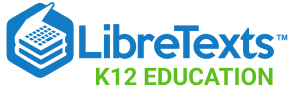
- school Campus Bookshelves
- menu_book Bookshelves
- perm_media Learning Objects
- login Login
- how_to_reg Request Instructor Account
- hub Instructor Commons
- Download Page (PDF)
- Download Full Book (PDF)
- Periodic Table
- Physics Constants
- Scientific Calculator
- Reference & Cite
- Tools expand_more
- Readability
selected template will load here
This action is not available.
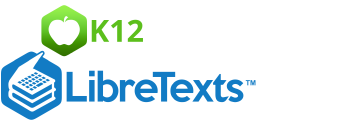
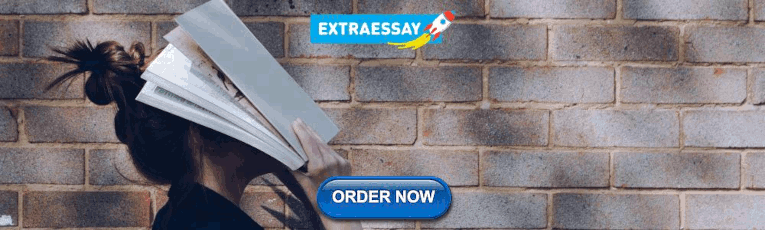
3.16: The Theory of Production
- Last updated
- Save as PDF
- Page ID 6810
The Theory of Production
The theory of production examines the relationship between the factors of production (land, labor, capital, entrepreneur) and the output of goods and services. The theory of production is based on the "short run" or a period of production that allows production to change the amount of variable input, in this case, labor. The "long run" is a period of production that is long enough for producers to adjust various inputs to analyze the best mix of the factors of production.
Universal Generalizations
- The theory of production deals with the relationship between the factors of production and the output of goods and services.
- The law of variable proportions can explain how increasing units of a single input will cause the output to vary.
Guiding Questions
- Why is it important for an owner of a company to understand the theory of production?
- What is the easiest factor of production to change in order to vary total product output?
Video: Diminishing Marginal Returns
Video: Economies of Scale
Law of Variable Proportion
The Law of Variable Proportion can be best illustrated by using the "production function" for the concept that describes the relationship between changes in output to different amounts of a single input while all other inputs are held constant. This concept helps a producer determine the best use of resources to effect output. The basic model used by economists is the hypothetical production schedule to determine output when the number of workers changes. In this scenario, the company can calculate the total product, or total output, that the firm will produce.
Generally, there are three stages of production. Each stage impacts returns. Stage 1 begins when the first worker is hired, but there are not enough workers to produce efficiently enough to create a positive return. Until the company hires enough workers to run all of the machinery, this stage results in increasing returns. As long as each new worker contributes to the total output than the worker before, total output rises faster and faster.
Unfortunately, a company cannot continue in Stage 1 because as soon as it is discovered that adding additional workers increases output, the company continues to hire additional employees. By Stage 2 production output continues to rise, but at small and smaller increments. Soon additional workers hired may be needed to do things other than produce, like stock shelves or answer phones. The total production is slowing down so this stage is no longer producing increasing returns, but now it is diminishing returns.
At Stage 3 the company has hired too many workers and now the output is considered producing in negative returns. Too many workers get in each other's way and do not produce as much as in Stage 1 or even Stage 2. By Stage 3 the marginal output becomes negative and the total factory output decreases. The exact number of workers needed by a company can only be ascertained when the cost of adding each new worker is calculated. If the cost is low, then more workers can be hired. If the cost is high, the factory will need to consider how to produce the highest output amount in Stage 2 for the least amount of money.
Once the output has been calculated the measure is known as "marginal product". Marginal product is the extra output or change in the total product caused by the addition of one more unit of variable input, in this case, the number of workers. In order to determine the optimal input used in production, the changes in marginal product are examined in the various stages of production. The stages of production analyze the increasing returns, diminishing returns, and the negative returns, to calculate the best use of resources and inputs to produce at an optimal level.
How Production Costs Affect Supply
A supply curve shows how quantity supplied will change as the price rises and falls, assuming ceteris paribus so that no other economically relevant factors are changing. If other factors relevant to supply do change, then the entire supply curve will shift. Just as a shift in demand is represented by a change in the quantity demanded at every price, a shift in supply means a change in the quantity supplied at every price.
In thinking about the factors that affect supply, remember what motivates firms: profits, which are the difference between revenues and costs. Goods and services are produced using combinations of labor, materials, and machinery, or what we call inputs or factors of production. If a firm faces lower costs of production while the prices for the good or service the firm produces remain unchanged, a firm’s profits go up. When a firm’s profits increase, it is more motivated to produce output, since the more it produces the more profit it will earn. So, when costs of production fall, a firm will tend to supply a larger quantity at any given price for its output. This can be shown by the supply curve shifting to the right.
Take, for example, a messenger company that delivers packages around a city. The company may find that buying gasoline is one of its main costs. If the price of gasoline falls, then the company will find it can deliver messages more cheaply than before. Since lower costs correspond to higher profits, the messenger company may now supply more of its services at any given price. For example, given the lower gasoline prices, the company can now serve a greater area and increase its supply.
Conversely, if a firm faces higher costs of production, then it will earn lower profits at any given selling price for its products. As a result, a higher cost of production typically causes a firm to supply a smaller quantity at any given price. In this case, the supply curve shifts to the left.
Consider the supply of cars, shown by curve S 0 in Figure 1. Point J indicates that if the price is $20,000, the quantity supplied will be 18 million cars. If the price rises to $22,000 per car, ceteris paribus, the quantity supplied will rise to 20 million cars, as point K on the S 0 curve shows. The same information can be shown in table form, as in Table 1.
Shifts in Supply: A Car Example
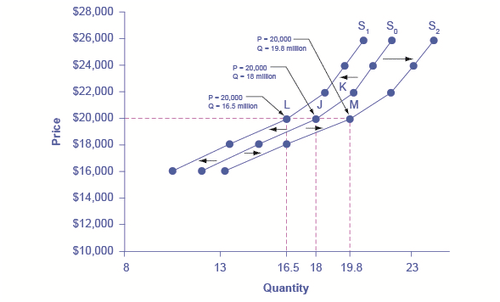
Decreased supply means that at every given price, the quantity supplied is lower, so that the supply curve shifts to the left, from S 0 to S 1 . Increased supply means that at every given price, the quantity supplied is higher, so that the supply curve shifts to the right, from S 0 to S 2 .
Imagine that the price of steel, an important ingredient in manufacturing cars, rises. Now producing a car has become more expensive. At any given price for selling cars, car manufacturers will react by supplying a lower quantity. This can be shown graphically as a leftward shift of supply, from S 0 to S 1 , which indicates that at any given price, the quantity supplied decreases. In this example, at a price of $20,000, the quantity supplied decreases from 18 million on the original supply curve (S 0 ) to 16.5 million on the supply curve S 1 , which is labeled as point L.
Conversely, if the price of steel decreases, producing a car becomes less expensive. At any given price for selling cars, car manufacturers can now expect to earn higher profits, so they will supply a higher quantity. The shift of supply to the right, from S 0 to S 2 , means that at all prices, the quantity supplied has increased. In this example, at a price of $20,000, the quantity supplied increases from 18 million on the original supply curve (S 0 ) to 19.8 million on the supply curve S 2 , which is labeled M.
Economists often use the ceteris paribus or “other things being equal” assumption: while examining the economic impact of one event, all other factors remain unchanged for the purpose of the analysis. Factors that can shift the demand curve for goods and services, causing a different quantity to be demanded at any given price, include changes in tastes, population, income, prices of substitute or complement goods, and expectations about future conditions and prices. Factors that can shift the supply curve for goods and services, causing a different quantity to be supplied at any given price, include input prices, natural conditions, changes in technology, and government taxes, regulations, or subsidies.
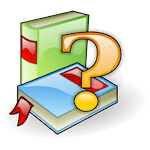
Self Check Questions
- What is the theory of production?
- The theory of production is generally based on the "short run". Which input is the variable that is changed? Why this one?
- What does the Law of Variable Proportions state? Give an example of this law.
- What is the production function? How can it be illustrated?
- How many stages of production are there? Which stage is the best one to produce in?
- Explain how marginal product changes in the stages of production.
- What are diminishing returns?

Want to create or adapt books like this? Learn more about how Pressbooks supports open publishing practices.
7.2 Theory of Production
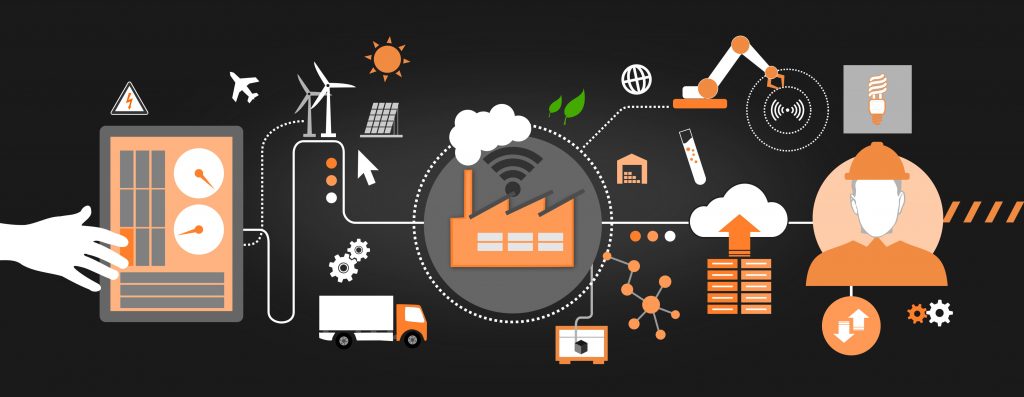
Production is the process (or processes) a firm uses to transform inputs (e.g. labour, capital, raw materials) into outputs, i.e. the goods or services the firm wishes to sell.
In perspective
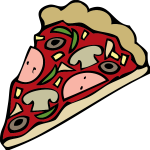
Consider pizza making. The pizzaiolo (pizza maker) takes flour, water, and yeast to make dough. Similarly, the pizzaiolo may take tomatoes, spices, and water to make pizza sauce. The cook rolls out the dough, brushes on the pizza sauce, and adds cheese and other toppings. The pizzaiolo uses a peel—the shovel-like wooden tool—to put the pizza into the oven to cook. Once baked, the pizza goes into a box (if it’s for takeout) and the customer pays for the goods.
We can summarize the ideas so far in terms of a production function , a mathematical expression or equation that explains the engineering relationship between inputs and outputs.
The production function gives the answer to the question, how much output can the firm produce given different amounts of inputs. We can describe inputs as either fixed or variable .
Definition: Fixed Inputs and Variable Inputs
Fixed inputs are those that can’t easily be increased or decreased in a short period of time. In the pizza example, the building is a fixed input. Once the entrepreneur signs the lease, he or she is stuck in the building until the lease expires. Fixed inputs define the firm’s maximum output capacity.
Variable inputs are those that can easily be increased or decreased in a short period of time. The pizzaiolo can order more ingredients with a phone call, so ingredients would be variable inputs. The owner could hire a new person to work the counter pretty quickly as well. So labor is a variable input.
Economists also differentiate between short and long-run production.
The short-run is the period of time during which at least one or more factors of production are fixed. During the period of the restaurant lease, the pizza restaurant is operating in the short run, because it is limited to using the current building (an example of capital) —the owner can’t choose a larger or smaller building. Plant size or capital is a fixed factor of production in the short run.
The long run is the period of time during which all factors are variable. Once the lease expires for the restaurant, the shop owner can move to a larger or smaller place.
Let’s explore production in the short run using a specific example: offering haircuts in a salon.
Since capital (the salon space) is fixed, the amount of output (e.g. haircuts per day) depends only on the amount of labour employed (e.g. number of barbers working). We can express this production function numerically as the table in Fig 7.1 below shows.
Total Product, Marginal Product, and Average Product of Labour
Note that we have introduced some new languages in the above example. We also call Output (Q) as Total Product (TP) . TP is the amount of output produced with a given amount of labour and a fixed amount of capital. In this example, one barber can give 15 haircuts in a day. Two barbers can produce 22 haircuts in a day and so on.
We should also introduce a critical concept: Marginal Product . Marginal product is the additional output of one more worker. Mathematically, Marginal Product is the change in total product divided by the change in labour: MP = ΔTP ÷ ΔL. In the table below (Fig 7.2), since 0 workers produce 0 trees, the marginal product of the first worker is eight haircuts per day, but the marginal product of the second worker is nine haircuts per day.
Short Run production
Why might the MPL be rising? To understand the reason behind this pattern, consider that a one-man barbershop is a very busy operation. The single barber needs to do everything: say hello to people, answer the phone, cut hair, clean up, and run the cash register. A second barber reduces the level of disruption from jumping back and forth between these tasks and allows a greater division of labour and specialization . The result can be greater increasing marginal returns. Suppose we add a third barber to the story. What will that person’s marginal product be? What will that person contribute to the salon? However, as other barbers are added, the advantage of each additional barber is less, since the specialization of labour can only go so far. The addition of a third or fourth or fifth barber will have less impact than the second one had. Given the fixed space inside the salon, as additional barbers are hired, they begin bumping elbows and getting in each other’s way. In this case, the addition of still more barbers would cause the output by each worker to decrease and result in falling marginal returns This is called the Law of Diminishing Marginal Product and it’s a characteristic of production in the short run. Why does diminishing marginal productivity occur? It’s because of fixed capital. If the salon manager is unable to lease a larger space but keeps hiring additional barbers, the rate at which TP rises starts to go down and MP decreases.
Below we see how the TP and the MP curves look graphically.
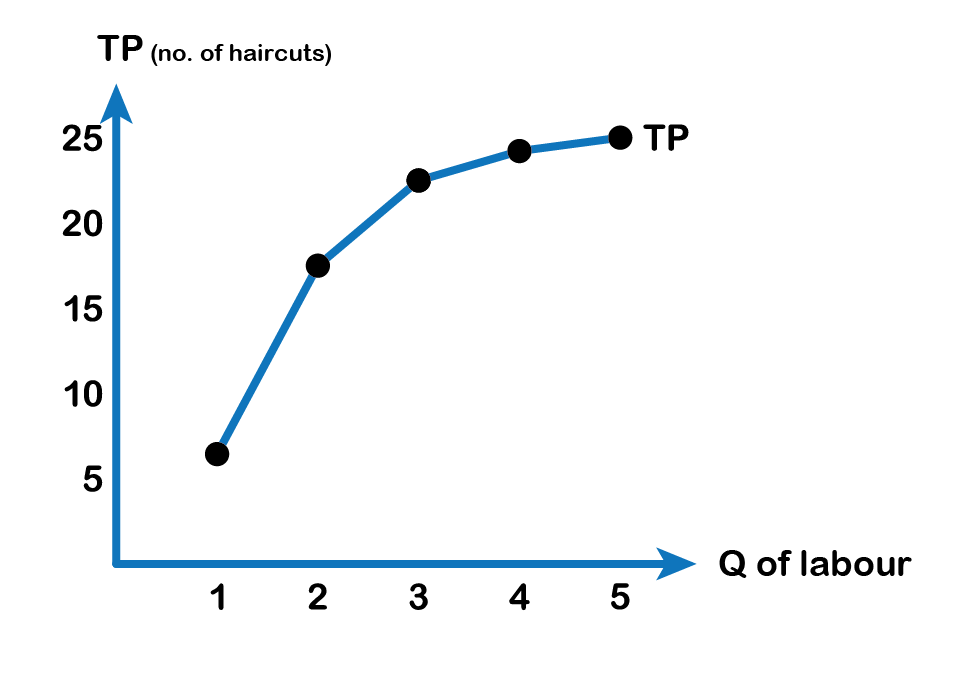
The average product of labour is the total product divided by the quantity of labour. AP=TP/L. What we observe from the third and fourth columns of Fig 7.2 is that, as the marginal product rises, so does the average product, and as the marginal product starts to diminish, the average product starts falling too. As a general rule as long as,
MP > AP, AP rises
MP < AP, AP falls
MP = AP , AP is maximum (maximum productivity of the business)
This relationship between MP and AP is mathematically true. Suppose, 5 friends plan to go for dinner, each carrying $20. In that case, the average amount that each friend is carrying is ($20 × 5 )÷ 5 = $20. Now suppose a sixth friend joins in for dinner who is carrying $25. Then the average rises to approximately $21. So the amount carried by the sixth friend is like the marginal product in our example, which is greater than the average product of $20, and that pulls up the average to about $21. Conversely, if the sixth friend carries $16, then the average changes to $19. Therefore, we find when the marginal ($16) falls below the average ($20), that pulls the average product down ($19). And when the sixth friend carries the same amount of $20 as the five others, the marginal and average both remain the same at $20.
The graph below shows the MP and the AP curves.
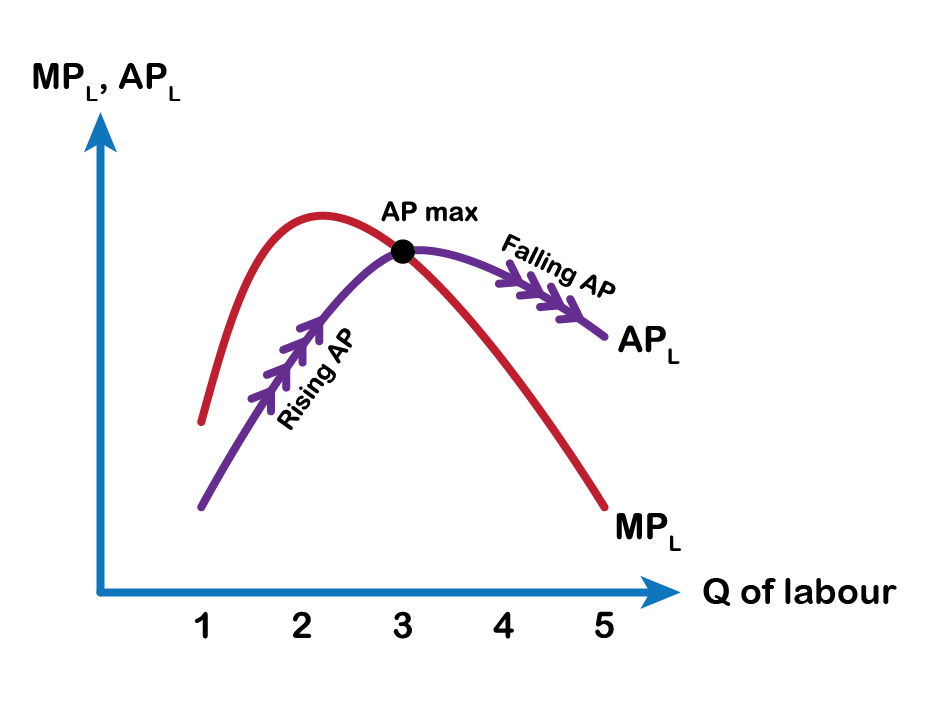
Attribution
“ 7.1 Building Producer Theory ” in Principles of Microeconomics by Dr. Emma Hutchinson, University of Victoria is licensed under a Creative Commons Attribution 4.0 International License , except where otherwise noted.
“ 7.1 Explicit and Implicit Costs, and Accounting and Economic Profits ” in Principles of Economics 2e by OpenStax is licensed under Creative Commons Attribution 4.0 International License .
“ Module 6: Firms and their Production Decisions ” in Intermediate Microeconomics by Patrick M. Emerson is licensed under a Creative Commons Attribution-NonCommercial-ShareAlike 4.0 International License , except where otherwise noted.
Principles of Microeconomics Copyright © 2022 by Sharmistha Nag is licensed under a Creative Commons Attribution-NonCommercial-ShareAlike 4.0 International License , except where otherwise noted.
Share This Book
Social Sciences
The copyright to this article and other articles from JournalQuest are maintained by the publishing journal and/or the individual authors according to agreements set forth by and between the publishing journal and the author. Other materials © 2024 Student Pulse, LLC. All rights reserved. ISSN: 2153-5760.
Disclaimer: content on this website is for informational purposes only. It is not intended to provide medical or other professional advice. Moreover, the views expressed here do not necessarily represent the views of Inquiries Journal or Student Pulse, its owners, staff, contributors, or affiliates.
Home | Current Issue | Blog | Archives | About The Journal | Submissions Terms of Use :: Privacy Policy :: Contact
Need an Account?
Forgot password? Reset your password »
ORIGINAL RESEARCH article
This article is part of the research topic.
Incentive Policies for Green Innovation, Energy Efficiency, Environmental Protection, and Sustainability
A Comparative Assessment of Policy Induced Diffusion Pathways for Utility Scale Solar PV: Case Study of Indonesia Provisionally Accepted
- 1 Imperial College London, United Kingdom
- 2 Centre for Environmental Policy, Faculty of Natural Sciences, Imperial College London, United Kingdom
The final, formatted version of the article will be published soon.
Lack of effective policies hinder the uptake of Utility-scale solar PV, even though they are projected to play a pivotal role in achieving Indonesia’s 2050 net-zero energy target. This study seeks to identify a cost-effective pathway to increase the capacity of utility-scale solar PV in Indonesia through supportive policies that ensure equitable cost distribution between the government and industry. A novel Market Penetration Optimization Model is developed and applied in simulation mode to assess existing policies, and optimization mode to determine new policy recommendations and compare three policy induced diffusion pathways. Results show that current price-based policies are insufficient to stimulate growth in the solar PV market, only covering approximately 13% of the investment cost required by the industry. Thus, necessitating a reactivation of Feed-in-Tariffs. The optimal tariffs rates required range from 0.39 to 1.47 cents /kWh for the most economic pathway during the initial ten-year post-construction period. The Innovation Diffusion Theory-based pathway necessitates the lowest initial investment cost while yielding the highest revenue from electricity sales, demonstrating its superior cost-effectiveness compared to both the supply-based and linear pathways. This study enriches the literature by exploring the financial implications of policy induced diffusion pathways.
Keywords: Utility scale solar PV, Indonesia energy policy, Feed-in Tariff, Innovation diffusion theory, market penetration optimization, clean technology uptake modelling Market Penetration Optimisation Model MW: Megawatt NDC: Nationally Determined Contribution PLN: Perusahaan Listrik Negara (State Electricity Company
Received: 06 Oct 2023; Accepted: 12 Apr 2024.
Copyright: © 2024 Oluleye and Syafina. This is an open-access article distributed under the terms of the Creative Commons Attribution License (CC BY) . The use, distribution or reproduction in other forums is permitted, provided the original author(s) or licensor are credited and that the original publication in this journal is cited, in accordance with accepted academic practice. No use, distribution or reproduction is permitted which does not comply with these terms.
* Correspondence: Dr. Gbemi Oluleye, Imperial College London, London, United Kingdom
People also looked at
Search Cornell
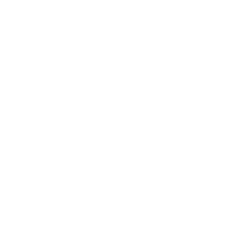
Class Roster
Section menu.
- Toggle Navigation
- Summer 2024
- Spring 2024
- Winter 2024
- Archived Rosters
Last Updated
- Schedule of Classes - April 11, 2024 7:34PM EDT
- Course Catalog - April 11, 2024 7:07PM EDT
SHUM 2437 Economy, Power, and Inequality
Course description.
Course information provided by the Courses of Study 2023-2024 . Courses of Study 2024-2025 is scheduled to publish mid-June.
How do humans organize production, distribution, exchange, and consumption? What social, political, environmental, and religious values underlie different forms of economic organization? And how do they produce racial, ethnic, class, gender, and sexual inequalities? This course uses a range of historical and contemporary case studies to address these questions, in the process introducing a range of analytic approaches including formalism, substantivism, Marxist and feminist theory, critical race studies, and science and technology studies. Course themes include gifts and commodities; the nature of money, markets, and finance; credit and debt relations; labor, property, and value; licit and illicit economies; capitalism and socialism; development and underdevelopment.
When Offered Spring.
Distribution Category (SBA-AS, SCD-AS, SSC-AS) Course Attribute (CU-SBY)
View Enrollment Information
Regular Academic Session. Choose one lecture and one discussion. Combined with: ANTHR 2437
Credits and Grading Basis
3 Credits Stdnt Opt (Letter or S/U grades)
Class Number & Section Details
19461 SHUM 2437 LEC 001
Meeting Pattern
- TR 10:10am - 11:00am To Be Assigned
- Aug 26 - Dec 7, 2024
Instructors
To be determined. There are currently no textbooks/materials listed, or no textbooks/materials required, for this section. Additional information may be found on the syllabus provided by your professor.
For the most current information about textbooks, including the timing and options for purchase, see the Cornell Store .
Additional Information
Instruction Mode: In Person
19462 SHUM 2437 DIS 201
- F 12:20pm - 1:10pm To Be Assigned
19463 SHUM 2437 DIS 202
- F 1:25pm - 2:15pm To Be Assigned
Or send this URL:
Available Syllabi
About the class roster.
The schedule of classes is maintained by the Office of the University Registrar . Current and future academic terms are updated daily . Additional detail on Cornell University's diverse academic programs and resources can be found in the Courses of Study . Visit The Cornell Store for textbook information .
Please contact [email protected] with questions or feedback.
If you have a disability and are having trouble accessing information on this website or need materials in an alternate format, contact [email protected] for assistance.
Cornell University ©2024
Why is nutrient cycling in food systems so limited? A case study from the North-Netherlands region
- Original Article
- Open access
- Published: 12 April 2024
Cite this article
You have full access to this open access article
- Durk W. Tamsma ORCID: orcid.org/0000-0002-3748-6660 1 ,
- Corina E. van Middelaar ORCID: orcid.org/0000-0002-6835-998X 2 ,
- Imke J. M. de Boer ORCID: orcid.org/0000-0002-0675-7528 2 ,
- Johannes Kros ORCID: orcid.org/0000-0003-1354-4990 3 ,
- Martin K. van Ittersum ORCID: orcid.org/0000-0001-8611-6781 1 &
- Antonius G. T. Schut ORCID: orcid.org/0000-0002-7512-728X 1
Identifying pathways to circular agriculture requires a profound understanding of nutrient flows and losses throughout the food system, and of interactions between biophysical conditions, land use, food production and food consumption. We quantified nitrogen (N) and phosphorus (P) flows of the food system of the North-Netherlands (NN) region and of its 30 subregions varying in biophysical and socio-economic conditions. The food system included agriculture, food processing, consumption, and waste processing. Nitrogen use efficiency (NUE), phosphorus use efficiency (PUE) and the nutrient cycling counts were calculated. Results show a low NUE (25%) and PUE (59%) of the food system. External inputs were used to maintain high yields and production. Nutrient cycling was very limited with losses from agriculture ranging from 143 to 465 kg N ha −1 y −1 and 4 to 11 kg P ha −1 y −1 . Food system losses ranged from 181 to 480 kg N ha −1 y −1 and from 7 to 31 kg P ha −1 y −1 and varied with biophysical conditions, population density and farming systems. Large losses were associated with livestock farming and farming on drained peat soils. Food system efficiency was strongly associated with the utilization of produce. We conclude that increasing circularity requires tailoring of agriculture to local biophysical conditions and food system redesign to facilitate nutrient recycling. Steps towards circularity in NN include: matching livestock production to feed supply from residual flows and lands unsuitable for food crops, diversifying crop production to better match local demand and increasing waste recovery.
Avoid common mistakes on your manuscript.
Introduction
Today’s food system has a significant impact on the planet (Crippa et al. 2021 ; Mueller et al. 2012 ; Pereira et al. 2010 ). Currently food systems heavily rely on external resources including energy, mined nutrients and chemicals (Kuokkanen et al. 2017 ). Various factors enhance this input dependency. The disconnection of food production and consumption leads to losses of nutrients in the system from urban areas. On-farm and regional specialization in agriculture with de-coupled crop and livestock production (Garrett et al. 2020 ; Martin et al. 2016 ; Schut et al. 2021 ) has resulted in inefficient use of nutrients (Tan et al. 2022 ) with manure excess in areas of intensive livestock production (Bai et al. 2022 ; de Vries et al. 2021 ). Furthermore, the utilization of less suitable soils for intensive agriculture comes with substantial nutrient losses and greenhouse gas (GHG) emissions, the latter in particular from degradation of organic soils (Crippa et al. 2021 ). The theory of circular agriculture provides a guideline to resolve several of these issues and is increasingly seen as an important means for a sustainable food future.
The concept of circularity has been applied to agriculture (de Boer and van Ittersum 2018 ), food systems (Jurgilevich et al. 2016 ) and the biobased economy (Muscat et al. 2021 ). Muscat et al. ( 2021 ) argue that the foremost principle of circular agriculture is to safeguard the health of (agro)ecosystems by not exceeding the regenerative capacity of natural resources. In addition, they emphasize the importance of efficient resource use by avoiding non-essential products and residual flows of essential products, prioritizing biomass use for human needs, and by recycling of unavoidable residual flows. Finally, they propose that the external energy requirement of the system can be decreased by working with nature and by maximising the utility of materials and recycling (Bergen et al. 2001 ; Muscat et al. 2021 ). By following these principles of circularity, food systems could in theory transition from a linear extract-consume-discard system to a more circular one in which resource loops are closed to prevent depletion of mineral and fossil resources and reduce emissions to the air, water and soil (Muscat et al. 2021 ).
Circularity of resource flows in the food system requires a food systems lens to quantify and eventually optimize flows between food system components in order to minimize resource use and environmental impacts at the level of the entire food system. This goes beyond resource use efficiency of individual agricultural sectors or production chains. Circular food systems also require spatial reconfiguration of production to enhance re-coupling of nutrient flows with limited biomass transport and to stay within local environmental carrying capacities (Bai et al. 2022 ; Koppelmäki et al. 2021 ). Furthermore, production may need to be coupled to food demand on more local or regional scales to limit nutrient imbalances between regions and to minimize energy use and GHG emissions from transportation. However, the feasibility of recoupling nutrient flows locally is strongly tied to the local biophysical production conditions which determine the suitability for and efficiency of food production. The scale at which striving for circularity in food systems is feasible is therefore determined by the biophysical context affecting the ability to produce food, but also by the processing capacity of agricultural products, food demand and the availability of consumer waste.
Several studies have quantified biomass and/or nutrient flows at farm (de Vries et al. 2018 ; Schröder et al. 2003 ), regional (Hanserud et al. 2016 ; Le Noë et al. 2017 ; Theobald et al. 2016 ; Vingerhoets et al. 2023 ), national (van Selm et al. 2022 ) or global (Crippa et al. 2021 ; Willett et al. 2019 ) levels with the aim of more circular resource use. However, such studies either focus on the entire food system with a subnational region as the smallest spatial unit, or on agriculture in its local context but with limited attention for the complexity of agriculture as part of the food system. Hence, it is still poorly understood how the biophysical production environment, type and productivity of production systems and local food demand interact with nutrient flows, losses and circularity of the food system.
To improve our understanding of these interrelationships, the objective of this study was twofold. First, we aimed to quantify nitrogen (N) and phosphorus (P) flows and to evaluate differences in efficiency between subsystems of the food system and between subregions within the ‘North-Netherlands’ (NN); and second to benchmark the circularity of the current food system and provide a deeper understanding of nutrient cycling, losses and inefficiencies in this highly specialized, food exporting region. We compared four subregions that strongly differed in soil type as a proxy for the biophysical conditions, production volumes of crop- and animal products as an indicator of specialization and population as a proxy for consumption. Three subregions had suitable sandy- and clay soils. Of these Achtkarspelen was characterized by predominantly intensive husbandry and low population density, Veendam-Pekela by arable farming and low population density and Assen by mixed farming and high population density. Subregion de Fryske Marren was characterized by predominantly peat soils with dairy farming and a low population density. Nitrogen use efficiency (NUE) and phosphorus use efficiency (PUE) were evaluated at subsystem level and for the entire food system. A cycling count indicator (van Loon et al. 2023 ) was used to evaluate the degree of nutrient recycling in the current food system and to identify opportunities to enhance circular resource use. We hypothesized that subregional differences in indicators of efficiency and circularity of the food system are directly linked to the biophysical characteristics of the production environment and associated agricultural specialization and to local food demand and thus to import and export.
A mass flow analysis was conducted to quantify nitrogen (N) and phosphorus (P) flows in the food system of the North Netherlands region and of constituent subregions for the years 2015–2019. Total flows and losses as well as nutrient use efficiency and the nutrient cycling count of the current food system were calculated.
Delineation of case study
Region description.
The North Netherlands (NN) region covers an area of 8,292 km 2 land and includes the provinces of Friesland, Groningen, and Drenthe with in total 1,7 million inhabitants. The population density of 216 inhabitants km −2 is much lower than the Dutch average of 517 inhabitants km −2 , yet is still far above the EU27 average of 118 inhabitants km −2 (CBS 2020 ; EUROSTAT 2021 ). However, NN is relatively land abundant with 0.31 ha of cultivated land per capita, which includes cropland, grassland, and horticulture (CBS 2022a , b ). This is way more than the 0.06 ha cultivated land per capita for the Netherlands and 0.22 ha cultivated land per capita for the EU27 (The World Bank 2020 ), highlighting the role of NN as a food producing region. About 70% of the land in NN is used for agriculture, including 45% for livestock farming and 19% for cropping (CBS 2018 , 2022b ). Main crops are: winter and spring wheat, barley, sugar beets and seed, ware and starch potatoes. The livestock sector consists mainly of dairy cattle with a smaller number of pig and poultry farms (Smit et al. 2017 ). Horticulture takes up about 1% of total agricultural land (Smit et al. 2017 ). There is an ongoing trend of specialization and increasing farm size, in line with wider European trends (Schut et al. 2021 ; Smit et al. 2017 ). The NN is a strong exporting region producing for the EU and world markets (CBS 2016 ). The region includes a wide variety of bio-physical conditions, with different soil types and ground water levels. NN is an important region for biodiversity conservation, and supports large populations of migratory birds (Reneerkens et al. 2005 ) and has unique habitats for various animal and plant species (Aptroot et al. 2012 ; Peeters and Reemer 2003 ). It also borders the Wadden sea, the largest tidal flats in the world that provide a unique habitat for numerous species (Wortelboer 2010 ).
We divided NN into 30 constituent subregions that contrasted in predominant soil type, agricultural specialization and population density (Fig. 1 ). Soil type was chosen as a proxy for variations in the biophysical production environment, as it is the most important determinant of the suitability for and the nutrient efficiency of the production of different crops in the region. Agricultural specialization determines what is produced, processed and exported from a subregion. Variation in population density is directly related to consumption and the availability of recyclable consumer waste. These proxies for the biophysical environment, specialization and consumption together give insight in the potential for and possible pathways to circularity in a local or regional food system.
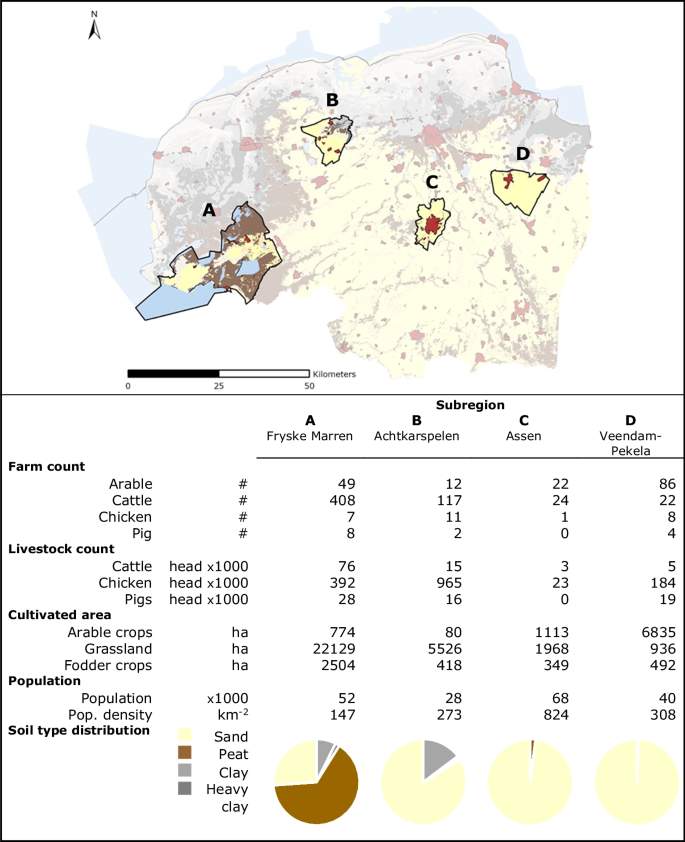
Map and summary statistics of four subregions in North Netherlands (NN). These subregions illustrate the local contrasts in soil types, agricultural specialization and population density found within the region. The presented statistics include farm- and livestock counts (CBS 2022b ), the cultivated area of arable and feed crops (CBS 2022b ) and population density (CBS 2022a ) and are average values for the years 2015–2019. The pie charts show the ratio between different soil types in each subregion
Definition of the food system and substance flow model
This case study focused on the food system within mainland NN. The subsystems included were: agriculture, food- and feed processing, (human) consumption and waste processing. A substance flow model was developed to analyse annual nitrogen (N) and phosphorus (P) in- and outflows of the system, including flows within and between the different subsystems (Supplementary Material; Fig. S1 ). The selection of flows was based on earlier studies on comparable food systems (Smit et al. 2010 ; van der Wiel et al. 2020 , 2021 ) and adapted to region-specific conditions. Where possible, flows were quantified by using publicly available data from national databases and research reports. Important sources included Statistics Netherlands (CBS), the Dutch Ministry of Infrastructure and Water Management (Rijkswaterstaat), the National Institute for Health and the Environment (RIVM) and the INITIATOR (Integrated Nutrient ImpacT Assessment Tool On a Regional scale) model developed by Wageningen Environmental Research. Gaps in the data were filled through interviews with experts from the region. Data was collected for the municipality level. Average data from the years 2015–2019 was used where possible to account for temporal variation. As the 2015–2019 period coincided with a reorganisation of municipalities in NN, the spatial entities changed. Therefore, subregions were created by merging municipalities to the level at which data was available for all years, creating the smallest possible spatial entities. For several flows no data was available at the subregional spatial level, most notably trade data for food and feed. In these cases a feed and food balance approach was used to estimate imports and exports (van der Wiel et al. 2021 ). Agricultural products were assumed to be processed in the subregion of origin, since flows of agricultural products to centralized processing facilities could not be tracked. Thus the systems presented here for NN and each subregion represent the food systems flows that are associated with the food production and consumption in NN and in each respective subregion.
Calculation of flows
A complete overview of all flows, their main data sources and calculation is given in the Supplementary Material (Fig. S1 ; Table S1 ). The entire calculation procedure has been made available online (DOI: https://doi.org/10.4121/8899e893-c68a-4fe1-813e-6af228eb0ef1 ). Below we provide an outline of the calculation procedure for the main components in the food system.
Agriculture
Agriculture was restricted to arable crop and livestock production, (greenhouse) horticulture was excluded. Livestock included dairy and beef cattle, pigs, poultry, and sheep. Livestock from hobby farms were excluded as these were not included in the agricultural census.
Most internal flows in the subsystem agriculture were calculated using the INITIATOR model (de Vries et al. 2023 ; Kros et al. 2019 ). INITIATOR calculates spatially explicit N, P and C fluxes in agriculture, including the supply of N, P and C in the form of fertilizer, animal manure, deposition and N fixation, the N and P discharge by the crop and the emissions of methane (CH 4 ), ammonia (NH 3 ), nitrous oxide (N 2 O) and nitrogen oxides (NO x ) to the atmosphere. The model uses detailed spatial data that largely came from available national datasets, such as the geographically explicit agricultural census data, including crop type, cropping area, livestock numbers and housing type at farms. INITIATOR was used to calculate manure excretion on farm level as well as gaseous N losses from animal housing and manure storage. Manure was distributed over the fields of the farm, up to the legal application limit for animal manure per hectare. Any excess manure was initially divided over the agricultural area within each (aggregated) municipality. Any remaining excess was subsequently exported to regions of NN with a manure shortage, i.e. where more manure could be applied within the legal limit. Similarly, if the legal application limit was not reached, manure was imported from other regions of the Netherlands with excess manure. In the Netherlands, legislation enforces that excess manure needs to be exported as manure or in processed form to farmers in manure deficient areas who get rewarded for manure application on cropland. Gaps between effective N and P applied with animal manure and the legal total effective application limits were assumed to be filled up with artificial fertilizers and compost. This is a reasonable assumption, because fertilizer inputs are cheap relative to product prices in the Netherlands and farmers utilize the entire application norm (Langeveld et al. 2007 ; Reijneveld et al. 2010 ). In addition N and P inputs from atmospheric deposition were taken into account. The INITIATOR soil module was used to calculate the amounts of nitrogen fixation and decomposition of organic matter as well as crop uptake and losses from manure storage, fertilizer application, leaching, runoff and denitrification per hectare. Loss fractions differed between soil types and groundwater levels (de Vries et al. 2023 ).
The crop offtake of kg N ha −1 and kg P ha −1 and crop area obtained from INITIATOR were multiplied to obtain production per subregion. Crops in INITIATOR output were grouped into: potatoes, sugar beet, wheat, other cereals, grass, silage maize and miscellaneous crops. We segregated the production of crop groups into individual crops by estimating for each subregion the production ratio between each crop in a group. To this aim crop areas and yields from (CBS 2022b ) and crop nutrient content obtained from experimental studies in the Netherlands were multiplied to provide N and P production per crop (de Ruijter et al. 2020 ; Ehlert et al. 2009 ). The total mass of N and P in consumed feed was calculated using the average fodder and feed concentrate consumption per animal per feed group reported by (CBS 2019 ) and the livestock numbers from the agricultural census (CBS 2022b ). It was assumed that all fodder was produced in the region. In most cases the production of fodder crops did not exactly match the calculated consumption by livestock. Such discrepancies were resolved by adjusting the grass nutrient content, as this was a major source of uncertainty. The feed concentrate requirement was filled with residual flows from the processing and waste processing industries and supplemented with feed imports if needed (see Sects. 2.2.3 and 2.2.5). Animal production was calculated by multiplying livestock numbers by production per animal and the N and P content of various animal products (CBS 2019 ). Nutrient balances were maintained, i.e. nutrients in feed supply exactly matched offtake with animal products and excreta.
Food processing
Food processing included processing of raw products from agriculture in each subregion and NN only. The fraction of each raw crop and animal product that was processed was derived from literature (Supplementary Material; Table S1 ). The remaining produce was assumed to be consumed in unprocessed form. Crop and animal products were converted into food products and residual flows using transfer coefficients (TCs). TCs were derived either directly from processing industries in the region or from Dutch sectorial sources (Supplementary Material; Table S1 ). The most common application of each residual flow was obtained from industry reports or directly from processing companies. Applications of processing output were grouped as: food, livestock feed, soil amendment and other flows that left the food system. In case flows had multiple applications a best-case scenario was assumed where application as livestock feed was preferred over application to soil, which in turn was preferred over applications outside the food system. Food, feed, and soil amendments that were not exported were designated as inputs to consumption and agriculture respectively (see 2.2.5). We assumed that seed potatoes and non-food cash crops (e.g. fiber hemp) were exported as whole products from the region without further processing. Losses from processing to the environment were not quantified because of data limitations.
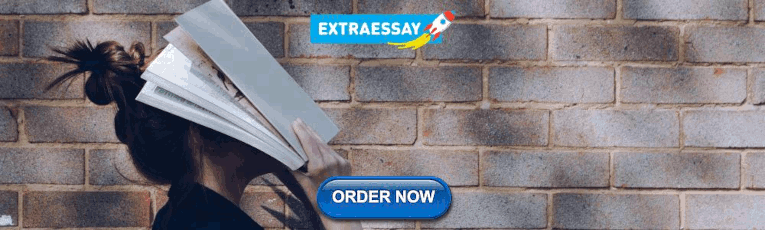
Food consumption
Consumption included the food consumption of the human population in the region, excluding their pets and recreational animals. Food consumption was calculated from regional and municipal demographic data (CBS 2022a ) and consumption per capita. Per capita consumption of products in different food groups was derived from the Dutch Food Consumption Survey (van Rossum et al. 2020 ). An overview of food groups is given in (van Rossum et al. 2020 ). One average diet was assumed for the entire population, based on the average diet of males and females between the ages of 1 and 79, weighed by the population composition of NN (van Rossum et al. 2020 ). The N and P contents per food item were obtained from the Dutch Food Composition Database and averaged for all products in a food group (RIVM 2021 ). The amount of food waste was estimated based on the food waste survey by (CREM Waste Management 2017 ). Human body mass changes were ignored, and it was therefore assumed that all consumed N and P in food was excreted.
Waste processing
Waste processing included organic waste arising from the food system only, thus including food waste and human excreta but excluding garden waste. All human excreta were assumed to be treated at a typical communal wastewater treatment plant (WWTP), since 99.7% of Dutch households is connected to the communal sewer (Oosterom and Hermans 2013 ). N and P from human excreta were allocated to sludge, emissions to the air and to surface water (Rijkswaterstaat 2019 ; WSBD 2018 ). In the Netherlands nutrients are currently not recovered from sludge in any significant quantity and were therefore considered lost from the system (Regelink et al. 2017 ). Food waste went to incineration, landfilling, livestock feed, composting or anaerobic digestion in ratios derived from (Soethoudt and Vollebregt 2020 ). All incinerated and landfilled food waste was considered lost from the food system. Compost, digestate and livestock feed were returned to agriculture.
Retail: Food and feed import- and export
A net balance approach was used to estimate food and feed imports and exports at subregional and at NN regional level. Livestock N and P requirements were met with local roughage and co-products from processing industries and waste processing and were supplemented with feed imports. Local food demand for each food group was filled with produce from the subregion or NN region and supplemented with food imports. Consumed horticulture products were part of the food imports, as horticulture was not included in this study due to its small acreage in the region. Products that were produced but not consumed were assumed to be exported. Summed totals of import and export were used to calculate the net import/export balance over all food groups. This is an approach that determines the highest possible degree of local consumption rather than actual local consumption, used to compensate for the lack of trade data at the sub-national level. Losses from retail were ignored as losses from supermarkets in the Netherlands are estimated at only 1.7% (CBL 2020 ).
Data reliability
Data uncertainty was accounted for using the approach of (van der Wiel et al. 2021 ). An uncertainty level was assigned to each flow based on the type of data source, as most sources did not provide uncertainty estimates. Flows were assigned the uncertainty level of the most uncertain data source used in their calculation, rather than the propagated uncertainty of all variables (van der Wiel et al. 2021 ). The level of aggregation of source data differed substantially between flows, meaning that uncertainty propagation would disproportionally affect flows based on less aggregated data. Each uncertainty level was associated with a relative uncertainty interval as specified by (van der Wiel et al. 2021 ) (Table 1 ). Subsequently, uncertainty ranges were calculated by multiplying each flow by their respective relative uncertainty interval. Flows in the subsystem agriculture were assigned the uncertainty level nearest to the uncertainties reported for each flow by (de Vries et al. 2003 ) and N losses were assigned the highest uncertainty level as the fate of N in the environment is not well documented. The uncertainty estimates of all flows can be found in the Supplementary Material (Table S1 ).
All flows, balances and indicators were computed for the NN region and for each subregion. Flows were expressed in megagram per year (Mg y −1 ) and converted to kilogramme per hectare of agricultural land per year (kg ha −1 y −1 ), using the total area used for agriculture in a (sub)region. Checks were done to ensure the law of mass conservation was observed. This was done for each subsystem and for the whole food system. The use efficiencies (UE) for N (NUE) and P (PUE) were determined for the whole food system and for each subsystem:
For NUE and PUE output and input are the N or P flows out of and into the respective (sub)system, excluding losses. An overview of the most important inputs and outputs into the system and subsystems is given in Fig. 2 . For example, inputs into subsystem livestock are feed from waste processing, feed from food processing, feed import and feed crops. Outputs of livestock are animal products and excreted manure with losses all resulting from manure storage. A complete overview of all individual flows is given in the Supplementary Material (Fig. S1 ). All flows with a purpose outside the food system or outside the (sub)region were considered outputs, thus including food exports, manure export (in the case of any excess) and residual flows of food processing, but excluding landfilled food waste which was considered a loss. To assess the effects of the assumption that manure is a useful export product, we also calculated UEs excluding manure export as useful output, as excess manure is of limited value compared to food exports. In this case excess manure was counted as a loss. Furthermore, we estimated the externalized losses from the production of feed imports, by dividing the amount of imported feed by the production efficiency of feed crops in NN. Depletion of N and P in the soil was counted as an input to the system and accumulation as an output.
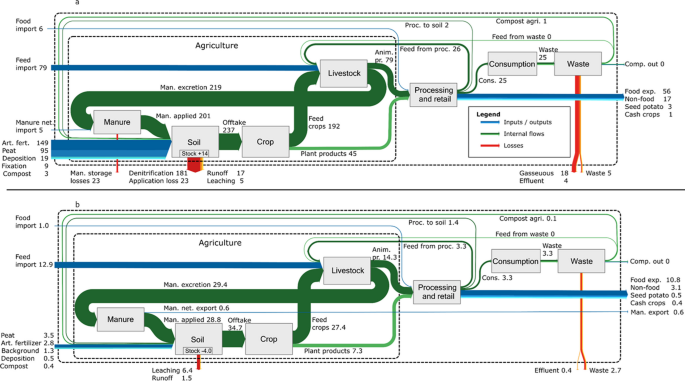
Nitrogen (panel a.) and phosphorus (panel b.) flows in the North Netherlands food system expressed in kilograms per hectare of agricultural land per year (kg ha −1 y. −1 ). System in- and outputs are shown in blue where inputs enter the system (and each subsystem) from the left, outputs leave from the right. Internal flows are shown in green and losses are shown as red arrows leaving the (sub)system from the bottom. Values are averages for the period 2015–2019. Note that all (sub)system balances are 0, which may appear differently in this figure due to rounding. The unrounded numbers for each flow are given in the Supplementary Material (Fig. S2 )
To assess the recycling of nutrients within the food system, we determined the cycle count indicator (CyCt) for nitrogen (CyCt N ) and phosphorus (CyCt P ) (van Loon et al. 2023 ). The CyCt quantifies the average number of times that inputs are cycled through the food system before being removed from the system as outputs or losses. It is defined as:
where A is the fraction of inputs that is removed from the food system per cycle and (1-A) indicates the fraction of inputs retained in the system after each cycle.
The flows, UE and CyCt of N and P in the NN region were compared to studies on regional nutrient flows to contextualize our results and thereby provided the baseline for subregional food system flows.
Nutrient flows in North Netherlands food system
In total, 99% of imported N and 96% of imported P went to agriculture and the rest was captured in imported food (Fig. 2 ). The largest N inputs were artificial fertilizer (41%), mineralisation of organic peat soils (26%) and imported animal feed (22%). The largest P inputs were imported animal feed (48%), soil stock change due to lowered yearly application limits (15%), mineralisation of organic peat soils (13%) and artificial fertilizer (10%). The main system outputs were the export of food, non-food biomass from processing and the production and export of seed potatoes. About 91% of N losses and 72% of P losses were from agriculture. The NUE and PUE were respectively 0.25 and 0.59 for the food system compared to 0.36 and 0.74 for agriculture (Table 2 ).
The largest flows in the food system were associated with agriculture (Fig. 2 ), as NN region is a net production region. Total crop offtake was 237 kg N ha −1 y −1 and 35 kg P ha −1 y −1 , of which 81% was in fodder, and 19% in food and feed crops for processing. The combined production of plant and animal products for processing and human consumption was 124 kg N ha −1 y −1 and 22 kg P ha −1 y −1 . Of this, 36% of N and 34% of P were in plant products and 64% of N and 66% of P in animal products. Losses from agriculture were the largest of the food system, with the majority of N losses being lost from the soil via denitrification (72%), fertilizer application losses consisting mainly of NH 3 (9%), runoff (7%) and leaching (2%). The remaining 9% was lost from manure storage, predominantly as NH 3 . P was only lost from the soil via leaching (76%) and runoff (24%). In total, 81% of harvested N and 79% of P was directly fed to livestock. Losses were largest in the subsystem soil. Hence, a large proportion of losses from agriculture can to attributed to feed production. Of all livestock feed 26% of N and 30% of P was imported from outside the region. The losses during the production of imported feed were not included in this analysis. Including such losses would increase the N and P losses associated to animal production even further.
Processing and retail
Of the total production of crop- and animal products in the region about 60% of N and P ended up in food products, 23% was returned to agriculture as feed or soil amendment and 14% ended up in products not used in the food system (Fig. 2 ). A substantial part of the flows leaving the food system was offal, of which a large part is used in the production of pet feed. Smaller flows leaving the system included seed potatoes, non-food cash crops and non-food/feed products of animal origin (Supplementary Material; Fig. S2 ). Losses from processing could not be accounted for due to a lack of data. However, 14% of N and P going into processing ended up in non-food products, that left the food system and were essentially lost. For instance, offal from animal carcases is processed into pet feed and is eventually excreted by pets and generally not recovered. The generation of non-food flows thus indicates an inefficiency in the use of food crops that could (partly) have been used to feed the human population.
Only 25% of N and 18% of P of locally produced food products was consumed within the region, with the rest being exported. This means that 75% of N losses and 82% of P losses from agriculture and food processing could be attributed to the production of food exports, when assuming uniform losses per kg of N and P across crops and animal products. As animal products made up a large share of exports, the percentage of losses associated with export is likely higher.
Consumption and waste
About 24% of N and 30% of P in purchased food was imported from outside the NN food system (Fig. 2 ). Of purchased food, 91% of N and 93% of P was consumed and ultimately disposed in wastewater. The remaining 9% of N and 7% of P was food waste, which is in line with the 10% reported by (Yahia and Mourad ( 2020 ) for European consumers.
Nitrogen in wastewater was lost as N 2 (64%) or N 2 O (1%). About 19% ended up in sewage sludge and 16% was lost to surface water. Phosphorus in wastewater went to sludge (86%) and to surface water (14%). Sludge is usually dried and burned or landfilled, and N and P in sludge was a loss from the system.
Food waste was either burned (35% of N and 40% of P) or composted (30% of N and 27% of P), digested (22% of N and 9% of P), landfilled (3% of N and 3% of P) or used as livestock feed (2% of N and 21% of P). Only compost, digestate and feed from waste were returned to the system, with the rest effectively being lost.
Subregional variation in specialization, soil type and N balances of agriculture
Within NN there is spatial heterogeneity in soil types (Fig. 3 ; panel a), population density (Fig. 3 ; panel b) and agricultural specialization, represented by the fraction of produced N in crop products (Fig. 3 .; panel d). Most areas dominated by sandy soils have a mixture of specialized crop and livestock farms. The eastern part of the region with sandy soils, with some oligotrophic peat residues that were left after peat excavation, is largely specialized in crop production. The fertile clay soils near the coast that were reclaimed from the sea are used by specialized crop farmers (light grey in Fig. 3 ; panel a), whereas the areas with heavy clay soils further inland (dark grey in Fig. 3 ; panel a) are dominated by dairy farmers. The south-(western) part of the region that is dominated by peat soils is largely livestock-oriented, specifically toward dairy farming. The spatially segregated specialized crop and livestock production in the northern part of the region is not distinguishable on these maps due to low spatial resolution of the available data, thus showing a ratio of crop to animal production close to 50%. Patterns of specialization largely follow patterns of soil type and groundwater levels. Urban centres are relatively small and population densities are diluted by low population density in the rest of the subregion.
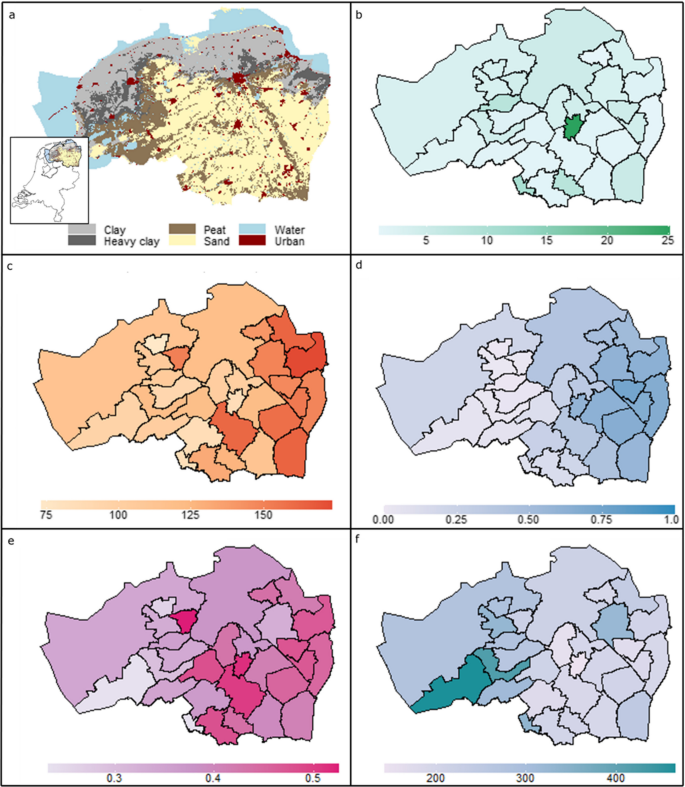
Maps of soil types and urban areas ( a. ), population density ( b. ), total N yield of crop and animal products ( c. ), proportion of N yield in crop products ( d. ), nitrogen use efficiency (NUE) of agriculture ( e. ) and nitrogen losses from agriculture ( f. ) per subregion in NN
The maps of NUE (Fig. 3 ; panel e) and losses (Fig. 3 ; panel f) show a similar pattern as specialization (Fig. 3 ; panel c). The highest NUEs and lowest losses were found in the eastern parts, whilst NUE was lower and losses were higher in the southern part of the region. In the cropping regions higher production levels of agricultural products (excl. feed) were achieved (Fig. 3 ; panels c and d) with lower losses (and less inputs). Most subregions with predominantly animal production had lower NUE and higher losses per hectare. However, there were several outliers to this pattern, which are explained below. The spatial correlation between soil type and NUE and losses was less clear than that between specialization and NUE. The exception was that losses on the south-western peat soils were notably higher than in the rest of the region.
Comparing nutrient flows in contrasting subregions
The N balances of the food system of four selected subregions, contrasting in specialization, soil type and population density are shown in Fig. 4 and NUE and PUE values of their subsystems in Table 2 . Additionally, an overview of N flows in the food systems of these subregions is given in the Supplementary Material (Fig. S3 ). These balances and flows are used to explain the patterns observed for NUE and PUE and specialization and soil type, as well as exceptions to patterns.
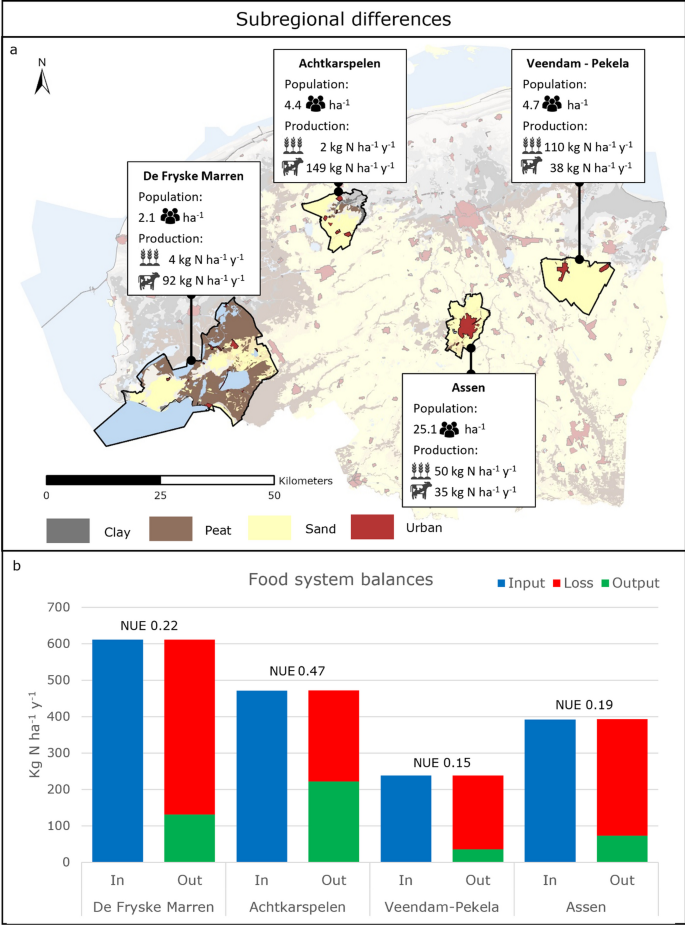
Overview of contrasting subregions in north-Netherlands (NN), varying in soil type, specialization, and population density ( a. ) and their respective food system N balances and nitrogen use efficiencies (NUE) ( b. ). Food system losses were especially large in areas of dairy farming on peat soils ( Fryske Marren ) and in more urbanized areas where losses were large from both agriculture and consumer waste ( Assen ). Intensive animal production had lower losses inside the region ( Achtkarspelen ), as losses from the production of imported feed and utilization of exported manure took place outside the region. Including such losses would drastically reduce the NUE. Arable farming was associated with higher yields per hectare and smaller losses, but as a large share of nutrients in crop products was processed into livestock feed, the food system NUE and outputs in areas dominated by arable farming were very low ( Veendam-Pekela )
De Fryske Marren
De Fryske marren is located on peat soil and is strongly specialized in dairy production (Fig. 4 ). There was a substantial input from decomposing peat of 335 kg N ha −1 y −1 . Agriculture in De Fryske Marren had an NUE of 0.23 and a PUE of 0.68, slightly lower than the average NUE of 0.36 and PUE of 0.74 for the NN region (Table 2 ). Losses from agriculture were 465 kg N ha −1 y −1 , 86% higher than the regional average of 250 kg N ha −1 y −1 . Production was 96 kg N ha −1 y −1 , 23% lower than the regional average of 124 kg N ha −1 y −1 . The excess N was mostly lost to the air via denitrification. De Fryske Marren also had a high feed self-sufficiency, as local feed production met 77% of demand. The losses associated with feed production were thus largely internalized. Of the N in harvested plant material, 99% was in fodder. As the largest part of consumed feed was converted into manure and not into food products, food production was low despite the large inputs and losses.
Achtkarspelen
Achtkarspelen is located on sandy soil (Fig. 4 ) and had the highest intensity of animal production in the region including landless poultry and pork production, yielding 149 kg N ha −1 y −1 in animal source food products, which was 89% higher than the regional average. The total production including crops was 151 kg N ha −1 y −1 , which was 22% above the regional average. At the same time, the NUE of agriculture of 0.53 and PUE of 0.88 were among the highest of all subregions (Table 2 ). Losses from agriculture were 219 kg N ha −1 y −1 , which was 13% lower than the regional average.
The apparently high efficiency of animal production in this subregion resulted from the externalization of some main sources of losses, i.e. feed production and manure application. Achtkarspelen had the largest feed imports and manure exports per hectare of all subregions (Supplementary Material; Fig. S2 ). About 40% of feed was imported from outside the subregion, thus externalizing a large part of the losses associated with growing feed crops. Also, manure export made up about a third of system output, but manure is of much lower utility than food products and its utilization causes losses elsewhere. Excluding manure export as system output reduced the NUE of this subregion from 0.53 to 0.38 and the PUE from 0.88 to 0.55 (Table 2 ). Also including the losses related to the production of imported feed would further reduce the efficiency (to about NUE 0.25).
Veendam-Pekela
Veendam-Pekela is situated on sandy soils with mostly arable farming of especially starch potatoes along with substantial areas of sugar beets and cereals (Fig. 4 ). The NUE of agriculture in Veendam-Pekela was 0.47 and the PUE 0.90, which was higher than the NUE of 0.36 and PUE of 0.74 for the NN region (Table 2 ). Losses were 169 kg N ha −1 y −1 , 32% lower than the regional average. Production was 147 kg N ha −1 y −1 , which was 19% higher than the regional average. About 74% of N outputs were in plant products and 26% in animal products. Starch potatoes and sugar beets are processed into starch and sugar products which mostly consist of carbohydrates, whilst most of the nutrients in the raw products end up in non-edible residual flows. Therefore only 27% of total N production ended up in food products; the other part was used for animal feed (58%), soil amendment (9%) or left the food system in non-food products (9%). Therefore, whilst the NUE and PUE of agriculture and productivity were higher than average, food export was lower than in less productive subregions as a large proportion of produce ended up in non-food flows. This resulted in an NUE of only 0.15 and PUE of 0.60 on food system level (Table 2 ).
With 25 persons ha −1 Assen is the subregion with the highest population density in the NN region (Fig. 4 ). Consumers purchased 188 kg N ha −1 y −1 in food, of which 87% was imported from outside the subregion. The largest leak of the system was consumer waste processing (178 kg N ha −1 y −1 ) rather than agriculture (143 kg N ha −1 y −1 ). The NUE of agriculture was 0.51 compared to 0.05 for waste processing (Table 2 ). The PUE of agriculture was 0.74, compared to 0.04 for waste processing. Therefore, subregions with higher population density generally had a lower food system NUE and PUE. However, per kg N in produced food products, 4.0 kg N ha −1 y −1 was lost from agriculture, while of each kg N in purchased food products 0.9 kg N ha −1 y −1 was lost after waste(water) processing. Thus, per kg N in food products losses from agriculture still outweighed losses from consumption. Even if all N lost through consumer waste in this urban subregion were returned to agriculture, it would not be sufficient to replace current inputs to agriculture. Agriculture in turn did not provide sufficient food to feed the local population.
Current circularity in NN and subregions
Nutrient cycling was very low in the food systems of NN and its subregions (Table 2 ), indicating that hardly any N inputs were recycled through the system. All calculated CyCt values were < 0.03, indicating that the vast bulk of N and P inputs did not complete a full cycle through the food system. This resulted from very little re-use of nutrients after consumption and large losses and exports from the system. Subregions with a high production to consumption ratio scored worse on the circularity indicator. Nutrients in exported food were lost from the region and not returned as recycled system inputs and therefore reduced the cycling count.
Nutrient flows and efficiencies varied strongly between subsystems and subregions. At system level NUE varied most strongly with agricultural specialization and soil type, whilst PUE varied most strongly with population density. The cycling count was very low for both N and P in all subregions, indicating that the current system heavily depends on external inputs with large losses from the system.
Nutrient dynamics of the food system and its subsystems
Food system performance in this export oriented region was mainly determined by the performance of the subsystem agriculture. The very limited recovery from consumer waste had an insignificant impact on the NN region as these flows were relatively small (Geertjes et al. 2016 ; Soethoudt and Timmermans 2013 ). The NUE of the current food system in the NN region was 0.25, which equals the NUE for the Netherlands (Erisman et al. 2018 ) and is higher than the reported NUE value (0.18) for Europe (Leip et al. 2022 ). The relatively high PUE of 0.59 was mostly due to small P surpluses in agriculture when compared to other regions (Einarsson et al. 2020 ) and partly due to the legacy of historically applied P (Sattari et al. 2012 ).
The low NUE (0.36) of the agriculture subsystem mainly resulted from the low N conversion efficiency of livestock, the cultivation of feed crops and mineralisation of peat soils. This NUE was lower than the 0.43 reported by CBS for agriculture in the Netherlands (CBS 2022c ). The lower NUE found here was mainly the result of counting the additional inputs provided by peat soil, which are usually omitted. In peatlands large nutrient surpluses were found. Strongly reducing application limits for peatlands would improve NUE, reduce N 2 O emission and limit P saturation of peatlands without production loss.
The cycle count (CyCt) of the NN food system was only 0.0069 for N and 0.0091 for P. These figures were lower than the CyCt of 0.02 found by van Loon et al. ( 2023 ) for the highly linear food system in the Flanders region (Belgium). The lower values for NN can be explained by larger inputs and larger losses per hectare in NN and especially by a larger share of products leaving the region in exports. Of all inputs into the subsystem agriculture only 7% of N was recycled from the NN food system. A similarly strong dependency on nutrient imports has also been reported in other regions with intensive agriculture (Chowdhury et al. 2018 ; Le Noë et al. 2018 ; Papangelou and Mathijs 2021 ). Increasing the CyCt and reducing the dependency on nutrient imports can be achieved by reducing exports and reducing losses, as the CyCt counts the proportion that remains in the system but does not distinguish between losses and useful flows that leave the system.
Differences in nutrient flows between subregions
Variation with soil type: large losses from organic soils.
Nutrient flows and losses varied substantially with soil type. Losses from peat soils were higher than from clay and sandy soils and consequently, UE and CyCt were low in subregions with mainly peat soils (Fig. 3 ; panels a. and f.). Groundwater tables in areas of peat soils in NL are typically lowered to facilitate intensive agriculture. The resulting aerobic conditions strongly enhance the mineralisation rate of organic matter stored in peat soils, estimated here at 335 kg N ha −1 y −1 and 38 kg P ha −1 y −1 for a subregion dominated by peat soils. Similar rates were found for one test site in the Netherlands (Pijlman et al. 2020 ), though most estimates for the Netherlands are somewhat lower, i.e. ca. 250 kg ha −1 y −1 (Pijlman et al. 2020 ; Vellinga and André 1999 ). Peat soils also receive substantial inputs of N and P from applications of animal manures and artificial fertilizers, resulting in a comparatively large surplus of 465 kg N ha −1 y −1 and 11 kg P ha −1 y −1 . Most of the N surplus is eventually lost through denitrification with a substantial amount of N 2 O (Velthof et al. 2009 ). The oxidation of peat soils is also one of the largest sources of GHGs from agriculture worldwide (Leifeld and Menichetti 2018 ; Lin et al. 2022 ; Tiemeyer et al. 2016 ). Peat soils in the Netherlands are frequently P saturated (Schoumans and Chardon 2015 ), which increases the potential risk of P leaching to water bodies (Lin et al. 2022 ; Schrier-Uijl et al. 2014 ).The large differences in NUE and PUE between soil types suggest that prioritizing the use of most suitable soils for agriculture will substantially increase efficiency and reduce losses from agriculture (Muscat et al. 2021 ; Netherlands Scientific Council for Government Policy 1992 ).
Nutrient use efficiency of agriculture is strongly associated with specialization
Spatial patterns of NUE, PUE and losses from agriculture largely matched patterns of specialization. In subregions more specialized in crop production the inputs were generally lower, but N and P yields were higher. Losses from animal production were substantial and resulted from losses in the field for feed crop production, manure storage and manure application. The efficiency of e.g., dairy fed mostly with local roughage was low (NUE 0.23). The N and P efficiency of intensive animal husbandry systems fed on imported feeds was much higher, i.e., up to NUEs of 0.53 and PUEs of 0.88. In this case losses during the production of imported feed and application of exported manure took place outside the subregion’s borders and are externalised. Externalizing losses may give a false sense of efficiency of the production systems in question (Quemada et al. 2020 ; Schröder et al. 2011 ). When accounting for the external losses that result from manure application elsewhere, the NUE of 0.25 of these subregions was similar to that of subregions with more local feed production, congruent with an NUE of 23% for pig farms (Quemada et al. 2020 ).
The apparently high NUE and PUE of agriculture in subregions specialised in crop production do not account for the larger losses at food system level. For example Veendam-Pekela mainly produces food crops for the processing industry, predominantly starch potato and sugar beet. During processing most nutrients in these crops end up in residual flows that are used as feed and for non-food purposes. Yet, animal food derived from these food crops comes with a substantially larger loss per kg food than food crops: only around 27% of N and 33% of P of nutrients in feed crops is converted into edible food. The rest of the N and P is recycled via manure with associated losses elsewhere. Hence, at system level the UE is much lower. Sectors should therefore be evaluated in a food system context to account for such externalized losses (van Loon et al. 2023 ).
Low efficiency and large losses from urban areas
Population density was negatively associated with UE and CyCt of the food system, as the UE of waste treatment was lower than that of agriculture and processing. For PUE this effect was stronger than for NUE, as relatively little P was lost from agriculture (PUE 0.74) whilst from waste processing most P was lost (PUE 0.04). The NUE for the subsystem waste processing was 0.05, still much lower than the NUE of 0.36 of the subsystem agriculture. Subregion Assen was the only subregion with a net-consumption, i.e., more food was consumed than produced. In this subregion, only 13% of the N that was consumed came from local products. This means recycling of N from consumer waste into agriculture also adds some external N to the farming system. However, about 49% of N going into agriculture is lost in agriculture and another part ends up as non-food product flows after processing. This means that even in a region with net food import, recycling consumer waste can only replace a very limited part of agricultural N inputs. Recycling P from waste processing to agriculture strongly reduces losses in the system, as the main P losses were from consumer waste whilst P losses from the agriculture subsystem were relatively small. Recycling the nutrients from waste flows requires the adoption of technologies for resource recovery into the waste management system, that until now has been geared toward pollution prevention rather than resource recovery (Coppens et al. 2016 ; Verger et al. 2018 ).
Towards circularity in NN
Improving circularity in nn.
The circularity of food systems can be improved by avoiding non-essential products and residual flows of essential products, prioritizing biomass use for human consumption, repurposing residual flows and minimizing energy use (Muscat et al. 2021 ). Applying these principles to the food system of NN could provide benefits to the environment (IFA 2022 ; Struik and Kuyper 2017 ) and human health (van Selm et al. 2021 ), but may also have other consequences. First, nutrient inputs would need to be reduced and internal cycling enhanced by limiting the currently large losses and by re-using residual flows. This could increase the currently low NUE (0.25) and PUE (0.59) of the food system. Reducing losses requires more efficient farming methods and technologies to recover unavoidable urban waste. Food exports should be limited, to maintain a neutral regional nutrient balance. Where possible, nutrients must also be recovered from non-food flows with applications outside the food system such as pet feed or biobased building materials. Second, limiting feed imports would mean that a higher share of the feed that is used in the region must be produced locally, preferably from land unsuitable for food crops and from residual flows from the food system. In NN heavy clay soils that are currently used as grasslands may be unsuited for cropping. Peat soils are not suitable for circular intensive agriculture, including feed production, as oxidation and associated N emissions from peat soils can only be restricted by restoring and maintaining high groundwater tables (Lin et al. 2022 ; Schrier-Uijl et al. 2014 ). Safeguarding peat soils would therefore limit their agricultural use to systems suited to wet conditions (Lin et al. 2022 ; Offermanns et al. 2023 ; Schoumans and Chardon 2015 ). The possible production of additional feed from available recyclable flows that are not yet used is very limited when compared to current feed imports. Therefore, adhering to the principles of circularity would strongly reduce current livestock production in NN. Third, to meet most of the food demand with food produced in NN requires that rotations are diversified and include more crop types, consequently diversifying current narrow crop rotations. Diversification of crop rotations has additional benefits, including reduced nutrient leaching (Nemecek et al. 2015 ), increased resilience to biotic and abiotic stress (Degani et al. 2019 ) and increased productivity (Mudgal et al. 2010 ). Lastly, a more local food production may lower energy use by reducing the need for transportation. However, there are trade-offs: local production may be less efficient and lead to larger losses (Schulte-Uebbing and De Vries 2021 ), more energy use and higher GHG emissions in agriculture when more land is needed for cultivation. In subregions with sandy and suitable clay soils for cropping it may be possible to provide a full diet locally, yet in subregions dominated by peat and heavy clay soils only few crops can be grown. In subregions with plenty suitable land but low population density, the exploitation of good soils would be limited by the availability of residual flows as circular inputs. Therefore, closing nutrient loops in NN on the subregional level is probably not feasible.
Consequences of circularity in NN
A more circular food system will have economic consequences for a strongly export-oriented region like NN. Required changes will affect exports (and associated inflow of foreign currency) and the income of farmers, but will at the same time reduce environmental costs that are currently not accounted for. Reducing food export to improve circularity in NN would affect food importing regions, including the nearby Randstad metropolitan area (with larger cities including Amsterdam) with insufficient suitable land. Reduced food production in exporting regions might affect food availability in food importing regions in the short term (Mayer et al. 2015 ; van Berkum 2021 ) when this cannot be compensated elsewhere. However, it can also be argued that maintaining the current system compromises global food security due to the strong food-feed competition of current intensive animal production systems (Mottet et al. 2017 ; Muscat et al. 2021 ). Increasing food security with limited environmental impact and reduced land requirements per person requires a shift towards more plant-based diets (Leip et al. 2022 ). Regardless of dietary composition, some regions may not have the capacity to produce enough food to fulfil local demand in a sustainable way (Schulte-Uebbing and De Vries 2021 ). For example, there is not much suitable soil for food crops in the Randstad area, located in a region with mostly peat soils unsuitable for most crops. In other areas, crop production may be less efficient than in NN. Shifting production to such an area is likely to lead to large losses with large impacts in those regions, and consequently increase resource use and GHG emissions at national or global level (Leifeld and Menichetti 2018 ; Tiemeyer et al. 2016 ). Hence a strict application principles of circularity may thus be detrimental for efficiency at larger scales. A circular food system at the appropriate scale increases the options to produce food where it can be done most efficiently (Billen et al. 2018 ), where the optimum scale is determined by trade-offs between the efficiency of production and use of recycled biomass flows including associated transport requirements. These environmental and socio-economic trade-offs of circularity on various scales and with varying land use intensities are still poorly understood (Koppelmäki et al. 2021 ; Muscat et al. 2020 ). Our study shows that integrating knowledge of the local context and the food system will be key for proper planning of circular systems with limited losses at larger geographical scales, such as the Netherlands including the Randstad metropolitan area.
Quantification of nutrient flows as a prerequisite for circular agriculture
The first step towards circularity is a clear understanding of nutrient flows in the current food system, by using the best available data. Yet, we acknowledge data limitations encountered for the NN region. Nutrient flows are currently poorly monitored, at both the farm and regional scales. Nutrient emissions from food processing and waste processing facilities were not readily available. Some of the most important flows in the food system, such as livestock feed composition and origin of ingredients, are currently unavailable (Koppelmäki et al. 2021 ; van der Wiel et al. 2021 ). We recommend open access of existing data sources and for regular sampling of nutrient contents of farm inputs and produce and of all processing flows including waste. This would also provide key insights in crop nutrient offtakes and hence direct options to improve soil nutrient management and reduce losses in the agricultural system in a local context (Silva et al. 2021 ; Sylvester-Bradley et al. 2022 ).
Large differences in flows and efficiencies between subregions with different soil types, specialization, and population density were found. In food system studies not accounting for the local context, such differences are masked. We also found that the efficiency of subsystems in the food system and specialized subregions within the region differ strongly. Food system efficiency strongly depends on how flows are utilized in the system. If flows are not utilized efficiently, food system efficiency will be very low, even though the efficiency of individual subsystems may be high. Hence, to improve efficiency and circularity, a food system lens is required to better tailor the agricultural system to local biophysical conditions and regional food demand. Follow-up research will be needed to address the question what a circular food system would look like in practice, or at what scale such systems should operate.
In the current NN food system the NUE and PUE were low and nutrient recycling was extremely limited with a cycling count close to zero. The system depends heavily on feed and fertilizer imports. A large proportion of the losses can be attributed to production for export. There is little scope to improve nutrient cycling by utilizing unused residues as these residual flows are small compared to the losses from the system. We conclude that reducing losses would therefore require substantial changes to the current food system, especially to the subsystem agriculture. Important steps towards circularity in production-oriented regions such as NN include: matching livestock production with the potential feed supply from residual flows and lands unsuitable for food crops, diversification of crop production to better match local demand and the adoption of technologies to facilitate recovery of nutrients from (consumer) waste. In more urbanized regions where consumption exceeds production, dietary change and the adoption of technologies for nutrient recovery from waste will be most important.
Aptroot A, Herk KV, Sparrius L (2012) Basisrapport voor de Rode Lijst Korstmossen. Buxbaumiella 92:1–117
Google Scholar
Bai Z, Fan X, Jin X, Zhao Z, Wu Y, Oenema O, Velthof G, Hu C, Ma L (2022) Relocate 10 billion livestock to reduce harmful nitrogen pollution exposure for 90% of China’s population. Nat Food 3(2):152–160. https://doi.org/10.1038/S43016-021-00453-Z
Article CAS PubMed Google Scholar
Bergen SD, Bolton SM, Fridley JL (2001) Design principles for ecological engineering. Ecol Eng 18(2):201–210. https://doi.org/10.1016/S0925-8574(01)00078-7
Article Google Scholar
Billen G, Lassaletta L, Garnier J, Noë J Le, Aguilera E, Sanz-Cobeña A (2018) Opening to distant markets or local reconnection of agro-food systems? Environmental consequences at regional and global scales. Agroecosyst Divers Reconciling Contemp Agric Environ Qual 391–413. https://doi.org/10.1016/B978-0-12-811050-8.00025-X
CBL (2020) Nederlandse supermarkten maken voedselverspilling inzichtelijk. https://www.cbl.nl/nederlandse-supermarkten-maken-voedselverspilling-inzichtelijk/ . Accessed 14 Oct 2022
CBS (2016) Export buiten EU groot in Friesland en Noord-Brabant. https://www.cbs.nl/nl-nl/nieuws/2016/14/export-buiten-eu-groot-in-friesland-en-noord-brabant . Accessed 23 Jan 2023
CBS (2018) Bodemgebruik; uitgebreide gebruiksvorm, per gemeente. https://www.cbs.nl/nl-nl/cijfers/detail/70262ned . Accessed 23 Feb 2023
CBS (2019) Dierlijke mest en mineralen 1990–2018. Centraal Bureau voor de Statistiek, p 127. https://www.cbs.nl/nl-nl/publicatie/2019/49/dierlijke-mest-en-mineralen-2018
CBS (2020) Regionale kerncijfers Nederland. https://opendata.cbs.nl/statline/#/CBS/nl/dataset/70072ned/table?dl=4BA5F . Accessed 9 Feb 2021
CBS (2022a) Bevolkingsontwikkeling; regio per maand. https://opendata.cbs.nl/statline/#/CBS/nl/dataset/37230NED/table?fromstatweb . Accessed 25 Feb 2023
CBS (2022b) Landbouw; gewassen, dieren en grondgebruik naar gemeente. https://opendata.cbs.nl/#/CBS/nl/dataset/80781ned/table . Accessed 25 Feb 2023
CBS (2022c) Mineralenbalans landbouw. https://opendata.cbs.nl/#/CBS/nl/dataset/83475NED/table . Accessed 25 Apr 2023
Chowdhury RB, Moore GA, Weatherley AJ (2018) A multi-year phosphorus flow analysis of a key agricultural region in Australia to identify options for sustainable management. Agric Syst 161(December 2016):42–60. https://doi.org/10.1016/j.agsy.2017.12.005
Coppens J, Meers E, Boon N, Buysse J, Vlaeminck SE (2016) Follow the N and P road: High-resolution nutrient flow analysis of the Flanders region as precursor for sustainable resource management. Resour Conserv Recycl 115(2016):9–21. https://doi.org/10.1016/j.resconrec.2016.08.006
CREM Waste Management (2017) Bepaling voedselverspilling in huishoudelijk afval Nederland 2016. https://www.voedingscentrum.nl/Assets/Uploads/voedingscentrum/Documents/Professionals/Pers/Persmappen/Verspilling%202017/Voedselverspilling%20via%20Huishoudelijk%20afval%202016_sorteeranalyse%20(CREMWM).pdf
Crippa M, Solazzo E, Guizzardi D, Monforti-Ferrario F, Tubiello FN, Leip A (2021) Food systems are responsible for a third of global anthropogenic GHG emissions. Nat Food 2(3):198–209. https://doi.org/10.1038/s43016-021-00225-9
de Boer IJM, van Ittersum MK (2018) Circularity in agricultural production. Wageningen University and Research. https://edepot.wur.nl/470625
de Ruijter FJ, van Dijk W, van Geel WCA, Holshof G, Postma R, Wilting P (2020) Actualisatie van stikstof- en fosfaatgehalten van akkerbouwgewassen met een groot areaal (Rapport / Stichting Wageningen Research, Wageningen Plant Research (WPR), Business unit Agrosysteemkunde; No. WPR-957). Wageningen Plant Research. https://doi.org/10.18174/520624
de Vries W, Kros J, Oenema O, de Klein J (2003) Uncertainties in the fate of nitrogen II: A quantitative assessment of the uncertainties in major nitrogen fluxes in the Netherlands. Nutr Cycl Agroecosyst 66(1):71–102. https://doi.org/10.1023/A:1023354109910
de Vries W, Kros J, Voogd JCH, van Duijvendijk K, Ros GH (2018) Kansen voor het sluiten van de mineralenbalansen in Noord-Nederland: Effecten op regionale schaal en bedrijfsschaal. (Wageningen Environmental Research rapport; No. 2925), Wageningen Environmental Research. https://doi.org/10.18174/467746
de Vries W, Kros J, Voogd JC, Ros GH (2023) Integrated assessment of agricultural practices on large scale losses of ammonia, greenhouse gases, nutrients and heavy metals to air and water. Sci Total Environ 857. https://doi.org/10.1016/j.scitotenv.2022.159220
de Vries W, Schulte-Uebbing L, Kros H, Voogd JC, Louwagie G (2021) Spatially explicit boundaries for agricultural nitrogen inputs in the European Union to meet air and water quality targets. Sci Total Environ 786:147283. https://doi.org/10.1016/J.SCITOTENV.2021.147283
Article PubMed Google Scholar
Degani E, Leigh SG, Barber HM, Jones HE, Lukac M, Sutton P, Potts SG (2019) Crop rotations in a climate change scenario: short-term effects of crop diversity on resilience and ecosystem service provision under drought. Agric Ecosyst Environ 285(May). https://doi.org/10.1016/j.agee.2019.106625
Ehlert PAI, Dekker PHM, van der Schoot JR, Visschers R, van Middelkoop JC, van der Maas MP, Pronk AA, van Dam AM (2009) Fosforgehalten en fosfaatafvoercijfers van landbouwgewassen. Alterra Rep 1773:125
Einarsson R, Pitulia D, Cederberg C (2020) Subnational nutrient budgets to monitor environmental risks in EU agriculture: calculating phosphorus budgets for 243 EU28 regions using public data. Nutr Cycl Agroecosyst 0123456789. https://doi.org/10.1007/s10705-020-10064-y
Erisman JW, Leach A, Bleeker A, Atwell B, Cattaneo L, Galloway J (2018) An integrated approach to a nitrogen use efficiency (NUE) indicator for the food production-consumption chain. Sustainability 10(4):1–29. https://doi.org/10.3390/su10040925
EUROSTAT (2021) Population density. https://ec.europa.eu/eurostat/databrowser/view/tps00003/default/bar?lang=en . Accessed 9 Feb 2021
Garrett RD, Ryschawy J, Bell LW, Cortner O, Ferreira J, Garik AVN, Gil JDB, Klerkx L, Moraine M, Peterson CA, Dos Reis JC, Valentim JF (2020) Drivers of decoupling and recoupling of crop and livestock systems at farm and territorial scales. Ecol Soc 25(1):24. https://doi.org/10.5751/ES-11412-250124
Geertjes K, Baas K, Verschuren S, Kaashoek R, Graveland C (2016) Stikstof in afvalwater en slib. Centraal Bureau voor de Statistiek. https://www.cbs.nl/-/media/_pdf/2016/12/stikstof-in-afvalwater.pdf
Hanserud OS, Brod E, Øgaard AF, Müller DB, Brattebø H (2016) A multi-regional soil phosphorus balance for exploring secondary fertilizer potential: the case of Norway. Nutr Cycl Agroecosyst 104(3):307–320. https://doi.org/10.1007/s10705-015-9721-6
Article CAS Google Scholar
IFA (2022) Reducing emissions from fertilizer use. https://www.systemiq.earth/wp-content/uploads/2022/11/Fertilizer_Report_Final.pdf . Accessed 23 Jan 2023
Jurgilevich A, Birge T, Kentala-Lehtonen J, Korhonen-Kurki K, Pietikäinen J, Saikku L, Schösler H (2016) Transition towards circular economy in the food system. Sustainability 8(1):1–14. https://doi.org/10.3390/su8010069
Koppelmäki K, Helenius J, Schulte RPO (2021) Nested circularity in food systems: A Nordic case study on connecting biomass, nutrient and energy flows from field scale to continent. Resour Conserv Recycl 164:105218. https://doi.org/10.1016/j.resconrec.2020.105218
Kros H, van Os J, Voogd JC, Groenendijk P, van Bruggen C, te Molder R, Ros G (2019) Ruimtelijke allocatie van mesttoediening en ammoniakemissie: beschrijving mestverdelingsmodule INITIATOR versie 5. (Wageningen Environmental Research rapport; No. 2939). Wageningen Environmental Research. https://doi.org/10.18174/474513
Kuokkanen A, Mikkilä M, Kuisma M, Kahiluoto H, Linnanen L (2017) The need for policy to address the food system lock-in: A case study of the Finnish context. J Clean Prod 140:933–944. https://doi.org/10.1016/j.jclepro.2016.06.171
Langeveld JWA, Verhagen A, Neeteson JJ, van Keulen H, Conijn JG, Schils RLM, Oenema J (2007) Evaluating farm performance using agri-environmental indicators: Recent experiences for nitrogen management in The Netherlands. J Environ Manage 82(3):363–376. https://doi.org/10.1016/j.jenvman.2005.11.021
Le Noë J, Billen G, Esculier F, Garnier J (2018) Long-term socioecological trajectories of agro-food systems revealed by N and P flows in French regions from 1852 to 2014. Agr Ecosyst Environ 265:132–143. https://doi.org/10.1016/j.agee.2018.06.006
Le Noë J, Billen G, Garnier J, Barcelo D (2017) How the structure of agro-food systems shapes nitrogen, phosphorus, and carbon fluxes: The generalized representation of agro-food system applied at the regional scale in France-NC-ND license ( http://creativecommons.org/licenses/by-nc-nd/4.0/ ). Sci Total Environ 586:42–55. https://doi.org/10.1016/j.scitotenv.2017.02.040
Leifeld J, Menichetti L (2018) The underappreciated potential of peatlands in global climate change mitigation strategies. Nat Commun 9(1071). https://doi.org/10.1038/s41467-018-03406-6
Leip A, Caldeira C, Corrado S, Hutchings NJ, Lesschen JP, Schaap M, de Vries W, Westhoek H, van Grinsven HJM (2022) Halving nitrogen waste in the European Union food systems requires both dietary shifts and farm level actions. Glob Food Sec 35:100648. https://doi.org/10.1016/J.GFS.2022.100648
Lin F, Zuo H, Ma X, Ma L (2022) Comprehensive assessment of nitrous oxide emissions and mitigation potentials across European peatlands. Environ Pollut 301. https://doi.org/10.1016/j.envpol.2022.119041
Martin G, Moraine M, Ryschawy J, Magne MA, Asai M, Sarthou JP, Duru M, Therond O (2016) Crop–livestock integration beyond the farm level: a review. Agron Sustain Dev 36(3):1–21. https://doi.org/10.1007/S13593-016-0390-X
Mayer A, Schaffartzik A, Haas W, Sepulveda AR (2015) Patterns of global biomass trade - Implications for food sovereignty and socio-environmental conflicts. EJOLT Report (20):106. https://doi.org/10.13140/2.1.1442.5128
Mottet A, de Haan C, Falcucci A, Tempio G, Opio C, Gerber P (2017) Livestock: on our plates or eating at our table? A new analysis of the feed/food debate. Glob Food Sec 14:1–8. https://doi.org/10.1016/j.gfs.2017.01.001
Mudgal S, Lavelle P, Cachia F, Somogyi D, Majewski EF, Laurence Bechini L, Debaeke P (2010) Environmental impacts of different crop rotations in the European Union (ENV.B.1/ETU/2009/0026). European Commission - DG ENV. https://www.researchgate.net/publication/363884907_Environmental_impacts_of_crop_rotations_in_the_EU
Mueller ND, Gerber JS, Johnston M, Ray DK, Ramankutty N, Foley JA (2012) Closing yield gaps through nutrient and water management. Nature 490(7419):254–257. https://doi.org/10.1038/nature11420
Muscat A, de Olde EM, de Boer IJM, Ripoll-Bosch R (2020) The battle for biomass: a systematic review of food-feed-fuel competition. Glob Food Sec 25(September):100330. https://doi.org/10.1016/j.gfs.2019.100330
Muscat A, de Olde EM, Ripoll-Bosch R, Van Zanten HHE, Metze TAP, Termeer CJAM, van Ittersum MK, de Boer IJM (2021) Principles, drivers and opportunities of a circular bioeconomy. Nat Food 2(8):561–566. https://doi.org/10.1038/s43016-021-00340-7
Nemecek T, Hayer F, Bonnin E, Carrouée B, Schneider A, Vivier C (2015) Designing eco-efficient crop rotations using life cycle assessment of crop combinations. Eur J Agron 65:40–51. https://doi.org/10.1016/j.eja.2015.01.005
Netherlands Scientific Council for Government Policy (1992) Ground for choices: four perspectives for the rural areas in the European Community. Sdu uitgeverij.
Offermanns L, Tiemeyer B, Dettmann U, Rüffer J, Düvel D, Vogel I, Brümmer C (2023) High greenhouse gas emissions after grassland renewal on bog peat soil. Agric For Meteorol 331(October 2022). https://doi.org/10.1016/j.agrformet.2023.109309
Oosterom E, Hermans R (2013) Riolering in beeld benchmark rioleringszorg 2013. Stichting Rioned. https://www.riool.net/documents/20182/326834/Riolering+in+Beeld+2013/691bd284-0772-4c3f-8a29-1e08b1af0237
Papangelou A, Mathijs E (2021) Assessing agro-food system circularity using nutrient flows and budgets. J Environ Manag 288. https://doi.org/10.1016/J.JENVMAN.2021.112383
Peeters TMJ, Reemer M (2003) Bedreigde en verdwenen bijen van Nederland (Apidae S.L.). Basisrapport met voorstel voor de Rode Lijst (EIS2003–02). – European Invertebrate Survey - Nederland, Leiden. https://repository.naturalis.nl/pub/220052
Pereira HM, Leadley PW, Proença V, Alkemade R, Scharlemann JPW, Fernandez-Manjarrés JF, Araújo MB, Balvanera P, Biggs R, Cheung WWL, Chini L, Cooper HD, Gilman EL, Guénette S, Hurtt GC, Huntington HP, Mace GM, Oberdorff T, Revenga C, … Walpole M (2010) Scenarios for global biodiversity in the 21st century. Science 330(6010):1496–1501. https://doi.org/10.1126/science.1196624
Pijlman J, Holshof G, van den Berg W, Ros GH, Erisman JW, van Eekeren N (2020) Soil nitrogen supply of peat grasslands estimated by degree days and soil organic matter content. Nutr Cycl Agroecosyst 117(3):351–365. https://doi.org/10.1007/s10705-020-10071-z
Quemada M, Lassaletta L, Jensen LS, Godinot O, Brentrup F, Buckley C, Foray S, Hvid SK, Oenema J, Richards KG, Oenema O (2020) Exploring nitrogen indicators of farm performance among farm types across several European case studies. Agric Syst 177. https://doi.org/10.1016/J.AGSY.2019.102689
Regelink I, Ehlert P, Römkens P (2017) Perspectieven voor de afzet van (fosfaat-verarmd) zuiveringsslib naar de landbouw. (Wageningen Environmental Research rapport; No. 2819). Wageningen Environmental Research. https://doi.org/10.18174/420057
Reijneveld JA, Ehlert PAI, Termorshuizen AJ, Oenema O (2010) Changes in the soil phosphorus status of agricultural land in the Netherlands during the 20th century. Soil Use Manag 26(4):399–411. https://doi.org/10.1111/J.1475-2743.2010.00290.X
Reneerkens J, Piersma T, Spaans B (2005) De Waddenzee als kruispunt van vogeltrekwegen; Literatuurstudie naar de kansen en bedreigingen van wadvogels in internationaal perspectief (NIOZ-REPORT 2005–4; Issues 2005–4). Koninklijk Nederlands Instituut voor Onderzoek der Zee. https://www.commissiemer.nl/docs/mer/p15/p1543/1543-83literatuurstudie.pdf
Rijkswaterstaat (2019) Effluenten RWZI’s (gemeten stoffen). https://www.emissieregistratie.nl/
RIVM (2021) NEVO-online versie 2021/7.0. https://nevo-online.rivm.nl/ . Accessed 12 Dec 2022
Sattari SZ, Bouwman AF, Giller KE, Van Ittersum MK (2012) Residual soil phosphorus as the missing piece in the global phosphorus crisis puzzle. Proc Natl Acad Sci USA 109(16):6348–6353. https://doi.org/10.1073/PNAS.1113675109/-/DCSUPPLEMENTAL/PNAS.201113675SI.PDF
Article CAS PubMed PubMed Central Google Scholar
Schoumans OF, Chardon WJ (2015) Phosphate saturation degree and accumulation of phosphate in various soil types in The Netherlands. Geoderma 237:325–335. https://doi.org/10.1016/j.geoderma.2014.08.015
Schrier-Uijl AP, Kroon PS, Hendriks DMD, Hensen A, Van Huissteden J, Berendse F, Veenendaal EM (2014) Agricultural peatlands: towards a greenhouse gas sink - A synthesis of a Dutch landscape study. Biogeosciences 11(16):4559–4576. https://doi.org/10.5194/bg-11-4559-2014
Schröder JJ, Aarts HFM, Ten Berge HFM, Van Keulen H, Neeteson JJ (2003) An evaluation of whole-farm nitrogen balances and related indices for efficient nitrogen use. Eur J Agron 20(1–2):33–44. https://doi.org/10.1016/S1161-0301(03)00070-4
Schröder JJ, Smit AL, Cordell D, Rosemarin A (2011) Improved phosphorus use efficiency in agriculture: a key requirement for its sustainable use. Chemosphere 84(6):822–831. https://doi.org/10.1016/j.chemosphere.2011.01.065
Schulte-Uebbing L, De Vries W (2021) Reconciling food production and environmental boundaries for nitrogen in the European Union. Sci Total Environ 786:147427. https://doi.org/10.1016/j.scitotenv.2021.147427
Schut AGT, Cooledge EC, Moraine M, Van De Ven GWJ, Jones, DL, Chadwick DR (2021) Reintegration of crop-livestock systems in Europe: an overview. Front Agric Sci Eng. https://doi.org/10.15302/J-FASE-2020373
Silva JV, van Ittersum MK, ten Berge HFM, Spätjens L, Tenreiro TR, Anten NPR, Reidsma P (2021) Agronomic analysis of nitrogen performance indicators in intensive arable cropping systems: An appraisal of big data from commercial farms. Field Crops Res 269(May). https://doi.org/10.1016/j.fcr.2021.108176
Smit AL, Van Middelkoop JC, Van Dijk W, Van Reuler H, De Buck AJ (2010) A quantification of phosphorus flows in the Netherlands through agricultural production, industrial processing and households. (Rapport / Plant Research International 364) - 55. Wageningen: Plant Research International. https://library.wur.nl/WebQuery/wurpubs/402102
Smit AB, Jager JH, Van der Meer RW, Verhoog D, Vogelzang TA, Dolman M, Kros H (2017) De Noord-Nederlandse agrosector en agrocluster in beeld. https://edepot.wur.nl/414084
Soethoudt H, Timmermans T (2013) Monitor Voedselverspilling: mid-term rapportage (Food & Biobased Research 1372). Wageningen UR Food & Biobased Research. https://www.wur.nl/upload_mm/e/2/d/ab1ed91f-5884-4ee4-ab50-e0e204a96877_2013-05-13%20Rapport%20monitor%20Voedselverspilling.pdf
Soethoudt H, Vollebregt M (2020) Monitor Voedselverspilling: update 2009–2018: Hoeveel kilo gaat er in Nederland verloren? (Rapport / Wageningen Food & Biobased Research; No. 2050). Wageningen Food & Biobased Research. https://doi.org/10.18174/522604
Struik PC, Kuyper TW (2017) Sustainable intensification in agriculture: the richer shade of green. A review. Agron Sustain Dev 37(5). https://doi.org/10.1007/s13593-017-0445-7
Sylvester-Bradley R, Roques S, Baxter C, Kendall S (2022) Nutrient harvests : the essential yardstick to transform crop nutrition. In: IFS Proceedings 874. International Fertiliser Society. https://fertiliser-society.org/store/nutrient-harvests-the-essential-yardstick-to-transform-crop-nutrition/
Tan M, Hou Y, Zhang L, Shi S, Long W, Ma Y, Zhang T, Oenema O (2022) Nutrient use efficiency of intensive dairy farms in China – Current situation and analyses of options for improvement. Agric Syst 203(September):103495. https://doi.org/10.1016/j.agsy.2022.103495
The World Bank (2020) Arable land (hectares per person) - European Union. https://data.worldbank.org/indicator/AG.LND.ARBL.HA.PC?locations=EU . Accessed 25 Apr 2023
Theobald TFH, Schipper M, Kern J (2016) Regional phosphorus flows Berlin-Brandenburg phosphorus flows in Berlin-Brandenburg, a regional flow analysis. Resour Conserv Recycl 112:1–14. https://doi.org/10.1016/j.resconrec.2016.04.008
Tiemeyer B, Albiac Borraz E, Augustin J, Bechtold M, Beetz S, Beyer C, Drösler M, Ebli M, Eickenscheidt T, Fiedler S, Förster C, Freibauer A, Giebels M, Glatzel S, Heinichen J, Hoffmann M, Höper H, Jurasinski G, Leiber-Sauheitl K, … Zeitz J (2016) High emissions of greenhouse gases from grasslands on peat and other organic soils. Glob Change Biol 22(12):4134–4149. https://doi.org/10.1111/GCB.13303
van Berkum S (2021) How trade can drive inclusive and sustainable food system outcomes in food deficit low-income countries. Food Secur 13(6):1541–1554. https://doi.org/10.1007/s12571-021-01218-z
Article PubMed PubMed Central Google Scholar
van der Wiel BZ, Weijma J, van Middelaar CE, Kleinke M, Buisman CJN, Wichern F (2020) Restoring nutrient circularity: A review of nutrient stock and flow analyses of local agro-food-waste systems. Resour Conserv Recycl X 3(August):100014. https://doi.org/10.1016/j.rcrx.2019.100014
van der Wiel BZ, Weijma J, van Middelaar CE, Kleinke M, Buisman CJN, Wichern F (2021) Restoring nutrient circularity in a nutrient-saturated area in Germany requires systemic change. Nutr Cycl Agroecosyst 121(2–3):209–226. https://doi.org/10.1007/s10705-021-10172-3
van Loon MP, Vonk WJ, Hijbeek R, van Ittersum MK, ten Berge HFM (2023) Circularity indicators and their relation with nutrient use efficiency in agriculture and food systems. Agric Syst 207. https://doi.org/10.1016/j.agsy.2023.103610
van Rossum CTM, Buurma-Rethans EJM, Dinnissen CS, Beukers MH, Brants HAM, Dekkers ALM, Ocké MC (2020) The diet of the Dutch - Results of the Dutch National Food Consumption Survey 2012–2016 (RIVM report 2020–0083). National Institute for Public Health and the Environment. https://www.rivm.nl/bibliotheek/rapporten/2020-0083.pdf
van Selm B, Frehner A, de Boer IJM, van Hal O, Hijbeek R, van Ittersum MK, Talsma EF, Lesschen JP, Hendriks CMJ, Herrero M, van Zanten HHE (2022) Circularity in animal production requires a change in the EAT-Lancet diet in Europe. Nat Food 3(1):66–73. https://doi.org/10.1038/s43016-021-00425-3
van Selm B, Frehner A, van Hal O (2021) The compatibility of animal-sourced food and circularity in healthy European diets. Res Square 1–15. https://doi.org/10.21203/rs.3.rs-147410/v1 License:
Vellinga THV, André G (1999) Sixty years of Dutch nitrogen fertiliser experiments, an overview of the effects of soil type, fertiliser input, management and developments in time. Neth J Agric Sci 47:215–241
Velthof GL, Oudendag D, Witzke HP, Asman WAH, Klimont Z, Oenema O (2009) Integrated assessment of nitrogen losses from agriculture in EU-27 using MITERRA-EUROPE. J Environ Qual 38(2):402–417. https://doi.org/10.2134/jeq2008.0108
Verger Y, Petit C, Barles S, Billen G, Garnier J, Esculier F, Maugis P (2018) A N, P, C, and water flows metabolism study in a peri-urban territory in France: the case-study of the Saclay plateau. Resour Conserv Recycl 137(April):200–213. https://doi.org/10.1016/j.resconrec.2018.06.007
Vingerhoets R, Spiller M, De Backer J, Adriaens A, Vlaeminck SE, Meers E, Moreira MT (2023) Detailed nitrogen and phosphorus flow analysis, nutrient use efficiency and circularity in the agri-food system of a livestock-intensive region. J Clean Prod 410:137278. https://doi.org/10.1016/j.jclepro.2023.137278
Willett W, Rockström J, Loken B, Springmann M, Lang T, Vermeulen S, Garnett T, Tilman D, DeClerck F, Wood A, Jonell M, Clark M, Gordon LJ, Fanzo J, Hawkes C, Zurayk R, Rivera JA, De Vries W, Majele Sibanda L, … Murray CJL (2019) Food in the Anthropocene: the EAT–Lancet Commission on healthy diets from sustainable food systems. Lancet 393(10170):447–492. https://doi.org/10.1016/S0140-6736(18)31788-4
Wortelboer FG (2010) Natuurkwaliteit en biodiversiteit van de Nederlandse zoute wateren (50040216/2010). Planbureau voor de Leefomgeving. https://www.pbl.nl/publicaties/natuurkwaliteit-en-biodiversiteit-van-de-nederlandse-zoute-wateren
WSBD (2018) Zuiveringsspiegel 2018. Waterschap Brabantse Delta. https://simcms.brabantsedelta.nl/_flysystem/media/zuiveringsspiegel_2018_-_waterschap_brabantse_delta.pdf
Yahia EM, Mourad M (2020) Preventing food losses and waste to achieve food security and sustainability, 1st edn. Burleigh Dodds Science Publishing, pp 341–366. https://doi.org/10.19103/as.2019.0053.14
Download references
Acknowledgements
This work was funded by the Dutch Research Council (NWO) Green III program (Grant number GROEN.2019.001) with co-funding from the Dutch Ministry of Agriculture, Nature and Food Quality; Rabobank; Agrifirm Noord-West Europa; and Meststoffen Nederland. The authors express their gratitude to all supporting parties.
Author information
Authors and affiliations.
Plant Production Systems Group, Wageningen University & Research, Wageningen, the Netherlands
Durk W. Tamsma, Martin K. van Ittersum & Antonius G. T. Schut
Animal Production Systems Group, Wageningen University & Research, Wageningen, the Netherlands
Corina E. van Middelaar & Imke J. M. de Boer
Wageningen Environmental Research, Wageningen, the Netherlands
Johannes Kros
You can also search for this author in PubMed Google Scholar
Contributions
Durk W. Tamsma, Corina E. van Middelaar, Imke J.M. de Boer, Martin K. van Ittersum and Antonius G.T. Schut wrote the main manuscript and contributed to the study design. Durk W. Tamsma prepared the figures. Durk W. Tamsma and Johannes Kros collected the data. Durk W. Tamsma and Antonius G.T. Schut prepared R scripts and analyzed the data. All authors reviewed the manuscript.
Corresponding author
Correspondence to Durk W. Tamsma .
Ethics declarations
Competing interests.
The authors declare no competing interests.
Additional information
Publisher's note.
Springer Nature remains neutral with regard to jurisdictional claims in published maps and institutional affiliations.
Supplementary Information
Below is the link to the electronic supplementary material.
Supplementary file1 (PDF 1.43 MB)
Rights and permissions.
Open Access This article is licensed under a Creative Commons Attribution 4.0 International License, which permits use, sharing, adaptation, distribution and reproduction in any medium or format, as long as you give appropriate credit to the original author(s) and the source, provide a link to the Creative Commons licence, and indicate if changes were made. The images or other third party material in this article are included in the article's Creative Commons licence, unless indicated otherwise in a credit line to the material. If material is not included in the article's Creative Commons licence and your intended use is not permitted by statutory regulation or exceeds the permitted use, you will need to obtain permission directly from the copyright holder. To view a copy of this licence, visit http://creativecommons.org/licenses/by/4.0/ .
Reprints and permissions
About this article
Tamsma, D.W., van Middelaar, C.E., de Boer, I.J.M. et al. Why is nutrient cycling in food systems so limited? A case study from the North-Netherlands region. Nutr Cycl Agroecosyst (2024). https://doi.org/10.1007/s10705-024-10352-x
Download citation
Received : 22 May 2023
Accepted : 12 March 2024
Published : 12 April 2024
DOI : https://doi.org/10.1007/s10705-024-10352-x
Share this article
Anyone you share the following link with will be able to read this content:
Sorry, a shareable link is not currently available for this article.
Provided by the Springer Nature SharedIt content-sharing initiative
- Food system
- Circular agriculture
- Nutrient use efficiency
Advertisement
- Find a journal
- Publish with us
- Track your research
Supply Chain Management
Creating resilient supply chains. Sustainable, flexible and autonomous.
Panasonic Connect Intros New and Enhanced Video Production Solutions at NAB 2024
New KAIROS mainframe, enhanced compact live switcher and software functionality to improve the efficiency and quality of video production.
Share this link via:
Or copy link:
Link has been copied
Wiesbaden, DE. 8th April 2024 – Panasonic Connect Europe today announced a series of new products and enhancements designed to improve the efficiency and quality of video production. The latest KAIROS mainframe, compact live switcher and enhanced software functionality will be on show at Panasonic Booth C3310 at the 2024 National Associations of Broadcasters (NAB) Show in Las Vegas from April 14 to 17.
New KAIROS mainframe and functionality
Panasonic is introducing the new mainframe KAIROS Core 200 AT-KC200L1 and the latest software update (version 1.7), including smart routing functionality. The AT-KC200L1, which supports SDI I/O as standard, and the new smart routing functionality [1] , provide improved video quality and productivity when using KAIROS for broadcasting, events, sports and in the corporate and education markets.
The AT-KC200L1 mainframe is equipped with similar functionality as the second-generation KAIROS Core 200 (AT-KC200) announced in June 2023. It supports 24 inputs / 12 outputs (FHD) as standard [2] but offers more flexible video production to customers using SDI-based video production systems. In addition, up to 32 inputs/16 outputs (FHD) can be supported by installing the optional product AT-KC20M1 SDI I/O Board, released in February 2024.
The 1.7 version software update is compatible with all KAIROS Mainframes (AT-KC2000/2000S1, AT-KC200/200L1 and AT-KC1000/KC100) and enables a new function called Smart Routing. It automatically imports video from the network switch [3] into KAIROS to enable the virtual handling of a larger number of input sources. As a result, video bandwidth limitations are removed when using a large number of inputs on KAIROS for video production.
[1] Optional equipment is required for IP in/out of the AT-KC200L1, future support. [2] IP input/output will be supported in the future. [3] For devices that have been confirmed to work with KAIROS Alliance Partners, please refer to the KAIROS Alliance Partner's webpage.
Live switcher enhancements
Panasonic has also announced the May 2024 release of exciting enhancements to its AV-HSW10 compact live switcher to improve the efficiency and quality of video production for webinars and other live events. The new AV-HSW10 will combine the stability of Panasonic's broadcast and professional hardware switchers with the scalability of a software solution. Updates include firmware version 2.00 for streamlined setup and the free AV-SF10 software control panel for efficient one-person operation and remote operation.
The updates will further enhance seamless video-production environments connected to Panasonic PTZ cameras, including support for various IP transmission standards designed for today's video-production needs. Also, integration with RP Link and tally control via IP connections will facilitate high-quality online communication.
Following the high-end AV-UHS500, the updated AV-HSW10 will also generate a moving colour-bar to expedite setup time at live events, especially those with LED walls and large displays. Colour bars available in moving and still picture mode will enable checks for missing dots in LEDs and displays and video output for IP connections, eliminating the need for an external test signal generator.
The new software control panel will allow switcher functions to be conveniently controlled from a PC or tablet (Windows 10/11 or MacOS Monterey/ Ventura/Sonoma). Detailed settings and confirmation of input/output sources, conventionally performed with buttons and knobs, can be easily executed with a PC keyboard or touch screen. Functions exclusive to a software control panel, such as sequencing of shot memory, direct write/replace of still memory will be possible for more efficient pre-shoot preparation and on-shoot review. The GUI will integrate the control screen, live video monitoring and multiviewer, similar to Panasonic’s popular large switchers, for easy switching between multiple video inputs/outputs. The intuitive GUI can be easily used by a single person, including operators with minimal experience.
New PTZ Camera and Teams certification
In addition, the NAB Show will be the first chance to see the latest AW-UE30 PTZ camera in operation. The UE30 is small, quiet, affordable and ideal for education and business meetings as it features built-in auto tracking The AW-UE4 PTZ camera will also be available for demonstration following its recent certification for use with Microsoft Teams.
Read more insights…
Panasonic demonstrates kairos at uk church sound & media summit.
Panasonic’s KAIROS live video production platform is at the heart of The UK Church Sound & Media Summit, showcasing the latest in broadcast technology.
What’s next for wireless connectivity investment in Europe?
Europe has always been at the heart of wireless technologies, from Marconi’s very first wireless transmission in 1897. Back then that initial radio transmission transformed the world and wireless connectivity continues to do so today.
Business Views Wireless Connectivity As A Route To Productivity But Challenges Remain
European businesses expect to see an average 32% boost in productivity in the three years after implementation of the latest wireless technology.
New Software-Based Media Production Suite for Intuitive and Efficient Video Production
Demonstrating at ISE 2024: New Media Production Suite and its Video Mixer plug-in that uses AI technology with the AI Keying function to eliminate the need for a green background, even when shooting outdoors.
Sorry there was an error... The files you selected could not be downloaded as they do not exist.
You selected items. Continue to select additional items or download selected items together as a zip file.
You selected 1 item. Continue to select additional items or download the selected item directly.
Select your language
- Asia-Pacific

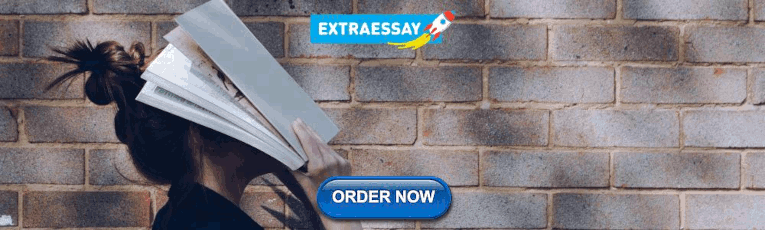
IMAGES
VIDEO
COMMENTS
The Theory of Production. The theory of production examines the relationship between the factors of production (land, labor, capital, entrepreneur) and the output of goods and services. The theory of production is based on the "short run" or a period of production that allows production to change the amount of variable input, in this case, labor. The "long run" is a period of production that ...
Mathematically, Marginal Product is the change in total product divided by the change in labour: MP = ΔTP ÷ ΔL. In the table below (Fig 7.2), since 0 workers produce 0 trees, the marginal product of the first worker is eight haircuts per day, but the marginal product of the second worker is nine haircuts per day. Short Run production.
A case study method should be considered when a study is focused on answering the question of 'how' (Yin, Citation 2003). Among the many types of case studies, an instrumental one is employed in this study. ... This study contributes to production management theory by proposing a new method for TOC implementation and a method for bottleneck ...
The central concept in the theory of production and costs is the production function. It relates quantities of inputs into a production process to the output of that process. The goods and services appearing in a production process are called factors of production; for any quantitative combination of these factors
This study provides the elasticities of capital and labour sector using production theor y in T NB, Malaysia. The Cobb -Douglas function was used to find out the sensitivity of electricit y
The traditional starting point of production theory is a set of physical technological possibilities, often represented by a production or transforma-tion function. The development of the theory par-allels the firm's objective (cost minimization or pro t maximization) and leads to input demands fi (and output supplies in the case of pro t ...
Section 4 also provides details on the use of the production theory approach for conducting resilience cost-benefit analysis, case study research, resilience scoring and macroeconomic analysis. Section 5 provides an assessment of extensions and complications of the production theory approach, and Section 6 concludes. 2. Background2.1.
Stewart, Paul, Mrozowicki, Adam, Danford, Andy, and Murphy, Ken. 2016. " Lean as Ideology and Practice: A Comparative Study of the Impact of Lean Production on the Working Life in Automotive Manufacturing in the United Kingdom and Poland. " Competition and Change. 20 ( 3 ): 147 - 165. Google Scholar.
Section 3 is divided into two parts: section 3.1 describes the case study and the problem formulation, while in section 3.2, new scenarios are proposed and assessed using Design of Experiment ... Because lean production theory calls for the production of parts according to customer demand, the ideal batch size is one. However, a batch size of ...
Accordingly, the technology-oriented theory of production constitutes, to some extent, a novel infinite - product environment. 5. Technology comprehension. Economic focus ( Type A - F, Dyckhoff ): Most production theories view production technology at a highly aggregated level, mainly focusing on economic aspects.
Production Function. Productions function is defined as "the technical relationship, which reveals the maximum amount of output capable of being produced by each and every set of inputs". In the traditional terms, economic theory speaks of four factors of production, land, labour, capital and entrepreneurship.
The eclectic theory of international production: A case study of the international hotel industry. John H. Dunning, John H. Dunning. Department of Economics, University of Reading, UK. ... and uses this data to provide empirical support for the eclectic theory of international production. Our understanding of the international hotel industry is ...
production systems - A systems theory approach, Concurrent E ngineering Researc h and Applications, 2011, 19(2), pp. 139-156, doi:10. 1177/1063293X11406751. ... Two case studies, a simple casing ...
A video presentation of case study about theory of production and costs. AC220B2A (ECO415)
The theory of production and cost defines the relation between input and output. A company can increase its output by changing variable factors, and the fixed variables remain the same. The cost of production theory of value says that short-term costs have short-term implications, giving a short output range.
Each case study will provide evidence for each director's production methods. Interviews and scenes will be referenced to provide examples of auteur, Schreiber, or collaborative production methods. This evidence will then be compared to theory to provide practical support for said theory.
In the past 100 years production management has evolved from a set of heuristic ideas to a portfolio of somewhat developed concepts and principles. "Just‐in‐time" and "total quality management" integrate most of the modern concepts and principles in the field. Furthermore, seminal studies carried out within production, such as the Gilbreth/Taylor, Hawthorne and Tavistock studies ...
The theory of constraints as a manufacturing strategy:a case study in a small manufacturing company Hamilton Pozo, ... In this case, the production costs are reduced while both the sales flow of products and the stock levels remain constant. A reduction in inventory levels has a direct
PDF | On Feb 8, 2018, RADOSLAW WOLNIAK and others published Application of the Theory of Constraints for Continuous Improvement of a Production Process-Case Study | Find, read and cite all the ...
Using TOC's thinking process, the core constraints that had limited the company's performance in the areas of production, distribution, supply group and projects were identified and eliminated. TOC's unique approach helped the company achieve a significant reduction in its finished goods, raw material and work-in-process inventories at various ...
This paper presents comprehensive data on the growth, structure and forms of involvement of multinational enterprises in the international hotel industry, and uses this data to provide empirical support for the eclectic theory of international production. Our understanding of the international hotel industry is that the ownership of a hotel often has the characteristics of portfolio investment ...
Even though this research is a case study in the beverage industry, any manufacturing industry can utilize TOC and other analysis methods used in this study to enhance production performance. To ensure the right identification of bottlenecks, multiple types of identification methods were utilized in this study.
The eco-agricultural park is a new comprehensive agricultural technology system integrating agricultural production, rural economic development, ecological environment protection, and efficient resource utilization. Therefore, an in-depth analysis of the ecosystem structure of eco-agricultural parks will help achieve the goal of coordinated symbiosis between human development and environmental ...
Lack of effective policies hinder the uptake of Utility-scale solar PV, even though they are projected to play a pivotal role in achieving Indonesia's 2050 net-zero energy target. This study seeks to identify a cost-effective pathway to increase the capacity of utility-scale solar PV in Indonesia through supportive policies that ensure equitable cost distribution between the government and ...
How do humans organize production, distribution, exchange, and consumption? What social, political, environmental, and religious values underlie different forms of economic organization? And how do they produce racial, ethnic, class, gender, and sexual inequalities? This course uses a range of historical and contemporary case studies to address these questions, in the process introducing a ...
Purpose: The theory of constraints (TOC) drum-buffer-rope methodology is appropriate when managing a production plant in complex environments, such as make-to-order (MTO) scenarios. However, some ...
The analysis showed that visitors' motivation and cave experience positively influenced their satisfaction. Moreover, satisfaction has an effect on visitors' revisit intention. This study is one of the first to attempt to reveal the nature of future behaviour of cave visitors within the context of self-determination theory.
Identifying pathways to circular agriculture requires a profound understanding of nutrient flows and losses throughout the food system, and of interactions between biophysical conditions, land use, food production and food consumption. We quantified nitrogen (N) and phosphorus (P) flows of the food system of the North-Netherlands (NN) region and of its 30 subregions varying in biophysical and ...
Wiesbaden, DE. 8th April 2024 - Panasonic Connect Europe today announced a series of new products and enhancements designed to improve the efficiency and quality of video production.The latest KAIROS mainframe, compact live switcher and enhanced software functionality will be on show at Panasonic Booth C3310 at the 2024 National Associations of Broadcasters (NAB) Show in Las Vegas from April ...
The study was positioned within the interpretivist paradigm, adopting a qualitative approach and an exploratory case study design. The interpretative approach gives the researcher an opportunity to study the environment in its natural settings and it endeavours to make sense of or interpret phenomena in terms of the meaning people bring to them.