74 vibration-analysis PhD scholarships
Filtered by.
- Scholarship
- vibration-analysis
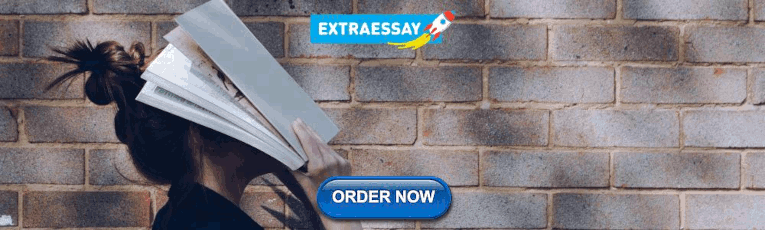
Refine Your Search
- Last-7-days 3
- Last-30-days 16
- United Kingdom 22
- Netherlands 19
- Australia 4
- Switzerland 3
- Amsterdam UMC 7
- ; University of Southampton 5
- Delft University of Technology 4
- University of Groningen 4
- Chalmers University of Technology 3
- University of Basel 3
- ; Cranfield University 2
- ; Swansea University 2
- ; University of Warwick 2
- Cranfield University 2
- Delft University of Technology (TU Delft) 2
- Eindhoven University of Technology 2
- Ghent University 2
- NTNU - Norwegian University of Science and Technology 2
- NTNU Norwegian University of Science and Technology 2
- Swansea University 2
- Technical University of Denmark 2
- University of Melbourne 2
- University of Nottingham 2
- ; Newcastle University 1
- ; University of Birmingham 1
- ; University of Essex 1
- ; University of Leeds 1
- Aarhus University 1
- Curtin University 1
- Fraunhofer-Gesellschaft 1
- Graz University of Technology 1
- Helmholtz 1
- Jagiellonian University 1
- Jagiellonian University in Krakow 1
- NORWEGIAN UNIVERSITY OF SCIENCE & TECHNOLOGY - NTNU 1
- University of Adelaide 1
- University of Antwerp 1
- University of Pardubice, Pardubice, Czech Republic 1
- University of Sheffield 1
- Uppsala University 1
- Computer Science 35
- Mathematics 15
- Engineering 8
- Chemistry 5
- Materials Science 3
- Earth Sciences 2
- Economics 2
- Education 2
- Electrical Engineering 2
- Linguistics 1
- Medical Sciences 1
PhD position Dynamic analysis and human vibration perception of hand held
PhD position Dynamic analysis and human vibration perception of hand held (V23.0732) « Back to the overview Job description We are looking for a candidate to undertake research in the field
Organisation Job description We are looking for a candidate to undertake research in the field of dynamics and vibration , with a particular focus on dynamic analysis and modelling of in hand
PhD Studentship: Novel Methods for the Characterisation of Helicopter Aero-acoustics
-mechanics, and aero-acoustics. This PhD project, situated within the Propulsion Engineering Centre, focuses on helicopter aerodynamic and aero-acoustic modelling. The objective is to develop a rapid analysis
PhD Uncertainties in the source and environment description: influence on noise impact assessment (M/F)
and proportionate underwater noise mitigation solutions, for improved know-how, decision-making and standards setting for a sustainable Blue Growth limiting the impact on marine wildlife. The project
PhD Acoustic metamaterials for transmission cancellation (M/W)
, multidisciplinary and international project METAVISION: “METAmaterials for VIbration and Sound reductION” (no. 101072415) funded under the Marie-Sklodowska-Curie Actions Doctoral Networks within the Horizon Europe
Codan Founders' PhD Scholarship
potential objects of interest in a geographic area for further inspection by a human or robot operator, and further performing a thorough analysis of a flagged location to confirm the presence of an object
PhD-position - CRC Project Computational Electric Machine Laboratory
Responsibilities Work on the FWF-project „FWF - Electric Machine Laboratory“, sub-project Noise and Vibration of Electric Drives: Space-Time Boundary Element Formulations: Simulation of noise and vibration with
PhD Studentship: Real-time gas sensing using terahertz quantum-cascade lasers
first potential satellite deployment of fast and low- noise THz receiver systems. Key objectives include: Real-time analysis of chemical reactions using THz radiation: The PGR will use a broadband TeraFET
PhD student position in Numerical modelling of vibrational effects..
--1-12606 Is the Job related to staff position within a Research Infrastructure? No Offer Description PhD student position in Numerical modelling of vibrational effects on buildings from blasting We
PhD Studentship: Space-based Quantum Sensing for Maritime Applications
advantages including suppression of significant noise sources while allowing operation with long measurement times that are not limited by the fall time of the atoms. It is therefore increasingly considered
Searches related to vibration analysis
- post doc dynamics vibration
- postdoctoral
- condition monitoring
- engineering
- vibration acoustic
- mechanical vibration
- structural health monitoring
- mechanical engineering
MIT Libraries home DSpace@MIT
- DSpace@MIT Home
- MIT Libraries
- Doctoral Theses
Visual vibration analysis
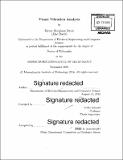
Other Contributors
Terms of use, description, date issued, collections.
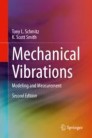
Mechanical Vibrations
Modeling and Measurement
- © 2021
- Tony L. Schmitz 0 ,
- K. Scott Smith 1
Mechanical, Aerospace, and Biomedical Engineering, University of Tennessee, Knoxville, Knoxville, USA
You can also search for this author in PubMed Google Scholar
Manufacturing Demonstration Facility, Machining and Machine Tools Research Group, Oak Ridge National Laboratory, Knoxville, USA
Presents a clear connection between continuous beam models and finite degree of freedom models
Includes MATLAB code to support numerical examples that are integrated into the text narrative
Uses mathematics to support vibrations theory and emphasizes the practical significance of the results
20k Accesses
12 Citations
1 Altmetric
This is a preview of subscription content, log in via an institution to check access.
Access this book
- Available as EPUB and PDF
- Read on any device
- Instant download
- Own it forever
- Compact, lightweight edition
- Dispatched in 3 to 5 business days
- Free shipping worldwide - see info
- Durable hardcover edition
Tax calculation will be finalised at checkout
Other ways to access
Licence this eBook for your library
Institutional subscriptions
Table of contents (10 chapters)
Front matter, introduction.
- Tony L. Schmitz, K. Scott Smith
Single Degree of Freedom Free Vibration
Single degree of freedom forced vibration, two degree of freedom free vibration, two degree of freedom forced vibration, model development by modal analysis, measurement techniques, continuous beam modeling, finite element introduction, receptance coupling, back matter.
- Receptance coupling
- Mechanical vibrations
- Modal analysis
- Modal testing
- System dynamics
- Equation of motion
- Differential equation
- Continuous beam models
About this book
- Presents a clear connection between continuous beam models and finite degree of freedom models;
- Includes MATLAB code to support numerical examples that are integrated into the text narrative;
- Uses mathematics to support vibrations theory and emphasizes the practical significance of the results.
“The book is suitable for undergraduate students, researchers, and practicing engineers who are interested in developing an understanding of essential concepts in vibration analysis of mechanical systems.” (IEEE Control Systems Magazine, Vol. 41 (2), April, 2021)
Authors and Affiliations
Tony L. Schmitz
K. Scott Smith
About the authors
Bibliographic information.
Book Title : Mechanical Vibrations
Book Subtitle : Modeling and Measurement
Authors : Tony L. Schmitz, K. Scott Smith
DOI : https://doi.org/10.1007/978-3-030-52344-2
Publisher : Springer Cham
eBook Packages : Engineering , Engineering (R0)
Copyright Information : Springer Nature Switzerland AG 2021
Hardcover ISBN : 978-3-030-52343-5 Published: 30 October 2020
Softcover ISBN : 978-3-030-52346-6 Published: 30 October 2021
eBook ISBN : 978-3-030-52344-2 Published: 29 October 2020
Edition Number : 2
Number of Pages : XII, 430
Number of Illustrations : 307 b/w illustrations, 28 illustrations in colour
Topics : Vibration, Dynamical Systems, Control , Solid Mechanics , Classical Mechanics
- Publish with us
Policies and ethics
- Find a journal
- Track your research
- University home
- For business
- Alumni and supporters
- Our departments
- Visiting us

PhDs in Vibration Engineering

Not your average PhD
As a phd researcher with the vibration engineering section, you can: .
Access the UK’s most advanced facilities for vibration testing, analysis and control
Bring your ideas to life beyond computer modelling: develop a prototype in our labs, test it and deploy it as a real-world solution, using our expertise and capabilities
- Work on industry-led projects and network with global engineering consultancy companies
Be supported by supervisors at the forefront of their field
Undertake helicopter escape training
Hear from our former PhD researchers
Funded PhD opportunities
There are no funded PhD opportunities available at this time.
Self-funded PhD studentships
Vibration Engineering academics can offer supervision for PhD Engineering candidates.
We are interested in hearing from candidates with an idea for a PhD project that relates to one or more of our research areas :
- Vibration control of structures
- Structural health monitoring
- Vibration serviceability of civil structures under human dynamic loads
- Human motion and biomechanics
Example project ideas
Below are some examples of the kinds of PhD topic areas we are interested in supervising. View our profiles to check if your idea fits with our research interests.
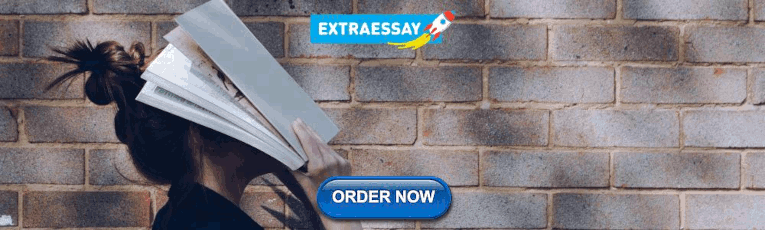
James Brownjohn
- Modelling and mitigating low level ground borne vibrations transmitted to vibration sensitive facilities
- Modelling behaviour of bridge bearings and expansion joints
- Monitoring human motion using wearable sensors to reduce risk of trips and falls
- Perception of vibration for stationary and moving people in objects of infrastructure
- In-situ monitoring and modelling effects of extreme events such as flooding, over-crowding and high winds on as-built structures
- Effects of vertical non-structural partitions and facade on vibration performance of building floors
Applying for a self-funded PhD
How to apply
- Read the information on the Engineering PhD programme page , and check you meet the entry criteria.
- Propose a project idea to one of our academics, following the instructions below.
- Browse our list of alternative funding sources and information on how to fund a PhD.
- Submit your application online, following the process explained on the postgraduate research website .
How to propose a PhD idea
- Before applying for a PhD, please prepare a 500-word outline of your proposed research project, and send this by email to the academic whose research specialism matches your project.
- If you receive a positive response, you can then plan to submit a formal application in the form of a research proposal. Please see the postgraduate research website for advice on writing a research proposal .
If your idea is not related to our specific research areas, please see the other Engineering research themes at the University, as another academic may be more suitable to supervise you.
PhDs part-funded by industry
If you work in an engineering-related field and want to enhance your knowledge and skills with a PhD, your employer may be able to sponsor you to undertake a PhD with us.
Likewise, if you are an employer interested in putting an employee forward for a PhD we would love to hear from you.
Email us for more information »
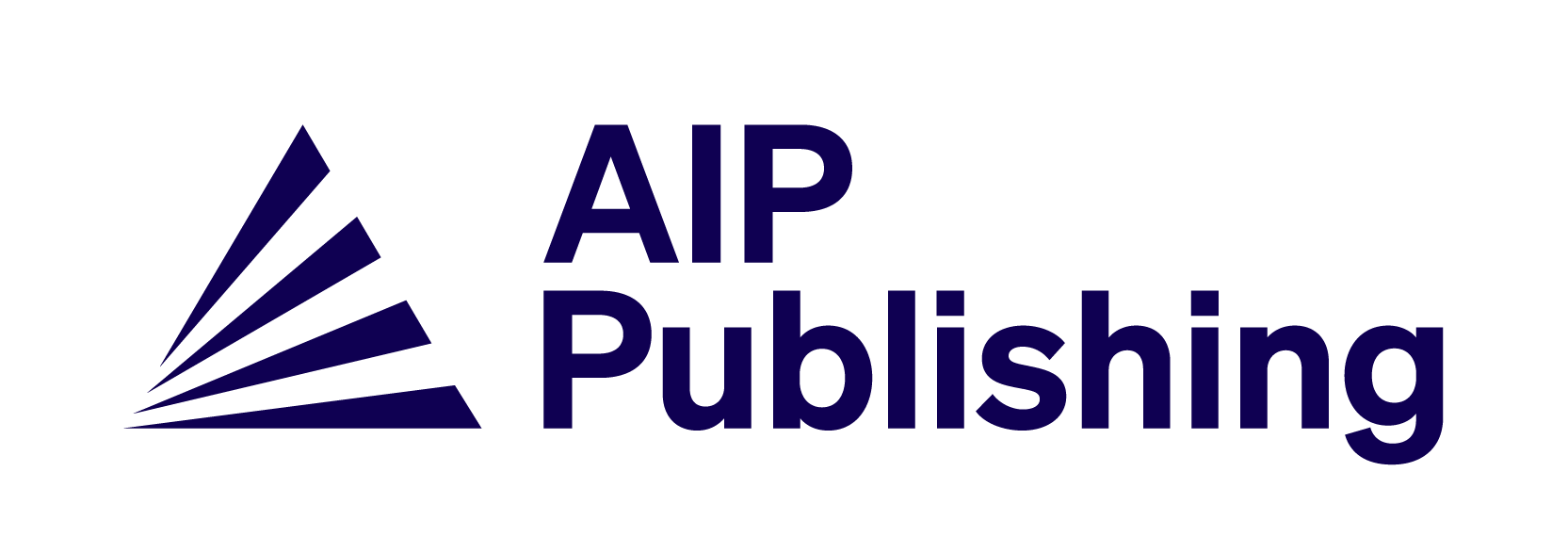
- Previous Article
Stress analysis of vibrating pipelines
Corresponding author: [email protected]
- Split-Screen
- Article contents
- Figures & tables
- Supplementary Data
- Peer Review
- Open the PDF for in another window
- Reprints and Permissions
- Cite Icon Cite
- Search Site
Janusz Zachwieja; Stress analysis of vibrating pipelines. AIP Conf. Proc. 3 March 2017; 1822 (1): 020017. https://doi.org/10.1063/1.4977691
Download citation file:
- Ris (Zotero)
- Reference Manager
The pipelines are subject to various constraints variable in time. Those vibrations, if not monitored for amplitude and frequency, may result in both the fatigue damage in the pipeline profile at high stress concentration and the damage to the pipeline supports. If the constraint forces are known, the system response may be determined with high accuracy using analytical or numerical methods. In most cases, it may be difficult to determine the constraint parameters, since the industrial pipeline vibrations occur due to the dynamic effects of the medium in the pipeline. In that case, a vibration analysis is a suitable alternative method to determine the stress strain state in the pipeline profile. Monitoring the pipeline vibration levels involves a comparison between the measured vibration parameters and the permissible values as depicted in the graphs for a specific pipeline type. Unfortunately, in most cases, the studies relate to the petrochemical industry and thus large diameter, long and straight pipelines. For a pipeline section supported on both ends, the response in any profile at the entire section length can be determined by measuring the vibration parameters at two different profiles between the pipeline supports. For a straight pipeline section, the bending moments, variable in time, at the ends of the analysed section are a source of the pipe excitation. If a straight pipe section supported on both ends is excited by the bending moments in the support profile, the starting point for the stress analysis are the strains, determined from the Euler–Bernoulli equation. In practice, it is easier to determine the displacement using the experimental methods, since the factors causing vibrations are unknown. The industrial system pipelines, unlike the transfer pipelines, are straight sections at some points only, which makes it more difficult to formulate the equation of motion. In those cases, numerical methods can be used to determine stresses using the kinematic inputs at a known vibration velocity amplitude and frequency. The study presents the method to determine the stresses.
Citing articles via
Publish with us - request a quote.
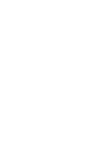
Sign up for alerts
- Online ISSN 1551-7616
- Print ISSN 0094-243X
- For Researchers
- For Librarians
- For Advertisers
- Our Publishing Partners
- Physics Today
- Conference Proceedings
- Special Topics
pubs.aip.org
- Privacy Policy
- Terms of Use
Connect with AIP Publishing
This feature is available to subscribers only.
Sign In or Create an Account
Thank you for visiting nature.com. You are using a browser version with limited support for CSS. To obtain the best experience, we recommend you use a more up to date browser (or turn off compatibility mode in Internet Explorer). In the meantime, to ensure continued support, we are displaying the site without styles and JavaScript.
- View all journals
- My Account Login
- Explore content
- About the journal
- Publish with us
- Sign up for alerts
- Open access
- Published: 08 April 2024
Mechanical characteristics analysis of high dimensional vibration isolation systems based on high-static-low-dynamic stiffness technology
- Bu-yun Li 1 , 2 ,
- Chang-geng Shuai 1 , 2 &
- Jian-guo Ma 1 , 2
Scientific Reports volume 14 , Article number: 8195 ( 2024 ) Cite this article
Metrics details
- Engineering
- Mechanical engineering
Large floating raft vibration isolation systems (FRVISs) based on high-static-low-dynamic stiffness (HSLDS) technology offer excellent low frequency vibration isolation performance with broad application prospects. However, the design process for these complex high-dimensional coupled nonlinear systems remains poorly developed, particularly when applied for ocean-going vessels that experience rolling and pitching motions. The present work addresses this issue by establishing a six-degree-of-freedom HSLDS vibration isolation model for FRVISs composed of eight isolators, and the model is applied to fully analyze the swing stability and multidimensional vibration isolation performance of these systems. The influence of nonlinearity on the mechanical properties of the vibration isolators is analyzed more clearly by assuming that each vibration isolator realizes nonlinear HSLDS characteristics in the z direction and linear characteristics in the x and y directions. The results demonstrate that the swing displacement responses of the system are greatly reduced under weak nonlinearity, which reflects the high static stiffness and high static stability characteristics of an HSLDS system. The multidimensional vibration isolation performance of the system is evaluated according to the impacts of nonlinearity, the installation height H z of the isolators, and the relative position D r of the two middle isolators. The results of analysis demonstrate that applying a value of H z = 0 produces the best vibration isolation performance overall under strong nonlinearity by avoiding unnecessary secondary peaks in the force transmission rate under harmonic mechanical excitation and ensuring a maximum high-frequency vibration isolation effect. However, applying a weak nonlinearity is better than a strong nonlinearity if H z is not zero. In contrast, D r has little effect on the vibration isolation effect of the raft in the x , y , and z directions. Therefore, an equidistant installation with D r = 0.5 would be considered ideal from the standpoint of installation stability.
Introduction
Floating raft vibration isolation systems (FRVISs) represent a state-of-the-art technology for minimizing the mechanical vibrations of ocean going vessels with a range of goals, such as for enhancing their acoustic stealth performance. Of particular importance in this regard is to maximize the low-frequency vibration isolation performance of these systems 1 . From a theoretical perspective, the low-frequency vibration isolation performance of these systems increases as their natural frequency decreases 2 . The primary means of reducing the natural frequency of an FRVIS is to reduce the stiffness of the vibration isolators applied therein. However, this strategy suffers from at least two problems. First, the lowest natural frequency of an isolator is limited by the physical properties, and cannot be reduced indefinitely. Second, system stability is increasingly compromised under decreasing stiffness because this also increases the deformation experienced by the system under the rolling and pitching conditions of the vessel.
These issues have been addressed in recent years by the development of high-static-low-dynamic stiffness (HSLDS) vibration isolation structures, which can reduce dynamic stiffness while ensuring static stability 3 , 4 . Ideally, the dynamic stiffness of this type of vibration isolation structure can approach a value of zero, and therefore represents quasi-zero stiffness (QZS) performance 5 . At present, a number of HSLDS vibration isolator structures have been designed, such as inclined spring structures 6 , 7 , 8 , 9 , 10 , cam-roller structures 11 , 12 , 13 , 14 , 15 , pneumatic structures 16 , 17 , 18 , magnetic structures 19 , 20 , 21 , 22 , and structures inspired by biological organisms 23 , 24 , 25 , 26 . Moreover, Li and Xu 27 , 28 were the first to design an FRVIS using QZS isolators, and the vibration isolation performance of the resulting system was analyzed. However, while the low-frequency vibration isolation performance of the system was demonstrated to be substantially improved via the use of QZS isolators, the model established was a little simple, and some factors affecting the vibration isolation effect of the system were not considered, such as the number of isolators, which can be very many in an FRVIS, and their installation positions. In addition, the influence of different excitation conditions and the nonlinearities of the isolators on the vibration isolation performance of FRVISs were also not considered. For example, the vibration isolation characteristics of the system were evaluated under non-eccentric excitations when the isolators realized QZS condition. However, these characteristics are not at all certain under all possible excitation conditions with non-QZS characteristics. The past research results inspired the research of this paper. This paper further studies the situations that have not been considered before to ensure engineering practicability.
Moreover, in contrast to land-based applications, ocean-going vessels experience rolling and pitching motions during operation, which introduce more rigorous stability requirements for HSLDS-FRVISs. However, the swing stability of these complex vibration isolation systems remains poorly evaluated. Meanwhile, some scholars have analyzed the swing stability of linear systems. For example, He et al. 29 analyzed the rolling stability of a vibration isolation system designed for a vessel propulsion system based on a single-layer linear vibration isolation model. However, the stability characteristics of such systems are quite different from those of highly nonlinear HSLDS systems. In addition, most studies focused on analyzing the dynamic stability of nonlinear systems have considered relatively simple HSLDS vibration isolator systems, including their nonlinear dynamic behaviors, such as jumping and bifurcation 30 , 31 , 32 . Accordingly, the design process for these complex high-dimensional coupled nonlinear systems remains poorly developed in ocean-going vessel applications. As a result, the design of efficient, safe, and stable HSLDS-FRVISs remains beyond the reach of the current state of the art.
The present work addresses this issue by establishing a six degrees-of-freedom (6-DOF) HSLDS-FRVIS model, and the model is applied to fully analyze the swing stability and multidimensional vibration isolation performance of these systems. Therefore, the current work lays a sound theoretical foundation for the subsequent design of HSLDS-FRVISs.
Simplified HSLDS-FRVIS model
For the convenience of analysis, it is assumed that the floating raft is rigid and supported only vertically by vibration isolators. For large floating rafts, vibration isolators will be symmetrically arranged at the four corners and the middle of the raft to ensure stability. According to the actual engineering needs, 6, 8, 10 or even more vibration isolators can be symmetrically installed. For general discussion, it is assumed that the floating raft is supported by eight vibration isolators, which is also a common isolator configuration for ship vibration isolation systems. Therefore, the simplified structure of the HSLDS-FRVIS investigated in the present study is illustrated schematically in Fig. 1 . As can be seen, the mechanical equipment is rigidly installed on top of the floating raft, and the floating raft is supported by eight HSLDS vibration isolators. The eight vibration isolators are numbered ① – ⑧ counterclockwise from the lower right corner. The global coordinate system OXYZ is located at the center of gravity jointly determined by the mechanical equipment and floating raft. The coordinate system of each vibration isolator coincides with the global coordinate system. The influence of nonlinearity on the mechanical properties of the vibration isolators is analyzed more clearly by assuming that each vibration isolator realizes nonlinear HSLDS characteristics in the z direction and linear characteristics in the x and y directions. The other model parameters include l c , b c , and h c , which are one-half of the length, width, and height of the raft, respectively. In addition, installation positions a x , a y 1 , and a z represent the coordinates of the vibration isolators in the coordinate system OXYZ , where \(\left| {a_{x} } \right| = l_{c}\) and \(\left| {a_{y1} } \right| = b_{c}\) . As can be seen, the positions of vibration isolators installed at the four corners of the floating raft structure are usually determined by the length and width of the floating raft. However, the positions of the middle isolators are arbitrarily adjustable. Therefore, we define a coordinate a y 2 , which represents the position of the middle vibration isolators (i.e., isolators ② , ③ , ⑥ , and ⑦ ) relative to the center of gravity along the y axis. In addition, while the installation heights of the isolators are arbitrarily adjustable, we apply a standard position of \(\left| {a_{z} } \right| = h_{c}\) herein unless otherwise specified. \(\left| {a_{z} } \right|\) is the distance between the upper end of the isolator with rated load and the center of gravity jointly determined by the mechanical equipment and floating raft. Therefore, a standard position of \(\left| {a_{z} } \right| = h_{c}\) means that the vibration isolators are installed on the bottom of the floating raft. Furthermore, the installation height of the isolators and the positions of the middle isolators are defined according to an installation height ratio \(H_{z} = \left| {{{a_{zi} } \mathord{\left/ {\vphantom {{a_{zi} } {h_{c} }}} \right. \kern-0pt} {h_{c} }}} \right|\) ( i = 1, 2, …, 8) and a distance ratio \(D_{r} = \left| {{{a_{ym} } \mathord{\left/ {\vphantom {{a_{ym} } {b_{c} }}} \right. \kern-0pt} {b_{c} }}} \right|\) ( m = 2, 3, 6, 7), respectively. The standard values of H z and D r applied are 1 and 0.5 respectively. Unless otherwise specified, the structural parameters applied herein are listed in Table 1 . The coordinates of an applied excitation force \({\mathbf{F}}\) are denoted as \((s_{x} ,s_{y} ,s_{z} )\) , where the absolute values of \(s_{x}\) , \(s_{y}\) , and \(s_{z}\) respectively represent the eccentric distances of F in the x , y , and z directions. If \(s_{x} = s_{y} = s_{z} = 0\) , the excitation force is located at the center of gravity of the system (i.e., at O ).
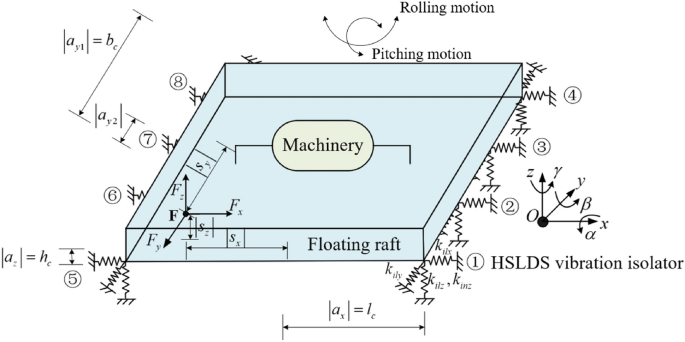
Schematic illustrating the simplified structure of a high-static-low-dynamic stiffness (HSLDS) floating raft vibration isolation system (FRVIS).
The dynamic equation of the system is given as follows 33 , 34 . The nonlinearity includes the linear stiffness term and the cubic nonlinear stiffness term, which is a part of a typical duffing equation.
Here, the operation \(\otimes\) is defined as the multiplications of elements in the same position of different matrices. \({\mathbf{M}}\) is a matrix containing the mass m , the moments of inertia \(I_{ii}\) ( i = x , y , z ), and the products of inertia \(I_{ij}\) ( i , j = x , y , z , \(i \ne j\) ) of the machinery and floating raft, which is defined as follows.
\({\mathbf{x}} = \left[ {\begin{array}{*{20}c} {x_{c} } & {y_{c} } & {z_{c} } & {\alpha_{c} } & {\beta_{c} } & {\gamma_{c} } \\ \end{array} } \right]^{{\text{T}}}\) is the displacement vector of the center of gravity, including translation terms ([ x c y c z c ] T ) and angle terms ([ α β γ ] T ), n i is the number of the isolators, \({\mathbf{G}}_{i} = \left[ {\begin{array}{*{20}c} {} & 0 & {a_{zi} } & { - a_{yi} } \\ {{\mathbf{E}}_{3 \times 3} } & { - a_{zi} } & 0 & {a_{xi} } \\ {} & {a_{yi} } & { - a_{xi} } & 0 \\ \end{array} } \right]\) is the position transformation matrix from the upper end of the i -th isolator to O , where \({\mathbf{E}}_{3 \times 3}\) is a \(3 \times 3\) identity matrix, and \(a_{\upsilon i}\) ( \(\upsilon = x,y,z\) ) are the above-defined vibration isolator coordinates, \({\mathbf{C}}_{i} = {\mathbf{T}}_{i}^{{\text{T}}} diag(c_{ix} ,c_{iy} ,c_{iz} ){\mathbf{T}}_{i}\) is the linear damping matrix, where \({\mathbf{T}}_{i}\) is an identity matrix because the coordinate system of each vibration isolator coincides with the global coordinate system, \({\mathbf{K}}_{il} = {\mathbf{T}}_{i}^{{\text{T}}} diag(k_{ilx} ,k_{ily} ,k_{ilz} ){\mathbf{T}}_{i}\) is the linear stiffness matrix, and \({\mathbf{K}}_{in} = {\mathbf{T}}_{i}^{{\text{T}}} diag(0,0,k_{inz} ){\mathbf{T}}_{i}\) is the nonlinear stiffness matrix. Accordingly, we can define the excitation force vector as \({\mathbf{F}} = \left[ {\begin{array}{*{20}c} {F_{x} } & {F_{y} } & {F_{z} } & {M_{x} } & {M_{y} } & {M_{z} } \\ \end{array} } \right]^{{\text{T}}}\) .
Introducing dimensionless parameters yields the following terms:
\(\hat{x}_{c} = \frac{{x_{c} }}{{l_{c} }}\) , \(\hat{y}_{c} = \frac{{y_{c} }}{{l_{c} }}\) , \(\hat{z}_{c} = \frac{{z_{c} }}{{l_{c} }}\) , \(\hat{\alpha }_{c} = \frac{{\alpha_{c} }}{\theta }\) , \(\hat{\beta }_{c} = \frac{{\beta_{c} }}{\theta }\) , \(\hat{\gamma }_{c} = \frac{{\gamma_{c} }}{\theta }\) , \(\omega_{n} = \sqrt {\frac{{8k_{z} }}{m}}\) , \(\tau = \omega_{n} t\) , \(\Omega = \frac{\omega }{{\omega_{n} }}\) , \(\xi_{\upsilon } = \frac{{c_{i\upsilon } \omega_{n} }}{{k_{z} }}\) , \(\hat{k}_{il\upsilon } = \frac{{k_{il\upsilon } }}{{k_{z} }}\) , \(\hat{k}_{inz} = \frac{{k_{inz} l_{c}^{2} }}{{k_{z} }}\) , \(\hat{F}_{\upsilon } = \frac{{F_{\upsilon } }}{{8k_{z} l_{c} }}\) , \(\hat{M}_{\upsilon } = \frac{{M_{\upsilon } }}{{8k_{z} l_{c}^{2} }}\) , and \(\hat{a}_{\upsilon } = \frac{{a_{\upsilon } }}{{l_{c} }}\) ( \(\upsilon = x,y,z\) ).
Here, \(\omega\) is the excitation frequency, \(\theta\) is a unit angle for rendering angle terms [ α β γ ] T dimensionless, τ is dimensionless time, ξ is the dimensionless damping ratio and k z is the static stiffness given by the static force divided by static displacement. It is assumed that the damping of an isolator is the same in all directions. For convenience, dimensionless parameters are still represented by original parameter variables except dimensionless time \(\tau\) and damping ratio \(\xi\) .
These dimensionless parameters transform Eq. ( 1 ) into a dimensionless dynamic equation of the system. It is further noted that the linear and nonlinear stiffness elements can be alternatively defined as \(k_{ilz} = 1 - 2\left( {\frac{{1 - \hat{l}}}{{\hat{l}}}} \right)\) and \(k_{inz} = \frac{{1 - \hat{l}^{2} }}{{\hat{l}^{3} }}\) 34 , 35 . The nonlinearity of the system can be conveniently represented by the dimensionless compression factor \(\hat{l}\) which is the ratio of the compressed length to the original length for springs, because k inz increases while k ilz decreases with increasing \(\hat{l}\) . Here, the system attains maximum nonlinearity when \(\hat{l} = 0.667\) , and is accordingly a QZS system. In contrast, a value \(\hat{l} = 1\) represents an equivalent linear system (ELS).
Static swing stability analysis
Swing motion includes rolling and pitching motions. Under rolling motion, the force exerted on the XOZ plane produces only translational displacements in the x and z directions and rotational displacements \(\beta\) around the y axis, as shown in Fig. 1 . Regardless of damping, the dimensionless equations defining the rolling motion of the 3-DOF system based on the 6-DOF system described in “ Simplified HSLDS-FRVIS model ” section are given as follows.
Here, k lx , k lz are the linear stiffness in the x , z directions respectively. k nz is the nonlinear stiffness in the z direction.
Under pitching motion, the force exerted on the YOZ plane produces only translational displacements in the y and z directions and rotational displacements \(\alpha\) around the x axis, as shown in Fig. 1 . Regardless of damping, the dimensionless equations defining the pitching motion of the corresponding 3-DOF system are defined as follows.
Here, k ly is the linear stiffness in the y direction.
The swing stability of an FRVIS is usually determined according to its static response because the swing frequency of a ship is quite small, and swing motion can therefore be regarded as a quasi-static process 29 . In addition, we assume that all vibration isolators realize QZS characteristics in the z direction (i.e., \(\hat{l} = 0.667\) ) because this enables us to obtain an analytical expression of the displacement response of the system that facilitates a clear analysis of the influence of system parameters on swing stability.
Ignoring the differential terms in Eq. ( 3 ) yields the following rolling displacement response of the FRVIS.
In contrast, the greater complexity of the equations of pitching motion in Eq. ( 4 ) make it impossible to obtain an explicit analytical pitching displacement response for the FRVIS. Therefore, the pitching displacement response of the system is given as follows. This equation can be solved using the solve function of the MATLAB ® software.
Similarly, the dimensionless rolling displacement responses \(x_{r - l}\) , \(z_{r - l}\) , and \(\beta_{r - l}\) obtained for an ELS ( \(\hat{l} = 1\) ) with only linear stiffness elements \({\mathbf{K}}_{il} = {\mathbf{T}}_{i}^{{\text{T}}} diag(k_{z} ,k_{z} ,k_{z} ){\mathbf{T}}_{i}\) and \({\mathbf{K}}_{in} = {\mathbf{0}}\) ( i = 1, 2, …,8) can be given as follows.
The dimensionless pitching displacement responses \(y_{p - l}\) , \(z_{p - l}\) , and \(\alpha_{{_{p - l} }}\) are also defined as follows.
To further simplify the analysis, we assume that the load is concentrated at the origin O of the global coordinate system (i.e., at the center of gravity of the system). This enables us to define the balance between the load and the support force of the vibration isolator during the rolling and pitching of the ship as being equivalent to applying the following rolling and pitching forces at O .
Here, \(G = \frac{{G_{a} }}{{8k_{z} l_{c} }}\) is the dimensionless load of the system, where \(G_{a}\) is the load, \(\rho = \frac{{\rho_{a} }}{\theta }\) is the dimensionless rolling angle, where \(\rho_{a}\) is the rolling angle, and \(\phi = \frac{{\phi_{a} }}{\theta }\) is the dimensionless pitching angle, where \(\phi_{a}\) is the pitching angle.
The impacts of ρ and G on the dimensionless displacement responses of the HSLDS-FRVIS ( \(\hat{l} = 0.667\) ) and the ELS ( \(\hat{l} = 1\) ) under rolling conditions are presented in Fig. 2 a and b as functions of ρ for G values of 1 and 10, respectively. Similarly, these impacts on the HSLDS-FRVIS and the ELS under pitching conditions are presented in Fig. 3 a and b as functions of φ for G values of 1 and 10, respectively. It is found that the responses of the HSLDS-FRVIS and ELS are nearly equivalent in the x and y directions under the swing conditions. This demonstrates that nonlinearity in the z direction has little influence on the system responses in the x and y directions. Under the rolling condition, the response of the HSLDS-FRVIS in the z direction is generally greater than that of the ELS with increasing ρ when G = 1, and is less than that of the ELS only at relatively large values of ρ . Similar behavior is observed under the pitching condition, except that the response of the HSLDS-FRVIS in the z direction is always greater than that of the ELS. However, this behavior changes when G = 10, where the responses of the HSLDS-FRVIS in the z direction are nearly always less than or equal to that of the ELS under both rolling or pitching conditions. Moreover, the gap between the two responses becomes increasingly obvious with increasing ρ or φ . This demonstrates that the displacement offset of the HSLDS-FRVIS is smaller than that of the ELS under large load and large swing angle conditions, which is beneficial for ensuring the stability of the vibration isolation system. This can be qualitatively explained from an analysis of Eqs. ( 5 ) and ( 7 ) combined with Eqs. ( 9 ) and ( 10 ) respectively, where we note that the load and swing angle have less influence on the responses of the HSLDS-FRVIS in the z direction than the ELS. Therefore, the HSLDS-FRVIS provides improved swing stability performance over that of the ELS.
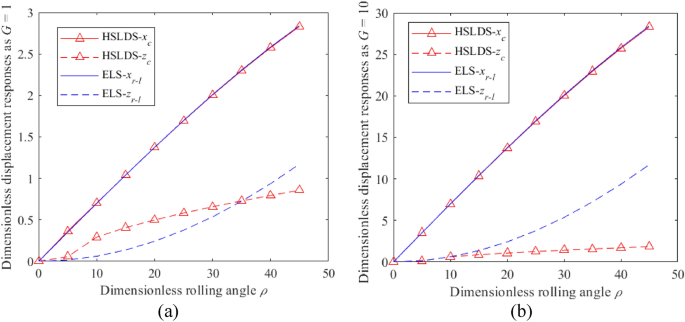
Dimensionless displacement responses obtained under rolling motion for the HSLDS-FRVIS ( \(\hat{l} = 0.667\) ) and the ELS ( \(\hat{l} = 1\) ) defined in Fig. 1 as functions of the dimensionless rolling angle ρ under different dimensionless loads G : ( a ) \(G = 1\) ; ( b ) \(G = 10\) .
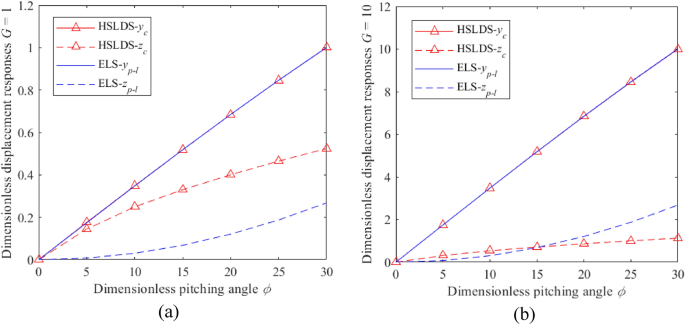
Dimensionless displacement responses obtained under pitching motion for the HSLDS-FRVIS and the equivalent linear system (ELS) as functions of the dimensionless pitching angle φ for different G : ( a ) \(G = 1\) ; ( b ) \(G = 10\) .
We also analyzed the influence of the degree of nonlinearity \(\hat{l}\) on the displacement responses of the HSLDS-FRVIS and the ELS in the z direction under swing motion for \(G = 10\) , and the results obtained under roll and pitch motions are presented as functions of ρ and φ in Fig. 4 a and b, respectively. It is found that even slight nonlinearity can greatly reduce the displacement response of the system and improve its swing stability under heavy load. In fact, the displacement response observed for \(\hat{l} = 0.9\) is little different from that obtained under the minimum displacement response at \(\hat{l} = 0.667\) , and is much less than that of the ELS. For example, the responses observed for \(\hat{l} = 0.9\) at the maximum rolling and pitching angles considered were about 12% and 30% those of the linear system ( \(\hat{l} = 1.0\) ), respectively. Accordingly, these results reflect the high static stiffness and high static stability characteristics of HSLDS technology.
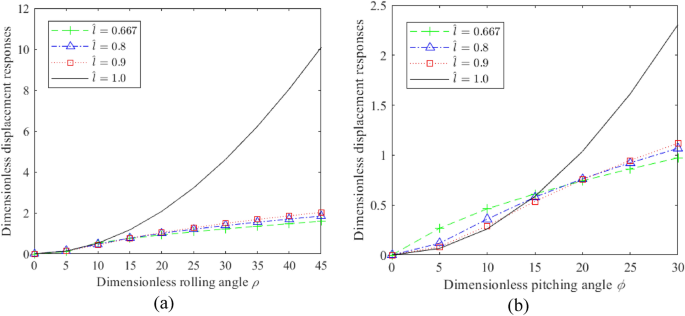
Dimensionless displacement responses obtained in the z direction under swing motions for the HSLDS-FRVIS and ELS as functions of ρ and φ for different degrees of nonlinearity \(\hat{l}\) with \(G = 10\) : ( a ) rolling motion; ( b ) pitching motion.
Taking rolling motion as an example, we further analyzed the influence of the installation height ratio H z of the vibration isolators on the displacement responses of the vibration isolation systems under rolling motion for \(G = 10\) , and the results obtained are presented for the HSLDS-FRVIS ( \(\hat{l} = 0.667\) ) and the ELS as functions of ρ in Fig. 5 a and b, respectively. It is found that different installation positions have relatively little effect on the displacement response of the HSLDS-FRVIS compared to that of the ELS. We further note that H z affects only the response of the ELS in the x direction, but has no effect on the response in the z direction. In addition, a value of H z = 0 (i.e., a z = 0) produces the minimum displacement response for the ELS in the x direction. This is more intuitively conveyed from an analysis of Eqs. ( 7 ) and ( 9 ). Therefore, nonlinear isolators weaken the influence of their installation position on the displacement response of the system compared with that of the ELS.
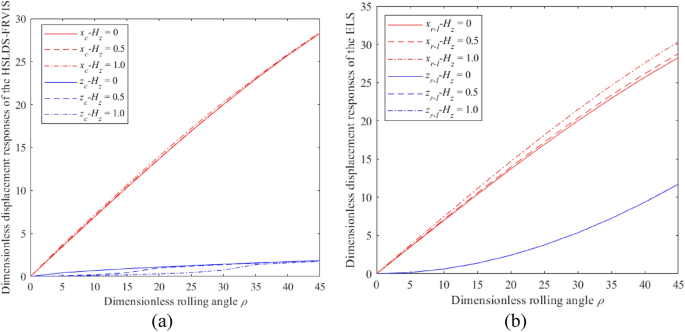
Dimensionless displacement responses obtained under rolling motion for different systems as functions of ρ with \(G = 10\) and different vibration isolator installation positions: ( a ) HSLDS-FRVIS ( \(\hat{l} = 0.667\) ); ( b ) ELS.
Multidimensional dynamic analysis
Under the established condition \(\xi = \xi_{x} = \xi_{y} = \xi_{z}\) , the dimensionless equations defining the dynamic motion of the 6-DOF system described in “ Simplified HSLDS-FRVIS model ” section are given as follows.
Past research has demonstrated that little difference is observed between solutions obtained by the harmonic balance analytical method and the numerical method in the higher excitation frequency range 27 . However, these solutions differ greatly in the low frequency range because the analytical solution includes a truncation error that arises as the solution is assumed to be first order, but the actual solution has infinite order terms. Therefore, the solution obtained by the numerical method is more accurate in the low frequency range. Moreover, it is difficult to obtain explicit analytical solutions for high-dimensional nonlinear systems. Collectively considering both accuracy and simplicity, the present work applies the numerical method to solve Eq. ( 11 ) by using the ode45 solver of the MATLAB ® software, which also facilitates a clear and accurate analysis of the influences of various parameters on the vibration isolation performance of the system.
In addition, the present work assumes that the external excitation is simple harmonic force excitation. Therefore, the vibration isolation effect of an FRVIS is analyzed in the x , y , and z directions according to the force transmission rate, which is defined as
Here, the function lg(∙) represents the logarithm with base 10 of its argument, the function RMS (∙) represents the root mean square of its argument, \(F_{t\upsilon }\) is the nonlinear force transmitted by the FRVIS to the foundation, and \(F_{\upsilon } = f_{\upsilon } \cos \Omega t\) is the external harmonic excitation force, where \(f_{\upsilon }\) is the dimensionless amplitude of the excitation force. According to Eq. ( 11 ), the forces transmitted to the foundation in the x , y , and z directions can be given as follows.
These forces can be analyzed further by considering the following two types of excitations.
Unidirectional excitation:
Multidirectional eccentric excitation:
As can be seen, the unidirectional excitation mode defined in Eq. ( 14 ) facilitates an analysis of the vibration isolation performance of a vibration isolation system in the z direction when the load is concentrated at the origin O of the global coordinate system (i.e., at the center of gravity of the system). We first analyze the influence of \(\hat{l}\) on the z -directional vibration isolation performance by plotting the force transmission rate T z obtained in the z direction as a function of Ω under various values of \(\hat{l}\) with \(\xi = 0.06\) in Fig. 6 . The values of Ω at which T z = 0 under the four values of \(\hat{l}\) are given as Ω 1 –Ω 4 . It is found that the vibration isolation effect of the system in the z direction increases with decreasing \(\hat{l}\) , and achieves a maximum vibration isolation performance at \(\hat{l} = 0.667\) . We also considered the differences between the Ω 1 –Ω 4 values relative to the vibration isolation frequency Ω 4 of the linear system, and the results were \({{(\Omega_{4} - \Omega_{1} )} \mathord{\left/ {\vphantom {{(\Omega_{4} - \Omega_{1} )} {\Omega_{4} }}} \right. \kern-0pt} {\Omega_{4} }} = 76.8\%\) , \({{(\Omega_{4} - \Omega_{2} )} \mathord{\left/ {\vphantom {{(\Omega_{4} - \Omega_{2} )} {\Omega_{4} }}} \right. \kern-0pt} {\Omega_{4} }} = 30.3\%\) and \({{(\Omega_{4} - \Omega_{3} )} \mathord{\left/ {\vphantom {{(\Omega_{4} - \Omega_{3} )} {\Omega_{4} }}} \right. \kern-0pt} {\Omega_{4} }} = 12.7\%\) . Accordingly, an increasing degree of nonlinearity is found to expand the range of vibration isolation frequencies and improve the low-frequency vibration isolation performance of the isolator. This can be explained from an analysis of Eq. ( 11 ), where we note that the nonlinear stiffness term is zero at \(\hat{l} = 1\) , such that the system response is mainly composed of the dominant harmonic solution. However, the proportion of the subharmonic solution increases with decreasing \(\hat{l}\) because this decreases the linear stiffness term and increases the nonlinear stiffness term, and the frequency corresponding to the peak system transmittance shifts to the left, resulting in an expanded vibration isolation frequency band.
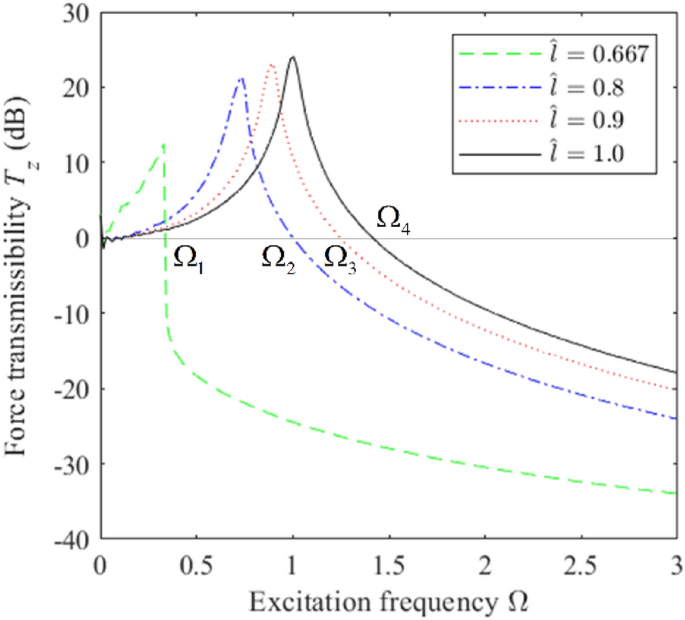
Force transmission rate T z obtained in the z direction for an HSLDS-FRVIS as a function of the dimensionless excitation frequency Ω under various values of \(\hat{l}\) with a dimensionless damping ratio \(\xi = 0.06\) .
In contrast to the above unidirectional excitation analysis, the multidirectional excitation mode defined in Eq. ( 15 ), which is common in practical engineering settings, enables the influences of nonlinearity and the installation positions of the vibration isolators on the vibration isolation performance of the HSLDS-FRVIS to be analyzed under eccentric loading at coordinates s x , s y , and s z . The eccentricity was set as \({{s_{x} }/{l_{c} }} = 0.8\) , \({{s_{y} }/{b_{c} }} = 0.8\) , and \({{s_{z} }/{h_{c} }} = - 1\) .
We first analyze the influence of \(H_{z}\) on the vibration isolation performance of an HSLDS-FRVIS with \(\hat{l}\) = 0.667 and otherwise standard parameters (i.e., those in Table 1 and \(\xi = 0.06\) ) by plotting the force transmission rates T x , T y , and T z obtained in the x , y , and z directions in Fig. 7 a–c, respectively, as functions of Ω under various values of \(H_{z}\) . Corresponding results are presented in Figs. 8 and 9 for \(\hat{l}\) = 0.8 and \(\hat{l}\) = 0.9, respectively. First of all, it is found that \(H_{z}\) has little effect on the vibration isolation performance of the raft in the z direction, and mainly affects the isolation performance in the x and y directions. The relative impacts of \(H_{z}\) in the x , y , and z directions can be explained from an analysis of Eq. ( 11 ), which indicates that \(H_{z}\) (i.e., \(a_{z}\) ) mainly affects the vibration response of the raft in the x , y , \(\alpha\) , and \(\beta\) directions. Moreover, we note that the apparent impact of \(H_{z}\) on the isolation performance in the x and y directions increases with increasing \(\hat{l}\) . In fact, the respective plots of T x and T y in Fig. 10 a and b as functions of Ω for different values of \(\hat{l}\) with \(H_{z} = 0\) demonstrate that the degree of nonlinearity has no effect on the response of the raft in the x and y directions under this isolator installation condition. However, the degree of nonlinearity clearly affects the response of the raft in the x and y directions for H z values of 0.5 and 1. In fact, secondary peaks are observed in the T x and T y spectra under these isolator installation conditions, which weakens the vibration isolation performance of the raft. Moreover, the magnitudes of these secondary peaks increase with increasing H z , which is an increasing detriment to the vibration isolation performance. In addition, the high-frequency vibration isolation effect of the raft in the x and y directions increases with decreasing H z . Therefore, an HSLDS-FRVIS design with \(H_{z} = 0\) provides an overall optimal vibration isolation effect by avoiding secondary peaks in the T x and T y spectra and ensuring the best high-frequency vibration isolation effect in the x and y directions.
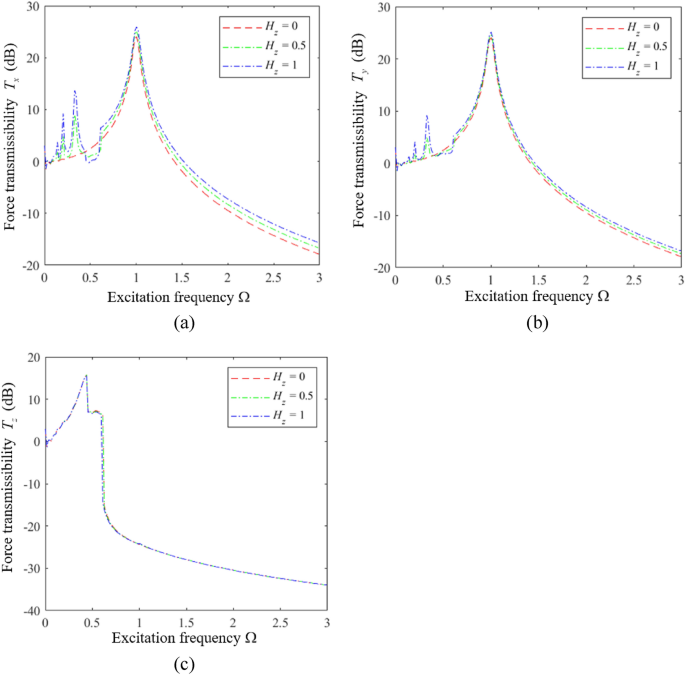
Force transmission rates T x , T y , and T z obtained for an HSLDS-FRVIS in the x , y , and z directions, respectively, as functions of Ω under various values of H z with \(\hat{l} = 0.667\) and otherwise standard conditions: ( a ) T x ; ( b ) T y ; ( c ) T z .
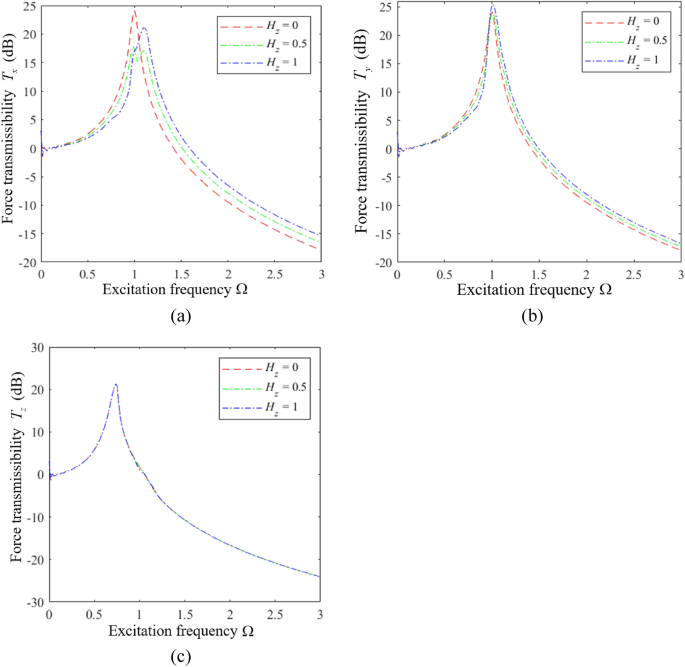
Force transmission rates obtained for an HSLDS-FRVIS as functions of Ω under various values of H z with \(\hat{l} = 0.8\) and otherwise standard conditions: ( a ) T x ; ( b ) T y ; ( c ) T z .
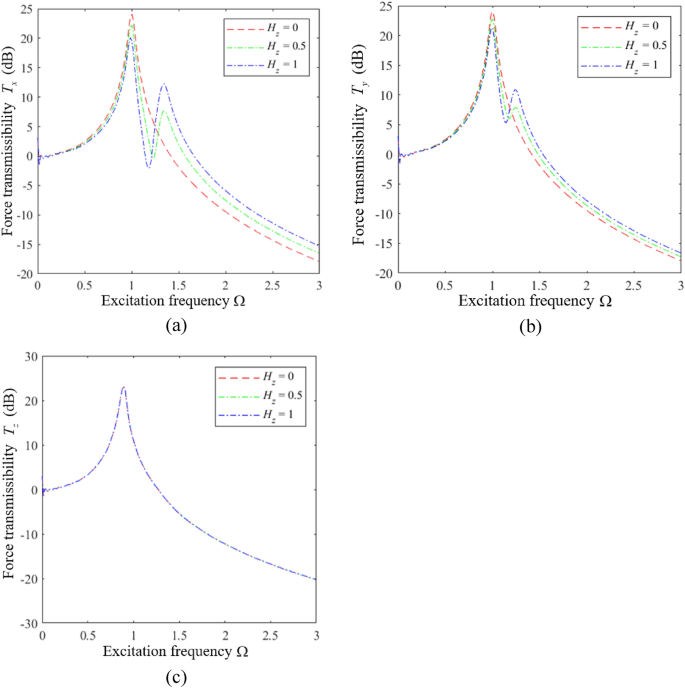
Force transmission rates obtained for an HSLDS-FRVIS as functions of Ω under various values of H z with \(\hat{l} = 0.9\) and otherwise standard conditions: ( a ) T x ; ( b ) T y ; ( c ) T z .
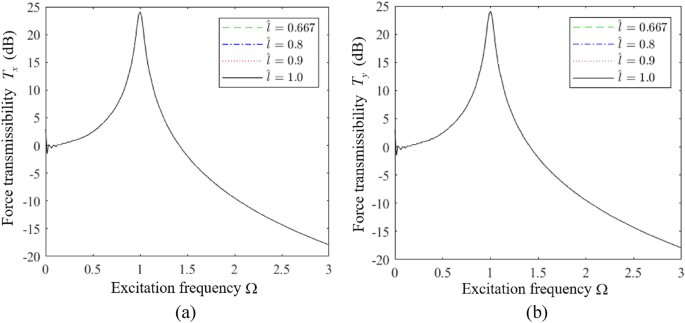
Force transmission rates obtained for an HSLDS-FRVIS as functions of Ω under various values of \(\hat{l}\) with \(H_{z} = 0\) and otherwise standard conditions: ( a ) T x ; ( b ) T y .
However, the mechanisms by which secondary peaks arise in the T x and T y spectra require some analysis. This can be ascertained based on the respective plots of T x and T y in Fig. 11 a and b as functions of Ω for different values of \(\hat{l}\) with \(H_{z} = 1\) as an example. We first consider the ELS ( \(\hat{l} = 1.0\) ), which yields two strong peaks in the T x and T y spectra. We must further note that, according to Eq. ( 11 ), the responses in the x and y directions are functions solely of a 2-DOF system. The response in the x direction is defined according to the following typical dynamic equations for a 2-DOF system.
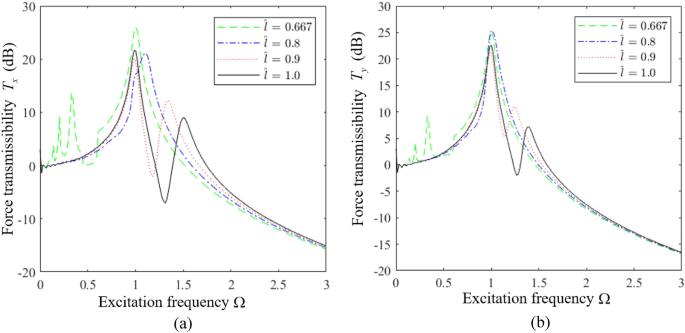
Force transmission rates obtained for an HSLDS-FRVIS as functions of Ω under various values of \(\hat{l}\) with \(H_{z} = 1\) and otherwise standard conditions: ( a ) T x ; ( b ) T y .
The y -direction responses are the same. Therefore, the system generates two transmission peaks in both the x and y directions. We further note that the strongly nonlinear QZS system ( \(\hat{l} = 0.667\) ) generates a large number of secondary spectra at frequencies less than the primary peak, which detracts from the low-frequency vibration isolation effect of the raft. These secondary spectra arise because the linear stiffness term in the z direction is zero when \(\hat{l} = 0.667\) , and the strong nonlinearity in the z direction leads to subharmonic solutions with large magnitudes in the x and y directions. As can be observed, these secondary peaks do not appear for weakly nonlinear systems ( \(\hat{l} = 0.8\) and \(\hat{l} = 0.9\) ), which either exhibit responses that represent a combination of the responses of the ELS and the QZS system for \(\hat{l} = 0.8\) , or responses that are quite similar to the ELS for \(\hat{l} = 0.9\) . This is because the nonlinear stiffness term of the system decreases with increasing \(\hat{l}\) , while the linear stiffness term increases, and this reduces the magnitude of the subharmonic responses obtained in the solution.
Finally, the influence of the distance ratio \(D_{r}\) on the vibration isolation performance of the HSLDS-FRVIS was analyzed according to the plots of T x , T y , and T z presented as functions of Ω under various values of \(D_{r}\) with \(\hat{l} = 0.667\) in Fig. 12 a–c, respectively. Corresponding results are presented in Fig. 13 for \(\hat{l}\) = 0.8. It can be found that \(D_{r}\) has little effect on the vibration isolation performance of the HSLDS-FRVIS in any direction. Therefore, an equidistant installation with \(D_{r} = 0.5\) would be considered ideal from the standpoint of installation stability.
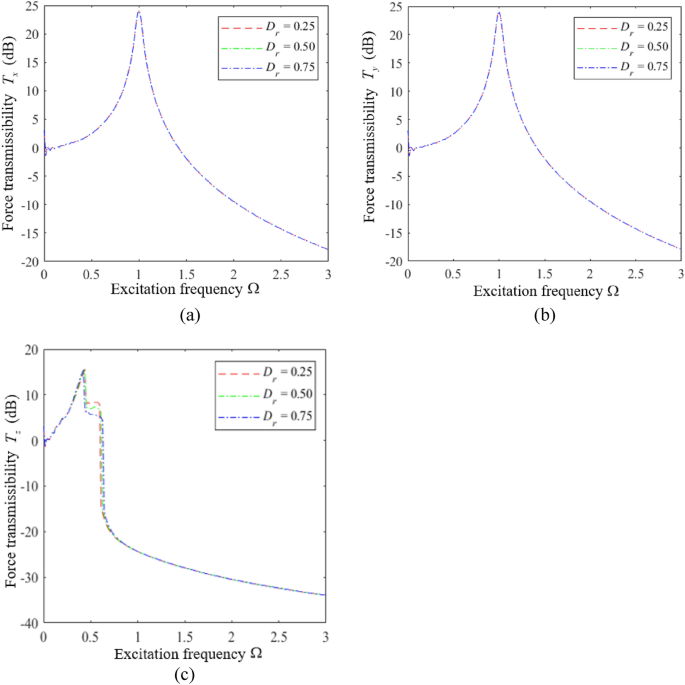
Force transmission rates obtained for an HSLDS-FRVIS as functions of Ω under various values of D r with \(\hat{l} = 0.667\) and otherwise standard conditions: ( a ) T x ; ( b ) T y ; ( c ) T z .
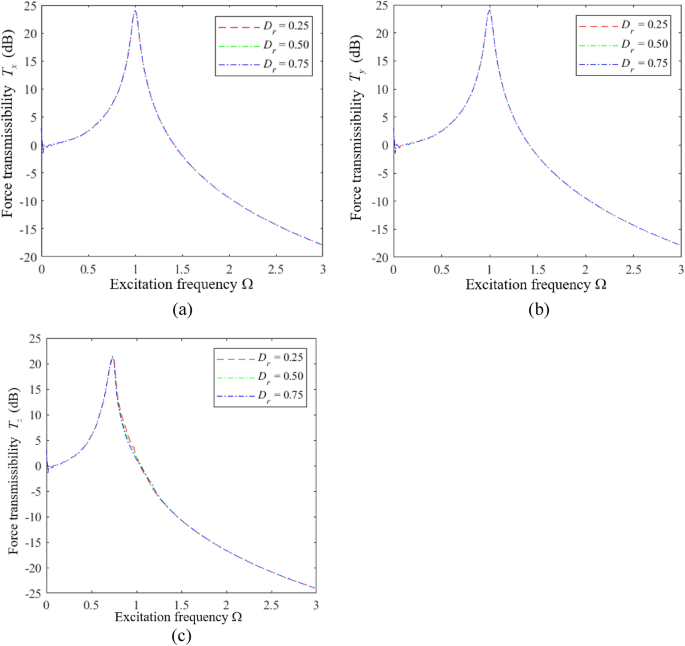
Force transmission rates obtained for an HSLDS-FRVIS as functions of Ω under various values of D r with \(\hat{l} = 0.8\) and otherwise standard conditions: ( a ) T x ; ( b ) T y ; ( c ) T z .
The present work addressed the poorly developed process for designing the complex high-dimensional HSLDS-FRVISs applied in ocean-going vessels by establishing a 6-DOF HSLDS-FRVIS model, and applying that model for fully analyzing the swing stability and multidimensional vibration isolation performance of these systems. The results of extensive analysis demonstrate the following conclusions.
Weak nonlinearity greatly reduces the swing displacement responses of the system and improves the swing stability under heavy loads and large swing angles. This reflects the high static stiffness and high static stability characteristics of HSLDS systems. In addition, applying different installation positions for the vibration isolators has little effect on the displacement responses of the HSLDS systems in comparison with that of the corresponding linear systems. Meanwhile, the influence of the installation position on the swing displacement responses of the system decreases with increasing nonlinearity.
In terms of the multidimensional vibration isolation performance of the systems, the low-frequency vibration isolation effect can increase with increasing nonlinearity. However, a strongly nonlinear QZS system is more sensitive to parameter changes than weakly nonlinear HSLDS systems. The results of analysis demonstrate that applying a value of H z = 0 produces the best vibration isolation performance overall under strong nonlinearity by avoiding unnecessary secondary peaks in the force transmission rate under harmonic mechanical excitation and ensuring a maximum high-frequency vibration isolation effect. However, applying a weak nonlinearity is better than a strong nonlinearity if H z is not zero. Therefore, a weakly nonlinear HSLDS system is preferred to a highly nonlinear QZS system in engineering design practice to ensure an optimal vibration isolation performance. Meanwhile, \(D_{r}\) has little impact on the vibration isolation effects of the system in x , y , and z directions. Therefore, applying an equidistant installation with \(D_{r} = 0.5\) would be considered ideal from the standpoint of installation stability.
Accordingly, the current work lays a sound theoretical foundation for the subsequent design of HSLDS-FRVISs.
Data availability
Some of the data and models generated during the study are available from the corresponding author by reasonable request.
Howard, C. Q. Recent developments in submarine vibration isolation and noise control. In Proceedings of 1st Submarine Science Technology and Engineering Conference, Adelaide, SA, Australia (2011).
Vane, F. A Guide for the Selection and Application of Resilient Mountings to Shipboard Equipment (Revised) . David Taylor Model Basin Report 880 (1958).
Carrella, A., Brennan, M. J. & Waters, T. P. Static analysis of a passive vibration isolator with quasi-zero-stiffness characteristic. J. Sound Vib. 301 , 678–689 (2007).
Article ADS Google Scholar
Carrella, A., Brennan, M. J., Waters, T. P. & Shin, K. On the design of a high-static–low-dynamic stiffness isolator using linear mechanical springs and magnets. J. Sound Vib. 315 , 712–720 (2008).
Alabuzhev, P. & Rivin, E. Vibration Protecting and Measuring Systems with Quasi-Zero Stiffness (Hemisphere Publishing Corporation, 1989).
Google Scholar
Gatti, G., Shaw, A. D., Gonçalves, P. J. P. & Brennan, M. J. On the detailed design of a quasi-zero stiffness device to assist in the realisation of a translational Lanchester damper. Mech. Syst. Signal Process. 164 , 108258 (2022).
Article Google Scholar
Shaw, A. D., Gatti, G., Gonçalves, P. J. P., Tang, B. & Brennan, M. J. Design and test of an adjustable quasi-zero stiffness device and its use to suspend masses on a multi-modal structure. Mech. Syst. Signal Process. 152 , 107354 (2021).
Ishida, S., Uchida, H., Shimosaka, H. & Hagiwara, I. Design and numerical analysis of vibration isolators with quasi-zero-stiffness characteristics using bistable foldable structures. J. Vib. Acoust. 139 , 031015 (2017).
Ishida, S., Suzuki, K. & Shimosaka, H. Design and experimental analysis of origami-inspired vibration isolator with quasi-zero-stiffness characteristic. J. Vib. Acoust. 139 , 051004–051012 (2017).
Xu, D., Zhang, Y., Zhou, J. & Lou, J. On the analytical and experimental assessment of the performance of a quasi-zero-stiffness isolator. J. Vib. Control 20 , 2314–2325 (2014).
Zhang, Y., Wei, G., Wen, H., Jin, D. & Hu, H. Design and analysis of a vibration isolation system with cam–roller–spring–rod mechanism. J. Vib. Control 28 , 1781–1791 (2022).
Article MathSciNet CAS Google Scholar
Yao, Y., Wang, X. & Li, H. Design and analysis of a high-static-low-dynamic stiffness isolator using the cam-roller-spring mechanism. J. Vib. Acoust. 142 , 021009 (2020).
Han, J., Meng, L. & Sun, J. Design and characteristics analysis of a nonlinear isolator using a curved-mount-spring-roller mechanism as negative stiffness element. Math. Probl. Eng. 2018 , 1359461 (2018).
MathSciNet Google Scholar
Wang, K., Zhou, J. & Xu, D. Sensitivity analysis of parametric errors on the performance of a torsion quasi-zero-stiffness vibration isolator. Int. J. Mech. Sci. 134 , 336–346 (2017).
Zhou, J., Xu, D. & Bishop, S. A torsion quasi-zero stiffness vibration isolator. J. Sound Vib. 338 , 121–133 (2015).
Vo, N. Y. P. & Le, T. D. Dynamic analysis of quasi-zero stiffness pneumatic vibration isolator. Appl. Sci. 12 , 2378 (2022).
Article CAS Google Scholar
Vo, N. Y. P., Nguyen, M. K. & Le, T. D. Analytical study of a pneumatic vibration isolation platform featuring adjustable stiffness. Commun. Nonlinear Sci. Numer. Simul. 98 , 105775 (2021).
Article MathSciNet Google Scholar
Shuai, C., Li, B. & Ma, J. A novel multi-directional vibration isolation system with high-static–low-dynamic stiffness. Acta Mech. 233 , 5199–5214 (2022).
Zhou, Z., Zhou, M., Dai, Z., Liu, X. & Li, Z. Design and experimental validation of a vibration isolator with high-static low-dynamic stiffness and operating point variable property. J. Vib. Control 28 , 1341–1350 (2022).
Jiang, Y., Song, C., Ding, C. & Xu, B. Design of magnetic-air hybrid quasi-zero stiffness vibration isolation system. J. Sound Vib. 477 , 115346 (2020).
Yuan, S. et al. Tunable negative stiffness spring using Maxwell normal stress. Int. J. Mech. Sci. 193 , 106127 (2021).
Yuan, S. et al. A tunable quasi-zero stiffness isolator based on a linear electromagnetic spring. J. Sound Vib. 482 , 115449 (2020).
Sun, B. & Jing, X. A tracked robot with novel bio-inspired passive “legs”. Robot. Biomim. 4 , 18 (2017).
Jiang, G., Jing, X. & Guo, Y. A novel bio-inspired multi-joint anti-vibration structure and its nonlinear HSLDS properties. Mech. Syst. Signal Process. 138 , 106552 (2020).
Jing, X., Zhang, L., Feng, X., Sun, B. & Li, Q. A novel bio-inspired anti-vibration structure for operating hand-held jackhammers. Mech. Syst. Signal Process. 118 , 317–339 (2019).
Dai, H., Jing, X., Wang, Y., Yue, X. & Yuan, J. Post-capture vibration suppression of spacecraft via a bio-inspired isolation system. Mech. Syst. Signal Process. 105 , 214–240 (2018).
Li, Y. & Xu, D. Vibration attenuation of high dimensional quasi-zero stiffness floating raft system. Int. J. Mech. Sci. 126 , 186–195 (2017).
Li, Y. & Xu, D. Force transmissibility of floating raft systems with quasi-zero-stiffness isolators. J. Vib. Control 24 , 3608–3616 (2018).
He, L., Xu, W., Bu, W. & Shi, L. Dynamic analysis and design of air spring mounting system for marine propulsion system. J. Sound Vib. 333 , 4912–4929 (2014).
Xu, X., Liu, H., Jiang, X. & Atindana, A. V. Uncertainty analysis and optimization of quasi-zero stiffness air suspension based on polynomial chaos method. Chin. J. Mech. Eng. 35 , 93 (2022).
Wang, X., Liu, H., Chen, Y. & Gao, P. Beneficial stiffness design of a high-static-low-dynamic-stiffness vibration isolator based on static and dynamic analysis. Int. J. Mech. Sci. 142–143 , 235–244 (2018).
Lu, Z., Chen, L., Brennan, M. J., Li, J.-M. & Ding, H. The characteristics of vibration isolation system with damping and stiffness geometrically nonlinear. J. Phys. Conf. Ser. 744 , 012115 (2016).
Harris’ Shock and Vibration Handbook . (McGraw-Hill, 2010).
Tang, B. & Brennan, M. J. On the shock performance of a nonlinear vibration isolator with high-static-low-dynamic-stiffness. Int. J. Mech. Sci. 81 , 207–214 (2014).
Carrella, A., Brennan, M. J., Waters, T. P. & Lopes, V. Force and displacement transmissibility of a nonlinear isolator with high-static-low-dynamic-stiffness. Int. J. Mech. Sci. 55 , 22–29 (2012).
Download references
Acknowledgements
This research was funded by the National Key Research and Development Program of China, Grant Number HJ2019C020499.
Author information
Authors and affiliations.
Institute of Noise & Vibration, Naval University of Engineering, Wuhan, 430030, People’s Republic of China
Bu-yun Li, Chang-geng Shuai & Jian-guo Ma
National Key Laboratory on Ship Vibration & Noise, Wuhan, 430030, People’s Republic of China
You can also search for this author in PubMed Google Scholar
Contributions
BL and CS initiated this study and proposed the model of this work; BL designed the structure, performed research, derived the modeling, and wrote the manuscript; JM assist with analysis. All authors read and approved the final manuscript.
Corresponding author
Correspondence to Jian-guo Ma .
Ethics declarations
Competing interests.
The authors declare no competing interests.
Additional information
Publisher's note.
Springer Nature remains neutral with regard to jurisdictional claims in published maps and institutional affiliations.
Rights and permissions
Open Access This article is licensed under a Creative Commons Attribution 4.0 International License, which permits use, sharing, adaptation, distribution and reproduction in any medium or format, as long as you give appropriate credit to the original author(s) and the source, provide a link to the Creative Commons licence, and indicate if changes were made. The images or other third party material in this article are included in the article's Creative Commons licence, unless indicated otherwise in a credit line to the material. If material is not included in the article's Creative Commons licence and your intended use is not permitted by statutory regulation or exceeds the permitted use, you will need to obtain permission directly from the copyright holder. To view a copy of this licence, visit http://creativecommons.org/licenses/by/4.0/ .
Reprints and permissions
About this article
Cite this article.
Li, By., Shuai, Cg. & Ma, Jg. Mechanical characteristics analysis of high dimensional vibration isolation systems based on high-static-low-dynamic stiffness technology. Sci Rep 14 , 8195 (2024). https://doi.org/10.1038/s41598-024-58469-x
Download citation
Received : 07 August 2023
Accepted : 29 March 2024
Published : 08 April 2024
DOI : https://doi.org/10.1038/s41598-024-58469-x
Share this article
Anyone you share the following link with will be able to read this content:
Sorry, a shareable link is not currently available for this article.
Provided by the Springer Nature SharedIt content-sharing initiative
- High-static-low-dynamic stiffness
- Quasi-zero stiffness
- High dimensional nonlinear system
- Floating raft vibration isolation system
- Swing stability
By submitting a comment you agree to abide by our Terms and Community Guidelines . If you find something abusive or that does not comply with our terms or guidelines please flag it as inappropriate.
Quick links
- Explore articles by subject
- Guide to authors
- Editorial policies
Sign up for the Nature Briefing newsletter — what matters in science, free to your inbox daily.

Academia.edu no longer supports Internet Explorer.
To browse Academia.edu and the wider internet faster and more securely, please take a few seconds to upgrade your browser .
Enter the email address you signed up with and we'll email you a reset link.
- We're Hiring!
- Help Center
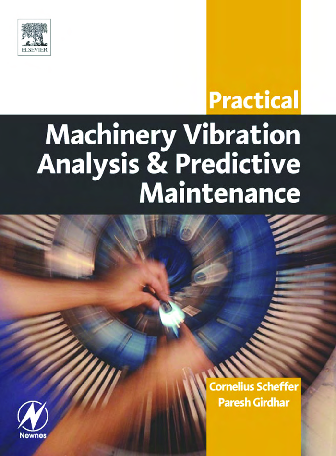
Practical Machinery Vibration Analysis and Predictive Maintenance

Related Papers
Ulises Reyes
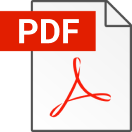
Yoseline Minero Villegas
Haseeb Mustafa
atolagbe olajide
Syed Tafazzul Mahmood
Maxim Zemsky
Ashman Noordin
mahervan p. boyce
VENGATESH RAJ
Adrian Petre
geeteu mech
RELATED PAPERS
Hussein Thamer Hameed
Sulo Lahdelma
Alifdot Shah
Krimo Kitouchi
arash araee
manoj badgujar
Penjani Kanyimbo
aqib bagong
SADIA] Carmela Wallace
Arthur Lees
Athanasios Chasalevris
M.Kalam Azat
Engineer Bilal Nasir
Samuel Inabuaye-omimi
Amnart Sathaporn249
World Applied Sciences Journal
Pss Srinivasan
GJESR Journal
International Journal of Rotating Machinery
Michele Russo
Mohammad Mohebbi
Abhijeet G Chavan , Dhananjay Thombare
Ilton M Pacheco
Seshagiri Rao Boddu
Pranay Gupta
IAEME Publication
(IJMER) www.ijmer.com International Journal of Modern Engineering Research
IJMER Journal
Applied Acoustics
Juan Chacon
American Journal of Mechanical Engineering
EMMANUEL ADIGIO
Wawan .Esideika
Sayal Gupta
Jiraphon Srisertpol
Felix L I P H W A N Munthe
IJSTE - International Journal of Science Technology and Engineering
JULIO CESAR ZAMBRANO REALPE
Unefalcons Uneng
Rajat Mishra
Noureddine Rida
IAEME OUBLICATION
RELATED TOPICS
- We're Hiring!
- Help Center
- Find new research papers in:
- Health Sciences
- Earth Sciences
- Cognitive Science
- Mathematics
- Computer Science
- Academia ©2024
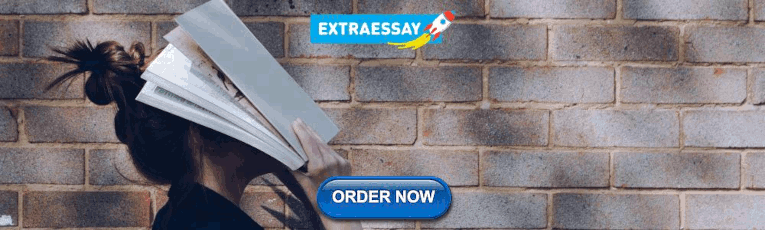
IMAGES
VIDEO
COMMENTS
Search Funded PhD Projects, Programmes & Scholarships in vibration. Search for PhD funding, scholarships & studentships in the UK, Europe and around the world. PhDs ; ... and Vibration analysis. Read more Supervisors: Prof R Mishra, Dr S Sikdar. 12 April 2024 PhD Research Project Competition Funded PhD Project (UK Students Only) More Details ...
PhD Nonlinear dynamics & vibration analysis for structural/condition monitoring (V23.0484) « Back to the overview Job description We are looking for a candidate to undertake research in the field. PhD Studentship: Low-noise Ducted Propulsion Systems for Next-Gen eVTOL Platforms
PhD position Dynamic analysis and human vibration perception of hand held (V23.0732) « Back to the overview Job description We are looking for a candidate to undertake research in the field PhD position Dynamic analysis and human vibration perception of hand held
Abstract. This dissertation shows how regular cameras can be used to record and analyze the vibrations of visible objects. Through careful temporal analysis, we relate subtle changes in video to the vibrations of recorded surfaces, and use that information to reason about the physical properties of objects and the forces that drive their motion.
PhD (1988) and dr.techn. (2003) in mechanical vibrations and dynamics; associate professor at the Technical University of Denmark, Dept. of Mechanical Engineering. ... Author of 100+ peer reviewed scientific publications, covering many aspects of theoretical and experimental vibration analysis, in particular nonlinear effects and analysis. 30 ...
PhD Scholarship in Vibration Analysis and Control of Electromechanical Systems: Reference: 21ITM_DH: Length of Scholarship: Up to 36 months, subject to satisfactory progression: Places: 1: ... On successful completion of the PhD, the students will be awarded a PhD degree from University of Nottingham. No reference will be made on the degree ...
About this book. Now in an updated second edition, this classroom-tested textbook describes essential concepts in vibration analysis of mechanical systems.The second edition includes a new chapter on finite element modeling and an updated section on dynamic vibration absorbers, as well as new student exercises in each chapter.
As a PhD researcher with the Vibration Engineering Section, you can: Access the UK's most advanced facilities for vibration testing, analysis and control. Bring your ideas to life beyond computer modelling: develop a prototype in our labs, test it and deploy it as a real-world solution, using our expertise and capabilities.
The main objective of this position is to develop an innovative methodology that combines vibration analysis, data-driven modeling (e.g. MIMO NARX modelling), and nonlinear frequency domain ...
A PhD training program is part of the agreement and you will be enrolled in the Graduate School of the Faculty of Science and Engineering. Consider our website for information about work at the ...
DEGREE OF DOCTORATE OF PHILOSOPHY (PHD) III ABSTRACT Centrifugal pumps are widely used in many different industrial processes, such as power ... Andrew Ball, A New Method of Vibration Analysis for the diagnosis of impeller in a centrifugal pump, University of Huddersfield, UK. Accepted for publication on ICSV 21 Beijing/China 2014.
SIMULATION AND EXPERIMENTAL STUDY. FOR VIBRATION ANALYSIS ON ROTATING. MACHINERY. Msc in Mechanical Engineering with Emphas is on. Structural Mechanic. MOHD SHAFIQ SHARHAN BIN ZAINAL. Supervisor ...
In that case, a vibration analysis is a suitable alternative method to determine the stress strain state in the pipeline profile. Monitoring the pipeline vibration levels involves a comparison between the measured vibration parameters and the permissible values as depicted in the graphs for a specific pipeline type. Unfortunately, in most cases ...
The results of analysis demonstrate that applying a value of H z = 0 produces the best vibration isolation performance overall under strong nonlinearity by avoiding unnecessary secondary peaks in ...
The effectiveness of the currently available vibration analysis techniques is investigated using in-flight vibration data from Royal Australian Navy helicopters and seeded fault data from a purpose built spur gear test rig. Detailed analysis of techniques for synchronous signal averaging of gear vibration data is
UKnowledge- University of Kentucky Libraries
2.1 Vibration Analysis Vibration analysis is a commonly used machine condition monitoring technique for fixed-plant rotating machinery, due to relatively fast data collection and interpretation when compared to other available off-line techniques (Ebersbach & Peng, 2008). The data is collected digitally, which enables further interpretation
To Increase the Fifth Mode - Increase the thickness of the middle section. 3. STANDARD NORMAL MODES ANALYIS. To see how this is applied in practice, we will run through an analysis project from start to finish (Normal Modes, Modal Frequency, PSD and Direct Transient). The model has been tweaked to protect the innocent.
Vibration analysis of spring-mass system Prepared by Semayat Fanta (Candidate PhD) Vibration analysis of spring-mass system 1: The motion of a spring-mass system As an example of simple harmonic motion, we first consider the motion of a block of mass m that can slide without friction along a horizontal surface. The mass is attached to a spring ...
Prof. Carmen Muller-Karger, PhD Figures and content adapted from Textbook: Mechanical Vibrations Singiresu S. Rao. Mechanical Vibration, Pearson sixth edition Brief history • People became interested in vibration when they created the first musical instruments ( as long as 4000 B.C.).
PhD Thesis-Khaldoon Brethee. September 2020; DOI:10.13140 ... condition monitoring based on vibration analysis has become an effective technique to detect faults in gearbox and provide valued ...
Learn how to perform practical machinery vibration analysis and predictive maintenance with this comprehensive and easy-to-follow guide. This book covers the theory and practice of vibration analysis, fault diagnosis, and corrective actions for various types of rotating equipment. It also includes case studies, exercises, and references to help you master the skills and techniques of vibration ...
Dr. Wael Salman is the Instructor for Machinery Vibration Analysis Course. His qualifications are: - PhD degree in Condition Monitoring of Rotating Machines - Dynamics and Vibrations, Faculty of Mechanical Engineering, Shoubra, Benha University. - Master degree in Solid Mechanics - Dynamics and Vibrations, Faculty of Aerospace and ...
This work uses modal acoustic transfer vector (MATV) technology in conjunction with vibration response analysis to determine optimal target optimization parameters for automobile integrated water tanks. The effects of square and hexagonal reinforcement honeycomb structures on the vibration damping performance of water tanks are analyzed, using ...