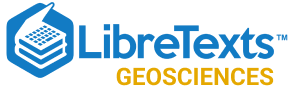
- school Campus Bookshelves
- menu_book Bookshelves
- perm_media Learning Objects
- login Login
- how_to_reg Request Instructor Account
- hub Instructor Commons
- Download Page (PDF)
- Download Full Book (PDF)
- Periodic Table
- Physics Constants
- Scientific Calculator
- Reference & Cite
- Tools expand_more
- Readability
selected template will load here
This action is not available.
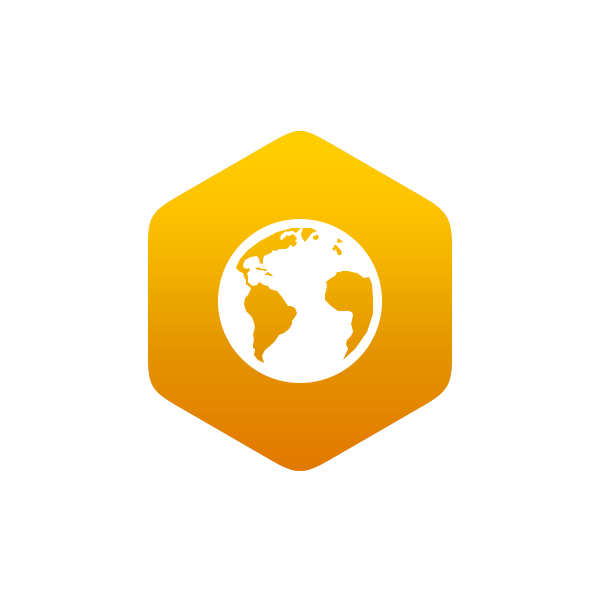
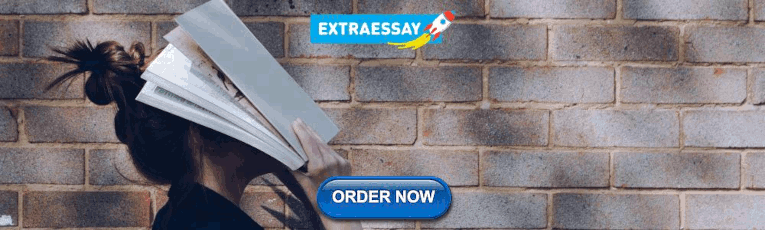
2.4: Representing Geographic Features
- Last updated
- Save as PDF
- Page ID 20562
Maps are a representation of the earth. Central to this representation is reducing the earth’s features of interest to a manageable size (i.e., map scale) and its transformation into a functional two-dimensional form (i.e., map projection). The choice of both map scale and, to a lesser extent, map projection will influence the content and shape of the map.
In addition to the objective decisions made by cartographers and GIS users regarding map scale and map, projections are those concerning what to include and what to omit from the map. The purpose of a map will certainly guide some of these decisions, but other choices may be based on space limitations, map complexity, and desired accuracy. Furthermore, decisions about how to classify, simplify, or exaggerate features and how to symbolize objects of interest simultaneously fall under the realms of art and science.
Moving from the real world to the world of maps is map abstraction . This process involves making choices about how to represent features. More concerning geographic information systems (GIS), we must be explicit, consistent, and precise in defining and describing geographical features of interest. Failure to be explicit, consistent, and precise will return incorrect, inconsistent, and error-prone maps, analyses, and decisions based on such maps and GIS.
One of the most pressing environmental issues facing the world is deforestation. Deforestation refers to the reduction of forest area. This is an essential issue because it has implications for climate change, global warming, biodiversity, and the earth’s water balance, among other things. In the last century, deforestation has increased at an alarming rate and is mainly attributed to human activity. Therefore, mapping forests regularly with a GIS is a logical way to monitor deforestation and has the potential to inform policies regarding forest conservation efforts. Easy enough, so let us get started.
So, what exactly is a forest? How do we know where a forest begins and where it ends? How can natural wildfires be differentiated from those started by humans? Can a forest exist in a swamp or wetland? For that matter, what is the difference between a swamp and a wetland? Such questions are not trivial in the context of mapping and GIS. Consistent and precise definitions of features like forests or swamps increase the reliability and efficiency of maps, mapping, and analysis with GIS.
The world comprises various features or entities within maps, cartography, and GIS. Such entities include but are not restricted to fire hydrants, caves, roads, rivers, lakes, hills, valleys, oceans, and the occasional barn. Moreover, such features have a form, and more precisely, a geometric form. For instance, fire hydrants and geysers are considered point-like features; rivers and streams are linear features; lakes, countries, and forests are areal features.
Features can also be categorized as either discrete or continuous. Discrete features are well defined and are easy to locate, measure, and count, and their edges or boundaries are readily defined. Examples of discrete features in a city include buildings, roads, traffic signals, and parks. Continuous features, on the other hand, are less well defined and exist across space. The most cited examples of continuous features are temperature and elevation. Changes in temperature and elevation tend to be gradual over relatively large areas.
Geographical features also have several characteristics, traits, or attributes that may or may not be attractive. For instance, to continue the deforestation example, determining whether a forest is a rainforest or whether a forest is in a protected park may be necessary. More general attributes may include measurements such as tree density per acre, average canopy height in meters, or proportions like percent palm trees or invasive species per hectare in the forest.
Notwithstanding the purpose of the map or GIS project, definitions of features must be clear and remain consistent. Similarly, it is essential that the attributes of features are also consistently defined, measured, and reported to generate accurate and valuable maps efficiently. Defining features and attributes of interest is often an iterative process of trial and error. Being able to associate a feature with a particular geometric form and determine the feature type are central to map abstraction, facilitating mapping, and GIS application.
Map Content and Generalization
The shape and content of maps vary according to purpose, need, and resources, among other factors. What is familiar to most maps and those within a GIS is that they are graphical representations of reality. Put another way, various graphical symbols represent geographical features or entities. Annotation or text is also commonly used on maps and facilitates map interpretation. Learning about map content and map generalization is essential because they serve as the building blocks for spatial data used within a GIS.
Building upon the previous discussion about the geometric form of geographic features, maps typically rely on three geometric objects: the point, the line, and the polygon or area. A point is defined by x and y coordinates, two points define a line, and a minimum of three points defines a polygon. The vital thing to note is that the definition of a point is analogous to a location that is defined by longitude and latitude. Furthermore, since lines and polygons are made up of points, location information (i.e., x and y, or longitude and latitude, coordinates) is intrinsic to points, lines, and polygons.
Simple and complex maps can be made using these three simple geometric objects. Additionally, by changing the graphical characteristics of each object, an infinite number of mapping possibilities emerge. Such changes can be made to the respective size, shape, color, and patterns of points, lines, and polygons. For instance, different sized points can reflect variations in population size. Line color or line size (i.e., thickness) can denote volume or the amount of interaction between locations. Furthermore, assorted colors and shapes can be used to reflect different values of interest.
Complementing the graphical elements described previously is annotation or text. Annotation is used to identify geographic features, such as cities, states, bodies of water, or other points of interest. Like the graphical elements, text can be varied according to size, orientation, or color. There are also numerous text fonts and styles that are incorporated into maps. For example, bodies of water are often labeled in italics.
Another map element that deserves to be mentioned and combines graphics and text is the map legend or map key. A map legend provides users with information about how geographic information is represented graphically. Legends usually consist of a title that describes the map and the various symbols, colors, and patterns used on the map. Such information is often vital to the proper interpretation of a map.
As more features and graphical elements are put on a given map, the need to generalize such features arises. Map generalization involves resolving conflicts associated with too much detail, too many features, or too much information and data to map. Generalization can take several forms:
- The simplification or symbolization of features for emphasis
- The masking or displacement of detail to increase clarity or legibility
- The selection of detail for inclusion or omission from the map
- The exaggeration of features for emphasis
Determining which aspects of generalization to use is mostly a matter of personal preference, experience, map purpose, and trial and error. Though there are general guidelines about map generalization, there are no universal standards or requirements concerning the generalization of maps and mapping. At this point that cartographic and artistic license, prejudices and biases, and creativity and design sense—or lack thereof—emerge to shape the map.
Making a map and, more generally, mapping involves a range of decisions and choices. From selecting the appropriate map scale and projection to deciding which features to map and omit, mapping is a complex blend of art and science. Many historical maps are indeed viewed as works of art, and rightly so. Learning about maps’ scale, shape, and content increases our understanding of maps and deepens our appreciation of maps and map-making. This increased geographical awareness and appreciation of maps promote the sound and effective use and application of a GIS.
Image maps , in large part derived from satellites, are ubiquitous. Such maps can be found on the news, on the Internet, in your car, and on your mobile phone. Moreover, such images are in living color and exceedingly high resolution. Not long ago, such image maps from satellites were the sole domain of meteorologists, local weather forecasters, and various government agencies. Public access to such images was limited to the evening news.
In conjunction with the commercialization of space flight, technological advances in imaging technology opened the door for companies like Mexar (which used to be called DigitalGlobe) to provide satellite imagery and maps to the masses at the turn of the twenty-first century. With online mapping services such as Google Earth providing free and user-friendly access to such images, a revolution in maps and mapping was born.
Image maps now provide a geographic context for nightly news stories worldwide, serve as a backdrop to local real estate searches and driving directions, and are also used for research purposes. The popularity and widespread use of such images speak not only to recent technological advances and innovations but also, more important, to the geographer in us all.

Want to create or adapt books like this? Learn more about how Pressbooks supports open publishing practices.
2 Chapter 2 Techniques of Geographic Analysis
R. Adam Dastrup
A map can be defined as a graphic representation of the real world. Because of the infinite nature of our Universe, it is impossible to capture all of the complexity found in the real world. For example, topographic maps abstract the three-dimensional real world at a reduced scale on a two-dimensional plane of paper.
Maps are used to display both cultural and physical features of the environment. Standard topographic maps show a variety of information including roads, land-use classification, elevation, rivers and other water bodies, political boundaries, and the identification of houses and other types of buildings. Some maps are created with particular goals in mind, with an intended purpose.
2.1 Understanding Maps
Most maps allow us to specify the location of points on the Earth’s surface using a coordinate system. For a two-dimensional map, this coordinate system can use simple geometric relationships between the perpendicular axes on a grid system to define spatial location. Two types of coordinate systems are currently in general use in geography: the geographical coordinate system and the rectangular (also called Cartesian ) coordinate system .

Geographical Coordinate System
The geographical coordinate system measures location from only two values, despite the fact that the locations are described for a three-dimensional surface. The two values used to define location are both measured relative to the polar axis of the Earth. The two measures used in the geographic coordinate system are called latitude and longitude.
Latitude is an angular measurement north or south of the equator relative to a point found at the center of the Earth. This central point is also located on the Earth’s rotational or polar axis. The equator is the starting point for the measurement of latitude. The equator has a value of zero degrees. A line of latitude or parallel of 30° North has an angle that is 30° north of the plane represented by the equator. The maximum value that latitude can attain is either 90° North or South. These lines of latitude run parallel to the rotational axis of the Earth.
Lines connecting points of the same latitude, called parallels , has lines running parallel to each other. The only parallel that is also a great circle is the equator. All other parallels are small circles. The following are the most important parallel lines:
- Equator, 0 degrees
- Tropic of Cancer, 23.5 degrees N
- Tropic of Capricorn, 23.5 degrees S
- Arctic Circle, 66.5 degrees N
- Antarctic Circle, 66.5 degrees S
- North Pole, 90 degrees N (infinitely small circle)
- South Pole, 90 degrees S (infinitely small circle)
Longitude is the angular measurement east and west of the Prime Meridian. The position of the Prime Meridian was determined by international agreement to be in-line with the location of the former astronomical observatory at Greenwich, England. Because the Earth’s circumference is similar to a circle, it was decided to measure longitude in degrees. The number of degrees found in a circle is 360. The Prime Meridian has a value of zero degrees. A line of longitude or meridian of 45° West has an angle that is 45° west of the plane represented by the Prime Meridian. The maximum value that a meridian of longitude can have is 180° which is the distance halfway around a circle. This meridian is called the International Date Line. Designations of west and east are used to distinguish where a location is found relative to the Prime Meridian. For example, all of the locations in North America have a longitude that is designated west.
At 180 degrees of the Prime Meridian in the Pacific Ocean is the International Date Line . The line determines where the new day begins in the world. Now because of this, the International Date Line is not a straight line, rather it follows national borders so that a country is not divided into two separate days.
Ultimately, when parallel and meridian lines are combined, the result is a geographic grid system that allows users to determine their exact location on the planet.
This may be an appropriate time to briefly discuss the fact that the earth is round and not flat.
Great and Small Circles

Much of Earth’s grid system is based on the location of the North Pole, South Pole, and the Equator. The poles are an imaginary line running from the axis of Earth’s rotation. The plane of the equator is an imaginary horizontal line that cuts the earth into two halves. This brings up the topic of great and small circles. A great circle is any circle that divides the earth into a circumference of two halves. It is also the largest circle that can be drawn on a sphere. The line connecting any points along a great circle is also the shortest distance between those two points.
Examples of great circles include the Equator, all lines of longitude, the line that divides the earth into day and night called the circle of illumination, and the plane of the ecliptic, which divides the earth into equal halves along the equator. Small circles are circles that cut the earth, but not into equal halves.
Universal Transverse Mercator System (UTM)
Another commonly used method to describe a location on the Earth is the Universal Transverse Mercator (UTM) grid system. This rectangular coordinate system is metric, incorporating the meter as its basic unit of measurement. UTM also uses the Transverse Mercator projection system to model the Earth’s spherical surface onto a two-dimensional plane. The UTM system divides the world’s surface into 60, six-degree longitude-wide zones that run north-south. These zones start at the International Date Line and are successively numbered in an eastward direction. Each zone stretches from 84° North to 80° South. In the center of each of these zones is a central meridian .
Location is measured in these zones from a false origin , which is determined relative to the intersection of the equator and the central meridian for each zone. For locations in the Northern Hemisphere, the false origin is 500,000 meters west of the central meridian on the equator. Coordinate measurements of location in the Northern Hemisphere using the UTM system are made relative to this point in meters in eastings (longitudinal distance) and northings (latitudinal distance). The point defined by the intersection of 50° North and 9° West would have a UTM coordinate of Zone 29, 500000 meters east (E), 5538630 meters north (N). In the Southern Hemisphere, the origin is 10,000,000 meters south and 500,000 meters west of the equator and central meridian, respectively. The location found at 50° South and 9° West would have a UTM coordinate of Zone 29, 500000 meters E, 4461369 meters N (remember that northing in the Southern Hemisphere is measured from 10,000,000 meters south of the equator).

The UTM system has been modified to make measurements less confusing. In this modification, the six degree wide zones are divided into smaller pieces or quadrilaterals that are eight degrees of latitude tall. Each of these rows is labeled, starting at 80° South, with the letters C to X consecutively with I and O being omitted. The last row X differs from the other rows and extends from 72 to 84° North latitude (twelve degrees tall). Each of the quadrilaterals or grid zones are identified by their number/letter designation. In total, 1200 quadrilaterals are defined in the UTM system.
The quadrilateral system allows us to define location further using the UTM system. For the location 50° North and 9° West, the UTM coordinate can now be expressed as Grid Zone 29U, 500000 meters E, 5538630 meters N.
Each UTM quadrilateral is further subdivided into a number of 100,000 by 100,000-meter zones. These subdivisions are coded by a system of letter combinations where the same two-letter combination is not repeated within 18 degrees of latitude and longitude. Within each of the 100,000-meter squares, one can specify a location to one-meter accuracy using a five-digit eastings and northings reference system.
The UTM grid system is displayed on all United States Geological Survey (USGS) and National Topographic Series (NTS) of Canada maps. On USGS 7.5-minute quadrangle maps (1:24,000 scale), 15-minute quadrangle maps (1:50,000, 1:62,500, and standard-edition 1:63,360 scales), and Canadian 1:50,000 maps the UTM grid lines are drawn at intervals of 1,000 meters. Both are shown either with blue ticks at the edge of the map or by full blue grid lines. On USGS maps at 1:100,000 and 1:250,000 scale and Canadian 1:250,000 scale maps a full UTM grid is shown at intervals of 10,000 meters.
2.2 Time Zones
Before the late nineteenth century, timekeeping was primarily a local phenomenon. Each town would set their clocks according to the motions of the Sun. Noon was defined as the time when the Sun reached its maximum altitude above the horizon. Cities and towns would assign a clockmaker to calibrate a town clock to these solar motions. This town clock would then represent “official” time, and the citizens would set their watches and clocks accordingly.
The ladder half of the nineteenth century was a time of increased movement of humans. In the United States and Canada, large numbers of people were moving west and settlements in these areas began expanding rapidly. To support these new settlements, railroads moved people and resources between the various cities and towns. However, because of the nature of how local time was kept, the railroads experience significant problems in constructing timetables for the various stops. Timetables could only become more efficient if the towns and cities adopted some standard method of keeping time.
In 1878, Canadian Sir Sanford Fleming suggested a system of worldwide time zones that would simplify the keeping of time across the Earth. Fleming proposed that the globe should be divided into 24 time zones, every 15 degrees of longitude in width. Since the world rotates once every 24 hours on its axis and there are 360 degrees of longitude, each hour of Earth rotation represents 15 degrees of longitude.
Railroad companies in Canada and the United States began using Fleming’s time zones in 1883. In 1884, an International Prime Meridian Conference was held in Washington D.C. to adopt the standardized method of timekeeping and determined the location of the Prime Meridian. Conference members agreed that the longitude of Greenwich, England would become zero degrees longitude and established the 24 time zones relative to the Prime Meridian. It was also proposed that the measurement of time on the Earth would be made relative to the astronomical measurements at the Royal Observatory at Greenwich. This time standard was called Greenwich Mean Time (GMT).
Today, many nations operate on variations of the time zones suggested by Sir Fleming. In this system, time in the various zones is measured relative the Coordinated Universal Time (UTC) standard at the Prime Meridian . Coordinated Universal Time became the standard legal reference of time all over the world in 1972. UTC is determined from atomic clocks that are coordinated by the International Bureau of Weights and Measures (BIPM) located in France. The numbers located at the bottom of the time zone map indicate how many hours each zone is earlier (negative sign) or later (positive sign) than the Coordinated Universal Time standard. Also, note that national boundaries and political matters influence the shape of the time zone boundaries. For example, China uses a single time zone (eight hours ahead of Coordinated Universal Time) instead of five different time zones.
2.3 Coordinate Systems and Projections
Distance on maps.
Depicting the Earth’s three-dimensional surface on a two-dimensional map creates a variety of distortions that involve distance, area, and direction. It is possible to create maps that are somewhat equidistance. However, even these types of maps have some form of distance distortion. Equidistance maps can only control distortion along either lines of latitude or lines of longitude. Distance is often correct on equidistance maps only in the direction of latitude.
On a map that has a large scale, 1:125,000 or larger, distance distortion is usually insignificant. An example of a large-scale map is a standard topographic map. On these maps measuring straight line distance is simple. Distance is first measured on the map using a ruler. This measurement is then converted into a real-world distance using the map’s scale. For example, if we measured a distance of 10 centimeters on a map that had a scale of 1:10,000, we would multiply 10 (distance) by 10,000 (scale). Thus, the actual distance in the real world would be 100,000 centimeters.
Measuring distance along map features that are not straight is a little more difficult. One technique that can be employed for this task is to use several straight-line segments. The accuracy of this method is dependent on the number of straight-line segments used. Another method for measuring curvilinear map distances is to use a mechanical device called an opisometer. This device uses a small rotating wheel that records the distance traveled. The recorded distance is measured by this device either in centimeters or inches.
Direction on Maps
Like distance, direction is difficult to measure on maps because of the distortion produced by projection systems. However, this distortion is quite small on maps with scales larger than 1:125,000. Direction is usually measured relative to the location of North or South Pole. Directions determined from these locations are said to be relative to True North or True South. The magnetic poles can also be used to measure direction. However, these points on the Earth are located in spatially different spots from the geographic North and South Pole. The North Magnetic Pole is located at 78.3° North, 104.0° West near Ellef Ringnes Island, Canada. In the Southern Hemisphere, the South Magnetic Pole is located in Commonwealth Day, Antarctica and has a geographical location of 65° South, 139° East. The magnetic poles are also not fixed over time and shift their spatial position over time.
Topographic maps typically have a declination diagram drawn on them. On Northern Hemisphere maps, declination diagrams describe the angular difference between Magnetic North and True North. On the map, the angle of True North is parallel to the depicted lines of longitude. Declination diagrams also show the direction of Grid North. Grid North is an angle that is parallel to the easting lines found on the Universal Transverse Mercator (UTM) grid system.
In the field, the direction of features is often determined by a magnetic compass which measures angles relative to Magnetic North. Using the declination diagram found on a map, individuals can convert their field measures of magnetic direction into directions that are relative to either Grid or True North. Compass directions can be described by using either the azimuth system or the bearing system. The azimuth system calculates direction in degrees of a full circle. A full circle has 360 degrees. In the azimuth system, north has a direction of either the 0 or 360°. East and West have an azimuth of 90° and 270°, respectively. Due south has an azimuth of 180°.
The bearing system divides direction into four quadrants of 90 degrees. In this system, north and south are the dominant directions. Measurements are determined in degrees from one of these directions.
Geography is about spatial understanding, which requires an accurate grid system to determine absolute and relative location. Absolute location is the exact x- and y- coordinate on the Earth. Relative location is the location of something relative to other entities. For example, when someone uses his or her GPS on his or her smartphone or car, they will put in an absolute location. However, as they start driving, the device tells them to turn right or left relative to objects on the ground.
2.4 Geospatial Technology
Data, data, data… data is everywhere. There are two basic types of data to be familiar with: spatial and non-spatial data. Spatial data , also called geospatial data , is data directly related to a specific location on Earth. Geospatial data is becoming “big business” because it is not just data, but data that can be located, tracked, patterned, and modeled based on other geospatial data. Census information that is collected every ten years is an example of spatial data. Non-spatial data is data that cannot be traced to a specific location, including the number of people living in a household, enrollment within a specific course, or gender information. However, non-spatial data can easily become spatial data if it can connect in some way to a location. Geospatial technology specialists have a method called geocoding that can be used to give non-spatial data a geographic location. Once data has a spatial component associated with it, the type of questions that can be asked dramatically changes.
Remote Sensing
Remote sensing can be defined as the collection of data about an object from a distance. Humans and many other types of animals accomplish this task with the aid of eyes or by the sense of smell or hearing. Geographers use the technique of remote sensing to monitor or measure phenomena found in the Earth’s lithosphere, biosphere, hydrosphere, and atmosphere. Remote sensing of the environment by geographers is usually done with the help of mechanical devices known as remote sensors. These gadgets have a significantly improved ability to receive and record information about an object without any physical contact. Often, these sensors are positioned away from the object of interest by using helicopters, planes, and satellites. Most sensing devices record information about an object by measuring an object’s transmission of electromagnetic energy from reflecting and radiating surfaces.
Remote sensing imagery has many applications in mapping land-use and cover, agriculture, soils mapping, forestry, city planning, archaeological investigations, military observation, and geomorphological surveying, among other uses. For example, foresters use aerial photographs for preparing forest cover maps, locating possible access roads, and measuring quantities of trees harvested. Specialized photography using color infrared film has also been used to detect disease and insect damage in forest trees.
Satellite Remote Sensing
The simplest form of remote sensing uses photographic cameras to record information from visible or near-infrared wavelengths. In the late 1800s, cameras were positioned above the Earth’s surface in balloons or kites to take oblique aerial photographs of the landscape. During World War I, aerial photography played an important role in gathering information about the position and movements of enemy troops. These photographs were often taken from airplanes. After the war, civilian use of aerial photography from airplanes began with the systematic vertical imaging of large areas of Canada, the United States, and Europe. Many of these images were used to construct topographic and other types of reference maps of the natural and human-made features found on the Earth’s surface.

The development of color photography following World War II gave a more natural depiction of surface objects. Color aerial photography also substantially increased the amount of information gathered from an object. The human eye can differentiate many more shades of color than tones of gray. In 1942, Kodak developed color infrared film, which recorded wavelengths in the near-infrared part of the electromagnetic spectrum. This film type had good haze penetration and the ability to determine the type and health of vegetation.
In the 1960s, a revolution in remote sensing technology began with the deployment of space satellites. From their high vantage-point, satellites have an extended view of the Earth’s surface. The first meteorological satellite, TIROS-1, was launched by the United States using an Atlas rocket on April 1, 1960. This early weather satellite used vidicon cameras to scan broad areas of the Earth’s surface. Early satellite remote sensors did not use conventional film to produce their images. Instead, the sensors digitally capture the images using a device similar to a television camera. Once captured, this data is then transmitted electronically to receiving stations found on the Earth’s surface.
In the 1970s, the second revolution in remote sensing technology began with the deployment of the Landsat satellites. Since this 1972, several generations of Landsat satellites with their Multispectral Scanners (MSS) have been providing continuous coverage of the Earth for almost 30 years. Current, Landsat satellites orbit the Earth’s surface at an altitude of approximately 700 kilometers. The spatial resolution of objects on the ground surface is 79 x 56 meters. Complete coverage of the globe requires 233 orbits and occurs every 16 days. The Multispectral Scanner records a zone of the Earth’s surface that is 185 kilometers wide in four wavelength bands: band 4 at 0.5 to 0.6 micrometers, band 5 at 0.6 to 0.7 micrometers, band 6 at 0.7 to 0.8 micrometers, and band 7 at 0.8 to 1.1 micrometers. Bands 4 and 5 receive the green and red wavelengths in the visible light range of the electromagnetic spectrum. The last two bands image near-infrared wavelengths. A second sensing system was added to Landsat satellites launched after 1982. This imaging system, known as the Thematic Mapper, records seven wavelength bands from the visible to far-infrared portions of the electromagnetic spectrum. Also, the ground resolution of this sensor was enhanced to 30 x 20 meters. This modification allows for significantly improved clarity of imaged objects.
The usefulness of satellites for remote sensing has resulted in several other organizations launching their own devices. In France, the SPOT (Satellite Pour l’Observation de la Terre) satellite program has launched five satellites since 1986. Since 1986, SPOT satellites have produced more than 10 million images. SPOT satellites use two different sensing systems to image the planet. One sensing system produces black and white panchromatic images from the visible band (0.51 to 0.73 micrometers) with a ground resolution of 10 x 10 meters. The other sensing device is multispectral capturing green, red, and reflected infrared bands at 20 x 20 meters. SPOT-5, which was launched in 2002, is much improved from the first four versions of SPOT satellites. SPOT-5 has a maximum ground resolution of 2.5 x 2.5 meters in both panchromatic mode and multispectral operation.
Radarsat-1 was launched by the Canadian Space Agency in November, 1995. As a remote sensing device, Radarsat is entirely different from the Landsat and SPOT satellites. Radarsat is an active remote sensing system that transmits and receives microwave radiation. Landsat and SPOT sensors passively measure reflected radiation at wavelengths roughly equivalent to those detected by our eyes. Radarsat’s microwave energy penetrates clouds, rain, dust, or haze and produces images regardless of the Sun’s illumination allowing it to image in darkness. Radarsat images have a resolution between 8 to 100 meters. This sensor has found important applications in crop monitoring, defense surveillance, disaster monitoring, geologic resource mapping, sea-ice mapping and monitoring, oil slick detection, and digital elevation modeling.
Today, the GOES (Geostationary Operational Environmental Satellite) system of satellites provides most of the remotely sensed weather information for North America. To cover the entire continent and adjacent oceans, two satellites are employed in a geostationary orbit. The western half of North America and the eastern Pacific Ocean is monitored by GOES-10, which is directly above the equator and 135° West longitude. The eastern half of North America and the western Atlantic are cover by GOES-8. The GOES-8 satellite is located overhead of the equator and 75° West longitude. Advanced sensors aboard the GOES satellite produce a continuous data stream so images can be viewed at any instance. The imaging sensor produces visible and infrared images of the Earth’s terrestrial surface and oceans. Infrared images can depict weather conditions even during the night. Another sensor aboard the satellite can determine vertical temperature profiles, vertical moisture profiles, total precipitable water, and atmospheric stability.
Principles of Object Identification
Most people have no problem identifying objects from photographs taken from an oblique angle. Such views are natural to the human eye and are part of our everyday experience. However, most remotely sensed images are taken from an overhead or vertical perspective and distances quite removed from ground level. Both of these circumstances make the interpretation of natural and human-made objects somewhat difficult. In addition, images obtained from devices that receive and capture electromagnetic wavelengths outside human vision can present views that are quite unfamiliar.
To overcome the potential difficulties involved in image recognition, professional image interpreters use some characteristics to help them identify remotely sensed objects. Some of these characteristics include:
- Shape : this characteristic alone may serve to identify many objects. Examples include the long linear lines of highways, the intersecting runways of an airfield, the perfectly rectangular shape of buildings, or the recognizable shape of an outdoor baseball diamond.
- Size : noting the relative and absolute sizes of objects are essential in their identification. The scale of the image determines the absolute size of an object. As a result, it is essential to recognize the scale of the image to be analyzed.
- Image Tone or Color : all objects reflect or emit specific signatures of electromagnetic radiation. In most cases, related types of objects emit or reflect similar wavelengths of radiation. Also, the types of the recording device and recording media produce images that are reflective of their sensitivity to a particular range of radiation. As a result, the interpreter must be aware of how the object being viewed will appear on the image examined. For example, on color, infrared images vegetation has a color that ranges from pink to red rather than the usual tones of green.
- Pattern : many objects arrange themselves in typical patterns. This is especially true of human-made phenomena. For example, orchards have a systematic arrangement imposed by a farmer, while natural vegetation usually has a random or chaotic pattern.
- Shadow : shadows can sometimes be used to get a different view of an object. For example, an overhead photograph of a towering smokestack or a radio transmission tower normally presents an identification problem. This difficulty can be overcome by photographing these objects at Sun angles that cast shadows. These shadows then display the shape of the object on the ground. Shadows can also be a problem to interpreters because they often conceal things found on the Earth’s surface.
- Texture : imaged objects display some degree of coarseness or smoothness. This characteristic can sometimes be useful in object interpretation. For example, we would typically expect to see textural differences when comparing an area of grass with field corn. Texture, just like object size, is directly related to the scale of the image.
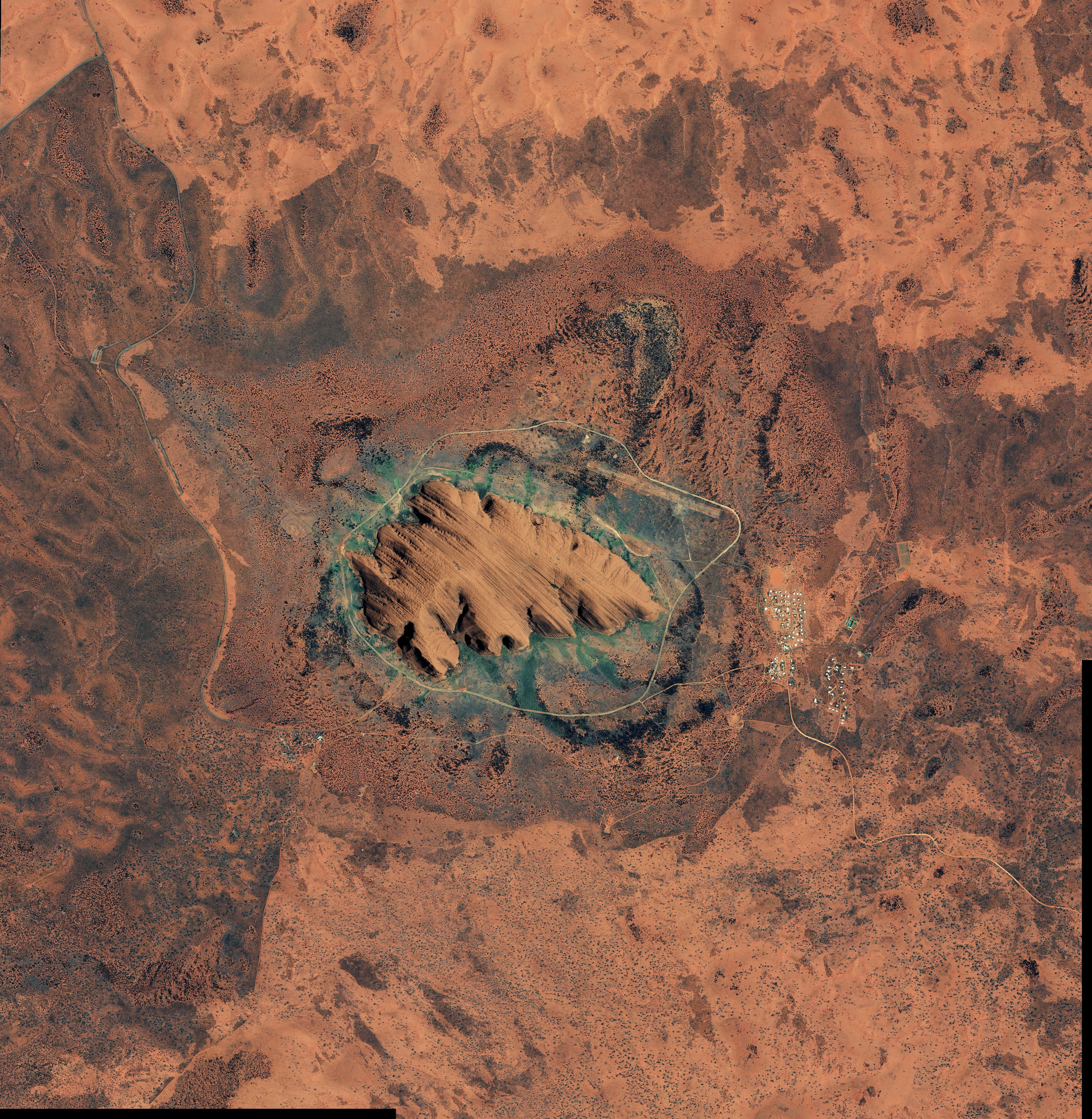
Global Positioning Systems
Another type of geospatial technology is global positioning systems (GPS) and a key technology for acquiring accurate control points on Earth’s surface. Now to determine the location of that GPS receiver on Earth’s surface, a minimum of four satellites is required using a mathematical process called triangulation. Usually, the process of triangulation requires a minimum of three transmitters, but because the energy sent from the satellite is traveling at the speed of light, minor errors in calculation could result in significant location errors on the ground. Thus, a minimum of four satellites is often used to reduce this error. This process using the geometry of triangles to determine location is used not only in GPS, but a variety of other location needs like finding the epicenter of earthquakes.
A user can use a GPS receiver to determine their location on Earth through a dynamic conversation with satellites in space. Each satellite transmits orbital information called the ephemeris using a highly accurate atomic clock along with its orbital position called the almanac. The receiver will use this information to determine its distance from a single satellite using the equation D = rt, where D = distance, r = rate or the speed of light (299,792,458 meters per second), and t = time using the atomic clock. The atomic clock is required because the receiver is trying to calculate distance, using energy that is transmitted at the speed of light. Time will be fractions of a second and requires a “time clock” up the utmost accuracy.
Determination of location in field conditions was once a difficult task. In most cases, it required the use of a topographic map and landscape features to estimate location. However, technology has now made this task very simple. Global Positioning Systems (GPS) can calculate one’s location to an accuracy of about 30-meters. These systems consist of two parts: a GPS receiver and a network of many satellites. Radio transmissions from the satellites are broadcasted continually. The GPS receiver picks up these broadcasts and through triangulation calculates the altitude and spatial position of the receiving unit. A minimum of three satellite is required for triangulation.
GPS receivers can determine latitude, longitude, and elevation anywhere on or above the Earth’s surface from signals transmitted by a number of satellites. These units can also be used to determine direction, distance traveled, and determine routes of travel in field situations.
Geographic Information Systems
The advent of cheap and powerful computers over the last few decades has allowed for the development of innovative software applications for the storage, analysis, and display of geographic data. Many of these applications belong to a group of software known as Geographic Information Systems (GIS). Many definitions have been proposed for what constitutes a GIS. Each of these definitions conforms to the particular task that is being performed. A GIS does the following activities:
- Measurement of natural and human-made phenomena and processes from a spatial perspective. These measurements emphasize three types of properties commonly associated with these types of systems: elements, attributes, and relationships.
- Storage of measurements in digital form in a computer database. These measurements are often linked to features on a digital map. The features can be of three types: points, lines, or areas (polygons).
- Analysis of collected measurements to produce more data and to discover new relationships by numerically manipulating and modeling different pieces of data.
- Depiction of the measured or analyzed data in some type of display – maps, graphs, lists, or summary statistics.
The first computerized GIS began its life in 1964 as a project of the Rehabilitation and Development Agency Program within the government of Canada. The Canada Geographic Information System (CGIS) was designed to analyze Canada’s national land inventory data to aid in the development of land for agriculture. The CGIS project was completed in 1971, and the software is still in use today. The CGIS project also involved a number of key innovations that have found their way into the feature set of many subsequent software developments.
From the mid-1960s to 1970s, developments in GIS were mainly occurring at government agencies and universities. In 1964, Howard Fisher established the Harvard Lab for Computer Graphics where many of the industries early leaders studied. The Harvard Lab produced a number of mainframe GIS applications including SYMAP (Synagraphic Mapping System), CALFORM, SYMVU, GRID, POLYVRT, and ODYSSEY. ODYSSEY was first modern vector GIS, and many of its features would form the basis for future commercial applications. Automatic Mapping System was developed by the United States Central Intelligence Agency (CIA) in the late 1960s. This project then spawned the CIA’s World Data Bank, a collection of coastlines, rivers, and political boundaries, and the CAM software package that created maps at different scales from this data. This development was one of the first systematic map databases. In 1969, Jack Dangermond, who studied at the Harvard Lab for Computer Graphics, co-founded Environmental Systems Research Institute (ESRI) with his wife, Laura. ESRI would become in a few years the dominant force in the GIS marketplace and create ArcInfo and ArcView software. The first conference dealing with GIS took place in 1970 and was organized by Roger Tomlinson (key individual in the development of CGIS) and Duane Marble (professor at Northwestern University and early GIS innovator). Today, numerous conferences dealing with GIS run every year attracting thousands of attendants.
In the 1980s and 1990s, many GIS applications underwent substantial evolution regarding features and analysis power. Many of these packages were being refined by private companies who could see the future commercial potential of this software. Some of the popular commercial applications launched during this period include ArcInfo, ArcView, MapInfo, SPANS GIS, PAMAP GIS, INTERGRAPH, and SMALLWORLD. It was also during this period that many GIS applications moved from expensive minicomputer workstations to personal computer hardware.
There is a technology that exists that can bring together remote sensing data, GPS data points, spatial and non-spatial data, and spatial statistics into a single, dynamic system for analysis and that is a geographic information system (GIS). A GIS is a powerful database system that allows users to acquire, organize, store, and most importantly analyze information about the physical and cultural environments. A GIS views the world as overlaying physical or cultural layers, each with quantifiable data that can be analyzed. A single GIS map of a national forest could have layers such as elevation, deciduous trees, evergreens, soil type, soil erosion rates, rivers and tributaries, major and minor roads, forest health, burn areas, regrowth, restoration, animal species type, trails, and more. Each of these layers would contain a database of information specific to that layer.
GIS combines computer cartography with a database management system. GIS consists of three subsystems: (1) an input system that allows for the collection of data to be used and analyzed for some purpose; (2) computer hardware and software systems that store the data, allow for data management and analysis, and can be used to display data manipulations on a computer monitor; (3) an output system that generates hard copy maps, images, and other types of output.
Two basic types of data are typically entered into a GIS. The first type of data consists of real-world phenomena and features that have some form of spatial dimension. Usually, these data elements are depicted mathematically in the GIS as either points, lines, or polygons that are referenced geographically (or geocoded) to some type of coordinate system. This type data is entered into the GIS by devices like scanners, digitizers, GPS, air photos, and satellite imagery. The other type of data is sometimes referred to as an attribute. Attributes are pieces of data that are connected or related to the points, lines, or polygons mapped in the GIS. This attribute data can be analyzed to determine patterns of importance. Attribute data is entered directly into a database where it is associated with feature data.
Within the GIS database, a user can enter, analyze, and manipulate data that is associated with some spatial element in the real world. The cartographic software of the GIS enables one to display the geographic information at any scale or projection and as a variety of layers which can be turned on or off. Each layer would show some different aspect of a place on the Earth. These layers could show things like a road network, topography, vegetation cover, streams and water bodies, or the distribution of annual precipitation received.
Nearly every discipline, career path, or academic pursuit uses geographic information systems because of the vast amount of data and information about the physical and cultural world. Disciplines and career paths that use GIS include: conservation, ecology, disaster response and mitigation, business, marketing, engineering, sociology, demography, astronomy, transportation, health, criminal justice and law enforcement, travel and tourism, news media, and the list could endlessly go on.
Now, GIS primarily works from two different spatial models: raster and vector. Raster models in GIS are images much like a digital picture. Each image is broken down into a series of columns and rows of pixels, and each pixel is georeferenced to somewhere on Earth’s surface is represents a specific numeric value – usually a specific color or wavelength within the electromagnetic spectrum. Most remote sensing images come into a GIS as a raster layer.
The other type of GIS model is called a vector model . Vector-based GIS models are based on the concept of points that are again georeferenced (i.e., given an x-, y-, and possibly z- location) to somewhere specific on the ground. From points, lines can be created by connecting a series of points and areas can be created by closing loops of vector lines. For each of these vector layers, a database of information can be attributed to it. So for example, a vector line of rivers could have a database associated with it such as length, width, stream flow, government agencies responsible for it, and anything else the GIS user wants to be connected to it. What these vector models represent is also a matter of scale. For example, a city can be represented as a point or a polygon depending on how zoomed in you are to the location. A map of the world would show cities as points, whereas a map of a single county may show the city as a polygon with roads, populations, pipes, or grid systems within it.

Chapter 2 Techniques of Geographic Analysis Copyright © by R. Adam Dastrup is licensed under a Creative Commons Attribution 4.0 International License , except where otherwise noted.
Share This Book
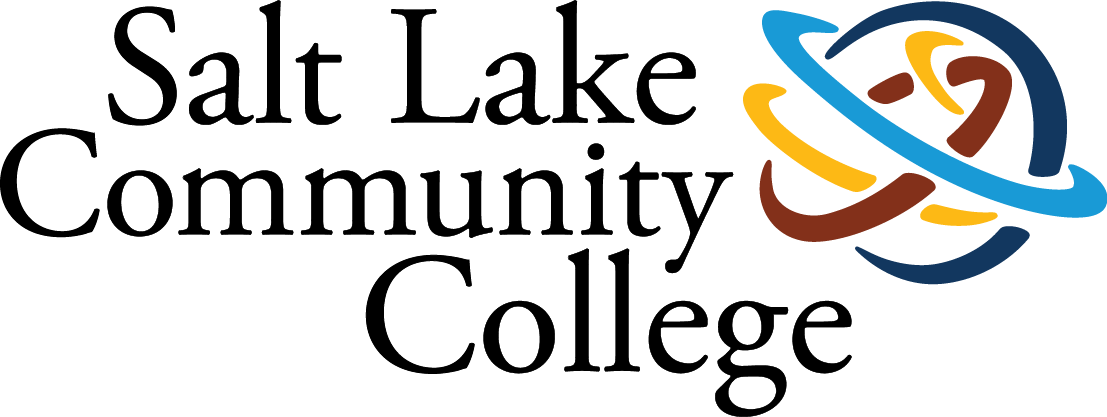
Want to create or adapt books like this? Learn more about how Pressbooks supports open publishing practices.
2.4 Representing Geographic Features
Maps are a representation of the earth. Central to this representation is reducing the earth’s features of interest to a manageable size (i.e., map scale) and its transformation into a functional two-dimensional form (i.e., map projection). The choice of both map scale and, to a lesser extent, map projection will influence the content and shape of the map.
In addition to the objective decisions made by cartographers and GIS users regarding map scale and map, projections concern what to include and omit from the map. The purpose of a map will certainly guide some of these decisions, but other choices may be based on space limitations, map complexity, and desired accuracy. Furthermore, decisions about how to classify, simplify, or exaggerate features and how to symbolize objects of interest simultaneously fall under the realms of art and science. Moving from the real world to the world of maps is map abstraction . This process involves making choices about how to represent features. Regarding geographic information systems (GIS), we must be explicit, consistent, and precise in defining and describing geographical features of interest. Failure to be explicit, consistent, and precise will return incorrect, inconsistent, error-prone maps, analyses, and decisions based on such maps and GIS.
One of the most pressing environmental issues facing the world is deforestation. Deforestation refers to the reduction of forest area. This is an essential issue because it has implications for climate change, global warming, biodiversity, and the earth’s water balance, among other things. Unfortunately, in the last century, deforestation has increased at an alarming rate and is attributed to human activity. Therefore, mapping forests regularly with a GIS is a logical way to monitor deforestation and has the potential to inform policies regarding forest conservation efforts. Easy enough, so let us get started.
So, what exactly is a forest? How do we know where a forest begins and where it ends? How can natural wildfires be differentiated from those started by humans? Can a forest exist in a swamp or wetland? For that matter, what is the difference between a swamp and a wetland? Such questions are not trivial in the context of mapping and GIS. Consistent and precise definitions of features like forests or swamps increase the reliability and efficiency of maps, mapping, and analysis with GIS.
Discrete and Continuous Features
The world comprises various features or entities within maps, cartography, and GIS. Such entities include but are not restricted to fire hydrants, caves, roads, rivers, lakes, hills, valleys, oceans, and the occasional barn. Moreover, such features have a form, more precisely, a geometric form. For instance, fire hydrants and geysers are considered point-like features; rivers and streams are linear features; lakes, countries, and forests are areal features. Features can also be categorized as either discrete or continuous . Discrete features are well defined, easy to locate, measure, and count, and their edges or boundaries are readily defined. Examples of discrete features in a city include buildings, roads, traffic signals, and parks. Continuous features , on the other hand, are less well defined and exist across space. The most cited examples of continuous features are temperature and elevation. Changes in temperature and elevation tend to be gradual over relatively large areas.
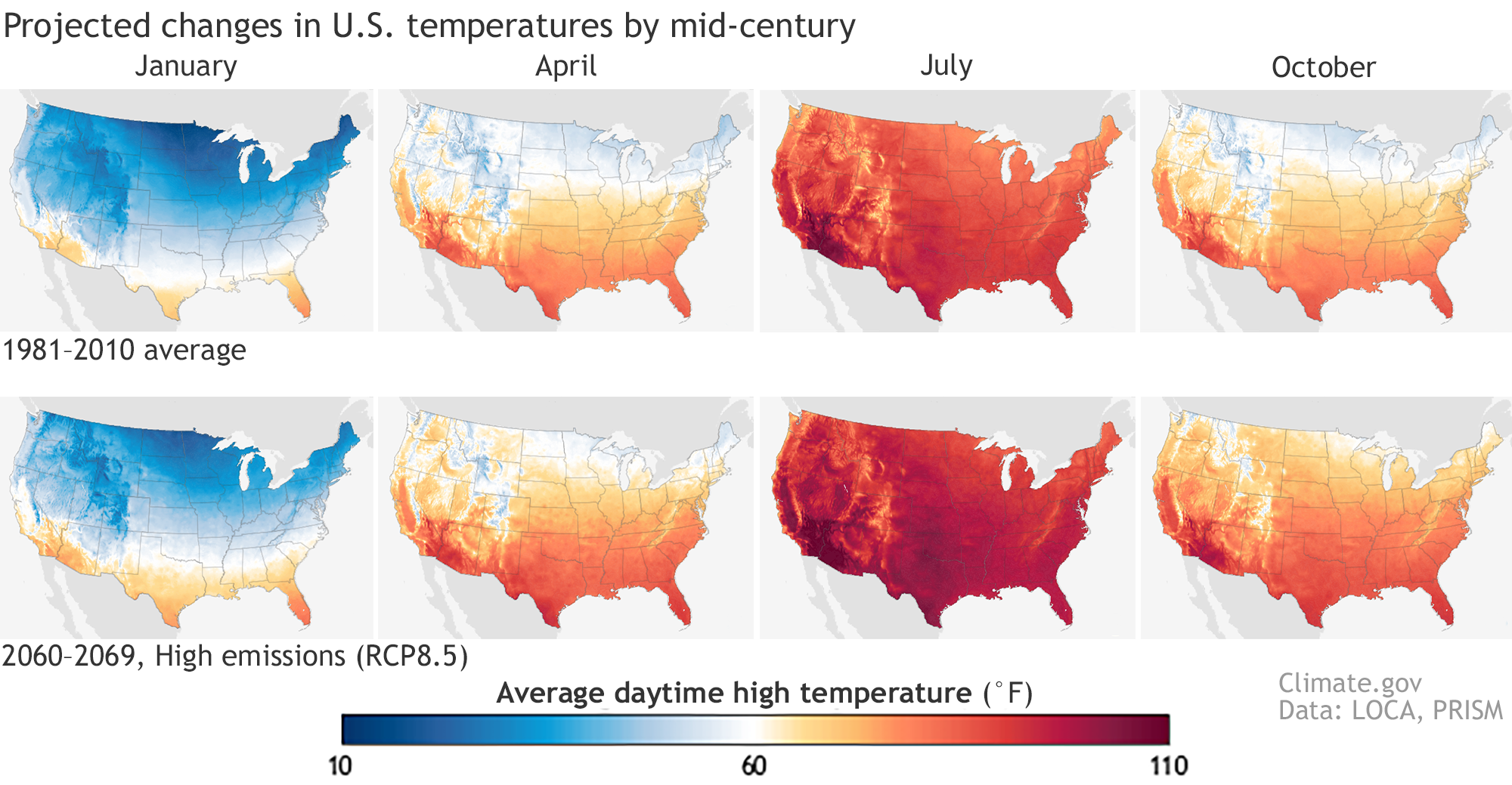
Geographical features also have several characteristics, traits, or attributes that may or may not be attractive. For instance, to continue the deforestation example, determining whether a forest is a rainforest or whether a forest is in a protected park may be necessary. More general attributes may include measurements such as tree density per acre, average canopy height in meters, or proportions like percent palm trees or invasive species per hectare in the forest.
Notwithstanding the map’s or GIS project’s purpose, definitions of features must be clear and consistent. Similarly, it is essential that the attributes of features are also consistently defined, measured, and reported to generate accurate and valuable maps efficiently. Defining features and attributes of interest is often an iterative process of trial and error. Accommodating a feature with a particular geometric form and determining the feature type is central to map abstraction, facilitating mapping, and GIS application.
Map Content and Generalization
The shape and content of maps vary according to purpose, need, and resources, among other factors. What is familiar to most maps and those within a GIS is that they are graphical representations of reality. Put another way, various graphical symbols represent geographical features . Annotation or text is also commonly used on maps and facilitates map interpretation. Learning about map content and generalization is essential because they serve as the building blocks for spatial data used within a GIS.
Building upon the previous discussion about the geometric form of geographic features, maps typically rely on three geometric objects: point , line , or a polygon .
- Point – the x and y coordinate of a feature. Geographic examples could include the location of capitals on a small-scale map, fire hydrants, or mountain peaks.
- Line – two geographic points connected together. Geographic examples could include rivers or streets.
- Polygon – a series of geographic points connected and enclosed. Geographic examples could include parcels, political boundaries, large bodies of water, or wildlife refuges.
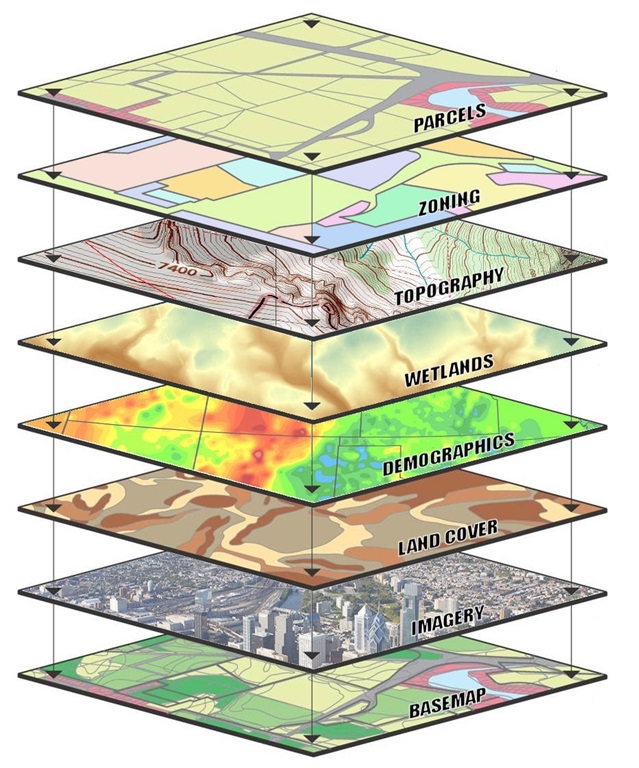
Simple and complex maps can be made using these three simple geometric objects. Additionally, by changing the graphical characteristics of each object, an infinite number of mapping possibilities emerge. Such changes can be made to the respective size, shape, color, and patterns of points, lines, and polygons. For instance, different sized points can reflect variations in population size. Line color or line size (i.e., thickness) can denote volume or the amount of interaction between locations. Furthermore, assorted colors and shapes can be used to reflect different values of interest.
Complementing the graphical elements described previously is annotation or text. Annotation is used to identify geographic features, such as cities, states, bodies of water, or other points of interest. Like the graphical elements, text can be varied according to size, orientation, or color. There are also numerous text fonts and styles that are incorporated into maps. For example, bodies of water are often labeled in italics.
Another map element that deserves to be mentioned and combines graphics and text is the map legend or map key . A map legend provides users with information about how geographic information is represented graphically. Legends usually consist of a title that describes the map and the various symbols, colors, and patterns used on the map. Such information is often vital to the proper interpretation of a map.
As more features and graphical elements are put on a given map, the need to generalize such features arises. Map generalization involves resolving conflicts associated with too much detail, too many features, or too much information and data to map. Generalization can take several forms:
- Simplification or symbolization of features for emphasis
- Masking or displacement of detail to increase clarity or legibility
- Selection of detail for inclusion or omission from the map
- Exaggeration of features for emphasis
Determining which aspects of generalization to use is mostly a matter of personal preference, experience, map purpose, and trial and error. Though there are general guidelines about map generalization, there are no universal standards or requirements concerning the generalization of maps and mapping. At this point that cartographic and artistic license, prejudices and biases, and creativity and design sense—or lack thereof—emerge to shape the map.
Making a map and, more generally, mapping involves various decisions and choices. From selecting the appropriate map scale and projection to deciding which features to map and omit, mapping is a complex blend of art and science. Many historical maps are indeed viewed as works of art, and rightly so. Learning about maps’ scale, shape, and content increases our understanding of maps and deepens our appreciation of maps and map-making. This increased geographical awareness and appreciation of maps promote the sound and effective use and application of a GIS.
Image maps , in large part derived from satellites, are ubiquitous. Such maps can be found on the news, on the Internet, in your car, and on your mobile phone. Moreover, such images are in living color and exceedingly high resolution. Not long ago, such satellite image maps were the sole domain of meteorologists, local weather forecasters, and various government agencies. Public access to such images was limited to the evening news.
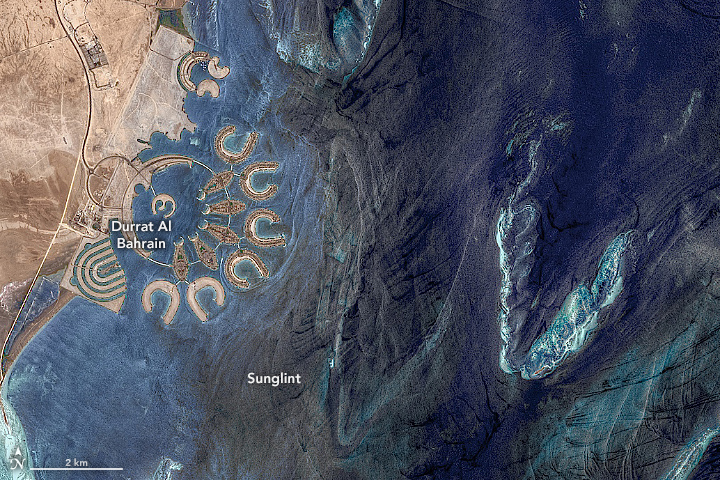
In conjunction with the commercialization of space flight, technological advances in imaging technology opened the door for companies like Mexar (which used to be called Digital Globe) to provide satellite imagery and maps to the masses at the turn of the twenty-first century. With online mapping services such as Google Earth providing free and user-friendly access to such images, a revolution in maps and mapping was born.
Image maps now provide a geographic context for nightly news stories worldwide, serve as a backdrop to local real estate searches and driving directions, and are also used for research purposes. The popularity and widespread use of such images speak not only to recent technological advances and innovations but also, more important, to the geographer in us all.
Click the “ Previous ” button on the lower left or the ‘ Next ” button on the lower right to navigate throughout the textbook.
Geographic Information Systems and Cartography Copyright © 2022 by adamdastrup is licensed under a Creative Commons Attribution-NonCommercial-ShareAlike 4.0 International License , except where otherwise noted.
Share This Book
Geography Department Penn State
- GIS Program
5. Graphic Map Scales

Another way to express map scale is with a graphic (or "bar") scale. Unlike representative fractions, graphic scales remain true when maps are shrunk or magnified.
If they include a scale at all, most maps include a bar scale like the one shown above left (Figure 2.6.1). Some also express map scale as a representative fraction. Either way, the implication is that scale is uniform across the map. In fact, except for maps that show only very small areas, scale varies across every map. As you probably know, this follows from the fact that positions on the nearly-spherical Earth must be transformed to positions on two-dimensional sheets of paper. Systematic transformations of this kind are called map projections . As we will discuss in greater depth later in this chapter, all map projections are accompanied by deformation of features in some or all areas of the map. This deformation causes map scale to vary across the map. Representative fractions may, therefore, specify map scale along a line at which deformation is minimal ( nominal scale ). Bar scales denote only the nominal or average map scale. Variable scales, like the one illustrated above right, show how scale varies, in this case by latitude, due to deformation caused by map projection.
A map is a symbolic representation of selected characteristics of a place, usually drawn on a flat surface
Geography, Geographic Information Systems (GIS)
Loading ...
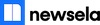
Learning materials
- National Geographic MapMaker
A map is a symbolic representation of selected characteristics of a place, usually drawn on a flat surface. Maps present information about the world in a simple, visual way. They teach about the world by showing sizes and shapes of countries, locations of features, and distances between places. Maps can show distributions of things over Earth, such as settlement patterns . They can show exact locations of houses and streets in a city neighborhood . Mapmakers, called cartographers , create maps for many different purposes. Vacationers use road maps to plot routes for their trips. Meteorologists —scientists who study weather —use weather maps to prepare forecasts . City planners decide where to put hospitals and parks with the help of maps that show land features and how the land is currently being used. Some common features of maps include scale , symbols , and grids . Scale All maps are scale models of reality. A map’s scale indicates the relationship between the distances on the map and the actual distances on Earth. This relationship can be expressed by a graphic scale , a verbal scale , or a representative fraction . The most common type of graphic scale looks like a ruler. Also called a bar scale , it is simply a horizontal line marked off in miles, kilometers, or some other unit measuring distance. The verbal scale is a sentence that relates distance on the map to distance on Earth. For example, a verbal scale might say, “one centimeter represents one kilometer” or “one inch represents eight miles.” The representative fraction does not have specific units. It is shown as a fraction or ratio —for example, 1/1,000,000 or 1:1,000,000. This means that any given unit of measure on the map is equal to one million of that unit on Earth. So, 1 centimeter on the map represents 1,000,000 centimeters on Earth, or 10 kilometers. One inch on the map represents 1,000,000 inches on Earth, or a little less than 16 miles. The size of the area covered helps determine the scale of a map. A map that shows an area in great detail, such as a street map of a neighborhood, is called a large-scale map because objects on the map are relatively large. A map of a larger area, such as a continent or the world, is called a small-scale map because objects on the map are relatively small. Today, maps are often computerized . Many computerized maps allow the viewer to zoom in and out, changing the scale of the map. A person may begin by looking at the map of an entire city that only shows major roads and then zoom in so that every street in a neighborhood is visible . Symbols Cartographers use symbols to represent geographic features. For example, black dots represent cities, circled stars represent capital cities, and different sorts of lines represent boundaries, roads, highways, and rivers. Colors are often used as symbols. Green is often used for forests, tan for deserts , and blue for water. A map usually has a legend , or key, that gives the scale of the map and explains what the various symbols represent. Some maps show relief, or changes in elevation. A common way to show relief is contour lines , also called topographic lines . These are lines that connect points that have equal elevation. If a map shows a large enough area, contour lines form circles. A group of contour line circles inside one another indicates a change in elevation. As elevation increases, these contour line circles indicate a hill . As elevation decreases, contour line circles indicate a depression in the earth, such as a basin . Grids Many maps include a grid pattern, or a series of crossing lines that create squares or rectangles. The grid helps people locate places on the map. On small-scale maps, the grid is often made up of latitude and longitude lines. Latitude lines run east-west around the globe , parallel to the Equator , an imaginary line that circles the middle of Earth. Longitude lines run north-south, from pole to pole. Latitude and longitude lines are numbered. The intersection of latitude and longitude lines, called coordinates , identify the exact location of a place. On maps showing greater detail, the grid is often given numbers and letters. The boxes made by the grid may be called A, B, C, and so on across the top of the map, and 1, 2, 3, and so on across the left side. In the map’s index , a park’s location might be given as B4. The user finds the park by looking in the box where column B and row 4 cross. Other Map Features: DOGSTAILS Along with scale, symbols, and grids, other features appear regularly on maps. A good way to remember these features is DOGSTAILS: date, orientation , grid, scale, title, author, index, legend, and sources. Title, date, author, and sources usually appear on the map though not always together. The map’s title tells what the map is about, revealing the map’s purpose and content. For example, a map might be titled “Political Map of the World” or “Battle of Gettysburg, 1863.” “Date” refers to either the time the map was made or the date relevant to the information on the map. A map of areas threatened by a wildfire, for instance, would have a date, and perhaps even a time, to track the progress of the wildfire. A historical map of the ancient Sumerian Empire would have a date range of between 5,000 B.C. and 1,000 B.C. Noting a map’s author is important because the cartographer’s perspective will be reflected in the content. Assessing accuracy and objectivity also requires checking sources. A map’s sources are where the author of the map got his or her information. A map of a school district may list the U.S. Census Bureau, global positioning system (GPS) technology, and the school district’s own records as its sources. Orientation refers to the presence of a compass rose or simply an arrow indicating directions on the map. If only an arrow is used, the arrow usually points north. A map’s index helps viewers find a specific spot on the map using the grid. A map’s legend explains what the symbols on a map mean. Map Projections Transferring information from the spherical , or ball-shaped, surface of Earth onto a flat piece of paper is called projection. A globe, a spherical model of Earth, accurately represents the shapes and locations of the continents. But if a globe were cut in half and each half were flattened out into a map, the result would be wrinkled and torn. The size, shape, and relative location of land masses would change. Projection is a major challenge for cartographers. Every map has some sort of distortion . The larger the area covered by a map, the greater the distortion. Features such as size, shape, distance, or scale can be measured accurately on Earth, but once projected on a flat surface only some, not all, of these qualities can be accurately represented. For example, a map can retain either the correct sizes of landmasses or the correct shapes of very small areas, but not both. Depending on the map’s purpose, cartographers must decide what elements of accuracy are most important to preserve. This determines which projection to use. For example, conformal maps show true shapes of small areas but distort size. Equal area maps distort shape and direction but show true relative sizes of all areas. There are three basic kinds of projections: planar, conical, and cylindrical. Each is useful in different situations. In a planar projection , Earth’s surface is projected onto a plane , or flat surface. Imagine touching a globe with a piece of cardboard, mapping that point of contact, then projecting the rest of map onto the cardboard around that point. Planar projections are most accurate at their centers, where the plane “touches” the globe. They are often used for maps of one of the poles. Imagine you wrapped a cone around Earth, putting the point of the cone over one of the poles. That is a conical projection . The cone intersects the globe along one or two lines of latitude. When the cone is unwrapped and made into a flat map, latitude lines appear curved in circles or semicircles. Lines of longitude are straight and come together at one pole. In conical projection, areas in the mid-latitudes—regions that are neither close to the Equator nor close to the poles—are represented fairly accurately. For this reason, conical projections are often used for maps of the United States, most of which lies in the mid-latitudes. For a cylindrical projection , imagine that Earth’s surface is projected onto a tube that is wrapped around the globe. The cylinder touches Earth along one line, most often the Equator. When the cylinder is cut open and flattened into a map, the regions near the Equator are the most accurate. Regions near the poles are the most distorted. Surveying and Remote Sensing Cartographers rely on survey data for accurate information about the planet. Surveying is the science of determining the exact size, shape, and location of a piece of land. Surveyors gather information from regions both above sea level and beneath bodies of water. Surveying can be done on foot. Surveyors use many instruments to measure the features, or topography , of the land. A compass , measuring device, and theodolites are often used by surveyors doing field work. A theodolite is an instrument that measures angles. A surveyor may calculate the angle of hills, valleys, and other features by using a theodolite, which is usually mounted on a tripod , or three-legged platform. Today, many surveyors use remote sensing to collect data about an area without actually physically touching it. Sensors that detect light or radiation emitted by objects are mounted to airplanes or space satellites , collecting information about places on Earth from above. One method of remote sensing is aerial photography , taking photographs of Earth from the air. Aerial photography has eliminated much of the legwork for surveyors and has allowed precise surveying of some places that are impossible to reach on foot. Satellites, spacecraft that orbit Earth, perform remote sensing. For example, Landsat , a satellite that circles Earth 14 times a day, transmits huge volumes of data to computers on Earth. The data can be used to quickly make or correct maps. How Maps Are Made Before making a map, cartographers decide what area they want to display and what type of information they want to present. They consider the needs of their audience and the purpose of the map. These decisions determine what kind of projection and scale they need, and what sorts of details will be included. The language of the map is one thing a cartographer must consider. A blind reader needs a map that has information in braille , for instance. The audience for a map can determine how widely a map is used. A map might use red and green symbols to show the location of maple and pine trees. This information might be easily displayed in a simple legend. However, such a map could not be used by people who are color-blind. Lines of latitude and longitude are mathematically plotted on a flat surface. Features are drawn in their appropriate location. Before the development of advanced computer and printing techniques, maps were drawn by hand. Cartographers would draw, or scribe , the map on a sheet of coated plastic with a special etching tool, scraping away the colored coating to leave clear, sharp lines. Several different sheets of plastic were layered on top of each other to add shading and place names. The plastic sheets were used to make a metal printing plate , or proof, for publishing the map. Today, most mapping is done with the help of computers. The coordinates of every point are entered into a computer. By feeding new data into the computer or deleting old data, map changes can be made quickly and easily. Colors can be changed, new roads added, and topographic features, such as the flow of a river, altered. The new map can then be printed out easily. Types of Maps Cartographers make many different types of maps, which can be divided into two broad categories: general reference maps and thematic maps . General reference maps show general geographic information about an area, including the locations of cities, boundaries, roads, mountains, rivers, and coastlines . Government agencies such as the U.S. Geological Survey ( USGS ) make some general reference maps. Many are topographic maps, meaning that they show changes in elevation. They show all the hills and valleys in an area. This is useful to everyone from hikers trying to choose a route to engineers trying to determine where to build highways and dams . Thematic maps display distributions, or patterns, over Earth’s surface. They emphasize one theme, or topic. These themes can include information about people, other organisms, or the land. Examples include crop production, people’s average income , where different languages are spoken, or average annual rainfall. Many thematic maps are now made with the help of geographic information system (GIS) technology. GIS are computer systems that capture, store, and display data related to positions on Earth’s surface. This technology combines information from maps with other data about people, the land, climate , farms, houses, businesses, and much more, allowing multiple sets of data to be displayed on a single map. Many industries and governments use GIS technology for analysis and decision making. For example, GIS data helps officials determine which streams are most in danger of being polluted . It can also help a business decide where to locate a new store. History of Mapmaking Through the ages, maps have taken many different forms. The earliest maps were probably sketches made on the ground that showed the surrounding area. People native to the Marshall Islands used palm fibers to show wave patterns between islands in the Pacific Ocean. They used seashells to represent islands. Inuit fishermen in the Arctic carved pieces of driftwood to show coastal features. One of the world’s oldest existing maps was found on a stone tablet in Spain. It dates back nearly 14,000 years. The ancient Greeks are usually considered the founders of scientific cartography. Greek scholars knew the general size and shape of Earth, and they developed the grid system of latitude and longitude. Eratosthenes , who lived from about 276 to 194 B.C., calculated the size of Earth using mathematics and observations of the sun. Claudius Ptolemaeus, or Ptolemy , was an astronomer , mathematician , and geographer in the second century A.D. He brought mapmaking to a level of precision that would not be seen again until the fifteenth century. He combined all his knowledge about the world into a book called Geography . In Europe during the Middle Ages , cartographers drew maps reflecting their religious beliefs. These maps were generally simple and sometimes fanciful . The city of Jerusalem, holy to Jews , Christians , and Muslims , was sometimes placed in the center. Many medieval European maps with Jerusalem at the center are called T&O maps. The mass of land was represented as a round wheel encircled with a single round ocean, the “O” of the T&O. The land encircled by the ocean was divided by a “T” into the three continents known by medieval European cartographers: Asia was the large land mass above the T, Africa and Europe were the two smaller sections on either side of the T, and Jerusalem was at the center. The T-shape splitting the continents was composed of the Mediterranean Sea (between Europe and Africa), the Nile River (between Africa and Asia) and the Don River (between Europe and Asia). The Nile and the Don meet in a single line to form the top of the T. During these Dark Ages in Europe, Arab scholars kept scientific cartography alive. They preserved the works of Ptolemy and translated them to Arabic . Arab cartographers produced the first reliable globe of the Western world. During the Islamic Golden Age , Arab cartographers used complicated mathematical and astronomical formulas to help them determine different map projections. In 1154, the scientist and cartographer al-Idrisi made a map of the world that was better than the world maps Europeans were producing. Al-Idrisi’s map included a representation of the entire continent of Eurasia , including Scandinavia , the Arabian Peninsula, the island of Sri Lanka, and the Black and Caspian Seas. In the fifteenth century, cartography in Europe improved. The development of printing and engraving meant maps that had previously been painted by hand could be copied more quickly. Around the same time, sailors began traveling farther on the oceans. They added newly discovered lands and more detailed coastlines to their maps. Explorers brought back descriptions of the interiors, as well as the coastlines, of continents. Europeans explored much of the Americas during the sixteenth century, Australia in the seventeenth century, and Antarctica was finally sighted in the early nineteenth century. At this point, fairly accurate maps of the entire world were beginning to be assembled . In the nineteenth century, cartography became more advanced with the development of a printing process called lithography . Lithography allowed cartographers to make many accurate copies of maps with less labor and expense. Photography, color printing, and computers all improved mapmaking even more. In just a few decades, the relationship between people and maps changed drastically. For example, instead of using paper street maps, many people navigate using GPS units that communicate with satellites to determine their exact location on Earth. Digital versions of maps can represent Earth in three dimensions, defying the limitations of the flat maps of the past. Almost the entire surface of Earth has been mapped with remarkable accuracy, and this information is available instantly to anyone with an internet connection.
Beyond Earth Using images taken from spacecraft, cartographers have created detailed maps of the surfaces of the moon and Mars. Astrocartographers have identified martian valleys, craters, and even dry riverbeds.
Eratosthenes Eratosthenes was an astronomer, librarian, mathematician, and poet. He also invented the discipline of geography in his spare time. Using the position of the sun, Eratosthenes was able to calculate the circumference of Earth without leaving Egypt, his home. He used the length of a stadium as his unit of distance. Because stadiums came in two different sizes in the world of ancient Greece, and we don't know which stadium Eratosthenes used, we can't know exactly what he calculated for the circumference of Earth. If he used the larger Greek stadium, his circumference would be larger than Earth by about 16 percent. If he used the smaller, so-called "Egyptian stadium," his calculation would still be largerbut only by 1 percent.
Misleading Maps A type of cylindrical projection called a Mercator projection shows direction well. It was long used to make charts that sailors could use to find their way around the globe. Like all cylindrical projections, a Mercator projection greatly distorts the size of land near the poles. In a Mercator projection, Greenland and Africa are about the same size. In reality, Africa is 14 times the size of Greenland.
Printing Pioneers The Chinese were skilled cartographers. The first map was printed in China in 1155 C.E., some 300 years before maps were printed in Europe.
Media Credits
The audio, illustrations, photos, and videos are credited beneath the media asset, except for promotional images, which generally link to another page that contains the media credit. The Rights Holder for media is the person or group credited.
Illustrators
Educator reviewer, last updated.
February 20, 2024
User Permissions
For information on user permissions, please read our Terms of Service. If you have questions about how to cite anything on our website in your project or classroom presentation, please contact your teacher. They will best know the preferred format. When you reach out to them, you will need the page title, URL, and the date you accessed the resource.
If a media asset is downloadable, a download button appears in the corner of the media viewer. If no button appears, you cannot download or save the media.
Text on this page is printable and can be used according to our Terms of Service .
Interactives
Any interactives on this page can only be played while you are visiting our website. You cannot download interactives.
Related Resources
Diagrammatic Representation of Geographical Data
- First Online: 30 November 2021
Cite this chapter
- Swapan Kumar Maity 3
Part of the book series: Advances in Geographical and Environmental Sciences ((AGES))
255 Accesses
Diagrammatic representation and visualization of geographical data is very simple, attractive and easy to understand and explain to the geographers as well as to the common literate people. It helps to explore the nature of data, the pattern of their spatial and temporal variations and understanding their relationships to accurately recognize and analyse features on or near the earth’s surface. This chapter focuses on the detailed discussion of various types of diagrams classified on a different basis. All types of one-dimensional (bar, pyramid etc.), two-dimensional (circular, triangular, square etc.), three-dimensional (cube, sphere etc.) and other diagrams (pictograms and kite diagram) have been discussed with suitable examples in terms of their appropriate data structure, necessary numerical (geometrical) calculations, methods of construction, appropriate illustrations, and advantages and disadvantages of their use. It includes all the fundamental geometric principles and derivation of formulae used for the construction of these diagrams. A step-by-step and logical explanation of their construction methods becomes helpful for the readers for an easy and quick understanding of the essence of the diagrams. Each diagram represents a perfect co-relation between the theoretical knowledge of various geographical events and phenomena and their proper practical application with suitable examples.
This is a preview of subscription content, log in via an institution to check access.
Access this chapter
- Available as PDF
- Read on any device
- Instant download
- Own it forever
- Available as EPUB and PDF
- Compact, lightweight edition
- Dispatched in 3 to 5 business days
- Free shipping worldwide - see info
- Durable hardcover edition
Tax calculation will be finalised at checkout
Purchases are for personal use only
Institutional subscriptions
Saksena RS (1981) A handbook of statistics. Indological Publishers & Booksellers
Google Scholar
Sarkar A (2015) Practical geography: a systematic approach. Orient Blackswan Private Limited, Hyderabad, Telengana, India. ISBN: 978-81-250-5903-5
Sharma PD (1975) Ecology and environment. Rastogi Publications, Gangitri, Shivaji Road, Meerut-250002, ISBN: 978–93–5078–122–7
Singh RL, Singh RPB (1991) Elements of practical geography. Kalyani Publishers, New Delhi
Download references
Author information
Authors and affiliations.
Department of Geography, Nayagram P.R.M. Government College, Jhargram, West Bengal, India
Swapan Kumar Maity
You can also search for this author in PubMed Google Scholar
Rights and permissions
Reprints and permissions
Copyright information
© 2021 The Author(s), under exclusive license to Springer Nature Singapore Pte Ltd.
About this chapter
Maity, S.K. (2021). Diagrammatic Representation of Geographical Data. In: Essential Graphical Techniques in Geography. Advances in Geographical and Environmental Sciences. Springer, Singapore. https://doi.org/10.1007/978-981-16-6585-1_3
Download citation
DOI : https://doi.org/10.1007/978-981-16-6585-1_3
Published : 30 November 2021
Publisher Name : Springer, Singapore
Print ISBN : 978-981-16-6584-4
Online ISBN : 978-981-16-6585-1
eBook Packages : Earth and Environmental Science Earth and Environmental Science (R0)
Share this chapter
Anyone you share the following link with will be able to read this content:
Sorry, a shareable link is not currently available for this article.
Provided by the Springer Nature SharedIt content-sharing initiative
- Publish with us
Policies and ethics
- Find a journal
- Track your research
- Topographic Map – Definition, Structure, Examples
Topographic Map
A topographic map refers to a detailed, graphical, and accurate representation of features that appear on the Earth’s surface.
Topographic maps are an essential part of the field of geology due to the comprehensive analysis of a particular surface. Students can explore more about the topographic map here.
Definition and Meaning of Topographic Map
A topographic map is a map that represents the locations of geographical features. Furthermore, these geographical features can be mountains, valleys, plain surfaces, water bodies and many more.
Topographic maps refer to maps at large and medium scales that incorporate a massive variety of information. All the components of topographic maps carry equal importance.
Topographic maps refer to a graphical representation of the three-dimensional configuration of the surface of the Earth. Moreover, such maps show the size, shape, and distribution of landscape features.
Also, such maps present the vertical and horizontal positions of those features whose representations take place. Most noteworthy makes use of contour lines so as to show different elevations on a map.
Structure of Topographic Map
Topographic maps have a detailed and compendious structure. The various aspects of a topographic map can be divided into three major groups:
Relief: The depiction of the relief aspect is with brown contour lines that represent the mountains , hills, valleys, plains, etc. The elevations are available in meters (or feet) above the mean sea level.
Furthermore, there are also spot elevations are shown in the black colour. Moreover, in these spot elevations, the marking of the lake level, the summit of a hill, or the road intersections takes place for the purpose of elevation.
Water: The depiction of the water aspects takes place in the blue colour. Moreover, the water aspects represent the oceans, rivers, streams, lakes, swamps etc.
Cultural: The depiction of these aspects takes place in the black colour. Furthermore, they are representative of all man-made features.
Above all, these man-made features include the roads, railroads, land boundaries, buildings, airports, urban development etc.
Contour line: A contour line refers to a type of isoline. In such a case, it is a line of equal elevation. Moreover, if one walks along a contour line, then one would not go uphill or downhill.
Mathematically speaking, a contour line refers to a curve in two dimensions on which the value of a function f(x,y) happens to be a constant.
Examples of Topographic Map
Below are some examples of topographic maps from different nations or countries:
Germany- Each federal state of Germany is in charge of producing official topographic maps. Moreover, the production and publishing of the maps which are between 1:5000 and 1:100000 take place by the land surveying offices of each federal state.
Also, the production and publishing of the maps between 1:200000 and 1:1000000 take place by a federal house(BKG) in Frankfurt am Main.
Israel- The Survey of Israel has the responsibility for carrying out the topographic and civilian mapping of Israel. Moreover, the standard map scales in Israel are 1:50000 and 1:100000. Also, one can access the 1:50000 map online. Above all, Israel is a country that contains many high elevation places.
Nepal- In Nepal, the surveying and publishing of a definitive series of large scale topographic maps took place from 1992 to 2000 through a joint project by the Government of Nepal Survey Department along with Finland’s Ministry for Foreign Affairs.
Topographic maps which are at 1:25000 scale and covering the 7.5 minutes latitude and longitude map the regions of Terai and Middle Mountain regions which have dense populations.
Solved Question For You
Q1 Which of the following is not an aspect of the topographic map structure?
A. Contour line B. Relief C. Natural disaster D. Cultural
A1 The correct option is C., ‘natural disaster.’ This is because there is usually no representation of natural disasters in the topographic map.
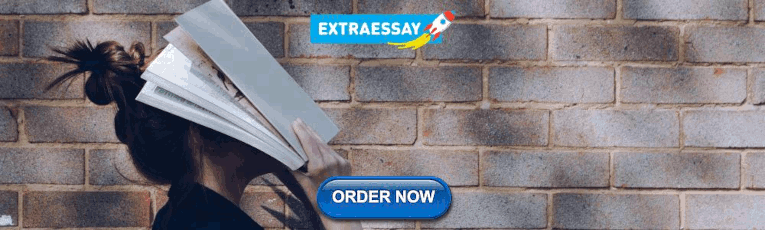
Customize your course in 30 seconds
Which class are you in.

Our Changing Earth
- Northern Hemisphere and Southern Hemisphere – An Overview
- Coastal Plains in India and How are They Formed?
- What Is Topography?
- How Is Temperature On Mars?
- Asthenosphere & the Lithosphere – Definition and Properties
- The Ever Changing Earth
One response to “The Ever Changing Earth”
Not helpfully
Leave a Reply Cancel reply
Your email address will not be published. Required fields are marked *
Download the App
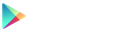
The Geography of Transport Systems
The spatial organization of transportation and mobility
A.5 – Graph Theory: Definition and Properties
Authors: dr. jean-paul rodrigue and dr. cesar ducruet.
Graph theory is a branch of mathematics concerned about how networks can be encoded, and their properties measured.
1. Basic Graph Definition
A graph is a symbolic representation of a network and its connectivity. It implies an abstraction of reality so that it can be simplified as a set of linked nodes. The origins of graph theory can be traced to Leonhard Euler, who devised in 1735 a problem that came to be known as the “Seven Bridges of Konigsberg”. In this problem, someone had to cross all the bridges only once, and in a continuous sequence, a problem the Euler proved to have no solution by representing it as a set of nodes and links. This led to the foundation of graph theory and its subsequent improvements. It has been enriched in the last decades by growing influences from studies of social and complex networks.
In transport geography, most networks have a prominent spatial foundation , namely road, transit, and rail networks, which tend to be defined more by their links than by their nodes. This is not necessarily the case for all transportation networks. For instance, since they are often unclear, maritime and air networks are more defined by their nodes than links . A telecommunication system can also be represented as a network, while its spatial expression can have limited importance and be difficult to represent. Mobile phone networks or the Internet, possibly the most complex graphs to be considered, are relevant cases of networks having a structure that can be difficult to symbolize. However, cellular phones and antennas can be represented as nodes, while the links could be individual phone calls. Servers, the core of the Internet, can also be represented as nodes within a graph, while the physical infrastructure between them, namely fiber optic cables, can act as links. Consequently, all transport networks can be represented by graph theory in one way or the other.
The following elements are fundamental to understanding graph theory:
Graph . A graph G is a set of vertices (nodes) v connected by edges (links) e . Thus G=(v, e) .
Vertex (Node). A node v is a terminal point or an intersection point of a graph. It is the abstraction of a location such as a city, an administrative division, a road intersection, or a transport terminal (stations, terminuses, harbors, and airports).
Edge (Link). An edge e is a link between two nodes. The link ( i , j ) is of initial extremity i and of terminal extremity j . A link is the abstraction of a transport infrastructure supporting movements between nodes. It has a direction that is commonly represented as an arrow. When an arrow is not used, it is assumed the link is bi-directional.
Sub-Graph. A sub-graph is a subset of a graph G where p is the number of sub-graphs. For instance, G’ = ( v’ , e’ ) can be a distinct sub-graph of G . Unless the global transport system is considered in its whole, every transport network is in theory a sub-graph of another. For instance, the road transportation network of a city is a sub-graph of a regional transportation network, which is itself a sub-graph of a national transportation network.
Buckle (Loop or self edge). A link that makes a node correspond to itself is a buckle.
Planar Graph . A graph where all the intersections of two edges are a vertex. Since this graph is located within a plane, its topology is two-dimensional. This is typically the case for power grids, road and railway networks, although great care must be inferred to the definition of nodes (terminals, warehouses, cities).
Non-planar Graph . A graph where there are no vertices at the intersection of at least two edges. Networks that can be considered in a planar fashion, such as roads, can be represented as non-planar networks. This implies a third dimension in the topology of the graph since there is the possibility of having a movement “passing over” another movement such as for air and maritime transport, or an overpass for a road. A non-planar graph has potentially much more links than a planar graph.
Simple graph . A graph that includes only one type of link between its nodes. A road or rail network are simple graphs.
Multigraph . A graph that includes several types of links between its nodes. Some nodes can be connected to one link type while others can be connected to more than one that are running in parallel. A graph depicting a road and a rail network with different links between nodes serviced by either or both modes is a multigraph.

2. Links and their Structures
A transportation network enables flows of people, freight, or information, which are occurring along its links. Graph theory must thus offer the possibility of representing movements as linkages, which can be considered over several aspects:
Connection . A set of two nodes as every node is linked to the other. Considers if a movement between two nodes is possible, whatever its direction. Knowing connections makes it possible to find if it is possible to reach a node from another node within a graph.
Path . A sequence of links that are traveled in the same direction. For a path to exist between two nodes, it must be possible to travel an uninterrupted sequence of links. Finding all the possible paths in a graph is a fundamental attribute in measuring accessibility and traffic flows.
Chain. A sequence of links having a connection in common with the other. Direction does not matter.
Length of a Link, Connection or Path . Refers to the label associated with a link, a connection or a path. This label can be distance, the amount of traffic, the capacity or any relevant attribute of that link. The length of a path is the number of links (or connections) in this path.
Cycle . Refers to a chain where the initial and terminal node is the same and that does not use the same link more than once is a cycle.
Circuit . A path where the initial and terminal node corresponds. It is a cycle where all the links are traveled in the same direction. Circuits are very important in transportation because several distribution systems are using circuits to cover as much territory as possible in one direction (delivery route).
Clique . A clique is a maximal complete subgraph where all vertices are connected.
Cluster . Also called community, it refers to a group of nodes having denser relations with each other than with the rest of the network. A wide range of methods are used to reveal clusters in a network, notably they are based on modularity measures (intra- versus inter-cluster variance).
Ego network . For a given node, the ego network corresponds to a sub-graph where only its adjacent neighbors and their mutual links are included.
Nodal region . A nodal region refers to a subgroup (tree) of nodes polarized by an independent node (which largest flow link connects a smaller node) and several subordinate nodes (which largest flow link connects a larger node). Single or multiple linkage analysis methods are used to reveal such regions by removing secondary links between nodes while keeping only the heaviest links.
Dual graph . A method in space syntax that considers edges as nodes and nodes as edges. In urban street networks, large avenues made of several segments become single nodes while intersections with other avenues or streets become links (edges). This method is particularly useful to reveal hierarchical structures in a planar network.
Common neighbor . For two or more nodes, the number of nodes that they are commonly connected two.

3. Basic Structural Properties
The organization of nodes and links in a graph conveys a structure that can be described and labeled. The basic structural properties of a graph are:
Symmetry and Asymmetry . A graph is symmetrical if each pair of nodes linked in one direction is also linked in the other. By convention, a line without an arrow represents a link where it is possible to move in both directions. However, both directions have to be defined in the graph. Most transport systems are symmetrical, but asymmetry can often occur as it is the case for maritime (pendulum) and air services. Asymmetry is rare on road transportation networks, unless one-way streets are considered.
Assortativity and disassortativity . Assortative networks are those characterized by relations among similar nodes, while disassortative networks are found when structurally different nodes are often connected. Transport (or technological) networks are often disassortative when they are non-planar, due to the higher probability for the network to be centralized into a few large hubs.
Completeness. A graph is complete if two nodes are linked in at least one direction. A complete graph has no sub-graph and all its nodes are interconnected.
Connectivity . A graph considered connected if for all its distinct pairs of nodes, there is a linking chain. Direction does not have importance for a graph to be connected but may be a factor for the level of connectivity. If p >1 the graph is not connected because it has more than one sub-graph (or component). There are various levels of connectivity, depending on the degree at which each pair of nodes is connected.
Complementarity . Two sub graphs are complementary if their union results in a complete graph. Multimodal transportation networks are complementary as each sub-graph (modal network) benefits from the connectivity of other sub-graphs.
Root . A node r where every other node is the extremity of a path coming from r is a root. Direction has an importance. A root is generally the starting point of a distribution system, such as a factory or a warehouse.
Trees . A connected graph without a cycle is a tree. A tree has the same number of links than nodes plus one. ( e = v-1 ). If a link is removed, the graph ceases to be connected. If a new link between two nodes is provided, a cycle is created. A branch of root r is a tree where no links are connecting any node more than once. River basins are typical examples of tree-like networks based on multiple sources connecting towards a single estuary. This structure strongly influences river transport systems .
Articulation Node . In a connected graph, a node is an articulation node if the sub-graph obtained by removing this node is no longer connected. It therefore contains more than one sub-graph ( p > 1). An articulation node is generally a port or an airport, or an important hub of a transportation network, which serves as a bottleneck. It is also called a bridge node.
Isthmus . In a connected graph, an isthmus is a link that is creating, when removed, two sub-graphs having at least one connection. Most central links in a complex network are often isthmuses, which removal by reiteration helps revealing dense communities (clusters).

Related Topics
- The Geography of Transportation Networks
- Graph Theory: Measures and Indices
- Geographic Information Systems for Transportation (GIS-T)
- Transportation and Accessibility
- Network Data Models
Bibliography
- Arlinghaus, S.L., W.C. Arlinghaus, and F. Harary (2001) Graph Theory and Geography: An Interactive View. New York: John Wiley & Sons.
- Garrison, W. and D. Marble (1974) “Graph theoretic concepts” in Transportation Geography: Comments and Readings, New York: McGraw Hill, pp. 58-80.
- Nystuen, J.D., and M.F.A. Dacey (1961) “A graph theory interpretation of nodal regions”. Papers of the Regional Science Association 7, 29-42. https://doi.org/10.1007/BF01969070
Share this:
Geography - Representation of Geographical Data | 12th Geography : Chapter 12 : Representation of Geographical Data
Chapter: 12th geography : chapter 12 : representation of geographical data.
Representation of Geographical Data

Learning Objectives
* Identify various types of Geographical data.
* Understand various methods of drawing geographical data.
* Analyse the various methods of constructing diagram suitable for particular data.
Introduction
Economists, statisticians, and geographers make use of statistical facts and data in their respective fields. Climatic data like temperature, pressure, rainfall, etc., may be represented by diagram such as isotherms, isobars and isohyets respectively. Besides the tabular form, the data may also be presented in some graphical or diagrammatic form. Population, climatic, and Socio economic data can be represented visually for better understanding in the form of graphs, diagrams, charts and maps. Thus, geographical data needs to be scientifically visualised for appropriate cartographic communication. Therefore, cartographic presentation of geographical data is important to explore the nature of data, their pattern of variations over time and space, to analyse the data set, and to identify and classify the real world objects.
We study climatic, economic and population data in geography. The numerical data or facts when collected in a systematic manner to serve some purpose and presented in a tabulated form they are called statistics.
The diagrams representing statistical data are known as statistical diagrams. Geometrical figures such as bars, rectangles, squares, circles, cubes, sphere, etc., and curves or lines are used to represent statistical data diagrammatically. These diagrams serve the following purposes:
1. They enable us to compare figures at a glance.
2. They cast easily memorable impression on the mind.
3. They enable us to analyse the data and draw inference easily.
Related Topics
Privacy Policy , Terms and Conditions , DMCA Policy and Compliant
Copyright © 2018-2024 BrainKart.com; All Rights Reserved. Developed by Therithal info, Chennai.
- School Guide
- Class 12 Syllabus
- Class 12 Revision Notes
- Maths Notes Class 12
- Physics Notes Class 12
- Chemistry Notes Class 12
- Biology Notes Class 12
- NCERT Solutions Class 12 Maths
- RD Sharma Solutions Class 12
- Methods of Relief Representation| Class 11 Geography
Graphical Representation of Data
- Diagrammatic and Graphic Presentation of Data
- Cartography: Class 11 Geography Notes- Practical Work
- Classification of Map Projection| Class 11 Geography
- Need and Elements of Map Projection| Class 11 Geography
- Types of Maps| Class 11 Geography Notes
- Class 9 RD Sharma Solutions - Chapter 23 Graphical Representation of Statistical Data - Exercise 23.2 | Set 1
- Class 9 RD Sharma Solutions - Chapter 23 Graphical Representation of Statistical Data - Exercise 23.1 | Set 1
- Class 11 Geography Introduction to Maps
- Interpretation of Topographical Maps| Class 11 Geography Notes
- Map Scale| Class 11 Geography Notes
- NCERT Solutions For Class 9 Geography Social Science Chapter 2: Physical Features of India
- Uses of Maps| Class 11 Geography Notes
- Class 8 RD Sharma Solutions - Chapter 24 Data Handling II (Graphical Representation of Data as Histograms) - Exercise 24.1 | Set 1
- NCERT Solutions For Class 10 Geography Chapter 3 : Water Resources
- Essentials of Map Making| Class 11 Geography
- History of Maps| Class 11 Geography
- Characteristics of data in geographical information system (GIS)
Graphical Representation of Data| Practical Work in Geography Class 12
Geography Class 12 Chapter 3 talks about the Graphical Representation of Data. It includes all types of representation processes of data through different types of graphs like line, bar, pie, dot, and isopleth maps. Graphical representation gives us a visual of the raw data which helps us to understand to analyze it through different numeric formations.
In this article, we are going to discuss the Practical Work in Geography Class 12 with the Chapter called Graphical Representation of Data.
Graphical Representation of Data means to analyze the numerical data sources through different types of graphs. It creates a relation between the data set with a diagram. The graphical representation is simple and easy to understand which is a part of the important learning technique. The Graphical Representation process is totally dependent process on data sources.
Let us discuss different types of Graphical Representation of Data as mentioned below.
- Line Graphs: Line graphs are one type of linear graph that examines the continuous data sources to predict the future.
- Histograms: The histograms use the bar formations to represent the data as the frequency of the numerical data sources. Here the intervals are present in an equal manner.
- Bar Graphs: The bar graphs are used to depict the different categories and compare the data by using solid bars of quantities.
- Frequency Table: The frequency table represents the data by following a proper time interval.
- Line Plot: The line plot shows the data in a manner of frequency that is written as a line number.
- Circle Graph: The circle graph is also known as the pie chart. It shows the relationships between the data parts. The circle holds 100 % data by mentioning the data portions in percentages.
- Scatter Plots: The scatter plots depict the data to establish the relationship between two data sets.
- Venn Diagram: The Venn diagram is a process graph where the set is important. The inner part of the circle makes and shows the representation of graphs.
- Stem and Leaf Plots: They are the representation of the least and highest value of a particular data set. The lowest value is known as the leaf and the highest value is the stem.
- Box and Whisker Plot: This is the process of summarizing the data into four different parts. It majorly represents the spread and median of the different data sets.
Rules for Graphical Representation of Data
There are some major rules to make the Graphical Representation of Data as mentioned below.
- Give a Title: Give a suitable title for the graph which presents the subject.
- Scale: The scale needs to be used efficiently in an accurate way.
- Mention the Measurement Units: It is important to mention the measurement units to represent the dataset as a graph in a proper way.
- Index Formation: It is needed to apply the different types of colors, shades, and designs to make the related graph more understanding with more information.
- Data Sources: Include the proper sources of data at the bottom of the graph when it is necessary to make it more authentic.
- Make It Simple: A simple graph is more understandable than a hard one. You need to make it more easy for the readers.
People Also Read:
- Spatial and Geographical data
Graphical Representation of Data- FAQs
What are the 4 different types of graphical representation.
There are four most widely used graphs namely histogram, pie diagram, frequency polygon, and ogive frequency graph.
What is a graphical form of representation?
Graphical Representation is a way of analysing numerical data. It exhibits the relation between data, ideas, information and concepts in a diagram. It is easy to understand and it is one of the most important learning strategies. It always depends on the type of information in a particular domain.
What are the graphical displays of data?
Two common types of graphic displays are bar charts and histograms. Both bar charts and histograms use vertical or horizontal bars to represent the number of data points in each category or interval.
What is a graphical display?
Graphical displays communicate comparisons, relationships, and trends. They emphasize and clarify numbers. To choose the appropriate type of display, first define the purpose of the report, and then identify the most effective display to suit that purpose. For example, you can use a multiline display to show trends.
What is a graphical representation called?
The method of presenting these numerical data is called a chart. There are different kinds of charts such as a pie chart, bar graph, line graph, etc, that help in clearly showcasing the data.
Why are statistical graphs important?
Raw data might contain hidden patterns and relationships that you cannot identify by just looking at the raw data. These will be revealed using a picture. A display of data will help you identify the most significant features of your data.
Please Login to comment...
Similar reads.
- School Geography
- Social Science
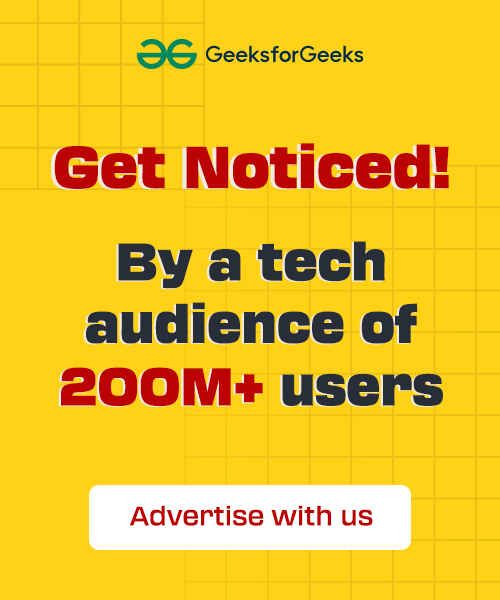
Improve your Coding Skills with Practice
What kind of Experience do you want to share?
Graphical Representation of Data
Graphical representation of data is an attractive method of showcasing numerical data that help in analyzing and representing quantitative data visually. A graph is a kind of a chart where data are plotted as variables across the coordinate. It became easy to analyze the extent of change of one variable based on the change of other variables. Graphical representation of data is done through different mediums such as lines, plots, diagrams, etc. Let us learn more about this interesting concept of graphical representation of data, the different types, and solve a few examples.
Definition of Graphical Representation of Data
A graphical representation is a visual representation of data statistics-based results using graphs, plots, and charts. This kind of representation is more effective in understanding and comparing data than seen in a tabular form. Graphical representation helps to qualify, sort, and present data in a method that is simple to understand for a larger audience. Graphs enable in studying the cause and effect relationship between two variables through both time series and frequency distribution. The data that is obtained from different surveying is infused into a graphical representation by the use of some symbols, such as lines on a line graph, bars on a bar chart, or slices of a pie chart. This visual representation helps in clarity, comparison, and understanding of numerical data.
Representation of Data
The word data is from the Latin word Datum, which means something given. The numerical figures collected through a survey are called data and can be represented in two forms - tabular form and visual form through graphs. Once the data is collected through constant observations, it is arranged, summarized, and classified to finally represented in the form of a graph. There are two kinds of data - quantitative and qualitative. Quantitative data is more structured, continuous, and discrete with statistical data whereas qualitative is unstructured where the data cannot be analyzed.
Principles of Graphical Representation of Data
The principles of graphical representation are algebraic. In a graph, there are two lines known as Axis or Coordinate axis. These are the X-axis and Y-axis. The horizontal axis is the X-axis and the vertical axis is the Y-axis. They are perpendicular to each other and intersect at O or point of Origin. On the right side of the Origin, the Xaxis has a positive value and on the left side, it has a negative value. In the same way, the upper side of the Origin Y-axis has a positive value where the down one is with a negative value. When -axis and y-axis intersect each other at the origin it divides the plane into four parts which are called Quadrant I, Quadrant II, Quadrant III, Quadrant IV. This form of representation is seen in a frequency distribution that is represented in four methods, namely Histogram, Smoothed frequency graph, Pie diagram or Pie chart, Cumulative or ogive frequency graph, and Frequency Polygon.
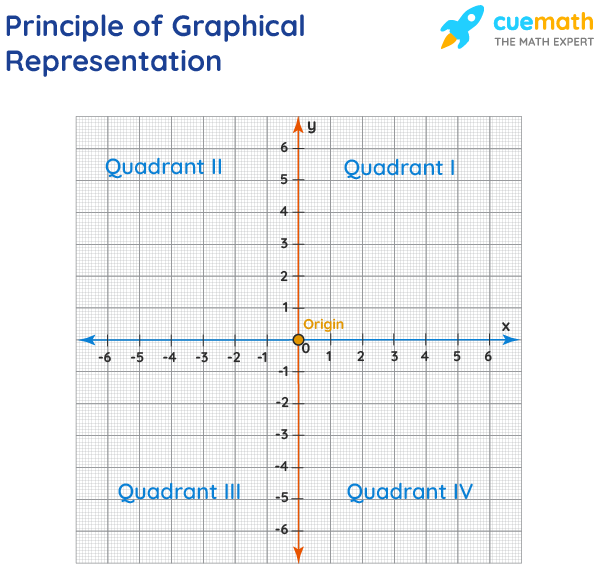
Advantages and Disadvantages of Graphical Representation of Data
Listed below are some advantages and disadvantages of using a graphical representation of data:
- It improves the way of analyzing and learning as the graphical representation makes the data easy to understand.
- It can be used in almost all fields from mathematics to physics to psychology and so on.
- It is easy to understand for its visual impacts.
- It shows the whole and huge data in an instance.
- It is mainly used in statistics to determine the mean, median, and mode for different data
The main disadvantage of graphical representation of data is that it takes a lot of effort as well as resources to find the most appropriate data and then represent it graphically.
Rules of Graphical Representation of Data
While presenting data graphically, there are certain rules that need to be followed. They are listed below:
- Suitable Title: The title of the graph should be appropriate that indicate the subject of the presentation.
- Measurement Unit: The measurement unit in the graph should be mentioned.
- Proper Scale: A proper scale needs to be chosen to represent the data accurately.
- Index: For better understanding, index the appropriate colors, shades, lines, designs in the graphs.
- Data Sources: Data should be included wherever it is necessary at the bottom of the graph.
- Simple: The construction of a graph should be easily understood.
- Neat: The graph should be visually neat in terms of size and font to read the data accurately.
Uses of Graphical Representation of Data
The main use of a graphical representation of data is understanding and identifying the trends and patterns of the data. It helps in analyzing large quantities, comparing two or more data, making predictions, and building a firm decision. The visual display of data also helps in avoiding confusion and overlapping of any information. Graphs like line graphs and bar graphs, display two or more data clearly for easy comparison. This is important in communicating our findings to others and our understanding and analysis of the data.
Types of Graphical Representation of Data
Data is represented in different types of graphs such as plots, pies, diagrams, etc. They are as follows,
Related Topics
Listed below are a few interesting topics that are related to the graphical representation of data, take a look.
- x and y graph
- Frequency Polygon
- Cumulative Frequency
Examples on Graphical Representation of Data
Example 1 : A pie chart is divided into 3 parts with the angles measuring as 2x, 8x, and 10x respectively. Find the value of x in degrees.
We know, the sum of all angles in a pie chart would give 360º as result. ⇒ 2x + 8x + 10x = 360º ⇒ 20 x = 360º ⇒ x = 360º/20 ⇒ x = 18º Therefore, the value of x is 18º.
Example 2: Ben is trying to read the plot given below. His teacher has given him stem and leaf plot worksheets. Can you help him answer the questions? i) What is the mode of the plot? ii) What is the mean of the plot? iii) Find the range.
Solution: i) Mode is the number that appears often in the data. Leaf 4 occurs twice on the plot against stem 5.
Hence, mode = 54
ii) The sum of all data values is 12 + 14 + 21 + 25 + 28 + 32 + 34 + 36 + 50 + 53 + 54 + 54 + 62 + 65 + 67 + 83 + 88 + 89 + 91 = 958
To find the mean, we have to divide the sum by the total number of values.
Mean = Sum of all data values ÷ 19 = 958 ÷ 19 = 50.42
iii) Range = the highest value - the lowest value = 91 - 12 = 79
go to slide go to slide
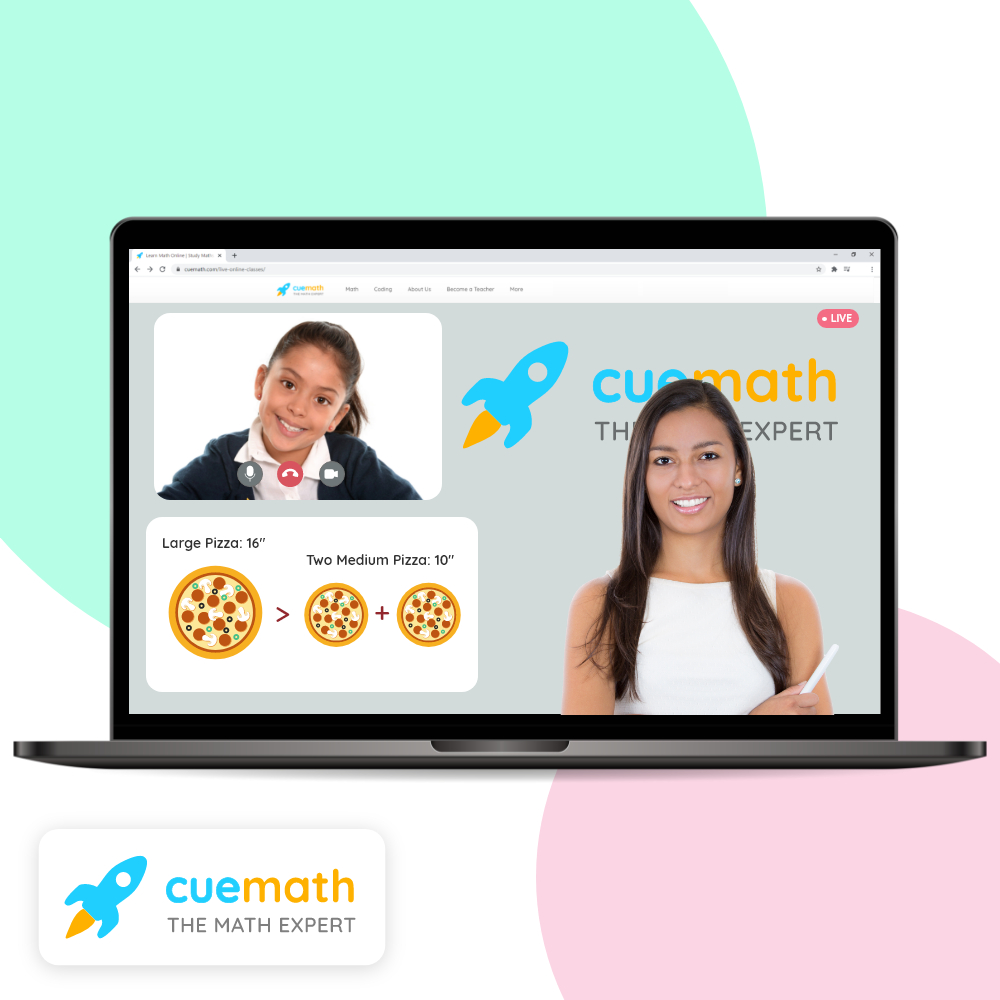
Book a Free Trial Class
Practice Questions on Graphical Representation of Data
Faqs on graphical representation of data, what is graphical representation.
Graphical representation is a form of visually displaying data through various methods like graphs, diagrams, charts, and plots. It helps in sorting, visualizing, and presenting data in a clear manner through different types of graphs. Statistics mainly use graphical representation to show data.
What are the Different Types of Graphical Representation?
The different types of graphical representation of data are:
- Stem and leaf plot
- Scatter diagrams
- Frequency Distribution
Is the Graphical Representation of Numerical Data?
Yes, these graphical representations are numerical data that has been accumulated through various surveys and observations. The method of presenting these numerical data is called a chart. There are different kinds of charts such as a pie chart, bar graph, line graph, etc, that help in clearly showcasing the data.
What is the Use of Graphical Representation of Data?
Graphical representation of data is useful in clarifying, interpreting, and analyzing data plotting points and drawing line segments , surfaces, and other geometric forms or symbols.
What are the Ways to Represent Data?
Tables, charts, and graphs are all ways of representing data, and they can be used for two broad purposes. The first is to support the collection, organization, and analysis of data as part of the process of a scientific study.
What is the Objective of Graphical Representation of Data?
The main objective of representing data graphically is to display information visually that helps in understanding the information efficiently, clearly, and accurately. This is important to communicate the findings as well as analyze the data.
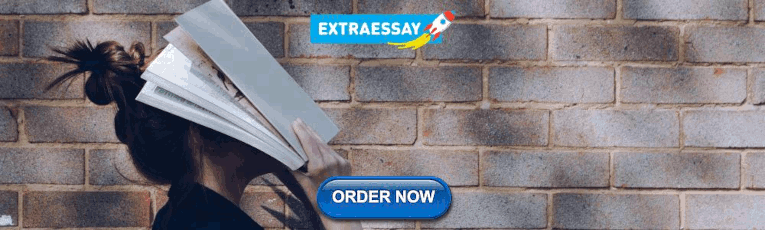
IMAGES
VIDEO
COMMENTS
map, graphic representation, drawn to scale and usually on a flat surface, of features—for example, geographical, geological, or geopolitical—of an area of the Earth or of any other celestial body. Globes are maps represented on the surface of a sphere. Cartography is the art and science of making maps and charts. globe. A globe.
2.4: Representing Geographic Features. Page ID. Maps are a representation of the earth. Central to this representation is reducing the earth's features of interest to a manageable size (i.e., map scale) and its transformation into a functional two-dimensional form (i.e., map projection). The choice of both map scale and, to a lesser extent ...
2 Chapter 2 Techniques of Geographic Analysis . R. Adam Dastrup. A map can be defined as a graphic representation of the real world. Because of the infinite nature of our Universe, it is impossible to capture all of the complexity found in the real world. For example, topographic maps abstract the three-dimensional real world at a reduced scale on a two-dimensional plane of paper.
4.3 Representing Geographic Features - Introduction to Geographic Information Systems. As previously discussed, maps are a representation of the earth. Central to this representation is the reduction of the earth and its features of interest to a manageable size (i.e., map scale) and its transformation into a useful two-dimensional form (i.e ...
2.4 Representing Geographic Features. Maps are a representation of the earth. Central to this representation is reducing the earth's features of interest to a manageable size (i.e., map scale) and its transformation into a functional two-dimensional form (i.e., map projection). The choice of both map scale and, to a lesser extent, map ...
Many other definitions of a map exist. For example, there is the definition found in The History of Cartography Encyclopedia where Woodward and Lewis (Citation 1998) define the maps as: 'graphic representations that facilitate a spatial understanding of things, concepts, conditions, processes, or events in the human world'. Interestingly ...
The passage from more schematic representations to the first traces of cartographies, as we understand them today, is due to the Greek culture and to Eratosthenes of Cyrene Footnote 2, who first introduced the term geography with the meaning of description of the Earth, and is considered the founder of mathematical geography.. Just with the Greek-Roman civilisation quality of geographical ...
However, Harley and Woodward , in the preface of their History of Cartography, gave a definition of map that does seems to embrace also this kind of representation: "Maps are graphic representations that facilitate a spatial understanding of things, concepts, conditions, processes, or events in the human world." In fact, in the history of ...
Geography is a scientific discipline which emphasizes on the collection, processing, suitable representation and logical and scientific interpretation of various types of primary and secondary data for better understanding and explanation of the spatial distributions and variations of different geographical features and phenomena on or near the surface of the earth.
The graphic representation of a town or city has always been studied in different disciplines. Geographers, Civil Engineers, Architects and Urban Planners have established ... criteria, whether due to the precise definition of an organized street or due to the application of the grid concept and a clear organization of public and private spaces ...
GRAPHIC REPRESENTATION IN GEOGRAPHY. I. GEOGRAPHY as taught in the schools today, and as presented. in our modern text-books, is almost a new subject. One of the. features of the so-called new geography is the prominence given. to relief and to the various landscape forms. The surface of. the land - that is, the relation of high lands to low ...
1. Introduction. The past four decades have witnessed a massive proliferation of geographical information system (GIS) data models, data structures, and discussions of geographic representation and ontology. Berry's geographic matrix (Berry 1964) and Sinton's three‐dimensional schema (Sinton 1978) provided early models of the geographic world ...
Representation in Geography When we talk about, write or teach geography, we are involved in the act of representation. In its broadest sense, geography is representation. The etymol-ogy of geography suggests this: the Greek origins of the word geography are geo (earth) and graphia (writing). Earth writing takes the world as we experience or
Figure 2.6.1 Graphic Scales. If they include a scale at all, most maps include a bar scale like the one shown above left (Figure 2.6.1). Some also express map scale as a representative fraction. Either way, the implication is that scale is uniform across the map. In fact, except for maps that show only very small areas, scale varies across ...
Maps. A map is a symbolic representation of selected characteristics of a place, usually drawn on a flat surface. Maps present information about the world in a simple, visual way. They teach about the world by showing sizes and shapes of countries, locations of features, and distances between places.
Representation Schemes for Spatial Phenomena Current Representation Schemes in Geography The most universal and well-known repre-sentation for geographic phenomena is the map. The map has a dual identity that has resulted in two very different areas of study regarding maps as representations of geographic space.
Diagram is another important form of visual representation of geographical data in which importance is laid on the basic facts of one selected element. In the diagram, data are represented in a very much abstract and conventionalized geometric form. All types of categorical and geographical data, including time series and spatial series data, can be easily represented in diagrams.
A topographic map refers to a detailed, graphical, and accurate representation of features that appear on the Earth's surface. Topographic maps are an essential part of the field of geology due to the comprehensive analysis of a particular surface. Students can explore more about the topographic map here. Definition and Meaning of Topographic Map
Graph theory is a branch of mathematics concerned about how networks can be encoded, and their properties measured. 1. Basic Graph Definition. A graph is a symbolic representation of a network and its connectivity. It implies an abstraction of reality so that it can be simplified as a set of linked nodes.
GCSE; WJEC; Graphical skills - WJEC Types of graphs in geography. Graphs and maps can be used to show geographical information. Choosing the correct method of data presentation is important.
Therefore, cartographic presentation of geographical data is important to explore the nature of data, their pattern of variations over time and space, to analyse the data set, and to identify and classify the real world objects. We study climatic, economic and population data in geography. The numerical data or facts when collected in a ...
Graphical Representation of Data means to analyze the numerical data sources through different types of graphs. It creates a relation between the data set with a diagram. The graphical representation is simple and easy to understand which is a part of the important learning technique. The Graphical Representation process is totally dependent ...
Graphical representation is a form of visually displaying data through various methods like graphs, diagrams, charts, and plots. It helps in sorting, visualizing, and presenting data in a clear manner through different types of graphs. Statistics mainly use graphical representation to show data.