Thank you for visiting nature.com. You are using a browser version with limited support for CSS. To obtain the best experience, we recommend you use a more up to date browser (or turn off compatibility mode in Internet Explorer). In the meantime, to ensure continued support, we are displaying the site without styles and JavaScript.
- View all journals
- My Account Login
- Explore content
- About the journal
- Publish with us
- Sign up for alerts
- Review Article
- Open access
- Published: 17 March 2021
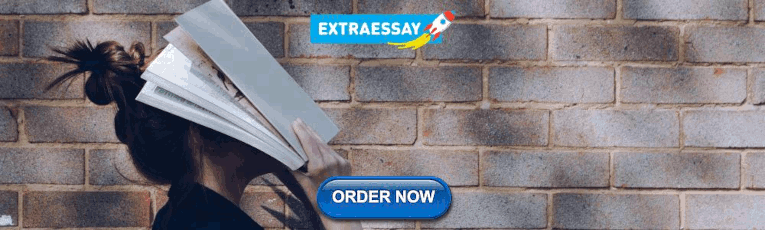
Portable and wearable self-powered systems based on emerging energy harvesting technology
- Chen Xu 1 ,
- Yu Song ORCID: orcid.org/0000-0002-4185-2256 2 ,
- Mengdi Han 3 &
- Haixia Zhang 1 , 2
Microsystems & Nanoengineering volume 7 , Article number: 25 ( 2021 ) Cite this article
33k Accesses
194 Citations
25 Altmetric
Metrics details
- Nanoscale devices
A self-powered system based on energy harvesting technology can be a potential candidate for solving the problem of supplying power to electronic devices. In this review, we focus on portable and wearable self-powered systems, starting with typical energy harvesting technology, and introduce portable and wearable self-powered systems with sensing functions. In addition, we demonstrate the potential of self-powered systems in actuation functions and the development of self-powered systems toward intelligent functions under the support of information processing and artificial intelligence technologies.
Similar content being viewed by others
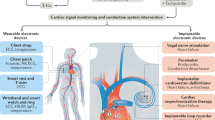
Self-powered cardiovascular electronic devices and systems
Qiang Zheng, Qizhu Tang, … Zhou Li
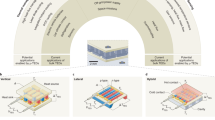
Micro-thermoelectric devices
Qihao Zhang, Kangfa Deng, … Kornelius Nielsch
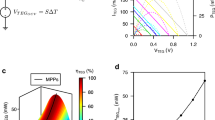
High-efficient energy harvesting architecture for self-powered thermal-monitoring wireless sensor node based on a single thermoelectric generator
Albert Álvarez-Carulla, Albert Saiz-Vela, … Pere Ll. Miribel-Català
Introduction
In recent years, portable and wearable electronic devices have been in a stage of rapid development 1 , 2 . Personalized electronic devices such as smart watches and smart glasses have sprung up, bringing much convenience to people’s life 3 , 4 . At the same time, with the promotion of flexible electronic technology 5 , big data technology 6 , 7 and artificial intelligence technology 8 , portable and wearable electronic devices have shown the development trend of flexibility, integration, and intellectualization, which have also facilitated rich applications such as health monitoring 9 , 10 , human–machine interaction 11 , 12 , and the Internet of Things 13 , 14 .
For portable and wearable electronic devices, the energy supply is a major obstacle to its flexible and integrated application. Replaceable batteries are now the common energy source of electronic devices. However, the rigid characteristics of these batteries limit the overall flexibility of electronic devices. The limited life of batteries and potential environmental pollution problems also do not conform to the principles of sustainable development. As a result, many efforts have been made to explore new environmentally friendly, renewable energy sources to power electronic devices.
Self-powered technology provides a solution for the sustainable energy supply of portable and wearable systems. Self-powered technology means that the device can maintain its own operation by collecting energy in the working environment without an external energy supply. The effective collection of various forms of energy in the working environment is the basis of self-powered technology.
The energy sources available for portable and wearable electronic devices, such as mechanical energy, thermal energy, chemical energy, and solar energy, are extensive. According to the characteristics of these forms of energy, energy harvesting systems suitable for collecting various forms of energy have gained substantial attention. For example, triboelectricity is generated during the contact-separation process of two different materials. Piezoelectricity is produced during the mechanical deformation of piezoelectric materials, and electromagnetic power comes from the conductor moving through a stationary magnetic field. Conversion from mechanical energy to electrical energy exists in all the above transduction mechanisms. Therefore, triboelectric 15 , 16 , piezoelectric 17 , 18 , and electromagnetic 19 , 20 energy harvesters are suitable for harvesting mechanical energy during human motion. Biofuel cells use enzymes or microbes as catalysts to convert chemical energy into electrical energy. The large amount of chemical energy contained in the biochemical environment of the human body can act as an ideal energy source for biofuel cells, making it a potential method for harvesting human chemical energy 21 , 22 . The human body temperature is constant, and there is a temperature difference with the outside world. Therefore, thermoelectric generators can convert the heat of the human body into usable electric energy as a stable source of energy 23 , 24 . Solar energy is also a kind of green renewable clean energy that is an ideal power source for wearable electronic devices 25 , 26 . Furthermore, hybrid energy harvesters that integrate capabilities of harvesting various forms of energy further improve the efficiency of energy harvesting and broaden the application scenarios 27 , 28 .
Sensing technology plays a decisive role in our life as an important way for electronic devices to obtain external information. With the development of self-powered technology, self-powered sensing systems that can sense important physical 29 , 30 , chemical 31 , 32 , and biochemical 33 , 34 information without an external energy supply have gradually come into people’s view. Since there are no issues such as battery replacement and environmental pollution, a self-powered sensing system is expected to be the major form of sensor nodes in the future Internet of Things era. There are two main ways to realize self-powered sensing. Active sensing can be realized by using the output electrical signal itself as the sensing signal. For example, a triboelectric signal can be used as a sensing signal to realize pressure sensing 35 . Using energy harvesting technology to provide energy to a sensor module is another way to realize self-powered sensing 36 . For instance, many self-powered systems based on piezoelectric 37 , thermoelectric 38 , wet electricity 39 , and redox electricity 40 have been studied to power sensor modules and realize active sensing.
Electronic devices such as actuators can assist humans in completing diverse and complex operations in specific scenarios. The development of self-powered technology makes it possible to realize various actuation functions without an external energy supply. For example, many researchers use electrical energy converted from other forms of energy as an excitation signal to realize the functions of automatic control 41 , 42 , microfluidics 43 , 44 , drug delivery and release 45 , and adjuvant therapy 46 , 47 .
From a long-term point of view, we will eventually witness human society entering the age of intelligence. The Internet of Things, artificial intelligence, and big data technology change our lives with each passing day. The relationship between human beings and electronic devices has also presented an unprecedented state. Electronic devices with a single function will no longer meet the functional requirements of portable electronic devices in the intelligent era. Portable and wearable self-powered intelligent systems are gradually replacing bulky computers as the interface of a new generation of intelligent human–machine interactions and playing an important role in intelligent identification 48 , intelligent control 49 , and other fields.
Self-powered systems are gradually becoming the mainstream trend in the development of electronic devices. Recently, some articles have summarized the latest development of self-powered systems. For example, Khalid et al. summarized the human-powered energy harvesting technology that can be used in smart electronic systems 50 . Dong et al. started with self-powered sensors and presented state-of-the-art works based on the synergy between wearable sensors and AI technology 51 . Wang et al. and Gunawardhana et al. focused on triboelectric energy harvesting technology and introduced in detail the development and application of wearable triboelectric nanogenerators (TENGs) in self-powered systems 52 , 53 . However, the integration of multiple energy harvesting methods and the diversification of system functions are inevitable development directions of self-powered systems in the future. Therefore, a comprehensive overview of multiple energy harvesting methods and the diverse applications of self-powered systems helps to further establish the connection between energy harvesting and self-powered applications. Starting from such a foothold, we covered a wide range of energy harvesters and diversified self-powered system functions in this review. We first focus on the development of self-powered systems based on emerging energy harvesting technology. In the second part, portable and wearable energy harvesting technology based on various principles is introduced. The third part presents some self-powered sensing systems based on various principles. The fourth part mainly introduces the representative self-powered actuation system. The fifth part mainly shows the self-powered intelligent system as the human–machine interaction interface, and the synergy between self-powered sensors and AI technology is presented. In the sixth part, we discuss our perspective of the development direction of this field.
Portable and wearable energy harvester
Energy harvesting is the basis of a self-powered system. Additionally, for consideration of convenience and environmental protection, we need sustainable, clean, and renewable energy to power portable and wearable devices. There are various forms of energy in the environment, including not only the energy produced by the human body itself but also the energy provided by the external environment. In daily life, human mechanical movements such as finger movement, walking, and running can produce considerable mechanical energy. However, due to the multimode and low-frequency characteristics of human mechanical movement, it is not easy to collect the mechanical energy of the human body effectively.
Triboelectric and piezoelectric generators are the two most common ways to collect mechanical energy generated by human motion. Triboelectric energy harvesting is based on the well-known principle of friction electrification. The contact of two different objects will induce static charges on the surface of the objects. Subsequently, the relative motion between the two charged objects will produce a potential difference, thus driving the flow of charges. Due to the advantages of a wide selection of materials, low operating frequency and high output power, TENGs have become the most common ways of collecting the mechanical energy of human motion.
In Fig. 1a , an arch-shaped TENG was proposed by Z. L. Wang’s research group in 2012 54 . The pyramid patterns on the surface of the TENG help increase the output of the TENG by increasing the contact area of the two triboelectric layers. The output voltage, current density, and energy volume density of the TENG reached 230 V, 15.5 μA cm −2 and 128 mW cm −3 , respectively. The energy conversion efficiency is as high as 10–39% and meets the demands of wireless sensor systems and mobile phones. This work demonstrates for the first time the potential of TENGs for driving personal mobile electronic devices and shows how TENGs can affect lifestyle.
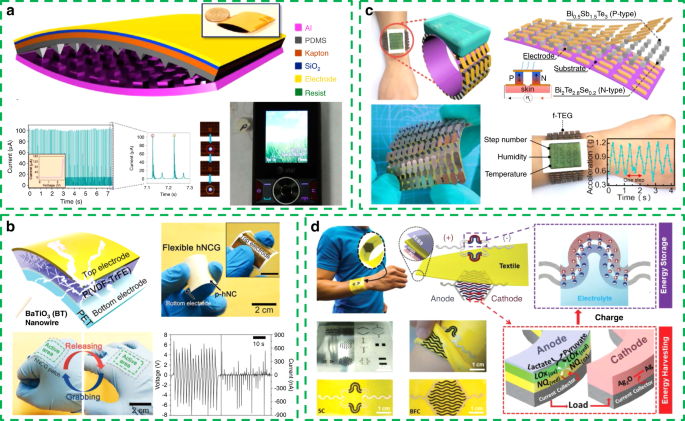
a Arch-shaped TENG as a power supply for mobile phones. Reprinted from ref. 54 with permission. b Hybrid nanocomposite generator (hNCG) for hand movement energy harvesting. Reprinted from ref. 68 with permission. c Flexible thermoelectric generator (f-TEG) for harvesting human thermal energy. Reprinted from ref. 73 with permission. d Wearable textile-based hybrid supercapacitor–biofuel cell (SC–BFC) system as a biochemical energy harvester. Reprinted from ref. 77 with permission.
Since invented by Wang in 2012, TENGs have been studied systematically in materials 55 , 56 , structure 57 , 58 , working mode 59 , 60 , 61 , 62 , and power management 63 , 64 , during which time, the output of TENGs has been greatly improved. As the most suitable energy harvesting method for human motion mechanical energy, TENGs still face some shortcomings that need to be overcome. For example, stability and reliability are the largest problems. The ability of reliable power management and tolerance to environmental factors such as humidity and temperature also need to be further studied.
As another common means of harvesting mechanical energy, piezoelectric generators have particularly attracted much attention because of their high energy density characteristics and clearer physical mechanism. Piezoelectric energy harvesting is based on the piezoelectric effect. Electrical energy can be generated by the mechanical deformation of piezoelectric materials. Common inorganic piezoelectric materials are hard materials 65 and are not suitable for portable and wearable devices. With the development of materials science, flexible polymer piezoelectric materials have entered people’s field of vision 66 , 67 . With excellent flexibility, polymer piezoelectric materials can conformally attach to the surface of the human body, greatly improving wearability and further promoting the development of piezoelectric energy harvesters for human body mechanical energy harvesting.
Jeong et al. developed a 1D–3D (1–3) fully piezoelectric nanocomposite using perovskite BaTiO3 (BT) nanowire-employed poly(vinylidene fluoride-co-trifluoroethylene) (P(VDF-TrFE)) for a high-performance hybrid nanocomposite generator (hNCG) device (Fig. 1b ). The output of the flexible hNCG reached 14 V and 4 μA when used for hand movement energy harvesting. Such output performance is higher than the current levels of even previous piezoceramic film-based flexible energy harvesters 68 .
In addition to collecting hand movement energy, piezoelectric energy harvesters also play important roles in intelligent shoes 69 , implantable devices 70 , and intelligent fabrics 71 for biomechanical energy harvesting. Compared with TENGs, piezoelectric energy harvesters may be limited by the range of material selection. However, the advantages of a higher output current, simple structure, and working mode still make piezoelectric generators an indispensable method for mechanical energy harvesting.
In addition to the mechanical energy produced by human motion, the heat energy of the human body is also valuable energy that can be collected and utilized. The body temperature is constant, and there is a temperature difference with the external environment. Therefore, the use of a thermoelectric generator can achieve continuous energy harvesting. The working principle of a thermoelectric generator is based on the Seebeck effect, that is, the diffusion of electrons and holes caused by a temperature gradient.
There have been many studies on flexible thermoelectric generators (f-TEG) using inorganic thin-film thermoelectric materials or organic compound materials 72 . However, the energy conversion efficiency of these thermoelectric generators is too low to meet the power demand of portable and wearable electronic devices.
To address this problem, Yuan et al. proposed a systematic optimization method for designing a f-TEG (Fig. 1c ) 73 . Through optimizing the number of thermoelectric grains, the fill factor and the series–parallel connection mode of the f-TEG, high energy efficiency and power matching with a wearable sensory system are achieved. Such a highly efficient f-TEG utilizing bismuth telluride grains assembled on a flexible polyimide substrate exhibits excellent power performance with power densities of 3.5 µW cm −2 and 12.3 µW g −1 and can drive the sensor module and finally form a self-powered wearable human body sensing system. Compared with mechanical energy harvesting technology, thermoelectric generators are passive energy harvesting technologies. A thermoelectric generator can achieve continuous and long-term energy collection without any movement of the human body because of the stable energy source. By further increasing the power output, thermoelectric generators may become an ideal energy supply method for portable and wearable self-powered devices in the future.
The human body is a complex physiological environment. In addition to biomechanical energy and heat energy, the human body also has available chemical energy. Biofuel cells are an energy harvesting technology that can collect chemical energy from the human body. Biofuel cells are mainly divided into enzyme-catalyzed biofuel cells and microbial cell catalytic fuel cells that use biofuels such as ethanol 74 or glucose 75 to realize the conversion of chemical energy to electric energy.
In the current research, the output power density of biofuel cells is approximately a few microwatts per square centimeter. This level of energy output makes the biofuel cell insufficient to supply sufficient energy in any actual scenario 76 .
Therefore, Lv et al. integrated biofuel cells into self-charging units and presented a wearable textile-based hybrid supercapacitor–biofuel cell (SC–BFC) system (Fig. 1d ) 77 . This kind of biofuel cell can scavenge biochemical energy from human sweat and store it in a supercapacitor module. A hybrid energy system integrated with an energy harvesting and energy storage module can solve the problem of the small output energy of biofuel cells and ensure a stable energy supply.
On the basis of single energy harvesting technology, a hybrid energy harvesting system integrated with multiple modes takes advantage of various energy harvesting methods and improves the energy efficiency. For example, biomechanical energy can only be collected when the human body maintains a specific motion posture, while the thermoelectric generator can continuously harvest energy regardless of the human state.
In Fig. 2a , Lee et al. fabricated a highly stretchable, hybrid energy-scavenging nanogenerator based on a micropatterned piezoelectric P(VDF-TrFE) polymer, a micropatterned polydimethylsiloxane (PDMS)–carbon nanotube composite and graphene nanosheets 78 . The P(VDF-TrFE) polymer has both a piezoelectric effect and excellent thermoelectric properties with pyroelectric coefficients up to ≈200 µCm −2 K −1 . This kind of hybrid energy harvester can realize the simultaneous collection of biomechanical energy and heat energy when attached to a human hand, a shoulder, an elbow, and other parts.
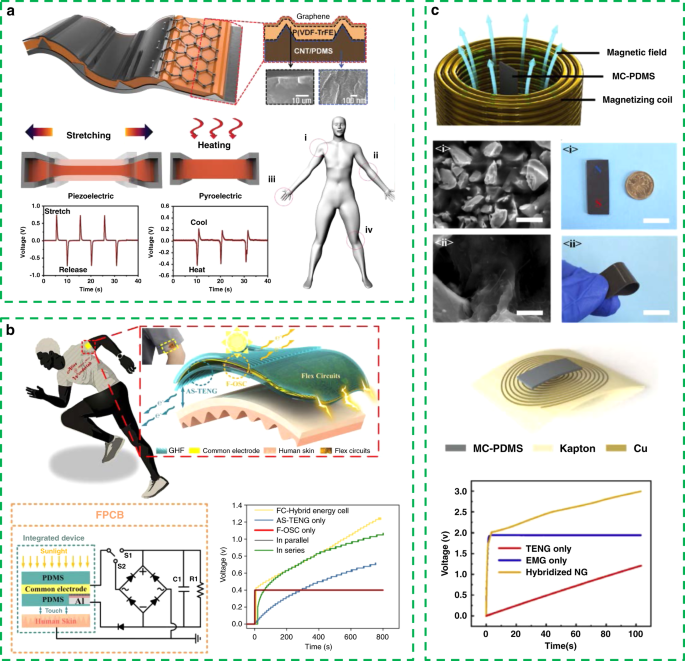
a Piezoelectric and thermoelectric hybrid energy-scavenging nanogenerator. Reprinted from ref. 78 with permission. b Solar-triboelectric hybrid energy harvesting system. Reprinted from ref. 79 with permission. c Hybrid energy harvester based on triboelectric and electromagnetic principles. Reprinted from ref. 80 with permission.
As a kind of sustainable clean energy, solar energy plays an important role in the field of energy harvesting. The simultaneous collection of solar energy and biomechanical energy is also regarded as an effective means to improve energy collection efficiency. H. X. Zhang’s group proposed a solar-triboelectric hybrid energy harvesting system (Fig. 2b ) 79 . Through the design of a common electrode structure and the introduction of an energy management module, this kind of hybrid energy harvester achieves a better charging effect than a single energy harvesting mode in the charging test of capacitors.
In addition, this research group has also proposed a hybrid energy harvester based on triboelectric and electromagnetic principles (Fig. 2c ) 80 . Compared with TENGs, electromagnetic generators are different in principle, output characteristics, and applicable frequency. Therefore, integration can achieve complementary advantages to adapt to a variety of applications. In this work, a flexible hybrid energy harvester is proposed based on magnetic and conductive PDMS material, which complements the large output voltage characteristics of TENGs and the large output current characteristics of electromagnetic generators. This hybrid energy harvester can charge a capacitance of 10 μf to 3 V in 110 s, which is superior to the TENG or EMG only.
Energy harvesting technology is the basis of self-powered systems, giving these systems the ability to achieve some functions without an external energy supply. As a necessary way for electronic devices to perceive the external environment, sensors are the cornerstone of the rich functions of electronic devices. Sensors used in wearable scenes can also act as an extension of five human sense organs, giving humans stronger environmental perception capabilities. Therefore, self-powered systems have great application potential in the wearable sensing field.
Portable and wearable self-powered sensing system
On the basis of energy harvesting technology, a variety of portable, wearable self-powered sensors for monitoring physical, chemical, and physiological information have been developed. There are two main ways to realize self-powered sensing. The first is active sensing, which uses the electrical signal itself as the sensing signal, where the output electrical signal will be affected by some external factors. Active sensing has been widely used in the monitoring of pressure 81 , humidity 82 , and temperature 83 .
In Fig. 3a , Liu et al. reported self-powered epidermal electronics with a tactile sensing function 84 . This kind of epidermal electronics can reflect the pressure on the skin, as well as the pressure distribution, through the triboelectric signal, which is of broad potential interest in wearable electronics.
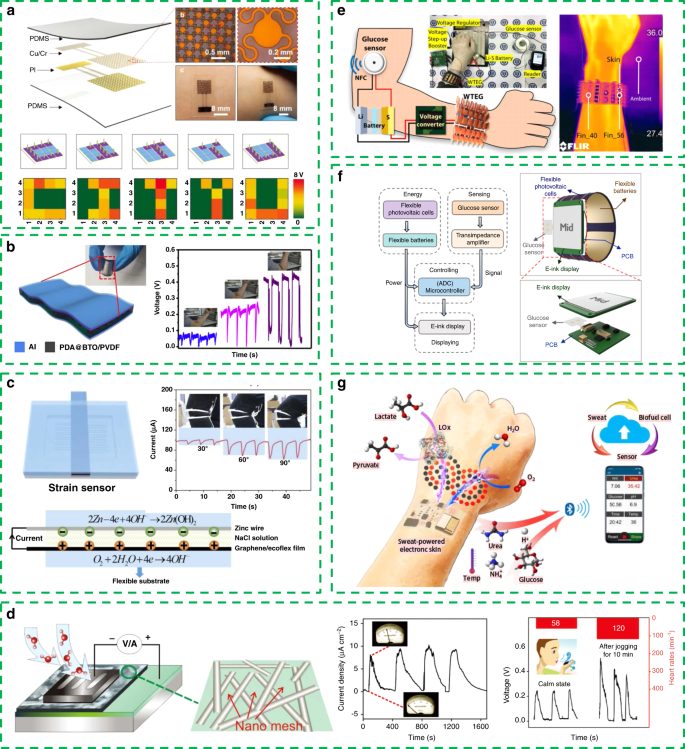
a Self-powered epidermal electronics with the tactile sensing function. Reprinted from ref. 84 with permission. b Flexible wearable pressure sensor based on piezoelectric materials. Reprinted from ref. 85 with permission. c The new kind of self-powered strain sensor based on redox electricity. Reprinted from ref. 86 with permission. d Active humidity sensor based on a moisture-driven electrical generator. Reprinted from ref. 87 with permission. e Self-powered thermoelectric glucose sensing system integrating the wearable thermoelectric generator (WTEG). Reprinted from ref. 88 with permission. f Self-powered glucose sensing smart watch based on photovoltaic cells. Reprinted from ref. 89 with permission. g Flexible perspiration-powered electronic skin (PPES). Reprinted from ref. 90 with permission.
The output of the piezoelectric material will be affected by its deformation degree, which allows these materials to realize the active sensing of human body postures.
Yang et al. showed a flexible wearable pressure sensor based on piezoelectric materials (Fig. 3b ) 85 . On the basis of organic/inorganic piezoelectric material BaTiO3(BTO)/polyvinylidene fluoride(PVDF) composites, polydopamine was introduced as a surface modifier to modify BTO, bringing the improved dispersion of BTO in the organic PVDF matrix. The pressure sensor can realize the active sensing of the elbow bending posture when placed on the elbow of the human body.
In addition, a new self-powered strain sensor based on redox electricity has been proposed using a graphene/Ecoflex film and meandering zinc wire (Fig. 3c ) 86 . In the state of stretching, the resistance of graphene/Ecoflex increases with increasing strain, which leads to a decrease in the redox current. This sensor has been utilized to realize the active motion detection of knee joints.
However, pressure sensors based on triboelectric or posture sensors based on piezoelectric and redox electricity are only small-scale laboratory devices as the primary prototype. To achieve a stable wearable active sensor that can cover the whole body of the human body, it is also necessary to solve key problems such as weak device stability and a single sensing mode.
In Fig. 3d , Shen et al. developed an active humidity sensor based on a moisture-driven electrical generator, which realizes the sensing of another physical quantity in addition to the common pressure and strain 87 . The output voltage of the generator is strongly dependent on the humidity of the ambient environment. This new type of device can play the role of self-powered wearable human breathing monitors and touch pads.
In addition to active sensing, using an energy harvester as an energy supply, driving the sensor module is another way to realize wearable self-powered sensing. This mode is mainly used for monitoring physiological indexes of the human body, such as glucose, urea, NH4+, and pH.
In Fig. 3e , Kim et al. proposed a self-powered thermoelectric glucose sensing system integrating a wearable thermoelectric generator (WTEG) and an emerging Li-S battery 88 . WTEG can provide a sufficient power output (378 μW) to drive the commercial glucose sensor and store the remaining energy in the Li-S battery. It can provide a stable energy supply even when the power supply and demand fluctuate greatly. This work demonstrates for the first time the feasibility of fully utilizing human body heat to drive commercial sensors.
Figure 3f presents a self-powered glucose sensing smart watch based on photovoltaic cells 89 . The energy collected by photovoltaic cells can be used to drive the sweat glucose sensor and enable real-time and in situ data analysis/display for driving e-ink screens.
The human body is a complex physiological system. In addition to glucose, the physiological indicators closely related to human health include urea, NH4+, pH, etc. Therefore, it is of great significance for real-time human metabolic health monitoring to realize the self-powered sensing of multiple physiological indicators at the same time.
As shown in Fig. 3g , W. Gao’s research group recently proposed a flexible perspiration-powered electronic skin that uses human sweat to generate electricity 90 . The unique integration of the zero- to three-dimensional nanomaterials helps the device reach a record breaking power density of 3.5 mW cm −2 for biofuel cells in untreated human body fluids (human sweat). This electronic skin has the ability to perform multiplexed metabolic sensing in situ and can wirelessly transmit the data to a user interface using Bluetooth technology. Such a self-powered sensor system with a high energy output and a multiple indicator monitoring capability has important application value in human metabolic monitoring, early diagnosis of diseases and other scenarios.
At present, the function of self-powered sensing systems has been greatly enriched. However, facing the obstacles of long-term stability, multimode sensing ability, and energy harvesting efficiency, the self-powered energy system has a long way to go before it can be used in large-scale applications.
Self-powered actuation system
In addition to wearable sensing functions, self-powered systems can also realize actuations such as object transportation, automatic control, drug delivery and release, adjuvant therapy, and microrobot control. In this section, some actuation applications of self-powered systems are briefly summarized.
Electrowetting refers to changing the wettability of droplets on the substrate by changing the voltage between the droplet and the insulating substrate, that is, changing the contact angle to make the droplets deform and shift. This process is often used to control the position and velocity of a fluid in microfluidic systems. Figure 4a shows a self-powered microfluidic transport system combining electrowetting with a TENG 91 . Here, a miniature vehicle composed of four droplets can transport small objects on the track electrodes when driven by a TENG. Such a self-powered transport system shows great applications in the fields of microsolid/liquid manipulators, drug delivery systems, microrobotics, etc.
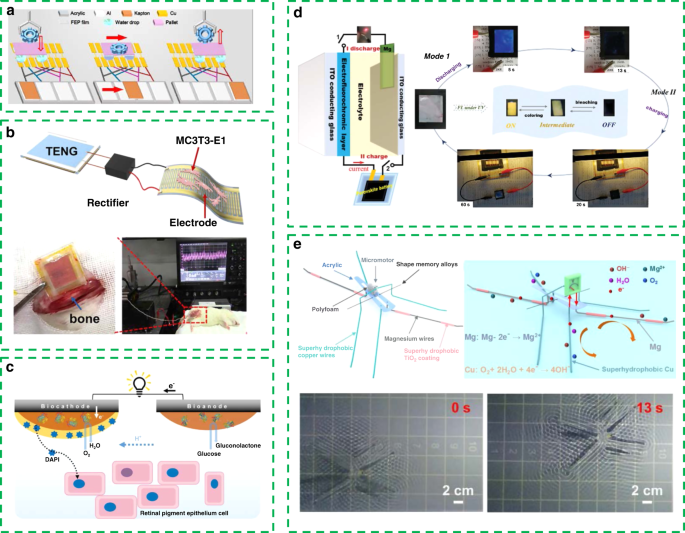
a Self-powered microfluidic transport system combining electrowetting with a TENG. Reprinted from ref. 91 with permission. b Flexible implantable electrical stimulator composed of a TENG and a flexible interdigital electrode. Reprinted from ref. 92 with permission. c Self-powered drug delivery system based on enzyme biofuel cells. Reprinted from ref. 93 with permission. d Self-powered electrofluorochromic devices in intelligent windows. Reprinted from ref. 94 with permission. e Micro self-powered robot. Reprinted from ref. 95 with permission.
In addition, TENGs also play an important role in clinical adjuvant therapy. Tian et al. presented a flexible implantable electrical stimulator composed of a TENG and a flexible interdigital electrode, as shown in Fig. 4b 92 . This electrical stimulation can significantly promote the adhesion, proliferation, and differentiation of osteoblasts and increase the intracellular Ca2+ level. Obviously, this self-powered electrical stimulation system shows great application potential in implantable medical devices and the clinical treatment of osteoporosis and osteoporosis-related fractures.
The transportation and release of drugs without an external power supply is also an important part of intelligent medicine. As shown in Fig. 4c , Xiao et al. proposed a self-powered drug delivery system based on enzyme biofuel cell 93 . Upon discharging the biofuel cell, the drug doped with a conductive polymer will be released rapidly. This work demonstrated the control and ex situ use of ibuprofen, fluorescein, and 4′,6-dimediyl-2-phenylindole (DAPI) and confirmed the feasibility of self-powered technology in drug transport and release scenarios.
Self-powered actuation systems also have important application value in smart homes. Figure 4d shows the application of self-powered electrofluorochromic devices in intelligent windows 94 . Perovskite solar cells can convert solar energy into electrical energy and rapidly color electrochromic materials.
The work in Fig. 4e shows a micro self-powered robot that can walk on the surface of water 95 . Superhydrophobic wires as artificial legs can obtain electric energy from water by redox reactions to supply power to the micromotor and drive the microrobot to move on water. Such a way of driving a robot could provide open prospects for developing self-powered microrobots in aquatic environments.
Self-powered actuation systems play important roles in medical, industrial, and household applications. With the development of processing technology, self-powered actuation systems are expected to undertake more sophisticated and complex functions in the future and further liberate human hands.
Portable and wearable self-powered smart system
In the era of big data and artificial intelligence technology, electronic devices are no longer limited to a single sensing or actuation function. It is an inevitable trend for portable and wearable electronic devices to develop toward the direction of intelligence 96 . In the future, wearable electronic devices with powerful data processing capabilities will become a new interface of human–machine interaction. In this section, we introduce the application of wearable self-powered systems in human–machine interaction scenarios and then show the synergy of wearable self-powered systems and machine learning technology.
In Fig. 5a , Guo et al. proposed a multifunctional electronic skin based on the synchronized triboelectrification and piezoelectric effect 97 . Through the design of network cross electrodes, the electronic skin can identify the motion trajectory in a full plane. A smart anti-counterfeiting signature system with the capability of recognizing the writing habits of people is realized and demonstrates its great application potential in a human–machine interaction interface and intelligent recognition.
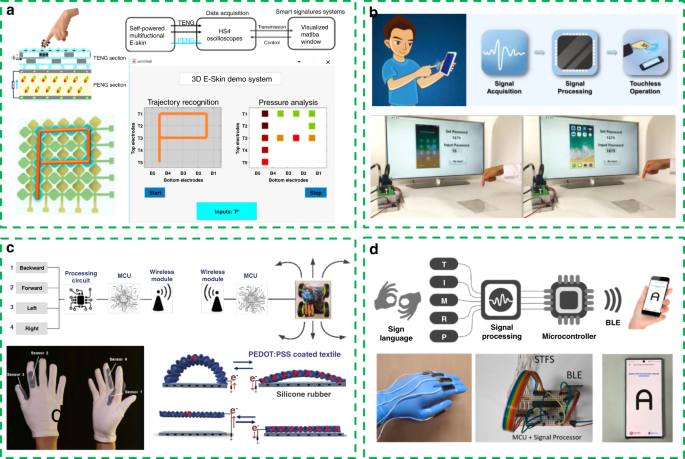
a Multifunctional electronic skin as a smart anti-counterfeiting signature system (SASS). Reprinted from ref. 97 with permission. b Triboelectric touch-free screen sensor (TSS). Reprinted from ref. 98 with permission. c Glove-based multifunctional human–machine interface based on TENG. Reprinted from ref. 99 with permission. d Self-powered triboelectric flex sensor (STFS) for sign language interpretation. Reprinted from ref. 100 with permission.
However, the human–machine interface based on the two-dimensional plane mentioned above can only detect limited kinds of gestures, such as touching and sliding. When using the three-dimensional space as the human–machine interaction interface, we can have an increased freedom of detection. Figure 5b shows an awesome concept of a noncontact human–machine interaction 98 . Here, a triboelectric touch-free screen sensor for recognizing various gestures in a noncontact mode shows the ability to detect multiple gestures, such as the drop and lift of a finger with different speeds, making a fist, opening a palm, and flipping the palm in different directions. In addition, this work shows a noncontact screen control system for smart phones, which presents a new touch-free design concept to develop the next generation of screen sensors.
The human hand is an important channel through which people express information. Through the development of wearable electronic devices that can be attached to the hand, it is possible to use rich hand movements to achieve a variety of interactive functions. Figure 5c shows a glove-based multifunctional human–machine interface based on TENG 99 . By matching the output signals under different gestures with specific functions, this human–machine interface is endowed with various functions, such as wireless car control, wireless drone control, minigame control, and VR game control.
In Fig. 5d , Maharjan et al. developed another self-powered triboelectric flex sensor that can be attached to a finger 100 . Additionally, based on the monitoring of a finger posture, this work shows a real-time application of sign language interpretation. It can be predicted that in the future, there will be more interactive functions beyond imagination, which can be realized through a self-powered human–machine interface.
Artificial intelligence technology represented by machine learning has infused vigor into the development of wearable electronic devices, which enables wearable electronic devices to realize more complex and accurate functions.
For example, a self-powered machine learning glove based on a superhydrophobic triboelectric fabric structure is proposed in Fig. 6a 101 . With the aid of a machine learning algorithm (convolutional neural network), the glove can achieve very accurate virtual reality/augmented reality (VR/AR) controls, including gun shooting, baseball pitching, and flower arrangement.
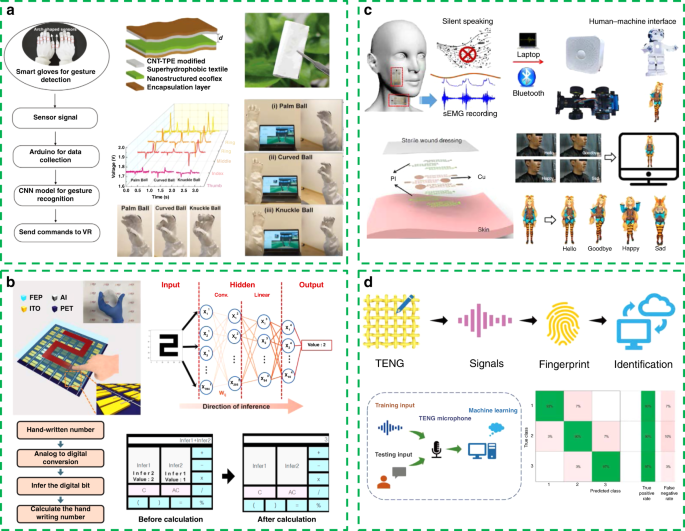
a Self-powered machine learning glove based on a superhydrophobic triboelectric fabric structure. Reprinted from ref. 101 with permission. b Self-powered triboelectricity-based touchpad (TTP). Reprinted from ref. 102 with permission. c Epidermal sEMG tattoo-like patch for silent speech recognition. Reprinted from ref. 103 with permission. d TENG-based smart electronics for both voice signal recognition and handwritten signal recognition. Reprinted from ref. 104 with permission.
The self-powered triboelectricity-based touchpad (TTP) presented in Fig. 6b 102 has the same basic function as the electronic skin based on the piezoelectric-triboelectric principle 97 . However, the introduction of a pretrained neural network greatly enhances the accuracy and real-time trajectory recognition. Therefore, the TTP can be implemented as the smart calculator by using graphical user interface modeling.
The next generation of the computer revolution is from a graphical interface to a voice user interface, so voice recognition technology is a very competitive development direction in human–machine interaction.
Surface electromyography (sEMG) generated from the jaw contains valuable voice information. Therefore, Liu et al. demonstrated an epidermal sEMG tattoo-like patch with the ability of sEMG recording for silent speech recognition, as shown in Fig. 6c 103 . With the aid of wavelet decomposition and pattern recognition, the average accuracy of action instructions can reach 89.04%, and the average accuracy of emotion instructions is as high as 92.33%. Based on this performance, this tattoo-like patch can even be expected to help aphasia patients regain the ability to communicate with others.
Similarly, the TENG-based smart electronics in Fig. 6d has the ability of both voice signal recognition and handwritten signal recognition 104 . The “medium Gaussian support vector machine” is used as the machine learning model, and a recognition accuracy of 93.5% is obtained.
In summary, the combination of a self-powered system and machine learning technology makes wearable electronic devices more intelligent. It is worth mentioning that in the current work, self-powered intelligent systems mainly possess the two functions of recognition (Figs. 5a , d and 6b , d ) and control (Figs. 5b, c and 6a , c ), playing the role of assisting humans. In future developments, we can even devote ourselves to empower traditional electronics to “think,” “analyze,” and “decide” and realize the real integration of electronic devices and the human body.
Summary and perspective
Self-powered systems show great potential in energy harvesting, sensing, actuating, and human–machine interaction applications and are expected to become the main form of electronic devices in the Internet of Things era. In this review, we give a comprehensive introduction to the development of portable and wearable self-powered systems. We started with portable and wearable energy harvesting technology as the basis of self-powered systems and then introduced the application of self-powered systems in the wearable sensing of a series of physical and physiological indicators. In addition, we also introduced typical self-powered systems with actuating functions. Intelligentization is an inevitable trend in the development of electronic equipment. Therefore, we focus on the state-of-the-art developments of portable and wearable self-powered systems as a new type of human–machine interaction interface and demonstrate the importance of machine learning in empowering self-powered systems with higher-level functions. At the same time, we also discussed the application characteristics of different forms of portable wearable self-powered systems in different scenarios.
However, before the portable and wearable self-powered system can move toward large-scale practical applications, there are still many problems that should be fully addressed in the near future (Fig. 7 ).
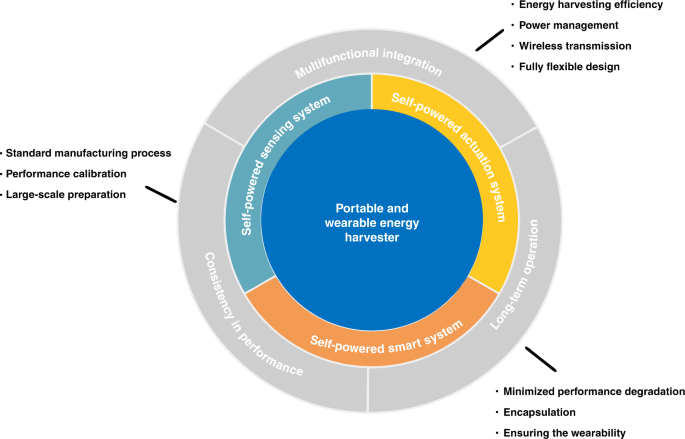
Challenges and prospects of portable and wearable self-powered systems.
Most wearable and portable self-powered systems are based on flexible materials, which experience device performance degradation during long-term operation. Therefore, in the future, we should study additional material and structural designs to improve the stability of the system under long-term work while ensuring the wearability of the device.
Currently, portable wearable self-powered electronic devices are mainly desktop laboratory devices, which only demonstrate a concept. Since there is no work to propose a standardized manufacturing process for portable and wearable self-powered electronic devices, it is impossible to achieve complete consistency with respect to the performance of two different devices. Therefore, in the future, we need to consider the issue of performance calibration between different devices, or we can develop standardized processes for portable wearable devices that can be mass produced.
Hybrid energy harvesting technology that integrates multiple transduction methods is most likely to act as a power source for future self-powered systems. However, the large differences in the frequency, amplitude, and waveform of electrical power converted through different transduction methods make it an unsolved problem to develop power management technologies suitable for different energy harvesting methods. Furthermore, an increasing number of functional modules are being integrated into self-powered systems. We need to rationally design power management circuits to improve the energy conversion efficiency and achieve energy distribution among various functional modules. In addition, a wireless module is needed to realize the transmission of information.
In summary, portable and wearable self-powered systems have made significant progress and may become the main form of the next generation of electronic devices. The rapid development of materials science, processing technology, and smart technology will pave the way for wearable self-powered systems in practical applications.
An, B. et al. Smart sensor systems for wearable electronic devices. Polymers 9 , 303 (2017).
Article Google Scholar
Stoppa, M. & Chiolerio, A. Wearable electronics and smart textiles: a critical review. Sensors 14 , 11957–11992 (2014).
Rawassizadeh, R., Price, B. A. & Petre, M. Wearables: has the age of smartwatches finally arrived? Commun. ACM 58 , 45–47 (2014).
Rauschnabel, P. A. & Ro, Y. K. Augmented reality smart glasses: an investigation of technology acceptance drivers. Int. J. Technol. Mark. 11 , 123–148 (2016).
Liu, Y., He, K., Chen, G., Leow, W. R. & Chen, X. Nature-inspired structural materials for flexible electronic devices. Chem. Rev. 117 , 12893–12941 (2017).
Jagadish, H. V. et al. Big data and its technical challenges. Commun. ACM 57 , 86–94 (2014).
Chen, M. et al. Wearable 2.0: enabling human-cloud integration in next generation healthcare systems. IEEE Commun. Mag. 55 , 54–61 (2017).
Shi, Q. et al. Progress in wearable electronics/photonics—moving toward the era of artificial intelligence and internet of things. InfoMat 2 , 1131–1162 (2020).
Majumder, S., Mondal, T. & Deen, M. J. Wearable sensors for remote health monitoring. Sensors 17 , 130 (2017).
Liu, Y., Pharr, M. & Salvatore, G. A. Lab-on-skin: a review of flexible and stretchable electronics for wearable health monitoring. ACS Nano 11 , 9614–9635 (2017).
Dong, B. et al. Wearable triboelectric–human–machine interface (THMI) using robust nanophotonic readout. ACS Nano 14 , 8915–8930 (2020).
Sim, K. et al. Metal oxide semiconductor nanomembrane–based soft unnoticeable multifunctional electronics for wearable human-machine interfaces. Sci. Adv. 5 , eaav9653 (2019).
Zaia, E. W., Gordon, M. P., Yuan, P. & Urban, J. J. Progress and perspective: soft thermoelectric materials for wearable and Internet‐of‐Things applications. Adv. Electron. Mater. 5 , 1800823 (2019).
Xiao, N., Yu, W. & Han, X. Wearable heart rate monitoring intelligent sports bracelet based on Internet of Things. Measurement 164 , 108102 (2020).
Xia, K. et al. Painting a high-output triboelectric nanogenerator on paper for harvesting energy from human body motion. Nano Energy 50 , 571–580 (2018).
Zou, Y., Raveendran, V. & Chen, J. Wearable triboelectric nanogenerators for biomechanical energy harvesting. Nano Energy 77 , 105303 (2020).
Mondal, S., Paul, T., Maiti, S., Das, B. K. & Chattopadhyay, K. K. Human motion interactive mechanical energy harvester based on all inorganic perovskite-PVDF. Nano Energy 74 , 104870 (2020).
Anwar, S. et al. Piezoelectric nylon-11 fibers for electronic textiles, energy harvesting and sensing. Adv. Funct. Mater. 31 , 2004326 (2021).
Maharjan, P. et al. High-performance cycloid inspired wearable electromagnetic energy harvester for scavenging human motion energy. Appl. Energy 256 , 113987 (2019).
Digregorio, G., Pierre, H., Laurent, P. & Redouté, J. M. Modeling and experimental characterization of an electromagnetic energy harvester for wearable and biomedical applications. IEEE Access 8 , 175436–175447 (2020).
Bandodkar, A. et al. Soft, stretchable, high power density electronic skin-based biofuel cells for scavenging energy from human sweat. Energy Environ. Sci. 10 , 1581–1589 (2017).
Huang, X. et al. Wearable biofuel cells based on the classification of enzyme for high power outputs and lifetimes. Biosens. Bioelectron. 124 , 40–52 (2019).
Zhao, X. et al. Fabrication of transparent paper-based flexible thermoelectric generator for wearable energy harvester using modified distributor printing technology. ACS Appl. Mater. Interfaces 11 , 10301–10309 (2019).
Nozariasbmarz, A. et al. Review of wearable thermoelectric energy harvesting: from body temperature to electronic systems. Appl. Energy 258 , 114069 (2020).
Hashemi, S. A., Ramakrishna, S. & Aberle, A. G. Recent progress in flexible-wearable solar cells for self-powered electronic devices. Energy Environ. Sci. 13 , 685–743 (2020).
Satharasinghe, A., Hughes‐Riley, T. & Dias, T. An investigation of a wash‐durable solar energy harvesting textile. Prog. Photovoltaics Res. Appl. 28 , 578–592 (2020) .
Ryu, H., Yoon, H. J. & Kim, S. W. Hybrid energy harvesters: toward sustainable energy harvesting. Adv. Mater. 31 , 1802898 (2019).
Zhang, T., Yang, T., Zhang, M., Bowen, C. R. & Yang, Y. Recent progress in hybridized nanogenerators for energy scavenging. iScience 23 , 101689 (2020).
Wang, Y. et al. Hierarchically patterned self-powered sensors for multifunctional tactile sensing. Sci. Adv. 6 , eabb9083 (2020).
Chen, X., Ren, Z., Guo, H., Cheng, X. & Zhang, H. Self-powered flexible and transparent smart patch for temperature sensing. Appl. Phys. Lett. 116 , 043902 (2020).
Chen, H. et al. Superior self‐powered room‐temperature chemical sensing with light‐activated inorganic halides perovskites. Small 14 , 1702571 (2018).
Kim, Y. et al. 2D transition metal dichalcogenide heterostructures for p‐and n‐type photovoltaic self‐powered gas sensor. Adv. Funct. Mater. 30 , 2003360 (2020).
Chen, Y., Ji, W., Yan, K., Gao, J. & Zhang, J. Fuel cell-based self-powered electrochemical sensors for biochemical detection. Nano Energy 61 , 173–193 (2019).
Kanik, M., Marcali, M., Yunusa, M., Elbuken, C. & Bayindir, M. Continuous triboelectric power harvesting and biochemical sensing inside poly (vinylidene fluoride) hollow fibers using microfluidic droplet generation. Adv. Mater. Technol. 1 , 1600190 (2016).
Wang, H. L. et al. Large‐area integrated triboelectric sensor array for wireless static and dynamic pressure detection and mapping. Small 16 , 1906352 (2020).
Sun, T. et al. Wearable textile supercapacitors for self-powered enzyme-free smartsensors. ACS Appl. Mater. Interfaces 12 , 21779–21787 (2020).
Yang, Y. et al. Flexible piezoelectric pressure sensor based on polydopamine-modified BaTiO3/PVDF composite film for human motion monitoring. Sens. Actuators A Phys. 301 , 111789 (2020).
Wang, Y. et al. Self-powered wearable pressure sensing system for continuous healthcare monitoring enabled by flexible thin-film thermoelectric generator. Nano Energy 73 , 104773 (2020).
Li, L. et al. Moisture-driven power generation for multifunctional flexible sensing systems. Nano Lett. 19 , 5544–5552 (2019).
Wang, Y. & Yang, Y. Superhydrophobic surfaces-based redox-induced electricity from water droplets for self-powered wearable electronics. Nano Energy 56 , 547–554 (2019).
Wu, J. et al. A wheeled robot driven by a liquid‐metal droplet. Adv. Mater. 30 , 1805039 (2018).
Wang, J. et al. Normally transparent tribo-induced smart window. ACS Nano 14 , 3630–3639 (2020).
Chen, G., Liu, X., Li, S., Dong, M. & Jiang, D. A droplet energy harvesting and actuation system for self-powered digital microfluidics. Lab Chip 18 , 1026–1034 (2018).
Guo, Z. H. et al. Self‐powered electrowetting valve for instantaneous and simultaneous actuation of paper‐based microfluidic assays. Adv. Funct. Mater. 29 , 1808974 (2019).
Liu, G. et al. Flexible drug release device powered by triboelectric nanogenerator. Adv. Funct. Mater. 30 , 1909886 (2020).
Liu, Z. et al. Human motion driven self-powered photodynamic system for long-term autonomous cancer therapy. ACS Nano 14 , 8074–8083 (2020).
Song, Y., Min, J. & Gao, W. Wearable and implantable electronics: moving toward precision therapy. ACS Nano 13 , 12280–12286 (2019).
Zhang, W. et al. Multilanguage-handwriting self-powered recognition based on triboelectric nanogenerator enabled machine learning. Nano Energy 77 , 105174 (2020).
Chen, T. et al. Intuitive-augmented human-machine multidimensional nano-manipulation terminal using triboelectric stretchable strip sensors based on minimalist design. Nano Energy 60 , 440–448 (2019).
Khalid, S., Raouf, I., Khan, A., Kim, N. & Kim, H. S. A review of human-powered energy harvesting for smart electronics: recent progress and challenges. Int. J. Precis. Eng. Man. GT. 6 , 1–31 (2019).
Google Scholar
Dong, B. et al. Technology evolution from self-powered sensors to AIoT enabled smart homes. Nano Energy 79 , 105414 (2021).
Wang, H., Han, M., Song, Y. & Zhang, H. Design, manufacturing and applications of wearable triboelectric nanogenerators. Nano Energy 81 , 105627 (2021).
Gunawardhana, K. S. D., Wanasekara, N. D. & Dharmasena, R. I. G. Towards truly wearable systems: optimising and scaling up wearable triboelectric nanogenerators. Iscience 23 , 101360 (2020).
Wang, S., Lin, L. & Wang, Z. L. Nanoscale triboelectric-effect-enabled energy conversion for sustainably powering portable electronics. Nano Lett. 12 , 6339–6346 (2012).
Yu, A., Zhu, Y., Wang, W. & Zhai, J. Progress in triboelectric materials: toward high performance and widespread applications. Adv. Funct. Mater. 29 , 1900098 (2019).
Kim, D. W., Lee, J. H., Kim, J. K. & Jeong, U. Material aspects of triboelectric energy generation and sensors. NPG Asia Mater. 12 , 1–17 (2020).
Lee, K. Y. et al. Hydrophobic sponge structure‐based triboelectric nanogenerator. Adv. Mater. 26 , 5037–5042 (2014).
Cheng, P. et al. Largely enhanced triboelectric nanogenerator for efficient harvesting of water wave energy by soft contacted structure. Nano Energy 57 , 432–439 (2019).
Wang, S. et al. Sliding-triboelectric nanogenerators based on in-plane charge-separation mechanism. Nano Lett. 13 , 2226–2233 (2013).
Wang, X. et al. Bionic single-electrode electronic skin unit based on piezoelectric nanogenerator. Acs Nano 12 , 8588–8596 (2018).
Wang, S., Xie, Y., Niu, S., Lin, L. & Wang, Z. L. Freestanding triboelectric‐layer‐based nanogenerators for harvesting energy from a moving object or human motion in contact and non‐contact modes. Adv. Mater. 26 , 2818–2824 (2014).
Yin, X. et al. Structure and dimension effects on the performance of layered triboelectric nanogenerators in contact-separation mode. ACS Nano 13 , 698–705 (2018).
Cheng, X. et al. High efficiency power management and charge boosting strategy for a triboelectric nanogenerator. Nano Energy 38 , 438–446 (2017).
Cheng, X. et al. Power management and effective energy storage of pulsed output from triboelectric nanogenerator. Nano Energy 61 , 517–532 (2019).
Priya, S. et al. A review on piezoelectric energy harvesting: materials, methods and circuit. Energy Harvesting Syst. 4 , 3–39 (2019).
Ramadan, K. S., Sameoto, D. & Evoy, S. A review of piezoelectric polymers as functional materials for electromechanical transducers. Smart Mater. Struct. 23 , 033001 (2014).
Mishra, S., Unnikrishnan, L., Nayak, S. K. & Mohanty, S. Advances in piezoelectric polymer composites for energy harvesting applications: a systematic review. Macromol. Mater. Eng. 304 , 1800463 (2019).
Jeong, C. K., Baek, C., Kingon, A. I., Park, K. I. & Kim, S. H. Lead‐free perovskite nanowire‐employed piezopolymer for highly efficient flexible nanocomposite energy harvester. Small 14 , 1704022 (2018).
Xin, Y. et al. Shoes-equipped piezoelectric transducer for energy harvesting: a brief review. Ferroelectrics 493 , 12–24 (2016).
Jiang, L. et al. Flexible piezoelectric ultrasonic energy harvester array for bio-implantable wireless generator. Nano Energy 56 , 216–224 (2019).
Kim, J. et al. Cost-effective and strongly integrated fabric-based wearable piezoelectric energy harvester. Nano Energy 75 , 104992 (2020).
Du, Y., Xu, J., Paul, B. & Eklund, P. Flexible thermoelectric materials and devices. Appl. Mater. Today 12 , 366–388 (2018).
Yuan, J. & Zhu, R. A fully self-powered wearable monitoring system with systematically optimized flexible thermoelectric generator. Appl. Energy 271 , 115250 (2020).
Selloum, D. & Tingry, S. Ethanol/Oxygene microfluidic biofuel cells. Mater. Biomater. Sci. 1 , 011–015 (2018).
Wang, C. et al. Sustainable and high-power wearable glucose biofuel cell using long-term and high-speed flow in sportswear fabrics. Biosens. Bioelectron. 169 , 112652 (2020).
Gamella, M., Koushanpour, A. & Katz, E. Biofuel cells–activation of micro-and macro-electronic devices. Bioelectrochemistry 119 , 33–42 (2018).
Lv, J. et al. Sweat-based wearable energy harvesting-storage hybrid textile devices. Energy Environ. Sci. 11 , 3431–3442 (2018).
Lee, J. H. et al. Highly stretchable piezoelectric‐pyroelectric hybrid nanogenerator. Adv. Mater. 26 , 765–769 (2014).
Ren, Z. et al. Wearable and self-cleaning hybrid energy harvesting system based on micro/nanostructured haze film. Nano Energy 67 , 104243 (2020).
Wan, J. et al. A flexible hybridized electromagnetic-triboelectric nanogenerator and its application for 3D trajectory sensing. Nano Energy 74 , 104878 (2020).
Zhao, X. et al. Polyimide/graphene nanocomposite foam‐based wind‐driven triboelectric nanogenerator for self‐powered pressure sensor. Adv. Mater. Technol. 4 , 1800723 (2019).
Su, Y. et al. Novel high-performance self-powered humidity detection enabled by triboelectric effect. Sens. Actuators B Chem. 251 , 144–152 (2017).
Xia, K., Zhu, Z., Zhang, H. & Xu, Z. A triboelectric nanogenerator as self-powered temperature sensor based on PVDF and PTFE. Appl. Phys. A 124 , 520 (2018).
Liu, Y. et al. Thin, skin‐integrated, stretchable triboelectric nanogenerators for tactile sensing. Adv. Electron. Mater. 6 , 1901174 (2020).
Wang, Y., Wang, Y. & Yang, Y. Graphene–polymer nanocomposite‐based redox‐induced electricity for flexible self‐powered strain sensors. Adv. Energy Mater. 8 , 1800961 (2018).
Shen, D. et al. Self‐powered wearable electronics based on moisture enabled electricity generation. Adv. Mater. 30 , 1705925 (2018).
Kim, J. et al. Self-charging wearables for continuous health monitoring. Nano Energy 79 , 105419 (2020).
Zhao, J. et al. A fully integrated and self-powered smartwatch for continuous sweat glucose monitoring. ACS Sens. 4 , 1925–1933 (2019).
Yu, Y. et al. Biofuel-powered soft electronic skin with multiplexed and wireless sensing for human-machine interfaces. Sci. Robot. 5 , eaaz7946 (2020).
Nie, J. et al. Self-powered microfluidic transport system based on triboelectric nanogenerator and electrowetting technique. Acs Nano 12 , 1491–1499 (2018).
Tian, J. et al. Self-powered implantable electrical stimulator for osteoblasts’ proliferation and differentiation. Nano Energy 59 , 705–714 (2019).
Xiao, X., Denis McGourty, K. & Magner, E. Enzymatic biofuel cells for self-powered, controlled drug release. J. Am. Chem. Soc. 142 , 11602–11609 (2020).
Sun, J. et al. Reversible self-powered fluorescent electrochromic windows driven by perovskite solar cells. Chem. Commun. 55 , 12060–12063 (2019).
Wang, Y., Jiang, Y., Wu, H. & Yang, Y. Floating robotic insects to obtain electric energy from water surface for realizing some self-powered functions. Nano Energy 63 , 103810 (2019).
Guo, H. et al. Self-powered multifunctional electronic skin for a smart anti-counterfeiting signature system. ACS Appl. Mater. Interfaces 12 , 22357–22364 (2020).
Tang, Y. et al. Triboelectric touch‐free screen sensor for noncontact gesture recognizing. Adv. Funct. Mater. 30 , 1907893 (2020).
He, T. et al. Self-powered glove-based intuitive interface for diversified control applications in real/cyber space. Nano Energy 58 , 641–651 (2019).
Maharjan, P. et al. A human skin-inspired self-powered flex sensor with thermally embossed microstructured triboelectric layers for sign language interpretation. Nano Energy 76 , 105071 (2020).
Wen, F. et al. Machine learning glove using self‐powered conductive superhydrophobic triboelectric textile for gesture recognition in VR/AR applications. Adv. Sci. 7 , 2000261 (2020).
Yun, J., Jayababu, N. & Kim, D. Self-powered transparent and flexible touchpad based on triboelectricity towards artificial intelligence. Nano Energy 78 , 105325 (2020).
Liu, H. et al. An epidermal sEMG tattoo-like patch as a new human–machine interface for patients with loss of voice. Microsyst. Nanoeng. 6 , 1–13 (2020).
Ji, X., Zhao, T., Zhao, X., Lu, X. & Li, T. Triboelectric nanogenerator based smart electronics via machine learning. Adv. Mater. Technol. 5 , 1900921 (2020).
Download references
Acknowledgements
This work was supported by the National Key R&D Project from the Minister of Science and Technology, China (2016YFA0202701, 2018YFA0108100) and the National Natural Science Foundation of China (Grant No. 61674004).
Author information
Authors and affiliations.
Academy for Advanced Interdisciplinary Studies, Peking University, Beijing, China
Chen Xu & Haixia Zhang
National Key Laboratory of Science and Technology on Micro/Nano Fabrication, Institute of Microelectronics, Peking University, Beijing, China
Yu Song & Haixia Zhang
Department of Biomedical Engineering, College of Future Technology, Peking University, Beijing, China
You can also search for this author in PubMed Google Scholar
Corresponding authors
Correspondence to Mengdi Han or Haixia Zhang .
Ethics declarations
Conflict of interest.
The authors declare no competing interests.
Rights and permissions
Open Access This article is licensed under a Creative Commons Attribution 4.0 International License, which permits use, sharing, adaptation, distribution and reproduction in any medium or format, as long as you give appropriate credit to the original author(s) and the source, provide a link to the Creative Commons license, and indicate if changes were made. The images or other third party material in this article are included in the article’s Creative Commons license, unless indicated otherwise in a credit line to the material. If material is not included in the article’s Creative Commons license and your intended use is not permitted by statutory regulation or exceeds the permitted use, you will need to obtain permission directly from the copyright holder. To view a copy of this license, visit http://creativecommons.org/licenses/by/4.0/ .
Reprints and permissions
About this article
Cite this article.
Xu, C., Song, Y., Han, M. et al. Portable and wearable self-powered systems based on emerging energy harvesting technology. Microsyst Nanoeng 7 , 25 (2021). https://doi.org/10.1038/s41378-021-00248-z
Download citation
Received : 09 November 2020
Revised : 09 January 2021
Accepted : 02 February 2021
Published : 17 March 2021
DOI : https://doi.org/10.1038/s41378-021-00248-z
Share this article
Anyone you share the following link with will be able to read this content:
Sorry, a shareable link is not currently available for this article.
Provided by the Springer Nature SharedIt content-sharing initiative
This article is cited by
Electrostatic generator enhancements for powering iot nodes via efficient energy management.
- Xiongying Ye
Microsystems & Nanoengineering (2024)
Intelligent block copolymer self-assembly towards IoT hardware components
- Geon Gug Yang
- Hee Jae Choi
- Sang Ouk Kim
Nature Reviews Electrical Engineering (2024)
High-performance hybrid nanogenerator for self-powered wireless multi-sensing microsystems
- Dan-Liang Wen
- Xiao-Sheng Zhang
Microsystems & Nanoengineering (2023)
Chicken skin based Milli Watt range biocompatible triboelectric nanogenerator for biomechanical energy harvesting
- Muhammad Umair Khan
- Eman Mohammad
- Baker Mohammad
Scientific Reports (2023)
Mechanical energy harvesters with tensile efficiency of 17.4% and torsional efficiency of 22.4% based on homochirally plied carbon nanotube yarns
- Mengmeng Zhang
- Wenting Cai
- Ray H. Baughman
Nature Energy (2023)
Quick links
- Explore articles by subject
- Guide to authors
- Editorial policies

- Search Menu
- Advance Articles
- Collections
- Author Guidelines
- Reviewer Guidelines
- Submission Site
- Open Access Options
- Self-Archiving Policy
- Publish with us
- About Oxford Open Energy
- Editorial Board
- Advertising & Corporate Services
- Journals on Oxford Academic
- Books on Oxford Academic

Article Contents
Introduction, photovoltaics-based solar cogeneration, power cycle-based solar cogeneration, comparison of energy and exergy efficiencies and system applications, conclusions, authors’ contributions, data availability, a. appendix.
- < Previous
Efficient approaches for harvesting solar energy in cogeneration: a review
- Article contents
- Figures & tables
- Supplementary Data
Jian Yao, Wenjie Liu, Yifan Jiang, Sihang Zheng, Yao Zhao, Yanjun Dai, Junjie Zhu, Vojislav Novakovic, Efficient approaches for harvesting solar energy in cogeneration: a review, Oxford Open Energy , Volume 1, 2022, oiab004, https://doi.org/10.1093/ooenergy/oiab004
- Permissions Icon Permissions
Stronger urbanization will increase the demand for power and thermal energy to meet the new energy service requirements, which often leads to higher fossil fuels use and emissions. Renewable energy utilization has high potential in urban context to reduce carbon emissions. Solar energy in particular has proved to be promising renewable source due to its ubiquity, abundance and sustainability. Efficient utilization of solar energy for cogeneration is an important application in the built environment, with wide applicability. This review provides a comprehensive state-of-the-art analysis of solar energy for combined heat and power supply based on the available literature. Different approaches to solar cogeneration are classified and critically reviewed. The review shows that efficient solar cogeneration methods could significantly improve the utilization efficiency of solar energy.

The increase of population and social progress has led to increased consumption of fossil fuel, causing higher carbon emissions and being the main reason for global climate change. Developing renewable energy is an important strategy to mitigate the energy and environmental crisis, but also effectively alleviate environmental pollution. For example, China has initiated policies to stabilize carbon emissions by 2030 and reach carbon neutrality by 2060 [ 1 ]. Many countries have also put forward targeted energy strategies on renewable energy for carbon emission reductions. For instance, the European Union has issued a new directive to promote renewable energy use, which sets a target of 32% of total final energy demand for renewable energy by 2030 [ 2 ]. According to the 2019 Federal Energy Regulatory Commission, renewable energy in the United States provided 23% of the electricity of the whole country, and it generated more electricity than coal for the first time [ 3 ]. Japan’s Energy Basic Plan aims to increase the share of renewable energy to 22–24% by 2030 and nuclear power to 20–22% and decrease the share of fossil fuels to 56% [ 4 ]. According to the Statistical Review of World Energy, 3.2 EJ (exajoule) of renewable energy was produced from renewable energy sources in 2019, the largest increase in all energy sources in 2019 [ 5 ].
The efficient utilization of renewable energy would significantly mitigate the detrimental impacts from fossil fuel consumption. Solar energy is the most promising source among the existing renewable energy sources due to its merits including ubiquity, abundance and sustainability [ 6 ]. Moreover, the environmental effect of using solar energy is minimal as it does not generate greenhouse gases or polluting gases. Its market share of global energy is positively increasing [ 7 ]. Electricity as commercial energy is the first target for renewable energy conversion. Examples of methods to convert solar energy into electricity are photovoltaics, combined power cycle with concentrating solar collectors (CSCs), photochemistry, etc. Some of the solar radiation is converted to waste heat during the electrical energy generation processes, e.g. in concentrated solar power (CSP) plants. The solar energy utilization efficiency could be maximized if the waste heat could be used properly, e.g. through combined heat and power schemes. On the other hand, the heat and power generated by solar energy could then meet higher shares of the energy demand in the built environment. Therefore, efficient solar cogeneration has a high potential for applications within the building sector.
Classification of approaches on solar cogeneration
Photovoltaic effect and solar power cycle are the mainstream approaches of solar-electricity conversion, and these two approaches are more efficient and mature than photochemistry methods. Thus, this review focuses on the efficient approaches to harvesting solar energy for solar cogeneration.
The efficient solar cogeneration process could be mainly divided into two categories: photovoltaic effect-based solar cogeneration and power cycle-based solar cogeneration.
The photovoltaic effect-based solar cogeneration technology refers to photovoltaic–thermal (PVT) technology. It could be classified into liquid-based PVT module, air-based PVT module and refrigerant-based PVT module. Moreover, the spectral beam splitting PVT module is recently proposed to utilize the full spectrum of solar irradiation according to the wavelength. The liquid-based PVT module could be further divided into water (including antifreeze), nanofluids and heat transfer oil-based PVT modules. The refrigerant-based PVT module includes the direct expansion PVT module and heat pipe-based PVT module. In addition, nanofluids-based spectral beam splitter, nano-film-based spectral beam splitter and semitransparent PV cell-based spectral beam splitter are the three sub-categories of the spectral beam splitting PVT module.
The power cycle-based solar cogeneration uses the turbine to generate electricity while the waste heat of the working medium could be used to generate useful thermal energy for domestic or industry applications [ 8 ]. According to the different power cycles, it could be classified as Brayton cycle, Stirling cycle and Rankine cycle-based solar cogeneration [ 9 ]. The driven temperatures are different of three kinds of power cycles, notwithstanding, the temperature demands are all above 100°C. Thus, the CSC is employed in these kinds of solar cogeneration systems to provide a high-temperature heat source. The linear concentrator, central receiver concentrator and dish mirrors are commonly adopted as CSC.
The classification of approaches for solar cogeneration is shown in Fig. 1 .

As shown in Fig. 2a , the photovoltaic effect could only be excited by the corresponding wavelength range of the solar spectrum (mainly the visible light) [ 10 ]. The rest of the solar irradiation would convert to waste heat and resulting in the temperature rise of the solar cells [ 11 ]. The high operating temperature of the solar cells would significantly diminish their electrical efficiency while causing deterioration in their service life [ 12 ]. Moreover, the waste heat absorbed by the PV module dissipates in the environment without appropriate usage. Thus, the proposal of PVT technology [ 13 ] could solve this problem as shown in Fig. 2b . The heat transfer medium could extract heat from the solar cells and transfer the waste heat to useful thermal energy. Meanwhile, the lower working temperature of the PV module would benefit its electrical efficiency. In this regard, the PVT module could realize solar cogeneration with high comprehensive solar energy utilization efficiency. The PVT technology could be classified into four categories based on the working medium, which would be reviewed specifically in the following subsections.

(a) Spectral utilization of the photovoltaic effect-based solar cogeneration. (b) Schematic diagram of the PVT module
![solar energy harvesting research papers (a) Upper and rear side of the water-based PVT module. (b) Photograph of the installation. [23] (APPLICABLE SOCIETY COPYRIGHT OWNER)](https://oup.silverchair-cdn.com/oup/backfile/Content_public/Journal/ooenergy/1/10.1093_ooenergy_oiab004/24/m_oiab004f3.jpeg?Expires=1714710160&Signature=prJeDJ-41S0ifWS01EDP1Cp08L3h8gngK~IDW17Vws7z2dB7uXACG0Cn2jWp3YwfNGs7eaQpPfYsrShaDXB8npLkqUH4EmLQBTAeEwjhj8XgYQBB0QslZOBYzFccZJSwkt5mR0czrRe4O97OLAEpfTmCRwkQSYO0UM6DXOX-Ks79E7rClP3ziR5~F9pingot67yzAJC8LS1o6JoWvf6lgEvXnKrZMGy-UPEQq1mxu4jYj3O1Y4wHSS6xWYs0w7IMZzVPD8dvSGY8WIkAZj0D79ryMpwKil9ifX0URjvFnotF9bDhxb2lwdxyO8lMMWt32NS~QEhmxEIgxlcB9TEKiA__&Key-Pair-Id=APKAIE5G5CRDK6RD3PGA)
(a) Upper and rear side of the water-based PVT module. (b) Photograph of the installation. [ 23 ] (APPLICABLE SOCIETY COPYRIGHT OWNER)
![solar energy harvesting research papers (a) Cross-section view of the structure of the water-based PVT module. (b–e) Four arrangements of PVT modules. [25] (APPLICABLE SOCIETY COPYRIGHT OWNER)](https://oup.silverchair-cdn.com/oup/backfile/Content_public/Journal/ooenergy/1/10.1093_ooenergy_oiab004/24/m_oiab004f4.jpeg?Expires=1714710160&Signature=UPz4nfEyijgc9MKzMR~Dp~4wuPsDssP4R~sOPNli5nRwXGHhutMkcfpMxYz6nU72Qnmd7ssi6uUk0erm3DJ9DKXTx0ig26qBD8J3gZMze6YMNSGL5qnD~n1lBH1-ojRuaENjd5YYMxmelPeO2~cj5TJvm86QailSmjsI6g~hFKbd49TNdW56Bfu0ez0rUC8SjgeCzTMFd-CkoEOXOlMgMx6iYbNDgdHsePS8Pte-Itz155lzz8w7IveAz-DngU~RtJH7TuN7YGDca2fqIqauEKaVbpLfbN74nh126qiq5CyBPFnI2ZuZVRHl-fUut4OB~3TYyPYAjeoPCIivPHXZNA__&Key-Pair-Id=APKAIE5G5CRDK6RD3PGA)
(a) Cross-section view of the structure of the water-based PVT module. (b–e) Four arrangements of PVT modules. [ 25 ] (APPLICABLE SOCIETY COPYRIGHT OWNER)
![solar energy harvesting research papers (a) Conductive layer. (b) Solar cell unit. (c) Structure of the building-integrated PVT module. [27] (APPLICABLE SOCIETY COPYRIGHT OWNER)](https://oup.silverchair-cdn.com/oup/backfile/Content_public/Journal/ooenergy/1/10.1093_ooenergy_oiab004/24/m_oiab004f5.jpeg?Expires=1714710160&Signature=gfrBFus4zXohIvpXBSwjBnfGmIqLeP8RAYkcQc455y21sKmgrAE~RvQmFH4kng5sT1nX~UF21qUZ4KBl2z9F7H8w-rzBnYF4-~e~eK3XgK60fVdU4MFI6oe4-eqpTM3B9gM775rztDjy80r8JvMTogmO6tZwmBHKN4y1YfX2z1jPF8~tG0ErstBL-BNmsGQNDYs~49539o-yTdrVhWLvGweRe4TjSUQKrAltCpn~ZOJ6w-XLSDg2vE~SKtw6d7-OSKG-cFrZQy943vkRFY56nYQk4wg5-tchP8tpsIwrCHR7pvfWvnzUmvZllMzZa9MRvcUiNcVRBMEF~zKmqD9rDA__&Key-Pair-Id=APKAIE5G5CRDK6RD3PGA)
(a) Conductive layer. (b) Solar cell unit. (c) Structure of the building-integrated PVT module. [ 27 ] (APPLICABLE SOCIETY COPYRIGHT OWNER)
![solar energy harvesting research papers Mini-channel PVT module. [28] (APPLICABLE SOCIETY COPYRIGHT OWNER)](https://oup.silverchair-cdn.com/oup/backfile/Content_public/Journal/ooenergy/1/10.1093_ooenergy_oiab004/24/m_oiab004f6.jpeg?Expires=1714710160&Signature=0Agtl4vXgbTPdGIE2GlK5LGer~6JJS1-w2Sk5NmQsmk~PDM8yrSqZEXtqB-OwvUkA26mFuJ251SQOOzDKjtGfqb0aiWqnNegKlaqfMbCf8hOgzMx1DuuB7iUUfHubhzBvt4zRQk3KWN5DpTYtSXFPhIkQBSyKA1PjbBKEuiPoArqneBM8S3YUxLIdIjng24sN6keSzaKXVpmUMsRqXirFbI9wLpSHWW3e1IQZlb2kGHtwnNq5wP9rZ4RGati6X9XpLV10M-IubreSRXjH8dbCMhwmrOpTI~qn8N33Uo7HeTc2L5TzVW3ba2W-zuCFXuCTsnpjcg4XVd~DuZAJFJQjw__&Key-Pair-Id=APKAIE5G5CRDK6RD3PGA)
Mini-channel PVT module. [ 28 ] (APPLICABLE SOCIETY COPYRIGHT OWNER)
![solar energy harvesting research papers The roll-bond panel-based water type PVT module. [29] (APPLICABLE SOCIETY COPYRIGHT OWNER)](https://oup.silverchair-cdn.com/oup/backfile/Content_public/Journal/ooenergy/1/10.1093_ooenergy_oiab004/24/m_oiab004f7.jpeg?Expires=1714710160&Signature=AJjbxYOWsdKzGJUrw5Z368mYe1D0wdsj-koQ1Jo71fLprAqJGba14fR-nAAyMSA2G98CsX2Yz2OfcMSN7H4vBtMGZLqD1R0cTZpW~Hl~PorXoIKcMoKqNql7xz33qM~x9ZEWx1kTvA1Id96obW90abBJCcD2rfwBX0v3JY3965mtS4owc8o0ISqqDaDktOfuJsMP0sK~-0Itx0NnOYyvbB7m4dndlH7NPBTpQAF548CFCgyKCJAQB~3XcXttdxTCWr2jDion--KG-Jnm7mHZgqdrrNcxOwVhnfFJainMInj4AKpJxgavHwLEuQhWQzye16oUIiOsd5fVAvFIa72Ung__&Key-Pair-Id=APKAIE5G5CRDK6RD3PGA)
The roll-bond panel-based water type PVT module. [ 29 ] (APPLICABLE SOCIETY COPYRIGHT OWNER)
![solar energy harvesting research papers (a) Schematic diagram of the three systems. (b) Photograph of the three systems. [30] (APPLICABLE SOCIETY COPYRIGHT OWNER)](https://oup.silverchair-cdn.com/oup/backfile/Content_public/Journal/ooenergy/1/10.1093_ooenergy_oiab004/24/m_oiab004f8.jpeg?Expires=1714710160&Signature=rboTd2oCDLyBlRot2RKgmhxGUA8xVQFBLMmE9zFNvhjPbSX87fp10UUN6OwtLyr2i2bNXjBkiJtAEJ55u1NOaMZNzTUBkioAzbSKHxloehraL5hokzPa1JTbVYP4O8-dZvJDCTArqSy-te1kHgzwPbFGAj8pal1DQBsIAqZH9nNQ81aWvWK-H0MGokR~-GRWo31GMP3YEkkwdEIhUBSQPbP8rEDeCNZfUyUpqDW9eD6tNlluFKPHhHrNEKc6Z2IUMiXC11ixIZFdYTM9cQOGY52FuS-5zfVl6nIl3yvLOX32BoTXxpIrezQcb2G3ptwK1Z~71w3ns9bb2eLhVpO~lw__&Key-Pair-Id=APKAIE5G5CRDK6RD3PGA)
(a) Schematic diagram of the three systems. (b) Photograph of the three systems. [ 30 ] (APPLICABLE SOCIETY COPYRIGHT OWNER)
Summary of water-based PVT systems
Liquid-based PVT
Water (including antifreeze), nanofluids and heat transfer oil are four working mediums of the liquid-based PVT module. These four cooling fluids have different characteristics and applications, thus, the structures of the liquid-based PVT would be diversified.
Water-based PVT module
The water-based PVT module uses water as the working medium, and water performs better in the heat transfer characteristic than air due to its large heat capacity [ 14 ]. However, the sealing requirements would be improved, which lead to an increasing system cost [ 15 ]. In addition, the usage of pure water would cause the freezing pipe bursting problem in cold climate regions [ 16 ]. In this regard, antifreeze (a solution of water and ethylene glycol) could be adopted to avoid the freezing problem [ 17 – 19 ]. Numerous researchers have proposed various structures of the water-based PVT module and their corresponding mathematical models to improve the thermal and electrical efficiencies [ 20 – 22 ].
The simplest structure of the water-based thermal collector is the sheet-and-tube type, the tube (copper tube is commonly used) is welded in the absorbing plate. Then, the sheet-and-tube collector is attached to the backside of the PV module to form the water-based PVT module. Bakker et al . [ 23 ] applied this kind of cooling component to improve the heat transfer between the solar cells and water. As shown in Fig. 3 , they installed 25 m 2 of water-based PVT module on the top of the roof for comparative experiments with the conventional PV module and solar collector. The experimental results indicated that this kind of system could meet the electricity and hot water demand of a single-family in Dutch. In terms of this kind of water-based PVT module, Vokas et al . [ 24 ] proposed a theoretical model to predict thermal and electrical performance. For a building with an installed area of 30 m 2 , the solar fraction of the PVT module could reach 47.79%. However, the thermal efficiency of the PVT module is lower than the conventional solar collector by 9%. Moreover, they investigated the payback period of the system is around 4.6 years.
![solar energy harvesting research papers (a) Linear-type fluid channel. (b) Spiral-type fluid channel. [46] (APPLICABLE SOCIETY COPYRIGHT OWNER)](https://oup.silverchair-cdn.com/oup/backfile/Content_public/Journal/ooenergy/1/10.1093_ooenergy_oiab004/24/m_oiab004f9.jpeg?Expires=1714710160&Signature=vOI0MCn5A-U792uYzaEPZeQ~ZcWjsmOal4-kNwhaA4u1UkCVW96A7a-F46psIC9I9xT1ZyrLnxRLfgzRnms17JnyXMjoNkGYijIrf2kqJj8RS8c7dLuRGfscVScnv6vsuNDPJnTLks6btQxFiWkazgSI3d2OqPfqGl2HUlBR6Wrz4SdkbbZfEh4QoanMiwTda5E-IHiqb7J2plPAwTAmx3268eXiDtY2r8s~2eKpCS7yKEgMrjeyob2gURhk6-l116OrGm4XVkwrwt5xKPnI4tq-h~5sAmXZBjUzxF57sLiTWdeEkHhftLmkTb-TTezSM1pCmDRs9KKpK3UO-mU0jw__&Key-Pair-Id=APKAIE5G5CRDK6RD3PGA)
(a) Linear-type fluid channel. (b) Spiral-type fluid channel. [ 46 ] (APPLICABLE SOCIETY COPYRIGHT OWNER)
![solar energy harvesting research papers Photograph of the PVT module, which uses copper oxide as nanoparticles. [48] (APPLICABLE SOCIETY COPYRIGHT OWNER)](https://oup.silverchair-cdn.com/oup/backfile/Content_public/Journal/ooenergy/1/10.1093_ooenergy_oiab004/24/m_oiab004f10.jpeg?Expires=1714710160&Signature=BRsgyMVWprqUMX7ATRBMuetVGgOaz6dCCHsmwfeuYBOhpBvcTM~4VIaZ3r5xJdh4ZUUGVJpgRqfsc3fLqT7a2IuYnh~dnAYJpTXm6ji3N9kLSyYjiwXibu~R0HYHl8MDG9TXoJLQ6Nh3f13LPKckGzZbnlPgcSRp2JzLVMD2Q68WSgfNI84bPB6Y03bhPOrmBLeEOeJJBZXjrkwOloxvk9nxmJ6WlKWzyeBaBpZEUcDMuXErVqZU2SGCPKcyMnaQwXpkUHHxnmZBJpDZAnZ83wduDAcgP~Ry6yZjx-QUB31-n8DnKn4CPCJXNT~BVKPMQO9-NLIZfGPtUcsbvS92JQ__&Key-Pair-Id=APKAIE5G5CRDK6RD3PGA)
Photograph of the PVT module, which uses copper oxide as nanoparticles. [ 48 ] (APPLICABLE SOCIETY COPYRIGHT OWNER)
The water-based PVT modules are commonly set in serial, different arrangements would influence the hydraulic, thermal and electrical performance of the system. Therefore, Dubey et al . [ 25 ] have done research about the arrangement style and fill factor of the PVT module. The structure of the PVT module and four different forms of PVT module arrangement are shown in Fig. 4 . The results show that the b-form arrangement is more suitable for the family that takes heat as the first demand while the levelized cost of heat is the lowest among the four arrangement forms. In the country, the e-form arrangement is more preferable for the family, which takes electricity as the first demand. This kind of system is applicable for the suburban and rural areas where both heat and electricity are needed. Based on the above system, Mishra et al . [ 26 ] analysed the energy conversion efficiency and exergy efficiency of the system under the constant water tank temperature mode. The simulation results showed that the annual average exergy efficiency of the system using the e-form is 39.16% higher than that of the traditional flat collector system.
Chen et al . [ 27 ] assembled a lab-scale sheet-and-tube PVT module, and its structure is shown in Fig. 5 . During the test of the components, it is found that higher irradiation conditions and greater mass flow of circulating water could improve the power generation efficiency. However, the heat collection efficiency of 620 W/m 2 solar radiation intensity is 4–8% higher than that of 1000 W/m 2 solar radiation intensity under different mass flow rates of circulating water. The highest power generation efficiency of the module is 15.82%, when the irradiation intensity is 1000 W/m 2 , the corresponding heat collection efficiency is 59.41%.
The mini-channel thermal collector is another kind of structure for the PVT module, and the mini-channel could enlarge the heat transfer area remarkably and thereby increase the thermal efficiency of the PVT module. Zhou et al . [ 28 ] evaluated the comprehensive performance of the mini-channel PVT module (as shown in Fig. 6 ) in summer mode. The experimental and simulated electrical efficiencies are 11.5% and 12.6%, respectively, while the thermal efficiencies are 46.8% and 48%, respectively. The outlet water temperature of the experiments could reach 59.3°C.
The demerit of the sheet-and-tube structure of the water-based PVT module is that the heat transfer area is limited due to the welding process. The low thermal conductive coefficient would have an adverse effect on the thermal performance of the water-based PVT module. Therefore, the heat transfer area between the working fluid and the absorbing plate should be extended. In this regard, the roll-bond thermal collector has been used in the PVT module for a higher heat transfer coefficient.
From this aspect, Aste et al . [ 29 ] proposed a roll-bond panel-based PVT module to improve the thermal efficiency of the water-based PVT module. As shown in Fig. 7 , the area of the PVT module is 1.62 m 2 , while the pump power is 230 W. The roll-bond panel has larger flow resistance than the sheet-and-tube PVT module. The experimental results indicated that the electrical efficiency of the PVT module is around 12.8–13.5% while the thermal efficiency is around 20.8–32.9%. The temperature uniformity of the water-based PVT module is not well, and in this case, the maximum temperature difference reached 30°C.
The operation of a traditional water-based PVT system needs a water pump to circulate the working fluid. The pump power is determined by the flow resistance of the fluid channel in the whole system, and it would be a significant power consumption of the PVT system. Thus, some researchers have proposed the PVT system without a water pump, the system has a natural circulation of water driven by gravity and density difference. As shown in Fig. 8 , He et al . [ 30 ] comparatively conducted experiments to evaluate the performance of conventional flat plate solar collectors, PV modules and the PVT module. The experimental results showed that the thermal efficiency of the PVT module is slightly lower than that of a conventional flat plate solar collector, and the total efficiency is much higher than that of a flat plate collector and single PV module (the type of single PV module is the same as the PVT module). The natural circulation PVT water collector has no moving parts and no power consumption. Its thermal efficiency fluctuates between 38% and 43.51% and its electrical efficiency fluctuates between 9.01% and 12.51%.
The summary of the water-based PVT systems has shown in Table 1 .
Nanofluids-based PVT module
The nanofluids have an outstanding heat transfer coefficient compared with water, which would improve the thermal efficiency of the PVT module significantly. The nanoparticles below 100 nm are added to the base fluid to form the nanofluids. It was firstly proposed by Choi et al . [ 39 ] and the characteristics of nanofluids have been studied and developed in the past decades. It was found that the type of nanoparticles [ 40 ], the volume fraction of nanoparticles [ 41 ], the particle size [ 42 ], the type of base liquid [ 43 ] and other factors [ 44 ] would influence the performance of the nanofluids to varying degrees [ 45 ].
Karami et al . [ 46 ] used the boehmite nanofluids to cool the solar cells and they designed two different fluid channel patterns (as shown in Fig. 9 ) to enhance the thermal efficiency. The average temperature of linear and spiral type decreases by 39.70% and 53.76%, respectively, when the nano-fluid concentration is 0.1 wt%. For linear and helical channels, the efficiency of the nanofluidic cooled PV modules increased by 20.57% and 37.67%, respectively. Sardarabadi et al . [ 47 ] found that the total energy efficiency of 1 wt% nanoparticles in the nanofluid increased by 3.6%, and that of 3 wt% nanoparticles increased by 7.9%. The thermal efficiency of 1 wt% and 3 wt% nanofluids increased by 7.6% and 12.8%, respectively. The total exergy energy in the PVT module of pure water, silicon/water nanofluids (1 wt%) and silicon/water nanofluids (3 wt%) were increased by 19.36%, 22.61% and 24.31%, respectively.
![solar energy harvesting research papers (a) Schematic diagram of the system. (b) Cross-section view of the magnetic nanofluids-based PVT module. [49] (APPLICABLE SOCIETY COPYRIGHT OWNER)](https://oup.silverchair-cdn.com/oup/backfile/Content_public/Journal/ooenergy/1/10.1093_ooenergy_oiab004/24/m_oiab004f11.jpeg?Expires=1714710160&Signature=xGAqg6EJ5TeDGuNv6Dk3qAk9A4DJm791TM2HP4lIfiShSGg2MnXjMvK-B3IP0wu0jp8bIElARLasSlIK8-SD8txpvzQFQ0K3l8x0Kn-xLkRygCmceLmt3uVxdpGcqFzr5eYemA6HjLtE0W8kN26tHJUWY7sYU~Rc0wonw4k09hgrDvZW5qmJv9ErbCnU1v-D3VrNSA76cclSrnM4dLE1wOkG1OflKgYlkHhKz7FvWu6LxgW0pzGbPPHoNxzYjmSTZzfHzZVdN51bCdUYSFw1H8tEcQpxiadoX~HeiabPjIay4~XBYtdySnYuZZLO7T9~NsY2xm6NE~I7vRfuKuwACg__&Key-Pair-Id=APKAIE5G5CRDK6RD3PGA)
(a) Schematic diagram of the system. (b) Cross-section view of the magnetic nanofluids-based PVT module. [ 49 ] (APPLICABLE SOCIETY COPYRIGHT OWNER)
![solar energy harvesting research papers (a) Photograph of the nanofluids-based PVT system. (b) Schematic diagram of the nanofluids-based PVT system. [50] (APPLICABLE SOCIETY COPYRIGHT OWNER)](https://oup.silverchair-cdn.com/oup/backfile/Content_public/Journal/ooenergy/1/10.1093_ooenergy_oiab004/24/m_oiab004f12.jpeg?Expires=1714710160&Signature=w4ViOQGWhX4r14Mn6-GqgdB8hVUJt5ZsluD1gCtlBNt-ijC3ix1LkIBbVNV0iK-rBbcPjAPxylNS~CP8R50UHs5d2-Ttuis86Hc3Vmr66p5u-lCI4S34g2ppfU-Un5yAeQai~S7BSLRoCdT5mJz4nakTJZ-WomP22UBeAAvXbQxYJWLHbDzyStW6Jvo7ymExdPnvkJVmfgSzjFjdQBl1bjeVCcqo2HWoR7zq2Oy2m1Ql-9J649LnOkMKGYVsSm3K0sQKpaNEucKPXiCZvAXGc6PYV3WtYM3W9tBgWZoIMU1dCqrNWC0boiXITjKJ6s5SqdKEhWKFpKYIYcdf5ouhqw__&Key-Pair-Id=APKAIE5G5CRDK6RD3PGA)
(a) Photograph of the nanofluids-based PVT system. (b) Schematic diagram of the nanofluids-based PVT system. [ 50 ] (APPLICABLE SOCIETY COPYRIGHT OWNER)
Michael et al . [ 48 ] directly embedded the absorber plate beneath the PV module to reduce the thermal conductive resistance between the solar cells and working fluid. The experimental rig is shown in Fig. 10 . They synthesized copper oxide nanoparticles and prepared copper oxide/water nanofluids with a volume concentration of 0.05%, and it could effectively reduce the temperature by 13.82%. The thermal efficiency is increased by 45% by using nanofluids as heat transfer fluid. The temperature of the PV module increases due to the high thermal conductivity of nanofluids. Thus, the output power and electrical efficiency of the PVT system decrease under high working temperatures.
Except for the traditional nanoparticles, Ghadiri et al . [ 49 ] proposed a magnetic nanoparticle (Fe 3 O 4 )-based nanofluid. The magnetic fluid could be magnetized by applying a magnetic field to the working fluid. The experimental setup has shown in Fig. 11 . The effect of magnetic nanofluids on the overall efficiency of the PVT system was studied by placing magnetic nanofluids under constant and variable magnetic fields. The overall efficiency of the PVT system could be increased by 50% and reach 45% when adding 3% ferric oxide compared with distilled water as the cooling liquid phase under the same conditions (50 Hz alternating frequency).
Rejeb et al . [ 50 ] numerically and experimentally investigated the thermoelectric properties of nanofluids-based PVT modules with sheet-and-tube structure collectors. The experimental rig and schematic diagrams have shown in Fig. 12 . They studied the combination of alumina and copper nanoparticles with water and glycol as the base solution. The effects of 0.1, 0.2 and 0.4 wt% concentration of nanoparticles on the performance of the PVT system were studied. It is found that the thermal and electrical properties of PVT modules are improved with the increase of the concentration of nanoparticles, and the performance of water-based nanofluids is better than that of ethylene glycol nanofluids.
![solar energy harvesting research papers Structure of the sheet-and-tube PVT module. [51] (APPLICABLE SOCIETY COPYRIGHT OWNER)](https://oup.silverchair-cdn.com/oup/backfile/Content_public/Journal/ooenergy/1/10.1093_ooenergy_oiab004/24/m_oiab004f13.jpeg?Expires=1714710160&Signature=gDr9Re1AZVlNhNxrfVKpd5BQORm5b0-FkLwkNP45KxMA47i5Vgj1t5MC8~9Ksjm1fCxouTdvJQlg3yLuovh78tnyjn2vRf61e13QLD2EgEUVTpaC66zlQSLS5~exbQwG5XDJI9892JhfYDNN6tVrUVAG1v4X~vvveSBbt6YjU3sMuhtxWFR7Tia82QQ-HOHblfQlg8sNXeyao-pGeeRREpE4KuZtw-QKBpPk3fjV0efTknX~QxgGGUG0qfnzRdduQNzRfm5GrPaSyqXHB3ANf51geIOf1ErKaHBFN3NPYiVtH6jyvLuVTrKc1EoJra2AO4Yrq7kv6tC8AI8mNC3RvQ__&Key-Pair-Id=APKAIE5G5CRDK6RD3PGA)
Structure of the sheet-and-tube PVT module. [ 51 ] (APPLICABLE SOCIETY COPYRIGHT OWNER)
![solar energy harvesting research papers Structure of the concentrating PVT module with directly cooling. [57] (APPLICABLE SOCIETY COPYRIGHT OWNER)](https://oup.silverchair-cdn.com/oup/backfile/Content_public/Journal/ooenergy/1/10.1093_ooenergy_oiab004/24/m_oiab004f14.jpeg?Expires=1714710160&Signature=mhANG8~gm0eVGzs7-aJD9-EHfp4Ye-uvNr3DiQySEiB8hofBx5kE3uvs0B~6UwCZvv6xLNMZmfyCwgqstr3Bx5jm4-C1gAQUBIUFij9iQNBN7XlBJ8~u4MZlTvTHY0OyltgZf0XUJI5TraIW8bysOBD8BXWP77opa5Z4D34xaFQQpVFHESEnzxv8ZcmF~SFeFAQn3LYj2djjMXHXFcEfo6cCsKsR83A9t3iJ1ozyMrX9stkkvqtzHNJYnshl2hRv~pscUL2DMx0pVAYE4w1RBXBcesHIVjTEfNbbyLp2ILaRjCPeKnR3Tpe5Pq-jaEHVyMMM3FInMcz2gpAZTt~SCA__&Key-Pair-Id=APKAIE5G5CRDK6RD3PGA)
Structure of the concentrating PVT module with directly cooling. [ 57 ] (APPLICABLE SOCIETY COPYRIGHT OWNER)
![solar energy harvesting research papers Photograph of the concentrating PVT module with directly cooling. [58] (APPLICABLE SOCIETY COPYRIGHT OWNER)](https://oup.silverchair-cdn.com/oup/backfile/Content_public/Journal/ooenergy/1/10.1093_ooenergy_oiab004/24/m_oiab004f15.jpeg?Expires=1714710160&Signature=qfptcKaPu5rZcmtsUVGfr18oHzNRlF29gtguVWAGsUWh-TgYzqA-smhL7ErPY~-cqC5PRRAIvsQCjMfOS-fMS2rwludpRcz1QyaI9oVC7e9IuOTawQdCvc-3lec7PXfeXAIexEo4jir07OsPoykbTrOSlOxWSO7bbu-XnMvvthpBkyY4El-h2NKslUx1DURCtm1DuEjjurP8E7YMLIStSXk8VHhYh5jvl4VVhP6E0xMNBZtN9V~S2POJ5DGHrBWXfmDSpYFB4h4YB~JMZcc~-Fq-Elr~2AfG6pr3zRzfqqvisYvTuvvUxPZXiJkOKkhPurEN756DI5jKtvfqyuNmbg__&Key-Pair-Id=APKAIE5G5CRDK6RD3PGA)
Photograph of the concentrating PVT module with directly cooling. [ 58 ] (APPLICABLE SOCIETY COPYRIGHT OWNER)

The simulation system of the heat transfer oil-based PVT module. (APPLICABLE SOCIETY COPYRIGHT OWNER)
Ag and Al 2 O 3 are other options to form the nanofluids, thus, Khanjari et al . [ 51 ] studied the performance of the Ag/water and Al 2 O3/water nanofluids. They investigated the effects of volume concentration of nanoparticles and inlet flow rate on efficiency and heat transfer enhancement. Figure 13 shows the structure of the sheet-and-tube PVT module. The results showed that the efficiency and heat transfer coefficient increase with the increase of the volume concentration of nanoparticles. Al 2 O 3 /water nanofluids and Ag/water nanofluids have the maximum enhancement of heat transfer coefficient of 12% and 43%, respectively. The heat transfer performance of Al 2 O 3 /water nanofluids and Ag/water nanofluids is 8–10% and 28–45% higher than that of pure water, respectively, when the volume fraction is 5%.
![solar energy harvesting research papers Surface morphology of synthesized MXene (Ti3C2). [60] (APPLICABLE SOCIETY COPYRIGHT OWNER)](https://oup.silverchair-cdn.com/oup/backfile/Content_public/Journal/ooenergy/1/10.1093_ooenergy_oiab004/24/m_oiab004f17.jpeg?Expires=1714710160&Signature=eqIVXOoW1BhO9H8GU1MkMJQJ67bDAi4GaxH4rHcIcIjk~6ArCgaRtlIf9mhhHYE2w5ALVi~5-0dHv-CIgm8OT8zczPv1CYribS4H2TLrgpYvuF0FIrp0YoVIouaxGcOjAnlZH2VU3D6Rnab5i1XFfacu9zlSON68tE5SQPRzNDVyedVvhDOb1~Eg4AOhsxy8VH8ev99Pxb5Eyr9Q9avPYmucbs0U-~G5-H7upx7HPR-SCnPVolsK7sZRyYxY2CbCHRuSVXoqAoK6nZapGUFoymzk9wut4LDVHs0Ykrob3g9GBkDveBmh~qlOssyHBKgHb0H8wsdcsgCooluhLd5N1g__&Key-Pair-Id=APKAIE5G5CRDK6RD3PGA)
Surface morphology of synthesized MXene (Ti3C2). [ 60 ] (APPLICABLE SOCIETY COPYRIGHT OWNER)
Table 2 summarizes the specific parameters of the nanofluids-based PVT system.
Summary of nanofluids-based PVT systems
Heat transfer oil-based PVT module
The heat transfer oil is another kind of working medium of the liquid-based PVT module. It is not commonly used as the cooling fluid of the PVT module due to its poor hydraulic behavior [ 55 , 56 ]. Notwithstanding, the heat transfer oil has its own merits including a wide working temperature range and low electric conductivity. Thus, the heat transfer oil-based PVT module is more suitable for the concentrating PVT module.
For instance, Ji et al . [ 57 ] proposed a transmissive concentrator PVT module that uses silicone oil to cool the solar cells and further realize solar cogeneration. As shown in Fig. 14 , the concentrating system would increase the temperature of solar cells significantly, thus, the conventional heat transfer fluid is not suitable. The heat transfer oil (silicon oil which used in this study) could stay steady when it absorbs heat from the high-temperature solar cells (around 119°C). The maximum temperature of the thermal receiver could reach 180°C and this kind of system could utilize 86.1% of the solar irradiation. Moreover, Codd et al . [ 58 ] experimentally tested the performance of the concentrating PVT module with directly cooling silicon oil (as shown in Fig. 15 ), and the results indicated that the total efficiency could reach 85.1% ± 3%, and 138 W of electrical power at 304 suns.
![solar energy harvesting research papers Schematic diagram of the typical CPVT system based on the silicon oil/MXene fluid. [61] (APPLICABLE SOCIETY COPYRIGHT OWNER)](https://oup.silverchair-cdn.com/oup/backfile/Content_public/Journal/ooenergy/1/10.1093_ooenergy_oiab004/24/m_oiab004f18.jpeg?Expires=1714710160&Signature=5Pj9RqBLPPwuLoxyN0gdZMUsWkIjZ7I4-f7jEbd5DUyGWnZAI-rB3H5MhLndnepxvZFqYVtyuqaGbqVCtv2lHRlBooAVFUhEoEQ5kUJcHTCQE5HFsaZ1fdcECNAlfRCa-zIgCFSUr21mGXkSrfirDcecMi0qdkrRP7ea91n8NdONUPv5nECu2NnTmKBWiKUoYFrxMRsiKaKuk8z7hvbMI---1wzrQ17vzXcoE4gIl2LVwoPUzRETHZj24Djj-pS6feiaoq1ApH-DsY8Cr~Xl00XUNiORrYj0cxf37KFOoElyIpMieUjXSJDPnF3DabFmfTg8ou-OPyiArwmpbnwo6Q__&Key-Pair-Id=APKAIE5G5CRDK6RD3PGA)
Schematic diagram of the typical CPVT system based on the silicon oil/MXene fluid. [ 61 ] (APPLICABLE SOCIETY COPYRIGHT OWNER)
Summary of heat transfer oil-based PVT systems
Samylingam et al . [ 59 ] proposed another heat transfer fluid based on olein palm oil (OPO), which could improve the thermal conductivity significantly compared to pure water (as shown in Fig. 16 ). They further added the MXene (Ti 3 C 2 ) into the OPO to enhance the heat transfer coefficient of the working fluid. It was found that the heat transfer coefficient could increase by 9% of the MXene-OPO fluid compared with the MXene-water fluid. Moreover, the employment of the novel heat transfer fluid could reduce the working temperature of the solar cells by 40% compared with the single PV module (the type of single PV module is the same as the PVT module).
Based on the abovementioned system, Rubbi et al . [ 60 ] optimized the performance of the PVT module through the Soybean oil/MXene heat transfer fluid. The CFD model was established to evaluate the thermal and electrical efficiencies of the novel PVT module. The thermal conductivity of the Soybean oil/MXene could be improved by 60.82% compared with the pure Soybean oil. The remarkable thermal performance of the heat transfer oil could decrease the working temperature of the solar cells and increase the electrical efficiency of the solar cells by 15.44% compared with the water/alumina fluid. The structure of the MXene particle is shown in Fig. 17 . Aslfattahi et al . [ 61 ] experimentally investigated the thermal and electrical performance of the Silicon oil/MXene-based PVT module. Figure 18 shows the schematic diagram of this system, they found that the maximum electrical efficiency could reach 17.8% while the thermal efficiency is 60%.
Table 3 presents the summary of the heat transfer oil-based PVT module.
![solar energy harvesting research papers Various structures of the air-based PVT module. [62] (APPLICABLE SOCIETY COPYRIGHT OWNER)](https://oup.silverchair-cdn.com/oup/backfile/Content_public/Journal/ooenergy/1/10.1093_ooenergy_oiab004/24/m_oiab004f19.jpeg?Expires=1714710160&Signature=s9z66R3vkQuuktXfY9oNDRHoBoFPwHh-mpmQNrwBfbUI9NCtqK5T82w~QgH1eJWTzd5zAKpgxT4b7h2qwFA6HFaP3F2deYl~JWJhYz6VgcsJKMqsHIHO90MxrtOZHTckASywfCy91-Z93VOvf6-ov7AufHai5xsvqRm5TGEaXcgbR5WXQv~4bBn4gtOY5xzwdUqWyMd9s8YFbu4LysbtpNUzHeVHbg5u8TYdS70QVeOVVFaHPfLUXvejVuM2wWbmIIwG7WMjeyzgQjxsSWni~CMAxy8SqHO-qK-vT1PoHU0QQaMwpKSKc1lB2xKY7qyAZTDY8f7Q7ZHfnihaS8BfGg__&Key-Pair-Id=APKAIE5G5CRDK6RD3PGA)
Various structures of the air-based PVT module. [ 62 ] (APPLICABLE SOCIETY COPYRIGHT OWNER)
![solar energy harvesting research papers (a) Cross-section view of the V-shape air-based PVT module. (b) Photograph of the V-shape fluid channel. [63] (APPLICABLE SOCIETY COPYRIGHT OWNER)](https://oup.silverchair-cdn.com/oup/backfile/Content_public/Journal/ooenergy/1/10.1093_ooenergy_oiab004/24/m_oiab004f20.jpeg?Expires=1714710160&Signature=pq7M3EW2hr1l2r7m0knXAMhHbq8y6sDdDkEg1nX2OEMN~iV2jSqKh~zgJHDb5J1IM7uy6TSqzYY24whoaNf2B0xtqBU8PIyo2~3rkshOz3SjyGKpCwWAzAEwUb5a19QS7btoC058UDfSx9aXQ73Gaqs1CvhuEb28ubkyTZIPtTVbbUGRkPTcWemyBV9f3knx05V26mmdBeO3~GJVcBgUGw4p3T~kZbFlIiL4aixMtkL4tolzg5Wf8n8nTl-qpqZ9zvzLzhUKxdSOuCJ-k6GC7lPRfqhhq9C96Ro1fshEcKhZlU6mjE8iZ42i~nuiELx-UfxwIeU1wPLYGCwrUcOhEA__&Key-Pair-Id=APKAIE5G5CRDK6RD3PGA)
(a) Cross-section view of the V-shape air-based PVT module. (b) Photograph of the V-shape fluid channel. [ 63 ] (APPLICABLE SOCIETY COPYRIGHT OWNER)
![solar energy harvesting research papers Four kinds of air-based bifacial PVT modules. [64] (APPLICABLE SOCIETY COPYRIGHT OWNER)](https://oup.silverchair-cdn.com/oup/backfile/Content_public/Journal/ooenergy/1/10.1093_ooenergy_oiab004/24/m_oiab004f21.jpeg?Expires=1714710160&Signature=K0AWkeWFkAVjGpd-OC~MgLL7G41sapd9lbHrRpCHO2JOEgPmtfRwrva4ReaonfaKm-3FRm4Ks9a4GozAj-xKFyiImPfMrCpkd1OLicr0g13UQtzrI20zS0qRGO9nZj2kUxC9OMSpWxcG03-M0oeYBoekBnTKj026EGIqleh86zFZq-9pogTW--5w~pQsXquezi3pAVDNV3XusaipBTlkf91qs5kybCnWF-4Cm8fnC57e8F1AwkoA-RYqHBhteJYZ9dFzdf9BPx3i01sDhDeJKrHBon8qwInLd~CYGCOfhkYtzyp3upLUfQqSfxdavbJbbJxUaDx36YYzoPrwqVAkyg__&Key-Pair-Id=APKAIE5G5CRDK6RD3PGA)
Four kinds of air-based bifacial PVT modules. [ 64 ] (APPLICABLE SOCIETY COPYRIGHT OWNER)
![solar energy harvesting research papers (a) Novel structured air-based PVT module. (b) Experimental setup of the system. [65] (APPLICABLE SOCIETY COPYRIGHT OWNER)](https://oup.silverchair-cdn.com/oup/backfile/Content_public/Journal/ooenergy/1/10.1093_ooenergy_oiab004/24/m_oiab004f22.jpeg?Expires=1714710160&Signature=dOzRWNlYOw8s7V0DqFDl~C~QP9owC1Ma5xewFn97hAAL2DxjEtwOl~u9rP91krQ150SR4gwsSUIuKx7UJWjXXd9hY-o7kjATJBlBCIx4IVLlfU320gzm-0~nPXZPQSWyvW1RZT9nQwsg41ZERmny1a~kOUaDb846zcyRZlFIEnC0KrmoJ772Vr2swxOFWYcyIlfjU7lZzD8Baor3n-Xazu2U8uRITQr~YB~i2sBxV0ZD3-aqrlccXFFSwfUU21Dx0rzK4Dc4G0rCjl8snN4hZUYTH0ckbkIiBfntbp~pczrzFzgFUSwSWBSOgVUAkwBGW6gje1lnCEKV0FdbN96q-A__&Key-Pair-Id=APKAIE5G5CRDK6RD3PGA)
(a) Novel structured air-based PVT module. (b) Experimental setup of the system. [ 65 ] (APPLICABLE SOCIETY COPYRIGHT OWNER)
Air-based PVT module
The air is free to acquire for the air-based PVT module, and the structure of this kind of PVT module is more simple and reliable. The sealing requirement of the airflow channel is lower than that of the liquid-based PVT module’s fluid channel, thus, the air-based PVT module has the advantage in the initial cost. In addition, the low heat capacity of the air could make a remarkable temperature rise of the air during the operation. Moreover, the air-based PVT module does not have the problem of freezing the working medium compared with the liquid-based PVT module. The high-temperature air could be used for space heating, solar dryers, dehumidification, and industry process preheating, etc. Numerous studies have been conducted in terms of mathematical models, boundary conditions and structures to evaluate and improve the performance of the air-based PVT module.
Tonui et al . [ 62 ] designed different structures of the air-based PVT module as shown in Fig. 19 , including glass-covered, uncovered, the channel with fins, thin metal sheet structure, etc. They established the corresponding mathematical models of each structure and experimentally validated their correctness. The adoption of fin and thin metal sheets could efficiently increase the heat transfer area between the air and the PV module and then improve the thermal efficiency of the PVT collector. The results indicated that the arrangement of fin and the thin metal sheet would not rise the initial cost significantly, but it could improve the comprehensive thermal and electrical efficiencies remarkably.
From the aspect of structure design, Fudholi et al . [ 63 ] developed a V-shape thermal collector for air-based PVT collectors. The cross-section view of the V-shape PVT module and its photograph have shown in Fig. 20 . The numerical model was proposed and verified through the experiments. It was found that the error between the simulation and the experiments was within 5.49%. The exergy efficiency of this kind of PVT module is 12.89%.
The bifacial PV module was adopted to form the PVT module by Ooshaksaraei et al . [ 64 ] as shown in Fig. 21 and experimentally studied the performance of different structures. The experimental results showed that the comprehensive conversion efficiency of the air-based bifacial PVT module with the double-channel in the same direction is 51–67%. The single-channel air-based bifacial PVT module has a minimum conversion efficiency of 28–49%. However, due to the use of dual flow channels, the fluid on the upper surface of the PV module and the cover plate will affect the direct radiation incident to the surface of the PV module. Therefore, it is recommended to use a single-flow channel PVT air collector if electricity is preferred, and the dual-flow channel air-based bifacial PVT module in the same direction should be adopted if heat generation is preferred. In addition, the single-channel air-based bifacial PVT module has the highest exergy efficiency of 8.2–8.4%, followed by the dual-channel co-directed air-based bifacial PVT module with the exergy efficiency of 7.2–8%.
To further increase the heat transfer coefficient of the air-based PVT module, Gholampour et al . [ 65 ] designed a novel structured air-based PVT module as shown in Fig. 22 . The air enters the channel from all sides for heat exchange with the back of the PV module, and the heated air enters the outlet channel through the suction effect of the fan to take heat away. Through CFD simulation and experimental approaches, it is found that the equivalent thermal efficiency is taken as the index to obtain the optimal value of air suction velocity and PV coverage under different conditions, which provides guidance for designers to design air-based PVT modules schemes in the future.
![solar energy harvesting research papers (a) Cross-section view of the multiple inlets air-based PVT module. (b) Photograph of the system. [66] (APPLICABLE SOCIETY COPYRIGHT OWNER)](https://oup.silverchair-cdn.com/oup/backfile/Content_public/Journal/ooenergy/1/10.1093_ooenergy_oiab004/24/m_oiab004f23.jpeg?Expires=1714710160&Signature=HCMSsoXIgtRz4126jiSZ1Nan1zIRS3N~Jerup5JN5EcgxFFESe22kh1eCO~giaNCx~p-Nl4oPyUrkIqvVoIMF31JJwfCllextHYMCg7-kzVvxZRYTj4a55QAXgHZocPCd9r-PZCtHVDXSiVGSmjLpoEilg2XA8KRVHJyL21RN9Z5SZVG1JzTIGO0SkKSiAo2i08cQtCWIpEJMtRpbo9qaQCl16T8mLdUJALrp7BcTuYKZEd6qAUAlefJ03foICIDz1J1MCh~KSzT8BQUKh~rlwAYMwsUDg3zVFlEiepm-uDRjc6OwXSgaE~QB3I0CkbrStNYLO5ZKfg3zjgJpDA1qw__&Key-Pair-Id=APKAIE5G5CRDK6RD3PGA)
(a) Cross-section view of the multiple inlets air-based PVT module. (b) Photograph of the system. [ 66 ] (APPLICABLE SOCIETY COPYRIGHT OWNER)
![solar energy harvesting research papers (a) Structure of the roof-type air-based PVT module. (b) Photograph of the PVT system. [68] (APPLICABLE SOCIETY COPYRIGHT OWNER)](https://oup.silverchair-cdn.com/oup/backfile/Content_public/Journal/ooenergy/1/10.1093_ooenergy_oiab004/24/m_oiab004f24.jpeg?Expires=1714710160&Signature=UxLnV41uI~C0ZzJ4kcY45rHfUNAz3mu4Mo~yI909qAFdWYZm~qhkw7~~riGDbvdW0OcfUoUvLLsOOEibCVlXLhwd2M0xHoNW1zehtCeG38OMN0FGQJDR56KpsYaySMZoTKWRlKZtmwgXKEi9y-tM6pFEVS2WGzrDoznmo3T1BvVlLCRzIri~uL8qdOYwIZ6A7V7RrWg3968pIgE7pb5QEa842GbYIGXB13bapF3xvuB1Qqz-o7~baus9XRBlKftfdQH4V~zcyjFQsg2ZP47Afgxox0CHh1WWH7ugXlHywTb7N2Pj~mxMhn6nrOrUc-rt0Bnj2S2juidUpnlGQ1F6rg__&Key-Pair-Id=APKAIE5G5CRDK6RD3PGA)
(a) Structure of the roof-type air-based PVT module. (b) Photograph of the PVT system. [ 68 ] (APPLICABLE SOCIETY COPYRIGHT OWNER)
![solar energy harvesting research papers (a) Photograph of the building integrated roof type air-based PVT module. (b) Cross-section view of the PVT module. (c) Schematic diagram of the roof type air-based PVT module. [73] (APPLICABLE SOCIETY COPYRIGHT OWNER)](https://oup.silverchair-cdn.com/oup/backfile/Content_public/Journal/ooenergy/1/10.1093_ooenergy_oiab004/24/m_oiab004f25.jpeg?Expires=1714710160&Signature=Ogv2I3Z~ZPGV~Xo2032WkaSfvJj72kJxA3KieI0x2kobn~FHYYY5vQjwJ1Tnp8Sxz4J8bA62HAEVeVgFIV37n2i3VkJDF3MaHwWrOdLt2r-zBY0IMLgorw~Cv4M7ryib~6TIPzGIJo-rCBgAZ5xGSdwCHUWQHm0p8WHHjZThsweHTYcolKy~PMV0D1mGSqO-1QGlHo6lFilGz5GjpcOScDJ~nPG2hJCjijuS1b2vReNDGfulwf8JZVdfBSgwQkUEnKr0ZFTVlMHZiHDyBXWoB0BaL9kgKOK4DhDQh2Uef9S8Lx2mAGb5saUm5t5XaHnYB2u~5twAagDavvMb2NeZTw__&Key-Pair-Id=APKAIE5G5CRDK6RD3PGA)
(a) Photograph of the building integrated roof type air-based PVT module. (b) Cross-section view of the PVT module. (c) Schematic diagram of the roof type air-based PVT module. [ 73 ] (APPLICABLE SOCIETY COPYRIGHT OWNER)
Yang et al . [ 66 ] improved on the basis of the original PVT air collector, multiple air inlets were set up at the assembly installation place and metal mesh heat collecting parts were installed at the outlet of the air flow passage. The cross-section view of the structure and photographs have shown in Fig. 23 . The experimental results revealed that the multi-inlet and metal mesh heat collecting parts could effectively improve the thermal efficiency, but also improve the working temperature of the air. Therefore, the working temperature of the PV module would also be increased and its electrical efficiency would be reduced. Thus, power generation or heat generation should be given priority according to different demands, and then the system should be designed and optimized accordingly. In addition, they [ 67 ] further studied the transmittance of this kind of PVT module. During the experiment, they found that the dual-inlet air-based PVT module with translucent PV modules improved the thermal efficiency by 7.6% compared with the dual-inlet air-based PVT module with opaque PV modules, and the dual-inlet air-based PVT module increased the thermal efficiency by 5% compared with the single-inlet air-based PVT module.
The air-based PVT module is suitable for building integration, Tiwari et al . [ 68 ] integrated the circular PV module with the air channel to form the roof air-based PVT module, and they used the PVT module to preheat the biogas. Figure 24a shows the structure of the roof-type air-based PVT module. The surface coverage is low due to the circular PV module unit, so more solar radiation could directly heat the air in the flow channel. Figure 24b shows the experimental rig. The experimental results showed that the thermal output of roof-type air-based PVT modules equipped with 1.27 square meters of components reaches 11.18 kWh under good irradiation conditions.
Figure 25 presents another kind of building integrated roof type air-based PVT module, the air flows from downside to upside and the tilt angle is 35 degrees. In the case of an effective building area of 65 m 2 , the annual electricity generation of the system is 16 209 kWh and the heat generation is 1531 kWh, and the average thermal efficiency is 53.7%. Based on this component structure form, Bambrook et al . [ 69 ] changed the mass flow rate of inlet air through experiments to explore the optimal mass flow rate of inlet air to achieve the highest comprehensive efficiency of the system. The experimental results showed that the power generation and thermal efficiency of the system are the highest at the mass flow rate of 0.03–0.05 kg/s. In addition, the electrical efficiency of the system fluctuates between 10.6% and 12.2%, and the thermal efficiency fluctuates between 28% and 55%. In addition to optimizing the operating parameters, Farshchimonfared et al . [ 70 , 71 ] optimized and analysed the physical structure parameters of air-based PVT modules. They used modules of 10, 15, 25 and 30 m 2 with ratios of 0.5, 1, 1.5 and 2, respectively. The optimal mass flow rate to component area ratio is 0.021 kg/s, and the optimal flow height is 0.026–0.09 m when the inlet and outlet temperature rises to 10°C. In addition, Singh et al . [ 72 ] optimized the performance of air-based PVT module by genetic algorithm, and analysed the effects of runner length and width, inlet velocity, inlet temperature, glass thickness and backplane thickness on component efficiency, respectively. The maximum power generation efficiency is 14.15%, and the thermal efficiency is 49.11% when the exergy efficiency is taken as the target.
Table 4 presents the summary of air-based PVT modules.
Summary of air-based PVT modules
![solar energy harvesting research papers Cross-section view of the direct expansion PVT module. [80] (APPLICABLE SOCIETY COPYRIGHT OWNER)](https://oup.silverchair-cdn.com/oup/backfile/Content_public/Journal/ooenergy/1/10.1093_ooenergy_oiab004/24/m_oiab004f26.jpeg?Expires=1714710160&Signature=AMBgwh-OnsXE~ptVqqtKF9t~Up6-ytSLOX9Xk5xF4CfR87goArquvDlmDtXlL7HE00uxXnXD9vAEE30bVTotoUNc00eYizvhA7eyrrX~OhbwURmequTaqh59faRw-u03-sUe0qdmQJBFvQXQwDhHcnedCAQTwaG1~msMBao4MlCAaKGwNFePaHrtPUiUq8LX9uNLyaWNhPnDpM3OAWaYzjOAMrhXMmB0xNNwPw5gIq1y~1L2FY1mF4PYSSFlmdGuSCHu0RwkZKU2~9DI4cqIQmuFVeThIkh5EiX14TJtxexbkN6onm6Nh5F8CpsnY1c24217wOw-7iyL~sEZhawRWQ__&Key-Pair-Id=APKAIE5G5CRDK6RD3PGA)
Cross-section view of the direct expansion PVT module. [ 80 ] (APPLICABLE SOCIETY COPYRIGHT OWNER)
Refrigerant-based PVT module
The liquid-based PVT module and air-based PVT module apply the sensible heat working fluid to collect heat, while the refrigerant-based PVT module applies the latent heat working fluid. The cooling ability of the latent heat working fluid is better than the sensible heat working fluid due to the higher heat transfer coefficient. In this subsection, the refrigerant-based PVT module would be reviewed accordingly.
![solar energy harvesting research papers (a) Cross-section view of the triangular tube-type sheet-and-tube collector. (b) Photograph of the system. [82] (APPLICABLE SOCIETY COPYRIGHT OWNER)](https://oup.silverchair-cdn.com/oup/backfile/Content_public/Journal/ooenergy/1/10.1093_ooenergy_oiab004/24/m_oiab004f27.jpeg?Expires=1714710160&Signature=dNk38cE0wMD-xZDAlnWSngmsvthdQfyBQPdY-GHHIMi7C1u~bYPyLk9TrDeiO3mCLgsVO4aZsI~i6Hv9vaagB3J1LRjtTNY2TEPsNsTeCnpfjgG~ihaJe5XX87hkn9wh~bIa83k4NYLffFFBuTguqHANYwJEulJyDK-JPRFwbGG9sh2Rqe~s01yBiPjHx5EI~dvBzpn8HZ6Q0dalh-8USGWb7AAR7Bn~Z90G6LB-iwAi1eSNAl90aYepyJ7cTxnWOVQ~gwpMD2Wj0ymiIAUn-PNB~GfIUl75zLUamayEz5rSxODtoEkmfasqLh2xo5Bg7gnup1RVT9gPkM2CcJ0log__&Key-Pair-Id=APKAIE5G5CRDK6RD3PGA)
(a) Cross-section view of the triangular tube-type sheet-and-tube collector. (b) Photograph of the system. [ 82 ] (APPLICABLE SOCIETY COPYRIGHT OWNER)
![solar energy harvesting research papers (a) Cross-section view of the novel evacuated PVT collector/evaporator. (b) Vertical view of the component. [83] (APPLICABLE SOCIETY COPYRIGHT OWNER)](https://oup.silverchair-cdn.com/oup/backfile/Content_public/Journal/ooenergy/1/10.1093_ooenergy_oiab004/24/m_oiab004f28.jpeg?Expires=1714710160&Signature=qDUgN5a2zIhlqsSLc3RnRpB0yBuCBRGlh9ma1SSJWMbCOO9ryeCzAgJ0I2AsS5n3fLvWctQ7-S-q5wB5jKB-0anzdqb5WOL3~BQnJ3EFvxy~YFlJAX8IDHCbkyPVvAcytobNA81JJtIiHKI4djULLErx-LM6jU4-5P9I-4bulYMQDul6OpsUGP-Ix9e-kl1pxtxNV7ftODKUV7CpV5T5MD2EGbL29tRmosdXBBCVQ6HDQN1om6lLLuC5-Ff4~y6MiV5ILClQFipGe0Fo2rYdOywJAGb3uhaCjRIeqMvR7D6qhIWyGX2YqQSC1dXl-ydyQ8YHZxdBENJ2EIarxBrfFw__&Key-Pair-Id=APKAIE5G5CRDK6RD3PGA)
(a) Cross-section view of the novel evacuated PVT collector/evaporator. (b) Vertical view of the component. [ 83 ] (APPLICABLE SOCIETY COPYRIGHT OWNER)
Direct expansion PVT module
The direct expansion PVT module means that the working medium (refrigerant) directly evaporates in the fluid channel and extracts heat from the solar cells through the phase transition process. The evaporation channel is attached to the PV module to form the direct expansion PVT module. Furthermore, the direct expansion PVT module is utilized as an evaporator. The solar-assisted PVT heat pump system consists of PVT module, compressor, expansion valve, and condenser. The refrigerant absorbs heat in the PVT module and then it would be compressed to a high-temperature and high-pressure state. Afterward, the refrigerant releases the heat to the heat storage medium [water, phase change material (PCM), etc.] during the condensation process. The condensed refrigerant enters the PVT collector/evaporator after cooling and reducing pressure through the expansion valve and then evaporates again through the heat absorption to complete the whole thermodynamic cycle. The thermal efficiency of the direct expansion PVT module is improved effectively due to the evaporation of refrigerants. However, the phase change process of the refrigerant is the two-phase flow, which would lead to a complex design of the fluid channel. The high flow resistance, uneven flow distribution and uneven temperature distribution would have adversely affected the performance of the PVT module. In this regard, various experiments and simulations have been studied to attain a higher comprehensive solar energy utilization efficiency.
Ji et al . [ 80 , 81 ] developed a sheet-and-tube PVT collector/evaporator, the fluid channel tube is welded to the heat-collecting aluminum metal sheet, which collects the heat from the back of the solar cells and transfers it to the copper tube through thermal conduction. The working medium in the copper tube collects the heat through the convection heat transfer process, the cross-section view of this kind of PVT module is shown in Fig. 26 . They coupled the component with the heat pump system to form the direct expansion solar-assisted PVT heat pump system. According to the experimental results, the maximum COP of the system could reach 8.4 while the average COP is 5.4, and the average electrical efficiency of the PVT module is about 13.4%. In addition, Ji et al . [ 81 ] developed a distributed dynamic model to describe the direct expansion solar-assisted PVT heat pump system. The model could calculate the refrigerant conditions, such as pressure, temperature, quality, enthalpy, etc. under given environmental conditions including ambient temperature, irradiation intensity, wind speed, etc. The simulation results showed that the electrical efficiency is 12% and the thermal efficiency is 50%.
The circular copper tube type sheet-and-tube collector has a demerit, which is that the heat transfer area between the copper tube and the absorbing plate is small. Therefore, Mohanraj et al . [ 82 ] designed a triangular tube-type sheet-and-tube collector and compared its performance with the conventional circular copper tube. Figure 27 shows the component structure and the experimental rig. In addition to experimental tests, they also used artificial neural network algorithms to make predictions about the system. In practical tests in India, it was found that the use of triangular flow channels resulted in significant improvements in system efficiency, such as a 3–7% increase in thermal efficiency, a 3–5% increase in COP and a 4–13% increase in electrical efficiency.
![solar energy harvesting research papers Structure of the flat-box-based PVT module. [84] (APPLICABLE SOCIETY COPYRIGHT OWNER)](https://oup.silverchair-cdn.com/oup/backfile/Content_public/Journal/ooenergy/1/10.1093_ooenergy_oiab004/24/m_oiab004f29.jpeg?Expires=1714710160&Signature=nRWNWIicFVoD5z7Wxbz9H~ZpwUL4Xr4VN8uEOGwt5teB2eRT~6RIRUiDXNZeUvf2pikrVySbFfECYrXBuEJxgUPBHdqjjvMsSXQoCiKhUyEJLoYDCr933uTm55LXUtMUsmMNQHG~GTRb1hx0mgPH4bfHVRPgFuXWD318~oNCczKPrZMgLBuXTV81cODbMTXgKldc9ehTQwUcwJDk5rou2b9S6SO-pw9joz3kFoXNT1M1HOy51Kl1dsTqKoAYGoTwc3Hrw97~acarCm3IlZhEplnfeox3Sn6B52e9RGkxReO1QXdIUi5MWB9OE-T~ArAlkMwspnkv7bE2EoIZ8uwasw__&Key-Pair-Id=APKAIE5G5CRDK6RD3PGA)
Structure of the flat-box-based PVT module. [ 84 ] (APPLICABLE SOCIETY COPYRIGHT OWNER)
![solar energy harvesting research papers (a–d) Four fluid channel patterns of the roll-bond panel. (e) Cross-section view of the roll-bond panel-based PVT module. [85] (APPLICABLE SOCIETY COPYRIGHT OWNER)](https://oup.silverchair-cdn.com/oup/backfile/Content_public/Journal/ooenergy/1/10.1093_ooenergy_oiab004/24/m_oiab004f30.jpeg?Expires=1714710160&Signature=N0-ORAFCLAE-Gfe1UcZKyd~BtRByzvdyh~uNB8zMtAa3j1d-D~Fc6vhb6c05aI6sDRiLaMRQEZJvEdLM3zQ25RZpqRopPoX9BAykhXLVlOxSyn4KfXP-UXzXCHuf50QIWIizpw5aU3ar~rpmJX3Kmj7iznxNt5pE2bSkw1a8clMLGPobS-zG5sTIUV3VxfNLd~JN7wpgd3V55jPeIqAjPzSvi9y~lx9bxAmNFYU9cMjgbWaTczB-osmqUmwTIDKd79Vxdz3ULYeD24AnzqY7D~CbnzgzvD3JcaHpMzZDu95~ndFD1XTKB0LhDocq6QZR4Py2UTLLywL665lz4jiGFw__&Key-Pair-Id=APKAIE5G5CRDK6RD3PGA)
(a–d) Four fluid channel patterns of the roll-bond panel. (e) Cross-section view of the roll-bond panel-based PVT module. [ 85 ] (APPLICABLE SOCIETY COPYRIGHT OWNER)
![solar energy harvesting research papers (a) Roof-type PVT module. [87] (b) Building integrating PVT module. [88] (APPLICABLE SOCIETY COPYRIGHT OWNER)](https://oup.silverchair-cdn.com/oup/backfile/Content_public/Journal/ooenergy/1/10.1093_ooenergy_oiab004/24/m_oiab004f31.jpeg?Expires=1714710160&Signature=HxI-h95DO5Yhzx7Ys5eMdxUDXszJhxqfinECjkx5puaCZ-yzJ92kQlRAL0YcI~P2q4P796uu06vbtHpXyW~P9yz7JJknjo8cWfinQC9On0UjRE27JdT~P7AqmCA8DOXPYfXB3XUciXgi7PtBpGBG8-p3-HEUx-5yCmLbhJyT8c5dcLcz-eNtmS0J1qcJGIHoH9Ncc0S6M69ySq95cI3AV6iuDitpf21MupIs67hJnEE509cHfGffsYUu5~BJIrTeKtdDHeowr3C-phY1E6TCS4dGK56bW9GpiehxCSFxEmR1kg1kr00IymS6gDuoO2iIV45duxI0iO3AL4urQ-vg7A__&Key-Pair-Id=APKAIE5G5CRDK6RD3PGA)
(a) Roof-type PVT module. [ 87 ] (b) Building integrating PVT module. [ 88 ] (APPLICABLE SOCIETY COPYRIGHT OWNER)
![solar energy harvesting research papers (a) [93] Heat pipe-based PVT module. (b) [94] (APPLICABLE SOCIETY COPYRIGHT OWNER)](https://oup.silverchair-cdn.com/oup/backfile/Content_public/Journal/ooenergy/1/10.1093_ooenergy_oiab004/24/m_oiab004f32.jpeg?Expires=1714710160&Signature=vCetcAK6DyRA4jCLBz-Jn9EDJ6FVfbTCzit~fGgA6cqxZ-W7xcVnPRVKi5OVRd1X6p3BcWUY6NmtIjmO-O~fCFqw4PmTqZSZbNWFRzY6r5h0wbfeg-zANS1~hPoL1gVWmunlX9XLX40vZ3MOG-W7raPB3YULR1XxaH~yeam1JAJ3J0ASA0ro8RIW0zt7MpbT753Zmy8oOb8Uwn-9iyooSExdX0BU60eh3DbuNoanZ1jOVIcLq3cryyrV9z9HTuvoR5vkekdGvFtEeCHQB9cOrDeEvos1V0~MrE~n0-LjCRXB7O1y0qmHAc1Kyhgr5IJcEVfBnn4Ekc3fkE0fX0Zcfw__&Key-Pair-Id=APKAIE5G5CRDK6RD3PGA)
(a) [ 93 ] Heat pipe-based PVT module. (b) [ 94 ] (APPLICABLE SOCIETY COPYRIGHT OWNER)
![solar energy harvesting research papers Novel structured heat pipe-based PVT module. [98] (APPLICABLE SOCIETY COPYRIGHT OWNER)](https://oup.silverchair-cdn.com/oup/backfile/Content_public/Journal/ooenergy/1/10.1093_ooenergy_oiab004/24/m_oiab004f33.jpeg?Expires=1714710160&Signature=O2cSHJCb8CNL-FYoL3bLQ9bbDg~EdfmRNop~nqfVwfjP8CncBb~GGT0wqu-s03dPWQxf5SGlWusuSh9dawgN9oh5httgeo1daMEq~AckKhJN8wvijpFl1Cr~aUXHAwqm7KfOtrhrzHr67xOXv7ZTKgmEWnMMidrhoXHk0yCUFA7uFQh6YDcZC4gW~ggExWuDy3ueW1EUvnWZisjCmzdPVgqNAeZP63PBQrha-yqJiZAdM7~CSbH2xSkxjhm2bpO~s4PjovkkLe9A-r11aw3DvAaa2UYYj0VMIcncaScb~x~rOic0Gbjs1OeGA2gbqEs8ksl4wD5sPZ7pWuBSU4QtbA__&Key-Pair-Id=APKAIE5G5CRDK6RD3PGA)
Novel structured heat pipe-based PVT module. [ 98 ] (APPLICABLE SOCIETY COPYRIGHT OWNER)
Summary of direct expansion PVT modules
To further improve the thermal efficiency of the PVT module, Chen et al . [ 83 ] developed a novel structured evacuated PVT collector/evaporator as shown in Fig. 28 . They adopted it to the solar-assisted heat pump system for water heating. The simulation results indicated that the average monthly thermal efficiency is 75.2% and the electrical efficiency is 15.5%. In addition, the average COP of the system is 5.35. The performance of the component decreases slightly when the irradiation intensity is low, so this kind of structure has a better application prospect in high latitude areas.
The flat-box absorber is another structure of the thermal collector, Mastrullo et al . [ 84 ] proposed a new structure of the PVT collector/evaporator with a flat-box absorber as shown in Fig. 29 . The arranged air gap could lower the thermal loss of the PVT module and then more waste heat could be absorbed by the working fluids. The simulated highest electrical efficiency could reach 14.2% while the thermal efficiency is 52.0%. The electrical efficiency is 13.7% when the highest thermal efficiency (84.3%) is attained. The temperature of the flat-box absorber would decrease with the increase of the fill factor of the solar cell units.
Except for the sheet-and-tube type and flat-box type thermal collector, the roll-bond panel type thermal collector is a promising method to realize high efficient thermal collection. The heat transfer area between the working fluids and the absorbing plate could be enlarged significantly due to its special structure. Yao et al . [ 85 ] proposed the roll-bond panel-based PVT module with an optimized fluid channel pattern. The various fluid channel patterns of the roll-bond panel and the cross-section view of the PVT module have shown in Fig. 30 . The temperature uniformity, electrical and thermal efficiencies and hydraulic performance have been evaluated numerically and comparatively. The simulation results indicated that the temperature uniformity of the fluid channel Pattern 2 is the best with the lowest flow resistance. It was found that the optimized fluid channel pattern could reduce the solar cells’ working temperature by up to 47.3°C and increase its electrical efficiency by 46.5% on a typical summer day. In this circumstance, the average system COP is 4.37, and the average temperature difference of the PVT module could be controlled within 4.6°C, while the average electrical and thermal efficiencies could reach 16.7% and 47.6%, respectively. Furthermore, they [ 86 ] conducted experiments to evaluate the performance of the PVT module, it was found that the average electrical and thermal efficiencies could reach 17.93% and 109.4%, respectively.
From the building integrating aspect, the roll-bond PVT module could also be used as a roof. For instance, Tsai et al . [ 87 ] integrated the direct expansion PVT module to the building façade as the roof as shown in Fig. 31a . The structure of the thermal collector is the sheet-and-tube type, and the refrigerant working medium evaporates and collects heat in the tube, taking away the heat of the PV module. The experimental results showed that the thermal efficiency of the component fluctuates between 73.6% and 74.2%, while the electrical efficiency fluctuates between 12.2% and 12.5% under dynamic operating conditions. The COP of the system fluctuates between 7.07% and 7.10 and the power produced by the PV module could be used by the compressor in self-operating mode. Similarly, Shao et al . [ 88 ] also developed a building attached type direct expansion solar-assisted PVT heat pump system, using the components as building roofs to provide power and heat for the building. Figure 31b shows the building integrated PVT collector/evaporator, and the collector type is roll-bond panel [ 89 ]. The advantages of the roll-bond panel are stable performance and high thermal efficiency. According to the experimental results, the average electrical efficiency and thermal efficiency of the system are 11.67% and 60.17%, respectively, and the average COP of the system is 3.7.
Table 5 presents the summary of the direct expansion PVT module and the specific parameters of the solar-assisted PVT heat pump system.
Heat pipe-based PVT module
A heat pipe encapsulates the working fluid (refrigerant) in the tube and the cold end would absorb heat through the evaporating process while the hot end would release the heat through the condensing process. The hot side is commonly linked to the heat exchanger and transfer the heat to heat storage material (water, air, PCM, etc.). The secondary heat exchange process would mildly decrease the thermal efficiency of the system. Notwithstanding, the useful heat could be used for space heating, domestic hot water usage, residential heating, etc. Moreover, few researchers have composed the heat pipe PVT module with the thermoelectric device for higher electricity output.
As shown in Fig. 32a , Wu et al . [ 93 ] developed a core type heat pipe-based PVT module, they attached the heat pipe to the absorb plate with thermal conductive glue, and the heat absorbed by the heat pipe was transferred through secondary heat exchange to realize heat generation. They developed a solution based on the Number of Transfer Units (NTU) (heat element) method and analysed the effects of medium inlet temperature, solar cells’ fill factor and mass flow rate of the medium inlet on system performance. The simulation results showed that the temperature difference between heat pipe-based PVT module and PV module is less than 2.5°C. Its thermal efficiency, electrical efficiency and exergy efficiency are 63.65%, 8.45% and 10.26%, respectively. Gang et al . [ 94 , 95 ] adopted a heat pipe with another structure as a primary heat collector, as shown in Fig. 32b . The thermal and electrical properties of the components under dynamic conditions were analysed by the finite element method and verified by experiments. They used water as the heat collecting medium for secondary heat transfer, and the results showed that the highest thermal and electrical efficiencies of its components are 41.9% and 9.4%, respectively. In addition, the components used in the system could also be integrated to the building [ 96 ].
This kind of heat pipe-based PVT module was coupled with PCM storage by Sweidan et al . [ 97 ] for water heating. They arranged the encapsulated PCM sphere in the water storage tank while the hot side of the heat pipe was also arranged in the tank. The working liquid in the heat pipe evaporates after collecting heat from the PV module and releases heat at the condensation end to heat the hot water in the water tank. The existence of PCM could stabilize the water temperature. They simulated the optimal number of PV modules and the quality of PCMs for building heating. The simulation results showed that the highest electrical efficiency is 12.23% and the highest thermal efficiency is 35.3% in January. Moreover, the payback period of the system is 13.7 years.
Moradgholi et al . [ 98 ] developed another kind of heat pipe-based PVT module and the structure has shown in Fig. 33 . Their siphon heat pipe absorbs the waste heat generated by a monocrystalline silicon solar cell module using a phase change process inside the pipe. In spring, the working medium with methanol as the system has a slope of 30°, and in summer, the working medium with acetone as the system has a slope of 40°. The temperature of the PV modules drops by up to 15°C in the developed heat pipe-based PVT system. The experimental results showed that the electrical efficiency and thermal efficiency are increased by 5.67% and 16.35% respectively in spring, and by 7.7% and 45.14% in summer. Compared with the single PV module system (the type of single PV module is the same as the PVT module), the total efficiency is 15.3% and 44.38% higher in spring and summer, respectively.
Hu et al . [ 99 ] experimentally studied the thermal and electrical properties of components at different inclinations for the performance of heat pipe-based PVT modules with wickless and wire-meshed heat pipes. Two heat pipe-based PVT systems were tested at inclination angles of 20° and 40°, as shown in Fig. 34 . The experimental results showed that under the angle of 20°, the heat transfer resistance of the wickless heat pipe is large, so its component performance is poor. The inclination angle has little effect on the performance of the PVT system with wire-meshed heat pipe. The thermal efficiency of PVT systems based on wickless heat pipe and PVT systems based on wickless heat pipe is 52.8% and 51.5%, respectively, when the tilt angle is 40°. The efficiency of the wickless heat pipe is higher at a latitude above 20°, and the efficiency of heat pipe with the core is higher at a latitude below 20°.
![solar energy harvesting research papers (a) Wickless heat pipe. (b) Wire-meshed heat pipe. [99] (APPLICABLE SOCIETY COPYRIGHT OWNER)](https://oup.silverchair-cdn.com/oup/backfile/Content_public/Journal/ooenergy/1/10.1093_ooenergy_oiab004/24/m_oiab004f34.jpeg?Expires=1714710160&Signature=iknhgrGUfc6Li7Z~hf~h2aPntBA8vsreTc9NrXy-f0zayByMi1VcyQUGXlwQURMJ4O8MNLOImyxWXBoUcu4bbIfu1ZWejEKgPNT6DT633QxfdPmsEzRG6XCpIprOezAB60TFNpIevxzZ5m2Pu7OEpxtydSQS6eGAsaLwOrXaBF0YjIacEHFKIkYz8DepVVJqAuWI8AGB~ZjAYu4xQj29oyxUUM0~Ibk4VcfE~TZ67dDQzR0VabFsusfhu4mVzm-YWx4qFcSi3CN3jGAMddWkgBmUASmswI1w2TR9K11HyzZ2EWVVQA1sTnpw4hNYVGb7saJvJq7Tox3Q78CZvaNCQQ__&Key-Pair-Id=APKAIE5G5CRDK6RD3PGA)
(a) Wickless heat pipe. (b) Wire-meshed heat pipe. [ 99 ] (APPLICABLE SOCIETY COPYRIGHT OWNER)
Except for the conventional circular type heat pipe, Deng et al . [ 100 ] adopted the microchannel heat pipe to form the heat pipe-based PVT module as shown in Fig. 35 . The microchannel contains micro fins, and its upper and lower surfaces are relatively flat, making it easier to fit with the PV module. The acetone acts as the working fluid of the heat pipe-based PVT module. The performance of the developed PVT system was tested under typical working conditions for four days. The results showed that the maximum electrical efficiency, thermal efficiency and average total efficiency are 14.65%, 33.07% and 45.38%, respectively.
![solar energy harvesting research papers Microchannel heat pipe-based PVT module. [100] (APPLICABLE SOCIETY COPYRIGHT OWNER)](https://oup.silverchair-cdn.com/oup/backfile/Content_public/Journal/ooenergy/1/10.1093_ooenergy_oiab004/24/m_oiab004f35.jpeg?Expires=1714710160&Signature=IMufcBRSidITzfkdDj-LlrAGH6w~LseznSujxsyEEyflR8tKpYbjAAKuqPgA2WYUqkr5oiBJX1WPCEUBGUNg~Vo4fml3vwQItdJO7lGV4Q40niDNNQJFi3UkorwZvrSavrSrOE4rD7uS5qmW7SKWBp1H2Rc~eiHCW~8S3FbIlXI4KRblOD5etnJLzJGSS21zumvmLvOxu~tnO9XRjDPZrysMw2X6-cWAKu4W8L2~HHf2ot0tqgTr8gIsGNgV-IobXJJC9TNQOO-h91vi5iAhf0rWDpr3tCiLoVM-9qEJCTVx-kI8RjzM0jTL3wuFE0HuQZgC87X7FQMOKbmVRMAk2w__&Key-Pair-Id=APKAIE5G5CRDK6RD3PGA)
Microchannel heat pipe-based PVT module. [ 100 ] (APPLICABLE SOCIETY COPYRIGHT OWNER)
The heat pipe-based PVT module could also be used for building integration. PVT component is combined with the building to form the building integrated heat pipe-based PVT module, which can not only be used as the building envelope but also increase the thermal power output of the building and realize energy self-supply. In this regard, Jouhara et al . [ 101 ] developed a building roof type heat pipe-based PVT module, as shown in Fig. 36 and the experimental tests were carried out. They tested the performance of the PVT system without PV, without cooling and with cooling. A mixture of 60% water and 40% ethylene glycol is used as the working liquid of the heat pipe. The thermal conversion rates were 50% and 64% for systems with and without PV layers, respectively, when tested on three identical systems. The electrical efficiency of the heat pipe-based PVT module was increased by 15%.
![solar energy harvesting research papers (a) Structure of the PVT heat mat. (b) Photograph of the roof-type heat pipe-based PVT module. [101] (APPLICABLE SOCIETY COPYRIGHT OWNER)](https://oup.silverchair-cdn.com/oup/backfile/Content_public/Journal/ooenergy/1/10.1093_ooenergy_oiab004/24/m_oiab004f36.jpeg?Expires=1714710160&Signature=R1ixtG8uYviHZ3riVuCY3YtCHQa4qTxmPNLTsDLsqff6BSF2~41wjhBHLUS5JcfCy0I3wacHnWwYJF~kSJXKAt-okpDhArNviroNIbU6L1vgAGRkl7nWtCyTHkSav1wLlqveQH7CmswZBQ1zuFYReZxHL~fyoQlgh0K8yE59QmVnl9EVqizwsM3TA1scvRaSTgJb0DBlJpZtmnXEMe3NXji7F5MpVzOqzLQh3~06mL-uOSNPk5cTgneHhDvq76XeF-Fp4ul0Pmc2vcMjuVwMvgHVGPExEPFrNmTrRrq~WlAH41s8uIDFhcl7g9FJNo5IBETOMZUKJk6p8OVFBw9Wsg__&Key-Pair-Id=APKAIE5G5CRDK6RD3PGA)
(a) Structure of the PVT heat mat. (b) Photograph of the roof-type heat pipe-based PVT module. [ 101 ] (APPLICABLE SOCIETY COPYRIGHT OWNER)
Table 6 presents a summary of heat pipe-based PVT modules.
Summary of heat pipe-based PVT modules
Spectral beam splitting PVT module
Different from the conventional PVT module, the spectral beam splitting PVT module adopts the splitter to utilize solar irradiation in different wavelengths. The solar energy would convert to electricity and waste heat (accumulated in solar cells) simultaneously of conventional PVT module, then the thermal collector absorbs heat from the solar cells to realize cogeneration. However, the spectral beam splitting PVT module could generate electricity and useful heat without convert to waste heat. The solar irradiation that could not excite the photovoltaic effect would convert to useful thermal energy directly due to the spectral beam splitter. The spectral beam splitting PVT module could decouple the photovoltaic and photothermal conversion process and realize efficient solar cogeneration. The spectral beam splitter could be mainly separated into three categories: nanofluids-based spectral beam splitter, nano-film-based spectral beam splitter and semitransparent PV cell-based spectral beam splitter [ 106 ]. The schematic diagrams of different spectral beam splitting PVT modules are shown in Fig. 37 .
![solar energy harvesting research papers Schematic diagrams of different spectral beam splitting PVT modules [106]. (APPLICABLE SOCIETY COPYRIGHT OWNER)](https://oup.silverchair-cdn.com/oup/backfile/Content_public/Journal/ooenergy/1/10.1093_ooenergy_oiab004/24/m_oiab004f37.jpeg?Expires=1714710160&Signature=uv2Ssod-DN1u3Y9GjFcCoKqTTOhXqJ8dYXNB540YGABE3I8d67~XrUJFEhjpGsWaR4Ki3kUHvaRa6Pttr9b7X5QC4NKv3jU~EGevxGa~LunRrhpigzpnJhneHiG80KgjTZ~r5SWF9WBm7-I3xv1voUlMTUrepQYhWmGdNAbvG5880HUA-QIcvH8~hW2vjH7M7c1BPHcM1r6ppbHq4V7r8EwqWw2Bm5QECG9XgbqiDiIEkQU0MRjRzXUqSMCTa9Woe0guEaBk0XXeMO0h4qQhd4O5sW1GrlT4VpIzETvIch5bP7DommISZazKymX21~tVjUJA-VyPblk1ZU3AMSR-ZQ__&Key-Pair-Id=APKAIE5G5CRDK6RD3PGA)
Schematic diagrams of different spectral beam splitting PVT modules [ 106 ]. (APPLICABLE SOCIETY COPYRIGHT OWNER)
Nanofluids-based spectral beam splitter
As shown in Fig. 37a , the nanofluids are employed as the spectral beam splitter as well as the heat collecting fluid. The infrared band of the solar irradiation (1109–2500 nm) would directly be absorbed by the nanofluids while the visible light (400–1109 nm) could penetrate the nanofluids and excite the photovoltaic effect. The nanofluids are arranged on the top of the PV module and this structure would decrease the electrical efficiency of the solar cells. The nanoparticles used in the nanofluids would cause sedimentation, leakage and pipe plugging problems. In this regard, numerous studies have been conducted to overcome the demerits of the nanofluids-based spectral beam splitter.
The typical structure of the nanofluids-based spectral beam splitter for PVT usage is shown in Fig. 38 . Ramdani and Ould-Lahoucine [ 107 ] numerically investigated the energy and exergy performance of this kind of PVT module. In their study, the water is regarded as the natural filter which could absorb infrared radiation. They developed the CFD model and conducted parametric analysis, the simulation results indicated that the thermal efficiency of the proposed PVT module could reach 17–40% while the electrical efficiency could reach 11.6–12.4%.
![solar energy harvesting research papers Cross-section view of the nanofluids beam splitter-based PVT module. [107] (APPLICABLE SOCIETY COPYRIGHT OWNER)](https://oup.silverchair-cdn.com/oup/backfile/Content_public/Journal/ooenergy/1/10.1093_ooenergy_oiab004/24/m_oiab004f38.jpeg?Expires=1714710160&Signature=wdXHV2Db1qgfo6tRUXvz1mTNVZdM9HcTJ6KCKE4zTPXfKt45jlLb7lTmcp9fEom4dio9bvOauZK5qolUDnfrecdBbSV23nXAk2fTqTvEAdq~e~M2X0LeonqB9IN2a20sULqvAwktuxKlEnk5OIoA6KtBE7qrdc9KBQv33CS7AM1mf1qgVj9vEO8DS7zEYJtzW7-Bse51kXhXeym3hP8Xwm5aPi3IBsRTOkp3qfYvuWfu30NjhvGjHze7a0Df3Ct4m1cQaSn~trGt8qOFu~FD6Bjo~vSU-eopEzT1HKAnLfd7XxndRu3geY4IbACf0YH0dKhw3BCaLQA9-tfgd1Rtyw__&Key-Pair-Id=APKAIE5G5CRDK6RD3PGA)
Cross-section view of the nanofluids beam splitter-based PVT module. [ 107 ] (APPLICABLE SOCIETY COPYRIGHT OWNER)
Moreover, Al-Shohani et al . [ 108 , 109 ] comparatively investigated the thermal and electrical performance of the PVT module with/without optical water filter as shown in Fig. 39 . Different thicknesses of the water filter would influence the energy efficiency of the PVT module. The experimental results showed that the thicker water filter could reduce the working temperature of the solar cells more remarkably, but also would cause higher optical loss. The electrical efficiency of the PVT module with a 5 cm water filter has the maximum thermal efficiency (42%), but its electrical efficiency is the minimum (8%) due to the absorption of visible light of the water filter, while the total efficiency could reach above 50%.
![solar energy harvesting research papers Photograph of the PVT module with and without an optical water filter. [108] (APPLICABLE SOCIETY COPYRIGHT OWNER)](https://oup.silverchair-cdn.com/oup/backfile/Content_public/Journal/ooenergy/1/10.1093_ooenergy_oiab004/24/m_oiab004f39.jpeg?Expires=1714710160&Signature=WfpU-~HaCo3r5afdfuFk1jd7p4r7Vc~jV2XPwd-iuEO4PQgTYZl7tmWj-W-EvtbNgnAp7r7S5j2qOPdZSqZdrlOMHH21Ub7IR~nkTCN2JD-SCJDrYYwL2dQbcSsuaXsG2bB2CWAmKna8Mmxg7f0kBtQXlMPVaA~Gs9L5vyoDyxzT0afeFsskNDeUpFBJlQTLkpzE7LEbGp2-tpA43~Hc1JdSJDKOd0MAVJJ~0fGbR1BkoR8BmLVZdrEHzCBNF9QEH~AlN6G4dt1zqw27ReKJDXzt9emQHAtBfZX11ZODV5QfX73DqpYyiRH0DKnVd4CSXFJm3dGVkAqB34Q0GzubUg__&Key-Pair-Id=APKAIE5G5CRDK6RD3PGA)
Photograph of the PVT module with and without an optical water filter. [ 108 ] (APPLICABLE SOCIETY COPYRIGHT OWNER)
![solar energy harvesting research papers Experimental rig for testing the PVT module. [110] (APPLICABLE SOCIETY COPYRIGHT OWNER)](https://oup.silverchair-cdn.com/oup/backfile/Content_public/Journal/ooenergy/1/10.1093_ooenergy_oiab004/24/m_oiab004f40.jpeg?Expires=1714710160&Signature=aNCLq~G8br-oMqmLX7QOng8YA5VsozaWywfkSrqGWN7MZnnSjaxvlU48zMijhxhU7JuRVNsl91R0WGsnZK9Szefjn62cwtwO-kYnMLqenGeNfsB2BP819-278cLBgquuHlyH~h9LxsR~4A-UeC8vZIGCt9VglQmWox3-t44qSj3PaPvMIoNRWWy~1m9fkl4fXY6k-QK8VQtsaJzUPrXRwVgIkoftuQiljKTboMIM52OYmAz4pUhZ8SDwaXseeV~0hGo3ZKujy2Ylehx4Neuwfqy95LXlM6F0RUxCES0Z-7TQp0zNhwzt4Ozo9tugE7HmrwNGUjeftl7zf0hAXrDzng__&Key-Pair-Id=APKAIE5G5CRDK6RD3PGA)
Experimental rig for testing the PVT module. [ 110 ] (APPLICABLE SOCIETY COPYRIGHT OWNER)
Pure water is easy to attain but its optical characteristics are not suitable for PVT usage. Thus, Han et al . [ 110 ] proposed five categories of nanofluids including inorganic aqueous salt, glycol, silicone oil, synthetic oil and mineral oil to improve the optical performance of the PVT module. The experimental rig has shown in Fig. 40 . Moreover, they adopted two types of solar cells (silicon cell and GaAs cell) for the PVT module. It was found that the electrical efficiency of the GaAs cell-based PVT module (13.1%) is lower than that of the silicon cell-based PVT module (15.7%) while the thermal efficiency is around 41%.
The fluid channel structure would influence the performance of the PVT module, thus, Rosa-Clot et al . [ 111 ] experimentally studied the S-shaped fluid channel type nanofluids beam splitter-based PVT module (as shown in Fig. 41 ). The field test revealed that the thermal efficiency varies from 30–60% while the electrical efficiency is around 13.19%. This kind of structure could improve the thermal and electrical efficiencies significantly while the maximum outlet temperature of the working fluid could reach 50°C.
To further improve the performance of the PVT module, Xiao et al . [ 112 ] investigated the influence of the S-shaped fluid channel’s structure on the system performance and temperature uniformity as shown in Fig. 42 . Model A has the best temperature uniformity under the same working conditions while its electrical and thermal efficiencies are 9.36% and 77.6%, respectively. However, the electrical efficiency of model C is the highest, which is 12.64%, but its thermal efficiency is the lowest, which is 71.15%. Therefore, different structures are recommended regarding the demand (electricity or heat).
![solar energy harvesting research papers Structure of the S-shaped fluid channel type nanofluids beam splitter-based PVT module. [111] (APPLICABLE SOCIETY COPYRIGHT OWNER)](https://oup.silverchair-cdn.com/oup/backfile/Content_public/Journal/ooenergy/1/10.1093_ooenergy_oiab004/24/m_oiab004f41.jpeg?Expires=1714710160&Signature=Gqvg1ZHgncfcnkPG4qSCu7nlkiyXLlbvOcmB6spPmsQFmMrbvBJ0CEv7kYVdqGNKLQKTfrQOc1FmZCkhR0y4TcM9S6Uqprr163OEFfOW7F9z-Hs-iBM9v0qa9028BV0~qRgYhWleMiDejlebaM9Vs-5rTxi52QN42A9y-YH5APE2DwN1cYmAGiu0cl86yrbh4-D4umg50OUpN~icNVBEjsIrfZ5Fg6JasYDgpY-tAgMCJ6iV3x9dOPKX9Go7q36PPJPZCsWQz4eF6RN20OO2Qy1cBlwLz1NHnn-nhzrvQkDxAykYYKbB15oxTUFu1IvLLDKeYM2OIbDKY0suf3ZzzQ__&Key-Pair-Id=APKAIE5G5CRDK6RD3PGA)
Structure of the S-shaped fluid channel type nanofluids beam splitter-based PVT module. [ 111 ] (APPLICABLE SOCIETY COPYRIGHT OWNER)
![solar energy harvesting research papers (a–b) Structure of the S-shaped PVT module. (c) Temperature distribution of different fluid channel structure-based PVT modules. [112] (APPLICABLE SOCIETY COPYRIGHT OWNER)](https://oup.silverchair-cdn.com/oup/backfile/Content_public/Journal/ooenergy/1/10.1093_ooenergy_oiab004/24/m_oiab004f42.jpeg?Expires=1714710160&Signature=c-yW8DidM3rVlRBeaGo5iAuYKS~Phtjcnpigwzhygc16y2IoN2VmhPdGjqhIaa-~dkEwgaEGslNUEYQI8WTjW38qwWeVgomevcopXst8~FBO8a62IUsfAks~uSIaToYkoj3PxMEPX8IBccIqXbi9w6-wxfRrRxcDoHukO3njUuUAF2sWmtk1OMz-Ri4SlaeHoJ80RFHvcBWL7glqwF0epvGof8nthmDSTCVaIH2LRmizQhkfhyjEFmAwhKAbrHJh9Afs9T2HZVB2CWYmoQIZXzPyJXZEKp6w5HZaHoXZzi2M-1SzISiK19DQa2IIJGaTWEs4hd5eZ5MIVsW4XqdCpw__&Key-Pair-Id=APKAIE5G5CRDK6RD3PGA)
(a–b) Structure of the S-shaped PVT module. (c) Temperature distribution of different fluid channel structure-based PVT modules. [ 112 ] (APPLICABLE SOCIETY COPYRIGHT OWNER)
![solar energy harvesting research papers Photograph of the nanofluids beam splitter-based PVT module. [113] (APPLICABLE SOCIETY COPYRIGHT OWNER)](https://oup.silverchair-cdn.com/oup/backfile/Content_public/Journal/ooenergy/1/10.1093_ooenergy_oiab004/24/m_oiab004f43.jpeg?Expires=1714710160&Signature=1T0N2PvbRH18X8K8a7t5rIgurYF-dkI8AhUjoFIqfJUhEo-Y8HHcf9KapGlYZA5uoNWbJZnOtVu0Fo4-jzk8aB9t8G8~IzrzjqzEhQ4ugqUWyXC1TpvbPfBchGYs08-LNzeTH5eqfOizlVMIJupO~nQjn19kmpJBzMyZFFaOk-Iy7J0YqfeL-Tam3VJU8W5RvpBrDTbEWrq4LSdZhbv7Pny7G31Ax3Il5~vmC3hz37v2GHIcIkm9Q70e5G22Wd2AlZqhd-qNKmTY-UbGjqHDRLUNqoSJF5Nkrj-qsRLIdDIPj60ifvC6CK6mxLTEKgXp1aOhktnuCvK9FWYGsYxVmQ__&Key-Pair-Id=APKAIE5G5CRDK6RD3PGA)
Photograph of the nanofluids beam splitter-based PVT module. [ 113 ] (APPLICABLE SOCIETY COPYRIGHT OWNER)
![solar energy harvesting research papers Prototype of the spectral splitting concentrating PVT system. [114] (APPLICABLE SOCIETY COPYRIGHT OWNER)](https://oup.silverchair-cdn.com/oup/backfile/Content_public/Journal/ooenergy/1/10.1093_ooenergy_oiab004/24/m_oiab004f44.jpeg?Expires=1714710160&Signature=B~XiOghpCmZE0elLY~l17QryNsQt1A0SKlkq6KrMoQbffKPcOuHpVfXN2YEOJsO-gsCj5sODML~xzW-2A4EHKJYS2OSaTBXSPZVJ7olXuzXewtmVuAni2yD5Y2EUxFj-Jw5waoN5iTG4LRfaFe6W~owOUvnHxbkd4oxaPTVD9Ovswb3XaVCbWcY3vs1nNKWFawAogEdmc-0dyo~UT2X0lPN5le9zpyYyJWA~Jmcb10UZHjVjQbxWhvuAFwi3lIGRoCr9Zfvzw-cH70fWGZMRmbzsJY2Z3586QGpbYnZfXH3KLziQ9cRwK2aKsexgLrdbIDHwVaHvnROQZTsUJxu8gg__&Key-Pair-Id=APKAIE5G5CRDK6RD3PGA)
Prototype of the spectral splitting concentrating PVT system. [ 114 ] (APPLICABLE SOCIETY COPYRIGHT OWNER)
![solar energy harvesting research papers The PCM/nanofluids beam splitter-based PVT module. [115] (APPLICABLE SOCIETY COPYRIGHT OWNER)](https://oup.silverchair-cdn.com/oup/backfile/Content_public/Journal/ooenergy/1/10.1093_ooenergy_oiab004/24/m_oiab004f45.jpeg?Expires=1714710160&Signature=pc94IzhsBcqhBdy~EEad5GTfkOBIM3cOv5512211xkarS5XQU1QG33w-Qh5R85lmXDFFdT63qWQshp9wur5SJ-BDYqQ~kOFNQMsR2X~RJwiqNgsA5AFQXNm-Q6STF5~8D3aZxxbt-ZHTyq47Y7fWRWgo4SFVwoUAWn~AvC7tvLBe6LpBEU4BETg5IF2luiVHiMaFyprSrxJtI-W7M55kpiIXyK5mVJjnKg6yVyfZrxam5pxoH7jOV5oI4lAznm8cn~fvswNXeo~93TO4Orophnfclqhjmook0~tB0-VYK6m3Ca2bGOGaJSJpEbA9pRnLZNnjuOSQbN4n1WDXWqEawQ__&Key-Pair-Id=APKAIE5G5CRDK6RD3PGA)
The PCM/nanofluids beam splitter-based PVT module. [ 115 ] (APPLICABLE SOCIETY COPYRIGHT OWNER)
![solar energy harvesting research papers The system configuration of the nano-film-based solar power generation system. [116] (APPLICABLE SOCIETY COPYRIGHT OWNER)](https://oup.silverchair-cdn.com/oup/backfile/Content_public/Journal/ooenergy/1/10.1093_ooenergy_oiab004/24/m_oiab004f46.jpeg?Expires=1714710160&Signature=t-lDMHmFNyaUN-jDKRab97nSikRvQD~HNr~32r7dWV71xNxi8~uPmGpdIz~xQ2fg9V-5kS1fFkRibW2D2vzaT16McdtiLVTh7QcjvtE3RDFY9~6GUZ4R~VcEBYxrYGmD6LrCRA7SbY3uvmmA7VU57qzHXuN2gOGayn09fS~MDJm~ZDzfbDkvi88LSMlzBlSkSHyJflw2DLBOy59GK317ZUhTT6om1uKMf~FMbYfQyShhtpcDRQE4oKJ4FVJ8TqkfNOCnxdE~gsUCYsCpQ2pWtGVmvHKRCaVdKsEvatVZ-Cpi9SbD1kTwdf2w6n55nsn~0FL7gN1jqwTpY7rFhS~~EQ__&Key-Pair-Id=APKAIE5G5CRDK6RD3PGA)
The system configuration of the nano-film-based solar power generation system. [ 116 ] (APPLICABLE SOCIETY COPYRIGHT OWNER)
![solar energy harvesting research papers Schematic diagram of the nano-film filter-based solar cogeneration system. [117] (APPLICABLE SOCIETY COPYRIGHT OWNER)](https://oup.silverchair-cdn.com/oup/backfile/Content_public/Journal/ooenergy/1/10.1093_ooenergy_oiab004/24/m_oiab004f47.jpeg?Expires=1714710160&Signature=uGdGe7f5lOBXRlOuEOx~KvWlGBnags0TJ~joyWa00Byw-KqCLetMtGauFhmGtFTJRg51JodfRsiMkVnrsmqeMY1jMjyPQPVASSCUo6AOexu5n2Ci8cWfWVvqSvFxwTJ0S2RhcTkhwwLfvnNqHw3eQNZ-dhZe8ryo4GICj4yoht0cCT39BGamVd6XDV-2IwnEVQbQWXYgnoMNER6hrmozdb37M4JU9kGrFECwdc-EIdGiwluVx3tBsEBnrN0JKvgTjGLaoDUMvOBsrdHKxnT1uwAbz4ry3Dy~MNPCPCsrRY4PK75h91Hk9WSyO8OG2nwTTrbdJDlQFHVwYE58EhNtyg__&Key-Pair-Id=APKAIE5G5CRDK6RD3PGA)
Schematic diagram of the nano-film filter-based solar cogeneration system. [ 117 ] (APPLICABLE SOCIETY COPYRIGHT OWNER)
![solar energy harvesting research papers Schematic diagram of the concentrated nano-film splitter-based PVT system. [118] (APPLICABLE SOCIETY COPYRIGHT OWNER)](https://oup.silverchair-cdn.com/oup/backfile/Content_public/Journal/ooenergy/1/10.1093_ooenergy_oiab004/24/m_oiab004f48.jpeg?Expires=1714710160&Signature=jUXtsYeo5rz5YbfosTneNCGMYRfMcjn9FSOV~u-qm8QWMeTWWclwlHMxMsR1jwRGgEUhZSIuVduZ9TrGHGOneTWx2UDFy7B~op5xxme-SU7FeDE6cHqg~sgObTapFwOvftiAprFghn3JLm38NaeYkO1wv-~IKswvoRrSABsUkFbDd861x~qoYOghF61PXo33ctRZMkA5eJ~RynIMw~mjRvR14VdoAyrTr4tpgq8hOcyo406EzJ-YNbtL5YJ-tc9Fnp68W75Zy7xk88Xb8dlmewElMD6Q3gWbQU9kyXjRPS99GfgukAFZiE24gJB4YrjBszSbpuHrbitG~6KgzsV4Tg__&Key-Pair-Id=APKAIE5G5CRDK6RD3PGA)
Schematic diagram of the concentrated nano-film splitter-based PVT system. [ 118 ] (APPLICABLE SOCIETY COPYRIGHT OWNER)
![solar energy harvesting research papers Schematic diagram of the solar CPVT system. [119] (APPLICABLE SOCIETY COPYRIGHT OWNER)](https://oup.silverchair-cdn.com/oup/backfile/Content_public/Journal/ooenergy/1/10.1093_ooenergy_oiab004/24/m_oiab004f49.jpeg?Expires=1714710160&Signature=WK~Ps-aT953kE583zLLZtjkY0WJUe1qsUxFkyej4MdOrx0jYL0eJT1Nbrc9OdUULGNGGLipdrYlY3GfeGAy-Z6mmcdOHCF8l0ROrR-ja29rPFnUKBm7FjLKpio5v64hO-b8AoiJ3sdLkq3SDTyBzm47uVoNB1w6zyT0JsCKBSfKZhXb6EILI5ah0PaTxGcJSQ0FkSrb18oQZKmG4aqoFnSWVzhUaoShqVVr3Ynh2nEE7IUGRXTAA4WZs7bOLF-CGLpzY~4WhT0f3bCjEHXFwTTQ4UuCxAMKNaF2~F2vtbP~We7a1GVWZbDxRprPzbzxgkdHJywlLHyoWXwVwim6Odw__&Key-Pair-Id=APKAIE5G5CRDK6RD3PGA)
Schematic diagram of the solar CPVT system. [ 119 ] (APPLICABLE SOCIETY COPYRIGHT OWNER)
![solar energy harvesting research papers (a) Cross-section view of the nano-film beam splitter-based PVT system. (b) Photograph of the system configuration. [120] (APPLICABLE SOCIETY COPYRIGHT OWNER)](https://oup.silverchair-cdn.com/oup/backfile/Content_public/Journal/ooenergy/1/10.1093_ooenergy_oiab004/24/m_oiab004f50.jpeg?Expires=1714710160&Signature=CBG9zkJYe45uqsIGzRVLT1Zq6oprpf6ONI6F4dBhFUA2IX-STbXieCsDs0yojBMmwR1kE6RKdUspkskxJ43-6Um7WmSeh-20weUStkLoQckhXW2aJKTBZt5LFRUwgmkA~d5R2Bv2dHf2AKtbAIdjgvQxyZ3nqp0Y9Vk23YLq7r~BhBfTigKyLXiMuX9PJ8F85G7gJ94BmZn8Knq0pn00hEk5Tz~F8Y8cB8chDW-gKtwOf9iWp-1jcIkYcux7tlYYM7PJgGbv-Jw3DFrpqfn2Gh6wcyKrX6ujJMos0FQr9q3sdYXFfc~SITfwym1AhzstoJ05NlcMdXh4IYlZ3qq44Q__&Key-Pair-Id=APKAIE5G5CRDK6RD3PGA)
(a) Cross-section view of the nano-film beam splitter-based PVT system. (b) Photograph of the system configuration. [ 120 ] (APPLICABLE SOCIETY COPYRIGHT OWNER)
![solar energy harvesting research papers Structure of the PV-TE system. [122] (APPLICABLE SOCIETY COPYRIGHT OWNER)](https://oup.silverchair-cdn.com/oup/backfile/Content_public/Journal/ooenergy/1/10.1093_ooenergy_oiab004/24/m_oiab004f51.jpeg?Expires=1714710160&Signature=QyFVYXUr9m2tWwMM4sqOJOC2ZS8NYWmGVSytU~oFXAqZaEnZnBq7M6t5pz6JitqufwRNkQgydTAnFXQt4nDMjK2Jfc7lMPOILD68y8Vsj9mjFI0qOcrFJPjSZRF4fmWzDKT6LJoSiRsnJYYhOF3hqiYZz3BbcAQNoRJLGGMHdBPOhgJf5DmbZCRNXwxXgt6vZMlZOUxSOZp79dktHuD3T~8iYrk9eF1EIZJRXOmkzxXfBe8pnq-6WHHiOTTmIp22iW3~j5ONo5g0cOFa4MqYlF1Z5BP7GM5drCAbKO0uvKHIY2p4IGXehkSLQ4suziVSg1F659NwjDBW-FeglTdMKg__&Key-Pair-Id=APKAIE5G5CRDK6RD3PGA)
Structure of the PV-TE system. [ 122 ] (APPLICABLE SOCIETY COPYRIGHT OWNER)

Configuration of typical power cycle-based solar cogeneration system
![solar energy harvesting research papers The configuration of the ORC with regenerator. [125] (APPLICABLE SOCIETY COPYRIGHT OWNER)](https://oup.silverchair-cdn.com/oup/backfile/Content_public/Journal/ooenergy/1/10.1093_ooenergy_oiab004/24/m_oiab004f53.jpeg?Expires=1714710160&Signature=fwOGs8XkBU2atc21m~jkguSclncrbtou1uQCMwDHvINmKfXDdorpxSY28levQpzpo4PgdLSS2eGOVN7FKbZRJReScTR9cfWrVCwzS5XhZ1xPXQALWrLNni-QC6pkCRt2oFUFraIYosdC1VgaOOh6XsfA7tWSkMFlENeeU3QBqA6W9b6F8WmfZYSoA~qQcKZVN0vlu1AttWyIIZ5h4mM7V3KpGJasbDuH0iE1jYCvMi2ezPOzl8CiX-4XFd2VE2~F23uHSH3U8PfiG3ZvUciElGA9nJ4KTa7XmtM2Dx77FM9akps41zBgLpGtIzk6AVc2UnaHW90BeVHniUaSML9nqg__&Key-Pair-Id=APKAIE5G5CRDK6RD3PGA)
The configuration of the ORC with regenerator. [ 125 ] (APPLICABLE SOCIETY COPYRIGHT OWNER)
![solar energy harvesting research papers The configuration of the ORC with regenerator. [125] (APPLICABLE SOCIETY COPYRIGHT OWNER)](https://oup.silverchair-cdn.com/oup/backfile/Content_public/Journal/ooenergy/1/10.1093_ooenergy_oiab004/24/m_oiab004f54.jpeg?Expires=1714710160&Signature=yF2IRlVMmdQPvwZk2cJxDd4RNxuPyqWO~G~4CbZmQDWCOX425WYDSB05lc8bgJ1GS-3PAW7dF3vChdq-kOIhJ-kAxuAv1NuZAUGgo1pTTx8fMNqRJiFJidKcbxMEYvqFbLbIMN3XF4pjkYkJsnJtcdKrjLmGBB-1ursY7E~lx4DxwWbaPZOVcVPlt76IO1danCWpGQ0rmjkUPrOWO63z2p7BaF30w0dx6tWa1CBPzkBRKdTtH4gchk35K3TFcTCbkuohXlWjfqwLtSm4dVTAa~4GIRr5c8MwSwYi1L0YVf1ymVYXCVUVe6~Pzx4R4Q-173FgjE0jQwNrlUmh3OaJ6g__&Key-Pair-Id=APKAIE5G5CRDK6RD3PGA)
ETC-FPC driven Rankine cycle-based solar cogeneration system. [ 128 ] (APPLICABLE SOCIETY COPYRIGHT OWNER)
![solar energy harvesting research papers Two kinds of solar-driven ORC cogeneration systems. [130] (APPLICABLE SOCIETY COPYRIGHT OWNER)](https://oup.silverchair-cdn.com/oup/backfile/Content_public/Journal/ooenergy/1/10.1093_ooenergy_oiab004/24/m_oiab004f56.jpeg?Expires=1714710160&Signature=uUJYhPCRUKqz83q2WwRcQkVVzX1FIndNZYU2XkwoAvdn0AZjtI5lV3xzYN9U86sXUAcffCrFZm7RmLhcXI-1mOOpl6dAGq9swUMm-Z~qOjYkRaH5P20NMRK3CrYJAmA3JKmioemEEklzPouZ0ukKIDQ7gJQ50bulA2D4CtCBfi5fhjXrJy0tbeWHnQI1MkQpbeR91B-B4wgydaIR4jZX2lhO88wiaDPl6MIUxtLkTJinulI-4gS4xaBXESxQgmgaLXDwh9fNGlAq5NqnsGJs3CIok4ZFdC8UJBGjAYgPnv1kralnaFaCJjW5xyDslzwDFeYSoVmAK9lswevn3qHPsQ__&Key-Pair-Id=APKAIE5G5CRDK6RD3PGA)
Two kinds of solar-driven ORC cogeneration systems. [ 130 ] (APPLICABLE SOCIETY COPYRIGHT OWNER)
![solar energy harvesting research papers Schematic of the proposed systems. (a) LHP-based solar CHP system with the single-stage turbine. (b) LHP-based solar CHP system with double stage turbine. [131] (APPLICABLE SOCIETY COPYRIGHT OWNER)](https://oup.silverchair-cdn.com/oup/backfile/Content_public/Journal/ooenergy/1/10.1093_ooenergy_oiab004/24/m_oiab004f57.jpeg?Expires=1714710160&Signature=hFV3Y5RzpeVpJ6m-cr~VM0avMUGsXyXbmVS7Ljt~moOSKji3lAWh~4pMQYunebeiIa9~2qe3e5UBXK8qCVlt0MOUAzy3yU9o87MbbgeKafEKM~QhTLQVgF7zIQcPuVuejGNit8dc2qwUPevdgfCyGTAsSJMd8FcAvEVS0ziqfOzVHUeznuJVri3Vtw2iDD0aNN8SX5avumAc1JzeINTLW5hntyqys5TmwUnMGuFcPItFMyj2lvD7Ne57IqnhzN5NtjObjODTNvvMD-JJJfeDBeGPCeCY9p2Ad5em0~6SkgV91NBQ7aSt5AfpGNIm1qy~Bp9VJNSd0LvFA4kGctMQVw__&Key-Pair-Id=APKAIE5G5CRDK6RD3PGA)
Schematic of the proposed systems. (a) LHP-based solar CHP system with the single-stage turbine. (b) LHP-based solar CHP system with double stage turbine. [ 131 ] (APPLICABLE SOCIETY COPYRIGHT OWNER)
![solar energy harvesting research papers Simplified schematic view of the three HPG configurations under comparison. [132] (APPLICABLE SOCIETY COPYRIGHT OWNER)](https://oup.silverchair-cdn.com/oup/backfile/Content_public/Journal/ooenergy/1/10.1093_ooenergy_oiab004/24/m_oiab004f58.jpeg?Expires=1714710160&Signature=xw2pz-8u6E~Ha8pogZdJXcKsB3YeB7L87dg0cCUOvxtwBo98YvZHeceRGKxNLkPzLvvFJa7gt~6sZUqGkt9MukUhol3vaKVy-n~j6hvLW1HcQCaoWlgsPz-t3jPtgjF3BLb18oeWi1DYmVf7uTLqEqJ-lUwtHW21nVBiM2TXbL7wAFadjDPxAPnMAMgcF~V6P3c7OzwAmtk1FhmQrjvfx-A4Zbz89x~LTSXVMMQZlEHheXLU1n~N9sWtZHDPepsV6Pyo9OAewq44aKc9H6876k4T6-~Y~ItX9MbW6ZLT5C4RRn9y-IAfomRraRC6fR35We4S2XT8n-xRqiXz-arB-Q__&Key-Pair-Id=APKAIE5G5CRDK6RD3PGA)
Simplified schematic view of the three HPG configurations under comparison. [ 132 ] (APPLICABLE SOCIETY COPYRIGHT OWNER)
![solar energy harvesting research papers Schematic of the solar dish Stirling micro-CHP system. [133] (APPLICABLE SOCIETY COPYRIGHT OWNER)](https://oup.silverchair-cdn.com/oup/backfile/Content_public/Journal/ooenergy/1/10.1093_ooenergy_oiab004/24/m_oiab004f59.jpeg?Expires=1714710160&Signature=MO~5AXVxIdwSgPkFpUfK0WrhWR~enJtXCaiK~G7qDBiGcGCQRhmq1JeykauToSWIsh0z4BMHHKsc2iYeCiLQeLKMukEqVYztjqLAztoUEf5Cl1p1eFX0PNhH0t3FdKaB6RiaeX~ZVv3mxM2ajqlJnD~MboWtS0h8oDcz4eb4JLh1gWrOXLaDrH-~9vDr0L49uy5-RPA7Db6wYUd9BGvrxR6KknGoMybneIs7ePRly5j3R-r9MWQCz9j8k4vZ~002kzbNHn~ijtkDDobpC6R3NVzjayUxJggp1SmZB49w22p2bBOiGIgOTzzzNjpEG6cpX0BCTBLS6lzk432rcsLB~g__&Key-Pair-Id=APKAIE5G5CRDK6RD3PGA)
Schematic of the solar dish Stirling micro-CHP system. [ 133 ] (APPLICABLE SOCIETY COPYRIGHT OWNER)
![solar energy harvesting research papers Schematic layout of solar-powered Stirling engine for micro-cogeneration. [134] (APPLICABLE SOCIETY COPYRIGHT OWNER)](https://oup.silverchair-cdn.com/oup/backfile/Content_public/Journal/ooenergy/1/10.1093_ooenergy_oiab004/24/m_oiab004f60.jpeg?Expires=1714710160&Signature=Px8B52GY~ds3nwWn8CymYTJVwANRJN-L9hN88g5HbppfaqGQvmtcvC3Ct47XKhvvK2OKXKnrqwoZG8vt3m5pR2TeKrccmOc0LB0KkmZutPJ3yVtyL0MeVEbBdQVGnqcRNcwlxvqmKz3F27yIymY4XqPVWon0V215Cyz83CpLgi7rOoieHkCAMth0VVwzIcSEIKHBCI2RIM58Zu~QZ84oKme0xbUJMNnZZDsVkUCCKtezLNdL5LvsoOx2137SZBjadUN6wxSimt1AYWDwH39ozdIVIAFrdlzOId4w5E22i2DtcJtP9WMSIn-6GYZ9EPzH-Hd7wpVxbKHRLQnCTRlX4w__&Key-Pair-Id=APKAIE5G5CRDK6RD3PGA)
Schematic layout of solar-powered Stirling engine for micro-cogeneration. [ 134 ] (APPLICABLE SOCIETY COPYRIGHT OWNER)
![solar energy harvesting research papers Schematic picture of the m-CHP system under development within the DiGeSPo project. [136] (APPLICABLE SOCIETY COPYRIGHT OWNER)](https://oup.silverchair-cdn.com/oup/backfile/Content_public/Journal/ooenergy/1/10.1093_ooenergy_oiab004/24/m_oiab004f61.jpeg?Expires=1714710160&Signature=D1B31Dw-h94P-QBjN-L-w3mHcA5nfSeNyXWRe0411y-2sWuRolkjYAt9sC1Q746GS~grvb3Mkh1begiAh74mGCJ7S~WERhzWDvB~LzZgpCKsBLCOPFPPFQz6kIn8zslRBBYUNaUZh0bJ5KdPtKeyhiQkGy3PlluLh5zXw9LO1B8liJEGx1hVZ60UDkY-a63yn4BtbDW9bkSQvL9gjwhysV1ZmzC5ydKuAnJDqfqyNJPmgDRIlLeOaRa4f5GpuViIS1ZKDraCjJ1pVFp-s4yJ3x4iZwx0FW1Db90cobMvyH20I3105TCuKzmg0O8lPIXwXb11bG~0~0c579k0vY6F~g__&Key-Pair-Id=APKAIE5G5CRDK6RD3PGA)
Schematic picture of the m-CHP system under development within the DiGeSPo project. [ 136 ] (APPLICABLE SOCIETY COPYRIGHT OWNER)
![solar energy harvesting research papers Schematic diagram of the new CCHP system with transcritical CO2 driven by solar energy. [139] (APPLICABLE SOCIETY COPYRIGHT OWNER)](https://oup.silverchair-cdn.com/oup/backfile/Content_public/Journal/ooenergy/1/10.1093_ooenergy_oiab004/24/m_oiab004f62.jpeg?Expires=1714710160&Signature=TeWzLCjFZ~pEMeAkeG95S49h72Kf5WErtm~i~TfrGIQTWDPx2KOyOJG7n5KMcRkOC~e6Ea9glSYZtviNa-ryvwycnayNkCojozPL-J~17ZGqD9pKoVSadeg9PYLKgTwtExyeyBBgnRBQ4vGNvxCFaL3euKtuLYYi1vBFUpxX2qBJPpgI~6RWJ7VIFDiJrl0yrA~5IRBxYM~ChkQDFHSBG-MIrcLBTjylgjny7XuqeBhzKcgU26Ab2r0z7~E81nlx6xXp92C~3sPh927bNS17w6mJbfiWcGUFU2O9s33I4b-~tq0QhdVVi2naEXkztqCLghfVOAn9orESuRrk7tqVaA__&Key-Pair-Id=APKAIE5G5CRDK6RD3PGA)
Schematic diagram of the new CCHP system with transcritical CO2 driven by solar energy. [ 139 ] (APPLICABLE SOCIETY COPYRIGHT OWNER)

Comparison of the electrical and thermal efficiencies of different solar cogeneration approaches

Comparison of the exergy efficiency and temperature of supply heating of different solar cogeneration approaches
Joshi and Dhoble [ 113 ] experimentally investigated theperformance of water, silicon oil and coconut oil-basedbeam splitter for PVT application. The experiment rig is shown in Fig. 43 . The maximum averagethermal and electrical efficiencies are 79% and 14%,respectively. It was found that the optical and thermalperformance of these three filters is similar for the PVTmodule.
To further improve the electricity and heat output, Zhang et al . [ 114 ] proposed and manufactured the spectral splitting concentrating PVT system and experimentally studied its performance. The structure of this prototype is shown in Fig. 44 . The maximum thermal and electrical efficiencies are 52% and 9.6%, respectively, when the diffuse irradiance ratios are 15.0%. Moreover, the adoption of the beam flitter could reduce the solar cells’ temperature by about 12°C. The outlet fluid temperature could reach 70°C when the concentration ratio is 15.
In another case, Yazdanifard et al . [ 115 ] employed the PCM as the first optical filter while the absorptive liquid as the second optical filter. The multi-layer structure of the proposed PVT module is shown in Fig. 45 . The numerical results showed that the electrical efficiency of the PVT module could reach 14.5% while the thermal efficiency of the PVT module is 46.5%. The nanofluids could maintain the temperature of the solar cells around 27°C while the temperature increase of the nanofluids is 7.2°C.
Table 7 summarizes the specific parameters of the nanofluids beam splitter-based PVT module.
Summary of nanofluids beam splitter-based PVT modules
Nano-film-based spectral beam splitter
As shown in Fig. 37b , the nano-film could realize spectral beam splitting benefit from its structure, and it could be used to separate the solar irradiation. The visible light (400–1109 nm) would be reflected by the nano-film when it is incident while the infrared light could penetrate the nano-film. Thus, the reflected visible light is used to generate electricity while the transmitted infrared light is used to generate heat. The PV module and thermal collector should be installed separately, which would cause a complex system arrangement. In this regard, the nano-film-based PVT module is not suitable for large-scale utilization due to the size limitation of the nano-film. The manufacturing process of the nano-film is not mature while the cost is high, which leads to poor economic performance. However, this kind of PVT module could realize high comprehensive solar energy utilization efficiency, and it has its suitable applications.
Shou et al . [ 116 ] developed a broadband TiO 2 /SiO 2 optical thin-film filter for solar power generation, the system configuration is shown in Fig. 46 . The thin-film filter could split the solar irradiation and reflect the visible light while penetrating the infrared light. The visible light could be used to generate electricity for the PV module, and the infrared light is also designed to generate electricity through the thermoelectric device. The maximum electrical efficiency of this system could reach 17.0% when the heterostructure cell is adopted, and the thermoelectric device could improve the overall electrical efficiency by 4.15%.
Crisostomo et al . [ 117 ] experimentally tested the performance of the SiN x /SiO 2 thin-film filters for PVT application. The test rig is shown in Fig. 47 . The linear Fresnel mirror is adopted to concentrate the incoming solar irradiation. The arrangement degree of the nano-film filter would affect the electrical efficiency of the solar cells. The testing maximum electrical efficiency of the solar cells could reach about 25% under 45 degrees arrangement while it is only 17% with 20 degrees. The total solar energy utilization efficiency could reach 85.6% of this system.
A similar structure as shown in Fig. 48 has been developed by Ling et al . [ 118 ] to generate heat and power simultaneously. The CdTe solar cell is adopted to generate electricity and it shows outstanding solar-to-electricity efficiency (39%, including thermochemical contribution). The electrical efficiency of the PV module is 15.5–19.5% while the thermal efficiency is around 60%. The cost analysis revealed that the specific cost of solar electricity is $0.20/kWh.
In another case, Wang et al . [ 119 ] designed and analysed the nano-film beam splitter-based PVT system as shown in Fig. 49 in terms of the thermodynamic aspect. They concluded that the overall optical efficiency is 66.2%. The thermodynamic analysis results revealed that the electrical efficiency of the PVT module could reach 26.6% while the overall efficiency could reach 30.5%.
Except for the linear concentration type, the point concentration Fresnel lens is adopted by Liang et al . [ 120 ] for small-scale usage as shown in Fig. 50 . The SiO2/TiO2 nano-film is used to split the solar irradiation, the experimental results showed that the electrical efficiency of the PV module is 13–16%, which is 9.4% higher than the conventional PV module. The overall energy efficiency and exergy efficiency of this study are 15.95% and 20.3%, respectively. Moreover, they [ 121 ] designed the two-axis sun tracking system for the nano-film beam splitter PVT module. The nano-film achieved a high reflectance (≥96.8%) for the visible light and a high transmittance (85%) at 1100–2500 nm. In this system, the overall energy efficiency could reach 22.72%.
Summary of nano-film beam splitter-based PVT modules
Table 8 presents the summary of nano-film beam splitter-based PVT modules.
Semitransparent PV cell-based spectral beam splitter
As shown in Fig. 37c , the semitransparent PV cell refers to the technology that utilizes PV cells with the transparent electrode (TCO) [ 106 ]. The semiconductor material shows transparent characteristics when the incoming photons could not excite the photovoltaic effect while it would strongly absorb the incident visible light and convert it to electricity. The long-wavelength spectrum of the incoming solar irradiation would transmit the semitransparent PV cell and be absorbed by the thermal collector. The initial cost of this kind of PVT module would significantly increase and the electrical efficiency of the solar cells could not maintain at a high level.
However, the research about the semitransparent PV cell-based spectral beam splitter is not rich. Several researchers coupled the semitransparent PV cell with the thermoelectric (TE) device to further improve the electrical efficiency of the system. For instance, Zhou et al . [ 122 ] proposed the concentrated PV-TE system as shown in Fig. 51 to realize power generation, and other researchers [ 123 , 124 ] have investigated the nano-structure of the PV module to improve the utilization efficiency of the incoming solar irradiation. Nevertheless, the usage of the semitransparent PV cell for PVT application is scarce.
Different from photovoltaics, CSP is another pathway using solar energy for electricity generation. Coupling CSCs with the boiler power cycle, typical CSP systems concentrate the solar irradiation to heat the working fluids. The heated working fluids (of which the temperature is higher than 100°C) directly or indirectly drive the power cycle to generate electricity. Besides, the lower-grade thermal energy released from the condenser can be retrieved for domestic or industrial uses, which enables solar cogeneration based on the power cycle.
As Fig. 52 illustrates, a typical power cycle-based solar cogeneration system consists of the solar field, thermal energy storage (TES) system and heat and power generation (HPG) section. The solar field is composed of an array of solar collectors to concentrate solar irradiation. TES system stores the thermal energy and conveys it to the HPG section, serving as an optional buffer to overcome the intermittency of solar irradiation. HPG sections, comprising power cycle and SPG subsections, generate thermal energy with lower grade (for direct use or driving absorption/adsorption heat pump) and electricity simultaneously.
The CSP systems differ in cycle type. The power cycles functioning in the power cycle-based solar cogeneration systems mainly include the Rankine cycle, Stirling cycle and Brayton cycle.
Rankine cycle-based solar cogeneration
Rankine cycle, especially organic Rankine cycle (ORC), is the dominant type of thermodynamic cycle functioning in the power cycle-based solar cogeneration systems. The concentrated solar irradiation heats the working fluid directly or indirectly (through a heat exchanger) and drives the Rankine cycle to generate electricity and heat.
Several types of solar collectors can serve in the solar field of Rankine cycle-based solar cogeneration system. Do Ango [ 125 ] et al . proposed a small-scale (electricity output <3 kW) solar cogeneration system ( Fig. 53 ), combining linear Fresnel collectors (as a solar field) and ORC with regenerator (as HPG sections). The test bench was manufactured ( Fig. 54 ), and an experiment was implemented to investigate the performance of the cogeneration system. The system offered an electrical efficiency of about 5% in the test condition.
Freeman et al . [ 126 ] proposed a small-scale solar ORC system for CHP with evacuated flat-plate collectors (EFPCs) or evacuated tube heat pipe collectors (ETHPCs) as a solar field. Freeman et al . simulated this proposed system with a collector area of 15 m 2 to access and compare the performance of systems with different collectors. The results presented the superiority of EFPC over ETHPC as a solar field and reported overall electrical efficiencies of 4.4–6.4% in the UK and of 6.3–7.3% in Cyprus under the simulation conditions. The system with EFPC was expected to have the potential to provide 3 h continuous 1-kW electricity output in Cyprus in January.
Borunda et al . [ 127 ] proposed a direct-feed solar cogeneration system coupling ORC and parabolic trough solar power plant. A case study (based on the meteorological data from Almeria) was conducted by TYNSYS to access the performance under different configurations. Under the simulation conditions, the electrical/thermal efficiency was estimated to be 6.79–8.35%/48.64–59.80% for different configurations, the overall exergy efficiency was estimated to be 24.87–30.58%.
Bellos et al . [ 128 ] designed an ORC solar cogeneration system with the combination of ETCs (evacuated tube collectors) and FPCs (flat plate collectors) as the solar field ( Fig. 55 ). A thermodynamic model based on EES was developed to investigate the performance variation of the system with the variation of heat production power. When the heating production varies from 5 kW to 35 kW, the overall energy efficiency was estimated to vary from 7.51% to 23.47%, while the exergy efficiency was ranged from 4.34% to 4.6%, respectively.
Eterafi et al . [ 129 ] conceptualized a solar cogeneration system combining dish collectors solar field and ORC for solar CHP. A TYNSYS-based simulation was implemented to evaluate the operation of the system daily and monthly. The required lowest inlet temperature was calculated at 266.1°C. After optimization, the average thermal and electric efficiency of the integrated system in July could reach 62.53% and 12.88%, respectively.
Zhang et al . [ 130 ] conducted an exergy analysis of two kinds of the solar cogeneration system, namely series mode and parallel mode ( Fig. 56 ), in Lhasa. Besides, three different collectors were investigated respectively based on different modes: FPC, ETC and PTC. The analysis was performed by Matlab and REFPROP to determine the optimal operation mode and collectors under different conditions.
HPG sections with different configurations are expected to influence the performance of the Rankine cycle-based solar cogeneration system. Beygzadeh et al . [ 131 ] conducted a thermodynamic comparison between solar ORC cogeneration systems (solar field: heat pipe collectors) with single-stage and double-stage turbines ( Fig. 57 ). The one-stage system was estimated to present a thermal/electrical/exergy efficiency of 63.39%/8.37%/11.22% with n-hexane as the working fluid for the single-stage system, and 63.36%/8.4%/11.26% for double stage system.
Cocco et al . [ 132 ] performed an exergy analysis by Matlab-based simulation to compare three different configurations ( Fig. 58 ) of HPG sections of an ORC solar cogeneration system with Linear Fresnel collectors as a solar field. The results suggested the superiority of HPG-A configuration for a small power-to-heat ratio and higher outlet water temperature.
Stirling cycle-based solar cogeneration
Stirling cycle, as a technology received renewed attention and investigation recently, also functions in some conception of power cycle-based solar cogeneration system. Stirling engine is especially suitable for distributed micro-CHP systems due to its advantages of miniaturization, adaptability to different heat sources and high efficiency.
Moghadam et al . [ 133 ] conceptualized a solar dish Stirling cogeneration system to provide energy demand for a residential building. Figure 59 briefly demonstrates the system configuration. 3E analysis was conducted to investigate and optimize the system performance. The conditions of fives Iran cities were discussed. The results estimated the highest electrical/overall efficiency of 34.5%/78.4% in Tabriz.
Ferreira et al . [ 134 ] proposed a micro solar cogeneration system equipped with a Stirling engine and dish collectors. Figure 60 briefly presents the system configuration. The proposed system was expected to output 1–5 kW of electricity and 2–35 kW of thermal power and to provide hot water with a temperature of 343 K. The system was estimated to offer an electrical/total efficiency of 26.2%/98.1% after optimization. Ferreira et al . [ 135 ] also proposed a solar-driven Stirling cycle cogeneration system for domestic use, reported an expected electrical/thermal efficiency of 23.91%/74.1%, with the output hot water temperature as 333 K.
Crema et al . [ 136 ] proposed and demonstrated a micro solar cogeneration system ( Fig. 61 ) combining parabolic trough collectors with a Stirling engine. Figure 61 presented the solar field and Stirling engine of the demonstration plant. The demonstration activities provided the best efficiency of 47%.
Brayton cycle-based solar cogeneration
Brayton cycle with supercritical carbon dioxide has higher thermal efficiency compared with Rankine cycle and is also reported to function in the power cycle-based solar cogeneration systems. Brayton cycle-based solar cogeneration system is suitable for large-scale CHP systems given the large volume of the devices and high working pressure.
The Brayton cycle could be utilized as the power cycle of the solar-assisted power generation system. Wang et al . [ 137 ] reported a Dish Brayton system for power generation with solar energy serving on preheating. However, the solar-driven Brayton cycle providing CHP/CCHP was rarely reported. Sharan et al . proposed a solar-driven Brayton cycle providing cogeneration of electricity and heat-driven desalination [ 138 ]. Wang et al . [ 139 ] conceptualized a solar-driven Brayton cycle combined with ejector refrigeration ( Fig. 62 ) for CCHP. Driven by trough collectors, this system provided a thermal efficiency of 53.0%, and net electricity output of 0.109 kW (the input solar irradiation was 135.277 kW).
Table 9 presents the summary of power cycle-based solar cogeneration systems.
Summary of power cycle-based solar cogeneration systems
The different approaches to harvesting solar energy for cogeneration have various energy and exergy efficiencies. In this regard, the applications for each technology method are differed according to the output temperature range. Thus, the comparison of energy and exergy efficiencies and suitable system applications is introduced in this section.
Energy and exergy efficiencies of different systems
Figure 63 illustrates the comparison results of the electrical and thermal efficiencies of different solar cogeneration approaches. The thermal efficiency of the liquid, air and spectral beam splitter-based PVT modules varies from 20% to 80% while the electrical efficiency is in the range of 5–18%, which is determined by the solar cells’ type and operating temperature. The liquid, air and spectral beam splitter-based PVT modules commonly adopt the working fluids that use sensible heat to collect heat. Thus, the heat transfer coefficient would be limited by the temperature difference between the solar cells and the working fluids. In this regard, the refrigerant-based PVT module that uses latent heat as a major method to extract heat performs better in thermal efficiency. Therefore, the higher thermal efficiency that is above 100% could be attained when the refrigerant evaporating temperature is lower than the ambient temperature. The electrical efficiency according to the field tests of the photovoltaic effect-based solar cogeneration system is generally below 20%. The conversion efficiency of the photovoltaic effect would limit the electrical efficiency of the PVT module, and the high operating temperature of the solar cells would have an adverse effect on its efficiency. In this regard, the power cycle-based solar cogeneration system shows better performance in electrical efficiency. For instance, the electrical efficiency of the Stirling cycle-based solar cogeneration system and Brayton cycle-based solar cogeneration system could reach around 35% and 42%, respectively, but the electrical efficiency of the Rankine cycle-based solar cogeneration system is around 12%. The thermal efficiency of the power cycle-based solar cogeneration system is around 50%. To be noted, the thermal efficiency of the Brayton cycle-based solar cogeneration system has not been reported clearly. Not all points within the rectangle range could be obtained. The rectangle range exists only to better demonstrate the electrical and thermal efficiencies of each technology.
The exergy efficiency and temperature of supply heating are shown in Fig. 64 . The power cycle-based solar cogeneration system has higher exergy efficiency than most photovoltaic effect-based solar cogeneration systems. Nevertheless, the exergy efficiency of the PVT module using CSC could be improved. The temperature of supply heating of power cycle-based solar cogeneration system could reach above 100°C while the outlet temperature of PVT module is generally below 80°C due to the limitation of the solar cells’ temperature. In this regard, the power cycle-based solar cogeneration system has better performance; however, the construction, initial cost and maintenance of this kind of system are much higher than the photovoltaic effect-based solar cogeneration system. To be noted, not all points within the rectangle range could be obtained. The rectangle range exists only to better demonstrate the exergy efficiency and temperature of supply heating of each technology.
System applications
The system applications would differ considering the system scale, installation area, temperature of thermal energy output, usage requirement, etc. Table 10 summarizes the system applications (focus on thermal energy usage) of different solar cogeneration approaches. Small scale system is suitable for household usage, while middle scale system is preferable for public buildings, factories, schools, etc., and a large-scale system is recommended for a district, village, community, etc.
Summary of system applications of various solar cogeneration approaches [ 11 ]
In this article, efficient approaches to harvesting solar energy for cogeneration have been reviewed. The photovoltaics-based solar cogeneration systems and power cycle-based solar cogeneration systems have been introduced, classified and analysed. Furthermore, the comparison of energy and exergy efficiencies and system applications identified suitable applications for each technology analysed.
The power cycle-based solar cogeneration system could reach higher exergy efficiency with high-grade thermal energy output. However, the initial cost, system installation, system safety and maintenance difficulty would limit the application of this technology. Therefore, the power cycle-based solar cogeneration system is recommended for middle and large-scale applications such as district heating, power supply, etc. On the contrary, the photovoltaic-based solar cogeneration systems (known as PVT) are more applicable for small-scale use. The compact system arrangement and flexible installation area make the PVT system a promising application in distributed use systems (households, single buildings, etc.). In terms of delivery temperature, the power cycle-based solar cogeneration system has a higher outlet temperature (>100°C) while the temperature of supply of the PVT system is generally below 80°C. Thus, the power cycle-based solar cogeneration system is more versatile and the high-grade thermal energy could also be used for industrial pre-heating, desalination, district heating, etc. The photovoltaic-based solar cogeneration system is more preferable for domestic hot water supply, residential heating, spacing heating, etc.
The photovoltaics-based solar cogeneration system is suitable for urban areas while the power cycle-based solar cogeneration system is preferable in suburbs, but each technology has its merits, depending on the perspective chosen. In the future, more efficient and lower-cost technologies could be developed to realize solar cogeneration, for instance, higher efficiency PV modules, efficient solar thermal systems, etc. Distributed solar energy utilization technologies could be further expanded in cities. Therefore, efficient solar cogeneration methods could significantly reduce the demand for fossil fuels usage, decrease carbon emissions and contribute to sustainable development.
STUDY FUNDING
This publication has been jointly written within the cooperative project ‘Key technologies and demonstration of combined cooling, heating and power generation for low-carbon neighborhoods/buildings with clean energy—ChiNoZEN’. The authors gratefully acknowledge the funding support from the Ministry of Science and Technology of China (MOST project number 2019YFE0104900) and from the Research Council of Norway (NRC project number 304191—ENERGIX).
CONFLICT OF INTEREST
None declared.
Jian Yao is responsible for the methodology, investigation, data collation and plotting and writing of the original draft. Wenjie Liu is responsible for the methodology, investigation and data collation and plotting. Yifan Jiang is responsible for the methodology and data collation and plotting. Sihang Zheng is responsible for the methodology and data collation and plotting. Yao Zhao is responsible for the methodology, investigation and data collation and plotting. Yanjun Dai is responsible for the conceptualization, supervision, funding acquisition and writing of the review and editing. Junjie Zhu is responsible for the supervision, methodology and writing of the review and editing. Vojislav Novakovic is responsible for the supervision, methodology and writing of the review and editing.
The data used to support the findings of this study are available from the corresponding author upon request.
Congress TtSMPs The Fourteenth Five-Year Plan of Shanghai Municipality for National Economic and Social Development and the Outline of the 2035 Long-Term Goals . China : Shanghai Municipal Government , 2021
Google Scholar
Google Preview
Guangyao Z . Current status and prospects of EU Laws and Policies on renewable energy . Sino-Glob Energy . 2020 ; 25 : 25 – 32
U.S. Renewable Energy Generation Report The World of Power Supply . 2019 : 46 .
Yin Xiaoping SP . Japan's new energy development and utilization policy and its enlightenment . China Manag Information . 2018 ; 21 : 102 – 5
BP Statistical Review of World Energy 2020 . 2020 .
Shahsavari A , Akbari M . Potential of solar energy in developing countries for reducing energy-related emissions . Renew Sust Energ Rev . 2018 ; 90 : 275 – 91
Kabir E , Kumar P , Kumar S et al. Solar energy: potential and future prospects . Renew Sust Energ Rev . 2018 ; 82 : 894 – 900
Dai Y , Chen J . Solar Cogeneration/Trigeneration. Encyclopedia of Sustainable Technologies . 2017 ; 357 – 66
Guney MS . Solar power and application methods . Renew Sust Energ Rev . 2016 ; 57 : 776 – 85
Imenes AG , Mills DR . Spectral beam splitting technology for increased conversion efficiency in solar concentrating systems: a review . Sol Energy Mater Sol Cells . 2004 ; 84 : 19 – 69
Joshi SS , Dhoble AS . Photovoltaic-thermal systems (PVT): technology review and future trends . Renew Sust Energ Rev . 2018 ; 92 : 848 – 82
Xu L , Liu W , Liu H et al. Heat generation and mitigation in silicon solar cells and modules . Joule . 2021 ; 5 : 631 – 45
Wolf M . Performance analyses of combined heating and photovoltaic power systems for residences . Energy Convers . 1976 ; 16 : 79 – 90
Assoa YB , Menezo C , Fraisse G et al. Study of a new concept of photovoltaic–thermal hybrid collector . Sol Energy . 2007 ; 81 : 1132 – 43
Khelifa A , Touafek K , Ben Moussa H et al. Modeling and detailed study of hybrid photovoltaic thermal (PV/T) solar collector . Sol Energy . 2016 ; 135 : 169 – 76
Nahar A , Hasanuzzaman M , Rahim NA . Numerical and experimental investigation on the performance of a photovoltaic thermal collector with parallel plate flow channel under different operating conditions in Malaysia . Sol Energy . 2017 ; 144 : 517 – 28
Hu M , Zhao B , Ao X et al. An analytical study of the nocturnal radiative cooling potential of typical photovoltaic/thermal module . Appl Energy . 2020 ; 277 :
Cirrincione L , Malara C , Marino C et al. Effect of the thermal storage dimensions on the performances of solar photovoltaic-thermal systems . Renew Energy . 2020 ; 162 : 2004 – 18
Pang W , Zhang Q , Cui Y et al. Numerical simulation and experimental validation of a photovoltaic/thermal system based on a roll-bond aluminum collector . Energy . 2019 ; 187 :
Boumaaraf B , Touafek K , Ait-cheikh MS et al. Comparison of electrical and thermal performance evaluation of a classical PV generator and a water glazed hybrid photovoltaic–thermal collector . Math Comput Simul . 2020 ; 167 : 176 – 93
Shyam TGN , Fischer O , Mishra RK et al. Performance evaluation of N-photovoltaic thermal (PVT) water collectors partially covered by photovoltaic module connected in series: an experimental study . Sol Energy . 2016 ; 134 : 302 – 13
Hissouf M , Mb F , Najim M et al. Performance of a photovoltaic-thermal solar collector using two types of working fluids at different fluid channels geometry . Renew Energy . 2020 ; 162 : 1723 – 34
Bakker M , Zondag HA , Elswijk MJ et al. Performance and costs of a roof-sized PV/thermal array combined with a ground coupled heat pump . Sol Energy . 2005 ; 78 : 331 – 9
Vokas G , Christandonis N , Skittides F . Hybrid photovoltaic–thermal systems for domestic heating and cooling—a theoretical approach . Sol Energy . 2006 ; 80 : 607 – 15
Dubey S , Tiwari GN . Analysis of PV/T flat plate water collectors connected in series . Sol Energy . 2009 ; 83 : 1485 – 98
Mishra RK , Tiwari GN . Energy and exergy analysis of hybrid photovoltaic thermal water collector for constant collection temperature mode . Sol Energy . 2013 ; 90 : 58 – 67
Chen F , Yin H . Fabrication and laboratory-based performance testing of a building-integrated photovoltaic-thermal roofing panel . Appl Energy . 2016 ; 177 : 271 – 84
Zhou J , Zhao X , Yuan Y et al. Mathematical and experimental evaluation of a mini-channel PV/T and thermal panel in summer mode . Sol Energy . 2021 ; 224 : 401 – 10
Aste N , Del Pero C , Leonforte F et al. Performance monitoring and modeling of an uncovered photovoltaic-thermal (PVT) water collector . Sol Energy . 2016 ; 135 : 551 – 68
He W , Zhang Y , Ji J . Comparative experiment study on photovoltaic and thermal solar system under natural circulation of water . Appl Therm Eng . 2011 ; 31 : 3369 – 76
Vaziri Rad MA , Kasaeian A , Mousavi S et al. Empirical investigation of a photovoltaic-thermal system with phase change materials and aluminum shavings porous media . Renew Energy . 2021 ; 167 : 662 – 75
Ul Abdin Z , Rachid A . Bond graph modeling of a water-based photovoltaic thermal (PV/T) collector . Sol Energy . 2021 ; 220 : 571 – 7
Salameh T , Tawalbeh M , Juaidi A et al. A novel three-dimensional numerical model for PV/T water system in hot climate region . Renew Energy . 2021 ; 164 : 1320 – 33
Li Z , Ji J , Yuan W et al. Experimental & numerical investigation and optimization on a novel flat-plate PV/T system using CdfTe thin-film solar modules of sandwich structure . Sol Energy . 2021 ; 223 : 261 – 77
Kaewchoothong N , Sukato T , Narato P et al. Flow and heat transfer characteristics on thermal performance inside the parallel flow channel with alternative ribs based on photovoltaic/thermal (PV/T) system . Appl Therm Eng . 2021 ; 185 :
Das D , Bordoloi U , Kamble AD et al. Performance investigation of a rectangular spiral flow PV/T collector with a novel form-stable composite material . Appl Therm Eng . 2021 ; 182 :
Colombini R , Molinaroli L , Simonetti R et al. Numerical analysis of different designs of roll-bond absorber on PV/T module and performance assessment . Appl Therm Eng . 2021 ; 192 :
Yu Y , Yang H , Peng J et al. Performance comparisons of two flat-plate photovoltaic thermal collectors with different channel configurations . Energy . 2019 ; 175 : 300 – 8
Choi SUS , Eastman J Enhancing Thermal Conductivity of Fluids With Nanoparticles , ASME International Mechanical Engineering Congress & Exposition, November 12-17, 1995, San Francisco, CA 1995
Verma SK , Tiwari AK . Progress of nanofluid application in solar collectors: a review . Energy Convers Manag . 2015 ; 100 : 324 – 46
Mahian O , Kianifar A , Kalogirou SA et al. A review of the applications of nanofluids in solar energy . Int J Heat Mass Transf . 2013 ; 57 : 582 – 94
Bozorgan N , Shafahi M . Performance evaluation of nanofluids in solar energy: a review of the recent literature . Micro Nano Syst Lett . 2015 ; 3 :
Al-Shamani AN , Yazdi MH , Alghoul MA et al. Nanofluids for improved efficiency in cooling solar collectors—a review . Renew Sust Energ Rev . 2014 ; 38 : 348 – 67
Elsheikh AH , Sharshir SW , Mostafa ME et al. Applications of nanofluids in solar energy: a review of recent advances . Renew Sust Energ Rev . 2018 ; 82 : 3483 – 502
Khanafer K , Vafai K . A review on the applications of nanofluids in solar energy field . Renew Energy . 2018 ; 123 : 398 – 406
Karami N , Rahimi M . Heat transfer enhancement in a PV cell using Boehmite nanofluid . Energy Convers Manag . 2014 ; 86 : 275 – 85
Sardarabadi M , Passandideh-Fard M , Zeinali HS . Experimental investigation of the effects of silica/water nanofluid on PV/T (photovoltaic thermal units) . Energy . 2014 ; 66 : 264 – 72
Michael JJ , Iniyan S . Performance analysis of a copper sheet laminated photovoltaic thermal collector using copper oxide—water nanofluid . Sol Energy . 2015 ; 119 : 439 – 51
Ghadiri M , Sardarabadi M , Pasandideh-fard M et al. Experimental investigation of a PVT system performance using nano ferrofluids . Energy Convers Manag . 2015 ; 103 : 468 – 76
Rejeb O , Sardarabadi M , Ménézo C et al. Numerical and model validation of uncovered nanofluid sheet and tube type photovoltaic thermal solar system . Energy Convers Manag . 2016 ; 110 : 367 – 77
Khanjari Y , Pourfayaz F , Kasaeian AB . Numerical investigation on using of nanofluid in a water-cooled photovoltaic thermal system . Energy Convers Manag . 2016 ; 122 : 263 – 78
Sangeetha M , Manigandan S , Ashok B et al. Experimental investigation of nanofluid based photovoltaic thermal (PV/T) system for superior electrical efficiency and hydrogen production . Fuel . 2021 ; 286 :
Hassani S , Saidur R , Mekhilef S et al. Environmental and exergy benefit of nanofluid-based hybrid PV/T systems . Energy Convers Manag . 2016 ; 123 : 431 – 44
Xu Z , Kleinstreuer C . Concentration photovoltaic–thermal energy co-generation system using nanofluids for cooling and heating . Energy Convers Manag . 2014 ; 87 : 504 – 12
Pang W , Cui Y , Zhang Q et al. A comparative analysis on performances of flat plate photovoltaic/thermal collectors in view of operating media, structural designs, and climate conditions . Renew Sust Energ Rev . 2020 ; 119 :
Sathe TM , Dhoble AS . A review on recent advancements in photovoltaic thermal techniques . Renew Sust Energ Rev . 2017 ; 76 : 645 – 72
Ji Y , Artzt LE , Adams W et al. A transmissive concentrator photovoltaic module with cells directly cooled by silicone oil for solar cogeneration systems . Appl Energy . 2021 ; 288 :
Codd DS , Escarra MD , Riggs B et al. Solar cogeneration of electricity with high-temperature process heat . Cell Rep Phys Sci . 2020 ; 1 :
Samylingam L , Aslfattahi N , Saidur R et al. Thermal and energy performance improvement of hybrid PV/T system by using olein palm oil with MXene as a new class of heat transfer fluid . Sol Energy Mater Sol Cells . 2020 ; 218 :
Rubbi F , Habib K , Saidur R et al. Performance optimization of a hybrid PV/T solar system using soybean oil/MXene nanofluids as a new class of heat transfer fluids . Sol Energy . 2020 ; 208 : 124 – 38
Aslfattahi N , Samylingam L , Abdelrazik AS et al. MXene based new class of silicone oil nanofluids for the performance improvement of concentrated photovoltaic thermal collector . Sol Energy Mater Sol Cells . 2020 ; 211 :
Tonui JK , Tripanagnostopoulos Y . Air-cooled PV/T solar collectors with low cost performance improvements . Sol Energy . 2007 ; 81 : 498 – 511
Fudholi A , Zohri M , Rukman NSB et al. Exergy and sustainability index of photovoltaic thermal (PVT) air collector: a theoretical and experimental study . Renew Sust Energ Rev . 2019 ; 100 : 44 – 51
Ooshaksaraei P , Sopian K , Zaidi SH et al. Performance of four air-based photovoltaic thermal collectors configurations with bifacial solar cells . Renew Energy . 2017 ; 102 : 279 – 93
Gholampour M , Ameri M . Energy and exergy analyses of photovoltaic/thermal flat transpired collectors: experimental and theoretical study . Appl Energy . 2016 ; 164 : 837 – 56
Yang T , Athienitis AK . A study of design options for a building integrated photovoltaic/thermal (BIPV/T) system with glazed air collector and multiple inlets . Sol Energy . 2014 ; 104 : 82 – 92
Yang T , Athienitis AK . Experimental investigation of a two-inlet air-based building integrated photovoltaic/thermal (BIPV/T) system . Appl Energy . 2015 ; 159 : 70 – 9
Tiwari S , Bhatti J , Tiwari GN et al. Thermal modelling of photovoltaic thermal (PVT) integrated greenhouse system for biogas heating . Sol Energy . 2016 ; 136 : 639 – 49
Bambrook SM , Sproul AB . Maximising the energy output of a PVT air system . Sol Energy . 2012 ; 86 : 1857 – 71
Farshchimonfared M , Bilbao JI , Sproul AB . Channel depth, air mass flow rate and air distribution duct diameter optimization of photovoltaic thermal (PV/T) air collectors linked to residential buildings . Renew Energy . 2015 ; 76 : 27 – 35
Farshchimonfared M , Bilbao JI , Sproul AB . Full optimisation and sensitivity analysis of a photovoltaic–thermal (PV/T) air system linked to a typical residential building . Sol Energy . 2016 ; 136 : 15 – 22
Singh S , Agarwal S , Tiwari GN et al. Application of genetic algorithm with multi-objective function to improve the efficiency of glazed photovoltaic thermal system for New Delhi (India) climatic condition . Sol Energy . 2015 ; 117 : 153 – 66
Agrawal B , Tiwari GN . Optimizing the energy and exergy of building integrated photovoltaic thermal (BIPVT) systems under cold climatic conditions . Appl Energy . 2010 ; 87 : 417 – 26
Shen C , Zhang Y , Zhang C et al. A numerical investigation on optimization of PV/T systems with the field synergy theory . Appl Therm Eng . 2021 ; 185 :116381
Akshayveer SAP , Kumar A , Singh OP . Effect of natural convection and thermal storage system on the electrical and thermal performance of a hybrid PV-T/PCM systems . Mater Today Proc . 2021 ; 39 : 1899 – 904
Wajs J , Golabek A , Bochniak R et al. Air-cooled photovoltaic roof tile as an example of the BIPVT system—an experimental study on the energy and exergy performance . Energy . 2020 ; 197 :
Kong D , Wang Y , Li M et al. Experimental study of solar photovoltaic/thermal (PV/T) air collector drying performance . Sol Energy . 2020 ; 208 : 978 – 89
Choi H-U , Kim Y-B , Son C-H et al. Experimental study on the performance of heat pump water heating system coupled with air type PV/T collector . Appl Therm Eng . 2020 ; 178 :
Arslan E , Aktaş M , Can ÖF . Experimental and numerical investigation of a novel photovoltaic thermal (PV/T) collector with the energy and exergy analysis . J Clean Prod . 2020 ; 276 :
Ji J , Liu K , Chow T-t et al. Performance analysis of a photovoltaic heat pump . Appl Energy . 2008 ; 85 : 680 – 93
Ji J , Pei G , Chow T-t et al. Experimental study of photovoltaic solar assisted heat pump system . Sol Energy . 2008 ; 82 : 43 – 52
Mohanraj M , Gunasekar N , Velmurugan V . Comparison of energy performance of heat pumps using a photovoltaic–thermal evaporator with circular and triangular tube configurations . Build Simul . 2016 ; 9 : 27 – 41
Chen H , Wei P . Numerical study on a novel photovoltaic/thermal heat pump system . Energy Procedia . 2011 ; 12 : 547 – 53
Mastrullo R , Renno C . A thermoeconomic model of a photovoltaic heat pump . Appl Therm Eng . 2010 ; 30 : 1959 – 66
Yao J , Liu W , Zhao Y et al. Two-phase flow investigation in channel design of the roll-bond cooling component for solar assisted PVT heat pump application . Energy Convers Manag . 2021 ; 235 :
Yao J , Dou P , Zheng S et al. Co-generation ability investigation of the novel structured PVT heat pump system and its effect on the “carbon neutral” strategy of Shanghai . Energy . 2022 ; 239 :
Tsai H-L . Design and evaluation of a photovoltaic/thermal-assisted heat pump water heating system . Energies . 2014 ; 7 : 3319 – 38
Shao N , Ma L , Zhang J . Experimental investigation on the performance of direct-expansion roof-PV/T heat pump system . Energy . 2020 ; 195 :
Zhou C , Liang R , Riaz A et al. Experimental investigation on the tri-generation performance of roll-bond photovoltaic thermal heat pump system during summer . Energy Convers Manag . 2019 ; 184 : 91 – 106
Vaishak S , Bhale PV . Investigation on the effect of different backsheet materials on performance characteristics of a photovoltaic/thermal (PV/T) system . Renew Energy . 2021 ; 168 : 160 – 9
Liang R , Pan Q , Wang P et al. Experiment research of solar PV/T cogeneration system on the building façade driven by a refrigerant pump . Energy . 2018 ; 161 : 744 – 52
Xu G , Zhang X , Deng S . Experimental study on the operating characteristics of a novel low-concentrating solar photovoltaic/thermal integrated heat pump water heating system . Appl Therm Eng . 2011 ; 31 : 3689 – 95
Wu S-Y , Zhang Q-L , Xiao L et al. A heat pipe photovoltaic/thermal (PV/T) hybrid system and its performance evaluation . Energy Build . 2011 ; 43 : 3558 – 67
Gang P , Huide F , Tao Z et al. A numerical and experimental study on a heat pipe PV/T system . Sol Energy . 2011 ; 85 : 911 – 21
Gang P , Huide F , Jie J et al. Annual analysis of heat pipe PV/T systems for domestic hot water and electricity production . Energy Convers Manag . 2012 ; 56 : 8 – 21
Long H , Chow T-T , Ji J . Building-integrated heat pipe photovoltaic/thermal system for use in Hong Kong . Sol Energy . 2017 ; 155 : 1084 – 91
Sweidan A , Ghaddar N , Ghali K . Optimized design and operation of heat-pipe photovoltaic thermal system with phase change material for thermal storage . J Renew Sust Energy . 2016 ; 8 :
Moradgholi M , Nowee SM , Abrishamchi I . Application of heat pipe in an experimental investigation on a novel photovoltaic/thermal (PV/T) system . Sol Energy . 2014 ; 107 : 82 – 8
Hu M , Zheng R , Pei G et al. Experimental study of the effect of inclination angle on the thermal performance of heat pipe photovoltaic/thermal (PV/T) systems with wickless heat pipe and wire-meshed heat pipe . Appl Therm Eng . 2016 ; 106 : 651 – 60
Deng Y , Quan Z , Zhao Y et al. Experimental research on the performance of household-type photovoltaic–thermal system based on micro-heat-pipe array in Beijing . Energy Convers Manag . 2015 ; 106 : 1039 – 47
Jouhara H , Milko J , Danielewicz J et al. The performance of a novel flat heat pipe based thermal and PV/T (photovoltaic and thermal systems) solar collector that can be used as an energy-active building envelope material . Energy . 2016 ; 108 : 148 – 54
Brahim T , Jemni A . Parametric study of photovoltaic/thermal wickless heat pipe solar collector . Energy Convers Manag . 2021 ; 239 :
Zhang T , Yan ZW , Xiao L et al. Experimental, study and design sensitivity analysis of a heat pipe photovoltaic/thermal system . Appl Therm Eng . 2019 ; 162 :
Zhang B , Lv J , Yang H et al. Performance analysis of a heat pipe PV/T system with different circulation tank capacities . Appl Therm Eng . 2015 ; 87 : 89 – 97
Zhang X , Zhao X , Shen J et al. Design, fabrication and experimental study of a solar photovoltaic/loop-heat-pipe based heat pump system . Sol Energy . 2013 ; 97 : 551 – 68
Liang H , Wang F , Yang L et al. Progress in full spectrum solar energy utilization by spectral beam splitting hybrid PV/T system . Renew Sust Energ Rev . 2021 ; 141 :
Ramdani H , Ould-Lahoucine C . Study on the overall energy and exergy performances of a novel water-based hybrid photovoltaic-thermal solar collector . Energy Convers Manag . 2020 ; 222 :
Al-Shohani WAM , Al-Dadah R et al. Reducing the thermal load of a photovoltaic module through an optical water filter . Appl Therm Eng . 2016 ; 109 : 475 – 86
Al-Shohani WAM , Sabouri A , Al-Dadah R et al. Experimental investigation of an optical water filter for photovoltaic/thermal conversion module . Energy Convers Manag . 2016 ; 111 : 431 – 42
Han X , Xue D , Zheng J et al. Spectral characterization of spectrally selective liquid absorption filters and exploring their effects on concentrator solar cells . Renew Energy . 2019 ; 131 : 938 – 45
Rosa-Clot M , Rosa-Clot P , Tina GM et al. Experimental photovoltaic-thermal power plants based on TESPI panel . Sol Energy . 2016 ; 133 : 305 – 14
Xiao L , Gan L-N , Wu S-Y et al. Temperature uniformity and performance of PV/T system featured by a nanofluid-based spectrum-splitting top channel and an S-shaped bottom channel . Renew Energy . 2021 ; 167 : 929 – 41
Joshi SS , Dhoble AS . Analytical approach for performance estimation of BSPVT system with liquid spectrum filters . Energy . 2018 ; 157 : 778 – 91
Zhang G , Wei J , Xie H et al. Performance investigation on a novel spectral splitting concentrating photovoltaic/thermal system based on direct absorption collection . Sol Energy . 2018 ; 163 : 552 – 63
Yazdanifard F , Ameri M , Taylor RA . Numerical modeling of a concentrated photovoltaic/thermal system which utilizes a PCM and nanofluid spectral splitting . Energy Convers Manag . 2020 ; 215 :
Shou C , Luo Z , Wang T et al. Investigation of a broadband TiO2/SiO2 optical thin-film filter for hybrid solar power systems . Appl Energy . 2012 ; 92 : 298 – 306
Crisostomo F , Taylor RA , Zhang T et al. Experimental testing of SiN x /SiO 2 thin film filters for a concentrating solar hybrid PV/T collector . Renew Energy . 2014 ; 72 : 79 – 87
Ling Y , Li W , Jin J et al. A spectral-splitting photovoltaic-thermochemical system for energy storage and solar power generation . Appl Energy . 2020 ; 260 :
Wang G , Yao Y , Lin J et al. Design and thermodynamic analysis of a novel solar CPV and thermal combined system utilizing spectral beam splitter . Renew Energy . 2020 ; 155 : 1091 – 102
Huaxu L , Fuqiang W , Ziming C et al. Performance study on optical splitting film-based spectral splitting concentrated photovoltaic/thermal applications under concentrated solar irradiation . Sol Energy . 2020 ; 206 : 84 – 91
Liang H , Han H , Wang F et al. Experimental investigation on spectral splitting of photovoltaic/thermal hybrid system with two-axis sun tracking based on SiO2/TiO2 interference thin film . Energy Convers Manag . 2019 ; 188 : 230 – 40
Zhou Y-P , Li M-J , Yang W-W et al. The effect of the full-spectrum characteristics of nanostructure on the PV-TE hybrid system performances within multi-physics coupling process . Appl Energy . 2018 ; 213 : 169 – 78
Yin E , Li Q , Xuan Y . One-day performance evaluation of photovoltaic-thermoelectric hybrid system . Energy . 2018 ; 143 : 337 – 46
Xu Y , Xuan Y . Photon management of full-spectrum solar energy through integrated honeycomb/cone nanostructures . Opt Commun . 2019 ; 430 : 440 – 9
Do Ango A-CM , Levêque G , Holaind N et al. Low temperature and power solar energy conversion for domestic use . Energy Procedia . 2019 ; 161 : 454 – 63
Freeman J , Guarracino I , Kalogirou SA et al. A small-scale solar organic Rankine cycle combined heat and power system with integrated thermal energy storage . Appl Therm Eng . 2017 ; 127 : 1543 – 54
Borunda M , Jaramillo OA , Dorantes R et al. Organic Rankine cycle coupling with a parabolic trough solar power plant for cogeneration and industrial processes . Renew Energy . 2016 ; 86 : 651 – 63
Bellos E , Tzivanidis C . Design of a solar-driven cogeneration system using flat plate collectors and evacuated tube collectors . Int J Energy Res . 2019 ; 43 : 5841 – 51
Eterafi S , Gorjian S , Amidpour M . Thermodynamic design and parametric performance assessment of a novel cogeneration solar organic Rankine cycle system with stable output . Energy Convers Manag . 2021 ; 243 :
Zhang H , Lei B , Yu T et al. Exergy analysis of two kinds of solar-driven cogeneration systems in Lhasa, Tibet, China . Int J Photoenergy . 2018 ; 2018 : 1 – 11
Beygzadeh V , Khalilarya S , Mirzaee I . Thermodynamic comparison of two novel combined systems based on solar loop heat pipe evaporator . Energy . 2020 ; 206 :
Cocco D , Petrollese M , Tola V . Exergy analysis of concentrating solar systems for heat and power production . Energy . 2017 ; 130 : 192 – 203
Moghadam RS , Sayyaadi H , Hosseinzade H . Sizing a solar dish Stirling micro-CHP system for residential application in diverse climatic conditions based on 3E analysis . Energy Convers Manag . 2013 ; 75 : 348 – 65
Ferreira AC , Nunes ML , Teixeira JCF et al. Thermodynamic and economic optimization of a solar-powered Stirling engine for micro-cogeneration purposes . Energy 2016 ; 111 :1– 17 .
Ferreira AC , Nunes ML , Teixeira JCF et al. Design of a solar dish Stirling cogeneration system: application of a multi-objective optimization approach . Appl Therm Eng . 2017 ; 123 : 646 – 57
Crema L , Alberti F , Wackelgard E et al. Novel system for distributed energy generation from a small scale concentrated solar power . Energy Procedia . 2014 ; 57 : 447 – 56
Wang W , Ragnolo G , Aichmayer L et al. Integrated design of a hybrid gas turbine-receiver unit for a solar dish system . Energy Procedia . 2015 ; 69 : 583 – 92
Sharan P , Neises T , McTigue JD et al. Cogeneration using multi-effect distillation and a solar-powered supercritical carbon dioxide Brayton cycle . Desalination . 2019 ; 459 : 20 – 33
Wang J , Zhao P , Niu X et al. Parametric analysis of a new combined cooling, heating and power system with transcritical CO2 driven by solar energy . Appl Energy . 2012 ; 94 : 58 – 64
A.1. The equations of electrical, thermal, overall and exergy efficiencies
Email alerts, citing articles via.
- Advertising & Corporate Services
- Journals Career Network
Affiliations
- Online ISSN 2752-5082
- Copyright © 2024 Oxford University Press
- About Oxford Academic
- Publish journals with us
- University press partners
- What we publish
- New features
- Open access
- Institutional account management
- Rights and permissions
- Get help with access
- Accessibility
- Advertising
- Media enquiries
- Oxford University Press
- Oxford Languages
- University of Oxford
Oxford University Press is a department of the University of Oxford. It furthers the University's objective of excellence in research, scholarship, and education by publishing worldwide
- Copyright © 2024 Oxford University Press
- Cookie settings
- Cookie policy
- Privacy policy
- Legal notice
This Feature Is Available To Subscribers Only
Sign In or Create an Account
This PDF is available to Subscribers Only
For full access to this pdf, sign in to an existing account, or purchase an annual subscription.
A simple and efficient solar energy harvesting for wireless sensor node
Ieee account.
- Change Username/Password
- Update Address
Purchase Details
- Payment Options
- Order History
- View Purchased Documents
Profile Information
- Communications Preferences
- Profession and Education
- Technical Interests
- US & Canada: +1 800 678 4333
- Worldwide: +1 732 981 0060
- Contact & Support
- About IEEE Xplore
- Accessibility
- Terms of Use
- Nondiscrimination Policy
- Privacy & Opting Out of Cookies
A not-for-profit organization, IEEE is the world's largest technical professional organization dedicated to advancing technology for the benefit of humanity. © Copyright 2024 IEEE - All rights reserved. Use of this web site signifies your agreement to the terms and conditions.
- Reference Manager
- Simple TEXT file
People also looked at
Review article, solar photovoltaic energy optimization and challenges.
- 1 Department of Electrical Engineering, Mehran University of Engineering and Technology, Jamshoro, Pakistan
- 2 Processes, Energy, Environment and Electrical Systems, University of Gabes, Gabes, Tunisia
- 3 Faculty of Electrical and Control Engineering, Gdańsk University of Technology, Gdańsk, Poland
- 4 Department of Materials Technologies, Silesian University of Technology, Gliwice, Poland
The study paper focuses on solar energy optimization approaches, as well as the obstacles and concerns that come with them. This study discusses the most current advancements in solar power generation devices in order to provide a reference for decision-makers in the field of solar plant construction throughout the world. These technologies are divided into three groups: photovoltaic, thermal, and hybrid (thermal/photovoltaic). As a result, this article begins by outlining the approach that will be employed to undertake this research. Following that, solar energy production methods are researched and their sub-classifications are described in order to establish their resource needs and features. Following that, a detailed conversation is held. Each technology’s environmental and economic performance will be evaluated. Furthermore, a statistical analysis is conducted to emphasize the efficiency and performance of each solar technology, as well as to identify their global rankings in terms of power output. Finally, research trends in the development of solar power plants are presented. The credibility of the Photovoltaic system, types and limitations is the discussion under study system makes use of sun’s energy to generate electricity with the help of varied procedural systems; stand-alone, hybrid or grid charged. Based on this research, it is possible to infer that the primary goals of optimization approaches are to reduce investment, operation and maintenance costs, and emissions in order to improve system dependability. This paper also includes a brief overview of several solar energy optimization problems and issues.
1 Introduction
Global warming is an element in climate change and explicitly refers to the influence of greenhouse gases on the overall surface temperature of the Earth. When describing extreme weather events produced by greenhouse gases; the label “global warming” is appropriate. When characterizing other long-term changes to the planet’s weather patterns, however, climate change is the most appropriate phrase. Opponents of climate change and global warming have noted out how the Earth’s temperature patterns have fluctuated for generations, and that current climatic changes are not as severe as stated, nor are they only the consequence of human activity.
The Earth’s atmosphere is made up of several gases that work as a layer, trapping heat from the sun and blocking it from escaping back into space. Human actions have contributed to rising global temperatures, according to 97% of active climate experts throughout the world. According to climate experts, such negativity arises from a fear of confronting the scale of the harm caused by human actions to the environment. Little fluctuations in the Earth’s orbit around the Sun enable the ice sheets to develop and disappear. Solar radiation levels fluctuate. Such changes have a wide range of consequences in space, the Earth’s atmosphere, and on the Earth’s surface ( Mohamed et al., 2014 ). Upshot changes in solar activity, according to current scientific opinion, have only a little role in the Earth’s temperature. The warming induced by increasing levels of man-made greenhouse gas emissions is several times more than any other factors: Recent changes in solar activity are to blame. In fact, solar energy is a lot more beneficial for human beings. As the modern technological world is getting updated day by day. There is dire need to find a credible energy source in order to ensure a promising ground. In terms of solar energy, the sun is the most major source which can turn into feasible means if it is used to produce photovoltaic energy. Photovoltaic energy can be produced with the help of solar energy and is converted into electricity with the aid of solar photovoltaic panels.
Many activities rely on solar energy. Pumping water is mostly used in agriculture. PV panels and electric batteries are utilized to power the electro-pumps, allowing the irrigation system to be completely self-sufficient. In the construction sector, solar energy is used for air conditioning, water heating, lighting, and refrigeration systems. Desalination of water is another key application of solar energy. Solar energy is utilized to extract low-salt water from saltwater in this technique. Telecommunications is another key sector that makes use of solar energy. Satellites’ electrical demands are met by solar panels installed on their spinning limbs. Solar energy is occasionally utilized as a backup power source for established telecommunications networks. Hydrogen generation and consumption by electrolysis of water is one of the most promising ways to achieving carbon neutrality by 2050. Figure 1 shows the typical Photovoltaic system. Solar energy has shown to be the most cost-effective and environmentally friendly option for electrolysis procedures. For power generation, three primary technologies are used, namely thermal, photovoltaic, and hybrid thermal photovoltaic. Numerous nations have already implemented similar systems in their electrical grids, including the United States, Spain, Morocco, India, China, and. Furthermore, in order to select the most appropriate technology for a specific country, a thorough examination and knowledge of the many solar technologies and their underlying challenges is required to assist responsible institutions in making decisions. As a result, a comprehensive assessment of all solar technologies for energy generation is required. As a result, the purpose of this study is to cover several research gaps in the literature, such as the absence of statistical analysis of existing solar power plants throughout the world. Moreover, decision-makers will be able to implement the most appropriate solar power technology for a specific geographic region. The second gap in the literature concerns a recent comprehensive study of solar energy technology for power generation. The third significant research gap is an in-depth comparison of the performance of the three primary solar technologies and their modifications, which, to the best of the authors’ knowledge, has yet to be addressed in any contemporary study.
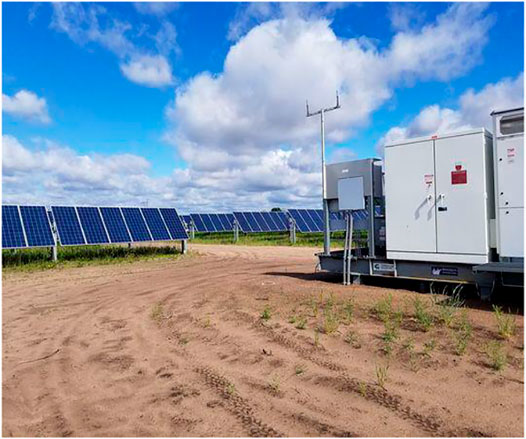
FIGURE 1 . Photovoltaic system (Flickr).
As a result, the following are the primary additions and innovations of the present article:
• A new summary of the three primary solar methods for generating power.
• Updated solar technology economic and environmental assessments.
• Audit of linear Fresnel reflectors, parabolic trough technology, Parabolic dish collectors, Heliostat field collectors, photovoltaic, and concentrated photovoltaic solar power plants.
• PV-CSP and PVT/CPVT are two hybrid systems for generating thermal solar electricity ( Getie et al., 2020 ).
The layout of this paper is as follows. Section methodology introduces the adopted methodology in this review paper. Section Technologies Overview for Generating Thermal Power describes the three main solar technologies for electricity production. The discussion and comparison of these technologies alongside future trends and evaluation of their environmental and economic aspects are conducted in section Discussions. Section Optimization Method discuses PV Base Hybrid System, PV Based Grid System and PV Based Standalone System. Section Utilization of Solar Photovoltaic Energy discusses application. Section Optimization Issues and Challenges highlight limitations, while Section Conclusion , provides the conclusion of this paper ( Bishoyi and Sudhakar, 2017 ).
2 Methodology
An accurate literature study was undertaken to assess the most recent relevant research and their conclusions in order to investigate solar technologies for power production. These latter have been investigated based on the accepted solar technologies, their working principle, their capabilities, and the environmental difficulties linked with them. Moreover, an analysis of the operating solar-powered power plants has been created. Finally, a comparison of all technologies is offered in terms of their advantages, efficiency, and resource needs. The paper covers an exact literature study to assess the most recent relevant research and their conclusions in directive to solar energy technology for electricity generation built on the solar techniques employed, their operating principles, and their performance. A list of all operational solar-powered power plants has also been established. A comparison of technologies in terms of their advantages, productivity, and reserve needs is presented as well. The review of literature is divided into three parts, the first of which was to gather the most recent information on the four essential technologies used in thermal solar stations ( Zhang et al., 2013 ) as follows:
• Parabolic trough collector (PTC) ( Ouagued et al., 2018 ).
• Linear Fresnel Reflector (LRF) ( Ghodbane et al., 2016 ).
• Heliostat field collector (HFC) ( Eddhibi et al., 2017 ).
• Parabolic dish collector (PDC) ( Chen et al., 2018 ).
Second part splits research into two areas based on the major technology used in solar power plants:
• Fundamentally as Photovoltaic (PV) ( Lokar and Virtic, 2020 ).
• Concentrated photovoltaic (CPV) ( Aqachmar et al., 2020 ).
Third part, the technologies used in hybrid thermal photovoltaic systems are investigated. Including:
• Photovoltaic thermal (PVT) ( Aqachmar et al., 2020 ).
• Concentrated photovoltaic thermal (CPVT) ( Bamisile et al., 2020 ).
Next section shows a detailed literature review in order to shed light on different optimization methods in terms of solar photo- voltaic energy. Furthermore, an overview on utilization of photovoltaic energy is presented. In the last step the cons of optimization methods are discussed in terms of challenges and issues to get a better understanding of debilitated points of this whole phenomenon.
2.1 Technologies Overview for Generating Thermal Power
DNI (Direct Normal Irradiance) is used to turn sunlight into electricity, solar thermal power uses the second principle of thermodynamics. This transition necessitates the use of two heat sources: a cold and a hot source. The heat transfer fluid (HTF) is employed as the hot source and water as the cold source in CSP power plants. Entropy is increased as a result of the natural heat exchange between water and HTF. After that, the HTF is heated using one of four different technologies: parabolic trough collectors, Fresnel reflectors, parabolic dish collectors, or solar power tower ( Alsaffar, 2015 ).
2.1.1 Plants Using PTC Technology
A solar field, a power block, and thermal energy storage (TES) are all parts of the PTC power plant. In the solar field, solar collectors with parabolic troughs and tubes filled with a heat transfer fluid (HTF) are employed. By way of it passes through the tubes, a reflected beam of solar light heats the HTF. The power block must be efficient and trustworthy because it is the core of the PTC system. As a result, Rankine or Hirn cycles are the most often used power blocks ( Zhar et al., 2021 ; Aqachmar et al., 2019 ). PTC power plants are already operational in 98 countries, with 43% in Spain, 7% in India and 17% in the United States. The capacity of these PTC plants between 0.15 MW in France to 2,474.5 MW in Spain ( Solar paces, 2019 ; Boukelia et al., 2017 ). As indicated in Table 1 , the LCOE of PTC power plants ranges from 0.07 to 0.23 USD/KWh, and is heavily impacted by the plant’s position (DNI), size (Capacity in MegaWatts), and TES time. This table covers a number of LCOE optimization studies were carried out for a number of PTC plants in various countries ( Boukelia, et al., 2017 ; Dowling et al., 2017 ; Aly et al., 2019 ; Achkari and El Fadar, 2020 ).
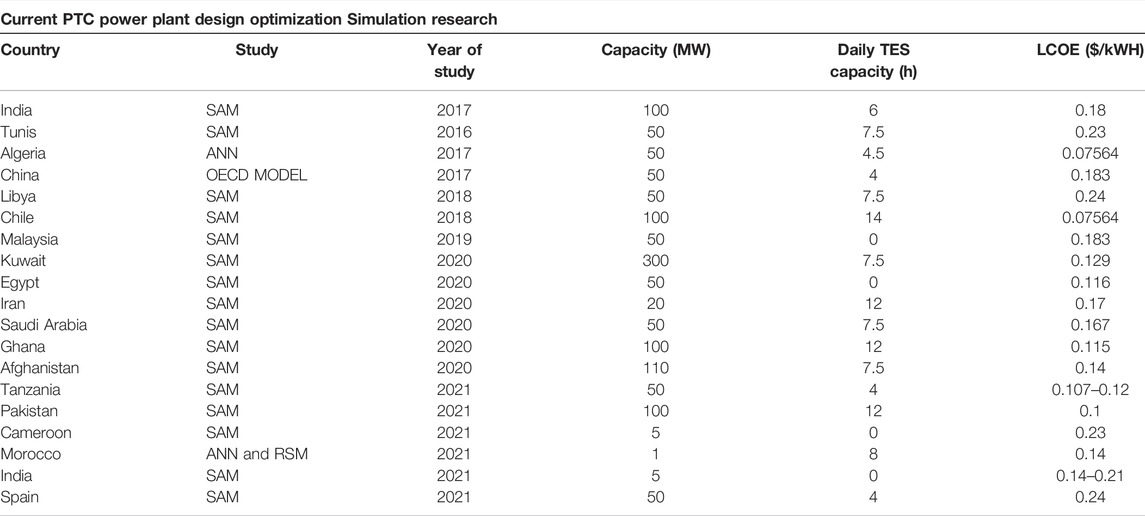
TABLE 1 . Current PTC power plant design optimization simulation research ( Zineb et al., 2021 ).
2.1.2 Heliostat Field Collector or Solar Power Tower
Large mirrors reflect the sun’s energy onto a receiver at the top of a tower in heliostat field collector power plants. Ceramics or any other physical substance that is stable at high temperatures is used to construct the receiver. The heat is subsequently transferred to the HTF, which, in turn, is used to generate electricity, when it reaches a particular temperature, activates the steam generating system. The focused solar radiation must reach the receiver at a rate of 200–1,000 kW/m 2 ( Simsek et al., 2018 ) to produce the required temperature for the procedure. In general, water, melted salt, sodium liquid or air, can be used as the HTF in SPT technology. Economic and technological research on SPT power plants have grown in popularity in recent years. This would include viability and optimization research, which necessitate an assessment of the power plant’s three components: the heliostat field (tower altitude, land-use factor, as well as the quantity, length, and width of separate mirrors (heliostats)), heat energy storing (area for storage, storage extent, storing capacity), and power generation, vessels, temperature levels), ( Chen et al., 2018 ; Simsek et al., 2018 ; Collado and Guallar, 2019 ; Zhuang et al., 2019 ; Awan et al., 2020a ; Agyekum and Velkin, 2020 ; Hakimi et al., 2020 ), and electricity cycle (thermal cycle, fluid transmit, effectiveness, boiler stress, etc.). Awan et al. (2020b) obtained a 35.6% gain when compared to the initial design, full load storage period (TES) required for multi-objective optimization, tower elevation, and SM resulted in a 35.6% increase in energy competence and a 16.9% drop in LCOE. Zhuang et al. (2019) showed a cost-benefit analysis of 100 MW SPT power stations in China utilizing various melted salts and anticipated that the LCOE in China will fall from 0.23 $/kWh in 2017 to 0.10 $/kWh by 2050. In a recent study from Chile looked at the impact of solar extinction on LCOE ( Marzo et al., 2021 ).
2.1.3 Solar Thermal Power Plant With a Linear Fresnel Solar
An absorber, a steam generation system (SGS), a tracking system, and an instrumentation system all employ a collection of Fresnel reflectors built of linear mirrors ( Ghodbane et al., 2019 ). LFR flat mirrors reflect the sun’s straight normal irradiance (or ray radiation) towards absorber surface ( Islam et al., 2018 ). As a result of the strong sun radiation, the water vaporizes. The steam turbine is spun by the evaporated water, which subsequently generator to produce electricity by rotating, thanks to the high pressure. As demonstrated in the study of a 120 MW LFR power plant in the El-Oued region ( Alotaibi et al., 2020 ) (LCOE 14 0, 0382$/kWh; avoided CO 2 14,420, 67 tCO 2 /year) and in India ( Bishoyi and Sudhakar, 2017 ) for countries with significant water stress, Fresnel reflector-based power production technology is a very promising and low-cost technology. However, when compared to other technologies, particularly PTC ( Bellos, 2019 ), in the solar industry, LFR power plants undergo from considerable optical losses. Sanda et al. (2019) gave a thorough review of thermal modeling and visual simulation tools for LFR power plant design. The prices of 50 MW LFR power plants are compared to PTC and SPC power plants in Table 2 with equivalent capacity in India’s diverse climatic zones ( Kumar et al., 2021 ). Several researches on various aspects of LFR power plants, the thermal energy storage system, for example, have been installed to improve the plant’s efficiency. By segregating the TES system from the rest of the system, into numerous modules ( Tascioni et al., 2020 ), established a fresh optimization strategy. This resulted in a 13% improvement in TES efficiency and a 30% reduction in solar field thermal loss. Lopez et al. (2020) were also able to improve the economic and energetic performance of an Iranian power plant by adopting phase change material (PCM) as the storage system.
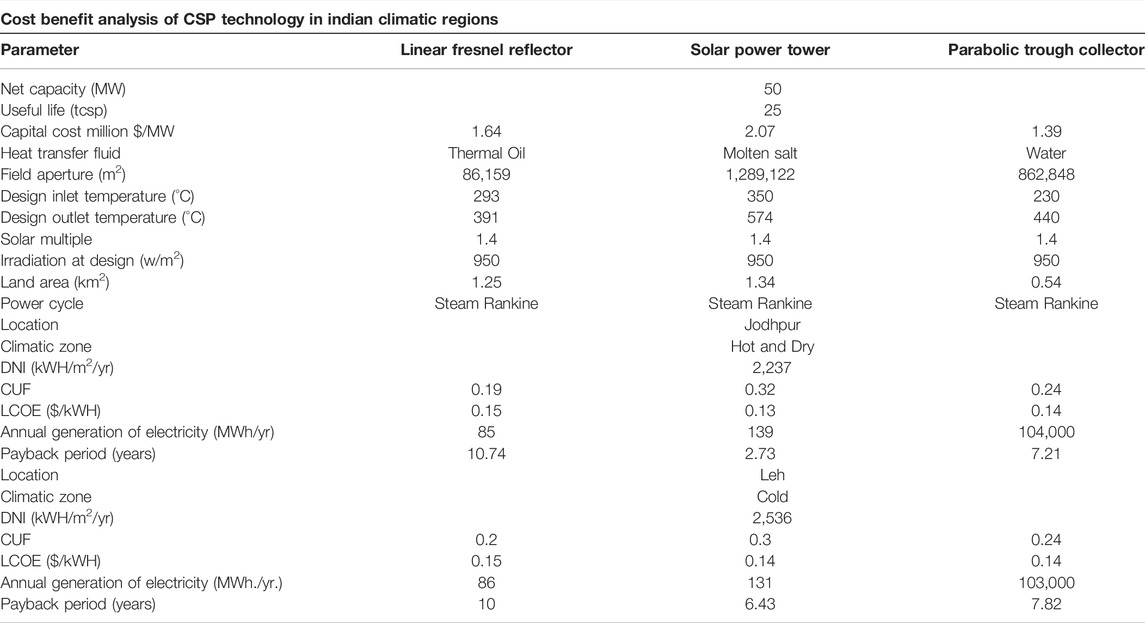
TABLE 2 . Cost-benefit analysis of CSP technology in Indian climatic regions ( Zineb et al., 2021 ).
2.1.4 Power Plants for Parabolic Dish Collectors
The parabolic dish collector (PDC) is a technique that directs solar energy beams gathered by a dish-shaped concentrator to a receiver at its focal point. Flat and cavity receivers are the two types of receivers. To track the direct normal irradiation, the concentrator uses a two-axis tracker. For optimal use of the obtained focused heat, at the focus point, an electrical generator with a Stirling-Brayton mechanism is placed ( Islam et al., 2019 ). When the ratio of concentrations surpasses 3000 ( Islam et al., 2018 ; Lopez et al., 2020 ), the pressure and temperature in the receiver might reach dangerous levels 200 bar and 700–750°C, respectively.
2.2 Photovoltaic Solar Energy Technologies Are Used to Generate Solar Power
2.2.1 pv technology.
PV technology is frequently used because to its simplicity in power generation. As seen in Figure 2 according to this, China (36%) leads the world in PV installed capacity, followed by the United States (13%), and Japan (11%) ( IEA, 2020 ). China is generating more than 175.01 GigaWatts of PV power, with the United States and Japan 62.2 GigaWatts, 55.5 GigaWatts coming in second and third, respectively.
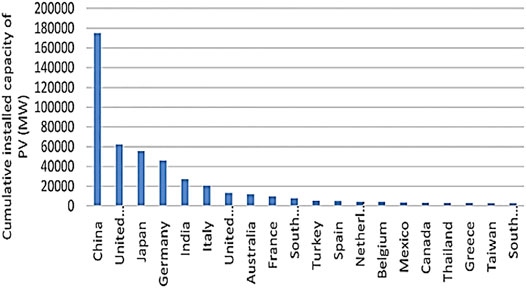
FIGURE 2 . Installed capacity of PV (MW) ( Zineb et al., 2021 ).
2.2.2 Concentrated PV Technology
Concentrated PV (CPV) cells were developed as a result of scientific advancements in the optical instruments field. The CPV focuses sunbeams onto PV cells with the help of utilizing an optical concentrator, such as a curved mirror or a lens. The solar cells’ efficiency improves as a result of the additional photons focused with the concentrator. Adding a concentrator to a cell, according to the literature, enhances the current generated by the cell and improves the efficiency of the cell operating voltage ( Gonzalez-Longatt, 2005 ; Luque and Hegedus, 2011 ). Appropriate concentration technology selection is critical because the performance of the CPV’s optics has a direct and significant impact on the CPV’s efficiency. As a result, Table 2 provides a detailed summary of various concentration schemes. Two (or more) concentration systems are sometimes combined to improve the efficiency of CPV systems. Figure 3 shows the total electricity generated by different countries utilizing CPV. CPV’s installed power ranges up till 2021 from 114 kW in Japan to 67.68 MW in China region. Concerned nations’ typical CPV output is around 22.43 MW. Moreover, China has half (50%) of the mounted CPV volume, tracked the United States having 21%. High concentrated PV (HCPV) accounts for 81% of installed CPV power plants, while low concentrated PV (LCPV) accounts for 19%. As a result, HCPV generates 93% of the produced electricity, whereas LCPV generates just 7%.
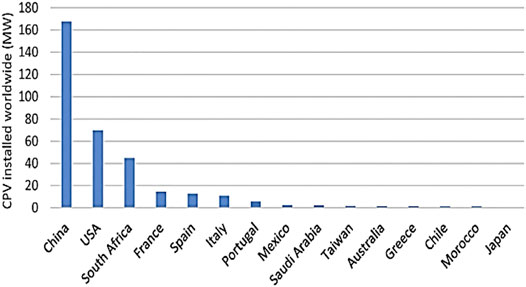
FIGURE 3 . Total Installed CPV worldwide ( Zineb et al., 2021 ).
2.3 Hybrid Solar Thermal Power Generating Technology
Decoupled Photovoltaic and connected PV-CPV/CSP are the two types of hybrid solar power systems. These are systems that combine the two technologies on one worksite. Photovoltaic thermal collector (PVT) or concentrated photovoltaic thermal collector (CPVT) systems are dense or coupled hybrid solar systems that combine CPV/PV and CSP into a sole system are the second type of hybrid solar systems.
2.3.1 Hybrid PV-CSP Technology
PV-CSP hybrid expertise is relatively different topic which has piqued the curiosity of scientists all over the world. By expanding hybrid power plants on large scale, this is especially promising, according to the IEA’s solar thermal energy technology roadmap. The fascinating properties of such a combo, that can enhance system stability, enhance energy quality, cut LCOE, limit heat losses, and increase efficiency of power plant, driving this decision. The strategy for hybrid PV-CSP power plants on a large scale includes a battery energy storage system i-e (BESS) plants in recent research. The expected drop in battery costs may make this option more realistic in future. Several modeling researches dedicated to hybrid decoupled PV/CSP systems are included in Table 3 ( Gaga et al., 2017 ; Ju et al., 2017 ; Moukhtar et al., 2021 ). Conventional PV-thermal hybrid solar systems. A standard photovoltaic module is combined by a thermal accumulator in the PVT solar system to harvest sun energy. Several investigations have been conducted into this ( Hissouf et al., 2020 ).
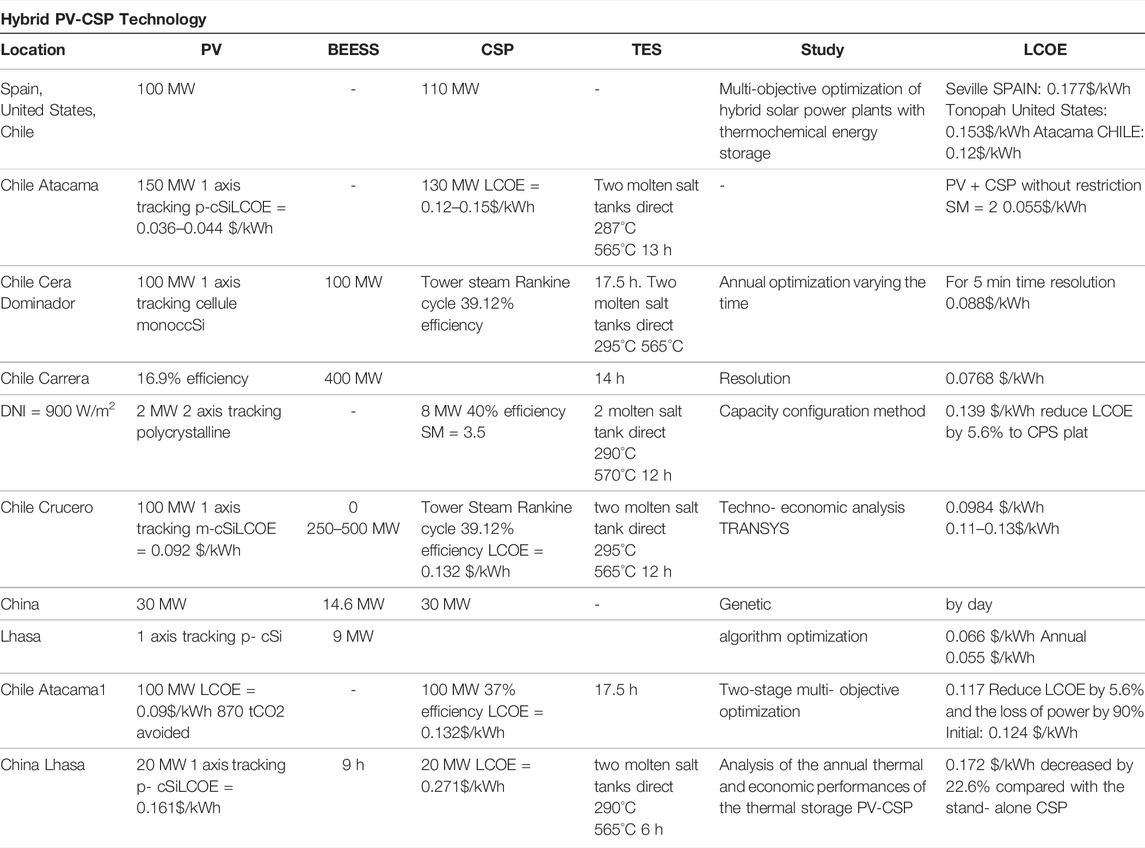
TABLE 3 . Hybrid PV-CSP technology ( Zineb et al., 2021 ).
PV-thermal hybrid solar systems with concentrated photovoltaic. CPV technology, despite its high conversion ratio, is not without flaws, has serious flaw: excessive PV cell heating. A cooling technique is essential to avoid this. In this case, incorporating a thermal procedure achieve dual goals of freezing the CPV cells while also producing valuable thermal heat. The development of hybrid CPV-thermal technology was sparked by this.
2.3.2 Discussion of Thermal Methods for Energy Generation
Thermal technology for energy generation may be split into four groups, according to the review: solar power tower, parabolic dish collector, parabolic trough collector, linear Fresnel reflector, and the parabolic dip is the most commonly used solar thermal technique, having 64 percent of all CSP installation units in operation. Following that is tower technology, which accounts for 31% of all CSP technology installed globally. On either side, because PDC and LFR are two separate entities, they are relatively new technologies with considerable obstacles to overcome, their contribution to the energy mix are fairly limited. Because the performance of each technology is influenced by a variety of elements such as geographic location and HTF used, selecting one technology over another for a given place should be based on these features. The conclusions of this study revealed the barriers to widespread CSP implementation. PDC, for example, is expensive; PTC has low conversion efficiency; and LFR devices have a restricted operating temperature. Furthermore, the significant both the SGS and the cooling process make use of water is a serious issue with CSP facilities. The focus on resolving these difficulties will improve CSP plant acceptability in the background of the global energy alteration.
2.3.3 Photovoltaic Technology Power Generation Discussion
Photovoltaic technologies for power generation are the focus of the present research. Depending on whether or not a concentrator is used, photovoltaic technology may be divided into two groups. Attempting to provide a simple summary of mounted CPV and PV systems across the world, regardless of the fact that CPV has a higher efficiency than PV, the accumulative capacity of CPV mounted globally is small, according to the statistics presented. The comparatively great prices of Concentrators and trackers for CPV explain this result ( Aqachmar et al., 2020 ) directed a viability evaluation of CPV large scale facilities and provided unique policy solutions for addressing the problem of high CPV device pricing. In addition ( Laarabi et al., 2021b ), looked at the soiling problem. In both Morocco and India, the authors reviewed a large amount of literature on PV soiling measurement methodologies, impacts, and cleaning approaches, further showed that soiling is a much localized process, with the position of the plants having a substantial influence on soiling.
2.4 Discussion of Hybrid Technologies to Produce Electricity
According to the findings of this study, hybrid photovoltaic thermal technology may be classed as either traditional PVT or concentrated PVT. The hybrid PV or CPV achieves a dual goal of cooling the PV cells, so increasing electrical output, and providing usable thermal heat for thermoelectric generators. It is worth noting that hybrid solar technologies, whether coupled or decoupled, are still in their infancy and will require more development before being utilized in large-scale power facilities. This covers, among other things, optimizing optical concentrators, water usage, and investment costs. Next section will cover solar photovoltaic energy system types and solar energy optimization method, issues and challenges ( Laarabi et al., 2021b ).
2.5 Types of Solar Photovoltaic Energy System
Figure 4 shows types of the solar photovoltaic systems which includes the most common configuration - a grid-connected PV system, which is used when customers want can reduce their energy costs, and the grid is accessible for using when the array PV is not generating electricity. A “Utility-Interactive PV System or Grid Tied PV System” is a PV-array without the need of a storage system; it is directly connected to the grid. Solar panels that generate part, if not all, of their power demands during the day while staying linked to the local electrical grid at night are included in these Connected Grid PV Systems. In most connected grid PV schemes, extra or surplus electricity is stored in batteries or sent back into the electrical grid. Solar energy can be utilized to meet some or all energy needs for those with a connected grid PV arrangement in their houses and buildings. Moreover, since this type of PV system is indefinitely linked to the grid, there is no need to calculate solar energy consumption or solar panel sizing, enabling for a variety of options, including a system as limited as 1.0 kiloWh on the tower to dramatically lessen your electricity bills, and a much bigger ground assembled array large enough just to totally eliminate your electricity costs completely. Hybrid PV systems are similar to stand-alone ones. The goal of a hybrid power system is to generate as much energy as possible from renewable sources while meeting load demand. An? AC or DC distribution system, a storing system, filters, converters, and a load management or supervisory system are all possible components of a hybrid system, in addition to energy sources. All of these elements related in a different ways. Depending on the system size, renewable energy sources can be linked to the DC bus. HPS systems can generate power ranging from just few watts for personal usage to very few megawatts for modest community electrification systems. As a result, DC loads are frequently supplied by hybrid systems used for extremely low-power applications. Commonly linked with more than 100 kW of power as well as an AC bus are intended to be a component of the system of massive interconnected networks. Furthermost hybrid systems like a UPS system, can serve as a backup power supply during a blackout due to their ability to store energy ( Georgescu-Roegen, 1979 ; Furkan and Mehmet Emin, 2010 ; Raturi, 2019 ). The word “hybrid” in the solar field refers to a system that employs a combination of solar and batteries and may interact with the power grid. The most cost-effective hybrid system employs a basic hybrid inverter, which includes a solar inverter and a battery inverter/charger, as well as smart controls that determine the most efficient practice of your available energy. PV system, stand-alone are suitable for sequestered rural areas and uses in which other sources of power are troublesome/nonexistent for powering lighting, applications, as well as additional equipment. This is frequently additional cost efficient for installing a solo stand-alone PV system to having local energy provider spread the power cables and lines directly towards the house as part of a grid-connected PV arrangement. A simple PV system is a self-contained solar expertise which produces electricity throughout the day to charge batteries for usage whenever the sun energy is absent at night. Rechargeable batteries are used to storing the electrical energy generated by panels (PV) or an arrangement in a stand-alone small-scale PV system ( Farh et al., 2018 ). The battery allows an independent photovoltaic system to run when the solar panel itself does not generate enough energy, because the size of the battery is proportional to the power previously consumed. The two main types of batteries used to store solar energy are deep cycle batteries and shallow cycle batteries. Batteries are necessary component on which every standalone self sufficient solar power system relies. It also transforms electric energy into chemical energy which then is stored for usage anytime the solar array is not generating electricity. The PV system provides direct electricity to the load during daylight hours, with any extra energy being stored in batteries for later use.
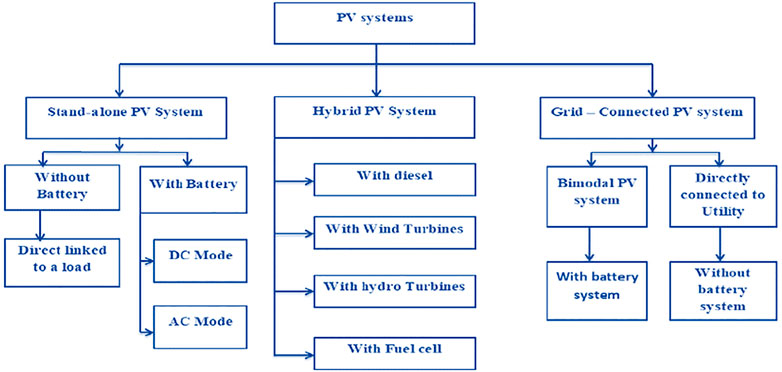
FIGURE 4 . Types of solar photovoltaic systems ( Assadeg et al., 2019 ).
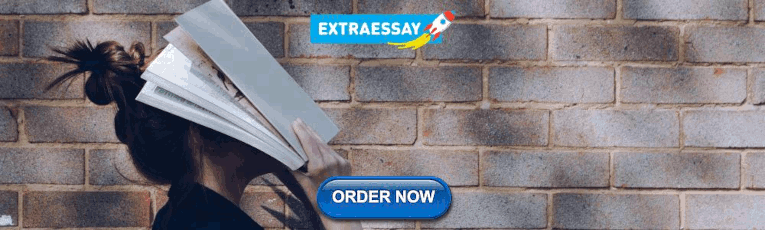
3 Overview of Solar Energy Optimization Method
Solar energy systems emit no noise and produce no pollutants during operation and maintenance. Photovoltaic cell technologies have less environmental dangers than other forms of electric energy sources ( Otero et al., 1998 ). Chemicals used in the manufacture of PV cells, on the other hand, might be discharged into the air, surface water, and groundwater in the production plant, installation site, and disposal or recycling facility. The solar collector storage system may provide energy at temperatures greater than the ambient outside air. A significant quantity of CO 2 is emitted by a PV power plant based on single crystalline silicon technology. There was no pressing necessity for optimizing the energy balance of the production process in the so far very modest PV sector. The analysis of the affecting solar energy system optimization, as well as operational characteristics, is critical aspects in improving power conversion efficiency. The climate has a considerable influence on the solar energy’s reliability systems. As a consequence, optimization tactics are crucial in boosting the solar system’s reliability and efficacy. To accomplish so, strategies for tackling challenging PV system optimization difficulties must be developed.
3.1 Optimization Method for PV Base Hybrid System
3.1.1 hybrid renewable energy system.
Wind turbines, photovoltaic, mini hydro, and/or anything else fossil-fuel-powered producers are all examples of hybrid power systems. Small systems that can power a single home to big systems that can power a colony or an island, these systems come in a variety of sizes. Many isolated locations, especially those in developing countries in which the grid operator is economically and technically non-viable, will benefit from hybrid power systems. In 1978, the first rural hybrid energy system systems, which included solar panels and diesel generators, were built in the United States. Until an electric grid was connected to the hamlet, the power generated by the system was used to power the communal laundry machine, refrigerator, stitching machine, lighting and water drives. Photovoltaic (PV), Micro hydropower (MHP) and tiny wind power bases are routinely used to provide electricity to clients in remote locations, with or without energy storage systems. Varied energy sources have different properties in terms of production, like as seasonal river flows, strong sunlight during the day rather than at night, and high wind speeds in the summer. Commercial PV or wind systems that operate they do not create power 24 h a day, 365 days a year. When PV and wind are combined, the battery bank capacity and fuel requirements (if a conventional generator is utilized as a backup) are reduced, among other benefits. However, in order for a hybrid PV-Wind system to work, the area must have a high potential for both solar and wind energy. Environmental conditions, PV capacity, wind generator capacity, storage device capacity, generating location, and other factors all have a significant impact on the hybrid PV/wind-diesel system’s operation, maintenance, and cost ( Prakash and Khatod, 2016 ).
Extra energy is stored in battery banks, which are then used to power the devices, load when the hybrid system is underpowered. The inverter (DC/AC) must convert to fulfill consumer load demand, the voltage is converted from DC to AC. The battery charger’s output terminal, the storage battery, and the input terminal of the (DC/AC) converter are all linked in equivalent. Because fluctuations in solar radiation and wind velocity have a significant impact on energy generation, hybrid systems must be carefully designed to ensure a consistent power supply to clients in changing climatic conditions. Similarly, to keep system costs low, a detailed design should be conducted.
3.1.2 Photovoltaic System
Solar photovoltaic is the world’s third-largest renewable energy source by installed capacity, after hydro and wind power. Solar panels transform the sun’s solar radiation directly into useful electrical energy ( Figure 5 ). California and the Agua Caliente Solar Project are the world’s largest standalone PV generating installations. The aggregate capacity of both power plants is more than 250 MWP. However, due to the high cost of solar panels, its use is limited to less than 1% of total global energy production. PV energy arrangements are supposed to be unique of the most economical alternatives to encounter rural needs of energy.
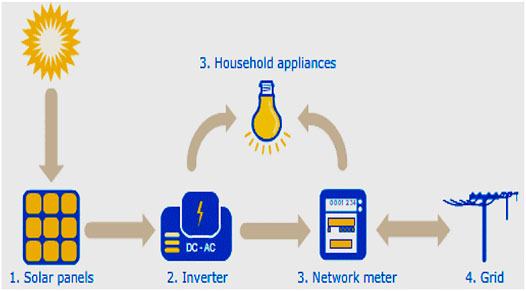
FIGURE 5 . Residential grid-tied solar Photovoltaic system diagram ( Wikimedia Commons, 2018 ).
For small communities of up to 100 homes, the economic feasibility has been built a hybrid PV system for decentralized power generation. The ideal mix can be determined using the hybrid PV system optimization approach based on the charge of energy produced, that is justified further by distance angle, tilt, and azimuth angle from the nearest power line. A PV hybrid system’s performance is measured in terms of electricity generation dependability across a wide range of load circumstances. The load and insolation were calculated using statistical methods. The output power of a PV panel is calculated using the equation below.
Where E stands for energy that is in (kWh). r shows solar panel yield which is in (percent). A stands for total covered panel area (m 2 ).
PR stands for performance ratio, a constant for losses (ranges lies between 0.5 and 0.9, showing default value = 0.75). H stands for solar radiation yearly average on slanted panels, and r is the solar panel return, which is computed by dividing one solar panel’s electrical power which is in kWp by its area.
3.1.3 Hydro System
Over the last four decades, global hydroelectric power output has gradually increased by an average of 3% every year. In 2011, hydropower from over 160 nations generated around 16% of global electricity. Water wheels are the forerunners of current turbines, which are used to transform hydraulic power into mechanical power, which is then converted into electrical power using a generator ( Li, 2021 ). Hydroelectric power, unlike solar and wind power that is fluctuating and constantly changing, is subject to a protracted seasonal cycle. The flow of water in rivers and streams fluctuates slowly as the seasons change.
3.1.4 Wind System
The area must have a high potential for wind energy throughout the year in order to operate a hybrid wind energy system successfully and affordably. Wind energy is currently captured utilizing a variety of small and large wind turbines of varying sizes and designs. It is one of the most rapidly increasing sources of alternative energy. It has a longer operational life than solar power and can generate electricity even on gloomy days and at night.
As a result, both wind and solar power systems require energy storage systems to store extra energy and use it when demand exceeds supply ( Zhang and Toudert, 2018 ; Zheng et al., 2018 ; Motahhir et al., 2020 ). The reassuring option, on the other hand, is that people can produce enough energy to satisfy their regular needs by setting up small solar or wind farms. Figure 6 shows the height to wind speed relation.
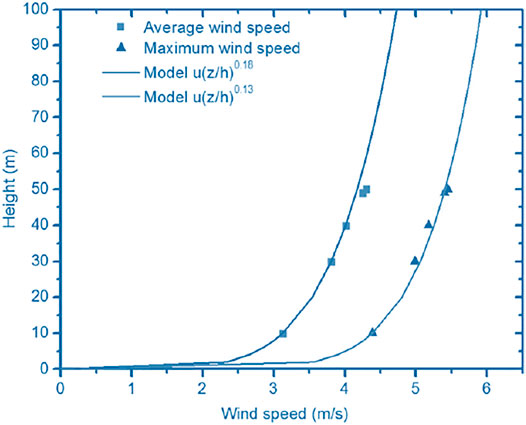
FIGURE 6 . Relation between Wind system and height ( Liu and Janajreh, 2012 ).
The generating capacity of a wind turbine is one of the most important factors to consider decisive criteria in selecting a certain kind of wind turbine for the chosen location is as an important component of a hybrid wind generators. Whenever feasible, turbine with best regular generating capacity recommended. A simplified technique for predicting yearly wind percentage was provided grounded based on the findings of an 8-year simulation using Wind statistics from five different places, hour by hour. Weibull wind speed distribution on a monthly basis data as input, other model factors such as the energy-to-load ratio, battery-to-load ratio, and others are used. Weibull devised the following equation to compute wind speed:
β = shape factor v = wind speed .
3.2 Optimization method for PV Based Grid System
The efficacy of grid-connected solar power is heavily dependent on the site’s solar irradiation, ambient temperature, load demand, and other factors in geographic area of installation. Identifying the best location and size for solar PV installation is a critical answer for improving radial distribution system performance. The design of the PV system to interface artificial intelligence techniques are used in the radial distribution network necessitates power system network analysis and mathematical modeling. The load flow analysis is performed in MATLAB simulation as shown in Figure 7 in conjunction with the SPSO to identify the placement and capacity of solar PV that will link to the Bahir Dar distribution network. By allowing for fluctuations in power demand over time, PV on-grid system integration is crucial for enhancing network capacity and system dependability. The utility grid is connected to an on-grid solar power system. The primary advantage of such a system is that electricity may be obtained from the utility grid, and when that power is unavailable, the PV system can step in. These on-grid systems might include or exclude battery storage. Batteries, a charge controller, solar modules, and inverters are used in these systems to improve the on-grid electrical system’s stability and offer long-term utilities service for a wide range of loads Electronic converters with high power play an important role in connecting a solar system to the grid by converting DC to AC and power conditioning ( Zakaria et al., 2020 ). A proposal is made for the current status of solar optimization study in a power system. This research looks on modeling approaches, restriction criteria, and optimization techniques. Because it is clean, ecologically friendly, and provides reliable power, the PV module system is full of potential. The effects of stand-alone and grid-installed solar generating on power system link, as well as their link to mandate answer, were researched. For calculating the location and size of solar generators, optimization approaches like as the genomic algorithm and swarm optimization were both introduced around the same time ( Getie et al., 2020 ) used the evolutionary algorithm and a geographical information system to integrate solar power with radial feeders. The genetic algorithm which was used to approximate the magnitude and point of penetration, and geographical information is utilized as data to decide where to install solar panels. As the multi objective function for photovoltaic integration, this study solely analyses real power loss and voltage profile.
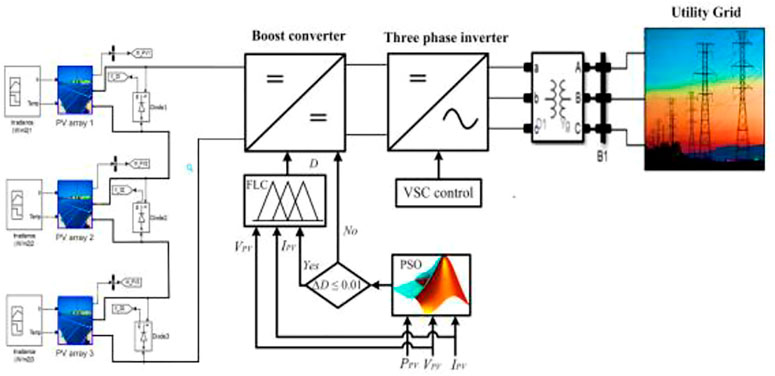
FIGURE 7 . PV based hybrid system MATLAB schematics ( Gonzalez-Longatt, 2005 ).
3.3 Optimization method for PV Based Stand Alone System
A stand-alone PV system should always be able to provide power to the load or energy consumption. The suggested technique, based on using hourly energy analysis, a curl (statistics) for battery bank (power, Wh), PV- array (space, m 2 ) proportions that satisfy the demand at all periods may be created. Since the solar power supply capacity varies, the battery store should be huge enough to provide enough power regardless of the number of cycles (discharge/charge) that the battery has to go concluded. This means that the solar grid will be backed up at sunset to meet the charging until more solar energy is available to start another charging cycle. Solar radiation, array size of PV, and storage volume are used to determine the efficiency of a PV system that is not connected to the grid. As a consequence, the scale of freestanding PV systems is essential to their dependability. Instinctive, analytical sizing methods and numerical are three types of sizing methods. Because it works with intuitive knowledge (without the use of cognitive processes), the first category of algorithms is highly imprecise and unreliable, and the risk of the end result being inaccurate is fairly high. The second is more precise, but accurate modeling requires series of solar radiation for a long term. There are ways in the third category that utilizes equations to describe the size of PV arrangement displaying as a result of dependability that is the focus of this study: boosting the output power of PV modules while lowering the system life cycle cost. Since most of the solar energy arrives in a straight line, solar panels or solar installations that point directly at the sun accumulate more energy by being perpendicular to the straight line between the panel and the sun. During the day, solar panels should face the earth’s equator (southern northern hemisphere or northern southern hemisphere) to capture as much solar energy as possible. The challenges and limitations of autonomous solutions to optimize the size of photovoltaic installations are highlighted to solve the problems of inaccurate parameter assumptions and poor demand performance evaluation of photovoltaic systems, which often lead to high material and installation costs. Along the same route, a new adaptation method was also proposed to improve the ability of photovoltaic generators to provide power to remote areas with pumping storage. Their research results show that zero power outages can be achieved at low energy costs, but the system does not use all the solar energy available in the area. Photovoltaic systems analysis refers to the concept of daily battery status to improve reliability while minimizing the possibility of power outages, excess energy, and cost constraints. However, priority must be given to strict compliance with the load profile. Another optimization strategy involves three steps. The first step is to calculate the photovoltaic power generation capacity connected to the grid with the help of 1-year solar energy data. It is believed that peak sunlight, ambient temperature, and cable and dust losses will affect the output energy of photovoltaic networks.
The quantity of stored energy, PV array output energy, load energy demand, battery efficiency, and inverter efficiency are used to compute the daily status of the battery storage in the second stage. In the third step, the chance of load loss is assessed, and the system cost is approximated using the costs of the PV array, batteries, and other components ( Lund and Mathiesen, 2009 ). On the other hand, the system cost equation is only partially derived and needs to be solved intuitively. The system simulation can be adapted to reduce the battery when the size of the solar photovoltaic device is not limited (very large). Since the solar photovoltaic device is huge, the system simulation can be repeated after determining the minimum battery size, but this time it is the smallest solar photovoltaic device. The size of the battery makes the battery sensitive to deep discharge when using this method and the size of the photovoltaic installation makes the system too large to be economically feasible. Therefore, the capacity of the battery pack can be increased, and each size can be simulated to find the smallest array. You can repeat this process to create a curve of the battery and PV array size pair, as shown in the results and discussion section. The accuracy of this method is determined by the simulation data, which includes both environmental parameters (such as sunlight exposure and ambient temperature) and loads. Therefore, hourly data obtained from reliable models such as those used in this study can guarantee availability and synchronization of exposure to solar radiation. To develop energy balances over time while taking into account real energy requirements for an energy efficient house as well as real radiation and ambient temperature data, a PV single system with dependencies on ambient temperature and other important factors was used in conjunction with inverter converter performance data. To ensure continuous functioning, the simulations first established a direct relationship between the area of the PV array and the capacity of the battery bank. One extreme of the connection is a high area of PV panels paired with a small battery capacity, resulting in low PV array effectiveness and a low battery consumption index. A small PV array, on the other hand, is the polar opposite. The tilt angle optimization approach was developed as a new optimization tool. Because of climatic and environmental conditions that fluctuate throughout the year, such as seasonal fluctuations, this optimization approach is focused at calculating effective tilt angles at various periods of the year. When compared to tracking systems, this strategy enhances collection efficiency while incurring no additional costs. Averaging the values of the solar geometry parameters for each mean solar day was used to do the calculations. The incident solar irradiances on a tilted surface, which include direct, diffuse, and reflected solar irradiances, may be expressed as a function of the global irradiance I on a horizontal surface by
The notion of the optimal periodical inclination angle was used to carry out this optimization, which allows for maximum incidence on the panels while simultaneously maximizing the use of the household’s actual energy usage. The inclination angle should be changed seven times per year, according to the computation of solar radiation for sloping surfaces using different solar geometry factors and incoming solar radiation, although total apparent power overestimates the load demand by 18%. On the other hand, these adjustments result in higher panel output and PV station reliability. The analysis of random load fluctuation demonstrates that the load profile must be followed notwithstanding the constraints. A power shortfall in the plant might occur from even slight increases in load demand.
3.3.1 Utilization of Solar Photovoltaic Energy
Photovoltaic systems power entire towns in distant places of the world. In the United States and Europe, a few utility companies operate “solar farms” to generate power ( Majidi et al., 2017 ). Photovoltaic cells have other industrial applications as well. These are often low-power applications in regions where regular electricity sources are cumbersome. Some emergency roadside phones use solar cells to charge their batteries. Some of the common applications are listed below.
3.3.2 Duffel Bags
The thin-film solar panels mounted on the outside of the backpack generate up to 4 W of power, which is enough to charge mobile phones, cameras and other electrical appliances while walking. External solar cells can also be added to briefcases and handbags. Students, hikers, and campers who need to keep their electronics charged while travelling or when they arrive at their destination would appreciate these backpacks.
3.3.3 Paint
Rather of utilizing standard silicon solar cells, polymers soaked in a solvent form “paint” or coat that may be put to any surface, include buildings, workplaces, and vehicles. It is low-cost and versatile. Instead of bulky solar photovoltaic panels, a solar paint employs thin-film nanoparticles as solar conductors rather than silicon. When small solar cells are placed to the surfaces of structure which confront the sun, they silently create pure, green power.
3.3.4 Solar Transportation
Photovoltaic (PV)-powered transportation is a novel technique to make the most of the sun’s energy. Solar energy can be used to power trains, subways, buses, airplanes, vehicles and even roads, and solar transportation is rapidly becoming a leading choice for renewable energy. A solar-powered aircraft has just completed a round-the-world voyage over the Pacific Ocean, capturing huge waves in unforgettable images. On the other hand, solar buses are helping China reduce its carbon footprint while ensuring efficient public transportation in densely populated areas such as Beijing. In the end, solar cars began to appear in racing competitions around the world, especially in Australia, where the solar spirit model aroused great interest. With these and other improvements, there is no doubt that solar energy is transforming the global transportation sector.
3.3.5 Refrigerators for Vaccines
As the entire world is experiencing the effects of the Corona Virus. Vaccine refrigerators are now required in all hospitals and clinics. There is no guarantee of 24-h electricity in developing countries, there is often no electrical infrastructure. Solar-powered vaccination coolers have been developed by private companies for use by healthcare workers in rural areas may provide crucial medication to individuals in need, according to Charlie Gay, Office of Energy Efficiency and Renewable Energy, Solar Energy Technologies Office. This technical solution has been saving lives for more than four decades.
3.3.6 Cell Phone Charger
After a few hours of ultraviolet radiation, the mobile phone USB charger can fully charge the mobile phone. These tablet-sized solar panels can power GPS trackers, tablets and even computers. They can be attached to backpacks and used to extract solar energy while walking, making them ideal for leisure activities ( Ming et al., 2017 ).
3.3.7 Solar Textile
Solar garments are a sort of solar textile that can be used for a variety of purposes. To generate useable solar power, solar cells are weaved into textile strands. According to Hicks, one variation, developed with faster than light (FTL) Solar, might remain erected like a camp to supply both electricity and shelter. Considering military service, safety missions, respite efforts, leisure activities, medical centers, and even makeshift housing as options. Solar fabric is the ideal answer for everywhere that need flexible and convenient solar power. According to Gay, roofs are one of hundreds of places somewhere solar panels create energy. We expect to see many more sites where solar technology is used to offer unconstrained, low-cost electricity as costs decrease and energy output rises.
3.3.8 Solar Water Pumps
Solar water pumps are used to promote water for irrigation, gardening, household use, drinking and other related purposes. These devices are suitable for areas where there is no electricity or limited power supply. The precisely crafted modules of the system are impact resistant and can withstand harsh weather conditions such as storms, rain, and dust.
3.3.9 Solar Tents
The solar tent is just a larger solar backpack. The built-in photovoltaic cells in the tent store solar energy throughout the day and are then used to illuminate the tent at night, as well as small electrical appliances such as charging or power electronics and radiators. The United States military uses a variant that can generate up to 2 kW of electricity during day.
3.3.10 Solar Buildings Technologies
Passive cooling and heating systems rely on the building’s design to satisfy specified thermal demand objectives with little or no mechanical support. Active heating systems use mechanical aid to provide hot water for space heating, while passive heating and cooling systems rely on the building’s architecture to satisfy set thermal demand objectives. Solar building technique is widely used in Pakistan ( Li and Zheng, 2019 ). However, there are no construction laws in place in the country that allow solar building systems installation.
3.3.11 Street Lights
Solar energy is increasingly being used to power streetlights around the world. The sun charges the batteries throughout the day, which power the light-emitting diodes (LEDs) that illuminate the streets at night. Smart sensors are being installed in streetlights in San Diego, which might direct motorists to open parking spots and assist first responders in an emergency. The combination of internet-connected sensors and solar-powered lamps saves both time and money ( Indra Gandhi et al., 2018 ).
3.3.12 Solar Ovens
Solar ovens, sometimes called solar cookers, use the sun’s energy to prepare food. Solar cookers either are parabolic or square shapes covered with a reflecting substance that focuses the amount of solar radiation into the box, warming the food equally. To assist focus the sun’s beams, the top lid is commonly made of glass. They are healthy to live with and are commonly employed in developing countries to reduce air pollution produced by fuel burning.
3.4 Optimization Issues
Solar energy confronts significant obstacles that might stymie its rapid expansion. These impediments can be characterized in terms of technology, politics, economics, and dependability. The adjustment of these issues, on the other hand, reduces the drawbacks and improves the solar energy system’s reliability. As a result, greater solar energy optimization can help to alleviate production uncertainty. PV power technology is being heavily invested in to improve efficiency and economic feasibility.
3.4.1 Extra Investment
Inverters and storage batteries must be purchased separately from PV cells. For use on the power grid, inverters convert direct current to alternating current. Storage batteries are important in on-grid connections for giving continuous power of electric power. On the other side, this higher spending could provide a solution to the PV cells’ intermittent problems ( Dong et al., 2019 ).
3.4.2 Issues With Intermittency
Solar energy and photovoltaic cells, like all other renewable energy sources, are prone to outages. It implies that it is not always available for power conversion, such as at night or when the weather is gloomy or damp. As a result, PV cells are unlikely to meet all of an electric power system’s demands.
3.4.3 Easily Broken
Solar PV has no upkeep or operating costs, it is vulnerable to damage due to its fragility. To protect your investment, there is a solution in the form of additional insurance.
3.4.4 Expensive
PV system market costs remain exorbitant and beyond of reach for many households. The higher production costs of non-conventional energy sources, combined with the availability of cheaper fossil fuel alternatives, entice customers and generate market rivalry for non-renewable technology ( Lagouir et al., 2019 ). The lack of economic models to support renewable energy technology prohibits small-scale PV systems from being scaled up to large-scale or commercial facilities. Subsidies are distributed more effectively to traditional fuel sources, giving them an unfair advantage over nonconventional sources. Governments must stimulate the market for PV technologies in order to reap the most advantage from renewable applications in the government market, government-driven market, and loan and cash market. PV plant input requirements, like as land and water, impede the installation of PV capacity.
3.4.5 Low Productivity Level in Future
In the near future, the technology will face another severe threat when the panels put during the early stages of the energy boom reach the end of their anticipated lifetime and are finally thrown in landfills. When the life duration of these panels reaches roughly 25 years, as indicated by the manufacturer, their productivity begins to decline. Recycling solar panels is a logical alternative for addressing the predicted worldwide PV waste, since retired PV panels may be reconditioned and redeployed. Recycling not only provides an effective method of recovering valuable elements from solar waste, but it also contributes to a better environment by using less energy to recover raw materials. The research and development work concerning solar PV recycling has already begun in countries such as Japan, the United States, India, Australia, and Europe.
3.4.6 Lack of Trained Professionals
The scarcity of trained personnel to teach, operate, and maintain non-conventional energy infrastructure, particularly in rural regions, has a detrimental impact on people’s desire to adopt these technologies. Geographic location is also important, because PV systems are only practical in certain places, and they face competition from alternative technologies that are better suited to the specific topography. Adopters are often concerned about systems failing during the rainy season and avoid purchasing PV systems owing to a lack of understanding. The absence of information between the supply and adopter sides further impedes the technology’s uptake. Because the generation and consuming sites are far apart, poor grid connection raises transportation costs and transmission losses. As a result, the majority of investors are hesitant to invest in technology.
3.4.7 Environmental Disadvantages
Due to various preliminary case studies, there is little understanding of the environmental and economic advantages of PV recycling technology. Although element recovery is advantageous, the energy required to collect valuable metals from discarded PV panels is greater than that required to gather, dismantle, and retrieve the modules. Although recycling operations are not viable, the cost of recycling PV panels is minimal ( Maulik and Das, 2018 ) According to research, the profit earned from selling the recycled material of copper indium gallium selenite solar panels (CIGS) is greater than the recovery price. However, the earned amount for c-Si and p-Si solar modules is less than the cost of solar panels. Despite the financial benefits of reselling recovered items and the environmental benefits of recycling, businesses prefer landfill disposal to recycling due to the lower initial cost of dumping.
4 Optimization Challenges
Table 4 shows the summary of different approaches which are used for optimization techniques and method. Non-conventional energy sources have been an important aspect of study and development among scholars since the early 20th century. Despite the remarkable technologies that have evolved in recent decades, the majority of developing countries have delayed the shift to renewable energy sources. Fossil fuels have boosted CO 2 emissions and contributed to global warming. Because of numerous types of hurdles, the majority of nations have exhibited reluctance to adopt renewable technologies. Based on the existing market, policy implementation is critical, which includes energy auctions, integration of PV technologies with non-conventional energy sources, and timely completion of PV projects. By allowing users to purchase or lease a portion of the shared PV system, community-shared solar projects aid in the creation of financial arrangements and the alleviation of financial constraints. These business models, in turn, contribute to the development of a PV system in the residential market, the stabilization of power prices, and the reduction of power bills. To reap the full benefits of an energy transition, policies must be strengthened through mobilizing financial investment, economic diversification, and information exchange. PV system market costs remain exorbitant and beyond of reach for many households. The lack of economic models to support renewable energy technology prohibits small-scale PV systems from being scaled up to large-scale or commercial facilities.
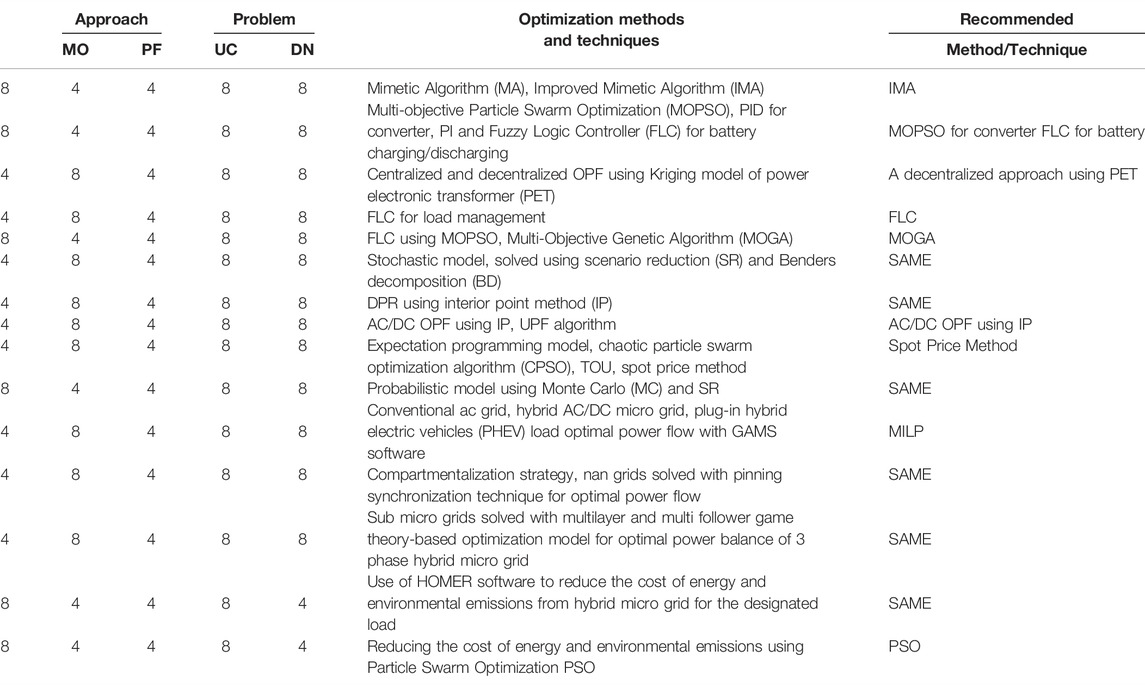
TABLE 4 . Summary of approach optimization technique and methods ( Li et al., 2016 ).
4.1 RES Optimization Challenges
To enhance efficiency, there is a significant investment in renewable energy technologies. The cost of producing renewable energy continues to plummet in 2017, according to an IRENA (2018) . The development and deployment of renewable energy technologies necessitate policies and expenditures that have not been thoroughly assessed. As a result, power quality is a metric that assesses a system’s capacity to offer users with continuous access to their electronic devices. Any irregularity or breakdown in the power grid that obstructs or interrupts electrical equipment’s operation indicates a lack of power quality or reliability. Renewable energy sources will save costs in both transmission and production. The most prevalent disadvantage of employing RESs is that they represent a constant challenge because to their fluctuating existence, which is entirely dependent on climate fluctuation and may result in load refusal in some places ( Maulik and Das, 2018 ). The power production of RESs has substantially grown as a consequence of the deployment of various optimization technologies in response to growing energy demand and improved performance. The entire power producing capacity has increased by about 9% since 2016 ( Zakaria et al., 2020 ). According to the reference, renewable energy accounted for 70% of net additions to global power capacity in 2017 ( Raturi, 2019 ). The key causes for this were the improved cost competitiveness of solar PV panels and wind turbine technology, as well as the availability of performance optimization technologies. Furthermore, the increased usage of renewable energy raises awareness of the need of energy efficiency and quality in power generation and distribution. The biggest disadvantage of adopting renewable resources is that they are intermittent nonetheless, one of the key advantages is the system reliability demonstrated by the operational parameters. Power generated from renewable sources will soon be less expensive than electricity generated from fossil fuels. General, the development of RESs has resolved various difficulties-optimization-in reducing energy costs, reducing costs, net and other optimizations related to costs, such as reducing life cycle costs (LCC). The optimal size and capacity of the trees is determined by providing the required reliability in terms of power supply, operating costs, and grid power and greenhouse gas emissions.
4.2 Energy Optimization Challenges
The uncertainty of how much of the sun’s rays it will get is an issue for solar PV because the weather might change at any time. As a result, determining how much energy to store for future use would be challenging. While power is still required, sunlight is rare during the night. Solar energy has significant obstacles that might limit its fast expansion. Technology, politics, economics, and reliability are the four areas that these hurdles fall into. On the other side, addressing these problems decreases the disadvantages and improves the solar energy system’s dependability. The researchers are also given information on the most recent developments in intelligent optimization in solar energy applications, as well as important research topics. Since the goal of optimization is to maximize benefits while reducing costs, it is critical to understand the advantages and disadvantages of the systems under consideration. In this setting, academics have begun to explore and propose strategies and models to maximize advantages while minimizing drawbacks. To overcome difficulties related to the design, operation, and process of renewable systems, several researches combine traditional optimization techniques with newer heuristic approaches ( Zhao T. et al., 2017 ; Zhao Zy. et al., 2017 ; Allam et al., 2018 ; Eajal et al., 2017 ). PV systems require precise and reliable performance data in order to precisely assess power output and capacity in current operating circumstances. The formulation of effective operational and control choices is aided by this dependable data. On the other hand, by examining the numerous aspects that impact performance and exploring potential ways to increase the power plant’s performance, the optimization and efficiency of a solar system may be improved. PV cells have a number of problems, including a halt in power output when the panel is not exposed to sunlight and a poor efficiency. This might result in the system’s original investment criteria not being met. As a result, solar energy storage devices have been proposed as a means of compensating for the lack of light and smoothing out power output. This technology is dependent on batteries, which are frequently bulky, huge, and heavy, take up a lot of space, and require maintenance or even replacement on a regular basis ( Li et al., 2016 ).
5 Conclusion
For policymakers all throughout the world, this document presented an in-depth review and relative analysis of solar technology for clean power generation.
According to the research results, there are two types of technologies: complex technologies, such as PTC, PV and STP, with a total installed capacity of 7,828.5 MW and an efficiency of 10–16%, LCEO is $0.1–0.24/kwh, which has broad prospects in terms of environmental impact and technical efficiency. There are also technologies that, although having a 390 MW installed capacity, look to be promising in terms of environmental implications and technological efficiency. Furthermore, CPVT and CPV, they have yet to be utilized in large-scale power facilities since they are still in the early stages of development. Nonetheless, Scientists from several nations are leading the charge in CPV and CPVT research. The use of solar energy to improve energy efficiency has been a concern due to the dynamic nature of solar energy, solar PV material, design, and challenging computation of optimization difficulties. As a result, this review looks into solar energy optimization in depth. The optimization techniques have shown excellent results in solar PV applications in terms of size, power production and capacity demand. Additionally, the enhancements to reduce operational expenses and power damages while also increasing peak power integration and controllability. The paper also looked at the primary roadblocks to solar PV optimization, emphasizing the importance of modern computers and objective function ( Qiu et al., 2019 ).
Author Contributions
AS, and AH, suggested the idea of this work, wrote the manuscript and made final improvement, whereas SC and PM provided help with alignment of the paper, proof reading, editing, improvement of the article. AI and MM provided the financial assistance.
Conflict of Interest
The authors declare that the research was conducted in the absence of any commercial or financial relationships that could be construed as a potential conflict of interest.
Publisher’s Note
All claims expressed in this article are solely those of the authors and do not necessarily represent those of their affiliated organizations, or those of the publisher, the editors and the reviewers. Any product that may be evaluated in this article, or claim that may be made by its manufacturer, is not guaranteed or endorsed by the publisher.
Achkari, O., and El Fadar, A. (2020). Latest Developments on TES and CSP Technologies - Energy and Environmental Issues, Applications and Research Trends. Appl. Therm. Eng. 167, 114806. doi:10.1016/j.applthermaleng.2019.114806
CrossRef Full Text | Google Scholar
Agyekum, E. B., and Velkin, V. I. (2020). Optimization and Techno-Economic Assessment of Concentrated Solar Power (CSP) in South-Western Africa: A Case Study on Ghana. Sustain. Energy Technol. Assessments 40, 100763. doi:10.1016/j.seta.2020.100763
Allam, M. A., Hamad, A. A., Kazerani, M., and El-Saadany, E. F. (2018). A Novel Dynamic Power Routing Scheme to Maximize Loadability of Islanded Hybrid AC/DC Microgrids under Unbalanced AC Loading. IEEE Trans. Smart Grid 9, 5798–5809. doi:10.1109/tsg.2017.2697360
Alotaibi, S., Alotaibi, F., and Ibrahim, O. M. (2020). Solar-assisted Steam Power Plant Retrofitted with Regenerative System Using Parabolic Trough Solar Collectors. Energy Rep. 6, 1 24–33. doi:10.1016/j.egyr.2019.12.019
Alsaffar, A. (2015). An Overview of Location Planning of Solar Generation. Available at: https://www.nrel.gov/docs/fy14osti/60240.pdf .
Google Scholar
Aly, A., Bernardos, A., Fernandez-Peruchena, C. M., Jensen, S. S., and Pedersen, A. B. (2019). Is Concentrated Solar Power (CSP) a Feasible Option for Sub-saharan Africa?: Investigating the Technoeconomic Feasibility of CSP in Tanzania. Renew. Energy 135, 12 24–40. doi:10.1016/j.renene.2018.09.065
Aqachmar, Z., Allouhi, A., Jamil, A., Gagouch, B., and Kousksou, T. (2019). Parabolic Trough Solar Thermal Power Plant Noor I in Morocco. Energy 178. doi:10.1016/j.energy.2019.04.160
Aqachmar, Z., Bouhal, T., and Lahrech, K. (2020). Energetic, Economic, and Environmental (3 E) Performances of High Concentrated Photovoltaic Large Scale Installations: Focus on Spatial Analysis of Morocco. Int. J. Hydrogen Energy 45, 10840–10861. doi:10.1016/j.ijhydene.2020.01.210
Assadeg, J., Sopian, K., and Fudholi, A. (2019). Performance of Grid-Connected Solar Photovoltaic Power Plants in the Middle East and North Africa. Int. J. Electr. Comput. Eng. (IJECE) 9, 3375. doi:10.11591/ijece.v9i5.pp3375-3383
Awan, A. B., Chandra Mouli, K. V. V., and Zubair, M. (2020a). Performance Enhancement of Solar Tower Power Plant: a Multi-Objective Optimization Approach. Energy Convers. Manag. 225, 113378. doi:10.1016/j.enconman.2020.113378
Awan, A. B., Zubair, M., and Chandra Mouli, K. V. V. (2020b). Design, Optimization and Performance Comparison of Solar Tower and Photovoltaic Power Plants. Energy 199, 117450. doi:10.1016/j.energy.2020.117450
Bamisile, O., Huang, Q., Dagbasi, M., and Adebayo, V. (2020). Thermo-environ Study of a Concentrated Photovoltaic Thermal System Integrated with Kalina Cycle for Multigeneration and Hydrogen Production. Int. J. Hydrogen Energy 45 (51), 267 16–32. doi:10.1016/j.ijhydene.2020.07.029
Bellos, E. (2019). Progress in the design and the applications of linear Fresnel reflectors e a critical review. Therm. Sci. Eng. Prog. 10, 1 12–37. doi:10.1016/j.tsep.2019.01.014
Bishoyi, D., and Sudhakar, K. (2017). Modeling and Performance Simulation of 100 MW LFR Based Solar Thermal Power Plant in Udaipur India. Resource-Efficient Technol. 3 (4), 365–377. doi:10.1016/j.reffit.2017.02.002
Boukelia, T. E., Arslan, O., and Mecibah, M. S. (2017). Potential Assessment of a Parabolic Trough Solar Thermal Power Plant Considering Hourly Analysis: ANN-Based Approach. Renew. Energy 105, 3 24–33. doi:10.1016/j.renene.2016.12.081
Chen, R., Rao, Z., and Liao, S. (2018). Determination of Key Parameters for Sizing the Heliostat Field and Thermal Energy Storage in Solar Tower Power Plants. Energy Convers. Manag. 177, 3 85–94. doi:10.1016/j.enconman.2018.09.065
Collado, F. J., and Guallar, J. (2019). Quick Design of Regular Heliostat Fields for Commercial Solar Tower Power Plants. Energy 178, 1 15–25. doi:10.1016/j.energy.2019.04.117
Dong, L., Zhang, T., Pu, T., Chen, N., and Sun, Y. (2019). A Decentralized Optimal Operation of AC/DC Hybrid Microgrids Equipped with Power Electronic Transformer. IEEE Access 7, 157946–157959. doi:10.1109/access.2019.2949378
Dowling, A. W., Zheng, T., and Zavala, V. M. (2017). Economic Assessment of Concentrated Solar Power Technologies: a Review. Renew. Sustain. energy Rev. 72, 1019–1032. doi:10.1016/j.rser.2017.01.006
Eajal, A. A., El-Saadany, E. F., and Ponnambalam, K. (2017). “Optimal Power Flow for Converter-Dominated AC/DC Hybrid Microgrids,” in Proceedings of the 2017 IEEE International Conference on Industrial Technology (ICIT) (Toronto, Canada: IEEE ), 603–608. doi:10.1109/icit.2017.7915427
Eddhibi, F., Ben Amara, M., Balghouthi, M., and Guizani, A. (2017). Design and Analysis of a Heliostat Field Layout with Reduced Shading Effect in Southern Tunisia. Int. J. Hydrogen Energy 42 (48), 289 73–96. doi:10.1016/j.ijhydene.2017.07.217
Farh, H. M. H., Eltamaly, A. M., and Othman,, M. F. (2018). Hybrid PSO-FLC for Dynamic Global Peak Extraction of the Partially Shaded Photovoltaic System. PLOS ONE 13, e0206171. doi:10.1371/journal.pone.0206171
PubMed Abstract | CrossRef Full Text | Google Scholar
Furkan, D., and Mehmet Emin, M. (2010). Critical Factors That Affecting Efficiency of Solar Cells. Smart Grid and Renewable Energy 1 (1), 47–50. doi:10.4236/sgre.2010.11007
Gaga, A., Benssassi, H., Errahimi, F., and Sbai, N. E. (2017). Battery State of Charge Estimation Using an Adaptive Unscented Kalman Filter for Photovoltaics Applications. Int. Rev. Autom. Control 10 (4), 3 49–58. doi:10.15866/ireaco.v10i4.11393
Georgescu-Roegen, N. (1979). Energy Analysis and Economic Valuation. South. Econ. J. 45, 1023–1058. doi:10.2307/1056953
Getie, E. M., Gessesse, B. B., and Workneh, T. G. (2020). Photovoltaic Generation Integration with Radial Feeders Using GA and GIS. Int. J. Photoenergy 2020, 8854711. doi:10.1155/2020/8854711
Ghodbane, M., Boumeddane, B., and Said, N. (2016). A Linear Fresnel Reflector as a Solar System for Heating Water: Theoretical and Experimental Study. Case Stud. Therm. Eng. 8, 176–186. doi:10.1016/j.csite.2016.06
Ghodbane, M., Boumeddane, B., Said, Z., and Bellos, E. (2019). A Numerical Simulation of a Linear Fresnel Solar Reflector Directedto Produce Steam for the Power Plant. J. Clean. Prod. 231, 494e508. doi:10.1016/j.jclepro.2019.05.201
Gonzalez-Longatt, F. M. (2005). Model of Photovoltaic Module in Matlab. Ii Cibelec 2005, 1–5.
Hakimi, M., Baniasadi, E., and Afshari, E. (2020). Thermo-economic Analysis of Photovoltaic, Central Tower Receiver and Parabolic Trough Power Plants for Herat City in Afghanistan. Renew. Energy 150, 8 40–53. doi:10.1016/j.renene.2020.01.009
Hissouf, M., Feddaoui, M., Najim, M., and Charef, A. (2020). Numerical Study of a Covered Photovoltaic-Thermal Collector (PVT) Enhancement Using Nanofluids. Sol. Energy 199, 1 15–27. doi:10.1016/j.solener.2020.01.083
IEA (2020). International Energy Agency. Available at: https://www.iea.org/ .
Indra Gandhi, V., Logesh, R., Subramaniyaswamy, V., Vijayakumar, V., Siarry, P., and Uden, L. (2018). Multi-Objective Optimization and Energy Management in Renewable Based AC/DC Microgrid. Comput. Electr. Eng. 70, 179–198.
IRENA (2018). Global Energy Transformation: A Roadmap to 2050 . International Renewable energy agency . Available at: https://www.irena.org/-/media/Files/IRENA/Agency/Publication/2018/Jul/IRENA_Renewable_Energy_Statistics_2018.pdf .
Islam, M. T., Huda, N., Abdullah, A. B., and Saidur, R. (2018). A Comprehensive Review of State-Of-The-Art Concentrating Solar Power (CSP) Technologies: Current Status and Research Trends. Renew. Sustain. Energy Rev. 91, 987–1018. doi:10.1016/j.rser.2018.04.097
Islam, M. T., Huda, N., and Saidur, R. (2019). Current Energy Mix and Techno-Economic Analysis of Concentrating Solar Power (CSP) Technologies in Malaysia. Renew. Energy 140, 789–806. doi:10.1016/j.renene.2019.03.107
Ju, X., Xu, C., Hu, Y., Han, X., Wei, G., and Du, X. (2017). A Review on the Development of Photovoltaic/concentrated Solar Power (PVCSP) Hybrid Systems. Sol. Energy Mater Sol. Cells 161 (12), 3 05–27. doi:10.1016/j.solmat.2016.12.004
Kumar, S., Agarwal, A., and Kumar, A. (2021). Financial Viability Assessment of Concentrated Solar Power Technologies under Indian Climatic Conditions. Sustain. Energy Technol. Assessments 43, 100928. doi:10.1016/j.seta.2020.100928
Laarabi, B., El Baqqal, Y., Dahrouch, A., Barhdadi, A., Deep Pizzi, S., Corbo, L., et al. (2021a). Fintech and SMEs Sustainable Business Models: Reflections and Considerations for a Circular Economy. J. Clean. Prod. 281, 125217.
Laarabi, B., El Baqqal, Y., Rajasekar, N., and Barhdadi, A. (2021b). Updated Review on Soiling of Solar Photovoltaic Systems Morocco and India Contributions. J. Clean. Prod. 311, 127608. doi:10.1016/j.jclepro.2021.127608
Lagouir, M., Badri, A., and Sayouti, Y. (2019). “An Optimal Energy Management System of Islanded Hybrid AC/DC Microgrid,” in Proceedings of the 2019 5th International Conference on Optimization and Applications (ICOA) (Kenitra, Morocco: IEEE ), 1–6.
Li, P., Hua, H., Di, K., and Zhou, J. (2016). “Optimal Operation of AC/DC Hybrid Microgrid under Spot Price Mechanism,” in Proceedings of the 2016 IEEE Power and Energy Society General Meeting (PESGM) (Boston, MA, USA: IEEE ), 1–5. doi:10.1109/pesgm.2016.7741670
Li, P., and Zheng, M. (2019). Multi-objective Optimal Operation of Hybrid AC/DC Microgrid Considering Source-Network-Load Coordination. J. Mod. Power Syst. Clean. Energy 7, 1229–1240. doi:10.1007/s40565-019-0536-3
Li, y. N. (2021). Renewable Power Generation Subsidies in china: An Economic Feasibility Analysis and Policy Recommendations . University of Tokyo . doctoral dissertation.
Liu, S., and Janajreh, I. (2012). “Wind Energy Assessment: Masdar City Case Study,” in 2012 8th International Symposium on Mechatronics and its Applications ( Springer ), 1–6. doi:10.1109/ISMA.2012.6215162
Lokar, J., and Virtic, P. (2020). The Potential for Integration of Hydrogen for Complete Energy Self-Sufficiency in Residential Buildings with Photovoltaic and Battery Storage Systems. Int. J. Hydrogen Energy 45, 345 66–78. doi:10.1016/j.ijhydene.2020.04.170
Lopez, O., Banos, A., and Arenas, A. (2020). On the Thermal Performance of Flat and Cavity Receivers for a Parabolic Dish Concentrator and Low/medium Temperatures. Sol. Energy 199, 91123. doi:10.1016/j.solener.2019.07.056
Lund, H., and Mathiesen, B. V. (2009). Energy System Analysis of 100% Renewable Energy Systems—The Case of Denmark in Years 2030 and 2050. Energy 34 (5), 524–531.
Luque, A., and Hegedus, S. (2011). Handbook of Photovoltaic Science and Engineering . John Wiley & Sons .
Majidi, M., Nojavan, S., Nourani Esfetanaj, N., Najafi-Ghalelou, A., and Zare, K. (2017). A Multi-Objective Model for Optimal Operation of a battery/PV/fuel Cell/grid Hybrid Energy System Using Weighted Sum Technique and Fuzzy Satisfying Approach Considering Responsible Load Management. Sol. Energy 144, 79–89. doi:10.1016/j.solener.2017.01.009
Marzo, A., Salmon, A., Polo, J., Ballestrín, J., Soto, G., Quiñones, G., et al. (2021). Solar Extinction Map in Chile for Applications in Solar Power Tower Plants, Comparison with Other Places from Sunbelt and Impact on LCOE. Renew. Energy 170, 197–211. doi:10.1016/j.renene.2021.01.126
Maulik, A., and Das, D. (2018). “Multi-Objective Optimal Dispatch of AC-DC Hybrid Microgrid,” in Proceedings of the 2018 IEEE PES Asia-Pacific Power and Energy Engineering Conference (APPEEC) (Kota Kinabalu, Malaysia: IEEE ), 82–87. doi:10.1109/appeec.2018.8566354
Ming, M., Wang, R., Zha, Y., and Zhang, T. (2017). Multi-objective Optimization of Hybrid Renewable Energy System Using an Enhanced Multi-Objective Evolutionary Algorithm. Energies 10 (5), 674. doi:10.3390/en10050674
Mohamed, A. F., Elarini, M. M., and Othman, A. M. (2014). A New Technique Based on Artificial Bee Colony Algorithm for Optimal Sizing of Stand-Alone Photovoltaic System. J. Adv. Res. 5 (3), 397–408. doi:10.1016/j.jare.2013.06.010
Motahhir, S., El Hammoumi, A., and El Ghzizal, A. (2020). The Most Used MPPT Algorithms: Review and the Suitable Low-Cost Embedded Board for Each Algorithm. J. Clean. Prod. 246, 118983. doi:10.1016/j.jclepro.2019.118983
Moukhtar, I., El Dein, A. Z., Elbaset, A. A., and Mitani, Y. (2021). “Penetration Characteristics of Hybrid CSP and PV Solar Plants Economic,” in Power Systems ( Springer Science and Business Media Deutschland GmbH ), 99–111. doi:10.1007/978-3-030-61307-5_5
Otero, A. F., Cidras, J., and Garrido, C. (1998). “Genetic Algorithm Based Method for Grounding Grid Design,” in 1998 IEEE International Conference on Evolutionary Computation Proceedings ( IEEE World Congress on Computational Intelligence, Cat. No. 98TH8360 ), 120–123.
Ouagued, M., Khellaf, A., and Loukarfi, L. (2018). Performance Analyses of CueCl Hydrogen Production Integrated Solar Parabolic Trough Collector System under Algerian Climate. Int. J. Hydrogen Energy 43 (6), 34 51–65. doi:10.1016/j.ijhydene.2017.11.040
Prakash, P., and Khatod, D. K. (2016). Optimal Sizing and Siting Techniques for Distributed Generation in Distribution Systems: A Review. Renew. Sustain. energy Rev. 57, 111–130. doi:10.1016/j.rser.2015.12.099
Qiu, H., Gu, W., Xu, Y., and Zhao, B. (2019). Multi-Time-Scale Rolling Optimal Dispatch for AC/DC Hybrid Microgrids with Day-Ahead Distributionally Robust Scheduling. IEEE Trans. Sustain. Energy 10 (4), 1653–1663. doi:10.1109/TSTE.2018.2868548
Raturi, A. K. (2019). Renewables 2019 Global Status Report. Available at: https://www.ren21.net/wp-content/uploads/2019/05/gsr_2019_full_report_en.pdf .
Sanda, A., Moya, S. L., and Valenzuela, L. (2019). Modeling and Simulation Tools for Direct Steam Generation in Parabolic-Trough Solar Collectors: a Review. Renew. Sustain. energy Rev. 113, 109226. doi:10.1016/j.rser.2019.06.033
Simsek, Y., Mata-Torres, C., Escobar, R., and Cardemil, J. M. (2018). Incentives and Financial Conditions Effect Analysis on Levelized Cost of Electricity (LCOE) and Government Cost for Concentrated Solar Power (CSP) Projects in Chile. AIP Conf. Proc. 2033. doi:10.1063/1.5067134
Solar paces (2019). Solar Paces NREL. Available at: https://solarpaces.nrel.gov/ .
Tascioni, R., Arteconi, A., Del Zotto, L., and Cioccolanti, L. (2020). Fuzzy Logic Energy Management Strategy of a Multiple Latent Heat Thermal Storage in a Small-Scale Concentrated Solar Power Plant. Energies 13 (11), 23–28. doi:10.3390/en13112733
Wikimedia Commons (2018). How Solar Power Works. Available at: https://commons.wikimedia.org/wiki/File:How_Solar_Power_Works.png .
Zakaria, A., Ismail, F. B., Lipu, M. S. H., and Hannan, M. A. (2020). Uncertainty Models for Stochastic Optimization in Renewable Energy Applications. Renew. Energy 145, 1543–1571. doi:10.1016/j.renene.2019.07.081
Zhang, H. L., Baeyens, J., Degreve, J., and Cac eres, G. (2013). Concentrated Solar Power Plants: Review and Design Methodology. Renew. Sustain. energy Rev. 22, 466e81. doi:10.1016/j.rser.2013.01.032
Zhang, H., and Toudert, J. (2018). Optical Management for Efficiency Enhancement in Hybrid Organic-Inorganic Lead Halide Perovskite Solar Cells. Sci. Technol. Adv. Mater. 19 (1), 411–424. doi:10.1080/14686996.2018.1458578
Zhao T, T., Xiao, J., Hai, K. L., and Wang, P. (2017). “Two-Stage Stochastic Optimization for Hybrid AC/DC Microgrid Embedded Energy Hub,” in Proceedings of the 2017 IEEE Conference on Energy Internet and Energy System Integration (EI2) (Beijing, China: IEEE ), 1–6. doi:10.1109/ei2.2017.8245648
Zhao Zy, Z. Y., Chen, Y. L., and Thomson, J. D. (2017). Levelized Cost of Energy Modeling for Concentrated Solar Power Projects: a China Study. Energy 120, 1 17–27. doi:10.1016/j.energy.2016.12.122
Zhar, R., Allouhi, A., Ghodbane, M., Jamil, A., and Lahrech, K. (2021). Parametric Analysis and Multi-Objective Optimization of a Combined Organic Rankine Cycle and Vapor Compression Cycle. Sustain. Energy Technol. Assessments 47, 101401. doi:10.1016/j.seta.2021.101401
Zheng, Y., Jenkins, B. M., Kornbluth, K., and Træholt, C. (2018). Optimization under Uncertainty of a Biomass-Integrated Renewable Energy Microgrid with Energy Storage. Renew. energy 123, 204–217. doi:10.1016/j.renene.2018.01.120
Zhuang, X., Xu, X., Liu, W., and Xu, W. (2019). LCOE Analysis of Tower Concentrating Solar Power Plants Using Different Molten-Salts for Thermal Energy Storage in China. Energies 12 (7), 1394. doi:10.3390/en12071394
Zineb, A., Hicham, B., Khadija, L., and Abdelfettah, B. (2021). Solar Technologies for Electricity Production: An Updated Review. nternational J. Hydrogen Energy 46 (60), 30790–30817. ISSN 0360-3199. doi:10.1016/j.ijhydene.2021.06.190
FPC Flat plate collector
CPV Concentrated photovoltaic
GaAs Gallium Arsenide
CPC Compound parabolic collector
GIS Geographic information system
HCPV High Concentrated photovoltaic
MWh Megawatt hour
LCPV Low Concentrated photovoltaic
CIGS Copper indium gallium selenide solar cells
LFR Linear Fresnel reflector
IEA International Energy Agency
HTF Heat transfer fluid
HFC Heliostat Field Collector
CdTe Cadmium telluride
BESS Battery Energy Storage System
MHP Micro hydropower
CSP Concentrated solar power
PTSTPP Parabolic Trough Solar Thermal Power Plant
PV Photovoltaic
ANN Artificial neural network
PVT Photovoltaic thermal collectors
CPVT Concentrated photovoltaic thermal collectors
SAM Solar advisor model
SBS Spectral beam splitting
SCR Solar Central Receiver
SGS Steam Generation System
TES Thermal energy storage
LCOE Levelized cost of electricity ($/kWh)
NREL National renewable energy laboratory
STP Standard temperature and pressure
PDC Parabolic dish collector
Keywords: photovoltaic, hybrid system, stand-alone system, grid system, energy, solar energy, renewable, clean energy optimization methods
Citation: Soomar AM, Hakeem A, Messaoudi M, Musznicki P, Iqbal A and Czapp S (2022) Solar Photovoltaic Energy Optimization and Challenges. Front. Energy Res. 10:879985. doi: 10.3389/fenrg.2022.879985
Received: 20 February 2022; Accepted: 24 March 2022; Published: 30 May 2022.
Reviewed by:
Copyright © 2022 Soomar, Hakeem, Messaoudi, Musznicki, Iqbal and Czapp. This is an open-access article distributed under the terms of the Creative Commons Attribution License (CC BY). The use, distribution or reproduction in other forums is permitted, provided the original author(s) and the copyright owner(s) are credited and that the original publication in this journal is cited, in accordance with accepted academic practice. No use, distribution or reproduction is permitted which does not comply with these terms.
*Correspondence: Arsalan Muhammad Soomar, [email protected] , [email protected]
This article is part of the Research Topic
Solar Photovoltaic System to Meet the Sustainable Development Goals
Academia.edu no longer supports Internet Explorer.
To browse Academia.edu and the wider internet faster and more securely, please take a few seconds to upgrade your browser .
- We're Hiring!
- Help Center
Solar Energy Harvesting
- Most Cited Papers
- Most Downloaded Papers
- Newest Papers
- Save to Library
- Energy Harvesting Follow Following
- finitie state machines using VHDL Follow Following
- BIO-MEMS Follow Following
- Energy Harvesting Devices Follow Following
- Broadband Energy Harvesting Follow Following
- Environmantal Policy Follow Following
- RF Energy Harvesting Follow Following
- Semiconductor thin films Follow Following
- Embedded Security Follow Following
- Functional Coatings Follow Following
Enter the email address you signed up with and we'll email you a reset link.
- Academia.edu Publishing
- We're Hiring!
- Help Center
- Find new research papers in:
- Health Sciences
- Earth Sciences
- Cognitive Science
- Mathematics
- Computer Science
- Academia ©2024
Predicting Active Solar Power with Machine Learning and Weather Data
- Original Paper
- Published: 04 October 2023
- Volume 5 , article number 15 , ( 2023 )
Cite this article
- Swikriti Khadke 1 ,
- Brindha Ramasubramanian 2 ,
- Pranto Paul 1 , 2 ,
- Raghavendra Lawaniya 1 ,
- Suma Dawn 3 ,
- Angana Chakraborty 4 ,
- Biswajit Mandal 5 ,
- Goutam Kumar Dalapati 1 , 2 ,
- Avishek Kumar 1 &
- Seeram Ramakrishna ORCID: orcid.org/0000-0001-8479-8686 2
180 Accesses
Explore all metrics
Artificial intelligence (AI) is crucial in optimizing energy consumption, improving renewable energy systems, enhancing efficiency, and enabling sustainability efforts and smart grid management. It facilitates the development of predictive models, like in the study, optimizing renewable energy use and reducing environmental impact. Leveraging AI helps us make informed decisions, reduce waste, and promote sustainable practices across industries for a greener future. The study’s goal was to develop a predicting model for solar photovoltaic (PV) systems that could account for weather unpredictability. To accomplish this goal, we collected data from both the plant inverter and the weather measurement system and used machine learning techniques like linear regression, random forest, principal component analysis, and support vector regression with RBF kernel to examine the data and create a model that can accurately predict the power output. The developed model may be used in any location for preliminary testing and estimating, allowing solar energy to be captured more successfully and consistently in the face of changing weather circumstances. We obtained 0.87 as the highest R 2 value with 0.002 as a mean square error.
This is a preview of subscription content, log in via an institution to check access.
Access this article
Price includes VAT (Russian Federation)
Instant access to the full article PDF.
Rent this article via DeepDyve
Institutional subscriptions
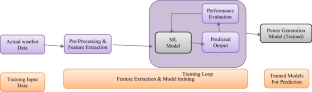
Similar content being viewed by others
Advanced Prediction of Solar Radiation Using Machine Learning and Principal Component Analysis
Exploring Machine Learning Models for Solar Energy Output Forecasting
Comparing Machine Learning Techniques for Hourly Solar Power Generation Prediction
Data availability.
The data and code used for the analysis in this study will be provided upon request to the corresponding author.
Abuella M, Chowdhury B (2017) Solar power forecasting using support vector regression. 2016 International Annual Conference of the American Society for Engineering Management, ASEM 2016
Adebayo TS, Rjoub H, Akinsola GD, Oladipupo SD (2021) The asymmetric effects of renewable energy consumption and trade openness on carbon emissions in Sweden: new evidence from quantile-on-quantile regression approach. Environ Sci Pollut Res:1–12. https://doi.org/10.1007/S11356-021-15706-4/FIGURES/4
Ardo S, Fernandez Rivas D, Modestino MA et al (2018) Pathways to electrochemical solar-hydrogen technologies. Energy Environ Sci 11:2768–2783. https://doi.org/10.1039/C7EE03639F
Article CAS Google Scholar
Baduge SK, Thilakarathna S, Perera JS et al (2022) Artificial intelligence and smart vision for building and construction 4.0: machine and deep learning methods and applications. Autom Constr 141:104440. https://doi.org/10.1016/J.AUTCON.2022.104440
Article Google Scholar
Barnhart CJ, Dale M, Brandt AR, Benson SM (2013) The energetic implications of curtailing versus storing solar- and wind-generated electricity. Energy Environ Sci 6:2804–2810. https://doi.org/10.1039/C3EE41973H
Bischl B, Binder M, Lang M et al (2023) Hyperparameter optimization: foundations, algorithms, best practices, and open challenges. Wiley Interdiscip Rev Data Min Knowl Discov 13:e1484. https://doi.org/10.1002/WIDM.1484
Chun H, Lee E, Nam K et al (2021) First-principle-data-integrated machine-learning approach for high-throughput searching of ternary electrocatalyst toward oxygen reduction reaction. Chem Catalysis 1:855–869. https://doi.org/10.1016/J.CHECAT.2021.06.001
Correa-Baena JP, Hippalgaonkar K, van Duren J et al (2018) Accelerating materials development via automation, machine learning, and high-performance computing. Joule 2:1410–1420. https://doi.org/10.1016/J.JOULE.2018.05.009
Ding T, Zhou Y, Ong WL, Ho GW (2021) Hybrid solar-driven interfacial evaporation systems: beyond water production towards high solar energy utilization. Mater Today 42:178–191. https://doi.org/10.1016/J.MATTOD.2020.10.022
Fang Y, Zou Y, Xu J et al (2021) Ambulatory cardiovascular monitoring via a machine-learning-assisted textile triboelectric sensor. Adv Mater 33:2104178. https://doi.org/10.1002/ADMA.202104178
Greenhill S, Rana S, Gupta S et al (2020) Bayesian optimization for adaptive experimental design: a review. IEEE Access 8:13937–13948. https://doi.org/10.1109/ACCESS.2020.2966228
Hayat MB, Ali D, Monyake KC et al (2019) Solar energy—a look into power generation, challenges, and a solar-powered future. Int J Energy Res 43:1049–1067. https://doi.org/10.1002/ER.4252
Houchins G, Viswanathan V (2020) An accurate machine-learning calculator for optimization of Li-ion battery cathodes. J Chem Phys 153:054124. https://doi.org/10.1063/5.0015872
Hu T, Zhang J, Whyte J et al (2022) Silicone oil nanofluids dispersed with mesoporous crumpled graphene for medium-temperature direct absorption solar-thermal energy harvesting. Sol Energy Mater Sol Cells 243:111794. https://doi.org/10.1016/J.SOLMAT.2022.111794
Isazadeh A, Ziviani D, Claridge DE (2023) Global trends, performance metrics, and energy reduction measures in datacom facilities. Renew Sust Energ Rev 174:113149. https://doi.org/10.1016/J.RSER.2023.113149
Jia J, Seitz LC, Benck JD et al (2016) Solar water splitting by photovoltaic-electrolysis with a solar-to-hydrogen efficiency over 30%. Nat Commun 7:1–6. https://doi.org/10.1038/ncomms13237
Kowal J, Ramasubramanian B, Rao RP et al (2022) Towards sustainable fuel cells and batteries with an AI perspective. Sustainability 14:16001. https://doi.org/10.3390/SU142316001
Kumar KK, Brindha R, Nandhini M et al (2019) Water-suspended graphene as electrolyte additive in zinc-air alkaline battery system. Ionics (Kiel) 25:1699–1706. https://doi.org/10.1007/S11581-019-02924-7/METRICS
Liu P, Zhan X, Wu X et al (2020a) Effect of weathering on environmental behavior of microplastics: properties, sorption and potential risks. Chemosphere 242:125193. https://doi.org/10.1016/J.CHEMOSPHERE.2019.125193
Liu Y, Guo B, Zou X et al (2020b) Machine learning assisted materials design and discovery for rechargeable batteries. Energy Storage Mater 31:434–450. https://doi.org/10.1016/J.ENSM.2020.06.033
Nourani V, Elkiran G, Abdullahi J, Tahsin A (2019) Multi-region modeling of daily global solar radiation with artificial intelligence ensemble. Nat Resour Res 28:1217–1238. https://doi.org/10.1007/S11053-018-09450-9/METRICS
Qi N, Yin Y, Dai K et al (2021) Comprehensive optimized hybrid energy storage system for long-life solar-powered wireless sensor network nodes. Appl Energy 290:116780. https://doi.org/10.1016/J.APENERGY.2021.116780
Ramasubramanian B, Reddy VS, Zhen Y et al (2023) Metal organic framework derived zirconia–carbon nanoporous mat for integrated strain sensor powered by solid-state supercapacitor. Adv Fiber Mater 5:1404–1416. https://doi.org/10.1007/S42765-023-00283-7/METRICS
Ramasubramanian B, Sundarrajan S, Chellappan V et al (2022b) Recent development in carbon-LiFePO4 cathodes for lithium-ion batteries: a mini review. Batteries 8:133. https://doi.org/10.3390/BATTERIES8100133
Ramasubramanian B, Subramanian S, Prasada Rayavarapu PR et al (2022a) Novel low-carbon energy solutions for powering emerging wearables, smart textiles, and medical devices. Energy Environ Sci. https://doi.org/10.1039/D2EE02695C
Sangster AJ (2016) Massive energy storage systems enable secure electricity supply from renewables. J Mod Power Syst Clean Energy 4:667–659. https://doi.org/10.1007/S40565-016-0204-9
Senthilkumar SH, Ramasubramanian B, Rao RP et al (2023) Advances in electrospun materials and methods for Li-ion batteries. Polymers 15:1622. https://doi.org/10.3390/POLYM15071622
Serras P, Ibarra-Berastegi G, Sáenz J, Ulazia A (2019) Combining random forests and physics-based models to forecast the electricity generated by ocean waves: a case study of the Mutriku wave farm. Ocean Eng 189:106314. https://doi.org/10.1016/J.OCEANENG.2019.106314
Shafizadeh-Moghadam H (2021) Fully component selection: an efficient combination of feature selection and principal component analysis to increase model performance. Expert Syst Appl 186:115678. https://doi.org/10.1016/J.ESWA.2021.115678
Sharma N, Sharma P, Irwin D, Shenoy P (2011) Predicting solar generation from weather forecasts using machine learning. 2011 IEEE International Conference on Smart Grid Communications. SmartGridComm 2011:528–533. https://doi.org/10.1109/SMARTGRIDCOMM.2011.6102379
Torabi M, Mosavi A, Ozturk P et al (2019) A Hybrid machine learning approach for daily prediction of solar radiation. Lect Notes Netw Syst 53:266–274. https://doi.org/10.1007/978-3-319-99834-3_35/COVER
Wang A, Zou Z, Wang D et al (2021) Identifying chemical factors affecting reaction kinetics in Li-air battery via ab initio calculations and machine learning. Energy Storage Mater 35:595–601. https://doi.org/10.1016/J.ENSM.2020.10.022
Wang X, Jin Y, Schmitt S, Olhofer M (2023) Recent advances in Bayesian optimization. ACM Comput Surv. https://doi.org/10.1145/3582078
Yao Z, Lum Y, Johnston A et al (2022) Machine learning for a sustainable energy future. Nat Rev Mater 8:202–215. https://doi.org/10.1038/s41578-022-00490-5
Download references
The authors would like to acknowledge the funding A-0000065-99-99 (WBS), Electrode for Green Hydrogen Production/Fuel Cells (Dr. Goutam Kumar Dalapati).
Author information
Authors and affiliations.
Sunkonnect Pte Ltd., Singapore, 637141, Singapore
Swikriti Khadke, Pranto Paul, Raghavendra Lawaniya, Goutam Kumar Dalapati & Avishek Kumar
Center for Nanotechnology & Sustainability, Department of Mechanical Engineering, College of Design and Engineering, National University of Singapore, 9 Engineering Drive 1, Singapore, 117575, Singapore
Brindha Ramasubramanian, Pranto Paul, Goutam Kumar Dalapati & Seeram Ramakrishna
Department of Computer Sciences, Jaypee Institute of Information Technology, Noida, 201309, India
Department of Computer Science and Engineering, Haldia Institute of Technology, Haldia, 721657, India
Angana Chakraborty
Department of Chemical Engineering, Haldia Institute of Technology, Haldia, 721657, India
Biswajit Mandal
You can also search for this author in PubMed Google Scholar
Contributions
S.K. and B.R., data analysis; S.K., B.R., S.D., A.C., and B.M., drafting the manuscript; R.L., S.R., A.K., G.K.D., concept, design, data collection, data analysis.
Corresponding author
Correspondence to Seeram Ramakrishna .
Ethics declarations
Conflict of interest.
No conflict of interest to declare. Prof Seeram Ramakrishna, one of the authors, is the Editor-in-Chief of Materials Circular Economy.
Rights and permissions
Springer Nature or its licensor (e.g. a society or other partner) holds exclusive rights to this article under a publishing agreement with the author(s) or other rightsholder(s); author self-archiving of the accepted manuscript version of this article is solely governed by the terms of such publishing agreement and applicable law.
Reprints and permissions
About this article
Khadke, S., Ramasubramanian, B., Paul, P. et al. Predicting Active Solar Power with Machine Learning and Weather Data. Mater Circ Econ 5 , 15 (2023). https://doi.org/10.1007/s42824-023-00087-5
Download citation
Received : 25 July 2023
Revised : 13 September 2023
Accepted : 19 September 2023
Published : 04 October 2023
DOI : https://doi.org/10.1007/s42824-023-00087-5
Share this article
Anyone you share the following link with will be able to read this content:
Sorry, a shareable link is not currently available for this article.
Provided by the Springer Nature SharedIt content-sharing initiative
- Hyperparameter tuning
- Find a journal
- Publish with us
- Track your research
Increasing the efficiency of eco-friendly solar cells by converting wind energy into high-voltage electricity
A research team led by Professor Lee Ju-hyuck of DGIST in the Department of Energy Science & Engineering has successfully developed an energy harvesting device that enhances solar energy efficiency by removing and preventing surface contamination on solar cells.
The device, developed through joint research with Dr. Seung Wan-chul of the Samsung Electronics Mechatronics & Manufacturing Technology Center, converts frictional force into electrical energy using a friction electrification power generator and applies it to an electrodynamic screen to prevent and remove contamination on the surface of solar cells, thus consistently maintaining their solar power generation efficiency.
Solar cells are being recognized as a sustainable energy source, but they demand regular maintenance due to the potential decline in energy production caused by surface contamination of the panels. Traditionally, human labor has been required to spray high-pressure water to clean dust from solar panels.
However, employing human workers in extreme environments such as deserts, mountainous regions, and even outer space is impractical. Therefore, research on an unmanned technology to prevent and remove surface contamination from solar panels has been ongoing.
Research has been underway on the utilization of an electrodynamic screen technology that displaces particles through the generation of high voltage in the form of alternating current on electrodes in a specific pattern. However, this technology incurs a loss in solar power generation due to the need for alternating current and high voltage.
To overcome this limitation, the research team innovated an energy harvesting technology that reuses consumed or discarded energy as electrodynamic screen technology. The team developed a friction electrification power generator that generates high-voltage energy from wind rotation.
To test its applicability, the researchers analyzed the relationship between wind speed and output based on changes in voltage output with respect to the device's rotation speed. The results showed that fast rotation due to high wind speed generated high voltage (up to 2,300 V). Further, the solar cells' output was restored by at least 90% when surface contamination was removed using the self-generating electrodynamic screen fabricated with the developed device.
Dr. Lee stated, "This research enabled us to develop the technology to prevent and remove surface contamination on solar cells by converting wind energy found in nature into electrical energy. We will continue researching further technological advancements to explore ways to maximize the efficiency of solar energy, which is a key future eco-friendly energy source."
The study is published in the journal Nano Energy .
More information: Minsu Heo et al, Self-powered electrodynamic dust removal for sustainable solar panels using triboelectric nanogenerators, Nano Energy (2024). DOI: 10.1016/j.nanoen.2024.109257
Provided by DGIST (Daegu Gyeongbuk Institute of Science and Technology)

Sustainable Energy & Fuels
Design and development of nanostructured photocatalyst for large scale solar green hydrogen generation.
The most intriguing research that offers hope for the future of solving world's energy hunger is known to be production of cleaner hydrogen through artificial photosynthesis. The evolution of green hydrogen by visible-light-driven photocatalysis is a challenging but feasible prospective. Photocatalytic solar power systems primarily rely on utilising the complete range of solar spectrum. The synthesis of an optimal photocatalyst should address all the influencing parameters with an efficient scaling method which remains unanswered despite several advancements in photocatalytic water-splitting applications. Real- time solutions are necessary to confine the issues that lacks photocatalytic efficacy of semiconducting nanomaterial in solar-powered systems. In addition to the proposal of designing solar-powered systems for hydrogen generation, this review paves the way in highlighting the difficulties associated with water reduction methods. It also offers some strategies to improve charge separation and migration in a semiconducting photocatalyst by encouraging light absorption and altering their band positions. In addition to that a cost-effective, ecologically safe, and photostable heterogeneous nano catalyst must be designed for visible light harvesting water-splitting processes. This article sketches various nanomaterials based photocatalysts, which act as base surface for photocatalytic solar water splitting. These include oxides, chalcogenides, and nitrides of metals, noble metals, plasmonic metals, ultrathin 2D Covalent-Organic-Frameworks (COFs), Metal-Organic-Frameworks (MOFs), and metal free polymeric graphitic carbon nitrides. Integration of multi-component nano-materials can be more appropriate than single-component photocatalysts to maximize their catalytic activity. Thin film photocatalysis is considered to be the most effective method for increasing hydrogen production rates in comparison to powder suspension-based photocatalysis. This article presents the latest advancements in thin-film based photocatalytic technology, outlining all the critical factors, prerequisites, and techniques for thin-film preparation. Future research in advanced photocatalysis focuses on harvesting green hydrogen for in situ carbon dioxide reduction, fine chemical synthesis, nitrogen fixation, and hydrogen peroxide synthesis. Experimentally photocatalytic solar powered systems utilize natural sun light. However, synthesis of ideal photocatalysts with effective scaling approaches remains a challenge. This paper advocates for solutions and a practical solar-powered system for green hydrogen production.
Article information
Download citation, permissions.

P. K. Sahu, A. Champati, A. Pradhan and B. Naik, Sustainable Energy Fuels , 2024, Accepted Manuscript , DOI: 10.1039/D4SE00056K
To request permission to reproduce material from this article, please go to the Copyright Clearance Center request page .
If you are an author contributing to an RSC publication, you do not need to request permission provided correct acknowledgement is given.
If you are the author of this article, you do not need to request permission to reproduce figures and diagrams provided correct acknowledgement is given. If you want to reproduce the whole article in a third-party publication (excluding your thesis/dissertation for which permission is not required) please go to the Copyright Clearance Center request page .
Read more about how to correctly acknowledge RSC content .
Social activity
Search articles by author.
This article has not yet been cited.
Advertisements
In Indonesia, deforestation is intensifying disasters from severe weather, climate change
- Show more sharing options
- Copy Link URL Copied!
Roads turned to murky brown rivers, homes were swept away by strong currents and bodies were pulled from mud during deadly flash floods and landslides after torrential rains hit West Sumatra in early March, marking one of the latest deadly natural disasters in Indonesia.
Government officials blamed the floods on heavy rainfall, but environmental groups have cited the disaster as the latest example of deforestation and environmental degradation intensifying the effects of severe weather across Indonesia.
“This disaster occurred not only because of extreme weather factors, but because of the ecological crisis,” Indonesian environmental rights group Indonesian Forum for the Environment wrote in a statement. “If the environment continues to be ignored, then we will continue to reap ecological disasters.”
A vast tropical archipelago stretching across the equator, Indonesia is home to the world’s third-largest rainforest, with a variety of endangered wildlife and plants, including orangutans, elephants, giant and blooming forest flowers. Some live nowhere else.
For generations the forests have also provided livelihoods, food and medicine while playing a central role in cultural practices for millions of Indigenous residents in Indonesia.
Since 1950, more than 285,700 square miles of Indonesian rainforest — an area twice the size of Germany — have been logged, burned or degraded for development of palm oil, paper and rubber plantations, mining and other commodities, according to Global Forest Watch.
Indonesia is the biggest producer of palm oil, one of the largest exporters of coal and a top producer of pulp for paper. It also exports oil and gas, rubber, tin and other resources. And it also has the world’s largest reserves of nickel — a crucial material for electric vehicles, solar panels and other goods needed for the green energy transition.
Toward a more sustainable California
Get Boiling Point, our newsletter exploring climate change, energy and the environment, and become part of the conversation — and the solution.
You may occasionally receive promotional content from the Los Angeles Times.
Indonesia has consistently ranked as one of the largest global emitters of plant-warming greenhouse gases, with its emissions stemming from the burning of fossil fuels, deforestation and peatland fires, according to the Global Carbon Project.
It’s also highly vulnerable to climate change impacts, including extreme events such as floods and droughts, long-term changes from sea level rise, shifts in rainfall patterns and increasing temperatures, according to the World Bank. In recent decades the country has already seen the effects of climate change: more intense rains, landslides and floods during rainy season, and more fires during a longer dry season.
But forests can help play a vital role in reducing the impact of some extreme weather events, said Aida Greenbury, a sustainability expert focusing on Indonesia.
Flooding can be slowed by trees and vegetation soaking up rainwater and reducing erosion. In dry season, forests release moisture that helps mitigate the effects of droughts, including fires.
But when forests diminish, those benefits do as well.
A 2017 study reported that forest conversion and deforestation expose bare soil to rainfall, causing soil erosion. Frequent harvesting activities — such as done on palm oil plantations — and the removal of ground vegetation lead to further soil compaction, causing rain to run off the surface instead of entering groundwater reservoirs. Downstream erosion also increases sediment in rivers, making them shallower and increasing flood risks, according to the research.
After the deadly floods in Sumatra in early March, West Sumatra Gov. Mahyeldi Ansharullah said there were strong indications of illegal logging around locations affected by floods and landslides. That, combined with extreme rainfall, inadequate drainage systems and improper housing development, contributed to the disaster, he said.
Experts and environmental activists have pointed to deforestation worsening disasters in other regions of Indonesia as well: In 2021 environmental activists partially blamed deadly floods in Kalimantan on environmental degradation caused by large-scale mining and palm oil operations. In Papua, deforestation was partially blamed for floods and landslides that killed more than 100 people in 2019.
There have been some signs of progress: In 2018 Indonesian President Joko Widodo, known as Jokowi, put a three-year freeze on new permits for palm oil plantations. And the rate of deforestation slowed in 2021-22, according to government data.
But experts warn that it’s unlikely deforestation in Indonesia will stop anytime soon as the government continues to move forward with new mining and infrastructure projects such as new nickel smelters and cement factories.
“A lot of land use and land-based investment permits have already been given to businesses, and a lot of these areas are already prone to disasters,” said Arie Rompas, an Indonesia-based forestry expert at Greenpeace.
President-elect Prabowo Subianto , who is scheduled to take office in October , has promised to continue Jokowi’s policy of development, include large-scale food estates, mining and other infrastructure development that are all linked to deforestation.
Environmental watchdogs also warn that environmental protections in Indonesia are weakening, including the passing of the controversial Omnibus Law, which eliminated an article of the Forestry Law regarding the minimum area of forest that must be maintained at development projects.
“The removal of that article makes us very worried [about deforestation] for the years to come,” Rompas said.
While experts and activists recognize that development is essential for Indonesia’s economy to continue to go, they argue that it should be done in a way that considers the environment and incorporates better land planning.
“We can’t continue down the same path we’ve been on,” said Greenbury, the sustainability expert. “We need to make sure that the soil, the land in the forest doesn’t become extinct.”
Milko writes for the Associated Press.
More to Read
At least 19 dead and 7 missing as landslide and flash floods hit Indonesia’s Sumatra island
March 10, 2024
How climate change contributes to wildfires like Chile’s
Feb. 6, 2024
Opinion: Why disappearing trees are so bad for our climate — and health
Jan. 5, 2024
Start your day right
Sign up for Essential California for news, features and recommendations from the L.A. Times and beyond in your inbox six days a week.
More From the Los Angeles Times
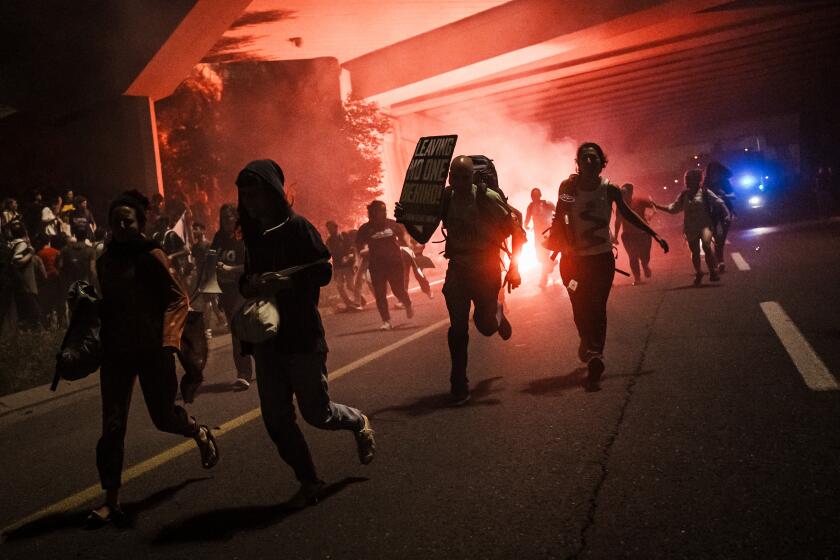
World & Nation
Israelis stage largest protest since war began to increase pressure on Netanyahu
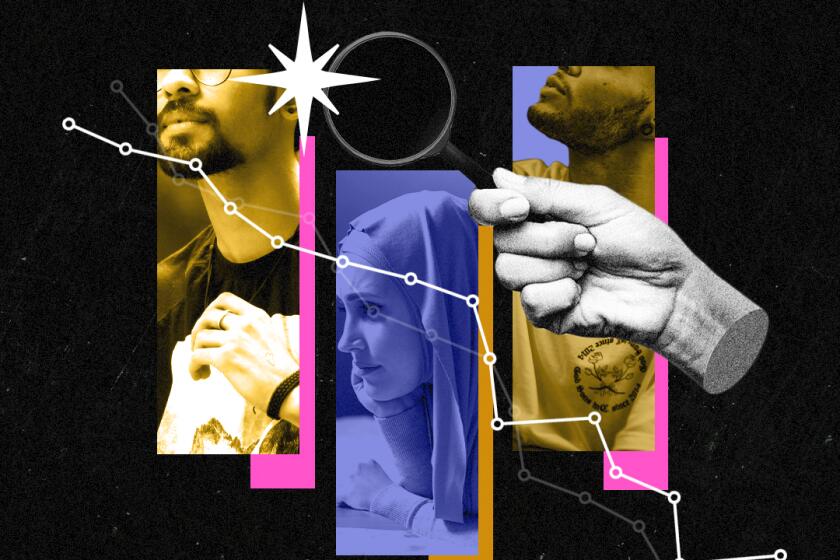
The Census is changing its categories on race and ethnicity. Here’s what that means for Latinos
March 31, 2024
Complicated task removing twisted steel, concrete from Baltimore bridge continues
Romania and Bulgaria join Europe’s Schengen travel zone but keep land border checks
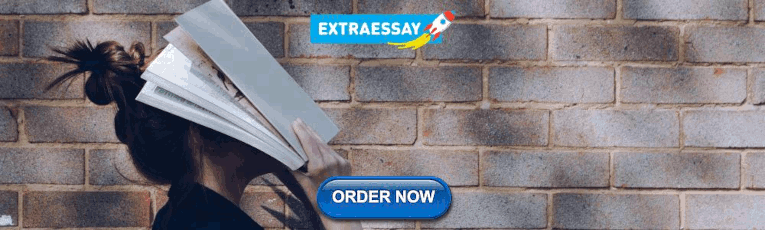
IMAGES
VIDEO
COMMENTS
Many researchers have conducted research on powering wearable devices by harvesting mechanical energy generated by human movement and external solar energy. Song et al. [ 53 ] presented a bracelet based on a hybrid self-charged power system for wearable electronics by harvesting body motion energy and solar energy to generate electricity.
Moreover, there are many types of solar cells, such as organic thin-film solar cells, 11,12) dye-sensitized solar cells 13) and perovskite solar cells. 14-16) The standardization of the method of evaluating the energy harvesting characteristics of each type of solar cell is essential for the promotion of research and development and the ...
Recent research focused on solar-tracking technology to maximize solar energy capture ... Several papers have been reviewed to select an efficient, low-cost, and reliable solar panel. ... Lim W (2018) Triple-axis tracking control algorithm for maximizing solar energy harvesting on a moving platform. ARPN J Eng Appl Sci 13:4617-4624
This study reviews solar energy harvesting (SEH) technologies for PV self-. powered applications. First, the PV power generation and scenarios of PV self-powered applications are. analyzed. Second ...
A self-powered system based on energy harvesting technology can be a potential candidate for solving the problem of supplying power to electronic devices. In this review, we focus on portable and ...
Renewable energy utilization has high potential in urban context to reduce carbon emissions. Solar energy in particular has proved to be promising renewable source due to its ubiquity, abundance and sustainability. Efficient utilization of solar energy for cogeneration is an important application in the built environment, with wide applicability.
Solar energy harvesting has already widely used in IoT applications. This paper reviews the key technologies in solar energy harvesting systems. Comparing the characteristics of several typical DC-DC converters, charge pump, especially, kinds of reconfigurable charge pump are designed to decrease the voltage gain discrete and extend conversion ratio matching for MPPT techniques. Besides, more ...
technologies related to solar energy harvesting in built environments. In particular, we focus on recent achievements in enabling the widespread distributed generation of electric energy assisted by energy capture in semi-transparent or even optically clear glazing systems and building wall areas.
To solve the problem of wireless sensor network (WSN) nodes' limited battery energy, this study's goal is to provide an effective solar energy harvesting method. Due to their short battery life, WSN nodes have a significant design limitation, so it's critical to look into solutions to supply a dependable and sustainable energy source for their continuous operation. The research's major ...
This Research Topic will focus on the technologies and design of materials for the challenge of sustainable renewable energy. It is dedicated to contributions in the development of new materials and devices for the conversion of solar energy into electrical and chemical energy. All areas of solar energy harvesting research are welcome ...
There are various forms of green energy available in the environment that can be extract, like thermal, mechanical, solar, acoustic, wind, etc and several techniques have been applied to harvest green energy from the environment source [1]. One of the methods is using solar PV cells to harvest the energy from sun. Various type of techniques have been developed i.e. stationary solar tracking ...
Feature papers represent the most advanced research with significant potential for high impact in the field. A Feature Paper should be a substantial original Article that involves several techniques or approaches, provides an outlook for future research directions and describes possible research applications. ... Interests: energy harvesting ...
This paper reviews the possibility of integrating photovoltaic (PV) and thermoelectric generators (TEG) in a PV-TEG hybrid system based on examining recent efforts in the field of PV-TEG creation. It also examines the efficiency improvement in PV-TEGs and their applications in recent years, offering a valuable guide for researchers and ...
In this research paper, a thermoelectric energy harvesting technique for solar panels is given. The unused thermal energy from photovoltage panels (PV) accessible to the sun's rays is used by this technique to power Photovoltaic monitoring devices in places where there is no other source of energy. Most PV panels only use 15-30% of the ...
Some initial success of the paper-based devices is being reported for solar energy harvesting as solar cells and current collectors. However, the progress is yet limited [2], [10], [11], [12]. The research related to paper devices for energy application is increasing continuously, as evident from Fig. 1 and their citations in diverse scientific ...
An increased use in wearable, mobile, and electronic textile sensing devices has led to a desire to keep these devices continuously powered without the need for frequent recharging or bulky energy storage. To achieve this, many have proposed integrating energy harvesting capabilities into clothing: solar energy harvesting has been one of the most investigated avenues for this due to the ...
Abstract. It is a basic description of solar pv cell and solar power harvest technology. This paper describes the scenario of this sector,from its very beginning to current developments. The basic ...
The study paper focuses on solar energy optimization approaches, as well as the obstacles and concerns that come with them. This study discusses the most current advancements in solar power generation devices in order to provide a reference for decision-makers in the field of solar plant construction throughout the world. These technologies are divided into three groups: photovoltaic, thermal ...
This paper is focused on a wireless energy harvesting system using a rectifying antenna (rectenna). The proposed device consists of a wideband cross-dipole antenna, a microwave low-pass filter and ...
Therefore, energy harvesting techniques have proved to be a promising solution for WSNs [3]. In this paper, an experiment-based path loss model for a WSN based on the TI eZ430-RF2500-SEH kit, which operates with solar energy harvesting, is obtained through measurements of RSS in an indoor environment.
1. Introduction. Energy harvesting is the conversion of ambient energy (in the form of light, vibrations, wind, etc.) into electrical energy. There has been an increase in investments in energy harvesting technologies across the world, and with that an increase in research in this field (Cao et al., 2021, Liu et al., 2018, Pei et al., 2020, Wang et al., 2018, Yang et al., 2018).
67. JRTE-2020. Solar Energy Technology. Sumedha R.G. Weliwaththage, Udara S.P.R. Arach chige. Faculty of Technology, University of Sri Jayewardenepura, Sri Lanka. Abstract Energy resources can ...
We combined ground-recorded solar PV plant inverter data from the previous two years (2019-2020) with meteorological data from the same plant. The inverter data contains characteristics such as active power, alternating current, alternating voltage, today's generation, direct current voltage, direct current power, and reactive power with 1 ...
A research team led by Professor Lee Ju-hyuck of DGIST in the Department of Energy Science & Engineering has successfully developed an energy harvesting device that enhances solar energy ...
The most intriguing research that offers hope for the future of solving world's energy hunger is known to be production of cleaner hydrogen through artificial photosynthesis. The evolution of green hydrogen by visible-light-driven photocatalysis is a challenging but feasible prospective. Photocatalytic solar
Indonesia is the biggest producer of palm oil, one of the largest exporters of coal and a top producer of pulp for paper. It also exports oil and gas, rubber, tin and other resources.