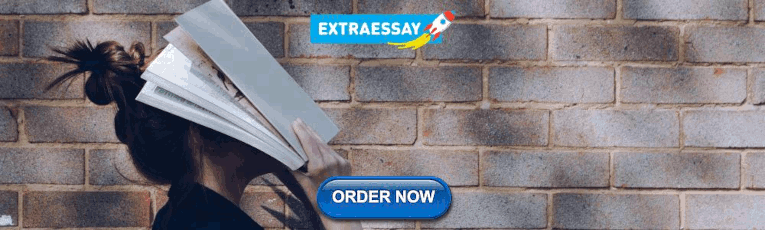
Renewable Energy Data, Analysis, and Decisions Viewed through a Case Study in Bangladesh: Preprint
- Accelerated Deployment and Decision Support
- Strategic Energy Analysis Center
Research output : Contribution to conference › Paper
NREL Publication Number
- NREL/CP-7A40-74147
- decision making
- developing countries
- renewable energy data
- Renewable Energy Data Explorer
- renewable energy resource data
This output contributes to the following UN Sustainable Development Goals (SDGs)
Access to Document
- https://www.nrel.gov/docs/fy19osti/74147.pdf
Fingerprint
- Renewable Energy Social Sciences 100%
- Decision Social Sciences 100%
- Data Analysis Social Sciences 100%
- Bangladesh Social Sciences 100%
- Case Study Social Sciences 100%
- Energy Sector Economics, Econometrics and Finance 100%
- Developing Countries Economics, Econometrics and Finance 100%
- Air Material Science 100%
T1 - Renewable Energy Data, Analysis, and Decisions Viewed through a Case Study in Bangladesh: Preprint
AU - Watson, Andrea
AU - Jacobson, Mark
AU - Cox, Sarah
N2 - Many developing countries around the world have signaled their intention to transition their energy sectors to rely on cleaner sources of electricity generation for a variety of reasons that may include complying with their Nationally Determined Contributions (in support of the Paris Climate Agreement), increasing energy security, or reducing air pollution. Renewable energy resources are increasingly a cost competitive option for new electricity generation; however, nations must consider renewable energy potential if they wish to increase these technologies in their electricity generation mix. Yet, goal setting, policymaking, grid modeling, and investment decisions that will enable renewable energy development all depend on the existence and quality of renewable energy resource data. This paper aims to summarize the relationship between renewable energy data, analysis, and decision making for developing countries seeking to transition their energy sector and to consider Bangladesh as a case study of a country that has worked with development organizations and the National Renewable Energy Laboratory to develop a national inventory of renewable energy resource data to enable critical decision making.
AB - Many developing countries around the world have signaled their intention to transition their energy sectors to rely on cleaner sources of electricity generation for a variety of reasons that may include complying with their Nationally Determined Contributions (in support of the Paris Climate Agreement), increasing energy security, or reducing air pollution. Renewable energy resources are increasingly a cost competitive option for new electricity generation; however, nations must consider renewable energy potential if they wish to increase these technologies in their electricity generation mix. Yet, goal setting, policymaking, grid modeling, and investment decisions that will enable renewable energy development all depend on the existence and quality of renewable energy resource data. This paper aims to summarize the relationship between renewable energy data, analysis, and decision making for developing countries seeking to transition their energy sector and to consider Bangladesh as a case study of a country that has worked with development organizations and the National Renewable Energy Laboratory to develop a national inventory of renewable energy resource data to enable critical decision making.
KW - analysis
KW - decision making
KW - developing countries
KW - renewable energy data
KW - Renewable Energy Data Explorer
KW - renewable energy resource data
T2 - 2019 Decision Analysis Conference
Y2 - 19 July 2019 through 21 July 2019
Case Studies in Energy Transitions: A Special Collection
Dustin Mulvaney, San Jose State University, and Maria Petrova, Georgetown University, USA
INTRODUCTION: The emphasis of this special collection of articles is case studies research and teaching activities about energy transitions—long term structural changes to energy systems, technologies, and patterns of use.
This curation of Case Studies in the Environment articles brings together papers that cover the core concepts, keywords, debates, best practices, techniques, tools, skills, and observations needed to improve our understandings of energy transitions. This special issue collection invites papers that engage with ideas and themes about energy transitions or that are incorporated into pedagogical activities. Examples topics in energy transitions include questions of temporal and spatial relevance on the magnitude of energy transitions, land use change, just transitions, life cycle assessment, finance/business, economics, behavioral concepts, socio-cultural change, policy tools and techniques, environmental justice issues, technological dependency, public participation, and carbon lock-in.
Green Energy from Garbage? A Case Study of Municipal Solid Waste’s Contested Inclusion in Maryland’s Renewable Portfolio Standard
Ingrid Behrsin, University of California, Davis, USA
Abstract: Renewable portfolio standards (RPSs) are powerful state-level climate policy tools that set minimum renewable energy targets. They have been adopted by 29 states, in the United States (U.S.) as well as Washington, D.C., and have fueled much of the growth in the U.S. renewable energy sector. However, because these policy tools are state-driven, the technologies and fuel types included in each state’s RPS vary. In this article, I discuss the inclusion of municipal solid waste in Maryland’s RPS, and a social movement for environmental justice that has emerged around this decision. Given the prominence of RPSs in both fueling renewable energy adoption in the U.S., as well as in encouraging particular technologies, it is increasingly important to interrogate the types of technologies and fuel sources that climate policies like RPSs incentivize, and how they are received by the communities for which they are proposed. Thus, this article’s objective is to inspire critical thought about the classification schemes that govern renewable energy production. Read more...
Renewable Energy on Tribal Lands: A Feasibility Study for a Biomass-to-Energy Plant on the Cocopah Reservation in Arizona
Lauren K. D’Souza, Renewable Resources Group LLC, Los Angeles, California, USA
William L. Ascher, Claremont McKenna College, Claremont, California, USA
Tanja Srebotnjak, Harvey Mudd College, Claremont, California, USA
Abstract: Native American reservations are among the most economically disadvantaged regions in the United States; lacking access to economic and educational opportunities that are exacerbated by “energy insecurity” due to insufficient connectivity to the electric grid and power outages. Local renewable energy sources such as wind, solar, and biomass offer energy alternatives but their implementation encounters barriers such as lack of financing, infrastructure, and expertise, as well as divergent attitudes among tribal leaders. Biomass, in particular, could be a source of stable base-load power that is abundant and scalable in many rural communities. This case study examines the feasibility of a biomass energy plant on the Cocopah reservation in southwestern Arizona. It considers feedstock availability, cost and energy content, technology options, nameplate capacity, discount and interest rates, construction, operation and maintenance (O&M) costs, and alternative investment options. Read more...
Barriers to the Uptake of Off-Grid Solar Lighting Products in Bihar
Sandeep Pai and Savannah Carr-Wilson, Central European University, Budapest, Hungary
Abstract: The federal government of India and the state government of Bihar, India’s least electrified state, have always focused on grid expansion to bring power to those living without grid access. However, grid expansion has been slow. In Bihar, 83% of people still live without electricity, relying on dangerous kerosene lamps to light their homes. In the 1980s, an alternative—a market for solar home systems and solar lanterns—started to develop in Bihar. Yet, this market has failed to thrive, despite three decades of intervention by the government and activity by private companies. Today, fewer than 4.2% of unelectrified Bihar households use a solar lighting product. Based on interviews with key stakeholders, this case study found that the biggest obstacle to market growth is the government kerosene subsidy, which halves the price of kerosene, and makes people less interested in solar lighting products. Read more...
Sidrap: A Study of the Factors That Led to the Development of Indonesia’s First Large-Scale Wind Farm
Martha Maulidia, University of Queensland, Brisbane, Australia
Paul Dargusch, University of Queensland, Brisbane, Australia
Peta Ashworth, University of Queensland, Brisbane, QLD, Australia
Agung Wicaksono, Institut Teknologi Bandung, Jakarta, Indonesia
Abstract: The first utility-scale (75 MW) wind farm facility in Indonesia (the “Sidrap” project) was launched in South Sulawesi in early 2018. In this case study, we assess how several factors contributed to the successful development of the Sidrap project including strong signals of support from the Indonesian Government; long-term local presence of private sector partners; familiarity of private sector partners with the risks and nuances of investing in Indonesia; and an innovative private-public sector partnership model. In the last 2 years, Indonesia’s electricity sector has changed much in terms of pricing policy and private sector involvement. Much effort has been directed toward the Indonesian Government meeting its renewable energy deployment target of 23% of the total energy mix by 2025. The question remains, however, on whether Indonesia will be able to develop additional renewable energy projects to Sidrap in the future, given the continuing changes and uncertainty in Indonesian’s renewable energy policy and politics. Read more...
Using Concepts from the Study of Social Movements to Understand Community Response to Liquefied Natural Gas Development in Clatsop County, Oregon
Trang Tran, University of Alaska, Anchorage, USA
Casey L. Taylor, University of Delaware, Newark, USA
Hilary S. Boudet, Oregon State University, Corvallis, USA
Keith Baker, SUNY College at Brockport, USA
Holly L. Peterson, Oregon State University, Corvallis, USA
Abstract: Shifts in natural gas supply and demand since the early 2000s have triggered proposals for import and export terminals in coastal locations around the United States. Demand for such facilities is likely to grow with increasing rates of natural gas exports. Clatsop County, Oregon, is one such location that experienced over 10 years of debate surrounding the development of these facilities. The first liquefied natural gas (LNG) facility was proposed in this area in 2004; the final was withdrawn in 2016. While residents expressed both support and opposition early on, opposition dominated by the end. Drawing on insights from the literature on social movements, we conduct a case study of community response to LNG proposals in Clatsop County. We show how opponents were able to successfully frame the potential risks of LNG in a manner that had strong community salience, allowing them to appropriate resources and create political opportunities to advance their cause and influence local and state decisions. Read more...
Closing Diablo Canyon Nuclear Power Plant, 2009–2018: Decision-Making on Energy Investments Relevant to Climate Change
John H. Perkins, The Evergreen State College (Emeritus), Washington, DC, USA
Abstract: Modern economies cannot function without electricity, and production of electric power affects citizens in many ways, including climate change. Production of electricity requires investments that easily reach billions of dollars, and streams of investment capital must be perpetual to procure fuel, build and maintain plants, and transmit electricity to customers. This case study addresses whether a California decision relevant to investments about generating electricity adequately considered competing concerns. In 2009, Pacific Gas and Electric (PG&E, a private, investor-owned utility) applied to renew the operating licenses of its two nuclear reactors at the Diablo Canyon Nuclear Power Plant (the “plant”). By 2016, PG&E had decided not to seek license renewal and asked the California Public Utilities Commission (CPUC) to approve a price increase for its electricity to pay for specified expenses in closing the plant, which generated 24% of PG&E’s electricity. Read more...
Pedagogy for the Ethical Dimensions of Energy Transitions from Ethiopia to Appalachia
Jen Fuller, Arizona State University, Tempe, AZ, USA
Sharlissa Moore, Michigan State University, East Lansing, MI, USA
Abstract: Education on energy ethics is a crucial part of engaging students in learning about energy systems and energy transitions that needs further development. This article describes the use of case studies and active learning tools to achieve learning outcomes related to the ethical and social dimensions of energy. It discusses a daylong workshop held for undergraduate and graduate students at Michigan State University in February 2017 and evaluates pre- and postlearning outcomes. Two case studies are described that highlight ethical trade-offs in energy transitions. An international case study on Ethiopia and the Grand Renaissance Dam illustrates the benefits and drawbacks of cross-border electricity trade related to energy access, economic growth, and the energy-water nexus. A domestic case study on coal miners and coal towns in Appalachia examines the layered influences of place attachment and the challenges of economic diversification post-peak coal extraction. Read more...
Socially Not Acceptable: Lessons from a Wind Farm Project in St-Valentin, Quebec
Louis Simard, University of Ottawa, Canada
Abstract: Social acceptability appears as a new public norm that major projects must meet in order to be authorized and realized. This article proposes to analyze the case of a wind farm project in the municipality of St-Valentin, Quebec, Canada near the border with Vermont, which was cancelled by the government due to lack of social acceptance, in order to illustrate the importance of this norm today. The project involved the construction of 25 turbines to generate 52 MW of power. Launched in 2006, the project was already significantly under way by 2008; however, in 2011, the government permanently shelved it. Through a combination of document analysis and 11 interviews, we identified the main reasons for the lack of social acceptability: lack of upstream consultation from the developer and wrong scale planned for the consultation process, controversies surrounding the public decision-making process, profound contradictions between the community’s values and interests and the nature of the project, and perceptions of the impacts on the landscape and conflicting uses. Read more...
Using a Community Vote for Wind Energy Development Decision-Making in King Island, Tasmania
R.M. Colvin, Australian National University, Canberra, Australia
G. Bradd Witt, The University of Queensland, Brisbane, Australia
Justine Lacey, Commonwealth Scientific and Industry Research Organisation (CSIRO), Brisbane, Australia
Abstract: In 2012, a large scale wind energy project was proposed for development in King Island, Tasmania, Australia. The project proponents adopted what they described as a ‘best practice’ approach to community engagement; an approach expected to achieve positive outcomes for developer and community by maximising community involvement in decision-making, limiting social conflict, and enhancing the potential of achieving the social licence to operate. Despite this, the community experience during the time of the proposal was one of conflict and distress, and the proposal was eventually cancelled due to exogenous economic factors. This case study explores a key element of the engagement process—holding a community vote—that caused significant problems for people and process. The vote appeared to be a democratic means to facilitate community empowerment in the decision-making process. However, in this study, we show that the vote resulted in an increase in conflict and polarisation, challenged the legitimacy of the consultative process and credibility of the proponents, and ultimately led to legal actions taken by opponents against the proponent. Factors including voter eligibility, the benchmark for success of the vote, campaigning, and responses to the outcome of the vote are examined to demonstrate the complexity of decision-making for renewable energy and land use change more generally. Read more...
Shooting for Perfection: Hawaii’s Goal of 100% Renewable Energy Use
Barry D. Solomon, Michigan Technological University, USA
Adam M. Wellstead, Michigan Technological University, USA
Abstract: In the United States, 29 states, Washington, D.C. and three territories have adopted a mandatory Renewable Portfolio Standard (RPS) for their electric power systems, while eight states and one territory have set renewable energy goals. Many foreign nations have adopted an RPS as well. Thus far, almost all RPSs across the United States have met their interim goals with targets and timetables that vary widely. Hawaii’s RPS is the most ambitious, with a 100% target set for 2045 (though Vermont set a 75% target for 2032). This paper provides a case study of the Hawai’i RPS. The paper focuses on geographical issues and perspectives that may tease out the course of the states’ electricity future: sensitivity to climate change, population distribution, interisland rivalries, as well as the need for greater energy storage and complementary policies. An important complexity is the challenge of meeting electricity demand on six separate Hawaiian Islands (because of the lack of an interisland transmission cable), although all of them have substantial renewable energy resources. Read more...
Evaluating Community Engagement and Benefit-Sharing Practices in Australian Wind Farm Development
Nina Lansbury Hall, The University of Queensland, Australia
Jarra Hicks, University of New South Wales, Australia
Taryn Lane, Embark, Victoria, Australia
Emily Wood, Independent Communications Contractor, Victoria, Australia
Abstract: The wind industry is positioned to contribute significantly to a clean energy future, yet the level of community opposition has at times led to unviable projects. Social acceptance is crucial and can be improved in part through better practice community engagement and benefit-sharing. This case study provides a “snapshot” of current community engagement and benefit-sharing practices for Australian wind farms, with a particular emphasis on practices found to be enhancing positive social outcomes in communities. Five methods were used to gather views on effective engagement and benefit-sharing: a literature review, interviews and a survey of the wind industry, a Delphi panel, and a review of community engagement plans. The overarching finding was that each community engagement and benefit-sharing initiative should be tailored to a community’s context, needs and expectations as informed by community involvement. This requires moving away from a “one size fits all” approach. This case study is relevant to wind developers, energy regulators, local communities and renewable energy-focused non-government organizations. It is applicable beyond Australia to all contexts where wind farm development has encountered conflicted societal acceptance responses. Read more...
Affiliations
- Recent Content
- All Content
- Special Collections
- Info for Authors
- Info for Reviewers
- Info for Librarians
- Editorial Team
- Prize Competition
- Online ISSN 2473-9510
- Copyright © 2024
Stay Informed
Disciplines.
- Ancient World
- Anthropology
- Communication
- Criminology & Criminal Justice
- Film & Media Studies
- Food & Wine
- Browse All Disciplines
- Browse All Courses
- Book Authors
- Booksellers
- Instructions
- Journal Authors
- Journal Editors
- Media & Journalists
- Planned Giving
About UC Press
- Press Releases
- Seasonal Catalog
- Acquisitions Editors
- Customer Service
- Exam/Desk Requests
- Media Inquiries
- Print-Disability
- Rights & Permissions
- UC Press Foundation
- © Copyright 2024 by the Regents of the University of California. All rights reserved. Privacy policy Accessibility
This Feature Is Available To Subscribers Only
Sign In or Create an Account
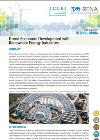
Renewable Energy Policy in Cities: Selected Case Studies
Browse by theme.
- Dezhou, China which has actively supported the establishment of renewable energy industries with the Dezhou Economic Development Zone for solar technology
- Chemnitz, Germany where the local government enabled the formulation of strategies to use renewable sources and in 2008 developed the Integrated Climate Protection Programme (Integriertes Klimaschutzprogramm).
- Belo Horizonte, Brazil which has reduced greenhouse gas (GHG) emission substantially and, since 2007, turning a closed landfill site into a waste-to-energy facility.
- Austin, US where the GreenChoice Program active since 2001 has stimulated the initial demand for renewable-based electricity, facilitating municipal and community procurement of renewable energy.
- Sydney, Australia, and Nagpur, India where energy efficiency and renewable energy have reduced emissions from public street lights.
- Sao Paolo, Brazil where a local regulation requires new residential, commercial and industrial buildings to install solar water heating systems (SWH) to cover at least 40% of the energy used for heating water.
- Malmo, Sweden which set targets significantly more ambitious than either the European Union target for Sweden (49% by 2020) or the national plan (50% by 2020), so that the city is expected to be climate neutral with municipal operations run on 100% renewable energy by 2030.
Additional analyses
100% renewable energy scenarios: supporting ambitious policy targets, green hydrogen for sustainable industrial development: a policy toolkit for developing countries, north africa: policies and finance for renewable energy, renewable energy market analysis: mano river union region, the breakthrough agenda report 2022, related content.
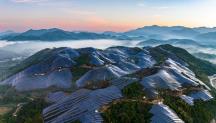
Record Growth in Renewables, but Progress Needs to be Equitable
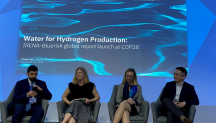
Innovative Policymaking is Crucial to Drive Green Hydrogen Market and Ensure its Sustainable Production
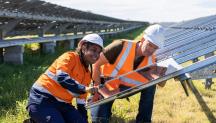
Renewables Jobs Nearly Doubled in Past Decade, Soared to 13.7 Million in 2022
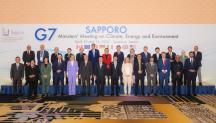
G7 Communiqué Echoes IRENA's Call for Rapid Deployment of Renewables
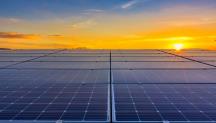
Under IRENA's ETAF, Masdar and AIIB Reach Financial Close on 3 Uzbek Solar Projects
Ørsted’s renewable-energy transformation
To stop climate change, companies in every industry must rapidly reduce their carbon emissions. That is no easy task, but a few businesses show it can be done. Ørsted, an energy company based in Denmark, stands out as an example. Twelve years ago, when it was called DONG Energy, the company earned most of its revenues by selling heat and power, 85 percent of which came from coal. Then, in 2009, management announced a major strategic shift: the company would seek to generate 85 percent of heat and power from renewable sources by 2040.
Ørsted invested aggressively in offshore wind and phased out coal. By 2019, it had become the world’s largest producer of offshore-wind energy. The company also raised its renewable-generation share to 86 percent—hitting its target 21 years ahead of schedule. In an interview with McKinsey, the CEO of Ørsted’s offshore-wind business, Martin Neubert, tells the story of the company’s transformation: the strategic decision that started it all, the changes it went through, and the outlook for the future. (The remarks below have been condensed and edited for clarity.)
McKinsey: Back in 2008, DONG Energy was a profitable and stable conventional-energy company. How did the idea of pivoting to renewables come up?
Martin Neubert: At that time, DONG Energy was largely a domestic Danish energy company. Eighty-five percent of our power and heat production was powered by coal, and 15 percent by renewables. For us, one key factor supporting the decision to rethink our strategy in favor of renewables was the failed attempt to develop a 1,600-megawatt coal-fired power plant project, called Lubmin, in Northeast Germany.
We had made substantial investments in this greenfield project during the more than six years we spent trying to develop it. And while the project was supported by the German federal government, we experienced strong local opposition against the idea of building a coal-fired power plant on the Mecklenburg-Vorpommern coastline. This was the first clear sign telling us that the world was beginning to move in a different direction, and we concluded that there was no sustainable way of realizing the project. Also, in 2009, the global renewable-energy agenda was positioned strongly at the United Nations COP15 [15th Conference of the Parties] climate summit in Copenhagen, supported both by the Danish government and by our board of directors.
McKinsey: How did management assess the company’s position and its ability to shift toward renewables?
Martin Neubert: In 2008–09, we formulated a new strategy and vision called 85/15, stating that we wanted to change our generation mix from 85 percent conventional, 15 percent renewable to 85 percent renewable, 15 percent conventional. The 85/15 split, which was decided on by executive management, reflected the ambition to conduct a complete turnaround of our generation mix. It also took into account that DONG Energy had spent three decades establishing itself as a company focused on the generation of conventional fuels. So the expectation was that such a turnaround would have to be completed within one generation, or the equivalent of 30 years.
At the time, I don’t think anyone thought we would turn our generation mix upside down within only ten years. But that was not the discussion then. Instead, we discussed what our future growth areas should be: areas where we had critical mass, where we had the right competences, and where we could differentiate ourselves. It became clear that one was wind power, which three of the six companies that merged to become DONG Energy in 2006 had already pursued.
Onshore wind was well established. We had a sizeable portfolio of projects in Poland and Sweden, and we had been involved in projects in Spain and Greece. As for offshore wind, we had early-stage operating projects in Denmark and the United Kingdom and large-scale development projects. That gave us critical mass in wind when we formulated our vision.
We also had a team of 50 or 60 people working on renewable-energy projects. Some had spent their careers on these technologies, particularly onshore wind. That gave us substantial in-house expertise, backed by a clear understanding of what it would take to develop wind power, technology-wise.
The 85/15 split, which was decided on by executive management, reflected the ambition to conduct a complete turnaround of our generation mix. It also took into account that DONG Energy had spent three decades establishing itself as a company focused on the generation of conventional fuels. So the expectation was that such a turnaround would have to be completed within one generation, or the equivalent of 30 years.
McKinsey: Back then, the technology landscape for offshore wind looked very different from what it looks like now. How did that factor into your thinking?
Martin Neubert: At the time, no offshore-wind projects bigger than 160 megawatts had been built. So we had to ask how we could build large-scale offshore-wind projects in a different way. Could we move from building one highly customized offshore-wind project every two or three years to building one or two more standardized projects every year? What would it take to go from handcrafting to serial production?
Answering that question involved a 360-degree review: the supply chain, our competencies, the financing models. We concluded that we could not do it alone. One challenge was installation. The installation companies in the market were small. We found a considerable risk that they could go bankrupt during a project. That led us to acquire A2SEA as an installation supplier.
We would also need strong partnerships with suppliers of turbines, foundations, and cables. Turbines were a particular issue. Since no purpose-built installation vessels existed, we reasoned that we would benefit from working with a manufacturer on the design, layout, and funding of second-generation installation vessels. Siemens quickly realized that offshore wind could develop into a large industry. We entered a partnership with them, which included the delivery of 500 3.6-megawatt turbines. At the time, it was one of the largest energy agreements Siemens had ever made.
McKinsey: How did executives and staff react to the decision to take the company in a new direction?
Martin Neubert: There was internal pressure to keep DONG Energy the same. It wasn’t unexpected, because we had spent three decades turning the company into a traditional fossil-fuel company. Fossil fuels were our core competence and the focus of our growth strategy. Our employees also perceived that we were the world’s best at running coal-fired power plants, and a benchmark for the industry. The skepticism was broad and profound.
Ultimately, though, internal skepticism receded. In 2012, when Henrik Poulsen had just joined as CEO, our portfolio of assets and activities had high exposure to gas and gas-fired power plants. As gas prices dropped in the United States, vast amounts of surplus American coal ended up in Europe, where it replaced gas as the preferred fuel for power generation. That caused us financial difficulties, which made it easier for people to accept the new focus on offshore wind and on the exploration and production of oil and gas, and the moves to divest noncore businesses.
We began implementing the new strategy by establishing a wind-power business unit. I think those of us who were asked to join this business unit saw it as the beginning of an interesting journey. A group of strong European utilities was active in UK offshore wind at the time. We all thought that something big was going on and that the UK would be the right place to pursue offshore-wind projects at industrial scale.
That proved to be the case when the UK government strengthened its support for offshore wind to help make these projects financially viable. If that hadn’t happened, I’m not sure that we would have progressed as fast as we did.
McKinsey: Getting into offshore wind required a multiyear effort to sell holdings and build up new assets. How did management secure the necessary capital even as the company was exiting businesses that were reliable sources of cash?
Martin Neubert: We had multiple new projects in the UK that needed funding. One model would have involved financing them with external debt and then divesting once the projects were operational. But raising debt for each project would not have worked well with our group-level funding strategy. Another approach, partnering with electric utilities, would have been too complicated, because these companies had their own asset portfolios and strategies.
We needed financial partners that could deliver capital and manage their investments while relying upon our experience constructing and operating offshore-wind projects. One structural issue, however, was that we did not want to use project financing, whereas many of our financial investors preferred or were even required to leverage their investment via project financing.
This led us to develop the “farm down” model, in which we could fund our half of a project on our balance sheet and partners could use project financing to fund the rest. With farm-downs happening before commissioning, we provided investors with turnkey project offerings, which would protect them from risks we can manage best, including development, construction, and operating risks. That model resonated with the Danish pension funds, and later with Dutch and Canadian pension funds and other investors.
Had we not developed the farm-down model, we couldn’t have funded all these projects in Europe. And the structure that we innovated became widely used in the industry.
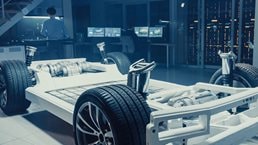
Powering up sustainable energy
McKinsey: What organizational changes took place as Ørsted’s portfolio shifted toward renewables?
Martin Neubert: By 2012, our wind-power business unit had grown to hundreds of employees. But it was still working like a start-up. To support new projects, we added whatever resources were needed, which led to inefficiencies. We lacked a proper organizational structure and operating model.
Correcting that was one of the key accomplishments of my predecessor, Samuel Leupold. He introduced our first real operating model, establishing global functions, clear project governance, and a product-line organization that systematically reduced the cost of offshore-wind electricity by eliminating ad hoc or project-specific sourcing and procurement.
During the past three years, Ørsted has also cultivated a “one company” approach spanning our business units. For example, we have established a management-team forum, consisting of all EVPs and SVPs, who meet four times a year to talk about our strategy and strategic enablers such as talent and digital. That forum facilitates open discussions to break silos, align our approach, and build a strong network among senior leaders. In addition, we have reestablished our leadership-forum meetings for our top 400 leaders.
McKinsey: Ørsted has made significant moves in recent years. Can you talk about those, and the rationale for them?
Martin Neubert: The strategic steps we’ve taken during the past three to five years have focused on turning Ørsted into a global renewable-energy major. The first step was divesting our oil and gas business, which concentrated our business almost entirely on renewables. We also invested in the conversion of our domestic heat and power plants, enabling them to move away from coal toward biomass. As a result, we will exit coal in 2023, and our power generation will be carbon neutral in 2025.
In 2016, we completed our IPO, and DONG Energy, which we were still called at the time, became a publicly listed company. The IPO provided us with the flexibility and access to equity that we need to fund growth. The IPO also gave institutional and retail investors an opportunity to take part in our green transition, while sharpening our profile as a renewable pure-play.
Within the past couple of years, we have reentered the onshore-wind market and moved into solar PV [photovoltaic] and storage solutions. These moves will help diversify our technology mix so we can better meet the demands of our customers. What’s important to note is that we are moving into these technologies at scale. North America, for example, is a large market for onshore wind and storage solutions, and we are investing there. Everything we do reflects our vision to create a world that runs entirely on green energy. And while offshore wind has the potential to power the world, we’re convinced that a broader technology mix will support the growth of our company even better.
Within the past couple of years, we have reentered the onshore-wind market and moved into solar PV and storage solutions. These moves will help diversify our technology mix so we can better meet the demands of our customers. Everything we do reflects our vision to create a world that runs entirely on green energy.
McKinsey: Ørsted’s transformation into an offshore-wind leader has been complete for some time. What opportunities do you see for growth in that market?
Martin Neubert: Our ambition is to remain the global leader in offshore wind. In the past two to three years, offshore wind has expanded from a predominantly European market to a global market. We’ve been a first mover as that shift has occurred. We were the first European developer that went into large-scale offshore wind in the US. We were also the first foreign offshore-wind developer to enter Taiwan. Within a few years, we have developed sizable project portfolios in both markets.
To support our growth, we recently reorganized our offshore-wind business and established four new regions. Moving closer to different markets is important for navigating their development. It also helps with commercial matters like owning wind farms. At the same time, we want to keep the scale advantages, leverage, and standards that our global operations and EPC [engineering, procurement, construction] functions deliver, and so they work closely with our regions.
McKinsey: New horizons for change in the energy sector are coming into view. How does management keep working hard to ensure that Ørsted remains a leader in offshore wind, while challenging itself to gain a strong position in the energy industry’s next evolutionary phase?
Martin Neubert: We ask ourselves that regularly. And I have been asked many times, by investors, by the media, and by people within our organization, if we are at risk, considering that bottom-fixed offshore wind is our bread and butter. We value our global leadership position in offshore wind, and we want to retain that. Obviously, we don’t want to miss out on major developments—for example, in floating offshore wind. But we must respond as the needs of our customers change.
The ability to reinvent ourselves has proven to be key. In 2006, DONG Energy consisted of some oil and gas licenses. Then it reinvented itself through the merger of six domestic energy companies. A few years later, the company reinvented itself again by establishing a wind-power business unit that became a global leader within a few years. Scanning new horizons and spotting new business areas are essential to Ørsted’s strategy and our ambition to become a global renewable-energy major.
Martin Neubert is executive vice president and CEO of offshore wind at Ørsted. This interview was conducted by Christer Tryggestad , a senior partner in McKinsey’s Oslo office.
This article was edited by Josh Rosenfield, an executive editor in the New York office.
Explore a career with us
Related articles.

How to decarbonize global power systems

Climate math: What a 1.5-degree pathway would take
- Help & FAQ
A case study of renewable energy development in Myanmar: challenges and potential solutions
Research output : Contribution to journal › Article › Research › peer-review
Myanmar has one of the lowest electrification rates in the world, and most of its inhabitants, who lack access to electricity, live off-grid in rural areas. Despite Myanmar having abundant sun and wind energy resources, which could potentially generate electricity for rural communities, renewable energy growth in Myanmar is stunted. In this article, we examine the case study of renewable energy development in Myanmar to better understand the factors that influence renewable energy development and deployment in a developing country context. Our analysis reveals that there are numerous reasons for the lack of solar, wind, and biomass energy growth in Myanmar, such as regressive electricity tariffs, problematic hydropower contracts, low levels of social acceptance and awareness of clean technologies, and a lack of institutional policy framework for renewable energy. To reduce energy poverty and increase the share of electricity generation from renewable resources in Myanmar, we recommend tariff reforms and the establishment of a transparent, effective national policy framework. Increasing access to financial resources in rural areas, gaining public trust and support from local communities, and promoting their involvement in decision making in renewable energy projects should also be prioritised. Disseminating knowledge on clean technologies is also recommended to increase environmental awareness and encourage long-term behavioural change.
- Developing countries
- Electrification
- Renewable energy development
- Solar energy
- Wind energy
This output contributes to the following UN Sustainable Development Goals (SDGs)
Access to Document
- 10.1525/cse.2020.1109204
Other files and links
- Link to publication in Scopus
T1 - A case study of renewable energy development in Myanmar
T2 - challenges and potential solutions
AU - Hlaing, Ei Hnin
AU - Dargusch, Paul
N1 - Publisher Copyright: © 2018 by the Regents of the University of California.
PY - 2020/10/28
Y1 - 2020/10/28
N2 - Myanmar has one of the lowest electrification rates in the world, and most of its inhabitants, who lack access to electricity, live off-grid in rural areas. Despite Myanmar having abundant sun and wind energy resources, which could potentially generate electricity for rural communities, renewable energy growth in Myanmar is stunted. In this article, we examine the case study of renewable energy development in Myanmar to better understand the factors that influence renewable energy development and deployment in a developing country context. Our analysis reveals that there are numerous reasons for the lack of solar, wind, and biomass energy growth in Myanmar, such as regressive electricity tariffs, problematic hydropower contracts, low levels of social acceptance and awareness of clean technologies, and a lack of institutional policy framework for renewable energy. To reduce energy poverty and increase the share of electricity generation from renewable resources in Myanmar, we recommend tariff reforms and the establishment of a transparent, effective national policy framework. Increasing access to financial resources in rural areas, gaining public trust and support from local communities, and promoting their involvement in decision making in renewable energy projects should also be prioritised. Disseminating knowledge on clean technologies is also recommended to increase environmental awareness and encourage long-term behavioural change.
AB - Myanmar has one of the lowest electrification rates in the world, and most of its inhabitants, who lack access to electricity, live off-grid in rural areas. Despite Myanmar having abundant sun and wind energy resources, which could potentially generate electricity for rural communities, renewable energy growth in Myanmar is stunted. In this article, we examine the case study of renewable energy development in Myanmar to better understand the factors that influence renewable energy development and deployment in a developing country context. Our analysis reveals that there are numerous reasons for the lack of solar, wind, and biomass energy growth in Myanmar, such as regressive electricity tariffs, problematic hydropower contracts, low levels of social acceptance and awareness of clean technologies, and a lack of institutional policy framework for renewable energy. To reduce energy poverty and increase the share of electricity generation from renewable resources in Myanmar, we recommend tariff reforms and the establishment of a transparent, effective national policy framework. Increasing access to financial resources in rural areas, gaining public trust and support from local communities, and promoting their involvement in decision making in renewable energy projects should also be prioritised. Disseminating knowledge on clean technologies is also recommended to increase environmental awareness and encourage long-term behavioural change.
KW - Developing countries
KW - Electrification
KW - Myanmar
KW - Renewable energy development
KW - Solar energy
KW - Wind energy
UR - http://www.scopus.com/inward/record.url?scp=85104191570&partnerID=8YFLogxK
U2 - 10.1525/cse.2020.1109204
DO - 10.1525/cse.2020.1109204
M3 - Article
AN - SCOPUS:85104191570
SN - 2473-9510
JO - Case Studies in the Environment
JF - Case Studies in the Environment
M1 - 1109204
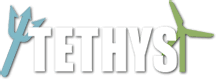
Energy justice & coastal communities: The case for Meaningful Marine Renewable Energy Development
Global climate change has prompted many national plans for rapid emissions reductions. For example, the United States recently committed to transitioning to 100% carbon-free electricity by 2035 and net-zero emissions economy-wide by 2050. Parallel to conversations surrounding emissions reductions is the call for energy justice, or the demand for more equitable distribution of energy-related burdens and benefits among communities. To date, energy justice has evolved as a mostly academic conversation, which may limit its utility to praxis. In response, we offer an interdisciplinary framework that aims to organize existing knowledge and lessons learned from energy development. Specifically, we developed the Meaningful Marine Renewable Energy (MRE) Development Framework and conducted a literature review using MRE as a case study. MRE was chosen because it is a nascent renewable energy technology in the US with projects mostly in demonstration stages and no commercial deployment, making it a useful case study to apply lessons learned from other energy sectors and other countries. Discussion of current resources being developed among the MRE community and their implications for furthering energy justice priorities are also explored. We conclude the review with a compiled list of questions meant to support stakeholders in translating theoretical concepts of Meaningful MRE Development to practice. Although the Meaningful MRE framework was developed using MRE as a use case, our interdisciplinary theoretical framework can be applied beyond MRE to other sustainable and renewable energy projects.
- Original article
- Open access
- Published: 05 September 2016
Smart energy systems for smart city districts: case study Reininghaus District
- Stephan Maier 1
Energy, Sustainability and Society volume 6 , Article number: 23 ( 2016 ) Cite this article
13k Accesses
29 Citations
2 Altmetric
Metrics details
Dense settlement structures in cities have high demands of energy. Usually, these demands exceed the local resource availability. Individually developed supply options to cover these demands differ from place to place and can also vary within the boundaries of a city. In a common sense of European governance, cities are pushed to save energy, increase renewables and reduce import dependency on fossil fuels. There are many innovative concepts and technologies available to tackle these needs. The paper provides a comprehensive methodology for planning and assessing the development of ‘smart’ energy systems leading to complex energy provision technology networks using different on-site as well as off-site resources.
The use of the P-graph (process-graph) method allows the optimisation of energy systems by using different energy sources for heating, storing and cooling. This paper discusses this method in the development of an urban brown field, the premises of the Reininghaus District , a former brewery in the city of Graz in Austria. The case study is interesting as it combines on-site energy sources (e.g. solar heat and photovoltaic) with nearby industrial waste heat and cooling at different temperatures and grid-based resources such as existing district heating, natural gas, and electricity. The case study also includes the competition between centralised technologies (e.g. large scale combined heat and power and heat pumps with district heating grids) and decentralised technologies (e.g. small scale combined heat and power, single building gas boilers, solar collectors, etc. in buildings).
Ecological assessment with the Energetic Long-Term Analysis of Settlement Structures (ELAS) calculator provides an evaluation of the ecological impact of the developed energy systems.
Different scenarios based on two building standards OIB (low energy house standard) and NZE (passive house standard) as well as different prices for key energy resources were developed for an urban development concept for the Reininghaus District. The results of these scenarios show a very wide spectrum of structures of the energy system with strong variations often caused by small changes in cost or prices. The optimisation shows that small changes in the setup of the price/cost structure can cause dramatic differences in the optimal energy system to supply a smart city district. However, decentralised systems with low-temperature waste heat and decentralised heat pumps in the building groups show the financially most feasible and, compared to alternatives, most ecological way to supply the new buildings.
Conclusions
The planning process for the development of the Reininghaus District is a complex and therefore lengthy process and shall be concretised over the next decades. Optimal energy technology networks and scenarios resulting from the application of the described methods support the framework energy plan. The accumulated knowledge can be used to form smart energy supply solutions as an integral part for the discussion of the stakeholders (investors, city department) to guide the forming of their action plan through the development of the city quarter.
Smart city approaches
Cities are the fastest growing form of settlement worldwide requiring sustainable energy systems to deal with their increasing density and size [ 1 ]. Although urban population growth in developed countries (0.5 %) is projected to be below population growth in less developed countries (2.3 %) from 2007 to 2025, there is a general shift from rural to urban areas; 60 % or 5 billion of the global population (8.4 billion people) will live in cities by 2030 [ 2 ]. This growth implicates a growing resource demand for buildings, infrastructure and energy supply in urban areas. City authorities are challenged worldwide because the demand for additional living and working space is rising.
The European Union provides different initiatives as well as funds and regulates by law how European cities should deal with these challenges in order to become smart cities [ 3 ]. The term ‘smart city’ was only developed quite recently. An exact approach to the definition of an optimal interpretation of ‘smart development’ and what ‘smart’ means for the city and its inhabitants is controversial. Every smart city design has a different focus on what ‘smart’ or ‘smarter city’ means and how to proceed with their specific development [ 4 ]. In this context, de Jong et al. gave a good overview about attributes which in the course of time have been attached to the word ‘city’ to name urban planning-related activities of researchers, decision makers and city planners, with definitions like liveable , green , intelligent , low carbon , sustainable , digital , information , knowledge , resilient , eco and ubiquitous [ 5 ]. They come to the conclusion that so far, most articles use the word sustainable city and since 2009, it seems to be replaced by the term smart city . From the organisational perspective, a differentiation of term smart cities was classified into the following two hierarchically counter-directed approaches [ 6 ]:
Top-down smart cities are usually initiated by city institutions, information and communication technology (ICT) and/or research facilities, and it is a straight forward planning concept.
Bottom-up smart cities are usually modelled by local inhabitants, and an innovative potential, societal knowledge and networks is used by the cities themselves to design the city.
A categorisation of a smart city so far is in many cases very technology-oriented putting expansion, improvement and integration of information and communication technologies in front of the attempt to smarten a city. However, with the difficulties that implementations of new technologies can pose also other aspects were added to the discussion. Some of the terms and ideas which are currently used in this relation are categorised [ 7 ]:
General improvement of urban energy and planning concepts
Environmental sustainability (sustainable resource use)
Social sustainability (realising social inclusion of different kinds of urban residents in public services, citizen democratisation/cultural and societal empowerment)
Higher quality of life through technical improvements in telecommunication infrastructure/administration/networks/living/mobility
Economic development/efficiency
Integrating private sector, business-oriented urban development
High-tech/creative industries in long-term growth
Social/relational capital in city development
Batty et al. classify the wide spectrum of terms and ideas which come up with the concept of ‘smart city’ and present and divide them into six functions [ 8 ]:
Smart economy (competitiveness)
Smart people (social and human capital)
Smart governance (participation)
Smart mobility (transport and ICT)
Smart environment (natural resources)
Smart living (quality of life)
Apart from the general observation of factual issues, it is the procedural setting which creates momentum in city development. Inside the functional framework, stakeholders can be identified as drivers in different institutions. Depending on their field of expertise, they have to deal with diverse contextual issues. In this relation, it is important to know which actor plays a role to which extent and how he or she is influencing the whole system of a city. In the Austrian research context, Saringer-Bory et al. categorise Smart City Actors in the SmartCityAkteursmatrix (smart city actors matrix) [ 9 ] as follows:
Institutional category (public science, government, non-governmental organisation (NGO), private businesses, etc.)
Field of expertise (spatial planning, architecture, urbanism, energy planning, mobility, climate research, social research, etc.)
Keywords (energy standard, consumption, resources, renovation, insulation, networks, logistics, etc.)
In this respect, technological expansions of city infrastructure do not simultaneously imply improvements neither regarding sustainability issues nor the reduction of energy demand and increase in quality of life, wealth and benefit for the whole community [ 10 ]. There may be the risk that a smart city development is interpreted one-sidedly from a technical-business perspective only, and social and environmental requirements can be missed again. Smart city planners must not forget that just letting grow new businesses to produce smart technologies can have rebound effects so that social and environmental requirements of a sustainable urban development can be missed again. Environmental savings and social justice can be outweighed by an additional implementation of technologies whose main goal is to increase quality of life.
From an ecological perspective, the European regulatory states clear legal framework conditions for the communities of the European Union. One goal of the united European governmental efforts is to reduce greenhouse gas emissions by 20 % below the 1990 level by 2020 [ 11 ] and achieve a 40 % reduction by 2030 [ 12 ]. According to the European Parliament, buildings account for 40 % of the total energy consumption and 36 % of CO 2 emissions in the EU [ 13 ]. Low energy buildings and zero energy buildings could contribute to energy savings and emission reductions [ 14 ]. Hence, the EU passed the Energy Performance of Buildings Directive 2010 [ 15 ] and in the Energy Efficiency Directive 2012 stating that all public buildings by 2018 and all new buildings by 2020 must be nearly zero energy buildings. The laws clearly state that smart initiatives must include more than just adding smart grid technologies and technical process automation to the existing infrastructure. Holistic and sustainable system thinking needs to permeate widely through different levels of society. This requires a critical discussion on sustainable development and innovation itself and how theoretical sustainable concepts can be realised [ 16 ].
New approaches to build a framework to optimise new city quarters or rehabilitate existing urban quarters provide a holistic view to find smart, economically feasible energy systems with social and ecological benefits for the society. Different examples of attempts to build frameworks to push innovative planning concepts can be discovered in often closely related developments. These developments reveal a basic tendency of trying to push spatial planning closer together with energy planning. In Austria, the OEROK ( Oesterreichische Raumordnungskonferenz /Austrian Conference on Spatial Planning) is an institution that was established by the federal government, the federal provinces and municipalities of Austria to coordinate spatial development on a national level. The OEROK started collecting and disseminating expert knowledge while raising awareness on the importance of Energieraumplanung (integrated spatial and energy planning) [ 17 ], as it is defined in the Oesterreichisches Raumentwicklungskonzept— OEREK [ 18 ]. The situation in Switzerland is similar to Austria: the programme EnergieSchweiz [ 19 ] provides a statement of requirements named Energierichtplanung (energy planning framework). In Germany, the term Energienutzungsplanung (energy utilisation planning), which is an energy usage plan to systematically develop future energy systems for municipalities according to specific local situations and renewable energy systems, first appeared in Bavaria in 2012 [ 20 ].
Across European approaches, an integration of energy and spatial planning is at different stages of development. Best practise examples and instruments for future-oriented and resource-efficient energy and spatial planning have already been defined and discussed by various authors [ 21 ]. An integrated spatial and energy planning process needs to integrate a reduction in the dependence on fossil fuels and an extended use of urban energy resources in comparison to the current state. Renewable energy technologies utilising industrial waste heat can be considered as they can provide positive impacts on local economy and reduce ecological burdens as well [ 22 ].
Planning and implementation of smart urban energy systems, however, involves a wide spectrum of stakeholders: from city administration to developers to energy providers to current as well as future inhabitants. The planning discourse between these stakeholders can be supported by reliable and comprehensive methods to design and evaluate complex energy systems. Such decision support methods
Provide answers to the different perceptions of the economic framework for the development of smart energy systems brought to the table by various stakeholders by creating reliable scenarios
Allow comparison of the scenarios by guaranteeing optimal energy systems generated by using different resource options and economic frameworks
Provide comprehensive ecological evaluation of the scenarios along with thorough economic and technical specification to enable a holistic planning process
An approach to support stakeholders and policy makers with a selection and an application of different methods for an integrated spatial and energy planning is provided by Stoeglehner et al. in a study which gives an overview about tools which cover analysis of energy savings, energy efficiency, renewable energy, spatial planning, mobility and evaluation and optimisation of planning schemes [ 23 ].
Smart energy planning
Energy planning that leads to ‘smart’ urban solutions requires integration of energy design into spatial planning and urban planning. This means that the design of new settlements, as well as the refurbishment of existing city quarters, requires an interdisciplinary planning approach that takes spatial and mobility planning, energy systems design, building and infrastructure design and the evaluation of ecological impacts into account [ 24 ]. Innovative approaches must therefore be applied. Electricity supply in urban areas has moved to the centre of debate on how to supply urban areas with renewable energy [ 25 ]. Heat integration and heat storage, the integration of industrial waste heat and solar thermal energy in supply networks have also become major aspects of smart city development [ 26 ]. The case study region of Reininghaus District has already been in the focus of scientific endeavours to merge all these aspects [ 27 ]. The following tools and methods have been applied to the framework energy plan for the Reininghaus District allowing for systemic energy system design and holistic ecological evaluation.
For the investigated area, total energy demands are needed as important basic information to optimise the energy technology network. A further downscale to the building level is essential to focus on the level of a city quarter. In Austria, the OIB-standard, set by the Austrian Institute of Construction Engineering [ 28 ], is the minimum requirement regulated by Austrian law. The maximum energy consumption is continuously being lowered to reach EU regulation goals within the next few years. To constantly decrease energy consumption per building, energy demand levels permitted by the current Austrian Low Energy Building Standard (OIB-standard) and the Nearly Zero Emission Buildings Standard (nZEB or NZE-standard) are under ongoing discussion. In the interest to guarantee a better readability in this work, the phrases ‘NZE-standard’ and ‘OIB-standard’ will be consistently used. To avoid misunderstandings, each part of the phrases is connected by a hyphen. Improvements which can be applied to save energy in buildings can be reached, for instance with energy efficiency measurements such as optimised insulation building retrofit [ 29 ]. The focus of the optimisation of a whole city quarter’s energy technology network needs to be extended from single house level to building groups. This is particularly important because small-scale modelling differs greatly from large-scale optimisation. Examples of how settlements could be designed and supplied with renewable energy often applied single technology approaches and partly hybrid renewable supply systems [ 30 ].
These approaches clearly highlight the importance and requirement for developing strategies and concepts to help city stakeholders with their burden of finding an optimal and secure future energy supply. Even though this paper is concerned with the challenges posed by these concepts, it also discusses an approach that is generally applicable to the selection of spatial planning processes. The paper considers the optimisation of renewable and fossil technologies along with specific conditions of the optimised city district, such as resource availability, competition and market prices.
Research problem
The research questions posed in this case study is the integration of available local energy sources into the energy system in order to meet future Reininghaus District demands. Three companies, which produce waste heat at different temperature levels, are located close to or even directly in the area: Steel plant Marienhütte Stahlwerk , which is already connected to the district heating grid of Graz, produces heat of approximately 80 °C, Linde Gas heat of around 20 °C and STAMAG Malzfabrik of around 20 °C. Additional waste heat in large amounts is available from the steel plant at a temperature of 30 °C. A cooling stream of 10 °C (which could also be used for upgrading in a heat pump) is available from groundwater wells left over from the former brewery within the district premises.
For a more precise approximation of different local differences, the Reininghaus District was split into 17 groups of buildings as they will be described in the case study description in chapter 3. After defining possible energy demands for the planned groups of buildings, the availability of local energy (e.g. waste heat, geothermal energy and rooftops for solar energy) and existing energy infrastructure to supply Reininghaus District was modelled. The district can easily be connected to the existing district heating grid of the city, currently supplied mainly by three large gas-, coal- and oil-fired combined heat and power (CHP) plants. The gas-fired plant (250 MW thermal power) is located within the city, and the coal (230 MW thermal power) and the oil-fired plant (230 MW thermal power) are located 20 km south of Graz [ 31 ]. Approximately 90 % of the district heat in Graz is generated from fossil fuels. Natural gas and electricity grid connection is also possible for the district. The model must provide consistent, economically optimal structures of the network between all possible sources, distribution within the quarters, conversion technologies and consumption. It takes seasonal variations into account. Subsequently, the optimal structures generated for different economical frameworks (scenarios) are ecologically evaluated.
Many approaches focus on smart energy system design and smart energy systems as a priori 100 % renewable systems (e.g. Lund et al. [ 32 ]). To keep the door open to compare existing energy regimes and infrastructure like fossil energy systems, the pre-definition of a specific target resource system was avoided in this work. The aim of this methodological framework is to provide information about optimal technology networks and the ecological and socio-economic evaluation of different options for future city developments. It consists of the Process Network Synthesis (PNS) and, inter alia, the tool Energetic Long-term Assessment of Settlement Structures (ELAS), the tool applying the Sustainable Process Index (SPI). In order to achieve the research goals raised in the framework plan to find smart and sustainable energy systems for cities, the PNS was chosen. With this method, it is possible to model complex systems and find optimal energy systems before studying the relevant matters in depth with the help of a modelling or design process. On the other hand, ELAS and SPI can deal with interdisciplinary issues of complex settlement structures and a comprehensive ecological evaluation. Process cycles of various energy systems can so be ecologically evaluated and provide usable information and a practical model for a stakeholder process.
Process Network Synthesis (PNS)
One method in particular that has proven its worth in planning tasks like integrated spatial and energy planning is PNS [ 33 ]. This method has been developed in the framework of process technology [ 34 ]. The mathematical structure behind has been discussed in several publications [ 35 ]. It uses a directed bipartite graph (p-graph or process-graph) method to describe process networks and employs combinatorial rules to find all feasible network solutions using all possible resources, intermediates and products as well as all relevant technologies processing these mass and energy flows (superstructure). Software employing this method (PNS Studio) is freely accessible from www.p-graph.com [ 36 ]. All data concerning flows and cost of technologies, pipes and transport can be provided in predefined material and operating units input tables of PNS Studio. Moreover, parameters like the required and maximum flows, lower bound and upper bound of capacity constraints for operating units can be set. Flows are split into resources, intermediates and products. Flows can then be set as input and output flows in the operating units to display conversion and production interdependencies between the technologies considered.
Figure 1 shows how the user-defined maximum technology structure is then the starting point for a rigorous evaluation of all feasible process networks linking resources to the desired products. The first step is the generation of a pre-optimisation of a feasible maximal process network (maximum structure) with the maximum structure generator (MSG), and in a second step, the optimal process network (optimum structure) is generated using a branch-and-bound optimisation included in the solver of PNS Studio.
Maximum structure and optimum structure of a technology network
In addition to its long tradition in process industry applications, PNS has also been successfully applied to regional resource utilisation problems [ 37 ]. A special software tool using the PNS method (RegiOpt) was recently developed [ 38 ] to provide a preliminary design for smart regional energy systems based on renewable resources and is freely available on the internet [ 39 ].
In this study, an in-depth modelling is carried out to generate scenarios for the optimal energy supply of the Reininghaus District using PNS. This method allows the optimisation of local energy and material demands and supplies situations represented in energy technology networks. Seasonal variations in resource provision and consumptions are treated by a multiperiodic option of the PNS [ 40 ].
ELAS calculator
ELAS was developed to analyse urban structures ranging from single houses to whole settlement structures regarding their energy situation and in particular their ecological performance. The calculator allows the evaluation of single buildings as well as whole settlements. It can be applied to existing structures as well as planning tasks and also allows the evaluation of refurbishing and extension plans to existing settlements [ 41 ].
The calculator takes site-specific data of residential settlements into account. This consists of energy consumption and supply, mobility induced by the location of the settlement as well as the distances to service provision. It uses a life cycle approach to the evaluation and accounts for the ecological impact of construction, use and disposal of all buildings and energy infrastructure in a settlement, such as roads, wastewater drains and lighting of public space.
Results of the ELAS calculator contain accumulated energy demand, ecological footprint (calculated with the SPI method) [ 42 ], CO 2 life cycle emissions and regional economic impact (turn over, value added, imports, jobs created or lost) of the settlements. The Sustainable Process Index is an ecological footprint method. It has recently been described and discussed in its international methodical context [ 43 ]. The SPI has been applied in several fields such as ecological evaluation of agricultural products [ 44 ] or collectively shown in the evaluation of energy technology systems based on renewable resources [ 45 ]. The ELAS calculator is an online tool that runs SPI evaluations in the background (along with other technical parameters and statistical variables) [ 46 ]. The ELAS calculator allows users to provide specific data via a GUI (graphical user interface).
ELAS provides municipalities a basis for sustainable energy supply and appropriate policy decisions or gives an overview of individual energy consumption and its economic and ecological effects. The tool is freely available online.
The result of the optimal structure of PNS obtained for a particular set of economic boundary conditions (a scenario) is used as input for ELAS evaluation. Amounts of resources (in this case, energy flows for the supply of the city quarter) resulting from PNS are one input for the assessment with ELAS calculator. In addition, the input parameters for ELAS are the site-specific data, building standards, infrastructure, induced mobility and energy, construction and mobility costs. Results are the ecological footprint (SPI), energy demand and CO 2 . The total sequence proceeds as shown in Fig. 2 .
Methodical framework
Case study description
Graz, the capital of the Austrian Federal State of Styria is a middle-sized city (approximately 280,000 inhabitants by 2016 [ 47 ]) that is estimated to reach approximately 325,000 inhabitants by 2030, which would result in an increasing demand of living and working space [ 48 ]. In order to meet the growing population’s needs, the city planning department is required to densify the city preferably in a vertical manner. A horizontal extension should be excluded from spatial planning. According to the City Government, a horizontal densification is allowed for new buildings only. Following this urban areas already dedicated as building land are currently under heavy exploitation. Some of the existing building ensembles have been designated a UNESCO heritage site, whereby they are subordinated by law according to the Dachlandschaftsverordnung [ 49 ]. This law protects the urban roofscape, particularly in central parts of the city. These old buildings must be conserved, meaning an addition of storeys is not permitted there.
Most of the Reininghaus District is green- and brownfield, measuring an area of 110 ha. Reininghaus District is located 1.8 km west of the historical city centre, and approximately 12,000 inhabitants are predicted to live there after completion of all developments. The development of this new city quarter is carried out by an interdisciplinary team, consisting of scientists, architects, developers and experts from various stakeholders. For the part of the scientific support, a consortium of Graz University of Technology is integrated in the project Energy City Graz, which is a sub-project of the flagship project Energy City Graz (ECR). The Reininghaus project is funded by the Austrian state research and technology programmes Building of Tomorrow and City of Tomorrow [ 50 ].
The case study carried out in this project is an integral part of the framework energy plan City Graz Reininghaus [ 51 ]. This framework plan covers:
The concept of a self-sufficient, in terms of energy, city district, the initiation and supervision of the development process for the energy-optimised and sustainable city district Graz-Reininghaus, the phrasing of guidelines, recommendations and a checklist for future energy optimised city developments in Graz and Styria
The development of specific energy values in private legal contracts between the city of Graz and future investors, promoted by incentive systems like bonus cubage and higher housing density for buildings
Concepts for the integration of the energy values in suitable manner in local plans and regulations (City Development Concept STEK Graz, City-District-Development-Concept Graz-Reininghaus and development plans for the city quarters on location)
Graz city officials have announced the intention of using the plan to define an innovative framework for the development of the Reininghaus District [ 52 ]. The outcome of the complete framework energy plan includes possible energy technology network solutions as well as architectural, mobility, environmental and infrastructural guidelines.
The forming of the optimal building characteristics by an interdisciplinary team played an important role to provide a basic model and let heat and electric experts calculate energy needs of the new quarter. Generally, a perimeter block development was chosen as a basic building concept for all groups of buildings. To guarantee individuality for the specification of the building characteristics for each group of buildings, an architectural competition was performed for the first groups and will be announced for the other groups of buildings over the next years or decades. Depending on side-related issues, the cubature and spatial planning aspects of each building and group of buildings were adapted related to the expected context (mixture of utilisation including open green areas, mobility, air quality issues, wind, shadowing effects, etc.). In coordination with the framework plan, superordinate goals such as for the whole district Reininghaus could so be followed. Apart from the energy technology network solutions, the other guidelines are not part of this work. Information directly important to form the context of this work is provided here. A detailed description of it can be found in the framework energy plan.
For the framework energy plan, the city district Reininghaus was divided into 17 groups of buildings consolidated in three geographical districts: North, East and South as shown in Fig. 3 . The group of buildings feature homogenous parameters, like energy standards for buildings, functional mixes, load profiles for heat and cooling, defined in the university consortium, which serves as the guideline for development. They are individual components in the model described in this study. Within these groups of buildings, average distribution distances for linking buildings to energy grids are assumed. For the larger geographical districts, it is necessary to define the overall structure of distribution grids for natural gas, district heating, electricity and wastewater collection systems as well.
Three geographical subareas N, E and S including 17 groups of city buildings
This paper presents a model that identifies the optimal technological network as well as locations for energy provision installations, which
Provide all groups of buildings with heat and electricity
Integrate locally available resources while generating the highest possible value added for the whole energy provision system of the total district Reininghaus
Take resource, investment and infrastructure costs as well as limitations of (and possible competition for) resources into account
This model may be used to develop consistent optimised scenarios for different economic framework configurations and evaluates them economically and ecologically. The scenarios serve as a solid basis for negotiations between the various stakeholders, whose interactions will shape the district’s future smart energy system. The goal of using this model is to provide stakeholders and decision makers with a factual basis for a discourse on the development and implementation of a smart energy system in this brownfield development project.
The energy aspects of the framework plan for the Reininghaus District brownfield development is based on the following premises:
The framework plan includes options for OIB-standard buildings (52.6 kWh/y.m 2 heating and 4.6 kWh/y.m 2 cooling demand) and NZE-standard buildings (11.2 kWh/y . m 2 heating and 11.4 kWh/y . m 2 cooling demand) as a basis for the calculation of the energy demand of the group of buildings.
Load profiles for heating and cooling are taken into account.
Existing sources of industrial waste heat shall be taken into consideration for optimal energy systems.
Additional infrastructure to utilise waste heat (e.g. distribution pipes) is accounted for.
A scenario presuming autarky (at least for cooling and heating) has to be provided for reference.
The model generating the scenarios forming the basis for the stakeholder discourse takes capacity limits and qualities of local energy sources into consideration.
The framework plan is the result of an intensive discourse between stakeholders in the Reininghaus District development, taking long-term trends in the economic and environmental framework for supplying and using energy in this district into account and was set as a requirement by the City of Graz and the Austrian Federal State of Styria provided to investors who want to develop the district.
Table 1 provides an overview of the existing heating/cooling sources in the district. Photovoltaic (PV) panels installed on roofs can supply a total of 1760 MWh/year; however, they compete with thermal solar collectors for roof space. The electricity grid can provide electricity with no practical capacity limit for the district. The natural gas grid is also assumed to cover any load necessary to supply the district. The maximum capacities and temperature levels of the waste heat and renewable energy sources range from 800 to 100,000 MWh/year and from 10 °C to almost 80 °C.
Definition of the maximal energy technology system
Besides quality and limitation of energy sources, the model must also consider seasonal variations in energy demand, in particular for heating and cooling. Respective demands were provided during the project by the Institute of Electrical Power Systems and the Institute of Thermal Engineering of Graz University of Technology and are shown in Figs. 4 and 5 as load functions for heating, cooling, warm water and electricity for the assumed scenarios of building standards for the district. In OIB and NZE standard cases, an electricity demand of 30 GWh/year (excluding electricity for providing heat via heat pumps) is assumed. The entire district requires 45.7 GWh/year of heat (warm water and heating) and 3.2 GWh/year of cooling for the OIB-standard scenario.
Load variation over time for the district assuming low energy house standard (OIB) in MWh [ 53 ]
Load variation over time for the district assuming passive house standard (NZE) in MWh [ 54 ]
For the NZE-standard scenario, the entire heat demand is with 16.6 GWh/year lower than the OIB-standard demand. On the other hand, NZE-buildings need 4.8 GWh/year more energy for cooling.
NZE-standard buildings can reduce heat demand by 63 % however with an increasing demand for cooling in summer (2.5 times more energy compared to OIB-standard) because the different construction needs more ventilation for cooling.
In order to align energy provision with these load profiles, three periods were defined to represent the time-dependent demand of the district. Months with similar energy demand levels were merged to three periods winter, midterm and summer (see Table 2 ).
The economic framework for the district’s development consists of the end-consumer prices and the cost of obtaining energy from different sources and the feed-in tariffs into distribution grids for surplus energy provided in the district. Additional file 1 : Table S1 provides energy prices and cost of this economic framework for the baseline scenario NZE. The prices and costs in this table are not fixed but subject to stakeholder negotiations and market price developments. The scenarios provided in the ‘ Results ’ section show how changes in this economic framework of prices and costs shape the complex structure of energy provision for the Reininghaus District.
Investment costs of the technologies (taking different sizes of installations into account) as well as costs of grid infrastructure within the district are based on industry information and experiences from other projects. They are summarised in Additional file 1 and remain constant in all scenarios.
There is a large portfolio of technologies that may be applied to provide heating, cooling and electricity for the Reininghaus District. This ranges from simply connecting the group of buildings to existing distribution grids for electricity or heat, to the utilisation of local heat sources to upgrade waste heat with heat pumps, or the collection of solar energy via thermal collectors or PV panels, to name just a few. Many of these technologies can be applied decentrally, meaning at the building site, or centrally, meaning at the site of the source. These options differ by the size of the installations and the distribution system (and distribution losses) as well. Low-temperature waste heat from an industrial source in the form of a water flow can, for example, be the input to a large heat pump at the source, which increases the temperature of the waste heat to the temperature level necessary for residential heating, subsequently distributing hot water via a district heating grid to the buildings. It can, however, also be distributed directly via a grid to the buildings and may locally be upgraded in smaller on-site heat pumps to heat the buildings. Investment costs and distribution losses will vary considerably for these cases and may also vary for different groups of buildings, given the differences in the length of the distribution pipes. This central/decentral option also applies to gas burners and CHP technologies based on gas. Lower prices for coal could influence the optimal energy technology system but a specific barrier to burning solid particles are air-quality restrictions within the city of Graz due to geographically related inversion problems with too high particulate accumulation.
Figure 6 shows the ‘maximum technology system’ of all suggested technologies used in the model with the exception of distribution grids. These distribution systems connect each sub-quarter with all energy sources available and are modelled in detail. This technology portfolio is the basis for the generation of scenarios provided in the subsequent chapter.
Maximum technology system for the energy system in detail.
Scenario section
The following describes the scenarios, generated with the PNS based on different economic framework conditions. They provide an example of the broad variation of structures for the energy system for the district, caused by often quite small differences in costs and relative prices of the energy sources in question. The total area of all roofs of the buildings was assumed for three kinds of use: 7 % for solar thermal, 1 % for photovoltaic panels (for energy installation) and 50 % for green planted roofs (for ecological cooling effects), and the remaining 42 % were not open to any purpose. The assumption for energy installations was set on a very low level for the following reasons. On the one hand, climate geographical analysis during the project showed that too much sealing would heat up passing air mass and so further deteriorate the micro-climate in Graz. On the other hand, architectural and constructional characteristic (especially different heights and alignments of buildings cause shadowing effects) as well as the Dachlandschaftsverordnung reduce the potential for solar use. This low energy potential was taken in order to be on the safe side with the assumptions.
The time frame for the realisation of the Smart City district Reininghaus is divided into two different segments. A first part consisting of two building blocks (see quarters 1, 4 and 4a in Fig. 3 ) will be realised within the next years while the remainder of the district will require a longer time to be realised. Therefore, the modelling was also split with one model containing only the two building blocks that will be realised soon and the comprehensive model that includes all building blocks. This arrangement also allows to analyse if the optimum of a subset of buildings leads to qualitatively different solutions than if the whole district is subject to the optimum. In all cases, the optimum was carried out for both OIB and NZE standards.
In a last step, the framework of all scenario groups underwent more specific changes. This was important for gaining an overview of how price changes in global market prices or considerations about autonomy and technical changes summarised below would influence the optimal energy technology system. The following parameter classes were defined:
Identification of sharp cost limits of available waste heat (reducing or increasing actual prices until technology structures start to switch)
Identification of cost limits for natural gas, existing district heat, cold water well, etc. are not economic anymore (reducing or increasing actual prices until technologies start to switch)
Autonomous heating and cooling supply options of Reininghaus
Each of the scenarios was also ecologically evaluated with ELAS calculator.
Table 3 describes scenario parameters. Value added per year is in this relation the main output of each optimal scenario and is the result of the total income from selling energy minus the sum of annualised investment cost, operating cost and resource cost. The optimal scenarios were calculated for the case of supplying all quarters of the case study area Reininghaus simultaneously. Results show that value added ranges, depending on changing of parameters, from 245,000 €/year to 641,000 €/year for the whole Reininghaus District and 17,000 €/year to 193,000 €/year for the subset of quarters 1, 4 and 4a. Cost variations were made starting from the basic purchase cost for energy from Additional file 1 : Table S1. Prices for selling the energy to the end-consumers were set as fixed market prices.
The following graphs are all based on the maximum technology system as defined in Fig. 6 . Sources and technologies that are part of the optimal structure under the designated economic framework are circled. Red circles mean heating, blue circles cooling and green circles identify technologies and sources used for electricity provision in the district. Broken circles represent sources and technologies that are only used for some groups of buildings, or only service a smaller part of the demand, supporting the main sources of energy. The baseline scenario and the scenarios with changed natural gas cost, changed district heat cost and heating autonomy will be shown here in further detail.
Figures 7 and 8 show the energy supply structure for the base line scenario for purchase cost, selling prices and feed-in tariffs defined in Additional file 1 : Table S1. The results of these two figures stand for all quarters.
Optimum energy technology system for base line scenario all quarters (OIB-standard)
Optimum energy technology system for base line scenario all quarters (NZE-standard)
Result overview
These figures show that under current economic conditions, providing heat with decentral natural gas burners in the buildings is optimal, with support from industrial waste heat. In the OIB-standard case (Fig. 7 ), gas furnaces and high temperature industrial waste heat from the steel plant Marienhütte Stahlwerk share the main burden of heat provision (of which 10 % comes from the industrial waste heat). Low-temperature industrial waste heat from the companies Marienhütte Stahlwerk and STAMAG Malzfabrik provide only a small fraction of the heat demand for quarters close to this source, with the waste heat from Marienhütte Stahlwerk being up-graded in de-centralized heat pumps, the STAMAG Malzfabrik waste heat will be used in a central heat pump at the site of the company. Cooling is provided by district cooling from the cold water sources located at the Erber estates. Electricity comes mainly from the grid, with local PV contributing 7 % of the demand. The picture is quite similar for the NZE-standard case (Fig. 8 ), with the exception that the share of the district heat covered by high-temperature waste heat from Marienhütte Stahlwerk is much larger at 30 %. Low-temperature heat from this steel plant will not be used. Cooling now will be provided by de-central air conditioners.
Results of structures for quarters 1, 4 and 4a show the same energy technology network but different amounts of energy described in Table 3 .
Figure 9 represents how dramatic changes in the overall structure can become if the cost for a certain source is changed even slightly. A 4 % (NZE-standard) to 16 % (OIB-standard) increase in the gas price will change the supply structure for the NZE-standard case from that given in Fig. 8 to that in Fig. 9 . Gas will no longer contribute to supplying heat to the district. It will be replaced by high-temperature industrial waste heat from the Marienhütte Stahlwerk, supported by de-central heat pumps fed by low-temperature waste heat from the Marienhütte Stahlwerk and Linde Gas. In this scenario, solar thermal collectors will also contribute to cover the heat demand, reducing the available area for PV panels to supply electricity.
Optimum energy technology system in the scenario natural gas all quarters with reduced gas price (OIB-standard and NZE-standard case)
The existing district heating system will only become part of the energy solution for the Reininghaus District if the cost per unit of energy is reduced by approximately 23 % (OIB-standard) to 26 % in the NZE-standard case.
Then, the optimal energy supply structure will change as seen in Fig. 10 . Although the main share of heat is still provided by de-centralized gas furnaces, a third of the group of buildings will be connected to the municipal district heating system. Small contributions for buildings close to the sources will be made by high-temperature waste heat from the steel plant Marienhütte Stahlwerk and by low-temperature waste heat from Linde Gas, upgraded to a central heat pump at the site of the company.
Optimum energy technology system in the scenario district heat all quarters with reduced district heating cost (OIB-standard and NZE-standard case)
Finally, the question of only supplying heat and cooling from local sources will be addressed in Fig. 11 . Full-energy autonomy is not a viable option for the district, as PV area is restricted to roofs of buildings (even with more intensive area use). This means that under this assumption, it will always be necessary to import electricity. Figure 9 already indicated that the heat load and as cooling may be supplied by local energy sources only (if electricity is not restricted). Figure 11 shows the most optimal energy system for autonomy regarding heat. In this case, the structures are quite similar for the OIB-standard and NZE-standard case. The main burden of heat supply will be taken by the high-temperature waste heat from the steel plant Marienhütte Stahlwerk, supported by de-central heat pumps fed by low-temperature waste heat from Marienhütte Stahlwerk and Linde Gas. This scenario requires an increase of imported electricity to power the heat pumps. It is interesting that the reduction of profit for this scenario is minor, with 13 % for the OIB-standard case and only 9 % for the NZE-standard case.
Optimum energy technology system for scenario heating autonomy all quarters (OIB-standard and NZE-standard case)
Apart from different amounts of energy, described in Table 3 , results of structures for quarters 1, 4 and 4a only show different energy technology networks. In this case (as well for OIB-standard as NZE-standard), instead of gas burners, the use of waste heat in combination with decentral heat pumps is the main part of the solution.
Within the optimal scenario where resources, infrastructure (a.o. pipes) and cost/prices are included, marginal prices of resources were calculated. Cost for energy on the local energy market can be tested for volatility. Natural gas becomes uneconomic at a purchase price more than 51 (NZE-standard) or 57 euro (OIB-standard) per MWh, existing district heat becomes economic up to a purchase price of 50 (NZE-standard) and 51 euro (OIB-standard) per MWh (Table 4 ) and cooling water from deep water wells for cooling processes becomes economic between 37 (NZE-standard) and 41 euro (OIB-standard) per MWh.
High resource costs of waste heat and cooling water favours decentralized technologies. These are primarily natural gas burners, solar thermal plants, heat pumps, photovoltaic systems, air conditioning and to a lesser extent cooling water and waste heat.
The scenario with the highest economic revenue considering both economic performance and low ecological impact leads to a scenario that is completely supplied by the locally available waste heat and cooling water. Total costs of approximately 2.9 million euros per year and product revenue around 3.6 million euros per year can be achieved in this way (Fig. 12 ). A total revenue of about 0.6 million euros per year could so be created with an optimal energy technology network in the OIB-standard case.
Total cost and revenue in Euro per year (OIB-standard case)
For a yearly-based calculation, the individual life cycle for all energy technologies has been taken into account. The resource cost can exceed investment and operating cost many times over (91 % resource cost of total cost, see Fig. 13 ).
Cost allocation in % per year (OIB-standard case)
All scenarios that are generated by optimising economic profit via the PNS model are also evaluated ecologically with the ELAS calculator. The results of this tool provide a broader picture than just the ecological impact of the energy system, also including the life cycle impact (evaluated with SPI) of buildings and urban infrastructure. By replacing fossil fuels with the use of waste heat, the environmental pressure decreases in both cases to a very low value compared to electricity (Fig. 14 ). Electricity remains constant because it is already supplied to a high extent with renewables, and the potential for local electricity production with photovoltaic systems was highly limited because of the respective scenario setting (PV in competition with solar and green biomass on roofs).
Comparison of ecological impact of scenarios evaluated with SPI for relation of heat to electricity, using the ELAS calculator (absolute)
Figure 15 provides a comparison of the ecological impact with the part of the energy system highlighted. The baseline optimum scenarios are diagrammed between two extreme scenarios. The advantage of using local waste energy sources is clearly visible by comparing the ‘gas free’ scenario and the heating autarky scenario with the others. The ecological performance of the NZE and OIB-standard overlap. In comparison to the 110 ha Reininghaus District, the ecological footprint of the OIB-standard is 4000 (100 % waste heat scenario) to 8000 times (100 % natural gas scenario) bigger. For the NZE-standard, it needs 5000 (100 % waste heat scenario) to 6000 (100 % natural gas scenario) times this area.
Comparison of total ecological impact of scenarios evaluated with SPI, using the ELAS calculator
The electricity demand is covered by the current Austrian mix (78.6 % renewable sources) and cannot be influenced much locally. But the ecological pressure for the heat production can be reduced by more than 60 % as Fig. 16 shows.
Comparison of ecological impact of scenarios evaluated with SPI for relation of heat to electricity, using the ELAS calculator (in %)
Due to less heat demand, NZE-buildings are already on a much lower level than OIB-buildings (about one third) with corresponding ecological pressure. Independently of the building standards, the substitution of fossil fuels in heat supply can reduce the ecological pressure in both cases.
Energy supply of the Reininghaus District is just a part of the total ecological footprint; therefore, it is suggested that other important factors be discussed further (e.g. mobility). Possible changes of ecological pressure due to heating and cooling (OIB-standard in comparison to NZE-standard) are strongly dependent to the technology network. The existing district heat network of Graz originates from approximately 90 % coal and natural gas supply, which implicates a high ecological pressure. This high value can be reduced if locally available waste heat is used (not including the internal energy demands of the companies Marienhütte Stahlwerk, Linde Gas and STAMAG Malzfabrik themselves). The import dependency on fossil fuels for direct energy supply for building demands can be reduced by almost 100 %.
In the search for and planning of a smart economy, optimal energy systems have been found with PNS. Results show that with current prices, decentral natural gas burners supported by industrial waste heat are the economically most feasible energy system. The testing of price variabilities in the scenarios showed that with an increase of the natural gas purchase price (16 % OIB-standard) and a slight increase (4 % NZE-standard), the option of burning gas is no longer a part of the energy system. Instead, an additional use of industrial waste heat in combination with de-central heat pumps is then financially feasible. To become financially feasible, the district heat purchase price must drop by 9 % in the OIB-standard case and by 26 % in the NZE-standard case, or alternatively, the price of fossils must increase. Depending on further parameters such as density of energy demand, also, solar thermal collectors can become financially feasible. The very cost-intensive energy system of the already existing district heat on location is not financially feasible at the current high price level.
Not less important than the financial issue in the definition of the background section, the need for a smart and, as a synonym for a far-reaching concept, a sustainable city was mentioned. This implies that an ecological evaluation of the discussed scenarios of energy technology systems can be supportive in the discussion and scaling of a well-balanced socio-ecological economic process.
After the process optimisation and the ecological evaluation, resource, financial and ecological aspects were overlapped to find the scenario which is most ecologically friendly at the lowest costs. In this consideration, decentralised systems with low temperature waste heat and decentralised heat pumps show the financially most feasible (revenue more than 640,000 €/year) and, compared to alternatives, most ecological way (more than 60 % reduction potential of the ecological pressures of heat) to supply the new quarter. This scenario has the highest financial revenue when energy cost for purchasing the cooling water at 10 °C from deep water wells is available at very low costs. This seemed to be a realistic option due to the fact that the investor and owner of the deep water wells was interested in independent implementation alternatives to provide a decentral energy system solution. A use of the local waste heat can reduce import dependency on fossil fuels by almost 100 % of the final energy consumption.
The general planning process for the Reininghaus District is still in progress. The scenario results were used to inform the involved stakeholders about how the ecological footprint could potentially be reduced and how financial issues of different developments of energy prices and energy technology supply options could be handled. This basic research about ecological aspects of optimal energy technology supply for the Reininghaus District further was compiled with other information collected in the project from project partners. This information consists of analyses concerning microclimate, city planning, construction materials and further modelling of concrete scenarios for the spatial planning and energy supply of the Reininghaus District. The framework energy plan City Graz Reininghaus can now evolve into a comprehensive guideline for an integrated city planning.
This work did not cover all discussed sustainable city or smart city aspects since it laid the focus on the application of the described methodology for the use in urban energy planning and environmental sustainability concepts. In this case, the field of application of the discussed methodology can be described as a top-down smart city approach initiated by institutions of all administrative levels of the state (municipality, province and federal ministry) and research facilities. From the perspective of a general smart city concept categorised into six smart city functions, the discussed methodology mainly considered the smart economy and smart environment functions.
However, it allowed to model the specific local context of a city quarter considering many factors in relation to urban energy planning like, e.g. available concerning energy consumption, available local and imported resources, energy standards, existing infrastructure, local stakeholder interventions, etc. Most of them were mentioned as keywords in the smart city actors matrix in the ‘ Background ’ section. With this contextual setting, the financial feasibility of well-established technologies could be identified by testing the elasticities of energy cost.
Applying PNS for resource and technology systems for the energy supply of city districts helped to discover optimal energy systems out of a setting of complex supply and demand options. In the case of the smart city district Reininghaus, Process Network Synthesis has been applied to guarantee that only optimal scenarios representing different economic boundary conditions considering cost variations for energy services and energy sources are compared.
The results of the scenario generation with the PNS method show that even small changes in the setup of the price/cost structure for cooling, heating and electricity can cause dramatic differences in the optimal energy system to supply a smart city district. Shifts between fossil and renewable systems already happen when cost for natural gas rise slightly (NZE-standard case). A shift to an integration of heat from the existing district heat system would need a significant reduction of the price for district heat. Integrating industrial waste heat may allow to cover the heat demand for buildings with NZE-standard and even OIB-standard with local sources at reasonable economic cost. Electricity supply however cannot be covered within the restriction of only applying PV panels on rooftops.
Besides the economic optimum, ecological performance is a major issue in planning smart cities. A comparison of the ecologic life cycle performance, including construction and operation of buildings as well as urban infrastructure and mobility, clearly indicates that energy plays a key role in the ecological impact of settlements. Using the comprehensive evaluation method of Sustainable Process Index (integrated in the ELAS-calculator), roughly two thirds of the ecological impact in the case of a smart city district within a middle-sized city can be attributed to different forms of energy supply (most of it for domestic heat purposes). Using local energy sources instead of imported fossil fuels, in particular industrial waste heat, can considerably reduce the ecological impact. In addition, this exposes a potential to stimulate regional value creation when reducing the import dependency on fossil fuels.
Applying the selected methods could provide useful information to give some answers to the challenges and research questions posed in the beginning of this case study. An integration of available local energy sources into an optimal energy system in order to meet future energy demands could be discussed with the generation of scenarios for a new development of a city district. These scenarios contain optimal solutions for energy systems considering resource limits and expectable changes of market prices. The analysis of settlement structures of the scenarios helped to find out the impact level for each energy system option from an ecological point of view. An energy system solution could be found with the lowest ecological impact and the highest financial revenue.
Abbreviations
°C, degrees Celsius; CHP, combined heat and power; €/year, currency of European Euros per year; ELAS, Energetic Long-term Analysis of Settlement Structures; Erber, investor, owning the estates where water wells are located in Reininghaus; GWh, gigawatt hours; GWh/year, gigawatt hours per year; ICT, information and communication technology; ID, identification number; kWh, kilowatt hour; Linde Gas, company for gas products; Marienhütte Stahlwerk, name of an industrial steel company; MWh, megawatt hour(s); MWh/year, megawatt hour(s) per year; NGO, non-governmental organisation; NZE-standard, Nearly Zero Emission standard (In the interest to guarantee a better readability in this work, the phrase ‘NZE-standard’ will be consistently used. To avoid misunderstandings, each part of the phrase is connected by a hyphen.); OIB-standard, Oesterreichisches Institut für Bautechnik (Austrian Institute for Construction Engineering), compulsory building standards (In the interest to guarantee a better readability in this work, the phrase ‘OIB-standard’ will be consistently used. To avoid misunderstandings, each part of the phrase is connected by a hyphen.); OEROK, Austrian Conference on Spatial Planning/Oesterreichische Raumordnungskonferenz; PNS, process network synthesis; PV, photovoltaic; STAMAG Malzfabrik, Stadtlauer Malzfabrik Aktiengesellschaft (malthouse); var., various
UN (2008) United Nations Expert Group meeting on population distribution, urbanization, internal migration and development, Population Division, Department of Economic and Social Affairs, UN Secretariat, 1-34, http://www.un.org/en/development/desa/population/events/pdf/expert/13/P01_UNPopDiv.pdf .
WHO (2014) Urban population growth, http://www.who.int/gho/urban_health/situation_trends/urban_population_growth_text/en/ , Download 2015/04/22.
European Smart Cities & Communities Initiative of the Strategic Energy Technology Plan (SET-Plan), http://ec.europa.eu/energy/en/topics/technology-and-innovation/strategic-energy-technology-plan . Download 2015/04/22.
Caragliu A, Del Bo C, Nijkamp P (2011) Smart cities in Europe. Journal of Urban Technology, (18/2):65-82, Special Issue: Creating Smart-er Cities, doi: 10.1080/10630732.2011.601117 .
de Jong M, Joss S, Schraven D, Zhan C, Weijnen M (2015) Sustainable–smart–resilient–low carbon–eco–knowledge cities: making sense of a multitude of concepts promoting sustainable urbanization. Journal of Cleaner Production, Available online 10 February 2015, ISSN 0959-6526, 190:25-38, doi: http://dx.doi.org/10.1016/j.jclepro.2015.02.004 .
Exner JP (2014) Smart Planning & Smart Cities. Proceedings / Tagungsband REAL CORP 2014, ISBN: 978-3-9503110-6-8 (CD-ROM); ISBN: 978-3-9503110-7-5 (Print), 39:603-610. http://www.corp.at/archive/CORP2014_39.pdf , Download 2015/11/30.
Jaekel M (2015) Smart City wird Realitaet: Wegweiser für neue Urbanitaeten in der Digitalmoderne. Springer Vieweg, Munich, Germany, ISBN 978-3-658-04454-1; ISBN 978-3-658-04455-8 (eBook), 1-312, doi: 10.1007/978-3-658-04455-8 .
Batty M, Axhausen K, Fosca G, Pozdnoukhov A, Bazzani A, Wachowicz M, Ouzounis G, Portugali Y (2012) Smart cities of the future. Eur PhysJ Special Topics 214:481–518. doi: 10.1140/epjst/e2012-01703-3
Article Google Scholar
Saringer-Bory B, Mollay U, Neugebauer W, Pol O (2012) SmartCitiesNet: Evaluierung von Forschungsthemen und Ausarbeitung von Handlungsempfehlungen für "Smart Cities", Smart City Akteursmatrix. Berichte aus Energie- und Umweltforschung, 38/2012, http://www.smartcities.at/assets/02-Stadtprojekte/endbericht-1238-smartcitiesnet.pdf , Download 2015/11/30.
Greenfield A (2013) Against the smart city: the city is here for you to use, part I. Do projects, New York City, ISBN 9780982438312, 1-153.
European Commission, 2020 climate and energy package, http://ec.europa.eu/clima/policies/strategies/2020/index_en.htm , Download 2015/04/22.
European Commission, 2030 framework for climate and energy policies, http://ec.europa.eu/energy/en/topics/energy-strategy/2030-energy-strategy , Download 2015/04/22.
Directive 2010/31/EU of the European Parliament and of the Council of 19 May 2010 on the energy performance of buildings, EUR-Lex, http://eur-lex.europa.eu/legal-content/EN/TXT/HTML/?uri=URISERV:en0021&from=EN&isLegissum=true , Introduction, Download 2015/04/22.
Angeliki K and Fokaides PA (2015) European smart cities: the role of zero energy buildings. Sustainable Cities and Society, (15/0):86–95, doi: 10.1016/j.scs.2014.12.003 .
Directive 2010/31/EU of the European Parliament and of the Council of 19 May 2010 on the energy performance of buildings, EUR-Lex, http://eur-lex.europa.eu/legal-content/EN/TXT/?uri=CELEX:32010L0031 , Article 9, Download 2015/04/22.
Baumgartner RJ (2011) Critical perspectives of sustainable development research and practice, J Cleaner Prod. (19/8):783-786, doi: 10.1016/j.jclepro.2011.01.005 .
Stoeglehner G, Neugebauer G, Erker S, Narodoslawsky M (2016) Integrated spatial and energy planning—supporting climate protection and the energy turn with means of spatial planning, Springer, 1-115, ISBN: 978-3-319-31868-4 (Print) 978-3-319-31870-7 (Online), doi: 10.1007/978-3-319-31870-7 .
Energieraumplanung in Austria—OEREK-Partnerschaft, Oesterreichisches Raumentwicklungskonzept (Austrian Conference of Spatial Planning), http://www.oerok.gv.at/raum-region/oesterreichisches-raumentwicklungskonzept/oerek-2011/umsetzung-oerek-partnerschaften/energieraumplanung.html , Download 2015/06/11.
Das Programm EnergieSchweiz / The SwissEnergy Programme, Schweizerische Eidgenossenschaft, http://www.bfe.admin.ch/energie/00458/index.html?lang=de , Download 2015/06/11.
Energienutzungsplanung in Germany, http://www.energieagentur.nrw.de/handbuch-klimaschutz/energienutzungsplanung-24679.asp , Download 2015/06/11.
Stoeglehner G, Haselsberger B, Hemis H, Bork H, Strasser H, Schatovich R, Stanzer G, Kanning H, Schaffer H, Dumke H, Piha S, Becker S, Wyss A, Arbach C, Klagge B, Wotha B, Rosner K, Christiner G, Fuchs S, Schneider U, Dell G, Stockinger F, Giffinger R, Zech S (2013) Energie und Raum. Forum Raumplanung, Oesterreichische Gesellschaft für Raumplanung. ISBN 978-3-643-50507-1, Technische Universität Wien, Lit Verlag Wien, 20:1-146.
Heinbach K, Aretz A, Hirschl B, Prahl A, Salecki S (2014) Renewable energies and their impact on local value added and employment. Energy, Sustainability and Society 2014, 4:1, 1-10, doi: 10.1186/2192-0567-4-1 .
Stoeglehner G, Erker S, Neugebauer G (2014) Tools für Energieraumplanung – Ein Handbuch für deren Auswahl und Anwendung im Planungsprozess, Oesterreichische Energieagentur / Austrian Energy Agency, Austrian Climate Initiative, klimaaktiv Dachmanagement, 1-66, www.klimaaktiv.at/dms/klimaaktiv/publikationen/mobilitaet/energieraumplanung/endbericht_tools_26-11-2014klein0/endbericht_tools_26-11-2014klein.pdf , Download 2015/11/30.
Stoeglehner G, N. Niemetz N, Kettl KH (2011) Spatial dimensions of sustainable energy systems: new visions for integrated spatial and energy planning. Energy, Sustainability and Society, 1:2, 1-9, www.energsustainsoc.com/content/1/1/2 , doi: 10.1186/2192-0567-1-2 .
Lund PD, Mikkola J, Ypyä J (2015) Smart energy system design for large clean power schemes in urban areas. J Cleaner Prod. 103:437-445, ISSN 0959-6526, doi: http://dx.doi.org/10.1016/j.jclepro.2014.06.005 .
Nemet A, Klemeš JJ, Varbanov PS, Kravanja Z (2012) Methodology for maximising the use of renewables with variable availability. Energy (44/1), 29-37 doi: dx.doi.org/10.1016/j.energy.2011.12.036 .
Maier S, Narodoslawsky M (2014) Optimal Renewable energy systems for smart cities, Computer Aided Chemical Engineering, (33):1849-1854, doi: http://dx.doi.org/10.1016/B978-0-444-63455-9.50143-4 .
Austrian Institute for Construction Engineering, http://www.oib.or.at/en , Download 2015/04/22.
Morrissey J, Dunphy N, MacSweeney R (2014) Energy efficiency in commercial buildings: capturing added-value of retrofit. J Prop Invest Financ. 32/4:396-414, doi: http://dx.doi.org/10.1108/JPIF-01-2014-0008 .
Baños R, Manzano-Agugliaro F, Montoya FG, Gil C, Alcayde A, Gómez J (2011) Optimization methods applied to renewable and sustainable energy: a review, Renewable and Sustainable Energy Reviews. (15/4):1753-1766, doi: 10.1016/j.rser.2010.12.008 .
Energie Graz (energy provider Graz), Information about district heat in Graz, http://www.energie-graz.at/energie/fernwaerme/dienstleistungen/was-ist-fernwaerme-wie-funktioniert-sie , Download 2016/04/26.
Lund H (2014) Renewable energy systems: a smart energy systems approach to the choice and modeling of 100 % renewable solutions. Academic Press, Elsevier, Massachusetts, USA, 978-0-12-410423-5
Google Scholar
Vance L, Heckl I, Bertok B, Cabezas H, Friedler F (2015) Designing sustainable energy supply chains by the P-graph method for minimal cost, environmental burden, energy resources input. J Cleaner Prod. 94:144-154, ISSN 0959-6526, doi: http://dx.doi.org/ 10.1016/j.jclepro.2015.02.011 .
Friedler F, Varga JB, Feher E, Fan LT (1996) Combinatorially accelerated branch-and-bound method for solving the MIP model of process network synthesis. Nonconvex Optimization and Its Applications, Computational Methods and Applications (Eds: C. A. Floudas and P. M. Pardalos), Kluwer Academic Publishers, Dordrecht. State of the Art in Global Optimization, Nonconvex Optimization and Its Applications. 7:609-626. doi: http://dx.doi.org/10.1007/978-1-4613-3437-8_35 .
Friedler F, Tarján F, Huang Y W, Fan LT (1992) Graph-theoretic approach to process synthesis: axioms and theorems. Chemical Engineering Science, (47/8):1973-1988, doi: http://dx.doi.org/10.1016/0009-2509(92)80315-4 .
Friedler et al. (2011) P-graph: p-graph.com/pnsstudio, PNS Software Version 3.0.4, 2011, www.p-graph.com , last accessed on 25/04/2016.
Narodoslawsky M, Niederl A, Halasz L (2008) Utilising renewable resources economically: new challenges and chances for process development. J Cleaner Prod. (16/2):164-170, doi: doi.org/10.1016/j.jclepro.2006.08.023 .
Niemetz N, Kettl KH, Eder M, Narodoslawsky M (2012) RegiOpt Conceptual Planner—identifying possible energy network solutions for regions, Chemical Engineering Transactions, 29:517-522, ISBN: 978-88-95608-20-4; ISSN: 1974-9791, doi: 10.3303/CET1229087 , http://www.aidic.it/cet/12/29/087.pdf .
Regional Optimiser (RegiOpt), free online access: http://regiopt.tugraz.at/ .
Heckl I, Halász L, Szlama A, Cabezas H, Friedler F (2015) Process synthesis involving multi-period operations by the P-graph framework, Computers & Chemical Engineering, 83:157-164, ISSN 0098-1354, doi: http://dx.doi.org/10.1016/j.compchemeng.2015.04.037 . ( http://dx.doi.org/10.1016/j.compchemeng.2015.04.037 ).
Stoeglehner G, Baaske W, Mitter H, Niemetz N, Kettl KH, Weiss M, Lancaster B, Neugebauer G (2014) Sustainability appraisal of residential energy demand and supply—a life cycle approach including heating, electricity, embodied energy and mobility, Energy, Sustainability and Society, (4/24):1-13. doi: dx.doi.org/ 10.1186/s13705-014-0024-6 , http://www.energsustainsoc.com/content/4/1/24 .
Narodoslawsky M, Krotscheck C (1995) The sustainable process index (SPI): evaluating processes according to environmental compatibility, Journal of Hazardous Materials, (41/2–3):383-397, ISSN 0304-3894, doi: http://dx.doi.org/10.1016/0304-3894(94)00114-V .
Narodoslawsky M (2015) Sustainable process index, Assessing and Measuring Environmental Impact and Sustainability, edited by Jiří Jaromír Klemeš, Butterworth-Heinemann, Oxford, 3:73-86, ISBN 9780127999685, doi: http://dx.doi.org/ 10.1016/B978-0-12-799968-5.00003-8 .
Kollmann R, Eder M, Narodoslawsky M (2014) Der oekologische Fußabdruck der konventionellen und biologischen Landwirtschaft im Vergleich, 15. Alpen-Adria Biosymposium „Bio auf dem Weg zur Schule“ - Bio-Landbau und die Bedeutung von Bio-Lebensmitteln, University of Maribor, Faculty of Agriculture and Life Sciences, Slovenia, 102-110, http://www.bioimpulse.eu/de/images/biosymposium/Biosymposium2014.pdf , Download 2016/01/19.
Kettl KH (2012) Evaluation of energy technology systems based on renewable resources, Dissertation, Institute for Process and Particle Engineering, Graz, Austria, 1-186, ( https://online.tugraz.at/tug_online/voe_main2.getVollText?pDocumentNr=264446&pCurrPk=66462 ).
ELAS calculator: energetic long-term assessment of settlement structures, 2011, www.elas-calculator.eu , last accessed on 27/08/2014.
Statistics Austria, Population of the city of Graz by 1. 1. 2016, http://www.statistik.at , Download 2016/04/19.
Stadt Graz - data.graz.gv.at, Statistik Austria (2015). Bevoelkerungsprognose Graz 2015 – 2034, Download 2016/04/19.
Federal Chancellery of Austria, Rechtsinformationssystem (legal information system), Landesrecht Steiermark: Gesamte Rechtsvorschrift für Erhaltung der Dachlandschaft im Schutzgebiet nach dem Grazer Altstadterhaltungsgesetz, Fassung vom 26.04.2016, https://www.ris.bka.gv.at/GeltendeFassung.wxe?Abfrage=LrStmk&Gesetzesnummer=20000897 , Download 2016/04/26.
Research and technology programme Building of Tomorrow, ECR Energy City Graz—subproject 2: Framework-Plan Energy City Graz-Reininghaus, BMVIT (Austrian Ministry for Transport, Innovation and Technology), https://nachhaltigwirtschaften.at/en/hdz/projects/ecr-energy-city-graz-reininghaus-urban-strategies-for-the-newconception-construction-operation-and-restructuring-of-an-energy-self-sufficient-city-district.php , Download 2015/04/22.
Rainer et al. (2015) ECR Energy City Graz—subproject 2: Framework-Plan Energy City Graz-Reininghaus, Rahmenplan Energie, final report.
Stadt Graz, Stadtteilentwicklung, Rahmenplan Graz-Reininghaus, http://www.stadtentwicklung.graz.at/cms/dokumente/10136566_2858034/4ab9da2e/Schlussbericht%20kurz_EULOGO_Text.pdf , Download 2015/06/12.
Calculation of thermal and electric energy demand for OIB-standard, Graz University of Technology, Institute of Electrical Power Systems, Institute of Thermal Engineering, Institute of Process and Particle Engineering (2015).
Calculation of thermal and electric energy demand for NZE-standard, Graz University of Technology, Institute of Electrical Power Systems, Institute of Thermal Engineering, Institute of Process and Particle Engineering (2015).
Download references
Acknowledgements
The author would like to thank for the funding by the BMVIT Building of the Future and City of the Future, municipality of Graz and the Land Steiermark, which made it possible to work in a new dimension of interdisciplinary teamwork between different departments throughout the University of Technology Graz and the Karl-Franzens-University Graz. Interdisciplinary hurdles within the project team were largely overcome thanks to a very constructive and open atmosphere for discussions.
Author’s contributions
The author has contributed to the Smart City project Energy City Graz Reininghaus. SM has written, read and approved the manuscript.
Author’s information
SM, born in 1983 in St. Andrä, Austria graduated in environmental system sciences from the University of Graz. He is currently working at the Institute for Process and Particle Engineering, Graz University of Technology. His research is focused on energy technology system optimisation in regions and rural and urban areas, holistic urban energy system planning and ecological evaluation.
Competing interests
The author declares that he has no competing interests.
Author information
Authors and affiliations.
Institute of Process and Particle Engineering, Graz University of Technology, Inffeldgasse 13/3, 8010, Graz/Gradec, Austria
Stephan Maier
You can also search for this author in PubMed Google Scholar
Corresponding author
Correspondence to Stephan Maier .
Additional file
Additional file 1:.
Cost and technology data. (DOCX 50 kb)
Rights and permissions
Open Access This article is distributed under the terms of the Creative Commons Attribution 4.0 International License ( http://creativecommons.org/licenses/by/4.0/ ), which permits unrestricted use, distribution, and reproduction in any medium, provided you give appropriate credit to the original author(s) and the source, provide a link to the Creative Commons license, and indicate if changes were made.
Reprints and permissions
About this article
Cite this article.
Maier, S. Smart energy systems for smart city districts: case study Reininghaus District . Energ Sustain Soc 6 , 23 (2016). https://doi.org/10.1186/s13705-016-0085-9
Download citation
Received : 12 December 2014
Accepted : 11 July 2016
Published : 05 September 2016
DOI : https://doi.org/10.1186/s13705-016-0085-9
Share this article
Anyone you share the following link with will be able to read this content:
Sorry, a shareable link is not currently available for this article.
Provided by the Springer Nature SharedIt content-sharing initiative
- Smart energy systems
- Urban energy systems
- Process synthesis
- Smart energy networks for urban areas
- Use of waste heat and renewable energy
- Sustainable Process Index
Energy, Sustainability and Society
ISSN: 2192-0567
- General enquiries: [email protected]
Thank you for visiting nature.com. You are using a browser version with limited support for CSS. To obtain the best experience, we recommend you use a more up to date browser (or turn off compatibility mode in Internet Explorer). In the meantime, to ensure continued support, we are displaying the site without styles and JavaScript.
- View all journals
- My Account Login
- Explore content
- About the journal
- Publish with us
- Sign up for alerts
- Open access
- Published: 15 March 2023
Green finance, renewable energy development, and climate change: evidence from regions of China
- Yunpeng Sun 1 ,
- Qun Bao 2 &
- Farhad Taghizadeh-Hesary ORCID: orcid.org/0000-0001-5446-7093 3
Humanities and Social Sciences Communications volume 10 , Article number: 107 ( 2023 ) Cite this article
5997 Accesses
17 Citations
2 Altmetric
Metrics details
In this study, using data from 2010 to 2021, and by utilizing the stochastic impacts by regression on population, affluence, and technology (STIRPAT) theory, and system generalized method of moments, the effect of green financing and deployment of renewable energy on carbon dioxide emissions in China and its provinces were analyzed. The results show that green financing reduces environmental pollution at the country level. Moreover, with a 1% increase in renewable energy consumption, carbon dioxide emission can be expected to decrease by 0.103%. It also demonstrates that green financing has a statistically significant coefficient only in provinces located in the eastern and western regions. Chinese policymakers should incentive policies for provinces in the eastern region of China in order to have a cleaner environment. The central region should be under supportive and pressure policies to move faster along the path to sustainable development.
Similar content being viewed by others
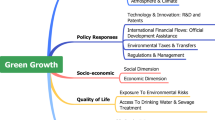
Comprehensive green growth indicators across countries and territories
Samuel Asumadu Sarkodie, Phebe Asantewaa Owusu & John Taden
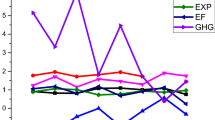
Influence of green technology, green energy consumption, energy efficiency, trade, economic development and FDI on climate change in South Asia
Gulzara Tariq, Huaping Sun, … Qasim Shah
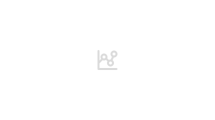
Pollution, income inequality and green finance in the new EU member states
Mihaela Simionescu & Beáta Gavurová
Introduction
Climate change is a complex concept in the literature on environmental economics and human challenge in the global context. Undoubtedly, climate change causes disturbances in agricultural production (risk to food security), global warming, droughts, and geopolitical tensions related to water. Some scholars believe that the threat of climate change is unsolvable in the short term because of its global nature; therefore, it requires global unity and policy to be solved. Li et al. ( 2021 ) have argued that climate change has no simple solution and necessitates global unity and alignment. Froehlich et al. ( 2022 ) have stated that the threat of climate change is a potential risk to food security, economic resilience, and global peace. Therefore, there is an urgent need for more international efforts to eliminate this threat.
However, it should be noted that efforts have been made, albeit small and integrated, to deal with climate change. These efforts (e.g., the U.N. Framework Convention on Climate Change of 1992, the Kyoto Protocol of 1992, and the Paris Agreement of 2015) have included international agreements to implement integrated and aligned policies to reduce the threat of climate change. Huang and Zhai ( 2021 ) have mentioned that global treaties are inefficient on their own, and countries’ commitment to these agreements must increase. Zhang et al. ( 2017 ) have highlighted the importance of countries’ alignment to make international agreements on climate change more efficient. They have mentioned the withdrawal of the U.S. from the Paris Agreement, which has lowered the validity of this global treaty. Other efforts have included regional cooperation and a plan to overcome the challenges of climate change. In this regard, the Regional Dialog on Carbon Pricing in ASEAN, the 2030 E.U. Climate Target Plan, and the Africa Clean Energy Corridor can be considered successful cases. Rabe ( 2022 ) has declared that regional planning to mitigate climate change threats can be considered a significant step toward global unification. In another study, Das et al. ( 2022 ) have confirmed that intra-regional planning for renewable energy sources can efficiently promote environmental protection and combat climate change. Other efforts have included local policies to promote environmental protection, green energy deployment, and lower carbon emissions.
Countries have implemented various policies to combat climate change. As a roadmap for implementing such policies, countries must have a long-term plan or clear vision of their sustainability goals. For example, in 2020, China announced its plans to achieve peak carbon by 2030 and carbon neutrality by 2060; in 2020, Japan declared its plan to implement net-zero emissions by 2050. Other plans have included CanREA’s 2050 Vision, UAE’s National Energy Strategy 2050, Qatar’s National Vision 2030, Turkish renewable energy vision 2023, Korea’s Energy Master Plan 2035, Germany’s landmark Renewable Energy Act 2021, and Singapore’s Green Plan 2030; all of these roadmaps have clear targets and set out intermediate, operational, and final goals.
Under these long-term plans, countries are implementing deterrent and incentive policies and tools based on their economies’ systems, weaknesses, and strengths. The most important policies are listed in Table 1 .
The critical point for implementing the policies listed in Table 1 is the development of related projects and sufficient capital to invest in them. Therefore, financing green projects has been one of the most critical and challenging topics among experts (e.g., Tolliver et al., 2020 ; see also Cicchiello et al., 2022 , Li et al., 2022 ; Han and Li, 2022 ) in recent years. Without an efficient green financing system, a country cannot advance its sustainable development goals or reduce climate-related threats. Green finance can be seen as a bridge to renewable energy deployment in countries, particularly where the government does not have sufficient capital to invest in green projects. Rasoulinezhad and Taghizadeh-Hesary ( 2022 ) have declared that green finance is a helpful instrument for governments that cannot invest significantly in renewable energy projects. On the one hand, green financing can increase the number of green projects implemented in countries; on the other hand, it will attract more capital from the private sector to green projects.
This study primarily examines the relationship between green finance, renewable energy deployment, and climate change in China. China is a leading Asian country in terms of establishing various plans to develop green energy consumption across various industries. As shown in Table 1 , China is among the countries that have implemented policies (green tax, green loans, green financing, green innovation, green jobs, and green transportation) to diversify pathways to reach its sustainability targets. In the last decade, financing green projects has become a priority for the Chinese government. According to The State Council of China ( 2021 ), the country provided over 2.5 trillion U.S. dollars in green loans by the end of 2021 (nearly 30% growth over 2020). It should be mentioned that in 2020, China ranked first in the world in providing green loans (USD 1.8 trillion) and second in the world in issuing green bonds (USD 125 billion). The development of green financial policies in China has become more critical after its vision to achieve peak carbon by 2030 and carbon neutrality by 2060. Wang et al. ( 2021 ) have emphasized the role of China’s two current climate change policies (peak carbon and carbon neutrality) as a pathway toward the era of the Chinese climate economy. Therefore, China should attempt to make its green financial system more efficient and attract more private capital to green economic projects.
Given the importance of studying the case of China regarding how green finance and renewable energy deployment can affect climate change, this research makes the following contributions to the existing literature:
It conducts a regional study of China that does not consider China’s country-level data. China has more than 1.4 billion people and a relatively wide geographical area (9,600,013 sq. km). Therefore, it should be regionally investigated. Therefore, in this study, data analysis of different regions of China was conducted as a panel data framework.
It uses the stochastic impacts by regression on population, affluence, and technology (STIRPAT) theoretical framework to explain the research problem and econometric modeling.
It uses carbon dioxide emissions per capita as a proxy for climate change and issued green bonds as a proxy for the green financing variable.
The results showed that green financing reduces environmental pollution at the country level. Additionally, with a 1% increase in renewable energy consumption, carbon dioxide emission can be expected to decrease by 0.103%. Therefore, green financing has a statistically significant coefficient only in provinces located in the eastern and western regions. Chinese policymakers should institute incentive policies in provinces in the eastern region of China. The central region should be under supportive and pressure policies to move faster along the path to sustainable development.
The remainder of this paper proceeds as follows. Section “Literature review” provides a brief literature review and clarifies the literature gaps this study tries to fill. The section “Green policies in China” provides information on green policies in China. Section “Data and model specification” discusses the data and model specifications, and the section “Empirical results” presents the empirical findings. The last section presents the concluding remarks, a discussion of practical policies, and recommendations for further research.
Literature review
Several earlier studies have drawn attention to the impacts of green finance and renewable energy deployment on climate change in China.
A group of scholars has focused on the importance of green finance in China’s sustainable development. Li et al. ( 2021 ) used the wavelet power spectrum approach to investigate the role of green finance in China after the COVID-19 pandemic. The major findings have confirmed the unidirectional causal relationship between renewable energy and green finance in China. In a recent study, Zhou and Xu ( 2022 ) evaluated the impact of green finance on regional ecological enhancement in China. The results of the estimations using the generalized method of moments (GMM) model revealed a U-shaped relationship between the two variables. Zhu et al. ( 2022 ) have discussed the role of green finance in terms of the development goals in China. Analyzing annual data from 1986 to 2019, they revealed a positive association between green finance and green development in China. Irfan et al. ( 2022 ) have investigated the relationship between green finance and green innovation at the regional level in China. Using the panel Vector Autoregression (VAR) technique and annual data from 2010 to 2019, they showed that green finance positively impacts green innovation across all regions of China. Lin et al. ( 2022 ) have explored the impact of green finance on CO 2 emissions reduction in China from 2007 to 2018. A dynamic spatial Durbin model has confirmed that green finance in China is efficient and can lower carbon dioxide emissions. Zhang et al. ( 2022 ) attempted to determine how green finance enhanced urban city-level green development in China from 2002 to 2019. The major results highlighted the role of green finance in lowering urban energy intensity. Hou et al. ( 2022 ) concentrated on five provinces in China in 2017 to explore the role of green financial policies in promoting environmental quality. They employed a difference-in-differences model and concluded that green finance has a heterogeneous effect among the examined provinces.
Previous studies have focused on the impact of green energy deployment on climate change in China. Qi et al. ( 2014 ) have attempted to identify a link between CO 2 emissions and renewable energy development in China from 2010 to 2020. The major findings confirmed the positive role of renewable power generation in reducing CO 2 emissions in the short term. Chen et al. ( 2019 ) employed the autoregressive distributed lag (ARDL) bounds testing approach using annual data from 1980 to 2014 to study the relationship between renewable energy and CO 2 emissions in China. They have concluded that any increase in green energy deployment leads to decreased carbon dioxide emissions in the short and long term. Recently, Wang ( 2022 ) used the ARDL approach to analyze annual data from 2007 to 2019 in China to determine how green energy can help the country achieve its decarbonization target. The results showed that, in the long term, green energy development could decrease carbon dioxide emissions in China. Dong et al. ( 2022 ) employed the STIRPAT approach to study carbon emissions mitigation in China. They have found a negative correlation between green energy deployment and carbon emissions. Abbasi et al. ( 2022 ) compared the impacts of green and fossil fuel energy resources on climate change in China. Employing dynamic ARDL and annual data from 1980 to 2018, they have concluded that green energy deployment has short- and long-term positive impacts on CO 2 mitigation. In another study, Lin and Qiao ( 2022 ) explored how green electricity can be adopted into people’s lifestyles to help China lower its environmental pollution. The major results revealed that reasonably priced green electricity could stimulate people to use it, leading to lower carbon emissions in China. Zhu et al. ( 2022 ) have addressed the role of green energy resources in mitigating fossil-fuel-based CO 2 emissions in rural China from 2007 to 2018. The findings have highlighted the significant long-term impact of green energy resource development on rural environmental protection in the country. Wang et al. ( 2023 ) studied net energy-related carbon dioxide emissions in China. The empirical findings have confirmed the positive role of green energy development in industrial production in the country. Zhang et al. ( 2023 ) also confirmed the positive effect of green energy resources on agricultural land expansion in China. Farmland expansion policy can be strengthened through the deployment of renewable energy sources.
Based on a summary of the most recent research, it can be said that many studies have been conducted on green finance in China; however, it seems that the results of such research are not useful to policymakers. Hou et al. ( 2022 ) have shown that the impact of green financing on climate threats in China is heterogeneous across different provinces. Additionally, the STIRPAT method, as a supporting theory of the econometric model, has rarely been used (see Dong et al., 2022 ). The selection of variables for the econometric model should have a clear theoretical basis. This study considered the effects of renewable energy consumption and green financing on China’s climate threat using a model. Furthermore, the selection of modeling variables was explained based on the structure of the STIRPAT theory. The analysis was conducted at the regional level in China. Each of the aforementioned cases represents a literature gap that this research will fill.
Green policies in China
The history of green policies in China dates back to 1972 when China attended the first United Nations Conference on the Human Environment. A decade later, in 1983, the Chinese government announced that protecting the country’s environment required government policy. In 1998, the State Environmental Protection Administration (SEPA) was established because of a flood disaster that became a national crisis. From its establishment in 1998 to 2002, the current administration has carried out essential actions in the environmental field. The SEPA policy of converting fields into forests in China during this period caused more than 7.7 million hectares of agricultural land to be converted to forest land. From 2002 to 2015, various green policies were developed in China, most of which were ineffective because of the need for fossil fuels to support China’s manufacturing industries. The signing of the Paris Agreement on December 12, 2015, by China and 195 other countries set a suitable road map for China to pay more attention to environmental issues. Additionally, the introduction of the Sustainable Development Goals by the United Nations on January 1, 2016, doubled China’s motivation to achieve sustainable development. In April 2019, China promoted the Belt and Road Initiative International Green Development Coalition as the first Chinese regional sustainable plan. In 2020, China launched its vision of peak carbon by 2030 and carbon neutrality by 2060 as two major projects aimed at improving air quality and environmental protection in the global context. China is a pioneer in implementing green financing to support green projects. Since March 2016, according to China’s 13th Five-Year Plan for Economic and Social Development, the country has established a green financing system that includes green credit, green bonds, and green development funds. The Agricultural Bank of China issued its first green bonds in late 2015. Recently, the Chinese government has supported these policies. In 2021, China’s green bond market was the second largest after that of the U.S. The country issued over 1643 green bonds valued at USD 270 billion in 2021. Regarding green loans, Chinese banks provided nearly USD 2.5 trillion in 2021, compared to around 1.8 trillion in 2020. China’s economic sector targets for developing green financing markets are green transportation, emerging industries, and industrial energy savings. In February 2021, the Chinese State Council published a roadmap for the transition pathway toward green and recycling development by 2025, and enhanced environmental protection in pursuit of the target of a “beautiful China” by 2035 (China briefing, 2022 ). In February 2022, the Chinese State Council announced guidelines to accelerate the establishment and enhancement of a green and renewable economic system.
Theoretical background
In this study, the supporting theory of econometric modeling is the STIRPAT theory, presented by Dietz and Rosa ( 1997 ). Given that this study aims to evaluate the impacts of green finance and renewable energy development on climate change, the effects of the variables on climate change could be addressed through the popular environmental STIRPAT pattern. This theory addresses the impact of human activity (I) on the quality of the environment. Human activity (I) is comprised of the following three factors: population (P), affluence (A), and technology (T). The advantages of employing this theory to construct the empirical model are as follows: (i) this theory can explain the channels of impacts of issued green bonds and renewable energy deployment on climate change; (ii) this theory is useful for hypothesis decomposition, which is necessary to obtain practical policies for countries; and (iii) anthropogenic factors such as urbanization, human development, and health can be evaluated through STIRPAT theory. This directly impacts the relationship between human activity and the environment.
The relationship between the environment and human activity in the STIRPAT framework can be represented by Eq. ( 1 ) as follows:
In Eq. ( 1 ), carbon dioxide emissions per capita are used as a proxy for the impact of climate change, whereas P , A , and T denote population (labor force as proxy), affluence (income per capita as proxy), and technology proxy with green finance.
Data and model specification
According to the theoretical framework, Eq. ( 1 ) can be transformed into an econometric Eq. ( 2 ), as follows:
Equation ( 2 ) in the logarithm form can be transformed into Eq. ( 3 ), as follows:
The extension of Eq. ( 2 ,) comprising covariate variables ( X ), can be written as Eq. ( 4 ), as follows:
In Eq. ( 4 ), s and μ represent unobserved factors of the error term, which have a normal distribution.
The covariate variables in this study were renewable energy deployment and inflation rates, which impact climate change.
Data on the variables (carbon emissions per capita, labor force, income per capita, green finance, inflation, and renewable energy deployment) were gathered from 2010 to 2021 for 25 Chinese provinces. The data were collected from China’s National Bureau of Statistics, the Statistical Yearbook of each province, and the China Statistical Yearbook on the environment. It should be noted that China’s first green bonds were printed in 2015, which is why we extracted the green financing variable of Chinese provinces since 2010 using the weighted average of green loans and green bonds.
To estimate the coefficients in Eq. ( 4 ), the system GMM approach proposed by Arellano and Bover ( 1995 ) is employed. The empirical model, in the form of the system GMM approach, is shown in Eq. ( 5 ) as follows:
where y is the dependent variable, y t −1 represents the lag term, and X represents the independent variables. It should be mentioned that the before–after estimation tests are conducted to explore the appropriate estimation technique and ensure the validation of the empirical findings.
Empirical results
In the first stage, it is necessary to perform tests to determine the most appropriate estimation technique. Accordingly, a first-generation panel unit root test (ADF test) is conducted. Table 2 reports the results of the stationarity test of the series.
It is argued that, in panel data studies, there may be cross-sectional dependency among the samples; therefore, it was necessary to carry out the second generation of panel unit root tests (CIPS and C.Z. tests). The results of the two tests are presented in Table 3 .
Based on the results of the first- and second-generation panel unit root tests, it can be concluded that all variables were stationary and stable processes.
Next, the coefficients were estimated using the system GMM technique. The results of the estimations are presented in Table 4 .
Table 4 shows that carbon dioxide emission per capita positively affects this variable in the present time, and this effect was statistically significant. The labor force has a positive coefficient, meaning that a 1% increase in China’s labor force leads to increased carbon dioxide emissions per capita. An increase in the labor force in a country will mean an increase in industrial production capacity and, subsequently, in the country’s economic growth. In a country like China, whose manufacturing industries depend on fossil fuels, the increase in economic growth from the growth of industrial production will result in more carbon dioxide emissions. China’s per capita income also positively affects carbon dioxide emissions. An increase in per capita income means an increase in people’s purchasing power and consumption of more goods. This consequently suggests an increase in fossil energy consumption and carbon dioxide emissions. Green financing has a small, negative impact coefficient. By increasing the volume of green financing in China, carbon dioxide emissions can decrease by 0.015%. The consumer price index (CPI) positively and significantly affects carbon dioxide emissions. Generally, with an increase in the CPI, the capital rental rate and acquisition of bank facilities increase; therefore, the price of green projects increases. As a result, investors will not desire to participate in such projects; therefore, the excessive consumption of fossil fuels will continue in China, causing more carbon dioxide emissions. Renewable energy consumption had a coefficient of 0.103. Therefore, a 1% increase in renewable energy consumption is expected to decrease CO 2 emissions by 0.103%.
Given the vast geographical area of China and the difference in the level of development across its provinces, the effectiveness of green financing and consumption of renewable energy varies from province to province. To obtain more practical findings, we categorized 25 selected provinces based on their geographical location into the following three regions: west, east, and center. The three-panel frameworks for these three regions were analyzed using the system GMM, as reported in Table 5 .
Lagged CO 2 emissions impacted only the provinces in the central and western regions of China, while the coefficient of lagged CO 2 emissions was not statistically significant for the eastern region’s provinces. The labor force across all three regions of China positively and significantly affected carbon dioxide emissions. The magnitude of this effect in the central region was greater than that in the other two regions, a major reason for which is the existence of many heavy industries in the provinces of the central region of China. Regarding income per capita, the estimation results showed that the effect of this variable on carbon dioxide emissions was positive in the central and western regions, and only the states located in the eastern region of China had a negative effect coefficient. This may be because the eastern provinces of China are more developed, and the higher the income levels in these provinces, the more the people are willing to change their lifestyles in a green way to protect the environment. Green financing had a statistically significant coefficient only in provinces in the eastern and western regions. Moreover, this variable’s influence on reducing carbon dioxide emissions was more significant in the eastern region of China. An important reason for this is that the eastern regions of China are more developed. Therefore, people in these regions have greater potential to participate in the green financing market and green projects. The use of renewable energy across all regions of China has had a positive effect on reducing carbon dioxide emissions. However, its influence in the eastern region has been greater than that in the other two regions. The CPI also had a significant coefficient and positive sign for all three regions. Therefore, in all provinces of China, an increase in the CPI is an obstacle to developing sustainable development policies.
To ascertain the validity of the empirical findings, a robustness check was employed. Accordingly, the dependent variable (CO 2 emissions per capita) was substituted using greenhouse gas emissions. The empirical model was re-estimated using the system GMM approach for Chinese regions. The results, as reported in Table 6 , confirmed that the signs of the coefficients were consistent with the earlier estimations (Table 5 ).
Conclusions and policy recommendations
Concluding remarks.
The threat of climate change has become a topic of concern for the international community in recent decades. Despite several meetings and discussions with experts and politicians, humankind has not been able to remove the shadow of this threat from life on the planet. Given its high volume of fossil fuel use, China has made a significant contribution to the creation and persistence of this threat. In this study, using data from 2010 to 2021, the STIRPAT theory, and the econometric method of panel data, the effect of green financing and deployment of renewable energy on the carbon dioxide emissions of China and its provinces have been analyzed and interpreted. The findings show that green financing has a slight negative impact coefficient on environmental pollution at the country level. By increasing the volume of green financing in China, carbon dioxide emissions can decrease by 0.015%. Moreover, renewable energy consumption has a coefficient of 0.103. This indicates that by increasing renewable energy consumption by 1%, CO 2 emissions can be expected to decrease by 0.103%. However, at the regional level of analysis, green financing has a statistically significant coefficient only for the provinces located in the eastern and western regions. Additionally, this variable’s influence on reducing carbon dioxide emissions is more significant in the eastern region of China. The critical reason for this is that the eastern regions of China (e.g., Shanghai) are more developed; therefore, the people in these regions have more potential to participate in the green financing market and green projects. The use of renewable energy across all regions of China has had a positive effect on reducing carbon dioxide emissions. However, the influence in the eastern region has been greater than that in the other two regions.
Practical policies
According to the obtained results, the issue of clean energy policies at the provincial and country levels in China is worth mentioning. The Chinese government should pay more attention to encouraging leading provinces in the field of renewable energy and supporting lagging provinces in the fight against environmental pollution. Chinese leaders must make strong decisions about the future of their country by supporting sustainable development. On September 22, 2020, the president of China announced that, in line with the Sustainable Development Goals, his country would try to reach peak emissions before 2030 and carbon neutrality before 2060. To achieve these two important targets, China should formulate and approve two separate policy groups at the national and provincial levels. At the macroeconomic level, China’s policymakers should try to create green jobs (to create a positive effect where increasing the labor force reduces carbon dioxide emissions), increasing the green basket of consumption in society (increasing the per capita income will improve environmental conditions), better development of the green financing market, wider financial support for green projects (to neutralize the negative effect of inflation on the development of green projects), and make more efforts to increase the consumption of renewable energy. However, at the regional and provincial levels, China’s policymakers should institute more incentive policies for the provinces in the eastern region so that they can move more easily along the path of sustainable development. Conversely, the central region should be under supportive policies (granting green loans and green subsidies) and pressure policies (carbon tax) to move faster toward sustainable development. The uniformity of applied policies toward different provinces and regions can become a factor in their ineffectiveness and the creation of inequality in the sustainable development of regions in the long term (Chen, 2022 ).
Future research recommendations
This study provides practical findings and innovative management policies for experts and policymakers in China and worldwide. It is important that China, as the largest contributor of greenhouse gas emissions and a major consumer of fossil fuels, creates a managed balance in its provinces in the field of sustainable development. The results of this study significantly contribute to achieving this goal. However, there were limitations (e.g., access to local data) in conducting this research. It is suggested that, in future research, field research and survey methods be used to obtain complementary results. Given the spread of COVID-19 since the end of 2019 and its transformation into a worldwide pandemic, future research should investigate the effect of this pandemic on the relationship between green financing and climate change. More advanced econometric methods, such as artificial neural networks, could be used to predict the relationships between variables in the future. Such research can help China reach its goals of peak carbon by 2030 and carbon neutrality by 2060.
Data availability
The datasets generated and analyzed in the current study are available from the corresponding author upon reasonable request.
Abbasi KR, Shahbaz M, Zhang J et al. (2022) Analyze the environmental sustainability factors of China: the role of fossil fuel energy and renewable energy. Renew Energy 187:390–402. https://doi.org/10.1016/j.renene.2022.01.066
Article CAS Google Scholar
Al-Qudah AA, Hamdan A, Al-Okaily M et al. (2022) The impact of green lending on credit risk: evidence from UAE’s banks. Environ Sci Pollut Res Int 1–13. https://doi.org/10.1007/s11356-021-18224-5
Arellano M, Bover O (1995) Another look at the instrumental variable estimation of error-components models. CEP Discussion Papers. J Econom 68:29–51. https://doi.org/10.1016/0304-4076(94)01642-D
Article MATH Google Scholar
Bowen A, Kuralbayeva K, Tipoe EL (2018) Characterising green employment: the impacts of ‘greening’ on workforce composition. Energy Econ 72:263–275. https://doi.org/10.1016/j.eneco.2018.03.015
Article Google Scholar
Chen S (2022) The inequality impacts of the carbon tax in China. Humanit Soc Sci Commun 9. https://doi.org/10.1057/s41599-022-01285-3
Chen Y, Wang Z, Zhong Z (2019) CO2 emissions, economic growth, renewable and non-renewable energy production and foreign trade in China. Renew Energy 131:208–216. https://doi.org/10.1016/j.renene.2018.07.047
China briefing (2022). What is China’s green and low-carbon plan and why is it relevant to foreign investors? https://www.china-briefing.com/news/what-is-chinas-green-and-low-carbon-plan-and-why-is-it-relevant-to-foreign-investors/ . Accessed 26 Jan 2023
Cicchiello AF, Cotugno M, Monferrà S et al. (2022) Which are the factors influencing green bonds issuance? Evidence from the European bonds market. Finance Res Lett 50:103190. https://doi.org/10.1016/j.frl.2022.103190
Das P, Kanudia A, Bhakar R et al. (2022) Intra-regional renewable energy resource variability in long-term energy system planning. Energy 245:123302. https://doi.org/10.1016/j.energy.2022.123302
Dell’Anna F (2021) Green jobs and energy efficiency as strategies for economic growth and the reduction of environmental impacts. Energy Policy 149:112031. https://doi.org/10.1016/j.enpol.2020.112031
Dietz T, Rosa EA (1997) Effects of population and affluence on CO2 emissions. Proc Natl Acad Sci USA 94:175–179. https://doi.org/10.1073/pnas.94.1.175 . 8990181
Article ADS CAS PubMed PubMed Central Google Scholar
Dong K, Wang B, Zhao J et al. (2022) Mitigating carbon emissions by accelerating green growth in China. Econ Anal Policy 75:226–243. https://doi.org/10.1016/j.eap.2022.05.011
Froehlich HE, Koehn JZ, Holsman KK et al. (2022) Emerging trends in science and news of climate change threats to and adaptation of aquaculture. Aquaculture 549:737812. https://doi.org/10.1016/j.aquaculture.2021.737812
Han Y, Li J (2022) Should investors include green bonds in their portfolios? Evidence for the USA and Europe. Int Rev Financ Anal 80:101998. https://doi.org/10.1016/j.irfa.2021.101998
Hao Y, Chen P (2022) Do renewable energy consumption and green innovation help to curb CO2 emissions? Evidence from E7 countries. Environ Sci Pollut Res Int 1–17. https://doi.org/10.1007/s11356-022-23723-0
Hou H, Zhu Y, Wang J et al. (2022) Will green financial policy help improve China’s environmental quality? The role of digital finance and green technology innovation. Environ Sci Pollut Res Int. https://doi.org/10.1007/s11356-022-22887-z
Huang M, Zhai P (2021) Achieving Paris Agreement temperature goals requires carbon neutrality by middle century with far-reaching transitions in the whole society. Adv Clim Change Res 12:281–286. https://doi.org/10.1016/j.accre.2021.03.004
Irfan M, Razzaq A, Sharif A et al. (2022) Influence mechanism between green finance and green innovation: exploring regional policy intervention effects in China. Technol Forecast Soc Change 182:121882. https://doi.org/10.1016/j.techfore.2022.121882
Kiani A (2017) Electric vehicle market penetration impact on transport-energy-greenhouse gas emissions nexus: a case study of United Arab Emirates. J Clean Prod 168:386–398. https://doi.org/10.1016/j.jclepro.2017.08.242
Li H, Wang X, Zhao X et al. (2021) Understanding systematic risk induced by climate change. Adv Clim Change Res 12:384–394. https://doi.org/10.1016/j.accre.2021.05.006
Li M, Hamawandy NM, Wahid F et al. (2021) Renewable energy resources investment and green finance: evidence from China. Resour Policy 74:102402. https://doi.org/10.1016/j.resourpol.2021.102402
Li Q, Zhang K, Wang L (2022) Where’s the green bond premium? Evidence from China. Fin Res Lett 48:102950. https://doi.org/10.1016/j.frl.2022.102950
Lin B, Qiao Q (2022) Exploring the acceptance of green electricity and relevant policy effect for residents of megacity in China. J Clean Prod 378:134585. https://doi.org/10.1016/j.jclepro.2022.134585
Lin Z, Liao X, Yang Y (2022). China’s experience in developing green finance to reduce carbon emissions: from spatial econometric model evidence. Environ Sci Pollut Res Int. https://doi.org/10.1007/s11356-022-23246-8
Meng X, Gu A, Wu X et al. (2021) Status quo of China hydrogen strategy in the field of transportation and international comparisons. Int J Hydrogen Energy 46:28887–28899. https://doi.org/10.1016/j.ijhydene.2020.11.049
Norouzi N, Fani M, Talebi S (2022) Green tax as a path to greener economy: a game theory approach on energy and final goods in Iran. Renew Sustain Energy Rev 156:111948. https://doi.org/10.1016/j.rser.2021.111968
Qi T, Zhang X, Karplus VJ (2014) The energy and CO2 emissions impact of renewable energy development in China. Energy Policy 68:60–69. https://doi.org/10.1016/j.enpol.2013.12.035
Rabe M (2022) Regional planning of distributed energy. Procedia Comput Sci 207:3073–3084. https://doi.org/10.1016/j.procs.2022.09.365
Rasoulinezhad E, Taghizadeh-Hesary F (2022) Role of green finance in improving energy efficiency and renewable energy development. Energy Effic 15(14):35529528. https://doi.org/10.1007/s12053-022-10021-4
Shahid M, Ullah K, Imran K et al. (2022) Economic and environmental analysis of green transport penetration in Pakistan. Energy Policy 166:113040. https://doi.org/10.1016/j.enpol.2022.113040
Tan X, Xiao Z, Liu Y et al. (2022) The effect of green credit policy on energy efficiency: evidence from China. Technol Forecast Soc Change 183:121924. https://doi.org/10.1016/j.techfore.2022.121924
Terneus Páez CF, Guayanlema V, Cabrera Mera AG (2022) Estimation of energy consumption due to the elimination of an environmental tax in Ecuador. Energy Sustain Dev 66:92–100. https://doi.org/10.1016/j.esd.2021.11.005
The State Council of China. China’s green loans maintain rapid growth in 2021. http://english.www.gov.cn/archive/statistics/202202/01/content_WS61f892e5c6d09c94e48a497b.html . Accessed 20 Oct 2022
Tolliver C, Keeley AR, Managi S(2020) Policy targets behind green bonds for renewable energy: do climate commitments matter? Technol Forecast Soc Change 157:120051. https://doi.org/10.1016/j.techfore.2020.120051
van der Ploeg F, Rezai A, Tovar Reanos M (2022) Gathering support for green tax reform: evidence from German household surveys. Eur Econ Rev 141:103966. https://doi.org/10.1016/j.euroecorev.2021.103966
Wang H, Qi S, Zhou C et al. (2022) Green credit policy, government behavior and green innovation quality of enterprises. J Clean Prod 331:129834. https://doi.org/10.1016/j.jclepro.2021.129834
Wang L (2022) Research on the dynamic relationship between China’s renewable energy consumption and carbon emissions based on ARDL model. Resour Policy 77:102764. https://doi.org/10.1016/j.resourpol.2022.102764
Wang Y, Guo C, Chen X et al. (2021) Carbon peak and carbon neutrality in China: goals, implementation path and prospects. China Geol 4:720–746
CAS Google Scholar
Wang Y, Jeong S, Hang Y et al. (2023) Determinants of net energy-related CO2 emissions in China: a source-to-sink decomposition analysis. Environ Impact Assess Rev 98:106979. https://doi.org/10.1016/j.eiar.2022.106979
Wang Y, Yu L (2021) Can the current environmental tax rate promote green technology innovation?—Evidence from China’s resource-based industries. J Clean Prod 278:123443. https://doi.org/10.1016/j.jclepro.2020.123443
Zhang D (2022) Does the green loan policy boost greener production?—Evidence from Chinese firms. Emerg Mark Rev 51:100882. https://doi.org/10.1016/j.ememar.2021.100882
Zhang D, Du P (2020) How China “Going green” impacts corporate performance? J Clean Prod 258:120604. https://doi.org/10.1016/j.jclepro.2020.120604
Zhang H, Dai H, Lai H et al. (2017) U.S. withdrawal from the Paris Agreement: reasons, impacts, and China’s response. Adv Clim Change Res 8:220–225. https://doi.org/10.1016/j.accre.2017.09.002
Article ADS CAS Google Scholar
Zhang H, Wang Y, Li R et al. (2022) Can green finance promote urban green development? Evidence from green finance reform and innovation pilot zone in China. Environ Sci Pollut Res Int 1–18. https://doi.org/10.1007/s11356-022-22886-0 ,:36103065
Zhang H, Xiong P, Yang S et al. (2023) Renewable energy utilization, green finance and agricultural land expansion in China. Resour Policy 80:103163. https://doi.org/10.1016/j.resourpol.2022.103163
Zhou H, Xu G (2022) Research on the impact of green finance on China’s regional ecological development based on system GMM model. Resour Policy 75:102454. https://doi.org/10.1016/j.resourpol.2021.102454
Zhou M, Li X (2022) Influence of green finance and renewable energy resources over the sustainable development goal of clean energy in China. Resour Policy 78:102816. https://doi.org/10.1016/j.resourpol.2022.102816
Zhu Y, Taylor D, Wang Z (2022) The role of renewable energy in reducing residential fossil energy-related CO2 emissions: evidence from rural China. J Clean Prod 366:132891. https://doi.org/10.1016/j.jclepro.2022.132891
Download references
Acknowledgements
We acknowledge the financial support of the Grant-in-Aid for Excellent Young Researchers of the Ministry of Education, Culture, Sports, Science and Technology of Japan, Grant-in-Aid for Young Scientists of the Japan Society for the Promotion of Science (JSPS) (No. 22K13432), and the Grant-in-Aid for Scientific Research (B) of the JSPS (No. 22H03816).
Author information
Authors and affiliations.
School of Economics, Tianjin University of Commerce, Tianjin, China
Yunpeng Sun
School of Economics, Nankai University, Tianjin, China
School of Global Studies, Tokai University, Tokyo, Japan
Farhad Taghizadeh-Hesary
You can also search for this author in PubMed Google Scholar
Corresponding authors
Correspondence to Yunpeng Sun or Farhad Taghizadeh-Hesary .
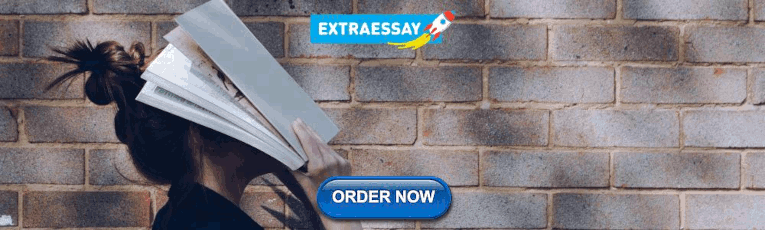
Ethics declarations
Competing interests.
The authors declare no competing interests.
Ethical approval
No human participants were involved.
Informed consent
Additional information.
Publisher’s note Springer Nature remains neutral with regard to jurisdictional claims in published maps and institutional affiliations.
Rights and permissions
Open Access This article is licensed under a Creative Commons Attribution 4.0 International License, which permits use, sharing, adaptation, distribution and reproduction in any medium or format, as long as you give appropriate credit to the original author(s) and the source, provide a link to the Creative Commons license, and indicate if changes were made. The images or other third party material in this article are included in the article’s Creative Commons license, unless indicated otherwise in a credit line to the material. If material is not included in the article’s Creative Commons license and your intended use is not permitted by statutory regulation or exceeds the permitted use, you will need to obtain permission directly from the copyright holder. To view a copy of this license, visit http://creativecommons.org/licenses/by/4.0/ .
Reprints and permissions
About this article
Cite this article.
Sun, Y., Bao, Q. & Taghizadeh-Hesary, F. Green finance, renewable energy development, and climate change: evidence from regions of China. Humanit Soc Sci Commun 10 , 107 (2023). https://doi.org/10.1057/s41599-023-01595-0
Download citation
Received : 28 October 2022
Accepted : 27 February 2023
Published : 15 March 2023
DOI : https://doi.org/10.1057/s41599-023-01595-0
Share this article
Anyone you share the following link with will be able to read this content:
Sorry, a shareable link is not currently available for this article.
Provided by the Springer Nature SharedIt content-sharing initiative
This article is cited by
Insight into energy production and consumption, carbon emissions and agricultural residues resources available for energy and environmental benefits in china.
Waste and Biomass Valorization (2024)
Connectedness of green financing, green energy and technological progress towards energy transition and climate goal: exposition of the best practise to achieve sustainable environmental development
- Edmund Ntom Udemba
- Burçin Çakır Gündoğdu
Stochastic Environmental Research and Risk Assessment (2024)
How do corporate social responsibility and green finance strategies impact sustainable development in China’s renewable energy sector?
Environmental Science and Pollution Research (2023)
Quick links
- Explore articles by subject
- Guide to authors
- Editorial policies

Sustainability Case Study: Renewable Energy
Link Copied
Brookfield Real Estate Is Committed to Executing Clean Energy Initiatives Across Its Portfolio, Driving Its Overall Carbon Footprint Reduction
As we execute our long-term strategy to deliver net-zero emissions across our business by 2050 or sooner, we are reducing our Scope 2 and 3 emissions by powering 100% of Brookfield’s U.S. Office portfolio with predominantly clean energy sources by 2026. Brookfield will predominantly leverage power purchase agreements (PPAs) to decarbonize its operations, ensuring that we source electricity from the same power grid in which we will use the electricity, further incentivizing the development of new clean energy sources. Clean energy sources for procurement include hydropower, solar, wind and nuclear power. Brookfield’s U.S. office portfolio will procure 600 GWh of clean electricity, reducing GHG emissions by 260,000 mtCO 2 e annually (the equivalent of avoiding burning 300 million pounds of coal). Clean Power Sources by City:
- Denver: Renewable electricity from local wind power facilities.
- Houston: Newly built, Texas-based solar power plant, with its construction initiated by Brookfield Properties
- Los Angeles: Newly built, California-based solar power plant, with its construction initiated by Brookfield Properties.
- New York: In-state, run-of-river hydropower facilities.
- San Francisco: Solar and wind farms through the CleanPowerSF SuperGreen program.
- Washington D.C.: Nuclear power facilities equipped by Brookfield’s Westinghouse Electric Corp.
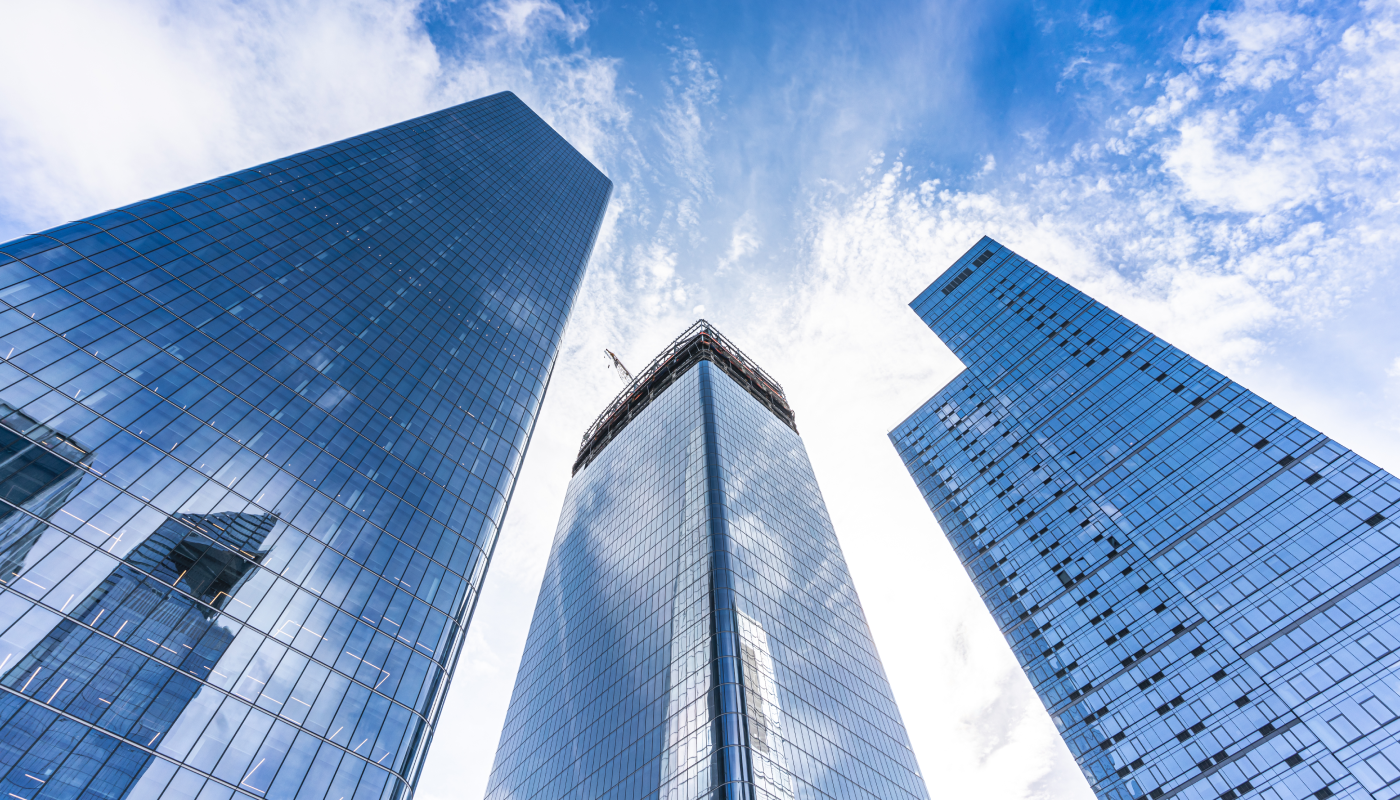
Accelerating our progress, in 2022, Brookfield Properties India committed to reach net-zero emissions by 2040 across its entire portfolio of 50 million square feet in India, which includes locations in which we have an operating presence. Brookfield Properties India’s strategy to achieve net zero is focused on energy efficiency, reducing water consumption, promoting recycling, and improving indoor air quality, which benefits all its tenants.
Brookfield Renewable will supply Brookfield Properties’ Canary Wharf with clean energy beginning in 2026, providing 80 GWh of annual electricity needed with power generated from the development of our new, onshore windfarm in Scotland.
Brookfield’s newly opened mixed-use complex in Shanghai, One East, uses renewable energy to collaborate on net zero-strategies with tenants. During construction, Brookfield installed solar panels on the roof of the complex’s retail area, which is visible to the tenants of both office towers. The solar panels generate 260 MWh of energy per year, which reduces the building’s GHG emissions by over 5,000 metric tons a year. Beyond supplying a portion of the complex’s energy, the solar panels are a conversation starter on how we develop sustainable real estate in alignment with China’s National Energy Administration policy.
Explore More Insights
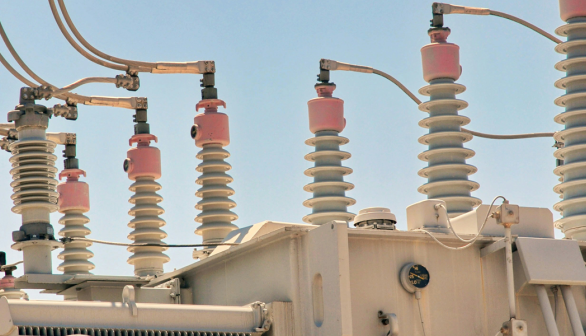
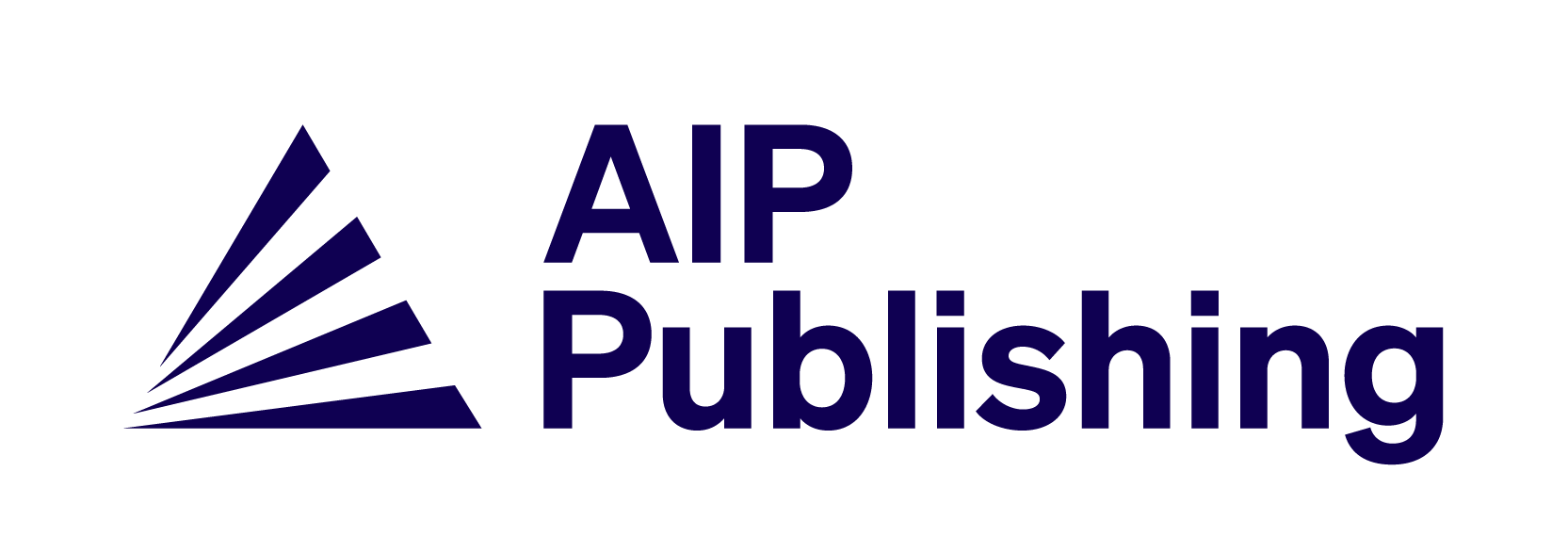
Requirements of sustainable renewable energy systems case study (Samawah city)
- Article contents
- Figures & tables
- Supplementary Data
- Peer Review
- Reprints and Permissions
- Cite Icon Cite
- Search Site
Hayder Lafta Albdiery , Suhad K. A. Al-Mosawy; Requirements of sustainable renewable energy systems case study (Samawah city). AIP Conf. Proc. 14 February 2024; 3009 (1): 030052. https://doi.org/10.1063/5.0190804
Download citation file:
- Ris (Zotero)
- Reference Manager
Renewable energy is a crucial and necessary means of attaining sustainable development. Nevertheless, relying on renewable energy sources is not always sustainable. These sources need advanced and expensive means and techniques to exploit them, in addition to a sophisticated technology that are sometimes monopolistic, and the raw materials needed for the manufacture and production of such equipment are much less prevalent than fossil fuel sources. Therefore, their monopoly is aggravated. Such as Nickel, Lithium, Cadmium, Zinc and other materials that are increasingly needed with the increase in the trend towards using renewable energies. Moreover, the increase in its supply will not meet the increase in demand for it, especially as it is subject to political fluctuations. Such as what happened during the war in Ukraine, where the price of lithium rose by 500% at the beginning of 2022. And the supply chains of photovoltaic equipment and wind turbines be governed by China by 80% and it witnessed great confusion because of what happened due to the closures to combat the Covid 19 epidemic. All of these requests for an extensive study of the requirements for the sustainability of operating and utilization of renewable energies, and this should be our top priority before moving forward on the path of transition to full dependence on renewable energies. Accordingly, we need to localize the industrial base associated with investing renewable energies as an introduction to reach sustainable investment and real solutions to energy issues. We have to determine accurately what is available to us from the elements of the renewable energy industry and what we can replace with local alternatives or provide from sustainable sources if it is not available locally. Only by doing this we will achieve a safe and sustainable transition from traditional energy sector to effective, efficient, sustainable renewable energies investment.
Sign in via your Institution
Citing articles via, publish with us - request a quote.
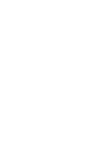
Sign up for alerts
- Online ISSN 1551-7616
- Print ISSN 0094-243X
- For Researchers
- For Librarians
- For Advertisers
- Our Publishing Partners
- Physics Today
- Conference Proceedings
- Special Topics
pubs.aip.org
- Privacy Policy
- Terms of Use
Connect with AIP Publishing
This feature is available to subscribers only.
Sign In or Create an Account
Sustainable Energy for Emerging Nations Development-A Case Study on Togo Renewable Energy
Ieee account.
- Change Username/Password
- Update Address
Purchase Details
- Payment Options
- Order History
- View Purchased Documents
Profile Information
- Communications Preferences
- Profession and Education
- Technical Interests
- US & Canada: +1 800 678 4333
- Worldwide: +1 732 981 0060
- Contact & Support
- About IEEE Xplore
- Accessibility
- Terms of Use
- Nondiscrimination Policy
- Privacy & Opting Out of Cookies
A not-for-profit organization, IEEE is the world's largest technical professional organization dedicated to advancing technology for the benefit of humanity. © Copyright 2024 IEEE - All rights reserved. Use of this web site signifies your agreement to the terms and conditions.

An official website of the United States government
The .gov means it’s official. Federal government websites often end in .gov or .mil. Before sharing sensitive information, make sure you’re on a federal government site.
The site is secure. The https:// ensures that you are connecting to the official website and that any information you provide is encrypted and transmitted securely.
- Publications
- Account settings
Preview improvements coming to the PMC website in October 2024. Learn More or Try it out now .
- Advanced Search
- Journal List
- v.5(1); 2019 Jan

Breaking barriers in deployment of renewable energy
Seetharaman.
a S P Jain School of Global Management, Singapore
Krishna Moorthy
b Faculty of Business and Finance, Universiti Tunku Abdul Rahman, Kampar Campus, Perak, Malaysia
Nitin Patwa
c S P Jain School of Global Management, Dubai, United Arab Emirates
d Taylors University, Malaysia
Associated Data
Several economic, institutional, technical and socio-cultural barriers hinder countries from moving from the high to the low emission pathway. The objective of this research is to find out the impacts of social, economic, technological and regulatory barriers in the deployment of renewable energy. Data were collected through an online questionnaire responded to by 223 professionals working in the energy sector all over the globe. This research shows that social, technological and regulatory barriers have a strong influence on the deployment of renewable energy, while economic barriers significantly influence it indirectly. By breaking research and development-related barriers, organizations will be able to invest greatly in developing advanced technologies that can optimize usage of renewable energy and make renewable energy appear more lucrative. With less polluting and lower tariff energy solutions being made available to local people, and higher profits for manufacturers, this will create an atmosphere where all stakeholders are satisfied.
1. Introduction
The world's population is growing at an unprecedented rate and that has necessitated a dramatic increase in energy demand globally. Matching supply with this surging demand is a principal and critical challenge for countries around the world. Currently, this demand is being met through the increased use of fossil fuels. The majority of the world's power is generated from the use of coal, oil and gas. These so-called fossil fuels, when burned, release heat energy which is then converted into electricity releasing into the atmosphere a lot of carbon dioxide (CO2), a greenhouse gas that contributes to the issue of global warming. A renewable energy supply offers a solution to both challenges. For economic growth and human advancement, energy has always been universally considered one of the most crucial measures ( Rawat and Sauni, 2015 ). There is a three-dimensional relationship alongside a bi-directional causal relationship between economy, the environment and energy ( Azad et al., 2014 ).
Globally, the population is growing at fast rate; however, the world's energy demand is likely to grow even more rapidly than the increase in the population. According to International Energy Outlook (2013), global energy demand will be increased by 56 per cent between 2010 and 2040 ( Azad et al., 2014 ). Currently, the majority of the world's energy consumption is satisfied by consuming energy created using fossil fuels. To satisfy the ever-increasing energy demand and to protect the climate, breakthrough advancements have been made in the past to design technologies that can control and harness power from alternative energy sources. As controlling carbon emissions is critical in dealing with climate change, renewable energy is an appropriate way to satisfy energy demand without degrading the ecosystem ( Jing, 2016 ). Apart from bringing environmental sustainability, renewable energy offers another advantage—the ability to provide power to even the most underprivileged people living in the remotest areas where traditional power is not yet available ( Rawat and Sauni, 2015 ).
Awareness of the need to encourage deployment of renewable energy has increased drastically in recent years. More countries, whether developed or developing, are promoting and changing policies to promote the deployment of renewable energy. In 2005, only 55 countries had taken steps to make renewable targets and create policies supporting renewable energy. This number had increased to 144 countries by 2013, with almost all the world understanding the need to reduce carbon emissions.
2. Background
Despite remarkable promotion and commitment from various nations, only a small percentage of energy is generated from renewable energy, especially in developing countries. This scenario is because of the numerous barriers that control the diffusion of renewable energy. These barriers prevent renewable energy from effectively competing with traditional energy and hamper achievement of the necessary large-scale deployment ( Nasirov et al., 2015 ). Penetration and scale-up of renewable require a strong political and regulatory framework which supports and promotes a continued focus on fossil fuels ( Karatayev et al., 2016 ).
A review of the literature shows that many studies have been conducted to identify barriers to the use of renewable energy. However, very few studies have grouped these barriers and discussed the impact of these barriers in the deployment of renewable energy. The variables which were identified from the literature review for use in future research were social barriers , economic barriers, technological barriers and regulatory barriers.
The objective of this research is to discover the impacts of breaking barriers in the deployment of renewable energy. This research tries to resolve the following questions to reach a solution which is in line with the objective of this research:
- a. What are the factors affecting the deployment of renewable energy and are they significant or not?
- b. What impact will breaking barriers have on the deployment of renewable energy?
- c. In the wake of breaking barriers, is Rogers' (2003) theory of diffusion (political and social) valid for renewable energy?
Theory of diffusion (technical, political & social) in the wake of breaking barriers.
Diffusion of innovation theory is one of the most important concepts in theorizing the changing format of energy provision, being concerned with the process of adoption of innovations by society ( Lacerda et al., 2014 ). Rogers (1983 : 11) defined diffusion as ‘the process by which innovation is communicated through certain channels over time among members of a social system’ and innovation as ‘an idea, practice or object that is perceived as new by an individual or other unit of adoption’ ( Sahin, 2006 ). Other types of diffusion include social diffusion and theories of change, going back to Lewin's description of the need to alter group standards to promote lasting individual change ( Lewin, 1951 ). The focus has since shifted towards external conditions that are likely to be more influential than group decisions ( Darnton, 2008 ). Political diffusion deals with the spread of policies and governance approaches across jurisdictional boundaries which come about because of external pressures and/or internal pressures relating to quests for legitimacy ( Weyland, 2005 ). More fundamentally, diffusion defines the often random movement of a characteristic. The theory of diffusion is used to understand the attitude and perception of people with regard to government policies.
4. Hypotheses
This literature review looks at the outcomes of penetration and deployment of renewable energy, which are affected by four major factors: social barriers, economic barriers, technological barriers and regulatory barriers.
4.1. Social barriers
The transition from conventional resources to renewable energy has encountered public resistance and opposition. This is due to a lack of awareness of the benefits of renewable energy, disruption of seascape, and acquisition of land which could have been used for agriculture, tourism, etc. ( Goldsmiths, 2015 ).
Public awareness and information barriers: Sustainable development stems from the satisfaction of human desires, through socially recognized technological systems and suitable policies and regulatory tools ( Paravantis et al., 2014 ). The main concerns with respect to public understanding are: 1) insufficient information regarding ecological and financial benefits; 2) inadequate awareness of renewable energy technologies (RET); and 3) uncertainties about the financial feasibility of RE installation projects ( Nasirov et al., 2015 ).
Not in my backyard’ (NIMBY) syndrome: According to NIMBY syndrome, people do support renewable energy generally, but not in their own neighbourhood. Renewable power project proposals often face opposition from individual citizens, political leaders, grassroots organizations, national interest groups and, in some cases, even environmental groups ( Jianjun and Chen, 2014 ). Public opposition occurs for a number of reasons, including landscape impact, environmental degradation and lack of consultation concerns among local communities ( Nasirov et al., 2015 ).
Loss of other/alternative income: A major issue with renewable plants (especially solar and wind farms) is the vast area of land required to produce an amount of energy equivalent to that which can be produced from a small coal fire power plant ( Chauhan and Saini, 2015 ).
To make a significant contribution to global energy consumption, there is a need to develop large scale renewable energy plants, but this requires vast areas of countryside. Enormous parts of the countryside, which includes farmland, need to be converted into buildings or roads or any other infrastructure to support a renewable energy power plant. In achieving this, often agriculture, tourism, fishing, etc. can be affected ( Nesamalar et al., 2017 ).
Lack of experienced professionals: Universal transition from fossil fuels to renewable energy sources requires the solid foundation of a skilled labour force. There is huge demand for skilled professionals to design, build, operate and maintain a renewable energy plant.
Incompetent technical professionals and lack of training institutes prevent renewable energy technologies from scaling new heights ( Ansari et al., 2016 ). There is a need to teach renewable energy courses and for proper training to be conducted to develop the skills required to install and operate renewable energy projects. The shortage of trained workforce to design, finance, build, operate and maintain renewable energy projects is considered a major obstacle to the wide penetration of renewable energy ( Karakaya and Sriwannawit, 2015 ).
Social barriers have a significant influence on the deployment of renewable energy.
Social barriers have a significant influence on economic barriers.
4.2. Economic barriers
Factors influencing economic and financial barriers are high initial capital, lack of financial institutes, lack of investors, competition from fossil fuels, and fewer subsidies compared to traditional fuel ( Raza et al., 2015 ). These factors have prevented renewable energy from becoming widespread.
Tough competition from fossil fuel: Fossil fuels will remain a dominant player in supplying energy in the future. A report by EIA's International Energy Outlook (2016) suggests that fossil fuels (oil, natural gas and coal) are expected to supply 78 per cent of the global energy used in 2040. Investment in fossil fuels (including supply and power generation) still accounts for 55 per cent of 2016 global energy investment, compared with 16 per cent for renewable energy. Coal is still a dominant fuel source in most counties because of its abundance, which makes it cheap and accessible ( Dulal et al., 2013 ). There have been huge changes in energy since summer 2014. Oil, as measured by the Brent crude contract, which was priced at $115.71/barrel in June 2014, fell to $27.10 on 20 January 2016, a huge drop of 76 per cent. Similarly, the ARA coal contract dropped from $84/tonne in April 2014 to $36.30 in February 2016. There was a huge decline in the price of natural gas, which slid from around $4.50/MMBtu in June 2014 to $1.91 in mid-February 2016. Due to falling prices and fossil fuel still emerging as a cheaper alternative to renewable energy, it is able to offer tough competition to renewable energy projects.
Government grants and subsidies: The amount of government subsidies provided to conventional energy is much higher than the subsidies awarded to renewable energy. This keeps renewable energy at a disadvantage. The subsidies provided by governments to generate electricity from fossil fuel sources is overshadowing the wide use of low emission technologies. For example, coal companies in Australia and Indonesia still receive government subsidies for mining and exploration ( Dulal et al., 2013 ).
Fewer financing institutions: Renewable energy developers and producers face severe difficulties in securing financing for projects at rates which are as low as are made available for fossil fuel energy projects ( Ansari et al., 2016 ). There are limited financial instruments and organizations for renewable project financing. This reflects that the investments are considered somewhat risky, thus demotivating investors ( Ohunakin et al., 2014 ).
High initial capital cost: Renewable energy projects require high initial capital cost and, because of the lower efficiency of renewable technology, the net pay back period is high, which in turn pushes investors on to the back foot ( Ansari et al., 2016 ). Both the users and the manufacturers may have very low capital and to install a plant they require capital financing. This problem is further highlighted by the strict lending measures that restrict access to financing even when funding is available for traditional energy projects ( Suzuki, 2013 ). High cost of capital, often lack of capital and most important with high payback period projects often becomes un-viable ( Painuly. J, 2001 ).
Intangible costs: Currently, in almost all countries, the total cost of fuel includes the cost of exploration, production, distribution and usage, but it does not include the cost of the damage it does to the environment and society. Despite severe effects on health and on the atmosphere, the unseen costs (externalities) which are connected with traditional fuels are not included in their price ( Arnold, 2015 ). Understanding these impacts is essential for evaluating the actual cost of utilizing fossil fuels for energy generation.
Economic barriers have a significant influence on the deployment of renewable energy.
4.3. Technological barriers
There are a number of legitimate technological barriers to the widespread deployment of renewable energy, including limited availability of infrastructure, inefficient knowledge of operations and maintenance, insufficient research and development initiatives, and technical complexities like energy storage and unavailability of standards ( Zhao et al., 2016 ).
Limited availability of infrastructure and facilities: There is limited availability of advanced technologies required for renewable energy, especially in developing countries, which acts as a factor preventing penetration of renewable energy. Even if this technology is available, the cost of procuring it is very high ( Dulal et al., 2013 ). Since renewable energy power plants are mostly placed in remote locations, they require additional transmission lines to connect to the main grid. Since most of the existing grids are not designed to integrate with renewable energy, these existing grids need to be upgraded or modified ( Izadbakhsh et al., 2015 ). Grid integration is amongst the biggest problems affecting the development of renewable energy projects.
Lack of operation and maintenance culture: Since renewable energy technology is comparatively new and not optimally developed, there is a lack of knowledge about operation and maintenance. Efficiency cannot be achieved if a plant is not optimally operated and if maintenance is not carried out regularly ( Sen and Bhattacharyya, 2014 ). Lack of availability of equipment, components and spare parts will require a substantial increase in the production costs, as these items need to be imported from other countries, therefore being procured at high prices and so increasing the overall cost ( Bhandari et al., 2015 ).
Lack of research and development (R&D) capabilities: Investment in research and development (R&D) is insufficient to make renewable energies commercially competitive with fossil fuel. Both governments and energy firms shy away from spending on R&D as renewable energy is in its development stage and risks related to this technology are high ( Cho et al., 2013 ).
Technology complexities: There are not enough standards, procedures and guidelines in renewable energy technologies in terms of durability, reliability, performance, etc. This prevents renewable energy from achieving large scale commercialization ( Nasirov et al., 2015 ). A major technical issue which renewable energy is facing today is the storage of energy. The supply of sun or wind is not continuous despite their infinite abundance and electricity grids cannot operate unless they are able to balance supply and demand. To resolve these issue, large batteries need to be developed which can compensate for the times when a renewable resource is not available ( Weitemeyer et al., 2014 ).
Technological barriers have a significant influence on the deployment of renewable energy.
Technological barriers have a significant influence on economic barriers.
4.4. Regulatory barriers
Factors like lack of national policies, bureaucratic and administrative hurdles, inadequate incentives, impractical government targets, and lack of standards and certifications have prevented renewable energy from expanding dramatically ( Stokes, 2013 ).
Ineffective policies by government: Strong regulatory policies within the energy industry are not only required for a nation's sustainable development, but also resolve the inconsistency between renewable and non-renewable energy. Lack of effective policies creates confusion among various departments over the implementation of the subsidies. Major issues such as unstable energy policy, insufficient confidence in RET, absence of policies to integrate RET with the global market and inadequately equipped governmental agencies act as barriers to the deployment of renewable energy projects ( Zhang et al., 2014 ).
Inadequate fiscal incentives: There have not been enough measures by governments to remove tax on imports of the equipment and parts required for renewable energy plants. Feed-in tariffs are the measures by which governments aim to subsidize renewable energy sources to make them cost-competitive with fossil fuel-based technologies, but the absence of these adequate financial incentives results in high costs that hinder the industry's development, operation and maintenance, and stagnate the future ( Sun and Nie, 2015 ).
Administrative and bureaucratic complexities: Obstacles arising in the deployment of renewable energy projects are manifold, including (and not limited to) administrative hurdles such as planning delays and restrictions. Lack of coordination between different authorities and long lead times in obtaining authorization unnecessarily increase the timeline for the development phase of the project. Higher costs are also associated with obtaining permission due to lobbying. All these factors prolong the project start-up period and reduce the motivation required to invest in renewable energy ( Ahlborg & Hammar, 2014 ).
Impractical government commitments: There is a gap between the policy targets set by governments and the actual results executed by implementation ( Goldsmiths, 2015 ). There is a lack of understanding of a realistic target and loopholes in the implementation process itself. The responsibility for overcoming these commitment issues lies with governments. Policies should be devised that can offer clear insight into important legislation and regulatory issues so that the industry can be promoted as stable and offering growth. Governments can fix this mismatch by becoming more responsive and reactive.
Lack of standards and certifications: Standards and certificates are required to ensure that the equipment and parts manufactured or procured from overseas are in alignment with the standards of the importing company. These certifications make sure that companies are operating the plant in compliance with local law. Absence of such standards creates confusion and energy producers have to face unnecessary difficulties ( Emodi et al., 2014 ).
Regulatory barriers have a significant influence on the deployment of renewable energy.
Regulatory barriers have a significant influence on economic barriers.
4.5. Breaking barriers in deployment of renewable energy
Deployment of renewable energy is crucial not only to meet energy demands but also to address concerns about climate change ( Byrnes et al., 2013 ). However, the barriers (social, economic, technological and regulatory) existing in this sector prevents the development and penetration of renewable energy globally.
User-friendly procedures: Bureaucratic procedures in the deployment of renewable energy are considered the biggest hindrance, and this demotivates investors and entrepreneurs from entering and investing in renewable energy. Government policies are not aligned at national and state level, thus failing to attract energy sector investment ( Nesamalar et al., 2017 ). Countries with excessively complicated administrative procedures have less penetration of renewable energy compared to countries with simple and straightforward procedures ( Huang et al., 2013 ).
Higher stakeholder satisfaction: Energy is the backbone of the socioeconomic development of any country ( Raza et al., 2015 ). By utilizing more renewable energy resources, nations can help fulfil energy deficiencies without damaging nature. The repercussions of this change would be the creation of more jobs in the designing, building, operation and maintenance of renewable energy project infrastructures. Higher levels of diffusion will help to achieve economies of scale, and that will bring down the costs and thus the price for the end user. This will improve investors' confidence and will trigger increased investments in renewable energy projects. Higher benefits can be reaped from the availability of green energy as there will not be severe environmental implications, and that can help in maintaining the earth's ecosystem.
Successful research and development (R&D) ventures: In a study conducted by Halabi et al. (2015) , it was pointed out that technological advancement to effectively generate, store and distribute renewable energy at lower costs is crucial. However, compared to conventional energy, insufficient R&D initiatives are undertaken. This is due to fact that organizations are unable to earn beneficial returns from R&D, and that makes the future of these initiatives look dull.
Cost savings: The biggest challenge that renewable energy faces is the competition from low cost fossil fuels ( El-katiri, 2014 ). Renewable energy projects require huge land areas to produce the amount of energy which a conventional plant can produce in a small area. Prohibitive costs are involved in establishing and running renewable energy projects, mainly due to the huge financial capital required to acquire a suitable piece of land, the costs associated with lobbying, and power losses due to inefficient energy storage capabilities.
5. Methodology
The research framework of this study is given in Fig. 1 below:

Research framework.
5.1. Data collection
The survey questionnaire (please see the questionnaire) was framed based on independent variables and their sub-variables. The questionnaire, a pretesting of the questionnaire was conducted to ensure that all the questions were relevant and understandable to respondents. Initially, the survey questionnaire was sent out to 33 energy industry experts and their feedbacks were collected. The insights generated from this pilot testing led to further refinement of the questionnaire and a final questionnaire was developed. The final survey form consisted of 26 main questions for both dependent and independent variables and another three questions to understand the demographics of the respondent. Each question consisted of five options (Likert scale) from which the respondent had to select the one which he/she thought suited the best, with ‘1’ as strongly disagree and ‘5’ as strongly agree.
5.2. Profile of respondents
The survey respondents were professionals in the energy industry (manufacturing of rigs, power generation, power distribution, oil and gas, mining and renewable energy). The participants were selected based on their familiarity with and knowledge of renewable energy sources and technology across America, Europe, Asia Pacific, Africa and Australia. The survey questionnaire was sent out to 645 potential respondents, of which only 223 practical survey responses were received. The response rate is calculated to be 34.5 per cent. The demographics of the respondents are provided in Table 1 .
Demographics of the respondents (n = 223) with respect to job level, region and industry sector across energy sector.
5.3. Data analysis
The data collected from the survey questionnaire were analysed using ADANCO 2.0.1 software. ADANCO software is used for this purpose as it is specialised for variance based structural equation modelling. It implements several limited information estimators such as partial least squares path modelling or ordinary least squares regression based on sum scores for testing the hypothesis and analysing research models ( Henseler et al., 2014 ). To verify the correlation and confidence in the hypotheses, ADANCO software works well as it does not enforce normality on the data. Data analysis was conducted by first gauging the modelling of the structural model and then measuring the reliability and validity of the model by estimating model parameters.
5.4. Reliability
Cronbach's alpha value was considered to determine the reliability of the model fit. Alpha values above 0.7 show a satisfactory level of reliability. Jöreskog's Rho value also confirms that the model is consistent and uniform: i.e. composite reliability is within the appropriate range ( Marshall, 2014 ). The figures for each construct are listed in Table 3 .
Discriminant validity: Fornell-Larcker criteria.
5.5. Convergent validity
Convergent validity can be defined as the degree to which two measures of constructs that theoretically should be related are in fact related ( Campbell and Fiske, 1959 ). The value of average variance extracted is required to be above 0.5 in order to be accepted. The convergent validity is shown in Table 2 below. The minimum AVE value obtained is 0.5042, which proves that the validity of this model is acceptable.
Overall Reliability of the construct and Convergent validity.
5.6. Discriminant validity
Discriminant validity is used to test if the models or concepts that are not in relation are unrelated. According to Fornell and Larcker's theory ( Cable et al., 2014 ), if the root of the average variance extracted (AVE) of one path is less than the average variance extracted (AVE) of the other path, then it is considered accepted. In Table 3 below, Fornell and Larcker's theory is successfully matched; thus the discriminant validity of this model is satisfactory.
5.7. Structural equation model (SEM)
Structural modelling through bootstrapping is provided in Fig. 2 . Path analysis is a special case of structural equation modelling and employs a causal modelling approach to explore the correlations within a defined network. This correlation is equated by calculating the sum of the contributions of the paths that connect all the variables. To evaluate the strength of each path, products of the path coefficients along the path are calculated ( Schreiber et al., 2015 ). The R-squared value of our research model is 0.545, which supports the research model.

Structural modelling through bootstrapping.
5.8. Hypothesis testing
ADANCO 2.0.1 is used to conduct hypothesis testing because it uses variance to model structural equations. The bootstrapping option can be selected in the ADANCO software to model unknown population data ( Sarstedt et al., 2011 ). The level of significance is measured by establishing the t-statistic. The outcomes of the hypothesis testing is given in Table 4 below:
Outcomes of the hypothesis testing.
Note: SB = social barriers; EB = economic barriers; TB = technological barriers; RB = regulatory barriers; RE = deployment of renewable energy.
In total, seven hypotheses were identified. Out of the seven hypotheses, six hypotheses are accepted as their path coefficient is either positively or significantly related. A detailed explanation of each hypothesis is given below.
Hypotheses H1 highlights the influence of social barriers on the deployment of renewable energy. The effect of social barriers is moderately significant with (t- value = 1.8749) and (β=0.1063, p < 0.01) thus hypothesis H1 got accepted. This shows that social barriers have a moderate influence on the deployment of renewable energy. Earlier studies ( Paravantis et al., 2014 ) have advised that future studies be conducted to determine whether renewable energy is socially accepted. In our study, the positively related t-value testifies to a positive level of significance, implying that social barriers are still a hindrance to the deployment of renewable energy. Fig. 3 below shows the Social barriers with associated path coefficients.

Social barriers with associated path coefficients.
Hypothesis H2 highlights the impact of social barriers on economic barriers. The effect of social barriers is highly significant with (t- value = 4.505) and (β=0.317, p < 0.01) was accepted. This indicates that the parameters, such as opportunity cost and opposition by residents, strongly influence economic parameters. Earlier studies ( Jianjun and Chen, 2014 ) have supported that social barriers impact economic parameters. However, the earlier studies did not conduct research to understand the strength of the impact. Through our survey, we have determined that social barriers do have a strong correlation with the economic barriers associated with the implementation of renewable energy.
Hypothesis H3 tested the influence of economic barriers on the deployment of renewable energy. The statistical results with (t- value = 0.4968) and (p > 0.01) as not supported. This indicates that the parameters of economic barriers do not influence the deployment of renewable energy directly. Previous studies ( Boie et al., 2014 ) have pointed out that financial and economic parameters act as hurdles in the wide usage of renewable energy. However, this research contradicts the earlier findings. Fig. 4 below depicts the Economic barriers with associated path coefficients.

Economic barriers with associated path coefficients.
Hypothesis H4 tested the effect of technological barriers on the deployment of renewable energy. The effect of technological barriers is moderately related (t- value = 1.6491) and (β=0.1317, p < 0.01) thus H4 is accepted. This indicates that technological barriers are moderately significant in the deployment of renewable energy. Earlier research ( Gullberg et al., 2014 ) has pointed out that lack of technology advancement has created obstacles for implementing renewable energy. This research paper corroborates the findings of previous studies. Fig. 5 shows the Technological barriers with associated path coefficients.

Technological barriers with associated path coefficients.
Hypothesis H5 examined the impact of technological barriers on economic barriers. The effect of technological barriers on economic barriers is highly significant, with a (t- value = 3.0797) and (β=0.2367, p < 0.01) thus hypothesis H5 is accepted. This indicates that the technological barriers have a highly significant impact on economic barriers. Earlier research ( Zyadin et al., 2014 ) pointed out that lack of research and development has kept the costs of renewable energy higher compared to energy produced from fossil fuels. This study validates the findings of earlier studies.
Hypothesis H6 examined the effects of regulatory barriers on the deployment of renewable energy. Once again, the effect of regulatory barriers on the deployment of renewable energy is highly significant, as the (t- value = 7.7281) and (β=0.5705, p < 0.01). This indicates that regulatory barriers have a significant impact on the implementation of renewable energy. Earlier studies ( Jing, 2016 ) discuss how government policies and administration affect the usage of renewable energy. However, the earlier studies were specific to a country. This study fills the gap by conducting research globally and taking all major countries into consideration. Fig. 6 shows the Regulatory barriers with associated path coefficients.

Regulatory barriers with associated path coefficients.
Hypothesis H7 argued for the effects of regulatory barriers on economic barrier parameters. The effect of regulatory barriers on economic barriers is once more highly significant with (t- value = 5.0687 ) and (β= 0.3249 , p < 0.01) thus supported strongly. This indicates that regulatory barriers have a highly significant impact on economic barriers regarding the deployment of renewable energy. Conversely, the earlier literature ( Harrison, 2015 ) discusses how regulatory and government policies affect the implementation of renewable energy. This research fills the gap by establishing a strong association between regulatory and economic barriers.
7. Discussion & conclusion
Research was conducted to understand the barriers associated with the deployment of renewable energy and the benefits of overcoming these barriers. This research answers all the questions identified as part of the research objective.
Firstly, the factors affecting the deployment of renewable energy were identified and grouped into social, economic, technological and regulatory barriers. This research shows that social, technological and regulatory barriers have a strong influence on the deployment of renewable energy, while economic barriers, though not directly influencing it, and significantly influence it indirectly. Fig. 7 indicates the Deployment of renewable energy and its path coefficients.

Deployment of renewable energy and its path coefficients.
Secondly, in the structural equation model above, the path coefficient of user-friendly procedures is 0.808, that of stakeholder satisfaction is 0.81, successful R&D ventures is 0.86 and cost savings is 0.80. Since the path coefficient for the entire four constructs is equal or greater than 0.80, this implies that breaking barriers in the deployment of renewable energy has a strong impact on all four constructs (user-friendly procedures, stakeholder satisfaction, successful R&D ventures and cost savings).
Finally, the research confirms that political implications have a big impact on the deployment of renewable energy. Technological barriers are preventing renewable energy from being efficient and preventing it from being cost effective. Social awareness and opposition also have a positive impact on the deployment of energy. These results are in line with the theory of diffusion and answer the third question of the research objective.
7.1. Implications for renewable energy industry
In our research, we have studied the impact of various barriers on the deployment of renewable energy. By breaking research and development-related barriers, organizations will be able to invest greatly in developing advanced technologies that can optimize usage of renewable energy and make renewable energy appear more lucrative. With less polluting and lower tariff energy solutions being made available to local people, and higher profits for manufacturers, this will create an atmosphere where all stakeholders are satisfied. Breaking red tape in government procedures will lead to generating interest among investors in renewable energy projects and, by breaking the barriers to the deployment of renewable energy, a greater number of projects will start up. This will help to achieve economies of scale and will bring down operation and maintenance costs. By supporting further innovative technological advancements, more efficient plants will be developed which may require smaller portions of land. Modern technologies will also make offshore wind/solar farms economically feasible.
Though renewable energy would prevent degradation of the environment, however, a small fraction of the ecosystem will still be affected: for example, in the case of offshore wind farms, underwater marine life might be disturbed.
7.2. Limitations and future research
In this research, we have considered the presence of four barriers as factors preventing the successful deployment of renewable energy globally; however, it is reasonable to expect that not all the barriers will be present in each country and there could be some new barriers that have not yet been conceptualized. Though this research has been conducted to understand the global perception, the data collected constituted only 9.8 per cent from Europe, 6.3 per cent from America, 5.9 per cent from the Middle East and Africa, and five per cent from Australia. The research conducted was mainly based on data collected from the Asia Pacific region. Cultural characteristics of Asians can be considered to be different from those of other countries; hence it is advised to practise caution when generalizing the findings in the context of renewable energy.
Finally, regarding future research, further study is required to understand and compare the impact of barriers to renewable energy in developing and developed countries.
7.3. Conclusion
In the long run, due to increasing awareness of environmental damage, conventional power generation based on exhaustible fuels (oil, coal and gas) is generally considered unsustainable. Alternative energies that have minimal impact on the environment and are inexhaustible, such as renewable energy, can be a solution to the long-fought sustainability problem. However, despite on-going awareness of the manifold advantages of renewable energy, the diffusion of renewable energy is limited globally. This restriction has been attributed to social, economic, technological and regulatory barriers.
This research presents the impact of social, economic, technological and regulatory barriers on the deployment of renewable energy and how these barriers are interrelated. Focusing on factors influencing barriers and the deployment of renewable energy, a research model was developed and tested by analysing the data collected from 223 respondents. Respondents were experienced professionals from the energy industry. The findings show that social barriers have a positive impact while technological and regulatory barriers have a very significant impact on the deployment of renewable energy. However, this research shows that economic barriers do not directly impact the deployment of renewable energy, but are interrelated with social, technological and regulatory barriers, thus indirectly affecting the deployment of renewable energy. The simultaneous increase in energy demand and the negative impact of fossil fuels on the environment underscores the need for energy production from renewable energy sources. Renewable energy sources strike a perfect balance between economic, technical and environmental considerations, and contribute to a more sustainable development that will favour future generations.
Declarations
Author contribution statement.
Seetharaman Conceived and designed the experiments.
Krishna Moorthy: Performed the experiments, Analyzed and interpreted the data, Wrote the paper.
Nitin Patwa: Performed the experiments.
Saravanan: Analyzed and interpreted the data.
Yash Gupta: Contributed reagents, materials, analysis tools or data.
Funding statement
This research did not receive any specific grant from funding agencies in the public, commercial, or not-for-profit sectors.
Competing interest statement
The authors declare no conflict of interest.
Additional information
No additional information is available for this paper.
Appendix A. Supplementary data
The following is the supplementary data related to this article:
- Ahlborg H., Hammar L. Drivers and barriers to rural electrification in Tanzania and Mozambique: Grid-extension, off-grid and renewable energy technologies. Renew. Energy. 2014; 61 :117–124. [ Google Scholar ]
- Ansari M.F., Kharb R.K., Luthra S., Shimmi S.L., Chatterji S. Analysis of barriers to implement solar power installations in India using interpretive structural modeling technique. Renew. Sustain. Energy Rev. 2016; 27 :163–174. [ Google Scholar ]
- Arnold U. Economic risk analysis of decentralized renewable energy infrastructures: A Monte Carlo simulation approach. Renew. Energy. 2015; 77 :227–239. [ Google Scholar ]
- Azad A.K., Rasul M.G., Khan M.M.K., Ahasan T., Ahmed S.F. Energy scenario: Production, consumption and prospect of renewable energy in Australia. J. Power Energy Eng. 2014; 2 :19–25. [ Google Scholar ]
- Bhandari B., Lee K., Lee G., Cho Y., Ahn S. Optimization of hybrid renewable energy power systems: A review. Int. J. Precis. Eng. Manuf. Green Technol. 2015; 2 (1):99–112. [ Google Scholar ]
- Boie I., Fernandes C., Frías P., Klobasa M. Efficient strategies for the integration of renewable energy into future energy infrastructures in Europe:An analysis based on transnational modeling and case studies for none European regions. Energy Policy. 2014; 67 :170–185. [ Google Scholar ]
- Byrnes L., Brown C., Foster J., Wagner L.D. Australian renewable energy policy: Barriers and challenges. Renew. Energy. 2013; 60 (1):711–721. [ Google Scholar ]
- Cable D.M., Cable D.M., Derue D.S. The convergent and discriminant validity of subjective fit perceptions. J. Appl. Psychol. 2014; 87 (5):875–884. [ PubMed ] [ Google Scholar ]
- Campbell D.T., Fiske D.W. Convergent and discriminant validation by the multitrait-multimethod matrix. Psychol. Bull. 1959; 56 (2):81. [ PubMed ] [ Google Scholar ]
- Chauhan A., Saini R.P. Renewable energy based off-grid rural electrification in Uttarakhand state of India: Technology options, modelling method, barriers and recommendations. Renew. Sustain. Energy Rev. 2015; 51 (December):662–681. [ Google Scholar ]
- Cho C., Yang L., Chu Y., Yang H. Renewable energy and renewable R&D in EU countries. Cointegrat. Anal. 2013; 2 (1):10–16. [ Google Scholar ]
- Darnton A. Government Social Research Behaviour Change Knowledge Review; UK: 2008. Reference report: An overview of behaviour change models and their uses. [ Google Scholar ]
- Dulal H.B., Shah K.U., Sapkota C., Uma G., Kandel B.R. Renewable energy diffusion in Asia: Can it happen without government support? Energy Policy. 2013; 59 (April):301–311. [ Google Scholar ]
- El-katiri L. 2014. A roadmap for renewable energy in the Middle East and North Africa. [ Google Scholar ]
- Emodi V.N., Yusuf S.D., Boo K. The necessity of the development of standards for renewable energy technologies in Nigeria. Smart Grid Renew. Energy. 2014; 5 (November):259–274. [ Google Scholar ]
- Goldsmiths K.R. 2015. Barriers and solutions to the development of renewable energy technologies in the Caribbean. (April) [ Google Scholar ]
- Gullberg A.T., Ohlhorst D., Schreurs M. Towards a low carbon energy future: Renewable energy cooperation between Germany and Norway. Renew. Energy. 2014; 68 (August):216–222. [ Google Scholar ]
- Halabi M.A., Al-qattan A., Al-otaibi A. Application of solar energy in the oil industry: Current status and future prospects. Renew. Sustain. Energy Rev. 2015; 43 :296–314. [ Google Scholar ]
- Harrison B. Expanding the renewable energy industry through tax subsidies using the structure and rationale of traditional energy tax subsidies. Univ. Muichigan J. Law Reform. 2015; 48 (3) [ Google Scholar ]
- Henseler J., Dijkstra T.K., Sarstedt M., Ringle C.M., Diamantopoulos A., Straub D.W., Calantone R.J. Common beliefs and reality about PLS: Comments on Rönkkö and Evermann (2013) Organ. Res. Methods. 2014; 17 (2):182–209. [ Google Scholar ]
- Huang S., Lo S., Lin Y. To re-explore the causality between barriers to renewable energy eevelopment: A case study of wind energy. Energies. 2013; 6 (9):4465–4488. [ Google Scholar ]
- Izadbakhsh M., Gandomkar M., Rezvani A., Ahmadi A. Short-term resource scheduling of a renewable energy based micro grid. Renew. Energy. 2015; 75 (March):598–606. [ Google Scholar ]
- Jianjun J., Chen D.S. Willingness to pay for renewable electricity: A contingent valuation study in Beijing, China. Energy Policy. 2014; 68 (May):340–347. [ Google Scholar ]
- Jing E. Development of renewable energy in Australia and China: A comparison of policies and status. Renew. Energy. 2016; 85 (January):1044–1051. [ Google Scholar ]
- Karakaya E., Sriwannawit P. Barriers to the adoption of photovoltaic systems: The state of the art. Renew. Sustain. Energy Rev. 2015; 49 :60–66. [ Google Scholar ]
- Karatayev M., Hall S., Kalyuzhnova Y., Clarke M.L. Renewable energy technology uptake in Kazakhstan: Policy drivers and barriers in a transitional economy. Renew. Sustain. Energy Rev. 2016; 66 :120–136. [ Google Scholar ]
- Lacerda J.S., van den Bergh J.C.J.M., Stern D.I. 2014. International diffusion of renewable energy innovations: Lessons from the lead markets for wind power in China, Germany and USA. [ Google Scholar ]
- Lewin K. 1951. Field theory in social science. [ Google Scholar ]
- Marshall A.L. 2014. International physical activity questionnaire: 12-country reliability and validity. [ PubMed ] [ Google Scholar ]
- Nasirov S., Silva C., Agostini C.A. Investors’ perspectives on barriers to the deployment of renewable energy sources in Chile. Energies. 2015; 8 (5):3794–3814. [ Google Scholar ]
- Nesamalar J.J.D., Venkatesh P., Raja S.C. The drive of renewable energy in Tamilnadu: Status, barriers and future prospect. Renew. Sustain. Energy Rev. 2017; 73 (June):115–124. [ Google Scholar ]
- Ohunakin O.S., Adaramola M.S., Oyewola O.M., Fagbenle R.O. Solar energy applications and development in Nigeria: Drivers and barriers. Renew. Sustain. Energy Rev. 2014; 32 :294–301. [ Google Scholar ]
- Painuly J.P. Barriers to renewable energy penetration; a framework for analysis. Renew. Energy. 2001; 24 (1):73–89. [ Google Scholar ]
- Paravantis J., Stigka E., Mihalakakou G. An analysis of public attitudes towards renewable energy in Western Greece. Renew. Sustain. Energy Rev. 2014; 32 (March 2015):100–106. [ Google Scholar ]
- Rawat D., Sauni P. Importance and prospects of renewable energy: Emerging issues in India. Int. J. Art Hum. Sci. 2015; 2 (4):11–18. [ Google Scholar ]
- Raza W., Saula H., Islam S.U., Ayub M., Saleem M., Raza N. Renewable energy resources: Current status and barriers in their adaptation for Pakistan. J. Bioprocess. Chem. Eng. 2015; 3 (3):1–9. [ Google Scholar ]
- Rogers Everett M. third ed. Free Press of Glencoe; New York: 1983. Diffusion of innovations. [ Google Scholar ]
- Rogers E.M. fifth ed. 2003. Diffusion of innovations. [ Google Scholar ]
- Sahin I. Detailed review of Rogers' diffusion of innovations theory and educational technology-related studies based on Rogers' theory. Turk. Online J. Educ. Technol. 2006; 5 (2):14–23. [ Google Scholar ]
- Sarstedt M., Henseler J., Ringle C.M. Multigroup analysis in partial least squares (PLS) path modeling: Alternative methods and empirical results. In: Sarstedt M., Schwaiger M., Taylor C.R., editors. Measurement and research methods in international marketing (Advances in international marketing, volume 22) Emerald Group Publishing Limited; 2011. pp. 195–218. [ Google Scholar ]
- Schreiber J.B., Nora A., Stage F.K., Barlow E.A., King J. Reporting modeling analysis and confirmatory results: Equation factor review. J. Educ. Res. 2015; 99 (6):323–337. [ Google Scholar ]
- Sen R., Bhattacharyya S.C. Off-grid electricity generation with renewable energy technologies in India: An application of HOMER. Renew. Energy. 2014; 62 :388–398. [ Google Scholar ]
- Stokes L.C. The politics of renewable energy policies: The case of feed-in tariffs in Ontario, Canada. Energy Policy. 2013; 56 :490–500. [ Google Scholar ]
- Sun P., Nie P. A comparative study of feed-in tariff and renewable portfolio standard policy in renewable energy industry. Renew. Energy. 2015; 74 (February):255–262. [ Google Scholar ]
- Suzuki M. 2013. What are the roles of national and international institutions to overcome barriers in diffusing clean energy technologies in Asia? Matching barriers in technology diffusion with the roles of institutions. http://cdn.intechopen.com/pdfs/43765/InTech-What_are_the_roles_of_national_and_international_institution s_to_ overcome_barriers_in_diffusing_clean_energy_technologies_in_asia_matching_barriers_in_technology_diffusion_with_the_roles_of_institutions.pdf Retrieved from: [ Google Scholar ]
- Weitemeyer S., Kleinhans D., Vogt T., Agert C. Integration of renewable energy sources in future power systems: The role of storage. Renew. Energy. 2014; 75 :14–20. [ Google Scholar ]
- Weyland K. Theories of policy diffusion lessons from Latin American pension reform. World Polit. 2005; 57 (02):262–295. [ Google Scholar ]
- Zhang H., Li L., Zhou D., Zhou P. Political connections, government subsidies and firm financial performance: Evidence from renewable energy manufacturing in. Renew. Energy. 2014; 63 :330–336. [ Google Scholar ]
- Zhao Z., Chang R., Chen Y. What hinders the further development of wind power in China ? A socio-technical barrier study. Energy Policy. 2016; 88 (January):465–476. [ Google Scholar ]
- Zyadin A., Halder P., Kähkönen T., Puhakka A. Challenges to renewable energy: A bulletin of perceptions from international academic arena. Renew. Energy. 2014; 69 (September):82–88. [ Google Scholar ]
Urban energy planning towards achieving an economically and environmentally optimized energy flow by 2050 based on different scenarios (a case study)
- Published: 05 April 2024
Cite this article
- Mohammad Hossein Jahangir ORCID: orcid.org/0000-0002-0991-7646 1 ,
- Reza Mokhtari 1 ,
- Fatemeh Salmanpour 1 &
- Hossein Yousefi 1
Explore all metrics
With the global population on the rise and the pressing challenges of energy crises and climate change, there is an ever-increasing need for innovative strategies to address these issues. Industrial cities play a pivotal role in these challenges, and prompt action is essential to mitigate their impacts. This research focuses on investigating Isfahan province, which serves as the industrial core of Iran and exhibits a substantial demand for electricity and natural gas. Currently, Iran relies solely on a national strategic energy plan for energy system development. This study aims to underscore the significance of formulating a comprehensive long-term urban energy plan tailored to regional resources. Such plans offer valuable insights to policymakers, aiding them in recognizing opportunities for energy system development and prioritizing infrastructure projects. To achieve this goal, an optimized energy planning process has been employed, targeting the reduction of both CO 2 emissions and total costs in Isfahan by the year 2050. Key decision variables considered in this planning process include the sizing of solar plants, wind farms, biogas facilities, combined heat and power (CHP) plants, and the extent of district heating coverage. The excess heat generated by industrial processes is harnessed and distributed through the district heating network to support residential heating needs. The findings of this study reveal that an optimized strategy could result in substantial benefits. Specifically, it is projected that this strategy could lead to a reduction of 9 million metric tons of CO 2 emissions compared to the basic strategy and save approximately 3.8 billion dollars in costs by 2050. Furthermore, the results emphasize that, despite being a relatively expensive solution, district heating has the potential to achieve significant savings in natural gas consumption.
This is a preview of subscription content, log in via an institution to check access.
Access this article
Price includes VAT (Russian Federation)
Instant access to the full article PDF.
Rent this article via DeepDyve
Institutional subscriptions
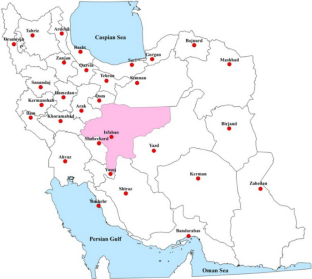
Data availability
There is no data availability.
Iran’s renewable Energy and Energy Efficiency Organization.
Abbasi, K. R., Hussain, K., Abbas, J., Adedoyin, F. F., Shaikh, P. A., Yousaf, H., et al. (2021). Analyzing the role of industrial sector's electricity consumption, prices, and GDP: A modified empirical evidence from Pakistan. Aims Energy , 9 (1), 29–49.
Article Google Scholar
Abbasi, M. R., Paighambari, S. Y., Pouladi, M., & Ghorbani, R. (2017). Catch composition, length frequency and biomass of commercial carps in Zayandehrud dam, Isfahan Province, Iran. Biodiversitas, 18 (3), 939–44. https://doi.org/10.13057/biodiv/d180310
Agricultural Statistics of Iran 2020.
Alavijeh, K. M., & MasihYaghmaei, S. (2016). Biochemical production of bioenergy from agricultural crops and residue in Iran. Waste Management, 52 , 375–394. https://doi.org/10.1016/j.wasman.2016.03.025
Article CAS Google Scholar
Alegre, S., Míguez, J. V., & Carpio, J. (2017). Modelling of electric and parallel-hybrid electric vehicle using matlab/simulink environment and planning of charging stations through a geographic information system and genetic algorithms. Renewable and Sustainable Energy Reviews, 74 (February), 1020–1027. https://doi.org/10.1016/j.rser.2017.03.041
Alves, M., Segurado, R., & Costa, M. (2020). On the road to 100% renewable energy systems in isolated islands. Energy, 198 , 117321. https://doi.org/10.1016/j.energy.2020.117321
Aman, J., Abbas, J., Mahmood., Nurunnabi, M., & Bano, S. (2019). The influence of islamic religiosity on the perceived socio-cultural impact of sustainable tourism development in Pakistan. A Structural Equation Modeling Approach Sustainability , 11 (11), 3039. https://doi.org/10.3390/su11113039
Google Scholar
Ardebili, S., & Mohammad, S. (2020). Green electricity generation potential from biogas produced by anaerobic digestion of farm animal waste and agriculture residues in Iran. Renewable Energy . https://doi.org/10.1016/j.renene.2020.02.102
Askeland, K., Rygg, B. J., & Sperling, K. (2020). The role of 4th generation district heating (4GDH) in a highly electrified hydropower dominated energy system–The Case of Norway. International Journal of Sustainable Energy Planning and Management, 27 , 17–34. https://doi.org/10.5278/ijsepm.3683
Bagheri, A., Bagheri, M., & Lorestani, A. (2020). Optimal reconfiguration and DG integration in distribution networks considering switching actions costs using tabu search algorithm. Journal of Ambient Intelligence and Humanized Computing . https://doi.org/10.1007/s12652-020-02511-z
Bellocchi, S., Manno, M., Noussan, M., Prina, M. G., & Vellini, M. (2020). Electrification of transport and residential heating sectors in support of renewable penetration: Scenarios for the Italian energy system. Energy, 196 , 117062. https://doi.org/10.1016/j.energy.2020.117062
Berthou, M., and D. Bory. (2012). Overview of waste heat in the industry in France In ECEE summer study on energy efficiency in industry .
Borghei, Moein, & Ghassemi, Mona. (2021). Optimal planning of microgrids for resilient distribution networks. International Journal of Electrical Power and Energy Systems, 128 , 106682. https://doi.org/10.1016/j.ijepes.2020.106682
Deb, K., Pratap, A., Agarwal, S., & Meyarivan, T. (2002). A fast and elitist multiobjective genetic algorithm: NSGA-II. IEEE Transactions on Evolutionary Computation. 10 (1109/4235), 996017.
Dehkordi, S. M., Jahromi, A. R., Ferdowsi, A., Shumal, M., & Dehnavi, A. (2020). Investigation of biogas production potential from mechanical separated municipal solid waste as an approach for developing countries (Case Study: Isfahan-Iran). Renewable and Sustainable Energy Reviews, 119 , 109586. https://doi.org/10.1016/j.rser.2019.109586
Caglar, E., & Abdullah. (2020). The importance of renewable energy consumption and FDI Inflows in reducing environmental degradation: Bootstrap ARDL bound test in selected 9 countries. Journal of Cleaner Production, 264 , 121663. https://doi.org/10.1016/j.jclepro.2020.121663
Energy, R. (2018). Energy efficiency organization (SATBA). Renewable energy in Iran. Retrieved May, 15. 2018.
Energypedia. 2021. Costs of a biogas plant. https://energypedia.info/wiki/Costs_of_a_Biogas_Plant#References
Galván, I. M., Valls, J. M., Cervantes, A., & Aler, R. (2017). Multi-objective evolutionary optimization of prediction intervals for solar energy forecasting with neural networks. Information Sciences, 418–419 , 363–382. https://doi.org/10.1016/j.ins.2017.08.039
Gasoline Prices. 2021. https://www.globalpetrolprices.com/gasoline_prices/
Global solar atlas. 2021. https://globalsolaratlas.info/
Global wind atlas. 2021. https://globalwindatlas.info/
Huang, Z., Hang, Yu., Peng, Z., & Zhao, M. (2015). Methods and tools for community energy planning: A review. Renewable and Sustainable Energy Reviews, 42 (4800), 1335–1348. https://doi.org/10.1016/j.rser.2014.11.042
Icaza-Alvarez, D., Jurado, F., & Tostado-Véliz, M. (2023). Long-term planning for the integration of electric mobility with 100% renewable energy generation under various degrees of decentralization: Case study Cuenca, Ecuador. Energy Reports , 9 , 4816–4829.
IEA-ETSAP. 2014. Energy technology system analysis programme hydrogen production & distribution.” Technology Brief P12 .
IEA, Coal. (2020). IEA, Paris https://Www.Iea.Org/Reports/Coal-2020
Iran office of energy, industry and mining studies. 2021.
Iranian Energy Balance Sheet [in Persian]. 2018.
Jahangir, M. H., Mokhtari, R., & Mousavi, S. A. (2021). Performance evaluation and financial analysis of applying hybrid renewable systems in cooling unit of data centers–A case study. Sustainable Energy Technologies and Assessments . https://doi.org/10.1016/j.seta.2021.101220
Kannan, D., Moazzeni, S., & Sobhan mostafayi Darmian, and Ahmadreza Afrasiabi. (2021). A hybrid approach based on MCDM methods and Monte Carlo simulation for sustainable evaluation of potential solar sites in East of Iran. Journal of Cleaner Production, 279 , 122368. https://doi.org/10.1016/j.jclepro.2020.122368
Karatayev, M., & Hall, S. (2020). Establishing and comparing energy security trends in resource-rich exporting nations (Russia and the Caspian Sea Region). Resources Policy, 68 (July), 101746. https://doi.org/10.1016/j.resourpol.2020.101746
Karatayev, M., Gaduš, J., & Lisiakiewicz, R. (2023). Creating pathways toward secure and climate neutral energy system through EnergyPLAN scenario model: The case of Slovak Republic. Energy Reports , 10 , 2525–2536.
Kiwan, S., & Al-Gharibeh, E. (2020). Jordan toward a 100% renewable electricity system. Renewable Energy, 147 , 423–436. https://doi.org/10.1016/j.renene.2019.09.004
Klemm, C., & Vennemann, P. (2021). Modeling and optimization of multi-energy systems in mixed-use districts: a review of existing methods and approaches. Renewable and Sustainable Energy Reviews, 135 , 110206. https://doi.org/10.1016/j.rser.2020.110206
Leopoldović, L. (2021). Blue energy potential assessment and their impact on the electricity system of the Republic of Croatia Doctoral Dissertation . University of Zagreb.
Li, J., Bo, Lu., Wang, Z., & Zhu, M. (2021). Bi-level optimal planning model for energy storage systems in a virtual power plant. Renewable Energy, 165 , 77–95. https://doi.org/10.1016/j.renene.2020.11.082
Lund, H., Thellufsen, J. Z., Østergaard, P. A., Sorknæs, P., Skov, I. R., & Mathiesen, B. V. (2021). EnergyPLAN–Advanced analysis of smart energy systems. Smart Energy, 1 , 100007. https://doi.org/10.1016/j.segy.2021.100007
McKenna, R. C., & Norman, J. B. (2010). Spatial modelling of industrial heat loads and recovery potentials in the UK. Energy Policy . https://doi.org/10.1016/j.enpol.2010.05.042
Mensour, O., Nait, B. E., Ghazzani, B. H., & Ihlal, A. (2017). Modeling of solar energy potential in Souss-Massa Area-Morocco, using intelligence artificial neural networks (ANNs). Energy Procedia, 139 , 778–784. https://doi.org/10.1016/j.egypro.2017.11.287
Moret, S. (2017). Strategic energy planning under uncertainty. Mechanical Engineering, 7961 (October), 268.
Mostafa, M. H., Aleem, S. H., Ali, S. G., Ali, Z. M., & Abdelaziz, A. Y. (2020). Techno-economic assessment of energy storage systems using annualized life cycle cost of storage (LCCOS) and levelized cost of energy (LCOE) metrics. Journal of Energy Storage, 29 , 101345. https://doi.org/10.1016/j.est.2020.101345
Mostafaeipour, A., Rezayat, H., & Rezaei, M. (2020). A thorough investigation of solar-powered hydrogen potential and accurate location planning for big cities: A case study. International Journal of Hydrogen Energy, 45 (56), 31599–31611. https://doi.org/10.1016/j.ijhydene.2020.08.211
Mousavi, S. A., Zarchi, R. A., Astaraei, F. R., Ghasempour, R., & Khaninezhad, F. M. (2021). Decision-making between renewable energy configurations and grid extension to simultaneously supply electrical power and fresh water in remote villages for five different climate zones. Journal of Cleaner Production . https://doi.org/10.1016/j.jclepro.2020.123617
NASA surface meteorology and solar energy. NASA Data Source 2018. Available from: https://Data.Nasa.Gov/Earth-Science/Surface-Meteorology-AndSolar-Energy/ .” n.d.
NASA surface meteorology and solar energy database. 2021.
Noorollahi, Y., Golshanfard, A., Ansaripour, S., Khaledi, A., & Shadi, M. (2021a). Solar energy for sustainable heating and cooling energy system planning in arid climates. Energy, 218 , 119421. https://doi.org/10.1016/j.energy.2020.119421
Noorollahi, Y., Itoi, R., Yousefi, H., Mohammadi, M., & Farhadi, A. (2017). Modeling for diversifying electricity supply by maximizing renewable energy use in Ebino City Southern Japan. Sustainable Cities and Society, 34 (June), 371–384. https://doi.org/10.1016/j.scs.2017.06.022
Noorollahi, Y., Lund, H., Nielsen, S., & Zinck, J. (2021b). Smart energy energy transition in petroleum rich nations: Case study of Iran. Smart Energy, 3 , 100026. https://doi.org/10.1016/j.segy.2021.100026
Prina, M. G., Lionetti, M., Manzolini, G., Sparber, W., & Moser, D. (2019). Transition pathways optimization methodology through EnergyPLAN software for long-term energy planning. Applied Energy, 235 (2018), 356–68. https://doi.org/10.1016/j.apenergy.2018.10.099
Prina, M. G., Moser, D., Vaccaro, R., & Sparber, W. (2020). EPLANopt optimization model based on EnergyPLAN applied at regional level: The future competition on excess electricity production from renewables. International Journal of Sustainable Energy Planning and Management, 27 , 35–50. https://doi.org/10.5278/ijsepm.3504
Qin, C., Yan, Q., & He, G. (2019). Integrated energy systems planning with electricity, heat and gas using particle swarm optimization. Energy, 188 , 116044. https://doi.org/10.1016/j.energy.2019.116044
Rad, M. A., Vaziri, R. G., Rahdan, P., Mousavi, S., & Arastounia, M. (2020). Techno-economic analysis of a hybrid power system based on the cost-effective hydrogen production method for rural electrification, a case study in Iran. Energy . https://doi.org/10.1016/j.energy.2019.116421
Rajabi, M., Golmehr, E., & Majid, D. (2011). Developing a TOPSIS-based model for hydropower dam site selection in Isfahan Province. Journal of Advanced Defense Science and Technology, 2 (4), 315–324.
Ravar, Z., Zahraie, B., Sharifinejad, A., Gozini, H., & Jafari, S. (2020). “System dynamics modeling for assessment of water–food–energy resources security and Nexus in Gavkhuni Basin in Iran. Ecological Indicators, 108 , 105682. https://doi.org/10.1016/j.ecolind.2019.105682
Rueda, R., Ruiz, L. G. B., Cuéllar, M. P., & Pegalajar, M. C. (2020). An ant colony optimization approach for symbolic regression using straight line programs. Application to energy consumption modelling. International Journal of Approximate Reasoning, 121 , 23–38. https://doi.org/10.1016/j.ijar.2020.03.005
Samadi, S. H., Ghobadian, B., & Nosrati, M. (2020). Prediction and estimation of biomass energy from agricultural residues using air gasification technology in Iran. Renewable Energy . https://doi.org/10.1016/j.renene.2019.10.109
Sorknæs, P., Lund, H., Skov, I. R., Djørup, S., Skytte, K., Morthorst, P. E., & Fausto, F. (2020). Smart energy markets—Future electricity, gas and heating markets. Renewable and Sustainable Energy Reviews . https://doi.org/10.1016/j.rser.2019.109655
Taghadomi-Saberi, S., & Razavi, S. J. (2019). Evaluating potential of artificial neural network and neurofuzzy techniques for global solar radiation prediction in Isfahan, Iran. Journal of Agricultural Science and Technology, 21 (2), 295–307.
Thellufsen, J. Z., Lund, H., Sorknæs, P., Østergaard, P. A., Chang, M., Drysdale, D., Nielsen, S., Djørup, S. R., & Sperling, K. (2020). Smart energy cities in a 100% renewable energy context. Renewable and Sustainable Energy Reviews . https://doi.org/10.1016/j.rser.2020.109922
Tsangas, M., Papamichael, I., & Zorpas, A. A. (2023). Sustainable energy planning in a new situation. Energies , 16 (4), 1626.
Vesta MAIS spatial energy model for the built environment. 2021. https://github.com/RuudvandenWijngaart/VestaDV .
Viesi, D., Luigi Crema, Md., Mahbub, S., Verones, S., Brunelli, R., Baggio, P., Fauri, M., et al. (2020). Integrated and dynamic energy modelling of a regional system: A cost-optimized approach in the deep decarbonisation of the province of Trento (Italy). Energy, 209 , 118378. https://doi.org/10.1016/j.energy.2020.118378
Woolley, E., Luo, Y., & Simeone, A. (2018). Industrial waste heat recovery: A systematic approach. Sustainable Energy Technologies and Assessments . https://doi.org/10.1016/j.seta.2018.07.001
Zhang, W., Maleki, A., Rosen, M. A., & Liu, J. (2018). Optimization with a simulated annealing algorithm of a hybrid system for renewable energy including battery and hydrogen storage. Energy, 163 , 191–207. https://doi.org/10.1016/j.energy.2018.08.112
Zoghi, M., Ehsani, A. H., Sadat, M., Javad Amiri, M., & Karimi, S. (2017). Optimization solar site selection by fuzzy logic model and weighted linear combination method in arid and semi-arid region: A case study Isfahan-IRAN”. Renewable and Sustainable Energy Reviews, 68 , 986–96. https://doi.org/10.1016/j.rser.2015.07.014
Download references
This research did not receive any specific grant from funding agencies in the public, commercial, or not-for-profit sectors.
Author information
Authors and affiliations.
Renewable Energies and Environmental Department, Faculty of New Sciences and Technologies, University of Tehran, Tehran, Iran
Mohammad Hossein Jahangir, Reza Mokhtari, Fatemeh Salmanpour & Hossein Yousefi
You can also search for this author in PubMed Google Scholar
Corresponding author
Correspondence to Mohammad Hossein Jahangir .
Additional information
Publisher's note.
Springer Nature remains neutral with regard to jurisdictional claims in published maps and institutional affiliations.
Rights and permissions
Springer Nature or its licensor (e.g. a society or other partner) holds exclusive rights to this article under a publishing agreement with the author(s) or other rightsholder(s); author self-archiving of the accepted manuscript version of this article is solely governed by the terms of such publishing agreement and applicable law.
Reprints and permissions
About this article
Jahangir, M.H., Mokhtari, R., Salmanpour, F. et al. Urban energy planning towards achieving an economically and environmentally optimized energy flow by 2050 based on different scenarios (a case study). Environ Dev Sustain (2024). https://doi.org/10.1007/s10668-024-04754-8
Download citation
Received : 17 April 2022
Accepted : 07 March 2024
Published : 05 April 2024
DOI : https://doi.org/10.1007/s10668-024-04754-8
Share this article
Anyone you share the following link with will be able to read this content:
Sorry, a shareable link is not currently available for this article.
Provided by the Springer Nature SharedIt content-sharing initiative
- Hybrid renewable energy systems
- Energy planning
- Decarbonization
- Zero energy buildings
Advertisement
- Find a journal
- Publish with us
- Track your research
Print Preview
U.s. department of energy - energy efficiency and renewable energy, alternative fuels data center.
- Printable Version
- Production & Distribution
- Research & Development
- Benefits & Considerations
- Laws & Incentives
Electric Vehicle Research and Development
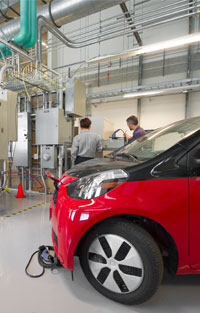
The U.S. Department of Energy (DOE) is working with its partners in the public and private sectors to research, develop, demonstrate, and deploy technologies that enhance the performance of electric-drive vehicles.
Environmental and Market Analysis
Electric vehicles (EVs) and hybrid electric vehicles (HEVs) offer reduced operating costs, fuel savings, and environmental benefits. For example, the National Renewable Energy Laboratory (NREL) and Idaho National Laboratory report, Levelized Cost of Charging Electric Vehicles in the United States , finds that driving an EV instead of a comparable conventional vehicle, can save a driver as much as $14,500 on fuel costs over 15 years. Additionally, Argonne National Laboratory’s (ANL) Autonomie model demonstrates that an all-electric vehicle is more than three times more efficient than its conventional counterpart. In Assessment of Light-Duty Plug-In Electric Vehicles in the United States, 2010-2019 , ANL estimates the electricity generation for the operation of an all-electric vehicle produces 53% less emissions than the tailpipe emissions from the operation of a gasoline vehicle.
Additionally, ANL’s An Extensive Study on Sizing, Energy Consumption, and Cost of Advanced Vehicle Technologies report demonstrates significant improvements in fuel economy over time. By 2045, HEVs could achieve a 43% to 81% improvement in fuel economy and PHEVs could achieve a 73% to 96% improvement in fuel economy. In its National Economic Value Assessment of Plug-In Electric Vehicles , NREL uses a scenario approach to estimate costs and benefits of increased EV market growth across the United States. Under various EV adoption scenarios, NREL estimates a 22% to 36% decrease in greenhouse gas emission reductions.
Further, the widespread adoption of EVs will require a robust network of charging stations, from home-based AC charging to DC powered extreme fast charging. Researchers are examining opportunities and impacts associated with a full range of charging technologies.
See the following resources for more information related to research in environmental and market analysis:
- Electric Vehicle Grid Integration
- Vehicle Electrification
- Transportation Energy Data Book: Edition 40
- National Economic Value Assessment of Plug-In Electric Vehicles
- An Extensive Study on Sizing, Energy Consumption, and Cost of Advanced Vehicle Technologies
- Cradle-to-Grave Lifecycle Analysis of U.S. Light-Duty Vehicle-Fuel Pathways: A Greenhouse Gas Emissions and Economic Assessment of Current (2015) and Future (2025-2030) Technologies
Maps & Data
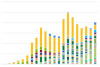
More Electricity Data | All Maps & Data
Case Studies
- Electric School Buses Clear the Air in the Mid-Atlantic
- Seattle Rideshare Fleet Adds EVs, Enjoys Success
- Massachusetts School Fleets Get Answers through Electric Bus Testing
More Electricity Case Studies | All Case Studies
Publications
- Electric Vehicles (Conceptos básicos sobre los vehículos eléctricos)
- Duluth Transit Authority Battery-Electric Bus Evaluation
- Foothill Transit Battery Electric Bus Demonstration Results: Second Report
More Electricity Publications | All Publications
- Laws and Incentives Search
- Electric Vehicle Charging Station Locations
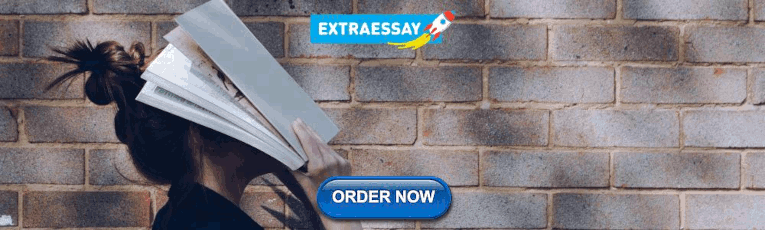
IMAGES
VIDEO
COMMENTS
climate, the world must increase its renewable energy development dramatically as the centerpiece of this expansion. However, all energy sources present trade-offs. Without careful planning of the world's needed energy development, the resulting "energy sprawl"—the amount of new land and water area needed to produce energy—could cause
Myanmar has one of the lowest electrification rates in the world, and most of its inhabitants, who lack access to electricity, live off-grid in rural areas. Despite Myanmar having abundant sun and wind energy resources, which could potentially generate electricity for rural communities, renewable energy growth in Myanmar is stunted. In this article, we examine the case study of renewable ...
[email protected]. McKinsey estimates that by 2026, global renewable-electricity capacity will rise more than 80 percent from 2020 levels (to more than 5,022 gigawatts). 1 Of this growth, two-thirds will come from wind and solar, an increase of 150 percent (3,404 gigawatts). By 2035, renewables will generate 60 percent ...
Herein, I methodically optimize a distributed energy resource in terms of the production, management, utilization, and/or transaction of renewable energies during the deployment process. I deliver ...
This paper aims to summarize the relationship between renewable energy data, analysis, and decision making for developing countries seeking to transition their energy sector and to consider Bangladesh as a case study of a country that has worked with development organizations and the National Renewable Energy Laboratory to develop a national ...
National Renewable Energy Laboratory 4. National Energy Technology Laboratory 5. Idaho National Laboratory ... These case studies can help inform the development of an interactive decision framework to guide transitions in energy-dependent communities. This is discussed in Chapter 9. The decision framework will look at
The United States, China, Australia, and the United Kingdom have all successfully developed renewable energy storage systems. Sun et al. conducted a study of these countries to determine the policies and market mechanisms that could help other countries promote their own energy storage deployments. "Energy storage development is an essential ...
About the authors. McKinsey estimates that between 2021 and 2030, planned global electricity generation from committed solar and on- and offshore wind projects (excluding China) will more than triple, from 125 gigawatts to 459 gigawatts (Exhibit 1). 1 This could further accelerate as countries seek to make renewables part of their strategy to ...
This requires moving away from a "one size fits all" approach. This case study is relevant to wind developers, energy regulators, local communities and renewable energy-focused non-government organizations. It is applicable beyond Australia to all contexts where wind farm development has encountered conflicted societal acceptance responses.
Renewable Energy Policy in Cities: Selected Case Studies. Chemnitz, Germany where the local government enabled the formulation of strategies to use renewable sources and in 2008 developed the Integrated Climate Protection Programme (Integriertes Klimaschutzprogramm). Belo Horizonte, Brazil which has reduced greenhouse gas (GHG) emission ...
impact of renewable energy on rural development. Drawing on case studies of renewable energy in 16 rural regions across Europe and North America, the report shows that renewable energy does not automatically create employment in rural regions. For renewable energy to trigger rural economic growth requires a coherent policy framework and the ...
By 2019, it had become the world's largest producer of offshore-wind energy. The company also raised its renewable-generation share to 86 percent—hitting its target 21 years ahead of schedule. In an interview with McKinsey, the CEO of Ørsted's offshore-wind business, Martin Neubert, tells the story of the company's transformation: the ...
In this article, we examine the case study of renewable energy development in Myanmar to better understand the factors that influence renewable energy development and deployment in a developing country context. Our analysis reveals that there are numerous reasons for the lack of solar, wind, and biomass energy growth in Myanmar, such as ...
In response, we offer an interdisciplinary framework that aims to organize existing knowledge and lessons learned from energy development. Specifically, we developed the Meaningful Marine Renewable Energy (MRE) Development Framework and conducted a literature review using MRE as a case study. MRE was chosen because it is a nascent renewable ...
The paper presents a case study of Crete, showcasing how the REV idea can work in real life. ... Renewable energy valleys (REVs) represent a transformative concept poised to reshape global energy ...
Smart city approaches. Cities are the fastest growing form of settlement worldwide requiring sustainable energy systems to deal with their increasing density and size [].Although urban population growth in developed countries (0.5 %) is projected to be below population growth in less developed countries (2.3 %) from 2007 to 2025, there is a general shift from rural to urban areas; 60 % or 5 ...
Renewable Energy and Inclusive Economic Development: An African Case Study. D. Yuni, N. Ezenwa, +2 authors. Khothalo Mohlori-Sepamo. Published in International Journal of… 30 October 2023. Environmental Science, Economics. Climate change constitutes a major challenge to economic growth and development across the globe as it negatively affects ...
Humanities and Social Sciences Communications - Green finance, renewable energy development, and climate change: evidence from regions of China. ... a case study of United Arab Emirates. J Clean ...
Regarding prevailing methods, 19 papers present literature reviews that seek to go beyond case studies to map trends in conceptual framings, methods, and empirical findings (Table 1). For example, Segreto et al. (2020) identify the key determinants of local and general social acceptance of renewable energy projects.
Brookfield's newly opened mixed-use complex in Shanghai, One East, uses renewable energy to collaborate on net zero-strategies with tenants. During construction, Brookfield installed solar panels on the roof of the complex's retail area, which is visible to the tenants of both office towers. The solar panels generate 260 MWh of energy per ...
Cost Savings: $300,000 (@$0.15/kWh) Cost: $12.25 million. The Housing Authority of the County of Santa Barbara (HACSB) has successfully implemented a portfolio-wide renewable energy strategy offsetting 100% of the electrical consumption at 21 properties and HACSB's administration buildings. The 1.7 mW project involved the installation of over ...
Renewable energy is a crucial and necessary means of attaining sustainable development. Nevertheless, relying on renewable energy sources is not always sus. ... Requirements of sustainable renewable energy systems case study (Samawah city) Hayder Lafta Albdiery; Hayder Lafta Albdiery a) 1. ...
The case study is based on Togo renewable energy sustainability and employs qualitative and quantitative research to achieve the result. The study stems from the fact that Togo like most African countries has a lot of natural resources such as solar, wind and hydro-electric power required to implement a nationwide sustainable energy system.
Barriers and solutions to the development of renewable energy technologies in the Caribbean. (April) [Google Scholar ... Lin Y. To re-explore the causality between barriers to renewable energy eevelopment: A case study of wind energy. Energies. 2013; 6 (9):4465-4488. [Google Scholar] Izadbakhsh M., Gandomkar M., Rezvani A., Ahmadi A. Short ...
Contested visions of sustainable development in conflicts over renewable energy, land, and human rights: A case study of Unión Hidalgo, Mexico ... however, suggest the polyvalence of human rights, but that they are highly contingent - in this case, critically, part of social mobilisation, domestic litigation, and extra-territorial ...
Renewable energy derived from natural resources, is less harmful to the environment than fossil fuels and serves as an alternative to traditional energy sources (Dey et al. 2022).Renewable energy in buildings refers to the integration of sustainable energy sources, such as solar, wind, geothermal, and biomass, into the full building life cycle of design, construction, operation, and ...
Energy is one of the most important aspects of urban development (Tsangas et al., 2023).However, Energy consumption, and in particular the burning of fossil fuels, is the main source of greenhouse gas emissions The participation of renewable energy can lead to emission reduction, reaching the sustainable development goals, diversing the energy basket and create a balance of demand and supply ...
The multi-generation systems with simultaneous production of power by renewable energy, in addition to polymer electrolyte membrane electrolyzer and fuel cell (PEMFC-PEMEC) energy storage, have become more and more popular over the past few years. The fresh water provision for PEMECs in such systems is taken into account as one of the main challenges for them, where conventional desalination ...
Development of renewable energy has become increasingly important in recent years due to the negative impact of fossil fuels on the environment. ... ensure access to this page indefinitely. Copy URL. Copy DOI. Potential Applications of Innovative Ai-Based Tool in Hydrogen Energy Development: A Case Study of Chatgpt. 13 Pages Posted: 6 Apr 2024 ...
By 2045, HEVs could achieve a 43% to 81% improvement in fuel economy and PHEVs could achieve a 73% to 96% improvement in fuel economy. In its National Economic Value Assessment of Plug-In Electric Vehicles, NREL uses a scenario approach to estimate costs and benefits of increased EV market growth across the United States.