
An official website of the United States government
Here's how you know
Official websites use .gov A .gov website belongs to an official government organization in the United States.
Secure .gov websites use HTTPS A lock ( ) or https:// means you’ve safely connected to the .gov website. Share sensitive information only on official, secure websites.
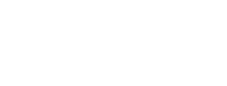
- Digg
Latest Earthquakes | Chat Share Social Media
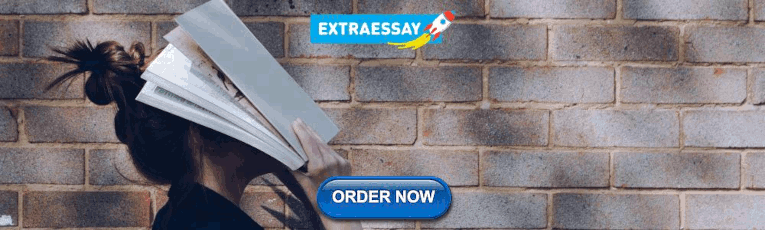
A global synthesis of multi-year drought effects on terrestrial ecosystems Active
Drought impacts on terrestrial ecosystems have increased globally in the 21st century, and droughts are expected to become more frequent, extreme, and spatially extensive in the future. Historical site-based observations are inadequate to predict how future extreme water deficits will affect the global terrestrial surface, because future droughts and their impacts may be more extreme than they have been historically and reach well beyond a single ecosystem. This USGS Powell Center working group will conduct the first comprehensive synthesis of ecosystem impacts across a coordinated, globally distributed drought experiment network of over 100 sites that have imposed an extreme multi-year drought. Knowledge about how sensitivity to drought changes across ecosystems and over time can reveal the mechanisms underlying drought impacts, improve forecasting, and assist land managers and policy-makers with short-term decision-making and long-term planning.
Principal Investigators: Melinda D. Smith (Colorado State University) Scott L. Collins (University of New Mexico) Seth M. Munson (USGS - Southwest Biological Science Center) Fellow: Kate Wilkins (Colorado State University) A networked drought experiment and Austin Rueda, hard at work.
- Source: USGS Sciencebase (id: 5efcef4282ce3fd7e8a5bae2 )
Seth Munson, Ph.D.
Research ecologist.
- Search Menu
- Advance Articles
- Collections
- Focus Collections
- Browse by cover
- High-Impact Research
- Author Guidelines
- Quick and Simple Author Support
- Focus Issues Call for Papers
- Submission Site
- Open Access Options
- Self-Archiving Policy
- Why Publish with Us?
- About Plant Physiology
- Editorial Board
- Advertising & Corporate Services
- Journals on Oxford Academic
- Books on Oxford Academic
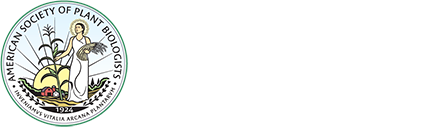
Article Contents
Materials and methods, acknowledgments, literature cited.
- < Previous
Molecular and Physiological Analysis of Drought Stress in Arabidopsis Reveals Early Responses Leading to Acclimation in Plant Growth
This work was supported by the Generation Challenge Project G4007.02 and the National Science Foundation (grant no. DBI–0922747).
Corresponding author; e-mail [email protected] .
The author responsible for distribution of materials integral to the findings presented in this article in accordance with the policy described in the Instructions for Authors ( www.plantphysiol.org ) is: Andy Pereira ( [email protected] ).
Some figures in this article are displayed in color online but in black and white in the print edition.
The online version of this article contains Web-only data.
Open Access articles can be viewed online without a subscription.
www.plantphysiol.org/cgi/doi/10.1104/pp.110.161752
- Article contents
- Figures & tables
- Supplementary Data
Amal Harb, Arjun Krishnan, Madana M.R. Ambavaram, Andy Pereira, Molecular and Physiological Analysis of Drought Stress in Arabidopsis Reveals Early Responses Leading to Acclimation in Plant Growth, Plant Physiology , Volume 154, Issue 3, November 2010, Pages 1254–1271, https://doi.org/10.1104/pp.110.161752
- Permissions Icon Permissions
Plant drought stress response and resistance are complex biological processes that need to be analyzed at a systems level using genomics and physiological approaches to dissect experimental models that address drought stresses encountered by crops in the field. Toward this goal, a controlled, sublethal, moderate drought (mDr) treatment system was developed in Arabidopsis ( Arabidopsis thaliana ) as a reproducible assay for the dissection of plant responses to drought. The drought assay was validated using Arabidopsis mutants in abscisic acid (ABA) biosynthesis and signaling displaying drought sensitivity and in jasmonate response mutants showing drought resistance, indicating the crucial role of ABA and jasmonate signaling in drought response and acclimation. A comparative transcriptome analysis of soil water deficit drought stress treatments revealed the similarity of early-stage mDr to progressive drought, identifying common and specific stress-responsive genes and their promoter cis-regulatory elements. The dissection of mDr stress responses using a time-course analysis of biochemical, physiological, and molecular processes revealed early accumulation of ABA and induction of associated signaling genes, coinciding with a decrease in stomatal conductance as an early avoidance response to drought stress. This is accompanied by a peak in the expression of expansin genes involved in cell wall expansion, as a preparatory step toward drought acclimation by the adjustment of the cell wall. The time-course analysis of mDr provides a model with three stages of plant responses: an early priming and preconditioning stage, followed by an intermediate stage preparatory for acclimation, and a late stage of new homeostasis with reduced growth.
Drought is a major environmental stress factor that affects the growth and development of plants. Drought or soil water deficit can be chronic in climatic regions with low water availability or random and unpredictable due to changes in weather conditions during the period of plant growth. The effects of drought are expected to increase with climate change and growing water scarcity. Water is an increasingly scarce resource given current and future human population and societal needs, putting an emphasis on sustainable water use ( Rosegrant and Cline, 2003 ). Thus, an understanding of drought stress and water use in relation to plant growth is of importance for sustainable agriculture.
Plants, being sessile, have evolved specific acclimation and adaptation mechanisms to respond to and survive short- and long-term drought stresses. Analysis of these protective mechanisms will contribute to our knowledge of tolerance and resistance to stress. The complex responses to environmental stress, from perception to transcriptional and physiological changes, need to be considered at a global systems biology level to study the multiple interactive components in this biological process ( Krishnan and Pereira, 2008 ).
In response to drought brought about by soil water deficit, plants can exhibit either drought escape or drought resistance mechanisms, with resistance further classified into drought avoidance (maintenance of tissue water potential) and drought tolerance ( Levitt, 1980 ; Price et al., 2002 ). Drought escape is described as the ability of plants to complete the life cycle before severe stress sets in. Drought avoidance is by maintenance of high tissue water potential despite a soil water deficit. Mechanisms such as improved water uptake under stress and the capacity of plant cells to hold acquired water and further reduce water loss confer drought avoidance. Plants respond to water deficit using mechanisms of avoidance by improved root traits ( Price et al., 2002 ) and by reducing water loss through reduced epidermal (stomatal and cuticular) conductance, reduced radiation absorption, and reduced evaporative surface (leaf area). Drought tolerance is the ability to withstand water deficit with low tissue water potential ( Ingram and Bartels, 1996 ). Plants under drought stress may survive by, among other mechanisms, maintaining cell turgor and reducing evaporative water loss by accumulating compatible solutes ( Yancey et al., 1982 ).
In recent years, much molecular information has been generated on the response of plants to environmental stresses. Plants respond to environmental stresses such as drought by the induction of both regulatory and functional sets of genes ( Ingram and Bartels, 1996 ; Ramanjulu and Bartels, 2002 ; Bartels and Sunkar, 2005 ). Very little is known about the early events in the perception of stress signals ( Urao et al., 1999 ; Ueguchi et al., 2001 ; Wohlbach et al., 2008 ). The common stress signaling pathways have been distinguished into abscisic acid (ABA) dependent and ABA independent ( Shinozaki and Yamaguchi-Shinozaki, 1997 , 2007 ). Most of the key genes in these pathways have been identified, such as transcription factors belonging to the class of DRE-binding protein (DREB)/C-repeat-binding factor (CBF), ABA-binding factor (ABF), MYC, and MYB ( Abe et al., 1997 ; Bartels and Sunkar, 2005 ; Sakuma et al., 2006 ), including the identification of the stress-responsive cis-elements ABA-responsive element (ABRE) and dehydration-responsive element (DRE; Yamaguchi-Shinozaki and Shinozaki, 1994 ; Ramanjulu and Bartels, 2002 ; Bartels and Sunkar, 2005 ). Downstream of the early signal perception events, signaling genes and molecules acting as secondary messengers have been identified, revealing the role of Ca + and reactive oxygen species (ROS) as secondary messengers ( Bartels and Sunkar, 2005 ). These regulatory mechanisms induce downstream functional genes, which are needed to establish new cellular homeostasis that leads to drought tolerance and/or resistance ( Yancey, 2001 ; Ramanjulu and Bartels, 2002 ).
Most of our knowledge of drought responses at the molecular level is based on plant responses to molecular laboratory experimental conditions of dehydration and/or osmotic treatments ( Yamaguchi-Shinozaki and Shinozaki, 1994 ; Abe et al., 1997 ; Oono et al., 2003 ; Umezawa et al., 2004 ). Although these conditions are far from the soil water deficit/drought met by plants under field conditions, there has been valuable knowledge gained from such studies. Dehydration studies revealed the common stress signaling pathways of ABA dependent and ABA independent, which have become a paradigm in plant stress biology ( Shinozaki and Yamaguchi-Shinozaki, 1997 , 2007 ). These pathways were discovered in Arabidopsis ( Arabidopsis thaliana ) as a model system, which paved the way for the discovery of parallel pathways in other crop plants such as in rice ( Oryza sativa ) as a model for monocot plants ( Nakashima et al., 2009 ).
A number of drought treatments have been used to test the response of plants for improved tolerance/resistance. One method is progressive drought (pDr), in which water is withheld for a certain period of time until symptoms of wilting are observed. Usually, this method of drought treatment has been used to determine survival rate or to monitor gene expression changes of wild-type plants or of plant genotypes overexpressing candidate genes for drought tolerance ( Sakuma et al., 2006 ; Catala et al., 2007 ; Nelson et al., 2007 ; Yu et al., 2008 ; Ning et al., 2010 ). These studies have shed some light on plant responses to drought at both the physiological and molecular levels. However, one of the drawbacks of pDr treatment, because of the uncontrolled soil water moisture, is that it cannot be used to compare the performance of different genotypes with different growth characteristics, such as smaller plants. In nature, drought often develops during a growing season and occurs for a short period, which tolerant plants can manage to survive and complete their growth cycle. Methods to simulate field-like conditions and quantify drought responses will provide a better understanding of drought resistance mechanisms.
Soil water deficit causing drought stress in crop plants has been recently tested in Arabidopsis using controlled soil moisture treatment that is not lethal. Controlled drought treatment, exposing plants to constant levels of soil moisture deficit, enables the evaluation between genotypes/ecotypes for plant responses to sublethal drought ( Granier et al., 2006 ; Bouchabke et al., 2008 ). PHENOPSIS has been developed as an automated controlled drought screen, which was used to compare the performance of different Arabidopsis ecotypes (accessions) and resulted in the identification of a resistant accession, An1 ( Granier et al., 2006 ). Controlled drought was also used to study the response of the Arabidopsis erecta mutant and ERECTA gene complementation ( Masle et al., 2005 ), the overexpression of the Arabidopsis ESKIMO1 gene ( Bouchabke-Coussa et al., 2008 ), and overexpression of the Pro biosynthesis gene in chickpea ( Cicer arietinum ; Bhatnagar-Mathur et al., 2009 ).
Comprehensive physiological and molecular studies have not yet been done on the response of plants to moderate drought (mDr). A transcriptome study in loblolly pine ( Pinus taeda ), treated for cycles of mild drought and recovery ( Watkinson et al., 2003 ; Vásquez-Robinet et al., 2010 ), showed a photosynthetic acclimation pattern in response to mild drought in contrast to photosynthesis inhibition under severe drought. A comprehensive understanding of the response of plants to mDr with physiological and molecular tools would provide us with a better understanding of the acclimation process. We present here an analysis of controlled mDr in Arabidopsis under soil water deficit treatment simulating field conditions of crop plants. A semiautomated, controlled mDr testing system was employed to compare with pDr treatment for physiological and molecular responses. This revealed differential gene reprogramming under the two drought treatments. The dissection of mDr treatment is presented using a time-course study to provide a picture of physiological and molecular responses toward acclimation in plant growth.
Plant Temporal Responses to mDr
To study the response of Arabidopsis to controlled soil water deficit drought, the effect of mDr ( Fig. 1 ) was tested at different vegetative developmental stages. Plants were grown under well-watered conditions, and drought stress was applied by withholding water at different growth stages to three batches of plants: at 25 d after sowing (DAS), 30 DAS, and 35 DAS. These growth stages, as defined for Arabidopsis ( Boyes et al., 2001 ), of drought initiation correspond to six-leaf (1.06), eight-leaf (1.08), and 10-leaf (1.10) stages when watering was withheld, respectively. Around 5 to 7 d after drought initiation and evapotranspirational water loss, mDr stress is achieved and then maintained by adding water daily to reach a soil moisture level of 2 g g −1 dry soil, with the plant stages for the three batches at drought initiation at eight leaf (1.08), 10 leaf (1.10), and 12 leaf (1.12), respectively ( Boyes et al., 2001 ). The soil moisture level was maintained at a level that was nonlethal and above the wilting point, at 30% field capacity, by replenishing the evapotranspired water, and the reduction in biomass was taken as a quantitative measure of growth calculated as described in “Materials and Methods.” Figure 2 shows that the highest relative reduction in biomass (RB) of mDr-treated compared with well-watered plants was at initiation of drought at the 30-DAS stage, with the 25-DAS treatment also significant and the 35-DAS treatment least responsive to the drought treatment ( Fig. 2A ). In addition, the duration of mDr treatment was tested, and 5 or 10 d of moderate drought (DMD) treatment gave similar RB (data not shown). The growth rate of Arabidopsis ecotype Columbia plants was determined for two developmental stages: 25 to 30 DAS and 30 to 35 DAS. The rate of growth (both in terms of biomass and leaf area) during the first developmental stage, 25 to 30 DAS, was higher than that during the second stage, 30 to 35 DAS ( Fig. 2B ).
![analysis and synthesis of drought Schematic illustration of the drought treatments and sampling in this study. For drought treatments, water was withheld at 25 DAS, and the progress of drought was monitored by soil moisture, shown here as percentage field capacity (FC). Two pDr treatments were done: wilting and prewilting (1 d before wilting, predicted on soil moisture content). Controlled mDr was used to study plant responses at physiological and molecular levels, with the sampling times indicated (−1, 0, 1, 2, and 3). [See online article for color version of this figure.]](https://oup.silverchair-cdn.com/oup/backfile/Content_public/Journal/plphys/154/3/10.1104_pp.110.161752/2/m_plphys_v154_3_1254_f1.jpeg?Expires=1714545761&Signature=P8ePFDRK1jnqFGAlFs4ms7eqQRIHTH2liOGNeRuLe~WXXl12HniAHJOwUoZAhvJqLPrRzpr~ZqFQ3LkxXl-WZLoEKJ3JjvD6XRuIpLQwyTztMIVaRi4ELNLYxTNeA5iWXNK4bYrRZ45j1xphhOnm4j1Pt07~XDNP78Ovd6cSWjb1dOyKIDSccRY1YphZUy5pFbiKAlFoAjwW00gXeXvR7PCgUPNHvbg3O1JSxCW93ZHaBPdinQb6kkUpnurZ6kk715m8ePs0MgllJHTi2hzW7kbtTP7C93-bTISmEx8Up2Su4o~W0akcrPE8w0lhvWI0odhLAPaSrn6anDgKauIIWA__&Key-Pair-Id=APKAIE5G5CRDK6RD3PGA)
Schematic illustration of the drought treatments and sampling in this study. For drought treatments, water was withheld at 25 DAS, and the progress of drought was monitored by soil moisture, shown here as percentage field capacity (FC). Two pDr treatments were done: wilting and prewilting (1 d before wilting, predicted on soil moisture content). Controlled mDr was used to study plant responses at physiological and molecular levels, with the sampling times indicated (−1, 0, 1, 2, and 3). [See online article for color version of this figure.]
![analysis and synthesis of drought Growth of Arabidopsis ecotype Columbia in response to mDr. A, Response of different developmental stages to mDr in terms of RB. The experiment was repeated twice (n = 7, P < 0.001). B, Relative growth rate (RGR), shown in biomass, and relative expansion rate (RER) in leaf area during two developmental stages, stage 1 (25–30 DAS) and stage 2 (30–35 DAS). Error bars represent se; asterisks indicate significant differences (n = 16, P < 0.01). C, LRWC (%) at different DMD under well-watered (WW) and drought (DRT) conditions. The experiment was repeated twice (n = 12, P < 0.0001). D, LRWC (%; n = 12) and corresponding soil water content (SWC%; n = 20) at different DMD. The experiments were repeated twice. [See online article for color version of this figure.]](https://oup.silverchair-cdn.com/oup/backfile/Content_public/Journal/plphys/154/3/10.1104_pp.110.161752/2/m_plphys_v154_3_1254_f2.jpeg?Expires=1714545761&Signature=FCTefuBtGpw6oD3OiKbR1YKQOBqyIiLW5xggYSmOFkdNfWf-bud0MgfkLV05sJqBLaovKgsIcOOUmrYd2zNlu6w6gaBjI6q8vexdBZUhdSV4DENM96DJUDjOfa0QRZ~imHuGZieVTLp-3b0zNMxNlimn4fHTTangpzAdoP8ANKu-i0HQZ9kXGmuKhvdOBup0wZdIFbyAYTgry5TVHDo4XVlRNIedZ1Xso6BEyM61SwTt17OLebGuTXyWVdv16zbWSXCu5JYyPGomeP9MnFnGgQa6~sjrp1O2YFd~6lg0151fguzdHlkp7yv1utJJGmgryZhQWejqteuDrat9zNVpfg__&Key-Pair-Id=APKAIE5G5CRDK6RD3PGA)
Growth of Arabidopsis ecotype Columbia in response to mDr. A, Response of different developmental stages to mDr in terms of RB. The experiment was repeated twice ( n = 7, P < 0.001). B, Relative growth rate (RGR), shown in biomass, and relative expansion rate (RER) in leaf area during two developmental stages, stage 1 (25–30 DAS) and stage 2 (30–35 DAS). Error bars represent se ; asterisks indicate significant differences ( n = 16, P < 0.01). C, LRWC (%) at different DMD under well-watered (WW) and drought (DRT) conditions. The experiment was repeated twice ( n = 12, P < 0.0001). D, LRWC (%; n = 12) and corresponding soil water content (SWC%; n = 20) at different DMD. The experiments were repeated twice. [See online article for color version of this figure.]
To determine at what time point plants start to sense drought stress, a time-course experiment was conducted and plant samples were taken starting at 2 d before mDr treatment (−2), 1 d before mDr (−1), and 0, 1, 2, and 3 DMD. The mDr stress (+1) is defined when the plants reach 2 g water g −1 dry soil and water supplemented (if needed) to maintain the controlled drought, as described in “Materials and Methods.” The relative water content in plant samples and in the soil was determined for each time point ( Fig. 2, C and D ). Leaf relative water content (LRWC) measurements showed that plants start to sense drought 1 d before mDr treatment is stabilized, designated as −1 ( Fig. 2C ), and further analysis was done beginning at this time point. At day 0 of mDr (beginning of mDr), the LRWC decreases, and it continues to decrease at day 1 of mDr ( Fig. 2, C and D ). However, at day 2, the LRWC starts to increase to a normal level like that of the well-watered control ( Fig. 2C ). The soil water content is held constant from day 1 until the end of mDr treatment ( Fig. 2D ).
Drought Responses of Hormonal Pathway Mutants
To validate the drought screening on genotypes known to be affected by drought, the response of ABA signaling and biosynthesis knockout mutants in two Arabidopsis backgrounds (Columbia and Landsberg erecta ) was tested under mDr conditions. The reduction in growth (measured as biomass) under controlled mDr stress compared with well-watered controls provides a parameter to compare different genotypes with the wild type and to distinguish genotypes with altered sensitivity/resistance to drought. The ABA signaling mutant ( ABA insensitive1 [ abi1 ]) and biosynthesis mutant ( aba1 ) show higher sensitivity to drought stress compared with their respective wild-type controls ( Fig. 3, A and B ).
![analysis and synthesis of drought Responses of ABA and JA mutants to mDr treatment. A, Biomass of ABA mutants under well-watered (WW) and drought (DRT) conditions. B, RB of ABA mutants. C, Biomass of JA mutants under well-watered and drought conditions. D, RB of JA mutants. Error bars represent se (n = 8, P < 0.001); asterisks indicate significant differences from the wild-type (WT) control. Col, Ecotype Columbia; Ler, ecotype Landsberg erecta. [See online article for color version of this figure.]](https://oup.silverchair-cdn.com/oup/backfile/Content_public/Journal/plphys/154/3/10.1104_pp.110.161752/2/m_plphys_v154_3_1254_f3.jpeg?Expires=1714545761&Signature=PCu8d2KIBkZ5y2cX16atSfjNlDDoXP~IQxyGV-ZxdiLPMWFIUHU7wa8phVP~-d~DjmU9X~~ebj1Yw4BWL41ho-8npS3fbFWqDpdb~N2~HdD8BnOxX5x1-DGtL2CMZQN6mXCC16igO0NP~MmeMUZ1eMbAWQzZmakhomS-vqTh-S4u8g1XnjDCHvXIgk2BmRI4CIkfFswq4S32pdZVREuWqyjAFJ1o7IMp~sZd~9A2tgXwxMZUenivZRroyvmAw0HxR9QhtcSdKfne59noI6mPHhpUIOJhkFWOp-uFhw6f1J0EpRPZNcYvE-olZ51qMpl4wyoB9AlfBS1h5VEsByoxHw__&Key-Pair-Id=APKAIE5G5CRDK6RD3PGA)
Responses of ABA and JA mutants to mDr treatment. A, Biomass of ABA mutants under well-watered (WW) and drought (DRT) conditions. B, RB of ABA mutants. C, Biomass of JA mutants under well-watered and drought conditions. D, RB of JA mutants. Error bars represent se ( n = 8, P < 0.001); asterisks indicate significant differences from the wild-type (WT) control. Col, Ecotype Columbia; Ler, ecotype Landsberg erecta . [See online article for color version of this figure.]
In other experiments, additional hormone response mutants were tested. The jasmonate response mutants coi1 and jin1 display significant drought resistance in the screen at 10 d of mDr ( Fig. 3, C and D ). The other jasmonate response mutant, jar1 , shows a drought response phenotype not significantly different from the wild type. The response of this mutant to jasmonate is also moderate compared with the coi1 and jin1 mutants, probably because this jar1 allele is not a complete gene knockout but an amino acid substitution mutant ( Staswick et al., 2002 ).
Gas-Exchange Parameter Changes in Response to mDr
Stomatal conductance showed a decrease at 1 d of mDr (1 DMD), reaching 59% of the well-watered control ( Fig. 4A ). It was reduced by almost 50% from that at day −1 and 0 d of mDr ( Fig. 4A ) and continued to decrease until day 2 of mDr, with about 40% reduction from that of the well-watered control. At day 3 of mDr, it increased to the level of the well-watered control. The same trend was shown for the internal CO 2 concentration ( Fig. 4A ). However, photosynthesis showed a different trend, as it did not decrease at day 1 of mDr and at day 2 it showed a 10% decrease compared with the well-watered control ( Fig. 4A ). Instantaneous water use efficiency (WUEi) was higher than in the well-watered control at days 1 and 2 of mDr ( Fig. 4B ). It was above 4 μmol mmol −1 at days 1 and 2 of mDr, while the well-watered WUEi was around 2 μmol mmol −1 . At days −1, 0, and 3, WUEi was the same as that of the well-watered control ( Fig. 4B )
![analysis and synthesis of drought Gas-exchange measurements and WUEi in a time course of mDr. A, Photosynthesis (Pn), stomatal conductance (gs), and internal CO2 (Ci). B, WUEi in a time course of mDr. n = 5 per treatment per time point, three leaves were measured per plant, the experiment repeated twice, and error bars represent se (P < 0.001). DRT, Drought; WW, well watered. [See online article for color version of this figure.]](https://oup.silverchair-cdn.com/oup/backfile/Content_public/Journal/plphys/154/3/10.1104_pp.110.161752/2/m_plphys_v154_3_1254_f4.jpeg?Expires=1714545761&Signature=lV9QNQvv~2bSAhzPiLZFbT5ZMY~f9y8~ynKm~sDbtMuoHecZep7JJxdrLmVdpobpuGI4Tx6N1mV3lfae0H09fgNy~G9SCT6eFHeXn3tMpbQO6Pr-h2JLBh6QP6iayZC-7cUWtgoVaYyCfmeoP71DlzwZD7rsZpnbNgq4DiI4mGbAsI4r07p3qpGvpya78UElfYSqD3pyJWUb-E5PeD2fut-3kCbr6VBrHBXLBvbn8cmZxKY5oqCr-BUnaqoUDFZUX7mAMWsydKjM~xygICV~PBTnDaruCqTkQjAV6oqliahnHDPPd~o1aJW-0MvROHtrCb5XxyMdWQQ4aJp9phX2Wg__&Key-Pair-Id=APKAIE5G5CRDK6RD3PGA)
Gas-exchange measurements and WUEi in a time course of mDr. A, Photosynthesis (Pn), stomatal conductance (gs), and internal CO 2 (Ci). B, WUEi in a time course of mDr. n = 5 per treatment per time point, three leaves were measured per plant, the experiment repeated twice, and error bars represent se ( P < 0.001). DRT, Drought; WW, well watered. [See online article for color version of this figure.]
A Portrait of Plant Transcriptional Response to Soil Water Deficit
In order to understand the global effects of drought stress on gene expression, microarrays were used to profile gene expression levels under mDr (Day01 and Day10) and pDr conditions with corresponding controls in samples from young leaves. Analysis of differential expression showed that a large number of genes (2,039) are significantly perturbed very early (Day01) in response to mDr. However, after a prolonged spell of moderate stress (Day10), a far lesser number of genes (728) show any response. Compared with both these responses, the backdrop of severe effects of drought on gene expression is revealed by the response to pDr (wilting): 7,648 differentially expressed genes, approximately 30% of the genome, replete with well-known stress response genes and processes.
Comparison of the three responses (mDr Day01, mDr Day10, and pDr) was carried out first at the gene level ( Fig. 5; Supplemental Table S1 ). The mDr and pDr treatments share a set of 178 differentially expressed genes (91 up-regulated and 87 down-regulated), while 1,083 (545 up-regulated and 538 down-regulated) genes are specific to mDr. All the drought response genes from each of these treatments were functionally characterized using enrichment analysis of gene sets, mostly as described by Gene Ontology (GO; Ashburner et al., 2000 ) biological process terms, but also including gene sets concerning ABA response obtained from previous publications ( Nemhauser et al., 2006 ). Results from these analyses are summarized in Figure 6 , and details are provided in Supplemental Table S2 . Among the genes up-regulated in both mDr Day01 and pDr (646 genes) are predominantly water deprivation response genes ( q value approximately 1E-15), with overlapping sets of genes known to respond to ABA stimulus ( q approximately 1E-12.6), osmotic ( q approximately 1E-8.1), cold ( q approximately 1E-4.1), and oxidative ( q approximately 1E-2.5) stresses. Expression dynamics of several of these genes have been verified using quantitative reverse transcription (qRT)-PCR (see below). Fundamental processes of the cell known to be grossly affected by drought, including DNA packaging ( q approximately 1E2.6), ribosome biogenesis ( q approximately 1E-2.9), and protein folding ( q approximately 1E-3.2), were concomitantly down-regulated in mDr Day01 and pDr. Thus, the plants are mounting an early response to mDr that is very similar to the classical response to pDr. However, severe effects, including down-regulation of photosynthesis ( q approximately 1E-20.7) and related processes, are restricted to pDr.
![analysis and synthesis of drought Gene expression analysis under mDr and pDr. Venn diagrams comparing up-regulated and down-regulated genes of pDr, mDr D01, and mDr D10 treatments are shown at top. Sequence logos of cis-elements derived by de novo promoter analysis with similarity to the ABRE element in the three drought treatments are shown at bottom. [See online article for color version of this figure.]](https://oup.silverchair-cdn.com/oup/backfile/Content_public/Journal/plphys/154/3/10.1104_pp.110.161752/2/m_plphys_v154_3_1254_f5.jpeg?Expires=1714545761&Signature=k2xJGaUdOPWQpID3ACsdq-BUWS~kC7WYp2NjkaHXopwoVMuiskWoGsqKkQ75MTVznae4ckK~wLmJ8rHdmHod090Q~Ff8U2O92v12SbDiiHOEBmvJbasjrq5wxpeX2sA2hPoa3zNflDmS4xc~wJmt-77cO48K2uPdYiH~KN3VBUGGnOlAkGGHVG~BRygJo2gGKNZvophumgAgOZnLztysmr0epauMf3CJOZLQDQ8nN230A6uFxxmLimpo6t7FuSa~oxAs7vzjek3HrOlEh96G~fXD2qbKpA8JHUNDfRdpV~kxdZMqnbuAavqk7HIUiPD7Y759dwMzs8DBetRI1XDinA__&Key-Pair-Id=APKAIE5G5CRDK6RD3PGA)
Gene expression analysis under mDr and pDr. Venn diagrams comparing up-regulated and down-regulated genes of pDr, mDr D01, and mDr D10 treatments are shown at top. Sequence logos of cis-elements derived by de novo promoter analysis with similarity to the ABRE element in the three drought treatments are shown at bottom. [See online article for color version of this figure.]

Differentially expressed mDr Day01 genes in relation to other factors. The up-regulated and down-regulated mDr Day01 genes compared with other gene sets, including mDr Day10, pDr, GO biological process, GO cellular components, ABA-related, and cis-element-based gene sets, are shown. Only gene set pairs with significant enrichment have been connected to each other. The key describes the identities of nodes, where colors refer to the type of gene set. Node size corresponds to the number of genes in that gene set (indicated in parentheses on each node), and edge thickness corresponds to the level of overlap between the gene sets connected.
The distinctive reaction of the plant to mDr Day01 is the activation of plant cell wall modification genes that underlie cell growth ( q approximately 1E-4.4), which is, in fact, down-regulated by pDr. This aspect of mDr response was pursued experimentally. On the other hand, expression of most of the typical “response to water deprivation” genes, found to be up-regulated by mDr Day01 and pDr, was similar to control, while some were even down-regulated ( q approximately 1E-2.8) as the plants grew under sustained mDr (mDr Day10). This “quenched” response is most probably due to acclimation of the plant to the continued stress. Among the genes up-regulated only at this stage are a few hormone- (ABA)-mediated signaling genes ( q approximately 1E-2.1), possibly mediating acclimation. Glucosinolate ( q approximately 1E-4), indole-3-acetic acid derivative ( q approximately 1E-2.9), jasmonic acid (JA; q approximately 1E-2.8), and very-long-chain fatty acid ( q approximately 1E-2) metabolism genes are among those solely down-regulated in mDr Day10. Some genes involved in cell wall thickening ( q approximately 1E-2.9) and a few others involved in the regulation of cell growth ( q approximately 1E-2.8) are also down-regulated in mDr Day10 only and mDr Day10 and pDr, respectively. This supports the idea that cell growth, in general, is hampered by prolonged water deficit.
cis-Regulation of Drought Response Genes
Several cis-regulatory elements (CREs) have been identified previously that are known to mediate responses to environmental stresses including drought. To identify CREs potentially mediating the transcriptional regulation of drought response genes identified here, we devised a CRE discovery pipeline: discover novel elements using the de novo motif discovery tool FIRE ( Elemento et al., 2007 ), compare the found elements with known cis-elements in prominent databases ( Higo et al., 1999 ; Davuluri et al., 2003 ; Galuschka et al., 2007 ; Mahony and Benos, 2007 ), and draw out sequence logos of CREs of interest ( Crooks et al., 2004 ). Applying this pipeline to mDr Day01, mDr Day10, and pDr (further separated into up-regulated and down-regulated) gene sets led to the identification of several known and novel CREs ( Supplemental Table S3 ). The CREs discovered to be associated with mDr and pDr are represented in Figure 7 . A consolidated view of the enrichment analyses showing cis-elements related to specific stresses and gene sets is provided in Figure 6 . Motifs discussed below are referred to by the name of the most similar “known” CRE (for the key, see the “PLACE motifs” table in Fig. 7 ).
![analysis and synthesis of drought cis-Regulatory elements identified in the upstream regions of mDr- and pDr-regulated genes. Each element identified along the rows was identified using de novo motif discovery to find short degenerate DNA sequences whose presence or absence in the 1-kb upstream regions of genes is highly informative about the expression of the given gene set (e.g. up-regulated genes in mDr Day01) given the background distribution of the sequence in the upstream sequences of all the genes in the genome. The colored matrix indicates which motifs were identified using genes regulated in which drought treatment, with yellow (reverse diagonal stripe) indicating down-regulation and blue (diagonal crosshatch) indicating up-regulation. Motifs informative about up-regulation and down-regulation together are indicated by green (dots). In the adjoining table, the sequences of the de novo motifs are given in the nucleotide International Union of Pure and Applied Chemistry nomenclature along with the Z-score of the information value of the motif, reflecting how far the observed value is, in number of sds, from the average random information (see “Materials and Methods”). Known elements in the PLACE database with significant matches to each de novo motif are presented in the PLACE motifs table in the form of the database identifier (ID), DNA sequence, and E-value of the sequence match with the de novo motif. Motifs with no match to any known element are novel putative regulatory elements. [See online article for color version of this figure.]](https://oup.silverchair-cdn.com/oup/backfile/Content_public/Journal/plphys/154/3/10.1104_pp.110.161752/2/m_plphys_v154_3_1254_f7.jpeg?Expires=1714545761&Signature=nMGD5IRhPEKaagWmgsxFFW95a9ZPrTA~VLgvmc-Ex2JLOz05ahpTo2bI7i~RPLt8nZxSTPidnfKkmRT~gxTgXuLMvCk1VsePeal6MCbY1AMPn5b2aenIO8N8r20zk47r~xxzJk20l7huLbV3py3xZx8ryKZGoNEHDZ0H1dw4VtyvNz8jdLYJZ2dYx~B1OqKLOUFdaHQ9~GBb7MKEN9wXaML5ZNF6vtLs3DEtukLuJC1Fec-tpJGxV~j6UU8rE6aj8BINKAshK7mM32oBONI0tzsP~s5sFMBaobrVyb-lX7whqUvzKNAYKxuWDODyLlanl9TiCeA5WYHZFOcjwJUTXQ__&Key-Pair-Id=APKAIE5G5CRDK6RD3PGA)
cis-Regulatory elements identified in the upstream regions of mDr- and pDr-regulated genes. Each element identified along the rows was identified using de novo motif discovery to find short degenerate DNA sequences whose presence or absence in the 1-kb upstream regions of genes is highly informative about the expression of the given gene set (e.g. up-regulated genes in mDr Day01) given the background distribution of the sequence in the upstream sequences of all the genes in the genome. The colored matrix indicates which motifs were identified using genes regulated in which drought treatment, with yellow (reverse diagonal stripe) indicating down-regulation and blue (diagonal crosshatch) indicating up-regulation. Motifs informative about up-regulation and down-regulation together are indicated by green (dots). In the adjoining table, the sequences of the de novo motifs are given in the nucleotide International Union of Pure and Applied Chemistry nomenclature along with the Z-score of the information value of the motif, reflecting how far the observed value is, in number of sd s, from the average random information (see “Materials and Methods”). Known elements in the PLACE database with significant matches to each de novo motif are presented in the PLACE motifs table in the form of the database identifier (ID), DNA sequence, and E-value of the sequence match with the de novo motif. Motifs with no match to any known element are novel putative regulatory elements. [See online article for color version of this figure.]
The CRE standing out among the genes up-regulated in mDr Day01 and pDr was one highly similar to the experimentally identified ACGT-containing ABRE motif ACGTG(G/T)C ( Fig. 5 ; Hattori et al., 2002 ). At position 6, the (G/T) degeneracy is exactly preserved in the pDr-ABRE, while it is strictly T in the mDr Day01-ABRE, thus, making it more similar to the GADOWN (for GA down-regulated) motif. Interestingly, an element very similar to the ABRE, A(A/C)(A/C)RCGTG, was found among genes down-regulated in mDr Day10, which is more similar to the functionally equivalent coupling element 3 ( Hobo et al., 1999 ). This class of ABRE-like CREs, hence, is probably mediating the ABA-dependent water deprivation response that is found to be up-regulated in mDr Day01 and pDr but down-regulated in mDr Day10.
Another element that supports this inverse regulation was one highly similar to the DRE/C-repeat (CRT) motif (A/G)CCGAC recovered from genes up-regulated in mDr Day01 and down-regulated in mDr Day10, intriguingly, with opposite orientation biases, backward and forward, respectively. DRE/CRT and ABRE have been found to be interdependent in the dehydration-responsive expression of the RESPONSIVE TO DEHYDRATION29A ( RD29A ) gene in Arabidopsis ( Narusaka et al., 2003 ).
Yet another classical stress response in early mDr, comprising slowing down of protein folding in the endoplasmic reticulum that triggers the unfolded protein response (UPR; Martínez and Chrispeels, 2003 ), is vindicated by the identification of the UPR element-like element among genes up-regulated in mDr Day01. In contrast, a UPR element-like element was identified among genes down-regulated by pDr. Here, it is important to note that “protein folding” was enriched among the down-regulated genes in mDr Day01 and pDr, supporting the fact that, in both treatments, protein folding is affected. As for the association of the UPR element, upset protein folding causes UPR involving the up-regulation of genes that help in remedying the situation, an early signal captured by the UPR element in mDr Day01 genes, while severe drought stress (pDr) pushes the system beyond repair, causing the down-regulation of the whole protein machinery. Moreover, several genes involved in cell elongation and division are down-regulated by UPR ( Martínez and Chrispeels, 2003 ), genes that are down-regulated in pDr but up-regulated in mDr Day01.
Two elements, both AT rich, were discovered among the up-regulated genes in mDr Day01 and among up-regulated and down-regulated genes in mDr Day10: the former similar to CREs present in photoresponsive genes (AT1BOX and CCA1 motif2; Terzaghi and Cashmore, 1995 ; Wang et al., 1997 ) and the latter similar to the Evening Element involved in the circadian control of gene expression in Arabidopsis ( Harmer et al., 2000 ). It is unclear how photoresponse and circadian cycling play a role in drought response. However, in the promoters of down-regulated genes in mDr Day10, the Evening Element was found to be significantly colocalizing with the DRE/CRT-like element.
A novel CRE, (G/T)(A/C)CAGCT(A/C/G)(A/T), has been identified to be uniquely enriched among genes down-regulated in mDr Day10 with as yet unknown function. Overall, the CREs discovered de novo corroborate our understanding about the various facets of drought response concerning the mDr and pDr treatments and point the way to several associations of processes and pathways perturbed in drought to their transcriptional regulation.
Cellular Metabolism under Drought Stress
Global gene expression analysis showed a substantial down-regulation of many photosynthetic genes under pDr wilting drought compared with a subtle change under mDr ( Supplemental Table S1 ). In Arabidopsis, more than 50% of the photosynthate is stored as starch ( Zeeman and Rees, 1999 ). Therefore, we examined the gene expression data for effects of both drought treatments on starch biosynthesis and degradation. Two enzymes in starch biodegradation, α-amylase and β-amylase, were induced under pDr with expression log 2 ratios of 1.5 and 3, respectively. Under mDr, only β-amylase was induced, with a log 2 ratio of 0.4. To validate these observations, plants were sampled for starch quantification from both drought treatments. The highest accumulation of starch in wild-type Arabidopsis plants was found to be in the late afternoon (at the end of the daily photoperiod; Caspar et al., 1985 ). Therefore, individual plants of the same age (30 DAS) were collected at the late afternoon from plants treated to 1 d of wilting and plants of 1 d of mDr. Starch analysis showed no accumulation of starch in the wilting plants, compared with normal starch accumulation in plants exposed to 1 d of mDr (data not shown).
Gas-exchange measurements in the time-course mDr treatment showed that plants have almost normal photosynthetic rates ( Fig. 4A ). Since Arabidopsis stores more than 50% of the photosynthate as starch, we wanted to confirm the gas-exchange measurements by quantifying starch accumulation under the time course of mDr. Starch concentration was determined at five time points, −1, 0, 1, 2, and 3 d of mDr, and showed no significant differences for these time points compared with their corresponding well-watered control (data not shown).
To test for evidence of osmotic adjustment under mDr treatment, Pro content was determined at days 3 and 4 of mDr. We found no significant change in Pro in drought-treated plants compared with well-watered controls (data not shown). Moreover, gas chromatography-mass spectrometry metabolomic analysis also showed no significant changes in Pro concentration over the time course of mDr (J. Shuman, personal communication).
Because ABA is the most important stress hormone, we also tested the change in ABA concentration in the time-course experiment of mDr. At day 0 of mDr, plants accumulated high ABA concentration, the concentration continued to increase until day 1 of mDr ( Fig. 8A ), and at day 2, the ABA concentration started to decrease.
![analysis and synthesis of drought Drought stress responses in ABA levels and ABA-related genes. A, ABA quantification (in percentage of well-watered control) from day 0 to day 2 of mDr (DMD). B, qRT-PCR analysis of stress signaling genes and stress marker genes under pDr prewilting (PPW) and mDr Day01 (MD). C to E, Time-course responses in DMD (x axis) of stress-related genes using qRT-PCR showing fold change (y axis). C, ABA signaling pathway genes NCED3 and ABF3. D, DREB2A (ABA-independent signaling pathway). E, Stress marker genes. For A to D, three replications with five plants pooled per replication were used; error bars represent se. [See online article for color version of this figure.]](https://oup.silverchair-cdn.com/oup/backfile/Content_public/Journal/plphys/154/3/10.1104_pp.110.161752/2/m_plphys_v154_3_1254_f8.jpeg?Expires=1714545761&Signature=zltrDklQJm3rv-jx6xZRs3QjBKksbQBCLex36OuxBaBb21GV2x5tGucfcR12j2TLGyUpg2aNNspCwjF6FCkEpZpw0tV1qsFWhnNqUYN4j-GqbB6PEKqL~4H2xzAdooVfGrQllnE-aSJ9MwREopgjPCIeZPJh-lwXxs39Glckj2ifDnEIMOr37OUgqM7KkV4ME3~etgZQDK6xOHFJZGxWeD~7ye0ih4EvOKiSSz9yyHLt0TjHzvHhVoVBw23TdgTnR4poHyXyKt-jOYmlsKHBQydLIZs1fWEswwYEPCLN2O5NeYe~2~D3LrnG31Shy2ntVor3qy7pWVw7eN62jp5BZA__&Key-Pair-Id=APKAIE5G5CRDK6RD3PGA)
Drought stress responses in ABA levels and ABA-related genes. A, ABA quantification (in percentage of well-watered control) from day 0 to day 2 of mDr (DMD). B, qRT-PCR analysis of stress signaling genes and stress marker genes under pDr prewilting (PPW) and mDr Day01 (MD). C to E, Time-course responses in DMD ( x axis) of stress-related genes using qRT-PCR showing fold change ( y axis). C, ABA signaling pathway genes NCED3 and ABF3 . D, DREB2A (ABA-independent signaling pathway). E, Stress marker genes. For A to D, three replications with five plants pooled per replication were used; error bars represent se . [See online article for color version of this figure.]
Expression of Stress Signaling Pathway Genes under Drought Stress
On exposure of plants to drought stress, ABA-dependent and ABA-independent signaling pathways have been shown to be induced ( Shinozaki and Yamaguchi- Shinozaki, 1997 ). To test the effect of soil water deficit achieved in two different ways, pDr prewilting (1 d before wilting) drought and controlled mDr Day01 treatments, on the response of the signaling pathways at the molecular level, the expression levels of key genes in the signaling pathways were quantified. The ABA biosynthesis gene NINE-CIS-EPOXYCAROTENOID DIOXYGENASE3 ( NCED3 ) was induced 4-fold more under pDr prewilting compared with mDr ( Fig. 8B ). The drought-responsive transcription factor DREB2A was induced at a higher level under pDr compared with mDr. Another gene showing differential expression between the two drought treatments is RD29B , which is induced almost 100-fold under pDr compared with a 20-fold change under mDr Day01 ( Fig. 8B ). The drought-responsive expression levels of the rest of the tested stress signaling genes were not significantly different between the two drought treatments ( Fig. 8B ).
The expression levels of key genes in the stress signaling pathways, ABA dependent and ABA independent, were quantified in the time-course analysis of mDr. NCED3, an important enzyme in ABA biosynthesis, was highly induced at days 0 and 1 of mDr compared with days −1, 2, and 3 ( Fig. 8C ). The expression of ABF3 (ABA-dependent pathway) was high at 0 d of mDr, and after that its expression started to decrease. In the ABA-independent pathway, DREB2A showed induction at day 0 and stayed induced at day 1 of mDr. After day 1, its expression was decreased ( Fig. 8D ).
Downstream of NCED3, ABF3, and DREB2A, there are many responsive genes that are recognized as stress marker genes: RD22 , RD29A , RD29B , and RESPONSIVE TO ABA18 ( RAB18 ). The expression profiles of these marker genes in a time course of mDr ( Fig. 8E ) showed induction at day 0 of mDr that continued at day 1 and then decreased.
Stomatal Responses in a Time-Course Analysis of mDr
To understand the stomatal responses to mDr treatment at the molecular level, a set of stomatally related genes were chosen based on our microarray data of mDr to study the kinetics of expression changes in the time-course study of mDr. For PLDα1 and GPA1 , two positive regulators of ABA signaling in the stomata, the expression under mDr started increasing from day −1 to peak at day 1 and then decreased thereafter ( Fig. 9A ). Since outward potassium channels have an important role in stomatal response to the surrounding environment, we quantified the expression of GORK , an outward K + channel gene. The highest induction of GORK was at day 1 of mDr, which was then reduced from day 2 onward ( Fig. 9A ).
![analysis and synthesis of drought Gene expression profiles of stomatally related genes during mDr treatment. The y axis shows the fold change using qRT-PCR, and the x axis shows the days of mDr. A, PLDα1, GPA1, and GORK. B, PP2Cs. C, RPK1. D, MYB60 transcription factor. For all panels, three replications with five plants pooled per replication were used; error bars represent se. [See online article for color version of this figure.]](https://oup.silverchair-cdn.com/oup/backfile/Content_public/Journal/plphys/154/3/10.1104_pp.110.161752/2/m_plphys_v154_3_1254_f9.jpeg?Expires=1714545761&Signature=oJoFSIMAxDBfBqZkuUYPRWXhEcgUpVUe-d~1ovA~DZIXCmQHcB~ok1px4jEv5zvyJtMVyE~IqX7ECBISw4Hp3RD1aNAcILDkxnl8jI1b0eH2RcgEfbnFKY4~CckttyjDGTyhUODgpryyy7Pc53nR3-z9OpnREnxcKqZzPfOdgjyM88PdUWKLaEFCcYgriWs0OQueAKq6C94X5Q9sGyHakgnm2B~6z8QgVdqYEUfpTOQ43UuqemsTC-AZeRRHtlrLimoffSr4aD77e5NHXM1TEr9G8o8TPTDAkV190XqrXJDNyFvTBWPlFWzwGf273HHUHZx6roiHfU1x7BYY7saTXA__&Key-Pair-Id=APKAIE5G5CRDK6RD3PGA)
Gene expression profiles of stomatally related genes during mDr treatment. The y axis shows the fold change using qRT-PCR, and the x axis shows the days of mDr. A, PLDα1 , GPA1 , and GORK . B, PP2Cs. C, RPK1 . D, MYB60 transcription factor. For all panels, three replications with five plants pooled per replication were used; error bars represent se . [See online article for color version of this figure.]
Another group of genes with a major role in stomatal response and ABA signaling belong to the family of protein phosphatases type C (PP2Cs). Therefore, we tested the expression profiles of three main PP2Cs: ABI1 , ABI2 , and HOMOLOGY TO ABI1 ( HAB1 ). The expression of ABI1 and ABI2 started to increase at day −1 of mDr, continued to day 1, and then decreased ( Fig. 9B ). HAB1 showed a decrease in expression at day −1, followed by an induction at day1 and a decrease thereafter ( Fig. 9B ). RECEPTOR-LIKE PROTEIN KINASE1 ( RPK1 ), described to be active in the early response to ABA signaling in the stomata ( Osakabe et al., 2005 ), was induced in our mDr microarray. In the mDr time-course analysis, RPK1 showed induction at day −1, which continued to day 1, followed by a decrease ( Fig. 9C ).
In our drought microarrays, we found MYB60 repressed at day 1 of mDr and under pDr. This gene has been found to be specifically expressed in guard cells, and its null mutant reduces stomatal opening ( Cominelli et al., 2005 ). Therefore, we tested the response during the time course of mDr, quantifying its expression at five time points. MYB60 was induced at days −1 and 0 of mDr ( Fig. 9D ) and repressed at day 1; it stabilized from day 2 onward to that of the well-watered control ( Fig. 9D ).
Expression of Photosynthesis and Antioxidant Genes under Drought Stress
The comparison of mDr and pDr microarrays revealed that many photosynthesis genes were significantly repressed under wilting, in contrast to the subtle effect of 1 d under mDr ( Supplemental Table S1 ). We selected a few photosynthesis genes whose proteins are part of PSI ( PQL2 , PSAH2 ) and PSII ( PSBW , PSBQA ) to profile their expression under the mDr time-course experiments ( Fig. 10A ). The expression of PSBW significantly decreased from day 1 to its lowest level at day 2 of mDr, while PSBQA expression was normal at days −1, 0, and 1 and started to decrease at day 2. The PSI subunit gene PQL2 showed normal expression levels at days −1, 0, 1, and 2, and at day 3 it started to decrease. PSAH2 was induced at day −1, reduced to a normal level at day 0, and then induced at days 1 and 2, followed by repression at day 3 of mDr ( Fig. 10A ).
![analysis and synthesis of drought Gene expression profiles of drought-responsive genes. Time course is in DMD (x axis) showing gene expression fold change (y axis). A, Photosynthesis-related genes. B, Antioxidant enzyme genes. For both panels, three replications with five plants pooled per replication were used; error bars represent se. [See online article for color version of this figure.]](https://oup.silverchair-cdn.com/oup/backfile/Content_public/Journal/plphys/154/3/10.1104_pp.110.161752/2/m_plphys_v154_3_1254_f10.jpeg?Expires=1714545761&Signature=eWEdPacH8XhmqHqBuYYMjMk7RkVkJiqjrCD-0iTDXanJ10IJ0jY7jK-v~XVE~Atu~Q6rHpdyAPWglePug93HQtKGPaotBtsLQU0svfo5KPRA5VWF-4HGi4keyknF-003rQuAdXEPYGmuoAqt2YaJKsSZvMNYzmrdEClvNUHPAmXmGxpRO~toseBgcqN~rIfOm9cNCw7sb3XU9fX3uaR-gEHeVS47ShWOqwViwvvhm3BrSa4nfnPau9SCPRgr6uyWHnbMrCPFKvV6-lX8HIgGWDi9Ez08nXWvGTCcM3o-YSwmKF8jYhuqEaadrPIajCri-cpyJRxccYD3~91RO~P2eQ__&Key-Pair-Id=APKAIE5G5CRDK6RD3PGA)
Gene expression profiles of drought-responsive genes. Time course is in DMD ( x axis) showing gene expression fold change ( y axis). A, Photosynthesis-related genes. B, Antioxidant enzyme genes. For both panels, three replications with five plants pooled per replication were used; error bars represent se . [See online article for color version of this figure.]
To test for oxidative stress-related molecular events in response to mDr, six enzymes with antioxidant activity were chosen: ascorbate peroxidase 1 (APX1), thioredoxin peroxidase 1 (TPX1), glutathione peroxidase 6 (GPX6), cytosolic copper/zinc dismutase (CSD1), chloroplastic copper/zinc dismutase (CSD2), and iron dismutase (FSD1). The expression of APX1 and TPX1 was slightly changed over the time-course period ( Fig. 10B ). GPX6 showed a drastic decrease in expression at day 1 of mDr. Both CSD1 and CSD2 were significantly repressed at days 1 and 2 of mDr, while FSD1 was slightly changed over the time-course period ( Fig. 10B ).
Expression of Cell Expansion Genes under Drought
The induction of cell expansion genes was specific to mDr, and the same genes were down-regulated or not differentially expressed in pDr. GO enrichment analysis of mDr differentially expressed genes showed the enrichment of cell expansion-related genes. In addition, the comparison of mDr and pDr microarray data with ABA-responsive genes showed that cell expansion was up-regulated in mDr compared with pDr wilting drought and ABA treatments. Therefore, we quantified the effect of pDr prewilting and mDr treatments on the expression levels of the EXPANSIN genes EXPA3 , EXPA4 , EXPA8 , EXPA10 , and EXPANSIN-LIKE B1 ( EXLB1 ; Fig. 11A ). After 1 d of mDr, most of the expansin genes were induced, while under pDr, EXPA3 and EXPA8 showed repression and EXPA4 remained unchanged ( Fig. 11A ).
![analysis and synthesis of drought Gene expression profiles of expansin genes in drought acclimation response. A, Expression of expansin genes under pDr prewilting (PPW) and mDr (MD) showing fold change (y axis). B, Expression profiles of expansin genes shown in fold change (y axis) in a time course of mDr (x axis). For both panels, three replications with five plants pooled per replication were used; error bars represent se. [See online article for color version of this figure.]](https://oup.silverchair-cdn.com/oup/backfile/Content_public/Journal/plphys/154/3/10.1104_pp.110.161752/2/m_plphys_v154_3_1254_f11.jpeg?Expires=1714545761&Signature=LsFJGW7jBOKTCvF1u8vytvKMmMcSYxvZEZyFHFWUHa0rx9cQ5iu8kA5gOnBP3ql3T~Hv3lONuKpg-sdddtykQcoiYvhVSFaFzHnZYqNJPv7dz0sqFxbNPGGJvyQURweGoHlZXaL7QpysqjvAwlEp6C8deq8mTaNn2vxTjIeSHT98vnwUPWoP4259rUf6yj2zwJL~D9jP8Hs~2PdVCbCm9u2JV2mjdw53aE2~Klugnmki5pfTJbg6rEWGCm1oFrgYcn-39kv2ELSCecgpaEx5BtM6ZMtNt2qs9TRoBZmHPA0LE929UjDuAkgObFcA9l7LOD0uJFwQ6B1Uin-2DZOY-Q__&Key-Pair-Id=APKAIE5G5CRDK6RD3PGA)
Gene expression profiles of expansin genes in drought acclimation response. A, Expression of expansin genes under pDr prewilting (PPW) and mDr (MD) showing fold change ( y axis). B, Expression profiles of expansin genes shown in fold change ( y axis) in a time course of mDr ( x axis). For both panels, three replications with five plants pooled per replication were used; error bars represent se . [See online article for color version of this figure.]
In the time-course analysis of mDr, the EXPA3 , EXPA4 , EXPA8 , and EXPA10 genes were repressed at day 0, induced at day 1, followed by a decrease at days 2 and 3 of mDr ( Fig. 11B ). EXLB1 showed a steady increase in expression until day 1 and then a gradual decline to day 3, reaching a normal expression level relative to well-watered control ( Fig. 11B ).
Growth Reduction under Drought Stress
The application of controlled mDr stress on Arabidopsis plants enabled us to evaluate many parameters in relation to the drought stress treatment as well as the response of plants at the physiological and molecular levels simultaneously. mDr, maintained by daily replenishing evapotranspired water, was applied at plant growth stages 1.08 to 1.10, corresponding to the eight- to 10-leaf stages ( Boyes et al., 2001 ), for 5 to 10 d and caused a significant reduction in growth, as observed by dry matter accumulation and leaf expansion. The reduction was dependent on the developmental stage of the plants: drought initiation at stages 1.08 and 1.10, corresponding to 25 and 30 DAS, showed a highly significant growth reduction compared with later developmental stages. Moreover, 5 or 10 d of drought both gave a significant reduction in growth. To understand the biological processes involved in the response of plants to drought that inhibit plant growth, gene expression analysis of plants treated to 10 d of mDr was done. The perturbation in expression was much reduced at the later time point, suggesting a stabilization or acclimation in responses. Our interest, therefore, was to find the causes of the reduction of growth due to drought stress, the time when the responses start, and the physiological, biochemical, and molecular changes responsible for the reduction of growth under drought.
Drought Transcriptome Analysis
A comparative transcriptome analysis of pDr wilting and mDr (1 and 10 d) revealed common drought-responsive processes. This was substantiated by GO analysis, which showed enrichment for genes involved in stress response processes such as desiccation, stress, and water deprivation in the two drought treatments ( Fig. 6 ). In addition, common stress-responsive cis-elements were enriched in promoters of genes up-regulated under pDr wilting and mDr Day01 and down-regulated under mDr Day10. Moreover, qRT-PCR analysis showed that most of the characteristic stress signaling and stress marker genes were similarly induced under pDr prewilting and mDr Day01 treatments. In conclusion, these expression analyses revealed regular drought responses at the early stage of mDr. Hence, Arabidopsis plants under mDr sense and respond to drought stress in a similar way to the more drastic progressive wilting or dry-down drought treatments, which lead to lethality. mDr treatment, therefore, is a good model system to dissect the response and resistance of plants to drought.
The analysis of the late stage of drought in mDr Day10 compared with the earlier mDr Day01 indicates the continuity of stress responses, which were examined for evidence of plant acclimation responses. Prominent among genes up-regulated early and late were the homeobox genes ATHB7 and ATHB12 , which have been shown to be involved as regulators of plant growth under drought stress ( Olsson et al., 2004 ). Mutants in these genes displayed reduced sensitivity to ABA, and overexpression showed ABA hypersensitivity and phenocopy of wild-type Arabidopsis under drought treatment. These previous results suggested that the ATHB7 and ATHB12 genes probably maintain the reduced growth of plants under drought, which is an acclimation response of plants to survive prolonged drought stress. Other genes coexpressed with these homeobox regulators are probably also involved in a similar role, such as transcription factors belonging to the NAC- and CCAAT-binding (CBF-B/NF-YA) family, protein phosphatases, and kinases. These expression studies, therefore, reveal a number of drought-responsive genes that might be important in protecting plants from drought stress and are candidates for future genetic analysis.
In our Affymetrix array analysis, the ABA up-regulated genes ( Nemhauser et al., 2006 ) made up 26% (944 of 3,625) of the pDr up-regulated genes, 31% (341 of 1,089) of mDr Day01, and 22% (93 of 416) of mDr Day10 differentially regulated genes ( Fig. 5 ). These results of mDr and pDr are consistent and reveal the similarity in significance of ABA- and non-ABA-related or -dependent pathways in drought responses. In genome-wide oligonucleotide microarray studies of Arabidopsis soil water deficit pDr responses ( Huang et al., 2008 ), a higher level of drought-regulated genes was found in comparison with ABA responses using a more response-eliciting ABA analog ( Huang et al., 2007 ).
Stress Perception and Signaling Are Transient and Occur at an Early Stage of Drought
Plant responses to different stresses have been shown to be mediated by ABA-dependent and ABA-independent stress signaling pathways ( Shinozaki and Yamaguchi- Shinozaki, 1997 ; Hirayama and Shinozaki, 2010 ). In addition, extensive studies on ABA signaling reveal the central role of ABA in response to different environmental stimuli ( Cutler et al., 2010 ; Kim et al., 2010 ). To assess the role of ABA in mDr stress treatment, ABA biosynthesis and signaling mutants were tested under mDr stress and showed significant reduction in growth (higher sensitivity) compared with the wild type. Therefore, ABA is needed for normal drought response, and any perturbation in ABA biosynthesis or signaling will negatively affect plant growth under drought.
To determine the time course of ABA accumulation under drought, ABA was quantified at three time points (days 0, 1, and 2) of mDr, showing highest concentrations at days 0 and 1. Consistent with this, the expression pattern of some characteristic genes in stress signaling pathways, DREB2A , ABF3 , and NCED3 , showed induction at an early stage of the drought stress. Moreover, the same expression pattern was shown for a group of downstream-regulated genes that are designated as stress markers: RD22 , RD29A , RD29B , and RAB18 . These experiments thus show that drought stress perception and signaling occur at an early stage of mDr treatment, and they enable a molecular genetic and physiological dissection of subsequent responses to the stress.
Drought Avoidance by Stomatal Closure at an Early Stage of Drought Stress
Stomatal closure under drought is an avoidance response/strategy adopted by plants to save water and maintain turgor ( Levitt, 1980 ; Chaves and Oliveira, 2004 ; Skirycz and Inze, 2010 ). Under mDr treatment, plants showed an early response with a drastic decrease in LRWC and stomatal conductance, but photosynthesis rate remained normal. Moreover, the expression pattern of stomatally related genes showed a peak at an early stage of drought stress. The α-subunit of the heterotrimeric G protein gene ( GPA1 ) and PLDα1 , which were found to play critical roles in the inhibition of stomatal opening ( Mishra et al., 2006 ; Nilson and Assmann, 2010 ; Zhang et al., 2009 ; Zhao et al., 2010 ), exhibit high expression, consistent with their roles in the inhibition of stomatal opening. Outward and inward potassium channels regulate the movement of K + across the membrane of guard cells in response to ABA signals ( Schroeder et al., 2001 ; Nilson and Assmann, 2007 ). Under stress, the outward channels are induced and inward channels are repressed. In agreement with this, the outward channel gene GORK showed the highest expression at day 1 of drought stress treatment.
Another important group of genes in ABA signaling in the guard cells are the PP2C genes, which act as negative regulators in ABA signaling in stomata ( Pedro, 1998 ; Gosti et al., 1999 ; Saez et al., 2006 ). Some of the PP2Cs were found to be induced under drought, salt stress, and low temperature ( Tähtiharju and Palva, 2001 ; Bray, 2004 ). Here, three PP2Cs, ABI1 , ABI2 , and HAB1 , were found to be induced early during drought treatment. Another gene, RPK1 , was found to be induced at an early stage of drought stress. This is consistent with its role in mediating an early response in ABA signaling and the regulation of guard cells under stress, with a function in the improvement of abiotic stress tolerance ( Osakabe et al., 2010 ). MYB60 has an important role in the regulation of guard cells, with the knockout mutation resulting in stomatal closure ( Cominelli et al., 2005 ). Under mDr time-course experiments, MYB60 expression is lowest at day 1, corresponding with the drastic decrease in stomatal conductance under drought treatment. The mediation of drought responses through the regulation of a stomatal drought response network, resulting in the induction of GPA1 , PLDα1 , GORK , and the PP2C s and repression of the transcription factor MYB60 , is consistent with available data.
Normal Photosynthesis and No Oxidative Stress under mDr
Photosynthesis rate determined by instantaneous gas-exchange measurements was not affected by the mDr stress treatment, which was supported by the expression profile of photosynthesis-related genes. Starch accumulation was also normal during mDr treatment (data no shown). In agreement with these observations, previous studies found that photosynthesis usually is not affected by mild drought and mDr ( Cornic and Massacci, 1996 ; Flexas and Medrano, 2002 ). Moreover, analysis of the publicly available expression profiling data under drought and salt stress showed a nonsignificant effect of mild drought on the expression of photosynthetic genes both qualitatively and quantitatively ( Chaves et al., 2009 ).
ROS are produced in different compartments of the plant cell, both under normal and stressful conditions ( Grene, 2002 ). When plants are challenged by drought or other abiotic stresses, ROS are generated as a result of the inhibition of photosynthesis and the predominance of photorespiration ( Noctor et al., 2002 ). ROS are found to have a dual function in plants: they are needed as signaling molecules, but a high concentration is detrimental ( Kwak et al., 2003 ; Slesak et al., 2007 ). High ROS concentration is hence a stress symptom, and plants have to maintain the ROS within a certain level that is required for normal cellular homeostasis. ROS concentration in the cell is maintained by the antioxidant system, which is made up of the antioxidant molecules ascorbate, glutathione, and α-tocopherol in addition to the antioxidant enzymes peroxidases, catalases, and dismutases ( Alscher et al., 2002 ; Grene, 2002 ). The induction of members of the antioxidant system is highly correlated with the severity of the stress. Under severe abiotic stresses such as high light, low temperature, high temperature, salt stress, severe drought, and a combination of stresses, antioxidant enzymes are differentially and highly induced ( Kliebenstein et al., 1998 ; Noctor et al., 2002 ; Rodriguez Milla et al., 2003 ; Miao et al., 2006 , ; Miller et al., 2010 ).
mDr did not exhibit acute oxidative stress, as shown by the nearly normal expression levels of three antioxidant enzyme genes, APX1 , TPX1 , and FSD1 , and the repression of the other tested antioxidant enzyme genes, GPX6 , CSD1 , and CSD2 . This is consistent with the normal photosynthesis rate under mDr, proven both at the physiological and molecular levels. However, GPX6 and TPX1 are slightly, yet significantly, up-regulated (approximately 2-fold) at the very early stage of drought stress, and these peroxidases might be involved in reducing very early ROS responses, partly from stress signaling ( Kwak et al., 2003 ; Pham and Desikan, 2009 ). Indeed, the promoter of GPX6 was found to have the common stress cis-elements (ABRE and CRT/DRE), and it was responsive to osmotic stress ( Rodriguez Milla et al., 2003 ). In conclusion, the mDr level is below the threshold required for the generation of a highly destructive concentration of ROS. Therefore, there was a net normal level of antioxidant enzymes except for the dismutases, which were repressed. The repression of the dismutases can be explained by the low concentration of superoxide and efficient photosynthesis, due to the incomplete closure of the stomata under stress conditions ( Cruz de Carvalho, 2008 ). In addition, studies on many crops showed a discrepancy regarding the expression of the antioxidant enzymes in response to drought. In some cases they were induced, but in other cases they were repressed ( Cruz de Carvalho, 2008 ), suggesting that different ROS balance and levels are required at different responses.
Acclimation to mDr by Cell Wall Adjustment
One of the first acclimation responses to drought is the decrease in leaf growth, which results in the maintenance of cell turgor and reduces the transpiration area ( Mathews et al., 1984 ; Neumann, 1995 ). In addition to cell turgor, cell wall biochemical and biophysical characteristics play an important role in cell growth ( Mathews et al., 1984 ; Neumann, 1995 ). In Arabidopsis, leaf size is a result of both cell division and cell expansion ( Horiguchi et al., 2006 ), and under mild drought, Arabidopsis leaves compensate for low expansion rate by the extension of expansion duration ( Aguirrezabal et al., 2006 ). Cell expansion is a process of cell wall modification and loosening catalyzed by enzymatic and nonenzymatic protein components of the cell wall ( Cosgrove, 2005 ), which is composed of cellulose and hemicelluloses in a matrix of pectins and proteins ( Cosgrove, 2005 ). Expansins are the key cell wall-loosening proteins, which act by the breakage of hydrogen (noncovalent) bonds between cellulose and the surrounding matrix, leading to slippage of the cell wall components under acidic pH (acid growth) and, consequently, the increase in extensibility of the cell wall ( McQueen-Mason et al., 1992 ).
Physical properties of the cell wall play a crucial role in the response of plants to water deficit ( Bacon, 1999 ). Transcriptome analysis of pDr showed the repression of many expansin genes ( Bray, 2004 ), while mild osmotic stress revealed the induction of expansin genes ( Skirycz et al., 2010 ). Cell expansion in response to drought was characterized in the maize ( Zea mays ) root system as an adaptation to low water potential ( Wu and Cosgrove, 2000 ). In addition, there are many studies on leaf growth under water deficit in maize leaves and other crop plants such as sunflower ( Helianthus annuus ) as well as in Arabidopsis ( Mathews et al., 1984 ; Aguirrezabal et al., 2006 ; Bouchabke et al., 2006 ; Granier and Tardieu, 2009 ). Despite the plethora of studies on cell expansion in response to drought, very little is known about the molecular basis of this process in plant responses to internal and external stimuli.
In this research, microarray and qRT-PCR analyses revealed the up-regulation of cell expansion genes under mDr treatment. In contrast, under pDr prewilting and pDr wilting drought treatments, most of the expansin genes were down-regulated. The expression profiles of four expansin genes in the time-course analysis of mDr showed a pattern of repression at the beginning of drought (day 0), induction at day 1, and repression thereafter. A fifth expansin gene, EXLB1 , has a different expression pattern, with a peak in expression at day 1 and a decrease starting at day 2. This early peak in expansin expression can be interpreted as an acclimation to mDr by cell wall adjustment. This is a common type of acclimation response, which can proceed by loosening and/or tightening of the cell wall structure depending on the species, organ, and tissue ( Neumann, 1995 ; Moore et al., 2008 ). A study of the resurrection plant ( Craterostigma plantagineum ) showed an increase in expansin expression and activity at an early stage of dehydration, resulting in a flexible cell wall as an adaptation to dehydration ( Jones and McQueen-Mason, 2004 ). Consistently in our study, there was no significant RB at an early stage of mDr compared with a later stage (day 5 and afterward), supported in part by the slight increase in expansin expression as an adaptation to stress that occurs as an early response. Differential spatial expression of expansins was shown in maize leaves, tomato ( Solanum lycopersicum ) shoot apex, and tomato embryos ( Chen et al., 2001 ; Vogler et al., 2003 ; Muller et al., 2007 ), and in Arabidopsis, the spatial expression of EXPA10 in leaf growth and development has been described ( Cho and Cosgrove, 2000 ). Therefore, the spatial and temporal patterns of expansin expression and activity need to be studied in response to drought.
The plant cell wall is required not only for mechanical support but for growth and adaptation to hostile environments. There is still a lot to be learned about cell wall modification under different abiotic stresses at the molecular, cellular, tissue, and whole-plant levels. Studies on the effects of overexpression of expansin genes show enhanced growth in rice and high sensitivity to hormones and salt stress in Arabidopsis ( Choi et al., 2003 ; Kwon et al., 2008 ). These suggest the important role of expansins in acclimation and adaptive responses of plants to abiotic stresses.
Drought Acclimation Processes at Late Stages of mDr
Gas-exchange measurements at the late stage of mDr (Day10) showed no significant differences in stomatal conductance and photosynthesis of drought-treated plants compared with the well-watered control (data not shown). Moreover, no stomatally related genes were differentially expressed at this stage. In contrast, microarray and qRT-PCR analyses of the early stage of mDr (Day01) showed that many stomatally related genes were either up-regulated or down-regulated. Hence, the early stage (Day01) showed reduced stomatal conductance, whereas normal stomatal conductance and reduced growth were shown at late stage of mDr (Day10).
JA biosynthesis and signaling were among the main enriched GO categories in the down-regulated genes at the late stage of mDr. Jasmonates have been found to have a potential role in response to drought stress in soybean ( Glycine max ), as they showed an early increase within 2 h of dehydration and a decrease in concentration afterward ( Creelman and Mullet, 1995 ). Moreover, jasmonates were found to cause stomatal closure ( Raghavendra and Reddy, 1987 ). This role was confirmed by the impaired stomatal response to exogenous jasmonates in jasmonate-insensitive mutants ( jar1 and coi1 ; Suhita et al., 2004 ; Munemasa et al., 2007 ). There is cross talk between jasmonates and ABA as they utilize a similar cascade of events to stimulate stomatal closure ( Suhita et al., 2004 ).
Under our mDr treatment, the coi1 and jin1 mutants were found to be significantly resistant (or insensitive to drought stress) compared with the wild type, with biomass accumulation under drought not different from the well-watered control. This suggests that the reduced growth as a response to drought stress, as a developmental program for acclimation, is not switched on in the absence of JA signal perception and response. This is supported by studies that show that the JA-mediated inhibition of seedling and root growth is suppressed in the coi1 mutant ( Xie et al., 1998 ).
In experiments on stomatal closure, a characteristic drought response, jasmonates induce closure that is suppressed in the coi1 mutant, which retains normal ABA responsiveness ( Munemasa et al., 2007 ). Likewise, studies on barley ( Hordeum vulgare ) genotypes and ABA-deficient tomato mutant plants revealed the role of JA in stomatal modulation through ABA ( Herde et al., 1997 ; Bandurska et al., 2003 ). In the tomato ABA-deficient mutant, exogenous ABA was sufficient to close stomata and reduce transpiration ( Herde et al., 1997 ). Since JA was also shown to repress photosynthesis genes ( Reinbothe et al., 1994 ), one can expect photosynthesis to be unaffected in JA-insensitive mutants through a JA-mediated signaling program, although the ABA response would still be active.
At the early stage of mDr (Day01), plants accumulate high ABA concentration with induction of ABA biosynthesis and signaling genes but with no significant differential expression of JA pathway genes. At the late stage of mDr (Day10), the ABA level is normal, with biosynthesis genes not up-regulated. However, JA signaling and biosynthesis genes are significantly down-regulated. The negative correlation in expression of the ABA and JA pathway genes is also seen in transcription profiling studies of methyl jasmonate-treated plants, which show repression of ABA/drought-responsive genes such as ATHB12 and ABF3 ( Devoto et al., 2005 ).
We propose that at the early stage of mDr, endogenous JA in combination with high ABA level is enough to stimulate the preparatory response needed for drought acclimation (e.g. stomatal closure and cell wall modification). JA is probably not required at high concentration under drought stress, and an increase in its concentration might negatively affect plant response in growth. Indeed, interaction between cellulose synthesis and a high concentration of JA revealed a negative effect of JA on cell wall modification and plant growth, which enhanced plant resistance to fungal pathogens ( Ellis et al., 2002 ). Moreover, JA induction in response to wounding and herbivory freezes the cell cycle, inhibits cell expansion, and results in stunted growth ( Zhang and Turner, 2008 ; Onkokesung et al., 2010 ). To minimize the inhibitory effect of JA on plant growth under prolonged drought (late mDr), the down-regulation of JA biosynthesis and signaling pathways can act in establishing new homeostasis in the acclimation process.
Model of Plant Responses to Drought
The response to mDr, extended over a period of time, can be distinguished into multiple stages, from early to intermediate to late ( Fig. 12 ). During the early priming or preconditioning stage, stress perception, signaling, and reprogramming of gene expression take place. Many of these immediate responses, such as ABA response genes and ROS scavengers, probably involved in signaling responses, are also observed in pDr. The drought response pathways can be traced by the expression pattern of individual genes of known function. The differentially expressed genes at the early stage are characterized by the induction of a set of enzymes, channel protein genes, and transcription factors, which interact to control the stomatal aperture in response to internal and external stimuli. RPK1, a receptor-like kinase, functions upstream in the ABA signaling pathway in the stomata. Downstream, PLDα1 interacts with GPA1 to inhibit stomatal opening and frees phosphatidic acid, which in turn interacts with the PP2C (ABI1) to stimulate stomatal closure ( Mishra et al., 2006 ). Moreover, the induced outward K+ channel (GORK) extrudes K+ outside the guard cells, resulting in the loss of turgor and stomatal closure. Another key protein is the transcription factor MYB60, which is repressed at the early stage of drought, regulating stomatal closure. In the intermediate stage responses, there is an onset of reduction in growth, although no changes in growth are measurable. However, cell wall adjustments take place as part of the acclimation response. At the late stage, plants reach a new homeostasis status, reaching an altered jasmonate-ABA pathway balance, having reduced growth with reduced levels of energy-consuming processes but with stabilized metabolism and physiology similar to the well-watered control.
![analysis and synthesis of drought Physiological, biochemical, and molecular plant responses to mDr. Plant responses to mDr are dissected into three stages: early priming (preconditioning) stage, in which all stress signaling and avoidance processes take place; intermediate stage, which is preparatory for acclimation, as plants modify and adjust cell walls for reprogrammed growth responses at later stages; and late stage, in which plants are set to a new homeostasis with altered hormonal signaling and reduction in energy-demanding processes, leading to acclimated plants with reduced growth. FC, Field capacity. [See online article for color version of this figure.]](https://oup.silverchair-cdn.com/oup/backfile/Content_public/Journal/plphys/154/3/10.1104_pp.110.161752/2/m_plphys_v154_3_1254_f12.jpeg?Expires=1714545761&Signature=EpuuZ5dQM6BVnnrBd1PsSxBVrGtJl-wyizWxyMgIYdn4e~6Fu9V1y3yPcsW63XZ9dPKas7j59lIKL-KQVuki6g8VpTfK2tiHpRhPt7Zuzu10xx9flQ7EGwcbpXexv2vUDnD9uKyHDtEZ3gyaV0CkQbq4IzSMS2pjqRz2z2yLgjM~Mrc5AMza4vFR2thPzyHvMbts7DBiY4c0UV29bMBT~GPdfHaGTXH9QjZYpq7qSyjfIi4RTgtfjCLMIVXCCii4nYAjrrnOXivQWvi-lRTdiEpk98KHp9L0bi0hStfaUKZrjWkKQnuJ~orD9KBQzsTkK3GcJXNlmKsV-Mqacg1Z3w__&Key-Pair-Id=APKAIE5G5CRDK6RD3PGA)
Physiological, biochemical, and molecular plant responses to mDr. Plant responses to mDr are dissected into three stages: early priming (preconditioning) stage, in which all stress signaling and avoidance processes take place; intermediate stage, which is preparatory for acclimation, as plants modify and adjust cell walls for reprogrammed growth responses at later stages; and late stage, in which plants are set to a new homeostasis with altered hormonal signaling and reduction in energy-demanding processes, leading to acclimated plants with reduced growth. FC, Field capacity. [See online article for color version of this figure.]
Growth Conditions and Drought Treatments
Arabidopsis ( Arabidopsis thaliana ecotype Columbia) seeds were sown in moistened peat pellets (Jiffy Products), stratified at 4°C for 2 d, and then transferred to a growth room kept at 10 h of light (100 μmol m −2 s −1 ) and 22°C. For drought treatment, pellets were weighed before sowing to determine the amount of water in pellets at the beginning of the experiment. Controlled mDr was maintained by giving plants water to keep the soil moisture level at 30% of field capacity, which is 200% or 2 g water g −1 dry soil. To do this, a semiautomated system was developed; a balance (GF-1000; A&D) was connected to the computer utilizing software for communication, which enabled entering of the weights directly into an Excel worksheet file. On the Excel worksheet file, a set of equations were used to calculate the water content in each weighed pellet, the required final water content, and the amount of water to be added. The pellets were weighed daily and were supplemented with the calculated amount of water to reach 30% of field capacity (mDr level).
The sensitivity of different developmental stages to mDr was tested utilizing the same drought treatment as described above. Water was withheld at 25 DAS for the first group, 30 DAS for the second group, and 35 DAS for the third group. After around 5 to 7 d, a mDr stress is achieved as a soil moisture level of 2 g g −1 dry soil is reached, and the plant stages for the three groups are as follows: eight leaf (1.08), 10 leaf (1.10), and 12 leaf (1.12), respectively ( Boyes et al., 2001 ). The mDr treatments are referred to by the initiation date (25, 30, 35 DAS) in the experiments reported. The three groups were exposed to mDr for 10 d, and then the sensitivity was assessed by calculation of the RB as follows: RB = ( B WW − B DRT )/ B WW , where B WW is biomass under well-watered conditions and B DRT is biomass under mDr conditions. The effect of the duration of mDr was also tested by harvesting plants at 5 DMD and at 10 DMD.
For pDr treatment, plants were grown in a growth room as described above, water was withheld at 35 DAS, and pellets were kept to dry and monitored by weighing the pellets until the required pDr level was reached. Two levels were tested in this study: wilting and 1 d before wilting (prewilting).
Measurement of Growth Rate during Vegetative Stages
The rate of growth of Arabidopsis ecotype Columbia at two different developmental stages was determined as follows. Plants were grown under normal growth conditions as described above and then harvested for biomass measurement at different stages. Plants were harvested at 25 DAS for group 1, 30 DAS for group 2, and 35 DAS for group 3. These dates are the actual dates of harvest, unlike for the drought treatments described above. The rate of growth during two developmental stages, 25 to 30 DAS and 30 to 35 DAS, was calculated using the following formula: relative growth rate = (ln W 2 − ln W 1 )/( t 2 − t 1 ) ( Hoffman and Poorter, 2002 ). The growth rate was assessed based on biomass and leaf area. Leaf area was determined using ImageJ (National Institutes of Health), which was used to analyze the scanned rosettes of the drought and well-watered treatment plants. Relative expansion rate was calculated as described for biomass.
ABA and JA Mutants under mDr Screens
ABA-deficient and ABA signaling mutants and JA signaling mutants were tested under our mDr conditions. The following mutants were ordered from the Arabidopsis Biological Resource Center at Ohio State University: abi1 (SALK_076309C), coi1 (SALK_095916C), jin1 (SALK_061267C), and jar1 (CS8072) in the Columbia background and abi1 (CS22) and aba1 (CS21) in the Landsberg erecta background. Eight replications per mutant and the corresponding wild type were tested, and the performance assessed by comparing the biomass under drought with that under well-watered treatment and calculation of the RB were done as mentioned above.
Gas-Exchange Measurements
Gas-exchange measurements were done under mDr conditions in a time-course study in which five time points of mDr were tested: −1, 0, 1, 2, and 3 DMD. For the gas-exchange measurements, a LICOR 6400XT and an Arabidopsis Extended chamber were used, and the following conditions were set for LICOR measurement: flow rate of 150 μmol s −1 , CO 2 at 400 μmol, and humidity of 50%.
Biochemical Analyses
Starch analysis was done on plants treated for drought and well-watered conditions. Samples were taken at different time points of drought treatment (−1, 0, 1, 2, and 3 DMD) and from well-watered controls at the same times and stored at −80°C. Sampling was done in the late afternoon, during the period of highest starch concentration ( Caspar et al., 1985 ). The samples were ground to a fine powder under liquid nitrogen, the weight of each sample was determined, and starch was quantified using the EnzymChrom starch assay kit (BioAssay Systems) following the instructions of the manufacturer.
For ABA quantification, plant samples were harvested at different times of mDr (0, 1, and 2 DMD) and were stored at −80°C. ABA was extracted from plant samples as described ( Bray and Beachy, 1985 ), and ABA was quantified using the Phytodetek ABA test kit (Agdia) following the manufacturer’s instructions. Pro was quantified in plant samples at 3 and 4 DMD as described ( Bates et al., 1973 ).
Analysis of Gene Expression Profiles
For each of the drought experiments, mDr Day01, mDr Day10, and pDr, raw data were background corrected, normalized, and summarized according to the custom chip definition file (CDF; see below) using robust multichip average ( Ihaka and Gentleman, 1996 ; Irizarry et al., 2003 ; Gentleman et al., 2004 ), followed by nonspecific filtering of genes that do not have enough variation (interquartile range) across samples less than median interquartile range) to allow reliable detection of differential expression. A linear model was then used to detect differential expression of the remaining genes ( Smyth, 2004 ). The P values from the moderated t tests were converted to q values to correct for multiple hypothesis testing ( Storey and Tibshirani, 2003 ), and genes with q < 0.1 were declared as differentially expressed in response to the drought treatments.
Reannotation of Arabidopsis GeneChip Probe-Gene Mapping
The mapping of Affymetrix ATH1 probe sets to Arabidopsis loci provided by The Arabidopsis Information Resource (TAIR) is arrived at using the following procedure (ftp://ftp.arabidopsis.org/Microarrays/Affymetrix/README on 7/30/09). The mapping to the TAIR8 transcripts was performed using the BLASTN program with E-value cutoff ≤ 9.9e-6. For the 25-mer oligonucleotide probes used on the Affymetrix chips, the required match length to achieve this E-value is 23 or more identical nucleotides. To assign a probe set to a given locus, at least nine of the probes included in the probe set were required to match a transcript at that locus. Disregarding probe sets that map to more than one locus, this procedure results in mapping 21,180 probe sets to 21,019 genes.
TAIR as a database will wish to preserve the probe-“probe set” definitions provided by Affymetrix for users to map probe sets to genes after performing microarray analysis using the default CDF. But strictly, there are two issues in this procedure that could lead to significant inaccuracies in the estimation of gene expression: (1) a probe set mapped to a locus can contain up to two probes that do not match the locus at all and other probes that do not match the locus uniquely; (2) since multiple probe sets can map to the same locus, during analysis one has to use an ad hoc procedure to either combine information from all the mapping probe sets or choose one of the probe sets based on an arbitrary criterion. Both choices have been used in previous studies frequently.
To get around these issues and improve the mapping generally, we sought to (1) increase the stringency of mapping a 25-mer probe from 23 or more identical nucleotides to a perfect match; (2) assign a probe to a locus only when it uniquely maps to that locus; and (3) combine all the probes that uniquely map to a given locus into a single probe set, identified after the locus. Previous studies have shown that such a reannotation procedure to achieve correct mapping of probes to genes in GeneChips leads to significantly altered quantification of gene expression ( Dai et al., 2005 ).
Thus, a high-quality CDF was built for the Arabidopsis ATH1 array by uniquely mapping 232,697 probe sequences ( http://www.affymetrix.com/analysis/downloads/data/ ) to 21,389 Arabidopsis (TAIR8; Swarbreck et al., 2008 ) gene-based probe sets in the following manner: (1) probes that have perfect sequence identity with a single target gene were selected; (2) probes mapping to reverse complements of genes were annotated separately as antisense probes (not used in the above counts); and finally, (3) probes were grouped into probe sets, each corresponding to a single gene, and probe sets with at least three probes were retained (more than 99% of probe sets have five or more probes). Note that these stringent criteria used to construct the CDF make it possible to reliably measure the expression values of members of multigene families (free from cross-hybridization between paralogs showing high sequence similarity). This new custom CDF is available from the National Center for Biotechnology Information with the Gene Expression Omnibus accession number GPL10948 .
Promoter Analysis
For analysis of potential promoter-resident CREs, FIRE ( Elemento et al., 2007 ) was used to discover motifs informative about the different sets of differentially expressed genes compared with the rest of the genes in the genome. Briefly, FIRE seeks to discover motifs whose patterns of presence/absence across all considered regulatory regions (motif profile) are most informative about the expression of the corresponding genes (expression profile). To measure these associations, FIRE uses mutual information (MI; Cover and Thomas, 2006 ). FIRE performs a randomization test and considers an observed MI value (for a motif-expression profile pair) to be significant only when it is greater than all the random MI values calculated by randomly assigning the expression values to genes. A Z-score reflecting how far the observed MI value is, in number of sd s, from the average random MI is calculated. These are the Z-scores presented for each motif in Figure 7 . Moreover, it also performs jack-knife resampling ( Efron, 1979 ), where, in each of 10 trials, the above randomization test is carried out. Only motifs that are statistically significant in at least six trials are reported. Newly discovered motifs were compared with known cis-elements in the PLACE, AGRIS, and AthaMap databases ( Higo et al., 1999 ; Davuluri et al., 2003 ; Galuschka et al., 2007 ) and with each other using STAMP ( Mahony and Benos, 2007 ). All upstream sequences were obtained from TAIR. This de novo approach was taken since (1) CREs could diverge far more quickly than coding sequences across species, making them difficult to find simply by searching, and (2) searching based on known elements in Arabidopsis is limited by the scope of experimental identification in a select set of genes, making identification of degenerate yet potentially functional positions in the element difficult.
Gene Functional Enrichment Analysis
Gene function descriptions and GO annotations were downloaded from TAIR (TAIR8; Swarbreck et al., 2008 ). For enrichment analysis, biological process (BP) and cellular component (CC) branches of GO were further used. Applying the true-path rule, a gene annotated with a particular GO term was also annotated with all its parents. To avoid very generic, noninformative terms for analysis, only terms annotating 500 or fewer genes (“specific GO terms”) were retained. Genes annotated with a given specific GO term were considered as a gene set. ABA response gene sets were obtained from Nemhauser et al. (2006) . Genes containing CREs discovered de novo were further included as additional gene sets.
To adjust for multiple comparisons, a Benjamini-Hochberg false discovery rate ( q value; Benjamini and Hochberg, 1995 ) was calculated from the P values, and a q value threshold of 0.01 was used for significance.
The results from the enrichment analysis were visualized in the form of a gene set graph, where pairs of significantly overlapping gene sets (nodes) are connected to each other by edges. The graph was augmented with information about gene set size (node size), source/type (node color), and extent of overlap between gene sets (edge width). The graph was visualized using Cytoscape ( Shannon et al., 2003 ).
Gene Expression Analysis by qRT-PCR
RNA was isolated using the RNeasy Kit (Qiagen). After that, genomic DNA was eliminated using DNase I (Qiagen) digestion. The first-strand cDNA was synthesized using the iScript cDNA Synthesis Kit (Bio-Rad). Bio-Rad SYBER Green was used to quantify the expression of the genes ( Supplemental Table S4 ). Fold change of expression was calculated relative to UBQ10 (At4g05320) and SAND (At2g28390) reference genes ( Czechowski et al., 2005 ) and relative to the corresponding well-watered control as described by Livak and Schmittgen (2001) .
The Arabidopsis Genome Initiative locus identifier numbers for the genes investigated in this study are as follows: ABI1 (At4g26080), ABI2 (At5g57050), APX1 (At1g07890), CSD1 (At1g08830), CSD2 (At2g28190), EXLB1 (At4g17030), EXPA10 (At1g26770), EXPA3 (At2g37640), EXPA4 (At2g39700), EXPA8 (At2g40610), FSD1 (At4g25100), GORK (At5g37500), GPA1 (At2g26300), GPX6 (At4g11600), HAB1 (At1g72770), MYB60 (At1g08810), NCED3 (At3g14440), PLDα1 (At3g15730), PQL2 (At3g01440), PSAH2 (At1g52230), PSBQA (At4g21280), PSBW (At2g30570), RAB18 (At5g66400), RD29A (At5g52310), RD29B (At5g52300), RPK1 (At1g69270), and TPX1 (At1g65980). The gene expression data reported here are available from the National Center for Biotechnology Information with the Gene Expression Omnibus accession number GSE24177 .
Supplemental Data
The following materials are available in the online version of this article.
Supplemental Table S1 . Differentially expressed genes identified using expression profiling of mDr and pDr treatments.
Supplemental Table S2 . GO enrichment analysis of mDr- and pDr-regulated genes.
Supplemental Table S3 . cis-Regulatory element analysis of mDr- and pDr-regulated genes.
Supplemental Table S4 . List of genes and primers used in this study.
We acknowledge the help of high school students Abhi Loganathan, Sherwood Lin, and Olivia Crasta for their patient and dedicated analysis of plant drought treatments. We are thankful to Dr. Joel Shuman for help in metabolomic analysis. We are also grateful to Dr. Erik Nilsen, Dr. Ruth Grene, and Dr. Nataraja Karaba for helpful discussions during the course of this research and to Dr. Arun Shanker for helpful comments on the manuscript.
Abe H Yamaguchi-Shinozaki K Urao T Iwasaki T Hosokawa D Shinozaki K ( 1997 ) Role of Arabidopsis MYC and MYB homologs in drought- and abscisic acid-regulated gene expression . Plant Cell 9 : 1859 – 1868
Google Scholar
Aguirrezabal L Bouchier-Combaud S Radziejwoski A Dauzat M Cookson SJ Granier C ( 2006 ) Plasticity to soil water deficit in Arabidopsis thaliana : dissection of leaf development into underlying growth dynamic and cellular variables reveals invisible phenotypes . Plant Cell Environ 29 : 2216 – 2227
Alscher RG Erturk N Heath LS ( 2002 ) Role of superoxide dismutases (SODs) in controlling oxidative stress in plants . J Exp Bot 53 : 1331 – 1341
Ashburner M Ball CA Blake JA Botstein D Butler H Cherry JM Davis AP Dolinski K Dwight SS Eppig JT et al. ( 2000 ) Gene Ontology: tool for the unification of biology . Nat Genet 25 : 25 – 29
Bacon M ( 1999 ) The biochemical control of leaf expansion during drought . Plant Growth Regul 29 : 101 – 112
Bandurska H Srtoinski A Kubis J ( 2003 ) The effect of jasmonic acid on the accumulation of ABA, proline and spermidine and its influence on membrane injury under water deficit in two barley genotypes . Acta Physiol Plant 25 : 279 – 285
Bartels D Sunkar R ( 2005 ) Drought and salt tolerance in plants . Crit Rev Plant Sci 24 : 23 – 58
Bates LS Waldren RP Teare ID ( 1973 ) Rapid determination of free proline for water-stress studies . Plant Soil 39 : 205 – 207
Benjamini Y Hochberg Y ( 1995 ) Controlling the false discovery rate: a practical and powerful approach to multiple testing . J R Statist Soc B 57 : 289 – 300
Bhatnagar-Mathur P Vadez V Devi M Lavanya M Vani G Sharma K ( 2009 ) Genetic engineering of chickpea ( Cicer arietinum L.) with the P5CSF129A gene for osmoregulation with implications on drought tolerance . Mol Breed 23 : 591 – 606
Bouchabke O Chang F Simon M Voisin R Pelletier G Durand-Tardif M ( 2008 ) Natural variation in Arabidopsis thaliana as a tool for highlighting differential drought responses . PLoS One 3 : e1705
Bouchabke O Tardieu F Simonneau T ( 2006 ) Leaf growth and turgor in growing cells of maize (Zea mays L.) respond to evaporative demand under moderate irrigation but not in water saturated soil . Plant Cell Environ 29 : 1138 – 1148
Bouchabke-Coussa O Quashie ML Seoane-Redondo J Fortabat MN Gery C Yu A Linderme D Trouverie J Granier F Téoulé E et al. ( 2008 ) ESKIMO1 is a key gene involved in water economy as well as cold acclimation and salt tolerance . BMC Plant Biol 8 : 1 – 27
Boyes DC Zayed AM Ascenzi R McCaskill AJ Hoffman NE Davis KR Görlach J ( 2001 ) Growth stage-based phenotypic analysis of Arabidopsis : a model for high throughput functional genomics in plants . Plant Cell 13 : 1499 – 1510
Bray EA ( 2004 ) Genes commonly regulated by water-deficit stress in Arabidopsis thaliana . J Exp Bot 55 : 2331 – 2341
Bray EA Beachy RN ( 1985 ) Regulation by ABA of β-conglycinin expression in cultured developing soybean cotyledons . Plant Physiol 79 : 746 – 750
Caspar T Huber SC Somerville C ( 1985 ) Alterations in growth, photosynthesis, and respiration in a starchless mutant of Arabidopsis thaliana (L.) deficient in chloroplast phosphoglucomutase activity . Plant Physiol 79 : 11 – 17
Catala R Ouyang J Abreu IA Hu Y Seo H Zhang X Chua NH ( 2007 ) The Arabidopsis E3 SUMO ligase SIZ1 regulates plant growth and drought responses . Plant Cell 19 : 2952 – 2966
Chaves MM Flexas J Pinheiro C ( 2009 ) Photosynthesis under drought and salt stress: regulation mechanisms from whole plant to cell . Ann Bot (Lond) 103 : 551 – 560
Chaves MM Oliveira MM ( 2004 ) Mechanisms underlying plant resilience to water deficits: prospects for water-saving agriculture . J Exp Bot 55 : 2365 – 2384
Chen F Dahal P Bradford KJ ( 2001 ) Two tomato expansin genes show divergent expression and localization in embryos during seed development and germination . Plant Physiol 127 : 928 – 936
Cho HT Cosgrove DJ ( 2000 ) Altered expression of expansin modulates leaf growth and pedicel abscission in Arabidopsis thaliana . Proc Natl Acad Sci USA 97 : 9783 – 9788
Choi D Lee Y Cho HT Kende H ( 2003 ) Regulation of expansin gene expression affects growth and development in transgenic rice plants . Plant Cell 15 : 1386 – 1398
Cominelli E Galbiati M Vavasseur A Conti L Sala T Vuylsteke M Leonhardt N Dellaporta SL Tonelli C ( 2005 ) A guard-cell-specific MYB transcription factor regulates stomatal movements and plant drought tolerance . Curr Biol 15 : 1196 – 1200
Cornic G Massacci A ( 1996 ) Leaf photosynthesis under drought stress . In Baker NR , ed , Photosynthesis and the Environment . Kluwer Academic , New York , pp 347–366
Cosgrove DJ ( 2005 ) Growth of the plant cell wall . Nat Rev Mol Cell Biol 6 : 850 – 861
Cover TM Thomas JA ( 2006 ) Elements of Information Theory, Ed 2 . Wiley-Interscience , Hoboken, NJ
Creelman RA Mullet JE ( 1995 ) Jasmonic acid distribution and action in plants: regulation during development and response to biotic and abiotic stress . Proc Natl Acad Sci USA 92 : 4114 – 4119
Crooks GE Hon G Chandonia JM Brenner SE ( 2004 ) WebLogo: a sequence logo generator . Genome Res 14 : 1188 – 1190
Cruz de Carvalho MH ( 2008 ) Drought stress and reactive oxygen species: production, scavenging and signaling . Plant Signal Behav 3 : 156 – 165
Cutler SR Rodriguez PL Finkelstein RR Abrams SR ( 2010 ) Abscisic acid: emergence of a core signaling network . Annu Rev Plant Biol 61 : 651 – 679
Czechowski T Stitt M Altmann T Udvardi MK Scheible WR ( 2005 ) Genome-wide identification and testing of superior reference genes for transcript normalization in Arabidopsis . Plant Physiol 139 : 5 – 17
Dai M Wang P Boyd AD Kostov G Athey B Jones EG Bunney WE Myers RM Speed TP Akil H et al. ( 2005 ) Evolving gene/transcript definitions significantly alter the interpretation of GeneChip data . Nucleic Acids Res 33 : e175
Davuluri RV Sun H Palaniswamy SK Matthews N Molina C Kurtz M Grotewold E ( 2003 ) AGRIS: Arabidopsis Gene Regulatory Information Server, an information resource of Arabidopsis cis-regulatory elements and transcription factors . BMC Bioinformatics 4 : 25
Devoto A Ellis C Magusin A Chang HS Chilcott C Zhu T Turner JG ( 2005 ) Expression profiling reveals COI1 to be a key regulator of genes involved in wound- and methyl jasmonate-induced secondary metabolism, defence, and hormone interactions . Plant Mol Biol 58 : 497 – 513
Efron BL ( 1979 ) Bootstrap methods: another look at the jackknife . Ann Stat 7 : 1 – 26
Elemento O Slonim N Tavazoie S ( 2007 ) A universal framework for regulatory element discovery across all genomes and data types . Mol Cell 28 : 337 – 350
Ellis C Karafyllidis I Wasternack C Turner JG ( 2002 ) The Arabidopsis mutant cev1 links cell wall signaling to jasmonate and ethylene responses . Plant Cell 14 : 1557 – 1566
Flexas J Medrano H ( 2002 ) Drought-inhibition of photosynthesis in C3 plants: stomatal and non-stomatal limitations revisited . Ann Bot (Lond) 89 : 183 – 189
Galuschka C Schindler M Bülow L Hehl R ( 2007 ) AthaMap Web tools for the analysis and identification of co-regulated genes . Nucleic Acids Res 35 : D857 – D862
Gentleman RC Carey VJ Bates DM Bolstad B Dettling M Dudoit S Ellis B Gautier L Ge Y Gentry J et al. ( 2004 ) Bioconductor: open software development for computational biology and bioinformatics . Genome Biol 5 : R80
Gosti F Beaudoin N Serizet C Webb AA Vartanian N Giraudat J ( 1999 ) ABI1 protein phosphatase 2C is a negative regulator of abscisic acid signaling . Plant Cell 11 : 1897 – 1910
Granier C Aguirrezabal L Chenu K Cookson SJ Dauzat M Hamard P Thioux JJ Rolland G Bouchier-Combaud S Lebaudy A et al. ( 2006 ) PHENOPSIS, an automated platform for reproducible phenotyping of plant responses to soil water deficit in Arabidopsis thaliana permitted the identification of an accession with low sensitivity to soil water deficit . New Phytol 169 : 623 – 635
Granier C Tardieu F ( 2009 ) Multi-scale phenotyping of leaf expansion in response to environmental changes: the whole is more than the sum of parts . Plant Cell Environ 32 : 1175 – 1184
Grene R ( 2002 ) Oxidative stress and acclimation mechanisms in plants . In Somerville CR Meyerowitz EM , eds, The Arabidopsis Book . American Society of Plant Biologists , Rockville, MD , doi/10.1199/tab.0036.1, http//aspb.org/publications/arabidopsis/
Harmer SL Hogenesch JB Straume M Chang HS Han B Zhu T Wang X Kreps JA Kay SA ( 2000 ) Orchestrated transcription of key pathways in Arabidopsis by the circadian clock . Science 290 : 2110 – 2113
Hattori T Totsuka M Hobo T Kagaya Y Yamamoto-Toyoda A ( 2002 ) Experimentally determined sequence requirement of ACGT-containing abscisic acid response element . Plant Cell Physiol 43 : 136 – 140
Herde O Pena-Cortes H Willmitzer L Fisahn J ( 1997 ) Stomatal responses to jasmonic acid, linolenic acid and abscisic acid in wild-type and ABA-deficient tomato plants . Plant Cell Environ 20 : 136 – 141
Higo K Ugawa Y Iwamoto M Korenaga T ( 1999 ) Plant cis-acting regulatory DNA elements (PLACE) database: 1999 . Nucleic Acids Res 27 : 297 – 300
Hirayama T Shinozaki K ( 2010 ) Research on plant abiotic stress responses in the post-genome era: past, present and future . Plant J 61 : 1041 – 1052
Hobo T Asada M Kowyama Y Hattori T ( 1999 ) ACGT-containing abscisic acid response element (ABRE) and coupling element 3 (CE3) are functionally equivalent . Plant J 19 : 679 – 689
Hoffman W Poorter H ( 2002 ) Avoiding bias in calculations of relative growth rate . Ann Bot (Lond) 80 : 37 – 42
Horiguchi G Ferjani A Fujikura U Tsukaya H ( 2006 ) Coordination of cell proliferation and cell expansion in the control of leaf size in Arabidopsis thaliana . J Plant Res 119 : 37 – 42
Huang D Jaradat MR Wu W Ambrose SJ Ross AR Abrams SR Cutler AJ ( 2007 ) Structural analogs of ABA reveal novel features of ABA perception and signaling in Arabidopsis . Plant J 50 : 414 – 428
Huang D Wu W Abrams SR Cutler AJ ( 2008 ) The relationship of drought-related gene expression in Arabidopsis thaliana to hormonal and environmental factors . J Exp Bot 59 : 2991 – 3007
Ihaka R Gentleman R ( 1996 ) R: a language for data analysis and graphics . J Comput Graph Statist 5 : 299 – 314
Ingram J Bartels D ( 1996 ) The molecular basis of dehydration tolerance in plants . Annu Rev Plant Physiol Plant Mol Biol 47 : 377 – 403
Irizarry RA Bolstad BM Collin F Cope LM Hobbs B Speed TP ( 2003 ) Summaries of Affymetrix GeneChip probe level data . Nucleic Acids Res 31 : e15
Jones L McQueen-Mason S ( 2004 ) A role for expansins in dehydration and rehydration of the resurrection plant Craterostigma plantagineum . FEBS Lett 559 : 61 – 65
Kim TH Böhmer M Hu H Nishimura N Schroeder JI ( 2010 ) Guard cell signal transduction network: advances in understanding abscisic acid, CO 2 , and Ca 2+ signaling . Annu Rev Plant Biol 61 : 561 – 591
Kliebenstein DJ Monde RA Last RL ( 1998 ) Superoxide dismutase in Arabidopsis: an eclectic enzyme family with disparate regulation and protein localization . Plant Physiol 118 : 637 – 650
Krishnan A Pereira A ( 2008 ) Integrative approaches for mining transcriptional regulatory programs in Arabidopsis . Brief Funct Genomics Proteomics 7 : 264 – 274
Kwak JM Mori IC Pei ZM Leonhardt N Torres MA Dangl JL Bloom RE Bodde S Jones JD Schroeder JI ( 2003 ) NADPH oxidase AtrbohD and AtrbohF genes function in ROS-dependent ABA signaling in Arabidopsis . EMBO J 22 : 2623 – 2633
Kwon YR Lee HJ Kim KH Hong SW Lee SJ Lee H ( 2008 ) Ectopic expression of Expansin3 or Expansinbeta1 causes enhanced hormone and salt stress sensitivity in Arabidopsis . Biotechnol Lett 30 : 1281 – 1288
Levitt J ( 1980 ) Responses of Plants to Environmental Stresses . Vol 2 . Water, Radiation, Salt and other Stresses . Academic Press , New York , pp 93–128
Livak KJ Schmittgen TD ( 2001 ) Analysis of relative gene expression data using real-time quantitative PCR and the 2(-Delta Delta C(T)) method . Methods 25 : 402 – 408
Mahony S Benos PV ( 2007 ) STAMP: a Web tool for exploring DNA-binding motif similarities . Nucleic Acids Res 35 : W253 – W258
Martínez IM Chrispeels MJ ( 2003 ) Genomic analysis of the unfolded protein response in Arabidopsis shows its connection to important cellular processes . Plant Cell 15 : 561 – 576
Masle J Gilmore SR Farquhar GD ( 2005 ) The ERECTA gene regulates plant transpiration efficiency in Arabidopsis . Nature 436 : 866 – 870
Mathews M Volkenburgh E Boyer J ( 1984 ) Acclimation of leaf growth to low water potentials in sunflower . Plant Cell Environ 7 : 199 – 206
McQueen-Mason S Durachko DM Cosgrove DJ ( 1992 ) Two endogenous proteins that induce cell wall extension in plants . Plant Cell 4 : 1425 – 1433
Miao Y Lv D Wang P Wang XC Chen J Miao C Song CP ( 2006 ) An Arabidopsis glutathione peroxidase functions as both a redox transducer and a scavenger in abscisic acid and drought stress responses . Plant Cell 18 : 2749 – 2766
Miller G Suzuki N Ciftci-Yilmaz S Mittler R ( 2010 ) Reactive oxygen species homeostasis and signalling during drought and salinity stresses . Plant Cell Environ 33 : 453 – 467
Mishra G Zhang W Deng F Zhao J Wang X ( 2006 ) A bifurcating pathway directs abscisic acid effects on stomatal closure and opening in Arabidopsis . Science 312 : 264 – 266
Moore JP Vicré-Gibouin M Farrant JM Driouich A ( 2008 ) Adaptations of higher plant cell walls to water loss: drought vs desiccation . Physiol Plant 134 : 237 – 245
Muller B Bourdais G Reidy B Bencivenni C Massonneau A Condamine P Rolland G Conéjéro G Rogowsky P Tardieu F ( 2007 ) Association of specific expansins with growth in maize leaves is maintained under environmental, genetic, and developmental sources of variation . Plant Physiol 143 : 278 – 290
Munemasa S Oda K Watanabe-Sugimoto M Nakamura Y Shimoishi Y Murata Y ( 2007 ) The coronatine-insensitive 1 mutation reveals the hormonal signaling interaction between abscisic acid and methyl jasmonate in Arabidopsis guard cells: specific impairment of ion channel activation and second messenger production . Plant Physiol 143 : 1398 – 1407
Nakashima K Ito Y Yamaguchi-Shinozaki K ( 2009 ) Transcriptional regulatory networks in response to abiotic stresses in Arabidopsis and grasses . Plant Physiol 149 : 88 – 95
Narusaka Y Nakashima K Shinwari ZK Sakuma Y Furihata T Abe H Narusaka M Shinozaki K Yamaguchi-Shinozaki K ( 2003 ) Interaction between two cis-acting elements, ABRE and DRE, in ABA-dependent expression of Arabidopsis rd29A gene in response to dehydration and high-salinity stresses . Plant J 34 : 137 – 148
Nelson DE Repetti PP Adams TR Creelman RA Wu J Warner DC Anstrom DC Bensen RJ Castiglioni PP Donnarummo MG et al. ( 2007 ) Plant nuclear factor Y (NF-Y) B subunits confer drought tolerance and lead to improved corn yields on water-limited acres . Proc Natl Acad Sci USA 104 : 16450 – 16455
Nemhauser JL Hong F Chory J ( 2006 ) Different plant hormones regulate similar processes through largely nonoverlapping transcriptional responses . Cell 126 : 467 – 475
Neumann P ( 1995 ) The role of cell wall adjustment in plant resistance to water deficits . Crop Sci 35 : 1258 – 1266
Nilson SE Assmann SM ( 2007 ) The control of transpiration: insights from Arabidopsis . Plant Physiol 143 : 19 – 27
Nilson SE Assmann SM ( 2010 ) The alpha-subunit of the Arabidopsis heterotrimeric G protein, GPA1, is a regulator of transpiration efficiency . Plant Physiol 152 : 2067 – 2077
Ning J Li X Hicks LM Xiong L ( 2010 ) A Raf-like MAPKKK gene DSM1 mediates drought resistance through reactive oxygen species scavenging in rice . Plant Physiol 152 : 876 – 890
Noctor G Veljovic-Jovanovic S Driscoll S Novitskaya L Foyer CH ( 2002 ) Drought and oxidative load in the leaves of C3 plants: a predominant role for photorespiration? Ann Bot (Lond) 89 : 841 – 850
Olsson AS Engström P Söderman E ( 2004 ) The homeobox genes ATHB12 and ATHB7 encode potential regulators of growth in response to water deficit in Arabidopsis . Plant Mol Biol 55 : 663 – 677
Onkokesung N Gális I von Dahl CC Matsuoka K Saluz HP Baldwin IT ( 2010 ) Jasmonic acid and ethylene modulate local responses to wounding and simulated herbivory in Nicotiana attenuata leaves . Plant Physiol 153 : 785 – 798
Oono Y Seki M Nanjo T Narusaka M Fujita M Satoh R Satou M Sakurai T Ishida J Akiyama K et al. ( 2003 ) Monitoring expression profiles of Arabidopsis gene expression during rehydration process after dehydration using ca 7000 full-length cDNA microarray . Plant J 34 : 868 – 887
Osakabe Y Maruyama K Seki M Satou M Shinozaki K Yamaguchi-Shinozaki K ( 2005 ) Leucine-rich repeat receptor-like kinase1 is a key membrane-bound regulator of abscisic acid early signaling in Arabidopsis . Plant Cell 17 : 1105 – 1119
Osakabe Y Mizuno S Tanaka H Maruyama K Osakabe K Todaka D Fujita Y Kobayashi M Shinozaki K Yamaguchi-Shinozaki K ( 2010 ) Overproduction of the membrane-bound receptor-like protein kinase 1, RPK1, enhances abiotic stress tolerance in Arabidopsis . J Biol Chem 285 : 9190 – 9201
Pedro R ( 1998 ) Protein phosphatase 2C (PP2C) function in higher plants . Plant Mol Biol 38 : 919 – 927
Pham J Desikan R ( 2009 ) ROS signaling in stomata . In del Río LA Puppo A , eds, Reactive Oxygen Species in Plant Signaling . Springer , Berlin , pp 55–71
Price AH Cairns JE Horton P Jones HG Griffiths H ( 2002 ) Linking drought-resistance mechanisms to drought avoidance in upland rice using a QTL approach: progress and new opportunities to integrate stomatal and mesophyll responses . J Exp Bot 53 : 989 – 1004
Raghavendra AS Reddy KB ( 1987 ) Action of proline on stomata differs from that of abscisic acid, G-substances, or methyl jasmonate . Plant Physiol 83 : 732 – 734
Ramanjulu S Bartels D ( 2002 ) Drought- and desiccation-induced modulation of gene expression in plants . Plant Cell Environ 25 : 141 – 151
Reinbothe S Mollenhauer B Reinbothe C ( 1994 ) JIPs and RIPs: the regulation of plant gene expression by jasmonates in response to environmental cues and pathogens . Plant Cell 6 : 1197 – 1209
Rodriguez Milla MA Maurer A Rodriguez Huete A Gustafson JP ( 2003 ) Glutathione peroxidase genes in Arabidopsis are ubiquitous and regulated by abiotic stresses through diverse signaling pathways . Plant J 36 : 602 – 615
Rosegrant MW Cline SA ( 2003 ) Global food security: challenges and policies . Science 302 : 1917 – 1919
Saez A Robert N Maktabi MH Schroeder JI Serrano R Rodriguez PL ( 2006 ) Enhancement of abscisic acid sensitivity and reduction of water consumption in Arabidopsis by combined inactivation of the protein phosphatases type 2C ABI1 and HAB1 . Plant Physiol 141 : 1389 – 1399
Sakuma Y Maruyama K Osakabe Y Qin F Seki M Shinozaki K Yamaguchi-Shinozaki K ( 2006 ) Functional analysis of an Arabidopsis transcription factor, DREB2A, involved in drought-responsive gene expression . Plant Cell 18 : 1292 – 1309
Schroeder JI Allen GJ Hugouvieux V Kwak JM Waner D ( 2001 ) Guard cell signal transduction . Annu Rev Plant Physiol Plant Mol Biol 52 : 627 – 658
Shannon P Markiel A Ozier O Baliga NS Wang JT Ramage D Amin N Schwikowski B Ideker T ( 2003 ) Cytoscape: a software environment for integrated models of biomolecular interaction networks . Genome Res 13 : 2498 – 2504
Shinozaki K Yamaguchi-Shinozaki K ( 1997 ) Gene expression and signal transduction in water-stress response . Plant Physiol 115 : 327 – 334
Shinozaki K Yamaguchi-Shinozaki K ( 2007 ) Gene networks involved in drought stress response and tolerance . J Exp Bot 58 : 221 – 227
Skirycz A De Bodt S Obata T De Clercq I Claeys H De Rycke R Andriankaja M Van Aken O Van Breusegem F Fernie AR et al. ( 2010 ) Developmental stage specificity and the role of mitochondrial metabolism in the response of Arabidopsis leaves to prolonged mild osmotic stress . Plant Physiol 152 : 226 – 244
Skirycz A Inze D ( 2010 ) More from less: plant growth under limited water . Curr Opin Biotechnol 21 : 1 – 7
Slesak I Libik M Karpinska B Karpinski S Miszalski Z ( 2007 ) The role of hydrogen peroxide in regulation of plant metabolism and cellular signalling in response to environmental stresses . Acta Biochim Pol 54 : 39 – 50
Smyth GK ( 2004 ) Linear models and empirical Bayes methods for assessing differential expression in microarray experiments . Stat Appl Genet Mol Biol 3 : Article 3
Staswick PE Tiryaki I Rowe ML ( 2002 ) Jasmonate response locus JAR1 and several related Arabidopsis genes encode enzymes of the firefly luciferase superfamily that show activity on jasmonic, salicylic, and indole-3-acetic acids in an assay for adenylation . Plant Cell 14 : 1405 – 1415
Storey JD Tibshirani R ( 2003 ) Statistical significance for genomewide studies . Proc Natl Acad Sci USA 100 : 9440 – 9445
Suhita D Raghavendra AS Kwak JM Vavasseur A ( 2004 ) Cytoplasmic alkalization precedes reactive oxygen species production during methyl jasmonate- and abscisic acid-induced stomatal closure . Plant Physiol 134 : 1536 – 1545
Swarbreck D Wilks C Lamesch P Berardini TZ Garcia-Hernandez M Foerster H Li D Meyer T Muller R Ploetz L et al. ( 2008 ) The Arabidopsis Information Resource (TAIR): gene structure and function annotation . Nucleic Acids Res 36 : D1009 – D1014
Tähtiharju S Palva T ( 2001 ) Antisense inhibition of protein phosphatase 2C accelerates cold acclimation in Arabidopsis thaliana . Plant J 26 : 461 – 470
Terzaghi WB Cashmore AR ( 1995 ) Light-regulated transcription . Annu Rev Plant Physiol Plant Mol Biol 46 : 445 – 474
Ueguchi C Koizumi H Suzuki T Mizuno T ( 2001 ) Novel family of sensor histidine kinase genes in Arabidopsis thaliana . Plant Cell Physiol 42 : 231 – 235
Umezawa T Sugiyama N Mizoguchi M Hayashi S Myouga F Yamaguchi-Shinozaki K Ishihama Y Hirayama T Shinozaki K Umezawa T et al. ( 2004 ) SRK2C, a SNF1-related protein kinase 2, improves drought tolerance by controlling stress-responsive gene expression in Arabidopsis thaliana . Proc Natl Acad Sci USA 101 : 17306 – 17311
Urao T Yakubov B Satoh R Yamaguchi-Shinozaki K Seki M Hirayama T Shinozaki K ( 1999 ) A transmembrane hybrid-type histidine kinase in Arabidopsis functions as an osmosensor . Plant Cell 11 : 1743 – 1754
Vásquez-Robinet C Watkinson JI Sioson AA Ramakrishnan N Heath LS Grene R ( 2010 ) Differential expression of heat shock protein genes in preconditioning for photosynthetic acclimation in water-stressed loblolly pine . Plant Physiol Biochem 48 : 256 – 264
Vogler H Caderas D Mandel T Kuhlemeier C ( 2003 ) Domains of expansin gene expression define growth regions in the shoot apex of tomato . Plant Mol Biol 53 : 267 – 272
Wang ZY Kenigsbuch D Sun L Harel E Ong MS Tobin EM ( 1997 ) A Myb-related transcription factor is involved in the phytochrome regulation of an Arabidopsis Lhcb gene . Plant Cell 9 : 491 – 507
Watkinson JI Sioson AA Vasquez-Robinet C Shukla M Kumar D Ellis M Heath LS Ramakrishnan N Chevone B Watson LT et al. ( 2003 ) Photosynthetic acclimation is reflected in specific patterns of gene expression in drought-stressed loblolly pine . Plant Physiol 133 : 1702 – 1716
Wohlbach DJ Quirino BF Sussman MR ( 2008 ) Analysis of the Arabidopsis histidine kinase ATHK1 reveals a connection between vegetative osmotic stress sensing and seed maturation . Plant Cell 20 : 1101 – 1117
Wu Y Cosgrove DJ ( 2000 ) Adaptation of roots to low water potentials by changes in cell wall extensibility and cell wall proteins . J Exp Bot 51 : 1543 – 1553
Xie DX Feys BF James S Nieto-Rostro M Turner JG ( 1998 ) COI1: an Arabidopsis gene required for jasmonate-regulated defense and fertility . Science 280 : 1091 – 1094
Yamaguchi-Shinozaki K Shinozaki K ( 1994 ) A novel cis-acting element in an Arabidopsis gene is involved in responsiveness to drought, low-temperature, or high-salt stress . Plant Cell 6 : 251 – 264
Yancey P ( 2001 ) Water stress, osmolytes and proteins . Am Zool 41 : 699 – 709
Yancey PH Clark ME Hand SC Bowlus RD Somero GN ( 1982 ) Living with water stress: evolution of osmolyte systems . Science 217 : 1214 – 1222
Yu H Chen X Hong YY Wang Y Xu P Ke SD Liu HY Zhu JK Oliver DJ Xiang CB ( 2008 ) Activated expression of an Arabidopsis HD-START protein confers drought tolerance with improved root system and reduced stomatal density . Plant Cell 20 : 1134 – 1151
Zeeman SC Rees T ( 1999 ) Changes in carbohydrate metabolism and assimilate export in starch-excess mutants of Arabidopsis . Plant Cell Environ 22 : 1445 – 1453
Zhang Y Turner JG ( 2008 ) Wound-induced endogenous jasmonates stunt plant growth by inhibiting mitosis . PLoS One 3 : e3699
Zhang Y Zhu H Zhang Q Li M Yan M Wang R Wang L Welti R Zhang W Wang X ( 2009 ) Phospholipase Dα1 and phosphatidic acid regulate NADPH oxidase activity and production of reactive oxygen species in ABA-mediated stomatal closure in Arabidopsis . Plant Cell 21 : 2357 – 2377
Zhao Z Stanley BA Zhang W Assmann SM ( 2010 ) ABA-regulated G protein signaling in Arabidopsis guard cells: a proteomic perspective . J Proteome Res 9 : 1637 – 1647
Author notes
Supplementary data, email alerts, citing articles via.
- Recommend to Your Librarian
- Advertising & Corporate Services
- Awards & Funding
- Plant Science Today
- Plant Biology Meeting
- Meeting Management Services
- Plant Science Research Weekly
- Taproot: A Plantae Podcast
Affiliations
- Online ISSN 1532-2548
- Print ISSN 0032-0889
- Copyright © 2024 American Society of Plant Biologists
- About Oxford Academic
- Publish journals with us
- University press partners
- What we publish
- New features
- Open access
- Institutional account management
- Rights and permissions
- Get help with access
- Accessibility
- Advertising
- Media enquiries
- Oxford University Press
- Oxford Languages
- University of Oxford
Oxford University Press is a department of the University of Oxford. It furthers the University's objective of excellence in research, scholarship, and education by publishing worldwide
- Copyright © 2024 Oxford University Press
- Cookie settings
- Cookie policy
- Privacy policy
- Legal notice
This Feature Is Available To Subscribers Only
Sign In or Create an Account
This PDF is available to Subscribers Only
For full access to this pdf, sign in to an existing account, or purchase an annual subscription.
Click through the PLOS taxonomy to find articles in your field.
For more information about PLOS Subject Areas, click here .
Loading metrics
Open Access
Peer-reviewed
Research Article
Meta-analysis of drought-tolerant genotypes in Oryza sativa : A network-based approach
Roles Conceptualization, Data curation, Formal analysis, Writing – original draft, Writing – review & editing
Affiliation Centre for Computational Natural Sciences and Bioinformatics, International Institute of Information Technology, Hyderabad, India
Roles Conceptualization, Supervision, Writing – original draft, Writing – review & editing
* E-mail: [email protected]

- Sanchari Sircar,
- Nita Parekh
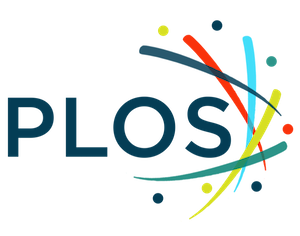
- Published: May 6, 2019
- https://doi.org/10.1371/journal.pone.0216068
- Reader Comments
Drought is a severe environmental stress. It is estimated that about 50% of the world rice production is affected mainly by drought. Apart from conventional breeding strategies to develop drought-tolerant crops, innovative computational approaches may provide insights into the underlying molecular mechanisms of stress response and identify drought-responsive markers. Here we propose a network-based computational approach involving a meta-analytic study of seven drought-tolerant rice genotypes under drought stress.
Co-expression networks enable large-scale analysis of gene-pair associations and tightly coupled clusters that may represent coordinated biological processes. Considering differentially expressed genes in the co-expressed modules and supplementing external information such as resistance/tolerance QTLs, transcription factors, network-based topological measures, we identify and prioritize drought-adaptive co-expressed gene modules and potential candidate genes. Using the candidate genes that are well-represented across the datasets as ‘seed’ genes, two drought-specific protein-protein interaction networks (PPINs) are constructed with up- and down-regulated genes. Cluster analysis of the up-regulated PPIN revealed ABA signalling pathway as a central process in drought response with a probable crosstalk with energy metabolic processes. Tightly coupled gene clusters representing up-regulation of core cellular respiratory processes and enhanced degradation of branched chain amino acids and cell wall metabolism are identified. Cluster analysis of down-regulated PPIN provides a snapshot of major processes associated with photosynthesis, growth, development and protein synthesis, most of which are shut down during drought. Differential regulation of phytohormones, e.g., jasmonic acid, cell wall metabolism, signalling and posttranslational modifications associated with biotic stress are elucidated. Functional characterization of topologically important, drought-responsive uncharacterized genes that may play a role in important processes such as ABA signalling, calcium signalling, photosynthesis and cell wall metabolism is discussed. Further transgenic studies on these genes may help in elucidating their biological role under stress conditions.
Currently, a large number of resources for rice functional genomics exist which are mostly underutilized by the scientific community. In this study, a computational approach integrating information from various resources such as gene co-expression networks, protein-protein interactions and pathway-level information is proposed to provide a systems-level view of complex drought-responsive processes across the drought-tolerant genotypes.
Citation: Sircar S, Parekh N (2019) Meta-analysis of drought-tolerant genotypes in Oryza sativa : A network-based approach. PLoS ONE 14(5): e0216068. https://doi.org/10.1371/journal.pone.0216068
Editor: Niranjan Baisakh, Louisiana State University, UNITED STATES
Received: December 15, 2018; Accepted: April 12, 2019; Published: May 6, 2019
Copyright: © 2019 Sircar, Parekh. This is an open access article distributed under the terms of the Creative Commons Attribution License , which permits unrestricted use, distribution, and reproduction in any medium, provided the original author and source are credited.
Data Availability: All relevant data are within the manuscript and its Supporting Information files.
Funding: The authors received no specific funding for this work.
Competing interests: The authors have declared that no competing interests exist.
Drought is an inevitable consequence of drastic climate change. A look at the World Drought Map for the year 2017 from the Global Drought Information System [ 1 ] reveals the severity and intensity of drought across the globe. At this juncture, meeting the nutritional demands for an ever-increasing population is a challenge for policy makers. Although drought and heat waves have destroyed nearly a tenth of the cereal harvests, populations across the world continue to depend on them for food.
Irrigated rice occupies half the world’s rice field and accounts for ~75% of the global rice supply. The yield of this water-intensive crop is highly susceptible to climate change. Abiotic stress conditions like drought induces a wide array of physiological, morphological and molecular changes which might provide cues towards drought-responsive/adaptive mechanisms. However, these approaches are limited by the fact that nearly ~50% of the rice genes lack functional annotation for biological processes [ 2 ]. Analysis of high-throughput data (e.g., microarrays, RNASeq, proteomics, etc.) by computational methods such as network-based approaches, comparative genomics, etc. can be effective in functional characterization of rice genes, reducing the search space for identification of novel candidate genes and elucidating biological processes under various environmental conditions. Various drought tolerant rice genotypes exist, such as upland indica variety Bala with a high stomatal sensitivity to water loss and upland japonica variety Azucena with thick and deep roots. The deep-rooted and stress tolerant aus rice cultivar Nagina 22 (N22) is known to have enhanced enzymatic activity, α-linolenic acid metabolism and heat shock proteins [ 3 , 4 ]. Studies performed with indigenous cultivar Dagad Deshi [ 5 ] also identified quantitative traits associated with grain yield. The drought tolerant upland variety IRAT109 is known for its superior yield and other parameters compared to the lowland Zhenshan97 under drought stress [ 6 ] and have been used for studying traits like osmotic potential [ 7 ]. Thus there has been a continuous effort in conventional breeding strategies to develop high-yielding drought-tolerant rice varieties.
Currently, a large number of gene-expression data corresponding to drought tolerant genotypes are available in public domain. Systematic meta-analysis of these datasets can help in increasing the reproducibility and provide new biological insights. Numerous methods are available to merge gene expression data and remove batch effects, viz ., Z-score standardization, mean centering [ 8 ], cross-platform normalization [ 9 ], empirical Bayes method for smaller batch size [ 10 ], etc. Network-based approaches help in summarizing large-scale data with thousands of genes into manageable clusters or functional modules. Further, these approaches help to prioritize important genes based on their topological parameters such as degree, betweenness and eigen-gene centralities, apart from fold-change and functional enrichment. For example, co-expression networks are popularly used to draw relationship between genes and identify tightly coordinated biological processes and functional characterization of novel genes [ 11 – 15 ]. An integrative approach combining co-expression networks with protein-protein interactions and metabolic pathway information can help in associating genotype to phenotype to a considerable extent [ 16 – 18 ].
In this study, we carried out meta-analysis of drought-tolerant rice genotypes under drought stress using network-based approach with the objective of identifying gene signatures and processes that are hallmark of drought tolerant species. For this analysis, from the six microarray datasets containing seven drought-tolerant genotypes (Azucena, Bala, Nagina, Dagad deshi, Vandana, IRAT109 and Oryza DK151), 57 samples corresponding to the shoot tissue and seedlings from whole plant (Affymetrix platform) are considered ( Table 1 ). Samples corresponding to drought-sensitive genotypes or root tissue in these datasets are not considered for the analysis (see S1 Table for dataset details). The R package, Weighted Gene Co-expression Network Analysis (WGCNA), is used to capture interaction patterns between genes [ 19 ] resulting in co-expressed gene modules. These co-expressed modules are associated with additional information such as enrichment of differentially expressed genes, transcription factors, known stress/tolerance associated QTLs, module-trait associations and gene ontology, to identify drought-responsive modules. The key driver genes in these modules are identified using topological network parameters and up- and down-regulated protein-protein interaction networks are constructed from known interaction of these genes. Tightly coupled gene clusters that may be associated to various signalling and metabolic processes likely to be affected due to drought are analyzed.
- PPT PowerPoint slide
- PNG larger image
- TIFF original image
https://doi.org/10.1371/journal.pone.0216068.t001
For this analysis, six microarray datasets containing seven drought-tolerant genotypes (Azucena, Bala, Nagina, Dagad deshi, Vandana, IRAT109 and Oryza DK151), corresponding to Affymetrix Rice Genome Array from NCBI (platform accession: GPL2025) and the EBI ArrayExpress Archive are considered. The details of the datasets are summarized in Table 1 . The validation of gene expression by real-time PCR has been reported for five out of six studies and shown to exhibit good correlation with the microarray data generated. The conditions, stages and tissues are given as follows:
- GSE41647 : Hydroponically grown 7-day-old seedlings of Dagad deshi, subjected to drought stress by placing them on 3 mm Whatmann sheets under light for 3 h and 6 h at 28±1°C. For control samples, seedlings were kept in RGM (root growth medium) for 6 h at 28±1°C.
- E-MEXP-2401 : Seedlings from Nagina-22 (N-22, tolerant) were grown side by side in plastic pots for 14 days at 28±1°C with a daily photoperiodic cycle of 14/10 h light/dark provided by fluorescent tubes. Sterile absorbent cotton soaked with Hoagland’s solution was used as seed bed for growing rice seedlings. Water stress was given to the plants by withholding water supply till visible leaf rolling appeared in the plants.
- GSE21651 : For drought stress, seeds of Vandana were sown in Hoaglands nutrient solution. After 14 days seedlings were blot dried and kept in air for 12 hrs and leaf samples were collected for RNA extraction.
- GSE26280 : Drought tolerant rice line, DK151 (an F7 line derived from a cross between two DT IR64 introgression lines (ILs), DGI 187 and DGI 74) were used gene expression patterns across leaves and roots at tillering stage and panicle elongation stage, leaves and young panicle at booting stage. Stress at three different stages were applied: 4-tiller (tillering) stage, panicle elongation stage, and booting stage. Plants were stressed until the leaf became fully rolled at noon (RWC: 65%-75%). It took three and two days for drought stress to become apparent at the tillering and panicle elongation stages and at booting stage, respectively. Both leaf and root samples were collected for the first two stages, and leaves and young panicle samples were collected at the booting stage. In the current study, only leaf tissue was considered.
- GSE24048 : two drought tolerant cultivars, Bala and Azucena were grown in 1.2 m2 plots under flooded conditions. Starting at 59 days after sowing, drought was imposed by withholding water, while a set of control plots had continued flooding conditions. The drought was imposed for 24 days during which time a small amount of water was added on 3 occasions to raise soil moisture to 30% by volume. After 24 days the second youngest fully expanded leaf was taken and gene expression analysis performed.
- GSE25176 : IRAT109 (drought-resistant) were grown in PVC pipes. For half of the plants, two drought-rehydration cycles were performed to each plant in PVC pipes at the booting stage (about 14 days before flowering). When all leaves of a stressed rice plant became completely rolled, watering was applied to the full capacity of the pipe, and the second cycle of drought stress was applied until all leaves became completely rolled again and watering was resumed for the rest of the lifecycle. Four samples with RWC in the range of 94%–95% (no stress, D0), 83%–88% (slight drought in which leaves were slightly rolled, D1), 74%–78% (moderate drought in which about half of each leaf was rolled, D2) and 65%–69% (severe drought in which all leaves were completely rolled, D3) were collected for expression profiling analysis. For the current study, DEGs at D3 vs . D0 were considered.
Data Pre-processing
A first step in any transcriptomic studies is to check the data quality. All the samples were found to pass quality check on using the package, ArrayQualityMetrics that assesses reproducibility, outlier arrays, batch effects and computes measures of signal-to-noise ratio [ 20 ]. Datasets were normalized using Robust Multiarray Averaging (RMA) package to remove systematic variations due to different experimental conditions, dye effects, uneven hybridizations, etc. [ 21 ]. In a meta-analytic approach, when multiple datasets from different experimental conditions are combined, batch-specific variations need to be eliminated. For this, COMBAT, an Empirical Bayes method in the R package inSilicoMerging, was used to merge the expression values across the datasets [ 10 ]. Probes having no gene annotations, mapping to more than one gene annotation (ambiguous probes), or having very low intensity values (< 20 across all samples) were removed. For multiple probes mapping to the same gene, the one exhibiting a higher coefficient of variation across the samples was considered. This resulted in 14,270 genes that were used for the construction of co-expression network.
Co-expression network construction
https://doi.org/10.1371/journal.pone.0216068.t002
Statistical and biological significance of network modules
Statistical significance of network modules..
To assess the statistical robustness of the co-expressed modules, module quality statistics were computed by re-sampling the dataset using the modulePreservation function in WGCNA. It randomly permutes the gene labels 200 times and computes various network quality statistics such as density, module membership, connectivity, etc. for each module. The log p -values and Z -scores for each module are summarized as psummary and Zsummary (see S3 Table ). The Z -score provides evidence that a module is preserved more significantly than a random sampling of genes and p -value gives the probability of seeing the module quality statistic in a random sampling of genes of the same size. It is observed that the psummary value is very low (∼ 0.0) and Zsummary > 10, providing strong evidence of network connectivity preservation and robustness of the co-expressed modules [ 24 ].
Biological relevance of network modules.
Gene ontology and enrichment analysis of the modules carried out using agriGO [ 25 ] indicates probable pathways captured by the module-genes. After merging the six datasets using inSilicoMerging, differentially expressed genes (DEGs) were identified using the criteria of average fold-change across 6 datasets ≥ |1.2| and a t -test with p -value ≤ 0.05. A total of 6441 DEGs were identified and mapped on to the co-expressed modules ( Table 3 ). We observe a clear demarcation in the distribution of up- and down-regulated genes on the modules, clearly showing the effect of constructing a ‘signed’ network. The Turquoise, Yellow, Tan and Brown modules are enriched with only up-regulated genes (3012) while Blue, Green, Red, Magenta, Salmon and Purple modules contain mainly down-regulated genes (3235) and these modules are likely to capture processes that are activated or repressed in response to drought, respectively.
Modules are ordered by their size.
https://doi.org/10.1371/journal.pone.0216068.t003
Construction of minimal drought-adaptive PPI networks
The modules obtained in the co-expression network are in general quite large and as such are not very useful. Also, fold-change alone does not say much about the systems-level significance of a gene. To address some of these issues, we additionally consider network topological measures such as intra-modular connectivity/degree centrality (k IM ), which help in identifying ‘hub’ genes and eigengene-based centrality (k ME ), which is a measure of module membership of a gene obtained by correlating its expression profile with eigengene of the corresponding module, to filter important stress-responsive genes. Top 20% genes ranked based on high k IM and k ME values that are also differentially expressed (fold-change ≥ |1.2|) were selected from each of the 4 up-regulated (Turquoise, Yellow, Tan and Brown) and 6 down-regulated (Blue, Green, Red, Magenta, Purple and Salmon) drought-responsive modules. This resulted in 754 up-regulated and 893 down-regulated genes. These stress-responsive genes were used as ‘seeds’ to search the database of protein-protein interaction networks, STRING [ 26 ] and a total of 939 interactions between 425 up-regulated genes and 8639 interactions between 640 down-regulated genes were identified. The remaining unmapped genes were queried in co-function networks, RiceNet [ 27 ] and their orthologs in AraNet [ 28 ], and additional interactions (between 76 up-regulated and 80 down-regulated genes respectively) that were conserved between both RiceNet and AraNet were retrieved. This resulted in two PPINs which we refer to as the up-regulated drought tolerant network (uDTN) consisting of 466 nodes and 1015 edges, and the down-regulated drought tolerant network (dDTN), consisting of 665 nodes and 8719 edges. The largest connected component in these two PPINs was considered for further analysis. The largest connected component of uDTN containing 422 nodes and 990 edges, and that of dDTN with 623 nodes and 8627 edges, was observed to follow an approximate power law degree distribution (with R 2 value of 0.85 and 0.76 respectively), indicating scale-invariant topology. These two components were then subjected to Markov Cluster Algorithm (MCL). Tightly coupled gene clusters (13 in uDTN and 14 in dDTN) of size ≥ 5 and showing significant functional enrichment in terms of pathway, GO or domain in STRING database were identified. We expect these gene clusters to provide insight into the biological processes that are activated or repressed in response to drought stress in drought tolerant varieties. The networks were visualized using Cytoscape [ 29 ] and topological analysis is carried out using NetworkAnalyzer plugin.
Identification of drought-adaptive modules
The signed weighted co-expression network of 14,270 genes resulted in 13 co-expressed modules. These modules are large, comprising hundreds and thousands of genes. To identify key drought-responsive processes we carry out various statistical operations discussed below.
Differential gene expression analysis and GO enrichment
GO enrichment analysis of the 13 co-expressed gene modules is carried out using agriGO [ 25 ] and the top enriched GO terms are given in Table 3 . The number of up- and down-regulated genes (DEGs) and known rice transcription factors (TFs) extracted from PlnTFDB [ 30 ] are identified and mapped on these modules. The results are summarized in Table 3 . The DEGs are identified based on fold-change (≥ |1.2|) and filtered using t -test with p -value (≤ 0.05). It is evident from Table 3 that Turquoise module has the highest number of up-regulated genes (2103). It harbors genes involved in the regulation of various cellular processes and transcription factors such as bZIPs, NACs and MYBs, which are known to be involved in drought stress. The next major up-regulated module is the Yellow module with 561 DEGs. Apart from being involved in transport and localization, this module also consists of genes involved in catabolic processes and energy generating processes. Blue module has a large number of down-regulated genes (1861), majority of which are involved in photosynthesis and associated metabolic processes known to be down-regulated in shoot tissue during drought [ 31 , 32 ]. Green and Magenta modules also contain a significant fraction of down-regulated genes (713 and 187 respectively) and are involved in protein synthesis and cellulose metabolism respectively. Both these processes are known to be hampered during drought as a result of growth retardation and damage to membranes [ 33 ]. The Red module contains 286 down-regulated genes involved in gene expression, ribosome biogenesis and RNA processing, indicating a finer regulatory mechanism controlling protein synthesis. Based on GO enrichment analysis and distribution of DEGs, we associate 4 modules (Turquoise, Yellow, Brown and Tan) with up-regulated processes and 6 modules (Blue, Green, Red, Magenta, Purple and Salmon) with down- regulated processes.
Transcription factors play an important role in translating stress-induced signals to cellular responses by binding to specific cis -elements of downstream target genes. A number of regulons, viz ., like AREB/ABF regulon, DREB1/DREB2 regulon, NAC regulon, MYB and WRKY TFs have been identified to play an important role in stress response. Interactions between the regulons such as AREB/ABFs and NACs have been reported where NAC TFs regulate ABA biosynthetic genes. NAC-induced regulation of ABA regulons and presence of ABRE elements in the promoter regions of SNAC TFs hints at complex cross talks between the regulons [ 34 – 36 ]. Similarly, MYB TFs have been reported to be involved in stomatal patterning, regulation of guard cells controlling stomatal movements indicating an overlap with the ABA signals [ 37 – 39 ]. The DREB genes have been shown to physically interact with AREB/ABFs [ 40 ]. We show that our co-expressed modules are able to capture this inter-dependence of TFs and genes shown to be involved in drought tolerance.
To identify the differentially expressed TFs associated with the regulons, we analyzed their distribution across the 13 co-expressed modules. For this analysis, known rice transcription factors (2385 genes) are downloaded from PlnTFDB (3.0) [ 30 ]. Of the 776 TFs that mapped to the co-expression network, 232 are up-regulated and 192 down-regulated. The distribution of these differentially expressed TFs on the co-expressed modules is summarized in Table 3 . We observe that 171 of the up-regulated TFs mapped to Turquoise, 43 to Yellow, and 8 and 7 to Tan and Brown modules respectively, while 99 of the down-regulated TFs mapped to Blue, 57 to Green, 9 to Salmon, 8 to Magenta, etc.
In Fig 1(A) and 1(B) , the total number of known members of various TF families that mapped to our co-expression network is depicted in ‘black’, while the ‘red’ and ‘blue’ bars depict the number of known up- and down-regulated TFs respectively. It is evident from Fig 1(A) that high numbers of up-regulated TFs belong to bZIP family followed by NAC, MYB-related and AP2-EREBP TFs, while from Fig 1(B) we observe that high numbers of HB, Orphans and bHLH are down-regulated. In plants, bZIP TFs regulate diverse processes including seed germination, flowering, photomorphogenesis, abiotic stress and ABA signalling [ 41 ]. Among the 21 up-regulated bZIP TFs, 17 are mapped to Turquoise module, including the well characterized OsABF1 (OsABI5/OREB1/OsbZIP10) TF. Recent study indicates that OsABF1 is a universal positive regulator of drought tolerance and ABA signalling in rice. It directly regulates OsbZIP23, OsbZIP46, and OsbZIP72 all of which are a part of Turquoise module [ 42 ]. It is also noted that both OsABF1 and OsbZIP23 are ‘hubs’ in the module (top 20% high degree genes). The NAC regulon plays an important role in stress response under various abiotic and biotic stress conditions as well as in various developmental programs such as lateral root development, flower development, formation of secondary walls, maintenance of secondary walls, etc. [ 43 ]. Among the 19 up-regulated NAC TFs, 14 mapped to Turquoise module, 4 to Yellow module and 1 to Brown module. For example, OsNAC52 which has been shown to be involved in drought tolerance [ 44 ] and LOC_Os07g48450 (yet to be characterized in drought stress) are observed to have a significant fold-change and high degree in the Turquoise module. The MYB-type TFs are one of the most abundant TFs in plants and play essential roles in development, growth and stress response and have been classified based on the number of MYB repeats [ 45 ]. We observe 18 MYB-related and 9 MYB TFs that are up-regulated, many of which are not yet functionally characterized for stress tolerance except for OsMYB55, reported to be involved in heat tolerance and amino acid metabolism and OsMYB91, in plant growth and salt tolerance [ 46 ]. We also other 8 MYB and 10 MYB-related TFs to be down-regulated ( Fig 1(B) ). Among these, the MYB TF LOC_Os01g44390 (OsRAD1), involved in floral development, is significantly down-regulated and is also a ‘hub’ gene in the Blue module. The other class of important TFs are AP2-EREBPs which are highly abundant and involved in development, sugar signalling, ethylene response, pathogen response and abiotic stress [ 47 , 48 ]. We observe 17 up-regulated AP2-EREBPs TFs in our co-expression network, of which 13 map to Turquoise module and 2 each to Yellow and Tan modules. The DREB genes of this TF family bind to dehydration responsive element (DRE)/C-repeat (CRT) regions and activate LEAs, dehydrins, starch-degrading enzymes, Hsps, etc. [ 49 ] and play a role in stress response pathway. In Fig 1(B) , we observe 17 homeobox genes to be down-regulated. These genes encode TFs which are important regulators for development, cell fate and body plan specification [ 46 ]. Majority of these TFs are part of the Blue module. Transcription factors (9 up-regulated and 14 down-regulated) belong to the Orphans category in PlnTFDB, which corresponds to TFs that could not be categorized into existing TF families. We observe 4 WRKY TFs in Green module, viz ., WRKY45 (associated with disease resistance [ 50 ]), WRKY47 (positive regulator drought in P SARK :: IPT plants [ 51 ]), WRKY68 and WRKY74, and WRKY69, WRKY109 part of Black and Blue module respectively. All these WRKYs are down-regulated.
In (A) top 20 up-regulated TF families (‘red’ bars), and (B) top 20 down-regulated TF families (‘blue’ bars) are shown. The vertical bars in ‘black’ depict the total number of members of the respective TF families in (A) and (B). The gene-ID-TF mapping is taken from PlnTFDB v3.0.
https://doi.org/10.1371/journal.pone.0216068.g001
Thus, we observe that the coordinated up-regulation of TFs corresponding to different regulons are part of the same modules (mainly Turquoise and Yellow), while TFs associated with disease resistance, flowering and development are part of the down-regulated (mainly Blue and Green) modules.
QTL genes mapping to Co-expressed modules
To further validate the biological significance of the 10 co-expressed modules in response to drought stress, we mapped known quantitative trait locus (QTL) genes on these modules. For this analysis, genes spanning QTLs that are associated with resistance/tolerance traits are obtained from Q-TARO database [ 52 ] and mapped on to the co-expressed modules. We identified 249 QTL genes in our co-expression network that are reported to be associated with resistance/tolerance traits in Q-TARO database. Of these, 73 QTL genes are up-regulated, 67 down-regulated and 109 are not differentially expressed.
As shown in Fig 2 , twenty seven of the 73 up-regulated genes are associated with drought tolerance and mapped to the up-regulated modules, Turquoise (23 genes), Yellow (3) and Tan (1), while thirteen of the 67 down-regulated genes are associated with drought and mapped to the down-regulated modules, Green (7), Blue (4) and Magenta (2). Some of these drought tolerance QTL genes that mapped to the Turquoise module are known transcription factors, viz ., OsHOX22, bZIP TFs (e.g., OsABF1, OsbZIP23 and ABI5-Like1(ABL1)), and NAC TFs (e.g., OsNAC6 and OsNAC2). Apart from these QTL genes, Turquoise module also harbors QTL genes associated with salinity (21), cold stress (7), other soil stress tolerance (8), blast resistance (8), bacterial blight (6), etc. This indicates common stress responsive mechanisms in abiotic and biotic stress conditions. Previous studies suggest that drought and salinity responses share common pathway components and regulators in the plant [ 53 ]. This seems to be well captured in our study with bZIP TFs involved in ABA-mediated stress response under drought stress, kinases (SnRK2-type SAPK4) with a role in ABA signalling and also implicated in the regulation of ion homeostasis and salinity tolerance [ 54 ], PLDα involved in ABA-regulated stomatal closure, zinc-finger proteins (e.g., ZOS3-21—C2H) involved in salt tolerance and ABA induced anti-oxidant defense, being up-regulated and co-expressed together in the same module. We also observe some of the QTL genes associated with biotic stress to be up-regulated and co-expressed with drought-responsive genes in Turquoise module. For example, selenium-binding gene OsSBP (associated with increased H 2 O 2 ), OsGH3.2 involved in modulating auxin and ABA as well as in pathogen defense by suppressing pathogen-induced IAA accumulation, OsWRKY31 (involved in auxin signalling) and OsNAC6 TFs (involved in broad spectrum of resistance including wounding and blast disease). Similarly, majority of the down-regulated genes associated with blast resistance such as the well characterized WRKY45 and OsNPRI involved in salicylic acid (SA) signalling pathway and defense response, some drought-associated QTLs, e.g., OsGSK1 associated with brassinosteroid (BR)-signalling and flowering, three AP2-EREBP TFs (OsDREB1E, OsDREB6, OsDREB1B) involved in ethylene singling are down-regulated and part of the Green module.
The solid bars represent modules with up-regulated genes, while striped bars represent modules with down-regulated genes. It may be noted that a significant number of DEGs from Turquoise, Yellow, Green and Blue modules are associated with osmotic stress (drought and salinity tolerance). The gene ID-QTL mapping is taken from the Q-TARO database.
https://doi.org/10.1371/journal.pone.0216068.g002
Analysis of minimal drought-adaptive modules
To understand the core drought-responsive signalling and metabolic changes, we discuss below the analysis of two PPI networks, uDTN (comprising 466 genes and 1015 interactions) and dDTN (comprising 665 nodes and 8719 interactions). These networks integrate protein-protein interactions with co-expressed gene profiles for the DEGs that are topologically significant (top 20% k IM and k ME values) in the co-expression network. As discussed in Section 1.4, protein-protein interactions for these gene sets are extracted from STRING database and co-function networks, RiceNet and AraNet ( S4 and S5 Tables).
Analysis of uDTN
To identify the processes that are induced in response to drought stress, we applied MCL algorithm to identify tightly coupled gene clusters of genes (size ≥ 5) that are functionally enriched in STRING database for domains, pathways, or GO terms. This resulted in 13 clusters summarized in Table 4 . Among the clusters shown here with a total of 151 genes, 83 edges have experimental evidences from model organisms (confidence score ≥ 0.4 in STRING DB). A brief description of the biological processes captured by these clusters is given below based on annotations in MapMan [ 55 ] and RGAP [ 56 ].
(TQ: Turquoise, Y: Yellow, BR: Brown, T: Tan).
https://doi.org/10.1371/journal.pone.0216068.t004
Cluster U1, U10 and U13: Components of ABA signalling, primary metabolism and biosynthesis of secondary metabolism
From our analysis, we observe that the three clusters, U1, U10, and U13, capture the abscisic acid (ABA) signal transduction process, and components of primary and secondary metabolism. It is known that on perceiving abiotic stress, the adaptive response of plants is controlled mainly by the phytohormone, ABA. Under drought stress, a sudden increase in ABA synthesis is induced accompanied by various physiological and morphological changes in the plant, namely, regulation of growth, stomatal closure, hydraulic conductivity, etc. However, mechanisms of fine-tuning the ABA-levels are not yet well understood. Earlier studies suggest that stress responsive genes mediate through at least two pathways: ABA-dependent and ABA-independent, with a possible crosstalk between their components. Calcium, which serves as a second messenger for various stresses, is proposed to be a strong candidate, mediating such cross talks. Recent progress in our understanding of ABA signal transduction indicates that the central signalling module comprises three protein classes: Pyracbactin Resistance/Pyracbactin resistance-like/Regulatory Component of ABA Receptor (PYR/PYL/RCARs) proposed to be the ABA receptors, Protein Phosphatase 2Cs (PP2Cs) which act as negative regulators, and SNF1-related protein kinases 2 (SnRKs) which are positive regulators. In the presence of ABA, the PYR/PYL/RCAR-PP2C complex formation leads to inhibition of PP2C activity, allowing activation of SnRKs which target membrane proteins, ion channels and transcription factors, and facilitate transcription of ABA-responsive genes (see Fig 3(A) , reproduced from KEGG [ 57 ].
(A) Components of ABA signalosome complex: PYR/PYL receptors, PP2Cs, SnRK2 kinases and ABF/bZIP transcription factors are depicted (reproduced from KEGG). (B) Subnetwork of Cluster U1 (‘blue’), Cluster U10 (‘green’) and Cluster U13 (‘pink’) obtained using MCL algorithm on up-regulated drought-tolerant network (uDTN) is seen to capture the crosstalk between the signalling components: PP2Cs, kinases, bZIP23, OsNAC6, SnRK2 kinases (Cluster U1), bZIPs (Cluster U1 and Cluster U13) with metabolic components of Cluster U1 via UMPS2. This clearly indicates the role of UMPS2 as a novel candidate for drought.
https://doi.org/10.1371/journal.pone.0216068.g003
We observe that the subnetwork comprising three gene clusters in Fig 3(B) : U1 (35 genes in blue), U10 (5 genes in green) and U13 (5 genes in pink) capture very well essential components of the ABA-signalling pathway ( Fig 3(A) ). Based on functional analysis, U1-cluster genes can be divided into two groups, one involved in signalling processes and the other involved in primary metabolism and biosynthesis of secondary metabolites. The genes involved in signalling processes include 10 kinases, 5 PP2Cs and a membrane-bound ankyrin-like protein (LOC_Os02g50270) with a probable role in calcium-mediated signalling. From AraNet we find that the Arabidopsis ortholog of LOC_Os02g50270 is co-expressed with calmodulin genes, CAM3, 6 and 7. These Ca 2+ sensors have been implicated in various developmental and environmental stimuli response [ 58 ]. The upstream promoter region of the ankyrin-like protein consists of ABRE elements, heat stress-responsive elements, and light responsive elements (e.g., G-box) indicating its possible role in stress. In our subnetwork we observe that its first neighbors are kinases involved in signalling and protein posttranslational modifications. The 10 kinases include cell-wall associated kinase 25, OsWAK25, involved in pathogenic resistance [ 59 ], chloroplast localized kinases (LOC_Os07g47270, LOC_Os01g71000 and LOC_Os03g06330) involved in redox regulation and phosphorylation [ 60 ], OsRPK1-receptor like kinase, a negative regulator of auxin transport [ 61 ] and receptor-like cytoplasmic kinase, OsRLCK-XV (LOC_Os06g07070) highly induced during drought and cold stress [ 62 ]. Topologically significant (high degree), the biologically relevant (co-clustered with ABA signalosome and connected to important kinases) along with ABA-responsive cis -elements in its promoter and potential role in calcium mediated signalling makes the ankyrin-like protein (LOC_Os02g50270) a likely novel candidate for drought stress. Cluster U1 also consists of two transcription factors (TFs), ABF-type OsbZIP23 and OsNAC6/SNAC2. The OsbZIP23 TF is associated with ABA signalling pathway and strongly induced by drought, high-salinity, polyethylene glycol (osmotic stress) and ABA-treatments. The interactions of OsbZIP23 with 7 genes of U10-cluster and 4 genes of U13-cluster in this subnetwork are in accordance with a genome-wide study using a ChIP assay by Zong et al [ 63 ]. Transgenic rice plants overexpressing OsNAC6/SNAC2 displayed increased sensitivity to ABA and tolerance to dehydration and high salinity stresses, suggesting its role as a transcriptional activator in both salinity and drought stresses [ 64 – 66 ]. Thus, we see that this subnetwork is able to capture apart from the ABRE regulon, role of NAC regulon in the transcriptional networks of abiotic stress response.
The rice SnRK2 kinases are shown to be over-expressed under abiotic stress with a probable role in phosphorylating ABF family of bZIP TFs for down-stream ABA signalling [ 67 , 68 ]. This association is well captured in our subnetwork exhibiting interactions of OsbZIP23 TF with SnRK2 kinases and PP2Cs (Cluster U1). The 5 ABF genes of Cluster U13 (red) are also seen to exhibit direct/indirect association with PP2Cs ( Fig 3(B) ) and their role in stress response is well documented. The OsbZIP66/TRAB1 gene encoding ABF-type TF (ABRE-binding bZIP factor) is reported to be up-regulated under dehydration and salt stress conditions [ 69 ]. The terminal or nearly terminal event of the primary ABA signal transduction pathway is phosphorylation of this gene [ 70 , 71 ]. The TFs OsbZIP45 and OsbZIP12 are also well characterized for their role in providing drought tolerance [ 72 , 73 ], while OsbZIP20/RISBZ3/RITA-1 is shown to be expressed in the late stages of seed development with a possibsle role in regulating seed-specific genes [ 74 , 75 ]. As both seed maturation and response to drought is co-regulated by ABA, the involvement of OsbZIP20 in drought tolerance can be further investigated. Similarly, OsbZIP86 being up-regulated and topologically significant in the co-expression network and its association with other stress-responsive bZIP TFs in the uDTN, suggest its possible role in stress response. These interactions suggest OsbZIP20 and OsbZIP86 transcription factors as probable novel candidates for drought stress.
The 10 kinase signalling genes (Cluster U1) are connected to 16 genes associated with primary metabolism and biosynthesis of secondary metabolites via Uridine 5'-monophosphate synthase, UMPS2 (LOC_Os01g72250). It is a precursor for pyrimidine nucleotides and is associated with the last step of de novo pathway of pyrimidine biosynthesis. Eleven of these 16 genes are involved in the biosynthesis of secondary metabolites associated with sugars and amino acids, and 5 genes belong to Pentose Phosphate Pathway (PPP). Role of PPP is to maintain redox potential to protect against oxidative stress by producing reductants such as NADPH [ 76 ]. To ensure these genes are possible targets of ABA-induced downstream signalling cascade, we carried out promoter analysis of these 17 genes (16 metabolic pathway genes+UMPS2). For this, 1 kb upstream region of these genes are analyzed using cis -acting regulatory elements database, PlantPAN (v2.0) [ 77 ]. The results indicate the presence of ABRE motifs like ‘ABRELATERD1’ in all the 17 genes upstream of the genes ( S6 Table ). The gene UMPS2 is up-regulated, has ABRE motifs in its promoter region and is topologically significant (‘hub’ gene) both in the co-expressed module and in uDTN. These results suggest the probable role of UMPS2 in relaying ABA-induced metabolic changes, hitherto unknown. To our knowledge there is only one proteomic study in which UMPS2 has been reported to be up-regulated in rice seedlings after ABA treatment followed by subsequent salt stress [ 78 ]. Thus, this analysis suggests UMPS2 as a potential drought-tolerant candidate gene in rice.
Cluster U2: Molecular chaperons and heat stress transcription factors
Almost all stresses induce the production of a group of proteins called heat-shock proteins (Hsps) or stress-induced proteins as adverse environmental conditions disrupt protein folding and lead to an increase in reactive oxygen species (ROS) and oxidative stress within the cell [ 79 , 80 ]. Their transcription is controlled by regulatory proteins called heat stress transcription factors (Hsfs) by binding to the highly conserved heat shock elements (HSEs) in the promoter regions. The Hsps function as molecular chaperones, regulating cellular homeostasis and preventing protein misfolding and aggregation, thus promoting survival under stressful conditions. Cluster U2 in uDTN clearly captures these interactions. It contains 19 genes of which 7 are heat shock proteins (5 DnaJ/Hsp40 family and 2 DnaK/HSP70 family), 4 HsFs (OsHsfA9, OsHsfA2e, OsHsfB2c and OsHsfA2c), MYB TF (LOC_Os04g30890), CDK5RAP3 and another molecular chaperon, ERD1 protein (chloroplast precursor, LOC_Os02g32520) and involved in heat stress. The Hsp70s, assisted by co-chaperone DnaJ-proteins and nucleotide exchange factor constitute a chaperone machine that participates in protein folding, prevention of protein aggregation, translocation of proteins across membranes, targeting proteins towards degradation, and regulation of translation initiation [ 81 , 82 ].
Promoter analysis of the four HSFs revealed presence of ABRE elements suggesting their role in ABA-dependent signal transduction. It has been suggested that Hsps and Hsfs might be important elements in crosstalk of different stress signal transduction networks [ 83 ]. The first-degree neighbors of these TFs (extracted from STRING database) mapped to 13 genes in our co-expression network, with 10 genes being common neighbors to all the 4 TFs. Promoter analysis of the up-regulated neighbors (8) was carried out to identify possible regulatory elements in these transcriptionally active genes under drought. Majority of these genes are Hsps (LOC_Os09g29840, two Hsp70s, Hsp90, Hsp40, Hsp81-3, dnak—LOC_Os03g11910) and an RNA recognition motif family protein, LOC_Os03g15890. All the 8 genes have ABRE elements in their promoters, 5 of the genes have heat shock elements (HSEs) and 6 of them also have cis -elements which are responsive to methyl jasmonate (MeJa). These results indicate that the Hsps and HSFs are induced in an ABA-dependent manner and at the same time, they are also responsive to jasmonates indicating complex interplay between the two phytohormones. In a study by [ 84 ], it has been shown that OsHsfA2c (part of Turquoise module) binds to OsClpB-cyt/Hsp100 promoter (also part of Turquoise module) and possibly play the role of transcriptional activator in heat stress. Our analysis show that OsHsfA2c exhibits highest fold change, followed by OsHsfB2c, OsHsfA2e and OsHsfA9. Also, both ABRE and HSE elements are present in the promoter of OsClpB/Hsp100 gene, and is up-regulated in our network. These results suggest OsHsfA2c may play the role of transcriptional activator even in drought stress and the HSFs, OsHsfA2c, OsHsfB2c and OsHsfA2e are probable biomarkers for drought tolerance.
Cluster U3, U4, U9 and U11: Components of amino acid metabolism, cell wall and TCA/Citric Acid Cycle and Mitochondrial ETC/ATP synthesis
Maintaining cellular elasticity and integrity by increased cellulose and hemicellulose synthesis is an important drought-tolerant trait [ 85 , 86 ]. We observe that these processes are captured by the subnetwork formed by Clusters U3 (Cell wall and amino acid metabolism), U4 (Amino acid degradation and mitochondrial ETC), U9 (TCA cycle) and U11 (Mitochondrial ETC/ATP synthesis), shown in Fig 4 .
It depicts the crosstalk between the components of Amino Acid Metabolism (Cluster U3 and U4), Cell Wall metabolism (Cluster U3), TCA/Citric Acid Cycle (Cluster U9) and ATP synthesis (Cluster U4). These interactions indicate link between structural integrity of plant and cellular energy generating processes as a drought-adaptive mechanism.
https://doi.org/10.1371/journal.pone.0216068.g004
It is known from literature that Proline accumulation occurs under various stress conditions and imparts beneficial role to plants by maintaining osmotic balance, stabilizing membranes (thereby preventing electrolyte leakage) and in scavenging of free radical and ROS species [ 87 , 88 ]. Proline is also known to be a key determinant of many cell wall proteins that play an important role in cell wall signal transduction cascades, plant growth and differentiation. The interactions of amino acid kinases P5CS (LOC_Os05g38150) and LOC_Os01g62900, involved in proline biosynthesis with 4 genes involved in cell wall synthesis, OsUGE1 (UDP-glucose 4-epimerase 1), MUR4 (UDP-arabinose 4-epimerase), and GHMP kinases (LOC_Os02g04840 and LOC_Os03g61710) in Cluster U3, capture the role of proline as an important component of the cell wall matrix, providing tensile strength to the cell walls [ 89 ]. In Fig 4 , we observe that the genes involved in proline biosynthesis in Cluster U3 is involved in crosstalks with clusters U4 (Amino acid degradation and mitochondrial ETC), U9 (TCA cycle) and U11 (Mitochondrial ETC/ATP synthesis).
Three genes of Cluster U3, glyoxalase family protein (LOC_Os02g07160), Fumarylacetoacetate (LOC_Os02g10310) and Homogentisate 1, 2-dioxygenase (LOC_Os06g01360) are involved in phenylalanine and tyrosine degradation. Fumarate (also a metabolite of citric acid cycle) and acetoacetate (3-ketobutyroate) are liberated in tyrosine catabolic process. Acetoacetate, on activation with succinyl-CoA, is converted into acetyl-CoA (LOC_Os01g02020 of Cluster U4), which in turn can be oxidized by citric acid cycle or used for fatty acid synthesis. This provides a crosstalk between genes of Clusters U3 (Cell wall and amino acid metabolism) and U4 (Amino acid degradation and mitochondrial ETC). Secondary metabolites such as lignins, flavonoids, isoflavonoids, etc. are usually derived from the catabolic pathway of tyrosine [ 90 ]. Accumulation of these phenolic compounds during drought is required for ROS scavenging. Moreover, increased lignin deposition, cell wall stiffness and reduced cell expansion is reported to inhibit plant growth [ 91 – 93 ]. Several stress-responsive genes such as hyperosmolality-gated calcium-permeable channels which act as osmosensors, OsOSCA1.2 (LOC_Os05g51630) and OsOSCA4.1 (LOC_Os03g04450) [ 94 ], MATE efflux family protein (LOC_Os03g42830) involved in transport [ 95 ], immutans protein (LOC_Os04g57320) involved in electron transport, and asparagine synthetase (LOC_Os03g18130) involved in nitrogen metabolism [ 96 ] are part of this subnetwork (Cluster U3) and connected to the amino acid kinases P5CS (LOC_Os05g38150) and LOC_Os01g62900, involved in proline biosynthesis suggesting transport and distribution of small metabolites.
The degradation of branched-chain amino acids (BCAAs) is elevated during stress to provide intermediates for TCA cycle and electron donors of the mitochondrial electron transport chain to generate cellular energy [ 97 – 99 ]. This process is captured by the six genes of Cluster U4, involved in the degradation of amino acids valine, leucine and isoleucine. The cluster also includes mitochondrial and peroxisomal genes, explaining the degradation of BCAAs occurring predominantly in these two cellular organelles [ 100 ]. Cluster U4 includes genes involved in electron carrier activity, viz ., EFTA (LOC_Os03g61920), electron transfer flavoprotein subunit alpha-mitochondrial precursor and LOC_Os10g37210, FAD-dependent oxidoreductase domain containing protein (involved in electron transfer to mitochondrial respiratory chain). Membrane-bound cytochrome P450 genes (LOC_Os07g45000 and LOC_Os01g08810) with oxidoreductase activity are up-regulated and part of this cluster. Four out of 5 genes of Cluster U11 are also involved in mitochondrial electron transport chain (ETC)/ATP synthesis. This cluster shows an interaction between rice alternative oxidases OsAOX1b (LOC_Os04g51160), OsAOX1c (LOC_Os02g47200), NADH-ubiquinone oxidoreductases (LOC_Os08g04630, LOC_Os01g61410) and putative erythronate-4-phosphate dehydrogenase domain containing protein (LOC_Os06g29180). A number of roles have been associated with AOXs such as optimization of respiratory mechanisms, replenishment of TCA cycle intermediates [ 101 ], preventing excess generation of ROS [ 102 ] and shown to be up-regulated in response to biotic and abiotic stress conditions [ 103 – 106 ]. The genes of NADH-ubiquinone oxidoreductase complex in mitochondria are entry point to the respiratory chain from TCA cycle and are involved in ATP synthesis. The up-regulation of ATP synthesis is probably to meet energy demands of the plant to sustain metabolism under drought stress [ 107 ]. The putative homolog of LOC_Os06g29180 is an NAD+ -dependent formate dehydrogenase in Arabidopsis with a probable role in NADH synthesis [ 108 ]. Here too, we observe crosstalk between genes of Clusters U3 and U11 via amino acid kinases P5CS (LOC_Os05g38150) and LOC_Os01g62900 and NADH-ubiquinone oxidoreductases (LOC_Os08g04630, LOC_Os01g61410) linking proline catabolism inside mitochondria with a probable role in ROS production [ 109 ]
The TCA cycle takes place in mitochondria in a series of reactions to generate ATP. Five genes of Cluster U9 viz ., citrate synthases (LOC_Os02g13840, LOC_Os02g10070), dehydrogenases (LOC_Os01g16900, LOC_Os04g40310) and aconitase (LOC_Os03g04410), are involved in the Citrate Cycle. Increase in the expression levels of these genes suggest an increase in ATP production as well as increase in reductants and substrates for non-essential amino acid synthesis like glutamine, proline, arginine, etc. [ 110 – 112 ]. Indeed, we do capture these interactions in the crosstalk between U4 (Amino acid degradation and mitochondrial ETC) and Cluster U9. The interactions between HMG-CoA (LOC_Os01g16350) and genes of the melavonate pathway (acetyl-CoA acetyltransferase (LOC_Os01g02020) and HMG-CoA synthase (LOC_Os08g43170)) in Cluster U4 with the genes of the TCA cycle (Cluster U9) provides a snapshot of the different metabolic fates of the acetyle-CoA, a central metabolite which can be incorporated into the TCA cycle for ATP generation in mitochondria, synthesis of amino acid carbon skeletons or metabolized in the cytosol to produce a plethora of phytochemicals required for growth, development and tolerance towards environmental stress [ 113 , 114 ].
Smaller uDTN clusters
Apart from the major processes discussed above, several other smaller clusters identified in uDTN revealed various processes up-regulated in drought-stress, and are summarized in Table 4 . For example, Cluster U5 is involved in RNA binding and harboring genes with RNA recognition motifs. RNA-processing via post-transcriptional modifications and alternative splicing are important components responsible for fine-tuning the regulatory mechanisms in response to environment stress [ 115 – 117 ]. Clusters U6 and U8 are involved in protein ubiquitination and degradation which are major posttranslational modifications, providing a dynamic and reversible control over processes such as degradation of misfolded proteins, trafficking, signal transduction and cell division [ 118 , 119 ]. Cluster U7 consists of genes associated with plant defense system. Other than stress-induced lipases and transporters, genes like CXE carboxylesterase (LOC_Os06g11090) and GID1L2-gibberellin receptors (LOC_Os11g13670 and LOC_Os05g33730) which are involved in biodegradation of xenobiotics (herbicides) are also present in this cluster. These genes are induced as a result of increased oxidative stress in the system [ 54 ] and may have a role in ROS scavenging in the plant. Starch synthesis and degradation is an important aspect regarding metabolic shifts during drought stress as seen in Cluster U13. It harbors alpha and beta-amylases involved in breakdown of starch and produce soluble sugars, OsDPE1 involved in transitory starch breakdown and AGPlar involved in starch synthesis. The increase in amylase activity in the shoot can be seen as an important drought tolerant trait, providing tensile strength and maintaining cellular membranes [ 120 , 121 ]. Thus we show that by using an integrated approach by combining co-expression and protein-protein interaction information we are able to capture some of the core biological processes in drought response. In the up-regulated PPIN, we are able to extract some of the key components of the ABA signalosome including the PP2Cs, the SnRK2 kinases and a number of ABF-type bZIP transcription factors. Along with the genes involved in signalling, we observe a crosstalk with the genes involved in metabolic pathways like Pentose Phosphate Pathway and biosynthesis of sugars and amino acids via the gene UMPS2, a novel candidate for drought. We also extracted a cluster comprising molecular chaperons and heat shock transcription factors as well as an MYB transcription factor co-clustered with these genes. The other major crosstalk is observed between processes, namely biosynthesis of proline which is an osmolyte and an important component of cell wall matrix, cell wall metabolism, degradation of branched chain amino acids to serve as intermediates for TCA cycle, enzymes of the TCA and finally, mitochondrial electron transport/ATP synthesis to generate cellular energy during stress. There is a re-partitioning of metabolites during drought stress as genes involved in starch biosynthesis as well as degradation are seen to be up-regulated. Finer regulatory mechanisms such as RNA-processing via post-transcriptional modifications and alternative splicing are captured in this network. Finally, we observe that protein ubiquitination and degradation is a prominent process in drought by which degradation of misfolded proteins due to stress, trafficking, signal transduction and cell division are dynamically controlled during stress.
Analysis of dDTN
A similar analysis is carried out on the down-regulated drought tolerant network, dDTN, to identify the processes down-regulated in response to drought stress by the proposed network-based approach. Seventeen tightly-coupled gene clusters are identified using MCL algorithm in dDTN. For the clusters shown in Table 5 with a total of 449 genes, 465 edges have experimental evidences from model organisms (confidence score ≥ 0.4 in StringDB). Functional annotation for biological processes associated with these gene clusters using MapMan and RGAP is summarized in Table 5 and is discussed below in detail.
(B: Blue, P: Purple, G: Green, M: Magenta, S: Salmon).
https://doi.org/10.1371/journal.pone.0216068.t005
Cluster D1: Photosynthesis and associated processes
Photosynthesis, a part of the primary metabolic process in plant, plays a central role in drought response. With decrease in carbon uptake due to stomatal closure, major metabolic shifts take place to re-partition the photo-assimilates between root and shoot, which lead to the cessation of shoot growth and maintenance of root growth under water deficit conditions [ 122 ]. Around ~23% of Cluster D1 genes are directly involved in photosynthesis, viz ., genes associated with photosynthetic light reaction such as ATP synthases and NADPH dehydrogenase subunits, chloroplast precursors and chlorophyll A-B binding proteins. Closely associated processes with photosynthesis such as carbon fixation, tetrapyrrole synthesis and carbohydrate metabolism are also components of this cluster. Closely clustered with the photosynthetic genes, around 30% genes are involved in protein synthesis, folding, targeting, posttranslational modifications and degradation. On closer look, a large majority of them are ribosomal proteins, many of which are located in chloroplast (21 genes). Genes associated with RNA processing (helicases), regulation and binding (52 genes) are also seen to be clustered together with photosynthetic processes. The crosstalk between these processes suggests down-regulation of photosynthesis and transcription and translation of plastidial genes.
Twenty one genes in this cluster have no functional annotation. These genes are queried in AraNet and the top functional predictions for each gene are listed in S6 Table . Of these, ~20 genes have predicted functions associated with photosynthetic machinery, pathogen response or both. Promoter analysis of these 21 uncharacterized genes using PlantPan database revealed presence of cis-elements like ‘GT1CONSENSUS’ and ‘CGACGOSAMY3’ indicating the presence of light-responsive elements like G-box in all the genes. These elements are known to regulate light-specific gene expressions and response to stimuli [ 123 ]. Other motifs like “SITEIOSPCNA” which is shown to be involved in gibberellin-responsive pathway in regulating photosynthesis [ 124 ] are also present. The above analyses indicate that the 21 uncharacterized genes of this cluster that are topologically significant and tightly clustered with photosynthetic genes, are likely to be regulated by light, respond to pathogen invasion/wounding and may be influenced by hormones such as gibberellin. We presume these genes to be important candidates for transgenic studies to elucidate their role in photosynthesis and drought-response.
Cluster D2, cell wall metabolism
Plant cell walls are of critical importance and provide cell shape and mechanical support to withstand the turgor pressure. However, during water deficit, aerial growth is limited due to reduced cell division in the meristematic zones [ 125 ] followed by reduced ability of cells to expand the polysaccharide network. Cluster D2 genes are found to be associated with cell wall metabolism, e.g., cellulose synthases OsCESA6, 7 and 8 and cellulose synthase-like genes OsCLSA1 and OsCLF6, and OsBC1L4/OsCOBRA involved in cellulose biosynthesis. While OsCESA6, 8 and OsBC1L4/OsCOBRA are reported to be involved in primary cell wall biosynthesis [ 126 ], OsCESA7 is a secondary cell wall-specific cellulose synthase [ 127 ]. The gene OsCLF6 is shown to mediate mixed-linkage glucans (MLG) synthesis in rice [ 128 ]. MLG is a cell wall polysaccharide, known to be involved in the regulation of cell wall expansion in young tissues [ 129 , 130 ]. This cluster also includes 3 Fasciclin-like arabinogalactan proteins (OsFLA6, 16 and 24) that are involved in cell wall adhesion, signalling, biosynthesis and remodeling and in the development of new shoot and root meristems [ 10 ]. Among the 5 uncharacterized genes, we observe that Arabidopsis orthologs of LOC_Os06g11990 and LOC_Os05g32500 are involved in cellulose synthesis while that of LOC_Os07g43990 has a role in transferring glycosyl groups ( S6 Table ). Since cell walls are also the first line of defense against pathogens, some of the genes, e.g., Harpin-induced protein 1 (LOC_Os02g33550) and CHIT1 (LOC_Os09g32080- Chitinase family protein) are down-regulated and are part of this cluster. Some of the kinases (LOC_Os02g43870, LOC_Os03g12250 and LOC_Os04g55620) have LRR domains and are important for protein-protein interactions, signalling and response to biotic stress. Thus, a low turgor pressure during drought stress leads to reduction of growth by the inhibition of cell wall elongation. The down-regulation of Cluster D2 genes clearly reveals the processes associated with cell wall biosynthesis that are down-regulated in leaves.
Cluster D3, D7 and D14: Down-regulation of biotic stress associated genes
The natural phenolic compound, salicylic acid (SA) is one of the main hormones involved in endogenous signalling in plant pathogen response and disease resistance [ 131 ]. In many studies drought tolerance and disease resistance pathways are reported to be antagonistic and the role of ABA in suppressing SA-signalling pathway [ 132 – 134 ]. A transcriptional activator NPR1 is an important regulator of SA-mediated defense signalling pathway and responsible for activation of a large number of downstream pathogen-responsive genes [ 135 , 136 ]. It is shown that ABA suppresses transcriptional up-regulation of WRKY45 and OsNPR1, the two important components of SA-signalling pathway [ 137 ]. Also, OsNPR1 has been implicated with reduced drought tolerance in rice [ 138 ]. Both WRKY45 and OsNPR1 (part of Cluster D3) are co-expressed in Green module and down-regulated in agreement with earlier studies, thus capturing the crosstalk between ABA and SA signalling pathways.
Majority of Cluster D3 genes (~ 23) are kinases including receptor-like kinases involved in signalling and post-translational modifications. Many of these genes are shown to be involved in plant immunity against pathogens and are potential pattern recognition receptors (PRRs), e.g., leucine-rich repeats (LRR) and ankyrin (ANK) repeats [ 139 – 142 ]. This module consists of three LRR repeat genes, Strubbelig-receptor FAMILY8 precursor (LOC_Os06g42800), a putative transmembrane protein kinase 1, a putative protein kinase (LOC_Os01g41870) and Brassinosteroid insensitive 1-associated receptor kinase 1 precursor (BAK1, LOC_Os11g39370). Previous studies indicate that these kinases influence cellular morphogenesis, orientation, cellular proliferation, size and shape [ 143 – 145 ]. Genes with ankyrin repeats include OsNPR1 (LOC_Os01g09800) a master regulator characterized for resistance to plant pathogens [ 146 – 148 ] and LOC_Os03g63480, are both involved in biotic stress and down-regulated in the network.
Three disease resistance proteins viz ., RGA3, RPM1 and LOC_Os12g25170 with NB-ARC domains containing LRR repeats and having ATPase and nucleotide binding activities are part of Cluster D14. These proteins are involved in pathogen resistance and help in discriminating between self and non-self (R-protein mediated response) [ 149 ]. Cluster D7 consists of 2 genes (OsGF14b and OsGF14e) that belong to 14-3-3 gene family. These signalling proteins are reported to be involved in biotic stress and probably associated with JA and SA pathways [ 150 , 151 ]. They interact with Beta-2- and Beta-5-tubulin proteins which play critical role in cell division, elongation and growth. Binding of 14-3-3-signalling proteins with those involved in cellular organization (tubulins) hint at finer regulations of cell cycle events [ 152 ]. Thus, the analysis of the subnetwork of Cluster D3, D7 and D14 indicate suppression of the defense system of plants under drought stress, that is, the vulnerability of the plant to pathogen infection under drought condition.
Cluster D4 and D5: Biogenesis of rRNA and tRNA genes
A number of clusters are associated with synthesis, post-translational modifications and processing of ribosomal proteins. Cluster D4 includes genes involved in pre-rRNA processing in nucleolus and cytoplasm (nucleolar protein 5A-LOC_Os03g22880, LOC_Os04g49580 and Gar2-LOC_Os08g09350), ribosomal proteins (LOC_Os08g41300, LOC_Os06g03790, LOC_Os06g02510 and LOC_Os03g41612), DEAD/DEAH box helicases and WD domain containing proteins, t-RNA synthetases (LOC_Os05g08990 and LOC_Os04g02730) and other RNA-binding genes. The rRNA processing and ribosome biogenesis is tightly coupled with growth and cell proliferation. Down-regulation of these pathways hint at conservation of energy by the plant [ 153 , 154 ]. Closely interacting with Cluster D4, is Cluster D5 with genes involved in amino-acyl tRNA biosynthesis (8 genes), genes involved in protein-processing like ubiquitin conjugating enzymes (LOC_Os01g46926, LOC_Os04g58800, LOC_Os06g44080 and LOC_Os03g03130), further indicating a decrease of protein turnover, cell proliferation and growth.
Cluster D6: Development and flowering
Cluster D6 consists of zinc finger domain containing proteins-LOC_Os04g46020, OsTIFY1b, OsBBX25, zinc finger protein-LOC_Os08g15050, MADs box genes (OsMADS50 and OsMADS55) and response-regulator genes (OsRR10 type-A and OsRR2 type-A). The TIFY family genes are involved in development and have been shown to be stress-responsive [ 155 ]. The MADs box and BBX genes are also involved in flowering and development [ 156 – 158 ]. Interestingly, rice response regulator (OsRR) genes are part of the cytokinin-dependent signal-transduction pathways which also affect development and morphological changes in the plant [ 159 , 160 ]. The down-regulation of genes in this cluster suggests a decrease in growth, development and delay in flowering of the plant under drought stress.
Cluster D8: α-Linolenic acid metabolism
The phytohormone Jasmonic Acid (JA) regulates a wide array of biological processes in plants including growth, development, secondary metabolism, pathogen defense, wounding as well as tolerance to abiotic stress [ 161 ]. Two genes of GH3 family, viz . OsJAR1 and OsJAR2 control the homeostasis of JA by forming bioactive JA-amino acid conjugates. They have been reported to be involved in wound and pathogen-induced JA-signalling [ 162 ]. Also, OsOPR7, LOX7 and OsAOS1 genes that are involved in JA synthesis [ 163 , 164 ] are down-regulated and part of this cluster. The wound inducible and leaf-specific gene, OsHPL3 of this cluster is reported to modulate the amount of JA by consuming common substrates [ 165 ]. As majority of the genes in JA biosynthesis are down-regulated, it can be inferred that during drought stress, there is an increased susceptibility to wounding and pathogen attack.
Other smaller dDTN clusters
Apart from some of the major clusters discussed above, several smaller clusters are detected ( Table 5 ). For example, Cluster D9 consists of six glutathione s-transferases (GSTs). The GST family of genes are widely involved in cellular detoxification and can act on substrates such as endobiotic and xenobiotic compounds [ 166 ]. However, studies have indicated that not all the GST genes express the same way across different stress, tissue and developmental conditions [ 167 ]. A similar behavior is observed in the present case with GSTs in D9 are down-regulated, but 12 other GSTs are up-regulated in the overall co-expression network, and are not part of uDTN. Cluster D10 consists of genes associated with serine metabolism and are down-regulated. Among these, two genes are phosphoserine phosphatase which catalyzes the last step in the plastidial phosphorylated pathway of serine biosynthesis. The other genes include serine hydroxymethyltransferase (SHMT) gene and three aminotransferases with a probable role in glycine cleavage system. The glycine cleavage system is required for photorespiration in C3 plants and closely interacts with serine SHMT for the methylation of glycine to serine. These amino acids have been associated with photorespiration and precursors for other metabolites involved in stress protection [ 168 ]. It has been shown that DNA repair mechanisms are affected by drought stress. This is captured by Cluster D11 genes involved in maintaining genome stability and repair mechanisms. With increase in ROS due to stress, plants undergo ABA-mediated growth retardation with increase in DNA damage, inhibition of DNA replication and cell division [ 169 – 171 ]. Genes involved in vitamin B6 metabolism are captured in Cluster D12. Lower levels of vitamin B6 levels have been attributed to impairment of flowering and reduced plant growth in Arabidopsis mutants [ 172 , 173 ]. The Cluster D13 consists of genes 3 major sperm proteins (MSPs) which have not been studied in the context of stress response. These MSPs have a probable role in cell vesicle transport and mediate protein-protein interactions within or between cells [ 167 ]
The fascinating complexity of biological processes in plants is reflected in the intricate interactions in co-expression networks. With the availability of a large number of transcriptomic datasets in public repositories, co-expression network analysis allows us to integrate this information and perform large-scale multi-genic studies. On one hand, we need this complexity to infer functional annotations of genes from tightly connected neighborhood (‘guilt-by-association’), while on the other hand, we need to dissect the networks to remove noisy connections and identify critical biological processes. Various approaches have been proposed, e.g., construction of condition-dependent co-expression networks with context-specific interactions [ 174 , 175 ], split co-expression networks into functional modules which may represent tightly coordinated biological processes [ 176 , 177 ], use of functional and comparative genomics [ 178 , 179 ], etc.
Here we propose a computational approach using network parameters that can help in identifying important drought specific processes. However, a typical meta-analytic study based on publicly available microarray datasets is likely to have heterogeneity in the data arising from various sources such as microarray platforms, tissues, developmental stages, experimental conditions, etc. Hence, for the analysis DEGs are restricted to only the 10 drought-responsive modules, which are further filtered based on topological measures, viz ., intramodular connectivity (K IM ) and eigengene-based centrality (K ME ) to identify biologically significant genes across the genotypes. We observe that greater than 50% of these topologically significant DEGs are well represented in majority of the drought-tolerant genotypes considered ( S10 Table ), clearly suggesting their relevance. Using this filtered set of DEGs, two PPI networks are constructed by extracting known protein-protein interactions (from STRING, AraNet and RiceNet) within the set of up-regulated and down-regulated genes. Tightly-coupled gene clusters identified are identified using Markov clustering: uDTN (466 nodes, 1015 edges) and dDTN (665 nodes, 8719 edges). It is confirmed that these gene clusters are well-represented across all the 9 data subsets considered ( S12 and S13 Tables).
Plant’s response to drought stress involves complex interactions across several layers leading to molecular, biochemical, physiological and morphological changes in the plant. By our proposed computational approach, we identified various up- and down-regulated genes/gene clusters that are likely to play an important role in drought responsive processes. Functional characterization of these gene clusters, aided by interaction databases (such as STRING DB, RiceNet and AraNet) and additional information curated from literature support the proposed systems-level model for drought stress response in Fig 5 . We observe that the key players in providing drought tolerance are bZIP TFs (mainly bZIP12 (U10), bZIP23 (U1), and bZIP46 (U13), ABA signalling machinery and interaction of its signalling components with metabolic pathways. From previous studies it is well known that ABA signalling pathway is triggered in response to drought stress [ 180 – 183 ]. The interaction between various bZIPs is seen to connect the three clusters U1, U10 and U13 (that are up-regulated across all the three stages of plant ( S12 Table ), and capture the ABA signalosome complex ( Fig 1 ).
Red nodes indicate key genes and clusters up-regulated and part of uDTN. Blue nodes indicate key genes and processes down-regulated and part of dDTN. Green nodes indicate key processes affected by uDTN genes. Nodes with ‘*’ indicate uDTN seed genes.
https://doi.org/10.1371/journal.pone.0216068.g005
In over-expression experiments, OsbZIP23 TF (U1) has been shown to regulate genes with roles in drought tolerance, such as Dehydrins (OsDhn1, RAB16C), OsbZIP40 (U13) and Oshox22 (involved in ABA biosynthesis [ 184 ]) and an increased concentration of osmolytes such as proline and soluble sugars observed [ 185 ]. These genes are all part of our predicted uDTN and provide drought-tolerant traits. The interactions between these genes represent the mechanisms providing drought tolerance are depicted in Fig 5 .
The OsbZIP12 (Cluster U10) regulates a large number of genes involved in ABA signalling and in metabolic pathways [ 186 ] and provides link between these two important mechanisms in drought response (depicted in Fig 5 ). It has been reported that its major targets are OsbZIP23 (U1), OsbZIP46 (U13) and OsGAD1 (associated with regulation of stomatal closure during drought stress [ 187 ], polyamine and proline accumulations [ 188 ]) and various PP2C genes (OsPP2C09, OsPP2C30 and OsPP2C49), in a study on transgenic rice lines using RNA-seq and Chip-seq assays by Zang et al . [ 42 ], further providing evidence to the interactions captured by our model. Metabolic pathway genes (4-alpha-glucanotransferase (DEP1) and beta-amylase (OsSTA246)) involved in degradation of starch into soluble sugars (Cluster U12) are known to play an important role in osmotic adjustments [ 189 ] and are also regulated by OsbZIP12 [ 42 ].
The bZIP TFs, OsbZIP12 and OsbZIP40 (a probable target of OsbZIP23), have been indicated as negative inhibitors of floral initiation [ 190 ]. This interaction is represented in Fig 5 , linking the bZIP TFs to the down-regulated Cluster D6, capturing flowering and developmental processes and provides a probable drought escape mechanism [ 191 ]. Stress avoidance mechanisms involve dynamic changes in protein and metabolite abundances. Although a direct correlation with transcriptomic changes is a challenge owing to the intervening post-translational and other regulatory mechanisms, snapshots of these events are indeed captured by our meta-analysis. For example, Arginine, Proline and Asparigine metabolisms are up-regulated (U3, U4) resulting in an increase in free amino acid level. This has been reported in many drought tolerant lines from various plant species [ 192 , 193 ]. A similar pattern of protein degradation and ubiquitination is observed (U6, U8) and protein synthesis likely to be affected due to down-regulation of ribosome biogenesis (D4, D5).
Carbohydrate metabolism (Glycolysis (U1), TCA cycle (U9) and Pentose Phosphate Pathway (U1)) plays a key role in drought response. These pathways aid in energy production under adverse conditions, sugar accumulation, providing carbon skeletons for primary and secondary metabolites, and have been reported to be drought-tolerant mechanisms [ 194 , 195 ]. From Fig 3 , their association with ABA signalling can be noticed. The oxidative branch of PEP links carbohydrate and fatty acid as well as purine and pyrimidine nucleotide metabolisms. The PEP pathway has been categorized as a “metabolic sensor” in response to oxidative stress and at the same time provides precursors for de novo biosynthesis of pyrimidines via PRPP [ 196 ], thereby linking UPMS2 to ABA signalling. The presence of ABRE cis elements in UMPS2 and its 16 neighbours of the metabolic components ( Fig 3 ) further confirm this fact. As such, it forms a ‘hub’ and can be explored further as a novel candidate for drought tolerance. Other protective mechanisms are provided by heat shock proteins and transcription factors (HSPs and HSFs). Presence of ABRE elements and cis -elements associated with methyl-jasmonate (MeJa) (U2) in these up-regulated proteins indicate a protective role in cellular homeostasis, ROS scavenging as well as in osmotic adjustments.
Photosynthesis is a key process of the primary metabolism. A number of associated processes such as stomatal closure with reduced CO 2 diffusion, induction of senescence by ABA, and rolling of leaves leading to reduced surface area contribute towards reduced photosynthetic rates [ 197 ]. In the present study, genes associated with photosynthetic light reaction such as ATP synthases and NADPH dehydrogenase subunits, chloroplast precursors and chlorophyll A-B binding proteins, etc. were found to be down-regulated as a result of drought stress. Antagonistic interactions between ABA and NPR1 and WRKY-45 dependent SA signalling pathway (D3) lead to the down-regulation of several disease resistance genes (D7, D14) and potential PRRs (kinases) with LRR and ANK repeats (D3). Additionally, genes involved in JA homeostasis are seen to be down-regulated, thereby making the plant susceptible to wounding and pathogen attack. Majority of the signalling and transport related genes (D7) down-regulated across all the 9 data subsets indicate susceptibility to biotic stress as well as growth arrests under drought stress. Finally, as expected, genes involved in cellulose synthesis and in primary and secondary metabolism (D2) are down-regulated under drought stress. At the same time, a compensatory mechanism between proline biosynthetic genes (P5CS) and cell wall-related genes (U3), and increase in secondary metabolites are observed in uDTN.
Thus, we see that the proposed integrated network-based approach is helpful in identifying important drought responsive processes that are well-represented in majority of the data subsets considered. Moreover, in silico functional annotations of uncharacterized genes, e.g., membrane-bound ankyrin-like protein (LOC_Os02g50270) associated with ABA signalling in uDTN, uncharacterized genes associated with known photosynthetic genes and light-responsive promoters, as well as those associated with cellulose synthesis are identified as important drought-responsive biomarkers. Further analysis of these genes using transgenic studies may help in elucidating their role in drought response.
In this study, a comprehensive analysis of the complex drought-responsive processes using an integrated, systems-level approach across seven drought tolerant rice genotypes is presented. Preliminary analysis of the co-expression network revealed that transcriptional regulatory processes, post-translational modifications followed by photosynthesis are at the forefront of drought response. With the integration of protein-protein interactions, a detailed view of the system is possible and critical processes which otherwise lay hidden are brought forth. The phytohormone abscisic acid is seen to play a central role in regulating the energy metabolism of cells. Protective mechanisms like the transcriptional activation of molecular chaperons, amino acid metabolism and cellular respiratory processes are enhanced. The down-regulation of photosynthetic machinery and cellulose-associated genes explains a cessation of aerial growth during drought stress. The antagonistic relationship observed between ABA and SA-dependent pathogen response pathways indicate increased susceptibility of plants to certain pathogens during drought.
The systems-based approach discussed above, apart from capturing important stress-responsive processes, also help in insilico functional annotation of uncharacterized genes. Based on neighborhood analysis in the co-expression and protein-protein interaction networks, important biomarkers with significant topological properties and connectivity to other stress-responsive genes have been identified. A systematic dataset-specific analysis revealed that certain processes are well-conserved across all genotypes/stages, while some exhibit genotype-specific variations. Drought resistance/tolerance is indeed a complex process with dependencies on many variables, viz ., soil, weather conditions, duration of stress, tissue-specific response, etc. Better designed experiments with larger sample sizes and taking these variables into consideration will further enhance the efforts in reliable detection of drought-tolerant biomarkers.
Supporting information
S1 table. dataset details..
https://doi.org/10.1371/journal.pone.0216068.s001
S2 Table. Co-expression network with genes and module labels.
https://doi.org/10.1371/journal.pone.0216068.s002
S3 Table. Module preservation statistics.
https://doi.org/10.1371/journal.pone.0216068.s003
S4 Table. Up-regulated PPIN (uDTN).
https://doi.org/10.1371/journal.pone.0216068.s004
S5 Table. Down-regulated PPIN (dDTN).
https://doi.org/10.1371/journal.pone.0216068.s005
S6 Table. Analysis of cis-elements for some of the clusters.
https://doi.org/10.1371/journal.pone.0216068.s006
S7 Table. Stage-specific DEGs across the datasets.
https://doi.org/10.1371/journal.pone.0216068.s007
S8 Table. Distribution of DEGs in the 6 microarray studies across 9 data subsets.
https://doi.org/10.1371/journal.pone.0216068.s008
S9 Table. Distribution of DEGs from up and down-regulated drought-responsive modules in the 6 microarray studies across 9 data subsets.
https://doi.org/10.1371/journal.pone.0216068.s009
S10 Table. Distribution of the DEGs (after screening for important genes) used for the construction of uDTN and dDTN shown across 9 data subsets.
https://doi.org/10.1371/journal.pone.0216068.s010
S11 Table. Distribution of uDTN and dDTN DEGs shown across 9 data subsets (after extracting PPIs).
https://doi.org/10.1371/journal.pone.0216068.s011
S12 Table. Distribution of DEGs in uDTN clusters.
https://doi.org/10.1371/journal.pone.0216068.s012
S13 Table. Distribution of DEGs in dDTN clusters.
https://doi.org/10.1371/journal.pone.0216068.s013
- 1. Current Conditions | Global Drought Information System [Internet]. [cited 5 Apr 2017]. https://www.drought.gov/gdm/current-conditions
- View Article
- PubMed/NCBI
- Google Scholar
- 91. Alvarez PJC (National AIC (Paraguay). D of G of BSC, Krzyzanowski FC, Mandarino JMG, Franca Neto JB. Relationship between soybean seed coat lignin content and resistance to mechanical damage. Seed Sci Technol. International Seed Testing Association; 1997;
- 101. Finnegan PM, Soole KL, Umbach AL. Alternative Mitochondrial Electron Transport Proteins in Higher Plants. Springer Netherlands; 2004. pp. 163–230.
- 134. Takatsuji H, Jiang C-J. Plant Hormone Crosstalks Under Biotic Stresses. Phytohormones: A Window to Metabolism, Signaling and Biotechnological Applications. New York, NY: Springer New York; 2014. pp. 323–350. https://doi.org/10.1007/978-1-4939-0491-4_11
- 193. Muttucumaru N, Powers SJ, Elmore JS, Mottram DS, Halford NG. Effects of Water Availability on Free Amino Acids, Sugars, and Acrylamide-Forming Potential in Potato. 2015;
Thank you for visiting nature.com. You are using a browser version with limited support for CSS. To obtain the best experience, we recommend you use a more up to date browser (or turn off compatibility mode in Internet Explorer). In the meantime, to ensure continued support, we are displaying the site without styles and JavaScript.
- View all journals
- My Account Login
- Explore content
- About the journal
- Publish with us
- Sign up for alerts
- Open access
- Published: 03 November 2021
RNASeq analysis of drought-stressed guayule reveals the role of gene transcription for modulating rubber, resin, and carbohydrate synthesis
- Chen Dong 1 na1 ,
- Grisel Ponciano 1 na1 ,
- Naxin Huo 1 ,
- Yong Gu 1 ,
- Daniel Ilut 2 &
- Colleen McMahan 1
Scientific Reports volume 11 , Article number: 21610 ( 2021 ) Cite this article
2035 Accesses
4 Citations
Metrics details
- Biotechnology
- Computational biology and bioinformatics
- Molecular biology
- Plant sciences
The drought-adapted shrub guayule ( Parthenium argentatum ) produces rubber, a natural product of major commercial importance, and two co-products with potential industrial use: terpene resin and the carbohydrate fructan. The rubber content of guayule plants subjected to water stress is higher compared to that of well-irrigated plants, a fact consistently reported in guayule field evaluations. To better understand how drought influences rubber biosynthesis at the molecular level, a comprehensive transcriptome database was built from drought-stressed guayule stem tissues using de novo RNA-seq and genome-guided assembly, followed by annotation and expression analysis. Despite having higher rubber content, most rubber biosynthesis related genes were down-regulated in drought-stressed guayule, compared to well-irrigated plants, suggesting post-transcriptional effects may regulate drought-induced rubber accumulation. On the other hand, terpene resin biosynthesis genes were unevenly affected by water stress, implying unique environmental influences over transcriptional control of different terpene compounds or classes. Finally, drought induced expression of fructan catabolism genes in guayule and significantly suppressed these fructan biosynthesis genes. It appears then, that in guayule cultivation, irrigation levels might be calibrated in such a regime to enable tunable accumulation of rubber, resin and fructan.
Similar content being viewed by others
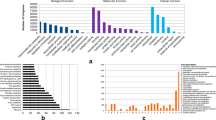
De-novo transcriptome analysis unveils differentially expressed genes regulating drought and salt stress response in Panicum sumatrense
Rasmita Rani Das, Seema Pradhan & Ajay Parida
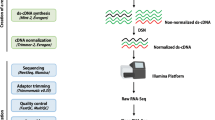
De novo transcriptome assembly and annotation for gene discovery in avocado, macadamia and mango
Tinashe G. Chabikwa, Francois F. Barbier, … Christine A. Beveridge
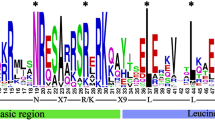
Genome-wide characterization of bZIP gene family identifies potential members involved in flavonoids biosynthesis in Ginkgo biloba L.
Huan Han, Feng Xu, … Jiabao Ye
Introduction
Guayule ( Parthenium argentatum A. Gray), the perennial desert shrub native to the Southwestern United States and northern Mexico, is a rubber-producing plant adapted to arid and semiarid regions 1 , 2 . Large scale guayule cultivation in the United States could provide a domestic source of natural rubber (NR, cis -1,4-polyisoprene) necessary for national defense, modern transportation, and medicine 3 , 4 . One challenge in guayule crop management is to maximize rubber yield while maintaining efficient irrigation water use. Techno-economic models for guayule cultivation point to water use as a major cost to growers 5 . However, high yields of rubber can be achieved with subsurface drip irrigation 6 . In that study, as in others 7 , 8 , 9 , 10 , 11 , guayule biomass and rubber yield responded positively to total water applied; however plant rubber content (wt%) consistently increased as irrigation water levels decreased. The possibility that drought stress may positively impact rubber biosynthesis is compelling, especially since water availability is an economic and environmental constraint in the Southwestern US.
In addition to NR, guayule produces other secondary metabolites in appreciable amounts such as resin (5–12% dry weight 12 ). Guayule resin constitutes a mixture of terpenoids, sterols, fatty acids, aromatic compounds, and low-molecular weight rubber, but the precise composition is much more complex 13 . Among the most abundant resin extractable compounds are argentatins (20–30%) which may have anti-cancer properties 14 and the guayulins (10–15%) known to have fungistatic and miticide activities 15 . The biological roles of individual resin compounds have likely evolved in response to biotic and abiotic stresses, and/or have ecological functions as is the case of many plant secondary metabolites 16 . Interestingly, the cellular locations of resin and NR production in guayule are in close proximity. The terpene components of resin are produced in resin canals in the bark parenchyma tissue and in the pith of stems, and NR is predominantly found in a single layer of epithelial cells surrounding the resin canal 17 . This intimate spatial distribution of the two metabolites makes it difficult to extract resin-free rubber (residual resin in the rubber extract compromises its stability 18 ), and vice-versa. Proposed commercial uses of guayule resin include in wood preservatives, paints and adhesives 19 , asphalt 20 , and pharmaceutical compounds 14 , 21 .
Another abundant metabolite produced by guayule is the carbohydrate fructan (up to ~ 10% dry weight 22 ), a biobased chemical feedstock. Fructans are fructose-based polymers with the predominant role of serving as carbon reserves when metabolic demand exceeds carbon availability 23 , but are also considered to be protective agents against abiotic stresses 24 , 25 , 26 . In guayule, fructans accumulate mainly in the root and stems and to lesser extent in leaves 22 . Fructan levels fluctuate seasonally 22 , 27 , and are highest at the onset of and during winter conditions parallel to rubber biosynthesis 28 .
The precise mechanism of in vivo NR synthesis remains to be elucidated, but in general the biosynthetic pathway comprises two main phases. First, the monomeric unit isopentenyl pyrophosphate (IPP), the rubber polymer-monomer, and the allylic initiator (typically farnesyl pyrophosphate, FPP) required for rubber biosynthesis, are produced by the cytosolic mevalonate pathway (MVA). Importantly, the IPP and FPP pools are substrates for biosynthesis of all downstream isoprenoids (terpenes, dolichols, sterols, etc.) many of which make up guayule resin. The second phase involves the actual NR synthesis by an enzymatic complex (rubber transferase, RuT) of unknown identity. The RuT is presumed to be localized on the surface of a vesicle derived from the endoplasmic reticulum and known as a rubber particle (RP). Current models propose FPP first binds to the RuT-binding site, and subsequently thousands of IPP undergo condensation reactions to produce the rubber molecule. As NR is synthesized it accumulates inside the RP while various distinctive proteins associate to stabilize it and/or modulate rubber biosynthesis 29 , 30 , 31 , 32 .
It is well established that cold stress elicits rubber biosynthesis in guayule stem bark tissues 33 , 34 , 35 , but water stress has also been reported to have a positive effect in guayule rubber content 6 , 11 , 36 . With the goal of identifying molecular players of drought-stressed guayule impacting rubber biosynthesis, we analyzed the transcriptome of field-grown plants under two irrigation water treatments: drought-like and fully irrigated control. A comprehensive transcriptome database was built using genome-guided 37 and de novo RNA-seq assembly. Our comparative analyses of the global transcription expression revealed that transcripts related to rubber biosynthesis were mostly down-regulated. Further, transcripts related to fructan biosynthesis (but not fructan catabolism) were also down-regulated. Expression regulation of genes encoding enzymes responsible for terpene resin biosynthesis was mixed.
Results and discussion
Transcriptome sequencing and read assembly.
To elucidate the molecular responses impacting secondary metabolite production in guayule during drought stress, six libraries from drought-treated and control stem tissue RNA were sequenced using Illumina HiSeq 2000 platform. In total, 196,861,972 raw pair-end reads with a read length of 2 × 150 bp were generated (Table 1 ). After quality trimming, 97.11% of cleaned reads were recovered and used for sequence assembly. A comprehensive transcriptome database was built using both genome-guided and Trinity de novo RNA-Seq assembly methods incorporated in the PASA pipeline 38 . A total of 229,190 unique contigs were obtained with non-redundant cut off at 95%, following a further filtration process to retain transcripts longer than 300 bp only. The most highly expressed transcripts that represent 84% of the total normalized expression data achieved an N50 of 1851 bps. The total accumulated size of the assembled transcripts was approximately 249 Mb, with length ranging from 300 to 17,875 bp. Additionally, 90.75% of all clean reads perfectly mapped back to the reference transcriptome, suggesting a strong representation of the read input and the quality of the assembly was sufficient for downstream analysis.
Differential gene expression and functional enrichment
To quantify abundances of each unigene, Kallisto 39 was used to generate TPM (transcripts per million), reflecting the relative molar concentration of transcripts in each sample. edgeR 40 was deployed to identify the differentially expressed transcripts with FDR < 0.05 and log2 fold change > 2. As a result, 1677 were found to be significantly differentially expressed, with 881 up-regulated and 796 down-regulated transcripts in drought-stressed tissue compared to the control (Fig. 1 ).
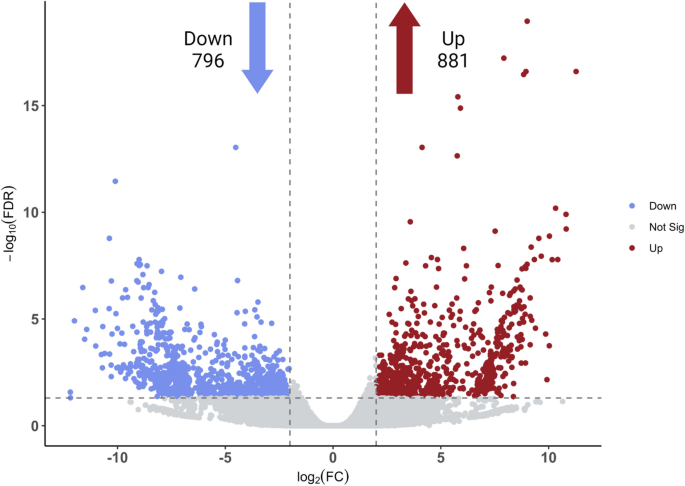
Volcano plot representation of differential expression. Red and blue points mark the transcripts with significantly increased or decreased expression in drought stressed plants versus control plants, respectively (FDR < 0.05). The x-axis shows log2 fold-changes in expression and the y-axis log10 false discovery rate of a transcript being differentially expressed.
To predict and analyze the function of the differentially expressed unigenes, we assessed the assembled transcripts using BLASTX search against databases listed in Table 1 . Among the 229,190 transcripts, 143,286 (62.52%) could be annotated in Nr (NCBI non-redundant protein sequences), 51,596 (47.94%) in Pfam (protein family database), 104,111 (45.43%) in Swiss-Prot (a manually annotated and reviewed protein sequences database), 34,502 (15.37%) in GO (Gene Ontology), and 40,917 (17.85%) in KEGG (Kyoto Encyclopedia of Genes and Genomes). Overall, 70.15% transcripts were significantly matched to known genes in the public databases mentioned above (Table 1 ), while those with no significant protein matches may represent novel proteins and long non-coding RNAs in guayule.
Transcription factors (TFs) regulate the transcription of genes and play key regulatory roles in plant growth, development, and response to environmental stress. Our analysis revealed that 2759 transcripts (1.20%) encode putative TFs that can be classified into 56 families (Supplementary Table S1 ). Most abundantly represented were the basic helix-loop-helix (bHLH) family (292, 10.58%), followed by cysteine2-histidine2 zinc finger (C2H2) family (179, 6.49%), basic leucine zipper (bZIP) family (166, 6.02%), no apical meristem/ATAF1–2/cup-shaped cotyledon (NAC) family (160, 5.80%), myeloblastosis (MYB) and related (296, 10.73%) and the cysteine3histidine (C3H) family (139, 5.04%). It is worth noting that MYB-related, bHLH and WRKY families are known to regulate secondary metabolism pathways in plants. In the rubber producing tree Hevea brasiliensis (Hevea), HbMYC2 was found to be highly expressed in bark and possibly positively regulating the RP associated gene HbSRPP 41 and to activate NR biosynthesis genes HbFPS1 and HbSRPP1 42 .
The three most enriched differentially expressed TF families were constans-like (2 transcripts up- and 7 down-regulated), MYB-related (9 transcripts up), golden2-like (1 transcript up- and 5 down-regulated) and bHLH (3 up- and 1 down-regulated). More than 60% of CO-like TF family transcripts (9 of 15) were significantly enriched under drought condition, among which more than 77% were down-regulated. The identification of this large set of TFs, along with their expression profiling under drought stress, provides a rich resource for future characterization of specific roles of TFs in rubber biosynthesis pathway under drought stress condition. Interestingly, we performed a comparative analysis of the transcriptome profile from greenhouse grown guayule subjected to cold 43 , and our drought stressed guayule transcriptome found a total of 58 significantly differentially expressed contigs under both stresses; sixteen of these encode transcription factors (Supplementary Table S2 ).
Gene Ontology assignments were used to determine the potential functions of the transcripts and classify them based on various biological processes. In total, 34,502 contigs were assigned to three major functional categories: biological process, molecular function, and cellular component (Fig. 2 ). The GO sub-categories with the largest transcripts were ‘cellular process’, ‘metabolic process’, ‘catalytic activity’, ‘binding’, and ‘cellular anatomical entity’.
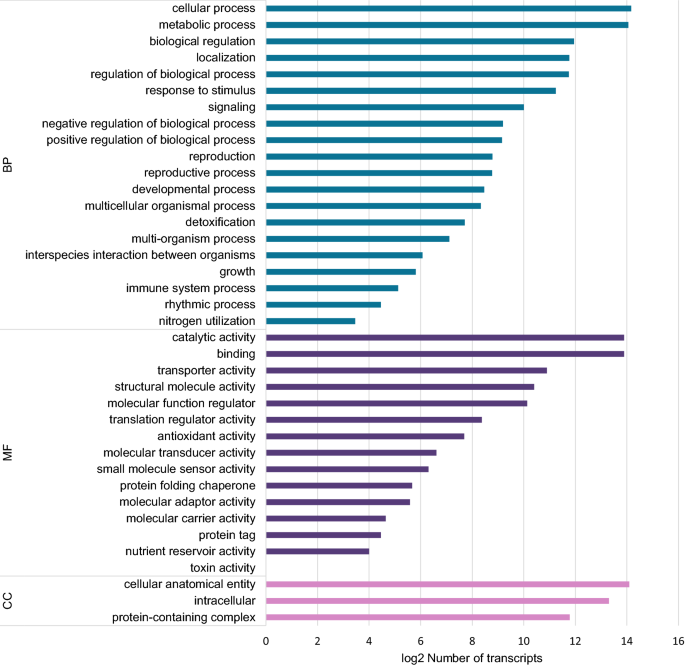
Gene Ontology (GO) analysis of RNA-seq. GO term classification of the entire transcriptome. BP biological process; CC cellular component; MF molecular function.
For GO enrichment analysis, we took consideration of correlations within a gene set with modest variability in expression but which may exhibit a general trend. The entire contig list, ranked based on the level of fold change without eliminating the ones considered non-significant, was supplied into Kolmogorov–Smirnov test. Overall, a total of 33 ontology terms (Supplementary Table S3 ) were enriched with FDR < 0.01. Interestingly, only one set involving terpene synthase activity (GO:0010333) was recognized as down-regulated in the drought stressed plants. Seven out of the remaining 32 up-regulated gene sets were, not surprisingly, related to redox activity grouped across both biological process and molecular function. We also found 5 enrichment sets of transcripts associated with proton transport coupled with ATP production and decomposition via oxidative phosphorylation and the tricarboxylic acid cycle as well as transcription and translation machinery, indicating that drought stressed plants were in a highly active energetic state through cellular respiration. Notably, glycolytic process (GO:0006096) producing pyruvate as end-product, which after oxidative decarboxylation fuels the carbon source for rubber biosynthesis 44 , is also significantly enriched in drought-stressed plants.
KEGG pathway analysis was performed to identify the active biological pathways in the annotated guayule sequences. Upon mapping the annotated sequences, 40,917 transcripts (17.85%) were identified and assigned to 353 metabolic pathways, including ‘cellular processes’, ‘environmental information processing’, ‘genetic information processing’, ‘metabolism’ and ‘organismal systems’ (Fig. 3 ). Among these pathways, signal transduction (18,565 transcripts), carbohydrate metabolism (8768), and transport and catabolism (6294) were the three most represented. Fisher’s exact test on the identified 1677 differentially expressed genes (DEG) was performed to explore the changes in metabolic pathways under drought treatment (Fig. 4 ). Environmental adaptation, not surprisingly, was the category with the most differentially expressed transcripts. Interestingly, 1314 contigs mapped pathways involved in metabolism of terpenoids and polyketides, including monoterpenoid, sesquiterpenoid and triterpenoid, and terpenoid backbone biosynthesis. The expression of transcripts in this category were the second most differentially expressed by irrigation treatment. Terpenoids are highly abundant components of guayule resin. Isoflavonoid biosynthesis and circadian rhythm were among the topmost enriched pathways. Plant hormone signal transduction also showed a high enrichment score. Finally, the most enriched gene count among all pathways in our assembly encode a family of protein transporters. Aquaporins are water channels important for maintaining salt and water homeostasis, especially under biotic stress 45 . In agreement with Nelson et al. 46 , PIP1-3 (PaTc_178810) is one of the most induced contigs (FC = 12.18) in drought stressed guayule.
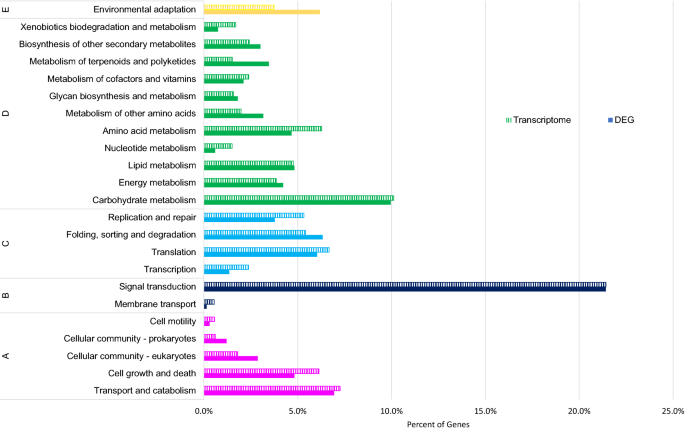
KEGG classification of unique sequences in both entire transcriptome (dashed line) and differentially expressed contigs (solid line). (A) Cellular processes; (B) Environmental information processing; (C) Genetic information processing; (D) Metabolism; and (E) Organismal systems. A total of 65,879 unique sequences were classified in the KEGG database.
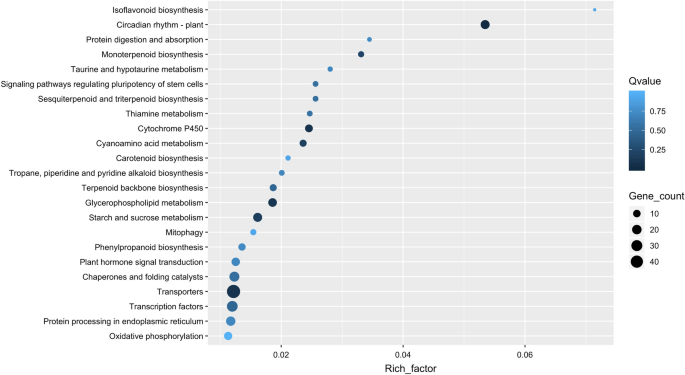
Scatter plot-enriched Kyoto Encyclopedia of Genes and Genomes (KEGG) pathways of differentially expressed genes (DEGs) in drought stressed plants compared to control plants in the field. The x-axis represents the gene ratio, which refers to the ratio of the DEG numbers annotated in the pathway term to all gene numbers annotated in the pathway term. The circle size indicates the number of DEGs that are associated with each significant pathway. The circle color indicates the significant level with the adjusted p-value using Benjamin Hochberg method.
Rubber and resin content in guayule are impacted differently by water irrigation levels
The guayule field irrigation study conducted in 2012–2015 in Maricopa, Arizona found consistently higher rubber content in plants treated with low (25% replacement of evapotranspiration (EVA), simulating drought conditions) irrigation water levels compared to the control (100% EVA) irrigation 6 (Sup Fig. 1 A). Stem subsamples from plants harvested in March 2015 were collected for the transcriptome analysis reported here. At that point drought-stressed plants had 8.6% rubber content, significantly higher than the 6.0% rubber in control plants (Sup Fig. 1 B). Higher rubber content for guayule subjected to drought stress has been reported in greenhouse 47 and field 6 , 36 studies. The mechanism is unknown, but water stress could create less cell expansion due to the reduced turgor pressure, and smaller cell volume concentrating solutes in the cell, including substrate for rubber biosynthesis, providing a possible explanation to the observed higher rubber content in drought-stressed plants. Remarkably, Reddy and Das 48 observed that low leaf water potential increased rubber transferase activity (and concomitant rubber content) in guayule.
In contrast, resin content was not correlated to irrigation level (Sup Fig. 1 A) and was not significantly different between the two treatments (Sup Fig. 1 B). Resin content in guayule is relatively insensitive to growing conditions such as seasonal temperature and water inputs 6 , 11 , 28 , 47 , 49 . It appears that, as a whole, unlike rubber, resin biosynthesis in guayule is less affected by the environment. However, guayule resin is a complex mixture 13 , 50 and it is possible that biosynthesis of individual resin components such as terpenes may be impacted by water inputs or other environmental factors. Our transcriptome analysis found that many resin biosynthesis related transcripts were differentially expressed by drought (see section below), and therefore may provide breeding and genetic engineering targets for guayule improvement.
The mevalonate pathway is mostly down-regulated in drought stressed guayule
All isoprenoids, including NR, are derived from the precursor IPP. In plants, IPP is synthesized by two independent pathways: the mevalonate pathway (MVA) located mainly in the cytoplasm and the methylerythritol phosphate pathway (MEP) in plastids. Previous studies have shown that MVA pathway is likely the main source of IPP for rubber production in plants 51 , 52 , 53 . All genes encoding the MVA pathway enzymes were represented in our transcriptome (Table 2 ) however most of them (70%) were down-regulated by drought (Fig. 5 ). The enzyme 3-hydroxy-3-methylglutaryl-coenzyme A reductase (HMRG) is considered the key regulatory step in cytosolic IPP synthesis 54 , 55 , 56 . Our analysis identified five putative HMGR transcripts. Two HMGR -like transcripts were statistically significantly down-regulated (PaTc_112447 and PaTc_099724 with adjusted p-value at 0.0004 and 0.000, respectively). Interestingly, isoform PaTc_112447 (HMGR1 in Fig. 5 ), corresponds to a previously identified isoform from a guayule cold acclimated EST library 57 . No correlation between HMGR expression and rubber transferase activity was found in that study. As for the other three putative isoforms, one was moderately down-regulated (PaTc_071662), one slightly up-regulated (PaTc_042319) and another showed no change in expression level associated with plant water status (PaTc_036263). Activity of one of these five HMGR isoforms tracked with rubber transferase activity corresponding to an increase in rubber formation 58 . Identification of this rubber biosynthesis associated HMGR isoform will be critical not only for a better understanding of rubber biosynthetic pathway, but also to target this isoform, alongside other genes, in metabolic engineering and breeding efforts to increase rubber content in guayule.
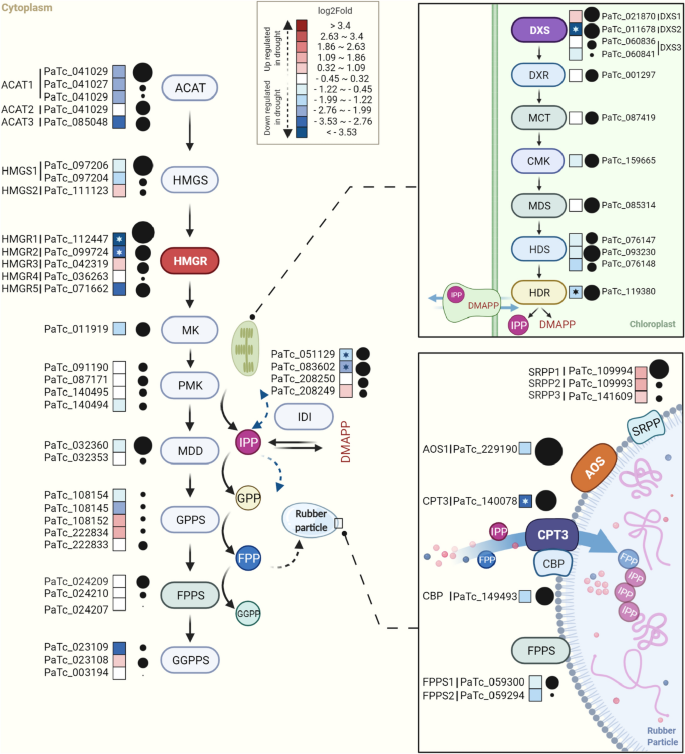
Expression of transcripts involved in mevalonate pathway (MVA), methylerythritol phosphate pathways (MEP) and rubber particle associated genes. Lower right, schematic model of the rubber biosynthetic machinery. Relative differential expression showed by a color gradient from low (blue) to high (red). Asterisks indicate significant differential expression (FDR < 0.05 and log2 fold-change > 2). Black dot sizes proportional to the expression level.
Interestingly, almost all MVA enzyme coding transcripts had at least one isoform unaffected by water treatment and/or another isoform slightly up-regulated. This diversity of transcriptional control suggests different MVA enzymes’ isoforms respond to different stimuli to exert specific metabolic control. The highest up-regulated transcript (PaTc_108152) encodes a geranyl pyrophosphate synthase (GPPS) isoform and the most down-regulated transcript encodes HMGR1 (Fig. 5 ). Overall our results indicate most of the MVA pathway in guayule is transcriptionally down-regulated by drought. It should be noted that isoprenoid pathway genes such as HMGR , 3-hydroxy-3-methylglutaryl-coenzyme A synthase ( HMGS ), phosphomevalonate kinase ( PMK ), isopentenyl diphosphate isomerase ( IDI ), and genaryl genaryl pyrophosphate synthase ( GGPPS ), have been reported to be down-regulated by drought in other plant species 59 , 60 , 61 .
Contigs coding all eight consecutive enzymes of the plastidic MEP pathway (Fig. 5 ) were identified (Supplementary Table S4 ). Of 129 contigs, 29 showed a higher expression trend under drought stress, however none was significant, while 55 showed a lower expression trend. Among the down-regulated contigs, 1-deoxy-D-xylulose 5-phosphate synthase (DXS), the rate limiting enzyme of MEP pathway was significantly down-regulated (Foldchange = − 5.49). Down-regulation of 4‐hydroxy‐3‐methylbut‐2‐enyl diphosphate reductase (HDR) was also significant. MEP pathway analysis under drought stress in grape showed down-regulation of genes regulating the early pathway steps 62 .
It appears that at the transcription level, both MVA and MEP pathways are depressed by prolonged drought in guayule. In conifers, metabolic flux analysis found the MEP pathway was reduced by drought but much less than photosynthesis and transpiration, suggesting alternative carbon sources feed this metabolic pathway under drought stress 63 . A similar situation could apply to drought-stressed guayule; that is, an alternative source of carbon may be activated to provide the necessary synthesis of IPP and subsequently rubber.
Is rubber biosynthesis under transcriptional control?
The RP is the site of NR production in plants and some fungi 64 , 65 , 66 ; the polymer is synthesized by the membrane-associated RuT enzymatic complex. Two potentially essential members of the guayule RuT complex are a cis -prenyltransferase (CPT3) and a CPT-binding protein (CBP) which are hypothesized to form an active heteromer 29 , 67 , 68 . In addition to these proteins, the RuT complex may include two other RP-associated proteins: the small rubber particle protein (SRPP), and allene oxide synthase (AOS), both of indeterminate function but with a recognized indirect role in rubber biosynthesis 69 , 70 , 71 , 72 . Transcripts of all the above RP associated proteins were identified in our transcriptome (Table 2 ) and found to be mostly down-regulated by drought, with the exception of SRPP . Interestingly, the transcript encoding CPT3 (PaTc_140078), the specific isoform involved in rubber biosynthesis 67 ( PaCPT3 ) was found to be highly abundant in stem tissue as expected, but statistically significantly down-regulated by drought, in spite of the fact that drought-stressed guayule plants had higher rubber content (Fig. 5 ). The two other guayule CPT transcripts not involved in rubber biosynthesis 67 ( PaCPT1 and PaCPT2 ) were of low abundance (Table 2 ), and were either slightly up-regulated ( PaCPT2 , PaTc_069159) or unaffected by plant water status ( PaCPT1 , PaTc_044561). These two CPTs are most likely involved in biosynthesis of dolichols 67 , 73 and/or plastidial polyprenols essential for photosynthesis 74 . Transcripts encoding CBP (PaTc_149493) were also down-regulated by drought, although not to the extent of CPT3 (Fig. 5 ). The AOS transcript PaTc_229190, encoding the most abundant protein associated with guayule RP 72 , 75 , 76 , not surprisingly had the highest expression levels among all other rubber biosynthesis related genes under both conditions (Table 2 ). Interestingly, this transcript was down-regulated 39% by drought despite the high rubber content. This negative correlation of AOS levels and rubber content was observed in AOS -silenced transgenic guayule lines, resulting in increased rubber content and higher RuT activity 72 . In that study, a structural role of AOS in guayule RP was proposed. Two other AOS isoforms (AOS-like, AOSL) of unknown function and localization have different expression profiles of slightly up-regulated ( AOSL2 , PaTc_122848) or no differential expression compared to control ( AOSL3 , PaTc_122845). The function of these AOSs could be the well-known role in jasmonic acid synthesis 77 , 78 , 79 .
The only RP-associated transcript found to be up-regulated was SRPP (PaTc_109994). SRPP is a stress response protein 80 , 81 , 82 so it is not surprising drought would result in its up-regulation. Only one guayule SRPP gene has been cloned 69 ( g uayule h omolog of S RPP, GHS); our assembly identified two additional putative SRPP isoforms of unknown function but also up-regulated by drought (Table 2 ). Kajiura et al. 17 antibodies used for RP proteins detection by western blot recognize an epitope present in GHS, and partially (92%) in the deduced protein sequences of the newly identified transcripts. The possibility of antibodies cross-reaction cannot be ruled out and therefore localization of the new SRPP isoforms remains to be determined. Although the role of SRPP in rubber biosynthesis remains to be elucidated, down-regulation of SRPP affected accumulation and quality of rubber in dandelion 70 , 71 . Dai et al. 32 propose SRPP (and a related protein, rubber elongation factor (REF)) are likely negatively charged in the electrically neutral environment of Hevea latex, thus allowing the RP to maintain a stable colloidal form. Additionally, they propose SRPP and REF associates to the growing RP, allowing it to enlarge for the accumulation of new rubber molecules. SRPP may be needed to stabilize growing rubber particles in drought-stressed, high rubber-producing guayule.
Farnesyl pyrophosphate, required for initiation of rubber biosynthesis, is synthesized by farnesyl pyrophosphate synthase (FPPS) through condensation of genaryl pyrophosphate (GPP) and IPP. Two guayule FPPS genes have been cloned, characterized and their corresponding proteins confirmed to localize on the RP surface (Pan et al. 1996). Expression of FPPS1 transcript (PaTc_059300) was slightly down-regulated by drought whereas that of FPPS2 (PaTc_024209) appears to be unaffected (Fig. 5 ). Both transcripts are moderately abundant in stem tissue compared to three other putative isoforms identified in our assembly (Table 2 ).
The fidelity of the in silico DEG predictions for rubber biosynthesis related genes was validated by quantitative reverse transcription PCR (qPCR). We analyzed the expression of CPT3 , CBP , AOS , and SRPP as well as three MVA pathway genes ( HMGS , HMGR , and FPPS , Fig. 6 ). In agreement with the DEG analysis, only SRPP was up-regulated under drought; all others were down-regulated compared to the control.
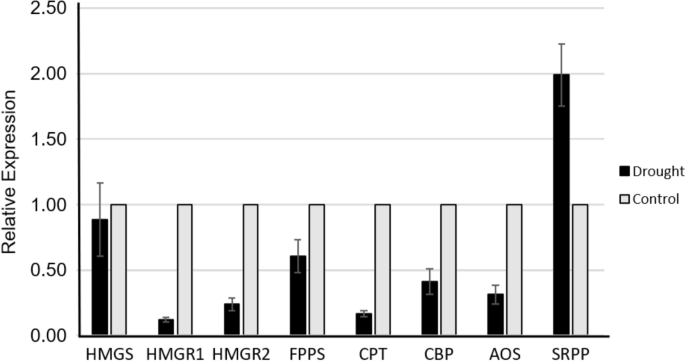
RT-PCR validation of expression levels. Selected guayule rubber biosynthesis related genes from stem tissues of plants grown under drought stress condition (black bars) relative to full irrigation control (grey bars). Values are the average of three biological replicates, error bars correspond to standard deviation error.
In summary, the low expression levels observed in rubber biosynthesis related transcripts (and the MVA pathway) contradict the high rubber content commonly found in drought-stressed guayule plants. These seemingly contradictory facts could be explained by the prospect that the rubber biosynthesis regulation is at the post-transcriptional, translational and/or post-translational levels. An earlier guayule field study 57 failed to find a direct correlation between gene expression and RuT activity, also implying the control point of rubber biosynthesis in guayule may not be at the transcriptional level. Interestingly, drought-responsive long non-coding RNAs have been identified in guayule 43 , including one (GFTW01168370.1) featuring a conserved binding site for miR166, a drought-responsive microRNA.
Previously, under a laboratory environment, cold stress elicited expression of RP encoding genes in guayule including AOS , CPT , FPPS and SRPP 43 . Although both stresses (drought and cold) in guayule promote rubber synthesis, it appears that different genes in the biosynthetic pathway are expressed differently under each type of stress. Additionally, evidence suggests the coarse control of the MVA pathway is at the transcriptional level while the fine-tuning control at the post-transcriptional and/or post-translational levels 56 . In Hevea, proteomic analysis of latex found phosphorylation of some REF and SRPP isoforms 83 following stimulation of rubber biosynthesis by ethylene treatment. Therefore, it is likely that both posttranscriptional and posttranslational mechanisms regulate rubber biosynthesis, as has been demonstrated for other plant secondary metabolites 84 , 85 .
Drought has a mixed effect on expression levels of resin biosynthesis genes
The largest class of compounds that make up guayule resin are terpenoids 12 synthesized by terpene synthases (TPS). Using the highly conserved amino- and carboxyl- terminal domains, PF01397 and PF03936 respectively, we searched our assembly and identified a total of 70 contigs (Supplementary Table S5 ). Phylogenetic analysis (Fig. 7 ) clustered these contigs into 5 subfamilies with TPS-a and TPS-b the most expanded groups (26 and 27 contigs, respectively), comprising about 75% of the total TPSs. This is in accordance with other plant TPS profiles 86 , 87 , 88 . Previous studies have shown that TPS genes exhibit distinct tempo-spatial expression patterns with and without stress 86 , 88 . Among the 70 identified TPS in guayule, 15 of them showed no detectable transcript level in both control and drought-stressed plants for meaningful differential expression comparison. These TPS contigs are either nonfunctional pseudogenes or they are preferentially expressed in other organs. Among the other 55 contigs, which showed a differential expression pattern under drought stress, 21 contigs were up-regulated in the range of 0.23–5.53-fold, with an average fold change of 1.59; only two (PaTc_112885, PaTc_180037; Fig. 7 ) were significant, both involved in sesquiterpene synthesis. These two putative β-caryophyllene synthases likely synthesize a volatile terpene known to be induced when plants are subjected to different stresses, including wounding and herbivore attack 89 .
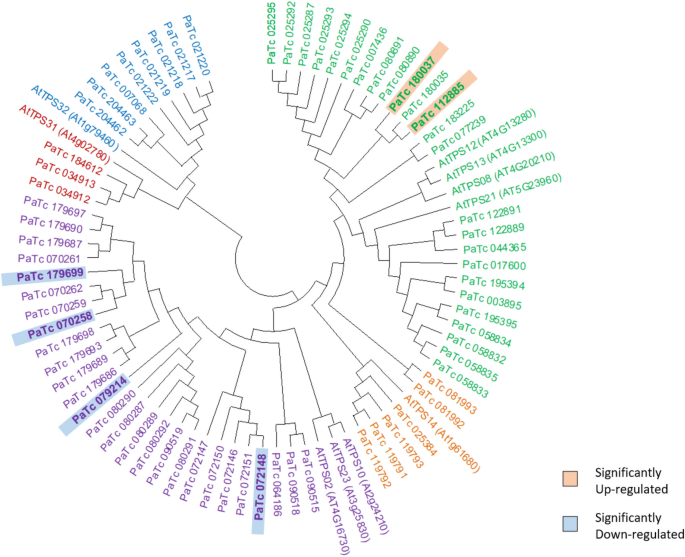
Molecular phylogenetic analysis of terpene synthases TPSs in stem tissue of field-grown guayule. The tree was constructed with MEGA X with maximum Likelihood method. Subfamilies highlighted by color: green (TPS-a), purple (TPS-b), red (TPS-c), blue (TPS-e/f) and orange (TPS-g) respectively. Significantly differentially expressed contigs in bold.
Thirty-four TPS contigs were suppressed under drought stress, in the range from 0.17 to 9.14-fold. Four of them (PaTc_070258, PaTc_079214, PaTc_179699, PaTc_072148; Fig. 7 ) were significantly down-regulated, all putative monoterpene synthases. These four contigs encode R-linalool synthase QH1 which synthesizes (3R)-linalool from GPP. Linalool is one of the most common monoterpenoids produced by plants and has been extensively investigated for its role in plant–insect interactions, including pollinator attraction 90 and defense 91 . Notably, studies in Solanum lycopersicum and Camellia sinensis both showed significant decrease of linalool under drought 88 , 92 .
The most dramatically suppressed gene expression among the TPSs occurred to PaTc_079214 encoding a previously reported putative terpene synthase 3 46 . Interestingly, this transcript is one of the most significantly down regulated among the DEG set identified in this study.
The commercial viability of guayule as a crop depends on the successful utilization of resin as a high-value co-product. Our transcriptome offers a searchable database that can be mined to identify biosynthetic enzymes of the most abundant and high-value compounds including the guayulins and argentatins.
Down-regulation of fructan synthesis by drought is accompanied by up-regulation of fructan depolymerization and other osmolytes biosynthesis genes
Fructan, a water-soluble polysaccharide, is the main reserve of carbohydrates in guayule 27 . Evidence shows fructans are actively involved in cold and drought stress response in plants by their capacity of maintaining cell membrane integrity through insertion in the lipid headgroup region of the membrane 25 , 26 . Two enzymes, sucrose:sucrose 1-fructosyl transferase (1-SST) and fructan:fructan 1-fructosyl transferase (1-FFT) are responsible for fructan synthesis, while the enzyme fructan 1-exohydrolase (1-FEH) catabolizes fructans 24 . In our assembly, contigs encoding 1Sst (PaTc_199271, PaTc_199269) and 1Fft (PaTc_016980) were both down-regulated significantly under drought stress, with an average fold change of 4.3 and 5.6 respectively, while 1-Feh (PaTc_110775) was significantly induced (FC = 3.5). This suggests that drought-stressed guayule likely activated fructan depolymerization (Supplementary Table S6 ). In cereals, drought stress likewise led to fructan degradation 93 , 94 . Notably, these three genes showed the same expression pattern in cold-induced guayule stem tissue 43 (Supplementary Table S6 ). Cold stress triggers rubber biosynthesis in guayule and the commonality of fructan metabolism transcriptional control in both drought and cold stress justifies a deeper analysis of fructans’ role in rubber biosynthesis as previously suggested by Benzioni and Mills 49 .
Interestingly, a similar gene expression pattern was observed in other carbohydrates metabolism (Supplementary Table S6 ). For example, fructose-bisphosphate aldolase (PaTc_033225), β-fructofuranosidase (PaTc_004249), GDP-L-galactose phosphorylase (PaTc_066454) and β-glucosidase (PaTc_068062), all of which are involved in hydrolyzing polysaccharides into simpler saccharides, were significantly induced under drought condition. On the other hand, glucose-1-phosphate adenylyltransferase (PaTc_166809) and raffinose synthase (PaTc_066497), both involved in polysaccharide synthesis, were down-regulated in stressed plants. Moreover, extensive studies have shown that polyamine (PA) is an important stress modulator in plants 95 , 96 . In our analyses, PA-synthesizing enzymes arginine decarboxylase (PaTc_082215) and S-adenosylmethionine decarboxylase (PaTc_065600, PaTc_003065, PaTc_118154) were heavily induced, while polyamine oxidase (PaTc_033142, PaTc_033141, PaTc_033146, PaTc_033145) were significantly down-regulated with average fold change of 3.5, suggesting a possible drought response strategy by maintaining a higher level of PA.
Taken together, it appears that osmotic stress in guayule triggers the accumulation of soluble sugars and PAs to help the plant cope with low water status. Additionally, drought generally suppresses photosynthesis and therefore there is less sugar available to support metabolic reactions such as rubber synthesis. Other sources of carbon such as carbohydrates’ catabolism could be providing the needed carbon for rubber synthesis.
Our transcriptome analyses introduce a new perspective to expression of gene families related to key metabolic pathways in guayule stem tissue under drought stress. Results revealed that transcriptional status of genes involved in biosynthesis of the three major compounds, natural rubber, terpene resin, and fructan, are affected differently. Under drought conditions, the cytosolic isoprenoid biosynthetic pathway genes (MVA) and transcripts of RP associated proteins (including putative components of the RT complex) are mostly down-regulated in stem tissue, the main site of rubber synthesis in guayule, despite higher overall rubber content. This suggests the rubber biosynthesis control point is at the post-transcriptional level and beyond. Future engineering strategies for guayule, and possibly other rubber-producing crops, should carefully consider the growing body of transcriptomic information beyond metabolic pathway genes, as evidence of more complex regulation schemes emerge. Guayule resin biosynthesis transcripts, on the other hand, appear to be variably affected by water status and transcriptional control of individual resin compounds’ synthesis is likely unique. Finally, carbohydrate metabolism (in response to drought) is shown to be under transcriptional control in guayule, as is the case under cold stress, and under both conditions leading to enhanced rubber content in guayule.
Plant material and treatments
The plant material used was Parthenium argentatum , guayule, line AZ-3 97 , provided by the United States Department of Agriculture as publicly-curated germplasm ( https://www.grin-global.org/),accession number PI 599676. Seeds were greenhouse planted, grown into seedlings, then transplanted into a field site at the University of Arizona, Maricopa Agricultural Center (MAC) and grown as described in Hunsaker et al. 6 . Following surface irrigation to establish the plants, subsurface drip irrigation was applied at 100% (control) and 25% (drought stress) of the soil water depletion, determined by field-calibrated, neutron moisture meters (Model 503, Campbell Pacific Nuclear, CPN, Martinez, CA). Plants were harvested in March 2015; rubber, resin, and biomass were quantified as per Hunsaker et al. 6 . Three biological replicates of bark tissue from stem were collected during the harvest. Tissues were immediately frozen in liquid nitrogen and stored at − 80 °C until RNA extraction. All germplasm sourcing, field operations, and laboratory procedures complied with relevant institutional, national, and international permissions, guidelines, and legislation.
Library preparation and RNA-sequencing
Stem bark tissue (~ 2 g) from 29-month old guayule plants was the source of RNA for sequencing and qPCR analysis. Total RNA was extracted following Laudencia et al. 98 protocol with the use of acid phenol:chloroform MB grade (Ambion, USA) instead of phenol:chloroform:isoamyl alcohol. The precipitated RNA was further cleaned with Qiagen RNeasy Plant Mini Kit (Qiagen, USA) and treated with DNA- free ™ kit (Ambion, USA). PolyA-RNA was prepared employing Qiagen RNeasy/QIAshredder protocols (Qiagen, USA). RNA concentration was quantified with Quant-iT™ RiboGreen™ RNA Assay Kit (Thermofisher Scientific, USA). RNA quality was analyzed using 2100 Bioanalyzer (Agilent Technologies, USA). RNAseq library construction was carried out with KAPA Stranded RNA-Seq Library Preparation Kit Illumina® platforms (Kapa Biosystems, USA) per the manufacturer's instructions. RNAseq libraries with insert sizes of 200–500 bp, and sequenced using Illumina HiSeq 2000 platform with paired-end (PE) reads of 150 bp.
De novo assembly and sequence processing
The raw reads were first cleaned by filtering out adaptor sequences and low-quality reads using Trimmomatic (v0.32). Trimmed reads, quality confirmed by running FastQC, were combined across six reads of both conditions. Both Trinity 99 (v2.9.0) de novo and Trinity genome-guided (diploid guayule genome 37 ) assemblies were supplied into the PASA pipeline 38 to generate the comprehensive transcriptome database. To remove the redundant sequences, CD-HIT package was used for further clustering with a 300-bp sequence length and 95% similarity cut-off values. High-quality reads were mapped back to the assembled transcriptome sequences for validation. Reads were aligned using Bowtie-2 with default parameters.
Gene function annotation
Gene functions were annotated against the Nr (NCBI non-redundant protein sequences), PFAM (protein family), Swiss-Prot (a manually annotated and reviewed protein sequence database), and TrEMBL (a computer-annotated protein sequence database) using local BLASTX program with an E-value threshold of 1e −10 . GO analysis was performed using Blast2GO with the same E-value cutoff. Metabolic pathway mapping of the transcripts was performed using the KEGG Automatic Annotation Server ( https://www.genome.jp/tools/kaas/ ). Plant transcription factors (TFs), transcriptional regulators (TRs) and protein kinases (PKs) were identified and classified into different gene families using standalone iTAK 100 (v 18.12).
Identification of differentially expressed genes (DEGs)
Differentially expressed transcripts under the two irrigation treatments were identified with edgeR 40 , using transcripts per million counts for each sample generated by Kallisto 39 . A two-fold change (FC ≥ 2) and FDR value < 0.05 were used to define the significant DEGs between treatment and control. GO and KEGG enrichment were performed on the transcripts identified as DEGs as well. For comparative analysis with cold stress guayule transcriptome, the raw data was acquired from NCBI BioProject PRJNA387289 with accession SRR5597223, SRR5597220, SRR5597221, SRR5597216, SRR5597215, SRR5597214, SRR5597224, SRR5597213, SRR5597212, SRR5597228, SRR5597231 AND SRR5597230 mapped back to TSA GFTW000000000.1 43 . Expression and differential expression analyses were completed in the same way as stated for the current drought study.
Quantitative RT-PCR (qRT-PCR)
Total RNA from ground (~ 100 mg) stem bark tissue was extracted with TRIzol® reagent (Life Technologies, USA), cleaned with RNeasy Mini Kit (Qiagen, USA), and traces of DNA removed with DNA-free™ Kit (Life Technologies, USA). Two micrograms of total RNA were the template for oligo (dT) 20 -generated cDNA with SuperScriptIII First-Strand Synthesis System for qPCR (Life Technologies, USA) following manufacturer instructions. The qPCR reactions were carried out using Applied Biosystems 7500 Fast Real Time PCR System and SYBR Green chemistry (Life Technologies, USA) in 20 µl volume reactions containing 400 ng of template cDNA, 900 nM of each forward and reverse primer, 10 µl of Fast SYBR® Green Master Mix, and water as needed. The primers used are listed in Supplementary Table S7 . Thermocycler temperature regime was: 95 °C for 20 s, followed by 40 cycles of 95 °C for 3 s and 60 °C for 30 s. Data were analyzed using the 7500 Fast System Detection Software (Life Technologies, USA) with manually set threshold. Expression of each target gene was calculated with the Livak and Schmittgen 101 method, normalized to expression of the endogenous reference gene eIF4a or 18S , and then to its expression in a calibrator (fully irrigated control plant). Three technical replicates reactions were run for each target gene, and the whole experiment was performed three times using the same RNA but freshly synthesized cDNA.
Data availability
The raw Illumina data generated in this study were deposited in the NCBI Sequence Read Archive (SRA) under the BioProject accession number PRJNA400611. The transcriptome assembly generated from the current study has been made available on the ARS Guayule genome website: https://probes.pw.usda.gov/Guayule/ .
McGinnies, W. G. & Haase, E. F. Guayule: A Rubber-producing Shrub for Arid and Semiarid Regions (ed. McGinnies, W. G.) 274 (University of Arizona, 1975).
Sulas, L. et al. Adaptation, biometric traits and performances of guayule lines grown in two Mediterranean environments. Agri 10 , 651. https://doi.org/10.3390/agriculture10120651 (2020).
Article CAS Google Scholar
Van Beilen, J. B. & Poirier, Y. Establishment of new crops for the production of natural rubber. Trends Biotech. 25 , 523–529. https://doi.org/10.1016/j.tibtech.2007.08.009 (2007).
Cornish, K. Alternative natural rubber crops: why should we care?. Tech. Innov. 18 , 245–256. https://doi.org/10.21300/18.4.2017.245 (2017).
Sproul, E. et al. Integrated techno-economic and environmental analysis of guayule rubber production. J. Cleaner Prod. 273 , 122811. https://doi.org/10.1016/j.jclepro.2020.122811 (2020).
Article Google Scholar
Hunsaker, D. J., Elshikha, D. M. & Bronson, K. F. High guayule rubber production with subsurface irrigation in the US desert Southwest. Agric. Water Manage. 220 , 1–12. https://doi.org/10.1016/j.agwat.2019.04.016 (2019).
Miyamoto, S. & Bucks, D. A. Water quantity and quality requirements of guayule: current assessment. Agric. Water Manage. 10 , 205–219. https://doi.org/10.1016/0378-3774(85)90012-5 (1985).
Bucks, D. B., Nakayama, F. S., French, O. F., Rasnick, B. A. & Alexander, W. L. Irrigated guayule-Plant growth and production. Agric. Water Manage. 10 , 81–93. https://doi.org/10.1016/0378-3774(85)90036-8 (1985).
Bucks, D. B., Nakayama, F. S., French, O. F., Legard, W. W. & Alexander, W. L. Irrigated guayule-production and water use relationships. Agric. Water Manage. 10 , 95–102. https://doi.org/10.1016/0378-3774(85)90037-X (1985).
Benzioni, A., Mills, D. & Forti, M. Effect of irrigation regimes on the water status, vegetative growth and rubber production of guayule plants. Expl. Agric. 25 , 189–197. https://doi.org/10.1017/S0014479700016690 (1989).
Hunsaker, D. J. & Elshikha, D. M. Surface irrigation management for guayule rubber production in the US desert Southwest. Agric. Water Manage. 185 , 43–57. https://doi.org/10.1016/j.agwat.2017.01.015 (2017).
Cheng, F. et al. Characterization and evaluation of guayule processing residues as potential feedstock for biofuel and chemical production. Ind. Crops Prod. 150 , 112311. https://doi.org/10.1016/j.indcrop.2020.112311 (2020).
Dehghanizadeh, M., Cheng, F., Jarvis, J. M., Holguin, F. O. & Brewer, C. E. Characterization of resin extracted from guayule ( Parthenium argentatum ): a dataset including GC–MS and FT-ICR MS. Data Brief 31 , 105989. https://doi.org/10.1016/j.dib.2020.105989 (2020).
Article PubMed Central PubMed Google Scholar
Tavarez-Santamaría, Z. et al. A higher frequency administration of the nontoxic cycloartane-type triterpene argentatin A improved its anti-tumor activity. Molecules 25 , 1780. https://doi.org/10.3390/molecules25081780 (2020).
Article CAS PubMed Central Google Scholar
Jara, F. M., Cornish, K. & Carmona, M. Potential applications of guayulins to improve geasibility of guayule cultivation. Agronomy 9 , 804. https://doi.org/10.3390/agronomy9120804 (2019).
Croteau R., Kutchan T. & Lewis, N. Biochemistry and Molecular Biology of Plants . (eds. Buchanan, B., Gruissem, W., Jones, R.) 1250–1268 (American Society of Plant Biologists, 2000).
Kajiura, H., Suzuki, N., Mouri, H., Watanabe, N. & Nakazawa, Y. Elucidation of rubber biosynthesis and accumulation in the rubber producing shrub, guayule ( Parthenium argentatum Gray). Planta 247 , 513–526. https://doi.org/10.1007/s00425-017-2804-7 (2018).
Article CAS PubMed Google Scholar
Keller, R. W., Winkler, D. S. & Stephens, H. L. Degradative effects of guayule resin on natural rubber. Rubber Chem. Tech. 54 , 115–123. https://doi.org/10.5254/1.3535786 (1981).
Nakayama, F. S. Guayule future development. Ind. Crops Prod. 22 , 3–13. https://doi.org/10.1016/j.indcrop.2004.05.006 (2005).
Lusher, S. M. & Richardson, D. N. Guayule plant extracts as recycling agents in hot mix asphalt with high reclaimed binder content. J. Mater. Civ. Eng. https://doi.org/10.1061/(ASCE)MT.1943-5533.0001238 (2015).
Pascual-Villalobos, M. J. & Lopez, M. D. New application of guayule resin in controlled release formulations. Ind. Crops Prod. 43 , 44–49. https://doi.org/10.1016/j.indcrop.2012.07.001 (2013).
Dong, N. et al. Fructan reduction by downregulation of 1-SST in guayule. Ind. Crops Prod. 107 , 609–617. https://doi.org/10.1016/j.indcrop.2017.04.034 (2017).
Ritsema, T. & Smeekens, S. Fructans: beneficial for plants and humans. Curr. Opin. Plant Biol. 6 , 223–230. https://doi.org/10.1016/S1369-5266(03)00034-7 (2003).
Van den Ende, W., De Coninck, B. & Van Laere, A. Plant fructan exohydrolases: a role in signaling and defense?. Trends Plant Sci. 9 , 523–528. https://doi.org/10.1016/j.tplants.2004.09.008 (2004).
Valluru, R. & Van den Ende, W. Plant fructans in stress environments: emerging concepts and future prospects. J. Exp. Bot. 59 , 2905–2916. https://doi.org/10.1093/jxb/ern164 (2008).
Livingston, D. P., Hincha, D. K. & Heyer, A. G. Fructan and its relationship to abiotic stress tolerance. Cell. Mol. Life Sci. 66 , 2007–2023. https://doi.org/10.1007/s00018-009-0002-x (2009).
Article CAS PubMed Central PubMed Google Scholar
Kelly, K. M. & Van Staden, J. A preliminary study of the carbohydrate metabolism in Parthenium argentatum. Bioresour. Technol. 35 , 127–132. https://doi.org/10.1016/0960-8524(91)90019-G (1991).
Salvucci, M. E., Barta, C., Byers, J. A. & Canarini, A. Photosynthesis and assimilate partitioning between carbohydrates and isoprenoid products in vegetatively active and dormant guayule: physiological and environmental constraints on rubber accumulation in a semiarid shrub. Physiol Plant. 140 , 368–379. https://doi.org/10.1111/j.1399-3054.2010.01409.x (2010).
Qu, Y. et al. A Lettuce ( Lactuca sativa ) homolog of human Nogo-B receptor interacts with cis -prenyltransferase and is necessary for natural rubber biosynthesis. J. Bio. Chem. 290 (4), 1898–1914. https://doi.org/10.1074/jbc.M114.616920 (2015).
Yamashita, S. et al. Identification and reconstitution of the rubber biosynthetic machinery on rubber particles from Hevea brasiliensis . Elife 5 , 19022. https://doi.org/10.7554/eLife.19022 (2016).
Brown, D. et al. Subcellular localization and interactions among rubber particle proteins from Hevea brasiliensis . J. Exp. Bot. 68 , 5045–5055. https://doi.org/10.1093/jxb/erx331 (2017).
Dai, L., Nie, Z., Kang, G., Li, Y. & Zeng, R. Identification and subcellular localization analysis of two rubber elongation factor isoforms on Hevea brasiliensis rubber particles. Plant Physiol. Biochem. 111 , 97–106. https://doi.org/10.1016/j.plaphy.2016.11.006 (2017).
Bonner, J. Effect of temperature on rubber accumulation in guayule plant. Bot. Gaz. 105 , 233–243. https://doi.org/10.1086/335212 (1943).
Cornish, K. & Backhaus, R. A. Induction of rubber transferase activity in guayule ( Parthenium argentatum Gray) by low temperatures. Ind. Crops Prod. 17 , 83–92. https://doi.org/10.1016/S0926-6690(02)00079-1 (2003).
Benedict, C. R., Greer, P. & Foster, M. A. The physiology and biochemical responses of guayule to the low temperature of the Chihuahuan desert in the biosynthesis of rubber. Ind. Crops Prod. 27 , 225–235. https://doi.org/10.1016/j.indcrop.2007.09.003 (2008).
Allen, S. G., Nakayama, F. S., Dierig, D. A. & Rasnick, B. A. Plant water relations, photosynthesis, and rubber content of young guayule plants during water stress. Agron. J. 79 , 1030–1035. https://doi.org/10.2134/agronj1987.00021962007900060016x (1987).
Valdes Franco, J. A. et al. Modular assembly of transposable element arrays by microsatellite targeting in the guayule and rice genomes. BMC Genomics 19 , 271. https://doi.org/10.1186/s12864-018-4653-6 (2018).
Haas, B. J. et al. Improving the Arabidopsis genome annotation using maximal transcript alignment assemblies. Nucleic Acids Res. 31 , 5654–5666. https://doi.org/10.1093/nar/gkg770 (2003).
Bray, N. L., Pimentel, H., Melsted, P. & Pachter, L. Near-optimal probabilistic RNA-seq quantification. Nat. Biotechnol. 34 , 525–527. https://doi.org/10.1038/nbt.3519 (2016).
Robinson, M. D., McCarthy, D. J. & Smyth, G. K. edgeR: a Bioconductor package for differential expression analysis of digital gene expression data. Bioinformatics 26 , 139–140. https://doi.org/10.1093/bioinformatics/btp616 (2010).
Guo, D., Li, H. L., Wang, Y., Zhu, J. H. & Peng, S. Q. A myelocytomatosis transcription factor from Hevea brasiliensis positively regulates the expression of the small rubber particle protein gene. Ind. Crops Prod. 133 , 90–97. https://doi.org/10.1016/j.indcrop.2019.01.052 (2019).
Deng, X. et al. Jasmonate signalling in the regulation of rubber biosynthesis in laticifer cells of rubber tree, Hevea brasiliensis . J. Exp. Bot. 69 , 3559–3571. https://doi.org/10.1093/jxb/ery169 (2018).
Stonebloom, S. H. & Scheller, H. V. Transcriptome analysis of rubber biosynthesis in guayule ( Parthenium argentatum gray ). BMC Plant Biol. 19 , 71. https://doi.org/10.1186/s12870-019-1669-2 (2019).
Alessandro, C. M., Luiz Edson, M. O., Paulo, M. & Nelson, D. F. Anatomical characteristics and enzymes of the sucrose metabolism and their relationship with latex yield in the rubber tree ( Hevea brasiliensis Muell. Arg.). Braz. J. Plant Physiol. 18 , 263–268. https://doi.org/10.1590/S1677-04202006000200002 (2006).
Kapilan, R., Vaziri, M. & Zwiazek, J. J. Regulation of aquaporins in plants under stress. Biol. Res. 51 , 4. https://doi.org/10.1186/s40659-018-0152-0 (2018).
Nelson, A. D. L. et al. Transcriptomic and evolutionary analysis of the mechanisms by which P. argentatum , a rubber producing perennial, responds to drought. BMC Plant Biol. 19 , 494. https://doi.org/10.1186/s12870-019-2106-2 (2019).
Veatch-Blohm, M., Ray, D. T. & McCloskey, W. B. Water stress-induced changes in resin and rubber concentration and distribution in greenhouse-grown guayule. Agron. J. 98 , 766–773. https://doi.org/10.2134/agronj2005.0203 (2006).
Reddy, A. R. & Das, V. S. R. Enhanced rubber accumulation and rubber transferase activity in guayule under water stress. J. Plant Physiol. 133 , 152–155. https://doi.org/10.1016/S0176-1617(88)80130-5 (1988).
Benzioni, A. & Mills, D. The effect of water status and season on the incorporation of 14 CO 2 and [ 14 C]-acetate into resin and rubber fractions in guayule. Physiol. Plant. 81 , 45–50. https://doi.org/10.1111/j.1399-3054.1991.tb01710.x (1991).
Schloman, W. W., Hively, R. A., Krishen, A. & Andrews, A. M. Guayule byproduct evaluation: extract characterization. J. Agric. Food Chem. 31 , 873–876. https://doi.org/10.1021/jf00118a050 (1983).
Hepper, C. M. & Audley, B. G. The biosynthesis of rubber from β-hydroxy-β methylglutaryl-Coenzyme A in Hevea brasiliensis latex. Biochem. J. 114 , 379–386. https://doi.org/10.1042/bj1140379 (1969).
Sando, T. et al. Cloning and characterization of the 2-C-methyl-D-erythritol 4-phosphate (MEP) pathway genes of a natura-rubber producing plant, Hevea brasiliensis . Biosci. Biotech. Bioch. 72 , 2903–2917. https://doi.org/10.1271/bbb.80387 (2008).
Tang, C. et al. The rubber tree genome reveals new insights into rubber production and species adaptation. Nat. Plants 2 , 16073. https://doi.org/10.1038/NPLANTS.2016.73 (2016).
Bach, T. J. Hydroxymethylglutaryl-CoA reductase, a key enzyme in phytosterol synthesis?. Lipids 21 , 82–88. https://doi.org/10.1007/BF02534307 (1986).
Vranova, E., Coman, D. & Gruissem, W. Structure and dynamics of the isoprenoid pathway network. Mol. Plant 5 , 318–333. https://doi.org/10.1093/mp/sss015 (2012).
Rodrıguez-Concepcion, M. & Boronat, A. Breaking new ground in the regulation of the early steps of plant isoprenoid biosynthesis. Curr. Opin. Plant Biol. 25 , 17–22. https://doi.org/10.1016/j.pbi.2015.04.001 (2015).
Ponciano, G. et al. Transcriptome and gene expression analysis in cold-acclimated guayule ( Parthenium argentatum ) rubber-producing tissue. Phytochemistry 79 , 57–66. https://doi.org/10.1016/j.phytochem.2012.04.007 (2012).
Ji, W., Benedict, C. R. & Foster, M. A. Seasonal variations in rubber biosynthesis, 3-hydroxy-3-methylglutaryl-coenzyme a reductase, and rubber transferase activities in Parthenium argentatum in the Chihuahuan Desert. Plant Physiol. 103 , 535–542. https://doi.org/10.1104/pp.103.2.535 (1993).
Singh, R. et al. Modulations in primary and secondary metabolic pathways and adjustment in physiological behaviour of Withania somnifera under drought stress. Plant Sci. 272 , 42–54. https://doi.org/10.1016/j.plantsci.2018.03.029 (2018).
Haider, M. S. et al. Insights into grapevine defense response against drought as revealed by biochemical, physiological and RNA-Seq analysis. Sci. Rep. 7 , 13134. https://doi.org/10.1038/s41598-017-13464-3 (2017).
Article ADS CAS PubMed Central PubMed Google Scholar
Shivhare, R., Asif, M. H. & Lata, C. Comparative transcriptome analysis reveals the genes and pathways involved in terminal drought tolerance in pearl millet. Plant Mol. Biol. 103 , 639–652. https://doi.org/10.1007/s11103-020-01015-w (2020).
Savoi, S. et al. Transcriptome and metabolite profiling reveals that prolonged drought modulates the phenylpropanoid and terpenoid pathway in white grapes ( Vitis vinifera L.). BMC Plant Biol. 16 , 67. https://doi.org/10.1186/s12870-016-0760-1 (2016).
Perreca, E. et al. Effect of drought on the methylerythritol 4-phosphate (MEP) pathway in the isoprene emitting conifer Picea glauca. Front. Plant Sci. 11 , 546295. https://doi.org/10.3389/fpls.2020.546295 (2020).
Stewart, W. D., Wachtel, W. L., Shipman, J. J. & Yanko, J. A. Synthesis of rubber by fungi. Science 122 , 1271–1272. https://doi.org/10.1126/science.122.3183.1271 (1955).
Article ADS CAS PubMed Google Scholar
Nakazawa, Y. et al. Production of eucommia-rubber from eucommia ulmoides oliv. (Hardy Rubber Tree). Plant Biotechnol. 26 , 71–79. https://doi.org/10.5511/plantbiotechnology.26.71 (2009).
Cherian, S., Ryu, S. B. & Cornish, K. Natural rubber biosynthesis in plants, the rubber transferase complex, and metabolic engineering progress and prospects. Plant Biotechnol. J. 17 , 2041–2061. https://doi.org/10.1111/pbi.13181 (2019).
Lakusta, A. M. et al. Molecular studies of the protein complexes involving cis -prenyltransferase in Guayule ( Parthenium argentatum ), an alternative rubber-producing plant. Front. Plant Sci. 10 , 165. https://doi.org/10.3389/fpls.2019.00165 (2019).
Epping, J. et al. A rubber transferase activator is necessary for natural rubber biosynthesis in dandelion. Nat. Plants 1 , 15048. https://doi.org/10.1038/nplants.2015.48 (2015).
Kim, I. J., Ryu, S. B., Kwak, Y. S. & Kang, H. A novel cDNA from Parthenium argentatum Gray enhances the rubber biosynthesis activity in vitro . J. Exp. Bot. 55 , 377–385. https://doi.org/10.1093/jxb/erh039 (2004).
Collins-Silva, J. et al. Altered levels of the Taraxacum kok-saghyz (Russian dandelion) small rubber particle protein, TkSRPP3, result in qualitative and quantitative changes in rubber metabolism. Phytochemistry 79 , 46–56. https://doi.org/10.1016/j.phytochem.2012.04.015 (2012).
Hillebrand, A. et al. Down-regulation of small rubber particle protein expression affects integrity of rubber particles and rubber content in Taraxacum brevicorniculatum . PLoS ONE 7 , e41874. https://doi.org/10.1371/journal.pone.0041874 (2012).
Placido, D. F. et al. Downregulation of a CYP74 rubber particle protein increases natural rubber production in Parthenium argentatum . Front Plant Sci. 10 , 760. https://doi.org/10.3389/fpls.2019.00760 (2019).
Brasher, M. I. et al. A two-component enzyme complex is required for dolichol biosynthesis in tomato. Plant J. 82 , 903–914. https://doi.org/10.1111/tpj.12859 (2015).
Akhtar, T. A. et al. Polyprenols are synthesized by a plastidial cis-prenyltransferase and influence photosynthetic performance. Plant Cell 29 , 1709–1725. https://doi.org/10.1105/tpc.16.00796 (2017).
Backhaus, R. A., Cornish, K., Chen, S. F., Huang, D. S. & Bess, V. H. Purification and characterization of an abundant rubber particle protein from guayule. Phytochemistry 30 , 2493–2497. https://doi.org/10.1016/0031-9422(91)85088-H (1991).
Pan, Z. et al. The major protein of guayule rubber particles is a cytochrome P450. Characterization based on cDNA cloning and spectroscopic analysis of the solubilized enzyme and its reaction products. J. Biol. Chem. 270 , 8487–8494. https://doi.org/10.1074/jbc.270.15.8487 (1995).
Harms, K. et al. Expression of a flax allene oxide synthase cDNA leads to increased endogenous jasmonic acid (JA) levels in transgenic potato plants but not to a corresponding activation of JA-responding genes. Plant Cell 7 , 1645–1654. https://doi.org/10.1105/tpc.7.10.1645 (1995).
Wang, C., Avdiushko, S. & Hildebrand, D. F. Overexpression of a cytoplasm- localized allene oxide synthase promotes the wound-induced accumulation of jasmonic acid in transgenic tobacco. Plant Mol. Biol. 40 , 783–793. https://doi.org/10.1023/a:1006253927431 (1999).
Wasternack, C. & Song, S. Jasmonates, biosynthesis, metabolism, and signaling by proteins activating and repressing transcription. J. Exp. Bot. 68 , 1303–1321. https://doi.org/10.1093/jxb/erw443 (2017).
Kim, E. Y., Seo, S. Y., Lee, H. & Kim, W. T. Constitutive expression of CaSRP1 , a hot pepper small rubber particle protein homolg, resulted in fast growth and improved drought tolerance om transgenic Arabidopsis plants. Planta 232 , 71–83. https://doi.org/10.1007/s00425-010-1149-2 (2010).
Kim, E. Y., Park, K. Y., Seo, Y. S. & Kim, W. T. Arabidopsis small rubber particle protein homolog SRPs play dual roles as positive factors for tissue growth and development and in drought stress responses. Plant Physiol. 170 , 2494–2510. https://doi.org/10.1104/pp.16.00165 (2016).
Laibach, N. et al. Small rubber particle proteins from Taraxacum brevicorniculatum promote stress tolerance and influence the size and distribution of lipid droplets and artificial poly( cis -1,4-isoprene) bodies. Plant J. 93 , 1045–1061. https://doi.org/10.1111/tpj.13829 (2018).
Wang, X. et al. Comprehensive proteomics analysis of laticifer latex reveals new insights into ethylene stimulation of natural rubber production. Sci. Rep. 5 , 13778. https://doi.org/10.1038/srep13778 (2015).
Article ADS PubMed Central PubMed Google Scholar
Hemmerlin, A. Post-translational events and modifications regulating plant enzymes involved in isoprenoid precursor biosynthesis. Plant Sci. 203–204 , 41–54. https://doi.org/10.1016/j.plantsci.2012.12.008 (2013).
Patra, B., Schluttenhofer, C., Wu, Y., Pattanaik, S. & Yuan, L. Transcriptional regulation of secondary metabolite biosynthesis in plants. Biochim. Biophys. Acta. 1829 , 1236–1247. https://doi.org/10.1016/j.bbagrm.2013.09.006 (2013).
Chen, F., Tholl, D., Bohlmann, J. & Pichersky, E. The family of terpene synthases in plants: a mid-size family of genes for specialized metabolism that is highly diversified throughout the kingdom. Plant J. 66 , 212–229. https://doi.org/10.1111/j.1365-313x.2011.04520.x (2011).
Zerbe, P. & Bohlmann, J. Plant diterpene synthases: exploring modularity and metabolic diversity for bioengineering. Trends Biotechnol. 33 , 419–428. https://doi.org/10.1016/j.tibtech.2015.04.006 (2015).
Zhou, H. C. et al. Analysis of terpene synthase family genes in camellia sinensis with an emphasis on abiotic stress conditions. Sci. Rep. 10 , 933. https://doi.org/10.1038/s41598-020-57805-1 (2020).
Muchlinski, A. et al. Biosynthesis and emission of stress-induced volatile terpenes in roots and leaves of switchgrass ( Panicum virgatum L.). Front. Plant Sci. 10 , 1144. https://doi.org/10.3389/fpls.2019.01144 (2019).
Reisenman, C. E., Riffell, J. A., Bernays, E. A. & Hildebrand, J. G. Antagonistic effects of floral scent in an insect-plant interaction. Proc. R. Soc. B. 277 , 2371–2379. https://doi.org/10.1098/rspb.2010.0163 (2010).
Xiao, Y. et al. Specific herbivore-induced volatiles defend plants and determine insect community composition in the field. Ecol Lett. 15 , 1130–1139. https://doi.org/10.1111/j.1461-0248.2012.01835.x (2012).
Asensio, D., Rapparini, F. & Peñuelas, J. AM fungi root colonization increases the production of essential isoprenoids vs. nonessential isoprenoids especially under drought stress conditions or after jasmonic acid application. Phytochemistry 77 , 149–161. https://doi.org/10.1016/j.phytochem.2011.12.012 (2012).
Clark, G. T., Zuther, E., Outred, H. A., McManus, M. T. & Heye, A. G. Tissue-specific changes in remobilisation of fructan in the xerophytic tussock species Festuca novae-zelandiae in response to a water deficit. Funct Plant Biol. 31 , 377–389. https://doi.org/10.1071/fp03230 (2004).
Yang, J., Zhang, J., Wang, Z., Zhu, Q. & Liu, L. Activities of fructan- and sucrose-metabolizing enzymes in wheat stems subjected to water stress during grain filling. Planta 220 , 331–343. https://doi.org/10.1007/s00425-004-1338-y (2004).
Cohen, S. S. A Guide to the Polyamines (Oxford University Press, 1998).
Google Scholar
Bouchereau, A., Aziz, A., Larher, F. & Martin-Tanguy, J. Polyamines and environmental challenges: recent development. Plant Sci. 140 , 103–125. https://doi.org/10.1016/S0168-9452(98)00218-0 (1999).
Ray, D. T., Dierig, D. A., Thompson, A. E. & Coffelt, T. A. Registration of six guayule germplasms with high yielding ability. Crop Sci. 39 , 300 (1999).
Laudencia-Chingcuanco, D. L. et al. Transcriptional profiling of wheat caryopsis development using cDNA microarrays. Plant Mol. Biol. 63 , 651–668. https://doi.org/10.1007/s11103-006-9114-y (2007).
Grabherr, M. G. A. et al. Full-length transcriptome assembly from RNA-seq data without a reference genome. Nat. Biotechnol. 29 , 644–652. https://doi.org/10.1038/nbt.1883 (2011).
Zheng, Y. et al. iTAK: a program for genome-wide prediction and classification of plant transcription factors, transcriptional regulators, and protein kinases. Mol. Plant 9 , 1667–1670. https://doi.org/10.1016/j.molp.2016.09.014 (2016).
Livak, K. J. & Schmittgen, T. D. Analysis of relative gene expression data using real-time quantitative PCR and the 2(-Delta Delta C(T)) method. Methods 25 , 402–408. https://doi.org/10.1006/meth.2001.1262 (2001).
Download references
Acknowledgements
The authors thank Yulex Corporation for providing the guayule seedlings for the study; Ms. Valerie Teetor, University of Arizona, for performing rubber and resin extraction analyses; Drs. Doug Hunsaker and Diaa Elshikha for field trial design and execution; the Maricopa Agricultural Center for providing field service operations. This project was supported by USDA-NIFA Biomass Research and Development Initiative Competitive Grant no. 2012-10006-19391 OH.
Author information
These authors contributed equally: Chen Dong and Grisel Ponciano.
Authors and Affiliations
USDA Agricultural Research Service, Western Regional Research Center, Albany, CA, 94710, USA
Chen Dong, Grisel Ponciano, Naxin Huo, Yong Gu & Colleen McMahan
Plant Breeding and Genetics Section, School of Integrative Plant Science, Cornell University, Ithaca, NY, 14853, USA
Daniel Ilut
You can also search for this author in PubMed Google Scholar
Contributions
C.M. devised the experimental design, collected plant tissue samples in the field, and wrote sections of the manuscript. C.D. performed all bioinformatics, data analysis, and wrote the manuscript. G.P. performed the RNA extractions, qPCR, data analysis, and wrote the manuscript. Y.Q.G and H.N. contributed to sequence acquisition. D.I. devised analysis and interpretation strategies. All authors reviewed and approved the manuscript.
Corresponding author
Correspondence to Colleen McMahan .
Ethics declarations
Competing interests.
The authors declare no competing interests.
Additional information
Publisher's note.
Springer Nature remains neutral with regard to jurisdictional claims in published maps and institutional affiliations.
Supplementary Information
Supplementary information., rights and permissions.
Open Access This article is licensed under a Creative Commons Attribution 4.0 International License, which permits use, sharing, adaptation, distribution and reproduction in any medium or format, as long as you give appropriate credit to the original author(s) and the source, provide a link to the Creative Commons licence, and indicate if changes were made. The images or other third party material in this article are included in the article's Creative Commons licence, unless indicated otherwise in a credit line to the material. If material is not included in the article's Creative Commons licence and your intended use is not permitted by statutory regulation or exceeds the permitted use, you will need to obtain permission directly from the copyright holder. To view a copy of this licence, visit http://creativecommons.org/licenses/by/4.0/ .
Reprints and permissions
About this article
Cite this article.
Dong, C., Ponciano, G., Huo, N. et al. RNASeq analysis of drought-stressed guayule reveals the role of gene transcription for modulating rubber, resin, and carbohydrate synthesis. Sci Rep 11 , 21610 (2021). https://doi.org/10.1038/s41598-021-01026-7
Download citation
Received : 28 May 2021
Accepted : 18 October 2021
Published : 03 November 2021
DOI : https://doi.org/10.1038/s41598-021-01026-7
Share this article
Anyone you share the following link with will be able to read this content:
Sorry, a shareable link is not currently available for this article.
Provided by the Springer Nature SharedIt content-sharing initiative
This article is cited by
New insights on the regulatory network of drought-responsive key genes in arabidopsis thaliana.
- Maryam Pasandideh Arjmand
- Habibollah Samizadeh Lahiji
- Mohammad Hassan Biglouei
Genetica (2023)
By submitting a comment you agree to abide by our Terms and Community Guidelines . If you find something abusive or that does not comply with our terms or guidelines please flag it as inappropriate.
Quick links
- Explore articles by subject
- Guide to authors
- Editorial policies
Sign up for the Nature Briefing: Translational Research newsletter — top stories in biotechnology, drug discovery and pharma.

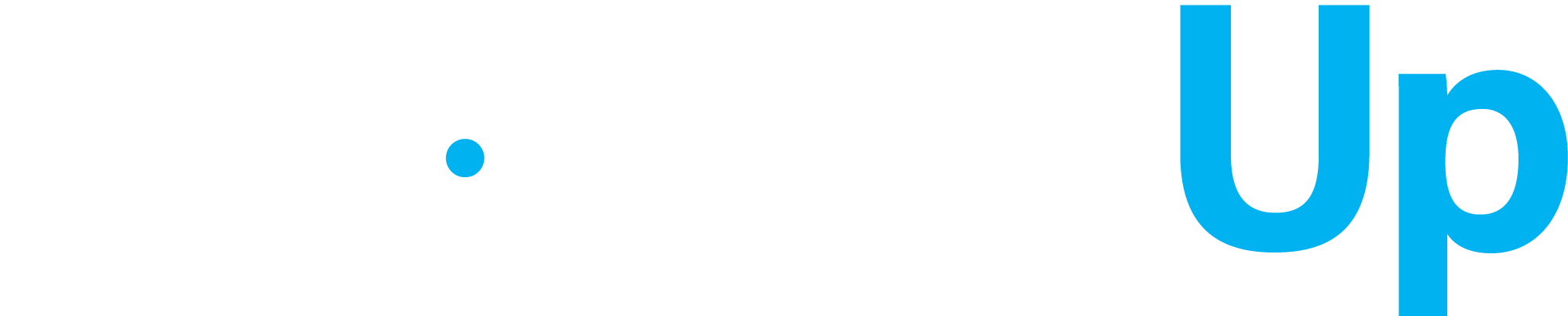
How severe is the drought? An analysis of the latest data
Facts are few, opinions plenty, on the cause of the water crisis.
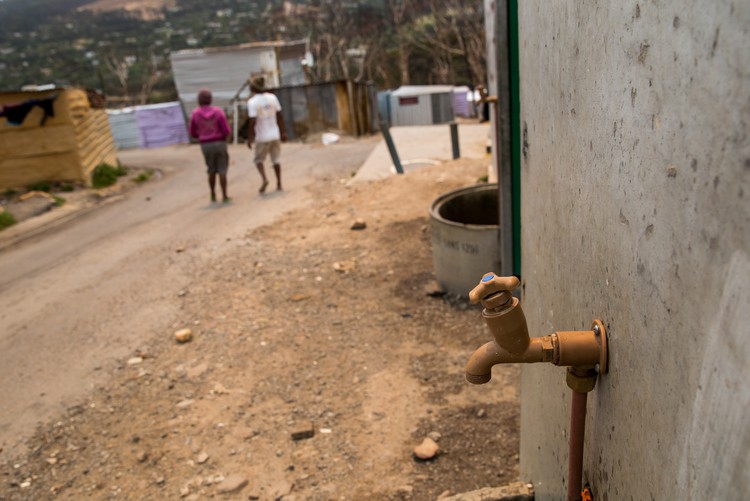
Editor’s summary
- Piotr Wolski, a water scientist at UCT, has used the latest rainfall and best available weather station data to compare the current drought to past ones.
- The data shows that the drought over the water catchment area is the worst on record over one- and three-year periods. Rainfall in 2017 was especially poor.
- This kind of drought is expected once in 300 years (90% confidence interval: 105 to 1,280 years).
- The drought in the city itself is severe, but not the worst on record. Very little of Cape Town’s water is sourced from in the city itself.
- There is a trend towards lower rainfall over the past 84 years in the catchment area. This may be due to human-caused climate change.
The causes of Cape Town’s water crisis are hotly contested. There is a drought, of course. But there are also other reasons brought up in the public discourse, particularly on social media, such as population and water demand growth, unreported agricultural use, invasive species sucking out water in catchments, poor planning and mismanagement of water supply system, and lack of foresight in development of new water sources. Are these significant contributors to the shortage? It’s very difficult to say without dedicated, comprehensive analyses. But facts are few, opinions plenty.
Few people question whether there is a drought: what is questioned is its severity. This has important implications. If the drought has been mild, then it should not have resulted in a major water crisis. If it has been severe, then it has just been a bad ticket on the climate lottery, and all the other factors would be at most aggravating factors but not the main cause. The issue is obviously socially tense, creating wedges between authorities and citizens, between those who institute water restrictions and those who have to bear the brunt of them.
In this article I look at the most up-to-date rainfall data to assess how severe the current drought is. I have tried to answer that question before . But that article was based on very limited data, and was carried out well before 2017’s rainy season had finished. Now I have a more comprehensive dataset, which provides an opportunity to perform a more robust assessment.
There are a number of stations that measure rainfall in the vicinity of the Western Cape Water Supply System (WCWSS) dams. Data for them are available from the Department of Water and Sanitation (DWS) website .
Not all stations in this dataset have good records; there are numerous gaps. It appears that nine stations have data available for recent years, but four are located in the region of the WCWSS dams, and have no significant gaps or systematic errors from 1981 through 2017. Those stations are: Vogel Vallij, Zacharashoek, Theewaterskloof and Kogel Baai.
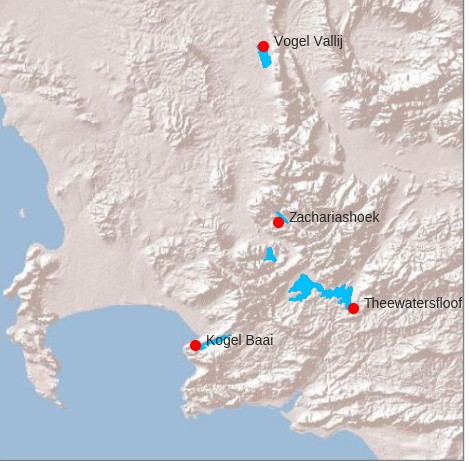
This is annual rainfall at these stations for 1981 to 2017:
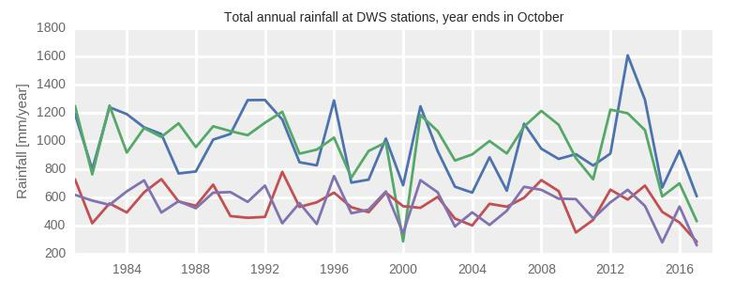
Note that the year in the above figures is taken to be between November and October. This is because October is more or less the end of rainy season, and the last month when an increase in dam levels may be recorded. Also, because at the time of writing this, data are available only till October 2017. Putting the end of the year in October allows me to use the 2017 data without estimating rainfall in November and December.
To make further analyses more robust, instead of analysing data from individual stations, I calculated their simple average. And to account for the fact that the drought is likely of a multi-year character, I created plots showing two-, three- and four-year average rainfall:
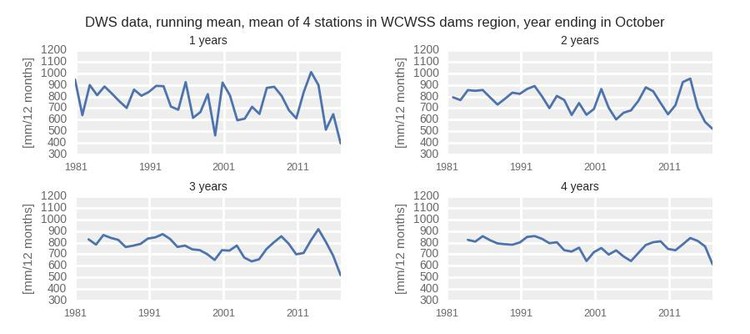
These plots tell a consistent story: 2017, as well as the preceding two-, three- and four-year average rainfall in the region are the lowest since 1981. What it means is that the 2017 drought was at least as rare as once in 36 years.
But looking at the last 36 years is not enough. We know that before that there were droughts in the 1920s and 1970s. We need to compare the current drought to the old ones. Can we go back further in time?
The DWS data do not span further back. But data from other sources do. The rainfall data from the South African Weather Service (SAWS) for some stations in the Western Cape go as far back as the late 1800s. Unfortunately, there isn’t any overlap between the DWS and SAWS rain stations, so we cannot simply extend the record of the four stations used above. We need to repeat the analyses on the entire SAWS dataset. If available SAWS data are screened in a similar manner as the DWS data were (i.e. for continuity and consistency of record), we get five stations with data covering the period 1933 to 2017. Only three are located in the WCWSS dam region: Vrugbaar, Rustfontein and Nuweberg.
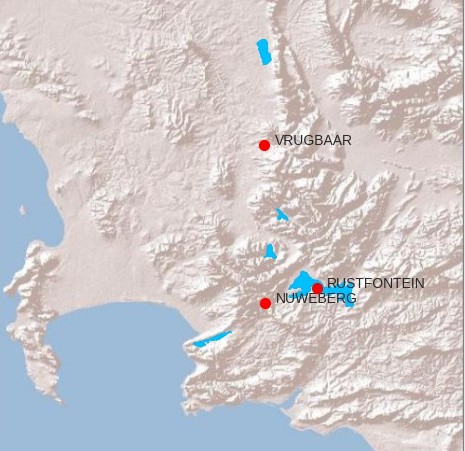
Similarly to the above analysis, I plot below the mean rainfall for the three stations:
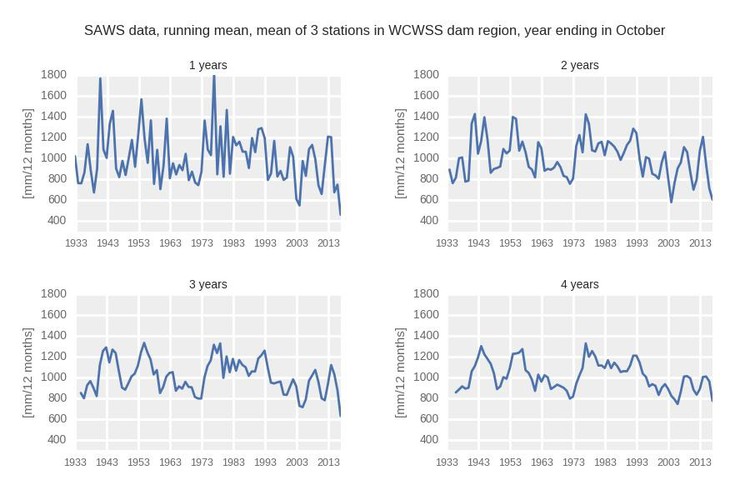
The figures show that 2017 was the lowest rainfall year since 1933. They also show that the mean rainfall in the three preceding years, 2015-2017, was unprecedented. However, the two-year mean as well as the four-year mean leading to 2017, were not the driest.
We can even go back further in time to 1920, but then we can rely on one station only (Vrugbaar). This is how plots for this station look:
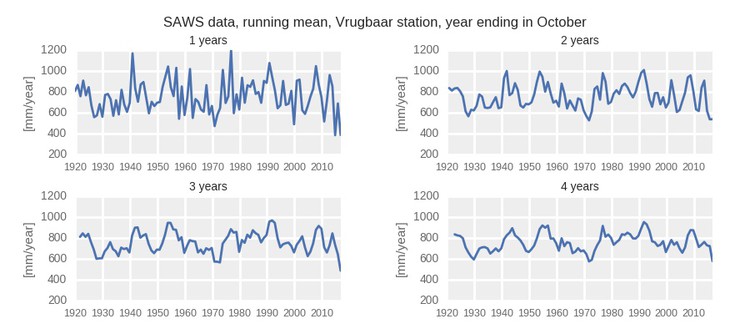
Again, the 2017 rainfall for that station, and importantly the mean of 3-years prior to 2017 were lower than in any period experienced by this station since 1920.
Interestingly, if you look at the long-term rainfall data for the stations located near the coast, the situation is slightly different:
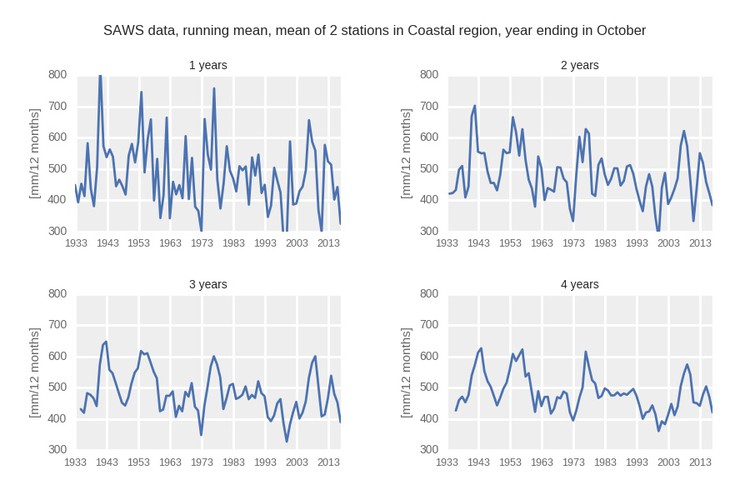
Here, although relatively bad, 2017 was not – either alone or in combination with preceding years — the driest on record. But this is not the area where most of our water is supplied from.
So the long-term SAWS data from the WCWSS dams region shows that 2017 and the period 2015 to 2017 were the driest since 1933. This translates into a drought return period of once in 84 years, possibly rarer. But how rare exactly? The statistical analysis I have done to calculate this are explained in my more detailed blog version of this article . My findings are that this kind of drought occurs once in 311 years with a 90% confidence that it falls between 105 and 1,280 years.
This is pretty rare and actually in the same range as the earlier estimates .
Of course, one may question that result in many ways. Are the stations used representative? Perhaps if we had a different set of observations we would get a different result? Does it make sense to use the average of several stations in the analysis? Perhaps there are errors in data?
I think this dataset is robust. I have taken care in the preparation of the dataset to find continuous, long-term data. Using averages of several stations reduces the chance that data errors affect the result.
The drought, as manifested by rainfall in the region of WCWSS dams, is indeed very rare, and very severe. Importantly, the analyses reveal that the drought was likely less severe in the coastal plains and in Cape Town itself. A possible reason for that might be a weaker penetration of cold fronts that bring winter rainfall to the region into the higher and distant inland regions.
Before I conclude this story – one more figure to think about:
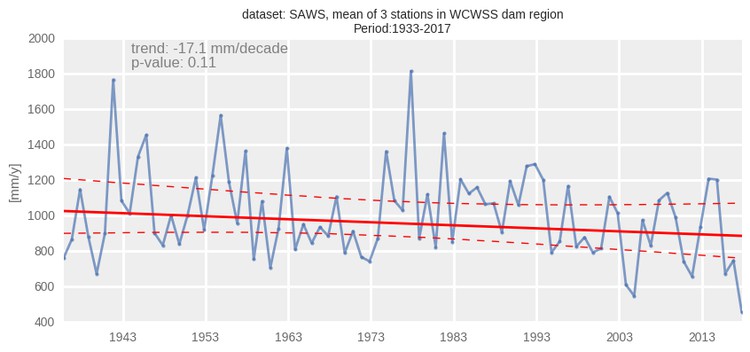
This figure shows a trend in rainfall in the WCWSS region over the last 84 years. That trend is towards lower rainfall and it has a relatively strong magnitude – 17mm per ten years. It is barely statistically significant, though. The important thing is that this trend may be an expression of human-caused climate change, and may be affecting the magnitude of droughts. Simply, if that trend was not there, the 2017 drought would likely be less severe.
In summary – the analyses presented above, based on the best rainfall data available at this time, show that the drought, manifested through low rainfall in 2015 to 2017, was very rare and severe.
Acknowledgements to SAWS and DWS for data, and Chris Jack and Stefaan Conradie (both CSAG/UCT) for valuable discussions.
A more detailed version of this analysis has been published.
Dr Wolski is a researcher with UCT’s Climate System Analysis Group.
More about Cape Town water crisis
- After the drought: Cape Town’s gushing water 07 September 2020
- Coca-Cola and Cape Town’s sweetheart Day Zero deal 08 May 2020
- Cape Town residents battle spring floods 28 October 2019
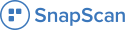
Next: No compensation for man after work accident
Previous: Soweto learners protest state’s failure to fix school
Can we forecast rain for 2018? Or is it totally random?
Dear Editor
Thank you for interesting analysis.
Just a couple of questions from this layman:
Can one forecast on past trends and on the data available (a) what the likelihood is of above average rainfall in the WC in 2018 and (b) if heavy rain is likely, from the monthly data how early in the year these heavy rains may fall following a three-year lower than average rainfall? Or is this totally random?
Sincerely Lorna Jones 25 Jan 2018
Revised data on drought crisis still not very useful
Thank you for publishing this, but I note that a lot of the data begins in 1933. This was the end of the 'great drought' experienced in Cape Town, from 1928-1933.
It would surely have been far more helpful to have data from 1928. The researcher has thus omitted the most important 'drought' period, except for one data set.
Furthermore, the current 'drought' isn't showing significantly lower rainfall that before, is it? It's a few percentage points at most. So when do semantics inform science? Wouldn't it be better to say, 'this is an average 12 year drought, maybe slightly worse than usual, but we can't say if it's really worse than the 1928 drought?'
Sincerely Mark J 25 Jan 2018
Predicting rainfall
GroundUp Editor's Response
In response to Lorna Jones, Piotr Wolski writes:
Extrapolation of a trend is possible, particularly if we know what is the driving force behind it. In this particular case we suspect that it is anthropogenic climate change, so it is not likely that the trend will get reversed in the next couple of years. However, the trend explains very little of the overall variance in data. So any prediction based on the trend will not be very accurate. In other words, the current drought has not been caused by the trend; the trend only magnified it. Similarly, the rainfall in the forthcoming season, and in the seasons following it, will be affected by the trend, but only so slightly. The actual magnitude of that rainfall will be determined by factors that are independent of the trend.
At this stage, we have a relatively good understanding of what atmospheric processes and factors affect the onset and magnitude of rains in the winter rainfall region, but a very limited ability to forecast them. Thus, from a practical point of view, at this stage we have to consider that indeed, it is a coin toss between early/late or wet/dry rainy season. Perhaps we will be able to tell more closer to the actual season, i.e. in May/June.
Sincerely GroundUp Editor 26 Jan 2018
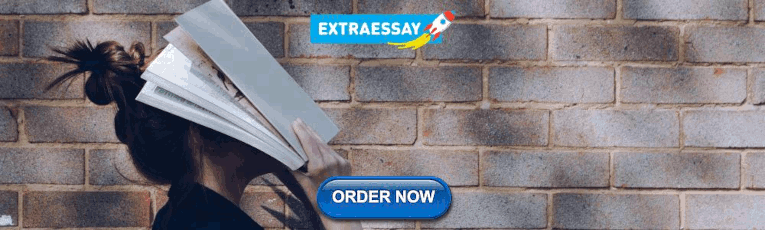
Learning from Perth's water catchment
I come from Perth, a city of 1.6 million on the south western tip of Australia - with a climate almost identical to Cape Town. Originally, our city relied 100% on rainfall flowing into our dams.
However, this all changed when we realised in the 1990's that our climate was changing permanently. We now only get 10% of the stream flow that was flowing into the dams for the first 70 years of last century.
The most illuminating graph can be found here: https://www.watercorporation.com.au/water-supply/rainfall-and-dams/streamflow/streamflowhistorical .
If Cape Town is experiencing a similar trend then this would mean a total change to where you get your water. I trust the lessons learnt in Perth can be applied to Cape Town.
James Marshall Water Engineer
Sincerely James Marshall 6 Feb 2018
Population growth affects water supply
Since Cape Town lies in an area prone to dry spells, one might presume that the city would be prepared for such eventualities. But that appear to be not the case. One factor should be obvious: population growth.
From worldpopulationreview.com :
Year Population Growth(%) Growth 2030 4,322,000 5.65% 231,000 2025 4,091,000 5.98% 231,000 2020 3,860,000 2.22% 84,000 2018 3,776,000 3.17% 116,000 2015 3,660,000 9.42% 315,000 2010 3,345,000 10.54% 319,000 2005 3,026,000 11.45% 311,000 2000 2,715,000 13.41% 321,000 1995 2,394,000 11.09% 239,000 1990 2,155,000 11.95% 230,000
Take a finite resource and distribute it to twice as many people without efforts to increase that finite resource and you might, just might find yourself in a world of hurt.
Sincerely Bruce Hall 16 Feb 2018

You may republish this article, so long as you credit the authors and GroundUp, and do not change the text. Please include a link back to the original article.
Most popular
28-year-old arrested after devastating fish hoek fire.
- 100 shacks demolished by City of Joburg on rainy weekend
- Suspected Fish Hoek arsonist released
- “Anti-vax” doctor’s disciplinary inquiry gets underway
- I’m a monster killer, Philippi extortionist tells GroundUp
Our answers to your questions
- What can I do if I was exempted from paying full school fees but don't have enough money to pay the outstanding amount?
- What visa can I get if my child is South African and I want to get a job?
- Can I reclaim South African citizenship after receiving citizenship of another country?
- How can I get my ID if my parent has multiple IDs?
- What does a ZEP holder need to claim UIF?
You might like
Elections 2024: what the major political parties say about the courts, palestinian supporters march to parliament.
- Open access
- Published: 16 March 2024
Physiological responses to drought stress of three pine species and comparative transcriptome analysis of Pinus yunnanensis var. pygmaea
- Feng Xiao 1 ,
- Yang Zhao 1 ,
- Xiurong Wang 1 ,
- Xueyan Jian 2 &
- Yao Yang 1
BMC Genomics volume 25 , Article number: 281 ( 2024 ) Cite this article
468 Accesses
1 Altmetric
Metrics details
Drought stress can significantly affect plant growth, development, and yield. Fewer comparative studies have been conducted between different species of pines, particularly involving Pinus yunnanensis var. pygmaea ( P. pygmaea ). In this study, the physiological indices, photosynthetic pigment and related antioxidant enzyme changes in needles from P. pygmaea , P. elliottii and P. massoniana under drought at 0, 7, 14, 21, 28 and 35 d, as well as 7 days after rehydration, were measured. The PacBio single-molecule real-time (SMRT) and Illumina RNA sequencing were used to uncover the gene expression differences in P. pygmaea under drought and rehydration conditions. The results showed that the total antioxidant capacity (TAOC) of P. pygmaea was significantly higher than P. massoniana and P. elliottii. TAOC showed a continuous increase trend across all species. Soluble sugar (SS), starch content and non-structural carbohydrate (NSC) of all three pines displayed a "W" pattern, declining initially, increasing, and then decreasing again. P. pygmaea exhibits stronger drought tolerance and greater recovery ability under prolonged drought conditions. Through the PacBio SMRT-seq, a total of 50,979 high-quality transcripts were generated, and 6,521 SSR and 5,561 long non-coding RNAs (LncRNAs) were identified. A total of 2310, 1849, 5271, 5947, 7710, and 6854 differentially expressed genes (DEGs) were identified compared to the control (Pp0D) in six pair-wise comparisons of treatment versus control. bHLH , NAC , ERF , MYB_related , C3H transcription factors (TFs) play an important role in drought tolerance of P. pygmaea. KEGG enrichment analysis and Gene set enrichment analysis (GSEA) analysis showed that P. pygmaea may respond to drought by enhancing metabolic processes such as ABA signaling pathway, alpha-linolenic acid. Weighted gene co-expression network analysis (WGCNA) revealed GST , CAT , LEC14B , SEC23 were associated with antioxidant enzyme activity and TAOC. This study provides a basis for further research on drought tolerance differences among coniferous species.
Peer Review reports
Introduction
Pinus massoniana Lamb. ( P. massoniana ), mainly distributed from 21°41′N to 33°56′N and 102°10′E to 123°14′E [ 1 ], plays a vital role in soil and water conservation, restoration, and pulpwood production. The broad distribution and diverse uses make P. massoniana pivotal for sustainable forestry production and ecological environment construction [ 2 ]. Compared with Pinus elliottii ( P. elliottii ), P. massoniana grows slower in the early stage (10–15 years) and faster in the later stage (> 15 years) [ 3 ]. P. elliottii is an important timber and resin-producing species grown worldwide [ 4 ]. In China, due to its strong adaptability, high resin yield, low resin crystallization rate, and high turpentine content, P. elliottii has become one of the main tree species for resin-tapping [ 5 ]. P. yunnanensis suffers from genetic degradation, with high proportion of twisted trunk, leading to the emergence of mutated variants such as the Pinus yunnanensis var. pygmaea ( P. pygmaea ) [ 6 ]. P. pygmaea maintains its dwarfism even growing in a suitable habitat [ 7 ]. Compared to angiosperms, gymnosperms are characterized by higher vulnerability to subsequent droughts and more evident lagged drought effects (“legacy effects”) [ 8 ]. The above-mentioned three pine species have a widespread distribution and significant economic value in China. However, there is limited research on the response to adversities and stress among different pine tree species.
Frequent natural abiotic stresses such as low temperature [ 9 ], drought [ 10 ], and low phosphorus [ 11 ] make us realize that the strength of tolerance is a key factor that affects and limits the normal growth into forests, and directly determines the survival rate and stand retention rate after afforestation. Due to climate change, drought will become increasingly frequent, severe, and longer [ 12 , 13 ]. Drought significantly reduces the photosynthetic rate, the accumulation of biomass, and inhibits plant growth [ 14 , 15 ]. Plants respond to drought stress through morphological and structural changes, expression of drought-resistant genes, hormone synthesis, and osmotic regulation substances [ 16 ]. Under continuous drought conditions, trees close stomata or reduce leaf area to reduce water lose [ 17 ]. For instance, P. palustris responded to drought treatment with greater stomatal control of plant water loss [ 18 ]. Plant hormones, especially ABA, are considered to be closely associated with drought responses. Stomatal closure is controlled by both active (abscisic acid(ABA)-mediated closure) and passive (hydraulic closure) regulation [ 19 ]. ABA-dependent stomatal closure is among the most rapid plant reactions to water stress [ 20 ]. ABA accumulates during drought stress and rapidly decreases upon rehydration [ 8 ]. Long-term water shortage induces the formation of stress memory that maintained a higher level of ABA accumulation in trees grown in more arid long-term conditions [ 21 ]. Osmoprotectants such as total soluble sugars and proteins were early accumulated in primed plants during the stress [ 22 ]. Meanwhile, antioxidant protection systems perform an essential function during drought stress [ 23 ]. Many cell components are damaged by reactive oxygen species (ROS) that are generated as a result of decreases in photosynthesis [ 24 ]. Antioxidant enzymes, such as catalase (CAT), peroxidase (POD) and superoxide dismutase (SOD) play a central role in plant adaptation to environmental changes [ 25 ]. Osmolarity-related compounds and antioxidant enzyme activity will increase under drought stress [ 26 ]. The high level of antioxidative enzyme activity is important for plants to tolerate stress for keeping good growth state and forming good quality [ 27 ]. Plants can adapt to prolonged drought by remodeling transcriptomes [ 28 ]. The recovery ability after rehydration is important for the successful adaptation of plants to arid environments [ 29 ]. Recovery of P. massoniana seedlings is more likely to be inhibited when plants experience increasing drought stress [ 30 ].
Third-generation Pacific BioSciences (PacBio) single-molecule real-time (SMRT) sequencing does not need to interrupt RNA fragments, and direct reverse transcription can be used to obtain full-length cDNA [ 31 , 32 ]. Full-length transcripts that do not require assembly can be used to detect genes with AS events, APA events, lncRNAs and fusion transcripts [ 33 ]. Pacbio SMRT sequence is widely used to obtain long reads and assemble a high quality reference transcriptome. PacBio-SMRT sequencing can provide a high-quality reference transcriptome for non-genomic species. RNA-seq is one of the most important and practical methods for exploring conifers genes [ 34 , 35 ]. RNA-seq helps to provide differential gene expression information under different stress conditions, time periods, and genotypes [ 36 ]. Resilience strategies differed among tree provenances: wet forests showed higher growth resistance to drought, while dry forests presented faster growth recovery [ 37 ]. The response to stress pressure varies inconsistently among different species. Pterocarya stenoptera and P. elliottii seedlings developed different adaptive strategies in response to continuous flooding [ 38 ]. Among P. elliottii , P. palustris and P. taeda , P. elliottii is the least drought-tolerant [ 39 ]. There has been extensive research on the drought response of P. massoniana . P. pygmaea has narrower niche and more adaption for extreme ecological conditions [ 40 ]. However, fewer comparative studies have been conducted between different species of pines, particularly involving P. pygmaea . The physiological and molecular mechanisms of P. pygmaea are unclear.
In this study, the needles of three pines ( P. pygmaea , P . elliottii , P. massoniana ) were used as the research material to measure the antioxidant enzyme activity and physiological responses after drought and rehydration. By combining PacBio SMRT seq and RNA-seq, the molecular responses of P. pygmaea were studied to identify key genes and pathways. This study provides a foundation for breeding drought-resistant pine trees, and comprehending the physiological and molecular mechanisms underlying drought response.
Physiological responses of three pine species under drought and rehydration
Physiological indicators were measured in the three pine species ( P. pygmaea , P. elliottii , P. massoniana ) under prolonged drought stress. As the drought time lengthened, total antioxidant capacity (TAOC) showed a continuous increase across all species. At the Re7D, P. pygmaea had significantly higher TAOC than P. massoniana and P. elliottii (Fig. 1 A). The soluble protein (SP) content of P. elliottii exhibited an initial decrease followed by an increase, with levels significantly higher than those of P. massoniana and P. pygmaea at several points between 14 and 35 days of drought stress (Fig. 1 B). The soluble sugar (SS) content (Fig. 1 C), starch content (Fig. 1 D), and Non-structural carbohydrate (NSC) (Fig. 1 E) of all three pines displayed a "W" pattern. Initially, they decreased under prolonged drought stress, then increased, and finally decreased again before recovering after rehydration. After rehydration, P. pygmaea 's POD enzyme activity was higher than the other two species (Fig. 1 F), and malondialdehyde (MDA) content was lower than P. elliottii and P. massoniana at Re7D (Fig. 1 G). During drought, the content of Chlorophyll a (Chla) and Chlorophyll b (Chlb) decreased continuously (Fig, 1H&I). After the drought treatments on three pine species, compared to the control (0d), TAOC showed an increasing trend, while chla and chlb exhibited a decreasing trend (Fig. 1 J). Principal component analysis (PCA) (Fig. 1 K) indicated that Re7D samples of the three pines were relatively similar, while the control (CK) samples of the pines were closed to each other.
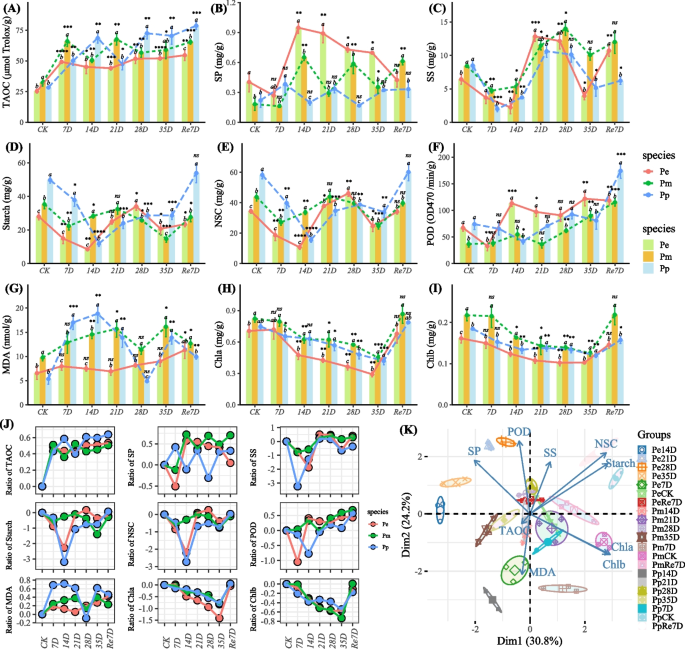
The physiological changes of three pines under drought and rehydration. A Total antioxidant capacity (TAOC); B Soluble proteins (SP); C Soluble sugars (SS); D Starch; E Non-structural carbohydrate (NSC); F Peroxidase (POD) activity; G Malondialdehyde (MDA) content; H Chlorophyll a (Chla); I Chlorophyll b (Chlb); J The relative change rate of indicators compared to CK at different time points (ratio = (Value (point) —Value (CK) )/Value (CK) ); K PCA analysis. Note: In (A–I), 7-35D represented drought treatment after 7 D, 14D, 21D, 28D, and 35D. Re7D represented 7 D after rehydration; error bars indicated the standard deviation, the number of seedlings measured for each species was n = 3; the left Y-axis represented value of physiological traitors, “CK” group of each species was set as a reference to compare the mean by the Student's t-Test, “ns” means no difference,”*” P ≤ 0.05;”**” P ≤ 0.01;”***” P ≤ 0.001;”****” P ≤ 0.0001. A least significant difference test (LSD) was used at a probability level of 0.05 to verify the significance between species at sample point
PacBio SMRT sequencing data analysis
The RNA extraction quality and concentration of all samples was satisfactory (A260/280 = 2.0–2.2; A260/230 = 1.8–2.2; 28S/18S = 1.4–2.7; Rin ≥ 8.0). The mixed samples of the P. pygmaea were sequenced using the PacBio SMRT platform. For PacBio SMRT-seq, a total of 33,095,230 subreads (75.1 Gb of bam file) with 1200.8 mean read length were obtained, 360,635 circular consensus (CCS) reads, 274,760 full-length Non-concatemer (FLNC), and 139,075 consensus reads were acquired. A total of 50,979 transcripts encoded by 42,919 genes were obtained after removing redundancy and clustering by CD-HIT. The mean length of the transcripts was 1,468.5 bp. 6,521 SSR candidates and 5,561 long non-coding RNA (LncRNA) candidates were identified. The Benchmarking Universal Single-Copy Orthologs (BUSCO) completeness score for nonredundant transcripts showed the final transcriptome was 59.3% (951) complete and 2.6% (41) fragmented. A total of 41,198 (80.81%) transcripts were annotated using the NR database and exhibited homology with known proteins of various species, including Picea sitchensis (36.09%), Asparagus officinalis (8.69%), Amborella trichopoda (5.05%). Using Uniprot, 41,295 (81%) transcripts were annotated. Identification of transcription factors found that the number of bHLH family was the most (2650), followed by NAC (1832) (Table S 2 ). Furthermore, 16,574 transcripts were identified in the Kyoto encyclopedia of genes and genomes (KEGG) database and grouped into 265 KEGG pathways, which were divided into five broad categories: genetic information processing (12,081), signal transduction (4601), and metabolism (4420) (Fig. 2 ).
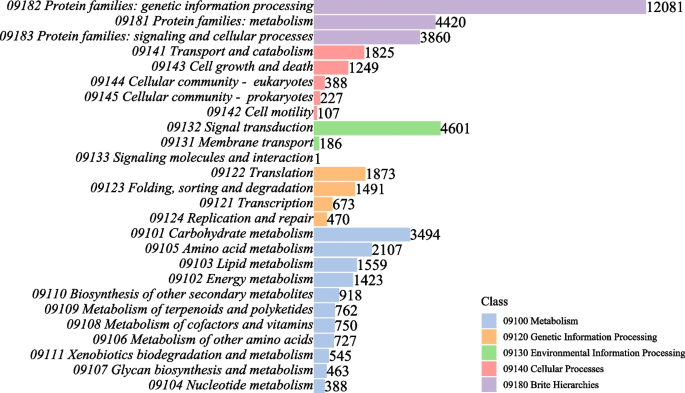
KEGG functional classifications of high-quality transcripts
Screening and functional annotation of differentially expressed genes (DEGs)
For Illumina RNA-seq, a total of 45 Gb raw reads were obtained, the Q20 and Q30 scores for all samples of clean data aboved 99%, and the average content of GC was 44.54%, indicating good sequencing quality. Then, the clean reads were successfully mapped back to the full-length transcripts of PacBio SMRT. PCA analysis showed that samples within the same group were close to each other, indicating good repeatability (Fig. 3 A). Based on the DEGs screening criteria, DEGs among all pairwise combinations were identified (Fig. 3 B). Among them, compared with the PpCK (Pp0D), the numbers of DEGs between Pp14D and Pp35D were 1,849 (1082 (58.52%) up-regulated and 767 (41.48%) down-regulated) and 7,710 (3,486 (45.21%) up-regulated genes and 4,224 (54.79%)). There were 541 DEGs commonly shared between all groups compared with the PpCK (Fig. 3 C), and 499 DEGs commonly shared between all groups compared with the Pp35D (Fig. 3 D). Cluster analysis of the DEGs shared by PpCK revealed that genes within C1 (2,708 gene) and C2 (1,564 gene) were highly expressed in PpCK, but lowly expressed in all other samples which were related to drought stress. Genes within C6 (1,960 gene) and C7 (1,218 gene) were highly expressed in PpRe7D and maybe related to rehydration (Fig. 3 E).
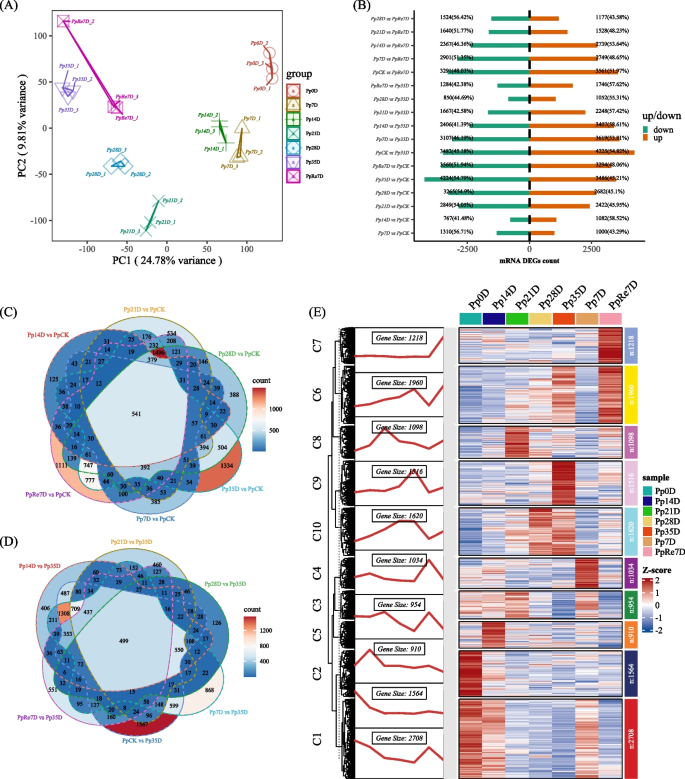
Transcriptome data analysis and differential expression gene quantity analysis. A PCA; B Summary of the identified DEGs; C Venn diagram of DEGs between PpCK and other groups; D Venn diagram of DEGs between Pp35D and other groups; E The clustered and heatmap analysis of DEGs
Short time-series expression miner analysis (STEM) and gene set enrichment analysis (GSEA)
STEM analysis identified 8 significant profiles. The Profile 18 (0, -1, 1, 1, 2, 2, 0) showed a high expression level in the later stages of drought (Fig. 4 A). The Profile 48 (0, 2, 2, 2, 2, 3, 1) showed a highest expression level at Pp35D (Fig. 4 B). The Profile 49 showed a lowest expression level at Pp14D and Pp35D (Fig. 4 C). The Profile 39 (0, 1, 2, 3, 4, 5, 6) showed a up-regulated trend (Fig. 4 D), the KEGG enrichment showed that “Glycolysis / Gluconeogenesis” (ko00010), “Galactose metabolism” (ko00052), “Alpha-Linolenic acid metabolism” (ko00592), “Pyruvate metabolism” (ko00620), Metabolism of xenobiotics by cytochrome P450 (ko00980), “Flavonoid biosynthesis” (ko00941), and others pathways were enriched. The GO enrichment showed “fatty acid catabolic process” (GO:0006633), “fatty acid beta-oxidation” (GO:0006635), “positive regulation of hormone metabolic process” (GO:0032352), “hormone metabolic process” (GO:0042445), and “response to oxygen radical” (GO:0000305) were enriched.
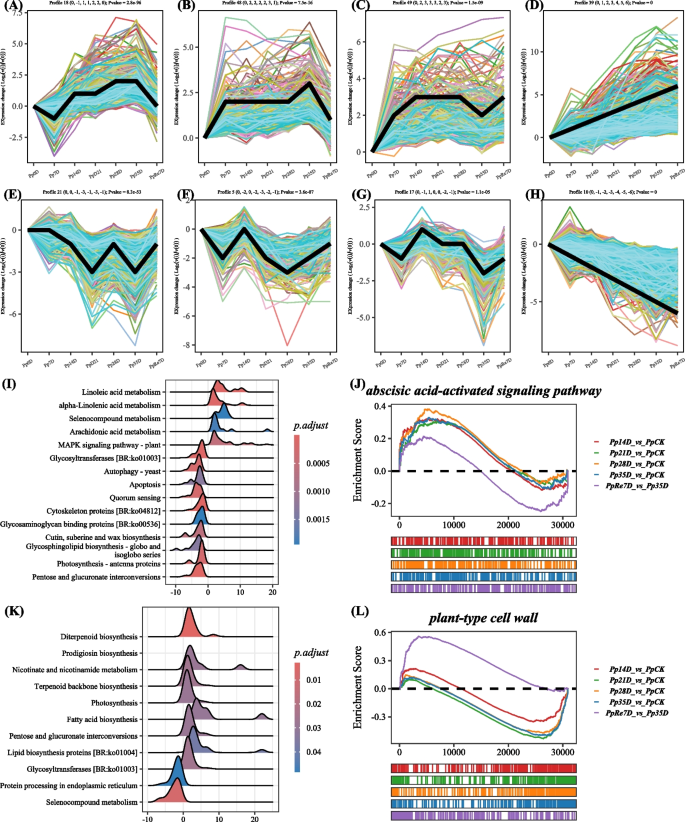
STEM analysis of the gene expression pattern and GSEA analysis. Note: In A-H , each subfigure was a significant profile. The upper part of the subfigure showed the number and corresponding trend of the modules. Black line represented the profile trendline. Each line represented the relative expression trend of a gene. In I , GSEA of KEGG pathway between Pp35D and Pp0D; In J , GSEA of the “abscisic acid-activated signaling pathway” among the four groups; In K , GSEA of KEGG pathway between PpRe7D and Pp35D; In L , GSEA of the “plant-type cell wall” among the four groups
The Profile 21 (0, 0, -1, -3, -1, -3, -1) showed a lowest expression at PpD21 and PpD35 (Fig. 4 E). The Profile 5 (0, -2, 0, -2, -3, -2, -1) showed a lowest expression at PpD28 (Fig. 4 F). The Profile 17 (0, -1, 1, 0, 0, -2, -1) showed a lowest expression at PpD28 (Fig. 4 G). The Profile 10 (0, -1, -2, -3, -4, -5, -6) showed a downregulated trend (Fig. 4 H). The KEGG enrichment of the Profile 10 genes showed that “Cutin, suberine and wax biosynthesis” (ko00073), “Photosynthesis proteins (ko00194), Photosynthesis” (ko00195), “Photosynthesis-antenna proteins” (ko00196), “Porphyrin and chlorophyll metabolism” (ko00860), “beta-Alanine metabolism” (ko00410) and others pathways were enriched.
The enrichment of GO terms and KEGG pathways by gene set enrichment analysis (GSEA) in the transcriptome, comparing Pp35D with Pp0D and PpRe7D. Results of the GSEA between Pp35D and Pp0D showed that “Linoleic acid metabolism”, “Alpha-Linolenic acid metabolism”, “Selenocompound metabolism”, “Arachidonic acid metabolism” and “MAPK signaling” pathway were upregulated in the Pp35D, “Cytoskeleton proteins”, “Glycosaminoglycan binding proteins”, “Photosynthesis-antenna proteins” were downgulated in the Pp35D (Fig. 4 I). The GO term of “abscisic acid-activated signaling pathway” was enriched in two drought term (Pp28D, Pp35D) compared with PpCK (Fig. 4 J). Compared with the Pp35D, “Diterpenoid biosynthesis”, “Prodigiosin biosynthesis, “Nicotinate and nicotinamide metabolism”, “Terpenoid backbone biosynthesis”, “Photosynthesis, “Fatty acid biosynthesis”, “Fatty acid biosynthesis”, “Lipid biosynthesis proteins”, “Glycosyltransferases” were upregulated in the PpRe7D (Fig. 4 K). The GO term of “plant-type cell wall” was inhibited under drought conditions and activated after rehydration (Fig. 4 L).
Identification of co-expression module
All filtered DEGs were retained for WGCNA analysis. The analysis identified 15 co-expression modules (expect for grey module) (Fig. 5 A), of which bisque4 module was positive correlation with POD (correlation coefficient ( r ) = 0.93, p = 1.3 × 10 –9 ), while salmon and white module was negative correlation with POD, with r of -0.55 ( p = 0.0093), -0.51 ( p = 0.019), respectively. The brown module was negative correlation with TAOC ( r = -0.75, p = 1 × 10 –4 ). The plum2 module was positive correlation with TAOC ( r = 0.7, p = 0.00037). The genes within the bisque4 module showed relatively lower expression during the term of rehydration (Fig. 5 B). The genes within the plum2 module showed a higher expression at Pp28D and PpRe7D (Fig. 5 C). The genes within the brown module showed a lower expression after Pp14D (Fig. 5 D), while the darkgreen module genes showed a highest expression at Pp28D (Fig. 5 E).
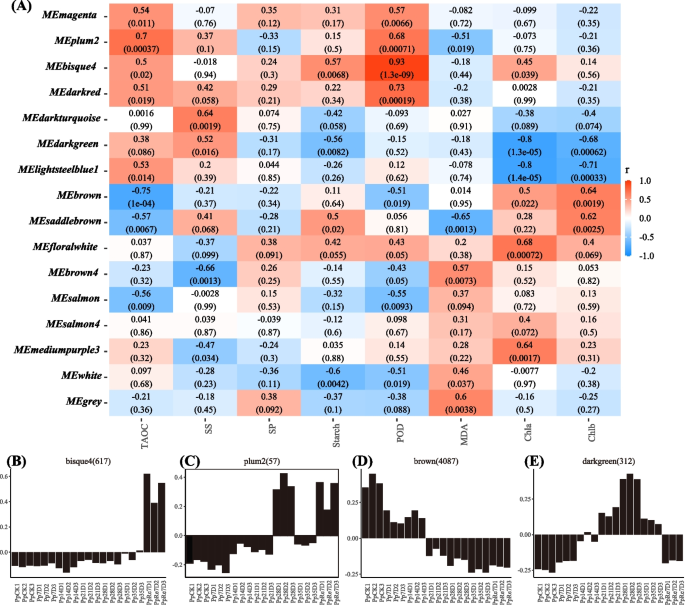
Weighted gene co-expression network analysis of the genes A Correlated heatmap of the adjacency of modules; B-E The eigengene expression of bisque4, plum2, brown, darkgreen module, respectively. Note: In a, each row represented a module, the color and number of each cell represented the correlation coefficient between modules and traits, the top number in the cell represented the correlation coefficient, the bottom number represented the p - value , the module name was shown on the y-axis, the color scale on the right shows module-trait correlation from −1 (blue) to 1 (red)
In the bisque4 module (Fig. 6 A), three GST gene (D1_transcript_10562, D1_transcript_110803, D1_transcript_99191) were enriched. Four Catalase (CAT) genes (D1_transcript_52135, D1_transcript_55430, D1_transcript_55990, D1_transcript_57940), protein transport SEC23 (D1_transcript_53479), LEC14B homolog (D1_transcript_43700) were enriched in the plum2 module (Fig. 6 B).
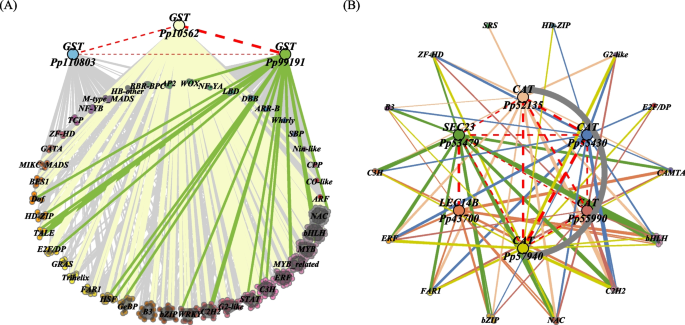
Partial of the co-expression network of module bwtween structure genes and transcription factors. A bisque4 module; B plum2 module. Note: In A , the top three genes which filled with different colors represented structural genes, with 'Pp' abbreviating 'D1_transcript_'. The TF families were shown below. In B , 'Pp' abbreviated 'D1_transcript_', and the genes which filled with different colors in the middle represented structural genes. The arc encompasses genes of the same class, with TF connected on the periphery. In both A and B , the points within each gene family were randomly distributed inside the circle, with the radius of the circle proportional to the number of distribution points. The outermost points were calculated and connected using the chull function of grDevices R Package. The connections between structural genes were mapped in 'red' color with a dashed linetype. The thickness of the lines were mapped based on the weight value
Validation of RNA-seq expression analysis
To validate the accuracy of RNA-seq data, seven genes were randomly selected for the quantitative real-time PCR (qRT-PCR) analysis. The qRT-PCR results showed that the expression patterns of genes were similar to their results in the RNA-seq (Fig. 7 A-G). A strong positive correlation ( r = 0.91, R 2 = 0.73) was obtained by a linear regression analysis (Fig. 7 H), suggesting that the transcriptome data was reliable.
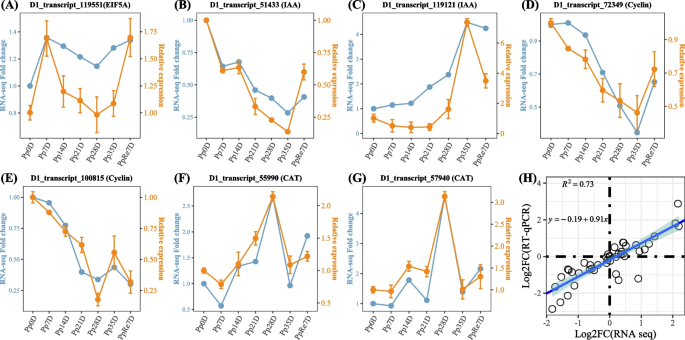
qRT-PCR verification of DEGs. Seven genes were randomly chosen for qRT-PCR validation. Data was shown as mean ± SD (standard deviation). The bars represented the standard deviation ( n = 3). The actin was used as an internal control to normalize the expression data. Note: In A-G the left of the double axis represented RNA-seq, the right of the double axis represented the qRT-PCR; In H , correlation between the relative expression of transcriptome and qRT-PCR
In the present study, we investigated the response of P. pygmaea , P. elliottii , and P. massoniana seedlings to drought and subsequent recovery. We measured changes in the antioxidant system and oxidative stress levels, and evaluated the physiological plasticity of these species following rehydration.
Plant varieties with drought resistance have armed with higher antioxidant enzyme activities [ 41 ]. Drought induced an increase in TAOC which is proportional to the duration and intensity of water deprivation [ 27 ]. The results showed that the TAOC of P. pygmaea was significantly higher than that of the other two pines (Fig. 1 A), which may have stronger drought tolerance. After rehydration, the TAOC of the three species remained at a relatively high level. This maintenance of high TAOC after rehydration is similar to other plants [ 42 ]. The SP of P. elliottii exhibited an initial decrease followed by an increase, with levels significantly higher than those of P. massoniana and P. pygmaea at several points between 14 and 35 days of drought stress (Fig. 1 B). Above-ground tissues of drought trees showed increases in both sucrose and starch [ 43 ]. NSC are major substrates for plant metabolism and have been implicated in mediating drought-induced tree mortality [ 44 ]. NSC are important “buffers” to maintain plant functions under drought conditions, the seedlings under the severe and moderate drought conditions tended to invest newly fixed C to starch storage and hydraulic repair instead of growth [ 45 ]. Once drought ceased, C allocation to storage was still prioritized at the expense of growth [ 46 ]. Severe drought treatment largely reduced photosynthetic carbon assimilation [ 47 ]. Plants may have allocated more NSC from source organs to roots [ 48 ]. After rehydration, the content of starch and NSC in P. pygmaea was higher than that of the other species, indicating a strong recovery ability (Fig. 1 B). The occurrence of drought stress can affect the decrease of photosynthetic parameters and chlorophyll fluorescence [ 27 ]. The SS content, starch content, and NSC (Fig. 1 C-E) of all three pines displayed a "W" pattern. Drought stress lasted for 15 days caused obvious photosynthesis inhibition in Atractylodes lancea [ 27 ]. In general, drought significantly reduce Chla and Chlb content [ 49 ]. During drought, the content of Chla and Chlb decreases continuously (Fig. 1 H&I). The activities of CAT and POD increased under drought stress [ 50 ], the activities of POD and SOD increased significantly compared with the control [ 51 ]. After rehydration, P. pygmaea 's POD activity was higher than the other two species (Fig. 1 F), and MDA content was lower than P. elliottii and P. massoniana at Re7D (Fig. 1 G). Most antioxidant enzyme indicators still fail to reach the control level after 7 days of rehydration, which may indicate that the 7 day period is insufficient to offset the impact of drought. Other study have shown that, the recovery of P. massoniana MAD needs to continue to decrease to the control level after 15 days of rehydration [ 52 ]. Long term drought still requires a period of recovery, this recovery time may be determined by the characteristics of species.
Through the PacBio SMRT-seq, approximately 75G of raw bam file was obtained, a total of 50,979 high-quality transcripts were generated. The number of DEGs gradually increased with the deepening of drought and decreased after rehydration, which was consistent with previous research [ 53 ]. Continuous drought can affect the reduction of photosynthetic related genes in plants [ 54 ], Trachycarpus fortunei reduced its light-capturing ability and composition of its photosynthetic apparatus, thereby reducing photosynthesis [ 55 ]. Drought induced a common reduction in the level of DEGs and differentially expressed proteins associated with photosynthesis [ 56 ]. The DEGs in Profile 10 (Fig. 4 H) involved in "Cutin, suberine and wax biosynthesis” (ko00073), “Photosynthesis proteins (ko00194), Photosynthesis” (ko00195), “Photosynthesis (antenna proteins)” (ko00196), “Porphyrin and chlorophyll metabolism” (ko00860), “beta-Alanine metabolism” (ko00410). "Starch and sucrose metabolism", “porphyrin and chlorophyll metabolism”, “photosynthesis (antenna protein)” and other pathways were enriched in the drought stress response of Nitraria tangutorum [ 57 ]. DEGs were enriched in soluble sugar-related and cell wall-related processes in P. massoniana under drought [ 58 ] . Drought stress inhibited the synthesis of Chla, Chlb and photosynthesis in Phoebe bournei [ 59 ]. In our study, the genes on the “Porphyrin and Chlorophyll metabolism” (ko00860) pathway were suppressed. “Glycolysis/Gluconeogenesis” (ko00010), “Galactose metabolism” (ko00052), “alpha-Linolenic acid metabolism” (ko00592), “Pyruvate metabolism” (ko00620), “Metabolism of xenobiotics by cytochrome P450” (ko00980), “Flavonoid biosynthesis” (ko00941) and others pathways were enriched in Profile 39 (0, 1, 2, 3, 4, 5, 6) (Fig. 4 D). TFs are widely recognized to be involved in plant growth, development, and biotic/abiotic stress responses. Four TFs ( MYB , AP2/ERF , WRKY , and bHLH ) mainly appeared in the roots and stems at D15 and D25 of drought stress in P. elliottii [ 60 ]. Through the STEM analysis, TFs with continuously increasing gene expression trends in Profile39 (Fig. 4 D) were identified, resulting in a total of 1064 TFs. Among them, bHLH (116), NAC (89), ERF (76), MYB_related (76), C3H (62) genes accounted for the majority. This indicates that bHLH , NAC , ERF , MYB_related , and C3H TFs play crucial roles in drought tolerance of P. pygmaea .
GSEA showed that “Linoleic acid metabolism”, “Alpha-Linolenic acid metabolism” were upregulated in the Pp35D (compared to the Pp0D). “Cytoskeleton proteins”, “Glycosaminoglycan binding proteins”, “Photosynthesis-antenna proteins” were downgulated in the Pp35D (Fig. 4 I). Alpha-Linolenic acid significantly increases under drought stress [ 61 ]. Compared with fully watered P. taeda seedlings, levels of some unsaturated fatty acids significantly reduced in needles of under water stress, such as palmitelaidic acid, erucic acid, and alpha-linolenic acid [ 62 ]. The needles of P. massoniana seedlings may respond to drought mainly through regulating ABA and JA hormone-related pathways [ 63 ]. ABA can accumulate 10- to 30-fold in plants under drought stress relative to unstressed conditions [ 64 ]. ABA signaling pathway is an important signal, induces stomatal closure and inhibition of transpiration under drought stress [ 65 , 66 ]. ABA-responsive genes were obviously upregulated at drought treatment for 7 days [ 67 ]. The GO term of “abscisic acid-activated signaling pathway” was enriched in two drought term (Pp28D, Pp35D) compared with PpCK (Fig. 4 J). Two SNRK2 (sucrose non-fermenting 1-related protein kinase 2) genes, five PYL genes, eight PP2C genes, seven ACAA1 genes and three ABF genes were enriched in the core enrichment of the between Pp35D and PpCK. PYR/PYLs are ABA receptors functioning at the apex of a negative regulatory pathway that controls ABA signaling by inhibiting PP2Cs [ 68 ]. PYLs/PP2Cs heterodimer blocks substrates binding to PP2Cs , and thus releases SnRK2s whose kinase activity is formerly inhibited by PP2Cs through physical interaction and dephosphorylation, finally allows the plants to adapt to the stress conditions [ 69 ]. TaABFs -regulated PYL gene ( TaPYL1-1B ) exhibited higher ABA sensitivity, photosynthetic capacity and water-use efficiency, all contributed to higher drought tolerance than that of wild-type [ 70 ]. Alpha-linolenic acid mostly participated alleviated drought stress through JA signaling [ 71 ]. As a precursor to the synthesis of JA, α-linolenic acid in lipid metabolism is related to drought stress [ 71 ]. Different strains of tobacco seedlings were enriched in functions about alpha-linolenic acid, and arginine and proline metabolisms under drought [ 72 ]. In the alpha-linolenic acid pathway, two alcohol dehydrogenase ( ADH1 ) genes, five Lipoxygenase ( LOX ) genes were enriched. LOX play essential roles in responding to biotic and abiotic stresses through oxidizing various lipids [ 73 ]. Overexpression of oriental melon CmLOX13 improved drought tolerance in Arabidopsis [ 74 ]. Compared with the Pp35D, “Diterpenoid biosynthesis”, “Prodigiosin biosynthesis”, “Nicotinate and nicotinamide metabolism”, “Terpenoid backbone biosynthesis”, “Photosynthesis”, “Fatty acid biosynthesis”, “Fatty acid biosynthesis”, “Lipid biosynthesis proteins”, “Glycosyltransferases” were upregulated in the PpRe7D (Fig. 4 K). These findings suggest that P. pygmaea may respond to drought by enhancing metabolic processes such as ABA signaling pathway, alpha-linolenic acid, which enhances overall antioxidant capacity.
The results of WGCNA showed that bisque4 module was positively associated with POD (Fig. 5 A). In the bisque4 module, “Flavonoid biosynthesis” (ko00941) and other pathways were enriched. Three GST gene were enriched in the bisque4 module. Overexpression of MsGSTU8 of Medicago sativa in Nicotiana tabacum resulted in maintained chlorophyll content, increased antioxidant enzyme activity, and soluble sugar levels after salt-alkali treatments [ 75 ]. These findings suggest that GST genes play an important role in scavenging reactive oxygen species and enhancing antioxidant enzyme activity. The brown module was negative correlation with TAOC. The genes in the brown module were significantly inhibited in expression after drought. “Cytoskeleton proteins” (ko04812), “Photosynthesis-antenna proteins” (ko00196), “Phenylpropanoid biosynthesis” (ko00940), “Porphyrin and chlorophyll metabolism” (ko00860) and others were enriched. This indicates that drought can inhibit the synthesis of genes involved in photosynthesis, chlorophyll, and phenylpropanoid biosynthesis. The plum2 was positively associated with TAOC. Four CAT genes, protein transport SEC23, LEC14B homolog were enriched in the plum2 module. The cultivars Coral Orange, Tendersweet and Solar Yellow were tolerant to drought stress, which was supported by their higher transcript levels of CAT , SOD genes [ 76 ]. The drought tolerant cultivars have higher accumulated of CAT gene [ 77 ]. LEC14B (JK340642) had significantly higher level of expression in drought-tolerant genotype (Tifway) than in drought-sensitive genotype (C299) at 10 d of drought, but no significant differences were detected between two genotypes at 5 of drought [ 78 ]. These suggests that CAT, LEC14B genes may enhance antioxidant capacity and suppress ROS. Sec23 / Sec24 are important cytosolic components of vesicle coat protein II (COPII) [ 79 ]. SEC23 is responsible for transporting newly synthesized proteins and lipids from the endoplasmic reticulum (ER) to the Golgi apparatus in cells, Steptoe SEC23 (A0A287NVF2) was down regulated under N deficiency, drought stress or both stress [ 80 ]. In our study, two SEC23 (D1_transcript_5780, D1_transcript_28060), two SEC13 , two SEC24 , three SEC31 were enriched in the brown modules, showing a relatively low expression trend in the later stages of drought (Fig. 5 D). But not all SEC genes expression were inhibited, the SEC23 (D1_transcript_53479) was one of the plum2 module and one of the Profile 39 (0, 1, 2, 3, 4, 5, 6) genes, showed a upregulated trends under drought stress, meaning SEC23 in P. pygmaea play important roles in regulating both trafficking and plasma membrane stability.
Considering the significance of the selected module hub genes, we will proceed to validate the functions of the related genes through gene cloning and overexpression. Furthermore, it is worth noting whether the drought-tolerant type of P. pygmaea , when used as a rootstock for grafting with P. massoniana scions, leading to changes in phenotypes such as scion height and resistance in the grafted plants. This study will provide a valuable direction for seedling nursery establishment, rootstock breeding, and reduction of artificial production costs in conifer orchards.
Conclusions
Different species exhibit inconsistent drought tolerance. Physiological assessments of drought and rehydration responses in three pine species ( P. pygmaea , P. elliottii and P. massoniana ) indicate that P. pygmaea exhibited a stronger drought response and a strong recovery ability under prolonged drought conditions. High-quality transcriptome of P. pygmaea was obtained using PacBio SMRT sequencing. Comparative transcriptome analysis indicates that drought suppresses pathways related to photosynthesis and chlorophyll synthesis metabolism. P. pygmaea may respond to drought by enhancing metabolic processes such as ABA signaling pathway, alpha-linolenic acid, which enhances overall antioxidant capacity. WGCNA revealed GST , CAT , LEC14B , SEC23 were associated with antioxidant enzyme activity and TAOC. qRT-PCR analysis correlated well with the data obtained by RNAseq. This study provides valuable insights into the drought response and regulatory processes of P. pygmaea .
Materials and methods
Plant material and drought treatment.
P. pygmaea exhibit a clear absence of apical dominance with an inconspicuous main trunk. The base is multi-branched, clumped, with a height range of 40–50 cm to 1–2 m. Leaves grow short and upright while its seed cones cluster together [ 3 ]. Tracheid length of P. pygmaea is shortened, resin canals increase and more or less concentrate in the late wood [ 7 ]. P. elliottii trees grow to 30 m tall. Through early seed resource investigation and collection, the seeds of half-sib progeny of P. pygmaea , P. massoniana and P. elliottii were obtained from the high-quality half-sibling progeny of Ma'anshan Seed Garden (26°16’ N and 107°31’ E), Duyun City, Guizhou Province, China. The seeds were planted in the greenhouse, using a humus: yellow loam soil mixture (1:3) as the soil medium. In order to obtain a detailed genetic background of P. pygmaea , different parts of a 5-year P. pygmaea were collected for PacBio SMRT sequencing, including the roots, needles, ovulate strobilus, and staminate strobilus. We selected three types of annual pine trees as the experimental subjects. Prior to the drought treatment, the three types of pine trees were irrigated normally. Sampling was conducted between 8:00–9:00 in the morning, mature needles were collected from the stem tips every seven days from new plants, labeled as 0D, 7D, 14D, 21D, and 28D during the drought treatment. A total of 35 days between the cessation of irrigation and the end of the severe drought. When the drought has lasted for 35 days, some seedlings began to die, and the drought treatment is stopped (labeled as 35D). After rehydration, the seedlings were left to grow for seven days. At the end of this period, samples were collected and labeled as Re7d. Three biological replicates were used for each treatment.
Determination of physiological indexes
The TAOC of needles was determined using 2,2'-azino-bis[3-ethylbenzothiazoline-6-sulfonic acid] diammonium salt (ABTS •+ ) method [ 81 ]. The frozen samples (about 2.0 g) were homogenized with 15 mL of 50% methanol and centrifuged at 10,000 rpm for 20 min at 4 °C, the supernatant was collected for ABTS analysis. SP content was measured by Coomassie Brilliant Blue G-250 staining [ 82 ]. Fresh needles (0.1 g) were cut and fully ground in phosphate buffer. Then added 5 mL of Coomassie Brilliant Blue G-250 solution. The mixture was then fully mixed and incubated for 2 min at room temperature. The absorbance at 595 nm was measured. SS content was determined using phenol method [ 83 ]. Fresh needles (0.5 g) was extracted in 10 mL of ddH 2 O for 20 min at 90 °C and centrifuged at 4000 rpm for 5 min. A combination of 1 mL of sample extract, 0.5 mL of anthrone-ethylacetate reagent, and 5 mL of concentrated sulfuric acid was homogenized and boiled for 5 min, and then rapidly cooled. The absorbance of the mixtures was detected at 630 nm. Starch content was measured by the anthrone colorimetric [ 84 ]. NSC was defined as the sum of soluble sugar and starch concentrations [ 85 ]. POD enzyme activity was determined using guaiacol method. The reaction mixture was as follows, 50 mmol/L Tris–HCl buffer (pH 7.0) containing 0.1 mmol/L EDTA, 10 mmol/L guaiacol, 5 mmol/L H 2 O 2 and 100 μL enzyme extract. After the enzyme solution was added to the reaction system, it was immediately incubated at 34 °C in a water bath for 3 min. The changes in absorbance values were measured at 470 nm. One unit of POD activity was expressed as units (μmol guaiacol decomposed per minute) per mg of fresh weight (FW). MDA content was determined using thiobarbituric acid (TBA) method [ 86 ]. Each fresh sample (about 0.5 g) was homogenized with 4 ml of 0.1% 0.1% (w/v) trichloroacetic acid (TCA) in ice bath and centrifuged at 4000 rpm for 10 min at 4 °C, for every 1 ml aliquot, 1 ml of 20% (w/v) TCA comprised of 0.5% (w/v) TBA was added. The mixture was heated at 95 °C for 30 min and then cooled immediately before centrifugation again. The absorbance of the supernatants was detected at 532, 450, and 600 nm. Fresh needles were cut into pieces and merged in 80% (v/v) acetone in the dark at room for 24 h to extract chlorophyll. Chla, Chlb were calculated by ultraviolet–visible spectrophotometer at 663, 646 and 470 nm [ 87 ]. Three biological replicates were used for the above experiments.
PacBio library construction,sequencing, and annotation
Total RNA was extracted according to the instruction manual for Trizol reagent (Invitrogen, Carlsbad, CA, USA). RNA integrity was assessed by agarose gel electrophoresis, RNA purity (OD260/280 and OD260/230), concentration and RNA integrity number (RIN), 28S/18S were detected using a NanoDrop 2000 spectrophotometer and an Agilent 2100 (Agilent Technologies, Santa Clara, California, USA). The mRNA was enriched with Oligo (dT) magnetic beads. After all RNA samples were qualified, the total RNA from different tissues was mixed at equal ratios. After the library was qualified, it was sequenced base on the PacBio platform (Menlo Park, CA, USA). The raw bam file had been deposited in the NCBI SRA database (BioProject acc. PRJNA994856). The raw seqencing subreads were filtered using SMRTLink v8.0 ( https://www.pacb.com/support/software-downloads/ ) with default parameters. The CCS was obtained by merging the subreads from the same polymerase reads, CCS were obtained by self-correction. FLNC sequences refer to a kind of CCS that contains both 5’ and 3’ primers and poly-A tail, without chimeric reads. After clustering the FLNC reads using the IsoSeq module, the consistent sequences in each cluster were further corrected. Finally, high-quality full-length transcripts (HQ) with an accuracy greater than 99% and low-quality (LQ) transcripts were obtained. Using CD-HIT software [ 88 ] to remove redundant sequences in transcripts and cluster the transcript sequences. BUSCO v5.3.2 [ 89 ] was used to assess the assembly quality, the BUSCO dataset of 1603 predefined single-copy genes dedicated to gymnosperms [ 90 ] were download and used. The transcripts were annotated using the NCBI non-redundant protein (NR) database, the Swiss-Prot protein ( http://www.expasy.org/sprot/ ), the Eukaryotic Orthologous Groups (KOG) database ( http://www.ncbi.nlm.nih.gov/COG/ ), the Pfam database ( http://pfam.sanger.ac.uk/ ), Kyoto Encyclopedia of Genes and Genomes (KEGG) ( http://www.genome.jp/kegg , cut-off e-value ≤ 1e-5) pathways, and the eggNOG database ( http://eggnog5.embl.de/#/app/home ) [ 91 ]. The SSRs were identified using the MISA software [ 92 ]. To identify long non-coding RNA (LncRNA), the transcripts with coding potential were filtered using four computational approaches, including the Coding Potential Assessment Tool (CPAT), Coding-Non-Coding Index (CNCI), Coding Potential Calculator (CPC), and Pfam protein structure domain analysis (Pfam).
RNA-seq library preparation, sequencing
The needles samples of different stages of P. pygmaea 's response to drought and rehydration were collected. The total RNA was isolated using the Trizol Reagent (Invitrogen, Carlsbad, CA, USA). RNA purity, concentration and integrity were assessed using agarose gel electrophoresis, NanoDrop 1000 spectrophotometer (NanoDrop Technologies Inc., Wilmington, DE, USA) and Agilent 2100 (Agilent Technologies, Santa Clara, California, USA). The mRNA was enriched with Oligo (dT) magnetic beads. The mRNA was added to fragmentation buffer and cut into short fragments. Using mRNA as a template, cDNA was reverse-transcribed using six-base random primers. The double-stranded cDNA samples were purified, end-repaired, added with poly(A) tails, and then ligated to sequencing adapters to create cDNA libraries, qualified libraries were sequenced using the NovaSeq 6000 system with paired-end reads. The raw reads generated from Illumina sequencing were deposited in the NCBI SRA database (BioProject acc. PRJNA972125).
Expression quantification, identification of DEGs
Fastp v0.20.1 was used for for quality control [ 93 ]. The non-redundant transcripts which obtained from Pacbio SMRT sequencing was used as background transcripts. Bowtie2 v2.4.3 [ 94 ] was used to align the sequenced paired-end transcriptomic data. The gene expression level was determined according to the fragments per kilobase of transcript per million mapped reads (FPKM) by the RSEM v1.2.12 [ 95 ]. The DEGs were identified based on the counts using DESeq2 v1.40.1 R package [ 96 ]. DEGs screening threshold was set to adjusted p-value cutoff < 0.05 and |foldchange|> 2. Gene Ontology (GO) and KEGG enrichment analysis were performed by the clusterProfiler v4.8.1 R package [ 97 ]. STEM software [ 98 ] was used to perform the trend clustering analysis. GSEA based on GO terms and KEGG pathways were conducted by the clusterProfiler, gene sets were considered statistically significant at an p.adjust of 0.05. Gene co-expression networks were constructed using the WGCNA v1.72.1 R package [ 99 ]. All the DEGs were filtered with at least one sample FPKM value greater than 5.
Quantitative real-time PCR analysis
In order to verify the accuracy of transcriptome, seven genes were randomly selected for qRT-PCR. The Primer Premier 5.0 software was used to design the primers (Table S 1 ). The first strand of the cDNA was synthesized from total RNA. qRT-PCR was performed on a real-time CFX96 Touch Real-Time PCR System (Bio-Rad, USA). Each 50 μL reaction included: 25 μL of 2X SYBR® Green PCR Master Mix, 1μL of Forward /Reverse primer (10 μM), 2.0 μL of cDNA template, and 21 μL of RNase-free water. The PCR reaction conditions were as follows: preheating at 95 °C for 30 s, 40 cycles of heat denaturation at 95 °C for 5 s, annealing at 60 °C for 34 s. The actin gene was used as a reference gene. The gene relative expression levels were calculated according to the 2 −ΔΔCt method [ 100 ].
Statistical analysis
The variance of the data was analyzed using the R v4.2.3 software, and the significance threshold was set at p < 0.05. LSD was used at a probability level of 0.05 to verify the significance between species. PCA was performed using the FactoMineR v2.8 R package [ 101 ].
Availability of data and materials
The raw reads generated from Illumina sequencing have been deposited in the NCBI SRA database (BioProject acc. PRJNA972125). The raw bam file from PacBio SMRT sequencing has been deposited in the NCBI SRA database (BioProject acc . PRJNA994856).
Abbreviations
Pinus massoniana
- Pinus yunnanensis var. pygmaea
Abscisic acid
Circular consensus sequencing
Correlation coefficient
Chlorophyll a
Chlorophyll b
Coding Potential Assessment Tool
Coding-Non-Coding Index
Coding Potential Calculator
Differentially expressed genes
Fragments per kilobase of transcript per million mapped reads
Full-length non-concatemer
Gene set enrichment analysis
High-quality full-length transcripts
Kyoto encyclopedia of genes and genomes
Long non-coding RNAs
Lipoxygenase
Least significant difference test
Malondialdehyde
Pacific BioSciences
Principal component analysis
Quantitative real-time PCR
RNA integrity number
Reactive oxygen species
Standard deviation
Soluble protein
Soluble sugar
Single-molecule real-time
Short time-series expression miner
The total antioxidant capacity
Weighted gene co-expression network analysis
Fan F, Wang Q, Li H, Ding G, Wen X. Transcriptome-wide identification and expression profiles of masson pine WRKY transcription factors in response to low phosphorus stress. Plant Mol Biol Rep. 2021;39:1–9.
Article CAS Google Scholar
Quan W, Ding G. Root tip structure and volatile organic compound responses to drought stress in Masson pine (Pinus massoniana Lamb.). Acta Physiol Plant. 2017;39:1–10.
Xiao F, Zhao Y, Wang X, Yang Y. Targeted metabolic and transcriptomic analysis of pinus yunnanensis var. pygmaea with loss of apical dominance. Curr Issues Mol Biol. 2022;44(11):5485–97.
Article CAS PubMed PubMed Central Google Scholar
Du B, Luan Q, Ni Z, Sun H, Jiang J. Radial growth and non-structural carbohydrate partitioning response to resin tapping of slash pine (Pinus elliottii Engelm. var. elliottii). J Forestry Res. 2022;33(2):423–33.
Lai M, Zhang L, Lei L, Liu S, Jia T, Yi M. Inheritance of resin yield and main resin components in Pinus elliottii Engelm at three locations in southern China. Industrial crops and products. 2020;144:112065.
Cai N, Xu Y, Li G, Deng L, Li W, Wang D. Research status and prospect of the crooked and twisted characteristics of P. yunnanensis stem. For Invent Plan. 2016;41:19–23.
Google Scholar
Zheng-li L, Yong-jun F, Ke-ming C. Comparative anatomical observations of wood structures of Pinus yunnanensis and P. yunnanensis var. pygmaea. J Integr Plant Biol. 1994;36(7):502–5.
Zlobin IE, Vankova R, Dobrev PI, Gaudinova A, Kartashov AV, Ivanov YV, Ivanova AI, Kuznetsov VV. Abscisic Acid and Cytokinins Are Not Involved in the Regulation of Stomatal Conductance of Scots Pine Saplings during Post-Drought Recovery. Biomol. 2023;13(3):523.
CAS Google Scholar
Shi Y, Ding Y, Yang S. Cold signal transduction and its interplay with phytohormones during cold acclimation. Plant Cell Physiol. 2015;56(1):7–15.
Article CAS PubMed Google Scholar
Ilyas M, Nisar M, Khan N, Hazrat A, Khan AH, Hayat K, et al. Drought tolerance strategies in plants: a mechanistic approach. J Plant Growth Regul. 2021;40:926–44.
Chen H, Quan W, Liu H, Ding G. Effects of Suillus luteus and S. bovinus on the physiological response and nutrient absorption of Pinus massoniana seedlings under phosphorus deficiency. Plant and Soil. 2022;471:577–90.
Stocker T, et al. Climate Change 2013: The Physical Science Basis. Contribution of Working Group I to the Fifth Assessment Report of the Intergovernmental Panel on Climate Change. Cambridge and New York: Cambridge University Press; 2013. p. 1535.
Mantova M, Herbette S, Cochard H, Torres-Ruiz JM. Hydraulic failure and tree mortality: from correlation to causation. Trends Plant Sci. 2022;27(4):335–45.
Dong S, Jiang Y, Dong Y, Wang L, Wang W, Ma Z, et al. A study on soybean responses to drought stress and rehydration. Saudi J Biol Sci. 2019;26(8):2006–17.
Article PubMed PubMed Central Google Scholar
Li LL, Li Y, Ding GJ. Research progress on effects of high temperature and drought on carbon metabolism in woody plants. J Mt Agric Biol. 2022;41(6):30–6.
Yang X, Lu M, Wang Y, Wang Y, Liu Z, Chen S. Response mechanism of plants to drought stress. Horticulturae. 2021;7(3):50.
Article Google Scholar
Nadal-Sala D, Grote R, Birami B, Knüver T, Rehschuh R, Schwarz S, Ruehr NK. Leaf shedding and non-stomatal limitations of photosynthesis mitigate hydraulic conductance losses in scots pine saplings during severe drought stress. Front Plant Sci. 2021;12:715127.
Samuelson LJ, Stokes TA, Ramirez MR, Mendonca CC. Drought tolerance of a Pinus palustris plantation. Forest Ecol Manage. 2019;451:117557.
Hasan MM, Gong L, Nie Z-F, Li F-P, Ahammed GJ, Fang X-W. ABA-induced stomatal movements in vascular plants during dehydration and rehydration. Environ Exp Bot. 2021;186:104436.
Pashkovskiy PP, Vankova R, Zlobin IE, Dobrev P, Kartashov AV, Ivanova AI, Ivanov VP, Marchenko SI, Nartov DI, Ivanov YV. Hormonal responses to short-term and long-term water deficit in native Scots pine and Norway spruce trees. Environ Exp Bot. 2022;195:104789.
Kartashov AV, Zlobin IE, Pashkovskiy PP, Pojidaeva ES, Ivanov YV, Ivanova AI, Ivanov VP, Marchenko SI, Nartov DI, Kuznetsov VV. Effects of drought stress memory on the accumulation of stress-protective compounds in naturally grown pine and spruce. Plant Physiol Biochem. 2023;200:107761.
Pérez-Oliver MA. González-Mas MdC, Renau-Morata B, Arrillaga I, Sales E: Heat-Priming during Somatic Embryogenesis Increased Resilience to Drought Stress in the Generated Maritime Pine (Pinus pinaster) Plants. Int J Mol Sci. 2023;24(11):9299.
Guo Y, Zhang S, Ai J, Zhang P, Yao H, Liu Y, Zhang X. Transcriptomic and biochemical analyses of drought response mechanism in mung bean (Vignaradiata (L.) Wilczek) leaves. Plos one. 2023;18(5):e0285400.
Fox H, Doron-Faigenboim A, Kelly G, Bourstein R, Attia Z, Zhou J, et al. Transcriptome analysis of Pinus halepensis under drought stress and during recovery. Tree Physiol. 2018;38(3):423–41.
Chutipaijit S. Changes in physiological and antioxidant activity of indica rice seedlings in response to mannitol-induced osmotic stress. Chilean J Agri Res. 2016;76(4):455–62.
Luo Y, Hu T, Huo Y, Wang L, Zhang L, Yan R. Transcriptomic and physiological analyses reveal the molecular mechanism through which exogenous melatonin increases drought stress tolerance in chrysanthemum. Plants. 2023;12(7):1489.
Zhang A, Liu M, Gu W, Chen Z, Gu Y, Pei L, Tian R. Effect of drought on photosynthesis, total antioxidant capacity, bioactive component accumulation, and the transcriptome of Atractylodes lancea. BMC Plant Biol. 2021;21(1):1–14.
Byeon S, Kim S, Hong J, Kim TK, Huh W, Kim K, et al. Drought hardening effect on improving transplant stress tolerance in Pinus densiflora. Environ Exp Bot. 2023;207:105222.
Zhou J, Chen S, Shi W, David-Schwartz R, Li S, Yang F, Lin Z. Transcriptome profiling reveals the effects of drought tolerance in Giant Juncao. BMC Plant Biol. 2021;21:1–20.
Li M, Wang H, Zhao X, Lu Z, Sun X, Ding G. Role of Suillus placidus in improving the drought tolerance of Masson Pine (Pinus massoniana Lamb.) seedlings. Forests. 2021;12(3):332.
Rhoads A, Au KF. PacBio sequencing and its applications. Genomics Proteomics Bioinformatics. 2015;13(5):278–89.
Li J, Harata-Lee Y, Denton MD, Feng Q, Rathjen JR, Qu Z, Adelson DL. Long read reference genome-free reconstruction of a full-length transcriptome from Astragalus membranaceus reveals transcript variants involved in bioactive compound biosynthesis. Cell Discov. 2017;3(1):1–13.
Li W, Fu Y, Lv W, Zhao S, Feng H, Shao L, Li C, Yang J. Characterization of the early gene expression profile in Populus ussuriensis under cold stress using PacBio SMRT sequencing integrated with RNA-seq reads. Tree Physiol. 2022;42(3):646–63.
Wei J, Pei X, Hu X, Sun S, Zhao C, Han R, et al. Applications of transcriptome in conifer species. Plant Cell Tissue Organ Cult (PCTOC). 2022;150(3):511–25.
Jiang C, Li X, Zou J, Ren J, Jin C, Zhang H, Yu H, Jin H. Comparative transcriptome analysis of genes involved in the drought stress response of two peanut (Arachis hypogaea L) varieties. BMC Plant Biol. 2021;21(1):1–14.
de María N, Guevara MÁ, Perdiguero P, Vélez MD, Cabezas JA, López-Hinojosa M, et al. Molecular study of drought response in the Mediterranean conifer Pinus pinaster Ait: differential transcriptomic profiling reveals constitutive water deficit-independent drought tolerance mechanisms. Ecol Evol. 2020;10(18):9788–807.
Sánchez-Salguero R, Camarero JJ, Rozas V, Génova M, Olano JM, Arzac A, et al. Resist, recover or both? Growth plasticity in response to drought is geographically structured and linked to intraspecific variability in Pinus pinaster. J Biogeography. 2018;45(5):1126–39.
Yang Y, Li C. Photosynthesis and growth adaptation of Pterocarya stenoptera and Pinus elliottii seedlings to submergence and drought. Photosynthetica. 2016;54(1):120–9.
Eberhardt TL, Samuelson LJ. Comparison of lignin and polysaccharide sugar contents for slash, longleaf, and loblolly pine growth rings formed during periods of soil moisture extremes. Wood Sci Technol. 2022;56(2):389–408.
Chen J, Zhang S, Luo T, Zheng W, Yang W, Li J, Wang Y, Wang S. Distribution patterns of Pinus yunnanensis and P yunnanensis var pygmaea and related key ecological factors. J Northeast For Univ. 2021;49:8–14.
Wang J, Yu Y, Jiang C, Sun Z, Wang X, Wang Z, Ren J, Wang Z, Wang X, Yang Z. Comparative analysis of physiology-anatomy and transcriptome-metabolome involving acute drought stress response of root between two distinct peanut cultivars at seedling stage. Environ Exp Bot. 2023;214:105442.
Talbi S, Rojas JA, Sahrawy M, Rodríguez-Serrano M, Cárdenas KE, Debouba M, Sandalio LM. Effect of drought on growth, photosynthesis and total antioxidant capacity of the saharan plant Oudeneya africana. Environ Exp Bot. 2020;176:104099.
Hartmann H, Ziegler W, Kolle O, Trumbore S. Thirst beats hunger–declining hydration during drought prevents carbon starvation in Norway spruce saplings. New Phytol. 2013;200(2):340–9.
Signori-Müller C, Oliveira RS, Barros FV, Tavares JV, Gilpin M, Diniz FC, Zevallos MJM, Yupayccana CAS, Acosta M, Bacca J, et al. Non-structural carbohydrates mediate seasonal water stress across Amazon forests. Nat Commun. 2021;12(1):2310.
Article ADS PubMed PubMed Central Google Scholar
Guo X, Peng C, Li T, Huang J, Song H, Zhu Q, Wang M. The effects of drought and re-watering on non-structural carbohydrates of Pinus tabulaeformis seedlings. Biology. 2021;10(4):281.
Galiano L, Timofeeva G, Saurer M, Siegwolf R, Martínez-Vilalta J, Hommel R, Gessler A. The fate of recently fixed carbon after drought release: towards unravelling C storage regulation in Tilia platyphyllos and Pinus sylvestris. Plant, Cell Environ. 2017;40(9):1711–24.
Chandrasekaran U, Byeon S, Kim K, Kim SH, Park CO, Lee Y-S, Kim HS. Short-term severe drought influences root volatile biosynthesis in eastern white pine (Pinus strobus L). Front Plant Sci. 2022;13:1030140.
Lemoine R, Camera SL, Atanassova R, Dédaldéchamp F, Allario T, Pourtau N, Bonnemain J-L, Laloi M, Coutos-Thévenot P, Maurousset L. Source-to-sink transport of sugar and regulation by environmental factors. Front Plant Sci. 2013;4:272.
Li XH, Xia ZL, Yu QW, Wang J, Wang ZW, Wu D, et al. Evaluation essay for drought tolerance of tobacco varieties at different developmental stages. J Mt Agric Biol. 2019;38(1):35–42.
Li P, Lin P, Zhao Z, Li Z, Liu Y, Huang C, Huang G, Xu L, Deng Z, Zhang Y. Gene co-expression analysis reveals transcriptome divergence between wild and cultivated sugarcane under drought stress. Int J Mol Sci. 2022;23(1):569.
Cheng S-B, Yang X-Z, Zou L, Wu D-D, Lu J-L, Cheng Y-R, Wang Y, Zeng J, Kang H-Y, Sha L-N. Comparative physiological and root transcriptome analysis of two annual ryegrass cultivars under drought stress. J Plant Physiol. 2022;277:153807.
Shao C, Duan H, Ding G, Luo X, Fu Y, Lou Q. Physiological and biochemical dynamics of Pinus massoniana Lamb seedlings under extreme drought stress and during recovery. Forests. 2022;13(1):65.
Su Y, Jiao M, Guan H, Zhao Y, Deji C, Chen G. Comparative transcriptome analysis of Saposhnikovia divaricata to reveal drought and rehydration adaption strategies. Mol Biol Rep. 2023;50(4):3493–502.
Chen Y, Li C, Yi J, Yang Y, Lei C, Gong M. Transcriptome response to drought, rehydration and re-dehydration in potato. Int J Mol Sci. 2019;21(1):159.
Feng X, Yang Z, Wang X. Tissue-specific transcriptome analysis of drought stress and rehydration in Trachycarpus fortunei at seedling. PeerJ. 2021;9:e10933.
Lei P, Liu Z, Li J, Jin G, Xu L, Ji X, Zhao X, Tao L, Meng F. Integration of the physiology, transcriptome and proteome reveals the molecular mechanism of drought tolerance in cupressus gigantea. Forests. 2022;13(3):401.
Liu C, Duan N, Chen X, Li H, Zhao X, Duo P, Wang J, Li Q. Metabolic pathways involved in the drought stress response of Nitraria tangutorum as revealed by transcriptome analysis. Forests. 2022;13(4):509.
Chen X, Chen H, Xu H, Li M, Luo Q, Wang T, Yang Z, Gan S. Effects of drought and rehydration on root gene expression in seedlings of Pinus massoniana Lamb. Tree Physiol. 2023;43(9):1619–40.
Li X, Liu L, Sun S, Li Y, Jia L, Ye S, Yu Y, Dossa K, Luan Y. Leaf-transcriptome profiles of phoebe bournei provide insights into temporal drought stress responses. Front Plant Sci. 2022;13:4170.
Zhang Y, Diao S, Ding X, Sun J, Luan Q, Jiang J. Transcriptional regulation modulates terpenoid biosynthesis of Pinus elliottii under drought stress. Ind Crops Prod. 2023;202:116975.
Rabeh K, Sbabou L, Rachidi F, Ferradouss A, Laghmari G, Aasfar A, Arroussi HE, Ouajdi M, Antry SE, Belkadi B. Lipidomic Profiling of Argania spinosa L (Skeels) Following Drought Stress. Appl Biochem Biotechnol. 2023;195(3):1781–99.
Wu C, Wang Y, Sun H. Targeted and untargeted metabolomics reveals deep analysis of drought stress responses in needles and roots of Pinus taeda seedlings. Front Plant Sci. 2022;13:1031466.
Xiao F, Zhao Y, Wang X-R, Liu Q, Ran J. Transcriptome analysis of needle and root of Pinus massoniana in response to continuous drought stress. Plants. 2021;10(4):769.
Joshi-Saha A, Valon C, Leung J. A brand new START: abscisic acid perception and transduction in the guard cell. Sci Signal. 2011;4(201):re4–re4.
Article PubMed Google Scholar
Agurla S, Gahir S, Munemasa S, Murata Y, Raghavendra AS. Mechanism of stomatal closure in plants exposed to drought and cold stress. Adv Exp Med Biol. 2018;1081:215–32.
Li W, Lee J, Yu S, Wang F, Lv W, Zhang X, Li C, Yang J. Characterization and analysis of the transcriptome response to drought in Larix kaempferi using PacBio full-length cDNA sequencing integrated with de novo RNA-seq reads. Planta. 2021;253:1–13.
Meng H-L, Sun P-Y, Wang J-R, Sun X-Q, Zheng C-Z, Fan T, Chen Q-F, Li H-Y. Comparative physiological, transcriptomic, and WGCNA analyses reveal the key genes and regulatory pathways associated with drought tolerance in Tartary buckwheat. Front Plant Sci. 2022;13:985088.
Park S-Y, Fung P, Nishimura N, Jensen DR, Fujii H, Zhao Y, Lumba S, Santiago J, Rodrigues A. Chow T-fF: Abscisic acid inhibits type 2C protein phosphatases via the PYR/PYL family of START proteins. Science. 2009;324(5930):1068–71.
Article ADS CAS PubMed PubMed Central Google Scholar
Jiang L, Zhang X., Chen Z. Structural Basis of ABA Perception by PYR/PYL/RCAR Receptors. In: Zhang DP, editor. Abscisic Acid: Metabolism, Transport and Signaling. Dordrecht: Springer; 2014. p. 117–35.
Mao H, Jian C, Cheng X, Chen B, Mei F, Li F, Zhang Y, Li S, Du L, Li T. The wheat ABA receptor gene TaPYL1-1B contributes to drought tolerance and grain yield by increasing water-use efficiency. Plant Biotechnol J. 2022;20(5):846–61.
Zi X, Zhou S, Wu B. Alpha-linolenic acid mediates diverse drought responses in maize (Zea mays l) at seedling and flowering stages. Molecules. 2022;27(3):771.
Yang H, Zhao L, Zhao S, Wang J, Shi H. Biochemical and transcriptomic analyses of drought stress responses of LY1306 tobacco strain. Sci Rep. 2017;7(1):17442.
Zhang Q, Zhao Y, Zhang J, Li X, Ma F, Duan M, Zhang B, Li H. The responses of the lipoxygenase gene family to salt and drought stress in foxtail millet (Setaria italica). Life. 2021;11(11):1169.
Xing Q, Zhang X, Li Y, Shao Q, Cao S, Wang F, Qi H. The lipoxygenase CmLOX13 from oriental melon enhanced severe drought tolerance via regulating ABA accumulation and stomatal closure in Arabidopsis. Environ Exp Bot. 2019;167:103815.
Du B, Zhao W, An Y, Li Y, Zhang X, Song L, Guo C. Overexpression of an alfalfa glutathione S-transferase gene improved the saline-alkali tolerance of transgenic tobacco. Biol Open. 2019;8(9):bio043505.
Junaid MD, Öztürk Gökçe ZN, Gökçe AF. Investigation of drought induced biochemical and gene expression changes in carrot cultivars. Mol Biol Rep. 2023;50(1):349–59.
Chaudhry UK, Gökçe ZNÖ, Gökçe AF. Drought and salt stress effects on biochemical changes and gene expression of photosystem II and catalase genes in selected onion cultivars. Biologia. 2021;76(10):3107–21.
Zhou P, An Y, Wang Z, Du H, Huang B. Characterization of gene expression associated with drought avoidance and tolerance traits in a perennial grass species. PLoS ONE. 2014;9(8):e103611.
Aboulela M, Nakagawa T, Oshima A, Nishimura K, Tanaka Y. The Arabidopsis COPII components, AtSEC23A and AtSEC23D, are essential for pollen wall development and exine patterning. J Exp Bot. 2018;69(7):1615–33.
Li L, Wang Y. Independent and combined influence of drought stress and nitrogen deficiency on physiological and proteomic changes of barley leaves. Environ Exp Bot. 2023;210:105346.
Re R, Pellegrini N, Proteggente A, Pannala A, Yang M, Rice-Evans C. Antioxidant activity applying an improved ABTS radical cation decolorization assay. Free Radic Biol Med. 1999;26(9–10):1231–7.
Zhang J, Huang D, Zhao X, Zhang M. Evaluation of drought resistance and transcriptome analysis for the identification of drought-responsive genes in Iris germanica. Sci Rep. 2021;11(1):16308.
Buysse J, Merckx R. An improved colorimetric method to quantify sugar content of plant tissue. J Exp Bot. 1993;44(10):1627–9.
Hansen J, Møller I. Percolation of starch and soluble carbohydrates from plant tissue for quantitative determination with anthrone. Anal Biochem. 1975;68(1):87–94.
Li Mh. Xiao Wf, Shi P, Wang Sg, Zhong Yd, Liu Xl, Wang Xd, Cai Xh, Shi Zm: Nitrogen and carbon source–sink relationships in trees at the Himalayan treelines compared with lower elevations. Plant, Cell Environ. 2008;31(10):1377–87.
Jin X, Yang X, Islam E, Liu D, Mahmood Q. Effects of cadmium on ultrastructure and antioxidative defense system in hyperaccumulator and non-hyperaccumulator ecotypes of Sedum alfredii Hance. J Hazard Mater. 2008;156(1–3):387–97.
Lichtenthaler HK, Wellburn AR. Determinations of total carotenoids and chlorophylls a and b of leaf extracts in different solvents. Biochem Soc Trans. 1983;11:591–2.
Li W, Godzik A. Cd-hit: a fast program for clustering and comparing large sets of protein or nucleotide sequences. Bioinformatics. 2006;22(13):1658–9.
Simão FA, Waterhouse RM, Ioannidis P, Kriventseva EV, Zdobnov EM. BUSCO: assessing genome assembly and annotation completeness with single-copy orthologs. Bioinformatics. 2015;31(19):3210–2.
Wu J-J, Han Y-W, Lin C-F, Cai J, Zhao Y-P. Benchmarking gene set of gymnosperms for assessing genome and annotation completeness in BUSCO. Horticulture Res. 2023;10(9):uhad165.
Huerta-Cepas J, Szklarczyk D, Heller D, Hernández-Plaza A, Forslund SK, Cook H, Mende DR, Letunic I, Rattei T, Jensen LJ. eggNOG 5.0: a hierarchical, functionally and phylogenetically annotated orthology resource based on 5090 organisms and 2502 viruses. Nucleic Acids Res. 2019;47(D1):D309–14.
Beier S, Thiel T, Münch T, Scholz U, Mascher M. MISA-web: a web server for microsatellite prediction. Bioinformatics. 2017;33(16):2583–5.
Chen S, Zhou Y, Chen Y, Gu J. fastp: an ultra-fast all-in-one FASTQ preprocessor. Bioinformatics. 2018;34(17):i884–90.
Langmead B, Salzberg SL. Fast gapped-read alignment with Bowtie 2. Nat Methods. 2012;9(4):357–9.
Li B, Dewey CN. RSEM: accurate transcript quantification from RNA-Seq data with or without a reference genome. BMC Bioinformatics. 2011;12:323.
Love MI, Huber W, Anders S. Moderated estimation of fold change and dispersion for RNA-seq data with DESeq2. Genome Biol. 2014;15(12):1–21.
Wu T, Hu E, Xu S, Chen M, Guo P, Dai Z, Feng T, Zhou L, Tang W, Zhan L, et al. clusterProfiler 4.0: A universal enrichment tool for interpreting omics data. Innovation (Camb). 2021;2(3):100141.
CAS PubMed PubMed Central Google Scholar
Ernst J, Bar-Joseph Z. STEM: a tool for the analysis of short time series gene expression data. BMC Bioinformatics. 2006;7:191.
Langfelder P, Horvath S. WGCNA: an R package for weighted correlation network analysis. BMC Bioinformatics. 2008;9(1):1–13.
Livak KJ, Schmittgen TD. Analysis of relative gene expression data using real-time quantitative PCR and the 2− ΔΔCT method. Methods. 2001;25(4):402–8.
Lê S, Josse J, Husson F. FactoMineR: an R package for multivariate analysis. J Stat Softw. 2008;25:1–18.
Download references
Acknowledgements
Not applicable.
This research was funded by National Natural Science Foundation of China (32060353); The Science and Technology Talent Platform Project of Guizhou Province [2018]5261.
Author information
Authors and affiliations.
Institute for Forest Resources and Environment of Guizhou, Key Laboratory of Forest Cultivation in Plateau Mountain of Guizhou Province, College of Forestry, Guizhou University, Guizhou, 550025, China
Feng Xiao, Yang Zhao, Xiurong Wang & Yao Yang
College of Continuing Education, Yanbian University, Jilin, 133002, China
Xueyan Jian
You can also search for this author in PubMed Google Scholar
Contributions
Conceptualization, Xiao F., Yang Y. and Zhao Y.; methodology, Xiao F.; software, Xiao F., Zhao Y.; validation, Xiao F., Zhao Y.; formal analysis, Wang X.; investigation, Xiao F.; resources, Xiao F., Zhao Y., Jian X.Y., Yang Y.; data curation, Zhao Y.; writing-original draft preparation, Xiao F.; writing-review and editing, Xiao F., Zhao Y., Jian X.Y.; visualization, Xiao F., Zhao Y.; supervision, Zhao Y.; project administration, Wang X.; funding acquisition, Wang X. All authors have read and agreed to the published version of the manuscript.
Corresponding author
Correspondence to Yang Zhao .
Ethics declarations
Ethics approval and consent to participate.
All plants samples were collected on Ma'anshan Seed Garden, we had obtained the permissions from that unit. Experimental research and field studies on plants including the collection of plant material are comply with relevant guidelines and regulation. All samples were identified by the Professor Yang Zhao and Xiurong Wang, and all voucher specimens were deposited in the forestry college of Guizhou University (voucher ID numbers: Pp202212 for P. pygmaea , Pe202212 for P. elliottii , Pm202212 for P. massoniana ).
Consent for publication
Competing interests.
The authors declare no competing interests.
Additional information
Publisher’s note.
Springer Nature remains neutral with regard to jurisdictional claims in published maps and institutional affiliations.
Supplementary Information
Supplementary material 1., rights and permissions.
Open Access This article is licensed under a Creative Commons Attribution 4.0 International License, which permits use, sharing, adaptation, distribution and reproduction in any medium or format, as long as you give appropriate credit to the original author(s) and the source, provide a link to the Creative Commons licence, and indicate if changes were made. The images or other third party material in this article are included in the article's Creative Commons licence, unless indicated otherwise in a credit line to the material. If material is not included in the article's Creative Commons licence and your intended use is not permitted by statutory regulation or exceeds the permitted use, you will need to obtain permission directly from the copyright holder. To view a copy of this licence, visit http://creativecommons.org/licenses/by/4.0/ . The Creative Commons Public Domain Dedication waiver ( http://creativecommons.org/publicdomain/zero/1.0/ ) applies to the data made available in this article, unless otherwise stated in a credit line to the data.
Reprints and permissions
About this article
Cite this article.
Xiao, F., Zhao, Y., Wang, X. et al. Physiological responses to drought stress of three pine species and comparative transcriptome analysis of Pinus yunnanensis var. pygmaea . BMC Genomics 25 , 281 (2024). https://doi.org/10.1186/s12864-024-10205-5
Download citation
Received : 29 December 2023
Accepted : 08 March 2024
Published : 16 March 2024
DOI : https://doi.org/10.1186/s12864-024-10205-5
Share this article
Anyone you share the following link with will be able to read this content:
Sorry, a shareable link is not currently available for this article.
Provided by the Springer Nature SharedIt content-sharing initiative
- Drought stress
- Rehydration
- Transcriptome
BMC Genomics
ISSN: 1471-2164
- Submission enquiries: [email protected]
- General enquiries: [email protected]

An official website of the United States government
The .gov means it’s official. Federal government websites often end in .gov or .mil. Before sharing sensitive information, make sure you’re on a federal government site.
The site is secure. The https:// ensures that you are connecting to the official website and that any information you provide is encrypted and transmitted securely.
- Publications
- Account settings
Preview improvements coming to the PMC website in October 2024. Learn More or Try it out now .
- Advanced Search
- Journal List
- Front Plant Sci
Transcriptome Profile Analysis of Arabidopsis Reveals the Drought Stress-Induced Long Non-coding RNAs Associated With Photosynthesis, Chlorophyll Synthesis, Fatty Acid Synthesis and Degradation
1 Department of Biotechnology, College of Life Science and Technology, Huazhong University of Science and Technology, Wuhan, China
2 Key Laboratory of Ecology of Rare and Endangered Species and Environmental Protection, College of Life Science, Guangxi Normal University, Guilin, China
3 School of Mechanical and Electrical Engineering, Guilin University of Electronic Technology, Guilin, China
Associated Data
The original contributions presented in the study are included in the article/ Supplementary Material , further inquiries can be directed to the corresponding author/s.
Long non-coding RNAs (lncRNAs) play an important role in the response of plants to drought stress. The previous studies have reported that overexpression of LEA3 and VOC could enhance drought tolerance and improve the oil content in Brassica napus and Arabidopsis thaliana , and most of the efforts have been invested in the gene function analysis, there is little understanding of how genes that involved in these important pathways are regulated. In the present study, the transcriptomic results of LEA3 and VOC over-expressed (OE) lines were compared with the RNAi lines, mutant lines and control lines under long-term and short-term drought treatment, a series of differentially expressed lncRNAs were identified, and their regulation patterns in mRNA were also investigated in above mentioned materials. The regulation of the target genes of differentially expressed lncRNAs on plant biological functions was studied. It was revealed that the mutant lines had less drought-response related lncRNAs than that of the OE lines. Functional analysis demonstrated that multiple genes were involved in the carbon-fixing and chlorophyll metabolism, such as CDR1 , CHLM , and CH1 , were regulated by the upregulated lncRNA in OE lines. In LEA-OE, AT4G13180 that promotes the fatty acid synthesis was regulated by five lncRNAs that were upregulated under both long-term and short-term drought treatments. The key genes, including of SHM1, GOX2 , and GS2 , in the methylglyoxal synthesis pathway were all regulated by a number of down-regulated lncRNAs in OE lines, thereby reducing the content of such harmful compounds produced under stress in plants. This study identified a series of lncRNAs related to the pathways that affect photosynthesis, chlorophyll synthesis, fatty acid synthesis, degradation, and other important effects on drought resistance and oil content. The present study provided a series of lncRNAs for further improvement of crop varieties, especially drought resistant and oil content traits.
Drought is one of the most common abiotic stresses that plants face, and which is a leading cause of crop failure globally ( Lesk et al., 2016 ). In recent years, various studies have focussed on the biology of drought tolerance and laid some foundations on the mechanism underlying the drought resistance of plants. Some important genes and pathways that related to drought stress have been identified, such as the dehydration response element binding proteins (DREBs) ( Agarwal et al., 2006 ) and abscisic acid signal transduction system ( Cai et al., 2017 ). Consequently, these multiple genes involved in a complex regulatory networks related to drought tolerance have posed challenges in the selection of drought tolerance traits in plant breeding. Late embryogenesis abundant group 3 ( LEA3 ) genes are part of a category that could function in the protection of membranes and proteins. Vicinal oxygen chelate ( VOC ) proteins are members of an enzyme superfamily with a common mechanistic attribute enabled by conserved active site residues. In our previous study, we found that overexpression of LEA3 and VOC genes could increase the drought resistance and oil content in Arabidopsis and B. napus ( Liang et al., 2019 ).
Long non-coding RNA (lncRNA) refers to the RNA that is longer than 200 nucleotides and is not capable of translation into protein. Some IncRNAs can take part in the regulation of response to abiotic stresses with genes ( Liu et al., 2013 ). A large number of lncRNAs have been identified in multiple species with the rapid development of high-throughput sequencing technology, and some of them could be classified as polyadenylic acid and non-polyadenylic acid ( Liu et al., 2012 , 2013 ). The lncRNA plays an important role in response to abiotic stress in plants. Previous studies have shown that the regulatory mechanism of lncRNA is very complex. For example, lncRNA could regulate the expression of protein-coding genes in cis or trans format and plays a regulatory role in modification of histones ( Wang et al., 2011 ; Kung et al., 2013 ). In cassava, 124 lncRNAs under drought treatment that exhibited significant effects on carbohydrate metabolism, the Calvin cycle, light response, and light signalling, have been identified ( Ding et al., 2019 ). Xiao et al. (2019) have identified two lncRNAs (LNC_001148 and LNC_000160) that related to drought resistance of tetraploid cassava, which mediate drought tolerance by regulating the stomatal density ( Xiao et al., 2019 ). Drought-responsive lncRNA has been discovered to play a role as a transcription regulator in tomato, and it has a certain regulatory effect on tolerance-related genes such as stimuli, signalling, and response to transporter activity ( Eom et al., 2019 ). A recent study in Arabidopsis thaliana also identified an important drought-induced lncRNA (lncRNA-DRIR) which had the key role in regulating plant tolerance to drought and salt stress ( Qin et al., 2017 ). In addition, a large number of lncRNAs related to stress have been identified in corn, grape, Tibetan wild barley and pepper ( Fei et al., 2019 ; Pang et al., 2019 ; Qiu et al., 2019 ; Wang et al., 2019 ).
B. napus (AACC, 2n = 38) is an allopolyploid species with a triplicated genome structure and many duplicated genes ( Chalhoub et al., 2014 ). Rapeseed is the third largest oil seed crop in the world, and many areas where it is planted are affected by drought. Thus, it is important to further study the exact roles of genes involved in oil accumulation and response to drought stress conditions.
LEA genes are part of a category that could function in the protection of membranes and proteins. Some studies have suggested that LEA-type proteins could act as water-binding molecules, playing important roles in macromolecule and membrane stabilisation and in ion sequestration ( Wang et al., 2003 ; Chakrabortee et al., 2012 ). These structural characteristics are related to the prediction of their function in response to desiccation stress ( Chakrabortee et al., 2007 ; Candat et al., 2014 ). VOC proteins are members of an enzyme superfamily with a common mechanistic attribute enabled by conserved active site residues. Glyoxalase I (GLYI) is a major member of the VOC family. It is a metalloenzyme that participates in the glyoxalase system, which has been reported to be a major pathway for the detoxification of methylglyoxal (MG) in living organisms. GLYI can use one molecule of glutathione (GSH) to convert MG to S-D-lactoylglutathione and functions in abiotic stress response ( Singla-Pareek et al., 2003 ; Mustafiz et al., 2014 ).
In our previous study, it was revealed that the LEA and VOC proteins were highly expressed in B. napus plants that had high oil content by proteomics analysis ( Gan et al., 2013 ). We also found that most of the BnLEA and BnVOC genes had higher expression levels under drought stress in B. napus ( Liang et al., 2016 , 2017 ). The overexpression (OE) of different copies of the drought response genes LEA3 and VOC enhanced both drought tolerance and oil content in B. napus and Arabidopsis . In contrast, oil content and drought tolerance were decreased in the AtLEA3 mutant ( atlea3 ) and AtVOC-RNAi of Arabidopsis and in both BnLEA-RNAi and BnVOC-RNAi B. napus RNAi lines. Hybrids between two lines with increased or reduced expression (LEA3-OE with VOC-OE, atlea3 with AtVOC-RNAi) showed corresponding stronger trends in drought tolerance ( Liang et al., 2019 ).
All these results showed that LEA3 and VOC genes can play an important role in drought response (not only AtLEA and AtVOC genes, but also BnLEA and BnVOC genes). And different transgenic plants lines which can be used for further study, including the OE lines, RNAi lines, knock-out mutants and hybrids. In the previous study, we have demonstrated the differential expression patterns of protein-coding genes and their corresponding effects in important pathways ( Liang et al., 2019 ). In the present study, the expression pattern of lncRNA in each sample, and their effect on the regulation of target genes on plant drought resistance and key pathways related to lipid synthesis were analysed in order to investigate the role of LEA and VOC genes in long-term and short-term drought stress. 2744 known lncRNAs and 1113 new lncRNAs in 56 samples were identified, and a large number of genes, especially in the metabolism of carbon and chlorophyll, that are regulated by lncRNA were observed. Among which, some single genes are regulated by multiple lncRNAs, such as CRD1 are trans-regulated by AT1G07993, AT1G09513, AT5G15845, AT2G08665, AT1G05207, AT3G08795, AT5G02095, and AT2G23672. The current study revealed that lncRNA plays an important role in the regulation of abiotic stress and enhances oil accumulation in Arabidopsis .
Materials and Methods
Data sources.
The RNA-seq data of 40 samples from leaf and silique tissues of multi genotypes of Arabidopsis in the present study were from our previous study, including the overexpression of AtLEA , AtVOC , RNAi of AtVOC , and AtLEA mutant ( Liang et al., 2019 ). Detailed sample information is listed in Table 1 . Based on these data, we explored the expression levels of known and newly predicted lncRNA, and the expression patterns of lncRNA in transgenic, overexpression, mutation, and RNAi-treated samples under long-term drought treatment and short-term drought treatment conditions were studied, as well as the regulation of the expression of protein-coding genes.
RNA-seq analysis codes for the drought treatments combining the genotype and sample tissues.
Data Processing
After removing sequencing adaptors and low-quality sequences using Cutadapt ( Martin, 2011 ), the RNA-seq clean data of each sample was aligned to the Arabidopsis reference genome using HiSAT2 ( Kim et al., 2015 ). RNA-seq alignment results of each sample were further assembled and merged by StringTie ( Pertea et al., 2015 ).
Identification of lncRNAs
To obtain qualified lncRNA candidates, we performed a preliminary filtering of transcripts and predicted the coding potential of the screened transcripts. Four principles were employed in the filtering step ( Jia et al., 2020 ): (1) Transcript length and exon number should be above 200 bp and 2, respectively. (2) Transcripts should be covered by at least five reads in all samples. (3) Transcripts aligned to known mRNAs and other non-coding RNAs (such as rRNA, tRNA, snoRNA, snRNA) of this species should be discarded. (4) The potential lncRNA, intronic lncRNA, and anti-sense lncRNA were identified according to class_code information (“u,” “i,” “x”). Lacking coding ability, the candidate lncRNA transcripts were further measured on coding potential using four methods. The coding-non-coding index (CNCI) ( Sun et al., 2013 ), coding potential calculator (CPC) ( Kong et al., 2007 ), and coding potential assessment tool (CPAT) ( Wang et al., 2013 ) were utilised to calculate the encoding and non-coding capabilities of transcripts. In addition, the protein domains in HMM library were searched in each candidate sequence using pfamscan 1 tool to screen out sequences with known protein domains.
Joint Analysis of lncRNA and mRNA
The DESeq ( Anders and Huber, 2010 ) was used for differential expression analysis, and genes with | log 2 ratio| ≥ 1 and q < 0.05 as a cut-off for significant differential expression were selected. The lncRNA mainly binds to regulatory regions of protein-coding genes and regulates gene expression in cis or trans formations. For differentially expressed lncRNAs, cis and trans regulation analyses were performed, and their function was indirectly predicted by the target genes. Cis regulation refers to the function of lncRNA that is related to the protein-encoding genes adjacent to it at the genomic locus. Therefore, the prediction method of the target gene for cis regulation is to screen out protein-encoding genes adjacent to lncRNA (upstream and downstream 50 Kb) as target genes. The trans -regulated genes were recognised according to the correlation coefficient of lncRNA and mRNA expression values (pearson correlation coefficient ≥ 0.9 or pearson correlation coefficient ≤ −0.9). Heatmaps of the differentially expressed lncRNA and mRNAs were plotted with the Complexheatmap package ( Gu et al., 2016 ). The qPCR were performed based by the methods in previous study ( Liang et al., 2019 ).
Function Analysis of Differentially Expressed lncRNA Target Genes
The target genes were analysed for enriched GO ( Harris et al., 2004 ) and KEGG categories ( Kanehisa and Goto, 2000 ) by applying hypergeometric tests. An adjusted P -value of 0.05 was used as a threshold for significant enrichment.
The Identification of lncRNAs and Their Expression Patterns
A total of 2,744 known lncRNAs were identified in 40 samples, 1,751, 2,344, 2,203, and 1,631 novel lncRNAs were identified by using CNCI, CPC, and CPAT software, and pfam database, respectively. Among these lncRNAs, 1113 new lncRNAs were shared in the results of all four methods. Compared to lncRNA, the mRNAs presented a larger exon number and a longer gene length ( Figures 1 , 2A,B ).

Numbers of novel lncRNAs in 56 samples (details in Table 1 ). Novel lncRNAs were identified by using CNCI, CPC, and CPAT software, and compared with the pfam database, respectively.

Comparison of IncRNA and mRNA. (A) Exon numbers of IncRNA and mRNA. (B) Length of IncRNA and mRNA. (C) JS score distribution of IncRNA and mRNA. (D) FPKM distribution of IncRNA and mRNA.
The FPKM of transcripts for mRNA and lncRNA in Arabidopsis were calculated in order to explore the expression patterns of identified lncRNAs, and a lower expression level of lncRNA (Wilcoxon rank sum test P < 0.001) than protein-coding genes were observed ( Figure 2D ). To quantitatively evaluate the expression specificity of each transcript, an entropy-based metric relying on the Jensen-Shannon (JS) distance was employed to calculate the expression-specific score for each transcript. For each transcript, the maximum JS score of each RNA was considered to be tissue-specific. A large proportion of lncRNA exhibited a higher maximal JS score ( Figure 2C ), indicating that the identified lncRNA carries a stronger tissue-specificity of expression.
Expression Level of lncRNA in Siliques and Leaves Under Long-Term Drought Treatment
For leaf samples, the RNA sequencing data of AtLEALL, AtVOCLL, AtVOCRNAiLL, mLEALL, AtLEALS, AtVOCLS, AtVOCRNAiLS, and mLEALS were analysed. Compared with WTLS, 209 and 5 differentially expressed lncRNAs in AtLEALS and mLEALS were detected, respectively. Compared with WTLL, 161 and 38 differentially expressed lncRNAs for AtLEALL and mLEALL under long-term treatment conditions were also detected ( Figures 3A,B , ,4A). 4A ). The present results indicated that the AtLEA over-expressed lines could induce more changes of lncRNA expression levels than mutant lines under both long-term and short-term drought treatment conditions.

Numbers of differentially expressed lncRNAs in samples. (A,E) Leaves samples in long-term drought; (B,F) leaves samples in short-term drought; (C,G) silique samples in long-term drought; (D,H) silique samples in short-term drought. For leaf samples, by using the wild type long-term treatment sample (WTLL) and wild type short-term treatment sample (WTLS) as controls (the first letter “L” represents leaf samples, the second letters “L” and “S” represent long-term and short-term drought treatments, respectively, the first letter “m” represents mutants). For siliques, using WTSL and WTSS (the first letter “S” represents the silique sample, and the second letter “L” and “S” indicate long-term and short-term drought treatments, respectively, the first letter “m” indicates mutants) as controls.

Heatmaps of differentially expressed lncRNAs in samples. (A) Leaves of LEA relative samples; (B) siliques of LEA relative samples; (C) leaves of VOC relative samples; (D) siliques of VOC relative samples.
For leaf samples focussing on VOC , 19 and 2 differentially expressed lncRNAs were identified from AtVOCLS and AtVOCRNAiLS under short-term drought treatment conditions compared with WTLS, respectively. Of these, only one lncRNA was shared. Under long-term drought treatment, 82 and 48 differentially expressed lncRNAs from AtVOCLL and AtVOCRNAiLL were identified compared with WTLL, respectively. Of which, 16 lncRNAs were shared ( Figures 3E,F , ,4C). 4C ). These results indicated that the changes of target genes of lncRNAs that effected by VOC was mainly occurred from long-term drought treatment.
For siliques, the sequencing sample data of AtLEASL, AtVOCRNAiSL, AtVOCSL, mLEASL, ATLEASS, AtVOCRNAiSS, AtVOCSS, and mLEASS were further analysed. For the LEA gene of siliques under short-term treatment, 55 and 51 differentially expressed lncRNAs in samples ATLEASS and mLEASS were detected compared with WTSS, respectively. 50 and 6 differentially expressed lncRNAs for AtLEASL and mLEASL were detected under long-term treatment conditions ( Figures 3C,D , ,4B). 4B ). The results indicated that more differentially expressed lncRNA were produced in the mutant lines of LEA under short-term drought treatments.
In silique samples focussing on the VOC gene under short-term treatment 22 and 8 differentially expressed lncRNAs for samples AtVOCRNAiSS and AtVOCSS were detected compared with WTSS, respectively. 175 and 342 differentially expressed lncRNAs in AtVOCRNAiSL and AtVOCSL under long-term treatment conditions were detected ( Figures 3G,H , ,4D 4D ).
Effects of lncRNA Expression Level on Lipid Metabolism and Photosynthetic Carbon Sequestration
It would be enhanced the biosynthesis and attenuated degradation of fatty acid (FA) in the LEA overexpression lines as revealed in our previous study ( Liang et al., 2019 ). KEGG pathway analysis showed that a number of key enzyme genes that involved in FA synthesis and degradation pathways were differentially expressed in these LEA -overexpressing plants. Interestingly, based on lncRNA target gene prediction results, we also discovered that several genes that differentially expressed in the FA synthesis and degradation pathways were regulated by lncRNAs ( Figure 5A ). These lncRNAs were also differentially expressed in LEA -overexpressing plants. For example, AT4G13180 [NAD (P) -binding Rossmann-fold superfamily protein] was regulated by five differentially expressed lncRNAs.

Key genes expression in crucial pathways. (A,B) Pathways of FA metabolism; (C) porphyrin and chlorophyll metabolism; (D) glyoxylate and dicarboxylate metabolism.
VOC genes were actively involved in FA degradation and FA elongation, and multiple genes related to FA degradation were downregulated in VOC over-expressed samples ( Liang et al., 2019 ). The lncRNA analysis revealed that only few FA-related genes were regulated by lncRNA in VOC over-expressed samples ( Figure 5B ). Ten differentially expressed lncRNAs exhibited a regulatory function on AT4G13180. Moreover, the regulation patterns of these lncRNAs on AT4G13180 were not consistent. The lncRNAs AT1G09627, AT3G24518, and AT2G35945 were downregulated in AtVOCSL, and lncRNA MSTRG.35793 was upregulated in AtVOCSL.
The KEGG pathway analysis of the lncRNA target genes also showed that the genes involved in carbon fixation and chlorophyll metabolism of photosynthetic organisms could be regulated by multiple lncRNAs. Most of the lncRNAs that regulate these genes were upregulated in LEA -overexpressing samples ( Figure 5C ). However, the expression level of lncRNAs in samples did not exhibit a significant difference under short-term drought conditions. In long-term drought conditions, lncRNAs in AtLEA overexpressing samples tended to play a regulatory role ( Figure 5C ) to assist the plants to obtain a stronger photosynthetic capacity and thus stronger drought resistance.
Metabolism of glyoxylic acid and dicarboxylic acid as well as plant hormone signal transduction, was vigorous in the leaves of transgenic rapeseed VOC gene overexpression samples. Additionally, the results of lncRNA target genes analysis indicated that the extensively downregulated lncRNAs were involved in the regulation of genes involved in the metabolism of glyoxylate, dicarboxylic acid, and transduction of plant hormone signals ( Figure 5D ). In the short-term drought treatment, AGT , GOX1 , GOX2 , and PMDH2 genes were regulated by several upregulated lncRNAs, which further revealed that glyoxylate metabolism and oxygen-related metabolic processes were affected by VOC genes ( Figure 5D ).
GO Enrichment of Target Genes of Differentially Expressed lncRNAs
GO analysis was performed on the target genes of differentially expressed lncRNAs. In the silique of LEA -overexpressing plants, genes regulated by upregulated lncRNA under short-term or long-term drought treatment were significantly enriched in transferase activity, transferring acyl groups, and acyl groups converted into alkyl groups on transfer ( Figures 6C,D ). Moreover, under short-term drought treatment, downregulated lncRNA also affected the genes that related to the regulation of endosperm development, lipid transport, and lipid localisation in LEA -overexpressing plants. In contrast, lncRNA of the mutant plants under long-term treatment did not exhibit a significant effect on the functions of these genes, but under short-term treatment, a downregulating effect on genes with functions such as regulation of endosperm development was observed.

GO enrichment of differentially expressed lncRNAs in samples. (A,E) Leaves samples in long-term drought; (B,F) leaves samples in short-term drought; (C,G) silique samples in long-term drought; (D,H) silique samples in short-term drought.
In leaves, overexpression of LEA affected multiple genes that related to photosynthesis and stress resistance ( Figures 6A,B ). Under short-term and long-term treatment conditions, the target genes of up-regulated lncRNA in the chloroplast envelope, chloroplast, photosynthetic membrane and chloroplast were enriched. Moreover, additional functions such as response to stress and oxygen-containing compounds were also regulated by lncRNA during short-term treatment. These results suggested that a number of genes related to stress resistance are rapidly regulated by lncRNA in Arabidopsis in early stage of treatment under the condition of LEA overexpression, while stress responses that are based on the lncRNA regulation are fewer in the mutant plants under short-term drought treatment.
Compared with LEA , the overexpression of VOC genes induced more lncRNAs in leaves (especially upregulated lncRNA) to participate in the regulation of photosynthesis and stress-related genes ( Figures 6E,F ). Under short-term treatment, the target genes in AtVOC over-expressing plants were mainly participated in the regulation of photosynthesis. Notably, in AtVOCRNAi plants, short-term treatment did not stimulate the regulation of stress and photosynthesis by lncRNA, but was consistent with AtVOCLS under long-term conditions ( Figures 6G,H ).
AtVOC over-expressing plants produced upregulated lncRNA mostly under long-term drought treatment, which significantly regulated the carbohydrate biosynthetic process, response to salicylic acid, and other functions. For AtVOCRNAi-treated plants, regardless of short-term or long-term drought treatment, down-regulated lncRNA were produced in response to some of the above functions, indicating that the suppression of the VOC gene had a negative effect on short-term stress and long-term adaptation of plants, which was not conducive to plant resistance to stress.
Specific Expression Pattern of lncRNA Trans-Regulatory Target Genes
The trans-regulatory target genes of the differentially expressed lncRNAs were predicted by the absolute values of correlation coefficient between lncRNA and mRNA expression values. A heatmap was used to show the expression pattern of differentially expressed lncRNAs and its target genes ( Figure 7 ). The figure indicates that the expression pattern of these target genes were line-specific. The trans-regulatory target genes were almost all high expressed in WTLS, mLEALS, AtVOCRNAiLS, and WTSS ( Figures 7A,C,D ). Meanwhile, as Figure 7 shows, the expression level of lncRNAs were lower than its trans-regulatory target genes.

Heatmaps of IncRNAs and target mRNAs. (A) Leaves of LEA relative samples; (B) siliques of LEA relative samples; (C) leaves of VOC relative samples; (D) siliques of VOC relative samples.
The stomata of LEA and VOC -OE transgenetic lines were regularly closed under drought conditions, which could maintain their normal shape and function, while the leaf surface of the wide type were possessed more wrinkles and tended to dry up under drought stress. Previous studies have shown that a number of drought marker genes, including RD29A , SnRK2.6 , and RbohD , were highly expressed in the OE line ( Liang et al., 2019 ), However, it was mainly focussed on the changes in the expression of coding genes and essential pathways, such as ABA signalling and photosynthesis pathways. In the present study, we focussed on the changes of lncRNA expression and the effects of these differentially expressed lncRNAs on the regulation of coding genes against drought. The previous study showed that VOC genes were able to affect late silique development under drought conditions ( Liang et al., 2019 ). The present results further suggest that lncRNA is more likely to participate in the regulation of VOC genes during late silique development. At the same time, the present results shown that compared to the lncRNA, the mRNAs had a larger exon number and a longer gene length ( Figures 1 , 2A,B ), which concurs with a previous study ( Lagarde et al., 2017 ). An expression difference between mRNA and lncRNA is also consistent with previous reports ( Deng et al., 2018 ). The identified lncRNA carries a stronger tissue-specificity of expression ( Figure 2C ), which is consistent with previous findings that the expression pattern is heterogeneous between lncRNA and mRNA ( Chen et al., 2018 ).
Studies on various plants, such as rice and cotton have shown that lncRNAs are essential to the resistance of plants to drought stress ( Chen et al., 2018 ; Deng et al., 2018 ). Predicting the function of lncRNA target genes could accelerate the study of lncRNAs. The predicated possible target genes of differentially expressed lncRNAs revealed that some target genes were significantly enriched in different pathways. Photosynthesis is fundamental in the synthesis of carbohydrates, which is a critical raw material for plants to further synthesise fatty acids. The photosynthesis-related genes analysis results indicated that the carbon fixation and chlorophyll metabolism of photosynthetic organisms were upregulated in LEA overexpression lines, and LEA was able to activate plants to improve photosynthesis capacity under drought stress ( Liang et al., 2019 ). Furthermore, under adverse environments, lncRNA participates in regulating photosynthetic pathways, which are essential for plants to resist stress ( Scherer et al., 1984 ; Colom and Vazzana, 2003 ; Xu et al., 2019 ; Zeng et al., 2019 ). When the genes are far away from lncRNAs, regulation occurs through trans-action, and then the expression levels of target genes were changed. These trans-action lncRNAs can guide ribonucleoprotein (RNP) complexes to specific genome locations or recruit chromatin-modifying enzymes to target genes ( Wu et al., 2020 ). This study revealed that, a large number of up-regulated lncRNAs were involved in trans-regulation of target genes involved in photosynthesis and chlorophyll synthesis pathways in LEA -overexpressing plants, among which, some genes were trans-regulated by multiple lncRNAs. CRD1 and CHLM are essential genes in the process of chlorophyll synthesis ( Kobayashi and Masuda, 2016 ; Herbst et al., 2018 ; Zubo et al., 2018 ), the present study revealed that the CRD1 was trans-regulated by eight lncRNAs including AT1G07993, AT1G09513, AT5G15845, AT2G08665, AT1G05207, AT3G08795, AT5G02095, and AT2G23672; and the CHLM was trans-regulated by six lncRNAs including AT1G07693, AT1G05207, AT5G07885, AT1G09927, AT5G02095, and AT2G23672. In Cucumis , the CHLM was regulated by LINC-chc01G00070-1 when the plant response to allopolyploidization, indicating that CHLM is involved in the lncRNA-mediated regulatory mechanisms ( Wang et al., 2020 ). The fact that a large number of upregulated lncRNAs were involved in the regulation of these genes, further reveals that lncRNA regulation of the photosynthesis and chlorophyll synthesis pathways is a key factor in trigging the stress response in plants. Pearson correlation analysis also showed that these lncRNAs were positively correlated with CRD1 and CHLM . In addition, CRD1 and CHLM were significantly upregulated in LEA -overexpressing lines. The maintenance of chlorophyll synthesis in leaves may assist plants to enhance photosynthesis in arid environments, thereby further promoting the accumulation of FA. The lncRNA in LEA -OE and VOC -OE strains exhibited a positive regulatory effect on photosynthetic efficiency, which could further enhance the drought resistance and promote lipid accumulation.
In the leaves of VOC- overexpressing plants, lncRNA also showed very interesting results in the regulation of methylglyoxal (MG). MG is a highly cytotoxic and mutagenic compound. Under normal growth conditions, the basal level of MG in plants is low, and it acts as an important signalling molecule. However, when the plant is subjected to abiotic stress, MG can accumulate, and acts as a toxic molecule under this situations, inhibiting different developmental processes, including seed germination, photosynthesis, and root growth ( Hoque et al., 2016 ; Li et al., 2017 ), a large number of down-regulated lncRNAs in VOC -OE were involved in the regulation of the MG synthesis pathway were observed. The key genes, including SHM1 , GOX2 , and GS2 , involved in the methylglyoxal synthesis pathway, were all regulated by multiple down-regulated lncRNAs, which were positively correlated with the expression levels of SHM1 , GOX2 , and GS2 . Through such regulation, the accumulation of MG was reduced. In another study, the GOX2 was trans-regulated by lncRNA MSTRG.14375 under infection of downy mildew in Chinese cabbage ( Zhang et al., 2021 ). That study shows that GOX2 is the target of lncRNA regulation in response to stress. These results indicated that when VOC is overexpressed in Arabidopsis , lncRNAs produced under drought stress were able to regulate the glyoxylate enzyme system to a certain extent, and thus could be a factor in the response to drought stress.
Previous studies ( Liang et al., 2019 ) have demonstrated that the oil content of LEA -OE and VOC -OE lines has increased. Under drought stress conditions, LEA -OE would drive seeds to form a stable and thick cell membrane to protect lipid synthesis in plants. In the siliques of the VOC and LEA overexpression lines, lncRNAs were not intensively involved in the regulation of the fatty acid synthesis pathway. However, but a few genes were still regulated by multiple differentially expressed lncRNAs, for example in LEA -OE, AT4G13180 was regulated by seven lncRNAs, of which five were upregulated, both under long-term and short-term drought treatments, and this promoted fatty acid synthesis.
In summary, the changes in lncRNA caused by the expression of LEA and VOC genes may be related to the drought tolerance and oil content of Arabidopsis thaliana , and the lines that overexpressed these two genes showed strong drought resistance and oil production. LEA and VOC increase photosynthetic efficiency and reduce reactive oxygen species under drought stress. For future breeding purposes, one of the regulatory methods to achieve this goal is to regulate the key genes involved in these two pathways through up-regulation and down-regulation of the related lncRNAs. Consequently, these drought-responsive lncRNAs not only played a role in the drought stress, but also promote oil accumulation, which requires further investigation. In short, this study provides a novel perspective for the study of the relationship between oil accumulation and drought resistance from the regulatory relationship between lncRNA and mRNA, offering a new approach to further promote the breeding of oil crops.
Data Availability Statement
Author contributions.
ML designed the experiments. YH and KC conducted the bioinformation analysis. CL performed the data collection. YL wrote the manuscript. All authors contributed to the article and approved the submitted version.
Conflict of Interest
The authors declare that the research was conducted in the absence of any commercial or financial relationships that could be construed as a potential conflict of interest.
Abbreviations
Funding. This work was supported by the Guangxi Science and Technology Base and Talent Project (Guike AD19245098; Guike AD19245141), the Guangxi Key Lab of Manufacturing System and Advanced Manufacturing Technology under Grant 19-050-44-005Z, Research Project of Key Laboratory of Ecology of Rare and Endangered Species and Environmental Protection (ERESEP2020Z09), and National Natural Science Foundation of China (31871656).
1 https://www.ebi.ac.uk/Tools/pfa/pfamscan/
Supplementary Material
The Supplementary Material for this article can be found online at: https://www.frontiersin.org/articles/10.3389/fpls.2021.643182/full#supplementary-material
Supplementary Figure 1
The qPCR results of some lines and time points, and it was compared with the RNA-seq data.
Supplementary Table 1
SRA accession number of RNA-seq data uploaded to the NCBI database.
Supplementary Table 2
LncRNAs and their trans-regulatory target genes in leaves of LEA relative samples.
Supplementary Table 3
LncRNAs and their trans-regulatory target genes in siliques of LEA relative samples.
Supplementary Table 4
LncRNAs and their trans-regulatory target genes in leaves of VOC relative samples.
Supplementary Table 5
LncRNAs and their trans-regulatory target genes in siliques of VOC relative samples.
Supplementary Table 6
Differentially expressed lncRNAs between AtLEALL and WTLL samples.
Supplementary Table 7
Differentially expressed lncRNAs between AtLEALS and WTLS samples.
Supplementary Table 8
Differentially expressed lncRNAs between AtLEASL and WTSL samples.
Supplementary Table 9
Differentially expressed lncRNAs between AtLEASS and WTSS samples.
Supplementary Table 10
Differentially expressed lncRNAs between AtVOCLL and WTLL samples.
Supplementary Table 11
Differentially expressed lncRNAs between AtVOCLS and WTLS samples.
Supplementary Table 12
Differentially expressed lncRNAs between AtVOCSL and WTSL samples.
Supplementary Table 13
Differentially expressed lncRNAs between AtVOCSS and WTSS samples.
Supplementary Table 14
Primers used in qPCR.
- Agarwal P. K., Agarwal P., Reddy M. K., Sopory S. K. (2006). Role of DREB transcription factors in abiotic and biotic stress tolerance in plants. Plant Cell Rep. 25 1263–1274. 10.1007/s00299-006-0204-8 [ PubMed ] [ CrossRef ] [ Google Scholar ]
- Anders S., Huber W. (2010). Differential expression analysis for sequence count data. Genome Biol. 11 :R106. 10.1186/gb-2010-11-10-r106 [ PMC free article ] [ PubMed ] [ CrossRef ] [ Google Scholar ]
- Cai S., Chen G., Wang Y., Huang Y., Marchant D. B., Wang Y., et al. (2017). Evolutionary conservation of ABA signaling for stomatal closure. Plant Physiol. 174 732–747. 10.1104/pp.16.01848 [ PMC free article ] [ PubMed ] [ CrossRef ] [ Google Scholar ]
- Candat A., Paszkiewicz G., Neveu M., Gautier R., Logan D. C., Avelange-Macherel M. H., et al. (2014). The ubiquitous distribution of late embryogenesis abundant proteins across cell compartments in Arabidopsis offers tailored protection against abiotic stress. Plant Cell 26 3148–3166. 10.1105/tpc.114.127316 [ PMC free article ] [ PubMed ] [ CrossRef ] [ Google Scholar ]
- Chakrabortee S., Boschetti C., Walton L. J., Sarkar S., Rubinsztein D. C., Tunnacliffe A. (2007). Hydrophilic protein associated with desiccation tolerance exhibits broad protein stabilization function. Proc. Natl. Acad. Sci. U.S.A. 104 18073–18078. 10.1073/pnas.0706964104 [ PMC free article ] [ PubMed ] [ CrossRef ] [ Google Scholar ]
- Chakrabortee S., Tripathi R., Watson M., Kaminski Schierle G. S., Kurniawan D. P., Kaminski C. F., et al. (2012). Intrinsically disordered proteins as molecular shields. Mol. Biosyst. 8 210–219. 10.1039/c1mb05263b [ PMC free article ] [ PubMed ] [ CrossRef ] [ Google Scholar ]
- Chalhoub B., Denoeud F., Liu S., Parkin I. A. P., Tang H., Wang X., et al. (2014). Early allopolyploid evolution in the post-neolithic Brassica napus oilseed genome. Science 345 950–953. 10.1126/science.1253435 [ PubMed ] [ CrossRef ] [ Google Scholar ]
- Chen L., Zhang Y. H., Pan X., Liu M., Wang S., Huang T., et al. (2018). Tissue expression difference between mrnas and lncrnas. Int. J. Mol. Sci. 19 :3416. 10.3390/ijms19113416 [ PMC free article ] [ PubMed ] [ CrossRef ] [ Google Scholar ]
- Colom M. R., Vazzana C. (2003). Photosynthesis and PSII functionality of drought-resistant and drought-sensitive weeping lovegrass plants. Environ. Exp. Bot. 49 135–144. 10.1016/S0098-8472(02)00065-5 [ CrossRef ] [ Google Scholar ]
- Deng P., Liu S., Nie X., Weining S., Wu L. (2018). Conservation analysis of long non-coding RNAs in plants. Sci. China Life Sci. 61 190–198. 10.1007/s11427-017-9174-9 [ PubMed ] [ CrossRef ] [ Google Scholar ]
- Ding Z., Tie W., Fu L., Yan Y., Liu G., Yan W., et al. (2019). Strand- specific RNA-seq based identification and functional prediction of drought-responsive lncRNAs in cassava. BMC Genomics 20 :214. 10.1186/s12864-019-5585-5 [ PMC free article ] [ PubMed ] [ CrossRef ] [ Google Scholar ]
- Eom S. H., Lee H. J., Lee J. H., Wi S. H., Kim S. K., Hyun T. K. (2019). Identification and functional prediction of drought-responsive long non-coding RNA in tomato. Agronomy 9 :629. 10.3390/agronomy9100629 [ CrossRef ] [ Google Scholar ]
- Fei X., Shi Q., Liu Y., Yang T., Wei A. (2019). RNA sequencing and functional analyses reveal regulation of novel drought-responsive, long-non-coding RNA in Zanthoxylum bungeanum Maxim. Plant Growth Regul. 90 425–440. 10.1007/s10725-019-00541-y [ CrossRef ] [ Google Scholar ]
- Gan L., Zhang C. Y., Wang X. D., Wang H., Long Y., Yin Y. T., et al. (2013). Proteomic and comparative genomic analysis of two Brassica napus lines differing in oil content. J. Proteome Res. 12 4965–4978. 10.1021/pr4005635 [ PubMed ] [ CrossRef ] [ Google Scholar ]
- Gu Z., Eils R., Schlesner M. (2016). Complex heatmaps reveal patterns and correlations in multidimensional genomic data. Bioinformatics 32 2847–2849. 10.1093/bioinformatics/btw313 [ PubMed ] [ CrossRef ] [ Google Scholar ]
- Harris M. A., Clark J., Ireland A., Lomax J., Ashburner M., Foulger R., et al. (2004). The Gene Oncology (GO) database and informatics resource. Nucleic Acids Res. 32 D258–D261. 10.1093/nar/gkh036 [ PMC free article ] [ PubMed ] [ CrossRef ] [ Google Scholar ]
- Herbst J., Girke A., Hajirezaei M. R., Hanke G., Grimm B. (2018). Potential roles of YCF54 and ferredoxin-NADPH reductase for magnesium protoporphyrin monomethylester cyclase. Plant J. 94 485–496. 10.1111/tpj.13869 [ PubMed ] [ CrossRef ] [ Google Scholar ]
- Hoque T. S., Hossain M. A., Mostofa M. G., Burritt D. J., Fujita M., Tran L. S. P. (2016). Methylglyoxal: an emerging signaling molecule in plant abiotic stress responses and tolerance. Front. Plant Sci. 7 :1341. 10.3389/fpls.2016.01341 [ PMC free article ] [ PubMed ] [ CrossRef ] [ Google Scholar ]
- Jia X., Tang L., Mei X., Liu H., Luo H., Deng Y., et al. (2020). Single-molecule long-read sequencing of the full-length transcriptome of Rhododendron lapponicum L. Sci. Rep. 10 :6755. 10.1038/s41598-020-63814-x [ PMC free article ] [ PubMed ] [ CrossRef ] [ Google Scholar ]
- Kanehisa M., Goto S. (2000). KEGG: kyoto encyclopedia of genes and genomes. Nucleic Acids Res. 28 27–30. 10.1093/nar/28.1.27 [ PMC free article ] [ PubMed ] [ CrossRef ] [ Google Scholar ]
- Kim D., Langmead B., Salzberg S. L. (2015). HISAT: a fast spliced aligner with low memory requirements. Nat. Methods 12 357–360. 10.1038/nmeth.3317 [ PMC free article ] [ PubMed ] [ CrossRef ] [ Google Scholar ]
- Kobayashi K., Masuda T. (2016). Transcriptional regulation of tetrapyrrole biosynthesis in Arabidopsis thaliana . Front. Plant Sci. 7 :1811. 10.3389/fpls.2016.01811 [ PMC free article ] [ PubMed ] [ CrossRef ] [ Google Scholar ]
- Kong L., Zhang Y., Ye Z. Q., Liu X. Q., Zhao S. Q., Wei L., et al. (2007). CPC: assess the protein-coding potential of transcripts using sequence features and support vector machine. Nucleic Acids Res. 35 W345–W349. 10.1093/nar/gkm391 [ PMC free article ] [ PubMed ] [ CrossRef ] [ Google Scholar ]
- Kung J. T. Y., Colognori D., Lee J. T. (2013). Long noncoding RNAs: past, present, and future. Genetics 193 651–669. 10.1534/genetics.112.146704 [ PMC free article ] [ PubMed ] [ CrossRef ] [ Google Scholar ]
- Lagarde J., Uszczynska-Ratajczak B., Carbonell S., Pérez-Lluch S., Abad A., Davis C., et al. (2017). High-throughput annotation of full-length long noncoding RNAs with capture long-read sequencing. Nat. Genet. 49 1731–1740. 10.1038/ng.3988 [ PMC free article ] [ PubMed ] [ CrossRef ] [ Google Scholar ]
- Lesk C., Rowhani P., Ramankutty N. (2016). Influence of extreme weather disasters on global crop production. Nature 529 84–87. 10.1038/nature16467 [ PubMed ] [ CrossRef ] [ Google Scholar ]
- Li Z. G., Duan X. Q., Min X., Zhou Z. H. (2017). Methylglyoxal as a novel signal molecule induces the salt tolerance of wheat by regulating the glyoxalase system, the antioxidant system, and osmolytes. Protoplasma 254 1995–2006. 10.1007/s00709-017-1094-z [ PubMed ] [ CrossRef ] [ Google Scholar ]
- Liang Y., Kang K., Gan L., Ning S., Xiong J., Song S., et al. (2019). Drought-responsive genes, late embryogenesis abundant group3 ( LEA3 ) and vicinal oxygen chelate, function in lipid accumulation in Brassica napus and Arabidopsis mainly via enhancing photosynthetic efficiency and reducing ROS. Plant Biotechnol. J. 17 2123–2142. 10.1111/pbi.13127 [ PMC free article ] [ PubMed ] [ CrossRef ] [ Google Scholar ]
- Liang Y., Wan N., Cheng Z., Mo Y., Liu B., Liu H., et al. (2017). Whole-genome identification and expression pattern of the vicinal oxygen chelate family in rapeseed ( Brassica napus L.). Front. Plant Sci. 8 :745. 10.3389/fpls.2017.00745 [ PMC free article ] [ PubMed ] [ CrossRef ] [ Google Scholar ]
- Liang Y., Xiong Z., Zheng J., Xu D., Zhu Z., Xiang J., et al. (2016). Genome-wide identification, structural analysis and new insights into late embryogenesis abundant (LEA) gene family formation pattern in Brassica napus . Sci. Rep. 6 :24265. 10.1038/srep24265 [ PMC free article ] [ PubMed ] [ CrossRef ] [ Google Scholar ]
- Liu J., Jung C., Xu J., Wang H., Deng S., Bernad L., et al. (2012). Genome-wide analysis uncovers regulation of long intergenic noncoding RNAs in arabidopsisC W. Plant Cell 24 4333–4345. 10.1105/tpc.112.102855 [ PMC free article ] [ PubMed ] [ CrossRef ] [ Google Scholar ]
- Liu T. T., Zhu D., Chen W., Deng W., He H., He G., et al. (2013). A global identification and analysis of small nucleolar RNAs and possible intermediate-sized non-coding RNAs in Oryza sativa . Mol. Plant 6 830–846. 10.1093/mp/sss087 [ PMC free article ] [ PubMed ] [ CrossRef ] [ Google Scholar ]
- Martin M. (2011). Cutadapt removes adapter sequences from high-throughput sequencing reads. EMBnet J. 17 10–12. 10.14806/ej.17.1.200 [ CrossRef ] [ Google Scholar ]
- Mustafiz A., Ghosh A., Tripathi A. K., Kaur C., Ganguly A. K., Bhavesh N. S., et al. (2014). A unique Ni 2 + -dependent and methylglyoxal-inducible rice glyoxalase I possesses a single active site and functions in abiotic stress response. Plant J. 78 951–963. 10.1111/tpj.12521 [ PubMed ] [ CrossRef ] [ Google Scholar ]
- Pang J., Zhang X., Ma X., Zhao J. (2019). Spatio-temporal transcriptional dynamics of maize long non-coding RNAs responsive to drought stress. Genes (Basel) 10 :138. 10.3390/genes10020138 [ PMC free article ] [ PubMed ] [ CrossRef ] [ Google Scholar ]
- Pertea M., Pertea G. M., Antonescu C. M., Chang T. C., Mendell J. T., Salzberg S. L. (2015). StringTie enables improved reconstruction of a transcriptome from RNA-seq reads. Nat. Biotechnol. 33 290–295. 10.1038/nbt.3122 [ PMC free article ] [ PubMed ] [ CrossRef ] [ Google Scholar ]
- Qin T., Zhao H., Cui P., Albesher N., Xionga L. (2017). A nucleus-localized long non-coding rna enhances drought and salt stress tolerance. Plant Physiol. 175 1321–1336. 10.1104/pp.17.00574 [ PMC free article ] [ PubMed ] [ CrossRef ] [ Google Scholar ]
- Qiu C. W., Zhao J., Chen Q., Wu F. (2019). Genome-wide characterization of drought stress responsive long non-coding RNAs in Tibetan wild barley. Environ. Exp. Bot. 164 124–134. 10.1016/j.envexpbot.2019.05.002 [ CrossRef ] [ Google Scholar ]
- Scherer S., Ernst A., Chen T. W., Böger P. (1984). Rewetting of drought-resistant blue-green algae: time course of water uptake and reappearance of respiration, photosynthesis, and nitrogen fixation. Oecologia 62 418–423. 10.1007/BF00384277 [ PubMed ] [ CrossRef ] [ Google Scholar ]
- Singla-Pareek S. L., Reddy M. K., Sopory S. K. (2003). Genetic engineering of the glyoxalase pathway in tobacco leads to enhanced salinity tolerance. Proc. Natl. Acad. Sci. U.S.A. 100 14672–14677. 10.1073/pnas.2034667100 [ PMC free article ] [ PubMed ] [ CrossRef ] [ Google Scholar ]
- Sun L., Luo H., Bu D., Zhao G., Yu K., Zhang C., et al. (2013). Utilizing sequence intrinsic composition to classify protein-coding and long non-coding transcripts. Nucleic Acids Res. 41 :e166. 10.1093/nar/gkt646 [ PMC free article ] [ PubMed ] [ CrossRef ] [ Google Scholar ]
- Wang K. C., Yang Y. W., Liu B., Sanyal A., Corces-Zimmerman R., Chen Y., et al. (2011). A long noncoding RNA maintains active chromatin to coordinate homeotic gene expression. Nature 472 120–124. 10.1038/nature09819 [ PMC free article ] [ PubMed ] [ CrossRef ] [ Google Scholar ]
- Wang L., Park H. J., Dasari S., Wang S., Kocher J. P., Li W. (2013). CPAT: coding-potential assessment tool using an alignment-free logistic regression model. Nucleic Acids Res. 41 :e74. 10.1093/nar/gkt006 [ PMC free article ] [ PubMed ] [ CrossRef ] [ Google Scholar ]
- Wang P., Dai L., Ai J., Wang Y., Ren F. (2019). Identification and functional prediction of cold-related long non-coding RNA (lncRNA) in grapevine. Sci. Rep. 9 1–15. 10.1038/s41598-019-43269-5 [ PMC free article ] [ PubMed ] [ CrossRef ] [ Google Scholar ]
- Wang P., Yu X., Zhu Z., Zhai Y., Zhao Q., Meng Y., et al. (2020). Global profiling of lncRNAs expression responsive to allopolyploidization in cucumis. Genes (Basel) 11 :1500. 10.3390/genes11121500 [ PMC free article ] [ PubMed ] [ CrossRef ] [ Google Scholar ]
- Wang W., Vinocur B., Altman A. (2003). Plant responses to drought, salinity and extreme temperatures: towards genetic engineering for stress tolerance. Planta 218 1–14. 10.1007/s00425-003-1105-5 [ PubMed ] [ CrossRef ] [ Google Scholar ]
- Wu L., Liu S., Qi H., Cai H., Xu M. (2020). Research progress on plant long non-coding RNA. Plants 9 :408. 10.3390/plants9040408 [ PMC free article ] [ PubMed ] [ CrossRef ] [ Google Scholar ]
- Xiao L., Shang X. H., Cao S., Xie X. Y., Zeng W. D., Lu L. Y., et al. (2019). Comparative physiology and transcriptome analysis allows for identification of lncRNAs imparting tolerance to drought stress in autotetraploid cassava. BMC Genomics 20 :514. 10.1186/s12864-019-5895-7 [ PMC free article ] [ PubMed ] [ CrossRef ] [ Google Scholar ]
- Xu L., Fan J., Wang Q. (2019). Omics application of bio-hydrogen production through green alga chlamydomonas reinhardtii. Front. Bioeng. Biotechnol. 7 :201. 10.3389/fbioe.2019.00201 [ PMC free article ] [ PubMed ] [ CrossRef ] [ Google Scholar ]
- Zeng M., He S., Hao L., Li Y., Zheng C., Zhao Y. (2019). Conjoint analysis of genome-wide lncrna and mrna expression of heteromorphic leavesin response to environmental heterogeneityin populus euphratica. Int. J. Mol. Sci. 20 :5148. 10.3390/ijms20205148 [ PMC free article ] [ PubMed ] [ CrossRef ] [ Google Scholar ]
- Zhang B., Su T., Li P., Xin X., Cao Y., Wang W., et al. (2021). Identification of long noncoding RNAs involved in resistance to downy mildew in Chinese cabbage. Hortic. Res. 8 1–15. 10.1038/s41438-021-00479-1 [ PMC free article ] [ PubMed ] [ CrossRef ] [ Google Scholar ]
- Zubo Y. O., Blakley I. C., Franco-Zorrilla J. M., Yamburenko M. V., Solano R., Kieber J. J., et al. (2018). Coordination of chloroplast development through the action of the GNC and GLK transcription factor families. Plant Physiol. 178 130–147. 10.1104/pp.18.00414 [ PMC free article ] [ PubMed ] [ CrossRef ] [ Google Scholar ]

An official website of the United States government
Official websites use .gov A .gov website belongs to an official government organization in the United States.
National Weather Service launches new website for water prediction and products
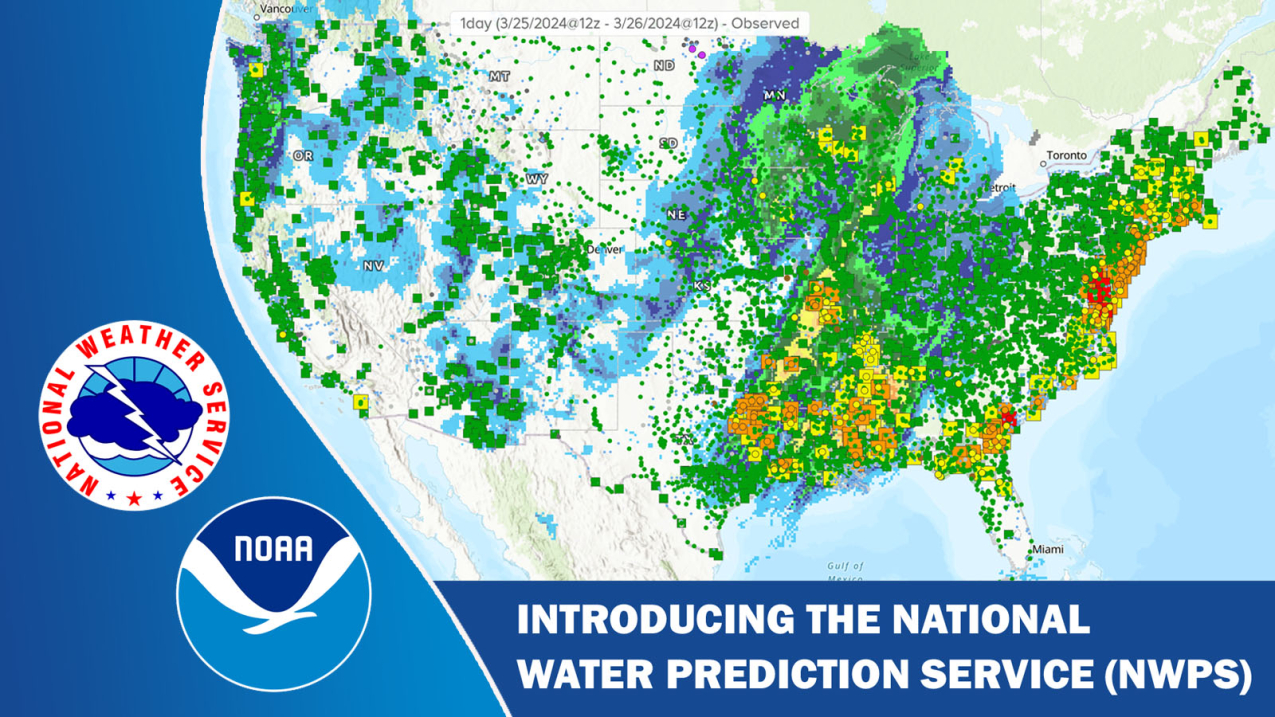
The National Weather Service (NWS) is introducing the new National Water Prediction Service (NWPS), transforming how water resources information and services are delivered, and providing a greatly improved user experience through enhanced displays. (Image credit: NOAA)
NOAA’s National Weather Service launched a new website today: The National Water Prediction Service . This new hub for water data, products and services combines local and regional forecasts with water data and new national level capabilities, such as flood inundation maps and the National Water Model.
"This online water hub is modern and flexible — providing information to help our partners and the public make sound decisions for water safety and management," said Ed Clark, director of NOAA’s National Water Center. “The new site leverages modern software, geospatial technology and cloud infrastructure, vastly improving the customer experience before, during and after extreme water events such as floods and droughts.”
Key features integrated into the National Water Prediction Service website
- A new, dynamic and seamless national map with flexible options and expansive layers available to help analyze water conditions anywhere in the country.
- Improved hydrographs that are frequently updated, depict water level observations over the past 30 days and provide river flood forecasts up to 10 days in advance.
- The National Water Model , which provides 24 hour coverage, seven days a week hydrologic forecast guidance along 3.4 million river miles across the U.S., including river segments, streams and creeks that have no river gauges.
- Real-time, comprehensive Flood Inundation Maps, which are being implemented in phases, will cover nearly 100% of the U.S. by October 2026.
- An Application Programming Interface (API) has been added to the traditional geographic information system (GIS) data, which will allow customers to flow water information into their own applications and services.
NWS continues to improve the essential support services it provides to communities locally and nationwide as part of its transformation into a more nimble, flexible and mobile agency that works hand-in-hand with decision makers. The National Water Prediction Service website provides tools that deliver actionable hydrologic information across all time scales to address the growing risk of flooding, drought and water availability, and enables partners and the American public to make smart water decisions.
Weather.gov Webstory - January 12, 2024
NWPS Product and Users Guide
Flood Inundation Mapping news release
Biden-Harris Administration announces $80 million to improve flood prediction capabilities
National Weather Service - Flood Safety Tips and Resources
Media Contact
Michael Musher, michael.musher@noaa.gov , (771) 233-1304
Related Features //
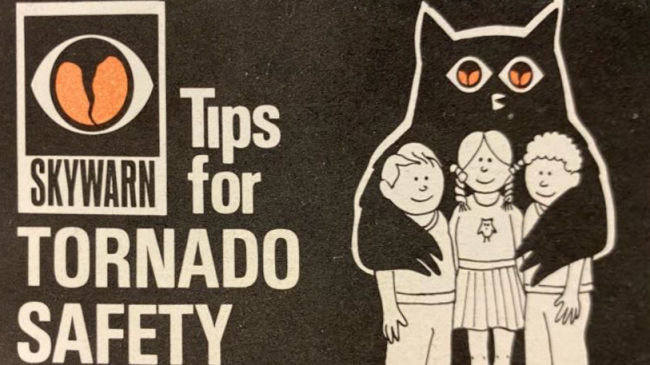
ORIGINAL RESEARCH article
Characterization of fba genes in potato (solanum tuberosum l.) and expression patterns in response to light spectrum and abiotic stress.

- 1 Country Center for Crop Biotechnology, College of Agriculture, Anhui Science and Technology University, Fengyang, China
- 2 Country College of Life Sciences, Shihezi University, Shihezi, China
Fructose-1, 6-bisphosphate aldolase (FBA) plays vital roles in plant growth, development, and response to abiotic stress. However, genome-wide identification and structural characterization of the potato ( Solanum tuberosum L.) FBA gene family has not been systematically analyzed. In this study, we identified nine StFBA gene members in potato, with six StFBA genes localized in the chloroplast and three in the cytoplasm. The analysis of gene structures, protein structures, and phylogenetic relationships indicated that StFBA genes were divided into Class I and II, which exhibited significant differences in structure and function. Synteny analysis revealed that segmental duplication events promoted the expansion of the StFBA gene family. Promoter analysis showed that most StFBA genes contained cis -regulatory elements associated with light and stress responses. Expression analysis showed that StFBA3 , StFBA8, and StFBA9 showing significantly higher expression levels in leaf, stolon, and tuber under blue light, indicating that these genes may improve photosynthesis and play an important function in regulating the induction and expansion of microtubers. Expression levels of the StFBA genes were influenced by drought and salt stress, indicating that they played important roles in abiotic stress. This work offers a theoretical foundation for in-depth understanding of the evolution and function of StFBA genes, as well as providing the basis for the genetic improvement of potatoes.
1 Introduction
Plants utilize various enzymes within their bodies to absorb solar energy and convert CO 2 into organic carbon in nature ( Wildman, 2002 ). Ribulose-1, 5-bisphosphate carboxylase/oxygenase (Rubisco) plays a crucial role in carbon fixation and regulates biomass synthesis during photosynthesis ( Ashida et al., 2019 ). However, the low catalytic efficiency of Rubisco remains a major limiting factor in photosynthesis ( Bracher et al., 2017 ). With the rise in global temperatures and CO 2 concentrations, Rubisco is susceptible to oxidation, resulting in substantial loss of photosynthetic products. Some plants conserve energy by reducing the synthesis of Rubisco proteins when grown in high CO 2 environments ( Jordan and Ogren, 1984 ; Long et al., 2004 ). Previous studies have shown that increasing the levels of enzymes associated with the regeneration phase of ribulose-1, 5-bisphosphate (RuBP) in the Calvin cycle, such as sedoheptulose-1, 7-bisphosphatase (SBP), transketolase, and aldolase, can enhance plant carbon assimilation efficiency ( Raines, 2003 ; Lefebvre et al., 2005 ). Among them, aldolase reaches its highest activity level under CO 2 saturation ( Haake et al., 1999 ). Overexpression of a gene-encoding plastid aldolase in transgenic tobacco enhanced the activity of the enzyme, accelerated the regeneration of RuBP, and the biomass significantly increased with increasing CO 2 levels ( Uematsu et al., 2012 ).
Fructose-1, 6-bisphosphate aldolase (FBA; EC 4.1.2.13) is an important enzyme involved in plant photosynthesis and energy metabolism ( Carrera et al., 2021 ). It catalyzes the condensation of glyceraldehyde-3-phosphate (GAP) and dihydroxyacetone phosphate (DHAP) to fructose-1, 6-bisphosphate (FBP) during glycolysis and its reversible reaction, which is ultimately used for the regeneration of RuBP ( Zgiby et al., 2000 ; Capodagli et al., 2014 ). FBA can be divided into Class I and Class II. Class I aldolase is primarily found in eukaryotes, while Class II aldolase is widely distributed in bacteria, saccharomycetes, and fungi. There are significant differences in the structure and function of these classes ( Marsh and Lebherz, 1992 ; Izard and Sygusch, 2004 ; Galkin et al., 2007 ). In higher plants, Class I aldolase is composed of homotetramers ( Lebherz et al., 1984 ; Perham, 1990 ) and its protein tertiary structure contains a typical conserved structural domain, called the TIM barrel ( Hester et al., 1991 ; Blom and Sygusch, 1997 ). The TIM barrel is a conserved protein fold pattern, which consists of eight repetitive α-helices and eight β-folds, which are arranged alternately, forming a characteristic α/β-barrel structure present in the protein’s main peptide segment ( Lorentzen et al., 2003 ; Frenkel and Trifonov, 2005 ). In addition, there are two subtypes of Class I aldolase: chloroplast aldolase, and cytoplasmic aldolase ( Pelzer-Reith et al., 1993 ; Tsutsumi et al., 1994 ). Chloroplast aldolase is a bifunctional enzyme in the Calvin cycle, involved in the synthesis of FBP and sedoheptulose-1, 7-bisphosphate (SuBP). It catalyzes the generation of metabolic products in the process of starch synthesis ( Sonnewald et al., 1994 ; Flechner et al., 1999 ). Furthermore, cytoplasmic aldolase is an important metabolic enzyme in the pathways of glycolysis and gluconeogenesis, where it catalyzes the production of FBP and participates in the synthesis of sucrose ( Gross et al., 1999 ; Fan et al., 2009 ). Mutations in Arabidopsis cytoplasmic aldolase AtFBA3 have been reported to affect the plastid glycolytic metabolism in the roots of plants, thereby limiting the synthesis of essential compounds such as amino acids ( Carrera et al., 2021 ).
Previous studies reported that a slight reduction in FBA activity significantly inhibited the photosynthetic and carbon assimilations of antisense transgenic plants, which affected the synthesis of starch and severely inhibited the growth of plants ( Jacquot et al., 1995 ; Haake et al., 1998 ; Bryant, 2000 ; Henkes et al., 2001 ), while reduced activity of FBA in tomato plants led to smaller seed size and slower plant growth ( Cai et al., 2018 ). Suppressing the expression of the chloroplast FBA gene affects the development of tomatoes ( Obiadalla-Ali et al., 2004 ). In rice, the OsALD - Y (fructose-1, 6-bisphosphate aldolase) encodes an FBA protein, which affects photosynthesis and sugar metabolism ( Zhang et al., 2016 ). Furthermore, the FBA gene is also involved in a variety of physiological and biochemical processes, such as the regulation of secondary metabolism ( Zeng et al., 2014 ), and regulation of plant growth and development ( Haake et al., 1998 ; Obiadalla-Ali et al., 2004 ; Mutuku and Nose, 2012 ), responding to various biotic and abiotic stress ( Lu et al., 2012 ; Cai et al., 2018 ), to regulate plant responses to hormones such as abscisic acid ( Osakabe et al., 2005 ) and gibberellin ( Konishi et al., 2004 ), as well as responses to environmental light signals ( Oelze et al., 2014 ). It is therefore possible that FBA genes play a crucial role in enhancing the photosynthetic efficiency of plants, to improve crop yield and quality.
Potato is one of the four largest food crops worldwide ( Zhang et al., 2017 ), and a significant crop for both food and as a vegetable in our country. The available genome sequences of potatoes ( Pham et al., 2020 ; Zhou et al., 2020 ; Zhang et al., 2021 ) facilitate their use in potato growth and development. Members of the FBA gene family have been identified in different species, with eight identified in Arabidopsis thaliana ( Lu et al., 2012 ), eight in Solanum lycopersicum ( Cai et al., 2016 ), 22 in Brassica napu s ( Zhao et al., 2019 ), 16 in Nicotiana tabacum ( Zhao et al., 2021 ), and 17 in cotton ( Li et al., 2021 ). When expression of potato chloroplast aldolase (Registry Number: Y10380) was inhibited using antisense repression of expression technology, the photosynthesis of plants was decreased to significantly affect the sugar and starch contents of potatoes ( Haake et al., 1999 ). However, there is still a lack of systematic genome-wide identification and functional investigation of potato FBA gene family members. In this study, we identified nine StFBA genes using bioinformatics tools, to systematically analyze gene structures, protein structures, chromosome distributions, phylogenetic relationships, synteny, collinearities, and cis -acting elements in promoter regions. Furthermore, we determined the expression patterns of StFBA in different tissues and in response to abiotic stress. The results provide a theoretical basis for the in-depth use of StFBA functions to improve photosynthetic efficiency and tuber development in potatoes.
2 Materials and methods
2.1 plant materials.
Potato (cv. Shepody ) was used as experimental material, provided by the Crop Biotechnology Research Center of Anhui University of Science and Technology. Under sterile conditions, potato plantlets were cut into 1–1.5 cm segments with leaves and then placed into medium in tissue culture bottles (inner diameter, 63 mm; height, 85 mm). Each bottle contained four stem segments and was placed in a tissue culture room with relative humidity of 65% ± 5%, daytime temperature of 22°C ± 2°C, night temperature of 18°C ± 2°C, photoperiod uniformly set to 8 h light/16 h dark, and photosynthetic photon flux density of 65 μmol m −2 s −1 . The medium used for propagation of plantlets in vitro was solid Murashige and Skoog (MS) medium (4%, w/v, sucrose, 0.9%, w/v, agar). The medium used for induction of microtubers was solid, higher sucrose MS medium (8%, w/v, sucrose, 0.9%, w/v, agar). Potato plantlets grown for 30 days were acclimated and transplanted to pots containing vegetative soil and vermiculite (1:1) and placed in a plant growth room where the relative humidity was 65% ± 5%, the temperature was 22°C ± 2°C, and the photoperiod was uniformly set at 12 h light/12 h dark. Light intensity was 200 μmol m −2 s −1 . The flower tissue, petal, stamen, and pistil at 45 days of growth, as well as the leaf, petiole, stem, stolon, root, and tuber at 60 days of growth were collected for tissue expression analysis of the StFBA gene.
2.2 Identification of StFBA members in potato
The whole genome sequence, protein and encode sequences of potato v6.1 were downloaded from the Phytozome v13 website ( https://phytozome.jgi.doe.gov/pz/portal.html ). The protein sequences of the Arabidopsis FBA gene family were sourced from the TAIR database ( http://www.arabidopsis.org ). Genomic data for rice, tomato, eggplant, tobacco, and wheat were acquired from the Rice Genome Annotation Project (RGAP, http://rice.plantbiology.msu.edu/ ) and Ensemble database ( http://plants.ensembl.org/index.html ), reserved for subsequent analysis. Algorithm based on BLASTP was performed using the amino acid sequences of A . thaliana FBA proteins as queries in the protein databases of S . tuberosum , with an E -value ≤ 1e-5 and other parameters as default values, and redundant sequences were manually deleted. The Hidden Markov Model (HMM) of Glycolytic (PF00274) and fructose-bisphosphate aldolase class II domain (PF01116) were downloaded from InterPro database ( https://www.ebi.ac.uk/interpro/search/sequence/ ) to identify all FBA proteins in the potato genome. To further verify the reliability of the candidate sequences, the structural domains of the candidate FBA protein sequences were identified in the Pfam database ( http://pfam.xfam.org/ ) and SMART database ( http://smart.embl-heidelberg.de ). The website Cell-Ploc 2.0 ( http://www.csbo.sjtu.edu.cn/bioinf/Cell-PLoc-2 ) was used to predict the subcellular localization of StFBA proteins. The online software ExPASy ( https://web.expasy.org/protparam/ ) was used to analyze the physicochemical properties of the StFBA members.
2.3 Phylogenetic, gene structure and conserved motifs analyses of StFBA
The nine StFBA protein sequences were subjected to multiple sequence alignment using ClustaW software ( Thompson et al., 2002 ) with default parameters. Neighbor-Joining (NJ) phylogenetic tree was constructed using MEGA11 software with 1,000 bootstrap replicates. The same method was used for constructing and analyzing the phylogenetic tree of potato FBA gene family and the FBA genes of eight in Arabidopsis ( Lu et al., 2012 ), eight in tomato ( Cai et al., 2016 ), seven in eggplant, 16 in tobacco ( Zhao et al., 2021 ), seven in rice ( Zhang et al., 2016 ) and 21 in wheat ( Lv et al., 2017 ). The exon-intron structures were visualized using the Gene Structure Display Server (GSDS, http://gsds.cbi.pku.edu.cn ) ( Hu et al., 2015 ). The motif prediction was performed on the MEME program ( http://meme-suite.org/ ) ( Bailey et al., 2009 ), with the motif length ranging from 10 to 60 amino acid residues and maximum motif number set to 20, with all other parameters being default.
2.4 Synteny analysis and chromosome localization
TBtools software ( Chen et al., 2020 ) was used to obtain information such as chromosome length and gene density in the potato genome and to draw the chromosome location maps. The synteny relationships of the FBA genes within and among different species were calculated using MCScanX, with a threshold of E < 1 × 10 −5 ( Wang et al., 2012 ).
2.5 Structure prediction of StFBA proteins
SOPMA software ( http://npsa-pbil.ibcp.fr/cgi-bin/npsa_automat.pl?page=npsa_sopma.html ) was used to predict the secondary structure of StFBA protein. Tertiary structure prediction of StFBA proteins was performed using the latest artificial intelligence algorithm, AlphaFold2 ( Jumper et al., 2021 ), which utilizes large-scale structural databases and deep-learning models that are able to provide more accurate protein structure prediction results. The tertiary structure visualization of StFBA proteins was performed with PyMOL2.5 software ( https://pymol.org/2/ ).
2.6 Analysis of cis -acting elements in the promoter of StFBA genes
The sequence spanning 2000 bp upstream of the initiation codon of each StFBA gene was submitted to PlantCARE ( http://bioinformatics.psb.ugent.be/webtools/plantcare/html/ ) ( Lescot et al., 2002 ) for predicting cis -elements. TBtools software was used to draw the cis -acting elements in the promoter region.
2.7 Abiotic stress treatments of potato plants
Two batches of potato plantlets with 30 days of healthy and uniform growth were selected. The middle parts of one batch of plantlets were cut into 1–1.5 cm stem segments with leaves and inoculated into glass tubes. They were first placed in dark conditions for cultivation and after 2 days, they were exposed to blue light at a wavelength of 460 nm, red light at a wavelength of 620 nm, and white light (control) by LEDs (Opt-run Biotechnology Co., Nanjing, China). Leaves and stolon of 35 days potato plantlets and 80 days tuber under different light conditions were then collected. Another batch of potato plantlets was transferred to hydroponic conditions for 1 week and then placed in 150 mM NaCl solution and 15% polyethylene glycol (PEG6000) solution for salt and drought stress treatments at 0, 3, 6, 12, and 24 h. Then, the leaves from the top of the potato plants were collected. All tissue samples collected were immediately placed in liquid nitrogen and stored at −80°C for later use, with each treatment group containing three biological replicates.
2.8 Quantitative real-time PCR analysis
RNA extraction and reverse transcription were referred to Huang et al. (2017) , and qRT-PCR was referred to Jin et al. (2019) method. PCR was performed using an ABIViiA7 real-time PCR instrument (Life Technologies, United States). Specific amplification primers for the StFBA gene were designed using NCBI Primer-BLAST ( https://www.ncbi.nlm.nih.gov/ ) with potato elongation factor - 1alpha ( EF1α ) as an internal control ( Supplementary Table S4 ) ( Tang et al., 2017 ). The qRT-PCR for three independent biological replicate experiments, with three replicates for each PCR reaction. The relative expression of StFBA genes in different tissues and different treatments was calculated using the 2 −ΔΔCT and 2 −ΔΔCT method, respectively ( Livak and Schmittgen, 2001 ). The one-way ANOVA of variance was used to conduct the statistical analyses of data results by SPSS software, and the significance of differences was analyzed using Duncan’s Multiple Range Test (DMRT) as a post hoc test ( p < 0.05). GraphPad software was used for statistical analysis and drawing of data.
3.1 Genome-wide identification of StFBA gene family members
A total of nine StFBA genes were identified from the potato genome using genome-wide analysis ( Table 1 ). The StFBA genes were randomly and unevenly distributed across seven chromosomes; they were named StFBA1 ∼ StFBA9 according to their order in the potato chromosome ( Supplementary Figure S1 ). Analysis of the amino acid characteristics of StFBA family proteins showed that the number of amino acids (aa) of StFBA proteins ranged from 311 (StFBA3) to 1,379 (StFBA4), where the sequence length of the amino acid sequences of class II was significantly longer than that of class I. This difference may be due to the variations in their structure and function. The protein molecular weights ranged from 33,653.82 (StFBA3) to 148,195.01 (StFBA4) Da, while the theoretical isoelectric point varied between 5.94 (StFBA6) to 8.76 (StFBA5). Prediction of subcellular localizations of proteins showed that StFBA1∼StFBA5 and StFBA8 were localized in chloroplasts, and StFBA6, StFBA7 and StFBA9 were localized in the cytoplasm.
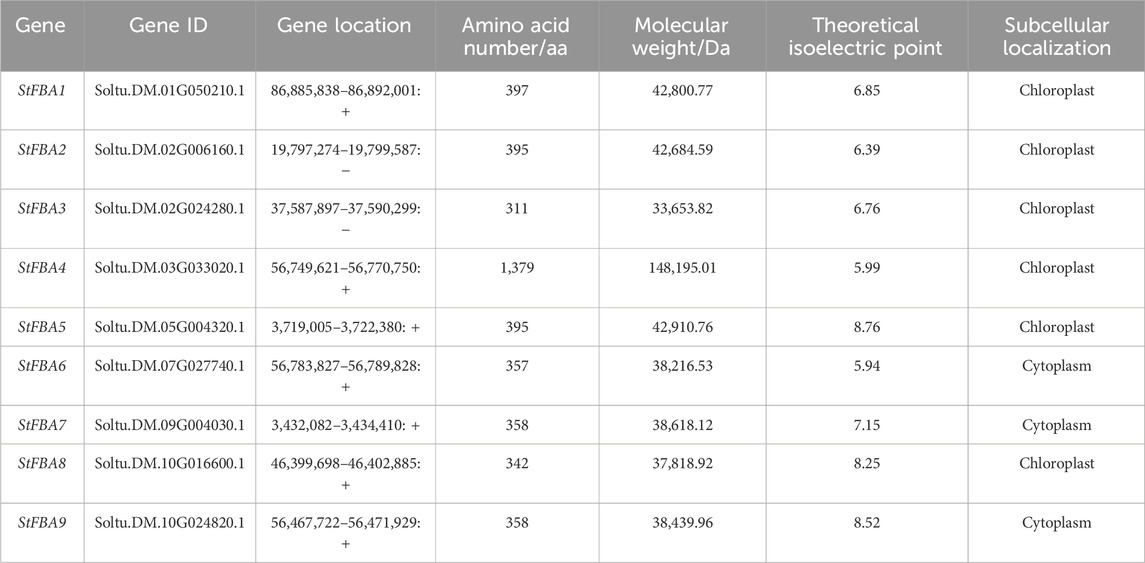
Table 1 . Profiles of the identified FBA gene family in S. tuberosum .
3.2 Phylogeny of StFBA and other plant FBA genes
To determine evolutionary relationships of the FBA gene family, 76 FBA protein amino acid sequences from seven species, including Arabidopsis , tomato, tobacco, rice, eggplant, wheat, and potato, were used to construct a phylogenetic tree ( Supplementary Table S1 ). The results indicated that the StFBA gene, like other species, was classified into class I and II subclades ( Figure 1 ). Class I could be further divided into four subclasses. Among the nine StFBA proteins, one was in Class I-a, two in Class I-b, one in Class I-c, four in Class I-d, and one in Class II. Of these, the identified wheat TaFBA19 - TaFBA21 belonged to Class II, which was consistent with the clustering characteristics of StFBA4 . The phylogenetic tree showed that FBA proteins were more closely related and similar between potato and other Solanaceae plants. In terms of evolutionary relationships, the FBA genes of potato and tomato were more closely related than those of other species, suggesting that they might have similar biological functions.
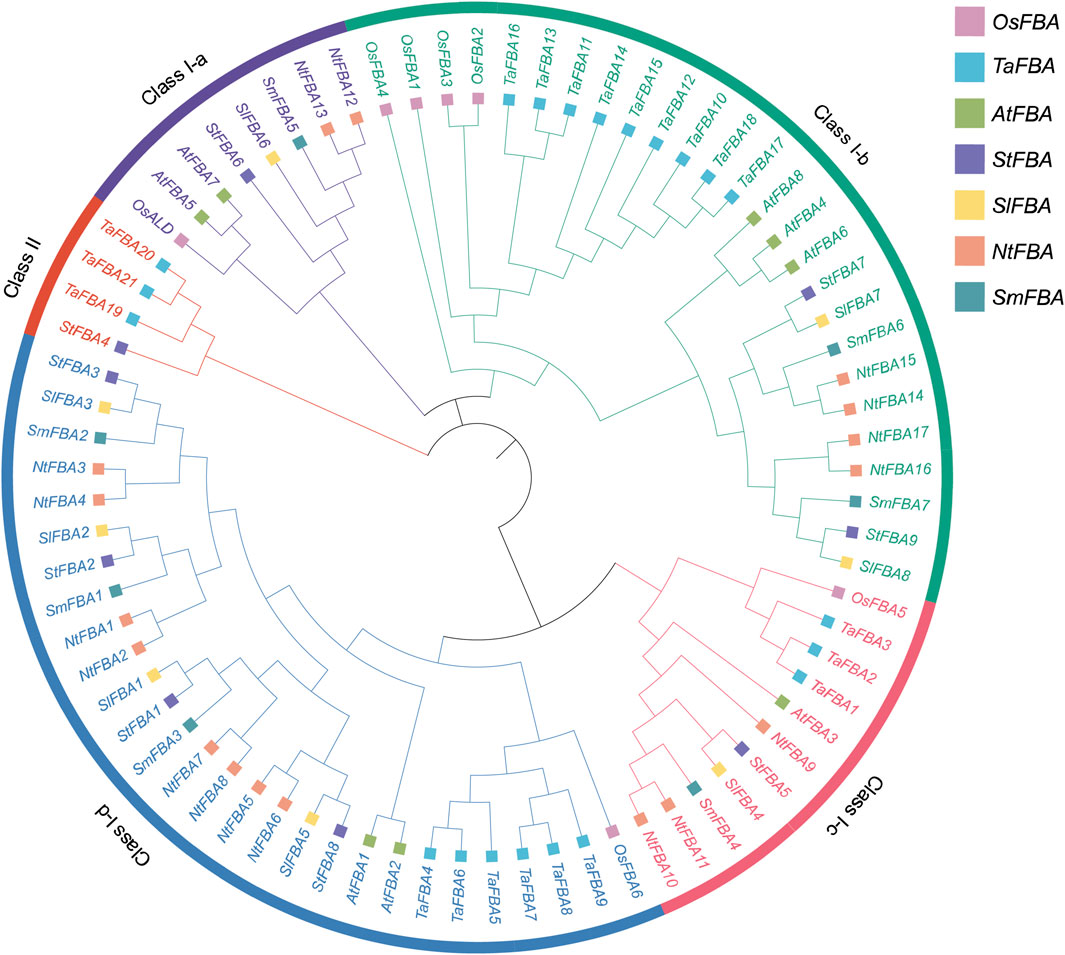
Figure 1 . Phylogenetic tree of FBA proteins from potato and other plant species. Abbreviations represent the following species: S. tuberosum ( St ), A. thaliana ( At ), S. lycopersicum ( Sl ), S. melongena ( Sm ), N. tabacum ( Nt ), Oryza sativa ( Os ) and Triticum aestivum ( Ta ). Different colored squares represented different species. Different colored arcs indicate different classes or subclasses.
3.3 Gene structure and protein motif analyses of the StFBA gene family
Phylogenetic analysis indicated that FBA was divided into two classes in potatoes. Eight StFBA genes were identified in Class I and only StFBA4 was identified in Class II ( Figure 2A ). Analyses of gene structures revealed significant variations in exon count and sequence length among different subgroups of StFBA genes ( Figure 2B ). Class I had 3–6 exons, with the fewest exons found in StFBA7 and StFBA9 , and the most in StFBA2 and StFBA5 . Class II contained 42 exons, which is significantly more than Class I members, suggesting that StFBA4 might have a more complex structure, potentially including functional domains or regulatory elements. The motif distributions in FBA proteins were analyzed, and 20 conserved motifs were predicted ( Supplementary Figure S2 ). Motif 1, motif 5, motif 6, and motif 10 were exclusive to Class I. StFBA1 and StFBA2 had 12 common motifs. StFBA6, StFBA7, and StFBA9 had 11 common motifs, with motif3 is a conserved motif specific to them. Moreover, some genes are missing more motifs, for example, StFBA8 contains only 6 motifs, suggesting that its function may be altered. Except for motif 9, the remaining eight motifs were specific to StFBA4 in Class II ( Figure 2C ). Overall, the type and distribution of conservative base sequences of Class I StFBA showed a high degree of uniformity, indicating that the structures of StFBA proteins were highly conserved in specific subclasses. In contrast, the gene structure and function of members of the potato FBA gene family might be varied between subclasses.
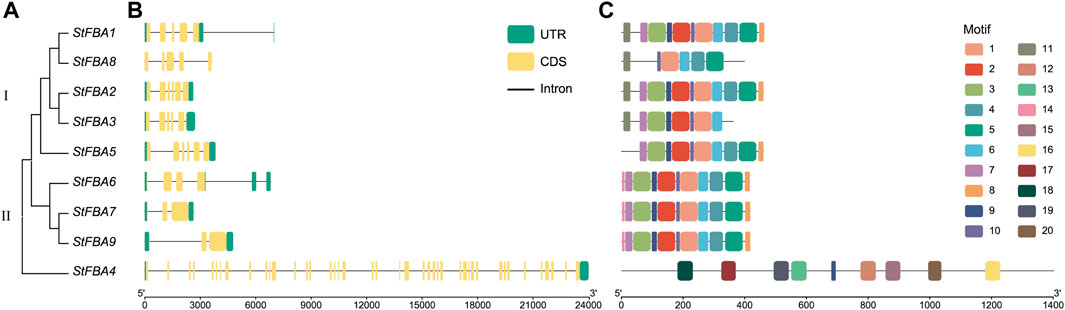
Figure 2 . Phylogenetic relationships, gene structure, and conserved motifs of the StFBA gene family: (A) Phylogenetic relationships of the nine StFBA genes. (B) Gene structures of StFBA . UTR, untranslated region; CDS, coding sequence. (C) Distributions of the conserved motifs within StFBA proteins.
3.4 Tertiary structure of the StFBA proteins
Analyses of tertiary structures revealed that the StFBA proteins mainly consisted of α-helices, β-turns, irregular coils, and extended chains. The percentage of α-helix was the highest (42.77%–53.22%), followed by irregular coils (27.17%–33.76%), while extended chains and β-folds accounted for lower percentages ( Supplementary Table S2 ). Comparison of the predicted tertiary structures of StFBA proteins revealed significant differences in the protein structures of different subclasses. The three-dimensional conformations of eight Class I StFBA gene family members were comparable ( Figure 3 ). StFBA1, StFBA2, and StFBA5 were predicted to have more consistent protein structures. Compared to other members, the StFBA3 protein had a simpler structure, with fewer α-helices and β-turns, implying possible changes in its function. The remaining members had subtle differences in protein structures. StFBA4 had a larger number of amino acid sequences and more complex protein tertiary structures, suggesting that it might be involved in more intricate biological functions.
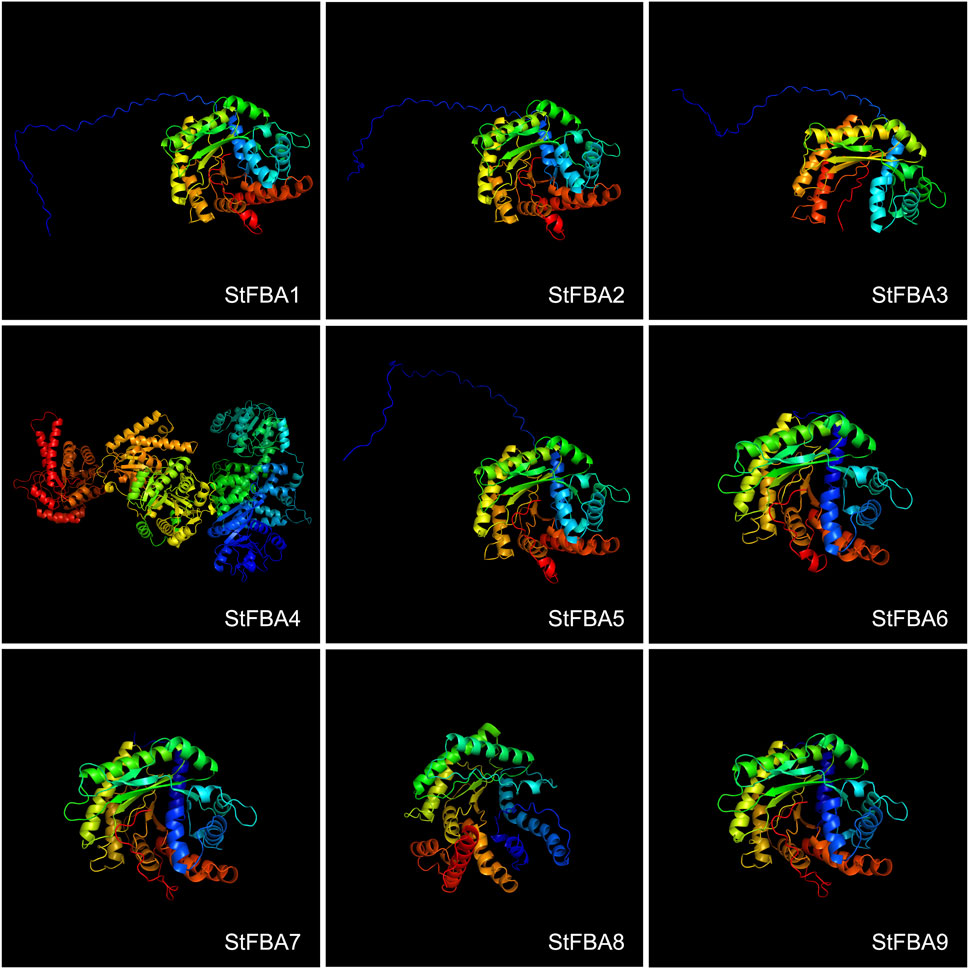
Figure 3 . Predicted tertiary structures of FBA proteins in S. tuberosum .
3.5 Synteny analysis of FBA gene family members
To study the contraction and expansion of StFBA gene family members during evolution, the FBA genes of potato, tomato, and Arabidopsis were compared using collinearity analyses ( Figure 4 ). The results showed that 11 StFBA members were found to have synteny with AtFBA , but StFBA2 , StFBA3, and StFBA6 had no homologous genes in Arabidopsis . There were 14 pairs of syntenic FBA genes between potatoes and tomatoes, suggesting that the FBA genes were more homologous and conserved in Solanaceae than in dicotyledons. It is noteworthy that we have found that StFBA4 is homologous to AT1G18270 in Arabidopsis and Solyc03g118640.3.1 in tomato. Although AT1G18270 (NCBI Reference Sequence: NP_001117303.1) is annotated as ketose-bisphosphate aldolase class-II family protein, and Solyc03g118640.3.1 (NCBI Reference Sequence: XP_004235744.1) is annotated as uncharacterized protein LOC101261901. They all contain fructose-bisphosphate aldolase class-II (PF01116) domain and is further assigned as fructose-bisphosphate aldolase. Thus, AT1G18270 and Solyc03g118640.3.1 are actually both fructose-bisphosphate aldolase class II family proteins. Additionally, there were four pairs of duplicate segments, StFBA1 - StFBA2 , StFBA2 - StFBA3 , StFBA1 - StFBA3, and StFBA1 -S tFBA8, in the potato genome region ( Supplementary Figure S3 ). Expansion of potato FBA family members was mainly associated with segmental duplication events ( Supplementary Table S3 ).
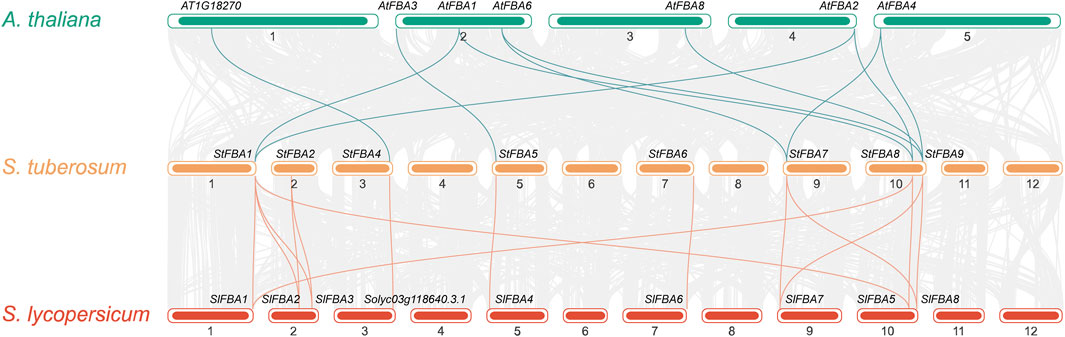
Figure 4 . Collinear relationships of FBA genes in S. tuberosum , A. thaliana , and S. lycopersicum . The gray lines in the background indicate collinearity between potato genes and those of other species, and the green and yellow lines indicate collinear FBA gene pairs.
3.6 Expression patterns of StFBA genes in different tissues
To further understand the potential function of StFBA genes, the expression levels of nine StFBA genes in a variety of tissues were analyzed ( Figure 5 ). It was found that some class I StFBA genes showed tissue specificities. For example, StFBA1 was highly expressed in leaves, StFBA2 was mainly expressed in green organs compared to other tissues, while StFBA5 was mainly expressed in floral organs. StFBA6 and StFBA9 were specifically expressed in petiole and had similar expression characteristics. StFBA3 was expressed at a lower level and was not expressed in many tissues. Most of the FBA genes were expressed in all tissues, indicating that they might be involved in the growth and development of potatoes. Specifically, StFBA7 was highly expressed in floral organs, tubers, and stolon, suggesting it might serve a pivotal function during flowering and tuber formation in potatoes. The class II StFBA4 was expressed in most tissues, but at a lower expression level.
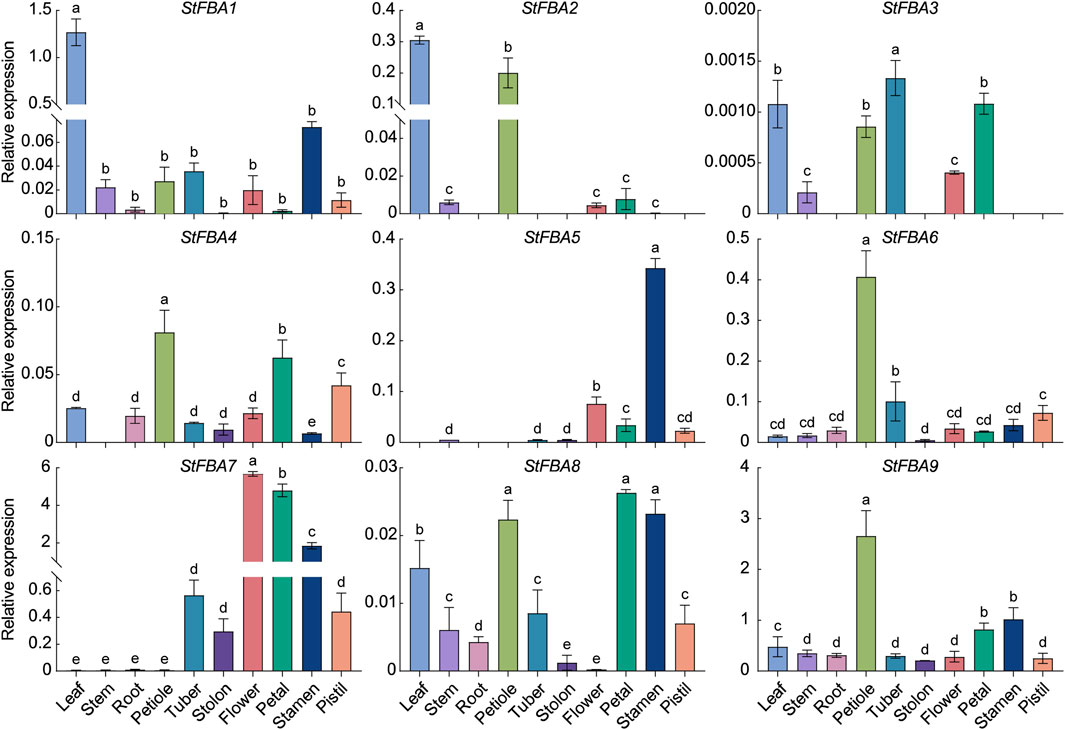
Figure 5 . Expression analyses of StFBA genes in different tissues. Significant differences among the groups were compared based on Duncan’s test ( p < 0.05). The data points represent the mean ± SD.
3.7 Expression patterns of StFBA genes in response to different light
Multiple cis -regulatory elements were detected in the promoter region of StFBA genes ( Figure 6 ). Based on functions of the cis -acting elements, the selected 20 elements were categorized into four groups: light response, stress response, hormone response and plant growth and development. Hormonal response elements included responses to auxin, abscisic acid, gibberellin, and salicylic acid; and stress included responses to drought and cold stress elements. We found that multiple light-responsive elements existed in the promoter regions of each StFBA gene, indicating that the StFBA genes were an important component of light responses in potatoes. Thus, the expression levels of StFBA in leaves, stolon, and microtubers were analyzed under different light treatments. The expression patterns of FBA genes exhibited discrepancies under different spectra. Due to monochromatic red light exposure, the leaves development of the microtubers was severely inhibited, hence there was no leaf sample under red light ( Figure 7A ). Under blue light, StFBA7 showed lower expression in leaves, but significantly increased expression in stolon and microtubers. Under red light, StFBA3 , StFBA7 , StFBA8, and StFBA9 showed higher expressions, when compared to under white light in stolon. In contrast, the expression levels of StFBA1 , StFBA2 , StFBA3, and StFBA9 were significantly higher than that of the other treatments in tubers. Class II StFBA4 gene showed lower expression in all tissues under red and blue light, suggesting that the gene might be spectrally insensitive. Moreover, the expression levels of StFBA1 and StFBA2 in all tissues were highly similar under various spectral treatments, suggesting that these genes might have similar regulatory mechanisms in response to different lighting conditions ( Figure 7B ).
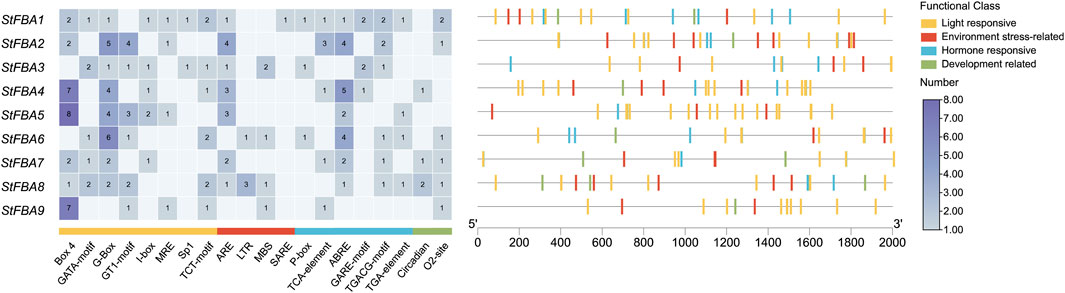
Figure 6 . Distribution of cis -elements in the promoter regions of StFBA genes. The heat map represents classifications and statistics of cis -acting components, the cis -elements were divided into four broad categories. Different colors represent different types of elements, and the ruler at the bottom indicates the direction and length of the sequence.
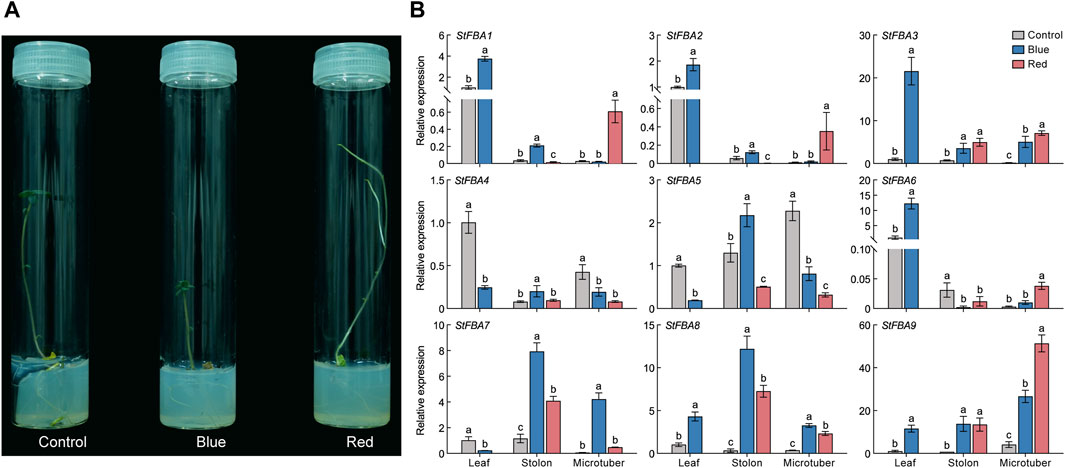
Figure 7 . Growth of potato plantlet under different light spectrum and expressions of nine StFBA genes in different tissues under red and blue light treatments: (A) Growth of potato plantlet under different light spectrum. Blue, 460 nm blue light; red, 620 nm red light; control, white light. (B) The qRT-PCR was performed to analyze the relative expression levels of StFBA genes. Error bars represent standard deviations of the means from three independent experiments. Significant differences among the groups were compared based on Duncan’s test ( p < 0.05). The data points represented the mean ± SD.
3.8 Expression profiles of the StFBA genes in response to abiotic stresses
The expression patterns of the StFBA gene ins response to abiotic stress such as drought and salt were determined, indicating that the expression levels of the nine StFBA genes varied to different degrees under different stress treatments. Under the 15% PEG6000 treatment, StFBA3 mainly showed a decreasing trend, while expression levels of StFBA5 and StFBA7 were significantly upregulated, so they might be important candidate genes involved in drought stress. StFBA4 expression was minimally affected. The expression levels of StFBA5 and StFBA7 were significantly upregulated ( p < 0.01). The expression patterns of the remaining genes were more complicated. For example, StFBA1, StFBA2, and StFBA6 were highly induced at 3 h, then decreased at 6 h, then finally increased again at 12 h treatment, StFBA8 and StFBA9 expression levels showed a tendency to decrease and then increase, and all of these genes gradually recovered to their untreated expression levels at 24 h ( Figure 8 ). Under salt conditions (150 mM NaCl), expression of StFBA3 significantly decreased. The expressions of StFBA1 , StFBA2 , StFBA4, and StFBA6 peaked at 3 h, while the expressions of StFBA5 , StFBA7 , StFBA8, and StFBA9 peaked at 6 h, then all of them showed a decreasing trend ( Figure 9 ). Together, the results further confirmed the possible role of the StFBA gene family in potato responses to various abiotic stresses.
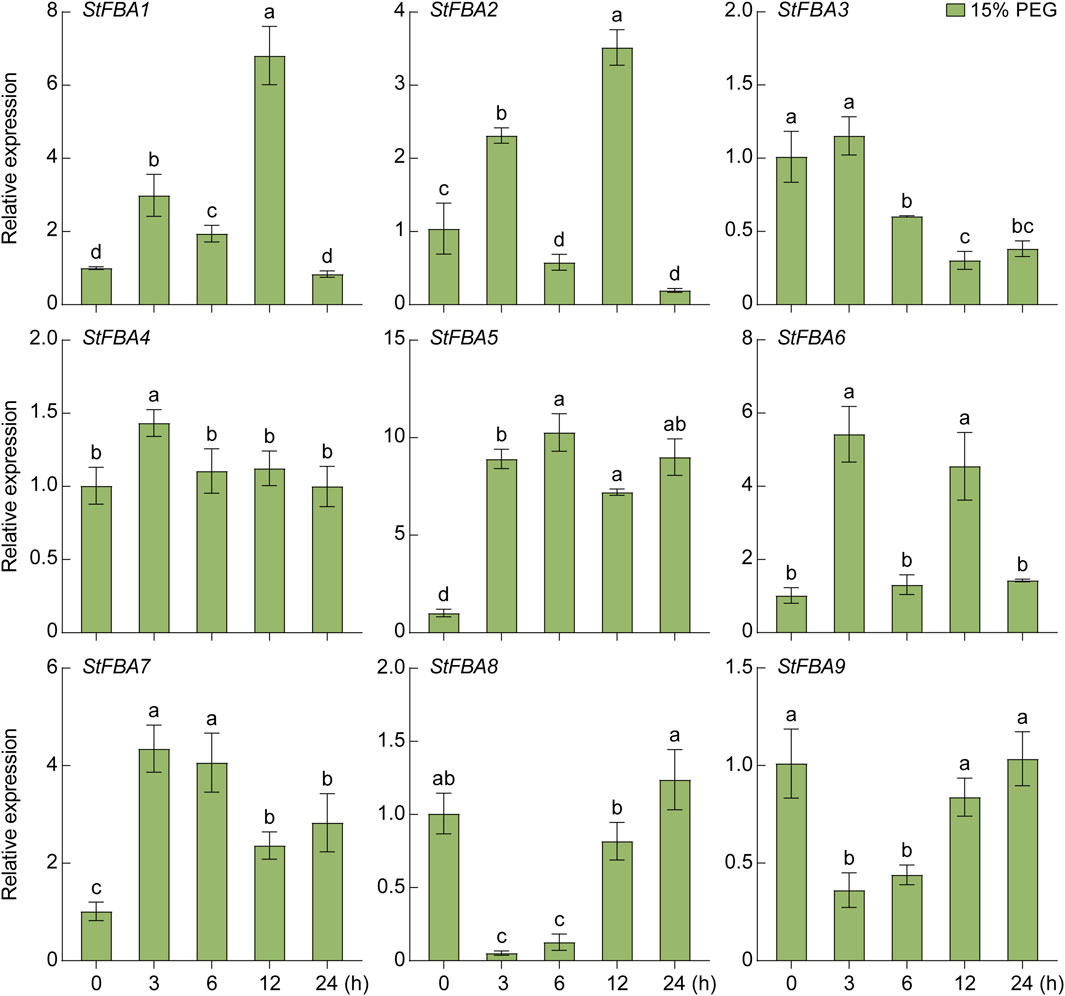
Figure 8 . Expression profiles of the StFBA genes after 15% PEG6000 simulated drought stress. PEG, polyethylene glycol. Significant differences among the groups were compared based on Duncan’s test ( p < 0.05). The data points represented the mean ± SD.
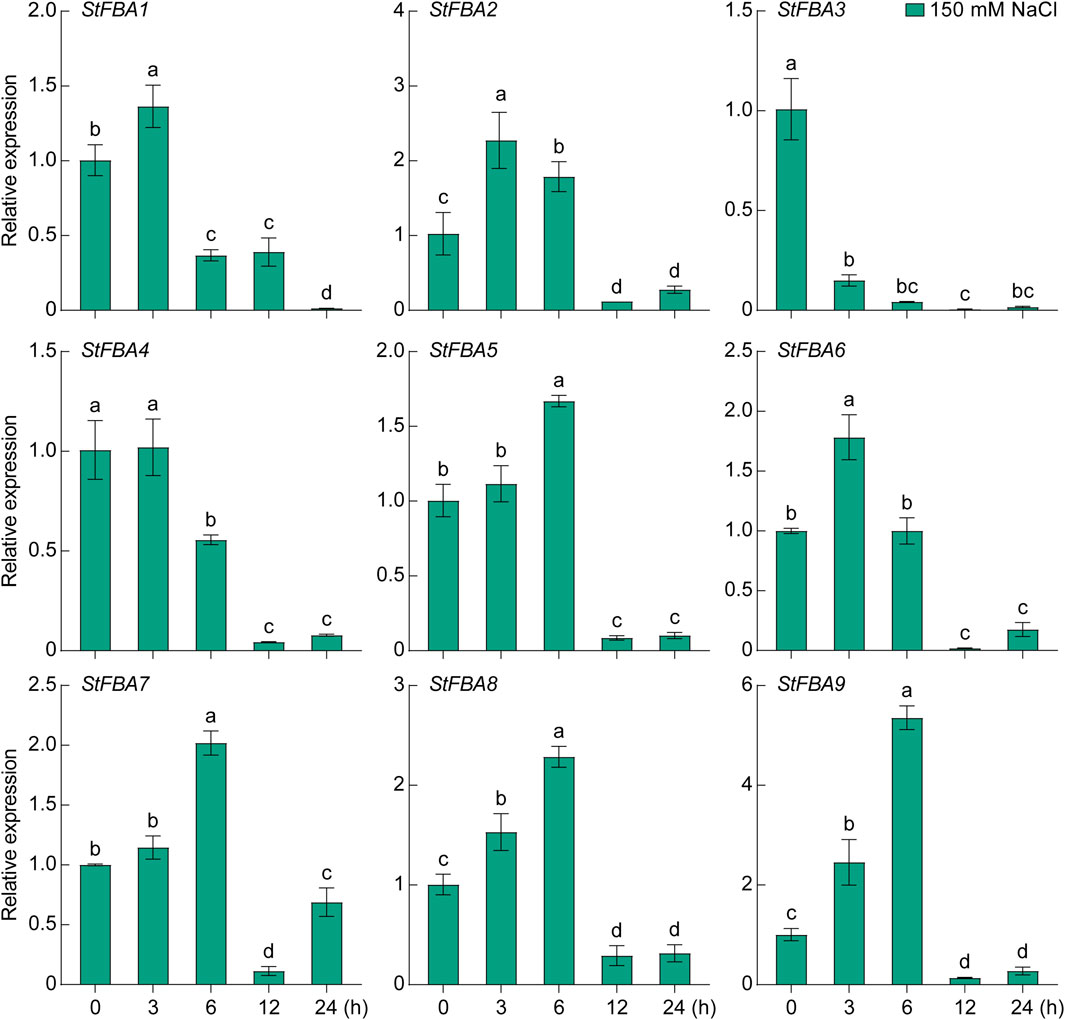
Figure 9 . Expression profiles of the StFBA genes under salt stress treatment using 150 mM NaCl. Significant differences among the groups were compared based on Duncan’s test ( p < 0.05). The data points represent the mean ± SD.
4 Discussion
Organic compounds produced by photosynthesis can promote the formation and enlargement of potatoes ( Fernie and Willmitzer, 2001 ). As a key enzyme in the Calvin cycle and glycolysis, FBA participates in the metabolic process of starch biosynthesis. Decreasing the activity of this enzyme can result in stunted potato growth, reduced photosynthetic efficiency, and an impact on tuber starch content, ultimately affecting potato yield and quality ( Kossmann et al., 1995 ). Hence, FBA plays a vital regulatory role in the growth and tuber yield of potatoes. Our study identified nine FBA genes in the complete genome sequence of potatoes, which were divided into Class I and Class II members, based on their two structural domains ( Table 1 ). Potatoes, like Arabidopsis and tomatoes, possess eight Class I members, indicating the conservation of Class I StFBA genes during their evolution. Class I and Class II FBA have no similarities in gene sequence, protein structure, and function, and are often considered to have evolved from different origins ( Schnarrenberger et al., 1990 ; Zgiby et al., 2000 ). We identified one Class II FBA gene in S . tuberosum , which has 1,379 amino acids and 42 exons, a significantly higher number than Class I. This finding aligns with observations in other plant species, three have been identified in Triticum aestivum ( Lv et al., 2017 ), two in B . napus ( Zhao et al., 2019 ), and four in cotton ( Li et al., 2021 ). The amino acid numbers of these genes ranged from 1,352 to 1,383, with exon numbers between 41 and 42. This indicates that Class II FBAs are conserved among different species and might have similar functions. According to the conserved motif analysis results of StFBA, we found that apart from StFBA1 and StFBA2, which contain all 10 motifs, other members exhibited a certain degree of motif loss, with the most loss in StFBA8, suggesting possible functional changes in this gene. Class II FBA shared fewer motifs in common with Class I ( Figure 2 ). Overall, members within the same subclass showed similarities in sequence, gene structure, and conserved motif composition, indicating that StFBA family members may have undergone similar genetic evolution processes.
Based on the phylogenetic tree, eight Class I StFBA members were further divided into four subclasses ( Figure 1 ), the Class I-d subclass had the most clustered StFBA genes. Closely related gene members in the phylogenetic tree may have similar structures and functions. Because both S . tuberosum and S . lycopersicum belong to the Solanaceae plant family, they were closer in the evolutionary tree. It is worth noting that SlFBA7 and StFBA7 clustered together, had the same number of amino acids, and were located in the cytoplasm. Overexpression of SlFBA7 can significantly increase the FBA activity and the expression level of other enzymes in the Calvin cycle of tomato seedling leaves, along with an increase in regeneration of RuBP ( Cai et al., 2016 ). Therefore, we speculate that StFBA7 may play a pivotal role in enhancing the photosynthetic efficiency of potatoes. At present, the functions of some FBA genes have been thoroughly studied in model plants such as A . thaliana and O . sativa ( Lu et al., 2012 ; Simkin et al., 2017 ). For example, AtFBA2 has the highest activity and expression level in leaves, and overexpression of AtFBA2 boosts the photosynthetic capacity and biomass of the plants. However, fba2 mutant plants are dwarfed and the starch level is significantly reduced. AtFBA3 is mainly expressed in the vascular tissues of leaves and roots, so the absence of AtFBA3 limits the biosynthesis of essential compounds such as amino acids. Similarly, the fba3 mutants grow more slowly and show a decrease in photosynthesis ( Carrera et al., 2021 ).
Collinearity analysis between S . tuberosum and A . thaliana revealed that AtFBA2 was homologous with StFBA1 and StFBA8 , while AtFBA3 shared homology with StFBA5 ( Figure 4 ). We speculate that StFBA1 , StFBA5, and StFBA8 might contribute to enhancing tuber yield. However, their specific functions and mechanisms of action still require further confirmation. In plants, gene duplication events primarily consist of tandem duplication and segmental duplication, which play key roles in biological evolution, gene family formation, and the transmission of genetic information ( Kong et al., 2007 ; Paterson et al., 2010 ). The members of the StFBA gene family were closely associated with segmental duplication events. The multiple occurrences of StFBA1 in repetitive events suggested that this gene may have played a significant role in driving the evolution of the FBA gene family, leading to gene amplifications and functional changes. The tertiary structure of proteins determines their function in cells and how they interact with other molecules ( Kuhlman and Bradley, 2019 ). Based on the physicochemical properties and predicted protein structure of StFBA, we showed that the structures of StFBA3 and StFBA8 were simpler than other members ( Figure 3 ). These two proteins exhibited the lowest number of amino acids and the smallest percentage of α-helices ( Supplementary Table S2 ). It is therefore possible that during the process of segmental duplication, these two genes underwent loss or alterations in some of their conserved domains and functions. StFBA6 , StFBA7, and StFBA9 were located in the cytoplasm, had very similar amino acid numbers, and showed a high degree of similarity in their secondary and tertiary structures, indicating that they may be homologous genes.
Through the analysis of transcriptome data and qRT-PCR validation, we obtained the expression levels of StFBA genes in different potato tissues. StFBA7 exhibited higher expressions in tubers, stolon, and flower organs, indicating that it may be a key gene regulating the processes of microtuber formation and expansion ( Figure 5 ). The expression patterns of StFBA family members in different tissues demonstrated that FBA genes had specific roles during potato growth and development. The cis -acting element analysis revealed an abundance of light-responsive elements within the StFBA gene family ( Figure 6 ). Throughout the growth and development of potatoes, light influenced tuber induction and expansion via the processes of the photoperiod, light intensity, and spectrum. Among them, light spectrum is one of the key signals regulating tuberization in potato plants. It has been reported that the transcription level of StBEL5, a primary signal for potato tuberization, was mostly affected by the light spectrum, with the red-blue spectrum inducing the initiation of StBEL5 synthesis ( Chatterjee et al., 2007 ). Further studies found that blue light at 460 nm wavelength aided tuber expansion, while 620 nm red light was more favorable for tuber induction ( Li et al., 2020 ). The microtubers under blue light were significantly larger than other spectra in our study ( Supplementary Figure S4 ). The qRT-PCR results indicated that StFBA genes were highly responsive to spectral changes, with StFBA3 , StFBA8, and StFBA9 showing significantly higher expression levels in leaf, stolon, and tuber, when compared to the white light control. These results implied that they may improve photosynthesis and play an important function in regulating the induction and expansion of microtubers ( Figure 7 ).
Potato is highly sensitive to drought and salt stress. Drought affects the aboveground plant height and leaf area index, leading to a reduction in photosynthetic efficiency and adversely affecting potato yield and quality through shortened growth cycles and decreased underground tuber quantity and size ( Deblonde and Jean-François, 2001 ). High salt stress disrupts the source-sink balance in potatoes, impairing the production of antioxidants and antioxidant enzyme activity, and accelerating the occurrence of plant diseases ( Albacete et al., 2014 ; Chourasia et al., 2021 ). Studies have shown that FBA genes played a role in responses to various abiotic stresses, including drought, high salt ( Yamada et al., 2000 ), and cold stress ( Liu et al., 2019 ). We found that under drought stress, the expression of StFBA5 significantly increased, and it maintained a high expression level even after 24 h ( Figure 8 ). When exposed to high salt stress, the expression levels of most StFBA genes showed an initial increase followed by a sharp decline ( Figure 9 ). These results indicated that StFBA members played a crucial role in responding to different environmental stress. In addition, FBA has been found to have a wide range of functions in other species. For example, AtFBA6 participates in the memory of high temperature stress in the apical meristem of Arabidopsis ( Olas et al., 2021 ). AtFBA8 responds to environmental humidity changes and interacts with actin, involving guard cell opening and closing ( Van der Linde et al., 2011 ). The soybean gmfbacgmfbac2 double mutant exhibits slow growth, narrowed leaves, dwarfed seedlings, and severe imbalance in carbon-nitrogen metabolism ( Qiu et al., 2023 ). It is hypothesized that the StFBA gene may have functional diversity, but the specific functional mechanism needs to be verified through future experiments.
5 Conclusion
In this study, eight class I and one class II StFBA gene members were identified using genome-wide analyses. We found that the FBA of potatoes and tomatoes were closely related in the evolutionary tree and in synteny relationships. StFBA1 was a key gene in the fragment duplication event within the gene family. StFBA6 , StFBA7, and StFBA9 were involved in growth and development of potatoes. StFBA3 , StFBA8, and StFBA9 may influence photosynthesis and play a critical function in regulating the induction and expansion of microtubers, while StFBA5 and StFBA9 played essential roles in responses to drought and salt stress. The results of this study provided a basis for understanding the potential functions of the StFBA gene family, and presented ideas for the improvement of photosynthetic efficiency and response to adversity stress of potatoes.
Data availability statement
The datasets presented in this study can be found in online repositories. The names of the repository/repositories and accession number(s) can be found in the article/ Supplementary Material .
Author contributions
TL: Data curation, Visualization, Writing–original draft. XnH: Visualization, Writing–original draft, Data curation. ZS: Data curation, Writing–original draft. BM: Data curation, Writing–original draft. XW: Data curation, Writing–original draft. TF: Supervision, Writing–review and editing. HA: Supervision, Writing–review and editing. XaH: Conceptualization, Project administration, Supervision, Writing–review and editing. RL: Conceptualization, Funding acquisition, Project administration, Supervision, Writing–review and editing.
The author(s) declare that financial support was received for the research, authorship, and/or publication of this article. This research was funded by the Natural Science Foundation of Anhui (No. 2108085QC125), the Key Project of Anhui Provincial Education Department (Nos 2022AH051627 and 2023AH051886), the Talent Introduction Start-up Fund Project of Anhui Science and Technology University (No. NXYJ202102), the National Innovation and Entrepreneurship Training Program for College Students (No. 202310879007), and the Provincial Graduate Full English/Bilingual Demonstration Course (No. 2022qyw/sysfkc040). The funding institution was not involved in the design of the study, collection, analysis, and interpretation of data, and in writing the manuscript.
Acknowledgments
We thank International Science Editing ( http://www.internationalscienceediting.com ) for editing this manuscript.
Conflict of interest
The authors declare that the research was conducted in the absence of any commercial or financial relationships that could be construed as a potential conflict of interest.
Publisher’s note
All claims expressed in this article are solely those of the authors and do not necessarily represent those of their affiliated organizations, or those of the publisher, the editors and the reviewers. Any product that may be evaluated in this article, or claim that may be made by its manufacturer, is not guaranteed or endorsed by the publisher.
Supplementary material
The Supplementary Material for this article can be found online at: https://www.frontiersin.org/articles/10.3389/fgene.2024.1364944/full#supplementary-material
Albacete, A. A., Martínez-Andújar, C., and Pérez-Alfocea, F. (2014). Hormonal and metabolic regulation of source-sink relations under salinity and drought: from plant survival to crop yield stability. Biotechnol. Adv. 32, 12–30. doi:10.1016/j.biotechadv.2013.10.005
PubMed Abstract | CrossRef Full Text | Google Scholar
Ashida, H., Mizohata, E., and Yokota, A. (2019). Learning RuBisCO's birth and subsequent environmental adaptation. Biochem. Soc. Trans. 47, 179–185. doi:10.1042/BST20180449
Bailey, T. L., Boden, M., Buske, F. A., Frith, M., Grant, C. E., Clementi, L., et al. (2009). MEME SUITE: tools for motif discovery and searching. Nucleic Acids Res. 37, W202–W208. doi:10.1093/nar/gkp335
Blom, N., and Sygusch, J. (1997). Product binding and role of the C-terminal region in class I D-fructose 1, 6-bisphosphate aldolase. Nat. Struct. Biol. 4, 36–39. doi:10.1038/nsb0197-36
Bracher, A., Whitney, S. M., Hartl, F. U., and Hayer-Hartl, M. (2017). Biogenesis and metabolic maintenance of Rubisco. Annu. Rev. Plant Biol. 68, 29–60. doi:10.1146/annurev-arplant-043015-111633
Bryant, B. A. (2000). The production and analysis of tobacco plants with reduced levels of the Calvin cycle enzyme sedoheptulose-1, 7-bisphosphatase . Doctoral dissertation, England: University of Essex .
Google Scholar
Cai, B., Li, Q., Liu, F., Bi, H., and Ai, X. (2018). Decreasing fructose-1,6-bisphosphate aldolase activity reduces plant growth and tolerance to chilling stress in tomato seedlings. Physiol. Plant 163, 247–258. doi:10.1111/ppl.12682
Cai, B., Li, Q., Xu, Y., Yang, L., Bi, H., and Ai, X. (2016). Genome-wide analysis of the fructose 1, 6-bisphosphate aldolase (FBA) gene family and functional characterization of FBA7 in tomato. Plant Physiol. Biochem. 108, 251–265. doi:10.1016/j.plaphy.2016.07.019
Capodagli, G. C., Sedhom, W. G., Jackson, M., Ahrendt, K. A., and Pegan, S. D. (2014). A noncompetitive inhibitor for Mycobacterium tuberculosis 's class IIa fructose 1, 6-bisphosphate aldolase. Biochemistry 53, 202–213. doi:10.1021/bi401022b
Carrera, D. Á., George, G. M., Fischer-Stettler, M., Galbier, F., Eicke, S., Truernit, E., et al. (2021). Distinct plastid fructose bisphosphate aldolases function in photosynthetic and non-photosynthetic metabolism in Arabidopsis . J. Exp. Bot. 72, 3739–3755. doi:10.1093/jxb/erab099
Chatterjee, M., Banerjee, A. K., and Hannapel, D. J. (2007). A BELL1 -like gene of potato is light activated and wound inducible. Plant Physiol. 145, 1435–1443. doi:10.1104/pp.107.105924
Chen, C., Chen, H., Zhang, Y., Thomas, H. R., Frank, M. H., He, Y. H., et al. (2020). TBtools: an integrative toolkit developed for interactive analyses of big biological data. Mol. Plant 13, 1194–1202. doi:10.1016/j.molp.2020.06.009
Chourasia, K. N., Lal, M. K., Tiwari, R. K., Dev, D., Kardile, H. B., Patil, V. U., et al. (2021). Salinity stress in potato: understanding physiological, biochemical and molecular responses. Life (Basel). 11, 545. doi:10.3390/life11060545
Deblonde, P. M. K., and Jean-François, L. (2001). Effects of moderate drought conditions on green leaf number, stem height, leaf length and tuber yield of potato cultivars. Eur. J. Agron. 14, 31–41. doi:10.1016/S1161-0301(00)00081-2
CrossRef Full Text | Google Scholar
Fan, W., Zhang, Z., and Zhang, Y. (2009). Cloning and molecular characterization of fructose-1, 6-bisphosphate aldolase gene regulated by high-salinity and drought in Sesuvium portulacastrum . Plant Cell Rep. 28, 975–984. doi:10.1007/s00299-009-0702-6
Fernie, A. R., and Willmitzer, L. (2001). Molecular and biochemical triggers of potato tuber development. Plant Physiol. 127, 1459–1465. doi:10.1104/pp.127.4.1459
Flechner, A., Gross, W., Martin, W. F., and Schnarrenberger, C. (1999). Chloroplast class I and class II aldolases are bifunctional for fructose-1, 6-biphosphate and sedoheptulose-1, 7-biphosphate cleavage in the Calvin cycle. FEBS Lett. 447, 200–202. doi:10.1016/s0014-5793(99)00285-9
Frenkel, Z. M., and Trifonov, E. N. (2005). Closed loops of TIM barrel protein fold. J. Biomol. Struct. Dyn. 22, 643–656. doi:10.1080/07391102.2005.10507032
Galkin, A., Kulakova, L., Melamud, E., Li, L., Wu, C., Mariano, P., et al. (2007). Characterization, kinetics, and crystal structures of fructose-1, 6-bisphosphate aldolase from the human parasite, Giardia lamblia . J. Biol. Chem. 282, 4859–4867. doi:10.1074/jbc.M609534200
Gross, W., Lenze, D., Nowitzki, U., Weiske, J., and Schnarrenberger, C. (1999). Characterization, cloning, and evolutionary history of the chloroplast and cytosolic class I aldolases of the red alga Galdieria sulphuraria . Gene 230, 7–14. doi:10.1016/s0378-1119(99)00059-1
Haake, V., Geiger, M., Walch-Liu, P., Of Engels, C., Zrenner, R., and Stitt, M. (1999). Changes in aldolase activity in wild-type potato plants are important for acclimation to growth irradiance and carbon dioxide concentration, because plastid aldolase exerts control over the ambient rate of photosynthesis across a range of growth conditions. Plant J. 17, 479–489. doi:10.1046/j.1365-313X.1999.00391.x
Haake, V., Zrenner, R., Sonnewald, U., and Stitt, M. (1998). A moderate decrease of plastid aldolase activity inhibits photosynthesis, alters the levels of sugars and starch, and inhibits growth of potato plants. Plant J. 14, 147–157. doi:10.1046/j.1365-313x.1998.00089.x
Henkes, S., Sonnewald, U., Badur, R., Flachmann, R., and Stitt, M. (2001). A small decrease of plastid transketolase activity in antisense tobacco transformants has dramatic effects on photosynthesis and phenylpropanoid metabolism. Plant Cell 13, 535–551. doi:10.1105/tpc.13.3.535
Hester, G., Brenner-Holzach, O., Rossi, F. A., Struck-Donatz, M., WinterhalterWinterhalter, K. H., Smit, J. D., et al. (1991). The crystal structure of fructose-1, 6-bisphosphate aldolase from Drosophila melanogaster at 2.5 A˚ resolution. FEBS Lett. 292, 237–242. doi:10.1016/0014-5793(91)80875-4
Hu, B., Jin, J., Guo, A. Y., Zhang, H., Luo, J., and Gao, G. (2015). GSDS 2.0: an upgraded gene feature visualization server. Bioinformatics 31, 1296–1297. doi:10.1093/bioinformatics/btu817
Huang, X., Yang, L., Jin, Y., Lin, J., and Liu, F. (2017). Generation, annotation, and analysis of a large-scale expressed sequence tag library from Arabidopsis pumila to explore salt-responsive genes. Front. Plant Sci. 8, 955. doi:10.3389/fpls.2017.00955
Izard, T., and Sygusch, J. (2004). Induced fit movements and metal cofactor selectivity of class II aldolases: structure of Thermus aquaticus fructose-1, 6-bisphosphate aldolase. J. Biol. Chem. 279, 11825–11833. doi:10.1074/jbc.M311375200
Jacquot, J. P., Lopez-Jaramillo, J., Chueca, A., Cherfils, J., Lemaire, S., Chedozeau, B., et al. (1995). High-level expression of recombinant pea chloroplast fructose-1, 6-bisphosphatase and mutagenesis of its regulatory site. Eur. J. Biochem. 229, 675–681. doi:10.1111/j.1432-1033.1995.tb20513.x
Jin, Y., Liu, F., Huang, W., Sun, Q., and Huang, X. (2019). Identification of reliable reference genes for qRT-PCR in the ephemeral plant Arabidopsis pumila based on full-length transcriptome data. Sci. Rep. 9, 8408. doi:10.1038/s41598-019-44849-1
Jordan, D. B., and Ogren, W. L. (1984). The CO 2 /O 2 specificity of ribulose 1, 5-bisphosphate carboxylase/oxygenase: dependence on ribulose bisphosphate concentration, pH and temperature. Planta 161, 308–313. doi:10.1007/BF00398720
Jumper, J., Evans, R., Pritzel, A., Green, T., Figurnov, M., Ronneberger, O., et al. (2021). Highly accurate protein structure prediction with AlphaFold. Nature 596, 583–589. doi:10.1038/s41586-021-03819-2
Kong, H., Landherr, L. L., Frohlich, M. W., Leebens-Mack, J., Ma, H., and DePamphilis, C. W. (2007). Patterns of gene duplication in the plant SKP1 gene family in angiosperms: evidence for multiple mechanisms of rapid gene birth. Plant J. 50, 873–885. doi:10.1111/j.1365-313X.2007.03097.x
Konishi, H., Yamane, H., Maeshima, M., and Komatsu, S. (2004). Characterization of fructose-bisphosphate aldolase regulated by gibberellin in roots of rice seedling. Plant Mol. Biol. 56, 839–848. doi:10.1007/s11103-004-5920-2
Kossmann, J., Sonnewald, U., and Willmitzer, L. (1995). Reduction of the chloroplastic fructose-1,6-bisphosphatase in transgenic potato plants impairs photosynthesis and plant growth. Plant J. 6, 637–650. doi:10.1046/j.1365-313X.1994.6050637.x
Kuhlman, B., and Bradley, P. (2019). Advances in protein structure prediction and design. Nat. Rev. Mol. Cell Biol. 20, 681–697. doi:10.1038/s41580-019-0163-x
Lebherz, H. G., Leadbetter, M. M., and Bradshaw, R. A. (1984). Isolation and characterization of the cytosolic and chloroplast forms of spinach leaf fructose diphosphate aldolase. J. Biol. Chem. 259, 1011–1017. doi:10.1016/S0021-9258(17)43558-7
Lefebvre, S., Lawson, T., Zakhleniuk, O. V., Lloyd, J. C., Raines, C. A., Fryer, M., et al. (2005). Increased sedoheptulose-1, 7-bisphosphatase activity in transgenic tobacco plants stimulates photosynthesis and growth from an early stage in development. Plant Physiol. 138, 451–460. doi:10.1104/pp.104.055046
Lescot, M., Déhais, P., Thijs, G., Marchal, K., Moreau, Y., Van de Peer, Y., et al. (2002). PlantCARE, a database of plant cis -acting regulatory elements and a portal to tools for in silico analysis of promoter sequences. Nucleic Acids Res. 30, 325–327. doi:10.1093/nar/30.1.325
Li, R. N., You, J., Miao, C., Kong, L., Long, J. H., Yan, Y. Z., et al. (2020). Monochromatic lights regulate the formation, growth, and dormancy of in vitro -grown Solanum tuberosum L. microtubers. Sci. Hortic. 261, 108947. doi:10.1016/j.scienta.2019.108947
Li, Z. Q., Zhang, Y., Li, H., Su, T. T., Liu, C. G., Han, Z. C., et al. (2021). Genome-wide characterization and expression analysis provide basis to the biological function of cotton FBA genes. Front. Plant Sci. 12, 696698. doi:10.3389/fpls.2021.696698
Liu, X., Fu, L., Qin, P., Sun, Y., Liu, J., and Wang, X. (2019). Overexpression of the wheat trehalose 6-phosphate synthase 11 gene enhances cold tolerance in Arabidopsis thaliana . Gene 710, 210–217. doi:10.1016/j.gene.2019.06.006
Livak, K. J., and Schmittgen, T. D. (2001). Analysis of relative gene expression data using real-time quantitative PCR and the 2 −∆∆CT Method. Methods 25, 402–408. doi:10.1006/meth.2001.1262
Long, S. P., Ainsworth, E. A., Rogers, A., and Ort, D. R. (2004). Rising atmospheric carbon dioxide: plants FACE the future. Annu. Rev. Plant Biol. 55, 591–628. doi:10.1146/annurev.arplant.55.031903.141610
Lorentzen, E., Pohl, E., Zwart, P., Stark, A., Russell, R. B., Knura, T., et al. (2003). Crystal structure of an archaeal class I aldolase and the evolution of (betaalpha)8 barrel proteins. J. Biol. Chem. 278, 47253–47260. doi:10.1074/jbc.M305922200
Lu, W., Tang, X., Huo, Y., Xu, R., Qi, S., Huang, J., et al. (2012). Identification and characterization of fructose 1, 6-bisphosphate aldolase genes in Arabidopsis reveal a gene family with diverse responses to abiotic stresses. Gene 503, 65–74. doi:10.1016/j.gene.2012.04.042
Lv, G. Y., Guo, X. G., Xie, L. P., Xie, C. G., Zhang, X. H., Yang, Y., et al. (2017). Molecular characterization, gene evolution, and expression analysis of the fructose-1, 6-bisphosphate aldolase (FBA) gene family in wheat ( Triticum aestivum L.). Front. Plant Sci. 8, 1030. doi:10.3389/fpls.2017.01030
Marsh, J. J., and Lebherz, H. G. (1992). Fructose-bisphosphate aldolases: an evolutionary history. Trends Biochem. Sci. 17, 110–113. doi:10.1016/0968-0004(92)90247-7
Mutuku, J. M., and Nose, A. (2012). Changes in the contents of metabolites and enzyme activities in rice plants responding to Rhizoctonia solani kuhn infection: activation of glycolysis and connection to phenylpropanoid pathway. Plant Cell Physio 53, 1017–1032. doi:10.1093/pcp/pcs047
Obiadalla-Ali, H., Fernie, A. R., Lytovchenko, A., Kossmann, J., and Lloyd, J. R. (2004). Inhibition of chloroplastic fructose 1, 6-bisphosphatase in tomato fruits leads to decreased fruit size, but only small changes in carbohydrate metabolism. Planta 219, 533–540. doi:10.1007/s00425-004-1257-y
Oelze, M. L., Muthuramalingam, M., Vogel, M. O., and Dietz, K. J. (2014). The link between transcript regulation and de novo protein synthesis in the retrograde high light acclimation response of Arabidopsis thaliana . BMC Genomics 15, 320. doi:10.1186/1471-2164-15-320
Olas, J. J., Apelt, F., Annunziata, M. G., John, S., Richard, S. I., Gupta, S., et al. (2021). Primary carbohydrate metabolism genes participate in heat-stress memory at the shoot apical meristem of Arabidopsis thaliana . Mol. Plant 14, 1508–1524. doi:10.1016/j.molp.2021.05.024
Osakabe, Y., Maruyama, K., Seki, M., Satou, M., Shinozaki, K., and Yamaguchi-Shinozaki, K. (2005). Leucine-rich repeat receptor-like kinase1 is a key membrane-bound regulator of abscisic acid early signaling in Arabidopsis . Plant Cell 17, 1105–1119. doi:10.1105/tpc.104.027474
Paterson, A. H., Freeling, M., Tang, H., and Wang, X. (2010). Insights from the comparison of plant genome sequences. Annu. Rev. Plant Biol. 61, 349–372. doi:10.1146/annurev-arplant-042809-112235
Pelzer-Reith, B., Penger, A., and Schnarrenberger, C. (1993). Plant aldolase: cDNA and deduced amino-acid sequences of the chloroplast and cytosol enzyme from spinach. Plant Mol. Biol. 21, 331–340. doi:10.1007/BF00019948
Perham, R. N. (1990). The fructose-1, 6-bisphosphate aldolases: same reaction, different enzymes. Biochem. Soc. Trans. 18, 185–187. doi:10.1042/bst0180185
Pham, G. M., Hamilton, J. P., Wood, J. C., Burke, J. T., Zhao, H. N., Vaillancourt, B., et al. (2020). Construction of a chromosome-scale long-read reference genome assembly for potato. Gigascience 9, giaa100. doi:10.1093/gigascience/giaa100
Qiu, Z., Bai, M., Kuang, H., Wang, X., Yu, X., Zhong, X., et al. (2023). Cytosolic fructose-1, 6-bisphosphate aldolases modulate primary metabolism and phytohormone homeostasis in soybean. Agronomy 13, 1383. doi:10.3390/agronomy13051383
Raines, C. A. (2003). The Calvin cycle revisited. Photosynth Res. 75, 1–10. doi:10.1023/A:1022421515027
Schnarrenberger, C., Jacobshagen, S., Müller, B., and Krüger, I. (1990). Evolution of isozymes of sugar phosphate metabolism in green algae. Prog. Clin. Biol. Res. 344, 743–764.
PubMed Abstract | Google Scholar
Simkin, A. J., Lopez-Calcagno, P. E., Davey, P. A., Headland, L. R., Lawson, T., Timm, S., et al. (2017). Simultaneous stimulation of sedoheptulose 1, 7-bisphosphatase, fructose 1, 6-bisphophate aldolase and the photorespiratory glycine decarboxylase-H protein increases CO 2 assimilation, vegetative biomass and seed yield in Arabidopsis . Plant Biotechnol. J. 15, 805–816. doi:10.1111/pbi.12676
Sonnewald, U., Lerchl, J., Zrenner, R., and Frommer, W. (1994). Manipulation of sink-source relations in transgenic plants. Plant Cell Environ. 17, 649–658. doi:10.1111/j.1365-3040.1994.tb00156.x
Tang, X., Zhang, N., Si, H., and Calderón-Urrea, A. (2017). Selection and validation of reference genes for RT-qPCR analysis in potato under abiotic stress. Plant Methods 13, 85. doi:10.1186/s13007-017-0238-7
Thompson, J. D., Gibson, T. J., and Higgins, D. G. (2002). Multiple sequence alignment using ClustalW and ClustalX. Curr. Protoc. Bioinforma. 2, Unit 2.3. Chapter. doi:10.1002/0471250953.bi0203s00
Tsutsumi, K., Kagaya, Y., Hidaka, S., Suzuki, J., Tokairin, Y., Hirai, T., et al. (1994). Structural analysis of the chloroplastic and cytoplasmic aldolase-encoding genes implicated the occurrence of multiple loci in rice. Gene 141, 215–220. doi:10.1016/0378-1119(94)90574-6
Uematsu, K., Suzuki, N., Iwamae, T., Inui, M., and Yukawa, H. (2012). Increased fructose 1, 6-bisphosphate aldolase in plastids enhances growth and photosynthesis of tobacco plants. J. Exp. Bot. 63, 3001–3009. doi:10.1093/jxb/ers004
Van der Linde, K., Gutsche, N., Leffers, H. M., Lindermayr, C., Müller, B., Holtgrefe, S., et al. (2011). Regulation of plant cytosolic aldolase functions by redox-modifications. Plant Physiol. Biochem. 49, 946–957. doi:10.1016/j.plaphy.2011.06.009
Wang, Y., Tang, H., Debarry, J. D., Tan, X., Li, J., Wang, X., et al. (2012). MCScanX: a toolkit for detection and evolutionary analysis of gene synteny and collinearity. Nucleic Acids Res. 40, e49. doi:10.1093/nar/gkr1293
Wildman, S. G. (2002). Along the trail from Fraction I protein to Rubisco (ribulose bisphosphate carboxylase-oxygenase). Photosynth Res. 73, 243–250. doi:10.1023/A:1020467601966
Yamada, S., Komori, T., Hashimoto, A., Kuwata, S., Imaseki, H., and Kubo, T. (2000). Differential expression of plastidic aldolase genes in Nicotiana plants under salt stress. Plant Sci. 154, 61–69. doi:10.1016/s0168-9452(00)00188-6
Zeng, Y., Tan, X., Zhang, L., Jiang, N., and Cao, H. (2014). Identification and expression of fructose-1, 6-bisphosphate aldolase genes and their relations to oil content in developing seeds of tea oil tree ( Camellia oleifera ). PLoS One. 9, e107422. doi:10.1371/journal.pone.0107422
Zgiby, S. M., Thomson, G. J., Qamar, S., and Berry, A. (2000). Exploring substrate binding and discrimination in fructose1, 6-bisphosphate and tagatose 1, 6-bisphosphate aldolases. Eur. J. Biochem. 267, 1858–1868. doi:10.1046/j.1432-1327.2000.01191.x
Zhang, C., Yang, Z., Tang, D., Zhu, Y., Wang, P., Li, D., et al. (2021). Genome design of hybrid potato. Cell 184, 3873–3883.e12. doi:10.1016/j.cell.2021.06.006
Zhang, F., Zhang, P., Zhang, Y., Wang, S., Qu, L., Liu, X., et al. (2016). Identification of a peroxisomal-targeted aldolase involved in chlorophyll biosynthesis and sugar metabolism in rice. Plant Sci. 250, 205–215. doi:10.1016/j.plantsci.2016.06.017
Zhang, H., Xu, F., Wu, Y., Hu, H. H., and Dai, X. F. (2017). Progress of potato staple food research and industry development in China. J. Integr. Agric. 16, 2924–2932. doi:10.1016/S2095-3119(17)61736-2
Zhao, W., Liu, H., Zhang, L., Hu, Z., Liu, J., Hua, W., et al. (2019). Genome-wide identification and characterization of FBA gene family in polyploid crop Brassica napus . Int. J. Mol. Sci. 20, 5749. doi:10.3390/ijms20225749
Zhao, Y., Jiao, F., Tang, H., Xu, H., Zhang, L., and Wu, H. (2021). Genome-wide characterization, evolution, and expression profiling of FBA gene family in response to light treatments and abiotic stress in Nicotiana tabacum . Plant Signal Behav. 16, 1938442. doi:10.1080/15592324.2021.1938442
Zhou, Q., Tang, D., Huang, W., Yang, Z. M., Zhang, Y., Hamilton, J. P., et al. (2020). Haplotype-resolved genome analyses of a heterozygous diploid potato. Nat. Genet. 52, 1018–1023. doi:10.1038/s41588-020–0699-x
Keywords: fructose-1, 6-bisphosphate aldolase, genome-wide identification, light spectrum, abiotic stress, gene expression, Solanum tuberosum
Citation: Li T, Hou X, Sun Z, Ma B, Wu X, Feng T, Ai H, Huang X and Li R (2024) Characterization of FBA genes in potato ( Solanum tuberosum L.) and expression patterns in response to light spectrum and abiotic stress. Front. Genet. 15:1364944. doi: 10.3389/fgene.2024.1364944
Received: 03 January 2024; Accepted: 29 March 2024; Published: 12 April 2024.
Reviewed by:
Copyright © 2024 Li, Hou, Sun, Ma, Wu, Feng, Ai, Huang and Li. This is an open-access article distributed under the terms of the Creative Commons Attribution License (CC BY). The use, distribution or reproduction in other forums is permitted, provided the original author(s) and the copyright owner(s) are credited and that the original publication in this journal is cited, in accordance with accepted academic practice. No use, distribution or reproduction is permitted which does not comply with these terms.
*Correspondence: Xianzhong Huang, [email protected] ; Ruining Li, [email protected]
† These authors have contributed equally to this work and share first authorship
Disclaimer: All claims expressed in this article are solely those of the authors and do not necessarily represent those of their affiliated organizations, or those of the publisher, the editors and the reviewers. Any product that may be evaluated in this article or claim that may be made by its manufacturer is not guaranteed or endorsed by the publisher.

Chemical Communications
Solvent-free synthesis and chiroptical properties of a c–n axially chiral cruciform dimer of benzo[ b ]phenoxazine.
A novel C–N axially chiral molecule composed of two tert-butyl-substituted benzo[ b ]phenoxazine ( BPO ) was synthesized via solvent-free reactions. The absolute configurations of the enantiomers were determined by X-ray single-crystal analysis. The enantiomers had a sufficiently high racemization barrier to ignore racemization at room temperature, and the solutions exhibited dual circularly polarized emissions stemming from fluorescence and phosphorescence.
Supplementary files
- Supplementary information PDF (1906K)
- Crystal structure data CIF (4787K)
Article information

Download Citation
Permissions.

S. Ishikawa, D. Sakamaki, M. Gon, K. Tanaka and H. Fujiwara, Chem. Commun. , 2024, Accepted Manuscript , DOI: 10.1039/D4CC00977K
This article is licensed under a Creative Commons Attribution-NonCommercial 3.0 Unported Licence . You can use material from this article in other publications, without requesting further permission from the RSC, provided that the correct acknowledgement is given and it is not used for commercial purposes.
To request permission to reproduce material from this article in a commercial publication , please go to the Copyright Clearance Center request page .
If you are an author contributing to an RSC publication, you do not need to request permission provided correct acknowledgement is given.
If you are the author of this article, you do not need to request permission to reproduce figures and diagrams provided correct acknowledgement is given. If you want to reproduce the whole article in a third-party commercial publication (excluding your thesis/dissertation for which permission is not required) please go to the Copyright Clearance Center request page .
Read more about how to correctly acknowledge RSC content .
Social activity
Search articles by author.
This article has not yet been cited.
Advertisements
- Election 2024
- Entertainment
- Newsletters
- Photography
- Personal Finance
- AP Investigations
- AP Buyline Personal Finance
- Press Releases
- Israel-Hamas War
- Russia-Ukraine War
- Global elections
- Asia Pacific
- Latin America
- Middle East
- Election Results
- Delegate Tracker
- AP & Elections
- March Madness
- AP Top 25 Poll
- Movie reviews
- Book reviews
- Personal finance
- Financial Markets
- Business Highlights
- Financial wellness
- Artificial Intelligence
- Social Media
Caitlin Clark’s next big move could be helping Indiana Fever end seven-year WNBA playoff drought
Iowa guard Caitlin Clark (22) drives around South Carolina guard Raven Johnson (25) during the second half of the Final Four college basketball championship game in the women’s NCAA Tournament, Sunday, April 7, 2024, in Cleveland. (AP Photo/Morry Gash)
Iowa guard Caitlin Clark sits on the bench at the end of the Final Four college basketball championship game against South Carolina in the women’s NCAA Tournament, Sunday, April 7, 2024, in Cleveland. South Carolina won 87-75. (AP Photo/Morry Gash)
Iowa head coach Lisa Bluder talks with guard Caitlin Clark (22) at the end of the Final Four college basketball championship game against South Carolina in the women’s NCAA Tournament, Sunday, April 7, 2024, in Cleveland. South Carolina won 87-75. (AP Photo/Morry Gash)
Iowa guard Caitlin Clark, center, questions a call during the second half of the Final Four college basketball championship game against South Carolina in the women’s NCAA Tournament, Sunday, April 7, 2024, in Cleveland. (AP Photo/Carolyn Kaster)
Iowa fan Grace Clucas cheers after a Caitlin Clark three during a watch party for women’s college Final Four championship game the NCAA Tournament in Cleveland, Sunday, April 7, 2024, at Carver-Hawkeye Arena in Iowa City, Iowa. (Geoff Stellfox/The Gazette via AP)
- Copy Link copied
INDIANAPOLIS (AP) — John Meinen has been an avid Iowa basketball fan for decades.
The 61-year-old former hoops player never lost touch with the sport, his home state or his beloved Hawkeyes’ men’s or women’s teams, even after moving to Indianapolis.
So with the Indiana Fever expected to draft Caitlin Clark with the No. 1 overall pick Monday night, Meinen can’t hide his excitement over seeing the potential of a game-changing player suddenly making the Fever relevant again after a seven-year playoff drought.
“She’s Bob Cousy meets Steph Curry. I mean there just hasn’t been anyone, certainly in the women’s game, who plays the way she does,” Meinen said. “I don’t know her, I’ve never met her, but she seems like a very nice person. The other piece of it is she’s got a little Taylor Swift in her because girls just look up to her.”
He’s not alone.
Fever guard Erica Wheeler told social media fans Clark was the real deal after attending one Iowa game in February 2023. Another Fever guard, Grace Berger already knows Clark’s scouting report after chasing her around Big Ten courts for three seasons.
Neither had any idea back then, though, that the Fever would win a second straight draft lottery that would allow them to team up with the 2023 unanimous rookie of the year Aliyah Boston and the greatest scorer in Division I history.
This young, star-studded combination suddenly makes last season’s 13-win team a must-watch team, a title Indiana last held during Tamika Catchings’ final season in 2016.
So fans in Indianapolis and around the Hoosier State are focused on how quickly Indiana can rise in the standings and become a legitimate contender.
“I think she’s in the right situation here,” Meinen said. “Indiana is all about basketball, they’re going to embrace her. They have a good young core. I think the future’s very, very bright for this team.”
Clark’s transformation from generational college superstar to becoming the face of a WNBA franchise has been eagerly anticipated since Clark and the Hawkeyes started selling out arenas while getting record TV ratings over the past two seasons.
That is expected to continue at the pro level.
While the Fever have declined to release ticket sales or merchandising information — or discuss expectations for Clark on the court — because she is not yet officially on the team’s roster, that has not stopped other teams from promoting their matchups against Clark.
Two-time defending WNBA champion Las Vegas already has announced the Indiana game on July 2 will be played at T-Mobile Arena, which seats about 6,000 more fans than its traditional home venue.
The Phoenix Mercury also have dubbed the June 30 date against the Fever as “ The GOAT vs. The Rook,” capitalizing on a seemingly thinly veiled rivalry between Diana Taurasi and Clark.
“Reality is coming,” Taurasi recently said on ESPN. “You look superhuman playing against some 18-year-olds, but you’re going to come play with some grown women that have been playing professional basketball for a long time.”
How quickly and smoothly Clark adapts to bigger, stronger, more experienced opponents is a serious concern.
While most evaluators believe Clark’s shooting and passing skills will travel to the WNBA, there are questions about her defense and how the 6-foot, 155-pound point guard will deal with established stars unlikely to give the newcomer the red carpet treatment.
She’ll also have only a short break between the end of a demanding 39-game schedule that included becoming the focal point of every opposing defense and a highly publicized record-breaking scoring quest, and the mid-May start of a 40-game WNBA schedule. Playing on the U.S. Olympic Team also remains a possibility, too.
“I know what’s next is soon,” Clark said after losing Sunday’s NCAA championship game, which Meinen watched on his phone just before the NBA’s Indiana Pacers and Miami Heat tipped off in Indy.
Life certainly is coming at Clark quickly.
Indiana opens preseason play May 3 at Dallas with the regular-season opener set for May 14 at Connecticut. If all goes as expected Monday, Clark’s home debut would come May 16 against New York, last season’s WNBA runner-up.
For women’s basketball fans, Clark’s pro career can’t start soon enough. And in Indy, Clark looks like the perfect answer for a franchise that’s had 58 wins in the past seven seasons.
She’ll learn the rookie ropes from a poised point guard such as Wheeler while teaming up with Boston, forward NaLyssa Smith, the No. 2 overall pick in 2022, and shooting guard Kelsey Mitchell, the No. 2 overall pick in 2018.
“I don’t know if they’ll make the playoffs right out of the box,” Meinen said. “She’s going to make them better. She’ll make them instantly more fun to watch. You can be really fun to watch, and it doesn’t mean that your game translates into wins. But I think she’s going to be really, really good. I just can’t imagine that she’s not going to be good.”
AP WNBA: https://apnews.com/hub/wnba-basketball
- FanNation FanNation FanNation
- SI.COM SI.COM SI.COM
- SI Swimsuit SI Swimsuit SI Swimsuit
- SI Sportsbook SI Sportsbook SI Sportsbook
- SI Tickets SI Tickets SI Tickets
- SI Showcase SI Showcase SI Showcase
- SI Resorts SI Resorts SI Resorts
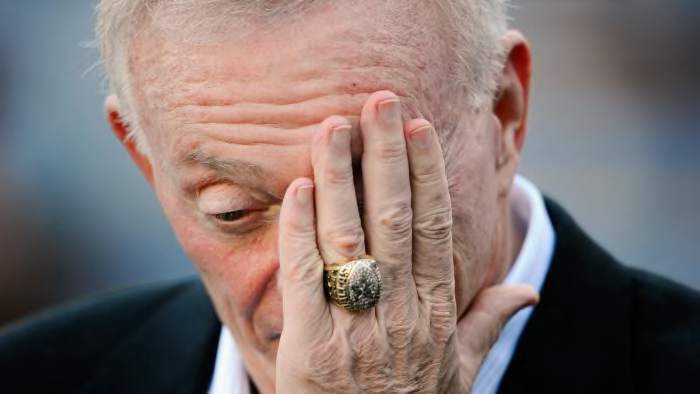
4th Fiddle? Dallas Cowboys' Drought Drags Them Down DFW Sports Rankings
When O.J. Simpson owned Dallas Cowboys' Texas Stadium, Deja Vu for Dallas Mavericks, Deja Boo for Texas Rangers and calling for a daily eclipse, all in this week's DFW sports notebook.
- Author: Richie Whitt
In this story:
WHITT'S END 4.12.24:
Whether you’re at the end of your coffee, your day, your week or even your rope, welcome to Whitt’s End …
*They’re popular. They’re profitable. But nothing about the Dallas Cowboys these days is productive. And “productive” I mean successful for the last – oh – 30ish years.
Was on vacation a couple weeks ago when a tourist said to me upon learning I was from Dallas: “Oh, Cowboys. Yeah. They’re always right up there.”
I mean … sorta.
They have three straight 12-win seasons and 36 regular-season wins, behind only the two-time Super Bowl champion Chiefs. But since winning Super Bowl XXX in the 1995 season, they are only 5-13 in the playoffs and haven’t even sniffed a return to the NFC Championship.
“Right up there” in revenue. But, no, not in results.
Matter of fact, this side of the Bills – who lost four consecutive Super Bowls in the 1990s – the Cowboys are the most successful unsuccessful team in the NFL. The Dallas Stars, Dallas Mavericks and Texas Rangers have all won championships since the Cowboys last played in a conference championship game.
Worst news: Nothing about this supposedly “All in!” offseason is doing diddly poo to change the narrative.
With only Eric Kendricks arriving a slew of players – Tyron Smith, Tyler Biadasz, Tony Pollard, etc. – departing, the Cowboys’ Super Bowl odds have actually decreased since the start of free agency. They now have only the NFL’s ninth-best odds to win Super Bowl LIX next February, behind the Eagles in their own division and the Texans in their own backyard.
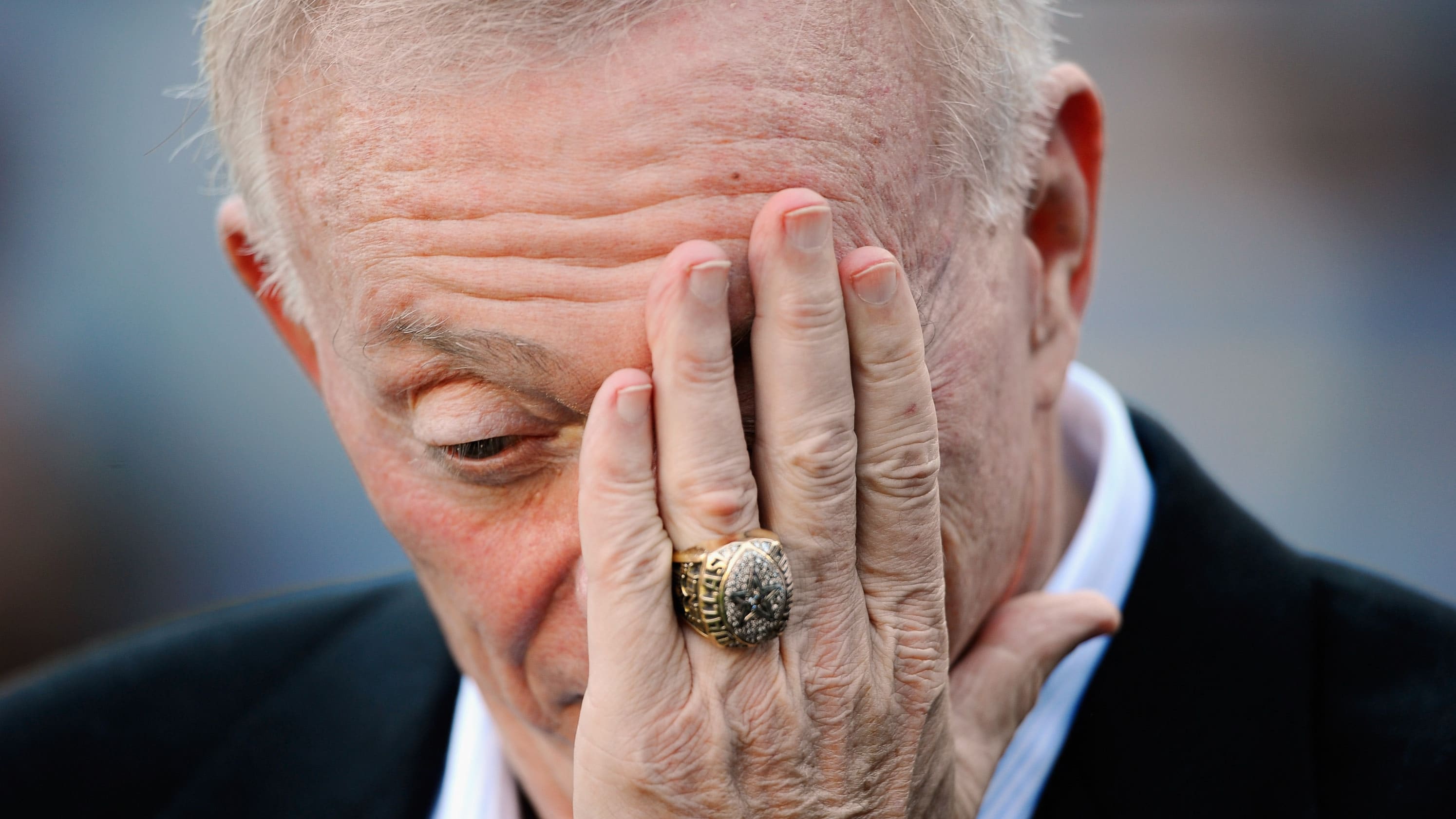
*Chiefs vs. 49ers in the Super Bowl. Biden vs. Trump for President. Why not run back another battle from 2020 in 2024: Mavericks vs. Los Angeles Clippers. No matter who owns the homecourt advantage in their impending first-round playoff series, Luka Doncic and the Mavs have unfinished business with the Clips.
In 2020 in the bubble, Doncic hit that miraculous buzzer-beating 3-pointer to cap a 43-point performance in Dallas’ Game 4 win. Bur the Mavs lost the next two games.
In 2021, the Mavs took series leads of 2-0 and 3-2 but couldn’t win a single game on their home court and wound up losing Game 7 in L.A.
Even four years later, some of the main characters will be familiar in the rivalry: Luka, Tim Hardaway Jr. and Maxi Kleber for Dallas; Kawhi Leonard, Paul George, Ivica Zubac and Terance Mann for L.A.
*Stop me if you’ve heard this one before: Jose Leclerc is a bum of a closer and the Rangers won’t win anything if they can’t beat the Oakland A’s! It was almost a year ago that I – and you – bemoaned the Rangers losing layup games to the Triple-A A’s. We know how 2023 turned out. But … this week Leclerc gave up a two-run, game-losing homer in the 9th inning Tuesday and Texas was almost no-hit Thursday in losing two of three.
*Not only was this week’s total solar eclipse a stunning visual, it did something I thought was no longer possible in the Divided States of America. It brought us together. All of us. For a glorious, magical four minutes – the length of “totality” – every American put down their tribal rhetoric, cultural differences and literally looked in the same direction. Up, at the moon/sun. I mean, then we immediately got in our cars and cut each other off in traffic and got on social media to criticize everyone who doesn’t look and act exactly like we do, but … we need an eclipse every damn day.
Next chance in the U.S.: August 2044. If you just can’t wait, head to Egypt in 2027 where there will be another total eclipse moving over the Pyramids.
*Whether you remember him as the host of Channel 8’s Bowling For Dollars, one of the original voices of the Dallas Cowboys or the signature voice of Augusta’s famed 16th hole, Verne Lundquist is a Dallas treasure. Ol’ Vern, now 83, is working his 40th and final Masters this weekend on CBS. Lundquist belongs on DFW’s Sports Media Mount Rushmore alongside Dale Hansen, Randy Galloway, Blackie Sherrod and Norm Hitzges.
* Hot .
* Not .
*Long before he was the most enigmatic suspect in American justice history, O.J. Simpson was an entertaining superstar. Simpson, who died of cancer this week at 76, went 0-3 against the Cowboys during his Hall-of-Fame career and never rushed for more than 78 yards in a game. He was, however, the MVP of a game played at Texas Stadium. O.J. ran for 112 yards and a touchdown to lead the AFC to a win in the 1973 Pro Bowl played on a 44-degree January afternoon at the Cowboys’ old home. Chasing him that day for the NFC defense: Cornell Green, Dick Butkus and Merlin Olsen.
*Fans of women’s college basketball are crowing that their National Championship Game drew a bigger television audience than the men’s game this week. Of course, the women played on ABC (available to 305 million) and the men on TBS (71 million), but don’t let facts get in the way of a blowhard argument. If you want to debate “popularity,” the men’s tournament generated $200 million revenue; the women $25 million. But instead of resurrecting 29-year-old Billie Jean King beating 55-year-old Bobby Riggs in 1973’s “Battle of the Sexes,” let me ask a serious question: If you really love something, why do you desperately seek other people’s validation of it? Popularity in no way mandates quality. McDonald’s as America’s best-selling hamburger and 2 Broke Girls having a six-year run on prime-time TV quickly come to mind.
*Aside from his insane ball-handling wizardry and clutch shooting, best thing Kyrie Irving is doing for the Mavs is making basketball fun again for Luka. After a timeout this week in Miami, Doncic sprinted the length of the floor to help Kyrie up after an acrobatic layup. There were times the last couple of seasons where – despite his MVP-caliber play – Luka never smiled.
*To no surprise, Texas was near the top for white supremacist events held in 2023 . Our state hosted 500 wanna-be Ku Klux Klan meetings. Shockingly, that wasn’t the most in the U.S. Congrats to … Virginia?
*I have two vivid O.J. memories: 1. Interviewing him at Valley Ranch in the early 1990s when he was a sideline reporter for NBC’s NFL coverage; 2. Covering a World Cup game at the Cotton Bowl in 1994 and huddling shoulder-to-shoulder with journalists from all over the world to watch O.J.’s white Bronco slow-speed chase.
*History with a strong Dallas accent: Only athlete to ever win an Olympic medal and a Super Bowl in the same calendar year: SMU’s Michael Carter (1984); Only athlete to ever win an Olympic gold medal and a Super Bowl? Cowboys’ Bob Hayes.
*If you thought the recent earthquakes and eclipse were ominous signs, here come the locusts. Check that, cicadas. Whew. Billions – with a b – will emerge from the ground in the next month as two giant broods converge. The swarms haven’t coincided since Thomas Jefferson was President and won’t again until 2245. They’re loud, but essentially harmless. Unlike locusts, they don't destroy farmers' crops.
* During last year’s epic Rangers run to the World Series it was Creed’s “ Higher ” that served as the musical motivational backdrop. Of course fans don’t want to ruin the mojo so this year they voted to hear after wins at Globe Life Field … Pat Green’s “I Like Texas”?
*One of golf’s hidden jewels resides just north at Tanglewood Resort. Just a 3-wood from Lake Texoma, it boasts a gorgeous layout with all the fixins. And next month they’ll celebrate Cinco de Mayo with a Tequila & Tacos soiree . Fine, stay and play in DFW. But guarantee you get three margaritas and an all-you-can-eat taco buffet for – wait for it - $25. See ya there?
* This Weekend? Saturday let’s take Big Brothers Big Sisters lil’ bro Ja to a second day “working” as a firefighter. Sunday let’s mix in watching The Masters with playing tennis. As always, don’t be a stranger.
Latest Cowboys News
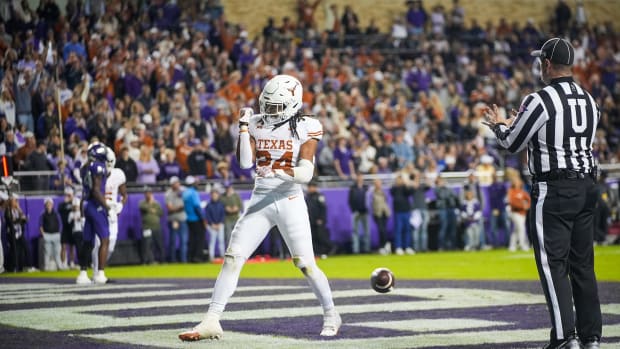
Cowboys Have ‘Intel’ on Brooks Injury, Set Draft Visit
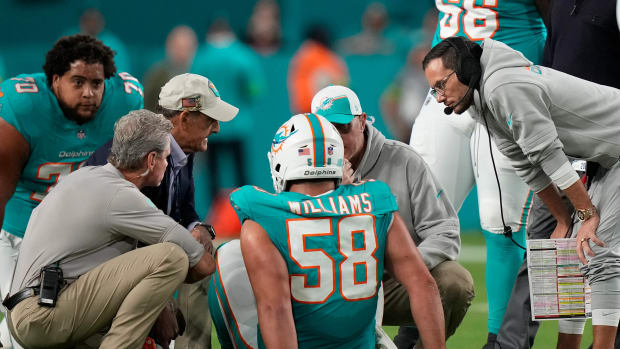
'Typical!' Cowboys Signing Injured Connor Williams?
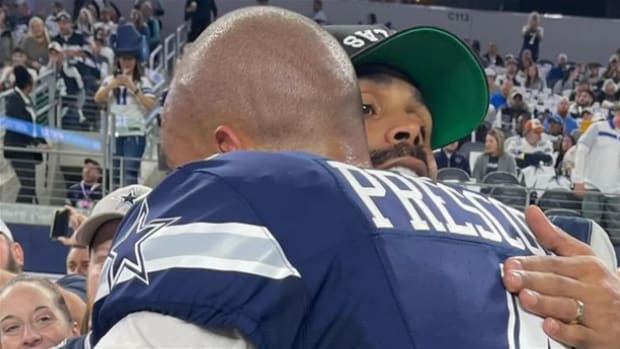
Cowboys' Prescott Ranked Second-Best NFL QB? By Whom?!
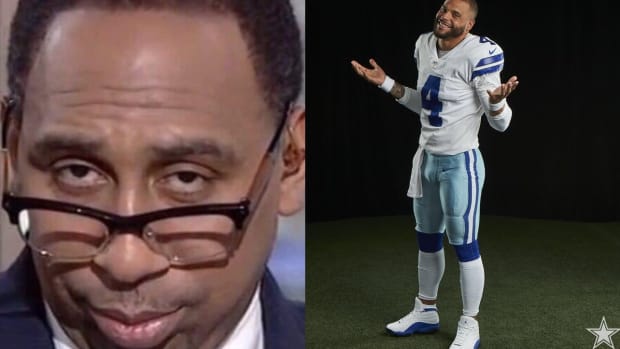
Cowboys vs. Dak Problem Solved - by Stephen A. Smith?!
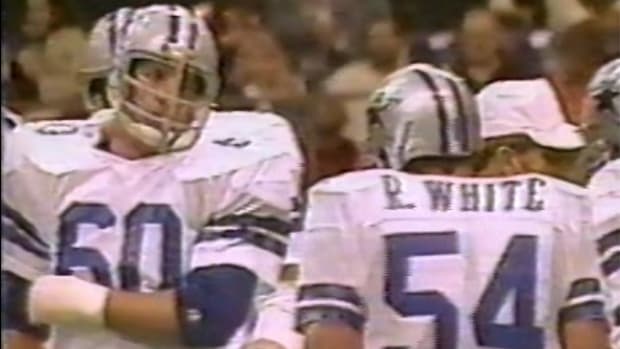
Cowboys Ex Lineman Dead At 66
Deep learning based synthesis of MRI, CT and PET: Review and analysis
Affiliations.
- 1 Department of Data Science and AI, Faculty of Information Technology, Monash University, Clayton VIC 3800, Australia. Electronic address: [email protected].
- 2 Monash Biomedical Imaging, Clayton VIC 3800, Australia.
- 3 Department of Medical Imaging and Radiation Sciences, Faculty of Medicine, Monash University, Clayton VIC 3800, Australia.
- 4 Bioengineering Department and Imperial-X, Imperial College London, W12 7SL, United Kingdom.
- 5 Department of Data Science and AI, Faculty of Information Technology, Monash University, Clayton VIC 3800, Australia.
- 6 Department of Data Science and AI, Faculty of Information Technology, Monash University, Clayton VIC 3800, Australia; Monash Biomedical Imaging, Clayton VIC 3800, Australia.
- PMID: 38052145
- DOI: 10.1016/j.media.2023.103046
Medical image synthesis represents a critical area of research in clinical decision-making, aiming to overcome the challenges associated with acquiring multiple image modalities for an accurate clinical workflow. This approach proves beneficial in estimating an image of a desired modality from a given source modality among the most common medical imaging contrasts, such as Computed Tomography (CT), Magnetic Resonance Imaging (MRI), and Positron Emission Tomography (PET). However, translating between two image modalities presents difficulties due to the complex and non-linear domain mappings. Deep learning-based generative modelling has exhibited superior performance in synthetic image contrast applications compared to conventional image synthesis methods. This survey comprehensively reviews deep learning-based medical imaging translation from 2018 to 2023 on pseudo-CT, synthetic MR, and synthetic PET. We provide an overview of synthetic contrasts in medical imaging and the most frequently employed deep learning networks for medical image synthesis. Additionally, we conduct a detailed analysis of each synthesis method, focusing on their diverse model designs based on input domains and network architectures. We also analyse novel network architectures, ranging from conventional CNNs to the recent Transformer and Diffusion models. This analysis includes comparing loss functions, available datasets and anatomical regions, and image quality assessments and performance in other downstream tasks. Finally, we discuss the challenges and identify solutions within the literature, suggesting possible future directions. We hope that the insights offered in this survey paper will serve as a valuable roadmap for researchers in the field of medical image synthesis.
Keywords: Generative deep-learning models; Medical image synthesis; Pseudo-CT; Synthetic MR; Synthetic PET.
Copyright © 2023 The Author(s). Published by Elsevier B.V. All rights reserved.
Publication types
- Deep Learning*
- Image Processing, Computer-Assisted / methods
- Magnetic Resonance Imaging
- Positron-Emission Tomography
- Tomography, X-Ray Computed
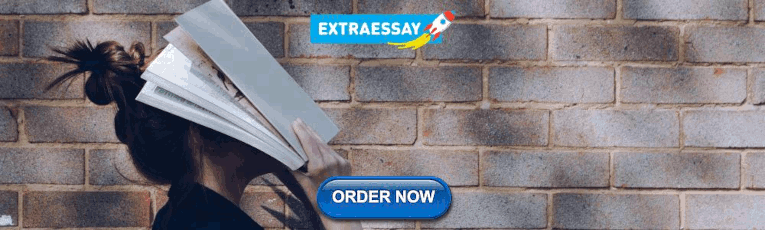
IMAGES
VIDEO
COMMENTS
This USGS Powell Center working group will conduct the first comprehensive synthesis of ecosystem impacts across a coordinated, globally distributed drought experiment network of over 100 sites that have imposed an extreme multi-year drought. Knowledge about how sensitivity to drought changes across ecosystems and over time can reveal the ...
Drought is a prominent feature of Hawaiʻi's climate. However, it has been over 30 years since the last comprehensive meteorological drought analysis, and recent drying trends have emphasized the need to better understand drought dynamics and multi-sector effects in Hawaiʻi. Here, we provide a comprehensive synthesis of past drought effects in Hawaiʻi that we integrate with geospatial ...
Our results have shown that the extent of yield reduction due to drought was largely determined by crop species. Within the cereal group, wheat demonstrated the lowest (i.e., <25%) yield reduction under similar water stress (i.e., ∼40% water deficit) compared to other species such as durum wheat, pearl millet, and maize (Fig. 1 A, B).Anaerobic rice, on the other hand, showed a greater yield ...
Through the analysis of global climate drought characteristics, it is believed that the CMIP6 inherits part of the advantages of CMIP5, and can show the future close to the real drought situation, which is more obvious under high emission scenarios. However, there are still few discussions related to CMIP6, and it cannot be simply assumed that ...
Here, we provide the first cross-species synthesis of tree drought mortality mechanisms. We used a standardized physiological framework to analyse drought-induced tree mortality across species and ...
We conducted a modeling analysis of the effects of extreme drought on two key ecosystem processes, production and respiration, and, to provide a broader context, we complemented this with a synthesis of published results that cover a wide variety of ecosystems. ... Knapp, A. K., and Luo, Y.: Differential effects of extreme drought on production ...
The engineering of key enzymes involved in the synthesis of drought-regulated metabolites have been proven successfully in producing drought tolerant plants 10,11,12,13. This strategy is an ...
drought in different ways and with different magnitudes, and that each measure responses a unique way (Luo et al. 2011). 2 Materials and methods 2.1 Synthesis methods and data analysis We searched the ISI web of science using these search strings: "(drought OR severe drought OR extreme drought) AND (ecosystem fluxes OR ecosystem carbon ...
Hence, Arabidopsis plants under mDr sense and respond to drought stress in a similar way to the more drastic progressive wilting or dry-down drought treatments, which lead to lethality. mDr treatment, therefore, is a good model system to dissect the response and resistance of plants to drought. The analysis of the late stage of drought in mDr ...
Drought is a prominent feature of Hawaiʻi's climate. However, it has been over 30 years since the last comprehensive meteorological drought analysis, and recent drying trends have emphasized ...
The analysis of drought risk is understood as the initial step to foster drought risk management at different scales and thematic foci. ... Furthermore, the practical implementation of drought risk analysis into risk maps is evaluated. Finally, a synthesis of the detected pattern gives guidance for future applications. 2. Definitions and ...
Synthesis. Forest-ANPP shows high sensitivity to long-term moderate drought, whereas grass-ANPP is more responsive to short-term drought. ... the responses of plant aboveground productivity and belowground respiration of terrestrial ecosystems to experimental drought via a meta-analysis. To do this, we compiled 610 comparisons of ANPP and the ...
Drought has been a major cause of agricultural disaster, yet how it affects the vulnerability of maize and wheat production in combination with several co-varying factors (i.e., phenological phases, agro-climatic regions, soil texture) remains unclear. Using a data synthesis approach, this study aim …
Drought has been a major cause of agricultural disaster, yet how it affects the vulnerability of maize and wheat production in combination with several co-varying factors (i.e., phenological phases, agro-climatic regions, soil texture) remains unclear. Using a data synthesis approach, this study aims to better characterize the effects of those co-varying factors with drought and to provide ...
Cluster analysis of down-regulated PPIN provides a snapshot of major processes associated with photosynthesis, growth, development and protein synthesis, most of which are shut down during drought. Differential regulation of phytohormones, e.g., jasmonic acid, cell wall metabolism, signalling and posttranslational modifications associated with ...
drought to agricultural impacts, with a focus on precipitation shortfalls, evapotranspiration differences,7 soil moisture deficits, reduced groundwater or reservoir levels, and other effects. The timing and severity of drought conditions and the types of plants involved may yield different agricultural impacts. Some
RNASeq analysis of drought-stressed guayule reveals the role of gene transcription for modulating rubber, resin, and carbohydrate synthesis
The results of the synthesis revealed a total of 26,221 articles from 45,329 authors and 3181 journals. The annual research production in drought-related research thus exhibited a 7.87% annual ...
The data shows that the drought over the water catchment area is the worst on record over one- and three-year periods. Rainfall in 2017 was especially poor. This kind of drought is expected once in 300 years (90% confidence interval: 105 to 1,280 years). The drought in the city itself is severe, but not the worst on record.
Abscisic acid (ABA), a phytohormone, and its analogs have been found to enhance plant resistance to various biotic and abiotic stresses, particularly drought, by activating the ABA signaling pathway. This study used a combination of structure-directed design and molecular docking screening methods to synthesize a novel series of opabactin (OP) analogs. Among them, compounds 4a-4d and 5a ...
Drought stress inhibited the synthesis of Chla, Chlb and photosynthesis in Phoebe bournei . In our study, the genes on the "Porphyrin and Chlorophyll metabolism" (ko00860) pathway were suppressed. ... Wu C, Wang Y, Sun H. Targeted and untargeted metabolomics reveals deep analysis of drought stress responses in needles and roots of Pinus ...
Long non-coding RNAs (lncRNAs) play an important role in the response of plants to drought stress. The previous studies have reported that overexpression of LEA3 and VOC could enhance drought tolerance and improve the oil content in Brassica napus and Arabidopsis thaliana, and most of the efforts have been invested in the gene function analysis, there is little understanding of how genes that ...
This work is focused on G-quadruplex-forming sites in two gene sets of interest: drought stress-responsive genes, and genes related to the production/biosynthesis of phenolic compounds in the model plant organism Arabidopsis thaliana. In addition, 20 housekeeping genes were analyzed as well, where the constitutive gene expression was expected ...
The National Water Prediction Service website provides tools that deliver actionable hydrologic information across all time scales to address the growing risk of flooding, drought and water availability, and enables partners and the American public to make smart water decisions. Resources. Weather.gov Webstory - January 12, 2024
The analysis of gene structures, ... Expression levels of the StFBA genes were influenced by drought and salt stress, ... -1, 5-bisphosphate carboxylase/oxygenase (Rubisco) plays a crucial role in carbon fixation and regulates biomass synthesis during photosynthesis (Ashida et al., 2019). However, ...
Further transcriptome analysis revealed distinct mechanisms of action between compound 4c and iso-PhABA in enhancing drought tolerance in A. thaliana. These findings highlight the application prospect of 4c and its analogs in agricultural cultivation, particularly in drought resistance. Additionally, they provide new insights into the ...
Solvent-free synthesis and chiroptical properties of a C-N axially chiral cruciform dimer of benzo[b]phenoxazine ... The absolute configurations of the enantiomers were determined by X-ray single-crystal analysis. The enantiomers had a sufficiently high racemization barrier to ignore racemization at room temperature, and the solutions ...
So with the Indiana Fever expected to draft Caitlin Clark with the No. 1 overall pick Monday night, Meinen can't hide his excitement over seeing the potential of a game-changing player suddenly making the Fever relevant again after a seven-year playoff drought. "She's Bob Cousy meets Steph Curry.
When O.J. Simpson owned Dallas Cowboys' Texas Stadium, Deja Vu for Dallas Mavericks, Deja Boo for Texas Rangers and calling for a daily eclipse, all in this week's DFW sports notebook.
Additionally, we conduct a detailed analysis of each synthesis method, focusing on their diverse model designs based on input domains and network architectures. We also analyse novel network architectures, ranging from conventional CNNs to the recent Transformer and Diffusion models. This analysis includes comparing loss functions, available ...