Science Activities for Critical Thinking
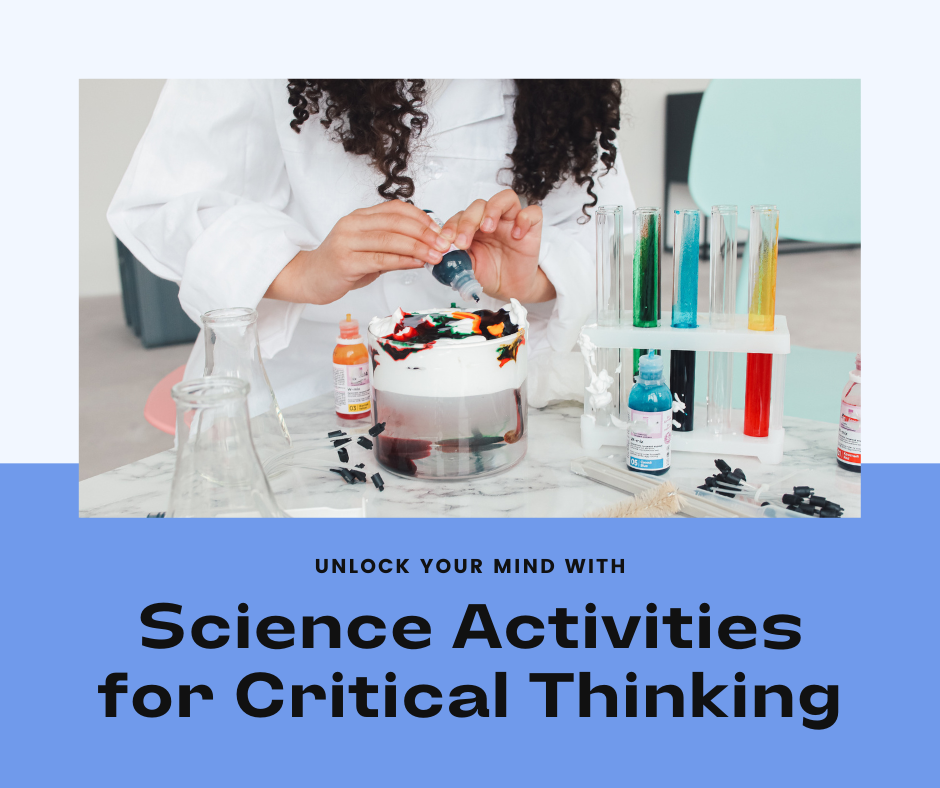
Are you ready for exciting science activities? Science and engineering are puzzles. They’re like adventures. You need curiosity to dive into details. As NGSS says, it’s all about getting into the heart of things.
…students cannot fully understand scientific and engineering ideas without engaging in the practices of inquiry and the discourses by which such ideas are developed and refined. At the same time, they cannot learn or show competence in practices except in the context of specific content. (NRC Framework, 2012, p. 218)
To understand science, you need to dive in. Roll up your sleeves! Get your hands dirty with activities. So, let’s start now! Here are some of my favorite science activities for critical thinking.
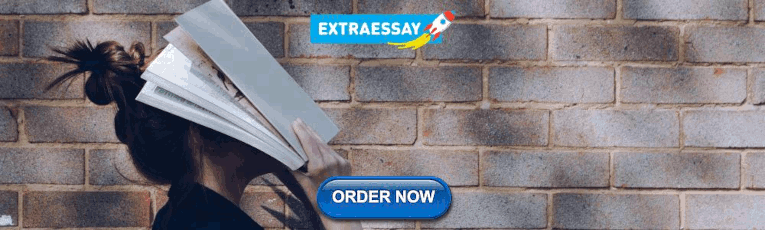
Why Teach Critical Thinking?
Wondering about the importance of teaching critical thinking through analysis, engineering, and exploration? It helps students:
- Understand Better: Grasp topics more easily.
- Solve Problems: Improve at tackling challenges and making wise decisions.
- Think Independently: Develop self-thinking, understanding, and openness to new ideas.
- Communicate Effectively: Enhance speaking skills through collaborative science.
- Prepare for the Future: Get ready for life’s challenges.
Each reason is crucial. Together, they highlight the need for teaching critical thinking. It profoundly impacts learners. Now, let’s explore those activities.
Activity #1: Fact Strainer Exercise
Need fast activities for your daughter? Check out Julie Bogart’s “ Raising Critical Thinkers. A Parent’s Guide to Growing Wise Kids in the Digital Age .” It’s full of challenges for parents and students.
An example? The “Fact Strainer” exercise in Chapter 2. Kids sift facts from stories. They find facts in news articles about the same event. They highlight where these facts appear, like at the beginning, middle, or end. Then, they list the facts on paper in the order they found them.
Discuss why facts are placed where they are. What was the author’s goal? The exercise teaches spotting facts first. It helps ignore the writer’s bias. Bogart’s book has even more ideas along these lines.
Activity #2: Science Buddies Activities
Imagine having a buddy who’s always up for some cool science experiments. Science Buddies has a treasure trove of fun activities. These will make you say, “Whoa, I didn’t know science could be this awesome!” Featured activities include:
- Build a Paper Roller Coaster
- Build a Balloon Car
- Turn Milk into Plastic
- Secret Messages with Invisible Ink!
- Make Ice Cream in a Bag
- Make a Lemon Volcano
Activity #3: Education Possible
Education Possible has a great list of Fun and Engaging Science Activities for middle school students. They prove science can be exciting! In this collection, you will find activities like making volcanoes erupt, chemical reactions, and how to create rainbow colors. With these science activities, you are in for a blast (not the explosive kind, of course!). These are split up into life science, physical science. miscellaneous, and more.
Activity #4: 55 Clever 7th Grade Science Fair Projects and Classroom Experiments
Think your students are too young? These projects work for any grade. You might become a star science fair facilitator. Here are my top ten favorite activities from this article:
- Balloon-Powered Car: Build a balloon-driven car. Test its speed.
- Geodesic Dome: Use newspaper and tape to construct a sturdy dome.
- Solar Oven: Create an oven that cooks with the sun. Learn about energy.
- Spherify Drinks: Turn drinks into tiny balls. A chemistry experiment.
- Purify Water with Charcoal: See how charcoal filters water.
- Wave Machine: Make a simple machine to understand waves.
- Water Clock: Build an ancient-style clock. Watch how it measures time.
- DIY Barometer: Construct a barometer. Predict weather changes.
- Hydraulic Power: Explore hydraulics. Create your hydraulic device.
- Grow and Experiment with Crystals: Learn about crystals. Grow them yourself.
Given those cool activities, which would you try first?
Activity #5: Little Bins for Little Hands Science Experiments
Get ready to find wonders with everyday items. Additional science activities on this site feature chemistry , earth sciences , physics , and STEM . You and your students can create amazing things. From their website, here’s a supermarket supply list:
Mason jars, plastic bottles, baking soda, salt, vinegar, zip-top bags, rubber bands, glue, hydrogen peroxide, food coloring (optional), and other common items. These make science easy for everyone.
Using such materials brings science closer to students. Involve your whole school in collecting these supplies.
But wait, there’s more!
Explore over 60 science activities and videos! It’s like a science museum in your hands. Don’t miss this chance to turn learning into an adventure! Which activity will you try out first?
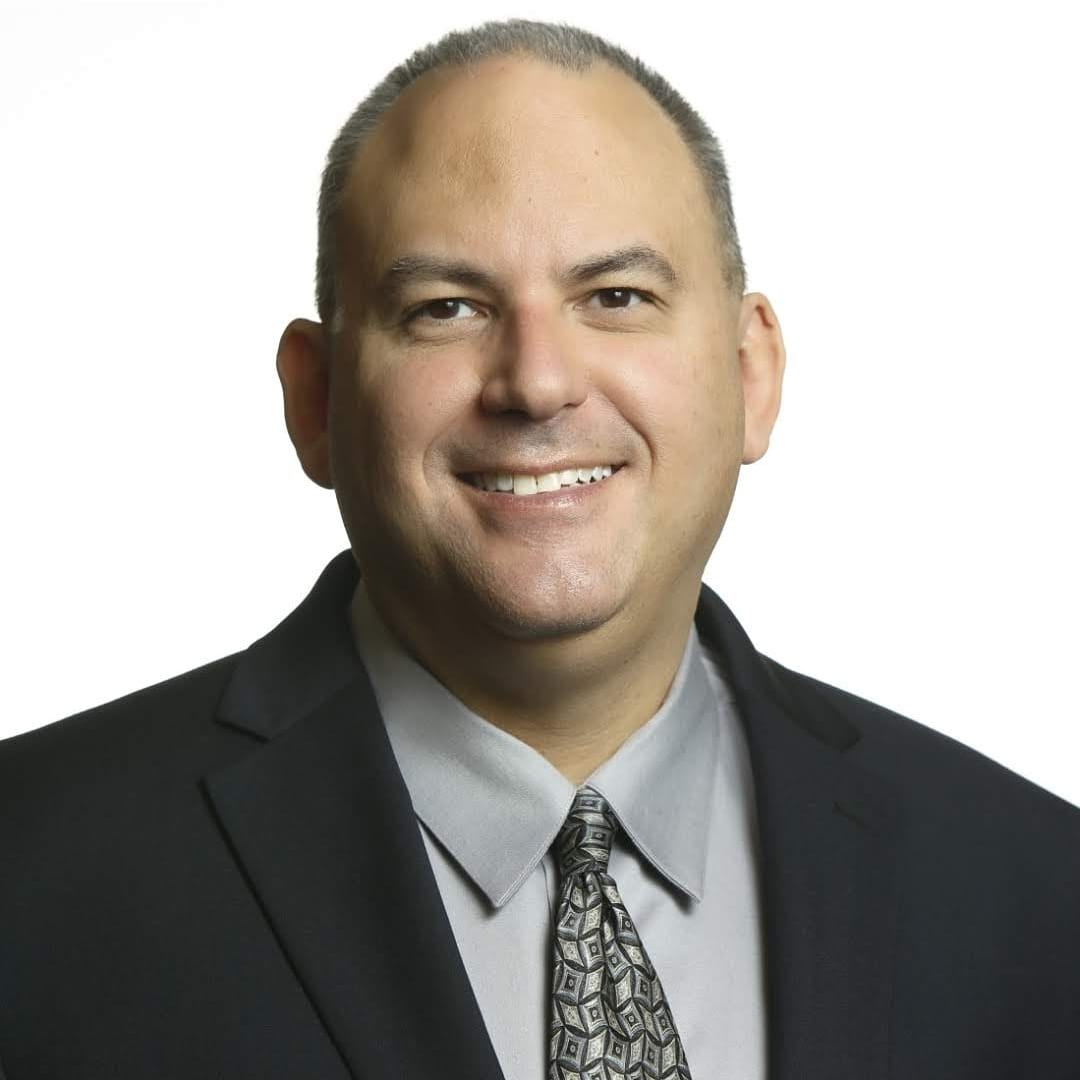
Miguel Guhlin
Transforming teaching, learning and leadership through the strategic application of technology has been Miguel Guhlin’s motto. Learn more about his work online at blog.tcea.org , mguhlin.org , and mglead.org /mglead2.org. Catch him on Mastodon @[email protected] Areas of interest flow from his experiences as a district technology administrator, regional education specialist, and classroom educator in bilingual/ESL situations. Learn more about his credentials online at mguhlin.net.
How to Choose Your 2024 Word of the Year
Five ways to make chatgpt your time-saving ai assistant, you may also like, earth day ideas to inspire young eco-heroes, four solar eclipse-themed activities, the new k-5 science teks and free, editable..., 20+ science books for middle and high school..., examples for teaching with fake news and pseudoscience, five video sources for science teachers, the four reading comprehension strategies of collaborative strategic reading..., solar eclipse activities, resources, and safety information, ai meme generators and classroom activities, resources for teaching graph making and analysis skills, leave a comment cancel reply.
Save my name, email, and website in this browser for the next time I comment.
You've Made It This Far
Like what you're reading? Sign up to stay connected with us.
*By downloading, you are subscribing to our email list which includes our daily blog straight to your inbox and marketing emails. It can take up to 7 days for you to be added. You can change your preferences at any time.
You have Successfully Subscribed!
By subscribing, you will receive our daily blog, newsletter, and marketing emails.

- MISSION / VISION
- DIVERSITY STATEMENT
- CAREER OPPORTUNITIES
- Kide Science
- STEMscopes Science
- Collaborate Science
- STEMscopes Math
- Math Nation
- STEMscopes Coding
- Mastery Coding
- DIVE-in Engineering
- STEMscopes Streaming
- Tuva Data Literacy
- NATIONAL INSTITUTE FOR STEM EDUCATION
- STEMSCOPES PROFESSIONAL LEARNING
- RESEARCH & EFFICACY STUDIES
- STEM EDUCATION WEBINARS
- LEARNING EQUITY
- DISTANCE LEARNING
- PRODUCT UPDATES
- LMS INTEGRATIONS
- STEMSCOPES BLOG
- FREE RESOURCES
- TESTIMONIALS
Critical Thinking in Science: Fostering Scientific Reasoning Skills in Students
ALI Staff | Published July 13, 2023 | Updated December 14, 2023
Thinking like a scientist is a central goal of all science curricula.
As students learn facts, methodologies, and methods, what matters most is that all their learning happens through the lens of scientific reasoning what matters most is that it’s all through the lens of scientific reasoning.
That way, when it comes time for them to take on a little science themselves, either in the lab or by theoretically thinking through a solution, they understand how to do it in the right context.
One component of this type of thinking is being critical. Based on facts and evidence, critical thinking in science isn’t exactly the same as critical thinking in other subjects.
Students have to doubt the information they’re given until they can prove it’s right.
They have to truly understand what’s true and what’s hearsay. It’s complex, but with the right tools and plenty of practice, students can get it right.
What is critical thinking?
This particular style of thinking stands out because it requires reflection and analysis. Based on what's logical and rational, thinking critically is all about digging deep and going beyond the surface of a question to establish the quality of the question itself.
It ensures students put their brains to work when confronted with a question rather than taking every piece of information they’re given at face value.
It’s engaged, higher-level thinking that will serve them well in school and throughout their lives.
Why is critical thinking important?
Critical thinking is important when it comes to making good decisions.
It gives us the tools to think through a choice rather than quickly picking an option — and probably guessing wrong. Think of it as the all-important ‘why.’
Why is that true? Why is that right? Why is this the only option?
Finding answers to questions like these requires critical thinking. They require you to really analyze both the question itself and the possible solutions to establish validity.
Will that choice work for me? Does this feel right based on the evidence?
How does critical thinking in science impact students?
Critical thinking is essential in science.
It’s what naturally takes students in the direction of scientific reasoning since evidence is a key component of this style of thought.
It’s not just about whether evidence is available to support a particular answer but how valid that evidence is.
It’s about whether the information the student has fits together to create a strong argument and how to use verifiable facts to get a proper response.
Critical thinking in science helps students:
- Actively evaluate information
- Identify bias
- Separate the logic within arguments
- Analyze evidence
4 Ways to promote critical thinking
Figuring out how to develop critical thinking skills in science means looking at multiple strategies and deciding what will work best at your school and in your class.
Based on your student population, their needs and abilities, not every option will be a home run.
These particular examples are all based on the idea that for students to really learn how to think critically, they have to practice doing it.
Each focuses on engaging students with science in a way that will motivate them to work independently as they hone their scientific reasoning skills.
Project-Based Learning
Project-based learning centers on critical thinking.
Teachers can shape a project around the thinking style to give students practice with evaluating evidence or other critical thinking skills.
Critical thinking also happens during collaboration, evidence-based thought, and reflection.
For example, setting students up for a research project is not only a great way to get them to think critically, but it also helps motivate them to learn.
Allowing them to pick the topic (that isn’t easy to look up online), develop their own research questions, and establish a process to collect data to find an answer lets students personally connect to science while using critical thinking at each stage of the assignment.
They’ll have to evaluate the quality of the research they find and make evidence-based decisions.
Self-Reflection
Adding a question or two to any lab practicum or activity requiring students to pause and reflect on what they did or learned also helps them practice critical thinking.
At this point in an assignment, they’ll pause and assess independently.
You can ask students to reflect on the conclusions they came up with for a completed activity, which really makes them think about whether there's any bias in their answer.
Addressing Assumptions
One way critical thinking aligns so perfectly with scientific reasoning is that it encourages students to challenge all assumptions.
Evidence is king in the science classroom, but even when students work with hard facts, there comes the risk of a little assumptive thinking.
Working with students to identify assumptions in existing research or asking them to address an issue where they suspend their own judgment and simply look at established facts polishes their that critical eye.
They’re getting practice without tossing out opinions, unproven hypotheses, and speculation in exchange for real data and real results, just like a scientist has to do.
Lab Activities With Trial-And-Error
Another component of critical thinking (as well as thinking like a scientist) is figuring out what to do when you get something wrong.
Backtracking can mean you have to rethink a process, redesign an experiment, or reevaluate data because the outcomes don’t make sense, but it’s okay.
The ability to get something wrong and recover is not only a valuable life skill, but it’s where most scientific breakthroughs start. Reminding students of this is always a valuable lesson.
Labs that include comparative activities are one way to increase critical thinking skills, especially when introducing new evidence that might cause students to change their conclusions once the lab has begun.
For example, you provide students with two distinct data sets and ask them to compare them.
With only two choices, there are a finite amount of conclusions to draw, but then what happens when you bring in a third data set? Will it void certain conclusions? Will it allow students to make new conclusions, ones even more deeply rooted in evidence?
Thinking like a scientist
When students get the opportunity to think critically, they’re learning to trust the data over their ‘gut,’ to approach problems systematically and make informed decisions using ‘good’ evidence.
When practiced enough, this ability will engage students in science in a whole new way, providing them with opportunities to dig deeper and learn more.
It can help enrich science and motivate students to approach the subject just like a professional would.
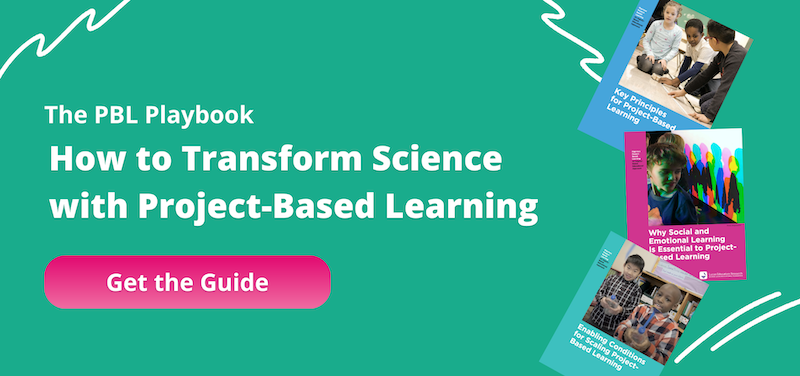
Share this post!
Related articles.
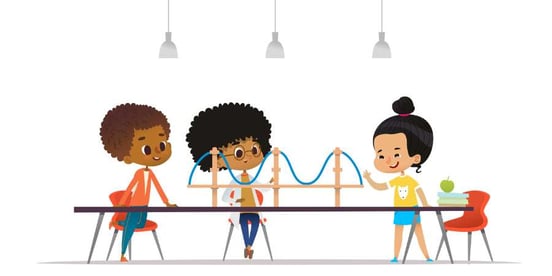
Engineering in Elementary School
Integrating engineering concepts into elementary education is a crucial topic in this day and age.
It's about...
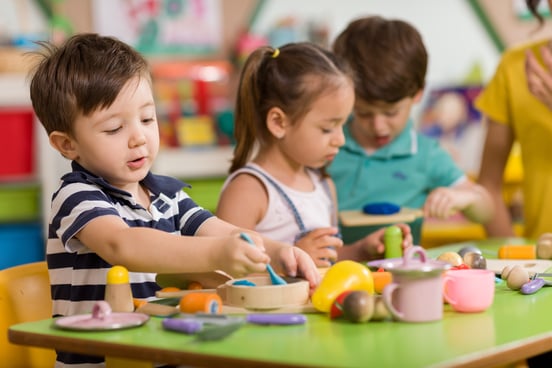
Top 7 Benefits of Play-Based Learning In Early Childhood Education
The idea of a play-based curriculum may sound counterintuitive.
“Play” suggests giving children free rein to explore...
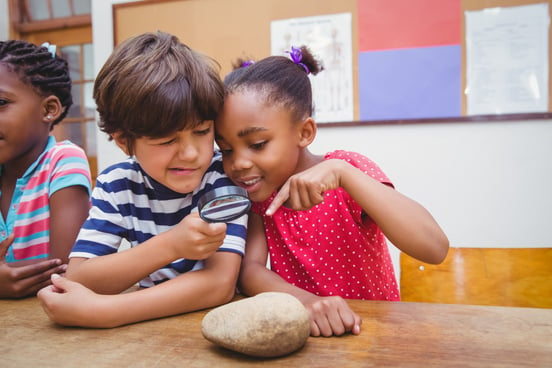
Exploring the Engineering Design Process for Kids
Introducing the Engineering Design Process for kids can sound like an intimidating concept.
As with any other framework...
STAY INFORMED ON THE LATEST IN STEM. SUBSCRIBE TODAY!
Which stem subjects are of interest to you.
STEMscopes Tech Specifications STEMscopes Security Information & Compliance Privacy Policy Terms and Conditions
© 2024 Accelerate Learning
Want a daily email of lesson plans that span all subjects and age groups?
Subjects all subjects all subjects the arts all the arts visual arts performing arts value of the arts back business & economics all business & economics global economics macroeconomics microeconomics personal finance business back design, engineering & technology all design, engineering & technology design engineering technology back health all health growth & development medical conditions consumer health public health nutrition physical fitness emotional health sex education back literature & language all literature & language literature linguistics writing/composition speaking back mathematics all mathematics algebra data analysis & probability geometry measurement numbers & operations back philosophy & religion all philosophy & religion philosophy religion back psychology all psychology history, approaches and methods biological bases of behavior consciousness, sensation and perception cognition and learning motivation and emotion developmental psychology personality psychological disorders and treatment social psychology back science & technology all science & technology earth and space science life sciences physical science environmental science nature of science back social studies all social studies anthropology area studies civics geography history media and journalism sociology back teaching & education all teaching & education education leadership education policy structure and function of schools teaching strategies back thinking & learning all thinking & learning attention and engagement memory critical thinking problem solving creativity collaboration information literacy organization and time management back, filter by none.
- Elementary/Primary
- Middle School/Lower Secondary
- High School/Upper Secondary
- College/University
- TED-Ed Animations
- TED Talk Lessons
- TED-Ed Best of Web
- Under 3 minutes
- Under 6 minutes
- Under 9 minutes
- Under 12 minutes
- Under 18 minutes
- Over 18 minutes
- Algerian Arabic
- Azerbaijani
- Cantonese (Hong Kong)
- Chinese (Hong Kong)
- Chinese (Singapore)
- Chinese (Taiwan)
- Chinese Simplified
- Chinese Traditional
- Chinese Traditional (Taiwan)
- Dutch (Belgium)
- Dutch (Netherlands)
- French (Canada)
- French (France)
- French (Switzerland)
- Kurdish (Central)
- Luxembourgish
- Persian (Afghanistan)
- Persian (Iran)
- Portuguese (Brazil)
- Portuguese (Portugal)
- Spanish (Argentina)
- Spanish (Latin America)
- Spanish (Mexico)
- Spanish (Spain)
- Spanish (United States)
- Western Frisian
sort by none
- Longest video
- Shortest video
- Most video views
- Least video views
- Most questions answered
- Least questions answered
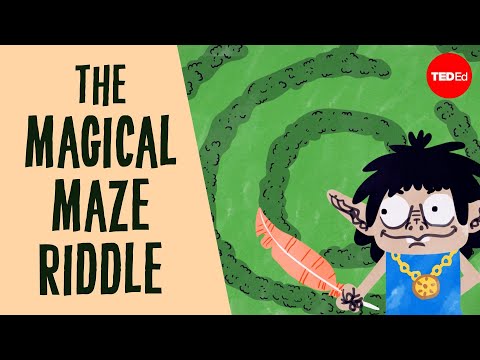
Can you solve the magical maze riddle?
Lesson duration 04:51
276,668 Views
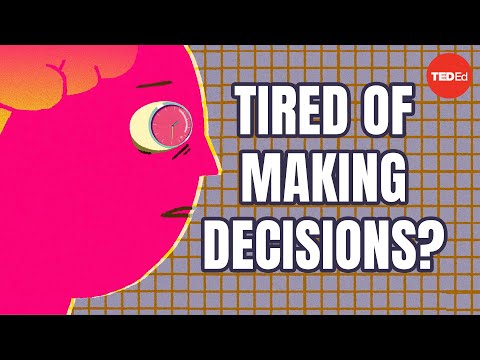
How to make smart decisions more easily
Lesson duration 05:16
962,316 Views

Can you solve a mystery before Sherlock Holmes?
Lesson duration 05:17
441,239 Views
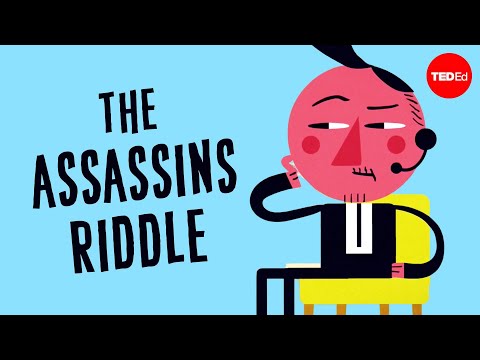
Can you solve the secret assassin society riddle?
Lesson duration 05:01
618,370 Views

How to overcome your mistakes
Lesson duration 04:52
844,412 Views
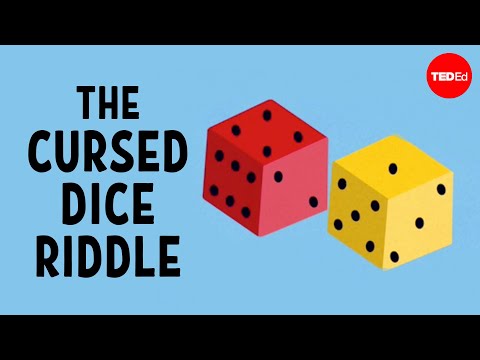
Can you solve the cursed dice riddle?
Lesson duration 04:31
631,626 Views
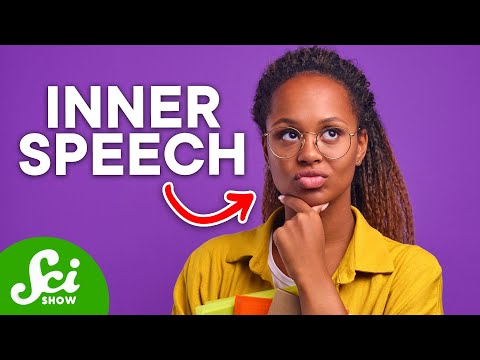
Why some people don't have an inner monologue
Lesson duration 12:03
2,612,474 Views
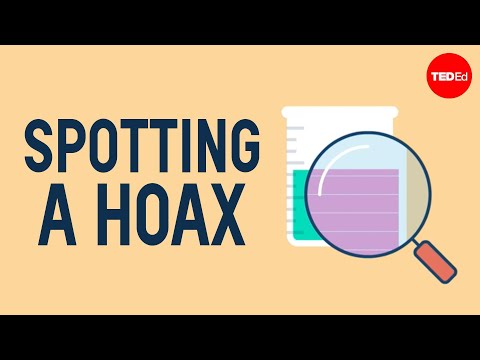
Science vs. Pseudoscience
Lesson duration 05:48
342,047 Views

Can you solve the time traveling car riddle?
Lesson duration 05:18
597,484 Views

This one weird trick will get you infinite gold
Lesson duration 05:08
917,563 Views
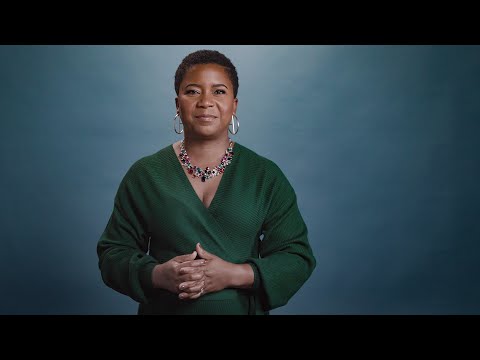
How to quit your job — without ruining your career - Gala Jackson
Lesson duration 06:13
100,472 Views
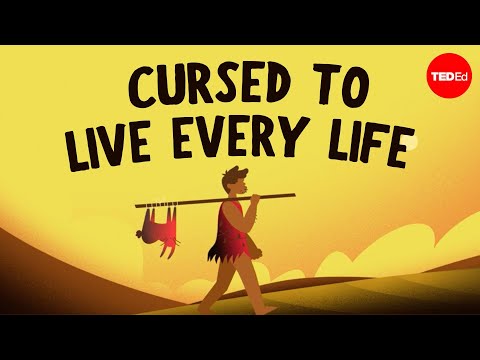
What if you experienced every human life in history?
Lesson duration 05:21
2,695,858 Views
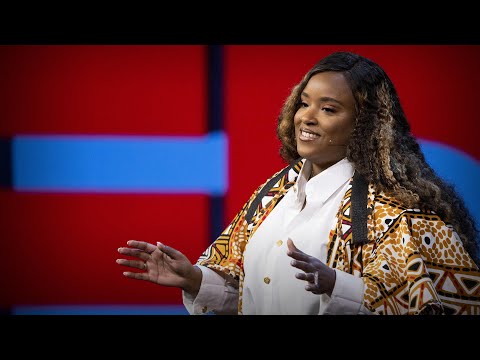
How to design climate-resilient buildings - Alyssa-Amor Gibbons
Lesson duration 14:12
42,514 Views

The case for free, universal basic services - Aaron Bastani
Lesson duration 19:09
79,436 Views
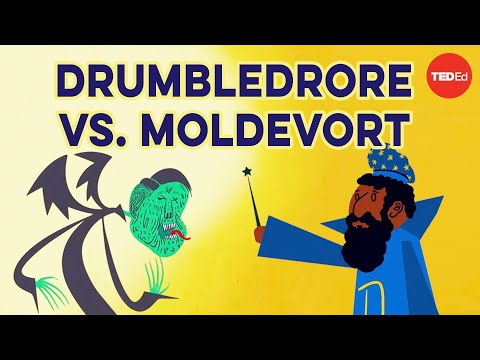
Can you steal the most powerful wand in the wizarding world?
Lesson duration 05:20
737,808 Views
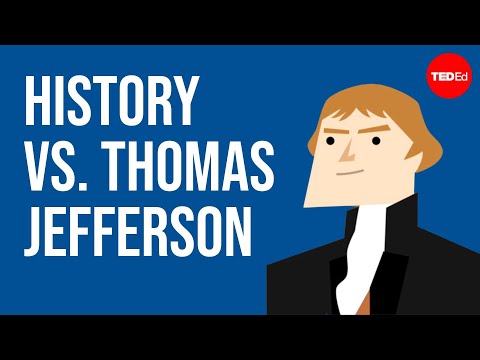
History vs. Thomas Jefferson
436,359 Views
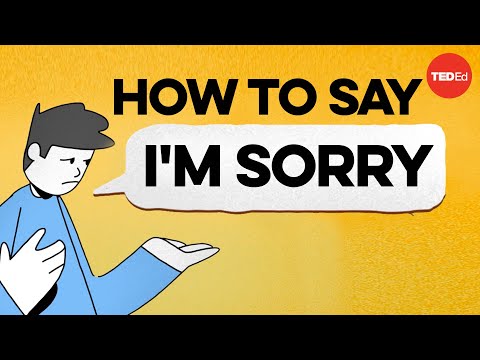
The best way to apologize (according to science)
Lesson duration 05:06
1,363,903 Views
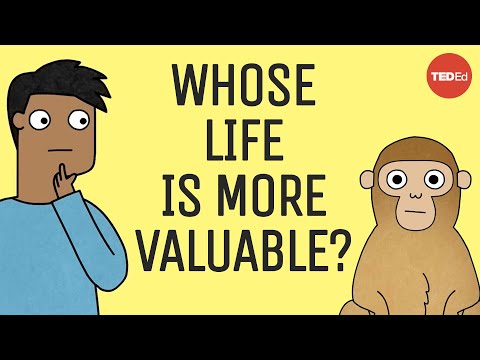
How do we determine the value of a life?
Lesson duration 06:06
634,559 Views

What’s the smartest age?
Lesson duration 04:53
1,508,113 Views

The Boltzmann brain paradox
Lesson duration 05:40
1,093,218 Views
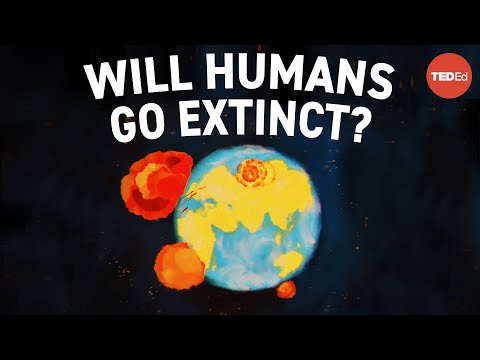
The 4 greatest threats to the survival of humanity
Lesson duration 05:24
482,262 Views
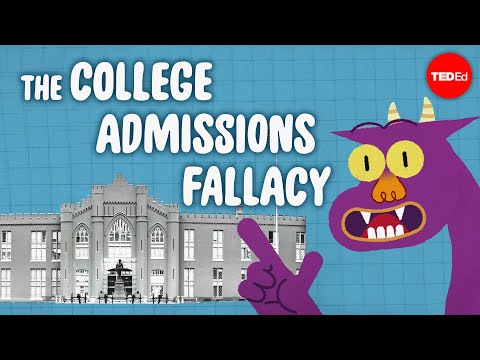
Can you outsmart the college admissions fallacy?
Lesson duration 06:17
803,962 Views
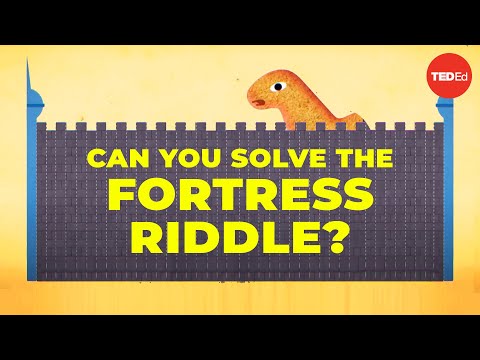
Can you solve the fortress riddle?
Lesson duration 05:23
1,171,362 Views
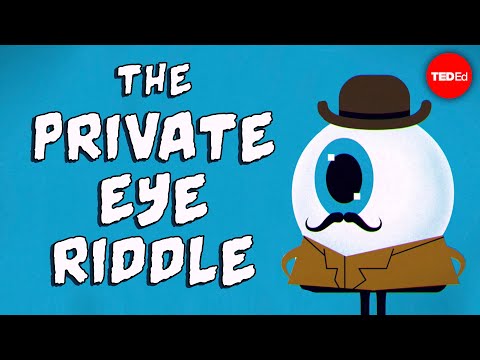
Can you solve the private eye riddle?
1,261,932 Views
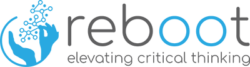
3. Critical Thinking in Science: How to Foster Scientific Reasoning Skills
Critical thinking in science is important largely because a lot of students have developed expectations about science that can prove to be counter-productive.
After various experiences — both in school and out — students often perceive science to be primarily about learning “authoritative” content knowledge: this is how the solar system works; that is how diffusion works; this is the right answer and that is not.
This perception allows little room for critical thinking in science, in spite of the fact that argument, reasoning, and critical thinking lie at the very core of scientific practice.
Argument, reasoning, and critical thinking lie at the very core of scientific practice.
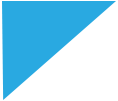
In this article, we outline two of the best approaches to be most effective in fostering scientific reasoning. Both try to put students in a scientist’s frame of mind more than is typical in science education:
- First, we look at small-group inquiry , where students formulate questions and investigate them in small groups. This approach is geared more toward younger students but has applications at higher levels too.
- We also look science labs . Too often, science labs too often involve students simply following recipes or replicating standard results. Here, we offer tips to turn labs into spaces for independent inquiry and scientific reasoning.
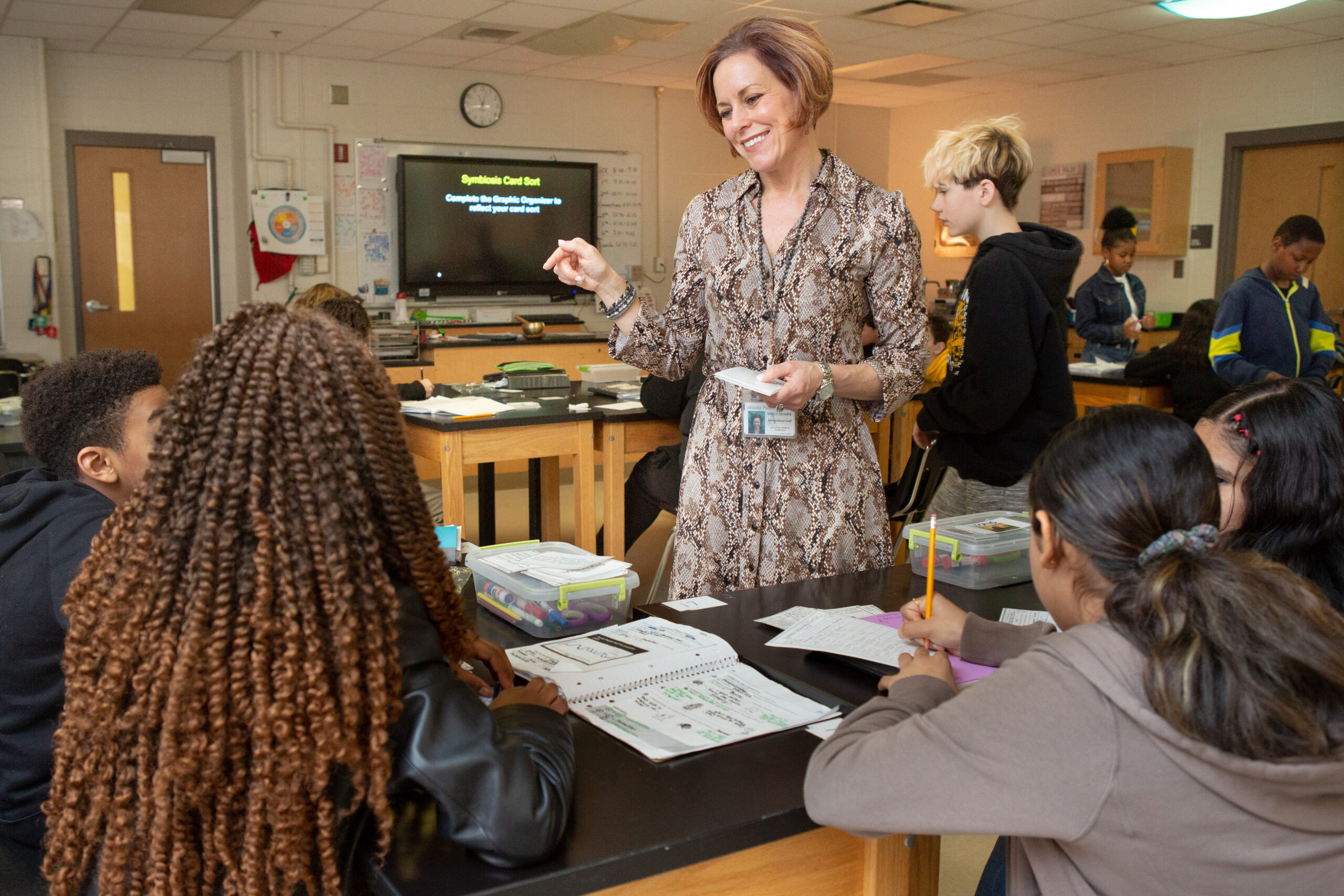
I. Critical Thinking in Science and Scientific Inquiry
Even very young students can “think scientifically” under the right instructional support. A series of experiments , for instance, established that preschoolers can make statistically valid inferences about unknown variables. Through observation they are also capable of distinguishing actions that cause certain outcomes from actions that don’t. These innate capacities, however, have to be developed for students to grow up into rigorous scientific critical thinkers.
Even very young students can “think scientifically” under the right instructional support.
Although there are many techniques to get young children involved in scientific inquiry — encouraging them to ask and answer “why” questions, for instance — teachers can provide structured scientific inquiry experiences that are deeper than students can experience on their own.
Goals for Teaching Critical Thinking Through Scientific Inquiry
When it comes to teaching critical thinking via science, the learning goals may vary, but students should learn that:
- Failure to agree is okay, as long as you have reasons for why you disagree about something.
- The logic of scientific inquiry is iterative. Scientists always have to consider how they might improve your methods next time. This includes addressing sources of uncertainty.
- Claims to knowledge usually require multiple lines of evidence and a “match” or “fit” between our explanations and the evidence we have.
- Collaboration, argument, and discussion are central features of scientific reasoning.
- Visualization, analysis, and presentation are central features of scientific reasoning.
- Overarching concepts in scientific practice — such as uncertainty, measurement, and meaningful experimental contrasts — manifest themselves somewhat differently in different scientific domains.
How to Teaching Critical Thinking in Science Via Inquiry
Sometimes we think of science education as being either a “direct” approach, where we tell students about a concept, or an “inquiry-based” approach, where students explore a concept themselves.
But, especially, at the earliest grades, integrating both approaches can inform students of their options (i.e., generate and extend their ideas), while also letting students make decisions about what to do.
Like a lot of projects targeting critical thinking, limited classroom time is a challenge. Although the latest content standards, such as the Next Generation Science Standards , emphasize teaching scientific practices, many standardized tests still emphasize assessing scientific content knowledge.
The concept of uncertainty comes up in every scientific domain.
Creating a lesson that targets the right content is also an important aspect of developing authentic scientific experiences. It’s now more widely acknowledged that effective science instruction involves the interaction between domain-specific knowledge and domain-general knowledge, and that linking an inquiry experience to appropriate target content is vital.
For instance, the concept of uncertainty comes up in every scientific domain. But the sources of uncertainty coming from any given measurement vary tremendously by discipline. It requires content knowledge to know how to wisely apply the concept of uncertainty.
Tips and Challenges for teaching critical thinking in science
Teachers need to grapple with student misconceptions. Student intuition about how the world works — the way living things grow and behave, the way that objects fall and interact — often conflicts with scientific explanations. As part of the inquiry experience, teachers can help students to articulate these intuitions and revise them through argument and evidence.
Group composition is another challenge. Teachers will want to avoid situations where one member of the group will simply “take charge” of the decision-making, while other member(s) disengage. In some cases, grouping students by current ability level can make the group work more productive.
Another approach is to establish group norms that help prevent unproductive group interactions. A third tactic is to have each group member learn an essential piece of the puzzle prior to the group work, so that each member is bringing something valuable to the table (which other group members don’t yet know).
It’s critical to ask students about how certain they are in their observations and explanations and what they could do better next time. When disagreements arise about what to do next or how to interpret evidence, the instructor should model good scientific practice by, for instance, getting students to think about what kind of evidence would help resolve the disagreement or whether there’s a compromise that might satisfy both groups.
The subjects of the inquiry experience and the tools at students’ disposal will depend upon the class and the grade level. Older students may be asked to create mathematical models, more sophisticated visualizations, and give fuller presentations of their results.
Lesson Plan Outline
This lesson plan takes a small-group inquiry approach to critical thinking in science. It asks students to collaboratively explore a scientific question, or perhaps a series of related questions, within a scientific domain.
Suppose students are exploring insect behavior. Groups may decide what questions to ask about insect behavior; how to observe, define, and record insect behavior; how to design an experiment that generates evidence related to their research questions; and how to interpret and present their results.
An in-depth inquiry experience usually takes place over the course of several classroom sessions, and includes classroom-wide instruction, small-group work, and potentially some individual work as well.
Students, especially younger students, will typically need some background knowledge that can inform more independent decision-making. So providing classroom-wide instruction and discussion before individual group work is a good idea.
For instance, Kathleen Metz had students observe insect behavior, explore the anatomy of insects, draw habitat maps, and collaboratively formulate (and categorize) research questions before students began to work more independently.
The subjects of a science inquiry experience can vary tremendously: local weather patterns, plant growth, pollution, bridge-building. The point is to engage students in multiple aspects of scientific practice: observing, formulating research questions, making predictions, gathering data, analyzing and interpreting data, refining and iterating the process.
As student groups take responsibility for their own investigation, teachers act as facilitators. They can circulate around the room, providing advice and guidance to individual groups. If classroom-wide misconceptions arise, they can pause group work to address those misconceptions directly and re-orient the class toward a more productive way of thinking.
Throughout the process, teachers can also ask questions like:
- What are your assumptions about what’s going on? How can you check your assumptions?
- Suppose that your results show X, what would you conclude?
- If you had to do the process over again, what would you change? Why?
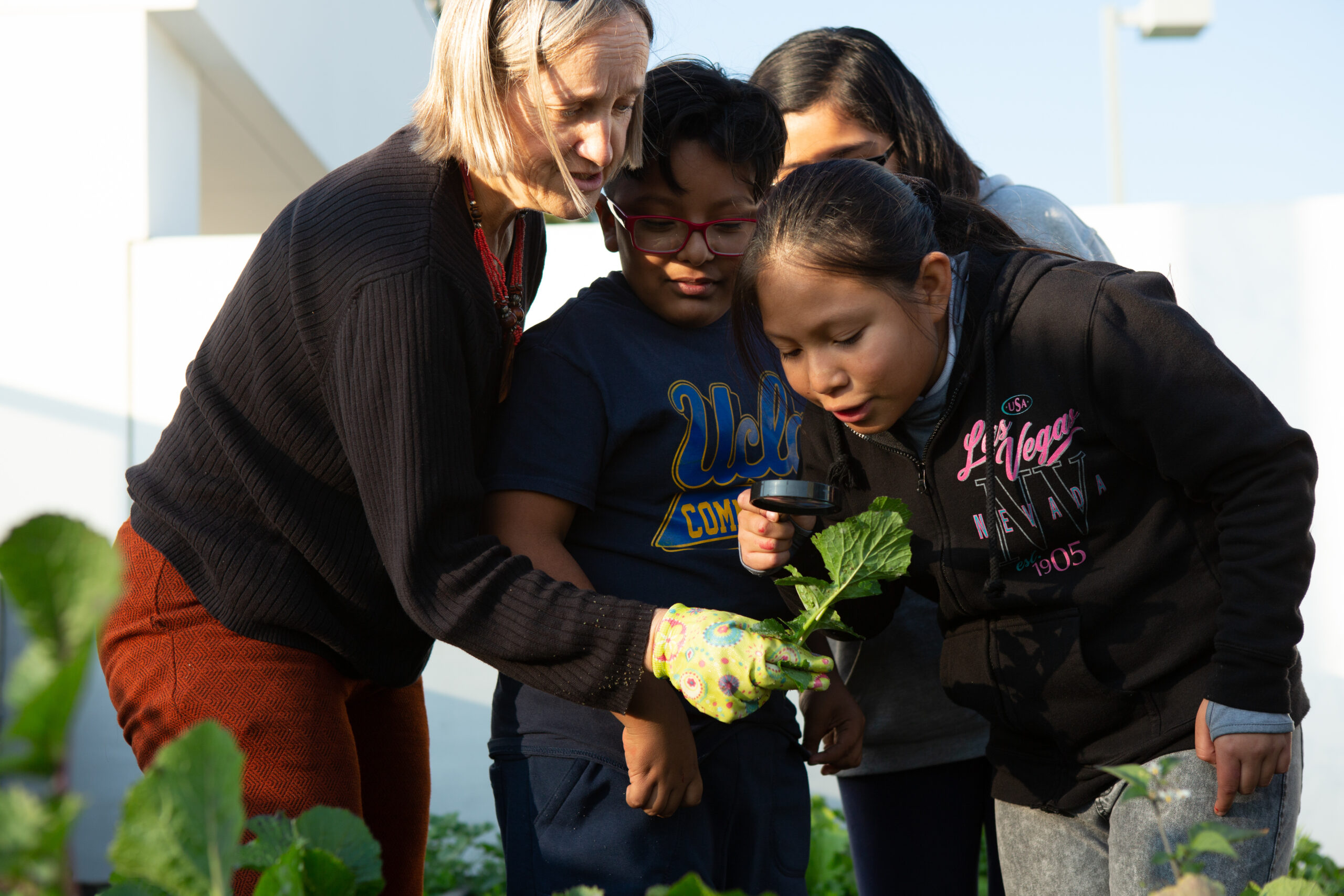
II. Rethinking Science Labs
Beyond changing how students approach scientific inquiry, we also need to rethink science labs. After all, science lab activities are ubiquitous in science classrooms and they are a great opportunity to teach critical thinking skills.
Often, however, science labs are merely recipes that students follow to verify standard values (such as the force of acceleration due to gravity) or relationships between variables (such as the relationship between force, mass, and acceleration) known to the students beforehand.
This approach does not usually involve critical thinking: students are not making many decisions during the process, and they do not reflect on what they’ve done except to see whether their experimental data matches the expected values.
With some small tweaks, however, science labs can involve more critical thinking. Science lab activities that give students not only the opportunity to design, analyze, and interpret the experiment, but re -design, re -analyze, and re -interpret the experiment provides ample opportunity for grappling with evidence and evidence-model relationships, particularly if students don’t know what answer they should be expecting beforehand.
Such activities improve scientific reasoning skills, such as:
- Evaluating quantitative data
- Plausible scientific explanations for observed patterns
And also broader critical thinking skills, like:
- Comparing models to data, and comparing models to each other
- Thinking about what kind of evidence supports one model or another
- Being open to changing your beliefs based on evidence
Traditional science lab experiences bear little resemblance to actual scientific practice. Actual practice involves decision-making under uncertainty, trial-and-error, tweaking experimental methods over time, testing instruments, and resolving conflicts among different kinds of evidence. Traditional in-school science labs rarely involve these things.
Traditional science lab experiences bear little resemblance to actual scientific practice.
When teachers use science labs as opportunities to engage students in the kinds of dilemmas that scientists actually face during research, students make more decisions and exhibit more sophisticated reasoning.
In the lesson plan below, students are asked to evaluate two models of drag forces on a falling object. One model assumes that drag increases linearly with the velocity of the falling object. Another model assumes that drag increases quadratically (e.g., with the square of the velocity). Students use a motion detector and computer software to create a plot of the position of a disposable paper coffee filter as it falls to the ground. Among other variables, students can vary the number of coffee filters they drop at once, the height at which they drop them, how they drop them, and how they clean their data. This is an approach to scaffolding critical thinking: a way to get students to ask the right kinds of questions and think in the way that scientists tend to think.
Design an experiment to test which model best characterizes the motion of the coffee filters.
Things to think about in your design:
- What are the relevant variables to control and which ones do you need to explore?
- What are some logistical issues associated with the data collection that may cause unnecessary variability (either random or systematic) or mistakes?
- How can you control or measure these?
- What ways can you graph your data and which ones will help you figure out which model better describes your data?
Discuss your design with other groups and modify as you see fit.
Initial data collection
Conduct a quick trial-run of your experiment so that you can evaluate your methods.
- Do your graphs provide evidence of which model is the best?
- What ways can you improve your methods, data, or graphs to make your case more convincing?
- Do you need to change how you’re collecting data?
- Do you need to take data at different regions?
- Do you just need more data?
- Do you need to reduce your uncertainty?
After this initial evaluation of your data and methods, conduct the desired improvements, changes, or additions and re-evaluate at the end.
In your lab notes, make sure to keep track of your progress and process as you go. As always, your final product is less important than how you get there.
How to Make Science Labs Run Smoothly
Managing student expectations . As with many other lesson plans that incorporate critical thinking, students are not used to having so much freedom. As with the example lesson plan above, it’s important to scaffold student decision-making by pointing out what decisions have to be made, especially as students are transitioning to this approach.
Supporting student reasoning . Another challenge is to provide guidance to student groups without telling them how to do something. Too much “telling” diminishes student decision-making, but not enough support may leave students simply not knowing what to do.
There are several key strategies teachers can try out here:
- Point out an issue with their data collection process without specifying exactly how to solve it.
- Ask a lab group how they would improve their approach.
- Ask two groups with conflicting results to compare their results, methods, and analyses.
Download our Teachers’ Guide
(please click here)
Sources and Resources
Lehrer, R., & Schauble, L. (2007). Scientific thinking and scientific literacy . Handbook of child psychology , Vol. 4. Wiley. A review of research on scientific thinking and experiments on teaching scientific thinking in the classroom.
Metz, K. (2004). Children’s understanding of scientific inquiry: Their conceptualizations of uncertainty in investigations of their own design . Cognition and Instruction 22(2). An example of a scientific inquiry experience for elementary school students.
The Next Generation Science Standards . The latest U.S. science content standards.
Concepts of Evidence A collection of important concepts related to evidence that cut across scientific disciplines.
Scienceblind A book about children’s science misconceptions and how to correct them.
Holmes, N. G., Keep, B., & Wieman, C. E. (2020). Developing scientific decision making by structuring and supporting student agency. Physical Review Physics Education Research , 16 (1), 010109. A research study on minimally altering traditional lab approaches to incorporate more critical thinking. The drag example was taken from this piece.
ISLE , led by E. Etkina. A platform that helps teachers incorporate more critical thinking in physics labs.
Holmes, N. G., Wieman, C. E., & Bonn, D. A. (2015). Teaching critical thinking . Proceedings of the National Academy of Sciences , 112 (36), 11199-11204. An approach to improving critical thinking and reflection in science labs. Walker, J. P., Sampson, V., Grooms, J., Anderson, B., & Zimmerman, C. O. (2012). Argument-driven inquiry in undergraduate chemistry labs: The impact on students’ conceptual understanding, argument skills, and attitudes toward science . Journal of College Science Teaching , 41 (4), 74-81. A large-scale research study on transforming chemistry labs to be more inquiry-based.
Privacy Overview
- Grades 6-12
- School Leaders
FREE Book Bracket Template. For March and Beyond!
5 Critical Thinking Activities That Get Students Up and Moving
More movement means better learning.
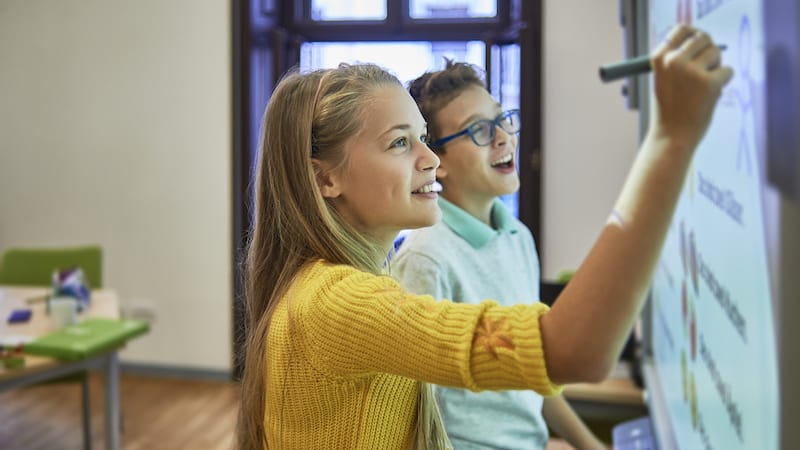
It’s easy to resort to having kids be seated during most of the school day. But learning can (and should) be an active process. Incorporating movement into your instruction has incredible benefits—from deepening student understanding to improving concentration to enhancing performance. Check out these critical thinking activities, adapted from Critical Thinking in the Classroom , a book with over 100 practical tools and strategies for teaching critical thinking in K-12 classrooms.
Four Corners
In this activity, students move to a corner of the classroom based on their responses to a question with four answer choices. Once they’ve moved, they can break into smaller groups to explain their choices. Call on students to share to the entire group. If students are persuaded to a different answer, they can switch corners and further discuss.
Question ideas:
- Which president was most influential: George Washington, Thomas Jefferson, John Adams, or Abraham Lincoln?
- Is Holden Caulfield a hero: Strongly Agree, Agree, Disagree, or Strongly Disagree?
Gallery Walk
This strategy encourages students to move around the classroom in groups to respond to questions, documents, images, or situations posted on chart paper. Each group gets a different colored marker to record their responses and a set amount of time at each station. When groups move, they can add their own ideas and/or respond to what prior groups have written.
Gallery ideas:
- Political cartoons
Stations are a great way to chunk instruction and present information to the class without a “sit and get.” Group desks around the room or create centers, each with a different concept and task. There should be enough stations for three to five students to work for a set time before rotating.
Station ideas:
- Types of rocks
- Story elements
- Literary genres
Silent Sticky-Note Storm
In this brainstorming activity, students gather in groups of three to five. Each group has a piece of chart paper with a question at the top and a stack of sticky notes. Working in silence, students record as many ideas or answers as possible, one answer per sticky note. When time is up, they post the sticky notes on the paper and then silently categorize them.
- How can you exercise your First Amendment rights?
- What are all the ways you can divide a square into eighths?
Mingle, Pair, Share
Take your Think, Pair, Share to the next level. Instead of having students turn and talk, invite them to stand and interact. Play music while they’re moving around the classroom. When the music stops, each student finds a partner. Pose a question and invite students to silently think about their answer. Then, partners take turns sharing their thoughts.
- How do organisms modify their environments?
- What is the theme of Romeo and Juliet ?
Looking for more critical thinking activities and ideas?
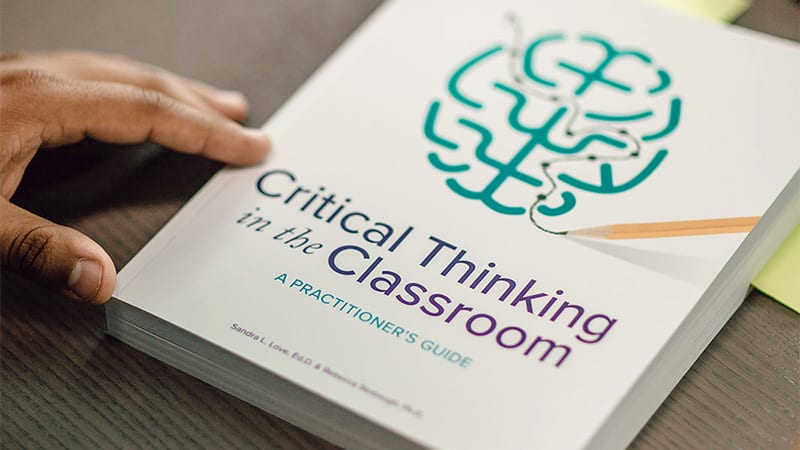
Critical Thinking in the Classroom is a practitioner’s guide that shares the why and the how for building critical thinking skills in K-12 classrooms. It includes over 100 practical tools and strategies that you can try in your classroom tomorrow!
Get Your Copy of Critical Thinking in the Classroom
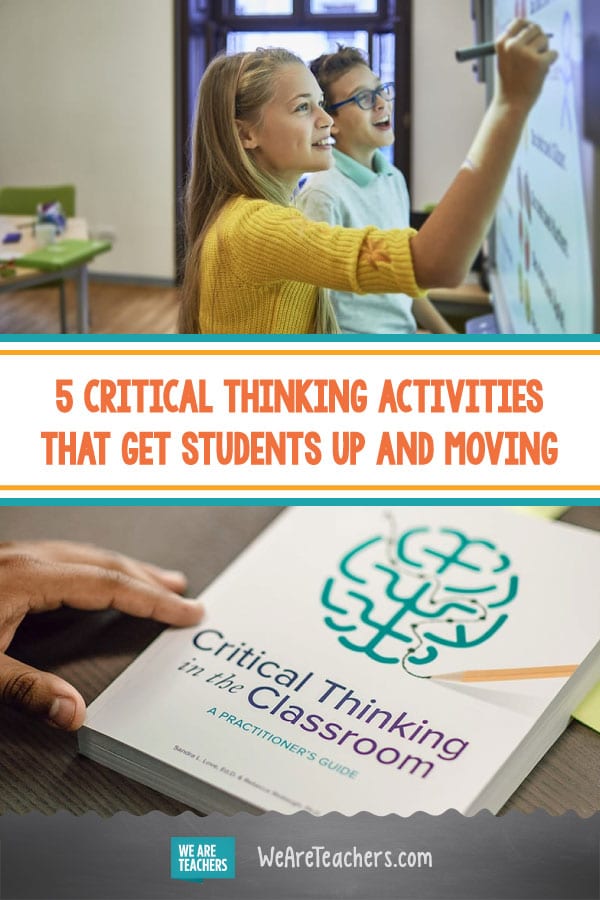
You Might Also Like
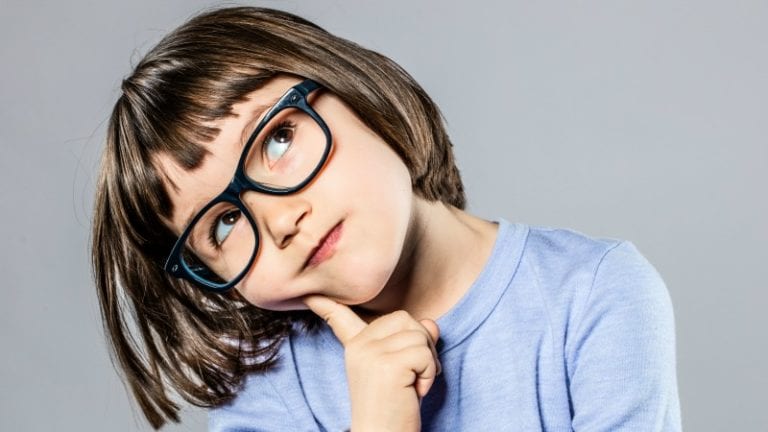
5 Activities To Help Students Improve Their Working Memory
Bell ringers that focus on what your students need most. Continue Reading
Copyright © 2023. All rights reserved. 5335 Gate Parkway, Jacksonville, FL 32256
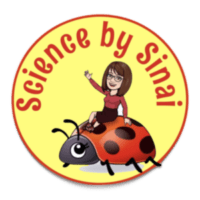
Science By Sinai
Middle School Science Tips, Ideas, and Resources
Boost Your Student’s Critical Thinking with CER in Science Class
CER (Claim, Evidence, Reasoning) is a critical thinking framework that encourages students to analyze evidence and draw conclusions that are thorough and appropriate .
I have pushed my students for years to think “out of the box“ while trying to pull data together. However, they have always struggled with the concept of “why?”. They would ramble on about the data but often not look at it deeply enough. I was getting beautiful lab reports with weak conclusion sections.
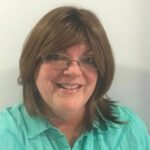
Karen Sinai
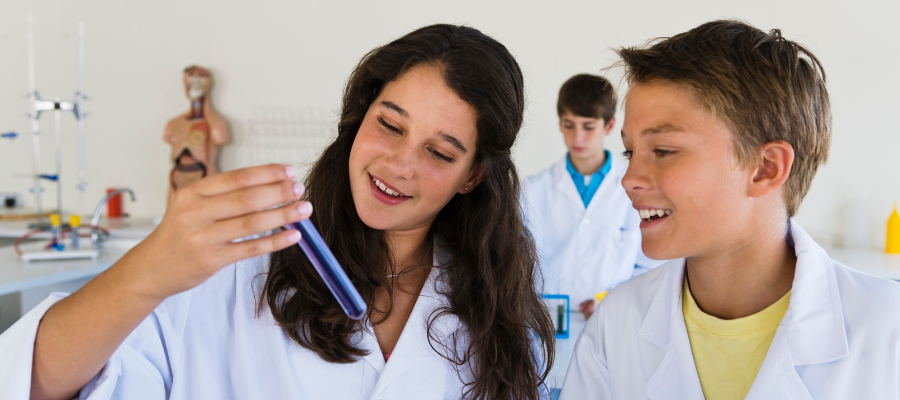
Updated July 9,2023
Using CER to Improve Writing in Science
A few summers ago I purchased a book called “Writing in Middle School Science,” by Scott Phillips. It really made me realize that the CER process was doable!
I had dabbled with it before but never really “named it” in my classroom. It was time that I got serious with the student’s writing! The premise behind the book was that students use only five or six sentences to lay out the claim, evidence and reasoning.
When I first introduced this process, my middle school students really liked the fact that they were doing minimum writing. However, after using this approach, I was amazed at the QUALITY that was going into those few sentences.
Early on, introducing critical thinking CER, students struggled with listing evidence without trying to do any reasoning yet. By using lots of examples, they started to understand that they must look at ONLY what they observe. Many teachers struggle with the reasoning part of CER. Scott Phillips discussed using the prompt “ In science we know that…therefore… ” This simple approach was a game changer with a lightbulb effect in my classroom. Students had to dig into the previous knowledge for a scientific principle or research it depending on their evidence.
Of course, this didn’t come quickly, so I needed to model, model, model.
Introducing CER with Inference TV Commercials
Not wanting to start the school year with heavy writing, I decided to lighten the atmosphere with a few inference TV commercials that are found on YouTube. I did find, however, that my seventh and eighth graders were better able to separate the “silly science” from the “real science” than my younger sixth graders.
I think most teachers who start CER using commercials, use the old favorite Audi commercial . The young girl states “I have evidence that my father is an alien”. It certainly lends itself to a very good discussion with well stated “evidence” for students to analyze.
Again, this was easier for my slightly older students as I asked them to take the approach of supporting the young girl’s evidence from HER point of view . I then had them refute the evidence with scientific principles.
More Favorite Inference Commercials
By far my favorite CER inference TV commercial is the Doritos “missing cat”. Students watching it for the first time do exactly what the commercial wants us to do.
This video is set up to have viewers jump to the conclusion that the dog has harmed the cat. In the dog’s guilt, he bribes his owner with bags of Doritos to keep him quiet. Students listed the evidence and we had very good discussions about “circumstantial evidence” in a court of law. I prompted them by asking them if they actually ever saw the cat. Could the dog just be digging and feel guilty about destroying the garden?
We discussed how evidence can sometimes be linked together to form a theory by filling in the missing parts with “imagination” or inferring. This is NOT the way we want to be doing science. This led into an analysis of what kind of evidence they could gather if they had the opportunity to find out the truth about this video. They came up with answers such as “we could go dig in the garden” or we could “find out if the cat is still missing”. Other useful commercials may be The Nolan’s Cheddar Cheese Mouse Trap , Gatorade Be Like Mike , Tabasco Mosquito Super Bowl Spot and Skechers Go Run Cheetah ad , Ice Cream and Golf Volkswagen
Templates for CER for Their Digital Science Notebooks
All of my students have digital science notebooks using templates I created. While we were watching the commercial videos, they used the colorful CER templates that I sent to them. I made a wide assortment of borders and layouts to appeal to different tastes. Students told me that they like the CER approach because it all fits onto one page. I love the critical thinking they are producing. True example of quality versus quantity!
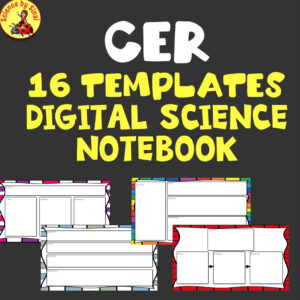
Critical Thinking CER Images for Deeper Discussions
After they did very well with the above activities, we moved onto the more difficult image prompts. I searched for a long time for images that were multifaceted.
After doing this activity with several classes, it was fascinating to find deeper levels of the photographs that I hadn’t noticed! For example, I have an image of apples and leaves on the ground and the apples are in different stages of decomposition. When I chose the photograph I wanted them to look at the signs of decomposition on the apples such as mold, shriveling, color change, worm holes, etc. My eighth graders noticed that the leaves in the photograph were also in different stages of decomposition, based on their colors and dryness. They also determined that some of the leaves were on top of the apples and some were under the apples.
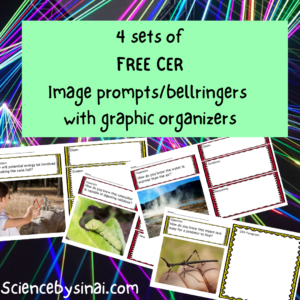
Using CER Prompts to Create Labs
Too often when we are looking for labs to do in the classroom they tend to be very cookie-cutter with a known result. These are useful at times to support a concept.
However, I love throwing a question out to the students to wrestle with coming up with their own methodology for finding an answer. I start with simple data collecting questions such as “What color M&Ms do people choose to eat first?”. Although it sounds silly, they can set up a legitimate testing experiment with good parameters to gather evidence.
Questions can be posed such as “which location in this classroom is best suited to germinate bean seeds?”, “from which direction does the wind blow across the schoolyard most often?”or “will balls of different masses roll different distances after they go down the same ramp?”.
Students put together a claim, design an experiment and gather at least three evidence sentences. They then use the prompts discussed earlier, “in science we know that… therefore…”, to pull everything together.
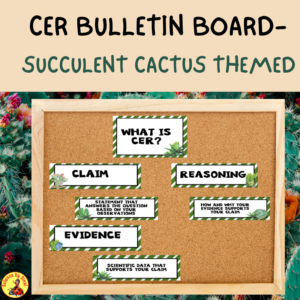
Using CER to Analyze Data Charts and Graphs
There are lots of sources online for finding data for the students to analyze and draw conclusions. You can give students a ready-made graph and have them interpret the data, or you can give them the raw data and have them graph and analyze it. It is quite easy to find lists of data such as the heart rates of animals, breathing rates , boiling points of liquids, top speed of animals, or the lifespans of different organisms. You can even find the data of the profits of Starbucks stores over time or the amount of Starbucks stores per state!
Having them analyzing line graphs, bar graphs and paragraphs is an important skill.
Using CER to Analyze Sort Cards
Many of the sorting sets and task cards that you already have may lend themselves well to a CER activity. Here are a few of my image prompts/sort card sets that I now use with CER templates.
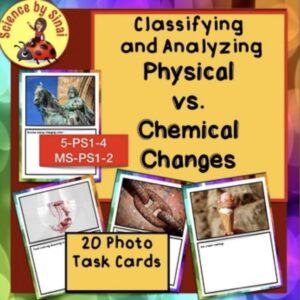
Using critical thinking with CER in my classroom has literally changed my students’ outlook in a very short time. I use the method as a stand alone activity or as the conclusion section of a standard lab report. My students seem much more confident with their new understanding of how to pull evidence together to write a good reasoning section.
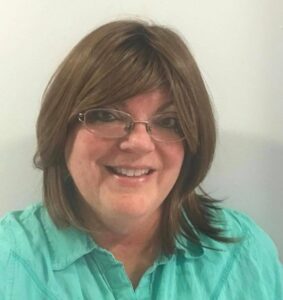
Similar Posts
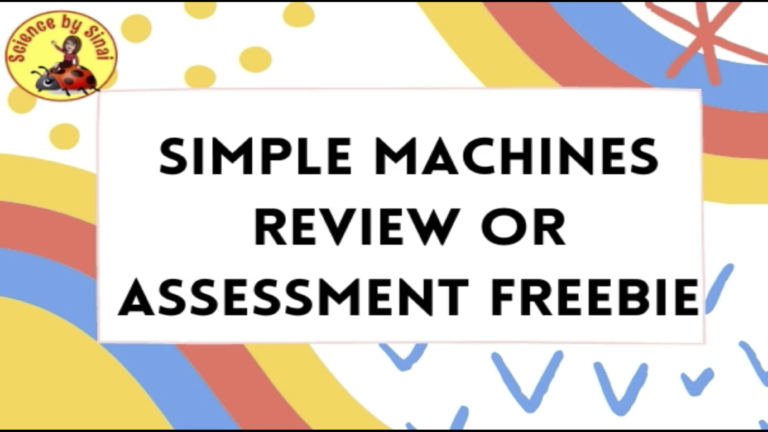
SIMPLE MACHINES REVIEW / ASSESSMENT
Here are three simple machine review slides with fill in the blanks for types of simple machines plus the types of levers. Great for introduction, review or assessment.
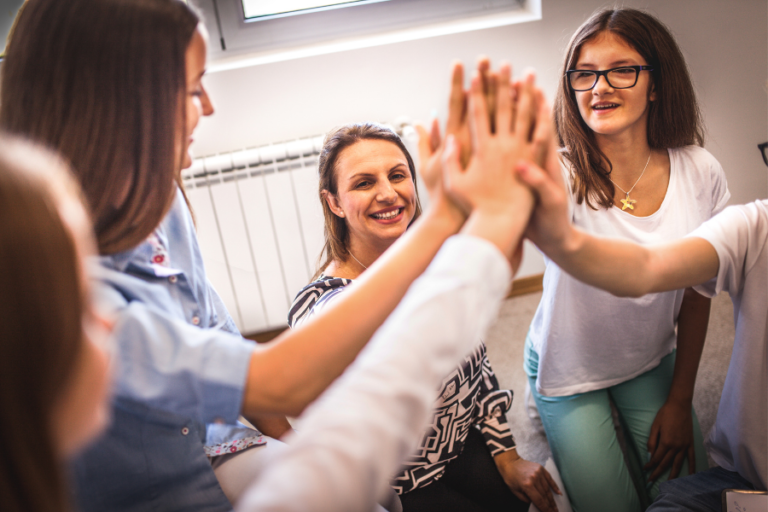
10 Tips to Prevent Discipline Problems in Your Classroom
10 Tips to Prevent Discipline Problems in Your Classroom Are you nervous about starting the school year teaching middle school and worried about having discipline problems? Does having the students out of their seats during hands on activities intimidate you? Here are some suggestions on ways to alleviate any major discipline issues BEFORE they develop. Discipline…
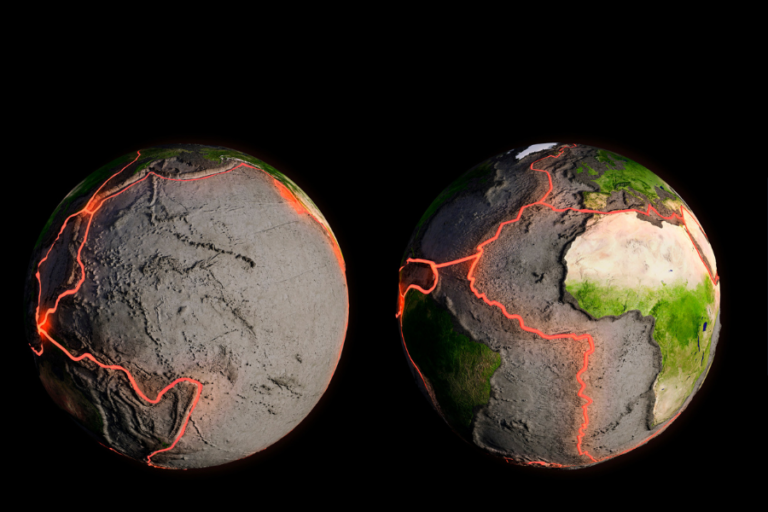
Move the Plates! Teaching Plate Tectonics is Fun
Move the Plates! Teaching Plate Tectonics is Fun Are you teaching plate tectonics and looking for a way for students to manipulate the plates while learning the types of boundaries? Would you like a digital component to add to your earth science unit? Utilizing plate tectonic drag and drop activities with Google Earth analysis helps…
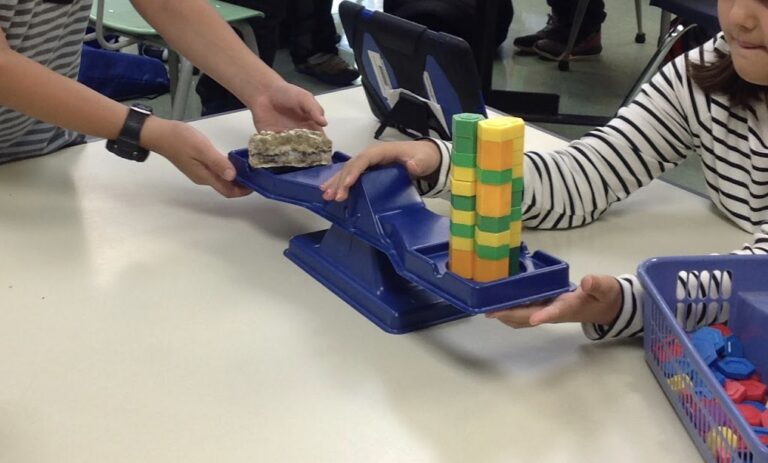
Give Your Students a Measurement Review with these 10 Fun Activities
Give Your Students a Measurement Review with these 10 Fun Activities Are you finding that middle school students seem to lack basic measurements skills in spite of us reviewing and reinforcing this as we head back to school every year? I can’t tell you how many times I’ve had middle schoolers measure from the end…
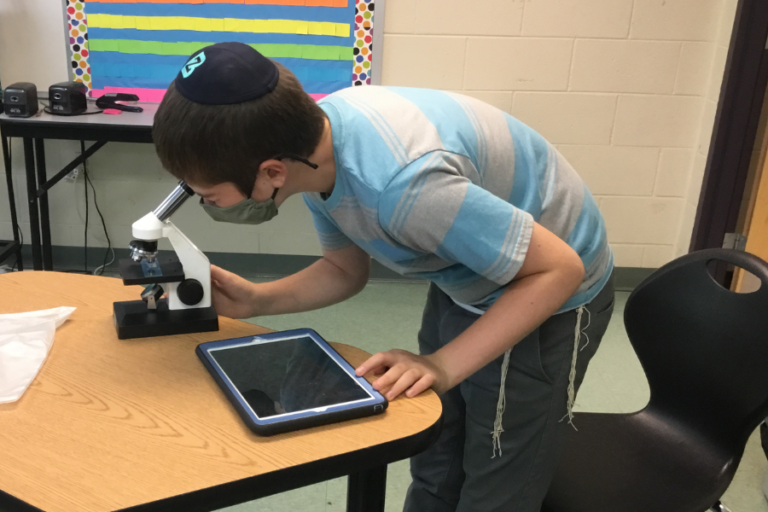
How to Introduce the Characteristics of Living Things
How to Introduce the Characteristics of Living Things Do you find that students struggle with being able to determine the basic characteristics of living things? Even in middle school, students have a hard time distinguishing the differences between a living versus a non-living structure. It is better to deal with any misconceptions first, using engaging activities,…
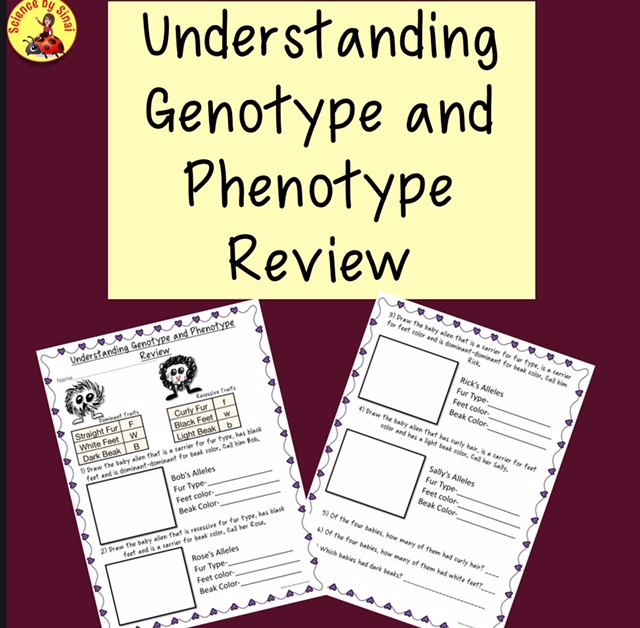
Understanding Genotype and Phenotype Review
Understanding Genotype and Phenotype Review Here’s a super set of two worksheets to make sure your students completely understand genotype and phenotypes. They look at the alien parents and decide on the appearance of the four babies based on their genotypes.
Leave a Reply Cancel reply
Your email address will not be published. Required fields are marked *
Don't subscribe All new comments Replies to my comments Notify me of followup comments via e-mail. You can also subscribe without commenting.
Science Literacy: Equipping Students with Critical Thinking for a Scientific World
Developing critical thinking is crucial for science education.
This article will explore practical strategies to promote scientific literacy and critical analysis in the classroom.
You'll learn the importance of critical thinking in science, activities to sharpen analysis skills, and methods to assess progress in scientific literacy.
The Importance of Critical Thinking in Science
Critical thinking is the objective analysis and evaluation of information to reach an unbiased judgment. It is essential for science literacy, as it empowers students to logically assess scientific evidence, challenge assumptions, and advance innovative ideas.
Defining Critical Thinking in Science
Critical thinking in science involves skills like:
- Careful examination of research data and scientific claims
- Identifying limitations, biases, or flaws in studies
- Determining the soundness of experimental design and methodology
- Drawing reasonable conclusions based on available evidence
- Recognizing inappropriate inferences or illogical reasoning
It enables the questioning of conventional wisdom in pursuit of factual truth.
The Role of Critical Thinking in Scientific Progress
Critical thinking is the engine that drives innovation in science. By scrutinizing accepted theories, scientists can identify gaps in knowledge and pave the way for new discoveries. For example, Einstein's skepticism of Newtonian physics led him to develop the groundbreaking theory of relativity. Critical analysis of research allows scientists to build on current ideas or develop entirely new ones. Cultivating critical thinking skills in students promotes more revolutionary thinkers who can advance science.
Developing Critical Thinking Skills in Science Learners
Critical ability to assess the credibility and implications of scientific claims is fundamental for students. Educators must go beyond rote memorization of facts and challenge learners to:
- Evaluate the soundness of experimental conclusions
- Identify questionable assumptions in hypotheses
- Assess the limits of interpretability of results
- Detect bias in the reporting of findings
Activities like analyzing real scientific studies, reviewing controversies, and discussing open-ended questions encourage the reflective skepticism vital for comprehension.
Research About Critical Thinking Skills
Studies demonstrate critical thinking allows deeper learning. Students taught to critically analyze scientific reports show better grasp of core concepts than those who only read excerpts passively. Research also reveals a high correlation between critical thinking abilities and academic performance in science. Implementing activities focused on interpretation, inference, and evaluation of research produces significant gains in student scientific literacy. These findings spotlight the pivotal role of analytical thinking in science education.
What is scientific literacy and critical thinking skills?
Scientific literacy refers to an individual's ability to understand key scientific concepts and processes required to make informed decisions about scientific issues. It involves the capacity to ask questions, acquire new knowledge, and identify and evaluate scientific information based on evidence.
Critical thinking encompasses the cognitive skills needed to analyze, interpret, evaluate, and integrate information. It includes skills such as:
- Identifying assumptions and biases
- Evaluating the quality and credibility of evidence
- Distinguishing facts from opinions
- Drawing reasonable conclusions
- Applying information to real-world contexts
Both scientific literacy and critical thinking are essential for navigating an increasingly complex world driven by science and technology.
Students who develop strong skills in these areas are better equipped to:
- Understand current events involving science, health, and the environment
- Evaluate the credibility of news reports and social media claims related to science
- Make informed decisions about issues such as nutrition, medical treatments, consumer products, energy solutions, and policies around innovation
Educators play a vital role in helping students build this knowledge and these skills. By integrating activities focused on scientific literacy and critical thinking across subjects and grade levels, teachers can empower students to analyze issues logically, question ideas, and make evidence-based decisions throughout their lives.
Why is critical thinking important in science education?
Critical thinking is a crucial skill for students to develop in science education. Here are some of the key reasons why:
Addressing Assumptions
One way critical thinking aligns so perfectly with scientific reasoning is that it encourages students to challenge assumptions. While students work with facts and data, there is still a risk of making unsupported assumptions. Critical thinking pushes them to question:
- What evidence supports this claim?
- What alternative explanations could there be?
- What assumptions am I making here?
Getting students into the habit of questioning assumptions strengthens their scientific analysis skills.
Evaluating Sources
Students also need to critically evaluate sources of information. With so much information available, it's vital they can judge which sources to trust. Critical thinking helps them develop skills to:
- Check an author's credentials and potential biases
- Evaluate the evidence presented
- Distinguish facts from opinions
- Identify gaps in an argument
Strengthening these source analysis abilities will lead to better scientific evaluations.
Applying Concepts
Critical thinking also involves applying concepts to new situations. In science education, students learn theories, processes, and models. But true learning comes when they can take that knowledge and apply it creatively to explain new phenomena. Critical thinking is key here.
In summary, critical thinking fundamentally aligns with core scientific skills - questioning assumptions, analyzing sources, and applying concepts. Nurturing critical thinking lays the foundation for stronger scientific reasoning.
How does scientific literacy influence science teaching?
Scientific literacy is crucial for equipping students with the critical thinking skills needed to understand and participate in an increasingly scientific world. As science educators, integrating literacy strategies into our teaching can profoundly shape how students develop scientific competencies.
Fostering Deep Understanding
Using reading and writing to teach key concepts allows students to engage with material in a meaningful way. Learning how to decode complex diagrams, data sets, and scientific papers facilitates comprehension of multifaceted ideas that may otherwise remain unclear.
Enabling Students to "Do Science"
Science is an active process of questioning, investigating, and communicating findings. By teaching students how to access, assess, and articulate scientific information, we empower them to fully participate in the scientific endeavor as young researchers.
Preparing Students for Real-World Application
Ultimately, scientific literacy prepares students to navigate scientific issues in their everyday lives. Learning how to critically evaluate media reports on science topics, for example, helps students make informed decisions regarding their health, society, and environment.
sbb-itb-bb2be89
How do you promote critical thinking in a science classroom.
Critical thinking is an essential skill for students to develop, especially in science education. Here are some effective strategies teachers can use to promote critical thinking in the science classroom:
Emphasize the scientific method
The scientific method inherently requires critical thinking. When students learn to ask thoughtful questions, research existing ideas, develop hypotheses, design experiments, collect and analyze data, draw conclusions, and communicate results, they build critical thinking capacities.
Teachers should model the scientific method through demonstrations and hands-on labs. Allow students to practice by designing their own experiments to test hypotheses. Critiquing the experimental designs of peers also develops evaluation abilities.
Facilitate open-ended discussions
Class discussions centered around open-ended scientific questions promote critical analysis, evaluation of evidence, and consideration of multiple perspectives. Teachers can introduce contradictory research, ethical dilemmas, or gaps in scientific understanding to stimulate discourse.
Allowing students to respectfully challenge each other's reasoning in a collaborative environment helps strengthen critical thinking and communication abilities.
Integrate real-world applications
Relating scientific concepts to current events, social issues, and students' own lives makes the content relevant and engaging. Analyzing real-world problems through a scientific lens requires weighing evidence, questioning assumptions, and synthesizing information across disciplines.
For example, discussing the science underlying climate change policies, medical treatments, or new technologies pushes students to think critically and make informed decisions.
Ask probing questions
Rather than simply testing content knowledge, teachers should ask probing questions that push students to justify their claims, make connections to prior knowledge, provide counterexamples, and consider alternative perspectives. This type of Socratic questioning challenges assumptions, forces deeper thinking, and promotes intellectual humility.
Open-ended questions allow for multiple, creative answers rather than fixed solutions, helping develop flexible thinking. Questions that spark curiosity also drive intrinsic motivation to analyze and understand scientific phenomena.
Critical Thinking Science Activities for Classroom Practice
Teachers can design impactful science critical thinking questions that promote analysis, evaluation, and application of concepts. ### Designing Science Critical Thinking Questions
- Ask open-ended questions that have multiple potential answers, requiring students to justify their reasoning. For example, "How might the climate be different if the Gulf Stream ocean current changed direction?"
- Present real-world scenarios and ask students to identify issues, state claims, provide evidence, assess assumptions, and consider alternative perspectives.
- Encourage metacognition by asking students to reflect on their own thought processes. "What previous knowledge or experiences influenced your ideas about this topic?"
Incorporating Real-World Examples in Teaching
Using relevant real-world examples in science instruction allows students to connect classroom learning to current events and their own lives. Teachers can find case studies on latest discoveries, emerging technologies, or socioscientific issues like climate change and genetic engineering. Analyzing authentic examples requires applying science knowledge critically.
Encouraging Healthy Skepticism through Activities
Teachers should promote constructive questioning, rather than blind acceptance of information. When introducing concepts, have students list their initial assumptions and re-examine them later. Evaluate sources together by looking at author expertise, potential biases, and type of evidence presented. Design labs for students to test claims themselves, rather than taking them at face value.
Hands-On Experiments and Critical Analysis
Lab activities present a meaningful opportunity to develop critical thinking skills. Have students design their own experiments to test hypotheses, ensuring they identify variables and controls. When interpreting results, prompt them to explain unexpected outcomes and errors, making evidence-based critiques of the experimental design. Require written lab reports, emphasizing sound scientific arguments.
Assessing Critical Thinking in Scientific Research
Critical thinking is an essential skill for students to develop scientific literacy. Assessing students' critical thinking progress allows teachers to gauge comprehension and identify areas needing improvement.
Rubrics Aligned to Critical Thinking Standards
Rubrics outline criteria for evaluating critical thinking in science. They act as a guide for students by clearly defining expectations. Rubrics should:
- Measure skills like interpreting data, identifying assumptions, and drawing conclusions
- Align to science education standards and learning objectives
- Use a rating scale (e.g. emerging, developing, proficient)
With well-designed rubrics, students understand what constitutes quality scientific thinking. Teachers can provide meaningful feedback tied to rubric dimensions.
Open-Ended Writing Prompts for Scientific Literacy
Open-ended questions prompt students to demonstrate scientific literacy skills. Well-crafted prompts allow students to:
- Explain science concepts in their own words
- Make connections between ideas
- Apply learning to real-world contexts
- Analyze study limitations and implications
Scoring guides assess writing on criteria such as accuracy, depth of explanation, and use of evidence.
Individual and Group Discussions on Scientific Topics
Class discussions reveal students' abilities to think critically about science. Teachers can facilitate conversations that require students to:
- Make claims backed by scientific reasoning
- Constructively critique peers' ideas
- Ask thoughtful questions that further understanding
- Adjust thinking based on new information
Tracking participation and exchanges helps gauge growth of critical analysis skills over time.
Peer Review and Feedback Loops
Peer review develops critical thinking by having students assess project drafts. Review criteria should map to scientific literacy goals. The process teaches students to:
- Provide constructive, actionable feedback
- Thoughtfully consider diverse perspectives
- Reflect on their own work for improvement
Iterative peer review and revision loops strengthen students' critical analysis abilities.
Overcoming Obstacles to Teaching Critical Thinking
Teaching critical thinking can be challenging, but with some strategic planning, it is possible to overcome common barriers.
Limited Class Time and Critical Thinking
- Prioritize critical thinking skills in lesson planning. Map out where you can integrate activities, questions, and discussions that build analysis and evaluation abilities.
- Use a flipped classroom model. Have students watch prerecorded lectures at home and use class time for open-ended investigations, debates, and inquiry-based labs. This maximizes active learning.
- Create assignments focused on interpreting data, weighing evidence, and making reasoned arguments rather than rote tasks. Stress depth over breadth of coverage.
Standardized Testing Pressures and Scientific Literacy
- Explicitly discuss with students how critical thinking skills prepare them for exams and life by building comprehension and problem-solving abilities.
- Design test prep lessons that incorporate analysis of graphs, experimental design, evidence evaluation, and argument formation. These mirror scientific literacy goals while reviewing content.
- Encourage students to explain their reasoning on exams rather than just state answers. Make critical thinking an assessed skill set.
Student Resistance to New Teaching Methods
- Communicate the relevance and utility of critical analysis for careers and situations students will encounter outside the classroom. Make connections explicit.
- Start with small, low-stakes activities focused on debate, evaluation, and decision-making before building up to larger critical thinking projects. This allows students to gain confidence with the approach.
- Be transparent about the pedagogical reasons for teaching critical thinking skills. Discuss with students how the approach trains habits of mind that serve them inside and outside school.
Adapting to Diverse Learning Styles
- Vary the formats used for critical thinking, including visual tools, verbal discussions, written reflections, group work, and hands-on investigations. This reaches different learning preferences.
- Structure activities so groups contain a mix of learning styles. Students can learn from observing how their peers approach analyzing and evaluating problems from different perspectives.
- Ask students to assess and give input on activities. Adjust approaches based on feedback about which critical thinking methods connect best with more students.
Conclusion and Key Takeaways
Critical thinking is a crucial skill for science students to develop scientific literacy. By teaching students how to think critically, educators equip them to evaluate claims, question assumptions, and make evidence-based decisions.
Critical Thinking as a Crucial Science Skill
Critical thinking allows students to analyze scientific information objectively. It empowers them to draw conclusions based on facts rather than assumptions. Mastering critical thought is key for students to succeed in science fields.
Implementing Critical Pedagogy in Science Education
To build critical thinking, educators should design activities that challenge assumptions and require objective analysis. Open-ended experiments, debates, and reflective writing prompts develop evaluative abilities.
Assessing Progress in Critical Thinking and Scientific Literacy
Rubrics, concept maps, and reflective writing provide insight on students' analytical skills. Educators should evaluate both content knowledge and ability to think critically about scientific concepts.
Embracing the Future of Science Education
As science and technology advance, critical thinking becomes increasingly vital. Educators should continuously adapt teaching methods to instill adaptable, rational thinking skills that prepare students to evaluate new information.
Related posts
- Teaching Resources for Teachers: Cultivate Critical Thinking
- Incorporating Global Perspectives in Lessons
- The Art of Questioning: Techniques to Promote Critical Thinking and Inquiry
- How to Develop Critical Thinking Skills in Students
Teachers Teaching Teachers: Tech Integration
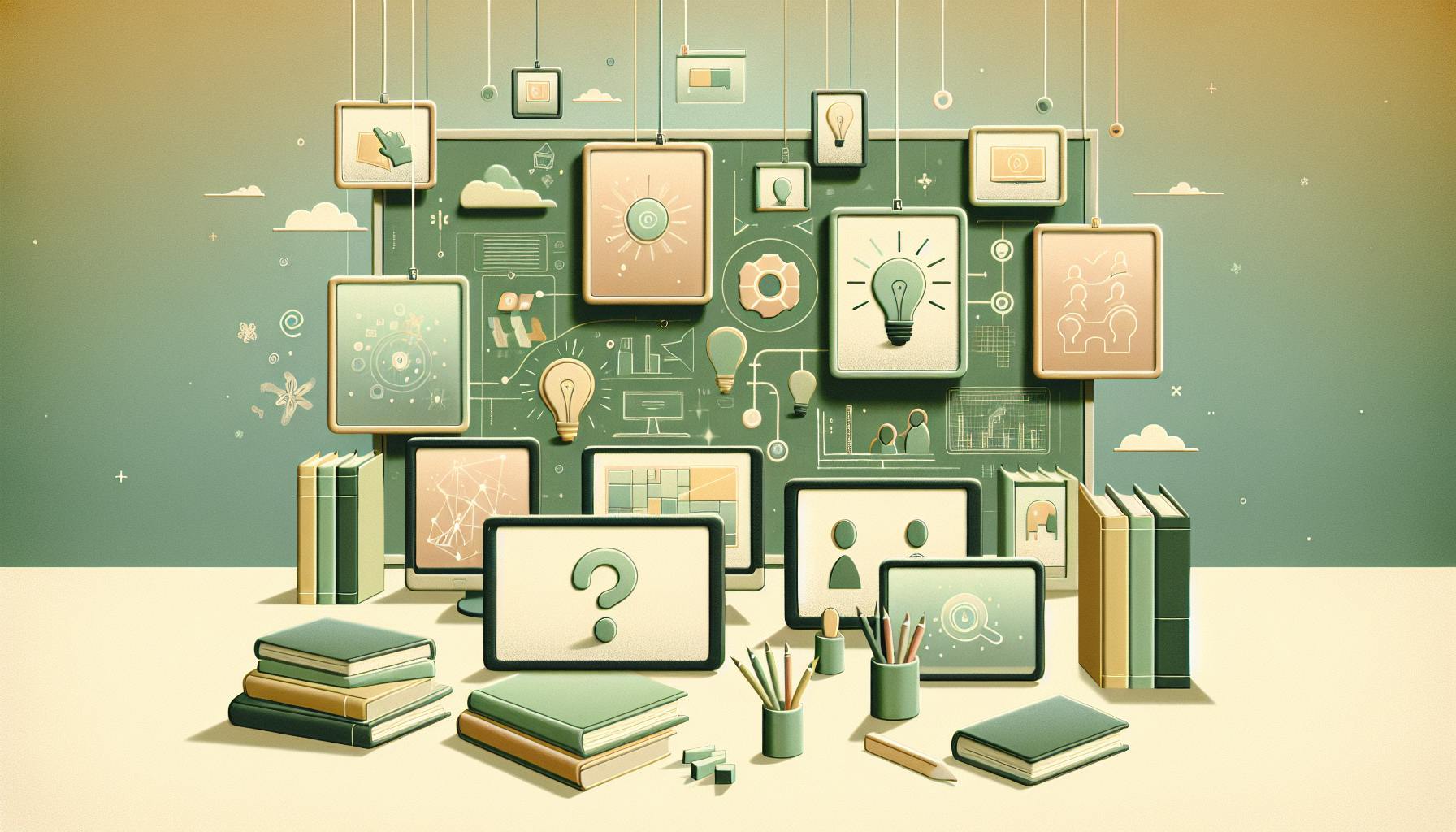
Strategies for Engaging Remote Students
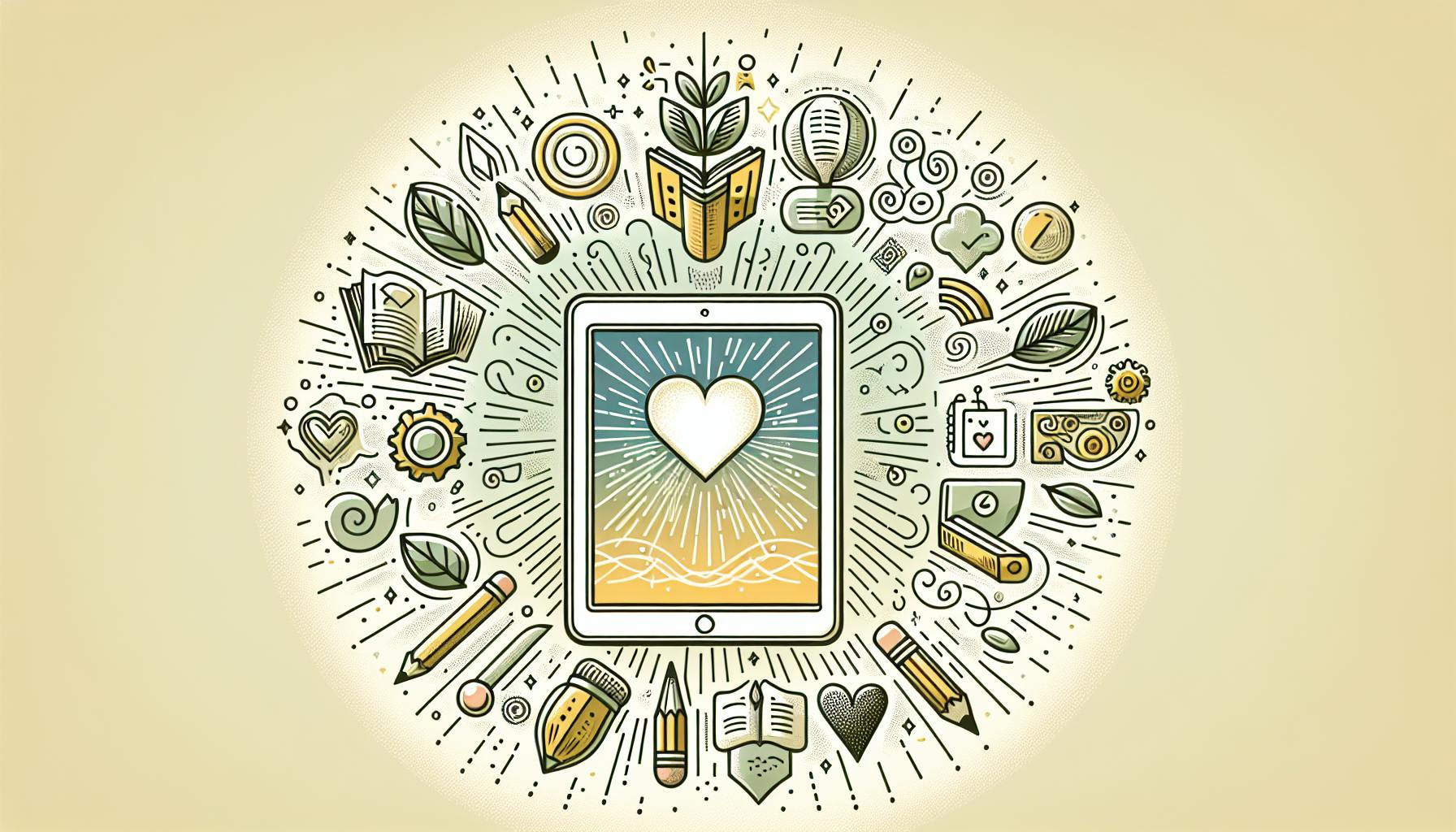
Assistive Technology Tools for Diverse Learning Needs
Become a buddy..
Join 500+ teachers getting free goodies every week. 📚

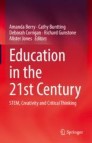
Education in the 21st Century pp 29–47 Cite as
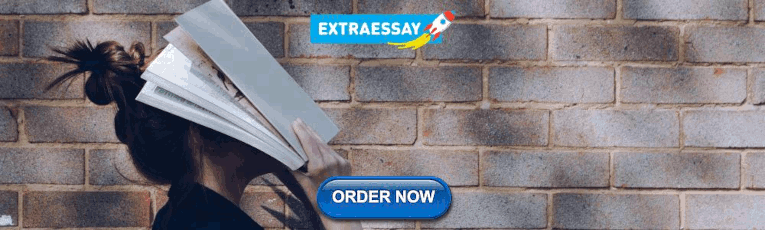
Fostering Students’ Creativity and Critical Thinking in Science Education
- Stéphan Vincent-Lancrin 6
- First Online: 31 January 2022
914 Accesses
1 Citations
What does it mean to redesign teaching and learning within existing science curricula (and learning objectives) so that students have more space and appropriate tasks to develop their creative and critical thinking skills? The chapter begins by describing the development of a portfolio of rubrics on creativity and critical thinking, including a conceptual rubric on science tested in primary and secondary education in 11 countries. Teachers in school networks adopted teaching and learning strategies aligned to the development of creativity and critical thinking, to these OECD rubrics. Examples of lesson plans and pedagogies that were developed are given, and some key challenges for teachers and learners are reflected on.
- Critical thinking
- Science education
- Innovation in education
- Lesson plans
The analyses given and the opinions expressed in this chapter are those of the author and do not necessarily reflect the views of the OECD and of its members.
Download chapter PDF
3.1 Introduction
What does it mean to redesign teaching and learning within existing science curricula (and learning objectives) so that students have more time and appropriate tasks to develop their creative and critical thinking skills?
The first difficulty to overcome is to operationalise the concepts of creativity and critical thinking so that each would be tangible and visible for science teachers. What do creativity and critical thinking mean in science education? What do these mean when students are not yet experts in their domain? To answer these questions, the OECD developed a portfolio of rubrics on creativity and critical thinking through a quick prototyping model, including a conceptual rubric on science that was tested in primary and secondary education in 11 countries between 2015 and 2019 (Vincent-Lancrin et al., 2019 ).
This chapter will show how teaching and learning strategies aligned to the development of creativity and critical thinking – and to those rubrics – could be used in science education. The first section will present the rubrics and how they related to theories of creativity and critical thinking in general. The second section will present two examples of lesson plans and pedagogies that were developed during the OECD project to foster and assess creativity and critical thinking in education. Beyond the above-mentioned rubrics, these lesson plans illustrate how the development of creativity and critical thinking skills can look in practice in a science unit. The chapter will also reflect on some key challenges for teachers and learners to make the development of creative and critical thinking skills possible. It will conclude by highlighting the importance of integrating similar approaches in other school subjects so that students experience enough opportunities to develop those skills.
3.2 How to Support Creativity and Critical Thinking in Science Education: Concepts and Rubrics
Most contemporary education systems include creativity and critical thinking as part of their list of key skills students should acquire in their schooling. Most curricula in OECD countries do include in one form or another critical thinking and creativity as expected learning outcomes. Their importance in education and higher education has become consensual worldwide (Fullan, Quinn & McEachen, 2018 ; Newton & Newton, 2014 ; Lucas & Spencer, 2017 ). The role of education in the development of critical thinking is also increasingly acknowledged within many countries, where a majority of the population believe that schools should help students to become “independent thinkers” rather than passive receivers of transmitted knowledge – or at least recognise the importance of such an objective (Fig. 3.1 ). Developing critical thinking and creativity leads to more independent thinking, which can thus be considered as a good proxy for those skills .
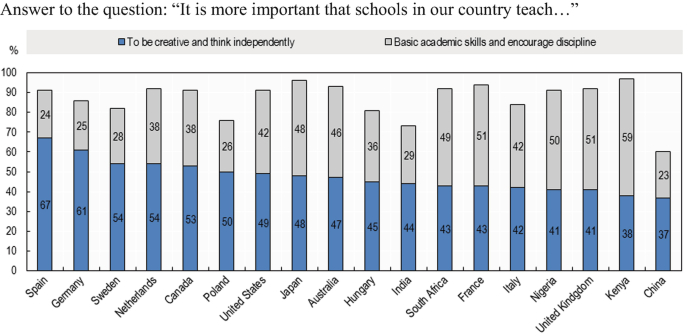
Many societies support the fostering of creativity and critical thinking in education. (Source: Pew Research Centre, Spring 2016 Global Attitudes Survey)
However, even though the importance of creativity and critical thinking is usually well accepted, it remains unclear to teachers what these terms actually mean and entail in education. In order to create a shared professional language on creativity and critical thinking in education, the OECD worked over five years with a network of schools and teachers in 11 countries (Vincent-Lancrin et al., 2019 ). (The countries are: Brazil, France, Hungary, India, the Netherlands, Slovak Republic, Russian Federation, Spain, Thailand, United States, United Kingdom [Wales].)
In addition to the lack of clarity on the definitions of those skills, another difficulty lies in the levels of teacher-friendliness of the language used. To this effect, a portfolio of rubrics was developed to help teachers be more informed, intentional and consistent in their efforts to develop their students’ creativity and critical thinking. A conceptual rubric for science education is part of this portfolio.
3.2.1 Creativity and Critical Thinking
Creativity and critical thinking are two distinct but related higher-order cognitive skills. As such, both require significant mental effort and energy; both are cognitively challenging. Creativity aims to create novel, appropriate ideas and products. Critical thinking aims to carefully evaluate and judge statements, ideas and theories relative to alternative explanations or solutions so as to reach a competent, independent position – possibly for action.
The research on creativity and research on critical thinking actually do not overlap much, even though critical thinking often plays an important role in creativity, and vice versa (see Ellerton & Kelly, Chap. 2 ). School curricula and educational rubrics are however prone to group the two together and to talk about “creative and critical thinking”. In the same spirit, Lucas and Spencer ( 2017 ) include critical thinking (as well as problem solving) under the concept of “creative thinking”.
Sternberg and Lubart ( 1999 ) proposed a simple definition of creativity: “creativity is the ability to produce work that is both novel (i.e., original, unexpected) and appropriate (i.e., useful, adaptive concerning tasks constraints” (p. 3). The use of “appropriate” in this definition reminds us that creativity happens within a system or context with its established standards; it is not just about doing something new. As Dennett ( 2013 ) puts it: “Being creative is not just a matter of casting about for something novel – anybody can do that, since novelty can be found in any random juxtaposition of stuff – but of making the novelty jump out of some system , a system that has become established, for good reason” (p. 45).
Emphasising both process and output, Lubart ( 2000 ) defines creativity as “a sequence of thoughts and actions that leads to novel, adaptive production” (p. 295). What is this sequence? Creativity research has explored the cognitive processes involved in creativity. Guilford ( 1950 ) emphasised two processes leading to creativity: divergent thinking (generating many ideas) and convergent thinking (choosing and developing a good one). Torrance ( 1970 ), distinguished four aspects of the creativity process: fluency (having many relevant ideas), flexibility (having different types of relevant ideas), originality (having statistically novel ideas) and elaboration (being able to elaborate one’s ideas). Most standardised tests of creativity or creative potential (e.g., Torrance, Wallach-Kogan, Guilford, Getzel-Jackson, Mednick, Runco) decompose the creative process along similar lines and focus on some of its aspects.
Critical thinking may be a step in the creative process, or may not: convergent thinking does not necessarily have to be “critical” (Runco, 2009 ). Critical thinking mainly aims at assessing the strength and appropriateness of a statement, theory or idea through a questioning and perspective-taking process – which may in turn result (or not) in a possibly novel statement or theory. Critical thinking need not lead to an original position to a problem: the most conventional one may be the most appropriate. However, it typically involves the examination and evaluation of different possible positions.
In education (including higher education), the theory of critical thinking has been developed by philosophers such as Ennis ( 1996 , 2018 ), Facione ( 1990 ) and McPeck ( 1981 ) (see Davies & Barnett, 2015 , and Hitchcock, 2018 , for overviews of the literature). Hitchcock ( 2018 ) summarises most conceptions by defining critical thinking as “careful goal-directed thinking” – another version of Ennis’ definition: “reasonable reflective thinking focused on deciding what to believe or do” (Ennis, 2018 , p. 165). In many cases, definitions of critical thinking emphasise logical or rational thinking, that is, the ability to reason, assess arguments and evidence, and argue in a sound way to reach a relevant and appropriate solution to a problem. However, critical thinking also includes a dimension of “critique” and “perspective-taking”. In addition to rational or logical thinking, critical thinking thus includes two other dimensions: the recognition of multiple perspectives (and/or the possibility of challenging a given one) and the recognition of the assumptions and limitations of any perspective, even when that perspective appears superior to all other available ones.
Many of the cognitive processes involved in creativity and critical thinking share commonalities. Both require prior knowledge in the domain of application. The sub-skills that need to be deployed for each skill involve imagining, inquiring, doing and reflecting. Creativity puts more emphasis on imagining (brainstorming, generating ideas and alternatives), while critical thinking places more emphasis on “inquiring”, including its more analytical and systematic dimensions (understanding and decomposing the problem, etc.). Critical thinking is primarily inquisitive, a detective way of thinking; creative thinking is more imaginative, an artist way of thinking. However, critical thinking does involve imagining alternative theories, counterfactuals, reasons, and results in an action (making a judgment); creativity does require making judgments and decisions about the alternative ideas generated in the imaginative process, and, more fundamentally, the examination of the assumptions of existing solutions and conventions. In this sense, creativity and critical thinking can be thought of as two ends of a continuum.
Both creativity and critical thinking require a certain level of openness and curiosity. Both may lead to challenges to authority, values or accepted norms; this is what may make them both valuable, and sometimes challenging. Critical thinking requires integrity; creativity requires discipline and judgment. When education is conceived as the mere transmission of socially accepted knowledge, there is little room for either. In fact, like most other skills, creativity and critical thinking only have to be exercised at some points; even if a world in which people would be creative all the time or critical all the time was concretely possible this world would be most dysfunctional. Students also need to learn when and about what they can or should think creatively or critically. In an educational context, both creative and critical thinking necessarily pursue the deeper understanding of knowledge and solutions, and thus deeper learning. Developing creativity and critical thinking is actually a way to improve learning and achievement – whether such thinking leads to the proposing of new knowledge and solutions or not.
Even though one can describe them at the conceptual level in a domain-general way, both creativity and critical thinking in practice are mainly domain-specific: each requires knowledge about a field or context to be practiced, and usually being a strong creative or critical thinker in a particular domain does not imply any transfer of those skills to another domain. The research literature overwhelmingly emphasises the “domain-specificity” of both, even though at the conceptual level each can be described in a domain-general way.
3.2.2 Rubrics to Support Creativity and Critical Thinking in Science Teaching and Learning
There is overall a common understanding among researchers on the key dimensions of creativity and of critical thinking. However, transferring the concepts to a consistent educational application requires further translation. This is where rubrics intervene. Rubrics are a way to simplify, translate and construct social representations of what creativity and critical thinking look like in the teaching and learning process, and so create a shared understanding of what each means in the classroom, and lead to common expectations among teachers, and among teachers and students. The function of rubrics is to simplify and elaborate the complex concepts of creativity and critical thinking so that they become relevant to teachers and learners in their actual educational activities. The rubrics also allow teachers to monitor and formatively assess whether their students develop those skills. Rubrics are a metacognitive tool that helps make learning visible and tangible, and teaching intentional.
Different types of rubrics serve different purposes. “Conceptual rubrics” are those that clarify “what counts” or “what teachers and students should particularly keep in mind”, while “assessment rubrics” articulate levels of progression or proficiency involved in the acquisition of creative and critical thinking skills. Both types were developed in the OECD project from which this chapter draws, here we will focus on only the conceptual rubrics.
The development of rubrics requires balancing between simplicity and complexity. To be useful for teachers and classrooms, rubrics have to be teacher-friendly (and possibly student-friendly), and have a language that is easily understandable by teachers at different school levels. On the one hand, the descriptors of the different key ideas have to relate sufficiently to the concepts as understood by experts in creativity and critical thinking. On the other hand, the descriptors have to be simple enough to be easily understood by teachers and students, and have to relate to skills and activities that are meaningful in school settings. Ideally, one would easily memorise some of the language used in the rubric so that this becomes internalised. Using a language inspired by the “five habits of mind” rubric developed by Lucas, Claxton and Spencer ( 2013 ), and a review of other existing rubrics, the OECD rubrics that are the focus in this chapter tried to capture different dimensions of both creativity and critical thinking through four high level and easily memorable descriptors (dimensions): imagining , enquiring , doing , reflecting . Each of those active words is then associated with some more specific descriptor(s) for creativity and for critical thinking.
Two domain-general conceptual rubrics were developed: a “comprehensive” rubric and “class-friendly” rubric. Domain-specific adaptations of those rubrics were also developed, including for science. Table 3.1 shows the “comprehensive” domain-general rubric, while Table 3.2 presents the “class-friendly” rubric for creativity and critical thinking in science education.
In the case of creativity, the four dimensions in the left hand column of Table 3.1 can be elaborated as follows:
Inquiring . This dimension of the creative cognitive process is close to scientific inquiry. Torrance ( 1966 ) highlights the importance of identifying problems, gaps in knowledge, missing knowledge and elements in the creative process. Because creativity cannot happen without knowledge about the field or problem investigated, looking for information, finding the problem and understanding its different possible dimensions are important aspects of the creative process. These can take different forms, depending on the problem, from feeling and empathising with people to a more objective approach of observing, describing and analysing from different possible perspectives what the issues and problems at stake are. Both curiosity and unconventional connections between different knowledge and problems matter in the creative inquiry process.
Imagining . Imagination refers to the ability to see and play with ideas and things in one’s mind. This ability allows people to get free from conventional reality and to pursue novel ideas and invent new stories, anticipate the future, pursue different scenarios, envision counterfactuals, simulate consequences of different ideas and solutions, etc. In the context of creativity, imagination is about a free and playful generation of ideas, theories and assumptions, with a certain level of intentionality. This can take the form of an independent generation of multiple ideas or association of ideas, either by seeing actual or sometimes metaphorical connections (Mednick, 1962 ; Runco, 2009 ). Being able to push ideas to their limits, or to explore unconventional (or even seemingly absurd) ideas without much actual risk, is one of the cognitive processes that creativity may involve.
Doing . Creativity implies the creation of something novel and appropriate, based on one’s inquiry and imagination. This is typically the convergent or integrative part of the creative process. This output production can take different forms based on the domain: it can be a product, a performance, an idea, a physical or mental model, etc. It implies the selection of some of the ideas that have been imagined and inquired, and thus some level of reflection and audacious decision-making to meet the two main aspects of creativity. While products can be associated with the final stage of the creative process, the creative process can also include some tinkering processes of trial and error, or the development of prototypes and models, and can intervene at different stages of the process.
Reflecting . Finally, intentionality and reflection are key aspects of creativity. Intentionality distinguishes creativity from random novelty, and sometimes from small children’s spontaneity. The level of intentionality and reflection can vary with age, but also with one’s level of creative proficiency. As noted above, reflection also occurs at different stages of the creative process as one decides which ideas to select and how to move forward.
While these different aspects of creativity do not necessarily come in a definite order, or are solicited at different points in the creative process, the four can easily be related to the design thinking method, which codifies the innovation or creativity process and aims to turn it into an art (Kelley, 2001 ; see Kelly and Ellerton, Chap. 2 ). For educational purposes, the d.school at Stanford University summarised the innovation process in five steps that can be looped: empathise, define, ideate, prototype, test. Many of those processes are included in the proposed rubrics.
In the case of critical thinking, in order to have a parallelism with creativity, the underlying cognitive processes or sub-skills can be described under the rubrics’ headings:
Inquiring . Determining and understanding the problem at hand, including its boundaries, is a first important dimension of critical thinking’s inquisitive process. Sometimes this includes wondering about why the problem is posed in a certain way, or examining whether the associated solutions or statements may be based on inaccurate facts or reasoning and identifying the knowledge gaps. This inquiry process partly concerns rational thinking (checking facts, observing, analysing the reasoning), but also includes a more “critical” dimension when it comes to identifying the possible limitations of the solution and challenging some of the underlying assumptions and interpretations, even when facts are accurate. In many cases, inquiring involves acquiring knowledge, verifying knowledge, and examining the components of the problem in detail as well as the problem as a whole.
Imagining . In critical thinking, imagination plays an important role as the mental elaboration of an idea – but any thinking involves some level of imagination. At a higher level, imagining is also about identifying and reviewing alternative, competing world views, theories and assumptions, so as to consider the problem from multiple perspectives. This allows for a better identification of the strengths and weaknesses of proposed evidence, arguments and assumptions, even though this evaluation also belongs to the inquisitive process. Imagination also plays a role in thought experiments, which can be a strong component of any good thinking and also a way to make a point when experimentation is not possible (Dennett, 2013 ).
Doing . The product of critical thinking is one’s position or solution to a problem (or judgment about others’ positions or solutions). This mainly implies careful inference, a balancing act between different ways of looking at the problem, and thus recognition of its (possible) complexities. As in any productive thinking, critical thinking implies the ability to argue and justify one’s position rationally, according to some existing perspectives and socially recognised ways of reasoning, or possibly some new ones.
Reflecting . Finally, even though one may consider one’s position or way of thinking superior to some alternatives, perhaps just because it embraces a wider view or is better supported by existing evidence, critical thinking implies some self-reflective process about the perspective one endorses, its possible limitations and uncertainties, and thus a certain level of humility and openness to other competing ideas. While one does not have to embrace ancient scepticism and suspend one’s judgment in all cases, this may sometimes be the most appropriate position.
The OECD rubrics for creativity and critical thinking were meant to be used by teachers working in real-life settings in different ways: (1) designing and revising lesson plans so that they would give students the opportunity to develop their creativity and critical thinking skills; (2) assessing student work and progression in the acquisition of these skills; (3) generating new aligned rubrics adapted to their local context or self-assessment tools. Field work showed that seven in ten teachers participating in the international network did on average use the OECD rubrics for those purposes. The rubrics have thus proven to be useful and well adopted by teachers in most of the countries in which the project was implemented.
3.2.3 Creativity and Critical Thinking in Science
While science education can be one of the many vehicles to develop students’ creativity and critical thinking in a school context, it is noteworthy that critical thinking and creativity are also at the core of scientific practice. When practiced by expert scientists, science is about creativity and critical thinking.
Scientists usually need to have creative or original ideas to receive grants and get published in scientific journals. Scientific awards (such as the Nobel Prizes) typically celebrate advances that bring some ideas or techniques that are “new to the world” (and in this sense, creative in the full meaning of the word). One aspect of scientific practice that is usually somewhat downplayed is “imagination”. It is nevertheless a key aspect of science as Nobel Prize winner and famous physicist Feynman ( 1963 ) noted:
Experiment is the sole judge of scientific “truth.” But what is the source of knowledge? Where do the laws that are to be tested come from? Experiment, itself, helps to produce these laws, in the sense that it gives us hints. But also needed is imagination to create from these hints the great generalizations—to guess at the wonderful, simple, but very strange patterns beneath them all, and then to experiment to check again whether we have made the right guess. This imagining process is so difficult that there is a division of labor in physics: there are theoretical physicists who imagine, deduce, and guess at new laws, but do not experiment; and then there are experimental physicists who experiment, imagine, deduce, and guess. (p. 1)
As for critical thinking, it is in fact at the heart of scientific progress – and one of the prerequisites of science. Science’s very core value is doubt, the possibility to question what authorities (including teachers and scientists) say. There is no science without a certain level of scepticism, as Feynman ( 1955 ) forcefully noted:
The scientist has a lot of experience with ignorance and doubt and uncertainty, and this experience is of very great importance [...] We have found it of paramount importance that in order to progress we must recognize our ignorance and leave room for doubt. Scientific knowledge is a body of statements of varying degrees of certainty - some most unsure, some nearly sure, but none absolutely certain. […] Our freedom to doubt was born out of a struggle against authority in the early days of science. It was a very deep and strong struggle: permit us to question - to doubt - to not be sure. I think that it is important that we do not forget this struggle and thus perhaps lose what we have gained. Herein lies a responsibility to society. […] It is our responsibility as scientists… to teach how doubt is not to be feared but welcomed and discussed; and to demand this freedom as our duty to all coming generations. (pp. 245–247)
Teaching and learning creativity and critical thinking in science education in schools is thus one way to “think like a scientist” and understand the values of science, even if, as for the technical skills in science (that is, the mastery of content and procedural knowledge), students are not necessarily expected to be as proficient as expert scientists – not to mention the most celebrated ones.
3.3 Creativity and Critical Thinking in Action in Science Education
Depending on the subject of the lesson and the learning outcomes they want to achieve, using a conceptual rubric while designing a lesson helps teachers to build in some assignments or tasks giving students the opportunity to develop at least some of the sub-skills of creativity or critical thinking. Some lessons may aim to develop just a few sub-skills, while others could cover the full range, with an emphasis on either creativity or critical thinking (or both). Existing lessons could be modified according to the same process, just adding one opportunity to develop a sub-skill here and another there through small changes to the lesson or its pedagogical delivery.
The conceptual rubrics also represent a key element of a quality assurance method: after decomposing their lessons or entire course into steps, teachers can identify when students were given the possibility or were requested to practice some of the skills identified in the rubric. Examples of lesson plans developed during the lessons/ course can thus include a mapping of the different steps of the lesson against the sub-skills of the conceptual rubrics.
Teams working on redesigning their science education courses implemented the OECD project that is the focus of this chapter in different ways. Two “signature pedagogies” were used by some of the teams (project-based and research-based learning), while most others just designed short projects or activities or improved more traditional lesson plans.
One example of lesson plans in science education grounded in project-based learning, and included in the OECD examples of courses, is now presented to give tangible ideas of how critical thinking and creativity can be developed in science education while also teaching technical skills of science (declarative and procedural knowledge).
3.3.1 What Controls My Health?
Developed by Adler et al. ( 2017 ), “What controls my health?” is a 20-lesson course engaging students in investigations to understand the importance of both genetic and environmental factors in their risk for disease. Students start the unit by experiencing the phenomenon of Type 2 diabetes through the eyes of a peer recently diagnosed with the disease. They develop an initial model to answer the driving question of the whole project: “What caused Monique’s diabetes?” The driving question is particularly relevant to the students for whom it was designed and who live in Detroit, a city which is predominantly African-American and where most students are likely to have relatives suffering from diabetes.
Throughout the unit, students learn that diabetes, like many common diseases, is caused by a combination of both genetic and environmental factors. They also investigate how lifestyle options for healthy foods and exercise help prevent or reduce Type 2 diabetes. One lesson includes several opportunities for students to construct, test, revise and share their models to explain the investigated phenomena, while performing experiments and using computer simulations. For their final assignment, students conduct an action research project, based on their scientific and technological knowledge and understanding, which aims to improve the health of their school or neighbourhood to help prevent or reduce diabetes.
A summary description of the course is now presented (a more elaborated outline is publicly available at Adler et al., 2017 ):
Periods 1 – 2: Why does Monique have diabetes? Students learn about Type 1 and Type 2 diabetes (video). They develop an initial model that explains a health phenomenon of their choice.
Periods 3–5 : How can we describe Monique’s diabetes? Students learn more (through reading), and share information about the cause, symptoms and treatment of both Type 1 and Type 2 diabetes. They perform a glucose tolerance test by analysing simulated blood plasma samples to determine if the person has Type 1 or Type 2 diabetes. They learn about the heart, as an example of an organ which may be affected by diabetes. They revisit the Driving Question Board Footnote 1 and reflect upon their learning. They revise their models and add the biological aspect of diabetes to their model.
Periods 6–9: How does Monique’s family affect her diabetes? Students examine pictures of a family to identify some genetic factors of characteristics that might be inherited. They collect data on tongue rolling and arm span, and use these data to explore the population variation of the inheritance patterns of single and multi-factorial genes. They use beads to simulate the inheritance of risk factors for diabetes. They identify the risk of diabetes in offspring based on the number and type of risk factors inherited during the simulation. They revisit the Driving Question Board and reflect upon their learning. They revise their models and add the effect of genetic factors on Monique’s diabetes.
Periods 10–12: How does where Monique lives and what she does affect her diabetes? Students study the influence of environment on living organisms through plant growth.
Periods 13–16: How do Monique’s characteristics and environment affect her diabetes? Through simulation, students consider how genetics and environment affect the health of sand rats.
Periods 17–18: What can Monique do to make her environment healthier? Students study the role of nutrition.
Periods 19–20: Community action projects: How can we work together to make our environment healthier? Students develop and choose their inquiry question, design and develop their research tools, then plan and carry out their investigations. They analyse the data and draw conclusions, share their findings with their peers and broader community, suggest solutions and potential actions based on their findings.
This sequence is a good example of how teachers could allow their students to learn about science technical skills while giving them opportunities to also develop their creativity and critical thinking (as well as some social and behavioural skills). In terms of technical skills, that is, the mastery of content and procedural scientific knowledge, students clearly learn about: diabetes; the heart as an organ; the growth of plants; genetics, the influence of environmental factors; nutrition; the multiple drivers of health; making tests and experiments, including through computer simulation, and interpreting them.
The main focus of the “What controls my health?” course is actually critical thinking as students: identify and question their assumptions or accepted ideas about diabetes and its causes (steps 1 and 7 above); consider several perspectives on the problem at hand (steps 3 to 6); explain both the strengths and limitations of their scientific solution (steps 6 and 7); and consistently reflect on the chosen scientific approaches that they consider relative to possible alternatives (steps 2, 3, 4 and 7).
The lessons also allow students to develop some creativity skills as they: are induced to make connections to other scientific concepts or ideas throughout the project and to use remote examples to better understand (heart, plants) (steps 2 and 5); generate and play with unusual ideas as they revisit the questions of the Driving Question Board and have to generate their own solution (steps 1, 4, 7); propose how to solve a scientific problem in a personally novel way (steps 1 and 7); and reflect on those steps at the end of the process (step 7).
3.3.2 Evaporative Cooling
Another 10-lesson science (chemistry) unit was developed as part of a US-Finland project on problem-based learning in science, showing how to craft optimal learning moments in science learning environments (Schneider et al., 2020 ). The unit was also contributed to the OECD bank of pedagogical resources and is called “Evaporative cooling” (Paddock et al., 2019 ). It engages students in investigating the following driving question: “when I am sitting by the pool, why do I feel colder when I am wet than when I am dry?”
Students learn about intermolecular forces and energy transfer between molecules during phase changes of matter. Students start by experiencing the phenomenon of evaporation and cooling of different liquids. Later activities include experiments to measure temperature and mass changes when liquids evaporate, and, using several computer-based simulations, to explore energy transfer, forces, and interactions between molecules in different phases. Throughout, and under the guidance and with the scaffolding of their teacher, students continuously build, use, evaluate, and revise their own computational and hand-drawn models to answer the driving question.
A summary description of the course is now presented (a more elaborated outline is publicly available at Paddock et al., 2019 ):
Lesson 1: Why does having wet skin makes you feel cooler? Students are introduced to the Driving Question, and construct and draw their initial model of the phenomenon in pairs so that they access their prior knowledge about this phenomenon. The purpose for the teacher is for formative assessment to inform planning for lesson 2. Student pairs explain their model to another pair in turns so that they can share ideas and begin working toward some consensus in understanding.
Lesson 2: Does evaporation depend on coverage? Students go to two stations that are designed to test the rate of evaporation of acetone, water, and ethanol and the temperature change during the process. Students observe temperature change across time while the liquid is covered and while uncovered. Students observe mass change across time (covered and uncovered), collect data and graph these. Following the activity, students answer questions to guide their noticing of patterns.
Lessons 3–4: Why does having wet skin makes you feel cooler? (a specific return to the driving question) Students review their drawn models from lesson 1 and learn how to use a new modelling tool, SageModeler (freely available at https://learn.concord.org/building-models ). They create an initial model of their experimental results using the modelling tool. Students use a computer simulation to compare properties of the states of matter. Properties include: spacing of molecules (which connects with potential energy) and kinetic energy.
Lesson 5: How does thermal energy work? Students learn that thermal energy can be transferred during phase changes. They develop a model to show how a system gains or loses thermal energy.
Lesson 6: Why does having wet skin makes you feel cooler? (a further deliberate return to the driving question) Students reflect on their drawn models. They review the learning from Lesson 5 and incorporate ideas from the lesson into their SageModeler model, and share their models to receive feedback.
Lessons 7–8: Do matter viscosity and intermolecular forces play a role? Students compare viscosity of water to acetone by observing how each chemical spreads on two different surfaces (a coin and wax paper). They investigate how the strength of the intermolecular forces in a substance effect the state of matter of a substance at a certain temperature using a computer simulation.
Lessons 9–10: Why does having wet skin makes you feel cooler? (a final return to the driving question) Students revisit their model, and, in pairs again, create a final draft of the model and evaluate this using a provided rubric. Students assess their peers’ models with the rubric, and make a final revision of their own model. They present their final models and explanations to the whole class and may take a unit test. Students then perform a final assessment of the unit to reflect on what has been learned.
Here, again, there are clear technical skills to be acquired, both in terms of scientific content and procedural knowledge. In terms of content knowledge, students learn about the structure of matter, the behaviour of particles, intermolecular forces, the position and arrangement of atoms, and about relationships between molecular motion, the position of molecules, and kinetic and potential energy. The model they build and revise seeks to identify a pattern between the structure of particles and the behaviour (evaporation and temperature) of particles. In terms of procedural knowledge, students learn to model a phenomenon, to experiment, to analyse data, and to revise their model.
Although the sequence of lessons is essentially science (chemistry), it also gives students more opportunities to develop their creativity - and this more than their critical thinking. Or, to put it another way, the sequence does not dwell on identifying and questioning assumptions and generally accepted ideas of a scientific explanation or approach to a problem. The lesson does not offer students as many different perspectives as the previous example sequence (“What controls my health?”), as that was organised to show how different branches of science and technology explain different interacting parts of the puzzle. Nevertheless, “evaporative cooling” does seek to help students understand that several aspects of matter have to be factored in to understand and explain the phenomenon.
Students exercise their scientific creativity by repeatedly imagining how the phenomenon under study can be explained, generating and playing with different ideas that are unusual and new to them, making connections with their life but also with other knowledge they have. They are given opportunities to put forward an explanation of this scientific problem in a personally novel way at different stages of the unit (lessons 3, 5 and 8). They have to imagine from the outset how the phenomenon could be explained, making connections with their own and new experiences of evaporation, and as they look for and are given new information and knowledge, they continue to play with new ideas and imagine new solutions that they revise throughout the unit. Whether they have room to play with unusual or radical ideas depends on the teacher, who could very well encourage them to go in that direction to possibly prove them wrong or help them understand what a scientific (falsifiable) statement looks like.
In the Evaporative cooling lessons students practice some of their critical thinking skills by considering several perspectives on the driving question, gradually enriching and adding new concepts within the theoretical frame in which they operate. They have considerable room throughout the lessons to reflect individually and collectively on the strengths and limitations of the successive models they elaborate, identifying gaps and looking for alternative models.
The lessons shows that, even when a unit is framed around one main “theory” or “knowledge” to learn, there is room for students to have some level of agency, to imagine possible solutions, inquire about them, craft experiments and models to test their ideas, and reflect on them.
3.3.3 Design Criteria for Good Lessons
While the conceptual rubrics presented above can support teachers to review their curriculum units and plan lessons that give students opportunities to develop the sub-skills identified by the rubrics, they do not provide guidance on all key dimensions of the pedagogical work. In fact, while creativity and critical thinking can be nurtured in any domain, within and outside of science (or other apsects of STEM), these do require giving students certain types of tasks and problems. A set of “design criteria” was thus developed by Vincent-Lancrin et al. ( 2019 ) to support teachers further, building on learning science principles, including motivation, cognitive activation, self-regulation and opportunities for formative assessment (see Table 3.3 ). These design criteria for good lesson plans represent another set of quality checks and new perspectives on how to approach pedagogical redesign to foster students’ creativity and critical thinking.
The “design criteria” highlight that tasks to develop and then demonstrate creativity and critical thinking skills in education share some general features: they seek to engage students, they may have a deliberately open nature, and they encourage students to explore multiple solutions to problems within parameters and constraints that clarify goals yet remain relatively flexible to allow students to address them with a certain level of agency.
The successful teaching of creativity and critical thinking also hinges critically on teachers’ attitude and in their ability to create learning environments where students feel safe to take risks in their thinking and expressions. This in turn presupposes a positive attitude towards mistakes and learner empowerment. A positive attitude among teachers towards student “mistakes” or “failure” can take the form of using these to trigger reflection about opportunities for learning, thus helping students to see misunderstandings and other matters too often labelled ‘failures’ as a chance for improvement (see Mansfield & Gunstone, Chap. 9 ). Choosing questions and tasks that teachers themselves cannot resolve can make it clear to students that the thinking process behind a problem can be as important as its answer. This is typically the role of the Driving Question Board in project-based learning (Schneider et al., 2020 ), something that demands a positive teacher attitude towards students’ questions, and also students’ explanations.
3.4 Concluding Remarks
To foster their students’ creative and critical thinking skills in science education, teachers have to be intentional – and thus clear about what creativity and critical thinking mean in an educational setting, what subskills they should have their students practice, and what they should observe and monitor in the classroom. This clarity could typically be provided by the use of rubrics on creativity and critical thinking, both to create a more accessible and better understanding of what creativity and critical thinking entail and to ensure that students have opportunities to practice these higher order skills during their class work.
Exemplars of lesson plans or curriculum units should typically supplement those rubrics and illustrate how to equip students with creativity and critical thinking skills while teaching and learning traditional science education subjects. It is noteworthy that resources such as rubrics and examples of lesson plans are just a second-best but a much cheaper and much more widely available option than direct professional development of teachers can ever be.
While science and STEM education can provide tasks that would allow students to develop both their critical thinking and their creativity, the consistent acquisition of creative and critical thinking skills must be reinforced by other disciplines as well. There are two main reasons for this. The first is time. It takes practice to develop any skill, and it is possible that science lessons in school cannot provide enough occasions (hours) for students to practice the creativity and critical thinking skills that have been highlighted in this chapter. Significant reinforcement and development of those skills implies that they are experienced in multiple subject areas. A second fundamental reason lies in the domain-specificity of creativity and of critical thinking. Even though creativity and critical thinking can be discussed in a general way at the conceptual level, as if the domain of their application did not matter, in practice the fact that each requires knowledge and some level of expertise in a particular field means that they have to be practiced over and over in different fields. If they were domain-general, one could teach them in special creativity or critical thinking classes, or, for example, one could have the visual arts teacher be in charge of creativity, and the science or philosophy teacher be in charge of critical thinking.
But this is not the case. Creativity and critical thinking need to become a key objective of all subjects taught in schools, something that is reflected in the general perspectives across all subject areas in many Twenty-first Century school curricula. These general curriculum perspectives are commonly intended to allow students to develop some habits of mind, but the realisation of such intentions does require a mainstreaming of those learning objectives in all subject areas of education. While science teachers might feel that they are somewhat in charge of critical thinking, as science often challenges common wisdom, they could still emphasise more the remaining uncertainty of scientific “truths” (Rennie, 2020 ), even though the methods through which such “truths” are established are robust. Even more so, teachers should keep in mind that science requires creativity and imagination, as what may currently appear as the most obvious and conventional scientific statement was initially created by a very imaginative scientist.
Many project-based science units/ courses initially develop “Driving Questions” to contextualise the unit and give learners opportunities to connect the unit to their own experiences and prior ideas.
Adler, L., Bayer, I., Peek-Brown, D., Lee, J., & Krajcik, J. (2017). What controls my health . https://www.oecd.org/education/What-Controls-My-Health.pdf
Davies, M. (2015). In R. Barnett (Ed.), The Palgrave handbook of critical thinking in higher education . Palgrave Macmillan.
Chapter Google Scholar
Dennett, D. C. (2013). Intuition pumps and other tools for thinking . England: Penguin.
Google Scholar
Ennis, R. (1996). Critical thinking . Upper Saddle River, NJ: Prentice-Hall.
Ennis, R. (2018). Critical thinking across the curriculum: A vision. Topoi, 37 (1), 165–184. https://doi.org/10.1007/s11245-016-9401-4 .
Article Google Scholar
Facione, P.A. (1990). Critical thinking: A statement of expert consensus for purposes of educational assessment and instruction . Research findings and recommendations prepared for the Committee on Pre-College Philosophy of the American Philosophical Association. Retrieved from http://www.eric.ed.gov/ERICWebPortal/detail?accno=ED315423
Feynman, R. (1963). The Feynman lectures on physics . (Volume I: The New Millennium Edition: Mainly Mechanics, Radiation, and Heat.). Basic Books.
Feynman, R. (1955). The value of science. In R. Leighton (Ed.), What do you care what other people think? Further adventures of a curious character (pp. 240–257). Penguin Books.
Fullan, M., Quinn, J., & McEachen, J. (2018). Deep learning: Engage the world, change the world . Corwin Press and Ontario Principals’ Council.
Guilford, J. P. (1950). Creativity. American Psychologist, 5 (9), 444–454. https://doi.org/10.1037/h0063487 .
Hitchcock, D. (2018). Critical thinking. In Zalta, E.N. (ed.), The Stanford encyclopedia of philosophy (Fall 2018 Edition). Retrieved from : https://plato.stanford.edu/archives/fall2018/entries/critical-thinking .
Kelley, T. (2001). The art of innovation: Lessons in creativity from IDEO . Currency: America’s leading design firm.
Lubart, T. (2000). Models of the creative process: Past, present and future. Creativity Research Journal, 13 (3–4), 295–308. https://doi.org/10.1207/S15326934CRJ1334_07 .
Lucas, B., Claxton, G., & Spencer, E. (2013). Progression in student creativity in school: First steps towards new forms of formative assessments. In OECD education working papers, 86 . Paris: OECD. https://doi.org/10.1787/5k4dp59msdwk-en .
Lucas, B., & Spencer, E. (2017). Teaching creative thinking: Developing learners who generate ideas and can think critically . England: Crown House Publishing.
McPeck, J. E. (1981). Critical thinking and education . New York: St. Martin’s.
Mednick, S. A. (1962). The associative basis of the creative process. Psychological Review, 69 (3), 220–232. https://doi.org/10.1037/h0048850 .
Newton, L. D., & Newton, D. P. (2014). Creativity in 21st century education. Prospects, 44 (4), 575–589. https://doi.org/10.1007/s11125-014-9322-1 .
Paddock, W., Erwin, S., Bielik, T., & Krajcik, J. (2019). Evaporative cooling . Retrieved from : https://www.oecd.org/education/Evaporative-Cooling.pdf
Rennie, L. (2020). Communicating certainty and uncertainty in science in out-of-school contexts. In D. Corrigan, C. Buntting, A. Jones, & A. Fitzgerald (Eds.), Values in science education: The shifting sands (pp. 7–30). Cham, Switzerland: Springer.
Runco, M. A. (2009). Critical thinking. In M. A. Runco & S. R. Pritzker (Eds.), Encyclopedia of creativity (pp. 449–452) . Academic.
Schneider, B., Krajcik, J., Lavonen, J., & Samela-Aro, K. (2020). Learning science: The value of crafting engagement in science environments . United States: Yale University.
Book Google Scholar
Sternberg, R. J., & Lubart, T. (1999). The concept of creativity: Prospects and paradigm. In R. J. Sternberg (Ed.), Handbook of creativity (pp. 3–14). England: Cambridge University.
Torrance, E. P. (1966). Torrance tests of creative thinking: Norms. Technical manual research edition; Verbal Tests, Forms A and B, Figural Tests, Forms A and B . Princeton, NJ: Personnel.
Torrance, E. P. (1970). Encouraging creativity in the classroom . United States: W.C. Brown.
Vincent-Lancrin, S., González-Sancho, C., Bouckaert, M., de Luca, F., Fernández-Barrerra, M., Jacotin, G., Urgel, J., & Vidal, Q. (2019). Fostering students’ creativity and critical thinking in education: What it means in school . Paris: OECD. https://doi.org/10.1787/62212c37-en .
Download references
Author information
Authors and affiliations.
Directorate for Education and Skills, Organisation for Economic Co-operation and Development, Paris, France
Stéphan Vincent-Lancrin
You can also search for this author in PubMed Google Scholar
Corresponding author
Correspondence to Stéphan Vincent-Lancrin .
Editor information
Editors and affiliations.
Monash University, Clayton, VIC, Australia
Amanda Berry
University of Waikato, Hamilton, New Zealand
Cathy Buntting
Deborah Corrigan
Richard Gunstone
Alister Jones
Rights and permissions
Reprints and permissions
Copyright information
© 2021 The Author(s), under exclusive license to Springer Nature Switzerland AG
About this chapter
Cite this chapter.
Vincent-Lancrin, S. (2021). Fostering Students’ Creativity and Critical Thinking in Science Education. In: Berry, A., Buntting, C., Corrigan, D., Gunstone, R., Jones, A. (eds) Education in the 21st Century. Springer, Cham. https://doi.org/10.1007/978-3-030-85300-6_3
Download citation
DOI : https://doi.org/10.1007/978-3-030-85300-6_3
Published : 31 January 2022
Publisher Name : Springer, Cham
Print ISBN : 978-3-030-85299-3
Online ISBN : 978-3-030-85300-6
eBook Packages : Education Education (R0)
Share this chapter
Anyone you share the following link with will be able to read this content:
Sorry, a shareable link is not currently available for this article.
Provided by the Springer Nature SharedIt content-sharing initiative
- Publish with us
Policies and ethics
- Find a journal
- Track your research
Educationise
11 Activities That Promote Critical Thinking In The Class
52 Critical Thinking Flashcards for Problem Solving
Critical thinking activities encourage individuals to analyze, evaluate, and synthesize information to develop informed opinions and make reasoned decisions. Engaging in such exercises cultivates intellectual agility, fostering a deeper understanding of complex issues and honing problem-solving skills for navigating an increasingly intricate world. Through critical thinking, individuals empower themselves to challenge assumptions, uncover biases, and constructively contribute to discourse, thereby enriching both personal growth and societal progress.
Critical thinking serves as the cornerstone of effective problem-solving, enabling individuals to dissect challenges, explore diverse perspectives, and devise innovative solutions grounded in logic and evidence. For engaging problem solving activities, read our article problem solving activities that enhance student’s interest.
What is Critical Thinking?
Critical thinking is a 21st-century skill that enables a person to think rationally and logically in order to reach a plausible conclusion. A critical thinker assesses facts and figures and data objectively and determines what to believe and what not to believe. Critical thinking skills empower a person to decipher complex problems and make impartial and better decisions based on effective information.
More Articles from Educationise
- 10 Innovative Strategies for Promoting Critical Thinking in the Classroom
- How to Foster Critical Thinking Skills in Students? Creative Strategies and Real-World Examples
- 9 Must-Have AI Tools for Teachers to Create Interactive Learning Materials
- The Future of Education: 8 Predictions for the Next Decade
- The Latest in EdTech: 5 Innovative Tools and Technologies for the Classroom
- 8 Free Math Problem Solving Websites and Applications
Critical thinking skills cultivate habits of mind such as strategic thinking, skepticism, discerning fallacy from the facts, asking good questions and probing deep into the issues to find the truth.
Importance of Acquiring Critical Thinking Skills
Acquiring critical thinking skills was never as valuable as it is today because of the prevalence of the modern knowledge economy. Today, information and technology are the driving forces behind the global economy. To keep pace with ever-changing technology and new inventions, one has to be flexible enough to embrace changes swiftly.
Read our article: How to Foster Critical Thinking Skills in Students? Creative Strategies and Real-World Examples
Today critical thinking skills are one of the most sought-after skills by the companies. In fact, critical thinking skills are paramount not only for active learning and academic achievement but also for the professional career of the students. The lack of critical thinking skills catalyzes memorization of the topics without a deeper insight, egocentrism, closed-mindedness, reduced student interest in the classroom and not being able to make timely and better decisions.
Benefits of Critical Thinking Skills in Education
Certain strategies are more eloquent than others in teaching students how to think critically. Encouraging critical thinking in the class is indispensable for the learning and growth of the students. In this way, we can raise a generation of innovators and thinkers rather than followers. Some of the benefits offered by thinking critically in the classroom are given below:
- It allows a student to decipher problems and think through the situations in a disciplined and systematic manner
- Through a critical thinking ability, a student can comprehend the logical correlation between distinct ideas
- The student is able to rethink and re-justify his beliefs and ideas based on facts and figures
- Critical thinking skills make the students curious about things around them
- A student who is a critical thinker is creative and always strives to come up with out of the box solutions to intricate problems
- Critical thinking skills assist in the enhanced student learning experience in the classroom and prepares the students for lifelong learning and success
- The critical thinking process is the foundation of new discoveries and inventions in the world of science and technology
- The ability to think critically allows the students to think intellectually and enhances their presentation skills, hence they can convey their ideas and thoughts in a logical and convincing manner
- Critical thinking skills make students a terrific communicator because they have logical reasons behind their ideas
Critical Thinking Lessons and Activities
11 Activities that Promote Critical Thinking in the Class
We have compiled a list of 11 activities that will facilitate you to promote critical thinking abilities in the students. We have also covered problem solving activities that enhance student’s interest in our another article. Click here to read it.
1. Worst Case Scenario
Divide students into teams and introduce each team with a hypothetical challenging scenario. Allocate minimum resources and time to each team and ask them to reach a viable conclusion using those resources. The scenarios can include situations like stranded on an island or stuck in a forest. Students will come up with creative solutions to come out from the imaginary problematic situation they are encountering. Besides encouraging students to think critically, this activity will enhance teamwork, communication and problem-solving skills of the students.
Read our article: 10 Innovative Strategies for Promoting Critical Thinking in the Classroom
2. If You Build It
It is a very flexible game that allows students to think creatively. To start this activity, divide students into groups. Give each group a limited amount of resources such as pipe cleaners, blocks, and marshmallows etc. Every group is supposed to use these resources and construct a certain item such as building, tower or a bridge in a limited time. You can use a variety of materials in the classroom to challenge the students. This activity is helpful in promoting teamwork and creative skills among the students.
It is also one of the classics which can be used in the classroom to encourage critical thinking. Print pictures of objects, animals or concepts and start by telling a unique story about the printed picture. The next student is supposed to continue the story and pass the picture to the other student and so on.
4. Keeping it Real
In this activity, you can ask students to identify a real-world problem in their schools, community or city. After the problem is recognized, students should work in teams to come up with the best possible outcome of that problem.
5. Save the Egg
Make groups of three or four in the class. Ask them to drop an egg from a certain height and think of creative ideas to save the egg from breaking. Students can come up with diverse ideas to conserve the egg like a soft-landing material or any other device. Remember that this activity can get chaotic, so select the area in the school that can be cleaned easily afterward and where there are no chances of damaging the school property.
6. Start a Debate
In this activity, the teacher can act as a facilitator and spark an interesting conversation in the class on any given topic. Give a small introductory speech on an open-ended topic. The topic can be related to current affairs, technological development or a new discovery in the field of science. Encourage students to participate in the debate by expressing their views and ideas on the topic. Conclude the debate with a viable solution or fresh ideas generated during the activity through brainstorming.
7. Create and Invent
This project-based learning activity is best for teaching in the engineering class. Divide students into groups. Present a problem to the students and ask them to build a model or simulate a product using computer animations or graphics that will solve the problem. After students are done with building models, each group is supposed to explain their proposed product to the rest of the class. The primary objective of this activity is to promote creative thinking and problem-solving skills among the students.
8. Select from Alternatives
This activity can be used in computer science, engineering or any of the STEM (Science, Technology, Engineering, Mathematics) classes. Introduce a variety of alternatives such as different formulas for solving the same problem, different computer codes, product designs or distinct explanations of the same topic.
Form groups in the class and ask them to select the best alternative. Each group will then explain its chosen alternative to the rest of the class with reasonable justification of its preference. During the process, the rest of the class can participate by asking questions from the group. This activity is very helpful in nurturing logical thinking and analytical skills among the students.
9. Reading and Critiquing
Present an article from a journal related to any topic that you are teaching. Ask the students to read the article critically and evaluate strengths and weaknesses in the article. Students can write about what they think about the article, any misleading statement or biases of the author and critique it by using their own judgments.
In this way, students can challenge the fallacies and rationality of judgments in the article. Hence, they can use their own thinking to come up with novel ideas pertaining to the topic.
10. Think Pair Share
In this activity, students will come up with their own questions. Make pairs or groups in the class and ask the students to discuss the questions together. The activity will be useful if the teacher gives students a topic on which the question should be based.
For example, if the teacher is teaching biology, the questions of the students can be based on reverse osmosis, human heart, respiratory system and so on. This activity drives student engagement and supports higher-order thinking skills among students.
11. Big Paper – Silent Conversation
Silence is a great way to slow down thinking and promote deep reflection on any subject. Present a driving question to the students and divide them into groups. The students will discuss the question with their teammates and brainstorm their ideas on a big paper. After reflection and discussion, students can write their findings in silence. This is a great learning activity for students who are introverts and love to ruminate silently rather than thinking aloud.
Read our next article: 10 Innovative Strategies for Promoting Critical Thinking in the Classroom
Share this:
4 thoughts on “ 11 activities that promote critical thinking in the class ”.
- Pingback: What is Growth Mindset? 50+ Motivational Quotes on Growth Mindset - Educationise
- Pingback: 6 Steps To Implement Project-Based Learning In The Classroom - Educationise
- Pingback: Engaging Problem-Solving Activities That Spark Student Interest - Educationise
Thanks for the great article! Especially with the post-pandemic learning gap, these critical thinking skills are essential! It’s also important to teach them a growth mindset. If you are interested in that, please check out The Teachers’ Blog!
Leave a Reply Cancel reply
Discover more from educationise.
Subscribe now to keep reading and get access to the full archive.
Type your email…
Continue reading
Your browser is not supported
Sorry but it looks as if your browser is out of date. To get the best experience using our site we recommend that you upgrade or switch browsers.
Find a solution
- Skip to main content
- Skip to navigation
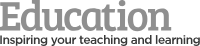
- Back to parent navigation item
- Collections
- Sustainability in chemistry
- Simple rules
- Teacher well-being hub
- Women in chemistry
- Global science
- Escape room activities
- Decolonising chemistry teaching
- Teaching science skills
- Post-lockdown teaching support
- Get the print issue
- RSC Education
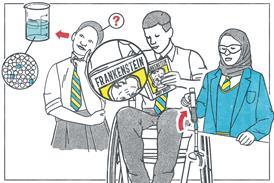
- More from navigation items
Critical thinking in the lab (and beyond)

- No comments
How to alter existing activities to foster scientific skills
Although many of us associate chemistry education with the laboratory, there remains a lack of evidence that correlates student learning with practical work. It is vital we continue to improve our understanding of how students learn from practical work, and we should devise methods that maximise the benefits. Jon-Marc Rodriguez and Marcy Towns, researchers at Purdue University, US, recently outlined an approach to modify existing practical activities to promote critical thinking in students, supporting enhanced learning. [1]
Although many of us associate chemistry education with the laboratory, there remains a lack of evidence that correlates student learning with practical work. It is vital we continue to improve our understanding of how students learn from practical work, and we should devise methods that maximise the benefits. Jon-Marc Rodriguez and Marcy Towns, researchers at Purdue University, US, recently outlined an approach to modify existing practical activities to promote critical thinking in students , supporting enhanced learning.
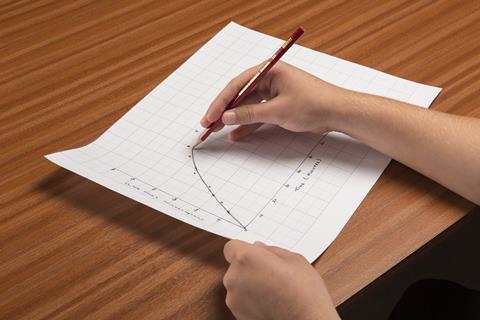
Source: © Science Photo Library
After an experiment, rather than asking a question, task students with plotting a graph; it’ll induce critical thinking and engagement with science practices
Jon-Marc and Marcy focused on critical thinking as a skill needed for successful engagement with the eight ‘science practices’. These practices come from a 2012 framework for science education published by the US National Research Council. The eight practices are: asking questions; developing and using models; planning and carrying out investigations; analysing and interpreting data; using mathematics and computational thinking; constructing explanations; engaging in argument from evidence; and obtaining, evaluating and communicating information. Such skills are widely viewed as integral to an effective chemistry programme. Practising scientists use multiple tools simultaneously when addressing a question, and well-designed practical activities that give students the opportunity to engage with numerous science practices will promote students’ scientific development.
The Purdue researchers chose to examine a traditional laboratory experiment on acid-base titrations because of its ubiquity in chemistry teaching. They characterised the pre- and post-lab questions associated with this experiment in terms of their alignment with the eight science practices. They found only two of ten pre- and post-lab questions elicited engagement with science practices, demonstrating the limitations of the traditional approach. Notably, the pre-lab questions included numerous calculations that were not considered to promote science practices-engagement. Students could answer the calculations algorithmically, with no consideration of the significance of their answer.
Next, Jon-Marc and Marcy modified the experiment and rewrote the pre- and post-lab questions in order to foster engagement with the science practices. They drew on recent research that recommends minimising the amount of information given to students and developing a general understanding of the underlying theory. [2] The modified set of questions were fewer, with a greater emphasis on conceptual understanding. They questioned aspects such as the suitability of the method and the central question behind the experiment. Questions were more open and introduced greater scope for developing critical thinking.
Next, Jon-Marc and Marcy modified the experiment and rewrote the pre- and post-lab questions in order to foster engagement with the science practices. They drew on recent research that recommends minimising the amount of information given to students and developing a general understanding of the underlying theory. The modified set of questions were fewer, with a greater emphasis on conceptual understanding. They questioned aspects such as the suitability of the method and the central question behind the experiment. Questions were more open and introduced greater scope for developing critical thinking.
In taking an existing protocol and reframing it in terms of science practices, the authors demonstrate an approach instructors can use to adapt their existing activities to promote critical thinking. Using this approach, instructors do not have to spend excessive time creating new activities. Additionally, instructors will have the opportunity to research the impact of their approach on student learning in the teaching laboratory.
Teaching tips
Question phrasing and the steps students should go through to get an answer are instrumental in inducing critical thinking and engagement with science practices. As noted above, simple calculation-based questions do not prompt students to consider the significance of the values calculated. Questions should:
- refer to an event, observation or phenomenon;
- ask students to perform a calculation or demonstrate a relationship between variables;
- ask students to provide a consequence or interpretation (not a restatement) in some form (eg a diagram or graph) based on their results, in the context of the event, observation or phenomenon.
This is more straightforward than it might first seem. The example question Jon-Marc and Marcy give requires students to calculate percentage errors for two titration techniques before discussing the relative accuracy of the methods. Students have to use their data to explain which method was more accurate, prompting a much higher level of engagement than a simple calculation.
As pre-lab preparation, ask students to consider an experimental procedure and then explain in a couple of sentences what methods are going to be used and the rationale for their use. As part of their pre-lab, the Purdue University research team asked students to devise a scientific (‘research’) question that could be answered using the data collected. They then asked students to evaluate and modify their own questions as part of the post-lab, supporting the development of investigative skills. It would be straightforward to incorporate this approach into any practical activity.
Finally, ask students to evaluate a mock response from another student about an aspect of the theory (eg ‘acids react with bases because acids like to donate protons and bases like to accept them’). This elicits critical thinking that can engage every student, with scope to stretch the more able.
These approaches can help students develop a more sophisticated view of chemistry and the higher order skills that will serve them well whatever their future destination.
[1] J-M G Rodriguez and M H Towns, J. Chem. Educ. , 2018, 95 , 2141, DOI: 10.1021/acs . jchemed.8b00683
[2] H Y Agustian and M K Seery, Chem. Educ. Res. Pract., 2017, 18 , 518, DOI: 10.1039/C7RP00140A
J-M G Rodriguez and M H Towns, J. Chem. Educ. , 2018, 95 , 2141, DOI: 10.1021/acs . jchemed.8b00683
H Y Agustian and M K Seery, Chem. Educ. Res. Pract., 2017, 18 , 518, DOI: 10.1039/C7RP00140A
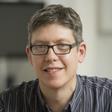
More from David Read
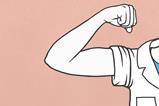
How building your subject knowledge bolsters your teaching confidence
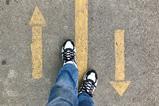
3 ways to boost knowledge transfer and retention
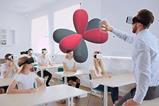
The science classroom of the future
- Acids and bases
- Education research
- Evidence-based teaching
- Secondary education
Related articles
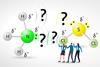
Understanding how students untangle intermolecular forces
2024-03-14T05:10:00Z By Fraser Scott
Discover how learners use electronegativity to predict the location of dipole−dipole interactions
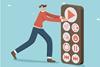
Why I use video to teach chemistry concepts
2024-02-27T08:17:00Z By Helen Rogerson
Helen Rogerson shares why and when videos are useful in the chemistry classroom
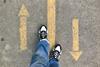
2024-02-20T05:00:00Z By David Read
Apply these evidence-informed cognitive processes to ensure your learners get ahead
No comments yet
Only registered users can comment on this article., more from education research.
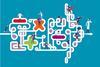
Boost maths skills to improve chemistry learning
2024-01-18T08:00:00Z By Fraser Scott
Use these evidence-based tips to help your learners get ahead with chemical calculations
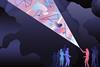
Support student sensemaking through directed dialogue
2023-12-19T09:27:00Z By David Read
Discover how to encourage effective classroom conversation to boost student understanding
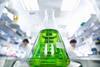
How to banish misconceptions with green chemistry
2023-11-23T05:00:00Z By Fraser Scott
Evidence-informed tips on how a greener approach can improve problem-solving abilities and address misconceptions
- Contributors
- Print issue
- Email alerts
Site powered by Webvision Cloud

An official website of the United States government
The .gov means it’s official. Federal government websites often end in .gov or .mil. Before sharing sensitive information, make sure you’re on a federal government site.
The site is secure. The https:// ensures that you are connecting to the official website and that any information you provide is encrypted and transmitted securely.
- Publications
- Account settings
Preview improvements coming to the PMC website in October 2024. Learn More or Try it out now .
- Advanced Search
- Journal List
- CBE Life Sci Educ
- v.17(1); Spring 2018
Understanding the Complex Relationship between Critical Thinking and Science Reasoning among Undergraduate Thesis Writers
Jason e. dowd.
† Department of Biology, Duke University, Durham, NC 27708
Robert J. Thompson, Jr.
‡ Department of Psychology and Neuroscience, Duke University, Durham, NC 27708
Leslie A. Schiff
§ Department of Microbiology and Immunology, University of Minnesota, Minneapolis, MN 55455
Julie A. Reynolds
Associated data.
This study empirically examines the relationship between students’ critical-thinking skills and scientific reasoning as reflected in undergraduate thesis writing in biology. Writing offers a unique window into studying this relationship, and the findings raise potential implications for instruction.
Developing critical-thinking and scientific reasoning skills are core learning objectives of science education, but little empirical evidence exists regarding the interrelationships between these constructs. Writing effectively fosters students’ development of these constructs, and it offers a unique window into studying how they relate. In this study of undergraduate thesis writing in biology at two universities, we examine how scientific reasoning exhibited in writing (assessed using the Biology Thesis Assessment Protocol) relates to general and specific critical-thinking skills (assessed using the California Critical Thinking Skills Test), and we consider implications for instruction. We find that scientific reasoning in writing is strongly related to inference , while other aspects of science reasoning that emerge in writing (epistemological considerations, writing conventions, etc.) are not significantly related to critical-thinking skills. Science reasoning in writing is not merely a proxy for critical thinking. In linking features of students’ writing to their critical-thinking skills, this study 1) provides a bridge to prior work suggesting that engagement in science writing enhances critical thinking and 2) serves as a foundational step for subsequently determining whether instruction focused explicitly on developing critical-thinking skills (particularly inference ) can actually improve students’ scientific reasoning in their writing.
INTRODUCTION
Critical-thinking and scientific reasoning skills are core learning objectives of science education for all students, regardless of whether or not they intend to pursue a career in science or engineering. Consistent with the view of learning as construction of understanding and meaning ( National Research Council, 2000 ), the pedagogical practice of writing has been found to be effective not only in fostering the development of students’ conceptual and procedural knowledge ( Gerdeman et al. , 2007 ) and communication skills ( Clase et al. , 2010 ), but also scientific reasoning ( Reynolds et al. , 2012 ) and critical-thinking skills ( Quitadamo and Kurtz, 2007 ).
Critical thinking and scientific reasoning are similar but different constructs that include various types of higher-order cognitive processes, metacognitive strategies, and dispositions involved in making meaning of information. Critical thinking is generally understood as the broader construct ( Holyoak and Morrison, 2005 ), comprising an array of cognitive processes and dispostions that are drawn upon differentially in everyday life and across domains of inquiry such as the natural sciences, social sciences, and humanities. Scientific reasoning, then, may be interpreted as the subset of critical-thinking skills (cognitive and metacognitive processes and dispositions) that 1) are involved in making meaning of information in scientific domains and 2) support the epistemological commitment to scientific methodology and paradigm(s).
Although there has been an enduring focus in higher education on promoting critical thinking and reasoning as general or “transferable” skills, research evidence provides increasing support for the view that reasoning and critical thinking are also situational or domain specific ( Beyer et al. , 2013 ). Some researchers, such as Lawson (2010) , present frameworks in which science reasoning is characterized explicitly in terms of critical-thinking skills. There are, however, limited coherent frameworks and empirical evidence regarding either the general or domain-specific interrelationships of scientific reasoning, as it is most broadly defined, and critical-thinking skills.
The Vision and Change in Undergraduate Biology Education Initiative provides a framework for thinking about these constructs and their interrelationship in the context of the core competencies and disciplinary practice they describe ( American Association for the Advancement of Science, 2011 ). These learning objectives aim for undergraduates to “understand the process of science, the interdisciplinary nature of the new biology and how science is closely integrated within society; be competent in communication and collaboration; have quantitative competency and a basic ability to interpret data; and have some experience with modeling, simulation and computational and systems level approaches as well as with using large databases” ( Woodin et al. , 2010 , pp. 71–72). This framework makes clear that science reasoning and critical-thinking skills play key roles in major learning outcomes; for example, “understanding the process of science” requires students to engage in (and be metacognitive about) scientific reasoning, and having the “ability to interpret data” requires critical-thinking skills. To help students better achieve these core competencies, we must better understand the interrelationships of their composite parts. Thus, the next step is to determine which specific critical-thinking skills are drawn upon when students engage in science reasoning in general and with regard to the particular scientific domain being studied. Such a determination could be applied to improve science education for both majors and nonmajors through pedagogical approaches that foster critical-thinking skills that are most relevant to science reasoning.
Writing affords one of the most effective means for making thinking visible ( Reynolds et al. , 2012 ) and learning how to “think like” and “write like” disciplinary experts ( Meizlish et al. , 2013 ). As a result, student writing affords the opportunities to both foster and examine the interrelationship of scientific reasoning and critical-thinking skills within and across disciplinary contexts. The purpose of this study was to better understand the relationship between students’ critical-thinking skills and scientific reasoning skills as reflected in the genre of undergraduate thesis writing in biology departments at two research universities, the University of Minnesota and Duke University.
In the following subsections, we discuss in greater detail the constructs of scientific reasoning and critical thinking, as well as the assessment of scientific reasoning in students’ thesis writing. In subsequent sections, we discuss our study design, findings, and the implications for enhancing educational practices.
Critical Thinking
The advances in cognitive science in the 21st century have increased our understanding of the mental processes involved in thinking and reasoning, as well as memory, learning, and problem solving. Critical thinking is understood to include both a cognitive dimension and a disposition dimension (e.g., reflective thinking) and is defined as “purposeful, self-regulatory judgment which results in interpretation, analysis, evaluation, and inference, as well as explanation of the evidential, conceptual, methodological, criteriological, or contextual considerations upon which that judgment is based” ( Facione, 1990, p. 3 ). Although various other definitions of critical thinking have been proposed, researchers have generally coalesced on this consensus: expert view ( Blattner and Frazier, 2002 ; Condon and Kelly-Riley, 2004 ; Bissell and Lemons, 2006 ; Quitadamo and Kurtz, 2007 ) and the corresponding measures of critical-thinking skills ( August, 2016 ; Stephenson and Sadler-McKnight, 2016 ).
Both the cognitive skills and dispositional components of critical thinking have been recognized as important to science education ( Quitadamo and Kurtz, 2007 ). Empirical research demonstrates that specific pedagogical practices in science courses are effective in fostering students’ critical-thinking skills. Quitadamo and Kurtz (2007) found that students who engaged in a laboratory writing component in the context of a general education biology course significantly improved their overall critical-thinking skills (and their analytical and inference skills, in particular), whereas students engaged in a traditional quiz-based laboratory did not improve their critical-thinking skills. In related work, Quitadamo et al. (2008) found that a community-based inquiry experience, involving inquiry, writing, research, and analysis, was associated with improved critical thinking in a biology course for nonmajors, compared with traditionally taught sections. In both studies, students who exhibited stronger presemester critical-thinking skills exhibited stronger gains, suggesting that “students who have not been explicitly taught how to think critically may not reach the same potential as peers who have been taught these skills” ( Quitadamo and Kurtz, 2007 , p. 151).
Recently, Stephenson and Sadler-McKnight (2016) found that first-year general chemistry students who engaged in a science writing heuristic laboratory, which is an inquiry-based, writing-to-learn approach to instruction ( Hand and Keys, 1999 ), had significantly greater gains in total critical-thinking scores than students who received traditional laboratory instruction. Each of the four components—inquiry, writing, collaboration, and reflection—have been linked to critical thinking ( Stephenson and Sadler-McKnight, 2016 ). Like the other studies, this work highlights the value of targeting critical-thinking skills and the effectiveness of an inquiry-based, writing-to-learn approach to enhance critical thinking. Across studies, authors advocate adopting critical thinking as the course framework ( Pukkila, 2004 ) and developing explicit examples of how critical thinking relates to the scientific method ( Miri et al. , 2007 ).
In these examples, the important connection between writing and critical thinking is highlighted by the fact that each intervention involves the incorporation of writing into science, technology, engineering, and mathematics education (either alone or in combination with other pedagogical practices). However, critical-thinking skills are not always the primary learning outcome; in some contexts, scientific reasoning is the primary outcome that is assessed.
Scientific Reasoning
Scientific reasoning is a complex process that is broadly defined as “the skills involved in inquiry, experimentation, evidence evaluation, and inference that are done in the service of conceptual change or scientific understanding” ( Zimmerman, 2007 , p. 172). Scientific reasoning is understood to include both conceptual knowledge and the cognitive processes involved with generation of hypotheses (i.e., inductive processes involved in the generation of hypotheses and the deductive processes used in the testing of hypotheses), experimentation strategies, and evidence evaluation strategies. These dimensions are interrelated, in that “experimentation and inference strategies are selected based on prior conceptual knowledge of the domain” ( Zimmerman, 2000 , p. 139). Furthermore, conceptual and procedural knowledge and cognitive process dimensions can be general and domain specific (or discipline specific).
With regard to conceptual knowledge, attention has been focused on the acquisition of core methodological concepts fundamental to scientists’ causal reasoning and metacognitive distancing (or decontextualized thinking), which is the ability to reason independently of prior knowledge or beliefs ( Greenhoot et al. , 2004 ). The latter involves what Kuhn and Dean (2004) refer to as the coordination of theory and evidence, which requires that one question existing theories (i.e., prior knowledge and beliefs), seek contradictory evidence, eliminate alternative explanations, and revise one’s prior beliefs in the face of contradictory evidence. Kuhn and colleagues (2008) further elaborate that scientific thinking requires “a mature understanding of the epistemological foundations of science, recognizing scientific knowledge as constructed by humans rather than simply discovered in the world,” and “the ability to engage in skilled argumentation in the scientific domain, with an appreciation of argumentation as entailing the coordination of theory and evidence” ( Kuhn et al. , 2008 , p. 435). “This approach to scientific reasoning not only highlights the skills of generating and evaluating evidence-based inferences, but also encompasses epistemological appreciation of the functions of evidence and theory” ( Ding et al. , 2016 , p. 616). Evaluating evidence-based inferences involves epistemic cognition, which Moshman (2015) defines as the subset of metacognition that is concerned with justification, truth, and associated forms of reasoning. Epistemic cognition is both general and domain specific (or discipline specific; Moshman, 2015 ).
There is empirical support for the contributions of both prior knowledge and an understanding of the epistemological foundations of science to scientific reasoning. In a study of undergraduate science students, advanced scientific reasoning was most often accompanied by accurate prior knowledge as well as sophisticated epistemological commitments; additionally, for students who had comparable levels of prior knowledge, skillful reasoning was associated with a strong epistemological commitment to the consistency of theory with evidence ( Zeineddin and Abd-El-Khalick, 2010 ). These findings highlight the importance of the need for instructional activities that intentionally help learners develop sophisticated epistemological commitments focused on the nature of knowledge and the role of evidence in supporting knowledge claims ( Zeineddin and Abd-El-Khalick, 2010 ).
Scientific Reasoning in Students’ Thesis Writing
Pedagogical approaches that incorporate writing have also focused on enhancing scientific reasoning. Many rubrics have been developed to assess aspects of scientific reasoning in written artifacts. For example, Timmerman and colleagues (2011) , in the course of describing their own rubric for assessing scientific reasoning, highlight several examples of scientific reasoning assessment criteria ( Haaga, 1993 ; Tariq et al. , 1998 ; Topping et al. , 2000 ; Kelly and Takao, 2002 ; Halonen et al. , 2003 ; Willison and O’Regan, 2007 ).
At both the University of Minnesota and Duke University, we have focused on the genre of the undergraduate honors thesis as the rhetorical context in which to study and improve students’ scientific reasoning and writing. We view the process of writing an undergraduate honors thesis as a form of professional development in the sciences (i.e., a way of engaging students in the practices of a community of discourse). We have found that structured courses designed to scaffold the thesis-writing process and promote metacognition can improve writing and reasoning skills in biology, chemistry, and economics ( Reynolds and Thompson, 2011 ; Dowd et al. , 2015a , b ). In the context of this prior work, we have defined scientific reasoning in writing as the emergent, underlying construct measured across distinct aspects of students’ written discussion of independent research in their undergraduate theses.
The Biology Thesis Assessment Protocol (BioTAP) was developed at Duke University as a tool for systematically guiding students and faculty through a “draft–feedback–revision” writing process, modeled after professional scientific peer-review processes ( Reynolds et al. , 2009 ). BioTAP includes activities and worksheets that allow students to engage in critical peer review and provides detailed descriptions, presented as rubrics, of the questions (i.e., dimensions, shown in Table 1 ) upon which such review should focus. Nine rubric dimensions focus on communication to the broader scientific community, and four rubric dimensions focus on the accuracy and appropriateness of the research. These rubric dimensions provide criteria by which the thesis is assessed, and therefore allow BioTAP to be used as an assessment tool as well as a teaching resource ( Reynolds et al. , 2009 ). Full details are available at www.science-writing.org/biotap.html .
Theses assessment protocol dimensions
In previous work, we have used BioTAP to quantitatively assess students’ undergraduate honors theses and explore the relationship between thesis-writing courses (or specific interventions within the courses) and the strength of students’ science reasoning in writing across different science disciplines: biology ( Reynolds and Thompson, 2011 ); chemistry ( Dowd et al. , 2015b ); and economics ( Dowd et al. , 2015a ). We have focused exclusively on the nine dimensions related to reasoning and writing (questions 1–9), as the other four dimensions (questions 10–13) require topic-specific expertise and are intended to be used by the student’s thesis supervisor.
Beyond considering individual dimensions, we have investigated whether meaningful constructs underlie students’ thesis scores. We conducted exploratory factor analysis of students’ theses in biology, economics, and chemistry and found one dominant underlying factor in each discipline; we termed the factor “scientific reasoning in writing” ( Dowd et al. , 2015a , b , 2016 ). That is, each of the nine dimensions could be understood as reflecting, in different ways and to different degrees, the construct of scientific reasoning in writing. The findings indicated evidence of both general and discipline-specific components to scientific reasoning in writing that relate to epistemic beliefs and paradigms, in keeping with broader ideas about science reasoning discussed earlier. Specifically, scientific reasoning in writing is more strongly associated with formulating a compelling argument for the significance of the research in the context of current literature in biology, making meaning regarding the implications of the findings in chemistry, and providing an organizational framework for interpreting the thesis in economics. We suggested that instruction, whether occurring in writing studios or in writing courses to facilitate thesis preparation, should attend to both components.
Research Question and Study Design
The genre of thesis writing combines the pedagogies of writing and inquiry found to foster scientific reasoning ( Reynolds et al. , 2012 ) and critical thinking ( Quitadamo and Kurtz, 2007 ; Quitadamo et al. , 2008 ; Stephenson and Sadler-McKnight, 2016 ). However, there is no empirical evidence regarding the general or domain-specific interrelationships of scientific reasoning and critical-thinking skills, particularly in the rhetorical context of the undergraduate thesis. The BioTAP studies discussed earlier indicate that the rubric-based assessment produces evidence of scientific reasoning in the undergraduate thesis, but it was not designed to foster or measure critical thinking. The current study was undertaken to address the research question: How are students’ critical-thinking skills related to scientific reasoning as reflected in the genre of undergraduate thesis writing in biology? Determining these interrelationships could guide efforts to enhance students’ scientific reasoning and writing skills through focusing instruction on specific critical-thinking skills as well as disciplinary conventions.
To address this research question, we focused on undergraduate thesis writers in biology courses at two institutions, Duke University and the University of Minnesota, and examined the extent to which students’ scientific reasoning in writing, assessed in the undergraduate thesis using BioTAP, corresponds to students’ critical-thinking skills, assessed using the California Critical Thinking Skills Test (CCTST; August, 2016 ).
Study Sample
The study sample was composed of students enrolled in courses designed to scaffold the thesis-writing process in the Department of Biology at Duke University and the College of Biological Sciences at the University of Minnesota. Both courses complement students’ individual work with research advisors. The course is required for thesis writers at the University of Minnesota and optional for writers at Duke University. Not all students are required to complete a thesis, though it is required for students to graduate with honors; at the University of Minnesota, such students are enrolled in an honors program within the college. In total, 28 students were enrolled in the course at Duke University and 44 students were enrolled in the course at the University of Minnesota. Of those students, two students did not consent to participate in the study; additionally, five students did not validly complete the CCTST (i.e., attempted fewer than 60% of items or completed the test in less than 15 minutes). Thus, our overall rate of valid participation is 90%, with 27 students from Duke University and 38 students from the University of Minnesota. We found no statistically significant differences in thesis assessment between students with valid CCTST scores and invalid CCTST scores. Therefore, we focus on the 65 students who consented to participate and for whom we have complete and valid data in most of this study. Additionally, in asking students for their consent to participate, we allowed them to choose whether to provide or decline access to academic and demographic background data. Of the 65 students who consented to participate, 52 students granted access to such data. Therefore, for additional analyses involving academic and background data, we focus on the 52 students who consented. We note that the 13 students who participated but declined to share additional data performed slightly lower on the CCTST than the 52 others (perhaps suggesting that they differ by other measures, but we cannot determine this with certainty). Among the 52 students, 60% identified as female and 10% identified as being from underrepresented ethnicities.
In both courses, students completed the CCTST online, either in class or on their own, late in the Spring 2016 semester. This is the same assessment that was used in prior studies of critical thinking ( Quitadamo and Kurtz, 2007 ; Quitadamo et al. , 2008 ; Stephenson and Sadler-McKnight, 2016 ). It is “an objective measure of the core reasoning skills needed for reflective decision making concerning what to believe or what to do” ( Insight Assessment, 2016a ). In the test, students are asked to read and consider information as they answer multiple-choice questions. The questions are intended to be appropriate for all users, so there is no expectation of prior disciplinary knowledge in biology (or any other subject). Although actual test items are protected, sample items are available on the Insight Assessment website ( Insight Assessment, 2016b ). We have included one sample item in the Supplemental Material.
The CCTST is based on a consensus definition of critical thinking, measures cognitive and metacognitive skills associated with critical thinking, and has been evaluated for validity and reliability at the college level ( August, 2016 ; Stephenson and Sadler-McKnight, 2016 ). In addition to providing overall critical-thinking score, the CCTST assesses seven dimensions of critical thinking: analysis, interpretation, inference, evaluation, explanation, induction, and deduction. Scores on each dimension are calculated based on students’ performance on items related to that dimension. Analysis focuses on identifying assumptions, reasons, and claims and examining how they interact to form arguments. Interpretation, related to analysis, focuses on determining the precise meaning and significance of information. Inference focuses on drawing conclusions from reasons and evidence. Evaluation focuses on assessing the credibility of sources of information and claims they make. Explanation, related to evaluation, focuses on describing the evidence, assumptions, or rationale for beliefs and conclusions. Induction focuses on drawing inferences about what is probably true based on evidence. Deduction focuses on drawing conclusions about what must be true when the context completely determines the outcome. These are not independent dimensions; the fact that they are related supports their collective interpretation as critical thinking. Together, the CCTST dimensions provide a basis for evaluating students’ overall strength in using reasoning to form reflective judgments about what to believe or what to do ( August, 2016 ). Each of the seven dimensions and the overall CCTST score are measured on a scale of 0–100, where higher scores indicate superior performance. Scores correspond to superior (86–100), strong (79–85), moderate (70–78), weak (63–69), or not manifested (62 and below) skills.
Scientific Reasoning in Writing
At the end of the semester, students’ final, submitted undergraduate theses were assessed using BioTAP, which consists of nine rubric dimensions that focus on communication to the broader scientific community and four additional dimensions that focus on the exhibition of topic-specific expertise ( Reynolds et al. , 2009 ). These dimensions, framed as questions, are displayed in Table 1 .
Student theses were assessed on questions 1–9 of BioTAP using the same procedures described in previous studies ( Reynolds and Thompson, 2011 ; Dowd et al. , 2015a , b ). In this study, six raters were trained in the valid, reliable use of BioTAP rubrics. Each dimension was rated on a five-point scale: 1 indicates the dimension is missing, incomplete, or below acceptable standards; 3 indicates that the dimension is adequate but not exhibiting mastery; and 5 indicates that the dimension is excellent and exhibits mastery (intermediate ratings of 2 and 4 are appropriate when different parts of the thesis make a single category challenging). After training, two raters independently assessed each thesis and then discussed their independent ratings with one another to form a consensus rating. The consensus score is not an average score, but rather an agreed-upon, discussion-based score. On a five-point scale, raters independently assessed dimensions to be within 1 point of each other 82.4% of the time before discussion and formed consensus ratings 100% of the time after discussion.
In this study, we consider both categorical (mastery/nonmastery, where a score of 5 corresponds to mastery) and numerical treatments of individual BioTAP scores to better relate the manifestation of critical thinking in BioTAP assessment to all of the prior studies. For comprehensive/cumulative measures of BioTAP, we focus on the partial sum of questions 1–5, as these questions relate to higher-order scientific reasoning (whereas questions 6–9 relate to mid- and lower-order writing mechanics [ Reynolds et al. , 2009 ]), and the factor scores (i.e., numerical representations of the extent to which each student exhibits the underlying factor), which are calculated from the factor loadings published by Dowd et al. (2016) . We do not focus on questions 6–9 individually in statistical analyses, because we do not expect critical-thinking skills to relate to mid- and lower-order writing skills.
The final, submitted thesis reflects the student’s writing, the student’s scientific reasoning, the quality of feedback provided to the student by peers and mentors, and the student’s ability to incorporate that feedback into his or her work. Therefore, our assessment is not the same as an assessment of unpolished, unrevised samples of students’ written work. While one might imagine that such an unpolished sample may be more strongly correlated with critical-thinking skills measured by the CCTST, we argue that the complete, submitted thesis, assessed using BioTAP, is ultimately a more appropriate reflection of how students exhibit science reasoning in the scientific community.
Statistical Analyses
We took several steps to analyze the collected data. First, to provide context for subsequent interpretations, we generated descriptive statistics for the CCTST scores of the participants based on the norms for undergraduate CCTST test takers. To determine the strength of relationships among CCTST dimensions (including overall score) and the BioTAP dimensions, partial-sum score (questions 1–5), and factor score, we calculated Pearson’s correlations for each pair of measures. To examine whether falling on one side of the nonmastery/mastery threshold (as opposed to a linear scale of performance) was related to critical thinking, we grouped BioTAP dimensions into categories (mastery/nonmastery) and conducted Student’s t tests to compare the means scores of the two groups on each of the seven dimensions and overall score of the CCTST. Finally, for the strongest relationship that emerged, we included additional academic and background variables as covariates in multiple linear-regression analysis to explore questions about how much observed relationships between critical-thinking skills and science reasoning in writing might be explained by variation in these other factors.
Although BioTAP scores represent discreet, ordinal bins, the five-point scale is intended to capture an underlying continuous construct (from inadequate to exhibiting mastery). It has been argued that five categories is an appropriate cutoff for treating ordinal variables as pseudo-continuous ( Rhemtulla et al. , 2012 )—and therefore using continuous-variable statistical methods (e.g., Pearson’s correlations)—as long as the underlying assumption that ordinal scores are linearly distributed is valid. Although we have no way to statistically test this assumption, we interpret adequate scores to be approximately halfway between inadequate and mastery scores, resulting in a linear scale. In part because this assumption is subject to disagreement, we also consider and interpret a categorical (mastery/nonmastery) treatment of BioTAP variables.
We corrected for multiple comparisons using the Holm-Bonferroni method ( Holm, 1979 ). At the most general level, where we consider the single, comprehensive measures for BioTAP (partial-sum and factor score) and the CCTST (overall score), there is no need to correct for multiple comparisons, because the multiple, individual dimensions are collapsed into single dimensions. When we considered individual CCTST dimensions in relation to comprehensive measures for BioTAP, we accounted for seven comparisons; similarly, when we considered individual dimensions of BioTAP in relation to overall CCTST score, we accounted for five comparisons. When all seven CCTST and five BioTAP dimensions were examined individually and without prior knowledge, we accounted for 35 comparisons; such a rigorous threshold is likely to reject weak and moderate relationships, but it is appropriate if there are no specific pre-existing hypotheses. All p values are presented in tables for complete transparency, and we carefully consider the implications of our interpretation of these data in the Discussion section.
CCTST scores for students in this sample ranged from the 39th to 99th percentile of the general population of undergraduate CCTST test takers (mean percentile = 84.3, median = 85th percentile; Table 2 ); these percentiles reflect overall scores that range from moderate to superior. Scores on individual dimensions and overall scores were sufficiently normal and far enough from the ceiling of the scale to justify subsequent statistical analyses.
Descriptive statistics of CCTST dimensions a
a Scores correspond to superior (86–100), strong (79–85), moderate (70–78), weak (63–69), or not manifested (62 and lower) skills.
The Pearson’s correlations between students’ cumulative scores on BioTAP (the factor score based on loadings published by Dowd et al. , 2016 , and the partial sum of scores on questions 1–5) and students’ overall scores on the CCTST are presented in Table 3 . We found that the partial-sum measure of BioTAP was significantly related to the overall measure of critical thinking ( r = 0.27, p = 0.03), while the BioTAP factor score was marginally related to overall CCTST ( r = 0.24, p = 0.05). When we looked at relationships between comprehensive BioTAP measures and scores for individual dimensions of the CCTST ( Table 3 ), we found significant positive correlations between the both BioTAP partial-sum and factor scores and CCTST inference ( r = 0.45, p < 0.001, and r = 0.41, p < 0.001, respectively). Although some other relationships have p values below 0.05 (e.g., the correlations between BioTAP partial-sum scores and CCTST induction and interpretation scores), they are not significant when we correct for multiple comparisons.
Correlations between dimensions of CCTST and dimensions of BioTAP a
a In each cell, the top number is the correlation, and the bottom, italicized number is the associated p value. Correlations that are statistically significant after correcting for multiple comparisons are shown in bold.
b This is the partial sum of BioTAP scores on questions 1–5.
c This is the factor score calculated from factor loadings published by Dowd et al. (2016) .
When we expanded comparisons to include all 35 potential correlations among individual BioTAP and CCTST dimensions—and, accordingly, corrected for 35 comparisons—we did not find any additional statistically significant relationships. The Pearson’s correlations between students’ scores on each dimension of BioTAP and students’ scores on each dimension of the CCTST range from −0.11 to 0.35 ( Table 3 ); although the relationship between discussion of implications (BioTAP question 5) and inference appears to be relatively large ( r = 0.35), it is not significant ( p = 0.005; the Holm-Bonferroni cutoff is 0.00143). We found no statistically significant relationships between BioTAP questions 6–9 and CCTST dimensions (unpublished data), regardless of whether we correct for multiple comparisons.
The results of Student’s t tests comparing scores on each dimension of the CCTST of students who exhibit mastery with those of students who do not exhibit mastery on each dimension of BioTAP are presented in Table 4 . Focusing first on the overall CCTST scores, we found that the difference between those who exhibit mastery and those who do not in discussing implications of results (BioTAP question 5) is statistically significant ( t = 2.73, p = 0.008, d = 0.71). When we expanded t tests to include all 35 comparisons—and, like above, corrected for 35 comparisons—we found a significant difference in inference scores between students who exhibit mastery on question 5 and students who do not ( t = 3.41, p = 0.0012, d = 0.88), as well as a marginally significant difference in these students’ induction scores ( t = 3.26, p = 0.0018, d = 0.84; the Holm-Bonferroni cutoff is p = 0.00147). Cohen’s d effect sizes, which reveal the strength of the differences for statistically significant relationships, range from 0.71 to 0.88.
The t statistics and effect sizes of differences in dimensions of CCTST across dimensions of BioTAP a
a In each cell, the top number is the t statistic for each comparison, and the middle, italicized number is the associated p value. The bottom number is the effect size. Correlations that are statistically significant after correcting for multiple comparisons are shown in bold.
Finally, we more closely examined the strongest relationship that we observed, which was between the CCTST dimension of inference and the BioTAP partial-sum composite score (shown in Table 3 ), using multiple regression analysis ( Table 5 ). Focusing on the 52 students for whom we have background information, we looked at the simple relationship between BioTAP and inference (model 1), a robust background model including multiple covariates that one might expect to explain some part of the variation in BioTAP (model 2), and a combined model including all variables (model 3). As model 3 shows, the covariates explain very little variation in BioTAP scores, and the relationship between inference and BioTAP persists even in the presence of all of the covariates.
Partial sum (questions 1–5) of BioTAP scores ( n = 52)
** p < 0.01.
*** p < 0.001.
The aim of this study was to examine the extent to which the various components of scientific reasoning—manifested in writing in the genre of undergraduate thesis and assessed using BioTAP—draw on general and specific critical-thinking skills (assessed using CCTST) and to consider the implications for educational practices. Although science reasoning involves critical-thinking skills, it also relates to conceptual knowledge and the epistemological foundations of science disciplines ( Kuhn et al. , 2008 ). Moreover, science reasoning in writing , captured in students’ undergraduate theses, reflects habits, conventions, and the incorporation of feedback that may alter evidence of individuals’ critical-thinking skills. Our findings, however, provide empirical evidence that cumulative measures of science reasoning in writing are nonetheless related to students’ overall critical-thinking skills ( Table 3 ). The particularly significant roles of inference skills ( Table 3 ) and the discussion of implications of results (BioTAP question 5; Table 4 ) provide a basis for more specific ideas about how these constructs relate to one another and what educational interventions may have the most success in fostering these skills.
Our results build on previous findings. The genre of thesis writing combines pedagogies of writing and inquiry found to foster scientific reasoning ( Reynolds et al. , 2012 ) and critical thinking ( Quitadamo and Kurtz, 2007 ; Quitadamo et al. , 2008 ; Stephenson and Sadler-McKnight, 2016 ). Quitadamo and Kurtz (2007) reported that students who engaged in a laboratory writing component in a general education biology course significantly improved their inference and analysis skills, and Quitadamo and colleagues (2008) found that participation in a community-based inquiry biology course (that included a writing component) was associated with significant gains in students’ inference and evaluation skills. The shared focus on inference is noteworthy, because these prior studies actually differ from the current study; the former considered critical-thinking skills as the primary learning outcome of writing-focused interventions, whereas the latter focused on emergent links between two learning outcomes (science reasoning in writing and critical thinking). In other words, inference skills are impacted by writing as well as manifested in writing.
Inference focuses on drawing conclusions from argument and evidence. According to the consensus definition of critical thinking, the specific skill of inference includes several processes: querying evidence, conjecturing alternatives, and drawing conclusions. All of these activities are central to the independent research at the core of writing an undergraduate thesis. Indeed, a critical part of what we call “science reasoning in writing” might be characterized as a measure of students’ ability to infer and make meaning of information and findings. Because the cumulative BioTAP measures distill underlying similarities and, to an extent, suppress unique aspects of individual dimensions, we argue that it is appropriate to relate inference to scientific reasoning in writing . Even when we control for other potentially relevant background characteristics, the relationship is strong ( Table 5 ).
In taking the complementary view and focusing on BioTAP, when we compared students who exhibit mastery with those who do not, we found that the specific dimension of “discussing the implications of results” (question 5) differentiates students’ performance on several critical-thinking skills. To achieve mastery on this dimension, students must make connections between their results and other published studies and discuss the future directions of the research; in short, they must demonstrate an understanding of the bigger picture. The specific relationship between question 5 and inference is the strongest observed among all individual comparisons. Altogether, perhaps more than any other BioTAP dimension, this aspect of students’ writing provides a clear view of the role of students’ critical-thinking skills (particularly inference and, marginally, induction) in science reasoning.
While inference and discussion of implications emerge as particularly strongly related dimensions in this work, we note that the strongest contribution to “science reasoning in writing in biology,” as determined through exploratory factor analysis, is “argument for the significance of research” (BioTAP question 2, not question 5; Dowd et al. , 2016 ). Question 2 is not clearly related to critical-thinking skills. These findings are not contradictory, but rather suggest that the epistemological and disciplinary-specific aspects of science reasoning that emerge in writing through BioTAP are not completely aligned with aspects related to critical thinking. In other words, science reasoning in writing is not simply a proxy for those critical-thinking skills that play a role in science reasoning.
In a similar vein, the content-related, epistemological aspects of science reasoning, as well as the conventions associated with writing the undergraduate thesis (including feedback from peers and revision), may explain the lack of significant relationships between some science reasoning dimensions and some critical-thinking skills that might otherwise seem counterintuitive (e.g., BioTAP question 2, which relates to making an argument, and the critical-thinking skill of argument). It is possible that an individual’s critical-thinking skills may explain some variation in a particular BioTAP dimension, but other aspects of science reasoning and practice exert much stronger influence. Although these relationships do not emerge in our analyses, the lack of significant correlation does not mean that there is definitively no correlation. Correcting for multiple comparisons suppresses type 1 error at the expense of exacerbating type 2 error, which, combined with the limited sample size, constrains statistical power and makes weak relationships more difficult to detect. Ultimately, though, the relationships that do emerge highlight places where individuals’ distinct critical-thinking skills emerge most coherently in thesis assessment, which is why we are particularly interested in unpacking those relationships.
We recognize that, because only honors students submit theses at these institutions, this study sample is composed of a selective subset of the larger population of biology majors. Although this is an inherent limitation of focusing on thesis writing, links between our findings and results of other studies (with different populations) suggest that observed relationships may occur more broadly. The goal of improved science reasoning and critical thinking is shared among all biology majors, particularly those engaged in capstone research experiences. So while the implications of this work most directly apply to honors thesis writers, we provisionally suggest that all students could benefit from further study of them.
There are several important implications of this study for science education practices. Students’ inference skills relate to the understanding and effective application of scientific content. The fact that we find no statistically significant relationships between BioTAP questions 6–9 and CCTST dimensions suggests that such mid- to lower-order elements of BioTAP ( Reynolds et al. , 2009 ), which tend to be more structural in nature, do not focus on aspects of the finished thesis that draw strongly on critical thinking. In keeping with prior analyses ( Reynolds and Thompson, 2011 ; Dowd et al. , 2016 ), these findings further reinforce the notion that disciplinary instructors, who are most capable of teaching and assessing scientific reasoning and perhaps least interested in the more mechanical aspects of writing, may nonetheless be best suited to effectively model and assess students’ writing.
The goal of the thesis writing course at both Duke University and the University of Minnesota is not merely to improve thesis scores but to move students’ writing into the category of mastery across BioTAP dimensions. Recognizing that students with differing critical-thinking skills (particularly inference) are more or less likely to achieve mastery in the undergraduate thesis (particularly in discussing implications [question 5]) is important for developing and testing targeted pedagogical interventions to improve learning outcomes for all students.
The competencies characterized by the Vision and Change in Undergraduate Biology Education Initiative provide a general framework for recognizing that science reasoning and critical-thinking skills play key roles in major learning outcomes of science education. Our findings highlight places where science reasoning–related competencies (like “understanding the process of science”) connect to critical-thinking skills and places where critical thinking–related competencies might be manifested in scientific products (such as the ability to discuss implications in scientific writing). We encourage broader efforts to build empirical connections between competencies and pedagogical practices to further improve science education.
One specific implication of this work for science education is to focus on providing opportunities for students to develop their critical-thinking skills (particularly inference). Of course, as this correlational study is not designed to test causality, we do not claim that enhancing students’ inference skills will improve science reasoning in writing. However, as prior work shows that science writing activities influence students’ inference skills ( Quitadamo and Kurtz, 2007 ; Quitadamo et al. , 2008 ), there is reason to test such a hypothesis. Nevertheless, the focus must extend beyond inference as an isolated skill; rather, it is important to relate inference to the foundations of the scientific method ( Miri et al. , 2007 ) in terms of the epistemological appreciation of the functions and coordination of evidence ( Kuhn and Dean, 2004 ; Zeineddin and Abd-El-Khalick, 2010 ; Ding et al. , 2016 ) and disciplinary paradigms of truth and justification ( Moshman, 2015 ).
Although this study is limited to the domain of biology at two institutions with a relatively small number of students, the findings represent a foundational step in the direction of achieving success with more integrated learning outcomes. Hopefully, it will spur greater interest in empirically grounding discussions of the constructs of scientific reasoning and critical-thinking skills.
This study contributes to the efforts to improve science education, for both majors and nonmajors, through an empirically driven analysis of the relationships between scientific reasoning reflected in the genre of thesis writing and critical-thinking skills. This work is rooted in the usefulness of BioTAP as a method 1) to facilitate communication and learning and 2) to assess disciplinary-specific and general dimensions of science reasoning. The findings support the important role of the critical-thinking skill of inference in scientific reasoning in writing, while also highlighting ways in which other aspects of science reasoning (epistemological considerations, writing conventions, etc.) are not significantly related to critical thinking. Future research into the impact of interventions focused on specific critical-thinking skills (i.e., inference) for improved science reasoning in writing will build on this work and its implications for science education.
Supplementary Material
Acknowledgments.
We acknowledge the contributions of Kelaine Haas and Alexander Motten to the implementation and collection of data. We also thank Mine Çetinkaya-Rundel for her insights regarding our statistical analyses. This research was funded by National Science Foundation award DUE-1525602.
- American Association for the Advancement of Science. (2011). Vision and change in undergraduate biology education: A call to action . Washington, DC: Retrieved September 26, 2017, from https://visionandchange.org/files/2013/11/aaas-VISchange-web1113.pdf . [ Google Scholar ]
- August D. (2016). California Critical Thinking Skills Test user manual and resource guide . San Jose: Insight Assessment/California Academic Press. [ Google Scholar ]
- Beyer C. H., Taylor E., Gillmore G. M. (2013). Inside the undergraduate teaching experience: The University of Washington’s growth in faculty teaching study . Albany, NY: SUNY Press. [ Google Scholar ]
- Bissell A. N., Lemons P. P. (2006). A new method for assessing critical thinking in the classroom . BioScience , ( 1 ), 66–72. https://doi.org/10.1641/0006-3568(2006)056[0066:ANMFAC]2.0.CO;2 . [ Google Scholar ]
- Blattner N. H., Frazier C. L. (2002). Developing a performance-based assessment of students’ critical thinking skills . Assessing Writing , ( 1 ), 47–64. [ Google Scholar ]
- Clase K. L., Gundlach E., Pelaez N. J. (2010). Calibrated peer review for computer-assisted learning of biological research competencies . Biochemistry and Molecular Biology Education , ( 5 ), 290–295. [ PubMed ] [ Google Scholar ]
- Condon W., Kelly-Riley D. (2004). Assessing and teaching what we value: The relationship between college-level writing and critical thinking abilities . Assessing Writing , ( 1 ), 56–75. https://doi.org/10.1016/j.asw.2004.01.003 . [ Google Scholar ]
- Ding L., Wei X., Liu X. (2016). Variations in university students’ scientific reasoning skills across majors, years, and types of institutions . Research in Science Education , ( 5 ), 613–632. https://doi.org/10.1007/s11165-015-9473-y . [ Google Scholar ]
- Dowd J. E., Connolly M. P., Thompson R. J., Jr., Reynolds J. A. (2015a). Improved reasoning in undergraduate writing through structured workshops . Journal of Economic Education , ( 1 ), 14–27. https://doi.org/10.1080/00220485.2014.978924 . [ Google Scholar ]
- Dowd J. E., Roy C. P., Thompson R. J., Jr., Reynolds J. A. (2015b). “On course” for supporting expanded participation and improving scientific reasoning in undergraduate thesis writing . Journal of Chemical Education , ( 1 ), 39–45. https://doi.org/10.1021/ed500298r . [ Google Scholar ]
- Dowd J. E., Thompson R. J., Jr., Reynolds J. A. (2016). Quantitative genre analysis of undergraduate theses: Uncovering different ways of writing and thinking in science disciplines . WAC Journal , , 36–51. [ Google Scholar ]
- Facione P. A. (1990). Critical thinking: a statement of expert consensus for purposes of educational assessment and instruction. Research findings and recommendations . Newark, DE: American Philosophical Association; Retrieved September 26, 2017, from https://philpapers.org/archive/FACCTA.pdf . [ Google Scholar ]
- Gerdeman R. D., Russell A. A., Worden K. J., Gerdeman R. D., Russell A. A., Worden K. J. (2007). Web-based student writing and reviewing in a large biology lecture course . Journal of College Science Teaching , ( 5 ), 46–52. [ Google Scholar ]
- Greenhoot A. F., Semb G., Colombo J., Schreiber T. (2004). Prior beliefs and methodological concepts in scientific reasoning . Applied Cognitive Psychology , ( 2 ), 203–221. https://doi.org/10.1002/acp.959 . [ Google Scholar ]
- Haaga D. A. F. (1993). Peer review of term papers in graduate psychology courses . Teaching of Psychology , ( 1 ), 28–32. https://doi.org/10.1207/s15328023top2001_5 . [ Google Scholar ]
- Halonen J. S., Bosack T., Clay S., McCarthy M., Dunn D. S., Hill G. W., Whitlock K. (2003). A rubric for learning, teaching, and assessing scientific inquiry in psychology . Teaching of Psychology , ( 3 ), 196–208. https://doi.org/10.1207/S15328023TOP3003_01 . [ Google Scholar ]
- Hand B., Keys C. W. (1999). Inquiry investigation . Science Teacher , ( 4 ), 27–29. [ Google Scholar ]
- Holm S. (1979). A simple sequentially rejective multiple test procedure . Scandinavian Journal of Statistics , ( 2 ), 65–70. [ Google Scholar ]
- Holyoak K. J., Morrison R. G. (2005). The Cambridge handbook of thinking and reasoning . New York: Cambridge University Press. [ Google Scholar ]
- Insight Assessment. (2016a). California Critical Thinking Skills Test (CCTST) Retrieved September 26, 2017, from www.insightassessment.com/Products/Products-Summary/Critical-Thinking-Skills-Tests/California-Critical-Thinking-Skills-Test-CCTST .
- Insight Assessment. (2016b). Sample thinking skills questions. Retrieved September 26, 2017, from www.insightassessment.com/Resources/Teaching-Training-and-Learning-Tools/node_1487 .
- Kelly G. J., Takao A. (2002). Epistemic levels in argument: An analysis of university oceanography students’ use of evidence in writing . Science Education , ( 3 ), 314–342. https://doi.org/10.1002/sce.10024 . [ Google Scholar ]
- Kuhn D., Dean D., Jr. (2004). Connecting scientific reasoning and causal inference . Journal of Cognition and Development , ( 2 ), 261–288. https://doi.org/10.1207/s15327647jcd0502_5 . [ Google Scholar ]
- Kuhn D., Iordanou K., Pease M., Wirkala C. (2008). Beyond control of variables: What needs to develop to achieve skilled scientific thinking? . Cognitive Development , ( 4 ), 435–451. https://doi.org/10.1016/j.cogdev.2008.09.006 . [ Google Scholar ]
- Lawson A. E. (2010). Basic inferences of scientific reasoning, argumentation, and discovery . Science Education , ( 2 ), 336–364. https://doi.org/10.1002/sce.20357 . [ Google Scholar ]
- Meizlish D., LaVaque-Manty D., Silver N., Kaplan M. (2013). Think like/write like: Metacognitive strategies to foster students’ development as disciplinary thinkers and writers . In Thompson R. J. (Ed.), Changing the conversation about higher education (pp. 53–73). Lanham, MD: Rowman & Littlefield. [ Google Scholar ]
- Miri B., David B.-C., Uri Z. (2007). Purposely teaching for the promotion of higher-order thinking skills: A case of critical thinking . Research in Science Education , ( 4 ), 353–369. https://doi.org/10.1007/s11165-006-9029-2 . [ Google Scholar ]
- Moshman D. (2015). Epistemic cognition and development: The psychology of justification and truth . New York: Psychology Press. [ Google Scholar ]
- National Research Council. (2000). How people learn: Brain, mind, experience, and school . Expanded ed. Washington, DC: National Academies Press. [ Google Scholar ]
- Pukkila P. J. (2004). Introducing student inquiry in large introductory genetics classes . Genetics , ( 1 ), 11–18. https://doi.org/10.1534/genetics.166.1.11 . [ PMC free article ] [ PubMed ] [ Google Scholar ]
- Quitadamo I. J., Faiola C. L., Johnson J. E., Kurtz M. J. (2008). Community-based inquiry improves critical thinking in general education biology . CBE—Life Sciences Education , ( 3 ), 327–337. https://doi.org/10.1187/cbe.07-11-0097 . [ PMC free article ] [ PubMed ] [ Google Scholar ]
- Quitadamo I. J., Kurtz M. J. (2007). Learning to improve: Using writing to increase critical thinking performance in general education biology . CBE—Life Sciences Education , ( 2 ), 140–154. https://doi.org/10.1187/cbe.06-11-0203 . [ PMC free article ] [ PubMed ] [ Google Scholar ]
- Reynolds J. A., Smith R., Moskovitz C., Sayle A. (2009). BioTAP: A systematic approach to teaching scientific writing and evaluating undergraduate theses . BioScience , ( 10 ), 896–903. https://doi.org/10.1525/bio.2009.59.10.11 . [ Google Scholar ]
- Reynolds J. A., Thaiss C., Katkin W., Thompson R. J. (2012). Writing-to-learn in undergraduate science education: A community-based, conceptually driven approach . CBE—Life Sciences Education , ( 1 ), 17–25. https://doi.org/10.1187/cbe.11-08-0064 . [ PMC free article ] [ PubMed ] [ Google Scholar ]
- Reynolds J. A., Thompson R. J. (2011). Want to improve undergraduate thesis writing? Engage students and their faculty readers in scientific peer review . CBE—Life Sciences Education , ( 2 ), 209–215. https://doi.org/10.1187/cbe.10-10-0127 . [ PMC free article ] [ PubMed ] [ Google Scholar ]
- Rhemtulla M., Brosseau-Liard P. E., Savalei V. (2012). When can categorical variables be treated as continuous? A comparison of robust continuous and categorical SEM estimation methods under suboptimal conditions . Psychological Methods , ( 3 ), 354–373. https://doi.org/10.1037/a0029315 . [ PubMed ] [ Google Scholar ]
- Stephenson N. S., Sadler-McKnight N. P. (2016). Developing critical thinking skills using the science writing heuristic in the chemistry laboratory . Chemistry Education Research and Practice , ( 1 ), 72–79. https://doi.org/10.1039/C5RP00102A . [ Google Scholar ]
- Tariq V. N., Stefani L. A. J., Butcher A. C., Heylings D. J. A. (1998). Developing a new approach to the assessment of project work . Assessment and Evaluation in Higher Education , ( 3 ), 221–240. https://doi.org/10.1080/0260293980230301 . [ Google Scholar ]
- Timmerman B. E. C., Strickland D. C., Johnson R. L., Payne J. R. (2011). Development of a “universal” rubric for assessing undergraduates’ scientific reasoning skills using scientific writing . Assessment and Evaluation in Higher Education , ( 5 ), 509–547. https://doi.org/10.1080/02602930903540991 . [ Google Scholar ]
- Topping K. J., Smith E. F., Swanson I., Elliot A. (2000). Formative peer assessment of academic writing between postgraduate students . Assessment and Evaluation in Higher Education , ( 2 ), 149–169. https://doi.org/10.1080/713611428 . [ Google Scholar ]
- Willison J., O’Regan K. (2007). Commonly known, commonly not known, totally unknown: A framework for students becoming researchers . Higher Education Research and Development , ( 4 ), 393–409. https://doi.org/10.1080/07294360701658609 . [ Google Scholar ]
- Woodin T., Carter V. C., Fletcher L. (2010). Vision and Change in Biology Undergraduate Education: A Call for Action—Initial responses . CBE—Life Sciences Education , ( 2 ), 71–73. https://doi.org/10.1187/cbe.10-03-0044 . [ PMC free article ] [ PubMed ] [ Google Scholar ]
- Zeineddin A., Abd-El-Khalick F. (2010). Scientific reasoning and epistemological commitments: Coordination of theory and evidence among college science students . Journal of Research in Science Teaching , ( 9 ), 1064–1093. https://doi.org/10.1002/tea.20368 . [ Google Scholar ]
- Zimmerman C. (2000). The development of scientific reasoning skills . Developmental Review , ( 1 ), 99–149. https://doi.org/10.1006/drev.1999.0497 . [ Google Scholar ]
- Zimmerman C. (2007). The development of scientific thinking skills in elementary and middle school . Developmental Review , ( 2 ), 172–223. https://doi.org/10.1016/j.dr.2006.12.001 . [ Google Scholar ]
- Skip to primary navigation
- Skip to main content
- Skip to primary sidebar
Teaching Expertise
- Classroom Ideas
- Teacher’s Life
- Deals & Shopping
- Privacy Policy
20 Critical Thinking Activities for Elementary Classrooms
April 1, 2023 // by Seda Unlucay
With the barrage of mainstream news, advertising, and social media content out there, it’s vital for students to think independently and learn to differentiate between fact and fiction.
This series of critical thinking activities, STEM-based design challenges, engaging Math puzzles, and problem-solving tasks will support students in thinking rationally and understanding the logical connection between concepts.
1. Teach Students How to Obtain Verifiable News
There’s probably no 21st-century skill more important than differentiating between real and fake sources of news. This editable PowerPoint bundle covers traditional media, social networks, and various target audiences and teaches students how to find verifiable facts.
Learn More: Teachers Pay Teachers
2. Watch and Discuss a Critical Reasoning Video
This kid-friendly video teaches students to break arguments down into claims, evidence, and reasoning. Armed with this lifelong learning tool, they will be able to make more informed decisions when consuming all types of information.
Learn More: Brain Pop
3. Complete a Critical Design Challenge
This science and designed-based classroom activity challenges students to find ways to prevent a falling egg from breaking. Pairing it with the classic Humpty Dumpty nursery rhyme is sure to inspire many creative ideas.
Learn More: Education
4. Critical Community Engagement Activity
This community engagement activity requires analytical skills to determine what items can be recycled in the classroom and in their neighborhood. By creating recycling bins from reusable cardboard boxes, students have an opportunity to contribute to the environmental well-being of their community while practicing social responsibility.
Learn More: Kaboom
5. Develop Logical Skills with a Then and Now Activity
We may no longer use candles for reading or quill pens for writing, but can your students identify the objects that have replaced them? This activity engages their writing, drawing, and logical skills while giving them a chance to reflect on all the changes in our modern world.
Learn More: Education
6. Play a Critical Thinking Game
This active learning activity requires students to use their critical thinking skills to make comparisons and create meaningful analogies. The fun animal safari theme is sure to inspire many funny and creative ideas!
7. Develop Social-Emotional Problem-Solving Skills
Through this lesson, students will understand that while conflicts are a normal part of life, it’s vital to have problem-solving skills to resolve them. This is also an excellent opportunity for developing their social awareness and relationship skills.
Learn More: ED Foundations
8. Desert Island Survival Game
This classic game is sure to inspire student engagement, as they use their critical thinking skills to survive being stranded on a desert island. Students have to watch out for ideological assumptions and question ideas in order to determine the appropriate items to bring.
9. Play a Problem-Solving Treasure Hunt Game
This exciting game for kids requires them to use key math skills to break a series of codes. With ample time, designated progress monitors, and sharp critical thinking skills, students are sure to find the hidden treasure.
Learn More: Twinkl
10. Use Writing to Increase Critical Empathy
This activity builds writing fluency while giving students a chance to show appreciation for each other. As they reflect emphatically on their classmates’ contributions and character, their base level of kindness and sense of ethical responsibility is bound to increase.
Learn More: Edutopia
11. Learn How to Make Logical Inferences
This activity for kids teaches the critical academic skill of making inferences from a series of texts. Students will surely enjoy playing the role of detective in order to draw their own logical conclusions.
Learn More: Study
12. Think Critically About Cultural Assumptions
This engaging activity for students challenges them to think critically about why people from a variety of cultures decorate their bodies. It helps them to break through cultural assumptions while comparing and contrasting the different forms of hand and body painting around the world.
Learn More: Harmony
13. Big Paper Silent Reflection Activity
After posing some open-ended questions, students silently write their responses with colored markers on large chart paper. After each group has circulated around the room, students can share their critical reflections and learn from the various perspectives of their classmates.
Learn More: Slideshare
14. Watch a TED Video About the Socratic Method
Socrates is one of the forefathers of critical thinking, who focused on making his students thinking visible by questioning their logic and reasoning. The accompanying quiz and discussion questions are an excellent way to reinforce student learning.
Learn More: Ted Ed
15. Brainstorm Ways to Help a Homeless Person
This lesson in civic responsibility teaches students about the causes of homelessness and guides them to find ways to help the homeless in their communities. It develops key problem-solving skills while building critical empathy.
Learn More: National Homeless.org
16. Guess the Object Game
This video features a series of twenty zoomed-in mystery objects. Students will love using their critical thinking skills to guess each one!
Learn More: Andy – The ESL Guy
17. Solve Some Challenging Math Brain Teasers
This abundant series of brain teasers is the perfect choice if you’re looking to test your children’s memory and problem-solving skills. Encourage them to use their knowledge of numbers to complete these tricky math problems that are not only designed to challenge your little brainiacs but are also compiled in an easy-to-use format.
Learn More: Mental Up
18. Complete a STEM Elevator Challenge
In this design and engineering-based lesson, students have to build a functional elevator that can carry an object to the top of a structure. It’s a terrific way to encourage cooperative learning while sharpening their problem-solving skills.
Learn More: Georgia Youth Science and Technology Centers
19. Create the Perfect Farm
There’s no better way to develop critical thinking skills than by solving real-world problems. This video encourages students to think about ways to feed a growing global population in an environmentally sustainable way.
20. Solve Logic Grid Puzzles
These logic grid puzzles will motivate students to use logical reasoning skills and the process of elimination to solve a series of clues. But be warned, they are highly addictive and difficult to put down once you get started!
Learn More: Puzzle Baron’s Logic Puzzles
10 Early Finisher Activities in Science: Igniting Curiosity and Critical Thinking
by Katrina | Nov 2, 2023 | Lesson Ideas
In every science classroom, there are students who zoom through tasks with lightning speed, leaving their peers in the dust. While their efficiency is commendable, it’s important to ensure that these early finishers remain engaged and challenged. That’s where a well-curated arsenal of early finisher activities comes into play.
In this blog post, we’ll explore some of the best early finisher activities in science that will not only keep these eager learners occupied but also foster a deeper understanding of scientific concepts.
Early Finisher Activities in Science

Disclaimer: This blog post, ‘Early Finisher Activities in Science’, may contain affiliate links or links to resources that I have created. Read full disclaimer here . activ
Best Early Finisher Activities in Science
1. crosswords: unraveling scientific terminology.
Crossword puzzles are a fantastic way to reinforce scientific vocabulary. By providing a grid filled with clues related to a particular topic (e.g., the periodic table or cell biology), early finishers get to apply their knowledge in a fun and engaging manner. Not only does this activity improve their grasp of terminology, but it also enhances their cognitive skills and critical thinking. Grab some ready to go crosswords here.
2. Thinking Puzzles/Einstein Puzzles: A Mental Workout
Einstein puzzles, known for their logic-based challenges, are an excellent way to stimulate critical thinking in students. These puzzles often involve a set of clues that lead to a single solution. Early finishers can spend their time dissecting the information, making connections, and honing their deductive reasoning skills. This activity not only entertains but also nurtures a scientific mindset. See an example of an Einstein Puzzle here.
3. Scientific Method Review: Inquiry
Engaging early finishers in a review of the scientific method is a fruitful endeavor. Provide students with experiment examples and challenge them to identify the steps of the scientific method involved. Encourage them to consider questions like: What is the hypothesis? What are the variables? How can the experiment be improved? This activity not only reinforces essential scientific skills but also encourages a deeper understanding of the scientific process.
My favorite no-prep early finisher activities

4. Design Your Own Experiment: Fostering Creativity and Inquiry
Encourage early finishers to step into the shoes of a scientist and design their own experiments. Provide them with a basic framework or allow them to choose a topic of interest. This activity empowers students to think critically about variables, controls, and methodologies, while also promoting creativity and independent inquiry. It’s a hands-on way to nurture their scientific curiosity.
5. Escape Rooms: Collaborative Problem-Solving
Bring an element of excitement and teamwork into the classroom by introducing science-themed escape rooms. These immersive challenges require students to solve a series of puzzles and riddles to “escape” from a scenario. Early finishers can work together, applying their scientific knowledge and problem-solving skills to crack codes, decipher clues, and ultimately succeed in the mission. This activity not only promotes collaboration but also reinforces scientific concepts in a dynamic and memorable way.
Grab a FREE digital escape room below by signing up to my email list!
Or grab some topic specific digital escape rooms here.
6. Practice scientific variable skills
Understanding scientific variables are so important for progression into senior sciences. Help students to review their understanding and practice identifying scientific variables in a variety of scenarios.
What to do:
- Provide students with a set of scenarios or experiments and ask them to identify and label the independent, dependent, and controlled variables. You can use real-world examples or create hypothetical situations for them to work through.
- Encourage them to think critically about how changing one variable affects the others. This can be done through written responses or discussions in pairs or small groups.
- Offer a mix of straightforward scenarios and more complex ones to challenge students at different levels of understanding.
- If you want a ready to go resource check this one out with a Train your pet dragon theme.

Want a FREE digital escape room?
Enter your email address here and I'll send you a FREE no-prep digital escape room on the topic 'Scientific method & science equipment'.
You have successfully joined our subscriber list.
We will not send you spam.
You can unsubscribe at any time.
7. Create a Kahoot or Quizlet
Have students create a Kahoot or Quizlet to test or reinforce the content. When its finished you can play it as a class!
- Explain to students that they will be responsible for creating a quiz to review the content covered in class. This can be done individually or in pairs/small groups.
- Provide clear guidelines for the quiz format and content. For example, specify the number of questions, the types of questions (multiple choice, true/false, short answer, etc.), and the topics to be covered.
- Have them include explanations or hints for each answer to ensure they understand the material.
- Once completed, allow the student(s) to share their Kahoot or Quizlet with the class for a fun and interactive review session.
8. Explore Virtual Labs
If available, direct them to online virtual labs or simulations related to the topic. PhET simulations or labXchange are a good place to start.
- Direct students to online platforms that offer virtual labs or simulations related to the current topic. PhET and labXchange are reliable sources for interactive science experiences.
- Provide specific instructions for what they should focus on during the virtual lab, such as observing specific phenomena, making predictions, or drawing conclusions based on the data they collect.
- Ask students to record their observations and findings in a lab report format, including variables, procedures, data, and conclusions.
9. Research project
Assign a mini research project related to the current topic. Students can investigate a specific aspect in more detail. This could be as small as answering one specific question, or creating a presentation or poster for a topic.
- Assign a focused research task related to the current topic. Provide clear guidelines on the scope of the project, including the specific question or aspect they should investigate.
- Encourage students to use reliable sources such as scientific journals, reputable websites, and books. Emphasize the importance of proper citation and referencing.
- Depending on the complexity of the topic, students can create a presentation, poster, or written report to share their findings with the class.
- Allow time for students to present their research to the class, fostering a culture of sharing and learning from peers.
Want some ideas? Here is a FREE resource containing 20 science research ideas for early finishers .
10. Peer Tutoring
Peer tutoring is a collaborative learning activity where students work together in pairs or small groups to help each other understand and master the material. In this case, students who finish early will have the opportunity to assist their peers who may be struggling with the concepts.
- Observe the class and identify students who may benefit from extra help in understanding the material. Pair them with students who have demonstrated proficiency in the topic.
- Clearly communicate the purpose and benefits of peer tutoring. Emphasize that both the tutor and the tutee can benefit from this collaborative learning experience.
- Provide guidelines for how the peer tutoring session should be conducted. For example, encourage active listening, clear explanations, and patience.
Don’t get stuck next time your student’s finish work early. Have these ideas ready to go so you’re students can keep learning and you don’t have to come up with ideas on the spot!
Comment below with your favorite early finisher activities in science.
About the Author

Katrina Harte is a multi-award winning educator from Sydney, Australia who specialises in creating resources that support teachers and engage students.
Recent posts.

Check out my best selling no-prep lesson activities!
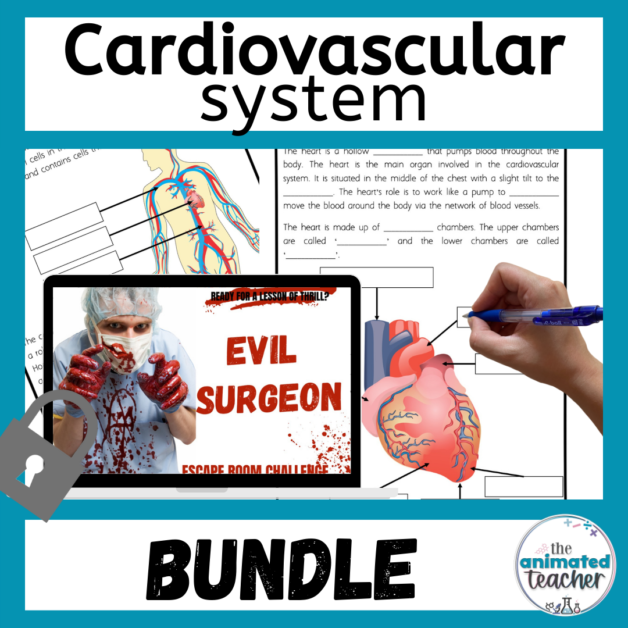
⭐ Texas educators: our K-5 Tech Apps curriculum is a state-approved instructional material. Learn More .
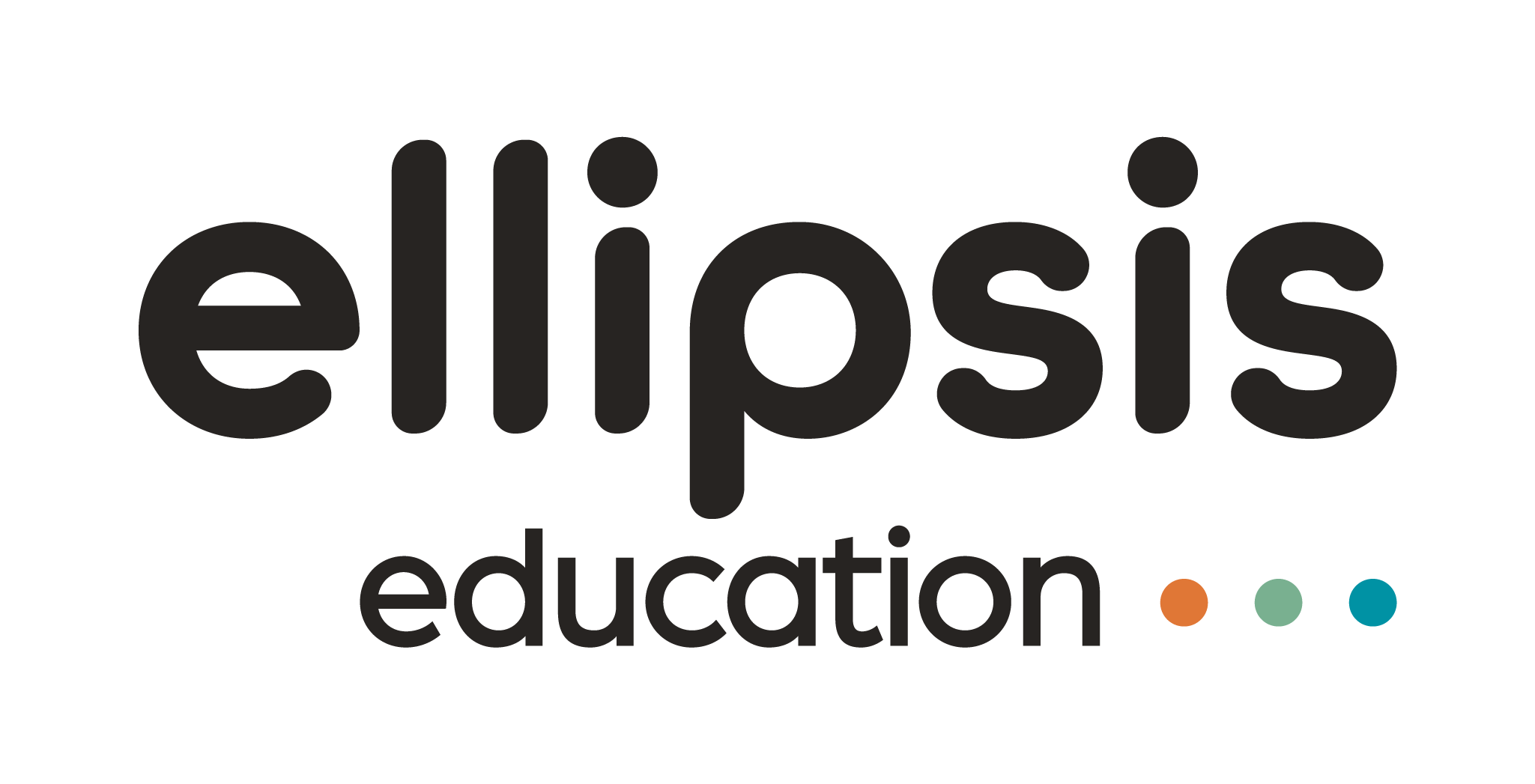
- Vision / Mission
- Leadership Team
- Computer Science Courses
- Curriculum Delivery Platform
- Texas Technology Applications
- Curriculum Training
- Professional Development Workshops
- Computer Science Resources
- Computer Science Lesson Plans
- Computer Science Webinars
- Computer Science Library
- Take a Look
- Schedule a Demo
Critical Thinking Activities for Students
Critical thinking is an important skill for students, and computer science is a great way to introduce it. Ellipsis Education has computer science curriculum for all age levels. We put learning in context so a teacher, not a machine, helps students connect apply technology skills in their lives.
- Critical Thinking Curriculum
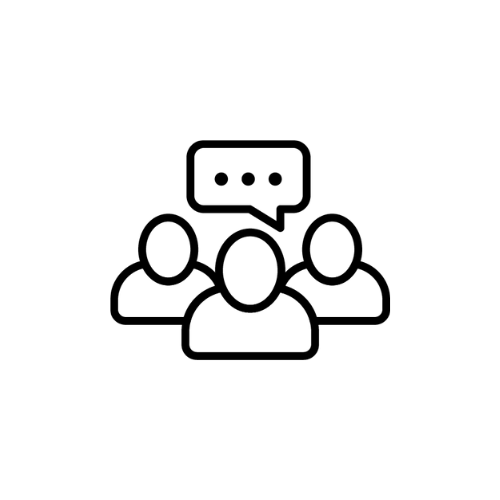
Any Teacher Can Teach
From scripted lesson plans to robust training to continuous learning, Ellipsis helps teachers build confidence and capacity.
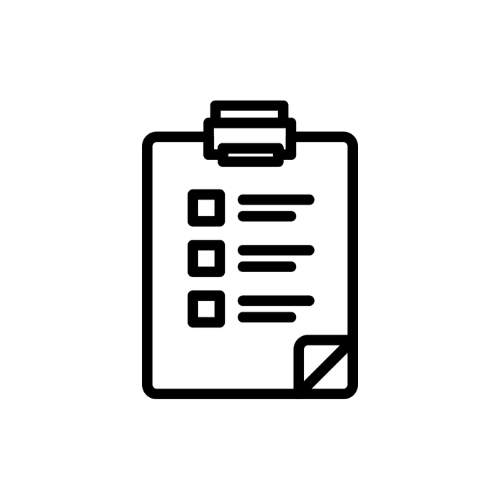
Everything in One Place
The Curriculum Delivery Platform houses all your computer science lessons – no more piecing together free resources.
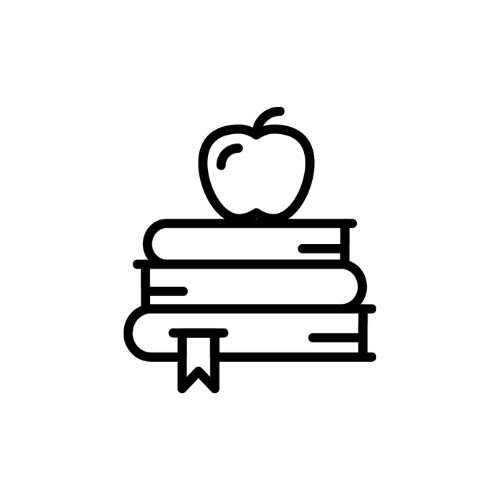
Teacher-Led
Just like any reading or math curriculum, Ellipsis leverages your best resource: teachers.
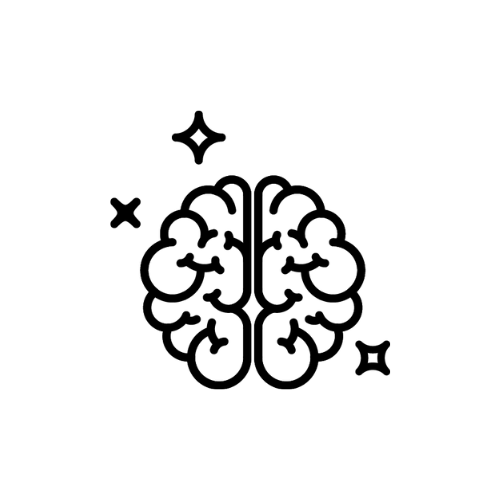
Beyond Coding
Ellipsis lessons develop the attitudes, knowledge, and skills necessary to thrive – in academic settings and beyond.
K-12 Computer Science Curriculum
Critical Thinking Activities
Download a free lesson plan from Ellipsis Education to use in your classroom.
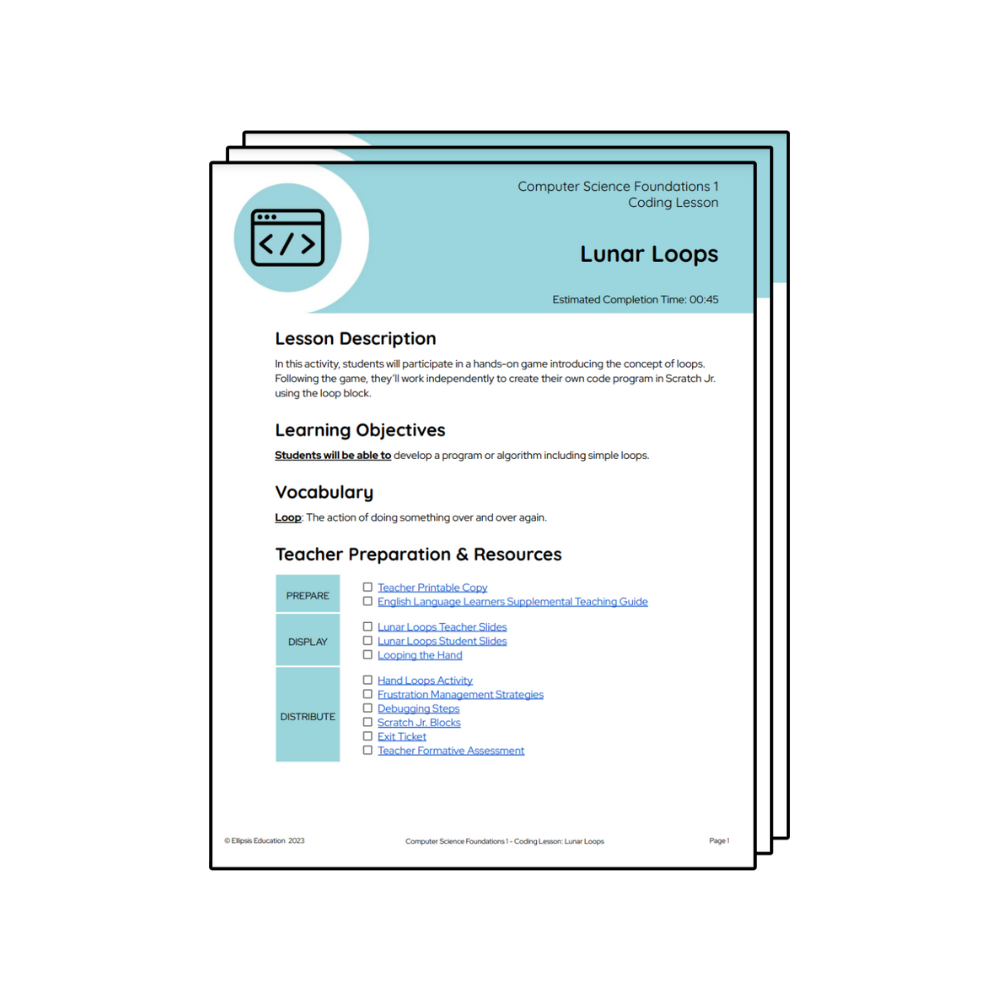
Lunar Loops
In Lunar Loops, students will participate in a hands-on game introducing the concept of loops.
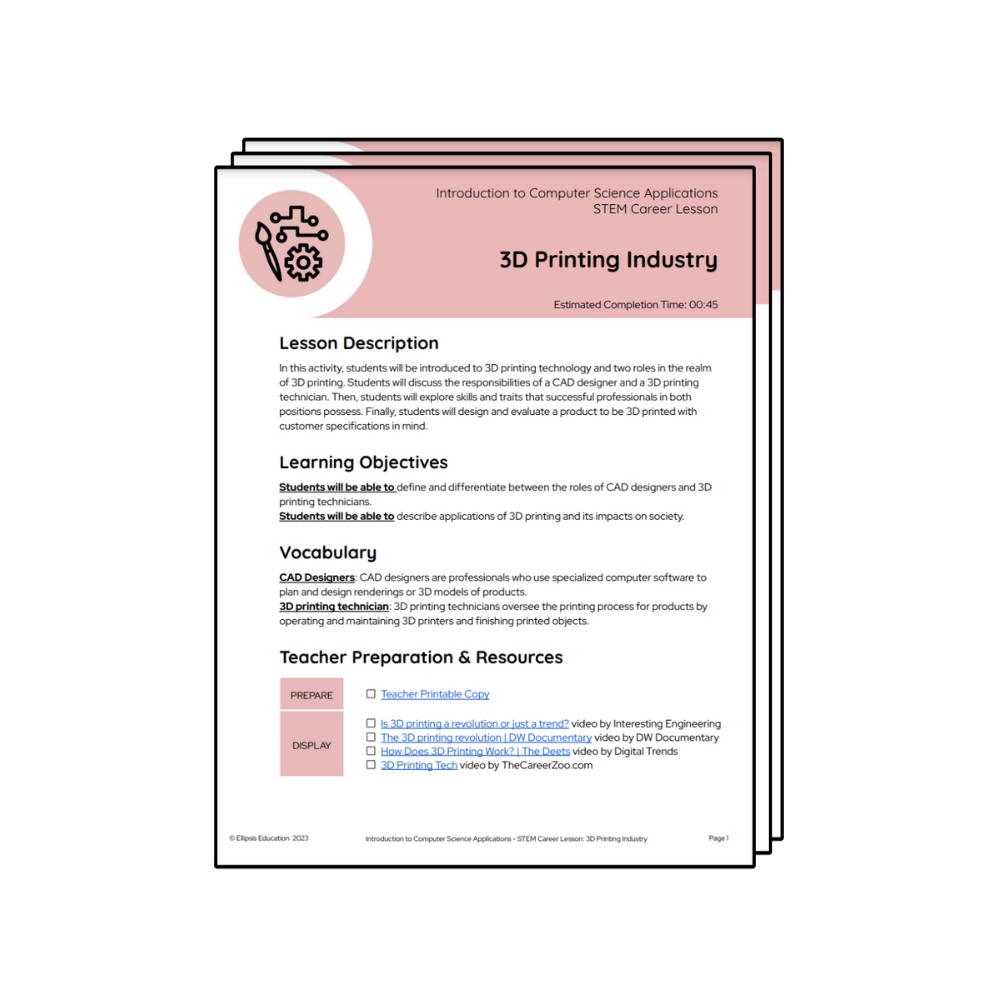
3D Printing Industry
In 3D Printing Industry, students explore roles within the industry and design & evaluate a product.
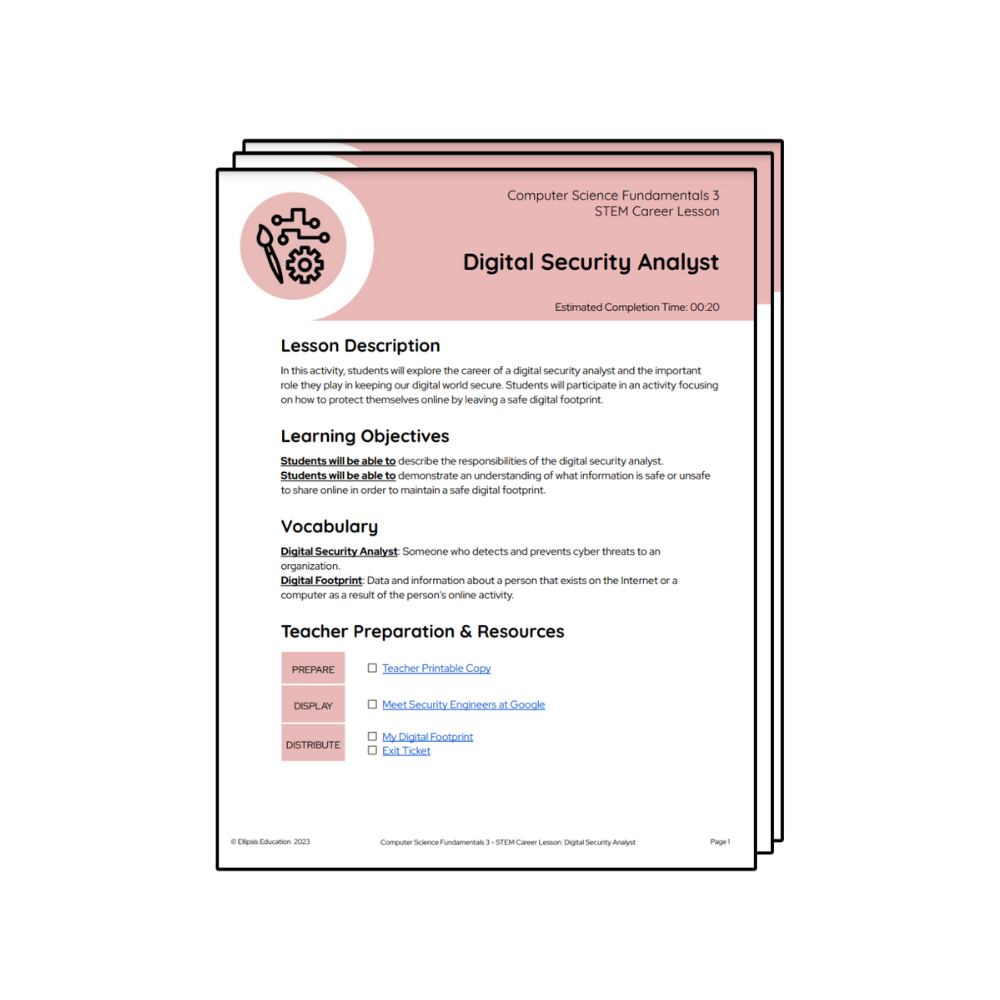
Digital Security Analyst
In Digital Security Analyst, students learn about Digital Security Analysts and how to leave a good digital footprint.
Ready to develop your students’ critical thinking skills?
Computer science courses from Ellipsis Education can help. We e nsure teachers have the curriculum, resources, and support they need to confidently teach computer science – and computational thinking.
Critical thinking is a skill that even the most advanced educators can struggle with teaching. However, it is a crucial part of learning, especially in an increasingly digital and interconnected world.
Training students to think critically can empower them to navigate academic and everyday life more effectively. With various strategies and practices at teachers’ disposal, fostering this in-demand skill can be an achievable goal within any classroom setting.
To help teachers implement critical thinking exercises in their curriculum, here are several critical thinking activities for students of all years.
Class Discussions
This encourages students to engage with their peers, analyze different perspectives, and deepen their understanding. Teachers can introduce diverse topics relevant to the curriculum or current affairs, provoking thought and discussion. You can find a comprehensive list of excellent class discussion topics here. This is one of the best critical thinking activities for students in the classroom, as it provides a safe space to explore significant issues.
Brainstorming Exercises
Similarly, brainstorming serves as an excellent platform for students to bounce ideas off each other, providing a sense of open-mindedness and enhancing problem-solving skills. Use a digital mind mapper like Popplet to allow your students to visualize problems during these critical thinking activities.
Case Studies
Utilizing case studies relevant to the course material can cultivate critical thinking by encouraging students to analyze, interpret, and apply knowledge. Professors can come up with hypothetical scenarios or use real-life examples. This is particularly relevant to critical thinking activities for university students in the classroom.
One of the best confidence-boosting critical thinking activities for students is debating. A structured argument where students defend their viewpoints can stimulate higher-level thinking. This practice nurtures critical thinking and cultivates public speaking and persuasive skills. Make sure to tailor debate topics for younger and older students.
Problem-Solving Activities
Strategic problem-based tasks can foster critical thinking by encouraging students to devise solutions to complex problems. Teachers can incorporate breakout sessions, puzzles, and riddles into these activities. Check out these great riddles you can use in the morning to get your students in the critical thinking mindset.
These interactive critical thinking activities place students in diverse scenarios and persuade them to think from a different perspective. It encourages empathy and improves problem-solving abilities. For inspiration, check out these great role-playing classroom games.
Along with the links provided, you can find these activities on various online educational platforms, such as Edutopia , along with practical guidance on how to implement them in the classroom. Additionally, the Critical Thinking Community shares a series of lessons and strategies designed to aid teachers in fostering this skill in their classroom.
Remember, embedding critical thinking activities into the curriculum is not an extra task but instead supports and enhances students’ understanding of the content dealt with. It is about teaching students to receive information and process, analyze, question, and apply it, giving them a solid foundation to navigate the world responsibly.
Nurturing critical thinking can result in a more engaged, confident, and continuous classroom learning environment. Whether through debates, problem-solving exercises, or role-play, each activity listed offers a unique chance to enrich the educational experience and inspire students to analyze, question, and solve.
What is Critical Thinking?
Critical thinking is an essential skill that plays a starring role in cognitive development. It signifies the capacity to analyze facts to form a judgment. It’s the cornerstone of rational thoughts, rational actions, and the ability to understand how all ideas and concepts connect.
In an educational context, especially in K-12 schools, fostering critical thinking skills in students holds immense value. The importance of critical thinking for students stretches beyond simply improving academic performance. It helps students to develop a versatile mind capable of comprehending complex concepts, making informed decisions, solving problems, and navigating the complexities of today’s information-heavy society.
Beyond academia, critical thinking empowers students to dissect various perspectives to discern the most rational views.
Unfortunately, there are common misconceptions surrounding teaching critical thinking, specifically within computer science education. For instance, some believe that computer science teaching can be delayed until high school. In reality, students require regular introductions to critical thinking-based computer science concepts from an early age.
It’s also a misconception that specialized knowledge or certifications are vital to teaching computer science. Truthfully, any competent educator equipped with an empathic approach, solid curriculum, and strong support can teach critical thinking through computer science appropriately.
The idea that computer science is exclusively for “gifted and talented” students is far from reality. Regardless of their status, every student stands to gain enormously from learning and incorporating critical thinking in their everyday life.
Lastly, computer science is not a code-dominated field. On the contrary, it’s about empowering students to apply critical thinking to navigate and understand a progressively digital world responsibly. And far from being just another demanding subject, it perfectly complements contemporary education priorities. Therefore, the inclusion of critical thinking through computer science in the K-12 curriculum is not only significant but indispensable.
Types of Critical Thinking Activities
When it comes to enhancing the minds of K-12 students, types of critical thinking activities play an essential role. These activities are not just haphazard games or exercises; they’re specially designed tools and strategies to prompt students to question, critique, ascertain, and create instead of just passively perceiving what they learn.
Teachers can incorporate plenty of easy critical thinking activity examples in the classroom. To help uncover the potential of critical thinking, we should analyze various types of critical thinking activities.
Critical Thinking Games
Educators have a rich array of options in the realm of critical thinking games. These games are designed to facilitate critical thinking while being engaging and enjoyable. For instance, games like chess and strategy-based board games push students to plan several steps ahead, understand opponents’ strategies, and adapt their moves accordingly. Puzzle games stimulate logical thinking and pattern identification, while deduction games like ‘Clue’ offer hypothesis testing and logical reasoning opportunities.
Problem-Solving
When it comes to problem-solving activities, educators can employ fun critical thinking activities to improve students’ abilities to make decisions. Engaging students in real-world problem-solving scenarios encourages them to analyze issues, consider alternative solutions, and make informed decisions. For instance, presenting students with complex puzzles challenges them to think logically and develop creative approaches to find solutions. Similarly, collaborative projects that require students to address multifaceted challenges provide a platform for them to apply problem-solving skills in a team setting.
Creativity and Innovation
Fostering creativity and innovation in students involves utilizing activities that stimulate unconventional thinking and idea generation. Implementing brainstorming sessions allows students to explore a variety of perspectives and generate novel ideas collectively. Creative problem-solving exercises encourage students to approach challenges with an open mind, fostering innovative thinking. By introducing structured creativity activities, teachers provide students with frameworks to think divergently and consider alternative solutions.
Collaboration
Collaboration is a cornerstone of critical thinking, and educators can enhance this skill through purposeful activities. Group projects allow students to work together, combining diverse perspectives to achieve common objectives. Jigsaw activities, where each group focuses on a specific aspect of a broader topic, encourage collaboration as students synthesize their collective knowledge. Engaging in collaborative critical thinking activities strengthens problem-solving skills and nurtures essential interpersonal skills.
Metacognition
Metacognition, the ability to reflect on one’s thinking processes, is one of the most powerful critical thinking exercises for students. Teachers can integrate metacognitive strategies into classroom activities to encourage self-awareness and continuous improvement. Self-assessment prompts students to evaluate their thinking strategies, identify strengths, and pinpoint areas for growth. Goal-setting exercises guide students in establishing specific objectives for their critical thinking development. By incorporating metacognitive elements into various activities, educators empower students to become more aware of their cognitive processes, fostering a habit of introspection and self-directed learning.
Teachers must remember that cultivating a culture of critical thinking extends beyond just academic benefits. Critical thinking activities induce adaptability, empathy, and resilience. These lifelong skills enable K-12 students to navigate academic challenges and life obstacles just as effectively. Educators can create an enriching learning environment that facilitates comprehensive skill development by employing critical thinking games, fun activities, and targeted exercises in the classroom.
Implementing Critical Thinking Activities in Education
Implementing critical thinking activities in education is a pivotal cornerstone in molding young minds, especially within the realm of computer science.
While classroom games are essential for critical thinking in schools, the addition of computer science brings a modern, relevant aspect that sets students up for the world beyond their education. Teaching critical thinking becomes a seamless addition to a powerful subject in a way that engages students meaningfully.
By weaving critical thinking into a comprehensive computer science curriculum, educators have the opportunity to sculpt a learning environment that nurtures analytical prowess and problem-solving acumen.
Embracing project-based learning, instructors can task students with real-world problems, beckoning them to apply coding skills to tangible scenarios. Adding ethical discussions, entwined with case studies as part of a critical thinking curriculum, encourages students to delve into the societal implications of technology. Collaborative software development projects mirror industry practices, nurturing teamwork and project management skills.
From debugging challenges that demand systematic issue resolution to simulations modeling real-world systems, students engage in hands-on problem-solving. Reflective journals and documentation instill the habit of introspection, while debates on programming paradigms encourage a critical evaluation of different approaches.
By thoughtfully integrating these activities of critical thinking in education, teachers shape a curriculum that not only imparts technical proficiency but cultivates a profound understanding of the ethical, collaborative, and systemic dimensions inherent in computer science.
At Ellipsis Education, we provide a robust platform for educators to integrate influential learning materials into their teaching regimen seamlessly. Our emphasis extends beyond just coding; we believe in nurturing students to become responsible digital citizens who navigate the continually evolving digital world with wisdom and forethought.
Any teacher can effectively impart computer science education through the right critical thinking curriculum and support.
Related Links
- Computational Thinking Elementary School
- Computational Thinking Examples
- Computational Thinking Curriculum
- Computational Thinking Questions
- Critical Thinking In Education
- Importance Of Critical Thinking For Students
- Computational Thinking Activities For Kindergarten
- Critical Thinking For Students
Computer science, taught by teachers.
JavaScript seems to be disabled in your browser. For the best experience on our site, be sure to turn on Javascript in your browser.
- Order Tracking
- Create an Account

200+ Award-Winning Educational Textbooks, Activity Books, & Printable eBooks!
- Compare Products
Reading, Writing, Math, Science, Social Studies
- Search by Book Series
- Algebra I & II Gr. 7-12+
- Algebra Magic Tricks Gr. 2-12+
- Algebra Word Problems Gr. 7-12+
- Balance Benders Gr. 2-12+
- Balance Math & More! Gr. 2-12+
- Basics of Critical Thinking Gr. 4-9
- Brain Stretchers Gr. 5-12+
- Building Thinking Skills Gr. Toddler-12+
- Building Writing Skills Gr. 3-7
- Bundles - Critical Thinking Gr. PreK-9
- Bundles - Language Arts Gr. K-8
- Bundles - Mathematics Gr. PreK-9
- Bundles - Multi-Subject Curriculum Gr. Toddler-12+
- Bundles - Test Prep Gr. Toddler-12+
- Can You Find Me? Gr. PreK-1
- Complete the Picture Math Gr. 1-3
- Cornell Critical Thinking Tests Gr. 5-12+
- Cranium Crackers Gr. 3-12+
- Creative Problem Solving Gr. PreK-2
- Critical Thinking Activities to Improve Writing Gr. 4-12+
- Critical Thinking Coloring Gr. PreK-2
- Critical Thinking Detective Gr. 3-12+
- Critical Thinking Tests Gr. PreK-6
- Critical Thinking for Reading Comprehension Gr. 1-5
- Critical Thinking in United States History Gr. 6-12+
- CrossNumber Math Puzzles Gr. 4-10
- Crypt-O-Words Gr. 2-7
- Crypto Mind Benders Gr. 3-12+
- Daily Mind Builders Gr. 5-12+
- Dare to Compare Math Gr. 2-7
- Developing Critical Thinking through Science Gr. 1-8
- Dr. DooRiddles Gr. PreK-12+
- Dr. Funster's Gr. 2-12+
- Editor in Chief Gr. 2-12+
- Fun-Time Phonics! Gr. PreK-2
- Half 'n Half Animals Gr. K-4
- Hands-On Thinking Skills Gr. K-1
- Inference Jones Gr. 1-6
- James Madison Gr. 8-12+
- Jumbles Gr. 3-5
- Language Mechanic Gr. 4-7
- Language Smarts Gr. 1-4
- Mastering Logic & Math Problem Solving Gr. 6-9
- Math Analogies Gr. K-9
- Math Detective Gr. 3-8
- Math Games Gr. 3-8
- Math Mind Benders Gr. 5-12+
- Math Ties Gr. 4-8
- Math Word Problems Gr. 4-10
- Mathematical Reasoning Gr. Toddler-11
- Middle School Science Gr. 6-8
- Mind Benders Gr. PreK-12+
- Mind Building Math Gr. K-1
- Mind Building Reading Gr. K-1
- Novel Thinking Gr. 3-6
- OLSAT® Test Prep Gr. PreK-K
- Organizing Thinking Gr. 2-8
- Pattern Explorer Gr. 3-9
- Practical Critical Thinking Gr. 9-12+
- Punctuation Puzzler Gr. 3-8
- Reading Detective Gr. 3-12+
- Red Herring Mysteries Gr. 4-12+
- Red Herrings Science Mysteries Gr. 4-9
- Science Detective Gr. 3-6
- Science Mind Benders Gr. PreK-3
- Science Vocabulary Crossword Puzzles Gr. 4-6
- Sciencewise Gr. 4-12+
- Scratch Your Brain Gr. 2-12+
- Sentence Diagramming Gr. 3-12+
- Smarty Pants Puzzles Gr. 3-12+
- Snailopolis Gr. K-4
- Something's Fishy at Lake Iwannafisha Gr. 5-9
- Teaching Technology Gr. 3-12+
- Tell Me a Story Gr. PreK-1
- Think Analogies Gr. 3-12+
- Think and Write Gr. 3-8
- Think-A-Grams Gr. 4-12+
- Thinking About Time Gr. 3-6
- Thinking Connections Gr. 4-12+
- Thinking Directionally Gr. 2-6
- Thinking Skills & Key Concepts Gr. PreK-2
- Thinking Skills for Tests Gr. PreK-5
- U.S. History Detective Gr. 8-12+
- Understanding Fractions Gr. 2-6
- Visual Perceptual Skill Building Gr. PreK-3
- Vocabulary Riddles Gr. 4-8
- Vocabulary Smarts Gr. 2-5
- Vocabulary Virtuoso Gr. 2-12+
- What Would You Do? Gr. 2-12+
- Who Is This Kid? Colleges Want to Know! Gr. 9-12+
- Word Explorer Gr. 6-8
- Word Roots Gr. 3-12+
- World History Detective Gr. 6-12+
- Writing Detective Gr. 3-6
- You Decide! Gr. 6-12+

Middle School Science
Key concepts, practice, and quizzes.
Grades: 6-8
Critical Thinking, Science
This 512 page book for Grades 6-8 provides a concise and useful understanding of middle school general science. It offers definitions, instruction, examples, practice reviews, and practice tests. It can be used as a comprehensive review, for tutoring, as a reference for helping students with homework, for test prep, or as a complete homework guide and supplement to another middle school general science textbook. It is also well suited for use in Adult Basic Education programs.
- Each concept, term, phrase, etc. has a clear definition followed by examples.
- Easily locatable key terms, definitions, properties, phrases, concepts,formulas, rules, etc.
- Clear step-by-step procedures for applying theorems.
- A concise study guide, that gets to the heart of each particular topic,providing students with a quick review before doing homework or preparing for a test.
- Clear and easy-to-understand written format and style. Materials are presented in both visual and color formats with less text and more tables, outlines, boxes, charts, etc.
- Tables that organize and summarize key terms, procedures, methods,equations, etc. clearly presenting information to make studying more effective.
- A summary at the end of each chapter conveniently emphasizes the key points in the chapter for students reviewing before exams.
- Practice sheets and quizzes are in each chapter; students can take the quiz to determine how much they know about the topic. If they do well, they may decide to move on to the next topic without reading the lesson.
Description and Features
All products in this series.
• Our eBooks digital, electronic versions of the book pages that you may print to any paper printer. • You can open the PDF eBook from any device or computer that has a PDF reader such as Adobe® Reader®. • Licensee can legally keep a copy of this eBook on three different devices. View our eBook license agreement details here . • You can immediately download your eBook from "My Account" under the "My Downloadable Product" section after you place your order.
- Add to Cart Add to Cart Remove This Item
- Special of the Month
- Sign Up for our Best Offers
- Bundles = Greatest Savings!
- Sign Up for Free Puzzles
- Sign Up for Free Activities
- Toddler (Ages 0-3)
- PreK (Ages 3-5)
- Kindergarten (Ages 5-6)
- 1st Grade (Ages 6-7)
- 2nd Grade (Ages 7-8)
- 3rd Grade (Ages 8-9)
- 4th Grade (Ages 9-10)
- 5th Grade (Ages 10-11)
- 6th Grade (Ages 11-12)
- 7th Grade (Ages 12-13)
- 8th Grade (Ages 13-14)
- 9th Grade (Ages 14-15)
- 10th Grade (Ages 15-16)
- 11th Grade (Ages 16-17)
- 12th Grade (Ages 17-18)
- 12th+ Grade (Ages 18+)
- Test Prep Directory
- Test Prep Bundles
- Test Prep Guides
- Preschool Academics
- Store Locator
- Submit Feedback/Request
- Sales Alerts Sign-Up
- Technical Support
- Mission & History
- Articles & Advice
- Testimonials
- Our Guarantee
- New Products
- Free Activities
- Libros en Español
- Understanding Classroom Layout and Design
- Inquiry-Based Learning: An Introduction to Teaching Strategies
- Creative Thinking Skills
- Problem-solving Skills: A Comprehensive Overview
- Classroom Management
- Behavior management techniques
- Classroom rules
- Classroom routines
- Classroom organization
- Assessment Strategies
- Summative assessment techniques
- Formative assessment techniques
- Portfolio assessment
- Performance-based assessment
- Teaching Strategies
- Active learning
- Inquiry-based learning
- Differentiated instruction
- Project-based learning
o2c-library/governance/arc-organisation-reports/final%20report.pdf
- Learning Theories
- Behaviorism
- Social Learning Theory
- Cognitivism
- Constructivism
- Critical Thinking Skills
- Analysis skills
- Creative thinking skills
- Problem-solving skills
- Evaluation skills
- Metacognition
- Metacognitive strategies
- Self-reflection and metacognition
- Goal setting and metacognition
- Teaching Methods and Techniques
- Direct instruction methods
- Indirect instruction methods
- Lesson Planning Strategies
- Lesson sequencing strategies
- Unit planning strategies
- Differentiated Instruction Strategies
- Differentiated instruction for English language learners
- Differentiated instruction for gifted students
- Standards and Benchmarks
- State science standards and benchmarks
- National science standards and benchmarks
- Curriculum Design
- Course design and alignment
- Backward design principles
- Curriculum mapping
- Instructional Materials
- Textbooks and digital resources
- Instructional software and apps
- Engaging Activities and Games
- Hands-on activities and experiments
- Cooperative learning games
- Learning Environment Design
- Classroom technology integration
- Classroom layout and design
- Instructional Strategies
- Collaborative learning strategies
- Problem-based learning strategies
- 9-12 Science Lesson Plans
- Life science lesson plans for 9-12 learners
- Earth science lesson plans for 9-12 learners
- Physical science lesson plans for 9-12 learners
- K-5 Science Lesson Plans
- Earth science lesson plans for K-5 learners
- Life science lesson plans for K-5 learners
- Physical science lesson plans for K-5 learners
- 6-8 Science Lesson Plans
- Earth science lesson plans for 6-8 learners
- Life science lesson plans for 6-8 learners
- Physical science lesson plans for 6-8 learners
- Science Learning
- Analysis Skills: Understanding Critical Thinking and Science Learning
This article provides an overview of the analysis skills necessary for critical thinking and science learning. It offers tips and strategies to help readers develop their analysis skills.
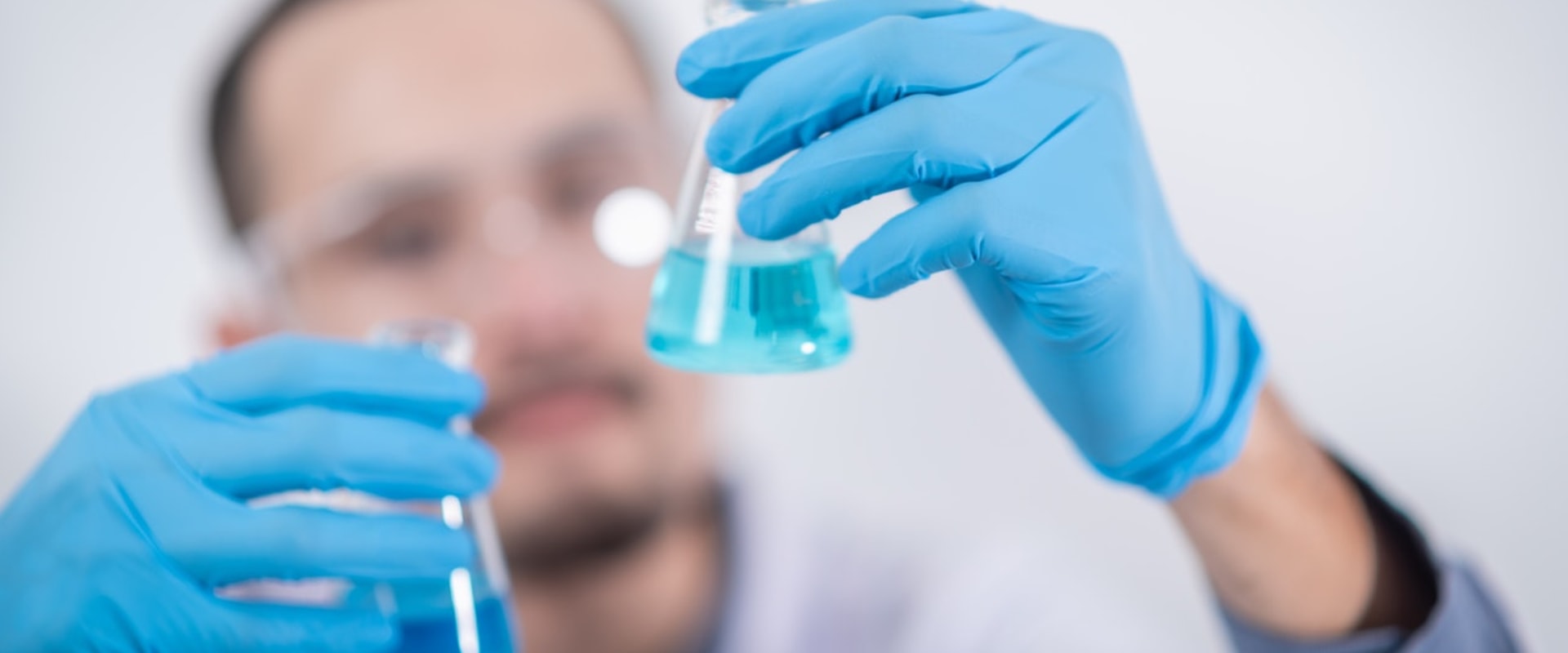
Having strong analysis skills is essential for success in any field, whether it's science, business, or any other field. Critical thinking and science learning are two key components of having these skills. In order to become an expert in any field, one must be able to analyze information and make informed decisions. This article will explore the importance of analysis skills and how to develop them through critical thinking and science learning.
Additionally, it will discuss the benefits of seeking help from Profs online stata tutors to further hone these skills. Critical thinking is the process of making rational judgments about a situation or problem. It involves gathering facts, weighing evidence, and forming conclusions based on what you learn. Science learning refers to the process of acquiring knowledge about scientific theories, experiments, and discoveries. Both of these skills are necessary for gaining a thorough understanding of any topic. This article will explain why it is important to develop both analysis skills and critical thinking.
It will also provide tips on how to use critical thinking and science learning to enhance your analysis skills. Finally, it will discuss the importance of using both skills together in order to achieve better results. Analysis skills are essential for critical thinking and science learning. They involve the ability to think logically, break down problems into smaller parts, identify patterns and relationships, and use data to draw conclusions. Analytical thinking is the process of examining information, breaking it down into smaller components, and understanding how the components are related.
Problem-solving skills involve using a systematic approach to identify solutions to complex problems. Data analysis involves gathering, organizing, and analyzing data in order to draw meaningful conclusions. Having strong analysis skills is important for making informed decisions, solving complex problems, and understanding complex topics. It can help you identify potential consequences of actions, develop alternative solutions, and think critically about different perspectives.
It can also be beneficial when learning new topics or researching different areas of study. There are several strategies that you can use to improve your analysis skills. To improve analytical thinking, try breaking down complex problems into smaller parts and using data to identify patterns or relationships. For problem-solving skills, try brainstorming potential solutions and using a systematic approach to evaluate the options.
To improve data analysis skills, practice organizing data into meaningful categories and interpreting the data to draw meaningful conclusions. Analysis skills can be applied in various contexts. In business, data analysis can help identify trends and make informed decisions. Analytical thinking can be used to solve complex problems or develop new products or services.
In science, problem-solving skills can be used to explore new ideas or research topics. There are a variety of resources available to help you further develop your analysis skills. Books such as The Art of Analyzing Data by John DeKok provide an introduction to data analysis and how to use data for decision-making. Online courses such as Analytical Thinking & Problem Solving by edX provide an in-depth exploration of how to develop problem-solving skills.
Analytical thinking
Data analysis requires the ability to collect, organize, and interpret data in order to draw meaningful conclusions. Having strong analysis skills is important for a variety of reasons. They can help us make better decisions by allowing us to evaluate different options and make informed choices. They can also help us understand complex topics by breaking them down into more manageable pieces.
Finally, they can help us solve complex problems by allowing us to identify patterns, draw connections between different elements, and develop creative solutions. To develop analysis skills, it is important to practice analytical thinking, problem-solving, and data analysis. To improve analytical thinking, it is helpful to break down problems into smaller parts and look for patterns or connections between different elements. To improve problem-solving skills, it is helpful to brainstorm potential solutions and use a systematic approach when evaluating them.
To improve data analysis skills, it is important to collect relevant data, organize it in a meaningful way, and interpret it accurately. Analysis skills can be applied in a variety of contexts. In business, for example, data analysis can be used to identify trends or insights about customer behavior . Analytical thinking can be used to evaluate complex problems and develop creative solutions.
Problem-solving skills can be used to develop new products or services or find ways to improve existing ones. In science learning, analysis skills are essential for understanding complex topics and developing hypotheses that can be tested through experimentation. There are many resources available for those interested in further developing their analysis skills. Books such as The Art of Analytical Thinking , Data Analysis for Beginners , and The Power of Problem-Solving provide useful tips and strategies for improving analytical thinking, problem-solving, and data analysis.
Examples of Analysis Skills
Analytical thinking:, problem-solving skills:, why are analysis skills important, what are analysis skills.
It involves breaking down a problem into its component parts, examining each part in detail, and then using evidence-based logic to solve the problem. Problem-solving is another type of analysis skill that involves identifying the root cause of a problem and developing an effective strategy to address it. Data analysis is another type of analysis skill that involves collecting, analyzing, and interpreting data in order to draw meaningful conclusions. Analysis skills are important because they enable a person to think critically and make informed decisions. These skills are also essential for science learning, as they enable a person to analyze data, identify patterns, and draw conclusions.
How to Develop Analysis Skills
Using data:, systematic approach:, resources for developing analysis skills.
Some books that may be of particular interest include “Analytical Thinking: A Guide to Critical Thinking and Problem Solving” by Paul C. Nuttal, “The Analytic Thinker: Mastering the Skills of Reasoning, Analysis, and Critique” by Michael Starbird, and “The Art of Thinking Clearly: Better Thinking, Better Decisions” by Rolf Dobelli. Online courses can also be a great resource for developing analysis skills. Coursera, edX, and Udemy offer a wide range of courses on analytical thinking, problem-solving, and critical thinking.
These courses can provide an in-depth look at the fundamentals of analytical thinking and how it can be applied in different contexts. Websites can also be a great source of information on analytical thinking and problem-solving. There are many sites dedicated to the subject, such as the Cognitive Science Lab at Stanford University, which offers resources on cognitive psychology, artificial intelligence, and more. The website Thinking Critically by Peter Facione offers a wealth of information on critical thinking, including tips and strategies for developing analysis skills.
This allows for more informed decision-making and analysis. Additionally, analyzing data can help identify patterns and draw conclusions from the information. When looking for patterns, it is important to consider the context in which the data was gathered and how it relates to the overall problem. To improve problem-solving skills, brainstorming can be an effective technique. Brainstorming involves coming up with multiple ideas, without judging them, and then assessing their viability.
Books can be an excellent resource for developing analytical thinking skills. Popular titles include Thinking, Fast and Slow by Daniel Kahneman, The Art of Thinking Clearly by Rolf Dobelli, Thinking Critically by John Chaffee, and The Power of Thinking Differently by Edward de Bono. Online courses are also available to help readers better understand analytical thinking. Popular options include Coursera's Introduction to Logic and Critical Thinking , edX's Analytical Thinking: A Strategic Skill for Leaders , and Udemy's Data Analysis & Visualization in R: Analytical Thinking . Websites can also provide helpful resources for developing analytical thinking skills.
Sites like Khan Academy, which offers free tutorials on topics like logic and problem solving, as well as websites like Brilliant.org, which offers free online courses on a variety of topics, can be great resources for honing analytical skills. Finally, there are many other materials that readers can use to further develop their analytical thinking skills. These can include podcasts, videos, articles, and more. All of these resources can provide insight into different aspects of analytical thinking and help readers hone their skills. Analysis skills are essential for critical thinking and science learning. This article has provided an overview of what analysis skills are, why they are important, and tips and strategies for developing these skills.
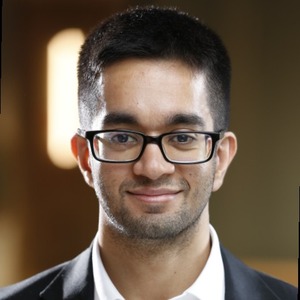
Shahid Lakha
Shahid Lakha is a seasoned educational consultant with a rich history in the independent education sector and EdTech. With a solid background in Physics, Shahid has cultivated a career that spans tutoring, consulting, and entrepreneurship. As an Educational Consultant at Spires Online Tutoring since October 2016, he has been instrumental in fostering educational excellence in the online tutoring space. Shahid is also the founder and director of Specialist Science Tutors, a tutoring agency based in West London, where he has successfully managed various facets of the business, including marketing, web design, and client relationships. His dedication to education is further evidenced by his role as a self-employed tutor, where he has been teaching Maths, Physics, and Engineering to students up to university level since September 2011. Shahid holds a Master of Science in Photon Science from the University of Manchester and a Bachelor of Science in Physics from the University of Bath.
New Articles
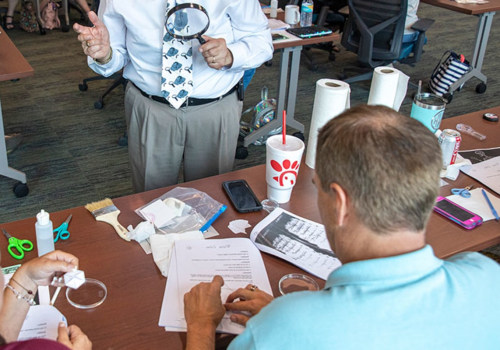
- Physical Science Lesson Plans for 9-12 Learners
Find out about physical science lesson plans for 9-12 learners, including what topics to cover and how to make them engaging and informative.
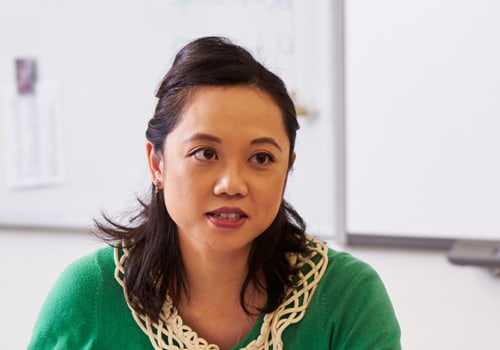
- Evaluation Skills: A Comprehensive Overview
This article provides an overview of evaluation skills, including what they are, why they are important, and how to develop them. It is written for anyone interested in improving their science learning and critical thinking skills.
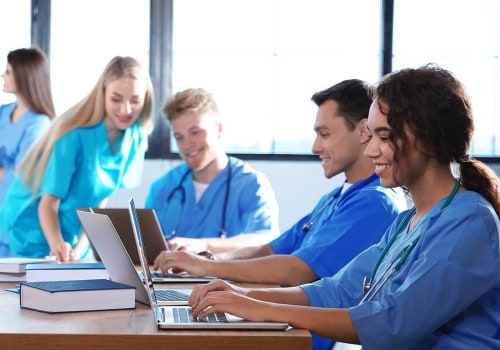
- Active Learning: A Comprehensive Overview
Learn what active learning is and how it can be used as an effective teaching strategy in the classroom.
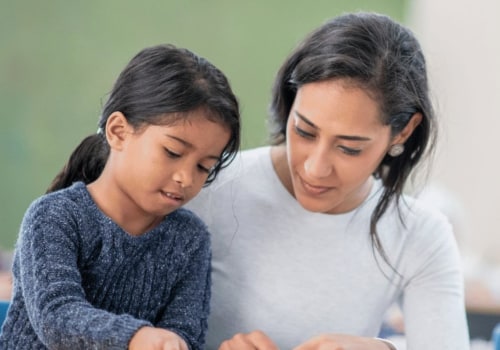
- Summative Assessment Techniques: An Overview
This article provides an overview of summative assessment techniques and how they can be used in the classroom.
Leave Reply
Your email address will not be published. Required fields are marked *
I agree that spam comments wont´t be published
- Behavior Management Techniques
- Behaviorism: A Comprehensive Overview
- Social Learning Theory Explained
- Formative Assessment Techniques
- Understanding Cognitivism: A Learning Theory
Constructivism: Exploring the Theory of Learning
- Classroom Rules - A Comprehensive Overview
- Exploring Portfolio Assessment: An Introduction
- Differentiated Instruction: A Comprehensive Overview
- Classroom Routines: A Comprehensive Overview
- Effective Classroom Organization Strategies for Science Teaching
- Project-Based Learning: An In-Depth Look
- Performance-Based Assessment: A Comprehensive Overview
- Understanding Direct Instruction Methods
- State Science Standards and Benchmarks
- Course Design and Alignment
- The Advantages of Textbooks and Digital Resources
- Engaging Hands-on Activities and Experiments
- An Overview of Metacognitive Strategies
- Backward Design Principles: Understanding Curriculum Design
- Engaging Cooperative Learning Games
- Integrating Technology into the Classroom
- Lesson Sequencing Strategies: A Comprehensive Overview
- Instructional Software and Apps: A Comprehensive Overview
- Understanding Curriculum Mapping
- Collaborative Learning Strategies
- Indirect Instruction Methods: A Comprehensive Overview
- Understanding National Science Standards and Benchmarks
- Exploring Problem-Based Learning Strategies
- Unit Planning Strategies
- Exploring Self-Reflection and Metacognition
- Exploring Goal Setting and Metacognition
- Life Science Lesson Plans for 9-12 Learners
- Earth Science Lesson Plans for K-5 Learners
- Differentiated Instruction for English Language Learners
- Life Science Lesson Plans for K-5 Learners
- Earth Science Lesson Plans for 6-8 Learners
- Earth Science Lesson Plans for 9-12 Learners
- Life Science Lesson Plans for 6-8 Learners
- Physical Science Lesson Plans for K-5 Learners
- Physical Science Lesson Plans for 6-8 Learners
Differentiated Instruction Strategies for Gifted Students
Recent posts.
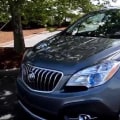
Which cookies do you want to accept?
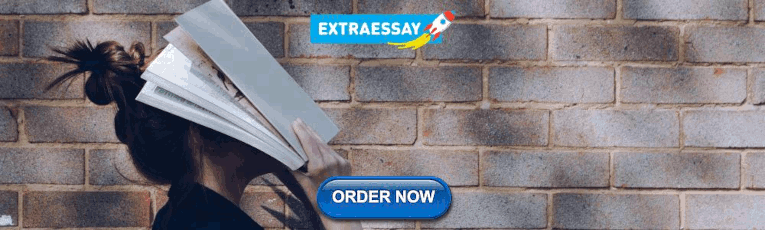
IMAGES
VIDEO
COMMENTS
To understand science, you need to dive in. Roll up your sleeves! Get your hands dirty with activities. So, let's start now! Here are some of my favorite science activities for critical thinking. Why Teach Critical Thinking? Wondering about the importance of teaching critical thinking through analysis, engineering, and exploration? It helps ...
8 Science-Based Strategies For Critical Thinking. 1. Challenge all assumptions. And that means all assumptions. As a teacher, I've done my best to nurture the students' explorative questions by modeling the objective scientific mindset. Regardless of our goals in the teaching and learning process, I never want to squelch the curiosity of ...
Critical thinking is essential in science. It's what naturally takes students in the direction of scientific reasoning since evidence is a key component of this style of thought. It's not just about whether evidence is available to support a particular answer but how valid that evidence is. It's about whether the information the student ...
TED-Ed lessons on the subject Critical Thinking. TED-Ed celebrates the ideas of teachers and students around the world. Discover hundreds of animated lessons, create customized lessons, and share your big ideas. ... The best way to apologize (according to science) Lesson duration 05:06 1,360,523 Views. 06:06. Philosophy How do we determine the ...
With some small tweaks, however, science labs can involve more critical thinking. Science lab activities that give students not only the opportunity to design, analyze, and interpret the experiment, but re-design, re-analyze, and re-interpret the experiment provides ample opportunity for grappling with evidence and evidence-model relationships ...
Scientific inquiry includes three key areas: 1. Identifying a problem and asking questions about that problem. 2. Selecting information to respond to the problem and evaluating it. 3. Drawing conclusions from the evidence. Critical thinking can be developed through focussed learning activities. Students not only need to receive information but ...
Check out these critical thinking activities, adapted from Critical Thinking in the Classroom , a book with over 100 practical tools and strategies for teaching critical thinking in K-12 classrooms. Four Corners. In this activity, students move to a corner of the classroom based on their responses to a question with four answer choices.
For many years, cognitive science has been interested in studying what scientific thinking is and how it can be taught in order to improve students' science learning (Klarh et al., 2019; Zimmerman & Klarh, 2018).To this end, Kuhn et al. propose taking a characterization of science as argument (Kuhn, 1993; Kuhn et al., 2008).They argue that this is a suitable way of linking the activity of ...
Boost Your Student's Critical Thinking with CER in Science Class. CER (Claim, Evidence, Reasoning) is a critical thinking framework that encourages students to analyze evidence and draw conclusions that are thorough and appropriate.. I have pushed my students for years to think "out of the box" while trying to pull data together.
Critical Thinking Science Activities for Classroom Practice. Teachers can design impactful science critical thinking questions that promote analysis, evaluation, and application of concepts. ### Designing Science Critical Thinking Questions. Ask open-ended questions that have multiple potential answers, requiring students to justify their ...
Students also learn and practice critical thinking through the application of the scientific method of investigation. Each activity is a 10- to 30-minute guided experiment in which students are prompted to verbalize their step-by-step observations, predictions, and conclusions. Reproducible pictures or charts are included when needed, but the ...
3.2.1 Creativity and Critical Thinking. Creativity and critical thinking are two distinct but related higher-order cognitive skills. As such, both require significant mental effort and energy; both are cognitively challenging. Creativity aims to create novel, appropriate ideas and products.
6. Start a Debate. In this activity, the teacher can act as a facilitator and spark an interesting conversation in the class on any given topic. Give a small introductory speech on an open-ended topic. The topic can be related to current affairs, technological development or a new discovery in the field of science.
3. Create science cartoon strips: Have students develop creative thinking skills through this writing activity. Examples: After discussing the earlier models of atomic structure, you could ask, "Create a comic strip bringing out the conversation that might have taken place between J.J. Thomson and Ernest Rutherford.".
Jon-Marc Rodriguez and Marcy Towns, researchers at Purdue University, US, recently outlined an approach to modify existing practical activities to promote critical thinking in students, supporting enhanced learning. Jon-Marc and Marcy focused on critical thinking as a skill needed for successful engagement with the eight 'science practices'.
Critical-Thinking Activities Engaging Activities and Reproducibles to Develop Kids' Higher-Level Thinking Skills by Laurie Rozakis ... Then have the class research general information on science, Activity Page Content Area Time Capsule 8 language arts Mind Squeeze 9 language arts Trivia Trackdown 10 math, science/social studies ...
This framework makes clear that science reasoning and critical-thinking skills play key roles in major learning outcomes; for example, "understanding the process of science" requires students to engage in (and be metacognitive about) scientific reasoning, and having the "ability to interpret data" requires critical-thinking skills.
This activity engages their writing, drawing, and logical skills while giving them a chance to reflect on all the changes in our modern world. 6. Play a Critical Thinking Game. This active learning activity requires students to use their critical thinking skills to make comparisons and create meaningful analogies.
Not only does this activity improve their grasp of terminology, but it also enhances their cognitive skills and critical thinking. Grab some ready to go crosswords here. 2. Thinking Puzzles/Einstein Puzzles: A Mental Workout. Einstein puzzles, known for their logic-based challenges, are an excellent way to stimulate critical thinking in students.
It makes you a well-rounded individual, one who has looked at all of their options and possible solutions before making a choice. According to the University of the People in California, having critical thinking skills is important because they are [ 1 ]: Universal. Crucial for the economy. Essential for improving language and presentation skills.
Implementing critical thinking activities in education is a pivotal cornerstone in molding young minds, especially within the realm of computer science. While classroom games are essential for critical thinking in schools, the addition of computer science brings a modern, relevant aspect that sets students up for the world beyond their education.
Critical Thinking, Science. This 512 page book for Grades 6-8 provides a concise and useful understanding of middle school general science. It offers definitions, instruction, examples, practice reviews, and practice tests. ... Critical Thinking Activities to Improve Writing Gr. 4-12+ Critical Thinking Coloring Gr. PreK-2 ; Critical Thinking ...
Analysis skills are essential for critical thinking and science learning, as they enable a person to analyze data, identify trends, and make well-reasoned arguments. Analytical thinking is a type of analysis skill that involves logical reasoning, problem-solving, and critical thinking.