
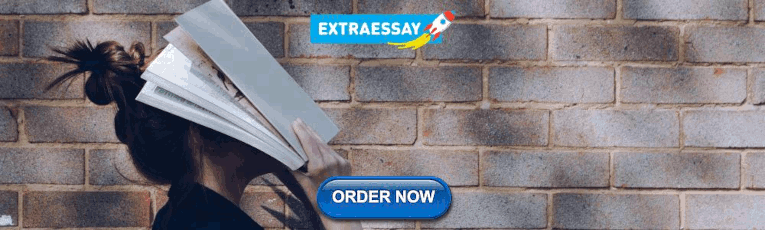
Essay on Minerals
Students are often asked to write an essay on Minerals in their schools and colleges. And if you’re also looking for the same, we have created 100-word, 250-word, and 500-word essays on the topic.
Let’s take a look…
100 Words Essay on Minerals
Introduction.
Minerals are solid substances found naturally in the earth. They have a definite chemical composition and crystal structure. Minerals are the building blocks of rocks.
Types of Minerals
There are over 3000 known minerals. They are categorized into two types: Macro-minerals and Trace minerals. Macro-minerals are needed in large amounts, like calcium and potassium. Trace minerals are required in smaller amounts, like iron and zinc.
Uses of Minerals
Minerals are vital for our daily life. They are used in construction, manufacturing, and even in our bodies for various functions like bone formation and carrying oxygen in blood.
Conservation
It’s essential to conserve minerals as they are non-renewable resources. Over-exploitation can lead to their depletion, affecting future generations.
250 Words Essay on Minerals
Minerals, the naturally occurring, inorganic substances with a definite chemical composition and ordered internal structure, are indispensable components of our everyday life. Their significance transcends various realms, including economic, environmental, and health sectors.
Classification and Formation
Minerals are classified based on their physical and chemical properties. The process of their formation is a complex interplay of geological activities like erosion, sedimentation, and volcanic eruptions. The formation process significantly influences the mineral’s characteristics, making each type unique.
Economic Importance
Minerals are the backbone of industrial development. They serve as raw materials in industries like construction, manufacturing, and technology. For instance, iron ore is crucial in steel production, while silicon is used in computer chips.
Environmental Implications
While minerals contribute to economic development, their extraction often has environmental implications. Mining activities can lead to habitat destruction, pollution, and climate change. Therefore, sustainable mining practices are imperative to mitigate these impacts.
Health Significance
Minerals also play a vital role in human health. Essential minerals like calcium, potassium, and iron are necessary for various bodily functions, from bone health to oxygen transport.
In conclusion, minerals are integral to our existence, contributing significantly to economic growth, technological advancement, and human health. However, their extraction must be balanced with environmental sustainability to ensure the wellbeing of our planet.
500 Words Essay on Minerals
Introduction to minerals.
Minerals are naturally occurring, inorganic substances that exist as solids. They have a defined chemical composition and crystal structure, exhibiting unique physical properties. These substances are integral to the Earth’s structure and vital for human survival, providing necessary nutrients for plants and animals, and raw materials for industries.
Classification of Minerals
Minerals are classified based on their chemical composition into major groups: silicates, carbonates, oxides, sulfates, sulfides, phosphates, and native elements. Silicates, the most abundant group, form the earth’s crust. Carbonates are often part of sedimentary rocks, while oxides include valuable ores like hematite and magnetite.
Formation of Minerals
Minerals form under various geological processes. They originate from magma cooling into igneous rocks, or from sediment accumulation forming sedimentary rocks. Metamorphic rocks bear minerals that form under intense heat and pressure. Minerals also form from hydrothermal fluids rich in dissolved elements, which cool to form mineral-rich deposits.
Minerals and Human Life
Minerals play a critical role in human life. They are essential components of our diet, supplying necessary elements for bodily functions. For instance, calcium from minerals forms our bones and teeth, while iron is vital for blood production. Moreover, minerals are indispensable in industries. We extract metals from mineral ores, use minerals in construction, and harness their unique properties in technology.
Environmental Impact of Mineral Extraction
However, the extraction and use of minerals also pose environmental challenges. Mining activities can lead to deforestation, soil erosion, and water pollution. Additionally, the processing of mineral ores releases greenhouse gases, contributing to climate change. Therefore, sustainable mining practices and efficient use of minerals are vital for environmental conservation.
Conclusion: The Future of Minerals
The future of minerals lies in sustainable practices and innovative technologies. As resources deplete, recycling and substitution will become increasingly important. Simultaneously, advancements in technology can lead to the discovery of new mineral deposits and more efficient extraction methods. It is crucial to strike a balance between our dependence on minerals and the need to preserve the environment, ensuring that future generations can also benefit from these invaluable resources.
In conclusion, minerals are more than just inanimate objects; they are the lifeblood of the Earth, supporting diverse ecosystems and human civilization. Their study and understanding are not only fascinating but also essential for our survival and progress.
That’s it! I hope the essay helped you.
If you’re looking for more, here are essays on other interesting topics:
- Essay on Milkha Singh
- Essay on Milk
- Essay on Microscope
Apart from these, you can look at all the essays by clicking here .
Happy studying!
Leave a Reply Cancel reply
Your email address will not be published. Required fields are marked *
Save my name, email, and website in this browser for the next time I comment.

- Metamorphic
- Sedimentary
How to Identify Minerals in 10 Steps (Photos)
Identifying minerals is a fundamental skill for geologists, gemologists, and rock enthusiasts. The ability to distinguish one mineral from another is essential for understanding the Earth's composition and unlocking the secrets of its history. In this comprehensive guide, we'll walk you through the 10 steps to confidently identify minerals and unlock the captivating world beneath the surface.
Step 1: Pick Your Mineral
Learning mineral identification is like learning to cook. You begin by following step-by-step procedures and looking up a lot of things. But after a while you notice regularities, become familiar with the usual suspects, make some productive mistakes, and get better at it until it becomes easy and fun.
Another way mineral identification is like cooking is that professionals can go to school, learn to use expensive equipment and master the subject fully, yet amateurs can handle nearly all the common possibilities using just a few simple tools. The first thing to do is to observe and test your mineral. Use the largest piece you can find, and if you have several pieces, make sure sure that they are all the same mineral. Examine your mineral for all of the following properties, writing down the answers. After that you'll be ready to take your information to the right place.
Step 2: Hardness
Hardness is a mineral's ability to resist being scratched. Minerals that are not easily scratched are hard. You test the hardness of a mineral by scratching its surface with a mineral of a known hardness. Mineralogists use Mohs Scale as a reference for mineral hardness. The scale lists common minerals in order of their relative hardness. You can use the minerals in the scale to test the hardness of an unknown mineral.
As you can see, diamond is a 10 on Mohs Scale. Diamond is the hardest mineral, which means that no other mineral can scratch a diamond. Quartz is a 7, so it can be scratched by topaz, corundum, and diamond. Quartz will scratch minerals, such as fluorite, that have a lower number on the scale. Suppose you tested a piece of pure gold for hardness. Calcite would scratch the gold, but gypsum would not because gypsum is a 2 and calcite is a 3. That would mean gold is between the hardness of gypsum and calcite, or 2.5 on the scale. A hardness of 2.5 means that gold is a relatively soft mineral. It is only about as hard as your fingernail.
Step 3: Luster

Luster describes the way light reflects off of the surface of the mineral. You might describe diamonds as sparkly or pyrite as shiny, but mineralogists have special terms to describe the luster of a mineral. They first divide minerals into metallic and non-metallic luster. Minerals like pyrite that are opaque and shiny have a metallic luster. Minerals with a non-metallic luster do not look like metals. There are many types of non-metallic luster, six of which are described in Table:

Step 4: Color
Color is probably the easiest property to observe. Unfortunately, you can rarely identify a mineral only by its color. One of the most important physical properties of minerals, reflecting the nature of the interaction of the electromagnetic radiation of the visible region with the electrons of the atoms, molecules, and ions of the crystals and with the electron system of the crystal as a whole. In mineralogy, color is one of the primary diagnostic properties of natural compounds, of great importance in geological prospecting for the identification of minerals.
The color of gems and semiprecious stones is one of their main qualitative (gem) characteristics. A distinction is made between the color of minerals in individual crystals and lumps of ore, the color of minerals in transparent thin sections (under the microscope), the color of minerals in polished sections (in reflected light), and the color of a mineral’s streak (the color of the fine powder of the mineral).
Three main groups of minerals are identified on the basis of the property of color: idiochromatic, allochromatic, and pseudochro-matic.
Idiochromatic minerals are "self colored" due to their composition. The color is a constant and predictable component of the mineral. Examples are blue Azurite, red Cinnabar, and green Malachite.
Allochromatic minerals are "other colored" due to trace impurities in their composition or defects in their structure. In this case, the color is a variable and unpredictable property of the mineral. Examples are the blue in Amazonite (orthoclase), yellow in Heliodor (spodumene) and the rose in rose quartz.
Pseudochromatic minerals are "false colored" due to tricks in light diffraction. In these cases, color is variable but a unique property of the mineral. Examples are the colors produced by precious opal and the shiller reflections of labradorite.
Step 5: Streak
Streak is the color of the powder of a mineral. To do a streak test, you scrape the mineral across an unglazed porcelain plate. The plate is harder than many minerals, causing the minerals to leave a streak of powder on the plate. The color of the streak often differs from the color of the larger mineral sample. If you did a streak test on the yellow-gold pyrite, you would see a blackish streak. This blackish streak tells you that the mineral is not gold because gold has a gold-colored streak.
Streak is a more reliable property than the color of the mineral sample. The color of a mineral may vary, but its streak does not vary. Also, different minerals may be the same color, but they may have a different color streak . For example, samples of hematite and galena can both be dark gray, but hematite has a red streak and galena has a gray streak.
Step 6: Crystal Form and Mineral Habit

The term crystal habit describes the favored growth pattern of the crystals of a mineral species, whether individually or in aggregate. It may bear little relation to the form of a single, perfect crystal of the same mineral, which would be classified according to crystal system.
Subtle evidence of the crystal system to which a mineral species belongs is, however, frequently observed in the habit of the crystals which a specimen displays.
The terminology used to describe crystal habit is not intended to replace the precise nomenclature of crystallography. Instead, it is intended as a supplement to this system. Discussions of crystal habit are more descriptive than precise; for this reason the terminology is suited to the discussion of mineral samples discovered in the field. Naturally formed specimens are rarely quantitatively perfect.
Step 7: Cleavage and Fracture
Cleavage is the way a mineral breaks. Many minerals break along flat planes, or cleavages—some in only one direction (like mica), others in two directions (like feldspar), and some in three directions (like calcite) or more (like fluorite). Some minerals, like quartz, have no cleavage. Cleavage is a profound property that results from a mineral's molecular structure, and cleavage is present even when the mineral doesn't form good crystals. Cleavage can also be described as perfect, good or poor.
Fracture is breakage that is not flat. The two main kinds of fracture are conchoidal (shell-shaped, as in quartz) and uneven. Metallic minerals may have a hackly (jagged) fracture. A mineral may have good cleavage in one or two directions but fracture in another direction.
To determine Cleavage and fracture, you'll need a rock hammer and a safe place to use it on minerals. A magnifier is also handy, but not required. Carefully break the mineral and observe the shapes and angles of the pieces. It may break in sheets (one cleavage), splinters or prisms (two cleavages), cubes or rhombs (three cleavages) or something else.
Step 8: Magnetism

Magnetism is a distinctive property in a few minerals. Magnetite is the prime example, but a few other minerals may be weakly attracted by a magnet, notably chromite (a black oxide) and pyrrhotite (a bronze sulfide). Use a strong magnet. The magnets I use came from the corners of an old plastic shower curtain. Another way to test magnetism is to see if the specimen attracts a compass needle.
Step 9: Other Mineral Properties
Taste is definitive for halite ( rock salt ), of course, but a few other evaporite minerals also have distinctive tastes. Just touch your tongue to a fresh face of the mineral and be ready to spit—after all it's called taste, not flavor. Don't worry about taste if you don't live in an area with these minerals.
Fizz means the effervescent reaction of certain carbonate minerals to the acid test. For this test, vinegar will do.
Heft is how heavy a mineral feels in the hand, an informal sense of density. Most minerals are about three times as dense as water, that is, they have a specific gravity of about 3. Make note of a mineral that is noticeably light or heavy for its size. Galena, on the right, is distinctly heavy. Sulfides and oxides tend to be dense
Step 10: Look It Up

Now you are ready for mineral identification. Once you have observed and noted these mineral properties, you can take your information to a book or to an online resource. Start with my table of the rock-forming minerals , because these are the most common and the ones you should learn first.
Each mineral's name is linked to a good photograph and notes to help you confirm the identification. If your mineral has metallic luster, go to my Minerals with Metallic Luster gallery to see the most likely minerals in this group. If your mineral is not one of these, try the sources in the Mineral Identification Guides category.
Popular This Week
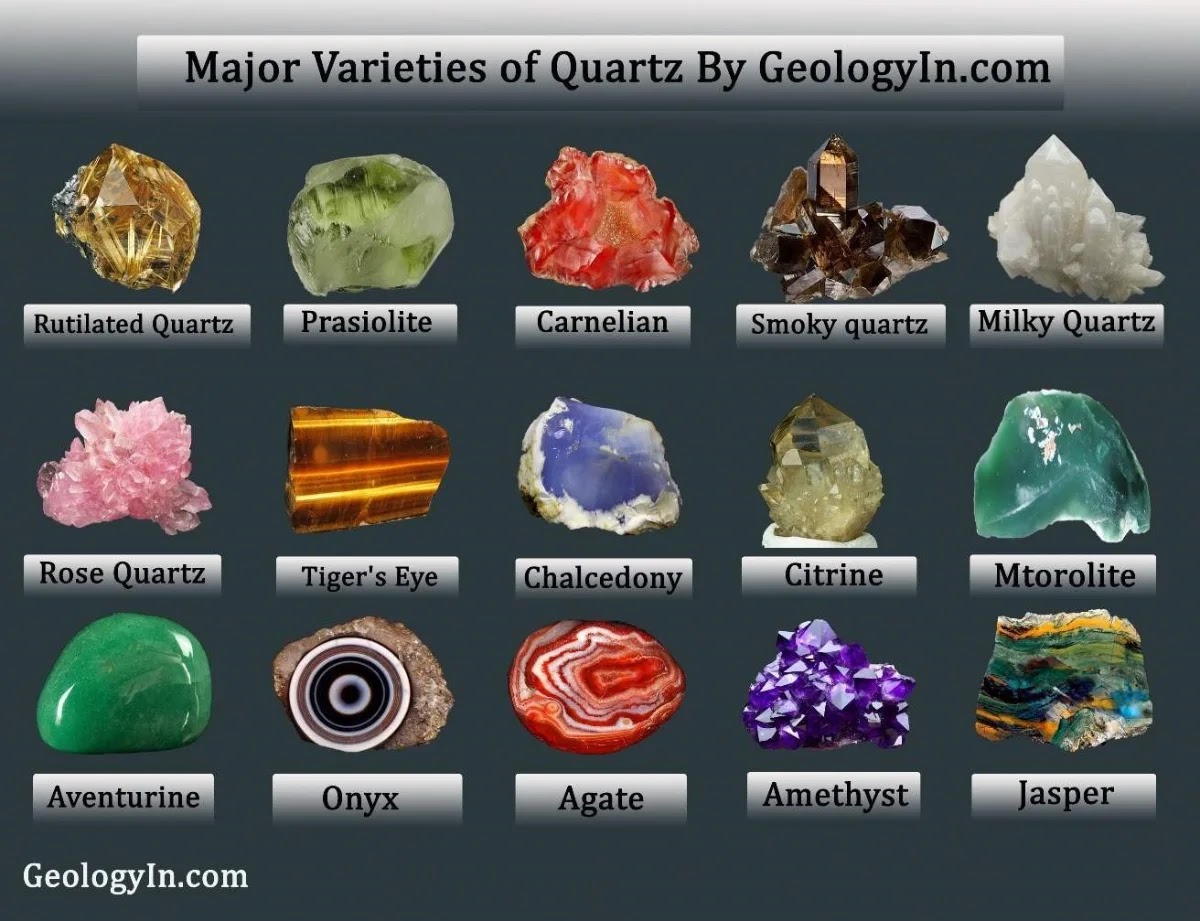
Quartz: Types and Varieties of Quartz (Photos)
%20(1).webp)
Types of Drainage Patterns
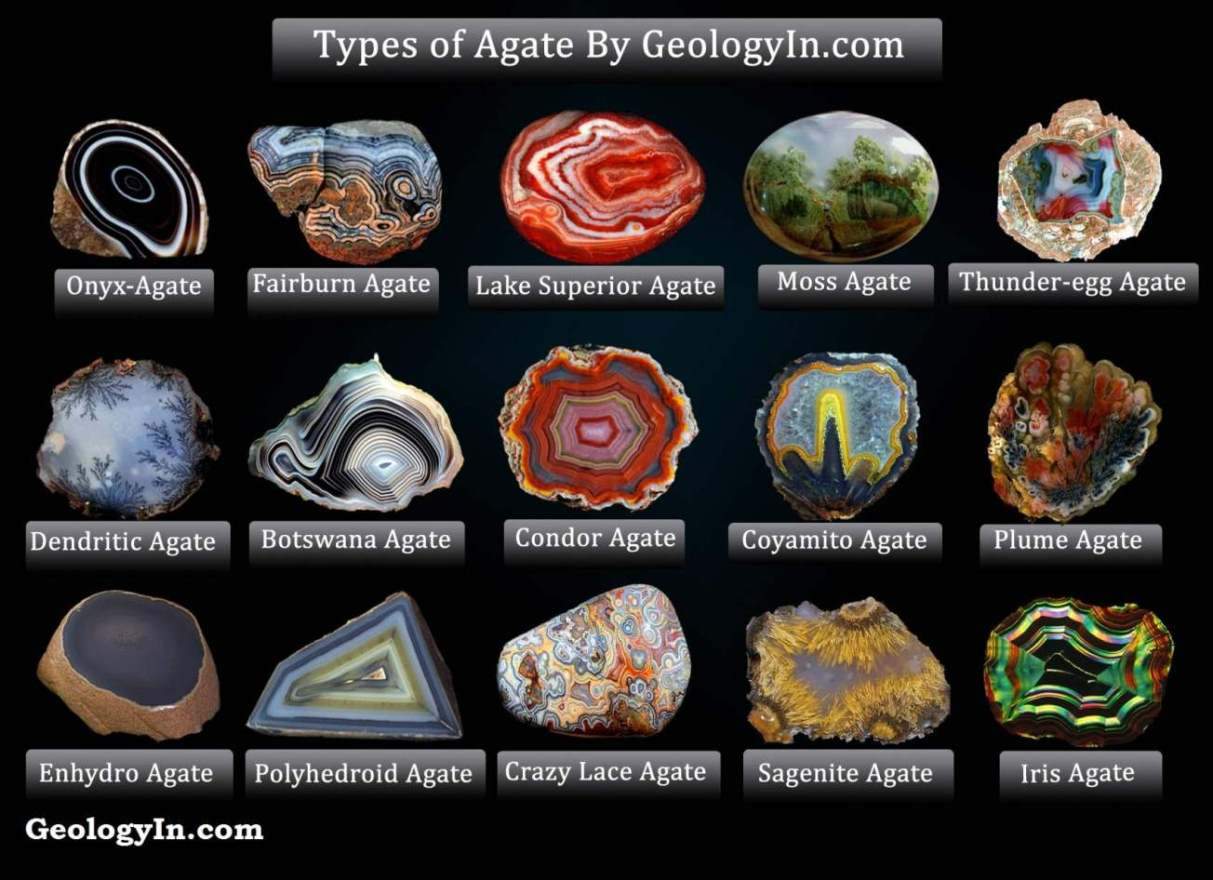
Types of Agate With Photos

The Most Expensive Fine Mineral Specimen, La Madona Rosa

Cave of Crystals "Giant Crystal Cave" at Naica - Mexico

Oldest Preserved Spider Web Dates Back to Dinosaurs
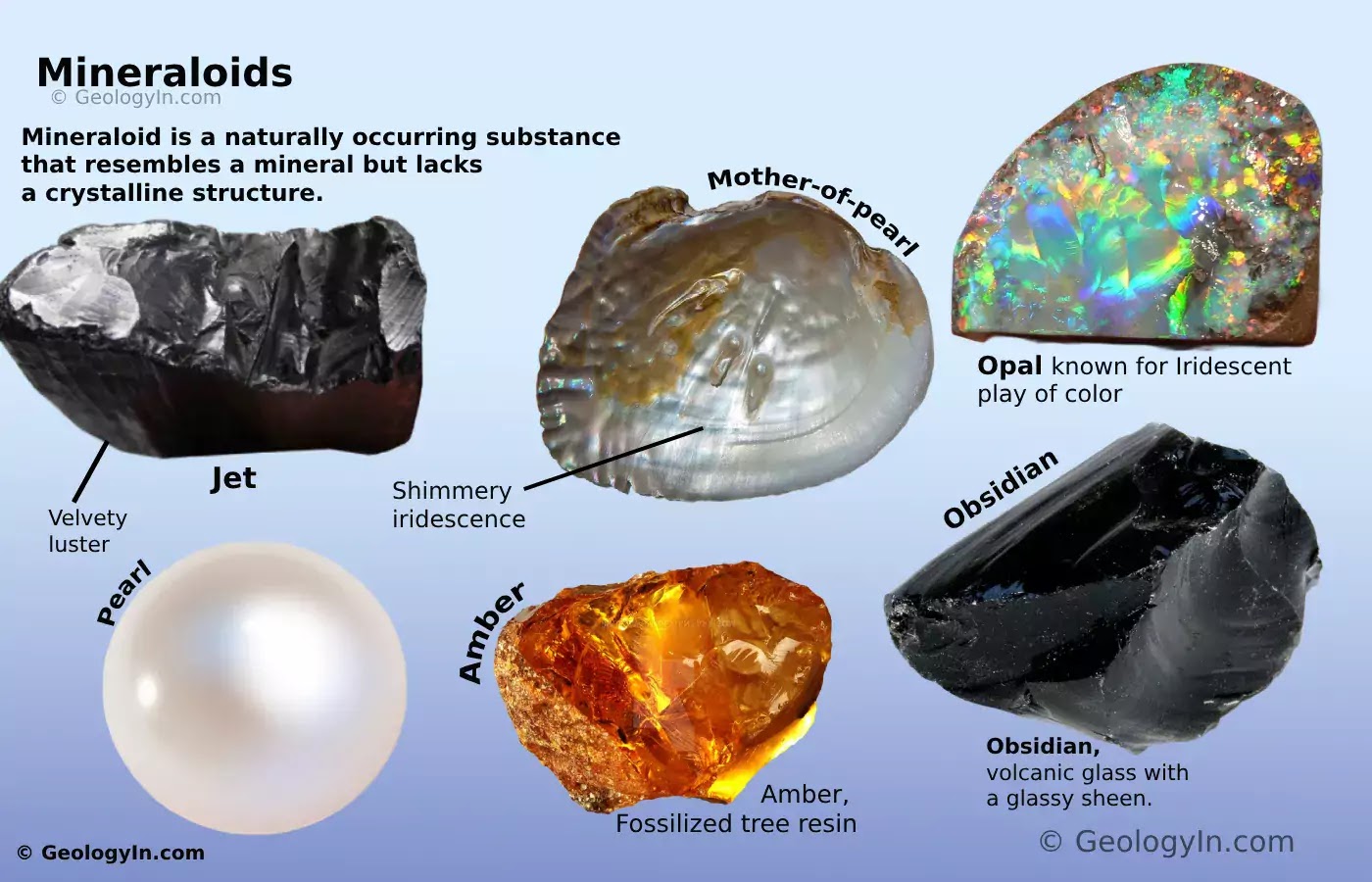
Mineraloids: Definition, Examples, Uses

Convergent Boundary: Definition, Types, Examples, Features
10 Steps for Easy Mineral Identification
Use a few simple tools and your own powers of observation
- Types Of Rocks
- Landforms and Geologic Features
- Plate Tectonics
- Weather & Climate
- B.A., Earth Sciences, University of New Hampshire
Almost all rocks are made of minerals. The exceptions are obsidian (which is made of volcanic glass) and coal (which is made of organic carbon.)
Learning the basics of mineral identification is easy. All you need are a few simple tools (like a magnet and a magnifying glass) and your own powers of careful observation. Have a pen and paper or a computer handy to record your notes.
Pick Your Mineral
Cyndi Monaghan/Getty Images
Use the largest mineral sample you can find. If your mineral is in pieces, bear in mind that they may not all be from the same rock. Finally, make sure your sample is free of dirt and debris, clean and dry. Now you're ready to begin identifying your mineral.
Luster describes the way a mineral reflects light. Measuring it is the first step in mineral identification. Always check for luster on a fresh surface; you may need to chip off a small portion to expose a clean sample. Luster ranges from metallic (highly reflective and opaque) to dull (nonreflective and opaque.) In between are a half-dozen other categories of luster that assess the degree of a mineral's transparency and reflectivity.
Hardness is measured on the 10-point Mohs scale , which is essentially a scratch test. Take an unknown mineral and scratch it with an object of known hardness (like a fingernail or a mineral like quartz.) Through trial and observation, you can determine your mineral's hardness, a key identification factor. For example, powdery talc has a Mohs hardness of 1; you could crumble it between your fingers. A diamond, on the other hand, has a hardness of 10. It's the hardest material known.
Color is important in mineral identification. You'll need a fresh mineral surface and a source of strong, clear light to examine it. If you have an ultraviolet light, check to see if the mineral has a fluorescent color. Make note if it displays any other special optical effects , such as iridescence or changes in color.
Color is a fairly reliable indicator in the opaque and metallic minerals like the blue of the opaque mineral lazurite or the brass-yellow of the metallic mineral pyrite. In translucent or transparent minerals, however, color is less reliable as an identifier because it is usually the result of a chemical impurity. Pure quartz is clear or white, but quartz can have many other colors.
Try to be precise in your identification. Is it a pale or deep shade? Does it resemble the color of another common object, like bricks or blueberries? Is it even or mottled? Is there one pure color or a range of shades?
Streak describes the color of a finely crushed mineral. Most minerals leave a white streak, regardless of their overall color. But a few minerals leave a distinctive streak that can be used to identify them. To identify your mineral, you'll need a streak plate or something like it. A broken kitchen tile or even a handy sidewalk can do.
Scratch your mineral across the streak plate with a scribbling motion, then look at the results. Hematite, for example, will leave a red-brown streak . Bear in mind that most professional streak plates have a Mohs hardness of about 7. Minerals that are harder will scratch the place and won't leave a streak.
Mineral Habit
A mineral's habit (its general form) can be especially useful for identifying some minerals. There are more than 20 different terms describing habit . A mineral with visible layers, like Rhodochrosite, has a banded habit. Amethyst has a drusy habit, where jagged projectiles line a rock's interior. Close observation and perhaps a magnifying glass are all you need for this step in the mineral identification process.
Cleavage and Fracture
Cleavage describes the way a mineral breaks. Many minerals break along flat planes or cleavages. Some cleave in only one direction (like mica), others in two directions (like feldspar ), and some in three directions (like calcite) or more (like fluorite). Some minerals, like quartz, have no cleavage.
Cleavage is a profound property that results from a mineral's molecular structure, and cleavage is present even when the mineral doesn't form good crystals. Cleavage can also be described as perfect, good, or poor.
Fracture is breakage that is not flat, and there are two types: conchoidal (shell-shaped, as in quartz) and uneven. Metallic minerals may have a hackly (jagged) fracture. A mineral may have good cleavage in one or two directions but fracture in another direction.
To determine cleavage and fracture, you'll need a rock hammer and a safe place to use it on minerals. A magnifier is also handy, but not required. Carefully break the mineral and observe the shapes and angles of the pieces. It may break in sheets (one cleavage), splinters or prisms (two cleavages), cubes or rhombs (three cleavages) or something else.
A mineral's magnetism can be another identifying characteristic in some instances. Magnetite, for example, has a strong pull that will attract even weak magnets. But other minerals have only a weak attraction, notably chromite (a black oxide) and pyrrhotite (a bronze sulfide.) You'll want to use a strong magnet. Another way to test magnetism is to see if your specimen attracts a compass needle.
Other Mineral Properties
Taste can be used to identify evaporite minerals (minerals formed by evaporation) like halite or rock salt because they have distinctive tastes. Borax , for instance, tastes sweet and slightly alkaline. Be careful, though. Some minerals can sicken you if ingested in sufficient quantities. Gently touch the tip of your tongue to a fresh face of the mineral, then spit it out.
Fizz refers to the effervescent reaction of certain carbonate minerals in the presence of an acid like vinegar. Dolomite, found in marble, will fizz actively if dropped in a small bath of acid, for example.
Heft describes how heavy or dense a mineral feels in the hand. Most minerals are about three times as dense as water; that is, they have a specific gravity of about 3. Make note of a mineral that is noticeably light or heavy for its size. Sulfides like Galena, which is seven times more dense than water, will have a notable heft.
The final step in mineral identification is to take your list of characteristics and consult an expert source. A good guide to rock-forming minerals should list the most common, including hornblende and feldspar, or identify them by a common characteristic like metallic luster . If you still can't identify your mineral, you may need to consult a more comprehensive mineral identification guide.
- How to Use Mineral Streak to Identify Rock Samples
- Identifying Black Minerals
- How to Distinguish Brown Minerals
- 10 Minerals That Have Metallic Luster
- Picture Guide to Common and Less-Common Minerals
- How to Identify 10 Red and Pink Minerals
- The Mohs Hardness Scale
- Rock Identification Made Easy
- Guide to Identifying Yellow Minerals
- What You Need to Know Before You Buy a Rock Collection
- The 12 Most Common Blue, Violet, and Purple Minerals
- How to Look at a Rock Like a Geologist
- Examples of Different Mineral Lusters
- Get to Know the 7 Delicate Sulfate Minerals
- Feldspar Distinctions, Characteristics, and Identification
- Sulfide Minerals
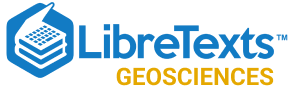
- school Campus Bookshelves
- menu_book Bookshelves
- perm_media Learning Objects
- login Login
- how_to_reg Request Instructor Account
- hub Instructor Commons
- Download Page (PDF)
- Download Full Book (PDF)
- Periodic Table
- Physics Constants
- Scientific Calculator
- Reference & Cite
- Tools expand_more
- Readability
selected template will load here
This action is not available.
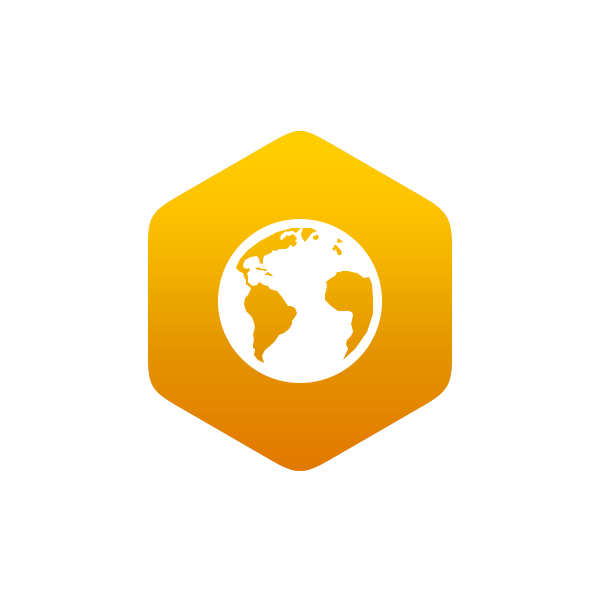
56.1: Mineral Properties and Identification
- Last updated
- Save as PDF
- Page ID 22848
Geologists identify minerals by their physical properties. In the field, where geologists may have limited access to advanced technology and powerful machines, they can still identify minerals by testing several physical properties: luster, color, streak, hardness, crystal habit, cleavage and fracture, and some special properties. Our Big Ten Minerals make up the majority of Earth’s lithosphere and are usually seen as small grains in the rock. Geologists rely on a magnifying hand lens as an essential tool for identifying most minerals in the field. Of the many properties that minerals can exhibit, we will consider only those most useful for identification as small grains surrounded by other minerals in rock.
Luster and Color
The first thing to notice about a mineral is its surface appearance, specifically luster and color. Luster describes how the mineral reflects light. Metallic luster looks like a shiny metal such as chrome, steel, silver, or gold. Metallic minerals are also always opaque. Non-metallic minerals may be shiny and reflect light, however, they do not look like a metal.
Surface color may be helpful in identifying minerals, although it can be quite variable even within a single mineral family. Mineral colors are affected by the main elements present, but also by impurities, inclusions, and defects in the crystal structure. Pressure and temperature conditions can also affect mineral color. Using the mineral quartz for example, in its purest form (SiO 2 ), it will be clear. However, impurities by trace amounts of iron can change the color from purple (amethyst) to yellow (citrine) or pink (rose quartz); tiny inclusions of the titanium mineral rutile can give quartz a blue color, and exposure to natural radiation within the crust can provide a grey (smoky) color.
There are some minerals that predominantly show a single color. However this is not true for our “Big Ten Minerals” or the other most common rock forming minerals. All of these minerals have a predictable range of colors due to element substitution within their crystalline structures. Feldspars, the most abundant minerals in the Earth’s crust, range in composition from calcium-rich to sodium-rich to potassium-rich varieties and many compositions in between. Feldspar colors including pink, white, green, gray and others. With notable exceptions, color is usually not a reliable diagnostic property of minerals. For identifying many minerals, a more reliable indicator is streak, which is the color of the powdered mineral.
Streak examines the color of a powdered mineral, and can be seen when a mineral sample is scratched or scraped on an unglazed porcelain streak plate. A paper page in a field notebook may also be used for the streak of some minerals. Minerals that are harder than the streak plate (or the paper) will not show streak, but will scratch the porcelain (and tear the paper!). For these minerals, a streak test can be obtained by powdering the mineral with a hammer and smearing the powder across a streak plate or notebook paper. Note the absence of a mineral streak via this method is also a useful observation to record.
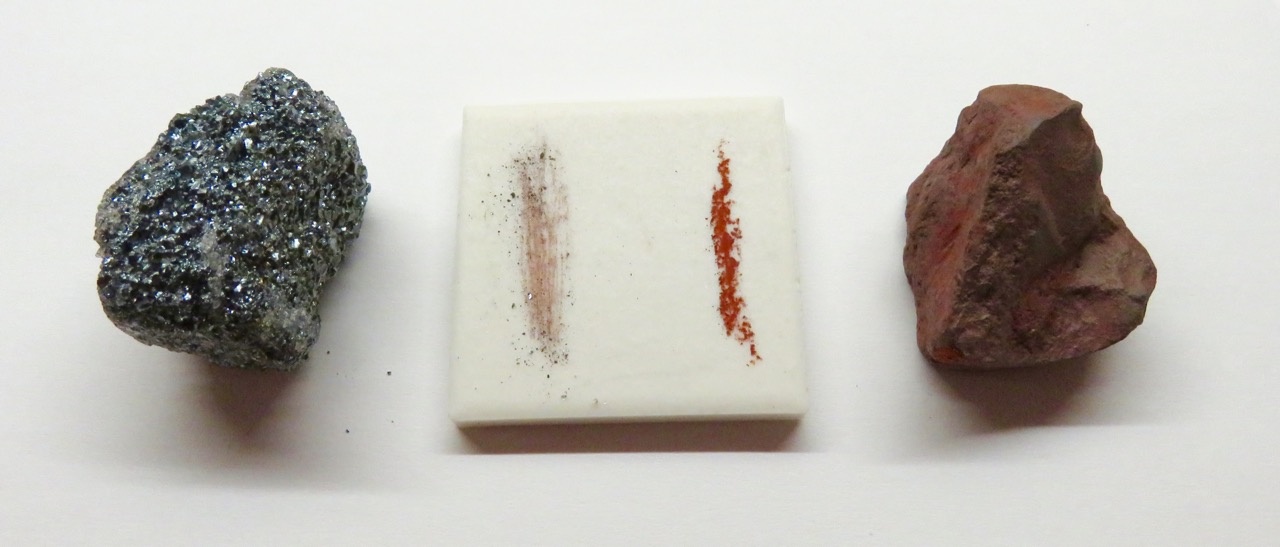
While mineral surface colors and appearances may vary, their streak colors can be diagnostically useful. An example of this property is seen in the iron-oxide mineral hematite. Hematite occurs in a variety of forms, colors and lusters, from shiny metallic silver to earthy red-brown, and different physical appearances. A hematite streak is consistently reddish brown, no matter what the original specimen looks like. Iron sulfide or pyrite, is a brassy metallic yellow. Commonly called fool’s gold, pyrite has a characteristic black to greenish-black streak.
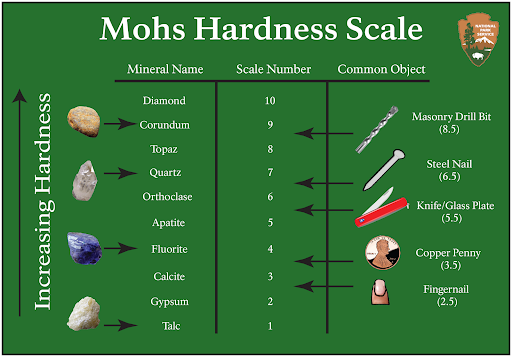
Hardness measures the resistance of a mineral to abrasion. Mohs Hardness Scale assigns a number between 1 and 10 indicating the relative scratch-resistance of minerals when compared to a standardized set of minerals of increasing hardness. Mohs scale was developed by German geologist Fredrick Mohs in the early 20th century, although the idea of identifying minerals by hardness goes back thousands of years. Mohs hardness values are determined by the strength of a mineral’s atomic bonds. The figure shows the minerals associated with specific hardness values, together with some common items readily available for use in field testing and mineral identification. A steel pocket knife blade, which has a hardness value of 5.5, distinguishes between hard and soft minerals on many mineral identification keys.
Cleavage and Fracture
Minerals often show characteristic patterns of breaking along specific cleavage planes or show characteristic fracture patterns. Cleavage planes are smooth, flat, parallel planes within the crystal. The cleavage planes may show as reflective surfaces on the crystal, as parallel cracks that penetrate into the crystal, or show on the edge or side of the crystal as a series of steps like rice terraces. Cleavage arises in crystals where the atomic bonds between atomic layers are weaker along some directions than others, meaning they will break preferentially along these planes.
Because they develop on atomic surfaces in the crystal, cleavage planes are optically smooth and reflect light, although the actual break on the crystal may appear jagged or uneven. In such cleavages, the cleavage surface may appear like rice terraces on a mountainside that all reflect sunlight from a particular sun angle. Some minerals have a strong cleavage, some minerals only have weak cleavage or do not typically demonstrate cleavage. But, don’t be fooled: Among mineralogists, a common saying is that “cleavage in a mineral is great, when you see it. But if you don’t, do not assume it is not there.” Fracture is different than cleavage in that the broken surface will not be flat or even. The breakage does not occur along a distinct plane within the crystal lattice. Fracture is typically uneven however can also be very distinctive. Several common minerals, quartz and olivine, will often display a conchoidal fracture similar to that seen in obsidian or broken glass.
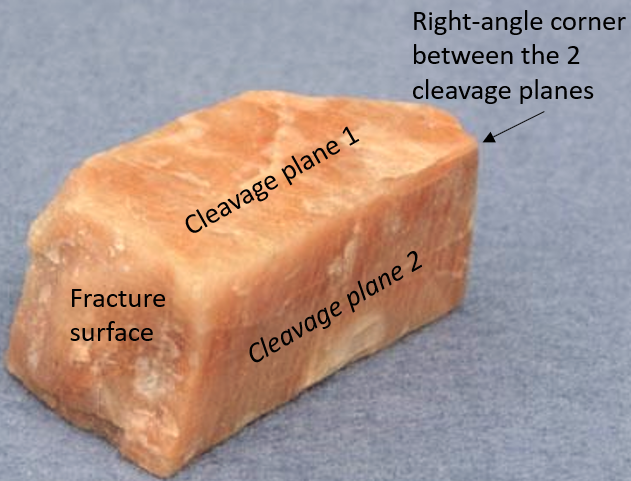
Special Properties
Special properties are unique and identifiable characteristics used to identify minerals or that allow some minerals to be used for special purposes. A simple identifying special property is taste, such as the salty flavor of halite or common table salt (NaCl).
A simple test for identifying calcite and dolomite is to drop a bit of dilute hydrochloric acid (10-15% HCl) on the specimen. If the acid drop effervesces or fizzes on the surface of the rock, the specimen is calcite. If not, and dolomite is suspected, the specimen can be scratched to make a small amount of powder. If the acid drop fizzes slowly on the powdered mineral, the specimen is dolomite. The video below compares these two minerals and their effervescence reaction. Geologists often carry a small dropper bottle of dilute HCl in their field kit.
Some iron-oxide minerals are magnetic and are attracted to magnets. Common magnetic minerals include magnetite (\(\ce{Fe3O4}\)) and ilmenite (\(\ce{FeTiO3}\)). Magnetite is strongly attracted to magnets. Ilmenite and some types of hematite are weakly magnetic. Geologists often carry a free-swinging magnet in their field kit to test for magnetism.
Review the following video to learn more about the basic properties of the rock forming minerals.
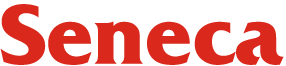
Want to create or adapt books like this? Learn more about how Pressbooks supports open publishing practices.
Nahgeib Miller
Learning Objectives
After completing this chapter, you should be able to:
- Define a mineral.
- Explain how minerals are formed.
- Describe the common rock-forming minerals, including silicates.
- Identify minerals based on their physical properties.
With an appreciation for the origin of Earth and the global macroscopic processes of plate tectonics, we understand that the materials that make up the interior and the crust are essentially the same (they exist in different proportions, though). However, the materials that make up the mantle are molten and the materials that make up the crust are in a solid state. The transformation of mantle materials to solid rock occurs when the materials are exposed at the surface, during an eruption, and are added to a plate. The change in temperature results in a change of energy that allows for the combination of the mantle materials in a variety of solid forms. These solid forms are called minerals and it is these minerals that come together to make rock.
3.1 An Introduction to Minerals
Minerals are the “Lego blocks” used to build rocks. That is, rocks are made of and from minerals. Think of it this way: minerals are to rocks as students are to a class. Many different students can make up a class just like many different minerals make up a rock. A rock can also be made up of many crystals of the same mineral, just like a class can be made up of just one student (that must be a bad teacher!).
A more scientific definition of minerals is that they are characterized by:
- Solid ity While minerals are chemical compounds, not all chemical compounds are minerals. Some chemical compounds exist in a liquid or gaseous state, while minerals must be in a solid state to be classified as such.
- Crystalline Structure This term is applied to particles or atoms that are arranged in a regular, repeating three-dimensional array. That is, the mineral that is visible to the eye is the result of millions of repeated three-dimensional stacks of the same microscopic mineral “block.”
- Ordered Internal Structure It is possible to have our solid microscopic mineral “block” stacked in a three-dimensional array with a chaotic pattern instead of a regular repeating one. Such a material is called a glass , not a mineral. A mineral must have an ordered arrangement of particles.
- Specific Chemical Composition The mineral building block that is arranged in a repeating three-dimensional array must also have a specific combination of atoms. Each unique mineral will have its own “recipe” of atomic combination ratios. For example, halite, or rock salt, has equal parts sodium (Na) and chlorine (Cl) to give NaCl.
- Inorganic Nature Any material derived from living tissue is not considered to be a mineral, with just one small catch. Organic material like coal and anthracite can be solid with a specific chemical composition; however, these materials are not crystalline and are therefore not considered to be minerals. Graphite, on the other hand, is crystalline and is considered a mineral even though it is derived from coal/anthracite. Technically, the requirement of an inorganic origin still holds because graphite is one step removed from living tissue.
- Natural Occurrence Minerals should occur in nature, which means that minerals created in a lab would not be considered as such. While artificial minerals would meet most of the criteria to be considered minerals, they would be of no use to geologists because no Earth processes would be recorded. That means we could not infer the crystallization environment, temperature of formation, weathering, erosion, transport mechanisms, or depositional environment.
3.2 A Brief Overview of Matter
In our previous example of halite as a mineral, sodium (Na) and chlorine (Cl) are elements that combined (reacted with) each other to create NaCl. The smallest part of an element that retains its physical and chemical properties is called an atom . An atom consists of a nucleus of protons and neutrons and a surrounding cloud of electrons that orbit the nucleus. Protons are positively charged, neutrons have no charge, and electrons are negatively charged. It is the electrostatic attraction between the protons in the nucleus and the electrons that is responsible for chemical reactions and bonding (Animation 3.1).
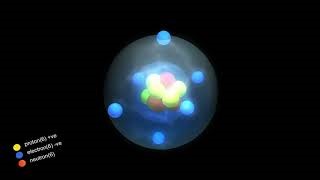
Usually, atoms of a specific element have the same number of neutrons as there are protons. However, there are small amounts of the same atom with different numbers of neutrons in the nucleus; these differing atoms are called isotopes . Let us take the element carbon as an example. Carbon generally has 6 protons and 6 neutrons in its nucleus, yielding an atomic number of 12 (in carbon-12, or C 12 ). However, small amounts of carbon also exist, with 7 or 8 neutrons, yielding isotopes of carbon with atomic numbers 13 (C 13 ) and 14 (C 14 ), respectively.
When we think back to our definition of a mineral, the criterion of having a specific chemical composition speaks to the interaction of two or more atoms of elements in a certain fixed ratio or proportion. This interaction is called a chemical reaction . That is, for example, the combination of one sodium atom with one chlorine atom to make sodium chloride (NaCl). Chemical reactions form chemical compounds by either sharing electrons or transferring electrons from one atom to the other. These compounds are held together by the attraction between the positively charged nucleus (specifically, the protons in the nucleus) and the negatively charged electrons.
Chemical reactions in which electrons are transferred are a result of ionic bonding , as in the formation of NaCl (Figure 3.1).
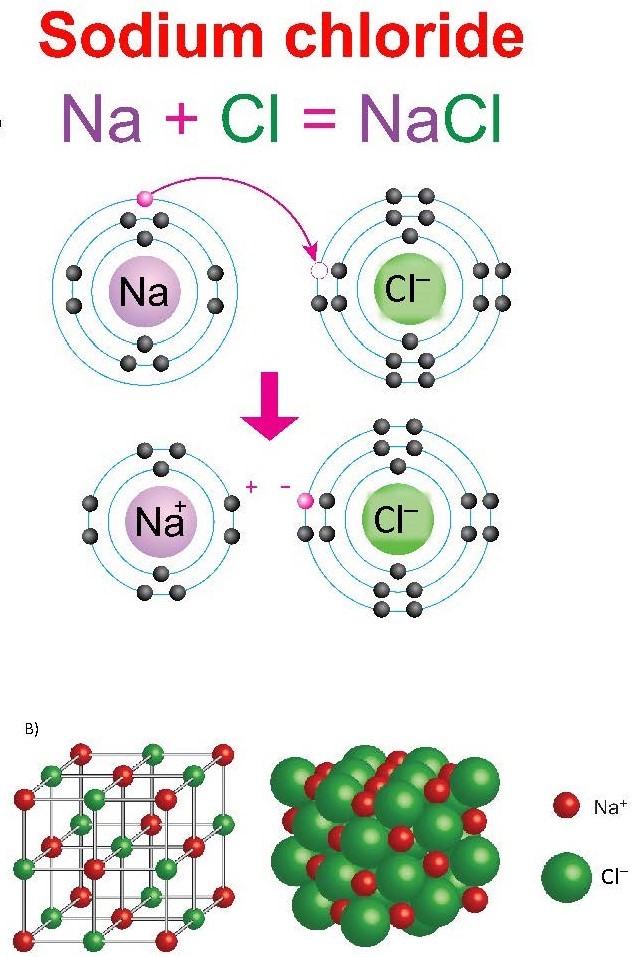
Chemical reactions in which electrons are shared are a result of covalent bonding , as in the formation of diamond (carbon; Figure 3.2).
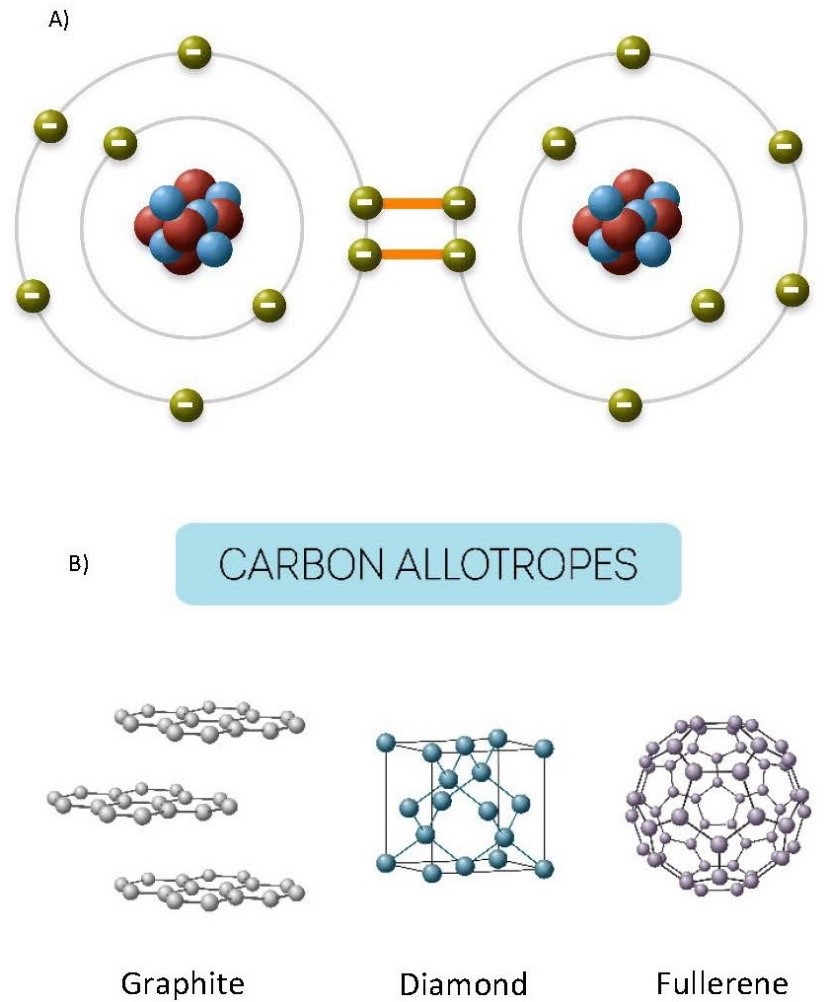
Covalent bonds are stronger than ionic bonding, manifesting in the mineral physical property of hardness, with covalently bonded minerals being harder than ionically bonded minerals. However, most minerals are ionically bonded.
Although we represent the atoms of elements in their stable form with an overall neutral charge (that is, an equal number of protons and electrons), in a chemical compound they actually exist as ions . Some atoms will want to lose an electron or two to become stable in the compound and some will want to gain an electron or two. When atoms give up electrons, they become positively charged overall (more protons than electrons) and are called cations . When atoms gain electrons, they become negatively charged overall (more electrons than protons) and are called anions . Anions and cations will combine based on their atomic size and charge. The larger an ion’s atomic charge, the more reactive the ion will be. Furthermore, the smaller the atomic size, the more reactive it will be (Figure 3.3). This is because the reaction or bonding between ions is due to the attraction between one ion’s nucleus and the other ion’s electrons. Therefore, the closer the nucleus can get to the other ion’s electrons, the stronger the potential for reaction and the stronger the bonds. Smaller atomic sizes allow the nucleus to get closer because the atomic radius is small. Hence the compound that would preferentially form would be between the smallest ions with the largest charges.
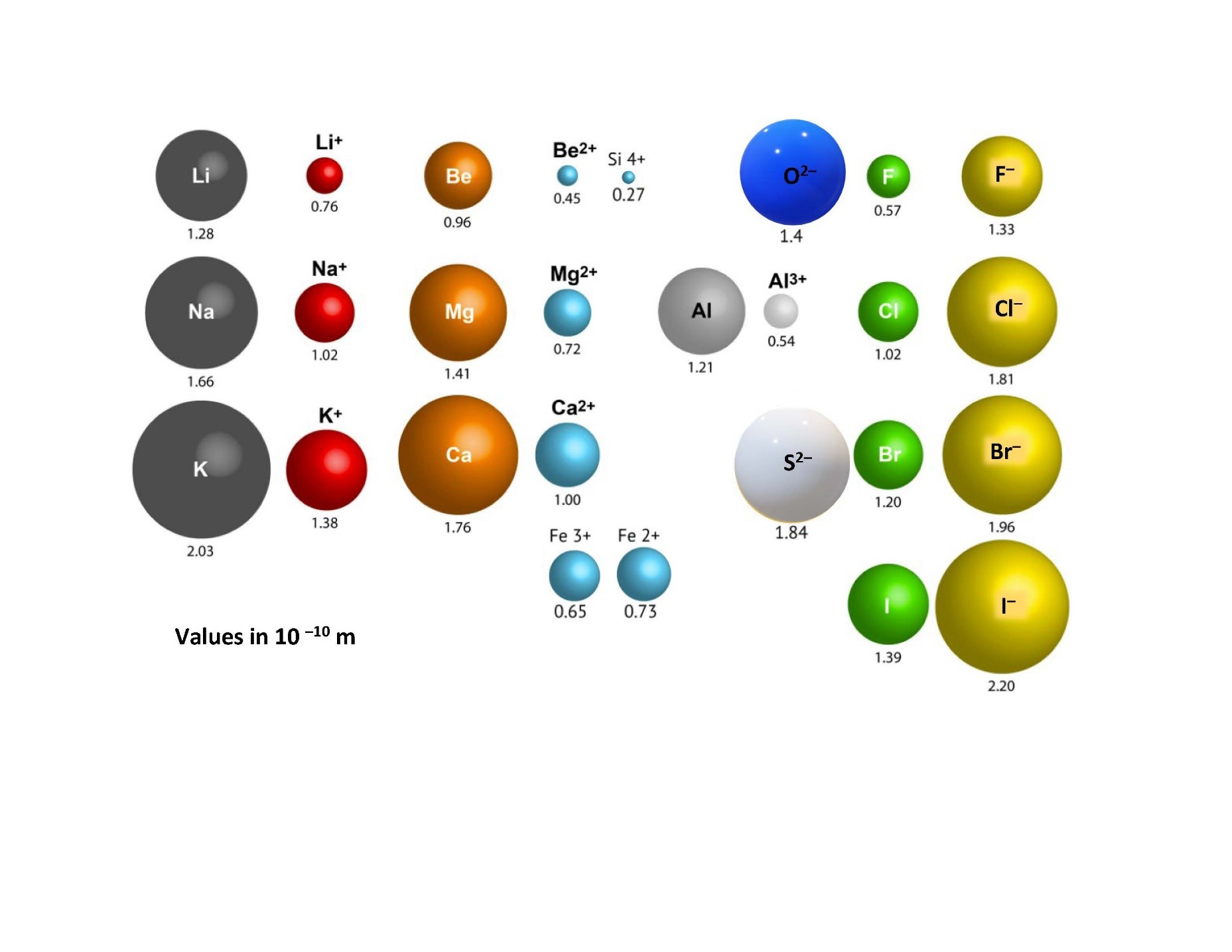
3.3 Mineral Formation
Minerals form in three ways:
- Crystallization from cooling molten rock As molten rock rises through Earth’s crust at spreading centres, convergences zones, and hotspots, it begins to cool and form minerals, which aggregate into rocks. Let us think of this molten rock as a “soup of potential” containing our common mineral-forming anions and cations. This “soup” is a high-energy environment that is about 1300 °C when the rock is completely molten; in this environment, the ions are not able to bond because they are vibrating too much. As the molten rock cools below 1300 °C, the roots of the first minerals start to form because the only ions that can bond are the ones that would make the strongest bonds. That is, they are able to form a stable bond in a high-energy environment. The smallest ions with the highest charge bond first. Referring to Figure 3.3, those ions would be silicon and oxygen, which would bond to form a larger silicate anion SiO 4 – 4 (see Figure 3.4). This silicate anion forms the basis of 95% of all minerals in the crust.
- Crystallization from water evaporation As water percolates through Earth’s crust and flows over rocks exposed at the surface, it dissolves soluble minerals, returning their ions to another type of “soup of potential.” As the water evaporates, it can no longer hold the ions in solution: the ions bond to form minerals that then fall out as sediments. These sediments eventually accumulate and turn into rock.
- Recrystallization from existing minerals due to changes in temperature and pressure Existing minerals can be changed into new minerals without melting when they are exposed to new temperatures and pressures. Upon exposure to new prevailing temperatures and pressures, minerals will rearrange their crystal structure to achieve stability under these new conditions. The rearrangement results in the creation of a new mineral entirely.
3.4 Common Rock-Forming Mineral Classes
The crystal arrangement of minerals depends on how the anions are packed and how the cations are packed between the anions. This is because the anions are larger than the cations and will take up the most space. Minerals with similar anions will have a similar crystal structure and, therefore, similar physical properties, which vary slightly depending on the type of cations bonded with them. Hence it is advantageous to classify minerals based on their anions, yielding seven mineral classes, as follows:
- Silicates These contain silicon (S) and oxygen (O) bonded in silicate tetrahedra (SiO 4 – 4 ).
- Carbonates These contain carbon (C) and oxygen (O) bonded in a carbonate anion (CO 3 – ).
- Oxides These result when an oxygen (O – 2 ) anion bonds with a metallic cation.
- Sulfides These contain a sulfur (S – ) anion bonded with a metallic cation.
- Sulfates In these minerals, cations are bonded with a sulfate anion (SO 4 2 – ), which contains sulfur and oxygen.
- Halides These minerals have cations bonded with bromide (Br – ), chloride (Cl – ), fluoride (F – ), and iodide (I – ) anions.
- Hydroxides These minerals have cations bonded with a hydroxyl anion (OH – ), which contains oxygen (O) and hydrogen (H).
Because the vast majority of rock-forming minerals are silicates (Figure 3.4), we will focus on this mineral class.
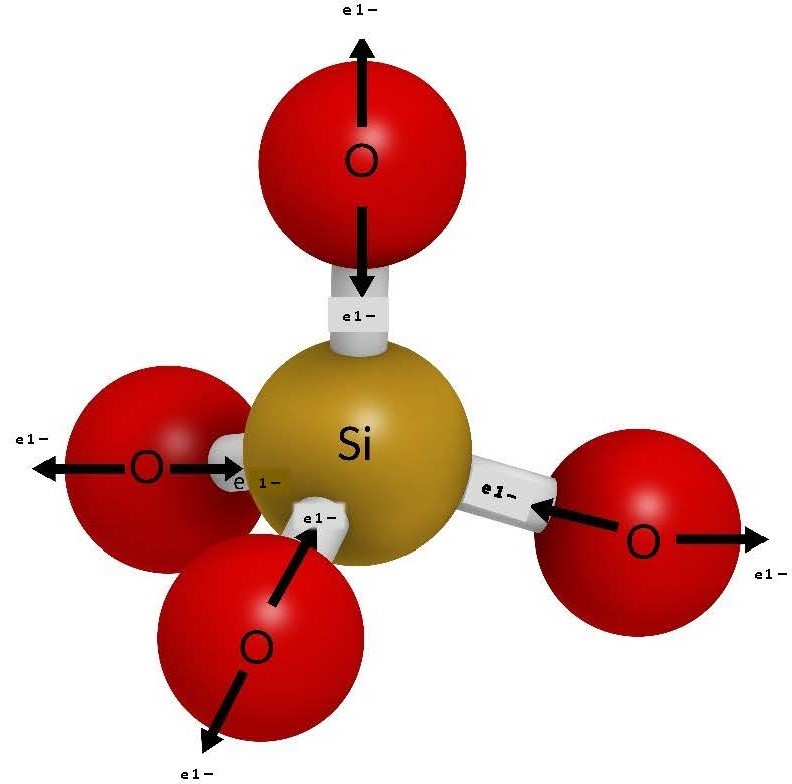
The silicates mineral class is based on the arrangement and packing of the silicate anion as the “soup of potential” cools. As previously stated, the first thing that forms in our high-energy soup of potential is the silicate anion, which then bonds with any available cations. Due to the high-energy environment, the only cations capable of bonding with the silicate anion are those that are small with high charges, such as iron (Fe 3+ , Fe 2+ ), magnesium (Mg 2+ ), and aluminum (Al 3+ ). The name of a mineral in each silicate group depends on the ratio of the bonded cations, where a mineral with 100% iron will be at one end of the group and a mineral with 100% magnesium will be at the other end. Note that most (if not all) of the silicon (the smallest cation with the biggest charge) is used up to create the silicate anion, which has an overall 4– charge due to the available electron each oxygen, bonded to the silicon, provides.
As the soup cools the arrangement of the silicate anions are in order as follows:
- Isolated T etrahedra Isolated tetrahedra form the olivine group of silicate minerals. As the soup of potential continues to cool, after the formation of the silicate tetrahedra, the Fe 3+ , Fe 2+ , and Mg 2+ cations are able to bond due to the reduction in energy of the environment. Therefore, all of the available electrons provided by the oxygens in the tetrahedron are bonded to a cation, which is in turn bonded to another oxygen in an adjacent tetrahedron (Figure 3.5a). Each tetrahedron is effectively cut off or isolated from the others because they are separated by a cation. This also creates one cleavage plane in the crystal structure (we will discuss this in the next section). The minerals in this group will fall somewhere between forsterite (100% Mg as the cation; Figure 3.5b) and fayalite (100% Fe as the cation). This variation in the composition of the mineral yields the chemical formula (Mg, Fe) 2 SiO 4 .
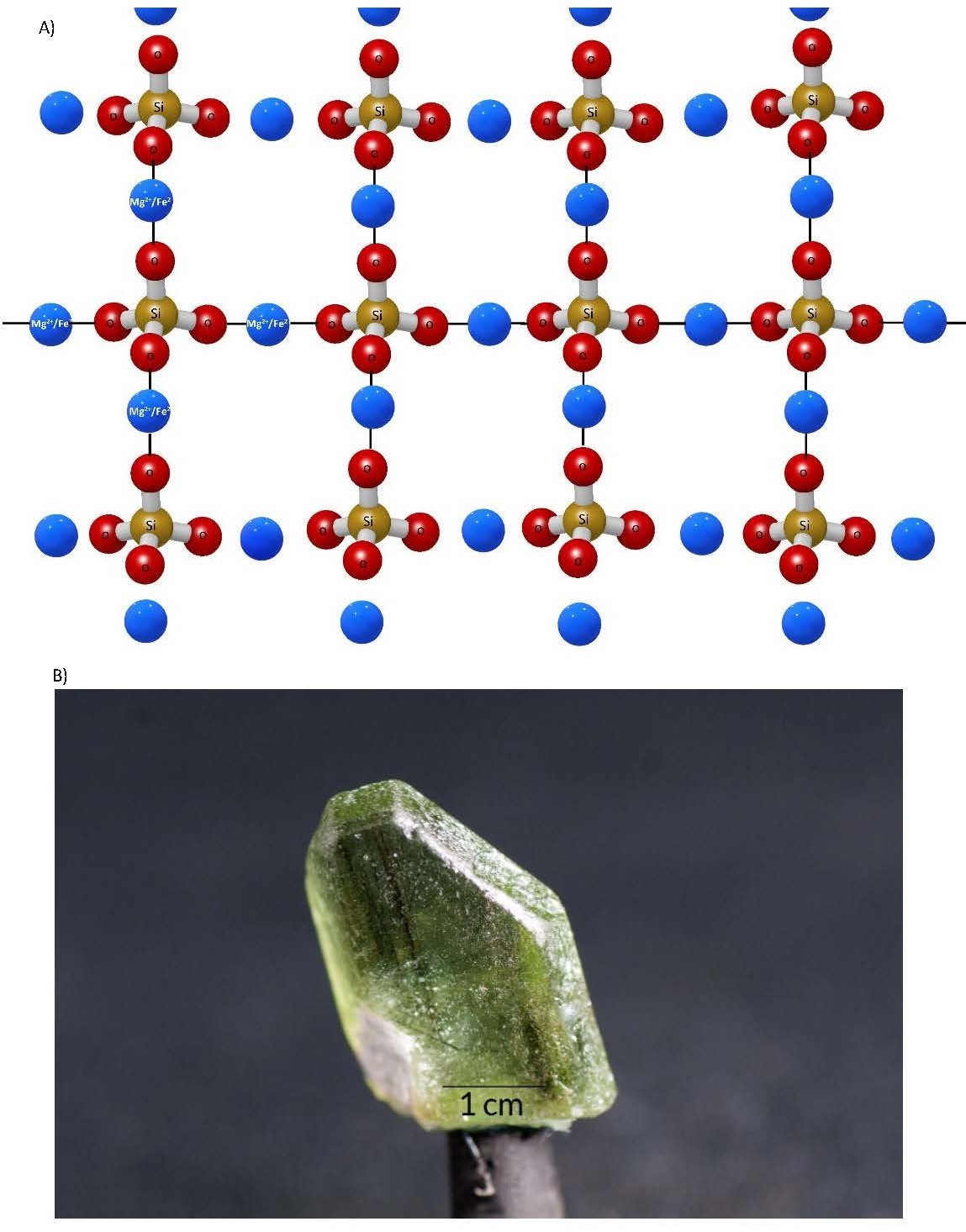
- Single – C hain T etrahedra Single-chain tetrahedra form the pyroxene group of silicate minerals. As cooling continues, the reduction of energy in the environment allows for adjacent tetrahedra to bond through a common oxygen. That is, one oxygen in a tetrahedron is bonded to the silicon in another tetrahedron, with the other three oxygens bonded to a cation. This creates a repeating link between adjacent tetrahedra, like a single chain — hence the name. The crystal structure that eventually results creates two cleavage planes at 90° to each other. A wide range of cation substitutions can occur, yielding a wide range of minerals, of which augite and diopside are the most common. The chemical formula for the pyroxene group is (Mg, Fe, Ca, Al)SiO 3 (Figure 3.6). Note that aluminum can substitute for the silicon.
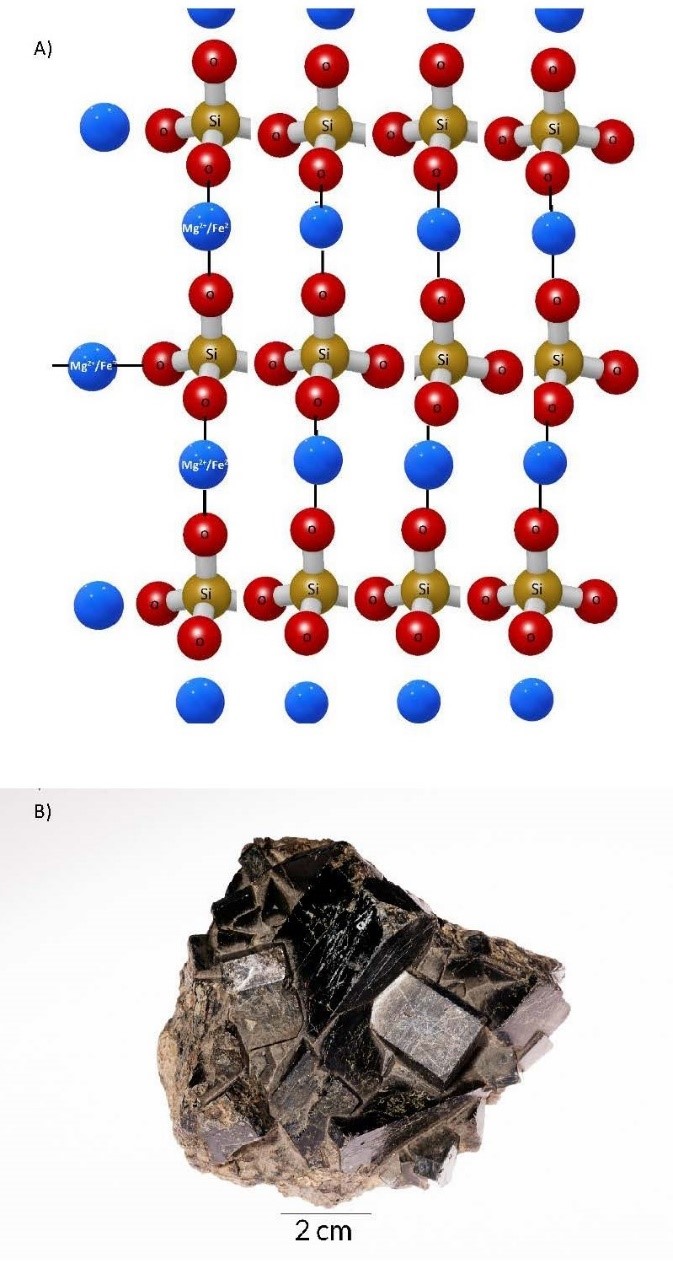
- Double – C hain T etrahedra Double-chain tetrahedra form the amphibole group of silicate minerals. As cooling continues below the temperature at which pyroxenes form, the lowered energy allows two of the oxygens in adjacent silicate tetrahedra to share the same silicon ion. This leaves only the other two oxygens to bond with a cation. The lowered energy allows for larger cations to bond in a more complex crystal structure that has two single chains stitched together to form a double chain. This crystal structure yields two cleavage planes, at 56°/124° to each other. A wide range of cation substitution yields numerous mineral names within the group, as reflected by the chemical formula (Na, Ca) 2 (Mg, Fe) 5 Si 8 O 22 (OH) 2 (Figure 3.7).
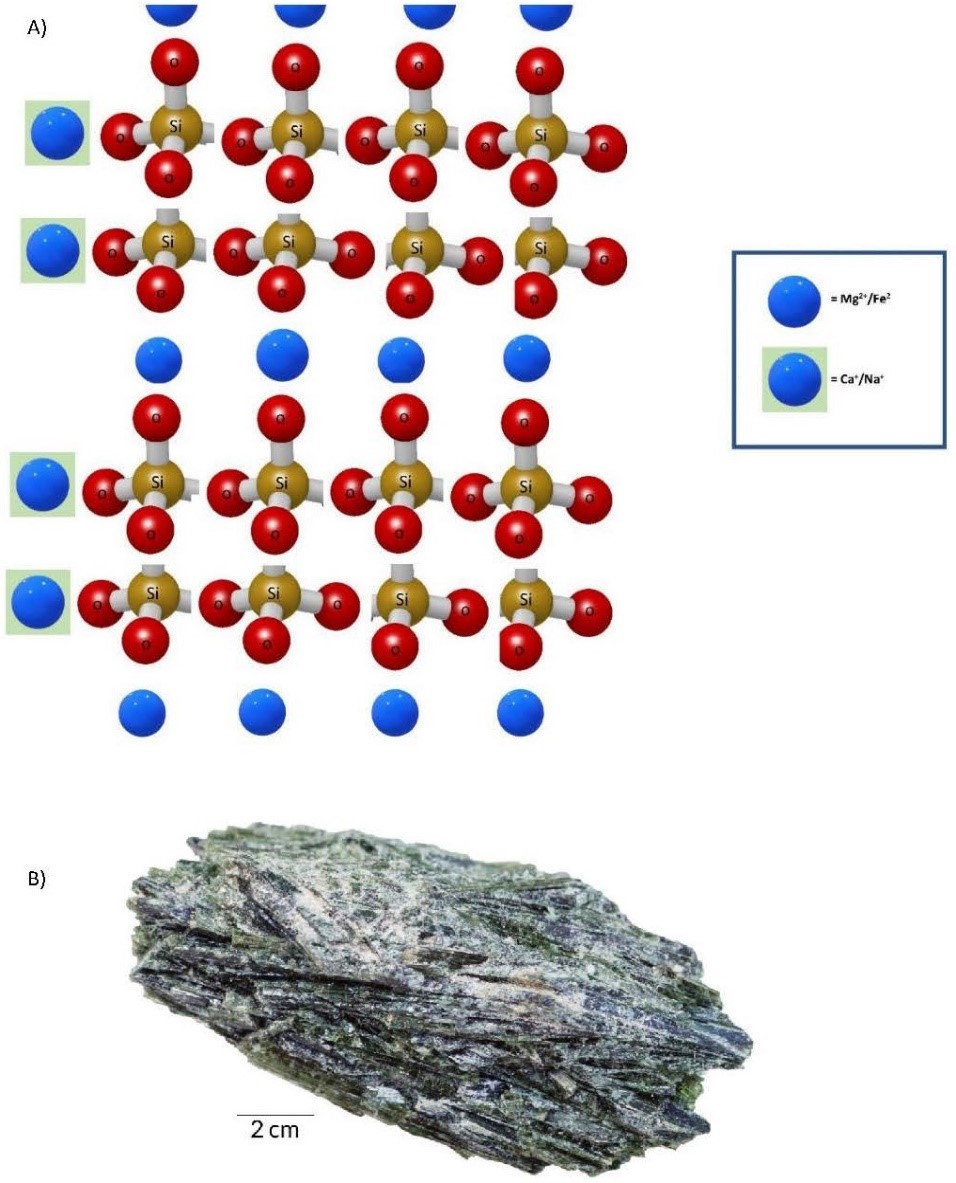
- Sheet T etrahedra Sheet tetrahedra form the mica group of minerals. Cooling of molten rock in our “soup of potential” to below that at which amphiboles form allows three of the four oxygens to bond with another silicon in an adjacent tetrahedron. Essentially, these are the three oxygens at the base of the tetrahedron, resulting in a sheetlike crystal structure (Figure 3.8). The lower temperature also allows larger cations to bond, yielding two types of chemical compositions: muscovite , KAl 2 (AlSi 3 O 10 )(OH) 2 , and biotite , K(Mg, Fe) 3 (AlSi 3 O 10 )(OH) 2 . The sheetlike crystal structure yields one perfect cleavage plane.
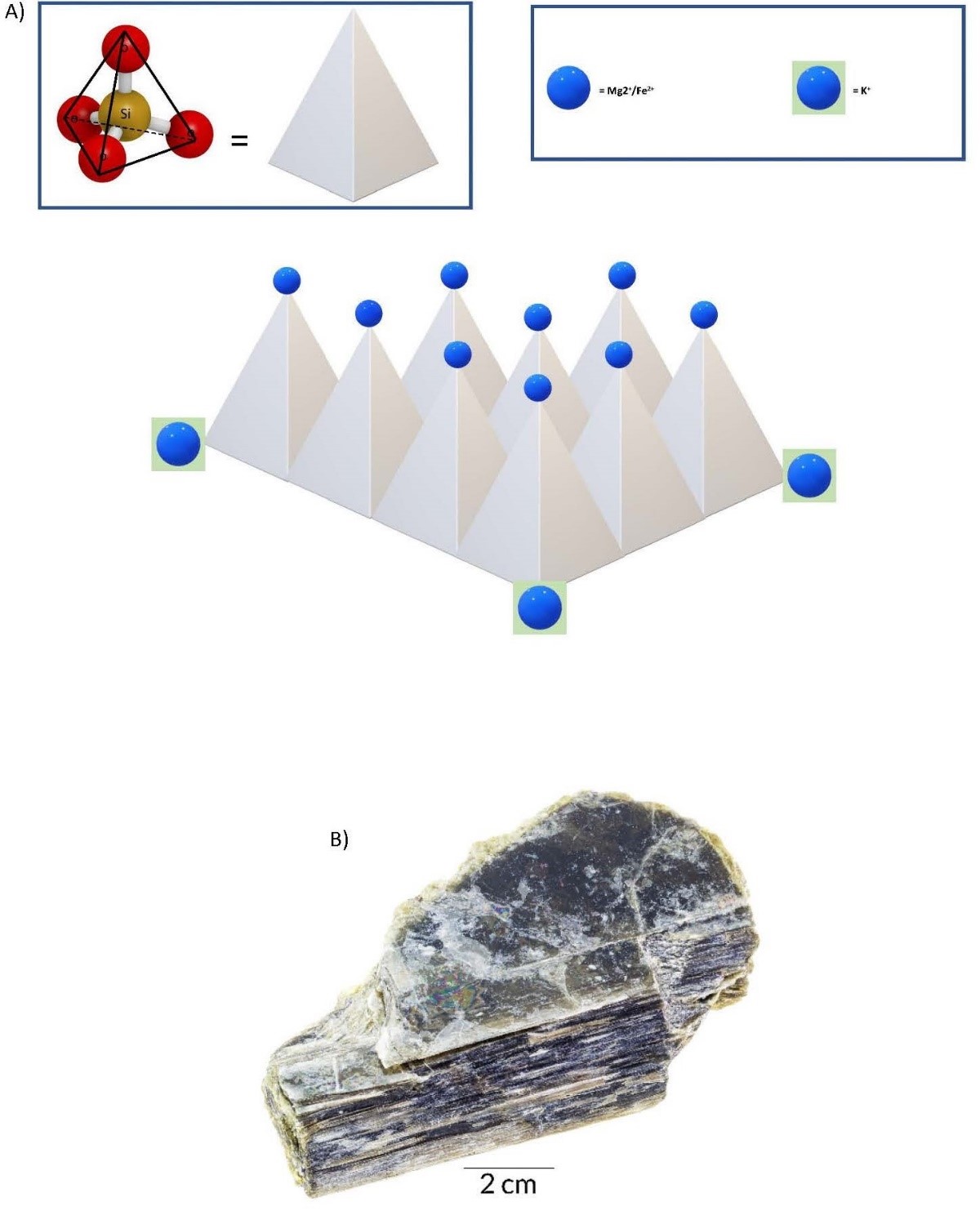
- Framework T etrahedra Framework tetrahedra form the feldspar group of minerals, which occur toward the end of the cooling phase, before complete solidification. In this lower-energy environment, most of the oxygens are bonded to a silicon in an adjacent tetrahedron with a few cation bonds. The cleavage planes would be arranged at 90° to each other along the cation bonds. The large, weakly charged cations of K, Ca, and Na now bond easily, yielding two types of chemical compositions. These are orthoclase feldspar , KAlSi 3 O 8 , and plagioclase feldspar , (Ca, Na) AlSi 3 O 8 . The cleavage planes are defined by the cation bonds and are arranged at 90° to each other. The last mineral to crystallize is pure silica, with no cation bonds, in which state it is called quartz . Because there are no cations, there are no natural planes of weakness, and thus quartz has no cleavage.
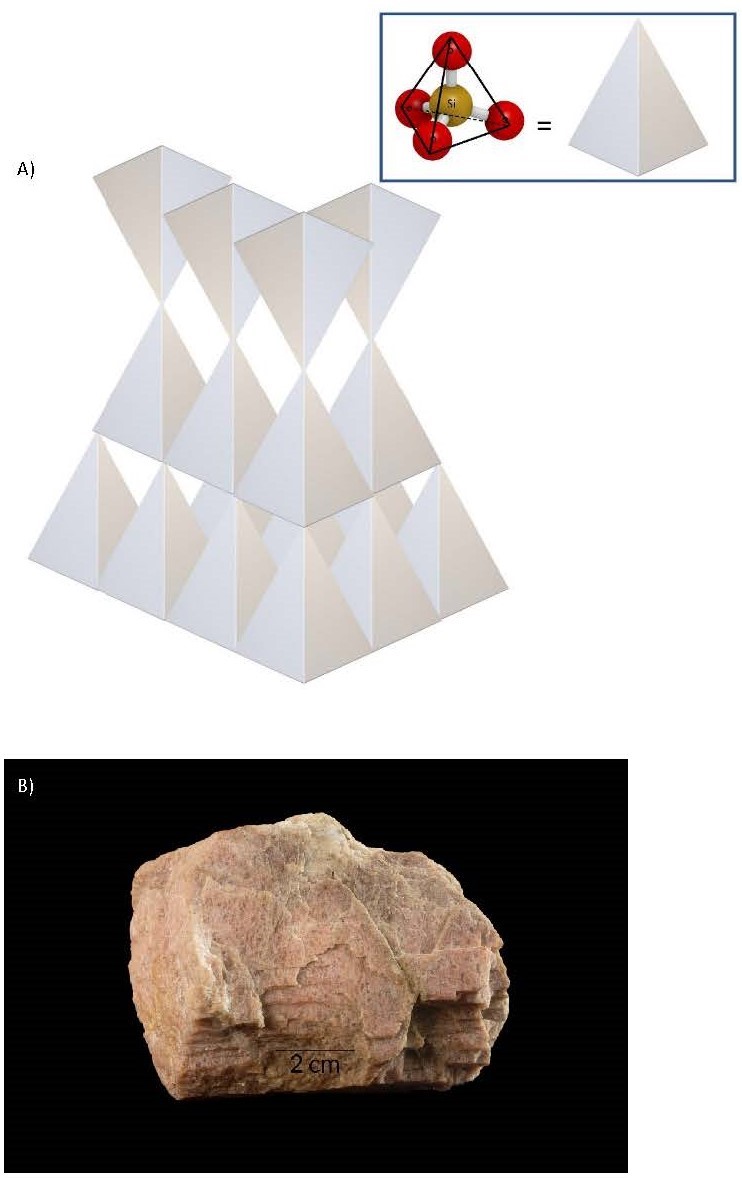
3.5 Mineral Identification Using Physical Properties
The chemical properties and crystal structure of a minerals are macroscopically expressed in its physical properties. These properties — colour, lustre, streak, hardness, cleavage, fracture, density, and crystal habit — can be used to differentiate between the main mineral groups, including the silicates. Let us first explore the individual mineral physical properties in order to understand how to use these to identify minerals.
The Physical Properties of Minerals
- Colour The colour of a mineral is strongly influenced by its cations, with metallic cations creating deeper, darker colours than non-metallic cations.
- Lust r e Lustre describes how the surface of a mineral reflects light. Not to be confused with colour, which is an internal expression of reflected light, lustre is a surface expression of how incident light bounces off the mineral. Lustre can be described as glassy/vitreous , or reflecting light like a glass (even if it has a dark colour!); dull , or without much reflection; metallic , or reflecting like a metal; greasy , or reflecting light like an oily surface would; or iridescent , or reflects light like the surface of a pearl. Ionically bonded minerals tend to be glassy, while covalently bonded minerals tend to have a more variable lustre.
- Streak Streak is the colour of the powdered form of a mineral, which is more representative. However, this state can be achieved only for minerals that are softer than the streak plate.
- Hardness Hardness is a measure of how resistant a mineral is to being scratched. The stronger the prevailing chemical bonds within the crystal structure, the harder the mineral. That means that ionically bonded minerals have a lower hardness than covalently bonded ones. Also, quartz will be harder than olivines because tetrahedron–tetrahedron bonds are stronger than tetrahedron–cation bonds. Minerals are identified with a hardness according to the relative scale of Moh’s Scale of Hardness .
Table 3.1. Moh’s Scale of Hardness
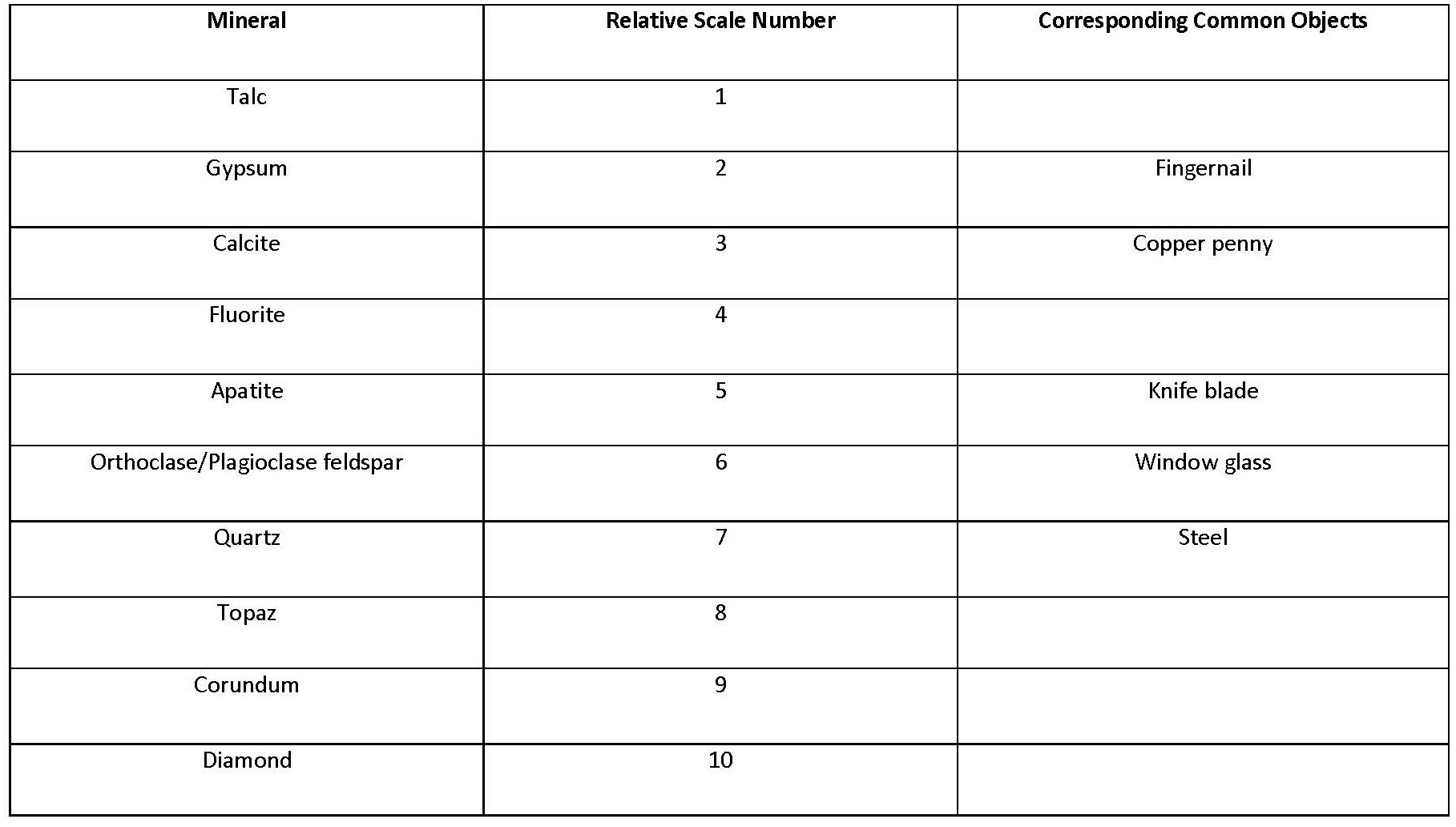
- Cleavage Cleavage is the tendency for a mineral to break along planar surfaces and is an expression of internal chemical bonds. Ionically bonded minerals tend to be weaker than covalently bonded minerals and therefore produce better cleavage planes, because weaker bonds are easier to break under an incident force. Furthermore, cation–tetrahedron bonds (olivines, pyroxenes, amphiboles, and micas) are weaker than tetrahedron–tetrahedron bonds (quartz) and tend to form better cleavage planes. The number of planes and the angles those planes make with each other depend on the crystal structure and chemical bonds and can be used to differentiate between minerals that have similar colour and hardness (Video 3.1).
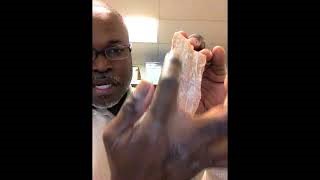
- Fracture The term fracture describes the tendency for a mineral to break along irregular surfaces and is also an expression of internal chemical bonds. Essentially, fracture is the opposite of cleavage because minerals that have good cleavage will not have many (or any) fracture surfaces. Another way to look at fracture is that tetrahedron–tetrahedron bonds (quartz) are stronger than cation–tetrahedron bonds (olivines, pyroxenes, amphiboles, and micas) and tend to form irregular fracture surfaces rather than cleavage planes (Video 3.2).
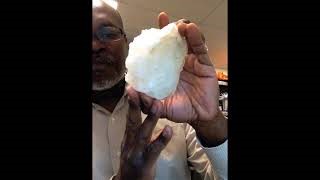
- Density A mineral’s density is a direct manifestation of the packing of the ions in the crystal structure as well as the atomic mass. Abundant smaller, heavier metallic cations will create a denser mineral than with larger, less massive ions. That is, minerals with higher metallic content, such as olivines, pyroxenes and amphiboles, are denser than minerals with low metallic content, such as quartz.
- Crystal Habit A mineral’s crystal habit is the geometric description of the overall shape of the completely developed mineral. A mineral’s crystal habit depends on the internal crystal structure that grows in a repeating three-dimensional array, along with the speed and prevailing direction of crystal growth (see Table 3.2).
Although the physical properties of hardness, cleavage, and colour are the most useful in differentiating between minerals, the use of all eight properties allows us to accurately identify minerals in the different silicate groups as well as minerals in the general mineral classes. Furthermore, a definitive identification for the mineral calcite is its effervescent reaction with dilute HCl (Video 3.3).
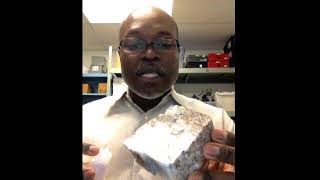
Table 3.2 Common minerals and their physical properties. A Density of 1–3 g/cm 3 is considered low; 3.1–4 g/cm 3 is considered medium density; above 4 g/cm 3 is considered high density.

A naturally occurring solid, inorganic, three-dimensional array of specific chemical composition with an ordered crystalline structure
A three-dimensional array of elements with a chaotic pattern
The smallest part of an element that retains its physical and chemical properties
The central, high-density portion of an atom consisting of protons and neutrons
Positively charged particles in an atom’s nucleus
Noncharged particles in an atom’s nucleus
Negatively charged particles that orbit an atom’s nucleus and that take part in atomic reactions
Atoms of an element with the same number of protons but a different number of neutrons
The interaction of two or more atoms of elements in a certain fixed ratio or proportion
A transfer of electrons from one atom to another
The sharing of electrons between atoms
An atom or molecule that has gained or lost an electron or electrons
Ions that have resulted from an atom giving up an electron or electrons and that are thus positively charged
Ions that have resulted from an atom gaining an electron or electrons and that are thus negatively charged
Minerals with a silicate anion
A silicate structure in which a silicate anion is separated from an adjacent anion by a cation
Silicates in which oxygens of adjacent tetrahedra bond to a shared cation
Silicates in which one oxygen in a tetrahedron bonds with the silicon of an adjacent tetrahedron
Silicates in which two oxygens in a tetrahedron bond with the silicon of an adjacent tetrahedron
Silicates in which three oxygens bond with the silicon of an adjacent tetrahedron
Silicates with few cation bonds
Solid silica with no cation bonds
A description of how the surface of a mineral reflects light
The colour of the powdered form of a mineral
A measure of how resistant a mineral is to being scratched
A range from 1 to 10 defining the relative degree to which a mineral resists being scratched
The tendency for a mineral to break along planar surfaces
The tendency for a mineral to break along irregular surfaces
The geometric description of the overall shape of a completely developed mineral
A Brief Introduction to Geology and Geomorphology Copyright © by Nahgeib Miller. All Rights Reserved.
Share This Book
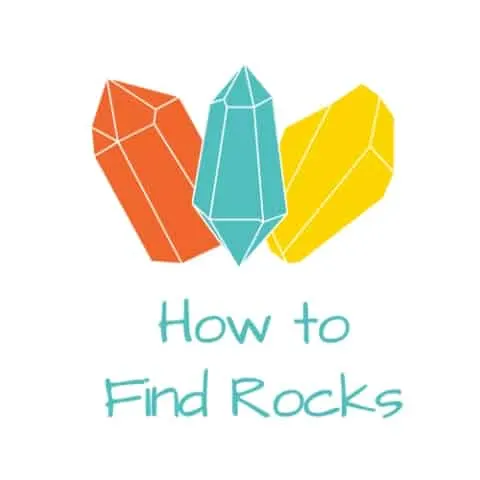
Easy Step-by-Step Mineral Identification (Expert Explains)
By Author Olena Rybnikova
Posted on Published: June 25, 2022 - Last updated: March 13, 2023
As an Amazon Associate, I earn from qualifying purchases with no additional costs for you.
Mineral identification is an integral part of work for both mineral collectors and scientists. Everybody wants to know what mineral they find during rock hunting, bought at a mineral show, or want to make research about. An approach to identifying minerals also varies for different types of stones and purposes.
Mineral identification is based on the testing of the physical properties and morphology of crystals. Luster, hardness, and color are helpful to identify minerals in the field. Geologists use electron microprobe, X-ray diffraction, and Raman spectroscopy to identify minerals on an advanced scientific level.
Some minerals can be easily identified at a first glance because of their unique properties, but others require state-of-the-art appliances. Today we are going to cover how to prepare your sample, how to choose the right method for diagnosis, what tools are needed for precise identification at home, and, as a bonus, how to identify the most common minerals. Prepare your samples – we are going to do some practical things today!
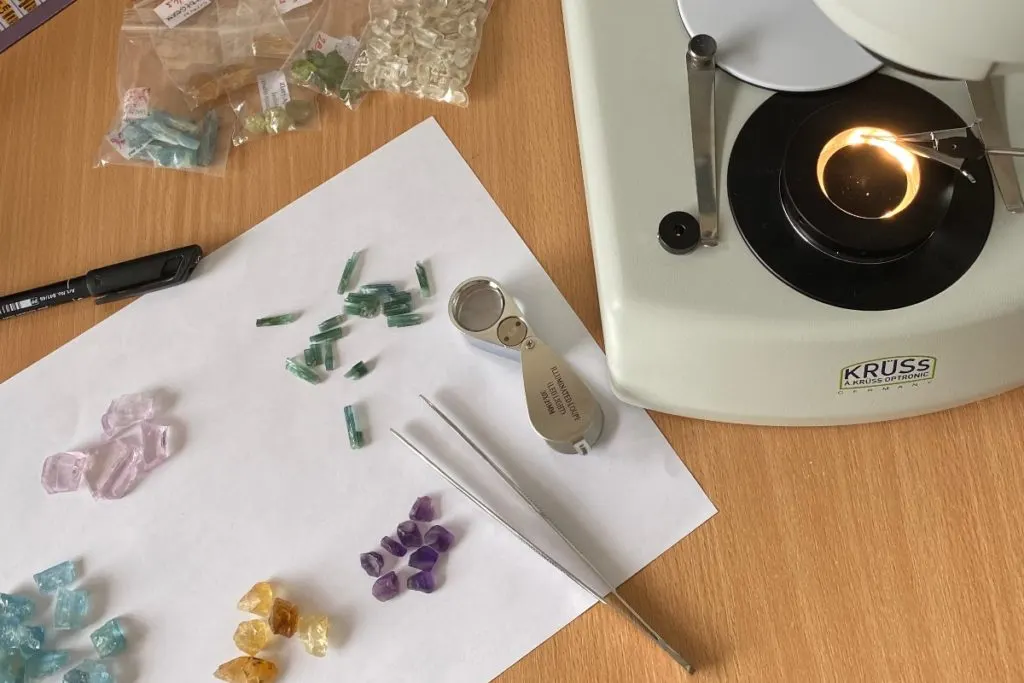
If you are interested in checking out the best books about rock and minerals identification you can find them by clicking here (Amazon link).
Create Your Mineral Identification Kit
A mineral identification kit is kind of a hand lab for geologists. If you work with minerals quite often, it is a good practice to have all the necessary instruments together. Mineral identification kits are available in online shops; however, it is quite easy to create your own kit.
A mineral identification kit is a set of instruments that allows testing of the various properties of minerals. The essential tools are copper wire, a pocket knife, a piece of glass, and a quartz crystal for hardness test; a streak plate, dilute HCl acid, UV light, a magnet, and a hand lens.
- Tools needed for hardness tests are non-mineral analogs of the Mohs scale members:
- Copper wire. Its hardness is around 3.5. This means that copper wire can scratch talc, gypsum, and calcite but cannot scratch fluorite.
Tip! Some sources can offer to use copper penny or copper coin. We offer to stick to the copper wire as a coin can be made of copper alloy with other metals, which distort the hardness, and also, different coins are used worldwide. So using copper wire is the best international option.
- Pocket knife. It is not necessary to have an expensive knife because, in this case, steel will be harder and can distort your measurements. A common inexpensive knife is the best option. The hardness of this tool is equivalent to 5.5 – 6.5 on the Mohs scale.
- A piece of glass. Glass hardness is around 5.5, so it lets us differentiate between apatite and orthoclase.
- A small quartz crystal. It is a very effective tool, as quartz is a common mineral, and it is easy to find an inexpensive well-formed crystal. Quartz hardness is 7, so it is helpful to understand whether a mineral sample is harder or softer than quartz on the Mohs scale.
- A streak plate . This tool is necessary for streak color identification. For example, it allows you to differentiate between gold and pyrite (fool’s gold). A streak plate can be any unglazed porcelain.
Tip! It is better to have a white and black streak plate for light and dark-colored minerals.
- Dilute HCl acid . This is the best tool to identify calcite, as a reaction between acid and mineral produces bubbles. It allows you to differentiate between calcite and gypsum or calcite and halite quickly.
- A magnet . Iron-bearing minerals can be identified and differentiated with the help of a magnet. Magnetite (iron oxide with the highest iron concentration) can attract metal pieces, pyrrhotite can be attracted by a magnet, and hematite does not react to a magnet.
- UV light . It’s an essential tool if you are looking for fluorite, calcite, and scheelite (tungsten ore).
- A hand lens . A hand lens allows you to check the form (habit) of tiny crystals and identify them (for example uvarovite garnet) or to check if faceted gemstones have any inclusions or fractures.
BTW: Do you want to know more about rock and mineral identification? The books listed below are the best ones you can find on the internet (Amazon links):
- Smithsonian Handbooks: Rocks & Minerals
- Gemstone & Crystal Properties (Quick Study Home)
- Ultimate Explorer Field Guide: Rocks and Minerals (National Geographic Kids)
Main Mineral Properties that Will Help You to Identify a Mineral
The mineral identification process is based on methodically checking the physical properties of minerals . The most significant advantage of these methods is that they can be easily held in the field, on mineral shows, or at home.
Mineral properties that are used for mineral identification are luster, hardness, color, transparency, streak, fracture, cleavage, specific gravity, magnetism, luminescence, reaction with hydrochloric acid, and morphology of crystals. Also, optical phenomena like birefringence and pleochroism can be tested.
So let’s check out the physical properties of minerals:
Color and Any Other Visible Optical Effect
Color is the first obvious property that we notice. Minerals can occur in any color, hue, saturation, and tone. No additional tools are needed to identify a color. The sample should be clean and not weathered.
TIP: Rock color is the first property we pay attention to. Do you know why rocks have different colors? Find out the complete explanation in the article below: Rock Colors: What Determines Color & Why Different Colors
Luster
For luster identification, no additional tools are needed too. Minerals have metallic and non-metallic (adamantine, glassy, matte, oily, waxy, mother-of-pearl, iridescent, and silky) luster.
Transparency
Transparency is the degree to which light is transmitted through a mineral. To differentiate between opaque, translucent, and transparent minerals, you may need a flashlight and a piece of printed text.
The streak is the color of the mineral in a powdered state. Sometimes the color of the mineral sample and streak are different. This property can help you to identify some minerals very precisely. Use a streak plate or piece of unglazed porcelain for this test.
Cleavage and fracture
Cleavage is the ability of crystalline minerals to split along planes of weakness in the mineral’s structure, while the fracture is a characteristic of the surface that is left after the splitting of a mineral.
No additional tool is needed to observe these properties if you don’t want to destroy your sample. However, if you want to initiate a fresh cleavage of fracture, you may need a hammer and chisel or a steel nail.
Hardness is a resistance of minerals to scratching. A relative hardness scale used in mineralogy is the Mohs hardness scale. To determine the hardness, you can use your fingernail copper wire, a piece of glass, a pocket knife, and a quartz crystal, as we have discussed in Create your mineral identification kit passage.
TIP: The advantage of the hardness test is that it can be easily done at home or even in the field while rockhounding. Check out the complete guide in the article below: DIY Guide: Testing Mineral’s Hardness (Explained by Expert)
Specific Gravity
Specific gravity is the ratio between the mass (weight) of a mineral and the mass (weight) of an equal volume of water. In the field, it is determined only by weighing on the hand.
Magnetism, Luminescence, Reaction with Hydrochloric Acid
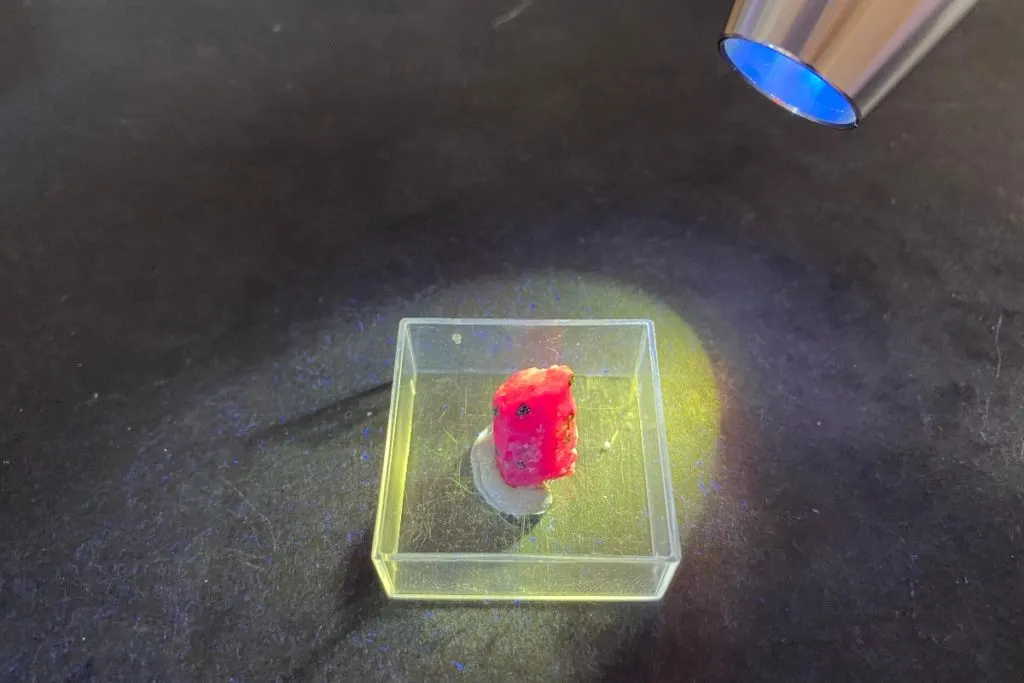
These properties are kind of exotic, and very few minerals have them, but they are crucial for minerals like magnetite, fluorite, and calcite, respectively.
Professional Mineral Identification
Today geologists use far more advanced methods to identify minerals. They mostly rely on chemical composition and crystal structure (atomic arrangement) rather than on physical properties.
Some methods are quick and non-destructive; others require powdering a part of a sample. Today it is possible to make precise identification of a few nanometers of mineral, which is invisible to the naked eye.
Professional geologists and scientists use refractometers, polarization microscopes, electron microprobe analyzers, X-ray fluorescence, X-ray diffraction, Raman, and Infrared spectrometers to identify minerals on an advanced level. Sometimes minerals should undergo a complicated sample preparation stage.
Sometimes it is quite easy to identify a mineral just by looking at it. For example, violet crystals of amethyst are quite obvious. It is harder when minerals are cut and flawless. They lack any crystal faces, natural fracture surfaces, and inclusions.
Also, it is unacceptable to destroy even a small part of a stone. That is why non-destructive spectroscopic methods are so important for gemologists.
Nowadays, any new mineral has been found under huge magnification (with the help of an electron microscope). It is a great exception when a new mineral is found in macro sizes. But miracles happen! The new material comes mostly from remote areas in Africa.
TIP: Every professional geologist do it. Licking rocks and minerals in geology is a common practice to define some kind of minerals and, of course, a bunch of fun. Find out more in the article below: Licking Rocks in Geology: Why & How (Answered by Geologist)
How to Prepare a Mineral for Identification
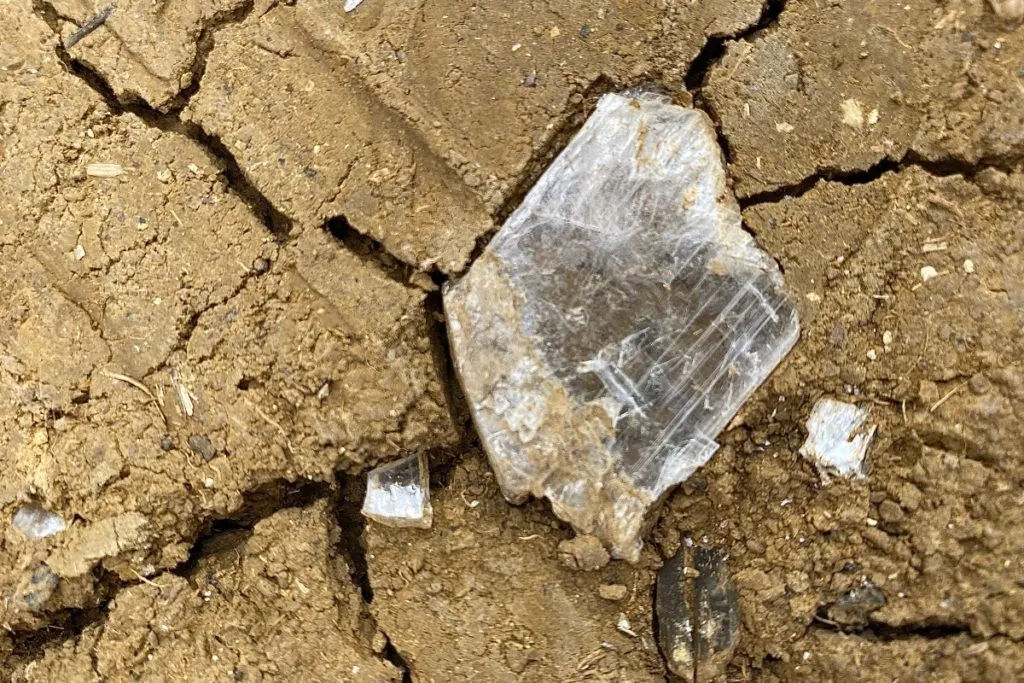
One of the most underestimated steps in mineral identification is sample preparation. That is why we decided to start with this stage, as many mistakes occur here.
We are going to discuss why it is important and how sample preparation differs for fresh minerals from the field, tumbled stones bought from mineral shows, faceted stones, and minerals that are going to be studied by scientists.
Before the identification of minerals, the sample should be checked and prepared. The studied sample should be carefully cleaned with water and dried. No weathered or altered surfaces should undergo testing of hardness and streak. Check if your sample is an individual crystal, mineral aggregate, or rock.
The most important rule for sample preparation is to clean the stone no matter if it is just a freshly dug sample, tumbled opaque stone, or faceted sparkling gemstone.
You need to see a true color (not covered by clay from earth or dust from a shelf) and luster. For example, dust can lower the stone’s luster from glassy to resinous or waxy.
It may seem obvious that freshly dug stone should be washed, but it is important to clean even faceted stone because the grease from hands left on the stone facets can distort refraction index measurements.
Mineral hunters need to pay attention to the freshness of the sample. Weathered surfaces have other physical properties: color is lighter, luster is more dull or absent, hardness is lower, and the fraction is earthy. The weathered surface should be removed. Physical properties should be tested on fresh surfaces only.
After cleaning the sample, observe it attentively. Try to respond to the next questions:
- Does it look like crystals with well-defined faces? For example, a prismatic quartz crystal or octahedral magnetite.
- Is it composed of one kind of mineral? Is it mineral aggregate? Like chalcedony, turquoise or malachite.
- Is it composed of different minerals? If yes, it is a rock. Lapis lazuli is a rock composed of 3 different types of minerals (lazurite, pyrite, calcite). If you have a rock sample, pay attention to what mineral you are going to test for physical properties.
For rock hunters, it is very useful to pay attention to country rocks where the mineral is found. The type of country rock (sedimentary, metamorphic, or igneous) can narrow down possible candidates for identification.
For example, if you have found white transparent crystals between fractures of clay, it will hardly be colorless topaz as it tends to pegmatite bodies. Most probably, it is gypsum or calcite. Check hardness and reaction to hydrochloric acid, and your identification is completed!
TIP: The best way to identify the mineral is to consult with a specialist or with a rocks and minerals book. Check out the best book for Identifying Rocks & Minerals in the article below: 5 Best Books for Identifying Rocks & Minerals You Must Read
Step-by-Step Identification of Mineral
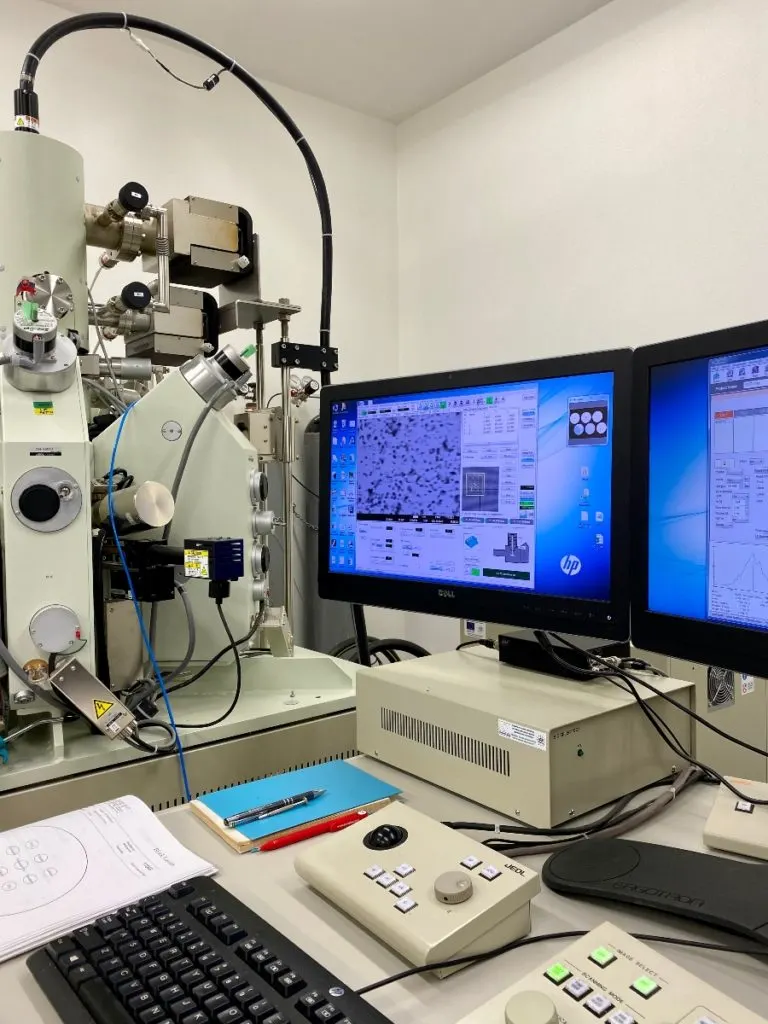
Let’s move to the identification. Follow the next 14 steps to identify your mineral sample. It’s going to be an interesting journey.
The most prominent way to identify minerals correctly is gradual and methodical testing of all physical properties. Check the essential ones first: hardness, luster, transparency, color, streak, cleavage, and fracture. Further, proceed with magnetism, luminescence, and morphology of crystals.
Step 1: Protection
During the mineral identification process, don’t forget to use gloves to protect your hand and something resistible, like a rubber mat or wooden board, to protect a surface where a test takes place. (tabletop or a floor).
Step 2: Choosing a Strategy
There are two approaches to mineral identification to narrow down possible candidates.
- The first one is based on luster (metallic or non-metallic). In most cases, it will place the unknown sample into the sulfide or iron oxides group (metallic luster) or the silicate or halide group (non-metallic) luster.
- The second approach is based on hardness. An unknown mineral is tested by a piece of glass (5 on the Mohs scale) and then placed into a less than 5 hardness group or more than 5 hardness group.
These are just two possible options for the first step, and it doesn’t mean that in the case of luster identification, the hardness test should be omitted or vice versa. It’s just solving different tasks.
Step 3: Luster
No additional tools are needed to observe a luster. Metallic luster means a studied mineral belongs to the sulfide or iron oxides group. In the case of non-metallic luster, specify the type: adamantine, glassy, matte, oily, waxy, mother-of-pearl, iridescent, or silky.
Step 4: Hardness Identification (Scratch Test)
Hardness identification is one of the most important tests. It is based on a comparison if your mineral sample is harder or softer than a tool used for scratching (with an equivalent hardness to the Mohs scale standards).
To test the hardness of minerals, try to scratch a piece of glass. It allows you to understand whether your sample is harder or softer than 5 and gives you a direction on how to choose the next tool.
Should it be copper wire or quartz crystal? This strategy lets you save some time and not move from the softest to the hardest tool or vice versa.
TIP: A scratch test is an essential method of mineral identification. It is widely used because of its simplicity. Check out the complete guide in the article below: Performing Scratch Test on Rocks (Follow These 8 Steps)
Step 5: Color Identification
To identify the color of the mineral, start with the most obvious color and then add a shade. For example, blue -> greenish-blue for aquamarine.
In the cases with bicolor or polychrome minerals like tourmaline or ametrine indicate two colors.
Step 6: Transparency
Transparency is also a quite obvious characteristic. In order to differentiate between opaque and translucent minerals, you can try to watch the sun through the stone or use a flashlight. Minerals with metallic luster (Step 3) are opaque in most cases.
Please, keep in mind that some minerals can occur as transparent and opaque, depending on the mineral formation process and environment.
Step 7: Streak Test
After completing the previous 5 steps, you already know what to expect from the streak test and whether it can be helpful in your situation or not.
- The streak test is a turning point in case the luster determined in Step 3 is metallic. The streak test allows differentiating between gold and fool’s gold (pyrite). Because of the discrepancy in the color of the mineral sample and the color of the streak, minerals like pyrite, sphalerite, galena, hematite, magnetite, and chromite can be identified and distinguished between each other.
- The streak test is important for minerals that can occur in different colors. For example, calcite and fluorite can be found in any color; however, the streak color will always be white.
- Even when we see that the streak test is inevitable and deciding for some minerals, it can be omitted for hard minerals (more than 6 on the Mohs scale), as the hardness of the streak plate is 5 -5.5. If you try to scratch a streak plate with a harder mineral, you can observe some line, but look closer, it is probably a scratch on the streak plate, and you observe a powder from the plate.
TIP: Minerals’ streak is one of the most important tests for mineral identification, especially while working with native metals and sulfide minerals. Check out the complete guide in the article below: DIY Guide: Testing Mineral’s Streak (Explained by Expert)
Step 8: Cleavage and Fracture
Observe your sample carefully. If it is a perfect undeformed crystal, there is no visible cleavage and fracture. At this stage, you have to decide if it is acceptable to destroy part of the sample or not.
If you can see distinct cleavage directions or fracture surfaces, try to classify them. Perfect, good, fair, poor for cleavage and granular, earthy, conchoidal, splintery, stepped, and uneven for fracture.
Cleavage is an important property for the identification of micas (biotite, muscovite, fuchsite). Conchoidal fracture, for instance, is typical for quartz.
Step 9: Specific Gravity
Specific gravity (SG) depends on the chemical composition and structure of the mineral. In the field, it is determined only by weighing on the hand. If a mineral feels light (like gypsum), its SG is around 2.5 g/cm 3 . Heavy minerals like galena have SG of more than 5 g/cm 3
The medium SG is more common (from 2.5 to 5 g/cm 3 and is typical for silicate minerals like quartz and feldspars.
Step 10: Magnetism, Luminescence, Reaction with Acid
At this stage, you can test and observe some non-typical properties like magnetism, luminescence, reaction with hydrochloric acid, and optical phenomena.
Checking of magnetism is needed if, in previous steps, the color of a mineral is indicated as black, luster is metallic and specific gravity is determined as heavy. Magnetism can help to differentiate between magnetite (which attracts a magnet) and hematite (which doesn’t interact with a magnet).
Luminescence can be tested with the help of UV light. Test your mineral in a dark room to obtain the best results. There is a list of minerals that glow under UV. The best examples are fluorite, calcite, corundum, scheelite, sodalite, and spodumene.
Reaction with hydrochloric acid can help you to identify calcite easily. After placing a few drops of dilute hydrochloric acid (HCl) on a surface of calcite, you will observe bubbles.
TIP: Minerals and rocks can completely change their colors by being subjected to UV light or black light. Check out the most common rocks and minerals that glow under UV light: 12 Rocks & Minerals That Glow Under UV Light & Black Light
Step 11: Optical Phenomena
Observe a sample carefully if it has a cat’s eye effect (typical for quartz, chrysoberyl, apatite), asterism (common for rubies and sapphires), does it have pleochroism (tourmalines, tanzanite, iolite), color change effect (alexandrite), or birefringence (calcite, zircon).
These optical phenomena can help you to identify a mineral sample.
Check 23 Main Properties of Rocks & Minerals Explained by Experts to see more minerals that have optical phenomena.
Step 12: Crystal Shape (Habit)
If your mineral sample has some even crystal faces or is a well-formed crystal, observe it attentively. Crystal habit can tell you the mineral species:
- Cubic form is typical for fluorite and pyrite
- Octahedron – for spinel, diamond, magnetite, and fluorite.
- Dodecahedron – for garnets
- Rhombohedral (tilted cube) and scalenohedron are typical for calcite
- Hexagonal – typical for beryls (emerald, aquamarine, heliodorus)
Step 13: Mineral associations
Sometimes mineral associations can be a game-changer. For example, lazurite and azurite both are blue minerals that usually occur as aggregates. Lazurite usually occurs together with golden specks of pyrite and white crystals of calcite, while azurite is commonly associated with malachite.
Step 14: Results
All these steps will help you to identify minerals or at least narrow down possible candidates and to decide on a group of minerals (oxides, sulfides, halides, silicates).
You can use the next resources to compare your obtained results and observations with tables of possible mineral candidates:
- Mineral Identification Table
- Mineral Identification Help Sheet
TIP: Do you know the difference between precious and semi-precious stones? Precious and semi-precious stone terms are surrounded by feelings of beauty, brilliance, and wealth. Find out more in the article below: Precious & Semi-Precious Gemstones: Explanation & Difference
Bonus! The Most Common Minerals Identification Tips
Here is a table with a list of very common minerals and their typical characteristics, which help to identify a sample quickly.
For example, pyrite’s unique properties that differ from any other minerals are metallic luster, greenish-black streak, and cubic shape of crystals.
Making an identification of minerals with discussed step-by-step instructions is easy and amusing. The approach is based on:
- testing the physical properties,
- the morphology of crystals,
- and minerals association.
Hardness, luster, cleavage, color, transparency, streak, fracture, and specific gravity are the main physical properties for mineral identification in the field or at home.
Optical phenomena, magnetism, luminescence, and reaction with acid can also come in handy with some kinds of crystals.
Note: This article is written by gemologist expert Olena ( LinkedIn profile ). She is a Ph.D. candidate (Mineralogy) holding an Applied Jewelry Professional diploma from GIA ( Gemological Institute of America ).
TIP: So you already know how to identify minerals. What about starting to find minerals on your own? Check out the ultimate guide on starting rockhounding in the article below: How to Start Rockhounding: The Ultimate Beginner’s Guide
Historical Geology
A free online textbook for Historical Geology courses
Earth Materials – Mineral Identification
Mineral properties and identification.
Geologists identify minerals by their physical properties. In the field, where geologists may have limited access to advanced technology and powerful machines, they can still identify minerals by testing several physical properties: luster, color, streak, hardness, crystal habit, cleavage and fracture, and some special properties. Our Big Ten Minerals make up the majority of Earth’s lithosphere and are usually seen as small grains in the rock. Geologists rely on a magnifying hand lens as an essential tool for identifying most minerals in the field. Of the many properties that minerals can exhibit, we will consider only those most useful for identification as small grains surrounded by other minerals in rock.
Luster and Color

There are some minerals that predominantly show a single color. However this is not true for our “Big Ten Minerals” or the other most common rock forming minerals. All of these minerals have a predictable range of colors due to element substitution within their crystalline structures. Feldspars, the most abundant minerals in the Earth’s crust, range in composition from calcium-rich to sodium-rich to potassium-rich varieties and many compositions in between. Feldspar colors including pink, white, green, gray and others. With notable exceptions, color is usually not a reliable diagnostic property of minerals. For identifying many minerals, a more reliable indicator is streak, which is the color of the powdered mineral.
Streak examines the color of a powdered mineral, and can be seen when a mineral sample is scratched or scraped on an unglazed porcelain streak plate. A paper page in a field notebook may also be used for the streak of some minerals. Minerals that are harder than the streak plate (or the paper) will not show streak, but will scratch the porcelain (and tear the paper!). For these minerals, a streak test can be obtained by powdering the mineral with a hammer and smearing the powder across a streak plate or notebook paper. Note the absence of a mineral streak via this method is also a useful observation to record.

While mineral surface colors and appearances may vary, their streak colors can be diagnostically useful. An example of this property is seen in the iron-oxide mineral hematite. Hematite occurs in a variety of forms, colors and lusters, from shiny metallic silver to earthy red-brown, and different physical appearances. A hematite streak is consistently reddish brown, no matter what the original specimen looks like. Iron sulfide or pyrite, is a brassy metallic yellow. Commonly called fool’s gold, pyrite has a characteristic black to greenish-black streak.

Hardness measures the resistance of a mineral to abrasion. Mohs Hardness Scale assigns a number between 1 and 10 indicating the relative scratch-resistance of minerals when compared to a standardized set of minerals of increasing hardness. Mohs scale was developed by German geologist Fredrick Mohs in the early 20th century, although the idea of identifying minerals by hardness goes back thousands of years. Mohs hardness values are determined by the strength of a mineral’s atomic bonds. The figure shows the minerals associated with specific hardness values, together with some common items readily available for use in field testing and mineral identification. A steel pocket knife blade, which has a hardness value of 5.5, distinguishes between hard and soft minerals on many mineral identification keys.
Cleavage and Fracture

Special Properties
Special properties are unique and identifiable characteristics used to identify minerals or that allow some minerals to be used for special purposes. A simple identifying special property is taste, such as the salty flavor of halite or common table salt (NaCl).
A simple test for identifying calcite and dolomite is to drop a bit of dilute hydrochloric acid (10-15% HCl) on the specimen. If the acid drop effervesces or fizzes on the surface of the rock, the specimen is calcite. If not, and dolomite is suspected, the specimen can be scratched to make a small amount of powder. If the acid drop fizzes slowly on the powdered mineral, the specimen is dolomite. The video below compares these two minerals and their effervescence reaction. Geologists often carry a small dropper bottle of dilute HCl in their field kit.
Some iron-oxide minerals are magnetic and are attracted to magnets. Common magnetic minerals include magnetite (Fe 3 O 4 ) and ilmenite (FeTiO 3 ). Magnetite is strongly attracted to magnets. Ilmenite and some types of hematite are weakly magnetic. Geologists often carry a free-swinging magnet in their field kit to test for magnetism.
Review the following video to learn more about the basic properties of the rock forming minerals.
Chapter Contents
- 1.1 Luster and Color
- 1.3 Hardness
- 1.4 Cleavage and Fracture
- 1.5 Special Properties
Your Article Library
Essay on minerals | geology.
ADVERTISEMENTS:
Read this essay to learn about Minerals. After reading this essay you will learn about:- 1. Introduction to Minerals 2. Formation of Minerals 3. Elements of Most Rock Forming Minerals 4. Origin 5. Identification 6. Utility 7. Distribution of Elements among Rock-Forming Minerals 8. Properties.
- Essay on the Properties of Minerals
Essay # 1. Introduction to Minerals:
A mineral is a naturally occurring inorganic crystalline solid having a definite chemical composition.
For a substance to be classified as a mineral, it should meet the following five requirements:
(i) It should be a naturally formed substance
(ii) It should be an inorganic substance
(iii) It should be a solid
(iv) It should have a specific chemical composition
(v) It should have a characteristic crystal structure.
A mineral should be a naturally formed substance. This means the material should be one, formed as a result of natural processes in or on the earth. It is not a material manufactured in a factory or synthesized in a laboratory. Steel, glass, plastic etc. are not minerals.
A mineral should be a solid. Liquids and gases and naturally occurring liquids like oil and natural gas are not minerals, since they are not solids. Ice present in a glacier is a mineral, but water in a stream is not a mineral though ice and water are made of the same compound.
Minerals are inorganic substances. Hence, twigs, leaves which originate from living organisms and contain organic compounds are not minerals. Coal is not also a mineral since it is derived from the remains of plant material.
Minerals should possess a specific chemical composition. They may exist either as simple chemical elements like gold, silver etc. or as compounds having specific chemical composition and formulae. Quartz having the formula SiO 2 is a mineral. Some minerals have complicated formulae. Ex: Phlogopite, KMg 3 AlSi 3 O 10 (OH) 2 .
A mineral should have a characteristic crystal structure. The atoms in minerals are organized in a regular repeated geometric pattern. A geometric pattern of the atoms in a mineral is called a crystal structure.
All specimens of a mineral have the unique characteristic of having identical crystal structure. With powerful ultra-high-resolution microscopes we can view the crystal structures of minerals and the orderly arrangement of atoms in the mineral.
Essay # 2. Formation of Minerals :
Minerals are formed under specific conditions, mainly the right temperature and pressure when certain elements are present. In most of the cases mineral formation depends on the rock family-igneous, sedimentary and metamorphic.
Igneous minerals generally crystallize from magma (molten rock) at temperatures between 600°C to 1200°C and at depth of about 30 kilometres. Ex: Quartz, biotite mica. Sedimentary minerals form through the evaporation of water.
Ex: halite or table salt, or by precipitation from water brought about a change in chemical condition. Ex: Chert, carbonates or through the deposition of hard parts like bones or shells of organisms, Ex : aragonite. Metamorphic minerals form within rocks, due to recrystallization in response to changes in heat and pressure.
Essay # 3 . Elements of Most Rock Forming Minerals :
Eight chemical elements make up most of the rock-forming minerals. The most abundant minerals are Oxygen and Silicon. These two elements bond together to become the basic building blocks of the most common mineral group called silicates.
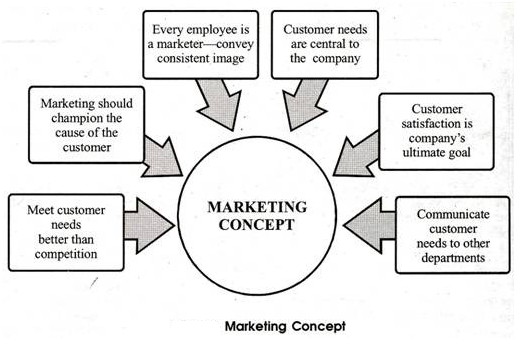
A brief description of these elements of most rock forming minerals is given below.
Oxygen is the active and aggressive constituent of the atmosphere. Combined with other elements, it is of great geological importance. The simpler forms of oxygen compounds are known as oxides and of these the oxide of hydrogen, water is by far the most common. Besides being so essential to human life, Oxygen is a very potent factor in the manifold changes which are constantly taking place in the regions of the earth’s crust.
ii. Silicon:
Silicon is next to oxygen among the most abundant of the constituents of the earth, though it exists only in combination either as an oxide (Silica) or with other elements to form silicates. In these two forms it is the predominating constituent in all but the calcareous rocks. In the form of Silica (SiO 2 ) or quartz, it forms one of the most indestructible of natural compounds. It is also found as the prevailing constituent in nearly all sands and soils.
iii. Aluminium:
Aluminium is the next element in the order of importance. It occurs mainly combined with silicon and oxygen forming an important series of minerals known as aluminous silicates. It is well known in corundum and bauxite as a sesquioxide.
Though iron is less abundant than oxygen and silicon, it ranks as an important constituent due to the variety of compounds of which it forms a part and also to the colours characteristic of its oxides and of the iron bearing silicates.
The most conspicuous forms of iron on the immediate surface of the earth are the oxides while carbonates, sulphides and silicates exist at greater depths. Though iron is so common combined with other elements it occurs but rarely free due to its affinity for oxygen.
v. Calcium:
Calcium is another important element of the earth’s crust. Its most conspicuous form of occurrence is in combination with carbon dioxide forming the mineral calcite (CaCO 3 ) or the rock limestone. In this form it is slightly soluble in water containing carbonic acid and this is the reason it has become the usual ingredient of natural waters. It is also an important constituent of many silicates.
vi. Sodium:
The most common and widespread form of the element sodium is the compound with chlorine namely sodium chloride (NaCl) or common salt. In this form it is the most abundant of the salts present in sea water. It also constitutes rock masses interstratified with other rocks of the earth’s crust. Combined with silica, lime and alumina, sodium is an important constituent of the soda-lime feldspars and many other silicate minerals.
vii. Potassium:
Combined with silica, potassium is an important element in many mineral silicates, as orthoclase, leucite and nepheline. In smaller amount it is present in silicates of mica, amphibole and pyroxene groups. As a chloride potassium is invariably present in sea water and as a nitrate it forms the mineral nitre or saltpetre.
viii. Magnesium:
Magnesium is found in combination with carbonic acid as carbonate forming an essential part of the mineral magnetite and the rock dolomite. The bitter taste of sea water and some mineral waters is due to the presence of salts of magnesia. In combination with silica it forms an essential part of such rocks like serpentine, soapstone and talc.
ix. Manganese:
Next to iron manganese is the abundant heavy metal. It occurs as an oxide, carbonate or in combination with two or more other elements as a silicate.
Barium is mainly combined with sulphuric acid to form the mineral barite. Sometimes it occurs as a carbonate and more rarely as a silicate.
xi. Phosphorus:
Though phosphorus exists in comparatively insignificant proportions, it is still an important element. In nature it occurs in combination with various bases mainly lime to form phosphates.
In this form it is found in bones of animals, the seeds of plants and constitutes the essential portions of the minerals apatite and phosphorite. Though small in proportions phosphorus is a very important constituent of fertile soils. Its chief source, in the older, crystalline rocks is the mineral apatite.
Carbon of the solid elements occurring free or uncombined carbon is the more abundant, being found in the form as diamond and graphite or when quite impure, as coal. In combination as a dioxide (CO 2 ) it forms carbonic acid gas which like oxygen is a powerful agent to bring about important changes in the rocks with which it comes into contact.
The physical properties of minerals depend on the chemical composition and in turn have a great influence on the characteristics of rocks formed by the minerals. For instance, the dark minerals rich in iron and magnesium generally have a higher melting point than the light coloured minerals.
Dark coloured minerals tend to form hot fluid magmas when in the molten stage. Consequently, the dark igneous rocks like basalt, in magma state are very hot and can flow far and fast whereas, the light coloured rocks like rhyolite (much silica and very little coloured minerals) are relatively at lower temperatures and viscous in the molten state flow little and with great difficulty.
The above properties have great influence on the behaviour of volcanoes. Volcanoes that erupt silica-poor lava form cones with gentle slopes. In contrast volcanoes that erupt silica-rich lava tend to have their throats plugged by viscous lava. Consequently the gas pressures inside the volcano rise until the plug is blown out in a catastrophic explosion.
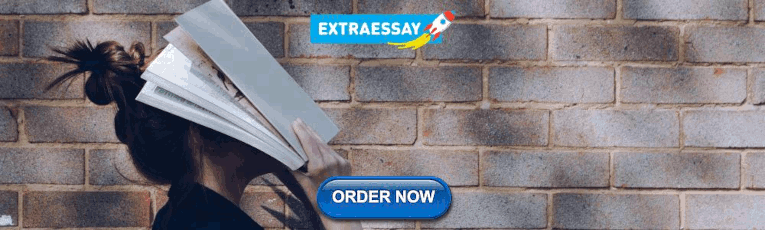
Essay # 4 . Origin of Minerals :
There are many ways in which minerals are formed. The same kind of mineral can be formed under different conditions. Most of the minerals take thousands of years to develop, others may need just a few years and in some special cases even a few hours.
The formation of minerals takes place either in the molten rock (magma) at or close to the earth’s surface or deep in the earth’s crust, as a consequence of transformations, i.e. metamorphic processes. Such origins are referred to as igneous, sedimentary and metamorphic origins.
i. Igneous Origin:
A large number of minerals are formed from the magma. For example, feldspar, mica and quartz form as the magma cools down at great depth in the earth’s crust at temperatures from 1100°C to 550°C. Other minerals form from exhalations during which gases escape from the magma.
As these gases cool down, a reaction takes place with the adjacent rock resulting in the formation of chloride, fluoride and sulphate minerals (as well as gold and silver). During the further cooling stage of magma to below 400°C substances separate out and these substances together with the infiltration of materials from the surrounding rock results in the formation of minerals.
ii. Sedimentary Origin:
At or close to the earth’s surface, minerals develop through the weathering of rock and the subsequent formation of new rock. The chief agents are water, carbon dioxide and the oxygen present in the air.
During this process, substances are dissolved in the upper layers, which seep downwards and interact with the groundwater to produce new minerals in certain specific areas which become rich in these minerals and are known as enrichment zones.
Silver and copper deposits are formed in this way. In areas of little rainfall and high temperatures, saline minerals are formed in salt lakes, salt swamps or cut off marine embayment’s. This takes place in a process of chemical precipitation as a consequence of the high rate of evaporation.
Numerous organisms directly or indirectly contribute to the formation of minerals, by supplying oxygen or removing carbonic acid through processes of bacterial decay or growth of calcareous shells or siliceous skeletons from substances present in solution.
iii. Metamorphic Origin:
As rocks are moved down into deeper parts of the earth’s crust, due to the increase in thickness of overlying sediments or by mountain building processes, new minerals form through the reconstruction of minerals which exist already.
This happens due to the high temperature prevailing in these areas along with the high pressures exerted. A similar metamorphic effect occurs though on a lesser scale when the molten magma forces its way up in Volcanic vents or along fissures and here they contact with the adjacent rock.
Essay # 5 . Identification of Minerals :
The properties of minerals depend on their composition and crystal structure. They are generally identified by their physical properties by some simple tests. Properties generally used to identify minerals are colour, luster, habit, cleavage, fracture, streak, hardness etc. For rarer minerals, laboratory tests are needed to identify them. With the use of blow pipe and some simple reagents tests may be performed and mineral powders or fine grained mixture can be investigated.
All types of silicates may be identified in the field, but actual determinations can be made using a polarizing microscope. In this method, the mineral specimen is ground into very thin slices and polarized light is passed through them. The effect on light of one transparent mineral differs from that of another transparent mineral. Minerals which are opaque like metal sulphides can be studied under microscope by reflected light.
In more scientific studies:
(i) We may use x-ray diffraction to study crystal structure,
(ii) We may make spectrographic analysis to identify chemical element components.
Simple physical properties of minerals used in the identification of minerals are briefly described below.
A. Colour :
Colour is the first property we note about any mineral. A mineral’s colour depends on the way the light waves interact with the electrons of the elements that make up the mineral. In short, colour is a function of composition. Some minerals have a single distinctive colour. For instance, sulphur is characterized by its yellow colour, whereas malachite (copper carbonate) is always green.
Among the common rock forming minerals those containing iron and magnesium (olivine, pyroxene, amphibole and biotite) dark in colour (typically black, brown or green) are referred to as mafic minerals. The light coloured rock forming minerals (feldspar and quartz) which are typically white, pink or colourless are referred to as felsic.
It should be noted that the colour of a mineral should be observed on a freshly exposed surface since a mineral surface is often discoloured by the action of weather. Colour alone is not a reliable property to identify a mineral. Some minerals may have the same colour and some minerals may also occur in different colours.
Quartz can exist as yellow quartz, pink quartz, white quartz. Olivine though distinctively green also exists in yellow colour. Garnet occurs in every colour and shade from black to colourless.
There are also minerals having a definite colour.
Malachite occurs in green colour. Azurite occurs in blue colour.
There are some properties of minerals that give them colour.
These are briefly described below:
(i) Idiochromatic:
These minerals are mainly self coloured having their colour due to their composition. Elements responsible for these colours are part of the mineral’s chemistry and even a small amount of the element can create a deep colour in the mineral. Cinnabar is usually red as iron is part of the mineral’s composition. Azurite appears blue due to copper part of the composition.
(ii) Allochromatic:
These minerals appear in different colours due to the presence of small amounts of impurities or defects in the mineral’s structure. In such a case colour becomes an unpredictable property for identification.
Different impurities cause fluorite to appear in every colour of the rainbow. Smoky quartz may appear almost black due to growth imperfections which interfere with light passing through the crystal.
Some minerals also get colours due to presence of minute air bubbles in them.
(iii) Pseudo-Chromatic:
The colour shown by this mineral is false. The colour appearing is neither due to the mineral nor the atomic properties. Instead the mineral is made up of layers or films that create colour by interference of light.
Precious opal, moonstone and labradorite reflect in a characteristic way but the colours are not true of the type of minerals.
(iv) Some Mineral Specimens Displaying Different Colours:
This property is exhibited by some minerals. The mineral specimen shows different colours when viewed from two different angles under the very same source of light. This property is called dichroism. If three colours appear when seen from different angles under the same source of light, the property is called trichroism. The above effect is caused by the varying light absorption patterns along different axes of the crystal.
B. Luminescence :
Luminescence is a collective term for the various ways in which a substance emits visible light under the influence of certain rays. Minerals which become luminescent when exposed to ultraviolet cathode or x-rays are fluorescent. Minerals such as fluorite, calcite, zircon, common opal, diamond can be optically excited when exposed to ultraviolet light.
This is called fluorescence. If the glow continues even after the ultraviolet is switched off, the phenomenon is called phosphorescence. In the darkness, these minerals glow in violet green and red which are different from the colours they glow in natural light.
C. Lustre :
The lustre of a mineral is its surface appearance and is dependent on the quantity and quality of the reflected light. Lustre is generally independent of the colour of the mineral. The intensity of any kind of lustre is determined by the transparency, reflectivity and surface structure of the mineral.
A transparent mineral such as quartz loses much of the incident light by transmission and so has a different lustre from an opaque metallic mineral such as chalcopyrites which reflects most of the incident light from the surface.
The surface configuration affects the intensity and perfection of the lustre. For example, a smooth crystal face such as the rhombohedral face of crystallized hematite will reflect more light better than a cleavage fragment of hornblende with its somewhat hacky surface, resulting from the cleavage.
Two chief types of lustre are recognized, namely metallic and non-metallic lustres.
(i) Metallic:
The luster seen in such metals like tin gold or copper. Such minerals are truly opaque, for no light is transmitted through even thin edges of the substances.
(ii) Non-Metallic:
All minerals other than metals display this type of lustre, and this group is subdivided into the five following lustres:
1. Adamantine – having the cold, hard brilliance of diamond. Minerals of this group have great hardness, high density and high refractive indices (1.9 – 2.6). Diamond, corundum and crystallized sulphur are typical of this type.
2. Vitreous – having the sort of lustre shown by broken glass. This lustre is typical of as many as 70 per cent of all minerals. Many are transparent or translucent and all have refractive indices between 1.3 and 1.9. Quartz, Garnet, Topaz. Barytes and Calcite illustrate this type.
3. Resinous – showing the type of lustre seen in resin. This is best seen in zinc sulphide, sphalerite.
4. Pearly – showing iridescence from either well developed cleavage planes or foliated minerals the former exemplified by selenite and the latter by talc. The surface shows appearance like that of pearls.
5. Silky – a lustre associated with fibrous structure and excellently shown by fibrous gypsum, asbestos, some serpentine.
Two different minerals with almost identical colour can have different lustres.
The degree or intensity of lustre may be described by the following terms:
Splendent – The surface gives brilliant reflection. Example: Rhombohedral faces of crystallized hematite.
Shining – Image is produced by reflection but it is rather indistinct. Example: Good crystals of celestite.
Glistening – There is a general reflection from the surface, but no image is seen. Example: Chalcopyrites.
Glimmering – Imperfect reflection as if from scattered points. Example: Fractured surface of flint.
Dull – No lustre. Lack of reflection. Gives earthy appearance. Example: Magnesite.
D. Transparency, Translucency and Opacity:
In contrast to lustre which describes the ability to reflect light transparency, translucency and opacity describe the capacity of a mineral to transmit light. If light can travel through a mineral (as it does through a window glass) then the mineral is transparent. If light cannot pass through a mineral, then the mineral is opaque.
Between these two extremes lie translucent minerals – light passes through such minerals, but we cannot see clearly through them. The minerals diamond, galena and garnet are transparent, opaque and translucent respectively.

E. Crystal Habit :
Crystal habit refers to a characteristic appearance of a mineral. Minerals grow in a variety of ways. Many crystals grow as simple prisms, which is a habit referred to as columnar or prismatic. Individual crystals may also be long and needle-like, i.e. acicular. They may be long and flat like a knife blade, i.e. bladed.
If they are like fine thread they are fibrous. Crystals often grow as groups or aggregates. In such cases the aggregates may be described as dendritic, i.e. diverging network of crystals, like branches of a tree. They are radiating when the elongate crystals grow out in different directions from a common point. The habit may be batryoidal when the mineral forms groups of fine radiating clumps that form spherical shapes resembling a bunch of grapes.
F. Structure :
Structure refers to the shape and form (state of aggregation) of minerals. The following terms are used to indicate the structures of minerals.
(i) Columnar Structure:
In this case the mineral is an aggregate of more or less parallel imperfect prismatic crystals. Example: Tourmaline.
(ii) Bladed Structure:
This term is used for a flat columnar aggregate having a knife-blade shape. Example: Kyanite.
(iii) Fibrous Structure:
This refers to an aggregate of fibres. The fibres may or may not be separable. Example: Asbestos, Gypsum.
(iv) Radiated Structure:
In this case columns or fibres diverge from central points. Example : Celestite.
(v) Lamellar Structure:
The mineral is like a platy aggregate made up of separable plates. Example: Chlorite.
(vi) Foliated Structure:
In this case the mineral can be easily separated into thin sheets or plates. Example: Mica.
(vii) Micaceous Structure:
This is an aggregate of mineral which can be split exceedingly thin sheets. Example: Hematite.
(viii) Granular Structure:
In this case the mineral is an aggregate of crystalline particles of nearly same size. Example: Dolomite.
(ix) Oolitic Structure:
This refers to an aggregate of small spheres. Example: Siliceous oolite.
(x) Pisolitic Structure:
This is an aggregate of larger spheres. Example: Bauxite.
(xi) Botryoidal Structure:
The mineral is an aggregate like a bunch of grapes. Example: Psilomelane.
(xii) Mammillary Structure:
This is an aggregate made up of low rounded prominences. Example: Chalcedony.
(xiii) Reniform Structure:
This refers to kidney shaped aggregate. Example: Hematite.
(xiv) Stalactitic Structure:
Cylindrical or conical form of minerals generally due to deposition by dripping water. Example: Calcite.
(xv) Nodular or Concretionary Structure:
This refers to irregular rounded or ellipsoidal lumps of mineral. Example: Azurite.
(xvi) Earthy Structure:
A uniform aggregate of exceedingly minute particles like china clay. Example: Magnesite.
(xvii) Dendritic Structure:
This is like a tree-like form or moss-like form usually produced by deposition of minerals from solutions by capillary action. Example: Manganese Oxiae (Wad)
(xviii) Coralloidal Structure:
Minerals exhibiting coral forms.
(xix) Massive Structure:
This term is used for a mineral or mineral aggregate without crystal faces, i.e. without regular boundaries.
G. Cleavage :
Cleavage is a very important mineralogical property. Cleavage of a mineral refers to the tendency of the mineral to break or separate easily along certain planes. This happens because in some minerals the bond between the layers of atoms aligned in a certain direction is weaker than bonds between other layers. Because of this, when a mineral with cleavage breaks, it splits, leaving a clean flat face parallel to the zone of weakness (called a cleavage plane).
For some minerals there may be a single plane of cleavage as in the mineral mica. Other minerals may have two to six cleavage planes. For example, halite and fluorite have four cleavage planes while calcite can split along three cleavage planes leaving a diamond shape called a rhombohedron.
The cleavage planes occur in specific angles with each other which are characteristic to the mineral and help in the identification of the mineral. The cleavage pattern is controlled by the internal arrangement of the atoms and its structure. The direction in which cleavage can occur is controlled by the mineral’s internal crystal structure. Cleavage occurs along such planes where the bonds between the atom layers are weak.
The table below shows some common minerals with prominent cleavage planes.

H. Crystal Symmetry :
By examining a perfect specimen of a mineral we find that it is of a complex geometric form completely bounded by plane surfaces. These plane surfaces are called crystal faces. Few mineral specimens are perfect. Most mineral grains, especially in the rocks may not reveal any of their own faces but will instead be bounded by surfaces developed as a result of growth impeded by the presence of other mineral grains.
Grains that have none of their own crystal faces are called anhedral. If a mineral has a few, but not all of its own crystal faces and the grain is said to be subhedral. In some rare cases, a grain is bounded completely by its own faces and is said to be euhedral. It should however be noted that as per the definition of a mineral irrespective of the shape of the mineral, the characteristic geometric packing of atoms is the same in all specimens of the mineral.
(i) Plane of Symmetry:
This is an imaginary plane dividing a crystal into two halves so that each half is a mirror image of the other. Some crystals (for example, in the shape of a cube) have as many as nine planes of symmetry, while some may not have any plane of symmetry at all.
(ii) Axis of Symmetry:
This is an imaginary straight line through a crystal about which the crystal can be rotated in such a way that the viewer will see identical faces repeated two or more times during a complete rotation. There are 2-, 3-, 4- and 6- fold axes of symmetry. Some crystals have several kinds of axes (a cube has six 2- fold axes, four 3-fold axes and three 4-fold axes). Others may have none.
(iii) Centre of Symmetry:
The centre of symmetry is present in a crystal if a straight line can be passed from any point on the surface through the centre to a similar point on the opposite side. Most crystals have a centre of symmetry.
I. Fracture :
If there are no planes of weakness or if the mineral is equally strong in all the directions such a mineral. Fractures irregularly, showing rough and uneven fractured surface. The way a mineral fractures leaving a rough uneven surface can be used for identification.
Some minerals fracture showing a smooth curved surface like that of a clamshell. Such a fracture is called a conchoidal fracture. Some fractures of minerals show distinctive patterns characteristic of the minerals. The generally observed patterns of fracture are given below.
(i) Uneven Fracture:
In this case the fractured surface is irregular. Barite, sulphur, anhydrite show this type of fracture.
(ii) Hacky Fracture:
The fracture surface shows sharp edges. Iron, silver, copper, nickel show this type of fracture.
(iii) Fibrous Fracture (or Splintery Fracture):
Fibers and long thin splinters are shown in this fracture. Asbestos, chrysotite, actinolite, tremolite, calcite show this fracture.
(iv) Conchoidal Fracture:
The fracture shows smooth curved surface. Quartz, gypsum, olivine, garnet show this fracture.
J. Streak :
The streak of a mineral is the colour of the mineral in powdered form and is characteristic and useful in its identification more than the colour of the mineral. The powder of the mineral can be easily obtained by crushing the mineral to a powder state or by marking the mineral over a slightly roughened white surface. A rough unglazed porcelain plate called a streak plate is used for this purpose.
The streak plate grinds off some part of the mineral into a powdered line or streak on the plate. Streak is an important character in identifying a mineral. Since it is nearly a constant feature of each coloured mineral. The streak left by chalk is white. Hematite which may be red, black or steel grey leaves a reddish brown streak.
Streak may not be very useful in the case of coloured silicate minerals most of which have a white streak, but many carbonates, phosphates, arsenates and sulphides do give a characteristic streak. Brass-yellow copper sulphide, chalco pyrites always give a greenish-black streak.
The three iron minerals magnetite, limonite and hematite are all blackish in the massive state, but magnetite has a black streak, limonite has a yellowish-brown streak and hematite has a cherry red streak. The lead ore galena has a metallic grey colour but has a black streak.
In case a mineral is harder than the streak plate, the mineral should be powdered and the colour of the powder is observed. Most of the harder minerals have pale streaks. For example, the mineral tourmaline which may be pink or green or even black, when powdered is pale or colourless.
K. Hardness :
Hardness is a very useful property to identify minerals. A mineral’s hardness is a measure of its ability to withstand abrasion or scratching by other substances. It is the resistance of the mineral in getting scratched. When the surface of a mineral is scratched the bonds between the atoms are broken. Hence, hardness can also be viewed as a measure of the strength of the bonds.
The hardness of a mineral is expressed as a number adopting Mohs’ scale of relative hardness which is given below. This scale is named after Friedrich Mohs (1773-1839), a German born mineralogist. This scale of hardness ranks the hardness of minerals in relation to one another.
The scale of hardness is given in the form of a table. The table is based on nothing more than the idea that a mineral with a lower hardness number can be scratched by a mineral with a higher hardness number.
Diamond the hardest mineral known (which is why it is used in certain saw blades, drills and grinding wheels) is arbitrarily assigned a hardness value of 10. In contrast we can easily scratch the mineral Talc with a finger nail and it being the softest mineral is assigned a hardness value of 1. The standard minerals on the scale range from softest (1) to hardest (10).

To find the hardness of a mineral sample in Mohs scale of hardness we find out which reference mineral it can scratch, and which reference mineral it cannot scratch. For example, halite can scratch gypsum or anything softer but it cannot scratch calcite and anything harder. Therefore the hardness of halite is between 2 and 3, say 2.5.
The point of an ordinary pocket knife blade (5.2) has a hardness of a little over 5 and fragments of common window glass have a hardness of about 5.5. Both a pocket knife and glass are useful aids in determining mineral hardness. For softer materials a copper coin with a hardness of 3.5 and a finger nail with a hardness of 2.5 are very helpful.
For one skilled in its use, hardness can be a particularly valuable property. With a little practice anyone can develop the necessary skills. Indeed it will soon become, that hardness testing can be performed with a knife blade alone. With a little practice one can estimate the hardness of any material at 5 or below simply by the ease with which it is scratched by the blade.
When applying the hardness test two precautions should be taken. First, we should be sure that the surface being tested is clean and fresh. Most surface contaminants and thin layers of alteration products are softer than the underlying mineral. Second, we must be sure that a single mineral grain is being tested. If the test is applied to an aggregate of small grains, the knife blade may simply separate the grains or even crush them, suggesting too low hardness.
The Mohs’ hardness scale is a relative hardness scale. With it one can only ascertain which mineral can scratch which mineral. No evidence is forthcoming about the absolute measure of increase in hardness within the scale. The Mohs’ hardness scale cannot be used as a truly scientific basis for hardness testing since it is only relative and is also too inexact.
Thus for such scientific purposes absolute hardness values are determined, which require the expenditure of great technical resources. In the table below the absolute hardness (grading hardness according to Rosival) is listed against Mohs’ values.
From this table it is possible to realize how unequal the span is within the individual Mohs’ hardness categories. Nevertheless the Mohs’ hardness scale is of great value to the collector since it is impossible for the collector to determine absolute hardness.

Other Scales of Hardness :
There are also other scales of hardness, but they are less practical for an average person to use. There are tests to measure the depth or area of indentation left by an object (i.e., the indenter) of a standard shape. The indentation is made by exerting a specific force on the mineral for a specified interval of time. The three common methods based on this principle are Brinnel, Vickers and Rockwell methods.
L. Specific Gravity:
The specific gravity of a mineral is the ratio of the weight of the mineral to the weight of an equal volume of water. This can be determined by using a Jolly balance, beam balance, pycnometer etc. Most of the familiar non-metallic minerals in the earths crust have a specific gravity of 2.5 to 3. Many of the commonly seen metallic minerals have a specific gravity over 5. A high value of specific gravity is indicative of close packing of atoms in the crystal structure.
If a mineral has an essentially fixed chemical composition, as for example quartz, SiO 2 , its specific gravity has a fixed value 2.65 and any departure from that value must be due to the presence of impurities. Most minerals however are solid solutions and therefore range considerably in chemical composition. In accordance with such variations, the specific gravity of such a mineral has a range of values.
The table below shows the specific gravities of some common minerals.

M. Magnetism :
This is applicable to magnetic minerals. Magnetic behaviour varies with minerals. There are minerals (magnetite) which themselves have a magnetic effect. There are minerals (pyrrhotite) which are attracted by magnets. There are also minerals which do not react magnetically at all.
Both type of magnetic behaviour can be clearly detected by a freely suspended compass needle. The magnetic needle reacts to any magnetic influence. A specimen can be brought near the stationary magnetic needle and how the needle is affected is observed.
We can find whether the specimen is a magnet or a magnetic material. A simple test for the presence of iron in a sample is to use a hand magnet. Minerals with high iron content like pyrrhotite get attracted by a hand magnet. Weak magnetic samples turn the compass needle.
N. Sense of Taste, Smell and Touch for Identification :
The following properties fall under this heading.
In order a mineral may be tested it must be water soluble. Salt tastes saline.
A few more descriptive terms are in use such as:
Astringent – taste of vitriol
Sweetish astringent – taste of alum
Alkaline – taste of soda
Bitter – taste of Epsom salt
Sour – taste of sulphuric acid
Unaltered minerals in the dry state have no characteristic smell, but some do give off an odour when struck, rubbed, breathed or heated.
The following terms are used to describe odours which may occur.
Sulphurous – smell of burning sulphur – smell sensed when iron pyrites is struck by hammer or when sulphide minerals are strongly heated.
Alliaceous – smell of onion. This is felt when arsenic compounds are strongly heated.
Argillaceous – smell of damp clay or earth. This may be sensed in any argillaceous rock, but the only common mineral which gives this smell is moistened serpentine.
Of the three senses mentioned, the sense of touch is very useful in identifying a mineral. Touch can be sensed in the following types.
Smooth – This refers to the sensation felt when fingers are passed across a glass pane. This is sensed in many well-formed crystals with plane-bounding faces.
Greasy – This sensation is felt when passing the fingers over a candle. Talc, serpentine and fibrous gypsum are greasy.
Harsh – This sensation is felt when fingers are passed on sand paper. Most minerals occurring in granular masses such as olivine present this sensation.
Other Simple Useful Properties :
Thin sheets of mica will bend and elastically snap back. Gold is malleable and can be easily shaped. Talc and graphite both have distinctive feels, Talc feels soapy and graphite feels greasy. Magnetite can be picked up by a magnet.
Some minerals exhibit special optical properties. For example, when a transparent piece of calcite is placed over printed material, the letters appear twice. This optical property is known as double refraction. In summary, a number of special physical and chemical properties are useful in identifying minerals.
These include, taste, smell elasticity, malleability, feel, magnetism, double refraction and chemical reaction with hydrochloric acid. It may be noted that every one of these properties depends on the composition (elements) of a mineral and its structure (how the atoms are arranged).
Blowpipe or Fusibility Test to Identify Minerals :
This is a simple test used to identify minerals. This test is based on the idea that every mineral has a definite melting point and can be compared to their mineral melting points. As the mineral is exposed to a flame, the observer must recognize the behaviour of the mineral.
Whether the sample was easy, normal or difficult to melt and whether it changes its colours, bubbles or expands on heating are observed. These observations are compared with properties already known about minerals.
Essay # 6 . Utility of Minerals :
Minerals are valuable and useful in many ways. Many minerals are valuable to humans. For thousands of years humans have used minerals (and rocks) to construct structures, to make tools and to make paint. Abrasives are vital to many industrial, drilling, cutting and smoothing operations and include diamonds, corundum, garnet and quartz.
Minerals are sources of raw materials for metallurgical, chemical and electronic industries. Minerals are used in the food industry as mineral supplements for human and other living organisms. Minerals are used in everything from metal to our lamps and light bulbs to quartz in our watches.
Minerals are of economic importance. Approximately about 100 minerals are of economic importance. Besides the well-known metallic minerals like gold, silver, copper, aluminium, molybdenum and cobalt, the energy minerals like coal, oil shale and uranium and industrial minerals for construction and agriculture, like sand, gravel, clay and limestone are all minerals of utility. There are also minerals valued as adornments like, diamond, emerald, sapphire and ruby. There are minerals which otherwise may not be of economic value are most sought after as gemstones.
Essay # 7 . Distribution of Elements among Rock-Forming Minerals:
Most of the rock forming minerals is silicates. The commonly found oxides in rock analysis are SiO 2 , Al 2 O 3 , FeO, Fe 2 O 3 , MgO, CaO, Na 2 O and K 2 O, together with TiO 2 , H 2 O, P 2 O 5 and MnO.
i. Silica, SiO 2 :
Silica appears in most of the minerals. This is the sole constituent of the mineral quartz. Rocks containing more than 66% silica are acid rocks, those containing 66% to 52% silica are intermediate rocks and rocks containing 52% to 45% are basic rocks and those with less than 45% are ultra basic rocks.
ii. Alumina, Al 2 O 3 :
This is a very important constituent of the feldspars, feldspathoids and some members of the amphibole family (Ex.: hornblende and basaltic hornblende) and the mica group.
iii. Ferrous Iron, FeO, Ferric Iron Fe 2 O 3 , Magnesia MgO :
These three constituents are present in the group of minerals known as ferromagnesian group which includes olivine, augite, hornblende and the dark mica biotite.
iv. Lime, CaO :
Lime is of great importance in the plagioclase feldspars. The feldspars are useful in drawing up a classification of igneous rocks. It is also an important constituent of some of the ferromagnesian minerals.
v. Potassium K 2 O, Soda Na 2 O:
These two oxides are described collectively as the alkalies and are important in the alkali feldspars – Orthoclase, micro-line and albite – minerals which form a large proportion of the more acid igneous rocks.
Essay # 8 . Properties of Minerals:
A. quartz group :.
Quartz and opal and the rare minerals Tridymite, Cristobalite, Coesite and Stishovite are members of the quartz group. These are made of silica.

The crystals generally appear as a six-sided prism with pyramidal termination. The vertical faces show horizontal striations. Very huge crystals have been observed.
Quartz is mechanically resistant and is most unlikely attacked chemically (soluble only in hydrofluoric acid). This (after feldspar) is the most abundant mineral present in the upper crust of the earth. It is the main constituent of the igneous and metamorphic rocks and also in most sands. It appears generally colourless or milky and turbid.
Quartz is the raw material for glass and ceramic manufacture. It has piezoelectric property. Thin slices of quartz are used for accurate frequency control in radio and radar transmission. Very beautiful coloured quartzes are popular gemstones.
(i) Doubly Terminated Quartz :
These are quartz crystals having pyramid ends at both ends. These are seen in carbonate rocks.
(ii) Sceptre Quartz :
These are big crystals capping a slender stem.
(iii) Ferruginous Quartz :
These are coloured quartz aggregate. Due to presence of iron oxide, they may be of yellow, brown or red colour.
(iv) Rock Quartz :
This is a colourless. It occurs all over the earth. It is used in costume jewellery and as diamond imitation.
(v) Amethystine Quartz – Amethyst :
This is a compact formation generally banded and streaked with milky quartz. When heated it presents yellow, brown and green and even colourless form.
(vi) Milky Quartz :
This has many micro-cavities and looks milky white.
(vii) Rose Quartz :
This is pink coloured mostly cloudy variety of quartz. The crystals are rare. Its colour can fade. When it is cut it presents a six-rayed star feature.
(viii) Smoky Quartz :
Colour varies from brown to black. In the trade it is misleadingly called smoky topaz. It loses its colour when heated to 300°C to 400°C. A black opaque variety is called Morion.
(ix) Blue Quartz:
This is a cloudy blue massive variety of quartz. Some crystals are also clear transparent to translucent. The colour is due to inclusion of crocidolite or rutile fibres.
(x) Tiger’s Eye:
This is a finely fibrous and opaque and a gem variety of quartzite. The golden yellow colour is due to the inclusion of limonite. When cut it shows surface iridescence (changing colours like a rainbow).
(xi) Cat’s Eye Quartz:
These are massive quartz aggregates which are white grey, greenish or brownish in colour with cat’s eye effect. This is due to inclusion of parallel oriented fine asbestos fibres which are all parallel oriented.
(xii) Agate – Moss Agate:
This is colourless and translucent chalcedony with green moss like hornblende. These are seen in fissure fillings and also as pebbles.
(xiii) Jasper:
This is a micro crystalline variety of quartz, a chalcedony. Its colour is determined by foreign material which exists to 20%. It is multi-coloured and striped and spotted. It has trade names Basanite (Black colour), Plasma (Green colour), Silex (Brown/red colour).
(xiv) Flint:
This is round chalcedonic form of silica not more than 10 cm in diameter. Its colour is from grey to black. The dark colour is due to organic impurities. Due to loss of water white patina or skin appears. In many cases remains of silicious sponges are embedded inside. This is believed to have been used as raw-material for weapons in the Stone Age. When subjected to blow it emits sparks of fire.
Belonging to the quartz group, opal is amorphous. It contains small quantity of finely crystalline cristobalite and tridymite which produce the rainbow iridescence. This is found as crusts and nodules. There are three varieties – opaque common opal opalescent precious opal and the orange red fire opal. It is used in jewellery and as gem stones.

B. Feldspar Group :
This group of minerals constitutes about 60 per cent of the earth’s crust.
These are aluminium silicates of two principal kinds:
(i) Potash or potash soda feldspar generally called orthoclase feldspar,
(ii) Soda-lime feldspar known as plagioclase feldspar. Though this is arbitrarily divided into six subspecies, the dark labradorite (which shows a play of colours) and Albite have distinctive appearance. We can recognize plagioclase by the fine striations on a cleavage surface.

C. Mica Group :
Micas are most easily identified by their highly perfect basal cleavage, allowing to be easily separated into thin tough elastic laminae. This most common members of the mica group are Muscovite mica (Muscovy glass) and Biotite mica.
Muscovite is hydrous potassium aluminium silicate KAl 3 Si 3 O 10 (OH) 2 which is white or colourless. It is used extensively for electrical insulation, in high grade cleavage sheets for electric irons and toasters. Mica in the form of sheet (isinglass) is used for glazing and in powder or flake form for filler, heat insulation, decoration.
Biotite is K(MgFe) 3 AlSi 3 O 10 (OH) 2 is also known as black mica.
Sheet mica is obtained from coarse textured igneous rocks like pegmatite along with large crystals of feldspar and quartz.
Other micas are phlogopite which is bronze coloured magnesium mica and Lepidolite which is lithium bearing mica.

D. Pyroxene Group :
Pyroxenes are complicated silicates containing Calcium, Magnesium, Aluminium, Iron, Sodium. The most frequently seen mineral in this group is Augite. It is seen as stubby crystals and also irregular masses. Other members in this group include Enstatite, Hypersthene and Diopside.
Pyroxenes crystallise in three different crystal systems. Enstatite and Hypersthene crystallize in orthorhombic system. Augite and Diopside crystallize in monoclinic system. The minerals Rhodonite and Babingtonite crystallise in Triclinic system.

E. Amphibole Group :
This series of minerals are common constituents of many rocks. Hornblende and Arfvedsonite occur in igneous rocks. Actinolite, Tremolite, Riebeckite, Glaucophane and Anthophyllite are present in metamorphic rocks.
The more commonly minerals’ properties are given below:
Hornblende :
This is the most common mineral of this group. It is a complex silicate of sodium, calcium, magnesium, iron and aluminium with a small percentage of hydroxyl ions. It is commonly seen in light coloured igneous rocks in association with feldspar and in some cases with quartz. It is abundantly found in diorites along with plagioclase feldspar.

F. Garnet Group :
Garnets are silicates of iron, calcium or magnesium. Garnets include Almandine Fe 3 Al 2 (SiO 4 ) 3 the common red garnet. Pyrope garnet Mg 3 Al 2 [SiO 4 ] 3 , Grossularite Ca 3 Al 2 (SiO 4 ) 3 an Andradite Ca 3 Fe 2 [SiO 4 ] 3 .
Garnets are generally red or brown having a vitreous or resinous luster. They are very hard – as hard as quartz. They do not have good cleavage. They break like quartz or glass.
Garnet is used as a coated abrasive on sand paper. It is also used as a gem and as watch jewel.

Related Articles:
- Rock Cleavage: Meaning, Types and Importance |Geology
- Crystals of Minerals | Geology
Science , Essay , Geology , Minerals , Essay on Minerals
Comments are closed.
Module 2: Rock Forming Minerals
Outcome: identifying minerals, identify and classify common rock forming minerals..
The solid earth is made of rocks, which are made of minerals. To understand rocks you need to become familiar with minerals and how they are identified. This outcome gives you the background needed to understand the terms used in identifying minerals.
This section will introduce you to minerals. You will learn the various techniques used by geologists to identify and classify minerals.
What You’ll Learn to Do
- Identify minerals based on their physical characteristics.
- Sort minerals into the correct mineral class.
Learning Activities
The learning activities for this section include the following:
- Reading: Physical Characteristics of Minerals
- Reading: Classifying Minerals
- Self Check: Identifying Minerals
Contribute!
Improve this page Learn More
- Authored by : Lumen Learning and SBCTC. License : CC BY: Attribution
- Basics - Minerals. Authored by : Ralph L. Dawes and Cheryl D. Dawes. Provided by : Wenatchee Valley College. Located at : http://commons.wvc.edu/rdawes/G101OCL/Basics/minerals.html . Project : Geology 101 - Introduction to Physical Geology. License : CC BY: Attribution
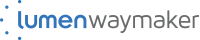

- Science Notes Posts
- Contact Science Notes
- Todd Helmenstine Biography
- Anne Helmenstine Biography
- Free Printable Periodic Tables (PDF and PNG)
- Periodic Table Wallpapers
- Interactive Periodic Table
- Periodic Table Posters
- How to Grow Crystals
- Chemistry Projects
- Fire and Flames Projects
- Holiday Science
- Chemistry Problems With Answers
- Physics Problems
- Unit Conversion Example Problems
- Chemistry Worksheets
- Biology Worksheets
- Periodic Table Worksheets
- Physical Science Worksheets
- Science Lab Worksheets
- My Amazon Books
What Is a Mineral? Definition and Examples

In geology, a mineral is a naturally occurring solid that has a well-defined chemical composition and crystal structure. Most minerals are inorganic , although some mineralogists allow for minerals that are organic compounds or else made by organisms rather than geological processes. The word mineral comes from the Medieval Latin word minera , which means ore or mine. Several thousand minerals are known. Of these, around one hundred are components of rocks.
- A mineral is a natural solid with a defined chemical composition and crystalline structure.
- Most minerals are inorganic and form from geological processes.
- A mineral can be a pure elements or a compound. It is not a mixture of two or more substances.
Examples of Minerals
Minerals include elements that occur in relatively pure form as solids in nature ( native elements ) and compounds. However, unlike most compounds in chemistry, minerals often contain two or more elements that occupy the same positions in a mineral’s crystal structure. For example, the formula for the mineral mackinawite is (Fe,Ni) 9 S 8 , so FeNi 8 S8 and Fe 2 Ni 6 S 8 both are possible.
Here are some examples of minerals and their chemical formulas:
- Apatite [Ca 5 (PO 4 ) 3 (OH,Cl,F)]
- Calcite (CaCO 3 )
- Copper (Cu)
- Corundum (Al 2 O 3 )
- Diamond (C)
- Fluorite (CaF 2 )
- Graphite (C)
- Olivine [(Mg,Fe) 2 SiO 4 ]
- Orthoclase feldspar (KAlSi 3 O 8 )
- Quartz (SiO 2 )
- Silicon (S)
- Topaz [Al 2 SiO 4 (OH,F) 2 ]
Difference Between a Rock and a Mineral
The terms “rock” and “mineral” don’t mean the same thing . A mineral is a natural solid with a definite composition and structure. A rock consists of one or more minerals or mineraloids. So, a rock can be a mineral, and vice versa. But, some samples of minerals are not rocks. Most rocks consist of multiple minerals and/or mineraloids. For example, granite (a rock) mainly contains the minerals quartz, feldspar, and plagioclase. An ore is a rock that is rich in certain minerals.
Difference Between a Mineral and a Gem
Most gems consist of minerals, but not all minerals are gems . A gem or gemstone is a cut and polished solid that is usually a mineral crystal. While all minerals have a crystalline structure, sometimes it is only visible as tiny grains in the overall solid. For example, the gemstones ruby and sapphire consist of the mineral corundum.
Mineraloids
Some natural solids are similar to minerals, but don’t meet all of the criteria for the definition. Mineraloids are natural mineral-like substances that don’t have the constant chemical composition of a mineral or else lack a crystal structure. Obsidian and opal are good example of mineraloid that are amorphous rather than crystalline. Pearl is a mineraloid because it contains organic matter bonded to calcite or aragonite in no definite proportion.
How to Identify a Mineral
Identifying a mineral is a complex task, as two minerals may look similar at first glance. Here are some the properties that distinguish different minerals.
- Chemical composition : Chemical composition gives the identity and ratio of elements in a mineral.
- Crystal structure : Crystal structure is the geometric arrangement of atoms in space.
- Hardness : Hardness is a mineral’s resistance to being scratched.
- Luster : Luster describes how light reflects from a sample’s surface.
- Diaphaneity : Diaphaneity is a measure of how transparent a mineral is.
- Color : Color actually isn’t that helpful, in part because trace impurities affect it.
- Streak: The streak test indicates the color left behind when you rub a mineral against a hard surface.
- Other Optical Properties : These include asterism, iridescence, chatoyancy, pleochroism, and tarnish.
- Cleavage : Cleavage describes the characteristic way a mineral breaks.
- Specific gravity : This is a measure of a mineral’s density .
- Other properties : Other characteristics include radioactivity, magnetism, acid resistance, odor, and flavor.
Types of Minerals
The two major types of minerals are silicates and non-silicates. The reason is because most of the Earth’s crust consists of silicates, which are minerals containing the elements silicon and oxygen.
Mineralogists classify silicates largely by their crystal structure, based on the [SiSO 4 ] 4- tetrahedron.
- Orthosilicates : Tetrahedra share no corners. Examples of orthosilicates include pyrope garnet, zircon, and topaz.
- Disilicates : Two tetrahedra share one oxygen atom. Epidote is a disilicate.
- Inosilicates : Single silicate chains share two corners, while double chains share two or three corners. The pyroxenes and amphiboles are inosilicates.
- Phyllosilicates : A sheet structure forms with three shared oxygen atoms. Mica is an example of a phyllosilicate mineral.
- Tectosilicates (framework silicates) : Tetrahedra share all four corners. Examples include quartz, feldspar, and zeolite.
- Cyclosilicates (ring silicates) : Tetrahedra share two corners, forming a cylinder. Beryl and tourmaline are cyclosilicates.
Non-Silicates
Some of the important classes of non-silicate minerals are carbonates, halides, oxides, phosphates, sulfates, and sulfides.
- Carbonates : Carbonates contain the carbonate anion (CO 3 ) combined with one more other elements. An example of a carbonate is calcite (CaCO 3 ).
- Halides : Halides all contain a halogen element (e.g., F, Cl, I). Halite (NaCl, salt) is an example of a halide.
- Oxide : The main element in an oxide is oxygen. An example of an oxide is chromite (FeCr 2 O 4 ).
- Phosphates : Phosphates contain the phosphate anion (PO 4 3- ). An example of a phosphate mineral is fluoroapatite [Ca 5 (PO 4 ) 3 F].
- Sulfates : Sulfates contain the sulfate anion (SO 4 2- ). These minerals occur in both hydrous (with water) and anhydrous forms. Examples include celestine (SrSO 4 ) and gypsum (CaSO 4 ⋅2H 2 O).
- Sulfides : Sulfides contain one or more metals or semimetals, bound to sulfur. Sphalerite (ZnS), galena (PbS), and cinnabar (HgS) are examples of sulfide minerals.
The native elements are another broad class of non-silicates. These include diamond, graphite, copper, iron, and gold.
The organic minerals are in their own class. These substances contain organic carbon (i.e., carbon bonded to hydrogen), yet form via geologic processes. An example of an organic mineral is whewellite (CaC 2 O 4 ⋅H 2 O). Whewellite deposits from hydrothermal vents.
- Chesterman, C.W.; Lowe, K.E. (2008). Field Guide to North American Rocks and Minerals . Toronto: Random House of Canada. ISBN 978-0394502694.
- Dyar, M.D.; Gunter, M.E. (2008). Mineralogy and Optical Mineralogy . Chantilly, VA: Mineralogical Society of America. ISBN 978-0939950812.
- Klein, Cornelis; Hurlbut, Cornelius S. Jr. (1993). Manual of Mineralogy (21st ed.). New York: Wiley. ISBN 047157452X.
- Lowenstam, H.A. (1981). “Minerals formed by organisms”. Science . 211 (4487): 1126–31. doi: 10.1126/science.7008198
- Rafferty, John P., ed. (2011): Minerals . In the series Geology: Landforms, Minerals, and Rocks . Rosen Publishing Group. ISBN 978-1615304899.
Related Posts
Identifying Minerals: Characterizing minerals' physical properties
by Anne E. Egger, Ph.D.
Teach with this
Supporting student learning.
- Assign the reading quiz
This module has a multiple-choice quiz associated with it that allows students to check their own answers and get feedback that helps them choose the correct answer. Ask them to report their score and reflect on the questions they missed.
- Use a just-in-time approach for formative assessment
In a just-in-time approach, students submit responses to questions at least an hour before class, and no more than 24 hours before. You review their responses before class begins and adjust in-class activities to build on their current understanding and ideas. A simple question like, “What surprised you or interested you from this reading?” can elicit student ideas and help them connect personally to the reading.
The goal of assigning these questions to be completed before class is to allow you to see responses ahead of time, summarize their responses, and modify your teaching accordingly. It is helpful to keep the grading very simple so that you can read and give points for them very quickly, in 20-30 minutes prior to class. You can use a very simple, 2-point rubric for these questions that encourages complete responses over finding the "right" answer:
- 2 points: Complete and thoughtful answer to the question
- 1.5 points: Complete answer but does not go beyond what is written in text
- 1 point: Partial answer, does not support response
- 0.5 points: Minimal answer, just a few words
- 0 points: No response
- For future teachers: Show NGSS annotations
Do you have future teachers in your course? They will need to be comfortable with the Next Generation Science Standards (NGSS) when they start teaching. You can help them gain confidence by encouraging them to use the toggle to show NGSS annotations in the modules, read the full annotations, and reflect on how the three dimensions of science and engineering practices, cross-cutting concepts, and disciplinary core ideas are integrated in the real process of science.
Deepening understanding
- Explore the data and figures
Help your students engage with the data-rich figures and animations in their readings. These are available at high resolution by clicking on them: you can project them in class or print them out on handouts to serve as the starting point for group discussions. Ask students to explain the figure to each other, or generate questions about the figure. See our module Using Graphs and Visual Data in Science to help students practice explaining graphs.
- Explore the scientists and their research
Help your students connect to scientists by further exploring the individuals they read about, or finding others who have worked on similar research.
You can also further explore their published papers with students. See our module Understanding Scientific Journals and Articles for ideas to help students read the literature.
Table of Contents
A Mineral Appearance and Physical Properties Essay
In nature, minerals occur as inorganic solids with defined chemical compositions as well as crystalline lattice structures. All minerals have unique physical and chemical characteristics that make them different from each other. While the chemical formula and the crystal lattice of a given mineral can be determined in a laboratory setup, it is possible to determine the identity of a mineral using its physical properties (Carmichael, 2017). Noteworthy, the physical properties of a mineral, which refers to the way in which a mineral appears, result from the inner chemical properties of that specific mineral (Ha et al., 2017). All minerals are identified using their physical properties. In essence, there is a relationship between what a mineral looks like and its physical properties.
Color is the most common characteristic that mineralogists look for when identifying a given mineral. Nevertheless, color is the least effective method of identifying minerals since many different minerals can have the same color. In the same manner, a specific mineral can assume more than one color (Ha et al., 2017). For instance, several minerals, such as epidote, olivine, and actinolite, are green in color (Carmichael, 2017). In the same manner, quartz can be pink, smoky, yellow, or purple, depending on the level of impurity in its chemical structure. Similar to other objects, minerals reflect, emit, and absorb light of different wavelengths, which is then perceived by the human eye and interpreted in the brain as a specific color (Carmichael, 2017). Depending on the state of oxidation, iron can produce more than one color, mostly red, black, or green.
The crystal form is one of the most effective ways of determining the color of a given mineral. In mineralogy, the internal atomic structure determines the external shape of a mineral crystal. The crystal form of a mineral is defined by the angular relationship between the faces of its crystals. For instance, halite and pyrite are salts with cubic forms, while tourmaline has a prismatic form. Minerals like malachite and azurite are both copper ores that do not have regular crystals but appear amorphous.
Hardness is a common characteristic of the objects used to determine a given mineral’s identity. The hardness of a substance is defined by its resistance to scratching and is the measure of the length of the structure of its internal crystals. The Mohs’ scale, developed by Fredrick Mohs in 1883, is a diagnostic tool that measures the degree of hardness of minerals. The scale runs from 1 to 10 depending on the level of hardness, wherein 1 indicates the softest while 10 indicates the hardest (Carmichael, 2017). The bonding of a crystal structure determines the degree of hardness of a mineral. In addition, the bonding of a crystal structure differs with directions, implying that a given mineral’s hardness can differ with crystallographic directions. As an example, the hardness of kyanite on Mohs’s scale is 4.5 when considered parallel to the chains of silica tetrahedra is 6.5 when considered in the direction of perpendicular to the chains (Ha et al., 2017). Consequently, hardness cannot be used as the sole diagnostic approach for minerals.
Minerals can be magnetic or non-magnetic depending on the elements that make their structure. For example, minerals with copper, nickel, cobalt, iron, and chromium can have magnetism because the elements tend to have magnetic fields in nature. In nature, minerals can be diamagnetic, paramagnetic, or ferromagnetic, depending on how they react to a magnet. Diamagnetic refers to the slight repulsion or tendency to move towards the regions of the weaker magnetic field (Carmichael, 2017). Paramagnetic characteristic refers to slight attraction in which materials tend to move towards regions of strong magnetic fields. Ferromagnetism is the tendency to have a strong attraction towards regions of strong fields.
Carmichael, R. S. (2017). Magnetic properties of minerals and rocks. In Handbook of physical properties of rocks (pp. 229-288). CRC Press.
Ha, W. N., Nicholson, T., Kahler, B., & Walsh, L. J. (2017). Mineral trioxide aggregate—a review of properties and testing methodologies. Materials , 10 (11), 1261. Web.
- Chicago (A-D)
- Chicago (N-B)
IvyPanda. (2023, May 3). A Mineral Appearance and Physical Properties. https://ivypanda.com/essays/a-mineral-appearance-and-physical-properties/
"A Mineral Appearance and Physical Properties." IvyPanda , 3 May 2023, ivypanda.com/essays/a-mineral-appearance-and-physical-properties/.
IvyPanda . (2023) 'A Mineral Appearance and Physical Properties'. 3 May.
IvyPanda . 2023. "A Mineral Appearance and Physical Properties." May 3, 2023. https://ivypanda.com/essays/a-mineral-appearance-and-physical-properties/.
1. IvyPanda . "A Mineral Appearance and Physical Properties." May 3, 2023. https://ivypanda.com/essays/a-mineral-appearance-and-physical-properties/.
Bibliography
IvyPanda . "A Mineral Appearance and Physical Properties." May 3, 2023. https://ivypanda.com/essays/a-mineral-appearance-and-physical-properties/.
- Magnetism and Magnetic Field
- Rocks Along the Beach and Their Characteristics
- Theories in Magnetic Resonance Imaging
- Atacamite Mineral: Physical and Chemical Structure
- Lattice Production Line Analysis
- Polarization in Electric Charges and Magnets
- Liquid Crystals: Fundamental Properties, and the Effects of External Factors
- Nuclear Energy: High-Entropy Alloy
- The Application of Curved Crystals for the Control of Particle Beam
- Peridot - Natural Sciences
- Extinction of Dinosaurs in North America and Texas
- Traveling With Geological Time Machine
- Integrated Ocean Drilling Program Expedition 342
- Geology and Formation of the Canary Islands
- Human Activity and Growing Number of Earthquakes

Minerals, Critical Minerals, and the U.S. Economy (2008)
Chapter: 6 conclusions and recommendations, chapter 6 conclusions and recommendations.
Minerals, or more specifically the mineral products derived from them, are essential to the functioning of modern processes and products. Some minerals are more essential than others, in the sense that they have few if any substitutes capable of providing similar functionality at similar costs.
The availability of these minerals is a function of geologic, technical, environmental and social, political, and economic factors. Some minerals are more prone than others to disruptive restrictions in supply.
It is this combination of importance in use and supply risk, and specifically the potential that an important mineral may be subject to supply restrictions, that motivated this study. The committee was charged to carry out a number of specific tasks identified in Chapter 1 :
Identify the critical minerals and mineral products that are essential for industry and emerging technologies in the domestic economy.
Assess the trends in the sources and production status of these critical minerals and mineral products worldwide.
Examine actual or potential constraints, including but not limited to geologic, technologic, economic, and political issues, on the availability of these minerals and mineral products for domestic applications.
Identify the impacts of disruptions in supply of critical minerals and mineral products on the domestic workforce and economy.
Describe and evaluate the current mineral and mineral product databases and other sources of information available for decision making on mineral policy issues.
Identify types of information and possible research initiatives that will enhance understanding of critical minerals and mineral products in a global context.
Chapters 2 through 5 have examined the various dimensions of the overall task, and each chapter concluded with principal findings. This chapter presents the committee’s principal conclusions, drawing on each previous chapter’s findings, and summarizes the committee’s recommendations following from these conclusions. Throughout its examination of these issues, the committee found it essential to consider minerals, and critical minerals, in the context of a global mineral and material cycle—from mineral ores at the mine to metallic and nonmetallic minerals in potentially recyclable materials and products.
The committee established parameters regarding a mineral’s importance in use and availability (supply risk) to apply the criticality matrix to 11 minerals or mineral groups: copper, gallium, indium, lithium, manganese, niobium, platinum group metals (PGMs), rare earth elements (REs), tantalum, titanium, and vanadium. The committee did not have the time or resources to evaluate all potentially critical minerals. Instead, the committee selected the minerals identified above on the basis of two considerations. First, the set of minerals the committee examined had to illustrate the range of circumstances that the matrix methodology accommodates and considers. For example, in its selection of the minerals examined in this report, the committee considered minerals used in large quantities throughout the economy in traditional applications and others used in limited quantities in a small number of (often emerging) applications, minerals produced largely as by-products, and other minerals for which recycling of scrap is an important source of supply. Second, the set of minerals had to consist of those that, in the professional judgment of committee members, would likely be included in a more comprehensive assessment of all potentially critical minerals. The committee used a combination of quantitative
measures and expert (qualitative) judgment in implementing the matrix methodology.
CONCLUSIONS
Defining criticality.
The committee concludes that all minerals and mineral products could be or could become critical to some degree, depending on their importance and availability —in the sense that the chemical and physical properties they provide are essential to a specific product or use or more broadly, that specific minerals are an essential input for a national priority (for example, national defense) or for an industry, or may be important (or have the potential to become important) to a region or the nation as a whole. Materials derived from minerals are essential to the performance of nearly all products and services we take for granted—cellular telephones, automobiles, home appliances, computers and other electronic products, and aircraft, for example. The degree of a mineral’s importance can vary considerably over time as technologies and the economy evolve and change.
The committee also concludes, however, that more useful from the federal perspective is the concept of a critical mineral as one that is both essential in use and subject to supply restriction . In other words, the key determinants of criticality here are importance in use and availability. Based on these determinants, the committee developed a methodology—a ‘criticality matrix’—for assessing the criticality of specific minerals and identified the information requirements for implementing this methodology. The matrix has two dimensions. The first (vertical axis) represents the degree of importance of a mineral or, equivalently, the impact of a supply restriction. The second dimension (horizontal axis) represents the degree of supply risk or the risk of a supply restriction.
This methodology emphasizes that criticality is a relative concept in that minerals are more or less critical, rather than critical or not critical. At any time, and for any organization or nation, some minerals will be more critical than others. Over time, the criticality of a specific mineral
can and likely will change as production technologies evolve and new products are developed.
Furthermore, the committee concludes that in implementing the methodology to assess criticality, it is important to distinguish among three time or adjustment periods . In the short term (period of a few months to a few years), mineral markets and in turn prices are influenced primarily by unexpected changes in mineral demand, such as the largely unanticipated increase in Chinese mineral demand over the last several years, and by unexpected shortfalls in production due to technical or other problems at existing mines and production facilities. In the short term, from the perspective of a mineral market as a whole, mineral users and producers are constrained by their existing production capacity, and therefore, unexpected changes in demand or supply are reflected largely in inventories held by producers, users, and commodity exchanges.
In the medium term (a few years, but no more than about a decade), markets respond to short-term developments but still in a relatively limited manner; for example, if a mineral’s availability has become restricted, mineral users make any easy substitution for this mineral, and mineral producers bring into production any easy-to-develop, higher-cost sources of the restricted mineral (e.g., higher-cost scrap that previously was not recycled; and higher-cost, known but underdeveloped mineral deposits). In the medium term, mineral users and producers are essentially limited by existing technologies and known primary and secondary mineral resources.
Over the long term (roughly a decade or more), mineral users and producers can respond more significantly to changes in mineral availability through conscious decisions about whether and to what degree to invest in innovative activities in mineral exploration, mine development, mineral processing, product design and manufacturing, and recycling technology and policy.
Understanding Importance in Use or the Impact of a Supply Restriction
Users demand minerals and mineral attributes for the functionality they provide—their chemical and physical properties in specific applications
such as strength, corrosion resistance, electrical conductivity, low density, and so on. As noted at the beginning of this chapter, some minerals are more essential than others in the sense that they have few if any substitutes capable of providing similar functionality at similar costs. The greater the difficulty, expense, or time it takes for material substitution to occur, the more critical a mineral is to a specific application or product—or analogously, the greater is the impact of a supply restriction.
The impact of a specific supply restriction, in other words, depends on the nature of the restriction. A supply restriction can occur in two general forms. First, demand can increase and outstrip existing production capacity (a demand shock). Second, in what normally would be considered a disruption, a material that previously was available becomes unavailable (a supply shock). In either case, it is possible that a mineral or mineral product becomes physically unavailable; in this situation, the product a user makes cannot be manufactured, sold, and then used by the prospective purchaser. More typically, however, a mineral or mineral product remains physically available, but at a higher price. In this situation, supply will be reallocated to those users willing to pay more for a mineral or mineral product and away from lower-valued uses.
The specific impact of a supply restriction will depend on circumstances: Is the mineral physically unavailable, or have prices increased? If prices rise, by how much? How flexible or inflexible is demand (that is, how easy or difficult is it to substitute for the restricted mineral)? Finally, time is important. In the short term, mineral users will be relatively limited in the degree to which they can adjust to physical unavailability or higher prices for a mineral or mineral product. Users are constrained by the flexibility of their production processes that use minerals as inputs. Most production processes are relatively inflexible in the short term. A facility that manufactures aluminum cans, for example, cannot immediately reduce the amount of aluminum it uses per can or convert itself into glass bottle making facility. In the medium term, users have somewhat more flexibility. An aluminum can-making facility might be able to invest in existing technology that uses less aluminum per can than its facility currently requires. Alternatively, it might decide to become a glass bottle-making facility. Over
the long term, users of minerals and mineral products will be relatively most flexible to respond to a supply restriction. There is time for a facility that manufactures aluminum cans to innovate and develop a process for using less aluminum per can than previously.
In any of these adjustment periods, the types of possible effects include impacts on:
Domestic production of minerals and mineral products: there may be opportunities for increased domestic production of the mineral or mineral product whose supply has been restricted (higher-cost but previously uneconomic primary or secondary production).
Domestic users of minerals or mineral products (typically producers of semifabricated products and manufacturers of final products):
Lost production due to lack of availability or higher costs (use will be concentrated in higher-valued uses of a mineral or mineral product);
Higher costs of production, which producers may or may not be able to pass along to consumers;
Slower growth than otherwise in emerging-use industries;
Less employment than otherwise in industries using minerals and mineral products as inputs;
Ultimately lower value added in those sectors using minerals and mineral products, and lower gross domestic product (GDP), although the impact on GDP of a supply disruption for any single mineral or mineral product will be small from the perspective of the national economy;
Higher costs or reduced availability of products related to national defense.
Domestic purchasers of goods containing minerals and mineral products: there may be fewer purchases or more expensive purchases because goods have become more expensive (in either case, purchasers are worse off than previously).
The committee did not attempt to quantify these effects. To do so would have required detailed and separate economic impact analyses for each specific circumstance, and the committee was not constituted with sufficient expertise to carry out this type of quantitative analysis. However, the committee notes that the largest impacts on national employment and GDP would come from supply restrictions on minerals and mineral products used in large quantities; of the minerals the committee examined using its criticality methodology, copper falls into this category, even though copper did not qualify as critical in the committee’s eyes because its supply risk is low. Other minerals that the committee believes would be evaluated similarly include iron ore, aluminum, and aggregates.
Understanding Availability and Supply Risk
Fundamentally, minerals are a primary resource in that we obtain them from the Earth’s crust. At any point in time, however, minerals—or more precisely the mineral products obtained from them—are available as secondary resources through recycling of obsolete or discarded products and materials. Finally, from the perspective of a nation, mineral products are available as tertiary resources embodied in imported products or imported scrap. The U.S. economy obtains minerals and mineral products in all three forms—primary, secondary, and tertiary. Although the United States has been and remains an important producer of primary and secondary minerals, it also relies on imports for a number of primary and tertiary minerals.
For primary production worldwide and in the United States, mineral exploration, mining, and mineral processing are sectors whose fortunes change significantly from year to year because of the strong link between mineral demand and economic growth. In periods of especially strong economic growth, mineral use in general expands more quickly than production capacity, tending to drive up mineral prices, whereas in periods of slower growth or recession, mineral use tends to grow more slowly than production capacity and prices tend to fall. Given the fragility of the balance between demand and supply, mineral prices tend to swing significantly
from one year to another. Since early in this decade, the mineral sector overall has experienced an extended boom (and relatively high mineral prices) due to a number of factors, including unexpectedly large increases in mineral demand in China and some other countries and unexpected interruptions in production at a number of mines due to technical problems and other factors.
The level and location of mine production today depend on the level and location of mineral exploration in the past. The level of exploration tends to follow changes in mineral prices, but usually with a short time lag. The composition of exploration activity varies with mineral prices. In recent years during a period of relatively high mineral prices, exploration by small exploration companies (termed “juniors”) in riskier and more remote locations has increased proportionately more than exploration by larger and more established mining companies. Conversely, when mineral prices fall, exploration by junior companies tends to fall proportionately more than that by larger companies, resulting in relatively less exploration in remote locations and more exploration in proximity to existing mines. The geographic location of exploration and mining also evolves over time. In recent years, relatively more exploration and mining has occurred outside the established areas of Australia, Canada, and the United States.
Turning from primary to secondary production, recycling tends to be concentrated close to semifabrication and metal manufacturing facilities and close to urban centers to take advantage of the creation of scrap when buildings are demolished and products are discarded. As a result, most metal recycling occurs in industrialized economies where the majority of metal use historically has occurred. Nevertheless, a significant amount of recycling occurs in developing economies, where perhaps a larger percentage of the available scrap is actually recycled than in industrialized economies. Given the long-term trend of increasing mineral use and low rates of recycling, recycled materials cannot presently meet a large proportion of demand for most materials. Over time, as products used in developing economies become available for recycling, we can expect scrap flows to increase and the location of recycling to become more geographically diverse than at present.
In considering supply risk and implementing the matrix methodology, as noted above, the committee found it essential to distinguish between short- and medium-term availability of minerals and mineral products, on the one hand, and long-term availability, on the other. In the short and medium term , there may be significant restrictions to supply for at least five reasons. First, demand may increase significantly , and if production already is occurring at close to capacity, then either a mineral may become physically unavailable or, more likely, its price will rise significantly—demand can increase more quickly than production capacity can respond. Second, an increase in demand due to growth in new applications of a mineral may be especially restrictive or disruptive if preexisting uses were small relative to the new use ( thin markets ). Third, supply may be prone to restriction if production is concentrated ; if concentrated in a small number of mines, supply may be prone to restriction if unexpected technical or labor problems occur at a mine; if concentrated in the hands of a small number of companies, supply may be prone to restriction by opportunistic behavior of companies with market power; if concentrated in the hands of a small number of producing countries, supply may be prone to restriction due to political decisions in the producing country. Fourth, if mine production comes predominantly in the form of by-product production , then the output over the short term (and perhaps even longer) may be insensitive to changes in market conditions for the by-product because the output of a by-product is largely a function of market conditions for the main product. Finally, the lack of available old scrap for recycling or of the infrastructure required for recycling makes a market more prone to supply restriction than otherwise.
An additional factor, import dependence, often is cited as an indicator of vulnerable supply and has carried the implication that imported supply may be less secure than domestic supply. The committee concludes that import dependence by itself is not a useful indicator of supply risk . In fact, import reliance may be good for the U.S. economy if an imported mineral has a lower cost than the domestic alternative. Rather, for imports to be vulnerable to supply restriction, some other factor must be present that makes them vulnerable to disruption—for example, supply is concentrated
in one or a small number of exporting nations with high political risk or in a nation with such significant growth in internal demand that formerly exported minerals may be redirected toward internal, domestic use. However, imports may be no less secure than domestic supply if they come from a diverse set of countries or firms or if they represent intracompany transfers within the vertical chain of a firm (for example, imported metal concentrate to be smelted and refined at a company’s domestic processing facilities).
Over the longer term , the availability of minerals and mineral prod ucts is largely a function of investment and the various factors that influ ence the level of investment and its geographic allocation and success. An important investment is that in education and research, and the committee suggests that the long-term availability of minerals and mineral products also requires continued investment in mineral education and research .
Education and research contribute to determining long-term mineral availability for both primary and secondary resources in all of their dimensions. For primary resources, the first important dimension is geologic availability (in what quantities, concentrations, and mineralogical forms does a mineral exist in Earth’s crust?). Education and research of course do not determine whether and in what form a mineral occurs in Earth’s crust; rather education and research determine our knowledge of Earth’s crust. The second determinant is technical availability (does the technology exist to extract and process the element or mineral?). Technical availability depends on investment in technological knowledge. The third determinant is environmental and social availability (can we mine and process minerals such that the consequences of these activities on local communities and on the natural environment are consistent with social preferences and requirements?). Environmental and social availability depends on investment in activities that appeal to social preferences and that develop means for carrying out mining and mineral processing in socially acceptable ways. The fourth determinant is political availability (to what extent do public policies influence mineral supply?). Political availability depends on investment in the design of public policy and on the political decisions governments make that influence the level and location of production. The fifth and
final determinant is economic availability (can we produce minerals and mineral products at prices that users are willing and able to pay?). In some sense, economic availability reflects the combined effects of the other four determinants of availability.
For secondary resources over the longer term, availability depends on four of the same above factors. Technology in the secondary resources sector is far behind that in the primary sector, and many gains are to be had by investing additional engineering time and effort. On the environmental and social front, recycling needs to occur with a greater degree of urgency, and making changes in this area is largely a social challenge. Politically, attention needs to be paid to understanding the national implications of resource scarcity, to providing the funds to better characterize the secondary resource, and to better evaluate opportunities for domestic recovery of secondary materials. Finally, it will be necessary to create economic incentives to make better use of the secondary resources now above the ground and in use, but often more costly to use at present than imported virgin material. Well-designed and competently directed research into improved recycling technologies may prove an effective tool in the reduction of our dependence on imports of critical minerals.
Implementing the Mineral Criticality Matrix
The committee applied its criticality matrix methodology to 11 minerals or mineral families it considered candidates for criticality. The committee acknowledges the existence of numerous other minerals that individuals, industrial sectors, organizations, or government officials might consider critical to their particular needs or requirements now or in the future. At a practical level, the committee did not have the resources for comprehensive analysis of all minerals using its methodology.
In evaluating these minerals or mineral families, the committee took a short- and a medium-term perspective—that is, within the next decade, what are the risks of a supply restriction, and how significant would the impact of restrictions be should they occur? Of the 11 minerals or mineral families the committee examined, those that exhibit the highest degree
of criticality at present are: indium, manganese, niobium, PGMs, and REs . The committee studied PGMs and REs in some depth, while it examined indium, manganese, and niobium in a more limited manner. Each of these minerals has a slightly different story in terms of importance in use (impact of a supply restriction) and availability (supply risk), the two dimensions of criticality.
PGMs—consisting primarily of platinum, palladium, and rhodium—are essential in automotive catalysts. Palladium can partially substitute for platinum in gasoline vehicles. Palladium cannot be substituted for platinum in diesel vehicles. Rhodium has no known substitutes in the control of NO x emissions. PGMs also are essential determinants of product quality in several industrial applications (the production of fertilizers, explosives, and petrochemicals). PGMs are mined almost exclusively in South Africa and Russia, and are typically mined as coproducts. The United States has two small PGM mines and a minor quantity of subeconomic PGM resources. Recycling occurs, primarily of spent automotive catalysts, but this amount is modest relative to annual use. The PGM market is relatively small, with annual worldwide mine production on the order of 200,000 kilograms.
REs are essential, with few if any good substitutes, in automotive catalytic converters, permanent magnets, and phosphors used in medical imaging devices, televisions, and computer monitors. The RE market is fragile because it is small—worldwide mine production in 2006 was on the order of 100,000 metric tons. U.S. manufacturers import REs predominantly from China. Very little recycling occurs. The United States has significant RE resources, but at present these resources are subeconomic.
Indium has no adequate substitutes for flat-panel displays. This use has experienced rapid growth in recent years. Worldwide mine production is small—some 500 metric tons in 2006, largely as a by-product of zinc mining and processing. The indium that U.S. manufacturers use comes primarily from China, Canada, Japan, and Russia. Very little indium is recovered through recycling.
Manganese has no satisfactory substitutes as a hardening element in various types of steel. It is not mined at present in the United States. The
majority of U.S. imports comes in the form of ore from Gabon and South Africa and ferromanganese from South Africa, China, Brazil, and France. U.S. manganese resources are subeconomic. Some manganese is recovered as a part of ferrous and nonferrous scrap recovery; almost none of this recovery is for manganese in particular but rather for the steel or other nonferrous metal of which manganese is a minor element.
Niobium is used in carbon, high-strength low-alloy (HSLA), and stainless steels. It also is used in superalloys for aircraft engines. Where substitution is technically possible, performance is sacrificed. Niobium use in HSLA steels has fallen considerably, but has increased in superalloys. Niobium is not mined in the United States, at least not in any significant quantity. U.S. users import the majority of their niobium from Brazil and to a lesser extent from Canada. The niobium market is small; estimated 2006 mine production was on the order of 60,000 metric tons. Known U.S. resources are very small and subeconomic. Significant recycling of niobium from niobium-containing steels and superalloys occurs; very little of this recycling is targeted at niobium in particular but rather for the steel or superalloy itself.
On the basis of these applications of the methodology, the committee concludes that the criticality matrix methodology is a useful conceptual framework for evaluating a mineral’s criticality in a balanced manner in a variety of circumstances that will be useful for decision makers in the public and private sectors . Decision makers should be prepared to reevaluate a mineral’s criticality whenever one of the underlying determinants of criticality changes or appears likely to change. In the short to medium term, the most likely factors to change are, first of all, demand, which could increase sharply if a new application is developed for a specific mineral and, second, the degree to which a mineral’s production is concentrated in a small number of companies or countries, which in turn might be prone to opportunistic behavior. A more nuanced and quantitative version of the matrix could be established and used as part of the federal program for mineral data collection, analysis, and dissemination.
Assessing Information and Research Needs
In the progress of this study, the committee has frequently compared the constrained scope and depth of information on minerals with the broad scope and great depth of financial information acquired and analyzed by the federal government. The usefulness of this financial information by governments, industries, and many other users suggests that an enhanced information program on minerals could be more broadly and deeply beneficial as well. The mineral information available at present is used widely but is also acknowledged to be considerably less detailed than is desirable. This is particularly the case for mineral information related to other countries, where high-quality data are essential for accurate determinations of criticality for U.S. industries and for the country as a whole.
A large number of government and nongovernmental, international, and domestic organizations collect and disseminate information and databases relevant for decision making on critical minerals and other mineral policy issues for public and private use. The consensus view of private, academic, and federal professionals is that the U.S. Geological Survey (USGS) Minerals Information Team is the most comprehensive, responsible, and responsive source of mineral information internationally, but that the quantity and depth of its data and analysis have fallen in recent years, due at least in part to reduced or static budgets and associated reductions in staff and data coverage.
In its evaluation of information and research needs, the committee concludes the following:
Decision makers in both the public and the private sectors need continuous, unbiased, and thorough mineral information pro vided through a federally funded system of information collec tion and dissemination.
The effectiveness of a government agency or program is de pendent on the agency’s or program’s autonomy, its level of resources, and its authority to enforce data collection. In the committee’s view, federal information gathering for minerals at
present does not have sufficient authority and autonomy to ap propriately carry out data collection, dissemination, and analy sis. In particular, the committee concludes that USGS Minerals Information Team activities are less robust than they might be, in part because it does not have status as a “principal” statistical agency.
More complete information needs to be collected, and more re search needs to be conducted, on the full mineral life cycle. The committee includes its specific recommendations in the following section. A common theme in these recommendations is the value of an investment in material flow accounting to better quantify stocks, flows, and uncertainty for primary, secondary, and tertiary resources.
RECOMMENDATIONS
Recognizing the dynamic nature of mineral supply and demand and of criticality, and in light of the conclusions above, the committee makes the following recommendations:
The federal government should enhance the types of data and infor mation it collects, disseminates, and analyzes on minerals and mineral products, especially as these data and information relate to minerals and mineral products that are or may become critical.
In particular, more attention than at present needs to be given to those areas of the mineral life cycle that are underrepresented in current information-gathering activities, including: reserves and subeconomic resources; by-product and coproduct primary production; stocks and flows of secondary material available for recycling; in-use stocks; material flows; international trade, especially of metals and mineral products embodied in imported and exported products; and related information deemed appropriate and necessary. Enhanced mineral analysis should include periodic assessment of mineral criticality over a wider range of minerals and in
greater depth than was possible for this committee to undertake, using the committee’s methodology or some other suitable method.
The federal government should continue to carry out the neces sary function of collecting, disseminating, and analyzing mineral data and information. The USGS Minerals Information Team, or whatever federal unit might later be assigned these responsibilities, should have greater authority and autonomy than at present. It also should have suf ficient resources to carry out its mandate, which would be broader than the Minerals Information Team’s current mandate if the committee’s recommendations are adopted. It should establish formal mechanisms for communicating with users, government and nongovernmental or ganizations or institutes, and the private sector on the types and quality of data and information it collects, disseminates, and analyzes. It should be organized to have the flexibility to collect, disseminate, and analyze additional, nonbasic data and information, in consultation with users, as specific minerals and mineral products become relatively more critical over time (and vice versa).
The Energy Information Administration provides a potential model for such an agency or administrative unit. The federal government should consider whether a comparable mineral information administration would have status as a principal statistical agency and, if not, what other procedures should be investigated and implemented to give an agency with the mandate to collect mineral data and information greater autonomy and authority, as well as sufficient resources to carry out its mandate. In the globalized mineral market, it is essential that the United States has a central authority through which to conduct outreach and exchange programs on mineral data with international counterparts and to collect and harmonize data from international sources. Combined U.S. government and foreign government efforts are likely to provide the most accurate, uniform, and complete data sets of this information over time and thereby provide adequate information to all communities concerned about future global mineral or material supply and demand trends.
Federal agencies, including the National Science Foundation, De partment of the Interior (including the USGS), Department of Defense, Department of Energy, and Department of Commerce, should develop and fund activities, including basic science and policy research, to en courage U.S. innovation in the areas of critical minerals and materials and to enhance understanding of global mineral availability and use.
Without renewed federal commitment to innovative mineral research and education, it is doubtful whether the recommended activities regarding mineral information will be sufficient for the nation to successfully anticipate and respond to possible short- to long-term restrictions in mineral markets.
The committee recommends the following additional initiatives in this regard:
Funded support for scientific, technical, and social scientific research focusing on the entire mineral life cycle, especially those specific areas identified in Recommendation 1; and
Cooperative programs involving academic organizations, industry, and government to enhance education and applied research.
This page intentionally left blank.
Minerals are part of virtually every product we use. Common examples include copper used in electrical wiring and titanium used to make airplane frames and paint pigments. The Information Age has ushered in a number of new mineral uses in a number of products including cell phones (e.g., tantalum) and liquid crystal displays (e.g., indium). For some minerals, such as the platinum group metals used to make cataytic converters in cars, there is no substitute. If the supply of any given mineral were to become restricted, consumers and sectors of the U.S. economy could be significantly affected. Risks to minerals supplies can include a sudden increase in demand or the possibility that natural ores can be exhausted or become too difficult to extract. Minerals are more vulnerable to supply restrictions if they come from a limited number of mines, mining companies, or nations. Baseline information on minerals is currently collected at the federal level, but no established methodology has existed to identify potentially critical minerals. This book develops such a methodology and suggests an enhanced federal initiative to collect and analyze the additional data needed to support this type of tool.
Welcome to OpenBook!
You're looking at OpenBook, NAP.edu's online reading room since 1999. Based on feedback from you, our users, we've made some improvements that make it easier than ever to read thousands of publications on our website.
Do you want to take a quick tour of the OpenBook's features?
Show this book's table of contents , where you can jump to any chapter by name.
...or use these buttons to go back to the previous chapter or skip to the next one.
Jump up to the previous page or down to the next one. Also, you can type in a page number and press Enter to go directly to that page in the book.
Switch between the Original Pages , where you can read the report as it appeared in print, and Text Pages for the web version, where you can highlight and search the text.
To search the entire text of this book, type in your search term here and press Enter .
Share a link to this book page on your preferred social network or via email.
View our suggested citation for this chapter.
Ready to take your reading offline? Click here to buy this book in print or download it as a free PDF, if available.
Get Email Updates
Do you enjoy reading reports from the Academies online for free ? Sign up for email notifications and we'll let you know about new publications in your areas of interest when they're released.
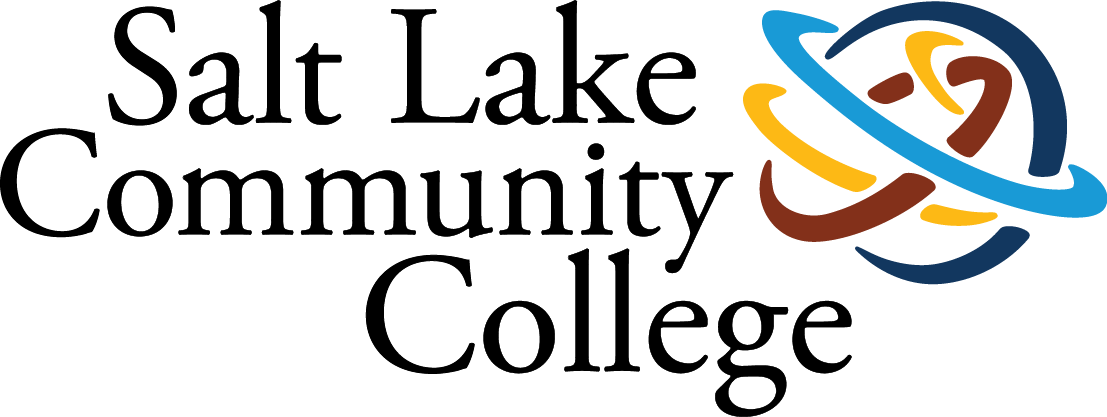
Want to create or adapt books like this? Learn more about how Pressbooks supports open publishing practices.
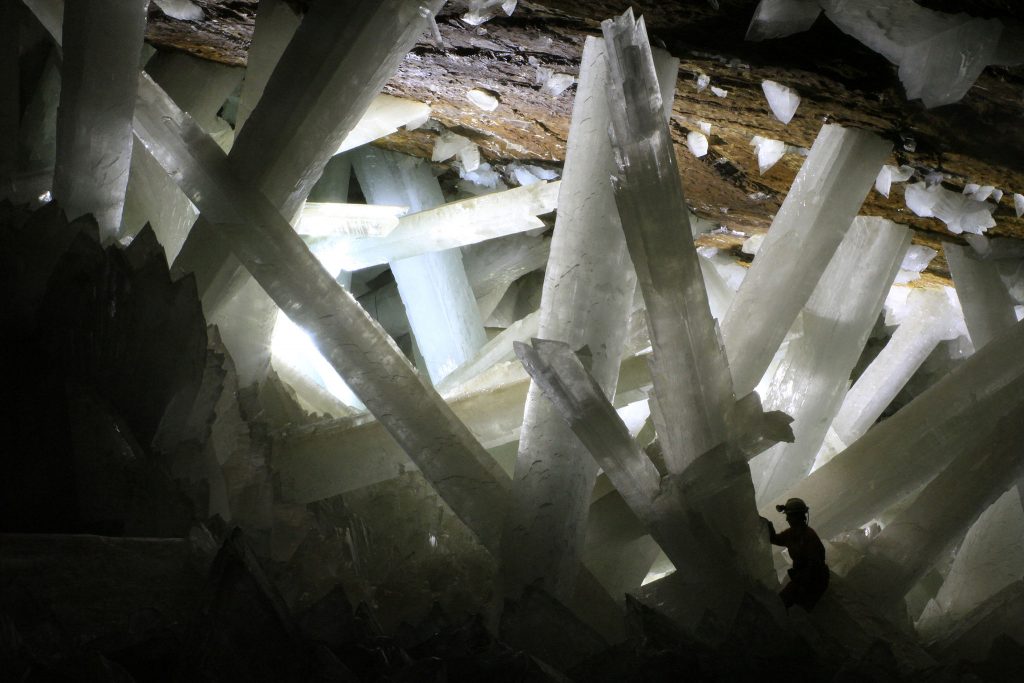
KEY CONCEPTS
At the end of this chapter, students should be able to:
- Define mineral .
- Describe the basic structure of the atom.
- Derive basic atomic information from the Periodic Table of Elements .
- Describe chemical bonding related to minerals .
- Describe the main ways minerals form.
- Describe the silicon-oxygen tetrahedron and how it forms common silicate minerals .
- List common non- silicate minerals in oxide , sulfide , sulfate , and carbonate groups.
- Identify minerals using physical properties and identification tables.
The term “ minerals ” as used in nutrition labels and pharmaceutical products is not the same as a mineral in a geological sense. In geology, the classic definition of a mineral is: 1) naturally occurring, 2) inorganic, 3) solid at room temperature , 4) regular crystal structure, and 5) defined chemical composition . Some natural substances technically should not be considered minerals , but are included by exception. For example, water and mercury are liquid at room temperature . Both are considered minerals because they were classified before the room- temperature rule was accepted as part of the definition. Calcite is quite often formed by organic processes, but is considered a mineral because it is widely found and geologically important. Because of these discrepancies, the International Mineralogical Association in 1985 amended the definition to: “A mineral is an element or chemical compound that is normally crystalline and that has been formed as a result of geological processes.” This means that the calcite in the shell of a clam is not considered a mineral . But once that clam shell undergoes burial, diagenesis , or other geological processes, then the calcite is considered a mineral . Typically, substances like coal , pearl, opal, or obsidian that do not fit the definition of mineral are called mineraloids.
A rock is a substance that contains one or more minerals or mineraloids. As is discussed in later chapters, there are three types of rocks composed of minerals : igneous (rocks crystallizing from molten material), sedimentary (rocks composed of products of mechanical weathering (sand, gravel, etc.) and chemical weathering (things precipitated from solution ), and metamorphic (rocks produced by alteration of other rocks by heat and pressure.
3.1 Chemistry of Minerals
Rocks are composed of minerals that have a specific chemical composition . To understand mineral chemistry, it is essential to examine the fundamental unit of all matter, the atom.
3.1.1 The Atom
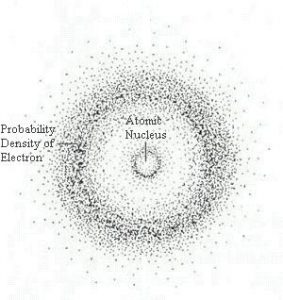
Matter is made of atoms. Atoms consists of subatomic particles— protons , neutrons , and electrons . A simple model of the atom has a central nucleus composed of protons, which have positive charges, and neutrons which have no charge. A cloud of negatively charged electrons surrounds the nucleus, the number of electrons equaling the number of protons thus balancing the positive charge of the protons for a neutral atom. Protons and neutrons each have a mass number of 1. The mass of an electron is less than 1/1000 th that of a proton or neutron, meaning most of the atom’s mass is in the nucleus.
3.1.2 Periodic Table of the Elements
Matter is composed of elements which are atoms that have a specific number of protons in the nucleus. This number of protons is called the Atomic Number for the element . For example, an oxygen atom has 8 protons and an iron atom has 26 protons. An element cannot be broken down chemically into a simpler form and retains unique chemical and physical properties. Each element behaves in a unique manner in nature. This uniqueness led scientists to develop a periodic table of the elements , a tabular arrangement of all known elements listed in order of their atomic number.
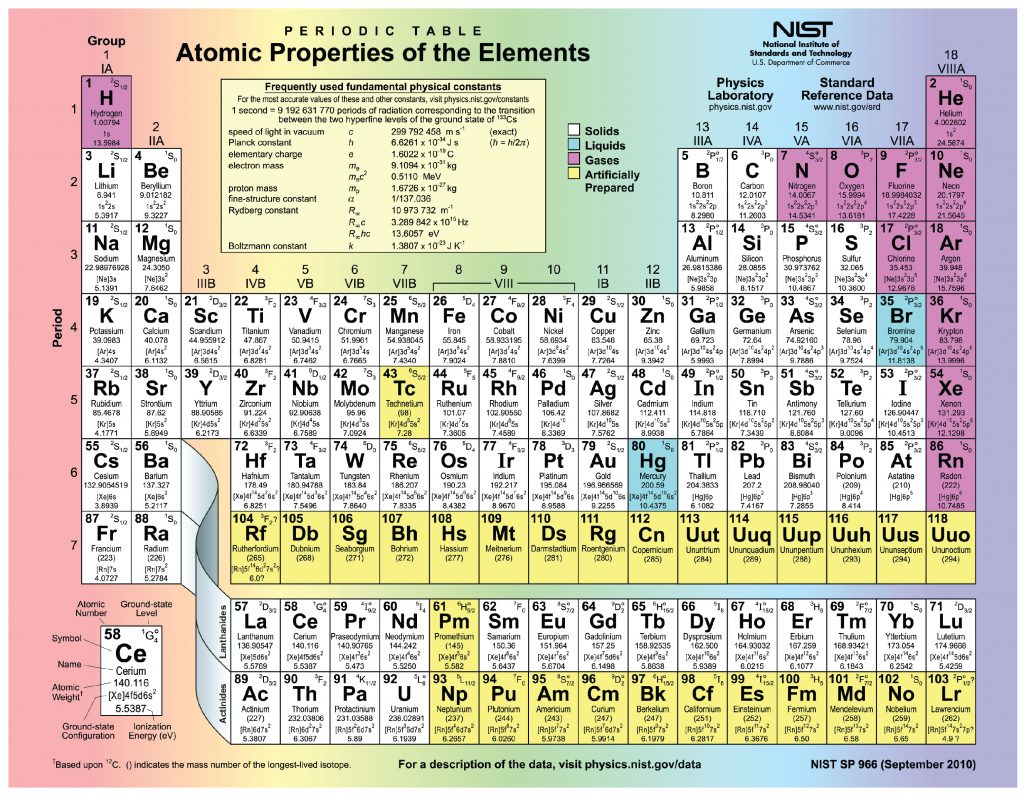
The first arrangement of elements into a periodic table was done by Dmitri Mendeleev in 1869 using the elements known at the time . In the periodic table, each element has a chemical symbol, name, atomic number, and atomic mass. The chemical symbol is an abbreviation for the element , often derived from a Latin or Greek name for the substance . The atomic number is the number of protons in the nucleus. The atomic mass is the number of protons and neutrons in the nucleus, each with a mass number of one. Since the mass of electrons is so much less than the protons and neutrons, the atomic mass is effectively the number of protons plus neutrons.
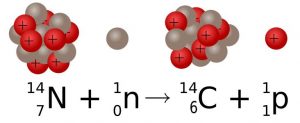
The atomic mass of natural elements represents an average mass of the atoms comprising that substance in nature and is usually not a whole number as seen on the periodic table, meaning that an element exists in nature with atoms having different numbers of neutrons. The differing number of neutrons affects the mass of an element in nature and the atomic mass number represents this average. This gives rise to the concept of isotope . Isotopes are forms of an element with the same number of protons but different numbers of neutrons. There are usually several isotopes for a particular element . For example, 98.9% of carbon atoms have 6 protons and 6 neutrons. This isotope of carbon is called carbon-12 ( 12 C). A few carbon atoms, carbon-13 ( 13 C), have 6 protons and 7 neutrons. A trace amount of carbon atoms, carbon-14 ( 14 C), has 6 protons and 8 neutrons.
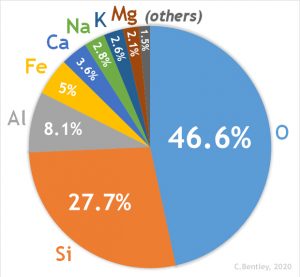
Among the 118 known elements , the heaviest are fleeting human creations known only in high energy particle accelerators, and they decay rapidly. The heaviest naturally occurring element is uranium, atomic number 92. The eight most abundant elements in Earth’s continental crust are shown in Table 1 . These elements are found in the most common rock forming minerals .
Table 1. Eight Most Abundant Elements in the Earth’s Continental Crust % by weight (source: USGS ). All other elements are less than 1%.
3.1.3 Chemical Bonding
Most substances on Earth are compounds containing multiple elements . Chemical bonding describes how these atoms attach with each other to form compounds, such as sodium and chlorine combining to form NaCl, common table salt. Compounds that are held together by chemical bonds are called molecules. Water is a compound of hydrogen and oxygen in which two hydrogen atoms are covalently bonded with one oxygen making the water molecule. The oxygen we breathe is formed when one oxygen atom covalently bonds with another oxygen atom to make the molecule O 2 . The subscript 2 in the chemical formula indicates the molecule contains two atoms of oxygen.
Most minerals are also compounds of more than one element . The common mineral calcite has the chemical formula CaCO 3 indicating the molecule consists of one calcium, one carbon, and three oxygen atoms. In calcite , one carbon and three oxygen atoms are held together by covalent bonds to form a molecular ion , called carbonate , which has a negative charge. Calcium as an ion has a positive charge of plus two. The two oppositely charged ions attract each other and combine to form the mineral calcite , CaCO3. The name of the chemical compound is calcium carbonate , where calcium is Ca and carbonate refers to the molecular ion CO 3 -2 .
The mineral olivine has the chemical formula (Mg,Fe) 2 SiO 4 , in which one silicon and four oxygen atoms are bonded with two atoms of either magnesium or iron. The comma between iron (Fe) and magnesium (Mg) indicates the two elements can occupy the same location in the crystal structure and substitute for one another.
3.1.3.1 Valence and Charge
The electrons around the atom’s nucleus are located in shells representing different energy levels. The outermost shell is called the valence shell . Electrons in the valence shell are involved in chemical bonding . In 1913, Niels Bohr proposed a simple model of the atom that states atoms are more stable when their outermost shell is full . Atoms of most elements thus tend to gain or lose electrons so the outermost or valence shell is full. In Bohr’s model, the innermost shell can have a maximum of two electrons and the second and third shells can have a maximum of eight electrons. When the innermost shell is the valence shell, as in the case of hydrogen and helium, it obeys the octet rule when it is full with two electrons. For elements in higher rows, the octet rule of eight electrons in the valence shell applies.
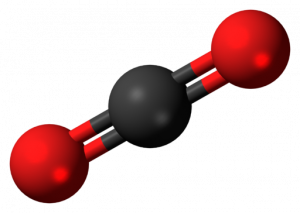
The rows in the periodic table present the elements in order of atomic number and the columns organize elements with similar characteristics, such as the same number of electrons in their valence shells. Columns are often labeled from left to right with Roman numerals I to VIII, and Arabic numerals 1 through 18. The elements in columns I and II have 1 and 2 electrons in their respective valence shells and the elements in columns VI and VII have 6 and 7 electrons in their respective valence shells.
In row 3 and column I, sodium (Na) has 11 protons in the nucleus and 11 electrons in three shells—2 electrons in the inner shell, 8 electrons in the second shell, and 1 electron in the valence shell. To maintain a full outer shell of 8 electrons per the octet rule , sodium readily gives up that 1 electron so there are 10 total electrons. With 11 positively charged protons in the nucleus and 10 negatively charged electrons in two shells, sodium when forming chemical bonds is an ion with an overall net charge of +1 .
All elements in column I have a single electron in their valence shell and a valence of 1. These other column I elements also readily give up this single valence electron and thus become ions with a +1 charge. Elements in column II readily give up 2 electrons and end up as ions with a charge of +2. Note that elements in columns I and II which readily give up their valence electrons, often form bonds with elements in columns VI and VII which readily take up these electrons. Elements in columns 3 through 15 are usually involved in covalent bonding . The last column 18 (VIII) contains the noble gases . These elements are chemically inert because the valence shell is already full with 8 electrons, so they do not gain or lose electrons. An example is the noble gas helium which has 2 valence electrons in the first shell. Its valence shell is therefore full. All elements in column VIII possess full valence shells and do not form bonds with other elements .
As seen above, an atom with a net positive or negative charge as a result of gaining or losing electrons is called an ion . In general the elements on the left side of the table lose electrons and become positive ions, called cations because they are attracted to the cathode in an electrical device. The elements on the right side tend to gain electrons. These are called anions because they are attracted to the anode in an electrical device. The elements in the center of the periodic table, columns 3 through 15, do not consistently follow the octet rule . These are called transition elements . A common example is iron, which has a +2 or +3 charge depending on the oxidation state of the element . Oxidized Fe +3 carries a +3 charge and reduced Fe +2 is +2. These two different oxidation states of iron often impart dramatic colors to rocks containing their minerals —the oxidized form producing red colors and the reduced form producing green.
3.1.3.2 Ionic Bonding
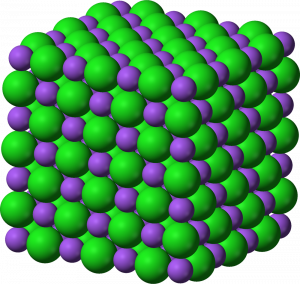
Ionic bonds , also called electron-transfer bonds , are formed by the electrostatic attraction between atoms having opposite charges. Atoms of two opposite charges attract each other electrostatically and form an ionic bond in which the positive ion transfers its electron (or electrons) to the negative ion which takes them up. Through this transfer both atoms thus achieve a full valence shell. For example one atom of sodium (Na +1 ) and one atom of chlorine (Cl -1 ) form an ionic bond to make the compound sodium chloride (NaCl). This is also known as the mineral halite or common table salt. Another example is calcium (Ca +2 ) and chlorine (Cl -1 ) combining to make the compound calcium chloride (CaCl 2 ). The subscript 2 indicates two atoms of chlorine are ionically bonded to one atom of calcium.
3.1.3.3 Covalent Bonding
Ionic bonds are usually formed between a metal and a nonmetal . Another type, called a covalent or electron-sharing bond , commonly occurs between nonmetals. Covalent bonds share electrons between ions to complete their valence shells. For example, oxygen (atomic number 8) has 8 electrons—2 in the inner shell and 6 in the valence shell. Gases like oxygen often form diatomic molecules by sharing valence electrons. In the case of oxygen, two atoms attach to each other and share 2 electrons to fill their valence shells to become the common oxygen molecule we breathe (O 2 ). Methane (CH 4 ) is another covalently bonded gas. The carbon atom needs 4 electrons and each hydrogen needs 1. Each hydrogen shares its electron with the carbon to form a molecule as shown in the figure.
Take this quiz to check your comprehension of this section.
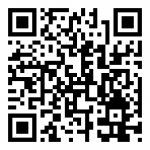
3.2 Formation of Minerals
Minerals form when atoms bond together in a crystalline arrangement. Three main ways this occurs in nature are: 1) precipitation directly from an aqueous (water) solution with a temperature change, 2) crystallization from a magma with a temperature change, and 3) biological precipitation by the action of organisms.
3.2.1 Precipitation from aqueous solution
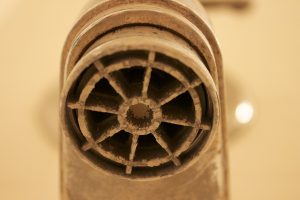
Solutions consist of ions or molecules, known as solutes, dissolved in a medium or solvent. In nature this solvent is usually water. Many minerals can be dissolved in water, such as halite or table salt, which has the composition sodium chloride, NaCl. The Na +1 and Cl -1 ions separate and disperse into the solution .
Precipitation is the reverse process, in which ions in solution come together to form solid minerals . Precipitation is dependent on the concentration of ions in solution and other factors such as temperature and pressure. The point at which a solvent cannot hold any more solute is called saturation . Precipitation can occur when the temperature of the solution falls , when the solute evaporates, or with changing chemical conditions in the solution . An example of precipitation in our homes is when water evaporates and leaves behind a rind of minerals on faucets, shower heads, and drinking glasses.
In nature, changes in environmental conditions may cause the minerals dissolved in water to form bonds and grow into crystals or cement grains of sediment together. In Utah, deposits of tufa formed from mineral -rich springs that emerged into the ice age Lake Bonneville. Now exposed in dry valleys, this porous tufa was a natural insulation used by pioneers to build their homes with a natural protection against summer heat and winter cold. The travertine terraces at Mammoth Hot Springs in Yellowstone Park are another example formed by calcite precipitation at the edges of the shallow spring -fed ponds.
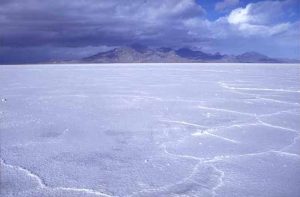
Another example of precipitation occurs in the Great Salt Lake, Utah, where the concentration of sodium chloride and other salts is nearly eight times greater than in the world’s oceans [zotpressInText item=”{DU5CMSHJ}” format=”%num%” brackets=”yes”] . Streams carry salt ions into the lake from the surrounding mountains. With no other outlet, the water in the lake evaporates and the concentration of salt increases until saturation is reached and the minerals precipitate out as sediments . Similar salt deposits include halite and other precipitates, and occur in other lakes like Mono Lake in California and the Dead Sea.
3.2.2 Crystallization from Magma
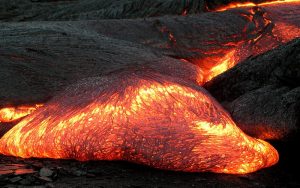
Heat is energy that causes atoms in substances to vibrate. Temperature is a measure of the intensity of the vibration. If the vibrations are violent enough, chemical bonds are broken and the crystals melt releasing the ions into the melt. Magma is molten rock with freely moving ions. When magma is emplaced at depth or extruded onto the surface (then called lava ), it starts to cool and mineral crystals can form.
3.2.3 Precipitation by Organisms
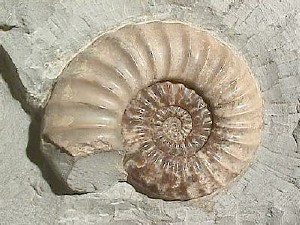
Many organisms build bones, shells, and body coverings by extracting ions from water and precipitating minerals biologically. The most common mineral precipitated by organisms is calcite , or calcium carbonate (CaCO3). Calcite is often precipitated by organisms as a polymorph called aragonite. Polymorphs are crystals with the same chemical formula but different crystal structures. Marine invertebrates such as corals and clams precipitate aragonite or calcite for their shells and structures. Upon death, their hard parts accumulate on the ocean floor as sediments , and eventually may become the sedimentary rock limestone . Though limestone can form inorganically, the vast majority is formed by this biological process. Another example is marine organisms called radiolaria, which are zooplankton that precipitate silica for their microscopic external shells. When the organisms die, the shells accumulate on the ocean floor and can form the sedimentary rock chert . An example of biologic precipitation from the vertebrate world is bone, which is composed mostly of a type of apatite, a mineral in the phosphate group. The apatite found in bones contains calcium and water in its structure and is called hydroxycarbonate apatite, Ca 5 (PO 4 ) 3 (OH). As mentioned above, such substances are not technically minerals until the organism dies and these hard parts become fossils .
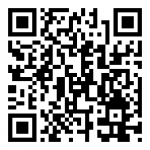
3.3 Silicate Minerals
Minerals are categorized based on their composition and structure. Silicate minerals are built around a molecular ion called the silicon-oxygen tetrahedron . A tetrahedron has a pyramid-like shape with four sides and four corners. Silicate minerals form the largest group of minerals on Earth, comprising the vast majority of the Earth’s mantle and crust . Of the nearly four thousand known minerals on Earth, most are rare. There are only a few that make up most of the rocks likely to be encountered by surface dwelling creatures like us. These are generally called the rock-forming minerals .
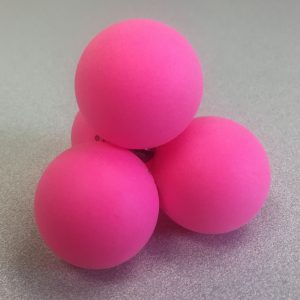
The silicon-oxygen tetrahedron (SiO 4 ) consists of a single silicon atom at the center and four oxygen atoms located at the four corners of the tetrahedron. Each oxygen ion has a -2 charge and the silicon ion has a +4 charge. The silicon ion shares one of its four valence electrons with each of the four oxygen ions in a covalent bond to create a symmetrical geometric four-sided pyramid figure. Only half of the oxygen’s valence electrons are shared, giving the silicon-oxygen tetrahedron an ionic charge of -4. This silicon-oxygen tetrahedron forms bonds with many other combinations of ions to form the large group of silicate minerals .
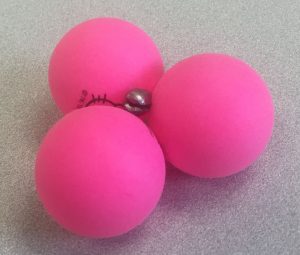
The silicon ion is much smaller than the oxygen ions (see the figures) and fits into a small space in the center of the four large oxygen ions, seen if the top ball is removed (as shown in the figure to the right). Because only one of the valence electrons of the corner oxygens is shared, the silicon-oxygen tetrahedron has chemically active corners available to form bonds with other silica tetrahedra or other positively charged ions such as Al +3 , Fe +2,+3 , Mg +2 , K +1 , Na +1 , and Ca +2 . Depending on many factors, such as the original magma chemistry, silica-oxygen tetrahedra can combine with other tetrahedra in several different configurations. For example, tetrahedra can be isolated, attached in chains, sheets, or three dimensional structures. These combinations and others create the chemical structure in which positively charged ions can be inserted for unique chemical compositions forming silicate mineral groups.
3.3.1 The dark ferromagnesian silicates
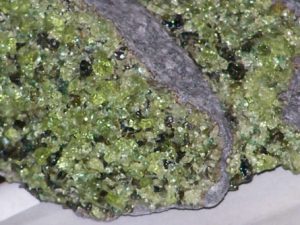
The Olivine Family
Olivine is the primary mineral component in mantle rock such as peridotite and basalt . It is characteristically green when not weathered. The chemical formula is (Fe,Mg) 2 SiO 4 . As previously described, the comma between iron (Fe) and magnesium (Mg) indicates these two elements occur in a solid solution . Not to be confused with a liquid solution , a solid solution occurs when two or more elements have similar properties and can freely substitute for each other in the same location in the crystal structure.
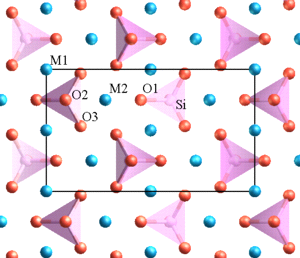
Olivine is referred to as a mineral family because of the ability of iron and magnesium to substitute for each other. Iron and magnesium in the olivine family indicates a solid solution forming a compositional series within the mineral group which can form crystals of all iron as one end member and all mixtures of iron and magnesium in between to all magnesium at the other end member. Different mineral names are applied to compositions between these end members. In the olivine series of minerals , the iron and magnesium ions in the solid solution are about the same size and charge, so either atom can fit into the same location in the growing crystals. Within the cooling magma , the mineral crystals continue to grow until they solidify into igneous rock . The relative amounts of iron and magnesium in the parent magma determine which minerals in the series form. Other rarer elements with similar properties to iron or magnesium, like manganese (Mn), can substitute into the olivine crystalline structure in small amounts. Such ionic substitutions in mineral crystals give rise to the great variety of minerals and are often responsible for differences in color and other properties within a group or family of minerals . Olivine has a pure iron end-member (called fayalite) and a pure magnesium end-member (called forsterite). Chemically, olivine is mostly silicon, oxygen, iron, and magnesium and therefore is grouped among the dark-colored ferromagnesian (iron=ferro, magnesium=magnesian) or mafic minerals , a contraction of their chemical symbols Ma and Fe. Mafic minerals are also referred to as dark-colored ferromagnesian minerals . Ferro means iron and magnesian refers to magnesium. Ferromagnesian silicates tend to be more dense than non-ferromagnesian silicates . This difference in density ends up being important in controlling the behavior of the igneous rocks that are built from these minerals : whether a tectonic plate subducts or not is largely governed by the density of its rocks, which are in turn controlled by the density of the minerals that comprise them.
The crystal structure of olivine is built from independent silica tetrahedra . Minerals with independent tetrahedral structures are called neosilicates (or orthosilicates). In addition to olivine , other common neosilicate minerals include garnet, topaz, kyanite, and zircon .
Two other similar arrangements of tetrahedra are close in structure to the neosilicates and grade toward the next group of minerals , the pyroxenes. In a variation on independent tetrahedra called sorosilicates, there are minerals that share one oxygen between two tetrahedra, and include minerals like pistachio-green epidote, a gemstone. Another variation are the cyclosilicates, which as the name suggests, consist of tetrahedral rings, and include gemstones such as beryl, emerald, aquamarine, and tourmaline
3.3.2 Pyroxene Family
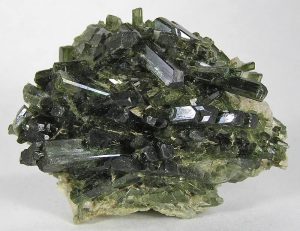
Pyroxene is another family of dark ferromagnesian minerals , typically black or dark green in color. Members of the pyroxene family have a complex chemical composition that includes iron, magnesium, aluminum, and other elements bonded to polymerized silica tetrahedra . Polymers are chains, sheets, or three-dimensional structures, and are formed by multiple tetrahedra covalently bonded via their corner oxygen atoms. Pyroxenes are commonly found in mafic igneous rocks such as peridotite , basalt , and gabbro , as well as metamorphic rocks like eclogite and blue schist .
Pyroxenes are built from long, single chains of polymerized silica tetrahedra in which tetrahedra share two corner oxygens. The silica chains are bonded together into the crystal structures by metal cations. A common member of the pyroxene family is augite, itself containing several solid solution series with a complex chemical formula (Ca,Na)(Mg,Fe,Al,Ti)(Si,Al) 2 O 6 that gives rise to a number of individual mineral names.
This single-chain crystalline structure bonds with many elements , which can also freely substitute for each other. The generalized chemical composition for pyroxene is XZ(Al,Si) 2 O 6 . X represents the ions Na, Ca, Mg, or Fe, and Z represents Mg, Fe, or Al. These ions have similar ionic sizes, which allows many possible substitutions among them. Although the cations may freely substitute for each other in the crystal, they carry different ionic charges that must be balanced out in the final crystalline structure. For example Na has a charge of +1, but Ca has charge of +2. If a Na + ion substitutes for a Ca +2 ion , it creates an unequal charge that must be balanced by other ionic substitutions elsewhere in the crystal. Note that ionic size is more important than ionic charge for substitutions to occur in solid solution series in crystals.
3.3.3 Amphibole Family
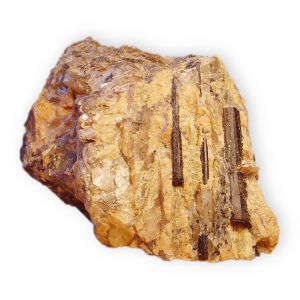
Amphibole minerals are built from polymerized double silica chains and they are also referred to as inosilicates. Imagine two pyroxene chains that connect together by sharing a third oxygen on each tetrahedra. Amphiboles are usually found in igneous and metamorphic rocks and typically have a long-bladed crystal habit . The most common amphibole , hornblende, is usually black; however, they come in a variety of colors depending on their chemical composition . The metamorphic rock , amphibolite, is primarily composed of amphibole minerals .
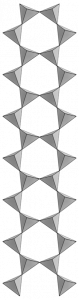
Amphiboles are composed of iron, magnesium, aluminum, and other cations bonded with silica tetrahedra . These dark ferromagnesian minerals are commonly found in gabbro , baslt, diorite , and often form the black specks in granite . Their chemical formula is very complex and generally written as (RSi 4 O 11 ) 2 , where R represents many different cations . For example, it can also be written more exactly as AX 2 Z 5 ((Si,Al,Ti) 8 O 22 )(OH,F,Cl,O) 2 . In this formula A may be Ca, Na, K, Pb, or blank; X equals Li, Na, Mg, Fe, Mn, or Ca; and Z is Li, Na, Mg, Fe, Mn, Zn, Co, Ni, Al, Cr, Mn, V, Ti, or Zr. The substitutions create a wide variety of colors such as green, black, colorless, white, yellow, blue, or brown. Amphibole crystals can also include hydroxide ions (OH – ) , which occurs from an interaction between the growing minerals and water dissolved in magma .
3.3.4 Sheet Silicates
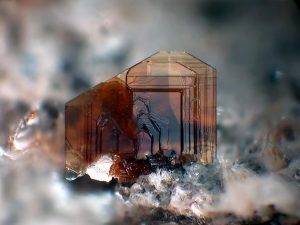
Sheet silicates are built from tetrahedra which share all three of their bottom corner oxygens thus forming sheets of tetrahedra with their top corners available for bonding with other atoms. Micas and clays are common types of sheet silicates , also known as phyllosilicates. Mica minerals are usually found in igneous and metamorphic rocks, while clay minerals are more often found in sedimentary rocks. Two frequently found micas are dark-colored biotite , frequently found in granite , and light-colored muscovite , found in the metamorphic rock called schist .
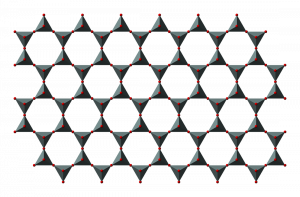
Chemically, sheet silicates usually contain silicon and oxygen in a 2:5 ratio (Si 4 O 10 ). Micas contain mostly silica, aluminum, and potassium. Biotite mica has more iron and magnesium and is considered a ferromagnesian silicate mineral . Muscovite micas belong to the felsic silicate minerals . Felsic is a contraction formed from feldspar , the dominant mineral in felsic rocks.
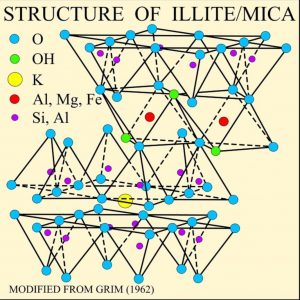
The illustration of the crystalline structure of mica shows the corner O atoms bonded with K, Al, Mg, Fe, and Si atoms, forming polymerized sheets of linked tetrahedra, with an octahedral layer of Fe, Mg, or Al, between them. The yellow potassium ions form Van der Waals bonds (attraction and repulsion between atoms, molecules, and surfaces) and hold the sheets together. Van der Waals bonds differ from covalent and ionic bonds , and exist here between the sandwiches, holding them together into a stack of sandwiches. The Van der Waals bonds are weak compared to the bonds within the sheets, allowing the sandwiches to be separated along the potassium layers. This gives mica its characteristic property of easily cleaving into sheets.
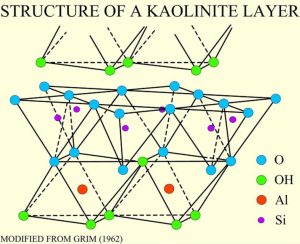
Clays minerals occur in sediments formed by the weathering of rocks and are another family of silicate minerals with a tetrahedral sheet structure. Clay minerals form a complex family, and are an important component of many sedimentary rocks. Other sheet silicates include serpentine and chlorite, found in metamorphic rocks.
Clay minerals are composed of hydrous aluminum silicates . One type of clay, kaolinite, has a structure like an open-faced sandwich, with the bread being a single layer of silicon-oxygen tetrahedra and a layer of aluminum as the spread in an octahedral configuration with the top oxygens of the sheets.
3.3.5 Framework Silicates
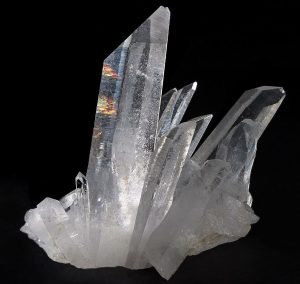
Quartz and feldspar are the two most abundant minerals in the continental crust . In fact, feldspar itself is the single most abundant mineral in the Earth’s crust . There are two types of feldspar , one containing potassium and abundant in felsic rocks of the continental crust , and the other with sodium and calcium abundant in the mafic rocks of oceanic crust . Together with quartz , these minerals are classified as framework silicates . They are built with a three-dimensional framework of silica tetrahedra in which all four corner oxygens are shared with adjacent tetrahedra. Within these frameworks in feldspar are holes and spaces into which other ions like aluminum, potassium, sodium, and calcium can fit giving rise to a variety of mineral compositions and mineral names.
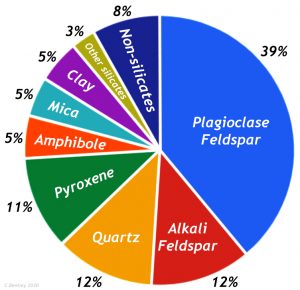
Feldspars are usually found in igneous rocks, such as granite , rhyolite , and basalt as well as metamorphic rocks and detrital sedimentary rocks. Detrital sedimentary rocks are composed of mechanically weathered rock particles, like sand and gravel. Quartz is especially abundant in detrital sedimentary rocks because it is very resistant to disintegration by weathering . While quartz is the most abundant mineral on the Earth’s surface, due to its durability, the feldspar minerals are the most abundant minerals in the Earth’s crust , comprising roughly 50% of the total minerals that make up the crust .
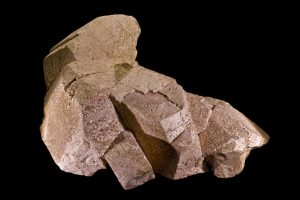
Quartz is composed of pure silica, SiO 2 , with the tetrahedra arranged in a three dimensional framework. Impurities consisting of atoms within this framework give rise to many varieties of quartz among which are gemstones like amethyst, rose quartz , and citrine. Feldspars are mostly silicon, oxygen, aluminum, potassium, sodium, and calcium. Orthoclase feldspar (KAlSi 3 O 8 ), also called potassium feldspar or K-spar , is made of silica, aluminum, and potassium. Quartz and orthoclase feldspar are felsic minerals . Felsic is the compositional term applied to continental igneous minerals and rocks that contain an abundance of silica. Another feldspar is plagioclase with the formula (Ca,Na)AlSi 3 O 8 , the solid solution (Ca,Na) indicating a series of minerals , one end of the series with calcium CaAl 2 Si 2 O 8 , called anorthite, and the other end with sodium NaAlSi 3 O 8 , called albite. Note how the mineral accommodates the substitution of Ca ++ and Na + . Minerals in this solid solution series have different mineral names.
Note that aluminum, which has a similar ionic size to silicon, can substitute for silicon inside the tetrahedra (see figure). Because potassium ions are so much larger than sodium and calcium ions, which are very similar in size, the inability of the crystal lattice to accommodate both potassium and sodium/calcium gives rise to the two families of feldspar , orthoclase and plagioclase respectively. Framework silicates are called tectosilicates and include the alkali metal-rich feldspathoids and zeolites.
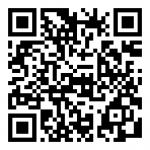
3.4 Non-Silicate Minerals
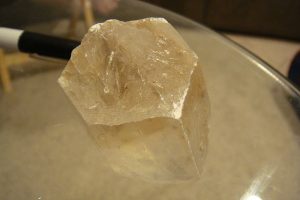
The crystal structure of non- silicate minerals (see table) does not contain silica-oxygen tetrahedra . Many non- silicate minerals are economically important and provide metallic resources such as copper, lead, and iron. They also include valuable non- metallic products such as salt, construction materials, and fertilizer.
Common non- silicate mineral groups.
3.4.1 Carbonates
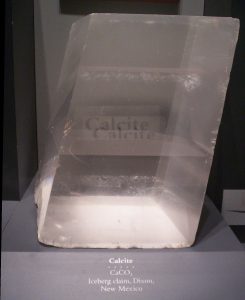
Calcite (CaCO 3 ) and dolomite (CaMg(CO 3 ) 2 ) are the two most frequently occurring carbonate minerals , and usually occur in sedimentary rocks, such as limestone and dolostone rocks, respectively. Some carbonate rocks, such calcite and dolomite, are formed via evaporation and precipitation . However, most carbonate -rich rocks, such as limestone , are created by the lithification of fossilized marine organisms. These organisms, including those we can see and many microscopic organisms, have shells or exoskeletons consisting of calcium carbonate (CaCO 3 ). When these organisms die, their remains accumulate on the floor of the water body in which they live and the soft body parts decompose and dissolve away. The calcium carbonate hard parts become included in the sediments , eventually becoming the sedimentary rock called limestone . While limestone may contain large, easy to see fossils , most limestones contain the remains of microscopic creatures and thus originate from biological processes.
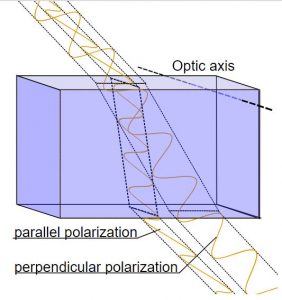
Calcite crystals show an interesting property called birefringence , meaning they polarize light into two wave components vibrating at right angles to each other. As the two light waves pass through the crystal, they travel at different velocities and are separated by refraction into two different travel paths. In other words, the crystal produces a double image of objects viewed through it. Because they polarize light, calcite crystals are used in special petrographic microscopes for studying minerals and rocks.
Many non- silicate minerals are referred to as salts. The term salts used here refers to compounds made by replacing the hydrogen in natural acids. The most abundant natural acid is carbonic acid that forms by the solution of carbon dioxide in water. Carbonate minerals are salts built around the carbonate ion (CO3 -2 ) where calcium and/or magnesium replace the hydrogen in carbonic acid (H 2 CO 3 ). Calcite and a closely related polymorph aragonite are secreted by organisms to form shells and physical structures like corals. Many such creatures draw both calcium and carbonate from dissolved bicarbonate ions (HCO 3 – ) in ocean water. As seen in the mineral identification section below, calcite is easily dissolved in acid and thus effervesces in dilute hydrochloric acid (HCl). Small dropper bottles of dilute hydrochloric acid are often carried by geologists in the field as well as used in mineral identification labs.
Other salts include halite (NaCl) in which sodium replaces the hydrogen in hydrochloric acid and gypsum (Ca[SO 4 ] • 2 H 2 O) in which calcium replaces the hydrogen in sulfuric acid. Note that some water molecules are also included in the gypsum crystal. Salts are often formed by evaporation and are called evaporite minerals .
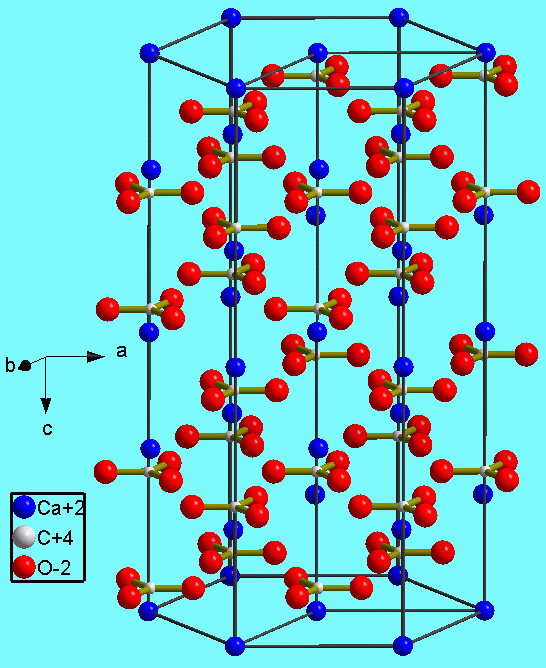
The figure shows the crystal structure of calcite (CaCO 3 ). Like silicon, carbon has four valence electrons. The carbonate unit consists of carbon atoms (tiny white dots) covalently bonded to three oxygen atoms (red), one oxygen sharing two valence electrons with the carbon and the other two sharing one valence electron each with the carbon, thus creating triangular units with a charge of -2. The negatively charged carbonate unit forms an ionic bond with the Ca ion (blue), which as a charge of +2.
3.4.2 Oxides, Halides, and Sulfides
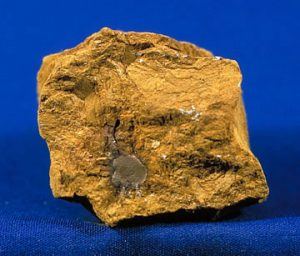
After carbonates , the next most common non- silicate minerals are the oxides , halides , and sulfides .
Oxides consist of metal ions covalently bonded with oxygen. The most familiar oxide is rust, which is a combination of iron oxides (Fe 2 O 3 ) and hydrated oxides . Hydrated oxides form when iron is exposed to oxygen and water. Iron oxides are important for producing metallic iron. When iron oxide or ore is smelted, it produces carbon dioxide (CO 2 ) and metallic iron.
The red color in rocks is usually due to the presence of iron oxides . For example, the red sandstone cliffs in Zion National Park and throughout Southern Utah consist of white or colorless grains of quartz coated with iron oxide which serve as cementing agents holding the grains together.
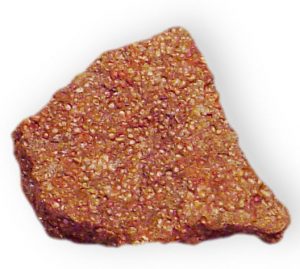
Other iron oxides include limonite, magnetite, and hematite. Hematite occurs in many different crystal forms. The massive form shows no external structure. Botryoidal hematite shows large concentric blobs. Specular hematite looks like a mass of shiny metallic crystals. Oolitic hematite looks like a mass of dull red fish eggs. These different forms of hematite are polymorphs and all have the same formula, Fe 2 O 3 .
Other common oxide minerals include:
- ice (H 2 O), an oxide of hydrogen
- bauxite (Al 2 H 2 O 4 ), hydrated oxides of aluminum, an ore for producing metallic aluminum
- corundum (Al 2 O 3 ), which includes ruby and sapphire gemstones.
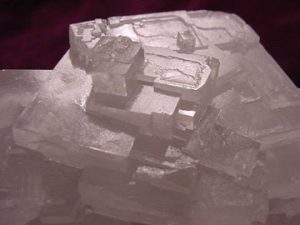
The halides consist of halogens in column VII, usually fluorine or chlorine, ionically bonded with sodium or other cations . These include halite or sodium chloride (NaCl), common table salt; sylvite or potassium chloride (KCl); and fluorite or calcium fluoride (CaF 2 ).
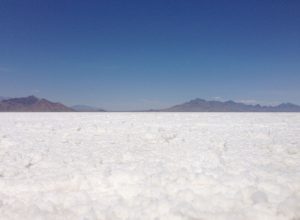
Halide minerals usually form from the evaporation of sea water or other isolated bodies of water. A well-known example of halide mineral deposits created by evaporation is the Bonneville Salt Flats, located west of the Great Salt Lake in Utah (see figure).
Many important metal ores are sulfides , in which metals are bonded to sulfur. Significant examples include: galena (lead sulfide ), sphalerite (zinc sulfide ), pyrite
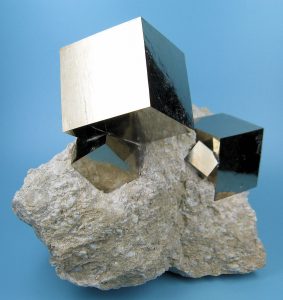
( iron sulfide , sometimes called “fool’s gold”), and chalcopyrite (iron-copper sulfide ). Sulfides are well known for being important ore minerals . For example, galena is the main source of lead, sphalerite is the main source of zinc, and chalcopyrite is the main copper ore mineral mined in porphyry deposits like the Bingham mine (see chapter 16 ). The largest sources of nickel, antimony, molybdenum, arsenic, and mercury are also sulfides .
3.4.3 Sulfates
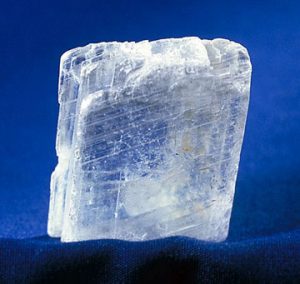
Sulfate minerals contain a metal ion , such as calcium, bonded to a sulfate ion . The sulfate ion is a combination of sulfur and oxygen (SO 4 – 2 ). The sulfate mineral gypsum (CaSO 4 ᐧ2H 2 O) is used in construction materials such as plaster and drywall. Gypsum is often formed from evaporating water and usually contains water molecules in its crystalline structure. The ᐧ2H 2 O in the formula indicates the water molecules are whole H 2 O. This is different from minerals like amphibole , which contain a hydroxide ion (OH – ) that is derived from water, but is missing a hydrogen ion (H + ). The calcium sulfate without water is a different mineral than gypsum called anhydrite (CaSO 4 ).
3.4.4 Phosphates
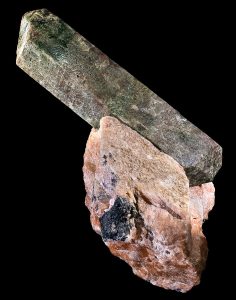
Phosphate minerals have a tetrahedral phosphate unit (PO 4 -3 ) combined with various anions and cations . In some cases arsenic or vanadium can substitute for phosphorus. Phosphates are an important ingredient of fertilizers as well as detergents, paint, and other products. The best known phosphate mineral is apatite, Ca 5 (PO 4 ) 3 (F,Cl,OH), variations of which are found in teeth and bones. The gemstone turquoise [CuAl 6 (PO 4 ) 4 (OH) 8 ·4H2O ] is a copper-rich phosphate mineral that, like gypsum , contains water molecules.
3.4.5 Native Element Minerals
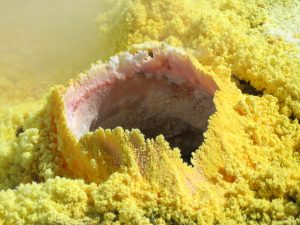
Native element minerals , usually metals, occur in nature in a pure or nearly pure state. Gold is an example of a native element mineral ; it is not very reactive and rarely bonds with other elements so it is usually found in an isolated or pure state. The non- metallic and poorly-reactive mineral carbon is often found as a native element , such as graphite and diamonds. Mildly reactive metals like silver, copper, platinum, mercury, and sulfur sometimes occur as native element minerals . Reactive metals such as iron, lead, and aluminum almost always bond to other elements and are rarely found in a native state.
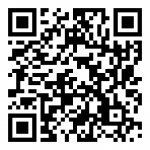
3.5 Identifying Minerals
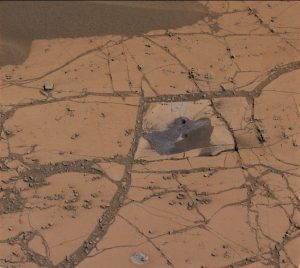
Geologists identify minerals by their physical properties. In the field, where geologists may have limited access to advanced technology and powerful machines, they can still identify minerals by testing several physical properties: luster and color, streak , hardness , crystal habit , cleavage and fracture , and some special properties. Only a few common minerals make up the majority of Earth’s rocks and are usually seen as small grains in rocks. Of the several properties used for identifying minerals , it is good to consider which will be most useful for identifying them in small grains surrounded by other minerals .
3.5.1 Luster and Color
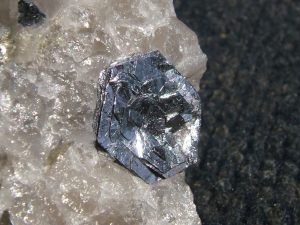
The first thing to notice about a mineral is its surface appearance, specifically luster and color. Luster describes how the mineral looks. Metallic luster looks like a shiny metal such as chrome, steel, silver, or gold. Submetallic luster has a duller appearance. Pewter, for example, shows submetallic luster .
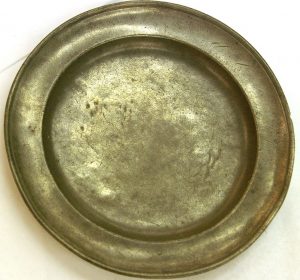
Nonmetallic luster doesn’t look like a metal and may be described as vitreous (glassy), earthy, silky, pearly, and other surface qualities. Nonmetallic minerals may be shiny, although their vitreous shine is different from metallic luster . See the table for descriptions and examples of nonmetallic luster .
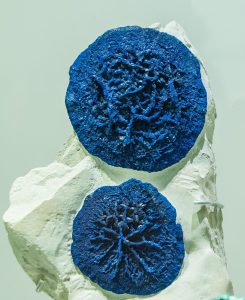
Surface color may be helpful in identifying minerals , although it can be quite variable within the same mineral family. Mineral colors are affected by the main elements as well as impurities in the crystals. These impurities may be rare elements —like manganese, titanium, chromium, or lithium—even other molecules that are not normally part of the mineral formula. For example, the incorporation of water molecules gives quartz , which is normally clear, a milky color.
Some minerals predominantly show a single color. Malachite and azurite are green and blue, respectively, because of their copper content. Other minerals have a predictable range of colors due to elemental substitutions, usually via a solid solution . Feldspars , the most abundant minerals in the earth’s crust , are complex, have solid solution series, and present several colors including pink, white, green, gray and others. Other minerals also come in several colors, influenced by trace amounts of several elements . The same element may show up as different colors, in different minerals . With notable exceptions, color is usually not a definitive property of minerals . For identifying many minerals . a more reliable indicator is streak , which is the color of the powdered mineral .
3.5.2 Streak
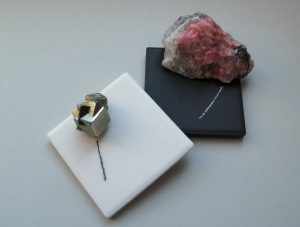
Streak examines the color of a powdered mineral , and can be seen when a mineral sample is scratched or scraped on an unglazed porcelain streak plate . A paper page in a field notebook may also be used for the streak of some minerals . Minerals that are harder than the streak plate will not show streak , but will scratch the porcelain. For these minerals , a streak test can be obtained by powdering the mineral with a hammer and smearing the powder across a streak plate or notebook paper.
While mineral surface colors and appearances may vary, their streak colors can be diagnostically useful. An example of this property is seen in the iron- oxide mineral hematite. Hematite occurs in a variety of forms, colors and lusters, from shiny metallic silver to earthy red-brown, and different physical appearances. A hematite streak is consistently reddish brown, no matter what the original specimen looks like. Iron sulfide or pyrite, is a brassy metallic yellow. Commonly named fool’s gold, pyrite has a characteristic black to greenish-black streak .
3.5.3 Hardness
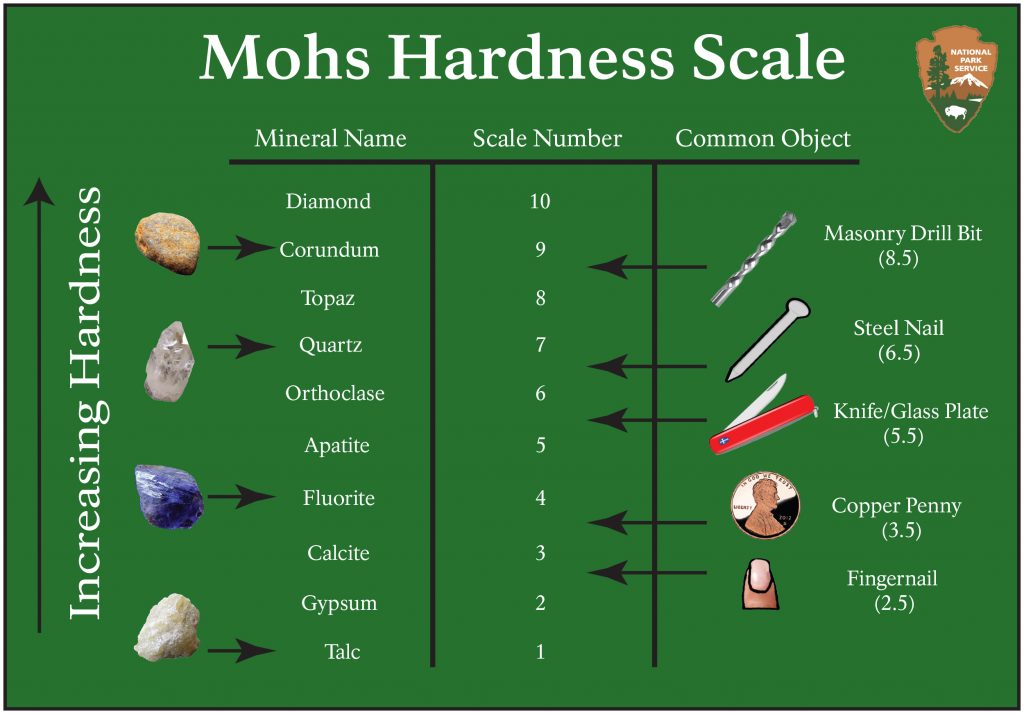
Hardness measures the ability of a mineral to scratch other substances. The Mohs Hardness Scale gives a number showing the relative scratch-resistance of minerals when compared to a standardized set of minerals of increasing hardness. The Mohs scale was developed by German geologist Fredrick Mohs in the early 20th century, although the idea of identifying minerals by hardness goes back thousands of years. Mohs hardness values are determined by the strength of a mineral ’s atomic bonds .
The figure shows the minerals associated with specific hardness values, together with some common items readily available for use in field testing and mineral identification. The hardness values run from 1 to 10, with 10 being the hardest; however, the scale is not linear. Diamond defines a hardness of 10 and is actually about four times harder than corundum, which is 9. A steel pocketknife blade, which has a hardness value of 5.5, separates between hard and soft minerals on many mineral identification keys.
3.5.4 Crystal Habit
Minerals can be identified by crystal habit , how their crystals grow and appear in rocks. Crystal shapes are determined by the arrangement of the atoms within the crystal structure. For example, a cubic arrangement of atoms gives rise to a cubic-shaped mineral crystal. Crystal habit refers to typically observed shapes and characteristics; however, they can be affected by other minerals crystallizing in the same rock. When minerals are constrained so they do not develop their typical crystal habit , they are called anhedral . Subhedral crystals are partially formed shapes. For some minerals characteristic crystal habit is to grow crystal faces even when surrounded by other crystals in rock. An example is garnet. Minerals grown freely where the crystals are unconstrained and can take characteristic shapes often form crystal faces. A euhedral crystal has a perfectly formed, unconstrained shape. Some minerals crystallize in such tiny crystals, they do not show a specific crystal habit to the naked eye. Other minerals , like pyrite, can have an array of different crystal habits, including cubic, dodecahedral, octahedral, and massive . The table lists typical crystal habits of various minerals .
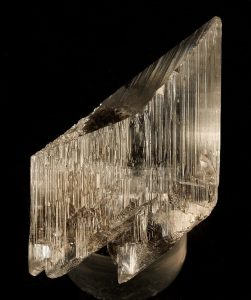
Another crystal habit that may be used to identify minerals is striations, which are dark and light parallel lines on a crystal face. Twinning is another, which occurs when the crystal structure replicates in mirror images along certain directions in the crystal.
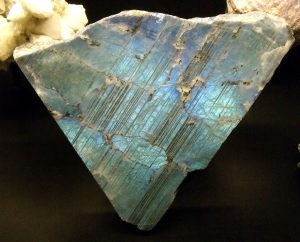
Striations and twinning are related properties in some minerals including plagioclase feldspar . Striations are optical lines on a cleavage surface. Because of twinning in the crystal, striations show up on one of the two cleavage faces of the plagioclase crystal.
3.5.5 Cleavage and Fracture
Minerals often show characteristic patterns of breaking along specific cleavage planes or show characteristic fracture patterns. Cleavage planes are smooth, flat, parallel planes within the crystal. The cleavage planes may show as reflective surfaces on the crystal, as parallel cracks that penetrate into the crystal, or show on the edge or side of the crystal as a series of steps like rice terraces . Cleavage arises in crystals where the atomic bonds between atomic layers are weaker along some directions than others, meaning they will break preferentially along these planes. Because they develop on atomic surfaces in the crystal, cleavage planes are optically smooth and reflect light, although the actual break on the crystal may appear jagged or uneven. In such cleavages, the cleavage surface may appear like rice terraces on a mountainside that all reflect sunlight from a particular sun angle. Some minerals have a strong cleavage, some minerals only have weak cleavage or do not typically demonstrate cleavage.
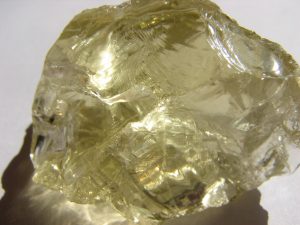
For example, quartz and olivine rarely show cleavage and typically break into conchoidal fracture patterns.
Graphite has its carbon atoms arranged into layers with relatively strong bonds within the layer and very weak bonds between the layers. Thus graphite cleaves readily between the layers and the layers slide easily over one another giving graphite its lubricating quality.
Mineral fracture surfaces may be rough and uneven or they may be show conchoidal fracture . Uneven fracture patterns are described as irregular, splintery, fibrous. A conchoidal fracture has a smooth, curved surface like a shallow bowl or conch shell, often with curved ridges. Natural volcanic glass, called obsidian , breaks with this characteristic conchoidal pattern
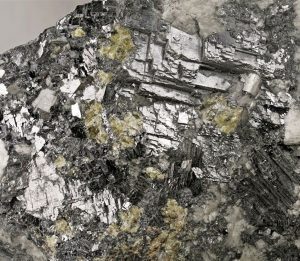
To work with cleavage, it is important to remember that cleavage is a result of bonds separating along planes of atoms in the crystal structure. On some minerals , cleavage planes may be confused with crystal faces. This will usually not be an issue for crystals of minerals that grew together within rocks. The act of breaking the rock to expose a fresh face will most likely break the crystals along cleavage planes. Some cleavage planes are parallel with crystal faces but many are not. Cleavage planes are smooth, flat, parallel planes within the crystal. The cleavage planes may show as parallel cracks that penetrate into the crystal (see amphibole below), or show on the edge or side of the crystal as a series of steps like rice terraces . For some minerals characteristic crystal habit is to grow crystal faces even when surrounded by other crystals in rock. An example is garnet. Minerals grown freely where the crystals are unconstrained and can take characteristic shapes often form crystal faces (see quartz below).
In some minerals , distinguishing cleavage planes from crystal faces may be challenging for the student. Understanding the nature of cleavage and referring to the number of cleavage planes and cleavage angles on identification keys should provide the student with enough information to distinguish cleavages from crystal faces. Cleavage planes may show as multiple parallel cracks or flat surfaces on the crystal. Cleavage planes may be expressed as a series of steps like terraced rice paddies. See the cleavage surfaces on galena above or plagioclase below. Cleavage planes arise from the tendency of mineral crystals to break along specific planes of weakness within the crystal favored by atomic arrangements. The number of cleavage planes, the quality of the cleavage surfaces, and the angles between them are diagnostic for many minerals and cleavage is one of the most useful properties for identifying minerals . Learning to recognize cleavage is an especially important and useful skill in studying minerals .
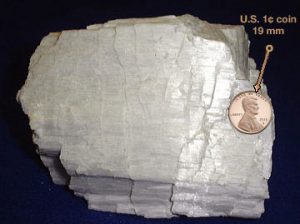
As an identification property of minerals , cleavage is usually given in terms of the quality of the cleavage (perfect, imperfect, or none), the number of cleavage surfaces, and the angles between the surfaces. The most common number of cleavage plane directions in the common rock-forming minerals are: one perfect cleavage (as in mica ), two cleavage planes (as in feldspar , pyroxene , and amphibole ), and three cleavage planes (as in halite , calcite , and galena). One perfect cleavage (as in mica ) develops on the top and bottom of the mineral specimen with many parallel cracks showing on the sides but no angle of intersection. Two cleavage planes intersect at an angle. Common cleavage angles are 60°, 75°, 90°, and 120°. Amphibole has two cleavage planes at 60° and 120°. Galena and halite have three cleavage planes at 90° (cubic cleavage). Calcite cleaves readily in three directions producing a cleavage figure called a rhomb that looks like a cube squashed over toward one corner giving rise to the approximately 75° cleavage angles. Pyroxene has an imperfect cleavage with two planes at 90°.
Cleavages on common rock-forming minerals
- Quartz —none ( conchoidal fracture )
- Olivine —none ( conchoidal fracture )
- Mica —1 perfect
- Feldspar —2 perfect at 90°
- Pyroxene —2 imperfect at 90°
- Amphibole —2 perfect at 60°/120°
- Calcite —3 perfect at approximately 75°
- Halite , galena, pyrite—3 perfect at 90°
3.5.6 Special Properties
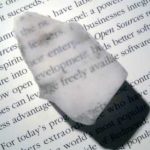
Special properties are unique and identifiable characteristics used to identify minerals or that allow some minerals to be used for special purposes. Ulexite has a fiber-optic property that can project images through the crystal like a high-definition television screen (see figure). A simple identifying special property is taste, such as the salty flavor of halite or common table salt (NaCl). Sylvite is potassium chloride (KCl) and has a more bitter taste.
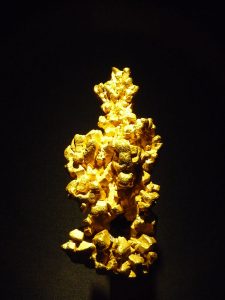
Another property geologists may use to identify minerals is a property related to density called specific gravity . Specific gravity measures the weight of a mineral specimen relative to the weight of an equal volume of water. The value is expressed as a ratio between the mineral and water weights. To measure specific gravity , a mineral specimen is first weighed in grams then submerged in a graduated cylinder filled with pure water at room temperature . The rise in water level is noted using the cylinder’s graduated scale. Since the weight of water at room temperature is 1 gram per cubic centimeter, the ratio of the two weight numbers gives the specific gravity . Specific gravity is easy to measure in the laboratory but is less useful for mineral identification in the field than other more easily observed properties, except in a few rare cases such as the very dense galena or native gold. The high density of these minerals gives rise to a qualitative property called “heft.” Experienced geologists can roughly assess specific gravity by heft, a subjective quality of how heavy the specimen feels in one’s hand relative to its size.
A simple test for identifying calcite and dolomite is to drop a bit of dilute hydrochloric acid (10-15% HCl) on the specimen. If the acid drop effervesces or fizzes on the surface of the rock, the specimen is calcite . If it does not, the specimen is scratched to produce a small amount of powder and test with acid again. If the acid drop fizzes slowly on the powdered mineral , the specimen is dolomite. The difference between these two minerals can be seen in the video. Geologists who work with carbonate rocks carry a small dropper bottle of dilute HCl in their field kit. Vinegar, which contains acetic acid, can be used for this test and is used to distinguish non- calcite fossils from limestone . While acidic, vinegar produces less of a fizzing reaction because acetic acid is a weaker acid.
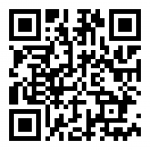
Some iron- oxide minerals are magnetic and are attracted to magnets. A common name for a naturally magnetic iron oxide is lodestone . Others include magnetite (Fe3O 4 ) and ilmenite (FeTiO 3 ). Magnetite is strongly attracted to magnets and can be magnetized. Ilmenite and some types of hematite are weakly magnetic.
Some minerals and mineraloids scatter light via a phenomenon called iridescence . This property occurs in labradorite (a variety of plagioclase ) and opal. It is also seen in biologically created substances like pearls and seashells. Cut diamonds show iridescence and the jeweler’s diamond cut is designed to maximize this property.
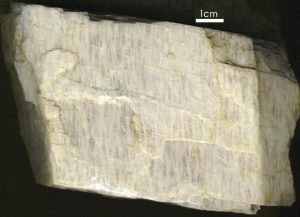
Striations on mineral cleavage faces are an optical property that can be used to separate plagioclase feldspar from potassium feldspar ( K-spar ). A process called twinning creates parallel zones in the crystal that are repeating mirror images. The actual cleavage angle in plagioclase is slightly different than 90 o and the alternating mirror images in these twinned zones produce a series of parallel lines on one of plagioclase ’s two cleavage faces. Light reflects off these twinned lines at slightly different angles which then appear as light and dark lines called striations on the cleavage surface. Potassium feldspar does not exhibit twinning or striations but may show linear features called exsolution lamellae , also known as perthitic lineation or simply perthite. Because sodium and potassium do not fit into the same feldspar crystal structure, the lines are created by small amounts of sodium feldspar (albite) separating from the dominant potassium feldspar ( K-spar ) within the crystal structure. The two different feldspars crystallize out into roughly parallel zones within the crystal, which are seen as these linear markings.
One of the most interesting special mineral properties is fluorescence . Certain minerals , or trace elements within them, give off visible light when exposed to ultraviolet radiation or black light. Many mineral exhibits have a fluorescence room equipped with black lights so this property can be observed. An even rarer optical property is phosphorescence. Phosphorescent minerals absorb light and then slowly release it, much like a glow-in-the-dark sticker.
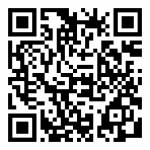
Minerals are the building blocks of rocks and essential to understanding geology. Mineral properties are determined by their atomic bonds . Most minerals begin in a fluid, and either crystallize out of cooling magma or precipitate as ions and molecules out of a saturated solution . The silicates are largest group of minerals on Earth, by number of varieties and relative quantity, making up a large portion of the crust and mantle . Based on the silicon-oxygen tetrahedra , the crystal structure of silicates reflects the fact that silicon and oxygen are the top two of Earth’s most abundant elements . Non- silicate minerals are also economically important, and providing many types of construction and manufacturing materials. Minerals are identified by their unique physical properties, including luster , color, streak , hardness , crystal habit , fracture , cleavage, and special properties.
Take this quiz to check your comprehension of this Chapter.
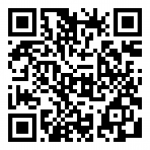
- Clarke, F.W.H.S.W., 1927, The Composition of the Earth’s Crust : Professional Paper, United States Geological Survey, Professional Paper.
- Gordon, L.M., and Joester, D., 2011, Nanoscale chemical tomography of buried organic-inorganic interfaces in the chiton tooth: Nature, v. 469, no. 7329, p. 194–197.
- Hans Wedepohl, K., 1995, The composition of the continental crust : Geochim. Cosmochim. Acta, v. 59, no. 7, p. 1217–1232.
- Lambeck, K., 1986, Planetary evolution: banded iron formations : v. 320, no. 6063, p. 574–574.
- metallic bond | chemistry.
- Scerri, E.R., 2007, The Periodic Table: Its Story and Its Significance: Oxford University Press, USA.
- Thomson, J.J., 1897, XL. Cathode Rays: Philosophical Magazine Series 5, v. 44, no. 269, p. 293–316.
- Trenn, T.J., Geiger, H., Marsden, E., and Rutherford, E., 1974, The Geiger-Marsden Scattering Results and Rutherford’s Atom, July 1912 to July 1913: The Shifting Significance of Scientific Evidence: Isis, v. 65, no. 1, p. 74–82.
Media Attributions
- Private: Cristales cueva de Naica © Alexander Van Driessche is licensed under a CC BY (Attribution) license
- Private: Electron cloud model of the atom © Louis de Broglie & Erwin Schrodinge is licensed under a All Rights Reserved license
- Private: Periodic Table © NIST
- Private: Carbon 14 formation and decay © C14_methode_physikalische_grundlagen.svg: Sgbeer adapted by NikNaks is licensed under a CC BY-SA (Attribution ShareAlike) license
- Private: elemental composition crust © Callan Bentley is licensed under a All Rights Reserved license
- H2O 2D labelled © Dan Craggs is licensed under a Public Domain license
- Private: Carbon dioxide 3D ball © Jynto is licensed under a CC0 (Creative Commons Zero) license
- Private: Sodium-chloride-3D-ionic © Benjah-bmm27 is licensed under a CC0 (Creative Commons Zero) license
- Private: Covalent © DynaBlast is licensed under a CC BY-SA (Attribution ShareAlike) license
- 3.1 Did I Get It QR Code
- Private: Hard Water Calcification © Bbypnda is licensed under a CC BY-SA (Attribution ShareAlike) license
- Private: Bonneville Salt Flats is licensed under a Public Domain license
- Private: Pahoehoe toe is licensed under a Public Domain license
- Private: Ammonite Asteroceras
- 3.2 Did I Get It QR Code
- Private: Tetrahedron © Cyp
- Private: Tetrahedron © Cameron Mosher is licensed under a All Rights Reserved license
- Private: Tetrahedron open © Cameron Mosher is licensed under a All Rights Reserved license
- Private: 03.12_Peridot_in_basalt © Vsmith (assumed) is licensed under a CC BY-SA (Attribution ShareAlike) license
- Private: Atomic structure of olivine 1 © Matanya (usurped) is licensed under a Public Domain license
- Private: Diopside-172005 © Rob Lavinsky, iRocks.com is licensed under a CC BY-SA (Attribution ShareAlike) license
- Private: Pyroxen-chain © Bubenik is licensed under a CC BY-SA (Attribution ShareAlike) license
- Private: Orthoclase Hornblende © Dave Dyet is licensed under a Public Domain license
- Private: Amphibole is licensed under a Public Domain license
- Private: Tremolite-chain © Bubenik is licensed under a CC BY-SA (Attribution ShareAlike) license
- Private: Biotite aggregate – Ochtendung, Eifel, Germany © Fred Kruijen
- MicaSheetUSGOV © "Minerals in Your World" project is licensed under a Public Domain license
- Private: Silicate-sheet-3D-polyhedra © Benjah-bmm27 is licensed under a Public Domain license
- Private: Crystal structure of mica is licensed under a Public Domain license
- Private: Illmenite mica sandwich © L. C. Mosher is licensed under a All Rights Reserved license
- Private: Kaolinite structure © US Geological Survey is licensed under a Public Domain license
- Private: Quartz, Tibet © JJ Harrison is licensed under a CC BY-SA (Attribution ShareAlike) license
- Private: min-crust-pie-chart © Callan Bentley is licensed under a All Rights Reserved license
- Private: OrthoclaseBresi © Didier Descouens is licensed under a CC BY-SA (Attribution ShareAlike) license
- Private: 23 feldspar structure © Used by permission of Justin Byron Richardson
- 3.3 Did I Get It QR Code
- Private: Hanksite © Matt Affolter(QFL247) is licensed under a CC BY-SA (Attribution ShareAlike) license
- Private: Calcite rhomb is licensed under a Public Domain license
- Private: Limestone etched section KopeFm new © Jstuby is licensed under a Public Domain license
- Private: Positively birefringent material © Mikael Häggström is licensed under a Public Domain license
- Private: Calcite © Materialscientist is licensed under a CC BY-SA (Attribution ShareAlike) license
- Private: LimoniteUSGOV © "Minerals in Your World" project is licensed under a Public Domain license
- Private: Hematite – oolitic with shale Iron Oxide Clinton, Oneida County, New York © Dave Dyet is licensed under a Public Domain license
- Private: Halite-249324 (1) is licensed under a Public Domain license
- Private: 2014-07-05 13 04 30 View across the Bonneville Salt Falts, Utah from ground level © Famartin is licensed under a CC BY (Attribution) license
- Private: FluoriteUV is licensed under a CC BY-SA (Attribution ShareAlike) license
- Private: 2780M-pyrite1 © CarlesMillan is licensed under a CC BY-SA (Attribution ShareAlike) license
- Private: SeleniteGypsumUSGOV © "Minerals in Your World" project is licensed under a Public Domain license
- Private: Apatite_Canada is licensed under a CC BY-SA (Attribution ShareAlike) license
- Private: Sulfer_Fumarola_Vulcano © brisk_g is licensed under a Public Domain license
- Private: Native_Copper (1) is licensed under a CC BY-SA (Attribution ShareAlike) license
- 3.4 Did I Get It QR Code
- Private: Curiosity_Mars_Rover_Finds_Mineral_Match © NASA is licensed under a Public Domain license
- Private: Molly Hill molybdenite © John Chapman (Pyrope) is licensed under a CC BY-SA (Attribution ShareAlike) license
- Private: Pewter Plate is licensed under a Public Domain license
- Quartz Brésil © Didier Descouens is licensed under a CC BY-SA (Attribution ShareAlike) license
- Private: earthy_luster_KaolinUSGOV (1) is licensed under a Public Domain license
- Private: Selenite Gips Marienglas © Ra'ike (see also: de:Benutzer:Ra'ike) is licensed under a CC BY (Attribution) license
- Private: pearly_luster_Mineral_Mica_GDFL006 © Luis Miguel Bugallo Sánchez is licensed under a CC BY-SA (Attribution ShareAlike) license
- Private: Sphalerite4 © Andreas Früh (Andel) is licensed under a CC BY-SA (Attribution ShareAlike) license
- Private: Azurite in siltstone, Malbunka mine NT © Graeme Churchard is licensed under a CC BY (Attribution) license
- Private: Streak_plate_with_Pyrite_and_Rhodochrosite (1) is licensed under a CC BY-SA (Attribution ShareAlike) license
- Private: Mohs Hardness Scale Print © National Park Service is licensed under a Public Domain license
- Private: Kyanite crystals © User:Aelwyn is licensed under a CC BY-SA (Attribution ShareAlike) license
- Private: Malachite_Kolwezi_Katanga_Congo © Didier Descouens is licensed under a CC BY-SA (Attribution ShareAlike) license
- Private: Ametyst-geode © Juppi66 is licensed under a Public Domain license
- Private: Cubic_Calcite-Galena-elm56c © Robert M. Lavinsky is licensed under a CC BY-SA (Attribution ShareAlike) license
- Private: Pyrite elbe © Didier Descouens is licensed under a CC BY-SA (Attribution ShareAlike) license
- Private: Dendrites01 © Wilson44691 is licensed under a Public Domain license
- Peridot2 © S kitahashi (assumed) is licensed under a CC BY-SA (Attribution ShareAlike) license
- Private: Tremolite Campolungo © Didier Descouens is licensed under a CC BY-SA (Attribution ShareAlike) license
- Private: Muscovite-Albite-122887 © Rob Lavinsky, iRocks.com is licensed under a CC BY-SA (Attribution ShareAlike) license
- Private: Calcite-Wulfenite-tcw15b © Rob Lavinsky is licensed under a CC BY-SA (Attribution ShareAlike) license
- Hanksite © Matt Affolter is licensed under a CC BY-SA (Attribution ShareAlike) license
- Private: Fluorite crystals (rotated 90) © Ryan Salsbury adapted by TCO is licensed under a CC BY-SA (Attribution ShareAlike) license
- Private: Tourmaline-22335 © Rob Lavinsky is licensed under a CC BY-SA (Attribution ShareAlike) license
- Private: Pyrophyllite-236595 © Rob Lavinsky is licensed under a CC BY-SA (Attribution ShareAlike) license
- Private: Tetrahedrite Chalcopyrite Sphalerite 251531 © Rob Lavinsky is licensed under a CC BY-SA (Attribution ShareAlike) license
- Private: GypsumStriations © Rob Lavinsky is licensed under a CC BY-SA (Attribution ShareAlike) license
- Private: Staurolite 62645 © Rob Lavinsky is licensed under a CC BY-SA (Attribution ShareAlike) license
- Private: Striations in plagioclase © Mike Beauregard from Nunavut, Canada (Eyeful of Sky) is licensed under a CC BY (Attribution) license
- Private: conchoidal_Citrine-sample2 is licensed under a CC BY (Attribution) license
- Private: Graphite © Saumitra R Mehrotra & Gerhard Klimeck is licensed under a CC BY (Attribution) license
- Private: Argentiferous Galena-458851 © Modris Baum adapted by CombineZP is licensed under a Public Domain license
- Private: Cleavage_steps_in_wollastonite is licensed under a Public Domain license
- Private: Amphibol © Zimbres (assumed) is licensed under a CC BY-SA (Attribution ShareAlike) license
- Private: Ulexit_Fernsehstein © Zeichner: Sec is licensed under a Public Domain license
- Private: Latrobe_gold_nugget_Natural_History_Museum © Gump Stump is licensed under a CC BY-SA (Attribution ShareAlike) license
- Calcite and Dolomite YouTube QR Code
- Private: Magnetite Lodestone © Ryan Somma is licensed under a CC BY-SA (Attribution ShareAlike) license
- Private: Perthitic feldspar Dan Patch SD © Jstuby is licensed under a Public Domain license
- 3.5 Did I Get It QR Code
- Ch.3 Review QR Code
A natural substance that is typically solid, has a crystalline structure, and is typically formed by inorganic processes. Minerals are the building blocks of most rocks.
A group of all atoms with a specific number of protons, having specific, universal, and unique properties.
Two or more atoms or ions that are connected chemically.
A anion structure of one silicon bonded to four oxygens, in the shape of a tetrahedron, with the silicon in the center and four oxygens at the corners of the structure. It has a net charge of -4, and can bond to cations to form silicate minerals.
Mineral group in which the silica tetrahedra, SiO4-4, is the building block.
Minerals in which ions are bonded to oxygen, such as in ice, H2O.
Minerals bonded via a sulfur (S-2) atom.
Minerals bonded via a sulfate ion, SO4-2.
Mineral group in which the carbonate ion, CO3-2, is the building block. This can also refer to the rocks that are made from these minerals, namely limestone and dolomite (dolostone).
The measure of the vibrational (kinetic) energy of a substance.
The mineral make up of a rock, i.e. which minerals are found within a rock.
CaCO3. Pure form is clear, but can take on many different colors with impurities. It is soft, fizzes in acid, and has three cleavages that are not at 90°. Thus, it can form slanted blocks, though it is visually common to be without any structure. Found in many sedimentary rocks from marine settings, rarely in igneous rocks, in the metamorphic rock marble, but is common as a secondary mineral throughout surface rocks.
Changes in sedimentary rocks due to increased (but low when compared to metamorphism) temperatures and pressures. This can include deposition of new minerals (e.g. limestone converting to dolomite) or dissolution of existing minerals.
Former swamp-derived (plant) material that is part of the rock record.
Dark colored volcanic glass, with extremely small microscopic crystals or no crystals. Typically form from felsic volcanism.
Rocks that are formed from liquid rock, i.e. from volcanic processes.
The physical breakdown (weathering) of bedrock by processes such as pressure, ice expansion, etc.
Breaking down of mineral material via chemical methods, like dissolution and oxidation.
The act of a solid coming out of solution, typically resulting from a drop in temperature or a decrease of the dissolving material.
The act of taking a solid and dissolving it into a liquid. This commonly occurs with salts and other minerals in water.
Rocks and minerals that change within the Earth are called metamorphic, changed by heat and pressure. Metamorphism is the name of the process.
An atom that has different number of neutrons but the same number of protons. While most properties are based on the number of protons in an element, isotopes can have subtle changes between them, including temperature fractionation and radioactivity.
The layers of igneous, sedimentary, and metamorphic rocks that form the continents. Continental crust is much thicker than oceanic crust. Continental crust is defined as having higher concentrations of very light elements like K, Na, and Ca, and is the lowest density rocky layer of Earth. Its average composition is similar to granite.
An atom or molecule that has a charge (positive or negative) due to the loss or gain of electrons.
(Fe,Mg)2SiO4. Typically translucent olive green and equant, with no cleavage. Common in mafic igneous rocks and in the mantle, but easily weathered in surface conditions. Structure is isolated silica tetrahedra. Known as peridot when a gem.
A rule that says the outer valence shell of electrons is complete when it contains 8 electrons.
A positively-charged ion. In geology, this commonly includes ions of the elements Ca+2, Na+1, K+1, Fe+2,+3, Al+3, and Mg+2.
A negatively-charged ion. In geology, this commonly includes elements and molecules like SiO4-4, S-2, SO4-4, and O-2.
Certain metallic elements (like iron) take in oxygen, causing reactions like rust.
Also known as rock salt, or table salt. 3 cleavages at 90°, cubic crystal habit. Typically clear or white, hardness of 3.
The process of liquid rock freezing into solid rock. Because liquid rock is made of many components, the process is complex as different components freeze at different temperatures.
Liquid rock within the Earth.
The process in which solids (like minerals) are disassociated and the ionic components are dispersed in a liquid (usually water).
A solution that has the maximum allowed dissolved component, and is unable to dissolve more.
Detached, free-falling rocks from very steep slopes.
Pieces of rock that have been weathered and possibly eroded.
Porous variety of carbonate that form in relatively unheated water, sometimes as towers and spires.
A period of cooler temperatures on Earth in which ice sheets can grow on continents.
Porous, concentric, or layered variety of carbonate that forms with often heated water in springs and/or caves.
An elevated erosional surface caused by glacial or fluvial action.
A place where pressurized groundwater flows onto the surface.
A channelled body of water.
Liquid rock on the surface of the Earth.
Minerals with the same composition and different crystal structures
Places that are under ocean water at all times.
Relatively flat ocean floor, which accumulates very fine grained detrital and chemical sediments.
Rocks that are formed by sedimentary processes, including sediments lithifying and precipitation from solution.
A chemical or biochemical rock made of mainly calcite.
A very fine grained version of silica deposited with or without microfossils.
Organisms that possess vertebrate or some form of a spinal column, including humans.
Minerals that are bonded with the phosphate anion, PO4+3.
Any evidence of ancient life.
Middle chemical layer of the Earth, made of mainly iron and magnesium silicates. It is generally denser than the crust (except for older oceanic crust) and less dense than the core.
The outermost chemical layer of the Earth, defined by its low density and higher concentrations of lighter elements. The crust has two types: continental , which is the thick, more ductile, and lowest density, and oceanic , which is higher density, more brittle, and thinner.
An intrusive ultramafic rock, which is the main component of the mantle. The minerals in peridotite are typically olivine with some pyroxene.
General name of a mafic rock that is extrusive. Generally has a black groundmass color.
Two or more elements that can easily substitute for each other, due to similarities in ionic size and charge.
Can refer to a volcanic rock with lower silica composition, or the minerals that make up those rocks, namely olivine, pyroxene, amphibole, and biotite. Mafic rocks are darker in color and contain more minerals that are dark in color, but can contain some plagioclase feldspar. Primary mafic rocks are basalt (extrusive) and gabbro (intrusive).
The theory that the outer layer of the Earth (the lithosphere) is broken in several plates, and these plates move relative to one another, causing the major topographic features of Earth (e.g. mountains, oceans) and most earthquakes and volcanoes.
A solid part of the lithosphere which moves as a unit, i.e. the entire plate generally moves the same direction at the same speed.
A process where an oceanic plate descends bellow a less dense plate, causing the removal of the plate from the surface. Subduction causes the largest earthquakes possible, as the subducting plate can lock as it goes down. Volcanism is also caused as the plate releases volatiles into the mantle, causing melting.
ZrSiO4. Relatively chemically inert with a hardness of 8.5. Common accessory mineral in igneous and metamorphic rocks, as well as detrital sediments. Uranium can substitute for zirconium, making zircon a valuable mineral in radiometric dating.
A qualitative measure of the amount of metamorphism that has occurred or the amount of a resource present in an ore.
XY(Al,Si)2O6, in which X typically equals Na, Ca, Mg, or Fe and Y typically equals Mg, Fe, or Al. Typically black to dark green, blocky, with two cleavages at ~90°. Common in mafic igneous rocks and some metamorphic rocks. Structure is a single chain of silica tetrahedra.
General name of a mafic rock that is intrusive. Has more mafic minerals than felsic minerals.
Rock more metamorphosed than phyllite, to the point that mica grains are visible. Larger porphyroblasts are sometimes present.
(RSi4O11)2, where R is a large number of different cations that can sub in. Can be many colors, but the common form, hornblende, is dark brown to black. Has two cleavages at 54° and 126°. Crystals are typically elongated needles or diamond shapes. Common in many igneous rocks and some metamorphic rocks. Structure is a double chain of silica tetrahedra.
The typical form or forms a crystal takes when it grows.
Rocks formed via heat and pressure which change the minerals within the rock.
General name of an intermediate rock that is intrusive. Has about the same amount of felsic minerals and mafic minerals.
General name of a felsic rock that is intrusive. Has more felsic minerals than mafic minerals.
X1A2-3Z4O10(OH, F)2, where commonly X=K, Na, Ca; A=Al, Mg, Fe; Z=Si, Al. Has two more-common occurrences, light-colored (translucent and pearly tan) muscovite, and dark colored biotite. Has one strong cleavage, and is typically seen as sheets, in stacks or "books." Common in many igneous and metamorphic rocks. Structure is two-dimensional sheets of silica tetrahedra in a hexagonal netowork.
Can refer to a volcanic rock with higher silica composition, or the minerals that make up those rocks, namely quartz, feldspar (both potassium feldspar and plagioclase feldspar), and muscovite mica. Felsic rocks are lighter in color and contain more minerals that are light in color, but can contain some biotite and amphibole. Primary felsic rocks are rhyolite (extrusive) and granite (intrusive).
Consisting of three end members: potassium feldspar (K-spar, KAlSi3O8), plagioclase with calcium (CaAl2Si2O8, called anorthite), and plagioclase with sodium (NaAlSi3O8, called albite). Commonly blocky, with two cleavages as ~90°. Plagioclase is typically more dull white and grey, and K-spar is more vibrant white, orange, or red. The most common mineral found within the crust, and a major component of almost all igneous rocks, some sedimentary rocks, and some metamorphic rocks. Structure is a three-dimensional framework of silica tetrahedra, with locations open for cations (K, Na, Ca).
Rock spire that is offshore and a remnant of a rock layer.
Breaking down rocks into small pieces by chemical or mechanical means.
SiO2. Transparent, but can be any color imaginable with impurities. No cleavage, hard, and commonly forms equant masses. Perfect crystals are hexagonal prisms topped with pyramidal shapes. One of the most common minerals, and is found in many different geologic settings, including the dominant component of sand on the surface of Earth. Structure is a three-dimensional network of silica tetrahedra, connected as much as possible to each other.
The thin, outer layer of the Earth which makes up the rocky bottom of the ocean basins. It is made of rocks similar to basalt, and as it cools, even become more dense than the upper mantle below.
General name of a felsic rock that is extrusive. Generally has a white, tan, or pink groundmass color.
Sedimentary rocks made of mineral grains weathered as mechanical detritus of previous rocks, e.g. sand, gravel, etc.
Minerals with a luster similar to metal and contain metals, including valuable elements like lead, zinc, copper, tin, etc.
Minerals made from just a single element, bonded to itself. Examples include gold, silver, copper, and diamond, which is a native version of carbon.
A highly weathered soil deposit that consists of aluminum ores.
Valuable material in the Earth, typically used for metallic mineral resources.
Minerals based on bonds to column 17 halogens, such as chlorine and fluorine.
An evaporite mineral, CaSo4•2H2O. Has one cleavage, hardness of 2. Typically clear or white.
The process of turning sediment into a sedimentary rocks, including deposition, compaction, and cementation.
Waves that change direction due to changing speeds, typically caused by a change in density of the medium.
An acid that forms from carbon dioxide and water. It is a large contributor to chemical weathering.
A chemical sedimentary rock that forms as water evaporates.
A rock primarily made of sand.
A feature with no internal structure, habit, or layering.
A specific chemical composition that forms different minerals and different temperatures and pressures. Quartz has several different polymorphs, including coesite, tridimite, and stishovite.
Place where material is extracted from the Earth for human use.
The shine a mineral takes on, based on the way light reflects off of a mineral. This is typically divided into two main categories: metallic (metal-like shine) and nonmetallic (non metal-like shine).
The color(s) that a mineral produces when powdered or rubbed against a hard surface, usually a porcelain tile.
The ease or difficulty in scratching a mineral, measured by the qualitative Mohs hardness scale, which ranges from soft talc (#1 on the scale) to hard diamond (#10 on the scale).
A break within a rock that has no relative movement between the sides. Caused by cooling, pressure release, tectonic forces, etc.
Minerals that have a luster that is not similar to metal, and typically do not contain valuable metals like copper, lead, zinc, tin, etc.
A mineral that shows no crystal habit, either because it is not prone to have a habit, or because it grew in a way that it was confined so it could not grow with its normal habit.
A mineral which only shows some characteristics of its true crystal habit, and is not perfectly grown.
A mineral that perfectly shows its true crystal habit.
Thin (less than 1 cm) beds of rock.
Stubby, not long in any direction.
Fractures that have a circular appearance.
Place where lava is erupted at the surface.
Related to density; the ratio of the weight of a mineral vs. the weight of an equal volume of water.
An observation which is based on non-numerical data. While these types of observations are not preferred, they can still be useful.
An observation which is influenced by the observer's personal bias.
Linear alignment of minerals within a rock.
A process of using 3D seismic arrays to get subsurface images.
A sedimentary rock that formed long ago as free oxygen changed the solubility of iron, causing layers of iron rich and iron-poor sediments to form in thin layers, or bands.
An extensive, distinct, and mapped set of geologic layers.
An Introduction to Geology Copyright © 2017 by Chris Johnson, Matthew D. Affolter, Paul Inkenbrandt, Cam Mosher is licensed under a Creative Commons Attribution-NonCommercial-ShareAlike 4.0 International License , except where otherwise noted.
Share This Book
- Password reminder
- Registration
The writers of PenMyPaper establish the importance of reflective writing by explaining its pros and cons precisely to the readers. They tend to ‘do my essay’ by adding value to both you (enhancing your knowledge) and your paper.

Finished Papers
Useful Links
- Request a call back
- Write For Us
Customer Reviews
Charita Davis
Johan Wideroos

We use cookies. By browsing the site, you agree to it. Read more »
Connect with the writers
Once paid, the initial draft will be made. For any query r to ask for revision, you can get in touch with the online chat support available 24X7 for you.

Finished Papers
Customer Reviews
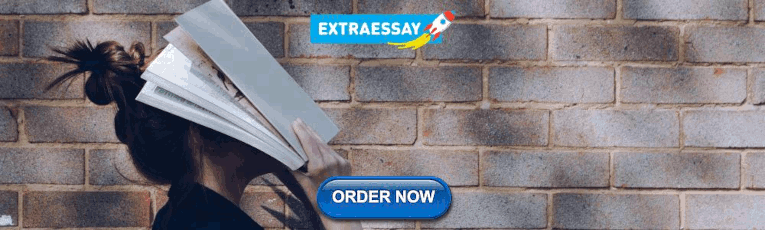
COMMENTS
Students are often asked to write an essay on Minerals in their schools and colleges. And if you're also looking for the same, we have created 100-word, 250-word, and 500-word essays on the topic. ... The process of their formation is a complex interplay of geological activities like erosion, sedimentation, and volcanic eruptions. ...
3.2: Mineral Identification. 3.1: Naming Minerals. 3.3: Crystal Shape. Dexter Perkins. University of North Dakota. Give a mineral specimen to a nongeologist and ask them to describe it. Generally, they mention the appearance, especially color, first. With a little prodding, they may go on to describe the shape and nature of visible crystals.
Step 2: Hardness. Kit of Mohs' Hardness Mineral Identification. Hardness is a mineral's ability to resist being scratched. Minerals that are not easily scratched are hard. You test the hardness of a mineral by scratching its surface with a mineral of a known hardness. Mineralogists use Mohs Scale as a reference for mineral hardness.
Figure 4.6.5 4.6. 5: Some minerals have different streaks than their visual color. Streak examines the color of a powdered mineral and can be seen when a mineral sample is scratched or scraped on an unglazed porcelain streak plate. A paper page in a field notebook may also be used for the streak of some minerals.
Scratch your mineral across the streak plate with a scribbling motion, then look at the results. Hematite, for example, will leave a red-brown streak. Bear in mind that most professional streak plates have a Mohs hardness of about 7. Minerals that are harder will scratch the place and won't leave a streak. 06.
One of the things mineralogists must do is identify and categorize minerals. While a mineralogist might use a high-powered microscope to identify some minerals, most are recognizable using physical properties. Figure 1. This mineral has shiny, gold, cubic crystals with striations, so it is pyrite. Check out the mineral in figure 1.
Figure 56.1.1 56.1. 1: Metallic Luster. The first thing to notice about a mineral is its surface appearance, specifically luster and color. Luster describes how the mineral reflects light. Metallic luster looks like a shiny metal such as chrome, steel, silver, or gold. Metallic minerals are also always opaque.
The same element may show up as different colors, in different minerals. With notable exceptions, color is usually not a definitive property of minerals. For identifying many minerals. a more reliable indicator is streak, which is the color of the powdered mineral. 3.5.2 Streak Some minerals have different streaks than their visual color
Minerals are the "Lego blocks" used to build rocks. That is, rocks are made of and from minerals. Think of it this way: minerals are to rocks as students are to a class. Many different students can make up a class just like many different minerals make up a rock. A rock can also be made up of many crystals of the same mineral, just like a ...
An approach to identifying minerals also varies for different types of stones and purposes. Mineral identification is based on the testing of the physical properties and morphology of crystals. Luster, hardness, and color are helpful to identify minerals in the field. Geologists use electron microprobe, X-ray diffraction, and Raman spectroscopy ...
Mineral Properties and Identification. Geologists identify minerals by their physical properties. In the field, where geologists may have limited access to advanced technology and powerful machines, they can still identify minerals by testing several physical properties: luster, color, streak, hardness, crystal habit, cleavage and fracture, and ...
Introduction. Minerals can be identified by their physical characteristics. The physical properties of minerals are related to their chemical composition and bonding. Some characteristics, such as a mineral's hardness, are more useful for mineral identification. Color is readily observable and certainly obvious, but it is usually less ...
Fill your container with water high enough to submerge the specimen. Place the container on the scale and 0 out the scale. Tie a string around the specimen. Hold the string and suspend the specimen in the water. Record the weight displayed on the scale. Divide the number from step 1 by the number in step 6.
Minerals are naturally occurring, inorganic solids with a definite chemical composition and a crystal lattice structure. Although thousands of minerals in the earth have been identified, just ten minerals make up most of the volume of the earth's crust—plagioclase, quartz, orthoclase, amphibole, pyroxene, olivine, calcite, biotite, garnet ...
Read this essay to learn about Minerals. After reading this essay you will learn about:- 1. Introduction to Minerals 2. Formation of Minerals 3. Elements of Most Rock Forming Minerals 4. Origin 5. Identification 6. Utility 7. Distribution of Elements among Rock-Forming Minerals 8.
Identify and classify common rock forming minerals. The solid earth is made of rocks, which are made of minerals. To understand rocks you need to become familiar with minerals and how they are identified. This outcome gives you the background needed to understand the terms used in identifying minerals. This section will introduce you to minerals.
Definition and Examples. A mineral is a natural inorganic solid with a defined chemical composition and crystal structure. In geology, a mineral is a naturally occurring solid that has a well-defined chemical composition and crystal structure. Most minerals are inorganic, although some mineralogists allow for minerals that are organic compounds ...
Explore the scientists and their research. Minerals are classified on the basis of their chemical composition, which is expressed in their physical properties. This module, the second in a series on minerals, describes the physical properties that are commonly used to identify minerals. These include color, crystal form, hardness, density ...
Color is the most common characteristic that mineralogists look for when identifying a given mineral. Nevertheless, color is the least effective method of identifying minerals since many different minerals can have the same color. In the same manner, a specific mineral can assume more than one color (Ha et al., 2017).
The committee concludes that all minerals and mineral products could be or could become critical to some degree, depending on their importance and availability—in the sense that the chemical and physical properties they provide are essential to a specific product or use or more broadly, that specific minerals are an essential input for a national priority (for example, national defense) or ...
In geology, the classic definition of a mineral is: 1) naturally occurring, 2) inorganic, 3) solid at room temperature, 4) regular crystal structure, and 5) defined chemical composition. Some natural substances technically should not be considered minerals, but are included by exception. For example, water and mercury are liquid at room ...
All of our writers are dedicated to their job and do their best to produce all types of academic papers of superior quality. We have experts even in very specific fields of study, so you will definitely find a writer who can manage your order. 1 (888)814-4206 1 (888)499-5521. 964.
We hire a huge amount of professional essay writers to make sure that our essay service can deal with any subject, regardless of complexity. Place your order by filling in the form on our site, or contact our customer support agent requesting someone write my essay, and you'll get a quote. Hire a Writer. User ID: 102732.