh, publications out of these countries remain disproportionately restricted behind paywalls. Contamination and phytoremediation, diarrheal disease, and water resources are the themes that characterize most of the research on water and health with the latter emerging as a key priority only in recent years.
The scientific literature on drinking water and health in LLMICs has grown, particularly since the start of the millennium, with publication volume increasing steeply since 2016. The sustained and robust production of research shown in the present study mirrors findings from similar investigations that evidence drinking water research as a developing field of study ( Fu et al. 2013 ; Wambu & Ho 2016 ) and is relevant to broader conclusions that previous scholars have established: health and water, the combined focus of the present study, are among the most researched sustainable development priorities ( Meschede 2020 ).
Following successful efforts to meet the water-related Millennium Development Goal (MDG) target, to reduce by half the proportion of the population without safe drinking water and sanitation, 2.6 billion people gained access to an improved source of drinking water, i.e. one that adequately protects from outside contamination, between 1990 and 2015 through supply programs and interventions ( WHO & UNICEF 2015 ). In 2015, the United Nations extended far beyond the MDGs through the adoption of the 2030 Agenda for Sustainable Development, a new global blueprint toward a more sustainable future. Included in the agenda is SDG 6, which aims to ensure the availability and sustainable management of water and sanitation for all ( UN DESA 2016 ). The universal clean water target (6.1) is particularly significant because it underscores several other SDGs ( Bhaduri et al. 2016 ). For example, an adequate supply of water is indispensable for and intricately connected to achieving good health and well-being (SDG 3), no poverty (SDG 1), and gender equality (SDG 5) ( UN-Water 2016 ). The growth of published drinking water research observed in the past 20 years is reflective of efforts to reduce drinking water inequalities through the MDGs and similarly, the acceleration of such research outputs since 2016 is likely a consequence of the international community's doubling down on drinking water priorities through the commitments to universal access outlined in the SDGs. The growth of the field can likely also be attributed to the expansion of internet access and telecommunications technologies, enhanced research capacity, and the improved ease of information sharing through digital channels over time. However, these conveniences have not been distributed equally across the globe, as some are better able to capitalize on technological tools and systems while others face barriers ( Shumba & Lusambili 2021 ). As will be discussed in the following sections, a necessary step in accelerating the production and application of water research is to reduce research inequalities by addressing enduring barriers to participation that exist within underrepresented regions.
Furthermore, it is relevant to evaluate recent progress on drinking water targets and assess current global events to predict whether research in this field will continue to expand in the future. Worldwide, progress toward the use of safely managed drinking water, which was set as the new indicator, has progressed at a rate of 0.63% per year between 2015 and 2020 ( WHO & UNICEF 2021 ). Yet, the world is not currently on track to achieve universal and equitable access to affordable drinking water by 2030 ( UN Water 2021 ). LLMICs continue to confront challenges in closing the gap in drinking water disparities, such as reaching rural communities, transitioning existing facilities (i.e. from wells to piped), and ensuring consistent monitoring ( WHO & UNICEF 2021 ). Likely to further challenge global progress on drinking water equality are emerging global threats associated with rapid population growth, viral pandemics, climate change, and conflict ( Hannah et al. 2020 ). These pressures have the capacity to place additional stress on global water supplies and potentially interrupt efforts toward achieving universal access. Modern-day challenges, combined with the new evidence needed to advance SDG 6, are the likely catalysts for the marked expansion of research outputs highlighted by the present study and are predicted to drive the continued expansion of the research field in years to come.
The present study assessed the key players in drinking water research, highlighting emerging influences at the institutional- and country-level. The results suggest that the United States and the United Kingdom have historically driven research, a finding that has been supported by similar literature analyzing the areas of drinking water and SDG 6 ( Basu et al. 2021 ; Roy et al. 2022 ). Although the United States and the United Kingdom presently remain important contributors, heightened LLMIC-affiliated research in recent years out of India and to a lesser extent, Pakistan, Iran, and Bangladesh are shifting the landscape of research production on drinking water and health in LLMICs. The growing influence of LLMIC-based research highlighted by the present study is at variance with other studies on SDG 6, such as one conducted by Dibbern et al. (2022) that did not determine a significant contribution from these regions on the field. This may, in part, be due to the explicit use of ‘health’ and ‘LLMICs’ in the present study's search strategy instead of ‘SDG 6,’ the latter of which may be utilized less often by LLMIC-based researchers despite similar driving research agendas (i.e. regarding the complexities surrounding what is considered global health versus public health ( Bozorgmehr 2010 )). Moreover, the present study revealed that the impact of publications out of LLMICs relative to the world and the proportion of research published in Q1 journals were notably lower than UMICs/HICs, pointing to a possible undervaluing of this scientific literature.
The United States and the United Kingdom are consistently evidenced as frontrunners in the production of global health research ( Cash-Gibson et al. 2018 ) and are among the top producers of research in several other scientific fields ( Sumathipala et al. 2004 ; Sweileh 2020 ). It is therefore unsurprising that these countries have contributed significantly to the literature on drinking water and health in LLMICs. Part of this dominance may be due to supportive national commitments to reducing drinking water inequalities and connected funding priorities. For example, the United Kingdom's Department for International Development (DFID) – now known as the Foreign, Commonwealth and Development Office (FCDO) – has supported numerous research initiatives and interventions to target drinking water in LLMICs in recent decades, with evidence pointing to 80 million people who gained access to WASH services from DFID projects between 2011 and 2019 ( Blaszczyk 2019 ). A push for greater assistance from governments and funding agencies in HICs to support global health research on drinking water, in addition to empowering LLMICs to participate in this research and drive agenda-setting, may facilitate the enhanced generation of impactful research in the coming years.
The growing representation of LLMICs in drinking water research in the past 5 years is promising, as India, Pakistan, Iran, and Bangladesh are now among the global leaders, while Nigeria and Ethiopia are also represented in the top 10 producers of literature. While other scholars have pointed India as the top LMIC contributor to health inequalities research in general ( Cash-Gibson et al. 2018 ), the present study reveals that India is now the new global leader in research on drinking water and health in LLMICs, recently surpassing the United States on scientific production. Despite this, evidence suggests that significant disparities exist within countries, such as India, suggesting that research capacity may be developing unevenly ( Kalita et al. 2015 ). For example, one study suggests that a heightened proportion of health research is produced by institutions in just four states in India, whereas the regions with the highest disease burdens are only minimally represented ( Kalita et al. 2015 ). As such, it is crucial that priority-setting highlights opportunities for underserved populations to become involved in research so that inequalities within countries do not escalate as global inequalities in research are reduced.
Although the increased representation of LLMICs on the list of the top 10 producers of research is encouraging, this does not represent the minuscule production and underrepresentation of many other LLMICs that face barriers to establishing research capacity. In the present study, for example, LICs are significantly less represented as producers of drinking water research than LMICs, meaning that while new research systems are strengthening in some regions, countries that possess the least resources remain at the periphery of global drinking water research, at times with more problems and without a voice to drive the research agenda. This implies that greater effort is needed to ensure the field of drinking water research becomes more representative of the LICs that are likely the ‘subjects’ or ‘recipients’ of the implications of such research. This speculation is consistent with a study conducted by Dimitris et al. (2021) that evidences uneven progress in the representation of authors from LMICs in global health research, despite greater representation as a whole. With this understanding, capacity-building and improved authentic partnering to encourage research out of low-resource settings should be prioritized to strengthen national research systems.
The continued presence of water-related diseases in the modern era is indicative of the complexities that underpin efforts to achieve universal access to clean drinking water. As solutions to drinking water challenges are often based on complex systems of unique, contextual factors, ‘silver bullet’ solutions fail to consider the changing landscapes that surround people's relationships with water in local settings. This is why it is important for drinking water research to include regional investigations, for example through implementation science, that are driven by principles of co-creation, local decision-making, and strengthened local research capacity ( Haque & Freeman 2021 ). Many scholars convey that barriers to reaching water and sanitation targets stem from a lack of local water governance, not simply a lack of funding, technology, or infrastructure ( Herrera 2019 ). Local researchers from LMICs are needed to guide context-specific research investigations and enhance considerations of organizational, political, social, and infrastructural circumstances that define the specific WASH needs of the community under study. Local involvement also facilitates the production of health research that aligns with processes that facilitate the greatest impact. The field of global health is predicated on eliminating health disparities between people, particularly focusing on empowering low-resource and marginalized groups through access to fundamental health opportunities. Similarly, there is a need for the field of global health to encourage research out of low-resource settings, which will not only foster publication equity but also empower actors who are better positioned to identify unique factors that may disrupt interventions from actually improving health outcomes.
The present study analyzed co-authorship to determine which countries have the strongest collaborative research ties and used bibliographic coupling to determine whether similar thematic areas emerge among organizations/countries. The visualizations in the present study suggest that the United States, England, Bangladesh, and India had the strongest international research collaborations. Partnerships were also strong between Bangladesh and Japan, and between Pakistan and China; however, these links were not as strong as connections between the United States and the following countries: Bangladesh, England, India, and Kenya. Heightened collaborative ties observed for the United States and England are consistent with previous findings by Basu et al. (2021 ); however, the strong international networks shown for Bangladesh and India are perhaps under-recognized in the literature. Moreover, findings suggest that similar research themes are pursued among European and American organizations, whereas Asian and Middle Eastern organizations tend to cluster together more.
In general, the network analysis in the present study depicts a rather robust map of international partnerships with several collaborative hubs. In particular, the well-established research partnerships between the United States and England and several other countries illustrate the continued role these two countries play in driving research in this field. The comparatively stronger ties between the United States/United Kingdom and certain LLMICs may have developed based on linguistic and historical happenings. As a large portion of global health research is driven by English-speaking HICs ( Cash-Gibson et al. 2018 ), it is likely that partnerships that were previously established based on the similar language spoken may have fostered collaborative research on drinking water as well. For example, strong ties between the United States/United Kingdom and English-speaking East African countries are particularly high. Bangladesh and India, also English-speaking LLMICs, are strong centers for international collaboration in their own right, which may further be explained by the heightened production of research out of these countries in general.
The strong collaborative ties between Japan and Bangladesh as well as China and Pakistan are notable. This is mirrored in the bibliographic coupling networks, which indicate Asian/Middle Eastern countries produce literature on different thematic areas than the rest of the world. This separation may be indicative of differing regional needs for drinking water research, apart from advancements in basic WASH that drive a significant amount of research on SDG 6. For example, China, India, Bangladesh, and Pakistan are among the leading producers of research on heavy metals, such as arsenic, in drinking water ( Abejon & Garea 2015 ; Han et al. 2020 ), which aligns with heightened concerns over contaminated waterways in populated Asian nations. Countries in the Middle East have differing agendas for advancing drinking water development, which requires research related to water scarcity and security ( World Bank 2018 ). Thus, it is speculated that clusters of co-authorship, in addition to bibliometric coupling networks are reflective of the pervasive research needs unique to each region.
Despite the well-established networks displayed in the present study, partnerships between LICs appear to be much fewer, with weaker link strengths. This highlights possible entry points for enhanced collaborative partnerships. International collaborations play a pivotal role in facilitating information sharing, enabling the transfer of technology, and widening the reach of research to affect global health outcomes ( Addo-Atuah et al. 2020 ). Such partnerships, however, must involve authentic partnering, inclusion, and shared benefits to ensure that power imbalances are not propagated in the process ( Plamondon & Bisung 2019 ).
Strengthening meaningful international partnerships across income groups and also between LLMICs can facilitate the advancement of drinking water targets through improved knowledge generation. The 2030 Agenda for Sustainable Development explicitly calls for expanded international co-operation and capacity-building to support low-resource settings with water and sanitation-related activities and programs (6.a) as well as supporting the participation of local communities in water management (6.b) ( UN DESA 2016 ). Not only is there a need to improve inter-country partnerships, but also, there is a growing call for water researchers to collaborate with actors in related sectors and partner with those working on connected development goals ( Bhaduri et al. 2016 ). In the face of contemporary global issues tied to rapid population growth and climate change, it is likely that inter-regional and inter-sectoral collaborations on water research will grow to explore the myriad of water-related challenges that flow through a range of development priorities. In the coming decade, collaborative research partnerships that transcend national borders will be crucial to attaining drinking water equity and minimizing the elevated water-related disease burden that weighs heavily on LLMICs.
The findings on OA trends in the present study are consistent with other studies, which point to publications becoming increasingly OA over time ( Kurata et al. 2013 ; Bosman & Kramer 2018 ). In the present study, UMICs/HICs exhibit a higher percentage of OA publications, with the United Kingdom demonstrating the highest rates across all years, likely due to funder mandates. Interestingly, in the past 5 years, East African countries had among the highest levels of OA content globally. Despite the progress realized in this region, several other LLMICs continue to lag behind the global average on OA indicators, particularly those emerging as leaders in the field, namely India, Iran, and Pakistan. These findings are consistent with conclusions by Iyandemye & Thomas (2019) that point to sub-Saharan Africa as having the highest rates of OA biomedical publications, with the Middle East and South Asia displaying among the lowest. Importantly, several countries with limited research capacity were left out of the analysis as they did not meet the minimum threshold of 100 publications in the field to be evaluated.
Since 2003, when the OA movement was declared a global priority at the UN's World Summit on Information Technology ( King & Tamber 2004 ), OA has been advocated as a tool to support the expansion of an equitable scientific knowledge base for social and economic development. The present study reveals a marked increase in OA content in the years following the announcement of the OA movement, which may illustrate the influence of international commitments to equitable information sharing on changing the nature of how research is accessed. Moreover, there are several possible explanations for the heightened rates of OA content in East Africa, and other low-income regions that the present study highlights. It is speculated that the role of OA fee waivers for low-income countries may be one possible reason. Further, heightened collaboration with countries with strong OA policies (i.e. UK) may have enabled a greater percentage of OA-published research to come out of LLMICs since publications with international and inter-regional collaboration tend to have considerably higher OA rates compared to single-country outputs ( Chan 2019 ). High OA rates in East Africa may also be explained by the heightened production of research into topics that are overrepresented in OA literature, such as poverty-related diseases ( Breugelmans et al. 2018 ). Finally, the expansion of OA repositories in countries such as Kenya, Tanzania, and Uganda in recent years, may have also contributed to heightened rates of OA publications ( Otando 2015 ).
In the present study, it is notable that India, Pakistan, and Iran have among the lowest percentages of OA publications, despite producing a large volume of literature on water and health. These findings may provide evidence of regions that can be better supported in the transition to OA research. There are numerous reasons why some countries and institutions are slow to adopt OA frameworks and practices. As Bosman & Kramer (2018) suggest, funder/publisher policies, institutional mandates, and repository infrastructure play influential roles in determining whether research will shift in the direction of OA. Further research into barriers that are restricting OA progress may be key to supporting regions slow to adopt OA priorities. Advocates of the OA movement suggest that it is a necessary mechanism for leveling the playing field between those who can afford to produce and access fee-based scientific literature and those who cannot ( Tennant et al. 2016 ).
The sustained production of research on both contamination and diarrheal disease is an indication of the global challenges that have driven long-term priorities in global water research. Chemical pollution from industrial discharge and untreated sewage remains a heightened area of concern in the modern, industrialized era since evidence points to a range of chronic diseases from exposure to contaminants found in water ( Villanueva et al. 2014 ; Hutton & Chase 2016 ). Moreover, diarrheal disease is consistently targeted as a research priority as it accounts for 829,000 deaths per year, representing one of the leading causes of death in children under five ( Prüss-Ustün et al. 2019 ). Scientific monitoring of contaminants in water and improved knowledge generation on diarrheal disease have the capacity to greatly reduce the associated health burdens, hence the continued effort to develop scientific literature on these topics.
In the present study, it is unsurprising that the greatest number of publications and most cited literature are associated with SDG 3 (good health and well-being) and SDG 6 (clean water and sanitation), since the search strategy explicitly included ‘health’ and ‘drinking water,’ both of which respectively represent these goals. Notably, SDG 14 (life below water) is the third most-published SDG in this study, aligning also with the increase in literature on the topic of ‘water resources’ in recent years. Such evidence may point to planetary health, a field dedicated to the relationships between human health and the earth's natural systems, as a growing global priority. The extension of the SDGs to incorporate efficient water resource allocation alongside environmental protection, rather than as a separate agenda item, is a likely explanation for the popular keywords ‘groundwater’ and ‘contamination’ highlighted in the searched health literature. The rise in publications related to water resources in the wake of announcements for the 2030 Agenda for Sustainable Development may therefore be reflective of a broader movement in the drinking water sector toward a more holistic, cross-sectoral integration of priorities on social and environmental development.
Interventions, policies, and research agendas are becoming increasingly multifaceted because of the recognition of how fundamental and interdependent water resources are for so many sectors. In 2016, water insecurity was named the greatest long-term risk facing society by the World Economic Forum ( WEF 2018 ). It is also evidenced that among other interconnected consequences, the growing strain on water supply would put global GDP and grain production at serious risk by 2050 ( WWAP 2016 ). As such, drinking water research has expanded to examine the interconnections between development goals and across targets listed within goals. Evidence from surveys of WASH professionals across 36 countries suggests that water research must further engage in multisectoral coordination to meet contemporary global needs ( Setty et al. 2020 ). It is predicted that collaborative approaches to water-related sustainable development, rather than fragmented ones, will continue to facilitate partnerships between actors across research fields and levels of society, which may lead to topics that reflect interdisciplinary priorities.
The patterns in publications that address social determinants of health, implementation science, or qualitative methods remain unexplored by the present study. There is growing recognition by health researchers, including those studying drinking water, of the relevance of social science perspectives to better understand how thoughts, attitudes, beliefs, and social norms shape behavior ( Lilje & Mosler 2017 ). For example, critical WASH technologies are rendered ineffective if psychosocial factors impede the rate of adoption by the receiving communities, such as beliefs among women that using latrines is strange or scary, compared to practicing open defecation ( Aiemjoy et al. 2017 ). Understanding how and why drinking water programs benefit communities or fail to reach intended outcomes is critical to reaching vulnerable populations most burdened by inadequate drinking water services ( Loevinsohn et al. 2015 ). Considerations of social determinants in drinking water research may therefore result in a more comprehensive understanding of the barriers associated with universal drinking water and lead to more effective interventions ( Dreibelbis et al. 2013 ). The possible movement toward the integration of social science perspectives in drinking water research is not captured by the present study; however, such investigations may make an interesting extension as it would further define the nature of research on drinking water and health in LLMICs.
Limitations
Although most of the literature on drinking water and health in LLMICs was captured in this review, this study has several limitations. First, only articles published in WOSCC were included, thereby excluding potentially relevant publications from local journals that are not indexed in this database. Moreover, papers from predatory journals may inevitably be indexed in reputable databases and due to the large number of papers included in this study, it was not feasible to screen out such papers. Thus, countries/institutions with a heightened volume of papers published in predatory journals may be inflated. Second, the search strategy does not include research on UMICs or low-resource settings within HICs, which may also contain disadvantaged groups that lack access to adequate drinking water but were not captured here. The search was also conducted in English, thereby missing records that were cataloged in different languages. This may have resulted in the underrepresentation of certain regions, such as China, that likely have contributed more to the field. Moreover, the search strategy included imprecise terms related to the ‘developing world,’ whose membership has changed throughout history or can be redefined based on different classifications. Third, there are numerous bibliometric indicators that can be utilized to evaluate a given field of research, and this study is limited by the indicators and visualization tools selected by the authors. For instance, VosViewer references ‘England’, without having the functionality to analyze the ‘United Kingdom,’ whereas data generated from Clarivate InCites are generally analyzed using ‘United Kingdom.’ Thus, international partnerships out of this particular region were likely underrepresented in network maps, as the ‘United Kingdom’ would have yielded greater international collaborative links. Finally, this study used citation-based metrics to examine the impact of publications in the field of drinking water research. However, a strong bibliometric impact may not always align with publications that yield the greatest societal impact, the latter of which may be better understood by evaluating policies and programs that were established as a result of published research articles. Despite these limitations, the current study provides a useful overview of global research on water and health in LLMICs.
As sustainable development priorities drive the continued expansion and globalization of research on drinking water and health, special efforts are needed to support the participation of low-resource and underrepresented researchers, particularly from LICs. Local voices and perspectives may be particularly significant for facilitating the translation of research into context-specific solutions and producing evidence-informed decisions that advance both policy and implementation efforts. In recognizing that information sharing is critically important to building research capacity, the OA movement, and policies that support low-resource researchers to access and publish accessible drinking water research may also play an important role in developing a more equitable scientific community. The present study may be used to inform future research priorities and decision-making processes, facilitate improved collaboration on research outputs, and identify where national research systems must be strengthened. This will enable gaps in global drinking water inequalities to be filled, while at the same time accelerating equitable, inclusive research in the realm of water and health.
All relevant data are available from an online repository or repositories (10.6084/m9.figshare.21944102).
The authors declare there is no conflict.
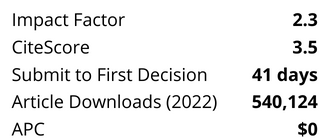
Affiliations
- ISSN 1477-8920 EISSN 1996-7829
- Open Access
- Collections
- Subscriptions
- Subscribe to Open
- Editorial Services
- Rights and Permissions
- Sign Up for Our Mailing List
- IWA Publishing
- Republic – Export Building, Units 1.04 & 1.05
- 1 Clove Crescent
- London, E14 2BA, UK
- Telephone: +44 208 054 8208
- Fax: +44 207 654 5555
- IWAPublishing.com
- IWA-network.org
- IWA-connect.org
- Cookie Policy
- Terms & Conditions
- Get Adobe Acrobat Reader
- ©Copyright 2021 IWA Publishing
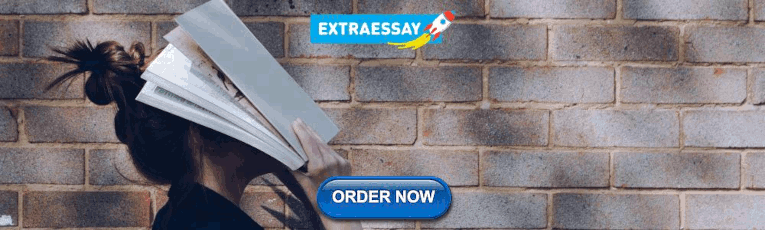
This Feature Is Available To Subscribers Only
Sign In or Create an Account
- Research article
- Open access
- Published: 17 July 2020
The quality of drinking and domestic water from the surface water sources (lakes, rivers, irrigation canals and ponds) and springs in cholera prone communities of Uganda: an analysis of vital physicochemical parameters
- Godfrey Bwire ORCID: orcid.org/0000-0002-8376-2857 1 ,
- David A. Sack 2 ,
- Atek Kagirita 3 ,
- Tonny Obala 4 ,
- Amanda K. Debes 2 ,
- Malathi Ram 2 ,
- Henry Komakech 1 ,
- Christine Marie George 2 &
- Christopher Garimoi Orach 1
BMC Public Health volume 20 , Article number: 1128 ( 2020 ) Cite this article
15k Accesses
30 Citations
1 Altmetric
Metrics details
Water is the most abundant resource on earth, however water scarcity affects more than 40% of people worldwide. Access to safe drinking water is a basic human right and is a United Nations Sustainable Development Goal (SDG) 6. Globally, waterborne diseases such as cholera are responsible for over two million deaths annually. Cholera is a major cause of ill-health in Africa and Uganda. This study aimed to determine the physicochemical characteristics of the surface and spring water in cholera endemic communities of Uganda in order to promote access to safe drinking water.
A longitudinal study was carried out between February 2015 and January 2016 in cholera prone communities of Uganda. Surface and spring water used for domestic purposes including drinking from 27 sites (lakes, rivers, irrigation canal, springs and ponds) were tested monthly to determine the vital physicochemical parameters, namely pH, temperature, dissolved oxygen, conductivity and turbidity.
Overall, 318 water samples were tested. Twenty-six percent (36/135) of the tested samples had mean test results that were outside the World Health Organization (WHO) recommended drinking water range. All sites (100%, 27/27) had mean water turbidity values greater than the WHO drinking water recommended standards and the temperature of above 17 °C. In addition, 27% (3/11) of the lake sites and 2/5 of the ponds had pH and dissolved oxygen respectively outside the WHO recommended range of 6.5–8.5 for pH and less than 5 mg/L for dissolved oxygen. These physicochemical conditions were ideal for survival of Vibrio. cholerae .
Conclusions
This study showed that surface water and springs in the study area were unsafe for drinking and had favourable physicochemical parameters for propagation of waterborne diseases including cholera. Therefore, for Uganda to attain the SDG 6 targets and to eliminate cholera by 2030, more efforts are needed to promote access to safe drinking water. Also, since this study only established the vital water physicochemical parameters, further studies are recommended to determine the other water physicochemical parameters such as the nitrates and copper. Studies are also needed to establish the causal-effect relationship between V. cholerae and the physicochemical parameters.
Peer Review reports
Water is the most abundant resource on the planet earth [ 1 ], however its scarcity affects more than 40% of the people around the world [ 2 ]. Natural water is an important material for the life of both animals and plants on the earth [ 3 ]. Consequently, access to safe drinking water is essential for health and a basic human right that is integral to the United Nations Resolution 64/292 of 2010 [ 4 ]. The United Nations set 2030 as the timeline for all countries and people to have universal access to safe drinking water; this is a Sustainable Development Goal (SDG) 6 of the 17 SDGs [ 5 ]. The availability of and access to safe water is more important to existence in Africa than it is elsewhere in the world [ 6 ]. Least Developed Countries (LDCs) especially in sub-Saharan Africa have the lowest access to safe drinking water [ 7 ]. In Africa, rural residents have far less access to safe drinking water and sanitation than their urban counterparts [ 8 ].
Natural water exists in three forms namely; ground water, rain water and surface water. Of the three forms, surface water is the most accessible. Worldwide, 144 million people depend on surface water for their survival [ 9 ]. In Uganda, 7% of the population depends on surface water (lakes, rivers, irrigation canal, ponds) for drinking water [ 10 ]. The same surface water is a natural habitat for many living organisms [ 11 ] some of which are responsible for transmission of infectious diseases such as cholera, typhoid, dysentery, guinea worm among others [ 12 ]. Surface water sources include lakes, rivers, streams, canals, and ponds. These surface water sources are often vulnerable to contamination by human, animal activities and weather (storms or heavy rain) [ 13 , 14 ]. Globally, waterborne diseases such as diarrheal are responsible for more than two million deaths annually. The majority of these deaths occur among children under-5 years of age [ 15 ].
Cholera, a waterborne disease causes many deaths each year in Africa, Asia and Latin America [ 16 ]. In 2018 alone, a total of 120,652 cholera cases and 2436 deaths were reported from 17 African countries to World Health Organization [ 17 ]. Cholera is a major cause of morbidity and mortality in Uganda [ 18 ]. The fishing communities located along the major lakes and the rivers in the African Great Lakes basin of Uganda constitutes 5% of the Uganda’s population, however these communities were responsible for the majority (58%) of the reported cholera cases during the period 2011–2015 [ 19 ]. Cholera outbreaks affect predominantly communities using the surface water and the springs. There is also high risk of waterborne disease outbreaks in the communities using these types of water [ 20 , 21 ]. Studies of the surface water from water sources located in the lake basins of the five African Great Lakes in Uganda identified Vibrio. cholerae [ 22 , 23 ] though no study isolated the toxigenic V. cholerae O1 or O139 that cause epidemic cholera. Cholera outbreaks in the African Great Lakes basins in Uganda have been shown to be propagated through water contaminated with sewage [ 20 , 24 ]. Cholera is one of the diseases targeted for elimination globally by the WHO by 2030 [ 25 ]. Hence, to prevent and control cholera outbreaks in these communities, promotion of use of safe water (both quantity and quality), improved sanitation and hygiene are the interventions prioritized by the Uganda Ministry of Health [ 26 ]. Most importantly, provision of adequate safe water is a major pillar of an effective cholera prevention program given that water is the main mode of V. cholerae transmission [ 27 , 28 ].
Availability of adequate safe water is essential for prevention of enteric diseases including cholera [ 29 ]. Therefore, access to safe drinking and domestic water in terms of quantity and quality is key to cholera prevention. Water quality is defined in terms of three key quality parameters namely, physical, chemical and microbiological characteristics [ 30 ]. A less common but important parameter is the radiological characteristics [ 31 ]. In regards to the physicochemical parameters, there are five parameters that are essential and impacts life (both flora and or fauna) within the aquatic systems [ 32 ]. These vital physicochemical parameters include pH, temperature, dissolved oxygen, conductivity and turbidity [ 32 ].
pH is a value that is based on logarithm scale of 0–14 [ 33 ]. Aquatic organisms prefer pH range of 6.5–8.5 [ 34 , 35 , 36 ]. Low pH can cause the release of toxic elements or compounds into the water [ 37 ]. The optimal pH for V. cholerae survival is in basic range (above 7). Vibrio cholerae may not survive for long in acidic pH [ 38 ]. A solution of pH below 4.5 will kill V.cholerae bacteria [ 39 ].
Most aquatic organisms are adapted to live in a narrow temperature range and they die when the temperature is too low or too high [ 34 ]. Vibrio cholerae , bacteria proliferate during algae bloom resulting in cholera outbreaks [ 40 , 41 ]. This proliferation could be due favourable warm temperature [ 42 ]. Relatedly, V. cholerae isolation from natural water in endemic settings is strongly correlated with water temperature above 17 °C [ 43 ].
Dissolved oxygen is the oxygen present in water that is available to aquatic organisms [ 34 ]. Dissolved oxygen is measured in parts per million (ppm) or milligrams per litre (mg/L) [ 35 ]. Organisms in water need oxygen in order to survive [ 44 ]. Decomposition of organic materials and sewage are major causes of low dissolved oxygen in water [ 12 ].
Water conductivity is the ability of water to pass an electrical current and is expressed as millisiemens per metre (1 mS m- 1 = 10 μS cm − 1 ) [ 29 ]. Most aquatic organisms can only tolerate a specific conductivity range [ 45 ]. Water conductivity increases with raising temperature [ 46 ]. There is no set standard for water conductivity [ 45 ]. Freshwater sources have conductivity of 100 – 2000μS cm − 1 . High water conductivity may be due to inorganic dissolved solids [ 46 ].
Turbidity is an optical determination of water clarity [ 47 ]. Turbidity can come from suspended sediment such as silt or clay [ 48 ]. High levels of total suspended solids will increase water temperatures and decrease dissolved oxygen (DO) levels [ 12 ]. In addition, some pathogens like V. cholerae, Giardia lambdia and Cryptosporidia exploit the high water turbidity to hide from the effect of water treatment agents and cause waterborne diseases [ 49 ]. Consequently, high water turbidity can promotes the development of harmful algal blooms [ 41 , 50 ].
Given the importance of the water physicochemical parameters, in order to ensure that they are within the acceptable limits, the WHO recommends that they are monitored regularly [ 51 ]. The recommended physicochemical parameters range for raw water are for pH of 6.5–8.5, turbidity of less than 5Nephlometric Units (NTU) and dissolved oxygen of not less than 5 mg/L [ 51 ]. Surface and spring water with turbidity that exceeds 5NTU should be treated to remove suspended matter before disinfection by either sedimentation (coagulation and flocculation) and or filtration [ 52 ].
Water chlorination using chlorine tablets or other chlorine releasing reagent is one of the most common methods employed to disinfect drinking water [ 53 , 54 ]. Chlorination is an important component of cholera prevention and control program [ 55 ]. In addition to disinfection to kill the pathogens, drinking water should also be safe in terms of physicochemical parameters as recommended by WHO [ 51 ]. However, to effectively make the water safe using chlorine tablets and other reagents, knowledge of the physicochemical properties of the surface and spring water being disinfected is important as several of the parameters affect the active component in the chlorine tablets [ 56 ]. For example, chlorine is not effective for water with pH above 8.5 or turbidity of above 5NTU [ 53 ].
Generally, there is scarcity of information about the quality and safety of drinking water in Africa [ 57 ]. Similarly, few studies exist on the physicochemical characteristics of the drinking water and water in general in Uganda. Furthermore, information from such studies is inadequate for use to increase safe water in cholera prone districts of Uganda where the need is greatest. The cholera endemic communities of Uganda [ 19 , 21 , 24 ] have adequate quantities of water that is often collected from the Great lakes, rivers and other surface water sources located within the lake basins. However, the water is of poor quality in terms of physicochemical and microbiological characteristics. Several studies conducted in Uganda have documented microbiological contamination of drinking water [ 20 , 24 , 58 , 59 ]. However, few studies exist on the physicochemical characteristics of these water. Furthermore, these studies focused on few water sources, for example testing the lakes and omitted the rivers, springs and ponds or testing the rivers and omitted the other water types. One such study was carried out on the water from the three lakes in western Rift valley and Lake Victoria in Uganda [ 23 ], This study did not assess the other common water sources such as the rivers, ponds and springs that were used by the communities for drinking and other household purposes. Other studies on water physicochemical characteristics assessed heavy metal water pollution of River Rwizi (Mbarara district, Western Uganda) [ 60 ] and of the drinking water (bottled, ground and tap water) in Kampala City (Central Uganda) [ 61 ] and Bushenyi district (Western Uganda) [ 62 ]. These studies found high heavy metal water pollution in the drinking water tested. The information gathered from such studies is useful in specific study area and is inadequate to address the lack of safe water in the cholera endemic districts of Uganda where the need for safe drinking water is greatest. Several epidemiological studies in Uganda have attributed cholera outbreaks to use of contaminated surface water [ 20 , 21 , 24 , 63 ]. Furthermore, studies conducted on the surface water focus on pathogen identification [ 63 , 64 ] leaving out the water physicochemical parameters which are equally important in the provision of safe drinking water [ 53 ] and are necessary for survival of all living organisms (both animals and plants) [ 44 ].
Therefore, the aim of this study was to determine the physicochemical characteristics of the surface water sources and springs located in African Great Lakes basins in Uganda so as to guide the interventions for provision of safe water to cholera prone populations [ 19 , 20 , 21 , 24 , 58 ] of Uganda. This study in addition has the potential to guide Uganda to attain the United Nations SDG 6 target of universal access to safe drinking water [ 2 ] and the WHO cholera elimination Roadmap [ 25 ] by 2030. Furthermore, these findings may guide future studies including those on causal-effect relationship between physicochemical parameters and infectious agents (pathogens).
This was a longitudinal study that was conducted between February 2015 and January 2016 in six districts of Uganda that are located in the African Great Lakes basins of the five lakes (Victoria, Albert, Kyoga, Edward and George). These districts had ongoing cholera outbreaks or history of cholera outbreaks in the previous five to 10 years (2005–2015). In addition, the selected study districts had border access to the following major water bodies (lakes: Victoria, Albert, Edward, George and Kyoga). The study area was purposively selected because the communities residing along these major lakes contributed most (58%) of the reported cholera cases and deaths in Uganda [ 19 , 65 ] and in the sub-Saharan Africa region [ 66 ] in the past 10 years. Water samples were collected monthly from 27 sites used by the communities for household purposes that included drinking. Water samples were then tested to determine the vital physicochemical parameters. The water samples were collected from lakes, rivers, springs, ponds and an irrigation canal that were located in the lake basins of the five African Great Lakes in Uganda. In one site, water was also collected from a nearby drainage channel and tested for V. cholerae [ 22 ] and physicochemical parameters. However, because the channel was not used for drinking the results were omitted in this article. Water samples were analysed to determine the pH, temperature, dissolve oxygen, conductivity and turbidity. The study sites were located in the districts of Kampala and Kayunga in central region of Uganda; Kasese and Buliisa districts in western Uganda; Nebbi and Busia districts in northern and eastern Uganda respectively. The study sites were the same as for the simultaneous bacteriological V. cholerae detection study [ 22 ] and are shown in Fig. 1 .
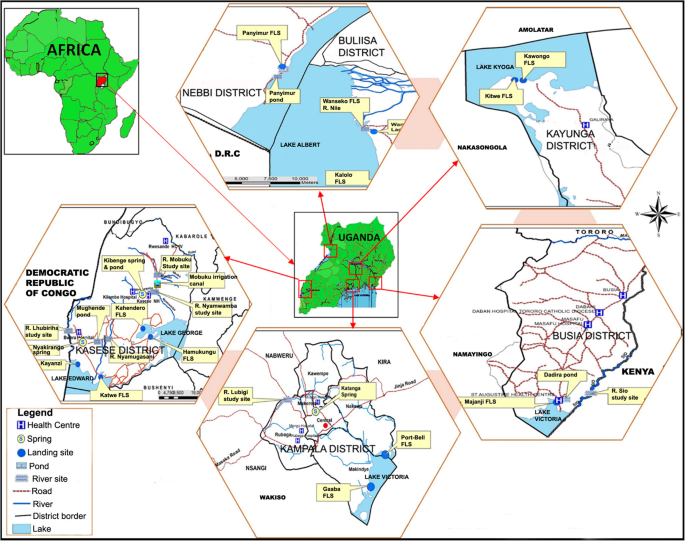
Map showing the location of Uganda, the study districts, major surface water sources and the study sites, February 2015 – January 2016. The blue shades are the African Great Lakes and their basins. (Map generated by ArcGIS version 10.2 [licenced] and assembled using Microsoft Office PowerPoint, Version 2016 [licenced] by the authors)
Rural-urban categorization of the study sites
The study sites were categorized as urban if they were found in Kampala district (the Capital City of Uganda) or rural if they were in the other five remote study districts (Kasese, Kayunga, Busia, Nebbi and Buliisa).
Identification of the study sites and water testing procedures
The sites for water testing were identified with the guidance of the local communities and after direct observation by the study team. Geo-coordinates of the sites were taken at the beginning of the study to ensure that subsequent water collection and measurements were done on water from specific points. Two water collection sites were selected on each of the African Great Lakes in Uganda. The selected sites were in different locations but within the communities with a history of cholera outbreaks in the previous 10 years prior to the study period. For each selected lake point, a site was also selected on a river, a spring and a pond located within the area and being used by the communities for domestic purposes that included drinking and preparation of food. A total of 27 sites, two of which were from each of the five lakes were selected and the water tested. The number of sites on each lake and their locations are shown in Additional file 1 .
Water samples were collected and tested monthly for 12 months by the research assistants who were health workers with background training in microbiology or environmental health. The research assistants received training on water collection and testing from a water engineer. The physicochemical parameters were measured by use of the digital meters namely the Hach meter HQ40d and digital turbidity meter.
Water samples were collected in five-litre containers, three litres were processed for V. cholerae detection by Polymerase Chain Reaction (PCR) test as previously described [ 67 ]. Vibrio cholerae Non O1/Non O139 pathogens were frequently detected in the water samples during the study period [ 22 ]. While the three litres of water were being processed for V. cholerae detection [ 22 ], the rest of the water (2 l), were simultaneously used for the onsite measurement of temperature, pH, conductivity and dissolved oxygen. The Hach meters , HQ40d used in the study, had three electrodes that were calibrated before each monthly testing according to the manufacturers’ manual [ 68 ]. The Hach meter calibrations were done using three specific standard buffer solutions that were for pH, dissolved oxygen and conductivity respectively. Turbidity (total suspended solids or water clarity) was measured using a turbidity meter according to previously published methods [ 49 ]. In addition, the research assistants were provided with Standard Operating Procedures (SOPs) and supervised monthly by the investigators before and during each scheduled monthly measurements.
Data management, analysis and statistical tests
Data were collected, entered, cleaned and stored in the spreadsheet. Errors in the recorded readings were removed using the correct records retrieved from the Hach meters’ HQ40d internal memory. Stata statistical package version 13 was used to analyse the data. Data were analysed to generate means and standard error of the mean for pH, temperature, dissolved oxygen (DO), conductivity (CD) and turbidity. Data were presented in the form of tables and graphs. Comparison for variations between the water samples were carried out using One-Way Analysis of Variance (ANOVA) test. Samples with significant One-Way ANOVA test were subjected to Turkey’s Post Hoc test to establish which of the variables were statistically significant.
The map was created using ArcGIS software, Version 10.2, licenced (ESRI, Redlands, California, USA). The graphs and figures were produced using Microsoft Excel and PowerPoints, Version 2016 (Microsoft, Redmond, Washington, USA). The administrative shapefiles used to create the map were obtained from open access domain, the Humanitarian Data Exchange: https://data.humdata.org/ . In order to generate the study locations on the map, Global Positioning System (GPS) coordinates for the study sites were converted to shapefiles that were combined with the administrative shapefiles corresponding to the locations.
A total of 318 water samples were tested from 27 sites as follows; lake water 40.9%, (130/318), rivers water 26.4% (84/318), ponds water 17.9% (57/318), spring water 11.0% (35/318) and canal water 3.8% (12/318).
Test results for the lake water collected at the fish landing sites (FLS)
The mean physicochemical test results for pH, temperature, dissolved oxygen, conductivity and turbidity are shown in Table 1 .
The mean physicochemical water characteristics of most of the sites were within the WHO recommended water safety range except for turbidity. Few sites had pH and dissolved oxygen outside the WHO recommended safety range.
Monthly variations of the lake water physicochemical characteristics
There were monthly variations in the physicochemical parameters between the water from the lake sites overtime. Most of the sites had steady pH overtime for the first half of the study period (February – August 2015). Thereafter, the pH reduced slightly during the second half (September, 2015 – January, 2016) of the study period. The highest pH fluctuations were in the months of October – December, 2015. The widest change in pH within the same site was observed at Gaaba Fish landing site, Lake Victoria basin, Kampala district.
There were differences in water temperature on the same lake but at different test sites. These differences were detectable mostly in the months of April, 2015. The lowest and highest water temperatures were both recorded on Lake Edward (Kasese district) at Kayanzi fish landing site of 18.9 °C and at Katwe FLS of 34 ° C in the period between April – August, 2015. Fluctuations in the dissolved oxygen were detectable throughout the study period. Kalolo Fish landing site on Lake Albert, Buliisa district showed the widest fluctuations in dissolved oxygen with the highest value of 10.73 mg/L and the lowest of 2.5 mg/L.
Most test sites had small conductivity fluctuations except for Panyimur and Kalolo both of which were located on Lake Albert in Nebbi and Buliisa districts These districts had high water conductivity fluctuations with arrange of 267.1 μS/cm – 2640 μS/cm at Kalolo (Buliisa district) FLS and 296 μS/cm – 2061 μS/cm at Panyimur (Nebbi district). Water turbidity for the majority of the sites changed overtime. Kahendero fish landing site (Lake George, Kasese district) had the highest turbidity which was most noticeable in the months of October 2015 to January 2016. Majanji fish landing site (Lake Victoria, Busia district) had the lowest and most stable water turbidity. Monthly variations of the lake water physicochemical parameters are shown in Fig. 2 .
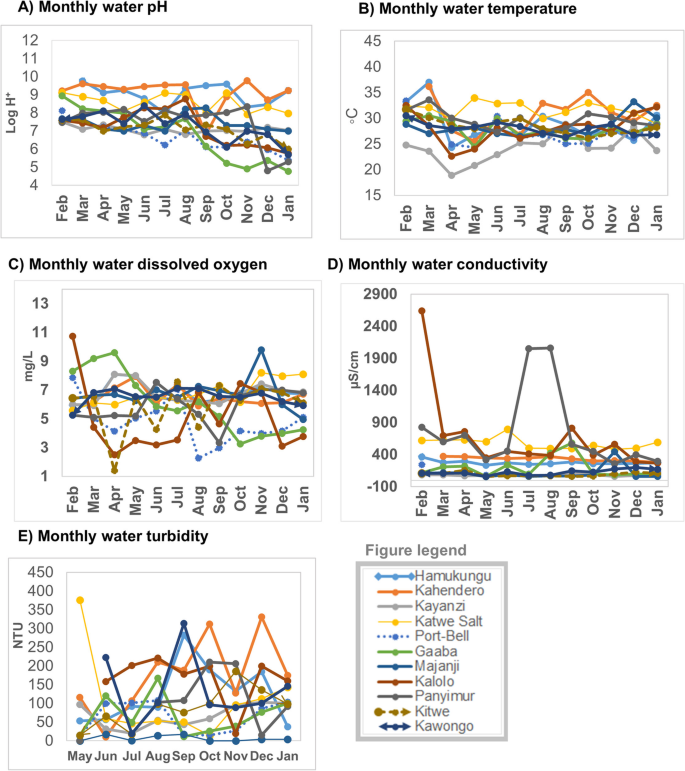
Monthly variations of lake water physicochemical characteristics (pH, temperature, dissolved oxygen, conductivity and turbidity), February 2015 – January 2016: Part a ) water pH variations; Part b ) water temperature variations; Part c ) water dissolved oxygen; Part d ) water conductivity variations; Part e ) water turbidity variations
River water physicochemical parameter test results
The mean physicochemical characteristics of water from the seven rivers studied are shown in Table 2 .
There were variations in the mean pH, temperature, dissolved oxygen and conductivity between study sites on the rivers. However, these mean parameter variations were in WHO acceptable drinking water safety limit except for River Lubigi, Kampala district which had mean dissolved oxygen below the recommended WHO range. At one time (February, 2015) River Lubigi had dissolved oxygen of 0.45 mg/L. The river water turbidity for all the test sites were above that recommended by WHO of less than 5NTU.
Monthly variations of the river water physicochemical characteristics
Monthly variations in the water physicochemical characteristics of the seven river test sites are shown in Fig. 3 .
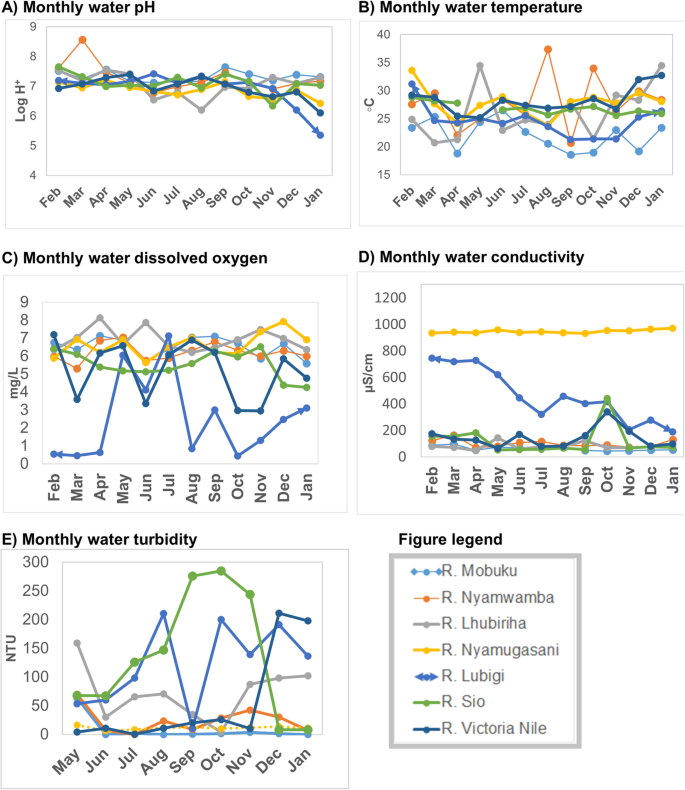
Monthly variations of the physicochemical characteristics of river water, February 2015 – January 2016: Part a ) water pH variations; Part b ) water temperature variations; Part c ) water dissolved oxygen variations; Part d ) water conductivity variations; Part e ) water turbidity variations
There were variations in the water physicochemical parameters between rivers and within the same river overtime. Most rivers showed fluctuations of water pH and temperature. Some rivers such as R. Nyamugasani and R. Lhubiriha both in Kasese district had wide temperature fluctuations. River Mobuku (Kasese district) had the lowest water temperature recorded over the study period. Fluctuations in dissolved oxygen were highest in R. Lubigi (Kampala district), Lake Victoria basin. Dissolved oxygen for R. Lubigi was below the recommended level of more than 5 mg/L for most of the study period. Seasonal variations of water dissolved oxygen were also more noticeable in R. Lubigi than the rest of the river sites. Relatively more dissolved oxygen was found during the rainy seasons (March – July, 2015, first rainy season and September – December, 2015, second rainy season) than in dry season.
There were small variations in the water conductivity in the majority of the rivers. Wide fluctuations in conductivity were observed for water samples of R, Lubigi (Kampala district). River Nyamugasani (Kasese district, Lake Edward basin) had steady but higher conductivity than all the other rivers. There were variations in turbidity within the same river overtime and between the different rivers. River Sio (Busia district) had the highest and the widest turbidity variations during the study period.
Water test results for the springs and ponds
The mean physicochemical characteristics of spring and pond water are shown in Table 3 .
The mean physicochemical characteristics of water from the springs and ponds showed variations between the sites. The majority of site means values were within the WHO accepted pH range. Two sites, Wanseko pond (Buliisa, district, Lake Albert basin) and Katanga spring (Kampala district, Lake Victoria basin) had mean water pH below the recommended WHO drinking water acceptable range at the acidic level of 5.73 and 6.19 respectively. Forty percent (40%, 2/5) of the ponds and 33% (1/3) of the springs had mean dissolved oxygen below the recommended WHO level. The ponds with the low dissolved oxygen were found within Lake Albert basin. Among the springs, Katanga spring (Kampala district, L. victoria basin) had mean dissolved oxygen that was below the WHO recommended level of 5 mg/L. Conductivities of the spring water were 89.81–3276.36 μS/cm and for ponds 55.99–3280.83 μS/cm. For both the springs and the ponds the differences between the lowest and the highest conductivities were wide.
Monthly variations of the springs and ponds water physicochemical characteristics
The monthly variations of spring and pond water physicochemical characteristics are shown in Fig. 4 .
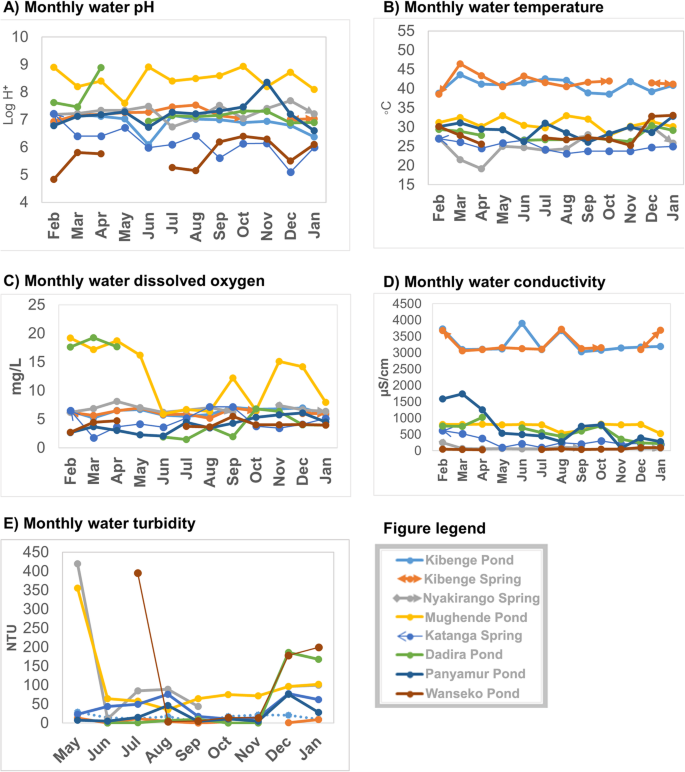
Monthly variations of the physicochemical characteristics of the spring and pond water, February 2015 – January 2016: Part a ) water pH variations; Part b ) water temperature variations; Part c ) dissolved oxygen variations; Part d ) conductivity variations and Part e ) water turbidity variations
There were variations in the water physicochemical characteristics of the spring and the pond water overtime. The variations in water (springs and ponds) were also present between the different sites. The springs had small monthly variations of the water physicochemical parameters while the ponds had wide variations. Mughende pond (Kasese district) had the highest pH for most of the study period. Katanga spring (Kampala district) had the lowest pH compared to other springs during the study period. Kibenge spring (Kasese district) had higher temperature than the rest of the two springs (Katanga spring, Kampala district and Nyakirango spring, Kasese district). Most springs and ponds had slight fluctuations in dissolved oxygen except for Mughende pond (Kasese district). Most springs and ponds except for Panyimur pond (Nebbi district) had small monthly fluctuations in water conductivity. Kibenge spring and pond (both located in Kasese district) had higher conductivity compared to the rest of the springs or ponds. Mughende spring and pond were outliers with higher conductivity than the rest of the water sites. There were variations in water turbidity with months for both the springs and the ponds. Apart from Mughende pond (Kasese district), the rest of the springs and ponds showed variations that had two peaks, the first peak (May – August, 2015) and the second peak (November – January, 2016).
Test results of the other surface water sources: Mobuku irrigation canal water
Mobuku irrigation canal water, water diverted from Mobuku River for irrigation purposes by the Mobuku irrigation scheme was tested because the local communities were using this water for domestic purposes including drinking. Apart from water turbidity which was above the WHO recommended standard of 5NTU, the rest of the water physicochemical parameters (pH, temperature, dissolved oxygen and conductivity) were in the WHO acceptable range as follow: pH of 7.93 ± Standard Error (SE) of 0.23, temperature of 26.57 °C ± SE of 1.25 °C, dissolved oxygen of 6.38 mg/L ± SE of 0.18 mg/L, conductivity of 69.06 ± SE of 2.57) and turbidity of 28.68 ± SE of 9.06NTU.
Monthly variations of physicochemical characteristics of Mobuku irrigation canal water
There were monthly variations in water physicochemical characteristics of Mobuku irrigation canal. The water pH and dissolved oxygen showed two peaks each. The first peak was in March – May, 2015 and the second peak, August – November, 2015. The variations of the Mobuku irrigation canal monthly water physicochemical parameters over the study period is shown in Fig. 5 .
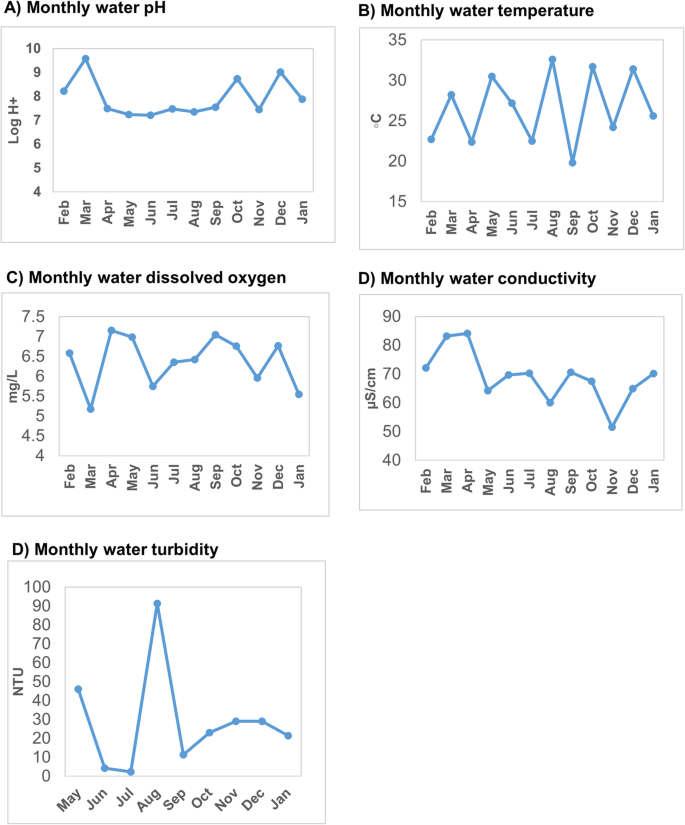
Monthly variations of the physicochemical characteristics of Mobuku irrigation canal water, February 2015 – January 2016: Part a ) water pH variations; Part b ) water temperature variations; Part c ) dissolved oxygen variations; Part d ) conductivity variations and Part e ) water turbidity variations
Results of statistical tests for the differences within sites overtime and between sites
One-Way ANOVA test.
There were no statistically significant differences within most of the study sites except for sites on the lakes and the rivers where the pH and temperature differences were statistically significantly within sites overtime. Statistically significant differences in the water physicochemical characteristics were observed between sites (all p -value < 0.05) as indicated in the additional file 2 .
Turkey’s post hoc test
There were statistically significant differences for all water physicochemical parameters for both the lake and river sites. For instance, Lake Edward had both the highest temperature (34 °C, May, 2015) which was registered at Katwe FLS (Kasese district) and the lowest temperature (18.9 °C, April, 2015) which was recorded at Kayanzi FLS (Kasese district). The results of the comparison of the physicochemical parameters of the various lake and river sites are shown in Table 4 .
Similarly, comparison of the springs or pond water showed statistically significant differences for most (80% of the total comparison) of the water parameters (pH, temperature, dissolved oxygen and conductivity) apart from the water turbidity. Turkey’s post Hoc test results for the comparison of springs and pond water physicochemical parameters are shown in Table 5 .
This study showed that water for drinking and domestic purposes from the surface water sources and springs in cholera affected communities/districts of Uganda were not safe for human use in natural form. The water samples from the water sources in the study area did not meet the WHO drinking water quality standards in terms of the important physicochemical parameters. In addition, all the surface water sources and the springs tested had turbidity above the WHO recommended level of 5NTU yet the same water were used for domestic purposes including drinking in the natural form by the households. The study also found variations in the other physicochemical parameters (pH, temperature, dissolved oxygen and conductivity) between study sites on the same lake and between the different water sources.
While the majority of the water sources had mean water physicochemical characteristics (excluding turbidity) in acceptable range, few water sources, mainly the sites on Lake George, including the springs and ponds had pH and dissolved oxygen outside the recommended WHO ranges. These water sources that did not meet the WHO drinking water standards could expose the users to harmful effects of unsafe drinking water including waterborne diseases such as cholera. The present study findings of high water turbidity if due to algae bloom could encourage pathogen persistence and infection spread, including V. cholerae bacteria [ 40 , 41 ] resulting in ill-health and cholera epidemics. In addition, the high water turbidity complicates water disinfection as it gives rise to significant chlorine demand [ 53 ]. The increased chlorine demand can be costly and difficult to ensure constant availability for disinfection of water since Uganda and several other developing countries need and receive supplementary donor support [ 69 ].
In regard to temperature, dissolved oxygen and conductivity, the majority of the surface water sources and springs tested met the recommended WHO drinking water standards. However, a few water sources such as River Lubigi in Kampala district had mean dissolved oxygen below the recommended WHO drinking water standards. Therefore, in order to ensure universal access to safe drinking water, the water sources that had vital physicochemical parameters outside the WHO drinking water range could be targeted for further studies.
There were statistically significant differences in the water physicochemical characteristics between the different sites and sources (lakes, rivers, springs and ponds). Despite these differences, the required approaches to ensure safe water access to the communities may not differ across sites. First and foremost, all sites and water types will need measures that reduce the high water turbidity to WHO acceptable levels. Secondly, in few instances, such as the water sources with pH in acidic range (Katanga spring in Kampala district, Lake Victoria Basin and Wanseko pond in Buliisa district, lake Albert basin) in addition to requiring further studies to identify the causes of the low pH (acidity), such water sources may also require the use of water treatment methods that neutralize the excess acidity [ 54 ]. Furthermore, since acidity is usually associated with increased solubility of toxic heavy metals (lead, arsenic and others) [ 34 ], testing such water for metallic contamination may be required. Heavy metal contamination of water causes ill-health due to chronic exposure which is cumulative and manifest late for correction to be done [ 70 ].
The findings of this study also highlight the differences in water quality between the urban surface water sources and springs (Kampala district) and the rural surface sources and springs (other study districts – Kasese, Kayunga, Busia, Nebbi and Buliisa) The water sources that met the WHO recommended drinking water quality standards [ 53 ] were mostly the rural springs and the rivers. However, these differences between the rural and the urban water sources do not alter the required approaches to ensure access to safe water which is by promoting measures that reduce the high water turbidity in combination with water disinfection to remove the pathogens. The relatively good quality of rural water sources compared to the urban ones could have been due to availability of plenty of vegetation in rural setting that filtered the water along the way downstream and possibly low level of pollution from industrial inputs in rural areas than in urban areas [ 71 , 72 ].
In relation to cholera outbreaks in the study communities, naturally, the physicochemical conditions for survival of V. cholerae O1 occur in an estuarine environment and other brackish waters [ 73 , 74 ]. In such circumstances, the favourable physicochemical conditions for V. cholerae isolation are the high water turbidity [ 49 ] and temperature of above 17 °C [ 43 ]. Interestingly, all the surface water sources and the springs tested had favourable physicochemical characteristics for the survival of V. cholerae in terms of these two parameters (high water turbidity and temperature of above 17 °C). Furthermore, two lakes sites (Kahendero FLS and Hamukungu FLS, Lake George, Kasese district) had also favourable mean pH for the survival of V. cholerae of 9.03 ± 0.17 and 9.13 ± 0.23 respectively. Favourable pH for V. cholerae survival in waters of Lake George was previously documented in the same area [ 23 ]. Hence, the frequent cholera outbreaks [ 19 , 20 , 21 , 24 ] in the study area could be attributed to both the favourable physicochemical water characteristics and use of unsafe water.
There were wide variations in conductivity between water sources and within the same source overtime. High water conductivities were recorded in the months of January to March 2015 (dry season), possibly due to high evaporation which increased the concentration of electrolytes present in water. Likewise, two rivers namely. River Lubigi (Kampala district) and Nyamugasani (Kasese district) had higher mean conductivities of 460.51 ± 57.83 μS/cm and 946.08 ± 3.63 μS/cm respectively than for typically unpolluted river of 350 μS/cm [ 75 ]. Consequently, given that the two rivers flow through areas of heavy metal mining (copper and cobalt mines in Kasese district by Kilembe Mines Limited and Kasese Cobalt Company Limited) and industrial activities (Kampala City), it is possible for the high water conductivity to be due to the heavy metal contamination as previously documented in drinking water in South-western Uganda [ 62 ] and Kampala City [ 61 ]. Thus, specific studies are required on water from the two rivers to determine the true cause of the high conductivity and to guide mitigation measures.
Hence, more efforts are required to promote safe water access in Uganda to attain the WHO cholera elimination target [ 25 ] and SDG 6 by 2030 since 26% (36/135) of mean physicochemical water tests did not meet WHO drinking water quality standards [ 53 ]. These findings together with those of the previous studies which demonstrated the presence of pathogenic V. cholerae in the same water sources [ 22 , 23 , 76 ] should guide stakeholders to improve access to safe water in the Great Lakes basins of Uganda holistically. Thus, measures such as promotion of use of safe water (using water disinfection), health education, sanitation improvement and hygiene promotion that address both the water bacteriological contents and physicochemical parameters should be considered in both the short and medium terms. However, long term plan to increase access to safe water by construction of permanent safe water treatment plants and distribution systems (pipes) should remain a top priority.
In the short and intermediate period, focusing on the measures that reduce water turbidity and disinfection of water (to kill microorganisms) should be prioritized so as to facilitate progress towards attainment of SDGs and cholera elimination in the study area. The basis for such prioritization lies in the fact that high water turbidity raises water temperature and prevents the disinfection effects of chlorine on water. These in return promote survival of the microorganisms and consequently cholera and other waterborne disease outbreaks. Furthermore, though boiling of water is feasible and recommended through technical guidelines [ 26 ] since it addresses both turbidity and kills the micro-organisms, it has issues of poor compliance due to lack of firewood which is the main cooking energy source in these communities [ 70 ]. Therefore, alternative safe water provision targeting reduction of high water turbidity and removal of microorganism by special filters such as decanting and sand filters and flocculation agents which do not need heat energy should be promoted [ 77 , 78 ]. Also, there is a need to explore the use of solar energy (solar water purifiers) [ 79 ] in these communities given their location in the tropics where sunshine is plenty. In the minority of situations, in addition to use of above methods to make water safe, there may be a need to employ different approaches of water purification depending on the water source. For example the water sources with lower or higher than recommended pH [ 53 ] (Wanseko pond, Hamukungu and Kahendero FLS on L. George), use of water treatment reagents that are affected by pH such as chlorine tablets should be reevaluated.
In additional to disinfection and turbidity corrective measures for all the water that were studied, each of the springs in the study area (Katanga in Kampala district and Nyakirango and Kibenge springs in Kasese district) will also need a sanitary survey (a comprehensive inspection of the entire water delivery system from the source to the mouth so as to identify potential problems and changes in the quality of drinking water) [ 80 ]. The findings of the sanitary survey should then guide the medium and long term interventions for water quality improvement in areas served by targeted springs. The following are some of the interventions that could be carried out after a sanitary survey: provision of a screen to prevent the entrance of animals, erecting a warning signs, digging of a diversion ditch located at the uphill end to keep rainwater from flowing over the spring area, establishment of an impervious barrier (a clay or a plastic liner) to prevent potential contaminants from entering into the water or and others measures described in the handbook for spring protection [ 81 ].
Furthermore, as a stopgap measure while access to safe water is scaled up, the communities in the study area should be protected from cholera using Oral Cholera Vaccines [ 82 ]. Protection of these communities is necessary since this study shows that favorable conditions for cholera propagation/transmission are present in the water in the study area. The favorable conditions that were documented in this study included the high water turbidity which makes it difficult to disinfect water [ 53 ] and the water temperature of above 17 °C which speeds up the multiplication of pathogens [ 43 ].
In addition, there were some other important study findings that were not fully understood. For example, some water sources (Kibenge spring and pond (located in Kasese district, western Uganda) had extreme vital physicochemical values for both conductivity and water temperature relative to the rest of above 40 °C and 3000 μS/cm respectively. It is possible that the extreme values were due to geochemical effects documented in water sources around Mount Rwenzori [ 83 ]. However, since there was copper and cobalt mining in Kasese district, high water conductivity could have been due to chemical contamination. Similarly, River Lubigi, Kampala district (central Uganda) had very low dissolved oxygen of less than 1 mg/L during some months (for example in January 2015, dissolved oxygen of 0.45 mg/L) which could have been due to organic pollutants from the communities in Kampala City [ 84 ] that used up the oxygen in the water. Also, Wanseko pond (Lake Albert basin, Buliisa district) had low pH of 4.84 in February 2015. Such water with low pH have the potential to increase the solubility of heavy metals some of which make water harmful when consumed [ 85 ]. Therefore, further studies will be required to better understand such extreme values.
Strength and limitations of this study
This study had several strengths. First, the longitudinal study design that employed repeated measurements of water physicochemical characteristics from the same site and source. This design reduced the likelihood of errors that could arise from one-off measurements seen in cross-sectional study designs resulting in increased validity of the study findings. Second, the inclusion of a variety of the water sources from which drinking and domestic water were collected namely, lakes, rivers, ponds, springs and a canal from different regions of Uganda made the findings representative of the water sources in study districts. Third, use of robust equipment, Hach meters, HQ40d [ 68 ] which automatically compensated for the weather changes (corrected for possible confounders and biases) for the parameters that had effect on each other such as raising water temperature impacting on the water conductivity and dissolved oxygen. Forth, purposive selection of the districts with frequent cholera outbreaks, an important waterborne disease that is targeted for elimination locally within Uganda and globally by WHO [ 25 ]. This meant that the findings had higher potential for used by stakeholders targeting to improve access to safe water and those for cholera prevention.
There were also some study limitations. First, though the study identified the favourable conditions (higher than recommended mean water turbidity and temperature of above 17 °C) for cholera in the study area, we could not report on causal-effect relationship between V. cholerae and the parameters studied. Vibrio cholera e pathogens were detected by use of multiplex Polymerase Chain Reaction (PCR). The results for PCR test were interpreted as positive or negative for V. cholerae O1, O139, non O1, and non O139 [ 22 ]. These data were not appropriate for establishment of causal-effect relationship Therefore, further studies using appropriate methods are recommended to establish such relationships.
Second, during some months of the study, water samples could not be obtained from some sources especially the ponds that had dried up during the dry season. The drying up reduced the number of samples collected from these points. However, since the months without water were few compared to the entire study period, the impact of the missing data could have been minimal.
Third, water samples were only tested for the five key physicochemical water characteristics, Vital Signs [ 32 ] however, there are many other parameters that effect survival and health of living things namely, nitrates, copper, lead, fluoride, phosphates, arsenic and others. Studies are therefore required to provide more information on these other parameters not addressed by the current study.
The study showed that surface and spring water for drinking and other domestic purposes in cholera prone communities in Great Lakes basins of Uganda were unsafe in terms of vital physicochemical water characteristics. These water sources had favourable physicochemical characteristics for transmission/propagation of waterborne diseases, including cholera. All test sites (100%, 27/27) had temperature above 17 °C that is suitable for V. cholerae survival and transmission and higher than the WHO recommended mean water turbidity of 5NTU. In addition, more than a quarter (27%) of lake sites and 40% of the ponds had pH and dissolved oxygen outside the WHO recommended range of 6.5–8.5 and less than 5 mg/L respectively. These findings complement bacteriological findings that were previously reported in the study area which found that use of this water increased their vulnerability to cholera outbreaks [ 22 ]. Therefore, in order for Uganda to attain the WHO cholera elimination and the United Nations SDG 6 target by 2030, stakeholders (the Ministry of Water and Environment, the local governments, Ministry of Health development partners and others) should embrace interventions that holistically improve water quality through addressing both physicochemical and biological characteristics. Furthermore, studies should be conducted to generate more information on the other physicochemical parameters not included in this study such as detection of the heavy metal contamination.
Availability of data and materials
The datasets generated and/or analysed during the current study are available in the Mendeley Data repository, https://doi.org/10.17632/57sw2w23tw.1 . The cholera incidence data used to identify the study area were from Uganda Ministry of Health and the district (Kasese, Busia, Nebbi, Buliisa and Kayunga) weekly epidemiological reports.
Abbreviations
Analysis of Variance
Conductivity
Central Public Health Laboratories
Dissolved Oxygen
Delivery of Oral Vaccines Effectively
Fish landing site
Institutional Review Board
Ministry of Health
Nephrometric units
Polymerase Chain Reaction
Sustainable Development Goal
Standard Operating Procedures
United States of America
World Health Organization
Walther J. Earth’s natural resources. Jones & Bartlett Learning; 2014. https://www.researchgate.net/publication/267327567_EARTH’S_NATURAL_RESOURCES/link/544e7df80cf2bca5ce90b65b/download .
UNDP. Sustainable Development GOALS 2030. 2015.
Nikanorov AM, Brazhnikova LV. Water chemical composition of Rivers, lakes and wetlands. Encycl Life Support Syst. 2009;2:42–80 https://www.eolss.net/Sample-Chapters/C07/E2-03-04-02.pdf .
Google Scholar
United Nations. International Decade for Action “Water for Life” 2005–2015. Focus Areas: The human right to water and sanitation1. UN. International Decade for Action “Water for Life” 2005–2015. Focus Areas: The human right to water and sanitation [Internet]. United Nati. United Nations. 2014. http://www.un.org/waterforlifedecade/human_right_to_water.shtml . Accessed 2 Dec 2019.
UNDP. Goal 6: Clean water and sanitation | UNDP. UNDP. 2015. https://www.undp.org/content/undp/en/home/sustainable-development-goals/goal-6-clean-water-and-sanitation.html . Accessed 29 Nov 2019.
Programme UNE. Africa water atlas. Nairobi, Kenya: United Nations Environment Programme; 2010. https://www.zaragoza.es/contenidos/medioambiente/onu/340-eng.pdf .
Dos Santos S, Adams EA, Neville G, Wada Y, de Sherbinin A, Mullin Bernhardt E, et al. Urban growth and water access in sub-Saharan Africa: progress, challenges, and emerging research directions. Sci Total Environ. 2017;607–8:497–508. https://doi.org/10.1016/j.scitotenv.2017.06.157 .
Walker C. Lack of safe water, sanitation spurs growing dissatisfaction with government performance. 2016. http://afrobarometer.org/countries/results-round . Accessed 9 July 2020.
WHO/UNICEF JMP. Progress on household drinking water , sanitation and hygiene I 2000-2017. Special Focus on Inequalities 2017. https://washdata.org . Accessed 3 Dec 2019.
Uganda Bureu of Statistics. Uganda Bureau of Statistics Education Sector Gender Statistics Profile November 2012. 2012; November:1–43.
University of Califonia Museum of Paleontology (UCMP). The Aquatic Biome. 2014;:1–4. http://www.ucmp.berkeley.edu/glossary/gloss5/biome/aquatic.html . Accessed 3 Aug 2017.
World Health Organization. Protecting Surface Water for Health. 2016. http://apps.who.int/iris/bitstream/10665/246196/1/9789241510554-eng.pdf?ua=1 . Accessed 3 Aug 2017.
Hunter PR. Climate change and waterborne and vector-borne disease. J Appl Microbiol Symp Suppl. 2003;94. https://doi.org/10.1046/j.1365-2672.94.s1.5.x .
Vitousek PM, Mooney HA, Lubchenco J, Melillo JM. Human domination of Earth’s ecosystems. In: Urban Ecology: An International Perspective on the Interaction Between Humans and Nature 2008.
World Health Organization. WHO | Water-related Diseases. https://www.who.int/water_sanitation_health/diseases-risks/diseases/diarrhoea/en/ . Accessed 9 July 2020.
World Health Organization. Weekly epidemiological record Relevé épidémiologique hebdomadaire. 2013;:321–36.
World Health Organization. Weekly epidemiological record Relevé épidémiologique hebdomadaire. 2019;94:561–80. https://extranet.who.int/iris/restricted/bitstream/handle/10665/330003/WER9448-eng-fre.pdf?ua=1 .
Bwire G, Malimbo M, Maskery B, Kim YE, Mogasale V, Levin A. The burden of cholera in Uganda. PLoS Negl Trop Dis. 2013;7:e2545. https://doi.org/10.1371/journal.pntd.0002545 .
Article PubMed PubMed Central Google Scholar
Bwire G, Munier A, Ouedraogo I, Heyerdahl L, Komakech H, Kagirita A, et al. Epidemiology of cholera outbreaks and socio-economic characteristics of the communities in the fishing villages of Uganda: 2011-2015. PLoS Negl Trop Dis. 2017;11.
Kwesiga B, Pande G, Ario AR, Tumwesigye NM, Matovu JKB, Zhu BP. A prolonged, community-wide cholera outbreak associated with drinking water contaminated by sewage in Kasese District, western Uganda. BMC Public Health. 2017;18. https://doi.org/10.1186/s12889-017-4589-9 .
Pande G, Kwesiga B, Bwire G, Kalyebi P, Riolexus AA, Matovu JKB, et al. Cholera outbreak caused by drinking contaminated water from a lakeshore water-collection site, Kasese District, South-Western Uganda, June-July 2015. PLoS One. 2018;13:e0198431. https://doi.org/10.1371/journal.pone.0198431 .
Article CAS PubMed PubMed Central Google Scholar
Bwire G, Debes AK, Orach CG, Kagirita A, Ram M, Komakech H, et al. Environmental surveillance of Vibrio cholerae O1/O139 in the five African Great Lakes and other major surface water sources in Uganda. Front Microbiol. 2018;9:1560. https://doi.org/10.3389/FMICB.2018.01560 .
Kaddumukasa M, Nsubuga D, Muyodi FJ. Occurence of Culturable Vibrio cholerae from Lake Victoria, and Rift Valley lakes Albert and George, Uganda. Lakes Reserv Res Manag. 2012;17:291–9.
Article CAS Google Scholar
Oguttu DW, Okullo A, Bwire G, Nsubuga P, Ario AR. Cholera outbreak caused by drinking lake water contaminated with human faeces in Kaiso Village, Hoima District, Western Uganda, October 2015. Infect Dis Poverty. 2017;66:146. https://doi.org/10.1186/s40249-017-0359-2 .
World Health Organization. Ending cholera: A global roadmap to 2030. 2017. http://www.who.int/cholera/publications/global-roadmap.pdf?ua=1 . Accessed 9 July 2020.
Ministry of Health Uganda. Prevention and Control of Cholera Operational Guidelines for the National and District Health Workers and Planners. 2017. http://www.health.go.ug/sites/default/files/Final CHOLERA GUIDELINES 2017_0.pdf. Accessed 13 Feb 2019.
Snow J. Mode of Communication of Cholera (John Snow, 1855). 1855;:1–38. http://www.ph.ucla.edu/epi/snow/snowbook.html .
Bingham P, Verlander NQ, Cheal MJ. John snow, William Farr and the 1849 outbreak of cholera that affected London: a reworking of the data highlights the importance of the water supply. Public Health. 2004;118:387–94.
United Nations Environment Programme and the World Health Organization. United Nations Environment Programme and the World Health Organization. 1996.
Daud MK, Nafees M, Ali S, Rizwan M, Bajwa RA, Shakoor MB, et al. Drinking Water Quality Status and Contamination in Pakistan 2017;2017.
Ford L, Bharadwaj L, Mcleod L, Waldner C. Human health risk assessment applied to rural populations dependent on unregulated drinking water sources : a scoping review. 2017.
Team CW. Vital Signs : The Five Basic Water Quality Parameters. 2010;:3–4.
Fondriest Environmental, Inc. “pH of Water” Fundamentals of Environmental Measurements. 2013. Web. https://www.fondriest.com/environmentalmeasurements/parameters/water-quality/ph/ . Accessed 7 July 2020.
Vancouver Water Resources Education Center. Water Quality: Temperature, pH and Dissolved Oxygen. 2019. www.middleschoolchemistry.com/lessonplans/ . Accessed 5 Dec 2019.
Fondriest Environmental, Inc. “Water Quality” Fundamentals of Environmental Measurements. 2013. Web. https://www.fondriest.com/environmentalmeasurements/parameters/water-quality/ . Accessed 7 July 2020.
Spellman FR. The Drinking Water Handbook 2017. doi: https://doi.org/10.1201/9781315159126 .
World Health Organization. Protecting Surface Water for health: Identifying, assessing and managing drinking-water quality risks in surface-water catchments. 2016. http://www.who.int . Accessed 4 July 2019.
Bliem R, Reischer G, Linke R, Farnleitner A, Kirschner A. Spatiotemporal dynamics of Vibrio cholerae in turbid alkaline lakes as determined by quantitative PCR. Appl Environ Microbiol. 2018;84:317–35. https://doi.org/10.1128/AEM.00317-18 .
U.S. Environmental Protection Agency. Drinking Water Treatability Database: Vinclozolin. 2009. https://iaspub.epa.gov/tdb/pages/contaminant/contaminantOverview.do%3FcontaminantId%3D10540 . Accessed 5 Dec 2019.
Sagir Ahmed M, Raknuzzaman M, Akther H, Ahmed S. The role of cyanobacteria blooms in cholera epidemic in Bangladesh. J Appl Sci. 2007;7:1785–9.
Article Google Scholar
Epstein PR. Algal blooms in the spread and persistence of cholera. BioSystems. 1993;31:209–21. https://doi.org/10.1016/0303-2647(93)90050-M .
Article CAS PubMed Google Scholar
Gil AI, Louis VR, Rivera ING, Lipp E, Huq A, Lanata CF, et al. Occurrence and distribution of Vibrio cholerae in the coastal environment of Peru. Environ Microbiol. 2004;6:699–706.
Nair G. B, Havelaar A. H, Bartram J, Jacob J, Hueb J et al. Vibrio cholerae 1. 1997;:119–42. http://www.who.int/water_sanitation_health/dwq/admicrob6.pdf .
Roberts MB V. Biology: a functional approach. 1986. https://books.google.co.ug/books?id=ASADBUVAiDUC&pg=PA232&lpg=PA232&dq=body+temperatures+of+microorganisms+the+same+as+surroundings+poikilothermic&source=bl&ots=hGQDzoXNw2&sig=ACfU3U3H3-n-YybOibjEg_Q_dOmSdu1L5Q&hl=en&sa=X&ved=2ahUKEwjAy5zerJ_mAhUICsAKHU5b . Accessed 5 Dec 2019.
Fondriest Environmental I. Conductivity, Salinity & Total Dissolved Solids - Environmental Measurement Systems. Fundamentals of Environmental Measurements, Fondriest Environmental, Inc. 2014;:Web. https://www.fondriest.com/environmental-measurements/parameters/water-quality/conductivity-salinity-tds/ . Accessed 19 Dec 2019.
Queensland G. Environmental protection (water) policy 2009 - monitoring and sampling manual physical and chemical assessment. 2018. https://environment.des.qld.gov.au/water/monitoring/sampling-manual/pdf/physical-and-chemical-assesssment-swab-sampling.pdf . Accessed 29 Nov 2019.
Fondriest Environmental, Inc. “Turbidity-total-suspended-solids-water-clarity” Fundamentals of Environmental Measurements. 2013. Web. https://www.fondriest.com/environmental-measurements/parameters/water-quality/turbiditytotal-suspended-solids-water-clarity/ . Accessed 7 July 2020.
Otieno OS. Physico-chemical and bacteriological quality of water from five rural catchment areas of Lake Victoria basin in Kenya 2015. doi: https://doi.org/10.1017/CBO9781107415324.004 .
Kelley CD, Krolick A, Brunner L, Burklund A, Kahn D, Ball WP, et al. An affordable open-source turbidimeter. Sensors (Switzerland). 2014;14:7142–55. https://doi.org/10.3390/s140407142 .
Sagir Ahmed M, Raknuzzaman M, Akther H, Ahmed S. The role of cyanobacteria blooms in cholera epidemic in Bangladesh. J Appl Sci. 2007;7:1785–9. https://doi.org/10.3923/jas.2007.1785.1789 .
Gorchev HG, Ozolins G. WHO guidelines for drinking-water quality 2011. doi: https://doi.org/10.1016/S1462-0758(00)00006-6 .
World Health Organization. Essential environmental health standards in health care. Non serial. Geneva: World Health Organization Press; 2008. https://books.google.co.ug/books?hl=en&lr=&id=QwVj87Mmz4sC&oi=fnd&pg=PA3&dq=Essential+environmental+health+standards+in+health+care.+World+Health++Organization&ots=qBUjaQCxQV&sig=CyG-4NLSRKIEZG-L0vDxuK5Ga-o&redir_esc=y#v=onepage&q=Essential%20environmental . Accessed 6 July 2020.
World Health Organization. Guidelines for Drinking Water Quality. Fourth Edi. 2017. https://apps.who.int/iris/bitstream/handle/10665/254636/9789241550017-eng.pdf;jsessionid=B0351A975B001E76D924D2B1183DE673?sequence=1 .
Sharma S, Bhattacharya A. Drinking water contamination and treatment techniques. Appl Water Sci. 2017;7:1043–67. https://doi.org/10.1007/s13201-016-0455-7 .
Centers for Disease Control and Prevention (CDC); Cholera prevention and control. Atlanta, USA. https://www.cdc.gov/cholera/pdf/five-basic-cholera-prevention-messages.pdf . Accessed 10 July 2020.
Luff R. Oxfam guidelines for water treatment in emergencies. 2001. http://ec.europa.eu/echo/files/evaluation/watsan2005/annex_files/OXFAM/OXF5 - Oxfam guidelines for water treatment in emergencies. PDF.
Iceland Kasozi K, Namubiru S, Kamugisha R, Daniel Eze E, Stuart Tayebwa D, Ssempijja F, et al. Safety of Drinking Water from Primary Water Sources and Implications for the General Public in Uganda 2019. doi: https://doi.org/10.1155/2019/7813962 .
Alajo S. Comparison of two communities affected by cholera in Kasese district in Uganda. Am J Trop Med Hyg. 2013;89:205 http://www.embase.com/search/results?subaction=viewrecord&from=export&id=L71312507%5Cnhttp://www.ajtmh.org/content/89/5_Suppl_1/153.full.pdf+html%5Cnhttp://vb3lk7eb4t.search.serialssolutions.com?sid=EMBASE&issn=00029637&id=doi:&atitle=Comparison+of+two+co.
Jutla A, Whitcombe E, Hasan N, Haley B, Akanda A, Huq A, et al. Environmental factors influencing epidemic cholera. Am J Trop Med Hyg. 2013;89:597–607. https://doi.org/10.4269/ajtmh.12-0721 .
Ojok W, Wasswa J, Ntambi E. Assessment of seasonal variation in water quality in river Rwizi using multivariate statistical techniques, Mbarara municipality, Uganda. J Water Resour Prot. 2017;09:83–97.
Semuyaba AS, Segawa I, Wamala A. Potential risk of Lead toxicity from bottled water in Uganda. MPJ. 2014;12:e13–20 https://chs.mak.ac.ug/content/potential-risk-lead-toxicity-bottled-water-uganda . Accessed 18 Dec 2019.
Kasozi KI, Namubiru S, Kamugisha R, Eze ED, Tayebwa DS, Ssempijja F, et al. Safety of drinking water from primary water sources and implications for the general public in Uganda. J Environ Public Health. 2019;2019. https://doi.org/10.1155/2019/7813962 .
Okello PE, Bulage L, Riolexus AA, Kadobera D, Kwesiga B, Kajumbula H, et al. A cholera outbreak caused by drinking contaminated river water, Bulambuli District, eastern Uganda, march 2016. BMC Infect Dis. 2019;19. https://doi.org/10.1186/s12879-019-4036-x .
Agensi A, Tibyangye J, Tamale A, Agwu E, Amongi C. Contamination potentials of household water handling and storage practices in Kirundo subcounty, Kisoro District, Uganda. J Environ Public Health. 2019;2019. https://doi.org/10.1155/2019/7932193 .
Bwire G, Ali M, Sack DA, Nakinsige A, Naigaga M, Debes AK, et al. Identifying cholera “hotspots” in Uganda: an analysis of cholera surveillance data from 2011 to 2016. PLoS Negl Trop Dis. 2017;11:e0006118. https://doi.org/10.1371/journal.pntd.0006118 .
Bompangue Nkoko D, Giraudoux P, Plisnier P-D, Tinda AM, Piarroux M, Sudre B, et al. Dynamics of cholera outbreaks in Great Lakes region of Africa, 1978-2008. Emerg Infect Dis. 2011;17:2026–34.
PubMed Google Scholar
Debes AK, Ateudjieu J, Guenou E, Lopez AL, Bugayong MP, Retiban PJ, et al. Evaluation in Cameroon of a novel, simplified methodology to assist molecular microbiological analysis of v. cholerae in resource-limited settings. PLoS Negl Trop Dis. 2016;10:4307. https://doi.org/10.1371/journal.pntd.0004307 .
Hach campany. HQ Series Portable Meters. 2006. www.hach.com . Accessed 4 Jan 2019.
Langan M. Budget support and Africa–European Union relations: free market reform and neo-colonialism? Eur J Int relations. 2015.
UNICEF. UNICEF Handbook On Water Quality. 2008. http://www.unicef.org/wes . Accessed 14 Dec 2019.
Ouma S, Ngeranwa JN, Juma KK, Mburu DN. Journal of environmental analytical seasonal variation of the physicochemical and bacteriological quality of water from five rural catchment areas of Lake Victoria Basin in Kenya. J Environ Anal Chem. 2016;3:1–7. https://doi.org/10.4172/2380-2391.1000170 .
Mapira J. River pollution in the City of Mutare (Zimbabwe) and its implications for sustainable development. J Sustain Dev Africa. 2011;13:181–94. https://pdfs.semanticscholar.org/a581/6beb20e79ea0590a7717de4a095c02dc14a5.pdf . Accessed 4 July 2019.
Colwell RR, Huq A. Chapter 9: Vibrios in the environment: viable but nonculturable Vibrio cholerae. In: Wachsmuth, Kaye I, Blake P, Olsvik Ø, editors. Vibrio cholerae and Cholera. Washington, DC: American Society of Microbiology; 1994. p. 117–33. https://www.asmscience.org/content/book/10.1128/9781555818364.chap9 . Accessed 9 July 2020.
Colwell RR, Spira WM. The ecology of Vibrio cholerae. In: Cholera. Boston, MA: Springer US; 1992. p. 107–27. https://doi.org/10.1007/978-1-4757-9688-9_6 .
Chapter Google Scholar
Koning N, Roos JC. The continued influence of organic pollution on the water quality of the turbid Modder River. Water SA. 1999;25:285–92.
CAS Google Scholar
Bagalwa M, Yalire M, Balole E, Karume K. A preliminary assessment of Physico-chemical and bacteriological characteristics of Lake Eduard and Majors tributaries Rivers, Democratic Republic of Congo. Sch Acad J Biosci. 2014;2:236–45 www.saspublisher.com . Accessed 29 July 2017.
Collin C. Biosand filtration of high turbidity water: modified filter design and safe filtrate storage. Massachusetts Institute of Technology; 2009. http://web.mit.edu/watsan/Docs/Student%20Theses/Ghana/2009/Thesis%20-%20Final,%20Clair%20Collin,%205-15-09.pdf . Accessed 9 July 2020.
Collin C. Biosand filtration of high turbidity water: modified filter design and safe filtrate storage. Massachusetts Institute of Technology; 2009. https://dspace.mit.edu/handle/1721.1/50623 . Accessed 7 July 2020.
Graf J, Togouet SZ, Kemka N, Niyitegeka D, Meierhofer R, Pieboji JG. Health gains from solar water disinfection (SODIS): Evaluation of a water quality intervention in Yaoundé, Cameroon. J Water Health. 2010;8:779–96. https://doi.org/10.2166/wh.2010.003 .
Open University. Hygiene and environmental health module: 16. Sanitary Survey of Drinking Water 2019. https://www.open.edu/openlearncreate/mod/oucontent/view.php?id=203&printable=1 . Accessed 6 Jun 2020.
Herrmann H, Bucksch H. spring protection. In: Dictionary Geotechnical Engineering/Wörterbuch GeoTechnik. 2014. p. 1290–1290.
World Health Organization. Oral Cholera Vaccine stockpile for cholera emergency response. 2013. http://www.who.int/cholera/vaccines/Briefing_OCV_stockpile.pdf .
Kato V, Kraml M. Geochemistry of Rwenzori hot spring waters. 2010; November:22–5. https://www.geothermal-energy.org/pdf/IGAstandard/ARGeo/2008/V.Kato_Rwenzori.pdf . Accessed 4 Jan 2019.
Kayima JK, Mayo AW, Nobert J. Ecological Characteristics and Morphological Features of the Lubigi Wetland in Uganda. Environ Ecol Res. 2018;6:218–28. https://doi.org/10.13189/eer.2018.060402 ..
Ozoko DC. Heavy metal geochemistry of acid mine drainage in Onyeama coal mine, Enugu, Southeastern Nigeria. J Environ Earth Sci. 2015.
Download references
Acknowledgements
The authors are grateful to the following: the district teams and the communities in Kasese, Kampala, Nebbi, Buliisa, Kayunga and Busia districts for the cooperation and support; the Ministry of Health, Makerere University School of Public Health, Dr. Asuman Lukwago, Dr. Jane Ruth Aceng and Prof. AK. Mbonye for technical guidance. The authors are grateful to Dunkin Nate from John Hopkins University for training of the field teams on water sampling and testing. The authors also thank Ambrose Buyinza Wabwire and to Damari Atusasiire for the support in creating the map and statistical guidance respectively. Special thanks to the laboratory teams in the district hospitals; CPHL (Kampala) and John Hopkins University (Maryland, USA) for carrying out the water tests.
This study was funded by the Bill and Melinda Gates Foundation, USA, through John Hopkins University under the Delivering Oral Vaccine Effectively (DOVE) project. (OPP1053556). The funders had no role in the implementation of the study and in the decision to publish the study findings.
Author information
Authors and affiliations.
Department of Community and Behavioral Sciences, Makerere University College of Health Sciences, School of Public Health, Kampala, Uganda
Godfrey Bwire, Henry Komakech & Christopher Garimoi Orach
Department of International Health, Johns Hopkins Bloomberg School of Public Health, Dove Project, Baltimore, MD, USA
David A. Sack, Amanda K. Debes, Malathi Ram & Christine Marie George
Uganda National Health Laboratory Services (UNHS/CPHL), Ministry of Health, Kampala, Uganda
Atek Kagirita
Department of Quality Control, Uganda National Drug Authority, Kampala, Uganda
Tonny Obala
You can also search for this author in PubMed Google Scholar
Contributions
GB, DAS, AKD and CGO conceived the idea. GB, CGO, AKD, MR, HK, AK, TO and CMG conducted the investigation. MR, HK and TO carried out data curation. MR, HK, GB, DAS, CMG, AKD and AK analysed data. GB, DAK, AKD, CGO, MR, AK, TO and CMG wrote the first draft. All authors read and approved the final manuscript.
Corresponding author
Correspondence to Godfrey Bwire .
Ethics declarations
Ethics approval and consent to participate.
This study was approved by the Makerere University School of Public Health Institution Review Board (IRB 00011353) and the Uganda National Council of Science and Technology. Cholera data used in selection of the water bodies and study communities were aggregated disease surveillance data from the Ministry of Health with no personal identifiers. The laboratory reports on the water sources found contaminated during the study period were shared immediately with the district team to ensure that preventive measures were instituted to protect the communities. In addition, the communities served by such water sources were educated on water treatment/purification (filtration, boiling, chlorination, use of Waterguard ).
Consent for publication
Not applicable.
Competing interests
The authors report no competing interests.
Additional information
Publisher’s note.
Springer Nature remains neutral with regard to jurisdictional claims in published maps and institutional affiliations.
Supplementary information
Additional file 1..
The number and the type of water sources in each of the lake basins in cholera prone communities of Uganda that were enrolled in the study, February 2015 – January 2016.
Additional file 2.
One Way ANOVA test results for the differences within the study sites overtime (February 2015 – January 2016) and between sites.
Rights and permissions
Open Access This article is licensed under a Creative Commons Attribution 4.0 International License, which permits use, sharing, adaptation, distribution and reproduction in any medium or format, as long as you give appropriate credit to the original author(s) and the source, provide a link to the Creative Commons licence, and indicate if changes were made. The images or other third party material in this article are included in the article's Creative Commons licence, unless indicated otherwise in a credit line to the material. If material is not included in the article's Creative Commons licence and your intended use is not permitted by statutory regulation or exceeds the permitted use, you will need to obtain permission directly from the copyright holder. To view a copy of this licence, visit http://creativecommons.org/licenses/by/4.0/ . The Creative Commons Public Domain Dedication waiver ( http://creativecommons.org/publicdomain/zero/1.0/ ) applies to the data made available in this article, unless otherwise stated in a credit line to the data.
Reprints and permissions
About this article
Cite this article.
Bwire, G., Sack, D.A., Kagirita, A. et al. The quality of drinking and domestic water from the surface water sources (lakes, rivers, irrigation canals and ponds) and springs in cholera prone communities of Uganda: an analysis of vital physicochemical parameters. BMC Public Health 20 , 1128 (2020). https://doi.org/10.1186/s12889-020-09186-3
Download citation
Received : 02 April 2019
Accepted : 01 July 2020
Published : 17 July 2020
DOI : https://doi.org/10.1186/s12889-020-09186-3
Share this article
Anyone you share the following link with will be able to read this content:
Sorry, a shareable link is not currently available for this article.
Provided by the Springer Nature SharedIt content-sharing initiative
- Drinking water
- Water quality
- Physicochemical parameter
- Water source
- Surface water
BMC Public Health
ISSN: 1471-2458
- Submission enquiries: [email protected]
- General enquiries: [email protected]
Accessibility Links
- Skip to content
- Skip to search IOPscience
- Skip to Journals list
- Accessibility help
- Accessibility Help
Click here to close this panel.
Purpose-led Publishing is a coalition of three not-for-profit publishers in the field of physical sciences: AIP Publishing, the American Physical Society and IOP Publishing.
Together, as publishers that will always put purpose above profit, we have defined a set of industry standards that underpin high-quality, ethical scholarly communications.
We are proudly declaring that science is our only shareholder.
The effect of drinking water quality on the health and longevity of people-A case study in Mayang, Hunan Province, China
J Lu 1 and F Yuan 1
Published under licence by IOP Publishing Ltd IOP Conference Series: Earth and Environmental Science , Volume 82 , 3rd International Conference on Water Resource and Environment (WRE 2017) 26–29 June 2017, Qingdao, China Citation J Lu and F Yuan 2017 IOP Conf. Ser.: Earth Environ. Sci. 82 012005 DOI 10.1088/1755-1315/82/1/012005
Article metrics
6773 Total downloads
Share this article
Author e-mails.
Author affiliations
1 Department of Engineering and Safety, UiT The Arctic University of Norway, N-9037 Tromsø, Norway
Buy this article in print
Drinking water is an important source for trace elements intake into human body. Thus, the drinking water quality has a great impact on people's health and longevity. This study aims to study the relationship between drinking water quality and human health and longevity. A longevity county Mayang in Hunan province, China was chosen as the study area. The drinking water and hair of local centenarians were collected and analyzed the chemical composition. The drinking water is weak alkaline and rich in the essential trace elements. The daily intakes of Ca, Cu, Fe, Se, Sr from drinking water for residents in Mayang were much higher than the national average daily intake from beverage and water. There was a positive correlation between Ni and Pb in drinking water and Ni and Pb in hair. There were significant correlations between Cu, K in drinking water and Ba, Ca, Mg, Sr in the hair at the 0.01 level. The concentrations of Mg, Sr, Se in drinking water showed extremely significant positive relation with two centenarian index 100/80% and 100/90% correlation. Essential trace elements in drinking water can be an important factor for local health and longevity.
Export citation and abstract BibTeX RIS
Content from this work may be used under the terms of the Creative Commons Attribution 3.0 licence . Any further distribution of this work must maintain attribution to the author(s) and the title of the work, journal citation and DOI.
- Open access
- Published: 21 January 2016
Drinking water quality assessment and its effects on residents health in Wondo genet campus, Ethiopia
- Yirdaw Meride 1 &
- Bamlaku Ayenew 1
Environmental Systems Research volume 5 , Article number: 1 ( 2016 ) Cite this article
111k Accesses
90 Citations
Metrics details
Water is a vital resource for human survival. Safe drinking water is a basic need for good health, and it is also a basic right of humans. The aim of this study was to analysis drinking water quality and its effect on communities residents of Wondo Genet.
The mean turbidity value obtained for Wondo Genet Campus is (0.98 NTU), and the average temperature was approximately 28.49 °C. The mean total dissolved solids concentration was found to be 118.19 mg/l, and EC value in Wondo Genet Campus was 192.14 μS/cm. The chloride mean value of this drinking water was 53.7 mg/l, and concentration of sulfate mean value was 0.33 mg/l. In the study areas magnesium ranges from 10.42–17.05 mg/l and the mean value of magnesium in water is 13.67 mg/l. The concentration of calcium ranges from 2.16–7.31 mg/l with an average value of 5.0 mg/l. In study areas, an average value of sodium was 31.23 mg/1and potassium is with an average value of 23.14 mg/1. Water samples collected from Wondo Genet Campus were analyzed for total coliform bacteria and ranged from 1 to 4/100 ml with an average value of 0.78 colony/100 ml.
On the basis of findings, it was concluded that drinking water of the study areas was that all physico–chemical parameters. All the Campus drinking water sampling sites were consistent with World Health Organization standard for drinking water (WHO).
Safe drinking water is a basic need for good health, and it is also a basic right of humans. Fresh water is already a limiting resource in many parts of the world. In the next century, it will become even more limiting due to increased population, urbanization, and climate change (Jackson et al. 2001 ).
Drinking water quality is a relative term that relates the composition of water with effects of natural processes and human activities. Deterioration of drinking water quality arises from introduction of chemical compounds into the water supply system through leaks and cross connection (Napacho and Manyele 2010 ).
Access to safe drinking water and sanitation is a global concern. However, developing countries, like Ethiopia, have suffered from a lack of access to safe drinking water from improved sources and to adequate sanitation services (WHO 2006 ). As a result, people are still dependent on unprotected water sources such as rivers, streams, springs and hand dug wells. Since these sources are open, they are highly susceptible to flood and birds, animals and human contamination (Messeret 2012 ).
The quality of water is affected by an increase in anthropogenic activities and any pollution either physical or chemical causes changes to the quality of the receiving water body (Aremu et al. 2011 ). Chemical contaminants occur in drinking water throughout the world which could possibly threaten human health. In addition, most sources are found near gullies where open field defecation is common and flood-washed wastes affect the quality of water (Messeret 2012 ).
The World Health Organization estimated that up to 80 % of all sicknesses and diseases in the world are caused by inadequate sanitation, polluted water or unavailability of water (WHO 1997 ). A review of 28 studies carried out by the World Bank gives the evidence that incidence of certain water borne, water washed, and water based and water sanitation associated diseases are related to the quality and quantity of water and sanitation available to users (Abebe 1986 ).
In Ethiopia over 60 % of the communicable diseases are due to poor environmental health conditions arising from unsafe and inadequate water supply and poor hygienic and sanitation practices (MOH 2011 ). About 80 % of the rural and 20 % of urban population have no access to safe water. Three-fourth of the health problems of children in the country are communicable diseases arising from the environment, specially water and sanitation. Forty-six percent of less than 5 years mortality is due to diarrhea in which water related diseases occupy a high proportion. The Ministry of Health, Ethiopia estimated 6000 children die each day from diarrhea and dehydration (MOH 2011 ).
There is no study that was conducted to prove the quality water in Wondo Genet Campus. Therefore, this study is conducted at Wondo Genet Campus to check drinking water quality and to suggest appropriate water treated mechanism.
Results and discussions
The turbidity of water depends on the quantity of solid matter present in the suspended state. It is a measure of light emitting properties of water and the test is used to indicate the quality of waste discharge with respect to colloidal matter. The mean turbidity value obtained for Wondo Genet Campus (0.98 NTU) is lower than the WHO recommended value of 5.00 NTU.
Temperature
The average temperature of water samples of the study area was 28.49 °C and in the range of 28–29 °C. Temperature in this study was found within permissible limit of WHO (30 °C). Ezeribe et al. ( 2012 ) reports similar result (29 °C) of well water in Nigeria.
Total dissolved solids (TDS)
Water has the ability to dissolve a wide range of inorganic and some organic minerals or salts such as potassium, calcium, sodium, bicarbonates, chlorides, magnesium, sulfates etc. These minerals produced un-wanted taste and diluted color in appearance of water. This is the important parameter for the use of water. The water with high TDS value indicates that water is highly mineralized. Desirable limit for TDS is 500 mg/l and maximum limit is 1000 mg/l which prescribed for drinking purpose. The concentration of TDS in present study was observed in the range of 114.7 and 121.2 mg/l. The mean total dissolved solids concentration in Wondo Genet campus was found to be 118.19 mg/l, and it is within the limit of WHO standards. Similar value was reported by Soylak et al. ( 2001 ), drinking water of turkey. High values of TDS in ground water are generally not harmful to human beings, but high concentration of these may affect persons who are suffering from kidney and heart diseases. Water containing high solid may cause laxative or constipation effects. According to Sasikaran et al. ( 2012 ).
Electrical conductivity (EC)
Pure water is not a good conductor of electric current rather’s a good insulator. Increase in ions concentration enhances the electrical conductivity of water. Generally, the amount of dissolved solids in water determines the electrical conductivity. Electrical conductivity (EC) actually measures the ionic process of a solution that enables it to transmit current. According to WHO standards, EC value should not exceeded 400 μS/cm. The current investigation indicated that EC value was 179.3–20 μS/cm with an average value of 192.14 μS/cm. Similar value was reported by Soylak et al. ( 2001 ) drinking water of turkey. These results clearly indicate that water in the study area was not considerably ionized and has the lower level of ionic concentration activity due to small dissolve solids (Table 1 ).
PH of water
PH is an important parameter in evaluating the acid–base balance of water. It is also the indicator of acidic or alkaline condition of water status. WHO has recommended maximum permissible limit of pH from 6.5 to 8.5. The current investigation ranges were 6.52–6.83 which are in the range of WHO standards. The overall result indicates that the Wondo Genet College water source is within the desirable and suitable range. Basically, the pH is determined by the amount of dissolved carbon dioxide (CO 2 ), which forms carbonic acid in water. Present investigation was similar with reports made by other researchers’ study (Edimeh et al. 2011 ; Aremu et al. 2011 ).
Chloride (Cl)
Chloride is mainly obtained from the dissolution of salts of hydrochloric acid as table salt (NaCl), NaCO 2 and added through industrial waste, sewage, sea water etc. Surface water bodies often have low concentration of chlorides as compare to ground water. It has key importance for metabolism activity in human body and other main physiological processes. High chloride concentration damages metallic pipes and structure, as well as harms growing plants. According to WHO standards, concentration of chloride should not exceed 250 mg/l. In the study areas, the chloride value ranges from 3–4.4 mg/l in Wondo Genet Campus, and the mean value of this drinking water was 3.7 mg/l. Similar value was reported by Soylak et al. ( 2001 ) drinking water of Turkey.
Sulfate mainly is derived from the dissolution of salts of sulfuric acid and abundantly found in almost all water bodies. High concentration of sulfate may be due to oxidation of pyrite and mine drainage etc. Sulfate concentration in natural water ranges from a few to a several 100 mg/liter, but no major negative impact of sulfate on human health is reported. The WHO has established 250 mg/l as the highest desirable limit of sulfate in drinking water. In study area, concentration of sulfate ranges from 0–3 mg/l in Wondo Genet Campus, and the mean value of SO 4 was 0.33 mg/l. The results exhibit that concentration of sulfate in Wondo Genet campus was lower than the standard limit and it may not be harmful for human health.
Magnesium (Mg)
Magnesium is the 8th most abundant element on earth crust and natural constituent of water. It is an essential for proper functioning of living organisms and found in minerals like dolomite, magnetite etc. Human body contains about 25 g of magnesium (60 % in bones and 40 % in muscles and tissues). According to WHO standards, the permissible range of magnesium in water should be 50 mg/l. In the study areas magnesium was ranges from 10.42 to 17.05 mg/l in Wondo Genet Campus and the mean value of magnesium in water is 13.67 mg/l. Similar value was reported by Soylak et al. ( 2001 ) drinking water of Turkey. The results exhibit that concentration of magnesium in Wondo Genet College was lower than the standard limit of WHO.
Calcium (Ca)
Calcium is 5th most abundant element on the earth crust and is very important for human cell physiology and bones. About 95 % of calcium in human body stored in bones and teeth. The high deficiency of calcium in humans may caused rickets, poor blood clotting, bones fracture etc. and the exceeding limit of calcium produced cardiovascular diseases. According to WHO ( 2011 ) standards, its permissible range in drinking water is 75 mg/l. In the study areas, results show that the concentration of calcium ranges from 2.16 to 7.31 mg/l in Wondo Genet campus with an average value of 5.08 mg/l.
Sodium (Na)
Sodium is a silver white metallic element and found in less quantity in water. Proper quantity of sodium in human body prevents many fatal diseases like kidney damages, hypertension, headache etc. In most of the countries, majority of water supply bears less than 20 mg/l, while in some countries the sodium quantity in water exceeded from 250 mg/l (WHO 1984 ). According to WHO standards, concentration of sodium in drinking water is 200 mg/1. In the study areas, the finding shows that sodium concentration ranges from 28.54 to 34.19 mg/1 at Wondo Genet campus with an average value of 31.23.
Potassium (k)
Potassium is silver white alkali which is highly reactive with water. Potassium is necessary for living organism functioning hence found in all human and animal tissues particularly in plants cells. The total potassium amount in human body lies between 110 and 140 g. It is vital for human body functions like heart protection, regulation of blood pressure, protein dissolution, muscle contraction, nerve stimulus etc. Potassium is deficient in rare but may led to depression, muscle weakness, heart rhythm disorder etc. According to WHO standards the permissible limit of potassium is 12 mg/1. Results show that the concentration of potassium in study areas ranges from 20.83 to 27.51 mg/1. Wondo Genet College with an average value of 23.14 mg/1. Present investigation was similar with reports made by other researchers’ study (Edimeh et al. 2011 ; Aremu et al. 2011 ). These results did not meet the WHO standards and may become diseases associated from potassium extreme surpassed.
Nitrate (NO 3 )
Nitrate one of the most important diseases causing parameters of water quality particularly blue baby syndrome in infants. The sources of nitrate are nitrogen cycle, industrial waste, nitrogenous fertilizers etc. The WHO allows maximum permissible limit of nitrate 5 mg/l in drinking water. In study areas, results more clear that the concentration of nitrate ranges from 1.42 to 4.97 mg/l in Wondo Genet campus with an average value of 2.67 mg/l. These results indicate that the quantity of nitrate in the study site is acceptable in Wondo Genet campus (Table 2 ).
Bacterial contamination
The total coliform group has been selected as the primary indicator bacteria for the presence of disease causing organisms in drinking water. It is a primary indicator of suitability of water for consumption. If large numbers of coliforms are found in water, there is a high probability that other pathogenic bacteria or organisms exist. The WHO and Ethiopian drinking water guidelines require the absence of total coliform in public drinking water supplies.
In this study, all sampling sites were not detected of faecal coliform bacteria. Figure 1 shows the mean values of total coliform bacteria in drinking water collected from the study area. All drinking water samples collected from Wondo Genet Campus were analyzed for total coliform bacteria and ranged from 1 to 4/100 ml with an average value of 0.78 colony/100 ml. In Wondo Genet College, the starting point of drinking water sources (Dam1), the second (Dam2) and Dam3 samples showed the presence of total coliform bacteria (Fig. 1 ). According to WHO ( 2011 ) risk associated in Wondo Genet campus drinking water is low risk (1–10 count/100 ml).
The mean values of total coliform bacteria in drinking water
According to the study all water sampling sites in Wondo Genet campus were meet world health organization standards and Ethiopia drinking water guideline. Figure 2 indicated that mean value of the study sites were under the limit of WHO standards.
Comparison of water quality parameters of drinking water of Wondo Genet campus with WHO and Ethiopia standards
Effect of water quality for residence health’s
Diseases related to contamination of drinking-water constitute a major burden on human health. Interventions to improve the quality of drinking-water provide significant benefits to health. Water is essential to sustain life, and a satisfactory (adequate, safe and accessible) supply must be available to all (Ayenew 2004 ).
Improving access to safe drinking-water can result in tangible benefits to health. Every effort should be made to achieve a drinking-water quality as safe as practicable. The great majority of evident water-related health problems are the result of microbial (bacteriological, viral, protozoan or other biological) contamination (Ayenew 2004 ).
Excessive amount of physical, chemical and biological parameters accumulated in drinking water sources, leads to affect human health. As discussed in the result, all Wondo Genet drinking water sources are under limit of WHO and Ethiopian guideline standards. Therefore, the present study was found the drinking water safe and no residence health impacts.
On the basis of findings, it was concluded that drinking water of the study areas was that all physico–chemical parameters in all the College drinking water sampling sites, and they were consistent with World Health Organization standard for drinking water (WHO). The samples were analyzed for intended water quality parameters following internationally recognized and well established analytical techniques.
It is evident that all the values of sodium (Na), potassium (K), calcium (Ca), magnesium (Mg), chloride (Cl), SO 4 , and NO 3 fall under the permissible limit and there were no toxicity problem. Water samples showed no extreme variations in the concentrations of cations and anions. In addition, bacteriological determination of water from College drinking water sources was carried out to be sure if the water was safe for drinking and other domestic application. The study revealed that all the College water sampling sites were not contained fecal coliforms except the three water sampling sites had total coliforms.
The study was conducted in Wondo Genet College of Forestry and Natural Resources campus, which is located in north eastern direction from the town of Hawassa and about 263 km south of Addis Ababa (Fig. 3 ). It lies between 38°37′ and 38°42′ East longitude and 7°02′ and 7°07′ north latitude. Landscape of the study area varies with an altitude ranging between 1600 and 2580 meters above sea level. Landscape of the study area varies with an altitude ranging between 1600 and 2580 meters above sea level.
Map of study area
The study area is categorized under Dega (cold) agro-ecological zone at the upper part and Woina Dega (temperate) agro-ecological zone at the lower part of the area. The rainfall distribution of the study area is bi-modal, where short rain falls during spring and the major rain comes in summer and stays for the first two months of the autumn season. The annual temperature and rainfall range from 17 to 19 °C and from 700 to 1400 mm, respectively (Wondo Genet office of Agriculture 2011).
Methodology
Water samples were taken at ten locations of Wondo Genet campus drinking water sources. Three water samples were taken at each water caching locations. Ten (10) water samples were collected from different locations of the Wondo Genet campus. Sampling sites for water were selected purposely which represents the entire water bodies.
Instead of this study small dam indicates the starting point of Wondo Genet campus drinking water sources rather than large dams constructed for other purpose. Taps were operated or run for at least 5 min prior to sampling to ensure collection of a representative sample (temperature and electrical conductivity were monitored to verify this). Each sample’s physico–chemical properties of water were measured in the field using portable meters (electrical conductivity, pH and temperature) at the time of sampling. Water samples were placed in clean containers provided by the analytical laboratory (glass and acid-washed polyethylene for heavy metals) and immediately placed on ice. Nitric acid was used to preserve samples for metals analysis.
Analysis of water samples
Determination of ph.
The pH of the water samples was determined using the Hanna microprocessor pH meter. It was standardized with a buffer solution of pH range between 4 and 9.
Measurement of temperature
This was carried out at the site of sample collection using a mobile thermometer. This was done by dipping the thermometer into the sample and recording the stable reading.
Determination of conductivity
This was done using a Jenway conductivity meter. The probe was dipped into the container of the samples until a stable reading will be obtained and recorded.
Determination of total dissolved solids (TDS)
This was measured using Gravimetric Method: A portion of water was filtered out and 10 ml of the filtrate measured into a pre-weighed evaporating dish. Filtrate water samples were dried in an oven at a temperature of 103 to 105 °C for \(2\frac{1}{2}\) h. The dish was transferred into a desiccators and allowed cool to room temperature and were weighed.
In this formula, A stands for the weight of the evaporating dish + filtrate, and B stands for the weight of the evaporating dish on its own Mahmud et al. ( 2014 ).
Chemical analysis
Chloride concentration was determined using titrimetric methods. The chloride content was determined by argentometric method. The samples were titrated with standard silver nitrate using potassium chromate indicator. Calcium ions concentrations were determined using EDTA titrimetric method. Sulphate ions concentration was determined using colorimetric method.
Microorganism analysis
In the membrane filtration method, a 100 ml water sample was vacuumed through a filter using a small hand pump. After filtration, the bacteria remain on the filter paper was placed in a Petri dish with a nutrient solution (also known as culture media, broth or agar). The Petri dishes were placed in an incubator at a specific temperature and time which can vary according the type of indicator bacteria and culture media (e.g. total coliforms were incubated at 35 °C and fecal coliforms were incubated at 44.5 °C with some types of culture media). After incubation, the bacteria colonies were seen with the naked eye or using a magnifying glass. The size and color of the colonies depends on the type of bacteria and culture media were used.
Statically analysis
All data generated was analyzed statistically by calculating the mean and compare the mean value with the acceptable standards. Data collected was statistically analyzed using Statistical Package for Social Sciences (SPSS 20).
Abbreviations
ethylene dinitrilo tetra acetic acid
Minstor of Health
nephelometric turbidity units
total dissolved solid
World Health Organization
Abebe L (1986) Hygienic water quality; its relation to health and the testing aspects in tropical conditions. Department of Civil Engineering, University of Tempere, Finland
Aremu MO et al (2011) Physicochemical characteristics of stream, well and borehole water sources in Eggon, Nasarawa State, Nigeria. J Chem Soc Nigeria 36(1):131–136
Google Scholar
Ayenew T (2004) Environmental implications of changes in the levels of lakes in the Ethiopian Rift since 1970. Reg Environ Chang 4:192–204
Article Google Scholar
Edimeh et al (2011) Physico-chemical parameters and some Heavy metals content of rivers Inachalo and Niger in Idah, Kogi State. J Chem Soc Nigeria 36(1):95–101
Ezeribe AL et al (2012) Physico-chemical properties of well water samples from some villages in Nigeria with cases of stained and mottle teeth. Sci World J 7(1):1–13
Jackson et al (2001) Water in changing world, Issues in Ecology. Ecol Soc Am, Washington, pp 1–16
Mahmud et al (2014) Surface water quality of Chittagong University campus, Bangladesh. J Environ Sci 8:2319-2399
Messeret B (2012) Assessment of drinking water quality and determinants of household potable water consumption in Simada district, ethiopia
MOH (2011) Knowledge, attitude and practice of water supply, environmental sanitation and hygiene practice in selected worked as of Ethiopia
Napacho A, Manyele V (2010) Quality assessment of drinking water in Temeke district (Part II): characterization of chemical parameters. Af J Environ Sci Technol 4(11):775–789
Sasikaran S et al (2012) Physical, chemical and microbial analysis of bottled drinking water. J Ceylon Medical 57(3):111–116
Soylak et al (2002) Chemical analysis of drinking water samples from Yozgat, Turkey. Polish J Environ Stud 11(2):151–156
WHO (1984) Guideline for drinking water quality. Health Criteria Support Inf 2:63–315
World Health Organization (1997) Basic Environmental Health, Geneva
World Health Organization (2004) Guidelines for drinking-water quality. World Health Organization, Geneva
World Health Organization (2006) In water, sanitation and health world health organization
WHO (2011) Guidelines for drinking-water quality, 4th edn. Geneva, Switzerland
Download references
Authors’ contributions
YM: participated in designing the research idea, field data collection, data analysis, interpretation and report writing; BA: participated in field data collection, interpretation and report writing. Both authors read and approved the final manuscript.
Authors’ information
Yirdaw Meride: Lecturer at Hawassa University, Wondo Genet College of Forestry and Natural Resources. He teaches and undertakes research on solid waste, carbon sequestration and water quality. He has published three articles mainly in international journals. Bamlaku Ayenew: Lecturer at Hawassa University, Wondo Genet College of Forestry and Natural Resources. He teaches and undertakes research on Natural Resource Economics. He has published three article with previous author and other colleagues.
Acknowledgements
Hawassa University, Wondo Genet College of Forestry and Natural Resources provided financial support for field data collection and water laboratory analysis. The authors thank anonymous reviewers for constructive comments.
Competing interests
The authors declare that they have no competing interests.
Author information
Authors and affiliations.
School of Natural Resource and Environmental Study, Wondo Genet College of Forestry and Natural Resources, Hawassa University, P.O. Box 128, Shashemene, Ethiopia
Yirdaw Meride & Bamlaku Ayenew
You can also search for this author in PubMed Google Scholar
Corresponding author
Correspondence to Yirdaw Meride .
Rights and permissions
Open Access This article is distributed under the terms of the Creative Commons Attribution 4.0 International License ( http://creativecommons.org/licenses/by/4.0/ ), which permits unrestricted use, distribution, and reproduction in any medium, provided you give appropriate credit to the original author(s) and the source, provide a link to the Creative Commons license, and indicate if changes were made.
Reprints and permissions
About this article
Cite this article.
Meride, Y., Ayenew, B. Drinking water quality assessment and its effects on residents health in Wondo genet campus, Ethiopia. Environ Syst Res 5 , 1 (2016). https://doi.org/10.1186/s40068-016-0053-6
Download citation
Received : 01 September 2015
Accepted : 06 January 2016
Published : 21 January 2016
DOI : https://doi.org/10.1186/s40068-016-0053-6
Share this article
Anyone you share the following link with will be able to read this content:
Sorry, a shareable link is not currently available for this article.
Provided by the Springer Nature SharedIt content-sharing initiative
- Drinking water
- Bacteriological
- Physico–chemical
Suggestions or feedback?
MIT News | Massachusetts Institute of Technology
- Machine learning
- Social justice
- Black holes
- Classes and programs
Departments
- Aeronautics and Astronautics
- Brain and Cognitive Sciences
- Architecture
- Political Science
- Mechanical Engineering
Centers, Labs, & Programs
- Abdul Latif Jameel Poverty Action Lab (J-PAL)
- Picower Institute for Learning and Memory
- Lincoln Laboratory
- School of Architecture + Planning
- School of Engineering
- School of Humanities, Arts, and Social Sciences
- Sloan School of Management
- School of Science
- MIT Schwarzman College of Computing
Desalination system could produce freshwater that is cheaper than tap water
Press contact :, media download.
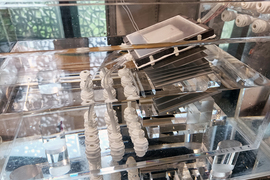
*Terms of Use:
Images for download on the MIT News office website are made available to non-commercial entities, press and the general public under a Creative Commons Attribution Non-Commercial No Derivatives license . You may not alter the images provided, other than to crop them to size. A credit line must be used when reproducing images; if one is not provided below, credit the images to "MIT."
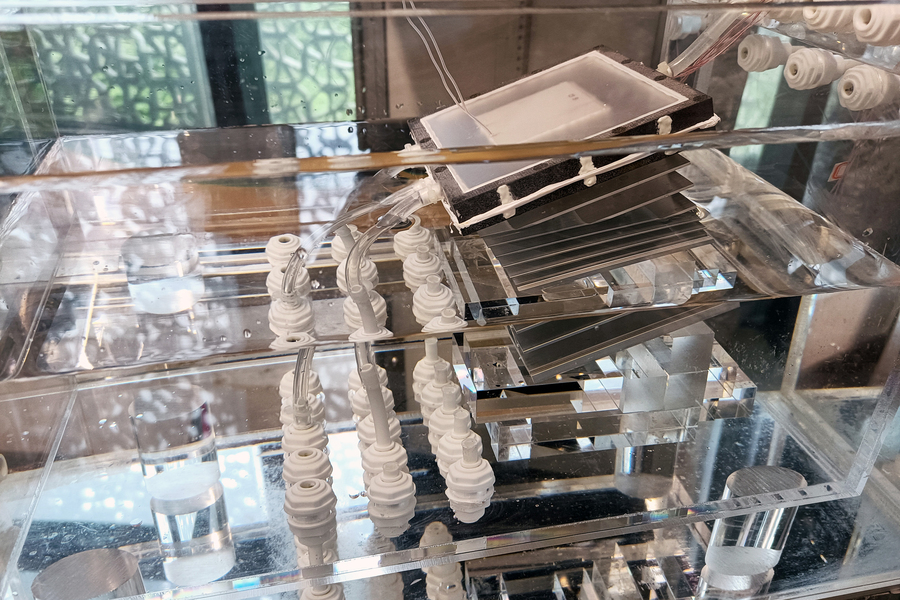
Previous image Next image
Engineers at MIT and in China are aiming to turn seawater into drinking water with a completely passive device that is inspired by the ocean, and powered by the sun.
In a paper appearing today in the journal Joule, the team outlines the design for a new solar desalination system that takes in saltwater and heats it with natural sunlight.
The configuration of the device allows water to circulate in swirling eddies, in a manner similar to the much larger “thermohaline” circulation of the ocean. This circulation, combined with the sun’s heat, drives water to evaporate, leaving salt behind. The resulting water vapor can then be condensed and collected as pure, drinkable water. In the meantime, the leftover salt continues to circulate through and out of the device, rather than accumulating and clogging the system.
The new system has a higher water-production rate and a higher salt-rejection rate than all other passive solar desalination concepts currently being tested.
The researchers estimate that if the system is scaled up to the size of a small suitcase, it could produce about 4 to 6 liters of drinking water per hour and last several years before requiring replacement parts. At this scale and performance, the system could produce drinking water at a rate and price that is cheaper than tap water.
“For the first time, it is possible for water, produced by sunlight, to be even cheaper than tap water,” says Lenan Zhang, a research scientist in MIT’s Device Research Laboratory.
The team envisions a scaled-up device could passively produce enough drinking water to meet the daily requirements of a small family. The system could also supply off-grid, coastal communities where seawater is easily accessible.
Zhang’s study co-authors include MIT graduate student Yang Zhong and Evelyn Wang, the Ford Professor of Engineering, along with Jintong Gao, Jinfang You, Zhanyu Ye, Ruzhu Wang, and Zhenyuan Xu of Shanghai Jiao Tong University in China.
A powerful convection
The team’s new system improves on their previous design — a similar concept of multiple layers, called stages. Each stage contained an evaporator and a condenser that used heat from the sun to passively separate salt from incoming water. That design, which the team tested on the roof of an MIT building, efficiently converted the sun’s energy to evaporate water, which was then condensed into drinkable water. But the salt that was left over quickly accumulated as crystals that clogged the system after a few days. In a real-world setting, a user would have to place stages on a frequent basis, which would significantly increase the system’s overall cost.
In a follow-up effort, they devised a solution with a similar layered configuration, this time with an added feature that helped to circulate the incoming water as well as any leftover salt. While this design prevented salt from settling and accumulating on the device, it desalinated water at a relatively low rate.
In the latest iteration, the team believes it has landed on a design that achieves both a high water-production rate, and high salt rejection, meaning that the system can quickly and reliably produce drinking water for an extended period. The key to their new design is a combination of their two previous concepts: a multistage system of evaporators and condensers, that is also configured to boost the circulation of water — and salt — within each stage.
“We introduce now an even more powerful convection, that is similar to what we typically see in the ocean, at kilometer-long scales,” Xu says.
The small circulations generated in the team’s new system is similar to the “thermohaline” convection in the ocean — a phenomenon that drives the movement of water around the world, based on differences in sea temperature (“thermo”) and salinity (“haline”).
“When seawater is exposed to air, sunlight drives water to evaporate. Once water leaves the surface, salt remains. And the higher the salt concentration, the denser the liquid, and this heavier water wants to flow downward,” Zhang explains. “By mimicking this kilometer-wide phenomena in small box, we can take advantage of this feature to reject salt.”
Tapping out
The heart of the team’s new design is a single stage that resembles a thin box, topped with a dark material that efficiently absorbs the heat of the sun. Inside, the box is separated into a top and bottom section. Water can flow through the top half, where the ceiling is lined with an evaporator layer that uses the sun’s heat to warm up and evaporate any water in direct contact. The water vapor is then funneled to the bottom half of the box, where a condensing layer air-cools the vapor into salt-free, drinkable liquid. The researchers set the entire box at a tilt within a larger, empty vessel, then attached a tube from the top half of the box down through the bottom of the vessel, and floated the vessel in saltwater.
In this configuration, water can naturally push up through the tube and into the box, where the tilt of the box, combined with the thermal energy from the sun, induces the water to swirl as it flows through. The small eddies help to bring water in contact with the upper evaporating layer while keeping salt circulating, rather than settling and clogging.
The team built several prototypes, with one, three, and 10 stages, and tested their performance in water of varying salinity, including natural seawater and water that was seven times saltier.
From these tests, the researchers calculated that if each stage were scaled up to a square meter, it would produce up to 5 liters of drinking water per hour, and that the system could desalinate water without accumulating salt for several years. Given this extended lifetime, and the fact that the system is entirely passive, requiring no electricity to run, the team estimates that the overall cost of running the system would be cheaper than what it costs to produce tap water in the United States.
“We show that this device is capable of achieving a long lifetime,” Zhong says. “That means that, for the first time, it is possible for drinking water produced by sunlight to be cheaper than tap water. This opens up the possibility for solar desalination to address real-world problems.”
“This is a very innovative approach that effectively mitigates key challenges in the field of desalination,” says Guihua Yu, who develops sustainable water and energy storage systems at the University of Texas at Austin, and was not involved in the research. “The design is particularly beneficial for regions struggling with high-salinity water. Its modular design makes it highly suitable for household water production, allowing for scalability and adaptability to meet individual needs.”
Funding for the research at Shanghai Jiao Tong University was supported by the Natural Science Foundation of China.
Share this news article on:
Press mentions, time magazine.
A number of MIT spinouts and research projects – including the MOXIE instrument that successfully generated oxygen on Mars, a new solar-powered desalination system and MIT spinout SurgiBox – were featured on TIME’s Best Inventions of 2023 list.
Insider reporter Katie Hawkinson explores how MIT researchers developed a new solar-powered desalination system that can remove the salt from seawater for less than the cost of U.S. tap water. Creating a device that relies on solar power, “eliminates a major financial barrier, especially for low-income countries experiencing water scarcity,” Hawkinson explains.
The Hill reporter Sharon Udasin writes that MIT researchers have developed a new solar-powered desalination device that “could last several years and generate water at a rate and price that is less expensive than tap water.” The researchers estimated that “if their model was scaled up to the size of a small suitcase, it could produce about 4 to 6 liters of drinking water per hour,” writes Udasin.
The Daily Beast
MIT researchers have developed a new desalination system that uses solar energy to convert seawater into drinkable water, reports Tony Ho Tran for the Daily Beast . The device could make it possible to, “make freshwater that’s even more affordable than the water coming from Americans’ kitchen faucets.”
Previous item Next item
Related Links
- Evelyn Wang
- Lenan Zhang
- Device Research Lab
- Department of Mechanical Engineering
Related Topics
- Desalination
- Mechanical engineering
- Sustainability
Related Articles
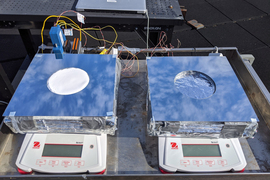
Passive cooling system could benefit off-grid locations
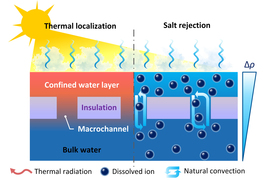
Solar-powered system offers a route to inexpensive desalination
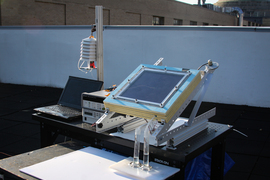
Solar-powered system extracts drinkable water from “dry” air
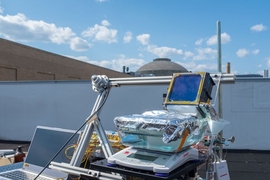
Simple, solar-powered water desalination
More mit news.
A biomedical engineer pivots from human movement to women’s health
Read full story →
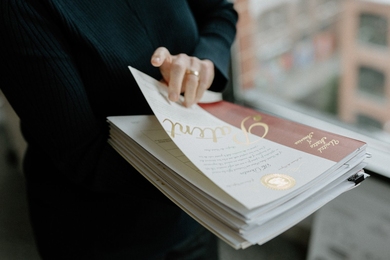
MIT tops among single-campus universities in US patents granted
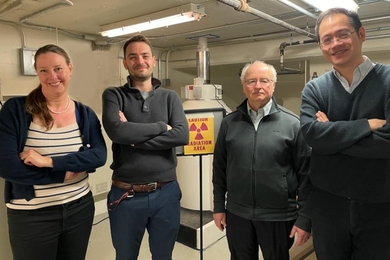
A new way to detect radiation involving cheap ceramics
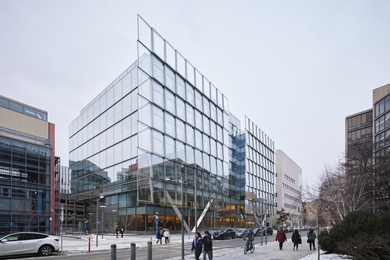
A crossroads for computing at MIT
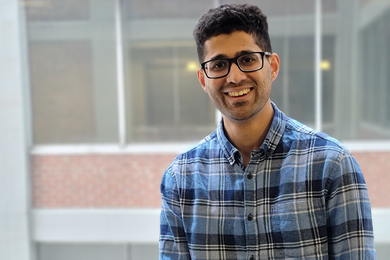
Growing our donated organ supply
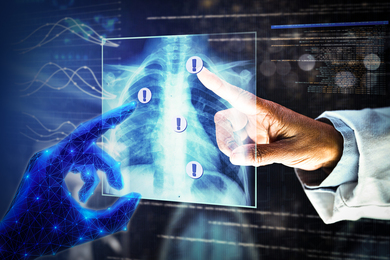
New AI method captures uncertainty in medical images
- More news on MIT News homepage →
Massachusetts Institute of Technology 77 Massachusetts Avenue, Cambridge, MA, USA
- Map (opens in new window)
- Events (opens in new window)
- People (opens in new window)
- Careers (opens in new window)
- Accessibility
- Social Media Hub
- MIT on Facebook
- MIT on YouTube
- MIT on Instagram

An official website of the United States government
The .gov means it’s official. Federal government websites often end in .gov or .mil. Before sharing sensitive information, make sure you’re on a federal government site.
The site is secure. The https:// ensures that you are connecting to the official website and that any information you provide is encrypted and transmitted securely.
- Publications
- Account settings
Preview improvements coming to the PMC website in October 2024. Learn More or Try it out now .
- Advanced Search
- Journal List
- Med Gas Res
- v.8(4); Oct-Dec 2018

Daily ingestion of alkaline electrolyzed water containing hydrogen influences human health, including gastrointestinal symptoms
Yoshinori tanaka.
1 Appliances Company, Panasonic Corporation, Shiga, Japan
Yasuhiro Saihara
Kyoko izumotani.
2 Osaka Municipal Health Promotion Center, Osaka, Japan
Hajime Nakamura
3 Osaka City University, Graduate School of Medicine, Osaka, Japan
Author contributions
In Japan, alkaline electrolyzed water (AEW) apparatus have been approved as a medical device. And for the patients with gastrointestinal symptoms, drinking AEW has been found to be effective in relieving gastrointestinal symptoms. But some users of AEW apparatus do not have abdominal indefinite complaint. Little attention has been given to the benefit for the users which have no abdominal indefinite complaint. The object of this study is to evaluate the effect on health, including gastrointestinal symptoms, when a person without abdominal indefinite complaint, etc ., drinks AEW on a daily basis. A double-blind, randomized controlled trial has been designed. Four-week period of everyday water drinking, PW drinking group: drink purified tap water as a placebo, AEW drinking group: drink alkaline electrolyzed water which made by electrolysis of purified tap water. Before the experiment and after the 4-week period of water drinking, Blood tests, physical fitness evaluations, and questionnaire evaluations is conducted. In this study, we did not specifically select patients with gastrointestinal symptoms. Sufficiently clear effect could not be confirmed. But the stools were more normal, and, as shown in the previous report, that drinking AEW is considered to contribute to intestinal normalization. In addition, when drinking AEW, a high proportion of the respondents said that they felt they were able to sleep soundly, and the proportion of subjects who answered that they felt good when awakening increased. The effect of reducing oxidative stress, thus allowing for improved sleep, was exhibited by drinking AEW containing hydrogen, which is considered to be an antioxidant substance. This research were approved by the Ethics Committee of the Osaka City University Graduate School of Medicine (No. 837) and were registered in the University Hospital Medical Information Network (UMIN) Clinical Trials Registry (UMIN ID: UMIN000031800) on March 22, 2018.
I NTRODUCTION
In Japan, water which is obtained on the cathode side by the electrolysis of tap water is called alkaline electrolyzed water (AEW) or reduced hydrogen water. 1 Improvement of gastrointestinal symptoms by ingesting AEW has been confirmed by Japanese researchers. For example, Naito et al. 2 reported the inhibitory effect of AEW ingestion on gastric mucosal disorder caused by aspirin, and Hayakawa et al. 3 reported the inhibitory effect of AEW ingestion on abnormal intestinal fermentation. Tashiro et al. 4 examined the effect of ingesting AEW or purified tap water (PW; as a placebo) at a rate of at 500 mL per day for 4 weeks in patients who had abdominal pain such as heartburn, stomach discomfort, abdominal bloating, diarrhea, constipation, etc ., and reported that the results of the AEW group were superior to those of the placebo group. 5 , 6 From these results, apparatus that produce AEW have been approved as medical devices by the Japanese Ministry of Health, Labour and Welfare. AEW is thought to be effective for functional gastrointestinal disorders. 5
Since AEW is produced by electrolyzing water, hydroxide ions, which are alkaline in nature, are generated. Hydrogen molecules are also generated on the electrode surface and dissolved in water. Therefore, AEW is alkaline water containing hydrogen. 1 In conventional efficacy studies, evaluations with respect to ingesting AEW have typically been conducted focusing on the alkalinity of the water. 2 , 3 , 4 , 5 In recent years, however, the assumed effectiveness of the antioxidant effect of dissolved hydrogen on various diseases has been reported. 7 , 8 , 9 , 10 , 11 , 12 , 13 , 14 , 15 Nevertheless, some users of AEW apparatus do not have any definite abdominal symptoms. In many cases, they are drinking AEW on a daily basis to improve their health, and many users also feel health benefits such as improvement in exercise capacity 12 . These may be thought to be due to the action of dissolved hydrogen. There have been no researched studies of these in detail. The object of this study is to evaluate the effect of daily ingestion of AEW on health, including gastrointestinal symptoms, in subjects without any definite abdominal symptoms.
P ARTICIPANTS AND M ETHODS
Participants.
Healthy men and women (20–60 years) who use the Osaka City Citizen Health Development Consultation Center were selected as test subjects to determine the health effect of daily AEW ingestion. It was aimed to clarify whether general subjects without gastrointestinal symptoms have another good effect besides gastrointestinal symptoms by drinking AEW which is good for gastrointestinal symptoms. We explained this purpose to the subjects and asked for research participation. Written informed consent was obtained from all subjects. All procedures used in this research were approved by the Ethics Committee of the Osaka City University Graduate School of Medicine (No. 837) and were registered in the University Hospital Medical Information Network (UMIN) Clinical Trials Registry (UMIN ID: UMIN000031800) on March 22, 2018. This study follows the Consolidated Standards of Reporting Trials (CONSORT) guidelines. A double-blind, randomized controlled trial has been designed, and the research design is shown in Figure 1 .

Research design.
Note: PW: Purified tap water; AEW: alkaline electrolyzed water.
Subjects were randomly divided into two groups, with an AEW group ( n = 30) and a PW group ( n = 30). Blood tests, physical fitness evaluations, and questionnaire evaluations were conducted before the experiment was initiated. Subjects were provided with AEW apparatus 1 that had been modified to produce only AEW or PW. They ingested 500 mL or more of freshly produced AEW or PW per day (they were required to ingest 200 mL immediately after awakening, and 300 mL or more during the rest of the day). After the end of the four-week period, blood tests, physical fitness evaluations, and questionnaire evaluations were conducted again to check whether the ingestion of AEW for four weeks had beneficial effects on the health of the subjects.
Blood sample/urinalysis
General blood test: Red blood cell count, white blood cell count, hemoglobin, hematocrit, and platelet count.
Blood biochemical examination: Total protein, albumin, glutamic oxaloacetic transaminase (GOT), glutamic pyruvic transaminase (GPT), γ-GTP, total cholesterol, high-density lipoprotein (HDL), cholesterol, low-density lipoprotein (LDL) cholesterol, neutral fat, uric acid, creatinine, and blood sugar.
Urinalysis: Urine sugar, urine protein, urine occult blood, and urine pH.
Physical measurements
Right/left grip strength, right/left leg muscle strength, vertical jump, whole body reaction time, standing time on one leg with eyes closed, sit-up, seated forward bend, and resting blood pressure.
Questionnaire variables
Gastrointestinal symptoms (stomachache, heartburn, heavy stomach, lower abdominal pain, bloated stomach), urinary frequency, condition of the stools (fecal properties and bowel movement), and physical condition (sleep quality and upon awakening).
Statistical analysis
In the blood data, the urinalysis and physical measurement values, the statistical significance of the average difference (before and after AEW, PW drinking) was analysed using a paired t -test (Statcel 4 Software [OMS Publishing, Saitama, Japan). The questionnaire data (before and after AEW, PW drinking) was analysed by the Wilcoxon signed-rank test using the same Statcel 4 software. Differences for which P values of < 0.05 and < 0.01 were inferred as significant.
Conditions of subjects and water quality
Subjects with abdominal symptoms such as heartburn, stomach discomfort, abdominal bloating, diarrhea, and constipation were used in the study previously performed. 4 , 5 For the current study, subjects aged 20 to 69 years were randomly selected among medical checkup examinees who visited the Osaka City Citizen Health Development Consultation Center, and then divided into two groups. One group ingested PW while the other ingested AEW. Neither the subjects nor the experimenters knew which group the subjects belonged to. Figure 2 shows that no significant differences were found in dispersion of mean values and distribution values.

Age distribution of subjects.
Each subject was provided with an AEW apparatus that had been modified to either produce or not produce AEW, and asked to install it at their home. In order to verify the quality of the drinking water, the water produced by the apparatus was taken into aluminum containers and collected when the subjects came in for measurement. Figure 3 shows the water quality distribution of each drinking water.

Water quality distribution of two types of drinking water.
Because we selected subjects who live in or around Osaka City, the tap water from either the same or a nearby water source was used for the evaluation. For this reason, the tests have been conducted using water of equivalent quality and which shows little bias in the distribution of ions.
Regarding the water before and after the electrolysis, the pH was 7.6 ± 0.2 for the PW group, and 9.2 ± 0.2 for the AEW group. Dissolved hydrogen concentration was not measurable at the subjects’ houses because hydrogen easily escapes water. However, for non-electrolyzed and electrolyzed tap water from the same water source and using the same water apparatus, the hydrogen concentration was confirmed as 0 mg/L in the PW group and 0.2 mg/L for the AEW group for the characteristics of the device.
Comparison of hematological values
The hematological data of subjects in the PW group and the AEW group were compared before and after the four-week period, but no significant differences were observed in both groups. This is consistent with the contents of the previous report. 5 However, the HDL cholesterol level, a newly measured value this time, of the AEW group showed a tendency to increase with P = 0.097, as shown in Figure 4 .

Change in HDL cholesterol before and after drinking.
Note: (A) alkaline electrolyzed water (AEW) drinking group, (B) purified tap water (PW) drinking group. HDL: High-density lipoprotein.
Comparison of data related to physical abilities
For the seated forward bend, vertical jump, right/left grip strength, and sit-up, there was no significant difference before and after the 4-week period for both the PW group and the AEW group.
Regarding the whole body reaction time, no significant differences were observed before and after the 4-week period in the case of the PW group, as seen in Figure 5B . However, a significant difference (decrease) ( P < 0.05) was observed in the AEW group, as seen in Figure 5A . As for standing time on one leg with eyes closed, longer times were observed in the AEW group ( P = 0.09), as seen in Figure 6A .

Change in whole body reaction time before and after drinking.
Note: (A) Alkaline electrolyzed water (AEW) drinking group; (B) purified tap water (PW) drinking group.

Changes in the standing time on one leg with eyes closed before and after drinking.
Note: (A) alkaline electrolyzed water (AEW) drinking group, (B) purified tap water (PW) drinking group.
Questionnaire to subjects
As for the questionnaire items, we asked the subjects to provide answers in 3 to 5 points about gastrointestinal symptoms ( Table 1 ), defecation and urination ( Table 2 ), and physical condition ( Table 3 ).
Gastrointestinal symptoms
Note: Scoring 1 to 4, where: Not at all = 1, and Very much = 4.
Defecation and urination
Physical conditionn
Note: Scoring 1 to 3, where: Good = 1, and Bad = 3.
First, as seen in Figures 7 7 to to 11 , 11 , as for gastrointestinal symptoms, sufficiently clear effect could not be confirmed in this study. Next, as seen in Figure 12 , the urinary frequency significantly increased in both groups, likely due to an increase in urine volume resulting from water ingestion. Regarding bowel movement, the stools slightly changed from slightly soft to normal or slightly hard, or from soft to normal ( P < 0.05) in the AEW group, as can be seen in Figure 13A . There was no difference between subjects of the two groups who had answered that they were in “good” or “bad” physical condition.

Change in stomach ache before and after drinking.
Note: Left side: alkaline electrolyzed water (AEW), and right side: purified tap water (PW).

Change in bloated stomach before and after drinking.

Change in urinary frequency before and after drinking.
Note: (A) Alkaline electrolyzed water (AEW) drinking group, and (B) purified tap water (PW) drinking group.

Changes in the condition of stools before and after drinking.

Change in heartburn before and after drinking.

Change in heavy stomach before and after drinking.

Change in lower abdominal pain before and after drinking.
Regarding sleep quality, there was a significant increase ( P < 0.01) in the number of AEW group subjects who responded that they were able to sleep well, as shown in Figure 14A , and there was a significant increase ( P < 0.05) in the number of subjects from the same group who said that they felt good upon awakening, as seen in Figure 15A .

Change in sleep quality before and after drinking.

Changes in the state of getting up before and after drinking.
D ISCUSSION
In Japan, AEW apparatus have been approved as medical devices, and ingesting AEW has been found to be effective in relieving gastrointestinal symptoms. A clinical evaluation of this effect was conducted with patients with gastrointestinal symptoms (heartburn, stomach discomfort, and abdominal symptoms such as abdominal bloating, diarrhea, and constipation). 5
Antioxidant action by hydrogen and gastric acid neutralization by alkaline pH have been considered. 6 In addition, recent studies have shown that the intestinal bacterial flora distribution changes. It seems that these are involved in the normalization of the gastrointestinal activity. 11 However, for this study, patients with gastrointestinal symptoms were not specifically selected. As for these as well as the previous results, in general, there was no difference in the hematological values between the PW group and the AEW group. 5 However, the newly measured HDL cholesterol value showed a tendency to increase with P = 0.097. The increase in HDL cholesterol by ingesting water containing hydrogen is reported by Gadek and colleagues. 16 The effect of hydrogen can be considered to have had an effect in the AEW group this time as well.
As for gastrointestinal symptoms—which showed a significant difference during the previous study (significant improvement of abdominal symptoms and improvement of abnormal bowel movement) 4 , 5 —sufficiently clear effect could not be confirmed by this study because the subjects did not show gastrointestinal symptoms, and very few of them responded that they had abnormal abdominal symptoms and bowel movement before participating in this study. Therefore, we believe this is the reason the answers of the subjects were the same before and after their participation in the study.
However, with respect to bowel movement, the stools slightly changed from soft to normal or slightly hard, or from loose to normal in the AEW group. This reflects that the stools are more normal, and, as shown in the previous report, that ingesting AEW is considered to contribute to intestinal normalization. 4 , 5 , 6 Regarding items other than the gastrointestinal tract, a high proportion of the respondents said that they felt they were able to sleep well, and the proportion of subjects who answered that they felt good when awakening increased. Various studies on the relationship between the ingestion of antioxidant substances and the condition of sleep have been undertaken, 17 and the effect of reducing oxidative stress, thus allowing for improved sleep quality, is exhibited by ingesting AEW containing hydrogen, which is considered an antioxidant substance.
Regarding sports performance, various reports on the effects of sleep on sports performance have concluded that willingly sleeping longer can lead to faster running, shortened reaction time, and improved motivation during practice and games. 18 Improved sleep quality by ingesting AEW is, therefore, believed to help reduce fatigue, ensure appropriate endurance recovery, and improve overall sports performance.
The findings of this study indicate that ingesting AEW on a daily basis improves health and exercise capacity, even in healthy people who do not have gastrointestinal symptoms.
Funding: The study was supported by a grant from Matsushita Electric Works Co., Ltd. Home Appliances R&D Center (to HN).
Conflicts of interest
The corresponding author (YT) is a salaried employee of the Panasonic Corporation. One of the authors (SY) was a salaried employee of the Panasonic Corporation. This study does not alter our adherence to Medical Gas Research policies on sharing data and materials. Another authors (KI and HN) report no conflict of interest related to this manuscript.
Financial support
The study was supported by a grant from Matsushita Electric Works Co., Ltd. Home Appliances R&D Center (to HW).
Institutional review board statement
All procedures used in this research were approved by the Ethics Committee of the Osaka City University Graduate School of Medicine (No. 837) and were registered in the University Hospital Medical Information Network (UMIN) Clinical Trials Registry (UMIN ID: UMIN000031800) on March 22, 2018.
Declaration of participant consent
The authors certify that they have obtained participant consent forms. In the form, participant have given their consent for their images andother clinical information to be reported in the journal. The patients understand that their names and initials not be published and due efforts will be made to conceal their identity, but anonymity cannot be guaranteed.
Reporting statement
This study follows the Consolidated Standards of Reporting Trials (CONSORT) guidelines.
Biostatistics statement
The statistical methods of this study were reviewed by the biostatistician of the Osaka City University, Osaka, Japan.
Copyright license agreement
The Copyright License Agreement has been signed by all authors before publication.
Data sharing statement
Individual participant data that underlie the results reported in this article, after deidentification (text, tables, figures, and appendices). Study protocol and informed consent form will be available immediately following publication, without end date. Results will be disseminated through presentations at scientific meetings and/or by publication in a peer-reviewed journal. Anonymized trial data will be available indefinitely at www.figshare.com .
Plagiarism check
Checked twice by iThenticate.
Peer review
Externally peer reviewed.
R EFERENCES
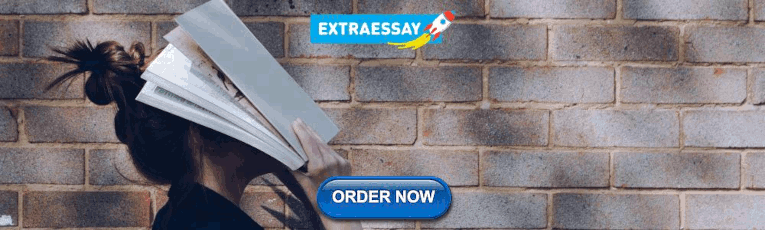
COMMENTS
The papers in this issue are interesting and cover many aspects of this research topic, and will be meaningful for the sustainable drinking water quality protection.
Exposure to chemicals in drinking water may lead to a range of chronic diseases (e.g., cancer and cardiovascular disease), adverse reproductive outcomes and effects on children's health (e.g., neurodevelopment), among other health effects [ 3 ]. Although drinking water quality is regulated and monitored in many countries, increasing knowledge ...
Water is a vital natural resource for human survival as well as an efficient tool of economic development. Drinking water quality is a global issue, with contaminated unimproved water sources and inadequate sanitation practices causing human diseases (Gorchev & Ozolins, 1984; Prüss-Ustün et al., 2019).Approximately 2 billion people consume water that has been tainted with feces ().
In association with the International Water Association Water Research has an open access companion journal Water Research X, sharing the same aims and scope, editorial team, submission system and rigorous peer review. Water Research publishes refereed, original research papers on all aspects of the science and technology of the anthropogenic water cycle, water quality, and its management ...
Using these two measures of poor water quality, we find 2.44% of community water systems, a total of 1165, were Safe Drinking Water Act Serious Violators and 3.37% of Clean Water Act permittees in ...
1. Parameter selection for measurement of water quality (Shah and Joshi 2017):. The selection is carried out based on the management objectives and the environmental characteristics of the research area (Yan et al. 2015).Many variables are recommended, since they have a considerable impact on water quality and derive from 5 classes namely, oxygen level, eutrophication, health aspects, physical ...
The quality of microplastic research has been debated recently (Burton, 2017; ... 2017), which is not covered in this paper that only addresses drinking water and its sources. The fact that high quality data are limited also has implications for human health risk assessment, which considers both exposure as well as health effects. Only four out ...
A summary of available peer-reviewed studies on POU water treatment technologies is provided in Table 11, where we compare contaminant types, specific contaminants, treatment technologies, and ...
This special issue features four review papers and three research papers that focus on solar desalination, atmospheric water harvesting and CDI for clean drinking water, which aim to further promote the development of related technologies. Direct solar desalination, which produces freshwater directly using solar energy with minimum carbon ...
Drinking water is considered as one possible medium for the introduction of MPs into the human body . There is a growing interest around the prevalence of MPs in drinking water underpinned by recent research but a systematic review of available evidence is lacking [29-35].
Water quality has been linked to health outcomes across the world. This study evaluated the physico-chemical and bacteriological quality of drinking water supplied by the municipality from source ...
The growth of published drinking water research observed in the past 20 years is reflective of efforts to reduce drinking water inequalities through the MDGs and similarly, the acceleration of such research outputs since 2016 is likely a consequence of the international community's doubling down on drinking water priorities through the ...
The terms "drinking water project" and "intervention" are used interchangeably in water research, and they commonly refer to improvement or protection of drinking water sources and distribution compared to a certain target level. ... we identified six psychosocial stressors related to inadequate drinking water services. This paper ...
Background Water is the most abundant resource on earth, however water scarcity affects more than 40% of people worldwide. Access to safe drinking water is a basic human right and is a United Nations Sustainable Development Goal (SDG) 6. Globally, waterborne diseases such as cholera are responsible for over two million deaths annually. Cholera is a major cause of ill-health in Africa and ...
New research papers, reviews, case reports and conference papers are welcome to this issue. Papers dealing with new approaches to derive drinking water standards or risk assessment and management are also welcome. Other manuscript types accepted include methodological papers, position papers, brief reports, and commentaries.
Two of the water sources which are community-based water supply systems recorded high levels of Al which exceeded the aesthetic permissible levels of drinking water; others fell within this limit. Similarly, the levels of iron (Fe) varied between 37.30-1354 mg/L and 35.21-1262 mg/L in the wet and the dry seasons, respectively ( Figure 9 ).
The drinking water is weak alkaline and rich in the essential trace elements. The daily intakes of Ca, Cu, Fe, Se, Sr from drinking water for residents in Mayang were much higher than the national average daily intake from beverage and water. There was a positive correlation between Ni and Pb in drinking water and Ni and Pb in hair.
192. Drinking Water Quality Assessment. Background: Drinking water quality is the great public health concern because it is a major risk factor for high. incidence of diarrheal diseases in Nepal ...
Water is a vital resource for human survival. Safe drinking water is a basic need for good health, and it is also a basic right of humans. The aim of this study was to analysis drinking water quality and its effect on communities residents of Wondo Genet. The mean turbidity value obtained for Wondo Genet Campus is (0.98 NTU), and the average temperature was approximately 28.49 °C.
1.2. Consumers of Bottled Water. Eighty-five million bottles of water are consumed in the United States every day and more than thirty billion bottles a year [].The adoption of a health preventive action like drinking bottled water is suggested to be influenced by perception of risk associated with drinking water [].The perception of risk is also thought to be closely related to the subjective ...
Microplastics (MPs) have entered drinking water (DW) via various pathways, raising concerns about their potential health impacts. This study provides a comprehensive review of MP-associated chemicals, such as oligomers, plasticizers, stabilizers, and ultraviolet (UV) filters that can be leached out during DW treatment and distribution. The leaching of these chemicals is influenced by various ...
The critical adaptations cross an array of species, including man. Without water, humans can survive only for days. Water comprises from 75% body weight in infants to 55% in elderly and is essential for cellular homeostasis and life. 1 Nevertheless there are many unanswered questions about this most essential component of our body and our diet ...
The Hill reporter Sharon Udasin writes that MIT researchers have developed a new solar-powered desalination device that "could last several years and generate water at a rate and price that is less expensive than tap water." The researchers estimated that "if their model was scaled up to the size of a small suitcase, it could produce about 4 to 6 liters of drinking water per hour ...
1. Introduction. Water is essential for life and is involved in virtually all functions of the human body [].It is important in thermoregulation, as a solvent for biochemical reactions, for maintenance of vascular volume, and as the transport medium for providing nutrients within and removal of waste from the body [].Deficits in body water can compromise our health if they lead to substantial ...
Four-week period of everyday water drinking, PW drinking group: drink purified tap water as a placebo, AEW drinking group: drink alkaline electrolyzed water which made by electrolysis of purified tap water. ... This research were approved by the Ethics Committee of the Osaka City University Graduate School of Medicine (No. 837) and were ...