- Open access
- Published: 28 May 2022
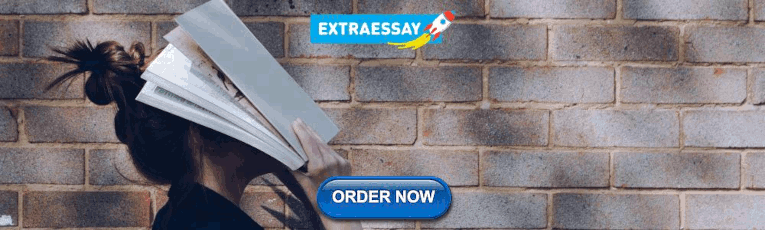
How to use biogas?: A systematic review of biogas utilization pathways and business models
- Anica Mertins ORCID: orcid.org/0000-0003-1462-6875 1 &
- Tim Wawer 2
Bioresources and Bioprocessing volume 9 , Article number: 59 ( 2022 ) Cite this article
6398 Accesses
11 Citations
1 Altmetric
Metrics details
There are many options for the utilization of biogas in different energy sectors (power, heat, mobility). The technical possibilities of using biogas are more diverse than the actual business models applied in the biogas industry. This paper shows the possible utilization pathways of biogas, divided into coupled power and heat generation, direct utilization and upgrading to a gas of a higher value. Subsequently, an overview of the business models discussed is given by a systematic literature review. The latter shows that the investigation of biogas business models is focused mainly on the last decade and has increased slightly over time. The regions of investigation can be found worldwide, with a clear focus on Europe. Direct use is studied mainly in the Asian and African regions. In the European context, a shift from investigating combined heat and power use to upgrading the biogas produced is evident.
Introduction
Over 90% of the biogas produced in the world was used for the production of power and heat in 2018, with only the remaining 9% being used as biomethane in the mobility sector or for injection into the natural gas grid (International Energy Agency 2020 ). However, the increasing number of biomethane plants suggests that the share of usage as biomethane will increase in the future (Banja et al. 2019 ). The utilization pathways differ between the countries, depending very much on the framework conditions of the country in which the biogas plant is operated (Capodaglio et al. 2016 ). Biogas plants in different countries have developed differently in terms of the substrate usage, pretreatment technology, plant size and utilization pathway of the biogas (Stürmer et al. 2021a ). Several regions throughout the world are striving for climate neutrality based on an energy system supplied 100% from renewable energies. This means that not only the power supply, but also the heat and fuel supply have to abandon fossil fuels. This raises the question what the future will look like and what role biogas technology will play in it.
In this paper, the utilization pathway is defined as a technical possibility for the use of biogas. This includes particularly converting biogas into usable energy and the subsequent possible fields of application. The term ‘business model’ is used to describe the options for monetizing biogas. In addition to the revenue streams, the business model specifies the utilization pathway and the profitability in a sales market, including costs.
This paper presents a systematic review of utilization pathways for biogas and evaluates the business models associated with each pathway. Conclusions can be drawn about the temporal course of research interest and geographic characteristics. The various business models that are conceivable based on the utilization pathways are presented. This approach reveals differences between the technical feasibility of a usage pathway and its actual implementation as part of a business model. These business models are examined independently of any specific region and should, therefore, be considered independently of local funding programs.
Several publications have already examined and compared different pathways of biogas, for example (Bystricky et al. 2010 ; Patrizio et al. 2015 ; Wu et al. 2016 ). Exemplarily, the use of biogas in a decentralized combined heat and power (CHP) plant, the injection of upgraded biomethane into the gas grid for off-site power and heat generation, and the use of biomethane as fuel are all under investigation. Business models for biogas have also already been investigated in the literature (Heffels et al. 2012 ; Horschig et al. 2019 ; Karlsson 2019 ). Focuses are, for example, on the direct marketing of power or different possibilities for biomethane becoming advantageous as a business model. To the best of our knowledge, no comprehensive analysis of biogas business models in the different pathways exists in the current literature. The novelty of this paper is, therefore, the analysis of the technical possibilities for the use of biogas combined with a detailed presentation of possible business models that are discussed in the literature. The specifics of each business model are discussed and the research strength in the area of the different business models is shown. In the context of the transformation of the energy system, it is relevant to reveal technical possibilities for energy generation that enable a profitability. The connection of technical utilization pathways with the consideration of possible business models plays a central role in the design of future energy supply, such as the use of biogas.
The paper is structured as follows: firstly, an overview of existing technical possibilities for biogas utilization is given. Subsequently, the method of literature review is presented. The results of the literature research on biogas business models are then presented in detail, subdivided according to the different utilization routes. The discussion of the results is followed by a conclusion.
Biogas utilization pathways
Biogas is a renewable energy that can be used in various ways, which is a major advantage of the technology. Possible utilization pathways are conceivable in all areas of consumption (Capodaglio et al. 2016 ), with three main distinctions:
Using the biogas in a CHP unit;
Using the biogas directly, for example, in machines or facilities in agricultural operations; and
Upgrading the biogas to a gas of a higher value.
The different pathways are shown in Fig. 1 and are explained in detail below.
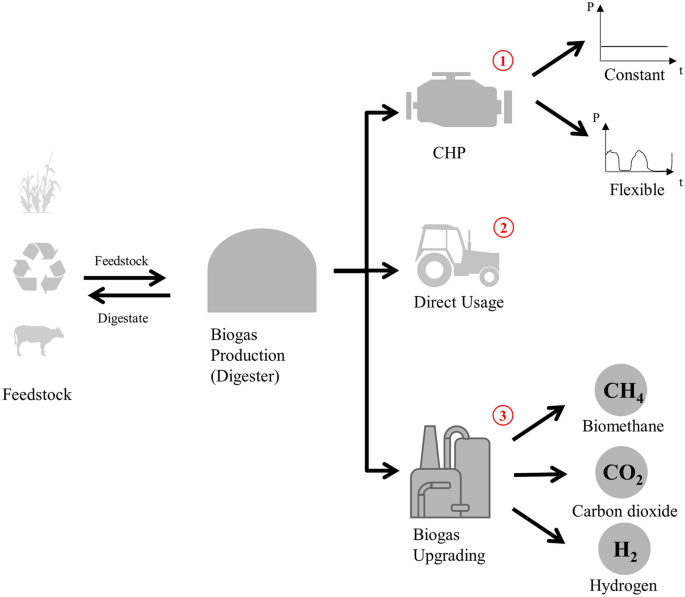
Potential utilization pathways for biogas
Pathway 1: CHP generation
The most commonly used pathway for biogas is in decentralized CHP units. The biogas produced is first dried, desulfurized and then used in a gas engine that drives a generator delivering power and heat. The power is normally used externally and, therefore, fed into the grid. Part of the generated heat is needed to operate the digester. The remaining heat can be used externally, for example, to heat buildings or for crop drying (Watter 2019 ). The electrical efficiency is usually between 30 and 40%, and the corresponding thermal efficiency is 35 to 55% (Lantz 2012 ). The highest mean fuel efficiency is achieved when all the heat produced can be used all year round (Fischer et al. 2016 ; Watter 2019 ).
A CHP unit can be operated in a base load mode, i.e., generates a constant amount of power and heat, or in a flexible mode. The latter enables the energy generation to be adapted to a demand for power or heat, or is driven by price signals. The digester is designed for a constant output, therefore, the flexibilization requires the adaptation of components (Liebetrau et al. 2015 ).
Regarding existing biogas plants, which were originally designed for base load operation, two retrofitting options for flexible generation are available: they can be adapted for flexible power production either by lowering the rated output or by increasing the power generation capacity. For the latter, a second CHP unit, a larger gas storage tank, facilities for external heat utilization and an adaptation of the connection to the power grid (e.g., transformer) are required. Alternative to the expansion of the gas storage, flexible biogas production can be achieved by changing the feedstock-feeding management (Daniel-Gromke et al. 2019 ).
One advantage of on-site use of a CHP unit is that the energy generated can be used to cover the internal energy demand of the anaerobic digestion process. The power demand for the digester and ancillary components in other utilization pathways must be covered from the power grid. Heat, on the other hand, can be generated by a gas boiler (Goulding and Power 2013 ).
Pathway 2: direct usage of biogas
In the second pathway, biogas is used in the immediate vicinity of the biogas plant. The aim of the on-site use of biogas is to reduce energy procurement costs or to reach energy self-sufficiency. Biogas can be used directly in households (Hamid and Blanchard 2018 ), for uncoupled heat generation, e.g., in heat boilers for use as process heat or steam or as fuel for machines on the farm (Lampinen 2004 ).
Another option is the direct utilization of biogas in tractors or agricultural machinery. Currently, biogas is not used in common practice for mobile internal combustion engines without upgrading (Owczuk et al. 2019 ). As untreated biogas does not meet the minimum specifications of a compressed natural gas (CNG) fuel due to the comparatively high proportion of carbon dioxide (CO 2 ), water vapor and hydrogen sulfide, it cannot be used in CNG-powered vehicles (Kruczyński et al. 2013 ). Compared to biomethane or natural gas, the energy content of biogas per unit mass is significantly lower. Large quantities of biogas can, thus, only be stored under high pressure, with the upgrading, storage and handling in this case leading to increased costs (Mihic 2004 ). On the other hand, biogas that has been separated from hydrogen sulfide and water is a significantly cheaper fuel than biomethane (Kruczyński et al. 2013 ). Another difficulty of using pure biogas as a fuel is to ensure a secure supply. Thus, periods with low biogas production or high consumption must be balanced out by storage (Redwanz and Walter 1984 ).
However, the use of biogas in a dual-fuel engine, together with the use of diesel, has been investigated. Different parameters are focused on here, for example, the concentration of different exhaust gases (Jaber et al. 2009 ; Owczuk et al. 2019 ), the effect of different fuel mixing ratios (Matuszewska et al. 2016 ). (Lampinen 2004 ) reported on a farm that is completely self-sufficient in energy (power, heat and fuel, and also fertilizers) through the biogas plant. Tractors using biogas as fuel were introduced in the early 2010s. The dual use of biogas and biodiesel to compensate for the low energy density of biogas saves up to 40% in fuel costs, according to one manufacturer (Kruczyński et al. 2013 ).
Pathway 3: upgrading of biogas to a gas of higher value
The third pathway is upgrading biogas to a gas of a higher value. Upgrading of biogas to biomethane is considered a mature technology (Verotti et al. 2016 ). The upgrading process mainly involves the separation of various impurities from the biogas. The most important part of the upgrading process is the separation of CO 2 , with the aim of increasing the methane content of the biogas (Bragança et al. 2020 ). The order of the individual steps for upgrading depends on the gas properties and the method of capturing the CO 2 (Deutsche Energie-Agentur GmbH 2019a ). Upgrading methods are water scrubbing, chemical scrubbing, physical scrubbing, pressure swing adsorption and membrane separation (Miltner et al. 2017 ). Biomethane provides the advantage of multiple applicability, thus, it can be used as a fuel in the mobility sector, as a natural gas substitute in the heating sector, or off-site in a CHP unit. Upgrading leads to higher costs, higher energy consumption and more consumption of material. In addition, methane slip can worsen the environmental footprint (Schmid et al. 2019 ).
The upgrading allows the injection of the gas into the existing gas grid. This provides the advantage that it can be used spatially and temporally independently of its production. This can help to make the use more efficient and, thus, increase sustainability, as a result of demand-orientated energy generation (Budzianowski and Budzianowska 2015 ). Another advantage is that the infrastructure for distributing and using the biomethane has already been established (Cavana and Leone 2019 ). Feeding biomethane into the grid is already a common practice in countries such as Germany, Sweden, the Netherlands, Switzerland and Austria (Korberg et al. 2020 ). The upgrading of biogas to biomethane becomes cheaper with increasing biogas volumes due to cost degression in investments costs; therefore, this pathway is particularly interesting for large biogas plants. There is the possibility of plant pooling, so that smaller biogas plants can also take advantage of the cost degression. Other biogas plants in the vicinity, which can cooperate for joint upgrading, are essential for this (Dotzauer et al. 2021 ). Proximity to the natural gas grid is important for the success of this utilization pathway (Pasini et al. 2019 ). One way to implement pooling is to sell raw biogas to the operator of the upgrading plant. This requires the construction of microgrids to connect the biogas plants to the upgrading plant. In this scenario, the biogas plant operator is therefore only responsible for the production of the biogas, which is subsequently upgraded by another market participant (Dotzauer et al. 2021 ).
In addition to upgrading following the biogas production (ex situ), it is also possible to produce biomethane in the digester, so-called in situ processes. Examples are CO 2 desorption, the pressure reactor, H 2 (hydrogen) addition and electromethanogenesis. For H 2 addition, for example, the H 2 is fed into the digester. The aim is to achieve biomethane of natural gas quality directly with the help of bacteria inside the digester (Aryal et al. 2018 ). Initial studies indicate that in situ methods can offer better profitability for small to medium-sized plants and achieve a methane content of 85%. However, in situ methods are still underdeveloped and currently mostly take place on a laboratory or microscale. Above all, research into the technology on a large scale needs to be improved (Sarker et al. 2018 ).
There is a possibility to further use the captured CO 2 , which is currently not a common practice (Billig et al. 2019 ). The biogenic CO 2 does not cause any climate-relevant emissions and is suitable for various utilization pathways itself (van Basshuysen 2016 ). Possible areas of usage would be the food, chemical and pharmaceutical industries (Horschig et al. 2019 ) or the production of synthetic fuels (Eggemann et al. 2020 ). Capture and subsequent storage of CO 2 , known as Bioenergy with Carbon Capture & Storage (BECCS), is one option for making biogas production CO 2 -negative (Rosa et al. 2021 ).
Under certain circumstances, it may be necessary to adjust the calorific value of the biomethane (e.g., by adding liquid gas). The biomethane must be compressed according to the pressure level of the grid for injection into the natural gas grid. In summary, regarding injection, the quality of the gas must comply with the provisions of the gas class and the deviations must not exceed the permissible limits (Deutsche Energie-Agentur GmbH 2019a ).
Another technical option is to upgrade biogas to green H 2 . Technical options for realization include reforming (steam, partial oxidation and autothermal reforming), biological processes (bio-photolysis, dark fermentation and photofermentation), thermochemical processes (pyrolysis, gasification, combustion and liquefaction) or water splitting (electrolysis, thermolysis and photolysis) (Nikolaidis and Poullikkas 2017 ). The advantage of reformation is that it is a proven technique, as it is conventionally an established process to produce syngas from natural gas (Wünning 2021 ).
Material and methods
A systematic literature analysis of possible business models and economically considered utilization pathways was carried out to obtain an overview of the established business models in the biogas industry. The EBSCOhost database was used for the systematic literature review. The main search term “biogas” had to be included in the title of all candidate papers. Various additional search keywords were used that had to be included in the title or abstract of the paper. The search terms include “business model”/“business case”/“economic”, “use”/“utilization”/“usage” and “pathway”/“path” in various combinations. The search was conducted between September 22 and October 18, 2021.
As a result of the systematic literature review, 242 scientific publications were found; after sorting out duplicate papers, 192 publications remained. The aim of the paper was determined by reviewing the title, abstract and keywords. On this basis, the relevance of the paper was determined for further investigation. Relevant for the selection of a publication was the clear consideration of the profitability of a business model. Publications addressing technical utilization paths or the ecological assessment were not considered further. Regarding the relevant papers, the literature utilized (backward search) and further developments in the research field (forward search) were examined for additional relevant publications. In conclusion, a set of 72 relevant papers remained for the detailed analysis.
Figure 2 shows that interest in biogas business models and utilization pathways is mainly centered in the European region (64% of the relevant papers). Most articles in Europe deal with Italy, Sweden and Germany. A good third of the papers do not deal with any specific country in their methodology.
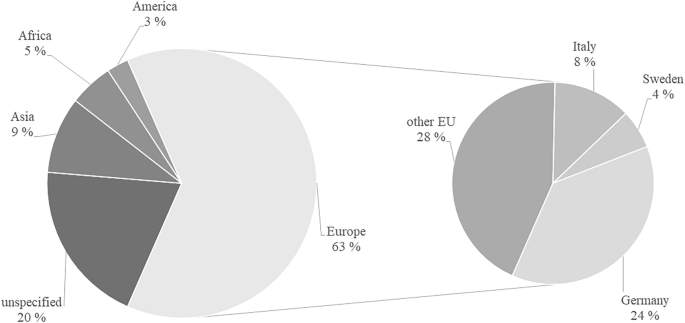
Distribution of papers by countries and regions considered
An initial classification of the publications into the three main biogas pathways: CHP usage, direct use or upgrading to a higher quality gas was made. If papers deal with several of these topics, they were included in more than one category. The papers in the three categories were examined regarding their publication date and the region under consideration and then classified again. The aim here is an evaluation according to the chronological course and geographical characteristics of individual usage pathways.
Different utilization pathways are covered in the papers, with a focus on CHP usage ( n = 30). Another utilization pathway that has been studied frequently is biomethane ( n = 26), with a focus on fuel production ( n = 16) and CHP off-site usage ( n = 6). In this context, the use of captured CO 2 was also investigated in seven papers. Five papers investigated the use of biogas to produce H 2 .
Figure 3 shows that the number of relevant papers has increased over the last few years. The research interest of direct utilization of biogas has remained relatively constant over time. The interest in CHP use has increased significantly until 2015, which has shifted over time to the field of biogas upgrading. While almost 40% of the publications address the CHP business model, as well as the upgrading of biogas, the share of direct use is comparatively low at 20%.
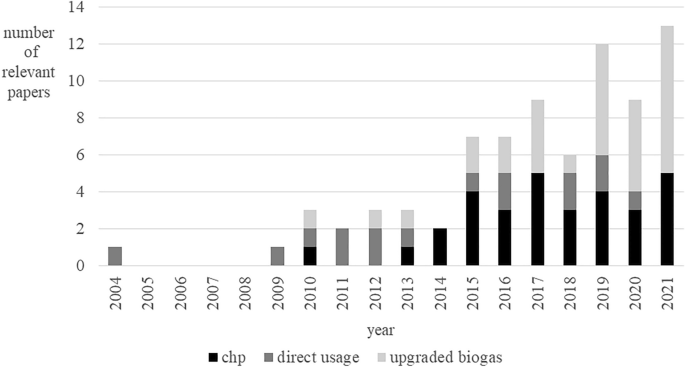
Development of relevant published papers by utilization pathway over time
The CHP generation is currently the biogas application pathway most widely discussed. It is treated in many publications in the literature. Thirty of the relevant 72 scientific papers deal with this utilization pathway. The first publication on biogas CHP usages dates back to 2010 (Bystricky et al. 2010 ), and since 2013, there has been an increasing number of publications in this field (Hahn et al. 2014 ; Szarka et al. 2013 ). The number of publications has been relatively constant since 2015. Research interest is concentrated in Europe, especially Germany ( n = 15, e.g., (Butemann and Schimmelpfeng 2020 ; Theuerl et al. 2019 )), Italy ( n = 3, including (Gandiglio et al. 2016 ; Patrizio et al. 2017 )) and Austria ( n = 3, including (Saracevic et al. 2018 ; Stürmer et al. 2021b )).
The aim of flexibilization of CHP units is primarily the power demand-orientated mode of operation, although various utilization pathways or mixed forms are also considered (Fig. 4 ). Hahn et al. ( 2014 ) and Häring et al. ( 2017 ) investigated the various options for flexible power production from biogas with the aim of meeting the requirements and demands of the power market. Grim et al. ( 2015 ) also compared the on-demand production of power to baseload operation and evaluates technical requirements and economic impacts of on-demand production. Dzene and Romagnoli ( 2015 ) showed the possibilities of using biogas to balance the volatile feed-in from wind energy, whereas Bär et al. ( 2020 ) pursued the goal of creating synergy effects from photovoltaics and biogas plants, with the aim of achieving a second-by-second balancing of volatile generation. The first publications on the subject of flexibilization deal with flexible production in Germany (Hahn et al. 2014 , 2015 ; Szarka et al. 2013 ), as incentives have been set to produce power demand-orientated in the EEG (Renewable Energy Act) 2012. However, the research interest in flexibilization in other countries started in 2015, for example, in Latvia (Dzene et al. 2014 ) and Sweden (Grim et al. 2015 ). Research interest in this utilization pathway exists primarily in Germany, as more than half of the publications present the utilization pathway using the German example (Fig. 5 ) (Güsewell et al. 2021 ; Häring et al. 2017 ; Hijazi et al. 2019 ).
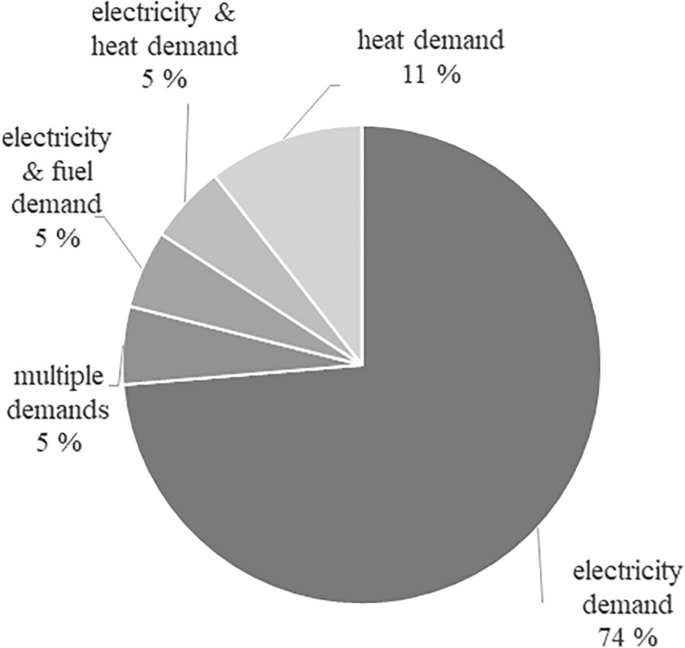
Aim of flexibilization of CHP plants investigated in studies, n = 19
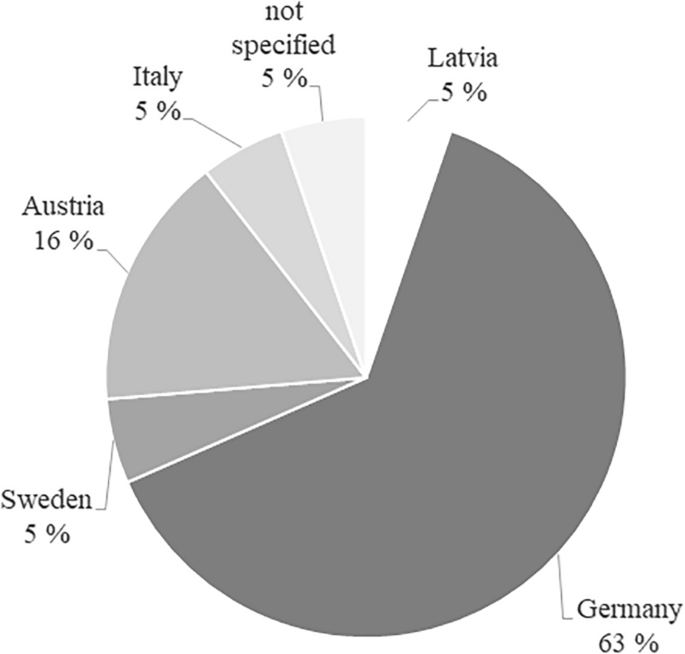
Allocation by country for studies on CHP flexibilization, n = 19
The direct use of biogas has been studied regularly in the literature since 2004. Seventeen relevant scientific publications were found in the literature search. They can be divided into two categories: the use of biogas in households, for example, for cooking or heating ( n = 10), or in tractors or agricultural machinery ( n = 7). The biogas for use in households is normally produced in a decentralized manner and used directly, for example, for cooking (Hamid and Blanchard 2018 ), but also to supply power or produce fertilizer for agricultural purposes (Das et al. 2016 ). Another goal of decentralized plants can be waste disposal, for example, of wastewater (Bensah and Brew-Hammond 2010 ) or to reduce greenhouse gas (GHG) emissions (Yuan et al. 2015 ). Research interest in biogas plants for household use is concentrated in Africa (Kenya (Hamid and Blanchard 2018 ), Ghana (Bensah et al. 2011 ; Bensah and Brew-Hammond 2010 ) and Africa in general (Kemausuor et al. 2018 )), and Asia (Bangladesh (Das et al. 2016 ), China (Chen et al. 2012 ; Yuan et al. 2015 ), Indonesia (Silaen et al. 2020 ), Bali (Bößner et al. 2019 ) and Pakistan (Yasmin and Grundmann 2019 )).
Twenty-six relevant papers were identified for the biogas upgrading pathway. The first publications date back to 2010 (Bystricky et al. 2010 ) and 2012 (Roose et al. 2012 ). An increasing research interest has been observed since 2015 (Budzianowski and Budzianowska 2015 ; Patrizio et al. 2015 ). The geographical focus is on European countries ( n = 16). There is a clustering for Italy ( n = 5, e.g., (Cavana and Leone 2019 ; Patrizio and Chinese 2016 )), Germany ( n = 4, e.g., (Billig et al. 2019 ; Theuerl et al. 2019 )) and Denmark ( n = 2 (Fenton and Kanda 2017 ; Korberg et al. 2020 )). Only three papers considered a region outside Europe: the USA (Murray et al. 2017 ), India (Bhatia et al. 2020 ) and Thailand (Wattanasilp et al. 2021 ). The research interest in Italy can be justified by the fact that the focus of biogas use has been set on biogas upgrading for use in the transport sector and a support program has been introduced since the Italian National Energy Strategy of 2017 (Murano et al. 2021 ).
There are different focuses on the upgrading of biogas in the literature, like the comparison of different biogas utilization pathways to the upgrading to biomethane (Horschig et al. 2019 ; Kalinichenko and Havrysh 2019 ; Wu et al. 2016 ), the potential role of biogas in the mobility sector of the future (Korberg et al. 2020 ) or the optimal process route, ranging from the upgrading technology to the transportation and utilization method, for biogas (Mohtar et al. 2021 ). The environmental impact can also be relevant to the profitability of the business model, CO 2 prices can lead to higher costs for fossil fuels, so that biomethane becomes more profitable in comparison due to high GHG savings (Patrizio et al. 2017 ). National subsidies that contribute to the profitability of biomethane business models are also explored in the literature (Budzianowski and Budzianowska 2015 ; Patrizio and Chinese 2016 ).
The literature search produced comparatively few hits on the business model of H 2 production. The five papers found are comparatively current from the years 2021 (Cvetković et al. 2021 ; Karaeva 2021 ; Wünning 2021 ) and 2020 (Antonini et al. 2020 ), only one publication dates back to 2013 (Wulf and Kaltschmitt 2013 ). No geographical focus of the research could be identified.
Biogas business models
In the energy industry, especially in oil and gas production, a distinction is made between upstream and downstream business models. The upstream business models involve the identification, extraction or production of raw materials, while the downstream business models follow on from production and primarily involve sales to consumers (Bern 2012 ). This analysis will be limited to the downstream business models. The profitability is considered for agricultural biogas plants, biogas production by wastewater or organic municipal waste is not included in detail.
Possible business models are evaluated starting from the structure given by the three utilization pathways presented in Biogas utilization pathways . Several business models within each pathway were identified in the literature.
The literature review revealed that CHP use can be divided into two basic modes of operation, constant and flexible generation. Following this categorization, the results of the literature review are presented below. Flexible generation can be further categorized according to the goal of the flexibilization. The focus is usually on power-led operation (Bär et al. 2020 ; Lauven et al. 2019 ; Szarka et al. 2013 ), but heat-led operation is also being investigated (Ertem and Acheampong 2018 ; Güsewell et al. 2021 ).
Business model 1.1: constant feed-in with heat concept
It is possible to generate a constant amount of energy in the CHP unit, the so-called baseload operation. Currently, about 75% of biogas plants in the EU use the biogas for CHP production (Calderón et al. 2021 ). The advantage of constant CHP generation over flexibilization is the low investment costs and the significantly lower effort required to operate the plant. However, the low costs do not usually lead to profitability, which would only be possible through subsidies (Lantz 2012 ). In comparison, the revenues in a flexible operation are always higher than in a baseload operation (Grim et al. 2015 ). As the European regulations show, the aim is not baseload operation as this is less beneficial to the energy system. Financial support is given to flexible power generation, with the aim of making the power from biogas usable for the system (Stürmer et al. 2021b ). The long-term viability of this business model is, therefore, questionable.
Business model 1.2: demand-oriented flexibilization
The second way to operate a CHP unit is to generate energy according to a demand or a price signal. Compared to other renewables that generate and feed in power in a volatile manner, biogas offers the possibility of being stored and thus generating energy flexibly and in accordance to demand (Szarka et al. 2013 ). In the case of flexibilization of biogas plants, the literature usually reports on flexibilization with the aim of its usage in the power market. By contrast, there is the possibility of a heat demand-oriented flexibilization of the biogas plant.
Thus, the goal is the balancing of volatile feed-in from other renewable energy sources such as wind turbines and PV plants (Güsewell et al. 2021 ), or the balancing of the residual load and the provision of system services (Szarka et al. 2013 ). In the energy system of the future, flexible power from biogas can reduce overall costs (Fleischer 2018 ) and can help operators generate additional revenue by selling power during periods of higher prices, potentially helping to make the plant profitable (Lauven et al. 2019 ). The revenues can be generated on the markets for ancillary services, e.g., as secondary or tertiary control reserve (Saracevic et al. 2018 ), or on the short-term markets, such as the day-ahead or spot market (Hochloff and Braun 2014 ).
The profitability of the business model is influenced primarily by the amount of the available overcapacities in comparison to the nominal load, schedule design and the amount of external heat use (Daniel-Gromke et al. 2019 ). For example, revenues can be increased by installing a thermal storage system (Wille-Haussmann et al. 2010 ). Furthermore, the amount of additional revenue is influenced by the size of the biogas storage capacity (Lauven et al. 2019 ). The business model of selling power is usually not profitable without subsidies due to declining income from power sales and the high investment costs in storage and CHP (Hochloff and Braun 2014 ; Lauven et al. 2019 ; Lee 2017 ). A positive net present value can be achieved with flexible operation of the existing CHP unit without an investment in new units under certain conditions (Grim et al. 2015 ).
The profitability of a CHP unit increases with high shares of heat utilization, e.g., by nearby industry or households (Goulding and Power 2013 ), so the idea of this utilization pathway is to raise the rate of heat utilization (of the externally available heat) to 100%, if possible (Güsewell et al. 2021 ). In the case of constant energy production by the CHP unit, a high utilization rate can only be achieved with a significantly higher heat demand than covered by the biogas plant (Güsewell et al. 2021 ). In the case of heat demand-oriented flexibilization, the biogas plant is designed and used in such a way that local heat sinks can be covered. Possible heat sinks can, for example, be owned by the biogas plant or neighboring agricultural companies, residential or public buildings, or agricultural drying processes (Herbes et al. 2018 ). The power that is generated simultaneously in the CHP unit is fed into the public power grid and remunerated according to the current tariffs (Daniel-Gromke et al. 2019 ). The actions to adapt an existing biogas plant to this utilization pathway are, firstly, the identification of potential heat sinks in the immediate proximity of the biogas plant, and, secondly, the adjustment of the generation profile to heat consumers. This can be realized by seasonal feeding, for example, adapted by the feedstock amounts used or substrates with different energy density. An alternative method is to use a biogas storage to compensate the fluctuations in the demand curve (Güsewell et al. 2021 ). The disadvantage is that many biogas plants are located in areas where heat utilization concepts are difficult to implement because potential consumers are too far away (Ertem and Acheampong 2018 ).
Short-term flexibilization increases profitability by exploiting higher power prices, while seasonal flexibilization additionally optimizes profitability by increasing heat utilization. However, both options currently do not lead to profitability in Germany under the current market conditions (Güsewell et al. 2021 ).
In addition to optimizing generation to meet an external demand, maximizing on-site energy use can also be a goal of flexibilization. Accordingly, it is necessary to adapt the generation profile of the biogas plant to the on-site load profile (Güsewell et al. 2020 ). The heat generated is currently already used for self-consumption or direct delivery. The idea of this utilization pathway is to consume the energy generated, i.e., power and heat, as completely as possible on-site or in the immediate vicinity. Possible sinks are the farm, residential houses or industrial plants. The aim here is to reduce energy purchase costs so that it is economically more advantageous to consume the energy produced by the biogas plant on-site instead of purchasing power from the grid or thermal energy from other external sources (Güsewell et al. 2020 ). The literature describes that favorable location factors are needed for this model to be profitable. These include low gas production costs and consumers close to the site with a permanently high demand for power. In addition, the possibility of the flexible control of consumer loads and a coupling with another depreciated renewable energy plant contribute to the advantageousness (Technische Hochschule Ingolstadt 2020 ).
The CO 2 price will have a further influence on the profitability of CHP use in the future. Compared to CNG upgrading, CHP use leads to higher GHG savings, which, under favorable conditions, can lead to profitability even with a comparatively low CO 2 price (Patrizio et al. 2015 ).
The direct use of biogas can be divided into two categories: the use of biogas in households, for example, for cooking or heating, or in tractors or agricultural machinery. The use of biogas in households is mainly conducted in Asia or Africa, these family plants mainly pursue the aim to supply households with the needed energy and to replace firewood and dung as energy source (Kemausuor et al. 2018 ). Thus, these biogas plants are especially intended to provide an affordable and reliable source of energy for households (Hamid and Blanchard 2018 ). The use of waste can also be a major goal of a biogas plant (Kemausuor et al. 2018 ).
The biogas utilization in the mobility sector is not common practice without upgrading (Owczuk et al. 2019 ). There is the possibility of utilization in a dual-fuel engine (Jaber et al. 2009 ; Matuszewska et al. 2016 ; Owczuk et al. 2019 ), however, the use of biogas in engines is very limited, making this utilization pathway not sustainable as a business model.
Biogas upgrading usage pathways can be divided into biomethane usage for CHP off-site usage, the heat market, mobility sector and on-site usage. Furthermore, there are the possibilities of CO 2 usage and H 2 generation. The central research interest has been the upgrading of biogas to biomethane, as shown in Fig. 6 . Within biomethane utilization, its use as fuel is currently a focus of research, while the other utilization pathways are under development. The use of captured CO 2 is a research interest that has emerged mainly in recent years.
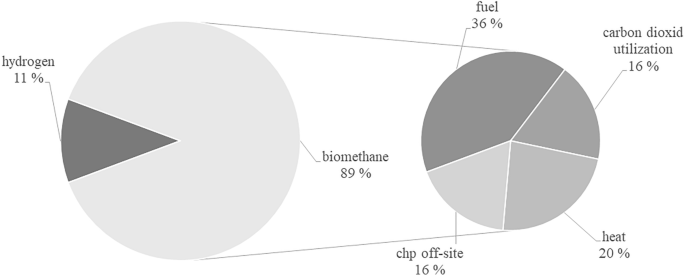
Share of the utilization pathways considered after biogas upgrading, n = 44
The investment required for upgrading biogas into biomethane is higher than that for on-site power generation due to the plant technology required for upgrading (Deutsche Energie-Agentur GmbH 2019a ). Upgrading biogas into biomethane is usually only an interesting business model when subsidies are involved since the costs for producing biomethane exceed the market price for natural gas (Budzianowski and Budzianowska 2015 ). However, rising natural gas prices may contribute to the better profitability of biomethane business models in the future (Banja et al. 2019 ). There are already various studies that evaluate the upgrading of biogas to biomethane as the best utilization path and assume that profitability can be achieved (Lee 2017 ).
Business model 3.1: biomethane feed-in for CHP off-site utilization
Basically, power and heat from off-site biomethane CHP units can serve the same markets as on-site CHP units, for example for demand-oriented use or balancing the fluctuating generation of wind and solar power (Budzianowski and Budzianowska 2015 ). The advantage of this option is that the utilization is independent of the location where the biogas is produced, which can lead to higher efficiency due to a higher heat utilization rate, as well as the possibility to use the natural gas grid as biomethane storage (Budzianowski and Budzianowska 2015 ; Horschig et al. 2016 ; Szarka et al. 2013 ). In Germany, off-site CHP use is the most favored utilization path for biomethane with over 85%. The advantage here is the subsidy from the EEG (Renewable Energy Law) for the power generated by the CHP, which can lead to profitability and was so far the best stimulation for biomethane expansion (Daniel-Gromke et al. 2017 ). The range of the CHP units in which the biomethane can subsequently be used extends from 1 kW el to 10 MW el . Thus, the use of biomethane is not only possible in an industrial context; mini CHP units in households are also available on the market (Fachagentur Nachwachsende Rohstoffe e. V. 2012 ). Since the price of biomethane is comparatively much higher than for natural gas and the infrastructure for its use causes the same costs, the substitution of natural gas by biomethane, e.g., in an industrial context, is not advantageous. Thus, profitability in comparison can only be achieved by the customer's decision to choose the more environmentally friendly option. In the future, pricing of CO 2 emissions or alternatively the sale of green gas certificates can contribute to profitability (Patrizio et al. 2015 ).
Business model 3.2: biomethane feed-in for the heat market
In addition to the use of heat from CHP units with a distribution via local heating networks, where the advantageousness depends on factors such as the proximity of the biogas plant to heat consumers and a suitable heat consumption profile, there is the possibility of providing heat via the use of biomethane (Banja et al. 2019 ). In this approach, biogas is upgraded to biomethane in upgrading plants and fed into the natural gas grid. Natural gas plays a central role in the heat supply particularly in countries with well-developed public natural gas grids, especially for supplying households and industry. This existing infrastructure can, thus, be used for the distribution of biomethane, since the latter can be substituted directly for the natural gas that is used throughout the country today (Cavana and Leone 2019 ). Since biomethane is a natural gas substitute, it can be used in conventional natural gas burners. Heating systems or gas stoves, therefore, do not have to be replaced (Banja et al. 2019 ; Fachagentur Nachwachsende Rohstoffe e. V. 2012 ).
In addition to its use in households, the use of biomethane is also interesting in industries that rely on the use of methane-based energy sources for heat generation, such as the iron and steel industry. Biomethane can reduce greenhouse gas emissions in these industries. However, according to current forecasts, an economic substitute is hardly possible even with rising CO 2 prices (Ahlström et al. 2020 ). The profitability of biomethane production depends on plant size, substrate input (Cucchiella and D’Adamo 2016 ) and grid connection costs (Pasini et al. 2019 ), whereby the profitability of different plant constellations could definitely be proven (Cucchiella and D’Adamo 2016 ). The success of biomethane in the heating sector depends primarily on the prices of substitute goods and suffers, above all, from low natural gas prices. However, the trend of rising natural gas prices since 2016 could contribute to better profitability in the future (Banja et al. 2019 ).
Biomethane is currently provided at a higher price than natural gas even under the best circumstances (Paturska et al. 2015 ). In the private sector, therefore, the end consumers' willingness to pay is particularly decisive for the market success of biomethane. For this reason, utilities are currently focusing on gas tariffs with an admixture of biomethane (Dunkelberg 2015 ). Since there is no subsidy for the provision of biomethane in the heat market, e.g., comparable to feed-in tariffs in the power market (Herbes et al. 2021 ), a market ramp-up in this area is hardly to be expected (Adler et al. 2014 ).
CO 2 prices will have a relevant impact on the success of biomethane in the future, especially in direct competition with natural gas. Compared to the direct use of biomethane in the mobility sector, the feed-in into the natural gas grid has the disadvantage that the GHG reduction is reduced by adding propane and thus can be monetized comparatively worse (Patrizio et al. 2015 ).
The issue of profitability is addressed in various studies, which conclude that the use of renewable gases by 2050 will be cheaper than full electrification of the heat sector (Cavana and Leone 2019 ). Other studies, including those which studied the use of biogas in gas boilers in Denmark and the EU, said that heat supply through district heating and individual electric heat pumps are better solutions compared to the use of biomethane. The reason is the more economical cost of the energy system and the biomass use being more economical in other sectors (Korberg et al. 2020 ).
Business model 3.3: biomethane feed-in for the mobility sector
Another utilization pathway for biomethane is its use in the mobility sector. Here, it is possible to feed the upgraded biomethane into the existing natural gas grid and then make it available virtually at natural gas filling stations. This utilization pathway is already common today; mixed products of biomethane and natural gas are usually sold at gas stations (Fachagentur Nachwachsende Rohstoffe e. V. 2012 ). Compared to gasoline, the use of biomethane can save GHG emissions, reducing them by 60% when produced from corn, 70% when produced from waste and about 80% when produced from manure (Banja et al. 2019 ).
The future development of this sales channel depends on the development of CNG vehicles (Fachagentur Nachwachsende Rohstoffe e. V. 2012 ). However, biomethane can be used not only in passenger cars; there are already other vehicles that run on natural gas. These include, for example, light commercial vehicles, trucks, buses (Natural & Bio Gas Vehicle Association 2019 ) and ships (Backman and Rogulska 2016 ). The number of natural gas vehicles in Europe has been steadily increasing in recent years, reaching 1.46 million in 2020, of which 1.25 million are passenger cars. The market share of natural gas cars is the largest in Italy with 2.49% of the total stock of cars. Italy has the largest absolute number with 981,000 CNG-powered cars. Germany has the second largest number of CNG-powered cars in absolute terms (83,000), but they account for only 0.17% of the total car population (European Alternative Fuels Observatory 2020 ). Sweden has by far the largest share of CNG-powered buses with 17.6%, while the Czech Republic (6.7%) and the Netherlands (6.0%) also use CNG-powered buses (European Automobile Manufacturers Association 2021 ). Due to the partial lack of infrastructure in the area of natural gas filling stations and the associated investment costs, as well as the remaining GHG emissions, it is partly assumed that the future of mobility will instead be shaped by electromobility (Banja et al. 2019 ).
It has been shown that various influencing factors determine the profitability of biomethane use in the transport sector. These include primarily subsidies, but also plant size and substrates. Larger plants are generally more likely to be profitable, as is the use of residual and waste materials (Cucchiella et al. 2018 ; D'Adamo et al. 2019 ). Lower taxes for biomethane also offer advantages for profitability (Browne et al. 2011 ). Several studies have shown that biomethane can already be competitive with other fossil fuels or liquid biofuels (Goulding and Power 2013 ). Compared to liquefaction, injection into the natural gas grid is generally cheaper if connection costs are low (Pasini et al. 2019 ).
There is also the possibility of liquefying the biomethane at the points of use to produce bio-LNG (Liquified Natural Gas) (Hönig et al. 2019 ). Bio-LNG can also be used in the transport sector and is particularly interesting for ships or heavy-duty transport due to its higher energy density. Again, a large biogas plant and the use of residual and waste materials as substrates tends to be more profitable (Deutsche Energie-Agentur GmbH 2019b ).
Business model 3.4: decentral biomethane filling stations
As an alternative to feeding the biomethane into the natural gas grid, there is the possibility of setting up a gas filling station near the biogas or upgrading plant. The aim here is also to use the biomethane as a fuel in the mobility sector. This way has been less established so far, but there are already examples in Germany (Fachagentur Nachwachsende Rohstoffe e. V. 2012 ). Customers for the biomethane fuel in the proximity who have their own machines and vehicles, but also logistics companies or bus fleet operators are relevant for the success of this sales channel. In addition, there should be sufficient space for the construction of the upgrading plant and the biomethane filling station, and good accessibility for the customers should be ensured. If there are bottlenecks in the supply of biomethane by the biogas plant, a connection to the natural gas grid should be established (Grösch et al. 2020 ). This can also be used to feed in biomethane during periods of lower sales. Alternatively, it is necessary to ensure an alternative use or storage (Hornbachner et al. 2009 ).
Business model 3.5: on-site usage of biomethane
In addition to the possibility of selling the biomethane produced, it can also be used on-site. The possibilities for use are similar to the on-site use of biogas (Pathway 2) and are primarily intended to contribute to reducing energy procurement costs and increasing the degree of energy self-sufficiency. Thus, biomethane can be used as a fuel in agriculture, replacing diesel and biodiesel. This has a high potential to reduce GHG emissions, contribute to a higher share of renewable energy in the agricultural sector and, thus, to a more sustainable agriculture (Bisaglia et al. 2018 ). Initially, there were tractors that were converted to use biomethane, and dual systems for the joint use of diesel and biomethane were also used (Bisaglia et al. 2018 ). In the meantime, a first series tractor that runs 100% on biomethane has reached market maturity (New Holland Agriculture UK 2021 ). However, the business model is not widespread, so there is no reliable information on profitability.
Business model 3.6: CO 2 utilization
Carbon dioxide is captured during the upgrading of biogas to biomethane. While the captured CO 2 is currently usually released into the atmosphere and not used further, it is also possible to use the captured CO 2 as well. This offers the opportunity in the field of carbon capture and utilization to make a further contribution to climate protection and replace fossil CO 2 generation (Billig et al. 2019 ). There are different ways to use and monetize CO 2 . Large amounts of dry ice are used, especially in the food industry, dry ice service companies and the chemical and pharmaceutical industries (Horschig et al. 2019 ). There are already initial studies showing that it is possible to produce CO 2 for food market utilization. Since the food market offers the most restrictive quality requirements, it would be therefore also conceivable to produce CO 2 for other markets. The CO 2 can also be used, for example, in Fischer–Tropsch synthesis for the production of cosmetic products or for the production of high-value chemicals and waxes (Horschig et al. 2019 ). It is also possible to use CO 2 to produce synthetic fuels. Power-to-fuel, for example, can use carbon to produce renewable fuels together with H 2 via methanol synthesis (Eggemann et al. 2020 ). By selling the CO 2 , the joint use pathway with biomethane can become profitable (Esposito et al. 2019 ). Alternatively, the capture of CO 2 can also lead to negative CO 2 emissions in biogas production. The BECCS process is considered profitable (Li et al. 2017 ). If regulations in the area of the carbon emission trading systems are strengthened, additional revenue can be generated through the negative CO 2 balance (Carranza-Abaid et al. 2021 ; Lisbona et al. 2021 ).
Business model 3.7: hydrogen production
Green H 2 is central in the discussion about energy transition. Comparing the possibilities of producing H 2 from biogas, steam reforming leads to twice the H 2 yield compared to power generation with subsequent electrolysis (Wünning 2021 ). The H 2 is currently transported via trucks as there is no existing H 2 grid. An alternative to this could be the decentralized production of biomethane with feed-in to the natural gas grid and subsequent decentralized upgrading to H 2 . On the other hand, decentralized production can also be an advantage for the nationwide production and distribution of H 2 for use in the transport sector (Wünning 2021 ). In the long term, it is being discussed whether the natural gas infrastructure can be used to distribute H 2 leading towards the elimination of the use of fossil fuels (Dodds and Demoullin 2013 ).
Due to the existing technology of the steam reforming, which already produces hydrogen by using natural gas, it is a market-ready and profitable technology. Compared to using natural gas, biomethane is more expensive but can also result in negative GHG emissions, which in turn can contribute to profitability (Braga et al. 2013 ). The payback period is less than 10 years (Braga et al. 2013 ; Montenegro Camacho et al. 2017 ) and the net present value is positive (Yao et al. 2017 ), making it possible to operate profitably over its lifetime. With production costs below 5 €/kg, biogenic hydrogen is considered competitive (Marcoberardino et al. 2018 ; Montenegro Camacho et al. 2017 ).
The sales markets for hydrogen are diverse and will continue to grow in the future. In industry, hydrogen is used, e.g., in refineries, in chemical production or in the future also in the steel and iron industry, as these will renounce the use of natural gas and coal in order to decarbonize. Furthermore, hydrogen is suitable for generating high-temperature heat (Noussan et al. 2021 ). In the transport sector, there are already passenger cars that run on hydrogen (Alves et al. 2013 ; Antonini et al. 2020 ). In the future, however, the need is seen more in applications that are difficult to electrify, such as trucks, buses, ships and aircraft. Hydrogen is also expected to play a relevant role in the energy system for flexible power generation in the future (Noussan et al. 2021 ).
There has been research interest in utilization pathways and business models in the field of biogas plants since 2004. However, this interest has increased steadily only since 2009. Considering that biogas plants were already being built in European countries in the 1980s (Demuynck and Nyns 1984 ), it is initially surprising that the research interest has only increased strongly in the last decade. However, during the period of this development, new requirements of the energy system have also emerged.
The systematic literature research has shown three basic ways of utilization: the utilization of biogas in a CHP plant, the direct utilization of biogas and the upgrading to a gas of a higher quality. These three utilization pathways contribute in different sectors. Flexible generation of biogas ( Business model 1.2: Demand-oriented flexibilization ), for example, could provide a system service in the power market by generating power from biogas during hours of low power generation from wind and photovoltaics. Many papers have already analyzed this business model extensively. However, compared to other renewables, biogas has high power generation costs and different studies conclude that it is unlikely to be competitive in the long term. On the other hand, it has also been shown that flexible power generation can be profitable under beneficial conditions, such as a high thermal and biogas storage. Another important influence are rising power prices due to rising costs of fossil fuels and rising CO 2 prices due to high GHG savings in CHP usage. Furthermore, the development of technologies to provide system services in the power market will have a relevant influence on the biogas usage in the future. A distinction has to be made between technologies for the short-term and long-term provision of flexible energy. It can be assumed that power storage and flexible generation from H 2 and biogas will complement each other.
Another option that has been considered in the literature is the direct use of biogas ( Pathway 2: Direct usage of biogas ). The option of using biogas in tractors and machinery does not seem very promising, as the lower energy density of biogas does not lend itself well to mobile applications. The use in households for heating, cooking and lighting is limited to low-income countries in Asia and Africa, where the energy supply is not sufficiently developed, especially in rural areas. In Europe, however, the focus is on CHP generation. Therefore, it is not expected that direct use of biogas will be a relevant business model in the future.
The last option is the upgrading of biogas ( Pathway 3: Upgrading of biogas to a gas of higher value ). The use of biomethane offers the advantage of versatility and distribution via an existing infrastructure. Many papers consider possibilities and potentials of the use of biomethane and compare it to alternative use pathways for biogas. A special focus is on the use in the mobility sector. The use of biomethane is of particular interest for vehicles that cannot be powered by power, such as ships or aircraft. The profitability of the use of biomethane in the various pathways currently depends on subsidies or the consumer decision for a green product. The future development in the area of GHG neutrality is relevant here. In a GHG-neutral energy system, biomethane can be relevant to cover methane-based needs in an industrial context. However, a carbon–neutral energy system of the future cannot work with natural gas, as it generates GHG emissions when used. Therefore, the long-term perspective of using the natural gas grid for biomethane is uncertain. In the long term, it could be advantageous for biogas plants to cover regional methane demands with biomethane, for example in the mobility sector in the form of filling stations or industrial demands.
Another advantage of upgrading biogas to biomethane is that the captured CO 2 can be made usable, thus, generate additional revenue. This CO 2 can be used as a substitute for fossil-generated CO 2 . A contribution in the area of carbon capture and storage would also be conceivable here, so, CO 2 could be specifically absorbed in cultivated plants, which would then be used in biogas plants. The CO 2 separated in the upgrading process could then be stored and, thus, removed from the environment. Consequently, biogas plants could contribute to energy production and the reduction of CO 2 in the atmosphere. The separation and utilization of CO 2 can contribute to the profitability of biomethane use in the future. This utilization pathway is influenced by the acceptance of use of biogenic CO 2 , e.g., in the food industry, and the costs and revenues of CO 2 storage.
The topic of generating H 2 from biogas ( Business model 3.7: hydrogen production ) is still of little consideration in the literature from the point of view of business models. However, since the technology for upgrading is already available, this utilization pathway would be feasible in the short term. Biogas could find a long-term sales market in a hydrogen based energy system. First studies show that biogenic H 2 can be profitable and competitive. Here, however, the competitiveness has to be investigated in detail.
A major unknown component for the future development of business models for biogas plants is national legislation. This can lead to a certain utilization pathway becoming financially more attractive. In the EU, for example, the Renewable Energy Directive 2018/2001 (RED II) was issued in 2018, which aims to achieve the climate protection targets—a GHG reduction in transport of 40 to 42% by 2030. Therefore, there should be a share of at least 14% renewable energy in fuel consumption by 2030, as well as a sub-quota of 3.5% for advanced biofuels from residues such as straw and manure (European Parliament and Council of the European Union 2018 ). Biomethane from residues can play a particularly important role in meeting the sub-quota. In addition, financial support for biogas plants in the form of feed-in tariffs for power or biomethane or investment subsidies is also conceivable. This financial support can be differentiated so that, for example, special substrates or technical processes are supported. Tax benefits are also conceivable, giving a competitive advantage to renewables over fossil alternatives, as it is already common practice in Sweden in the mobility and heating sectors. These tax benefits may be tied to minimum GHG reduction standards, for example.
A major influence in the future will be the CO 2 price, which will contribute to the profitability of various potential biogas utilization paths in the long term. On the one hand, the CO 2 price can ensure that fossil alternatives become financially less attractive compared to biogas and, on the other hand, additional revenues can be achieved in the production and sale of biogas through the sale of certificates. Accordingly, the further development of legislation is a major factor influencing the development of the biogas industry.
The use of biogas and associated business models are discussed worldwide, but with a clear dominance of the European perspective. Outside of Europe, the focus is on basic potential analyses of the use of biogas, and in Asia and Africa, also on the use of biogas in households. This business model is not discussed for Europe. The majority of the papers was published within the last decade. During this time, the number of publications has increased steadily, but not explosively.
Furthermore, a development in the focus of the discussion of business models has emerged. The consideration of the direct use of biogas has been relatively constant over the years at a comparatively low level. In Europe, CHP generation is currently the dominant utilization pathway, with an increasing focus on flexibilization since 2015. The dominant aim of flexibilization was to satisfy a power demand. Research on business models for biogas utilization has evolved since 2019, and the focus is now particularly on the upgrading of biogas. Regarding upgrading, many new utilization pathways and business models for biogas have arisen in Europe, after a long period of mainly on-site CHP use. Biogas is becoming more valuable and even more diverse in its possible uses through upgrading. Upgrading biogas to H 2 still represents a small part of the relevant research literature. It is not surprising that research into the field of business models for upgrading biogas to H 2 has been increasing in recent years especially with the increasing demand for H 2 expected in the future.
The systematic literature research has shown that no business model proves profitable under all circumstances, so attention must generally be paid to the individual case of the biogas plant. Direct use is only a partially interesting business model in regions without a well-developed gas distribution infrastructure. The possibilities of flexible CHP use and upgrading appear promising in the literature. Future use of CHP is only viable with high flexibility. If fossil options for flexible generation are abandoned in the future, the synergies of different flexibility options can be used to provide system services. Thus, biogas plants can play a crucial role, especially in the power sector of the future. On the other hand, upgrading biogas to biomethane offers the advantage of multiple uses. Especially in applications where there are no other green alternatives, biomethane could play a decisive role in the future. For example, biomethane can meet methane-based needs in industry or serve as a fuel for heavy-duty transportation. In the future, however, biomethane upgrading should always be accompanied by CO 2 capture and use or storage, with the goal of increasing profitability and environmental benefits. Upgrading of biogas to hydrogen has not been investigated much yet, but could play a significant role in the future. Further investigations are needed to determine whether upgrading to hydrogen has any advantages. Factors influencing the profitability of a given business model thus include the size of the biogas plant, since upgrading biogas becomes more cost-effective especially as volumes increase, and the availability of local customers for heat or generated biomethane.
The development of the CO 2 price will be relevant for biogas plants in the future. On the one hand, this has an influence on the amount of revenues by trading certificates for the reduction of GHG emissions and, on the other hand, on the competition with fossil fuels, which become comparatively more expensive due to a higher CO 2 price, resulting in a competitive advantage for biogas. However, as various studies have shown, political support instruments are particularly relevant for a development of a country's biogas plant stock. Thus, the promotion of a certain business model leads to an increasing implementation of this utilization pathway. In the interest of the national GHG reduction strategies, it would be useful to set clear signals for the future development of existing plants, so that plant operators can develop a clear long-term strategy for the use of their plants.
The utilization pathways for biogas have become more diverse and each of these pathways has advantages. However, there is competition between the individual pathways since biogas as an energy resource is finite. The future political framework is a particularly relevant influencing factor on the development of the biogas industry. It has already led to the biogas industry developing differently in different countries as a result of various support programs. It needs a consistent political framework for a target-oriented, further development of the industry in the next few decades. The system utility of the use pathways can be a major argument for use in a particular energy sector. The environmental impact of biogas use will also have a greater influence on utilization decisions in the future; the goal of integrating it into a circular economy is already being explored.
Availability of data and materials
The datasets used and/or analyzed during the current study are available from the corresponding author on reasonable request.
Adler P, Billig E, Brosowski A, Daniel-Gromke J, Falke I, Fischer E (eds) (2014) Leitfaden Biogasaufbereitung und -einspeisung, 5., vollständig überarbeitete Auflage. Fachagentur für Nachwachsende Rohstoffe e. V. (FNR), Gülzow-Prüzen.
Ahlström JM, Zetterholm J, Pettersson K, Harvey S, Wetterlund E (2020) Economic potential for substitution of fossil fuels with liquefied biomethane in Swedish iron and steel industry—Synergy and competition with other sectors. Energy Convers Manage 209:112641. https://doi.org/10.1016/j.enconman.2020.112641
Article Google Scholar
Alves HJ, Bley Junior C, Niklevicz RR, Frigo EP, Frigo MS, Coimbra-Araújo CH (2013) Overview of hydrogen production technologies from biogas and the applications in fuel cells. Int J Hydrogen Energy 38:5215–5225. https://doi.org/10.1016/j.ijhydene.2013.02.057
Article CAS Google Scholar
Antonini C, Treyer K, Streb A, van der Spek M, Bauer C, Mazzotti M (2020) Hydrogen production from natural gas and biomethane with carbon capture and storage—a techno-environmental analysis. Sust Energy Fuels 4:2967–2986. https://doi.org/10.1039/d0se00222d
Aryal N, Kvist T, Ammam F, Pant D, Ottosen LDM (2018) An overview of microbial biogas enrichment. Bioresour Technol 264:359–369. https://doi.org/10.1016/j.biortech.2018.06.013
Article CAS PubMed Google Scholar
Backman M, Rogulska M (2016) Biomethane use in Sweden. Arch Automot Eng 71:7–19. https://doi.org/10.14669/AM.VOL71.ART1
Banja M, Jégard M, Motola V, Sikkema R (2019) Support for biogas in the EU electricity sector—A comparative analysis. Biomass Bioenergy 128:105313. https://doi.org/10.1016/j.biombioe.2019.105313
Bär K, Wageneder S, Solka F, Saidi A, Zörner W (2020) Flexibility potential of photovoltaic power plant and biogas plant hybrid systems in the distribution grid. Chem Eng Technology 43:1571–1577. https://doi.org/10.1002/ceat.202000025
Bensah EC, Brew-Hammond A (2010) Biogas technology dissemination in Ghana: history, current status, future prospects, and policy significance. Int J Energy 1(2):277–294
Google Scholar
Bensah EC, Mensah M, Antwi E (2011) Status and prospects for household biogas plants in Ghana—lessons, barriers, potential, and way forward. Int J Energy Environ 2(5):887–898
Bern G (2012) Investing in energy: a primer on the economics of the energy industry Bloomberg financial series. Wiley, Hoboken
Book Google Scholar
Bhatia RK, Ramadoss G, Jain AK, Dhiman RK, Bhatia SK, Bhatt AK (2020) Conversion of waste biomass into gaseous fuel: present status and challenges in India. Bioenerg Res 13:1046–1068. https://doi.org/10.1007/s12155-020-10137-4
Billig E, Decker M, Benzinger W, Ketelsen F, Pfeifer P, Peters R, Stolten D, Thrän D (2019) Non-fossil CO2 recycling—The technical potential for the present and future utilization for fuels in Germany. J CO2 Util 30:130–141. https://doi.org/10.1016/j.jcou.2019.01.012
Bisaglia C, Brambilla M, Cutini M, Fiorati S, Howell M (2018) Methane/ gasoline bi-fuel engines as a power source for standard agriculture tractors: development and testing activities. Appl Eng Agric 34:365–375. https://doi.org/10.13031/aea.12262
Bößner S, Devisscher T, Suljada T, Ismail CJ, Sari A, Mondamina NW (2019) Barriers and opportunities to bioenergy transitions: an integrated, multi-level perspective analysis of biogas uptake in Bali. Biomass Bioenerg 122:457–465. https://doi.org/10.1016/j.biombioe.2019.01.002
Braga LB, Silveira JL, Da Silva ME, Tuna CE, Machin EB, Pedroso DT (2013) Hydrogen production by biogas steam reforming: a technical, economic and ecological analysis. Renew Sustain Energy Rev 28:166–173. https://doi.org/10.1016/j.rser.2013.07.060
Bragança I, Sánchez-Soberón F, Pantuzza GF, Alves A, Ratola N (2020) Impurities in biogas: analytical strategies, occurrence, effects and removal technologies. Biomass Bioenerg 143:105878. https://doi.org/10.1016/j.biombioe.2020.105878
Browne J, Nizami A-S, Thamsiriroj T, Murphy JD (2011) Assessing the cost of biofuel production with increasing penetration of the transport fuel market: a case study of gaseous biomethane in Ireland. Renew Sustain Energy Rev 15:4537–4547. https://doi.org/10.1016/j.rser.2011.07.098
Budzianowski WM, Budzianowska DA (2015) Economic analysis of biomethane and bioelectricity generation from biogas using different support schemes and plant configurations. Energy 88:658–666. https://doi.org/10.1016/j.energy.2015.05.104
Butemann H, Schimmelpfeng K (2020) Long-term electricity production planning of a flexible biogas plant considering wear and tear. J Bus Econ 90:1289–1313. https://doi.org/10.1007/s11573-019-00937-2
Bystricky M, Knödlseder T, Weber-Blaschke G, Faulstich M (2010) Comparing environmental impacts of electricity, heat and fuel from energy crops: evaluating biogas utilization pathways by the basket of benefit methodology. Eng Life Sci 10:570–576. https://doi.org/10.1002/elsc.201000072
Calderón C, Avagianos I, Jossart J-M (2021) Bioenergy Europe Statistical Report-Biogas. Bioenergy Europe, Brussels, Belgium
Capodaglio AG, Callegari A, Lopez MV (2016) European framework for the diffusion of biogas uses: emerging technologies, acceptance, incentive strategies, and institutional-regulatory support. Sustainability 8:298. https://doi.org/10.3390/su8040298
Carranza-Abaid A, Wanderley RR, Knuutila HK, Jakobsen JP (2021) Analysis and selection of optimal solvent-based technologies for biogas upgrading. Fuel 303:121327. https://doi.org/10.1016/j.fuel.2021.121327
Cavana M, Leone P (2019) Biogas blending into the gas grid of a small municipality for the decarbonization of the heating sector. Biomass Bioenergy 127:105295. https://doi.org/10.1016/j.biombioe.2019.105295
Chen S, Chen B, Song D (2012) Life-cycle energy production and emissions mitigation by comprehensive biogas-digestate utilization. Bioresour Technol 114:357–364. https://doi.org/10.1016/j.biortech.2012.03.084
Cucchiella F, D’Adamo I (2016) Technical and economic analysis of biomethane: a focus on the role of subsidies. Energy Convers Manage 119:338–351. https://doi.org/10.1016/j.enconman.2016.04.058
Cucchiella F, D’Adamo I, Gastaldi M, Miliacca M (2018) A profitability analysis of small-scale plants for biomethane injection into the gas grid. J Clean Prod 184:179–187. https://doi.org/10.1016/j.jclepro.2018.02.243
Cvetković SM, Radoičić TK, Kijevčanin M, Novaković JG (2021) Life cycle energy assessment of biohydrogen production via biogas steam reforming: case study of biogas plant on a farm in Serbia. Int J Hydrog Energy 46:14130–14137. https://doi.org/10.1016/j.ijhydene.2021.01.181
D’Adamo I, Falcone PM, Ferella F (2019) A socio-economic analysis of biomethane in the transport sector: the case of Italy. Waste Manag 95:102–115. https://doi.org/10.1016/j.wasman.2019.06.005
Article PubMed Google Scholar
Daniel-Gromke J, Rensberg N, Denysenko V, Trommler M, Reinholz T, Völler K, Beil M, Beyrich W (2017) Anlagenbestand Biogas und Biomethan–Biogaserzeugung und -nutzung in Deutschland, DBFZ Report Nr. 30. Deutsches Biomasseforschungszentrum (DBFZ), Leipzig, Germany
Daniel-Gromke J, Kornatz P, Dotzauer M, Stur M, Denysenko V, Stelzer M, Hahn H, Krautkremer B, Bredow Hv, Antonow K (2019) Leitfaden Flexibilisierung der Strombereitstellung von Biogasanlagen (LF Flex)-Schlussbericht, Deutsches Biomasseforschungszentrum
Das CK, Ehsan MA, Kader MA, Alam MJ, Shafiullah GM (2016) A practical biogas based energy neutral home system for rural communities of Bangladesh. J Renew Sustain Energy. https://doi.org/10.1063/1.4942783
Demuynck M, Nyns E-J (1984) Biogas plants in Europe. Int J Solar Energy 2:477–485. https://doi.org/10.1080/01425918408909945
Edel M, Kühnel C (2019) biogaspartner – gemeinsam einspeisen. Biogaseinspeisung und -nutzung in Deutschland und Europa Markt, Technik und Akteure, Deutsche Energie-Agentur GmbH, Berlin
Edel M, Jegal J, Siegemund S, Schmidt P, Weindorf W (2019) dena-STUDIE: Bio-LNG–eine erneuerbare und emissionsarme Alternative im Straßengüter- und Schiffsverkehr: Potenziale, Wirtschaftlichkeit und Instrumente. Deutsche Energie-Agentur, Berlin
Dodds PE, Demoullin S (2013) Conversion of the UK gas system to transport hydrogen. Int J Hydrog Energy 38:7189–7200. https://doi.org/10.1016/j.ijhydene.2013.03.070
Dotzauer M, Schering K, Barchmann T, Oehmichen K, Schmieder U, Steubing M, Wern B, Matschoss P, Pertagnol J, Eltrop L, Gouya S, Zielonka S, Böckmann A (2021) Bioenergie–Potentiale, Langfristperspektiven und Strategien für Anlagen zur Stromerzeugung nach 2020 (BE20plus)-Schlussbericht. Deutsches Biomasseforschungszentrum (DBFZ), Leipzig, Germany
Dunkelberg E (ed) (2015) Biomethan im Energiesystem: Ökologische und ökonomische Bewertung von Aufbereitungsverfahren und Nutzungsoptionen ; gefördert durch das Bundesministerium für Wirtschaft und Energie im Rahmen des Projektes "Optimierung des innovativen Einsatzes eines Membranverfahrens zur Aufbereitung von Biogas zur Einspeisung ins öffentliche Erdgasnetz (MEGAS)". Schriftenreihe des IÖW, Bd. 207. Institut für ökologische Wirtschaftsforschung (IÖW), Berlin
Dzene I, Romagnoli F (2015) Assessment of the potential for balancing wind power supply with biogas plants in Latvia. Energy Procedia 72:250–255. https://doi.org/10.1016/j.egypro.2015.06.036
Dzene I, Romagnoli F, Seile G, Blumberga D (2014) Comparison of different biogas use pathways for Latvia: biogas use in CHP vs. biogas upgrading. The 9th Conference Environmental Engineering 184. https://doi.org/10.3846/enviro.2014.017
Eggemann L, Escobar N, Peters R, Burauel P, Stolten D (2020) Life cycle assessment of a small-scale methanol production system: a power-to-fuel strategy for biogas plants. J Clean Prod. https://doi.org/10.1016/j.jclepro.2020.122476
Ertem FC, Acheampong M (2018) Impacts of demand-driven energy production concept on the heat utilization efficiency at biogas plants: heat waste and flexible heat production. Process Integr Optim Sustain 2:1–16. https://doi.org/10.1007/s41660-017-0024-z
Esposito E, Dellamuzia L, Moretti U, Fuoco A, Giorno L, Jansen JC (2019) Simultaneous production of biomethane and food grade CO 2 from biogas: an industrial case study. Energy Environ Sci 12:281–289. https://doi.org/10.1039/C8EE02897D
European Alternative Fuels Observatory (2020) Countries overview of AF infrastructure. https://www.eafo.eu/countries/european-union-efta-turkey/23682/countries-compared . Accessed 17 Aug 2021
ACEA (2021) Vehicles in use Europe. https://www.acea.auto/files/report-vehicles-in-use-europe-january-2021-1.pdf . Accessed 17 Aug 2021
European Parliament and Council of the European Union (2018) Directive (EU) 2018/2001 of the European Parliament and of the Council of 11 December 2018 on the Promotion of the Use of Energy from Renewable Sources (Recast)
Fachagentur Nachwachsende Rohstoffe e. V. (2012) Biomethan
Fenton P, Kanda W (2017) Barriers to the diffusion of renewable energy: studies of biogas for transport in two European cities. J Environ Plan Manag 60:725–742. https://doi.org/10.1080/09640568.2016.1176557
Fischer E, Postel J, Ehrendreich F, Nelles M (2016) Energetische Bewertung von landwirtschaftlichen Biogasanlagen mithilfe des mittleren Brennstoffausnutzungsgrades. 139–154 Seiten / LANDTECHNIK, Bd. 71 Nr. 4 (2016). https://doi.org/10.15150/LT.2016.3132
Fleischer B (2018) Systemeffekte von Bioenergie in der Elektrizitäts- und Fernwärmewirtschaft: Eine modellgestützte Analyse langfristiger Energiewendeszenarien in Deutschland. Dissertation, Universität Stuttgart
Gandiglio M, Drago D, Santarelli M (2016) Techno-economic analysis of a solid oxide fuel cell installation in a biogas plant fed by agricultural residues and comparison with alternative biogas exploitation paths. Energy Procedia 101:1002–1009. https://doi.org/10.1016/j.egypro.2016.11.127
Goulding D, Power N (2013) Which is the preferable biogas utilisation technology for anaerobic digestion of agricultural crops in Ireland: biogas to CHP or biomethane as a transport fuel? Renew Energy 53:121–131. https://doi.org/10.1016/j.renene.2012.11.001
Grim J, Nilsson D, Hansson P-A, Nordberg Å (2015) Demand-orientated power production from biogas: modeling and simulations under Swedish conditions. Energy Fuels 29:4066–4075. https://doi.org/10.1021/ef502778u
Grösch N, Trox C, Saidi A, Zörner W, Grüner V, Baumkötter D, Brügging E, Wetter C, Glötzl M, Kilburg U, Gleich J, Wagner R, Vogt R (2020) Biogas nach dem EEG–(wie) kann´s weitergehen?: Handlungsmöglichkeiten für Anlagenbetreiber, Technische Hochschule Ingolstadt
Güsewell J, Bahret C, Eltrop L (2020) Auswirkungen von veränderten energie- und umweltrelevanten Rahmenbedingungen und Technologiefortschritt auf die Entwicklung sächsischer Biogasanlagen - AuRaSa
Güsewell J, Eltrop L, Hufendiek K (2021) Seasonal flexibilisation: a solution for biogas plants to improve profitability. Adv Appl Energy 2:100034. https://doi.org/10.1016/j.adapen.2021.100034
Hahn H, Krautkremer B, Hartmann K, Wachendorf M (2014) Review of concepts for a demand-driven biogas supply for flexible power generation. Renew Sust Energ Rev 29:383–393. https://doi.org/10.1016/j.rser.2013.08.085
Hahn H, Hartmann K, Bühle L, Wachendorf M (2015) Comparative life cycle assessment of biogas plant configurations for a demand oriented biogas supply for flexible power generation. Bioresour Technol 179:348–358. https://doi.org/10.1016/j.biortech.2014.12.007
Hamid RG, Blanchard RE (2018) An assessment of biogas as a domestic energy source in rural Kenya: developing a sustainable business model. Renew Energy 121:368–376. https://doi.org/10.1016/j.renene.2018.01.032
Häring G, Sonnleitner M, Bär K, Brown N, Zörner W (2017) Demonstration of Controllable Electricity Production via Biogas Plants. Chem Eng Technol 40:298–305. https://doi.org/10.1002/ceat.201600195
Heffels T, McKenna R, Fichtner W (2012) Direct marketing of electricity from biogas and biomethane: an economic analysis of several business models in Germany. J Manag Control 23:53–70. https://doi.org/10.1007/s00187-012-0153-z
Herbes C, Halbherr V, Braun L (2018) Factors influencing prices for heat from biogas plants. Appl Energy 221:308–318. https://doi.org/10.1016/j.apenergy.2018.03.188
Herbes C, Rilling B, Ringel M (2021) Policy frameworks and voluntary markets for biomethane – How do different policies influence providers’ product strategies? Energy Policy 153:112292. https://doi.org/10.1016/j.enpol.2021.112292
Hijazi O, Tappen S, Effenberger M (2019) Environmental impacts concerning flexible power generation in a biogas production. Carbon Resour Convers 2:117–125. https://doi.org/10.1016/j.crcon.2019.05.001
Hochloff P, Braun M (2014) Optimizing biogas plants with excess power unit and storage capacity in electricity and control reserve markets. Biomass Bioenerg 65:125–135. https://doi.org/10.1016/j.biombioe.2013.12.012
Hönig V, Prochazka P, Obergruber M, Smutka L, Kučerová V (2019) Economic and Technological Analysis of Commercial LNG Production in the EU. Energies 12:1565. https://doi.org/10.3390/en12081565
Hornbachner D, Kryvoruchko V, Gikopoulos C, Dos Santos M, Targyik-Kumer L, Adler R, Klein E (2009) Wirtschaftliche Chancen der Biogas-Versorgung netzferner Gas-Tankstellen gegenüber konventioneller Erdgas-Versorgung: Bundesministerium für Verkehr, Innovation und Technologie
Horschig T, Adams PW, Röder M, Thornley P, Thrän D (2016) Reasonable potential for GHG savings by anaerobic biomethane in Germany and UK derived from economic and ecological analyses. Appl Energy 184:840–852. https://doi.org/10.1016/j.apenergy.2016.07.098
Horschig T, Welfle A, Billig E, Thrän D (2019) From Paris agreement to business cases for upgraded biogas: analysis of potential market uptake for biomethane plants in Germany using biogenic carbon capture and utilization technologies. Biomass Bioenergy 120:313–323. https://doi.org/10.1016/j.biombioe.2018.11.022
International Energy Agency (2020) Key World Energy Statistics 2020
Jaber N, Noguchi N, Wakabayashi S, Tsukamoto T (2009) The development of control algorithm for the dual-fuel engine of a biogas tractor - biogas flow optimization based on engine parameters. JSAM:4–74
Kalinichenko A, Havrysh V (2019) Feasibility study of biogas project development: technology maturity, feedstock, and utilization pathway. Arch Environ Prot 45:68–83. https://doi.org/10.24425/aep.2019.126423
Karaeva JV (2021) Hydrogen production at centralized utilization of agricultural waste. Int J Hydrog Energy 46(69):34089–96. https://doi.org/10.1016/j.ijhydene.2021.08.004
Karlsson NP (2019) Business models and business cases for financial sustainability: Insights on corporate sustainability in the Swedish farm-based biogas industry. Sustain Prod Consum 18:115–129. https://doi.org/10.1016/j.spc.2019.01.005
Kemausuor F, Adaramola MS, Morken J (2018) A review of commercial biogas systems and lessons for Africa. Energies 11:2984. https://doi.org/10.3390/en11112984
Korberg AD, Skov IR, Mathiesen BV (2020) The role of biogas and biogas-derived fuels in a 100% renewable energy system in Denmark. Energy 199:117426. https://doi.org/10.1016/j.energy.2020.117426
Kruczyński SW, Pawlak G, Wojs MK, Wołoszyn R (2013) Biogas as a perspective fuel for agriculture tractors. Zeszyty Naukowe Instytutu Pojazdów 5(5):151–156
Lampinen A (2004) Biogas farming: an energy self-sufficient farm in Finland. Refocus 5:30–32
Lantz M (2012) The economic performance of combined heat and power from biogas produced from manure in Sweden—a comparison of different CHP technologies. Appl Energy 98:502–511. https://doi.org/10.1016/j.apenergy.2012.04.015
Lauven L-P, Geldermann J, Desideri U (2019) Estimating the revenue potential of flexible biogas plants in the power sector. Energy Policy 128:402–410. https://doi.org/10.1016/j.enpol.2019.01.007
Lee D-H (2017) Evaluation the financial feasibility of biogas upgrading to biomethane, heat, CHP and AwR. Int J Hydrogen Energy 42:27718–27731. https://doi.org/10.1016/j.ijhydene.2017.07.030
Li H, Tan Y, Ditaranto M, Yan J, Yu Z (2017) Capturing CO2 from biogas plants. Energy Procedia 114:6030–6035. https://doi.org/10.1016/j.egypro.2017.03.1738
Liebetrau J, Daniel-Gromke J, Jacobi F (2015) Flexible power generation from biogas. In: Thrän D (ed) Smart bioenergy. Springer International Publishing, Cham, pp 67–82
Lisbona P, Pascual S, Pérez V (2021) Evaluation of synergies of a biomass power plant and a biogas station with a carbon capture system. Energies 14:908. https://doi.org/10.3390/en14040908
Marcoberardino G, Vitali D, Spinelli F, Binotti M, Manzolini G (2018) Green hydrogen production from raw biogas: a techno-economic investigation of conventional processes using pressure swing adsorption unit. Processes 6:19. https://doi.org/10.3390/pr6030019
Matuszewska A, Owczuk M, Zamojska-Jaroszewicz A, Jakubiak-Lasocka J, Lasocki J, Orliński P (2016) Evaluation of the biological methane potential of various feedstock for the production of biogas to supply agricultural tractors. Energy Convers Manag 125:309–319. https://doi.org/10.1016/j.enconman.2016.02.072
Mihic S (2004) Biogas for internal combustion engines. Ann Fac Eng Hunedoara 2(3):179–190
Miltner M, Makaruk A, Harasek M (2017) Review on available biogas upgrading technologies and innovations towards advanced solutions. J of Clean Prod 161:1329–1337. https://doi.org/10.1016/j.jclepro.2017.06.045
Mohtar A, Ravi A, Ho WS, Choy CW, Hashim H, Muis Ab, Zarina Yunus NA, Hassim MH, Mah AXY (2021) Mathematical optimisation of biogas production and utilisation. Chem Eng Trans 83:445–450. https://doi.org/10.3303/CET2183075
Montenegro Camacho YS, Bensaid S, Piras G, Antonini M, Fino D (2017) Techno-economic analysis of green hydrogen production from biogas autothermal reforming. Clean Techn Environ Policy 19:1437–1447. https://doi.org/10.1007/s10098-017-1341-1
Murano R, Maisano N, Selvaggi R, Pappalardo G, Pecorino B (2021) Critical issues and opportunities for producing biomethane in Italy. Energies 14:2431. https://doi.org/10.3390/en14092431
Murray BC, Galik CS, Vegh T (2017) Biogas in the United States: estimating future production and learning from international experiences. Mitig Adapt Strateg Glob Change 22:485–501. https://doi.org/10.1007/s11027-015-9683-7
Natural & Bio Gas Vehicle Association (2019) Vehicle Catalogue
New Holland Agriculture UK (2021) New Holland Agricultural Tractors T6 METHANE POWER Overview. https://agriculture.newholland.com/eu/en-uk/equipment/products/agricultural-tractors/t6-methane-power . Accessed 23 Aug 2021
Nikolaidis P, Poullikkas A (2017) A comparative overview of hydrogen production processes. Renew Sust Energ Rev 67:597–611. https://doi.org/10.1016/j.rser.2016.09.044
Noussan M, Raimondi PP, Scita R, Hafner M (2021) The role of green and blue hydrogen in the energy transition—a technological and geopolitical perspective. Sustainability 13:298. https://doi.org/10.3390/su13010298
Owczuk M, Matuszewska A, Kruczyński S, Kamela W (2019) Evaluation of using biogas to supply the dual fuel diesel engine of an agricultural tractor. Energies 12:1071. https://doi.org/10.3390/en12061071
Pasini G, Baccioli A, Ferrari L, Antonelli M, Frigo S, Desideri U (2019) Biomethane grid injection or biomethane liquefaction: a technical-economic analysis. Biomass Bioenerg 127:105264. https://doi.org/10.1016/j.biombioe.2019.105264
Patrizio P, Chinese D (2016) The impact of regional factors and new bio-methane incentive schemes on the structure, profitability and CO2 balance of biogas plants in Italy. Renew Energy 99:573–583. https://doi.org/10.1016/j.renene.2016.07.047
Patrizio P, Leduc S, Chinese D, Dotzauer E, Kraxner F (2015) Biomethane as transport fuel—a comparison with other biogas utilization pathways in northern Italy. Appl Energy 157:25–34. https://doi.org/10.1016/j.apenergy.2015.07.074
Patrizio P, Leduc S, Chinese D, Kraxner F (2017) Internalizing the external costs of biogas supply chains in the Italian energy sector. Energy 125:85–96. https://doi.org/10.1016/j.energy.2017.01.033
Paturska A, Repele M, Bazbauers G (2015) Economic assessment of biomethane supply system based on natural gas infrastructure. Energy Procedia 72:71–78. https://doi.org/10.1016/j.egypro.2015.06.011
Redwanz M, Walter H (1984) Biogas und Äthanol als alternative Kraftstoffe für landwirtschaftliche Motoren. Tropenlandwirt 85:15–27
Roose A, Reinsoo K, Oja A, Varžinskas V (2012) Underdog or bulldog: introducing biogas technologies in Estonia. Clean Techn Environ Policy 14:1085–1093. https://doi.org/10.1007/s10098-012-0513-2
Rosa L, Sanchez DL, Mazzotti M (2021) Assessment of carbon dioxide removal potential via BECCS in a carbon-neutral Europe. Energy Environ Sci 14:3086–3097. https://doi.org/10.1039/D1EE00642H
Saracevic E, Woess D, Theuretzbacher F, Friedl A, Miltner A (2018) Techno-economic assessment of providing control energy reserves with a biogas plant. Front Chem Sci Eng 12:763–771. https://doi.org/10.1007/s11705-018-1776-x
Sarker S, Lamb JJ, Hjelme DR, Lien KM (2018) Overview of recent progress towards in-situ biogas upgradation techniques. Fuel 226:686–697. https://doi.org/10.1016/j.fuel.2018.04.021
Schmid C, Horschig T, Pfeiffer A, Szarka N, Thrän D (2019) Biogas upgrading: a review of national biomethane strategies and support policies in selected countries. Energies 12:3803. https://doi.org/10.3390/en12193803
Silaen M, Taylor R, Bößner S, Anger-Kraavi A, Chewpreecha U, Badinotti A, Takama T (2020) Lessons from Bali for small-scale biogas development in Indonesia. Environ Innov Soc Transit 35:445–459. https://doi.org/10.1016/j.eist.2019.09.003
Stürmer B, Leiers D, Anspach V, Brügging E, Scharfy D, Wissel T (2021a) Agricultural biogas production: a regional comparison of technical parameters. Renew Energy 164:171–182. https://doi.org/10.1016/j.renene.2020.09.074
Stürmer B, Theuretzbacher F, Saracevic E (2021b) Opportunities for the integration of existing biogas plants into the Austrian electricity market. Renew Sust Energ Rev 138:110548. https://doi.org/10.1016/j.rser.2020.110548
Szarka N, Scholwin F, Trommler M, Fabian Jacobi H, Eichhorn M, Ortwein A, Thrän D (2013) A novel role for bioenergy: a flexible, demand-oriented power supply. Energy 61:18–26. https://doi.org/10.1016/j.energy.2012.12.053
Technische Hochschule Ingolstadt (2020) Repoweringmaßnahmen hinsichtlich zukünftiger Aufgaben von Biogasanlagen: Schlussbericht
Theuerl S, Herrmann C, Heiermann M, Grundmann P, Landwehr N, Kreidenweis U, Prochnow A (2019) The future agricultural biogas plant in Germany: a vision. Energies 12:396. https://doi.org/10.3390/en12030396
van Basshuysen R (2016) Natural gas and renewable methane for powertrains. Springer International Publishing, Cham
Verotti M, Servadio P, Bergonzoli S (2016) Biogas upgrading and utilization from ICEs towards stationary molten carbonate fuel cell systems. Int J Green Energy 13:655–664. https://doi.org/10.1080/15435075.2015.1018992
Wattanasilp C, Songprakorp R, Nopharatana A, Khompatraporn C (2021) Techno-cost-benefit analysis of biogas production from industrial cassava starch wastewater in Thailand for optimal utilization with energy storage. Energies 14:416. https://doi.org/10.3390/en14020416
Watter H (2019) Regenerative energiesysteme. Springer Fachmedien, Wiesbaden
Wille-Haussmann B, Erge T, Wittwer C (2010) Decentralised optimisation of cogeneration in virtual power plants. Sol Energy 84:604–611. https://doi.org/10.1016/j.solener.2009.10.009
Wu B, Zhang X, Shang D, Bao Di, Zhang S, Zheng T (2016) Energetic-environmental-economic assessment of the biogas system with three utilization pathways: combined heat and power, biomethane and fuel cell. Bioresour Technol 214:722–728. https://doi.org/10.1016/j.biortech.2016.05.026
Wulf C, Kaltschmitt M (2013) Life cycle assessment of biohydrogen production as a transportation fuel in Germany. Bioresour Technol 150:466–475. https://doi.org/10.1016/j.biortech.2013.08.127
Wünning JG (2021) Grüner Wasserstoff aus biogas. gwf Gas+Energie 4:37–40
Yao J, Kraussler M, Benedikt F, Hofbauer H (2017) Techno-economic assessment of hydrogen production based on dual fluidized bed biomass steam gasification, biogas steam reforming, and alkaline water electrolysis processes. Energy Convers Manage 145:278–292. https://doi.org/10.1016/j.enconman.2017.04.084
Yasmin N, Grundmann P (2019) Pre- and post-adoption beliefs about the diffusion and continuation of biogas-based cooking fuel technology in Pakistan. Energies 12:3184. https://doi.org/10.3390/en12163184
Yuan D, Wang J, Huang X, Mao X-F, Hong Z, Cao Y, Li J (2015) Emission reduction effects of biogas micro-digesters: a case study of rural households in Kaixian China. Fresenius Environ Bull 24(4b):1530–1536
CAS Google Scholar
Download references
Acknowledgements
We thank the three anonymous reviewers at Bioresources and Bioprocessing whose comments improved and clarified this manuscript significantly.
Open Access funding enabled and organized by Projekt DEAL. This research was funded by the German Federal Environmental Foundation, grant number AZ 34663.
Author information
Authors and affiliations.
Osnabrück University of Applied Sciences, Osnabrück, Germany
Anica Mertins
Osnabrück University of Applied Sciences, Lingen (Ems), Germany
You can also search for this author in PubMed Google Scholar
Contributions
The concept for this paper was developed by AM and TW. AM analyzed the published data, AM and TW have both interpreted the data. AM has drafted the work. All authors read and approved the final manuscript.
Corresponding author
Correspondence to Anica Mertins .
Ethics declarations
Ethics approval and consent to participate.
Not applicable.
Consent for publication
Competing interests.
The authors have no relevant financial or non-financial interests to disclose.
Additional information
Publisher's note.
Springer Nature remains neutral with regard to jurisdictional claims in published maps and institutional affiliations.
Rights and permissions
Open Access This article is licensed under a Creative Commons Attribution 4.0 International License, which permits use, sharing, adaptation, distribution and reproduction in any medium or format, as long as you give appropriate credit to the original author(s) and the source, provide a link to the Creative Commons licence, and indicate if changes were made. The images or other third party material in this article are included in the article's Creative Commons licence, unless indicated otherwise in a credit line to the material. If material is not included in the article's Creative Commons licence and your intended use is not permitted by statutory regulation or exceeds the permitted use, you will need to obtain permission directly from the copyright holder. To view a copy of this licence, visit http://creativecommons.org/licenses/by/4.0/ .
Reprints and permissions
About this article
Cite this article.
Mertins, A., Wawer, T. How to use biogas?: A systematic review of biogas utilization pathways and business models. Bioresour. Bioprocess. 9 , 59 (2022). https://doi.org/10.1186/s40643-022-00545-z
Download citation
Received : 06 January 2022
Accepted : 30 April 2022
Published : 28 May 2022
DOI : https://doi.org/10.1186/s40643-022-00545-z
Share this article
Anyone you share the following link with will be able to read this content:
Sorry, a shareable link is not currently available for this article.
Provided by the Springer Nature SharedIt content-sharing initiative
- Business models
- Utilization pathways
- Direct usage
- Biogas upgrading
- Hydrogen from biomass

An official website of the United States government
The .gov means it’s official. Federal government websites often end in .gov or .mil. Before sharing sensitive information, make sure you’re on a federal government site.
The site is secure. The https:// ensures that you are connecting to the official website and that any information you provide is encrypted and transmitted securely.
- Publications
- Account settings
Preview improvements coming to the PMC website in October 2024. Learn More or Try it out now .
- Advanced Search
- Journal List
- Int J Environ Res Public Health
- PMC10094619

Analysing the Impact on Health and Environment from Biogas Production Process and Biomass Combustion: A Scoping Review
Marco tamburini.
1 Unit of Biostatistics and Clinical Epidemiology, Department of Public Health, Experimental and Forensic Medicine, University of Pavia, 27100 Pavia, Italy
Roberta Pernetti
2 Unit of Occupational Medicine, Department of Public Health, Experimental and Forensic Medicine, University of Pavia, 27100 Pavia, Italy
Manuela Anelli
Enrico oddone.
3 Hospital Occupational Medicine Unit (UOOML), ICS Maugeri IRCCS, 27100 Pavia, Italy
Anna Morandi
Adam osuchowski, simona villani, cristina montomoli, maria cristina monti, associated data.
Not applicable.
The increasing demand for renewable energy production entails the development of novel green technologies, among them the use of biomass for energy generation. Industrial processes raise new issues regarding emerging risks for the health of people working in biogas plants and of nearby communities. The potential epidemiological and environmental impacts on human health related to biogas plants were assessed by means of a review of the available literature. Nineteen papers published between 2000 and 2022 were identified through electronic database search using search strings. The selected works are epidemiological studies and environmental monitoring studies, which aimed at investigating what are the health risk factors for biogas plant workers and for people living in the surrounding communities. The results of the epidemiological studies revealed a potential exposure to endotoxins and fungi that are associated with respiratory symptoms. Furthermore, the results from the environmental monitoring studies showed significant concentrations of particulate matter, microbial agents, endotoxins, and VOCs in occupational settings. In conclusion, the results of this literature review suggest that further analyses through an integrated approach combining environmental and health data are necessary for a comprehensive understanding of the potential risks associated with the uptake of biogas technology.
1. Introduction
The European Commission aims to reach the target of net-zero greenhouse gas emission by 2050 [ 1 ]. To comply with this objective, there is a need for significant energy transition based on both the improvement of energy efficiency and the uptake of renewable energy [ 1 ]. The significant need for increasing energy production from renewable sources, leads to the development of innovative “green” technologies. Biogas, is recognised by the European Commission as one of the key solutions for both existing buildings and several industrial applications [ 1 ]. Biogas production is based on anaerobic digestion (induced by specific mixture of bacteria and additives) of a biomass feedstock. The employed feedstock generally belongs to one of these categories: agricultural products or residues, wooden by-products, manure from livestock rearing, by-products of wastewater treatment, and urban organic waste [ 2 ]. In particular, the employed feedstock represents the main factor affecting the level of sustainability of biogas production in terms of both greenhouse gas (GHG) emissions [ 3 ] and life cycle assessment (LCA) of plants [ 4 , 5 , 6 ].
Several studies analysed biogas production technology in terms of costs and benefits, as well as potential environmental impact of biogas. These assessments highlighted a significant reduction in terms of carbon emissions associated with the production and application of biogas energy compared to fossil fuels, but the production may entail the release of potential pollutants that should be further evaluated and studied [ 7 , 8 , 9 ]. In this regard, the characterization of pollutant emissions, as well as the analysis of potential health implications at different levels (i.e., for workers and nearby communities), represent strategic outcomes to support the increase in social acceptance of this technology across Europe [ 5 , 6 ].
To study the main features of the process, it is possible to break down the operations into three macro-phases that deal with biogas production and biomass handling processing of digestate and biogas or biomass combustion for energy production ( Figure 1 ).

Overview of the main process and monitored activities within the included studies (modified from [ 10 ]).
The development of novel technologies and industrial processes presents new technical and organizational variables that can generate traditional occupational risks, which are usually managed through proven standards and existing requirements, as well as new emerging risks, which should be further assessed and deserve targeted analysis [ 11 ]. In a biogas plant, in addition to the traditional risks connected with manual handling of loads and, in particular, when handling feedstock and the produced digestate, there may be an exposure to both biological and chemical pollutants during the different phases of the process [ 12 , 13 , 14 ]. The novelty of the technologies, the limited amount of research available on the topic, the high number of processable feedstocks, the specific design of a facility, and the variety of implemented processes have led to a general difficulty in providing a clear statement on the potential exposure at occupational and residential levels. Therefore, novel technologies need a detailed assessment for the identification and quantification of potential risks to promote the implementation of safe working procedures and to identify variables that need to be controlled to limit the potential threats to workers as well as to the surrounding communities.
This paper aims to identify the potential effects of exposure to chemical and biological pollutants on workers and nearby communities of a biogas plant according to the processed feedstock and technology implemented. The complexity of this process entails several uncertainties that may have a significant impact when modelling the impact of a biogas plant [ 15 ]. Therefore, the added value provided by this review relies on the presentation of potential exposure through quantitative data from real biogas plants. In fact, one of the main requirements for the inclusion in the list of selected papers was the availability of data either from on-field monitoring or from an epidemiological study, aiming to collect benchmarks and reference values and to provide a characterization of potential emissions and related effects from existing plants at both occupational and community levels. This is crucial to derive clear statements on the potential risks associated with biogas production plants at both occupational and community levels and, on the other hand, to identify potential benchmarks for the assessment of further sites.
The results reported here provide an initial assessment of the main hazards to be further evaluated on the field.
2. Materials and Methods
The analysis was based on the workflow of an electronic database search and followed the Preferred Reporting Items for Systematic Reviews and Meta-Analyses (PRISMA) guidelines [ 16 ].
2.1. Inclusion/Exclusion Criteria
The research question used for the review was “What are the risk factors for the health of the people working in biogas plants and of the surrounding communities?” Starting from the research question, the inclusion criteria were defined. The framework of this review was based on an analysis of studies that present actual data from on-field environmental monitoring—dealing with biological and chemical pollution due to particulates, gases and volatile organic compounds (VOCs) released by the processes—and epidemiological investigation on populations that are potentially exposed. Moreover, given the limited amount of research currently available on biogas plants, the authors included studies regarding other types of bioenergy technology (namely biomass combustion plants and biorefineries) that also involve the use of biomass feedstock employed in biogas production.
In order to organize the included studies and provide a homogeneous description, the following classification criteria were adopted:
- Epidemiological studies: studies carried out on a general or specific population (both workers and surrounding communities) to assess the outcome frequency and its potential association with exposure;
- Environmental monitoring studies: studies with pollutant measurements carried out in the workplace or in the surroundings of biogas production sites. The pollutants include biological components, VOCs, and gases and emissions from biogas plants as analysed in Life Cycle Assessment (LCA) analyses;
- Primary studies written in English and published between 2000 and 2022.
- Furthermore, the following exclusion criteria were applied:
- Lack of quantitative data from modelling, monitoring, or epidemiological evaluations available;
- Papers focused solely on pollutant emissions from plants producing energy through biomass combustion or other sources;
- Works presenting analyses on domestic plants, as the focus of this research was on the industrial setting.
2.2. Information Sources and Search Strategy
The literature search was carried out by means of diverse electronic sources, i.e., PubMed, Web of Science (WoS), and ScienceDirect (SD). The search strategy was carefully designed to retrieve the most relevant results. Due to the specificity of the three databases employed, for each one, a different search string was built:
PubMed: (health OR human) AND (biomass OR biogas OR anaerobic digestate OR biofuel) AND (hazard OR risk OR exposure OR impact) AND (worker OR resident OR community OR population). Number of results: 1403.
- Web of Science: (ALL = (health)) OR ALL = (human) AND (((ALL = (biomass)) OR ALL = (biogas)) OR ALL = (anaerobic digestate)) OR ALL = (biofuel) AND (((ALL = (hazard)) OR ALL = (risk)) OR ALL = (exposure)) AND ALL = (impact) AND (((ALL = (worker)) OR ALL = (resident)) OR ALL = (community)) OR ALL = (population). Number of results: 845.
- ScienceDirect: (health) AND (biomass OR biogas OR anaerobic digestate) AND (risk OR impact) AND (worker OR community OR population). Number of results: 722.
Primary studies published in English between 2000 and 2022 were eligible for inclusion, and no regional restrictions were applied. Table 1 summarizes the key aspects considered in this review, namely focus, technology, topic, and exposed subjects, and the keywords adopted for the literature search.
Key aspects taken into consideration in the review.
The electronic database search was conducted in March 2022. From the screening of eligible studies, the reasons for the exclusion of studies were also reported.
Studies were also identified through citation searching. These articles were regarded as relevant even though they were not identified through the search strings.
2.3. Selection Process
Titles, abstracts, and full texts were screened by the research team, i.e., each member of the team screened a part of the whole set of studies. In case of doubt about the selection of a study, a collective decision on the selection was taken.
2.4. Data Extraction
For each category, a standardized data extraction sheet using Microsoft Excel 2016 was prepared, where the main information of the studies was collected (e.g., first author’s name, study title, publication year, DOI, country, biomass type, methodology, population involved, exposure, outcome and results, and additional notes).
3.1. Study Selection
Through the comprehensive literature search, 19 studies were included in this literature review. Eleven of them were identified via the database searches, and eight were identified via citation searching ( Figure 2 ).

Study selection process. For more information on this flowchart, see [ 16 ].
The number of the search records retrieved from the three database searches was 2970; additionally, 12 records were identified through cited reference searching. The number of the screened titles/abstracts after duplicate removal was 2527. One hundred seventy-nine full-text papers were analysed, among which 26 were assessed for eligibility. The rest of the papers do not report analyses dealing with potential occupational exposure or impact on the surrounding communities of biogas production plants; thus, they were excluded. Finally, 19 papers were included in the review, as indicated in Figure 2 .
3.2. Study Characteristics
As shown in Figure 3 , the analysed studies were conducted in different geographical contexts: most of them were carried out in Europe (n = 12), Asia (n = 4), and North America (n = 3). Each geographic context shows specific features in terms of level of technical development, availability of feedstock, and technical standards for plant construction and operation; these peculiarities were considered in the analysis of the results. Among the included works, 4 studies are classified as epidemiological studies and 15 as environmental monitoring studies.

Geographical distribution of the selected studies around the world and in the European countries. Red diamonds: environmental studies; black dots: epidemiological studies.
3.3. Epidemiological Studies
On the basis of the results of the literature search, the health impact of industrial biogas facilities seems to be a scarcely investigated topic from an epidemiological viewpoint. In particular, among the four epidemiological studies included in this section, none of them properly deals with plants where biogas is the sole final product. However, each study addresses certain aspects that may apply to the biogas production process. More specifically, the four epidemiological studies investigate the correlation between biomass process exposures and health conditions that are not strictly connected to the phases of a biogas plant.
3.3.1. Residential Setting Studies
Of the two studies carried out in residential settings, one is a cross-sectional study from Thailand [ 17 ], which aimed at exploring the impact of air pollution from the activity of two biomass power plants on nearby residents’ health. Both plants employ the same biomass type, i.e., rice husks. This study was performed on a population of 392 residents (households aged ≥ 15 years). Exposure was determined by the distance between the residential communities and the biomass power plants. Accordingly, three groups were identified: Group 1, residents living at a distance of 0–0.5 km from the plants; Group 2, residents living 0.5–1.0 km away from the plants, and Group 3, residents living at >1 km distance who were regarded as the reference category. The households reported their own health symptoms during the past week and answered questions about chronic diseases for each member of the household, resulting in 1254 participants. People working in the plants were excluded from the survey.
Air quality was measured by means of air monitoring stations; the pollutants considered were dust, total suspended particulate (TSP), particulate matter with a diameter less than 10 µm (PM 10 ), nitrogen dioxide (NO 2 ), sulphur dioxide (SO 2 ), and ozone (O 3 ). The outcomes were represented as chronic diseases (allergy, asthma, heart disease, chronic obstructive pulmonary disease (COPD), tuberculosis, and cancer) and health symptoms (itching/rash, eye irritation, cough, stuffy nose, allergic symptoms, sore throat, and difficulty breathing). The results showed ( Table S1 ) that living near the biomass power plants was significantly associated with all health symptoms for residents living at a distance of 0–0.5 km. For the second level of exposure (i.e., residents living at 0.5–1.0 km from the plants) the relationship was statistically significant only for difficulty breathing (detailed results are shown in Table S1 ). With regard to the relationship between “living near biomass power plants and chronic diseases”, the only significant association was related to allergy for the first exposure group when compared to the reference group. This study has some limitations: First, it is unclear if a standardized questionnaire was used to collect data about health symptoms, which presents a risk of information bias. Secondly, the study relied on self-reported information about chronic diseases and health symptoms, which were not confirmed by a clinician and might have been subsequently overestimated. Thirdly, in reporting their symptoms, the subjects might have demonstrated a recall bias. Finally, the analyses were conducted without taking into account possible confounders.
The other study is an up-to-date cross-sectional study conducted in New York State, USA [ 18 ], which aimed at assessing the potential connection between residential proximity to biorefineries or biorefinery-related emissions and respiratory morbidity. The study population was represented by New York State (NYS) residents aged 1–85 living within 20 km from biorefineries (regarded as “biorefinery sites”) and from the reference areas who received Emergency Department (ED) visits (n = 547,437) for lower respiratory diseases between January 2011 and December 2015. The study areas included 15 biorefineries located in NYS and using different biomass types: corn (n = 2), soybean (n = 2), and wood (n = 11). Fifteen reference areas with no biorefineries were also identified. These areas shared similar socio-demographic characteristics with the biorefinery sites (median income, age distribution, and percentage of African Americans). The outcomes were lower airway diseases, including asthma, chronic bronchitis, emphysema, and chronic airway obstruction. Exposure was represented by both residential proximity to biorefineries and air dispersion-modelled concentrations of multiple pollutants. Regarding proximity, distances from refineries were expressed as 0–5, >5–10, >10–15, and >15–20 km. In a second phase, analyses were carried out within 10 km, with this distance being identified as a threshold of health risk. Among the air pollutants, particulate matter with a diameter less than 2.5 µm (PM 2.5 ), SO 2 , and NO 2 were considered. The daily concentrations of air pollutant were estimated by using the American Meteorological Society and the U.S. EPA (AMS/EPA) Regulatory Model (AERMOD) over the period between 2011 and 2015. Possible confounders (i.e., age, race, sex, county-level smoking rate, meteorological variables, and annual mean concentrations of air pollutants) were taken into account in the analyses. The results show the importance of taking SO 2 and NO 2 into consideration for future studies, given their contribution to respiratory ED visits. Additionally, residential proximity (within 5 km) to plants emitting PM 2.5 , SO 2 , and NO 2 , such as biorefineries, was associated with an increased risk of emphysema. The health risk seemed to be greater for residents living near corn and soybean biorefineries than for those living close to wood biorefineries; the average PM 2.5 and NO 2 emission rates (g/s) from corn and soybean biorefineries were 1.5−3 times higher than the average PM 2.5 and NO 2 emission rates from wood biorefineries. Associations between biorefinery activity and respiratory health were higher during spring and winter compared to those during fall and summer (with the lowest risk). The detailed results are shown in Tables S2 and S3 . This study presents some limitations: only severe cases were considered, leaving aside mild cases; the study population included only health insurance owners; a selection bias might exist due to the geographical locations of the study population (residents of lower socio-economic status); unmeasured confounders, such as indoor exposure and pattern activity, might have introduced confounding bias; and the results might show ecological fallacy because of the aggregated nature of the data.
Table 2 shows the main features and the results of the two included studies in residential settings.
Main features of the included epidemiological studies in residential settings.
3.3.2. Occupational Setting Studies
Both studies related to occupational settings were carried out in Denmark [ 19 , 20 ].
The study by Schlünssen et al. (2011) [ 19 ] focused on a possible association between working in a biomass-fuelled plant and an increased risk of respiratory diseases compared to working in a conventional power plant. This cross-sectional study was conducted on 85 Danish heating and power plants using woodchip or straw and 11 heating and power plants using conventional fuel. In both types of facilities, the workers had similar tasks. The study investigated the exposure to dust, endotoxins, cultivable fungi, and Aspergillus fumigatus (Fresenius 1863). The workers were divided into three exposure groups (most, moderately, and least exposed) based on personal mean exposure to bioaerosols, as measured through job-exposure matrices. The data on respiratory diseases were collected through the European Community Respiratory Health Survey (ECRHS) short questionnaire, in which further questions about allergy, cough, asthma, rhinitis, smoking, toxic pneumonitis, and occupational history were added. The questionnaire was self-administered to the workers. Smoking and atopy were regarded as confounders. The results showed that working either in a heating or in a power plant using biofuel did not expose the workers to any considerable additional risk for respiratory diseases; however, the level of micro-organisms ( Aspergillus fumigatus ) had an impact on the occurrence of respiratory symptoms among the biofuel workers, despite a low exposure level. It follows that preventive precautions should be taken in power plants using biofuel to keep bioaerosol exposure as low as possible (detailed results are shown in Table S4 ). This study presents the following limitations: the low response rate (59%) among the reference workers, especially within the least exposed reference group, might be a source of differential misclassification; respiratory diseases were self-assessed with subjective single questions; and the self-reporting of data on respiratory diseases might produce information bias.
The study by Basinas et al. (2012) [ 20 ] addressed endotoxin exposure in diverse occupational settings, combining the results of four studies carried out in Denmark and the Netherlands. For the scope of our research, we deal with the first of the four studies: a cross-sectional study performed on Danish biofuel plant workers (n = 176) aimed at exploring the relationship between bioaerosol exposure and allergy and respiratory symptoms in plants using either woodchip or straw. Four exposure groups were identified: low, low mediate, high mediate, and highly exposed ( Table 3 ). The data about health outcomes were collected by means of a self-reported questionnaire. The confounders were gender, age, farm childhood, atopic predisposition, and smoking habits. The results showed that endotoxin exposure was statistically significantly associated with chronic bronchitis for the moderately exposed group and with wheezing for the most exposed group (detailed results are shown in Table S5 ). This study has some limitations: respiratory diseases were self-assessed with subjective single questions, and the self-reporting of data on respiratory diseases might produce information bias.
Main features of the included epidemiological studies in occupational settings.
3.4. Environmental Monitoring Studies
The studies included in the environmental monitoring section reported the results of on-field measurements with quantitative assessment of emissions associated with biogas production, or with technologies with processes that are partially overlapped with biogas production ones. As shown in Table 4 , it is possible to identify three main levels of monitoring:
- Occupational level (n = 10) reporting analyses dealing with the potential exposure and risk assessment for workers;
- Community level (n = 1) aiming to analyse air quality around biogas plants and to identify potential atmospheric pollution that can be released by biogas plants according to the context;
- Other emissions (n = 4), including two Life Cycle Assessment evaluations that identify green gas house emission and acidification potentially associated with biogas plants, and two studies about the features of digestate.
List of included papers identified as environmental monitoring studies. The papers are listed chronologically.
Most of the analysed plants produce biogas through the anaerobic digestion process that, in some cases, is coupled with energy production using biogas in a co-generator. Moreover, these plants adopt different feedstocks that can be classified into four categories, as reported in Table 5 : crop residues (CR), organic fraction of municipal solid waste (MSW), animal manure (AM), and wastewater sludge (WWS).
Overview of the plants analysed in the included studies (BG= biogas plants implementing anaerobic digestion, and BC= power generation through biogas combustion).
Considering the process of biogas production shown in Figure 1 , Table 6 summarises the monitoring approach of the included works, including identifying the monitored phases, the approach for the sampling measurements, and the feedstocks employed in the plants, where applicable.
Overview of the monitoring setup in the analysed plants. FT: Feedstock transport—including operation of handling within the plant facilities before initial treatment; PT: Pre-treatment—initial phases before anaerobic digestion; AD: Anaerobic digestion—sequence of processes by which microorganisms break down biodegradable material in the absence of oxygen; DT: Digestate treatments—operations for processing digestate; DS: Digestate storage—storage of digestate before distribution; CHP: Combined heat and power production through biogas.
1 With pulverised coal.
Regarding the contents of the analysed papers, the authors decided to organize the results according to the type of monitored pollutants:
- 4. Identification of biological pollutants in biomass and/or in aerosol;
- 5. VOCs and emitted gases;
- 6. Particulate matter and nanoparticles.
In addition, a fourth section reports the results of the LCA and digestate analysis.
3.4.1. Biological Pollutants in Biomass and/or in Aerosol
Despite numerous benefits related to the use of anaerobic digestion as an alternative source of energy, the handling of biomass could potentially create a biological risk due to exposure to harmful microbiological agents, which could cause respiratory problems.
Selected studies found several groups of bacteria and fungi in the air near different areas of biogas plants. These organisms came from different types of biomasses (e.g., forest and agricultural residues, sludge from wastewater treatment plants, and food waste). Any differences found in the exposure levels among the plants might partly be due to differences in the process equipment, tasks, and biofuel handled.
Since the same type of biomasses may be used in both biogas- and biomass-powered plants, studies conducted in both kinds of plants were included ( Table 6 ).
Madsen (2006) conducted a monitoring on workers’ exposure to organic dust in different working areas (e.g., storage areas and offices) of five biofuel plants in Denmark, which employed bark chips with salt water, straw, forest chips, and industry chips ( Table 6 ) [ 21 ]. GSP inhalable samplers were mounted with Teflon filters (pore size 1 mm) for endotoxin and NAGase (N-acetyl-beta- d -glucosaminidase, an enzyme mainly produced by fungi) analysis, and with polycarbonate filters (pore size 1 mm) for quantification of total number and cultivable units of bacteria and fungi. In addition, outdoor references were sampled upwind of the plants on each sampling day. Personal exposure to inhalable endotoxins resulted in a range between 2 and 119,000 EU/m 3 (EU: endotoxin unit), and 34% of the measured subjects were exposed to >150 EU/m 3 . The highest exposure was found for an employee who worked with a straw shredder for 90 min out of 6 working hours, while the lowest exposure was found for a worker working partly in an office and partly as a painter. In the working areas, the median endotoxin concentration was 66 EU/m 3 (average = 429; max = 21,000; and n = 88). Personal exposures to concentrations > 104 cfu/m 3 of mesophilic fungi were found for 81% of the workers, and 68% of them were exposed to concentrations of fungal spores > 105 m −3 . Aspergillus fumigatus , a potential pathogenic fungus species, was found in most areas, and the highest exposure was found for a person who cleaned a chip pit for 1 out of 7 working hours and did office work for the remaining 6 h.
In a Finnish plant using kitchen waste for digestion ( Table 6 ), Tolvanen and Hanninen (2006) determined the concentrations of mesophilic and thermophilic bacteria, fungi, and actinomycetes, including both viable and non-viable micro-organisms, by using a six-stage impactor and by collecting airborne micro-organisms using nucleopore filters via an estimation and analysis (CAMNEA) filter collection method [ 23 ]. They sampled five locations and activities: (I) pre-treatment and crushing of waste; (II) maintenance work in the pre-treatment hall; (III) maintenance work in the bioreactor hall; (IV) bioreactor hall during normal operation; and (V) drying hall. The level of microbes and endotoxins was a problem in the plant, especially during waste crushing, and the authors suggested the use of a respirator mask for the workers during dusty working phases. Nevertheless, the occupational hygiene of the plant was as good as that of plants treating biowaste aerobically.
Madsen et al. (2009) carried out a monitoring of organic dust in 14 Danish biofuel plants, with one plant mainly using straw and wood chips ( Table 6 ) [ 25 ]. Twenty-nine samples of airborne particles were taken using a Triplex cyclone. Moreover, they sampled total dust using 25 mm closed-face cassettes. They assessed the concentration of microorganisms in PM 1 dust and fungi in total dust using a modified CAMNEA method. This sampling was performed in the straw storage room at 1.5 m above floor level (i.e., the workers’ breathing zone). Cultivable mesophilic fungi and “total fungal spores” were found in 6 and 22 of the 29 samples, respectively. However, the median concentration of cultivable fungi was below the detection level, and no thermotolerant fungi were found. Moreover, for the first time, the authors found high concentrations of β- d -glucan (a polymer produced by most fungi and with a possible negative impact on health) in PM 1 dust sampled from the straw storage room where the workers handled straw.
Ławniczek-Wałczyk et al. (2012) investigated exposure to harmful microbiological agents during the handling of biomass in a Polish power plant in which forest and agricultural biomass is co-combusted with pulverised coal ( Table 6 ) [ 31 ]. Ten sampling stations were designated, including workplaces in the technological line (seven stations), a control laboratory where biomass quality was tested for its physical parameters (two stations), and one “background” station, to assess the reference baseline. Moreover, the measuring instruments were placed at the workers’ breathing zone height, and the microorganisms isolated from the air samples were identified down to the genus and/or species level. The concentration of bacterial aerosol ranged from 5.1 × 10 2 –2.3 × 10 4 cfu/m 3 in the workplaces in the technological line to 5.6 × 10 2 –3.3 × 10 3 cfu/m 3 in the laboratory, while the fungal aerosol concentration varied from 2.2 × 10 2 –2.0 × 10 4 cfu/m 3 in the workplaces to 1.1 × 10 3 –8.0 × 10 3 cfu/m 3 in the lab. A significantly higher concentration of bacteria and fungi in the air in the workplaces in the technological line, compared to those in the laboratory, was found. In general, the results of this study indicate that both workers employed in the technological line for biomass combustion and laboratory workers were exposed to bioaerosols containing potentially pathogenic bacteria and fungi. The qualitative analysis of the air microorganisms in the designated workplaces indicated the presence of bacterial and fungal specimens that, according to the Ordinance of the Polish Minister of Health, are classified into risk group 2, which means a possible adverse health effect (e.g., allergic reaction) for people with an impaired immune system.
Traversi et al. (2015) evaluated airborne exposure among anaerobic digestion workers at two Italian plants (S–plant and M–Plant) [ 27 ]. These plants used both agricultural and livestock biomasses ( Table 6 ). Microbiological sampling was performed during the input and output operations of both plants, and eight variables were measured: total bacteria as an environmental contamination indicator; total bacteria as an animal/human contamination indicator; total thermophilic bacteria; fungi and yeasts; Pseudomonadaceae as a biofilm formation indicator; Clostridia spp. as an indicator to evaluate possible anaerobic digestion selection; Enterobacteriaceae as a gut contamination indicator; and Actinomycetes as another environmental microbiological component most likely linked to the investigated biomasses. Moreover, the authors sampled endotoxins to evaluate the concentration level of this component of breathable particulate. The authors found that environmental total bacteria were in the range of those for mesophilic bacteria observed in composting facilities and were equal to 10 2 –10 8 cfu/m 3 , and the same evidence was observable for thermophilic Actinomycetes and moulds. Endotoxin concentration ranged from 5 to 3220 EU/mg, with a mean value of 428 EU/mg, which was a low range compared to those observed in other waste collection and treatment plants. In conclusion, the authors asserted that biological risk has to be carefully quantified and managed, particularly in indoor environments, and additional specific assessments may be necessary for emerging pathogens, such as viruses.
Laitinen et al. (2016) carried out occupational hygiene measurements at three biofuel-powered plants ( Table 6 ) in Finland in order to measure the exposure of employees to biological agents [ 30 ]. They collected air samples during the unloading and processing of solid biofuels, and in the control room and outdoor (for a reference baseline). They collected both viable bacteria and fungi and biologically active endotoxins in the workers’ breathing zone. The authors found that, while unloading biomasses, the workers were highly exposed to organic dust, which was mainly composed of bioaerosols, and a high concentration of endotoxins was found. The concentrations of airborne endotoxins often exceeded the limit value of 90 EU/m 3 (suggested by the Nordic and Dutch Expert Group), which might cause adverse health effects for workers over short- or long-term occupational exposure. Moreover, microbial concentrations during unloading operations were higher than 10 4 cfu/m 3 , which might be a threat to the workers’ health. The authors concluded that more attention should be paid to health and safety issues when developing a bioenergy supply chain, and employee exposure should be diminished by means of technical solutions and use of appropriate protective clothing and respirators.
Ławniczek-Wałczyk et al. (2017) monitored bacterial pathogens in workplaces in a Polish biomass-powered plant fed by wood chips and agricultural waste ( Table 6 ) [ 32 ]. The sampling points were in the workplaces associated with reloading, separation, sizing, storage, and conveyor’s transport of biomass. In each of the workplaces, bioaerosol, used respiratory masks, and swab samples from the workers’ hands were collected. The bacterial aerosol concentrations ranged from 5.6 × 10 4 to 4.1 × 10 6 cfu/m 3 . The highest concentrations were recorded in the workplaces involved in the conveyor’s transport of biomass, while the lowest concentrations were noted at the background sampling points. The number of bacteria in the swabs and respiratory masks reached a high value of 1.5 × 10 4 cfu/mL and 1.9 × 10 3 cfu/cm 2 , respectively. Moreover, the authors suggested the use of polymerase chain reaction (PCR)-based methods as a tool for a fast and precise typing of bacterial strains isolated from different sources in an occupational environment in order to implement appropriate prophylactic procedures and minimize the transmission of infectious agents at work sites
Mbareche et al. (2018) assessed fungal bioaerosols in two biomethanisation facilities (BF) in Quebec, Canada, using a next-generation sequencing approach combined with real-time PCR [ 33 ]. These plants employed both water sludge and organic industrial food waste as feedstocks for anaerobic digestion ( Table 6 ). Air samples were collected during work activities potentially associated with aerosol exposure at several plant sites (e.g., reception, biomass treatment area, and storage hall). An outdoor control air sample was also collected outside each plant as the reference baseline. The authors focused their study on Penicillium / Aspergillus spp. and Aspergillus fumigatus because of their potentially hazardous effects. The results are reported as number of ITS genes/m 3 , i.e., genic sequences belonging to the internal transcribed spacer of the selected fungus specimens. The concentrations of Penicillium / Aspergillus spp. ranged from 6.4 × 10 2 to 1.2 × 10 4 ITS genes/m 3 . All measured concentrations were higher in the summer than in the winter for both plants. For BF1, the reception, storage, and output sites had the highest concentrations of Penicillium / Aspergillus spp. (10 4 ITS genes/m 3 ). For BF2, similar concentrations were noted at the reception/shredding site and were higher than the concentrations identified at the mixing site. Aspergillus fumigatus was detected in the samples from all sampling sites in both facilities. The concentrations ranged from 9.6 × 10 1 to 1.2 × 10 4 ITS genes/m 3 . Comparisons between the facilities during the summer and winter showed trends that were similar to the Penicillium / Aspergillus spp. results. The highest concentrations (10 3 ITS genes/m 3 ) of Aspergillus fumigatus were found in the storage and output sites in BF1 during summer. For BF2, the reception/shredding site had the highest concentration (1.2 × 10 4 ITS genes/m 3 ) of Aspergillus fumigatus during summer, but a lower concentration (2.7 × 10 2 ITS genes/m 3 ) during winter. The differences between the concentrations of Penicillium/Aspergillus spp. and Aspergillus fumigatus from each sampling site were approximately one log, which indicates a dominance of Aspergillus fumigatus in the bioaerosol samples. The outdoor control samples collected during each visit were below the detection limit, indicating a very low presence of fungal bioaerosols in the air. The broad spectrum of fungi detected in this study included many known pathogenic agents, and the authors found fungal signatures associated with the type of waste treated. This study highlighted the importance of using a high-throughput sequencing method combined with a real-time qPCR assay for quantification and an in-depth characterization of fungal diversity in bioaerosols to assess occupational exposure. Moreover, the authors strongly recommended taking action to monitor workers’ exposure to these aerosols. Better air exchange rates, better confinement, and source ventilation may be suitable organizational measures to limit workers’ exposure. Otherwise, skin and respiratory protection for workers may be useful to reduce continuous exposure to harmful fungi present in bioaerosols.
Traversi et al. (2018) analysed workers’ bioaerosol exposure in several Italian plants that used agricultural and livestock biomasses (n = 3); food and feed producing by-products and food waste (n = 1); and wastewater sludge (n = 1; Table 6 ) [ 29 ]. Bioaerosol sampling was performed in these plants, and twelve microbiological variables were measured (bacterial environmental total count at 22° C; bacterial total count at 37 °C; thermophilic total count at 55 °C; yeasts/fungi; Pseudomonadaceae; Bacillus spp.; Clostridia; Gram-negative bacteria; Salmonella spp. and Shigella spp.; Actinomycetes; Enterobacteriaceae; and Staphylococcus spp.). In decreasing order, the authors found staphylococci, bacilli, enterococci, and Clostridia. Moreover, the levels of Gram-negative Pseudomonadaceae, Salmonella spp., and Shigella spp. were very limited.
The authors asserted that the management of biological risk deserves specific attention, especially in indoor areas, where organic wastes are treated. In these settings, bacterial and mould contamination is quite remarkable, and the presence of various pathogens is also shown to be dispersed into the air as part of the bioaerosol. Moreover, the maintenance technicians and workmen involved in the cleaning procedure near the biomasses showed bioaerosol exposure. The estimated occupational risk is considerable not only for bioaerosol, but also for endotoxin and particulate exposure. Nevertheless, the authors claimed that such problems cannot be an obstacle to the diffusion of anaerobic digestion treatment of organic waste and biomasses.
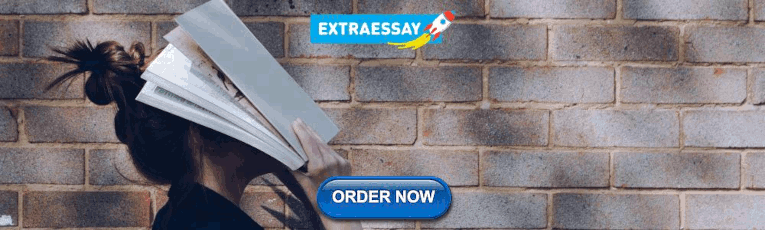
3.4.2. Volatile Organic Compounds and Gases Emitted by the Processes
Besides the assessment of biological risk, chemical characterization of pollutant emissions during the production and use of biogas is essential for a comprehensive evaluation of potential exposures. The results identified in the literature are organized according to the scope of the measurement and the monitored phases: occupational risk analysis of VOCs emitted during transport and handling raw materials, i.e., by-products of wood manufacturing and wastewater treatment [ 30 ]; occupational risk analysis of VOCs emitted by the anaerobic digestion process, i.e., food waste processing [ 34 ]; and environmental analysis of gas and particulate concentration by agricultural waste and biomasses [ 22 ].
Laitinen et al. (2016) reported a detailed personal monitoring of VOCs, dusts, and biological pollutants, focusing on workers responsible for transporting and unloading raw materials from trucks [ 30 ]. Although in a facility implementing a different technology, the results of the analysis are also relevant for biogas plants processing the same biomasses (i.e., forest chips, whole-tree chips, stem wood chips, wood processing industry residues, stumps, bask, wastewater sludge, and milled peat) since transport and handling of raw feedstock are included in the process. They demonstrated that employees were exposed to a huge amount of organic dusts and VOCs during the unloading of indigenous fuels (i.e., biomasses processed by the plant). The highest measured concentration of total VOCs during the unloading of fuels was six times the reference value recommended by the Finnish Institute of Occupational Health (18,000 mg/m 3 vs. 3000 mg/m 3 ). Table 7 shows the breakdown of the main detected VOCs, which were identified as being originated from pine and spruce softwood: α-pinene, Δ3-carene, β-pinene, limonene, and other monoterpenes (C 10 H 16 ). Moreover, an analysis of the reception hall highlighted a significant concentration of acetone and sulphur dioxide, which, according to the authors, was due to the emissions from trucks powered by diesel gasoline. As a conclusion, the authors highlighted the main work tasks that possibly caused exposure, namely unloading, screening, crushing, conveying of fuels, and handling of biomass in silos.
Main detected VOCs identified as being originated from pine and spruce softwood.
Zheng et al. (2020) analysed VOCs emitted by a plant with a treatment capacity of 300 tons/day of food waste [ 34 ]. The sampling points corresponded to four main working units: the sorting/crushing room (SR), the hydrothermal hydrolysis unit (HH), the anaerobic digestion unit (AD), and the biogas production unit (BP); different seasons were analysed along the one-year monitoring period. Table 7 summarises the main results of the monitoring. In particular, the analysis showed the highest cumulative emissions in spring (9.54 × 10 4 μg/m 3 ), while the lowest was in winter (1.39 × 10 4 μg/m 3 ), depending on the outdoor conditions and the composition of the feedstock. The working units showed different VOC concentration, and in particular, the HH unit presented a concentration significantly higher than other sampling points (3.49 × 10 4 μg/m 3 ), followed by the SR unit (8.97 × 10 3 μg/m 3 ), the anaerobic digestion unit (6.21 × 10 3 μg/m 3 ), and the biogas production unit (2.01 × 10 3 μg/m 3 ).
With regard to environmental analysis, the study by Merico et al. (2020) aimed to assess the potential influence of biogas plant emissions on local air quality, focusing on a plant for the production and combustion of biogas from agricultural wastes and biomasses in Italy [ 22 ]. The monitoring took place at 100 m from the boundaries of the biogas plant, which annual production accounts for 4,106,250 Nm 3 of biogas that is converted into energy through a co-generator working 24 h/day. Table 8 shows the overview of the main results in terms of emitted gases and particulate of the monitored sites (values in grey lines) in comparison with the limits set by the European Directive 2008/50/CE [ 35 ]. Although some significant peaks that can be attributed to the biogas plant were detected, the general results show average gas concentrations within the thresholds identified by the European Directive [ 35 ].
Annual average and maximum hourly values in comparison to the limits prescribed by the European Directive 2008/50/CE [ 35 ].
The authors found that vehicular traffic and biomass combustion (from agricultural activities and domestic heating) significantly influenced CO and nitrogen oxides [ 36 ], while NO emissions could be ascribed to the biogas production and combustion plant. SO 2 concentration was predominantly due to transport from a nearby industrial zone. However, a second local contribution compatible with the emissions of the biogas production and combustion plant was found.
3.4.3. Digestate Analysis
Digestate is one by-product of the anaerobic digestion process, and it is often employed as a fertilizer in agriculture due to its high nutritional value in terms of nitrogen and phosphorous concentrations. The application of this product in agriculture should always be preceded by an analysis of its safeness from a health point of view. The two studies investigating this topic found through the database search were conducted in California in 2017 and in China in 2022.
In a Californian plant, Kuo and Dow (2017) analysed some variables of the digestate obtained from the anaerobic digestion of fats, oils, grease, food waste, and wastewater sludge [ 24 ]. They found that all these variables were in the narrow ranges; thus, the anaerobic digesters of this plant could be considered as operating under stable conditions. Moreover, the ammonium concentration (1137 ± 83 mg/L) did not seem to inhibit biological activities.
In 2022, Ke and colleagues investigated the composition of digestate produced from 5 different feedstocks collected from 31 biogas plants in China [ 28 ]. More specifically, thirteen plants used swine manure as digester feedstock, seven used cattle manure, four used straw–manure mixture, four used chicken manure, and three used kitchen waste. Digestate samples were obtained from the drainage pipeline of the digesters and analysed using ion chromatography, liquid chromatography–tandem mass spectrometry, and inductively coupled plasma–tandem mass spectrometry. These analyses assessed chemical oxygen demand (COD), nitrogen and phosphorous concentrations, concentration of antibiotics and hormones, and concentration of heavy metals. Furthermore, the authors employed these analyses to calculate the eutrophication potential of digestate—combining the values of COD, nitrogen, and phosphorous—and the potential ecological risk index, using the concentration of heavy metals. The eutrophication potential of digestate was calculated for each feedstock, considering the potential of COD, phosphorous, and nitrogen. The eutrophication potential of digestate from chicken manure proved to be the most concerning for eutrophication threat, although this digestate contained the highest nutrient concentration for agricultural purposes. On the other hand, the digestate from cattle manure showed the lowest eutrophication potential. The antibiotic concentration was the highest in swine manure digestate. The risk indices calculated for heavy metal concentration showed that most digestates had a moderate potential ecological risk, except for the cattle manure digestate, which values were lower. These results indicated that the potential threat from digestate might be independent of the feedstock. The potential ecological risks of As and Hg were particularly high.
4. Life Cycle Assessment (LCA)
LCA deals with the estimation of potential environmental impact in terms of carbon footprint, CO 2 emissions, and global warming potential, representing indicators that are relevant for the potential health impact of biogas generation.
The two identified studies aimed to analyse the life cycle assessment of anaerobic treatment of human waste in the European and Chinese contexts. In particular, Iordan (2016) evaluated the impact of producing 1 MJ of energy through biogas derived from the digestion of urban waste at a Norwegian plant [ 10 ], while Duan et al. (2020) focused on the impact of an optimised anaerobic digestion process in terms of LCA and identified scenarios for energy system development in China [ 26 ].
Iordan (2016) assessed the LCA indexes of a process for treating the quantity of human waste required to generate 1 MJ of electricity through a CHP [ 10 ]. The main calculated indices were Global Warming Potential (GWP) with a time horizon of 20 and 100 years, and Global Temperature Change Potential with a time horizon of 20 and 100 years. The indices were reported considering the breakdown of N 2 O, CH 4 , and CO 2 emissions and including Near-Term Climate Forcers (NTCFs).
These species include the following pollutants: nitrogen oxides (NO x ), carbon monoxide (CO), volatile organic compounds (VOCs), black carbon (BC), organic carbon (OC), and sulphur oxides (SO x ). NTCFs may present a negative impact on GWP and GTP, resulting in a cooling contribution (represented as a negative value of gCO 2 –eq). The LCA for producing biogas energy and for managing digestate was then compared with the LCA for the reference scenario, based on the current energy mix in Norway [ 37 , 38 ]. A significant reduction in terms of CO 2 due to energy production from biogas was identified, which, on the other hand, causes an increase in CH 4 and NO 2 emissions when compared to the reference scenario. This increase is associated with digestate management (mainly due to the open storage) and methane (CH 4 ) losses during the anaerobic co-digestion. Moreover, terrestrial acidification potential (TAP), photochemical oxidant formation potential (POFP), and particulate matter formation potential (PMFP) were evaluated. The POFP of the biogas system is 10% lower than the reference system. On the other hand, the TAP and PMFP performance of the reference system is better when compared to the biogas system. This is mainly due to the large influence of digestate management.
This work also assessed the contribution of the different phases and the factors mainly affecting the environmental impact of a biogas plant, namely N 2 O emissions from storage, CH 4 leakages from the anaerobic tanks, and transport distances for the four feedstock types. In particular, closed storage was the scenario with the best GWP100 result, with 43 g CO 2 –eq/MJ, which represented a 41% improvement from the base scenario. The largest contributor was the anaerobic digestion process due to CH 4 losses, with a 33% share, followed by feedstock transport, with a 14% share, and digestate transport and spreading, with a 12% share. In this case, CO 2 contributed to 52% of the total GWP100, followed by CH 4 emissions, at 46%, and N 2 O, at 2%. When the transport distances for all four feedstock types were doubled, the GWP100 increased by 9%, at 79 g CO 2 –eq./MJ, with CO 2 and N 2 O contributing at 35% and CH 4 at 30%. This parameter was not as sensitive as CH 4 losses or digestate storage. The analysis showed that the most sensitive parameter was digestate storage, which had the highest impact on the LCA; specifically, closed storage might be regarded as the preferable digestate management option [ 3 ].
Duan et al. (2020) analysed a process based on anaerobic digestion of human waste, which aimed at producing biogas either for electricity generation through a combined heat and power production (CHP) plant or for conversion in biomethane [ 26 ]. The initial inputs of the LCA were the evaluation of the amount of human waste available for anaerobic digestion treatment and the potential of daily electricity production that could be generated through biogas combustion in the CHP plant at the national level in China. The authors estimated that the potential energy generated by biogas from available human waste has the potential to reduce the annual emissions of around 142 kt/CO 2 –eq at the energy system level.
5. Discussion
This work provides a comprehensive scientific overview that outlines the topic of electricity, biogas, and heat and biofuel generation through biomass use and the resulting health impacts on humans, both in occupational and residential settings. The aim was to answer the following research question: “What are the risk factors for the health of the people working in biogas plants and of the surrounding communities?” As a matter of fact, in the diverse research fields taken into consideration, i.e., environmental monitoring, epidemiology, toxicology, and human biomonitoring, an extremely heterogeneous terminology is employed. It follows that the keywords selected to build the search strings might prove to be adequate for certain fields, but not for others, where some relevant studies might have been missed. As a result, among the four research areas that were originally considered, only two were included due to the scarce number of results displayed in the others. Additionally, due to the small number of primary studies identified through the search strings and employed according to the inclusion criteria, twelve studies were identified through citation searching. Nineteen studies were, therefore, included in the review. Among them, 10 studies focused on biogas production through the anaerobic digestion of biomasses, and 9 studies dealt with biomass combustion.
Most of the studies were conducted in Europe, followed by North America and Asia. In regard to the four epidemiological studies, they were cross-sectional studies from Europe (Denmark, two studies), USA (New York State, one study) and Asia (Thailand, one study). Within this group, two were carried out in residential and two in occupational settings. The outcomes of interest were mainly respiratory symptoms and diseases, allergic reactions, and skin complaints.
We remind that the epidemiological studies investigated the correlation between biomass process exposures and health conditions not strictly connected to the phases of the biomass power plant. It is worth noted that the pollution (e.g., emission of S02 and NO2) and medical conditions related to the presence of the biorefinery cannot be reconducted directly to the phases that are in common with a biogas plant as well as endotoxins might be in common with a biogas plant, the respiratory and allergy conditions might be influenced by the biomass combustion activity.
The 15 environmental studies, mainly conducted in Europe, were classified into 3 categories: most of them (10) monitored power plants from an occupational point of view; one study monitored air quality at a community level; and the last category, “other studies”, consisted of two studies focusing on LCA analyses and two studies focusing on digestate analysis.
5.1. Epidemiological Studies
5.1.1. residential setting studies.
Both residential setting studies analysed the impact of living near industrial facilities using biomass on residents’ respiratory diseases and health symptoms. As mentioned before, the studies by Juntarawijit (2013) and Lee et al. (2021) appeared markedly different, especially because the former was carried out in two plants producing electricity from the same biomass type (rice husks) but by means of two distinct technologies (steam turbine and gasification with an internal combustion engine, respectively) [ 17 , 18 ]. The latter, instead, analysed 15 biorefineries producing ethanol from three biomass types: corn, wood and soybean. Despite these striking differences, both studies focused on the association between living in the vicinity of the facilities under study and the risk of respiratory morbidity (as well as other health symptoms) for the nearby communities. Additionally, the air pollutants taken into consideration were similar: particular matter (PM 10 by Juntarawijit and PM 2.5 by Lee et al.), sulphur dioxide, nitrogen dioxide, and ozone only in Juntarawijit (2013) [ 17 ]. The health effects of these pollutants are often well known [ 39 ]. PM exposure has been linked to cardiovascular and respiratory negative outcomes, as well as cancer. In particular, exposure to PM 2.5 has raised wide concern regarding mortality and premature death due to both long- [ 40 , 41 , 42 ] and short-term exposures [ 43 , 44 ]. Ozone is an oxidative gas with strong irritative effects on airways, with proven association to hospital admission [ 45 ] and mortality [ 46 ], although the health effects of long-term exposures still remain a matter of debate [ 47 ]. Exposure to nitrone dioxide has also been observed to be linked to respiratory, cardiovascular, and metabolism-related diseases [ 48 , 49 , 50 ], resulting in an increased mortality among those exposed [ 46 ]. Moreover, exposure to sulphur dioxide seems to exert its health effects on respiratory diseases and related mortality [ 51 ].
Consistent with these observations, the results of the study from Thailand revealed that living in proximity of the two biomass power plants was associated with an increased risk of suffering from respiratory diseases and other health symptoms.
Similar results are shown in the study by Lee et al. (2021), which dealt with the health impact of living in the vicinity of biorefineries using different types of biomasses [ 18 ]. We decided to include this study in our review not only because one of the stages involved in the biofuel production is represented by biomass fermentation, but also because of the high methodological quality of the study itself. In fact, in this study, information about respiratory diseases was retrieved from objective hospital data. Additionally, the large sample size analysed increased the statistical validity of the analysis.
Secondly, the association between biorefinery exposures and respiratory diseases was investigated according to two exposure indicators, that is, residential proximity and AERMOD-modelled air pollutant concentrations, to validate the findings.
Thirdly, seasonal difference was taken into consideration in the association between residential exposure and lower airway diseases: as a matter of fact, respiratory ED visit rates among residents living within 10 km of the biorefineries were significantly higher than those living in the reference areas in spring and winter. These data contribute to the high methodological quality of the study, even though some parameters must be taken into account: (I) increasing air pollution levels during spring and winter can be due to photochemical decomposition rates of air pollutants at cold temperatures; (II) during cold seasons, there are higher air pollution concentrations due to the stagnation of air pollutants; and (III) tree and grass pollens cause respiratory symptoms, such as asthma, in spring.
In conclusion, the high-level methodology adopted by Lee et al. (2021) should be considered as a reference model for other residential setting studies: the same methodological approach may also be used for the assessment of health risk among workers in industrial facilities, with the administration of an ad hoc, standardised, and validated questionnaire to investigate participants’ lifestyle [ 18 ]. If this study has an occupational setting counterpart, a complete model about human health risk will be available.
5.1.2. Occupational Setting Studies
Both studies exploring the health risks connected to biomass-based power generation in an occupational setting were carried out in Danish plants fuelled by straw and woodchips. While the study by Schlünssen et al. (2011) dealt with a generally formulated exposure to dust, fungi, airborne endotoxins, and Aspergillus fumigatus [ 19 ], the study by Basinas et al. (2012) only focused on endotoxin exposure [ 20 ].
The results of both studies confirm that exposure to endotoxins and fungi is significantly associated with respiratory symptoms. As mentioned before, due to the inherent characteristics of the biomasses in question (straw and woodchips), workers may be exposed during the pre-combustion phase to bioaerosols, including dust, microorganisms, and endotoxins, released during biomass handling, transport, storage, and agitation. Thus, the risk of bioaerosol formation should be limited, e.g., minimising storage times and avoiding conditions that favour mould development (for instance, accumulation of biomass in warm conditions).
Additionally, the association between exposure to certain fungal types, namely Aspergillus fumigatus , and respiratory symptoms, such as work-related asthma/wheeze and work-related rhinitis, implies the need for adequate control measures in order (I) to reduce workers exposure to fungal spores, and (II) to implement health surveillance to identify workers who may have a predisposition to health effects caused by the exposure.
Unlike traditional fuels, biomass tends to decompose, thus creating diverse exposure conditions and requiring different handling, transport, and storage methods in order to limit microbial growth (e.g., spore formation and endotoxin release), as well as emissions of VOCs or other gases. Furthermore, proactive training on biomass handling practices and health surveillance that focuses on workers’ respiratory health may represent a good starting point in that they would provide data on monitoring, occupational exposure, and risk assessment.
In conclusion, even though the environmental monitoring studies proved the harmfulness of particular matter (e.g., dust), microbial agents (e.g., fungi, bacteria), endotoxins, and VOCs in occupational settings, there is a need for epidemiological studies that focus on the effects that these substances have on workers’ health. An integrated approach combining environmental data with health data to investigate if they are significantly associated should be adopted.
5.2. Environmental Monitoring Studies
5.2.1. biological pollutants in biomass and/or in aerosol.
Biological pollutants (e.g., bacteria, fungi, and endotoxins) present in industrial biomasses can be harmful when inhaled by humans. In particular, exposure to endotoxins may represent an important threat to human health. In fact, endotoxins are high-molecular-weight lipopolysaccharides present in the outer layer of the cell wall of Gram-negative bacteria [ 52 ], with a high biological activity and an important resistance to heat. Thus, endotoxins cannot be removed by thermic treatments or disinfection procedures, resulting in respiratory diseases as well as immunotoxic effects [ 53 ] in workers exposed to them.
Thus, assessing the concentration of these pollutants in plants where biomasses are stocked and handled is useful for the protection of workers’ health. Each of the included studies monitored different biological pollutants in different sampling zones of the plant. Thus, a general summary of the results cannot be outlined. On the other hand, a few considerations can be drawn.
First of all, the CAMNEA (collection of airborne micro-organisms on nucleopore filters, estimation, and analysis) method [ 54 ] was employed in several studies.
Two studies [ 21 , 33 ] assessed the concentration of Aspergillus fumigatus , a potentially toxic fungus species. Thus, monitoring the concentration of this species and other congeneric species may lead to an improvement in the health of workers at biomass power plants.
Traversi et al. (2015) conducted a study in an attempt to provide reference guidelines [ 27 ]. In their study, they provided a list of safety limits for microbiological risk and for endotoxin inhalation ( Table 9 ). For microbiological risk, the Global Index of Microbial Contamination (GIMC) and the index of Mesophilic Bacterial Contamination (MBC) proposed by Dacarro et al. (2000) were considered as the reference values [ 55 ]. The GIMC is calculated as the sum of the values of the total microbial count determined for mesophilic bacteria, psychrophilic bacteria, and fungi in all sampled areas. The MBC is obtained by calculating the ratio between the cfu/m 3 value measured for mesophilic and psychrophilic bacteria in the same sampling point [ 55 ]. These indexes may be adopted in the monitoring of biological pollutants at biogas production plants. For endotoxin inhalation, two limit values were proposed, namely a punctual value by Feron et al. (1998) and a range value proposed by Duquenne et al. (2014) [ 56 , 57 ].
GIMC and MBC indexes. Adapted from [ 27 ].
5.2.2. Volatile Organic Compounds and Gases Emitted by the Processes
The literature analysis shows a limited number of studies, including an on-field monitoring of biogas plants, that are not homogeneous in terms of adopted feedstock, boundary conditions, technical process, and operation. Therefore, the results do not lend themselves to generalisation and may not be adopted as a direct reference for perfecting the processes and working procedures of each type of biogas plants. Moreover, the results cannot be adopted to exclude occupational and community potential risks that can be attributed to biogas plants. Nevertheless, the reported indications can be used as a preliminary reference for identifying key aspects to be controlled, parameters to be monitored, and conservative safety measures to be introduced for workers of a biogas plant. Long-term exposure to high concentrations of VOCs may entail a chronic or carcinogenic human health threat. Therefore, knowing the typology of VOCs and quantifying their emission are essential for adopting the needed control measures to reduce the potential impact on workers and communities.
Although the number of analysed works is limited, some interesting considerations can be derived as preliminary inputs of the potential risks to be evaluated in a biogas plant and promising fields for further investigations.
In fact, there are some common VOCs identified by both studies dealing with VOCs in occupational settings [ 30 , 34 ] despite the adoption of different feedstocks, which were wooden by-products and food waste, respectively. One of the common VOCs is limonene, which is classified by the European Chemical Agency (ECHA) as a skin sensitizer, causing skin irritation and possible allergic skin reaction. Although it is not classified as a cancerogenic substance for humans, the ECHA highlights that limonene presents a property of concern for chronic exposures. This information is important, especially for controlling the potential exposure of workers, whose skin may be in contact with limonene during the handling of both raw feedstocks and products of anaerobic digestion.
Another important result from Zheng et al. (2020) is the detection of high concentration of 1,2-dichloroethane during the process, especially during anaerobic digestion [ 34 ]. The ECHA has classified this compound as a “substance of very high concern” for both short-term and long-term exposure. In fact, on the one hand, it is harmful if swallowed and toxic in case of inhalation, and it may cause serious eye and skin irritation and respiratory inflammation. On the other hand, it has a possible carcinogenic effect, being classified in the group 2B by the International Agency for Research on Cancer. Therefore, the release of 1,2-dichloroethane during anaerobic digestion needs to be monitored and effectively controlled for the safety of the operators and in all the phases of the process. This would also be important for avoiding potential impacts on the community. In fact, the analysis of cancerogenic risk in Zheng et al. (2020) was performed by measuring the pollutant concentration at the plant boundary to identify the potential threat to the nearby community [ 34 ]. The results exceed the safety threshold of negligible cancer risk and indicate that the monitored release of VOCs from the analysed plant can threaten the health of the surrounding communities.
In regard to environmental analysis, the study by Merico et al. (2020) presented a comprehensive overview of the potential impact of a biogas plant processing agricultural waste at the community level, with a detailed analysis for apportioning the contribution of different sources (e.g., other surrounding industries, traffic, and heating systems) [ 22 ]. The monitoring shows gaseous (CO, NO, NO 2 , NO x , SO 2 , and O 3 ) and particle emission values that are below the annual thresholds provided by the Directive 2008/50/CE ( Table 8 ). Considering the annual average (calculated by extrapolating the results from the monitoring performed during the period 30 January–28 March 2018), the measured values do not exceed 50% of the limit values, for both the environmental limits to the more restrictive reference values for human health, namely ranging from 1% for SO 2 to 42% for NO x . On the other hand, the hourly monitored values are closer to the thresholds of the Directive 2008/50/CE, reaching 64% of the environmental limit for ozone, while for the limits for human health, the values are 55% for CO and 71% for NO 2 . The results of this study cannot be adopted as a reference for all biogas plants processing agricultural waste since the environmental analysis is related to the specific features of the plant, in particular to the context and the meteorological conditions. Nevertheless, this work demonstrated that, under specific conditions, the impact of a biogas plant can contribute to a significant increase in gaseous concentration in the environment, which can reach the thresholds indicated in the Directive 2008/50/CE. Therefore, the characterisation of gaseous emissions from a biogas plant would require on-field monitoring.
5.2.3. Digestate Analysis
The number of studies addressing the analysis of digestate was very low (n = 2). Nevertheless, these two studies provided useful information about the usage of digestate produced by the fermentation of different biomasses (e.g., agricultural and livestock residues, and kitchen waste).
The usage of digestate should be promoted as a positive reuse of resources, but strict health and environmental controls must be made.
The two analysed studies reported different conclusions. In the Californian plant [ 24 ], the only substance monitored in the digestate—ammonium—showed a low concentration, which could not inhibit biological activities. On the other hand, in the Chinese plants [ 28 ], the digestate was analysed for several substances, ranging from antibiotics to heavy metals, in order to assess the quality and health security of the digestate. The use of this digestate did not guarantee health nor environmental security, but the peculiarity of the study context, including the origin of the biomasses and the Chinese regulation on these topics, does not allow a comparison between this kind of digestate and the one produced in EU plants, where digestate use is regulated by the Commission Regulation (EU) No142/2011.
From an environmental point of view, the digestate produced by chicken manure should be used in agriculture, considering its very high eutrophication potential.
5.2.4. LCA Analysis
The evaluation of the LCA indicators does not provide a direct measure of pollutants emitted by a biogas plant, since the assessment is not based on direct monitoring but calculated according to standard reference procedures. Nevertheless, these indicators represent an indirect quantification of the potential impact on workers and on surrounding communities, providing estimations of the global warming potential and the pollutant emissions that can be associated with biogas production. The selected works analysed the life cycle of the energy production through biogas, from the transport of raw material to the co-generator and the inlet in the electrical grid, and compared the results with the traditional energy mix of the country under study. As a general consideration, the adoption of biogas for electric power generation would allow a significant reduction in CO 2 emissions. On the other hand, Iordan (2016) highlighted a significant increase in methane emissions derived from the anaerobic digestion, which needs to be addressed to reduce the negative impact [ 10 ]. The authors recommended reducing these emissions by means of direct interventions on the leakages of anaerobic digestion tanks. In conclusion, despite the adopted selection process identified in the two studies, the outlooks implied by the results highlight that electricity production through biogas has a positive impact in terms of LCA and reduction in CO 2 emissions.
6. Conclusions
This literature review highlights that the available knowledge on the potential occupational and community hazards associated with biogas plants is fragmented. On the one hand, there is a limited number of studies including on-field monitoring due to the novelty of the technology. On the other hand, the heterogeneity of biogas plants in terms of processed feedstock, technical features of the monitored sites, specificities of the national standards, and differences from other bioenergy technologies do not enable a direct comparison among different studies. Therefore, the results cannot be used to define consistent benchmarks but provide an overview of different aspects of biogas generation, with the purpose of stimulating the discussion on this technology both at the scientific and policy levels.
Workers and populations living near biogas plants could be potentially exposed to different pollutants, namely PM 10 and PM 2.5 , ozone, nitrogen dioxide, sulphur dioxide, some bacteria and fungi, endotoxins, and some VOCs.
The health effects of short-term exposures to PMs, ozone, nitrogen dioxide, and sulphur dioxide are generally well known, although the effects of long-term exposures are not always completely understood. Thus, environmental monitoring should not be neglected in these populations.
Some health issues could also arise from microbiological exposure to bacteria, fungi, and endotoxins. In particular, endotoxins could expose workers to some health risks, considering their high biological activity and thermic resistance.
Even though VOCs are compounds which toxicity is generally well known, some exposures could occur in biogas plant workers, and thus, environmental monitoring should not be neglected.
Through environmental measurements, the monitored biogas plants included in the study highlighted the release of VOCs, gaseous pollutants, and particulate. This may be due to the substances used in the process. In certain cases, the control of the emissions of potential hazardous VOCs may be necessary. The following compounds have been detected in the plants analysed in the literature: 1,2-dichloroethane, benzene, ethylbenzene, 1,1,2-trichloroethane, tetrachloroethylene, styrene, tetrachloromethane, and limonene.
Monitoring requires real-time measurement and aims to identify the boundary conditions and phases, as well as the settings characterised by the highest pollutant release. This monitoring allows the creation of background knowledge that can be used to improve the management of biogas plants and control emissions. Intervention in biogas plants’ technical features is regarded as a solution to reduce pollutant emissions. Furthermore, the main outcome of the literature analysis is that methane emissions can be diminished by controlling the leakages of anaerobic digestion tanks, a simple intervention that can be easily replicated.
In regard to the epidemiological analysis, the studies highlighted the importance of setting up a health surveillance program on workers and residents regarding their lifestyle (smoking, physical activity, diet, alcohol consumption, and health status) in order to effectively monitor the potential effects of occupational exposure in biogas plants.
With regard to the biological risk, monitoring sessions of airborne micro-organisms in different work areas of biogas plants through the CAMNEA method should be programmed. Finally, the monitoring of the eutrophication potential of digestate produced by different feedstocks, starting from sample plants, and the control of heavy metal concentration in digestate should be promoted.
Supplementary Materials
The following supporting information can be downloaded at: https://www.mdpi.com/article/10.3390/ijerph20075305/s1 , Table S1: Residential setting: Juntarawijit 2013—Results [ 17 ]; Table S2: Residential setting: Lee et al. 2021—Results (a) [ 18 ]; Table S3: Residential setting: Lee et al. 2021—Results (b) [ 18 ]; Table S4: Occupational setting: Schlünssen et al. 2011—Results [ 19 ]; Table S5: Occupational setting: Basinas et al. 2012—Results [ 20 ].
Funding Statement
This research was funded by the ENI S.p.A. The APC was funded by the University of Pavia.
Author Contributions
Conceptualization, M.A., M.C.M., C.M., A.M., E.O., R.P. and S.V.; methodology, M.C.M., C.M., E.O., R.P. and S.V.; validation, C.M.; formal analysis, M.A., M.C.M., C.M., A.M. and M.T.; investigation, A.O., R.P. and M.T.; data curation, M.T.; writing—original draft preparation, M.A., A.M., R.P. and M.T.; writing—review and editing, M.A., M.C.M., C.M., A.M., E.O. and S.V.; visualization, M.T.; supervision, C.M.; project administration, C.M.; funding acquisition, C.M. All authors have read and agreed to the published version of the manuscript.
Institutional Review Board Statement
Informed consent statement, data availability statement, conflicts of interest.
The authors declare no conflict of interest.
Disclaimer/Publisher’s Note: The statements, opinions and data contained in all publications are solely those of the individual author(s) and contributor(s) and not of MDPI and/or the editor(s). MDPI and/or the editor(s) disclaim responsibility for any injury to people or property resulting from any ideas, methods, instructions or products referred to in the content.
Biogas purification via optimal microalgae growth: A literature review
Affiliations.
- 1 Departamento de Ciencias Ambientales., División de Ciencias de la Vida del campus Irapuato-Salamanca, Universidad de Guanajuato, Irapuato, Mexico.
- 2 Departamento de Ingeniería Química, División de Ciencias Naturales y Exactas del campus Guanajuato., Universidad de Guanajuato, Guanajuato, Mexico.
- 3 C. A. Telemática, Departamento de Ingeniería Electrónica., División de Ingenierías del campus Irapuato-Salamanca, Universidad de Guanajuato, Salamanca, Mexico.
- PMID: 30294887
- DOI: 10.1002/btpr.2686
In this review, we compare works from the current decade that address the CO 2 -removal from biogas by means of microalgae. The microalgae culture process acts as a biochemical absorption process; it is potentially competitive with respect to classical and commercial absorption methods due to its additional benefits such as availing CO 2 for the production of valuable microalgae biomass and being an environmentally friendly technique. Nevertheless, the low yield of biogas purification translates into the need to use optimal operation strategies that render the whole biogas production process economically feasible. A class of these strategies requires models capable of reproducing key traits of the dynamical behavior of microalgae growth. Thus, without overlooking the classical physico-chemical methods for biogas purification, our literature review addresses: (i) biogas purification via microalgae and different microalgae growth conditions, (ii) approaches that maximize microalgae growth, in order to increase CO 2 -consumption, and (iii) different models that describe the representative characteristics of microalgae growth. This investigation traces a pathway to future considerations on optimal biogas purification alternatives by microalgae culture processes. © 2018 American Institute of Chemical Engineers Biotechnol. Prog., 34:1513-1532, 2018.
Keywords: biogas purification; dynamical models; microalgae; optimization.
© 2018 American Institute of Chemical Engineers.
Publication types
- Research Support, Non-U.S. Gov't
- Microalgae / metabolism*
Advertisement
Relating biogas technology and environmental impact assessment: a roadmap towards clean energy for environmental sustainability
- Research Article
- Published: 13 May 2023
- Volume 30 , pages 72832–72853, ( 2023 )
Cite this article
- Shahid Ali 1 ,
- Qingyou Yan 1 , 2 ,
- Muhammad Irfan ORCID: orcid.org/0000-0003-1446-583X 3 , 4 &
- Shah Fahad 5
315 Accesses
3 Citations
Explore all metrics
Atmosphere contamination and fast weather change have compelled scientists and entrepreneurs to seek eco-friendly solutions for saving the earth. Increasing energy consumption depletes limited natural resources and harms the climate and ecology. In this regard, biogas technology contributes in two ways: satisfying energy needs and saving plants. Pakistan is a farming nation with enormous biogas-based energy generating potential. The primary objectives of this study are to identify the most significant barriers to farmers’ investment in biogas technology. Non-probability sampling (purposive sampling) was utilized to establish the sample size. Ninety-seven investors and farmers were systematically sampled in biogas technology engaged in this survey. The planned questionnaire was practiced to obtain key facts via online interviews. The partial least square structural equation modeling (PLS-SEM) was used to evaluate designated hypotheses. The current research concludes that entire autonomous variables are substantial and interrelated with investment in biogas machinery, dropping energy disasters, and accomplishing environmental, financial, and maintenance government support objectives. The results also revealed that electronic and social media play a moderating influence. This conceptual model is considerably and positively affected by the chosen factors and their moderation. This study concludes that the primary tools for attracting farmers and investors in biogas technology are proper biogas technology awareness with relevant experts, financial and maintenance government responsibility, user efficiency and environmental concern of biogas plants, and the role of electronic and social media. The results also advised that the government should implement an incentive and maintenance policy to entice new farmers and investors in Pakistan to build biogas technology. Finally, the study’s limitations and recommendations for additional studies are highlighted.
This is a preview of subscription content, log in via an institution to check access.
Access this article
Price includes VAT (Russian Federation)
Instant access to the full article PDF.
Rent this article via DeepDyve
Institutional subscriptions
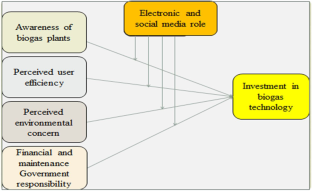
Similar content being viewed by others
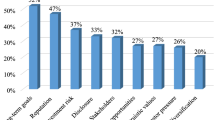
Environmental-, social-, and governance-related factors for business investment and sustainability: a scientometric review of global trends
Hadiqa Ahmad, Muhammad Yaqub & Seung Hwan Lee
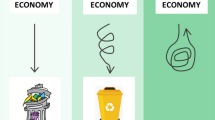
Circular economy strategies for combating climate change and other environmental issues
Mingyu Yang, Lin Chen, … Pow-Seng Yap
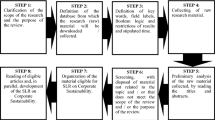
A systematic literature review on corporate sustainability: contributions, barriers, innovations and future possibilities
Ualison Rébula de Oliveira, Rodolfo Pombo Menezes & Vicente Aprigliano Fernandes
Data availability
The statistics supporting the outcomes of this investigation are available upon reasonable request from the first author.
Aamir M, Ahmad H, Javaid Q, Hasan SM (2018) Waste not, want not: a case study on food waste in restaurants of Lahore, Pakistan. J Food Prod Mark 24:591–610. https://doi.org/10.1080/10454446.2018.1472695
Article Google Scholar
Adrita UW, Mohiuddin MF (2020) Impact of opportunity and ability to translate environmental attitude into ecologically conscious consumer behavior. J Mark Theory Pract 28:173–186. https://doi.org/10.1080/10696679.2020.1716629
Afridi ZUR, Qammar NW (2020) Technical challenges and optimization of biogas plants. ChemBioEng Rev 7:119–129. https://doi.org/10.1002/cben.202000005
Article CAS Google Scholar
Afridi ZUR, Jing W, Younas H (2019) Biogas production and fundamental mass transfer mechanism in anaerobic granular sludge. Sustainability 11:4443. https://doi.org/10.3390/su11164443
Ahmar M, Ali F, Jiang Y, Alwetaishi M, Ghoneim SSM (2022) Households’ energy choices in rural Pakistan. Energies 15:3149. https://doi.org/10.3390/en15093149
Ahmed N, Li C, Khan A, Qalati SA, Naz S, Rana F (2020) Purchase intention toward organic food among young consumers using theory of planned behavior: role of environmental concerns and environmental awareness. J Environ Plan Manag 64:796–822. https://doi.org/10.1080/09640568.2020.1785404
Ahmed N, Li C, Khan A, Qalati SA, Naz S, Rana F (2021) Purchase intention toward organic food among young consumers using theory of planned behavior: role of environmental concerns and environmental awareness. J Environ Plan Manag 64:796–822. https://doi.org/10.1080/09640568.2020.1785404
Akbar A, Ali S, Ahmad MA, Akbar M, Danish M (2019) Understanding the antecedents of organic food consumption in pakistan: moderating role of food neophobia. Int J Environ Res Public Health 16:4043. https://doi.org/10.3390/ijerph16204043
Akther T, Nur T (2022) A model of factors influencing COVID-19 vaccine acceptance: a synthesis of the theory of reasoned action, conspiracy theory belief, awareness, perceived usefulness, and perceived ease of use. PLoS ONE 17:1–20. https://doi.org/10.1371/journal.pone.0261869
Ali S, Yan Q, Hussain MS, Irfan M, Ahmad M, Razzaq A, Dagar V, Işık C (2021) Evaluating green technology strategies for the sustainable development of solar power projects : evidence from Pakistan. Sustainability 13:12997. https://doi.org/10.3390/su132312997
Ali S, Yan Q, Irfan M, Ameer W, Atchike DW (2022) Green investment for sustainable business development : the influence of policy instruments on solar technology adoption. Front Energy Res 10:874824. https://doi.org/10.3389/fenrg.2022.874824
Ali S, Yan Q, Irfan M, Chen Z (2022a) Evaluating barriers on biogas technology adoption in China : the moderating role of awareness and technology understanding. Front Environ Sci 10:1–16. https://doi.org/10.3389/fenvs.2022.887084
Ali S, Yan Q, Irfan M, Chen Z (2022b) Evaluating barriers on biogas technology adoption in China: the moderating role of awareness and technology understanding. Front Environ Sci - Environ Econ Manag. 16648714, 290. https://doi.org/10.3389/fenvs.2022.887084
Ali S, Yan Q, Razzaq A, Khan I, Irfan M (2022d) Modeling factors of biogas technology adoption : a roadmap towards environmental sustainability and green revolution. Environ Sci Pollut Res 30:11838–11860. https://doi.org/10.1007/s11356-022-22894-0
Amir SM, Liu Y, Shah AA, Khayyam U, Mahmood Z (2019) Empirical study on influencing factors of biogas technology adoption in Khyber. Energy Environ 31:308–329. https://doi.org/10.1177/0958305X19865536
Anum S (2021) Development of an indigenous scale on altruism in Urdu. Pakistan Soc Sci Rev. 5:515–526. https://doi.org/10.35484/pssr.2021(5-III)38
Arshad M, Bano I, Khan N, Imran M, Younus M (2018) Electricity generation from biogas of poultry waste : an assessment of potential and feasibility in Pakistan. Renew Sustain Energy Rev 81:1241–1246. https://doi.org/10.1016/j.rser.2017.09.007
Bao Z, Shang B (2021) Self-efficacy and continuance intention of Web 2.0 platforms: a meta-analysis. Data Technol Appl 55:511–526. https://doi.org/10.1108/DTA-02-2020-0047
Bates MN, Pope K, Ram T, Pokhrel AK, Pillarisetti A, Lam NL, Verma SC (2019) Household fuel use and pulmonary tuberculosis in western Nepal : a case- control study. Environ Res 168:193–205. https://doi.org/10.1016/j.envres.2018.09.036
Baurzhan S, Jenkins GP (2016) Off-grid solar PV: Is it an affordable or appropriate solution for rural electrification in Sub-Saharan African countries? Renew Sustain Energy Rev 60:1405–1418. https://doi.org/10.1016/j.rser.2016.03.016
Bretter C, Unsworth KL, Russell SV, Quested TE, Doriza A, Kaptan G (2022) Don’t put all your eggs in one basket: testing an integrative model of household food waste. Resour Conserv Recycl 185:106442. https://doi.org/10.1016/j.resconrec.2022.106442
Breves P, Schramm H (2021) Bridging psychological distance: the impact of immersive media on distant and proximal environmental issues. Comput Human Behav 115:106606. https://doi.org/10.1016/j.chb.2020.106606
Chodkowska-Miszczuk J (2021) A new narrative for sustainability: exploring biogas plants as ‘first movers’ in raising energy awareness. Aust J Environ Educ 38:152–167
Clemens H, Bailis R, Nyambane A, Ndung’u V (2018) Africa Biogas Partnership Program: a review of clean cooking implementation through market development in East Africa. Energy Sustain Dev 46:23–31. https://doi.org/10.1016/j.esd.2018.05.012
Cohen J (1988) Statistical power analysis for the behavioral sciences 2nd edition. New York. NY Acad
Collier JE (2020) Applied Structural Equation Modeling Using AMOS, Applied structural equation modeling using AMOS: basic to advanced techniques. Routledge. Milton Park, Abingdon, Oxfordshire. https://doi.org/10.4324/9781003018414
D’Souza C (2022) Game meats: consumption values, theory of planned behaviour, and the moderating role of food neophobia/neophiliac behaviour. J Retail Consum Serv 66:102953. https://doi.org/10.1016/j.jretconser.2022.102953
Dai J, Yang X, Wen L (2018) Development of wind power industry in China: a comprehensive assessment. Renew Sustain Energy Rev 97:156–164. https://doi.org/10.1016/j.rser.2018.08.044
de Almeida MA, Colombo R (2021) Production Chain of first-generation sugarcane bioethanol: characterization and value-added application of wastes. BioEnergy Res 16:924–939. https://doi.org/10.1007/s12155-021-10301-4
Eid R, Agag G, Shehawy YM (2021) Understanding guests’ intention to visit green hotels. J Hosp Tour Res 45:494–528. https://doi.org/10.1177/1096348020947800
Esteves J, Valogianni K, Greenhill A (2021) Online social games: the effect of social comparison elements on continuance behaviour. Inf Manag 58:103452. https://doi.org/10.1016/j.im.2021.103452
Fu L, Sun Z, Zha L, Liu F, He L, Sun X, Jing X (2020) Environmental awareness and pro-environmental behavior within China’s road freight transportation industry: moderating role of perceived policy effectiveness. J Clean Prod 252:119796. https://doi.org/10.1016/j.jclepro.2019.119796
Govender I, Thopil GA, Inglesi-Lotz R (2019) Financial and economic appraisal of a biogas to electricity project. J Clean Prod 214:154–165. https://doi.org/10.1016/j.jclepro.2018.12.290
Green SM, Turner D, Logan RG (2018) Exploring the effect of sharing common facebook friends on the sexual risk behaviors of tinder users. Cyberpsychology Behav Soc Netw 21:457–462. https://doi.org/10.1089/cyber.2017.0581
Grigoryan L, Seo S, Simunovic D, Hofmann W (2022) WITHDRAWN: Helping the ingroup versus harming the outgroup: evidence from morality-based groups. J Exp Soc Psychol 101:104339. https://doi.org/10.1016/j.jesp.2022.104339
Hair JF, Risher JJ, Sarstedt M, Ringle CM (2019) When to use and how to report the results of PLS-SEM. Eur Bus Rev 31:2–24. https://doi.org/10.1108/EBR-11-2018-0203
Hair JF, Sarstedt M, Ringle CM (2019) Rethinking some of the rethinking of partial least squares. Eur J Mark 53:566–584. https://doi.org/10.1108/EJM-10-2018-0665
Hair Jr JF, Hult GTM, Ringle CM, Sarstedt M, Danks NP, Ray S (2021) Partial least squares structural equation modeling (PLS-SEM) using R: A workbook. https://doi.org/10.1007/978-3-030-80519-7
Hall CAS, Klitgaard K (2018) Energy and the wealth of nations, energy and the wealth of nations: an introduction to biophysical economics. Springer International Publishing, Cham. https://doi.org/10.1007/978-3-319-66219-0
Book Google Scholar
Han R, Xu J (2020) A comparative study of the role of interpersonal communication, traditional media and social media in pro-environmental behavior: a China-based study. Int J Environ Res Public Health 17:1883. https://doi.org/10.3390/ijerph17061883
Hartmann C, Lazzarini G, Funk A, Siegrist M (2021) Measuring consumers’ knowledge of the environmental impact of foods. Appetite 167:105622. https://doi.org/10.1016/j.appet.2021.105622
Havrysh V, Kalinichenko A, Mentel G, Olejarz T (2020) Commercial biogas plants : lessons for Ukraine. Energies 13:2668. https://doi.org/10.3390/en13102668
Hoang AT, Nižetić S, Olcer AI, Ong HC, Chen W-H, Chong CT, Thomas S, Bandh SA, Nguyen XP (2021) Impacts of COVID-19 pandemic on the global energy system and the shift progress to renewable energy: opportunities, challenges, and policy implications. Energy Policy 154:112322
Hull L, Goulding L, Khadjesari Z, Davis R, Healey A, Bakolis I, Sevdalis N (2019) Designing high-quality implementation research: development, application, feasibility and preliminary evaluation of the implementation science research development (ImpRes) tool and guide. Implement Sci 14:20. https://doi.org/10.1186/s13012-019-0897-z
Hussain M, Tahir S, Ishak D, Sarwar G, Haroon M, Ahmad W, Younas T, Tahir H (2019) Hybrid energy sources status of Pakistan : an optimal technical proposal to solve the power crises issues. Energy Strateg Rev 24:132–153. https://doi.org/10.1016/j.esr.2019.02.001
Ilyas SZ, Hassan A, Mufti H (2021) Review of the renewable energy status and prospects in Pakistan. Int J Smart grid 5(4):167–173. https://doi.org/10.20508/ijsmartgrid.v5i4.220.g174
Ingrao C, Bacenetti J, Adamczyk J, Ferrante V, Messineo A, Huisingh D (2019) Investigating energy and environmental issues of agro-biogas derived energy systems: a comprehensive review of Life Cycle Assessments. Renew Energy 136:296–307. https://doi.org/10.1016/j.renene.2019.01.023
Iqbal T, Dong CQ, Lu Q, Ali Z, Khan I, Hussain Z, Abbas A (2018) Sketching Pakistan’s energy dynamics: prospects of biomass energy. J Renew Sustain Energy 10(2):023101. https://doi.org/10.1063/1.5010393
Irfan M, Zhao ZY, Rehman A, Ozturk I, Li H (2021) Consumers’ intention-based influence factors of renewable energy adoption in Pakistan: a structural equation modeling approach. Environ Sci Pollut Res 28:432–445. https://doi.org/10.1007/s11356-020-10504-w
Jabeen G, Yan Q, Ahmad M, Fatima N, Jabeen M, Li H, Qamar S (2020) Household-based critical influence factors of biogas generation technology utilization: a case of Punjab province of Pakistan. Renew Energy 154:650–660. https://doi.org/10.1016/j.renene.2020.03.049
Jaiswal D, Singh B (2018) Toward sustainable consumption: investigating the determinants of green buying behaviour of Indian consumers. Bus Strateg Dev 1:64–73. https://doi.org/10.1002/bsd2.12
Jarrar L, Ayadi O, Al Asfar J (2020) Techno-economic aspects of electricity generation from a farm based biogas plant. J Sustain Dev Energy Water Environ Syst. 8:476–492. https://doi.org/10.13044/j.sdewes.d7.0302
Jha P, Schmidt S (2021) State of biofuel development in sub-Saharan Africa: how far sustainable? Renew Sustain Energy Rev 150:111432. https://doi.org/10.1016/j.rser.2021.111432
Joshi Y, Uniyal DP, Sangroya D (2021) Investigating consumers’ green purchase intention: examining the role of economic value, emotional value and perceived marketplace influence. J Clean Prod 328:129638. https://doi.org/10.1016/j.jclepro.2021.129638
Kabel TS, Bassim M (2020) Reasons for shifting and barriers to renewable energy: a literature review. Int J Energy Econ Policy 10:89–94. https://doi.org/10.32479/ijeep.8710
Kamran M (2018) Current status and future success of renewable energy in Pakistan. Renew Sustain Energy Rev 82:609–617. https://doi.org/10.1016/j.rser.2017.09.049
Karim AB, Avro SS, Shahriar S (2018) Prospect of geothermal energy resources in Bangladesh. Int J Renew Energy Resour 8:22–28
Google Scholar
Komasilovs V, Bumanis N, Kviesis A, Anhorn J, Zacepins A (2021) Development of the digital matchmaking platform for international cooperation in the biogas sector. Agron Res 19:809–818. https://doi.org/10.15159/AR.21.018
Kumar GA (2021) Framing a model for green buying behavior of Indian consumers: from the lenses of the theory of planned behavior. J Clean Prod 295:126487. https://doi.org/10.1016/j.jclepro.2021.126487
Kumar A, Prakash G, Kumar G (2021) Does environmentally responsible purchase intention matter for consumers? A predictive sustainable model developed through an empirical study. J Retail Consum Serv 58:102270. https://doi.org/10.1016/j.jretconser.2020.102270
La Barbera F, Ajzen I (2021) Moderating role of perceived behavioral control in the theory of planned behavior: a preregistered study. J Theor Soc Psychol 5:35–45. https://doi.org/10.1002/jts5.83
Lakens D, Caldwell AR (2021) Simulation-based power analysis for factorial analysis of variance designs. Adv Methods Pract Psychol Sci 4:251524592095150. https://doi.org/10.1177/2515245920951503
Larramendy M, Soloneski S (2019) Organic fertilizers: history, production and applications.
Le MH, Nguyen PM (2022) Integrating the theory of planned behavior and the norm activation model to investigate organic food purchase intention: evidence from Vietnam. Sustainability 14:816. https://doi.org/10.3390/su14020816
Leo S. Di, Caramuta P, Curci P, Cosmi C (2020) Regression analysis for energy demand projection: an application to TIMES-Basilicata and TIMES-Italy energy models. Energy 196:117058. https://doi.org/10.1016/j.energy.2020.117058
Loebnitz N, Frank P, Otterbring T (2022) Stairway to organic heaven: the impact of social and temporal distance in print ads. J Bus Res 139:1044–1057. https://doi.org/10.1016/j.jbusres.2021.10.020
Lohan SK, Dixit J, Kumar R, Pandey Y, Khan J, Ishaq M, Modasir S, Kumar D (2015) Biogas: a boon for sustainable energy development in India׳s cold climate. Renew Sustain Energy Rev 43:95–101. https://doi.org/10.1016/j.rser.2014.11.028
Lowe RJ, Drummond P (2022) Solar, wind and logistic substitution in global energy supply to 2050 – barriers and implications. Renew Sustain Energy Rev 153:111720. https://doi.org/10.1016/j.rser.2021.111720
Manesh MHK, Rezazadeh A, Kabiri S (2020) A feasibility study on the potential, economic, and environmental advantages of biogas production from poultry manure in Iran. Renew Energy 159:87–106. https://doi.org/10.1016/j.renene.2020.05.173
Marie M, Yirga F, Alemu G, Azadi H (2021) Status of energy utilization and factors affecting rural households’ adoption of biogas technology in north-western Ethiopia. Heliyon 7(3):e06487. https://doi.org/10.1016/j.heliyon.2021.e06487
Mazzanti M, Modica M, Rampa A (2021) The biogas dilemma: an analysis on the social approval of large new plants. Waste Manag 133:10–18. https://doi.org/10.1016/j.wasman.2021.07.026
Memon MATR, Cheah J-H, Ting H, Chuah F, Cham TH (2021) PLS-SEM statistical programs: a review. J Appl Struct Equ Model 5(1):1–14. https://doi.org/10.47263/JASEM.5(1)06
Mikhail J, Gallego-schmid A, Stamford L, Azapagic A (2020) Environmental sustainability of cooking fuels in remote communities : life cycle and local impacts. Sci Total Environ 713:136445. https://doi.org/10.1016/j.scitotenv.2019.136445
Mir KA, Park C, Purohit P, Kim S (2021) Comparative analysis of greenhouse gas emission inventory for Pakistan: Part II agriculture, forestry and other land use and waste. Adv Clim Chang Res 12:132–144. https://doi.org/10.1016/j.accre.2021.01.003
Mohsin M, Waqas H, Atif M, Sajjad M, Samad A (2021) Assessing the impact of transition from nonrenewable to renewable energy consumption on economic growth-environmental nexus from developing Asian economies. J. Environ. Manage. 284:111999. https://doi.org/10.1016/j.jenvman.2021.111999
Mohsin M, Taghizadeh-Hesary F, Iqbal N, Saydaliev HB (2022) The role of technological progress and renewable energy deployment in green economic growth. Renew Energy 190:777–787. https://doi.org/10.1016/j.renene.2022.03.076
Morgunova MO, Solovyev DA, Nefedova LV, Gabderakhmanova TS (2020) Renewable energy in the Russian Arctic: environmental challenges, opportunities and risks. J Phys Conf Ser 1565(1):012086. https://doi.org/10.1088/1742-6596/1565/1/012086
Mufidah I, Jiang B, Lin S-C, Chin J, Rachmaniati Y, Persada S (2018) Understanding the consumers’ behavior intention in using green ecolabel product through pro-environmental planned behavior model in developing and developed regions: lessons learned from Taiwan and Indonesia. Sustainability 10:1423. https://doi.org/10.3390/su10051423
Nagaraj S (2021) Role of consumer health consciousness, food safety & attitude on organic food purchase in emerging market: a serial mediation model. J Retail Consum Serv 59:102423. https://doi.org/10.1016/j.jretconser.2020.102423
Naz F, Oláh J, Vasile D, Magda R (2020) Green purchase behavior of University Students in Hungary: an empirical study. Sustainability 12:10077. https://doi.org/10.3390/su122310077
Ozturk I (2016) Biofuel, indicators’ nexus in the pansustainability, and forest el generalized method of moments estimation: evidence from 12 developed and developing countries Ilhan. Biofuels. Bioprod Biorefining 10:150–163. https://doi.org/10.1002/bbb.1628
Ozturk I (2017) Measuring the impact of alternative and nuclear energy consumption, carbon dioxide emissions and oil rents on specific growth factors in the panel of Latin American countries. Prog Nucl Energy 100:71–81. https://doi.org/10.1016/j.pnucene.2017.05.030
Ozturk I, Acaravci A (2013) The long-run and causal analysis of energy, growth, openness and financial development on carbon emissions in Turkey. Energy Econ 36:262–267. https://doi.org/10.1016/j.eneco.2012.08.025
Ozturk I, Al-Mulali U (2015) Investigating the validity of the environmental Kuznets curve hypothesis in Cambodia. Ecol Indic 57:324–330. https://doi.org/10.1016/j.ecolind.2015.05.018
Ozturk I, Aslan A, Altinoz B (2021) Investigating the nexus between CO2 emissions, economic growth, energy consumption and pilgrimage tourism in Saudi Arabia. Econ Res Istraz 35(1):3083–3098. https://doi.org/10.1080/1331677X.2021.1985577
Pandyaswargo AH, Gamaralalage PJD, Liu C, Knaus M, Onoda H, Mahichi F, Guo Y (2019) Challenges and an implementation framework for sustainable municipal organic waste management using biogas technology in emerging Asian countries. Sustain 11(22):6331. https://doi.org/10.3390/su11226331
Peterson RA (2021) Finding optimal normalizing transformations via bestNormalize. R J. 13:310
Pilloni M, Hamed TA, Joyce S (2020) Assessing the success and failure of biogas units in Israel: social niches, practices, and transitions among Bedouin villages. Energy Res Soc Sci 61:101. https://doi.org/10.1016/j.erss.2019.101328
Popp J, Kovács S, Oláh J, Divéki Z, Balázs E (2021) Bioeconomy: biomass and biomass-based energy supply and demand. N Biotechnol 60:76–84. https://doi.org/10.1016/j.nbt.2020.10.004
Raza MA, Khatri KL, Ul Haque MI, Shahid M, Rafique K, Waseer TA (2022) Holistic and scientific approach to the development of sustainable energy policy framework for energy security in Pakistan. Energy Rep 8:4282–4302. https://doi.org/10.1016/j.egyr.2022.03.044
Rehman A, Ma H, Ozturk I, Ahmad M, Rauf A, Irfan M (2021) Another outlook to sector-level energy consumption in Pakistan from dominant energy sources and correlation with economic growth. Environ Sci Pollut Res 28:33735–33750. https://doi.org/10.1007/s11356-020-09245-7
Rehman Zia UU, de Rashid T, Awan WN, Hussain A, Ali M (2020) Quantification and technological assessment of bioenergy generation through agricultural residues in Punjab (Pakistan). Biomass and Bioenergy 139:105612. https://doi.org/10.1016/j.biombioe.2020.105612
Saghir M, Zafar S, Tahir A, Ouadi M, Siddique B, Hornung A (2019) Unlocking the potential of biomass energy in Pakistan. Front Energy Res 7:1–18. https://doi.org/10.3389/fenrg.2019.00024
Sahakian M, Rau H, Grealis E, Godin L, Wallenborn G, Backhaus J, Friis F, Genus AT, Goggins G, Heaslip E, Heiskanen E, Iskandarova M, Louise Jensen C, Laakso S, Musch A-K, Scholl C, Vadovics E, Vadovics K, Vasseur V, Fahy F (2021) Challenging social norms to recraft practices: a Living Lab approach to reducing household energy use in eight European countries. Energy Res Soc Sci 72:101881. https://doi.org/10.1016/j.erss.2020.101881
Sarker SA, Wang S, Adnan KMM, Sattar MN (2020) Economic feasibility and determinants of biogas technology adoption: evidence from Bangladesh. Renew Sustain Energy Rev 123:109766. https://doi.org/10.1016/j.rser.2020.109766
Sarstedt M, Ringle CM, Hair JF (2022) Partial least squares structural equation modeling. Handbook of Market Research. Springer International Publishing, Cham, pp 587–632. https://doi.org/10.1007/978-3-319-57413-4_15
Chapter Google Scholar
Shah AALI, Sahito AR (2017) Appraisal of biogas potential of biogas from animal dung in Saeedabad. Pakistan 36:707–718
Shahzad HU, Mustafa MF, Afridi ZU (2020) Challenges and potential to adopt biogas technology: a case study of Faisalabad. Pakistan Int J Agric Ext 8:207–217. https://doi.org/10.33687/ijae.008.03.3391
Sheng G, Xie F, Gong S, Pan H (2019) The role of cultural values in green purchasing intention: empirical evidence from Chinese consumers. Int J Consum Stud 43:315–326. https://doi.org/10.1111/ijcs.12513
Silva HL de C e, Luisa H, Huamán Córdova ME, Barros RM, Tiago Filho GL, Silva Lora EE, Moreira Santos AH, dos Santos IFS, de Oliveira Botan MCC, Pedreira JR, Flauzino BK (2022) Lab-scale and economic analysis of biogas production from swine manure. Renew Energy 186:350–365. https://doi.org/10.1016/j.renene.2021.12.114
Simeone M, Scarpato D (2020) Sustainable consumption: how does social media affect food choices? J Clean Prod 277:124036. https://doi.org/10.1016/j.jclepro.2020.124036
Situmorang YA, Zhao Z, Yoshida A, Abudula A, Guan G (2020) Small-scale biomass gasification systems for power generation (<200 kW class): a review. Renew Sustain Energy Rev 117:109. https://doi.org/10.1016/j.rser.2019.109486
Smith JG (2020) Theoretical advances in our understanding of network effectiveness. Perspect Public Manag Gov 3:167–182. https://doi.org/10.1093/ppmgov/gvz032
Sreen N, Yadav R, Kumar S, Gleim M (2021) The impact of the institutional environment on green consumption in India. J Consum Mark 38:47–57. https://doi.org/10.1108/JCM-12-2019-3536
Su CC, Chan NK (2017) Predicting social capital on Facebook: the implications of use intensity, perceived content desirability, and Facebook-enabled communication practices. Comput Human Behav 72:259–268. https://doi.org/10.1016/j.chb.2017.02.058
Sun Y, Anwar A, Razzaq A, Liang X, Siddique M (2022) Asymmetric role of renewable energy, green innovation, and globalization in deriving environmental sustainability: evidence from top-10 polluted countries. Renew Energy 185:280–290. https://doi.org/10.1016/j.renene.2021.12.038
Taufique KMR, Islam S (2021) Green marketing in emerging Asia: antecedents of green consumer behavior among younger millennials. J Asia Bus Stud 15:541–558. https://doi.org/10.1108/JABS-03-2020-0094
Tian H, Wang X, Lim EY, Lee JTE, Ee AWL, Zhang J, Tong YW (2021) Life cycle assessment of food waste to energy and resources: centralized and decentralized anaerobic digestion with different downstream biogas utilization. Renew Sustain Energy Rev 150:111489. https://doi.org/10.1016/j.rser.2021.111489
Tillmanns S, Krafft M (2022) Logistic regression and discriminant analysis. Handbook of Market Research. Springer International Publishing, Cham, pp 329–367. https://doi.org/10.1007/978-3-319-57413-4_20
Trypolska G, Kyryziuk S, Krupin V, Wąs A, Podolets R (2021) Economic feasibility of agricultural biogas production by farms in Ukraine. Energies 15:87. https://doi.org/10.3390/en15010087
Tsallis C (2022) Enthusiasm and skepticism: two pillars of science—a nonextensive statistics case. Physics (College. Park. Md) 4:609–632. https://doi.org/10.3390/physics4020041
Tumusiime E, Kirabira JB, Musinguzi WB (2019) Long-life performance of biogas systems for productive applications : The role of R & D and policy. Energy Rep 5:579–583. https://doi.org/10.1016/j.egyr.2019.05.002
Ullah I, Rehman A, Khan FU, Shah MH, Khan F (2020) Nexus between trade, CO2 emissions, renewable energy, and health expenditure in Pakistan. Int J Health Plann Manage 35:818–831. https://doi.org/10.1002/hpm.2912
Valasai GD, Uqaili MA, Memon HR, Samoo SR, Mirjat NH, Harijan K (2017) Overcoming electricity crisis in Pakistan: a review of sustainable electricity options. Renew Sustain Energy Rev 72:734–745
Valdez RX, Peterson MN, Stevenson KT (2017) How communication with teachers, family and friends contributes to predicting climate change behaviour among adolescents. Environ Conserv 45:183–191. https://doi.org/10.1017/S0376892917000443
vom Brocke J, Schmid AM, Simons A, Safrudin N (2021) IT-enabled organizational transformation: a structured literature review. Bus Process Manag J 27:204–229. https://doi.org/10.1108/BPMJ-10-2019-0423
Wang Z, Ali S, Akbar A, Rasool F (2020) Determining the influencing factors of biogas technology adoption intention in Pakistan: the moderating role of social media. Int J Environ Res Public Health 17:2311. https://doi.org/10.3390/ijerph17072311
Wang Z-Y, Guo R, Shi X, Liu X, Qin H, Liu Y (2020) The superior performance of CoMnO x catalyst with ball- fl owerlike structure for low-temperature selective catalytic reduction of NO x by NH 3. Chem Eng J 381:122753. https://doi.org/10.1016/j.cej.2019.122753
Wu H, Li Y, Hao Y, Ren S, Zhang P (2020) Environmental decentralization, local government competition, and regional green development: evidence from China. Sci Total Environ 708:135085. https://doi.org/10.1016/j.scitotenv.2019.135085
Wu H, Xu L, Ren S, Hao Y, Yan G (2020) How do energy consumption and environmental regulation affect carbon emissions in China? New evidence from a dynamic threshold panel model. Resour Policy 67:101678. https://doi.org/10.1016/j.resourpol.2020.101678
Xia T, Rezaei M, Dampage U, Alharbi SA, Nasif O, Borowski PF, Mohamed MA (2021) Techno-economic assessment of a grid-independent hybrid power plant for co-supplying a remote micro-community with electricity and hydrogen. Processes 9:1375. https://doi.org/10.3390/pr9081375
Yang H-T, Yen G-F (2018) Consumer responses to corporate cause-related marketing. Eur J Mark 52:2105–2127. https://doi.org/10.1108/EJM-07-2017-0468
Yang H-T, Yen GF (2018) Consumer responses to corporate cause-related marketing: a serial multiple mediator model of self-construal, empathy and moral identity. Eur J Mark 52:2105–2127. https://doi.org/10.1108/EJM-07-2017-0468
Yaqoo H, Teoh YH, Din ZU, Sabah NU, Jamil MA, Mujtaba MA, Abid A (2021) The potential of sustainable biogas production from biomass waste for power generation in Pakistan. J Clean Prod 307:127. https://doi.org/10.1016/j.jclepro.2021.127250
Yasar A, Nazir S, Rasheed R, Tabinda AB, Nazar M (2017) Economic review of different designs of biogas plants at household level in Pakistan. Renew Sustain Energy Rev 74:221–229. https://doi.org/10.1016/j.rser.2017.01.128
Yasmin I, Akram W, Adeel S, Chandio AA (2022) Non-adoption decision of biogas in rural Pakistan: use of multinomial logit model. Environ Sci Pollut Res 29(35):53884–53905. https://doi.org/10.1007/s11356-022-19539-7
Yeh S-S, Guan X, Chiang T-Y, Ho J-L, Huan T-CT (2021) Reinterpreting the theory of planned behavior and its application to green hotel consumption intention. Int J Hosp Manag 94:102. https://doi.org/10.1016/j.ijhm.2020.102827
Yin J, Shi S (2021) Social interaction and the formation of residents’ low-carbon consumption behaviors: an embeddedness perspective. Resour Conserv Recycl 164:105. https://doi.org/10.1016/j.resconrec.2020.105116
Zhang S, Huang Q, Liu H, Wang Y (2022) Team social media usage and intra-team competition and cooperation: a social information processing perspective. Inf Technol People 35:410–434. https://doi.org/10.1108/ITP-02-2020-0058
Zhao C, Zhang M, Wang W (2019) Exploring the influence of severe haze pollution on residents’ intention to purchase energy-saving appliances. J Clean Prod 212:1536–1543. https://doi.org/10.1016/j.jclepro.2018.12.134
Download references
Author information
Authors and affiliations.
School of Economics and Management, North China Electric Power University, Beijing, 102206, China
Shahid Ali & Qingyou Yan
Beijing Key Laboratory of New Energy and Low-Carbon Development, North China Electric Power University, Beijing, 102206, China
Qingyou Yan
School of Economics, Beijing Technology and Business University, Beijing, 100048, China
Muhammad Irfan
Faculty of Management Sciences, Department of Business Administration, ILMA University, Karachi, 75190, Pakistan
School of Economics and Management, Leshan Normal University, Leshan, 614000, China
You can also search for this author in PubMed Google Scholar
Contributions
S.A: conceptualization, writing—original draft, formal analysis, data handling, conceptualization, variable construction, and methodology. Q.Y: supervision, funding acquisition. M.I: variable construction, writing—review and editing. S.F: writing—review and editing. All authors have read and approved the published form of the document.
Corresponding author
Correspondence to Muhammad Irfan .
Ethics declarations
Ethical approval.
The present study was directed according to the Declaration of Helsinki’s guiding principles. The Official Assessment Board of North China Electric Power University has demonstrated Pakistan (protocol 743–6 on 13 May 2022).
Consent to participate
All participants in this research study provided their informed permission.
Consent for publication
We give our consent for the publication.
Informed consent
Informed consent was obtained from all subjects involved in the study.
Competing interests
The authors declare no competing interests.
Additional information
Responsible Editor: Ilhan Ozturk
Publisher's note
Springer Nature remains neutral with regard to jurisdictional claims in published maps and institutional affiliations.
Rights and permissions
Springer Nature or its licensor (e.g. a society or other partner) holds exclusive rights to this article under a publishing agreement with the author(s) or other rightsholder(s); author self-archiving of the accepted manuscript version of this article is solely governed by the terms of such publishing agreement and applicable law.
Reprints and permissions
About this article
Ali, S., Yan, Q., Irfan, M. et al. Relating biogas technology and environmental impact assessment: a roadmap towards clean energy for environmental sustainability. Environ Sci Pollut Res 30 , 72832–72853 (2023). https://doi.org/10.1007/s11356-023-27553-6
Download citation
Received : 22 December 2022
Accepted : 07 May 2023
Published : 13 May 2023
Issue Date : June 2023
DOI : https://doi.org/10.1007/s11356-023-27553-6
Share this article
Anyone you share the following link with will be able to read this content:
Sorry, a shareable link is not currently available for this article.
Provided by the Springer Nature SharedIt content-sharing initiative
- Renewable energy
- Biogas technology
- Biogas potential
- Electronic and social media
- Find a journal
- Publish with us
- Track your research
Help | Advanced Search
Computer Science > Cryptography and Security
Title: large language models for blockchain security: a systematic literature review.
Abstract: Large Language Models (LLMs) have emerged as powerful tools in various domains involving blockchain security (BS). Several recent studies are exploring LLMs applied to BS. However, there remains a gap in our understanding regarding the full scope of applications, impacts, and potential constraints of LLMs on blockchain security. To fill this gap, we conduct a literature review on LLM4BS. As the first review of LLM's application on blockchain security, our study aims to comprehensively analyze existing research and elucidate how LLMs contribute to enhancing the security of blockchain systems. Through a thorough examination of scholarly works, we delve into the integration of LLMs into various aspects of blockchain security. We explore the mechanisms through which LLMs can bolster blockchain security, including their applications in smart contract auditing, identity verification, anomaly detection, vulnerable repair, and so on. Furthermore, we critically assess the challenges and limitations associated with leveraging LLMs for blockchain security, considering factors such as scalability, privacy concerns, and adversarial attacks. Our review sheds light on the opportunities and potential risks inherent in this convergence, providing valuable insights for researchers, practitioners, and policymakers alike.
Submission history
Access paper:.
- Download PDF
- HTML (experimental)
- Other Formats
References & Citations
- Google Scholar
- Semantic Scholar
BibTeX formatted citation

Bibliographic and Citation Tools
Code, data and media associated with this article, recommenders and search tools.
- Institution
arXivLabs: experimental projects with community collaborators
arXivLabs is a framework that allows collaborators to develop and share new arXiv features directly on our website.
Both individuals and organizations that work with arXivLabs have embraced and accepted our values of openness, community, excellence, and user data privacy. arXiv is committed to these values and only works with partners that adhere to them.
Have an idea for a project that will add value for arXiv's community? Learn more about arXivLabs .
Advertisement
Supported by
Henry Louis Gates Jr. Unpacks Black Literature’s ‘Black Box’
In his latest book, the Harvard scholar shows how African American writers have used the written word to shape their reality despite constraints imposed on them from outside.
- Share full article

By Tope Folarin
Tope Folarin is a writer and critic. He is the executive director of the Institute for Policy Studies and the author of the novel “A Particular Kind of Black Man.”
THE BLACK BOX: Writing the Race , by Henry Louis Gates Jr.
By way of explaining the metaphor that serves as the title of his latest book, “The Black Box,” the Harvard scholar Henry Louis Gates Jr. transcribes a conversation he had with his son-in-law after the birth of his granddaughter 10 years ago:
“Did you check the box?” I asked, apropos of nothing we had just discussed. Without missing a beat, my good son-in-law responded, “Yes, sir. I did.” “Very good,” I responded, as I poured a second shot of Pappy Van Winkle.
The box that Gates’s son-in-law checked on a birth registration form indicates that his granddaughter is Black, even though his daughter’s genetic admixture is 75 percent European, and his son-in-law is 100 percent European. In other words, as Gates notes, his granddaughter “will test about 87.5 percent European when she spits in the test tube.”
Gates offers this anecdote to suggest the arbitrariness of racial categories, and to focus our attention on the image of the box — a container that can function simultaneously as a “circumscribed enclosure” and a zone in which the confined can create a thriving “social and cultural world.”
For Gates, the box is a supple concept. Not only does it appear with surprising frequency in literature by Black Americans — from the fugitive slave author Henry Box Brown (who escaped slavery in a box) to Booker T. Washington (who described the box as a barrel) to the contemporary poet Terrance Hayes — but Gates extends the metaphor to other kinds of boxes that relate to Black experience, to ordeals withstood and survived. He cites an airplane’s flight recorder box, a device that “preserves a record of the truth amid disastrous circumstances,” and the slave ship, before arriving at his thesis: African Americans have consistently relied on the written word to express and shape their reality despite the constraints imposed on them from outside, which they have endured since they were first brought to this continent.
“The Black Box” is based on lectures Gates has delivered for many years in his Introduction to African American Studies class at Harvard. From the beginning, he shows, African Americans have turned to literary forms to validate their humanity. He quickly sketches the childhood of Phillis Wheatley — her journey to America via slave ship, her rapid mastery of English — and the varied responses to her poetry, which she began to publish as a precocious teenager.
Wheatley’s success undermined the prevailing sentiment that Black Americans were less intelligent than their white counterparts, and in response to her art some thinkers, such as Voltaire, revised their formerly negative perspectives on Black people, while others, including Thomas Jefferson, remained steadfast in their views. (Jefferson on Wheatley: “The compositions published under her name are below the dignity of criticism.”)
Gates traces the history of slave narratives, a genre that “always told the story” of how enslaved authors “learned to read and write, and always, always, of course, of their escape to freedom.” And he describes the intense conversations within the Black American community around self-definition: “They stood on uncertain ground. Were they Africans, Americans, both, or neither?” In each of these instances, as throughout his book, Gates adroitly demonstrates how literature served as a site of self-interrogation and a pathway to liberation.
In other chapters, Gates writes about the manifold contributions of W.E.B. Du Bois; the boldness and flair of the Harlem Renaissance generation; the fierce debate between Zora Neale Hurston and Richard Wright over the proper way to represent the Black experience in literature; and the politics of passing.
Many of these ideas will be familiar to readers — Gates has written about them before. The allure of this book, and the reason for its existence, are the narrative links he draws among these people and events, and his insistence that a survey of African American history is incomplete without a special consideration of how writing has undergirded and powered it. This is a literary history of Black America, but it is also an argument that African American history is inextricable from the history of African American literature.
“The Black Box” arrives at an auspicious moment. This year marks the 100th anniversary of the birth of a great man of American and African American letters: James Baldwin. Next year marks the 100th anniversary of the publication of “The New Negro,” an anthology of fiction, poetry, art and essays edited by Alain Locke that is widely regarded as a defining text of the Harlem Renaissance.
This is also a moment when many politicians are hard at work revising our shared history in order to justify present-day policy agendas. Gates addresses this phenomenon in his concluding chapter, portions of which appeared in The New York Times a year ago. He invokes the story of Mildred Lewis Rutherford, the historian general of the United Daughters of the Confederacy, who argued throughout her career that the Civil War was simply “the War Between the States,” and had nothing to do with slavery.
As Gates notes, it is important to repeat the truth about history as often as possible, and to repel efforts to redefine it, because “what is inscribed on the blackboard translates directly to social practices unfolding on the street.”
THE BLACK BOX : Writing the Race | By Henry Louis Gates Jr. | Penguin Press | 262 pp. | $30
Explore More in Books
Want to know about the best books to read and the latest news start here..
You never know what’s going to go wrong in these graphic novels, where Circus tigers, giant spiders, shifting borders and motherhood all threaten to end life as we know it .
When the author Tommy Orange received an impassioned email from a teacher in the Bronx, he dropped everything to visit the students who inspired it.
A few years ago, Harvard acquired the archive of Candida Royalle, a porn star turned pioneering director. Now, the collection has inspired a new book , challenging the conventional history of the sexual revolution.
Gabriel García Márquez wanted his final novel to be destroyed. Its publication this month may stir questions about posthumous releases.
Do you want to be a better reader? Here’s some helpful advice to show you how to get the most out of your literary endeavor .
Each week, top authors and critics join the Book Review’s podcast to talk about the latest news in the literary world. Listen here .
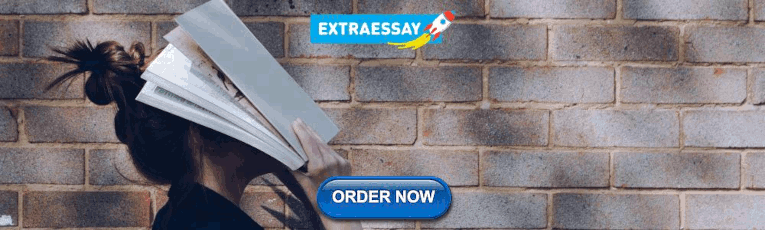
IMAGES
VIDEO
COMMENTS
Published research papers and data on biogas sources, production, and applications are collected from the literature. These sources cover the years from 1997 till 2020 to summarize the current situation and development relevant to biogas. A review of policies and regulations on national and international levels is presented.
Biogas is the end product of a biological mediated process, the so called anaerobic digestion, in which different microorganisms, follow diverse metabolic pathways to decompose the organic matter ...
Subsequently, an overview of the business models discussed is given by a systematic literature review. The latter shows that the investigation of biogas business models is focused mainly on the last decade and has increased slightly over time. The regions of investigation can be found worldwide, with a clear focus on Europe.
This review aims to present conventional and novel biogas production systems, as well as purification and upgrading technologies, nowadays applicable on a large scale, with a special focus on the CO 2 and H 2 S removal. It also gives an overview of feedstock and the parameters important for biogas production, together with digestate utilization ...
The literature review found that governmental involvement remains essential. In many cases, there is a lack of political support and specific programs to promote biogas technologies [see e.g., [57, 84, 123]]. The energy sector has not received significant attention in policy debates within developing countries [17].
Biogas production is a well-established technology primarily for the generation of renewable energy and also for the valorization of organic residues. Biogas is the end product of a biological mediated process, the so called anaerobic digestion, in which different microorganisms, follow diverse metabolic pathways to decompose the organic matter. The process has been known since ancient times ...
Biogas production is rising in the context of fossil fuel decline and the future circular economy, yet raw biogas requires purification steps before use. Here, we review biogas upgrading using physical, chemical and biological methods such as water scrubbing, physical absorption, pressure swing adsorption, cryogenic separation, membrane separation, chemical scrubbing, chemoautotrophic methods ...
A systematic literature review was performed to obtain the most relevant literature on modeling approaches for biogas production and its environmental impact, as shown
This literature review highlights that the available knowledge on the potential occupational and community hazards associated with biogas plants is fragmented. On the one hand, there is a limited number of studies including on-field monitoring due to the novelty of the technology.
The literature review shows that substantial research and development of models for biogas production was carried out in the last 18 years. Regarding biogas modeling on a process level (anaerobic digestion), these models differ substantially from modeling biogas for potential analysis, GHG emissions and in an energy system.
Literature review. The comprehensive review of the research work related to the biogas generation can be divided into the following subgroups for the purpose of better classification and better understanding. 2.1. Review based on the different feedstocks used for biogas production. Cow dung has been used traditionally for biogas production.
The social acceptance of biogas is often hampered by environmental and health concerns. In this study, the current knowledge about the impact of biogas technology is presented and discussed. The survey reports the emission rate estimates of the main greenhouse gases (GHG), namely CO 2, CH 4 and N 2 O, according to several case studies conducted ...
Literature Review on Biogas and its application in Rural Areas Gursimran Singh Sadana1 Hakkam Singh2 Ishaan Pokhariyal3 Raunak Kankaria4 Siddhant Mhatre5 1,2,3,4,5Symbiosis Institute of Technology, Pune, Maharashtra, India Abstract— The extensive use of fossil fuel and increased energy demand has escalated the need for renewable energy
Literature study on the engine characteristics of biogas fuelled SI engines. ... Techno-economic review of biogas cleaning technologies for small scale off-grid solid oxide fuel cell applications. Fuel Process Technol, 197 (2020), Article 106215. View PDF View article View in Scopus Google Scholar
This review showcases a comprehensive analysis of studies that highlight the different conversion procedures attempted across the globe. The resources of biogas production along with treatment methods are presented. The effect of different governing parameters like feedstock types, pretreatment approaches, process development, and yield to enhance the biogas productivity is highlighted. Biogas ...
Based on the literature, 14 biogas is an important fuel in producing clean and sustainable electric power. ... In this article, a review of biogas applications for electricity production is reported. Recent developments of installed biogas production and utilization plants are presented. A comprehensive study is performed on biogas-driven ...
This literature review highlights that the available knowledge on the potential occupational and community hazards associated with biogas plants is fragmented. On the one hand, there is a limited number of studies including on-field monitoring due to the novelty of the technology.
In this review, we compare works from the current decade that address the CO 2-removal from biogas by means of microalgae.The microalgae culture process acts as a biochemical absorption process; it is potentially competitive with respect to classical and commercial absorption methods due to its additional benefits such as availing CO 2 for the production of valuable microalgae biomass and ...
Therefore, the present study aims to conduct a systematic literature review of high impact research on LCA of electricity from biogas to investigate trends in this body of literature. To that end, the Methodi Ordinatio was applied to the raw portfolio to identify the most relevant, high impact, studies in the area.
In this review, we compare works from the current decade that address the CO 2-removal from biogas by means of microalgae.The microalgae culture process acts as a biochemical absorption process; it is potentially competitive with respect to classical and commercial absorption methods due to its additional benefits such as availing CO 2 for the production of valuable microalgae biomass and ...
Literature Review of Recent Research on Biogas and its Usage in Diesel Engines. Farm based waste digestion plants return higher profit if the emanating methane (CH4) rich biogas is purified and used in agricultural vehicles, as it is used to generate heat and electricity. For this reason, ignition process of diesel engines, the prime movers in ...
1. Introduction. Biogas is one of the most economically viable and environmentally-friendly renewable energy resources (Deublein and Steinhauser, 2011).This renewable biofuel on one hand can play a vital role in decreasing the concerns associated with the rapid increases in energy demands and on the other hand the resultant greenhouse gas (GHG) emissions and the downstream catastrophic ...
The following section investigates the literature review, investigation procedure, research strategy, and the construction of suppositions, as well as the literature review and conceptual model. ... Ndung'u V (2018) Africa Biogas Partnership Program: a review of clean cooking implementation through market development in East Africa. Energy ...
The rest of the review covers the regulatory challenges and responses emergent in the wake of AI's speedy deployment. We start by enumerating the rationales for regulation that have attracted most at-tention in the literature and regulatory action to date: market competition; privacy concern; intellectual
Large Language Models (LLMs) have emerged as powerful tools in various domains involving blockchain security (BS). Several recent studies are exploring LLMs applied to BS. However, there remains a gap in our understanding regarding the full scope of applications, impacts, and potential constraints of LLMs on blockchain security. To fill this gap, we conduct a literature review on LLM4BS. As ...
We review the literature on the effects of Artificial Intelligence (AI) adoption and the ongoing regulatory efforts concerning this technology. Economic research encompasses growth, employment, productivity, and income inequality effects, while regulation covers market competition, data privacy, copyright, national security, ethics concerns, and financial stability.
Post-splenectomy sepsis: a review of the literature. Cureus, 12 (2) (2020;), Article e6898. Google Scholar [5] V Ravikumar, G Rajamani, V Raju, R Sundar, S Ravikumar, R. Maniam. Teratoma arising from hepato duodenal ligament in the newborn with transection of portal vein, hepatic artery and common bile duct: A surgical challenge.
Wheatley's success undermined the prevailing sentiment that Black Americans were less intelligent than their white counterparts, and in response to her art some thinkers, such as Voltaire ...