Maps and Mapping
Whether your map is paper or digital, mastering the basics of reading it are vital to finding your way around and understanding how the world works. Maps are fantastic visual tools that can help us communicate spatial concepts and patterns, tell stories, and analyze data. However, there are some challenges to translating Earth onto a flat surface without adding bias or inaccuracies. Fortunately, cartographers have the training to minimize these issues.
Maps have been a part of the National Geographic Society since the beginning. Gilbert H. Grosvenor, the first full-time editor of National Geographic, described maps as “the greatest of all epic poems. It's lines and colors show the realization of great dreams.” Learn more about the basics of reading a map, creating a map, and choosing the best map projection.
Geography, Geographic Information Systems (GIS)
Academia.edu no longer supports Internet Explorer.
To browse Academia.edu and the wider internet faster and more securely, please take a few seconds to upgrade your browser .
Enter the email address you signed up with and we'll email you a reset link.
- We're Hiring!
- Help Center
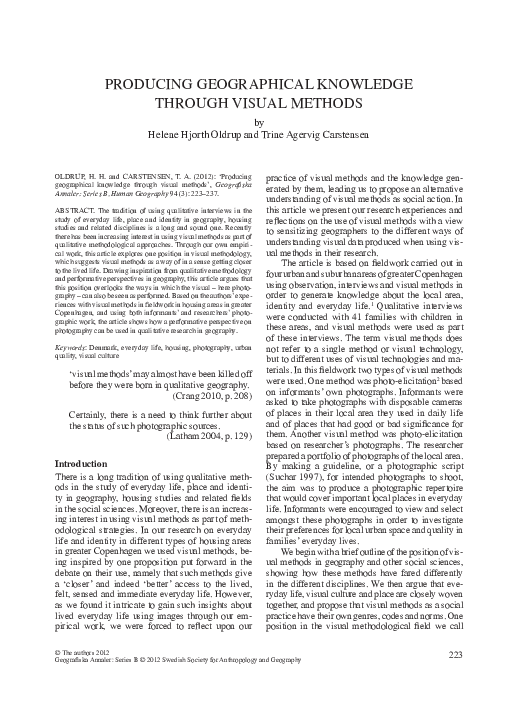
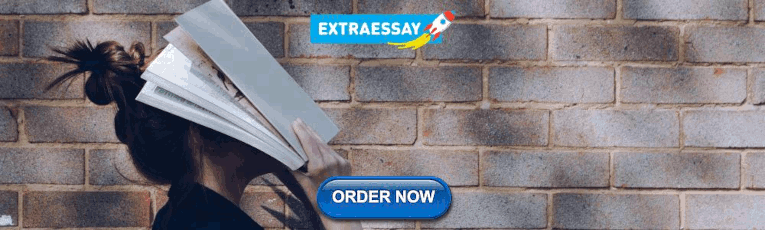
Producing Geographical Knowledge Through Visual Methods

2012, Geografiska Annaler: Series B, Human Geography
Related Papers
Michael Crang
In this chapter my aim is to suggest that an engagement with visuality is worthwhile, may be even necessary, for qualitative methods in geography. In doing this I want to push the case for these methods when despite sometimes warm words there are relatively few examples of their use. Indeed if one were to look at the methods in qualitative textbooks in geography, then the overwhelming dominance is of linguistic sources – be they written and/or spoken. I will focus upon methods connected to the production of what we might call visual ethnographies. In doing this I want to highlight not a set of techniques, as though they were some items on an a la carte menu, but also paradoxes in the ways visual material is treated in geographical work. That is I want to highlight an ambivalence around visuality and its treatment in geography, and point to some theoretical critiques and slippages. I shall effectively focus upon photographic and video work. Partly my aim is to focus on visual media at a time when they are proliferating in society, and thus may form either (and I would argue both) a topic for study and a means for studies. It is also a time when visual ways of knowing have come under intense and refined critique within the discipline. My starting point is a sense that ‘visual methods’ may almost have been killed off before they were born in qualitative geography by powerful arguments about the problematic elements of visual knowledge – and in geography especially. A variety of visual methods, and especially the long reliance on modes of observational practice in landscape work and visual tropes for truth and knowledge across the discipline, have been criticised for assumptions of detachment and objectivity of knower leading to objectification of the known. Recently the issue of representational knowledge has been challenged tout court – and the visual seems perhaps inescapably bound to the representational. Vision is positioned as the problem both in how geographers know and a powerful locus of practice within the discipline. And yet, as I browse through geographical journals, I am not exactly overwhelmed by the deployment of visual media. My contention is that we have allowed one sense of visuality, with a troubling past, to rather dominate our critical understanding of what visual methods might comprise or what they might do. This chapter will begin with a review of some of the classic heritages of visual knowledge in geography, and their politics and legacies. It will develop an account of some of the deployments of visual methods, and different modes of visuality therein. The chapter will examine visual ethnographies that seek to offer an engaged, participatory form of seeing and set it against a more ironic and perhaps even alienated, critical forms of seeing. It will conclude by trying to refigure how we think of seeing as representing rather than a medium of connecting and making present. It will thus ask about the how we might show what is not seen, when it cannot be pictured and how we might think about vision not as the antithesis of touch but through a haptic register
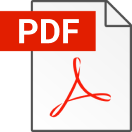
Phoebe Crisman
Isabelle Schoepfer
This paper seeks to present and discuss a specific methodological approach based on photography, which draws on a method that combines auto-photography during photo-walks with photo-elicitation interviews. The method was used in the framework of a study that explored how families, living in the city of Geneva, Switzerland, make sense of their everyday practiced space, and how this informs us on knowledge about neighbourhood images in the context of lived space. The paper discusses the roles, benefits and challenges of the combined use of words and pictures, as well as the practice of taking pictures as a research tool and practice.
Journal of Urban Design 15:3 293-324
fahriye sancar
Science, Technology and Arts Research Journal
Ali Asadpour
Terence Heng
The use of images, particularly photography, has been steadily gaining popularity in academia, but there has not yet been a book that deals with the act and process of photo-taking in the field. Drawing upon 21 years of photographic experience and sociological research, Terence Heng’s immersive and narrative style will: 1. introduce photography as a qualitative method; 2. discuss the intricacies of, challenges in and opportunities for using a camera in the field; 3. explore common themes and topics in social science research, including photographing rituals, space, people and objects; 4. advise on navigating the always evolving technological landscapes of traditional, digital and mobile photography. Visual Methods in the Field: Photography for the Social Sciences is a photography guide written for researchers by a researcher. Using in-depth ethnographic case studies from research done in various urban environments, this book will act as a crucial bridge for students in geography, sociology, education, media studies and other social sciences to incorporate photography into their research repertoire.
Gabrielle Bendiner-Viani
This article poses a methodological argument for the use of expressive photographs in fostering more mindful discussions of the experience of everyday places. The article builds on the author’s ‘guided tours’ methodology of walk/make/talk, which involves asking inhabitants to take the researcher on tours of their everyday places, the researcher making creative visual representations of those places and then, bringing those images back to residents to talk about how the photographs are similar, or in contrast, to lived experience. The article uses examples from research in Brooklyn, NY, and Oakland, CA, to specifically explore the ‘making’ and ‘talking’ part of the process, and to argue for the critical distance gained when a participant has the opportunity to see their most commonplace and often unremarked worlds brought back to them through another pair of eyes. The paper considers distinctions with other photo-elicitation methods and discusses how this model of bringing people’s worlds back to them often allowed the jolt of a new perspective to suspend the taken-for-grantedness of the world, creating a time and opportunity to consider the everyday in more depth. It further discusses how the method enabled people to clarify three aspects of everydayness that are hard to pin down: the feel of place, emotional responses, and seeing past time. The paper challenges conceptions of photographs as ‘data’, champions expressive work in research, and proposes that photographs made by a range of authors can enable new ways of seeing, challenging and discussing the everyday urban environment.
Dr John Hillman
[ ] With Design: Reinventing Design Modes
Valentina Nisi
British Sociological Association Annual Conference
RELATED PAPERS
Nuclear Physics A
Structural Concrete
Nasir Shafiq
The Journal of The Institute of Image Information and Television Engineers
Marius I Dan
RAY-I CHANG
Jurnal Riset dan Inovasi Peternakan (Journal of Research and Innovation of Animals)
safira huwaida
Abdul Kadir Sulaiman
Natalia Loghin-Oprea
Mountain Valley Holidays
Revista Tempo do Mundo
Pedro Silva Barros
International Journal of Environmental Research and Public Health
EAS journal of radiology and imaging technology
Proceedings of the 11th international ACM SIGACCESS conference on Computers and accessibility
Dimitris Metaxas
Interspeech 2014
Isidoros Rodomagoulakis
Springer eBooks
Roger Rothon
قصة أصحاب الجنة في ضوء سورة القلم دروس وأحكام
سامية المعبدي
Palgrave Macmillan UK eBooks
Gladys Teitelman
Roland Küstermann
Naukovij vìsnik Nacìonalʹnogo gìrničogo unìversitetu
Andrii Koveria
Művészettörténeti értesítő (Nyomtatott)
Péter Farbaky
tufhfgd hfdfsd
Journal of Genetics and Genomics
Emil Kofod-Olsen
2015 Brazilian Conference on Intelligent Systems (BRACIS)
Wolfgang Banzhaf
Phosphorus Research Bulletin
Masaru Murata
RELATED TOPICS
- We're Hiring!
- Help Center
- Find new research papers in:
- Health Sciences
- Earth Sciences
- Cognitive Science
- Mathematics
- Computer Science
- Academia ©2024
- Subject List
- Take a Tour
- For Authors
- Subscriber Services
- Publications
- African American Studies
- African Studies
- American Literature
- Anthropology
- Architecture Planning and Preservation
- Art History
- Atlantic History
- Biblical Studies
- British and Irish Literature
- Childhood Studies
- Chinese Studies
- Cinema and Media Studies
- Communication
- Criminology
- Environmental Science
- Evolutionary Biology
- International Law
- International Relations
- Islamic Studies
- Jewish Studies
- Latin American Studies
- Latino Studies
- Linguistics
- Literary and Critical Theory
- Medieval Studies
- Military History
- Political Science
- Public Health
- Renaissance and Reformation
- Social Work
- Urban Studies
- Victorian Literature
- Browse All Subjects
How to Subscribe
- Free Trials
In This Article Expand or collapse the "in this article" section Visualizations
Introduction, general overviews.
- Representation
- Interactivity
- Space-Time Visualizations
- Perception, Cognition, User-Centered Design
- Analytical Reasoning and Visual Analytics
- Design Patterns
- Data Quality and Uncertainty Representation
- Big Data & Visualizations
- Visualization Context, 3D and Extended Reality
- Critical Reflections on Visualization, Ethics
- Application Examples
Related Articles Expand or collapse the "related articles" section about
About related articles close popup.
Lorem Ipsum Sit Dolor Amet
Vestibulum ante ipsum primis in faucibus orci luctus et ultrices posuere cubilia Curae; Aliquam ligula odio, euismod ut aliquam et, vestibulum nec risus. Nulla viverra, arcu et iaculis consequat, justo diam ornare tellus, semper ultrices tellus nunc eu tellus.
- Cartography
- Geographic Methods: Visual Analysis
- Photographic and Video Methods in Geography
- Social Media Analytics
Other Subject Areas
Forthcoming articles expand or collapse the "forthcoming articles" section.
- GeoCapabilities in Geography Education
- Geography Faculty Development
- Urban Greening
- Find more forthcoming articles...
- Export Citations
- Share This Facebook LinkedIn Twitter
Visualizations by Arzu Çöltekin , Amy Griffin , Anthony Robinson LAST REVIEWED: 24 February 2021 LAST MODIFIED: 24 February 2021 DOI: 10.1093/obo/9780199874002-0224
Visualizations (i.e., thinking in images internally in the human mind) or externally expressing a concept via graphical means—such as documenting an observation in a hand-drawn or digital visuospatial sketch, or creating a visual output from data—have always been an integral part of scientific inquiry and communication. One might argue that the ‘graphy’ part of ‘geo graphy ’ refers to visually and spatially (i.e., visuospatially) documenting the world. For the vast majority of people, a significant part of human experience is shaped by sight, and the human visual system occupies a large chunk of human cognitive processing capacity. Given that, one can speculate that comprehension and communication through visuospatial means could be ‘second nature’ to people. There is ample evidence to support this line of thinking: As opposed to written words or a large list of numbers, visualizations allow us to see patterns and anomalies quickly, sometimes even at a glance. However, the power of visualizations depends on a number of factors including the details of their design , the abilities and background of the human viewing them, and the context in which a visualization is used. This power must also be critically viewed from an ethics perspective. These three factors are elaborated under various subsections. However, first, a fundamental question needs to be asked: Is visualization a product or a process ? The word visualization is commonly used as a noun for a visual product (e.g., a map or a plot is a visualization). However, both mental and external visualizations are processes, and the term ‘visualization’ as it was introduced into scientific discourse refers to a process . The process aspect is important to remember, because this is viewed as a key factor that distinguishes using visualizations to explain what is already known versus exploring the unknown. With the latter activity, visualizing things becomes a part of the scientific inquiry as an active tool that helps build hypotheses and thus facilitate thinking and reasoning, in addition to explaining what is already known. Whether the goal is to explain or to explore, the design and use of visualizations needs to be intentional and not arbitrary. To create and read visualizations intentionally, a certain level of visual literacy built on design, technology, and knowledge of human visuospatial cognition is necessary. This manuscript identifies scholarly resources to help all scientists and aspiring scientists, especially those in spatial sciences, to build, refresh their knowledge of, and learn or teach about visualizations.
The foundations of visualization in geography grew from the earlier work of statisticians (e.g., exploratory data analysis), and interacted with the work of computer scientists and graphic designers. The building blocks of graphics lie at the core of any visualization, and have been defined in terms of graphical variables (e.g., in Bertin 1967 ) as well as mathematical formulas ( Wilkinson 2005 ). Furthermore, knowledge about human vision and how people perceive information from graphics can help them make smart design decisions and recognize potential limitations of both humans and visualizations. The potential tasks that can be supported by visualization have been thoroughly explored in geographical as well as nongeographical contexts in seminal works such as Dykes, et al. 2005 ; Andrienko and Andrienko 2006 ; and Munzner 2014 . Distilled guidelines, such as those in Heer, et al. 2010 , Munzner 2014 , and Field 2018 exist to help visualization creators make better decisions about designing representations and interactivity, whereas theoretical works that frame geographic visualization as a cognitive and semiotic system, such as the seminal work MacEachren 2004 , ground the field in knowledge generated by previous generations. In spite of a great deal of scientific activity in this subfield of geography as exemplified in Dykes, et al. 2005 and Nöllenburg 2007 , Çöltekin, et al. 2017 demonstrates that key research challenges remain persistent.
Andrienko, N., and G. Andrienko. Exploratory Analysis of Spatial and Temporal Data: A Systematic Approach . Berlin: Springer Science & Business Media, 2006.
Emphasizing the exploratory potential for visualization, this work is particularly strong in its explanation of a wide range of possible analytical task types and for defining visualization design principles that connect tasks to functional implementation in systems.
Bertin, J. Semiology of Graphics: Diagrams, Networks, Maps . Paris: Gauthier Villars, 1967.
Foundational work from 1967 reprinted in 2010 (Redlands, CA: ESRI) on the ways in which graphics can be constructed to represent information. Bertin introduced the concept of visual variables in this volume with the intent to help provide guidelines that can be generally applied for representing a wide range of data types.
Çöltekin, A., S. Bleisch, G. Andrienko, and J. Dykes. “Persistent Challenges in Geovisualization: A Community Perspective.” International Journal of Cartography 3.suppl. 1 (2017): 115–139.
DOI: 10.1080/23729333.2017.1302910
Based on expert inputs, the research challenges posed in a number of previous research agenda articles are compared to what the geovisualization community believes are persistent problems in the field today. Three major areas emerged: the need to define geovisualization and its connection to allied fields, the need to develop a better understanding of human factors in geovisualization, and the need to develop practical guidelines for geovisualization design and implementation.
Dykes, J., A. M. MacEachren, and M. J. Kraak. Exploring Geovisualization . Amsterdam: Elsevier, 2005.
Spans the gamut of research in geovisualization, including core theoretical perspectives, computational frameworks, application areas, and evaluation methods. Also introduces key research challenges for the field, many of which remain relevant today.
Field, K. Cartography . Redlands, CA: Esri Press, 2018.
An extensively illustrated primer on map design, presented in a condensed format and dispensed in a nonlinear, nonacademic fashion. One of the easiest and most engaging ways to dive into cartographic design, and quite useful as a reference for experienced cartographers too.
Heer, J., M. Bostock, and V. Ogievetsky. “ A Tour through the Visualization Zoo .” Communications of the ACM 53.6 (2010): 59–67.
DOI: 10.1145/1743546.1743567
A concise and visual guide to visualization methods, including those commonly applied as well as those that are not typical but may be well-suited to particular situations. Written in a way to inspire the reader to learn the basics about each approach, its intent is to whet the appetite in a few pages rather than serve as a deep reference.
MacEachren, A. M. How Maps Work: Representation, Visualization, and Design . New York: Guilford Press, 2004.
Recognized as a key work in the field, this monograph expands on MacEachren’s earlier work to develop connections between cartography, cognition, and semiotics. It advances and develops a new theoretical framework for cartography as an engine of discovery beyond its use for communication.
Munzner, T. Visualization Analysis and Design: Principles, Techniques, and Practice . AK Peters Visualization Series. Boca Raton, FL: CRC Press, 2014.
DOI: 10.1201/b17511
Blends together principles for visualization design with core theoretical frameworks and synthesized results from perceptual studies to provide a comprehensive reference. A unique aspect of the book compared to other references is that it also delves into motivations for visualization and offers helpful rules of thumb.
Nöllenburg, M. “Geographic Visualization.” In Human-Centered Visualization Environments: Research Seminar at Dagstuhl Castle, Germany, 5–8 March 2006 . Edited by Andreas Kerren, Achim Ebert, and Jörg Meyer, 257–294. Berlin: Springer, 2007.
Offers a broad overview of geovisualization, ranging from its development as a subdiscipline of cartography to its implementation in various systems and its intersections with human-centered computing.
Wilkinson, L. The Grammar of Graphics . Berlin: Springer Science & Business Media, 2005.
Proposes a complete system whereby graphics can be constructed from mathematical and conceptual parts, including their aesthetic affordances as well. A particularly useful and general guide for anyone who seeks to implement visualizations in software.
back to top
Users without a subscription are not able to see the full content on this page. Please subscribe or login .
Oxford Bibliographies Online is available by subscription and perpetual access to institutions. For more information or to contact an Oxford Sales Representative click here .
- About Geography »
- Meet the Editorial Board »
- Abortion, Geographies of
- Accessing and Visualizing Archived Weather and Climate Dat...
- Activity Space
- Actor Network Theory (ANT)
- Age, Geographies of
- Agent-based Modeling
- Agricultural Geography
- Agricultural Meteorology/Climatology
- Animal Geographies
- Anthropocene and Geography, The
- Anthropogenic Climate Change
- Applied Geography
- Arctic Climatology
- Arctic, The
- Art and Geography
- Assessment in Geography Education
- Atmospheric Composition and Structure
- Automobility
- Aviation Meteorology
- Beer, Geography of
- Behavioral and Cognitive Geography
- Belt and Road Initiative
- Biodiversity Conservation
- Biodiversity Gradients
- Biogeography
- Biogeomorphology and Zoogeomorphology
- Biometric Technologies
- Biopedoturbation
- Body, Geographies of the
- Borders and Boundaries
- Brownfields
- Carbon Cycle
- Carceral Geographies
- Cartography, History of
- Cartography, Mapping, and War
- Chicago School
- Children and Childhood, Geographies of
- Citizenship
- Climate Literacy and Education
- Climatology
- Community Mapping
- Comparative Urbanism
- Conservation Biogeography
- Consumption, Geographies of
- Crime Analysis, GIS and
- Crime, Geography of
- Critical GIS
- Critical Historical Geography
- Critical Military Geographies
- Cultural Ecology and Human Ecology
- Cultural Geography
- Cultural Landscape
- Cyberspace, Geography of
- Desertification
- Developing World
- Development, Regional
- Development Theory
- Disability, Geography of
- Disease, Geography of
- Drones, Geography of
- Drugs, Geography of
- Economic Geography
- Economic Historical Geography
- Edge Cities and Urban Sprawl
- Education (K-12), Geography
- El Niño Southern Oscillation (ENSO)
- Elderly, Geography and the
- Electoral Geography
- Empire, Geography and
- Energy, Geographies of
- Energy, Renewable
- Energy Resources and Use
- Environment and Development
- Environmental Electronic Sensing Systems
- Environmental Justice
- Ethics, Geographers and
- Ethics, Geography and
- Ethnography
- Ethnonationalism
- Everyday Life, Geography and
- Extreme Heat
- Family, Geographies of the
- Feminist Geography
- Film, Geography and
- Finance, Geography of
- Financial Geographies of Debt and Crisis
- Fluvial Geomorphology
- Folk Culture and Geography
- Future, Geographies of the
- Gender and Geography
- Gentrification
- Geocomputation in Geography Education
- Geographic Information Science
- Geographic Methods: Archival Research
- Geographic Methods: Discourse Analysis
- Geographic Methods: Interviews
- Geographic Methods: Life Writing Analysis
- Geographic Thought (US)
- Geographic Vulnerability to Climate Change
- Geographies of Affect
- Geographies of Diplomacy
- Geographies of Education
- Geographies of Resilience
- Geography and Class
- Geography, Gramsci and
- Geography, Legal
- Geography of Biofuels
- Geography of Food
- Geography of Hunger and Famine
- Geography of Industrialization
- Geography of Public Policy
- Geography of Resources
- Geopolitics
- Geopolitics, Energy and
- Geospatial Artificial Intelligence (GeoAI)
- GIS and Computational Social Sciences
- GIS and Health
- GIS and Remote Sensing Applications in Geomorphology
- GIS and Virtual Reality
- GIS applications in Human Geography
- GIS, Geospatial Technology, and Spatial Thinking in Geogra...
- GIS, Historical
- GIS, History of
- GIS, Space-Time
- Glacial and Periglacial Geomorphology
- Glaciers, Geography of
- Globalization
- Health Care, Geography of
- Hegemony and Geographic Knowledge
- Historical Geography
- Historical Mobilities
- Histories of Protest and Social Movements
- History, Environmental
- Homelessness
- Human Dynamics, GIScience of
- Human Geographies of Outer Space
- Human Trafficking
- Humanistic Geography
- Human-Landscape Interactions
- Humor, Geographies of
- Hydroclimatology and Climate Variability
- Identity and Place
- "Imagining a Better Future through Place": Geographies of ...
- Immigration and Immigrants
- Indigenous Peoples and the Global Indigenous Movement
- Informal Economy
- Innovation, Geography of
- Intelligence, Geographical
- Islands, Human Geography and
- Justice, Geography of
- Knowledge Economy: Spatial Approaches
- Knowledge, Geography of
- Labor, Geography of
- Land Use and Cover Change
- Land-Atmosphere Interactions
- Landscape Interpretation
- Literature, Geography and
- Location Theory
- Marine Biogeography
- Marine Conservation and Fisheries Management
- Media Geography
- Medical Geography
- Migration, International Student
- Military Geographies and the Environment
- Military Geographies of Popular Culture
- Military Geographies of Urban Space and War
- Military Geography
- Moonsoons, Geography of
- Mountain Geography
- Mountain Meteorology
- Music, Sound, and Auditory Culture, Geographies of
- National Assessment of Educational Progress (NAEP) in Geog...
- Nations and Nationalism
- Natural Hazards and Risk
- Nature-Society Theory
- Neogeography
- New Urbanism
- Non-representational Theory
- Nuclear War, Geographies of
- Nutrition Transition, The
- Orientalism and Geography
- Participatory Action Research
- Peace, Geographies of
- Pedagogical Content Knowledge in Geography Education
- Perspectives in Geography Internships
- Phenology and Climate
- Physical Geography
- Polar Geography
- Policy Mobilities
- Political Ecology
- Political Geography
- Political Geology
- Popular Culture, Geography and
- Population Geography
- Ports and Maritime Trade
- Postcolonialism
- Postmodernism and Poststructuralism
- Pragmatism, Geographies of
- Producer Services
- Psychogeography
- Public Participation GIS, Participatory GIS, and Participa...
- Qualitative GIS
- Qualitative Methods
- Quantitative Methods in Human Geography
- Questionnaires
- Race and Racism
- Refugees, Geography of
- Religion, Geographies of
- Retail Trade, Geography of
- Rural Geography
- Science and Technology Studies (STS) in Geography
- Sea-Level Research, Quaternary
- Security and Securitization, Geographies of
- Segregation, Ethnic and Racial
- Service Industries, Geography of
- Settlement Geography
- Sexuality, Geography of
- Slope Processes
- Social Justice
- Soils, Diversity of
- Sonic Methods in Geography
- Spatial Analysis
- Spatial Autocorrelation
- Sports, Geography of
- Sustainability Education at the School Level, Geography an...
- Sustainability Science
- Sustainable Agriculture
- Synoptic Climatology
- Technological Change, Geography of
- Telecommunications
- Teleconnections, Atmospheric
- Terrestrial Snow, Measurement of
- Territory and Territoriality
- Terrorism, Geography of
- The Climate Security Nexus
- The Voluntary Sector and Geography
- Time, Geographies of
- Time Geography
- Time-Space Compression
- Tourism Geography
- Touristification
- Transnational Corporations
- Unoccupied Aircraft Systems
- Urban Geography
- Urban Heritage
- Urban Historical Geography
- Urban Meteorology and Climatology
- Urban Planning and Geography
- Urban Political Ecology
- Urban Sustainability
- Visualizations
- Vulnerability, Risk, and Hazards
- Vulnerability to Climate Change
- War on Terror, Geographies of the
- Weather and Climate Damage Studies
- Whiteness, Geographies of
- Wine, Geography of
- World Cities
- Young People's Geography
- Privacy Policy
- Cookie Policy
- Legal Notice
- Accessibility
Powered by:
- [66.249.64.20|185.147.128.134]
- 185.147.128.134
- Open access
- Published: 19 July 2015
The role of visual representations in scientific practices: from conceptual understanding and knowledge generation to ‘seeing’ how science works
- Maria Evagorou 1 ,
- Sibel Erduran 2 &
- Terhi Mäntylä 3
International Journal of STEM Education volume 2 , Article number: 11 ( 2015 ) Cite this article
73k Accesses
78 Citations
13 Altmetric
Metrics details
The use of visual representations (i.e., photographs, diagrams, models) has been part of science, and their use makes it possible for scientists to interact with and represent complex phenomena, not observable in other ways. Despite a wealth of research in science education on visual representations, the emphasis of such research has mainly been on the conceptual understanding when using visual representations and less on visual representations as epistemic objects. In this paper, we argue that by positioning visual representations as epistemic objects of scientific practices, science education can bring a renewed focus on how visualization contributes to knowledge formation in science from the learners’ perspective.
This is a theoretical paper, and in order to argue about the role of visualization, we first present a case study, that of the discovery of the structure of DNA that highlights the epistemic components of visual information in science. The second case study focuses on Faraday’s use of the lines of magnetic force. Faraday is known of his exploratory, creative, and yet systemic way of experimenting, and the visual reasoning leading to theoretical development was an inherent part of the experimentation. Third, we trace a contemporary account from science focusing on the experimental practices and how reproducibility of experimental procedures can be reinforced through video data.
Conclusions
Our conclusions suggest that in teaching science, the emphasis in visualization should shift from cognitive understanding—using the products of science to understand the content—to engaging in the processes of visualization. Furthermore, we suggest that is it essential to design curriculum materials and learning environments that create a social and epistemic context and invite students to engage in the practice of visualization as evidence, reasoning, experimental procedure, or a means of communication and reflect on these practices. Implications for teacher education include the need for teacher professional development programs to problematize the use of visual representations as epistemic objects that are part of scientific practices.
During the last decades, research and reform documents in science education across the world have been calling for an emphasis not only on the content but also on the processes of science (Bybee 2014 ; Eurydice 2012 ; Duschl and Bybee 2014 ; Osborne 2014 ; Schwartz et al. 2012 ), in order to make science accessible to the students and enable them to understand the epistemic foundation of science. Scientific practices, part of the process of science, are the cognitive and discursive activities that are targeted in science education to develop epistemic understanding and appreciation of the nature of science (Duschl et al. 2008 ) and have been the emphasis of recent reform documents in science education across the world (Achieve 2013 ; Eurydice 2012 ). With the term scientific practices, we refer to the processes that take place during scientific discoveries and include among others: asking questions, developing and using models, engaging in arguments, and constructing and communicating explanations (National Research Council 2012 ). The emphasis on scientific practices aims to move the teaching of science from knowledge to the understanding of the processes and the epistemic aspects of science. Additionally, by placing an emphasis on engaging students in scientific practices, we aim to help students acquire scientific knowledge in meaningful contexts that resemble the reality of scientific discoveries.
Despite a wealth of research in science education on visual representations, the emphasis of such research has mainly been on the conceptual understanding when using visual representations and less on visual representations as epistemic objects. In this paper, we argue that by positioning visual representations as epistemic objects, science education can bring a renewed focus on how visualization contributes to knowledge formation in science from the learners’ perspective. Specifically, the use of visual representations (i.e., photographs, diagrams, tables, charts) has been part of science and over the years has evolved with the new technologies (i.e., from drawings to advanced digital images and three dimensional models). Visualization makes it possible for scientists to interact with complex phenomena (Richards 2003 ), and they might convey important evidence not observable in other ways. Visual representations as a tool to support cognitive understanding in science have been studied extensively (i.e., Gilbert 2010 ; Wu and Shah 2004 ). Studies in science education have explored the use of images in science textbooks (i.e., Dimopoulos et al. 2003 ; Bungum 2008 ), students’ representations or models when doing science (i.e., Gilbert et al. 2008 ; Dori et al. 2003 ; Lehrer and Schauble 2012 ; Schwarz et al. 2009 ), and students’ images of science and scientists (i.e., Chambers 1983 ). Therefore, studies in the field of science education have been using the term visualization as “the formation of an internal representation from an external representation” (Gilbert et al. 2008 , p. 4) or as a tool for conceptual understanding for students.
In this paper, we do not refer to visualization as mental image, model, or presentation only (Gilbert et al. 2008 ; Philips et al. 2010 ) but instead focus on visual representations or visualization as epistemic objects. Specifically, we refer to visualization as a process for knowledge production and growth in science. In this respect, modeling is an aspect of visualization, but what we are focusing on with visualization is not on the use of model as a tool for cognitive understanding (Gilbert 2010 ; Wu and Shah 2004 ) but the on the process of modeling as a scientific practice which includes the construction and use of models, the use of other representations, the communication in the groups with the use of the visual representation, and the appreciation of the difficulties that the science phase in this process. Therefore, the purpose of this paper is to present through the history of science how visualization can be considered not only as a cognitive tool in science education but also as an epistemic object that can potentially support students to understand aspects of the nature of science.
Scientific practices and science education
According to the New Generation Science Standards (Achieve 2013 ), scientific practices refer to: asking questions and defining problems; developing and using models; planning and carrying out investigations; analyzing and interpreting data; using mathematical and computational thinking; constructing explanations and designing solutions; engaging in argument from evidence; and obtaining, evaluating, and communicating information. A significant aspect of scientific practices is that science learning is more than just about learning facts, concepts, theories, and laws. A fuller appreciation of science necessitates the understanding of the science relative to its epistemological grounding and the process that are involved in the production of knowledge (Hogan and Maglienti 2001 ; Wickman 2004 ).
The New Generation Science Standards is, among other changes, shifting away from science inquiry and towards the inclusion of scientific practices (Duschl and Bybee 2014 ; Osborne 2014 ). By comparing the abilities to do scientific inquiry (National Research Council 2000 ) with the set of scientific practices, it is evident that the latter is about engaging in the processes of doing science and experiencing in that way science in a more authentic way. Engaging in scientific practices according to Osborne ( 2014 ) “presents a more authentic picture of the endeavor that is science” (p.183) and also helps the students to develop a deeper understanding of the epistemic aspects of science. Furthermore, as Bybee ( 2014 ) argues, by engaging students in scientific practices, we involve them in an understanding of the nature of science and an understanding on the nature of scientific knowledge.
Science as a practice and scientific practices as a term emerged by the philosopher of science, Kuhn (Osborne 2014 ), refers to the processes in which the scientists engage during knowledge production and communication. The work that is followed by historians, philosophers, and sociologists of science (Latour 2011 ; Longino 2002 ; Nersessian 2008 ) revealed the scientific practices in which the scientists engage in and include among others theory development and specific ways of talking, modeling, and communicating the outcomes of science.
Visualization as an epistemic object
Schematic, pictorial symbols in the design of scientific instruments and analysis of the perceptual and functional information that is being stored in those images have been areas of investigation in philosophy of scientific experimentation (Gooding et al. 1993 ). The nature of visual perception, the relationship between thought and vision, and the role of reproducibility as a norm for experimental research form a central aspect of this domain of research in philosophy of science. For instance, Rothbart ( 1997 ) has argued that visualizations are commonplace in the theoretical sciences even if every scientific theory may not be defined by visualized models.
Visual representations (i.e., photographs, diagrams, tables, charts, models) have been used in science over the years to enable scientists to interact with complex phenomena (Richards 2003 ) and might convey important evidence not observable in other ways (Barber et al. 2006 ). Some authors (e.g., Ruivenkamp and Rip 2010 ) have argued that visualization is as a core activity of some scientific communities of practice (e.g., nanotechnology) while others (e.g., Lynch and Edgerton 1988 ) have differentiated the role of particular visualization techniques (e.g., of digital image processing in astronomy). Visualization in science includes the complex process through which scientists develop or produce imagery, schemes, and graphical representation, and therefore, what is of importance in this process is not only the result but also the methodology employed by the scientists, namely, how this result was produced. Visual representations in science may refer to objects that are believed to have some kind of material or physical existence but equally might refer to purely mental, conceptual, and abstract constructs (Pauwels 2006 ). More specifically, visual representations can be found for: (a) phenomena that are not observable with the eye (i.e., microscopic or macroscopic); (b) phenomena that do not exist as visual representations but can be translated as such (i.e., sound); and (c) in experimental settings to provide visual data representations (i.e., graphs presenting velocity of moving objects). Additionally, since science is not only about replicating reality but also about making it more understandable to people (either to the public or other scientists), visual representations are not only about reproducing the nature but also about: (a) functioning in helping solving a problem, (b) filling gaps in our knowledge, and (c) facilitating knowledge building or transfer (Lynch 2006 ).
Using or developing visual representations in the scientific practice can range from a straightforward to a complicated situation. More specifically, scientists can observe a phenomenon (i.e., mitosis) and represent it visually using a picture or diagram, which is quite straightforward. But they can also use a variety of complicated techniques (i.e., crystallography in the case of DNA studies) that are either available or need to be developed or refined in order to acquire the visual information that can be used in the process of theory development (i.e., Latour and Woolgar 1979 ). Furthermore, some visual representations need decoding, and the scientists need to learn how to read these images (i.e., radiologists); therefore, using visual representations in the process of science requires learning a new language that is specific to the medium/methods that is used (i.e., understanding an X-ray picture is different from understanding an MRI scan) and then communicating that language to other scientists and the public.
There are much intent and purposes of visual representations in scientific practices, as for example to make a diagnosis, compare, describe, and preserve for future study, verify and explore new territory, generate new data (Pauwels 2006 ), or present new methodologies. According to Latour and Woolgar ( 1979 ) and Knorr Cetina ( 1999 ), visual representations can be used either as primary data (i.e., image from a microscope). or can be used to help in concept development (i.e., models of DNA used by Watson and Crick), to uncover relationships and to make the abstract more concrete (graphs of sound waves). Therefore, visual representations and visual practices, in all forms, are an important aspect of the scientific practices in developing, clarifying, and transmitting scientific knowledge (Pauwels 2006 ).
Methods and Results: Merging Visualization and scientific practices in science
In this paper, we present three case studies that embody the working practices of scientists in an effort to present visualization as a scientific practice and present our argument about how visualization is a complex process that could include among others modeling and use of representation but is not only limited to that. The first case study explores the role of visualization in the construction of knowledge about the structure of DNA, using visuals as evidence. The second case study focuses on Faraday’s use of the lines of magnetic force and the visual reasoning leading to the theoretical development that was an inherent part of the experimentation. The third case study focuses on the current practices of scientists in the context of a peer-reviewed journal called the Journal of Visualized Experiments where the methodology is communicated through videotaped procedures. The three case studies represent the research interests of the three authors of this paper and were chosen to present how visualization as a practice can be involved in all stages of doing science, from hypothesizing and evaluating evidence (case study 1) to experimenting and reasoning (case study 2) to communicating the findings and methodology with the research community (case study 3), and represent in this way the three functions of visualization as presented by Lynch ( 2006 ). Furthermore, the last case study showcases how the development of visualization technologies has contributed to the communication of findings and methodologies in science and present in that way an aspect of current scientific practices. In all three cases, our approach is guided by the observation that the visual information is an integral part of scientific practices at the least and furthermore that they are particularly central in the scientific practices of science.
Case study 1: use visual representations as evidence in the discovery of DNA
The focus of the first case study is the discovery of the structure of DNA. The DNA was first isolated in 1869 by Friedrich Miescher, and by the late 1940s, it was known that it contained phosphate, sugar, and four nitrogen-containing chemical bases. However, no one had figured the structure of the DNA until Watson and Crick presented their model of DNA in 1953. Other than the social aspects of the discovery of the DNA, another important aspect was the role of visual evidence that led to knowledge development in the area. More specifically, by studying the personal accounts of Watson ( 1968 ) and Crick ( 1988 ) about the discovery of the structure of the DNA, the following main ideas regarding the role of visual representations in the production of knowledge can be identified: (a) The use of visual representations was an important part of knowledge growth and was often dependent upon the discovery of new technologies (i.e., better microscopes or better techniques in crystallography that would provide better visual representations as evidence of the helical structure of the DNA); and (b) Models (three-dimensional) were used as a way to represent the visual images (X-ray images) and connect them to the evidence provided by other sources to see whether the theory can be supported. Therefore, the model of DNA was built based on the combination of visual evidence and experimental data.
An example showcasing the importance of visual representations in the process of knowledge production in this case is provided by Watson, in his book The Double Helix (1968):
…since the middle of the summer Rosy [Rosalind Franklin] had had evidence for a new three-dimensional form of DNA. It occurred when the DNA 2molecules were surrounded by a large amount of water. When I asked what the pattern was like, Maurice went into the adjacent room to pick up a print of the new form they called the “B” structure. The instant I saw the picture, my mouth fell open and my pulse began to race. The pattern was unbelievably simpler than those previously obtained (A form). Moreover, the black cross of reflections which dominated the picture could arise only from a helical structure. With the A form the argument for the helix was never straightforward, and considerable ambiguity existed as to exactly which type of helical symmetry was present. With the B form however, mere inspection of its X-ray picture gave several of the vital helical parameters. (p. 167-169)
As suggested by Watson’s personal account of the discovery of the DNA, the photo taken by Rosalind Franklin (Fig. 1 ) convinced him that the DNA molecule must consist of two chains arranged in a paired helix, which resembles a spiral staircase or ladder, and on March 7, 1953, Watson and Crick finished and presented their model of the structure of DNA (Watson and Berry 2004 ; Watson 1968 ) which was based on the visual information provided by the X-ray image and their knowledge of chemistry.
X-ray chrystallography of DNA
In analyzing the visualization practice in this case study, we observe the following instances that highlight how the visual information played a role:
Asking questions and defining problems: The real world in the model of science can at some points only be observed through visual representations or representations, i.e., if we are using DNA as an example, the structure of DNA was only observable through the crystallography images produced by Rosalind Franklin in the laboratory. There was no other way to observe the structure of DNA, therefore the real world.
Analyzing and interpreting data: The images that resulted from crystallography as well as their interpretations served as the data for the scientists studying the structure of DNA.
Experimenting: The data in the form of visual information were used to predict the possible structure of the DNA.
Modeling: Based on the prediction, an actual three-dimensional model was prepared by Watson and Crick. The first model did not fit with the real world (refuted by Rosalind Franklin and her research group from King’s College) and Watson and Crick had to go through the same process again to find better visual evidence (better crystallography images) and create an improved visual model.
Example excerpts from Watson’s biography provide further evidence for how visualization practices were applied in the context of the discovery of DNA (Table 1 ).
In summary, by examining the history of the discovery of DNA, we showcased how visual data is used as scientific evidence in science, identifying in that way an aspect of the nature of science that is still unexplored in the history of science and an aspect that has been ignored in the teaching of science. Visual representations are used in many ways: as images, as models, as evidence to support or rebut a model, and as interpretations of reality.
Case study 2: applying visual reasoning in knowledge production, the example of the lines of magnetic force
The focus of this case study is on Faraday’s use of the lines of magnetic force. Faraday is known of his exploratory, creative, and yet systemic way of experimenting, and the visual reasoning leading to theoretical development was an inherent part of this experimentation (Gooding 2006 ). Faraday’s articles or notebooks do not include mathematical formulations; instead, they include images and illustrations from experimental devices and setups to the recapping of his theoretical ideas (Nersessian 2008 ). According to Gooding ( 2006 ), “Faraday’s visual method was designed not to copy apparent features of the world, but to analyse and replicate them” (2006, p. 46).
The lines of force played a central role in Faraday’s research on electricity and magnetism and in the development of his “field theory” (Faraday 1852a ; Nersessian 1984 ). Before Faraday, the experiments with iron filings around magnets were known and the term “magnetic curves” was used for the iron filing patterns and also for the geometrical constructs derived from the mathematical theory of magnetism (Gooding et al. 1993 ). However, Faraday used the lines of force for explaining his experimental observations and in constructing the theory of forces in magnetism and electricity. Examples of Faraday’s different illustrations of lines of magnetic force are given in Fig. 2 . Faraday gave the following experiment-based definition for the lines of magnetic forces:
a Iron filing pattern in case of bar magnet drawn by Faraday (Faraday 1852b , Plate IX, p. 158, Fig. 1), b Faraday’s drawing of lines of magnetic force in case of cylinder magnet, where the experimental procedure, knife blade showing the direction of lines, is combined into drawing (Faraday, 1855, vol. 1, plate 1)
A line of magnetic force may be defined as that line which is described by a very small magnetic needle, when it is so moved in either direction correspondent to its length, that the needle is constantly a tangent to the line of motion; or it is that line along which, if a transverse wire be moved in either direction, there is no tendency to the formation of any current in the wire, whilst if moved in any other direction there is such a tendency; or it is that line which coincides with the direction of the magnecrystallic axis of a crystal of bismuth, which is carried in either direction along it. The direction of these lines about and amongst magnets and electric currents, is easily represented and understood, in a general manner, by the ordinary use of iron filings. (Faraday 1852a , p. 25 (3071))
The definition describes the connection between the experiments and the visual representation of the results. Initially, the lines of force were just geometric representations, but later, Faraday treated them as physical objects (Nersessian 1984 ; Pocovi and Finlay 2002 ):
I have sometimes used the term lines of force so vaguely, as to leave the reader doubtful whether I intended it as a merely representative idea of the forces, or as the description of the path along which the power was continuously exerted. … wherever the expression line of force is taken simply to represent the disposition of forces, it shall have the fullness of that meaning; but that wherever it may seem to represent the idea of the physical mode of transmission of the force, it expresses in that respect the opinion to which I incline at present. The opinion may be erroneous, and yet all that relates or refers to the disposition of the force will remain the same. (Faraday, 1852a , p. 55-56 (3075))
He also felt that the lines of force had greater explanatory power than the dominant theory of action-at-a-distance:
Now it appears to me that these lines may be employed with great advantage to represent nature, condition, direction and comparative amount of the magnetic forces; and that in many cases they have, to the physical reasoned at least, a superiority over that method which represents the forces as concentrated in centres of action… (Faraday, 1852a , p. 26 (3074))
For giving some insight to Faraday’s visual reasoning as an epistemic practice, the following examples of Faraday’s studies of the lines of magnetic force (Faraday 1852a , 1852b ) are presented:
(a) Asking questions and defining problems: The iron filing patterns formed the empirical basis for the visual model: 2D visualization of lines of magnetic force as presented in Fig. 2 . According to Faraday, these iron filing patterns were suitable for illustrating the direction and form of the magnetic lines of force (emphasis added):
It must be well understood that these forms give no indication by their appearance of the relative strength of the magnetic force at different places, inasmuch as the appearance of the lines depends greatly upon the quantity of filings and the amount of tapping; but the direction and forms of these lines are well given, and these indicate, in a considerable degree, the direction in which the forces increase and diminish . (Faraday 1852b , p.158 (3237))
Despite being static and two dimensional on paper, the lines of magnetic force were dynamical (Nersessian 1992 , 2008 ) and three dimensional for Faraday (see Fig. 2 b). For instance, Faraday described the lines of force “expanding”, “bending,” and “being cut” (Nersessian 1992 ). In Fig. 2 b, Faraday has summarized his experiment (bar magnet and knife blade) and its results (lines of force) in one picture.
(b) Analyzing and interpreting data: The model was so powerful for Faraday that he ended up thinking them as physical objects (e.g., Nersessian 1984 ), i.e., making interpretations of the way forces act. Of course, he made a lot of experiments for showing the physical existence of the lines of force, but he did not succeed in it (Nersessian 1984 ). The following quote illuminates Faraday’s use of the lines of force in different situations:
The study of these lines has, at different times, been greatly influential in leading me to various results, which I think prove their utility as well as fertility. Thus, the law of magneto-electric induction; the earth’s inductive action; the relation of magnetism and light; diamagnetic action and its law, and magnetocrystallic action, are the cases of this kind… (Faraday 1852a , p. 55 (3174))
(c) Experimenting: In Faraday's case, he used a lot of exploratory experiments; in case of lines of magnetic force, he used, e.g., iron filings, magnetic needles, or current carrying wires (see the quote above). The magnetic field is not directly observable and the representation of lines of force was a visual model, which includes the direction, form, and magnitude of field.
(d) Modeling: There is no denying that the lines of magnetic force are visual by nature. Faraday’s views of lines of force developed gradually during the years, and he applied and developed them in different contexts such as electromagnetic, electrostatic, and magnetic induction (Nersessian 1984 ). An example of Faraday’s explanation of the effect of the wire b’s position to experiment is given in Fig. 3 . In Fig. 3 , few magnetic lines of force are drawn, and in the quote below, Faraday is explaining the effect using these magnetic lines of force (emphasis added):
Picture of an experiment with different arrangements of wires ( a , b’ , b” ), magnet, and galvanometer. Note the lines of force drawn around the magnet. (Faraday 1852a , p. 34)
It will be evident by inspection of Fig. 3 , that, however the wires are carried away, the general result will, according to the assumed principles of action, be the same; for if a be the axial wire, and b’, b”, b”’ the equatorial wire, represented in three different positions, whatever magnetic lines of force pass across the latter wire in one position, will also pass it in the other, or in any other position which can be given to it. The distance of the wire at the place of intersection with the lines of force, has been shown, by the experiments (3093.), to be unimportant. (Faraday 1852a , p. 34 (3099))
In summary, by examining the history of Faraday’s use of lines of force, we showed how visual imagery and reasoning played an important part in Faraday’s construction and representation of his “field theory”. As Gooding has stated, “many of Faraday’s sketches are far more that depictions of observation, they are tools for reasoning with and about phenomena” (2006, p. 59).
Case study 3: visualizing scientific methods, the case of a journal
The focus of the third case study is the Journal of Visualized Experiments (JoVE) , a peer-reviewed publication indexed in PubMed. The journal devoted to the publication of biological, medical, chemical, and physical research in a video format. The journal describes its history as follows:
JoVE was established as a new tool in life science publication and communication, with participation of scientists from leading research institutions. JoVE takes advantage of video technology to capture and transmit the multiple facets and intricacies of life science research. Visualization greatly facilitates the understanding and efficient reproduction of both basic and complex experimental techniques, thereby addressing two of the biggest challenges faced by today's life science research community: i) low transparency and poor reproducibility of biological experiments and ii) time and labor-intensive nature of learning new experimental techniques. ( http://www.jove.com/ )
By examining the journal content, we generate a set of categories that can be considered as indicators of relevance and significance in terms of epistemic practices of science that have relevance for science education. For example, the quote above illustrates how scientists view some norms of scientific practice including the norms of “transparency” and “reproducibility” of experimental methods and results, and how the visual format of the journal facilitates the implementation of these norms. “Reproducibility” can be considered as an epistemic criterion that sits at the heart of what counts as an experimental procedure in science:
Investigating what should be reproducible and by whom leads to different types of experimental reproducibility, which can be observed to play different roles in experimental practice. A successful application of the strategy of reproducing an experiment is an achievement that may depend on certain isiosyncratic aspects of a local situation. Yet a purely local experiment that cannot be carried out by other experimenters and in other experimental contexts will, in the end be unproductive in science. (Sarkar and Pfeifer 2006 , p.270)
We now turn to an article on “Elevated Plus Maze for Mice” that is available for free on the journal website ( http://www.jove.com/video/1088/elevated-plus-maze-for-mice ). The purpose of this experiment was to investigate anxiety levels in mice through behavioral analysis. The journal article consists of a 9-min video accompanied by text. The video illustrates the handling of the mice in soundproof location with less light, worksheets with characteristics of mice, computer software, apparatus, resources, setting up the computer software, and the video recording of mouse behavior on the computer. The authors describe the apparatus that is used in the experiment and state how procedural differences exist between research groups that lead to difficulties in the interpretation of results:
The apparatus consists of open arms and closed arms, crossed in the middle perpendicularly to each other, and a center area. Mice are given access to all of the arms and are allowed to move freely between them. The number of entries into the open arms and the time spent in the open arms are used as indices of open space-induced anxiety in mice. Unfortunately, the procedural differences that exist between laboratories make it difficult to duplicate and compare results among laboratories.
The authors’ emphasis on the particularity of procedural context echoes in the observations of some philosophers of science:
It is not just the knowledge of experimental objects and phenomena but also their actual existence and occurrence that prove to be dependent on specific, productive interventions by the experimenters” (Sarkar and Pfeifer 2006 , pp. 270-271)
The inclusion of a video of the experimental procedure specifies what the apparatus looks like (Fig. 4 ) and how the behavior of the mice is captured through video recording that feeds into a computer (Fig. 5 ). Subsequently, a computer software which captures different variables such as the distance traveled, the number of entries, and the time spent on each arm of the apparatus. Here, there is visual information at different levels of representation ranging from reconfiguration of raw video data to representations that analyze the data around the variables in question (Fig. 6 ). The practice of levels of visual representations is not particular to the biological sciences. For instance, they are commonplace in nanotechnological practices:
Visual illustration of apparatus
Video processing of experimental set-up
Computer software for video input and variable recording
In the visualization processes, instruments are needed that can register the nanoscale and provide raw data, which needs to be transformed into images. Some Imaging Techniques have software incorporated already where this transformation automatically takes place, providing raw images. Raw data must be translated through the use of Graphic Software and software is also used for the further manipulation of images to highlight what is of interest to capture the (inferred) phenomena -- and to capture the reader. There are two levels of choice: Scientists have to choose which imaging technique and embedded software to use for the job at hand, and they will then have to follow the structure of the software. Within such software, there are explicit choices for the scientists, e.g. about colour coding, and ways of sharpening images. (Ruivenkamp and Rip 2010 , pp.14–15)
On the text that accompanies the video, the authors highlight the role of visualization in their experiment:
Visualization of the protocol will promote better understanding of the details of the entire experimental procedure, allowing for standardization of the protocols used in different laboratories and comparisons of the behavioral phenotypes of various strains of mutant mice assessed using this test.
The software that takes the video data and transforms it into various representations allows the researchers to collect data on mouse behavior more reliably. For instance, the distance traveled across the arms of the apparatus or the time spent on each arm would have been difficult to observe and record precisely. A further aspect to note is how the visualization of the experiment facilitates control of bias. The authors illustrate how the olfactory bias between experimental procedures carried on mice in sequence is avoided by cleaning the equipment.
Our discussion highlights the role of visualization in science, particularly with respect to presenting visualization as part of the scientific practices. We have used case studies from the history of science highlighting a scientist’s account of how visualization played a role in the discovery of DNA and the magnetic field and from a contemporary illustration of a science journal’s practices in incorporating visualization as a way to communicate new findings and methodologies. Our implicit aim in drawing from these case studies was the need to align science education with scientific practices, particularly in terms of how visual representations, stable or dynamic, can engage students in the processes of science and not only to be used as tools for cognitive development in science. Our approach was guided by the notion of “knowledge-as-practice” as advanced by Knorr Cetina ( 1999 ) who studied scientists and characterized their knowledge as practice, a characterization which shifts focus away from ideas inside scientists’ minds to practices that are cultural and deeply contextualized within fields of science. She suggests that people working together can be examined as epistemic cultures whose collective knowledge exists as practice.
It is important to stress, however, that visual representations are not used in isolation, but are supported by other types of evidence as well, or other theories (i.e., in order to understand the helical form of DNA, or the structure, chemistry knowledge was needed). More importantly, this finding can also have implications when teaching science as argument (e.g., Erduran and Jimenez-Aleixandre 2008 ), since the verbal evidence used in the science classroom to maintain an argument could be supported by visual evidence (either a model, representation, image, graph, etc.). For example, in a group of students discussing the outcomes of an introduced species in an ecosystem, pictures of the species and the ecosystem over time, and videos showing the changes in the ecosystem, and the special characteristics of the different species could serve as visual evidence to help the students support their arguments (Evagorou et al. 2012 ). Therefore, an important implication for the teaching of science is the use of visual representations as evidence in the science curriculum as part of knowledge production. Even though studies in the area of science education have focused on the use of models and modeling as a way to support students in the learning of science (Dori et al. 2003 ; Lehrer and Schauble 2012 ; Mendonça and Justi 2013 ; Papaevripidou et al. 2007 ) or on the use of images (i.e., Korfiatis et al. 2003 ), with the term using visuals as evidence, we refer to the collection of all forms of visuals and the processes involved.
Another aspect that was identified through the case studies is that of the visual reasoning (an integral part of Faraday’s investigations). Both the verbalization and visualization were part of the process of generating new knowledge (Gooding 2006 ). Even today, most of the textbooks use the lines of force (or just field lines) as a geometrical representation of field, and the number of field lines is connected to the quantity of flux. Often, the textbooks use the same kind of visual imagery than in what is used by scientists. However, when using images, only certain aspects or features of the phenomena or data are captured or highlighted, and often in tacit ways. Especially in textbooks, the process of producing the image is not presented and instead only the product—image—is left. This could easily lead to an idea of images (i.e., photos, graphs, visual model) being just representations of knowledge and, in the worse case, misinterpreted representations of knowledge as the results of Pocovi and Finlay ( 2002 ) in case of electric field lines show. In order to avoid this, the teachers should be able to explain how the images are produced (what features of phenomena or data the images captures, on what ground the features are chosen to that image, and what features are omitted); in this way, the role of visualization in knowledge production can be made “visible” to students by engaging them in the process of visualization.
The implication of these norms for science teaching and learning is numerous. The classroom contexts can model the generation, sharing and evaluation of evidence, and experimental procedures carried out by students, thereby promoting not only some contemporary cultural norms in scientific practice but also enabling the learning of criteria, standards, and heuristics that scientists use in making decisions on scientific methods. As we have demonstrated with the three case studies, visual representations are part of the process of knowledge growth and communication in science, as demonstrated with two examples from the history of science and an example from current scientific practices. Additionally, visual information, especially with the use of technology is a part of students’ everyday lives. Therefore, we suggest making use of students’ knowledge and technological skills (i.e., how to produce their own videos showing their experimental method or how to identify or provide appropriate visual evidence for a given topic), in order to teach them the aspects of the nature of science that are often neglected both in the history of science and the design of curriculum. Specifically, what we suggest in this paper is that students should actively engage in visualization processes in order to appreciate the diverse nature of doing science and engage in authentic scientific practices.
However, as a word of caution, we need to distinguish the products and processes involved in visualization practices in science:
If one considers scientific representations and the ways in which they can foster or thwart our understanding, it is clear that a mere object approach, which would devote all attention to the representation as a free-standing product of scientific labor, is inadequate. What is needed is a process approach: each visual representation should be linked with its context of production (Pauwels 2006 , p.21).
The aforementioned suggests that the emphasis in visualization should shift from cognitive understanding—using the products of science to understand the content—to engaging in the processes of visualization. Therefore, an implication for the teaching of science includes designing curriculum materials and learning environments that create a social and epistemic context and invite students to engage in the practice of visualization as evidence, reasoning, experimental procedure, or a means of communication (as presented in the three case studies) and reflect on these practices (Ryu et al. 2015 ).
Finally, a question that arises from including visualization in science education, as well as from including scientific practices in science education is whether teachers themselves are prepared to include them as part of their teaching (Bybee 2014 ). Teacher preparation programs and teacher education have been critiqued, studied, and rethought since the time they emerged (Cochran-Smith 2004 ). Despite the years of history in teacher training and teacher education, the debate about initial teacher training and its content still pertains in our community and in policy circles (Cochran-Smith 2004 ; Conway et al. 2009 ). In the last decades, the debate has shifted from a behavioral view of learning and teaching to a learning problem—focusing on that way not only on teachers’ knowledge, skills, and beliefs but also on making the connection of the aforementioned with how and if pupils learn (Cochran-Smith 2004 ). The Science Education in Europe report recommended that “Good quality teachers, with up-to-date knowledge and skills, are the foundation of any system of formal science education” (Osborne and Dillon 2008 , p.9).
However, questions such as what should be the emphasis on pre-service and in-service science teacher training, especially with the new emphasis on scientific practices, still remain unanswered. As Bybee ( 2014 ) argues, starting from the new emphasis on scientific practices in the NGSS, we should consider teacher preparation programs “that would provide undergraduates opportunities to learn the science content and practices in contexts that would be aligned with their future work as teachers” (p.218). Therefore, engaging pre- and in-service teachers in visualization as a scientific practice should be one of the purposes of teacher preparation programs.
Achieve. (2013). The next generation science standards (pp. 1–3). Retrieved from http://www.nextgenscience.org/ .
Google Scholar
Barber, J, Pearson, D, & Cervetti, G. (2006). Seeds of science/roots of reading . California: The Regents of the University of California.
Bungum, B. (2008). Images of physics: an explorative study of the changing character of visual images in Norwegian physics textbooks. NorDiNa, 4 (2), 132–141.
Bybee, RW. (2014). NGSS and the next generation of science teachers. Journal of Science Teacher Education, 25 (2), 211–221. doi: 10.1007/s10972-014-9381-4 .
Article Google Scholar
Chambers, D. (1983). Stereotypic images of the scientist: the draw-a-scientist test. Science Education, 67 (2), 255–265.
Cochran-Smith, M. (2004). The problem of teacher education. Journal of Teacher Education, 55 (4), 295–299. doi: 10.1177/0022487104268057 .
Conway, PF, Murphy, R, & Rath, A. (2009). Learning to teach and its implications for the continuum of teacher education: a nine-country cross-national study .
Crick, F. (1988). What a mad pursuit . USA: Basic Books.
Dimopoulos, K, Koulaidis, V, & Sklaveniti, S. (2003). Towards an analysis of visual images in school science textbooks and press articles about science and technology. Research in Science Education, 33 , 189–216.
Dori, YJ, Tal, RT, & Tsaushu, M. (2003). Teaching biotechnology through case studies—can we improve higher order thinking skills of nonscience majors? Science Education, 87 (6), 767–793. doi: 10.1002/sce.10081 .
Duschl, RA, & Bybee, RW. (2014). Planning and carrying out investigations: an entry to learning and to teacher professional development around NGSS science and engineering practices. International Journal of STEM Education, 1 (1), 12. doi: 10.1186/s40594-014-0012-6 .
Duschl, R., Schweingruber, H. A., & Shouse, A. (2008). Taking science to school . Washington DC: National Academies Press.
Erduran, S, & Jimenez-Aleixandre, MP (Eds.). (2008). Argumentation in science education: perspectives from classroom-based research . Dordrecht: Springer.
Eurydice. (2012). Developing key competencies at school in Europe: challenges and opportunities for policy – 2011/12 (pp. 1–72).
Evagorou, M, Jimenez-Aleixandre, MP, & Osborne, J. (2012). “Should we kill the grey squirrels?” A study exploring students’ justifications and decision-making. International Journal of Science Education, 34 (3), 401–428. doi: 10.1080/09500693.2011.619211 .
Faraday, M. (1852a). Experimental researches in electricity. – Twenty-eighth series. Philosophical Transactions of the Royal Society of London, 142 , 25–56.
Faraday, M. (1852b). Experimental researches in electricity. – Twenty-ninth series. Philosophical Transactions of the Royal Society of London, 142 , 137–159.
Gilbert, JK. (2010). The role of visual representations in the learning and teaching of science: an introduction (pp. 1–19).
Gilbert, J., Reiner, M. & Nakhleh, M. (2008). Visualization: theory and practice in science education . Dordrecht, The Netherlands: Springer.
Gooding, D. (2006). From phenomenology to field theory: Faraday’s visual reasoning. Perspectives on Science, 14 (1), 40–65.
Gooding, D, Pinch, T, & Schaffer, S (Eds.). (1993). The uses of experiment: studies in the natural sciences . Cambridge: Cambridge University Press.
Hogan, K, & Maglienti, M. (2001). Comparing the epistemological underpinnings of students’ and scientists’ reasoning about conclusions. Journal of Research in Science Teaching, 38 (6), 663–687.
Knorr Cetina, K. (1999). Epistemic cultures: how the sciences make knowledge . Cambridge: Harvard University Press.
Korfiatis, KJ, Stamou, AG, & Paraskevopoulos, S. (2003). Images of nature in Greek primary school textbooks. Science Education, 88 (1), 72–89. doi: 10.1002/sce.10133 .
Latour, B. (2011). Visualisation and cognition: drawing things together (pp. 1–32).
Latour, B, & Woolgar, S. (1979). Laboratory life: the construction of scientific facts . Princeton: Princeton University Press.
Lehrer, R, & Schauble, L. (2012). Seeding evolutionary thinking by engaging children in modeling its foundations. Science Education, 96 (4), 701–724. doi: 10.1002/sce.20475 .
Longino, H. E. (2002). The fate of knowledge . Princeton: Princeton University Press.
Lynch, M. (2006). The production of scientific images: vision and re-vision in the history, philosophy, and sociology of science. In L Pauwels (Ed.), Visual cultures of science: rethinking representational practices in knowledge building and science communication (pp. 26–40). Lebanon, NH: Darthmouth College Press.
Lynch, M. & S. Y. Edgerton Jr. (1988). ‘Aesthetic and digital image processing representational craft in contemporary astronomy’, in G. Fyfe & J. Law (eds), Picturing Power; Visual Depictions and Social Relations (London, Routledge): 184 – 220.
Mendonça, PCC, & Justi, R. (2013). An instrument for analyzing arguments produced in modeling-based chemistry lessons. Journal of Research in Science Teaching, 51 (2), 192–218. doi: 10.1002/tea.21133 .
National Research Council (2000). Inquiry and the national science education standards . Washington DC: National Academies Press.
National Research Council (2012). A framework for K-12 science education . Washington DC: National Academies Press.
Nersessian, NJ. (1984). Faraday to Einstein: constructing meaning in scientific theories . Dordrecht: Martinus Nijhoff Publishers.
Book Google Scholar
Nersessian, NJ. (1992). How do scientists think? Capturing the dynamics of conceptual change in science. In RN Giere (Ed.), Cognitive Models of Science (pp. 3–45). Minneapolis: University of Minnesota Press.
Nersessian, NJ. (2008). Creating scientific concepts . Cambridge: The MIT Press.
Osborne, J. (2014). Teaching scientific practices: meeting the challenge of change. Journal of Science Teacher Education, 25 (2), 177–196. doi: 10.1007/s10972-014-9384-1 .
Osborne, J. & Dillon, J. (2008). Science education in Europe: critical reflections . London: Nuffield Foundation.
Papaevripidou, M, Constantinou, CP, & Zacharia, ZC. (2007). Modeling complex marine ecosystems: an investigation of two teaching approaches with fifth graders. Journal of Computer Assisted Learning, 23 (2), 145–157. doi: 10.1111/j.1365-2729.2006.00217.x .
Pauwels, L. (2006). A theoretical framework for assessing visual representational practices in knowledge building and science communications. In L Pauwels (Ed.), Visual cultures of science: rethinking representational practices in knowledge building and science communication (pp. 1–25). Lebanon, NH: Darthmouth College Press.
Philips, L., Norris, S. & McNab, J. (2010). Visualization in mathematics, reading and science education . Dordrecht, The Netherlands: Springer.
Pocovi, MC, & Finlay, F. (2002). Lines of force: Faraday’s and students’ views. Science & Education, 11 , 459–474.
Richards, A. (2003). Argument and authority in the visual representations of science. Technical Communication Quarterly, 12 (2), 183–206. doi: 10.1207/s15427625tcq1202_3 .
Rothbart, D. (1997). Explaining the growth of scientific knowledge: metaphors, models and meaning . Lewiston, NY: Mellen Press.
Ruivenkamp, M, & Rip, A. (2010). Visualizing the invisible nanoscale study: visualization practices in nanotechnology community of practice. Science Studies, 23 (1), 3–36.
Ryu, S, Han, Y, & Paik, S-H. (2015). Understanding co-development of conceptual and epistemic understanding through modeling practices with mobile internet. Journal of Science Education and Technology, 24 (2-3), 330–355. doi: 10.1007/s10956-014-9545-1 .
Sarkar, S, & Pfeifer, J. (2006). The philosophy of science, chapter on experimentation (Vol. 1, A-M). New York: Taylor & Francis.
Schwartz, RS, Lederman, NG, & Abd-el-Khalick, F. (2012). A series of misrepresentations: a response to Allchin’s whole approach to assessing nature of science understandings. Science Education, 96 (4), 685–692. doi: 10.1002/sce.21013 .
Schwarz, CV, Reiser, BJ, Davis, EA, Kenyon, L, Achér, A, Fortus, D, et al. (2009). Developing a learning progression for scientific modeling: making scientific modeling accessible and meaningful for learners. Journal of Research in Science Teaching, 46 (6), 632–654. doi: 10.1002/tea.20311 .
Watson, J. (1968). The Double Helix: a personal account of the discovery of the structure of DNA . New York: Scribner.
Watson, J, & Berry, A. (2004). DNA: the secret of life . New York: Alfred A. Knopf.
Wickman, PO. (2004). The practical epistemologies of the classroom: a study of laboratory work. Science Education, 88 , 325–344.
Wu, HK, & Shah, P. (2004). Exploring visuospatial thinking in chemistry learning. Science Education, 88 (3), 465–492. doi: 10.1002/sce.10126 .
Download references
Acknowledgements
The authors would like to acknowledge all reviewers for their valuable comments that have helped us improve the manuscript.
Author information
Authors and affiliations.
University of Nicosia, 46, Makedonitissa Avenue, Egkomi, 1700, Nicosia, Cyprus
Maria Evagorou
University of Limerick, Limerick, Ireland
Sibel Erduran
University of Tampere, Tampere, Finland
Terhi Mäntylä
You can also search for this author in PubMed Google Scholar
Corresponding author
Correspondence to Maria Evagorou .
Additional information
Competing interests.
The authors declare that they have no competing interests.
Authors’ contributions
ME carried out the introductory literature review, the analysis of the first case study, and drafted the manuscript. SE carried out the analysis of the third case study and contributed towards the “Conclusions” section of the manuscript. TM carried out the second case study. All authors read and approved the final manuscript.
Rights and permissions
Open Access This article is distributed under the terms of the Creative Commons Attribution 4.0 International License ( https://creativecommons.org/licenses/by/4.0 ), which permits use, duplication, adaptation, distribution, and reproduction in any medium or format, as long as you give appropriate credit to the original author(s) and the source, provide a link to the Creative Commons license, and indicate if changes were made.
Reprints and permissions
About this article
Cite this article.
Evagorou, M., Erduran, S. & Mäntylä, T. The role of visual representations in scientific practices: from conceptual understanding and knowledge generation to ‘seeing’ how science works. IJ STEM Ed 2 , 11 (2015). https://doi.org/10.1186/s40594-015-0024-x
Download citation
Received : 29 September 2014
Accepted : 16 May 2015
Published : 19 July 2015
DOI : https://doi.org/10.1186/s40594-015-0024-x
Share this article
Anyone you share the following link with will be able to read this content:
Sorry, a shareable link is not currently available for this article.
Provided by the Springer Nature SharedIt content-sharing initiative
- Visual representations
- Epistemic practices
- Science learning
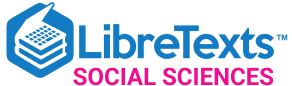
- school Campus Bookshelves
- menu_book Bookshelves
- perm_media Learning Objects
- login Login
- how_to_reg Request Instructor Account
- hub Instructor Commons
- Download Page (PDF)
- Download Full Book (PDF)
- Periodic Table
- Physics Constants
- Scientific Calculator
- Reference & Cite
- Tools expand_more
- Readability
selected template will load here
This action is not available.
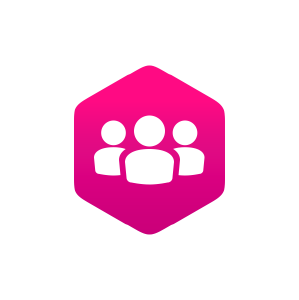
1.3: Maps- Place, Power, and Representation
- Last updated
- Save as PDF
- Page ID 147497
Learning Objectives
- Describe cartography, map types, and how geospatial technologies aid contemporary map-making.
- Critique the appropriateness of map scales, projections, and orientations for various purposes.
- Explain and exemplify maps as tools and representations of power.
- Describe countermapping and participatory making and their various applications.
- Imagine cartography from non-conventional and Indigenous perspectives.
Cartography
The use of maps to illustrate, explain, and analyze spatial phenomena is a distinguishing characteristic of the discipline of geography. Maps as we know them are a result of thousands of years of humans seeking to understand the Earth. Early geographical endeavors were often centered around cartography , the art and science of mapmaking. Many early geographers practiced a form of Earth writing through maps. A map attempts to represent human knowledge of the Earth's lands and peoples, and mapping continues to be a central element of the geography discipline.
Given the importance of spatial awareness in human history, it is difficult to attribute the beginning of geography or cartography to one founding figure or document. Geographical knowledge has been an essential part of the story of humans, a species that over thousands of years migrated out of Africa to occupy most corners of the world. Humans have always had the need and desire to explore and understand their surroundings, expand their influence over new lands (in ways ranging from life-affirming to destructive), and then to communicate and represent knowledge, whether in maps or stories (or both). Humanity’s geographical knowledge is both ancient and diverse, and certainly not entirely understood or represented in academic geography today. With that in mind, the historical maps and documents that survive provide us with a sense of early geographical explorations that can help us appreciate the beginnings of geography as an academic discipline.
The earliest known world map was inscribed in clay tablets by the Babylonians more than two thousand years ago. It centers Babylon (in modern-day Iraq) bisected by the Euphrates River and includes descriptive text in cuneiform, the earliest known writing system. The Babylonians were also the first to divide a map into quadrants, indicating a coordinate system (East/West/North/South). Many academic geographers, especially in the West, attribute the beginnings of the discipline of geography to the Greeks. In their maps we find detailed geographical knowledge and important improvements in the accuracy of geographical depictions of the Earth. Crates created the first globe (200 BCE), still the most accurate way to represent the Earth. It was Eratosthenes (ca. 285-205 BC), however, who likely coined the term “geographia” to describe the field of study. Eratosthenes was the first to calculate the Earth's circumference (with remarkable accuracy) and his publication Geographika maps over four hundred places in the world, connecting them through a system of parallels and meridians and scaled distances [1] [2] . Other important achievements include that of Arab-Islamic geographers, notably Muhammad al-Idrisi (circa 1100–66) who was commissioned by King Roger II of Sicily to produce a highly regarded historical map, Tabula Rogeriana. This map stood as a valuable reference of geographical knowledge for hundreds of years. Its inclusion of detailed geographical knowledge was crowdsourced, meaning that al-Idrisi interviewed merchants and travelers to compile detailed information from the major population centers of the known world. [3]
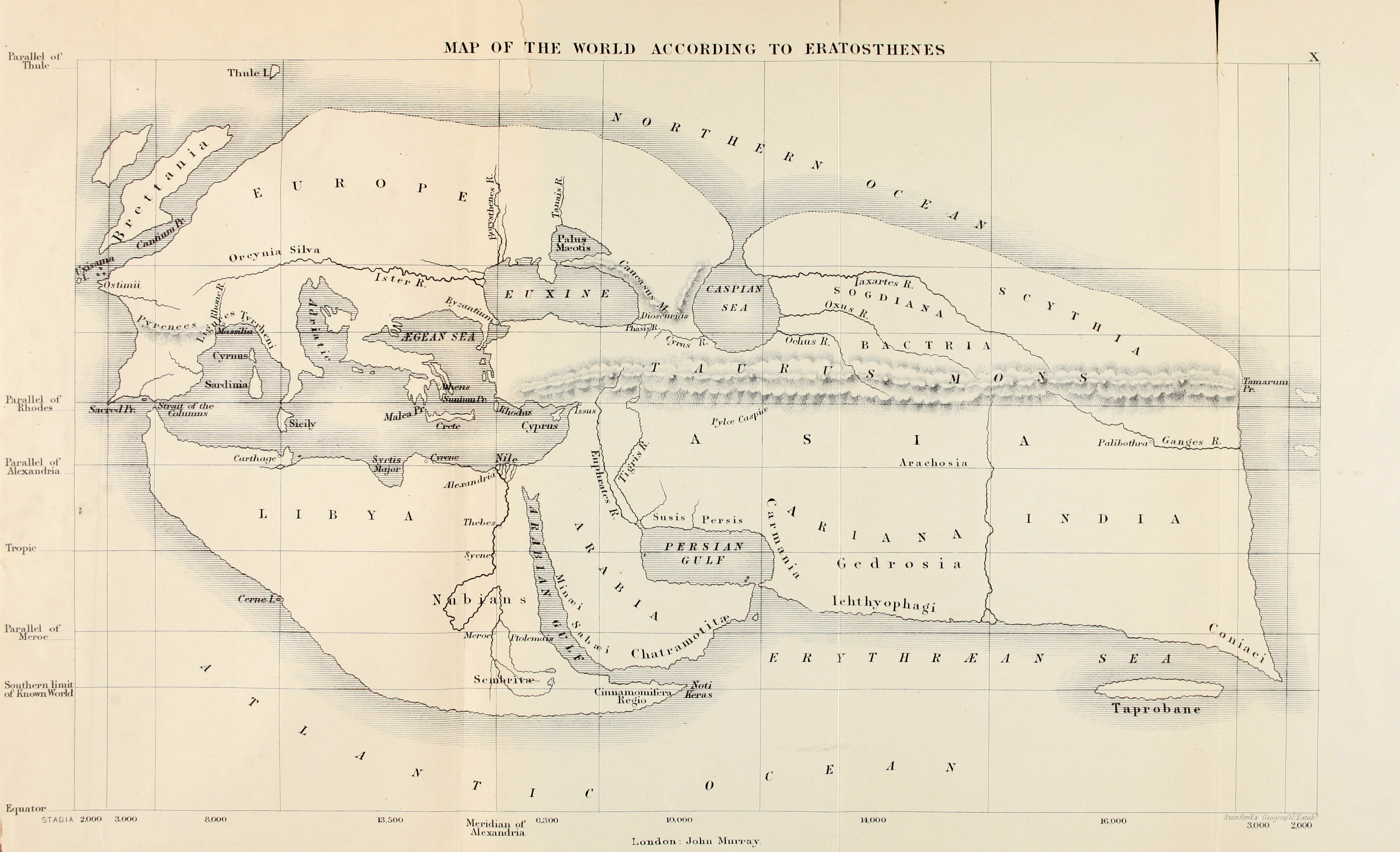
Reference maps: where we are
A reference map helps us learn where we are . Reference maps are used as navigational tools, as a reference for the absolute and relative locations of human and physical features. Developing an understanding of the Earth sufficient enough to create an accurate representation of it challenged cartographers for centuries. The early years of the discipline of geography were dedicated to understanding the shape, size, and distances of continents, a strenuous task before the development of geo-spatial tools such as GPS, remote sensing, and GIS. Today, technological innovations enable us to access zoomable and detailed reference maps on our smartphones that allow us to locate places and navigate the world. When you open your map app for navigation, you are accessing (and contributing data to) a reference map.
Thematic maps: who we are
A political map of the world emphasizes political borders that divide territories. They tell us very little about the world, except for the territorial delineations of countries, a reference map of where countries are in relation to other places. Political maps do not tell you where people live, the characteristics of places, or how people and places are connected. We need thematic maps for that. Thematic maps communicate information that help us learn who we are . These are maps that help us understand what places might be like. For example, a map of global transportation and communications shifts the emphasis from how we divide the world to how we use the world, showing us functional lines (like cables and rails) that dissolve political borders and connect us daily. A global map of biomes and topography gives us a greater sense of the natural world. A population density map conveys the number of people per area (often in square kilometers or square miles) and gives us a sense of patterns of human settlement. There is limitless information that thematic maps can communicate. Using maps as communication devices is a key element of contemporary geography, as thematic maps serve as visualizations and analytical tools for presenting and understanding geographical patterns.
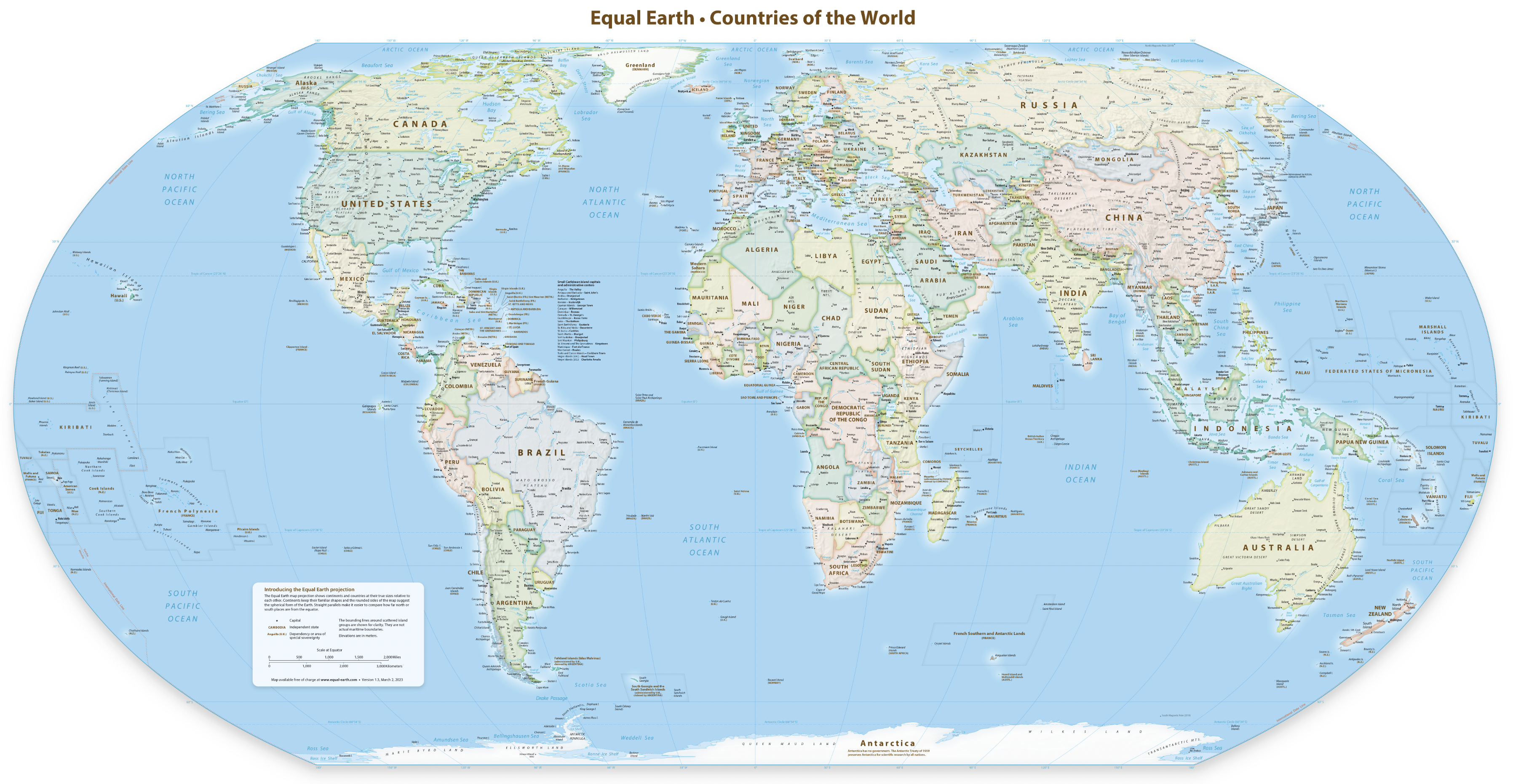
Geospatial Technologies
The ability to connect maps to data has revolutionized many facets of decision-making in our society. Spatial data , also called geospatial data , are data directly related to a specific location on Earth. Non-spatial data are data that cannot be traced to a specific location, like the number of people enrolled in an online course. Non-spatial data can easily become spatial data if they can be linked in some way to a location, like the address of each student enrolled in that course. Geospatial technology specialists have a method called geocoding they use to place non-spatial data at a particular geographic location (latitude and longitude). Once data become associated with a location, we can ask more complex questions. For example, at Fullerton College, geographers mapped the student population to better understand the geographical contexts of students amidst the covid-19 pandemic. The study showed that most students enrolled in online classes still lived within the vicinity of the college, and that despite distance education, students were choosing their colleges based on geographical proximity. More significantly, the study revealed that thousands of students lived in neighborhoods with poor access to green spaces and fresh foods, a specially challenging geographical context given the loss of access to campus resources due to the covid-19 state of emergency closures. This enhanced geographical understanding broadened the support for students’ basic needs in various areas of institutional planning.
Imagine, for example, levels of air pollution being collected at multiple stations - these data can then be mapped, and therefore help decision-makers understand disproportionate concentrations of pollution. When combining various data in layers of information about places, cartographers can identify populations most vulnerable to high levels of toxicity and what factors they have in common. The California Environmental Protection Agency has created a tool called EnviroScreen which does just that: it compiles a multilayer map that helps decision-makers correlate pollution to other demographic information such as income and race and ethnicity. Most commonly, we find that in California (and elsewhere in the US) low income and ethnic/racial minorities are disproportionately exposed to high levels of pollution. California knows this only because of the geospatial data it collects at various points throughout the state.
The efforts by the California Environmental Protection Agency to identify these areas of disproportionate impact rely on important technological innovations in cartography. Geographic Information Systems (GIS) is a combination of computer hardware and software that captures, stores, queries, and displays geospatial data. GIS has revolutionized mapmaking for its ability to produce maps that layer various data sets, thus revealing important correlations (such as the correlation between pollution, race, and income). Nearly all cartography today is done using GIS and its uses are limitless: Firefighters use GIS to monitor wildfires and to establish and communicate evacuation zones, conservation biologists to establish high priority areas for conservation, public health professionals to determine areas of high risk for infectious diseases, and insurance companies to identify proximities to geographical risks such as flooding and earthquakes. Given the heavy lifting potential of GIS to store, display, and analyze big spatial data for various purposes, the job prospects for GIS analysts continue to grow.
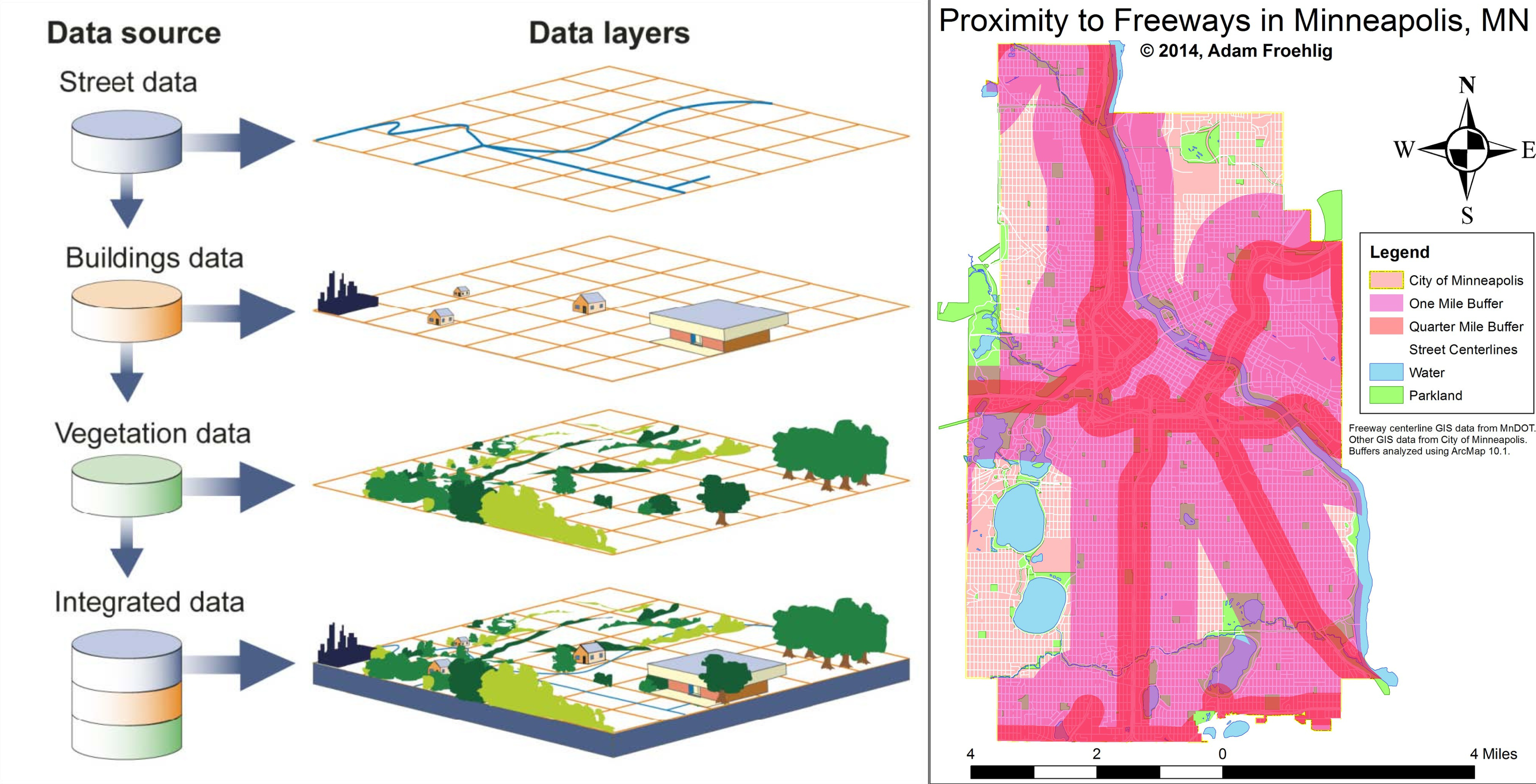
Geographers have developed a variety of ways to gather images and other data related to the Earth. One prominent technique is remote sensing, the collection of data about an object from a distance, usually done with the help of mechanical devices known as remote sensors which may be placed in planes or satellites. These sensors receive and record information about places/objects by measuring the transmission of electromagnetic energy from reflecting and radiating surfaces. These devices allow tropical ecologists to investigate the rainforest floor from half a world away, oceanographers to research the depths of the ocean, and students to count the trees in their backyards, all without leaving their desks. Remotely sensed images allow geographers to identify, understand, or explain a particular landscape, determine land use, and otherwise research places across the Earth. Remotely sensed images often serve as important components in the cartographic (map-making) process and can provide the means to examine and analyze changes on the earth’s surface caused by natural or human forces. Google Earth is an excellent example of a freely available online tool that collects and organizes remotely sensed images of the Earth. It now covers images for over 97 percent of the world’s surface, effectively compressing an interactive world map that fits in our phones.
Maps Are Not Mirrors
Maps are depictions of the Earth’s surface or a portion of it. They are not a reflection of the world but representations of it. All maps are imperfect representations, and the map is not the territory. What makes a "good map” varies depending upon its ability to fulfill an intended purpose. As noted above, a map designed for navigation might not tell us anything about the characteristics of places. Similarly, a map designed to communicate the population density of East Asia may also be useless for finding specific locations in Asia. Cartographers, or mapmakers, first and foremost consider what the map will be used for prior to designing it, then make important decisions to produce maps to fulfill that purpose. After determining the purpose of a map, cartographers face important decisions to convey geospatial data as objectively, accurately, and clearly as possible. Let’s explore some of these decisions.
Zoom In or Zoom Out?
Scale is the relationship of distance in the map versus the distance in the real world. A 1:1000 scale map, for example, would mean that 1 meter on the map equals 1000 meters, or 1 kilometer, on Earth’s surface. Scale can sometimes be a confusing concept, so it’s important to remember that it refers to a ratio. “Large” scale and “small” scale refer to the ratio, not to the size of the landmass on the map. 1 divided by 5,000 is 0.0002, which is a larger number than 1 divided by 50,000,000 (which is 0.00000002). Thus, a 1:5,000 scale map is considered “large” scale while 1:50,000,000 is considered “small” scale. In other words, small scale maps depict larger areas (ex: a world map) and large-scale maps smaller areas (ex: a map of your neighborhood).
Decisions pertaining to scale are important because geographic patterns vary depending on the scale of the map. Typically, as scale decreases (or as you zoom out), so too does the number of different features and the detail with which they are represented. Not only printed maps, but also digital geographic data sets that cover extensive areas, tend to be more generalized than datasets that cover limited areas. The scale of a map can be used to omit or reveal detail, depending on the message it intends to convey. Scale choices can significantly alter what the maps communicate.
A Distorted View
Maps turn a three-dimensional spherical Earth into a flat representation of it. This makes it inevitable that all maps are inaccurate in one way or another as ‘flattening the Earth’ always involves some form(s) of distortion. A map projection , how the spherical Earth is laid out on a flat plane, always distorts at least one these four properties: area, shape, distance, and direction. Some projections preserve as many as three of these properties while significantly distorting another. Other projections might distort all properties but to a smaller degree. In other words, cartographers make difficult compromises in mapmaking, giving up accuracy of some properties over others. See the video below:
Some familiar maps are distorted in ways that help perpetuate erroneous conceptions about the world. As the video discussed above, the wide adoption of the Mercator projection, which is used in Google maps and most other websites and online mapping platforms, materialized a common mental map that exaggerates the size of Europe in relation to Africa and other tropical and sub-tropical territories. Since humans often associate larger landmasses with prominence, such distortions may contribute to a mental map of the world that diminishes many of the territories formerly colonized by Europeans. Given the extensive ways in which European colonialism carved up and remade the world, it is essential to deconstruct maps and expose their biased geographic conceptions if we are to seek a greater understanding of the world.
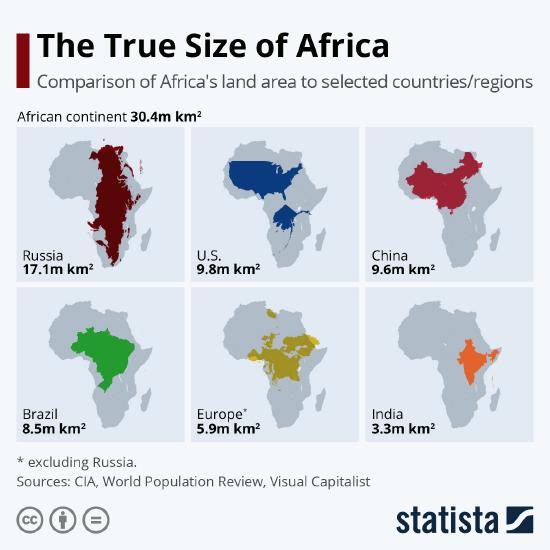
Flipping the Map
Throughout history, cartographers have made maps with varying orientation , meaning the map’s directionality or relationship to the four directions of the compass. Historically, the North of the map was rarely oriented to the top of the page. Recall the Tabula Rogeriana by Muslim cartographer Muhammad Al-Idrisi (Figure 1.3.1). The orientation of the total map places Mecca, the center of the Musim world in modern Saudi Arabia, at the center of the map, and with the South at the top of the page. Why did Al-Idrisi orient a map that remained the world’s most accurate map for three hundred years with South facing up? Well, why not? Most Westerners are conditioned by the last 500 years of cartography when most of the maps, produced mainly in North America and Europe, have been oriented with the North at the top. The perspective of North American and European cartographers emphasized the geography of the Northern Hemisphere while placing other continents “at the bottom” of the page, an arbitrary political decision that has casually become the norm.
The orientation of a map does not affect its accuracy (Arabic, Farsi, Hebrew, and Urdu are read from right to left). There is no scientific reason for a magnetic north to be perceived as the top of the world. Keep in mind that the Earth is an oblate spheroid spinning through space; there is no accurate “up” or “down.” Where the magnetic north should be represented depends on the cartographer’s relative position—from where they draw the map and to where they imagine distant places. What is North to the Inuit people of the Arctic looking out to the world, when everywhere is South in relation to their communities? What is a map of the world's continents for Polynesian cultures rooted in Pacific Islands? By diversifying map orientations to center the Southern Hemisphere, the Polar worlds, or the Pacific Ocean, for example, cartographers challenge (often Eurocentric) conventions and help better represent the kaleidoscopic geographical perspectives of the world – flipping the script by flipping the map. Take for example América Invertida (1929) by Uruguayan cartographer Joaquín Torres, illustrated below. The map is a cartographic manifesto: “Our North is the South.” Like all maps, this depiction carries and conveys political and social messages.
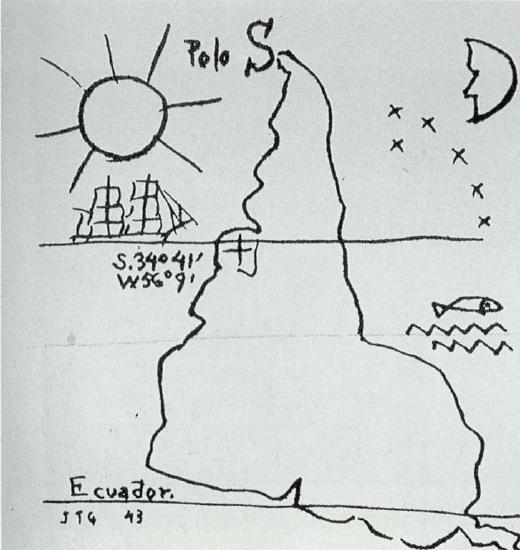
Maps and Power
“Cartography has a troubled history as a technology of power. The production and distribution of maps, often understood to be ideological representations that support the interests of their developers, have served as tools of colonization, imperialism, and global development, advancing Western notions of space and place at the expense of Indigenous peoples and other marginalized communities.” – Bjørn Sletoo [4]
“As a political technology, mapping has long played a key role in the world-making practices of colonialism through the appropriation, demarcation, naming, and partitioning of territory as part of the process of colonization and assertion of imperial rule over peoples and places.” - Rose-Rosewood et al [5]
Maps and colonization
How do you think about maps? Even though many of us think of maps as impartial documents that reflect the objective reality of the world, maps are instead subjective political projects that actively reimagine and remake the world. As introduced in the section above, political maps of the world hanging in most geography classrooms (in North America and elsewhere) are filled with an array of arbitrary decisions based on Western biases: they display an orientation that centers Europe, a projection (Mercator) that distorts the relative size of dispossessed territories, and political borders and place names superimposed by colonizers. These not-so-subtle cues make a familiar world map Eurocentric .
Our most familiar maps have evolved as tools and representations of power. [6] Throughout the rise and fall of so many empires, elites have cultivated the art and science of cartography – like the religious rulers of dynastic Egypt and Christian medieval Europe, the Greek and Roman philosophers, the Sultans of the Ottoman Empire, and India’s Mogul emperors. From the 16 th Century on, cartography as we know it had become closely intertwined with European imperialism, insomuch that the processes of colonialism and mapmaking each reinforced the other. [7] Take for example Gerardus Mercator (1512–94) whose widely influential projection we discussed earlier. Mercator also produced earlier remarkable works in cartography, including one of the first European maps of the Americas and the first to name it as such. His early map depicts the earth in two heart-shaped spheres as seen from the North and South poles. This spherical representation was an attempt to mediate distortions and provide a picture-like representation of the Earth. However, this type of map was impractical for navigational purposes and eventually led Mercator to create a different projection. The 1569 Mercator map of the world provided a squared grid that could be used for navigation, maintaining accuracy in distance and direction around the equator, regions Europeans were actively colonizing. Mercator thus adapted his cartography to facilitate European (con)quests. Despite its distortions of the size of landmasses moving away from the Equator, his 1569 map, actually a navigational chart, remains one of the most influential.
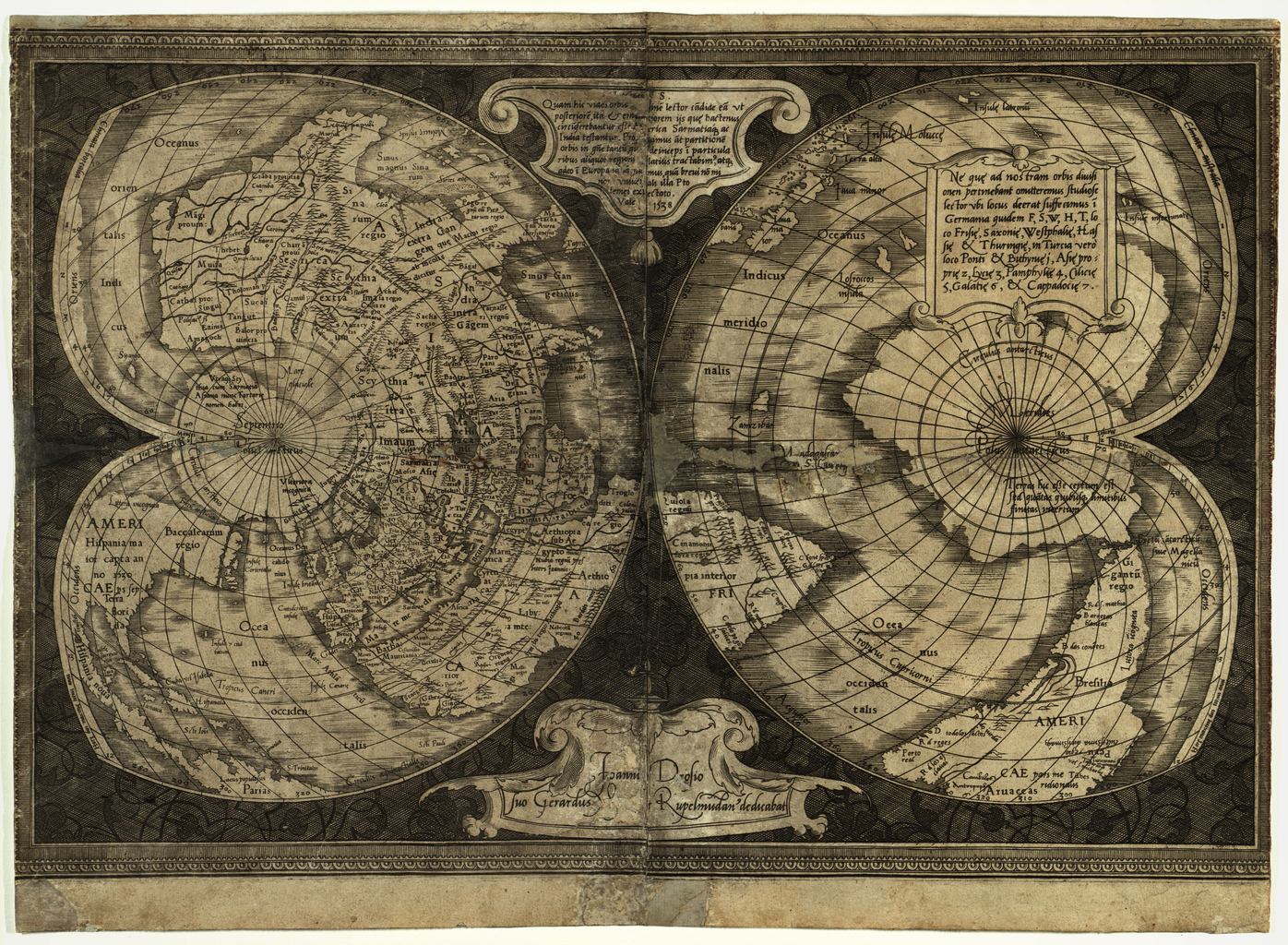
But maps were not only mere navigational tools for exploration. Like arms, germs, and ships, maps were a weapon of colonization as they allowed invaders to expropriate territories and displace Indigenous communities with arbitrary lines drawn on paper. [8] Take for example the 1494 Tordesillas Treaty, an agreement between the monarchs of Spain and Portugal that aimed to settle their squabbles over claims to foreign lands. By placing a line on a map through what was thought to be the middle of the Atlantic Ocean, but what was actually eastern South America, the treaty declared territories to its West for the Spanish and territories to its East for the Portuguese (see Figure 1.3.6). Imposed by distant monarchs, this line fundamentally shaped European colonialism in the Americas and remade the world. Even though European navigators had no means to accurately calculate longitude and thus locate the treaty's line. Still, maps based on the Line of Tordesillas documented European claims to lands across the Earth and set in motion processes of expropriation and colonization that unfolded over the next several centuries. The accidental placement of the Line of Tordesillas led the Portuguese to colonize the lands that became Brazil, Goa in India, and Macau in East Asia. Meanwhile, the Spanish received authority to colonize the Americas and the Caribbean west of Portuguese America. As such, these maps became weapons of power and plunder that divided and reproduced the world into territories of European expansion. [9]
.jpeg?revision=1&size=bestfit&width=1080&height=506)
From the 16 th to 20 th centuries, the materialization of colonial political borders from paper to reality continued to carve the world into European spheres of influence. Created by colonizers in support of their own interests, these colonial borders continue to shape contemporary geographies. The so-called “Scramble for Africa” is probably the most well-known example of how cartographic strokes have fragmented Indigenous homelands and their political organizations and environments. This legacy of colonial cartography and its arbitrary lines continues to sever African peoples and lands in problematic ways (discussed in Chapter 3). The 1947 British partitioning of India serves as another example of colonial impositions, leading to one of the greatest forced migrations and displacements in history and determining life and death for millions of people in the Indian subcontinent (discussed in Chapter 7). To this day, the border between India and Pakistan is marked by violence and military tension, creating one of the most dangerous political borders in the world. Similarly, the colonial legacy of the partition is the root cause for tensions and violence along the eastern Indian border with neighboring Bangladesh.
The United States is no exception as we can point to many historical (and ongoing) examples of the many ways that maps wield power, helping to perpetuate systemic racism and the disenfranchisement of Black, Indigenous, and other communities of color. For example, “redlining” maps funded by the US government prevented African Americans and immigrants from getting mortgages to buy homes and helped to solidify the racially segregated and inequitable cities that continue to plague the country (explained in Chapter 10). Similarly, electoral maps and voting district boundaries have been drawn and redrawn with the intent to diminish electoral power and representation of Black and Latinx voters – a type of cartographic manipulation known as gerrymandering. Even in the creation of US national parks, famously referred to as “America’s Best Idea,” territorial delineation for conservation dispossessed and displaced Indigenous peoples from their ancestral lands, imposing exclusionary ecological museums and institutionalizing Western conceptions of wilderness , an inaccurate way of seeing the natural world as disconnected from Indigenous communities and histories. All over the world, the making and remaking of territories begins on, and takes shape through, maps and cartography.
Guerilla Cartography
Powerful organizations and companies are far from the only groups that wield power with maps and cartography. Maps are also used as tools to convey and carve our world from the ground up, by people from all backgrounds and social classes. They can be venues for performance, expression, memory, and art. They can be imaginary and visionary. And, most importantly, mapping need not to be exclusionary. As mentioned in the opening of this chapter, every single one of us is enveloped in geographic experience. Representing, imagining, and envisioning our worlds in cartographic forms is something we all do; it could never be restricted to academics or elites. Maps today are more accessible than ever before. Even without formal training, producing and publishing paper or digital maps is easier today than ever before. Individuals and communities can engage in mapmaking for a cause, for art, for fun, for recollection, for navigation, for representation, and so much more.
Cartography is one way to critique, disrupt, and reclaim power. We can use maps as important visual communicators to confront injustice, demarcate and protect Indigenous territories, share placed-based community experience, and rename places, to name just a few examples. Many contemporary cartographers, artists, and activists use maps to challenge power and promote “bottom-up” approaches to mapmaking, in their own terms. This is because maps can be powerful tools for marginalized peoples to reimagine and reconstruct their worlds in avoidance of, or resistance to, the structures of authority. This is called countermapping , or a political practice of mapping back. It is mapping against tradition or prevailing power or knowledge systems, guerilla style. Much of countermapping is done via participatory mapmaking , a practice of co-creating maps with or by the communities most closely associated with the places or territories being mapped. In other words, participatory map-making is a method for collective cartography that allows community members to become mappers rather than passive subjects of maps.
Countermapping is rooted in self-determination and resistance. It can be traced back to the struggles of First Nations political organizations in Canada and Alaska in the 1970s and has played a crucial part in the First Nations campaigns for autonomy in North America. The maps created from these foundational struggles eventually led to the establishment of Nunavut, a self-governed Inuit territory of two million square kilometers in northern Canada. This success initiated the spread of Indigenous countermapping all over the world. Countermapping has also been an important part of the African American struggle for freedom. Civil rights leader W.E.B Du Bois, for example, prepared a variety of maps to deconstruct racist stereotypes and highlight Black cultural and economic achievements. Civil rights activists also utilized maps to record, tabulate, and map lynchings throughout the United States as a form of gathering and displaying scientific information to promote anti-lynching legislation. Activists of the women’s suffrage movement of the 20 th Century, too, used suffrage maps in flyers and pamphlets as a persuasive tool on the struggle for the right to vote.
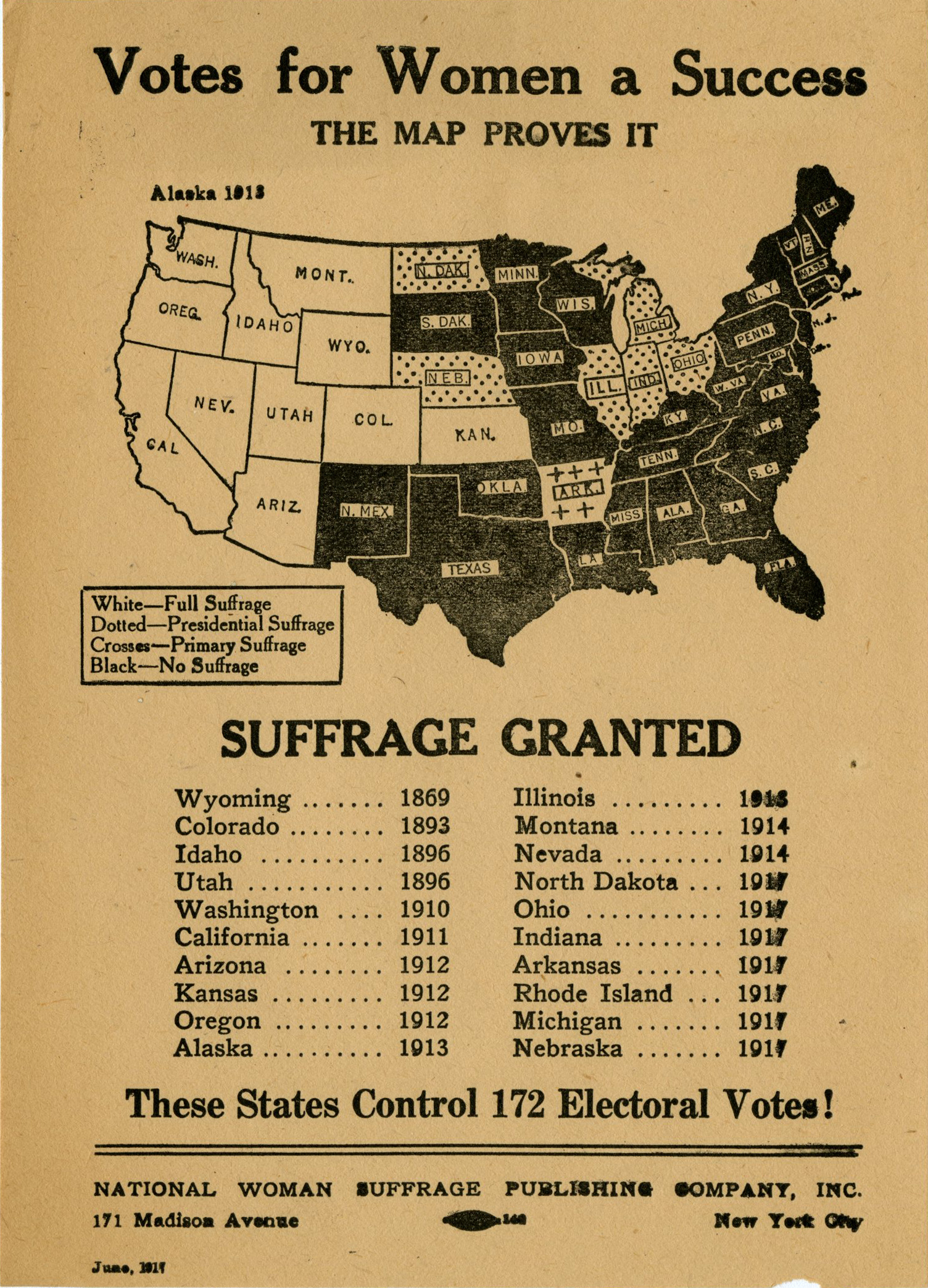
These few examples illustrate how cartography can be a practice for equality, liberation, and world-making. Today people everywhere participate in countermapping. This Is Not an Atlas compiles many powerful examples of counter-cartographies from all over the world illustrating the deep connections between maps and political struggle. In a related venture, mapmakers with Guerilla Cartography promote “decentralized mapping” that combine diverse methodologies, perspectives, and expressive forms to create innovative thematic maps on topics such as water, shelter, food, and migration. Decolonial Atlas publishes maps that challenge our conceptions of mapmaking and our relationships to lands, people, and the state. Each of these cartographic repositories help us expand our perspectives through the power of maps.
Towards A Safer City: Participatory Mapping of Sexual Harassment in Cairo, Egypt
Sexual harassment is misunderstood and underreported all over the world. Stigma and shame prevent many women from talking about it or reporting it, and data on the problem is scarce. New web and mobile technologies have, however, opened new possibilities to overcome some of the barriers to documentation and data collection. HarassMap.com offers an innovative tool for reporting and generating data on sexual harassment, and has enhanced debates and discussions around the issue in Egypt and worldwide.
HarassMap uses web and mobile technologies to crowdsource and map incidents of sexual harassment from all over Egypt. Anyone who experiences or witnesses sexual harassment can anonymously submit a report through the web interface (or through Facebook and Twitter). Reports that include basic geographical information (location and date/time of the incident) are automatically mapped using Google Maps and made publicly available on the HarassMap website. Each report appears as a red dot on the map that, when clicked, displays the full information of the report in its original language (Arabic or English). Besides the location and the date/time, each report also includes a narrative description of the incident, the type(s) of sexual harassment (for example comments, stalking, or following). Each report receives a response with information on how to access free legal services and psychological counseling.
HarassMap has gathered information that can be used to tailor advocacy campaigns and educational programs and serves as an important tool for motivating the public to report and stand up against sexual harassment. Immediately after launching the map in 2010, HarassMap received a large number of reports of sexual harassment, and over the years the crowdsourced data has helped to reformulate the discussion on sexual harassment in Egypt. It has also helped to challenge stereotypes and misinformation about the issue.
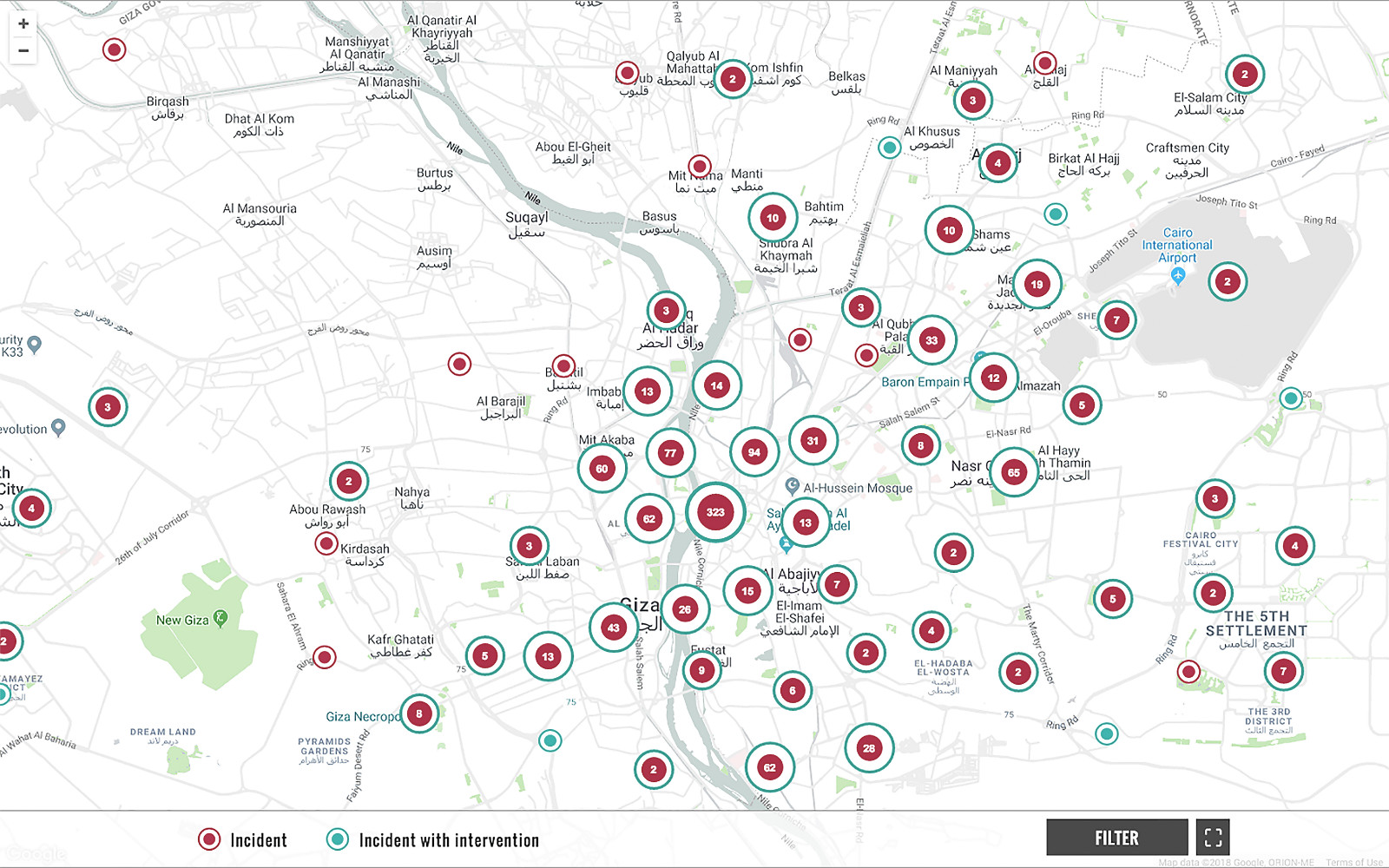
“Writing versus Righting”
“In essence, maps are images designed to represent spatial relationships, connecting land to other beings in one way or another... Maps are storytelling devices" – Annita Lucchesi
Cartography is an ancestral practice, well-rooted in Indigenous traditions all over the world. Consider the rich Mayan libraries containing many complex astronomical charts and maps, many of which were burned down by Spanish intruders. Or consider the Polynesian wayfinders who navigated one quarter of the Earth’s surface without instruments or Western-style maps, well before Europeans managed to cross the Atlantic. Over several centuries in Kaallit Nunaat (an Indigenous name for Greenland), Inuit peoples carved portable maps into driftwood to aid in navigating the rugged Arctic coastlines. These and countless other cases of Indigenous cartographic wisdom challenge the Western canon of geographical knowledge and its relatively narrow perceptions of maps. [11] They help us understand cartography as ancestral, dynamic, communal, and multi-dimensional.
These examples also help us reflect on the notion of a map as more than writing, or even the physical inscription of symbols. Most of the world’s languages are unwritten. Yet, everywhere in the world humans convey and build on geographic knowledge. History, traditions, and wisdom have been transmitted orally for thousands of years. Similarly, the practices of and possibilities for “writing the Earth” extend far beyond the illustrated map. Communities represent their relationships to land and navigation in an astounding variety of ways, each of them valid and valuable in their own ways. Indigenous languages, both with and without writing systems, often convey land and its uses orally, describing territory as a living fabric that weaves communities to their environments, free of definite borders. Indigenous scholar and organizer Maquiades Cruz describes Zapotec traditions of sharing of spatial knowledge in oral maps in Mexico’s Ricon de la Sierra Norte region:
“The story that the elders tell is a history they know from memory. It is not an object that can be tucked away in a museum like any old artifact. It is an oral map that circulates by word of mouth, contrasting with textual maps that circulate from hand to hand, eye to eye… Language allows us to relate people and things, weaving a fabric from their relations… The semiotic relationship between textile, fabric, and text (textile, tejido y texto) helps us reflect on the webs of knowledge and exchange that we try to make when we weave things together through contact, collaboration, knowledge, experience, sources, production, and circulation, creating the fabric of community.” [12]
These community-land weavings embody Indigenous traditions and ancestral wisdom through toponyms, or place names. Toponyms vary depending on perspective. An endonym is the name ascribed to a place by the people who live there. Endonyms reflect the language and cultural traditions of places. In contrast, exonyms are place names superimposed by outsiders. For example, “America” is an exonym placed on a landmass by a German cartographer, Martin Waldseemüller, who had never been there, and who named it for another European cartographer, Amerigo Vespucci. There are, however, many endonyms for “America,” including Turtle Island (See Figure 1.3.11).
Many efforts have been made to restore and reclaim Indigenous place names as a way of combating the invisibility of Indigenous communities and their knowledge, an endeavor some researchers refer to as “decolonizing the map.” As part of this effort, Native-Land.ca , an Indigenous-led non-profit organization, offers an interactive participatory map displaying various Indigenous claims to North American lands, as defined by the Indigenous groups themselves. The depth of oral traditions, however, means that the mere attribution of Indigenous place names remains insufficient because it lacks the interpretive storytelling content and practices necessary to convey the meaning and the history attached to places. To address this shortcoming of two-dimensional maps, some groups are creating multimedia “Indigital story maps” to accompany the conventional graphic map with narratives and other forms of storytelling, allowing Indigenous communities to incorporate their own voices, languages, toponyms, and stories. [13]
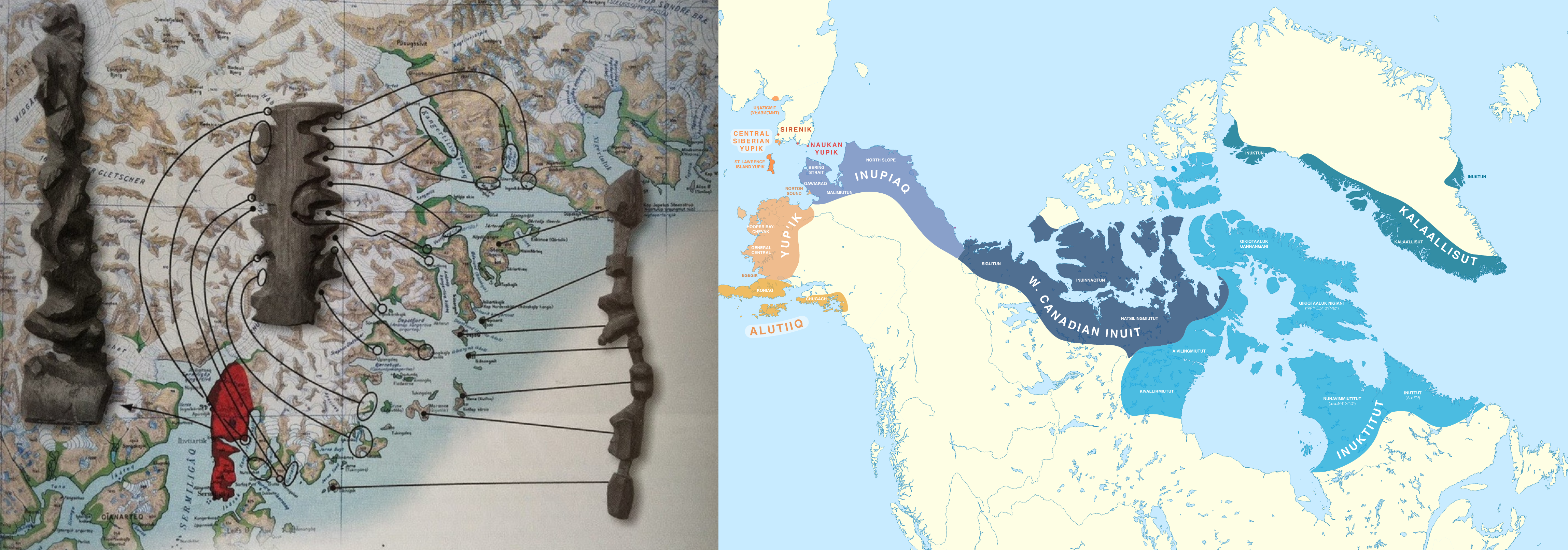
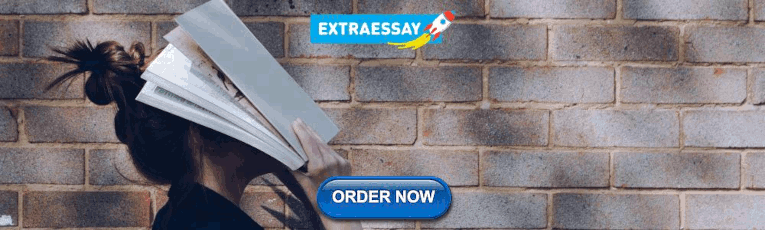
Open maps: A note from the authors
Perhaps in a geography course you could expect a long-winded lesson about maps. In this book, we accept maps to be neither impartial nor standard. They are valuable visuals that can be construed from diverse perspectives and for diverse purposes. We encourage you to apply critical scrutiny when examining maps, evaluating not only their technical features and functions but also their perspectives and contributions that might deepen our understanding of the world. Throughout the book, we have curated maps from the creative commons and public domain, meaning those we are permitted to publish in an open textbook. For example, you might notice that many of our images come from Wikimedia Commons or from Flickr. These are common publication venues not only for cartographers wishing to share their work freely but also national and international governmental agencies that share professional cartography with the public. We made an effort to source reliable and effective maps, examining their sources, perspectives, and their ability to communicate valid and valuable geographic information. In curating images, we embraced the power of maps as visual supplements to the topics discussed throughout the book. Still, all maps are limited and exclusive, some more than others, and even in ways that the authors may have missed. Read the maps in this book, and everywhere else, with care and critical appraisal.
References:
[1] ROLLER, D. W. (2010). Eratosthenes’ “Geography.” Princeton University Press.
[2] White, J. (2017). Geography and Empire .
[3] Pastuch, L. 2022. Al-Idrisi’s Masterpiece of Medieval Geography. Library of Congress.
[4] Sletto, B., Bryan, J., Wagner, A., and Hale, C., (eds). 2020. Radical Cartographies: Participatory Mapmaking from Latin America. Austin: University of Texas Press.
[6] White, J. (2017). Geography and Empire .
[7] Harley, B. 1988. Maps, Knowledge, and Power . In: D. Cosgrove and S. Daniels (Eds), The Iconography of Landscape . Cambridge: Cambridge University Press, 1988, pp. 277–312
[8] Harley, B. 1988. Maps, Knowledge, and Power . In: D. Cosgrove and S. Daniels (Eds), The Iconography of Landscape . Cambridge: Cambridge University Press, 1988, pp. 277–312
[9] Based on ideas of Pickles about maps preceding and forming territories, not just being representations of territory. As discussed on the literature review presented by Kitchin, R. and Dodge, R. 2007. Rethinking Maps . Progress in Human Geography 31(3), pp. 331-344.
[10] Alderman, D., Inwood, J. and Bottone, E. 2021. The mapping behind the movement: On recovering the critical cartographies of the African American Freedom Struggle. Geoforum, volume 120, pp. 67-78.
[11] Lucchesi, A. 2018. “Indians Don’t Make Maps”: Indigenous Cartographic Traditions and Innovations. American Indian Culture and Research Journal. 2018. Also recommended: Simpson, L. 2014. Land As Pedagogy: Nishnaabeg intelligence and rebellious transformation. Decolonization: Indigeneity, Education & Society 3 (3), pp 1-25.
[12] Maquiades (Kiado), C. 2020. Oral Narratives in the Ricon Zapoteco (pp. 22 & 26). In: Sletto, B., Bryan, J., Wagner, A., and Hale, C., (eds). 2020. Radical Cartographies: Participatory Mapmaking from Latin America. Austin: University of Texas Press.
Attributions:
“The Spatial Perspective” by Caitlin Finlayson, CC BY-NC-SA 4.0 .
“Map and Scale Accuracy” by David DiBiase & John A Dutton, CC BY-NC-SA 4.0 .
“Sub-fields of geography” and "Geospatial Technologies" is adapted from “Introduction to Geographic Science” by Darstrup via, Open Geography Education , CC-BY-4.0 .
This is Not An Atlas , editorial by Severin Halder and Boris Michael and " Mapping Sexual Harassment in Egypt " by HarassMap, CC-BY-4.0 .
A map is a symbolic representation of selected characteristics of a place, usually drawn on a flat surface
Geography, Geographic Information Systems (GIS)
Loading ...
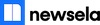
Learning materials
- National Geographic MapMaker
A map is a symbolic representation of selected characteristics of a place, usually drawn on a flat surface. Maps present information about the world in a simple, visual way. They teach about the world by showing sizes and shapes of countries, locations of features, and distances between places. Maps can show distributions of things over Earth, such as settlement patterns . They can show exact locations of houses and streets in a city neighborhood . Mapmakers, called cartographers , create maps for many different purposes. Vacationers use road maps to plot routes for their trips. Meteorologists —scientists who study weather —use weather maps to prepare forecasts . City planners decide where to put hospitals and parks with the help of maps that show land features and how the land is currently being used. Some common features of maps include scale , symbols , and grids . Scale All maps are scale models of reality. A map’s scale indicates the relationship between the distances on the map and the actual distances on Earth. This relationship can be expressed by a graphic scale , a verbal scale , or a representative fraction . The most common type of graphic scale looks like a ruler. Also called a bar scale , it is simply a horizontal line marked off in miles, kilometers, or some other unit measuring distance. The verbal scale is a sentence that relates distance on the map to distance on Earth. For example, a verbal scale might say, “one centimeter represents one kilometer” or “one inch represents eight miles.” The representative fraction does not have specific units. It is shown as a fraction or ratio —for example, 1/1,000,000 or 1:1,000,000. This means that any given unit of measure on the map is equal to one million of that unit on Earth. So, 1 centimeter on the map represents 1,000,000 centimeters on Earth, or 10 kilometers. One inch on the map represents 1,000,000 inches on Earth, or a little less than 16 miles. The size of the area covered helps determine the scale of a map. A map that shows an area in great detail, such as a street map of a neighborhood, is called a large-scale map because objects on the map are relatively large. A map of a larger area, such as a continent or the world, is called a small-scale map because objects on the map are relatively small. Today, maps are often computerized . Many computerized maps allow the viewer to zoom in and out, changing the scale of the map. A person may begin by looking at the map of an entire city that only shows major roads and then zoom in so that every street in a neighborhood is visible . Symbols Cartographers use symbols to represent geographic features. For example, black dots represent cities, circled stars represent capital cities, and different sorts of lines represent boundaries, roads, highways, and rivers. Colors are often used as symbols. Green is often used for forests, tan for deserts , and blue for water. A map usually has a legend , or key, that gives the scale of the map and explains what the various symbols represent. Some maps show relief, or changes in elevation. A common way to show relief is contour lines , also called topographic lines . These are lines that connect points that have equal elevation. If a map shows a large enough area, contour lines form circles. A group of contour line circles inside one another indicates a change in elevation. As elevation increases, these contour line circles indicate a hill . As elevation decreases, contour line circles indicate a depression in the earth, such as a basin . Grids Many maps include a grid pattern, or a series of crossing lines that create squares or rectangles. The grid helps people locate places on the map. On small-scale maps, the grid is often made up of latitude and longitude lines. Latitude lines run east-west around the globe , parallel to the Equator , an imaginary line that circles the middle of Earth. Longitude lines run north-south, from pole to pole. Latitude and longitude lines are numbered. The intersection of latitude and longitude lines, called coordinates , identify the exact location of a place. On maps showing greater detail, the grid is often given numbers and letters. The boxes made by the grid may be called A, B, C, and so on across the top of the map, and 1, 2, 3, and so on across the left side. In the map’s index , a park’s location might be given as B4. The user finds the park by looking in the box where column B and row 4 cross. Other Map Features: DOGSTAILS Along with scale, symbols, and grids, other features appear regularly on maps. A good way to remember these features is DOGSTAILS: date, orientation , grid, scale, title, author, index, legend, and sources. Title, date, author, and sources usually appear on the map though not always together. The map’s title tells what the map is about, revealing the map’s purpose and content. For example, a map might be titled “Political Map of the World” or “Battle of Gettysburg, 1863.” “Date” refers to either the time the map was made or the date relevant to the information on the map. A map of areas threatened by a wildfire, for instance, would have a date, and perhaps even a time, to track the progress of the wildfire. A historical map of the ancient Sumerian Empire would have a date range of between 5,000 B.C. and 1,000 B.C. Noting a map’s author is important because the cartographer’s perspective will be reflected in the content. Assessing accuracy and objectivity also requires checking sources. A map’s sources are where the author of the map got his or her information. A map of a school district may list the U.S. Census Bureau, global positioning system (GPS) technology, and the school district’s own records as its sources. Orientation refers to the presence of a compass rose or simply an arrow indicating directions on the map. If only an arrow is used, the arrow usually points north. A map’s index helps viewers find a specific spot on the map using the grid. A map’s legend explains what the symbols on a map mean. Map Projections Transferring information from the spherical , or ball-shaped, surface of Earth onto a flat piece of paper is called projection. A globe, a spherical model of Earth, accurately represents the shapes and locations of the continents. But if a globe were cut in half and each half were flattened out into a map, the result would be wrinkled and torn. The size, shape, and relative location of land masses would change. Projection is a major challenge for cartographers. Every map has some sort of distortion . The larger the area covered by a map, the greater the distortion. Features such as size, shape, distance, or scale can be measured accurately on Earth, but once projected on a flat surface only some, not all, of these qualities can be accurately represented. For example, a map can retain either the correct sizes of landmasses or the correct shapes of very small areas, but not both. Depending on the map’s purpose, cartographers must decide what elements of accuracy are most important to preserve. This determines which projection to use. For example, conformal maps show true shapes of small areas but distort size. Equal area maps distort shape and direction but show true relative sizes of all areas. There are three basic kinds of projections: planar, conical, and cylindrical. Each is useful in different situations. In a planar projection , Earth’s surface is projected onto a plane , or flat surface. Imagine touching a globe with a piece of cardboard, mapping that point of contact, then projecting the rest of map onto the cardboard around that point. Planar projections are most accurate at their centers, where the plane “touches” the globe. They are often used for maps of one of the poles. Imagine you wrapped a cone around Earth, putting the point of the cone over one of the poles. That is a conical projection . The cone intersects the globe along one or two lines of latitude. When the cone is unwrapped and made into a flat map, latitude lines appear curved in circles or semicircles. Lines of longitude are straight and come together at one pole. In conical projection, areas in the mid-latitudes—regions that are neither close to the Equator nor close to the poles—are represented fairly accurately. For this reason, conical projections are often used for maps of the United States, most of which lies in the mid-latitudes. For a cylindrical projection , imagine that Earth’s surface is projected onto a tube that is wrapped around the globe. The cylinder touches Earth along one line, most often the Equator. When the cylinder is cut open and flattened into a map, the regions near the Equator are the most accurate. Regions near the poles are the most distorted. Surveying and Remote Sensing Cartographers rely on survey data for accurate information about the planet. Surveying is the science of determining the exact size, shape, and location of a piece of land. Surveyors gather information from regions both above sea level and beneath bodies of water. Surveying can be done on foot. Surveyors use many instruments to measure the features, or topography , of the land. A compass , measuring device, and theodolites are often used by surveyors doing field work. A theodolite is an instrument that measures angles. A surveyor may calculate the angle of hills, valleys, and other features by using a theodolite, which is usually mounted on a tripod , or three-legged platform. Today, many surveyors use remote sensing to collect data about an area without actually physically touching it. Sensors that detect light or radiation emitted by objects are mounted to airplanes or space satellites , collecting information about places on Earth from above. One method of remote sensing is aerial photography , taking photographs of Earth from the air. Aerial photography has eliminated much of the legwork for surveyors and has allowed precise surveying of some places that are impossible to reach on foot. Satellites, spacecraft that orbit Earth, perform remote sensing. For example, Landsat , a satellite that circles Earth 14 times a day, transmits huge volumes of data to computers on Earth. The data can be used to quickly make or correct maps. How Maps Are Made Before making a map, cartographers decide what area they want to display and what type of information they want to present. They consider the needs of their audience and the purpose of the map. These decisions determine what kind of projection and scale they need, and what sorts of details will be included. The language of the map is one thing a cartographer must consider. A blind reader needs a map that has information in braille , for instance. The audience for a map can determine how widely a map is used. A map might use red and green symbols to show the location of maple and pine trees. This information might be easily displayed in a simple legend. However, such a map could not be used by people who are color-blind. Lines of latitude and longitude are mathematically plotted on a flat surface. Features are drawn in their appropriate location. Before the development of advanced computer and printing techniques, maps were drawn by hand. Cartographers would draw, or scribe , the map on a sheet of coated plastic with a special etching tool, scraping away the colored coating to leave clear, sharp lines. Several different sheets of plastic were layered on top of each other to add shading and place names. The plastic sheets were used to make a metal printing plate , or proof, for publishing the map. Today, most mapping is done with the help of computers. The coordinates of every point are entered into a computer. By feeding new data into the computer or deleting old data, map changes can be made quickly and easily. Colors can be changed, new roads added, and topographic features, such as the flow of a river, altered. The new map can then be printed out easily. Types of Maps Cartographers make many different types of maps, which can be divided into two broad categories: general reference maps and thematic maps . General reference maps show general geographic information about an area, including the locations of cities, boundaries, roads, mountains, rivers, and coastlines . Government agencies such as the U.S. Geological Survey ( USGS ) make some general reference maps. Many are topographic maps, meaning that they show changes in elevation. They show all the hills and valleys in an area. This is useful to everyone from hikers trying to choose a route to engineers trying to determine where to build highways and dams . Thematic maps display distributions, or patterns, over Earth’s surface. They emphasize one theme, or topic. These themes can include information about people, other organisms, or the land. Examples include crop production, people’s average income , where different languages are spoken, or average annual rainfall. Many thematic maps are now made with the help of geographic information system (GIS) technology. GIS are computer systems that capture, store, and display data related to positions on Earth’s surface. This technology combines information from maps with other data about people, the land, climate , farms, houses, businesses, and much more, allowing multiple sets of data to be displayed on a single map. Many industries and governments use GIS technology for analysis and decision making. For example, GIS data helps officials determine which streams are most in danger of being polluted . It can also help a business decide where to locate a new store. History of Mapmaking Through the ages, maps have taken many different forms. The earliest maps were probably sketches made on the ground that showed the surrounding area. People native to the Marshall Islands used palm fibers to show wave patterns between islands in the Pacific Ocean. They used seashells to represent islands. Inuit fishermen in the Arctic carved pieces of driftwood to show coastal features. One of the world’s oldest existing maps was found on a stone tablet in Spain. It dates back nearly 14,000 years. The ancient Greeks are usually considered the founders of scientific cartography. Greek scholars knew the general size and shape of Earth, and they developed the grid system of latitude and longitude. Eratosthenes , who lived from about 276 to 194 B.C., calculated the size of Earth using mathematics and observations of the sun. Claudius Ptolemaeus, or Ptolemy , was an astronomer , mathematician , and geographer in the second century A.D. He brought mapmaking to a level of precision that would not be seen again until the fifteenth century. He combined all his knowledge about the world into a book called Geography . In Europe during the Middle Ages , cartographers drew maps reflecting their religious beliefs. These maps were generally simple and sometimes fanciful . The city of Jerusalem, holy to Jews , Christians , and Muslims , was sometimes placed in the center. Many medieval European maps with Jerusalem at the center are called T&O maps. The mass of land was represented as a round wheel encircled with a single round ocean, the “O” of the T&O. The land encircled by the ocean was divided by a “T” into the three continents known by medieval European cartographers: Asia was the large land mass above the T, Africa and Europe were the two smaller sections on either side of the T, and Jerusalem was at the center. The T-shape splitting the continents was composed of the Mediterranean Sea (between Europe and Africa), the Nile River (between Africa and Asia) and the Don River (between Europe and Asia). The Nile and the Don meet in a single line to form the top of the T. During these Dark Ages in Europe, Arab scholars kept scientific cartography alive. They preserved the works of Ptolemy and translated them to Arabic . Arab cartographers produced the first reliable globe of the Western world. During the Islamic Golden Age , Arab cartographers used complicated mathematical and astronomical formulas to help them determine different map projections. In 1154, the scientist and cartographer al-Idrisi made a map of the world that was better than the world maps Europeans were producing. Al-Idrisi’s map included a representation of the entire continent of Eurasia , including Scandinavia , the Arabian Peninsula, the island of Sri Lanka, and the Black and Caspian Seas. In the fifteenth century, cartography in Europe improved. The development of printing and engraving meant maps that had previously been painted by hand could be copied more quickly. Around the same time, sailors began traveling farther on the oceans. They added newly discovered lands and more detailed coastlines to their maps. Explorers brought back descriptions of the interiors, as well as the coastlines, of continents. Europeans explored much of the Americas during the sixteenth century, Australia in the seventeenth century, and Antarctica was finally sighted in the early nineteenth century. At this point, fairly accurate maps of the entire world were beginning to be assembled . In the nineteenth century, cartography became more advanced with the development of a printing process called lithography . Lithography allowed cartographers to make many accurate copies of maps with less labor and expense. Photography, color printing, and computers all improved mapmaking even more. In just a few decades, the relationship between people and maps changed drastically. For example, instead of using paper street maps, many people navigate using GPS units that communicate with satellites to determine their exact location on Earth. Digital versions of maps can represent Earth in three dimensions, defying the limitations of the flat maps of the past. Almost the entire surface of Earth has been mapped with remarkable accuracy, and this information is available instantly to anyone with an internet connection.
Beyond Earth Using images taken from spacecraft, cartographers have created detailed maps of the surfaces of the moon and Mars. Astrocartographers have identified martian valleys, craters, and even dry riverbeds.
Eratosthenes Eratosthenes was an astronomer, librarian, mathematician, and poet. He also invented the discipline of geography in his spare time. Using the position of the sun, Eratosthenes was able to calculate the circumference of Earth without leaving Egypt, his home. He used the length of a stadium as his unit of distance. Because stadiums came in two different sizes in the world of ancient Greece, and we don't know which stadium Eratosthenes used, we can't know exactly what he calculated for the circumference of Earth. If he used the larger Greek stadium, his circumference would be larger than Earth by about 16 percent. If he used the smaller, so-called "Egyptian stadium," his calculation would still be largerbut only by 1 percent.
Misleading Maps A type of cylindrical projection called a Mercator projection shows direction well. It was long used to make charts that sailors could use to find their way around the globe. Like all cylindrical projections, a Mercator projection greatly distorts the size of land near the poles. In a Mercator projection, Greenland and Africa are about the same size. In reality, Africa is 14 times the size of Greenland.
Printing Pioneers The Chinese were skilled cartographers. The first map was printed in China in 1155 C.E., some 300 years before maps were printed in Europe.
Media Credits
The audio, illustrations, photos, and videos are credited beneath the media asset, except for promotional images, which generally link to another page that contains the media credit. The Rights Holder for media is the person or group credited.
Illustrators
Educator reviewer, last updated.
February 20, 2024
User Permissions
For information on user permissions, please read our Terms of Service. If you have questions about how to cite anything on our website in your project or classroom presentation, please contact your teacher. They will best know the preferred format. When you reach out to them, you will need the page title, URL, and the date you accessed the resource.
If a media asset is downloadable, a download button appears in the corner of the media viewer. If no button appears, you cannot download or save the media.
Text on this page is printable and can be used according to our Terms of Service .
Interactives
Any interactives on this page can only be played while you are visiting our website. You cannot download interactives.
Related Resources
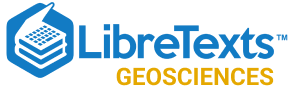
- school Campus Bookshelves
- menu_book Bookshelves
- perm_media Learning Objects
- login Login
- how_to_reg Request Instructor Account
- hub Instructor Commons
- Download Page (PDF)
- Download Full Book (PDF)
- Periodic Table
- Physics Constants
- Scientific Calculator
- Reference & Cite
- Tools expand_more
- Readability
selected template will load here
This action is not available.
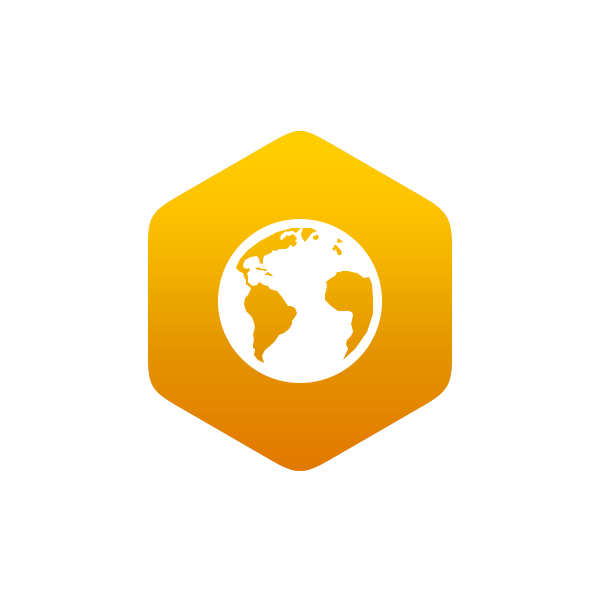
4: Design and Symbolization
- Last updated
- Save as PDF
- Page ID 5547

- Steven Manson
- University of Minnesota via University of Minnesota Libraries
“Design is so simple, that’s why it is so complicated.” Paul Rand, graphic designer
The difference between an ordinary map and one that is persuasive and interesting depends on how well the cartographer incorporates principles of good design and symbolization. Data, in isolation, cannot tell a story. It is up to the cartographer to make the data convincing by turning mundane information into artistic expressions. The concepts in this chapter are fairly basic to understand, yet they take years to put to good practice. Map design requires patience, trial and error, and careful attention to detail.
This chapter will introduce you to:
- Basic map elements: key elements, such as a title or source, are found on most maps and understanding their purpose will give you a solid foundation for cartography.
- Design principles: there are many principles of good design, but we start with the two of hierarchy and balance, which make the difference between boring maps and maps that pop out at the reader.
- Symbolization: cartographers have many tools to make data more memorable and convincing, and among these are symbolization basics including geometry and visual variables.
By the end of this chapter, you should understand basic principles of design and symbolization, and tools you can use to make interesting, aesthetically pleasing, and effective maps.
4.1 Map Elements
Maps are composed of a varying number of elements. There are potentially dozens of map elements but we focus here on a few key ones.
- Figure. The thing or place being mapped is called the figure of the map. In the map below, the figure of the map is the continental United States.
- Ground. The figure is juxtaposed against the ground of the map, or in other words, the background. In this case, it is the countries outside of the United States and the oceans.
- Frame. Maps often have frame , a line drawn around the figure and ground, that acts like a picture frame does for a picture. The more technical term neatline is also used.
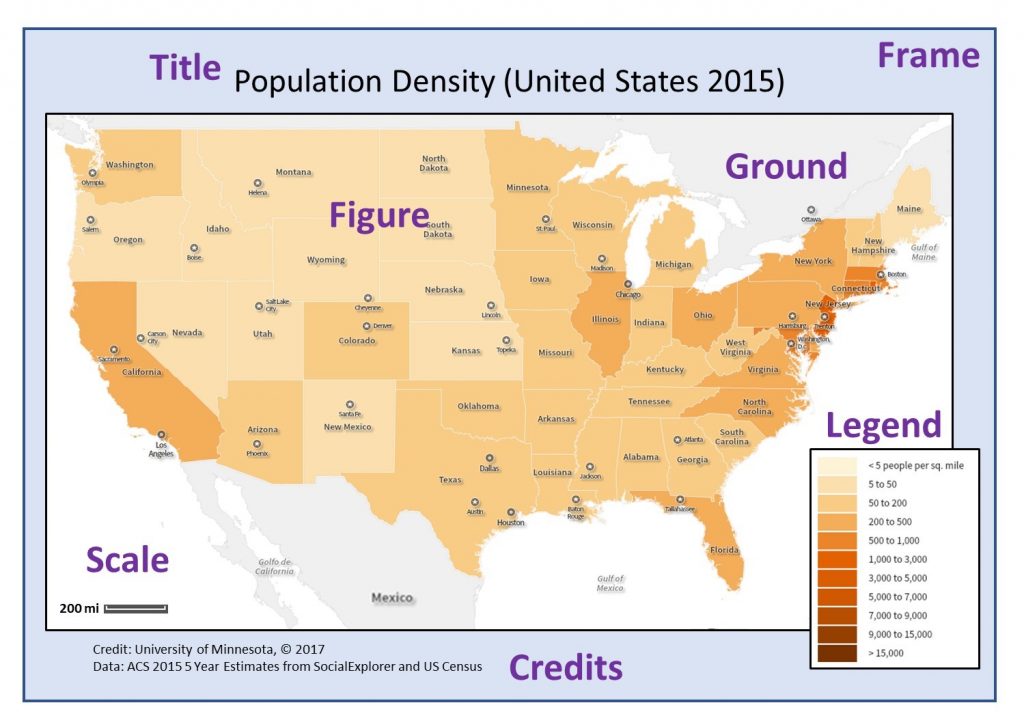
Other elements are informative enough to be used almost always, including a title, scale, legend, and source information.
- Title. The title is a short, descriptive text typically at or near the top of the map. A good title should provide some detail about the map’s content without being too wordy or descriptive. Map titles often include all or some of the following components: the subject of the map, the year, the spatial extent, the mapping resolution, and the data source.
- Legend. The legend lists the symbols used and explains what is being mapped. It is important to include the symbols on the legend exactly as they appear on the map. Legends include both text and graphics, and they sometimes contain additional details such as the source of the data or the year the data were collected. Labeling the units in the legend is important so the audience can properly understand the data being mapped. If your map of counties shows the number of bicycles per thousand people, then the legend should say so.
- Source/Credits. Think back to the data chapter and recall how important it is to know where the mapping data come from and what sort of biases are inherent in the data. For transparency purposes, it is necessary to provide attribution to the source of the data— the place, company, person or agency that made the data. These are also known more generally as credits . Including the source makes it possible for the audience to explore the data further if necessary. Knowing who made the map can help the reader better gauge the accuracy and reliability of the data. Sometimes, the mapmaker is highly regarded for accurate and unbiased use of data; other times, he or she is known for having a political agenda that would raise doubts about data accuracy. Source attribution can be as simple as a short text annotation near the bottom of the map, or it can include more detailed information.
- Scale. Almost all maps include a scale because maps are smaller than the reality being mapped. Providing the map audience with information about what scale you are using is important so they can have some understanding of relative size and distance. The scale is often provided in a less prominent part of the map and is typically displayed with graphics or text. There are times when the scale is so obvious that it is omitted from a map. For example, a map of the entire world does not typically have a scale bar on it, because everyone has a general understanding of how large the world is. Keep in mind from the section on projections, however, that many maps distort area, so the sizes that you see on a map might not always be spatially accurate. In this case, listing the projection with the scale is helpful.
There are many other possible elements that cartographers may include and that you may see used in maps. These other elements, while not as frequently included, often represent good mapmaking practice, especially if you want the reader to understand how the map was created. These elements include the cartographer’s name or company, the production date, and the projection. Some elements of the map, such as the north arrow, follow conventions, or commonly understood rules. Typically, we equate the orientation “up” with “north,” so cartographers will often omit the north arrow on a map unless the map breaks that convention.
4.2 Design Principles
While a title, legend, source, and scale are necessary elements of all maps, they need be applied according to map design principles in order to properly convey the information. Poorly designed maps, like the one below, can be both difficult to read and confusing.
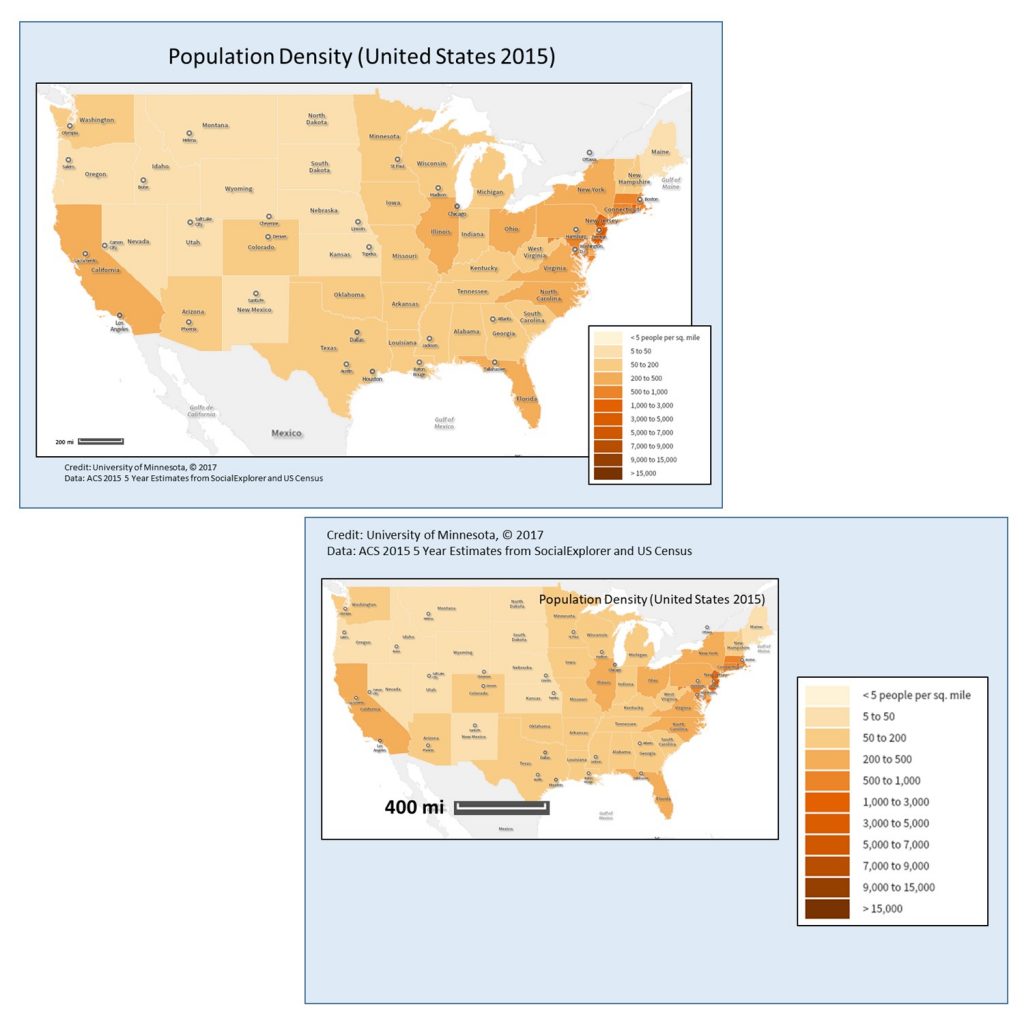
Designing maps to be visually appealing is an art form, and there are entire courses in cartography devoted to these principles. Two of the foundational elements of design that all good maps must incorporate are hierarchy and balance.
4.2.1 Hierarchy
Not all information on a map is of equal importance. A title is more important than the north arrow, so making them the same size would lead to inadequate emphasis on the title. It also does not make sense if a legend is so large that it overwhelms the actual figure that you are mapping. Visual hierarchy is the design principle that takes these factors into consideration. A map with good hierarchy emphasizes important information and figures by positioning them strategically on the map and by using visual variables appropriately. Typically, the top of the map is a good place for important information like the title. Size and color are two other important tools in creating strong visual hierarchy, as the more important text and figures can be larger in size.
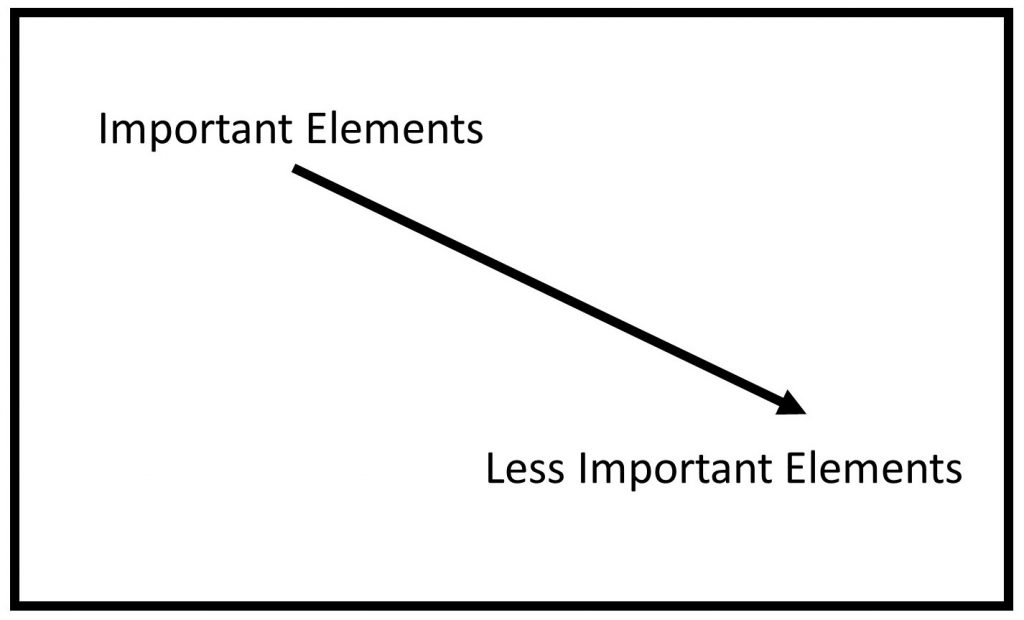
4.2.2 Balance
Not everything that is important can be in large text at the top of the map. After you satisfy requirements of visual hierarchy, the next step is to make sure that your map looks balanced . This entails aligning text with other text or elements so everything looks neat and organized. If your map is too cluttered it will be difficult to see the information, so maintaining a sufficient amount of white space is helpful.
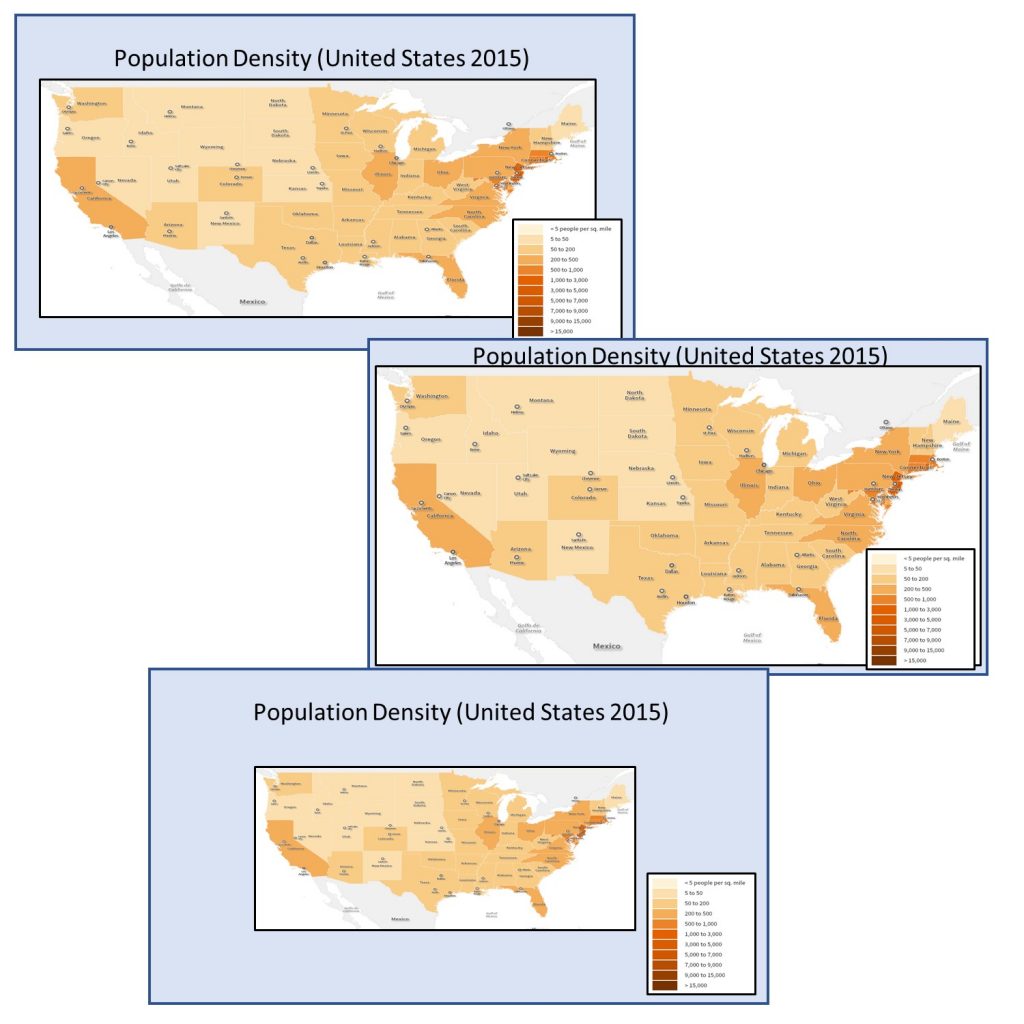
4.3 Symbolization
Symbolization is the term used to describe how the features on a map are visualized. Unless you are looking at an aerial photographic image, everything on a map is a symbol for something in the real world. A mapmaker must constantly decide how a map feature should be symbolized in a way that helps the reader to quickly understand what it represents and its degree of importance relative to other features in the map.
Before we continue, we need to consider a basic distinction between kinds of data, namely whether they are quantitative or qualitative.
- Qualitative data deal with descriptions of a real-world phenomenon that relate to the quality (which is where ‘qualitative’ comes from), or in other words, differences in kind or existence .
- Quantitative data are those that deal in measurements (or quantities, which gives us the term ‘quantitative’) that deal with differences in amount .
A qualitative map of cities, for example, would show whether a city exists or not in a given place, while a quantitative map would show the location of the city as well as some measurement, such as the number of people living there. A qualitative map of wildlife could indicate where different kinds of animals live, while a quantitative map would show the amounts of different animals.
We look at two key components of symbolization: geometry and visual variables. Per the figure below, these components all work together to make map symbolization.
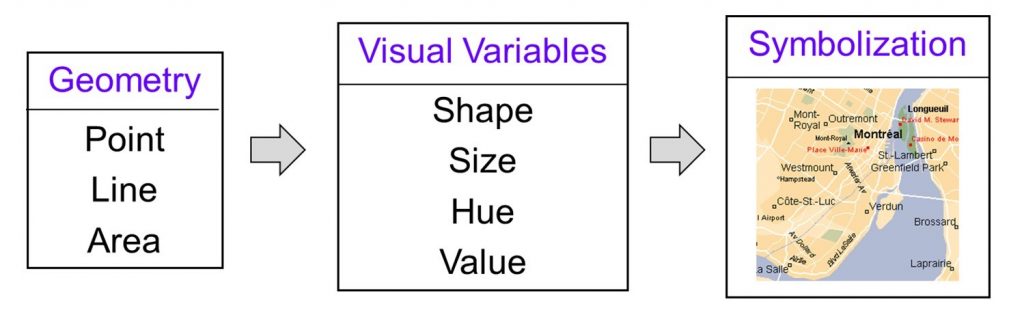
4.3.1. Geometry
Most map symbols are essentially variations on three simple geometric features: the point, the line, and the polygon.

Points are typically used for discrete features, such as a tree or the location of a store. Lines are often used to represent roads, telephone lines, or other long, continuous features. Areas or polygons are typically used to represent boundaries such as counties or parks, but they can also be used to help generalize data such as the concentration of many individual animals. It is very common for all three of these geometric objects to be used in a single map, such as the map of Montreal below which contains the locations of roads (lines), cities (points), and parks (areas).
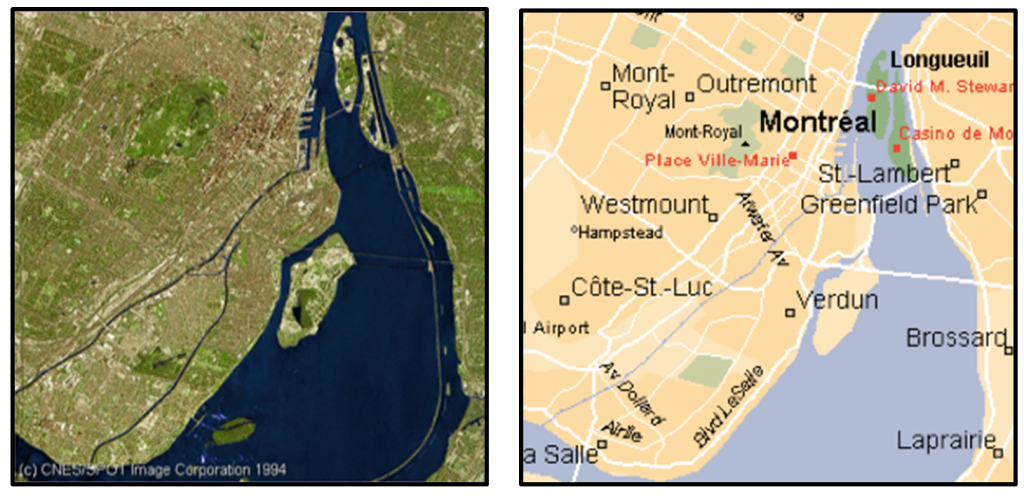
Power of symbolization. Satellite Image on the left compared to a map incorporating point, line, and area symbolization on the right. [8]
4.3.2 Visual Variables
We can modify the appearance of basic geometries of maps (points, lines, and polygons) in many ways. The modifications or variation of these points, lines, and polygons are referred to as visual variables because they describe how a given visual entity, like a line for example, varies from dark to light or big to small. Visual variables are important in displaying both qualitative and quantitative data. The four main visual variables that are commonly manipulated on maps are: shape, size, hue, and value. The figure below highlights the main tenants of these visual variables as well as what types of data they are best suited for.
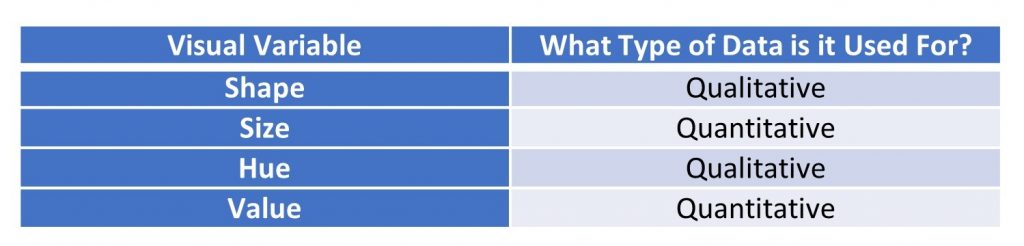
Shape. Shapes often indicate differences in kind, so they are good for qualitative data. There are certain conventions of shape use, such as an airplane for an airport, or a cross for a hospital. Shapes can also have much more specific representations. A wildlife map might show simple pictures of different species of animals to show the location of their habitats. Corporate logos can also be used, indicating the locations of dealerships for different models like Honda or Ford, or someone looking for a nearby fast food restaurant can easily distinguish between the locations of a McDonald’s or a Burger King.
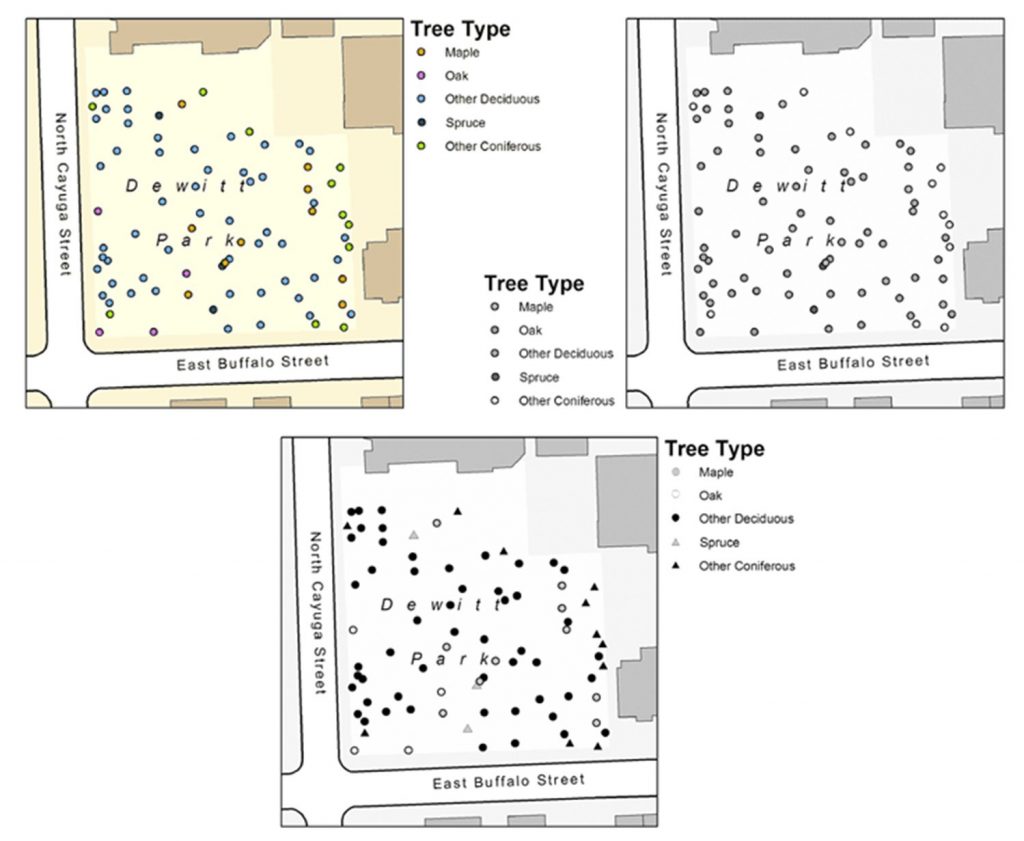
Qualitative data and shape. This map uses shapes to indicate different kinds of tree species. [10]
Size. Size is a visual variable especially well-suited for quantitative attributes. Large sizes are often understood to represent something of high value or importance, while small sizes represent low or less important values. Size is limited by the ability of the map audience to estimate the difference between sizes.
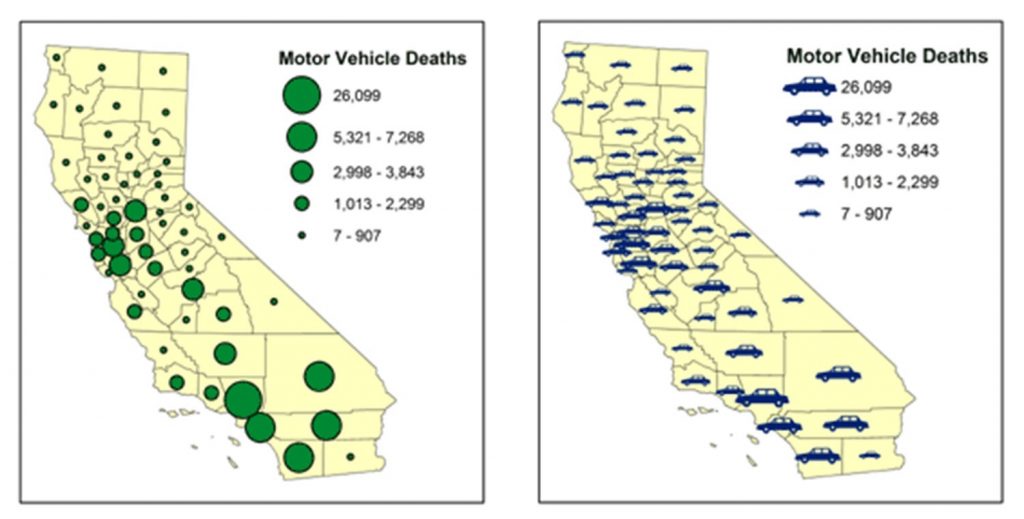
Hue. When people speak about color on a map they usually refer to what is technically known as hue. Hue is most commonly used to distinguish between qualitative data. Decisions are based on psychological and social factors because we associate certain colors with certain real-world things: blue (water), green (forest), and red (fire or heat). It is also common for red to be used to signify intensity or importance while calming colors such as blue or grey help to signify something of less intensity.
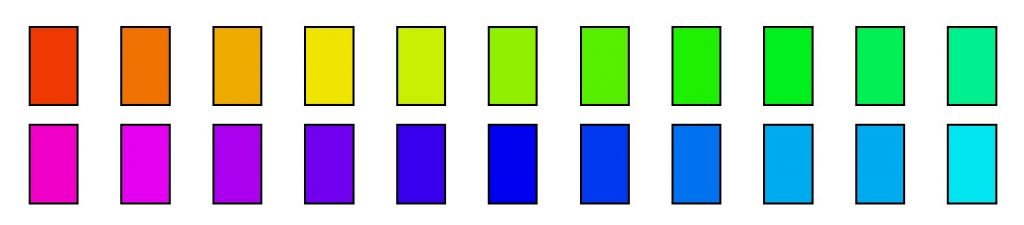
Value. Value refers to the lightness or darkness of a hue. It is most commonly used for quantitative data as it can demonstrate relative importance or amount on a continuous scale. While the distinction between light and dark is fairly obvious, be careful not to use too many different values on a map. The human eye has difficulty distinguishing more than about eight values on a map.

4.3.3 Recap
In very general terms, we use size and value for quantitative data and we represent qualitative data with hue and shape. This applies to points, lines, and areas, per this figure.
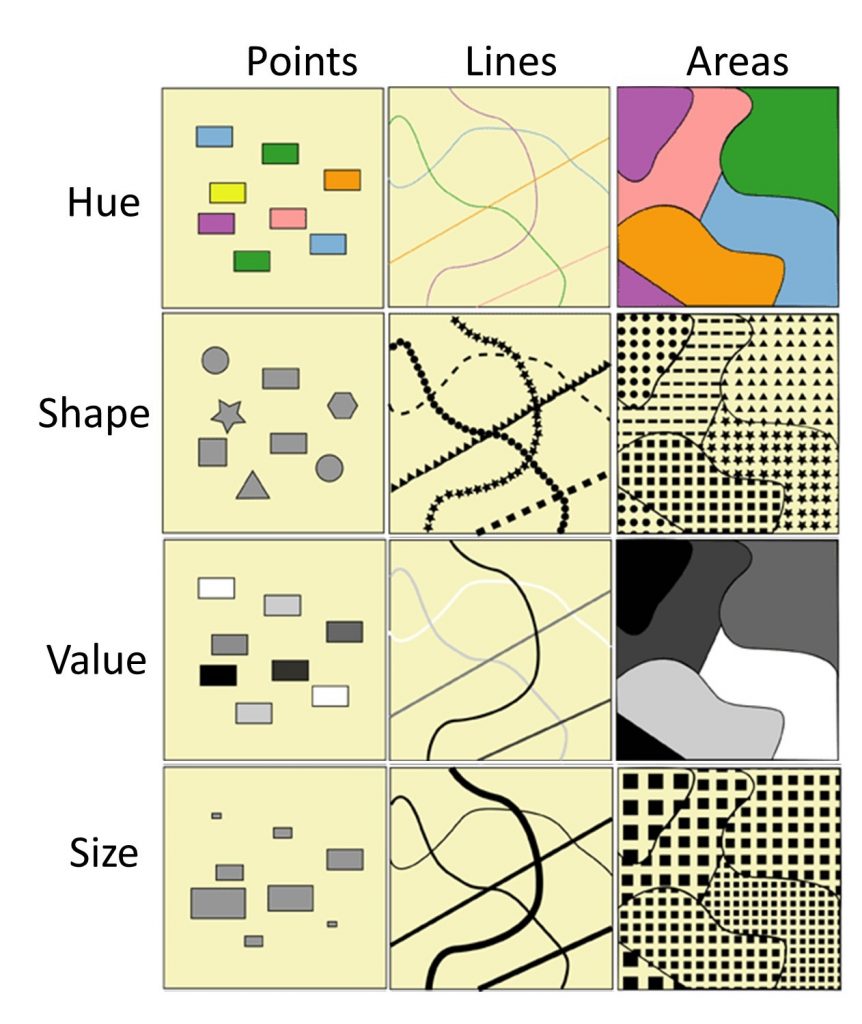
Note that the kinds of data, qualitative or quantitative, makes a difference in color choice. Consider the two maps below, which show internet users per 100 people by country. The first uses a single hue – purple – and different values. This first one is easier to understand because darker colors indicate higher values. The second one uses different hues – such as green or red or orange – to indicate different values. This one does not work as well because you have to study it intently to assess which places are high and low in terms of internet users.
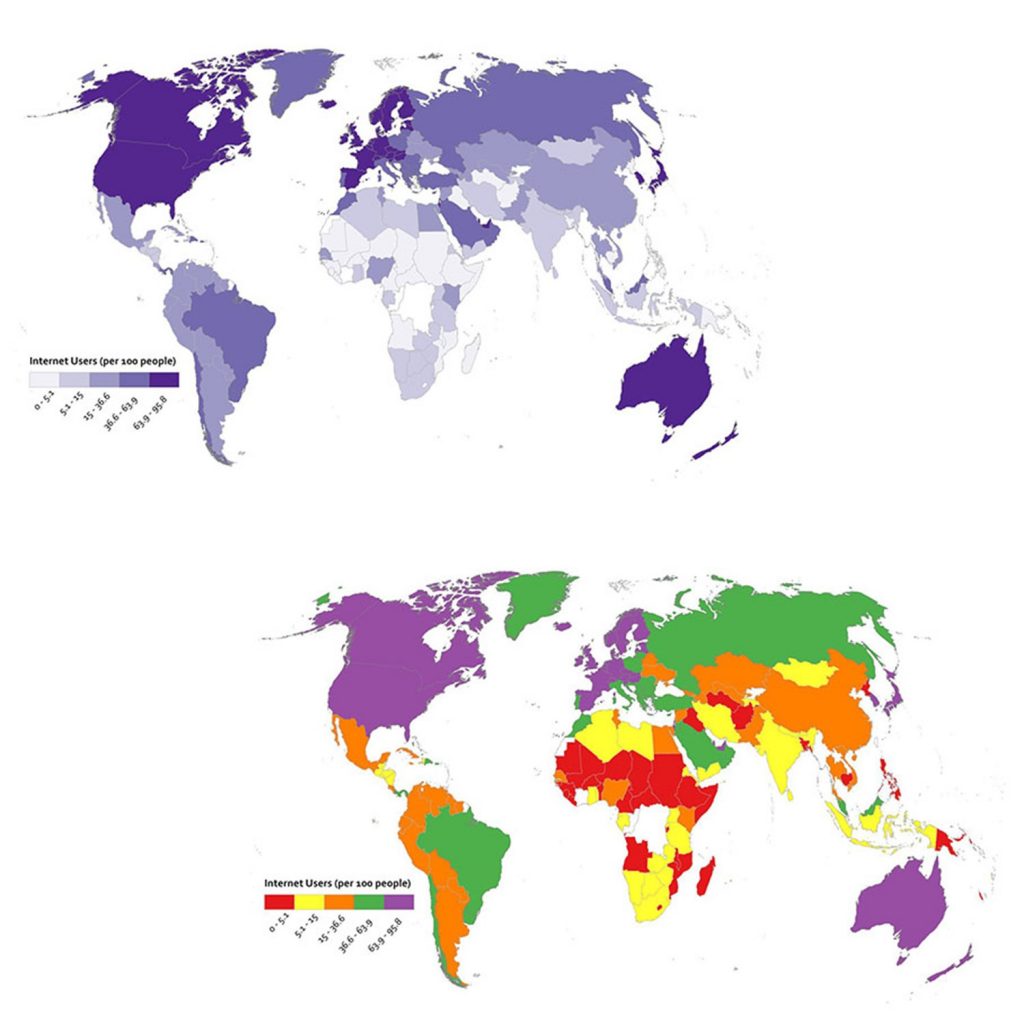
Symbolization choices may also be tied to the potential uses of your map. You may want to design a map in color but your map users may want to reproduce it in black-and-white, as when a color map gets photocopied on a black-and-white photocopier or printed on a monochrome printer. If you know that your color map may become reproduced in black-and-white, you may choose to adjust your color design so that important features do not become lost in the translation. The figure below explores these transitions for tree mapping.
4.4 Conclusion
In this chapter, we have looked at the basics of symbolization. Basic map elements include the figure, ground, and frame as well as ones commonly seen on maps such as title, legend, scale, and source. These elements are then integrated with design principles, particularly hierarchy and balance, and the tools of symbolization, such as geometry and visual variables including shape, size, hue, and value.
For more information about symbolization:
- Basic Elements of Map Composition at University of Colorado
- Visual Variables at University of Muenster
For more information on cartography:
- The Geographer’s Craft at University of Colorado
Portions of section 4.3 were adapted from Adrienne Gruver (2016). Cartography and Visualization and David DiBiase and Jim Sloan (2016). Mapping Our Changing World.
- CC BY-NC-SA 4.0. Steven Manson 2017. Data from SocialExplorer and US Census ↵
- Steven Manson 2017 ↵
- CC BY-NC-SA 3.0. Steven Manson 2017. Adapted from Paul Cote (2009). Fundamentals of GIS. http://www.pbcgis.com/courses ↵
- CC BY-NC-SA 4.0. Steven Manson 2013 ↵
- CC BY-NC-SA 4.0. Steven Manson 2017. Image on right from MapQuest 2011 ↵
- CC BY-NC-SA 4.0. Eric Deluca 2015. ↵
- CC BY-NC-SA 3.0. Adapted from Adrienne Gruver (2016). Cartography and Visualization. www.e-education.psu.edu/geog486/node/1859↵
- CC BY-NC-SA 3.0. Adapted from Anthony C. Robinson. Maps and the Geospatial Revolution. www.e-education.psu.edu/maps/l5_p5.html↵
- CC BY-NC-SA 3.0. Adapted from Adrienne Gruver (2016). Cartography and Visualization. www.e-education.psu.edu/geog486/l1_p7.html↵
- Privacy Policy
- Terms and Conditions
- GIS Analysis
- Maps & Cartography
- GIS Software
- GIS Mapping
What Tools Do Geographers Use: Exploring Their Role In Geography
- June 20, 2023 June 11, 2023
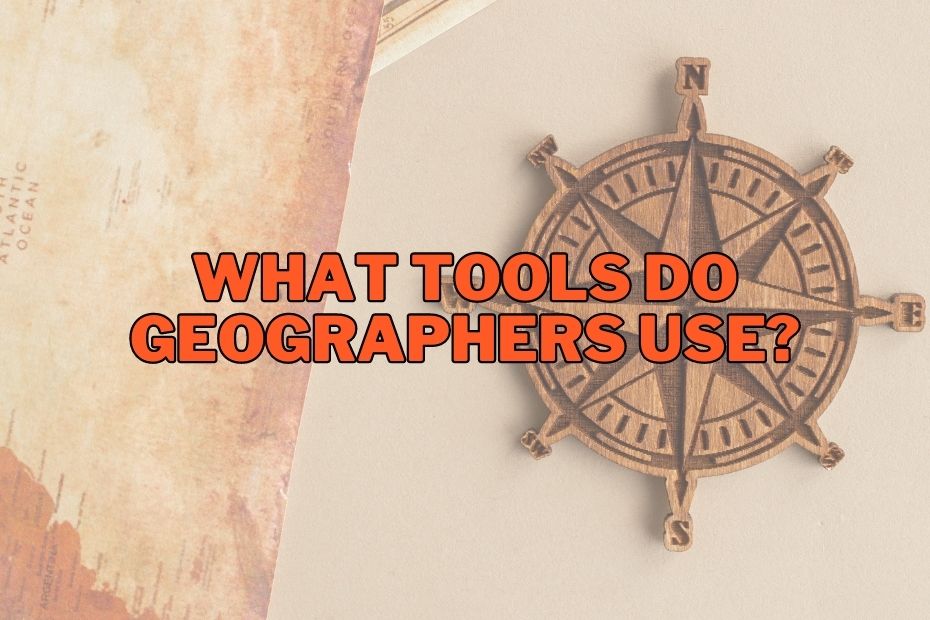
Geography is a fascinating field that involves the study of the Earth’s physical features, climate patterns, and human activities. Geographers rely on a variety of tools to collect, analyze, and interpret spatial data. In this article, we will explore: What Tools Do Geographers Use and how they contribute to our understanding of the world.
Geographers use various tools including Geographic Information Systems (GIS), remote sensing technologies like satellite imagery, aerial photographs, GPS devices, compasses, and surveying equipment. These tools enable them to analyze, interpret, and visualize geographic information effectively.
Table of Contents
What Tools Do Geographers Use?
Geography is not only about studying various locations on Earth, but it’s also about understanding the relationship between people and their environment.
It’s an extensive field that covers a lot, from the arrangement of continents to the impact of climate change on human societies. To effectively study these elements, geographers use a variety of tools.
1. Maps and Globes
Maps and globes are foundational tools in the field of geography. They provide a visual representation of the earth’s surface, helping geographers to interpret and understand spatial patterns and relationships. As geographical instruments, maps offer a detailed view of specific locations, showcasing physical features like rivers, mountains, and forests, as well as human-made structures like cities and roads.
Conversely, globes provide a more comprehensive view of the world, illustrating the spherical shape of the earth, and presenting a true picture of the size and position of continents and oceans. They are especially useful for understanding global phenomena.
An in-depth exploration on the importance of maps for geographers .
2. Map Projections
The earth is a three-dimensional object, and map projections are used to depict it on a two-dimensional surface, such as a piece of paper or a computer screen. This process inevitably introduces some degree of distortion. Map projections come in many different types, each with their unique attributes and uses.
Some map projections preserve areas, some maintain accurate shapes, while others ensure accurate distances or directions. The choice of projection is therefore highly dependent on the task at hand, as it can significantly affect the interpretation of geographical data.
3. Cartography
Cartography is the art and science of map-making , another key tool for geographers. It involves the gathering, processing, and representation of geographical data, ultimately producing detailed and informative maps. With the rise of digital technology, modern cartography has extended beyond traditional hand-drawn maps to include sophisticated computer-generated maps that can be interactively explored.
Cartographers play a vital role in geography, presenting complex geographical data in a form that is easily understandable. They need a deep understanding of spatial data and design principles, as well as expertise in the use of cartographic tools.
4. Geographic Information System (GIS)
Geographic Information System (GIS) is a powerful tool that allows geographers to analyze and interpret spatial data. It’s a computer-based system that can capture, store, manipulate, and display geographical information. By using GIS, geographers can visualize data in several ways, making patterns, trends, and relationships more apparent.
GIS provides a means to integrate different kinds of data, overlay maps, and carry out complex spatial analyses. Its applications span various fields, from urban planning and environmental management to transport and logistics.
5. Global Positioning System (GPS)
GPS is a satellite-based navigation system that provides location and time information in all weather conditions, anywhere on or near the earth. For geographers, GPS is an invaluable tool, allowing them to collect precise location data quickly and efficiently.
GPS helps geographers gather the accurate, real-time data they need to better understand our world. Geographers use GPS devices to track the movement of people, animals, and vehicles, as well as to map out geographic features accurately.
6. Satellite Imagery
Satellite imagery offers a bird’s eye view of the Earth’s surface, providing geographers with a wealth of information about our planet. Satellite imagery is essential for studying large-scale phenomena such as weather patterns, vegetation cover, urban growth, and changes in land use.
By analyzing this imagery, geographers can identify patterns and trends, monitor changes over time, and make predictions about future developments. This makes satellite imagery an indispensable tool for geographers.
7. Spatial Database
A spatial database is a database that is optimized to store and query data that represents objects defined in a geometric space. In geography, spatial databases are used to store and manage vast amounts of data, such as the coordinates of physical features or the boundaries of political regions.
Spatial databases allow geographers to conduct complex spatial queries, such as finding all the locations within a certain distance of a point, or determining whether a point lies within a specified area.
8. Surveying Equipment
Surveying equipment is another crucial tool used by geographers. These tools, including total stations, theodolites, and GPS units , are used to accurately measure terrestrial or three-dimensional positions of points and the distances and angles between them. This data is used to create detailed and precise maps and land surveys.
Surveying equipment enables geographers to gather accurate data on the physical features of the earth, which can then be used in a wide variety of applications, including urban planning, construction, and environmental management.
9. Field Notebooks
Field notebooks are indispensable tools for geographers working in the field. These notebooks allow geographers to record observations, measurements, and sketches during their research.
Field notebooks help geographers document their findings accurately and serve as a reference for further analysis.
10. Compass
A compass is a simple yet essential tool for geographers. It helps determine direction and navigate across landscapes. Geographers often use compasses in the field to orient themselves and measure angles accurately. This tool is particularly useful when conducting surveys or creating maps.
In addition to traditional magnetic compasses, modern geographers may also use digital or electronic compasses that incorporate sensors and technology to provide precise readings of direction and sometimes even additional information such as altitude, temperature, and GPS coordinates. These advancements have further enhanced the accuracy and versatility of compasses as indispensable tools for geographers.
11. Weather Instruments
Understanding weather patterns is vital for many geographers. Weather instruments, including thermometers, barometers, and anemometers, are used to collect meteorological data. Geographers use this data to analyze climate patterns, study weather phenomena, and predict changes in the environment.
Weather instruments help geographers gather accurate and reliable data, contributing to the field of meteorology and improving our understanding of weather patterns and climate dynamics.
Drones have become increasingly popular tools in geography. They offer a unique perspective and allow geographers to collect aerial data efficiently. Drones are equipped with cameras and sensors that capture high-resolution images and other valuable information. Geographers use drones to map landscapes, monitor changes, and conduct surveys in hard-to-reach areas.
By using drones, geographers can create detailed and accurate maps, including orthophotos and digital elevation models (DEMs) . These maps are crucial for understanding landscape patterns, land-use planning, and natural resource management. Drones also assist in assessing environmental conditions, such as monitoring pollution levels, identifying vegetation health, or detecting changes in water bodies.
13. Laser Scanners
Laser scanners, also known as LiDAR (Light Detection and Ranging) systems, are used to measure distances and create detailed 3D representations of landscapes. Geographers use laser scanners to capture precise elevation data, study landforms, and assess changes in terrain over time.
Moreover, laser scanners play a vital role in geospatial mapping and surveying . They enable the creation of highly detailed and precise maps, which are valuable for urban planning, land management, and environmental monitoring. The ability to capture data quickly and accurately makes laser scanners a preferred tool for geographers working in fields such as cartography, remote sensing, geomorphology, and natural resource management.
Conclusion: What Tools Do Geographers Use?
From maps and compasses to sophisticated software programs, the tools used by geographers are as diverse as the field itself. They serve as the backbone for understanding the complex interactions of the physical and human world. With the advancements in technology, geographical tools continue to evolve, offering new opportunities and challenges for geographers and for anyone with an interest in understanding our world.
FAQs: What Tools Do Geographers Use?
What are some traditional tools used in geography.
Traditional tools include maps, globes, compasses, and atlases.
Why are tools like GIS important in geography?
GIS is crucial in geography because it allows for the collection and analysis of spatial data. It enables geographers to visualize and understand patterns and relationships in a way that wasn’t possible before.
What are some examples of software tools used in geography?
Some examples of software tools include GIS software , remote sensing software , and statistical analysis software.
How do geographers use aerial photography?
Geographers use aerial photography to study landscapes, analyze landforms, and identify land use patterns. It provides a unique perspective that helps geographers gain a broader understanding of the geographic features they are studying.
Leave a Reply Cancel reply
Your email address will not be published. Required fields are marked *
Save my name, email, and website in this browser for the next time I comment.
This site uses Akismet to reduce spam. Learn how your comment data is processed .
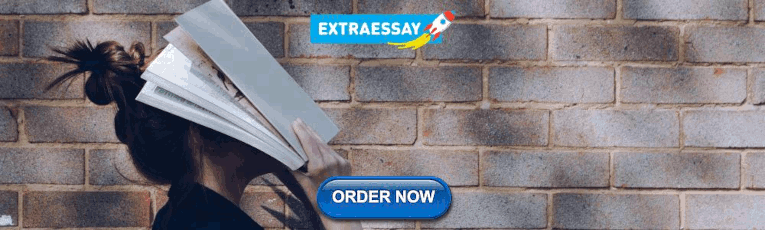
IMAGES
VIDEO
COMMENTS
This article brings together texts that have a focus on analyzing visual representations, visual technologies, and the acts of viewing and of producing visual media, highlighting the differences between methodologies used and the theoretical work they are informed by or most linked with. ... Cosgrove, Denis E. Geography and Vision: Seeing ...
Geography Compass is an authoritative and accessible geography journal publishing peer-reviewed surveys with a primary focus on human geography. ... discuss the politics of visibility and invisibility alongside a detailed unpacking of audio-visual self-representations. Exploring the aesthetic, for them, means paying attention to the sensory ...
The use of visuals as means of communication and depiction of both everyday and exceptional life situations dates back long into history (see Kress & van Leeuwen, 1990, pp. 3-4). As human communication developed, so did the use of images. Nowadays it is possible to use numerous means of expression to represent various phenomena (e.g.
Abstract. This paper forwards a hauntological approach to the study of visual images in human geography, providing a nuanced understanding of what images can do: their power, meanings, and our responses to them. Like ghosts, visual images have an undecidable, 'in-between' status, haunting between material and immaterial, real and virtual.
Introduction. Photographic and video methods (often referred to collectively as visual research methods) have undoubtedly gained increased prominence within many social science disciplines since the 1990s. This has also been true within the literatures of human geography, with a number of geographers having either argued for the significance ...
Abstract This paper suggests a number of questions which can be used to structure a small‐group discussion about the interpretation of visual images. As many geographers now are demonstrating, geographical knowledges are very often visualised. However, there is currently little on offer methodologically to help students approach the interpretation of visual images critically. This paper ...
However, the abstraction introduced requires more effort to interpret the visual representation. 9 D4: Interactivity (INTERACT) In addition to the construction of the visual representation through geography, network and their composition as described in the previous sections, interactivity can be essential for visual exploration.
Maps and Mapping. Whether your map is paper or digital, mastering the basics of reading it are vital to finding your way around and understanding how the world works. Maps are fantastic visual tools that can help us communicate spatial concepts and patterns, tell stories, and analyze data. However, there are some challenges to translating Earth ...
In the following we will look at the status of the visual in geography to understand the marginal position of visual methods in this discipline, as well as how visual methods have been used in the social sciences. ... Seeing visual methods - the use of representations as well as visual technologies - as a mode of social action means that it ...
Visualizations (i.e., thinking in images internally in the human mind) or externally expressing a concept via graphical means—such as documenting an observation in a hand-drawn or digital visuospatial sketch, or creating a visual output from data—have always been an integral part of scientific inquiry and communication. One might argue that ...
Others take a more human-centered view and describe geovisualization as "the creation and use of visual representations to facilitate thinking, understanding, and knowledge construction about geospatial data" [513] or as "the use of visual geospatial displays to explore data and through that exploration to generate hypotheses, develop ...
The use of visual representations (i.e., photographs, diagrams, models) has been part of science, and their use makes it possible for scientists to interact with and represent complex phenomena, not observable in other ways. Despite a wealth of research in science education on visual representations, the emphasis of such research has mainly been on the conceptual understanding when using ...
1.3: Maps- Place, Power, and Representation is shared under a CC BY-NC-SA 4.0 license and was authored, remixed, and/or curated by Aline Gregorio. Maps have always been central to geography. But maps are not matter-of-fact impartial documents as they are commonly thought to be.
Maps. A map is a symbolic representation of selected characteristics of a place, usually drawn on a flat surface. Maps present information about the world in a simple, visual way. They teach about the world by showing sizes and shapes of countries, locations of features, and distances between places.
Geography textbooks have many visuals, such as maps, graphs, tables, photographs, and illustrations, which makes geography a highly visual school subject. However, previous studies show that geography students can pay surprisingly little attention to textbook visuals. In this article, it is asked what sorts of visuals are there in geography textbooks if they do not attract students' attention.
This paper forwards a hauntological approach to the study of visual images in human geography, providing a nuanced understanding of what images can do: their power, meanings, and our responses to them. Like ghosts, visual images have an undecidable, 'in-between' status, haunting between material and immaterial, real and virtual. Both are theorized as dead and alive, representation and ...
On the Need to Ask How, Exactly, Is Geography "Visual"? Gillian Rose, Gillian Rose. The Open University, Milton Keynes, UK. Search for more papers by this author. Gillian Rose, Gillian Rose. ... Use the link below to share a full-text version of this article with your friends and colleagues. Learn more. Copy URL. Share a link. Share on ...
In terms of visual and art-based creative mapping approaches, the participating students' collages push the limit of the cartographic conventions of mapping to the esthetic and generative potent alternatively representing the lived experience of space (See, Fig. 5 for the use of collage maps as a visual research method). This collapses space ...
Finally, prospects of spatial and visual thinking in Geography are discussed. Their scarce use causes a devaluation of the opportunities that geospatial technology makes available to us in the twenty-first century. ... Visual and spatial thinking are intimately ingrained at cartographic representation. 3.2 Visual Analysis. Maps are one of the ...
A great resource for all geography teachers.' Professor Martin Evans, University of Manchester 'Geography, the ill-informed often quip, is about colouring in. In this wonderful book, Luke Tayler, shows us why good drawing matters and how effective visual representation of natural process and landforms can enhance geographical education and ...
In short, a revolution is underway in the way we. review of the visual basis of g eography as a discipline, not least at 'A' Level. geography. In fact it misses altogether the 'visual turn ...
Different visual variables are suited to quantitative data and qualitative data. [9] Shape. Shapes often indicate differences in kind, so they are good for qualitative data. There are certain conventions of shape use, such as an airplane for an airport, or a cross for a hospital. Shapes can also have much more specific representations.
To effectively study these elements, geographers use a variety of tools. 1. Maps and Globes. Maps and globes are foundational tools in the field of geography. They provide a visual representation of the earth's surface, helping geographers to interpret and understand spatial patterns and relationships. As geographical instruments, maps offer ...
Reprints & Permissions. View PDF View EPUB. While maps have been generally associated with geography, the use of maps in Geography education has been typically described within the context of mainstream school education. Maps certainly have utility beyond the geographical classroom, from wayfinding to planning and even for military purposes.