
An official website of the United States government
The .gov means it’s official. Federal government websites often end in .gov or .mil. Before sharing sensitive information, make sure you’re on a federal government site.
The site is secure. The https:// ensures that you are connecting to the official website and that any information you provide is encrypted and transmitted securely.
- Publications
- Account settings
Preview improvements coming to the PMC website in October 2024. Learn More or Try it out now .
- Advanced Search
- Journal List
- HHS Author Manuscripts

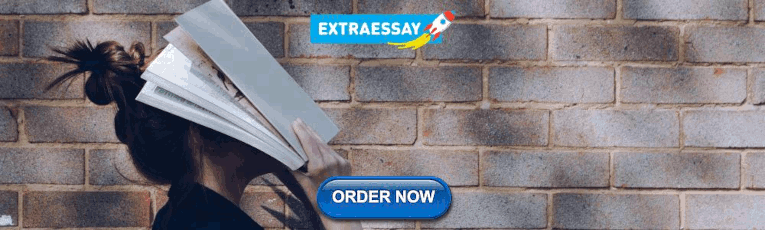
The benefits and risks of stem cell technology
The potential impact of stem cell technology on medical and dental practice is vast. Stem cell research will not only provide the foundation for future therapies, but also reveal unique insights into basic disease mechanisms. Therefore, an understanding of stem cell technology will be necessary for clinicians in the future. Herein, we give a basic overview of stem cell biology and therapeutics for the practicing clinician.
Introduction
Stem cell technology will transform medical practice. While stem cell research has already elucidated many basic disease mechanisms, the promise of stem cell–based therapies remains largely unrealized. In this review, we begin with an overview of different stem cell types. Next, we review the progress in using stem cells for regenerative therapy. Last, we discuss the risks associated with stem cell–based therapies.
Stem cell types
There are three major types of stem cells as follows: adult stem cells (also called tissue-specific stem cells), embryonic stem (ES) cells, and induced pluripotent stem (iPS) cells.
Adult stem cells
A majority of adult stem cells are lineage-restricted cells that often reside within ‘niches’ of their tissue of origin. Adult stem cells are characterized by their capacity for self-renewal and differentiation into tissue-specific cell types. Many adult tissues contain stem cells including skin, muscle, intestine, and bone marrow ( Gan et al , 1997 ; Artlett et al , 1998 ; Matsuoka et al , 2001 ; Coulombel, 2004 ; Humphries et al , 2011 ). However, it remains unclear whether all adult organs contain stem cells. Adult stem cells are quiescent but can be induced to replicate and differentiate after tissue injury to replace cells that have died. The process by which this occurs is poorly understood. Importantly, adult stem cells are exquisitely tissue-specific in that they can only differentiate into the mature cell type of the organ within which they reside ( Rinkevich et al , 2011 ).
Thus far, there are few accepted adult stem cell–based therapies. Hematopoietic stem cells (HSCs) can be used after myeloablation to repopulate the bone marrow in patients with hematologic disorders, potentially curing the underlying disorder ( Meletis and Terpos, 2009 ; Terwey et al , 2009 ; Casper et al , 2010 ; Hill and Copelan, 2010 ; Hoff and Bruch-Gerharz, 2010 ; de Witte et al , 2010 ). HSCs are found most abundantly in the bone marrow, but can also be harvested at birth from umbilical cord blood ( Broxmeyer et al , 1989 ). Similar to the HSCs harvested from bone marrow, cord blood stem cells are tissue-specific and can only be used to reconstitute the hematopoietic system ( Forraz et al , 2002 ; McGuckin et al , 2003 ; McGuckin and Forraz, 2008 ). In addition to HSCs, limbal stem cells have been used for corneal replacement ( Rama et al , 2010 ).
Mesenchymal stem cells (MSCs) are a subset of adult stem cells that may be particularly useful for stem cell–based therapies for three reasons. First, MSCs have been isolated from a variety of mesenchymal tissues, including bone marrow, muscle, circulating blood, blood vessels, and fat, thus making them abundant and readily available ( Deans and Moseley, 2000 ; Zhang et al , 2009 ; Lue et al , 2010 ; Portmann-Lanz et al , 2010 ). Second, MSCs can differentiate into a wide array of cell types, including osteoblasts, chondrocytes, and adipocytes ( Pittenger et al , 1999 ). This suggests that MSCs may have broader therapeutic applications compared to other adult stem cells. Third, MSCs exert potent paracrine effects enhancing the ability of injured tissue to repair itself. In fact, animal studies suggest that this may be the predominant mechanism by which MSCs promote tissue repair. The paracrine effects of MSC-based therapy have been shown to aid in angiogenic, antiapoptotic, and immunomodulatory processes. For instance, MSCs in culture secrete hepatocyte growth factor (HGF), insulin-like growth factor-1 (IGF-1), and vascular endothelial growth factor (VEGF) ( Nagaya et al , 2005 ). In a rat model of myocardial ischemia, injection of human bone marrow-derived stem cells upregulated cardiac expression of VEGF, HGF, bFGF, angiopoietin-1 and angiopoietin-2, and PDGF ( Yoon et al , 2005 ). In swine, injection of bone marrow-derived mononuclear cells into ischemic myocardium was shown to increase the expression of VEGF, enhance angiogenesis, and improve cardiac performance ( Tse et al , 2007 ). Bone marrow-derived stem cells have also been used in a number of small clinical trials with conflicting results. In the largest of these trials (REPAIR-AMI), 204 patients with acute myocardial infarction were randomized to receive bone marrow-derived progenitor cells vs placebo 3–7 days after reperfusion. After 4 months, the patients that were infused with stem cells showed improvement in left ventricular function compared to control patients. At 1 year, the combined endpoint of recurrent ischemia, revascularization, or death was decreased in the group treated with stem cells ( Schachinger et al , 2006 ).
Embryonic stem cells are derived from the inner cell mass of the developing embryo during the blastocyst stage ( Thomson et al , 1998 ). In contrast to adult stem cells, ES cells are pluripotent and can theoretically give rise to any cell type if exposed to the proper stimuli. Thus, ES cells possess a greater therapeutic potential than adult stem cells. However, four major obstacles exist to implementing ES cells therapeutically. First, directing ES cells to differentiate into a particular cell type has proven to be challenging. Second, ES cells can potentially transform into cancerous tissue. Third, after transplantation, immunological mismatch can occur resulting in host rejection. Fourth, harvesting cells from a potentially viable embryo raises ethical concerns. At the time of this publication, there are only two ongoing clinical trials utilizing human ES-derived cells. One trial is a safety study for the use of human ES-derived oligodendrocyte precursors in patients with paraplegia (Genron based in Menlo Park, California). The other is using human ES-derived retinal pigmented epithelial cells to treat blindness resulting from macular degeneration (Advanced Cell Technology, Santa Monica, CA, USA).
In stem cell research, the most exciting recent advancement has been the development of iPS cell technology. In 2006, the laboratory of Shinya Yamanaka at the Gladstone Institute was the first to reprogram adult mouse fibroblasts into an embryonic-like cell, or iPS cell, by overexpression of four transcription factors, Oct3/4, Sox2, c-Myc, and Klf4 under ES cell culture conditions ( Takahashi and Yamanaka, 2006 ). Yamakana's pioneering work in cellular reprogramming using adult mouse cells set the foundation for the successful creation of iPS cells from adult human cells by both his team ( Takahashi et al , 2007 ) and a group led by James Thomson at the University of Wisconsin ( Yu et al , 2007 ). These initial proof of concept studies were expanded upon by leading scientists such as George Daley, who created the first library of disease-specific iPS cell lines ( Park et al , 2008 ). These seminal discoveries in the cellular reprogramming of adult cells invigorated the stem cell field and created a niche for a new avenue of stem cell research based on iPS cells and their derivatives. Since the first publication on cellular reprogramming in 2006, there has been an exponential growth in the number of publications on iPS cells.
Similar to ES cells, iPS cells are pluripotent and, thus, have tremendous therapeutic potential. As of yet, there are no clinical trials using iPS cells. However, iPS cells are already powerful tools for modeling disease processes. Prior to iPS cell technology, in vitro cell culture disease models were limited to those cell types that could be harvested from the patient without harm – usually dermal fibroblasts from skin biopsies. However, mature dermal fibroblasts alone cannot recapitulate complicated disease processes involving multiple cell types. Using iPS technology, dermal fibroblasts can be de-differentiated into iPS cells. Subsequently, the iPS cells can be directed to differentiate into the cell type most beneficial for modeling a particular disease process. Advances in the production of iPS cells have found that the earliest pluripotent stage of the derivation process can be eliminated under certain circumstances. For instance, dermal fibroblasts have been directly differentiated into dopaminergic neurons by viral co-transduction of forebrain transcriptional regulators (Brn2, Myt1l, Zic1, Olig2, and Ascl1) in the presence of media containing neuronal survival factors [brain-derived neurotrophic factor, neurotrophin-3 (NT3), and glial-conditioned media] ( Qiang et al , 2011 ). Additionally, dermal fibroblasts have been directly differentiated into cardiomyocyte-like cells using the transcription factors Gata4, Mef2c, and Tb×5 ( Ieda et al , 2010 ). Regardless of the derivation process, once the cell type of interest is generated, the phenotype central to the disease process can be readily studied. In addition, compounds can be screened for therapeutic benefit and environmental toxins can be screened as potential contributors to the disease. Thus far, iPS cells have generated valuable in vitro models for many neurodegenerative (including Parkinson's disease, Huntington's disease, and amyotrophic lateral sclerosis), hematologic (including Fanconi's anemia and dyskeratosis congenital), and cardiac disorders (most notably the long QT syndrome) ( Park et al , 2008 ). iPS cells from patients with the long QT syndrome are particularly interesting as they may provide an excellent platform for rapidly screening drugs for a common, lethal side effect ( Zwi et al , 2009 ; Malan et al , 2011 ; Tiscornia et al , 2011 ). The development of patient-specific iPS cells for in vitro disease modeling will determine the potential for these cells to differentiate into desired cell lineages, serve as models for investigating the mechanisms underlying disease pathophysiology, and serve as tools for future preclinical drug screening and toxicology studies.
Regenerative therapy
Cardiovascular.
Despite substantial improvements in therapy, cardiovascular disease remains the leading cause of death in the industrialized world. Therefore, there is a particular interest in cardiovascular regenerative therapies. The potential of diverse progenitor cells to repair damaged heart tissue includes replacement (tissue transplant), restoration (activation of resident cardiac progenitor cells, paracrine effects), and regeneration (stem cell engraftment forming new myocytes) ( Codina et al , 2010 ). It is unclear whether the heart contains resident stem cells. However, experiments show that bone marrow mononuclear cells (BMCs) can repair myocardial damage, reduce left ventricular remodeling, and improve heart function by myocardial regeneration ( Hakuno et al , 2002 ; Amado et al , 2005 ; Dai et al , 2005 ; Schneider et al , 2008 ). The regenerative capacity of human heart tissue was further supported by the detection of the renewal of human cardiomyocytes (1% annually at the age of 25) by analysis of carbon-14 integration into human cardiomyocyte DNA ( Bergmann et al , 2009 ). It is not clear whether cardiomyocyte renewal is derived from resident adult stem cells, cardiomyocyte duplication, or homing of non-myocardial progenitor cells. Bone marrow cells home to the injured myocardium as shown by Y chromosome-positive BMCs in female recipients ( Deb et al , 2003 ). On the basis of these promising results, clinical trials in patients with ischemic heart disease have been initiated primarily using bone marrow-derived cells. However, these small trials have shown controversial results. This is likely due to a lack of standardization for cell harvesting and delivery procedures. This highlights the need for a better understanding of the basic mechanisms underlying stem cell isolation and homing prior to clinical implementation.
Although stem cells have the capacity to differentiate into neurons, oligodendrocytes, and astrocytes, novel clinical stem cell–based therapies for central and peripheral nervous system diseases have yet to be realized. It is widely hoped that transplantation of stem cells will provide effective therapy for Parkinson's disease, Alzheimer's disease, Huntington's Disease, amyloid lateral sclerosis, spinal cord injury, and stroke. Several encouraging animal studies have shown that stem cells can rescue some degree of neurological function after injury ( Daniela et al , 2007 ; Hu et al , 2010 ; Shimada and Spees, 2011 ). Currently, a number of clinical trials have been performed and are ongoing.
Dental stem cells could potentially repair damaged tooth tissues such as dentin, periodontal ligament, and dental pulp ( Gronthos et al , 2002 ; Ohazama et al , 2004 ; Jo et al , 2007 ; Ikeda et al , 2009 ; Balic et al , 2010 ; Volponi et al , 2010 ). Moreover, as the behavior of dental stem cells is similar to MSCs, dental stem cells could also be used to facilitate the repair of non-dental tissues such as bone and nerves ( Huang et al , 2009 ; Takahashi et al , 2010 ). Several populations of cells with stem cell properties have been isolated from different parts of the tooth. These include cells from the pulp of both exfoliated (children's) and adult teeth, the periodontal ligament that links the tooth root with the bone, the tips of developing roots, and the tissue that surrounds the unerupted tooth (dental follicle) ( Bluteau et al , 2008 ). These cells probably share a common lineage from neural crest cells, and all have generic mesenchymal stem cell-like properties, including expression of marker genes and differentiation into mesenchymal cells in vitro and in vivo ( Bluteau et al , 2008 ). different cell populations do, however, differ in certain aspects of their growth rate in culture, marker gene expression, and cell differentiation. However, the extent to which these differences can be attributed to tissue of origin, function, or culture conditions remains unclear.
There are several issues determining the long-term outcome of stem cell–based therapies, including improvements in the survival, engraftment, proliferation, and regeneration of transplanted cells. The genomic and epigenetic integrity of cell lines that have been manipulated in vitro prior to transplantation play a pivotal role in the survival and clinical benefit of stem cell therapy. Although stem cells possess extensive replicative capacity, immune rejection of donor cells by the host immune system post-transplantation is a primary concern ( Negro et al , 2012 ). Recent studies have shown that the majority of donor cell death occurs in the first hours to days after transplantation, which limits the efficacy and therapeutic potential of stem cell–based therapies ( Robey et al , 2008 ).
Although mouse and human ES cells have traditionally been classified as being immune privileged, a recent study used in vivo , whole-animal, live cell-tracing techniques to demonstrate that human ES cells are rapidly rejected following transplantation into immunocompetent mice ( Swijnenburg et al , 2008 ). Treatment of ES cell-derived vascular progenitor cells with inter-feron γ (to upregulate major histocompatibility complex (MHC) class I expression) or in vivo ablation of natural killer (NK) cells led to enhanced progenitor cell survival after transplantation into a syngeneic murine ischemic hindlimb model. This suggests that MHC class I-dependent, NK cell-mediated elimination is a major determinant of graft survivability ( Ma et al , 2010 ). Given the risk of rejection, it is likely that initial therapeutic attempts using either ES or iPS cells will require adjunctive immunosuppressive therapy. Immunosuppressive therapy, however, puts the patient at risk of infection as well as drug-specific adverse reactions. As such, determining the mechanisms regulating donor graft tolerance by the host will be crucial for advancing the clinical application of stem cell–based therapies.
An alternative strategy to avoid immune rejection could employ so-called ‘gene editing’. Using this technique, the stem cell genome is manipulated ex vivo to correct the underlying genetic defect prior to transplantation. Additionally, stem cell immunologic markers could be manipulated to evade the host immune response. Two recent papers offer alternative methods for ‘gene editing’. Soldner et al (2011) used zinc finger nuclease to correct the genetic defect in iPS cells from patients with Parkinson's disease because of a mutation in the α-Synuclein (α-SYN) gene. Liu et al (2011) used helper-dependent adenoviral vectors (HDAdV) to correct the mutation in the Lamin A (LMNA) gene in iPS cells derived from patients with Hutchinson–Gilford Progeria (HGP), a syndrome of premature aging. Cells from patients with HGP have dysmorphic nuclei and increased levels of progerin protein. The cellular phenotype is especially pronounced in mature, differentiated cells. Using highly efficient helper-dependent adenoviral vectors containing wild-type sequences, they were able to use homologous recombination to correct two different Lamin A mutations. After genetic correction, the diseased cellular phenotype was reversed even after differentiation into mature smooth muscle cells. In addition to the potential therapeutic benefit, ‘gene editing’ could generate appropriate controls for in vitro studies .
Finally, there are multiple safety and toxicity concerns regarding the transplantation, engraftment, and long-term survival of stem cells. Donor stem cells that manage to escape immune rejection may later become oncogenic because of their unlimited capacity to replicate ( Amariglio et al , 2009 ). Thus, ES and iPS cells may need to be directed into a more mature cell type prior to transplantation to minimize this risk. Additionally, generation of ES and iPS cells harboring an inducible ‘kill-switch’ may prevent uncontrolled growth of these cells and/or their derivatives. In two ongoing human trials with ES cells, both companies have provided evidence from animal studies that these cells will not form teratomas. However, this issue has not been thoroughly examined, and enrolled patients will need to be monitored closely for this potentially lethal side effect.
In addition to the previously mentioned technical issues, the use of ES cells raises social and ethical concerns. In the past, these concerns have limited federal funding and thwarted the progress of this very important research. Because funding limitations may be reinstituted in the future, ES cell technology is being less aggressively pursued and young researchers are shying away from the field.
Stem cell technology is unique in that it possesses both diagnostic and therapeutic potential. iPS cells and their derivatives have provided excellent in vitro models of disease processes. Moreover, patient-specific iPS cell models will serve as an invaluable tool for drug discovery and toxicology studies. The therapeutic potential of these cells to regenerate functional tissue and replace damaged tissue has vast potential. Additionally, stem cell–based therapies may enhance the body's own ability to repair itself. As previously mentioned, MSCs exert potent paracrine effects that are thought to be beneficial. For these reasons, the potential of stem cells to cure disease is virtually limitless. Nonetheless, major obstacles to safely implement these therapies remain and must be systematically addressed. Most immediate among these is the identification of stem cell populations that can be maintained and expanded in culture to provide the large numbers needed to be therapeutically useful. Additionally, the potential for cancerous transformation and immuno-logic rejection is largely unexplored. These issues highlight the potential danger of implementing stem cell therapies before they have been adequately studied in vitro and in vivo in animal models. More intensive characterization is required to avoid the possible lethal complications of this type of therapy. Although the current therapeutic potential of stem cell technology is in its infancy, basic research discoveries in the standardization of stem cell derivation, culture, and differentiation techniques, together with improved transplantation, engraftment, and survival strategies, will aid in the development of safer and more effective stem cell–based therapies in the future.
Author contributions
A. Leventhal, G. Chen and A. Negro wrote separate parts of the review. M. Boehm did overall coordination and final review.
- Amado LC, Saliaris AP, Schuleri KH, et al. Cardiac repair with intramyocardial injection of allogeneic mesenchymal stem cells after myocardial infarction. Proc Natl Acad Sci U S A. 2005; 102 :11474–11479. [ PMC free article ] [ PubMed ] [ Google Scholar ]
- Amariglio N, Hirshberg A, Scheithauer BW, et al. Donor-derived brain tumor following neural stem cell transplantation in an ataxia telangiectasia patient. PLoS Med. 2009; 6 :e1000029. [ PMC free article ] [ PubMed ] [ Google Scholar ]
- Artlett CM, Smith JB, Jimenez SA. Identification of fetal DNA and cells in skin lesions from women with systemic sclerosis. N Engl J Med. 1998; 338 :1186–1191. [ PubMed ] [ Google Scholar ]
- Balic A, Aguila HL, Caimano MJ, Francone VP, Mina M. Characterization of stem and progenitor cells in the dental pulp of erupted and unerupted murine molars. Bone. 2010; 46 :1639–1651. [ PMC free article ] [ PubMed ] [ Google Scholar ]
- Bergmann O, Bhardwaj RD, Bernard S, et al. Evidence for cardiomyocyte renewal in humans. Science. 2009; 324 :98–102. [ PMC free article ] [ PubMed ] [ Google Scholar ]
- Bluteau G, Luder HU, De Bari C, Mitsiadis TA. Stem cells for tooth engineering. Eur Cell Mater. 2008; 16 :1–9. [ PubMed ] [ Google Scholar ]
- Broxmeyer HE, Douglas GW, Hangoc G, et al. Human umbilical cord blood as a potential source of transplantable hematopoietic stem/progenitor cells. Proc Natl Acad Sci U S A. 1989; 86 :3828–3832. [ PMC free article ] [ PubMed ] [ Google Scholar ]
- Casper J, Wolff D, Knauf W, et al. Allogeneic hematopoietic stem-cell transplantation in patients with hematologic malignancies after dose-escalated treosulfan/fludarabine conditioning. J Clin Oncol. 2010; 28 :3344–3351. [ PubMed ] [ Google Scholar ]
- Codina M, Elser J, Margulies KB. Current status of stem cell therapy in heart failure. Curr Cardiol Rep. 2010; 12 :199–208. [ PMC free article ] [ PubMed ] [ Google Scholar ]
- Coulombel L. Identification of hematopoietic stem/progenitor cells: strength and drawbacks of functional assays. Oncogene. 2004; 23 :7210–7222. [ PubMed ] [ Google Scholar ]
- Dai W, Hale SL, Martin BJ, et al. Allogeneic mesenchymal stem cell transplantation in postinfarcted rat myocardium: short- and long-term effects. Circulation. 2005; 112 :214–223. [ PubMed ] [ Google Scholar ]
- Daniela F, Vescovi AL, Bottai D. The stem cells as a potential treatment for neurodegeneration. Methods Mol Biol. 2007; 399 :199–213. [ PubMed ] [ Google Scholar ]
- Deans RJ, Moseley AB. Mesenchymal stem cells: biology and potential clinical uses. Exp Hematol. 2000; 28 :875–884. [ PubMed ] [ Google Scholar ]
- Deb A, Wang S, Skelding KA, Miller D, Simper D, Caplice NM. Bone marrow-derived cardiomyocytes are present in adult human heart: a study of gender-miatched bone marrow transplantation patients. Circulation. 2003; 107 :1247–1249. [ PubMed ] [ Google Scholar ]
- Forraz N, Pettengell R, Deglesne PA, McGuckin CP. AC133+ umbilical cord blood progenitors demonstrate rapid self-renewal and low apoptosis. Br J Haematol. 2002; 119 :516–524. [ PubMed ] [ Google Scholar ]
- Gan OI, Murdoch B, Larochelle A, Dick JE. Differential maintenance of primitive human SCID-repopulating cells, clonogenic progenitors, and long-term culture-initiating cells after incubation on human bone marrow stromal cells. Blood. 1997; 90 :641–650. [ PubMed ] [ Google Scholar ]
- Gronthos S, Brahim J, Li W, et al. Stem cell properties of human dental pulp stem cells. J Dent Res. 2002; 81 :531–535. [ PubMed ] [ Google Scholar ]
- Hakuno D, Fukuda K, Makino S, et al. Bone marrow-derived regenerated cardiomyocytes (CMG Cells) express functional adrenergic and muscarinic receptors. Circulation. 2002; 105 :380–386. [ PubMed ] [ Google Scholar ]
- Hill BT, Copelan EA. Acute myeloid leukemia: when to transplant in first complete remission. Curr Hematol Malig Rep. 2010; 5 :101–108. [ PubMed ] [ Google Scholar ]
- Hoff NP, Bruch-Gerharz D. The role of hematopoietic stem-cell transplantation in cutaneous T-cell lymphoma. G Ital Dermatol Venereol. 2010; 145 :345–359. [ PubMed ] [ Google Scholar ]
- Hu BY, Weick JP, Yu J, et al. Neural differentiation of human induced pluripotent stem cells follows developmental principles but with variable potency. Proc Natl Acad Sci U S A. 2010; 107 :4335–4340. [ PMC free article ] [ PubMed ] [ Google Scholar ]
- Huang GT, Gronthos S, Shi S. Mesenchymal stem cells derived from dental tissues vs. those from other sources: their biology and role in regenerative medicine. J Dent Res. 2009; 88 :792–806. [ PMC free article ] [ PubMed ] [ Google Scholar ]
- Humphries A, Graham TA, McDonald SA. Stem cells and inflammation in the intestine. Recent Results Cancer Res. 2011; 185 :51–63. [ PubMed ] [ Google Scholar ]
- Ieda M, Fu JD, Delgado-Olguin P, et al. Direct reprogramming of fibroblasts into functional cardiomyocytes by defined factors. Cell. 2010; 142 :375–386. [ PMC free article ] [ PubMed ] [ Google Scholar ]
- Ikeda E, Morita R, Nakao K, et al. Fully functional bioengineered tooth replacement as an organ replacement therapy. Proc Natl Acad Sci U S A. 2009; 106 :13475–13480. [ PMC free article ] [ PubMed ] [ Google Scholar ]
- Jo YY, Lee HJ, Kook SY, et al. Isolation and characterization of postnatal stem cells from human dental tissues. Tissue Eng. 2007; 13 :767–773. [ PubMed ] [ Google Scholar ]
- Liu GH, Suzuki K, Qu J, et al. Targeted gene correction of laminopathy-associated LMNA mutations in patient-specific iPSCs. Cell Stem Cell. 2011; 8 :688–694. [ PMC free article ] [ PubMed ] [ Google Scholar ]
- Lue J, Lin G, Ning H, Xiong A, Lin CS, Glenn JS. Transdifferentiation of adipose-derived stem cells into hepatocytes: a new approach. Liver Int. 2010; 30 :913–922. [ PubMed ] [ Google Scholar ]
- Ma M, Ding S, Lundqvist A, et al. Major histocompatibility complex-I expression on embryonic stem cell-derived vascular progenitor cells is critical for syngeneic transplant survival. Stem Cells. 2010; 28 :1465–1475. [ PMC free article ] [ PubMed ] [ Google Scholar ]
- Malan D, Friedrichs S, Fleischmann BK, Sasse P. Cardiomyocytes obtained from induced pluripotent stem cells with long-QT syndrome 3 recapitulate typical disease-specific features in vitro. Circ Res. 2011; 109 :841–847. [ PubMed ] [ Google Scholar ]
- Matsuoka S, Tsuji K, Hisakawa H, et al. Generation of definitive hematopoietic stem cells from murine early yolk sac and paraaortic splanchnopleures by aorta-gonad-mesonephros region-derived stromal cells. Blood. 2001; 98 :6–12. [ PubMed ] [ Google Scholar ]
- McGuckin CP, Forraz N. Umbilical cord blood stem cells – an ethical source for regenerative medicine. Med Law. 2008; 27 :147–165. [ PubMed ] [ Google Scholar ]
- McGuckin CP, Pearce D, Forraz N, Tooze JA, Watt SM, Pettengell R. Multiparametric analysis of immature cell populations in umbilical cord blood and bone marrow. Eur J Haematol. 2003; 71 :341–350. [ PubMed ] [ Google Scholar ]
- Meletis J, Terpos E. Transplantation strategies for the management of patients with myelodysplastic syndromes. J Buon. 2009; 14 :551–564. [ PubMed ] [ Google Scholar ]
- Nagaya N, Kangawa K, Itoh T, et al. Transplantation of mesenchymal stem cells improves cardiac function in a rat model of dilated cardiomyopathy. Circulation. 2005; 112 :1128–1135. [ PubMed ] [ Google Scholar ]
- Negro A, St. Hilaire C, Boehm M. Cell-based regenerative therapies: role of major histocompatibility Complex-1 antigen. In: Hayat MA, editor. Stem Cells and Cancer Stem Cells. Vol. 3. Springer; Dordrecht: 2012. pp. 173–178. [ Google Scholar ]
- Ohazama A, Modino SA, Miletich I, Sharpe PT. Stem-cell-based tissue engineering of murine teeth. J Dent Res. 2004; 83 :518–522. [ PubMed ] [ Google Scholar ]
- Park IH, Arora N, Huo H, et al. Disease-specific induced pluripotent stem cells. Cell. 2008; 134 :877–886. [ PMC free article ] [ PubMed ] [ Google Scholar ]
- Pittenger MF, Mackay AM, Beck SC, et al. Multilineage potential of adult human mesenchymal stem cells. Science. 1999; 284 :143–147. [ PubMed ] [ Google Scholar ]
- Portmann-Lanz CB, Schoeberlein A, Portmann R, et al. Turning placenta into brain: placental mesenchymal stem cells differentiate into neurons and oligodendrocytes. Am J Obstet Gynecol. 2010; 202 :294e291–294e211. [ PubMed ] [ Google Scholar ]
- Qiang L, Fujita R, Yamashita T, et al. Directed conversion of Alzheimer's disease patient skin fibroblasts into functional neurons. Cell. 2011; 146 :359–371. [ PMC free article ] [ PubMed ] [ Google Scholar ] Retracted
- Rama P, Matuska S, Paganoni G, Spinelli A, De Luca M, Pellegrini G. Limbal stem-cell therapy and long-term corneal regeneration. N Engl J Med. 2010; 363 :147–155. [ PubMed ] [ Google Scholar ]
- Rinkevich Y, Lindau P, Ueno H, Longaker MT, Weissman IL. Germ-layer and lineage-restricted stem/progenitors regenerate the mouse digit tip. Nature. 2011; 476 :409–413. [ PMC free article ] [ PubMed ] [ Google Scholar ]
- Robey TE, Saiget MK, Reinecke H, Murry CE. Systems approaches to preventing transplanted cell death in cardiac repair. J Mol Cell Cardiol. 2008; 45 :567–581. [ PMC free article ] [ PubMed ] [ Google Scholar ]
- Schachinger V, Erbs S, Elsasser A, et al. Intracoronary bone marrow-derived progenitor cells in acute myocardial infarction. N Engl J Med. 2006; 355 :1210–1221. [ PubMed ] [ Google Scholar ]
- Schneider C, Krause K, Jaquet K, et al. Intramyocardial transplantation of bone marrow-derived stem cells: ultrasonic strain rate imaging in a model of hibernating myocardium. J Card Fail. 2008; 14 :861–872. [ PubMed ] [ Google Scholar ]
- Shimada IS, Spees JL. Stem and progenitor cells for neurological repair: minor issues, major hurdles, and exciting opportunities for paracrine-based therapeutics. J Cell Biochem. 2011; 112 :374–380. [ PMC free article ] [ PubMed ] [ Google Scholar ]
- Soldner F, Laganiere J, Cheng AW, et al. Generation of isogenic pluripotent stem cells differing exclusively at two early onset Parkinson point mutations. Cell. 2011; 146 :318–331. [ PMC free article ] [ PubMed ] [ Google Scholar ]
- Swijnenburg RJ, Schrepfer S, Govaert JA, et al. Immunosuppressive therapy mitigates immunological rejection of human embryonic stem cell xenografts. Proc Natl Acad Sci U S A. 2008; 105 :12991–12996. [ PMC free article ] [ PubMed ] [ Google Scholar ]
- Takahashi K, Yamanaka S. Induction of pluripotent stem cells from mouse embryonic and adult fibroblast cultures by defined factors. Cell. 2006; 126 :663–676. [ PubMed ] [ Google Scholar ]
- Takahashi K, Tanabe K, Ohnuki M, et al. Induction of pluripotent stem cells from adult human fibroblasts by defined factors. Cell. 2007; 131 :861–872. [ PubMed ] [ Google Scholar ]
- Takahashi C, Yoshida H, Komine A, Nakao K, Tsuji T, Tomooka Y. Newly established cell lines from mouse oral epithelium regenerate teeth when combined with dental mesenchyme. In Vitro Cell Dev Biol Anim. 2010; 46 :457–468. [ PMC free article ] [ PubMed ] [ Google Scholar ]
- Terwey TH, Kim TD, Arnold R. Allogeneic hematopoietic stem cell transplantation for adult acute lymphocytic leukemia. Curr Hematol Malig Rep. 2009; 4 :139–147. [ PubMed ] [ Google Scholar ]
- Thomson JA, Itskovitz-Eldor J, Shapiro SS, et al. Embryonic stem cell lines derived from human blastocysts. Science. 1998; 282 :1145–1147. [ PubMed ] [ Google Scholar ]
- Tiscornia G, Monserrat N, Belmonte JC. Modelling long QT syndrome with iPS cells: be still, my beating heart. Circ Res. 2011; 108 :648–649. [ PubMed ] [ Google Scholar ]
- Tse HF, Siu CW, Zhu SG, et al. Paracrine effects of direct intramyocardial implantation of bone marrow derived cells to enhance neovascularization in chronic ischaemic myocardium. Eur J Heart Fail. 2007; 9 :747–753. [ PubMed ] [ Google Scholar ]
- Volponi AA, Pang Y, Sharpe PT. Stem cell-based biological tooth repair and regeneration. Trends Cell Biol. 2010; 20 :715–722. [ PMC free article ] [ PubMed ] [ Google Scholar ]
- de Witte T, Hagemeijer A, Suciu S, et al. Value of allogeneic versus autologous stem cell transplantation and chemotherapy in patients with myelodysplastic syndromes and secondary acute myeloid leukemia. Final results of a prospective randomized European Intergroup Trial. Haematologica. 2010; 95 :1754–1761. [ PMC free article ] [ PubMed ] [ Google Scholar ]
- Yoon YS, Wecker A, Heyd L, et al. Clonally expanded novel multipotent stem cells from human bone marrow regenerate myocardium after myocardial infarction. J Clin Invest. 2005; 115 :326–338. [ PMC free article ] [ PubMed ] [ Google Scholar ]
- Yu J, Vodyanik MA, Smuga-Otto K, et al. Induced pluripotent stem cell lines derived from human somatic cells. Science. 2007; 318 :1917–1920. [ PubMed ] [ Google Scholar ]
- Zhang Q, Shi S, Liu Y, Uyanne J, Shi Y, Le AD. Mesenchymal stem cells derived from human gingiva are capable of immunomodulatory functions and ameliorate inflammation-related tissue destruction in experimental colitis. J Immunol. 2009; 183 :7787–7798. [ PMC free article ] [ PubMed ] [ Google Scholar ]
- Zwi L, Caspi O, Arbel G, et al. Cardiomyocyte differentiation of human induced pluripotent stem cells. Circulation. 2009; 120 :1513–1523. [ PubMed ] [ Google Scholar ]
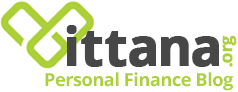
11 Stem Cell Research Pros and Cons
Stem cell research can be classified into two specific areas: embryonic stem cells and non-embryonic stem cells. Amniotic, induced pluripotent, and adult stem cells do not involve the creation or destruction of a human embryo to have them collected.
Even embryonic stem cells can be collected, to some extent, without the destruction of an embryo. Modern collection techniques include using stem cells that are found in the umbilical cord, in breast milk, or even in bone marrow.
The primary benefit of stem cell research is its clear potential. Since 1868, the idea of using stem cells as a medical treatment has been contemplated in one way or another, especially as we began to understand their full potential. With stem cell therapies, we have the potential to treat injuries, degenerative conditions, or even a genetic disease or disorder.
As for the primary disadvantage of stem cell research, the ethics of collecting embryonic stem cells tends to dominate the conversation. To some people, the idea of destroying an embryo to harvest cells equates to murder. For others, they see the hundreds of thousands of frozen embryos, many of which are simply thrown away after being stored for too long, as wasted potential.
Here are some additional stem cell research pros and cons to review.
List of the Pros of Stem Cell Research
1. It could treat several conditions that are virtually untreatable right now. Stem cell research opens numerous avenues for treatments or a cure to be found for several conditions that are either untreatable or without a cure today. Everything from Alzheimer’s disease to Parkinson’s disease to ALS could be improved. People who have a spinal cord injury could receive an injection of stem cells and potentially start the recovery process. Even mental health issues, such as schizophrenia, could one day be treated with stem cell applications.
2. It provides us with greater knowledge. By researching stem cells, we understand more about the growth process of humans. We learn more about how cells form and interact with one another. We can examine pluripotent cells, both induced and embryonic, to see what information is required for them to turn into a specific tissue cell. With a greater understanding of this micro-environment, we can learn more about who we are at our very core.
3. It offers new methods of testing. When new medical treatments are proposed, they must go through multiple stages of testing. This includes animal trials and human trials, which may or may not be successful. As our knowledge of stem cells grows, we could transition testing methods so that only cell populations are examined for a response instead of an innocent animal or a paid human research contributor. That may improve safety, reduce fatalities, and even speed up the approval process.
4. It reduces the risk of rejection. Many stem cell therapies today use the cells that are collected from a patient’s body. Because the cells are their own, the risk of rejection is reduced or even eliminated. If stem cells could be induced to form into organ tissues, such as a kidney, then the science of organ transplantation could be forever changed. Imagine growing a kidney that is a genetic match instead of trying to find a donor organ that could be rejected, even if a direct match is found. That is the potential of this medical research.
5. It could stop birth defects and mutations before they happen. By understanding the process of stem cell development, it could be possible to change the embryonic development process. Chromosomal concerns, birth defects, and other errors in development could be corrected before birth, giving more newborns a real chance to experience the gift of life. At the same time, the risks of pregnancy loss and health risks to new mothers could be decreased.
List of the Cons of Stem Cell Research
1. We have no idea about long-term side effect issues. According to the Canadian Cancer Society, there are several common short-term side effects that are associated with stem cell therapies. They may include infection, bleeding, skin or hair problems, unexplained pain, organ problems, or even the development of a secondary cancer. Every medical treatment provides some risk of a side effect, but this medical technology is so new that we have no idea what the long-term health effects might be.
2. It provides a health risk to everyone involved. Collecting stem cells from an adult carries a medical risk with it. Something could go wrong during the collection process that may reduce the quality of life for the patient. Their life could even be placed at-risk. For embryonic collection, the destruction of the blastocytes that are formed during egg fertilization is required. Since the embryo is technically a different form of human life, there will always be the chance of rejection occurring since the cells are not one’s own.
3. Adult stem cells offer limited potential. Our current stem cell research findings indicate that adult stem cells that have already transitioned into specific tissues or formats because of their body location will stay that way. That means stem cells taken from muscle tissue would only be able to create additional muscle tissues. Even if they are induced to be pluripotent, the end result tends to be duplication instead of identification because they have a determined type.
4. It is still an unproven medical technology. There is a lot of hope for stem cell treatments. Hematopoietic stem cell transplantation is performed about 50,000 times annually around the world and the success rate for the treatment is climbing above 90%. Because some forms of stem cell research are classified as illegal or immoral in the United States, however, progress to improve treatments or prove the effectiveness of this medical technology are not as advanced as their potential.
5. It isn’t cheap. Stem cell therapies are far from affordable. Because most health insurers classify this type of treatment as “experimental,” it is rarely a covered procedure. Most treatments that are approved for use in the US cost more than $10,000 per procedure. Some treatment options are six figures. Even the cost of harvesting stem cells from an embryo is a couple thousand dollars. Access to this technology is restricted to socioeconomic means globally and to almost everyone in the United States.
6. Opportunities are limited. Although stem cell research isn’t technically forbidden in the US, there are just 19 stem cell lines available for government grants and funding thanks to legislative restrictions that are enacted in 2001. Certain states have begun to draft legislation to completely ban stem cell research, or at least embryonic stem cell research, or at least place major restrictions on the process.
We should examine the ethics of embryonic stem cell research, but we should also examine the benefits it may provide. Adult stem cells, collected from consenting parties, should have no criticism whatsoever. As we move forward in this research, new pros and cons may also require additional contemplation. One thing is for certain: these stem cell research pros and cons show us that humanity is complex, beautiful, and wonderful in many ways.
Appointments at Mayo Clinic
Stem cells: what they are and what they do.
Stem cells offer promise for new medical treatments. Learn about stem cell types, current and possible uses, and the state of research and practice.
You've heard about stem cells in the news, and perhaps you've wondered if they might help you or a loved one with a serious disease. Here are some answers to frequently asked questions about stem cells.
What are stem cells?
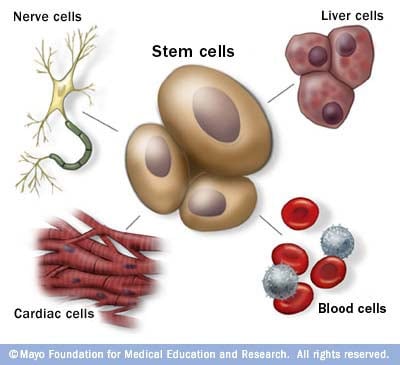
Stem cells: The body's master cells
Stem cells are the body's master cells. All other cells arise from stem cells, including blood cells, nerve cells and other cells.
Stem cells are a special type of cells that have two important properties. They are able to make more cells like themselves. That is, they self-renew. And they can become other cells that do different things in a process known as differentiation. Stem cells are found in almost all tissues of the body. And they are needed for the maintenance of tissue as well as for repair after injury.
Depending on where the stem cells are, they can develop into different tissues. For example, hematopoietic stem cells reside in the bone marrow and can produce all the cells that function in the blood. Stem cells also can become brain cells, heart muscle cells, bone cells or other cell types.
There are various types of stem cells. Embryonic stem cells are the most versatile since they can develop into all the cells of the developing fetus. The majority of stem cells in the body have fewer abilities to give rise to cells and may only help maintain and repair the tissues and organs in which they reside.
No other cell in the body has the natural ability to generate new cell types.
Why is there such an interest in stem cells?
Researchers are studying stem cells to see if they can help to:
- Increase understanding of how diseases occur. By watching stem cells mature into cells in bones, heart muscle, nerves, and other organs and tissue, researchers may better understand how diseases and conditions develop.
Generate healthy cells to replace cells affected by disease (regenerative medicine). Stem cells can be guided into becoming specific cells that can be used in people to regenerate and repair tissues that have been damaged or affected by disease.
People who might benefit from stem cell therapies include those with leukemia, Hodgkin disease, non-Hodgkin lymphoma and some solid tumor cancers. Stem cell therapies also might benefit people who have aplastic anemia, immunodeficiencies and inherited conditions of metabolism.
Stem cells are being studied to treat type 1 diabetes, Parkinson's disease, amyotrophic lateral sclerosis, heart failure, osteoarthritis and other conditions.
Stem cells may have the potential to be grown to become new tissue for use in transplant and regenerative medicine. Researchers continue to advance the knowledge on stem cells and their applications in transplant and regenerative medicine.
Test new drugs for safety and effectiveness. Before giving drugs in development to people, researchers can use some types of stem cells to test the drugs for safety and quality. This type of testing may help assess drugs in development for toxicity to the heart.
New areas of study include the effectiveness of using human stem cells that have been programmed into tissue-specific cells to test new drugs. For the testing of new drugs to be accurate, the cells must be programmed to acquire properties of the type of cells targeted by the drug. Techniques to program cells into specific cells are under study.
Where do stem cells come from?
There are several sources of stem cells:
Embryonic stem cells. These stem cells come from embryos that are 3 to 5 days old. At this stage, an embryo is called a blastocyst and has about 150 cells.
These are pluripotent (ploo-RIP-uh-tunt) stem cells, meaning they can divide into more stem cells or can become any type of cell in the body. This allows embryonic stem cells to be used to regenerate or repair diseased tissue and organs.
- Adult stem cells. These stem cells are found in small numbers in most adult tissues, such as bone marrow or fat. Compared with embryonic stem cells, adult stem cells have a more limited ability to give rise to various cells of the body.
Adult cells altered to have properties of embryonic stem cells. Scientists have transformed regular adult cells into stem cells using genetic reprogramming. By altering the genes in the adult cells, researchers can make the cells act similarly to embryonic stem cells. These cells are called induced pluripotent stem cells (iPSCs).
This new technique may allow use of reprogrammed cells instead of embryonic stem cells and prevent immune system rejection of the new stem cells. However, scientists don't yet know whether using altered adult cells will cause adverse effects in humans.
Researchers have been able to take regular connective tissue cells and reprogram them to become functional heart cells. In studies, animals with heart failure that were injected with new heart cells had better heart function and survival time.
Perinatal stem cells. Researchers have discovered stem cells in amniotic fluid as well as umbilical cord blood. These stem cells can change into specialized cells.
Amniotic fluid fills the sac that surrounds and protects a developing fetus in the uterus. Researchers have identified stem cells in samples of amniotic fluid drawn from pregnant women for testing or treatment — a procedure called amniocentesis.
Why is there controversy about using embryonic stem cells?
The National Institutes of Health created guidelines for human stem cell research in 2009. The guidelines define embryonic stem cells and how they may be used in research and include recommendations for the donation of embryonic stem cells. Also, the guidelines state that embryonic stem cells from embryos created by in vitro fertilization can be used only when the embryo is no longer needed.
Where do these embryos come from?
The embryos being used in embryonic stem cell research come from eggs that were fertilized at in vitro fertilization clinics but never implanted in women's uteruses. The stem cells are donated with informed consent from donors. The stem cells can live and grow in special solutions in test tubes or petri dishes in laboratories.
Why can't researchers use adult stem cells instead?
Progress in cell reprogramming and the formation of iPSCs has greatly enhanced research in this field. However, reprogramming is an inefficient process. When possible, iPSCs are used instead of embryonic stem cells since this avoids the ethical issues about use of embryonic stem cells that may be morally objectionable for some people.
Although research into adult stem cells is promising, adult stem cells may not be as versatile and durable as are embryonic stem cells. Adult stem cells may not be able to be manipulated to produce all cell types, which limits how adult stem cells can be used to treat diseases.
Adult stem cells are also more likely to contain irregularities due to environmental hazards, such as toxins, or from errors acquired by the cells during replication. However, researchers have found that adult stem cells are more adaptable than was first thought.
What are stem cell lines, and why do researchers want to use them?
A stem cell line is a group of cells that all descend from a single original stem cell and are grown in a lab. Cells in a stem cell line keep growing but don't become specialized cells. Ideally, they remain free of genetic defects and continue to create more stem cells. Clusters of cells can be taken from a stem cell line and frozen for storage or shared with other researchers.
What is stem cell therapy (regenerative medicine), and how does it work?
Stem cell therapy, also known as regenerative medicine, promotes the repair response of diseased, dysfunctional or injured tissue using stem cells or their derivatives. It is the next chapter in organ transplantation and uses cells instead of donor organs, which are limited in supply.
Researchers grow stem cells in a lab. These stem cells are manipulated to specialize into specific types of cells, such as heart muscle cells, blood cells or nerve cells.
The specialized cells can then be implanted into a person. For example, if the person has heart disease, the cells could be injected into the heart muscle. The healthy transplanted heart muscle cells could then contribute to repairing the injured heart muscle.
Researchers have already shown that adult bone marrow cells guided to become heart-like cells can repair heart tissue in people, and more research is ongoing.
Have stem cells already been used to treat diseases?
Yes. Doctors have performed stem cell transplants, also known as bone marrow transplants, for many decades. In hematopoietic stem cell transplants, stem cells replace cells damaged by chemotherapy or disease or serve as a way for the donor's immune system to fight some types of cancer and blood-related diseases. Leukemia, lymphoma, neuroblastoma and multiple myeloma often are treated this way. These transplants use adult stem cells or umbilical cord blood.
Researchers are testing adult stem cells to treat other conditions, including some degenerative diseases such as heart failure.
What are the potential problems with using embryonic stem cells in humans?
For embryonic stem cells to be useful, researchers must be certain that the stem cells will differentiate into the specific cell types desired.
Researchers have discovered ways to direct stem cells to become specific types of cells, such as directing embryonic stem cells to become heart cells. Research is ongoing in this area.
Embryonic stem cells also can grow irregularly or specialize in different cell types spontaneously. Researchers are studying how to control the growth and development of embryonic stem cells.
Embryonic stem cells also might trigger an immune response in which the recipient's body attacks the stem cells as foreign invaders, or the stem cells might simply fail to function as expected, with unknown consequences. Researchers continue to study how to avoid these possible complications.
What is therapeutic cloning, and what benefits might it offer?
Therapeutic cloning, also called somatic cell nuclear transfer, is a way to create versatile stem cells independent of fertilized eggs. In this technique, the nucleus is removed from an unfertilized egg. This nucleus contains the genetic material. The nucleus also is removed from the cell of a donor.
This donor nucleus is then injected into the egg, replacing the nucleus that was removed, in a process called nuclear transfer. The egg is allowed to divide and soon forms a blastocyst. This process creates a line of stem cells that is genetically identical to the donor's cells — in essence, a clone.
Some researchers believe that stem cells derived from therapeutic cloning may offer benefits over those from fertilized eggs because cloned cells are less likely to be rejected once transplanted back into the donor. And it may allow researchers to see exactly how a disease develops.
Has therapeutic cloning in people been successful?
No. Researchers haven't been able to successfully perform therapeutic cloning with humans despite success in a number of other species.
Researchers continue to study the potential of therapeutic cloning in people.
There is a problem with information submitted for this request. Review/update the information highlighted below and resubmit the form.
From Mayo Clinic to your inbox
Sign up for free and stay up to date on research advancements, health tips, current health topics, and expertise on managing health. Click here for an email preview.
Error Email field is required
Error Include a valid email address
To provide you with the most relevant and helpful information, and understand which information is beneficial, we may combine your email and website usage information with other information we have about you. If you are a Mayo Clinic patient, this could include protected health information. If we combine this information with your protected health information, we will treat all of that information as protected health information and will only use or disclose that information as set forth in our notice of privacy practices. You may opt-out of email communications at any time by clicking on the unsubscribe link in the e-mail.
Thank you for subscribing!
You'll soon start receiving the latest Mayo Clinic health information you requested in your inbox.
Sorry something went wrong with your subscription
Please, try again in a couple of minutes
- Stem cell basics. National Institutes of Health. https://stemcells.nih.gov/info/basics/stc-basics/#stc-I. Accessed March 21, 2024.
- Lovell-Badge R, et al. ISSCR guidelines for stem cell research and clinical translation: The 2021 update. Stem Cell Reports. 2021; doi:10.1016/j.stemcr.2021.05.012.
- AskMayoExpert. Hematopoietic stem cell transplant. Mayo Clinic; 2024.
- Stem cell transplants in cancer treatment. National Cancer Institute. https://www.cancer.gov/about-cancer/treatment/types/stem-cell-transplant/. Accessed March 21, 2024.
- Townsend CM Jr, et al. Regenerative medicine. In: Sabiston Textbook of Surgery: The Biological Basis of Modern Surgical Practice. 21st ed. Elsevier; 2022. https://www.clinicalkey.com. Accessed March 21, 2024.
- Kumar D, et al. Stem cell based preclinical drug development and toxicity prediction. Current Pharmaceutical Design. 2021; doi:10.2174/1381612826666201019104712.
- NIH guidelines for human stem cell research. National Institutes of Health. https://stemcells.nih.gov/research-policy/guidelines-for-human-stem-cell-research. Accessed March 21, 2024.
- De la Torre P, et al. Current status and future prospects of perinatal stem cells. Genes. 2020; doi:10.3390/genes12010006.
- Yen Ling Wang A. Human induced pluripotent stem cell-derived exosomes as a new therapeutic strategy for various diseases. International Journal of Molecular Sciences. 2021; doi:10.3390/ijms22041769.
- Alessandrini M, et al. Stem cell therapy for neurological disorders. South African Medical Journal. 2019; doi:10.7196/SAMJ.2019.v109i8b.14009.
- Goldenberg D, et al. Regenerative engineering: Current applications and future perspectives. Frontiers in Surgery. 2021; doi:10.3389/fsurg.2021.731031.
- Brown MA, et al. Update on stem cell technologies in congenital heart disease. Journal of Cardiac Surgery. 2020; doi:10.1111/jocs.14312.
- Li M, et al. Brachyury engineers cardiac repair competent stem cells. Stem Cells Translational Medicine. 2021; doi:10.1002/sctm.20-0193.
- Augustine R, et al. Stem cell-based approaches in cardiac tissue engineering: Controlling the microenvironment for autologous cells. Biomedical Pharmacotherapy. 2021; doi:10.1016/j.biopha.2021.111425.
- Cloning fact sheet. National Human Genome Research Institute. https://www.genome.gov/about-genomics/fact-sheets/Cloning-Fact-Sheet. Accessed March 21, 2024.
- Dingli D (expert opinion). Mayo Clinic. Nov. 17, 2023.
Products and Services
- A Book: Living Medicine
- Sign up for Email: Get Your Free Resource – Coping with Cancer
- Give today to find cancer cures for tomorrow
- Acute lymphocytic leukemia
- Acute myelogenous leukemia
- Adjuvant therapy for cancer
- Amyloidosis
- Aplastic anemia
- Atypical cells: Are they cancer?
- Biopsy procedures
- Blood Cancers and Disorders
- Bone marrow transplant
- Cancer blood tests
- Myths about cancer causes
- Infographic: Cancer Clinical Trials Offer Many Benefits
- Cancer diagnosis: 11 tips for coping
- Cancer-related fatigue
- Cancer pain: Relief is possible
- Cancer risk: What the numbers mean
- Cancer surgery
- Cancer survival rate
- Cancer survivors: Care for your body after treatment
- Cancer survivors: Late effects of cancer treatment
- Cancer survivors: Managing your emotions after cancer treatment
- Cancer treatment myths
- Chemotherapy side effects: A cause of heart disease?
- Chronic lymphocytic leukemia
- Chronic myelogenous leukemia
- Curcumin: Can it slow cancer growth?
- What is type 1 diabetes? A Mayo Clinic expert explains
- Type 1 diabetes FAQs
- Cancer-related diarrhea
- DiGeorge syndrome (22q11.2 deletion syndrome)
- Eating during cancer treatment: Tips to make food tastier
- Epidermolysis bullosa
- Gaucher disease
- Heart cancer: Is there such a thing?
- High-dose vitamin C: Can it kill cancer cells?
- Hodgkin's lymphoma (Hodgkin's disease)
- Hodgkin's vs. non-Hodgkin's lymphoma: What's the difference?
- Low blood counts
- Measles Virus as a Cancer Fighter
- Monoclonal antibody drugs
- Mort Crim and Cancer
- Mouth sores caused by cancer treatment: How to cope
- Multiple myeloma
- Infographic: Multiple Myeloma
- Myelofibrosis
- Neuroblastoma
- No appetite? How to get nutrition during cancer treatment
- Non-Hodgkin's lymphoma
- Scleroderma
- Self-Image During Cancer
- Sickle cell anemia
- Sisters' Bone Marrow Transplant
- Small cell, large cell cancer: What this means
- Stem Cells 101
- Thalassemia
- Tumor vs. cyst: What's the difference?
- Type 1 diabetes
- Stem cell transplant
- How cancer spreads
- PICC line placement
- When cancer returns: How to cope with cancer recurrence
Mayo Clinic does not endorse companies or products. Advertising revenue supports our not-for-profit mission.
- Opportunities
Mayo Clinic Press
Check out these best-sellers and special offers on books and newsletters from Mayo Clinic Press .
- Mayo Clinic on Incontinence - Mayo Clinic Press Mayo Clinic on Incontinence
- The Essential Diabetes Book - Mayo Clinic Press The Essential Diabetes Book
- Mayo Clinic on Hearing and Balance - Mayo Clinic Press Mayo Clinic on Hearing and Balance
- FREE Mayo Clinic Diet Assessment - Mayo Clinic Press FREE Mayo Clinic Diet Assessment
- Mayo Clinic Health Letter - FREE book - Mayo Clinic Press Mayo Clinic Health Letter - FREE book
- Stem cells What they are and what they do
Your gift holds great power – donate today!
Make your tax-deductible gift and be a part of the cutting-edge research and care that's changing medicine.

Embryonic and Adult Stem Cell Research - Addressing the Facts and the Issues: Pros and Cons of Stem Cell Research
- Pros and Cons of Stem Cell Research
- Stem Cells - Some Basics for Review
- News About Stem Cell Research and Developments
Positions in Favor of Stem Cell Research - Embryonic and/or Adult
- Examining the Ethics of Embryonic Stem Cell Research Source - Harvard Stem Cell Institute publication Stem Cell LInes
- The Case for Embryonic Stem Cell Research: An Interview with Jonathan Moreno Moreno is the David and Lyn Silfen University Professor and Professor of Medical Ethics and of History and Sociology of Science at the University of Pennsylvania as well as a senior fellow at the Center for American Progress in Washington, D.C. Previously, he was president of the American Society for Bioethics and Humanities and served as a senior staff member for two presidential advisory committees.
Overviews (Pros and Cons) of Ethical Issues
- Debating Pros and Cons Stem Cell Research from The Journal of the American Medical Association, August 9, 2000-Vol 284, No. 6: 681-682. Retrieved from the MAC Library's print collection
- Ethical Issues in Stem Cell Research Article from the research journal Endocrine Reviews authored by Bernard Lo and Lindsay Parham, 2009 May, 30(3): 204-213 Accessed through PubMed Central
- Ethics of Stem Cell Research Stanford Encyclopedia of Philosophy
- Pros and Cons of Stem Cell Research This article is from The Balance, a group of websites offering advice primarily on personal finance. The information appears to be objective and presented for educational purposes.
- Stem Cells Hastings Center Bioethics Briefs for Journalists, Policy Makers, and Educators
- Stem Cell Research as Innovation: Expanding the Ethical and Policy Consideration This article is from the research journal The Journal of Law, Medicine & Ethics authored by Rebecca Dresser, 2010 Summera, 38(2): 332-341. Accessed through PubMed Central.
The Pew Research Center - Religion and Public Life
- Religious Groups' Official Positions on Stem Cell Research
- Stem Cell Research at the Crossroads of Religion and Politics
- Stem Cell Research Around the World
- Religion and Stem Cell Research
About Pew Research Center Pew Research Center is a nonpartisan fact tank that informs the public about the issues, attitudes and trends shaping the world. We conduct public opinion polling, demographic research, content analysis and other data-driven social science research. We do not take policy positions.
Our Mission We generate a foundation of facts that enriches the public dialogue and supports sound decision-making. We are nonprofit, nonpartisan and nonadvocacy. We value independence, objectivity, accuracy, rigor, humility, transparency and innovation.
The Ethics of Stem Cells
Positions Not in Favor of Stem Cell Research - Embryonic and/or Adult
- Stem Cell Research.org Stem cell research promises great good and is a worthy scientific priority as long as we pursue it ethically. Obtaining stem cells from people without seriously harming people in the process can be ethical. However, obtaining stem cells from human embryos cannot be ethical because it necessarily involves destroying those embryos.
- Stem Cell Research from The Center for Bioethics & Human Dignity: Exploring the Nexus of Biomedicine, Biotechnology, & Our Common Humanity (Trinity International University)
- The Case Against Embryonic Cell Research: An Interview with Yuval Levin Yuval Levin is the author of Tyranny of Reason. Previously, Levin was the executive director of the President’s Council on Bioethics. Currently, he is the Hertog Fellow at the Ethics and Public Policy Center in Washington, D.C., where he also directs the center’s Bioethics and American Democracy program.
- Bishops Campaign Against Embryonic Stem Cells from The National Catholic Reporter
Concerns About the Uses of Stem Cell Therapies
- The Trouble with Stem Cell Therapy A new industry is booming. But critics worry that the treatments are ineffective and dangerous. Here's how to protect yourself. from Consumer Reports
- A Slow Road for Stem Cells from the journal Nature: International Journal of Science
- Regulating the Stem Cell Industry: Needs and Responsibilities Bulletin of the World Health Organization
- Current Safety Considerations in Stem Cell Therapy Article from the research journal Cell Journal authored by M. Mousavinejad, P.W.Andrews, & E.K. Shoraki, 2106 Jul-Sep, 18(2): 281-287. Accessed through PubMed Centarl
Normal Stem Cells

This file is licensed under the Creative Commons Attributed-Share Alike 3.0 Unported license. Malymajo [CC BY-SA 3.0 (https://creativecommons.org/licenses/by-sa/3.0)]
- << Previous: Home
- Next: Stem Cells - Some Basics for Review >>
- Last Updated: Apr 26, 2021 6:16 PM
- URL: https://libguides.mtaloy.edu/stem-cell-research

- Types of Stem Cell
- History of Stem Cell Use
- Importance of Stem Cells
- Pros and Cons
- Stem Cell FAQs
- Stem Cells at UNMC
Pros and Cons of Using Various Stem Cells
- Abundant somatic cells of donor can be used
- Issues of histocompatibility with donor/recipient transplants can be avoided
- Very useful for drug development and developmental studies
What is Stem Cell Research and Pros and Cons about
Learn about stem cell research, including the benefits and drawbacks, as well as the numerous types of stem cells accessible.
Stem Cell Therapy enhances the body’s natural healing processes, and is one of the most exciting breakthroughs in recent regenerative medicine. It offers incredible treatment for a wide range of illnesses for which doctors previously had no acceptable treatments.
In this Mind The Graph article, you will learn about stem cell research: pros and cons , what is it, and the various types of stem cells available. This article will also provide you with successful stories to better understand the topic.
What are stem cells?
The body has original raw material in the form of stem cells. These stem cells are responsible for the development of all other cells with specific roles. When given the right conditions, either in the body or in a laboratory, stem cells divide to produce new cells known as daughter cells.
The daughter cells have the ability to transform into more stem cells or cells with a specific function, such as blood cells, brain cells, heart muscle cells, or bone cells. There is no other cell in the body that can naturally generate new cell types like a stem cell.
Types of stem cells
Embryonic stem cells.
Embryonic stem cells are extracted from the blastocyst, a mostly empty ball of cells that develops three to five days after an egg cell is fertilized by a sperm in humans. Embryonic stem cells are pluripotent, which means they can give rise to all cell types in a fully developed organism, save the placenta and umbilical cord.
These cells that have been separated from the inner cell mass will evolve into more specialized cells, which will give rise to all of the body’s tissues and organs. When scientists remove the inner cell mass and cultivate it in a particular laboratory environment, the cells maintain the qualities of embryonic stem cells.
These cells are extremely significant because they provide a sustainable supply for researching normal development and illness, as well as evaluating medicines and other treatments.
Tissue-specific stem cells
Tissue-specific stem cells (most known as adult stem cells) have a higher level of specialization than embryonic stem cells. Typically, these stem cells may produce a wide range of cell types for the tissue or organ in which they dwell.
Tissue-specific stem cells are rare to detect in the human body, and they do not appear to self-renew in culture as easily as embryonic stem cells. However, research on these cells has expanded our overall understanding of normal development, how aging affects us, and what occurs in the event of an injury and disease.
Mesenchymal stem cells
Cells derived from stroma, the connective tissue that surrounds other tissues and organs, are referred to as “mesenchymal stem cells” or MSC. Many scientists refer to cells with this designation as “stromal cells.”
These cells were initially found in the bone marrow and were demonstrated to be capable of producing bone, cartilage, and fat cells. They have now been produced from various tissues such as fat and cord blood. Various MSCs are assumed to have stem cell and even immunomodulatory qualities, and they are being explored as therapies for a wide range of illnesses, although there is no evidence that they are useful yet. Scientists do not know for certain if these cells are stem cells or what sorts of cells they can generate. They do agree that not all MSCs are the same and that their properties vary depending on where they come from in the body and how they are separated and produced.
Induced pluripotent stem cells
Induced pluripotent stem (iPS) cells are lab-engineered cells that have been transformed from tissue-specific cells, such as skin cells and into cells that act like embryonic stem cells. IPS cells are important tools for scientists to understand more about developmental stages, illness initiation, and progression, as well as create and test new medications and treatments.
While iPS cells possess a lot of the same properties as embryonic stem cells, such as the potential to give birth to all cell types in the body, they are not identical. Scientists are trying to figure out what these distinctions are and what they represent. For one thing, the first iPS cells were created by inserting additional copies of genes into tissue-specific cells using viruses. Researchers are exploring a variety of methods for creating iPS cells, to eventually use them as a source of cells or tissues for medicinal therapies.
Development in Stem Cell Research: successful stories
Before delving into the pros and cons of stem cell research , a few successful tales must be mentioned in this article.
Multiple Sclerosis
Reema Sandhu’s stem cell success story began in 2014 when she was diagnosed with multiple sclerosis, according to DailyMail. The disease can affect the brain and spinal cord, causing a variety of life-altering symptoms such as severe disabilities, muscular spasms, and memory issues. She got an autologous stem cell transplant after years of frustration, in which her own stem cells were extracted from her blood and infused back into her body through intravenous infusion. Major improvements in Reema’s brain function were immediately evident. Her vision recovered two months after the transplant, and she went back to work. These encouraging results indicate that Reema’s MS has slowed as a result of her stem cell transplant.
Heart attack
According to DailyMail, Dave Randle was left with serious heart failure and a grim warning from specialists after suffering a heart attack in 2016. However, after learning that stem cells may be used to heal damaged hearts, Dave enrolled in therapy and received shots for 5 days in a row that pushed his bone marrow to allow stem cells to enter his circulation. These cells were then extracted and reintroduced into his heart. Dave’s stem cell success story had a happy ending weeks after the transplant – he began to feel stronger and physicians detected significant changes.
In 2013, a woman was diagnosed with HIV. According to the Journal, she was also diagnosed with acute myeloid leukemia in March 2017, making her a candidate for a stem cell transplant. She had a stem cell transplant via two blood transfusions: one from an older relative and the other from an unrelated baby. The baby, a partially matched donor, has a mutation in the CCR5 gene, which inhibits HIV’s capacity to infiltrate host cells. The woman stopped taking her HIV medication in 2020, and she hasn’t shown any measurable indications of the infection ever since. She has also been in remission from leukemia for almost four years.
Stem Cell Research: Pros and Cons
- Improve understanding of illness etiology: Researchers may gain a better understanding of how illnesses and ailments emerge by monitoring stem cells grow into cells in bones, heart muscle, neurons, and other organs and tissue.
- Generate healthy cells to replace diseased cells (regenerative medicine): Stem cells can be manipulated to become particular cells that can be employed in humans to regenerate and heal tissues that have been damaged or impaired by illness.
- Test novel pharmaceuticals for safety and efficacy: Before administering investigational drugs to humans, researchers can utilize certain types of stem cells to assess the drugs’ effectiveness and safety.
- Minimal rejection risk: Adult stem cells are less likely to be rejected in transplants and can be reprogrammed. Adult stem cell success has also been established in a variety of therapeutic applications.
- Hard to grow: Adult stem cells are difficult to cultivate for an extended length of time and there is currently no technique available to create large amounts of adult stem cells.
- Embryonic stem cells are generally rejected: When employed in transplants, embryonic stem cells may not be accepted.
- New technology: Stem cell treatment is still being researched, and there are a lot of things that need to be established. The therapy’s long-term adverse effects are yet unknown.
- Concerns about ethics: stem cell treatment uses embryonic stem cells from a human embryo. Many conservatives argue that “human life begins at conception” and that stem cell research results in the death of living babies.
Make scientifically accurate infographics in minutes
Use the power of infographics to help your scientific job succeed. Mind The Graph tool can assist you by giving amazing templates and allowing you to browse through 40 000+ scientifically accurate images in 80+ popular fields.
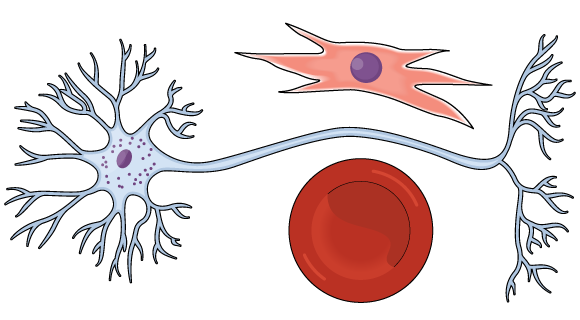
Subscribe to our newsletter
Exclusive high quality content about effective visual communication in science.
About Jessica Abbadia
Jessica Abbadia is a lawyer that has been working in Digital Marketing since 2020, improving organic performance for apps and websites in various regions through ASO and SEO. Currently developing scientific and intellectual knowledge for the community's benefit. Jessica is an animal rights activist who enjoys reading and drinking strong coffee.
Content tags


Stem Cell Research — What Are The Pros And Cons?
Stem cell research has been a topic of intense debate and discussion for many years. This is because it is a field that holds great promise for the future of medicine but also raises ethical concerns. Stem cells are unique cells that have the potential to develop into many different types of cells in the body. They can be used to replace damaged or diseased cells, and have the potential to treat a wide range of conditions, including cancer, diabetes, and neurological disorders.
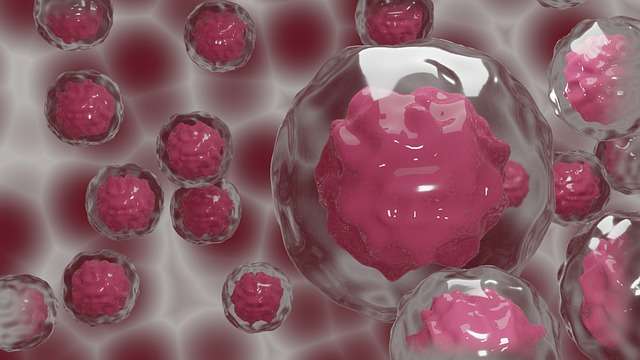
However, the use of stem cells, particularly those derived from embryos, has been controversial due to ethical concerns surrounding the destruction of embryos. In this article, we will explore the pros and cons of stem cell research, and discuss the potential benefits and drawbacks of this groundbreaking field.
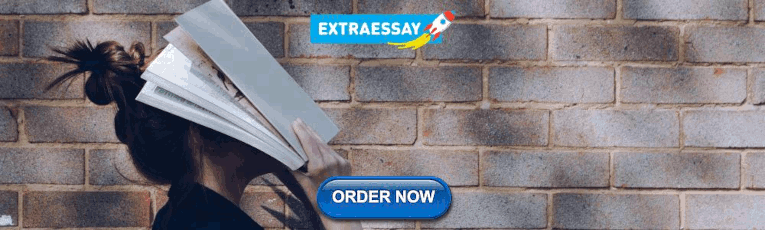
Pros of Stem Cell Research
1. potential to treat a wide range of diseases and conditions.
One of the most significant benefits of stem cell research is its potential to treat a wide range of diseases and conditions. Stem cells have the ability to differentiate into various types of cells, which means they can potentially be used to replace damaged or diseased cells in the body. This could lead to new treatments for conditions such as Parkinson’s disease, Alzheimer’s disease, spinal cord injuries, and heart disease, among others.
For example, researchers have been working on developing stem cell therapies for spinal cord injuries. By injecting stem cells into the injured area, they hope to stimulate the regeneration of damaged nerve cells and restore function to the affected area. This could potentially help patients regain movement and sensation, improving their quality of life.
2. Potential to Revolutionize Organ Transplants
Stem cell research could also revolutionize the field of organ transplantation. Currently, patients in need of an organ transplant must wait for a suitable donor, which can take months or even years. In some cases, patients may not survive the wait. However, with stem cell research, it may be possible to create organs in the lab using a patient’s own stem cells. This would eliminate the need for donor organs and reduce the risk of organ rejection, as the new organ would be genetically identical to the patient’s own tissue.
3. Advancements in Drug Testing and Development
Stem cell research can also play a crucial role in drug testing and development says the Scorpius bio company. Currently, new drugs are tested on animals before being tested on humans. However, this process can be time-consuming, and expensive, and may not always accurately predict how a drug will affect humans. By using stem cells to create human tissue in the lab, researchers can test new drugs on human cells, potentially speeding up the drug development process and reducing the need for animal testing.
4. Improved Understanding of Human Development and Disease
Stem cell research can also help scientists better understand human development and the progression of diseases. By studying how stem cells differentiate into various types of cells, researchers can gain insight into the genetic and environmental factors that influence development. This knowledge could potentially lead to new treatments and preventative measures for a variety of conditions.
Cons of Stem Cell Research
1. ethical concerns surrounding the use of embryonic stem cells.
One of the primary concerns surrounding stem cell research is the use of embryonic stem cells. These cells are derived from embryos that are just a few days old, and their extraction results in the destruction of the embryo. This has led to ethical debates, as some people believe that the destruction of an embryo is morally wrong, as it represents the destruction of potential human life.
2. Potential for Exploitation and Misuse
Another concern related to stem cell research is the potential for exploitation and misuse. For example, some people worry that the ability to create organs in the lab could lead to a market for designer organs, where wealthy individuals could pay for organs with specific traits or enhancements. Additionally, there is concern that stem cell research could be used for reproductive cloning, which is widely considered to be unethical.
3. Limited Availability of Stem Cells
While stem cells can be derived from various sources, including adult tissue and umbilical cord blood, the most versatile and potent stem cells are embryonic stem cells. However, the availability of these cells is limited, as they can only be derived from embryos. This has led to concerns about the potential for a shortage of stem cells, which could limit the progress of research and the development of new treatments.
4. Risk of Tumor Formation
One potential risk associated with stem cell therapy is the possibility of tumor formation. Because stem cells have the ability to divide and differentiate into various types of cells, there is a concern that they could form tumors if they are not properly controlled. While this risk is relatively low, it is still a concern that must be addressed in the development of stem cell therapies.
In conclusion, stem cell research holds great promise for the future of medicine, with the potential to treat a wide range of diseases and conditions and revolutionize organ transplantation.
Comments are closed.
- ARM microcontrollers
- AVR controlled signal generator
- AVR Microcontrollers
- Biomedical Engineering
- Construction
- Cool Gadgets
- DSP Lessons
- Electronics
- Electronics Circuits
- Electronics Tutorial
- Embedded General
- Energy Industry
- Engineering
- IoT and Home Automation
- Machine Learning and AI
- PCB Technology
- Programming
- Raspberry Pi
- Português Br
- Journalist Pass
Study documents safety, improvements from stem cell therapy after spinal cord injury
Susan Barber Lindquist
Share this:
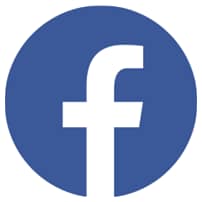
ROCHESTER, Minn. — A Mayo Clinic study shows stem cells derived from patients' own fat are safe and may improve sensation and movement after traumatic spinal cord injuries . The findings from the phase 1 clinical trial appear in Nature Communications . The results of this early research offer insights on the potential of cell therapy for people living with spinal cord injuries and paralysis for whom options to improve function are extremely limited.
In the study of 10 adults, the research team noted seven participants demonstrated improvements based on the American Spinal Injury Association (ASIA) Impairment Scale. Improvements included increased sensation when tested with pinprick and light touch, increased strength in muscle motor groups, and recovery of voluntary anal contraction, which aids in bowel function. The scale has five levels, ranging from complete loss of function to normal function. The seven participants who improved each moved up at least one level on the ASIA scale. Three patients in the study had no response, meaning they did not improve but did not get worse.
"This study documents the safety and potential benefit of stem cells and regenerative medicine," says Mohamad Bydon, M.D. , a Mayo Clinic neurosurgeon and first author of the study. "Spinal cord injury is a complex condition. Future research may show whether stem cells in combination with other therapies could be part of a new paradigm of treatment to improve outcomes for patients."
No serious adverse events were reported after stem cell treatment. The most commonly reported side effects were headache and musculoskeletal pain that resolved with over-the-counter treatment.
In addition to evaluating safety, this phase 1 clinical trial had a secondary outcome of assessing changes in motor and sensory function. The authors note that motor and sensory results are to be interpreted with caution given limits of phase 1 trials. Additional research is underway among a larger group of participants to further assess risks and benefits.
The full data on the 10 patients follows a 2019 case report that highlighted the experience of the first study participant who demonstrated significant improvement in motor and sensory function.
Watch: Dr. Mohamad Bydon discusses improvements in research study
Journalists: Broadcast-quality sound bites are available in the downloads at the end of the post. Please courtesy: "Mayo Clinic News Network." Name super/CG: Mohamad Bydon, M.D./Neurosurgery/Mayo Clinic.
Stem cells' mechanism of action not fully understood
In the multidisciplinary clinical trial, participants had spinal cord injuries from motor vehicle accidents, falls and other causes. Six had neck injuries; four had back injuries. Participants ranged in age from 18 to 65.
Participants' stem cells were collected by taking a small amount of fat from a 1- to 2-inch incision in the abdomen or thigh. Over four weeks, the cells were expanded in the laboratory to 100 million cells and then injected into the patients' lumbar spine in the lower back. Over two years, each study participant was evaluated at Mayo Clinic 10 times.
Although it is understood that stem cells move toward areas of inflammation — in this case the location of the spinal cord injury — the cells' mechanism of interacting with the spinal cord is not fully understood, Dr. Bydon says. As part of the study, researchers analyzed changes in participants' MRIs and cerebrospinal fluid as well as in responses to pain, pressure and other sensation. The investigators are looking for clues to identify injury processes at a cellular level and avenues for potential regeneration and healing.
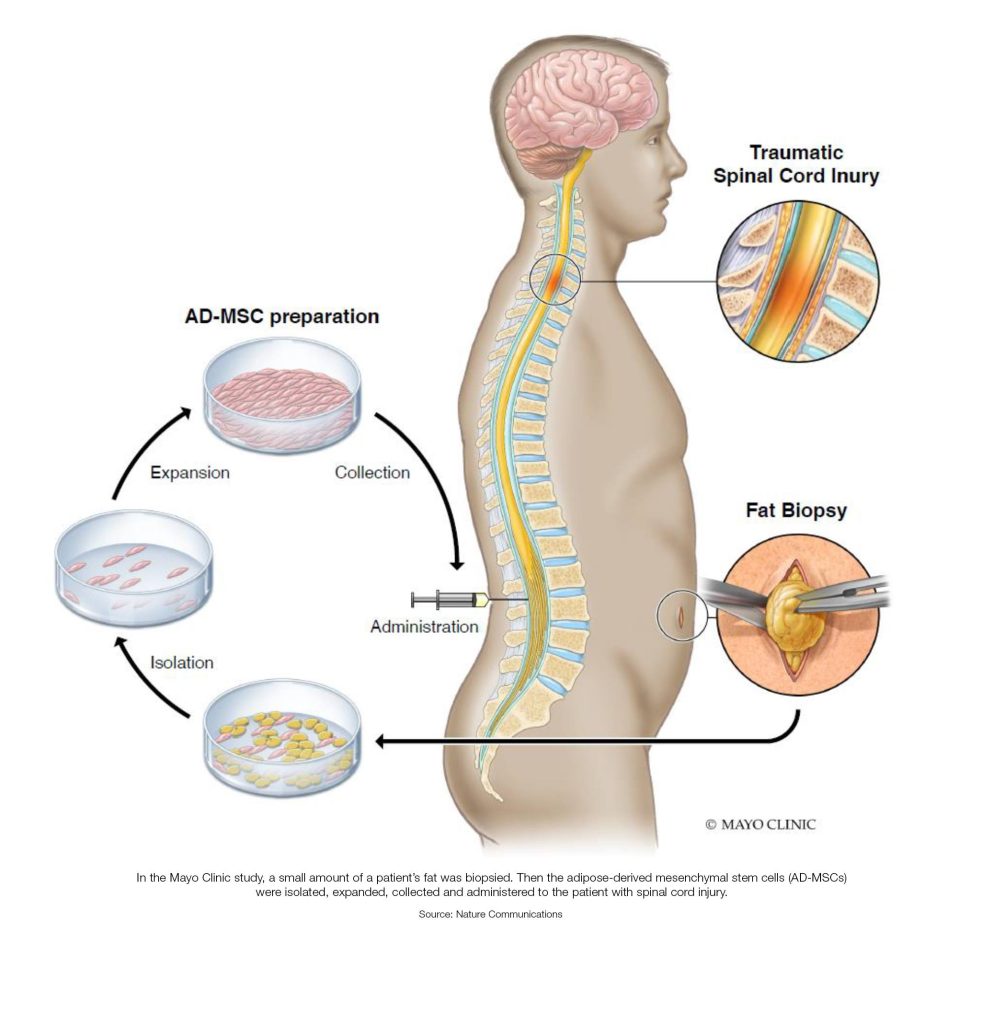
The spinal cord has limited ability to repair its cells or make new ones. Patients typically experience most of their recovery in the first six to 12 months after injuries occur. Improvement generally stops 12 to 24 months after injury. In the study, one patient with a cervical spine injury of the neck received stem cells 22 months after injury and improved one level on the ASIA scale after treatment.
Two of three patients with complete injuries of the thoracic spine — meaning they had no feeling or movement below their injury between the base of the neck and mid-back — moved up two ASIA levels after treatment. Each regained some sensation and some control of movement below the level of injury. Based on researchers' understanding of traumatic thoracic spinal cord injury, only 5% of people with a complete injury would be expected to regain any feeling or movement.
"In spinal cord injury, even a mild improvement can make a significant difference in that patient's quality of life," Dr. Bydon says.
Research continues into stem cells for spinal cord injuries
Stem cells are used mainly in research in the U.S., and fat-derived stem cell treatment for spinal cord injury is considered experimental by the Food and Drug Administration.
Between 250,000 and 500,000 people worldwide suffer a spinal cord injury each year, according to the World Health Organization .
An important next step is assessing the effectiveness of stem cell therapies and subsets of patients who would most benefit, Dr. Bydon says. Research is continuing with a larger, controlled trial that randomly assigns patients to receive either the stem cell treatment or a placebo without stem cells.
"For years, treatment of spinal cord injury has been limited to supportive care, more specifically stabilization surgery and physical therapy," Dr. Bydon says. "Many historical textbooks state that this condition does not improve. In recent years, we have seen findings from the medical and scientific community that challenge prior assumptions. This research is a step forward toward the ultimate goal of improving treatments for patients."
Dr. Bydon is the Charles B. and Ann L. Johnson Professor of Neurosurgery. This research was made possible with support from Leonard A. Lauder, C and A Johnson Family Foundation, The Park Foundation, Sanger Family Foundation, Eileen R.B. and Steve D. Scheel, Schultz Family Foundation, and other generous Mayo Clinic benefactors. The research is funded in part by a Mayo Clinic Transform the Practice grant.
Review the study for a complete list of authors and funding.
About Mayo Clinic Mayo Clinic is a nonprofit organization committed to innovation in clinical practice, education and research, and providing compassion, expertise and answers to everyone who needs healing. Visit the Mayo Clinic News Network for additional Mayo Clinic news.
Media contact:
- Susan Barber Lindquist, Mayo Clinic Communications, [email protected]
- Personal journey shapes unique perspective Mayo Clinic scientists pioneer immunotherapy technique for autoimmune diseases
Related Articles
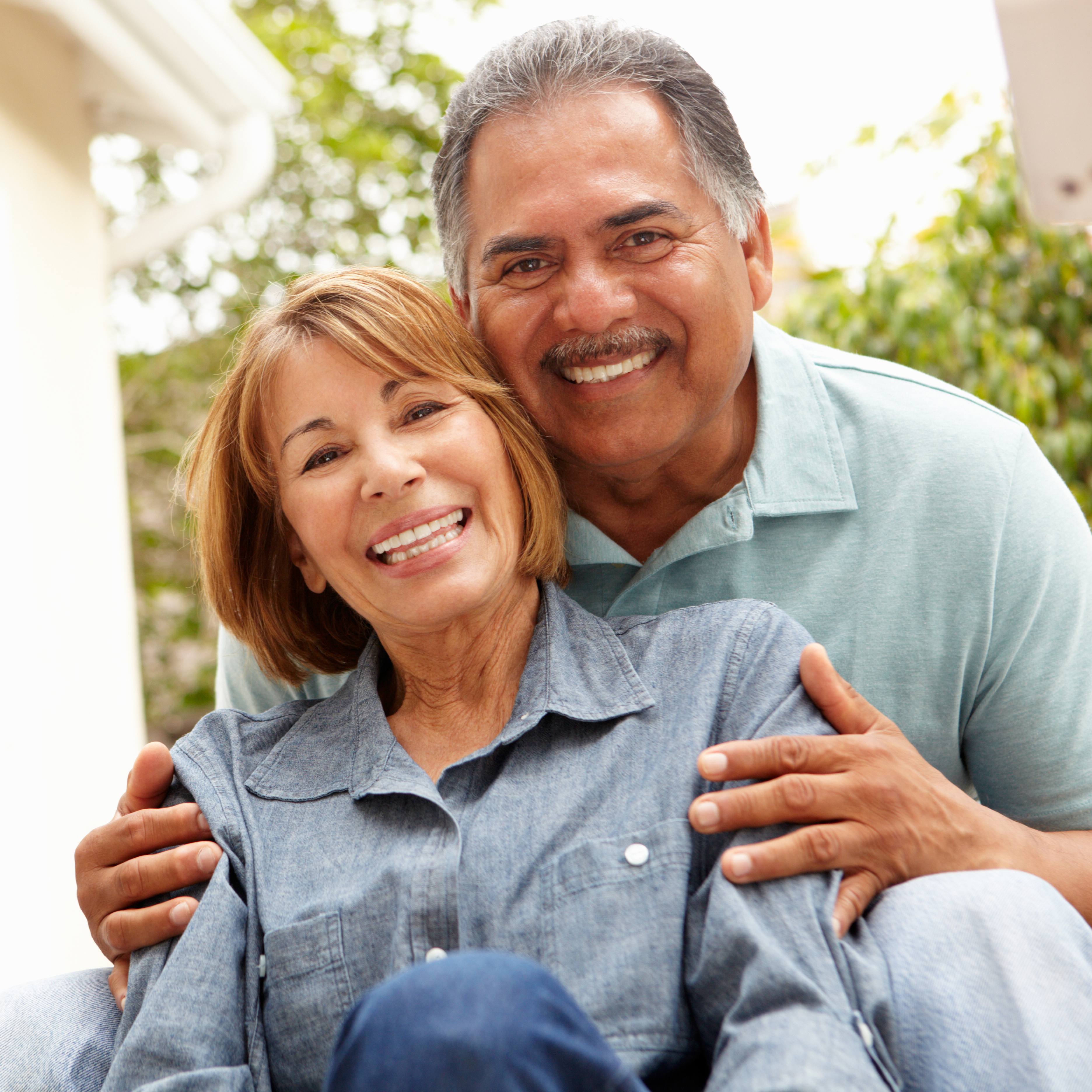

Connecting lab-grown brain cells provides insight into how our own brains work
The idea of growing a functioning human brain-like tissues in a dish has always sounded pretty far-fetched, even to researchers in the field. Towards the future goal, a Japanese and French research team has developed a technique for connecting lab-grown brain-mimicking tissue in a way that resembles circuits in our brain.
It is challenging to study exact mechanisms of the brain development and functions. Animal studies are limited by differences between species in brain structure and function, and brain cells grown in the lab tend to lack the characteristic connections of cells in the human brain. What's more, researchers are increasingly realizing that these interregional connections, and the circuits that they create, are important for many of the brain functions that define us as humans.
Previous studies have tried to create brain circuits under laboratory conditions, which have been advancing the field. Researchers from The University of Tokyo have recently found a way to create more physiological connections between lab-grown "neural organoids," an experimental model tissue in which human stem cells are grown into three-dimensional developmental brain-mimicking structures. The team did this by linking the organoids via axonal bundles, which is similar to how regions are connected in the living human brain.
"In single-neural organoids grown under laboratory conditions, the cells start to display relatively simple electrical activity," says co-lead author of the study Tomoya Duenki. "when we connected two neural organoids with axonal bundles, we were able to see how these bidirectional connections contributed to generating and synchronizing activity patterns between the organoids, showing some similarity to connections between two regions within the brain."
The cerebral organoids that were connected with axonal bundles showed more complex activity than single organoids or those connected using previous techniques. In addition, when the research team stimulated the axonal bundles using a technique known as optogenetics, the organoid activity was altered accordingly and the organoids were affected by these changes for some time, in a process known as plasticity.
"These findings suggest that axonal bundle connections are important for developing complex networks," explains Yoshiho Ikeuchi, senior author of the study. "Notably, complex brain networks are responsible for many profound functions, such as language, attention, and emotion."
Given that alterations in brain networks have been associated with various neurological and psychiatric conditions, a better understanding of brain networks is important. The ability to study lab-grown human neural circuits will improve our knowledge of how these networks form and change over time in different situations, and may lead to improved treatments for these conditions.
- Brain Tumor
- Nervous System
- Psychology Research
- Birth Defects
- Brain-Computer Interfaces
- Intelligence
- Brain Injury
- Neuroscience
- Neural network
- Brain damage
- Brain tumor
- Psycholinguistics
- Human brain
Story Source:
Materials provided by Institute of Industrial Science, The University of Tokyo . Note: Content may be edited for style and length.
Journal Reference :
- Tatsuya Osaki, Tomoya Duenki, Siu Yu A. Chow, Yasuhiro Ikegami, Romain Beaubois, Timothée Levi, Nao Nakagawa-Tamagawa, Yoji Hirano, Yoshiho Ikeuchi. Complex activity and short-term plasticity of human cerebral organoids reciprocally connected with axons . Nature Communications , 2024; 15 (1) DOI: 10.1038/s41467-024-46787-7
Cite This Page :
Explore More
- Quantum Effects in Electron Waves
- Star Trek's Holodeck Recreated Using ChatGPT
- Cloud Engineering to Mitigate Global Warming
- Detecting Delayed Concussion Recovery
- Genes for Strong Muscles: Healthy Long Life
- Brightest Gamma-Ray Burst
- Stellar Winds of Three Sun-Like Stars Detected
- Fences Causing Genetic Problems for Mammals
- Ozone Removes Mating Barriers Between Fly ...
- Parkinson's: New Theory On Origins and Spread
Trending Topics
Strange & offbeat.
- Open access
- Published: 03 June 2022
Characteristics of the cancer stem cell niche and therapeutic strategies
- Feng Ju 1 na1 ,
- Manar M. Atyah 2 , 3 na1 ,
- Nellie Horstmann 1 ,
- Sheraz Gul 4 , 5 ,
- Razi Vago 6 ,
- Christiane J. Bruns 1 ,
- Yue Zhao ORCID: orcid.org/0000-0002-6790-3402 1 ,
- Qiong-Zhu Dong 7 , 8 &
- Ning Ren 2 , 3 , 7 , 8
Stem Cell Research & Therapy volume 13 , Article number: 233 ( 2022 ) Cite this article
7250 Accesses
43 Citations
11 Altmetric
Metrics details
Distinct regions harboring cancer stem cells (CSCs) have been identified within the microenvironment of various tumors, and as in the case of their healthy counterparts, these anatomical regions are termed “niche.” Thus far, a large volume of studies have shown that CSC niches take part in the maintenance, regulation of renewal, differentiation and plasticity of CSCs. In this review, we summarize and discuss the latest findings regarding CSC niche morphology, physical terrain, main signaling pathways and interactions within them. The cellular and molecular components of CSCs also involve genetic and epigenetic modulations that mediate and support their maintenance, ultimately leading to cancer progression. It suggests that the crosstalk between CSCs and their niche plays an important role regarding therapy resistance and recurrence. In addition, we updated diverse therapeutic strategies in different cancers in basic research and clinical trials in this review. Understanding the complex heterogeneity of CSC niches is a necessary pre-requisite for designing superior therapeutic strategies to target CSC-specific factors and/or components of the CSC niche.
Definitions and background
Stem cells are undifferentiated cells that have the capacity to self-renew and proliferate for longer than non-stem cells as well as having the ability to generate multiple types of cells in the body [ 1 , 2 ]. Regardless of their proliferation potential, stem cells are usually quiescent, remaining in an inactive dormant state (G1, G0) and are protected from cellular damage or mutations [ 3 , 4 ].
In general, stem cells exhibit various levels of differentiation potential, starting with totipotency (greatest differentiation potential), pluripotency, multipotency, oligopotency and finally unipotency/monopotency (stem cells can generate only one cell type) [ 4 ]. The survival of stem cells is ensured at two levels: cellular asymmetrical division and population asymmetrical renewal. With asymmetrical division, one stem cell gives rise to a stem cell and one differentiating cell. With population asymmetry, one stem cell produces two stem cells or two differentiating cells. As the frequency of these two processes are similar, both result in a comparable amount of stem cells and differentiating cells [ 5 ].
Some types of tissues have higher turnover and renewal rates than others (such as gastric and intestinal tissues and bone marrow as compared to brain or liver tissues), making the capability of stem cell self-renewal essential for their replenishment and providing an explanation for their broad presence in such tissues [ 6 ]. Many studies have explored whether the stemness of these cells is a result of an independent mechanism or the interaction with other cells and their surrounding environment and it has been established that these interactions are vital for the survival of stem cells [ 7 ].
Due to the inherent nature of stem cells, their use in regenerative medicine and stem cell-based therapy has been widely explored. However, to date, clinical applications of such therapeutic approaches are only available in hematopoietic malignancies and limited immune deficiencies [ 4 ].
Similar to normal stem cells, Cancer stem cells (CSCs) are also able to self-renew and differentiate into tumor cells [ 8 ]. With the multipotent capacity of CSCs, heterogeneous lineages of different kinds of cancer cells can be generated [ 9 ]. The ability of CSCs to initiate and reconstitute tumor lesions together with features such as differentiation and chemo/radiotherapy resistance has been widely investigated [ 10 ]. Although many features of CSCs are similar to normal stem cells, an important difference is that normal stem cells are usually dormant during adulthood until their regeneration ability is required, whereas this ability of CSCs is active. This leads to the possibility of detecting CSC based on markers found specifically in active stem cells but not dormant ones, such as PSF1 which has been identified in upregulated hematopoietic stem cells (HSCs) [ 7 ]. Many technologies and assays have been proposed for the identification of CSCs including microsphere assay, serial dilution assay, side population assay and aldehyde dehydrogenase activity assay, the gold standard for the CSC identification is the sphere colony formation assay and in vivo xeno transplantation of tumor cells into immunodeficient mouse models. Although each of these methods has limitations, they assist in identifying CSCs in several tumors such as breast cancer, brain cancer, liver cancer, stomach and colon cancer [ 10 ].
The fundamental mechanism by which CSCs function in cancer progression is unclear. A number of proposals have been made with regards to this, such as CSCs derived from normal somatic cells regain stemness due to cancerous changes and acquired genetic/epigenetic mutations [ 11 ]. For this, two models prevail, namely the hierarchical and stochastic models. In the hierarchical model, tumor initiation begins with stem cells that escape normal growth control and regulation. Therefore, they can transform into CSCs which give rise to a distinct population of cells forming the biological basis of tumors. This model has been validated clinically and would explain why only a full elimination of CSCs can prevent the relapse of cancers. Nevertheless, this model is unable to explain the interaction between CSCs and other differentiated cancer cells. In contrast, the stochastic model suggests that under suitable conditions all tumor cells (differentiated or not) can initiate further lesions. However, this model has limitations in explaining the relation between tumor heterogeneity and the capacity of initiating tumors. These findings have led to the concept of cellular plasticity, where both models are merged. Based on the genetic/epigenetic and microenvironmental signals, it is suggested that cancer cells have the ability to shift between stemness and differentiation states [ 12 ].
The microenvironment of CSCs is essential for their function and due to their complex nature and interactions with other components and factors, is referred to as the CSC niche. The CSC niche usually includes niche cells, such as cancer cells, stromal and endothelial cells extracellular matrix (ECM), signaling molecules, intrinsic factors, blood vessels and other cellular and acellular components such as exosomes. The components and structure of the niche can vary among organisms and different types of tissues in order to provide distinct functions in response to the needs of the tumor (Fig. 1 ) [ 13 , 14 , 15 , 16 ]. CSC niches have been identified in many locations including intestinal, tissues and neural tissues, aiding the regulation and maintenance of stem cell renewal and differentiation [ 6 , 17 ].
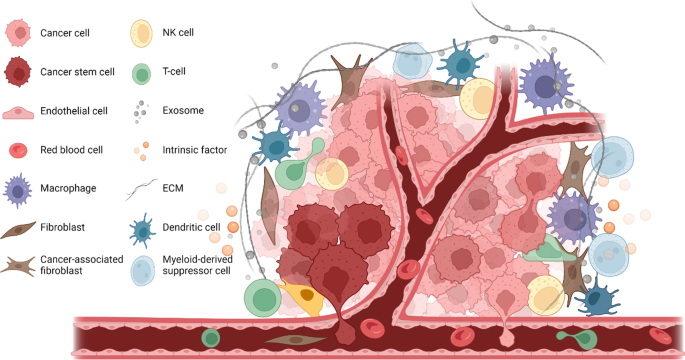
CSC niche in solid tumor. CSC niche has a complex microenvironment, it usually includes cancer cells, cancer stem cells, stromal cells, endothelial cells, fibroblasts, cancer-associated fibroblasts, ECM, exosomes and intrinsic factors. These components together contribute to the CSC renewal and maintain tumor malignancy
Due to the rarity of stem cells (compared to non-stem cells), it is challenging to clearly elucidate the mechanisms in the CSC niche [ 1 ]; however, some advances have been made with regards to this. The regulation mechanisms in the niche includes intrinsic mechanisms (associated with transcription factors expressed by cells), and extrinsic mechanisms (based on the signaling of the microenvironment and the connection to ECM). Disruption or interference in these mechanisms can lead to phenotypic changes that alter homeostasis of the niche [ 18 ]. Since the niche can contain more than one type of stem cells, competition between different stem cells within the niche is considered to control its function by factors such as E-cadherin [ 19 ], where the strength of the stem cell-niche connection can directly affect the fate of stem cells [ 14 ]. Even when only one type of stem cells are present in a niche, they still may lose their connection and, therefore, be replaced due to limited space or competition for occupancy [ 5 ] and this concept is crucial with regards to CSC niches.
Another important CSC feature is their ability to change and modify nearby stroma by CSC secreted proteins and molecular components, such as ECM proteins. The mechanism of ECM regulation has already been identified in different mammalian stem cells. In return, the niche can effectively regulate the biochemical status of keeping CSC in dormant. This is essential for the fate and plasticity of CSC and plays a role in their resistance to conventional therapies [ 15 ]. In order to better understand the characteristics of the CSC niche, we summarized the molecular features and mechanisms of the niche and reviewed the different therapeutic strategies targeting the CSC niche in various cancers from both basic and clinical aspects.
Conditions of the CSC niche
Hypoxia and its role in cscs.
Hypoxia is known for its role in the maintenance of the undifferentiated status of CSCs. The location of CSCs in hypoxic niches allows them to easily keep their slow cell cycling status with reduced proliferation, compared to a cycle with normal levels of oxygen. It is suggested that the rapid expansion of tumor mass and its vascular supply system might be the reason for tumor hypoxia that is responsible for the activation of several signaling pathways required in CSC functions. In solid tumors, the rapid growth of tumor tissues leads to a decrease in the quality of vascularization which affects the perfusion of blood and diffusion of substances it carries. That can be a cause for the poor outcome and the resistance of hypoxic niches against chemotherapy [ 20 ]. The hypoxic state of tumors is also linked to therapeutic resistance and local invasion [ 21 , 22 ]. It has also been shown that HSCs under hypoxic conditions can enhance the stemness of HSCs and shift cells from a quiescence to renewal phase [ 23 ]. Low levels of oxygen can also protect cells from DNA damage caused by oxidative stress which are common in aerobic metabolism. Studies have shown that rates of cellular damage are significantly higher at 20% O 2 relative to 3% O 2 . Low oxygen tension of 1% has also been shown to maintain the pluripotency of stem cells; therefore, hypoxia is essential for maintaining CSC characteristics [ 16 ].
In contrast to aerobic environments, hypoxic tissues use anaerobic glycolysis to metabolize glucose into lactate which produces much less ATP than in normal metabolism. This explains why cancer cells use higher rates of glucose compared to normal cells in aerobic conditions. However, reduced mitochondrial function is required, which is one way in which metabolic needs are down regulated in cancer cells and hypoxic niches. Such a difference between cancer cells and normal cells is usually controlled by the changes of oncogenes and tumor suppressor mutation profiles [ 20 ].
Many elements and factors participate in the control of hypoxic niches and related pathways such as mammalian target of rapamycin (mTOR) and the endoplasmic reticulum (ER) stress response. Examples of important transcription factors involved in the niche mechanisms are hypoxia inducible transcription factors (HIFs). Belonging to bHLH–PAS family of transcriptional factors, HIFs regulate many genes and play a role in oxygen homeostasis, glucose and iron metabolism and erythropoiesis [ 24 , 25 , 26 ]. These factors are also considered to be involved in several mechanisms such as the survival of cells under hypoxic conditions, cellular transcriptional responses, and activation of several signaling pathways. For example, expression of HIF-1α, CSC can promote tumor progression and metastasis. This transcription factor also affects genes involved in the regulation of the Notch and Oct4 pathways, as well as genes responsible for angiogenesis such as VEGF and GLUT-1 [ 1 ] by binding to HIF-1β and translocating to the nucleus where it can activate gene transcription. HIF signaling pathway can activate the angiogenic switch during tumor progression which is needed for the maintenance of oxygen homeostasis during the growth of tumor mass [ 27 ] (Fig. 2 ).
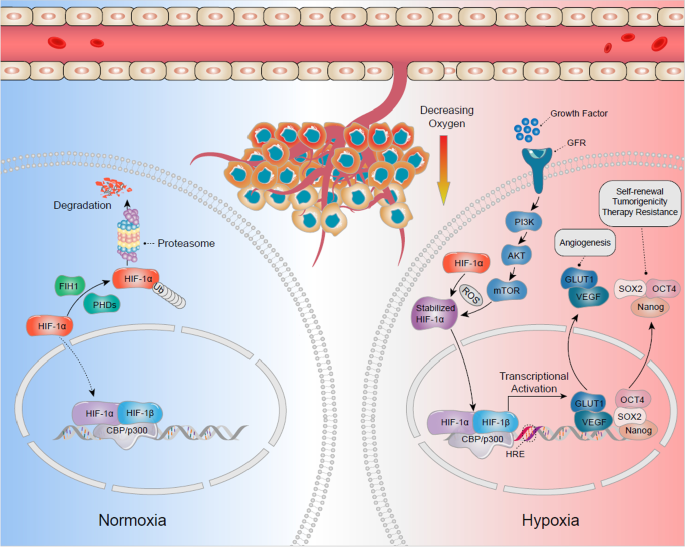
The hypoxic cancer stem cells (CSC) niches on CSC regulation. HIF-1α plays an important role in hypoxic process. Under normoxic conditions, HIF-1α is hydroxylated and inactivated by the function of poly1 hydroxylase domain-containing enzymes (PHDs) and Factor Inhibiting HIF (FIH1), and finally results in degradation. Under hypoxic conditions, both PHDs and FIH1 are inactivated, and HIF-1α stabilizes with the function of Reactive Oxygen Spices (ROS). HIF-1α could also be activated via activation of the PI-3 kinase/Akt-signaling pathway, which is induced by growth factor signaling. The stabilized HIF-1α translocates from cytoplasm to nucleus, where it dimerizes with HIF-1β and bind to Hypoxia Response Element (HRE). The heterodimer activates the downstream target genes transcription at these sites upon cofactor (CBP/p300) recruitment. These target genes in hypoxic niche, including Vascular Endothelial Growth Factor (VEGF), Glucose Transporter 1 (GLUT1), SOX2, Nanog and Octamer-binding Transcription Factor 4 (OCT4), may determine the fate of CSCs such as angiogenesis, self-renewal, tumorigenicity and induced therapy resistance
HIFs also play a role in regulating CSC proliferation, self-renewal, and tumorigenicity. The surface markers of CSC such as CD133 and CD44 show increased expression in hypoxic conditions [ 28 , 29 ]. With regards to therapy response, radiation has been found to double HIF-1 activity within 24–48 h following exposure, imposing further limitations on the outcome of such therapeutic approaches in cancer patients. Even after HIF activity is lost (usually occurring within minutes of activation), the targets may still show certain activities [ 20 ].
Metastasis of the CSC
Metastasis of CSCs was originally studied in hematopoietic malignancies followed by solid tumors which exhibited a similar phenomenon in brain, colon, breast, skin and pancreas cancers [ 30 , 31 , 32 , 33 , 34 ]. Most of these cancers are associated with specific metastasis-related genes as well as epigenetic amplification of cellular survival and renewal mechanisms than depend on driver mutations. Although migration occurs at an early stage of tumor progression, metastasis appears to take longer, due to the fact that not every migrated cell can initiate a tumor in distant locations and form a metastatic colony. Many conditions and mechanisms are required in both the migrated cells and the novel home tissues for this to occur, for example migrated cells must overcome many obstacles including lethal signals from reactive stroma that can downregulate the anti-apoptotic pathways in CSCs during the journey to the new environment [ 35 ]. CSCs must also survive the lack of growth factors and other environmental factors that are used to support their stemness and regulate their proliferation in the original niche [ 12 , 36 , 37 ]. Finally, after reaching the new site, the survivor CSCs need to induce specific niche factors to modulate the new niche according to their requirements.
It has also been found that one of the important stromal factors is POSTN (periostin) that normally plays a role in extracellular matrix formation and the development and function of teeth, bones, and heart tissues. In primary lesions, fibroblasts are responsible for POSTN expression; therefore, migrated tumor cells have to induce this factor in secondary lesions in order to successfully infiltrate into the new organs. POSTN is therefore a potential target for anti-metastasis therapies as it is considered to play a role in cancer stem cell maintenance. However, its deficiency only affects metastasis of tumors, but not normal cells nor primary lesions. Therefore, combination therapies may be necessary, but targeting POSTN alone may still prevent metastasis [ 38 ].
Following invasion of a new site, CSCs form a new niche to ensure their survival in the new environment where they face an aggressive immune response and a lack of survival signals and supportive stroma. Although most organs and tissues fend off CSC metastasis, some of these target tissues may be more susceptible than others. This creates an opportunity for CSCs to modulate the surrounding environment and build the metastatic niche (a novel niche in the new site that contains CSCs, niche components produced by CSCs themselves, and components produced by surrounding stromal cells) [ 12 , 35 ].
Epithelial to mesenchymal transition
During the development of tissues, some epithelial cells exhibit changes in their characteristics (such as a loss of adhesion contact with other cells and cellular polarity) and transform into a mesenchymal type with improved ability to relocate into different tissues. This phenomenon is termed the epithelial to mesenchymal transition (EMT) [ 1 ]. In cancer niches, transcription factors (e.g., Snail, Twist, Six1, Slug, Cripto, Zeb 1–2, E12-46, and others) can affect the connection of cells by activating EMT and downregulating important molecules such as E-cadherin and catenins. These molecules play an essential role in cellular adherens junctions (AJs) [ 9 ]. EMT is important during the development of organisms due to its impact on tissue morphogenesis; however, it can become a cause of concern in cancer tissues due to the risk of metastasis. In addition, cells having undergone EMT are associated with stronger invasiveness and resistance against apoptosis. They usually acquire features similar to CSCs and recent studies to suggest that the CSC itself may be a result of EMT (since all signals and conditions needed to initiate EMT can come from tumor microenvironment) [ 15 ]. It has also been reported that the de-differentiation of cancer cells into CSCs occurs after those cells have gone through EMT and migrate to other locations, thus indicating the important role of EMT in this process [ 39 ]. The interactions within the niche between microenvironment cells and components can also initiate EMT, with participation of cytokines and growth factors. Such interactions were also found to play a role in maintaining stemness of CSCs in several cancers such as breast and oral squamous cancers [ 15 ] (Fig. 3 ).
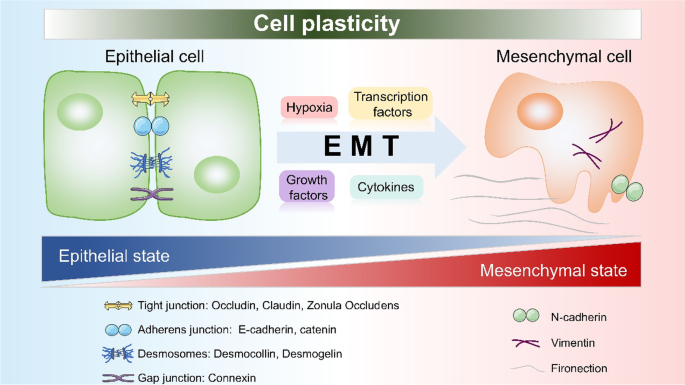
EMT and CSC niche. The crucial events in EMT are the dissolution of the epithelial cell–cell junctions, which include tight junction, adherens junction, desmosomes and gap junction. In CSC niche, those junctions start to break down via the induction of hypoxia, transcription factors, growth factors and cytokines. Then, the cells switch to a spindle-shaped mesenchymal morphology, as well as express markers (N-cadherin, vimentin and fibronectin) which would help to maintain the mesenchymal cell state
Additional studies found that EMT indeed strongly correlates with hypoxia, with HIF-1α activating several EMT-related transcription factors such as Snail and Twist. Hypoxia can also elevate the CSC self-renewal genes expression levels and activate related signaling pathways such as Wnt and Notch [ 40 , 41 ]. By activating EMT, hypoxic niches begin a chain of steps that eventually lead to metastasis, which also includes extracellular matrix modulation, intravasation, circulation, extravasation, homing, pre-metastatic niche formation, and mesenchymal epithelial transition (MET) [ 20 ].
Molecular mechanisms in the CSC niche
Essential molecules in the niche.
The homeostasis of the CSC niche is based on the interaction between different cells and components of the microenvironment. In order to maintain this homeostasis, many complicated mechanisms and molecules are required. Some of these molecules have been mentioned already in this review. In this section, we discuss other important molecules in the CSC niche modulation such as chemokines, cytokines and adhesion molecules.
Chemokines are common components in niche interactions, with one of the important chemokines discovered being SDF-1a and its receptor CXCR4. Studies in HSC found CXCR4 as essential for the retention, maintenance, and homing of tumor cells. The receptor is also important for the regulation of the CSCs’ renewal capacity. Mesenchymal stem cells (MSCs) are considered as a major source of the SDF-1 production whose expression is linked to metastasis in solid tumors (e.g., breast, liver, and lung cancers). Therefore, this chemokine has been suggested to play a contributing role in the changes that migrated CSCs apply on the surrounding environment during the initiation of metastasis colonization [ 1 , 6 , 15 ]. Another important component is reactive oxygen species (ROS) which usually affects the hypoxic conditions of the cancer niche in a dynamic manner. This can lead to DNA damage and changes in protein expression which are required in DNA repair [ 20 ]. Altering ROS levels in the cell also affects cellular stress responses which may favor tumor cells over normal ones. By increasing the levels of several factors (e.g., HIF-1α, HGF, EGF, TGF-β, TNF-α, TPA, MMP-3, and even micro-RNA), ROS can enhance and help the EMT and promote the metastasis of tumor cells [ 42 ].
Another family of molecules required for CSC metastasis is the cytokine family. CSCs, together with other tumor cells, can secrete different cytokines in the niche. Such cytokines and chemokines can further recruit several types of immune cells to create immunosuppression (e.g., tumor-associated neutrophils (TAN), tumor-associated macrophages (TAM), and myeloid-derived suppressor cells (MDSC)). Other cells involved in the niche region and are affected by CSCs include cancer-associated fibroblasts (CAFs), which are known to increase proliferation, enhance ECM production and secret essential factors such as CXCL12, VEGF, PDGF, and HGF [ 12 ].
A crucial ability of stem cell niches is the self-renewal of cells and cell–cell adhesion. Such adhesion relies on several molecular mechanisms and factors expressed in both the niche cells and stem cells. E-cadherin is a well-known example of such a cell adhesion molecule. In general, cadherins can modulate cell adhesion by interacting with intra- and extracellular domains, linking cells to cytoskeleton-associated proteins and nearby cells [ 43 ]. In doing so, cadherins can form strong AJs between cells. Cadherins have been found in stem cells in several types of tissues including brain stem cells and HSCs. Some cadherins can even regulate gene expression and signaling pathways by interacting with certain proteins and receptors. For example, N-cadherin can regulate the signaling by interacting with FGF receptors and also affect HSCs into quiescence by a similar mechanism.
In addition to cadherins, stem cells can also express integrins which are transmembrane molecules that modulate ECM interactions. Integrins can bind to ECM through certain proteins (e.g., collagen and laminin) and bind to surface adhesion molecules (e.g., CD54) and vascular cell adhesion molecules (e.g., CD106). Due to their ability to modulate cell adhesions, both cadherins and integrins are essential for spindle orientation of certain tissues (e.g., skin and brain) where the spindle of stem cells determines the type of division (symmetric or asymmetric division). It has been shown that the removal of such molecules can easily translate into randomized orientations. Other cellular mechanisms such as cell polarity, maintenance and signaling pathways can also be affected by adhesion molecules; therefore, it is difficult to draw a clear link between cellular adhesion and other cellular events. Other molecules that play a role in stem cells adhesion include Connexin 43 (a junction protein that support HSCs in stroma and affect CXCL12 secretion) and Vcam 1 [ 14 ].
Cancer-induced inflammation is a hallmark in solid tumor progression and considered to be essential in modifying their microenvironment. Although chemokines and cytokines, tumor surrounding cells (e.g., endothelial, mesenchymal, pericytes and fibroblast cells) can communicate directly with immune cells such as macrophages, neutrophiles, NK cells, T and B lymphocytes and other immune cells. Such interactions and the level of activity of each type of cell determine the resulting immune responses (pro or anti-tumor). For example, difference with tumor-induced inflammation is that it takes the profile of chronic inflammatory responses and uses cytokines and chemokines not only to communicate but also to recruit immune elements in order to resemble an unhealed wound. Consequently, the niche would remain in tumor favoring conditions such as hypoxia and EMT to enhance the survival and functioning of cells [ 9 ].
A complicated network of molecules is therefore responsible for the inflammatory response in the niche, and some of these are also involved in other pathways and functions. IL-6, IL-8, TGF-β, NFκ and TNF-α are some of the elements shown to play a role in the tumor-induced inflammatory environment. IL-6 is an important cytokine linked to proliferation, differentiation, and maturity of cells, making this molecule a promising target for many anti-tumor therapies. In CSCs, IL-6 has been found to play a role in Notch-3 regulation that leads to the malignant behavior of cells in human ductal breast carcinoma. The role of IL-6 in regulating CSCs was also studied in other types of human cancer (e.g., glioblastoma), where targeting it led to cellular apoptosis and reduction in tumor growth. NFκB pathway has also been found to be activated by inflammatory cytokines in the CSC niche. This pathway plays a role in modulating EMT factors Twist, Snail, and Slug. Therefore, NFκB is important for CSC migration and invasion in human cancers (e.g., pancreas, skin, and ovarian cancer) [ 1 ].
Current CSC-associated studies focus on their molecular features and differences and discovered novel markers including CD44, CD133, and CD24 [ 28 , 29 , 44 ]. However, markers specificity is still a challenge, since many are shared with normal stem cells and progenitors [ 3 ]. Several markers have already been linked to certain types of CSCs (breast cancer: CD44 + /CD24 − /ALDH + ; leukemia: CD34 + /CD8 − ; liver: CD90 + ; pancreas: CD44 + /CD24 + /ESA + ; epidermal surface antigen) [ 11 ]. Therefore, more efforts are required to identify CSC markers as there are contradicting reports regarding the exact role of markers in CSCs [ 6 ].
Genetics and epigenetic modulations in the CSC niche
The genetic diversity and heterogeneity of cancers has led to exploration of their genetic background and importance in CSC niches. Differences in genetic features or mutations among CSC clones can determine their phenotype (such as the level of activeness or dormancy, which can directly affect the survival of CSCs against chemotherapies) [ 45 ]. With the bidirectional relation between CSCs and their niches, genetic alterations on both sides can affect or determine the fate of CSCs or their link to the niche. This shows the advantage of the slow cycling of CSCs which helps avoid genetic mutations (which might not fit with the niche requirements) [ 3 , 46 ]. So far, two models prevail in explaining the intratumor heterogeneity, namely the hierarchical model which proposes that CSCs are originally organized in their niches in a cellular hierarchy similar to normal niches and the clonal evolution model which suggests that different somatic mutations and epigenetic alterations are acquired by different sub-clones of CSCs during tumor evolution, leading to molecular and biological differences among clones [ 8 ]. CSC niche conditions also influence the DNA profile and stability; for example, severe hypoxia leads to DNA replication arrests, which introduces different biological actions within the cells and adds to the intratumor heterogeneity [ 47 , 48 ]. As mentioned earlier, several transcriptional factors and signaling pathways can also affect the genetic profile of CSCs [ 49 ].
DNA methylation is a type of common epigenetic modification, and evidence is accumulating, suggesting that DNA methylation is a critical epigenetic reprogramming mechanism that may play a crucial role in CSCs biology [ 50 ]. BEX1, under the regulation of DNA methyltransferases 1 (DNMT1), was shown to have differential expression levels in Hepatoblastoma, CSC-hepatocellular carcinoma (HCC), and non-CSC HCC patients. This is essential for the self-renewal and maintenance of liver CSCs through activation of Wnt/β-catenin signaling [ 51 ]. Huang W et al. performed whole-genome bisulfite sequencing on tumor-repopulating cells (TRC), which are cancer stem cell (CSC)-like cells with highly tumorigenic and self-renewing abilities, their results showed that CSCs markers were biased toward altering their methylation in non-CG methylation and enriched in the gene body region, indicating non-CG DNA methylation plays a vital role in TRC selection [ 52 ].
Ubiquitination is also an important post-translational modification for CSCs self-renewal, maintenance, differentiation and tumorigenesis [ 53 ]. A recent study showed that targeting MYH9 could block HBX-induced GSK3β ubiquitination to activate the β-catenin destruction complex and then further suppress cancer stemness and EMT in hepatocellular carcinoma [ 54 ].
Besides, miRNAs and lncRNAs are also important modulating elements in the renewal and differentiation of CSCs. It has been shown that the loss of some miRNAs or lncRNAs can lead to the failure of the CSCs’ functioning (including the ability of CSCs to regulate stem cell markers, transcriptional factors, and binding proteins). Such changes affect the stemness and, therefore, the identity of CSCs. Interference with miRNAs or lncRNAs affects the role of cytokines and chemokines in CSCs, risks the whole balance of the CSC niche and influences the fate of those cells [ 11 , 55 , 56 , 57 , 58 ].
Signaling pathways regulating the CSC niche
The CSC niche contains many components that could maintain CSCs in a quiescence state and regulate the cell plasticity and quiescence by induction of several signaling pathways. Such signaling pathways in the CSC niche are the map or the network of all the interactions among cells and components in the niche. The importance of signaling pathways covers most characteristics of CSCs (e.g., self-renewal, proliferation, differentiation, metastasis, cell cycling, angiogenesis, and tumorigenesis) [ 15 , 59 ]. It has already been shown that several pathways in various types of niches such as Wnt, TGFβ, BMP, JAK-STAT, PI3K, and cell cycle pathways [ 60 ]. Signaling pathways in the CSC niche depend on the activation of other niche components (such as growth factors). In addition, many of these pathways act in the short term, thus requiring the CSCs to stay in the niche in order to benefit from the signaling [ 14 ]. Here, we highlight the common pathways often observed in the CSC niche (Fig. 4 ).
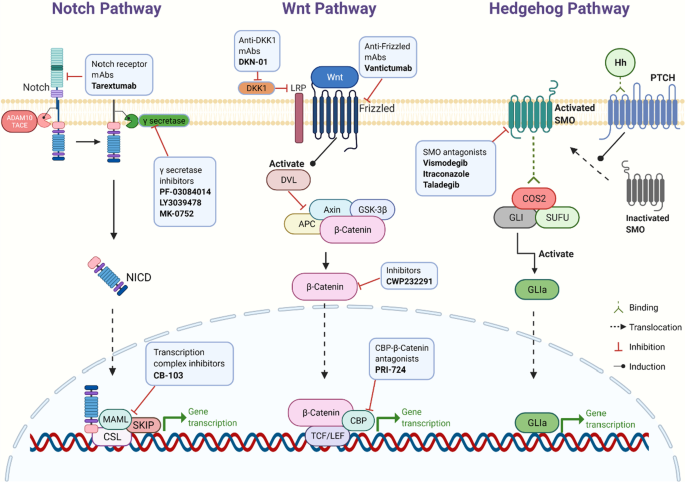
Key signaling pathways and related targeted inhibitors in the CSC niche. Notch pathway: After twice cleavages by ADAM10/TACE and γ-secretase, respectively, Notch intracellular domain (NICD) is finally released. NICD could translocate into the nucleus and interact with the transcription complex, promoting Notch target gene expression. Wnt pathway: When Wnt proteins bind to the Frizzled family receptors, dishevelled protein (DVL) is activated. DVL would inactivate the multiprotein destruction complex (including APC, Axin, and GSK-3β), which could lead β-catenin to degradation. With the DVL activation, β-catenin starts to accumulate in the cytoplasm. Part of β-catenin enters the nucleus to interact with TCF/LEF and CBP, promoting the expression of target genes. Hedgehog pathway: Binding PTCH with Hh would translocate smoothened (SMO) to the cell membrane and activate it. The activated SMO could easily bind to the COS2/GLI/SUFU complex, then GLI releases from the complex, referred to as GLIa. GLIa activates the transcription of downstream target genes
The Notch pathway is a well-known example in the interactions within the CSC niche. In this pathway, Notch binds to certain ligands that eventually translocate to the nucleus to activate cycling inhibitors (e.g., p21) [ 9 ]. Although the Notch pathway is responsible for the development of normal cells and maintenance of cellular proliferation and apoptosis, it is altered in several cancers (e.g., HCC) and can activate tumor initiation and progression. It has been shown that the Notch pathway is regulated by several genes including the tumor suppressor gene RUNX3, which explains the relation between Notch and tumor growth [ 59 ]. The Notch pathway is involved in the adhesion of CSCs in the niche, making it difficult to draw a clear line between the role of Notch in signaling and adhesion, especially in niches such as those in skin tissues [ 61 , 62 ]. All these functions of the Notch pathway make it a promising target of anti-tumor therapeutic approaches, regardless of its non-specific CSC nature. For example, blocking Notch-4 in breast cancer affected the ability of new cells formation [ 15 ].
The Wnt is another pathway and is similar to Notch as it is involved in the development and a functioning regulation of normal stem cells and therefore strongly correlates with CSCs. The hyper activation of Wnt has been shown to initiate cancer in the pituitary gland in animal models and increases the CSCs’ symmetrical division [ 63 , 64 , 65 ]. In HCC in particular, the Wnt/β-catenin pathway activation increases CSC activity and cells proliferation. Such an activation is controlled by PTEN, which is a tumor suppressor gene. Studies have shown that controlling or inhibiting the Wnt/β-catenin pathway can elevate the efficiency of drugs and other therapeutic approaches [ 66 , 67 , 68 ]. This pathway is also a potential target for future molecular therapies, since it depends on the concentration of β-catenin within the cytoplasm [ 69 ]. Together with Notch, Wnt is also involved in stemness stimulation in CSCs, which is essential for the identity of these cells [ 37 , 70 ].
The Hedgehog (Hh) pathway also plays a pivotal role in the CSC niche. In addition to its involvement in the development of cancer, it plays a role in determining the invasiveness and histological differentiation in tumors. Studies found that the simultaneous activation of the Hh pathway and EMT may be a reason for the chemoresistance that HCC cells usually exhibit [ 59 ]. Many drugs have been found to target Hh pathway (e.g., vismodegib, itraconazole, cyclopamine, and PTCN antibodies) to regulate CSC functions and inhibit tumor growth [ 11 , 15 ].
Another CSC controlling pathway is TGF-β. A recent study showed that tumor-initiating cells play a crucial role in creating a CSC niche microenvironment, which is required for cancer progression and therapy resistance [ 37 ]. Tumor cells release the interleukin-33 cytokine, which promotes the differentiation of the myeloid cells into macrophages. In turn, these macrophages further send TGF-β signals to CSCs, promoting their malignant progression and therapy resistance [ 71 ]. Other important pathways include TF pathway [ 72 ], Hippo pathway [ 73 ], and cell cycle pathways [ 74 ].
The CSC niche in different cancers
CSC niches have already been identified in several types of human cancers including brain, liver, breast, lung, head and neck, prostate, melanoma, gastric, pancreatic, renal, ovarian, esophageal and colorectal cancers [ 75 , 76 , 77 , 78 , 79 , 80 , 81 , 82 , 83 , 84 , 85 , 86 , 87 ]. Various studies have focused on the similarities and differences among the niches in different cancers and how cancer specific microenvironments can affect the formation and the development of CSC niches [ 4 , 6 , 88 , 89 , 90 ]. Here, we review some of the important examples of CSC niches in major cancers in order to further understand the interaction between tumors and CSC niches, and how the previously mentioned molecules and mechanisms participate in different tumors.
In general, the complexity of hepatic cancers posed a challenge for researchers since different tissues showed different sets of CSC markers and expression patterns [ 91 , 92 ]. To initiate a tumor in the liver, a CSC needs to survive the journey to the liver through the hepatic microvesiculation where it faces several intravascular death risks. CSCs therefore have to inhibit the liver immune responses to successfully complete clinical metastasis. In order to succeed, CSCs require several mechanisms and changes in the liver microenvironment, such as the inhibition of proteases by TIMP-1 which enables the formation of pre-metastatic niches and the expansion of metastasis. Hypoxia and HIF-1 can also activate HGF and its receptor Met which play a role in the development of liver metastasis. It has also been found that Kupffer cells and neutrophils in the niche increase the production of TNF-α, IL-1, and MMP-9 [ 68 ]. EGFR, VEGF, PDGF, TNF, SDF1, and other angiogenic factors enhance the growth and survival of hepatic cancer stem cells (HCSCs) and their resistance to radio- and chemotherapies [ 93 , 94 , 95 , 96 ].
So far, several HCSCs markers have been proposed (e.g., CD133, CD90, CD24, CD13, EpCAM, ALDH, and OV6) and current research efforts aim to link different combinations of these markers in HCSCs to the differences in phenotypes among CSC populations. For example, CD90 + cells have been found to upregulate genes related to inflammation and drug resistance while CD133 + cells have Akt/PKB pathway activation which translate into suppression of apoptosis and resistance to radiotherapy [ 59 ].
Studies showed that post surgery, any remaining HCSCs may be re-activated and even invade the vascular system leading to recurrence and metastasis of the primary tumor [ 97 ]. As for chemotherapy, HCSCs are more resistant to anti-tumor drugs including doxorubicin and 5-flouracil (5-FU). Such drugs can also alter the expression of certain genes (e.g., ABCG2) which increases the proliferation rate of HCSCs. Many agents are being developed to target certain molecules and markers of HCSCs, including AKT1 inhibitors, CD44 neutralizing antibodies, exogenous BMP4, pimozide, cylopamine, and other agents. Markers including CD133 and EpCAM also show great potential in the creation of specific targeted therapies as well as novel strategies targeting the crosstalk between the stroma and the tumor [ 59 ].
Gastric cancer is another type of malignancy with increasing incidence and mortality. Gastric cancer stem cells (GCSCs) have already been identified in different cell lines with markers including CD44, CD54, CD24, and CD71. Interestingly, many reports indicate the interaction between Helicobacter Pylori (HP) infection and GCSCs, such infection leads to modulation of stem cells into GCSCs in animal models [ 88 , 98 ]. The chronic inflammation caused by such an infection is also helpful for the GCSC niche. Since treatments such as surgery, chemotherapy, radiation, and immunotherapy are often challenged with the problem of tumor relapse, GCSCs have been proposed as potential targets for drugs that inhibit their stemness and functions. For example, Trastuzumab targets CD90 + GCSC and decreases the growth of gastric cancer tissues (when used with chemotherapy) [ 99 ]; Salinomycin targets ALDH + GCSC (a cell population which is resistant to chemotherapy drugs such as 5-FU and CDDP) [ 100 ].
In the case of breast cancer, it has been found that different populations of breast CSCs (BCSCs) can generate different lines of tumor cells. Yet, the genotype of the new cells may not completely resemble the original CSC genetic profile, which may indicate a number of mutations along the way [ 8 ]. It has been shown that BRCA1 expression (well-known tumor suppressor gene in breast cancer) is decreased by transcription factors such as Slug, while at the same time Slug stability is reduced by BRCA1 gene expression. This negative feedback-based balance is a key element in maintaining a normal growth of tumor and determining the level of stemness of niche cells [ 101 , 102 ]. Pluripotency factors including SOX are also over expressed in BCSC niches and other than their role in EMT and metastasis, the SOX family upregulates the expression of EZH2 which is important for histone methylation for several genes and can activate Raf1-β-catenin pathway [ 103 , 104 ]. With the identification of drugs that can specifically target BCSCs, essential pathways including Wnt can be inhibited. BCSCs resistance to radiotherapy can also be solved by advanced approaches including gold Nano-shell-mediated hyperthermia therapy [ 105 ].
In hematopoietic malignancies, HCSCs (also known as leukemia stem cells, LSCs) have been identified in acute myeloid leukemia (AML) in the 1990s, with specific markers such as CD34 + /CD38 − [ 45 , 106 ]. Although the interaction and communication mechanism between LSCs and their niche is not as clear as in solid tumors, targeting the niche may still be useful as it can affect the mobility of LSCs before escaping the original niche. This theory is supported by the expression of certain chemokines and receptors in the niche such as CXCR4 and CXCL12 [ 107 ]. In order to prevent relapse of primary cancer, targeting the niche might be of major therapeutical importance, as it provides a sanctuary for LSCs against conventional therapies [ 108 ].
The chemotherapeutic drugs cytarabine and daunorubicin have been the standard choices for many years, despite the risks of relapse or therapeutic resistance. As a result, there is an urgent need for novel therapies addressing the potential role of LSCs. In animal models, G-CSF treatment has shown promising results as it stimulates dormant LSCs and activated their cell cycle, leading to increased sensitivity to chemotherapy. Such treatments still require more development, but they certainly create new hope in this field [ 107 ].
In other examples of cancer, malignant bone tumors usually include osteosarcoma, multiple myeloma, and solid tumor metastasis. Bone metastasis pose a significant challenge as it is in general incurable and is associated with resistance to chemotherapy. Other than migrated tumor cells, the bones are also the source of HSCs. It has been shown that these two different populations of cells compete for the same niches in the bones, suggesting that stem cell niches may hold a great potential in the future as a target of treating metastatic tumors [ 109 ]. Although tumor stem cells can alter the microenvironment of the bone and the existing hematopoietic niches, the niche can still distinguish a normal stem cell from a CSC. Consequently, modulating the niche in favor of normal SC compared to CSC can prevent the invasion of migrated cells [ 110 ].
Therapeutic strategies targeting the CSC niche
Therapy resistance and tumor relapse are two major obstacles in the clinical management of solid cancers [ 111 ]. There is evidence that many residual tumors are enriched with CSCs after therapy [ 69 , 112 ]. Thus far, this phenomenon has been explained by the theory that even after a radical resection of tumor lesions ( R 0) by surgery and applying chemotherapy and radiotherapy, some CSCs still manage to escape and survive due to their therapy resistance [ 11 , 113 ]. CSCs are usually quiescent and slow cycling and have an active anti-apoptotic machinery [ 114 ], efficient DNA repairing systems (DNA checkpoint kinases) and stabilized stemness features, all of which may contribute to their resistance [ 115 ]. To date, many chemoresistance mechanisms have been found across distinct cancer types such as hypoxia, EMT and the signaling pathways which regulate CSC function, for instance Notch, Wnt and Hedgehog signaling. The recent clinical trials targeting CSCs or CSC-associated pathways are shown in Table 1 .
As described before, EMT is closely associated with the biological features of the CSC, including stemness, immune escape and resistance to chemotherapy [ 116 ]. Recent studies have shown that EMT plays a major role in therapy resistance [ 117 , 118 , 119 ]. Diverse factors and cytokines are involved in regulation of EMT, complicating targeted therapy approaches. For instance, responses to chemotherapy in a large group of breast cancer patients revealed a close association between chemoresistance and increased stromal gene expression, and this transcriptional upregulation appeared to be caused by activation of the EMT, which suggested anti-stromal agents may offer a new strategy to overcome therapy resistance [ 120 ]. A Phase I/II clinic trial showed that Plerixafor, as an anti-stromal agent which is an important inhibitor of CRCX4, combined with bortezomib restored the chemosensitivity in relapsed multiple myeloma, which suggested that the novel therapy may target the tumor microenvironment and overcome resistance to therapy [ 121 ]. In addition, Kari et al. established an EMT lineage-tracking system in breast-to-lung metastasis mice models, using a mesenchymal-specific Cre-mediated fluorescent marker switch system. They found out that EMT cells significantly contribute to recurrent lung metastasis formation after chemotherapy [ 122 ]. According to these capacities of the EMT, creating more effective treatment strategies to directly target the EMT program is promising and urgently needed.
Moreover, TGFβ signaling is one of the characterized pathways involved in EMT induction, as enhanced TGFβ signaling in cancer accelerates EMT program and maintains a highly proliferative phenotype [ 123 ]. Accumulating research studies have demonstrated that TGFβ-mediated EMT may initiate and facilitate therapy resistance, which is inhibited by blockade of TGFβ [ 124 , 125 , 126 ]. Fresolimumab, a TGFβ blocking antibody, showed an effective clinical application which was demonstrated in many clinical trials [ 127 , 128 , 129 ]. Furthermore, two doses of fresolimumab were explored in metastatic breast cancer in a prospective randomized trial, patients receiving the higher fresolimumab dose had a better systemic immune response and longer median overall survival compared to the lower dose group [ 130 ]. Therefore, TGFβ blockade might be a feasible and promising strategy for a CSC targeted therapy.
Additionally, another potential therapeutic target for preventing the induction of EMT is hepatocyte growth factor (HGF)-HGR receptor (HGFR, MET) signaling, which contributes to cancer pathogenesis, as exemplified by its frequent activation, often by point mutation or amplification of the MET, in many cancer types [ 131 ]. Therefore, targeting HGR or HGR-MET signaling is a promising therapeutic method which may also regulate CSCs. Recently, many studies show that anti-MET antibodies prevent HGF binding to MET and, subsequently, inhibit cancer progression and restore the chemoresistance, as well as improve the therapeutic efficiency [ 132 , 133 , 134 ].
In addition, hypoxia contributes to therapy resistance via a number of mechanisms, including maintaining CSC stemness, function of HIFs, drug transporters and affecting EMT [ 135 ]. Targeting these key factors is considered as a promising strategy for anti-CSC therapy. Acriflavine, a potent inhibitor of HIF-1α dimerization, could disturb glucose metabolism in melanoma regardless of the hypoxic condition [ 136 ]. Chetomin, another inhibitor of HIF-1α, could suppress the transcriptional activity via targeting p300 recruitment [ 137 ]. Furthermore, other agents, such as wortmannin, temsirolimus and camptothecin, have been proved to inhibit HIF-1α protein translation by targeting corresponding genes [ 138 ]. In addition, hypoxia can also mediate chemoresistance of CSCs by drug efflux through drug transporters [ATP-binding cassette (ABC) family membrane transporters]. ABCB1 and ABCG2 (BCRP), as the well-known ABC family transporters, have been reported to be induced in hypoxia [ 139 , 140 ]. A recent study showed that vatalanib, a multitargeted tyrosine kinase inhibitor for all isoforms of VEGFR, PDGFR and c-Kit, makes ABCB1 and ABCG2-overexpressing multidrug-resistant colon cancer cells sensitive to chemotherapy in hypoxic microenvironment. Utilizing the combination of vatalanib with conventional anti-tumor drugs might be a promising tool to restore the chemosensitivity in colon cancer treatment [ 141 ]. Similar mechanism is found in ovarian cancer stem cells: Hypoxia-induced HIF-2α overexpression endows ovarian cancer stem cells with resistance to adriamycin by promoting BCRP expression and adriamycin efflux [ 139 ].
We have described the Notch, Wnt and Hedgehog signaling pathways in CSC regulations before, which are widely explored in current CSC target therapies (Fig. 4 ). γ-secretase inhibitor (GSI), as a major clinical method used to inhibit Notch signaling, has been proved in many clinical trials. Combining trastuzumab plus a GSI has been reported to reduce recurrence rate for ErbB-2-positive breast tumors [ 142 ]. Another clinical trial shows that pharmacologic inhibition of the Notch pathway can reduce CSCs in breast cancer models [ 143 ]. A phase I study shows that ipafricept (OMP-54F28, a Decoy Receptor for Wnt Ligands) can be safely administered with manageable toxicities [ 144 ]. In addition, HH ligands, SMO and GLI transcription factors are three main targets of Hh pathway antagonists [ 60 ], whose effectiveness in basal cell carcinoma and prostate cancer was already shown in many trials [ 145 , 146 , 147 ].
In the past few years, CSC-directed immunotherapy has emerged. Besides the antibodies targeting CSC-related signaling pathways mentioned above, novel anti-CSC immunotherapeutic approaches, such as cancer vaccines, checkpoint inhibitors and chimeric antigen receptor T-cell (CAR-T cell) therapies, have been developed [ 148 , 149 ]. One personalized neoantigen dendritic cell vaccine was chosen to treat metastatic lung cancer (NCT02956551). In this trial, the disease control rate was 75% and the median overall survival was 7.9 months; the combination therapy of immune checkpoint inhibitors and the vaccines had a better progression free survival (2.2 vs 12.2 months) and overall survival (7.6 vs 11.2 months) trend; this study provided new evidence for cancer vaccine therapy and promising therapeutic opportunities for lung cancer treatment [ 150 ]. In addition, as a novel therapeutic strategy, CAR-T cell therapy is widely used in many types of cancer, especially against hematologic malignancies. CD19/CD22/CD33, been detected in leukemia patients, is a noteworthy target for immune-cellular therapy against AML [ 151 , 152 , 153 , 154 ]. The ongoing clinical trials of CAR-T cell therapy are listed in Table 2 .
The current studies have confirmed that CSCs are considered as the root of cancer relapse, metastasis and therapy resistance, while further research is required for the development of more effective strategies to eradicate CSCs.
Recent studies have expanded our knowledge regarding CSCs and their niche. The novel markers and mechanisms in CSC niches as greatly improved our understanding of cancer and its microenvironment. It also changed the proposition that metastasis is a late-stage complication of cancer and explains the long-standing dilemma of why a tumor may relapse even after a complete surgical removal, chemotherapy or radiotherapy. Regardless to the improvements in this field, we still need more efforts to annotate CSC niches regarding molecular interactions and mechanisms controlling their stemness capacity and relationship with dormancy and chemo-immuno resistance. More challenges are ahead before we can move forward from basic to translational research and further clinical applications; nonetheless, such findings may bring a great benefit for millions of patients and guide future anti-cancer therapeutic strategies.
Availability of data and materials
Not applicable.
Abbreviations
ATP-binding cassette sub-family G member 2
A disintegrin and metalloproteinase domain-containing protein 10
Adherens junctions
RAC-alpha serine/threonine-protein kinase
Aldehyde dehydrogenate
Acute myeloid leukemia
Adenomatous polyposis coli
Adenosine triphosphate
Axis inhibition protein
B-cell acute lymphoblastic leukemia
Breast cancer stem cells
Basic helix loop helix Per Arnt Sim
Bone morphogenetic proteins
BReast-CAncer susceptibility gene 1
Cancer-associated fibroblasts
CREB-binding protein
Cluster of differentiation
- Cancer stem cells
Colony stimulating factor 1
C-X-C motif chemokine 12 also known as SDF1
C-X-C chemokine receptor type 4
Dishevelled protein
Extracellular matrix
Epidermal growth factor
Epithelial cell adhesion molecule
Endoplasmic reticulum
Epidermal surface antigen
Enhancer of zeste homolog 2
Fibroblast growth factor
5-Flouracil
Gastric cancer stem cells
Glioma-associated oncogene
Glucose transporter 1
Glycogen synthase kinase 3 beta
Hepatocellular carcinoma
Hepatic cancer stem cells
Hepatocyte growth factor
Hedgehog pathway
Hypoxia inducible transcription factor
Helicobacter pylori
Hematopoietic stem cells
Insulin-like growth factor-1
Interleukin-6
Janus kinases
Leukemia stem cells
Myeloid-derived suppressor cell
Mesenchymal epithelial transition
Hepatocyte growth factor receptor
Matrix metalloproteinase 3
Mesenchymal stem cell
Mammalian target of rapamycin
Nuclear factor Kappa B
Notch intracellular domain
Natural killer cell
Octamer-binding transcription factor 4
Platelet derived growth factor
Phosphoinositide 3-kinase
Protein patched homolog
Partner of SLD five 1
Phosphatase and tensin homolog
Reactive oxygen species
Runt-related transcription factor 3
Sal-like protein 4
Stromal cell-derived factor-1
Sineoculis homeobox homolog 1
Zinc finger protein SNAI1
Signal transducer and activator of transcription proteins
Tumor necrosis factor-α converting enzyme
Tumor-associated macrophages
Tumor-associated neutrophils
T cell factor/lymphoid enhancer factor
Tissue factor
Transforming growth factor-beta
Tissue inhibitor of metalloproteinases 1
Tumor necrosis factor alpha
Tissue polypeptide antigen
Vascular cell adhesion molecule 1
Vascular endothelial growth factor
Zinc finger E-box binding homeobox
Cabarcas SM, Mathews LA, Farrar WL. The cancer stem cell niche–there goes the neighborhood? Int J Cancer. 2011;129(10):2315–27.
Article CAS PubMed PubMed Central Google Scholar
Kordes C, Häussinger D. Hepatic stem cell niches. J Clin Invest. 2013;123(5):1874–80.
Sottocornola R, Lo CC. Dormancy in the stem cell niche. Stem Cell Res Ther. 2012;3(2):10.
Lacina L, Plzak J, Kodet O, Szabo P, Chovanec M, Dvorankova B, Smetana K Jr. Cancer microenvironment: what can we learn from the stem cell niche. Int J Mol Sci. 2015;16(10):24094–110.
Article PubMed PubMed Central Google Scholar
Stine RR, Matunis EL. Stem cell competition: finding balance in the niche. Trends Cell Biol. 2013;23(8):357–64.
Ribatti D. Cancer stem cells and tumor angiogenesis. Cancer Lett. 2012;321(1):13–7.
Article CAS PubMed Google Scholar
Takakura N. Formation and regulation of the cancer stem cell niche. Cancer Sci. 2012;103(7):1177–81.
Guo W. Concise review: breast cancer stem cells: regulatory networks, stem cell niches, and disease relevance. Stem Cells Transl Med. 2014;3(8):942–8.
Shigdar S, Li Y, Bhattacharya S, O’Connor M, Pu C, Lin J, Wang T, Xiang D, Kong L, Wei MQ, et al. Inflammation and cancer stem cells. Cancer Lett. 2014;345(2):271–8.
Singh SR. Cancer stem cells: recent developments and future prospects. Cancer Lett. 2013;338(1):1–2.
Yu Z, Pestell TG, Lisanti MP, Pestell RG. Cancer stem cells. Int J Biochem Cell Biol. 2012;44(12):2144–51.
Plaks V, Kong N, Werb Z. The cancer stem cell niche: how essential is the niche in regulating stemness of tumor cells? Cell Stem Cell. 2015;16(3):225–38.
Batlle E, Clevers H. Cancer stem cells revisited. Nat Med. 2017;23(10):1124–34.
Chen S, Lewallen M, Xie T. Adhesion in the stem cell niche: biological roles and regulation. Development. 2013;140(2):255–65.
Yi SY, Hao YB, Nan KJ, Fan TL. Cancer stem cells niche: a target for novel cancer therapeutics. Cancer Treat Rev. 2013;39(3):290–6.
Mohyeldin A, Garzón-Muvdi T, Quiñones-Hinojosa A. Oxygen in stem cell biology: a critical component of the stem cell niche. Cell Stem Cell. 2010;7(2):150–61.
Liu L, Michowski W, Kolodziejczyk A, Sicinski P. The cell cycle in stem cell proliferation, pluripotency and differentiation. Nat Cell Biol. 2019;21(9):1060–7.
Watt FM, Huck WT. Role of the extracellular matrix in regulating stem cell fate. Nat Rev Mol Cell Biol. 2013;14(8):467–73.
Janiszewska M, Primi MC, Izard T. Cell adhesion in cancer: beyond the migration of single cells. J Biol Chem. 2020;295(8):2495–505.
Peitzsch C, Perrin R, Hill RP, Dubrovska A, Kurth I. Hypoxia as a biomarker for radioresistant cancer stem cells. Int J Radiat Biol. 2014;90(8):636–52.
Jing X, Yang F, Shao C, Wei K, Xie M, Shen H, Shu Y. Role of hypoxia in cancer therapy by regulating the tumor microenvironment. Mol Cancer. 2019;18(1):157.
Alsaab HO, Sau S, Alzhrani RM, Cheriyan VT, Polin LA, Vaishampayan U, Rishi AK, Iyer AK. Tumor hypoxia directed multimodal nanotherapy for overcoming drug resistance in renal cell carcinoma and reprogramming macrophages. Biomaterials. 2018;183:280–94.
Rovida E, Peppicelli S, Bono S, Bianchini F, Tusa I, Cheloni G, Marzi I, Cipolleschi MG, Calorini L, Sbarba PD. The metabolically-modulated stem cell niche: a dynamic scenario regulating cancer cell phenotype and resistance to therapy. Cell Cycle. 2014;13(20):3169–75.
Choudhry H, Harris AL. Advances in hypoxia-inducible factor biology. Cell Metab. 2018;27(2):281–98.
Ai Z, Lu Y, Qiu S, Fan Z. Overcoming cisplatin resistance of ovarian cancer cells by targeting HIF-1-regulated cancer metabolism. Cancer Lett. 2016;373(1):36–44.
Haase VH. Regulation of erythropoiesis by hypoxia-inducible factors. Blood Rev. 2013;27(1):41–53.
Schito L, Semenza GL. Hypoxia-inducible factors: master regulators of cancer progression. Trends Cancer. 2016;2(12):758–70.
Article PubMed Google Scholar
Won C, Kim BH, Yi EH, Choi KJ, Kim EK, Jeong JM, Lee JH, Jang JJ, Yoon JH, Jeong WI, et al. Signal transducer and activator of transcription 3-mediated CD133 up-regulation contributes to promotion of hepatocellular carcinoma. Hepatology. 2015;62(4):1160–73.
Bai J, Chen WB, Zhang XY, Kang XN, Jin LJ, Zhang H, Wang ZY. HIF-2α regulates CD44 to promote cancer stem cell activation in triple-negative breast cancer via PI3K/AKT/mTOR signaling. World J Stem Cells. 2020;12(1):87–99.
Lah TT, Novak M, Breznik B. Brain malignancies: glioblastoma and brain metastases. Semin Cancer Biol. 2020;60:262–73.
de Sousa e Melo F, Kurtova AV, Harnoss JM, Kljavin N, Hoeck JD, Hung J, Anderson JE, Storm EE, Modrusan Z, Koeppen H, et al. A distinct role for Lgr5(+) stem cells in primary and metastatic colon cancer. Nature. 2017;543(7647):676–80.
Article PubMed CAS Google Scholar
Carvalho R, Paredes J, Ribeiro AS. Impact of breast cancer cells’ secretome on the brain metastatic niche remodeling. Semin Cancer Biol. 2020;60:294–301.
Marzagalli M, Raimondi M, Fontana F, Montagnani Marelli M, Moretti RM, Limonta P. Cellular and molecular biology of cancer stem cells in melanoma: possible therapeutic implications. Semin Cancer Biol. 2019;59:221–35.
Lytle NK, Ferguson LP, Rajbhandari N, Gilroy K, Fox RG, Deshpande A, Schürch CM, Hamilton M, Robertson N, Lin W, et al. A Multiscale map of the stem cell state in pancreatic adenocarcinoma. Cell. 2019;177(3):572-86.e22.
Oskarsson T, Batlle E, Massagué J. Metastatic stem cells: sources, niches, and vital pathways. Cell Stem Cell. 2014;14(3):306–21.
Barbato L, Bocchetti M, Di Biase A, Regad T. Cancer stem cells and targeting strategies. Cells. 2019;8(8):926.
Article CAS PubMed Central Google Scholar
Oshimori N. Cancer stem cells and their niche in the progression of squamous cell carcinoma. Cancer Sci. 2020;111(11):3985–92.
Malanchi I, Santamaria-Martínez A, Susanto E, Peng H, Lehr HA, Delaloye JF, Huelsken J. Interactions between cancer stem cells and their niche govern metastatic colonization. Nature. 2011;481(7379):85–9.
Mani SA, Guo W, Liao MJ, Eaton EN, Ayyanan A, Zhou AY, Brooks M, Reinhard F, Zhang CC, Shipitsin M, et al. The epithelial-mesenchymal transition generates cells with properties of stem cells. Cell. 2008;133(4):704–15.
Zhang Z, Han H, Rong Y, Zhu K, Zhu Z, Tang Z, Xiong C, Tao J. Hypoxia potentiates gemcitabine-induced stemness in pancreatic cancer cells through AKT/Notch1 signaling. J Exp Clin Cancer Res. 2018;37(1):291.
Article PubMed PubMed Central CAS Google Scholar
Jiang N, Zou C, Zhu Y, Luo Y, Chen L, Lei Y, Tang K, Sun Y, Zhang W, Li S, et al. HIF-1ɑ-regulated miR-1275 maintains stem cell-like phenotypes and promotes the progression of LUAD by simultaneously activating Wnt/β-catenin and Notch signaling. Theranostics. 2020;10(6):2553–70.
Wang Z, Li Y, Sarkar FH. Signaling mechanism(s) of reactive oxygen species in epithelial–mesenchymal transition reminiscent of cancer stem cells in tumor progression. Curr Stem Cell Res Ther. 2010;5(1):74–80.
Kaszak I, Witkowska-Piłaszewicz O, Niewiadomska Z, Dworecka-Kaszak B, Ngosa Toka F, Jurka P. Role of cadherins in cancer-a review. Int J Mol Sci. 2020;21(20):7624.
Ooki A, VandenBussche CJ, Kates M, Hahn NM, Matoso A, McConkey DJ, Bivalacqua TJ, Hoque MO. CD24 regulates cancer stem cell (CSC)-like traits and a panel of CSC-related molecules serves as a non-invasive urinary biomarker for the detection of bladder cancer. Br J Cancer. 2018;119(8):961–70.
Minami Y. Overview: cancer stem cell and tumor environment. Oncology. 2015;89(Suppl 1):22–4.
Scadden DT. Nice neighborhood: emerging concepts of the stem cell niche. Cell. 2014;157(1):41–50.
Bristow RG, Hill RP. Hypoxia and metabolism. Hypoxia, DNA repair and genetic instability. Nat Rev Cancer. 2008;8(3):180–92.
Pires IM, Bencokova Z, Milani M, Folkes LK, Li JL, Stratford MR, Harris AL, Hammond EM. Effects of acute versus chronic hypoxia on DNA damage responses and genomic instability. Cancer Res. 2010;70(3):925–35.
Ruiz-Vela A, Aguilar-Gallardo C, Simón C. Building a framework for embryonic microenvironments and cancer stem cells. Stem Cell Rev Rep. 2009;5(4):319–27.
French R, Pauklin S. Epigenetic regulation of cancer stem cell formation and maintenance. Int J Cancer. 2021;148(12):2884–97.
Wang Q, Liang N, Yang T, Li Y, Li J, Huang Q, Wu C, Sun L, Zhou X, Cheng X, et al. DNMT1-mediated methylation of BEX1 regulates stemness and tumorigenicity in liver cancer. J Hepatol. 2021;75(5):1142–53.
Huang W, Hu H, Zhang Q, Wang N, Yang X, Guo AY. Genome-wide DNA Methylation enhances stemness in the mechanical selection of tumor-repopulating cells. Front Bioeng Biotechnol. 2020;8:88.
Deng L, Meng T, Chen L, Wei W, Wang P. The role of ubiquitination in tumorigenesis and targeted drug discovery. Signal Transduct Target Ther. 2020;5(1):11.
Lin X, Li AM, Li YH, Luo RC, Zou YJ, Liu YY, Liu C, Xie YY, Zuo S, Liu Z, et al. Silencing MYH9 blocks HBx-induced GSK3β ubiquitination and degradation to inhibit tumor stemness in hepatocellular carcinoma. Signal Transduct Target Ther. 2020;5(1):13.
Yang Q, Zhao S, Shi Z, Cao L, Liu J, Pan T, Zhou D, Zhang J. Chemotherapy-elicited exosomal miR-378a-3p and miR-378d promote breast cancer stemness and chemoresistance via the activation of EZH2/STAT3 signaling. J Exp Clin Cancer Res. 2021;40(1):120.
McCabe EM, Rasmussen TP. lncRNA involvement in cancer stem cell function and epithelial-mesenchymal transitions. Semin Cancer Biol. 2021;75:38–48.
He Y, Jiang X, Duan L, Xiong Q, Yuan Y, Liu P, Jiang L, Shen Q, Zhao S, Yang C, et al. LncRNA PKMYT1AR promotes cancer stem cell maintenance in non-small cell lung cancer via activating Wnt signaling pathway. Mol Cancer. 2021;20(1):156.
Ni H, Qin H, Sun C, Liu Y, Ruan G, Guo Q, Xi T, Xing Y, Zheng L. MiR-375 reduces the stemness of gastric cancer cells through triggering ferroptosis. Stem Cell Res Ther. 2021;12(1):325.
Sukowati CH, Tiribelli C. The biological implication of cancer stem cells in hepatocellular carcinoma: a possible target for future therapy. Expert Rev Gastroenterol Hepatol. 2013;7(8):749–57.
Clara JA, Monge C, Yang Y, Takebe N. Targeting signalling pathways and the immune microenvironment of cancer stem cells: a clinical update. Nat Rev Clin Oncol. 2020;17(4):204–32.
Totaro A, Castellan M, Battilana G, Zanconato F, Azzolin L, Giulitti S, Cordenonsi M, Piccolo S. YAP/TAZ link cell mechanics to Notch signalling to control epidermal stem cell fate. Nat Commun. 2017;8:15206.
Totaro A, Castellan M, Di Biagio D, Piccolo S. Crosstalk between YAP/TAZ and notch signaling. Trends Cell Biol. 2018;28(7):560–73.
Kim JH, Park SY, Jun Y, Kim JY, Nam JS. Roles of wnt target genes in the journey of cancer stem cells. Int J Mol Sci. 2017;18(8):1604.
Article PubMed Central CAS Google Scholar
Gaston-Massuet C, Andoniadou CL, Signore M, Jayakody SA, Charolidi N, Kyeyune R, Vernay B, Jacques TS, Taketo MM, Le Tissier P, et al. Increased Wingless (Wnt) signaling in pituitary progenitor/stem cells gives rise to pituitary tumors in mice and humans. Proc Natl Acad Sci U S A. 2011;108(28):11482–7.
Le Grand F, Jones AE, Seale V, Scimè A, Rudnicki MA. Wnt7a activates the planar cell polarity pathway to drive the symmetric expansion of satellite stem cells. Cell Stem Cell. 2009;4(6):535–47.
Katoh M. Canonical and non-canonical WNT signaling in cancer stem cells and their niches: cellular heterogeneity, omics reprogramming, targeted therapy and tumor plasticity (Review). Int J Oncol. 2017;51(5):1357–69.
Eyre R, Alférez DG, Santiago-Gómez A, Spence K, McConnell JC, Hart C, Simões BM, Lefley D, Tulotta C, Storer J, et al. Microenvironmental IL1β promotes breast cancer metastatic colonisation in the bone via activation of Wnt signalling. Nat Commun. 2019;10(1):5016.
Yang L, Shi P, Zhao G, Xu J, Peng W, Zhang J, Zhang G, Wang X, Dong Z, Chen F, et al. Targeting cancer stem cell pathways for cancer therapy. Signal Transduct Target Ther. 2020;5(1):8.
Najafi M, Farhood B, Mortezaee K. Cancer stem cells (CSCs) in cancer progression and therapy. J Cell Physiol. 2019;234(6):8381–95.
Zhan T, Rindtorff N, Boutros M. Wnt signaling in cancer. Oncogene. 2017;36(11):1461–73.
Taniguchi S, Elhance A, Van Duzer A, Kumar S, Leitenberger JJ, Oshimori N. Tumor-initiating cells establish an IL-33-TGF-β niche signaling loop to promote cancer progression. Science. 2020;369(6501):eaay1813.
Markopoulos GS, Roupakia E, Marcu KB, Kolettas E. Epigenetic regulation of inflammatory cytokine-induced epithelial-to-mesenchymal cell transition and cancer stem cell generation. Cells. 2019;8(10):1143.
Zhao B, Li L, Guan KL. Hippo signaling at a glance. J Cell Sci. 2010;123(Pt 23):4001–6.
Talukdar S, Bhoopathi P, Emdad L, Das S, Sarkar D, Fisher PB. Dormancy and cancer stem cells: an enigma for cancer therapeutic targeting. Adv Cancer Res. 2019;141:43–84.
Gimple RC, Bhargava S, Dixit D, Rich JN. Glioblastoma stem cells: lessons from the tumor hierarchy in a lethal cancer. Genes Dev. 2019;33(11–12):591–609.
Li Y, Tang T, Lee HJ, Song K. Selective anti-cancer effects of plasma-activated medium and its high efficacy with cisplatin on hepatocellular carcinoma with cancer stem cell characteristics. Int J Mol Sci. 2021;22(8):3956.
Lin X, Chen W, Wei F, Xie X. TV-circRGPD6 Nanoparticle suppresses breast cancer stem cell-mediated metastasis via the miR-26b/YAF2 axis. Mol Ther. 2021;29(1):244–62.
Raniszewska A, Vroman H, Dumoulin D, Cornelissen R, Aerts J, Domagała-Kulawik J. PD-L1(+) lung cancer stem cells modify the metastatic lymph-node immunomicroenvironment in nsclc patients. Cancer Immunol Immunother. 2021;70(2):453–61.
Liu C, Billet S, Choudhury D, Cheng R, Haldar S, Fernandez A, Biondi S, Liu Z, Zhou H, Bhowmick NA. Bone marrow mesenchymal stem cells interact with head and neck squamous cell carcinoma cells to promote cancer progression and drug resistance. Neoplasia. 2021;23(1):118–28.
Hagiwara M, Yasumizu Y, Yamashita N, Rajabi H, Fushimi A, Long MD, Li W, Bhattacharya A, Ahmad R, Oya M, et al. MUC1-C activates the BAF (mSWI/SNF) complex in prostate cancer stem cells. Cancer Res. 2021;81(4):1111–22.
Hsu MY, Yang MH, Schnegg CI, Hwang S, Ryu B, Alani RM. Notch3 signaling-mediated melanoma-endothelial crosstalk regulates melanoma stem-like cell homeostasis and niche morphogenesis. Lab Invest. 2017;97(6):725–36.
Hayakawa Y, Ariyama H, Stancikova J, Sakitani K, Asfaha S, Renz BW, Dubeykovskaya ZA, Shibata W, Wang H, Westphalen CB, et al. Mist1 expressing gastric stem cells maintain the normal and neoplastic gastric epithelium and are supported by a perivascular stem cell niche. Cancer Cell. 2015;28(6):800–14.
Nimmakayala RK, Leon F, Rachagani S, Rauth S, Nallasamy P, Marimuthu S, Shailendra GK, Chhonker YS, Chugh S, Chirravuri R, et al. Metabolic programming of distinct cancer stem cells promotes metastasis of pancreatic ductal adenocarcinoma. Oncogene. 2021;40(1):215–31.
Fendler A, Bauer D, Busch J, Jung K, Wulf-Goldenberg A, Kunz S, Song K, Myszczyszyn A, Elezkurtaj S, Erguen B, et al. Inhibiting WNT and NOTCH in renal cancer stem cells and the implications for human patients. Nat Commun. 2020;11(1):929.
Jain S, Annett SL, Morgan MP, Robson T. The cancer stem cell niche in ovarian cancer and its impact on immune surveillance. Int J Mol Sci. 2021;22(8):4091.
Yuan Y, Wang L, Ge D, Tan L, Cao B, Fan H, Xue L. Exosomal O-GlcNAc transferase from esophageal carcinoma stem cell promotes cancer immunosuppression through up-regulation of PD-1 in CD8(+) T cells. Cancer Lett. 2021;500:98–106.
Sphyris N, Hodder MC, Sansom OJ. Subversion of niche-signalling pathways in colorectal cancer: what makes and breaks the intestinal stem cell. Cancers (Basel). 2021;13(5):1000.
Singh SR. Gastric cancer stem cells: a novel therapeutic target. Cancer Lett. 2013;338(1):110–9.
Zhou C, Fan N, Liu F, Fang N, Plum PS, Thieme R, Gockel I, Gromnitza S, Hillmer AM, Chon SH, et al. Linking cancer stem cell plasticity to therapeutic resistance-mechanism and novel therapeutic strategies in esophageal cancer. Cells. 2020;9(6):1481.
Pinho S, Frenette PS. Haematopoietic stem cell activity and interactions with the niche. Nat Rev Mol Cell Biol. 2019;20(5):303–20.
Colombo F, Baldan F, Mazzucchelli S, Martin-Padura I, Marighetti P, Cattaneo A, Foglieni B, Spreafico M, Guerneri S, Baccarin M, et al. Evidence of distinct tumour-propagating cell populations with different properties in primary human hepatocellular carcinoma. PLoS ONE. 2011;6(6):e21369.
Wilson GS, Hu Z, Duan W, Tian A, Wang XM, McLeod D, Lam V, George J, Qiao L. Efficacy of using cancer stem cell markers in isolating and characterizing liver cancer stem cells. Stem Cells Dev. 2013;22(19):2655–64.
Nakamura T, Sakai K, Nakamura T, Matsumoto K. Hepatocyte growth factor twenty years on: much more than a growth factor. J Gastroenterol Hepatol. 2011;26(Suppl 1):188–202.
Lau CK, Yang ZF, Ho DW, Ng MN, Yeoh GC, Poon RT, Fan ST. An Akt/hypoxia-inducible factor-1alpha/platelet-derived growth factor-BB autocrine loop mediates hypoxia-induced chemoresistance in liver cancer cells and tumorigenic hepatic progenitor cells. Clin Cancer Res. 2009;15(10):3462–71.
Wang X, Sun W, Shen W, Xia M, Chen C, Xiang D, Ning B, Cui X, Li H, Li X, et al. Long non-coding RNA DILC regulates liver cancer stem cells via IL-6/STAT3 axis. J Hepatol. 2016;64(6):1283–94.
Fangmann L, Teller S, Stupakov P, Friess H, Ceyhan GO, Demir IE. 3D cancer migration assay with schwann cells. Methods Mol Biol. 2018;1739:317–25.
Dong HH, Xiang S, Liang HF, Li CH, Zhang ZW, Chen XP. The niche of hepatic cancer stem cell and cancer recurrence. Med Hypotheses. 2013;80(5):666–8.
Houghton J, Stoicov C, Nomura S, Rogers AB, Carlson J, Li H, Cai X, Fox JG, Goldenring JR, Wang TC. Gastric cancer originating from bone marrow-derived cells. Science. 2004;306(5701):1568–71.
Jiang J, Zhang Y, Chuai S, Wang Z, Zheng D, Xu F, Zhang Y, Li C, Liang Y, Chen Z. Trastuzumab (herceptin) targets gastric cancer stem cells characterized by CD90 phenotype. Oncogene. 2012;31(6):671–82.
Zhi QM, Chen XH, Ji J, Zhang JN, Li JF, Cai Q, Liu BY, Gu QL, Zhu ZG, Yu YY. Salinomycin can effectively kill ALDH(high) stem-like cells on gastric cancer. Biomed Pharmacother. 2011;65(7):509–15.
Proia TA, Keller PJ, Gupta PB, Klebba I, Jones AD, Sedic M, Gilmore H, Tung N, Naber SP, Schnitt S, et al. Genetic predisposition directs breast cancer phenotype by dictating progenitor cell fate. Cell Stem Cell. 2011;8(2):149–63.
Wu ZQ, Li XY, Hu CY, Ford M, Kleer CG, Weiss SJ. Canonical Wnt signaling regulates slug activity and links epithelial-mesenchymal transition with epigenetic breast Cancer 1, early onset (BRCA1) repression. Proc Natl Acad Sci USA. 2012;109(41):16654–9.
Tiwari N, Tiwari VK, Waldmeier L, Balwierz PJ, Arnold P, Pachkov M, Meyer-Schaller N, Schübeler D, van Nimwegen E, Christofori G. Sox4 is a master regulator of epithelial-mesenchymal transition by controlling Ezh2 expression and epigenetic reprogramming. Cancer Cell. 2013;23(6):768–83.
Chang CJ, Yang JY, Xia W, Chen CT, Xie X, Chao CH, Woodward WA, Hsu JM, Hortobagyi GN, Hung MC. EZH2 promotes expansion of breast tumor initiating cells through activation of RAF1-β-catenin signaling. Cancer Cell. 2011;19(1):86–100.
Atkinson RL, Zhang M, Diagaradjane P, Peddibhotla S, Contreras A, Hilsenbeck SG, Woodward WA, Krishnan S, Chang JC, Rosen JM. Thermal enhancement with optically activated gold nanoshells sensitizes breast cancer stem cells to radiation therapy. Sci Transl Med. 2010;2(55):55ra79.
Lapidot T, Sirard C, Vormoor J, Murdoch B, Hoang T, Caceres-Cortes J, Minden M, Paterson B, Caligiuri MA, Dick JE. A cell initiating human acute myeloid leukaemia after transplantation into SCID mice. Nature. 1994;367(6464):645–8.
Park D, Sykes DB, Scadden DT. The hematopoietic stem cell niche. Front Biosci (Landmark Ed). 2012;17:30–9.
Article CAS Google Scholar
Krause DS, Scadden DT, Preffer FI. The hematopoietic stem cell niche–home for friend and foe? Cytom B Clin Cytom. 2013;84(1):7–20.
Colmone A, Amorim M, Pontier AL, Wang S, Jablonski E, Sipkins DA. Leukemic cells create bone marrow niches that disrupt the behavior of normal hematopoietic progenitor cells. Science. 2008;322(5909):1861–5.
Chirgwin JM. The stem cell niche as a pharmaceutical target for prevention of skeletal metastases. Anticancer Agents Med Chem. 2012;12(3):187–93.
Idowu T, Samadder P, Arthur G, Schweizer F. Amphiphilic modulation of glycosylated antitumor ether lipids results in a potent triamino scaffold against epithelial cancer Cell lines and BT474 cancer stem cells. J Med Chem. 2017;60(23):9724–38.
Cazet AS, Hui MN, Elsworth BL, Wu SZ, Roden D, Chan CL, Skhinas JN, Collot R, Yang J, Harvey K, et al. Targeting stromal remodeling and cancer stem cell plasticity overcomes chemoresistance in triple negative breast cancer. Nat Commun. 2018;9(1):2897.
O’Leary DP, O’Leary E, Foley N, Cotter TG, Wang JH, Redmond HP. Effects of surgery on the cancer stem cell niche. Eur J Surg Oncol. 2016;42(3):319–25.
Lee KM, Giltnane JM, Balko JM, Schwarz LJ, Guerrero-Zotano AL, Hutchinson KE, Nixon MJ, Estrada MV, Sánchez V, Sanders ME, et al. MYC and MCL1 cooperatively promote chemotherapy-resistant breast cancer stem cells via regulation of mitochondrial oxidative phosphorylation. Cell Metab. 2017;26(4):633-47.e7.
Steinbichler TB, Dudás J, Skvortsov S, Ganswindt U, Riechelmann H, Skvortsova II. Therapy resistance mediated by cancer stem cells. Semin Cancer Biol. 2018;53:156–67.
Najafi M, Mortezaee K, Majidpoor J. Cancer stem cell (CSC) resistance drivers. Life Sci. 2019;234:116781.
Galle E, Thienpont B, Cappuyns S, Venken T, Busschaert P, Van Haele M, Van Cutsem E, Roskams T, van Pelt J, Verslype C, et al. DNA methylation-driven EMT is a common mechanism of resistance to various therapeutic agents in cancer. Clin Epigenetics. 2020;12(1):27.
Xu J, Liu D, Niu H, Zhu G, Xu Y, Ye D, Li J, Zhang Q. Resveratrol reverses doxorubicin resistance by inhibiting epithelial-mesenchymal transition (EMT) through modulating PTEN/Akt signaling pathway in gastric cancer. J Exp Clin Cancer Res. 2017;36(1):19.
Jin H, He Y, Zhao P, Hu Y, Tao J, Chen J, Huang Y. Targeting lipid metabolism to overcome EMT-associated drug resistance via integrin β3/FAK pathway and tumor-associated macrophage repolarization using legumain-activatable delivery. Theranostics. 2019;9(1):265–78.
Farmer P, Bonnefoi H, Anderle P, Cameron D, Wirapati P, Becette V, André S, Piccart M, Campone M, Brain E, et al. A stroma-related gene signature predicts resistance to neoadjuvant chemotherapy in breast cancer. Nat Med. 2009;15(1):68–74.
Ghobrial IM, Liu CJ, Zavidij O, Azab AK, Baz R, Laubach JP, Mishima Y, Armand P, Munshi NC, Basile F, et al. Phase I/II trial of the CXCR4 inhibitor plerixafor in combination with bortezomib as a chemosensitization strategy in relapsed/refractory multiple myeloma. Am J Hematol. 2019;94(11):1244–53.
Fischer KR, Durrans A, Lee S, Sheng J, Li F, Wong ST, Choi H, El Rayes T, Ryu S, Troeger J, et al. Epithelial-to-mesenchymal transition is not required for lung metastasis but contributes to chemoresistance. Nature. 2015;527(7579):472–6.
David CJ, Huang YH, Chen M, Su J, Zou Y, Bardeesy N, Iacobuzio-Donahue CA, Massagué J. TGF-β tumor suppression through a lethal EMT. Cell. 2016;164(5):1015–30.
Hua W, Ten Dijke P, Kostidis S, Giera M, Hornsveld M. TGFβ-induced metabolic reprogramming during epithelial-to-mesenchymal transition in cancer. Cell Mol Life Sci. 2020;77(11):2103–23.
Calon A, Espinet E, Palomo-Ponce S, Tauriello DV, Iglesias M, Céspedes MV, Sevillano M, Nadal C, Jung P, Zhang XH, et al. Dependency of colorectal cancer on a TGF-β-driven program in stromal cells for metastasis initiation. Cancer Cell. 2012;22(5):571–84.
Ahmadi A, Najafi M, Farhood B, Mortezaee K. Transforming growth factor-β signaling: tumorigenesis and targeting for cancer therapy. J Cell Physiol. 2019;234(8):12173–87.
den Hollander MW, Bensch F, Glaudemans AW, Oude Munnink TH, Enting RH, den Dunnen WF, Heesters MA, Kruyt FA, Lub-de Hooge MN, Cees de Groot J, et al. TGF-β antibody uptake in recurrent high-grade glioma imaged with 89Zr-fresolimumab PET. J Nucl Med. 2015;56(9):1310–4.
Trachtman H, Fervenza FC, Gipson DS, Heering P, Jayne DR, Peters H, Rota S, Remuzzi G, Rump LC, Sellin LK, et al. A phase 1, single-dose study of fresolimumab, an anti-TGF-β antibody, in treatment-resistant primary focal segmental glomerulosclerosis. Kidney Int. 2011;79(11):1236–43.
Lacouture ME, Morris JC, Lawrence DP, Tan AR, Olencki TE, Shapiro GI, Dezube BJ, Berzofsky JA, Hsu FJ, Guitart J. Cutaneous keratoacanthomas/squamous cell carcinomas associated with neutralization of transforming growth factor β by the monoclonal antibody fresolimumab (GC1008). Cancer Immunol Immunother. 2015;64(4):437–46.
Formenti SC, Hawtin RE, Dixit N, Evensen E, Lee P, Goldberg JD, Li X, Vanpouille-Box C, Schaue D, McBride WH, et al. Baseline T cell dysfunction by single cell network profiling in metastatic breast cancer patients. J Immunother Cancer. 2019;7(1):177.
Shibue T, Weinberg RA. EMT, CSCs, and drug resistance: the mechanistic link and clinical implications. Nat Rev Clin Oncol. 2017;14(10):611–29.
Huang X, Gan G, Wang X, Xu T, Xie W. The HGF-MET axis coordinates liver cancer metabolism and autophagy for chemotherapeutic resistance. Autophagy. 2019;15(7):1258–79.
Singh A, Settleman J. EMT, cancer stem cells and drug resistance: an emerging axis of evil in the war on cancer. Oncogene. 2010;29(34):4741–51.
Armstrong AJ, Freedland SJ, Garcia-Blanco M. Epithelial-mesenchymal transition in prostate cancer: providing new targets for therapy. Asian J Androl. 2011;13(2):179–80.
Sun X, Lv X, Yan Y, Zhao Y, Ma R, He M, Wei M. Hypoxia-mediated cancer stem cell resistance and targeted therapy. Biomed Pharmacother. 2020;130:110623.
Martí-Díaz R, Montenegro MF, Cabezas-Herrera J, Goding CR, Rodríguez-López JN, Sánchez-Del-Campo L. Acriflavine, a potent Inhibitor of HIF-1α, disturbs glucose metabolism and suppresses ATF4-protective pathways in melanoma under non-hypoxic conditions. Cancers (Basel). 2020;13(1):102.
Min S, Wang X, Du Q, Gong H, Yang Y, Wang T, Wu N, Liu X, Li W, Zhao C, et al. Chetomin, a Hsp90/HIF1α pathway inhibitor, effectively targets lung cancer stem cells and non-stem cells. Cancer Biol Ther. 2020;21(8):698–708.
Soni S, Padwad YS. HIF-1 in cancer therapy: two decade long story of a transcription factor. Acta Oncol. 2017;56(4):503–15.
He M, Wu H, Jiang Q, Liu Y, Han L, Yan Y, Wei B, Liu F, Deng X, Chen H, et al. Hypoxia-inducible factor-2α directly promotes BCRP expression and mediates the resistance of ovarian cancer stem cells to adriamycin. Mol Oncol. 2019;13(2):403–21.
Pinzón-Daza ML, Cuellar-Saenz Y, Nualart F, Ondo-Mendez A, Del Riesgo L, Castillo-Rivera F, Garzón R. Oxidative stress promotes doxorubicin-induced Pgp and BCRP expression in colon cancer cells under hypoxic conditions. J Cell Biochem. 2017;118(7):1868–78.
To KK, Poon DC, Wei Y, Wang F, Lin G, Fu LW. Vatalanib sensitizes ABCB1 and ABCG2-overexpressing multidrug resistant colon cancer cells to chemotherapy under hypoxia. Biochem Pharmacol. 2015;97(1):27–37.
Pandya K, Meeke K, Clementz AG, Rogowski A, Roberts J, Miele L, Albain KS, Osipo C. Targeting both Notch and ErbB-2 signalling pathways is required for prevention of ErbB-2-positive breast tumour recurrence. Br J Cancer. 2011;105(6):796–806.
Schott AF, Landis MD, Dontu G, Griffith KA, Layman RM, Krop I, Paskett LA, Wong H, Dobrolecki LE, Lewis MT, et al. Preclinical and clinical studies of gamma secretase inhibitors with docetaxel on human breast tumors. Clin Cancer Res. 2013;19(6):1512–24.
Jimeno A, Gordon M, Chugh R, Messersmith W, Mendelson D, Dupont J, Stagg R, Kapoun AM, Xu L, Uttamsingh S, et al. A first-in-human phase I study of the anticancer stem cell agent ipafricept (OMP-54F28), a decoy receptor for wnt ligands, in patients with advanced solid tumors. Clin Cancer Res. 2017;23(24):7490–7.
Basset-Séguin N, Hauschild A, Kunstfeld R, Grob J, Dréno B, Mortier L, Ascierto PA, Licitra L, Dutriaux C, Thomas L, et al. Vismodegib in patients with advanced basal cell carcinoma: primary analysis of STEVIE, an international, open-label trial. Eur J Cancer. 2017;86:334–48.
Antonarakis ES, Heath EI, Smith DC, Rathkopf D, Blackford AL, Danila DC, King S, Frost A, Ajiboye AS, Zhao M, et al. Repurposing itraconazole as a treatment for advanced prostate cancer: a noncomparative randomized phase II trial in men with metastatic castration-resistant prostate cancer. Oncologist. 2013;18(2):163–73.
Bendell J, Andre V, Ho A, Kudchadkar R, Migden M, Infante J, Tiu RV, Pitou C, Tucker T, Brail L, et al. Phase I study of LY2940680, a smo antagonist, in patients with advanced cancer including treatment-naïve and previously treated basal cell carcinoma. Clin Cancer Res. 2018;24(9):2082–91.
Dianat-Moghadam H, Mahari A, Salahlou R, Khalili M, Azizi M, Sadeghzadeh H. Immune evader cancer stem cells direct the perspective approaches to cancer immunotherapy. Stem Cell Res Ther. 2022;13(1):150.
Walcher L, Kistenmacher AK, Suo H, Kitte R, Dluczek S, Strauß A, Blaudszun AR, Yevsa T, Fricke S, Kossatz-Boehlert U. Cancer stem cells-origins and biomarkers: perspectives for targeted personalized therapies. Front Immunol. 2020;11:1280.
Ding Z, Li Q, Zhang R, Xie L, Shu Y, Gao S, Wang P, Su X, Qin Y, Wang Y, et al. Personalized neoantigen pulsed dendritic cell vaccine for advanced lung cancer. Signal Transduct Target Ther. 2021;6(1):26.
Ying Z, Huang XF, Xiang X, Liu Y, Kang X, Song Y, Guo X, Liu H, Ding N, Zhang T, et al. A safe and potent anti-CD19 CAR T cell therapy. Nat Med. 2019;25(6):947–53.
Singh N, Frey NV, Engels B, Barrett DM, Shestova O, Ravikumar P, Cummins KD, Lee YG, Pajarillo R, Chun I, et al. Antigen-independent activation enhances the efficacy of 4–1BB-costimulated CD22 CAR T cells. Nat Med. 2021;27(5):842–50.
Fathi E, Farahzadi R, Sheervalilou R, Sanaat Z, Vietor I. A general view of CD33(+) leukemic stem cells and CAR-T cells as interesting targets in acute myeloblatsic leukemia therapy. Blood Res. 2020;55(1):10–6.
Wang QS, Wang Y, Lv HY, Han QW, Fan H, Guo B, Wang LL, Han WD. Treatment of CD33-directed chimeric antigen receptor-modified T cells in one patient with relapsed and refractory acute myeloid leukemia. Mol Ther. 2015;23(1):184–91.
Download references
Acknowledgements
Figures were composed with the assistance of Servier Medical Art ( https://smart.servier.com/ ) and Biorender ( https://biorender.com/ ).
Open Access funding enabled and organized by Projekt DEAL. This work was supported by grants from the National Natural Science Foundation of China (81472672), International Science and Technology Cooperation Project of Shanghai (18410721900, 21490713700), Leading Talent Program of Minhang District (2017), the National Key Research and Development Program of China (2017YFC1308604), Köln Fortune Program/Faculty of Medicine, University of Cologne (457/2020), Medical Engineering Project of Fudan University (yg2021-017) and the Program of Shanghai Academic Research Leader (20XD1400900).
Author information
Feng Ju and Manar M. Atyah contributed equally to this work
Authors and Affiliations
General, Visceral and Cancer Surgery, University Hospital of Cologne, Kerpener Straße 62, Cologne, Germany
Feng Ju, Nellie Horstmann, Christiane J. Bruns & Yue Zhao
Department of Liver Surgery and Transplantation, Liver Cancer Institute, Zhongshan Hospital, Fudan University, No. 180 Fenglin Road, Shanghai, 200032, China
Manar M. Atyah & Ning Ren
Key Laboratory of Carcinogenesis and Cancer Invasion of Ministry of Education, Shanghai, China
Fraunhofer Institute for Translational Medicine and Pharmacology ITMP, 22525, Hamburg, Germany
Fraunhofer Cluster of Excellence for Immune-Mediated Diseases CIMD, 22525, Hamburg, Germany
Avram and Stella Goldstein-Goren, Department of Biotechnology Engineering, Ben-Gurion University of the Negev, Beer-Sheva, Israel
Institute of Fudan-Minhang Academic Health System, Minhang Hospital, Fudan University, Shanghai, 201199, China
Qiong-Zhu Dong & Ning Ren
Key Laboratory of Whole-Period Monitoring and Precise Intervention of Digestive Cancer of Shanghai Municipal Health Commission, Shanghai, 201199, China
You can also search for this author in PubMed Google Scholar
Contributions
Mr. FJ contributed to the writing and figure of the manuscript. Dr. MM. A participated in the reviewing of the literature and contributed to the writing and editing of the manuscript. Ms. NH participated in the reviewing and editing of the manuscript. Dr. SG and Prof. RV participated in the reviewing and editing of the manuscript. Prof. Dr. CJ Burns contributed to the reviewing and editing of the manuscript. Prof. Q-ZD, Dr. YZ and Prof. NR contributed to the construction of the manuscript and supervised the completion of the review. All authors read and approved the final manuscript.
Corresponding authors
Correspondence to Yue Zhao or Ning Ren .
Ethics declarations
Ethics approval and consent to participate, consent for publication.
All the authors have read and approved the paper and declare no potential conflicts of interest in the paper.
Competing interests
The authors declare that they have no competing interests.
Additional information
Publisher's note.
Springer Nature remains neutral with regard to jurisdictional claims in published maps and institutional affiliations.
Rights and permissions
Open Access This article is licensed under a Creative Commons Attribution 4.0 International License, which permits use, sharing, adaptation, distribution and reproduction in any medium or format, as long as you give appropriate credit to the original author(s) and the source, provide a link to the Creative Commons licence, and indicate if changes were made. The images or other third party material in this article are included in the article's Creative Commons licence, unless indicated otherwise in a credit line to the material. If material is not included in the article's Creative Commons licence and your intended use is not permitted by statutory regulation or exceeds the permitted use, you will need to obtain permission directly from the copyright holder. To view a copy of this licence, visit http://creativecommons.org/licenses/by/4.0/ . The Creative Commons Public Domain Dedication waiver ( http://creativecommons.org/publicdomain/zero/1.0/ ) applies to the data made available in this article, unless otherwise stated in a credit line to the data.
Reprints and permissions
About this article
Cite this article.
Ju, F., Atyah, M.M., Horstmann, N. et al. Characteristics of the cancer stem cell niche and therapeutic strategies. Stem Cell Res Ther 13 , 233 (2022). https://doi.org/10.1186/s13287-022-02904-1
Download citation
Received : 30 July 2021
Accepted : 16 May 2022
Published : 03 June 2022
DOI : https://doi.org/10.1186/s13287-022-02904-1
Share this article
Anyone you share the following link with will be able to read this content:
Sorry, a shareable link is not currently available for this article.
Provided by the Springer Nature SharedIt content-sharing initiative
- Niche/microenvironment
Stem Cell Research & Therapy
ISSN: 1757-6512
- Submission enquiries: Access here and click Contact Us
- General enquiries: [email protected]
MINI REVIEW article
This article is part of the research topic.
Editors Showcase 2023: Insights in Stem Cell Research
The progress of induced pluripotent stem cells derived from pigs: A mini review of recent advances Provisionally Accepted
- 1 University of Calgary, Canada
The final, formatted version of the article will be published soon.
Pigs (Sus scrofa) are widely acknowledged as an important large mammalian animal model due to their similarity to human physiology, genetics, and immunology. Leveraging the full potential of this model presents significant opportunities for major advancements in the fields of comparative biology, disease modeling, and regenerative medicine. Thus, the derivation of pluripotent stem cells from this species can offer new tools for disease modeling and serve as a steppingstone to test future autologous or allogeneic cell-based therapies. Over the past few decades, great progress has been made in establishing porcine pluripotent stem cells (pPSCs), including embryonic stem cells (pESCs) derived from pre-and peri-implantation embryos, and induced pluripotent stem cells (piPSCs) using a variety of cellular reprogramming strategies. However, the stabilization of pPSCs was not as straightforward as directly applying the culture conditions developed and optimized for murine or primate PSCs. Therefore, it has historically been challenging to establish stable pPSC lines that could pass stringent pluripotency tests. Here, we review recent advances in the establishment of stable porcine PSCs. We focus on the evolving derivation methods that eventually led to the establishment of pESCs and transgene-free piPSCs, as well as current challenges and opportunities in this rapidly advancing field.
Keywords: Porcine pluripotent stem cells, cellular reprogramming, Induced Pluripotent Stem Cells, Embryonic Stem Cells, Transgene-free
Received: 16 Jan 2024; Accepted: 10 Apr 2024.
Copyright: © 2024 Neira, Conrad, Rusteika and Chu. This is an open-access article distributed under the terms of the Creative Commons Attribution License (CC BY) . The use, distribution or reproduction in other forums is permitted, provided the original author(s) or licensor are credited and that the original publication in this journal is cited, in accordance with accepted academic practice. No use, distribution or reproduction is permitted which does not comply with these terms.
* Correspondence: Mx. Li-Fang (Jack) Chu, University of Calgary, Calgary, T2N 1N4, Alberta, Canada
People also looked at
Thank you for visiting nature.com. You are using a browser version with limited support for CSS. To obtain the best experience, we recommend you use a more up to date browser (or turn off compatibility mode in Internet Explorer). In the meantime, to ensure continued support, we are displaying the site without styles and JavaScript.
- View all journals
- Explore content
- About the journal
- Publish with us
- Sign up for alerts
- 10 April 2024
How to supercharge cancer-fighting cells: give them stem-cell skills
- Sara Reardon 0
Sara Reardon is a freelance journalist based in Bozeman, Montana.
You can also search for this author in PubMed Google Scholar
A CAR T cell (orange; artificially coloured) attacks a cancer cell (green). Credit: Eye Of Science/Science Photo Library
Bioengineered immune cells have been shown to attack and even cure cancer , but they tend to get exhausted if the fight goes on for a long time. Now, two separate research teams have found a way to rejuvenate these cells: make them more like stem cells .
Both teams found that the bespoke immune cells called CAR T cells gain new vigour if engineered to have high levels of a particular protein. These boosted CAR T cells have gene activity similar to that of stem cells and a renewed ability to fend off cancer . Both papers were published today in Nature 1 , 2 .
The papers “open a new avenue for engineering therapeutic T cells for cancer patients”, says Tuoqi Wu, an immunologist at the University of Texas Southwestern in Dallas who was not involved in the research.
Reviving exhausted cells
CAR T cells are made from the immune cells called T cells, which are isolated from the blood of person who is going to receive treatment for cancer or another disease. The cells are genetically modified to recognize and attack specific proteins — called chimeric antigen receptors (CARs) — on the surface of disease-causing cells and reinfused into the person being treated.
But keeping the cells active for long enough to eliminate cancer has proved challenging, especially in solid tumours such as those of the breast and lung. (CAR T cells have been more effective in treating leukaemia and other blood cancers.) So scientists are searching for better ways to help CAR T cells to multiply more quickly and last longer in the body.
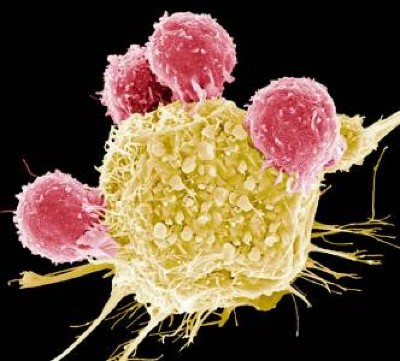
Cutting-edge CAR-T cancer therapy is now made in India — at one-tenth the cost
With this goal in mind, a team led by immunologist Crystal Mackall at Stanford University in California and cell and gene therapy researcher Evan Weber at the University of Pennsylvania in Philadelphia compared samples of CAR T cells used to treat people with leukaemia 1 . In some of the recipients, the cancer had responded well to treatment; in others, it had not.
The researchers analysed the role of cellular proteins that regulate gene activity and serve as master switches in the T cells. They found a set of 41 genes that were more active in the CAR T cells associated with a good response to treatment than in cells associated with a poor response. All 41 genes seemed to be regulated by a master-switch protein called FOXO1.
The researchers then altered CAR T cells to make them produce more FOXO1 than usual. Gene activity in these cells began to look like that of T memory stem cells, which recognize cancer and respond to it quickly.
The researchers then injected the engineered cells into mice with various types of cancer. Extra FOXO1 made the CAR T cells better at reducing both solid tumours and blood cancers. The stem-cell-like cells shrank a mouse’s tumour more completely and lasted longer in the body than did standard CAR T cells.
Master-switch molecule
A separate team led by immunologists Phillip Darcy, Junyun Lai and Paul Beavis at Peter MacCallum Cancer Centre in Melbourne, Australia, reached the same conclusion with different methods 2 . Their team was examining the effect of IL-15, an immune-signalling molecule that is administered alongside CAR T cells in some clinical trials. IL-15 helps to switch T cells to a stem-like state, but the cells can get stuck there instead of maturing to fight cancer.
The team analysed gene activity in CAR T cells and found that IL-15 turned on genes associated with FOXO1. The researchers engineered CAR T cells to produce extra-high levels of FOXO1 and showed that they became more stem-like, but also reached maturity and fought cancer without becoming exhausted. “It’s the ideal situation,” Darcy says.
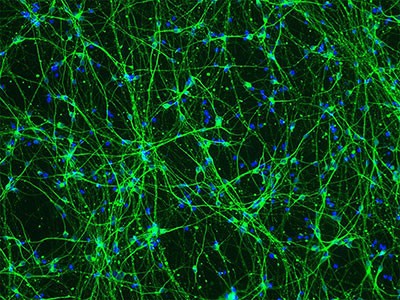
Stem-cell and genetic therapies make a healthy marriage
The team also found that extra-high levels of FOXO1 improved the CAR T cells’ metabolism, allowing them to last much longer when infused into mice. “We were surprised by the magnitude of the effect,” says Beavis.
Mackall says she was excited to see that FOXO1 worked the same way in mice and humans. “It means this is pretty fundamental,” she says.
Engineering CAR T cells that overexpress FOXO1 might be fairly simple to test in people with cancer, although Mackall says researchers will need to determine which people and types of cancer are most likely to respond well to rejuvenated cells. Darcy says that his team is already speaking to clinical researchers about testing FOXO1 in CAR T cells — trials that could start within two years.
And Weber points to an ongoing clinical trial in which people with leukaemia are receiving CAR T cells genetically engineered to produce unusually high levels of another master-switch protein called c-Jun, which also helps T cells avoid exhaustion. The trial’s results have not been released yet, but Mackall says she suspects the same system could be applied to FOXO1 and that overexpressing both proteins might make the cells even more powerful.
doi: https://doi.org/10.1038/d41586-024-01043-2
Doan, A. et al. Nature https://doi.org/10.1038/s41586-024-07300-8 (2024).
Article Google Scholar
Chan, J. D. et al. Nature https://doi.org/10.1038/s41586-024-07242-1 (2024).
Download references
Reprints and permissions
Related Articles
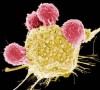
- Medical research
ROS-dependent S-palmitoylation activates cleaved and intact gasdermin D
Article 10 APR 24
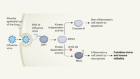
Blocking cell death limits lung damage and inflammation from influenza
News & Views 10 APR 24
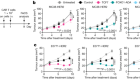
FOXO1 enhances CAR T cell stemness, metabolic fitness and efficacy
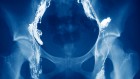
Biological age surges in survivors of childhood cancer
Research Highlight 11 APR 24
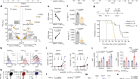
FOXO1 is a master regulator of memory programming in CAR T cells
The PARTNER trial of neoadjuvant olaparib in triple-negative breast cancer
Article 08 APR 24
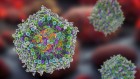
mRNA drug offers hope for treating a devastating childhood disease
News 03 APR 24
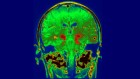
Diabetes drug slows development of Parkinson’s disease
Junior Group Leader Position at IMBA - Institute of Molecular Biotechnology
The Institute of Molecular Biotechnology (IMBA) is one of Europe’s leading institutes for basic research in the life sciences. IMBA is located on t...
Austria (AT)
IMBA - Institute of Molecular Biotechnology
Open Rank Faculty, Center for Public Health Genomics
Center for Public Health Genomics & UVA Comprehensive Cancer Center seek 2 tenure-track faculty members in Cancer Precision Medicine/Precision Health.
Charlottesville, Virginia
Center for Public Health Genomics at the University of Virginia
Husbandry Technician I
Memphis, Tennessee
St. Jude Children's Research Hospital (St. Jude)
Lead Researcher – Department of Bone Marrow Transplantation & Cellular Therapy
Researcher in the center for in vivo imaging and therapy.
Sign up for the Nature Briefing newsletter — what matters in science, free to your inbox daily.
Quick links
- Explore articles by subject
- Guide to authors
- Editorial policies
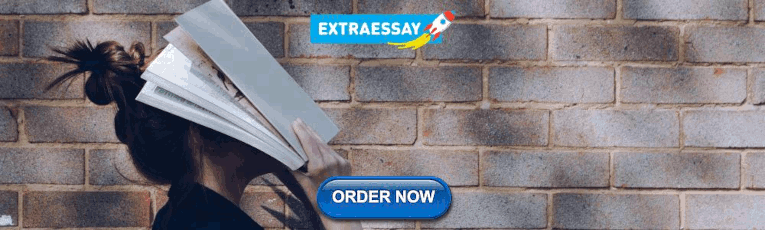
IMAGES
COMMENTS
Abstract. The potential impact of stem cell technology on medical and dental practice is vast. Stem cell research will not only provide the foundation for future therapies, but also reveal unique insights into basic disease mechanisms. Therefore, an understanding of stem cell technology will be necessary for clinicians in the future.
Since stem cells were first discovered, researchers have identified distinct stem cell populations in different organs and with various functions, converging on the unique abilities of self ...
In recent years, stem cell therapy has become a very promising and advanced scientific research topic. The development of treatment methods has evoked great expectations. This paper is a review focused on the discovery of different stem cells and the potential therapies based on these cells. The genesis of stem cells is followed by laboratory steps of controlled stem cell culturing and derivation.
Stem-cell research articles from across Nature Portfolio. Stem-cell research is the area of research that studies the properties of stem cells and their potential use in medicine. As stem cells ...
Stem Cell Research & Therapy (2023) Despite many reports of putative stem-cell-based treatments in genetic and degenerative disorders or severe injuries, the number of proven stem cell therapies ...
In the study published by Sun et al., a systematic review and meta-analysis illustrated the advantageous of stem cell therapy in diabetic foot and can improve the quality of life of patients. Nevertheless, the... Yan Bai and Fan Zhang. Stem Cell Research & Therapy 2024 15 :85.
An international peer-reviewed journal, it publishes high-quality open access research articles with a special emphasis on basic, translational and clinical research into stem cell therapeutics and regenerative therapies, including animal models and clinical trials. The journal also provides reviews, viewpoints, commentaries, reports and methods.
Here are some additional stem cell research pros and cons to review. List of the Pros of Stem Cell Research. 1. It could treat several conditions that are virtually untreatable right now. Stem cell research opens numerous avenues for treatments or a cure to be found for several conditions that are either untreatable or without a cure today.
Stem cells are noted for their ability to self-renew and differentiate into a variety of cell types. Some stem cells, described as totipotent cells, have tremendous capacity to self-renew and differentiate. Embryonic stem cells have pluripotent capacity, able to form tissues of all 3 germ layers but unable to form an entire live being. Research with embryonic stem cells has enabled ...
Stem-Cell Aging and Pathways to Precancer Evolution. C.H. Jamieson and I.L. WeissmanN Engl J Med 2023;389:1310-1319. Stem cells can renew themselves without differentiating. Aging, inflammation ...
Stem cells: The body's master cells. Stem cells are a special type of cells that have two important properties. They are able to make more cells like themselves. That is, they self-renew. And they can become other cells that do different things in a process known as differentiation. Stem cells are found in almost all tissues of the body.
Stem cell research promises great good and is a worthy scientific priority as long as we pursue it ethically. Obtaining stem cells from people without seriously harming people in the process can be ethical. However, obtaining stem cells from human embryos cannot be ethical because it necessarily involves destroying those embryos.
Stem Cell Research is dedicated to publishing high-quality manuscripts focusing on the biology and applications of stem cell research. Submissions to Stem Cell Research, may cover all aspects of stem cells, including embryonic stem cells, tissue-specific stem cells, cancer stem cells, developmental …. View full aims & scope.
Pros and Cons. Pros and Cons of Using Various Stem Cells. Limitations on ASC ability to differentiate are still uncertain; currently thought to be multi or unipotent. Unsure whether they would be rejected if used in transplants. Methods for ensured reproducibility and maintenance, as differentiated tissues are not certain.
Stem cells are found in multi-cellular organisms. These cells are crucial for the growth of an organism, as they have the capacity to renew themselves, enabling them to divide and perform specialized functions. In mammals, there are two types of stem cells - embryonic and adult. Stem cell research is carried out on these two types of cells.
Stem cells articles from across Nature Portfolio. Stem cells are cells that have the capacity to self-renew by dividing and to develop into more mature, specialised cells. Stem cells can be ...
Before delving into the pros and cons of stem cell research, a few successful tales must be mentioned in this article. Multiple Sclerosis. Reema Sandhu's stem cell success story began in 2014 when she was diagnosed with multiple sclerosis, according to DailyMail. The disease can affect the brain and spinal cord, causing a variety of life ...
Millman hopes that these stem cell-derived beta cells could be ready for research in humans within 3-5 years. Stem cells could also have vast potential in developing other new therapies. Using ...
However, the use of stem cells, particularly those derived from embryos, has been controversial due to ethical concerns surrounding the destruction of embryos. In this article, we will explore the pros and cons of stem cell research, and discuss the potential benefits and drawbacks of this groundbreaking field. Pros of Stem Cell Research 1.
ROCHESTER, Minn. — A Mayo Clinic study shows stem cells derived from patients' own fat are safe and may improve sensation and movement after traumatic spinal cord injuries.The findings from the phase 1 clinical trial appear in Nature Communications.The results of this early research offer insights on the potential of cell therapy for people living with spinal cord injuries and paralysis for ...
Summary: Researchers have developed a technique to connect lab-grown neural 'organoids' (three-dimensional developmental brain-like structures grown from human stem cells) using axonal bundles ...
Stem cells are undifferentiated cells that have the capacity to self-renew and proliferate for longer than non-stem cells as well as having the ability to generate multiple types of cells in the body [1, 2].Regardless of their proliferation potential, stem cells are usually quiescent, remaining in an inactive dormant state (G1, G0) and are protected from cellular damage or mutations [3, 4].
1 Department of Endocrinology, Union Hospital, Tongji Medical College, Huazhong University of Science and Technology, Wuhan, China; 2 Hubei Provincial Clinical Research Center for Diabetes and Metabolic Disorders, Wuhan, China; Stem cell-based therapies exhibit considerable promise in the treatment of diabetes and its complications. Extensive research has been dedicated to elucidate the ...
Pigs (Sus scrofa) are widely acknowledged as an important large mammalian animal model due to their similarity to human physiology, genetics, and immunology. Leveraging the full potential of this model presents significant opportunities for major advancements in the fields of comparative biology, disease modeling, and regenerative medicine. Thus, the derivation of pluripotent stem cells from ...
The bioengineered immune players called CAR T cells last longer and work better if pumped up with a large dose of a protein that makes them resemble stem cells. The papers "open a new avenue for ...
This pilot study aimed to evaluate the immunomodulatory effect of placental mesenchymal stem/stromal cells (MSCs) on peripheral blood mononuclear cells (PBMCs) from patients with hidradenitis suppurativa (HS). Blood samples were collected from 3 healthy and 3 patients with HS. Isolated PBMCs were stained with carboxyfluorescein succinimidyl ester (CFSE) and stimulated with phorbol 12-myristate ...