- Forum article
- Open access
- Published: 18 May 2017
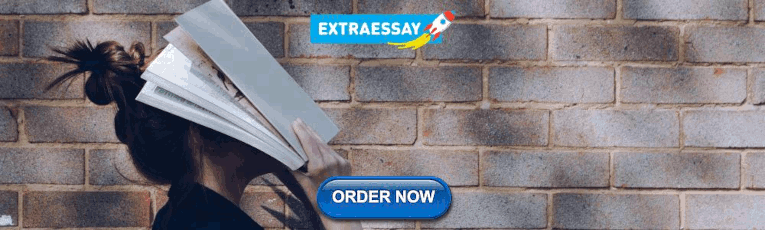
Biofuel research: perceptions of power and transition
- Lena Partzsch 1
Energy, Sustainability and Society volume 7 , Article number: 14 ( 2017 ) Cite this article
13k Accesses
8 Citations
1 Altmetric
Metrics details
Whether biofuels represent a sustainable innovation, a creative alternative, or a gold rush, very much depends on our perception of power and change with regard to sustainability. This article provides an overview of existing understandings of power in the research on biofuels, including positive perceptions that often lead to more optimistic evaluations of biofuels. It exposes the diversity with which one can understand power through three ideal type concepts: “power with,” “power to,” and “power over”. Integrating these concepts in one power framework allows for examining how the three dimensions interrelate with each other and developing the contours of a power lens on biofuel governance and research. With the 2007–2008 food price crisis, critics re-politicized the governance of biofuels. Several farmer associations have completely turned against biofuels. The article argues that this rejection of biofuels is due to a limited perception of power as a coercion and manipulation (power over). While the current governance of biofuels basically reproduces systems and positions, we should start to more seriously and intensively ask questions of where, when, and how the governance of biofuels may also allow for “green” resistance (power to) and collective empowerment (power with).
Introduction
Whether biofuels represent a sustainable innovation, a creative alternative or a gold rush [ 1 ], very much depends on our perception of power and change with regard to sustainability. This leads to the challenge of how to conceptualize these understandings. I gather diverse perceptions of power and illustrate them for biofuel research. The aim is to initiate a broader, more comprehensive debate across ontological and epistemological differences in this field of research. To begin the discussion, I introduce key components of the debate by identifying different perceptions of power that are common to research on biofuels along three ideal type conceptions:
Power with means collective empowerment through convincing and learning with and from each other. It refers to processes of developing shared values, finding common ground, and generating collective strengths [ 2 ]. Based on this understanding of power, biofuels can potentially be a sustainable innovation that serves the common good (climate protection, energy security, regional development, etc.) (e.g., [ 3 , 4 ]).
Power to corresponds to the ability of agents “to get things done” [ 5 ]. While Pitkin [ 6 ] defines power to as non-relational, Barnett and Duvall [ 7 ] define power to as tied to social relations of constitution that define who the actors are, along with their capacities and practices. Footnote 1 Scholars, who take a perspective of power to, may highlight the agency of producing biofuels as a creative alternative in hitherto fossil fuel-dependent societies (e.g., [ 8 , 9 ]).
Power over describes the direct and indirect ability of powerful actors, structures, and discourses to influence the actions and even the thoughts of others. It is based on power concepts by Dahl [ 10 ], Bachrach and Baratz [ 11 ], and Lukes [ 12 ], among others. I also discuss concepts of discursive power under this category (e.g., [ 13 , 14 ]), while I am aware that these concepts partly fall under the category of power to [ 7 ]. From a perspective of power over, biofuels can be seen as a gold rush: While everybody expected sudden wealth in this new field, there are very few winners and many losers (e.g. [ 15 , 16 ]).
I chose this tripartite approach as a framework for my article, because it is most comprehensive and makes an extension of the power discussion on biofuels possible. At the same time, the framework allows for the discussion of the well-known grouping of the four “faces of power” under the category of power over [ 17 , 18 ]. I will argue that in the research on biofuels, the understandings of power as power with and power to tend to prevail, even when they are not made explicit. This means that scholars have overemphasized the potential of biofuels as a creative alternative to fossil fuels and sustainable innovation for rural development. Concepts of power over have only more recently been applied, specifically since research has started to explicitly issue power. This has, in particular, been used to explain why any process of governing biofuels (biofuel governance) did not lead to urgent sustainability transitions, and why the biofuel boom should rather be seen as a gold rush. Scholars have demonstrated that the development of biofuels markets benefitted large companies and conglomerates [ 19 ]. Critical and post-structuralist perspectives have helped to understand this development by exploring structures and discourses favoring them [ 20 ]. Scholars have used Foucault’s concepts to outline how scientific knowledge practices render the very essence of problems (and solutions) raised on the biofuel agenda [ 21 , 22 ].
This article involves first of all implicit and explicit understandings of power (how do biofuel researchers think and talk about power?). These understandings are expressed in empirical research, as I will demonstrate below, and they hence also allow for an illustration of the practice of biofuel governance (how is power exercised in and through biofuel governance?). This makes the article also relevant for political practice. We should understand, not only in theoretical but also in practical terms, how we effectuate or prevent changes towards a more sustainable supply of energy and transport fuel. As in analytical heuristics, it is not possible to offhand separate power with , power to , and power over in empirical research. These categories shine multiple lights on different aspects of the same empirical phenomena. In practice, these forms of power exercise are mostly interrelated. My less concern is to weigh and compare the pros and cons of each perspective, but rather to outline an agenda for a multidimensional analysis of all three mechanisms of power and their interrelations.
In order to get the full picture of how change happens, we should understand how different perspectives add on to each other (besides overlaps and contradictions). To do this, I will begin by describing each perspective in itself. Based on a survey on biofuel research, I will give references for each perspective. These references are only illustrative. Then, I will exemplify the interrelations between each of these perspectives with respect to biofuel research. I explain how power imbalances can affect processes of power with and power to . Again, scholars have demonstrated how large conglomerates have manipulated biofuel governance in their favor, and why therefore the biofuels boom should be considered as a gold rush. However, I argue that interrelations may also work the other way around, and this is particularly relevant to the main argument of this article. Biofuels as a creative alternative and a sustainable innovation may also provoke changes in existing relations of power over and contribute to address asymmetries and inequalities in agrifood and transport systems. We need a multidimensional power approach to explore these interrelations.
Biofuel: sustainable innovation (power with)
Research on biofuel governance and other studies in the field of sustainability are most often based on a positive perception of power in the sense of power with . Power with is a term that refers to processes of developing shared values, finding common ground, and generating collective strengths [ 2 ]. This conception does not necessarily refer to the diffusion of already existing (predefined) norms. Rather, power with implies learning processes that allow actors to question self-perceptions and to actively build up a new awareness of individuals or groups [ 23 , 24 ]. In this vein, with regard to biofuels, scholars have assumed that collective empowerment and solidarity are possible and that biofuel technologies as a “sustainable innovation” can pave the way to post-carbon societies [ 25 , 26 ].
Power with is often linked to Arendt’s definition of power [ 27 ]. Footnote 2 According to Arendt, power always refers to a group or to a collective of individuals:
Power corresponds to the human ability not just to act but to act in concert. Power is never the property of an individual; it belongs to a group and remains in existence only so long as the group keeps together. When we say of somebody that he is ‘in power’ we actually refer to his being empowered by a certain number of people to act in their name ([ 28 ]: 44). Footnote 3
Research on environmental leadership (e.g., [ 29 ]) in pioneer countries, such as Germany and France in the biofuel sector [ 3 , 30 ], most obviously reflects such an understanding of power. Leaders or pioneers are empowered to act in the name of others from this perspective (while they dominate others from a perspective of power over , see below). In this sense, (Young [ 31 ]: 285) defines leadership in the interest of common welfare:
Leadership (…) refers to the actions of individuals who endeavor to solve or circumvent the collective action problems that plague the efforts of parties seeking to reap joint gains in processes of institutional bargaining.
Leaders and pioneers do not enforce their own interests against or over others; rather they seek “to reap joint gains” of environmentalism. Environmental leadership studies, based on such an understanding of power, usually follow the discourse of Ecological Modernization that highlights flexible and cost-efficient problem solving. Ecological modernization outlines a win-win storyline of environmental protection that benefits green (biofuel) business as much as the environment [ 32 , 33 ]. From this perspective, those who are neither leaders nor pioneers are considered free-riders or laggards , rather than subordinates. Non-leaders also benefit, at least in the long run, from power (with), since biofuels are expected to tackle common problems, such as climate change, enhance energy security, and to contribute to regional development [ 3 , 34 ]. Policies promoting biofuels are hence per se seen to be desirable since, from this perspective, they serve everybody’s interest.
Scholars have extensively analyzed the emergence, diffusion, efficiency, and effectiveness of policies promoting biofuels, with the (at least implicit) aim to foster their adoption and implementation [ 30 , 35 ]. In this context, policy learning and experiments have been gaining momentum [ 9 , 26 ]. Deliberative processes, including third-party certification schemes, were initiated and observed with the aim to introduce sustainable biofuel production schemes that would integrate those formerly excluded stakeholders with new technology; in everyday practice, every actor in the field would then become a winner [ 4 , 36 ].
Scholars who share this perspective of power as power with do not think in dichotomies such as winners - losers or good-bad . Instead, they understand power (or similar concepts, such as leadership) as serving the common good (climate protection, energy security, and sustainability). As there are no subordinates from this power perspective, no imperative follows to empower or to resist. The empowerment of non-leaders is not an issue because scholars assume that, in principle, they are also interested in developing sustainable innovations and that they likewise benefit from respective leadership efforts.
Biofuel: creative alternative and “green” resistance (power to)
While power with pertains to collective empowerment and solidarity, power to refers to single actors and separate groups, such as farmers, co-operatives, and individual processors who were initially key players in pioneering biofuel regions [ 19 ]. Accordingly, biofuels are often seen as an opportunity to empower green ideas and values. Pitkin [ 6 ] emphasizes how power can be non-relational, since an actor may have the power to accomplish something all by him- or herself. This understanding of power is related to the development of an individual identity; self-confidence and consciousness raising [ 23 ]. It is here where Nussbaum’s and Sen’s [ 37 ] capability approach comes in, which defines power as “a capability to act upon one’s environment” [ 38 ]. For example, an individual farmer can simply start to produce and use biomass-based fuels without any permission or interference from another actor, such as the petrol industry. However, constructivist research has demonstrated how every actor or group is defined through socially constituted relations that, at least indirectly, shape the actions of individuals [ 7 ]: only a farmer who receives knowledge about alternative technologies may effectively implement them.
Power to can be linked to Parsons’ definition of power as the ability “to get things done” [ 5 ]. It highlights a productive agency, especially in the cases where actors’ goals are opposed or resisted. Biofuel research by small farmers and rural communities is often based on this perception of power [ 9 , 39 ]. Scholars highlight the potential of biofuels for rural development by providing new markets for agricultural production. They assume that through the introduction of radically new technologies in niches, farmers are able to empower themselves in an attempt of an “agro-ecological revolution” [ 8 ]. They highlight the self-empowering agency of hitherto marginalized people to become “energy sheiks” [ 40 ], based on biomass production.
Scholars, who take a perspective of power to , focus on the productive agency of the biofuel sector. They are interested in the empowerment of alternative ideas and values which, in the case of biofuels, allow for transforming fossil fuel-dependent societies. These alternative agents criticize the practices or the authority of the dominant, carbon-intense system and refuse to reproduce their own positions in this system. Their non-conformism is perceived to serve the common good as they develop alternative technologies required by everyone in a world beyond petrol. From a perspective of power to and in difference to a perspective of power with , there are only a limited number of transformational agents: not everybody in the field is assumed to be a “winner” in the first place; there are only a few “energy sheiks”. However, scholars see an imperative to act based on normatively prior “green” values, for example, climate protection and sustainability (and everybody benefits from the realization of these values).
Biofuel: gold rush (power over)
Scholars who explicitly issue power in the context of biofuels usually perceive power as asymmetric. Biofuel governance is seen as a zero-sum game which produces winners and losers. From this perspective, powerful actors, structures, and discourses in the field of biofuel governance influence the actions and even the thoughts of others. In the following, I will illustrate this perspective, further differentiating the “four faces” of power over (see Table 1 ): visible , hidden , invisible , and unconscious power [ 2 , 41 ]. (the fourth dimension does not understand power as a zero-sum game and can also be added to power to , see the first footnote.)
In the first dimension, agents exercise visible power when they directly influence political decision-makers based on their material and ideational resources [ 42 ]. What is visible is not the power as such, but rather its physical means such as lobbying activities, party financing, and armed force. (Dahl [ 10 ]: 201) defines: “A has power over B to the extent that he can get B to do something that B would not otherwise do” (emphasis added). Any kind of state force implementing objectives of sustainability by top downregulation means exercising direct power. Non-state actors may also play a role in this game. Coase [ 43 ] explains this for business firms. Also when Pilgrim and Harvey [ 44 ] demonstrate how NGO lobbying significantly affected biofuel policy changes and sustainability regulation in the UK and in Europe, they assume that NGOs enforce their ideas against others in an arena of obviously competing demands.
The second dimension of hidden power refers to power not obviously opposed by anyone. Bachrach and Baratz [ 11 ] speak of “two faces of power” emphasizing that some issues never even make it onto the political agenda and are dismissed before observable negotiations start. For a long time, the EU issued biofuels only in the context of climate change, completely neglecting aspects of competing food demands and land use change in the Global South [ 45 , 46 ]. Scholars demonstrating such hidden aspects apply this second dimension of power over to analyze biofuel governance.
The traditional conception of structural (hidden) power in international relations aims to address the coercion resulting from the capital mobility of transnational corporations. Threats to shift investments abroad do not even need to be voiced in order to influence policies in their favor [ 42 , 47 ]. More recent studies point to the fact that businesses also exercise structural power by self-regulation and public-private partnerships; these types of governance allow business actors to actively set rules, for example, for the “sustainable” production of biofuels at the expense of state actors [ 42 , 48 ]. In addition, as public authorities have faced challenges in facilitating the implementation of their sustainability criteria outside their jurisdictions, the EU has started to use these private schemes to verify compliance with sustainability criteria in biofuel production outside its own territory [ 49 , 50 ]. As a result, following this perspective, power in the global political economy has been diffused, leaving biofuel conglomerates with considerable power over others [ 51 ].
Further, scholars are increasingly focusing on power relations linked to latent conflicts of interest. In the third dimension, invisible power comes to play as a result of norms and ideas [ 41 ]. Research analyzes discourses, communication practices, cultural values and institutions, which all work to shape relevant thoughts and actions [ 12 ]. With regard to biofuels, Munro [ 22 ] has shown how, in the United States, a powerful coalition of agricultural interests manipulated the governance of biofuels by linking it to public concerns about climate change and energy security. In consequence, corn biofuel received political support, tax reductions, and subsidies. Likewise, Puttkammer and Grethe [ 52 ] have found a coalition of biofuel advocates to dominate the public discourse in Germany, while scientists who doubted the efficiency of biofuels could not make their voice heard. The discourse only shifted with the 2007–2008 food price crisis when scholars demystified the “ethanol bubble” [ 53 ] and outlined potentially devastating implications for global poverty and food security. Experts, NGOs, and business actors who have challenged the sustainability of biofuels on many fronts began to be heard [ 20 , 22 ].
For the most part, these discourse scholars blame other scholars who apply a perspective of power with for neglecting and postponing important questions of social justice linked to biofuel production [ 21 , 54 ]. Win-win rhetoric is demonstrated to manifest global power asymmetries rather than to contribute to more ecology and fairness [ 22 , 53 ]. From this perspective, pioneers and leaders, whose role Young [ 31 ] and Bernard and Prieur [ 30 ], among others, consider to be positive, only serve dominant interests and prevent a more fundamental social transformation to sustainability. With reference to the International Political Economy, most scholars deny a simple confrontation of biofuel proponents (or pioneers) and opponents (or laggards). In this vein, Levidow [ 55 ] outlines how the EU can continue “its global plunder of resources” because it pursues global leadership for sustainable biofuels. Silva-Castaneda [ 56 ] demonstrates how, in Indonesia, some NGOs decided to participate in the Roundtable on Sustainable Palm Oil (RSPO), a certification process initiated by the WWF, among others. The local NGOs managed to include important clauses regarding indigenous and land rights in the RSPO standard. In practice, however, auditors rarely recognize as valid evidence the forms of proof put forward by local communities, and global conglomerates could even use the standards to increase their primacy vis-à-vis local farmers [ 56 ]. These examples reveal power over within multi-stakeholder processes.
Studies demonstrate that the expansion of biofuels in countries of the Global South was only possible through the partial neglect (simplification) of their cultural and ecological diversity [ 57 ]. Nygren [ 58 ] illustrates how leading retailers, in negotiation with environmental organizations, have guided consumers’ expectations of certified Southern forest products by building images of Southern community forest producers as authentic and exotic others . She concludes that certification as a market-based form of governance has only had a limited impact on altering the unequal relationship characteristic of global networks of production and consumption.
With reference to Foucault [ 13 ] and Bourdieu [ 59 ], we can capture links between knowledge, power, and politics in a fourth dimension of power over [ 17 ]. Critical and (post-) structuralist approaches understand power in a way that everything is socially constructed. Scholars analyze the normative impact on (supposed) losers, such as farmers in the Global South, as well as on (supposed) winners, such as major agribusiness actors. All actors work to mainly reproduce systems and positions [ 60 ]. With regard to biofuels, several studies have highlighted the central role of knowledge and framing [ 15 , 16 , 21 ]. Drawing on Foucault, Kuchler and Linnér [ 21 ] have analyzed the discursive practices of the three major international organizations focused on food and agriculture, energy, and climate with regard to biofuels over the last 20 years: the UN Food and Agriculture Organization (FAO), the International Energy Agency (IEA), and the Intergovernmental Panel on Climate Change (IPCC). They found that, in contrast to pro and contra accounts, the arguments of all three organizations reflected a policy consensus based on the mainstream notion of industrial agricultural production, promoting the intensification and expansion of rural production. The biofuel discourse has further constituted a concatenation of the three issues of agricultural production, energy security, and climate change mitigation. When the discourse shifted with the 2007–2008 food price crisis, all the three major organizations adapted to this shift [ 21 ]. Instead of exercising power over by manipulating discourses on biofuels according to specific pro or contra interests, the organizations were found to rather reproduce hegemonic discourses and their own positions.
The gold rush metaphor is used a lot to describe the situation of biofuels from a power over perspective [ 1 ]. Biofuel production, like gold mining, is unprofitable for most farmers, just like it was for diggers and mine owners. Both biofuel production and gold mining can in addition have very negative environmental effects. While, however, people are made to believe that everyone can become abundantly wealthy (“energy sheiks”), only some few investors make large fortunes. Applying discursive approaches of power over , we can argue that even such investors and major businesses are subject to and not only conscious manipulators of discourses of agricultural intensification and economic growth. The analysis of power over helps to understand why change to more sustainable transport and agricultural systems does not happen. However, as I argue in this article, it falls short on explaining when and why there also sometimes is disruptive change and empowerment.
Power to change: interrelations between power with, power to, and power over
While the perspectives of power with and power to (over-) emphasize the potential for change with regard to biofuels, scholars with understandings of power over often exaggerate their negative impacts. The tripartite framework allows for the combining of different analytical perspectives and to examine their interrelations. While the three categories are first of all analytical heuristics, they also stand for different mechanisms of the exercise of power (see Fig. 1 ). Power over affects what is considered a “sustainable innovation” and “creative alternative”. Research has demonstrated this. However, I argue that it is also possible the other way round: there are situations in which power with and power to can address power imbalances and prevent a situation in which there are only a few winners and many losers as a result of biofuel governance.
Agent-based power
As shown in Fig. 1 , besides considering material and ideational sources of power, we also need to consider different mechanisms of power (over/to/with), since they lead to different results of power (leading to a new distribution of sources in a circular process, see the arrow at the bottom of Fig. 1 ). Biofuels per se are neither a sustainable innovation, a creative alternative nor a gold rush. The three metaphors exemplify three different results of power: the exercise of power over leads to a gold rush situation. So, if scholars only ask for power over , they will always find winners and losers. By contrast, if we ask for the exercise of power to , we may find that biofuels are creative alternative. Finally, the exercise of power with can be exemplified by a case of finding an agreement on sustainability criteria of biofuel production. To demonstrate overlaps, especially, in terms of the results of power, I used dashed lines in Fig. 1 .
When, in the field of biofuels, scholars explicitly issue power, they generally use concepts of power over to explain why governance and research in this field have a blind spot for power asymmetries [ 49 , 53 ]. Biofuel opponents may have accomplished a shift in the biofuel discourse after the 2007–2008 food price crisis [ 20 , 22 ]. However, overriding power asymmetries have prevented a structural change in both the energy/transport and the agricultural sectors. The trend is now definitely towards large companies and conglomerates [ 49 , 50 ].
However, the fact that biofuels have caused no structural change and have disadvantaged rather than empowered small farmers in the Global South, does not mean that a structural change is impossible. What I want to argue in this article is that exercising consensual forms of power (power with) as well as self-empowerment and resistance (power to) can also eclipse and overcome power asymmetries (power over). Empirical research on deliberative processes suggests that communication and common action never happen among equals and that they are never free from any form of power over [ 36 , 61 ]. Hence, we need to understand power with as a form of exercising power, which is strategic (bargaining) as well as communicative (arguing). A crucial part of this process is the orientation of agents involved in processes of biofuel governance. If actors are open to changing their positions and developing shared understandings, transitions to sustainability can follow from dialogues [ 61 , 62 ].
Following this perspective, even if small farmers in the Global South have fewer capabilities compared to conglomerates from the EU and the United States, this does not mean that they have no possibility to act independently from them. For example, sugar is costly to establish, and thus is economically most efficient at large plantation scales. However, Jatropha can more readily be produced through outgrower schemes as it is less capital intensive [ 9 , 49 ]. While currently almost all bio-ethanol is produced from grain or sugarcane and therefore competes with food purposes, other efficient and economically viable technologies for ethanol production are available [ 63 ]. The production of perennial energy crops, such as grasses and trees, and crop residues, such as straw, are seen to require fewer inputs and less prime land [ 64 ].
Under specific conditions, empowerment is possible; processes of power with and power to can have a (positive) impact on unwanted relations of power over . For example, processes of stakeholder dialogue and certification demonstrate that an agreement beyond the lowest common denominator is possible. In addition, they can weaken the perceived legitimacy of powerful actors that are producing biofuels unsustainably. The critical discourse on biomass certification has issued consumers’ accountability for harmful social and environmental effects in countries of production [ 55 , 65 ]. When the legitimacy of unconditional import as well as of private certification schemes was put into question [ 50 ], transnational conglomerates lost ideational and material resources on which their power over others was based. In the agrifood sector, we can clearly see that certification has become a new normative obligation [ 66 ].
We can observe various kinds of empowerment and resistance related to biofuels. While Nygren [ 58 ] argues that certification schemes reproduce (inferior) positions of southern producers as authentic and exotic others, she does not completely deny that certification had a positive impact on altering asymmetries in global networks of production and consumption. Silva-Castaneda’s [ 56 ] study discloses new ways in which local communities can legally prove their land rights, for instance, by video documentation to replace missing formal documents or destructed land marks.
Scholars have described movements, such as Via Campesina, in terms of exercising power over and opposing transnational agriculture corporations [ 67 ]. In terms of reducing and overcoming power asymmetries, however, what is most striking is the fact that small farmers within this movement exercise power to by doing healthy and sustainable agriculture independently of the major agribusinesses to which, from a power over perspective, they would only be subordinated. At the same time, when producing organically, small farmers do not reproduce the system of industrial agricultural production (and their inferior positions within that system). So, their way of farming can be considered as a creative alternative and as a way of resistance. Moreover, within this movement of Via Campesina, despite widely different internal cultures, farmers also exercise power with by (re-) constituting a new shared peasant identity. From a perspective of power with, we can argue that, in the long run, everybody, even from outside this movement, may benefit and share norms and values developed here such as sustainability in farming. The movement delegitimizes the acquisition of land by established conglomerates (“land grabbing”), whose ideational sources of power shrink in consequence. The visible result is a new, more equal, and just distribution of (power) resources through land reforms.
Conclusions
This article should not only encourage a debate on power issues with regard to biofuels, but moreover, develop the debate more comprehensively. When political power has been analyzed in the context of biofuels, this has happened so far through using confrontational or structuralist and discursive approaches that are based on an understanding of power over . Respective scholars have accused other researchers of neglecting “real power concentrations” in the biofuels industries. Often quite rightly: biofuel research has neglected the limits of win-win for a very long time. Scholars have taken sides and normatively inflated their own pro biofuel position, while they have dispatched their adversaries as laggards with regard to the future of transport and agriculture. Of course, not every (supposedly) sustainable innovation is necessarily good in the sense that it is completely uncontroversial (even if there is no visible opposition as in the case of biofuels for a long time). In this context, the question of power essentially addresses the re-politicization of decisions perceived to be urgent and without alternative. With the 2007–2008’s shift in discourse, critics re-politicized the governance of biofuels. Several farmer associations have completely turned against biofuels. I argue that this rejection of biofuels is due to a limited perception of power as power over .
Why does it make sense to complement such a perception of power over ? Why does a multidimensional power framework make more sense? Naming different perspectives, as done here, with one and the same term—“power”—means, first, to put them on one normative level. Gold rush (power over) is a term with strongly negative connotations, on the one hand, and leads to normatively inflating sustainable innovations (power with) and creative resistance (power to), on the other. This is often unjustified because the exercise of power with and power to are not per se more legitimate forms of achieving social change. For example, preventing greenhouse gas emissions “from above” can be quite legitimate.
Secondly, as illustrated in this article, all three conceptions of power are already used in research on biofuels (although sometimes only implicitly; this should change). My hope is that this article addresses diverse communities and overcomes boundaries between them with this multidimensional power approach (in particular, between those who still celebrate biofuels as a “sustainable innovation” and those scholars who completely condemn them because of related power asymmetries). Especially those whose research is (implicitly) based on understandings of power as power with and power to could take stronger reference to researchers taking a critical viewpoint on their studies (power over)—in particular, through showing how consensual forms of power exercise (power with) and resistance and empowerment (power to) not only reproduce power asymmetries but also help overcome them. If we look at the gold rush metaphor from a perspective of power to , we may see that there is a lot of entrepreneurship involved in the discovery of gold deposits. From the perspective of power with , we may also see that people in the field of gold mining as well as of biofuel production find common ground among diverse interests and organize with each other.
Third, convincing and learning (power with) as well as creative ability (power to) and coercion and manipulation (power over) do not completely capture concrete change processes. The analytical categories applied in this paper help to cluster the various understandings of power in biofuel research, but they also reflect different mechanisms of power in reality. Power with perspectives focus on the benefits of biofuels (sustainable innovation); power to focuses on how new actors develop alternatives to fossil (and nuclear)-based economies; power over points to the limits of change because of the dominance of specific actors, structures, and discourses. The common terminology allows that the three perspectives on power are not considered as mutually exclusive (different interpretations of the same phenomenon), but as supplementary (different aspects of a change process). It becomes possible to examine their interrelations and their supplementary potential. With this article, I hope to have given an impetus for further research in this direction. A comprehensive analysis of power in diverse parts of biofuel research and governance is definitely a prerequisite for more seriously and intensively exploring questions of where, when, and how the governance of biofuels may also allow for “green” resistance and collective empowerment.
If actors create (reproduce) discourses and structures, I call this power to . Most constructivist studies however deal with identifying dominant (hegemonic) structures and discourses over others that are unconsciously reproduced, i.e., power over .
Power with is not identical to Arendt’s understanding of power or its empirical operationalization hardly accomplishes Arendt’s demands. So deliberative theories of democracy build upon her understanding of power without finding it comprehensively implemented in reality [ 61 , 68 , 69 ]. In difference to deliberative processes, power with encompasses communicative as well as common action.
An example, to which Arendt refers in a footnote to her definition of power, is the student protests at Berkeley and elsewhere at the end of the 1960s. She contrasts the power of the students—“obviously the strongest power on every campus simply because of the students’ superior number” ([ 28 ]: 44)—to the violence of the university authorities. An individual student leader ‘in power’ would speak on behalf of the movement.
Balkema A, Pols AJ (2015) Biofuels: sustainable innovation or gold rush? Identifying responsibilities for biofuel innovations. In: Koops B-J, Oosterlaken I, Romijn H et al (eds) Responsible innovation 2: concepts, approaches, and applications. Springer, New York, pp 283–303
Google Scholar
Partzsch L (2015) Kein Wandel ohne Macht–Nachhaltigkeitsforschung braucht ein mehrdimensionales Machtverständnis. GAIA 24(1):48–56
Article Google Scholar
Sordaa G, Banseb M, Kemfert C (2010) An overview of biofuel policies across the world. Energy Policy 38(11):6977–6988
Vermeulen S, Dufey A, Vorley B (2008) Biofuels: making tough choices. The International Institute for Environment and Development (IIED) (2)., pp 1–2
Parsons T (1963) On the concept of political power. Proc Am Philos Soc 107(3):232–262
Pitkin HF (1985) Wittgenstein and justice: on the significance of Ludwig Wittgenstein for social and political thought. University of California Press, Berkeley
Barnett M, Duvall R (2005) Power in global governance. In: Barnett MN, Duvall R (eds) Power in global governance. Cambridge University Press, Cambridge, pp 1–32
Altieri MA, Toledo VM (2011) The agroecological revolution in Latin America: rescuing nature, ensuring food sovereignty and empowering peasants. J Peasant Stud 38(3):587–612
van Eijcka J, Romijn H (2008) Prospects for Jatropha biofuels in Tanzania: an analysis with strategic niche management. Energy Policy 36(1):311–325
Dahl RA (1957) The concept of power. Behav Sci 2(3):201–215
Bachrach P, Baratz MS (1962) Two faces of power. Am Polit Sci Rev 4(56):947–952
Lukes S (1974) Power: a radical view. Macmillan, London
Book Google Scholar
Foucault M (1982) The subject and power. Crit Inq 8(4):777–795
Laclau E, Mouffe C (1985) Hegemony and socialist strategy: towards a radical democatic politics. Verso, London
Baka J (2014) What wastelands? A critique of biofuel policy discourse in South India. Geoforum 54(6):315–323
Hunsberger C (2010) The politics of Jatropha-based biofuels in Kenya: convergence and divergence among NGOs, donors, government officials and farmers. J Peasant Stud 37(4):939–962
Digeser P (1992) The fourth face of power. J Polit 54(4):977–1007
Haugaard M (2012) Rethinking the four dimensions of power: domination and empowerment. J Polit Power 5(1):33–54
Hunt SC, Sawin JL, Stair P (2006) Cultivating renewable alternatives to oil. In: Worldwatch Institute (ed) State of the world 2006: Special focus: India and China. Earthscan, London, pp 61–77
Sengers F, Raven R, van Venrooij A (2010) From riches to rags: biofuels, media discourses, and resistance to sustainable energy technologies. Energy Policy 38(9):5013–5027
Kuchler M, Linnér B-O (2012) Challenging the food vs. fuel dilemma: genealogical analysis of the biofuel discourse pursued by international organizations. Food Policy 37(5):581–588
Munro B (2015) The lost innocenece of ethanol: Power, knowledge, discourse, and the U.S. biofuel policy: Doctoral dissertation. Kansas State University, Kansas
Eyben R, Harris C, Pettit J (2006) Introduction: exploring power for change. IDS Bull 37(6):1–10
Gaard G (2010) Women, water, energy: an ecofeminist approach. In: Brown PG, Schmidt JJ (eds) Water ethics: foundational readings for students and professionals. Island Press, Washington, DC, pp 59–75
Balat M, Balat H (2009) Recent trends in global production and utilization of bio-ethanol fuel. Appl Energy 86(11):2273–2282
Nill J, Kemp R (2009) Evolutionary approaches for sustainable innovation policies: from niche to paradigm? Res Policy 38(4):668–680
Allen A (1998) Rethinking power. Hypatia 13(1):21–40
Arendt H (1970) On violence. Harcourt, Brace & World, New York
Skodvin T, Andresen S (2006) Leadership revisited. Glob Environ Polit 6(3):13–27
Bernard F, Prieur A (2007) Biofuel market and carbon modeling to analyse French biofuel policy. Energy Policy 35:5991–6002
Young OR (1991) Political leadership and regime formation: on the development of institutions in international society. Int Organ 45(3):281–308
Huber J (2000) Towards industrial ecology: sustainable development as a concept of ecological modernization. J Environ Policy Plan 2(4):269–285
Jänicke M (2008) Ecological modernisation: new perspectives. J Clean Prod 16(5):557–565
Bomb C, Deurwaarder E, Kaberger T (2007) Biofuels for transport in Europe: lessons from Germany and the UK. Energy Policy 35(4):2256–2267
Foxona T, Pearson P (2008) Overcoming barriers to innovation and diffusion of cleaner technologies: some features of a sustainable innovation policy regime. J Clean Prod 16(1):S148–S161
Longstaff H, Secko DM (2014) Assessing the quality of a deliberative democracy mini-public event about advanced biofuel production and development in Canada. Public Understanding of Science (online)., pp 1–10
Nussbaum M, Sen AK (1987) Internal criticism and Indian rationalist traditions. World Institute for Development Economics Research of the United Nations University, Helsinki
Scholtes F (2009) Analysing and explaining power a capability perspective, Bonn
Malik US, Ahmed M, Sombilla MA et al (2009) Biofuels production for smallholder producers in the Greater Mekong Sub-region. Appl Energy 86(S1):S58–S68
Rathke G-W, Diepenbrock W (2006) Mit Biomasse zum ‘Energie-Scheich’?: Stand, Ertragspotenziale und Perspektiven. Chancen für Landwirtschaft und angrenzende Industriezweige. In: DLG (ed) (Deutsche Landwirtschafts-Gesellschaft) Zukunftsstandort Deutschland: Strategien Für Die Landwirtschaft. DLG-Verlag, Frankfurt a.M
Gaventa J (2006) Finding the spaces for change: a power analysis. IDS Bull 37(6):23–33
Fuchs D (2005) Understanding business power in global governance. Nomos, Baden-Baden
Coase RH (1988) The firm, the market, and the law. University of Chicago Press, Chicago
Pilgrim S, Harvey M (2010) Battles over biofuels in Europe: NGOs and the politics of markets. Sociol Res Online 13(3):4
Stattman SL, Gupta A (2015) Negotiating authority in the global biofuel governance: Brazil and the EU in the WTO. Glob Environ Polit 15(1):41–59
Afionis S, Stringer LC (2012) European Union leadership in biofuels regulation: Europe as a normative power? J Clean Prod 32(1):114–123
Altvater E, Mahnkopf B (1999) Grenzen der Globalisierung: Ökonomie, Ökologie und Politik in der Weltgesellschaft, 4th edn. Westfälisches Dampfboot, Münster
Levin K, Cashore B, Koppell J (2009) Can non-state certification systems bolster state-centered efforts to promote sustainable development through the clean development mechanism? Wake Forest Law Review 44(1):777–798
Dauvergne P, Neville KJ (2010) Forests, food, and fuel in the tropics: the uneven social and ecological consequences of the emerging political economy of biofuels. J Peasant Stud 37(4):631–660
Ponte S, Daugbjerg C (2015) Biofuel sustainability and the formation of transnational hybrid governance. Environ Polit 24(1):96–114
Henriksen LF (2015) The global network of biofuel sustainability standards-setters. Environ Polit 24(1):115–137
Puttkammer J, Grethe H (2015) The public debate on biofuels in Germany: who drives the discourse? Ger J Agric Econ 64(4):263–273
Runge CF, Senauer B (2007) How biofuels could starve the poor. Foreign Aff 86(3):41–53
Horlings LG, Marsden TK (2011) Towards the real green revolution? Exploring the conceptual dimensions of a new ecological modernisation of agriculture that could ‘feed the world’. Glob Environ Chang 21(2):441–452
Levidow L (2013) EU criteria for sustainable biofuels: accounting for carbon, depoliticizing plunder. Geoforum 44(1):211–223
Silva-Castaneda L (2012) A forest of evidence: third-party certification and multiple forms of proof—a case study of oil palm plantations in Indonesia. Agric Hum Values 29(3):361–370
Selfa T, Bain C, Moreno R et al (2015) Interrogating social sustainability in the biofuels sector in Latin America: tensions between global standards and local experiences in Mexico, Brazil, and Colombia. Environmental Management
Nygren A (2015) Governance and images: representations of certified southern producers in high-quality design markets. Environ Values 24(3):391–412
Bourdieu P (1987) Sozialer Sinn: Kritik der theoretischen Vernunft. Suhrkamp, Frankfurt a.M
Guzzini S (1993) Structural power: the limits of neorealist power analysis. Int Organ 47(3):443–478
Dryzek JS (2000) Deliberative democracy and beyond: liberals, critics, contestations. Oxford University Press, New York
Prittwitz V (ed) (1996) Verhandeln und Argumentieren. Leske + Budrich, Opladen
Mussatto SI, Dragone G, Guimarães PM et al (2010) Technological trends, global market, and challenges of bio-ethanol production. Biotechnol Adv 28(6):817–830
Shortall O (2014) Rethinking bioenergy from an agricultural perspective: ethical issues raised by perennial energy crop and crop residue production for energy in the UK and Denmark: PhD thesis. University of Nottingham, Nottigham
Partzsch L (2011) The legitimacy of biofuel certification. Agric Hum Values 28(3):413–425
Kalfagianni A (2015) ‘Just food’: the normative obligations of private agrifood governance. Glob Environ Chang 31(1):174–186
Martínez-Torres ME, Rosset PM (2010) La Vía Campesina: the birth and evolution of a transnational social movement. J Peasant Stud 37(1):149–175
Habermas J (1998) Between facts and norms: contributions to a discourse theory of law and democracy. MIT Press, Cambridge
Young IM (2001) Activist challenges to deliberative democracy. Polit Theo 29(5):670–690
Partzsch L, Fuchs DA (2012) Philanthropy: power with in international relations. J Polit Power 5(3):359–376
Guzzini S (2007) The concept of power: a constructivist analysis. In: Berenskoetter F, Williams MJ (eds) Power in world politics. Routledge, New York, pp 23–42
Download references
Acknowledgements
The research received no funding.
Competing interests
The author declares that she has no competing interests.
Publisher’s Note
Springer Nature remains neutral with regard to jurisdictional claims in published maps and institutional affiliations.
Author information
Authors and affiliations.
Sustainability Governance, Institute of Environmental Social Sciences and Geography, University of Freiburg, Tennenbacher Strasse 4, D-79106, Freiburg, Germany
Lena Partzsch
You can also search for this author in PubMed Google Scholar
Corresponding author
Correspondence to Lena Partzsch .
Rights and permissions
Open Access This article is distributed under the terms of the Creative Commons Attribution 4.0 International License ( http://creativecommons.org/licenses/by/4.0/ ), which permits unrestricted use, distribution, and reproduction in any medium, provided you give appropriate credit to the original author(s) and the source, provide a link to the Creative Commons license, and indicate if changes were made.
Reprints and permissions
About this article
Cite this article.
Partzsch, L. Biofuel research: perceptions of power and transition. Energ Sustain Soc 7 , 14 (2017). https://doi.org/10.1186/s13705-017-0116-1
Download citation
Received : 01 February 2017
Accepted : 04 May 2017
Published : 18 May 2017
DOI : https://doi.org/10.1186/s13705-017-0116-1
Share this article
Anyone you share the following link with will be able to read this content:
Sorry, a shareable link is not currently available for this article.
Provided by the Springer Nature SharedIt content-sharing initiative
Energy, Sustainability and Society
ISSN: 2192-0567
- General enquiries: [email protected]
- Frontiers in Plant Science
- Plant Biotechnology
- Research Topics
Biofuels and Bioenergy
Total Downloads
Total Views and Downloads
About this Research Topic
Biofuels have gained popularity because of the growing concern about global warming caused by carbon dioxide emissions and the expected rise in oil demand and prices. Biofuels can be directly or indirectly derived from plants or microalgae through the process of photosynthesis. Feedstock for biomass ...
Keywords : Biofuels, Bioenergy, Biomass, Plant Cell Wall, Bioengineering
Important Note : All contributions to this Research Topic must be within the scope of the section and journal to which they are submitted, as defined in their mission statements. Frontiers reserves the right to guide an out-of-scope manuscript to a more suitable section or journal at any stage of peer review.
Topic Editors
Topic coordinators, recent articles, submission deadlines.
Submission closed.
Participating Journals
Total views.
- Demographics
No records found
total views article views downloads topic views
Top countries
Top referring sites, about frontiers research topics.
With their unique mixes of varied contributions from Original Research to Review Articles, Research Topics unify the most influential researchers, the latest key findings and historical advances in a hot research area! Find out more on how to host your own Frontiers Research Topic or contribute to one as an author.
- Our Unique Approach
- Leadership Team
- Research Advisory Board
- Regional Advisory Board
- Research Papers
- Sustainable CO2 Reduction and Climate Change Adaptation
- Sustainable Transportation
- Sustainable Energy Systems
- Sustainable Water and Agricultural Systems
- Sustainable Cities and Their Built Environment
- PUBLICATIONS
- Event Calendar
- Past Events
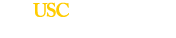
USC Center for Sustainability Solutions
Research papers: biofuels.
By Antonio M. Bento, Richard Klotz
Environmental Science & Technology
Lifecycle analysis (LCA) metrics of greenhouse gas emissions are increasingly being used to select technologies supported by climate policy. However, LCAs typically evaluate the emissions associated with a technology or product, not the impacts of policies. Here, we show that policies supporting the same technology can lead to dramatically different emissions impacts per unit of technology added, due to multimarket responses to the policy. Using a policy-based consequential LCA, we find that the lifecycle emissions impacts of four US biofuel policies range from a reduction of 16.1 gCO 2 e to an increase of 24.0 gCO 2 e per MJ corn ethanol added by the policy. The differences between these results and representative technology-based LCA measures, which do not account for the policy instrument driving the expansion in the technology, illustrate the need for policy-based LCA measures when informing policy decision making.
Research Areas
Research topics.

- Articles in Press
- Current Issue
Journal Archive
Join our Community on:
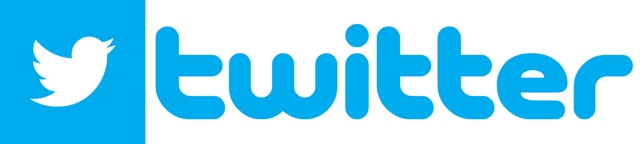
Biofuel Research Journal`s Official Channel on WeChat (in Mandarin Chinese)
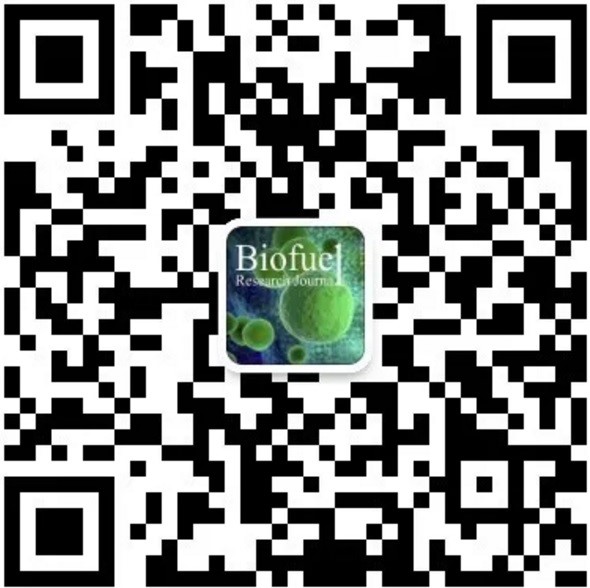
Latest News
Biofuel Research Journal
has been accepted to be indexed by Inspec!
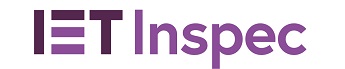
"Inspec, produced by The
Institution of Engineering
and Technology , has been
the definitive engineering
and physics research database
for over 50 years"
Read more about Inspec on Clarivate TM
has been accepted to be indexed by Ei Compendex (Engineering Village)!

Read more here !
Read the Editor`s Pick: Biogas Collection here !
is now indexed by ProQuest!
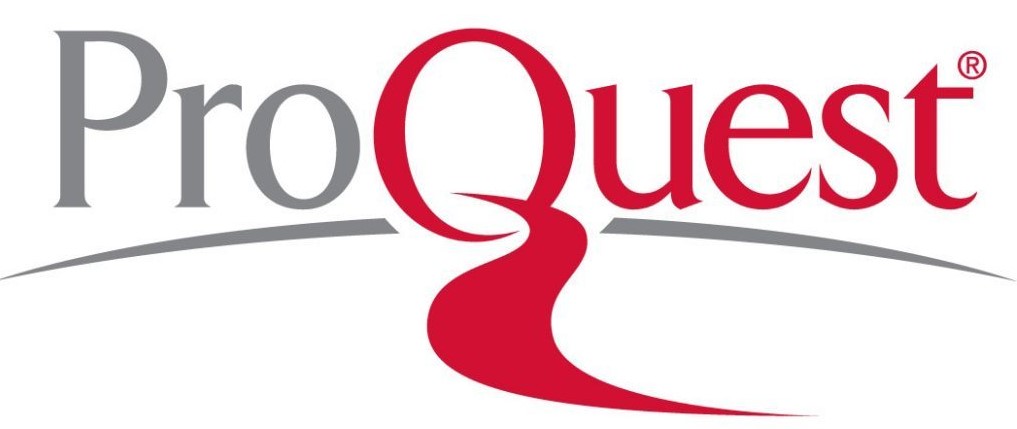
We are delighted to announce .... Read more here !
Celebrating a year of growth
for Biofuel Research Journal !
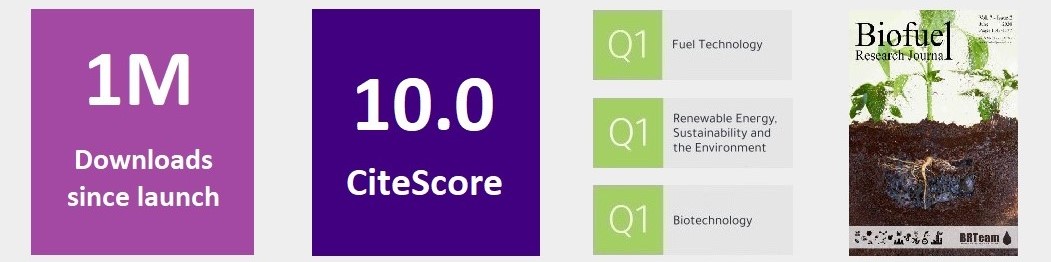
This year marks a year of growth .... Read more here !
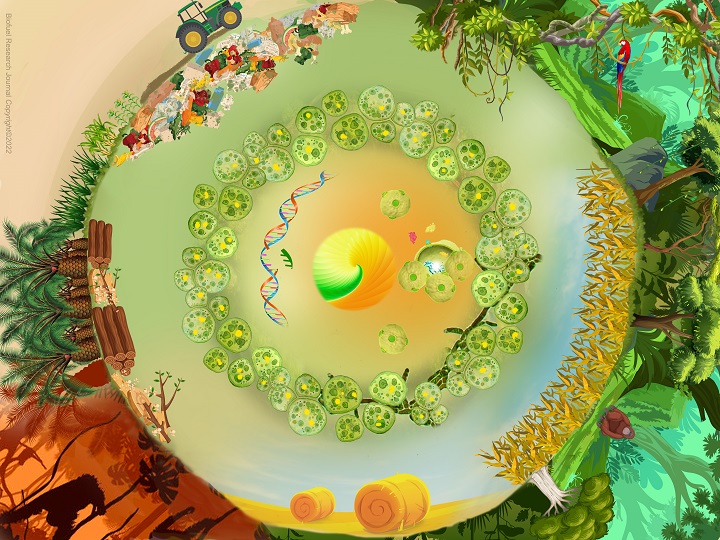
Biofuel Research Journal (BRJ) is a leading, peer-reviewed academic journal dedicated to publishing high-quality research on biofuels, bioproducts, and related biomass-derived materials and technologies. BRJ is an open-access online journal and completely free-of-charge, aiming to advance knowledge and understanding of sustainable energy solutions, environmental protection, and the circular economy through cutting-edge research and innovative applications. The journal welcomes original articles, review papers, case studies, short communications, and hypotheses in the following areas:
- Biofuels and Bioproducts: Exploring the production, characterization, and application of various biofuels, such as biodiesel, bioalcohols (bioethanol, biobutanol), biogas (biomethane, biohydrogen), algal biofuels, and other emerging biofuel sources. Additionally, BRJ encourages research on bioproducts, including biocomposites, bio-based smart materials, and innovative applications in the fields of food, pharmaceuticals, and beyond.
- Biomass Valorization: Investigating efficient and sustainable methods for biomass conversion, including biorefineries, bioprocesses, and advanced biomass utilization techniques to maximize energy and value-added product yields.
- Biomass-Derived Materials for Energy and Storage Systems: Advancing research on biomass-derived materials with potential applications in energy conversion and storage systems, including fuel cells, batteries, supercapacitors, and photovoltaic modules.
- Biomass-Derived Materials for Environmental Sustainability: Exploring using biomass-derived catalysts and materials for carbon dioxide capture and conversion to mitigate greenhouse gas emissions. Additionally, researching biomass-derived catalysts for air, soil, and water pollution mitigation contributes to improved environmental quality.
- Biomass-Derived Materials for Sustainable Applications: Investigating the use of biomass-derived materials in food applications, such as sustainable packaging materials or functional food ingredients, as well as their potential in biomedical applications, drug delivery systems, tissue engineering, and regenerative medicine.
- Biomass-Derived Materials for Catalytic Applications: Advancing the understanding and development of biomass-derived materials with catalytic properties, enabling green and sustainable manufacturing processes and chemical transformations.
- Techno-Economic and Environmental Assessments: Analyzing the techno-economic feasibility and environmental sustainability (life cycle assessment, exergy, emergy, risk assessment) of biofuel, bioproduct, and biomass-derived materials production and application pathways, ensuring their compatibility with international standards (e.g., PAS 2050:2011 and ISO 14040:2006).
- Climate Change and Sustainability: Investigating the impacts of biofuel and bioproduct production and consumption on climate change and promoting innovative, low-carbon pathways for their production and use. Articles focusing on strategies for limiting greenhouse gas emissions and promoting circular economy principles in the bioenergy sector are encouraged.
- Novel Processing and Integrated Systems: Highlighting innovative approaches and integrated systems for biofuel and bioproduct processing, as well as energy audits for production plants to enhance efficiency and sustainability.
- Artificial Photosynthesis for Biofuels Production: Exploring cutting-edge research on artificial photosynthesis as a potential green route for biofuels production.
- Promotion of Biofuels and Bioproducts in Developing Economies: Encouraging research on the promotion and adoption of biofuels and bioproducts in developing economies to foster indigenous development and sustainable energy solutions.
- Biofuels and Bioproducts in Circular Economy: Addressing the role of biofuels and bioproducts in the circular economy framework, focusing on resource efficiency, waste valorization, and sustainability.
- Biofuels and Bioproducts Finance: Exploring financial aspects related to biofuels and bioproducts, including investment strategies, funding mechanisms, and economic considerations for the development and commercialization of sustainable bioenergy solutions.
BRJ aims to foster interdisciplinary collaborations among researchers, engineers, scientists, policymakers, and industry experts to accelerate the adoption of sustainable energy solutions and foster a greener future. The journal is committed to maintaining the highest standards of peer review and editorial integrity, ensuring that only high-quality and impactful research is published.
Biofuel Research Journal is indexed in Scopus and Web of Science .
BRJ currently has no fees. Learn More .
Editor-in-Chief: Vijai Kumar Gupta
> View Full Editorial Board
Editorial Board
10.18331/BRJ2024.11.1.1
- PDF 190.06 K
Research Paper
Critical impacts of energy targeting on the sustainability of advanced biobutanol separation.
Pages 1999-2012
10.18331/BRJ2024.11.1.2
Keikhosro Karimi; Benyamin Khoshnevisan; Joeri F.M. Denayer
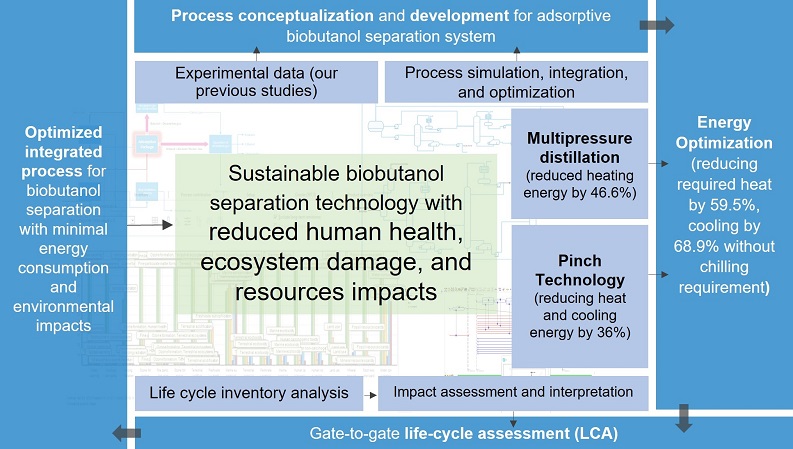
- View Article
Artificial humic substances as sustainable carriers for manganese: Development of a novel bio-based microfertilizer
Pages 2013-2024
10.18331/BRJ2024.11.1.3
Alexander Volikov; Helen Schneider; Nadezda V. Tarakina; Nader Marzban; Markus Antonietti; Svitlana Filonenko
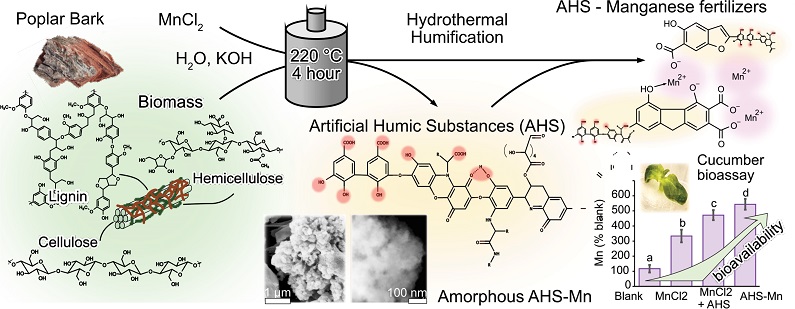
Lime-assisted hydrothermal humification and carbonization of sugar beet pulp: Unveiling the yield, quality, and phytotoxicity of products
Pages 2025-2039
10.18331/BRJ2024.11.1.4
Mona Ghaslani; Reza Rezaee; Omid Aboubakri; Ehsan Sarlaki; Thomas Hoffmann; Afshin Maleki; Nader Marzban
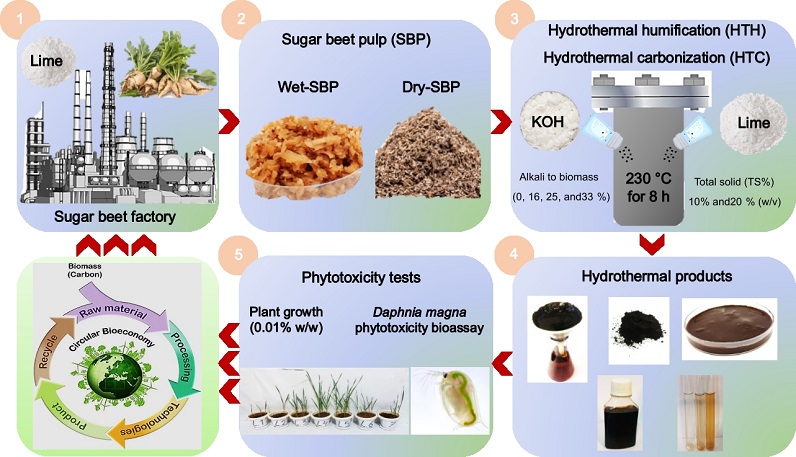
Review Paper
New trends in microbial lipid-based biorefinery for fermentative bioenergy production from lignocellulosic biomass.
Pages 2040-2064
10.18331/BRJ2024.11.1.5
Salauddin Al Azad; Meysam Madadi; Guojie Song; Chihe Sun; Fubao Sun
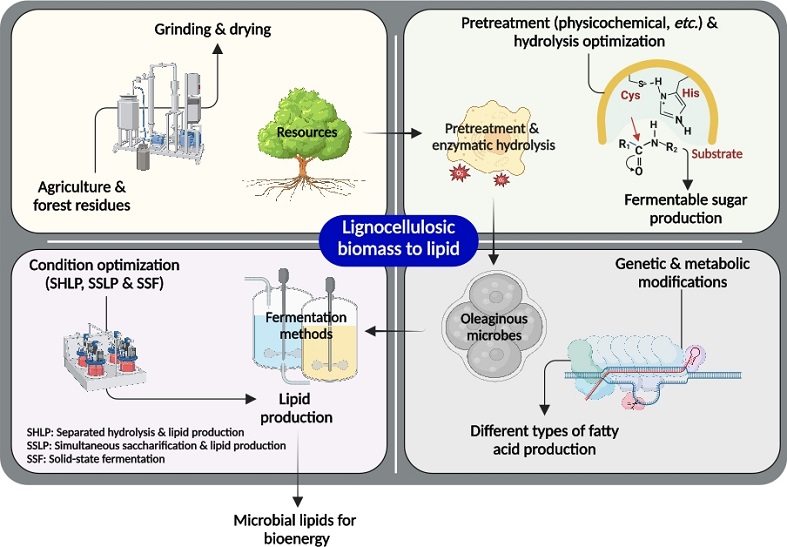
Publication Information
Indexing and abstracting.
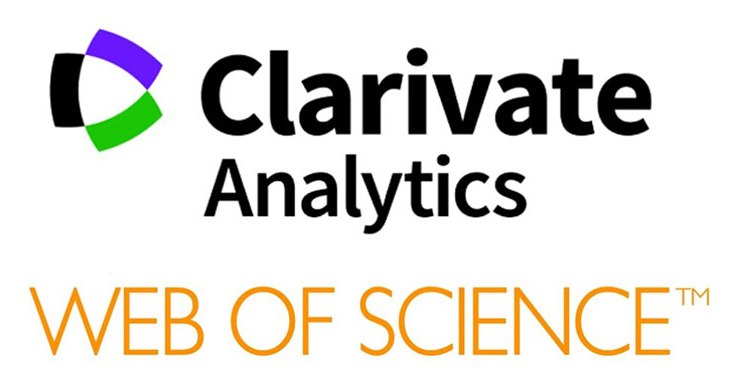

An official website of the United States government
The .gov means it’s official. Federal government websites often end in .gov or .mil. Before sharing sensitive information, make sure you’re on a federal government site.
The site is secure. The https:// ensures that you are connecting to the official website and that any information you provide is encrypted and transmitted securely.
- Publications
- Account settings
Preview improvements coming to the PMC website in October 2024. Learn More or Try it out now .
- Advanced Search
- Journal List
- Proc Math Phys Eng Sci
Environmental sustainability of biofuels: a review
Harish k. jeswani.
1 Department of Chemical Engineering and Analytical Science, The University of Manchester, Manchester M13 9PL, UK
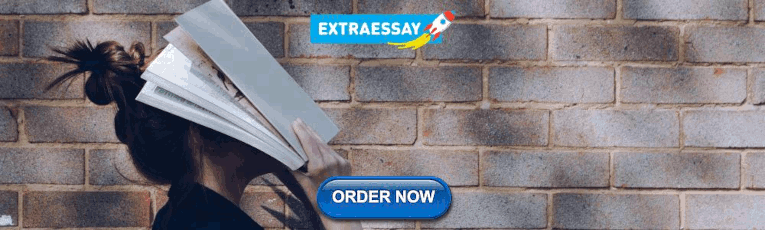
Andrew Chilvers
2 Royal Academy of Engineering, 3 Carlton House Terrace, London SW1Y 5DG, UK
Adisa Azapagic
Associated data.
This article does not contain any additional data.
Biofuels are being promoted as a low-carbon alternative to fossil fuels as they could help to reduce greenhouse gas (GHG) emissions and the related climate change impact from transport. However, there are also concerns that their wider deployment could lead to unintended environmental consequences. Numerous life cycle assessment (LCA) studies have considered the climate change and other environmental impacts of biofuels. However, their findings are often conflicting, with a wide variation in the estimates. Thus, the aim of this paper is to review and analyse the latest available evidence to provide a greater clarity and understanding of the environmental impacts of different liquid biofuels. It is evident from the review that the outcomes of LCA studies are highly situational and dependent on many factors, including the type of feedstock, production routes, data variations and methodological choices. Despite this, the existing evidence suggests that, if no land-use change (LUC) is involved, first-generation biofuels can—on average—have lower GHG emissions than fossil fuels, but the reductions for most feedstocks are insufficient to meet the GHG savings required by the EU Renewable Energy Directive (RED). However, second-generation biofuels have, in general, a greater potential to reduce the emissions, provided there is no LUC. Third-generation biofuels do not represent a feasible option at present state of development as their GHG emissions are higher than those from fossil fuels. As also discussed in the paper, several studies show that reductions in GHG emissions from biofuels are achieved at the expense of other impacts, such as acidification, eutrophication, water footprint and biodiversity loss. The paper also investigates the key methodological aspects and sources of uncertainty in the LCA of biofuels and provides recommendations to address these issues.
1. Introduction
Greenhouse gas (GHG) emissions from transport have been increasing at a faster rate than from any other sector [ 1 ]. The sector relies heavily on fossil fuels, which accounted for 96.3% of all transportation fuels in 2018 [ 2 ]. Transport is also responsible for 15% of the world's GHG emissions and 23% of total energy-related CO 2 emissions [ 1 ]. To reduce dependence on petroleum-based fuels, as well as to mitigate climate change, biofuels are viewed widely as promising alternative transportation fuels.
Biofuels have been used since the early days of the automotive industry. For instance, Rudolph Diesel tested his first engine on peanut oil [ 3 ] after pulverized coal was found to be unsuitable. Until the 1940s, biofuels were seen as viable transport fuels and bioethanol blends, such as Agrol, Discol and Monopolin, were commonly used in the USA, Europe and other regions [ 3 ]. Further development of bioethanol ceased after the Second World War as petroleum-derived fuel became cheaper. During the oil crisis in the 1970s, many countries showed renewed interest in production of commercial biofuels; however, only Brazil started to produce ethanol at a large scale as part of the National Ethanol Programme ‘Proálcool’ [ 4 ]. During the late 1990s, with the rise in crude oil prices and concerns over energy security, the USA and many nations in Europe developed policies in support of domestic biofuel industries [ 5 ]. The interest in biofuels further increased in the past decade with the development of policies on climate change mitigation and strategies to reduce GHG emissions from the transport sector. More than 60 countries have since launched biofuel programmes and set targets for blending biofuels into their fuel pools [ 6 ]. The most notable are Renewable Fuel Standard (RFS) [ 7 ] in the USA and the Renewable Energy Directive (RED) in Europe [ 8 ].
Owing to these policies, world bioethanol production has increased by 67%, from 67 to 110.4 billion litres, over the decade of 2008–2018 [ 2 ]. During the same period, biodiesel production increased more than threefold, from 12 to 41 billion litres. Currently, biofuels account for about 3.4% of total transportation fuels worldwide [ 2 ]. The global production of biofuels is dominated by the USA and Brazil—producing 69% of all biofuels in 2018—followed by Europe (EU-28) with 9% [ 9 ]. Production of bioethanol in the USA is almost exclusively from corn, whereas in Brazil, it is from sugarcane. In Europe, the main feedstocks are corn, wheat and sugar beet for bioethanol, while rapeseed and used cooking oil (UCO) are used for biodiesel production [ 10 ]. Argentina, Brazil and the USA also produce significant quantities of biodiesel, predominantly from soya bean, while Malaysia and Indonesia produce biodiesel from palm oil. Several international and national organizations have made mid- and long-term projections for global production of biofuels. These projections provide wide-ranging estimates of potential future increases in liquid biofuels for transport globally. The International Energy Agency (IEA) estimates that as much as one-third of all transportation fuel could come from biofuels by 2050 [ 11 ], while organizations, such as the OECD and BP, project approximately a 7% share of biofuels by 2030 [ 12 ]. A recent assessment [ 13 ] also suggests that the IEA projections could be impossible to achieve, estimating the maximum potential of transport biofuels by 2050 to be at least 30% lower than those projected by the IEA.
Biofuels can be differentiated according to a number of key characteristics, including feedstock type, conversion process, technical specification of the fuel and its use. Owing to this multitude of possible distinctions, various definitions are in use for biofuel types. Two commonly used typologies are ‘first, second and third generation’ and ‘conventional and advanced’ biofuels. Biofuels produced from food or animal feed crops are referred to as first-generation biofuels. Since first-generation biofuels are produced through well-established technologies and processes, such as fermentation, distillation and transesterification, they are also commonly referred to as ‘conventional biofuels'. A key characteristic for second-generation biofuels is that they are derived from non-food feedstocks, such as dedicated energy crops (e.g. Miscanthus , switchgrass, short rotation coppice (SRC) and other lignocellulosic plants), agricultural residues, forest residues and other waste materials (e.g. UCO and municipal solid waste). Biodiesel produced from microalgae through conventional transesterification or hydro-treatment of algal oil is commonly known as third-generation biofuel. Second- and third-generation biofuels are often referred to as ‘advanced biofuels’ as their production techniques or pathways are still in the research and development, pilot or demonstration phase. In this paper, the terminology ‘first, second and third generation’ has been selected and followed throughout. An overview of different biofuel types, their feedstocks and conversion routes can be seen in figure 1 .

An overview of feedstocks and production processes for different biofuels, also showing the life cycle of fuels from cradle to gate (well to tank) and cradle to grave (well to wheel). Adapted from [ 14 ]. The figure has been simplified and other feedstocks, production routes, products/by-products and uses are possible. The italic font denotes the focus of this review, i.e. bioethanol and biodiesel used for transportation. DDGS, dark distillers grain with solids. (Online version in colour.)
Biofuels offer both advantages and disadvantages in terms of environmental, economic and social sustainability [ 14 ]. On the one hand, reduction in GHG emissions, energy security and rural development are the most important drivers for biofuels globally. On the other hand, there are concerns related to increasing the production of biofuels, such as upward pressure on food prices, the risk of increase in GHG emissions through direct and indirect land-use change (LUC) from production of biofuel feedstocks, as well as the risks of degradation of land, forests, water resources and ecosystems [ 15 ]. The use of first-generation feedstocks, such as corn, has become a particularly contentious issue, largely owing to competition with food production and concerns over diverting agricultural land into fuel production. A growing demand for agricultural produce risks an increase in deforestation and use of land with a high biodiversity value to meet this demand, as well as associated usage of freshwater, fertilizers and pesticides, with negative consequences on the environment. Some of these issues could be addressed by using second-generation feedstocks; however, the economic viability of some second generation of biofuels remains doubtful in the current economic context, largely because of the low oil prices [ 16 – 18 ]. Third-generation (algal) biofuels could also avoid the issue of food competition and land use because microalgae can be grown on non-arable land and in wastewater, saline or brackish water and they grow extremely rapidly. However, the production of biofuels from microalgae is energy-intensive and at present economically unviable [ 19 ].
To encourage sustainable development of biofuels, regulatory policies, such as the RED and RFS, stipulate various sustainability criteria for biofuels. One of the main criteria is related to life cycle GHG emissions. The RED stipulates that biofuels should have at least 50% lower emissions than their fossil fuel alternatives for installations in operation before October 2015 and 60% for installations starting after this date, rising to 65% lower for biofuel plants commencing operation after 1 January 2021 [ 8 ]. RFS requires producers of advanced biofuels to reduce GHG emissions by at least 50%, while standard biofuels have to achieve a 20% reduction in GHG emissions [ 7 ]. The climate change impact related to GHG emissions and other sustainability aspects of biofuels should be evaluated on a life cycle basis via life cycle assessment (LCA) to avoid shifting burdens from one part of the life cycle or supply chain to another.
Numerous LCA studies have considered the potential of biofuels to achieve reductions in life cycle GHG emissions by estimating their potential impact on climate change. However, their findings are often conflicting, with a wide variation in the estimates. A number of review papers have also discussed LCA of biofuels, but these focused on a particular aspect, such as a region, feedstock or type of biofuel. For example, Shonnard et al . [ 20 ] reviewed LCA studies of biofuels in the Pan American region. Morales et al . [ 21 ] and Roy et al . [ 22 ] concentrated on LCA studies of lignocellulosic bioethanol, while Menten et al . [ 23 ] focused on advanced biofuels. Sieverding et al . [ 24 ] conducted a review of soya bean-based biodiesel and van Eijck et al . [ 25 ] reviewed issues pertaining to biodiesel from Jatropha .
However, a comprehensive review of all types of biofuels is not available in the literature. Besides, many more LCA studies on biofuels have been published since the publication of the above-mentioned reviews. Thus, this review paper aims to close this gap by analysing and synthesizing the latest information concerning LCA of biofuels. The main objective is to provide a greater clarity and understanding of the environmental sustainability of different liquid biofuels with the aim of informing future policy. A further objective is to examine the state-of-the-art knowledge on environmental issues associated with the production and consumption of biofuels. The next section provides an overview of the reviewed LCA studies in terms of their coverage with regard to biofuel type, geographical location and their approaches to handling critical methodological aspects in LCA. Section 3 presents results for the climate change impact, energy and water use and other environmental issues associated with different types of biofuels. The key methodological aspects and sources of uncertainty in assessing the environmental impacts of biofuels are investigated and discussed in detail in §4. The paper ends with conclusions and recommendations on addressing the key issues related to sustainability of biofuels.
2. Study methodology and coverage
A systematic literature search was performed in different databases (Science Direct, Web of Science, Scopus and relevant academic journals) to identify academic, peer-reviewed studies on the environmental sustainability of biofuels. To avoid outdated information, the review of the literature predominantly focused on the articles published in the period from 2009 to 2020. Some important earlier publications cited frequently in the literature were also taken into account. In total, 179 articles were primary (original) LCA studies, combining between them 613 assessments of different types of biofuels, all of which are included in this review. In addition, further publications focusing on environmental issues not usually included in LCA studies, such as water footprint, biodiversity and LUC, as well as discussing various methodological aspects were also reviewed. These studies covered a wide spectrum of first-, second- and third-generation biofuels produced from more than 20 types of feedstock. Table 1 provides an overview of the LCA studies related to the types of fuel, feedstock and geographical coverage. Among them, 52% assessed first generation, 38% considered second generation and the remaining 10% assessed third-generation biofuels. Regarding the type of biofuel, 56% of studies were for bioethanol and the rest for biodiesel. Geographically, 36% of studies were based in Europe, 26% in North America, 20% in Asia, 12% in South America, 4% in Africa and 1% in Australia. An overview of how different studies approached some critical LCA methodological aspects, including type of LCA, goal and scope of the study, definition of the functional unit, allocation methods and estimation of the impacts, is provided below.
Table 1.
An overview of the number of LCA studies by biofuel type, feedstock, location and land-use change.
a The total number of studies or analyses, rather than the number of papers published, as some papers included several studies or analyses.
(a) Type of life cycle assessment: attributional versus consequential
Two general types of LCA studies are distinguished: attributional (ALCA) and consequential (CLCA). They address different questions and follow different methodologies, and will normally have very different results. ALCA accounts for impacts directly related to the system of interest, attributing them to the activities within the system; hence, the term ‘attributional’. For biofuels, it is used mainly as an ‘accounting’ tool for estimating environmental impacts of various activities in the supply chain, comparisons of alternative systems and identification of environmental hotspots that can be targeted for improvements. CLCA, in addition to direct, also examines potential indirect consequences of the system under study by considering various ‘what if’ scenarios that could arise owing to this system; examples include changes in demand for the product of interest or technological improvements. For instance, CLCA can consider potential impacts of biofuel feedstock cultivation on other land-using sectors and the effect this might have on the food production system and LUC elsewhere in the global economy [ 26 , 27 ]. As such, CLCA is more suited for policy applications.
CLCA is still under development and, consequently, most of the LCA studies on biofuels found in the literature are attributional. Nevertheless, both the ALCA and CLCA are considered in this review.
(b) Goal and scope of studies
Goal and scope definition is an important initial step in LCA studies as the specific methodological approaches depend strongly on the specific goal, scope and question being addressed. The goal and scope of the study influence the definition of the system boundary and determine what activities and life cycle stages will be considered [ 28 ]. LCA studies of biofuels have addressed a wide range of goals and research questions, including:
- — What are the environmental impacts of the biofuel system under examination?
- — How do biofuels compare with fossil fuels?
- — What are the environmental hotspots in the life cycle of particular biofuel systems under study?
- — What are the improvement options to optimize the supply chain under study?
- — What are the environmental implications of biofuel policies?
Although the ISO 14040 LCA standard [ 28 ] requires clear definition of the goal and scope, a lack of or unclear definition of goal and scope is a common problem in LCA studies of biofuels. This can also mean that the study method and rationale can be unclear, making comparability of results difficult [ 29 ].
Two types of system boundaries were used in the reviewed LCA studies of biofuels: ‘cradle to gate’ (or ‘well to tank’) and ‘cradle to grave’ (or ‘well to wheel’); figure 1 . However, the latter system boundary is more appropriate as it is important to include the use of fuels to enable comparisons of biofuels with their fossil substitutes, since the combustion performance and associated emissions of biofuels can significantly differ from their fossil substitutes for the same type of vehicle [ 30 , 31 ]. Around half (48%) of the LCA studies reviewed considered a cradle to grave system boundary to compare environmental impacts of biofuels with fossil fuels, while the rest were from cradle to gate. Other inconsistencies include the omission in some studies of various inputs (such as enzymes, pesticides, fertilizers, etc.) and co-products. These differences are often important enough to influence the results significantly.
(c) Functional unit
In LCA, the term ‘functional unit’ describes the function of the system under study and represents the unit of analysis on which the study is based. The choice of the functional unit is driven by the goal of the study and must be representative of the system(s) studied and their main purpose (function). Biofuels regulations, such as the RED [ 8 ] and RFS [ 7 ], use the energy content of biofuels (MJ) as the functional unit. While this functional unit was often used in the reviewed literature, others include the distance travelled by a vehicle (vehicle . km) [ 21 , 32 ], volume (litre) [ 33 , 34 ] and mass (kilogram or tonne) [ 17 , 35 ] of biofuels. Some studies also used the mass of biofuel feedstock [ 36 , 37 ], agricultural land area [ 38 , 39 ] and annual operation of refinery [ 40 ]. The use of such a wide array of functional units makes comparisons of LCA studies challenging.
(d) Allocation methods
Biofuel production processes often produce several co-products, such as animal feed, heat, electricity and biochemicals. Therefore, to determine the impacts from the biofuel of interest, it is necessary to allocate the impacts between the biofuel and its co-products. The ISO 14040 and 14044 standards recommend that, if possible, allocation should be avoided through subdivision of processes, or by system expansion. The latter involves expanding the system boundary to include alternative ways of producing the co-products. The production system is then credited for displacing production of the co-products in the alternative systems by subtracting their impacts from the impacts of the biofuel production system. Hence, this method is also known as ‘substitution’ or the ‘avoided burden’ approach. If allocation cannot be avoided, the impacts can be apportioned between the biofuel and the co-products using allocation factors based on physical or economic relationships. Mass and energy content of biofuels and co-products are often used to derive allocation factors based on physical relationships. Economic allocation is based on the assumptions that the market prices are the driver for the production process and the impacts are apportioned in proportion to the economic value (cost or price) of the biofuel and the co-products. In LCA of biofuels, the most common approaches used to allocate the impacts are system expansion and allocation by the energy content. This perhaps reflects the regulatory requirements in the USA and Europe: RFS [ 7 ] prefers system expansion, while the RED [ 8 ] favours allocation based on the energy content of biofuels.
(e) Land-use change
LUC is an important source of GHG emissions that contributed 660 ± 290 Gt CO 2 to the atmospheric CO 2 in the period from 1750 and 2011 [ 41 ]. The majority of LUC is driven by demand for food, fibre and fuel [ 42 ]. Converting natural vegetation or forest to cultivate biofuel feedstocks releases a significant amount of carbon from soil and plant biomass, creating a ‘carbon debt’ that can take years to repay [ 43 , 44 ]. Furthermore, cultivation of biofuel feedstocks on land that has high soil carbon content, such as peat land, leads to a considerable increase in GHG emissions [ 45 ]. Besides increasing GHG emissions, changes in land use can have other environmental consequences, such as soil erosion, nutrient depletion, water consumption and loss of biodiversity [ 46 ]. LUC related to biofuels can occur in two ways: direct (DLUC) or indirect (ILUC). DLUC refers to the direct transformation of previously uncultivated areas (such as grasslands and forests) into croplands for biofuel feedstock production. ILUC occurs when additional demand for biofuel feedstock induces displacement of food and feed crop production to new land areas previously not used for cultivation. Only 25% of the reviewed LCA studies took LUC into account.
(f) Environmental impacts
GHG emissions and savings in comparison to fossil fuels are the centre of attention in most LCA studies on biofuels. Other environmental impact categories considered in biofuel LCA studies include acidification, eutrophication, photochemical smog, human toxicity and eco-toxicity. However, the number of studies that have assessed a wider set of impact categories is still limited: of the 179 (primary) LCA studies reviewed, only 40% of such studies were found in the literature. These are discussed in the next section, starting with the climate change impact, or global warming potential (GWP) as often referred to in LCA, and following on to discuss energy and water use, biodiversity and other impacts.
3. Environmental impacts of biofuels
(a) global warming potential.
For this impact, the LCA studies present contradictory results, ranging from favourable to unfavourable, even for the same type of feedstock. This is a result of the differences in the assumptions, data sources, allocation methods and LUC. The influence of these aspects is discussed in detail in §4. The GWP of biofuels reported in the reviewed LCA studies is summarized in figures 2 – 6 and discussed below for different types of biofuel. For further details, see electronic supplementary material, figures S1–S7.

GWP of first-generation biofuels without land-use change. Based on data from [ 24 , 32 , 34 , 47 – 118 ]. For the box plot legend, see electronic supplementary material, figure S1 and for the data used to plot this graph, see electronic supplementary material, figure S2. ‘Fossil fuel (reference)’ is the average carbon intensity of petrol and diesel supplied in the EU (94 g CO 2 eq. MJ −1 ) as specified in the RED [ 8 ]. ‘ A ’ refers to the number of LCA articles found in the literature and ‘ n ’ denotes the total number of analyses. (Online version in colour.)

GWP of microalgae biodiesel. Based on data from [ 19 , 85 , 97 , 113 , 141 , 167 , 190 – 209 ]. The negative values are due to the credits for co-products and avoided processes, such as wastewater treatment. For the box plot legend, see electronic supplementary material, figure S1 and for the data used to plot this graph, see electronic supplementary material, figure S6. ‘Fossil fuel (reference)’ is the average carbon intensity of petrol and diesel supplied in the EU (94 g CO 2 eq. MJ −1 ) as specified in the RED [ 8 ]. ‘ A ’ refers to the number of LCA articles found in the literature and ‘ n ’ denotes the total number of analyses. (Online version in colour.)
(i) First-generation biofuels
As first-generation biofuels may involve LUC, which in turn can affect significantly the total GWP, the results reported in the literature are discussed first for the cases without and then with LUC.
GWP without land-use change . As can be observed in figure 2 , the GWP of first-generation bioethanol from different food crops vary considerably, ranging from 3 to 162 g CO 2 eq. MJ −1 (see electronic supplementary material, figure S2). Figure 2 also shows that the average GWP of bioethanol is lower than that of petrol for all the feedstocks (23–59 versus 94 g CO 2 eq. MJ −1 ). However, only bioethanol from sugarcane can meet the RED requirement of 60% reduction in GHG emissions relative to petrol. The average reductions in emissions from the other four feedstocks—corn, wheat, molasses and sugar beet—are not sufficient to meet this requirement. The main reasons that bioethanol from sugarcane can meet the 60% reduction requirement are relatively lower inputs of agro-chemicals and higher yields of sugarcane crops as well as the credits for electricity produced as a co-product in a biorefinery.
The GHG emissions for first-generation biodiesel also show a large variation across the LCA studies, with the GWP ranging between 3 and 111 g CO 2 eq. MJ −1 (electronic supplementary material, figure S2). However, as shown in figure 2 , the average GWP of biodiesel from all the feedstocks considered is lower than that of fossil diesel. Nevertheless, only biodiesel from palm oil meets the RED requirement for 60% reduction of the GWP relative to diesel (average value). Rapeseed and soya bean also come close to fulfilling this requirement, but sunflower biodiesel cannot meet even the 35% reduction.
The large variability in the GWP of first-generation biofuels shown in figure 2 is due to several reasons. For example, the LCA study on corn ethanol and soya bean biodiesel production in China found that the GWP of corn ethanol and soya bean biodiesel were 40 and 20% higher than petrol and diesel, respectively, owing to the relatively higher use of fertilizers, higher process energy consumption and the coal-dominated energy mix of China [ 47 ]. Low or no GHG savings (0–20%) compared to the fossil fuels were reported for South African sugar beet bioethanol as well as rapeseed, soya bean and sunflower biodiesel due to water scarcity affecting crop yields [ 48 , 49 ]. On the other hand, owing to higher yield and lower farming inputs, soya bean biodiesel produced in Brazil, the USA and Argentina achieve more than 60% GHG savings relative to the fossil fuels [ 50 ]. Studies on palm [ 51 , 52 ], rapeseed [ 53 , 54 ] and sunflower [ 55 ] biodiesel have also found the significant effect on GHG emissions of different locations, farming practices and usage of fertilizers. In the case of palm oil, the emissions vary with the options for handling of methane emissions from the treatment of palm oil milling effluent [ 56 ]. The influence of the fuel used in the biorefinery was noted in the case of wheat ethanol, with the GHG savings varying from 4 to 85% depending on whether straw or distillers' dried grains are used as a fuel [ 57 ]. Similarly, another study on molasses-based ethanol found that the use of bagasse instead of fuel oil would reduce GHG emissions of bioethanol from 112 to 51 g CO 2 eq. MJ −1 [ 58 ]. A number of studies on biofuels from different feedstocks have also found that the emissions are highly influenced by the utilization of by-products and the allocation method [ 52 , 59 – 62 ]. The effects of allocation methods and other factors are discussed in more detail in §4.
GWP with land-use change . As shown in figure 3 , if LUC is involved and considering the average GWP values, bioethanol cannot meet the 60% GHG reduction requirement regardless of the type of feedstock [ 62 , 63 , 119 , 120 ]. The increasing demand for bioethanol from sugarcane in Brazil has led to a continuous expansion of land used for sugarcane cultivation [ 44 , 210 ]. If this involves deforestation of tropical rainforest, the GWP of bioethanol from sugarcane can be up to 60% higher than that of petrol [ 58 ]. Similarly, expansion of soya bean cultivation in Central and South America is driving both direct and indirect LUC [ 121 , 211 ]. Furthermore, palm cultivation in Malaysia and Indonesia is associated with deforestation and drainage of peat lands. As a consequence, biodiesel from palm oil on peat and forest lands can have 3–40 times higher GHG emissions than diesel [ 64 ]. A recent study assessing the LUC impact of biofuels consumed in Europe [ 212 ] also found that the GWP of palm oil and soya bean diesel are almost two times higher than that of diesel. The same study also estimated the GWP of biodiesel from rapeseed and sunflower to be 20 to 40% higher than from conventional diesel ( figure 3 ).

GWP of first-generation biofuels with land-use change. Based on data from [ 32 , 35 , 48 , 51 , 56 , 58 , 62 – 64 , 66 – 72 , 79 , 86 , 87 , 91 , 108 , 116 , 119 – 127 ]. For the box plot legend, see electronic supplementary material, figure S1 and for the data used to plot this graph, see electronic supplementary material, figure S3. ‘Fossil fuel (reference)’ is the average carbon intensity of petrol and diesel supplied in the EU (94 g CO 2 eq. MJ −1 ) as specified in the RED [ 8 ]. ‘ A ’ refers to the number of LCA articles found in the literature and ‘ n ’ denotes the total number of analyses. (Online version in colour.)
The significant variability shown in figure 3 for the GWP related to LUC is due to several reasons. For instance, some studies considered only ILUC [ 62 , 63 , 119 , 120 ] or DLUC [ 58 , 65 – 67 ], while others included both [ 68 , 122 ]. Furthermore, some studies applied partial equilibrium models and counterfactual (what if) scenarios to estimate ILUC emissions [ 62 , 119 ], whereas others used ILUC factors recommended by the US EPA [ 120 ]. The former tended to obtain higher ILUC emissions (34–155 g CO 2 eq. MJ −1 ) [ 62 , 119 , 123 ] than the latter (5–16 g CO 2 eq. MJ −1 ) [ 63 , 68 , 122 ]. For DLUC, several studies focused only on soil organic carbon (SOC) changes [ 65 , 122 ], but others also considered changes in the carbon stock related to the removal of biomass, both above and below the ground [ 58 , 67 , 68 ]. DLUC emissions also depend on the type of converted land and its previous use. For example, studies assuming palm oil cultivation on tropical forest and/or peat land in Malaysia and Indonesia estimated DLUC emissions in the range of 150–530 g CO 2 eq. MJ −1 [ 64 , 69 , 70 , 124 ]. On the other hand, studies on palm oil in Colombia and Thailand considered increase in the carbon stock due to LUC, assuming that the expansion of oil palm cultivation would occur in shrublands, savannahs, paddy fields and other agricultural lands [ 52 , 56 , 71 ]. In the case of sugarcane, molasses and soy, LUC emission reported in the literature varied from 30 to 200 g CO 2 eq. MJ −1 depending on the previous land use [ 35 , 58 , 69 , 72 , 121 ].
(ii) Second-generation biofuels
Figures 4 and and5 5 indicate that in most of the studies, the GWP of second-generation biofuels is considerably lower than that of fossil fuels. However, there is a large variation among different studies and feedstocks, with the values ranging from −115 to 173 g CO 2 eq. MJ −1 for bioethanol and −88 to 150 g CO 2 eq. MJ −1 for biodiesel (see electronic supplementary material, figures S4 and S5). These variations reflect the diversity of feedstocks and production routes, technology assumptions and methodological differences. Furthermore, some studies also considered emissions from ILUC [ 119 ] and SOC sequestration [ 68 , 128 ] associated with the production of SRC and perennial grasses as well as the reductions in SOC with removal of agricultural residues used as biofuel feedstocks [ 68 , 129 ]; for further discussion on SOC, see §4f. It should also be noted that the uncertainties related to technologies plays a particularly important role in the assessment of advanced biofuels as these are yet to be fully commercialized. Therefore, the quality of the available data is not as robust as in the case of the well-established first-generation biofuels.

GWP of second-generation bioethanol. Based on data from [ 17 , 18 , 33 , 34 , 40 , 66 , 68 , 119 , 120 , 102 , 104 , 105 , 115 , 117 , 128 – 163 ]. The negative values are due to the credits for co-products, such as heat and chemicals. For the box plot legend, see electronic supplementary material, figure S1 and for the data used to plot this graph, see electronic supplementary material, figure S4. ‘Fossil fuel (reference)’ is the average carbon intensity of petrol and diesel supplied in the EU (94 g CO 2 eq. MJ −1 ) as specified in the RED [ 8 ]. ‘ A ’ refers to the number of LCA articles found in the literature and ‘ n ’ denotes the total number of analyses. (Online version in colour.)

GWP of second-generation biodiesel. Based on data from [ 20 , 47 , 89 , 90 , 97 , 108 , 164 – 189 ]. The negative values are due to the credits for co-products. For the box plot legend, see electronic supplementary material, figure S1 and for the data used to plot this graph, see electronic supplementary material, figure S5. ‘Fossil fuel (reference)’ is the average carbon intensity of petrol and diesel supplied in the EU (94 g CO 2 eq. MJ −1 ) as specified in the RED [ 8 ]. ‘ A ’ refers to the number of LCA articles found in the literature and ‘ n ’ denotes the total number of analyses. (Online version in colour.)
In general, lignocellulosic bioethanol from agricultural and forest residues has a lower GWP than bioethanol from energy crops ( figure 4 ). This is largely due to N 2 O emitted during the cultivation of energy crops, related to the use of fertilizers. The latter are avoided in the case of residues as they are assumed to have no environmental burdens, which are all allocated to the original crop from which the waste is derived. In lignocellulosic bioethanol studies, the residual lignin is assumed to co-generate heat and power to meet the energy needs of the process, with surplus electricity exported to the grid. The biofuel production system is thus credited for avoiding the GHG emissions from the equivalent amount of grid electricity. For some feedstocks (SRC, forest residue, straw and corn stover), the credits for electricity generation and other co-products are higher than the total emissions from the biofuel production. Consequently, these studies report negative GWP, indicating the avoidance (saving) of GHG emissions. Some studies on energy crops also considered the increase in the carbon stock on the land that was converted to produce these crops, which led to the total net-negative GHG emissions [ 68 , 119 ]. On the other hand, harvesting of agricultural and forest residues can result in reduction of the land carbon stock [ 213 – 215 ], thus increasing GHG emissions [ 214 , 215 ]; however, most of the studies did not account for these changes. In the case of bioethanol from agricultural residues, other factors, such as the consideration of agricultural emissions [ 115 ], pre-treatment methods [ 130 ] and source of energy for the biorefinery [ 33 , 131 ], have a significant influence on GHG emissions.
While the LCA literature on second-generation bioethanol covers a wide range of feedstocks, the studies of biodiesel are more limited, focusing largely on three feedstocks: Jatropha, Camelina and UCO/tallow. As can be seen in figure 5 , the GWP of Jatropha and Camelina varies widely because of variations in the yield in different regions and differences in processes and assumptions, especially with respect to co-product allocation. For example, the yield of Jatropha oil seeds varies in different studies by a factor of 30, from 0.4 to 12 t ha −1 yr −1 [ 25 ]. The influence of allocation is also significant: using system expansion according to the US EPA methodology results in the GWP of Jatropha biodiesel of −88 g CO 2 eq. MJ −1 , while energy allocation as per the RED approach leads to GHG emissions of 15–20 g CO 2 eq. MJ −1 [ 20 ].
Although a majority of the studies of biodiesel from UCO report that GHG savings are greater than the RED reduction target of 60%, some studies also estimate that the GHG savings from this type of biodiesel are not sufficient to meet the target ( figure 5 ). This is due to some specific assumptions. For example, Intarapong et al . [ 90 ] considered pyrolysis for conversion of UCO to biodiesel, which is more energy-intensive than transesterification. Similarly, another study [ 47 ] assumed only a 5% biodiesel production yield, which is very low compared to more than 90% considered in other studies. Furthermore, Escobar et al . [ 171 ], who used consequential LCA methodology, considered indirect impacts, such as changes in the production of palm oil, soya bean and animal feed, found that the GWP of UCO biodiesel would be only 25% lower than that of diesel if ILUC and other indirect market impacts are considered.
(iii) Third-generation biofuels
In total, 27 LCA studies have estimated the GWP of third-generation algal biodiesel. However, they have all used very different approaches, process designs, system boundaries, methodologies and assumptions for feedstocks, nutrients and co-product management. As a result of the variation in these choices, the GWP differs widely between the studies, ranging from −2400 to 2880 g CO 2 eq. MJ −1 ( figure 6 ; electronic supplementary material, figure S6). These results would suggest that microalgae diesel can either reduce or increase GHG emissions significantly, relative to diesel, depending on the assumptions. However, a majority of the studies conclude that, at present state of development, algal biodiesel has higher life cycle GHG emissions than that of fossil diesel. The main reasons for higher emissions include lower algal yield [ 19 , 190 ] and high energy use in the cultivation, harvesting and drying stages [ 191 – 193 ].
Some studies which reported the high savings of GHG in comparison to diesel are based on the best-case assumptions that may not be feasible for large-scale implementation. These include the use of CO 2 from cement plants as a feedstock [ 216 ], cane sugar as a nutrient/feedstock [ 217 ] and recycling of nutrients from anaerobic digestion plants [ 194 ] or wastewater [ 190 ].
(b) Energy use
Various indicators have been used in LCA studies to quantify energy use in the life cycle of biofuels, including fossil energy consumption, primary, secondary or cumulative energy demand and net energy ratio [ 218 ]. However, many focused on fossil energy consumption, given that energy security and reducing dependence on fossil fuels are key objectives of national policies on biofuels, in addition to climate change mitigation.
As indicated in figure 7 , most of the studies estimate that the fossil energy consumption for first- and second-generation biofuels is below 0.5 MJ MJ −1 . However, there is a wide variation across different types of biofuel, ranging from 0.04 to 0.86 MJ MJ −1 for first generation and from −0.57 to 0.87 MJ MJ −1 for second-generation biofuels (see electronic supplementary material, figure S7), where negative values are due to energy credits for co-products, such as electricity and heat. These variations are due to several factors, including differences in feedstock productivity, agricultural practices, conversion technologies and allocation methods. The results are also affected by the assumption on the type of energy (biomass or fossil) used in the conversion process.

Fossil energy use in the life cycle of biofuels. Based on data from [ 17 – 19 , 40 , 47 , 48 , 54 , 55 , 57 , 58 , 60 , 68 , 76 , 77 , 80 , 85 , 89 , 92 , 93 , 95 , 96 , 98 , 108 , 113 – 115 , 120 , 121 , 129 , 132 , 133 , 136 , 138 , 139 , 143 , 144 , 147 , 152 – 154 , 156 , 159 , 161 , 162 , 164 – 167 , 169 – 171 , 175 , 176 , 179 – 181 , 187 , 190 – 192 , 195 – 199 , 204 , 206 , 208 , 219 – 223 ]. For the box plot legend, see electronic supplementary material, figure S1 and for the data used to plot this graph, see electronic supplementary material, figure S7. The value for third-generation biodiesel should be multiplied by 10 to obtain the actual value. ‘ A ’ refers to the number of LCA articles found in the literature and ‘ n ’ denotes the total number of analyses. (Online version in colour.)
The range of estimates for fossil fuel demand in the life cycle of algal biodiesel is even wider, ranging from 0.15 to 40.5 MJ MJ −1 ( figure 7 ; electronic supplementary material, figure S7). Like the GWP, the reasons for these variations are technological uncertainties and the diversity of potential feedstocks and production systems. However, most studies agree that algal biofuels are not energetically viable because of high energy requirements for pumping, dewatering, lipid extraction and thermal drying [ 141 , 191 , 224 – 226 ]. In general, algae cultivation in raceway ponds has lower energy demand than photo-bioreactors, with some studies suggesting that the former can have energy demand below 1 MJ MJ −1 [ 225 ].
(c) Water use
Water use in the production of feedstocks can be high, particularly for first-generation biofuels [ 5 , 227 , 228 ]. This is of concern where requirements for irrigation water for certain feedstocks might compete with water used for other purposes, such as food production. With increased agricultural biomass production for biofuels, the total global water consumption could increase significantly by 2050 [ 229 ] and, in areas that are already water stressed, additional water demand has a potential to substantially increase the overall environmental impacts of biofuels.
Water use is usually not included in LCA studies of biofuels, but there are numerous studies that have specifically focused on this aspect of biofuels production. Most of these provide a volumetric usage of water, such as the amount of green (soil moisture) and blue (surface) water. This is not sufficient to assess local environmental impacts of water consumption as these are highly dependent on the level of water availability in the local area and the specific characteristics of the hydrological cycle, even if the quantity used is the same for a particular product [ 230 ]. Furthermore, consideration of green water results in very large total water use for most agricultural crops. Since the local hydrological cycle may in reality be affected little by the use of green water in agriculture, inclusion of green water could overestimate the actual impact of water use for biofuels [ 231 ].
A more recent study [ 232 ] that assessed the water footprint of first-generation biofuels consumed in Europe suggests that blue water consumption of biofuels is very diverse, depending on the underlying crop and country ( figure 8 ). Bioethanol from sugar beet and wheat has lower water consumption because many countries produce crops using little or no irrigation. By contrast, the production of bioethanol from corn in Portugal consumes 86 m 3 GJ −1 . Furthermore, while no irrigation is needed to cultivate crops for biodiesel in the UK, Poland and Germany, in Spain, on average 90 m 3 of irrigation water is consumed to produce 1 GJ of crop-based biodiesel [ 232 ].

Blue water consumption for biofuels consumed in Europe. Based on data from [ 232 ]. Data labels represent the average values. (Online version in colour.)
As indicated in figure 8 , the average blue water consumption of bioethanol and biodiesel consumed in Europe is 3.3 m 3 GJ −1 and 1.9 m 3 GJ −1 , respectively—this is 40 and 60 times higher compared to their respective fossil alternatives. If regional water stress is taken into account, as opposed to just the volume of water consumed, biofuels have water footprints a factor of 55–246 higher than fossil fuels [ 232 ]. This is a result of a large share of water consumption in the production of biofuels occurring in relatively water-stressed countries.
The blue water consumption of algae-based biofuels depends on the geographical location, production systems and conversion routes [ 233 ]. For example, the blue water consumption for biofuels produced in a closed photo-reactor in the Netherlands is estimated at 8 m 3 GJ −1 , while it can be as high as 193 m 3 GJ −1 if algae are cultivated in open pond systems in Hawaii [ 233 ]. There is also a difference between dry and wet conversion, with the blue water consumption being higher for the latter.
(d) Biodiversity
Biofuels have the potential to contribute to loss of biodiversity through habitat loss and degradation, excessive nutrient load and other forms of pollution, over-exploitation and unsustainable use of land, as well as the cultivation of invasive alien species used as feedstocks [ 234 ]. The impact of biofuel production on biodiversity depends on the feedstock used and scale of production, management practices and LUC [ 235 ].
Intensive cultivation and use of agro-chemicals in the feedstock production for first-generation biofuels can create direct threats for local biodiversity [ 236 , 237 ]. LUC resulting from increased biofuel production exacerbates the risk of losing biodiversity through the direct loss of wildlife habitats, such as tropical rainforests [ 5 , 15 ].
Compared to first generation, second-generation biofuels are considered to have fewer negative impacts on biodiversity and could even have a positive effect [ 238 ]. For plant-based lignocellulosic feedstocks, this is because of their long growth cycle, low requirement for fertilizers and pesticides and less human intervention needed during the growth period. For example, large-scale SRC willow can provide benefits for some bird species, butterflies and flowering plants [ 239 ]. Furthermore, if degraded land is used for cultivation of feedstocks, the diversity of species might be enhanced. Similarly, perennial grasslands used for biomass production may enhance avian diversity, including migratory species. However, large energy crop monocultures can be detrimental to local biodiversity, particularly through habitat loss and the expansion of invasive species [ 5 ]. Eucalyptus, switchgrass and some Miscanthus species exhibit some traits of invasiveness [ 240 ].
The use of forest and agricultural residues as biofuel feedstock is expected to have a lower negative impact on biodiversity than dedicated energy crops [ 238 ]. Some of the impact on biodiversity associated with the use of forestry residues includes reduction in the amount of decaying wood—a niche habitat—and disturbance of wildlife caused by increased forest access. Excessive removal of agricultural residue from fields would also be a concern as it may increase weed growth, which could lead to the increased use of herbicides and thus affect local biodiversity.
For algal biofuels, the impact on biodiversity is uncertain. The large-scale cultivation of algae can bring significant risk to coastal biodiversity through invasion by algal species of coastal shallow ecosystems, such as mud flats, salt marshes, mangroves, sea grass bed and coral reefs [ 236 ].
Although the biodiversity loss is identified as one of the current key environmental concerns, it is only seldom included as an impact category in LCA studies of bioenergy systems [ 241 ]. Preserving biodiversity or avoiding biodiversity loss from biofuels is one of the criteria in sustainability certification schemes. However, biodiversity loss is difficult to measure and there are no standard ways of identifying and measuring systems that promote biodiversity.
(e) Other environmental impacts
The LCA studies on biofuels have used different impact assessment methods to estimate the other environmental impacts. Therefore, it is difficult to compare them and provide a meaningful range of impacts for different biofuels. Furthermore, the studies differ in scope, with some considering the cradle-to-gate and others cradle-to-grave system boundary. Results of the latter studies also depend on assumptions regarding the type of vehicle in which biofuels are used. Nonetheless, several studies suggest that reduction in GHG emissions from biofuels compared to fossil fuels is carried out at the expense of other impacts, such as acidification and eutrophication [ 32 , 54 , 76 , 81 , 83 , 88 , 121 , 129 , 139 , 148 , 216 , 242 – 244 ].
These two impacts are compared in table 2 for different feedstocks relative to fossil fuels. As can be seen, first-generation bioethanol has up to three times higher acidification and 3–20 times higher eutrophication. Similarly, first-generation biodiesel has 30–70% higher acidification and 3–14 times greater eutrophication than the fossil alternative. These impacts are largely due to the use of fertilizers and associated emissions of acid gases and nutrients to air and water.
Table 2.
Acidification and eutrophication of biofuels relative to fossil fuels.
a The values represent the ratio of impacts from biofuels over fossil fuels and are dimensionless.
Lignocellulosic bioethanol from SCR performs better for acidification, but bioethanol from switchgrass and straw is worse than petrol for both impact categories. However, biodiesel from UCO has lower acidification and eutrophication than fossil diesel. These two impacts are also higher for algal biodiesel than for the fossil equivalent [ 216 ]. However, as mentioned earlier, the absence of full-scale plant data, large variability in production parameters and various assumptions lead to high uncertainty in the LCA estimates for algal biofuels [ 197 ].
4. Discussion
While many studies on biofuels have examined multiple scenarios and conducted sensitivity analyses, only a few have conducted comprehensive uncertainty analyses [ 63 , 197 , 245 ], demonstrating that the variability in results can be large. It is also clear from the findings discussed above that the outcomes of LCA studies are highly situational and dependent on many factors, including assumptions, data variation and gaps, models, methodology and software tools used. The outcomes of the study are also affected by the choice of allocation method, system boundaries and the cut-off criteria for auxiliary inputs. Especially in relation to GWP, there are significant uncertainties in models for estimating soil N 2 O emissions, direct and indirect LUC and the extent and duration of changes in soil and vegetation carbon stocks. The effects of these aspects on LCA results are discussed below.
The problems related to data availability and quality are inherent to LCA. It is always preferable to use site-specific inventory data for developing LCA models of biofuels. However, data availability is often limited, particularly for second- and third-generation biofuels that, along with the associated process technologies, are still under development. As such, the use of unrepresentative data or assumptions to fill data gaps becomes a source of uncertainty [ 29 ]. There is also a great deal of technical, spatial and temporal variability associated with agronomic practices, such as fertilizer inputs, cultivation intensities and yields, as well as with biofuel conversion processes. LCA results are highly sensitive to variations in crop yields, use of nitrogen fertilizer and energy sources for biofuel conversion processes. Original and measured field data are still scarce and many studies rely on secondary data. There is also a room for improvement in existing LCA databases and a need to develop better, open access databases with common assumptions. Many data in common usage are reportedly out-of-date and finding new data is often difficult and time-consuming.
(b) Methodological approaches
As mentioned earlier, ALCA and CLCA are different techniques that follow different methodologies and will normally have very different results that must be interpreted carefully based on the goal and scope of the study. For example, Searchinger et al . [ 246 ] found that using ALCA resulted in a 20% saving in GHG emissions from US corn ethanol compared to petrol. However, following a CLCA approach and considering the increase in output required by the US Energy Independence and Security Act lead to a 47% increase in emissions relative to petrol. This increase was related to LUC induced by higher prices of corn, soya bean and other grains as a consequence of the additional demand for corn for ethanol production.
As also mentioned earlier, CLCA is more suited for policy applications. However, the use of CLCA for policy is still in infancy and its application to biofuels is controversial and subject to criticism [ 29 , 247 ]. One of the main reasons is that consequential analysis is highly complex, being dependent on future projections, formulation of possible ‘what if’ scenarios and counterfactual circumstances, economic models of relationships between demand for inputs, price elasticities, supply and market effects of co-products, all of which can be highly uncertain [ 26 , 248 , 249 ]. There is also a real challenge in defining meaningful scenarios for how the world would develop with a biofuels policy or production in place. This is true for individual feedstocks all the way up to the economic and energy system models incorporated into CLCA studies. Therefore, caution should be exercised with the interpretation of CLCA results [ 249 ]. Furthermore, unlike ALCA, there is still no internationally agreed methodology for CLCA, making it difficult to carry out and compare different studies.
(c) Allocation methods
Allocation is one of the most controversial issues in LCA. Both system expansion and allocation are subject to shortcomings: for system expansion, the difficulty is to estimate various substitution effects (similar to the related consequential issues in CLCA), while different allocation methods produce very different results. For instance, allocation by mass could result in the majority of impacts being allocated to the co-products rather than the biofuel which is the main (economic) product, while allocation by product cost/price leads to changes in the estimates of environmental impacts over time with variations in costs/prices without any other changes in the system. Several studies considered more than one allocation approach and found that the results were highly affected. For instance, some authors [ 17 , 132 ] showed that biofuels had significantly lower environmental impacts when using system expansion instead of allocation. In some cases, system expansion can lead to the negative values, suggesting net savings in environmental impacts, including in GHG emissions. However, studies assessing uncertainty in LCA of biofuels showed that system expansion also results in higher uncertainties [ 63 , 65 ]. Other authors found that environmental impacts were higher if economic allocation was used instead of mass and energy allocation [ 250 ]. For some biofuels, the co-products are sufficiently substantial that choice of allocation procedure can tip the balance between net benefit and net impact.
(d) Emissions of soil nitrous oxide
Emissions of N 2 O arise from application of nitrogen fertilizer and decomposition of organic matter in soil. N 2 O is a potent GHG with a GWP 265 times higher than CO 2 [ 41 ]; hence, its emission can have a significant effect on the GHG balance of biofuels. The N 2 O emissions are particularly significant for first-generation biofuel crops since fertilization rates are larger for these than for second-generation biofuels from perennial energy crops, which are usually grown without fertilizers, except during the initial establishment of the crop [ 251 , 252 ].
LCA studies often use the ‘Tier 1’ methodology developed by the Intergovernmental Panel for Climate Change (IPPC) to estimate N 2 O emissions from fertilizers [ 253 ]. According to this method, 1–1.5% of nitrogen in synthetic fertilizer applied to crops is emitted as N 2 O [ 253 ]. Since in reality, the occurrence and level of N 2 O emissions depend on many factors, including soil characteristics and local weather following fertilizer application on the soil, the default IPCC emission factors represent an uncertain estimate [ 23 ]. For example, a study by Crutzen et al . [ 254 ] suggested that N 2 O emissions in feedstock production can be three to five times higher than those estimated based on the IPCC methodology. Inclusion of these variable N 2 O rates leads to dramatically different estimates of GHG emissions in the life cycles of biofuels. For instance, for corn ethanol, the nitrogen conversion of 5% instead of 1.5% could change its GHG savings relative to petrol from around 40% to zero [ 255 ].
Conversely, a recent study in the UK concluded that N 2 O emissions averaged across arable land in the UK are below those determined by following the IPCC guidelines [ 256 ]. Compared to the default IPCC emissions factor of 1% (of the amount of nitrogen applied), direct N 2 O emissions from soil related to the use of fertilizers on crops for first-generation biofuels were estimated to be, on average, 0.46% of the nitrogen applied. However, the study noted that any one instance of fertilizer application is subject to interacting effects of rainfall and soil type, such that fertilizer-induced emissions could also be larger than the default IPCC emission factors in the wetter regions of the UK. Thus, in summary, the estimates of N 2 O emissions are highly variable and uncertain and should be treated with caution when interpreting the results.
An increasing global demand for biofuels highlighted the potential for the competition for land between cropland and natural ecosystems. Early LCA studies on biofuels, which excluded LUC, concluded that first-generation biofuels, such as corn ethanol, had lower GWP than petrol [ 257 ]. However, when attempts were made to account for the LUC effects of the expansion of first-generation biofuels, these findings came under question [ 43 , 246 ]. Since then, several other studies have cast doubt on the ability of first-generation biofuels to meet mandatory GHG savings targets if LUC is involved [ 119 , 258 ].
From an LCA perspective, DLUC is relatively straightforward and easy to include in the assessment, although the uncertainty remains high. However, estimating ILUC related to biofuels remains difficult, complex and highly uncertain [ 259 , 260 ]. The latter is exemplified by that fact that estimates of GHG emissions from ILUC range widely, from very small to very large [ 261 ]. For instance, a study on the ILUC associated with US corn ethanol found that the ILUC emissions varied from 10 to 340 g CO 2 eq. MJ −1 [ 262 ]. For these reasons, the effects of ILUC and how to account for them in assessing the sustainability of biofuels are key areas requiring further research and consensus building [ 42 , 260 ]. Part of the challenge is constructing and analysing credible counterfactual scenarios. Another challenge is the economic (equilibrium) models used for consequential modelling [ 247 , 263 ] and the assumed yield-price elasticities for crops [ 26 ]. ILUC models make various assumption to estimate how much indirect change might be induced up to 20 years into the future under prescribed scenarios. Therefore, such estimates would only apply for the assumed conditions and must be interpreted with caution [ 264 ]. The lack of transparency in ILUC models, many of which are proprietary, is also problematic.
There is an ongoing question about how policymakers should respond to the growing evidence on ILUC from biofuel production. The blanket application of ‘ILUC factors’ according to feedstock type is unpopular as it offers producers no opportunity to improve the performance of their individual supply chains [ 265 ]. Moreover, there are many other drivers of LUC besides biofuels, such as demand for food and timber, urban development and infrastructure, leading some to argue that it is unfair to consider ILUC only for biofuels [ 247 , 266 ].
(f) Soil organic carbon
SOC is one of the largest carbon pools in the terrestrial ecosystem [ 267 ]. Its balance is affected because of agricultural activities and LUC. Depending on various soil characteristics and agricultural practices, soil can act as either a sink or a source of carbon emissions. Soils may lose SOC by mineralization through cultivation, emitting CO 2 to the atmosphere. Alternatively, SOC may increase through cropping or from repeated addition of crop residues or organic manures [ 268 ].
When biomass is left to decay in the soil, a part of the carbon in the biomass is sequestered into soil. Therefore, assuming biomass would have otherwise been left to decay in the soil, harvesting it decreases SOC and this may affect significantly the GHG balance of a biofuel [ 269 , 270 ]. For example, a study that included the effects of the removal of corn residue across the US corn belt concluded that the GWP of corn stover ethanol may exceed that of conventional petrol [ 271 ]. Another study on wheat-straw ethanol suggested that there is only a 30% probability that its GHG emissions will be 35% lower than that of petrol if SOC changes are included in the analysis [ 272 ]. A study on sugarcane ethanol claimed that the GHG balance of sugarcane ethanol could be significantly higher if the impacts on SOC from pre-harvest burning were considered [ 210 ]. The burning of biomass in the field, which is often carried out prior to a sugarcane harvest to help manual harvest, means that far less crop residues are left on the land to be incorporated into the soil.
Changes in SOC can also have a major influence on GHG emissions from LUC associated with biofuel feedstock production [ 267 , 273 ]. For example, reversal of grassland, woodland and perennial crops back to arable lands could reduce soil carbon by 0.6–1.7 t C ha −1 yr −1 , which would be emitted to the atmosphere as CO 2 (2.2–6.2 t ha −1 yr −1 ). On the other hand, cultivation of perennial energy crops, such as SRC and Miscanthus , could sequester CO 2 from the atmosphere into the soil at the rate of 2.2 t CO 2 ha −1 yr −1 [ 267 ]. However, the sequestration potential is very site-specific and highly dependent on former and current agronomic practices, previous land use, as well as climate and soil characteristics [ 17 , 40 , 267 , 274 – 276 ]. Therefore, quantifying changes in SOC storage is an important factor in estimating GHG emissions of biofuels [ 277 ]. However, most LCA studies do not account for potential SOC changes from biomass cropping systems. This is probably due to inherent complexity of soil science, the high degree of intra- and inter-site variability, substantial data uncertainties and the challenges of linking biomass feedstock supply to specific soils [ 46 ]. Furthermore, there is no consensus in LCA on how to account for SOC change of agricultural activities and delayed GHG emissions [ 278 ]. However, the work on developing models to estimate SOC emissions related to biofuels is ongoing [ 273 – 275 ].
(g) Biogenic carbon
In the context of biofuels, the term biogenic carbon refers to CO 2 that is sequestered from the atmosphere during the growth of feedstocks and subsequently released during the combustion of the biofuel. ‘Carbon neutrality’ is achieved when CO 2 sequestered and subsequently released are in balance. However, carbon neutrality cannot be claimed if there is a potential imbalance or a time delay between the amount of CO 2 taken up during feedstock growth and the amount released through biofuel production and use. Since many bioenergy products—including annual crops and perennial grasses—have relatively short lifespans, carbon neutrality is commonly assumed in LCA standards and regulations. Hence, most LCA studies of biofuels assume that biogenic CO 2 emissions, both from end-use combustion and the burning biomass to produce energy for conversion processes, are fully balanced by CO 2 uptake during feedstock growth. While this assumption is reasonable for fuels from annual crops and perennial grass feedstocks, it is open to challenge in relation to biofuel production from feedstocks with harvest cycles of more than a few years—such as longer-lived lignocellulosic feedstocks from forestry [ 26 , 279 ]. For such feedstocks, it is important to consider the balance of carbon sequestered during feedstock growth versus that which is emitted during biofuel production and use, together with the overall time profile of biogenic carbon storage, emission and re-sequestration [ 279 ].
Different approaches to account for the temporal impact of carbon emissions are suggested in the literature; for example, carbon payback period, carbon discounting and time-integrated accounting of biogenic carbon [ 279 , 280 ]. Where accounting for the carbon storage in other, more long-lived bio-based products is required, there are various standards and methods [ 46 ] and these contain significant procedural differences. For example, GHG Protocol [ 281 ], PAS 2050 [ 282 ] and ISO 14067 [ 283 ] require reporting of emissions and removal of GHG emissions from biogenic carbon sources, while regulations the RED [ 8 ] and RFS [ 7 ] do not require such reporting. Furthermore, the time between the production of the product (storage of biogenic carbon) and its end of life (release of biogenic carbon), referred to as ‘delayed emissions’, varies among the standards. For instance, in PAS 2050 [ 282 ], all emissions that occur within a 100-year period are quantified and treated as if they occurred at the beginning of the time period. By contrast, ISO 14067 [ 283 ] makes a distinction between emissions released within and after the first 10 years.
(h) Other environmental impacts
Production and use of biofuel generate emissions of various air pollutants, including particulate matter (PM), carbon monoxide (CO), nitrogen oxides (NO x ), hydrocarbons and volatile organic compounds (VOCs). Unburned hydrocarbons, VOCs and NO x are precursors for the formation of summer smog and ground-level ozone. These pollutants are associated with increased morbidity and mortality from cardiovascular and respiratory diseases and certain cancers [ 284 , 285 ]. Air quality modelling studies show that life cycle emissions of some pollutants may be higher for biofuels when compared with fossil fuels, largely resulting from the emissions associated with feedstock production and biofuel processing [ 284 , 286 ]. For example, in the case of sugarcane ethanol in Brazil, burning of straw in fields is the common practice in certain areas and is the predominant source of PM [ 284 , 286 ]. Studies on health impacts of sugarcane ethanol in Brazil suggest that there is strong evidence that burning straw in sugarcane fields causes substantial respiratory diseases, such as asthma and pneumonia, in sugarcane fieldworkers and local populations [ 284 , 286 – 289 ]. These effects are often ignored in LCA studies.
In cradle-to-grave LCA studies, assessing impacts of vehicular exhaust emissions is another challenge as they are affected by many different parameters, including the type of engine and how it is run (the operational drive cycle), vehicle age and maintenance, the quality of the base fuel and exhaust after treatment [ 290 ]. Vehicular exhaust emissions of bioethanol blends vary with blend strength. However, in general, lower bioethanol blends (E5–E15) have lower CO and PM emissions compared to petrol [ 290 , 291 ]. Beer et al . [ 291 ] suggest that lower PM emissions from low-ethanol blends used in spark-ignition vehicles have slight health benefits over petrol. However, they lead to significantly higher emissions of acetaldehyde, which is one of the precursor VOCs involved in ground-level ozone formation. Similarly, higher ethanol blends (E85) lead to comparable, or slightly lower, levels of PM, NO x and CO emissions than petrol, but 5–10 times higher acetaldehyde emissions [ 290 , 292 , 293 ].
Compared to fossil diesel, biodiesel has generally lower exhaust emissions of PM, CO, hydrocarbons and VOCs, but higher NO x emissions [ 294 , 295 ]. These differences are small for 5–20% biodiesel blends and would lead to negligible or non-measurable impacts on air quality [ 294 ], but increase with higher blends [ 290 ]. On the other hand, Larcombe et al . [ 296 ] argue that, despite having lower PM emissions, biodiesel exhaust emissions could potentially be more harmful to human health because of higher proportion of ultra-fine particles (less than 100 nm diameter) compared to diesel exhaust. This is due to the fact that smaller particles remain suspended in the air for longer, are more easily inhaled and are able to penetrate more deeply into the lungs. However, other assessments on the potential human health implications of biodiesel suggest that the use of biodiesel fuel blends compared to fossil diesel results in minimal changes in health impacts [ 294 , 295 ]. Thus, the topic of human health impacts from biofuels remains open to debate, requiring further research and evidence.
Besides air pollution, production of liquid biofuels could affect human health directly through water and soil pollution and occupational hazards [ 284 ]. However, these effects are scarcely discussed in the literature and should be explored further to understand whether there are risks that need to be addressed.
5. Conclusion and recommendations
LCA is widely used as a tool to estimate GWP and other environmental impacts of biofuels. However, as evident from this review, the estimates vary widely among the studies owing to a wide range of methodological choices in LCA and various uncertainties. Despite this, the existing evidence base is instructive. Firstly, it shows that, if no LUC is involved, first-generation biofuels can—on average—have lower GHG emissions than fossil fuels, but GHG savings for most of the feedstocks are not sufficient to meet those required by the RED. Secondly, in general, second-generation biofuels have a greater potential than first generation to reduce GHG emissions, again provided there is no LUC. However, the development of second-generation biofuels will take time and is likely to depend on the continued support of first-generation fuels to give the industry the confidence to invest. Thirdly, it is also clear that, at present state of development, third-generation biofuels from algae are unlikely to make a contribution to the transport sector as their GHG emissions are higher than those from fossil fuels. Moreover, they are unproven and expensive to produce and, as such, the algal feedstock will continue to be restricted to high-value markets, such as cosmetics and dietary supplements.
LCA is a complex tool that lies at the interface between science, engineering and policy. Despite this complexity, it is often perceived as a tool that can give a definitive answer to multifaceted questions. As the findings in this review demonstrate clearly, there are no definitive answers. Even focusing only on the GWP of biofuels—one of the main drivers for their development—brings with it a host of uncertainties. Moreover, almost every aspect related to biofuels is dynamic in nature across different scales, which adds to the complexity. Examples include changes in soil carbon content over time (micro-scale); time needed to replace vegetation used as feedstock for biofuels (meso-scale); and development of global biofuel supply chains (macro-scale). Considering these dynamic aspects and their interconnections presents a considerable challenge. There are also significant uncertainties in the models for estimating direct and indirect LUC, changes in SOC stocks and N 2 O emissions. It is important to recognize these limitations and interpret the results accordingly.
In addition to the environmental impacts, there are many other sustainability issues that must be considered when assessing the sustainability of biofuels. These include: costs of production and competitiveness with fossil fuels; food, energy and water security; employment provision; rural development; and human health impacts. It is essential that the sustainability aspects of biofuels be evaluated on a life cycle basis across full supply chains to avoid shifting the burdens from one part of the life cycle or supply chain to another. It is also important to note that LCA and wider sustainability assessments are of little use if the results cannot be trusted. Therefore, strong auditing of biofuel supply chains is vital to prevent negative socio-economic effects as well as to ensure traceability of the fuels and to mitigate the risk of fraud. Moreover, improving transparency, data availability and sharing are key if LCA is to be trusted and useful for policy. This could be achieved through development of open national and global databases, in a similar way that national inventories have been developed for GHG reporting under the Kyoto Protocol. It is also important to ensure that the data and models from different disciplines that are used in LCA preserve reasonable levels of transparency, rigour and robustness to avoid misuse and misinterpretation.
ALCA studies, which account for the direct impacts, should follow the ISO 14040 and 14044 standards more rigorously. For CLCA, both methodological and practical aspects need improvements. For the former, further work is required towards the standardization of CLCA methodology. As part of that, there is a need to improve development of counterfactual (what if) scenarios and ILUC models. Involvement of multiple stakeholders can help to build consensus on the definition of the scenarios and to improve the transparency of ILUC models, their assumptions and the associated uncertainty. In addition to improving the CLCA methodology, much work is required in its application in practice. Specifically, there is a need to validate ILUC models with empirical evidence; empirical methods to test alternative hypotheses also require attention. Further work is also needed on the development of models and empirical evidence of changes in soil and plant carbon stocks as well as emissions of nitrous oxide related to the application of fertilizers. Research is also needed on estimations of biogenic carbon, particularly changes in the forest carbon stock that may be affected by an increase in biofuels demand.
It is also important to take into account that biofuels do not exist in isolation but are part of much wider systems, including energy, agriculture and forestry. Like other production systems with which they interact, biofuels impact on various ecosystem services, such as land, water and food. It is, therefore, essential to take an integrated, systems view to developing future policy to ensure that biofuels are not disadvantaged relative to other sectors or that progress made in this sector is not undone by unsustainable practices in others. Analysis and, ultimately, policies based on ecosystem services and natural capital at a landscape level are needed to make the best overall use of land. This would, in turn, optimize ecosystem services, such as carbon storage, biodiversity, reductions of agricultural run-off and increases in water quality and flood risk management. Complete value chains rather than single bioenergy products should be analysed together to understand the interactions across sectors and land uses with the goal of identifying opportunities where collective benefits can be realized.
Supplementary Material
Acknowledgements.
This review was originally carried out as part of the Royal Academy of Engineering study on Sustainability of liquid biofuels and subsequently fully updated as part of a Research Councils UK project (EP/K011820/1). The suggestions and comments by members of the Academy's expert working group, the Academy's Engineering Policy Committee as well as external stakeholders are gratefully acknowledged.
Data accessibility
Authors' contributions.
H.K.J.: literature review, analysis and presentation of results, paper writing. A.C.: literature reivew, paper drafting. A.A.: conceptualization, supervision, paper writing.
Competing interests
We declare we have no competing interests.
The study was funded by Department for Business, Energy and Industrial Strategy (BEIS), Department for Transport and Research Councils UK.

We use cookies. By browsing the site, you agree to it. Read more »
Niamh Chamberlain
If you can’t write your essay, then the best solution is to hire an essay helper. Since you need a 100% original paper to hand in without a hitch, then a copy-pasted stuff from the internet won’t cut it. To get a top score and avoid trouble, it’s necessary to submit a fully authentic essay. Can you do it on your own? No, I don’t have time and intention to write my essay now! In such a case, step on a straight road of becoming a customer of our academic helping platform where every student can count on efficient, timely, and cheap assistance with your research papers, namely the essays.
Finished Papers
Customer Reviews
Is buying essays online safe?
Shopping through online platforms is a highly controversial issue. Naturally, you cannot be completely sure when placing an order through an unfamiliar site, with which you have never cooperated. That is why we recommend that people contact trusted companies that have hundreds of positive reviews.
As for buying essays through sites, then you need to be as careful as possible and carefully check every detail. Read company reviews on third-party sources or ask a question on the forum. Check out the guarantees given by the specialists and discuss cooperation with the company manager. Do not transfer money to someone else's account until they send you a document with an essay for review.
Good online platforms provide certificates and some personal data so that the client can have the necessary information about the service manual. Service employees should immediately calculate the cost of the order for you and in the process of work are not entitled to add a percentage to this amount, if you do not make additional edits and preferences.
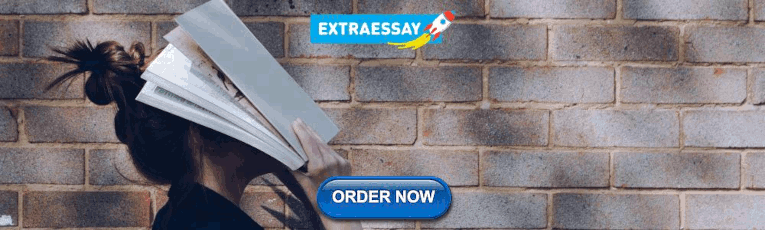
IMAGES
VIDEO
COMMENTS
However, scientific research has shown that various biofuels differ massively in the greenhouse gas balance when compared with petrol despite the potential advantages. Based on the techniques used for processing the fuel and production of the feedstock, certain crops may also emit more greenhouse gases than fossil fuels do [52], [53]. 3.2.
Biofuels are fuels produced from hydrocarbon-rich living organisms (biomass) — such as plants or microalgae — by thermal, chemical or biochemical conversion processes. As with fuels, biofuels ...
Abstract. Bioethanol, a renewable and sustainable b iofuel, has eme rged as a promising. solution to address environmental and energy challenges. This comprehensive. review explores the historical ...
Second- and third-generation biofuels are often referred to as 'advanced biofuels' as their production techniques or pathways are still in the research and development, pilot or demonstration phase. In this paper, the terminology 'first, second and third generation' has been selected and followed throughout.
Explore the latest full-text research PDFs, articles, conference papers, preprints and more on BIOFUEL PRODUCTION. Find methods information, sources, references or conduct a literature review on ...
Predominantly, biofuels are produced from photosynthetic organisms. such as photosynthetic bacteria, micro- and macro-algae and vascular land plants. The. primary products of biofuel may be in a ...
Biofuel technology has evolved through several generations of significant advancements. The predominant problem with first-generation biofuels is that they are derived from food crops (e.g., corn and sugar cane), which require fertilization, water, and soil, and thus directly compete with food production. ... This is a novel research area that ...
Whether biofuels represent a sustainable innovation, a creative alternative, or a gold rush, very much depends on our perception of power and change with regard to sustainability. This article provides an overview of existing understandings of power in the research on biofuels, including positive perceptions that often lead to more optimistic evaluations of biofuels. It exposes the diversity ...
In this Research Topic, we aim to make a cohesive collection of the knowledge on the current progress on the area of plant biotechnology for bioenergy production. This will hopefully bring together valuable information for researchers and practitioners in the biofuels and bioenergy industry chain. We will integrate several of the key components ...
Biofuels can be utilizing as fuel additives or in their pure form. Further, biofuels are commonly classified into bioethanol and biodiesel [5].The liquid biofuels can be utilized as an alternative source for conventional fuels in the transportation sector, contributing to approximately 18% of primary energy consumption [1], [6].Today, approximately 80% of liquid biofuel is manufactured in ...
Biofuels are a source of cleaner energy to promote a cleaner environment. India initiated bio-fuel production nearly a decade ago to reduce its dependence on imported oil and thus improve energy ...
Biofuels as a Key Renewable Energy Source: a Review of Life Cycle Assessment Studies in South Africa. Adaku Jane Echendu, Chima Cyril Hampo, Dare Olatunde, Judith Isioma Obasih, Oluwatosin Oni, Damilola Ojo, Musa Mathew, Modupeoluwa Adediji & Seun Oladipo. Published online: 29 Sep 2023.
Biofuels can be considered upon as a way of energy security which stands as a substitute of fossil fuels that are limited in availability, as well as environmental-friendly fuel, since biomass is considered a carbon-neutral or greenhouse gas neutral (GHG neutral) fuel [34]. Figure 6.8. Biomass types.
Here, we show that policies supporting the same technology can lead to dramatically different emissions impacts per unit of technology added, due to multimarket responses to the policy. Using a policy-based consequential LCA, we find that the lifecycle emissions impacts of four US biofuel policies range from a reduction of 16.1 gCO 2 e to an ...
Cover art by BiofuelResJ. ©2024. Biofuel Research Journal (BRJ) is a leading, peer-reviewed academic journal dedicated to publishing high-quality research on biofuels, bioproducts, and related biomass-derived materials and technologies. BRJ is an open-access online journal and completely free-of-charge, aiming to advance knowledge and ...
Certainly! Here are a few potential essay topics related to biofuel: 1. The environmental impact of biofuel production and use compared to traditional fossil fuels. 2. The economic benefits and challenges of transitioning to biofuel as a primary energy source. 3.
Second- and third-generation biofuels are often referred to as 'advanced biofuels' as their production techniques or pathways are still in the research and development, pilot or demonstration phase. In this paper, the terminology 'first, second and third generation' has been selected and followed throughout.
Despite the critical need and the encouraging prospects, the commercialization of biofuel products is hampered by challenges of sustainable production capacity [9] and relatively higher costs of production [109].This study seeks to highlight the status of the current conceptualization, antecedents and research trajectory towards a sustainable planet, with emphasis on the emerging gaps in the ...
W orld Bank Policy Research W orking Paper No. 4341. W ... Join ResearchGate to discover and stay up-to-date with the latest research from leading experts in Biofuels and many other scientific topics.
Biofuel Research Paper Topics - 741 Orders prepared. I accept. Terima kasih telah menghubungi Professional Development Center of Tourism & Hospitality, BINA MUTU BANGSA. Khusus seputar kursus/pendidikan serta penempatan kerja, dapat menghubungi WA : +62 812 4458 4482. Enter Requirements.
They tend to 'do my essay' by adding value to both you (enhancing your knowledge) and your paper. View Sample. ID 4817. 964 ... Biofuel Research Paper Topics, Custom Essays Writing Websites For University, Programme Associate Cover Letter, Freshers Resume For Mechanical Students, Acknowledgement Of Receipt Of Resume Letter, Against ...
An Overview of Biofuel. Muhammad Arshad, Muhammad Anjum Zia, Farman Ali Shah. and Mushtaq Ahmad. Abstract Fossil fuels applications are linked with current widely held environ-. mental issues. The ...
Microalgae or seaweed (Macroalgae) are considered superior compared with terrestrial plants - in terms of solar energy storage, nutrient assimilation and potential for biofuel production - due to ...