Copper/Zinc Ratio in Childhood and Adolescence: A Review
Affiliations.
- 1 Faculty of Medicine, University of Valladolid, Avenida Ramón y Cajal, 7, 47005 Valladolid, Spain.
- 2 Department of Analytical Chemistry, Science Faculty, Campus Miguel Delibes, University of Valladolid, Calle Paseo de Belén, 7, 47011 Valladolid, Spain.
- 3 Science Faculty, University of Cadiz, Paseo de Carlos III, 28, 11003 Cádiz, Spain.
- 4 Department of Chemistry, Science Faculty, University of Burgos, Plaza Misael Bañuelos sn, 09001 Burgos, Spain.
- 5 Department of Pediatrics, Faculty of Medicine, University of Valladolid, Section of Gastroenterology and Pediatric Nutrition, University Clinical Hospital of Valladolid, Avenida Ramón y Cajal, 7, 47005 Valladolid, Spain.
- PMID: 36677007
- PMCID: PMC9862945
- DOI: 10.3390/metabo13010082
Both copper (Cu) and zinc (Zn) are crucial micronutrients for human growth and development. This literature review covered the last five years of available evidence on the Cu/Zn ratio in children and adolescents. We searched PubMed, Web of Science, Google Scholar, Cochrane Library, and Science Direct for publications between 2017 and 2022, especially in English, although publications in other languages with abstracts in English were included. The main terms used were "copper", "zinc", "copper-zinc", and "zinc-copper" ratios. Cu and Zn determinations made in blood, plasma, or serum were included. This review comprises several cross-sectional and case-control studies with substantial results. The bibliographic search generated a compilation of 19 articles, in which 63.2% of the studies mostly reported a significantly higher Cu/Zn ratio, and 57.9% of them informed significantly lower levels of Zn. We conclude that children and adolescents with acute and chronic conditions are at greater risk of developing elevated Cu/Zn ratios, related to altered nutritional, infectious, and inflammatory status.
Keywords: chronic diseases; inflammation; nutritional status; oxidative stress; zinc-to-copper ratio.
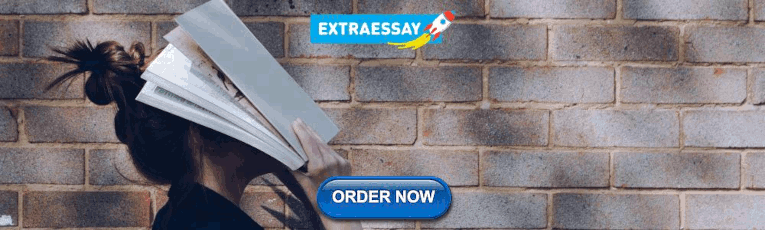
Publication types
Grants and funding.
Advertisement
A Literature Review of Taste Change and Zinc Deficiency After Bariatric Surgery: Could There Be a Causal Link?
- Open access
- Published: 19 November 2022
- Volume 33 , pages 313–331, ( 2023 )
Cite this article
You have full access to this open access article
- Boshra Mozaffar 1 , 2 &
- Iskandar Idris ORCID: orcid.org/0000-0002-7548-8288 1
2238 Accesses
2 Citations
1 Altmetric
Explore all metrics
In this review, we collated evidence relating to taste change and zinc deficiency in relation to bariatric surgery (BS) and effects of zinc replacement on taste perception and speculate on the possible role of zinc deficiency to induce taste change after BS. A literature search was conducted (33 studies, N = 3264). We showed that taste change and zinc deficiency are frequent complications after BS, which both typically occurred at 6 months post-surgery. Our analysis did not support a causal link between the two, but similar onset of incidences indirectly indicates a link. Supplementation with 45–50 mg of zinc sulphate, higher than current recommendation, was effective in improving taste. Further studies are required to establish the causal link between the two in the context of BS.
Similar content being viewed by others
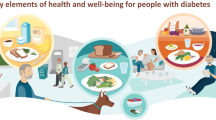
Evidence-based European recommendations for the dietary management of diabetes
The Diabetes and Nutrition Study Group (DNSG) of the European Association for the Study of Diabetes (EASD)
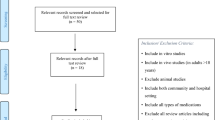
Modified medication use in dysphagia: the effect of thickener on drug bioavailability—a systematic review
Jayne Atkin, Christopher Devaney, … David Smithard
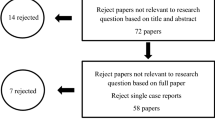
Impact of soft drinks to health and economy: a critical review
J. F. Tahmassebi & A. BaniHani
Avoid common mistakes on your manuscript.
Introduction
Bariatric surgery (BS) has emerged as the most cost-effective treatment to help patients with obesity to lose and maintain weight [ 1 ]. Over recent decades, the incidence of BS has increased globally, with an estimated 468,609 surgeries performed in 2013 [ 2 ]. In the UK, about two-thirds of all hospital admissions in 2016 and 2017 were due to obesity. A total of 6,760 consultant sessions for BS were completed between 2016 and 2017 [ 3 ].
Taste change is a common side effect reported by patients after BS [ 4 ]. Despite this, the evidence for the incidence of taste change following BS and the mechanism for this is limited. The few studies that have examined it have provided information regarding the prevalence of taste change following BS. For example, one study has found that taste change affected 73% of patients who underwent Roux-en-Y gastric bypass (RYGBP) [ 5 ], while another study reported 82% of laparoscopic Roux-en-Y gastric bypass (LRYGB) patients and 46% of laparoscopic adjustable gastric banding (LAGB) patients experience taste change [ 6 ].
In the USA, around 200,000 patients visit doctors each year complaining of either a taste or smell change [ 7 ], while about 240,000 patients were diagnosed with taste disorders in Japan [ 8 ] in 2003. Although causes of taste change is multifactorial—e.g., radiation therapy for cancers of the head and neck; surgery to the nose, ear, and/or throat; use antibiotic of antihistamines exposure to some chemicals [ 7 ]—the exact cause of taste change after BS remains unclear. Since zinc is an important element for developing taste buds in healthy people [ 9 ] and BS can lead to reduce dietary intake as well as zinc deficiency [ 10 ], we speculate a link between zinc deficiency as a cause of taste change following BS. Due to the high risk of zinc deficiency following BS, the British Obesity and Metabolic Surgery Society (BOMSS, 2020) recommends a minimum of 2 mg of copper and a ratio of 8–15 mg zinc. The tolerable upper intake of zinc level is the maximum daily intake unlikely to cause harmful effects on health 40 mg daily for all males and females ages 19 years and above [ 11 ]. Patients who undergo biliopancreatic diversion (BPD)/duodenal switch (DS) meanwhile need higher zinc supplementation than that for SG or RYGB; the optimal level for zinc supplementation is not known but recommends starting with at least 30 mg oral zinc daily [ 12 ].
While the mechanism of taste changes following BS remains poorly understood, evidence shows that zinc deficiency causes changes in the levels of gustin concentration and salivary flow [ 13 ]. Gustin is the major zinc-containing protein in the human parotid; low levels of gustin have been linked with growth and development disturbances of the taste buds [ 13 ], and taste change therefore may be due to low levels of total parotid saliva zinc. In an animal study, authors found that zinc deficiency induces the degeneration of soft palate taste buds on microscopy observations [ 14 ]. Although many studies have reported taste changes following BS, none has discussed the effect of zinc deficiency in taste change following BS.
The aim of this review is to collate evidence on zinc deficiency and taste change following BS. Thereafter, we plan to investigate if there is a link between the two in the context of BS. This will be undertaken by discussing evidence on the association between taste change and BS, association between zinc deficiency and BS, and evidence relating to zinc supplementation on taste perception in general and specifically in relation to BS.
Identifying Relevant Studies (Literature Search)
A literature search was conducted using the four electronic bibliographical databases of EMBASE, PubMed, AMED, and MEDLINE. Article bibliographies were also searched and yielded additional relevant studies. The following keywords were used: taste change, taste disorder, disguise, BS, sleeve gastrectomy, gastric bypass, banding and duodenal switch, biliopancreatic diversion, zinc, zinc sulphate or Zn, deficiency, supplementation, micronutrient deficiencies, vitamin and mineral supplementation, and nutritional deficiencies. There were no restrictions on publication date to facilitate the collection and identification of all available and relevant articles published before 30 February 2021.
Selecting Relevant Studies
After duplicates and irrelevant articles were removed, the literature search produced 690 articles. Of these, 523 articles were eliminated due to their irrelevance to the research question after the title and abstract were screened. The other 167 articles were assessed for eligibility, and the full texts were reviewed to determine which sources fulfilled the inclusion criteria . Thirty-three papers were found to fulfil the inclusion criteria (Fig. 1 ).
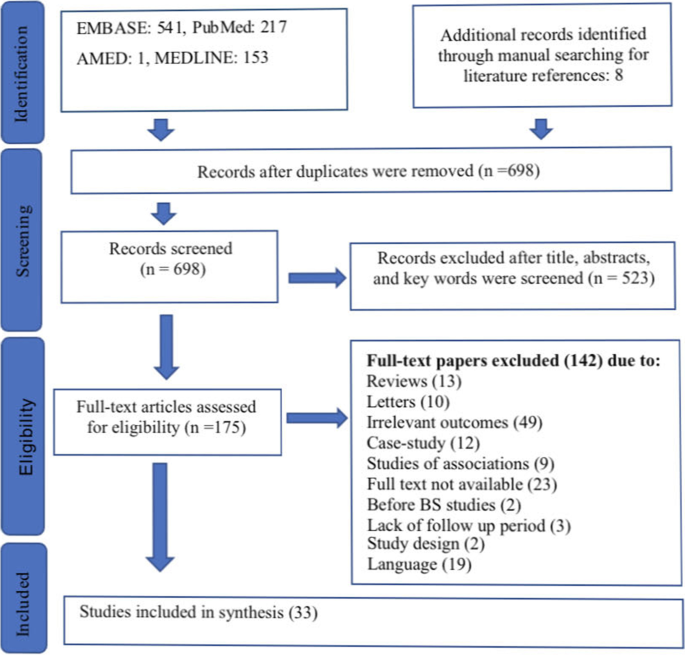
Flow diagram of study inclusions
The inclusion criteria were as follows:
Randomized controlled trials (RCTs) and cohort studies
Studies that focused on zinc deficiency and/or taste change after BS (such as sleeve gastrectomy (SG), gastric bypass (GBP), banding, duodenal switch, biliopancreatic diversion, or gastroplasty)
Studies populations consisting of adults ≥ 18 years
Studies regarding taste changes and zinc outcomes
Articles in the English language
Human studies
The exclusion criteria were as follows:
Study populations ≤ 17 years
Studies for which the full text was unavailable
Studies that did not include zinc or taste change outcomes
Studies not in the English language
Cross-sectional studies and case control studies
Animal studies
Data Extraction
The included studies were assessed and extracted, depending on three aspects. Table 1 describes studies about taste change following BS and classified according to author(s), year of publication, study location, study design and duration, population, type of BS, time since surgery, method, and taste change outcomes. Table 2 describes studies about zinc deficiency following BS—which are classified according to author(s), year and country, study design and duration, sample size and age, type of BS intervention and period, weight reduction science surgery, zinc dose, percentage of zinc absorption, and effectiveness of zinc supplementation. Table 3 describes the studies of the effectiveness of zinc in taste disorders. This table is classified into author(s), year and country, study design and duration, sample size, age, disease or case, methods, zinc dose/day, and treatment period.
Only one study was found regarding the role of zinc in taste change following BS [ 15 ], during data extraction. Meanwhile, many studies were found related to the effectiveness of zinc in taste change treatment.
Quality Assessment
The quality of the papers included was evaluated, using the Newcastle–Ottawa scale for randomized control trials and cohort studies. The quality assessment for the randomized controlled trial comprised three categories (“selection” contains four questions, “comparability” contains one question, and “exposure” contains three questions). The quality assessment for the cohort studies also included three categories (“selection” contains four questions, “comparability” contains one question, and “outcome” contains three questions). Each study can be given a maximum of one star for each numbered item within the categories. A maximum of two stars can be given for comparability. Thresholds for converting the Newcastle–Ottawa scales to AHRQ standards (good, fair, and poor) are the following: good quality, 3 or 4 stars in the selection domain AND 1 or 2 stars in the comparability domain AND 2 or 3 stars in the outcome/exposure domain; fair quality, 2 stars in the selection domain AND 1 or 2 stars in the comparability domain AND 2 or 3 stars in the outcome/exposure domain; and poor quality, 0 or 1 star in the selection domain OR 0 stars in the comparability domain OR 0 or 1 stars in the outcome/exposure domain.
Randomized Controlled Trial (RCT) Studies’ Quality Assessment
Twelve studies were deemed to be of good quality [ 33 , 34 , 35 , 36 , 37 , 38 , 39 , 41 , 42 , 43 , 44 , 45 ]: Three studies were of fair quality [ 21 , 27 , 40 ] ( see Table 4 ) .
Cohort Studies’ Quality Assessment
Nine studies were of good quality [ 17 , 19 , 20 , 22 , 25 , 28 , 29 , 31 , 32 ]. Eight studies were of fair quality [ 5 , 6 , 16 , 18 , 23 , 24 , 26 , 30 ]. One study was poor quality [ 15 ] ( see Table 5 ).
Association Between Bariatric Surgery with Taste Change: Qualitative Studies
A sample of 103 patients who underwent RYGB, 75% reported taste changes in a period of less than 12 months and that the foods that most commonly tasted different were meat (33%) and sweet flavours [ 5 ]. In a comparison between BS procedures, authors found that 35.5% of RYGB and 34% of laparoscopic sleeve gastrectomy (LSG) patients reported a taste change in commonly consumed food, in a mean time of 10 ± 6.7 months after surgery [ 16 ]. While there was no significant difference between the two surgeries in term of taste change, patients presenting with food aversion experienced higher excessive weight loss percentage (%EWL) compared with those without aversion, (73.3 ± 19.7 vs 65.8 ± 19.4%; p = 0.046) [ 16 ]. In a further study, a comparison was made between RYGB and laparoscopic adjustable gastric banding (LAGB) in a sample of 127 patients, of whom 82 underwent RYGB and 28 underwent LAGB. Decreased intensity of taste was reported in 82% of RYGB patients and 46% of LAGB patients ( p < 0.05), and 83% of RYGB patients and 69% of LAGB patients agreed that loss of taste led to better weight loss [ 6 ]. A recent study of 100 patients who underwent LSG found a decrease in preferences for core tastes, 6 months after surgery ( p < 0.002); in this study, the decrease in preferences did not influence significantly the % of total body weight loss except for salty taste a higher decrease in salty preferences correlated with a higher % of total body weight loss ( p = 0.02) [ 20 ]. In all four included studies that used questionnaires, the most important decline in taste preference was observed for sweet food [ 5 , 6 , 16 , 20 ] (Table 1 ).
Association Between Bariatric Surgery with Changes in Sensory-Discriminative Component: Quantitative Studies
The evaluation of taste perception includes assessment of taste quality (sweet, salty, bitter, sour, umami) and taste sensitivity. The four included studies measured detection thresholds (the minimum concentration a subject must taste to identify a taste stimulus as being different from water) or recognition thresholds (the minimum concentration that a subject need to recognize the taste quality of the stimulus). Burge, Schaumburg [ 15 ] found an increase in sweet taste sensitivity (decreased thresholds for sucrose detection and recognition) at 6 weeks post-RYGB ( p < 0.05). In contrast, Nance, Eagon [ 19 ] found no change in taste sensitivity after 1 year of LSG and RYGB p > 0.05. El Labban, Safadi [ 18 ] did not find a significant change in sweet sensitivity ( p > 0.05), but did find a significant change in sourness thresholds, among subjects who had undergone RYGB p = 0.0045. In a pilot functional magnetic resonance imaging (fMRI) study, the authors examined taste testing and fMRI for 13 subjects pre- and post-BS and compared to controls in response to sweet and salty solutions; the author found a significant decrease in brain activation in the reward system responding to all sweet tastes compared to pre surgery; however, the same effect appears in non-surgical controls ( p < 0.001) [ 17 ]. In contrast, significant increase in brain activation in the reward system responds to salty tastes after surgery compared to controls [ 17 ].
Association Between Bariatric Surgery with Zinc Deficiency
Twelve studies have investigated zinc status following BS. A randomized controlled trial of zinc absorption and zinc status after RYGB found that zinc absorption decreased significantly, from 23.3 to 13.6%, during the first 6 months after surgery. Patients’ zinc levels thus diminished, despite zinc supplementation at 15 mg/day. However, there was a slight improvement of zinc absorption at the end of the study (18 months) [ 21 ]. In Papamargaritis [ 22 ]’s study, the author found that zinc deficiency doubled from 7 to 15%—before and after surgery, respectively. The percentage of zinc deficiency peaked at 6 months after surgery ( n = 24; 13.8%). The percentage then decreased slightly throughout the follow-up period, to reach 7.1% by 36 months after surgery [ 22 ]. In contrast, Rojas, Carrasco [ 27 ] found zinc concentration levels to increase after 6 months of RYGB, despite the significant reduction in dietary intake of zinc and regardless of the supplementation group ( p < 0.001). Ruz, Carrasco [ 21 ] and Papamargaritis [ 22 ] found in cohort studies of zinc deficiency after SG, RYGB, and duodenal switch (DS) that zinc deficiency was common in 42.5% of patients, out of a sample of 324 patients at 12 months after surgery. Zinc deficiency was more prevalence in DS patients, with a percentage of 91.7% despite zinc supplementation at 22 mg/day [ 24 ]. Another cohort study compared serum zinc levels in 52 RYGB and 89 BPD patients. Zinc levels were significantly low in both groups ( p < 0.0001); however, zinc deficiency was more frequent in BPD patients—ranging from 44.9 to 74.9% deficiency Balsa [ 23 ]. Further studies [ 29 ] have supported the findings from Ruz, Carrasco [ 21 ] which showed that the peak effect of plasma zinc concentrations after RYGB occurred at ~ 6 months after surgery ( p < 0.05) [ 21 , 25 , 26 , 28 , 29 , 30 ]. There was however some discordance on the effects of LSG on zinc levels; i.e., Pech, Meyer [ 31 ] did not find significant zinc deficiency following LSG, while Katsogridaki, Tzovaras [ 32 ] found significant zinc deficiency after LSG ( p < 0.001) (Table 2 ).
Effectiveness of Zinc Replacement to Improve Taste Change
Thirteen studies have examined the effectiveness of zinc replacement as a treatment to taste change. All studies are randomized controlled trials, and none was performed in BS. Five studies have examined the effectiveness of 45–50 mg of zinc sulphate supplementation either 2 or 3 times per day, in cancer patients with head and neck cancer or chemotherapy, over a treatment period ranging from 1 to 8 months [ 33 , 34 , 36 , 37 , 45 ].
A study in 2019 study Khan [ 33 ] found that zinc sulphate was not effective in the treatment of taste alterations induced by chemoradiation ( p = 0.04) [ 33 ]. In contrast, an older study conducted in 1998, authors found a significant improvement in taste—which was measured at 64% in the zinc-treated group, compared with 22% in the placebo group, after receiving 45 mg of zinc 3 times per day over a 1-month period [ 34 ]. Both authors used detection thresholds to measure taste. Halyard, Jatoi [ 36 ] introduced 45 mg of zinc sulphate 3 times per day in a sample of 169 patients with head and neck cancer for a period of 6 months. The investigator found no significant difference in taste improvement, in either the zinc-treated or the placebo group ( p = 0.09) [ 36 ]. Similarly, Lyckholm [ 37 ] did not find a significant improvement in 58 patients after introducing 50 mg of zinc sulphate for 3 months, and both authors used a taste questionnaire to identify taste change. Najafizade, Hemati [ 45 ] found a slight increase in the threshold for the perception of salty taste ( p = 0.046). Two studies Ikeda [ 40 ] examined the effectiveness of zinc supplementation in taste disorder treatment in elderly patients. Ikeda [ 40 ] studied a large number of participants—408 patients, who received 17 mg of zinc—and found their zinc levels to increase significantly after 1 month of the treatment, from 69 mg/dl or lower to 91.0 mg/dl ( p , 0.001). Stewart-Knox, Simpson [ 44 ]’s study also showed significant improvements in taste after receiving 15–30 mg of zinc over 6 months.
Six studies involved participants with taste dysfunction. All these studies reported significantly high improvements in zinc levels after zinc supplementation. They found that taste dysfunction either improved or was cured—in a treatment period ranging from 3 to 6 months with a zinc dose ranging from 20 to 50 mg/day [ 35 , 38 , 39 , 41 , 42 , 43 ] (Table 3 ).
Numerous studies have been carried out on taste change following BS. Investigators have used different methods to determine said taste change. In this review, we have found that the results differed depending on the methods used to assess taste change. For instance, survey studies found that a considerable percentage of patients claimed to have taste change following BS, at a mean time of 6 months post-surgery [ 5 , 6 , 16 , 20 ], consistent with a review by Ahmed, Penney [ 4 ]. However, studies that examined taste change via experimental methods like recognition thresholds, fMRI, and the sweetness acceptability test did not find a significant change in taste following BS [ 18 , 19 ]. There was however one exception, where a study found an increase in sweet taste sensitivity, 6 months after RYGB surgery [ 15 ]. It is suggested that the recognition threshold methods used in the above-mentioned studies do not reflect current changes in taste intensity, as the concentrations are more closely related to our food experiences. While the discordance between findings from self-reported compared with experimental studies may not be overtly surprising, we believe that conclusion from self-reported studies would still play an important role in investigating the effects of BS on taste change, as this may still play an important role in patients eating behaviour. A study to validate self-reported survey with experimental study would be important to resolve future discordance findings between the two.
We suggest several reasons for taste change after BS. First, it may be associated with the rate of salivary flow after BS; Marsicano, Grec [ 46 ] found a reduction in the salivary flow rate, 3 months after surgery ( p < 0.05). Saliva is essential to dissolve food particles and stimulate taste receptor cells on the taste buds, located on the tongue papillae. Saliva dissolves some tastants, which then diffuse to the taste receptor sites. Taste sensitivity is related to the composition of saliva, in a complex process. For example, salivary bicarbonate ions can reduce the concentration of free hydrogen ions and thereby affect the sour taste. Proline‐rich proteins can affect the bitter taste. The other taste stimuli (sweet, salty, and umami) are likewise affected by different elements in saliva. Thus, reduced salivary flow affects the taste threshold following BS. Second, changing levels of gustin concentration may also induce taste changes. As noted, gustin is the major zinc-containing protein in the human parotid; changes therein may be linked with zinc deficiency. Shatzman [ 13 ] found that patients with hypogeusia had low concentrations of zinc in their saliva—administering zinc to these patients was associated with increased salivary zinc content and gustin concentrations. Third, nutritional deficiencies such as vitamin B12 deficiency which may manifest as a smooth, red tongue may lead to a loss of taste perception [ 47 ].
This review also offers insight via the evidence showing that zinc deficiency was clearly reported 6 months after BS [ 21 , 22 , 25 , 26 , 28 , 29 , 30 ]. One study showed paradoxical results, in which patients’ levels of zinc concentration increased after 6 months of RYGB [ 27 ]. These results should be interpreted with caution. The authors suggest that inflammation decreases zinc levels and that reduced inflammation was observed in their subjects. RYGB patients were particularly affected by zinc deficiency, because of the restrictive and malabsorptive nature of this surgery. The exclusion of the interior part of the stomach, duodenum, and proximal jejunum after RYGB causes a malabsorption of zinc—which is primarily absorbed in the duodenum—along with low dietary intake [ 48 , 49 ]. Yet, in a comparison between different BS procedures, Salle (2010) found that zinc deficiency was more frequent after DS and SG than after RYGB [ 24 ]. Another cohort found that zinc deficiency was higher in BPD patients, compared to RYGB [ 23 ]; however, Ruiz-Tovar [ 50 ] found that zinc level was in normal range one year after SG; zinc = 86.9 µg/dl.
Despite differences in the prevalence of zinc deficiency following different BS procedures, zinc deficiency frequently occurs in all types of BS procedures. However, studies by Balsa [ 23 ] and Salle [ 24 ] reported that the number of patients undergoing each procedure was not equal, which may influenced the reported percentage of individuals affected by zinc deficiency [ 23 , 24 ]. Furthermore, most studies( 29 ) focused on one type of BS procedure, RYGB. They likewise studied just a small number of patients [ 21 , 25 , 26 , 28 , 29 , 30 ].
Studies have reported zinc deficiency, even when multivitamins and mineral supplementation were prescribed to patients following surgery. There are several possible explanations for this. First, zinc absorption decreases significantly after surgery. Ruz, Carrasco [ 21 ] found a significantly decrease of zinc absorption from 32.3 to 13.6% at 6 months, after RYGBP, and to 21% at 18 months after surgery (see Table 2 ). To the best of our knowledge, no previous studies have specifically evaluated zinc absorption after BS, except from that of Ruz, Carrasco [ 21 ]. Second, the level of zinc dosage is too low to have a significant impact on absorption. It is suggested that the standard multivitamins and mineral supplementation prescribed by surgeons in current clinical practice—at between 8 and 15 mg of zinc—are not effective for avoiding zinc deficiency following surgery. Additional studies should be conducted to evaluate zinc absorption after BS.
Many high-quality papers have found a significant association between taste change and zinc deficiency. While causal link between taste and zinc deficiency after BS cannot be concluded from this review, the onset of both at approximately 6 months may suggest an indirect link between the two. Further interventional studies are required to confirm causal link. Importantly, current evidence has shown improvements in taste function, for patients with taste dysfunction, after receiving 30–45 mg of zinc supplementation daily over 6 months [ 35 , 38 , 39 , 40 , 41 , 42 , 43 , 45 ]. This is twice the dosage usually prescribed to patients following BS. This suggests that zinc is an effective treatment for taste change, in many cases of taste disorder induced by different diseases. Yet studies into taste change in cancer patients found no significant improvement after similar doses of zinc supplementation. This may be explained by several interpretations. First, a small sample size makes it difficult to detect a significant difference between the placebo and intervention groups. Second, a lack of follow-up assessment may influence the outcome. Third, the absence of a formal taste test may impact the results. Fourth, mucositis and oral infections may also influence taste changes—as may other medications taken by cancer patients—and may also be important factors in delayed zinc intervention. It is also suggested that routine zinc supplementation after BS is not effective to avoid taste change and that patients require a double dosage, based on above-mentioned studies. We therefore believe that results from this review are in agreement with another review [ 9 ], stating that zinc supplementation may potentially be used to treat taste disorders.
A high-quality RCT of 40 subjects with obesity found that 30 mg/day of zinc supplementation for 15 weeks with a restricted calorie diet of almost 300 kcal lower than the estimated energy requirements had a positive impact in weight reduction and appetite; supplemented group with zinc had lower appetite and more weight reduction comparing with placebo group which follow only restricted calorie diet [ 51 ].
This review also found that patients who experiences taste change had higher % EWL comparing with those with no taste change. In agreement with these findings, Makaronidis, Neilson [ 52 ] found a significant correlation between taste change and percent of weight loss following RYGB: 27.8%, n = 3, comparing to 23.1%, n = 35, with no taste change, confounding factors including procedure and patient’s selection and questionnaire design may have influence these findings [ 52 ]. Further studies on the relationship between taste change and % EWL with comparison between different procedures are needed. A recent study suggests that subjects with high % EWL had a significantly decrease preference to sweet-tasting food following surgery [ 53 ]. Taste alteration also has been linked to ageing. Around the age of 60, the ability to taste gradually decreased [ 54 ]. In this review, elderly people with taste disorder showed improvements of taste sensitivity after receiving zinc supplementation [ 40 , 44 ]. However, the age-related taste change after bariatric surgery has not previously been described in the current studies. This may constitute the object of future studies.
Strengths and Limitations
This review objectively examines the effects of zinc deficiency on taste change after BS. Several limitations need to be highlighted which may influence the interpretation derived from studies obtained from this review. One of the limitations of this study is the heterogeneity of the studies’ design, including most of the cohort and RCT studies. Another limitation is the lack of studies directly examining the effect of zinc on taste change, following BS. Lastly there are some caveats to interpreting circulating zinc level. All the studies measure plasma/serum level of zinc. While this may provide marker of patients circulating zinc level, it may not accurately reflect patients zinc status, especially in non-fasting state [ 55 ]. In addition, zinc concentrations are depressed by infection and inflammation [ 56 ]. Thus, plasma zinc concentrations will potentially overestimate the extent of zinc deficiency in the setting of infection or inflammation.
Recommendations and Implications
Future studies into zinc deficiency following BS should examine zinc absorption and compare the degree of absorption in different types of BS.
More RCTs are needed to examine the effectiveness of zinc in taste change treatment following bariatric surgery to obtain more reliable results.
Studies to link taste change with zinc levels to weight loss or weight regain
More RCTs are required to examine the association between taste change and saliva flow rate, leading to more reliable results.
Time, dosage, and duration of supplementation need further investigation. RCTs into the effectiveness of zinc in taste change treatment used zinc supplementation of around 45–50 mg, 2 or 3 times per day, and found it effective in taste change recovery. However, in current clinical practice, patients still receive only between 8 and 15 mg of zinc supplementation.
In conclusion, although causal link cannot be established, zinc deficiency appears to be linked with taste change following bariatric surgery. Supplementation with much higher doses of zinc—45–50 mg—has been effective in taste change treatment for a period of 3–6 months, for many cases of taste disorder. The results of this review are in agreement with the updated BOMSS guidelines that BS patients need high dose of zinc supplements and that zinc deficiency occurs most frequently after duodenal switch (DS). Yet routine multivitamin and mineral supplementation prescribed to patients following BS, in current clinical practice, are currently ineffective at avoiding zinc deficiency following BS. It is therefore also ineffective at avoiding taste change. Overall, taste change is more frequent after RYGB procedure comparing to other types of BS procedures. More research is crucial, to explore the association between zinc deficiency and taste change following BS—as well as the effect of BS in zinc absorption—and to ensure that BS patients are being given the level of zinc supplementation they require.
Abbreviations
Adjustable gastric banding
Biliopancreatic diversion
- Bariatric surgery
Duodenal switch
Excessive weight loss percentage
Gastric bypass
Laparoscopic adjustable gastric banding
Laparoscopic Roux-en-Y gastric bypass
Laparoscopic sleeve gastrectomy
National Health Service
Roux-en-Y gastric bypass
Sleeve gastrectomy
British obesity and metabolic surgery society
Picot J, Jones J, Colquitt JL, et al. The clinical effectiveness and cost-effectiveness of bariatric (weight loss) surgery for obesity: a systematic review and economic evaluation. Health Technol Assess. 2009;13(41):1–190 ( 215-357, iii-iv ).
Article CAS Google Scholar
Angrisani L, Santonicola A, Iovino P, et al. Bariatric surgery worldwide 2013. Obes Surg. 2015;25(10):1822–32.
Commission CQ. 2018 Adult inpatient survay: statistical release. UK: www.cqc.org.uk ; 2019. Acessed 9/9/2022
Ahmed K, Penney N, Darzi A, et al. Taste changes after bariatric surgery: a Systematic Review. Obes Surg. 2018;28(10):3321–32.
Article Google Scholar
Graham L, Murty G, Bowrey DJ. Taste, smell and appetite change after Roux-en-Y gastric bypass surgery. Obes Surg. 2014;24(9):1463–8.
Tichansky DS, Boughter JD Jr, Madan AK. Taste change after laparoscopic Roux-en-Y gastric bypass and laparoscopic adjustable gastric banding. Surgery for obesity and related diseases : official journal of the American Society for Bariatric Surgery. 2006;2(4):440–4.
NIDCD. Taste Disorders [Internet]. NIDCD; 2017 [updated May 12, 2017. https://www.nidcd.nih.gov/health/taste-disorders . Accessed 18 Nov 2022
Ikeda M, Hirai R, Shigihara S, et al. Taste disorders and zinc deficiency. International Journal of Oral-Medical Sciences. 2008;6(3):105–11.
Yagi T, Asakawa A, Ueda H, et al. The role of zinc in the treatment of taste disorders. Recent Pat Food Nutr Agric. 2013;5(1):44–51.
Mahawar KK, Bhasker AG, Bindal V, et al. Zinc deficiency after gastric bypass for morbid obesity: a systematic review. Obes Surg. 2017;27(2):522–9.
Gibson RS, King JC, Lowe N. A review of dietary zinc recommendations. Food Nutr Bull. 2016;37(4):443–60.
O’Kane M, Parretti HM, Pinkney J, et al. British Obesity and Metabolic Surgery Society guidelines on perioperative and postoperative biochemical monitoring and micronutrient replacement for patients undergoing bariatric surgery-2020 update. Obes Rev. 2020;21(11): e13087.
Shatzman AR, Henkin RI. Gustin concentration changes relative to salivary zinc and taste in humans. Proc Natl Acad Sci USA. 1981;78(6):3867–71.
Naganuma M, Ikeda M, Tomita H. Changes in soft palate taste buds of rats due to aging and zinc deficiency–scanning electron microscopic observation. Auris Nasus Larynx. 1988;15(2):117–27.
Burge JC, Schaumburg JZ, Choban PS, et al. Changes in patients’ taste acuity after Roux-en-Y gastric bypass for clinically severe obesity. J Am Diet Assoc. 1995;95(6):666–70.
Zerrweck C, Zurita L, Álvarez G, et al. Taste and olfactory changes following laparoscopic gastric bypass and sleeve gastrectomy. Obes Surg. 2016;26(6):1296–302.
Wang J-L, Yang Q, Hajnal A, et al. A pilot functional MRI study in Roux-en-Y gastric bypass patients to study alteration in taste functions after surgery. Surg Endosc. 2016;30(3):892–8.
El Labban S, Safadi B, Olabi A. Effect of Roux-en-Y gastric bypass and sleeve gastrectomy on taste acuity and sweetness acceptability in postsurgical subjects. Nutrition (Burbank, Los Angeles County, Calif). 2016;32(11–12):1299–302.
Nance K, Eagon JC, Klein S et al. Effects of sleeve gastrectomy vs. Roux-en-Y gastric bypass on eating behavior and sweet taste perception in subjects with obesity. Nutrients. 2017;10(1):18.
Gero D, Dib F, Ribeiro-Parenti L, et al. Desire for core tastes decreases after sleeve gastrectomy: a single-center longitudinal observational study with 6-month follow-up. Obes Surg. 2017;27(11):2919–26.
Ruz M, Carrasco F, Rojas P, et al. Zinc absorption and zinc status are reduced after Roux-en-Y gastric bypass: a randomized study using 2 supplements. Am J Clin Nutr. 2011;94(4):1004–11.
Papamargaritis D, Aasheim ET, Sampson B, le Roux CW. Copper, selenium and zinc levels after bariatric surgery in patients recommended to take multivitamin-mineral supplementation. 2015;31: 167–172).
Balsa JAB-C, Gomez-Martin JI, Peromingo JM, et al. Copper and zinc serum levels after derivative bariatric surgery: differences between Roux-en-Y gastric bypass and biliopancreatic diversion. Obesity Surgery. 2011;21(6):744–50.
Salle AD, Poirier D, Lelievre AL, et al. Zinc deficiency: a frequent and underestimated complication after bariatric surgery. Obesity Surgery. 2010;20(12):1660–70.
Billeter AT, Probst P, Fischer L, et al. Risk of malnutrition, trace metal, and vitamin deficiency post roux-en-Y gastric bypass–a prospective study of 20 patients with BMI < 35 kg/m2. Obes Surg. 2015;25(11):2125–34.
Madan AK, Orth WS, Tichansky DS, et al. Vitamin and trace mineral levels after laparoscopic gastric bypass. Obes Surg. 2006;16(5):603–6.
Rojas P, Carrasco F, Codoceo J, et al. Trace element status and inflammation parameters after 6 months of Roux-en-Y gastric bypass. Obes Surg. 2011;21(5):561–8.
Gobato RC, Seixas Chaves DF, Chaim EA. Micronutrient and physiologic parameters before and 6 months after RYGB. Surgery for obesity and related diseases : official journal of the American Society for Bariatric Surgery. 2014;10(5):944–51.
Pires LV, Martins LM, Geloneze B, et al. The effect of Roux-en-Y gastric bypass on zinc nutritional status. Obes Surg. 2007;17(5):617–21.
Dalcanale L, Oliveira CP, Faintuch J, et al. Long-term nutritional outcome after gastric bypass. Obes Surg. 2010;20(2):181–7.
Pech N, Meyer F, Lippert H, et al. Complications, reoperations, and nutrient deficiencies two years after sleeve gastrectomy. J Obes. 2012;2012:828737.
Katsogridaki G, Tzovaras G, Sioka E, et al. Hair loss after laparoscopic sleeve gastrectomy. Obes Surg. 2018;28(12):3929–34.
Khan AHS, Siddiqui J, Siddiqui SU. Efficacy of zinc sulfate on concurrent chemoradiotherapinduced taste alterations in oral cancer patients: a double blind randomized controlled trial. Pakistan Journal of Medical Sciences. 2019;35(3):624–9.
Ripamonti CZ, Brunelli E, Fulfaro C, et al. A randomized, controlled clinical trial to evaluate the effects of zinc sulfate on cancer patients with taste alterations caused by head and neck irradiation. Cancer. 1998;82(10):1938–45.
Sakai FY, Endo SS, Tomita H. Double-blind, placebo-controlled trial of zinc picolinate for taste disorders. Acta Oto-Laryngologica, Supplement. 2002;546:129–33.
Halyard MY, Jatoi A, Sloan JA, et al. Does zinc sulfate prevent therapy-induced taste alterations in head and neck cancer patients? Results of phase III double-blind, placebo-controlled trial from the North Central Cancer Treatment Group (N01C4). Int J Radiat Oncol Biol Phys. 2007;67(5):1318–22.
Lyckholm LH, Parker SP, Coyne G, et al. A randomized, placebo controlled trial of oral zinc for chemotherapy-related taste and smell disorders. J Pain Palliat Care Pharmacother. 2012;26(2):111–4.
Mahajan SK, Prasad AS, Lambujon J, et al. Improvement of uremic hypogeusia by zinc: a double-blind study. Am J Clin Nutr. 1980;33(7):1517–21.
Sakagami MI, Tomita M, Ikui H, et al. A zinc-containing compound, Polaprezinc, is effective for patients with taste disorders: randomized, double-blind, placebo-controlled, multi-center study. Acta Oto-Laryngologica. 2009;129(10):1115–20.
Ikeda MI, Komiyama A, Kobayashi A, et al. Causative factors of taste disorders in the elderly, and therapeutic effects of zinc. J Laryngol Otol. 2008;122(2):155–60.
Henkin RI, Schecter PJ, Friedewald WT, et al. A double blind study of the effects of zinc sulfate on taste and smell dysfunction. Am J Med Sci. 1976;272(3):285–99.
Heckmann SM, Hujoel P, Habiger S, et al. Zinc gluconate in the treatment of dysgeusia–a randomized clinical trial. J Dent Res. 2005;84(1):35–8.
Atkin-Thor E, Goddard BW, O’Nion J, et al. Hypogeusia and zinc depletion in chronic dialysis patients. Am J Clin Nutr. 1978;31(10):1948–51.
Stewart-Knox BJ, Simpson EE, Parr H, et al. Taste acuity in response to zinc supplementation in older Europeans. Br J Nutr. 2008;99(1):129–36.
Najafizade N, Hemati S, Gookizade A, et al. Preventive effects of zinc sulfate on taste alterations in patients under irradiation for head and neck cancers: A randomized placebo-controlled trial. J Res Med Sci. 2013;18(2):123–6.
Google Scholar
Marsicano JA, Grec PG, Belarmino LB, et al. Interfaces between bariatric surgery and oral health: a longitudinal survey. Acta Cir Bras. 2011;26(Suppl 2):79–83.
Kang B-J, Shin S-H, Ye M-K. Taste disorder caused by vitamin B<sub>12</sub> Deficiency. Journal of Clinical Otolaryngology Head and Neck Surgery. 2020;31(1):45–50.
Poitou Bernert C, Ciangura C, Coupaye M, et al. Nutritional deficiency after gastric bypass: diagnosis, prevention and treatment. Diabetes Metab. 2007;33(1):13–24.
Krebs NF. Overview of zinc absorption and excretion in the human gastrointestinal tract. J Nutr. 2000;130(5S Suppl):1374s-s1377.
Ruiz-Tovar JLC, Zubiaga L, Boix E. Maintenance of multivitamin supplements after sleeve gastrectomy. Obesity Facts. 2016;9:311.
Khorsandi H, Nikpayam O, Yousefi R, et al. Zinc supplementation improves body weight management, inflammatory biomarkers and insulin resistance in individuals with obesity: a randomized, placebo-controlled, double-blind trial. Diabetol Metab Syndr. 2019;11:101.
Makaronidis JM, Neilson S, Cheung WH, et al. Reported appetite, taste and smell changes following Roux-en-Y gastric bypass and sleeve gastrectomy: effect of gender, type 2 diabetes and relationship to post-operative weight loss. Appetite. 2016;107:93–105.
Papasavas P, Swede H, Stone A, et al. Successful weight loss post bariatric surgery associates with greater affinity for healthy dietary and activity behaviors—preliminary support from a case-controlled study. Surgery for Obesity and Related Diseases. 2015;11(6):S170–1.
Schiffman SS. Taste and smell losses in normal aging and disease. JAMA. 1997;278(16):1357–62.
Abdulla M. How adequate is plasma zinc as an indicator of zinc status? Prog Clin Biol Res. 1983;129:171–83.
CAS Google Scholar
Wieringa FT, Dijkhuizen MA, West CE, et al. Estimation of the effect of the acute phase response on indicators of micronutrient status in Indonesian infants. J Nutr. 2002;132(10):3061–6.
Download references
Acknowledgements
This study was supported by the Medical Research Council (grant numbers MR/R502364/1, MR/P021220/1) and the National Institute for Health Research (NIHR), Nottingham Biomedical Research Centre (BRC).
Author information
Authors and affiliations.
MRC-Versus Arthritis Centre for Musculoskeletal Ageing Research, National Institute for Health Research Nottingham Biomedical Research Centre, Clinical, Metabolic and Molecular Physiology, University of Nottingham, Royal Derby Hospital, Uttoxeter Road, Derby, DE22 3DT, UK
Boshra Mozaffar & Iskandar Idris
Applied Medical Sciences, Clinical Nutrition Department, Jazan University, Jazan, Kingdom of Saudi Arabia
Boshra Mozaffar
You can also search for this author in PubMed Google Scholar
Contributions
Both II and BM were responsible for the design and final contents. BM drafts the initial manuscript, and all authors have read and approved the final version of the manuscript.
Corresponding author
Correspondence to Iskandar Idris .
Ethics declarations
Informed consent.
Not applicable as this is a review article.
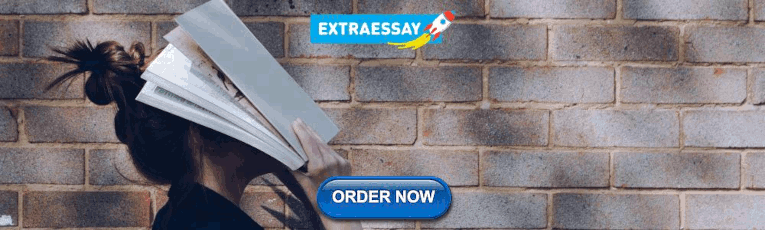
Human and Animal Rights and Informed Consent
Not applicable.
Conflict of Interest
The authors declare no competing interests.
Additional information
Publisher's note.
Springer Nature remains neutral with regard to jurisdictional claims in published maps and institutional affiliations.
Taste change is a common complication after bariatric surgery. However, the causes of this change still not clear. Zinc deficiency may play important role in taste change after bariatric surgery.
1. Taste change is a common side effect after bariatric surgery (BS); however, the mechanism is unclear.
2. Zinc deficiency is another side effect reported after BS.
3. Numerous investigators have examined the effect of zinc replacement to improve taste change. We therefore hypothesize a possible connection between taste change and zinc deficiency after bariatric surgery.
4. Our analysis did not support a causal link, but worth further research on the same topic to be undertaken to establish this possible association.
Rights and permissions
Open Access This article is licensed under a Creative Commons Attribution 4.0 International License, which permits use, sharing, adaptation, distribution and reproduction in any medium or format, as long as you give appropriate credit to the original author(s) and the source, provide a link to the Creative Commons licence, and indicate if changes were made. The images or other third party material in this article are included in the article's Creative Commons licence, unless indicated otherwise in a credit line to the material. If material is not included in the article's Creative Commons licence and your intended use is not permitted by statutory regulation or exceeds the permitted use, you will need to obtain permission directly from the copyright holder. To view a copy of this licence, visit http://creativecommons.org/licenses/by/4.0/ .
Reprints and permissions
About this article
Mozaffar, B., Idris, I. A Literature Review of Taste Change and Zinc Deficiency After Bariatric Surgery: Could There Be a Causal Link?. OBES SURG 33 , 313–331 (2023). https://doi.org/10.1007/s11695-022-06197-4
Download citation
Received : 13 April 2022
Revised : 30 June 2022
Accepted : 30 June 2022
Published : 19 November 2022
Issue Date : January 2023
DOI : https://doi.org/10.1007/s11695-022-06197-4
Share this article
Anyone you share the following link with will be able to read this content:
Sorry, a shareable link is not currently available for this article.
Provided by the Springer Nature SharedIt content-sharing initiative
- Taste change
- Taste disorder
- Zinc sulphate or Zn
- Supplementation
- Micro-nutrient deficiencies
- Find a journal
- Publish with us
- Track your research

An official website of the United States government
The .gov means it’s official. Federal government websites often end in .gov or .mil. Before sharing sensitive information, make sure you’re on a federal government site.
The site is secure. The https:// ensures that you are connecting to the official website and that any information you provide is encrypted and transmitted securely.
- Publications
- Account settings
Preview improvements coming to the PMC website in October 2024. Learn More or Try it out now .
- Advanced Search
- Journal List
- v.35; 2020 Aug
Zinc at the crossroads of exercise and proteostasis
Juan diego hernández-camacho.
a Centro Andaluz de Biología del Desarrollo, CSIC-UPO-JA, Universidad Pablo de Olavide, Sevilla, 41013, Spain
b CIBERER, Instituto de Salud Carlos III, Madrid, 28000, Spain
Cristina Vicente-García
Douglas s. parsons.
c Boston University School of Medicine, Boston, MA, 02118, USA
Ignacio Navas-Enamorado
Zinc is an essential element for all forms of life, and one in every ten human proteins is a zinc protein. Zinc has catalytic, structural and signalling functions and its correct homeostasis affects many cellular processes. Zinc deficiency leads to detrimental consequences, especially in tissues with high demand such as skeletal muscle. Zinc cellular homeostasis is tightly regulated by different transport and buffer protein systems. Specifically, in skeletal muscle, zinc has been found to affect myogenesis and muscle regeneration due to its effects on muscle cell activation, proliferation and differentiation. In relation to skeletal muscle, exercise has been shown to modulate zinc serum and urinary levels and could directly affect cellular zinc transport. The oxidative stress induced by exercise may provide the basis for the mild zinc deficiency observed in athletes and could have severe consequences on health and sport performance. Proteostasis is induced during exercise and zinc plays an essential role in several of the associated pathways.
- • Zinc deficiency could be a crucial issue in sport performance for athletes.
- • Exercise could modulate zinc serum and cellular homeostasis.
- • Zinc is part of proteostatic systems critical during exercise.
1. Introduction
1.1. zinc in human health and disease.
The first indication of the importance of zinc for biological systems dates back to 1869, when Jules Raulin demonstrated that zinc is required for the growth of Aspergillus niger [ 1 ]. Decades later, it was shown that zinc was also essential in plants [ 2 ] and animals ([ 3 ]), including humans [ 4 ]. Zinc is the second most abundant ‘trace’ metal in the human body after iron, with an average 70 kg human containing 2–3 g of zinc, with only 0.1% being replenished daily through dietary intake [ 5 ]. However, it is not homogenously distributed in the body, with the majority contained in skeletal muscle (~60%) and bone (30%) [ 6 , 7 ]. The tissue concentration of total zinc is also highly variable, as zinc is abundant in skeletal muscle, bone, prostate and pancreas (200 μg/g) [ 7 ], whereas heart, brain and plasma contain a lower concentration (1–23 μg/g). Only ~0.1% of bodily zinc is contained in plasma, where it is predominantly bound by albumin (~80%) and α2-macroglobulin (~20%) [ 8 , 9 ].
Zinc has structural and catalytic roles in proteins and cellular signalling functions [ 10 , 11 ]. Confirmation as a structural cofactor in proteins came in 1938 when insulin was crystallised with zinc [ 12 ], while the first catalytic function of zinc was described for carbonic anhydrase in 1939 [ 13 ]. Zinc has since been shown to be a cofactor in all six enzyme classes [ 14 ]. Additionally, zinc has been shown to regulate the activity of different proteins, for instance in protein tyrosine phosphatases [ 15 ]. The effects of cellular zinc signalling are an active research area given its wide implications in cell signalling.
The identification of zinc-finger domains in Xenopus laevis transcription factor TFIIIA [ 16 , 17 ] laid the foundation to search genomes for zinc proteins. Indeed, bioinformatics analyses predict that zinc proteins make up 4–5% of bacterial and archaeal proteomes [ 18 ], while mammals express more than 3000 zinc proteins, accounting for at least 9–10% of the total proteome [ 19 ]. Considering the vast amount of proteins that bind this cation, it is not surprising that zinc is involved in multiple physiological processes, including nucleic acid and protein synthesis, transcriptional regulation, glucose metabolism and hormone production, storage and secretion [ 10 ].
Zinc deficiency has detrimental consequences for many aspects of human health [ 7 ] and affects two billion humans worldwide [ 20 ]. Severe zinc deficiency leads to generalized poor growth concomitant with multiple disorders including loss of appetite, immune abnormalities, dwarfism, hypogonadism, mental lethargy and rough skin [ 4 , 21 ]. Due to its proliferative nature, the immune system is particularly susceptible to zinc deficiency [ 20 ]. Zinc regulates several crucial processes in innate immunity, including phagocytosis, intracellular killing and cytokine production, while in the adaptive immune system, zinc deficiency results in reduced T cell development and function and B cell antibody production [ 22 ]. Zinc deficiency has been also associated with development of cardiovascular diseases [ 23 ], anemia [ 24 , 25 ] and damages in neurogenesis during early development [ 26 ]. Zinc supplementation can improve these symptoms, but it must be accompanied by copper, otherwise the excess zinc can lead to copper deficiency [ 27 , 28 ]. Importantly, plasma zinc concentration cannot be used as a reliable marker of zinc status because it does not necessarily correlate with cellular zinc concentration [ 29 ]. Under multiple disorders associated with zinc deficiency, clinical improvement of these disorders associated with zinc administration could be used as indicator of zinc status and patient recovery due to plasma zinc could not be totally reliable [ 20 , 24 , 25 , 30 ].
1.2. Zinc cellular homeostasis
The human body does not have a tissue reservoir for zinc, nor does it have a protein analogous to ferritin, which stores iron and releases it when needed [ 31 ]. Therefore, an adequate dietary supply is required to prevent zinc deficiency unless a genetic condition is the root of the problem [ 32 ]. In this sense, zinc deficiency can result as symptom of diseases which impair either intestinal control of zinc uptake, for example in acrodermatitis enteropathica or cystic fibrosis [ 33 ], or intestinal release, for example in inflammatory bowel diseases such as Crohn's disease [ 34 ]. The myriad of biological processes in which zinc is required makes zinc homeostasis to be tightly controlled at the organism, tissue and cellular levels. Dietary zinc absorption is regulated by the duodenum and jejunum [ 35 ], while excretion of excess zinc is achieved through gastrointestinal secretion, sloughing of mucosal cells and integument, and renal excretion [ 36 ]. Zinc ingestion is dependent on the quantity and bioavailability of zinc in food [ 28 ]. Foods rich in zinc include red meat and pulses, while cereals containing non-digestible plant ligands such as phytate reduce zinc bioavailability [ 37 , 38 ].
Bone zinc accounts for approximately 29% of whole-body zinc, but the largest zinc pool in the body is muscle, which contains approximately 57% [ 9 ]. However, zinc is not homogeneously distributed in all muscles: it has been reported that red oxidative muscle can contain up to four-fold zinc than white glycolytic muscles [ 39 ]. The zinc pool mainly responsible for this difference is located in myofibrils and the nucleus. Intriguingly, muscle zinc levels are maintained under conditions of dietary zinc deficiency [ 6 ] except in soleus, a red muscle with high zinc content [ 40 ]. Interestingly, although growth is severely retarded in rats on a zinc-deficient diet, they do undergo an increase in muscle mass, which therefore requires an increase in total muscle zinc content [ 41 ]. This is possible because the organism can mobilize zinc from tissues with lower requirements, mainly bone, to others with higher requirements, especially muscle. This does not imply that the bone is the body reservoir for zinc, but that the organism is able prioritize in cases of extreme necessity [ 41 ].
Zinc complexes are second only to copper in their relative stability as described by the Irving-Williams series of transition divalent metal ions [ 42 ]. The high affinity of structural and catalytic protein-zinc complexes is one of the reasons for the large disparity between cellular total and free zinc; while the average total cellular zinc content is hundreds of micromolar, the free cytosolic zinc concentration is approximately picomolar [ 43 , 44 ]. The tight control of cytosolic zinc(II) ion concentrations in mammalian cells underpins the significance of zinc as a signalling ion akin to calcium and magnesium [ 45 ]. At the cellular level, the main components of zinc homeostasis regulation are zinc-binding proteins, zinc sensors, and zinc transporters. The critical function of this repertoire is to be able to deal with both long and short-term zinc stimuli [ 11 , 46 ].
1.3. Zinc-binding proteins and zinc sensors
Metallothioneins (MTs) are small, cysteine-rich cytosolic proteins [ 47 ] that in mammals can bind up to seven zinc(II) ions with differing affinity, linking the zinc occupancy of MTs with the zinc status of the cell. Indeed, during cytosolic zinc deficiency the zinc bound to low affinity sites in MTs will become displaced and available, whereas during high cytosolic zinc concentrations, zinc will occupy these low affinity sites, reducing the cytosolic free zinc. Thus, MTs can function as temporary storage for zinc [ 44 , 48 , 49 ]. Therefore, a major cytosolic store of zinc is bound by cysteine residues, meaning that cellular zinc homeostasis is inextricably linked to the redox state of the cell [ 11 ], despite zinc itself being redox neutral. In humans, MT-1 and MT-2 are ubiquitously expressed and their expression levels respond to different stimuli such as metal ions, cytokines, glucocorticoids and oxidative stress. MT-3 and MT-4 are found only in the central nervous system and epithelia respectively [ 47 , 50 ]. Special mention should be made to metal response element-binding transcription factor-1 or MTF-1, to date the only zinc sensor described in mammals [ 48 ]. When intracellular zinc concentration is high, MTF-1 suffers a conformational change upon zinc binding to its zinc-fingers. This allows MTF-1 binding to dedicated regulatory elements called MREs (metal response elements) located at the promoters of specific genes inducing their expression. MTs are among these genes and therefore, they can respond rapidly to changes in cellular zinc status [ 51 , 52 ].
1.4. Zinc transporters
Zinc transporters are proteins which enable the flux of Zn 2+ across biological membranes. The two families of zinc transporters expressed in mammals are the Zrt/Irt-like proteins (ZIPs; SLC39A ) and the zinc transporters (ZnTs; SLC30A ), which function to transport zinc into and out of the cytosol respectively [ 53 ]. In humans there are 14 ZIPs and 10 ZnTs, each with specific tissue expression and subcellular localisation [ 54 ].
2. Zinc and skeletal muscle
2.1. effects of zinc deficiency in skeletal muscle.
As stated above, zinc levels are maintained in skeletal muscle even if zinc intake is low. However, zinc deficiency does have a negative impact on muscle function, highlighting the biological relevance of small changes in the status of this cation. The first evidence towards this fact was reported in the late 197 0s, when a study found that zinc deficiency per se in post-weanling rats had little effect on the number and size of fibers, except for the soleus muscle, which showed an overall reduction in weight as a result of increased fiber loss [ 40 ]. The authors hypothesized that soleus muscles might be more susceptible to changes in zinc concentration because of their higher zinc content in comparison to more glycolytic muscles [ 40 ]. This result was confirmed by a second study, which also found that the proportion of fast to slow fiber types was increased in rats fed on a zinc-deficient diet with respect to ad libitum or pair-fed controls on a normal zinc diet [ 55 ]. This suggests that this phenotype would not be merely caused by the reduced food intake induced by inadequate zinc levels. Importantly, rat neonate soleus muscles contain a high proportion of fast fibers that turn into the slow type as the animal grows [ 55 ]. Thus, the authors hypothesized that zinc deficiency could specifically cause a defect in development or differentiation [ 55 ]. Similarly, the diaphragms of the zinc-deficient group also showed altered fiber type composition compatible with a perturbation in development [ 55 ]. It is important to mention that the development of the mature muscle fiber type composition partially depends on motor neuron input during pre- and post-natal development [ 56 , 57 ], and zinc may impinge in this process. Indeed, motor neurons differentiated from human induced stem cells under low zinc levels exhibit altered synaptic function [ 58 ]. Whether zinc also plays a role in motor neuron development in vivo remains to be elucidated. Still, animal models of zinc deficiency show apparent stiffness of the joints and abnormal gait, specially the growing guinea pig [ 59 ] and chick ([ 60 ]), as well as severely deficient rats [ 61 ]. These phenotypes could derive from defects in muscle, nerve or the neuromuscular junction (NMJ) alone or in combination. Thus, the alterations in fiber type composition observed upon dietary zinc deficiency in rats might be partially due to defects in motor neuron-muscle communication.
Zinc deficiency reduces the activity of some, but not all, metalloenzymes [ 10 ]. Further, the same metalloenzyme could be affected in some tissues but not in others. For example, lactic dehydrogenase activity was found to be significantly reduced in heart, kidney and gastrocnemius muscle of zinc deficient rats, whereas no changes were observed in pancreas or lung [ 62 ]. In the same experiment, the activity of mitochondrial glutamic dehydrogenase was not altered in any of the tissues analysed [ 62 ]. Thus, zinc deficiency does not affect all zinc-proteins equally. The decrease in the activity of zinc metalloenzymes could be due to the absence of appropriate amounts of zinc to use as a cofactor, or simply because the concentration of these proteins is reduced, either due to a reduction in their gene expression or to an increase in their degradation rate [ 63 ]. Therefore, even if zinc levels are mostly maintained in muscle in conditions of dietary zinc deficiency, muscle function is impaired to some extent due to the decline in the activity of specific zinc proteins.
Indeed, after the realization that zinc is necessary for the correct functioning of thousands of proteins in the organism, most experiments in the postgenomics era rely on the removal or downregulation of specific genes to unravel their function. Only in this manner, specific molecular mechanisms can be deciphered. This is in contrast with the majority of the experiments described above in the 70s and 80s, which characterize the general effects dietary zinc deficiency exerts on muscle biology and function as a whole. In accordance, these type of experiments are only applied nowadays when the experimenter is interested in the final outcome of performance of the organism, as in the case of the effect of zinc diet on exercise (see below).
2.2. Zinc functions in relation to skeletal muscle
Ex vivo muscle testing has been extensively used over decades to assess muscle properties, such as contractility, more accurately than with experiments in vivo . With this technique, it was found that zinc reversibly blocks synaptic transmission at the NMJ [ 64 ], and prolongs the active state of the frog's sartorius muscle but has no effect on its maximal intensity [ 65 ]. Additionally, it increases the absolute refractory period of the most excitable fibers: it takes them four times longer to initiate a second contraction than in the absence of zinc in the medium [ 65 ]. Considering the shape of the action potentials, the authors hypothesized that zinc probably delays the exit of K + ions during repolarization [ 65 ], which was later confirmed in the same experimental setting [ 66 ]. We now know that zinc regulates the activity of several ion channels, including acetylcholine signalling [ 67 ], causing the activation or inhibition of the corresponding ion currents, depending on zinc concentration and binding location [ 68 , 69 ].
Zinc has been shown to play a major role in myogenesis in vitro . For example, in the C2C12 mouse skeletal muscle cell line it was shown that addition of zinc in the growth medium promoted the proliferation and activation of myoblasts [ 70 , 71 ], as well as the differentiation and maturation of myofibers later in the process [ 70 ]. This raises the interesting possibility that the release of zinc from muscle upon damage in vivo could contribute to the activation and proliferation of muscle satellite cells to repair the damage [ 71 ]. Mechanistically, zinc would exert these functions through a cascade of events that initiates with the entry into the cell through dedicated ZIP transporters. Once in the cytosol, it would induce the phosphorylation and subsequent activation of Zip7, a zinc transporter localized to the endoplasmic reticulum. Activated ZIP7 releases Zn 2+ from the ER, promoting Akt phosphorylation and myogenic differentiation [ 70 ].
More molecular insight into how zinc impinges on myogenesis came recently from Padilla-Benavides’ group, who sought to fully characterize the zinc content and the expression of zinc transporters throughout the course of differentiation of C2C12 cells [ 72 ]. They showed that zinc content is dynamic during myoblast differentiation: it initially decreases but starts recovering after 12 hpd (hours post-differentiation) until it reaches a maximum in mature myotubes at 72 hpd. This dynamism is also observed in the expression of zinc transporters. Regarding the influx transporters, while the expression patterns of Zip5 and Zip6 exhibit a single discrete peak at 12 hpd, Zip3 and Zip8 are upregulated throughout the whole process. Of note, it has been shown that Zip8-mediated zinc influx promotes MTF-1 transcriptional activity, which in turn upregulates the expression of matrix metalloproteinases (MMPs) in chondrocytes [ 73 ]. The same mechanism could take place during myogenesis as MMPs are required for myotube fusion [ 74 ]. In relation to ZnT efflux proteins, it is interesting to note that the expression of ZnT4 , which is localized in endosomes and secretory vesicles, and of ZnT7 , which is localized in the Golgi apparatus, peak at 12 hpd. Importantly, the activity of the insulin-signalling pathway is downregulated in mouse skeletal muscle deficient for ZnT7 [ 75 ]. Furthermore, ZnT8 was the sole transporter to become downregulated after the onset of myogenesis, and its expression was only restored after differentiation [ 76 ]. Interestingly, ZnT8 is involved in the regulation of insulin secretion in pancreatic beta cells [ 76 ]. Hence, data on ZnT7 and ZnT8 can provide a link between zinc and insulin signalling pathways during myogenesis. Intriguingly, Zip11 accumulates late, at 72 hpd [ 76 ]. This is the only zinc transporter known to be localized to the nuclear membrane (it can also be found in the Golgi apparatus) , so it would be interesting to explore the potential influence of zinc inside the nucleus in mature myotubes in the future.
Further evidence accumulates for zinc biology contributing to myogenesis and the control of muscle mass. The ablation of MT-1 and MT-2 in mice causes a hypertrophic phenotype that is more pronounced in fast-twitch muscles and that correlates with an increase in muscle strength [ 77 ]. Yet, total intracellular zinc content remained constant in these mice [ 77 ] MT-1 and MT-2 are also upregulated in skeletal muscle atrophy models [ 78 ] and in sarcopenia [ 77 ], where total intracellular zinc content is increased as well. It is important to mention that none of these studies measured the concentration of free intracellular zinc. A change in zinc content could lead to the deregulation of specific signalling pathways and explain the observed phenotypes. Indeed, while the measurement of total intracellular zinc (free zinc and that bound to proteins) is relatively straightforward, the quantification of the free zinc pool, which relies on fluorescent approaches, is much more challenging [ 45 ]. Although these data suggest that MTs and zinc control muscle mass, the exact mechanisms remain unknown due to the complex interplay between zinc binding to MTs, which depends on zinc concentration and the redox state of the cell, the fact that zinc induces the expression of these proteins, the potential appearance of compensatory mechanisms for zinc buffering when the expression of MTs is compromised and the difficulty in measuring free intracellular zinc content. On the other hand, it was recently shown that the zinc transporter Zip14 is upregulated in cancer-associated atrophic muscles (cachexia), which leads to an excess total intracellular zinc content [ 79 ]. By employing muscle progenitor cells in vitro , the authors demonstrated that the Zip14-mediated increase in zinc uptake directly promoted muscle wasting through myosin heavy chain loss and impaired regeneration [ 79 ]. Altogether, these findings suggest that MT-1/2 and Zip14 could be used as targets to revert or ameliorate those conditions that involve loss of skeletal muscle mass.
Regarding cellular metabolism, zinc acts as an insulin mimetic agent leading to increased total glucose consumption in mouse, rat and human skeletal muscle cells in vitro [ 80 , 81 ]. Mechanistically, Zip7 could contribute to this function. Indeed, its expression is induced by glucose and repressed in conditions mimicking insulin resistance in C2C12, as well as in vivo in mice fed on a high fat diet [ 82 ]. Further, Zip7 levels have important consequences for cellular metabolism. Its silencing in vitro causes the downregulation of the expression of genes involved in glucose and glycogen metabolism, as well as to a decrease in Akt-mediated signalling [ 82 , 83 ]. As a result, in the absence of Zip7, cells become insulin resistant. On the contrary, Zip7 overexpression triggers the induction of the expression genes involved in insulin signalling and glucose metabolism, including insulin itself [ 82 , 83 ]. Taking these data together, Zip7 contributes to glycemic control in skeletal muscle cells. However, whether Zip7 expression levels really influence zinc cytoplasmic content remains to be explored.
Cardiotoxin (CTX) is a cytolytic toxin from snake venom that is widely used to study muscle regeneration in animal models. CTX selectively kills myofibers while leaving satellite cells unaffected [ 84 ]. After CTX-induced injury, satellite cells are activated, proliferate, differentiate and fuse to restore muscle structure and function. In mice , both marginal zinc deficiency or excessive zinc intake (8 or 190 mg Zn2+/kg diet, respectively, relative to a zinc-adequate diet of 35 mg Zn2+/kg diet) delay muscle recovery from CTX-induced injury [ 85 ]. The authors hypothesized that this delay was the result of the reduction in muscle protein synthesis observed under a zinc-deficient or zinc-supplemented diet [ 63 ]. However, given that zinc promotes the proliferation and activation of C2C12 cells in vitro [ 70 , 71 ], it would be worth exploring whether satellite cells are affected in this setting. Altogether, this finding could have profound implications for the general population but especially for athletes, who often incur muscle injuries: it is important to take appropriate amounts of zinc to prevent the impairment of muscle regeneration.
3. Zinc and its importance in exercise
Skeletal muscle is a tissue whose main function is contraction, force and movement production and involves a complex mix of compositions, structures and functions. It is constituted of several bundles of highly vascularized and innervated myofibers [ 86 ]. Skeletal muscle has been defined as a malleable tissue that is modulated by exercise where reactive oxygen and nitrogen species regulate adaptation training responses [ 87 ]. Physical exercise is considered a critical tool to prevent and treat muscle abnormalities [ 88 ]. Additionally, exercise is considered to be a polypill for health maintenance and recovery due to its potential to reduce risks and damage from chronic diseases, its easy availability and lack of adverse effects [ 89 ]. Furthermore, exercise helps to maintain muscle mass promoting healthy aging and reduced sarcopenia and has been proven to be a perfect toolkit for prevention and treatment of a wide variety of diseases [ 90 ].
3.1. Modulation of serum and urine zinc by exercise
Exercise has been proven to activate different metabolic pathways modulating the levels of many metabolites and minerals including zinc ( Table 1 ). Acute aerobic endurance and muscular strength exercise decrease zinc serum levels while increasing urinary zinc, especially in case of exercise until exhaustion [ [91] , [92] , [93] ]. These changes in zinc levels have been found during the recovery period, so it could be related with the muscle repairing processes that take part during this phase. However, immediately after exercise, increased zinc serum levels can be observed [ 95 ].
Effect of exercise on zinc homeostasis in serum and urine.
In contrast, after a chronic exercise intervention zinc serum levels were increased in humans [ 101 ] and animal models [ 102 ]. It is obvious that modulation of zinc levels by exercise are influenced by the duration of exercise intervention (chronic or acute). Even living an active or sedentary lifestyle influences basal zinc serum levels [ 104 ].
Zinc levels fluctuations during exercise could be related with changes in the antioxidant response stimulated by exercise. Zinc is involved in the antioxidant response to exercise through changes in expression of copper zinc superoxide dismutase (CuZnSOD) [ 105 ] and this response could be modulated by exercise and resting [ 105 , 106 ]. The antioxidant response promoted by exercise could vary in function of the sport modality due to differences in erythrocyte zinc, CuZnSOD activity and MT [ 107 ]. Zinc could be playing an essential role in antioxidant response to exercise by inhibiting free radical production, increasing antioxidant activity and preventing muscle exhaustion [ 108 ]. Zinc administration has been examined in pulmonary rehabilitation [ 109 ] in combination with exercise obtaining an increase in muscle strength and a tendency of higher type I fibre proportion. Zinc administration in combination with exercise could improve lipid profile decreasing total cholesterol, LDL-cholesterol and triglycerides and increase HDL cholesterol [ 110 ].
It has been proposed that zinc deficiency could be leading to higher levels of lipid peroxidation in different tissues while zinc administration produces an increase in glutathione levels in different tissues resulting in a higher antioxidant capacity [ 111 ]. Moreover, zinc deficiency has been related with a lower DNA integrity, more oxidative stress and altered DNA repair capacity [ 103 ]. Particularly in sport performance zinc deficiency has been associated with a lower peak oxygen uptake, carbon dioxide output and respiratory exchange ratio [ 112 ] and with a reduction in total work capacity of skeletal muscle [ 113 ]. Zinc deficiency in physically active men leads to a reduction carbonic anhydrase activity in red blood cells, impairing metabolic responses during exercise, and contributing to a lower physical performance observed in conditions of dietary zinc deficiency [ 112 ]. Zinc depletion in humans leads to a decrease in total work capacity for knee and shoulder extensors and flexors and a reduction in physical performance that correlates with decreased plasma zinc [ 113 ]. Zinc levels could have been maintained in muscle due to the compensatory effects from high zinc content tissues where zinc does not play a key role such as bone, highlighting the capacity of the organism to prioritize and mobilize zinc from different tissues to others [ 41 ]. The reason by which muscle performance was not maintained under zinc depletion could be related to lactic acid accumulation due to lactate dehydrogenase activity being reduced under zinc depletion leading to lactic acid increase and muscle fatigue [ 113 ]. Zinc deficiency is common in athletes in different training periods [ 114 ].
3.2. Effectiveness of zinc supplementation on exercise
Zinc deficiency constitutes a crucial issue for professional athletes because it could affect sport performance and health. Although zinc deficiency is a serious issue, there is not experimental evidence that support the possible benefits on zinc administration beyond recommended dietary intakes as a potential supplement for athletes, thus extra zinc for athletes would not report more beneficial effects [ 115 , 116 ] or enhance training adaptations [ 117 ]. Consequently, athletes should be discouraged to ingest extra zinc supplementation because studies that determine the effect of zinc supplementation in athletes beyond their recommended daily intakes levels in sport performance are lacking. The effect of extra zinc administration in this specific situation remains unclear further long human trials studies are required [ 51 ]. In agreement, it has been shown that the organism is able to make some adjustments in zinc excretion, in order to maintain a balanced supply to all tissues according to their specific demands, therefore extra zinc would be excreted [ 118 ]. Moreover, overdose of zinc could trigger side effects including nausea, abdominal cramping, vomiting, tenesmus, diarrhea, copper deficiency, even teratogenic or lethal effect during early embryogenesis [ 28 ].
Taking all these data into account, zinc could be involved in antioxidant response activated by exercise, therefore zinc deficiency in athletes could have consequences for metabolic adaptations modulated by exercise and it could induce negative effects to sport performance, exercise adaptation and affecting the long-term health of the athlete.
3.3. Modulation on cellular zinc levels by exercise
Exercise could modulate changes in serum and urine zinc levels. However, it should also be considered that these changes in zinc serum levels do not necessarily mean the same regulation in cellular free zinc levels. Both finding appropriate zinc biomarkers and measuring the approximately picomolar intracellular free zinc 2+ concentrations are ongoing challenges in zinc biochemistry [ 45 ]).
Particularly in cardiac cells [ 119 ], exercise induces RNA levels of metallothioneins (MT1 and MT2), while mice lacking MT1/2 exhibit cardiac dysfunction during exercise. Exercise could also stimulate MT levels in aortic tissues [ 120 ], even an increase has been reported specifically in muscle due to exercise [ 121 ].
Moreover, the effect of exercise on zinc homeostasis extend beyond muscle, stimulation of MT1 and MT2 by exercise has been shown using an in vitro model applying electric pulse stimulation in hepatocytes [ 122 ]. Additionally, exercise improved the spatial learning abilities of rats [ 123 ]. Interestingly, an upregulation of different ZnTs (ZnT2, ZnT4, ZnT5, ZnT6 and ZnT7) in hippocampus was reported indicating a potential effect of exercise on zinc cellular homeostasis [ 123 ]. Exercise could enhance the transport of cytosolic zinc to cellular organelles. Moreover, they also reported an increase in zinc intracellular transporters, specifically ZIP transporters (ZIP6 and ZIP7) was found, while MT1 and MT3 levels were also increased in hippocampus [ 123 ]. Additionally, exercise has been shown to promote activation of MTs, especially MT1, MT2 and MT3 in mouse spinal cord [ 124 ]. After two weeks of running 30 min per day, an increase in mRNA and proteins levels of MTs in spinal cord were found compared with sedentary controls [ 124 ]. Another study reported an increase in MTs levels in response to exercise in rat renal tubes suggesting a protective role against oxidative stress and apoptosis in proximal tubular cells [ 125 ].
Exercise is associated with oxidative stress production in muscle producing lipids and proteins oxidation [ 126 ]. As it has been discussed above, exercise could lead to a cytosolic zinc levels increase that could also promote zinc accumulation in mitochondria [ 127 ]. An abnormal mitochondrial zinc accumulation associated with over-exercise could be associated with higher oxidative stress production resulting in mitochondrial dysfunction in vascular smooth muscle cells [ 127 , 128 ]. Zinc accumulation could be regulating mitochondrial function affecting ZnTs, ZIPs and MTs homeostasis [ 127 ]. Consequently, zinc levels changes related to exercise could be involved in oxidative stress production.
On balance, exercise could stimulate zinc cellular pathways promoting zinc uptake into the cell and zinc storage in different proteins and cellular organelles (through ZnTs and MTs regulation) as it is shown in Fig. 1 thus this process is altered in athletes that present zinc deficiency leading to alterations that could affect health and sport performance.

Cellular Zinc homeostasis modulated by exercise.
An overview of Zinc cellular homeostasis modulated by exercise. Exercise induces Zn2+ celular input through ZIP transporters, upregulating Zn2+ input to nucleus and different organelles. High celular Zn2+ levels incite MTs Zn accumulation.
4. Zinc and protein degradation
4.1. proteostasis.
Proteostasis is the collective term for the mechanisms mediating the correct synthesis, folding, trafficking and degradation of proteins. These networks are comprised of various protein chaperones, degradation complexes and stress-response pathways. The balance between protein synthesis and protein degradation determines whether muscle increases or decreases in mass. A key component of muscle metabolism is the correct recognition and repair or degradation of misfolded or otherwise damaged proteins, allowing muscle remodelling, training adaptation and increased muscle mass and as Fig. 2 illustrates exercise could influence protein turnover. Proteolysis provides amino acid precursors for maintenance of proteostasis and is mediated through the autophagy-lysosome, calpain, caspase, and ubiquitin-proteasome system (UPS). These systems are interlinked and act together to regulate muscle proteolysis in response to nutrition and mechanical force during exercise [ 129 ]. Moderate exercise training improves muscle atrophy induced by impaired skeletal muscle autophagy and proteostasis [ 130 ]. Additionally, exercise enhanced protein turnover even when mechanisms to maintain proteostasis and protein degradation are impaired [ 131 ].

Proposed and reviewed effects of exercise on the proteasomal machinery. The bibliography reviewed suggests that there is more protein turnover with exercise to facilitate recovery and zinc may be implicated in the exercise-induced up regulation of degradation pathways.
4.2. Autophagy
Autophagy is a system of protein degradation that is the primary mechanism through which larger proteins, protein aggregates and organelles are degraded by the cathepsin protease family in the lumen of lysosomes. These proteases can degrade specific muscle proteins (e.g. troponin T, myosin heavy chain and tropomyosin) [ 132 ]. While animal models suggest that the majority of muscle protein degradation occurs via the UPS [ 129 ], autophagy is purported to be responsible for degrading membrane-bound receptor proteins which are critical for muscle remodelling [ 133 ]. Exercise has shown to increase markers of autophagy and autophagy capacity in skeletal muscle during the recovery period, therefore exercise could modulate autophagy [ 133 ]. During autophagy, translocation of substrates into the lysosome lumen is mediated through macroautophagy, microautophagy or chaperone-mediated autophagy.
Zinc is critical for basal and induced autophagy. In vitro studies have shown that supplementing culture medium with zinc enhances basal [ 134 , 135 ] and induced autophagy in cultured cells, particularly induced by various exogenous stressors including hydrogen peroxide in astrocytes [ 136 ], ethanol in human hepatoma cells [ 135 ], and tamoxifen in MCF7 breast cancer cells [ 137 ]. These studies also showed a decrease in basal and induced autophagy when cells were incubated with the zinc chelator TPEN or Chelex-100.
Zinc is involved in regulating both the initiation of autophagy (early autophagy) and the protein degradation in the lumen of the autolysosome (late autophagy). The mechanisms through which zinc may regulate early autophagy are not fully elucidated.
However, zinc regulation of phosphorylation of extracellular-signal-regulated kinases (ERK1/2) is required for its effects on autophagy [ 135 , 137 ], zinc supplementation in cells induced ERK activation, autophagy and cell death while chelation of zinc2+ blocks the increase in ERK phosphorylation reducing cell death and autophagic vacuoles formation [ 137 ]. Particularly, zinc administration that promotes ERK activity could modulate Beclin expression inducing autophagy [ 138 ]. ERK1/2 has been shown to promote the dissociation of the mTORC1 from the ULK1/2 complex through the Beclin1-PI3K complex [ 139 ], where PI3K is specifically induced by zinc in a dose dependent manner [ 140 ]. Exercise has shown to increase phosphorylation of AMPK Thr172 and ULK Ser317 in skeletal muscle along with different markers of autophagy while phosphorylation of mTORSer 2448 and ULK Ser757 was unaffected in skeletal muscle [ 133 ]. Particularly, zinc administration in macrophages has shown to decrease mTOR phosphorylation levels and activate apoptosis markers [ 141 ]. Moreover, an increase or a decrease in a zinc finger protein in breast cancer cells actives or inhibits autophagy associated to cell death through activation or inhibition of mTOR pathway [ 142 ]. Exercise stimulates zinc incorporation into the cell as it has been discussed above promoting ERK activation and autophagy induction through ULK1/2 complex and mTORC1 dissociation.
Both Beclin1-PI3K and ULK1/2 are involved in the formation of the phagophore in mammalian cells [ 143 , 144 ]. Beclin1 protein levels were increased in skeletal muscle following moderate intensity exercise [ 133 , 145 ].
Peroxisome proliferator-activated receptor alpha (PPARα) is a nuclear receptor that works as a transcription factor that is activated in energy deficiency conditions. Exercise can upregulate autophagy markers such as Beclin1 and LC311 and induce upregulation of PPARα triggering autophagy [ 146 ]. In addition, zinc administration can stimulate PPARα under autophagy activation conditions inducing lipolysis by autophagy-mediated lipophagy through the MTF-1 DNA binding at PPARα promoter region [ 144 ].
There are also predicted to be several MTF1 binding sites in the promoter regions of ATG7 and DFCP1 [ 147 ]. Regulation by MTF1 implicates the redox-sensitive zinc binding protein metallothionein as linking oxidative stress with autophagy induction [ 147 ]. Autophagy related gene 7 (ATG7) is involved in cell degradation and recycling, the knocked-out mice for this autophagy gene develop muscular atrophy and show that autophagy is necessary during exercise to eliminate damaged mitochondria and affected muscle fibers [ 148 ] and ATG7 autophagy function could be modulated by zinc [ 149 ]. DFCP1 is involved in membrane trafficking and cell signalling during autophagy, particularly in phagophore formation from omegasome compartments [ 150 ].
In late autophagy, there is evidence that zinc is necessary for lysosomal protein degradation. Zinc-deficient rats accumulated lipofuscin in the retina, a marker of incomplete lysosomal degradation of photoreceptor proteins [ 151 ]. Lipofuscin accumulation was also noted in astrocytes extracted from MT3 knockout mice [ 136 ]. These cells also had reduced activity of acid phosphatase and neuraminidase and reduced expression of cathepsin D and L [ 136 ]. Additionally, zinc depletion induced by TPEN treatment of hepatoma cells impaired degradation of lysosomal cargo [ 135 ]. Even, high zinc doses improved autophagy in mice exposed to ethanol while zinc deficiency impaired autophagy proposing the idea that zinc is necessary for a proper autophagy [ 149 ]. Conversely, in yeast, non-specific autophagy was found to be induced under conditions of zinc depletion [ 49 ]). The authors found that the autophagic response was a protective mechanism to recycle Zn 2+ ions for use in the cell, suggesting that the relationship between cellular zinc homeostasis and autophagy is a two-way street [ 49 , 152 ].
5. Calpain/caspase
In addition, the calpain and caspase families both also contribute to muscle proteolysis [ 153 , 154 ]. Both families cleave larger myofibrillar proteins into smaller actomyosin fragments, which are then able to be degraded by the UPS [ 154 , 155 ]. These families have muscle-specific specialisation with calpain-3 (aka p94, CAPN3) highly expressed in skeletal muscle [ 156 ] and caspase-3 activity increased in skeletal muscle following a modest, short-term energy deficit [ 157 ]. In muscle, protein and gene expression levels of calpain/caspase proteolytic system components such as calpain 1 and cathepsin D have been found to be increased after exercise [ 158 ]. Additionally, calpain 3 has been identified as a required element for muscle growth after exercise [ 159 ]. In mouse muscle caspase-3 cleaves the Rpt2 and Rpt6 subunits of the 19S proteasome, increasing proteasomal activity [ 160 ]. Interestingly, caspases-3, -6, and -8 are inhibited by physiologically relevant concentrations of Zn 2+ (i.e. low nanomolar) and bind inhibitory Zn 2+ with differing stoichiometries and at different sites [ 161 ], suggesting that Zn 2+ may be liberated from caspase-3 following an energy deficit. In retinal pigment epithelial cells, zinc deficiency produces a decrease in cells viability with an activation of calpain-1, caspase-9 and caspase 3 [ 162 ] while zinc administration generates a normalization in elevated calpain and caspase 3 activities [ 163 ]. Additionally, zinc chelator used in retinal pigment epithelium human cells to induce zinc deficiency lead to calpain activation [ 164 ]. Besides, zinc administration could promote caspase activation [ 165 ]. On balance, muscle proteolysis from calpain and caspase are related with zinc.
The UPS, another system of protein turnover, is responsible for degradation of more than 80% of intracellular proteins [ 166 ], and it is the primary mechanism through which actomyosin fragments are degraded in healthy muscle. Specific motifs in protein substrates are covalently tagged with activated ubiquitin by a ubiquitin (E3) ligase, further ubiquitin molecules are conjugated to the first and the protein is transferred to the 26S proteasome for degradation [ 166 , 167 ]. The proteasome then utilises multiple enzymatic and non-enzymatic tools to unfold, deubiquitinate and lyse the target protein. Hundreds of different E3 ligases provide specificity through motif recognition. The muscle-specific E3 ligases are MAFbx and muscle RING finger-1 (MuRF-1), which are upregulated in response to stressors including oxidative stress and malnutrition [ 167 ].
Most of the proteins are degraded by a large multi-subunit 26S proteasome that is constituted of two supercomplexes; a regulatory supercomplex (19S) and a core supercomplex (20S). The regulatory particle (RP) modifies and directs the proteins to the core particle (CP) where the substrates are degraded [ 168 ]. Zinc influences on the turnover of proteins that present susceptibility to proteasome-dependent proteolysis [ 168 , 169 ]. Several proteins in the 26S proteasome require zinc for their structure and function [ 170 ]. The 19S RP recognises polyubiquitinated proteins and the deubiquitinating enzymes Rpn11 and Ubp6 remove the polyubiquitin chain [ 170 ]. Rpn11 is a zinc metalloproteinase and mutation of the zinc-coordinating residues in the active site render the enzyme inactive [ 171 ]. Additionally, a member of the ubiquitous ZFAND protein family, ZFAND5/ZNF216 contains two zinc finger domains, is induced during muscle atrophy, and stimulates overall UPS protein degradation [ 172 ].
6. Zinc and oxidative stress
Oxidative stress and inflammation are associated with proteostasis induction and exercise and they are hallmarks of metabolic disorders including obesity, metabolic syndrome, diabetes mellitus type II and cardiovascular diseases. Zinc is a metal ion that binds to numerous proteins in the cell contributing to their stability and to enzymes regulating their catalytic activities. This connection with proteins makes zinc an important factor involved in multiple metabolic and transcriptomic pathways mostly related to prevent oxidative stress and inflammation [ 173 ].
The antioxidant function of zinc is related to multiple activities [ 174 ]. At an initial step, zinc upregulates the antioxidant system through the activation of the nuclear factor erythroid 2-related factor 2 (Nrf2) transcription factor [ 175 ] and prevents the production of reactive oxygen and nitrogen species [ 176 , 177 ]. Although Zn 2+ ions do not have a direct action on redox reactions, Zn 2+ is a cofactor of cytosolic Cu, Zn-superoxide dismutase (SOD1) and can also activate glutathione peroxidase (GPx) providing enzyme mechanisms of antioxidant protection against toxic molecules [ 178 , 179 ]. Furthermore, regular exercised individuals have shown higher serum levels of SOD-3 and endothelial nitric oxide synthase (eNOS) than aged-matched sedentary individuals, suggesting a protective role of exercise in antioxidant defence [ 180 ]. Additionally, zinc inhibits the activation of NF-кB through the activation of (PPARs-α) and zinc-finger protein A20 [ 181 ]. These regulatory functions of transcription factors by zinc are determinant in its anti-inflammatory properties causing a decrease of pro-inflammatory cytokine production [ 182 ]. Interestingly, low levels of zinc have been associated with increased inflammatory markers in either obese humans with metabolic syndrome or patients with type II diabetes, and inflammatory markers were decreased with zinc supplementation [ 100 , 183 , 184 ].
7. Conclusion
In conclusion, zinc deficiency could have serious consequences on health, particularly in athletes affecting sport performance. While the evidence presented here argues the benefits of meeting daily zinc requirements, there is no evidence to support that excess zinc ingestion would be beneficial beyond daily recommended intakes. In relation to skeletal muscle, zinc could be involved in skeletal muscle synthesis and regeneration that take part after exercise. More significantly, zinc seems to be involved in the different proteostatic systems modulated after exercise, but further studies would be necessary to support it. Zinc-proteins and their relation to exercise and physical performance is an understudied area of research and further investigation into the requirement of zinc for the benefits of exercise is warranted.
This work was supported by the Association Française contre les Myopathies (AFM) through fellowship grant #22450 to C.V.-G., and Spanish Ministry of Education, Culture and Sports [FPU16/03264] for J.D.H.C.
Declaration of competing interest
The authors declare that they have no known competing financial interests or personal relationships that could have appeared to influence the work reported in this paper.

Green Chemistry
Progress on stabilizing zinc anodes for zinc-ion batteries using electrolyte solvents engineering.
With the growing demand for scalable and cost-effective electrochemical energy storage systems, aqueous Zn-ion batteries (ZIBs) have attracted attention as cheap, efficient, and safe energy storage devices. However, practical applications of ZIBs are hampered by some challenges, such as Zn dendrite growth, corrosion, passivation, byproducts, cathodic dissolution, and harmful electrostatic interactions. These problems are directly associated with the electrolytes of ZIBs, which are in contact with both the cathode and anode. Recently, several studies have been conducted to optimize ZIB electrolytes. The electrolyte system includes the electrolyte and solvent. The solvent ensures stable operation of the electrolyte and improves the electrochemical efficiency of the cathode and anode, thus improving the electrochemical performance and stability of ZIBs. However, to date, the effect of solvents on the stability of Zn anodes remains unclear. Therefore, there is a need for detailed studies on solvents for ZIB electrolytes. Herein, we review the research progress on the stabilization of Zn anodes using various solvents, including alcohols, amines, acetonitrile, lipids, ethers, and dimethyl sulfoxide. In addition, we discuss the effects of different solvents on the stabilization mechanism of Zn anodes. Finally, the challenges and prospects of solvents used for stabilizing Zn anodes in electrolytes are analyzed, and future research directions for ZIB electrolyte dissolution are proposed.
Article information
Download citation, permissions.

L. Li, Y. Shi, S. Jia, C. Wang, H. Qiu, Y. Ji, M. Cao and D. Zhang, Green Chem. , 2024, Accepted Manuscript , DOI: 10.1039/D4GC00283K
To request permission to reproduce material from this article, please go to the Copyright Clearance Center request page .
If you are an author contributing to an RSC publication, you do not need to request permission provided correct acknowledgement is given.
If you are the author of this article, you do not need to request permission to reproduce figures and diagrams provided correct acknowledgement is given. If you want to reproduce the whole article in a third-party publication (excluding your thesis/dissertation for which permission is not required) please go to the Copyright Clearance Center request page .
Read more about how to correctly acknowledge RSC content .
Social activity
Search articles by author.
This article has not yet been cited.
Advertisements
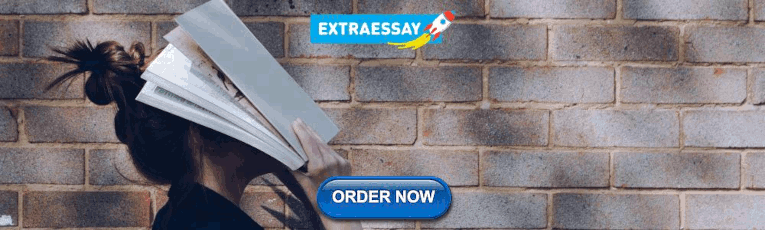
IMAGES
VIDEO
COMMENTS
INTRODUCTION. Zinc essentiality was established in 1869 for plants, in 1934 for experimental animals and in 1961 for humans.[] A syndrome of anemia, hypogonadism and dwarfism was reported in a 21-year-old Iranian farmer in 1961 who was subsisting on a diet of unrefined flat bread, potatoes, and milk.[] Shortly after, a similar syndrome was observed in Egyptian adolescents who had similar ...
Umbrella Review and Literature Search. Umbrella review is characterized as an integrative review of eligible meta-analyses and (or) systematic reviews, designated to provide a broader overview about the related topic. ... A very recent review showed that zinc supplemented human was more likely to meet a higher level of alkaline phosphatase (MD ...
Zinc is an essential trace element for living organisms and their biological processes. Zinc plays a key role in more than 300 enzymes and it is involved in cell communication, proliferation, differentiation and survival. ... This review of the literature on the possible linkage between zinc levels (especially in serum and plasma) and ...
However, critical insight regarding the effects of dietary vs. medicinal doses of zinc is lacking. Therefore, this review aims to provide an overview regarding the posology of zinc and its variability from both foodstuffs and supplements, and attempts to draw inferences for practical application. 2. Free and bound zinc.
Zinc is an essential micronutrient which is required for many important bodily functions. It has a critical role in cellular metabolism and exerts antioxidant and anti-inflammatory effects [6].It also plays a significant role in the determination and regulation of apoptosis [7].In the normally functioning liver, zinc is used in many enzymatic reactions such as in the activation of ornithine ...
Trace elements are minerals that are present in very low concentrations in the human body and yet are crucial for a wide range of physiological functions. Zinc, the second most abundant trace element, is obtained primarily from the diet. After being taken up in the intestine, zinc is distributed to various target organs, where it plays key roles in processes such as immunity, protein folding ...
This review of the literature on the possible linkage between zinc levels (especially in serum and plasma) and autoimmune diseases has revealed a huge amount of studies on this subject, although the selection due to meta-analysis methods has narrowed the final analysis to 62 publications, temporally distributed as shown in Figure S1 ...
In total, the umbrella review included 43 articles and identified 11 outcomes for dietary zinc intake and 86 outcomes for supplementary zinc intake. Dietary zinc intake in the highest dose would ...
Background Diabetes mellitus is a leading cause of morbidity and mortality worldwide. Studies have shown that Zinc has numerous beneficial effects in both type-1 and type-2 diabetes. We aim to evaluate the literature on the mechanisms and molecular level effects of Zinc on glycaemic control, β-cell function, pathogenesis of diabetes and its complications. Methods A review of published studies ...
Low levels of zinc have been reported in a range of cancers, with increasing evidence suggesting that low zinc levels in serum, plasma, and tissues may be linked to carcinogenesis [17, 18]. In this review, we will present the recent evidence that low zinc levels in a variety of situations are a hallmark of various cancers.
This review aims to describe the role of zinc in several different pathological states that have been in the limelight, including cancer, COVID-19, neurological disorders such as Alzheimer's disease, as well as its action in the gut microbiome. ... There is a huge literature addressing the problem if deficiency or excess of Zn 2+ is related to AD.
A literature review within the scope of this research area was conducted using articles available in PubMed (including MEDLINE), Web of Science and Cochrane Library databases. An analysis of available studies has shown that zinc improves hepatic lipid metabolism and has an impact on the lipid profile.
Zinc is a trace element essential to the gastrointestinal, immune, integumentary, reproductive, and central nervous systems. ... Zinc deficiency and its management in the pediatric population: a literature review and proposed etiologic classification J Am Acad Dermatol. 2013 Oct;69(4):616-624.e1. doi: 10.1016/j.jaad.2013.04.028.
Finally, a literature review, conducted in 2014, examined whether topical zinc-based products were effective in the treatment of chronic venous leg ulcers, and provided a summary of these findings. 2 Eleven articles were included in the analysis and described a range of studies using zinc-based dressings to heal chronic venous leg ulcers.
The importance of zinc in the proper functioning of human skin and its possible links to the pathogenesis and clinical advancement of atopic dermatitis are resented. Introduction and objective. Atopic dermatitis is a chronic inflammatory disease that significantly reduces the quality of life of the affecte patients. The disease is characterized by the occurrence of eczema, increased pruritus ...
Both copper (Cu) and zinc (Zn) are crucial micronutrients for human growth and development. This literature review covered the last five years of available evidence on the Cu/Zn ratio in children and adolescents. We searched PubMed, Web of Science, Google Scholar, Cochrane Library, and Science Direct for publications between 2017 and 2022 ...
1. Introduction. Zinc (Zn) is a very important trace element, and maintaining its optimal level in the human body is very important for healthy growth and development [1,2].In the human body, zinc is found in muscles (60%), bones (30%), and skin (5%) [].Its functions include its involvement in the activation of various enzymes and proteins []; it also contributes to the absorption of vitamin A ...
Recognize the limitations of the research studies in the literature review on topical zinc dressings. ABSTRACT Venous leg ulcers (VLUs) are chronic leg wounds that can have a debilitating effect on the physical and psychological health of patients. Older patients, who are a vulnerable group, suffer from VLUs more frequently, and the prevalence ...
In this review, we collated evidence relating to taste change and zinc deficiency in relation to bariatric surgery (BS) and effects of zinc replacement on taste perception and speculate on the possible role of zinc deficiency to induce taste change after BS. A literature search was conducted (33 studies, N = 3264). We showed that taste change and zinc deficiency are frequent complications ...
The zinc load modeling assessment conducted by Verschoor (2007) suggests that synthetic turf made from crumb rubber may translate to an average load of 800 mg/m2/year (600-1,000 mg/m2/year). Zinc loads from crumb rubber were 12-20 times greater than loads from agricultural sources.
1.2. Zinc cellular homeostasis. The human body does not have a tissue reservoir for zinc, nor does it have a protein analogous to ferritin, which stores iron and releases it when needed [].Therefore, an adequate dietary supply is required to prevent zinc deficiency unless a genetic condition is the root of the problem [].In this sense, zinc deficiency can result as symptom of diseases which ...
Literature Review on Synthesis of ZnO Nano Particles Using Natural and Synthetic Methods. Anup A Teragundi. Dr T S Nanjundeswaraswamy. Nowadays many efforts have been made for achieving ZnO ...
However, to date, the effect of solvents on the stability of Zn anodes remains unclear. Therefore, there is a need for detailed studies on solvents for ZIB electrolytes. Herein, we review the research progress on the stabilization of Zn anodes using various solvents, including alcohols, amines, acetonitrile, lipids, ethers, and dimethyl sulfoxide.