Our websites may use cookies to personalize and enhance your experience. By continuing without changing your cookie settings, you agree to this collection. For more information, please see our University Websites Privacy Notice .
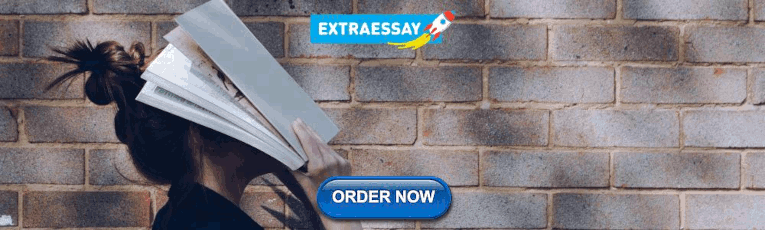
UConn Today
- School and College News
- Arts & Culture
- Community Impact
- Entrepreneurship
- Health & Well-Being
- Research & Discovery
- UConn Health
- University Life
- UConn Voices
- University News
September 7, 2021 | Combined Reports - UConn Communications
Study Shows the Impacts of Deforestation and Forest Burning on Biodiversity in the Amazon
Since 2001, between 40,000 and 73,400 square miles of Amazon rainforest have been impacted by fires

Ring of fire: Smoke rises through the understory of a forest in the Amazon region. Plants and animals in the Amazonian rainforest evolved largely without fire, so they lack the adaptations necessary to cope with it. (Credit: Paulo Brando)
A new study, co-authored by a team of researchers including UConn Ecology and Evolutionary Biology researcher Cory Merow provides the first quantitative assessment of how environmental policies on deforestation, along with forest fires and drought, have impacted the diversity of plants and animals in the Amazon. The findings were published in the Sept. 1 issue of Nature .
Researchers used records of more than 14,500 plant and vertebrate species to create biodiversity maps of the Amazon region. Overlaying the maps with historical and current observations of forest fires and deforestation over the last two decades allowed the team to quantify the cumulative impacts on the region’s species.
They found that since 2001, between 40,000 and 73,400 square miles of Amazon rainforest have been impacted by fires, affecting 95% of all Amazonian species and as many as 85% of species that are listed as threatened in this region. While forest management policies enacted in Brazil during the mid-2000s slowed the rate of habitat destruction, relaxed enforcement of these policies coinciding with a change in government in 2019 has seemingly begun to reverse this trend, the authors write. With fires impacting 1,640 to 4,000 square miles of forest, 2019 stands out as one of the most extreme years for biodiversity impacts since 2009, when regulations limiting deforestation were enforced.
“Perhaps most compelling is the role that public pressure played in curbing forest loss in 2019,” Merow says. “When the Brazilian government stopped enforced forest regulations in 2019, each month between January and August 2019 was the worse month on record (e.g. comparing January 2019 to previous January’s) for forest loss in the 20-year history of available data. However, based on international pressure, forest regulation resumed in September 2019, and forest loss declined significantly for the rest of the year, resulting in 2019 looking like an average year compared to the 20-year history. This was big: active media coverage and public support for policy changes were effective at curbing biodiversity loss on a very rapid time scale.”
The findings are especially critical in light of the fact that at no point in time did the Amazon get a break from those increasing impacts, which would have allowed for some recovery, says senior study author Brian Enquist, a professor in UArizona’s Department of Ecology and Evolutionary Biology .
“Even with policies in place, which you can think of as a brake slowing the rate of deforestation, it’s like a car that keeps moving forward, just at a slower speed,” Enquist says. “But in 2019, it’s like the foot was let off the brake, causing it to accelerate again.”
Known mostly for its dense rainforests, the Amazon basin supports around 40% of the world’s remaining tropical forests. It is of global importance as a provider of ecosystem services such as scrubbing and storing carbon from the atmosphere, and it plays a vital role in regulating Earth’s climate. The area also is an enormous reservoir of the planet’s biodiversity, providing habitats for one out of every 10 of the planet’s known species. It has been estimated that in the Amazon, 1,000 tree species can populate an area smaller than a half square mile.
“Fire is not a part of the natural cycle in the rainforest,” says study co-author Crystal N. H. McMichael at the University of Amsterdam. “Native species lack the adaptations that would allow them to cope with it, unlike the forest communities in temperate areas. Repeated burning can cause massive changes in species composition and likely devastating consequences for the entire ecosystem.”
Since the 1960s, the Amazon has lost about 20% of its forest cover to deforestation and fires. While fires and deforestation often go hand in hand, that has not always been the case, Enquist says. As climate change brings more frequent and more severe drought conditions to the region, and fire is often used to clear large areas of rainforest for the agricultural industry, deforestation has spillover effects by increasing the chances of wildfires. Forest loss is predicted reach 21 to 40% by 2050, and such habitat loss will have large impacts on the region’s biodiversity, according to the authors.
“Since the majority of fires in the Amazon are intentionally set by people, preventing them is largely within our control,” says study co-author Patrick Roehrdanz, senior manager of climate change and biodiversity at Conservation International. “One way is to recommit to strong antideforestation policies in Brazil, combined with incentives for a forest economy, and replicate them in other Amazonian countries.”
Policies to protect Amazonian biodiversity should include the formal recognition of Indigenous lands, which encompass more than one-third of the Amazon region, the authors write, pointing to previous research showing that lands owned, used or occupied by Indigenous peoples have less species decline, less pollution and better-managed natural resources.
The authors say their study underscores the dangers of continuing lax policy enforcement. As fires encroach on the heart of the Amazon basin, where biodiversity is greatest, their impacts will have more dire effects, even if the rate of forest burning remains unchanged.
The research was made possible by strategic investment funds allocated by the Arizona Institutes for Resilience at UArizona and the university’s Bridging Biodiversity and Conservation Science group. Additional support came from the National Science Foundation’s Harnessing the Data Revolution program . Data and computation were provided through the Botanical Information and Ecology Network , which is supported by CyVerse , the NSF’s data management platform led by UArizona.
Recent Articles

March 19, 2024
Searching for Data in DNA with CRISPR
Read the article

UConn Law Graduate Taps His Retirement Fund to Boost Scholarship

UConn Senior Offers Recipes for Success

- ORIENTATION
- ASSIGNMENTS
- Program Home Page
- LIBRARY RESOURCES
- Getting Help
- Engaging Course Concepts
Case Study: The Amazon Rainforest

The Amazon in context
Tropical rainforests are often considered to be the “cradles of biodiversity.” Though they cover only about 6% of the Earth’s land surface, they are home to over 50% of global biodiversity. Rainforests also take in massive amounts of carbon dioxide and release oxygen through photosynthesis, which has also given them the nickname “lungs of the planet.” They also store very large amounts of carbon, and so cutting and burning their biomass contributes to global climate change. Many modern medicines are derived from rainforest plants, and several very important food crops originated in the rainforest, including bananas, mangos, chocolate, coffee, and sugar cane.
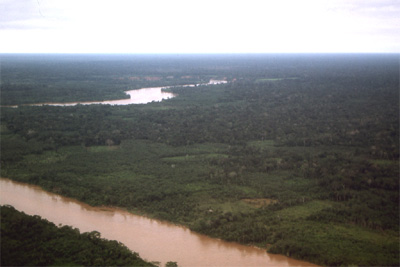
In order to qualify as a tropical rainforest, an area must receive over 250 centimeters of rainfall each year and have an average temperature above 24 degrees centigrade, as well as never experience frosts. The Amazon rainforest in South America is the largest in the world. The second largest is the Congo in central Africa, and other important rainforests can be found in Central America, the Caribbean, and Southeast Asia. Brazil contains about 40% of the world’s remaining tropical rainforest. Its rainforest covers an area of land about 2/3 the size of the continental United States.
There are countless reasons, both anthropocentric and ecocentric, to value rainforests. But they are one of the most threatened types of ecosystems in the world today. It’s somewhat difficult to estimate how quickly rainforests are being cut down, but estimates range from between 50,000 and 170,000 square kilometers per year. Even the most conservative estimates project that if we keep cutting down rainforests as we are today, within about 100 years there will be none left.
How does a rainforest work?
Rainforests are incredibly complex ecosystems, but understanding a few basics about their ecology will help us understand why clear-cutting and fragmentation are such destructive activities for rainforest biodiversity.
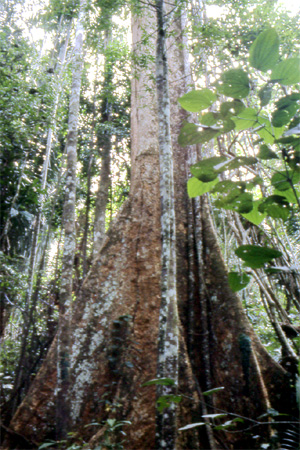
High biodiversity in tropical rainforests means that the interrelationships between organisms are very complex. A single tree may house more than 40 different ant species, each of which has a different ecological function and may alter the habitat in distinct and important ways. Ecologists debate about whether systems that have high biodiversity are stable and resilient, like a spider web composed of many strong individual strands, or fragile, like a house of cards. Both metaphors are likely appropriate in some cases. One thing we can be certain of is that it is very difficult in a rainforest system, as in most other ecosystems, to affect just one type of organism. Also, clear cutting one small area may damage hundreds or thousands of established species interactions that reach beyond the cleared area.
Pollination is a challenge for rainforest trees because there are so many different species, unlike forests in the temperate regions that are often dominated by less than a dozen tree species. One solution is for individual trees to grow close together, making pollination simpler, but this can make that species vulnerable to extinction if the one area where it lives is clear cut. Another strategy is to develop a mutualistic relationship with a long-distance pollinator, like a specific bee or hummingbird species. These pollinators develop mental maps of where each tree of a particular species is located and then travel between them on a sort of “trap-line” that allows trees to pollinate each other. One problem is that if a forest is fragmented then these trap-line connections can be disrupted, and so trees can fail to be pollinated and reproduce even if they haven’t been cut.
The quality of rainforest soils is perhaps the most surprising aspect of their ecology. We might expect a lush rainforest to grow from incredibly rich, fertile soils, but actually, the opposite is true. While some rainforest soils that are derived from volcanic ash or from river deposits can be quite fertile, generally rainforest soils are very poor in nutrients and organic matter. Rainforests hold most of their nutrients in their live vegetation, not in the soil. Their soils do not maintain nutrients very well either, which means that existing nutrients quickly “leech” out, being carried away by water as it percolates through the soil. Also, soils in rainforests tend to be acidic, which means that it’s difficult for plants to access even the few existing nutrients. The section on slash and burn agriculture in the previous module describes some of the challenges that farmers face when they attempt to grow crops on tropical rainforest soils, but perhaps the most important lesson is that once a rainforest is cut down and cleared away, very little fertility is left to help a forest regrow.
What is driving deforestation in the Amazon?
Many factors contribute to tropical deforestation, but consider this typical set of circumstances and processes that result in rapid and unsustainable rates of deforestation. This story fits well with the historical experience of Brazil and other countries with territory in the Amazon Basin.
Population growth and poverty encourage poor farmers to clear new areas of rainforest, and their efforts are further exacerbated by government policies that permit landless peasants to establish legal title to land that they have cleared.
At the same time, international lending institutions like the World Bank provide money to the national government for large-scale projects like mining, construction of dams, new roads, and other infrastructure that directly reduces the forest or makes it easier for farmers to access new areas to clear.
The activities most often encouraging new road development are timber harvesting and mining. Loggers cut out the best timber for domestic use or export, and in the process knock over many other less valuable trees. Those trees are eventually cleared and used for wood pulp, or burned, and the area is converted into cattle pastures. After a few years, the vegetation is sufficiently degraded to make it not profitable to raise cattle, and the land is sold to poor farmers seeking out a subsistence living.
Regardless of how poor farmers get their land, they often are only able to gain a few years of decent crop yields before the poor quality of the soil overwhelms their efforts, and then they are forced to move on to another plot of land. Small-scale farmers also hunt for meat in the remaining fragmented forest areas, which reduces the biodiversity in those areas as well.
Another important factor not mentioned in the scenario above is the clearing of rainforest for industrial agriculture plantations of bananas, pineapples, and sugar cane. These crops are primarily grown for export, and so an additional driver to consider is consumer demand for these crops in countries like the United States.
These cycles of land use, which are driven by poverty and population growth as well as government policies, have led to the rapid loss of tropical rainforests. What is lost in many cases is not simply biodiversity, but also valuable renewable resources that could sustain many generations of humans to come. Efforts to protect rainforests and other areas of high biodiversity is the topic of the next section.
Deforestation, warming flip part of Amazon forest from carbon sink to source
- July 14, 2021
The study area, which represents about 20 percent of the Amazon basin, has lost 30 percent of its rainforest
New results from a nine-year research project in the eastern Amazon rainforest finds that significant deforestation in eastern and southeastern Brazil has been associated with a long-term decrease in rainfall and increase in temperature during the dry season, turning what was once a forest that absorbed carbon dioxide into a source of planet-warming carbon dioxide emissions.
The study, published in the journal Nature , explored whether these changes had altered how much carbon the Amazon stored in its vast forests.
“Using nearly 10 years of CO 2 (carbon dioxide ) measurements, we found that the more deforested and climate-stressed eastern Amazon, especially the southeast, was a net emitter of CO 2 to the atmosphere, especially as a result of fires,” said John Miller, a scientist with NOAA’s Global Monitoring Laboratory and a co-author. “On the other hand, the wetter, more intact western and central Amazon, was neither a carbon sink nor source of atmospheric CO 2 , with the absorption by healthy forests balancing the emissions from fires.”
In addition to storing vast amounts of carbon, Amazonia is also one of the wettest places on Earth, storing immense amounts of water in its soils and vegetation. Transpired by leaves, this moisture evaporates into the atmosphere, where it fuels prodigious rainfall, averaging more than seven feet per year across the basin. For comparison the average annual rainfall in the contiguous U.S. is two and half feet. Several studies have estimated that water cycling through evaporation is responsible for 25 to 35 percent of total rainfall in the basin.
But deforestation and global warming over the last 40 years have affected rainfall and temperature with potential impacts for the Amazon’s ability to store carbon. Conversion of rainforest to agriculture has caused a 17 percent decrease in forest extent in the Amazon, which stretches over an area almost as large as the continental U.S.. Replacing dense, humid forest canopies with drier pastures and cropland has increased local temperatures and decreased evaporation of water from the rainforest, which deprives downwind locations of rainfall. Regional deforestation and selective logging of adjacent forests further reduces forest cover, amplifying the cycle of drying and warming. This, in turn, can reduce the capacity of the forests to store carbon, and increase their vulnerability to fires.
The 2.8 million square miles of jungle in the Amazon basin represents more than half of the tropical rainforest remaining on the planet. The Amazon is estimated to contain about 123 billion tons of carbon above and below ground, and is one of Earth’s most important terrestrial carbon reserves. As global fossil-fuel burning has risen, the Amazon has absorbed CO 2 from the atmosphere, helping to moderate global climate. But there are indications from this study and previous ones that the Amazon’s capacity to act as a sink may be disappearing.
Over the past several decades, intense scientific interest has focused on the question of whether the combined effects of climate change and the ongoing conversion of jungle to pasture and cropland could cause the Amazon to release more carbon dioxide than it absorbs.
In 2010, lead author Luciana Gatti, who led the international team of scientists from Brazil, the United Kingdom, New Zealand and the Netherlands, set out to explore this question. During the next nine years, Gatti, a scientist with Brazil’s National Institute for Space Research and colleagues obtained airborne measurements of CO 2 and carbon monoxide concentrations above Brazilian Amazonia. Analysis of CO 2 measurements from over 600 aircraft vertical profiles, extending from the surface to around 2.8 miles above sea level at four sites, revealed that total carbon emissions in eastern Amazonia are greater than those in the west.
“The regions of southern Pará and northern Mato Grosso states represent a worst-case scenario,” said Gatti.
The southeast region, which represents about 20 percent of the Amazon basin, and has experienced 30 percent deforestation over the previous 40 years. Scientists recorded a 25 percent reduction in precipitation and a temperature increase of at least 2.7 degrees Fahrenheit during the dry months of August, September and October, when trees are already under seasonal stress. Airborne measurements over nine years revealed this region was a net emitter of carbon, mainly as a result of fires, while areas further west, where less than 20 percent of the forest had been removed, sources balanced sinks. The scientists said the increased emissions were likely due to conversion of forest to cropland by burning, and by reduced uptake of CO 2 by the trees that remained.
These findings help scientists better understand the long-term impacts of interactions between climate and human disturbances on the carbon balance of the world’s largest tropical forest.
“The big question this research raises is if the connection between climate, deforestation, and carbon that we see in the eastern Amazon could one day be the fate of the central and western Amazon, if they become subject to stronger human impact,” Miller said. Changes in the capacity of tropical forests to absorb carbon will require downward adjustments of the fossil fuel emissions compatible with limiting global mean temperature increases to less than 2.0 or 1.5 degrees Celsius, he added.
This research was supported by NOAA’s Global Monitoring Laboratory and by funding from the State of Sao Paulo Science Foundation, UK Environmental Research Council, NASA, and the European Research Council.
For more information, contact Theo Stein, NOAA Communications: [email protected]
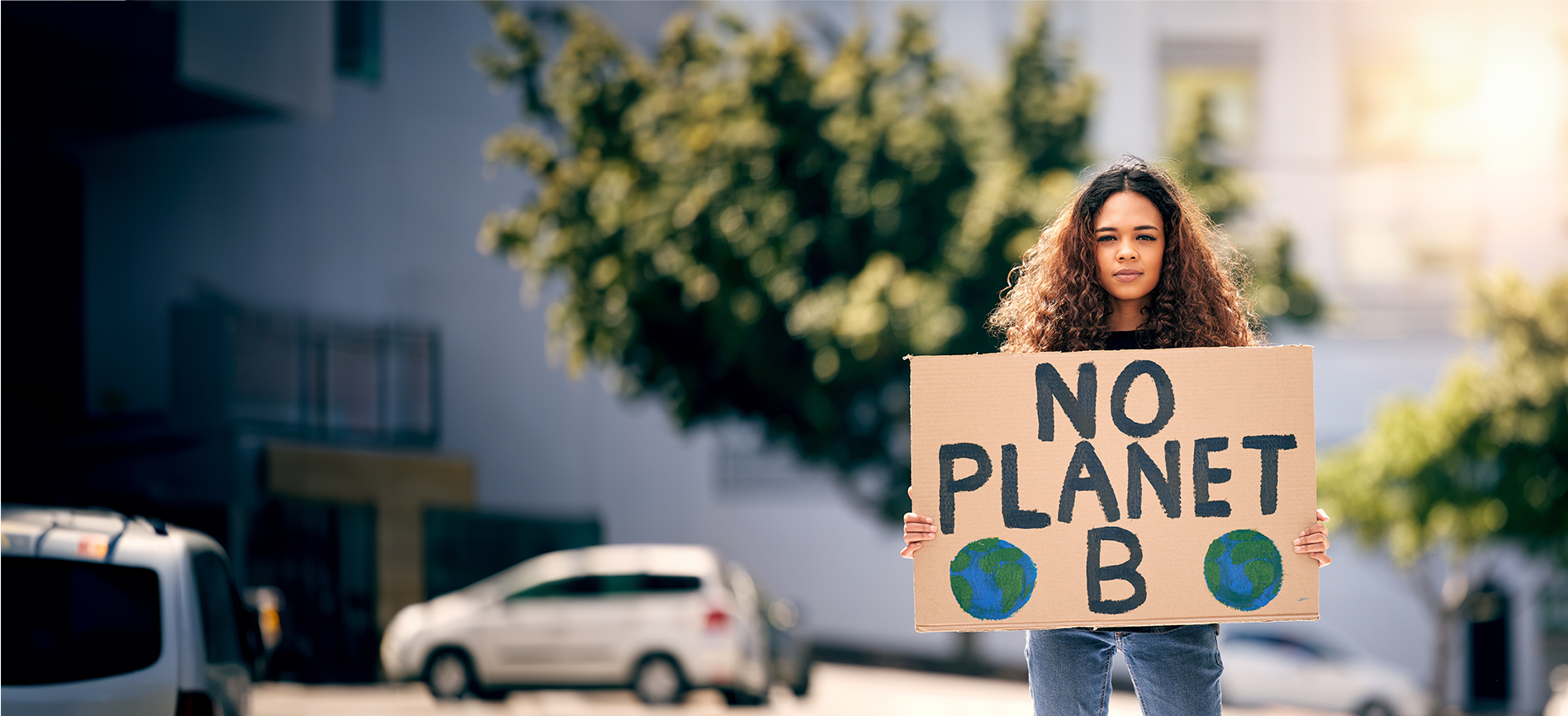
How social science helps us combat climate change
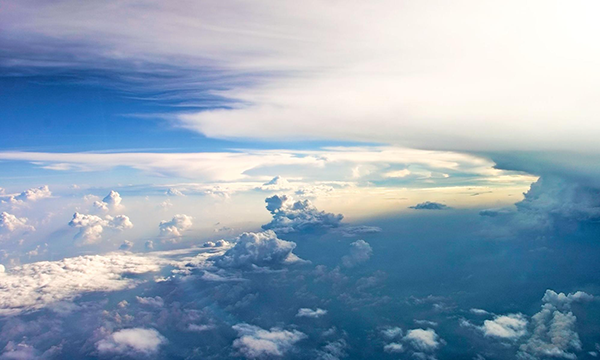
Could drying the stratosphere help cool the planet?
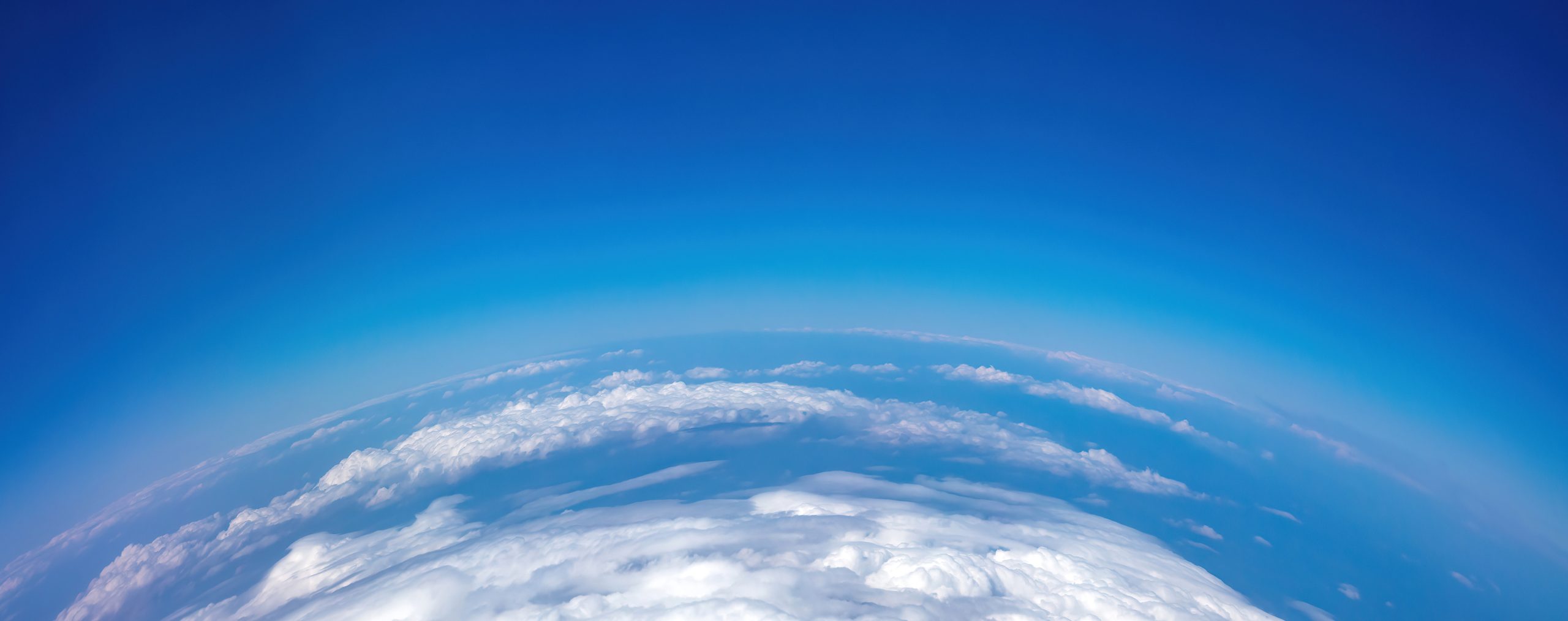
50 Years of Getting ENSO Predictions *Mostly* Correct
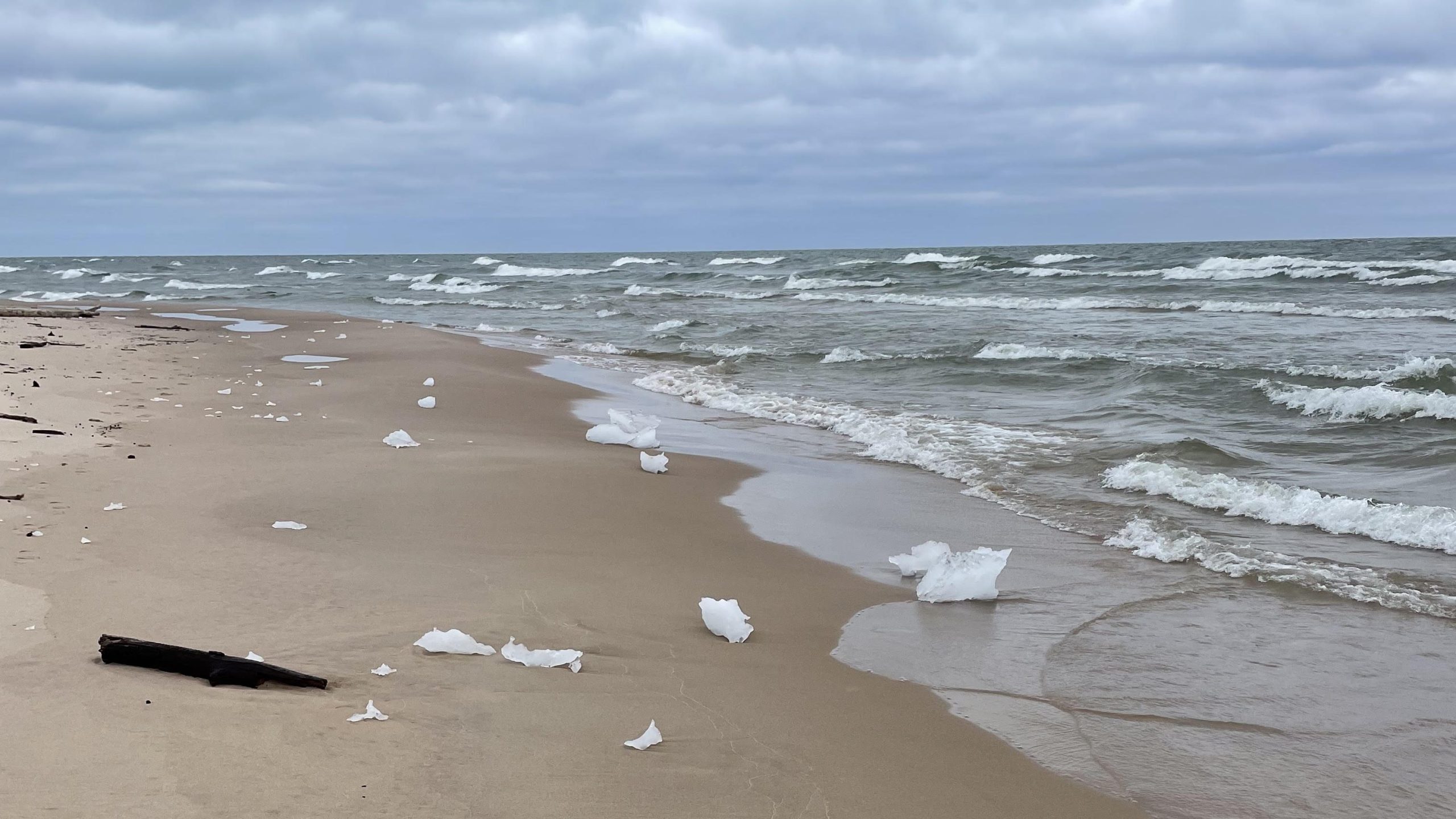
Great Lakes ice coverage reaches historic low
Popup call to action.
A prompt with more information on your call to action.
Jump to navigation
- Directory / Phonebook
Search form

Study Shows Impacts of Deforestation and Forest Burning on Amazon Biodiversity Combining extensive species mapping with remote sensing records of deforestation and fire reveals that the Amazon's biodiversity is extraordinarily vulnerable to changes in forest management and policy enforcement.
1_dsc01610.jpg.
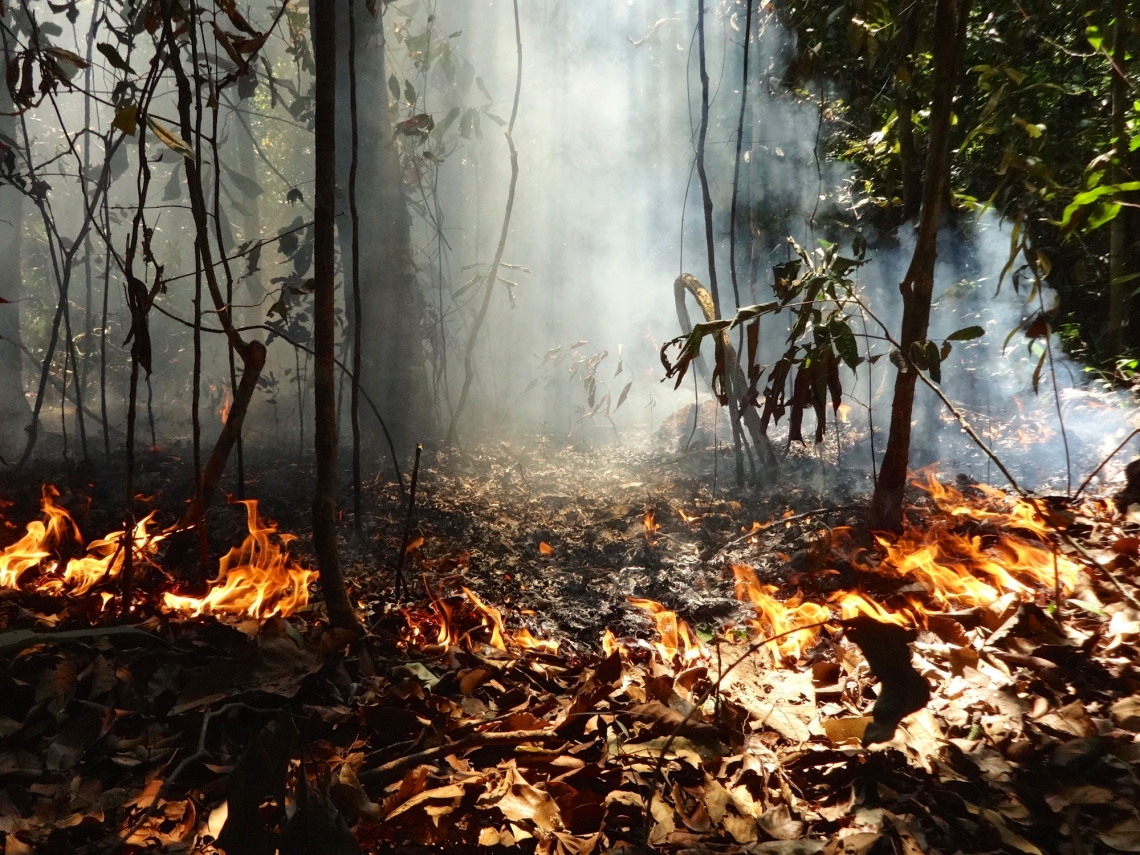
A new study , co-authored by University of Arizona researchers and published in the Sept. 1 issue of Nature, provides the first quantitative assessment of how environmental policies on deforestation, along with forest fires and drought, have impacted the diversity of plants and animals in the Amazon.
Researchers used records of more than 14,500 plant and vertebrate species to create biodiversity maps of the Amazon region. Overlaying the maps with historical and current observations of forest fires and deforestation over the last two decades allowed the team to quantify the cumulative impacts on the region's species.
They found that since 2001, between 40,000 and 73,400 square miles of Amazon rainforest have been impacted by fires, affecting 95% of all Amazonian species and as many as 85% of species that are listed as threatened in the region. While forest management policies enacted in Brazil during the mid-2000s slowed the rate of habitat destruction, relaxed enforcement of those policies coinciding with a change in government in 2019 has seemingly begun to reverse the trend, the authors write. With fires impacting 1,640 to 4,000 square miles of forest, 2019 stands out as one of the most extreme years for biodiversity impacts since 2009, when regulations limiting deforestation were enforced.
"We show how policy has had a direct and enormous influence on the pace at which biodiversity across the entire Amazon has been affected," said senior study author Brian Enquist , a professor in UArizona Department of Ecology and Evolutionary Biology . He added that the findings are especially critical in light of the fact that at no point in time did the Amazon get a break from those increasing impacts, which would have allowed for some recovery.
"Even with policies in place, which you can think of as a brake slowing the rate of deforestation, it's like a car that keeps moving forward, just at a slower speed," Enquist said. "But in 2019, it's like the foot was let off the brake, causing it to accelerate again."
"Assessments based on remote sensing of burned and deforested areas had been done before, but we haven't had a detailed record of what impact those have on the species diversity of the region," said Xiao Feng, the study's first author, who conducted most of the work while he was a postdoctoral researcher at UArizona and is now an assistant professor at Florida State University. "This has been a huge collection of data and an unprecedented informatics effort associated with biodiversity data, and that allowed us to make these interconnections visible."
1_DSCF6707.jpeg.jpg
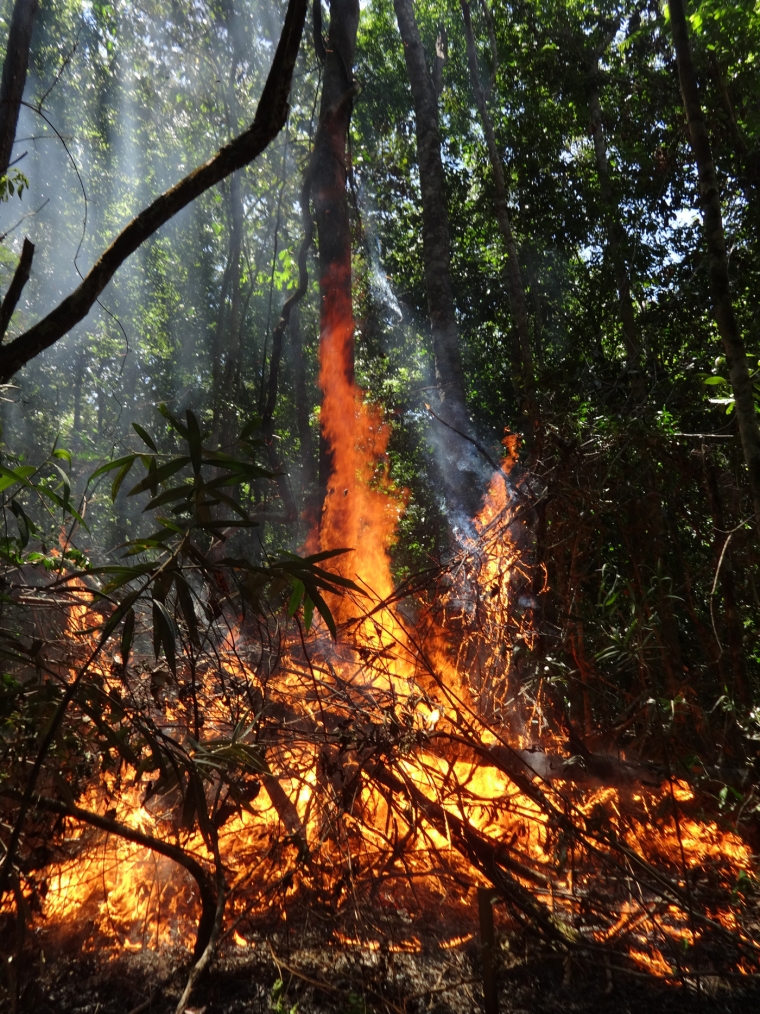
Known mostly for its dense rainforests, the Amazon basin supports around 40% of the world's remaining tropical forests. It is of global importance as a provider of ecosystem services such as scrubbing and storing carbon from the atmosphere, and it plays a vital role in regulating Earth's climate. The area also is an enormous reservoir of the planet's biodiversity, providing habitats for one out of every 10 of the planet's known species. It has been estimated that in the Amazon, 1,000 tree species can populate an area smaller than half a square mile.
"This is important in light of the fact that biodiversity goes hand in hand with ecosystem functioning," Enquist said, adding that species with the smallest ranges tend to suffer the most. "Species can become virtually extinct even before they lose their entire range of habitat."
"Fire is not a part of the natural cycle in the rainforest," said study co-author Crystal N. H. McMichael at the University of Amsterdam. "Native species lack the adaptations that would allow them to cope with it, unlike the forest communities in temperate areas. Repeated burning can cause massive changes in species composition and likely devastating consequences for the entire ecosystem."
Since the 1960s, the Amazon has lost about 20% of its forest cover to deforestation and fires. While fires and deforestation often go hand in hand, that has not always been the case, Enquist said. As climate change brings more frequent and more severe drought conditions to the region, and fire is often used to clear large areas of rainforest for the agricultural industry, deforestation has spillover effects by increasing the chances of wildfires. Forest loss is predicted reach 21% to 40% by 2050, and such habitat loss will have large impacts on the region's biodiversity, according to the authors.
"What's nasty about fire in that region is once it starts to encroach on areas that have not been burned, it degrades them irreparably and makes them more susceptible to burning in the future," he said.
"Since the majority of fires in the Amazon are intentionally set by people, preventing them is largely within our control," said study co-author Patrick Roehrdanz, senior manager of climate change and biodiversity at Conservation International. "One way is to recommit to strong anti-deforestation policies in Brazil, combined with incentives for a forest economy, and replicate them in other Amazonian countries."
1_Brando_Canarana_Churras_2016-29.jpg
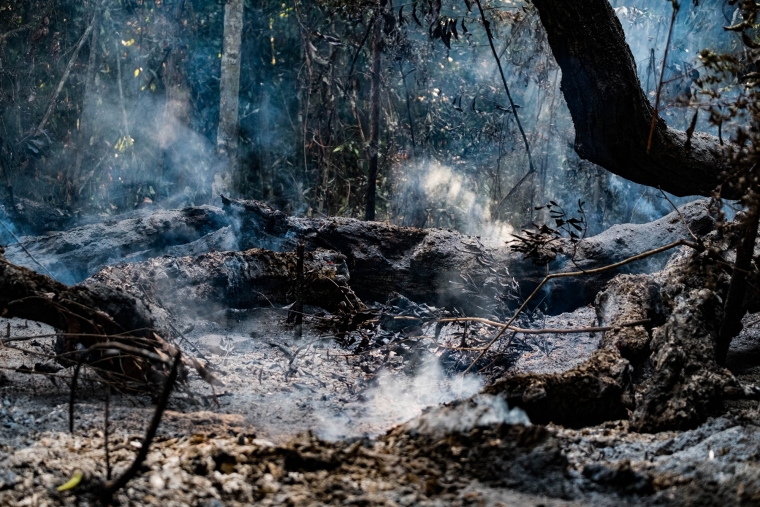
Policies to protect Amazonian biodiversity should include the formal recognition of Indigenous lands, which are more than one-third of the Amazon region, the authors write, pointing to previous research showing that lands owned, used or occupied by Indigenous peoples have less species decline, less pollution and better-managed natural resources.
The authors say their study underscores the dangers of continuing lax policy enforcement. As fires encroach on the heart of the Amazon basin, where biodiversity is greatest, their impacts will have more dire effects, even if the rate of forest burning remains unchanged.
"We have to remember that it took decades to reduce Amazon deforestation, but it may take just a few years to destroy the conservation policy pillars of conservation," said co-author Paulo Brando, assistant professor in the Department of Earth System Science at the University of California Irvine. "The recent reverse in deforestation and fires trends, and their impacts on Amazon biodiversity, should be a huge cause of concern."
The research was made possible by strategic investment funds allocated by the Arizona Institutes for Resilience at UArizona and the university's Bridging Biodiversity and Conservation Science group. Additional support came from the National Science Foundation's Harnessing the Data Revolution program . Data and computation were provided through the Botanical Information and Ecology Network , which is supported by CyVerse , the NSF's data management platform led by UArizona.
Resources for the media
Media contact(s).
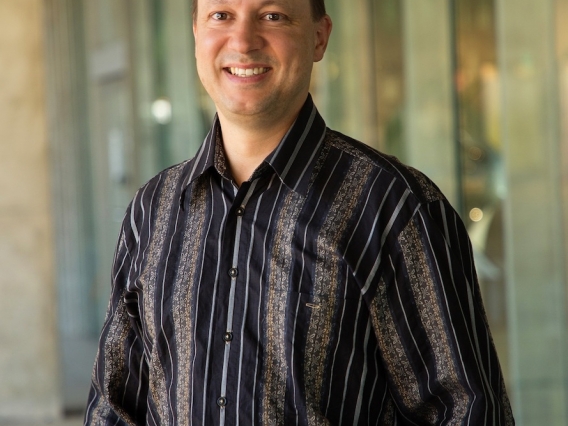
Daniel Stolte
Science Writer, University Communications
Researcher contact(s)
Brian enquist.
Department of Ecology and Evolutionary Biology
University of Arizona in the News
Subscribe to UANow
Get the latest University of Arizona news delivered in your inbox. Or, stay in the loop using our Amazon Alexa skill.
Get Alexa alerts

An official website of the United States government
The .gov means it’s official. Federal government websites often end in .gov or .mil. Before sharing sensitive information, make sure you’re on a federal government site.
The site is secure. The https:// ensures that you are connecting to the official website and that any information you provide is encrypted and transmitted securely.
- Publications
- Account settings
Preview improvements coming to the PMC website in October 2024. Learn More or Try it out now .
- Advanced Search
- Journal List
- v.9(24); 2019 Dec
Avian ecological succession in the Amazon: A long‐term case study following experimental deforestation
Cameron l. rutt.
1 Biological Dynamics of Forest Fragments Project, Instituto Nacional de Pesquisas da Amazônia (INPA), Manaus Brazil
2 School of Renewable Natural Resources, Louisiana State University and Louisiana State University AgCenter, Baton Rouge LA, USA
Vitek Jirinec
Mario cohn‐haft.
3 Coleções Zoológicas – INPA, Manaus Brazil
William F. Laurance
4 Centre for Tropical Environmental and Sustainability Science, College of Science and Engineering, James Cook University, Cairns Qld, Australia
Philip C Stouffer
Associated data.
- Rutt, C. L. , Jirinec, V. , Cohn‐Haft, M. , Laurance, W. E. , & Stouffer, P. C. (2019). Data from: Avian ecological succession in the Amazon: A long‐term case study following experimental deforestation Dryad Data Repository . 10.5061/dryad.xpnvx0kb2 [ CrossRef ]
A list of all species that we included in the regional species pool is archived at the Dryad Digital Repository https://doi.org/10.5061/dryad.xpnvx0kb2 . We annotated this list with the habitat associations for all species and designated which of these have already been detected at the BDFFP, as well as the more restricted subset that we consider to be members of the core avifauna.
- Approximately 20% of the Brazilian Amazon has now been deforested, and the Amazon is currently experiencing the highest rates of deforestation in a decade, leading to large‐scale land‐use changes. Roads have consistently been implicated as drivers of ongoing Amazon deforestation and may act as corridors to facilitate species invasions. Long‐term data, however, are necessary to determine how ecological succession alters avian communities following deforestation and whether established roads lead to a constant influx of new species.
- We used data across nearly 40 years from a large‐scale deforestation experiment in the central Amazon to examine the avian colonization process in a spatial and temporal framework, considering the role that roads may play in facilitating colonization.
- Since 1979, 139 species that are not part of the original forest avifauna have been recorded, including more secondary forest species than expected based on the regional species pool. Among the 35 species considered to have colonized and become established, a disproportionate number were secondary forest birds (63%), almost all of which first appeared during the 1980s. These new residents comprise about 13% of the current community of permanent residents.
- Widespread generalists associated with secondary forest colonized quickly following deforestation, with few new species added after the first decade, despite a stable road connection. Few species associated with riverine forest or specialized habitats colonized, despite road connection to their preferred source habitat. Colonizing species remained restricted to anthropogenic habitats and did not infiltrate old‐growth forests nor displace forest birds.
- Deforestation and expansion of road networks into terra firme rainforest will continue to create degraded anthropogenic habitat. Even so, the initial pulse of colonization by nonprimary forest bird species was not the beginning of a protracted series of invasions in this study, and the process appears to be reversible by forest succession.
We examined how nonprimary forest birds colonized a large‐scale deforestation experiment in the central Amazon across nearly 40 years, focusing on two roads that may act as corridors to facilitate species' arrivals. A disproportionate number of widespread generalists associated with secondary forest colonized quickly following deforestation, but these species remained restricted to anthropogenic habitats and did not infiltrate old‐growth forests nor displace forest birds. This suggests that the initial pulse of colonization by nonprimary forest birds was not the beginning of a protracted series of invasions in this study, and the process appears to be reversible by forest succession.

1. INTRODUCTION
Deforestation rates in the Amazon increased dramatically in the early 1970s, rose during the late 1990s to the highest absolute rates in the world, and accelerated once again during the early 2000s, before diminishing to the lowest rates in three decades (2012: Fearnside, 2005 ; INPE, 2019 ; Laurance, Albernaz, & Da Costa, 2001 ; Laurance, Cochrane, et al., 2001 ). In the past 4 years, however, that trend has reversed itself, with Amazon deforestation again growing to the highest rates in a decade (8,000 km 2 in 2018; Artaxo, 2019 ; INPE, 2019 ). Roads have consistently been implicated as direct and indirect drivers of Amazon deforestation (Barber, Cochrane, Souza, & Laurance, 2014 ; Barni, Fearnside, & Graca, 2015 ; Fearnside, 2015 ; Fearnside & Graca, 2006 ; Laurance, Albernaz, et al., 2001 ; Laurance et al., 2002 ; Nepstad et al., 2001 ; Soares‐Filho et al., 2006 ). When both highways and secondary roads are taken into account, 94% of regional deforestation occurred within 5.5 km of a road; together, this network and buffer covers nearly a third (31.7%) of the Brazilian Amazon (Barber et al., 2014 ). Among the diverse array of deleterious effects that roads exert on the flora and fauna of tropical forests (reviewed in Laurance, Goosem, & Laurance, 2009 ), road networks may act as corridors to facilitate species invasions (Gascon et al., 1999 ; Laurance et al., 2018 ). However, we are not aware of any long‐term studies in Amazonia that have examined vertebrate species invasions in the context of roads and land‐use change.
For Amazonian birds, a considerable body of research has shown the toll that deforestation (including partial deforestation characterized by forest fragments) and existing roads take on the forest bird community (e.g., Ahmed et al., 2014 ; Develey & Stouffer, 2001 ; Ferraz et al., 2003 ; Laurance, 2004 ; Laurance, Stouffer, & Laurance, 2004 ; Lees & Peres, 2006 , 2009 ; Mahood, Lees, & Peres, 2012 ; Stouffer, Johnson, Bierregaard, & Lovejoy, 2011 ). However, little attention has focused on these deforested landscapes and how ecological succession alters avian communities following anthropogenic change. After deforestation, early‐successional habitats could be populated by either local, preexisting forest species or colonized by foreign species from disjunct habitats, which could eventually infiltrate primary forest. Furthermore, the timing of arrival, persistence (temporary or permanent), and eventual turnover of these colonists remain poorly understood. Unfortunately, to date, most previous research has focused on short‐term, contemporary studies, which provide a static snapshot in this continual process. But due to the magnitude of Brazil's ongoing deforestation crisis, it is critically important to characterize the long‐term avifaunal changes in and adjacent to deforested regions.
To examine avian arrivals following deforestation, we chose a large‐scale experiment in the central Amazon that possesses a unique series of long‐term ornithological research—the Biological Dynamics of Forest Fragments Project (BDFFP). We employed three historical avian inventories, spread across four decades (1979–2017), to make inferences about the long‐term colonization and accumulation of species that were not part of the original forest avifauna (Cohn‐Haft, Whittaker, & Stouffer, 1997 ; Rutt et al., 2017 ; Stotz & Bierregaard, 1989 ). More specifically, we were interested in how patterns of avian arrivals relate to deforestation locally and along two roads leading north from the Manaus metropolitan area, a potential source for colonizing birds. Prior to the late 1970s, the region was continuous forest, but today the BDFFP represents a mosaic of regenerating second‐growth, small forest fragments, and continuous forest (Cohn‐Haft et al., 1997 ). Here, we (a) use the regional species pool to identify possible colonists to the BDFFP and estimate the expected proportion of arrivals by habitat type, (b) describe the chronosequence and source habitat of all birds added to the core avifauna (sensu Cohn‐Haft et al., 1997 ), (c) plot the location and habitat of all first detections since 1995, and (d) assess the contribution of landscape change, both locally and along two road corridors, to the process of colonization. We predict that new arrivals at the BDFFP are disproportionately represented by species from separate early‐successional habitats (e.g., second‐growth and riverine vegetation) and that these additions reflect changes in regional access via roads and not local landscape changes.
2.1. Study area
The BDFFP (2°20′S, 60°W) is located ~80 km north of Manaus, Amazonas, Brazil (Figure (Figure1). 1 ). Before the project was initiated in 1979, the entire site and much of the surrounding region consisted of continuous primary terra firme forest. Development on three ~15,000 ha cattle ranches at the BDFFP began in the late 1970s, and forest clearing was largely complete by the mid‐1980s (Cohn‐Haft et al., 1997 ; Stotz & Bierregaard, 1989 ). These cattle ranches, however, were gradually abandoned or operated at low production levels, providing a mosaic of open pastures, second growth of various ages (from 3 to >30 years), and forest fragments embedded in a region that continues to be dominated by primary forest. To this day, regional disturbance is still minimal, except for the lands between Manaus and the BDFFP. The largest city in Amazonia, Manaus is home to >2.1 million people (July 2017), representing more than half the estimated population for the state (IBGE, 2017 ). Only four major roads, all paved and operational year‐round, lead outward from Manaus and connect to adjacent cities. Two of these are federal highways (BR‐174 and BR‐319) and the other two are state highways (AM‐010 and AM‐070). Here, we focus on the two paved highways that leave Manaus heading north (BR‐174 and AM‐010) toward the BDFFP (Figure (Figure1 1 ).

Study area, showing the Biological Dynamics of Forest Fragments Project (BDFFP; represented by a 10‐km buffer around a control reserve and three ranches), as well as 2‐km buffers around two putative avian dispersal corridors that lead north from the city of Manaus, Brazil, and the confluence of the Rio Negro and the Rio Solimões (BR‐174 on the left and AM‐010/ZF‐7 on the right). Gray background represents land cover in 2017 that was classified as closed‐canopy forest in our analyses, whereas white indicates nonforest (roads, pastures, agriculture, scrub, etc.). Symbols refer to locations where each of the most recent 19 species added to the BDFFP core avifauna was first detected. Although some have appeared in very small areas of disturbance, the vast majority of these additions are from the major disturbed areas of the ranches
2.2. Generating the habitat associations for the regional species pool
Neotropical birds discriminate among different vegetation types and can broadly be categorized by habitat, thus allowing us to distinguish the primary avian habitats of Amazonia (Parker, Stotz, & Fitzpatrick, 1996 ). Terra firme forests are the dominant forest type in both area and species richness (Parker et al., 1996 ). In Amazonia, other main vegetation types include floodplain forests (e.g., várzea in seasonally flooded forests along “whitewater” rivers and igapó along “blackwater” rivers), river island scrub, and white sand forest, each with their own distinct avifauna and local contribution to Amazonian biodiversity (Borges, 2004 ; Parker et al., 1996 ; Remsen & Parker, 1983 ; Rosenberg, 1990 ). Secondary forests, on large scales almost exclusively created by anthropogenic disturbance, are increasingly becoming an important component in Amazonia and are occupied by more broadly distributed, habitat‐generalist birds (Parker et al., 1996 ; Perz & Skole, 2003 ). Major rivers also divide closely related species, leading to areas of endemism that further increase Amazonian biodiversity (Capparella, 1991 ; Cracraft, 1985 ). Collectively, this results in a distinct regional species pool that can be characterized by habitat types and separated by interfluves, giving us the opportunity to evaluate how habitat affinities of birds in a regional species pool contribute to the avifauna of an altered site.
We first developed a list that we consider to be the “regional species pool”—those species that might reasonably be expected to occur at the study site. This seemingly arbitrary task of deciding which species are most likely was based on meeting relatively simple requirements. First, the species must have been previously recorded somewhere in the Amazon (total ~1,300 spp.), thereby assuming that the Amazonian avifauna as a whole is well‐characterized despite knowledge gaps at a regional scale. Second, species known to be limited to upland ( terra firme ) forest (see below for habitat classifications) were only included if they occur within the Guiana area of endemism, that is, north of the Amazon River and east of the lower Rio Negro. This is because these large rivers are believed to delimit distributions for terra firme species and, empirically, because no terra firme species has been found at the study site that does not also occur elsewhere within the Guiana area (see Cohn‐Haft et al., 1997 and Section 3 ), even if those other terra firme species are normally found within a few kilometers of the Guiana area, but in adjacent areas of endemism (south of the Amazon River and west of the lower Rio Negro). Third, because species from other habitats are not known to exhibit the same degree of endemism as terra firme birds, we relaxed our criteria, included them if previously known from within a 500 km radius if nonmigratory (resident) and a 1,000 km radius if migratory. We then curated the list by hand, adding or removing species to ensure the final product matched current knowledge. The resulting list necessarily includes all species already detected within the study area.
Using the Parker et al. ( 1996 ) databases, we added habitat associations for all birds in the regional species pool. We used the first (primary) habitat type when appropriate; however, we made adjustments, accepting secondary or tertiary habitat codes when available, if the primary code suggested the species occurred in habitat not found in central Amazonia (e.g., montane forest and temperate grassland). We collapsed these 22 categories (21 distinct habitats plus “Edge”) for the regional species pool into a more manageable seven that adequately captured habitat diversity in the immediate vicinity of the BDFFP: aquatic, primary forest, riverine, secondary forest, white sand, palm, and grassland/pasture (see Appendix A ). We elected to use the term “riverine” to refer to terrestrial birds that occur in floodplain forests, river‐edge forest, and on river islands.
For those birds in the regional species pool ( n = 725), we first categorized species into two groups: those that have been recorded at the BDFFP and those that have not. Within the species that had been recorded, we categorized species as those that are part of the core forest avifauna and those that are not. The core avifauna is defined as all species that occupy primary terra firme forest at a relative abundance of rare, uncommon, or common (i.e., species regularly found in appropriate habitat, but not occasionally dispersing or wandering individuals; Remsen, 1994 ). This assemblage is a well‐characterized baseline after >35 years of ornithological coverage (Cohn‐Haft et al., 1997 ; Rutt et al., 2017 ). Those listed species that are not part of the core avifauna are presumed to have appeared following local landscape change. Three successive inventories then allowed us document the chronosequence of arriving colonists and migrants/vagrants, roughly covering the 1980s (Stotz & Bierregaard, 1989 ), the late 1980s to mid‐1990s (Cohn‐Haft et al., 1997 ), and the mid‐1990s to the present (Rutt et al., 2017 ). We distinguish between these two groups of noncore species by abundance, considering species that have reached a relative abundance of “uncommon” or “common” (species that occur in most or all appropriate habitats) in Cohn‐Haft et al. ( 1997 ) or Rutt et al. ( 2017 ) to have colonized and become established. Sampling has been systematic in continuous forest, fragments, and fragment borders but opportunistic in all other habitats. Taxonomy follows the South American Classification Committee (Remsen et al., 2018 ).
2.3. Location of recent additions
To verify that published habitat preferences match where a species first appears at a novel site, we plotted the approximate GPS coordinates for the first detection of each of the most recent 19 species added to the BDFFP (Figure (Figure1; 1 ; Rutt et al., 2017 ); no comparable raw data were available for additions before 1997. These locations were overlaid onto satellite imagery that allowed classification by coarse habitat types, which we combined with habitat descriptions from each of the species accounts in Rutt et al. ( 2017 ) to contextualize the local habitat at the time of detection.
2.4. Assessing long‐term changes in forest cover at the BDFFP and along two road corridors
For our purposes, we define the BDFFP study area as a 10‐km buffer around the 11 experimental fragments plus a 1,000‐ha control reserve known as Km 41 (Bierregaard, Gascon, Lovejoy, & Mesquita, 2001 ). For the two road corridors (BR‐174 and AM‐010/ZF‐7), we delineated 2 km buffers around each of these roads between the northern urban limits of Manaus and the southern extent of the BDFFP buffer. For all three zones, we used Landsat satellite imagery in 1987, 1997, 2007, and 2017 to quantify the extent of forest cover across 30 years. We selected cloud‐free imagery within our study area that resulted in all samples being taken during the dry season: 29 August 1987 (Landsat 5), 21 June 1997 (LS 5), 4 August 2007 (LS 5), and 30 July 2017 (LS 8). Land cover classification was conducted in GIS (ArcMap 10.5; ESRI) at 30‐m resolution for all imagery. We first generated false‐color images by combining spectral bands that create contrast between land classes of interest (bands 2, 3, 4 for LS 5, bands 3, 4, 5 for LS 8). We then classified multiband images into closed‐canopy “forest” (primary forest or mature regrowth) and “other” using ArcMap's interactive supervised classification, which employs user‐selected training samples. For “forest” training samples, we selected areas that were known to contain continuous forest that was at least 30 years old, whereas for “other” we chose bare soil, roads, clearcuts, open water, pastures, and housing. Training samples for both land cover categories were identical across the four time periods (e.g., areas that were always forest and roads). Because classified forest imagery contained many small holes, likely due to natural gap dynamics, we filled interior gaps ≤0.27 ha (3 pixels) before we calculated total forest cover.
2.5. Data analysis
To determine whether habitat associations of colonists and migrants/vagrants are disproportional to habitat associations of available birds in the regional species pool (i.e., excluding the core avifauna), we used G tests of independence. We similarly performed G tests to determine whether habitat associations of noncore species that appeared early (1979–86) and late (1987–2017) differed significantly from the regional species pool. If an overall G test was significant, we then ran post hoc tests with a Bonferroni correction—each nominal variable against the sum of all others (additional 2 × 2 contingency tables)—to identify habitat(s) that were disproportionately contributing colonists or additions (Figure (Figure2 2 ).

The number of observed (gray bars) and expected (empty bars) bird species per habitat added to the core avifauna at the Biological Dynamics of Forest Fragments Project (BDFFP) in the state of Amazonas, Brazil. Expected proportions were derived by assuming that species would filter passively in numbers proportional to the habitat associations of the Manaus regional species pool and, together, would sum to 139 species, the total number of birds added to the core avifauna of the BDFFP
3.1. Community structure and habitat associations
Our regional species pool of 725 species included more than half of all known Amazonian bird species (see Rutt, Jirinec, Cohn‐Haft, Laurance, & Stouffer, 2019 ). From that pool, 407 (56%) have been recorded at the BDFFP (Rutt et al., 2017 ). The core avifauna at the BDFFP typifies the forest community prior to disturbance and comprises 268 species (Rutt et al., 2017 ). Since 1979, 139 species that are not a part of the core avifauna have been recorded at the BDFFP: 85 were added by 1986, another 35 by 1994, and the final 19 by 2017 (Table (Table1). 1 ). The vast majority of these additions are considered rare or vagrants at the BDFFP (99 species; 71% of additions) or are regular austral or boreal migrants (5 species; 4%). We considered the remaining 35 species to be established permanent residents (Table (Table2). 2 ). We found no species endemic to areas west of the Rio Negro or south of the Amazon River. All primary terra firme forest birds at the BDFFP are widespread in the Guianan region.
Time period of first detection by habitat association for bird species added to the core avifauna at the Biological Dynamics of Forest Fragments Project (BDFFP) in the state of Amazonas, Brazil
Total species possible enumerates the regional species pool (minus the already identified core avifauna; see Section 2 ) and the final column those that have never been recorded at the BDFFP. Numbers in parentheses designate how many species of a particular habitat colonized during that interval; the balance refers to migrants and vagrants.
Bold cell values indicate statistically significant deviations from expected values given the total species possible (first column).
Thirty‐five bird species that colonized the Biological Dynamics of Forest Fragments Project in the state of Amazonas, Brazil, along with the interval during which each species was first detected on site and its habitat affiliation according to the Parker et al. ( 1996 ) databases
A species was considered to have colonized and become established if it was not a part of the original core avifauna and it reached a relative abundance of “uncommon” or “common” in 1994 or 2017 (Cohn‐Haft et al., 1997 ; Rutt et al., 2017 ).
Taken altogether, habitat associations of the 139 species of colonists and migrants/vagrants at the BDFFP were not representative of habitat associations for available birds in the regional species pool ( G = 27.11, df = 6, p < .001, Figure Figure2). 2 ). Excluding those habitats with very few species (sand and palm), post hoc tests with a Bonferroni correction ( p = .01) revealed that more secondary forest species ( G = 12.28, df = 1, p < .001) and fewer riverine species ( G = 18.87, df = 1, p < .001; Figure Figure2) 2 ) appeared than would have been expected from the regional species pool. The pattern was identical in the restricted subset of colonists ( G = 26.38, df = 6, p < .001), with more secondary forest species ( G = 17.91, df = 1, p < .001) and fewer riverine species ( G = 7.56, df = 1, p = .006) than predicted by the regional species pool. This difference in habitat association, however, was only evident for the 85 species added during the 1980s (Table (Table1; 1 ; G = 35.40, df = 6, p < .001) and was not significant for the subsequent 54 additions that accumulated from the late 1980s through the 2000s ( G = 4.41, df = 6, p = .62). Only during the 1980s did more secondary forest species ( G = 10.04, df = 1, p = .002) and fewer riverine species ( G = 27.83, df = 1, p < .001) appear than were expected from the regional species pool.
3.2. Location of recent additions
With only one exception, all of the 19 species whose preferred habitat can be found at the BDFFP (i.e., primary forest, secondary forest, or aquatic) were first detected in that habitat. The lone exception was Scaled Pigeon ( Patagioenas speciosa ; primary forest), which was first detected in mature secondary forest; however, this species' local and published habitat affinities actually include a variety of shorter and sparser forests, and it does not typically occupy primary forest here (see species account in Rutt et al., 2017 ). Species that Parker et al. ( 1996 ) classified as grassland (Upland Sandpiper [ Bartramia longicauda ]), riverine (Cinnamon Attila [ Attila cinnamomeus ], White‐throated Kingbird [ Tyrannus albogularis ]), and sand (Yellow‐crested Manakin [ Heterocercus flavivertex ]) birds—habitats not present at the BDFFP—first appeared in the closest on‐site analogs: pasture, a moriche palm ( Mauritia flexuosa ) swamp and forest pond, and stunted secondary forest, respectively. Two additional riverine species (Black‐chinned Antbird [ Hypocnemoides melanopogon ] and Yellow‐rumped Cacique [ Cacicus cela ]) were found at primary forest sites, but one was in a small camp clearing and the other in a small (10‐ha) forest fragment, suggesting association with local disturbance.
3.3. Temporal landscape changes
As delineated by our binary landscape classification, the BDFFP has been predominantly covered by closed‐canopy forest across all four time periods (90.0%–94.8%; Figure Figure3), 3 ), becoming more forested from 1987 to 2017. Although the majority of the two road buffers was also comprised of closed‐canopy forest (an average of 73.1% along BR‐174 and 75.5% along AM‐010/ZF‐7), nonforest habitat was much more uniformly distributed and prevalent, remaining between 21.6% and 30.6% of the total area of each road buffer during all four time periods. There were no clear temporal trends in the extent of forest cover along the two road corridors, as both had similar proportions of closed‐canopy forest in 1987 and 2017 (Figure (Figure3 3 ).

Results of land cover classification as closed‐canopy forest (primary forest or mature regrowth; black) and nonforest (white) using Landsat imagery across 30 years at the Biological Dynamics of Forest Fragments Project (BDFFP) and along two highways that connect the city of Manaus, Brazil, to the BDFFP. The percent of forest within the BDFFP and along each of the two corridors (BR‐174 to the west and AM‐010/ZF‐7 to the east) is illustrated during all four time periods
4. DISCUSSION
Our long‐term data allow us to describe the accumulation of novel species into an Amazon forest bird community following deforestation. In all, 139 species that are not part of the core avifauna have been added during the past ~40 years (1979–2017), representing 34% of the present BDFFP list (Rutt et al., 2017 ). Thirty‐five species are considered to have colonized and since become established at the BDFFP, a nontrivial addition to the local species assemblage—13% of the core avifauna (Rutt et al., 2017 ). Furthermore, because we can relate the detection of these species across time as well as to large‐scale temporal landscape changes, this study offers insight into the process of avian colonization and ecological species invasions (hereafter, “invasions”).
4.1. Invasions happen quickly
Despite relatively unchanging land cover at both the BDFFP and along two road corridors, novel species arrived quickly following deforestation and creation of pastures. Most additions to the original forest avifauna (61%; 85/139) were detected during the 1980s. Similarly, almost all colonists (94%; 33/35)—species that presumably established new breeding populations—first appeared during the 1980s.
4.2. Colonists were mostly widespread generalist species
Ubiquitous, widespread generalists associated with secondary forest appeared in greater numbers than were expected by passive filtering according to the regional species pool. Furthermore, of the noncore species that colonized and became established at the BDFFP, a disproportionate number (22 species; 63%) are classified as secondary forest birds (Table (Table2). 2 ). Assuming that all species evolved in natural, nonanthropic habitats, the bulk of these habitat generalists now able to exploit anthropogenic second growth likely originated from river‐edge habitat in the region (Terborgh & Weske, 1969 ). As classified here, however, riverine species are largely comprised of more habitat specialists, and fewer riverine species appeared at the BDFFP or colonized than were expected by chance. Thus, it seems that the most specialized riverine birds (true floodplain forest species and river island obligates) rarely disperse far inland, even along river‐like road disturbances, or colonize new sites such as the BDFFP. Further evidence of this is the fact that the avifauna of the city of Manaus is dominated by floodplain forest birds (M. Cohn‐Haft, pers. obs.), but many of these have not progressed farther inland nor reached the BDFFP, even though the city would seem to be a reasonable source for colonizing birds. Instead, primarily generalist species that are today associated with secondary forest actively dispersed into and colonized the BDFFP. Furthermore, despite >35 years of ornithological coverage, we never detected a single forest species from adjacent areas of endemism (west of the Rio Negro or south of the Amazon River).
4.3. Exotic species did not colonize
Interestingly, no truly exotic species (non‐Amazonian or non‐South American) have become established in our study area. The only such species found anywhere nearby are Cattle Egrets ( Bubulcus ibis ), Rock Pigeons ( Columba livia ), House Sparrows ( Passer domesticus ), and Common Waxbills ( Estrilda astrild ). The egrets are known as accidental at our sites and have probably not become established simply because the cattle ranches have all failed (Laurance et al., 2018 ). The other three species are present in the city of Manaus (pers. obs., Borges, Pacheco, & Whittaker, 1996 ), but have not been found away from dense human populations. This appears to attest to the resistance of Amazonian primary forest to invasion by exotic species, as well as the apparent resistance of disturbed, secondary forests.
4.4. Colonizers are not infiltrating old‐growth forests
Those species that have colonized the BDFFP only rarely penetrate primary forest or the interior of large fragments, and none have colonized these habitats. Out of the 35 colonists, 16 (46%) have been captured at least once during long‐term bird banding effort, for a total of 656 captures in our >69,000 capture dataset. However, most of these records are from very small forest fragments (1‐ha) or from nets placed along the border of larger fragments. Excluding captures within ~100 m of a forest border at all other sites leaves only 44 captures of 7 species (predominantly White‐bearded Manakin [ Manacus manacus ], House Wren [ Troglodytes aedon ], Silver‐beaked Tanager [ Ramphocelus carbo ], and Chestnut‐bellied Seed‐Finch [ Sporophila angolensis ]). Furthermore, only four of 35 species (Variable Chachalaca [ Ortalis motmot ], Common Pauraque [ Nyctidromus albicollis ], Silver‐beaked Tanager, and Blue‐gray Tanager [ Thraupis episcopus ]) were detected eight times in a 100‐ha continuous forest plot during an intensive whole‐community inventory (Johnson, Stouffer, & Vargas, 2011 ). Thus, invading birds largely represent nonforest taxa restricted to anthropogenic habitats in the matrix and rarely penetrate closed‐canopy forests, consistent with the earlier suggestion that intact rainforests are generally resistant to species invasions (Laurance & Bierregaard, 1997 ). Therefore, we believe that these additions and invaders have a minimal ecological impact on the intact forest (e.g., seed dispersal of pioneer plant species and nest parasitism), although they could be playing nontrivial roles in matrix and disturbed habitats, including the potential introduction of novel pathogens (Altizer, Bartel, & Han, 2011 ). Similarly, no primary forest birds colonized early‐successional habitat following disturbance.
4.5. Some species are still trickling in whereas others are retreating in response to forest succession
The appearance of novel species at the BDFFP is far from random and includes many species that were predicted to eventually arrive (Cohn‐Haft et al., 1997 ). Despite considerably less fieldwork at Reserva Ducke—near the juncture of BR‐174 and AM‐010 along the outskirts of Manaus—Willis ( 1977 ) found 30 species not reported at the BDFFP during the first inventory (Stotz & Bierregaard, 1989 ). Within the following decade, however, 14 of those 30 species had appeared at the BDFFP (Cohn‐Haft et al., 1997 ), and another six were detected between 1995 and 2017 (Rutt et al., 2017 ). Additional secondary forest species are still trickling in and may be in the early stages of colonization (Tropical Screech‐Owl [ Megascops choliba ], Yellow‐bellied Elaenia [ Elaenia flavogaster ], Boat‐billed Flycatcher [ Megarynchus pitangua ], Brown‐crested Flycatcher [ Myiarchus tyrannulus ], and White‐lined Tanager [ Tachyphonus rufus ]). At the same time, a number of established colonists have become rarer as their preferred habitat at the BDFFP decreased between 1997 and 2017 (e.g., ground‐doves [ Columbina spp.], House Wren, Yellow‐browed Sparrow [ Ammodramus aurifrons ], Blue‐black Grassquit [ Volatinia jacarina ], Chestnut‐bellied Seedeater [ Sporophila castaneiventris ], Thraupis spp., and Red‐breasted Meadowlark [ Sturnella militaris ]). Capture data reveal similar trends; for instance, there were 36 captures of House Wren between 1981 and 1993, but none thereafter. Similarly, Silver‐beaked Tanager was captured 232 times during that interval and only 21 times thereafter. Many of these early‐successional species were previously characterized as common and are obvious and familiar avian components around human habitation in the region. Although early‐successional species appear to be largely declining, however, some secondary forest species seem to be increasing (e.g., Dusky Antbird [ Cercomacroides tyrannina ], White‐bearded Manakin, and Buff‐throated Saltator [ Saltator maximus ]).
4.6. Are roads to blame?
Given that roads are both direct and indirect drivers of Amazonian deforestation (Barber et al., 2014 ; Barni et al., 2015 ; Fearnside, 2015 ; Fearnside & Graca, 2006 ; Laurance et al., 2002 ; Laurance, Cochrane, et al., 2001 ; Nepstad et al., 2001 ; Soares‐Filho et al., 2006 ), it is apparent that roads are promoting species invasions both directly (as invasion corridors) and indirectly (by promoting land‐use changes). Cohn‐Haft et al. ( 1997 ) first proposed the idea that roads visually resemble rivers, including adjacent successional vegetation, and may serve as biological conveyor belts to transport species from extensive areas of disturbance near Manaus into previously undisturbed rainforest. Roads have been specifically implicated as catalysts for some invasions and range expansions in Amazonia, in particular, the advancement of House Sparrows, a species exclusively associated with humans (Smith, 1973 , 1980 ). At our site, Cohn‐Haft et al. ( 1997 ) described watching Swallow‐winged Puffbirds ( Chelidoptera tenebrosa ) progress incrementally farther north from Manaus along BR‐174 until it was eventually detected (1991) at the BDFFP itself. We cannot confirm that roads have been the conduit for colonization, although the continuous extension of disturbed vegetation they have consistently presented over time is likely to have benefited many of the colonizing species we detected. On the other hand, in spite of a river‐like disturbance corridor leading outward from the city of Manaus, long‐range dispersal of true floodplain forest specialists has been very limited.
4.7. Natural habitat succession can remove potential colonists
Our data also suggest that if land abandonment and forest recovery are shielded from further disturbance and allowed to proceed unimpeded—especially while sufficiently connected to primary forest—regenerating secondary forests offer another advantage: the ability to weed out invading species over time. Our data indicate that where forest cover has recuperated over time, the presence of early‐successional bird species has diminished. This is similar to the well‐documented trend of increasing rarity of open‐country birds with the reforestation and afforestation of the eastern United States (Askins, 2000 ; Brennan & Kuvlesky, 2005 ). Although debate continues about the conservation value of secondary forests (Brook, Bradshaw, Koh, & Sodhi, 2006 ; Wright & Muller‐Landau, 2006a , 2006b ), the extent of secondary forests in the Brazilian Amazon is increasing (Neeff, Lucas, dos Santos, Brondizio, & Freitas, 2006 ; Perz & Skole, 2003 ). We believe that natural forest regeneration can further serve as an effective tool to eliminate new, distinct communities of invading colonists, providing further opportunity for the original forest avifauna to recover.
4.8. Species richness alone is an inappropriate indicator of habitat quality for partially disturbed sites
Although a commonly used metric in conservation assessments, our synthesis of these historical avian inventories also illustrates how species richness itself fails to capture landscape degradation. Total species richness increased by >100 species over the past nearly four decades—due to the foreign contribution of predominantly secondary forest birds—despite the project area losing ~10% of primary forest when the cattle ranches were clearcut beginning in 1979. Of course, this would be expected with the appearance of novel habitats and their associated avifauna, but we nonetheless believe this is worth highlighting because a greater number of species is typically synonymous with greater conservation value. The apparent increase in species richness, however, is inconsequential, as regional conservation measures should be aimed at species dependent on primary forest (habitat specialists), not widespread habitat generalists able to exploit anthropogenic disturbances. These latter species are simply a natural byproduct of disturbance and ecological succession in degraded landscapes. Thus, it is critical that we guard against these sorts of singular species richness assessments and instead focus on the constituent members of an identified community.
5. CONCLUSIONS
Approximately 20% of the Brazilian Amazon has now been deforested (Artaxo, 2019 ), including what amounts to a region of deforestation larger than the state of California since 1988 (INPE, 2019 ). Furthermore, the recent return to increasing deforestation rates seems likely to continue as a newly appointed administration led by President Jair Bolsonaro (inaugurated 1 January 2019) threatens to expand mining, pasture, and agricultural activities in the Amazon (Artaxo, 2019 ; Escobar, 2018 ). Amazon road networks are also anticipated to continue expanding (Ahmed, Ewers, & Smith, 2013 ; Ahmed et al., 2014 ; Ahmed, Souza, Riberio, & Ewers, 2013 ; Laurance et al., 2014 ). A recent analysis across the entire Brazilian Amazon found that, on average, nearly 17,000 km of roads were added every year between 2004 and 2007 (Ahmed, Souza, et al., 2013 ). In all, more than 260,000 km of roads (>70% unofficial or illegal) crisscross the Brazilian Amazon, enough to stretch more than two‐thirds the distance between the Earth and the moon (Barber et al., 2014 ). Given continued deforestation and habitat degradation in the Amazon, it is imperative that conservationists not only describe the quality of anthropogenic habitat for forest‐dependent birds, but also the biotic interchange and potential species interactions cultivated by these distinct habitats. Our unique, site‐specific data from one of the very few long‐term study areas in the Amazon provide an important benchmark to describe the processes of avian species invasions and ecological succession, as well as the separation of these anthropogenic avian communities.
We believe that the pattern of species accumulation and colonization of widespread generalists that we describe here is likely generalizable across Amazonia following deforestation, agricultural use, and eventual abandonment. The addition and establishment of 35 bird species to a once undisturbed tract of rainforest over about 40 years offers some of the strongest, large‐scale documentation of vertebrate species invasions in Amazonia following anthropogenic disturbance. The dire consequences of deforestation for primary forest birds, however, cannot be overlooked. Following deforestation, the two coexisting local communities—primary forest and pasture—largely remained segregated, and those new colonists did not invade intact habitat nor displace forest birds. Only a long‐term study site such as the BDFFP would be capable of describing this protracted process and monitoring changing communities over time, both of which would remain hidden in short‐term research. It will, however, take a much longer period of time to detect the possibility of eventual recovery and stability of the original avian community in these degraded habitats (Powell, 2013 ). Finally, we look forward to the results of future long‐term research to determine whether our results are applicable across other taxa and regions in Amazonia.
CONFLICT OF INTEREST
None declared.
AUTHOR CONTRIBUTIONS
CLR and PCS conceived of the study. MCH and CLR generated the regional species pool. VJ conducted the temporal landscape analysis. CLR and PCS analyzed the contingency tables. All authors contributed to the interpretation of the results. CLR wrote the manuscript with support and input from all coauthors.
ACKNOWLEDGMENTS
We thank the many mateiros , assistants, and banders for their help collecting these data, particularly those that contributed and documented new avian arrivals at the Biological Dynamics of Forest Fragments Project. Additionally, we thank Gonçalo Ferraz for valuable logistic support and help generating funding. Additional logistical support from the staff of the BDFFP made this research possible. The BDFFP is managed and supported by Brazil's Instituto Nacional de Pesquisas da Amazônia and the Smithsonian Institution. Funding for this research was provided by the US National Science Foundation (LTREB 0545491 and 1257340), Conservation International's TEAM program (through a grant from the Gordon and Betty Moore Foundation), the National Geographic Society, and the National Institute of Food and Agriculture, US Department of Agriculture, McIntire Stennis projects #94098 and #94327. Finally, we would like to thank Marco Antonio Rêgo for providing expert GIS input and J. V. Remsen, Jr. and Hollis R. Jones for improving previous versions of this manuscript. This is publication No. 774 of the BDFFP Technical Series and No. 48 of the Amazonian Ornithology Technical Series of the INPA Collections Program. The manuscript was approved by the Director of the Louisiana State University Agricultural Center as manuscript number 2019‐241‐34166.
APPENDIX A.
The seven categories that characterize habitat diversity at the Biological Dynamics of Forest Fragments Project (BDFFP) and the 22 categories that these were derived from in the Parker et al. databases ( 1996 ).
Rutt CL, Jirinec V, Cohn‐Haft M, Laurance WF, Stouffer PC. Avian ecological succession in the Amazon: A long‐term case study following experimental deforestation . Ecol Evol . 2019; 9 :13850–13861. 10.1002/ece3.5822 [ CrossRef ] [ Google Scholar ]
DATA AVAILABILITY STATEMENT
- Ahmed, S. E. , Ewers, R. M. , & Smith, M. J. (2013). Large scale spatio‐temporal patterns of road development in the Amazon rainforest . Environmental Conservation , 41 ( 3 ), 253–264. 10.1017/s0376892913000520 [ CrossRef ] [ Google Scholar ]
- Ahmed, S. E. , Lees, A. C. , Moura, N. G. , Gardner, T. A. , Barlow, J. , Ferreira, J. , & Ewers, R. M. (2014). Road networks predict human influence on Amazonian bird communities . Proceedings of the Royal Society B‐Biological Sciences , 281 ( 1795 ), 20141742 10.1098/rspb.2014.1742 [ PMC free article ] [ PubMed ] [ CrossRef ] [ Google Scholar ]
- Ahmed, S. E. , Souza, C. M. , Riberio, J. , & Ewers, R. M. (2013). Temporal patterns of road network development in the Brazilian Amazon . Regional Environmental Change , 13 ( 5 ), 927–937. 10.1007/s10113-012-0397-z [ CrossRef ] [ Google Scholar ]
- Altizer, S. , Bartel, R. , & Han, B. A. (2011). Animal migration and infectious disease risk . Science , 331 ( 6015 ), 296–302. [ PubMed ] [ Google Scholar ]
- Artaxo, P. (2019). Working together for Amazonia . Science , 363 ( 6425 ), 323 10.1126/science.aaw6986 [ PubMed ] [ CrossRef ] [ Google Scholar ]
- Askins, R. A. (2000). Restoring North America's birds: Lessons from landscape ecology . New Haven, CT: Yale University Press. [ Google Scholar ]
- Barber, C. P. , Cochrane, M. A. , Souza, C. M. , & Laurance, W. F. (2014). Roads, deforestation, and the mitigating effect of protected areas in the Amazon . Biological Conservation , 177 , 203–209. 10.1016/j.biocon.2014.07.004 [ CrossRef ] [ Google Scholar ]
- Barni, P. E. , Fearnside, P. M. , & Graca, P. M. L. A. (2015). Simulating deforestation and carbon loss in Amazonia: Impacts in Brazil's Roraima state from reconstructing Highway BR‐319 (Manaus‐Porto Velho) . Environmental Management , 55 ( 2 ), 259–278. 10.1007/s00267-014-0408-6 [ PubMed ] [ CrossRef ] [ Google Scholar ]
- Bierregaard, R. O. Jr , Gascon, C. , Lovejoy, T. E. , & Mesquita, R. (2001). Lessons from Amazonia: The ecology and conservation of a fragmented forest . New Haven, CT: Yale University Press. [ Google Scholar ]
- Borges, S. H. (2004). Species poor but distinct: Bird assemblages in white sand vegetation in Jaú National Park, Brazilian Amazon . Ibis , 146 ( 1 ), 114–124. 10.1111/j.1474-919X.2004.00230.x [ CrossRef ] [ Google Scholar ]
- Borges, S. H. , Pacheco, J. F. , & Whittaker, A. (1996). New records of the House Sparrow ( Passer domesticus ) in the Brazilian Amazon . Ararajuba , 4 ( 2 ), 116–117. [ Google Scholar ]
- Brennan, L. A. , & Kuvlesky, W. P. (2005). North American grassland birds: An unfolding conservation crisis? The Journal of Wildlife Management , 69 ( 1 ), 1–13. [ Google Scholar ]
- Brook, B. W. , Bradshaw, C. J. A. , Koh, L. P. , & Sodhi, N. S. (2006). Momentum drives the crash: Mass extinction in the tropics . Biotropica , 38 ( 3 ), 302–305. 10.1111/j.1744-7429.2006.00141.x [ CrossRef ] [ Google Scholar ]
- Capparella, A. P. (1991). Neotropical avian diversity and riverine barriers In Bell B. D. (Ed.), Acta XX Congressus Internationalis Ornithologici (Vol. 20 , pp. 307–316). Wellington, New Zealand: Congress Trust Board. [ Google Scholar ]
- Cohn‐Haft, M. , Whittaker, A. , & Stouffer, P. C. (1997). A new look at the “species‐poor” central Amazon: The avifauna north of Manaus, Brazil . Ornithological Monographs , 48 , 205–235. 10.2307/40157535 [ CrossRef ] [ Google Scholar ]
- Cracraft, J. (1985). Historical biogeography and patterns of differentiation within the South American avifauna: Areas of endemism . Ornithological Monographs , 36 , 49–84. 10.2307/40168278 [ CrossRef ] [ Google Scholar ]
- Develey, P. F. , & Stouffer, P. C. (2001). Effects of roads on movements by understory birds in mixed‐species flocks in central Amazonian Brazil . Conservation Biology , 15 ( 5 ), 1416–1422. 10.1111/j.1523-1739.2001.00170.x [ CrossRef ] [ Google Scholar ]
- Escobar, H. (2018). Scientists, environmentalists brace for Brazil's right turn . Science , 362 ( 6412 ), 273–274. [ PubMed ] [ Google Scholar ]
- Fearnside, P. M. (2005). Deforestation in Brazilian Amazonia: History, rates, and consequences . Conservation Biology , 19 ( 3 ), 680–688. 10.1111/j.1523-1739.2005.00697.x [ CrossRef ] [ Google Scholar ]
- Fearnside, P. M. (2015). Highway construction as a force in the destruction of the Amazon forest In van der Ree R., Smith D. J., & Grilo C. (Eds.), Handbook of road ecology (pp. 414–424). West Sussex, UK: John Wiley & Sons Ltd. [ Google Scholar ]
- Fearnside, P. M. , & Graca, P. M. L. A. (2006). BR‐319: Brazil's Manaus‐Porto Velho highway and the potential impact of linking the arc of deforestation to central Amazonia . Environmental Management , 38 ( 5 ), 705–716. 10.1007/s00267-005-0295-y [ PubMed ] [ CrossRef ] [ Google Scholar ]
- Ferraz, G. , Russell, G. J. , Stouffer, P. C. , Bierregaard, R. O. , Pimm, S. L. , & Lovejoy, T. E. (2003). Rates of species loss from Amazonian forest fragments . Proceedings of the National Academy of Sciences of the United States of America , 100 ( 24 ), 14069–14073. 10.1073/pnas.2336195100 [ PMC free article ] [ PubMed ] [ CrossRef ] [ Google Scholar ]
- Gascon, C. , Lovejoy, T. E. , Bierregaard, R. O. Jr , Malcolm, J. R. , Stouffer, P. C. , Vasconcelos, H. L. , … Borges, S. (1999). Matrix habitat and species richness in tropical forest remnants . Biological Conservation , 91 ( 2–3 ), 223–229. 10.1016/s0006-3207(99)00080-4 [ CrossRef ] [ Google Scholar ]
- IBGE . (2017). Estimativas de População . Instituto Brasileiro de Geografia e Estatística. [ Google Scholar ]
- INPE . (2019). Projecto prodes: Monitoramento da flororesta Amazonica Brasileira por satelite . Instituto Nacional de Pesquisas Espaciais. [ Google Scholar ]
- Johnson, E. I. , Stouffer, P. C. , & Vargas, C. F. (2011). Diversity, biomass, and trophic structure of a central Amazonian rainforest bird community . Revista Brasileira de Ornitologia , 19 ( 1 ), 1–16. [ Google Scholar ]
- Laurance, S. G. W. (2004). Responses of understory rain forest birds to road edges in central Amazonia . Ecological Applications , 14 ( 5 ), 1344–1357. 10.1890/03-5194 [ CrossRef ] [ Google Scholar ]
- Laurance, S. G. W. , Stouffer, P. C. , & Laurance, W. E. (2004). Effects of road clearings on movement patterns of understory rainforest birds in central Amazonia . Conservation Biology , 18 ( 4 ), 1099–1109. 10.1111/j.1523-1739.2004.00268.x [ CrossRef ] [ Google Scholar ]
- Laurance, W. F. , Albernaz, A. K. M. , & Da Costa, C. (2001). Is deforestation accelerating in the Brazilian Amazon? Environmental Conservation , 28 ( 4 ), 305–311. 10.1017/s0376892901000339 [ CrossRef ] [ Google Scholar ]
- Laurance, W. F. , Albernaz, A. K. M. , Schroth, G. , Fearnside, P. M. , Bergen, S. , Venticinque, E. M. , & Da Costa, C. (2002). Predictors of deforestation in the Brazilian Amazon . Journal of Biogeography , 29 ( 5–6 ), 737–748. 10.1046/j.1365-2699.2002.00721.x [ CrossRef ] [ Google Scholar ]
- Laurance, W. F. , & Bierregaard, R. O. (1997). Tropical forest remnants: Ecology, management, and conservation of fragmented communities . Chicago, IL: University of Chicago Press. [ Google Scholar ]
- Laurance, W. F. , Camargo, J. L. C. , Fearnside, P. M. , Lovejoy, T. E. , Williamson, G. B. , Mesquita, R. C. G. , … Laurance, S. G. W. (2018). An Amazonian rainforest and its fragments as a laboratory of global change . Biological Reviews , 93 ( 1 ), 223–247. 10.1111/brv.12343 [ PubMed ] [ CrossRef ] [ Google Scholar ]
- Laurance, W. F. , Clements, G. R. , Sloan, S. , O'Connell, C. S. , Mueller, N. D. , Goosem, M. , … Arrea, I. B. (2014). A global strategy for road building . Nature , 513 ( 7517 ), 229–232. 10.1038/nature13717 [ PubMed ] [ CrossRef ] [ Google Scholar ]
- Laurance, W. F. , Cochrane, M. A. , Bergen, S. , Fearnside, P. M. , Delamônica, P. , Barber, C. , … Fernandes, T. (2001). The future of the Brazilian Amazon . Science , 291 ( 5503 ), 438–439. 10.1126/science.291.5503.438 [ PubMed ] [ CrossRef ] [ Google Scholar ]
- Laurance, W. F. , Goosem, M. , & Laurance, S. G. W. (2009). Impacts of roads and linear clearings on tropical forests . Trends in Ecology & Evolution , 24 ( 12 ), 659–669. 10.1016/j.tree.2009.06.009 [ PubMed ] [ CrossRef ] [ Google Scholar ]
- Lees, A. C. , & Peres, C. A. (2006). Rapid avifaunal collapse along the Amazonian deforestation frontier . Biological Conservation , 133 ( 2 ), 198–211. 10.1016/j.biocon.2006.06.005 [ CrossRef ] [ Google Scholar ]
- Lees, A. C. , & Peres, C. A. (2009). Gap‐crossing movements predict species occupancy in Amazonian forest fragments . Oikos , 118 ( 2 ), 280–290. 10.1111/j.1600-0706.2008.16842.x [ CrossRef ] [ Google Scholar ]
- Mahood, S. P. , Lees, A. C. , & Peres, C. A. (2012). Amazonian countryside habitats provide limited avian conservation value . Biodiversity and Conservation , 21 ( 2 ), 385–405. 10.1007/s10531-011-0188-8 [ CrossRef ] [ Google Scholar ]
- Neeff, T. , Lucas, R. M. , dos Santos, J. R. , Brondizio, E. S. , & Freitas, C. C. (2006). Area and age of secondary forests in Brazilian Amazonia 1978–2002: An empirical estimate . Ecosystems , 9 ( 4 ), 609–623. 10.1007/s10021-006-0001-9 [ CrossRef ] [ Google Scholar ]
- Nepstad, D. , Carvalho, G. , Cristina Barros, A. , Alencar, A. , Paulo Capobianco, J. , Bishop, J. , … Prins, E. (2001). Road paving, fire regime feedbacks, and the future of Amazon forests . Forest Ecology and Management , 154 ( 3 ), 395–407. 10.1016/s0378-1127(01)00511-4 [ CrossRef ] [ Google Scholar ]
- Parker, T. A. , Stotz, D. F. , & Fitzpatrick, J. W. (1996). Ecological and distributional databases In Stotz D. F., Fitzpatrick J. W., Parker T. A., & Moskovits D. (Eds.), Neotropical birds: Ecology and conservation (pp. 113–407). Chicago, IL: University of Chicago Press. [ Google Scholar ]
- Perz, S. G. , & Skole, D. L. (2003). Secondary forest expansion in the Brazilian Amazon and the refinement of forest transition theory . Society & Natural Resources , 16 ( 4 ), 277–294. 10.1080/08941920390178856 [ CrossRef ] [ Google Scholar ]
- Powell, L. L. (2013). Recovery of understory bird movement in post‐pasture Amazonia . Dissertation, Louisiana State University, Baton Rouge, Louisiana, USA. [ Google Scholar ]
- Remsen, J. V. (1994). Use and misuse of bird lists in community ecology and conservation . The Auk , 111 ( 1 ), 225–227. 10.2307/4088531 [ CrossRef ] [ Google Scholar ]
- Remsen, J. V. Jr , Cadena, C. D. , Jaramillo, A. , Nores, M. , Pacheco, J. F. , Pérez‐Emán, J. , … Zimmer, K. J. (2018). A classification of the bird species of South America . American Ornithologists' Union; http://www.museum.lsu.edu/~Remsen/SACCBaseline.htm [ Google Scholar ]
- Remsen, J. V. , & Parker, T. A. (1983). Contribution of river‐created habitats to bird species richness in Amazonia . Biotropica , 15 ( 3 ), 223–231. 10.2307/2387833 [ CrossRef ] [ Google Scholar ]
- Rosenberg, G. H. (1990). Habitat specialization and foraging behavior by birds of Amazonian river islands in northeastern Peru . Condor , 92 ( 2 ), 427–443. 10.2307/1368240 [ CrossRef ] [ Google Scholar ]
- Rutt, C. L. , Jirinec, V. , Johnson, E. I. , Cohn‐Haft, M. , Vargas, C. F. , & Stouffer, P. C. (2017). Twenty years later: An update to the birds of the Biological Dynamics of Forest Fragments Project, Amazonas, Brazil . Revista Brasileira de Ornitologia , 25 ( 4 ), 259–278. [ Google Scholar ]
- Smith, N. J. H. (1973). House sparrows ( Passer domesticus ) in the Amazon . Condor , 75 ( 2 ), 242–243. [ Google Scholar ]
- Smith, N. J. H. (1980). Further advances of House Sparrows into the Brazilian Amazon . Condor , 82 , 109–111. [ Google Scholar ]
- Soares‐Filho, B. S. , Nepstad, D. C. , Curran, L. M. , Cerqueira, G. C. , Garcia, R. A. , Ramos, C. A. , … Schlesinger, P. (2006). Modelling conservation in the Amazon basin . Nature , 440 ( 7083 ), 520–523. 10.1038/nature04389 [ PubMed ] [ CrossRef ] [ Google Scholar ]
- Stotz, D. F. , & Bierregaard, R. O. Jr (1989). The birds of the fazendas Porto Alegre, Esteio and Dimona north of Manaus, Amazonas, Brazil . Revista Brasileira de Biologia , 49 ( 3 ), 861–872. [ Google Scholar ]
- Stouffer, P. C. , Johnson, E. I. , Bierregaard, R. O. Jr , & Lovejoy, T. E. (2011). Understory bird communities in Amazonian rainforest fragments: Species turnover through 25 years post‐isolation in recovering landscapes . PLoS ONE , 6 ( 6 ), e20543 10.1371/journal.pone.0020543 [ PMC free article ] [ PubMed ] [ CrossRef ] [ Google Scholar ]
- Terborgh, J. , & Weske, J. S. (1969). Colonization of secondary habitats by Peruvian birds . Ecology , 50 ( 5 ), 765–782. 10.2307/1933691 [ CrossRef ] [ Google Scholar ]
- Willis, E. O. (1977). Lista preliminar das aves da parte noroeste e áreas vizinhas da Reserva Ducke, Amazonas, Brasil . Revista Brasileira de Biologia , 37 ( 3 ), 585–601. [ Google Scholar ]
- Wright, S. J. , & Muller‐Landau, H. C. (2006a). The future of tropical forest species . Biotropica , 38 ( 3 ), 287–301. [ Google Scholar ]
- Wright, S. J. , & Muller‐Landau, H. C. (2006b). The uncertain future of tropical forest species . Biotropica , 38 ( 4 ), 443–445. 10.2307/30044025 [ CrossRef ] [ Google Scholar ]
- Publications
- Conferences & Events
- Professional Learning
- Science Standards
- Awards & Competitions
- Daily Do Lesson Plans
- Free Resources
- American Rescue Plan
- For Preservice Teachers
- NCCSTS Case Collection
- Partner Jobs in Education
- Interactive eBooks+
- Digital Catalog
- Regional Product Representatives
- e-Newsletters
- Bestselling Books
- Latest Books
- Popular Book Series
- Prospective Authors
- Web Seminars
- Exhibits & Sponsorship
- Conference Reviewers
- National Conference • Denver 24
- Leaders Institute 2024
- National Conference • New Orleans 24
- Submit a Proposal
- Latest Resources
- Professional Learning Units & Courses
- For Districts
- Online Course Providers
- Schools & Districts
- College Professors & Students
- The Standards
- Teachers and Admin
- eCYBERMISSION
- Toshiba/NSTA ExploraVision
- Junior Science & Humanities Symposium
- Teaching Awards
- Climate Change
- Earth & Space Science
- New Science Teachers
- Early Childhood
- Middle School
- High School
- Postsecondary
- Informal Education
- Journal Articles
- Lesson Plans
- e-newsletters
- Science & Children
- Science Scope
- The Science Teacher
- Journal of College Sci. Teaching
- Connected Science Learning
- NSTA Reports
- Next-Gen Navigator
- Science Update
- Teacher Tip Tuesday
- Trans. Sci. Learning
MyNSTA Community
- My Collections
The Deforestation of the Amazon
A Case Study in Understanding Ecosystems and Their Value
By Philip Camill
Share Start a Discussion
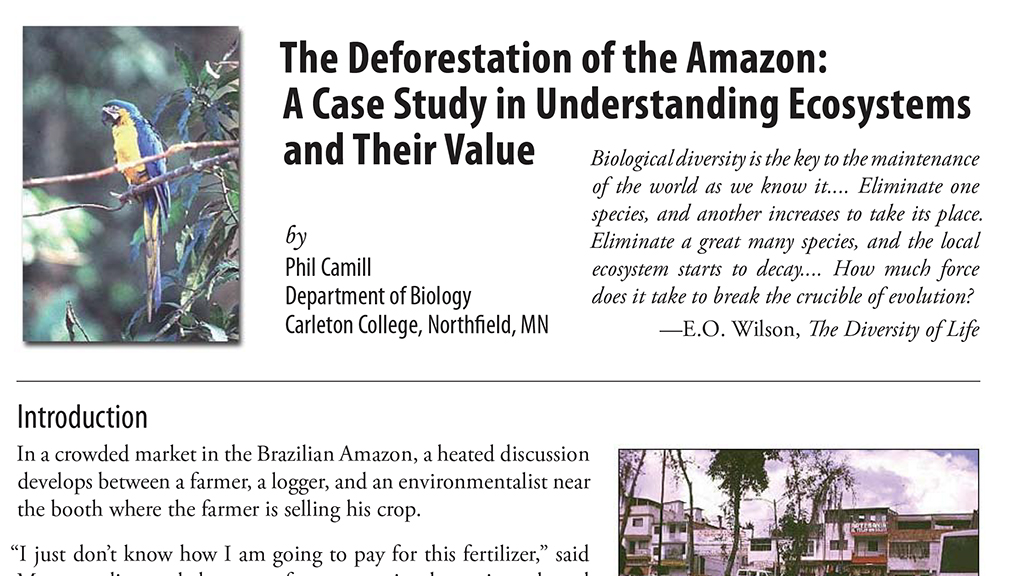
In this case study, students examine tropical deforestation in the Amazon from the perspective of three dominant stakeholders in the region: a peasant farmer, logger, and environmentalist. As part of the exercise, students perform a cost-benefit analysis of clearing a plot of tropical forest in the Amazon from the perspective of one of these stakeholder groups. Developed for a course in global change biology, this case could also be used in courses in general ecology, environmental science, environmental ethics, environmental policy, and environmental/ecological economics.
Download Case
Date Posted
- Understand the political, cultural, and economic history leading to tropical deforestation in Amazonia. Understand issues facing the major stakeholders in the Amazon.
- Understand the concern for such a large loss in biodiversity.
- Understand the concepts of market and non-market valuation of ecosystems, benefit-cost analysis, and opportunity cost.
- Perform a cost-benefit analysis of clearing a plot of tropical forest in the Amazon, from the perspective of a peasant farmer, logger, and environmentalist.
- Critically evaluate economic vs. ethical valuation of ecosystems.
- Appreciate the political, social, economic, and ecological complexity of tropical deforestation.
- Appreciate how difficult decisions must me made in the face of limited or nonexistent data.
Deforestation; Amazon; tropical forest; rainforest; ecosystem; biodiversity; bioprospecting; ecotourism; ecological economics; cost-benefit analysis; tropics; developing world; South America
Subject Headings
EDUCATIONAL LEVEL
High school, Undergraduate lower division, Undergraduate upper division
TOPICAL AREAS
Ethics, Policy issues, Social issues, Social justice issues
TYPE/METHODS
Teaching Notes & Answer Key
Teaching notes.
Case teaching notes are protected and access to them is limited to paid subscribed instructors. To become a paid subscriber, purchase a subscription here .
Teaching notes are intended to help teachers select and adopt a case. They typically include a summary of the case, teaching objectives, information about the intended audience, details about how the case may be taught, and a list of references and resources.
Download Notes
Answer Keys are protected and access to them is limited to paid subscribed instructors. To become a paid subscriber, purchase a subscription here .
Download Answer Key
Materials & Media
Supplemental materials, you may also like.
Web Seminar
School and district leaders are invited to join us on Thursday, April 11, 2024, from 7:00 PM to 8:00 PM ET, to learn about NSTA’s School and Distric...
Struggling with materials management in your district? Want to learn tips and tricks from the experts at ECA Science Kit Services? Join us on Th...
Join us on Tuesday, April 23, 2024, from 7:00 PM to 8:15 PM ET for a sponsored web seminar presented by Vernier Science Education....

- Deforestation
- Going Green
- Reforestation
- Privacy Policy

Deforestation: Case Studies
Deforestation is putting our planet at risk, as the following case studies exemplify. It is responsible for at least 10 per cent of global greenhouse gas emissions 1 and wipes out 137 species of plants, animals and insects every day 2 . The deplorable practice degenerates soil, losing half of the world’s topsoil over the past 150 years. 3 Deforestation also leads to drought by reducing the amount of water in the atmosphere. 4
Since the 1950s, deforestation has accelerated significantly, particularly in the tropics. 5 This is primarily due to rapid population growth and a resultant increase in demand for food and resources. 6 Agriculture drives about 80 per cent of deforestation today, as land is cleared for livestock, growing animal feed or other crops. 7 The below deforestation case studies of Brazil’s Amazon rainforest and the Congo Basin provide further insights into modern deforestation.
Deforestation case study: Brazil
Nearly two-thirds of the Amazon rainforest – the largest rainforest in the world – is within Brazil’s national borders. 8 Any examination of deforestation case studies would be incomplete without considering tree felling in Brazil.
History of deforestation in Brazil
Humans first discovered the Amazon rainforest about 13,000 years ago. But, it was the arrival of Europeans in the late 15th century that spurred the conversion of the forest into farmland. Nevertheless, the sheer size of the Amazon meant that the rainforest remained largely intact until the early 20th century. It was in the latter half of the 20th century that things began to change. 9

Industrial activities and large-scale agriculture began to eat away the southern and eastern fringes of the Amazon, from the 1950s onwards. 10 Deforestation in Brazil received a significant boost in 1964 when a military dictatorship took power and declared the jungle a security risk. 11 By the 1970s, the government was running television ads encouraging land conversion, provoking millions to migrate north into the forest. 12 Settlements replaced trees, and infrastructure began to develop. Wealthy tycoons subsequently bought the land for cattle ranches or vast fields of soy. 13
By the turn of the 21st century, more than 75 per cent of deforestation in the Amazon was for cattle ranching. But, environmentalists and Indigenous groups drew international attention to the devastation caused and succeeded in curtailing it by 2004. Between 2004 and the early 2010s, annual forest cover loss in Brazil reduced by about 80 per cent. The decline is attributed to “increased law enforcement, satellite monitoring, pressure from environmentalists, private and public sector initiatives, new protected areas, and macroeconomic trends”. 14
Brazil’s deforestation of the Amazon rainforest since 2010
Unfortunately, however, efforts to curtail deforestation in Brazil’s Amazon have stalled since 2012. 15 Tree felling and land conversion have been trending upwards ever since. The economic incentive for chopping the rainforest down has overcome the environmental benefits of leaving it standing. 16 Political movements and lax government legislation have leveraged this to their advantage. President Jair Bolsonaro won the 2018 election with a promise to open up the Amazon to business. 17 Since his inauguration, the rate of deforestation has leapt by as much as 92 per cent. 18
However, there is still hope for the Amazon rainforest. Bolsonaro’s principal international ally was US President Trump. Now that environmentally-conscious Joe Biden has replaced him in the White House, international pressure regarding deforestation will increase heavily. 19 Biden has made this clear with a promise of USD $20 billion to protect the Amazon. 20
The impact of continued deforestation in Brazil
For its three million plant and animal species and one million Indigenous inhabitants, it is imperative that Amazonian deforestation is massively and immediately reduced. 21 As much as 17 per cent of the Amazon has been lost already. 22 If this proportion increases to over 20 per cent, a tipping point will be reached. 23 This will irreversibly break the water cycle, and at least half of the remaining forest will become savannah. 24
Impact on climate change
Losing the Amazon would also mean losing the fight against climate change. Despite the rampant deforestation in recent years, the remaining Amazon rainforest still absorbs between 5 to 10 per cent of all human CO2 emissions. 25 Cutting trees down increases anthropogenic emissions. When felled, burned or left to rot, trees release sequestered carbon. 26 A combination of reducing greenhouse gas emissions and preserving existing forests is crucial to preventing dangerous levels of global warming. 27
Deforestation case study: The Congo Basin
The Congo Basin is the second-largest rainforest in the world. 28 It has been described as the ‘second lungs’ of the Earth because of how much carbon dioxide it absorbs and how much oxygen it produces. 29 But, just as the world’s first lungs – the Amazon – is being destroyed by humans, the Congo’s rainforest is also suffering heavy casualties. 30
60 per cent of the Congo Basin is located within the Democratic Republic of the Congo (DRC). 31 The DRC is one of the world’s largest and poorest countries, though it has immense economic resources. 32 Natural resources have fuelled an ongoing war that has affected all the neighbouring countries and claimed as many as six million lives. 33 The resultant instability combined with corruption and poor governance have led to an ever-increasing rate of deforestation within the DRC’s borders. 34
Deforestation in the Democratic Republic of the Congo (DRC)
Compared to the Amazon and Southeast Asia, deforestation in the Congo Basin has been low over the past few decades. 35 Nevertheless, great swathes of primary forest have been lost. Between 2000 and 2014, an area of forest larger than Bangladesh was destroyed. 36 From 2015 until 2019, 6.37 million hectares of tree cover was razed. 37 In 2019 alone, 475,000 hectares of primary forest disappeared, placing the DRC second only to Brazil for total deforestation that year. 38 Should the current rate of deforestation continue, all primary forest in the Congo Basin will be gone by the end of the century. 39
Drivers of deforestation in the DRC’s Congo Basin
Over the past 20 years, the biggest drivers of deforestation in the DRC has been small-scale subsistence agriculture. Clearing trees for charcoal and fuelwood, urban expansion and mining have also contributed to deforestation. Industrial logging is the most common cause of forest degradation. It opens up deeper areas of the forest to commercial hunting. There has been at least a 60 per cent drop in the region’s forest elephant populations over the past decade due to hunting and poaching. 40

Between 2000 and 2014, small-scale farming contributed to about 90 per cent of the DRC’s deforestation. This trend has not changed in recent years. The majority of small-scale forest clearing is conducted with simple axes by people with no other livelihood options. The region’s political instability and ongoing conflict are therefore inciting the unsustainable rate of deforestation within the Congo Basin. 41
In future, however, industrial logging and land conversion to large-scale agriculture will pose the greatest threats to the Congo rainforest. 42 There are fears that demand for palm oil, rubber and sugar production will promote a massive increase in deforestation. 43 The DRC’s population is also predicted to grow to almost 200 million people by 2050. 44 This increase will threaten the remaining rainforest further, as they try to earn a living in a country deprived of opportunities. 45
The impact of deforestation in the Congo Basin
80 million people depend upon the Congo Basin for their existence. It provides food, charcoal, firewood, medicinal plants, and materials for building and other purposes. But, this rainforest also indirectly supports people across the whole of sub-Saharan Africa. Like all forests, it is instrumental in regulating rainfall, which can affect precipitation hundreds of miles away. The Congo Basin is a primary source of rainfall for the Sahel region, doubling the amount of rainfall in the air that passes over it. 46
The importance of the Congo Basin’s ability to increase precipitation cannot be understated. Areas such as the Horn of Africa are becoming increasingly dry. Drought in Ethiopia and Somalia has put millions of people on emergency food and water rations in recent years. Destroying the DRC’s rainforest would create the largest humanitarian crisis on Earth. 47
It would also be devastating for biodiversity. The Congo Basin shelters some 10,000 animal species and more than 600 tree species. 48 They play a hugely important role in the forest, which has consequences for the entire planet. For instance, elephants, gorillas, and other large herbivores keep the density of small trees very low through predation. 49 This results in a high density of tall trees in the Congo rainforest. 50 Larger trees store more carbon and therefore help to prevent global warming by removing this greenhouse gas from the atmosphere. 51
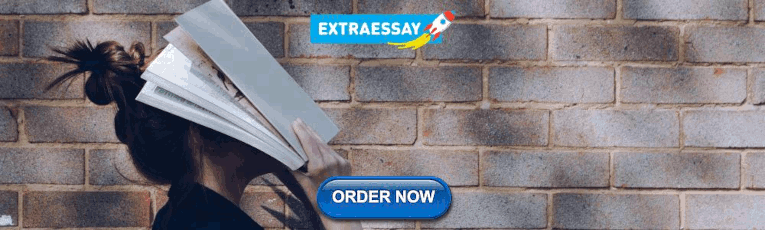
Preserve our forests
Preserving the Amazon and Congo Basin rainforests is vital for tackling climate change, as these deforestation case studies demonstrate. We must prioritise protecting and enhancing our existing trees if we are to limit the global temperature increase to 1.5°C, as recommended by the IPCC. 52
- Rainforest Alliance. (2018). What is the Relationship Between Deforestation And Climate Change? [online] Available at: https://www.rainforest-alliance.org/articles/relationship-between-deforestation-climate-change.
- www.worldanimalfoundation.com. (n.d.). Deforestation: Clearing The Path For Wildlife Extinctions. [online] Available at: https://www.worldanimalfoundation.com/advocate/wild-earth/params/post/1278141/deforestation-clearing-the-path-for-wildlife-extinctions#:~:text=Seventy%20percent%20of%20the%20Earth.
- World Wildlife Fund. (2000). Soil Erosion and Degradation | Threats | WWF. [online] Available at: https://www.worldwildlife.org/threats/soil-erosion-and-degradation.
- Butler, R.A. (2001). The impact of deforestation. [online] Mongabay. Available at: https://rainforests.mongabay.com/09-consequences-of-deforestation.html.
- The Classroom | Empowering Students in Their College Journey. (2009). The History of Deforestation. [online] Available at: https://www.theclassroom.com/the-history-of-deforestation-13636286.html.
- Greenpeace USA. (n.d.). Agribusiness & Deforestation. [online] Available at: https://www.greenpeace.org/usa/forests/issues/agribusiness/.
- Yale.edu. (2015). The Amazon Basin Forest | Global Forest Atlas. [online] Available at: https://globalforestatlas.yale.edu/region/amazon.
- Time. (2019). The Amazon Rain Forest Is Nearly Gone. We Went to the Front Lines to See If It Could Be Saved. [online] Available at: https://time.com/amazon-rainforest-disappearing/.
- Butler, R. (2020). Amazon Destruction. [online] Mongabay.com. Available at: https://rainforests.mongabay.com/amazon/amazon_destruction.html.
- the Guardian. (2020). Amazon deforestation surges to 12-year high under Bolsonaro. [online] Available at: https://www.theguardian.com/environment/2020/dec/01/amazon-deforestation-surges-to-12-year-high-under-bolsonaro.
- Earth Innovation Institute. (2020). Joe Biden offers $20 billion to protect Amazon forests. [online] Available at: https://earthinnovation.org/2020/03/joe-biden-offers-20-billion-to-protect-amazon-forests/.
- Brazil’s Amazon: Deforestation “surges to 12-year high.” (2020). BBC News. [online] 30 Nov. Available at: https://www.bbc.co.uk/news/world-latin-america-55130304.
- Carbon Brief. (2020). Guest post: Could climate change and deforestation spark Amazon “dieback”? [online] Available at: https://www.carbonbrief.org/guest-post-could-climate-change-and-deforestation-spark-amazon-dieback.
- Union of Concerned Scientists (2012). Tropical Deforestation and Global Warming | Union of Concerned Scientists. [online] www.ucsusa.org. Available at: https://www.ucsusa.org/resources/tropical-deforestation-and-global-warming#:~:text=When%20trees%20are%20cut%20down.
- Milman, O. (2018). Scientists say halting deforestation “just as urgent” as reducing emissions. [online] the Guardian. Available at: https://www.theguardian.com/environment/2018/oct/04/climate-change-deforestation-global-warming-report.
- Bergen, M. (2019). Congo Basin Deforestation Threatens Food and Water Supplies Throughout Africa. [online] World Resources Institute. Available at: https://www.wri.org/blog/2019/07/congo-basin-deforestation-threatens-food-and-water-supplies-throughout-africa.
- www.esa.int. (n.d.). Earth from Space: “Second lungs of the Earth.” [online] Available at: https://www.esa.int/Applications/Observing_the_Earth/Earth_from_Space_Second_lungs_of_the_Earth [Accessed 26 Feb. 2021].
- Erickson-Davis, M. (2018). Congo Basin rainforest may be gone by 2100, study finds. [online] Mongabay Environmental News. Available at: https://news.mongabay.com/2018/11/congo-basin-rainforest-may-be-gone-by-2100-study-finds/.
- Mongabay Environmental News. (2020). Poor governance fuels “horrible dynamic” of deforestation in DRC. [online] Available at: https://news.mongabay.com/2020/12/poor-governance-fuels-horrible-dynamic-of-deforestation-in-drc/ [Accessed 26 Feb. 2021].
- DR Congo country profile. (2019). BBC News. [online] 10 Jan. Available at: https://www.bbc.co.uk/news/world-africa-13283212.
- Butler, R.A. (2001). Congo Deforestation. [online] Mongabay. Available at: https://rainforests.mongabay.com/congo/deforestation.html.
- Mongabay Environmental News. (2020). Poor governance fuels “horrible dynamic” of deforestation in DRC. [online] Available at: https://news.mongabay.com/2020/12/poor-governance-fuels-horrible-dynamic-of-deforestation-in-drc/.
- Butler, R. (2020). The Congo Rainforest. [online] Mongabay.com. Available at: https://rainforests.mongabay.com/congo/.
- Editor, B.W., Environment (n.d.). Large trees are carbon-storing giants. www.thetimes.co.uk. [online] Available at: https://www.thetimes.co.uk/article/large-trees-are-more-valuable-carbon-stores-than-was-thought-k8hnggzs8#:~:text=The%20world [Accessed 26 Feb. 2021].
- IPCC (2018). Summary for Policymakers — Global Warming of 1.5 oC. [online] Ipcc.ch. Available at: https://www.ipcc.ch/sr15/chapter/spm/.
Related posts:
- Top 10 Facts about Deforestation
- Deforestation Debate
- When a Tree Falls It Makes More Than a Sound
- What is Deforestation?
Related Posts

10 Facts About Deforestation in 2021
The following facts about deforestation in 2021 show that our planet is in a precarious position. We must leave our...

Where Is Deforestation Happening in 2021?
To combat climate change, it is important to know where deforestation is happening in 2021. Deforestation currently accounts for at...

The History of Deforestation: The Past and Origins
In many ways, the history of deforestation is the same as the history of agriculture. When humans began to farm...

The Economic Impact of Deforestation
Deforestation is the permanent removal of forests. Agriculture usually replaces the woodland.Youmatter (2020). Deforestation - What Is It? What Are...

Silviculture and Timber Production
Over the past decade, millions of trees have been dying at a frightening pace across the US and Canada. Some...

How Does Planting Trees Affect Climate Change?
While climate change can be a politically controversial topic, tree planting rarely is. Planting trees and climate change are directly...

10 Causes of Deforestation: The Roots of Forest Degradation

How Much CO2 Does a Tree Absorb?

The Effect of Oil Sands Tailings Ponds on Climate Change
- Privacy & Policy
© 2020 Climate Transform
Privacy Overview
Thank you for visiting nature.com. You are using a browser version with limited support for CSS. To obtain the best experience, we recommend you use a more up to date browser (or turn off compatibility mode in Internet Explorer). In the meantime, to ensure continued support, we are displaying the site without styles and JavaScript.
- View all journals
- My Account Login
- Explore content
- About the journal
- Publish with us
- Sign up for alerts
- Open access
- Published: 10 May 2021
Deforestation reduces rainfall and agricultural revenues in the Brazilian Amazon
- Argemiro Teixeira Leite-Filho ORCID: orcid.org/0000-0002-8480-4254 1 ,
- Britaldo Silveira Soares-Filho ORCID: orcid.org/0000-0002-7703-946X 1 ,
- Juliana Leroy Davis 1 ,
- Gabriel Medeiros Abrahão ORCID: orcid.org/0000-0003-0336-6246 2 &
- Jan Börner 3
Nature Communications volume 12 , Article number: 2591 ( 2021 ) Cite this article
39k Accesses
111 Citations
646 Altmetric
Metrics details
- Attribution
- Environmental impact
It has been suggested that rainfall in the Amazon decreases if forest loss exceeds some threshold, but the specific value of this threshold remains uncertain. Here, we investigate the relationship between historical deforestation and rainfall at different geographical scales across the Southern Brazilian Amazon (SBA). We also assess impacts of deforestation policy scenarios on the region’s agriculture. Forest loss of up to 55–60% within 28 km grid cells enhances rainfall, but further deforestation reduces rainfall precipitously. This threshold is lower at larger scales (45–50% at 56 km and 25–30% at 112 km grid cells), while rainfall decreases linearly within 224 km grid cells. Widespread deforestation results in a hydrological and economic negative-sum game, because lower rainfall and agricultural productivity at larger scales outdo local gains. Under a weak governance scenario, SBA may lose 56% of its forests by 2050. Reducing deforestation prevents agricultural losses in SBA up to US$ 1 billion annually.
Similar content being viewed by others
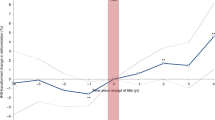
Impacts of a large-scale titling initiative on deforestation in the Brazilian Amazon
Benedict Probst, Ariel BenYishay, … Tiago N. P. dos Reis
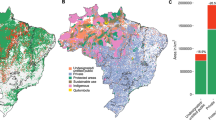
Land tenure drives Brazil’s deforestation rates across socio-environmental contexts
Andrea Pacheco & Carsten Meyer
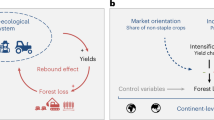
Agricultural intensification, Indigenous stewardship and land sparing in tropical dry forests
Marie Pratzer, Álvaro Fernández-Llamazares, … Tobias Kuemmerle
Introduction
Mounting evidence from model-based and empirical research indicates that the Amazon forest influences the spatiotemporal patterns and amount of rainfall 1 , 2 , 3 , 4 . Among the multiple forest ecosystem services, rainfall regulation is key to sustain agriculture in the region and beyond 5 , where an important share of Brazil’s soy and beef is produced. Despite this well-documented function, deforestation in the Brazilian Amazon is on the rise again. As of 2020, PRODES 6 reported 11,000 km 2 of forest loss, a 143% increase from 2012, the lowest deforestation rate on record. As forest loss accumulates, impacts on rainfall patterns may critically affect agriculture, especially in the Southern Brazilian Amazon (SBA), where forest losses already amount to 30% 6 . SBA is one of the most dynamic land-use frontiers in the world 7 and accounts for the lion’s share of croplands and pastures in the Brazilian Amazon (Fig. 1 ).
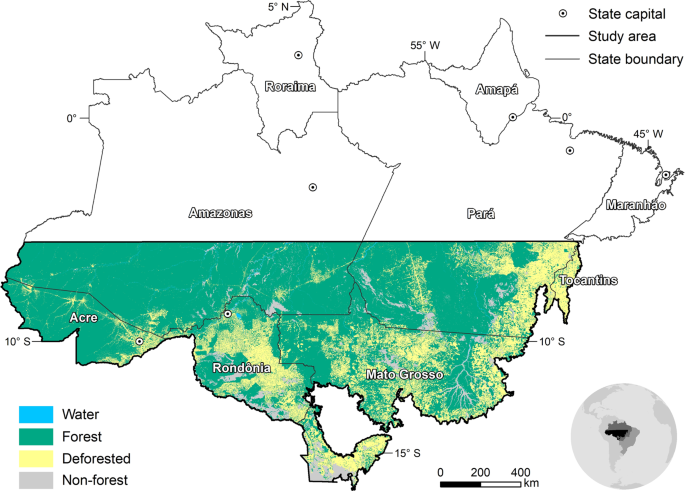
Southern Brazilian Amazon encompasses 1.9 million km² of the Amazon biome. Deforested area by 2019 according to data from the Program to Calculate Deforestation in the Amazon (PRODES).
Despite extensive research on greenhouse gas (GHG)-induced climate change 8 , few studies have addressed regional climate change in response to extensive land use and cover change (LUCC), such as deforestation. Forest conversion to pasture and croplands (forest loss) affects moisture cycling and energy balance 9 , 10 and may change rainfall patterns 3 , 4 , 11 , 12 . Hence, the regional climate could respond as much as or even stronger to LUCC than to global warming 13 , 14 . In the Amazon, the estimated impact on the annual radiative budget due to surface albedo-change is approximately six times higher than that of aerosol emissions, and projected impacts on agriculture from future deforestation-induced changes in climate are of the same magnitude as those from global climate change under the RCP 8.5 scenario 15 .
Studies point to a reliance of the Amazon regional rainfall regimes on the forest 16 , 17 , 18 . Although the effects of biome-wide deforestation affecting forest moisture recycling and irreversible biome transition are still relatively uncertain 19 , 20 , 21 , at smaller geographical scales, some studies suggest a negative linear response of rainfall to forest loss 22 , 23 , 24 , while others indicate a nonlinear response 2 , 25 , 26 , 27 . According to the latter, small-scale, patchy, heterogeneous deforestation patterns drive changes in mesoscale circulation of moisture flow from forests into the atmosphere that can enhance precipitation over nearby deforested areas 20 , 27 , 28 , 29 . However, as forest loss progresses, the region eventually reaches a critical threshold beyond which this relationship reverses with additional forest loss rapidly reducing rainfall 9 , 25 , 30 , 31 .
At regional scales (≈200–600-km grid-cell size), modelling experiments indicate that the critical threshold of forest loss beyond which rainfall progressively decreases lies between 30 and 50% of forest loss, depending on the spatial patterns of deforestation 25 , 30 . But to date, no study has empirically identified this critical threshold and the consequences of crossing it by using observed rainfall data.
In this work, we fill this gap by detrending the effects of geographic location, elevation and interannual variability on a satellite-derived rainfall time series to correlate the residual anomalies with forest loss at different geographical scales (28–224-km grid-cell sizes). To gauge future critical thresholds of forest loss and associated agricultural economic losses, we use projections of agricultural expansion in the region under weak and strong environmental governance scenarios and estimates of soybean and pasture yield losses due to rainfall reduction caused by deforestation across the Brazilian Amazon.
Results and discussion
Relationship between deforestation and rainfall at different geographical scales.
The relationship between forest loss fractions and annual mean rainfall anomalies at 28-, 56- and 112-km grid cells cannot be explained by using a single linear regression; the best fit was obtained by adjusting a multivariate adaptive regression spline (MARS) composed of two piecewise linear segments (Fig. 2a–f ). In turn, the best fit for the 224 × 224-km grid cells was attained by a single linear regression.
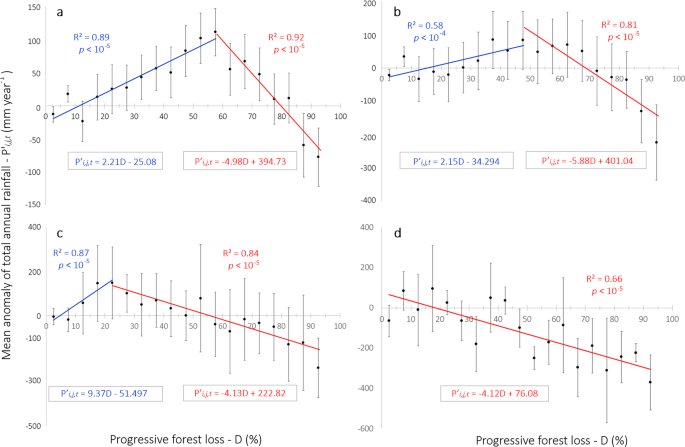
Two piecewise linear segments from MARS algorithm: a D < 57.5% (blue line) and D > = 57.5% (red line) for 28-km grid cells. b D < 47.5% (blue line) and D > = 47.5% (red line) for 56-km grid cells. c D < 27.5% (blue line) and D > = 27.5% (red line) for 112-km grid cells. d Best-fit linear model (dashed red line) for 224-km grid cells. Error bar represents the standard error of the mean rainfall anomaly for each forest loss interval. P ’ i,j,t are the residual annual rainfall anomalies (in mm/year), where the subscripts i and j represent space dimensions and the subscript t represents time dimension. D represents the progressive forest loss fraction (in percentage).
Forest loss affects rainfall differently, depending upon the geographical scale (Fig. 2a–d ). At the original spatial resolution of the TRMM data (28 × 28-km grid cell), there is a dual effect of forest loss, with rainfall increasing with forest loss up to ≈58% and then decreasing afterwards (Fig. 2a ). The gain before the critical threshold is of +22.1 ± 9.3 mm of precipitation per 10% of additional forest loss. However, beyond 57.5% of forest loss, precipitation reduces precipitously, with each 10% of additional forest loss inducing a net reduction in annual rainfall of −49.2 ± 11.3 mm.
We tested for correlation between forest loss and the mean long‐term trend in rainfall using t tests ( Supplementary Section 1.4 ). The test comparing the annual rainfall between cells with smaller forest loss and cells that experienced larger forest loss within two separated periods: 1999–2009 and 2010–2019 (Supplementary Table 6 ) demonstrated that the cells with smaller historical forest loss (below 55–60%) experienced an increase in annual rainfall by +96.9 ± 12.65 mm. Conversely, cells with larger forest losses (exceeding 55–60%) exhibited an annual decrease of −306.4 ± 42.77 mm (Supplementary Table 7 ). Both statistical inferences were statistically significant with P < 0.05.
Aggregating both forest loss and rainfall data to a coarser scale (56 × 56-km grid cell), the critical threshold dropped to ≈48% (Fig. 2b ). For 112 × 112-km grid cells, both the positive and negative effects were attenuated and the threshold reduced even more to ≈23% of forest loss (Fig. 2c ). This dual response of rainfall to forest loss is consistent with the theory and climate modelling experiments 26 , 30 . At smaller geographical scales (28 and 56 km), the reduction in rainfall after the critical threshold was more than twice as fast as the increase before the threshold. However, at larger geographical scales, this effect attenuated until we found a constant linear reduction at 224 × 224-km grid cells (Fig. 2d ).
Our multiscale analysis supports the argument that, if neighbouring grid cells also have high levels of forest loss, the critical threshold of forest loss kicks in at a lower level of forest loss. Therefore, widespread deforestation results in a negative-sum game where total reduction in rainfall outdoes local gains. In this sense, the critical thresholds we found within smaller grid cells are conservative because as regional deforestation progresses, local gains diminish and eventually cancel out.
At the scale from 28 to 56 km, the land-cover heterogeneity forms anomalous meteorological vertical cells, with enhanced convection and hence increased rainfall over part of the deforested patch and rainfall suppression over the surrounding forested regions, what is known as rainfall dipoles 20 . The location, size, strength and net effect of the rainfall dipoles are heavily influenced by the size of the deforested patch, large-scale winds and surrounding forested area 20 , 31 .
On the other hand, the reduction in rainfall at a larger geographical scale (224 × 224 km) is caused by a combination of the higher albedo of deforested surfaces and the smaller year-round evapotranspiration of crops and pastures relative to natural vegetation 27 . The former effects in addition to reduced surface roughness, which in turn diminishes the vertical transport of turbulence by horizontal winds 27 , 32 , 33 , decrease atmospheric moisture available for precipitation. In this respect, Sena et al. 15 indicate that precipitable water above deforested areas is ≈7% lower than that above the Amazon forest. Thus, the critical threshold is the point after which the combined effects of the higher albedo, smaller evapotranspiration and reduced surface roughness due to forest loss become stronger than the deforestation breeze effect, which weakens with forest loss. The net reduction we find after the critical threshold is much steeper than the prior net gains, which is consistent with climate modelling results 27 , 31 .
The quantitative effects of forest loss on rainfall are for the study region as a whole. Particular grid cells may be more or less affected according to their locations. The smallest interannual variability is verified in grid cells located in the south and southwest of the Amazon ( Supplementary Fig. 7 ), where the annual rainfall is lower ( Supplementary Fig. 1 ). Consequently, rainfall reduction due to forest loss is proportionally larger in those regions, currently reaching up 48% (Supplementary Fig. 8 ). This has economic implications for agriculture since the areas most affected by rainfall reduction are major soy production zones or regions where agriculture is likely to expand in the future (Supplementary Fig. 9 ). Crop yields and the success of double-cropping systems already vary significantly from year to year in SBA because of the interannual climate variability 34 , 35 . The effect of forest loss on rainfall superimposed to the interannual variability thus poses an additional risk of crop failure in dry or drought years, which tend to become more frequent and intense as deforestation progresses alongside climate change 36 ( Supplementary Section 2.1 ).
Additional evidence for forest loss causation of rainfall reduction
The relationship between forest loss and rainfall presented here is correlational. To demonstrate that this relationship is significantly greater than a potentially reverse causal effect, i.e., more severe deforestation systematically taking place in drier (or drying) parts of the forest, we superimposed a map of the effects of Maximum Climatological Water Deficit on deforestation 36 , 37 over our study region. We found that only 12 cells of a total 86 non-null cells (14% of our study region) showed a statistically significant effect that more severe forest loss tends to take place in drier (or drying) parts (Supplementary Fig. 11 ). However, the signal in these few grid cells was near zero. Although the feedback between drought and forest loss is notable in more humid regions of the Amazon that lack a marked dry season 37 , this is not the case for SBA, where the dry season is longer than 150 days 38 , hence sufficiently long to dry out the chopped-down understory vegetation to completely clear-cut the forest. In addition, empirical evidence suggests that the length of the rainy season decreases ≈0.9 ± 0.34 days for each additional 10% of forest loss 4 ( Supplementary Section 2.2 ).
Finally, we measured the spatial association between rainfall anomalies and forest loss by calculating the Cramer’s V 39 and the Spearman Rank Order Correlation coefficient 40 . After detrending the other signals, the resulting anomalies exhibited a spatial variability that correlated only with forest loss (Supplementary Fig. 10 ), which corroborates the hypothesized causal direction from forest loss to rainfall reduction in SBA ( Supplementary Section 2.2 ).
Future critical forest loss thresholds and associated agricultural economic losses
To assess the impacts of future regional rainfall reduction on agriculture, we simulated deforestation across SBA. To do so, we ran Otimizagro 41 , 42 , a countrywide LUCC model for Brazil, from 2015 to 2050 under two alternative policy scenarios, i.e., weak and strong environmental governance 42 ( Supplementary Section 1.5 ). The weak governance scenario (WEG) assumed the continued dismantling of Brazil’s conservation policies along with strong political support for environmentally damaging agricultural practices and implicit economic incentives for illegal deforestation ( Supplementary Section 1.5.1 ). The strong governance (SEG) scenario incorporated effective enforcement of conservation policies based on sustained political support for the environmental agenda in Brazil, including the full implementation of the Forest Code and additional conservation incentives 42 , 43 ( Supplementary Section 1.5.2 ).
As of 2019, 25% of SBA had already reached the 55–60% threshold of forest loss within 28 × 28-km grid cells. This critical threshold under the WEG would occur in 36% and 55% of the region’s grid cells by 2030 and 2050, respectively (Fig. 3 ). The realization of the SEG scenario could abate by 24% the number of grid cells that would reach the critical threshold by 2050 under the WEG. At coarser scales (56 km and 112 km), the critical threshold of forest loss ( D ≥ 47.5% and D ≥ 27.5%) under the WEG would occur in 61% and 69% of the region’s grid cells by 2050, respectively. Again, the full implementation of the SEG scenario could abate by 28% and 16% the number of 56- and 112-km grid cells, respectively, that would reach the critical threshold of forest loss by 2050 under the WEG.
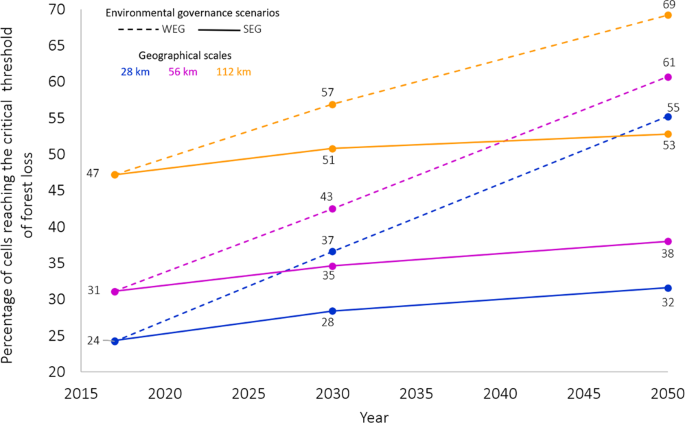
Percentage of different grid-cell sizes crossing the critical threshold of forest loss in southern Brazilian Amazon over time under the two environmental governance scenarios: weak scenario (WEG) and strong scenario (SEG) within 28-km, 56-km and 112-km grid cells.
Similar to other tropical regions, agriculture in the Amazon is mostly rainfed 44 , and as such, decreases in annual rainfall in response to forest loss will reduce yields or shift agriculture either away from the region or towards more drought-resistant crops. Forest suppression also delays the onset of the rainy season 3 , 4 , 45 , prepones its end 4 , 45 and shortens its length 4 , 46 . All of these rainy season characteristics are essential for the highly productive double-cropping systems in the region 34 , 35 . Hence, continued deforestation imposes major challenges for agricultural production in SBA, especially in regions that have already reached the critical threshold of forest loss within the 28 × 28-km grid cells, such as the north-eastern and south-eastern regions of Pará State, West of Maranhão State, the central part of Rondônia and, most notably, the northern soybean belt in Mato Grosso State (Fig. 4d–f ).
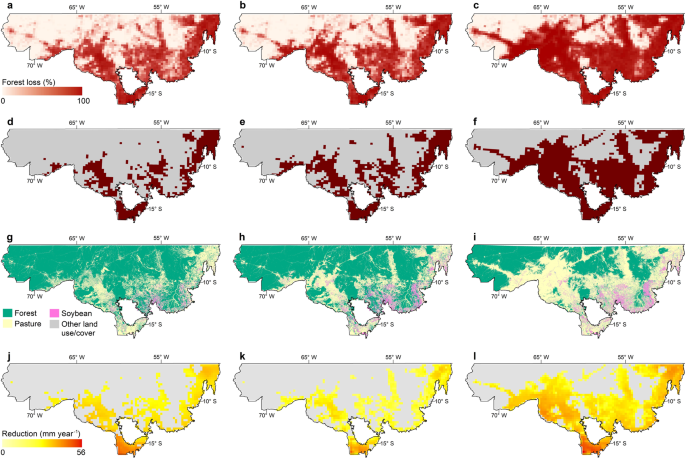
Percentage of forest loss: a by 2019. b Simulated for 2050 for SEG and c WEG. In all, 28 × 28-km grid cells reaching the critical forest loss threshold: d by 2019. e Simulated by 2050 for SEG. f WEG scenario. Land use/cover: g by 2019, h simulated by 2050 for SEG i and WEG. Rainfall reduction: j by 2019. Simulated by 2050 for k SEG and l WEG.
To gauge the potential agricultural economic losses implied by our two scenarios, we used the projections of agricultural expansion in the region for the SEG and WEG scenarios from the Otimizagro model 42 and estimates of soybean and pasture yield losses due to rainfall reduction caused by deforestation across the Brazilian Amazon 47 . Our goal was to explore whether the hydrological zero-sum game outlined above equivalently applies to the economics of agricultural expansion in the region. If so, the opportunity costs, in terms of foregone revenues from converting less forest to crop and pasturelands in the SEG scenario, would have to be outperformed by losses in crop and pasture productivity across SBA due to changes in rainfall patterns under the WEG ( Supplementary Section 1.6 ).
Considering only revenues from soy cultivation and beef production in SBA, we find that productivity losses and associated revenues under WEG (US$ 5.6 billion for soy and US$ 180.8 billion for beef production by 2050 in net present values—NPV) dwarf the conservation opportunity costs of US$ 19.5 billion in NPV under SEG. In other words, Brazil may have passed a threshold at which further Amazon deforestation translates into direct economic damage. Deforestation does not only result in CO 2 emissions and irreversible loss of globally valued biodiversity, it also imposes massive productivity losses worth up U$ 1 billion annually (Equivalent Annual Annuity; Supplementary Section 1.6 ) on the region’s agribusiness. It is unlikely, at least in the short or medium term, that accounting for adaptation costs and potential benefits would tip the balance in favour of agricultural expansion.
Anticipating the impacts of deforestation on the region’s ecosystem services, especially rainfall regulation for agriculture, is paramount to convince policymakers and other stakeholders to act before it is too late. Indeed, acknowledging those risks could help steer Brazil back to a course that sustainably integrates agricultural production and environmental conservation. Brazil’s agribusiness and their global partners are testing the limits of nature by expanding into natural forests at the risk of reducing the rainfall that sustains its productivity. The current land-use trajectory in the Brazilian Amazon, therefore, puts the largely rainfed agricultural systems of the country on an unsustainable pathway.
We analyzed the quantitative linkage between annual rainfall and Amazon forest loss from 1999 to 2019. Using a multiscale approach, we empirically determined the level of forest loss (the critical threshold) beyond which the effect of forest loss on rainfall reverses. Our approach sheds light on the unresolved question as to how continued forest loss affects annual rainfall at different geographical scales. We also explored when and which regions in SBA may reach the critical threshold of forest loss under two environmental governance scenarios (SEG and WEG) 42 .
SBA covers 1.9 million km². This region historically underwent agricultural and logging expansion from the south and east of Brazil.
Rainfall data
We used the rainfall data between 1999 and 2019 from the Tropical Rainfall Measuring Mission satellite (TRMM) 3B43 product (version 7). Monthly rainfall was aggregated to obtain yearly rainfall. TRMM data are originally at ≈28 × 28-km spatial resolution. This rainfall dataset has been extensively verified and validated for the Amazon biome 48 , 49 .
Deforestation data
We used data from PRODES (Program to Calculate Deforestation in the Amazon) 6 , originally released at ≈30-m spatial resolution. We aggregated PRODES data into time-series maps of percentages of forest loss per grid-cell sizes from 28 × 28 km, 56 × 56, 112 × 112 to 224 × 224 km.
Anomalies of annual rainfall
Rainfall in the region has a marked spatial gradient (Supplementary Fig. 1 ) and interannual variability. Thus, to minimize omitted variable bias in our analysis, we first removed the effects of factors other than deforestation that may affect rainfall across both time and space. To do so, we calculated anomalies of annual rainfall using a three-step procedure (Supplementary Fig. 4 ), based on a conceptual methodology developed for analyzing the onset, end and length of the rainy season in Southern Amazon 34 . We calculated anomalies of annual rainfall (P′ i,j,t ) using the three-step procedure summarized in Eqs. ( 1 ), ( 2 ) and ( 3 ) and Supplementary Fig. 4 ( Supplementary Sections 1.1 and 1.2). Notations are as follows: i, j, t are the subscripts representing space ( i, j ) and time ( t ), \({{\bf{P}}}_{{\boldsymbol{i}},{\boldsymbol{j}},{\boldsymbol{t}}}\) are the annual rainfall values in mm, \({\hat{\bf{P}}}_{{\boldsymbol{i}},{\boldsymbol{j}}}\) are the estimated values of rainfall due to geographical location and elevation, \({\bf{P}}_{{\boldsymbol{i}},{\boldsymbol{j}},{\boldsymbol{t}}}\) is the difference between observed annual rainfall values and the estimated ones due to geographical location and elevation, \({\bar{{\bf{P}}}}_{{\boldsymbol{t}}}\) are the annual averages of rainfall calculated throughout the study region calculated from 1999 to 2019, \({{\bf{P}}}_{{\boldsymbol{i}},{\boldsymbol{j}},{\boldsymbol{t}}}^{\prime}\) are the residual annual rainfall anomalies and \({\boldsymbol{\varphi }},{\boldsymbol{\lambda }},{\boldsymbol{\zeta }}\) are latitude, longitude (in degrees) and elevation (in metres).
To estimate the spatial pattern of annual rainfall, we used a second-degree model ( r ² = 0.72, P > 10 −5 ) to compute estimated values of rainfall due to this geographical pattern so that
To remove the climatological trend related to geographic location and elevation, we calculated the difference between raw values of observed rainfall in each year and the estimated values due to geographical position obtained from Eq. ( 1 ), so that
To remove the signal of large-scale factors, such as that of the ENSO, we subtracted the mean annual rainfall for the whole study region from the outputs of Eq. ( 2 )
The residual is assumed to be an “anomaly” that is not explained by the geographic location, elevation or large-scale time-varying factors.
LUCC modelling and associated agricultural economic impact
Otimizagro is a spatially explicit model that simulates land use, land-use change, forestry, deforestation and regrowth under various scenarios of agricultural land demand and deforestation policies for Brazil 41 . The model simulates nine annual crops (including single and double cropping), five perennial crops, and plantation forests. The model framework, developed using the Dinamica EGO platform (dinamicaego.com), is structured in four spatial levels: (i) Brazil’s biomes, (ii) IBGE micro-regions, (iii) Brazilian municipalities and (iv) a raster grid of 25 ha spatial resolution. Future demand for crops and deforestation, and regrowth rates are exogenous to the model. We assumed constant agricultural practices and prices over time under both governance scenarios. Opportunity costs are calculated as follows:
(I) We performed a simulation of deforestation in the Brazilian Amazon, as well as soybean and pasture expansion under the SEG and WEG scenarios 42 . (II) We calculated the average productivity using regional estimates of soybean and pasture yield losses due to rainfall reduction caused by Amazon biome-wide deforestation 47 ( Supplementary Section 1.6 ). (III) We projected productivity change until 2050 adjusting future soybean yield projections (3.7 ton/hectare) 50 and pasture productivity projections 51 (2.9 arroba/hectare) 51 by the average productivity losses calculated in step II. (IV) We computed annual revenues in US$ per hectare using current soybean and cattle arroba prices (US$ 302.58 per ton and US$ 201.50 per arroba, respectively) and projected soybean and pasture productivity for each of the two deforestation scenarios with and without decreases in yields. Under each scenario, total annual revenues are calculated by weighting soy and pasture revenues using the simulated future area under crops and pastures. (V) We calculated the net present value (NPV, Supplementary equation (14) ) of future revenues for SBA using as the discount rate the Selic interest rate of 3.75% (the Selic is determined by Brazil’s Central Bank) and converted NPV into an equivalent annual annuity (EAA, Supplementary equation 15 ). (VI) The difference between the total NPV of revenues under the WEG versus the SEG scenario is the opportunity cost of the SEG scenario. Similarly, the difference between EAA of revenues under the WEG versus the SEG scenario is the annual opportunity cost of the SEG scenario.
Data availability
All the data that support the findings of this study are obtained from publicly available sources. Annual deforestation data are obtained from the Program to Calculate Deforestation in the Amazon (PRODES) ( http://terrabrasilis.dpi.inpe.br/app/map/deforestation?hl=pt-br ). Precipitation data use the TRMM (TMPA/3B43) Rainfall Estimate L3 1 month 0.25-degree × 0.25-degree V7 from the U.S. National Aeronautics and Space Administration ( https://disc.gsfc.nasa.gov/datasets/TRMM_3B43_7/summary ). Elevation data come from the Shuttle Radar Topography Mission (SRTM) from the National Aeronautics and Space Administration of U.S. ( https://www.usgs.gov/centers/eros/science/usgs-eros-archive-digital-elevation-shuttle-radar-topography-mission-srtm-non?qt-science_center_objects=0#qt-science_center_objects ).
Code availability
All analytical and simulation models were developed using either Dinamica EGO (dinamicaego.com) or R (r-project.org). EGO models and R codes are available at https://doi.org/10.6084/m9.figshare.14192519.v1 .
Costa, M. H. & Pires, G. F. Effects of Amazon and Central Brazil deforestation scenarios in the duration of the dry season in the arc of deforestation. Int. J. Climatol. 30 , 1970–1979 (2010).
Article Google Scholar
Boers, N., Marwan, N., Barbosa, H. & Kurths, J. A deforestation-induced tipping point for the South American monsoon system. Sci. Rep. 7 , 41489 (2017).
Article ADS CAS PubMed PubMed Central Google Scholar
Leite-Filho, A. T., Sousa Pontes, V. Y. & Costa, M. H. Effects of deforestation on the onset of the rainy season and the duration of dry spells in southern Amazonia. J. Geophys. Res.: Atmospheres 124 , 5268–5281 (2019).
Article ADS Google Scholar
Leite‐Filho, A. T., Costa, M. H. & Rong, F. The southern Amazon rainy season: the role of deforestation and its interactions with large‐scale mechanisms. Int. J. Climatol. 40 , 2328–2341 (2019).
Costa, M. et al. Climate risks to Amazon agriculture suggest a rationale to conserve local ecosystems. Front. Ecol. Environ. 17 , 584–590 (2019).
Instituto Nacional de Pesquisas Espaciais (INPE). PRODES—Monitoramento da Floresta Amazônica Brasileira por Satélite. INPE database. http://www.obt.inpe.br/prodes/index.php (2020).
FAO. Global Forest Resources Assessment 2005: Progress Towards Sustainable Forest Management (Food and Agriculture Organization, United Nations, 2006).
Intergovernmental Panel on Climate Change (IPCC). Climate Change 2014: Synthesis Report. Contribution of Working Groups I, II and III to the Fifth Assessment Report of the Intergovernmental Panel on Climate Change (IPCC, 2015).
Costa, M. H., Yanagi, S. N. M., Souza, P. J. O. P., Ribeiro, A. & Rocha, E. J. P. Climate change in Amazonia caused by soybean cropland expansion as compared to caused by pastureland expansion. Geophys. Res. Lett. 34 , L07706 (2007).
ADS Google Scholar
Sheil, D. & Murdiyarso, D. How forests attract rain: an examination of a new hypothesis. BioScience 59 , 341–347 (2009).
Agudelo, J., Arias, P. A., Vieira, S. C. & Martínez, J. A. Influence of longer dry seasons in the Southern Amazon on patterns of water vapor transport over northern South America and the Caribbean. Clim. Dyn. 52 , 2647–2665 (2019).
Molina, R. R., Salazar, J. F., Martínez, J. A., Villegas, J. C. & Arias, P. A. Exponential growth of precipitation along a “forest-fed moisture convey or belt” above the Amazon. J. Geophys. Res.-Atmospheres 124 , 2589–2599 (2019).
Pongratz, J., C. Reick, H., Raddatz, T. & Claussen, M. Bio-geophysical versus biogeochemical climate response to historical anthropogenic land cover change. Geophys. Res. Lett. 37 , L08702 (2010).
Noblet, N. D. E. et al. Determining robust impacts of land-use-induced land cover changes on surface climate over North America and Eurasia: results from the first set of LUCID experiments. J. Clim. 25 , 3261–3281 (2012).
Sena, E., Artaxo, P. & Correia, A. Spatial variability of the direct radiative forcing of biomass burning aerosols and the effects of land use change in Amazonia. Atmos. Chem. Phys. 13 , 1261–1275 (2013).
Article ADS CAS Google Scholar
Fu, R. & Li, W. The influence of the land surface on the transition from dry to wet season in Amazonia. Theor. Appl. Climatol. 78 , 97 (2004).
Makarieva, A. M. & Gorshkov, V. G. Biotic pump of atmospheric moisture as driver of the hydrological cycle on land. Hydrol. Earth Syst. Sci. 11 , 1013–1033 (2007).
Ramos-da-Silva, R., Gandu, A., Sá, L. & Dias, M. Cloud streets and land-water interactions in the Amazon. Biogeochemistry 105 , 201–211 (2011).
Bagley, J. E., Desai, A. R., Harding, K. J., Snyder, P. K. & Foley, J. A. Drought and deforestation: has land cover change influenced recent precipitation extremes in the Amazon? J. Clim. 27 , 345–361 (2014).
Khanna, J., Medvigy, D., Fueglistaler, S. & Walko, R. Regional dry-season climate changes due to three decades of Amazonian deforestation. Nat. Clim. Change 7 , 200–204 (2017).
Swann, A. L. S., Longo, M., Knox, R. G., Lee, E. & Moorcroft, P. R. Future deforestation in the Amazon and consequences for South American climate. Agric. For. Meteorol. 214–215 , 12–24 (2015).
Akkermans, T., Thiery, W. & Lipzig, N. The regional climate impact of a realistic future deforestation scenario in the Congo Basin. J. Clim. 27 , 2714–2734 (2014).
Wu, H., Adler, R. F., Tian, Y., Gu, G. & Huffman, G. Evaluation of quantitative precipitation estimations through hydrological modeling in IFloodS river basins. J. Hydrometeorol. 18 , 529–553 (2017).
Zemp, D. C. et al. Self-amplified Amazon forest loss due to vegetation-atmosphere feedbacks. Nat. Commun. 8 , 14681 (2017).
Sampaio, G. et al. Regional climate change over eastern Amazonia caused by pasture and soybean cropland expansion. Geophys. Res. Lett. 34 , L17709 (2007).
Da Silva, R. R., Werth, D. & Avissar, R. Regional impacts of future land-cover changes on the Amazon basin wet-season climate. J. Clim. 21 , 1153–1170 (2008).
Lawrence, D. & Vandecar, K. Effects of tropical deforestation on climate and agriculture. Nat. Clim. Change 5 , 27–36 (2015).
Makarieva, A. M., Gorshkov, V. G. & Li, B. L. Precipitation on land versus distance from the ocean: evidence for a forest pump of atmospheric moisture. Ecol. Complex. 6 , 302–307 (2009).
Spracklen, D. V., Arnold, S. R. & Taylor, C. M. Observations of increased tropical rainfall preceded by air passage over forests. Nature 489 , 282–286 (2012).
Article ADS CAS PubMed Google Scholar
Nobre, P., Malagutti, M., Urbano, D. F., de Almeida, R. A. F. & Giarolla, E. Amazon deforestation and climate change in a coupled model simulation. J. Clim. 22 , 5686–5697 (2009).
Saad, S. I., da Rocha, H. R., Silva Dias, M. A. & Rosolem, R. Can the deforestation breeze change the rainfall in Amazonia? A case study for the BR-163 highway region. Earth Interact. 14 , 18 (2010).
Dickinson, R. E. & Kennedy, P. Impacts on regional climate of Amazon deforestation. Geophys. Res. Lett. 19 , 1947–1950 (1992).
D’Almeida, C. et al. The effects of deforestation on the hydrological cycle in Amazonia: a review on scale and resolution. Int. J. Climatol. 27 , 633–647 (2007).
Cohn, A. S., VanWey, L. K., Spera, S. A. & Mustard, J. F. Cropping frequency and area response to climate variability can exceed yield response. Nat. Clim. Change 6 , 601–604 (2016).
Abrahão, G. M. & Costa, M. H. Evolution of rain and photoperiod limitations on the soybean growing season in Brazil: the rise (and possible fall) of double cropping systems. Agric. For. Meteorol. 256–257 , 32–45 (2018).
Nobre, C. et al. Land-use and climate change risks in the Amazon and the need of a novel sustainable development paradigm. Proc. Natl Acad. Sci. USA 113 , 10759–10768 (2016).
Staal, A. et al. Feedback between drought and deforestation in the Amazon. Environ. Res. Lett. 15 , 044024 (2020).
Agudelo, J., Arias, P., Vieira, S. & Martinez, A. Influence of longer dry seasons in the Southern Amazon on patterns of water vapor transport over northern South America and the Caribbean. Clim. Dyn. 52 , 2647–2665 (2019).
Cramér, H. Mathematical Methods of Statistics , Ch. 21 (Princeton University Press, 1946).
Spearman, C. The proof and measurement of association between two things. Am. J. Psychol. 15 , 72–101 (1904).
Soares-Filho, B. S. et al. Brazil’s market for trading forest certificates. PLoS ONE 11 , e0152311 (2016).
Article PubMed PubMed Central CAS Google Scholar
Rochedo, P. R. R. et al. The threat of political bargaining to climate mitigation in Brazil. Nat. Clim. Change 8 , 695–698 (2018).
Rajão, R. et al. The rotten apples of Brazil’s agribusiness. Science 369 , 246 (2020).
Article ADS PubMed CAS Google Scholar
Sumila, T. C. A., Pires, G. F., Fontes, V. F. & Costa, M. H. Sources of water vapour to economically relevant regions in Amazonia and the effect of deforestation. J. Hydrometeorol. 18 , 1643–1655 (2017).
Debortoli, N. S. et al. Detecting deforestation impacts in Southern Amazonia rainfall using rain gauges. Int. J. Climatol. 37 , 2889–2900 (2016).
Barichivich, J. et al. Recent intensification of Amazon flooding extremes driven by strengthened Walker circulation. Sci. Adv. 4 , eaat8785 (2018).
Article ADS PubMed PubMed Central Google Scholar
Strand, J. et al. Spatially explicit valuation of the Brazilian Amazon Forest’s Ecosystem Services. Nat. Sustainability 1 , 657–664 (2018).
Aragão, L. E. O. C. et al. Spatial patterns and fire response of recent Amazonian droughts. Geophys. Res. Lett. 34 , L07701 (2007).
Ballari, D., Castro, E. & Campozano, L. Validation of satellite precipitation (TRMM 3B43) in Ecuadorian coastal plains, Andean highlands and Amazonian rainforest. ISPRS - Int. Arch. Photogramm., Remote Sens. Spat. Inf. Sci. XLI-B8 , 305–311 (2016).
Ministério da Agricultura, Pecuária e Abastecimento (MAPA). Projeções do Agronegócio 2018/19 a 2028/29, Brasília, Distrito Federal (2019).
Batista, E. et al. Large-scale pasture restoration may not be the best option to reduce greenhouse gas emissions in Brazil. Environ. Res. Lett. 14 , 125009 (2019).
Download references
Acknowledgements
This work was supported by Brazilian National Council for Scientific and Technological Development (CNPq grant 140147/2019-5 and CNPq/AWS 032/2019) and by the project “Development of systems to prevent forest fires and monitor vegetation cover in the Brazilian Cerrado” (World Bank Project no. P143185) – Forest Investment Program (FIP). B.S. and J.B. were supported by Alexander von Humboldt Foundation. B.S. also receives support from CNPq and Climate and Land Use Alliance (CLUA). J.B. also receives support from Deutsche Forschungsgemeinschaft (DFG, German Research Foundation) under Germany’s Excellence Strategy—EXC 2070—390732324.
Author information
Authors and affiliations.
Centre for Remote Sensing, Federal University of Minas Gerais, Belo Horizonte, Brazil
Argemiro Teixeira Leite-Filho, Britaldo Silveira Soares-Filho & Juliana Leroy Davis
Department of Agricultural Engineering, Federal University of Viçosa, Viçosa, Brazil
Gabriel Medeiros Abrahão
Centre for Development Research, University of Bonn, Bonn, Germany
You can also search for this author in PubMed Google Scholar
Contributions
A.L.-F. conceived and performed the experiment, and wrote the paper. B.S.F. ran LUCC scenarios and wrote the paper. J.D. ran LUCC scenarios. G.A. reviewed the climate results and discussion. J.B. calculated the opportunity costs and edited the paper. All authors provided critical feedback and helped shape the research, analysis and paper.
Corresponding author
Correspondence to Argemiro Teixeira Leite-Filho .
Ethics declarations
Competing interests.
The authors declare no competing interests.
Additional information
Peer review information Nature Communications thanks Henrique Barbosa, Leila Carvalho, and Arie Staal for their contribution to the peer review of this work.
Publisher’s note Springer Nature remains neutral with regard to jurisdictional claims in published maps and institutional affiliations.
Supplementary information
Supplementary information, rights and permissions.
Open Access This article is licensed under a Creative Commons Attribution 4.0 International License, which permits use, sharing, adaptation, distribution and reproduction in any medium or format, as long as you give appropriate credit to the original author(s) and the source, provide a link to the Creative Commons license, and indicate if changes were made. The images or other third party material in this article are included in the article’s Creative Commons license, unless indicated otherwise in a credit line to the material. If material is not included in the article’s Creative Commons license and your intended use is not permitted by statutory regulation or exceeds the permitted use, you will need to obtain permission directly from the copyright holder. To view a copy of this license, visit http://creativecommons.org/licenses/by/4.0/ .
Reprints and permissions
About this article
Cite this article.
Leite-Filho, A.T., Soares-Filho, B.S., Davis, J.L. et al. Deforestation reduces rainfall and agricultural revenues in the Brazilian Amazon. Nat Commun 12 , 2591 (2021). https://doi.org/10.1038/s41467-021-22840-7
Download citation
Received : 14 June 2020
Accepted : 31 March 2021
Published : 10 May 2021
DOI : https://doi.org/10.1038/s41467-021-22840-7
Share this article
Anyone you share the following link with will be able to read this content:
Sorry, a shareable link is not currently available for this article.
Provided by the Springer Nature SharedIt content-sharing initiative
This article is cited by
Amazon savannization and climate change are projected to increase dry season length and temperature extremes over brazil.
- Marcus Jorge Bottino
- Paulo Nobre
- Carlos Afonso Nobre
Scientific Reports (2024)
Near-term projection of Amazon rainfall dominated by phase transition of the Interdecadal Pacific Oscillation
npj Climate and Atmospheric Science (2024)
The time since land-use transition drives changes in fire activity in the Amazon-Cerrado region
- Andreia F. S. Ribeiro
- Lucas Santos
- Paulo M. Brando
Communications Earth & Environment (2024)
Effect of climate change and deforestation on populations of Penelope pileata (Galliformes-Cracidae)
- Erikson Bruno Loseiro Ferreira
- Gabriela Silva Ribeiro Gonçalves
- Marcos Pérsio Dantas Santos
Journal of Ornithology (2024)
Trends and correlation between deforestation and precipitation in the Brazilian Amazon Biome
- Rodrigo Martins Moreira
Theoretical and Applied Climatology (2024)
By submitting a comment you agree to abide by our Terms and Community Guidelines . If you find something abusive or that does not comply with our terms or guidelines please flag it as inappropriate.
Quick links
- Explore articles by subject
- Guide to authors
- Editorial policies
Sign up for the Nature Briefing newsletter — what matters in science, free to your inbox daily.

Living World - Amazon Case Study
The Amazon is the largest tropical rainforest on Earth. It sits within the Amazon River basin, covers some 40% of the South American continent and as you can see on the map below includes parts of eight South American countries: Brazil, Bolivia, Peru, Ecuador, Colombia, Venezuela, Guyana, and Suriname. The actual word “Amazon” comes from river.

Amazing Amazon facts; • It is home to 1000 species of bird and 60,000 species of plants • 10 million species of insects live in the Amazon • It is home to 20 million people, who use the wood, cut down trees for farms and for cattle. • It covers 2.1 million square miles of land • The Amazon is home to almost 20% of species on Earth • The UK and Ireland would fit into the Amazon 17 times!
The Amazon caught the public’s attention in the 1980s when a series of shocking news reports said that an area of rainforest the size of Belgium was being cut down and subsequently burnt every year. This deforestation has continued to the present day according to the Sao Paulo Space Research Centre. In 2005 they had lost 17% of Amazon rainforest or 650000 square kilometres. Their satellite data is also showing increased deforestation in parts of the Amazon. The process of deforestation The Amazon helps a Newly Emerging Economy(NEE), Brazil, to make money. They build roads into the forest, logging firms then go in and take out valuable hard woods such as mahogany and cedar, worth thousands of pounds in richer economies like Europe. Then farmers, often cattle ranchers from big companies, burn the rest to make way for cattle pasture. 75% of cleared areas are used in this way. This is clearly shown on the map on figure 22 in red. Many of the deforested areas follow roads and branch off from there. Deforestation is also worse in the South and South East of the Amazon basin, closer to major centres of population in Brazil.

© WWF Source Used with permission.
The causes of deforestation 1. Subsistence and commercial farming – subsistence farming is where poor farmers occupy plots of the forest to grow food to feed themselves and their families. They clear forest and then burn it, hence the name slash and burn. They grow crops until the soil is exhausted and then move on. This contributes to deforestation but not as much as commercial farming (Farming to sell produce for a profit to retailers or food processing companies). The Brazilian region of Mato Grosso was affected by deforestation in the 1980s and 1990s. 43% of rainforest losses were in this region, and area almost ½ the size of France. It has been replaced by fields for grain and cattle. This has allowed Brazil to overtake Australia as the largest exporter of beef in the world. The land is also flat and easy to farm. It also has high temperatures and lots of rainfall.
2. Logging – This involves cutting down trees for sale as timber or pulp. The timber is used to build homes, furniture, etc. and the pulp is used to make paper and paper products. Logging can be either selective or clear cutting. Selective logging is selective because loggers choose only wood that is highly valued, such as mahogany. Clear-cutting is not selective. Loggers are interested in all types of wood and therefore cut all of the trees down, thus clearing the forest, hence the name- clear-cutting.
3. Road building – trees are also clear for roads. Roads are an essential way for the Brazilian government to allow development of the Amazon rainforest. However, unless they are paved many of the roads are unusable during the wettest periods of the year. The Trans Amazonian Highway has already opened up large parts of the forest and now a new road is going to be paved, the BR163 is a road that runs 1700km from Cuiaba to Santarem. The government planned to tarmac it making it a superhighway. This would make the untouched forest along the route more accessible and under threat from development.
4. Mineral extraction – forests are also cleared to make way for huge mines. The Brazilian part of the Amazon has mines that extract iron, manganese, nickel, tin, bauxite, beryllium, copper, lead, tungsten, zinc and gold!
The Belo Monte dam site under construction, copyright Used with the kind permission of Phil Clarke-Hill - His website is amazing, click here to see it.
5. Energy development – This has focussed mainly on using Hydro Electric Power, and there are 150 new dams planned for the Amazon alone. The dams create electricity as water is passed through huge pipes within them, where it turns a turbine which helps to generate the electricity. The power in the Amazon is often used for mining. Dams displace many people and the reservoirs they create flood large area of land, which would previously have been forest. They also alter the hydrological cycle and trap huge quantities of sediment behind them. The huge Belo Monte dam started operating in April 2016 and will generate over 11,000 Mw of power. A new scheme the 8,000-megawatt São Luiz do Tapajós dam has been held up because of the concerns over the impacts on the local Munduruku people.

6. Settlement & population growth – populations are growing within the Amazon forest and along with them settlements. Many people are migrating to the forest looking for work associated with the natural wealth of this environment. Settlements like Parauapebas, an iron ore mining town, have grown rapidly, destroying forest and replacing it with a swath of shanty towns. The population has grown from 154,000 in 2010 to 220,000 in 2012. The Brazilian Amazon’s population grew by a massive 23% between 2000 and 2010, 11% above the national average.
Impacts of deforestation – economic development, soil erosion, contribution to climate change. • Every time forest is cleared species are lost – so we lose BIODIVERSITY • Climate Change - Burning the forest releases greenhouse gasses like CO2. This contributes to the warming of our planet via climate change and global warming. In addition, the loss of trees prevents CO2 being absorbed, making the problem worse. The Amazon also helps to drive the global atmospheric system. There is a lot of rainfall there and changes to the Amazon could disrupt the global system. • Economic development – Brazil has used the forests as a way to develop their country. The forest has many natural riches that can be exploited. In addition, Brazil has huge foreign debt and lots of poor people to feed, so they want to develop the forest. May Brazilians see deforestation as a way to help develop their country and improve people’s standard of living. • Soil erosion - the soils of the Amazon forest are not fertile and are quickly exhausted once the forest is cleared. The farmers now artificially fertilise the soil when in the past the nutrient cycle would have done this naturally. In addition, the lack of forest cover means that soils are exposed to the rainfall. This washes huge amounts of soil into rivers in the process of soil erosion.
NEXT TOPIC - Living World - Sustainable Forest Management
©2015 Cool Geography
- Copyright Policy
- Privacy & Cookies
- Testimonials
- Feedback & support

- Environment
Fires Imperil the Future of the Amazon Rainforest
“you might even look and think it’s really a beautiful forest, but it’s not so healthy.”, quentin septer.
- Share on Facebook
- Share on Twitter
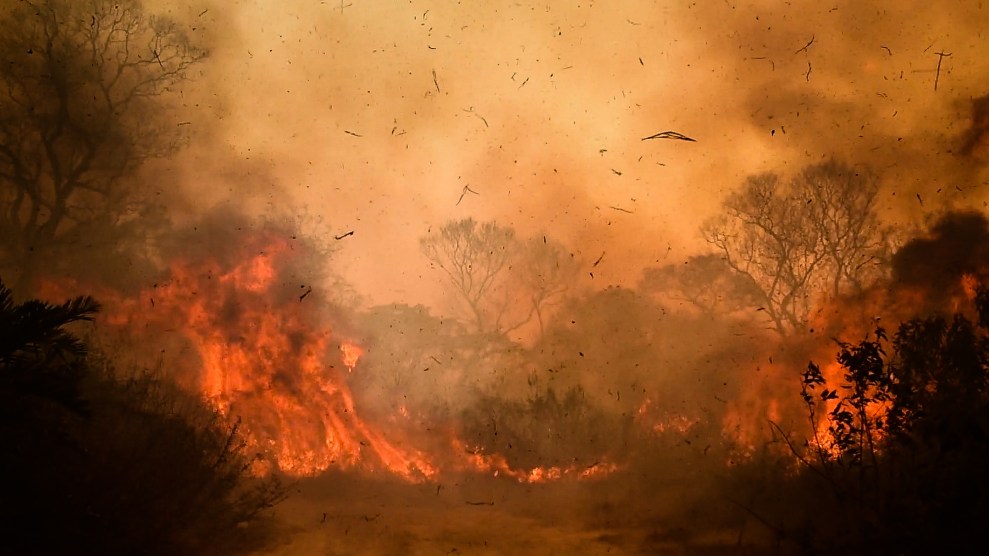
An August 2020 fire in the Brazilian state of Mato Grosso. Gustavo Basso/NurPhoto/Zuma
This story was originally published by Wired and is reproduced here as part of the Climate Desk collaboration.
The Amazon Rainforest is on fire. Or much of it, at least. On February 28, Brazil’s National Institute for Space Research announced that 2,940 fires had burned in the Brazilian Amazon over the course of that month—a record-breaking number for a February. Many of them are still blazing.
Real-time satellite monitoring shows that so far in 2024, more than 10,000 wildfires have ripped across 11,000 square kilometers of the Amazon, across multiple countries. Never have this many fires burned so much of the forest this early in the year. Scientists worry this is pushing the region closer and closer to a tipping point, where widespread degradation and repeated burning of the forest will become unstoppable.
“Fire is a contagious process,” says Bernando Flores, a researcher at Brazil’s Federal University of Santa Catarina, who studies changes in the Amazon. “If nothing is done to prevent fire from penetrating remote areas of the Amazon, the system may eventually collapse from megafires and become trapped in a persistently flammable, open-vegetation state.”
The Amazon Rainforest spans 6.7 million square kilometers, accounting for more than half the planet’s remaining rainforest. It is home to 10 percent of the planet’s terrestrial biodiversity, and plays a vital role in stabilizing both local and global climates. The Amazon stores between 15 and 20 years’ worth of global CO 2 emissions, and it has a cooling effect on the world thanks to the moisture that its plants store and transpire into the atmosphere.
Since the 1980s, the Amazon Basin has been warming at an average of 0.27 degrees Celsius per decade. Some parts, including the forest’s central and southeast regions, have been warming even faster, at a rate of 0.6 degrees per decade. Today, dry season temperatures are, on average, 2 degrees higher than they were 40 years ago.
These trends have come to a head this year. A study published in late January by researchers from World Weather Attribution, a scientific collaboration that tracks the influence of climate change on weather, found that the drought that has afflicted the Amazon Basin since the middle of last year is primarily being driven by climate change. This historic drought, the most severe ever recorded in the Amazon, has also been amplified by the El Niño weather phenomenon —a cyclical climate pattern defined by unusually warm temperatures over the central and eastern Pacific Ocean, which typically lasts for 9 to 12 months.
”When we have El Niño, or drought, the forest is drier and more susceptible to wildfire,” says Dolors Armenteras Pascual, an ecologist and professor at the National University of Colombia. Record-high temperatures and this extreme drought have sapped the Amazon’s trees of their moisture, leaving the forest understory littered with woody debris that has grown dry, primed to ignite. Amid this heat and dry air, the trees of the Amazon also struggle to draw the water they need from parched soil. They are “degraded” as a result.
A recent paper published by Flores and colleagues in the journal Nature finds that patterns of rainfall have also changed. In the southern Bolivian Amazon, for example, annual rainfall has declined 20 millimeters per year since the 1980s. The Amazon has become “more flammable” as a result, Flores says.
“Degradation means that you still have standing forest, but you are losing some of the structure, some of the functioning,” says Armenteras Pascual. “You might even look and think it’s really a beautiful forest, but it’s not so healthy.”
Being degraded also makes a forest more prone to wildfire. And once a part of the Amazon burns, it’s more likely to catch fire again. “When a forest burns, trees die, releasing organic matter above the soil and opening the canopy,” says Flores. “Hence, more fuel is available and more sunlight and wind can desiccate this fuel, causing the ecosystem to become more flammable. The consequence is that burnt forests are much more likely to burn again.”
When considering the impacts of human disturbance and extreme drought over recent decades, as much as 38 percent of what remains of the Amazon Rainforest may already be degraded, Flores and his colleagues found.
By considering all of the factors contributing to the degradation of the Amazon—climate change, drought, deforestation, wildfires—the team also developed models projecting heat, degradation, and fire trends into the future. The findings are gloomy. By 2050, their models show, temperatures over the Amazon Basin are expected to be 2 to 4 degrees Celsius warmer than they are today, depending on greenhouse gas emissions over the next two and a half decades. By 2050, the Amazon’s dry season may be a month longer than it is now. Wildfires are expected to increase in frequency and severity.
As a result, they estimate nearly half the Amazon may reach a “tipping point” by 2050, when it will cease being a forest at all and transition into savannah and grassland.
The impacts of this would be devastating on local and global levels. A 2021 report from the Science Panel for the Amazon found that 10,000 of the rainforest’s plant and animal species are at risk of extinction due to climate change and habitat destruction. A widespread collapse like this may well push these species over the edge. Many of the Amazon’s 40 million human inhabitants may be displaced by unbearable heat, and Indigenous peoples in particular would lose their livelihoods, ways of life, and knowledge systems.
As alarmist as this might sound, Armenteras Pascual thinks the warnings of Flores and his colleagues are, if anything, understated. “It’s not like half of the Amazon will collapse and the other half will go on just fine,” she says. “The whole system might collapse—the whole system in terms of hydrology, which is probably the most important role of the Amazon globally, its role in cooling the climate.”
If the Amazon were to undergo a “large-scale collapse” by 2050, as Flores and his colleagues warn, it may emit as much as 120 billion tons of carbon dioxide into the atmosphere, currently equivalent to about 3.5 years of global CO 2 emissions. Global temperatures may rise 0.3 degrees Celsius as a result .
For now, the unusually high number of fires are expected to continue burning through the month of April, when the rainy season begins. “It’s fire season,” Armenteras Pascual, who is working with the Colombian government to monitor emissions from fires in the Northern Amazon, says. “Just this past week we had 7,000 hectares burning that no one is talking about, in one of the nature preserves that we have near the border with Venezuela.”
“There are some fires here in Colombia as well,” she adds. Data from the satellites shows that just over 1,000 fires burned in the Colombian Amazon in the first week of March. “Fires are burning,” Armenteras Pascual says, “and they’re picking up.”
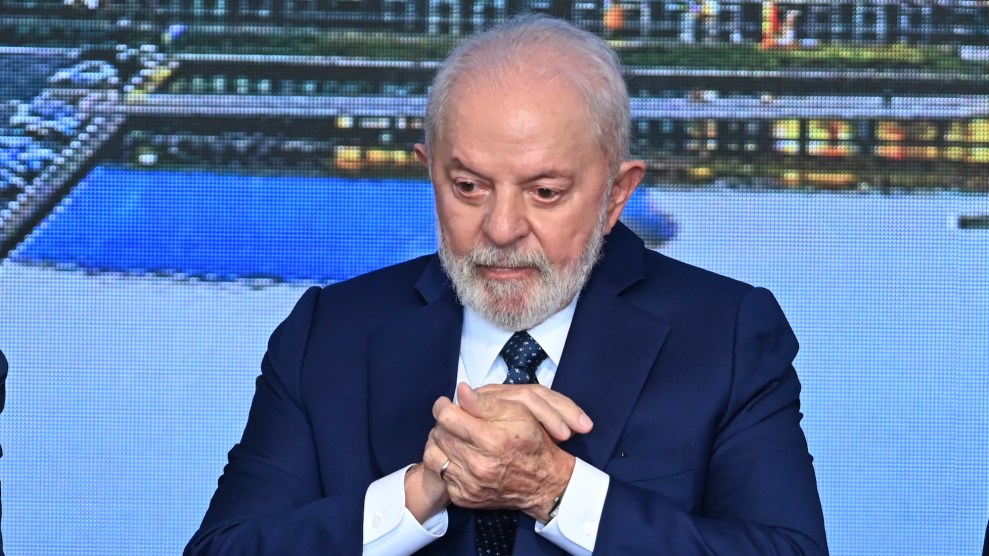
Vanishing Ants, Waning Forests, and Fading Hope for Brazil’s Amazon
Jonathan Watts
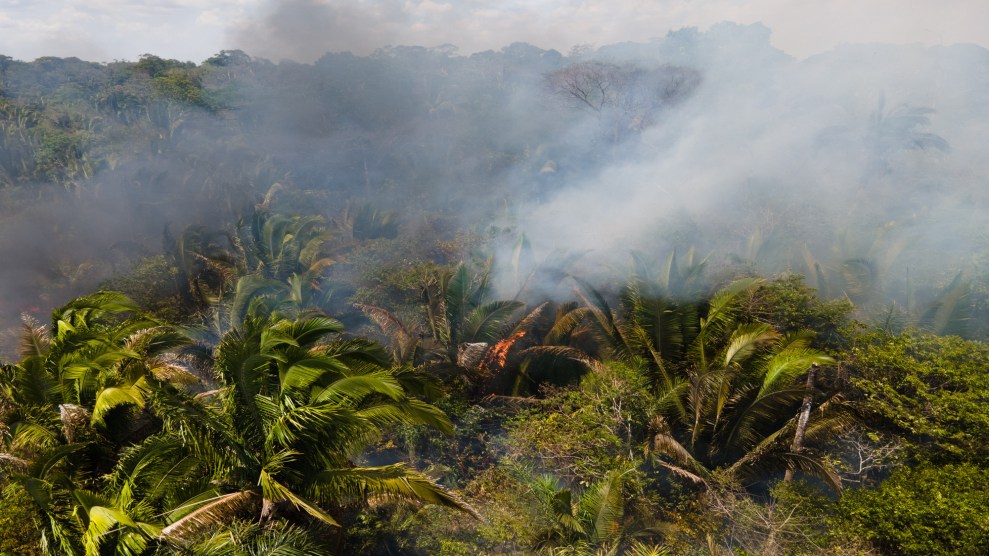
Witness to Paradise Lost: My Year in the Dying Amazon
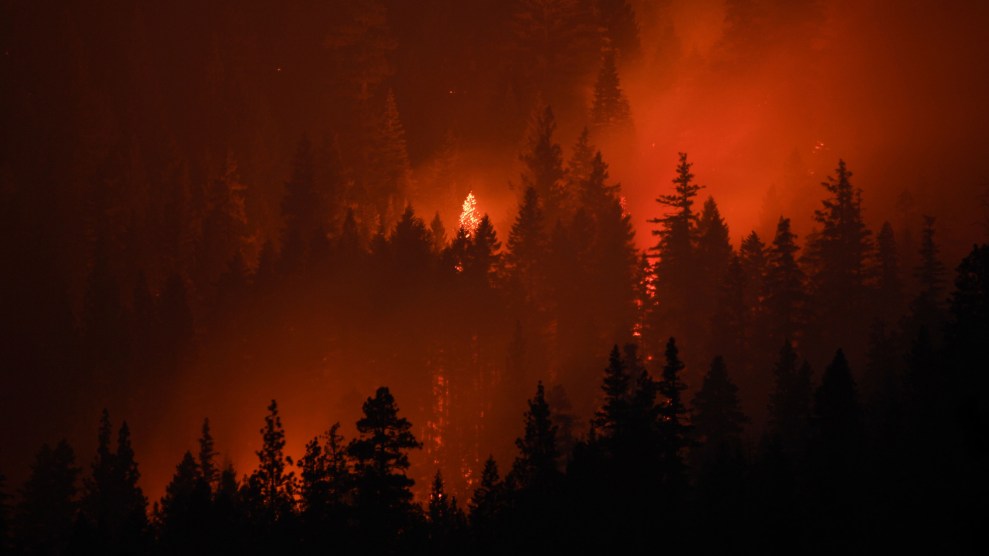
The Science of How Wildfires Got So Hellish
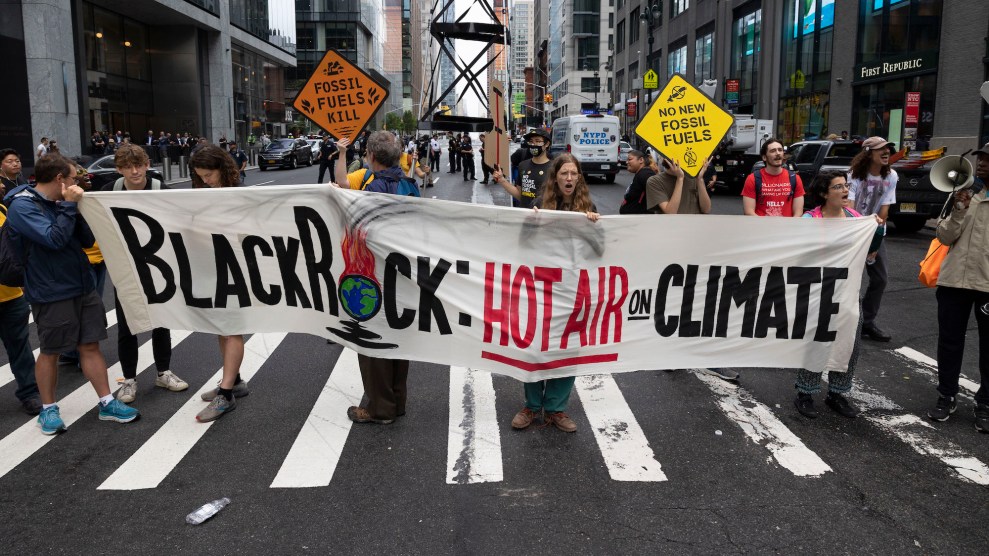
Firms That Once Called Themselves “Sustainable” Are Now “Greenhushing”
Kiley Price
We Recommend
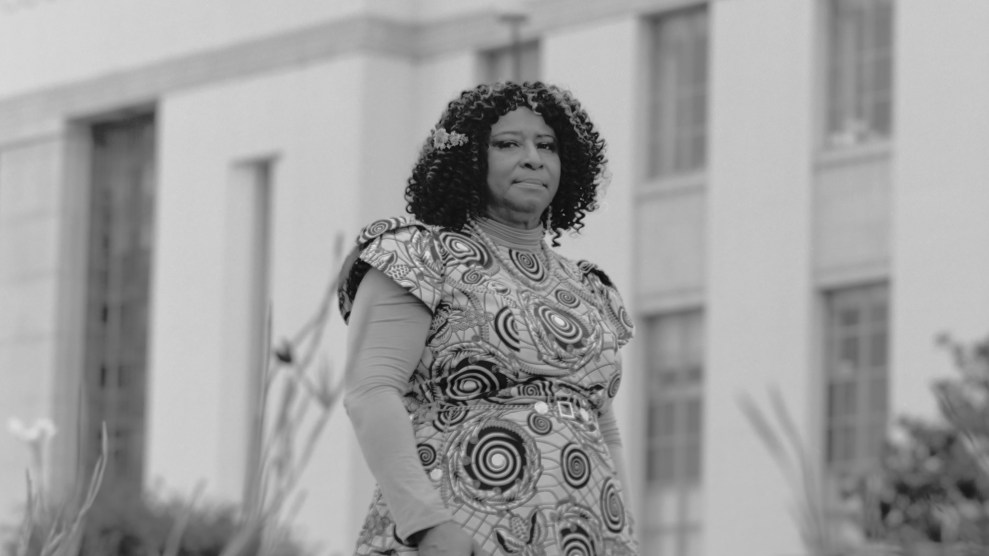
Oakland’s Progressive DA Just Wants to Do Her Job. In an Age of Recalls, That Job Is Changing.
Samantha Michaels
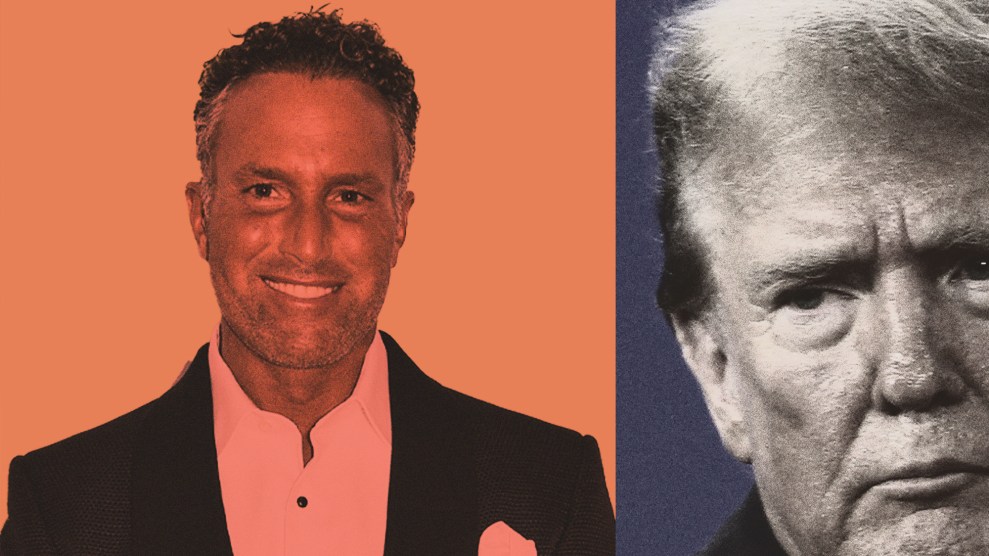
If Trump Wants to Curb Medicare Fraud, Why Did He Free This Fraudster From Prison?
Michael Mechanic
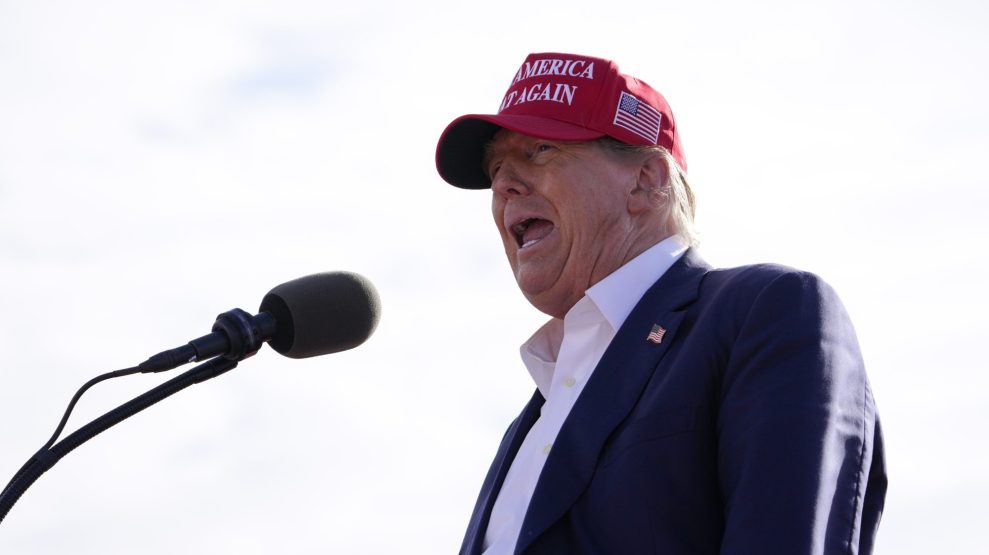
Trump Can’t Afford to Post Bond in His Fraud Case
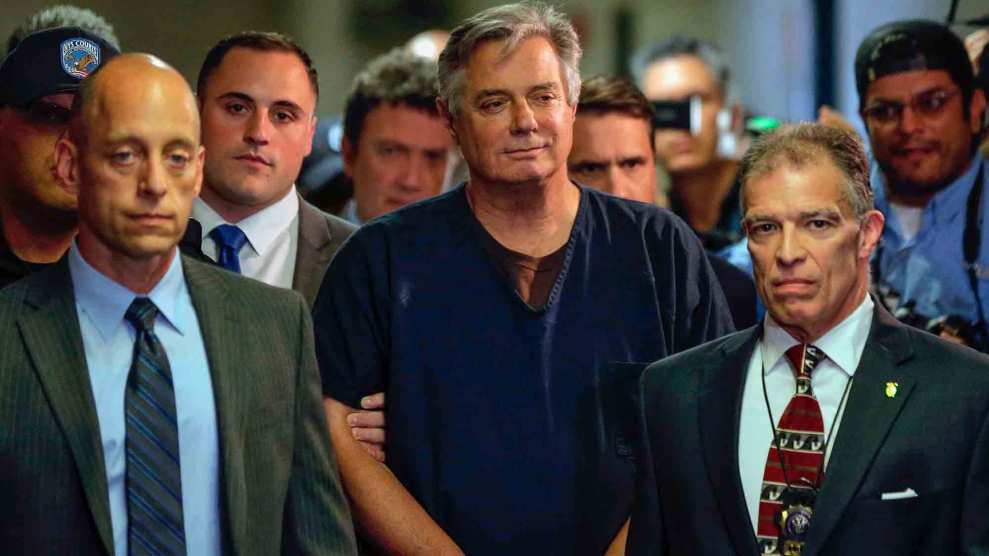
Trump Considers Adding Paul Manafort—a “Grave Counterintelligence Threat”—to His Campaign
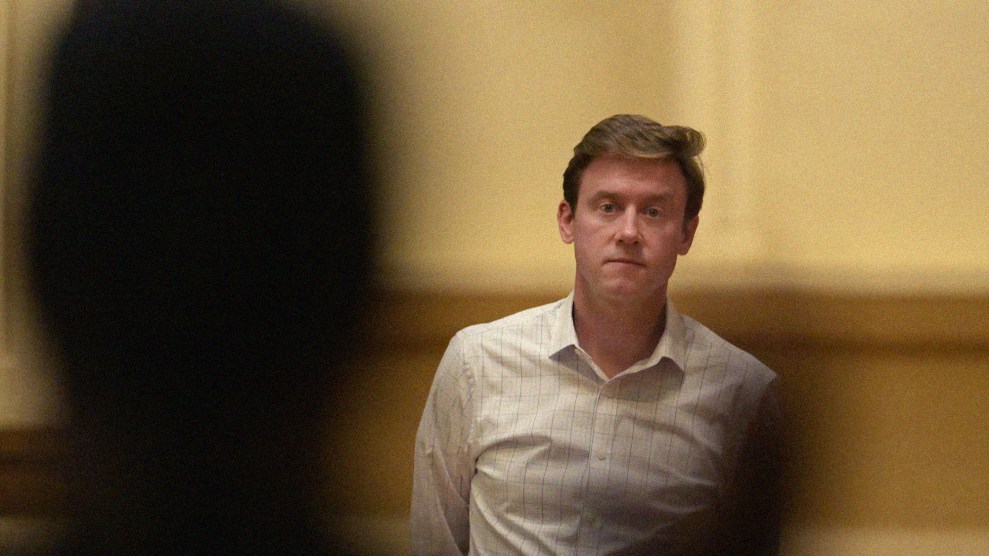
This Is a “Solvable” Crisis: Denver’s Mayor on How the City Is Handling Migrant Arrivals
Isabela Dias
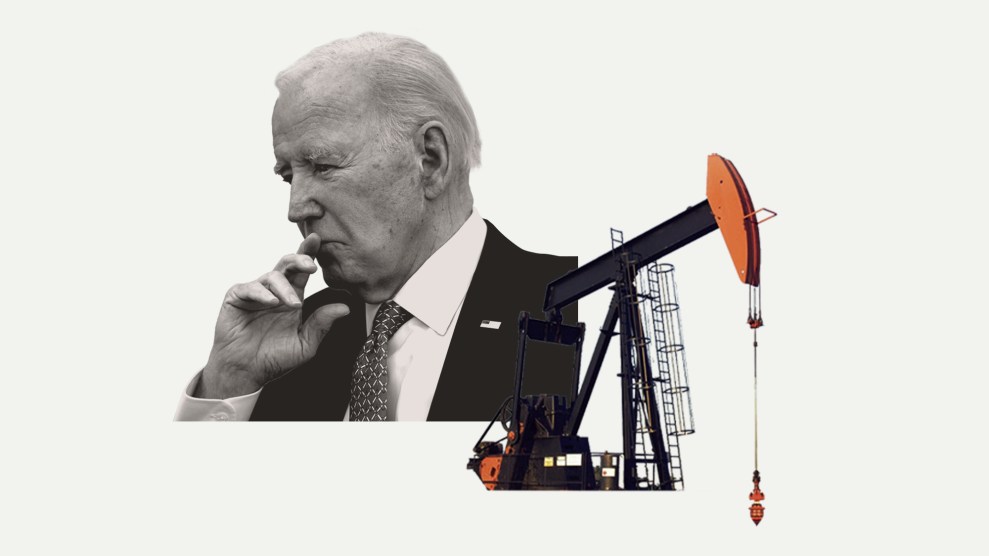
Biden Promised Not to Finance Fossil Fuels. So Why Is the US Backing a Huge Gas Project?
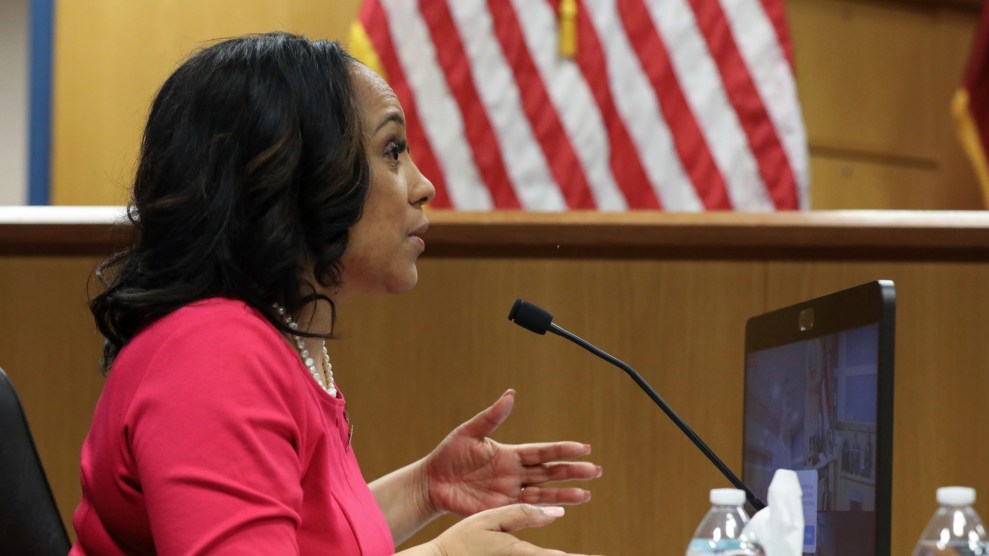
What the Fani Willis Ruling Says About the Criminal Justice System
Jeremy Schulman
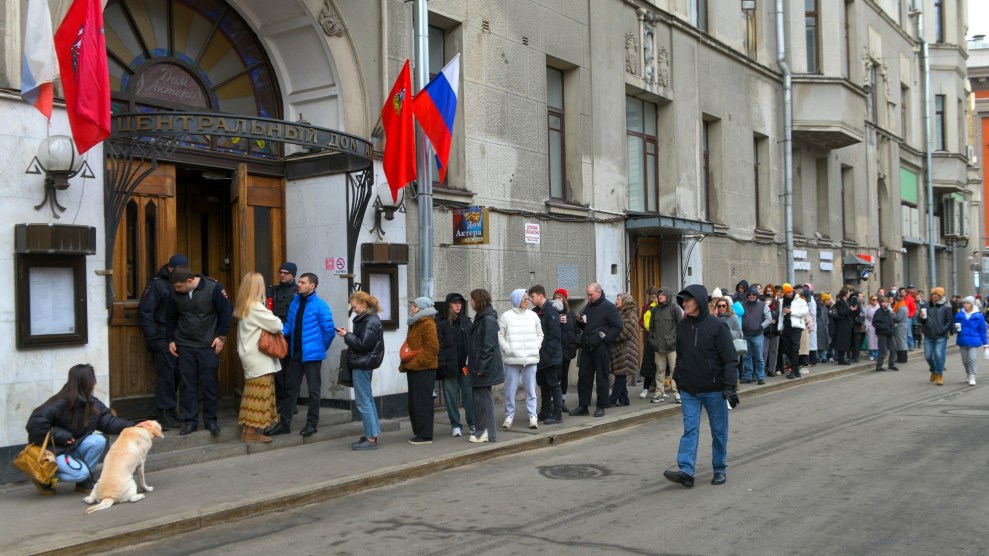
Russians Found a Different Way to Make Their Votes Matter
Sign up for our free newsletter.
Subscribe to the Mother Jones Daily to have our top stories delivered directly to your inbox.
By signing up, you agree to our privacy policy and terms of use , and to receive messages from Mother Jones and our partners.
Get our award-winning magazine
Save big on a full year of investigations, ideas, and insights.
Support our journalism
Help Mother Jones ' reporters dig deep with a tax-deductible donation.
Independent. In print. In your mailbox.
Inexpensive, too! Subscribe today and get a full year of Mother Jones for just $14.95.
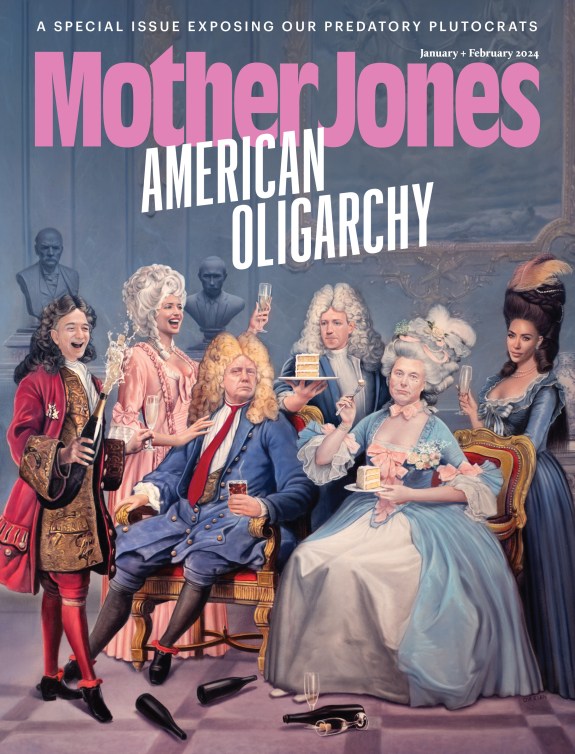
Bold. Brave. Beautiful.
Award-winning photojournalism. Stunning video. Fearless conversations.
Looking for news you can trust?
We noticed you have an ad blocker on..
Can you pitch in a few bucks to help fund Mother Jones' investigative journalism? We're a nonprofit (so it's tax-deductible), and reader support makes up about two-thirds of our budget.
We noticed you have an ad blocker on. Can you pitch in a few bucks to help fund Mother Jones' investigative journalism?
Don't let an algorithm decide what news you see.
Sign up for the free Mother Jones Daily newsletter and follow the news that matters.
Scientists warn Amazon Rainforest is in more danger than previously thought: 'This tipping point is closer than other studies estimated'
T he Amazon rainforest was hit with a historic drought in 2023, raising concerns that the complex ecosystem was on the verge of collapse. Scientists are now warning that there may be less time to turn things around than initially believed.
What's happening?
New research published in the journal Nature found that 10% of the Amazon was highly vulnerable to transitioning into a degraded state or grasslands. Meanwhile, as much as 47% of the forest was at "moderate" risk (or "transition potential"), including remote parts of the jungle with less human interference.
Unlike other major studies, which mostly looked at deforestation, researchers zeroed in on the "cumulative effects of a range of threats" while poring through the data, as reported by the New York Times. Some of the factors taken into account were legal protections, forest cover, rainfall patterns, and the threat of extreme weather events.
"This study adds it all up to show how this tipping point is closer than other studies estimated," Brazilian Earth systems scientist Carlos Nobre, an author of the study, told the outlet.
Why is this important?
It is difficult to map exactly what a collapse of the Amazon would mean, as some intersecting factors are still being investigated, but it would certainly be heavily disruptive to not only the world but also the millions of people who live there.
The Times wrote that as much as 20 years' worth of plant-warming carbon pollution could be released by a partial or total collapse.
A loss of the trees could also negatively impact global temperatures and rainfall patterns in ways that are unclear. According to the World Wildlife Fund , the forest sends roughly 22 billion tons of water into the atmosphere every day.
What can be done to help?
The study concluded that at least 5% of the Amazon should be restored, and large-scale deforestation should be eliminated. Limiting the warming of our planet to 2.7 degrees Fahrenheit above preindustrial levels is also key.
Over the summer, as reported by CNBC, Bolivia, Brazil, Ecuador, Colombia, Peru, Guyana, Venezuela, and Suriname signed a pact to support sustainable development and end illegal deforestation in the Amazon.
Some other nations outside of South America, including the United States , have also invested in Amazon conservation efforts.
However, the work is ongoing .
Making your voice heard in elections, holding corporations accountable by supporting eco-friendly brands, and choosing less polluting modes of transportation when possible are several ways to contribute to the protection of the forest directly or indirectly.
Join our free newsletter for cool news and cool tips that make it easy to help yourself while helping the planet.
Scientists warn Amazon Rainforest is in more danger than previously thought: 'This tipping point is closer than other studies estimated' first appeared on The Cool Down .
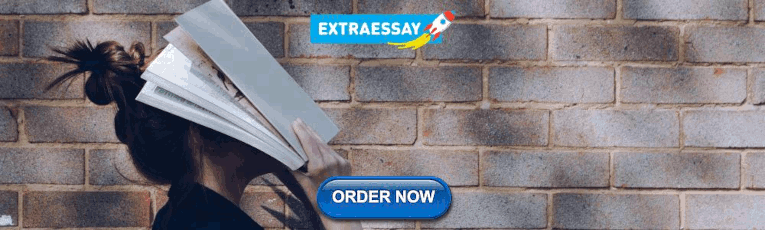
IMAGES
COMMENTS
A new study quantifies how environmental policies on deforestation, along with forest fires and drought, have affected the diversity of plants and animals in the Amazon region over the last two decades. The researchers found that since 2001, between 40,000 and 73,400 square miles of Amazon rainforest have been lost, affecting 95% of all Amazonian species and as many as 85% of species that are listed as threatened.
The Amazon in context. Tropical rainforests are often considered to be the "cradles of biodiversity.". Though they cover only about 6% of the Earth's land surface, they are home to over 50% of global biodiversity. Rainforests also take in massive amounts of carbon dioxide and release oxygen through photosynthesis, which has also given ...
A study in Nature reveals that deforestation and climate change have reduced the capacity of the Amazon forest to store carbon and emit CO 2 emissions. The study found that the eastern and southeastern Amazon, where deforestation is more intense, is a net source of CO 2, while the western and central Amazon is a net sink.
The planet is on track to reach 2.5 °C of warming by 2100, according to a report released by the United Nations last October. Nobre now wonders whether his earlier study was too conservative ...
Abstract. Biodiversity contributes to the ecological and climatic stability of the Amazon Basin 1, 2, but is increasingly threatened by deforestation and fire 3, 4. Here we quantify these impacts ...
A new study found that deforestation in the Amazon doesn't just raise temperatures in the areas where the deforestation took place but rather throughout the entire region. Researchers analyzed ...
Abstract. Amazonia hosts the Earth's largest tropical forests and has been shown to be an important carbon sink over recent decades 1, 2, 3. This carbon sink seems to be in decline, however, as ...
A new study, co-authored by University of Arizona researchers and published in the Sept. 1 issue of Nature, provides the first quantitative assessment of how environmental policies on deforestation, along with forest fires and drought, have impacted the diversity of plants and animals in the Amazon.. Researchers used records of more than 14,500 plant and vertebrate species to create ...
Based on estimates of 1% annual tropical forest loss, the Amazon may be losing as many as 11 to 16 species per day (Wilson 1989), and the resulting ecosystems are often highly degraded (Buschbacher 1986). Te deforestation of Amazonia presents a challenging study of the interactions among people, their values, and the environment.
In the case of the Amazon Protected Areas Program (ARPA), which beginning in 2002 created and fortified a series of conservation units to meet an objective of protecting 600,000 km 2 of Amazonian forest, the reserves have been shown to imply a reduction in deforestation (Nepstad et al., 2006; Soares-Filho, Moutinho, Nepstad, Anderson, Rodrigues ...
Deforestation risks pushing that system past a 'tipping point', which would trigger a collapse in precipitation across the South American continent. ... Study points to which Amazon regions ...
Amazon deforestation. Human presence in the Amazon can be traced back thousands of years, when indigenous people settled in a geographically complex organization throughout the entire area Citation [12].However, in the past 40 years the region has experienced drastic changes in the land cover and use, fostered, mainly, by the replacement of native vegetation for cattle ranching and subsistence ...
Avian ecological succession in the Amazon: A long‐term case study following experimental deforestation. ... In the past 4 years, however, that trend has reversed itself, with Amazon deforestation again growing to the highest rates in a decade (8,000 km 2 in 2018; Artaxo, 2019; INPE, 2019).
Amazon Deforestation: A Regional Conservation Case Study. GIS analysis of select strictly protected areas supported by the Amazon Region Protected Areas Program (ARPA)
Several studies have suggested that deforestation 8 and anthropogenic global warming 9,10, especially in combination, could push the Amazon rainforest past critical thresholds 11,12 where positive ...
100 billion tonnes of carbon are stored in the wood of the trees in the Amazon. If the Amazon were completely deforested, it would release the 100bn tonnes and also reduce the amount of carbon dioxide taken out of the atmosphere by 2bn tons each year. Trees anchor soil in the ground, bound to their roots. Deforestation damages the topsoil and ...
Amazon's record drought driven by climate change. One of our planet's most vital defences against global warming is itself being ravaged by climate change. It was the main driver of the Amazon ...
Abstract. In this case study, students examine tropical deforestation in the Amazon from the perspective of three dominant stakeholders in the region: a peasant farmer, logger, and environmentalist. As part of the exercise, students perform a cost-benefit analysis of clearing a plot of tropical forest in the Amazon from the perspective of one ...
Pop-Up Case: Stopping Deforestation in the Amazon Deforestation in the Amazon rainforest contributes to climate change, and as the practice continues, the Amazon is nearing a tipping point that ... While assigning individual roles for a brief case study is complicated, you could assign opinions. For example, assign one-third of the class to be ...
Deforestation case study: The Congo Basin The Congo Basin is the second-largest rainforest in the world. 28 It has been described as the 'second lungs' of the Earth because of how much carbon dioxide it absorbs and how much oxygen it produces. 29 But, just as the world's first lungs - the Amazon - is being destroyed by humans, the ...
Deforestation in the Amazon region has suggested to influence precipitation in a non-linear way. ... Studies point to a reliance of the Amazon regional rainfall regimes on the ... A case study for ...
Living World - Amazon Case Study The Amazon is the largest tropical rainforest on Earth. It sits within the Amazon River basin, covers some 40% of the South American continent and as you can see on the map below includes parts of eight South American countries: Brazil, Bolivia, Peru, Ecuador, Colombia, Venezuela, Guyana, and Suriname.
The Amazon stores between 15 and 20 years' worth of global CO 2 emissions, and it has a cooling effect on the world thanks to the moisture that its plants store and transpire into the atmosphere.
Link Copied! CNN's Bill Weir breaks down the possible effects of the collapse of the Amazon rainforest. Gen Alpha is more interested in skincare than toys. A dermatologist explains the issue ...
The study concluded that at least 5% of the Amazon should be restored, and large-scale deforestation should be eliminated. Limiting the warming of our planet to 2.7 degrees Fahrenheit above ...