
An official website of the United States government
The .gov means it’s official. Federal government websites often end in .gov or .mil. Before sharing sensitive information, make sure you’re on a federal government site.
The site is secure. The https:// ensures that you are connecting to the official website and that any information you provide is encrypted and transmitted securely.
- Publications
- Account settings
Preview improvements coming to the PMC website in October 2024. Learn More or Try it out now .
- Advanced Search
- Journal List
- v.15(2); 2023 Feb

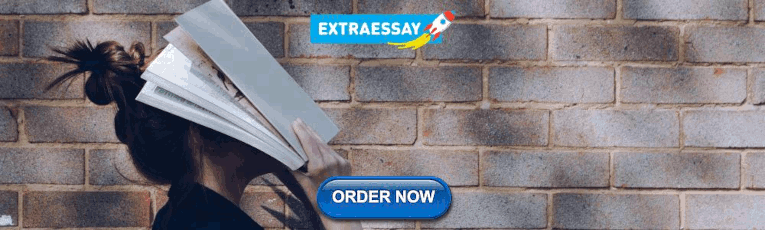
Biomedical Waste Management and Its Importance: A Systematic Review
Himani s bansod.
1 Community Medicine, Jawaharlal Nehru Medical College, Datta Meghe Institute of Higher Education and Research, Wardha, IND
Prasad Deshmukh
2 Head and Neck Surgery, Jawaharlal Nehru Medical College, Datta Meghe Institute of Higher Education and Research, Wardha, IND
The waste generated in various hospitals and healthcare facilities, including the waste of industries, can be grouped under biomedical waste (BMW). The constituents of this type of waste are various infectious and hazardous materials. This waste is then identified, segregated, and treated scientifically. There is an inevitable need for healthcare professionals to have adequate knowledge and a proper attitude towards BMW and its management. BMW generated can either be solid or liquid waste comprising infectious or potentially infectious materials, such as medical, research, or laboratory waste. There is a high possibility that inappropriate management of BMW can cause infections to healthcare workers, the patients visiting the facilities, and the surrounding environment and community. BMW can also be classified into general, pathological, radioactive, chemical, infectious, sharps, pharmaceuticals, or pressurized wastes. India has well-established rules for the proper handling and management of BMW. Biomedical Waste Management Rules, 2016 (BMWM Rules, 2016) specify that every healthcare facility shall take all necessary steps to ensure that BMW is handled without any adverse effect on human and environmental health. This document contains six schedules, including the category of BMW, the color coding and type of containers, and labels for BMW containers or bags, which should be non-washable and visible. A label for the transportation of BMW containers, the standard for treatment and disposal, and the schedule for waste treatment facilities such as incinerators and autoclaves are included in the schedule. The new rules established in India are meant to improve the segregation, transportation, disposal methods, and treatment of BMW. This proper management is intended to decrease environmental pollution because, if not managed properly, BMW can cause air, water, and land pollution. Collective teamwork with committed government support in finance and infrastructure development is a very important requirement for the effective disposal of BMW. Devoted healthcare workers and facilities are also significant. Further, the proper and continuous monitoring of BMW is a vital necessity. Therefore, developing environmentally friendly methods and the right plan and protocols for the disposal of BMW is very important to achieve a goal of a green and clean environment. The aim of this review article is to provide systematic evidence-based information along with a comprehensive study of BMW in an organized manner.
Introduction and background
The amount of daily biomedical waste (BMW) produced in India is enormous [ 1 ]. People from all segments of society, regardless of age, sex, ethnicity, or religion, visit hospitals, which results in the production of BMW, which is becoming increasingly copious and heterogeneous [ 2 ]. BMW produced in India is about 1.5-2 kg/bed/day [ 3 ]. BMW include anatomical waste, sharps, laboratory waste, and others and, if not carefully segregated, can be fatal. Additionally, inappropriate segregation of dirty plastic, a cytotoxic and recyclable material, might harm our ecosystem [ 4 ]. Earlier, BMW was not considered a threat to humans and the environment. In the 1980s and 1990s, fears about contact with infectious microorganisms such as human immunodeficiency virus (HIV) and hepatitis B virus (HBV) prompted people to consider the potential risks of BMW [ 5 ]. BMW is hazardous in nature as it consists of potential viruses or other disease-causing microbial particles; it may be present in human samples, blood bags, needles, cotton swabs, dressing material, beddings, and others. Therefore, the mismanagement of BMW is a community health problem. The general public must also take specific actions to mitigate the rising environmental degradation brought on by negligent BMW management. On July 20, 1998, BMW (Management and Handling) Rules were framed. On March 28, 2016, under the Environment (Protection) Act, 1986, the Ministry of Environment and Forest (MoEF) implemented the new BMW Rules (2016) and replaced the earlier one (1988). BMW produced goes through a new protocol or approach that helps in its appropriate management in terms of its characterization, quantification, segregation, storage, transport, and treatment.
According to Chapter 2 of the Medical Waste Management and Processing Rules, 2016, “The BMW could not be mixed with other wastes at any stage while producing inside hospitals, while collecting from hospitals, while transporting, and should be processed separately based on classification.” The COVID-19 pandemic has now transformed healthy societies worldwide into diseased ones, resulting in a very high number of deaths. It also created one significant problem: improper handling of the medical waste produced in the testing and treatment of the disease [ 6 ]. In India, BMW generated due to COVID-19 contributed to about 126 tonnes per day out of the 710 tonnes of waste produced daily [ 7 ].
The basic principle of the management of BMW is Reduce, Reuse, and Recycle-the 3Rs. Out of the total amount of BMW generated, 85% is general (non-hazardous) waste, and the remaining 15% is hazardous. As BMW contains sharps and syringes, the pathogens can enter the human body through cuts, abrasions, puncture wounds, and other ways. There might also be chances of ingestion and inhalation of BMW, which can lead to infections. Some examples of infections are Salmonella, Shigella, Mycobacterium tuberculosis, Streptococcus pneumonia, acquired immunodeficiency syndrome (AIDS), hepatitis A, B, and C, and helminthic infections [ 8 ]. This systematic review is conducted to obtain essential, up-to-date information on BMW for the practical application of its management. The highlight of the management of BMW is that the “success of BMW management depends on segregation at the point of generation” [ 9 ].
The findings have been reported following the principles and criteria of the Preferred Reporting Items for Systematic Review and Meta-Analysis (PRISMA). The systematic review has been conducted according to these standards and principles.
Search Sources/Search Strategy
We used the MeSH strategy to obtain articles from PubMed and ResearchGate employing the following terms: (“Biomedical/waste” [Majr] OR “Biomedical Waste/source” [Majr] OR “Biomedical Waste/hazards” [Majr] OR “Biomedical Waste/segregation” [Majr] OR “Biomedical Waste/rules” [Majr] OR “Biomedical Waste/laws” [Majr] OR “Biomedical Waste/environment” [Majr]). Specifically, for management-related studies, the search terms (“Management/steps” [Majr] OR “Management/handling” [Majr] OR “Management/coding” [Majr] OR “Color coding/segregation” [Majr] OR “Treatment/method” [Majr] OR “Autoclaving/waste” [Majr] OR “Incineration/waste” [Majr]) were used. We obtained the most pertinent research papers and used them in different arrangements using the Boolean operators “AND” and “OR.”
Inclusion and exclusion criteria
We focused on papers written in the English language, published within the last decade, relevant to the central questions of this review article, and that are systematic reviews such as randomized clinical trials and observational studies. We, however, excluded papers published in languages other than English, irrelevant to the questions, and related to topics other than BMW.
Search outcomes
After the initial screening, we narrowed the search results down to 264 papers. A total of 42 duplicate papers were removed. Subsequently, publications were refined by the title/abstract, and we eliminated a few studies due to the lack of full text and/or related articles. Finally, after assessing 27 items for eligibility, we included 11 papers in our review. Figure Figure1 1 is the flow chart for article selection formulated on PRISMA.

PRISMA: Preferred Reporting Items for Systematic Review and Meta-analysis, PMC: PubMed Central
Need for BMW management in hospitals
BMW threatens the health of medical staff, hospital-visiting patients, and people in the nearby community. Improper disposal leads to severe hospital-acquired diseases along with an increased risk of air and water pollution. Due to open-space waste disposal practices, animals and scavengers might get infected, leading to the scattering of waste and the spreading of infections. In countering such activities, four major principle functions of BMW management are applicable: the placement of bins at the source of generation of BMW, segregation of BMW, removal or mutilation of the recyclable waste, and disinfection of the waste [ 10 ]. BMW management methods aim predominantly to avoid the generation of waste and, if generated, then recover as much as possible [ 11 ].
BMW management rules in India
On March 28, 2016, under the Environment (Protection) Act, 1986, the MoEF notified the new BMW Rules, 2016 and replaced the earlier Rules (1988). BMW produced goes through a new protocol or approach which helps in the appropriate management of waste, i.e., its characterization, quantification, segregation, storage, transport, and treatment, all of which aim to decrease environmental pollution [ 12 ]. Problems with the improper management of BMW also shed light on the scavengers who, for recycling, segregate the potentially hazardous BMW without using gloves or masks. Strict rules have been implemented to ensure that there is no stealing of recyclable materials or spillage by some humans or animals and that it is transported to the common BMW treatment facility [ 10 ]. The first solution to stop the spread of hazardous and toxic waste was incineration. Incineration is required in all hospitals and healthcare facilities that produce BMW. However, due to the absence of services that provide certified incinerators in a few countries, BMW has to be sent to landfills, which leads to land contamination and harms the environment [ 13 ]. Incinerators used for disposal might also lead to environmental pollution. Numerous toxins are formed during incineration, which are the products of incomplete combustion. Thus, some new standards have been issued to resolve this problem and safeguard the environment and public health [ 14 ].
Steps in the management of BMW
BMW management needs to be organized, as even a single mistake can cause harm to the people in charge. There are six steps in the management of BMW [ 15 ]: surveying the waste produced; segregating, collecting, and categorizing the waste; storing, transporting, and treating the waste. Segregation is the separation of different types of waste generated, which helps reduce the risks resulting from the improper management of BMW. When the waste is simply disposed of, there is an increased risk of the mixture of waste such as sharps with general waste. These sharps can be infectious to the handler of the waste. Further, if not segregated properly, there is a huge chance of syringes and needles disposed of in the hospitals being reused. Segregation prevents this and helps in achieving the goal of recycling the plastic and metal waste generated [ 16 ]. According to Schedule 2, waste must be segregated into containers at the source of its generation, and according to Schedule 3, the container used must be labeled. The schedules of BMW (Management and Handling) Rules, 1998, which were initially ten in number, have now been reduced to four [ 17 ]. The collection of BMW involves the use of different colors of bins for waste disposal. The color is an important indicator for the segregation and identification of different categories of waste into suitable-colored containers. They must be labeled properly based on the place they have been generated, such as hospital wards, rooms, and operation theatres. It is also very important to remember that the waste must be stored for less than 8-10 hours in hospitals with around 250 beds and 24 hours in nursing homes. The storage bag or area must be marked with a sign [ 16 ].
Figure Figure1 1 shows the biohazard signs that symbolize the nature of waste to the general public.

Biohazards are substances that threaten all living things on earth. The biohazard symbol presented in Figure Figure1 1 was remarked as an important public sign, signaling the harms and hazards of entering the specified zone or room [ 18 ]. Along with the biohazard sign, the room door must have a label saying “AUTHORISED PERSONNEL ONLY.” The temporary storage room must always be locked and away from the general public's reach. The waste is then collected by the vehicles daily. A ramp must be present for easy transportation. The waste collected is then taken for treatment. The loading of wastes should not be done manually. It is very vital to properly close or tie the bag or the container to avoid any spillage and harm to the handlers, the public, and the environment. The transport vehicle or trolley must be properly covered, and the route used must be the one with less traffic flow [ 19 ].
BMW handling staff should be provided with personal protective equipment (PPE), gloves, masks, and boots. BMW retrievers must be provided with rubber gloves that should be bright yellow. After usage, the importance of disinfecting or washing the gloves twice should be highlighted. The staff working in or near the incinerator chamber must be provided with a non-inflammable kit. This kit consists of a gas mask that should cover the nose and mouth of the staff member. The boots should cover the leg up to the ankle to protect from splashes and must be anti-skid [ 16 ]. According to the revised BMW management rules, 2016, it is mandatory to provide proper training to healthcare facility staff members on handling BMW. The training should be mandatorily conducted annually. Along with the management step of the color coding for segregation, it is also important for the staff to be trained in record keeping. This practice of record-keeping helps track the total amount of waste generated and the problems that occurred during the management process, thus helping improve segregation, treatment, and disposal [ 20 ].
Color coding for segregation of BMW
Color coding is the first step of BMW management. Different wastes are classified into different types, and therefore, they must be handled and disposed of according to their classification. The bins used for waste disposal in all healthcare facilities worldwide are always color-coded. Based on the rule of universality, bins are assigned a specific color, according to which the waste is segregated. This step helps avoid the chaos that occurs when all types of waste are jumbled, which can lead to improper handling and disposal and further result in the contraction of several diseases [ 21 ]. The different kinds of categories of waste include sharp waste such as scalpels, blades, needles, and objects that can cause a puncture wound, anatomical waste, recyclable contaminated waste, chemicals, laboratory waste such as specimens, blood bags, vaccines, and medicines that are discarded. All the above-mentioned wastes are segregated in different colored bins and sent for treatment [ 22 ]. Yellow bins collect anatomical waste, infectious waste, chemical waste, laboratory waste, and pharmaceutical waste, covering almost all types of BMW. Different bins and various types of sterilization methods are used depending on how hazardous the waste is. The best tools for sterilization are autoclaves. Red bins collect recyclable contaminated wastes, and non-chlorinated plastic bags are used for BMW collection. Blue containers collect hospital glassware waste such as vials and ampoules. White bins are translucent where discarded and contaminated sharps are disposed of. Sharp wastes must always be disposed of in puncture-proof containers to avoid accidents leading to handlers contracting diseases [ 23 , 24 ].
Figure Figure3 3 illustrates the different colored bins used for the segregation of BMW.

BMW management refers to completely removing all the hazardous and infectious waste generated from hospital settings. The importance of waste treatment is to remove all the pathogenic organisms by decontaminating the waste generated. This helps in the prevention of many severe health-related issues that can be caused because of the infective waste. It is a method used to prevent all environmental hazards [ 25 ].
Methods for the treatment of BMW
There are many methods that are used for the treatment of BMW. One of the most economical ways of waste treatment is incineration, which is just not some simple “burning” but the burning of waste at very high temperatures ranging from 1800℉ to 2000℉ to decrease the total mass of decontaminated waste by converting it into ash and gases, which is then further disposed of in landfills [ 25 , 26 ]. Important instructions associated with the use of incinerators are as follows: chlorinated plastic bags must not be put inside the incinerators as they can produce dioxin [ 26 ]. Metals should not be destroyed in an incinerator. The metals present in BMW are made of polyvinyl chloride. When these metals are burned, they produce a huge amount of dioxin. Dioxins are very toxic chlorinated chemical compounds, as dioxins, when released into the environment, can lead to environmental pollution and a higher incidence of cancer and respiratory manifestations [ 14 ].
Autoclaving is an alternate method of incineration. The mechanism of this process involved sterilization using steam and moisture. Operating temperatures and time of autoclaving is 121℃ for 20-30 minutes. The steam destroys pathogenic agents present in the waste and also sterilizes the equipment used in the healthcare facility [ 25 ]. Autoclaving has no health impacts and is very cost-friendly. It is recommended for the treatment of disposables and sharps, but the anatomical, radioactive, and chemical wastes must not be treated in an autoclave [ 27 ]. Chemical methods are the commonest methods that include chemicals such as chlorine, hydrogen peroxide, and Fenton’s reagent. They are used to kill the microorganisms present in the waste and are mainly used for liquid waste, such as blood, urine, and stool. They can also be used to treat solid waste and disinfect the equipment used in hospital settings and surfaces such as floors and walls [ 28 ]. Thermal inactivation is a method that uses high temperatures to kill the microorganisms present in the waste and reduce the waste generated in larger volumes. The temperature differs according to the type of pathogen present in the waste. After the treatment is done, the contents are then discarded into sewers [ 29 ].
Very serious environmental and health hazards can be triggered if hospital waste is mixed with normal garbage, which can lead to poor health and incurable diseases such as AIDS [ 30 ]. The needle sticks can be highly infectious if discarded inappropriately. Injury by these contaminated needles can lead to a high risk of active infection of HBV or HIV [ 31 ]. The groups at increased risk of getting infected accidentally are the medical waste handlers and scavengers. Sharps must properly be disposed of in a translucent thin-walled white bin. If sharps are discarded in a thin plastic bag, there is a high chance that the sharps might puncture the bag and injure the waste handler [ 32 ]. It can also be the main cause of severe air, water, and land pollution. Air pollutants in BMW can remain in the air as spores. These are known as biological air pollutants. Chemical air pollutants are released because of incinerators and open burning. Another type of threat is water pollutants. BMW containing heavy metals when disposed of in water bodies results in severe water contamination. The landfills where the disposal takes place must be constructed properly, or the waste inside might contaminate the nearby water bodies, thus contaminating the drinking water. Land pollution is caused due to open dumping [ 33 ]. BMW must also be kept away from the reach of rodents such as black rats and house mice, which can spread the pathogens to the people living nearby [ 34 ].
Many promising steps were taken to minimize the volume of waste discarded from the source, its treatment, and disposal. The 3R system encourages the waste generators to reuse, reduce, and recycle. Everyone must be aware of the 3Rs because this approach can help achieve a better and cleaner environment [ 35 ]. Unfortunately, most economically developing countries cannot correctly manage BMW. Very few staff members of healthcare facilities are educated about proper waste management. The waste handlers are also poorly educated about the hazards of waste [ 36 ]. Every member helping in the waste management process must be made aware of the dangers of BMW to avoid accidents that harm the environment and living beings [ 37 ].
Conclusions
BMW is generated by healthcare facilities and can be hazardous and infectious. Improper handling can lead to health hazards. Collection, segregation, transportation, treatment, and disposal of BMW are important steps in its management. The color coding of bins, the use of technologies such as incineration and autoclaving, and attention to environmental impacts are also highly crucial. BMW management aims to reduce waste volume and ensure proper disposal. All those involved should strive to make the environment safer.
The content published in Cureus is the result of clinical experience and/or research by independent individuals or organizations. Cureus is not responsible for the scientific accuracy or reliability of data or conclusions published herein. All content published within Cureus is intended only for educational, research and reference purposes. Additionally, articles published within Cureus should not be deemed a suitable substitute for the advice of a qualified health care professional. Do not disregard or avoid professional medical advice due to content published within Cureus.
The authors have declared that no competing interests exist.
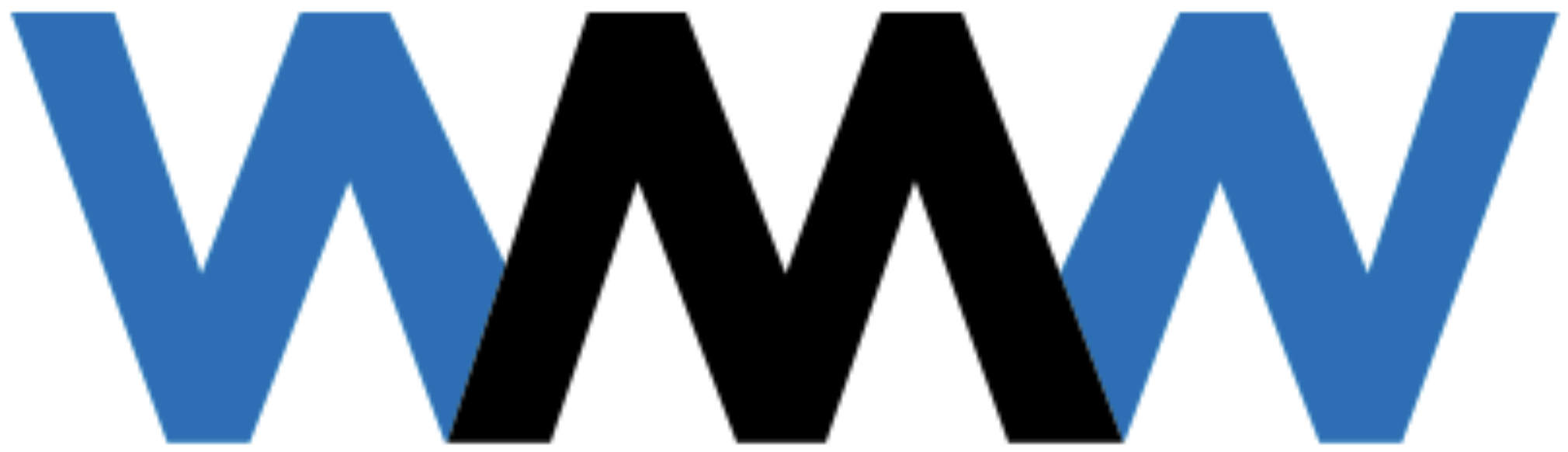
Reuse : System Change to Circular Economy and its Impact on the Waste Management Sector
The European Union has set ambitious climate change mitigation goals which it aims to achieve through measures that include the development of its economic system into a circular economy. Recycling has been misunderstood as the main pillar of a circular economy and therefore the importance of the waste management sector has been overestimated. Recent research by the author in a publicly funded project by the German Environmental Agency (Umweltbundesamt) ‘Weiße Ware Wiederverwenden’ (Reuse of White Goods) shows that while the waste management sector has a role to play, the groundwork towards a circular economy must be done by industry, especially by the producers of all kind of products. Their designs will determine the role of waste management in the future. After use, products will remain in newly developed CER twigs to direct the invested environmental costs in kg CO2e back to earlier points of the life cycle, following the pathways of the lowest emissions. Therefore, less and different waste will reach the traditional waste sector streams. The role of the waste management sector of the future will depend on changes in the system boundaries. Until this is decided, waste management must deal with the waste of yesterday and adapt to the waste of tomorrow.
Introduction: Green Deal and Circular Economy
With the European Green Deal the European Union and its Member States are striving to become the first climate-neutral continent in the near future. To reach this aim, ‘The European Commission has adopted a set of proposals to make the EU's climate, energy, transport and taxation policies fit for reducing net greenhouse gas emissions by at least 55% by 2030, compared to 1990 levels.’ (European Commission 2021) The reduction of these emissions should be done intelligently and not just in the form of radical restrictions on consumption. The EU wants to be climate-neutral by 2050, which means reducing CO2 emissions dramatically. (European Commission 2019)
There can be no ‘business as usual’ and merely cosmetic measures will not help to stop climate change and thus not prevent damage that is devastating to humanity. Policies alone are not sufficient to reach the goals. Significant efforts by all social groups, authorities and all economic sectors are necessary to achieve the reduction goal. Specifically, the Commission and the Member States intend to implement a circular economy that fulfils the task of reducing emissions as a new form of economy. (Directorate-General for Environment 2023a)
Producers, consumers and the waste management sector must play their part (climate change mitigation) in order for this to be successful and progress is not yet fast enough, according to the European Commission. (Directorate-General for Environment 2023b)
If we look at the detail, however, the situation is not that easy. We still lack knowledge of exactly how to manage products in circles so that products or parts of products follow the life cycle paths that cause the lowest emissions. Research on how to establish a circular economy for white goods determined that this would result in a large number of circles going back to different points in a life cycle for each product. (Ludwig et al. 2023) In the current discussion on how to realise a circular economy, ‘ecological design’ and ‘recycling’ are well-known keywords, while other important methods for creating a circular economy are hardly considered.
This means that totally new industries must be developed to close the circles. If that is so, the question is how this would change the current market players like the waste management sector. There was a slight misunderstanding caused by the EU’s Circular Economy Action Plan (CEAP) amending the EU Waste Directive in 2015 (European Parliament 2008), where the waste management sector would be the main player in a circular economy and therefore the recycling of materials was seen as a synonym for a circular economy. (Directorate-General for Environment 2023b) Even though the ‘waste hierarchy’ mentioned ‘prevention’ and ‘preparing for re-use’ as processes for handling products before they lose the functionality of their intended use, the directive, its amendments and the communication of the European Commission and the European Parliament always refer directly to the waste management sector and material recycling targets. This is a mistake that is also made in relation to communication about the circular economy. (European Parliament 2023)
Related article: Do a circular economy and fast fashion go together?
Discover now
- The Global Waste Management Outlook 2024 Apr 5, 2024
- More with less Apr 5, 2024
- Partitalia, interconnected IoT solutions for PAYT at IFAT 2024 Apr 4, 2024
- Take on Trash: global waste management TV #1 Jul 5, 2023
- #ohneFilter - Die Runde der Chefredakteur:innen Mar 24, 2022
- Cesaro Mac - Way of the Tiger Jan 4, 2022
However, ‘recycling’ is just one of the lower levels of a circular economy. (Ludwig et al. 2023:58) Recycling means that invested environmental costs which were necessary to create a product are lost forever. Environmental costs are not calculated in euros, their ‘currency’ is (at the moment mainly) kg CO2e. It is beyond doubt that recycled materials often have fewer environmental costs compared to newly mined materials, taken directly from nature and processed industrially to obtain usable pure materials, like aluminium or copper. (Franken, Vasters 2000:7) But this fact does not justify focusing mainly on recycling when talking about a circular economy. Furthermore, calculations as to how much material industry would obtain in the future from the recycling sector do not take into account the system change a circular economy really implies, instead merely calculating the hunger for input materials in current industrial production and expected growth in the years to come. (European Aluminium 2020) Producers of industrial input materials, whether they produce e.g. aluminium from bauxite or scrap, have the opinion that a circular economy means just obtaining more scrap in purer fractions and they assign the role of ensuring purer fractions to the waste management sector (complaining at the same time that they themselves will need to invest a huge amount of money, which is in reality just investing in the growth of their business model and not an investment in a circular economy). (Siebel 2022)
This might all be very true if recycling would be the main and most important measure for realising a circular economy. But it isn’t. Why? Because if it is not done at the right position in a circular economy, recycling wipes out environmental investment. At present (in 2023), recycling takes place within the traditional linear economy. Recycling is generally a good idea, but if implemented within an otherwise linear economy it hinders the development of a circular economy. Still, policymakers continue to favour recycling.
Related article: UN report: World must move beyond waste era and turn rubbish into resource
The advantages for policymakers to mainly favour legislation for recycling are apparent:
- Recycling already takes place; the technology exists and recycling machine producers promise to develop and produce even more effective sorting machines etc. for waste markets.
- Recycling rates are low, which means that growth could be achieved easily by forcing the market players to organise higher recycling rates, so that new laws show an impact relatively quickly.
- Recycling is one of the easiest methods to fulfil obligations concerning extended producer responsibility (EPR). With EPR, policymakers intend to make ‘producers responsible for the entire life cycle of the products that they introduce on the market, from their design until end of life (including waste collection and recycling)’. (OECD 2016) Producers do not need to do much to really be responsible for the circularity of their products. They just transfer money to the waste management sector to collect and recycle 1 . Increased recycling rates could be achieved and be shown as a success by policymakers, even though this does not mean a shift away from linear to circular economy.
- Recycling can be carried out by the waste management sector; no real system change is needed.
- Policymakers could always find positive examples of recycling to show to the public to give the impression of closing circles, though this might even for policymakers be a form of self-deception. The huge amount of material prevents them from seeing the real scale of the problem and enables them to ignore or defer it.
- Recycling is an easy option, especially for the packaging waste that people are faced with daily. For policymakers, recycling efforts are therefore an easy way to show people that they care and to tell the story that, even though nothing has really changed, people can consume and carry on like always with a less guilty conscience.
- Recycling packaging waste addresses the pollution problem of plastic waste, creating a win-win situation where two big public problems are tackled at once, avoiding resentment by the public.
- Recycling production waste in the same production line is easy to realise and shows incredible recycling rates. This kind of waste can usually be sorted in pure fractions more easily compared to the mixed waste after consumption of a product when its life ends.
1 Like paying for the ‘Green Dot’ in Germany or elsewhere.
The eight points above might give the impression that recycling would not be such a good idea, but this is not the case. Recycling has a place in a circular economy, and within a circular economy it should be handled efficiently with very good methods for collection, sorting and processing, when the product or parts of it actually become waste. Furthermore, research and development by the producers of recycling machines, collection systems and secondary raw material production, like pellet making or chemical recycling, is both necessary and useful.
The point of the matter is that any introduction of recycling methods should not hinder economic players when it comes securing as much environmental investment as possible during the life cycles of a product. In other words, recycling gives those affected the feeling that they have already done the best they can, which prevents them from fulfilling their real responsibility (EPR). Recycling activities should also avoid cherry-picking, namely removing just the ‘interesting’ fractions from waste and leaving the rubbish behind (on the streets), which is nowadays seen as a problem in plastics recycling projects, e.g. in Western Africa. 2
2 E.g. in Ghana. At the ISWA Germany/GIZ Prevent event in Eschborn, several organisations mentioned this problem.
In fact, recycling is just one of the many Circular Economy Recirculation twigs (CER twigs). As mentioned above, there are many circles that start at different points in a product's life cycle and go back to different points in the same product's life cycle. In a project financially supported by the German Environmental Agency and the Federal Ministry for the Environment, Nature Conservation, Nuclear Safety and Consumer Protection (BMUV) with the participation of the author of this article concerning white goods, these closing-the-loop parts of circles were named in German as ‘KWR-Kreise’ (abbreviation for Kreislaufwirtschaftsrückführungs-Kreise) (Ludwig et al. 2023:58) In this article, it is proposed that they be given the English name ‘CER twigs’ (Circular Economy Recirculation twigs).
Related article: The EU wants to fight the deluge of textile waste
The simple circular economy model is derived from nature. The idea behind it is that a plant grows and when it dies it decays and gives its material back to nature to feed a new plant exactly at the location where it lived. In an economy with production, trade, import and export, the life cycles of products are not that simple. There are many more cycles, branching off from the main cycle at various phases within the life of a product. Furthermore, the use of products interacts with the use of other products or resources. Returning to the main idea of a plant, the plant consumes water, releases it again and can reuse it when it enters the soil. The water could also be used by other plants or animals. In addition, excretions from animals could be used as fertilisers for plants, and so on, indicating a high level of interaction.
The crucial difference between the circularity of a plant and an industrial product is that parts of an already used product could be used again in a similar product to repair a broken product of the same kind or to create a new product (or the same product). In nature, a leaf could not be used again as a leaf; it must decay in order to be reused by the plant, which is a kind of a recycling, a production of secondary materials. In the context of industrial products, a leaf could be used again as a leaf, a concept nature does not foresee.
The advantage of the CER twigs concept is that the resource expenditure for creating functional units is minimised. How does it work and how could it be implemented given that the life cycles of industrial products are complex and that there are various CER twigs that the product or parts of it can go through?
First of all, the possible CER twigs need to be identified and new business models then need to be implemented in order to combine business requirements with environmental (CO2e) calculations. This was done in the aforementioned white goods project for washing machines (see Figure 1).
Figure 1: CER twigs for washing machines (WeWaWi project)
The model shows several possible CER twigs just for the product ‘washing machine’. The product life in this simple model ends after the use phase with the consumer. In order to become a product usable for the consumer, environmental costs in kg CO2e had to be paid during the production phase. 3 Once invested, the environmental costs (environmental spending) for the product remain the same at the end of life of the first use phase by the consumer. If e.g. 100 kg CO2e were paid for a new product, then a big part of this value will remain even in the afterlife of this product. In terms of money, e.g. in euros, the product has virtually no value anymore and consumers might even have to pay to dispose of it. However, this does not affect the invested environmental costs. Nowadays, in the traditional linear model the invested environmental costs are going to be wiped out by the waste management sector by killing every aspect of the functionality of the product and of all parts of it. Although material might be recycled, which is OK, the corresponding CER twig has nothing to do with the original product and is furthermore associated with a lot of entropy. In the CER twigs leading to second-hand machines or leading to the reuse of spare parts in new or in old machines or even in preliminary products, up to 90% of the environmental costs can be saved. 4 Investing just 10 kg CO2e to get a functional ‘new’ product of a value of 100 kg CO2e is a great environmental win. This win cannot be achieved by the recycling CER twig alone.
3 Usually, producers in the EU must arrange life-cycle assessments (LCAs) for each of their products. These LCAs are a good starting point for the environmental costs in kg CO2e of a given product. Unfortunately, the explanatory power of the unit kg CO2e for the real environmental impact does not cover all possible impacts on the environment like ozone depletion, terrestrial ecotoxicity, water consumption or marine eutrophication, amongst others. However, kg CO2e measures this damage indirectly because in all processes, exploiting the environment also causes emissions of CO2, and the avoidance of kg CO2e emissions in particular addresses the main and most important environmental problem: climate change. Therefore, the unit is chosen for the calculation of environmental costs.
4 In phase 3 of the WeWaWi project, evaluation of the environmental cost savings on the various CER twigs and the total environmental impact of a system change was started. The results will be published at the end of 2023.
Related article: Innovative product cycles for a circular economy
Recycling ist just one of many Circular Economy Recirculation Twigs.
In the aforementioned white goods project for washing machines (washing machines as an example for many industrial products), numerous CER twigs were identified and the authors developed monetarily profitable business models. (Ludwig et al. 2023:58)
These business models must handle large masses, as around 3.5 million washing machines are thrown away each year in Germany alone. This means that for a circular economy the European Union must establish a dismantling industry with a high degree of specialisation. Therefore, the Green Deal implies huge investment in new industries of a kind we have never seen before.
In this respect, dealing with mass flows in a circular economy differs significantly from a technical and organisational perspective from the way mass flows are currently handled in waste management.
A system conversion of today's economies into a circular economy will require a complex system of CER twigs to close as many loops as possible. The task at hand is the organisation of a macroeconomic avoidance of misallocations, respectively the organisation of a correct allocation of products and parts of products in the mass flows of the corresponding CER twigs.
Product Design
Implementation of a circular economy requires the identification of CER twigs for all products. For some products this is easier than it is for others. Some products must be managed in CER twigs (such as washing machines), while for other products perhaps only their packaging or other products needed to produce and use them have to be managed in CER twigs (e.g. for orange juice).
The main problem with the implementation of a circular economy at present is that we are confronted with products already produced in the past. These products are rarely or not at all designed to be managed in circles. Only recycling, as pointed out before, as one of the many possible CER twigs works (to an extent). This is because recycling is material-related and not product-related. We could set up some additional CER twigs for products that were produced in the past and e.g. repair some washing machines and sell these then on second-hand markets. However, the environmental effects would not be strongly enough related to climate change and the sourcing problem we face. This is because it would not affect the design of new products and would not reduce the number of new products using primary raw materials. We might end up with additional products but we would not reduce the environmental footprint of the industry.
If we want to significantly reduce the climate footprint of an industry the industry needs to produce as many ‘new’ machines as possible by using the whole mix of used spare parts, refurbished preliminary products and recycled materials. (Regenfelder, Slovak 2017) At the same time, the industry must reduce the number of new machines produced using only primary raw materials or new preliminary products. This can only be achieved through intelligent product design. Product design must take into account the use of as many CER twigs as possible. Designing new products merely with a view to the actually less important CER twig ‘recycling’ will slow us down or even restrict us on the path to a circular economy.
Product design must, of course, keep recycling in mind, but must not forget all other possible CER twigs and the design of the required dismantling industry for each CER twig. Producers will have to find ways to integrate the output of the different CER twigs into their new products in such a way that the quality of their products will be as good as if they had used only primary raw materials or preliminary products produced using only primary raw materials.
Waste Management and Circular Economy
There will always be traditional waste, but the question is in what quantities and at which point in time? This depends on how successful new CER twigs could be realised as described above and how much of the stream of product parts will be absorbed by them. This is not in the hands of the waste management sector. The waste management sector will be a part of the future circular economy, but its role will be relatively small if the system boundaries remain constant. The waste management sector can handle large mass flows of materials, but the traditional waste management players lack the knowledge required to set up CER twigs. New players will close life cycles and minimise the streams of waste transferred to the waste management sector for recycling, disposal or incineration.
The EU Green Deal brings about a transition period for the economic system. During this period, the waste management sector must continue to handle the waste of yesterday while at the same time organising the treatment of the waste of tomorrow. However, without any knowledge regarding the products of tomorrow, the waste management sector will keep the traditional system running and stick to their ideas to achieve higher recycling rates or better fulfil their clean-up operations. Engagement of the sector is both valuable and helpful and will still be needed for decades to come, but it has nothing to do with circular economy. This is because of the lack of any interaction or cooperation with producers. The PREVENT Waste Alliance created a mapping of digital solutions across the circular economy which showed that so called ‘digital actors in the circular economy’ from all over the world, who are trying to improve circular economy activities, are mainly active in the life cycle of products in the fields of collection and recycling while not a single actor deals with design at all. (Prevent Waste Alliance 2023) Taking this into account, it is to be expected that the waste management sector will not be the driver or even a main player of a future circular economy. This result of the above considerations is in stark contradiction to the political expectations as described above in connection with recycling. To achieve the goals of the Green Deal, politicians need to rethink. And producers, industry and trade must begin to play a much more active role.
Concomitant Research
It should be mentioned that to change the system, environmental spending for the change needs to be done in an efficient way in order to prevent unnecessary spending. Such spending can easily happen if the focus is on the creation of CER twigs for a certain product or product group while interacting CER twigs of other products are ignored. Therefore, some concomitant research will be needed to avoid such effects and some aspects of this are mentioned here.
Activities and real investment in new industries will depend on the stream volume within any CER twig. Some marketplaces might not be big enough to close loops close by. Therefore, e.g. small islands or regions might have to discuss which kind of investments would be reasonable for them. An island situation, for instance, is different to a highly populated continental setting. Since logistics are costly in terms of both money and environmental costs, it should be discussed which life cycles could be closed within such regions and what do to with the streams that could not be handled close by. This is a technical issue which must be resolved by looking at all aspects of the situation.
Furthermore, waste management needs to be integrated into the life cycles of products. Even the transformation process should consider preserving (saving) as much of the environmental spending already made as possible. Producers of production machines in particular need to look at the older machines they already sold and how these machines could be modified to contribute to a circular economy. This aspect is especially important for the German machine tool industry that serves many customers worldwide and therefore would have a high impact on the world’s future green production. This might include machines for the production of single-use plastic packaging. Avoiding these plastics should not lead to the wiping out of the environmental investment made into the machines, but the machines could be reused for the production of new, environmentally friendly multi-use products.
Waste management needs to be integrated into the life cycles of products.
Producers could also provide a list of components from disposed-of machines that could be used to create totally different machines for totally different products. This is possible because many different products use the same parts. During the coronavirus pandemic, for instance, producers of new products salvaged washing machines to get enough microchips for their own production needs. (Kempf 2022)
Last but not least, the change to a circular economy needs to address the shortage of skilled labour. No change or restructuring will be successful without the people who can do the work. The mobilisation of people with expertise as lateral-entry employees, even on a part-time basis, is one possible solution. Another is the expectation that reducing the production of new machines with primary raw materials could free up a workforce to meet the needs of the system change.
Summary and Conclusion
A system change from a linear economy to a circular economy means that invested environmental costs have to be preserved to the greatest extent possible. Therefore, so-called CER twigs must be identified for each product and corresponding business models must be created. The main driver for this change must be the product designer, not the waste management sector. The waste management sector must nevertheless still contribute to a circular economy and adapt to the new system. New industries must be created to handle the processes of the CER twigs. Policymakers must support this development.
Bibliography
1 European Commission (2021): COMMUNICATION FROM THE COMMISSION TO THE EUROPEAN PARLIAMENT, THE COUNCIL, THE EUROPEAN ECONOMIC AND SOCIAL COMMITTEE AND THE COMMITTEE OF THE REGIONS EMPTY - 'Fit for 55': delivering the EU's 2030 Climate Target on the way to climate neutrality. Brussels.
2 European Commission (2019): What is the European Green Deal? Luxembourg Publications Office.
3 Directorate-General for Environment (2023a): Circular economy . (last seen 26 July 2023)
4 Directorate-General for Environment (2023b): Circular economy: Faster progress needed to meet EU resource-efficiency targets, ensure sustainable use of materials and enhance strategic autonomy . (last seen 26 July 2023)
5 Ludwig, Dr Volker, Ebelt, Stefan, Schomerus, Prof Dr Thomas, Lösing, Felix, Alcantara, Helena, Raap, Fabienne (2023): Endbericht - Wiederverwendung von Haushaltsgroßgeräten in Deutschland steigern und neue Wege der Haushaltsgroßgeräte-Sammlung beschreiten mit neuen Geschäftsmodellen zwischen Handel und Werkstätten in Kooperation mit dem Hersteller. PDF here (last seen 26 July 2023)
6 European Parliament (2008): Directive 2008/98/EC of the European Parliament and of the Council of 19 November 2008 on waste and repealing certain Directives. Brussels.
7 European Parliament (2023): Circular economy: definition, importance and benefits. (last seen 26 July 2023)
8 Franken, Gudrun, Vasters, Jürgen (2000): Aluminium - Informationen zur Nachhaltigkeit (2020). Hannover: Bundesanstalt für Geowissenschaften und Rohstoffe.
9 European Aluminium (2020): EUROPEAN ALUMINIUM | A strategy for achieving aluminium’s full potential for circular economy by 2030. Brussels.
10 Siebel, Thomas (2022): So will die Aluminiumindustrie klimaneutral werden . In: Springer Professional. (last seen 26 July 2023)
11 OECD (2016): Extended Producer Responsibility Updated Guidance for Efficient Waste Management. Paris: OECD Publishing.
12 Regenfelder, Max, Andre P. Slovak (2017): Creating value, not wasting resources: sustainable innovation strategies. In: Innovation: The European Journal of Social Science Research. 30, 4, p. 455 – 475. DOI: 10.1080/13511610.2016.1192990 (last seen 26 July 2023)
13 Prevent Waste Alliance (2023): Digitalization for a Circular Economy, Summary notes of a practitioners’ exchange about digital circular solutions. (last seen 26 July 2023)
14 Kempf, Andreas (2022): Weil Mikro-Chips weiter fehlen, schlachten Unternehmen jetzt schon Waschmaschinen aus. In: Focus Online. (last seen 26 July 2023)
- # circular economy
- # collection and handling
- # legislation
- # recycling
United Nations
Office on drugs and crime, press release, global efforts needed to combat waste trafficking to southeast asia, new research by unodc and unep reveals , bangkok (thailand), 2 april 2024.
A first-ever mapping of waste trafficking trends from Europe to Southeast Asia has been published today. Produced by the UN Office on Drugs and Crime (UNODC) and the UN Environment Programme (UNEP), the new research sheds light on how criminal actors exploit legal trade and regulatory and enforcement loopholes for financial gain. It also explores the negative impact this crime has on the global circular economy.
Southeast Asia remains a key destination for illicit waste shipments, the report reveals, with Europe, North America, and Asia identified as primary regions of origin. Common tactics include false declarations, a lack of or incorrect notifications to circumvent regulations and avoid controls, along with missing or inadequate licenses or documents.
“In today’s globalized world, waste management has become an increasingly pressing concern in which production, consumption habits, waste crime, waste trafficking, corruption, organized crime, money laundering, and the circular economy are intertwined,” said Masood Karimipour, UNODC Regional Representative for Southeast Asia and the Pacific. “The crime of waste trafficking is taking away the value that legal, well-regulated waste trade brings to sustainable economies.”
Data collected from four Southeast Asian countries, three major European Union ports, and international enforcement operations highlight efforts in tackling illegal waste shipments by both origin and destination countries. However, despite regulatory and enforcement measures implemented by countries in which illegal waste ends up — such as Indonesia, Malaysia, Thailand, and Viet Nam — waste trafficking continues to pose a major challenge in the region.
“Waste trafficking is a crime that has a profound impact on the environment, bringing high profits and low risks to perpetrators. If we are to fight this crime, we must change this by closing regulatory gaps, increasing enforcement, and strengthening cooperation at home and abroad,” said Preeyaporn Suwannaked, Director General of the Pollution Control Department of the Ministry of Natural Resources and Environment of Thailand.
The report, titled Turning the Tide: A Look Into the European Union-to-Southeast Asia Waste Trafficking Wave, is a flagship within a series of publications that explore corruption, cybercrime, and legal loopholes as causes behind the problem. It is part of a comprehensive project ( Unwaste ) to address waste trafficking and its impact on the global circular economy.
“The environmental impacts of waste trafficking are contributing to the pollution crisis and need to be addressed. To do this, we must pursue good environmental governance and robust environmental rule of law. Projects such as Unwaste are critical in tackling issues through a multi-sector, multi-disciplinary approach. UNEP is proud to be part of the project, which advances solutions aimed at ensuring a healthy planet and a sustainable future,” said Patricia Kameri-Mbote, Director of the Law Division in UNEP.
Key types of waste trafficked include plastic, e-waste, metal, and paper, with mixed materials, textiles, vehicle parts, industrial, and medical waste also frequently encountered. Upon arrival at destination, take-back or repatriation procedures are a major challenge as shipments often cannot be traced to their countries of origin. Abandoned or unclaimed containers at ports exacerbate the issue, further complicating enforcement and investigation efforts. As a result, most waste ends up in illegal landfills, the ocean, or burnt in the open.
Often, penalties are disproportionately low compared to the potential environmental and health damage inflicted on destination countries. The research also shows a concerning lack of available data to assess the full scale of waste trafficking and identify the connections between criminal actors involved.
The report, which has been financed by the European Union, stresses the urgent need for further regulatory reforms, enhanced international cooperation, capacity development, research, and data along with stricter enforcement measures to combat waste trafficking effectively.
Click here to access the report series.
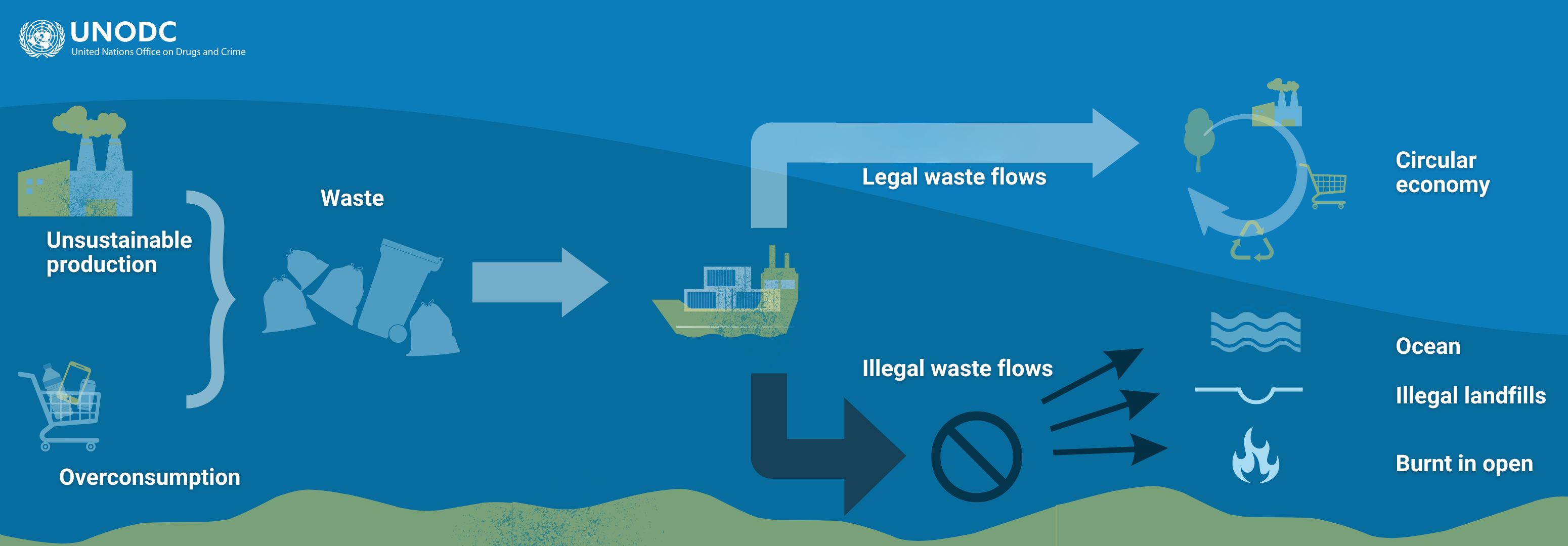
Waste trafficking leads to most waste ending up in illegal landfills, the ocean, or burnt in the open, thwarting any ambitions towards a circular economy
For media enquiries, please contact:
Laura Gil UNODC Communications Officer laura.gil[at]un.org
- Fraud Alert
- Legal Notice
Advertisement
Concepts of circular economy for sustainable management of electronic wastes: challenges and management options
- Review Article
- Published: 28 February 2023
- Volume 30 , pages 48654–48675, ( 2023 )
Cite this article
- Arun Lal Srivastav 1 ,
- Markandeya 2 ,
- Naveen Patel 3 ,
- Mayank Pandey 4 ,
- Ashutosh Kumar Pandey 5 ,
- Ashutosh Kumar Dubey 1 ,
- Abhishek Kumar 6 ,
- Abhishek Kumar Bhardwaj 7 &
- Vinod Kumar Chaudhary 8
7385 Accesses
18 Citations
Explore all metrics
The electronic and electrical industrial sector is exponentially growing throughout the globe, and sometimes, these wastes are being disposed of and discarded with a faster rate in comparison to the past era due to technology advancements. As the application of electronic devices is increasing due to the digitalization of the world (IT sector, medical, domestic, etc.), a heap of discarded e-waste is also being generated. Per-capita e-waste generation is very high in developed countries as compared to developing countries. Expansion of the global population and advancement of technologies are mainly responsible to increase the e-waste volume in our surroundings. E-waste is responsible for environmental threats as it may contain dangerous and toxic substances like metals which may have harmful effects on the biodiversity and environment. Furthermore, the life span and types of e-waste determine their harmful effects on nature, and unscientific practices of their disposal may elevate the level of threats as observed in most developing countries like India, Nigeria, Pakistan, and China. In the present review paper, many possible approaches have been discussed for effective e-waste management, such as recycling, recovery of precious metals, adopting the concepts of circular economy, formulating relevant policies, and use of advance computational techniques. On the other hand, it may also provide potential secondary resources valuable/critical materials whose primary sources are at significant supply risk. Furthermore, the use of machine learning approaches can also be useful in the monitoring and treatment/processing of e-wastes.
In 2019, ~ 53.6 million tons of e-wastes generated worldwide.
Discarded e-wastes may be hazardous in nature due to presence of heavy metal compositions.
Precious metals like gold, silver, and copper can also be procured from e-wastes.
Advance tools like artificial intelligence/machine learning can be useful in the management of e-wastes.
Graphical abstract
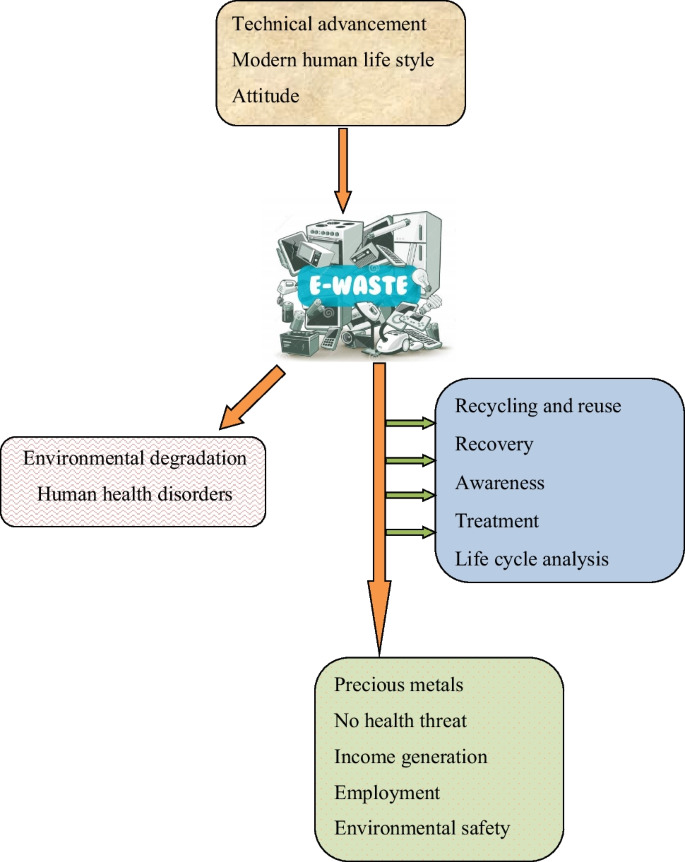
Similar content being viewed by others
Management of e-Waste: Technological Challenges and Opportunities
Management of E-Waste: Technological Challenges and Opportunities
E-Waste Management: Challenges and Opportunities
Avoid common mistakes on your manuscript.
Introduction
E-waste can be defined as unwanted, not working, or at the end of the useful life of any equipment which operates on an electromagnetic field and electrical currents (UNEP 2007 ). It conceals wide ranges of electronic devices, varying from hefty domestic appliances to equipment used in ITs and telecom sectors. It also encompasses appliances from the medical, automobile, sports, and toy sectors (Li et al. 2007 ; Chen et al. 2015 ). Different parts of electric and electronic equipment (EEE), like used batteries, electric wires, printed circuit boards (PCBs), plastic casings, cathode ray tubes (CRTs), poly(p-phenylene terephthalamide, activated glass, and lead capacitors, are also categorized as e-waste (Lambert et al. 2015 ; Ilankoon et al. 2018 ; Mazrouaa et al. 2019 ). The material design of EEE is very complex, as 69 elements from the periodic table can be found in EEE, including precious metals (like platinum, gold, rhodium, silver, copper, iridium, ruthenium, and osmium), Critical Raw Materials (CRM) (like indium, cobalt, bismuth, palladium, antimonyand germanium), and noncritical metals, such as iron and aluminum. Figure 1 shows the proportions of different types of metals which are expected to be present in e-wastes.
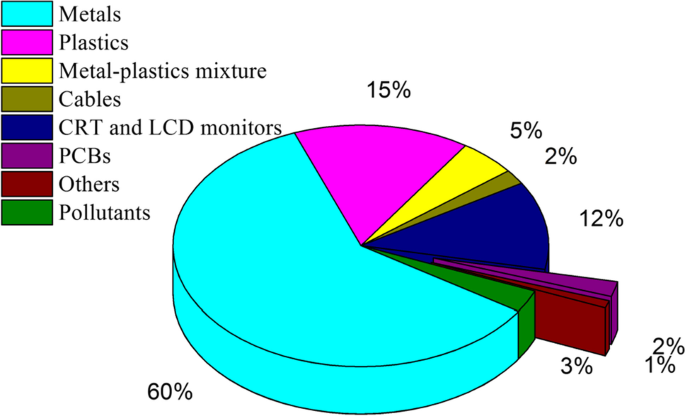
Generalized material composition in e-waste [data sources: Ongondo et al. ( 2011 ) and Mmereki et al. ( 2016 )]
From Fig. 1 , it is clear that categories of precious metals can be procured from the e-wastes including gold, silver, and copper. Due to the speedy evolution of technology and the rising need of consumers all over the world, there is enhanced consumption of natural resources which ultimately leads to greater production of electronic waste (Mmereki et al. 2016 ; Dhir et al. 2021 ). In 2019, e-waste generated global sales hit a record 53.6 MT, but only 17.4% was collected and recycled and it is growing up environmental concerns over the large volumes of e-waste. An enormous loss of recyclable material resources has always forced for developing an effective obsolescence management strategy that specifically targets e-waste to combat inappropriate practices such as dangerous landfill or home storage (Deng et al. 2023 ). In most developing nations, especially low-income and middle-income nations, a major fraction of e-waste is unscientifically getting disposed over landfill sites and it is not good for public health. Kuang et al. ( 2023 ) reported children-related health disorders due to waste recycling in China. Similarly, recycling e-waste in an informal way is also broadly accomplished. The plastic of wires is used to get burnt directly in the atmosphere for the recovery of copper. Similarly, for the extraction of valuable metals like palladium, gold, platinum, and silver from electronic equipment, the use of acid and extraction process are the commonly practiced methodologies in countries like China, India, Pakistan, and Vietnam. In countries like Nigeria and Ghana, e-waste is getting handled unscientifically by adopting a basic methodology to recuperate precious metals. People involved in these services neither have the amenities to protect themselves nor the environment. At present, India having the second largest population is among the top 4 countries for e-waste generation in the world (Forti et al. 2020 ; Arya and Kumar 2020 ). Figure 2 shows per-capita e-waste generation in different developed as well as developing countries.
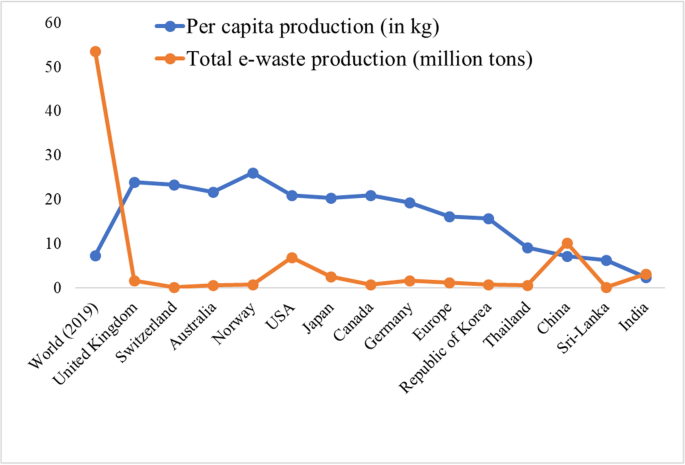
E-waste generation/capita in some countries (data sources: Ilankoon et al. 2018 ; Islam and Huda 2020 ; Tutton et al. 2022 )
From Fig. 2 , it is visible that in developed countries, e-waste generation is very high as compared to developing countries. In 2019, globally, 53.6 MT of e-waste was generated and this quantity may be exceeded up to 74 MT in 2030 and close to 59.4 MT by 2022 (Forti et al. 2020 ). Over the past decades, this worldwide rapid growth of e-waste has become a major area of concern and its immediate solution is requisite at this time (Dhir et al. 2021 ). In the present era, due to improvements in lifestyle and progressive evolution in technology, the use of electronics and electrical appliances in day-to-day life has enhanced manifold. These electrical and electronics equipment has become an essential and integrated part of our daily life, and we cannot imagine our life without them. The malicious metals used in these appliances are not only toxic to human beings, but also to the atmosphere which leads to grave consequences. Day by day, the problem of e-waste is increasing as the volumes of e-waste are being generated and the content of both toxic and valuable materials is also increasing (Babu et al. 2007 ). The “Solving the E-waste Problem (StEP)” initiative forecasts that the world will produce 60 MT or 33% more e-waste by 2022. Asia’s leading producer, China, produces about 12.2 MT of e-waste, followed by the USA with 13.1 MT (Forti et al. 2020 ). A report related to e-waste by the Associated Chambers of Commerce of India (ASSOCHAM) found that e-waste is growing at a compounded annual growth rate of about 25% in India (Garlapati 2016 ). In India, Mumbai is on the top with 1.2 MT in e-waste generation annually followed by Delhi (0.98 MT/year) and Bangalore (0.92 MT/year) (Kumar and Dixit 2018 ; Srivastava and Pathak 2020 ). In today’s world, the electronic market is among the most growing sectors in developing countries. There is also prompt financial growth due to an increase in demand and consumption of electronics products. This speedy progress of the electronic sector is also due to rapid urbanization, the quick transformation of technologies, the price drop of electronic goods, and the replacement of old electronic goods with new ones. This gigantic growth in the production of electronic gadgets also results in a colossal upsurge of hazardous electronic waste (Dwivedy and Mittal 2010 ). Improper handling of these wastes will not only cost for the exhaustion of resources but also causes serious environmental and economic crisis (Menikpura et al. 2014 ; Brindhadevi et al. 2023 ; Shi et al. 2023 ). Particularly in developing nations, proper handling and disposal of e-wastes is still a major area of concern. It is estimated that Brazil generated 2.2 MT of WEEE, i.e., 9.1 kg/capita in 2019. This is less than Mexico’s generation rate (1.2 MT) and Canada (0.77 MT) but it is more than the USA (6.9 MT) in Northern America (de Souza et al. 2016 ). Figure 3 shows the generation of e-waste in different continents, along with their utilization.
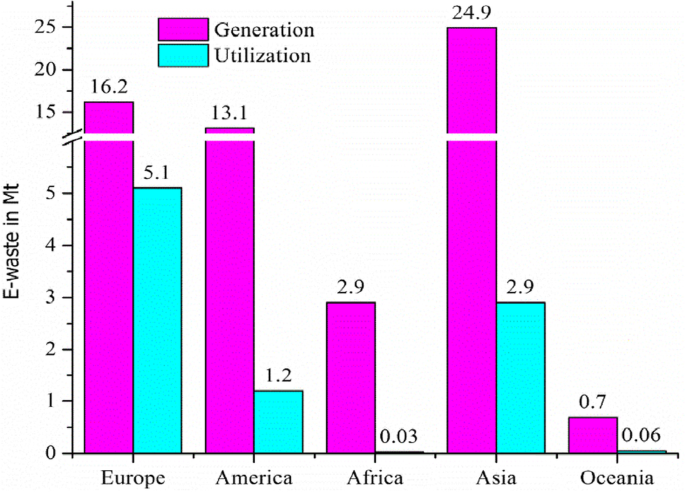
Generation and reuse of e-wastes in different continents (data source: Global E-waste 2020)
Based on Fig. 3 , relatively European countries are on the top in the generation of e-wastes as well as their reuse than other continents. Seitz ( 2014 ) studied the current e-waste management practices in 10 countries and observed that the management of e-waste is a major area of concern among these nations. It has also been found that there is very little awareness among people regarding jeopardy associated with human health and the environment due to the mismanagement of e-waste (Ikhlayel 2018 ). D’Adamo et al. ( 2022 ) found that among the waste management practices, the highest priority was given to e-waste management especially for electrical and electronic equipment and discarded vehicles. But, society is more inclined to manage municipal solid wastes only. Most of the environmental problems lie within human behavior; therefore, by changing our behavior towards the environment and with the help of innovative technologies, environmental sustainability can be achieved (Parajuly et al. 2020 ). For resource sustainability, electrical and electronic products (e-products) are a key area of concern as they may retain many types of rare earth metals (Breivik et al. 2014 ; Habib et al. 2015 ). As per the current pattern of increasing e-waste volume, it is expected to get doubled by 2045 (Parajuly et al. 2020 ). E-waste requires cautious treatment as it is composed of precious resources and noxious elements. E-products became abundant in day-to-day life, but their disposal is still far behind the proper scientific methodologies. This causes potential risks to the resource and negative impacts on the environment as well as human health (Wang et al. 2016 ; Taneja et al. 2021 ). As per the global e-waste monitoring report 2020, India is the only country in Southern Asia that is considering legislation with e-waste legislation, although several other countries besides Southern Asia are considering such legislation. Laws to manage e-waste have been already framed in India since the year 2011, mandating that only authorized dismantlers and recyclers collect e-waste. The generation of e-waste in India was 3.23 MT (per capita 2.4 kg) in 2019 (Nisa 2014 ). However, in India, due to the shortage of proper infrastructure for the management of e-waste, a major chunk of recyclable e-waste is getting disposed of directly to open landfill sites which is a grave threat to humans and the environment (Kumar and Dixit 2018 ). Singh et al. ( 2022 ) analyzed the importance of critical success factors in the designing of an e-waste collection policy in India using the fuzzy DEMATEL approach. Authors found that some critical factors like green practices, technology involvement, certification and licensing, environmental programs, public ethics, and stakeholder awareness of the circular economy are having a major influence on the collection of e-wastes in India. From the perspective of developing nations like India, China, Malaysia, Thailand, and Vietnam, very few studies have been conducted for providing practical solutions in e-waste treatment facilities (Awasthi and Li 2017 ; Milovantseva and Fitzpatrick 2015 ; Shumon et al. 2014 ; Zhang et al. 2012 ). In earlier studies, no emphasis has been given to the inter-relationship among the obstacles affecting the execution of e-waste management (Kumar and Dixit 2018 ). Reduce, Reuse, Recycle (3Rs) is a technology platform that connects markets to promote a circular economy and aims to encourage consumers to dispose of their e-waste. In developed countries, high priority is given to e-waste management, whereas, it is difficult for developing countries to fully adopt or replicate e-waste management in developed countries and many related problems, including a lack of investment and skilled human resources. Furthermore, the lack of infrastructure and inappropriate laws for e-waste management. In addition, there is another shortcoming which is the lack of awareness about the roles and responsibilities of stakeholders and institutions involving e-waste management etc. In the present paper, emphasis has been given to provide information for the effective management of the e-wastes through a meticulous literature review so that concepts of sustainable development goals and circular economy can be attained. Extractions of precious metals and the possibilities of developing a business venture using e-wastes have also been discussed. Moreover, it would be a great effort to conserve the environment along with social health protection.
Types of e-wastes and their possible sources
Different types of e-wastes are being generated in the environment. Possible sources of e-wastes may be our homes, IT industries, automobile industries, medical sector, and other electrical and electronic materials. There are 10 categories of e-wastes as per the European regulatory bodies of e-waste management (Garlapati 2016 ). Discarded e-wastes may cause severe problems as they may contain many harmful inorganic and/or organic contaminants. Some common categories of e-wastes such as computer accessories, toys, Internet materials, air conditioners, automobiles, microwave ovens, refrigerators, lamps, washing machines, phones, and printers have toxic heavy metals which are very harmful. In chips and batteries and medical and laboratory instruments, persistent organic contaminants and electrolytes and X-ray and radioactive chemicals are used respectively. Many harmful effects can be caused by these e-wastes (Kofi Adanu et al. 2020 ), and the advancement in technology, less life span of a material, and policy change are among the most responsible reasons for the generation of e-wastes (Amankwah-Amoah 2016 ). However, harmful materials may include toxic heavy metals like lead, mercury, chromium, and persistent organic contaminants (Ilankoon et al. 2018 ).
Methodology adopted to write this review paper
The relevant research papers, book chapters, conference papers, websites of regulatory bodies, and government reports were collected from different electronic databases which are very authentic for writing this paper. Usually, the research papers (journals and conferences) were accessed from Science direct, Pubmed, Springer, Taylor & Francis, Google scholar, etc. as depicted in Fig. 4 . Moreover, relevant book chapters and books were also searched for the same.
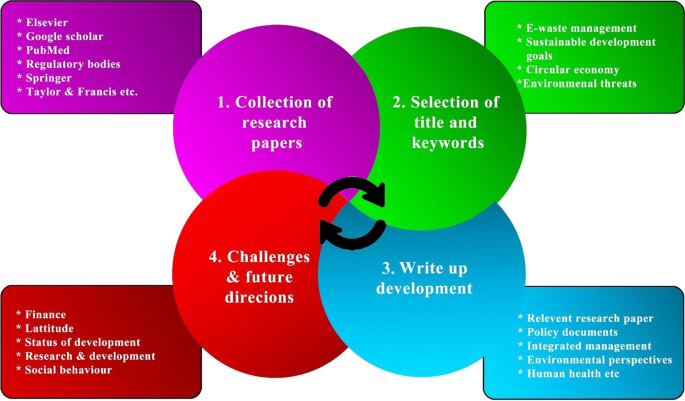
Adopted frameworks for writing present review paper
The title and framework of the paper were decided on the basis of different types of keywords such as e-waste-related problems (for example, environmental concern, social health), sustainable development goals, concept of circular economy in e-waste management, expected challenges, future scope, and application of machine intelligence. Management of e-waste was the central focus during the paper writing. It also ensured to evaluate all the critical factors such as recent publications, novelty and cost-effective methods of e-waste management, application of computational tools, and possible green processes. It was the brainchild of all the co-authors who were also involved in the brainstorming during the writing of this paper to ensure the maximum possible quality.
Impacts of e-wastes on human health and environment
Discarded e-wastes can cause many harmful impacts on the environment and human lives, if they have been mishandled such as chronic health disorders and environmental degradation (in terms of air, water, and soil contaminations) (Amankwah-Amoah 2016 ). Some specific section is illustrated in the following sections regarding e-waste-induced problems.
Impact on the environment
E-waste leads to environmental contamination including water, air, soil, and other biota afterwards, mainly due to poor disposal activities. E-waste disposal sites and recycling centers are reported releasing heavy metals and particulate matters into the environment (Chatterjee 2008 ). Substances liberated from e-waste have been categorized into two types; hazardous and non-hazardous. Hazardous substances include heavy metals, polycyclic aromatic hydrocarbons, poly dibenzofurans, brominated diphenyl ethers, and polychlorinated dibenzo-p-dioxins, whereas non-hazardous substances include metals Cu, Se, Pt, and Ag among others (Awasthi et al. 2016 ; Zeng et al. 2014 ; Zhang et al. 2013 ). Both the categories of pollutants released from e-waste cause ill effects on the environment and human health when their presence is above the permissible limits. The impacts of e-waste depend on the age of the specific e-waste, and hence, it varies for the same type of e-wastes. Recycling is a process in which pollutants are removed or delayed, but the large quantity of e-waste still causes the accumulation of enormous amounts of pollutants in landfills and the environment. Developing countries like India, Nigeria, Pakistan, and China use unscientific methods for e-waste recycling which releases heavy metals and other recalcitrant pollutants into the environment (Pradhan and Kumar 2014 ; Sthiannopkao and Wong 2013 ). Release of these hazardous contaminants into the environment gets easily associated with various processes like bioaccumulation, contamination of food, and widespread exposure to ecological processes and hence causing widespread risk (Sepúlveda et al. 2010 ; Chan and Wong 2013 ; Chen et al. 2011 ).
Impact on air quality
Several types of e-waste contaminants get dispersed as dust or fumes into the air. Ingestion, inhalation, and skin absorption are all primary routes of exposure in human beings (Mielke and Reagan 1998 ). Children and infants are considered to be the most susceptible to e-waste mishandling because of a high frequency of breathing, high chances of in utero-exposure, frequent breast-feeding, extreme hand-to-mouth activity, frequent food intake, and low efficiency of toxicants removal rate (Guo et al. 2012 ; Xu et al. 2012 , 2018 ). Various hazardous primary e-wastes including Hg, Pb, Cd, PCBs, and other secondary e-waste toxicants, including PAHs, dioxins, and difurans, are produced in the environment during the processing and recycling of e-waste at Guiyu, China; Agbogbloshie, Ghana; the National Capital Territory (NCT) region, India; Shershah (Karachi), Pakistan; Lagos, Nigeria; and Dhaka, Bangladesh (Sthiannopkao and Wong 2013 ). However, the presence of contaminants in the surrounding atmosphere may vary depending upon the type of work that is performed in the nearby area. For example, recycling and dumping sites which are often associated with electrical components are responsible for the release of substantial quantities of Pb and Cu into the atmosphere (Brigden 2005 ). The atmosphere acts as a mediator for the dispersion of these metals from one place to another place as these contaminants can stay for a long time and also can travel for greater distances (Brigden 2005 ). These metals are suspended in the form of particulate matters and also get deposited to the soil surface and open water resources and, hence, can be bio-accumulated in the lives of water, soil, and plants and will cause serious ill effects on the consumption of contaminated water or food product.
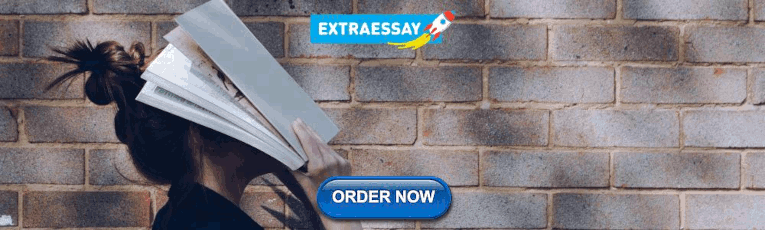
Impact on water quality
Waste effluents are also responsible for water pollution as reported in many studies too (Awual et al. 2019 ; Awual 2019 ). Many studies have been carried out recently on the removal of different types of heavy metals and toxic dyes from water (Awual et al. 2015 , 2016 , 2020 ; Awual 2015 ; Naushad et al. 2019 ; Khandaker et al. 2021 ; Yeamin et al. 2021 ; Kubra et al. 2021 ; Hasan et al. 2021 ; Teo et al. 2022 ). Contaminants from e-waste enter into the aquatic environment, both into groundwater and surface water via leaching and dissipating processes from electrical and electronic equipment dumping industries and other e-waste-related activities. A study performed by Luo et al. ( 2011 ) had suggested that poly brominated diphenyl ethers (PBDE) level in the sediment samples from the Nanyang River near Guiyu town had increased significantly and they also reported that carp from this river has great potential to bioaccumulate. According to a study conducted by Wu et al. ( 2008 ), on snakes, top predators present in an aquatic environment nearby an e-waste recycling plant consumed ~ 1091 ng/g PBDE and 16,512 ng/g PCBs on a wet weight basis. A study on water flow from downstream areas in Pearl River Delta had shown an elevated level of PBDE and PCBs (Luo et al. 2009 ). Besides PBDE, brominated flame retardants namely decabromodiphenyl ethane and1, 2-bis (2,4,6-tribromophenoxy) ethane, etc. were reported in down streams of Pearl River Delta (Shi et al. 2009 ). According to a study reported by Dharini et al. ( 2017 ), water resources present in the nearby area having e-waste recycling and detention facilities do not fulfill the general water quality standards in terms of pH, total dissolved solids, hardness, chlorides, and conductivity .
Impact on soil health
In recent decades, the sudden increase in human activities leads to the release of certain amounts of hazardous contaminants into the soil and hence causing pollution in farmland soil as well (Zhang et al. 2015 ). Waste recycling plants are considered one of the prime sources responsible for the contamination of soil mainly due to the release of metals and other contaminants in higher concentrations. However, the management of e-waste by using primitive methods has become one of the most problematic reasons for e-waste contamination of the soil (Awasthi et al. 2016 ). Plants, soil, and snails of Guiyu town were reported to have a higher concentration of PCBs and PBDEs (Liu et al. 2008 ). Luo et al. ( 2011 ) reported that the burning of metallic chips and electric circuits is one of the reasons for elevated metals like Cd, Cu, and Pb in soil. Luo et al. ( 2009 ) reported a higher value of PBDE in agricultural soils within 2 km of range from an e-waste recycling workshop. In another study, soil from the same range was reported to have high concentrations of polychlronateddibenzo-p-dioxins and dibenzofurans (PCDD/Fs), PCBs, and PAHs (Shen et al 2009 ). Several soil samples studied from the area of the e-waste recycling center in India located at Loni were found to have extremely high metal concentrations. Almost all of the soil samples examined were beyond their permissible levels, with the highest Pb content (~ 174 times greater) than the guidelines. Apart from that, excessive amounts of Cd, Hg, Cr, and Zn have been identified in this region also (Link 2014 ; Weerasundara et al. 2020 ). Plant roots can easily assimilate the harmful toxins including heavy metals present in the soil and transmit them to various parts of the plant including stem, leaf, and fruit. Hence, e-waste-contaminated soil is considered one of the major sources of crop and vegetable contamination (Meng et al. 2014 ).
Impact on the human health
Improper handling, processing, and application of poor recycling methods related to e-waste pose life-threatening impacts on human health (Orlins and Guan 2016 ). Exposure to hazardous substances released from the e-waste can enter the human being either via ingestion, inhalation, or dermal contact (Awasthi et al. 2018 ). Workers associated with e-waste handling or recycling have high chances of direct occupation exposure mainly due to a lack of safety measures (Chen et al. 2011 ). E-waste exposure identification is very complicated due to variety of exposure sources, routes, exposed individual characteristics, including body weight, age, sex and immunological status, and non-identical time periods of exposure (Alabi and Bakare 2017 ). Contaminated water, air, soil, and food are the main reason for the widespread risk associated to e-wastes in humans (Vaish et al. 2020 ). Children may have greater risks after having exposure to the e-wastes present in their surroundings or food items (Pronczuk-Garbino 2005 ; Kuang et al. 2023 ). As humans get exposed to e-waste contaminants, hazardous substances will be released into the human body and stored in fatty tissues and pose severe threats to human health (Liu et al 2018 ). Metals can enter the body of a human either by inhalation, ingestion, or dermal contact. Oral exposure includes the consumption of water and food contaminated with e-waste (Zheng et al. 2013 ; Fu et al. 2008 ). It has been found that heavy metal gets exposed to the human body mainly due to ingestion (90%) (Weerasundara et al. 2020 ). Heavy metals can also be bio-accumulated into the plant tissues from water resources, contaminated soil, or air deposition (Singh et al. 2010 ; Bi et al. 2009 ). Heavy metal–contaminated soil–grown food items are mostly responsible for the increase of heavy metal concentrations in meat-based products (González-Weller et al. 2006 ). Through a number of studies performed by various researchers around the globe, it had been reported that heavy metal leads to various kinds of disorders in humans, including the nervous system, urinary, cardiovascular, blood, liver, kidney, learning inability, cancer, and urine (Thomas et al. 2009 ; Bhutta et al. 2011 ; Yan et al. 2013 ). Therefore, it is necessary for local bodies and government organizations to perform an assessment study related to risk and exposure in the areas of e-waste processing and recycling. Through reports, it had been found that the e-waste recycling center located at Guiyu in China has led to an increase in the level of dioxins in humans above the recommended maximum limit set by WHO (Chatterjee 2007 ). The presence of dioxins above than prescribed limit has been found in the sample of human milk, hair, and placentas hence showing that dioxins are entering the human body from water, air, or via foodstuffs further posing serious ill effects (Chan et al. 2007 ). A study performed by Ha et al. ( 2009 ) reported that soil and water from the areas around the Guiyu e-waste recycling center had an elevated level of heavy metals and that poses serious ill effects on workers and residents living in these nearby areas (Chan et al. 2013 ; Sjödin et al. 1999 ; Guo et al. 2010 ; Wu et al. 2010 ; Huo et al. 2007 ; Xing et al. 2009 ; Li et al. 2008 ). Studies were performed by various researchers related to e-waste in Bangalore, India; China; Ghana; it has been found that the workers and residents were found to be exposed with an elevated level of heavy metal, polybrominated diphenyl ethers, dioxins, polychlorinated biphenyl, etc. (Asante et al. 2012 ; Zheng et al. 2011 ; Song and Li 2014 ; Ha et al. 2009 ; Alabi and Bakare 2017 ). Based on the above discussion, it is clear that toxic contaminants present in e-waste items may cause huge toxicity (Table 1 ), if they are mishandled or discarded openly.
Based on the above discussion, it is clear that toxic contaminants present in e-waste items may cause huge toxicity (Table 1 ), if they are mishandled or discarded openly.
Management strategies for the e-wastes
Advancement of technology is one of the fast-growing sectors because of the rise in the requirements of the consumer’s perception. Consequently, the quantities of e-wastes are increasing day by day across the world (Mmereki et al. 2016 ; Dhir et al. 2021 ). E-wastes may contain some hazardous elements such as cadmium, lead, mercury, polybrominated diphenyl esters, etc., and hence, their high rate of generation and mismanagement may create many disturbances to the environment and living entities. Therefore, safe management practices for the e-wastes need to be explored on a priority basis (Awasthi et al. 2016 ; Garlapati 2016 ; Kumar and Dixit 2018 ). Figure 5 shows a general framework of e-waste material (life cycle) since its production to disposal. It can also be correlated with the practices required to achieve the concept of circular economy in which waste materials are used up to maximum resource utilization.
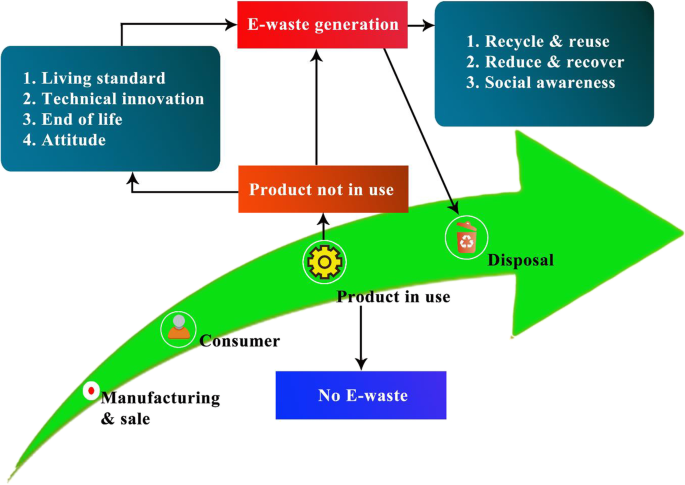
Framework of e-waste from production to disposal along with some management activities
From the above figure, it is apparent that technical advancement and human behavior are mostly responsible for the generation of e-wastes in the society. Moreover, policy formulation and implementation can also play a major role in the management of e-wastes as already are being adopted by some developed nations of the world (Table 2 ). Table 2 shows some practices of effective e-waste management in four developed countries including Japan, the UK, the USA, and Switzerland.
Based on the above table, it has been observed that better e-waste management can be achieved by inculcating the attitude of taking responsibility by the consumer, retailer, management authorities, and policy makers as well. Furthermore, some e-waste management options are proposed by worldwide researchers as discussed below:
Recovery of precious metals from the e-wastes
E-waste can be a source of income for individuals or industries. For example, in Bangalore (India), around 18,000 MT of e-wastes is generated annually. These e-wastes do have some precious metals like gold, silver, platinum, copper, aluminum, and rare earth metals and these elements can be reused. Furthermore, new commercial benefits from them can also be explored as opportunities (Garlapati 2016 ; Dias et al. 2018 ; Dhir et al. 2021 ; Xavier et al. 2023 ).
Recycling of e-wastes
Like other wastes, some parts of the e-wastes can also be recycled such as capacitors, circuit boards, and plastics. Energy production is also possible after incineration of the e-wastes as the plastic of the e-wastes a feedstock for pyrolysis (CII 2006 ). However, it will require extra setups for controlling toxic emissions (CII 2006 ).
Thermo-chemical approaches to generating energy using waste materials have attracted researchers across the world as it is also helping in the recycling of waste materials (Garlapati 2016 ). Additionally, new materials can also be manufactured from the recovered materials from the e-wastes (Baxter et al. 2016 ) as the prime objective of recycling is to procure worthy materials so that the threats to the environment and human health could be avoided. Furthermore, recycling may also create several opportunities for the society in terms of employment, business, etc. (Schluep et al. 2009 ). As per the studies of the United Nations University ( 2017 ), recycling e-waste would be beneficial for environmental conservation as well as society. However, people’s attitudes, knowledge, and behaviors are very important issues to determine the accomplishment of proper recycling as well as management of e-wastes (Echegaray and Hansstein 2017 ; Kumar 2019 ).
Arya and Kumar ( 2020 ) have found that India has taken many steps regarding the better management of its e-waste items as shown in Fig. 6 .
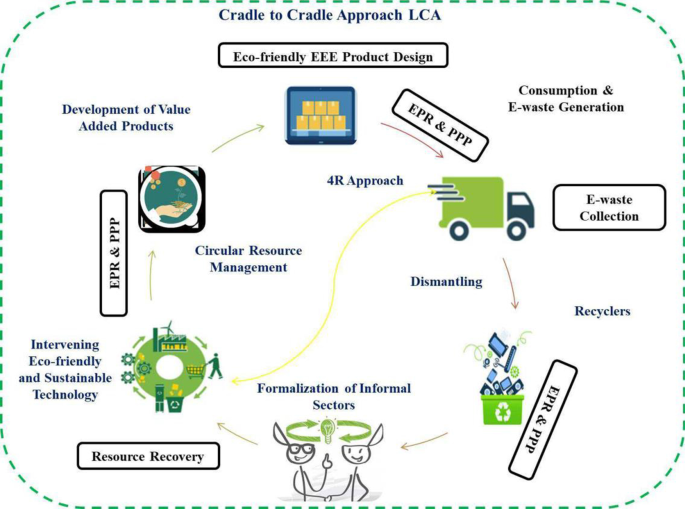
An Indian integrated approach for e-waste management (source: Arya and Kumar 2021: Reprinted from Arya, S., Kumar, S. (2021). E-waste in India at a Glance: Current Trends, Regulations, Challenges and Management Strategies. Journal of Cleaner Production, https://doi.org/10.1016/j.jclepro.2020.122707 with permission from Elsevier)
However, Indian authorities and citizens still need to do a lot regarding every environmental problem including waste management (municipal, electronic, plastic, hazardous, etc.). Recycling waste materials (including e-wastes) is one of the best practices to manage them. It can be achieved by using them as either developing building materials or some other valued products through a process or material alteration (Garlapati 2016 ). Furthermore, recycling e-wastes can also reduce the chance of secondary contamination in the different ecosystems. Therefore, it is recommended to develop an efficient collection, storage, transportation, processing, recovery of value-added products, treatment, and safe disposal of the e-wastes at local as well as national levels in consultation with regulatory bodies (Cucchiella et al. 2015 ). Furthermore, de Souza et al. ( 2016 ) observed that recycling and recovery of precious metals present in the e-wastes could be beneficial options to reduce their harmful impacts on the living world along with restricting burden on the landfills. Moreover, such practices will be helpful in the generation of employment and maintaining environmental safety guidelines as it may also discourage mining along with less emissions of greenhouse gases. Therefore, it can be inferred that recycling and recovery of wastes could also be helpful to cope with global warming and ultimately climate change.
Microrecycling concept for recycling of e-waste
Metal recovery from e-waste is an attractive opportunity of business for private enterprises and transportation of bulk materials of such waste would create high costs. Microrecycling units for material recovery can be established in smaller areas (around 50 m 2 ) using small cost. The microrecycling concept deals with the collection and segregation of e-waste materials from local communities at small-scale factories which helps to reduce the cost of transportation, consumption of raw materials, saving of fossil fuels, and environment same time able to produce value-added recycled materials for further use (Sahajwalla and Hossain 2020 ). These microfactories have various processes including microrecycling units for obtaining materials using the process of fractional heating and separation of various metals, polymers, and ceramics from e-waste. Furthermore, enabling multiple reactions using selective thermal transformation to obtain vigilant separation of valuable metals and alloys. Recently, it has been found that valuable materials can be obtained via novel and fundamental pathways including the generation of metals, metal nanoparticles, alloys, and nanoceramics from the e-waste (Singh et al. 2021a , b ). The main purpose of this concept is to process and recycle e-waste efficiently, safely, and sustainably at a smaller level which would be able to supply value-added materials for the utility of nearby industries.
Development of the effective policies for e-waste management
Effective policies have always been a remarkable tool to achieve success in many disciplines. If we take the example of India, e-waste management in its early stage as the country did not have effective policies, despite being a great manufacturing hub of electronic devices (Borthakur and Singh 2020 ; Garlapati 2016 ). However, the Indian environment ministry (i.e., MoEFCC-Ministry of Environment, Forest and Climate Change) has issued some guidelines for the effective management of e-wastes (Arain et al. 2020 ). Indian decision-makers have developed many waste management rules/guidelines between the years 1989 to 2016. In 2009, first-time e-waste handling rules were drafted, whereas in 2011, e-waste rules were provided along with plastic waste rules. In India, e-waste management–related policies are not well developed, and hence, it requires serious revision to provide safety for both the environment and human health (Garlapati 2016 ). But, it requires rigorous revisions for the effective management of e-wastes (Kumar and Agrawal 2020 ). Similarly, China has also formulated many waste management policies but most of them are focused on municipal solid waste management (Jin et al. 2021 ). European Union (EU) in the early 2000s had performed an important role in setting the guidelines to regulate e-waste management after the debates raised in the 1989 Basel Convention. The waste electrical and electronic equipment (WEEE) Directive of 2002 was the first important regulation in order to establish targets to ensure the recycling, recovering, and treatment of these types of waste (Isernia et al. 2019 ; Stonewell 2013 ). This directive in Europe was used for further elaboration of different legislations in the next years (Lopes dos Santos and Jacobi 2022 ). This directive was further used for developing national legislation in countries like Canada, the USA, Japan, and Australia (Bandyopadhyay 2008 ; Gough 2016 ; Kumar et al. 2017 ). Bibliometric studies can also be helpful in the development of fruitful policies for a particular subject as reported by Taneja et al. ( 2021 ) in the waste management discipline. Recently, Grab et al. (2023) and Asokan et al. ( 2023 ) stated that principles of extended producer responsibility could be a better option for e-waste management, if implemented in the framing of e-waste management policies and executed for the recycling and collection of the e-wastes.
Imparting professional training to the workers of waste management authorities
Waste management is a multi-functional process as it involves processing, use of instruments, safety measures, monitoring, supervision, etc. Hence, trained manpower could be a great asset for waste management authorities because these skilled persons can play significant roles in the management of wastes (Gaidajis et al. 2010 ; Heeks et al. 2014 ). Different types of stakeholders can be involved in the training for successful waste management including academic institutes, research organizations, ministries, municipalities, local public, technical persons, etc. (Srivastav and Kumar 2020 ; Arya and Kumar 2020 ). A similar hierarchy can also be followed for effective e-waste management including regulatory bodies, government organizations (local and national), non-governmental organizations, corporate world, society, etc.
Imposing heavy fines to the violators
A heavy fine may be imposed on the violators of waste management. The provision of the penalty should be elaborated by the regulatory authorities for all the stakeholders. The responsibilities of every stakeholder (individuals, institutions, industries, etc.) should be defined well so that it can be implemented honestly and efficiently. Furthermore, rules can be made very strict especially for the violators of e-waste management as it may have some dangerous elements too. Examples follow.
Rewarding with lucrative incentive to the best performers by the waste management authorities
Apart from imposing fines, there must be a provision of rewarding incentives to the best-performing firms, societies, institutions, or industries by the regulatory bodies on the basis of annual waste audit report. Therefore, it will create some positivity among the different stakeholders of the waste generators and their management (Ravindra et al. 2019 ). Cucchiella et al. ( 2015 ) reported that NGOs and manufacturers need to be encouraged for the different activities (collection, storage, recycling, etc.) related with e-waste management at different administrative levels.
To ensure the concepts of circular economy in waste management
Recently, the assimilation of concepts of circular economy (Ellen MacArthur Foundation 2019 ; Parajuly et al. 2020 ) and sustainable development goals are among the important objectives for every organization/institution. According to Sauvé et al. ( 2016 ), recycling of the materials and maximum resource recovery from wastes should be ensured to restrict future environmental pollution. Meaning, thereby, maximum use of the materials must be ensured to reduce waste generation (Ellen MacArthur Foundation 2019 ) and it can be achieved by adopting eco-friendly technologies and green policy developments as well as inculcating fruitful innovative corporate cultures (Ghisellini et al. 2016 ). Figure 7 is the representation of the concepts of circular economy in e-waste management.
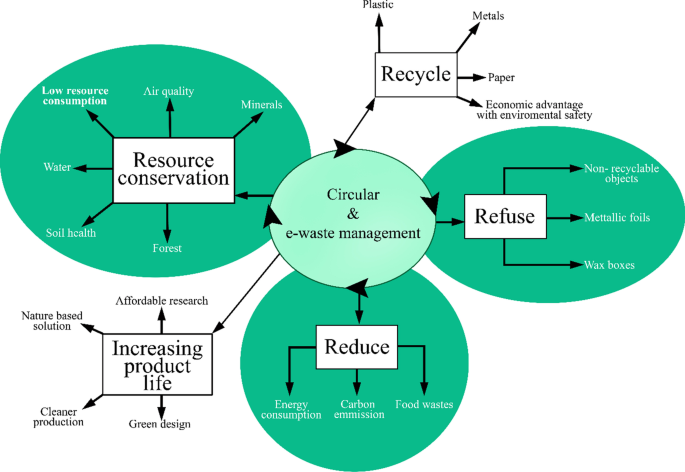
Concept of circular economy to be followed for effective e-waste management
Figure 7 shows that environmental and economical sustainabilities can be achieved in waste management in any nation/region after following the concepts of the circular economy. The above practices (mentioned in the figure) can be adopted to achieve better e-waste management through the concept of circular economy and sustainable development goals. However, wise consumption of the materials may depend on awareness, behavior, lifestyle, morality, culture, environmental ethics, psychology, etc. (Knussen and Yule 2008 ; Parajuly et al. 2020 ).
Role of machine intelligence techniques in e-waste management
The discarded electronic devices are generally termed e-waste (Bhutta et al. 2011 ; Mor et al. 2021 ; Umair et al. 2016 ). There is a serious concern regarding the unsafe handling of these devices (Bhutta et al. 2011 ; Mor et al. 2021 ; Umair et al. 2016 ). It may cause harm to human health and the environment as these scraps may contain cadmium, lead, beryllium, zinc, etc. (Bhutta et al. 2011 ; Mor et al. 2021 ; Umair et al. 2016 ). Several works have already been done by researchers in the domain of disposal and the management of e-waste but the challenges are so many. Awasthi et al. ( 2016 ) discussed the rapid growth in e-waste. They observed that the common methods of dealing with e-waste like acid baths and open burning may release furans, dioxins, and other heavy metals which may lead to harmful effects on the surroundings. Figure 7 shows a layout of artificial intelligence–based e-waste management system. All the information relevant to e-waste has been gathered and transferred to the cloud server. The cloud server matches the gathered e-waste sources from the existing database. The database category based on the review has already been maintained on the cloud server. The components from the e-waste product have been separated based on the classification. The automated machine learning–based category advisor can be capable of the classification of the components (Vimala et al. 2022 ; Thakur et al. 2022 ; Dubey et al. 2021 ). The components are then filtered and the IoT devices will accept the filtered component and provide a name and send an alert message to the server. It is already attached to the server for the demand of the individual component. It can be synchronized with the received e-waste resources. IoT-enabled devices help in the product assignment and set it in the cloud server with the updated product list. So, any request for these sources can be fulfilled properly from the server automatically. The machine intelligence approaches will be helpful in the reassignment, automation, and learning from history. So that the detection of the reusable components will be improved automatically. It will be helpful in gathering, filtration, and reusability and improving product usability based on the automated guidelines of the model.
Rahman et al. ( 2020 ) studied waste management. They have proposed an architecture for waste management based on deep learning and the Internet of Things (IoT). They devised a smart trash bin concept with multiple sensors. For data monitoring purposes, IoT and Bluetooth technology have been used. They achieved the convolutional neural network (CNN) accuracy of 91.3% and 86% of the system usability scale (SUS). Agarwal et al. ( 2020 ) studied the growth of e-waste. It has been discussed in terms of developing economies. They have reviewed and analyzed the artificial intelligence prospective in the literature from 2015 to 2020. They found that the computational technique may be helpful in the segregation of e-wastes. Kang et al. ( 2020 ) analyzed e-waste management in terms of precious base metals and found that smart e-waste collection management can be done by using smart techniques. Their approach has the automatic notification and scheduling of the e-waste management mechanism system as it was developed in Malaysia. Senthilselvi et al. ( 2020 ) studied the impacts of technological development in the case of e-waste worldwide. They have suggested the challenges in the disposal of electronic equipment. Their aim was to provide automation in metal purification measurement by the use of machine learning techniques. For the metal separator from the mobile phones, they used magnetic separation, eddy current, and pyro-metallurgical and hydrometallurgical processes. The noise removal from the images of the extracted metal was performed by CNN. It is helpful in feature extraction and classification. For validation from the learned features, they used and rectified linear units. They successfully extracted the metal which can be used in any other manufacturing process. Singh et al. ( 2021a , b ) studied the disposal of e-waste. They proposed an IoT-based collection vendor machine for e-waste management. It was associated with the QR code for the related information. It contains the alert system based on the capacity (threshold point) along with the secure disposal technique. Chen et al. ( 2021a , b ) discussed e-waste in terms of a tremendous increase in environmental issues due to the toxic substances that can adversely affect health as well as the environment. They proposed an artificial intelligence technique for the smart analysis of hazardous components. Abou et al. ( 2021 ) analyzed the growing need for automated e-waste recycling and management. They used AlexNet layers for e-waste sorting and management. Yu et al. ( 2021 ) discussed the challenges in waste disposal. They have discussed the mechanism of reducing, reusing, recycling, and recovering. They have proposed an automated artificial intelligence framework. Their framework was capable of specific information gathering, planning, and management. Li et al. ( 2021 ) utilized the machine learning technique in e-waste management. They used a gradient-boosting regression tree and neural network for the monitoring of urban sub-segments. The arrangement was improved based on machine learning techniques with an exactness of 99.1%. Islam et al. ( 2021 ) discussed teleworking. They suggested that teleworking has increased the generation of e-waste. They mainly focused on the collection of metals. The recovery was performed based on gravity, density, and integrated approaches. Aswani et al. ( 2021 ) suggested that in the current Indian scenario e-waste is a huge problem. They have suggested machine learning techniques to handle the recycling procedure of metals from the e-waste. Ramya et al. ( 2021 ) studied an advanced waste managing scheme based on long-range (LoRa) protocol and the TensorFlow framework. The communication protocol was managed by LoRa. Object detection was performed by the TensorFlow framework. It performs actual time object detection. The TensorFlow framework was used for actual time object detection and organization of waste data. Radio-frequency identification (RFID) was also used for the identification of e-waste. Khan et al. ( 2021 ) discussed the role of machine learning and IoT in e-waste collection. For effective e-waste management, they proposed an approach using machine learning and IoT. They used an Arduino UNO microcontroller, ultrasonic sensor, and moisture sensor. Their main aim is to measure the dumping ground waste index. Elangovan et al. ( 2021 ) studied the increase in e-waste. They used deep learning for the classification of the metal present in e-waste.
The major challenges found are as follows:
There are different levels of challenges in e-waste management like collection, extraction, reusing, and recycling based on the extracted patterns.
There is the need for applicability of machine intelligence methods based on the requirement levels.
There is the need for a hybrid framework with integrated approaches for the purpose of analysis, extraction, feature engineering, labelling of e-waste, automation of devices without human interference, and alert system with enhanced connectivity.
There is also the need for data handling mechanisms to achieve cost savings, flexibility, quality control, and data storage, increase collaboration, and automate software updates.
There is also the need for automated control flow in all levels.
A framework has been proposed as shown in Fig. 8 , to fulfill the abovementioned challenges. Our suggested framework is divided into four parts:
Optimized data collection and processing : in the present framework, cloud computing has been used for data collection and processing. This phase is responsible for surveying, monitoring, reporting, and notification system on e-waste. It accepts the request for e-waste collection from different users using mobile applications. It is responsible for the data gathering, identification, and other details like the requester, and information on the e-waste products including the metal and reusable components.
Machine intelligence approaches: in this phase, machine intelligence approaches like machine learning, deep learning, and machine vision approaches can be used for the purpose of analysis, extraction, feature engineering, labelling, and classification with the approach automation.
In the third phase, an IoT-based e-waste container has been proposed for handling the sensing and notification mechanism based on the collected data. This framework is also capable of filtering reusable components along with the precious metal.
The final phase is the automated notification for the requestor, if any need arises for the components received initially.
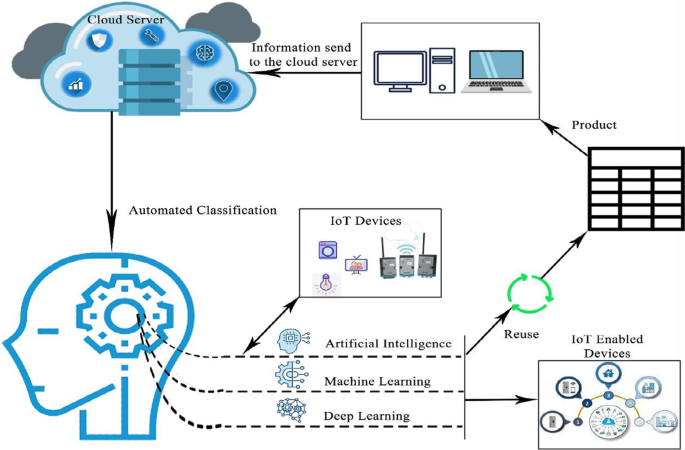
Framework for e-waste management based on machine intelligence approach
The above framework is capable of handling e-waste management in an efficient way in accordance with proper reuse and recycle mechanisms.
Miscellaneous recommendations for effective e-waste management
It is highly recommended that the concept of 4Rs (reduce, reuse, recycle, and recovery) must be entertained in e-waste management practices for a better future. In Spain, a well-illustrated recycling procedure was developed to manage e-wastes so that it can reach the suppliers after being discarded by the consumers (Queiruga et al. 2012 ) and such type of frameworks may be one of the best practices to endure maximum recycling of electronic waste products. Non-measurement of e-waste generation (as a separate account) is not being effectively implemented so it has been observed as a major hurdle for e-waste management. Hence, for effective management of the e-wastes at individual as well as municipal levels, it should ensure to develop an inventory containing harmful impacts on the environment and living creatures (Debnath et al. 2016 ). For better e-waste management, some common recommendations may be emphasized such as:
Public awareness (especially among consumers) about the negative impacts of e-waste on the environment and human beings and also their guidelines (Garlapati 2016 )
Introducing fees for recycling purposes (Garlapati 2016 )
Mandatory segregation of the e-wastes from the domestic wastes at the individual level.
Endeavors should be made to limit the use of electronic/electrical appliances up to the maximum extent or their lesser use should be encouraged. For example, a paperless provision in banks/offices may reduce the application printers along with saving papers.
Exploring the possibility of digitalization in every system (e-governance), especially traditional systems.
Monitors of computers should be energy-efficient so less consumption of energy can be ensured.
Electronic appliances should be switched off, when not in use.
Electronic parts of the computers (or any electronic devices) should be reused, if possible based on their life and condition.
Research activities should be increased for better e-waste management.
Eco-friendly electronic appliances should be developed as reported by Asus—a reputed company in Taiwan (Debnath et al. 2016 ).
For e-waste management, basically, two approaches are under practice in developed and developing nations. In developing nations, normally, e-wastes are not under proper recycling, and moreover, their proper disposal is also not being followed. Furthermore, developing countries are also involved in the extraction of precious metallic content from the e-wastes imported from the developed nations (Manhart 2011 ). Hence, it seems that sustainable management of e-waste could be achieved through developing proficient techniques for e-waste recycling, providing specialized training to the workers, and devising powerful policies. Furthermore, the policies, responsibilities of administration, waste management authorities, and electronic appliances manufacturing industries must be defined and monitored regularly (Esenduran et al. 2019 ). For example, since the year 2003, in Switzerland, the roles of production units and importing industries have been set to follow e-waste management guidelines as described in the policies namely Extended Producer Responsibility as well as the Advance Recycling Fee (Sinha-Khetriwalet al. 2005 ). Furthermore, Japan has also developed Consumer Pays model in which users can give back electronic gadgets (supposed as waste) to the retailers along with prescribed fees (Widmer et al. 2005 ). Similarly, the US Environmental Protection Agency (USEPA) is responsible for the better management of the e-wastes generated in the country under National Electronic Action Plans (Gaidajis et al. 2010 ). Some developing nations are also trying to manage their e-wastes like Peru which initiated sustainable approaches to e-waste management by developing skills to collect followed by recycling the same for protecting human beings and the environment. Columbia was able to recycle ~ 185 tons of cell phones generated from 30 cities during 2007–2014 (Kofi Adanu et al. 2020 ).
Anticipated challenges during e-waste management
Apart from the availability of the above management practices, there are many challenges that may come on the ground during e-waste management. If we take the example of Europe, most of the population is well aware of the wise use of resources and also knows that their exploitation is not good for the environment (European Union, 2014 ). Moreover, people claim that they are well involved in waste segregation, reuse, and also earning revenues from the wastes (Europeet al. 2018 ). Similarly, developing countries (China, Malaysia, Thailand, and India) are importing e-waste from the Organization for Economic Co-operation and Development (OECD) group of countries in the name of manufacturing and reuse (Kumar and Dixit 2018 ). Furthermore, social acceptance is another issue regarding the reuse and development of business models using e-wastes (vanWeelden et al. 2016 ). To ensure the proper recycling of the e-wastes, behavior of the individual person and right decision are also having a direct impact (Parajuly et al. 2020 ).
Furthermore, some commonly observed challenges faced by the management authorities regarding e-waste management include a lack of guidelines, negative public perception, lack of green initiatives, financial constraints, and inadequate infrastructure.
These challenges (as shown in Fig. 9 ) may be a lack of citizen’s knowledge about recycling (Godfrey et al. 2013 ; Kumaret al. 2016 ), no adequate guidelines (Chaturvedi et al. 2007 ; Wath et al. 2010 ), non-implementation of green initiatives (Ravi and Shankar 2014 ), financial crisis (Kumar and Dixit 2018 ), inadequate infrastructures of collection and recycling (Kumar et al. 2017 ), confusion among the producers and consumers regarding accountability (Manomaivibool and Vassanadumrongdee 2011 ; Kiddee et al. 2013 ), etc. Moreover, we are living in a digital age and the world is observing technological advancement at an unprecedented rate. The durability of electrical and electronic equipment (EEE) has decreased tremendously simply because of the frequent hardware and software up-gradation. Globally, this trend is contributing a voluminous amount of waste electrical and electronic equipment (WEEE) or e-waste. Studies predict that the total volume of e-waste will cross 74 million tons by 2030 as it is increasing at the rate of 3–5 percent per annum. China (10.1 MT/year), the USA (6.9 MT/year), India (3.2 MT/year), Japan (2.1 MT/year), Germany (1.6 MT/year), and Brazil (2.1 MT/year) are the biggest producers of WEEE (Andeobu et al. 2021 ; Shittu et al. 2021 ). E-waste is adversely affecting the biotic and abiotic components of the environment. Hence, in order to achieve the UN sustainable development goals (SDGs), circular economy and resource recovery, and efficiency, standardized and effective e-waste management procedures are indispensable. Materials present in the WEEE have been classified into five categories viz . ferrous metals, non-ferrous metals, glass, plastics, and other materials by different workers (Shittu et al. 2021 ). Scarcity and recovery of resources (minerals, rare earth metals, heavy metals, polymers, etc.) and energy are other dimensions which the globe is observing with the exponential rise in the manufacturing of electronic products. The biggest challenge before the world is to design and adopt standardized recycling procedures. Researchers suggest that only a small fraction of the e-waste is recycled by organized sectors (having required expertise and infrastructure) while a large chunk (nearly 82 percent) is tackled by the informal sector which creates environmental and occupational health hazards. China, India, and Ghana can be considered the hub of informal e-waste recycling while countries like Australia, Japan, and Canada have skilled workforce and infrastructure to scientifically recycle the waste (Rautela et al. 2021 ). Studies have suggested e-waste urban mining to effectively tackle the problem and emphasized the issues and challenges related with it. Urban mining helps in the recovery (rare earth metals, plastic and polymer, heavy metals, etc.) and recycling of e-waste in a phased manner (mechanical separation, hydrometallurgy, and pyrometallurgy), which in turn boost up the circular economy. However, in developing countries like India, there are serious challenges associated with urban mining, mainly the lack of coordination and integration between thee-waste collectors, dismantlers, recyclers, etc. (Ikhlayel 2018 ; Sharma et al. 2021 ). Due to this un-coordinated and unskilled approach, a huge volume of e-waste gets treated and discarded using different informal methods like dismantling and incinerating in open. Also, a large fraction of e-waste goes to landfill sites which is again a hazardous practice in the long run. The role and application of artificial intelligence, machine, and deep learning should also be explored in e-waste management (Chen et al. 2021a , b ).
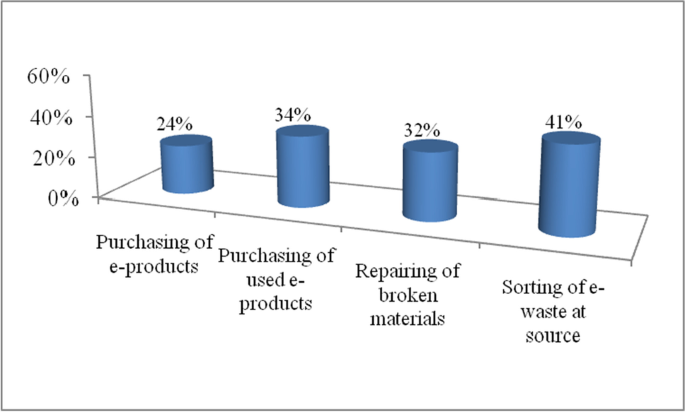
Actual gap in the claim and practice of European people (data extracted from Parajuly et al. 2020 )
However, the actual scenario is quite different from people’s claims as the gap in the claim and practice of European people (Parajuly et al. 2020 ) is shown in Fig. 9 .
Therefore, based on the above graph, a general opinion may change as can be seen in the case of European society (being considered one of the most civilized societies).
In the future, the focus should be given to spreading awareness among the local citizens (or all the stakeholders) to change their behavior so that e-waste management or any other environmental crisis can be overcome easily. Thus, it appears that principles of circular economy can be significantly ensured up to a maximum extent after public participation. Knowledge of relevant technologies, economical issues, principles of circular economy, and environmental concerns should also be discussed together as it may increase the better output in the recycling/reuse of goods (Makov et al. 2018 ). It has also been observed that environmental ethics, laws, and education have significantly elevated the consciousness of environmental protection among the public (Solomon 2010 ). Some prescribed amounts of money can also be paid by the consumers without any force; however, it may vary according to gender, wedding conditions, convenient recycling process, and the toxicity of the e-wastes (Saphores et al. 2012 ). In India, e-waste management is not an easy task because of knowledge gaps regarding environmental conservation and recycling processes along with incompetent policies, financial issues, etc. (Jindal and Sangwan 2011 ). Economical profit and societal acceptance can assist in better e-waste management after coordinating with all the stakeholders. Furthermore, strict laws and policies can also play significant roles in e-waste management (Ravi and Shankar 2014 ; Kumar and Dixit 2018 ). Similarly, there are no any legal frameworks in African countries for the recycling of e-wastes. However, they are good at reusing (~ 85%) electronic or electrical devices and it is around 2–3 times more in comparison to rich countries. Australia has a well-defined provision for recycling television and computers with the collaboration of government regulatory authorities and industries. China, India, Japan, South Korea, and Taiwan are the only Asian countries who have formulated e-waste management policies. However, Taiwan is at the top with 82% e-waste recycling, whereas Japan and South Korea have achieved 75% e-waste recycling. In other countries of the world, policies regarding recycling and standard development are not adequate (Garlapati 2016 ). Hence, other countries need to develop proper policies particularly for appropriate e-waste management. Most importantly, employment generation through developing business modules can also be performed using e-wastes as these wastes can be used for the production of fuels (energy) through pyrolysis and procurement of precious metals like gold, silver, platinum, copper, and aluminum, and are reusable (capacitors, circuit boards, plastics, etc.), and recyclable materials (CII 2006 ).
For effective and efficient management of high volumes of e-waste, they need to be addressed on a priority basis. A few of the challenges and tangible measures are given below:
Creating awareness about the hazardous impacts of e-waste and recycling of the same.
Accurate and real-time measurement and quantification of e-waste being generated.
Developing a skilled workforce and expertise to transform an unorganized recycling sector into an organized one and create a new organized recycling setup.
Developing a state-of-the-art infrastructure where e-waste can be recycled with minimum environmental and health hazards.
Incentivizing the recycling sector by incorporating various economic and financial tools like easy loaning to create recycling infrastructure, tax relaxation, extended producers’ responsibility, and public–private partnership (PPP).
Develop materials and technologies so that maximum recovery can be done after recycling.
Recovery of resources and energy is a crucial aspect which can be achieved via urban mining.
Informal and unscientific methods should be immediately checked.
Frequent monitoring and stringent policy should be adopted in order to mitigate any adverse impact on the environment.
Recent events showed that the organized and informal sector related to e-waste management has been severely affected by unprecedented and unalarmed situations like the COVID-19 pandemic outbreak. Therefore, policy formulators should consider such challenges also. Moreover, the feasibility of advance computational tools may also be explored especially for e-waste management so that it can be beneficial for the environment and public health. Cost–benefit analysis of the available techniques of e-waste management should be carried out appropriately which can provide a better direction for future studies.
Worldwide, large amounts of e-waste are produced as a result of purported modernization efforts. These e-wastes may also include some harmful elements in addition to certain valuable and recyclable items. Therefore, their careless handling and open disposal practices could also be harmful to the environment and biosphere. E-waste is frequently mismanaged since the general population is ignorant about their importance and also degrees of threat. Radioactive substances may also be present in e-wastes which can damage the plant tissues and ultimately inhibit plant growth. Radioactive materials in the soil can hinder the uptake capacity of the plants for nutrients. Apart from awareness, lack of responsible consumption and infrastructure facilities, financial crisis, etc. are also some major challenges to effective e-waste management. However, some good practices can reduce the levels of these challenges which include motivation of society, change in behaviors, availability of adequate funds/incentives, proper availability of e-waste management facilities, and adoption of green practices. The application of advanced computational tools is also playing crucial roles in multiple disciplines, and hence, better e-waste management practices can also be done by using techniques like artificial intelligence and machine learning. Furthermore, economic feasibility can also be maintained by applying such approaches. Moreover, it will also help in the achievement of many sustainable development goals either partial or complete along with accomplishing the concepts of a circular economy.
Data availability
Not applicable .
Abou BN, Szabo-Müller P, Handmann U (2021) Transfer learning-based method for automated e-waste recycling in smart cities. EAI endorsed transactions on smart cities, 1–9
Adanu SK, Gbedemah SF, Attah MK (2020) Challenges of adopting sustainable technologies in e-waste management at Agbogbloshie Ghana. Heliyon 6(8):e04548
Article Google Scholar
Agarwal V, Goyal S, Goel S (2020) Artificial intelligence in waste electronic and electrical equipment treatment: opportunities and challenges. InInternational Conference on Intelligent Engineering and Management pp. 526–529
Alabi AO, Bakare AA (2017) Genetic damage induced by electronic waste leachates and contaminated underground water in two prokaryotic systems. Toxicol Mech Methods 27(9):657–665
Article CAS Google Scholar
Stonewell A (2013) Environmental risk-the impact of the UK WEEE Regulation-an incentive to change occupational practice?
Amankwah-Amoah J (2016) Global business and emerging economies: towards a new perspective on the effects of e-waste. Technol Forecast Soc Change 105:20–26
Andeobu L, Wibowo S, Grandhi S (2021) An assessment of e-waste generation and environmental management of selected countries in Africa, Europe and North America: a systematic review. Sci Total Environ 792:148078
Arain AL, Pummill R, Adu-Brimpong J, Becker S, Green M, Ilardi M, Van Dam E, Neitzel RL (2020) Analysis of e-waste recycling behavior based on survey at a Midwestern US University. Waste Manag. 105, 119e127. https://doi.org/10.1016/j.wasman.2020.02.002
Arya S, Kumar S (2020) E-waste in India at a glance: current trends, regulations, challenges and management strategies. J Clean Prod. https://doi.org/10.1016/j.jclepro.2020.122707
Asante KA, Agusa T, Biney CA, Agyekum WA, Bello M, Otsuka M, Tanabe S (2012) Multi-trace element levels and arsenic speciation in urine of e-waste recycling workers from Agbogbloshie Accra in Ghana. Sci Total Environ 424:63–73
Asokan VA, Teah HY, Kawazu E, Hotta Y (2023) Ambitious EV policy expedites the e-waste and socio-environmental impacts in India. Resour Conserv Recycl 190:106829
Aswani T, Maurya A, Naik G, Perla M (2021) Automated e-waste disposal using machine learning. VIVA-Tech Intl J Res Innov 1:1–7
Google Scholar
Awasthi AK, Li J (2017) Management of electrical and electronic waste: a comparative evaluation of China and India. Renew Sustain Energy Rev 76:434–447
Awasthi AK, Wang M, Awasthi MK, Wang Z, Li J (2018) Environmental pollution and human body burden from improper recycling of e-waste in China: a short-review. Environ Pollut 243:1310–1316
Awasthi AK, Zeng X, Li J (2016) Environmental pollution of electronic waste recycling in India: a critical review. Environ Pollut 211:259–270
Awual MR, Hasan MM, Islam A, Rahman MM, Asiri AM, Khaleque MA, Sheikh MC (2019) Offering an innovative composited material for effective lead(II) monitoring and removal from polluted water. J Cleaner Prod 231:214–223
Awual MR (2019) Novel ligand functionalized composite material for efficient copper(II) capturing from wastewater sample. Composites Part B: Eng 172:387–396
Awual MR, Yaita T, Kobayashi T, Shiwaku H, Suzuki S (2020) Improving cesium removal to clean-up the contaminated water using modified conjugate material. J Environ Chem Eng 8(2):103684
Awual MR, Hasan MM, Shahat A, Naushad M, Shiwaku H, Yaita T (2015) Investigation of ligand immobilized nano-composite adsorbent for efficient cerium(III) detection and recovery. Chem Eng J 265:210–218
Awual MR (2015) A novel facial composite adsorbent for enhanced copper(II) detection and removal from wastewater. Chem Eng J 266:368–375
Awual MR, Hasan MM, Eldesoky GE, Khaleque MA, Rahman MM, Naushad M (2016) Facile mercury detection and removal from aqueous media involving ligand impregnated conjugate nanomaterials. Chem Eng J 290:243–251
Babu R, Parande AK, Basha AC (2007) Electrical and electronic waste: a global environmental problem. Waste Manag Res 25:307–318
Bandyopadhyay A (2008) A regulatory approach for e-waste management: a cross-national review of current practice and policy with an assessment and policy recommendation for the Indian perspective. Int J Environ Waste Manage 2(1–2):139–186
Baxter J, Lyng KA, Askham C, Hanssen OJ (2016) High-quality collection and disposal of WEEE: environmental impacts and resultant issues. Waste Manage 57:17–26
Bhutta MKS, Omar A, Yang X (2011) Electronic waste: a growing concern in today’s environment. Econ. Res. Int. 2011, 474230. https://doi.org/10.1155/2011/474230
Bi X, Feng X, Yang Y, Li X, Shin GP, Li F, Fu Z (2009) Allocation and source attribution of lead and cadmium in maize (Zea mays L) impacted by smelting emissions. Environ Pollut. 157(3):834–839
Borthakur A, Singh P (2020) The journey from products to waste: a pilot study on perception and discarding of electronic waste in contemporary urban India. Environ Sci Pollut Control Ser https://doi.org/10.1007/s11356-020-09030-6
Breivik K, Armitage JM, Wania F, Jones KC (2014) Tracking the global generation and exports of e-waste. Do existing estimates add up? Environ Sci Technol 48:8735–8743
Brigden K (2005) Recycling of electronic wastes in China and India: workplace and environmental contamination. http://www.greenpeace.org/raw/content/china/en/press/reports/recycling-of-electronic-wastes.pdf.2005 . Accessed 14 July 2022
Brindhadevi K, Barceló D, Chi NTL, Rene ER (2023) E-waste management, treatment options and the impact of heavy metal extraction from e-waste on human health: scenario in Vietnam and other countries. Environ Res 217:114926
Chan JK, Xing GH, Xu Y, Liang Y, Chen LX, Wu SC, Wong MH (2007) Body loadings and health risk assessment of polychlorinated dibenzo-p-dioxins and dibenzofurans at an intensive electronic waste recycling site in China. Environ Sci Technol 41(22):7668–7674
Chan JKY, Man YB, Wu SC, Wong MH (2013) Dietary intake of PBDEs of residents at two major electronic waste recycling sites in China. Sci Total Environ 463:1138–1146
Chan JKY, Wong MH (2013) A review of environmental fate, body burdens, and human health risk assessment of PCDD/Fs at two typical electronic waste recycling sites in China. Sci Total Environ 463:1111–1123
Chatterjee, P., 2008. Health costs of recycling. BMJ. 337.
Chatterjee R (2007) E-waste recycling spews dioxins into the air. Environ Sci Technol 41(16):5577–5577
CAS Google Scholar
Chaturvedi A, Arora R, Khatter V, Kaur J (2007). E-waste assessment in India–specific focus on Delhi, MAIT-GTZ study. Available at: https://nswai.com/docs/e-Waste%20Assessment%20in%20India%20-%20Specific%20Focus%20on%20Delhi.pdf (accessed on 16.09.2021)
Chen J, Huang S, BalaMurugan S, Tamizharasi GS (2021a) Artificial intelligence based e-waste management for environmental planning. Environ Impact Assess Rev 87:106498
Chen A, Dietrich KN, Huo X, Ho SM (2011) Developmental neurotoxicants in e-waste: an emerging health concern. Environ Health Perspect 119(4):431–438
Chen J, Huangb S, Murugan SB, Tamizharasi GS (2021b) Artificial intelligence-based e-waste management for environmental planning. Environ Impact Assess Rev 87:106498. https://doi.org/10.1016/j.eiar.2020.106498
Chen M, Huang J, Ogunseitan OA, Zhu N, Wang YM (2015) Comparative study on copper leaching from waste printed circuit boards by typical ionic liquid acids. Waste Manage 41:142–147
CII (2006) E-waste management, green business opportunities. Delhi: Confederation
Cucchiella F, D’Adamo I, Lenny Koh SC, Rosa P (2015) Recycling of WEEEs: an eco-nomic assessment of present and future e-waste streams. Renew Sustain Energy Rev 51:263–272
D’Adamo I, Mazzanti M, Morone P, Rosa P (2022) Assessing the relation between waste management policies and circular economy goals. Waste Manage 154:27–35
de Souza RG, Clímaco JC, Sant’Anna AP, Rocha TB, do Valle RD, Quelhas OL (2016) Sustainability assessment and prioritisation of e-waste management options in Brazil. Waste manage. 57, 46-56
Debnath B, Roychoudhuri R, Ghosh SK (2016) E-waste management–a potential route to green computing. Procedia Environ Sci 35:669–675
Deng S, Xiao Z, Zhang W, Noble A, Das S, Yih Y, Sutherland JW (2023) Economic analysis of precious metal recovery from electronic waste through gas-assisted microflow extraction. Resour Conserv Recycl 190:106810
Dharini K, Cynthia JB, Kamalambikai B, Celestina JAS, Muthu D (2017) Hazardous E-waste and its impact on soil structure. In IOP Conference Series: Earth and Environmental Science (Vol. 80, No. 1, p. 012057). IOP Publishing
Dhir A, Koshta N, Goyal RK, Sakashita M, Almotairi M (2021) Behavioral reasoning theory (BRT) perspectives on e-waste recycling and management. J Clean Prod 280:124269
Dias P, Bernardes AM, Huda N (2018) Waste electrical and electronic equipment (WEEE) management: an analysis on the Australian e-waste recycling scheme. J Clean Prod 197:750–764
Dubey A, Gupta U, Jain S (2021) Medical data clustering and classification using TLBO and machine learning algorithms. Comput Mater Continua 70(3):4523–4543
Dwivedy M, Mittal RK (2010) Future trends in computer waste generation in India. Waste Manage 30:2265–2277
Echegaray F, Hansstein FV (2017) Assessing the intention-behavior gap in electronic waste recycling: the case of Brazil. J Clean Prod 142:180–190
Elangovan S, Sasikala S, Kumar SA, Bharathi M, Sangath EN, Subashini TA (2021) Deep learning based multiclass segregation of e-waste using hardware software co-simulation. In Journal of Physics: Conference Series, p. 012039
Ellen MacArthur Foundation, 2019. Circular Economy. https://www.ellenmacarthurfoundation.org/circular-economy . Accessed 25 Aug 2022
Esenduran G, Atasu A, Wassenhove LN (2019) Valuable e-waste: implications for extended producer responsibility. IISE Trans 51(4):382396
Europe L. E., V. V. A. Europe, Ipsos Opinion-Infometrie, ConPolicy GmbH, and B. V. Trinomics. Behavioural study on consumers’ engagement in the circular economy. Publications Office of the European Union, 2018. Avaialabale at https://ec.europa.eu/info/sites/default/files/ec_circular_economy_final_report_0.pdf (accessed on 21.10.2021)
European Union (2014) Flash Eurobarometer 388 “Attitudes of Europeans towards waste
Forti V, Balde CP, Kuehr R, Bel G (2020) The Global E-waste Monitor 2020: quantities, flows and the circular economy potential. United Nations University (UNU)/United Nations Institute for Training and Research (UNITAR) – co-hosted SCYCLE Programme, International Telecommunication Union (ITU) & International Solid Waste Association (ISWA), Bonn/Geneva/Rotterdam. Available at- https://www.itu.int (accessed on 26.09.2021)
Fu J, Zhou Q, Liu J, Liu W, Wang T, Zhang Q, Jiang G (2008) High levels of heavy metals in rice (Oryzasativa L.) from a typical e-waste recycling area in southeast China and its potential risk to human health. Chemosphere 71(7):1269–1275
Gaidajis G, Angelakoglou K, Aktsoglou D (2010) E-waste: environmental problems and current management. J Eng Sci Technol Rev 3(1):193–199
Gangwar C, Choudhari R, Chauhan A, Kumar A, Singh A, Tripathi A (2019) Assessment of air pollution caused by illegal e-waste burning to evaluate the human health risk. Environ Int 125:191–199
Garb Y, Davis JM (2023) Hub-driven policy packages as a basis for e-waste reform: rationales and a case study. Environmental Politics, pp.1–21
Garlapati VK (2016) E-waste in India and developed countries: management, recycling, business and biotechnological initiatives. Renew Sustain Energy Rev 54:874–881
Ghisellini P, Cialani C, Ulgiati S (2016) A review on circular economy: the expected transition to a balanced interplay of environmental and economic systems. J Clean Prod 114:11–32
Godfrey L, Scott D, Trois C (2013) Caught between the global economy and local bureaucracy: the barriers to good waste management practice in South Africa. Waste Manag Res 31(3):295–305
González-Weller D, Karlsson L, Caballero A, Hernández F, Gutiérrez A, González-Iglesias T, Hardisson A (2006) Lead and cadmium in meat and meat products consumed by the population in Tenerife Island Spain. Food Addit Contam 23(8):757–763
Gough M (2016) Australian laws lag on electronic waste management. Accessed: Mar, 25, 2022
Grant K, Goldizen FC, Sly PD, Brune MN, Neira M, van den Berg M, Norman RE (2013) Health consequences of exposure to e-waste: a systematic review. Lancet Glob Health 1(6):e350–e361
Guo Y, Huo X, Li Y, Wu K, Liu J, Huang J, Xu X (2010) Monitoring of lead, cadmium, chromium and nickel in placenta from an e-waste recycling town in China. Sci Total Environ 408(16):3113–3117
Guo Y, Huo X, Wu K, Liu J, Zhang Y, Xu X (2012) Carcinogenic polycyclic aromatic hydrocarbons in umbilical cord blood of human neonates from Guiyu China. Sci Total Environ 427:35–40
Ha NN, Agusa T, Ramu K, Tu NPC, Murata S, Bulbule KA, Tanabe S (2009) Contamination by trace elements at e-waste recycling sites in Bangalore India. Chemosphere 76(1):9–15
Habib K, Parajuly K, Wenzel H (2015) Tracking the flow of resources in electronic waste - the case of end-of-Life computer hard disk drives. Environ Sci Technol 49:12441–12449
Hasan MN, Shenashen MA, Hasan MM, Znad H, Awual MR (2021) Assessing of cesium removal from wastewater using functionalized wood cellulosic adsorbent. Chemosphere 270:128668
Heeks R, Subramanianab L, Jones C (2014) Understanding e-waste management in developing countries: strategies, determinants, and policy implications in the indian ICT sector. Inf. Technol. Dev. 1–17
Huo X, Peng L, Xu X, Zheng L, Qiu B, Qi Z, Piao Z (2007) Elevated blood lead levels of children in Guiyu, an electronic waste recycling town in China. Environ Health Perspect 115(7):1113–1117
Ikhlayel M (2018) An integrated approach to establish e-waste management systems for developing countries. J Clean Prod 170:119–130
Ilankoon IM, Ghorbani Y, Chong MN, Herath G, Moyo T, Petersen J (2018) E-waste in the international context–a review of trade flows, regulations, hazards, waste management strategies and technologies for value recovery. Waste Manage 82:258–275
Isernia R, Passaro R, Quinto I, Thomas A (2019) The reverse supply chain of the e-waste management processes in a circular economy framework: evidence from Italy. Sustainability 11(8):2430
Islam MT, Huda N (2020) E-waste management practices in Australia. In: Prasad, M.N.V., Vithanage, M., Borthakur, A. (Eds.), Handbook of Electronic Waste Management. Butterworth-Heinemann, pp. 553e576
Islam A, Swaraz AM, Teo SH, Taufiq-Yap YH, Vo DV, Ibrahim ML, Abdulkreem-Alsultan G, Rashid U, Awual MR (2021) Advances in physiochemical and biotechnological approaches for sustainable metal recovery from e-waste: a critical review. Journal of Cleaner Production, 129015
Jin C, Sun S, Yang D, Sheng W, Ma Y, He W, Li G (2021) Anaerobic digestion: an alternative resource treatment option for food waste in China. Sci Total Environ 146397
Jindal A, Sangwan KS (2011) Development of an interpretive structural model of barriers to reverse logistics implementation in Indian industry. In Glocalized solutions for sustainability in manufacturing (pp. 448–453). Springer, Berlin, Heidelberg
Kang KD, Kang H, Ilankoon IM, Chong CY (2020) Electronic waste collection systems using Internet of Things (IoT): household electronic waste management in Malaysia. J Clean Prod 252:119801
Khan R, Kumar S, Srivastava AK, Dhingra N, Gupta M, Bhati N, Kumari P (2021) Machine learning and IoT-based waste management model. Computational Intelligence and Neuroscience
Khandaker S, Chowdhury MF, Awual MR, Islam A, Kuba T (2021) Efficient cesium encapsulation from contaminated water by cellulosic biomass based activated wood charcoal. Chemosphere 262:127801
Kubra KT, Salman MS, Hasan MN, Islam A, Teo SH, Hasan MM, Sheikh MC, Awual MR (2021) Sustainable detection and capturing of cerium(III) using ligand embedded solid-state conjugate adsorbent. J Mol Liquid 338:116667
Kumar A, Holuszko M, Espinosa DCR (2017a) E-waste: an overview on generation, collection, legislation and recycling practices. Resour Conserv Recycl 122:32–42
Kuang HX, Li MY, Li LZ, Li ZC, Wang CH, Xiang MD, Yu YJ (2023) Co-exposure levels of volatile organic compounds and metals/metalloids in children: implications for e-waste recycling activity prediction. Sci Total Environ 863:160911
Kiddee P, Naidu R, Wong MH (2013) Electronic waste management approaches: an overview. Waste Manage 33(5):1237–1250
Knussen C, Yule F (2008) “I’m not in the habit of recycling”: the role of habitual behavior in the disposal of household waste. Environ Behav 40:683–702
Kumar A (2019) Exploring young adults’ e-waste recycling behaviour using anextended theory of planned behaviour model: a cross-cultural study. Resour Conserv Recycl 141:378–389
Kumar A, Agrawal A (2020) Recent trends in solid waste management status, challenges, and potential for the future Indian cities–a review. Curr Opin Environ Sustain 2:100011
Kumar A, Dixit G (2018) An analysis of barriers affecting the implementation of e-waste management practices in India: a novel ISM-DEMATEL approach. Sustain Prod Consum 14:36–52
Kumar A, Dixit G, Prabhakar D (2016) Analyzing the factors affecting the sustainable municipal solid waste management (MSWM). Indian J Sci Technol 9(47):1–7
Kumar U, Singh D (2014) Electronic waste: concerns & hazardous threats. Int J Curr -Eng Technol 4:802–811
Lambert F, Gaydardzhiev S, Leonard G, Lewis G, Bareel PF (2015) Copper leaching from waste electric cables by biohydrometallurgy. Miner Eng 76:38–46
Li H, Jin Z, Krishnamoorthy S (2021) E-waste management using machine learning. In International Conference on Big Data and Computing, pp. 30–35
Li J, Lu H, Guo J, Xu Z, Zhou Y (2007) Recycle technology for recovering resources and products from waste printed circuit boards. Environ Sci Technol 41:1995–2000
Li W, Achal V (2020) Environmental and health impacts due to e-waste disposal in China–A review. Sci Total Environ 737:139745
Li Y, Xu X, Wu K, Chen G, Liu J, Chen S, Huo X (2008) Monitoring of lead load and its effect on neonatal behavioral neurological assessment scores in Guiyu, an electronic waste recycling town in China. J Environ Monit 10(10):1233–1238
Link T (2014) Impact of e-waste recycling on water and soil. New Delhi, India
Liu H, Zhou Q, Wang Y, Zhang Q, Cai Z, Jiang G (2008) E-waste recycling induced polybrominated diphenyl ethers, polychlorinated biphenyls, polychlorinateddibenzo-p-dioxins and dibenzo-furans pollution in the ambient environment. Environ Int 34(1):67–72
Liu Y, Huo X, Xu L, Wei X, Wu W, Wu X, Xu X (2018) Hearing loss in children with e-waste lead and cadmium exposure. Sci Total Environ 624:621–627
Lopes dos Santos K, Jacobi PR (2022) Alignments between e‐waste legislation and the Sustainable Development Goals: the United Kingdom, Brazil, and Ghana case studies. Geo: Geography and Environment, 9(1), e00104
Luo C, Liu C, Wang Y, Liu X, Li F, Zhang G, Li X (2011) Heavy metal contamination in soils and vegetables near an e-waste processing site, south China. J Hazard Mater 186(1):481–490
Luo Q, Wong M, Cai Z (2007) Determination of polybrominated diphenyl ethers in freshwater fishes from a river polluted by e-wastes. Talanta 72(5):1644–1649
Luo Y, Luo XJ, Lin Z, Chen SJ, Liu J, Mai BX, Yang ZY (2009) Polybrominated diphenyl ethers in road and farmland soils from an e-waste recycling region in Southern China: concentrations, source profiles, and potential dispersion and deposition. Sci Total Environ 407(3):1105–1113
Makov T, Fishman T, Chertow MR, Blass V (2018) What affects the secondhand value of smartphones: evidence from eBay. J Ind Ecol 23(3):549–559
Mazrouaa AM, Mansour NA, Abed MY, Youssif MA, Shenashen MA, Awual MR (2019) Nano-composite multi-wall carbon nanotubes using poly(p-phenylene terephthalamide) for enhanced electric conductivity. J Environ Chem Eng 7(2):103002
Manhart A (2011) International cooperation for metal recycling from waste electrical and electronic equipment: an assessment of the ‘‘best-of-twoworlds” approach. J Ind Ecol 15(1):13–30
Manomaivibool P, Vassanadumrongdee S (2011) Extended producer responsibility in Thailand. J Ind Ecol 15(2):185–205
Meng M, Li B, Shao JJ, Wang T, He B, Shi JB, Jiang GB (2014) Accumulation of total mercury and methylmercury in rice plants collected from different mining areas in China. Environ Pollut 184:179–186
Menikpura SNM, Santo A, Hotta Y (2014) Assessing the climate co-benefits from waste electrical and electronic equipment (WEEE) recycling in Japan. J Cleaner Prod 74:183–190
Mielke HW, Reagan PL (1998) Soil is an important pathway of human lead exposure. Environ Health Perspect 106(1):217–229
Milovantseva N, Fitzpatrick C (2015) Barriers to electronics reuse of transboundary e-waste shipment regulations: an evaluation based on industry experiences. Resour Conserv Recy 102:170–177
Mmereki D, Li B, Baldwin A, Hong L (2016) The generation, composition, collection, treatment and disposal system, and impact of E-waste. E-Waste Transit Pollut Resour 29:65–93
Mor RS, Sangwan KS, Singh S, Singh A, Kharub M (2021) E-waste management for environmental sustainability: an exploratory study. Procedia CIRP 98:193–198
Naushad M, Alqadami AA, Al-Kahtani AA, Ahamad T, Awual MR, Tatarchuk T (2019) Adsorption of textile dye using para-aminobenzoic acid modified activated carbon: kinetic and equilibrium studies. J Mol Liquid 296:112075
Nisa M (2014) E-waste management. J Nanosci Nanotechnol 2(1):766–768
Ongondo FO, Williams ID, Cherrett TJ (2011) How are WEEE doing? A global review of the management of electrical and electronic wastes. Waste Manage 31:714–730
Orlins S, Guan D (2016) China’s toxic informal e-waste recycling: local approaches to a global environmental problem. J Clean Prod 114:71–80
Pacyniak EK, Cheng X, Cunningham ML, Crofton K, Klaassen CD, Guo GL (2007) The flame retardants, polybrominated diphenyl ethers, are pregnane X receptor activators. Toxicol Sci 97(1):94–102
Parajuly K, Fitzpatrick C, Muldoon O, Kuehr R (2020) Behavioral change for the circular economy: a review with focus on electronic waste management in the EU. Resour Conserv Recycl:x 6:100035
Pavithra KG, Rajan PSS, Balaji D, Gopinath KP (2020) Sustainable electronic-waste management: implications on environmental and human health. In E-waste Recycling and Management (pp. 201–218). Springer, Cham
Pradhan JK, Kumar S (2014) Informal e-waste recycling: environmental risk assessment of heavy metal contamination in Mandoli industrial area, Delhi India. Environ Sci Pollut Res Int 21(13):7913–7928
Pronczuk-Garbino J (2005) Children’s health and the environment: a global perspective-a resource manual for the health sector. World health organization
Queiruga D, Benito JG, Lannelongue G (2012) Evolution of the electronic waste management system in Spain. J Clean Prod 24:56–65
Quinteros FA, Machiavelli LI, Miler EA, Cabilla JP, Duvilanski BH (2008) Mechanisms of chromium (VI)-induced apoptosis in anterior pituitary cells. Toxicology 249(2–3):109–115
Rahman MW, Islam R, Hasan A, Bithi NI, Hasan MM, Rahman MM (2020) Intelligent waste management system using deep learning with IoT. Journal of King Saud University-Computer and Information Sciences
Ramya P, Ramya V, Rao MB (2021) An e-waste collection system based on IoT using LoRa open-source machine learning framework. In Smart Technologies in Data Science and Communication, 97–105
Rautela R, Arya S, Vishwakarma S, Lee J, Kime K-H, Kumar S (2021) E-waste management and its effects on the environment and human health. Sci Total Environ 73:145623
Ravi V, Shankar R (2014) Reverse logistics: insights from sectoral analysis of Indian manufacturing industries. Int J Logist Syst Manag 17(2):234–259
Ravindra K, Singh T, Mor S (2019) Emissions of air pollutants from primary crop residue burning in India and their mitigation strategies for cleaner emissions. J Clean Prod 208:261–273
Sahajwalla V, Hossain R (2020) The science of microrecycling: a review of selective synthesis of materials from electronic waste. Mater Today Sustain 9:100040
Saphores JDM, Ogunseitan OA, Shapiro AA (2012) Willingness to engage in a pro-environmental behavior: an analysis of e-waste recycling based on a national survey of US households. Resour Conserv Recy 60:49–63
Sauvé S, Bernard S, Sloan P (2016) Environmental sciences, sustainable development and circular economy: alternative concepts for trans-disciplinary research. Environ Dev 17:48–56
Schluep M, Hagelüken C, Meskers C, Magalini F, Wang F, Müller E, Kuehr R, Maurer C, Sonnemann G (2009) Market potential of innovative e-waste recycling technologies in developing countries. InR’09 World Congress, Davos, Switzerland
Secretariat RS (2011) E-waste in India. India Research Unit (Larrdis), Rajya Sabha Secretariat, New Delhi
Seitz J (2014) Analysis of existing e-waste practices in MENA countries. In: The regional solid waste exchange of information and expertise network in Mashreq and Maghreb countries, Tunis (SWEEP-Net)
Senthilselvi A, Sellam V, Alahmari SA, Rajeyyagari S (2020) Accuracy enhancement in mobile phone recycling process using machine learning technique and MEPH process. Environ Technol Innov 20:101137
Sepúlveda A, Schluep M, Renaud FG, Streicher M, Kuehr R, Hagelüken C, Gerecke AC (2010) A review of the environmental fate and effects of hazardous substances released from electrical and electronic equipments during recycling: examples from China and India. Environ Impact Assess Rev 30(1):28–41
Shamim A, Mursheda AK, Rafiq I (2015) E-waste trading impact on public health and ecosystem services in developing countries. J Waste Resour 5(4):1–18
Sharma M, Joshi S, Govindan K (2021) Issues and solutions of electronic waste urban mining for circular economytransition: an Indian context. J Environ Manage 290:112373
Shen C, Chen Y, Huang S, Wang Z, Yu C, Qiao M, Lin Q (2009) Dioxin-like compounds in agricultural soils near e-waste recycling sites from Taizhou area, China: chemical and bioanalytical characterization. Environ Int 35(1):50–55
Shi J, Xiang L, Luan H, Wei Y, Ren H, Chen. (2019) The health concern of polychlorinated biphenyls (PCBs) in a notorious e-waste recycling site. Ecotoxicol Environ Saf 186:109817
Shi T, Chen SJ, Luo XJ, Zhang XL, Tang CM, Luo Y, Mai BX (2009) Occurrence of brominated flame retardants other than polybrominated diphenyl ethers in environmental and biota samples from southern China. Chemosphere 74(7):910–916
Shi Y, Chen S, Yan M, Cheng Z, Zhao L, Liu Y, Zhang B, Zhu H, Zhang T, Kannan K (2023) Elevated levels of biomarkers of oxidative stress and renal injury linked to nitrogenous flame retardants exposure in e-waste dismantling site: a case study in China. Chemosphere, 137747
Shittu OS, Williams ID, Shaw PJ (2021) Global e-waste management: can WEEE make a difference? A review ofe-waste trends, legislation, contemporary issues and future challenges. Waste Manage 120:549–563
Shumon MRH, Ahmed S, Islam MT (2014) Electronic waste: present status and future perspectives of sustainable management practices in Malaysia. Environ Earth Sci 72(7):2239–2249
Siddiqi MA, Laessig RH, Reed KD (2003) Polybrominated diphenyl ethers (PBDEs): new pollutants–old diseases. Clin Med Res 1(4):281–290
Singh MP, Bhardwaj AK, Bharati K, Singh RP, Chaurasia SK, Kumar S, Singh RP, Shukla A, Naraian R, Vikram K (2021a) Biogenic and non-biogenic waste utilization in the synthesis of 2D materials (graphene, h-BN, g-C2N) and their applications. Frontiers in Nanotechnology, p.53
Singh K, Arora G, Singh P, Gupta A (2021b) IoT-based collection vendor machine (CVM) for e-waste management. J Reliab Intell Environ 7:35–47
Singh A, Sharma RK, Agrawal M, Marshall FM (2010) Health risk assessment of heavy metals via dietary intake of foodstuffs from the wastewater irrigated site of a dry tropical area of India. Food Chem Toxicol 48(2):611–619
Singh S, Dasgupta MS, Routroy S (2022) Analysis of critical success factors to design e-waste collection policy in India: a fuzzy DEMATEL approach. Environ Sci Pollut Res 29(7):10585–10604
Sinha-Khetriwal D, Kraeuchi P, Schawninger M (2005) A comparison of electronic waste recycling in Switzerland and in India. Environ Impact Assess Rev 25(5):492–504
Sjödin A, Hagmar L, Klasson-Wehler E, Kronholm-Diab K, Jakobsson E, Bergman A (1999) Flame retardant exposure: polybrominated diphenyl ethers in blood from Swedish workers. Environ Health Perspect 107(8):643–648
Solomon UU (2010) A detailed look at the three disciplines, environmental ethics, law and education to determine which plays the most critical role in environmental enhancement and protection. Environ Dev Sustain 12(6):1069–1080
Song Q, Li J (2014) A systematic review of the human body burden of e-waste exposure in China. Environ Int 68:82–93
Srivastav AL, Kumar A (2020) Review-An endeavor to achieve sustainable development goals through floral waste management: a short review. J Clean Prod 283:124669
Srivastava RR, Pathak P (2020) Policy issues for efficient management of E-waste in developing countries. In Handbook of Electronic Waste Management (pp. 81–99). Butterworth-Heinemann
Sthiannopkao S, Wong MH (2013) Handling e-waste in developed and developing countries: initiatives, practices, and consequences. Sci Total Environ 463:1147–1153
Taneja A, Dutt I, Srivastav AL (2021) Advances of waste management practices in India and China along with bibliometric assessment of their research outcomes. Environ Sci Pollut. 1–11
Teo SH, Ng CH, Islam A, Abdulkareem-Alsultan G, Joseph CG, Janaun J, Taufiq-Yap YH, Khandaker S, Islam GJ, Znad H, Awual MR (2022) Sustainable toxic dyes removal with advanced materials for clean water production: a comprehensive review. J Cleaner Prod 332:130039
Thakur B, Kumar N, Gupta G (2022) Machine learning techniques with ANOVA for the prediction of breast cancer. Intl J Adv Technol Eng Explor 9(87):232
Tharappel JC, Lehmler HJ, Srinivasan C, Robertson LW, Spear BT, Glauert HP (2008) Effect of antioxidant phytochemicals on the hepatic tumor promoting activity of 3, 3′, 4, 4′-tetrachlorobiphenyl (PCB-77). Food Chem Toxicol 46(11):3467–3474
Thomas LD, Hodgson S, Nieuwenhuijsen M, Jarup L (2009) Early kidney damage in a population exposed to cadmium and other heavy metals. Environ Health Perspect 117(2):181–184
Tutton CG, Young SB, Habib K (2022) Pre-processing of e-waste in Canada: case of a facility responding to changing material composition, Resources, Environ. Sustainability 9, 100069. https://doi.org/10.1016/j.resenv.2022.100069
Umair S, Anderberg S, Potting J (2016) Informal electronic waste recycling in Pakistan. J Solid Waste Technol Manag 42:222–235
UNEP (2007) E-waste volume I and II: inventory assessment manual. United Nations Environment Protection
United Nations University (2017) E-waste in east and South-East Asia jumps 63% infive years. Retrieved from. https://unu.edu/media-relations/releases/e-wastein-east-and-south-east-asia-jumps-63-percent-in-five-years.html . Accessed 06 Oct 2022
Vaish B, Sharma B, Singh P, Singh RP (2020) E-waste and their implications on the environment and human health. In E-waste Recycling and Management (pp. 219–232). Springer, Cham
vanWeelden E, Mugge R, Bakker C (2016) Paving the way towards circular consumption: exploring consumer acceptance of refurbished mobile phones in the Dutch market. J Clean Prod 113:743–754
Vimala S, Madhusudhana Rao TV, Balaji A, Maram B (2022) Taylor-student psychology based optimization integrated deep learning in IoT application for plant disease classification. Wireless Networks, 1–21
Wang Z, Zhang B, Guan D (2016) Take responsibility for electronic-waste disposal. Nature News 536:23–25
Wath SB, Vaidya AN, Dutt PS, Chakrabarti T (2010) A roadmap for development of sustainable E-waste management system in India. Sci Total Environ 409(1):19–32
Weerasundara L, Mahatantila K, Vithanage M (2020) E-waste as a challenge for public and ecosystem health. In Handbook of Electronic Waste Management (pp. 101–117). Butterworth-Heinemann
Widmer R, Oswald-Krapf H, Sinha-Khetriwal D, Schnellmann M, Boni H (2005) Global perspectives on e-waste. Environ Impact Assess Rev 25:436458
Wu JP, Luo XJ, Zhang Y, Luo Y, Chen SJ, Mai BX, Yang ZY (2008) Bioaccumulation of polybrominated diphenyl ethers (PBDEs) and polychlorinated biphenyls (PCBs) in wild aquatic species from an electronic waste (e-waste) recycling site in South China. Environ Int 34(8):1109–1113
Wu K, Xu X, Liu J, Guo Y, Li Y, Huo X (2010) Polybrominated diphenyl ethers in umbilical cord blood and relevant factors in neonates from Guiyu China. Environ Sci Technol 44(2):813–819
Wu Q, Leung JY, Du Y, Kong D, Shi Y, Wang Y, Xiao T (2019) Trace metals in e-waste lead to serious health risk through consumption of rice growing near an abandoned e-waste recycling site: comparisons with PBDEs and AHFRs. Environ Pollut 247:46–54
Xavier LH, Ottoni M, Abreu LPP (2023) A comprehensive review of urban mining and the value recovery from e-waste materials. Resour Conserv Recycl 190:106840
Xing GH, Chan JKY, Leung AOW, Wu SC, Wong MH (2009) Environmental impact and human exposure to PCBs in Guiyu, an electronic waste recycling site in China. Environ Int 35(1):76–82
Xu X, Liao W, Lin Y, Dai Y, Shi Z, Huo X (2018) Blood concentrations of lead, cadmium, mercury and their association with biomarkers of DNA oxidative damage in preschool children living in an e-waste recycling area. Environ Geochem Health 40(4):1481–1494
Xu X, Yang H, Chen A, Zhou Y, Wu K, Liu J, Huo X (2012) Birth outcomes related to informal e-waste recycling in Guiyu China. Reprod Toxicol 33(1):94–98
Yan, C. H., Xu, J., Shen, X.M., 2013. Childhood lead poisoning in China: challenges and opportunities. A294-A295
Yeamin MB, Islam MM, Chowdhury A, Awual MR (2021) Efficient encapsulation of toxic dyes from wastewater using several biodegradable natural polymers and their composites. J Cleaner Prod 291:125920
Yu KH, Zhang Y, Li D, Montenegro-Marin CE, Kumar PM (2021) Environmental planning based on reduce, reuse, recycle and recover using artificial intelligence. Environ Impact Assess Rev 86:106492
Zeng X, Li J, Singh N (2014) Recycling of spent lithium-ion battery: a critical review. Crit Rev Environ Sci Technol 44(10):1129–1165
Zhang K, Schnoor JL, Zeng EY (2012) E-waste recycling: where does it go from here? Environ Sci Technol 46(20):10861–10867
Zhang K, Wei YL, Zeng EY (2013) A review of environmental and human exposure to persistent organic pollutants in the Pearl River Delta South China. Sci Total Environ 463:1093–1110
Zhang X, Zhong T, Liu L, Ouyang X (2015) Impact of soil heavy metal pollution on food safety in China. PLoS ONE 10(8):e0135182
Zheng J, Chen KH, Yan X, Chen SJ, Hu GC, Peng XW, Yang ZY (2013) Heavy metals in food, house dust, and water from an e-waste recycling area in South China and the potential risk to human health. Ecotoxicol Environ Saf 96:205–212
Zheng J, Luo XJ, Yuan JG, He LY, Zhou YH, Luo Y, Yang ZY (2011) Heavy metals in hair of residents in an e-waste recycling area, South China: contents and assessment of bodily state. Arch Environ Contam Toxicol 61(4):696–703
Download references
Author information
Authors and affiliations.
Chitkara University School of Engineering and Technology, Chitkara University, Solan, Himachal Pradesh, 174103, India
Arun Lal Srivastav & Ashutosh Kumar Dubey
Ex-Department of Civil Engineering, Indian Institute of Technology (BHU), Varanasi, 221005, India
Department of Civil Engineerin, IET, Dr. RammanoharLohia Avadh University, Uttar Pradesh, Ayodhya, India
Naveen Patel
Department of Environmental Studies, P.G.D.A.V. College (Evening), University of Delhi, Delhi, 110065, India
Mayank Pandey
Department of Earth Sciences, Banasthali Vidyapith, Radha Kishnpura, P. O. Banasthali, Rajasthan, 304022, India
Ashutosh Kumar Pandey
Department of Computer Science and Engineering, Chandigarh University, Gharuan, Mohali, Punjab, India
Abhishek Kumar
Amity School of Life Sciences, Department of Environmental Science, Amity University, Madhya Pradesh, Gwalior, 474001, India
Abhishek Kumar Bhardwaj
Department of Environmental Sciences, Dr. Rammanohar Lohia Avadh University, Ayodhya, Uttar Pradesh, India
Vinod Kumar Chaudhary
You can also search for this author in PubMed Google Scholar
Contributions
Arun Lal Srivastav: conceptualization and drafting; Markandeya: through literature review; Naveen Patel: impact analyses and literature review; Mayank Pandey: investigation on challenges; Ashutosh Kumar Pandey: methodology development; Ashutosh Kumar Dubey: editing and critical analysis; Abhishek Kumar: drafting and value addition; Abhishek Kumar Bhardwaj: drafting and editing; Vinod Kumar Chaudhary: supervision and critical review.
Corresponding author
Correspondence to Ashutosh Kumar Dubey .
Ethics declarations
Ethics approval.
Not applicable.
Consent to participate
Consent for publication.
All authors agree with publishing.
Competing interests
The authors declare no competing interests.
Additional information
Responsible Editor: Philippe Garrigues
Publisher's note
Springer Nature remains neutral with regard to jurisdictional claims in published maps and institutional affiliations.
Rights and permissions
Springer Nature or its licensor (e.g. a society or other partner) holds exclusive rights to this article under a publishing agreement with the author(s) or other rightsholder(s); author self-archiving of the accepted manuscript version of this article is solely governed by the terms of such publishing agreement and applicable law.
Reprints and permissions
About this article
Srivastav, A.L., Markandeya, Patel, N. et al. Concepts of circular economy for sustainable management of electronic wastes: challenges and management options. Environ Sci Pollut Res 30 , 48654–48675 (2023). https://doi.org/10.1007/s11356-023-26052-y
Download citation
Received : 16 November 2022
Accepted : 17 February 2023
Published : 28 February 2023
Issue Date : April 2023
DOI : https://doi.org/10.1007/s11356-023-26052-y
Share this article
Anyone you share the following link with will be able to read this content:
Sorry, a shareable link is not currently available for this article.
Provided by the Springer Nature SharedIt content-sharing initiative
- Electronic wastes
- Environmental health
- Recycling and recovery
- Circular economy
- Sustainable development goals
- Find a journal
- Publish with us
- Track your research
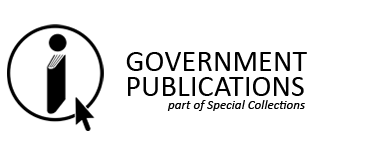
- Special Collections Home
- Databases A-Z
- Digital Collections
- Institutional Repositories
- Find items on the shelf
- Theses & Dissertations
- Subject Guides
- Government by Subject – International and Foreign Countries
- SA Government by Subject
- Useful Guides
- South African Government Links
- Searching the Catalogue
- Referencing Help
- UCT Libraries
- Bolus Herbarium Library
- Brand van Zyl Law Library
- Built Environment Library
- Government Publications
- Bongani Mayosi Health Sciences Library
- Hiddingh Hall Library
- Institute of Child Health Library
- WH Bell Music Library
- Special Collections
- Publication Archive
National Household Hazardous Waste Management Strategy
The Department of Forestry, Fisheries and the Environment has published the National Household Hazardous Waste Management Strategy for implementation. "To address issues associated with improved handling of HHW a strategic governance model has been developed with the objective to assist local governments with recommendations and guidance for introducing HHW collection."
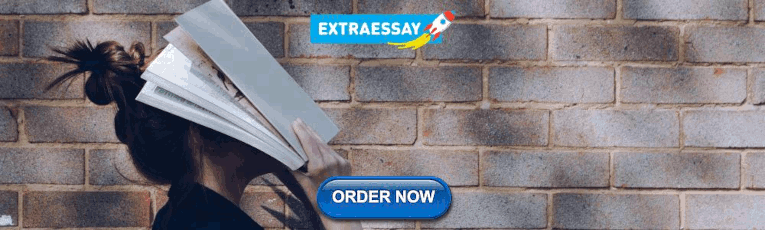
IMAGES
VIDEO
COMMENTS
Waste Management & Research: The Journal for a Sustainable Circular Economy (WM&R) publishes peer-reviewed articles relating to both the theory and practice of waste management and research. Published on behalf of the International Solid Waste Association (ISWA) topics include: wastes (focus on solids), processes and technologies, management systems and tools, and policy and regulatory ...
Waste Management Bulletin, a companion journal of Waste Management, is an international peer-reviewed and Gold Open Access journal, publishing original research on waste management and relevant fields. WMB is devoted to the presentation of data and information related to waste treatment, …. View full aims & scope.
Solid waste management (SWM) continues to dominate as a major societal and governance challenge, especially in urban areas overwhelmed by the high rate of population growth and garbage generation. ... The present paper utilizes a desktop research method of collecting and analyzing relevant data from the existing literature, as utilized in some ...
Solid waste management (SWM) is an integral part of an environmental management system. SWM approaches have been modified into a more practical and effective option to establish sustainability based on the "reduce", "reuse", and "recycle" (3R) principles. This review provides an overview of a wide range of existing SWM strategies ...
This research was conducted using the search keywords related to modern technologies for waste management. This paper presents a spectrum of selected modern solutions that changed in recent years and how they have impacted waste management. It also discusses challenges and future directions of waste management in the context of the circular ...
Waste management is the main challenge in the transition away from the linear "take-make-dispose" economy. Incorporating the principles of circularity in waste management would facilitate the achievement of Sustainable Development Goals. This paper aims to provide state-of-the-art research about circular waste management in the fulfillment of the 2030 Agenda. For this purpose, bibliometric ...
The waste composition has a significant impact on waste management practices. High-income groups utilise more packaged products, resulting in higher volumes of paper, glass, metals, plastics, and textiles as compare to low-income groups ( Sridevi et al., 2012 ).
2. Research Methods. In recent years, researchers have increasingly used quantitative and qualitative research (mixed methods) techniques to expand the scope and improve the analytic power of their studies [29,30].Quantitative research method is a statistical and interpretive technique used to describe or explain the meaning and relationships of a phenomenon under investigation.
Based on the considered 178 research papers, this paper provides the opportunities brought about by the Internet of Things, machine learning, Global Positioning System, and Blockchain in various waste management techniques, their application scenarios, real-time tracing and tracking of waste, reliable channelization and compliance with waste ...
Introduction to Waste Management. Waste disposal leads to direct an d in direct environmental impacts, such as land. occupation, resource depletion, a mplification of global warming due to methane ...
To summarize, this paper provides an overview of waste types, their generation, and associated issues, as well as explores various applications of artificial intelligence in waste management. ... Artificial intelligence-based models in waste management research are commonly categorized by the forecast period duration: short term (days to months ...
glass and plastics make up a significant percentage of the total municipal solid waste. Dixon & Jones (2005) reported that, the co mpositions of municipal solid include m aterials such as soil ...
1. Efficient waste management practices: A review. George Halkos and Kle oniki Natalia Petrou. Laboratory of Operations Research, Department of Economics, University of Thessaly. Abstract ...
Biomedical Waste Management Rules, 2016 (BMWM Rules, 2016) specify that every healthcare facility shall take all necessary steps to ensure that BMW is handled without any adverse effect on human and environmental health. ... We obtained the most pertinent research papers and used them in different arrangements using the Boolean operators "AND ...
However, the existing research mainly focused on the catalytic pyrolysis behavior of reagent grade or pure material without considering the effects of impurities in real-world plastics. In reality, biomass such as paper or cloths often co-exist with plastics in municipal solid waste (Ding et al., 2021, Kusenberg et al., 2022). With the booming ...
The European Union has set ambitious climate change mitigation goals which it aims to achieve through measures that include the development of its economic system into a circular economy. Recycling has been misunderstood as the main pillar of a circular economy and therefore the importance of the waste management sector has been overestimated.
Science. 28 Mar 2024. Vol 383, Issue 6690. pp. 1418 - 1420. DOI: 10.1126/science.adl0677. People living in urban and industrialized societies, which are expanding globally, spend more than 90% of their time in the indoor environment, breathing indoor air (IA). Despite decades of research and advocacy, most countries do not have legislated ...
A first-ever mapping of waste trafficking trends from Europe to Southeast Asia has been published today. Produced by the UN Office on Drugs and Crime (UNODC) and the UN Environment Programme (UNEP), the new research sheds light on how criminal actors exploit legal trade and regulatory and enforcement loopholes for financial gain.
The Theory on Waste Management (2004) by E. Pongrácz, P. S. Phillips, and R. L. Keiski is used as a reference through the progression of the study. ... This research paper was completed with the ...
Based on Fig. 3, relatively European countries are on the top in the generation of e-wastes as well as their reuse than other continents.Seitz studied the current e-waste management practices in 10 countries and observed that the management of e-waste is a major area of concern among these nations.It has also been found that there is very little awareness among people regarding jeopardy ...
03 Apr 2024. The Department of Forestry, Fisheries and the Environment has published the National Household Hazardous Waste Management Strategy for implementation. "To address issues associated with improved handling of HHW a strategic governance model has been developed with the objective to assist local governments with recommendations and ...
News. Research led by the Hazardous Waste Management Program in King County played a pivotal role in a groundbreaking state law prohibiting the sale of cookware tainted with lead and positioning Washington as a leader in public health protection.. The impetus for this legislative milestone stems from rigorous research conducted by the Hazardous Waste Management Program in collaboration with ...
WASTE MANAGEMENT & RESEARCH is an international journal that publishes studies of the theory and practice of waste management. These three journals have led the development of research in the SWM field, and the papers they have published have been widely read, discussed, and cited, making outstanding contributions to SWM research.
report, the current amount of waste produc ed in the. Philippine cities wi ll increase by approximately. 165% in 2025 - from abo ut 29,315 to 77, 776 tons. per day (Ng, 2012). The increasing ...
Waste Management & Research. Articles & Issues. Menu. Articles & Issues. Latest issue; All issues; Special issues and article collections; Submit search. ... WASTE MINIMIZATION AND RE-USE OF PAPER SLUDGES IN LANDFILL COVERS: A CASE STUDY. Horace K. Moo-Young, Jr., Thomas F. Zimmie. December 1997 View PDF.