Thank you for visiting nature.com. You are using a browser version with limited support for CSS. To obtain the best experience, we recommend you use a more up to date browser (or turn off compatibility mode in Internet Explorer). In the meantime, to ensure continued support, we are displaying the site without styles and JavaScript.
- View all journals
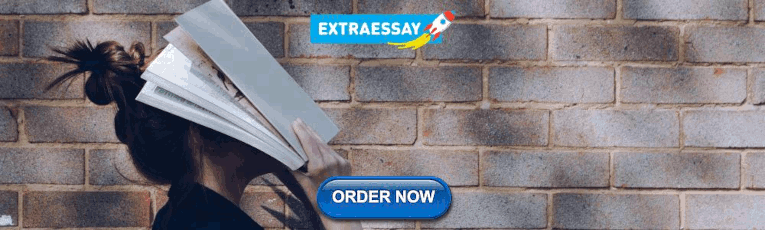
Biomedical engineering articles from across Nature Portfolio
Biomedical engineering is a branch of engineering that applies principles and design concepts of engineering to healthcare. Biomedical engineers deal with medical devices such as imaging equipment, biocompatible materials such as prostheses or therapeutic biologicals, or processes such as regenerative tissue growth, for example.
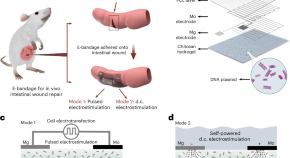
A flexible bioelectronic bandage for accelerated repair of intestinal wounds
A flexible, biodegradable and self-powered electronic bandage is designed to deliver dual-mode electrical stimulation, which can synergistically accelerate local intestinal wound healing. This approach also shows promise for reducing postoperative complications and could have broad potential for application in other tissues and organs.
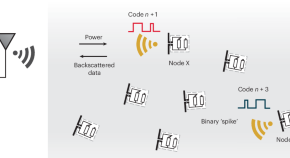
Wireless radiofrequency network of distributed microsensors
Distributed sensing of a dynamic environment is typically characterized by the sparsity of events, such as neuronal firing in the brain. Using the brain as inspiration, an event-driven communication strategy is developed that enables the efficient transmission, accurate retrieval and interpretation of sparse events across a network of thousands of wireless microsensors.
Latest Research and Reviews
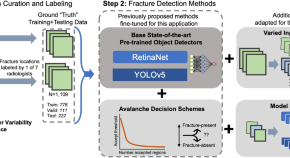
High sensitivity methods for automated rib fracture detection in pediatric radiographs
- Jonathan Burkow
- Gregory Holste
- Adam Alessio
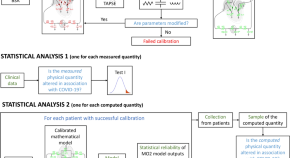
A mathematical model to assess the effects of COVID-19 on the cardiocirculatory system
- Andrea Tonini
- Christian Vergara
- Alfio Quarteroni
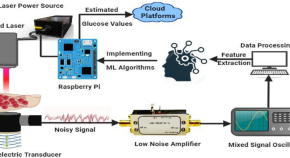
Augmenting authenticity for non-invasive in vivo detection of random blood glucose with photoacoustic spectroscopy using Kernel-based ridge regression
- P. N. S. B. S. V. Prasad V
- Ali Hussain Syed
- Pradyut Kumar Sanki
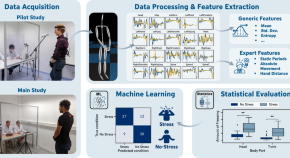
Machine learning-based detection of acute psychosocial stress from body posture and movements
- Robert Richer
- Veronika Koch
- Nicolas Rohleder
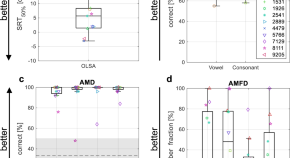
Psychoacoustic and electroencephalographic responses to changes in amplitude modulation depth and frequency in relation to speech recognition in cochlear implantees
- Waldo Nogueira
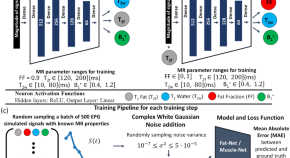
A deep learning approach for fast muscle water T2 mapping with subject specific fat T2 calibration from multi-spin-echo acquisitions
- Marco Barbieri
- Melissa T. Hooijmans
- Valentina Mazzoli
News and Comment
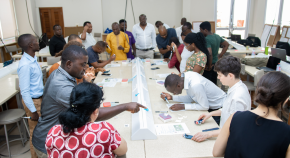
From equitable access to equitable innovation: rethinking bioengineering for global health
What does global health equity mean? In bioengineering, ‘equity’ is often interpreted as global ‘access’ to technologies, thereby neglecting wider structural inequalities. Here we suggest that concepts of equity need to be expanded to incorporate principles of equitable representation and recognition within the innovation ecosystem.
- Alice Street
- Maïwenn Kersaudy Kerhoas
- Zibusiso Ndlovu
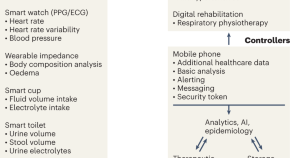
The potential of wearable sweat sensors in heart failure management
Wearable sweat sensors could be used to monitor patients with heart failure, providing a route to personalized and automated patient management in hospitals and at home.
- Noé Brasier
- Ole Frobert
- Roozbeh Ghaffari
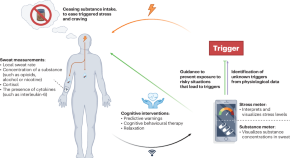
Towards on-skin analysis of sweat for managing disorders of substance abuse
A patient-centred system that leverages the analysis of sweat via wearable sensors may better support the management of patients with substance-use disorders.
- Noe Brasier
- Juliane R. Sempionatto
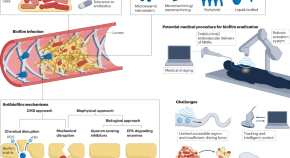
Micro- and nanorobots for biofilm eradication
Micro- and nanorobots present a promising approach for navigating within the body and eliminating biofilm infections. Their motion can be remotely controlled by external fields and tracked by clinical imaging. They can mechanically disrupt the biofilm matrix and kill the dormant bacterial cells synergistically, thereby improving the effectiveness of biofilm eradication.
- Staffan Kjelleberg
Quick links
- Explore articles by subject
- Guide to authors
- Editorial policies

Research on Biomedical Engineering
Research on Biomedical Engineering is an online, peer-reviewed journal dedicated to all fields of Biomedical Engineering.
- Multidisciplinary in nature, catering to readers and authors interested in developing tools based on engineering and physical sciences to solve biological and medical problems.
- Publishes Original Research Articles, Reviews, and Technical Communications.
- Covers a wide range of contributing areas including, metrology and biomedical engineering, health technology, health informatics and telemedicine, biotechnology, artificial organs, implants and biomaterials, proteomics, genomics and bioinformatics, biomedical instrumentation, biomechanics, rehabilitation engineering and assistive technologies, biomedical signal processing, modelling of physiological systems, use of laser in health, use of ultrasound in health, use of radiation in health and medical imaging.
- The official journal of the Brazilian Society of Biomedical Engineering.
- Alcimar Barbosa Soares
Societies and partnerships
- The Brazilian Society of Biomedical Engineering (opens in a new tab)
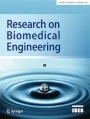
Latest issue
Volume 39, Issue 4
Latest articles
Antibacterial coating on magnesium alloys by mao for biomedical applications.
- Pralhad Pesode
- Shivprakash Barve
- Shailendra Dayane
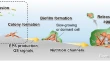
Effect of porogen agent on the size, shape, and structure of porous hydroxyapatite scaffolds
- Ika Maria Ulfah
- Nendar Herdianto
- Masruroh Masruroh
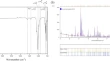
Usability evaluation of high-fidelity simulation manikin for cardiopulmonary resuscitation training for medical students
- Lucas de Brito Silva
- Fabrícia Torres Gonçalves
- Fernando Pasquini Santos
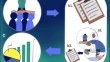
CADNet: cardiac arrhythmia detection and classification using unified principal component analysis and 1D-CNN model
- Subba Reddy Borra
- Dasari Ramesh Gari Amrutha Nayana
- Voriganti Sahithi
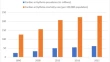
On the use of power-based connectivity between EEG and sEMG signals for three-weight classification during object manipulation tasks
- C.D. Guerrero-Mendez
- C. F. Blanco-Díaz
- A. F. Ruiz-Olaya
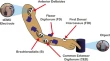
Journal updates
Special issue: 'emerging technologies for fighting covid-19, special issue form and guest editor guidelines.
A Special Issue Form and specific information for Guest Editors can be found here.
Top 10 Downloaded Articles 2023
Follow us on facebook, x, and linkedin.
Springer Engineering is on Facebook, X, and LinkedIn. Follow us and keep up to date with the latest journal news, research highlights, and more.
Journal information
- EI Compendex
- Google Scholar
- Japanese Science and Technology Agency (JST)
- OCLC WorldCat Discovery Service
- TD Net Discovery Service
- UGC-CARE List (India)
Rights and permissions
Springer policies
© Sociedade Brasileira de Engenharia Biomedica
- Find a journal
- Publish with us
- Track your research
- Search by keyword
- Search by citation
Page 1 of 44
Development and validation of an ultrasound-based deep learning radiomics nomogram for predicting the malignant risk of ovarian tumours
The timely identification and management of ovarian cancer are critical determinants of patient prognosis. In this study, we developed and validated a deep learning radiomics nomogram (DLR_Nomogram) based on u...
- View Full Text
Strategies to enhance the ability of nerve guidance conduits to promote directional nerve growth
Severely damaged peripheral nerves will regenerate incompletely due to lack of directionality in their regeneration, leading to loss of nerve function. To address this problem, various nerve guidance conduits ...
A deep learning framework for identifying and segmenting three vessels in fetal heart ultrasound images
Congenital heart disease (CHD) is one of the most common birth defects in the world. It is the leading cause of infant mortality, necessitating an early diagnosis for timely intervention. Prenatal screening us...
Noninvasive spinal stimulation improves walking in chronic stroke survivors: a proof-of-concept case series
After stroke, restoring safe, independent, and efficient walking is a top rehabilitation priority. However, in nearly 70% of stroke survivors asymmetrical walking patterns and reduced walking speed persist. Th...
Classification of vasovagal syncope from physiological signals on tilt table testing
The diagnostic test for vasovagal syncope (VVS), the most common cause of syncope is head-up tilt test (HUTT) assessment. During the test, subjects experienced clinical symptoms such as nausea, sweating, pallo...
Effects of immediate loading directionality on the mechanical sensing protein PIEZO1 expression and early-stage healing process of peri-implant bone
The reduced treatment time of dental implants with immediate loading protocol is an appealing solution for dentists and patients. However, there remains a significant risk of early peri-implant bone response f...
Enhancing automated lower limb rehabilitation exercise task recognition through multi-sensor data fusion in tele-rehabilitation
Tele-rehabilitation is the provision of physiotherapy services to individuals in their own homes. Activity recognition plays a crucial role in the realm of automatic tele-rehabilitation. By assessing patient m...
A novel in-bed body posture monitoring for decubitus ulcer prevention using body pressure distribution mapping
Decubitus ulcers are prevalent among the aging population due to a gradual decline in their overall health, such as nutrition, mental health, and mobility, resulting in injury to the skin and tissue. The most ...
Construction of in vitro liver-on-a-chip models and application progress
The liver is the largest internal organ of the human body. It has a complex structure and function and plays a vital role in drug metabolism. In recent decades, extensive research has aimed to develop in vitro...
Evaluating imaging repeatability of fully self-service fundus photography within a community-based eye disease screening setting
This study aimed to investigate the imaging repeatability of self-service fundus photography compared to traditional fundus photography performed by experienced operators.
Three-dimensional visualization of thyroid ultrasound images based on multi-scale features fusion and hierarchical attention
Ultrasound three-dimensional visualization, a cutting-edge technology in medical imaging, enhances diagnostic accuracy by providing a more comprehensive and readable portrayal of anatomical structures compared...
Effects of hyperbaric oxygen combined cabin ventilator on critically ill patients with liberation difficulty after tracheostomy
Critically ill patients undergoing liberation often encounter various physiological and clinical complexities and challenges. However, whether the combination of hyperbaric oxygen and in-cabin ventilator thera...
Diagnostic value of bedside lung ultrasound and 12-zone score in the 65 cases of neonatal respiratory distress syndrome and its severity
To explore the predictive value of bedside lung ultrasound score in the severity of neonatal respiratory distress syndrome (NRDS) and mechanical ventilation and extubation.
Designing and validating an experimental protocol to induce airway narrowing in older adults with and without asthma
Persons with asthma may experience excessive airway narrowing due to exercise or exposure to cold air, worsening their daily functionality. Exercise has several benefits for asthma control, but it may induce a...
Application of visual transformer in renal image analysis
Deep Self-Attention Network (Transformer) is an encoder–decoder architectural model that excels in establishing long-distance dependencies and is first applied in natural language processing. Due to its comple...
A concept for human use of real-time and remote monitoring of diabetic subjects using intermittent scanned continuous glucose measurement
Flash glucose monitoring systems like the FreeStyle Libre (FSL) sensor have gained popularity for monitoring glucose levels in people with diabetes mellitus. This sensor can be paired with an off-label convert...
HM_ADET: a hybrid model for automatic detection of eyelid tumors based on photographic images
The accurate detection of eyelid tumors is essential for effective treatment, but it can be challenging due to small and unevenly distributed lesions surrounded by irrelevant noise. Moreover, early symptoms of...
Simulating impaired left ventricular–arterial coupling in aging and disease: a systematic review
Aortic stenosis, hypertension, and left ventricular hypertrophy often coexist in the elderly, causing a detrimental mismatch in coupling between the heart and vasculature known as ventricular−vascular (VA) cou...
Non-invasive parameters of autonomic function using beat-to-beat cardiovascular variations and arterial stiffness in hypertensive individuals: a systematic review
Non-invasive, beat-to-beat variations in physiological indices provide an opportunity for more accessible assessment of autonomic dysfunction. The potential association between the changes in these parameters ...
Kinematic difference and asymmetries during level walking in adolescent patients with different types of mild scoliosis
Adolescent idiopathic scoliosis (AIS), three-dimensional spine deformation, affects body motion. Previous research had indicated pathological gait patterns of AIS. However, the impact of the curve number on th...
The effectiveness of simple heuristic features in sensor orientation and placement problems in human activity recognition using a single smartphone accelerometer
Human activity Recognition (HAR) using smartphone sensors suffers from two major problems: sensor orientation and placement. Sensor orientation and sensor placement problems refer to the variation in sensor si...
StairNet: visual recognition of stairs for human–robot locomotion
Human–robot walking with prosthetic legs and exoskeletons, especially over complex terrains, such as stairs, remains a significant challenge. Egocentric vision has the unique potential to detect the walking en...
Feasibility of using a depth camera or pressure mat for visual feedback balance training with functional electrical stimulation
Individuals with incomplete spinal-cord injury/disease are at an increased risk of falling due to their impaired ability to maintain balance. Our research group has developed a closed-loop visual-feedback bala...
Screening ovarian cancer by using risk factors: machine learning assists
Ovarian cancer (OC) is a prevalent and aggressive malignancy that poses a significant public health challenge. The lack of preventive strategies for OC increases morbidity, mortality, and other negative conseq...
Deep neural networks for wearable sensor-based activity recognition in Parkinson’s disease: investigating generalizability and model complexity
The research gap addressed in this study is the applicability of deep neural network (NN) models on wearable sensor data to recognize different activities performed by patients with Parkinson’s Disease (PwPD) ...
Abnormal interlimb coordination of motor developmental delay during infant crawling based on kinematic synergy analysis
Previous studies have reported that abnormal interlimb coordination is a typical characteristic of motor developmental delay (MDD) during human movement, which can be visually manifested as abnormal motor post...
Detecting bulbar amyotrophic lateral sclerosis (ALS) using automatic acoustic analysis
Automatic speech assessments have the potential to dramatically improve ALS clinical practice and facilitate patient stratification for ALS clinical trials. Acoustic speech analysis has demonstrated the abilit...
Advantages of transformer and its application for medical image segmentation: a survey
Convolution operator-based neural networks have shown great success in medical image segmentation over the past decade. The U-shaped network with a codec structure is one of the most widely used models. Transf...
Beyond timing and step counting in 360° turning-in-place assessment: a scoping review
Turning in place is a challenging motor task and is used as a brief assessment test of lower limb function and dynamic balance. This review aims to examine how research of instrumented analysis of turning in p...
Protocol for metadata and image collection at diabetic foot ulcer clinics: enabling research in wound analytics and deep learning
The escalating impact of diabetes and its complications, including diabetic foot ulcers (DFUs), presents global challenges in quality of life, economics, and resources, affecting around half a billion people. ...
Joint angle estimation during shoulder abduction exercise using contactless technology
Tele-rehabilitation, also known as tele-rehab, uses communication technologies to provide rehabilitation services from a distance. The COVID-19 pandemic has highlighted the importance of tele-rehab, where the ...
A dry polymer nanocomposite transcutaneous electrode for functional electrical stimulation
Functional electrical stimulation (FES) can be used in rehabilitation to aid or improve function in people with paralysis. In clinical settings, it is common practice to use transcutaneous electrodes to apply ...
Left main coronary artery morphological phenotypes and its hemodynamic properties
Atherosclerosis may be linked to morphological defects that lead to variances in coronary artery hemodynamics. Few objective strategies exit at present for generalizing morphological phenotypes of coronary art...
Multimodal diagnosis model of Alzheimer’s disease based on improved Transformer
Recent technological advancements in data acquisition tools allowed neuroscientists to acquire different modality data to diagnosis Alzheimer’s disease (AD). However, how to fuse these enormous amount differen...
Pulse wave-based evaluation of the blood-supply capability of patients with heart failure via machine learning
Pulse wave, as a message carrier in the cardiovascular system (CVS), enables inferring CVS conditions while diagnosing cardiovascular diseases (CVDs). Heart failure (HF) is a major CVD, typically requiring exp...
Effects of workload and saddle height on muscle activation of the lower limb during cycling
Cycling workload is an essential factor in practical cycling training. Saddle height is the most studied topic in bike fitting, but the results are controversial. This study aims to investigate the effects of ...
A neural network with a human learning paradigm for breast fibroadenoma segmentation in sonography
Breast fibroadenoma poses a significant health concern, particularly for young women. Computer-aided diagnosis has emerged as an effective and efficient method for the early and accurate detection of various s...
Unsupervised corneal contour extraction algorithm with shared model for dynamic deformation videos: improving accuracy and noise resistance
In this study, an automatic corneal contour extraction algorithm with a shared model is developed to extract contours from dynamic corneal videos containing noise, which improves the accuracy of corneal biomec...
Bioelectricity in dental medicine: a narrative review
Bioelectric signals, whether exogenous or endogenous, play crucial roles in the life processes of organisms. Recently, the significance of bioelectricity in the field of dentistry is steadily gaining greater a...

Effect of test duration and sensor location on the reliability of standing balance parameters derived using body-mounted accelerometers
Balance parameters derived from wearable sensor measurements during postural sway have been shown to be sensitive to experimental variables such as test duration, sensor number, and sensor location that influe...
Cycling using functional electrical stimulation therapy to improve motor function and activity in post-stroke individuals in early subacute phase: a systematic review with meta-analysis
Stroke necessitates interventions to rehabilitate individuals with disabilities, and the application of functional electrical stimulation therapy (FEST) has demonstrated potential in this regard. This study ai...
A weakly supervised deep learning model integrating noncontrasted computed tomography images and clinical factors facilitates haemorrhagic transformation prediction after intravenous thrombolysis in acute ischaemic stroke patients
Haemorrhage transformation (HT) is a serious complication of intravenous thrombolysis (IVT) in acute ischaemic stroke (AIS). Accurate and timely prediction of the risk of HT before IVT may change the treatment...
RAGE plays key role in diabetic retinopathy: a review
RAGE is a multiligand receptor for the immunoglobulin superfamily of cell surface molecules and is expressed in Müller cells, vascular endothelial cells, nerve cells and RPE cells of the retina. Diabetic retin...
Assessment of the functional severity of coronary lesions from optical coherence tomography based on ensembled learning
Atherosclerosis is one of the most frequent cardiovascular diseases. The dilemma faced by physicians is whether to treat or postpone the revascularization of lesions that fall within the intermediate range giv...
Artificial intelligence in glaucoma: opportunities, challenges, and future directions
Artificial intelligence (AI) has shown excellent diagnostic performance in detecting various complex problems related to many areas of healthcare including ophthalmology. AI diagnostic systems developed from f...
Radiotranscriptomics of non-small cell lung carcinoma for assessing high-level clinical outcomes using a machine learning-derived multi-modal signature
Multi-omics research has the potential to holistically capture intra-tumor variability, thereby improving therapeutic decisions by incorporating the key principles of precision medicine. The purpose of this st...
Wireless capsule endoscopy multiclass classification using three-dimensional deep convolutional neural network model
Wireless capsule endoscopy (WCE) is a patient-friendly and non-invasive technology that scans the whole of the gastrointestinal tract, including difficult-to-access regions like the small bowel. Major drawback...
Prediction of non-perfusion volume ratio for uterine fibroids treated with ultrasound-guided high-intensity focused ultrasound based on MRI radiomics combined with clinical parameters
Prediction of non-perfusion volume ratio (NPVR) is critical in selecting patients with uterine fibroids who will potentially benefit from ultrasound-guided high-intensity focused ultrasound (HIFU) treatment, a...
Comparison of plugin and redundant marker sets to analyze gait kinematics between different populations
Gait model consists of a marker set and a segment pose estimation algorithm. Plugin marker set and inverse kinematic algorithm (IK.) are prevalent in gait analysis, especially musculoskeletal motion analysis. ...
Effects of vibration therapy for post-stroke spasticity: a systematic review and meta-analysis of randomized controlled trials
The efficacy of vibration therapy (VT) in people with post-stroke spasticity (PSS) remains uncertain. This study aims to conduct a comprehensive meta-analysis to assess the effectiveness of VT in PSS.
- Editorial Board
- Manuscript editing services
- Instructions for Editors
- Sign up for article alerts and news from this journal
- ISSN: 1475-925X (electronic)
BioMedical Engineering OnLine
ISSN: 1475-925X
- Submission enquiries: [email protected]
biomedical engineering Recently Published Documents
Total documents.
- Latest Documents
- Most Cited Documents
- Contributed Authors
- Related Sources
- Related Keywords
Ophthalmological instruments of Al-Halabi fill in a gap in the biomedical engineering history
Virus-like particles: revolutionary platforms for developing vaccines against emerging infectious diseases.
Virus-like particles (VLPs) are nanostructures that possess diverse applications in therapeutics, immunization, and diagnostics. With the recent advancements in biomedical engineering technologies, commercially available VLP-based vaccines are being extensively used to combat infectious diseases, whereas many more are in different stages of development in clinical studies. Because of their desired characteristics in terms of efficacy, safety, and diversity, VLP-based approaches might become more recurrent in the years to come. However, some production and fabrication challenges must be addressed before VLP-based approaches can be widely used in therapeutics. This review offers insight into the recent VLP-based vaccines development, with an emphasis on their characteristics, expression systems, and potential applicability as ideal candidates to combat emerging virulent pathogens. Finally, the potential of VLP-based vaccine as viable and efficient immunizing agents to induce immunity against virulent infectious agents, including, SARS-CoV-2 and protein nanoparticle-based vaccines has been elaborated. Thus, VLP vaccines may serve as an effective alternative to conventional vaccine strategies in combating emerging infectious diseases.
Biomedical Engineering Technologies
Biocybernetics and biomedical engineering – current trends and challenges, deep learning models principles applied to biomedical engineering, deep learning models evolution applied to biomedical engineering, ieee transactions on biomedical engineering (t-bme), biomedical engineering and occupational therapy approach in technologies for enhancement human labor and defense abilities, artificial intelligence models applied to biomedical engineering, introduction to cognitive science, cognitive computing, and human cognitive relation to help in the solution of artificial intelligence biomedical engineering problems, export citation format, share document.
Biomedical Research Paper Topics

This page offers students an extensive list of biomedical research paper topics , expert advice on how to choose these topics, and guidance on how to write a compelling biomedical research paper. The guide also introduces the services of iResearchNet, an academic assistance company that caters to the unique needs of each student. Offering expert writers, custom-written works, and a host of other features, iResearchNet provides the tools and support necessary for students to excel in their biomedical research papers.
100 Biomedical Research Paper Topics
Biomedical research is a vibrant field, with an extensive range of topics drawn from various sub-disciplines. It encompasses the study of biological processes, clinical medicine, and even technology and engineering applied to the domain of healthcare. Given the sheer breadth of this field, choosing a specific topic can sometimes be overwhelming. To help you navigate this rich landscape, here is a list of biomedical research paper topics, divided into ten categories, each with ten specific topics.
Academic Writing, Editing, Proofreading, And Problem Solving Services
Get 10% off with 24start discount code.
1. Genetics and Genomics
- Role of genetics in rare diseases
- Advances in gene editing: CRISPR technology
- Human genome project: findings and implications
- Genetic basis of cancer
- Personalized medicine through genomics
- Epigenetic modifications and disease progression
- Genomic data privacy and ethical implications
- Role of genetics in mental health disorders
- Prenatal genetic screening and ethical considerations
- Gene therapy in rare genetic disorders
2. Bioengineering and Biotechnology
- Tissue engineering in regenerative medicine
- Bioprinting of organs: possibilities and challenges
- Role of nanotechnology in targeted drug delivery
- Biosensors in disease diagnosis
- Bioinformatics in drug discovery
- Development and application of biomaterials
- Bioremediation and environmental cleanup
- Biotechnology in agriculture and food production
- Therapeutic applications of stem cells
- Role of biotechnology in pandemic preparedness
3. Neuroscience and Neurology
- Pathophysiology of Alzheimer’s disease
- Advances in Parkinson’s disease research
- Role of neuroimaging in mental health diagnosis
- Understanding the brain-gut axis
- Neurobiology of addiction
- Role of neuroplasticity in recovery from brain injury
- Sleep disorders and cognitive function
- Brain-computer interfaces: possibilities and ethical issues
- Neural correlates of consciousness
- Epigenetic influence on neurodevelopmental disorders
4. Immunology
- Immune response to COVID-19
- Role of immunotherapy in cancer treatment
- Autoimmune diseases: causes and treatments
- Vaccination and herd immunity
- The hygiene hypothesis and rising allergy prevalence
- Role of gut microbiota in immune function
- Immunosenescence and age-related diseases
- Role of inflammation in chronic diseases
- Advances in HIV/AIDS research
- Immunology of transplantation
5. Cardiovascular Research
- Advances in understanding and treating heart failure
- Role of lifestyle factors in cardiovascular disease
- Cardiovascular disease in women
- Hypertension: causes and treatments
- Pathophysiology of atherosclerosis
- Role of inflammation in heart disease
- Novel biomarkers for cardiovascular disease
- Personalized medicine in cardiology
- Advances in cardiac surgery
- Pediatric cardiovascular diseases
6. Infectious Diseases
- Emerging and re-emerging infectious diseases
- Role of antiviral drugs in managing viral diseases
- Antibiotic resistance: causes and solutions
- Zoonotic diseases and public health
- Role of vaccination in preventing infectious diseases
- Infectious diseases in immunocompromised individuals
- Role of genomic sequencing in tracking disease outbreaks
- HIV/AIDS: prevention and treatment
- Advances in malaria research
- Tuberculosis: challenges in prevention and treatment
7. Aging Research
- Biological mechanisms of aging
- Impact of lifestyle on healthy aging
- Age-related macular degeneration
- Role of genetics in longevity
- Aging and cognitive decline
- Social aspects of aging
- Advances in geriatric medicine
- Aging and the immune system
- Role of physical activity in aging
- Aging and mental health
8. Endocrinology
- Advances in diabetes research
- Obesity: causes and health implications
- Thyroid disorders: causes and treatments
- Role of hormones in mental health
- Endocrine disruptors and human health
- Role of insulin in metabolic syndrome
- Advances in treatment of endocrine disorders
- Hormones and cardiovascular health
- Reproductive endocrinology
- Role of endocrinology in aging
9. Mental Health Research
- Advances in understanding and treating depression
- Impact of stress on mental health
- Advances in understanding and treating schizophrenia
- Child and adolescent mental health
- Mental health in the elderly
- Impact of social media on mental health
- Suicide prevention and mental health services
- Role of psychotherapy in mental health
- Mental health disparities
10. Oncology
- Advances in cancer immunotherapy
- Role of genomics in cancer diagnosis and treatment
- Lifestyle factors and cancer risk
- Early detection and prevention of cancer
- Advances in targeted cancer therapies
- Role of radiation therapy in cancer treatment
- Cancer disparities and social determinants of health
- Pediatric oncology: challenges and advances
- Role of stem cells in cancer
- Cancer survivorship and quality of life
These biomedical research paper topics represent a wide array of studies within the field of biomedical research, providing a robust platform to delve into the intricacies of human health and disease. Each topic offers a unique opportunity to explore the remarkable advancements in biomedical research, contributing to the ongoing quest to enhance human health and wellbeing.
Choosing Biomedical Research Paper Topics
The selection of a suitable topic for your biomedical research paper is a critical initial step that will largely influence the course of your study. The right topic will not only engage your interest but will also be robust enough to contribute to the existing body of knowledge. Here are ten tips to guide you in choosing the best topic for your biomedical research paper.
- Relevance to Your Coursework and Interests: Your topic should align with the courses you have taken or are currently enrolled in. Moreover, a topic that piques your interest will motivate you to delve deeper into research, resulting in a richer, more nuanced paper.
- Feasibility: Consider the practicality of your proposed research. Do you have access to the necessary resources, including the literature, laboratories, or databases needed for your study? Ensure that your topic is one that you can manage given your resources and time constraints.
- Novelty and Originality: While it is essential to ensure your topic aligns with your coursework and is feasible, strive to select a topic that brings a new perspective or fresh insight to your field. Originality enhances the contribution of your research to the broader academic community.
- Scope: A well-defined topic helps maintain a clear focus during your research. Avoid choosing a topic too broad that it becomes unmanageable, or so narrow that it lacks depth. Balancing the scope of your research is key to a successful paper.
- Future Career Goals: Consider how your chosen topic could align with or benefit your future career goals. A topic related to your future interests can provide an early start to your career, showcasing your knowledge in that particular field.
- Available Supervision and Mentoring: If you’re in a setting where you have a mentor or supervisor, choose a topic that fits within their area of expertise. This choice will ensure you have the best possible guidance during your research process.
- Ethical Considerations: Some topics may involve ethical considerations, particularly those involving human subjects, animals, or sensitive data. Make sure your topic is ethically sound and you’re prepared to address any related ethical considerations.
- Potential Impact: Consider the potential impact of your research on the field of biomedical science. The best research often addresses a gap in the current knowledge or has the potential to bring about change in healthcare practices or policies.
- Literature Gap: Literature review can help identify gaps in the existing body of knowledge. Choosing a topic that fills in these gaps can make your research more valuable and unique.
- Flexibility: While it’s essential to start with a clear topic, remain open to slight shifts or changes as your research unfolds. Your research might reveal a different angle or a more exciting question within your chosen field, so stay flexible.
Remember, choosing a topic should be an iterative process, and your initial ideas will likely evolve as you conduct a preliminary literature review and discuss your thoughts with your mentors or peers. The ultimate goal is to choose a topic that you are passionate about, as this passion will drive your work and make the research process more enjoyable and fulfilling.
How to Write a Biomedical Research Paper
Writing a biomedical research paper can be a daunting task. However, with careful planning and strategic execution, the process can be more manageable and rewarding. Below are ten tips to help guide you through the process of writing a biomedical research paper.
- Understand Your Assignment: Before you begin your research or writing, make sure you understand the requirements of your assignment. Know the expected length, due date, formatting style, and any specific sections or components you need to include.
- Thorough Literature Review: A comprehensive literature review allows you to understand the current knowledge in your research area and identify gaps where your research can contribute. It will help you shape your research question and place your work in context.
- Clearly Define Your Research Question: A well-defined research question guides your research and keeps your writing focused. It should be clear, specific, and concise, serving as the backbone of your study.
- Prepare a Detailed Outline: An outline helps organize your thoughts and create a roadmap for your paper. It should include all the sections of your research paper, such as the introduction, methods, results, discussion, and conclusion.
- Follow the IMRaD Structure: Most biomedical research papers follow the IMRaD format—Introduction, Methods, Results, and Discussion. This structure facilitates the orderly and logical presentation of your research.
- Use Clear and Concise Language: Biomedical research papers should be written in a clear and concise manner to ensure the reader understands the research’s purpose, methods, and findings. Avoid unnecessary jargon and ensure that complex ideas are explained clearly.
- Proper Citation and Reference: Always properly cite the sources of information you use in your paper. This not only provides credit where it’s due but also allows your readers to follow your line of research. Be sure to follow the citation style specified in your assignment.
- Discuss the Implications: In your discussion, go beyond simply restating your findings. Discuss the implications of your results, how they relate to previous research, and how they contribute to the existing knowledge in the field.
- Proofread and Edit: Never underestimate the importance of proofreading and editing. Checking for grammatical errors, punctuation mistakes, and clarity of language can enhance the readability of your paper.
- Seek Feedback Before Final Submission: Before submitting your paper, seek feedback from peers, mentors, or supervisors. Fresh eyes can often spot unclear sections or errors that you may have missed.
Writing a biomedical research paper is a significant academic endeavor, but remember that every researcher started where you are right now. It’s a process that requires time, effort, and patience. Remember, the ultimate goal is not just to get a good grade but also to contribute to the vast body of biomedical knowledge.
iResearchNet’s Custom Writing Services
Navigating the process of writing a biomedical research paper can be complex and demanding. At iResearchNet, we understand these challenges and strive to offer a stress-free, seamless solution to support your academic journey. With our roster of highly skilled, degree-holding writers, we are committed to delivering top-quality, custom-written papers tailored specifically to your individual requirements and desired outcomes.
- Expert Degree-Holding Writers: iResearchNet takes pride in our team of knowledgeable and experienced writers who hold advanced degrees in diverse fields. These writers are not only academic experts but are also keenly in tune with the complex landscape of biomedical research. This breadth and depth of expertise ensure that your paper benefits from a thorough understanding of the topic, resulting in a well-informed, academically credible document.
- Custom Written Works: We appreciate the unique academic goals and distinct requirements of each student. That’s why iResearchNet specializes in providing custom-written papers. Our aim is to capture your individual academic voice and perspective, blending it with our professional acumen to create a paper that reflects your specific academic needs and aspirations.
- In-Depth Research: Every paper that we produce is founded on the bedrock of extensive and in-depth research. Our writers are committed to exploring a wide range of credible and reputable sources to enrich your paper with diverse viewpoints and comprehensive information. This dedication to rigorous research ensures that your paper is not only thoroughly informed but also accurately referenced, adding to its academic integrity.
- Custom Formatting: Academic institutions often require different formatting styles. Be it APA, MLA, Chicago/Turabian, or Harvard, our writers are adept at all these academic formatting styles. We strive to adhere strictly to your specified formatting style, contributing to the polished and professional presentation of your paper.
- Top Quality: Quality is the cornerstone of our services at iResearchNet. We believe that each paper we craft should demonstrate a high standard of scholarship. Our writers dedicate their skills and effort to ensure every aspect of your paper, from clarity of language to depth of analysis and precision of information, reflects top-quality work.
- Customized Solutions: Recognizing that each research paper brings a distinct set of challenges and requirements, we offer customized solutions. Our approach is to thoroughly understand your specific needs and shape our writing services accordingly. We ensure that every aspect of your paper, from its overarching structure to the smallest details, aligns with your expectations.
- Flexible Pricing: We believe that high-quality academic writing services should be accessible. That’s why we offer our top-quality services at competitive prices, striking a careful balance between affordability and excellence. We provide a range of pricing options designed to cater to various budget needs without compromising on the quality of our services.
- Short Deadlines: Facing a fast-approaching deadline can be nerve-wracking. But with iResearchNet, you need not worry. Our expert writers are skilled at delivering high-quality papers under tight deadlines, even as short as three hours. Despite the time pressure, we ensure that the quality of your paper remains top-notch.
- Timely Delivery: At iResearchNet, we understand the critical importance of adhering to deadlines in the academic world. We commit to the timely delivery of all orders, ensuring that you are always able to submit your work on time. With our service, you can put aside worries about late submissions.
- 24/7 Support: Academic queries or concerns can arise at any time, and we are here to assist you around the clock. We have a dedicated support team ready to answer your questions, address your concerns, or simply provide guidance about your project, at any time of the day or night.
- Absolute Privacy: Your privacy is of utmost importance to us. All personal and financial information you share with us is handled with the highest level of confidentiality and security. Our strict privacy policies ensure that your details remain safe and private.
- Easy Order Tracking: We believe in providing a seamless experience for our clients. With our user-friendly platform, you can track your order’s progress easily and stay updated on your paper’s status. This feature provides real-time status reports, giving you peace of mind and assurance about the progress of your work.
- Money Back Guarantee: Your satisfaction is our ultimate goal. We strive to meet your expectations, but if for any reason the final work falls short, we offer a money-back guarantee. This policy is a testament to our confidence in the quality of our services and our commitment to your academic success.
At iResearchNet, we strive to be more than just a writing service provider. We aspire to be a trusted academic partner, providing support and expertise to help you navigate the complexities of writing a biomedical research paper. With our combination of expert knowledge, high commitment to quality, and excellent customer service, we are the ideal choice for all your academic writing needs.
Start Your Journey to Academic Success with iResearchNet Today!
Are you ready to elevate your academic journey and achieve your full potential? At iResearchNet, we are prepared to be your trusted partner every step of the way. Our team of expert writers, experienced in biomedical research, are ready and waiting to transform your academic vision into a top-quality, custom-written biomedical research paper that meets all your requirements.
Navigating the complexities of biomedical research can be overwhelming, but with iResearchNet, you don’t have to do it alone. Our dedicated team of professionals is committed to taking the stress out of the writing process, allowing you to focus on your learning. Imagine the relief of knowing your assignment is in the hands of experienced, degree-holding experts who are passionate about your success. With our meticulous research and thorough understanding of biomedical topics, we guarantee a paper that not only meets but surpasses your expectations.
From in-depth research and custom formatting to a final product that reflects the highest academic standards, iResearchNet provides a comprehensive solution for your academic needs. And it’s not just about delivering excellent papers. Our commitment extends to providing an exceptional experience marked by 24/7 support, absolute privacy, and a transparent order tracking system.
The clock is ticking, and your academic success is just a click away. Don’t let the opportunity to excel in your biomedical research paper slip through your fingers. Reach out to us today to start your journey with iResearchNet. You bring your academic aspirations, and we’ll bring our expertise and commitment. Together, let’s make your academic dreams come true.
ORDER HIGH QUALITY CUSTOM PAPER

Enjoy a completely custom, expertly-written dissertation. Choose from hundreds of writers, all of whom are career specialists in your subject.
45 Biomedical Research Topics for You
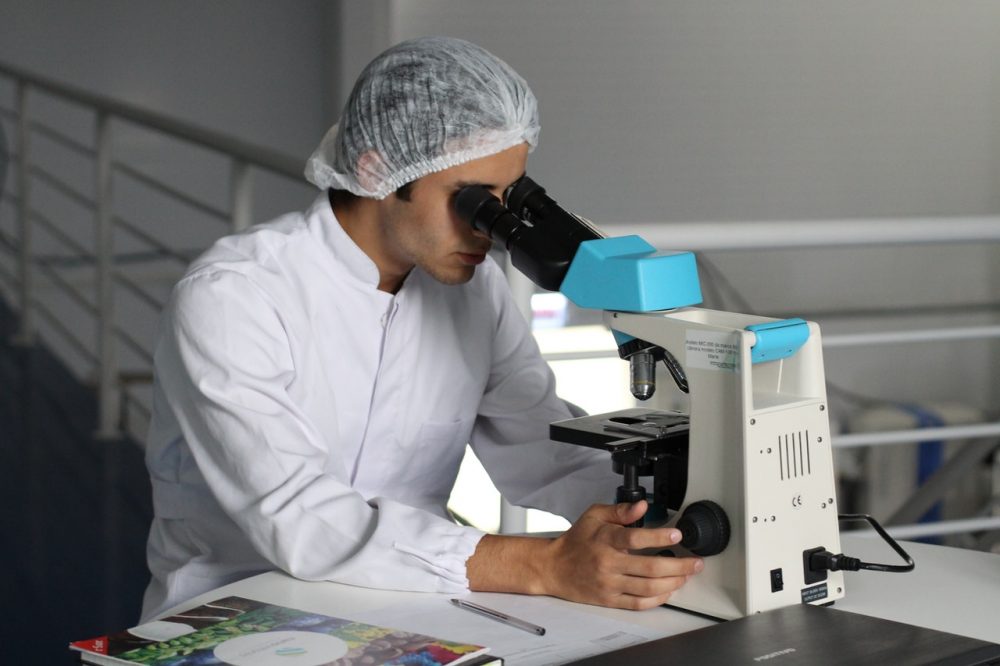
Although choosing relevant biomedical research topics is often an arduous task for many, it shouldn’t be for you. You no longer have to worry as we have provided you with a list of topics in biomedical science in this write-up.
Biomedical research is a broad aspect of science, and it is still evolving. This aspect of science involves a variety of ways to prevent and treat diseases that lead to illness and death in people.
This article contains 45 biomedical topics. The topics were carefully selected to guide you in choosing the right topics. They can be used for presentations, seminars, or research purposes, as the case may be.
So, suppose you need topics in biomedical ethics for papers or biomedical thesis topics for various purposes. In that case, you absolutely have to keep reading! Are you ready to see our list of biomedical topics? Then, let’s roll.
Biomedical Engineering Research Topics
Biomedical engineering is the branch of engineering that deals with providing solutions to problems in medicine and biology. Biomedical engineering research is an advanced area of research. Are you considering taking up research in this direction?
Research topics in this area cannot just be coined while eating pizza. It takes a lot of hard work to think out something meaningful. However, we have made a list for you! Here is a list of biomedical engineering topics!
- How to apply deep learning in biomedical engineering
- Bionics: the latest discoveries and applications
- The techniques of genetic engineering
- The relevance of medical engineering today
- How environmental engineering has affected the world
Biomedical Ethics Topics
There are ethical issues surrounding healthcare delivery, research, biotechnology, and medicine. Biomedical Ethics is fundamental to successful practice experience and is addressed by various disciplines. If you want to research this area, then you do not have to look for topics. Here’s a list of biomedical ethics for paper that you can choose from:
- The fundamentals of a physician-patient relationship
- How to handle disability issues as a health care sector
- Resource allocation and distribution
- All you need to know about coercion, consent, and or vulnerability
- Ethical treatment of subjects or animals in clinical trials
Relevant Biomedical Topics
Topics in Biomedical science are numerous, but not all are relevant today. Since biomedical science is constantly evolving, newer topics are coming up. If you desire in your topic selection, read on. Here is a list of relevant biomedical topics just for you!
- The replacement of gene therapy by gene editing
- Revolution of vaccine development by synthetic biology
- Introduction of artificial blood – the impact on the health sector
- Ten things know about artificial womb
- Transplanted reproductive organs and transgender birth
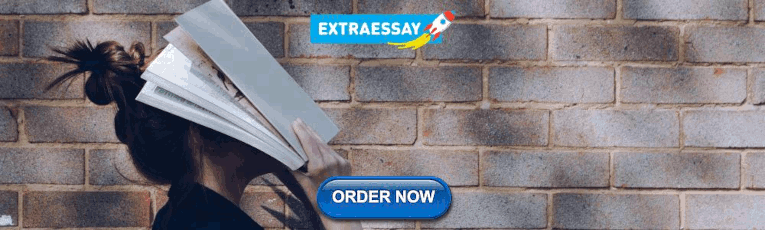
Biomedical Science Topics
Biomedical science is the aspect of scientific studies that focuses on applying biology and chemistry to health care. This field of science has a broad range of disciplines. If you intend to do research in this field, look at this list of research topics in biomedical science.
- The role of biomechanics in health care delivery
- Importance of biomaterials and regeneration engineering
- The application of cell and molecular engineering to medicine
- The evolution of medical instrumentation and devices
- Neural engineering- the latest discoveries
Seminar Topics for Biomedical Instrumentation
Biomedical science is constantly making progress, especially in the aspect of biomedical instrumentation. This makes it worthy of a seminar presentation in schools where it is taught. However, choosing a biomedical research topic for a biomedical instrumentation seminar may not come easy. This is why we have collated five brilliant topics for biomedical instrumentation just for you. They include:
- Microelectrode in neuro-transplants
- Hyperbaric chamber for oxygen therapy
- How concentric ring electrodes can be used to manage epilepsy
- How electromagnetic interference makes cochlear implants work
- Neuroprosthetics Management using Brain-computer interfaces (BCI)
Biomedical Engineering Topics for Presentation
One of the interesting aspects of biomedical science in biomedical engineering. It is the backbone that gives the biomedical science structure. Are you interested in making presentations about biomedical engineering topics? Or do you need biomedical engineering topics for paper? Get started here! We have compiled a list of biomedical engineering topics for you. Here they are:
- In-the-ear device to control stuttering: the basis of its operation
- How to implement the magnetic navigated catheterization
- Semiconductor-cell interfaces: the rudiments of its application
- The benefits of tissue engineering of muscle
- The benefits of sensitive artificial skin for prosthetic arms
Hot Topics in Biomedical Research
Biomedical research is fun because it is often relatable. As interesting as it seems, choosing a topic for research doesn’t come easy at all. Yet, there are also a lot of trending events around biomedical topics. To simplify your selection process, we have written out a few of them here.
Here are some hot biomedical research topics below.
- What is immunology, and what is the relevance today?
- Regenerative medicine- definition, importance, and application
- Myths about antibiotic resistance
- Vaccine development for COVID-19
- Infectious diseases now and before
Biomedical Research Topics
Biomedical research is an extensive process. It requires a lot of time, dedication, and resources. Getting a topic shouldn’t be added to that list. There are biomedical thesis topics and research topics in biomedical science for you here:
- Air pollution- sources, impact, and prevention
- Covid-19 vaccination- the effect on life expectancy
- Hyper insomnia- what is responsible?
- Alzheimer’s disease- newer treatment approaches
- Introduction of MRI compatible infusion pump
Biomedical Nanotechnology Topics
Biomedical research topics and areas now include nanotechnology. Nanotechnology has extended its tentacles to medicine and has been used to treat cancer successfully. This makes it a good research area. It is good for seminar presentations. Here are some biomedical nanotechnology topics below.
- The uses of functional particles and nanomaterials
- Nanoparticles based drug delivery system
- The incorporation of nanoporous membranes into biomedical devices
- Nanostructured materials for biological sensing
- Nanocrystals- imaging, transportation, and toxicity features
Seeking professional assistance to write your biomedical research or thesis? Look no further! At our reputable writing service, our experienced writers specialize in providing tailored support for the complexities of biomedical research. When you say, “ do my thesis for me ” we’re here to guide you through formulating research questions, conducting literature reviews, and analyzing data sets. Entrust the writing process to our experts while you focus on exploring the frontiers of biomedical research. Contact us today for a meticulously crafted thesis that enhances your chances of success.
We believe you have been thoroughly equipped with a list of biomedical topics. This way, you wouldn’t have to go through the stress of choosing a topic for research, seminars, or other educational purposes. Now that you have the topics at your fingertips make your choice and enjoy!
Frequently Asked Questions
Richard Ginger is a dissertation writer and freelance columnist with a wealth of knowledge and expertise in the writing industry. He handles every project he works on with precision while keeping attention to details and ensuring that every work he does is unique.
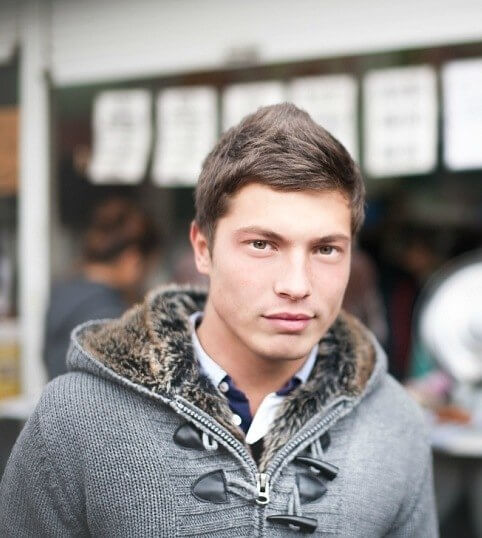
Succeed With A Perfect Dissertation
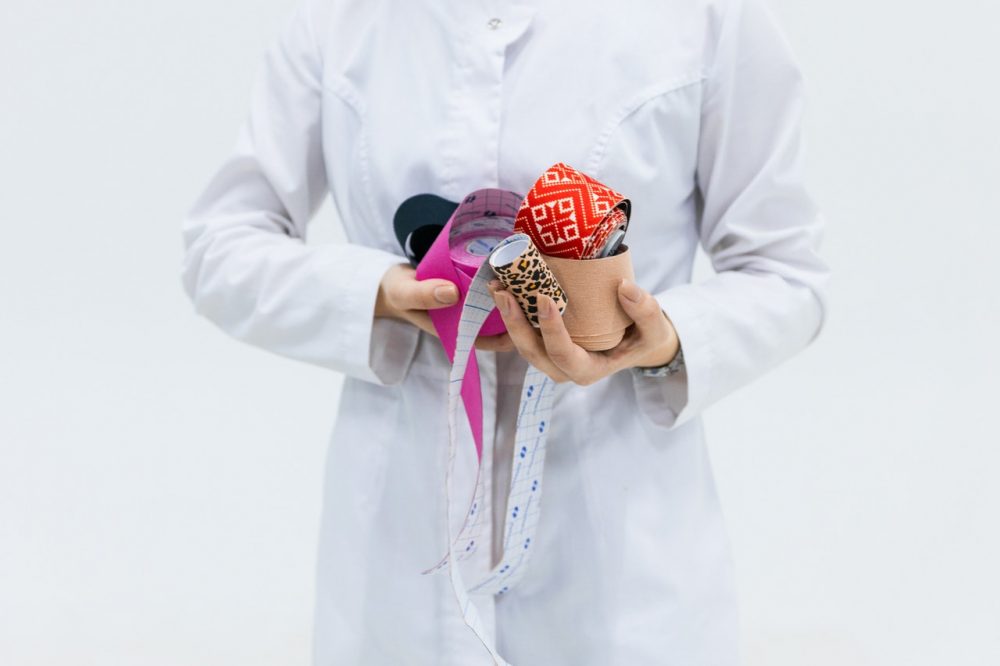
Leave a Reply Cancel reply
Your email address will not be published. Required fields are marked *
Save my name, email, and website in this browser for the next time I comment.
As Putin continues killing civilians, bombing kindergartens, and threatening WWIII, Ukraine fights for the world's peaceful future.
Ukraine Live Updates
- Jacobs School of Medicine and Biomedical Sciences
- School of Engineering and Applied Sciences
- UB Directory
- Department of Biomedical Engineering >
- Research >
Research Areas
Our research is spread across four broad areas. Our faculty work within and across these specific areas, and our students explore topics throughout them.
Molecular, Cellular and Tissue Engineering
As our understanding of how cells and tissues function at the molecular level improves, we are able to attempt to use this knowledge to develop radically different approaches to treating disease. For instance, if a patient is suffering from a faulty liver, tissue engineering may one day allow a new liver to be grown from the patient's own cells. This research includes developing and functionalizing new materials to grow cells, and eventually developing regenerated tissues and organs.
- Barbara Rita Alevriadou , PhD
- Ramkumar T. Annamalai , PhD
- Jonathan Lovell , PhD
- Debanjan Sarkar , PhD
- Jing Wang , PhD
- Yun Wu , PhD
- Ruogang Zhao , PhD
Biomedical Devices, Sensors, Instrumentation and Diagnostics
Improved understanding and prevention of disease can come from developing better systems to detect various analytes such as DNA, bacteria or functional molecules such as oxygenated blood in the body. New biomaterials can be engineered to replace damaged bones and joints. Advances in micro- and nano-electronics is enabling new devices for disease detection and monitoring in humans. By interfacing medical problems with molecular signatures and cutting-edge hardware detection, we can engineer new systems for detection of a huge range of molecules and processes. Our research in this area includes orthopedics, microfluidics, biomedical instrumentation, photoacoustic tomography, and DNA molecular detectors.
- Mark Ehrensberger , PhD
- Ciprian N. Ionita , PhD
- Filip Stefanovic , PhD
- Albert H. Titus , PhD
- Jun Xia , PhD
- Xiaoliang Zhang , PhD
Biomedical Artificial Intelligence, Computational Engineering and Modeling
Past decades have seen unbelievable improvements in computer processing power. This opens up a world of "in silico" possibilities for finding new solutions to challenging biomedical problems. Our research in this field involves developing novel data processing approaches, including biomedical artificial intelligence (AI), to improve medical diagnostics and healthcare. For example, biomedical AI, such as machine leaning and deep learning, enhances MRI diagnoses through fast imaging and reliable biomarker quantification, and assists surgeries with realistic tissue vascular models.
- Junghun Cho , PhD
- Leslie (Lei) Ying , PhD
Medical Imaging and Analysis
Imaging continues to play an important role in all aspects of medicine. We are developing new approaches to obtain and process data from multiple imaging modalities. For example, magnetic resonance imaging (MRI) is an appealing imaging modality that can, non-invasively, provide unprecedented levels of information about functional and pathological events that occur in the human body. Other modalities continue to evolve and improve. An example of this is X-ray imaging which is benefiting from solid-state detectors with improved sensitivities that can achieve better images with lower dosages. Also, new software analysis algorithms are required to keep up with the rapid pace of development in medical imaging hardware. Research in the department includes developing improved MRI image processing algorithms and X-ray imaging.
- Jungeun (Jenny) Won , PhD
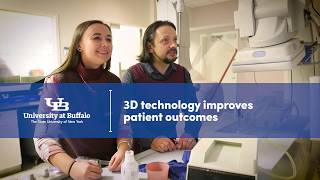
Combining knowledge of the human system and engineering-based quantitative problem-solving skills, biomedical engineering is at the forefront of research and development today. Spanning both the Jacobs School of Medicine and Biomedical Science and the School of Engineering and Applied Sciences, our faculty and students work on projects without limitations or departmental boundaries.
- 12/1/23 Learn more about our research.

Our core courses provide hands-on engineering experiences that involve design and modeling. Our students are encouraged to explore particular interests in greater depth. Students have numerous opportunities to participate in research and watch medical discoveries move from bench to bedside.
- 9/1/23 Learn what sets UB BME apart.

Our researchers developed a new drug delivery method to target cancer cells — not the entire body — and limit the side effects of chemotherapy. This image shows a nanoballoon before (left) and after (right) being hit by a red laser. The laser causes the balloon to pop open and release the anti-cancer drugs directly at a tumor. Credit: Jonathan Lovell.
- Learn more about the new drug delivery method.

We provide the environment for you to excel. Our faculty members work closely with students as part of their research activities, providing them with the skills and knowledge needed for them to become successful researchers themselves.
- 12/1/23 Learn more about our research areas.

Search form
Research focus areas.
Focused on solving some of the toughest problems facing Georgia, the nation, and the world.
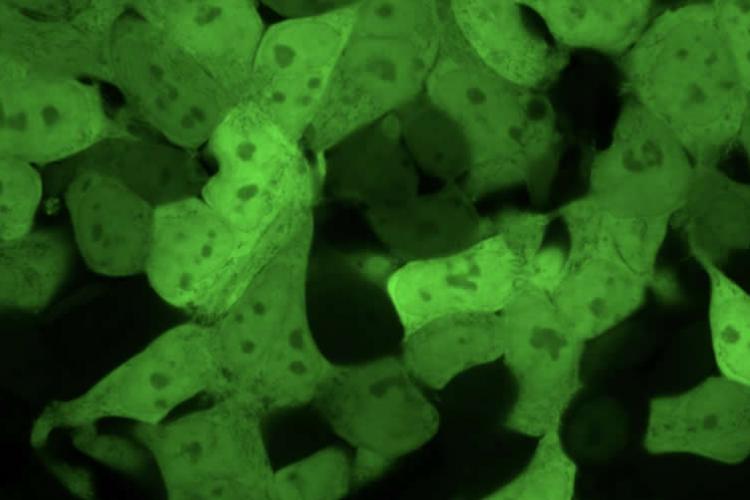
Latest BME Research News
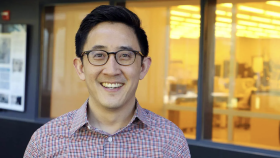
Tim Cope and Nick Housley unravel the neural pathways behind complex sensory and motor side effects of chemotherapy
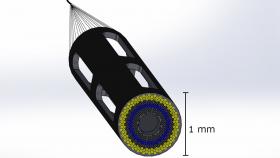
Meet our outstanding faculty members who are leading the way for biomedical research.
Our researchers are the best and the brightest, building a better world for medicine.
Essential members of the department, conducting research with our faculty and engaging with our students.
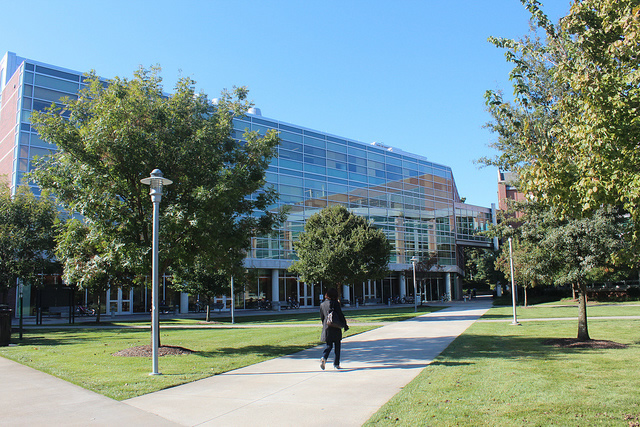
Our world-class facilities help advance the boundaries of biomedical engineering research.
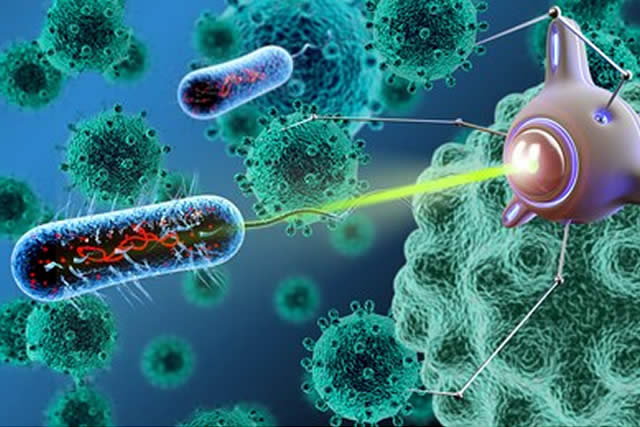
Building a better world for medicine. Explore our research .
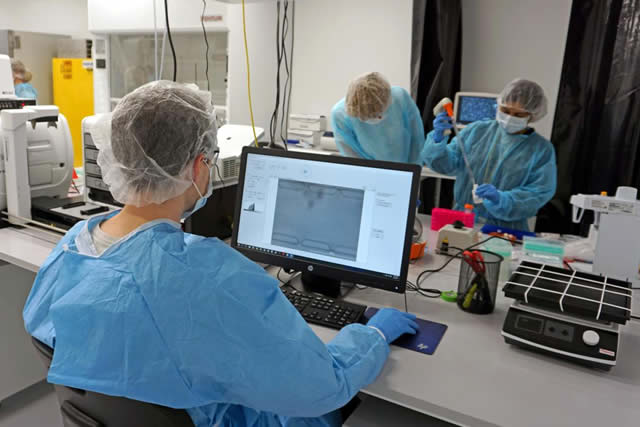
Biolocity provides Emory and Georgia Tech innovators with resources to commercialize their new technologies, therapies, and diagnostics.
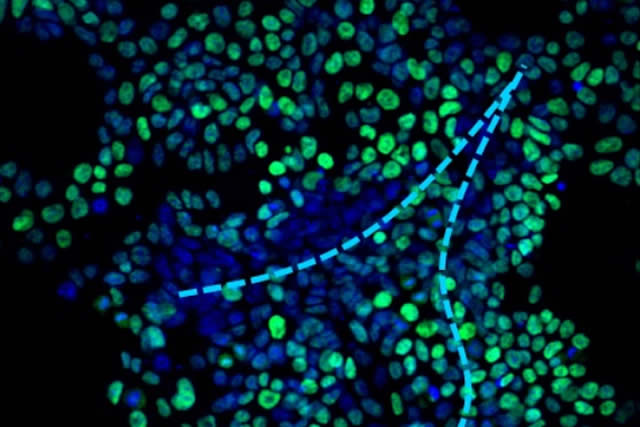
Connecting the best minds for a better world.
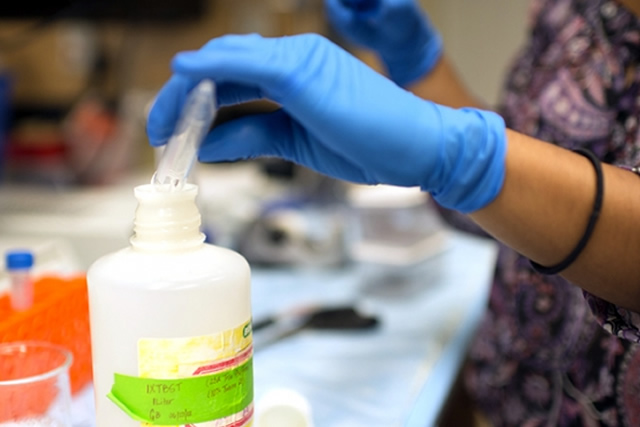
Finding breakthrough solutions for improving life. Let's collaborate .
You can actually invent things here.

An official website of the United States government
The .gov means it’s official. Federal government websites often end in .gov or .mil. Before sharing sensitive information, make sure you’re on a federal government site.
The site is secure. The https:// ensures that you are connecting to the official website and that any information you provide is encrypted and transmitted securely.
- Publications
- Account settings
Preview improvements coming to the PMC website in October 2024. Learn More or Try it out now .
- Advanced Search
- Journal List
- Int J Mol Sci

Mathematical Modelling in Biomedicine: A Primer for the Curious and the Skeptic
1 Laboratory of Systems Tumor Immunology, Comprehensive Cancer Center Erlangen and Deutsches Zentrum Immuntherapie (DZI), Department of Dermatology, FAU Erlangen-Nürnberg, Universitätsklinikum Erlangen, 91054 Erlangen, Germany; [email protected] (C.L.); [email protected] (X.L.); [email protected] (M.E.)
Christopher Lischer
Momchil nenov.
2 Institute of Mechanics, Bulgarian Academy of Sciences, Acad. G. Bonchev Str., bl. 4, 1113 Sofia, Bulgaria; [email protected] (M.N.); [email protected] (S.N.)
Svetoslav Nikolov
Martin eberhardt, associated data.
In most disciplines of natural sciences and engineering, mathematical and computational modelling are mainstay methods which are usefulness beyond doubt. These disciplines would not have reached today’s level of sophistication without an intensive use of mathematical and computational models together with quantitative data. This approach has not been followed in much of molecular biology and biomedicine, however, where qualitative descriptions are accepted as a satisfactory replacement for mathematical rigor and the use of computational models is seen by many as a fringe practice rather than as a powerful scientific method. This position disregards mathematical thinking as having contributed key discoveries in biology for more than a century, e.g., in the connection between genes, inheritance, and evolution or in the mechanisms of enzymatic catalysis. Here, we discuss the role of computational modelling in the arsenal of modern scientific methods in biomedicine. We list frequent misconceptions about mathematical modelling found among biomedical experimentalists and suggest some good practices that can help bridge the cognitive gap between modelers and experimental researchers in biomedicine. This manuscript was written with two readers in mind. Firstly, it is intended for mathematical modelers with a background in physics, mathematics, or engineering who want to jump into biomedicine. We provide them with ideas to motivate the use of mathematical modelling when discussing with experimental partners. Secondly, this is a text for biomedical researchers intrigued with utilizing mathematical modelling to investigate the pathophysiology of human diseases to improve their diagnostics and treatment.
1. Science: A World of Systems (and Models)
A good portion of science relies on the cascades of models that represent our reality at different spatial and temporal scales. A model is a simplified and often abstract representation of a complex natural system. Models are used in science to help understand, hypothesize about, or simulate the behavior of the natural systems they represent. Since a model is an abstraction of the natural system, it often includes only those elements, interactions, and processes of the system that are required to investigate the hypotheses in question. By exploiting the innate ability of the human brain to work with abstractions, models make highly complex phenomena accessible to study. In this sense, they can be used as a tool to develop hypotheses and to conceive and execute experiments to test those hypotheses. Models also provide an overview of the current knowledge on the natural system in question, facilitating the exchange of up-to-date information between researchers working on the same topic. Moreover, models can be used to simulate aspects of the natural system. A simulation is a rule-based recapitulation of the natural system’s behavior under relevant conditions using the model.
Since the features of a model are tightly linked to the purpose we want to give to it, there are multiple types of models. Models that are common to any branch of science are semantic models ; these consist in verbalization of the natural system’s features and the hypothesis using natural language. This is the type of model that underlies the results and discussion sections in most scientific papers.
More important for our discussion are the “lab bench” models and the “mathematical” models ( Figure 1 ). A lab bench model is a simplified or analogous version of a natural system employed in experiments under controlled conditions. In molecular and cell biology, lab bench models are usually units of life that can be conveniently propagated and studied in experiments to understand a given biological phenomenon. The consensus is that discoveries made via experimentation with the lab bench model provide insights into the behavior of similar phenomena in other organisms, especially humans. This is the case for cell lines, organoids, and mouse or rat strains with given genotypes or phenotypes, which have been used consistently in biomedicine as models for many human diseases.

Science employs different types of models to represent natural systems. Let us suppose that we are interested in investigating the properties and potential vulnerabilities of a melanoma metastasis (here, the “ natural system ”, visualized with MELC microscopy as in Ostalecki et al. 2017 [ 1 ]). One can represent the natural system with a semantic model , that is, the verbalization in natural language of the key compounds and processes as well as the hypotheses about a melanoma micrometastasis. Under some simplifying assumptions, a melanoma metastasis can be studied with lab bench models . For example, if one is interested in the interplay between cancer and immune cells, it is possible to co-culture tumor cells with relevant types of immune cells in vitro, like in Vescovi et al. 2019 [ 2 ]. In most cases, an alternative option is mathematical models , that is, sets of parametric equations that encode the key properties of metastasis and the hypotheses. The mathematical model is the basis for computational simulations to design experiments or to formulate or explore hypotheses like in Santos et al. 2016 [ 3 ].
A mathematical model is a set of parametric equations or other mathematical entities that encode the basic properties of the investigated natural system and that can be used to perform computational simulations. The same way that there are lab bench models with different features and purpose, there are different classes of mathematical models. One can classify them based on their treatment of the system’s dynamics as static models , which describe the system’s state at a point in time; comparative static models , which compare the properties of the system at different points in time; and dynamic models , which follow changes in the system over time. One can also classify models based on the mathematical apparatus they employ or the knowledge they exploit. There are mathematical models grounded in statistics that are used to process, analyze, and impute quantitative data generated in lab bench experiments or obtained from patient samples. All hypothesis tests and estimators of statistical correlation or inference between biological data sets are essentially (bio)statistical models.
However, there are also mathematical models that encode a mechanistic description of the natural system. This means the model equations and variables account for the interactions between the system’s key biological components and can be used for computational simulation of molecular and cellular processes. There are many subclasses of these models. A significant number of them reside in biophysics, developed to allow physically accurate simulations of atomic movement and molecular interactions in biomolecules including protein–protein interactions, DNA folding, or biomembrane dynamics. Other mechanistic models describe biochemical processes that shape cell phenotypes and cell-to-cell interactions. These are inspired by the mathematical models built in chemistry to understand and predict the kinetics of chemical reactions. In any case, just as one cannot elucidate all the mysteries of modern biomedicine with a single experimental technique, say confocal microscopy, a single subclass of mathematical model is not useful for every purpose. Every problem or hypothesis requires a carefully selected mathematical modelling approach.
2. The Scientific Method and the Role of Mathematical Modelling in It
Contemporary science consists in application of the scientific method and careful examination of the results one obtains with it. In the classical view, the scientific method is composed of three steps: observation of a natural phenomenon, elaboration of a hypothesis based on the observation, and design of an adequate experiment to test the hypothesis. If this is the way one would conceive scientific work nowadays, we would be strictly following the approaches employed by Galileo Galilei, Johannes Kepler, and Isaac Newton at the inception of modern science [ 4 ].
However, if you are a 21st century scientist, you are probably implementing a subtle variation of this method, which we will call the Einstein-grade scientific method. Here, the work is not performed in long-term isolation of a scientific ivory tower. Rather, the scientist continuously interacts with a community of peers. We retain the three basic steps mentioned above, but after the experimental test, one (more or less) immediately communicates the results in a booklet-length scientific publication. Early communication allows for your peers to try and reproduce your results. When experimental evidence accumulates and the underlying hypotheses are accepted, they are integrated in the scientific corpus of the field, that is, the set of theories, data, and hypotheses commonly accepted by the majority of researchers. Furthermore, new observations and experiments are continuously cross-checked against the scientific corpus in a way such that the method has an actual cyclic structure.
In the Einstein-grade scientific method, mathematical and computational modelling plays a pivotal role in many different manners ( Figure 2 ). Firstly, today’s “observations” in the majority of fields of natural sciences, including biomedicine, come as quantitative data that have to be processed, assessed, and analyzed with statistical models. In biomedicine, this is especially true in the case of sequencing data for the detection of common patterns in the sequence or the expression of genes in large cohorts of patients, a task impossible without sophisticated statistical and bioinformatics methods [ 5 ]. The features of these special mathematical models have a significant impact on the conclusions one can derive from sequencing data.

Sketch of the Einstein-grade scientific method (grey boxes) and the place that mathematical modelling occupies in it (blue boxes): the photograph of Albert Einstein is modified from the photo “Albert Einstein colorized” by Michael W. Gorth as stored in Wikimedia (CC-BY-SA-4.0, accession date: 10.08.2020; https://commons.wikimedia.org/wiki/File:Albert_Einstein_colourised_portrait.jpg ).
Secondly, computational models can be utilized to derive, ponder on, or substantiate hypotheses. Mathematical modelling has been employed to design validation experiments or to evaluate whether the results of the experiment agree with the expectations derived from the hypothesis and the scientific corpus . Finally, models can be used to achieve a formalized and unambiguous description of a field’s accepted knowledge in which theories, data, and hypotheses are organized and interconnected through equations. A remarkable example of this power is contained in a recent study by Meyer-Hermann and colleagues, in which a comprehensive mathematical model was used to summarize and elaborate around the contemporary knowledge on B-cell differentiation and maturation in germinal centers [ 6 ]. In one sense, Meyer-Hermann’s model is conceptually close to the equations that make up large physical theories, such as electromagnetism. In Supplementary Table S1 , we include and comment on select publications in which the different manners to deploy models are illustrated for biomedicine.
There are a few more issues to mention and discuss about the role of mathematical models in the scientific method. The formal language of a mathematical model enhances precision and clarity compared with natural-language descriptions in the semantic models usually preferred by biomedical experimentalists. Everybody with adequate training can develop an identical understanding of a statement formulated by an equation, for example, v = v max * S /( K M + S ). However, statements made in natural languages can be vague and can provoke misunderstandings. For example, is there a difference between “transcription factor X activates the expression of gene Y” and “transcription factor X promotes the expression of gene Y”?
The Einstein-grade method constitutes a collective endeavor. This means that it is common to find several researchers from different research institutions and locations and even with backgrounds complementing each other completing one cycle of the scientific method. For example, a German theoretical biologist, a sort of mathematical modeler, can publish a hypothesis based on his modelling efforts which will years later pique the interest of an Indonesian molecular biologist to perform experimental validation. Such occurrences are rather common in physics or chemistry, with a world record in some predictions made by Albert Einstein hundreds of years ago that have been experimentally validated only recently (which is why we use the term Einstein-grade here). However, they can also happen in biomedicine: Hodgkin and Huxley established in the first half of the 20th century a long-lasting scientific collaboration to elucidate the biophysical and biochemical mechanisms behind the initiation and propagation of action potential in nerve cell axons, a key phenomenon in understanding the ability of the nervous system to process and store information. The remarkable culmination of this collaboration was the amalgamation of their discoveries into a mathematical model which formalizes, explains, and quantifies the electrical excitability in nerve cells (see their seminal paper in Alan Hodgkin and Andrew Huxley 1952 [ 7 ] and a historical analysis in Schwiening 2012 [ 8 ]). Interestingly, some of the assumptions and simulations in the Hodgkin–Huxley model led Hodgkin’s team to predict already in 1955 that potassium ion channels can be occupied by multiple ions simultaneously. This prediction was confirmed using X-ray crystallography only in 1998, more than 40 years later, in a paradigmatic instance of the Einstein-grade scientific method in neurobiology [ 9 ].
Lastly, the scientific method is nowadays not monolithic and there are multiple variations of the general workflow commented on here [ 10 ]. This plurality of scientific methods may correspond to the different phases of discovery in diverse disciplines, the nature of the problems tackled, or even the styles of thinking belonging to different scientific communities. This notion applies also to mathematical modelling in biology and biomedicine [ 11 ].
3. The Love-and-Hate Relationship of Biology and Mathematics
The story of mathematics and modelling in biology is rather long, which might come as a surprise to many researchers. Biostatistical modelling and analysis have always been a key method in fields like evolutionary biology. In the beginning of the 20th century and right after the rediscovery of the Mendelian inheritance theory, there were many fundamental contributions to biology from statisticians that tried to bridge the gap between Mendelian genetics and Darwin’s theory of evolution. For example, the journal Biometrika [ 12 ] was established as early as 1901 by several founding fathers of modern statistics like Francis Galton and Karl Pearson with the intention of promoting biometrics, that is, the application of statistics to the analysis of biological data. Nowadays, it is difficult to dive into advanced concepts of evolutionary biology and population genetics without an understanding of mathematical modelling [ 13 ]. We also point out the seminal works of K.L. von Bertalanffy, P.A. Weiss, or M.D. Mesarovic in the application of the general systems theory to organisms dating back to the first part of 20th century (see Drack and Wolkenhauer [ 14 ] for detailed discussion).
In parallel to the search for the link between genetics and evolution theory, a new branch of science originating in physical chemistry was created by researchers interested in the dynamics of chemical reactions in living cells. This field became known as biochemistry, and already in 1913, biochemists made use of mathematical modelling to understand the mechanisms behind enzymatic catalysis. The Michaelis–Menten equation, taught in biochemistry courses throughout the world, is the first-ever mathematical model describing the dynamics of a biochemical reaction (see an updated translation of the original paper in Michaelis et al. 2011 [ 15 ]). We find in this field scientists like Jacques Monod and his team, who used mathematical modelling to understand sophisticated features of enzyme activity, such as allosteric regulation (see the seminal contribution in Monod et al. 1965 [ 16 ] and a 50-year retrospective analysis in Changeaux 2012 [ 17 ]). Fascinated by the elegance of these results, for several decades, a myriad of biochemists devoted their efforts to enzymology, that is, elucidation of the mechanisms of reaction, quantification, and modelling of the enzyme catalysis.
If mathematical modelling is necessary to immerse oneself in the connection between genes, phenotypes, and evolution as well as to understand the inner workings of catalytic proteins, how is it that certain modern biomedical researchers are reluctant to incorporate mathematical and theoretical approaches into their work? In the 1970s and 1980s, new experimental techniques were invented allowing the targeted mutation of selected genes [ 18 ]. These techniques became so fundamental in the hunt for the link between genes, proteins, and cellular functions that they led to the inception of modern molecular biology. An experimental approach based on targeted mutation followed by assessment of their effects has dominated the field since then. It is an approach that relies on advanced experimental skills, trial and error, intuition, and small-scale studies, and perhaps, that was what taught several generations of biomedical researchers that mathematics and formalized systems were somehow unnecessary in biology.
However, nowadays, two developments are turning this trend. On the one hand, newly discovered techniques for producing quantitative high-throughput data on whole classes of biomolecules (the omics revolution) required statistical methodologies for processing and analyzing these massive amounts of data. Additionally, mathematical and computational methods are indispensable in finding insights and connections between genes in these data [ 19 ]. This is the rationale behind medical genomics, the field that scans quantitative high-throughput data to find the genetic causation of diseases. On the other hand, there is mounting evidence that proteins and genes in cells do not work in isolation but rather organize into tightly interconnected networks which are often disturbed in pathological conditions [ 20 ]. These networks contain feedback loops, feedforward loops, or network hubs and gene regulatory circuits that can induce nonlinear behavior like homeostasis, self-sustained oscillations, or biostability [ 21 ]. There features, rather than anecdotic, are intrinsic and necessary to many vital cellular processes, e.g., the cell cycle [ 22 ].
The situation in 2020 is peculiar. We are rapidly conjuring biomedicine that necessarily relies more and more on quantitative high-throughput data, advanced statistics, bioinformatics, and computational modelling [ 23 ]. However, a significant fraction of its practitioners have insufficient mathematical and computational skills, probably ones worse than the generation that was initiated into the quantification of enzyme catalysis through kinetic equations and has recently retired. In line with this and to substantiate further discussion, we will now introduce the basics of mathematical modelling of biochemical networks.
4. A Primer on Mechanistic Modelling of Biochemical Systems
There are mathematical and computational models that encode a mechanistic description of the natural system in which equations and variables account for the interactions between the key biological components of the system, for example, signaling proteins and transcription factors. Among them, there is a family of models that are conceived to integrate the topology of biochemical networks and the kinetics of their molecular interactions with their ability to control cell phenotypes. These models are inspired by the models built in chemistry to understand and predict the kinetics of chemical reactions. These mathematical and computational models are formulated, characterized, and utilized following a well-established procedure common to several branches of physics, chemistry, and engineering, which we name here as the modelling workflow . In a nutshell, the workflow includes the following sequential operations ( Figure 3 ):

The modelling workflow in biomedicine: during model derivation, the biological knowledge and hypotheses about the studied system are encoded in a mathematical model. In model calibration, quantitative experimental data are added to characterize the mathematical model and to give values to the model parameters. In model validation, the ability of the model to make predictions is assessed by judging the agreement between new quantitative data and equivalent simulations of the calibrated model. In model analysis, a validated model is used to investigate the system using computer simulations or other tools like stability analysis.
Model derivation: Biomedical information from scientific literature is surveyed to select relevant biomolecules and interactions for the investigated hypothesis. With this information, a graphical depiction of the network of interacting molecules or cells is sketched. Under some formal or heuristic rules, a mathematical model is derived from the network graph. The mathematical model consists of mathematical equations (i.e., ordinary, partial differential, or integrodifferential equations) or other computational entities (i.e., Boolean-logic networks or Petri nets).
Model calibration: To ensure that the model mimics the behavior of the natural system in a given biological scenario, one has to attribute values to free parameters in the model. In some cases, it is possible to discern these from published quantitative data. More often, however, one has to design and perform biological experiments that produce adequate quantitative data. Later, the mathematical model and the quantitative data are integrated using a computational process which assigns optimal values to the model parameters while minimizing the mismatch between experimental observations and corresponding model simulations.
Model validation: The ability of the model to predict the system’s behavior is judged based on the alignment between quantitative data from a different experiment not used for calibration and the corresponding simulations of the calibrated model. A mismatch between data and simulation leads to reformulation of the hypothesis or the model’s structure, which is reflected in a modification of its mathematical equations and a re-iteration of the entire procedure.
Model analysis: A validated model can be used to design and perform predictive simulations, that is, simulations of the system’s behavior under new biological scenarios. This type of simulations has been successfully deployed to detect potential drug targets or to identify biomarkers for diagnosis in cancer and other multifactorial diseases (see Table S1 for selected examples). Furthermore, tools like stability analysis and bifurcation analysis can uncover nonlinear properties of the investigated network, delineating regions in the system’s phase space with distinctive stability or critical values of the model parameters provoking qualitative changes in the system’s behavior. Despite all the power that mathematical models bring to the table, however, it goes without saying that any prediction will require further experimental validation with lab bench models.
5. Frequent Unfounded Criticisms to Mathematical Modelling in Biomedicine
Now, we will list and discuss misconceptions about mathematical modelling in biomedicine which one can hear rather often when talking to experimental researchers in seminars and conferences (see also Table 1 for a summary).
Top misunderstandings on modelling and how to fight them.
5.1. Mathematical Models Cannot Reproduce the Complexity of Biology
When applied to biology in contrast to other natural sciences like physics or chemistry, this statement is a rather elaborate instance of magical thinking. Cells and physicochemical systems are governed by the same thermodynamic laws as any other natural system, laws that have been formulated in mathematical terms. The complexity of stellar systems’ dynamics is at least comparable with that of physiological systems, yet mathematical modelling is the standard tool to postulate hypotheses, to design experiments, and to formulate theories in astrophysics. The dynamics of the Earth’s atmosphere and climate are governed by the same laws of chemical kinetics and reaction-diffusion that apply to biochemical reactions. However, sophisticated mathematical modelling is behind the daily weather forecast or the recommendations of the Intergovernmental Panel on Climate Change. Besides nineteenth-century holistic thinking, there is not a single solid argument to support the notion that mathematical models cannot reproduce the complexity of biochemical and physiological systems.
5.2. Your Model Is Not Physiological. The Real System Is More Complex Than Your Mathematical Model
It may look like a softer version of the previous statement, but here, the emphasis is different. The idea is that modelling may be a valid option, but the current model does not contain sufficient detail to meaningfully represent the physiological context. When inspected more closely, this statement does not actually criticize mathematical modelling in particular but rather the fundamental act of utilizing any sort of model in biology. As we said above, any model, whether mathematical or experimental, is an abstraction that, rather than contemplating every detail of the system, includes the elements, interactions, and processes necessary to investigate the natural system under the hypothesis in question. This implies an intentional attempt at simplification by the researcher that is a shared feature of mathematical models and cell lines, organoids, or mouse models. This criticism can be countered by stating that the model must be as complex as necessary to capture the hypothesis, a notion equally valid for mathematical and lab bench models.
5.3. You Should Employ Data in Your Mathematical Model
A mathematical model in the sense discussed here always relies on quantitative data. As indicated above, model calibration is only possible with experimental data. Thus, a well-formulated mathematical model is based on quantitative data, which makes the above objection moot. Moreover, the interplay between model and quantitative data can come in different flavors. While usually one both calibrates and validates the model with data, one can also forego calibration in favor of analytical tools like stability analysis and can derive qualitative predictions about the system’s dynamics which are accessible to further experimental validation. Bar-or et al. 2000 [ 24 ] is a classic example of the ability of mathematical models to make qualitative predictions about the regulation of gene circuits from data. In the paper, the authors collected and synthesized all the information available at the time about the interplay between the TF p53 and its transcriptional target and repressor Mdm2 to hypothesize a) that they form a negative feedback loop gene circuit and b) that, under DNA damage, the system displayed oscillations in the expression of its components. They derived a qualitative mathematical model based on these hypotheses and found out that the model simulations predicted actual oscillations in p53 and Mdm2 levels in some experimental scenarios. They further validated this model-based prediction utilizing in vitro experiments. Interestingly, this “design principle” associated to oscillating gene circuits with TFs and their targets and repressors has been found in other master regulator TFs also by integrating quantitative data and mathematical modelling (see the case of NFkB in inflammation in Nelson et al. 2004 [ 25 ]).
5.4. Your Predictions Are Not Experimentally Validated
As explained above, once you perform an Einstein-grade version of the scientific method, it is not mandatory that a single paper conveys all the steps of the method. One team of researchers can formulate and investigate a hypothesis with mathematical modelling and simulations, and another team can follow up with validation experiments once technology and effort allow it (see the case of the neuronal action potential above). This is not to say that modelers are generally exempt from the need to validate, though. They should attempt to engage with experimental collaborators to see their own work come to fruition. An important aspect here is to provide ways of facilitating communication between modelers and experimentalists. In this sense, scientific papers on modelling and simulation should be written in a manner that allows the design of experiments to validate their hypotheses and predictions. Ultimately, this requires using common scientific language understandable for both mathematical modelers and experimental researchers.
5.5. I Do Not See the Clinical Relevance of Your Predictions
Very little of the scientific research in biomedicine delivers immediate clinical relevance, be it results obtained via mathematical modelling or through experimentation. However, at the same time, all basic research has an unpredictable long-term potential for enhancing clinical practice. To illustrate its potential, let us analyze a series of results obtained in the context of miRNA regulation and cancer. MicroRNAs exert posttranscriptional repression of selected gene targets [ 26 ] and play a pivotal role in some cell phenotypes subverted in cancer [ 27 ]. Lai et al. [ 28 ] utilized mathematical modelling to investigate the overarching hypothesis that different miRNAs can cooperate in the repression of some of their targets, a prediction that they experimentally validated for miR-572′s and miR-93′s joint repression of CDKNA1, a key cell cycle protein deregulated in cancer. In a continuation of this work, Schmitz et al. [ 29 ] used hybridization and molecular dynamics simulations of the binding of two cooperative miRNAs on their target mRNA to illustrate the general biophysical feasibility of this mechanism and to elucidate how it works at the molecular level. Moreover, they performed a human genome-wide exploration to systematically look for this type of joint miRNA regulation. Based on these results and further computational simulations, they hypothesized that miRNA cooperativity and its modelling can predict drug targets in cancer. They tested the hypothesis in a case study on cooperativity between miR-205-5p and miR-342-3p and its capacity to repress E2F1-mediated chemoresistance in cancer [ 30 ]. Their model-based prediction was confirmed experimentally. Finally, Lai et al. [ 31 ] extended this approach to the whole genome and systematically identified pairs of miRNAs that cooperatively target upregulated genes in metastatic melanoma. In summary, this series of interconnected papers illustrates how mathematical modelling can lead the way from hypothesis formulation and basic research to identifying potential clinical applications.
6. Rules to Build Mathematical Models That Can Be Understood by Experimentalists
To conclude, we elaborate on a few recommendations for biomedical modelers for when they conceive and implement their mathematical models, which hopefully will help bridge the cognitive chasm between them and experimentalists.
6.1. Know Your Problem
A good modeler should become an expert in the biomedical system that they plan to model. This is the best warranty that the structure and the hypotheses behind the mathematical model make sense and are consistent with the current biomedical knowledge. In addition, becoming an expert on the topic will help in choosing the right model assumptions, data, and hypotheses to be tested. Complementary to this, the best models emerge from constant interaction between competent biomodelers and experimental researchers. Remember, though, that collaboration is productive when communication is fluent, and this is only possible when a common language is spoken. In the present, this common language is the one that modelers need to learn when diving into the biology of the system they want to model. We refer again to Meyer-Hermann et al. 2012 [ 6 ] and their ability as modelers to acquire a deep understanding of B-cell biology and how they translated it into their mathematical model. However, we postulate that, in the long-term, biomedical researchers need in turn to rediscover the more precise language that math offers, which will also help them to quickly grasp advances in their own field of interest. There is an in-between methodology that could rekindle the growth of mathematical skills in experimentalists: network biology. In silico reconstruction, visualization, and modelling of intracellular biochemical networks provide a framework for connecting genes and molecules quantitatively to phenotypes and hence understanding the function and dynamics of cellular systems [ 32 ]. The network biology approach relies on mathematical concepts from graph theory, statistics, and mathematical modelling but is yet intuitive enough to allow a fluent discussion between wet- and dry-lab biologists. This has been, for example, advantageously used by yeast biologists to connect their experimentally detected biological interactions with their effect in cell phenotypes.
6.2. Select the Right Type of Mathematical Model, and Select It Early
The features of the mathematical model largely depend on the aim of the study; the scale and structural complexity of the investigated system; and the quantity, quality, and nature of the available experimental data. A model to investigate the nonlinearity associated with a feedback loop circuit has completely different requirements than a model of quantitative drug dosage in humans. This affects in particular the selection of the mathematical framework in which the model is derived and simulated. There is no single best modelling framework for every biomedical system or purpose, and therefore, the choice of model often relies on a trade-off between several requirements. We want to mention here (a) the computational demand and scalability, (b) the nature and necessary amount of calibration data, and (c) the way time and space are handled in the simulations. Sometimes, standard modelling frameworks are not suited for the problem in question and hybrid computational models of different types need to be considered (Chiam et al. 2006 illustrate how this type of hybridization can be done in the context of signaling pathways [ 33 ]; a discussion of this issue in the context of bacterial infection can be found in Cantone et al. 2017 [ 34 ]). When trying to facilitate the communication between modelers and experimentalists, one interesting approach is rule-based modelling. Compared to more math-heavy methods, rule-based modelling allows compact representations of reaction networks with a language-oriented structure; this makes them similar to semantic models and hence closer to the way of thinking of experimentalists (as a case study, see the epidermal growth factor receptor signaling network built in [ 35 ]).
6.3. Build on Preceding Efforts
To start the derivation of a mathematical model totally from scratch makes sense only when there is no alternative. A prudent modeler should reuse, extend, and adapt preexisting models when possible. In some cases, this will not be possible because the available models are based on different experimental conditions, formulated for a different biological scenario, or derived using an unsuitable modelling framework. In these cases, even if the model is found to be partially incompatible, its assessment will help judge the validity and portability of its assumptions and hypotheses in the context of one’s own modelling effort. If the problem lies in the modelling framework, it is sometimes worth translating the model into one’s chosen framework. In the ideal case, the model authors should have uploaded a fully annotated version of their model to a public repository (e.g., Biomodels [ 36 ]), which facilitates the work of incorporating the model into one’s own. Some tools even allow the semiautomatic translation of models from one formalism to another (see for example OdiFy [ 37 ]). As an example of this idea of building on preceding modelling efforts, in Csikász-Nagy 2009, one can find a comprehensive overview of the cascade of increasingly detailed mathematical models constructed since 1991 to understand the regulation of the cell cycle and how mathematical models are based on or have benefitted from the results obtained with previously developed models [ 38 ].
6.4. The Size Does Not Always Matter
There is nowadays a tendency to moon-shoot everything in biomedicine. This has translated also to biomodelling, and some researchers think that the quality of a mathematical model is measured in terms of the number of model variables as well as the required computational power and the complexity of the simulation algorithms. However, quality in modelling is primarily achieved through the biological precision of the assumptions encoded in the model equations. Thus, models can look simple and be small in terms of their number of equations but can actually possess the right features for the purpose of the specific modelling effort. This is somewhat similar to a BIC ballpoint pen. This is the simplest and cheapest ballpoint pen one can buy, but it actually displays a number of easy-to-overlook sophisticated features conceived to optimize its design in economic, ergonomic, and safety terms. One can also formulate BIC-like mathematical models, in which one gives priority to the description of the biological context and hypothesis and its planned utilization rather than to unnecessary size, complexity, or levels of detail ( Figure 4 ).

BIC pen-like mathematical models: prioritizing the purpose of the model instead of its detailedness . BIC pens look like the simplest and cheapest ballpoint pen one can buy, but their apparent simplicity conceals features conceived to optimize them in economic, ergonomic, and safety terms. In Santos and coworkers 2016 [ 3 ], a similar strategy was followed to build a mathematical model accounting for anticancer dendritic cell (DC) vaccination composed of only two ordinary differential equations, far simpler than other published models [ 41 ]. ( A ) Simplicity in design: BIC pens have a characteristically simple hexagonal structure; this apparently naïve choice significantly reduces the material consumption of the pen and minimizes the required space for storage. An important aspect to consider in DC vaccine modelling is the bioavailability of the cells after their injection. There are much elaborated models describing this process [ 42 ], but for our purpose, it was sufficient to model DC bioavailability with a cyclic piecewise linear function that mimics the known overall behavior of injected DCs. ( B ) Mathematics behind design principles: compared to standard circular pens, BIC pens hardly roll on the surface of a table. This feature was explicitly desired when drafting their design. In Santos et al., we wanted a simple enough model that was still able to mimic the interaction between the tumor and both innate and adaptive immunity; to this end, the model contained two nonlinear kinetic rates in a single equation, which are still able to mimic the basics of the interplay between the tumor and the two branches of immunity. ( C ) Ability to solve problems: in the end, simplicity has to be reconciled with effectiveness. The design of a BIC pen, for example, integrates more characteristics like minimizing the risk of suffocation when swallowing the cap. The predictions made in Santos et al. (2016) in terms of which phenotypic features sensitize the tumor to the therapy were aligned with patient data from clinical trials; furthermore, the model predicted alternative phenotypes that promote therapy resistance. The figures about DC vaccine modelling are adapted from Santos et al. 2016 under the conditions of an open access publication (CC BY 4.0). The figures about the BIC pen were inspired by the content of the webpage www.bicworld.com . Fight by doing: A route map to good mathematical modelling in biomedicine.
6.5. Set Your Results in Stone
One should prepare one’s own models in such a way that they can be understood and reused by other researchers, thereby closing the loop inherent to the scientific method. This is a frequently disregarded aspect in many fields in mathematical biomedicine, in which the number of modeler teams can be counted on two hands, and consequently, any model should have a high potential for reuse, adaptation, or integration. A model that is difficult to reproduce or understand by other modelers is guaranteed to collect virtual dust on its publication shelf. Thus, it is advantageous to implement equations and simulations in standard formats [ 39 ]. Further, whenever possible, the models and their supporting experimental data should be uploaded to repositories [ 36 ]. In closing, we emphasize the importance of the careful manual curation and annotation of mathematical models. In line with this, whenever possible, one should give preference to frameworks that allow for fast, simple, and standardized dissemination and model exchange [ 40 ].
7. The Best of Both Worlds—A Final Note on Mathematics, Models, Big Data, and Experimental Biology
The approach based on semantic and lab bench models has been very successful in past decades in describing many fundamental regulatory pathways in molecular biology. However, due to the massive amount of data produced nowadays, we are quickly reaching its limits. This is especially true (and dramatic) in the description of dynamic systems like heterogeneous cell populations, genomic regulation, or multifactorial diseases like cancer, for which this approach is clearly inadequate. There is no semantic solution to properly characterize multi-variable states, events, or diseases.
There is a clear understanding in the wet-lab biology community that advanced high throughput data analysis approaches are an urgent necessity for them, but in the opinion of many wet-lab biologists, data analysis and modelling are often conceived as a mere press of the button on computers that leads to publication-ready figures and plots within minutes. This has led to an entire industry producing “clickable” data analysis and modelling tools that make little demands on the knowledge of the operator. We think that this can lead to many problems, like reproducibility issues or the inability to critically judge the results produced by those tools, especially since most of them use proprietary algorithms. Additionally, the ability to generate an output from a purchased software or analysis suite might not automatically mean that this output is correct or sensible. Hence, an understanding of the underlying data and modelling theory is important and is often neglected in some wet-lab settings.
In the future, we as a community of biomedical researchers should strive to recognize that we need both sides of the coin, giving them equal weight in considerations like funding or time investment. Biology should orient itself towards the other two major natural science disciplines, physics and chemistry, and try to give their students a well-rounded education in mathematical understanding, data analysis, and modelling as well as in computer programming. Until that time, we need to make sure that we find ways to communicate between the two areas in a way that furthers productive collaboration.
Acknowledgments
Some of the misconceptions about modelling come from actual discussions with experimental and clinical researchers at the Department of Dermatology of the FAU Erlangen-Nürnberg, to whom we are grateful for their honest though at times scathing disagreement. Some parts of the text are based on a presentation given by J.V. at the Heidelberger Institut für Theoretische Studien (HITS), and others are from exchanges of ideas within the laboratory of Systems Tumor Immunology. J.V. also thanks Olaf Wolkenhauer (University of Rostock) for 15 years of continuous discussion on the foundations of systems biology.
Supplementary Materials
The following are available online at https://www.mdpi.com/1422-0067/22/2/547/s1 , Table S1: Real cases of mathematical modeling in biomedicine with additional bibliography and further reading.
Author Contributions
Initial draft: J.V.; Figures: J.V.; Final draft: J.V., M.E., C.L., S.N., M.N.; revision of the draft: J.V., X.L. All authors have read and agreed to the published version of the manuscript.
J.V.’s work in mathematical modelling applied to biomedicine has been generously funded over the years by the German Ministry of Education and Research (BMBF) through the initiatives FORSYS [CALSYS-FORSYS 0315264], e:Bio [e:Bio-miRSys 0316175A, e:Bio-MelEVIR 031L0073A], and e:Med [e:Med-CAPSyS 01ZX1304F, e:Med-MelAutim 01ZX1905A] and targeted initiatives in artificial intelligence [KI-VesD, 031L0244A] as well as by the German Research Foundation (DFG) [Ve642/1-1 in SPP1757] and the Bavarian Government [Gaminfection-UKER]. We also acknowledge support by Deutsche Forschungsgemeinschaft and Friedrich-Alexander-Universität Erlangen-Nürnberg within the funding program Open Access Publishing.
Conflicts of Interest
The authors declare no conflict of interest.
Publisher’s Note: MDPI stays neutral with regard to jurisdictional claims in published maps and institutional affiliations.
- Open access
- Published: 30 January 2019
BMC Biomedical Engineering: a home for all biomedical engineering research
- Alexandros Houssein ORCID: orcid.org/0000-0001-6993-4301 1 ,
- Alan Kawarai Lefor 2 ,
- Antonio Veloso 3 ,
- Zhi Yang 4 ,
- Jong Chul Ye 5 ,
- Dimitrios I. Zeugolis 6 &
- Sang Yup Lee 7
BMC Biomedical Engineering volume 1 , Article number: 1 ( 2019 ) Cite this article
8513 Accesses
3 Citations
3 Altmetric
Metrics details
This editorial accompanies the launch of BMC Biomedical Engineering , a new open access, peer-reviewed journal within the BMC series, which seeks to publish articles on all aspects of biomedical engineering. As one of the first engineering journals within the BMC series portfolio, it will support and complement existing biomedical communities, but at the same time, it will provide an open access home for engineering research. By publishing original research, methodology, database, software and review articles, BMC Biomedical Engineering will disseminate quality research, with a focus on studies that further the understanding of human disease and that contribute towards the improvement of human health.
Introduction
Biomedical engineering is a multidisciplinary field that integrates principles from engineering, physical sciences, mathematics and informatics for the study of biology and medicine, with the ultimate goal of improving human health and quality of life.
Biomedical engineering is not a new concept; however, it was not until the 1900s when rapid technological advancements in the chemical, physical and life sciences influenced breakthroughs in the prevention, diagnosis and treatment of disease. The invention of the electrocardiograph, the concept of x-ray imaging, the electron microscope, the mechanical heart valve and human genome sequencing, are just a few examples of technological innovations that revolutionised science and medicine and changed the approach to human healthcare. Current biomedical engineering technologies are a growing part of clinical decision making, which can now be influenced from multiscale observations, ranging from the nano to the macro-scale.
Today, the need for innovation in health technologies is ever more prominent. The annual global healthcare spending has seen continued growth and is projected to reach a staggering $8.7 trillion by 2020 [ 1 ]. Global health challenges are becoming more complex, wide spread and difficult to control. Resources are scarce and with a growing population, our society has a need for affordable, portable and sustainable solutions. The World Health Organisation has pledged to make a billion lives healthier by 2023 [ 2 ], a goal that will require widespread commitment by governments, funding agencies, researchers and clinicians. Biomedical engineers will be at the heart of this movement and face a responsibility for continuous innovation. Biomedical engineering research is expected to create health technologies that will drastically improve the prevention, diagnosis and treatment of disease, as well as patient rehabilitation. As an example, the NIH 2016–2020 strategic plan focuses on point of care and precision medicine technologies including genetic engineering, microfluidics, nanomedicine, imaging, digital/mobile-Health and big data [ 3 ].
BMC Biomedical Engineering will strive to complement these efforts and provide an open access venue for the dissemination of all biomedical engineering research. As part of the BMC series, a portfolio of journals serving communities across all sciences, the Journal will act as a resource for a wide range of disciplines. It aims to support scientists, engineers and clinicians by making their research openly and permanently available, irrespective of their location or affiliation.
Aims and scope
BMC Biomedical Engineering considers articles on all aspects of biomedical engineering, including fundamental, translational and clinical research. It combines tools and methods from biology and medicine with mathematics, physical sciences and engineering towards the understanding of human biology and disease and the improvement of human health. The Journal will publish a range of article types, including research, methodology, software, database and review articles.
As part of the BMC series, a collection of open access, peer-reviewed and community focused journals covering all areas of science, editorial decisions will not be made on the basis of the interest of a study or its likely impact. Studies must be scientifically valid. For research articles this includes a scientifically sound research question, the use of suitable methods and analysis, and following community-agreed standards relevant to the research field.
BMC Biomedical Engineering aims to publish work that undergoes a thorough peer review process by appropriate peer-reviewers and is deemed to be a coherent and valid addition to the scientific knowledge. It aims to provide an open access venue which allows for immediate and effective dissemination of research and enables our readers to explore and understand the latest developments, trends and practices in biomedical engineering. We believe that open access and the Creative Commons Attribution License [ 4 ] are essential to this, allowing universal and free access to all articles published in the Journal and allowing them to be read and the data re-used without restrictions. BMC Biomedical Engineering will work closely with the rest of the journals in the BMC series portfolio [ 5 ] to help authors find the right home for their research. We will highlight selected journal content through various promotional channels to ensure the research reaches its target audience and receives the attention it deserves.
Editorial sections
Many new technologies that have revolutionised biomedical engineering require the coalition of previously independent communities. 3D bioprinting of tissues and organs brings together methods from cell biology, biomaterials, nanotechnology and engineering and is being used for the transplantation of tissues, including skin, bone, muscle, soft tissue, cartilage and others [ 6 , 7 ]. The concept of tissue and disease modelling is being driven towards drug discovery and toxicology studies, aiming to increase the yield of drug testing by tackling limitations of current cell and animal models [ 8 ].
New approaches in natural and synthetic biomaterials have redefined bioelectronics. Silk fibroins and other unconventional interfaces can form flexible electronics and challenge the use of silicon-based technologies. For biomedical applications, these new approaches present advantages not only due to their biocompatibility and low cost, but also for their electromechanical and optical virtues [ 9 ]. Implantable probes are being redesigned so that they facilitate long term stability and high resolution, without perturbing the biological system or creating an immune response. Such technologies are now able to facilitate recordings of single neurons in vivo, in a chronically stable manner, with applications to the restoration of vision and retinal prosthetics [ 10 ].
For many years biomedical imaging has been connecting microscopic discoveries with macroscopic observations. Photoacoustic tomography (PAT) is now able to image large spatial scales, from organelles to small animals, at very high speeds [ 11 ]. In fact, single-shot real-time imaging can operate at 10 trillion frames per second and is finding applications in breast cancer diagnosis [ 12 , 13 ].
In the field of medical robotics, new approaches combine machine learning and artificial intelligence to strengthen the clinician’s decision making. Others are leveraging augmented reality (AR) to facilitate better immersion and more natural surgical workflows for computer assisted orthopaedic surgery [ 14 ].
BMC Biomedical Engineering celebrates the interdisciplinary nature of the field. In order to navigate the wide range of biomedical engineering research, the Journal is structured in six editorial sections.
Biomaterials, nanomedicine and tissue engineering
Medical technologies, robotics and rehabilitation engineering
Biosensors and bioelectronics
Computational and systems biology
Biomechanics
Biomedical Imaging
We are delighted to welcome our founding Section Editors along with a growing international group of Editorial board Members [ 15 , 16 ]. The Journal is supported by an expert Editorial Advisory group of senior engineers and scientists, which is chaired by Distinguished Professor Sang Yup Lee. Together with the in-house Editor, this group will provide academic leadership and expertise and will work together to transverse into multiple clinical and engineering disciplines. The Editorial Board will keep growing and developing to reflect and adapt to the nature of this diverse community.
Biomaterials, nanomedicine and tissue engineering section
This section primarily focuses on the development of biofunctional tissue substitutes, which possess the highest level of biomimicry, through recapitulation of nature’s innate sophistication and thorough processes. It considers research, methods, clinical trials, leading opinion and review articles on the development, characterisation and application of nano- and micro- biofunctional biomaterials, cell-assembled tissue substitutes, diagnostic tools, microfluidic devices and drug/gene discovery and delivery methods. Manuscripts focusing on permanently differentiated, engineered and stem cell biology and application are welcome. This section will place a substantial focus on clinical translation and technologies that advance the current status-quo. As such, articles that enhance the scalability and robustness of tissue engineering methodologies, or that enable new and improved industrial or clinical applications of biomedical engineering discoveries, tools and technologies are strongly encouraged.
Medical technologies, robotics and rehabilitation engineering section
This section seeks to represent research in engineering that encompasses a wide range of interests across medical specialties, including orthopaedic, cardiovascular, musculoskeletal, craniofacial, neurological, urologic and other medical technologies. It will consider research on medical robotics, computer assisted technologies, medical devices, e/m-health and other medical instrumentation. It aims to improve the prevention, diagnosis, intervention and treatment of injury or disease and it welcomes articles that represent new approaches to engineering that may be useful in the care of patients. Technical and practical aspects of rehabilitation engineering, from concept to clinic and papers on improving the quality of life of patients with a disability are encouraged. The section also seeks to represent clinically important research that is based on new and emerging technologies. This could include clinical studies of new approaches to robotic-assisted surgery, clinical studies of new devices, or other studies that are close to patient care or rehabilitation.
Biosensors and bioelectronics section
This section considers articles on the theory, design, development and application on all aspects of biosensing and bioelectronics technologies. The section will consider approaches that combine biology and medicine with sensing and circuits and systems technologies on a wide variety of subjects, including lab-on-chips, microfluidic devices, biosensor interfaces, DNA chips and bioinstrumentation. It also considers articles on the development of computational algorithms (such as deep learning, reinforcement learning, etc.) that interpret the acquired signals, hardware acceleration and implementation of the algorithms, brain-inspired or brain-like computational schemes, and bioelectronics technologies that can have a wide impact in the research and clinical community. Articles on implantable and wearable electronics, low-power, wireless and miniaturised imaging systems, organic semiconductors, smart sensors and neuromorphic circuits and systems are strongly encouraged.
Computational and systems biology section
Computational, integrative and systemic approaches are at the heart of biomedical engineering. This section considers papers on all aspects of mathematical, computational, systems and synthetic biology that result in the improvement of patient health. Integrative and multi-scale approaches, in the network and mechanism-based definition of injury and disease, or its prevention, diagnosis and treatment are welcome. Papers on high precision, interactive and personalised medicine, on digital/mobile health, on complex/big data analytics and machine learning, or on systemic and informatics approaches in a healthcare or clinical setting are encouraged.
Biomechanics section
This section represents the interdisciplinary field of biomechanics and investigates the relationship of structure with function in biological systems from the micro- to the macro- world. It considers papers on all aspects of analytical and applied biomechanics at all scales of observation, that improve the diagnosis, therapy and rehabilitation of patients or that advance their kinetic performance. The topics of interest range from mechanobiology and cell biomechanics to clinical biomechanics, orthopaedic biomechanics and human kinetics. Articles on the mechanics and wear of bones and joints, artificial prostheses, body-device interaction, musculoskeletal modelling biomechanics and solid/fluid computational approaches are strongly encouraged.
Biomedical imaging section
Biomedical imaging has been connecting microscopic discoveries with macroscopic observations for the diagnosis and treatment of disease and has seen considerable advances in recent years. This section will consider articles on all biomedical imaging modalities including medical imaging (MRI, CT, PET, ultrasound, x-ray, EEG/MEG), bio-imaging (microscopy, optical imaging) and neuroimaging across all scales of observation. Its primary focus will be to foster integrative approaches that combine techniques in biology, medicine, mathematics, computation, hardware development and image processing. Articles on new methodologies or on technical perspectives involving novel imaging concepts and reconstruction methods, machine learning, sparse sampling and statistical analysis tool development are encouraged.
The motivation for the launch of BMC Biomedical Engineering is to create an authoritative, unbiased and community-focused open access journal. We are committed to working together with our authors, editors and reviewers to provide an inclusive platform for the publication of high-quality manuscripts that span all aspects of biomedical engineering research. We welcome articles from all over the world and we will devote our efforts to ensure a robust and fair peer-review process for all. We believe in continuous improvement and we encourage the community to get in touch with us to provide ideas and feedback on how to improve the Journal and serve the community better.
We hope you will find the first group of articles an interesting and valuable read, and we look forward to working with you all to disseminate research into the exciting field of biomedical engineering.
Abbreviations
Augmented Reality
Computed Tomography
Electroencephalogram
Magnetoencephalography
Photoacoustic Tomography
Positron Emission Tomography
Deloitte. 2018 Global health care outlook 2018. https://www2.deloitte.com/xe/en/pages/life-sciences-and-healthcare/articles/global-health-care-sector-outlook.html# #.
Google Scholar
World Health Organisation . Thirteenth general programme of work, 2019-2023. 2018. http://apps.who.int/gb/ebwha/pdf_files/WHA71/A71_4-en.pdf?ua=1
National Institutes of Health. The NIH-wide strategic plan, fiscal years 2016–2020: turning discovery into health. 2015. https://www.nih.gov/sites/default/files/about-nih/strategic-plan-fy2016-2020-508.pdf
Creative Commons Attribution License, Creative Commons Attribution License. [Online]. Available: http://creativecommons.org/licenses/by/4.0/ .
BMC-series journals. Available https://www.biomedcentral.com/p/the-bmc-series-journals .
Murphy SV, Atala A. 3D bioprinting of tissues and organs. Nat Biotechnol. 2014;32(8):773–85.
Article Google Scholar
Zhang YS, Yue K, Aleman J, et al. 3D bioprinting for tissue and organ fabrication. Ann Biomed Eng. 2016;45(1):148–63.
Ronaldson-Bouchard K, Vunjak-Novakovic G. Organs-on-a-Chip: A fast track for engineered human tissues in drug development. Cell Stem Cell. 2018;22(3):310–24.
Zhu B, Wang H, Leow WR, et al. Silk fibroin for flexible electronic devices. Adv Mater. 2016;28(22):4250–65.
Hong G, Fu TM, Viveros RD, et al. A method for single-neuron chronic recording from the retina in awake mice. Science. 2018;360(6396):1447–51.
Wang LV, Yao J. A practical guide to photoacoustic tomography in the life sciences. Nat Methods. 2016;13(8):627–38.
Liang JY, Zhu LR, Wang LV. Single-shot real-time femtosecond imaging of temporal focusing. Nature. 2018;7(1):42.
Lin L, Hu P, Shi J, et al. Single-breath-hold photoacoustic computed tomography of the breast. Nat Comms. 2018;9(1):2352.
Liu H, Auvinet E, Giles J, et al. Augmented reality based navigation for computer assisted hip resurfacing: a proof of concept study. Ann Biomed Eng. 2018;46(10):1595–605.
BMC Biomedical Engineering Section Editors and Chair [Online] Available: https://bmcbiomedeng.biomedcentral.com/about/editorial-board/editor-profiles .
BMC Biomedical Engineering Editorial Board [Online] Available: https://bmcbiomedeng.biomedcentral.com/about/editorial-board .
Download references
Acknowledgements
Not applicable.
Availability of data and materials
Author information, authors and affiliations.
Springer Nature, 4 Crinan Street, London, N1 9XW, UK
Alexandros Houssein
Department of Surgery, Jichi Medical University, Shimotsuke, Tochigi, Japan
Alan Kawarai Lefor
Laboratory of Biomechanics and Functional Morphology, Faculty of Human Kinetics, Lisbon, Portugal
Antonio Veloso
Department of Biomedical Engineering, University of Minnesota, Minneapolis, MN, USA
Department of Bio and Brain Engineering, Korea Advanced Institute of Science and Technology, Daejeon, Republic of Korea
Jong Chul Ye
Regenerative, Modular and Developmental Engineering Laboratory (REMODEL), Biomedical Sciences Building, National University of Ireland Galway (NUI Galway), Galway, Ireland
Dimitrios I. Zeugolis
Department of Chemical and Biomolecular Engineering, Korea Advanced Institute of Science and Technology, Daejeon, Republic of Korea
Sang Yup Lee
You can also search for this author in PubMed Google Scholar
Contributions
AH wrote the introduction, aims and scope and conclusion. AH, AKL, AV, ZY, JCY, DIZ and SYL wrote the editorial sections. All authors read and approved the final version of the manuscript.
Corresponding author
Correspondence to Alexandros Houssein .
Ethics declarations
Ethics approval and consent to participate, consent for publication, competing interests.
AH is the Editor of BMC Biomedical Engineering and an employee of Springer Nature. AL, AV, ZY, JY, DZ and SL are members of the Editorial Board of BMC Biomedical Engineering .
Publisher’s Note
Springer Nature remains neutral with regard to jurisdictional claims in published maps and institutional affiliations.
Rights and permissions
Open Access This article is distributed under the terms of the Creative Commons Attribution 4.0 International License ( http://creativecommons.org/licenses/by/4.0/ ), which permits unrestricted use, distribution, and reproduction in any medium, provided you give appropriate credit to the original author(s) and the source, provide a link to the Creative Commons license, and indicate if changes were made. The Creative Commons Public Domain Dedication waiver ( http://creativecommons.org/publicdomain/zero/1.0/ ) applies to the data made available in this article, unless otherwise stated.
Reprints and permissions
About this article
Cite this article.
Houssein, A., Lefor, A.K., Veloso, A. et al. BMC Biomedical Engineering: a home for all biomedical engineering research. BMC biomed eng 1 , 1 (2019). https://doi.org/10.1186/s42490-019-0004-1
Download citation
Received : 07 December 2018
Accepted : 03 January 2019
Published : 30 January 2019
DOI : https://doi.org/10.1186/s42490-019-0004-1
Share this article
Anyone you share the following link with will be able to read this content:
Sorry, a shareable link is not currently available for this article.
Provided by the Springer Nature SharedIt content-sharing initiative
BMC Biomedical Engineering
ISSN: 2524-4426
- Submission enquiries: [email protected]
- General enquiries: [email protected]
- Open access
- Published: 25 September 2021
Biomedical engineering and ethics: reflections on medical devices and PPE during the first wave of COVID-19
- Alessia Maccaro 1 , 2 na1 ,
- Davide Piaggio ORCID: orcid.org/0000-0001-5408-9360 1 na1 ,
- Concetta Anna Dodaro 3 &
- Leandro Pecchia 1 , 4 , 5
BMC Medical Ethics volume 22 , Article number: 130 ( 2021 ) Cite this article
15k Accesses
6 Citations
2 Altmetric
Metrics details
In March 2019, the World Health Organization (WHO) declared that humanity was entering a global pandemic phase. This unforeseen situation caught everyone unprepared and had a major impact on several professional categories that found themselves facing important ethical dilemmas. The article revolves around the category of biomedical and clinical engineers, which were among those most involved in dealing with and finding solutions to the pandemic. In hindsight, the major issues brought to the attention of biomedical engineers have raised important ethical implications, such as the allocation of resources, the responsibilities of science and the inadequacy and non-universality of the norms and regulations on biomedical devices and personal protective equipment. These issues, analyzed one year after the first wave of the pandemic, come together in the appeal for responsibility for thought, action and, sometimes, even silence. This highlights the importance of interdisciplinarity and the definitive collapse of the Cartesian fragmentation of knowledge, calling for the creation of more fora, where this kind of discussions can be promoted.
Peer Review reports
Since early 2020, the current SARS-CoV-2 pandemic has challenged all the fields of knowledge, increasing the need for their interconnection. Medicine, science, politics, and more specialized sectors such as biomedical engineering (BME), faced crucial ethical issues, which can no longer be underestimated.
Biomedical engineers design medical devices, raising many ethical dilemmas in ordinary times, which become compelling during such a crisis. The authors of this manuscript had the privilege of different points of view thanks to the President of the European Society of BME (i.e., EAMBES) and Secretary General of the global society of BME and medical physics (IUPESM).
Three pivotal themes emerged in the global BME community:
The dilemma of identifying criteria for the allocation of medical devices
Responsibilities of science and technology
Inadequacy of regulations and norms, which lack universality
This manuscript does not follow the traditional structure of scientific papers (e.g., methods, results etc.), rather it is a critical analysis, revolving around the 3 above-mentioned pillars. The first pillar focuses on the surfacing of ethical dilemmas in times of pandemic (e.g., scarce resource allocation), delving into examples from Italy, where the new decision-making criteria often clashed against the existing constitutional and moral principles during the first wave.
The second pillar retrospectively reports how the medical device sector was affected by the current pandemic, touching on hazardous amatorial attempts of the general public to face the scarcity of resources and the urgent needs, and on the crisis-related challenges that surfaced for manufacturers, exacerbated by the lack of dialogue with decision-makers. In the same pillar, the theoretical debate among science and politics is addressed, referring the case of the “intended use” of a medical device, from two different philosophical perspectives, i.e., substantialism and utilitarianism. The former underlines the fundamentality of substances as ontological categories [ 1 ], suggesting that the intended use of a medical device should always be respected. The latter stresses the importance of maximizing the overall good, authorizing different uses, depending on the relativistic utility or, in exceptional times of crisis, on the emergency.
This invites the reader to a subsequent reflection on the inadequacy of the existing regulations on medical devices, in the third pillar. In respect to this, the manuscript proposes to offer a hermeneutic perspective close to the situational ethics that authorizes negotiations and mediations between the generality of principles and norms, and the specificity of the context.
Overall, the ethical considerations made in this manuscript, should be considered a valuable lesson for the future of crisis management. If and only if ethics and bioethics will be considered as effective support for science and scientists (doctors, biomedical engineers, etc.), the Cartesian separation of knowledge [ 2 ] could be overcome, establishing an interdisciplinary dialogue that involves peoples and emphasizes the public relevance of such issues.
In this way, such dilemmas could be anticipated by establishing a framework that could provide guidance and appropriate methodology to address arising urgent issues, without having to resort to specialists for questions that concern everyone and need a multidisciplinary approach.
Allocation of medical devices: clinical and ethical principles
Due to limited resources, decision-makers have had to compromise among all the potential useful interventions. Also in the past 10 years, the most public National health systems were massively privatized, resulting in a significant reduction of prevention services and a great reduction of Intensive Care Unit (ICU) beds. The rapid spread of the SARS-CoV-2 resulted in an unprecedented need of sub-intensive and ICU beds, which overcame the capacity of the most advanced national healthcare services. Within a few weeks, the available resources (i.e., medical devices, doctors, nurses) proved to be insufficient to cover the care needs of the multitude of COVID-19 patients, beyond the ordinary needs of other patients. Consequently, doctors and healthcare structures ended up pondering and making difficult ethical choices in a short time and identifying priority principles that could guide them.
National [ 3 , 4 , 5 , 6 ] and international [ 7 , 8 , 9 ] ethics committees, scientific societies [ 10 , 11 ], and experts [ 12 , 13 , 14 , 15 ] soon expressed their opinion on the matter [ 16 ], identifying requirements that respect human dignity and fundamental ethical principles, enshrined in the Charters of both National and International Rights [ 17 ].
The case of Italy, the first country significantly affected outside China, is emblematic. According to the Italian Constitution, health is a "fundamental right of the individual" and a "collective interest" (article 32). In addition, article 2 recognizes the personalist principle and the duty of solidarity, and article 3 establishes the principle of equality. Accordingly, the law founding the Italian National Health Services (NHS) (n. 883/1978) prescribes that care must be ensured according to the principles of universality, equality and fairness.
Leveraging on these fundamental principles, the Italian National Bioethics Committee [ 18 ] remarked the need to allocate medical devices and other resources based only on a clinical criterion, and without considering criteria such as age, gender, social attributes, ethnicity, disability and costs, in compliance with the principles of "justice, equity, solidarity". The envisaged method was that of "triage in a pandemic emergency", based on what the World Health Organization defines as "preparedness" (WHO) as a premise, and on two key concepts, i.e., "clinical appropriateness" and "actuality", identified by the healthcare professional on the basis of clinical criteria [ 19 ].
This point of view, which values the person and opposes attempts of objectification in a series of “pre-established criteria” (except for the point of view of the clinician), is well expressed by J. Habermas. In an interview with the newspaper Le Monde, Habermas underlined the inadequacy, in moral terms, of an “objective quantification” of patients and insisted on the essential issue of the “recognition” of the individual: “when addressing a second person (you, you), the other person's self-determination must either be respected or denied, that is, either accepted or ignored” [ 20 ].
On the other hand, the Italian society of anesthesiology (SIAARTI) introduced the identification of an age limit for accessing intensive care in case of necessity [ 21 ]. SIAARTI’s document explained that COVID-19 created a scenario, in which criteria for accessing ICU may be needed beyond the clinical appropriateness and proportionality of care, but also in distributive justice and the appropriate allocation of limited healthcare resources, which means privileging those with the "greatest life expectancy" [ 22 ]. Even if the choice of whom to admit to treatment is a terrible reality, it must be discussed by ethicists and bioethicists to further identify solutions that support medical doctors in taking these decisions. “This is not to deny their clinical authority and responsibility, but rather to urge a commitment to give such question public relevance" so that in the future the "public health perspective" [ 23 ] to follow is clear from the beginning.
Responsible thinking, responsible actions, responsible silence
COVID-19 created a global lack of essential medical devices (e.g., pulmonary ventilators) and personal protective equipment (PPE, such as masks, respirators) [ 24 ]. This led to an unprecedented amount of do-it-yourself (DIY) solutions, which were fomented on media worldwide. Consequently, ordinary individuals started producing PPE at home with 3D printers and everyday materials, and manufacturers converted their production facilities to develop medical devices and PPE.
Unfortunately, although very admirable, this approach is not feasible in critical sectors such as medical devices or PPE, which require postgraduate education, years of experience and deep knowledge of relevant international standards and norms, in order to ensure appropriate levels of safety, efficacy and resilience. Thus, only 7 manufacturers in the world are producing pulmonary ventilators. In fact, during a pandemic, we do not only need to ensure the usual standard of quality, but we should also consider making those devices more resilient, because, if hospitals fail, we will need to safely operationalize these devices in field hospitals, tent-like structures, and any other relevant setting. Therefore, experience, safe-by-design approaches, and the knowledge of additional standards (e.g., military standards) become relevant too.
Minimal scientific evidence exists on how harmful this DIY wave has been, but few facts can be clearly reported referencing major newspapers. In mid-March 2020, manufacturers were called upon to help to tackle medical devices and PPE crisis. Footnote 1 Footnote 2 Many responded, certainly moved by the noblest principle and willingness to help. Unfortunately, learning to manufacture complicated and highly regulated pulmonary ventilators cannot be done in a few weeks. By mid-April 2020, new productions of ventilators were stopped in Spain, Footnote 3 UK suspended orders of BlueSky ventilators Footnote 4 and France followed. Footnote 5 Once again, a virtuous example came from Italy, where the only Italian manufacturer of ventilators (i.e., Siare Engineering International Group l.t.d.) was supported by the Italian Government, which offered 25 highly specialized army engineers, by the former FIAT (now FCA), who supported producing electro-mechanic components, in addition to Ferrari providing electronic components. With this collaborative effort, Siare increased the production of top-of-the-range ventilators from 160 to 500 units per month, respecting the highest quality standards. In conclusion, the rise of useless and potentially harmful DIY approaches to PPE and medical devices could have been easily avoided at the start of the pandemic by decision-makers initially consulting with domain experts, such as biomedical and clinical engineers.
In this regard, the belief that disciplinary competence is to be sectorized and not interconnected, very often, leads to a further separation of knowledge that is detrimental to people. In fact, it could be good practice that politicians had a solid scientific background in order to legislate about scientific matters, above all if they involve public health. On the other hand, scientists should "grovel in the dirt of the city of Romulus" [ 25 ] keeping their related studies as tangible and accessible as possible, and acquire a more solid political culture and a growing awareness of their social role. After all, the relationship between science, policy-making and politics has been controversial since the dawns of civilization: people like Aristarchus of Samos (i.e., one of the fathers of an early Heliocentrism), censored by sectaries such as Cleanthes (i.e., the prince of stoics at that age), or like Socrates, accused, “censored”, and sentenced to death for being “unorthodox” by one of the most open and democratic societies of the times, the Polis of Athens [ 26 ], or like Galileus, who was condemned for radically opposing to the Sacred Scriptures- and Church-approved Geocentric model, are just a few stark examples. Although much progress has been made since then, there is still an ongoing debate among a Weberian distinction between science and politics and a Habermasian and Marcusean dichotomy between the technocratic and decisionist models of scientific advice to politics. Nonetheless, it is evident that we are transitioning towards ever more present democratization of science, and not without associated risks. In fact, how can this be achieved without compromising the epistemic quality of knowledge [ 27 ]?
In particular, the COVID-19-related debate, involving politicians, scientists (especially biomedical engineers) and ethics experts, is based on two distinct currents of thought, referring to two different philosophical matrices, i.e., utilitarianism and substantialism . In fact, some people believe that any kind of emergency-ready response that can make up for the shortage of PPE and medical devices is “better than nothing”, even at the expense of the safety and efficiency normally guaranteed by the standards. This way of thinking is in line with Utilitarianism’s conception of maximizing happiness and overall gains for all the affected individuals. However, the “better than nothing approach” is dubious and is a well-known logical fallacy, that of the relative privation [ 28 ]. This kind of fallacious way of reasoning also justifies the misuse of something that does meet the standards but was intended for completely different purposes. In fact, the intended purpose is key, at least in the world of medical devices, and it is what safeguards the manufacturers in case their products fail if they are used “off label”. In this case, the liability falls with the individual who misused the product in the first place [ 29 ]. This concept is well portrayed by what happened in Harrow, Footnote 6 , Footnote 7 where some nurses, after denouncing their precarious working conditions and the lack of PPE in the fight against COVID-19, had started using bin bags as a replacement of the unavailable PPE. In this case, the beneficial objective was given priority and the collective benefit was maximised. However, also the risk to the wearer had rapidly increased to the extent that it was not possible to predict its consequences (even negative).
Conversely, other people believe that the intended purpose of an object should be respected, in line with Substantialism’s theories, which attribute absolute value to an idea. According to this perspective, for example, a bin bag would be designed, tested and marketed to contain rubbish, not to protect healthcare workers from diseases (in this case the design principles and the tests will be different and stricter). Thus, these people tend towards a minimisation of the risk, but, at the same time, their precautional approach hinders the possible benefit underlying the other “less safe” alternatives. In this regard, it is necessary to recall the philosophy of Hans Jonas who, faced with an indeterminate and potential risk, the consequences of which cannot be estimated, introduced the imperative of responsibility in defence of future generations and based on the precautionary principle. His "heuristics of fear" implies foresight and ability to predict and adequately assess the consequences of collective activities in contemporary societies. Such principle implies to “act so that the effects of your action are compatible with the permanence of genuine life” and, in our present choices, to “include the future wholeness of Man among the objects of your (our) will” [ 30 ].
Consequently, this responsibility goes beyond the personal one of engineers, as it also includes the responsibility they partially assume if and when they do not limit reckless or inadequately considered actions, guided by the above-mentioned utilitarian approach. In fact, the compliance with international standards and the consequent CE marking does not only guarantee the quality, safety, efficiency, and efficacy of a product, but also the protection of manufacturers and users. In fact, as afore-mentioned from a general point of view, those to be blamed for the possible failure of bin bags used as PPE for the prevention of COVID-19 and the consequent infection and potential death of the healthcare workers using them are not the manufacturers of such items, but whomever decided to use this amateur substitution to other certified means of protection, and, to a certain extent, the biomedical engineers who did not respect their duty to identify the limits regarding the unintended uses.
Overall, two theoretical orientations are at the basis of these dichotomous approaches. However, in order to better frame them, it is necessary to analyse them in view of the extraordinary condition of necessity begotten by the pandemic. The dilemma revolves around the "intended use", or rather the purpose for which something (e.g., PPE or medical devices) was originally designed for: on the one hand there are those who assert that, in conditions of necessity, the contingent purpose, i.e., the social functionality that overcomes the intended use, ought to be preferred despite being “off label”. In fact, they firmly believe that it is preferable to maximize the current benefit while assuming an undefined risk. Although it is not easy to relate this trend to a specific current of thought, it certainly shares some points in common with utilitarianism, starting from the Benthamian one [ 31 ], if not with pragmatism (e.g., Dewey [ 32 ]).
On the other hand, the position of those who consider the “intended use” or rather the intrinsic purpose for which the product was manufactured tested and marketed, a priority, would seem evocative of Aristotelian substantialism or eschatology. In this case, the risks are limited by compliance with the law and the relevant standards, which also guarantee the achievement of the benefits. The refusal of this immediately relieves the manufacturer and the regulator from any responsibility related to the misuse of the object, leaving every possible and unforeseeable risk to the individual.
Here lies the crux of the problem. Using what is available and certified, albeit designed for a different “intended use”, seems more “reasonable” than not protecting oneself to everyone. However, we cannot refrain from asking ourselves the following questions: what is the limit within which it is possible to say, “better than nothing”? To what extent can science and policymakers put people's lives at risk in order to have a prompt, but probably unsafe answer in the wake of the "better than nothing" principle?
Regulatory frameworks and standards should be reviewed in this regard.
Beyond the DIY solutions, low-quality outputs have been affecting scientific production too. In fact, the high demand for information caused an acceleration in reporting scientific results, with many journals being overwhelmed with unprecedented numbers of papers, which challenged the capability of editors and reviewers to scrutinise articles.
The unprecedented high number of retracted papers can be a proxy for the high number of low-quality research on COVID-19. For this reason, a rapid search for papers regarding COVID-19 or SARS-CoV-2, and the previous epidemic/pandemics (i.e., SARS, MERS, Swine Flu) as a comparison, was performed both on OvidSP and the Retraction Watch Database. As regards COVID-19 publications, there were 124 retracted papers out of 264,530, i.e., 4.68 per 10,000 papers (compared to 1.16 per 10,000 papers concerning the previous pandemics/epidemics). Although this proxy is to be taken with a grain of salt, it should be a wake-up call for further investigations. Similar levels of confusion could be observed also among scientists and experts invited by media to interpret available scientific evidence and technical guidance.
The above-mentioned issues contributed to beget and feed an infodemic , defined by the United Nation as “an over-abundance of information—some accurate and some not—that makes it hard for people to find trustworthy sources and reliable guidance when they need it” [ 33 ], which is inducing an unprecedented need for responsible silence too.
Inadequacy of regulatory frameworks and norms
The pandemic creates a generalized condition of resource limited settings (RLSs), i.e., environments lacking means, specific knowledge, specialized personnel, medical devices and drugs within inappropriate medical locations. While this condition was already familiar to low- and middle-income countries, COVID-19 has overwhelmingly created RLS conditions in high-income countries, such as Europe, the USA and Japan, for the first time since World War II. This demonstrates how regulatory frameworks for medical devices and PPE are inadequate to RLS conditions. In fact, these regulations usually take into consideration standards that are too stringent and generic, proving impossible to fulfil in RLSs and, in times of the pandemic, difficult to adhere to universally. For example, the numerous tests and verifications required to assess the conformity of market respiratory protective equipment or eye protection equipment for healthcare purposes wasted time. One reason for this is that these standards are influenced by big manufacturers interested in having the largest market share. As a result, commercial standards for PPEs require testing in conditions that are not relevant for hospital workers (e.g., high temperature typical of heavy metals industry). Hence, international standards and norms followed the principle of generalism, losing universality and creating unnecessary burdens for small manufacturers [ 34 ]. In this regard, the WHO has published, for the first time, technical guidance on PPE specifically relevant for healthcare settings [ 35 , 36 ]. Differently to ISO standards for masks and respirators, the WHO guidance focuses on essential parameters, such as filtering capability, fit and breathability for masks.
Recalling the two aforementioned ethical perspectives, considering existing medical device regulation too generic to be universal, does not mean adhering to the utilitarian-pragmatic current tout court. There may be a contextualised response, regulated on the basis of tests, complying with flexible standards, or rather standards that are purposely designed to take into account different niche conditions. However, the use of any object must be certified and not random, and subject to tests relating to its specific intended use. Only in this way, people and their rights can be safeguarded, and science can prove to think deeply, act consciously and remain silent, when appropriate.
Contextualism is the basis of situational ethics [ 37 ], which seems to be the most adequate response to the specific needs of everyone and be able to face emergencies. In fact, it starts from the particular situation and tries to find universalizable answers, applying a heuristic and inductive method, progressive negotiations and interdisciplinary exchanges.
Conclusions
One year after the start of the pandemic, the need for ethic guidance is still tangible in everyday circumstances and essential during crisis or in RLSs. Respecting fundamental ethical principles while negotiating among different criteria (hospitalization demands vs available ICUs, generalism vs particularism, action vs responsible-action) requires clear guidance, deep knowledge, and peer-to-peer discussion among experts of different disciplines. The need for extreme specialization should never result in the fragmentation of knowledge.
Exactly a century, i.e., the Short Twentieth Century , separates COVID-19 from the last pandemic, the so-called "Spanish Flu", which flagellated Europe in 1918–1919. According to Hobsbawm, “ no period in history has been more penetrated by and more dependent on the natural sciences ” and “ yet no period, since Galileo's recantation, has been less at ease with it ”. This chasm between scientists and the general public is still open and, in some cases, fomented by populisms, which leverage on people's fears evoking war atmospheres, which have nothing to do with the catastrophic failure of many national healthcare systems’ response to this crisis. After a century, the dependence of medicine on biomedical science and engineering is evident, while their contribution to the definition of effective policies and norms is still negligible. Finally, the Cartesian fragmentation of knowledge, or rather “thinking in silos”, has persisted across the last century, calling for the creation of more fora where multidisciplinary discussions can be promoted. Three main needs emerged clearly: the need for responsible thinking, the need for responsible action and the need for responsible silence, when required and appropriate [ 38 ].
Availability of data and materials
Not applicable.
https://www.ft.com/content/491a4af4-66e7-11ea-a3c9-1fe6fedcca75 .
https://www.nytimes.com/2020/03/20/us/politics/trump-coronavirus-supplies.html .
https://www.lavanguardia.com/vida/20200411/48413026980/seat-detiene-produccion-respidradores-emergencia-descongestion-uci.html .
https://uk-mobile-reuters-com.cdn.ampproject.org/c/s/uk.mobile.reuters.com/article/amp/idUKKCN21U0UI .
https://www.franceinter.fr/coronavirus-8-500-respirateurs-produits-pour-rien .
https://www.bbc.co.uk/news/health-52145140 .
https://www.telegraph.co.uk/news/2020/04/08/exclusive-three-nurses-forced-wear-bin-bags-ppe-shortage-test/ .
Abbreviations
World Health Organization
Biomedical Engineering
European Alliance for Medical and Biological Engineering & Science
International Union for Physical and Engineering Sciences in Medicine
Intensive Care Unit
Società Italiana di Anestesia Analgesia Rianimazione e Terapia Intensiva
National Health Service
Personal Protective Equipment
French S. Between factualism and substantialism: Structuralism as a third way. Int J Philos Stud. 2018;26(5):701–21.
Article Google Scholar
Morin E. From the concept of system to the paradigm of complexity. J Soc Evolut Syst. 1992;15(4):371–85.
Department for Health of Ireland. Ethical framework for decision-making in a pandemic. https://assets.gov.ie/72072/989943ddd0774e7aa1c01cc9d428b159.pdf (2020). Accessed 19 June 2020.
The Nuffield Council of Bioethics. Responding to the COVID-19 Pandemic: Ethical Considerations. https://www.nuffieldbioethics.org/news/responding-to-the-covid-19-pandemic-ethical-considerations (2020). Accessed 19 June 2020.
Comité De Bioética De España. Informe Del Comité De Bioética De España Sobre Los Aspectos Bioéticos De La Priorización De Recursos Sanitarios En El Contexto De La Crisis Del Coronavirus. http://assets.comitedebioetica.es/files/documentacion/Informe CBE-Priorizacion de recursos sanitarios-coronavirus CBE.pdf (2020). Accessed 19 June 2020.
Deutscher Ethikrat. Solidarity and responsibility during the coronavirus crisis. https://www.ethikrat.org/en/press-releases/2020/solidarity-and-responsibility-during-the-coronavirus-crisis/ (2020). Accessed 19 June 2020.
UNESCO. Ethics in Research in Times of Pandemic COVID-19. https://en.unesco.org/news/ethics-research-times-pandemic-covid-19 (2020). Accessed 19 June 2020.
UNESCO. Statement on COVID-19: ethical considerations from a global perspective. https://unesdoc.unesco.org/ark:/48223/pf0000373115 (2020). Accessed 19 June 2020.
WHO. Ethical standards for research during public health emergencies: distilling existing guidance to support COVID-19 R&D. https://www.who.int/blueprint/priority-diseases/key-action/liverecovery-save-of-ethical-standards-for-research-during-public-health-emergencies.pdf?ua=1 (2020). Accessed 19 June 2020.
Berlinger N, Wynia M, Powell T, Hester DM, Milliken A, Fabi R, et al. Ethical framework for health care institutions responding to novel Coronavirus SARS-CoV-2 (COVID-19) guidelines for institutional ethics services responding to COVID-19. Safeguarding communities, guiding practice, 2; 2020.
Meyfroidt G, Vlieghe E, Biston P, De Decker K, Wittebole X, Collin V, et al. Ethical principles concerning proportionality of critical care during the COVID-19 pandemic: advice by the Belgian Society of IC medicine. Retrieved 2 April 2020. https://www.hartcentrumhasselt.be/professioneel (2020).
Emanuel EJ, Persad G, Upshur R, Thome B, Parker M, Glickman A, et al. Fair allocation of scarce medical resources in the time of Covid-19. Waltham: Mass Medical Soc.; 2020.
Book Google Scholar
Smith MJ, Ahmad A, Arawi T, Dawson A, Emanuel EJ, Garani-Papadatos T, et al. Top five ethical lessons of COVID-19 that the world must learn. Wellcome Open Res. 2021;6:17.
Smith MJ, Upshur RE. Learning lessons from COVID-19 requires recognizing moral failures. J Bioeth Inq. 2020;17(4):563–6.
Smith MJ, Upshur RE, Emanuel EJ. Publication ethics during public health emergencies such as the COVID-19 pandemic. Am J Public Health. 2020;110(7):947–8.
WHO. Resources on ethics and COVID-19. https://www.who.int/ethics/topics/outbreaks-emergencies/covid-19/en/ (2020). Accessed 19 June 2020.
WHO. Addressing human rights as key to the COVID-19 response. https://www.who.int/publications-detail/addressing-human-rights-as-key-to-the-covid-19-response (2020). Accessed 19 June 2020.
Comitato nazionale per la bioetica. Covid 19: clinical decision-making in conditions of resource shortage and the "pandemic emergency triage criterion". http://bioetica.governo.it/media/3987/p136_2020_covid-19-la-decisione-clinica-in-condizioni-di-carenza-di-risorse-e-il-criterio-del-triage-in-emergenza-pandemica.pdf (2020). Accessed 19 June 2020.
WHO Preparedness. https://www.who.int/environmental_health_emergencies/preparedness/en/ (2020). Accessed 19 June 2020.
The only cure is solidarity from Jürgen Habermas. https://www.classlifestyle.com/news/42575/kura-e-vetme-eshte-solidariteti-nga-jrgen-habermas/eng (2020). Accessed 19 June 2020.
Vergano M, Bertolini G, Giannini A, Gristina GR, Livigni S, Mistraletti G, et al. Clinical ethics recommendations for the allocation of intensive care treatments in exceptional, resource-limited circumstances: the Italian perspective during the COVID-19 epidemic. Berlin: Springer; 2020.
Google Scholar
Rosenbaum L. Facing COVID-19 in Italy—ethics, logistics, and therapeutics on the epidemic’s front line. N Engl J Med. 2020;382(20):1873–5.
Nicoli F, Gasparetto A. Italy in a time of emergency and scarce resources: the need for embedding ethical reflection in social and clinical settings. J Clin Ethics. 2020;31(1):92–4.
Ranney ML, Griffeth V, Jha AK. Critical supply shortages—the need for ventilators and personal protective equipment during the COVID-19 pandemic. N Engl J Med. 2020;382(18):e41.
Sokolov P, Ivanova J. 17th century political cartesianism and its opponents, or imaging the state from point fixe. Higher School of Economics Research Paper No. BRP, 8. 2012.
Jones D. Censorship: a world encyclopedia. London: Routledge; 2001.
Maasen S, Weingart P. Democratization of expertise? Exploring novel forms of scientific advice in political decision-making, vol. 24. Berlin: Springer; 2006.
Bennett B. Logically fallacious: the ultimate collection of over 300 logical fallacies (Academic Edition). EBookIt.com. 2017.
Regulation EU 2017/745 of the European Parliament and of The Council of 5 April 2017 on medical devices, amending Directive 2001/83/EC, Regulation (EC) No 178/2002 and Regulation (EC) No 1223/2009 and repealing Council Directives 90/385/EEC and 93/42/EEC (2017). In: The European parliament and the council of the European Union (Ed.).
Jonas H. The imperative of responsibility: In search of an ethics for the technological age. 1984.
Bentham J, Mill JS. Utilitarianism and other essays. London: Penguin; 2004.
Dewey J. The essential Dewey: pragmatism, education, democracy, vol. 1. Bloomington: Indiana University Press; 1998.
WHO. Novel coronavirus (2019-nCoV) Situation Report-13; 2020.
Pecchia L, Piaggio D, Maccaro A, Formisano C, Iadanza E. The inadequacy of regulatory frameworks in time of crisis and in low-resource settings: personal protective equipment and COVID-19. Health Technol. 2020;10:1375–83.
WHO. Rational use of personal protective equipment for COVID-19 and considerations during severe shortages: interim guidance, 23 December 2020. World Health Organization; 2020.
WHO. Technical specifications of personal protective equipment for COVID-19.
Fletcher JF. Situation ethics: the new morality. Louisville: Westminster John Knox Press; 1997.
Maccaro A, Piaggio D, Pagliara S, Pecchia L. The role of ethics in science: a systematic literature review from the first wave of COVID-19. Health Technol. 2021;3:1–9.
Download references
Acknowledgements
The authors would like to acknowledge Hardip Boparai (PhD student at the University of Warwick, UK) and Katy Stokes (PhD student at the University of Warwick, UK) for proofreading this manuscript and for their valuable suggestions.
DP and LP received support from the University of Warwick with two Warwick Impact Found Grants supported by the EPSRC Impact Accelerator Award (EP/K503848/1 and EP/R511808/1). AM’s Fellowship is supported by the WIRL COFUND – Marie Sklodowska Curie Actions, Institute of Advanced Study, University of Warwick (UK). DP, LP, and AM also received support from Health Global Research Priorities of the University of Warwick. By supporting the researchers, the funders indirectly supported all the parts of this study.
Author information
Alessia Maccaro and Davide Piaggio contributed equally to this work.
Authors and Affiliations
School of Engineering, University of Warwick, Coventry, CV47AL, UK
Alessia Maccaro, Davide Piaggio & Leandro Pecchia
Institute of Advanced Study, University of Warwick, Coventry, CV47AL, UK
Alessia Maccaro
Department of Advanced Biomedical Sciences, University of Naples Federico II, Naples, Italy
Concetta Anna Dodaro
European Alliance of Medical and Biological Engineering and Science (EAMBES), Leuven, Belgium
Leandro Pecchia
IUPESM, York, UK
You can also search for this author in PubMed Google Scholar
Contributions
Conceptualization (AM and LP), data curation (AM, DP), Funding acquisition (LP), investigation (AM, DP, CAD), Project administration (LP), Supervision (LP, CAD), Writing original draft (AM, DP), Writing review and editing (AM, DP, LP, CAD). All authors reviewed the manuscript and agreed with the final version.
Corresponding author
Correspondence to Davide Piaggio .
Ethics declarations
Ethics approval and consent to participate, consent for publication, competing interests.
The authors declare that they have no conflict of interest.
Additional information
Publisher's note.
Springer Nature remains neutral with regard to jurisdictional claims in published maps and institutional affiliations.
Rights and permissions
Open Access This article is licensed under a Creative Commons Attribution 4.0 International License, which permits use, sharing, adaptation, distribution and reproduction in any medium or format, as long as you give appropriate credit to the original author(s) and the source, provide a link to the Creative Commons licence, and indicate if changes were made. The images or other third party material in this article are included in the article's Creative Commons licence, unless indicated otherwise in a credit line to the material. If material is not included in the article's Creative Commons licence and your intended use is not permitted by statutory regulation or exceeds the permitted use, you will need to obtain permission directly from the copyright holder. To view a copy of this licence, visit http://creativecommons.org/licenses/by/4.0/ . The Creative Commons Public Domain Dedication waiver ( http://creativecommons.org/publicdomain/zero/1.0/ ) applies to the data made available in this article, unless otherwise stated in a credit line to the data.
Reprints and permissions
About this article
Cite this article.
Maccaro, A., Piaggio, D., Dodaro, C.A. et al. Biomedical engineering and ethics: reflections on medical devices and PPE during the first wave of COVID-19. BMC Med Ethics 22 , 130 (2021). https://doi.org/10.1186/s12910-021-00697-1
Download citation
Received : 17 August 2020
Accepted : 12 July 2021
Published : 25 September 2021
DOI : https://doi.org/10.1186/s12910-021-00697-1
Share this article
Anyone you share the following link with will be able to read this content:
Sorry, a shareable link is not currently available for this article.
Provided by the Springer Nature SharedIt content-sharing initiative
- Biomedical engineering
- Regulations
- Responsibility
BMC Medical Ethics
ISSN: 1472-6939
- General enquiries: [email protected]
- Browse Works
- Engineering
- Biomedical Engineering
Biomedical Engineering Research Papers/Topics
A survey in the different designs and control systems of powered exoskeleton for lower extremities.
Abstract: In this paper, previous studies in powered exoskeleton and their contributions in the field of robotics technology are presented, together with their corresponding control system. Specific problems and issues that were encountered and the solutions made to resolve the problems will be discussed. Gait cycle analysis and human body dynamic model will also be covered in the study to understand the biomechanics and the dynamics behind human walking.
Electroosmosis modulated peristaltic biorheological flow through an asymmetric microchannel: mathematical model
Abstract: A theoretical study is presented of peristaltic hydrodynamics of an aqueous electrolytic non-Newtonian Jeffrey bio-rheological fluid through an asymmetric microchannel under an applied axial electric field. An analytical approach is adopted to obtain the closed form solution for velocity, volumetric flow, pressure difference and stream function. The analysis is also restricted under the low Reynolds number assumption (Stokes flow) and lubrication theory approximations (large wavele...
Magnetic field effect on a fractionalized blood flow model in the presence of magnetic particles and thermal radiations
Abstract: The presence of magnetic particles is considered in a magneto-hydrodynamic blood flow through a circular cylinder. The fluid inside the tube is acted by an oscillating pressure gradient and an external constant magnetic field. The blood temperature is assumed to change with the blood and particle velocities, and the whole study is based on a mathematical model that includes Caputo fractional-order derivatives. Solutions for the particle and blood velocities, and blood temperature d...
Sweat sensors in the smart wearables era - A Review
In recent years, there has been significant interest in developing wearable devices to mimic the integrated sensing of life forms, which enhances their performance and survival capabilities. Progress in the development of physical sensors and wearable electronics has been promising, leading to numerous consumer products that measure activity, posture, heart rate, respiration rate, and blood oxygen level. Despite the challenges in retrieving and processing bodily fluids, wearable chemical sens...
Effect of Magnetic field on Micro-organisms
This study uses the model organism, C. elegans, to investigate its sensitivity and response to static magnetic fields. Wild-type C. elegans are put into microfluidic channels and exposed to permanent magnets for five cycles of thirty-second time intervals at field strengths ranging from 5 milli Tesla to 120 milli Tesla. Recorded and analyzed with custom software, the results of the worm's movement - the average velocity, turning and curling percentage - were compared to control experiments...
Worm Egg Counting using Machine learning
To evaluate the level of infestation of the soybean cyst nematode (SCN), Heterodera glycines, in the field, egg population densities are determined from soil samples. Sucrose centrifugation is a common technique for separating debris from the extracted SCN eggs. We have developed a procedure, however, that employs OptiPrep as a density gradient medium, with improved extraction and recovery of the eggs compared to the sucrose centrifugation technique. Also, we have built computerized methods t...
Paralysis modes of worms in drugs
The emergence of new drugs is often driven by the escalating resistance of parasites to existing drugs and the accessibility of more advanced technology platforms. Microfluidic devices have allowed for quicker testing of compounds, regulated sampling/sorting of entire animals, and automated behavioral pattern detection. In the majority of microfluidic devices, the effects of drugs on small animals (e.g. Caenorhabditis elegans)elegant are quantified by an endpoint, dose response curve that sho...
Modes of paralysis of worms in anthelmintic drugs
Microfluidic chip for culturing gene-edited bacteria.
By utilizing a low-cost engineering tool, we have created a microfluidic platform to study bacteria at the single cell level, allowing us to unlock insights into microbial physiology and genetics that would otherwise not be possible. The platform is composed of 3D devices made of adhesive tapes, an agarose membrane as the resting substrate, a temperature-controlled environmental chamber, and an autofocusing module. With this technology, we have been able to observe Escherichia coli morphologi...
Wearable devices at Work
The effects of the workplace on the safety, health, and productivity of personnel can be seen at various levels. To protect and boost general worker health, innovative hardware and software tools have been developed for the detection, elimination, substitution, and regulation of occupational hazards. Wearable technologies make it possible for constant tracking of workers and their environment, whereas connected worker solutions provide contextual information and support for decision-making. H...
Video Capsule Endoscopy : A Review of Technologies
In this review, we focus on the hardware and software technologies used for the purpose of gastrointestinal tract monitoring in a safe and comfortable manner. We review the FDA guidelines for ingestible wireless telemetric medical devices, and the features incorporated in capsule systems such as microrobotics, closed-loop feedback, physiological sensing, nerve stimulation, sampling and delivery, panoramic imaging and rapid reading software. Both experimental and commercialized capsule systems...
Animal Behavior Sensing Electronics
We propose a remote monitoring device for measuring behavioral indicators like posture, gait, vocalization, and external temperature which can help in evaluating the health and welfare of pigs. The multiparameter electronic sensor board was tested in a laboratory and on animals. Machine learning algorithms and decision support tools can be used to detect lameness, lethargy, pain, injury, and distress. The roadmap for technology adoption, potential benefits, and further challenges are discusse...
Electrical field effects on micro-organisms
We present the NERV, a nonmechanical, unidirectional valve, to control the locomotion of Caenorhabditis elegant (C. elegans) in microfluidic devices. This valve is created by establishing a region of lateral electric field which can be toggled between on and off states. We observed that C. elegans do not prefer to advance into this region when the field lines are facing their movement, so when they reach the boundary of the NERV, they partway enter the field, retreat, and switch direction. We...
Anthelmintic resistance in nematodes
It is becoming more essential to identify and recognize the phenotypes of anti-parasitic drug-resistant isolates. Current molecular methods of doing so are restricted. In this paper, we showcase a microfluidic bioassay to measure phenotype using parameters of nematode locomotion, using larvae of the animal parasite Oesophagostomum dentatum. Parameters of sinusoidal movement, including propagation speed, wavelength, wave amplitude, and oscillation frequency, were dependent on the levamisole-se...
Robotic Manipulator using an Inductive Sensor
This project involves the use of an inductive sensor to control a robotic arm system. The signals generated in the induction coil will help in controlling the manipulator to perform certain gripping and grabbing actions. Also, the report describes the method used to acquire and process these induced signals before they are sent (induced signals) to the manipulator through the help of two programmed microcontrollers.
Popular Papers/Topics
Study of blood glucose measurement based on blood resistivity, design for real time heart sounds recognition system, design of a hybrid artificial hip joint using finite element analysis, design of a programmed oxygen delivery system, early detection of stomach pain using reflexology, design of management programme for a clinical engineering department, adaptive techniques for cardiac arrhythmia detection by using artificial neural network, improvement u-net for mri brain tumour segmentation by searching for suitable activation function, a study of optical and thermal effectsof gold nanoparticles, noise reducing using hybrid technique in magnetic resonances image, central medical waste treatment facility, detection of breast cancer using artificial neural networks (ann), short messages technique in health level 7(hl7), designing a tele-pediatric system based on the internet, heart beat rate variability analysis using statistical methods.
Privacy Policy | Refund Policy | Terms | Copyright | © 2024, Afribary Limited. All rights reserved.
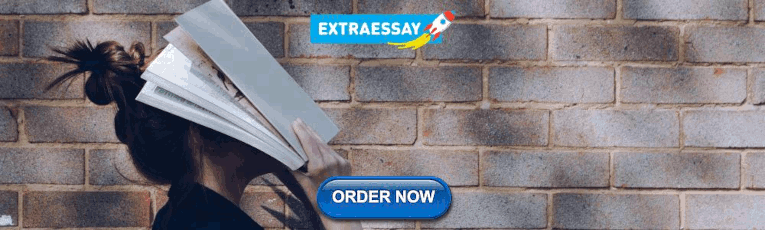
IMAGES
VIDEO
COMMENTS
Biomedical engineering is a branch of engineering that applies principles and design concepts of engineering to healthcare. Biomedical engineers deal with medical devices such as imaging equipment ...
Learn more about our seven focus areas: Biomedical Data Science. Extract knowledge from biomedical datasets of all sizes to understand and solve health-related problems. Learn More. Computational Medicine. Generate solutions in personalized medicine by building and utilizing computational models of health and disease.
Overview. Research on Biomedical Engineering is an online, peer-reviewed journal dedicated to all fields of Biomedical Engineering. Multidisciplinary in nature, catering to readers and authors interested in developing tools based on engineering and physical sciences to solve biological and medical problems. Publishes Original Research Articles ...
Aims and scope. BMC Biomedical Engineering considers articles on all aspects of biomedical engineering, including fundamental, translational and clinical research. It combines tools and methods from biology and medicine with mathematics, physical sciences and engineering towards the understanding of human biology and disease and the improvement of human health.
Biomedical Engineering and Computational Biology is an international, peer reviewed, open access journal that covers topics in biomedical engineering, bioinformatics, systems biology, and computational biology, in particular papers using computational methods to address complex problems in today's medicine and biology. The journal aims to provide a forum to bridge experimental research and ...
To explore the predictive value of bedside lung ultrasound score in the severity of neonatal respiratory distress syndrome (NRDS) and mechanical ventilation and extubation. Peipei Huang, Deng Chen, Xiuxiang Liu, Xiang Zhang and Xiazi Song. BioMedical Engineering OnLine 2024 23 :29. Research Published on: 6 March 2024.
With the recent advancements in biomedical engineering technologies, commercially available VLP-based vaccines are being extensively used to combat infectious diseases, whereas many more are in different stages of development in clinical studies. Because of their desired characteristics in terms of efficacy, safety, and diversity, VLP-based ...
100 Biomedical Research Paper Topics. Biomedical research is a vibrant field, with an extensive range of topics drawn from various sub-disciplines. It encompasses the study of biological processes, clinical medicine, and even technology and engineering applied to the domain of healthcare. Given the sheer breadth of this field, choosing a ...
We need an ethically aligned and driven healthcare system and sustainability. This topic, "Applied System on Biomedical Engineering, Healthcare and Sustainability", includes five journals, Journal of Clinical Medicine, Sustainability, Electronics, Healthcare, and Bioengineering, to publish excellent papers about relative fields.
We have compiled a list of biomedical engineering topics for you. Here they are: In-the-ear device to control stuttering: the basis of its operation. How to implement the magnetic navigated catheterization. Semiconductor-cell interfaces: the rudiments of its application. The benefits of tissue engineering of muscle.
Innovative Microphysiological Mimicries of Human Obstructive and Restrictive Pulmonary Disorders. Kambez H Benam. Anne M. Van Der Does. Sean Vincent Murphy. 108 views. A multidisciplinary journal that accelerates the development of biological therapies, devices, processes and technologies to improve our lives by bridging the gap between ...
See More. Johns Hopkins Biomedical Engineering. Contact BME. Homewood Campus. 3400 N. Charles StreetWyman Park BuildingSuite 400 WestBaltimore, MD 21218. (410) 516-8120. East Baltimore Campus. 720 Rutland AvenueBaltimore, MD 21205. (410) 955-3132.
By interfacing medical problems with molecular signatures and cutting-edge hardware detection, we can engineer new systems for detection of a huge range of molecules and processes. Our research in this area includes orthopedics, microfluidics, biomedical instrumentation, photoacoustic tomography, and DNA molecular detectors. Mark Ehrensberger, PhD.
Research Focus Areas. Focused on solving some of the toughest problems facing Georgia, the nation, and the world. Biomaterials and Regenerative Technologies. Biomaterials and Regenerative Technologies. Biomedical Imaging and Instrumentation. Biomedical Imaging and Instrumentation. Biomedical Informatics and Systems Modeling.
This manuscript was written with two readers in mind. Firstly, it is intended for mathematical modelers with a background in physics, mathematics, or engineering who want to jump into biomedicine. We provide them with ideas to motivate the use of mathematical modelling when discussing with experimental partners.
The paper further identified and discussed significant applications of Biomedical engineering for healthcare. Biomedical engineering is a fascinating field of life science that can change ...
The motivation for the launch of BMC Biomedical Engineering is to create an authoritative, unbiased and community-focused open access journal. We are committed to working together with our authors, editors and reviewers to provide an inclusive platform for the publication of high-quality manuscripts that span all aspects of biomedical engineering research.
The unprecedented high number of retracted papers can be a proxy for the high number of low-quality research on COVID-19. For this reason, a rapid search for papers regarding COVID-19 or SARS-CoV-2, and the previous epidemic/pandemics (i.e., SARS, MERS, Swine Flu) as a comparison, was performed both on OvidSP and the Retraction Watch Database.
Biomedical Engineering Research Papers/Topics . A survey in the different designs and control systems of powered exoskeleton for lower extremities. Abstract: In this paper, previous studies in powered exoskeleton and their contributions in the field of robotics technology are presented, together with their corresponding control system. ...
Regularized Extreme Learning Machine. R-CNN. PCA-Random Forest. Deep Wavelet Auto Encoder DNN. Support Vector Machine. PhD Research Topics in Biomedical Engineering is your remote to change your research end. To begin with, our team also knows a lot of methods for sure. In view of this, we have listed the methods for your view.