Sports Medicine Research
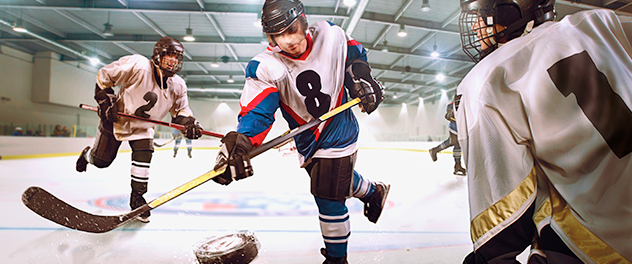
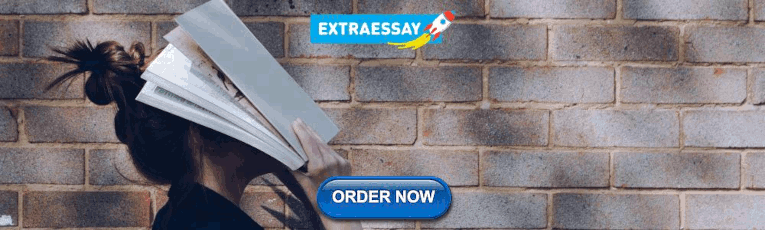
Fair Play rules make ice hockey safer
Fair Play (PDF) is an initiative developed and implemented by Mayo Clinic Sports Medicine Research to reduce the incidence of concussions and make hockey a safer sport.
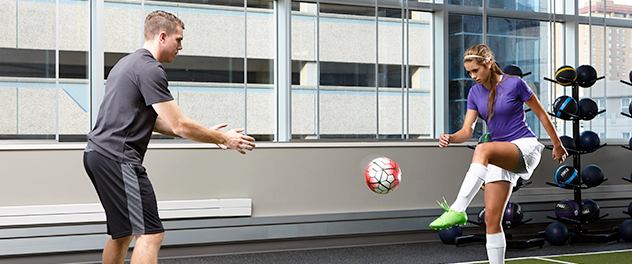
Research improves all aspects of sports mechanics
Mayo Clinic Sports Medicine Research is using research discoveries to improve strength, power, agility and speed and prevent common injuries such as ACL tears in athletes.
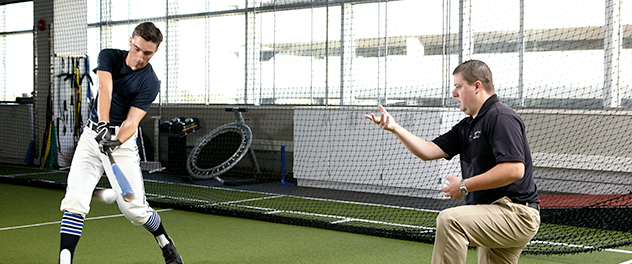
Biomechanics breaks down movement to prevent injury
Mayo Clinic Sports Medicine Research is using biomechanics research to show how pitching and swing mechanics can put an athlete at risk for injury.
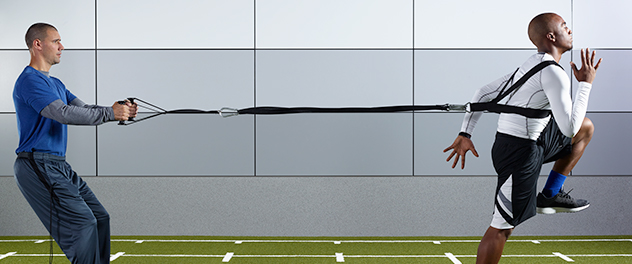
Striving to improve athletic performance
Mayo Clinic Sports Medicine Research: Preventing and treating sports injuries to improve and enhance athletic performance.
Mayo Clinic Sports Medicine Research performs leading-edge research to define the mechanism of injury and utilizes these findings to implement educational programs and therapeutic interventions. This work helps prevent injuries, enhances athletic performance and increases injury prevention during play.
Sports Medicine Research investigates all aspects of sports injury evaluation, treatment and prevention to provide optimal care to those involved in sports- or fitness-related activities. Recent work has addressed neuromuscular interventions to prevent anterior cruciate ligament (ACL) injuries, the use of subsymptom exercise to return athletes who've had concussions to a pre-head-trauma state and the modeling of lower extremity athletic joint injuries.
Research focus areas
Mayo Clinic Sports Medicine Research focuses on the following areas for optimal care and results for athletes:
- Mechanisms that may identify the anatomical and structural causes of injuries
- Screening using algorithms to identify athletes at a high risk of injury
- Intervention and prevention through neuromuscular training protocols
- Treatment strategies (surgical and nonsurgical) to optimize outcomes after injury
Comprehensive care comes from bringing research directly into practice. A complete team of surgeons, physicians, researchers, specialists and therapists work together to return people to physical activity as soon as possible.
- Twitter @mayoclinicsport - Connect Twitter @mayoclinicsport
- Facebook - Connect Facebook
- Instagram #mayoclinicsportsmedicine - Connect Instagram #mayoclinicsportsmedicine
- Contacts - Connect Contacts
Mayo Clinic Sports Medicine
Learn more about clinical services, sports performance training and how to make an appointment.
Focus Areas
Research is focused into specialized areas to diagnose, treat and prevent diseases or conditions that affect athletic performance for the professional and recreational athlete.
Clinical Research Areas
Clinical research areas in ACL, concussion, hip, knee and shoulder apply scientific discoveries to diagnose and treat athletes for the best outcomes.
More about research at Mayo Clinic
- Research Faculty
- Laboratories
- Core Facilities
- Centers & Programs
- Departments & Divisions
- Clinical Trials
- Institutional Review Board
- Postdoctoral Fellowships
- Training Grant Programs
- Publications
Mayo Clinic Footer
- Request Appointment
- About Mayo Clinic
- About This Site
Legal Conditions and Terms
- Terms and Conditions
- Privacy Policy
- Notice of Privacy Practices
- Notice of Nondiscrimination
- Manage Cookies
Advertising
Mayo Clinic is a nonprofit organization and proceeds from Web advertising help support our mission. Mayo Clinic does not endorse any of the third party products and services advertised.
- Advertising and sponsorship policy
- Advertising and sponsorship opportunities
Reprint Permissions
A single copy of these materials may be reprinted for noncommercial personal use only. "Mayo," "Mayo Clinic," "MayoClinic.org," "Mayo Clinic Healthy Living," and the triple-shield Mayo Clinic logo are trademarks of Mayo Foundation for Medical Education and Research.
- Access Member Benefits
- Exercise is Medicine
Now Available: IDEA & ACSM Health & Fitness Summit Online Content
20 brand new, online-exclusive courses, need to know.
- ACSM Member Exclusives
- Policy Corner
- Clinical & Medicine
- Education in Action
New ACSM Member Hub Now Available!
ACSM members can now access all of the member benefits in the new Member Hub! all of your exclusive content, partner discounts, grant and travel award applications, mentor forms and so much more can be found in one place. Check out the Member Hub *Must be logged in with ACSM ID to access.
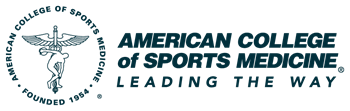
ACSM Supports Funding for National Concussion Surveillance System and Physical Activity Alliance Releases New Report
Last month, ACSM joined more than 30 other organizations in signing a letter requesting at least $2 million to fund the National Concussion Surveillance System. The funding would allow the Centers for Disease Control and Prevention (CDC) to develop an initial system that will collect necessary data to determine the incidence of sports-related concussions. This letter will go to House and Senate leadership and both the House and Senate Chairs and Ranking Members of the Appropriations Labor-HHS-Education Subcommittee. The Physical Activity Alliance (PAA), of which ACSM is a founding member, just released a new report: “Advancing Key Actions to Enhance Physical Activity Surveillance in the United States.” The report summarizes actions taken to advance surveillance in four areas: children and youth, workplace, health care and community support for physical activity. It also lays out steps to advance tracking physical activity engagement as a key public health priority and underscores the benefits of systematic data collection to drive policy and programming that supports equitable physical activity opportunities across the U.S. Read the report.
Team Physicians Update Guidance on Musculoskeletal Injuries
A group of delegates from ACSM, the American Academy of Family Physicians, American Academy of Orthopaedic Surgeons, American Medical Society for Sports Medicine, American Orthopaedic Society for Sports Medicine and the American Osteopathic Academy of Sports Medicine recently met to write updated guidelines on the care and treatment of select musculoskeletal injuries as part of the annual Team Physician Consensus Conference (TPCC). The TPCC Executive Committee, consisting of ACSM Fellows Stan Herring, M.D.; Ben Kibler, M.D.; and Margot Putukian, M.D., along with delegates from the partner organizations, reviewed the current scientific literature and clinical evidence to write the new evidence-based statement for clinicians. The resulting document, which will be published in 2022, will provide guidance and serve as a teaching tool for physicians, residents and fellows working in the sports medicine field. Learn More About TPCC
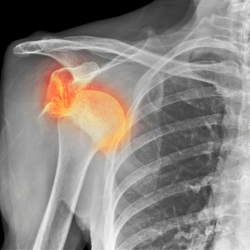
The Female Athlete Triad
MYTH: It's "normal" for female athletes to lose their period, or stop menstruating. REALITY: Amenorrhea due to working out is unhealthy. Mary Jane De Souza, Ph.D., FACSM, and Nancy Williams, Sc.D., FACSM, both researchers and professors at Pennsylvania State University, have spent many years studying the Female Athlete Triad.
Built For You. Your Career, Your Choice.
Whether it's building knowledge, building networks or building careers, ACSM can get you there . Together, we can make change happen. Enjoy the benefits.

ACSM Spotlight
Read stories of how ACSM members and certified professionals are leading change, breaking barriers and impacting lives around the world and across generations. Read the Stories
Get & Stay Certified
Dedicated to improving lives.
This is where passion, knowledge and skill come together. ACSM certified professionals improve lives by guiding patients and clients to better health, function and performance.
ACSM Certifications
Feature post, top news articles of 2023.
Top news articles featuring ACSM published in 2023.
Recent Blog Posts
- Recent Jobs
Career Resources
- ACSM Foundation
- ACSM Journals
See All Jobs
Free Webinars
ACSM experts provide career guidance on a variety of possible career choices.
Career Coaching
Coming from a variety of professional backgrounds, our certified coaches have the experience, training, and expertise needed to help you achieve your career goals.
More Resources
Endowments and Funds
At your request, you may designate your gift as restricted funds for one or more available endowments. Find Out More
Access Your ACSM Journals Here
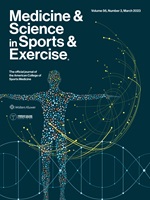
Go to Journals
Discover Free ACSM Journal Content

Highlighted Free Article
Video Abstract
- 2024 Election Results
- News Archive
- Certification Task Force
- Advertise with ACSM
- Current Partners
- Partner Development
- American Fitness Index
- National Youth Sports Health & Safety Institute
- Historical Timeline
- Annual Report 2022
- Annual Report 2023
- Honor & Citation Awards
- Student Membership
- Alliance Membership
- Professional-in-Training Membership
- Professional Membership
- Renew Membership
- Regional Chapter Challenge
- Member Code of Ethics
- Group Exercise Instructor
- ACSM Personal Trainer Prep
- ACSM Exercise Physiologist Prep
- ACSM Clinical Exercise Physiologist Prep
- Beijing Institute of Sports Medicine
- Wellness Academy
- Frequently Asked Questions
- Recertification FAQs
- Find an ACSM Certified Professional
- Certified Professional of the Year
- Wellcoaches
- Certified Professional Discounts
- Hire ACSM Certified Professionals
- Specialty Certificate Programs
- ceOnline FAQs
- Approved Providers
- Current Sports Medicine Reports
- Exercise and Sport Sciences Reviews
- Exercise, Sport, and Movement
- Health & Fitness Journal
- Medicine & Science in Sports & Exercise
- Translational Journal
- Paper of the Year Awards
- ACSM's Guidelines for Exercise Testing and Prescription
- ACSM's Resources for the Personal Trainer
- ACSM's Resources for the Exercise Physiologist
- ACSM's Clinical Exercise Physiology
- ACSM's Resources for the Group Exercise Instructor
- ACSM's Certification Review
- ACSM's Foundations of Strength Training and Conditioning
- ACSM's Nutrition Exercise Science
- ACSM's Essentials of Youth Fitness
- ACSM's Introduction to Exercise Science
- ACSM's Health/Fitness Facility Standards and Guidelines
- ACSM’s Body Composition Assessment
- ACSM's Complete Guide to Fitness and Health
- Preparticipation Physical Evaluation (PPE) Monograph, 5th Edition
- ACSM's Fitness Assessment Manual
- ACSMs Exercise Testing and Prescription
- Textbook Adoption
- Translated Position Stands
- ACSM Official Statements
- Team Physician Consensus Statements
- Resource Library
- Fitness Faceoff
- Autism and Exercise
- Sudden Cardiac Arrest
- Mental Health
- Physical Activity Guidelines
- Reducing Sedentary Behavior
- Faculty Resources
- EIM Clinical Resources
- Black History Month
- COVID-19 Reopening and Return to Play Resources
- Staying Active at Home
- ACSM's Brown Bag Series (archived)
- Sex Differences
- ACSM Podcast
- Emerging Physician Leaders Pilot Program
- Annual Meeting
- IDEA & ACSM Health & Fitness Summit
- Advanced Team Physician Course
- Sports Medicine Essentials
- Integrative Physiology of Exercise Conference
- ACSM World Heart Games
- International Team Physician Course
- Regional Chapter Meetings
- Meeting Exhibits and Sponsors
- Research & Program Grants
- Howard G. "Skip" Knuttgen Scholar Award
- Travel and Research Awards
- ACSM Research Grant Recipients
- Dedicated Endowments & Funds
- Planned Giving / Discovery Society
British Journal of Sports Medicine
(BJSM) is the leading, peer-reviewed journal in sports medicine, with additional multimedia resources.
Impact Factor: 18.6 Citescore: 25.1 All metrics >>
British Journal of Sports Medicine (BJSM) is a Plan S compliant Transformative Journal .
British Journal of Sports Medicine (BJSM) is a multimedia portal for authoritative original research, systematic reviews, consensus statements and debate in sport and exercise medicine (SEM). We define sport and exercise medicine broadly. BJSM’s web, print, video and audio material serves the international sport and exercise medicine community which includes 25 clinical societies who have over 13,000 members. You can access BJSM on Facebook , Twitter , Instagram and Youtube as well as via our podcasts , Stitcher and blog.
7th IOC World Conference on Prevention of Injury & Illness in Sport - 29th February - 2nd March 2024, please see here for more information >>
Meet the new Editors! For more information, please visit the landing page>>
BMJ and BJSM are fully committed to ensure best practice in publication ethics. Please see our joint statement for further information >>

Topical Issues

A collection of key resources, providing an overview of the current medical issues and research landscape in #GolfMedicine

a focus on the latest multidisciplinary research in hip and groin pathology

A global SEM team tackles some of sport’s toughest topics: Abuse, Racism, Ableism, Sexism, and Transphobia

“Men are from Mars, Women are from Venus” – answering the call for an increased focus on female-specific SEM research
Most Read Articles
Systematic review :
29 September 2023
Editorial :
14 June 2023
6 October 2023
Original research :
8 March 2024
Consensus statement :
Latest Articles
10 April 2024
Correction :
9 April 2024
Video Abstract
Consensus statement on concussion in sport: the 6th international conference on concussion in sport–amsterdam, october 2022.
For over two decades, the Concussion in Sport Group has held meetings and developed five international statements on concussion in sport. This 6th statement summarises the processes and outcomes of the 6th International Conference on Concussion in Sport held in Amsterdam on 27–30 October 2022 and should be read in conjunction with the (1) methodology paper that outlines the consensus process in detail and (2) 10 systematic reviews that informed the conference outcomes. Over 3½ years, author groups conducted systematic reviews of predetermined priority topics relevant to concussion in sport. The format of the conference, expert panel meetings and workshops to revise or develop new clinical assessment tools, as described in the methodology paper, evolved from previous consensus meetings with several new components.
BJSM accepts submissions of a wide range of article types, including original research, education articles and systematic reviews.
The Author Information section provides specific article requirements to help you turn your research into an article suitable for BJSM.
Information is also provided on editorial policies and open access
BMJ is delighted to announce the 2023 dates for BJSM Live . In partnership with the British Journal of Sports Medicine (BJSM), we will share the latest evidence and cutting-edge research, to give you expert advice on managing patients in your practice. For more information please click the link below.
Current Issue
2 April 2024


Recent Posts
Safe return following testing positive.
by Kyle P. Harris | Dec 6, 2023
Elite-level athletes with a history of COVID-19 had cardiac measurements similar to unexposed elite athletes. Hence, return to sports participation is safe for athletes following COVID-19.
Eliminating Body Checking has Been Positive; However, Female Ice Hockey Players are Still at Risk!
by Jane McDevitt | Nov 29, 2023
Youth ice hockey policy eliminating body checking decreases the risk of concussion; however, concussion rates are still high among female ice hockey players.
The Biological Basis of Sex Differences in Athletic Performance: Consensus Statement for the American College of Sports Medicine
by Jeffrey B. Driban | Nov 6, 2023
Quick Links
The American Sports Medicine Institute (ASMI) is a national and international leader in sports medicine research related to clinical and surgical outcomes, biomechanics, and rehabilitation. The foci of ongoing studies at ASMI includes both clinical and biomechanical research, and our team includes researchers with expertise in motion capture biomechanics, cadaver joint biomechanics, outcomes research, clinical trials, biostatistics, and human anatomy.
ASMI’s mission is to improve the understanding, prevention, and treatment of sports-related injuries through research and education. In our strive for this mission, ASMI studies are regularly published in high-impact sports medicine journals, presented at scientific meetings, publicized in the media, and utilized in bodies creating sports safety policies.
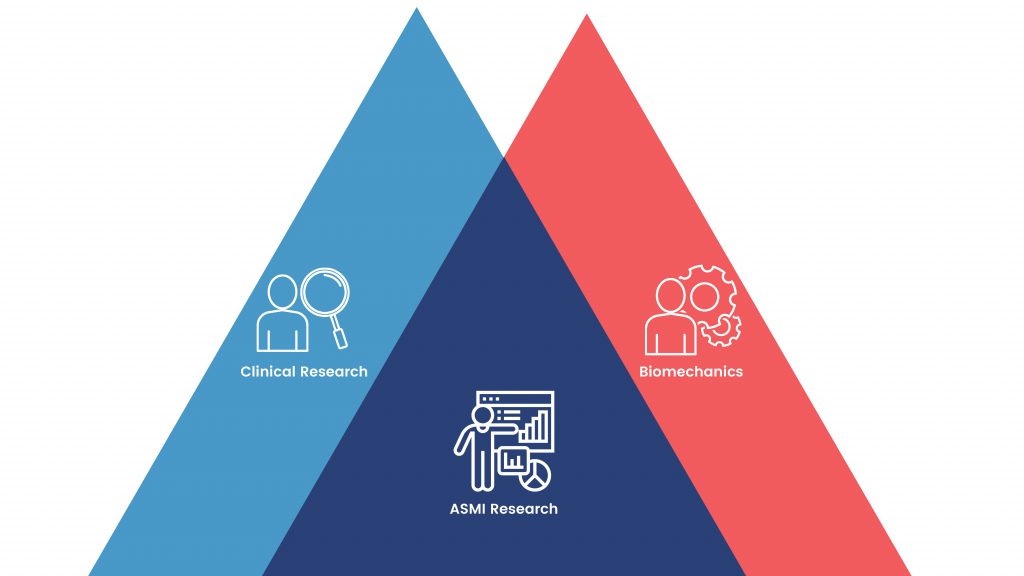
Frequently Asked Questions
- What is sports medicine? Sports medicine is a field of medicine concerned with the prevention and treatment of injuries and disorders that are related to participation in sports.
- Does your research only include athletes? The mission of ASMI is to improve the understanding, prevention, and treatment of sports-related injuries through research and education. This includes everyone from youth to professional athletes, as well as recreational adult athletes and people working to be healthy and fit. Our research team also explores treatment outcomes of non-athletes, particularly those with orthopaedic injuries or surgeries.
- What is the difference between biomechanical research and clinical research? ASMI’s biomechanics studies the motions and forces to minimize injury risk and maximize performance in sports. Other biomechanical studies at ASMI investigate the strength of human joints after surgery compared to their natural, undamaged state. Clinical research at ASMI involves both observational studies and clinical trials. Observational studies generally include examination of what is already occurring (no intervention), whereas clinical trials are carefully designed experiments that involve a specific treatment or intervention. Specifically, our clinical team works to evaluate surgical outcomes, rehabilitation efficacy, as well as injury mechanisms and prevention to improve treatment of sports-related and orthopaedic injuries. Importantly, our biomechanical and clinical research teams work closely together to fulfill the mission of ASMI.
- How can I get involved in research at ASMI? Being involved in ASMI research means contributing to knowledge that directly improves the prevention and treatment of sports-related injuries. We work closely with our local physician and surgeon partners and other collaborators around the country and world to achieve these impactful research goals. Opportunities to participate in research at ASMI’s center in Birmingham are available for students and scholars of all levels. Learn more about how to get involved here .
Biomechanical Research
Our Biomechanical Research department works extensively to further our goal of preventing injury in sports.
Clinical Research
Our Clinical Research department is instrumental in improving treatment for sports related injuries.
Student Researcher Program
The Student Researcher Program is an opportunity for college undergraduate students, graduate students, and others to participate in research at ASMI for thesis or school credit.
Submaximal Fitness Tests in Team Sports: A Theoretical Framework for Evaluating Physiological State
- Review Article
- Open access
- Published: 11 July 2022
- Volume 52 , pages 2605–2626, ( 2022 )
Cite this article
You have full access to this open access article
- Tzlil Shushan ORCID: orcid.org/0000-0002-0544-1986 1 ,
- Shaun J. McLaren ORCID: orcid.org/0000-0003-0480-3209 2 , 3 ,
- Martin Buchheit 4 , 5 , 6 , 7 ,
- Tannath J. Scott ORCID: orcid.org/0000-0003-4336-2370 8 , 9 ,
- Steve Barrett ORCID: orcid.org/0000-0002-6751-9937 10 &
- Ric Lovell ORCID: orcid.org/0000-0001-5859-0267 1
12k Accesses
8 Citations
55 Altmetric
Explore all metrics
Team-sports staff often administer non-exhaustive exercise assessments with a view to evaluating physiological state, to inform decision making on athlete management (e.g., future training or recovery). Submaximal fitness tests have become prominent in team-sports settings for observing responses to a standardized physical stimulus, likely because of their time-efficient nature, relative ease of administration, and physiological rationale. It is evident, however, that many variations of submaximal fitness test characteristics, response measures, and monitoring purposes exist. The aim of this scoping review is to provide a theoretical framework of submaximal fitness tests and a detailed summary of their use as proxy indicators of training effects in team sports. Using a review of the literature stemming from a systematic search strategy, we identified five distinct submaximal fitness test protocols characterized in their combinations of exercise regimen (continuous or intermittent) and the progression of exercise intensity (fixed, incremental, or variable). Heart rate-derived indices were the most studied outcome measures in submaximal fitness tests and included exercise (exercise heart rate) and recovery (heart rate recovery and vagal-related heart rate variability) responses. Despite the disparity between studies, these measures appear more relevant to detect positive chronic endurance-oriented training effects, whereas their role in detecting negative transient effects associated with variations in autonomic nervous system function is not yet clear. Subjective outcome measures such as ratings of perceived exertion were less common in team sports, but their potential utility when collected alongside objective measures (e.g., exercise heart rate) has been advocated. Mechanical outcome measures either included global positioning system-derived locomotor outputs such as distance covered, primarily during standardized training drills (e.g., small-sided games) to monitor exercise performance, or responses derived from inertial measurement units to make inferences about lower limb neuromuscular function. Whilst there is an emerging interest regarding the utility of these mechanical measures, their measurement properties and underpinning mechanisms are yet to be fully established. Here, we provide a deeper synthesis of the available literature, culminating with evidence-based practical recommendations and directions for future research.
Similar content being viewed by others
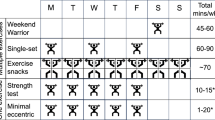
Resistance Exercise Minimal Dose Strategies for Increasing Muscle Strength in the General Population: an Overview
James L. Nuzzo, Matheus D. Pinto, … Kazunori Nosaka
The Mental Health of Elite Athletes: A Narrative Systematic Review
Simon M. Rice, Rosemary Purcell, … Alexandra G. Parker
The Importance of Muscular Strength: Training Considerations
Timothy J. Suchomel, Sophia Nimphius, … Michael H. Stone
Avoid common mistakes on your manuscript.
1 Background
Monitoring the training process and its outcomes within team-based sports requires a systematic approach that: (1) is grounded on a rigorous conceptual framework; (2) can be implemented pragmatically on a frequent basis; (3) uses proxy outcome measures possessing sufficient measurement properties; and (4) is sensitive to identify acute (e.g., post-match) and chronic (e.g., post-training program) training effects [ 1 , 2 , 3 , 4 ]. Such an approach can be used to inform decision making around athlete and training management, including future programming, adjustments to training delivery, or the implementation of recovery interventions [ 1 ].
Assessing aerobic-oriented training effects has traditionally been made via distinct maximal-effort exhaustive tests. For example, improvements denoted in maximal intermittent field tests (e.g., 30–15 Intermittent Fitness Test) can infer improved aerobic capacity (amongst other systems), whereas decreased values may be interpreted as a negative response or de-training [ 5 , 6 ]. However, given the nature of the in-season phase common to professional teams, which frequently experiences fixture congested periods, it is considered less feasible to expose athletes to serial exhaustive assessments [ 7 , 8 ]. With regard to neuromuscular function, a variety of test protocols are administered to quantify chronic training effects on athletic qualities such as strength and power, but more frequently as indicators of acute and transient responses (e.g., post-match recovery kinetics) [ 7 , 9 ]. Similarly, the practicability of such assessments in the team-sports environment is challenged by different factors such as the number of athletes, the time available for discreet testing protocols, and the numerous contextual and individual elements that may undermine inferences derived from the data (e.g., motivation, physical qualities, season stage) [ 1 , 7 , 8 ].
Submaximal fitness tests (SMFT) have been proposed to deliver a feasible alternative to evaluate an athlete’s physiological state, presumably because of their time-efficient nature, low physical/physiological burden, and relative ease of administration [ 10 ]. In essence, SMFT provide a pragmatic and systematic approach of observing response(s) to a standardized physical stimulus [ 11 , 12 ]. Such assessments have been investigated since the late 1940s [ 13 ] and were mainly adopted among clinicians diagnosing health conditions or physical fitness in non-athletic populations, whereby exposure to maximal or exhaustive activities was thought to be ill-advisable because of the health risk it posed to patients [ 13 , 14 ]. Over the years, a number of walking [ 15 , 16 , 17 , 18 , 19 ], cycling [ 14 , 20 , 21 , 22 ], and running [ 23 , 24 , 25 ] SMFT have been administered among clinical and healthy populations. These tests involve single or multiple continuous steady-state protocols, with some prescribing an absolute standardized intensity, while others include relative intensity ranges, or self-paced protocols (refer to File 1 in the Electronic Supplementary Material [ESM]).
1.1 Elite Sports
The implementation of SMFT in elite sports has been traditionally used for quantifying relevant physiological transitions between exercise intensity domains (e.g., anaerobic threshold [i.e., the threshold indicates an equal rate of lactate production and disposal] and the onset of blood lactate accumulation [4-mmol·L −1 lactate threshold]) [ 26 , 27 ], often administered to inform training prescription or determine exercise economy. However, these tests generally necessitate a laboratory environment, are resource intensive and obtrusive, and therefore considered less feasible in the day-to-day field context, particularly with large cohorts of athletes [ 7 ].
Individual endurance sport practitioners were the first to develop and implement SMFT as part of their training monitoring processes [ 28 , 29 ]. Throughout the years, a broad range of cycling and running SMFT have been adopted across a variety of endurance sport athletes such as cyclists [ 28 , 29 , 30 , 31 , 32 ], runners [ 33 , 34 , 35 ], and triathletes [ 29 , 36 , 37 ]. Extensively used SMFT in endurance sports include exercise tasks prescribed by fixed internal intensities (% of an individual heart rate [HR] maximum) [ 32 , 34 ], while the outcome measures are considered both external (e.g., speed [ 34 ], cadence, power [ 32 ] collected throughout the test) and internal responses (HR recovery [HRR] [ 38 , 39 ], ratings of perceived exertion [RPE] [ 32 ] collected immediately post-exercise). Alternatively, researchers have adopted tests using standardized external intensities (usually via absolute running speed values) [ 33 , 37 ]. Initially, the primary purpose of these SMFT was to predict performance (e.g., time trial) or physiological capacities (i.e., maximal oxygen uptake) [ 32 ]; however, more recently, they have been used to identify impaired performance (e.g., functional overreaching) [ 40 , 41 ].
Because of the simplicity of implementing SMFT, their non-exhaustive nature, and their potential to provide information regarding both positive and negative training effects, SMFT have become common in team sports. Indeed, the adoption of SMFT in team-sports research [ 8 , 10 , 42 ] and practice [ 43 , 44 ] has increased substantially over the last decade. However, given the broad range of SMFT adopted, including various protocols (continuous vs intermittent) [ 45 , 46 ], activity modes (running vs cycling) [ 47 , 48 ], intensities (fixed vs incremental, absolute vs individualized) [ 12 , 48 ], outcome measures (cardiorespiratory/metabolic, subjective, or mechanical) [ 12 , 49 , 50 ], and purposes (monitoring positive vs negative effects) [ 47 , 51 ], a synthesis appears warranted. Accordingly, the aims of this scoping review were to (1) develop an operational definition of SMFT and protocol taxonomy, (2) identify previously used SMFT in the team-sports research and discuss their conceptual and methodical aspects, (3) provide an audit of outcome measures, collection methods, and analytical processes, as well as evaluate the theoretical rationale underpinning their inclusion, (4) provide a narrative synthesis of the available research on SMFT as indicators of training effects in team sports, and (5) conclude with practical recommendations and future directions.
Systematic searches of the electronic databases MEDLINE, Scopus, and Web of Science were used to identify relevant studies. From 2170 records identified in the original searches, we accepted 87 team-sport studies meeting our inclusion criteria. A detailed description of the searching strategy, screening process, and the inclusion–exclusion criteria are provided in File 2 in the ESM.
3.1 Submaximal Fitness Test Definition
A table presenting the characteristics of the included studies is provided in File 3 in the ESM. Based on the available literature, we defined SMFT as a short exercise bout, undertaken at a standardized intensity that is intended to be non-exhausting, and performed with the purpose of inferring an athlete’s physiological state through the monitoring of relevant outcome measures. In this regard:
Exercise is typically a cyclic activity involving large muscle groups. In team-sports settings, this is often administered as running activities, however, cycling has also been featured.
Standardized intensity refers to a threshold(s) that is standardized based on an internal response or external intensity parameter, and can be either fixed for all athletes (i.e., absolute) or individualized to a capacity anchor (relative; e.g., fraction of HR maximum or maximal aerobic speed).
Non-exhausting generally excludes frequent or prolonged ‘all-out’ maximal intensities, intensities that would cause voluntary cessation, or intensities that elicit an excessive training stimulus beyond that originally intended. From a practical standpoint, in team sports, the test should not have negative carry-over effects for the subsequent training session (for instance, if administered during the warm-up), or elsewhere it is implemented as an integrated standardized training component within the session plan (see for example, Sect. 3.2 ; intermittent-variable category).
Physiological state can be defined as a particular condition or function of an individual’s physiological system, or a combination of systems—primarily, cardiovascular, respiratory, nervous, and muscular—at a specific point in time. In the context of SMFT, it may be used to infer an athlete’s current (physical) performance capacity or training effects (i.e., training responses).
Training effects [ 4 ] indicate the direction (i.e., positive, negative) and the time course of the effect. Considering the challenges of dichotomizing time course criteria, we opted to use commonly referenced durations [ 4 , 42 ] that align with the context and design of the included studies in our review, classified as acute (i.e., immediate [ 49 ] and up to a 1-week duration [ 47 , 52 ]), short term (typically 1–3 weeks; e.g., congested or intensified periods [ 53 , 54 ], training camps [ 54 , 55 ], exposure to extreme environments [ 56 , 57 ], season break [ 58 ]), and chronic (usually established over several weeks or months of training; e.g., pre-season [ 51 , 59 ], training intervention [ 60 , 61 ]). The first two are commonly referred as transient effects, while the latter typically indicates more ‘persistent’ or ‘enduring’ changes [ 4 ].
Outcome measures include cardiorespiratory/metabolic, subjective, mechanical, or a combination, and are used as proxy (surrogate) measures that reflect (either directly or indirectly) the physiological systems they intend to assess. These are collected continuously within exercise and then aggregated into a summary metric (e.g., mean HR, accumulated ground impacts estimated via accelerometery-derived data), or measured immediately post-exercise (e.g., HRR, blood lactate, RPE).
3.2 Protocol Taxonomy
Information on SMFT protocols was extracted and categorized in reference to two main levels of classification: (1) exercise regimen (continuous or intermittent) and (2) manipulation of exercise intensity (fixed, incremental, or variable). Regarding exercise regimen characteristics, continuous activity represents a constant load exercise bout (typically for at least several minutes), without frequent alterations in velocity or rest periods [ 14 , 62 ]. Alternatively, intermittent is defined as an activity that is interrupted and restarts after a particular time span, characterized by alternated loads and rest intervals [ 63 , 64 ]. Considering all possible combinations of these categories, we subsequently identified five distinct SMFT categories from the available literature (Fig. 1 ), with each category further sub-divided based on the activity mode (running or cycling), movement pattern (linear, change of direction, or multi-directional), and exercise environment (closed, semi-open, or open):
Continuous-fixed category represents a fixed-intensity exercise bout that remains constant for the entire SMFT and intends to elicit a steady state (e.g., 4 min running at 12 km·h −1 ) [ 65 , 66 ].
Continuous-incremental category is characterized by a progressive increase in intensity within (single) or between (multiple) exercise bout(s), whereas each bout lasts for several minutes (e.g., 4 min running with progressive increases in speed, 3 sets × 3-min bouts at 10, 11, and 12 km·h −1 , interceded by 1-min rest periods) [ 11 , 67 ].
Intermittent-fixed category involves reoccurring activities performed at a constant intensity and rest intervals (e.g., four running bouts × 50–60 m at 18–22.5 km·h −1 , separated by 30 s of recovery) [ 49 , 68 ].
Intermittent-incremental category predominantly involves fixed rest periods, while intensity is increased between exercise bouts (e.g., 30-s shuttle runs at 10–14 km·h −1 , alternated by a 15-s rest period and with a-0.5 km·h −1 increment after each bout) [ 59 , 69 ].
Intermittent-variable category represents specific and non-specific standardized drills, and therefore locomotive demands fluctuate during the exercise (i.e., multi-directional movements). This category can be further categorized into drill-based and game-based exercises. Drill-based exercises refer to exercises that do not include competition features (e.g., passing drills) [ 70 ], whereas game-based exercises are characterized with competition features (small-sided games [SSG]) [ 71 , 72 ].
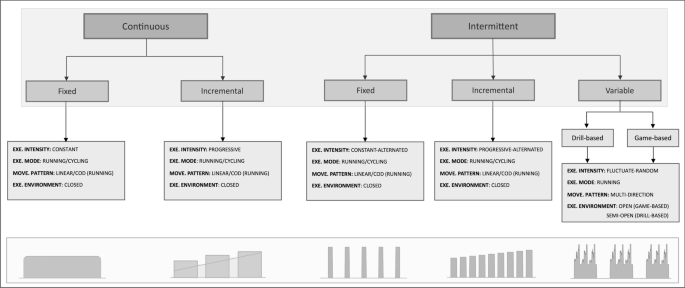
Submaximal fitness tests (SMFT) protocol taxonomy. Each protocol category consists of two levels: (1) exercise (EXE) intensity intermittency (continuous or intermittent) and (2) manipulation of exercise intensity (fixed, incremental, or variable), together yielding five distinct SMFT protocol categories ( shaded areas ). Intermittent-variable can be further categorized into drill-based and game-based formats. Each category can be further manipulated based on the movement (MOVE) pattern (linear, change of direction [CoD], and multi-direction), activity mode (running or cycling), and exercise environment (closed, semi-open, or open)
3.2.1 Application of the Taxonomy to the SMFT Team-Sports Literature
From the 87 included team-sport studies, we identified 100 independently described SMFT. As illustrated in Fig. 2 , the majority of studies in the literature adopted continuous-fixed SMFT (37%), followed by intermittent-incremental (34%), intermittent-variable (15%), continuous-incremental (8%), and intermittent-fixed (6%). Table 1 provides a summary of these SMFT as described in these studies. Continuous-fixed protocols were administered in both running and cycling exercise modes and include linear and change of direction (running protocols) movement patterns performed at absolute or relative standardized intensities. Continuous-incremental protocols comprised incremental exercises that were terminated when a specific internal (e.g., HR) or external (e.g., speed) intensity was achieved. Intermittent-fixed SMFT solely involved short-duration (8–12 s), high-intensity standardized bursts (~ 50–60 m). Intermittent-incremental SMFT incorporated shorter versions of the most common intermittent shuttle fitness tests, such as the Yo-Yo Intermittent Recovery Tests (Yo-YoIR1&2) [ 46 ] and 30–15 Intermittent Fitness Test [ 5 ]. Finally, intermittent-variable SMFT were mostly administered as game-based practices, including non-specific (handball, touchdown games) and specific (SSG of the sport) exercises, while drill-based practices included a variety of passing exercises (Table 1 ).
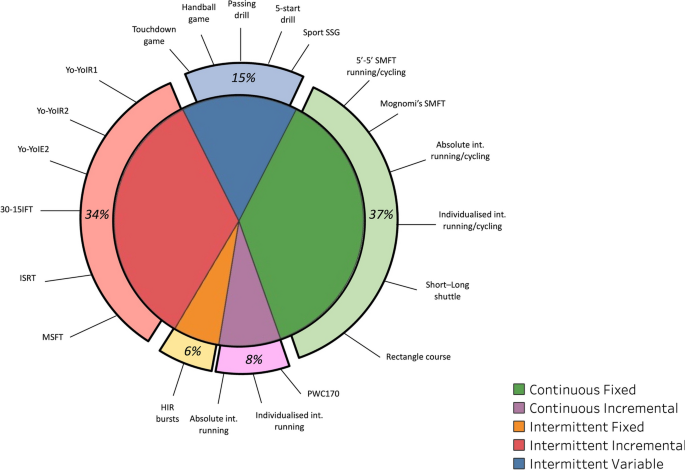
Frequency of submaximal fitness test (SMFT) categories and their highlighted individual tests as identified in the team-sports literature. A detailed description of these tests is highlighted in Table 1 . 30-15IFT 30–15 Intermittent Fitness Test, HIR high-intensity runs, Int. intensity, ISRT interval shuttle run test, MSFT multi-stage fitness test, PCW physical capacity work, Yo-YoIE2 yo-yo intermittent endurance level 2, Yo-YoIR1 yo-yo intermittent recovery test level 1, Yo-YoIR2 yo-yo intermittent recovery test level 2
4 Outcome Measures
We identified 202 total outcome measures used in previous team-sports research. As shown in Fig. 3 , cardiorespiratory/metabolic were the most used outcome measures (66%), followed by mechanical (28%) and subjective (6%). The following sections present the outcome measures corresponding to each response type, discuss their putative underlying mechanisms, and synthesize the current available evidence examining their changes within the SMFT framework.
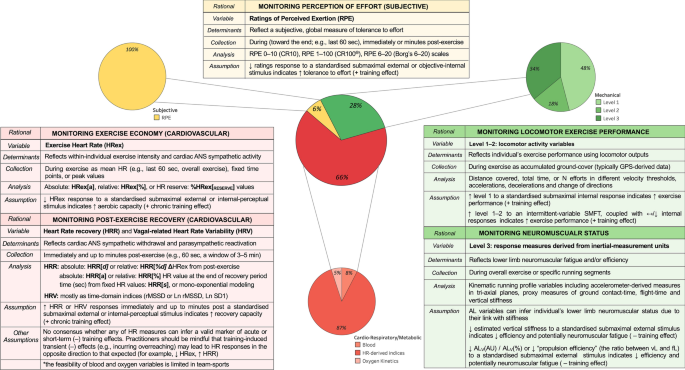
Frequency of submaximal fitness test (SMFT) outcome measures as identified in the team-sports literature. Heart rate (HR)-derived indices are the most common cardiorespiratory/metabolic outcome measures and include variables representing exercise intensity (HRex) and recovery (HRR and HRV). Level 1–2 mechanical outcome measures represent locomotor activity variables collected during SMFT that are standardized by an internal stimulus or intermittent-variable exercise such as small-sided games (SSG). Level 3 mechanical outcome measures are response measures derived from inertial measurement units (micro-electrical mechanical systems [MEMS]) for monitoring neuromuscular status. Subjective outcome measures represent tolerance to effort and have been monitored solely via ratings of perceived exertion (RPE). AL accelerometry-load, AL V (AU) the vertical vector magnitude component of tri-axial AL, AL V (%) percentage contribution of the vertical vector magnitude component to tri-axial AL, ANS autonomic nervous system, CT contact time, Force-load (fL) sum of estimated ground-reaction forces during all foot impacts, GPS global positioning system, Ln rMSSD natural log of rMSSD, Ln SD1 log-transformed standard deviation of successive R spikes measured from Poincaré plots, rMSSD root mean square of the sum of all differences between successive normal heartbeats, Velocity-load (vL) sum of distance covered weighted by the speed of displacement (in SMFT refers to the actual mean velocity), + positive, − negative
4.1 Cardiorespiratory/Metabolic Outcome Measures
In team-sports settings, which can include a large number of individuals who may possess different aerobic capacities [ 8 , 12 ], it is difficult to implement SMFT that are standardized by internal intensity variables. Accordingly, the majority of the tests were applied by standardizing the external intensity and measuring the corresponding internal responses [ 10 , 73 ]. A variety of cardiorespiratory/metabolic outcome measures have been used in the literature, with the most common being HR-derived indices (Fig. 3 ). These include variables collected during (exercise HR [HRex]), immediately after (HRR) the SMFT, and soon after (HR variability [HRV, vagal-related HRV]) the SMFT. Other measures include blood markers (e.g., blood lactate) [ 67 , 74 , 75 , 76 , 77 , 78 , 79 , 80 ] and oxygen consumption-related parameters (e.g., oxygen uptake) [ 75 , 76 , 81 , 82 ]. As blood and oxygen uptake outcome measures are time consuming, expensive, and obtrusive, their viability to provide standardized and repeatable response measures is considered limited, particularly in team sports [ 11 , 83 ]. Accordingly, we focused on HR-derived indices.
4.1.1 Exercise HR
Exercise HR is collected during the SMFT and is analyzed in absolute (beats per minute; HRex[a]) [ 47 , 59 , 66 , 69 , 74 , 75 , 76 , 81 , 82 , 84 , 85 , 86 , 87 , 88 , 89 , 90 , 91 , 92 , 93 , 94 , 95 , 96 , 97 , 98 , 99 , 100 , 101 , 102 , 103 , 104 ], relative to maximal HR (HRex[%]) [ 6 , 11 , 12 , 45 , 46 , 48 , 51 , 54 , 56 , 57 , 59 , 60 , 61 , 66 , 67 , 70 , 79 , 83 , 84 , 89 , 91 , 105 , 106 , 107 , 108 , 109 , 110 , 111 , 112 , 113 , 114 , 115 , 116 , 117 , 118 , 119 , 120 ], or HR reserve (HRex[reserve]) [ 53 ] values. A variety of methods are used to derive HRex, with the majority calculating the mean HR during the last 10–60 s of the test [ 11 , 12 , 45 , 47 , 48 , 51 , 53 , 54 , 55 , 56 , 57 , 58 , 59 , 60 , 61 , 66 , 69 , 71 , 74 , 75 , 82 , 83 , 85 , 86 , 87 , 89 , 92 , 93 , 96 , 97 , 103 , 105 , 106 , 107 , 108 , 111 , 113 , 114 , 115 , 117 , 119 , 120 , 121 ]. Other approaches calculate the mean HR during the overall test (particularly during intermittent-variable protocols such as SSG) [ 70 , 71 , 79 , 94 , 98 , 101 , 104 , 116 ], specific fixed timepoints [ 6 , 46 , 59 , 60 , 84 , 91 , 95 , 99 , 105 , 109 , 110 , 112 , 122 ], or peak values observed [ 61 , 90 ].
4.1.2 HR Recovery
Heart rate recovery can be defined as the rate at which HR declines after exercise cessation [ 10 , 39 ] and may be collected with athletes lying supine [ 123 ], sitting upright [ 12 , 45 ], standing [ 89 , 121 ], or walking [ 95 ]. Similarly, HRR can be assessed as the absolute (HRR[ d ]) [ 45 , 47 , 48 , 53 , 54 , 57 , 59 , 61 , 66 , 88 , 100 , 103 , 107 , 114 , 119 , 121 , 123 , 124 ] or relative (HRR[% d ]) [ 12 , 47 , 53 , 60 , 66 , 102 , 104 , 107 , 113 , 114 , 121 ] difference between HRex and HR value observed between 10 and 180 s post-test. Alternative approaches include the actual HR value observed at the end of the designated recovery period in absolute (HRR[a]) [ 66 , 74 , 89 , 95 , 99 , 121 , 125 ] or relative (HRR[%]) [ 66 , 89 , 109 , 110 , 113 ] values, or the overall mean HR during a variety of fixed time intervals [ 90 , 97 , 107 ]. Other approaches calculate time-based variables such as the time required to decrease from between fixed HR values (HRR[ s ]; e.g., time between 80 and 70% HR maximum) [ 79 ], or the time constant of HRR derived from mono-exponential modeling [ 61 , 126 ].
4.1.3 HR Variability
Vagal-related HRV is defined as the variability in the time intervals (usually in milliseconds) between adjacent heartbeats and reflects the regulation of cardiac autonomic nervous system (ANS) balance [ 10 , 127 ]. Heart rate variability indices are commonly collected in a seated or supine position and resting state in a laboratory or quiet room [ 10 , 42 ]; however, as the aim in SMFT is to monitor the response during and after a given submaximal workload, only HRV parameters observed immediately or soon after the test are relevant for SMFT in team sports. These measures are usually analyzed within a window of 3–5 min post-test cessation, and predominantly calculated as time domain-related variables such as the square root of the mean of the sum of the squares of all differences between successive normal heartbeats (rMSSD) [ 61 , 107 ] or its natural log (Ln rMSSD) [ 45 , 47 , 53 , 59 , 100 , 106 , 114 ], natural log of standard deviation of successive ‘R spikes’ (the peak of the QRS complex, reflective of ventricular depolarization, recorded from an electrocardiogram wave) measured from Poincaré plots (Ln SD1) [ 57 , 106 ], and the standard deviation of mean interval differences between normal heartbeats (SDNN) [ 61 , 107 ].
4.1.4 Putative Mechanisms
Heart rate-derived indices are commonly used to inform chronic aerobic-oriented training effects, attributed to the linear relationship between HRex and oxygen uptake during an intended steady-state activity [ 10 , 42 ]. A reduction in HRex to a standardized submaximal stimulus may represent improved exercise economy, which may translate to the development of aerobic fitness [ 51 , 120 ], whereas an increment of HRex is considered to reflect a negative response (de-training of the cardiorespiratory system) [ 87 ], likely due to central adaptations (i.e., left-ventricular function) [ 128 ]. In addition, an increment of HRR or vagal-related HRV measures is considered a positive effect [ 59 , 60 , 107 ], reflecting the reactivation of the parasympathetic system and hemodynamic adjustments post-exercise [ 10 ]. That said, in addition to being more time consuming, these measures may be influenced by preceding exercise, with higher intensities eliciting increased blood acidosis that simulate the metaboreflex, and therefore may reduce HR decay post-exercise and alter HRR and vagal-related HRV results [ 10 , 53 ].
The use of HR-derived indices to infer negative transient training effects is inconclusive in the SMFT research. The theoretical basis for their inclusion is due to the potential influence of various training-induced physiological processes that originate in central (i.e., ‘central command’) and peripheral (e.g., afferent feedback from skeletal muscles) body regions to alter cardiac ANS function (i.e., the balance between the sympathetic and parasympathetic systems), and subsequently HR activity [ 10 , 38 , 42 ]. It has been hypothesized that training-induced fatigue or an incomplete recovery might result in a greater muscle activation at a given intensity [ 129 ], promoting increased oxygen demands [ 129 ] and yielding accelerated cardiac sympathetic activity that consequently increase HRex, and reduce HRR and HRV [ 114 , 129 ]. In contrast, previous research has proposed that increased training stress (leading at least to an overreaching state) may cause opposite responses — increased parasympathetic activity or blood plasma volume, consequently lowering HRex and increasing HRR and HRV [ 37 , 38 , 130 ].
4.1.5 Inferring Physiological State
Generally, across a standard micro-cycle, HR measures tend to stay relatively stable [ 45 , 47 ]. For example, studies among youth and senior athletes have observed no changes in the day-to-day variability of SMFT HRex [ 45 , 47 , 75 ] and HRR [ 45 , 47 ] derived from multiple SMFT administered throughout the week, despite substantial variations in training loads. Whilst vagal-related HRV indices are considered to provide a better insight into the cardiac ANS [ 10 , 53 ], daily variations in training loads were associated with stable [ 45 , 47 ] and lower [ 53 ] responses. The disparity across studies may be due to the differences in training loads and HRV variables [ 53 ], an athlete’s fitness levels [ 45 ], or simply reflective of measurement ‘noise’ (either measurement errors or biological variations) [ 10 , 61 , 107 ], thus challenging between-study comparisons.
The current research suggests that the use of HR-derived indices appears more relevant after acute (3–7 days) and short-term (~ 2 weeks) altered training stress, albeit it may cause misleading interpretations. In team sports, 3–4 consecutive days of accumulated training loads have been associated with both increased [ 114 ] and decreased [ 54 ] SMFT HRex. Lower SMFT HRex values have been also observed after 2 weeks of intensified training [ 84 ], but also following a substantial decline in training loads due to season breaks [ 58 ]. Whilst the information in team-sports settings is limited, studies among various cohorts of individual endurance athletes (e.g., cyclists, triathletes) provide encouraging evidence that short-term intensified periods (1–3 weeks) of training-induced fatigue (incurring functional overreaching) lowered SMFT HRex [ 36 , 38 , 130 ] and increased SMFT HRR [ 36 , 37 , 39 ]. These responses likely reflect a complex interplay between acute cardiac ANS function (usually referred to as larger parasympathetic activity) and increased plasma volume [ 37 , 54 , 104 ], leading to an enhanced stroke volume for a similar cardiac output [ 10 , 131 ]. In summary, the use of HR-derived indices (especially HRex and HRR) to infer transient training effects associated with cardiac ANS dysfunction is currently questionable (at least, not straightforward), with no consensus around the underlying mechanisms and conflicting results in the literature.
Overall, SMFT HRex has small to very large inverse relationships with performance indicators (i.e., maximal oxygen uptake, intermittent endurance capacity) when measured concurrently (lower HRex is associated with higher test results) [ 46 , 48 , 105 ], suggesting its validity as an indicator of an individual’s current endurance capacity. Indeed, it has been highlighted that a chronic exposure to internal (e.g., HR-based training impulse, session RPE) [ 86 , 90 ] and external (e.g., total distance covered, force load) [ 71 , 120 ] loads is associated with reduced SMFT HRex. However, the studies examining training effects within athletes have reported contrasting findings. For example, studies in soccer players [ 46 , 91 ] have observed moderate to very large relationships between SMFT HRex and intermittent endurance performance at different timepoints across the season, albeit with no interaction between the magnitude of these effects. Likewise, improved [ 91 ] or maintained [ 87 ] intermittent running ability did not necessarily coincide with reduced or stable SMFT HRex, respectively. In contrast, studies conducted on a variety of team sports and age groups have observed significant relationships between changes in similar markers from pre-season to in-season [ 51 , 59 ], and across a full season [ 107 ]. Improved SMFT HRex were also largely correlated with the changes in running speed at 4 mmol·L −1 blood lactate [ 11 , 83 ] or a reduced oxygen uptake at fixed submaximal intensities (i.e., exercise economy) [ 81 ].
Research observations are more consistent where SMFT HRex has been administered to evaluate the adaptation time course to changes in extreme environments (heat and altitude) during training camps or competitions [ 55 , 56 , 57 , 93 , 104 , 106 , 132 ]. Collectively, HRex displayed substantial deteriorations upon arrival and up to days of exposure [ 56 , 57 , 93 , 104 ], and generally return to baseline values within 6–10 [ 55 , 106 ] or 14 days [ 56 ], with quicker adaptations among highly trained individuals or across repeated exposures within individuals [ 133 , 134 ]. Taken together, whilst it appears that SMFT HRex has the potential to serve as a valid and sensitive marker of positive training-induced effects, it remains questionable whether it can be used as a surrogate measure of within-athlete changes in maximal aerobic capacity. There is, however, considerable evidence suggesting its use during exposure to changes in environments for monitoring the athlete’s acclimatization.
The magnitude of correlations between cardiac parasympathetic-related variables (HRR and vagal-related HRV) and performance indicators is less clear and ranged from no correlation to a very large relationship [ 48 , 66 , 100 ]. The disparity could be the consequence of varied protocol intensities [ 10 , 35 , 42 , 45 ], collection time (e.g., 60 s and 180 s post-exercise) [ 48 ], analysis approaches [ 66 ], and fitness criterion measures [ 45 , 79 ]. Importantly, inferences regarding their long-term validity as proxy measures of chronic training effects can be somewhat impacted by SMFT intensity (i.e., HRex) [ 10 ]. The rate of the sympathetic withdrawal and parasympathetic reactivation post-exercise is altered when the recovery period starts from different intensities. As an example, different absolute HRR values (HRR[ d ]) may be expected 60 s post-SMFT if the exercise intensity varies (e.g., 90% vs 75% HR maximum). Hence, in theory, a significant reduction in HRex might influence the concurrent interpretation of post-exercise outcomes. Although this issue has been addressed by analyzing HRR in relative values (HRR[% d ]) [ 60 , 114 ], the time necessary to decrease from two fixed HR values (HRR[ s ]) [ 79 ], or employing individualized intensity protocols [ 12 ], these are not yet fully understood. In support of this, regardless of the HRR analysis used, authors examining training-induced changes in both HRex and HRR did not always find congruent trends [ 60 , 61 , 102 ]. Other studies observed a lack of association between changes in HRR and endurance performance, despite significant relationships with HRex [ 59 , 107 ]. However, increased SMFT vagal-related HRV has been shown to be more appropriate, with studies reporting its validity for monitoring endurance-oriented training effects [ 59 , 61 , 107 ].
4.1.6 Considerations
Of critical importance when using HR-derived indices as proxies of training effects is the range of confounding factors, such as environmental (e.g., temperature), habitual (e.g., sleep, diet), circadian (time during the day), and psychological (e.g., emotions, stress). These could all contribute to the error of measurement of SMFT HR measures — HRex (coefficient of variation (CV): 1.0–3.5%) [ 12 , 45 , 48 ], HRR (CV: 2.8–13.8%) [ 45 , 48 , 66 ], and vagal-related HRV (CV: 6.6–19.0%) [ 45 , 61 , 106 ] — and should be considered (or standardized where pragmatic) when interpreting changes in HR-derived indices responses to SMFT [ 10 , 42 ].
Further research is warranted to explore the use of all HR-derived measures to infer acute and short-term effects, in particular, verifying the interaction between temporary changes in cardiac ANS function and plasma volume responses. In this respect, it should be highlighted that HR responses may still be less appropriate to denote peripheral neuromuscular fatigue, which are considered more important to monitor delayed recovery and injury risk mitigation in team sports [ 49 , 135 ]. In the longer term, HRex is probably the easiest to collect and most reliable HR measure, and its utility in observing positive changes in aerobic capacity has stronger empirical support. Accordingly, the utility of adding post-exercise (HRR, vagal-related HRV) SMFT HR responses to infer chronic effects may be redundant. In order to enhance interpretations, future research should first determine meaningful changes in SMFT HRex (i.e., smallest worthwhile change) in reference to variations in physiological states.
Finally, when monitoring the responses to intermittent-variable SMFT (e.g., SSG), an athlete’s HRex may be influenced by their locomotor activity. These are likely to differ between tests and should be accounted for when interpreting data. Therefore, we recommend quantifying intermittent-variable SMFT locomotor activity for consideration. Given the reasonable association between internal and external measures during field-based sessions [ 136 ], it is also possible to standardize HRex to a given (fixed) external intensity parameter. Whilst some have attempted to achieve this by dividing the former by the latter, creating a ratio [ 137 ], there are complex statistical properties and assumptions associated with such indexes, presenting as a major validity concern [ 138 ]. To appropriately examine HRex while holding external intensity parameters constant, we recommend linear regression techniques, which do not violate statistical assumptions and achieve the desired outcome ratios [ 139 ].
4.2 Subjective Outcome Measures
Subjective measures are recognized by their ability to serve as gestalt measures that can be used across different exercise typologies, given their feasibility and low cost [ 140 ]. These are commonly applied to quantify an athlete’s perception of intensity and training effects. The former is derived solely from RPE, while the latter are commonly referred to as athlete-reported outcome measures [ 141 ] of latent response constructs such as readiness, wellness, and stress. [ 4 , 142 ]. Accordingly, RPE are the only subjective outcome measures that can be applied to SMFT, a notion supported by their exclusivity (albeit the limited number of studies) in the team-sport SMFT literature (Fig. 3 ). Among the available studies, different scales such as the Category-Ratio 10 (CR10 deciMax) [ 50 , 56 , 57 , 67 , 70 , 98 , 108 ], 100 (CR100® centiMax) [ 91 ], and 6–20 (Borg’s 6–20) [ 75 , 76 ] have been adopted, using a variety of collection protocols (during the last 180 s, immediately, and up to 5 minutes post-exercise).
4.2.1 Putative Mechanisms
Perception of effort is defined as the ‘conscious sensation of how hard or strenuous a physical task is’ [ 142 ], and mainly depends on how easy or hard it is to breathe and drive the working muscles during exercise [ 143 ]. As part of SMFT, the athlete provides a retrospective appraisal of perceived effort to a standardized stimulus that can be prescribed by either an objective internal [ 32 ] or external [ 91 ] means. Because RPE is strongly associated with cardiorespiratory, metabolic, and neuromuscular measures of exercise intensity [ 144 , 145 ], and influenced by the mental state [ 142 ], changes in RPE may reflect positive [ 32 , 91 ] or negative [ 37 , 41 ] alterations in the psycho-physiological state. However, given their gestalt nature, it is perhaps difficult to align RPE as a proxy to a single physiological system during SMFT. For example, RPE has been empirically associated with HRex during a continuous exercise [ 144 ] and might therefore be used as a cardiorespiratory proxy measure. However, spinal or supraspinal motoneuron inhibition, which is a neuromuscular phenomenon, can increase central motor command and subsequently RPE [ 146 ]. This is not to say that RPE cannot be used to infer a physiological state during SMFT, but rather the mechanisms may be less precise. In addition, given that RPE has also been used to regulate exercise intensity [ 147 ], RPE can be used as an anchoring intensity variable (i.e., running or cycling at fixed RPE), whereby external outcomes such as velocity or power output are used as response measures [ 148 ]. However, to our knowledge, there is no published team-sports research evaluating the theoretical basis of such SMFT and their actual utility.
4.2.2 Inferring Physiological State
Whilst some evidence supports the use of SMFT RPE [ 58 , 91 , 98 ], it is difficult to support or refute their utility to determine training effects, given the limited data available in team sports. In endurance athletes, RPE have been shown to detect negative transient effects associated with functional overreaching and disturbances in endurance performance [ 30 , 37 , 41 ], while their sensitivity to chronic positive effects is less certain [ 34 , 40 ]. In the team-sports context, one study [ 91 ] showed that reduced SMFT RPE (albeit maintained HRex[a]) was accompanied with enhanced intermittent running performance (Yo-YoIR1) and soccer match high-intensity running [ 91 ]. Another study [ 50 ] in professional soccer players did not observe any significant relationships between RPE collected immediately after an individualized SMFT and athlete-reported outcome measures across 6 in-season standard weeks. Despite the conflicting results, researchers have suggested the potential usefulness of SMFT RPE when measured concomitantly with other objective outcome measures (e.g., HRex) as part of a multivariate monitoring approach [ 37 , 42 , 130 ].
4.2.3 Considerations
It is noteworthy to highlight some of the challenges associated with collecting and interpreting RPE in team sports, where interpretation is challenged by the presence of the coach and peers biasing ratings, the application of unvalidated collection tools, lack of or inappropriate athlete familiarization/education, and their gestalt nature [ 142 , 146 , 149 ]. Moreover, RPE may be confounded by other sensations associated with exercise, such as mood, discomfort, pain, and enjoyment [ 142 ]. In view of these challenges, researchers may consider other perceptual measures such as ratings of fatigue [ 150 ], or techniques such as numerically blinded [ 149 ] and differential [ 151 ] RPE, alongside the implementation of rigorous familiarization processes to facilitate authentic and sensitive perceptual ratings associated with SMFT.
With this in mind, a benefit of SMFT in team sports is to provide an assessment that can be seamlessly integrated into the training session such as the warm-up or standardized drills. The need to collect RPE from all athletes individually (~ 20–40) under controlled conditions is likely to be disruptive and impractical, which is perhaps why there are few studies using this practice. It is probably reasonable to assume that unless SMFT are completed as a discreet activity, with smaller groups, or RPE collection procedures are made more accessible (e.g., mobile devices, human resources), these outcome measures may be less pragmatic or sustainable in team sports.
4.3 Mechanical Outcome Measures
Within the current monitoring schemes in sport, there is a broad classification of external load parameters. These indices represent kinematic outputs [ 152 ] performed by an athlete throughout an exercise bout/session [ 153 ] and have been classified into three distinct levels, which we will use here to audit their implementation in the context of SMFT [ 8 , 154 ].
4.3.1 Level 1–2 Measures
Level 1 variables are typically the locomotor performance outputs including distance covered, time spent, or the count of efforts in different velocity zones, whilst Level 2 variables reflect changes in velocity such as accelerations and decelerations (i.e., change of directions) [ 8 ]. Such kinematic parameters are routinely collected using global positioning systems (GPS) or other tracking technologies (i.e., semi-automated pixel tracking, local positioning systems). In the team-sports SMFT framework, the use of level 1–2 mechanical outcome measures can occur in two scenarios: (1) monitoring the speed achieved to a submaximal exercise that is standardized by internal intensity responses [ 50 , 155 ] and (2) monitoring the changes in these variables during intermittent-variable standardized drills [ 54 , 58 , 70 , 71 , 72 , 79 , 93 , 98 , 106 , 116 , 132 , 156 ], as they are standardized by a variety of other parameters such as duration, sets, recovery, and unique constraints (e.g., number of players, rules modifications). Whilst the former is considered less practical in team sports for many pragmatic reasons, the latter are implemented as a part of the training plan, encompassing sport-specific actions and are perhaps the most feasible to apply routinely [ 8 , 131 ]. Conceptually, higher values (e.g., accumulated distance covered), coupled with stable or lowered internal responses are indicative of positive effects (i.e., improved exercise performance) [ 8 , 58 , 98 ]. In fact, studies examining level 1–2 variables during drill (passing) and game (SSG) exercises have highlighted their pragmatic advantages to deliver information related to an athlete’s performance [ 58 , 71 , 72 , 98 ]. However, it should be noted that intermittent-variable SMFT are influenced by a variety of individual and contextual factors such as technical level, motivation, and tactics [ 8 , 72 ], and have a higher degree of variation (test–retest reliability) compared with other SMFT modalities [ 70 , 72 , 156 , 157 ], and therefore, should not necessarily be interpreted in the aforementioned simplistic manner [ 8 ].
4.3.2 Level 3 Measures
Level 3 external load variables are derived from inertial-measurement units such as tri-axial accelerometers, magnetometers, and gyroscopes [ 8 ] (collectively referred to as micro-electrical mechanical systems [MEMS] [ 158 , 159 ]). Unlike level 1–2 variables, these outcome measures can be used for the majority of SMFT applied in the team-sport context and have been proposed to provide an insight into an athlete’s neuromuscular system, given their potential link with lower limb vertical stiffness [ 135 , 154 , 160 , 161 , 162 , 163 ]. Vertical stiffness is considered to affect several athletic parameters, including elastic energy storage and utilization (i.e., stretch shortening cycle) [ 164 ] and has traditionally been measured through a variety of jump assessments (counter-movement, hopping, and drop jumps) using variables such as jump height, contact time, and flight time [ 52 , 165 , 166 ]. However, because of the limited viability of these assessments in field conditions and their lack of specificity (jumping activities may be less sensitive to detect changes in running strategies) [ 52 , 161 ], researchers and practitioners have started to collect proxy variables required to estimate vertical stiffness derived from MEMS during SMFT [ 49 , 52 , 55 , 65 , 68 , 72 , 167 , 168 , 169 ].
To date, studies have adopted accelerometer-derived vector magnitudes (collectively termed in this review as accelerometery load [AL]) [ 49 , 52 , 72 ] and individual vector components (vertical AL [AL V ], antero-posterior AL [AL AP ], and medio-lateral AL [AL ML ]) using MEMS-embedded accelerometers [ 49 , 52 , 68 , 167 , 168 ], predominantly collected during intermittent-fixed protocols comprising high-intensity running bursts [ 49 , 52 , 55 , 68 , 168 ]. Generally, reduced AL in the vertical plane (AL V [arbitrary units; AU]), or the percentage contribution of the AL V to the overall tri-axial vector magnitude (AL V [%]) during SMFT have been postulated as an indicator of reduced leg vertical stiffness and subsequently inferred a degree of lower limb neuromuscular fatigue [ 167 , 170 ]. Theoretically, reduced vertical stiffness may lead to reduced efficiency for the same speed through altered kinetic and kinematic parameters, such as reduced vertical ground-reaction forces and increased ground-contact time [ 161 , 170 ], which likely lead to decreased stride length [ 52 , 72 ] and elevated energy cost [ 162 , 170 ].
4.3.3 Inferring Physiological State
Studies investigating acute effects on mechanical outcome measures during SMFT are scarce, and those available are quite disparate in terms of protocols, variables, and their analytical processes [ 49 , 52 , 65 ]. Nonetheless, there is an emerging agreement from these studies that suggest AL measures can provide sensitive indicators of an athlete’s neuromuscular fatigue and efficiency. In a group of professional soccer players who performed an intermittent-fixed SMFT (4 × ~ 60-m runs, alternated by an ~ 30 s recovery) before and immediately after a training session, Buchheit et al. [ 49 ] found that various AL variables respond differently to different training modes (strength, speed, endurance-oriented conditioned sessions). Estimated vertical stiffness slightly increased after all training modes, whereas propulsion efficiency (the ratio between velocity loads and force load; refer to Fig. 3 for variables) was session dependent (largely increased after strength, small and moderate decreases after endurance and speed, respectively), suggesting its sensitivity to detect changes in running strategies (hypothetically, horizontal force application capability) [ 8 , 49 ]. A study [ 52 ] using a similar SMFT protocol and outcome measures among university rugby union players reported large relationships between the vector magnitude and vertical accelerometer components derived from the constant phase of the run, versus leg stiffness measured more directly via submaximal hopping test performed on a force platform [ 52 ]. Whilst only trivial effects were observed in leg stiffness over the week, the changes in SMFT AL data were large [ 52 ].
Similar trends were also found in a study investigating the alterations in triaxial AL data collected before, 48 h, and 96 h after an Australian football match [ 68 ]. A main finding reported in this latter study was that AL V (AU) and AL ML (AU) derived from the constant phase of the SMFT were still impaired 96 h post-match among players who were classified as ‘fatigued’ (> 8% reduced counter-movement jump at a 48 h post-match) [ 68 ]. Finally, a within-individual longitudinal study among soccer players [ 72 ] showed reductions in AL m·min −1 and AL slow·min −1 collected during a standardized SSG (5v5 + 5) 1 day before a match were concomitant with a reduction in neuromuscular function (flight-time:contact-time ratio measured from counter-movement jump) and an altered match running profile—increased AL ML (%) and decreased AL V (%) contribution to AL—indicative of potential neuromuscular fatigue [ 72 , 167 ]. Collectively, it appears that specific mechanical level 3 metrics may be useful for identifying acute variations in performance (neuromuscular fatigue and efficiency) associated with changes in lower limb function.
Inferring longer term training effects from mechanical variables ascertained during SMFT has received limited attention, and insights have been drawn exclusively from tracking locomotor outputs during intermittent-variable SMFT in the form of SSG. For example, moderate to very large positive relationships have been reported between higher level 1–2 outputs during SSG and intermittent running capacity [ 98 ]. In addition, during intensified training camps (1–2 weeks), within-individual increases in running parameters (e.g., total and high-speed running distance) measured during intermittent-variable SMFT were also concordant with improved intermittent running capacity [ 54 , 106 , 132 ]. Of note, the utility of all mechanical outcome measures (levels 1, 2, and 3) derived during SMFT in detecting chronic training effects in neuromuscular function such as improved running efficiency (enhanced muscle–tendon unit recoil) is unknown.
4.3.4 Considerations
Most studies adopting mechanical outcome measures to denote acute neuromuscular effects have administered SMFT characterized by intermittent high-intensity bursts. These outcome measures are often sampled from the constant running velocity phases of the SMFT and using the vertical accelerometer component [ 52 , 68 , 167 ], perhaps owing to an enhanced association with vertical stiffness [ 52 , 68 ]. Typically, such techniques have demonstrated an inferior degree of reliability (CV: 6.7–17.5%, standardized TE: small to moderate [ 49 , 52 , 68 ]) versus maximal and non-running-based assessments of neuromuscular function (jump and force indices; CV: 2.9–6.1%, standardized TE: small [ 49 , 65 , 171 ]). One study [ 65 ] using a continuous-fixed SMFT and lower running intensity (mean velocity of 12 km·h −1 compared to 18–22.5 km·h −1 ) reported lower measurement noise (CV: 2.1–8.0%, standardized TE rated as small). Additionally, the changes found in AL V (%) [decreased] and AL ML (AU) [increased] 24 h after a strenuous soccer training session were greater than smallest worthwhile change (signal-to-noise ratio > ± 1) [ 167 ]. Although these findings indicate enhanced reliability and sensitivity for SMFT involving lower running speeds in a more continuous manner, this is based on only one study and the utility of different SMFT protocols has not yet been compared in the available literature, and barriers to implementation should also be considered. Similarly, the questionable reliability of locomotor outputs recorded during intermittent-variable SMFT in the form of SSG (total distance CV: 2.3–11.7%, high-speed thresholds CV: 8.1–83.0% [ 70 , 72 , 94 , 98 , 156 , 157 ], small to moderate in magnitude [ 70 , 156 , 157 ], perhaps limits their utility to denote moderate-to-large effects only (i.e., larger CV may decrease the signal-to-noise ratio).
Although studies have suggested that changes in AL variables may reflect effects on lower limb stiffness [ 162 , 172 , 173 ], few have directly assessed stiffness [ 52 , 174 ]. Moreover, these studies have typically used MEMS mounted between the scapulae, which may be influenced by upper-body kinematics during running or dampening of ground-contact vibrations [ 172 , 175 ]. Whilst unit placement may have limited the impact under standardized conditions, intermittent-variable SMFT may be more susceptible to positioning noise as changes in orientation of the MEMS devices are not considered in the quantification of accelerometer metrics. In addition to positioning, users should be cautious of other extraneous factors such as movement artifacts within the device harness, running surface (e.g., ground stiffness), and footwear [ 158 ].
Future work should address the overall convergent validity of MEMS-derived data to obtain an accurate estimation of running strategy characteristics such as vertical stiffness. It is also necessary to investigate the theoretical framework for the sensitivity of these measures and their potential mechanisms (i.e., with respect to human tissue and gait mechanics). Furthermore, more research is required to examine whether protocol characteristics (e.g., exercise regimen, running intensity) and unit placement (e.g., center of mass, foot-mounted MEMS unit) can enhance measurement properties, and therefore facilitate inferences regarding lower limb stiffness and ultimately neuromuscular fatigue or efficiency.
5 Summary and Conclusions
Our review provides an overview of the literature regarding SMFT in team sports, including the development of the SMFT definition, protocol categorization, and a systematic audit of protocols and outcome measures. We also provide a narrative synthesis of the applications of SMFT within the training continuum of sport teams and future research directions (outlined in Table 2 ). In summary, SMFT have the potential to serve as time-efficient, non-exhaustive, and feasible standardized tests that can be administered to a group of athletes simultaneously as a part of the warm-up and using specific drill(s) during the training session. Multivariate outcome measures such cardiorespiratory/metabolic (e.g., HR-derived indices), subjective (e.g., RPE), and mechanical (GPS and MEMS-derived data) can be collected simultaneously, and in theory, provide a multifactorial evaluation for athlete monitoring in team sports. Collectively, the literature suggests that several outcome measures collected during and immediately post-SMFT can inform on an athlete’s physiological state. Heart rate-derived indices seem more appropriate to denote positive chronic training effects on endurance performance, whereas their role in detecting negative transient effects associated with variations in ANS function is questionable. Despite the lack of knowledge about the underlying mechanisms and the inconsistent findings between studies, their sensitivity appears to improve after days or over short periods that are characterized by substantial alterations in training stress, seemingly caused by an interaction between cardiac ANS status and plasma volume responses. Subjective outcome measures are less common in team-based sports and only global RPE have been adopted thus far. Although their validity and practicability have yet to be established, researchers have proposed their utility when measured concomitantly with other objective measures (e.g., HRex) as part of a multivariate monitoring system. Mechanical outcome measures are relatively novel and have been mostly investigated using intermittent-variable and intermittent-fixed protocols, whereby the former primarily involves GPS-derived kinematic variables (levels 1–2) to monitor exercise performance, while the latter includes response measures derived from inertial measurement units (level 3) to monitor lower limb neuromuscular function. Whilst monitoring locomotor outputs during standardized training drills is more feasible and has shown to provide valuable data on an athlete’s performance, practitioners should consider the large influence of various individual and contextual factors (e.g., technical/tactical level, motivation) that may undermine their interpretations. Accelerometery load parameters can provide sensitive indicators of acute changes in lower limb function and therefore neuromuscular fatigue and efficiency, albeit the overall validity of these outcome measures and the physiological mechanisms underpinning their changes have not yet been fully evaluated. Moreover, there is an absence of information on the use of all mechanical metrics (levels 1–3) to monitor chronic training effects. Finally, future research should also examine the methodical elements (e.g., protocol characteristics, collection, and analytical processes) related to SMFT to derive the most appropriate protocol to capture reliable, valid, and sensitive outcome measures that provide useful inferences regarding an athlete’s physiological state.
Impellizzeri FM, Menaspà P, Coutts AJ, et al. Training load and its role in injury prevention, part I: back to the future. J Athl Train. 2020;55:885–92. https://doi.org/10.4085/1062-6050-500-19 .
Article PubMed PubMed Central Google Scholar
Kalkhoven JT, Watsford ML, Impellizzeri FM. A conceptual model and detailed framework for stress-related, strain-related, and overuse athletic injury. J Sci Med Sport. 2020;23:726–34. https://doi.org/10.1016/j.jsams.2020.02.002 .
Article PubMed Google Scholar
Vanrenterghem J, Nedergaard NJ, Robinson MA, et al. Training load monitoring in team sports: a novel framework separating physiological and biomechanical load-adaptation pathways. Sports Med. 2017;47:2135–42. https://doi.org/10.1007/s40279-017-0714-2 .
Jeffries AC, Marcora SM, Coutts AJ, et al. Development of a revised conceptual framework of physical training for use in research and practice. Sports Med. 2022;52(4):709–24. https://doi.org/10.1007/s40279-021-01551-5 .
Buchheit M, Rabbani A. The 30–15 intermittent fitness test versus the Yo-Yo intermittent recovery test level 1: relationship and sensitivity to training. Int J Sports Physiol Perform. 2014;9:522–4. https://doi.org/10.1123/ijspp.2012-0335 .
Bradley PS, Bendiksen M, Dellal A, et al. The application of the Yo-Yo Intermittent Endurance Level 2 Test to elite female soccer populations: Yo-Yo IE2 testing in female soccer players. Scand J Med Sci Sports. 2014;24:43–54. https://doi.org/10.1111/j.1600-0838.2012.01483.x .
Article CAS PubMed Google Scholar
Carling C, Lacome M, McCall A, et al. Monitoring of post-match fatigue in professional soccer: welcome to the real world. Sports Med. 2018;48:2695–702. https://doi.org/10.1007/s40279-018-0935-z .
Lacome M, Simpson BM, Buchheit M. Monitoring training status with player-tracking technology: still on the road to Rome. Aspetar Sports Med J. 2018;7:54–63.
Google Scholar
Norris D, Joyce D, Siegler J, et al. Considerations in interpreting neuromuscular state in elite level Australian Rules football players. J Sci Med Sport. 2021;24:702–8. https://doi.org/10.1016/j.jsams.2021.02.007 .
Buchheit M. Monitoring training status with HR measures: do all roads lead to Rome? Front Physiol. 2014;5:73. https://doi.org/10.3389/fphys.2014.00073 .
Buchheit M, Simpson BM, Lacome M. Monitoring cardiorespiratory fitness in professional soccer players: is it worth the prick? Int J Sports Physiol Perform. 2020;15:1437–41. https://doi.org/10.1123/ijspp.2019-0911 .
Scott TJ, McLaren SJ, Caia J, et al. The reliability and usefulness of an individualised submaximal shuttle run test in elite rugby league players. Sci Med Football. 2018;2:184–90. https://doi.org/10.1080/24733938.2018.1448937 .
Article Google Scholar
Bruce RA, Pearson R. Variability of respiratory and circulatory performance during standardized exercise. J Clin Invest. 1949;28:1431–8. https://doi.org/10.1172/jci102208 .
Article CAS PubMed PubMed Central Google Scholar
Åstrand P-O, Ryhming I. A nomogram for calculation of aerobic capacity (physical fitness) from pulse rate during submaximal work. J Appl Physiol. 1954;7:218–21. https://doi.org/10.1152/jappl.1954.7.2.218 .
Bassey EJ, Fentem PH, MacDonald IC, et al. Self-paced walking as a method for exercise testing in elderly and young men. Clin Sci Mol Med. 1976;51:609–12.
CAS PubMed Google Scholar
Guyatt GH, Sullivan MJ, Thompson PJ, et al. The 6-minute walk: a new measure of exercise capacity in patients with chronic heart failure. Can Med Assoc J. 1985;132:919–23.
CAS PubMed PubMed Central Google Scholar
McGavin CR, Gupta SP, McHardy GJ. Twelve-minute walking test for assessing disability in chronic bronchitis. Br Med J. 1976;1:822–3. https://doi.org/10.1136/bmj.1.6013.822 .
Kline GM, Porcari JP, Hintermeister R, et al. Estimation of VO2max from a one-mile track walk, gender, age, and body weight. Med Sci Sports Exerc. 1987;19:253–9.
Article CAS Google Scholar
Ebbeling CB, Ward A, Puleo EM, et al. Development of a single-stage submaximal treadmill walking test. Med Sci Sports Exerc. 1991;23:966–73.
Broman S, Wigertz O. Transient dynamics of ventilation and heart rate with step changes in work load from different load levels. Acta Physiol Scand. 1971;81:54–74. https://doi.org/10.1111/j.1748-1716.1971.tb04877.x .
Haber P, Schlick W, Schmid P, et al. Estimation of the performance spectrum of healthy adolescents by using the PWC 170 (Physical Work Capacity). Acta Med Austriaca. 1976;3:164–6.
Franks BD. YMCA youth fitness test manual. 6th ed. Champaign (IL): YMCA of the USA; 1989.
Singh SJ, Morgan MD, Scott S, et al. Development of a shuttle walking test of disability in patients with chronic airways obstruction. Thorax. 1992;47:1019–24. https://doi.org/10.1136/thx.47.12.1019 .
Bruce RA. Exercise testing of patients with coronary heart disease: principles and normal standards for evaluation. Ann Clin Res. 1971;3:323–32.
Bruce RA, Kusumi F, Hosmer D. Maximal oxygen intake and nomographic assessment of functional aerobic impairment in cardiovascular disease. Am Heart J. 1973;85:546–62. https://doi.org/10.1016/0002-8703(73)90502-4 .
Ghosh AK. Anaerobic threshold: its concept and role in endurance sport. Malays J Med Sci. 2004;11:24–36.
PubMed PubMed Central Google Scholar
Wasserman K. The anaerobic threshold: definition, physiological significance and identification. Adv Cardiol. 1986;35:1–23.
Barbeau P, Serresse O, Boulay MR. Using maximal and submaximal aerobic variables to monitor elite cyclists during a season. Med Sci Sports Exerc. 1993;25:1062–9.
Urhausen A, Gabriel H, Weiler B, et al. Ergometric and psychological findings during overtraining: a long-term follow-up study in endurance athletes. Intl J Sports Med. 1998;19:114–20. https://doi.org/10.1055/s-2007-971892 .
Halson SL, Bridge MW, Meeusen R, et al. Time course of performance changes and fatigue markers during intensified training in trained cyclists. J Appl Physiol. 2002;93:947–56. https://doi.org/10.1152/japplphysiol.01164.2001 .
Jeukendrup A, Hesselink M, Snyder A, et al. Physiological changes in male competitive cyclists after two weeks of intensified training. Int J Sports Med. 1992;13:534–41. https://doi.org/10.1055/s-2007-1021312 .
Lamberts RP, Swart J, Noakes TD, et al. A novel submaximal cycle test to monitor fatigue and predict cycling performance. Br J Sports Med. 2011;45:797–804. https://doi.org/10.1136/bjsm.2009.061325 .
Wallace LK, Slattery KM, Coutts AJ. A comparison of methods for quantifying training load: relationships between modelled and actual training responses. Eur J Appl Physiol. 2014;114:11–20. https://doi.org/10.1007/s00421-013-2745-1 .
Vesterinen V, Nummela A, Äyrämö S, et al. Monitoring training adaptation with a submaximal running test under field conditions. Int J Sports Physiol Perform. 2016;11:393–9. https://doi.org/10.1123/ijspp.2015-0366 .
Vesterinen V, Nummela A, Laine T, et al. A submaximal running test with postexercise cardiac autonomic and neuromuscular function in monitoring endurance training adaptation. J Strength Cond Res. 2017;31:233–43. https://doi.org/10.1519/JSC.0000000000001458 .
Aubry A, Hausswirth C, Louis J, et al. The development of functional overreaching is associated with a faster heart rate recovery in endurance athletes. PLoS ONE. 2015;10: e0139754. https://doi.org/10.1371/journal.pone.0139754 .
Le Meur Y, Buchheit M, Aubry A, et al. Assessing overreaching with heart-rate recovery: what is the minimal exercise intensity required? Int J Sports Physiol Perform. 2017;12:569–73. https://doi.org/10.1123/ijspp.2015-0675 .
Bosquet L, Merkari S, Arvisais D, et al. Is heart rate a convenient tool to monitor over-reaching? A systematic review of the literature. Br J Sports Med. 2008;42:709–14. https://doi.org/10.1136/bjsm.2007.042200 .
Daanen HAM, Lamberts RP, Kallen VL, et al. A systematic review on heart-rate recovery to monitor changes in training status in athletes. Int J Sports Physiol Perform. 2012;7:251–60. https://doi.org/10.1123/ijspp.7.3.251 .
Capostagno B, Lambert MI, Lamberts RP. Analysis of a submaximal cycle test to monitor adaptations to training: implications for optimizing training prescription. J Strength Cond Res. 2021;35:924–30. https://doi.org/10.1519/jsc.0000000000003227 .
Decroix L, Lamberts RP, Meeusen R. Can the Lamberts and Lambert Submaximal Cycle Test reflect overreaching in professional cyclists? Int J Sports Physiol Perform. 2018;13:23–8. https://doi.org/10.1123/ijspp.2016-0685 .
Schneider C, Hanakam F, Wiewelhove T, et al. Heart rate monitoring in team sports: a conceptual framework for contextualizing heart rate measures for training and recovery prescription. Front Physiol. 2018;9:639. https://doi.org/10.3389/fphys.2018.00639 .
Akenhead R, Nassis GP. Training load and player monitoring in high-level football: current practice and perceptions. Int J Sports Physiol Perform. 2016;11:587–93. https://doi.org/10.1123/ijspp.2015-0331 .
Taylor K-L, Chapman DW, Cronin JB, et al. Fatigue monitoring in high performance sport: a survey of current trends. J Aust Strength Cond. 2012;20:12–23.
Buchheit M, Mendez-Villanueva A, Quod MJ, et al. Determinants of the variability of heart rate measures during a competitive period in young soccer players. Eur J Appl Physiol. 2010;109:869–78. https://doi.org/10.1007/s00421-010-1422-x .
Mohr M, Krustrup P. Yo-Yo intermittent recovery test performances within an entire football league during a full season. J Sports Sci. 2014;32:315–27. https://doi.org/10.1080/02640414.2013.824598 .
Thorpe RT, Strudwick AJ, Buchheit M, et al. Tracking morning fatigue status across in-season training weeks in elite soccer players. Int J Sports Physiol Perform. 2016;11:947–52. https://doi.org/10.1123/ijspp.2015-0490 .
Veugelers KR, Naughton GA, Duncan CS, et al. Validity and reliability of a submaximal intermittent running test in elite Australian football players. J Strength Cond Res. 2016;30:3347–53. https://doi.org/10.1519/jsc.0000000000001441 .
Buchheit M, Lacome M, Cholley Y, et al. Neuromuscular responses to conditioned soccer sessions assessed via GPS-embedded accelerometers: insights into tactical periodization. Int J Sports Physiol Perform. 2018;13:577–83. https://doi.org/10.1123/ijspp.2017-0045 .
Haddad M, Chaouachi A, Wong DP, et al. Influence of fatigue, stress, muscle soreness and sleep on perceived exertion during submaximal effort. Physiol Behav. 2013;119:185–9. https://doi.org/10.1016/j.physbeh.2013.06.016 .
Hulin BT, Gabbett TJ, Johnston RD, et al. Sub-maximal heart rate is associated with changes in high-intensity intermittent running ability in professional rugby league players. Sci Med Football. 2019;3:50–6. https://doi.org/10.1080/24733938.2018.1475748 .
Leduc C, Tee J, Lacome M, et al. Convergent validity, reliability, and sensitivity of a running test to monitor neuromuscular fatigue. Int J Sports Physiol Perform. 2020;15:1067–73. https://doi.org/10.1123/ijspp.2019-0319 .
Thorpe RT, Strudwick AJ, Buchheit M, et al. Monitoring fatigue during the in-season competitive phase in elite soccer players. Int J Sports Physiol Perform. 2015;10:958–64. https://doi.org/10.1123/ijspp.2015-0004 .
Malone S, Hughes B, Roe M, et al. Monitoring player fitness, fatigue status and running performance during an in-season training camp in elite Gaelic football. Sci Med Football. 2017;1:229–36. https://doi.org/10.1080/24733938.2017.1361040 .
Buchheit M, Cholley Y, Lambert P. Psychometric and physiological responses to a preseason competitive camp in the heat with a 6-hour time difference in elite soccer players. Int J Sports Physiol Perform. 2016;11:176–81. https://doi.org/10.1123/ijspp.2015-0135 .
Buchheit M, Simpson BM, Garvican-Lewis LA, et al. Wellness, fatigue and physical performance acclimatisation to a 2-week soccer camp at 3600 m (ISA3600). Br J Sports Med. 2013;47:i100–6. https://doi.org/10.1136/bjsports-2013-092749 .
Buchheit M, Voss SC, Nybo L, et al. Physiological and performance adaptations to an in-season soccer camp in the heat: associations with heart rate and heart rate variability: monitoring responses to training in the heat. Scand J Med Sci Sports. 2011;21:e477–85. https://doi.org/10.1111/j.1600-0838.2011.01378.x .
Buchheit M, Morgan W, Wallace J, et al. Physiological, psychometric, and performance effects of the Christmas break in Australian football. Int J Sports Physiol Perform. 2015;10:120–3. https://doi.org/10.1123/ijspp.2014-0082 .
de Freitas VH, Pereira LA, de Souza EA, et al. Sensitivity of the Yo-Yo Intermittent Recovery Test and cardiac autonomic responses to training in Futsal players. Int J Sports Physiol Perform. 2015;10:553–8. https://doi.org/10.1123/ijspp.2014-0365 .
Aoki MS, Ronda LT, Marcelino PR, et al. Monitoring training loads in professional basketball players engaged in a periodized training program. J Strength Cond Res. 2017;31:348–58. https://doi.org/10.1519/jsc.0000000000001507 .
Buchheit M, Millet GP, Parisy A, et al. Supramaximal training and postexercise parasympathetic reactivation in adolescents. Med Sci Sports Exerc. 2008;40:362–71. https://doi.org/10.1249/mss.0b013e31815aa2ee .
DiffSense. Continuous vs intermittent: what is the difference? 2021. Available from: https://diffsense.com/diff/continuous/intermittent . [Accessed 15 Jan 2021].
Christensen EH, Rune H, Bengt S. Intermittent and continuous running (a further contribution to the physiology of intermittent work.). Acta Physiol Scand. 1960;50:269–86. https://doi.org/10.1111/j.1748-1716.1960.tb00181.x .
Encyclopedia.com. Exercise, intermittent. 2021. Available from: https://www.encyclopedia.com/sports/sports-fitness-recreation-and-leisure-magazines/exercise-intermittent . [Accessed 15 Jan 2021].
Fitzpatrick JF, Hicks KM, Russell M, et al. The reliability of potential fatigue-monitoring measures in elite youth soccer players. Strength Cond Res. 2021;35:3448–52. https://doi.org/10.1519/JSC.0000000000003317 .
Rabbani A, Kargarfard M, Twist C. Reliability and validity of a submaximal warm-up test for monitoring training status in professional soccer players. J Strength Cond Res. 2018;32:326–33. https://doi.org/10.1519/jsc.0000000000002335 .
Castagna C, Manzi V, Impellizzeri F, et al. Validity of an on-court lactate threshold test in young basketball players. J Strength Cond Res. 2010;24:2434–9. https://doi.org/10.1519/JSC.0b013e3181e2e1bf .
Garrett J, Graham SR, Eston RG, et al. A novel method of assessment for monitoring neuromuscular fatigue in Australian Rules football players. Int J Sports Physiol Perform. 2019;14:598–605. https://doi.org/10.1123/ijspp.2018-0253 .
Buchheit M, Lefebvre B, Laursen PB, et al. Reliability, usefulness, and validity of the 30–15 Intermittent Ice Test in young elite ice hockey players. J Strength Cond Res. 2011;25:1457–64. https://doi.org/10.1519/jsc.0b013e3181d686b7 .
Iacono AD, Beato M, Unnithan V. Comparative effects of game profile-based training and small-sided games on physical performance of elite young soccer players. J Strength Cond Res. 2019;9. https://doi.org/10.1519/jsc.0000000000003225 .
Lacome M, Simpson B, Broad N, et al. Monitoring players’ readiness using predicted heart-rate responses to soccer drills. Int J Sports Physiol Perform. 2018;13:1273–80. https://doi.org/10.1123/ijspp.2018-0026 .
Rowell AE, Aughey RJ, Clubb J, et al. A standardized small sided game can be used to monitor neuromuscular fatigue in professional A-League football players. Front Physiol. 2018;9:1011. https://doi.org/10.3389/fphys.2018.01011 .
Scott TJ. Testing, prescribing and monitoring training in team sports: the efficiency and versatility of the 30–15 Intermittent Fitness Test. Sport Perform Sci Rep. 2018;5.
Delisle-Houde P, Reid RER, Insogna JA, et al. Seasonal changes in physiological responses and body composition during a competitive season in male and female elite collegiate ice hockey players. J Strength Cond Res. 2019;33:2162–9. https://doi.org/10.1519/jsc.0000000000002338 .
Dillern T. Markers of the aerobic energy-delivery system as measures of post-match fatigue and recovery in soccer; a repeated measures design. Asian J Sports Med. 2017;8. https://doi.org/10.5812/asjsm.14425 .
Dobbin N, Moss SL, Highton J, et al. An examination of a modified Yo-Yo test to measure intermittent running performance in rugby players. Eur J Sport Sci. 2018;18:1068–76. https://doi.org/10.1080/17461391.2018.1475509 .
Ferioli D, Bosio A, La Torre A, et al. Different training loads partially influence physiological responses to the preparation period in basketball. J Strength Cond Res. 2018;32:790–7. https://doi.org/10.1519/jsc.0000000000001823 .
Iaia FM, Fiorenza M, Larghi L, et al. Short- or long-rest intervals during repeated-sprint training in soccer? PLoS ONE. 2017;12: e0171462. https://doi.org/10.1371/journal.pone.0171462 .
Reinhardt L, Schulze S, Kurz E, et al. An investigation into the relationship between heart rate recovery in small-sided games and endurance performance in male, semi-professional soccer players. Sports Med Open. 2020;6:43. https://doi.org/10.1186/s40798-020-00273-8 .
Rampinini E, Donghi F, Martin M, et al. Impact of COVID-19 lockdown on Serie A soccer players’ physical qualities. Int J Sports Med. 2021;2:917–23. https://doi.org/10.1055/a-1345-9262 .
Owen AL, Wong DP, Paul D, et al. Effects of a periodized small-sided game training intervention on physical performance in elite professional soccer. J Strength Cond Res. 2012;26:2748–54. https://doi.org/10.1519/jsc.0b013e318242d2d1 .
Signorelli GR, Perim RR, Santos TM, et al. A pre-season comparison of aerobic fitness and flexibility of younger and older professional soccer players. Int J Sports Med. 2012;33:867–72. https://doi.org/10.1055/s-0032-1311597 .
Altmann S, Neumann R, Härtel S, et al. Using submaximal exercise heart rate for monitoring cardiorespiratory fitness changes in professional soccer players: a replication study. Int J Sports Physiol Perform. 2021;16:1096–102. https://doi.org/10.1123/ijspp.2020-0554 .
Bradley PS, Mohr M, Bendiksen M, et al. Sub-maximal and maximal Yo–Yo intermittent endurance test level 2: heart rate response, reproducibility and application to elite soccer. Eur J Appl Physiol. 2011;111:969–78. https://doi.org/10.1007/s00421-010-1721-2 .
Brink MS, Visscher C, Coutts AJ, et al. Changes in perceived stress and recovery in overreached young elite soccer players: stress and recovery in overreaching. Scand J Med Sci Sports. 2012;22:285–92. https://doi.org/10.1111/j.1600-0838.2010.01237.x .
Brink MS, Nederhof E, Visscher C, et al. Monitoring load, recovery, and performance in young elite soccer players. J Strength Cond Res. 2010;24:597–603. https://doi.org/10.1519/jsc.0b013e3181c4d38b .
Brink MS, Visscher C, Schmikli SL, et al. Is an elevated submaximal heart rate associated with psychomotor slowness in young elite soccer players? Eur J Sport Sci. 2013;13:207–14. https://doi.org/10.1080/17461391.2011.630101 .
Dobbin N, Highton J, Moss SL, et al. The effects of in-season, low-volume sprint interval training with and without sport-specific actions on the physical characteristics of Elite Academy rugby league players. Int J Sports Physiol Perform. 2020;15:705–13. https://doi.org/10.1123/ijspp.2019-0165 .
Doncaster G, Scott M, Iga J, et al. Reliability of heart rate responses both during and following a 6 min Yo-Yo IR1 test in highly trained youth soccer players. Sci Med Football. 2019;3:14–20. https://doi.org/10.1080/24733938.2018.1476775 .
Dubois R, Lyons M, Paillard T, et al. Influence of weekly workload on physical, biochemical and psychological characteristics in professional rugby union players over a competitive season. J Strength Cond Res. 2020;34:527–45. https://doi.org/10.1519/jsc.0000000000002741 .
Fanchini M, Schena F, Castagna C, et al. External responsiveness of the Yo-Yo IR Test Level 1 in high-level male soccer players. Int J Sports Med. 2015;36:735–41. https://doi.org/10.1055/s-0035-1547223 .
Fanchini M, Castagna C, Coutts AJ, et al. Are the Yo-Yo intermittent recovery test levels 1 and 2 both useful? Reliability, responsiveness and interchangeability in young soccer players. J Sports Sci. 2014;32:1950–7. https://doi.org/10.1080/02640414.2014.969295 .
Garvican LA, Hammond K, Varley MC, et al. Lower running performance and exacerbated fatigue in soccer played at 1600 m. Int J Sports Physiol Perform. 2014;9:397–404. https://doi.org/10.1123/ijspp.2012-0375 .
Hulka K, Weisser R, Belka J, et al. Stability of internal response and external load during 4-a-side football game in an indoor environment. Acta Gymnica. 2015;45:21–5. https://doi.org/10.5507/ag.2015.003 .
Hulse M, Morris J, Hawkins R, et al. A field-test battery for elite, young soccer players. Int J Sports Med. 2012;34:302–11. https://doi.org/10.1055/s-0032-1312603 .
Ingebrigtsen J, Bendiksen M, Randers MB, et al. Yo-Yo IR2 testing of elite and sub-elite soccer players: performance, heart rate response and correlations to other interval tests. J Sports Sci. 2012;30:1337–45. https://doi.org/10.1080/02640414.2012.711484 .
Natera AOW, Jennings J, Oakley AJ, et al. Influence of environmental conditions on performance and heart rate responses to the 30–15 Incremental Fitness Test in rugby union athletes. J Strength Cond Res. 2019;33:486–91. https://doi.org/10.1519/jsc.0000000000001865 .
Owen AL, Newton M, Shovlin A, et al. The use of small-sided games as an aerobic fitness assessment supplement within elite level professional soccer. J Hum Kinet. 2020;71:243–53. https://doi.org/10.2478/hukin-2019-0086 .
Owen C, Jones P, Comfort P. The reliability of the submaximal version of the Yo-Yo intermittent recovery test in elite youth soccer. J Train. 2017;6:31–4.
Pereira LA, Abad CCC, Leiva DF, et al. Relationship between resting heart rate variability and intermittent endurance performance in novice soccer players. Res Q Exerc Sport. 2019;90:355–61. https://doi.org/10.1080/02701367.2019.1601666 .
Younesi S, Rabbani A, Manuel Clemente F, et al. Session-to-session variations of internal load during different small-sided games: a study in professional soccer players. Res Sports Med. 2021;29:462–74. https://doi.org/10.1080/15438627.2021.1888103 .
Cornforth DJ, Robinson DJ, Spence I, et al. Heart rate recovery in decision support for high performance athlete training schedules. IJIKM. 2014;9:193–207.
Moreira A, Machado DG da S, Moscaleski L, et al. Effect of tDCS on well-being and autonomic function in professional male players after official soccer matches. Physiol Behav. 2021;233:113351. https://doi.org/10.1016/j.physbeh.2021.113351 .
Meylan CMP, Bowman K, Stellingwerff T, et al. The efficacy of heat acclimatization pre-World Cup in female soccer players. Front Sports Act Living. 2021;3: 614370. https://doi.org/10.3389/fspor.2021.614370 .
Araújo M, Baumgart C, Freiwald J, et al. Contrasts in intermittent endurance performance and heart rate response between female and male soccer players of different playing levels. Biol Sport. 2019;36:323–31. https://doi.org/10.5114/biolsport.2019.88755 .
Buchheit M, Racinais S, Bilsborough JC, et al. Monitoring fitness, fatigue and running performance during a pre-season training camp in elite football players. J Sci Med Sport. 2013;16:550–5. https://doi.org/10.1016/j.jsams.2012.12.003 .
Buchheit M, Simpson MB, Al Haddad H, et al. Monitoring changes in physical performance with heart rate measures in young soccer players. Eur J Appl Physiol. 2012;112:711–23. https://doi.org/10.1007/s00421-011-2014-0 .
Buchheit M, Simpson BM, Schmidt WF, et al. Predicting sickness during a 2-week soccer camp at 3600 m (ISA3600). Br J Sports Med. 2013;47:i124–7. https://doi.org/10.1136/bjsports-2013-092757 .
Deprez D, Fransen J, Lenoir M, et al. The Yo-Yo intermittent recovery test level 1 is reliable in young high-level soccer players. Biol Sport. 2015;32:65–70. https://doi.org/10.5604/20831862.1127284 .
Deprez D, Coutts AJ, Lenoir M, et al. Reliability and validity of the Yo-Yo intermittent recovery test level 1 in young soccer players. J Sports Sci. 2014;32:903–10. https://doi.org/10.1080/02640414.2013.876088 .
Francini L, Rampinini E, Bosio A, et al. Association between match activity, endurance levels and maturity in youth football players. Int J Sports Med. 2019;40:576–84. https://doi.org/10.1055/a-0938-5431 .
Lignell E, Fransson D, Krustrup P, et al. Analysis of high-intensity skating in top-class ice hockey match-play in relation to training status and muscle damage. J Strength Cond Res. 2018;32:1303–10. https://doi.org/10.1519/jsc.0000000000001999 .
Rabbani A, Kargarfard M, Twist C. Fitness monitoring in elite soccer players: group vs. individual analyses. J Strength Cond Res. 2020;34:3250–7. https://doi.org/10.1519/jsc.0000000000002700 .
Thorpe RT, Strudwick AJ, Buchheit M, et al. The influence of changes in acute training load on daily sensitivity of morning-measured fatigue variables in elite soccer players. Int J Sports Physiol Perform. 2017;12:S2–107-S2–113. https://doi.org/10.1123/ijspp.2016-0433 .
Vigh-Larsen JF, Beck JH, Daasbjerg A, et al. Fitness characteristics of elite and subelite male ice hockey players: a cross-sectional study. J Strength Cond Res. 2019;33:2352–60. https://doi.org/10.1519/jsc.0000000000003285 .
Younesi S, Rabbani A, Clemente FM, et al. Relationships between aerobic performance, hemoglobin levels, and training load during small-sided games: a study in professional soccer players. Front Physiol. 2021;12: 649870. https://doi.org/10.3389/fphys.2021.649870 .
Nakamura FY, Pereira LA, Moraes JE, et al. Physical and physiological differences of backs and forwards from the Brazilian national rugby union team. J Sports Med Phys Fitness. 2017;57:1549–56. https://doi.org/10.23736/s0022-4707.16.06751-7 .
Hulin BT, Gabbett TJ, Pickworth NJ, et al. Relationships among PlayerLoad, high-intensity intermittent running ability, and injury risk in professional rugby league players. Int J Sports Physiol Perform. 2020;15:423–9. https://doi.org/10.1123/ijspp.2019-0139 .
Ryan S, Pacecca E, Tebble J, et al. Measurement characteristics of athlete monitoring tools in professional Australian football. Int J Sports Physiol Perform. 2019;1–7. https://doi.org/10.1123/ijspp.2019-0060 .
Rago V, Krustrup P, Martín-Acero R, et al. Training load and submaximal heart rate testing throughout a competitive period in a top-level male football team. J Sports Sci. 2020;38:1408–15. https://doi.org/10.1080/02640414.2019.1618534 .
Fox R, Patterson SD, Waldron M. The relationship between heart rate recovery and temporary fatigue of kinematic and energetic indices among soccer players. Sci Med Football. 2017;1:132–8. https://doi.org/10.1080/24733938.2017.1329590 .
Ingebrigtsen J, Brochmann M, Castagna C, et al. Relationships between field performance tests in high-level soccer players. J Strength Cond Res. 2014;28:942–9. https://doi.org/10.1519/jsc.0b013e3182a1f861 .
Harry K, Booysen MJ. Faster heart rate recovery correlates with high-intensity match activity in female field hockey players: training implications. J Strength Cond Res. 2020;34:1150–7. https://doi.org/10.1519/jsc.0000000000003073 .
Starling LT, Nellemann S, Parkes A, et al. The sensitivity of the Fatigue and Fitness Test for Teams (FFITT) to measure the demands of a rugby match. Eur J Sport Sci. 2021;21:803–10. https://doi.org/10.1080/17461391.2020.1780327 .
Prajapat A, Ahmad I, Khan Z, et al. Cardiac autonomic profile of soccer, field hockey and basketball players: a comparative study. Asian J Sports Med. 2018;9. https://doi.org/10.5812/asjsm.62492 .
Vachon A, Berryman N, Mujika I, et al. Tapering and repeated high-intensity effort ability in young elite rugby union players: influence of pretaper fatigue level. Int J Sports Physiol Perform. 2021;16:993–1000. https://doi.org/10.1123/ijspp.2020-0639 .
Ernst G. Heart-rate variability: more than heart beats? Front Public Health. 2017;5:240. https://doi.org/10.3389/fpubh.2017.00240 .
Stone NM, Kilding AE. Aerobic conditioning for team sport athletes. Sports Med. 2009;39:615–42. https://doi.org/10.2165/00007256-200939080-00002 .
Kuipers H, Keizer HA. Overtraining in elite athletes: review and directions for the future. Sports Med. 1988;6:79–92. https://doi.org/10.2165/00007256-198806020-00003 .
ten Haaf T, Foster C, Meeusen R, et al. Submaximal heart rate seems inadequate to prescribe and monitor intensified training. Eur J Sport Sci. 2019;19:1082–91. https://doi.org/10.1080/17461391.2019.1571112 .
Laursen P, Buchheit M, editors. Science and application of high-intensity interval training. Champaign, IL, USA: Human Kinetics; 2019.
Racinais S, Buchheit M, Bilsborough J, et al. Physiological and performance responses to a training camp in the heat in professional Australian football players. Int J Sports Physiol Perform. 2014;9:598–603. https://doi.org/10.1123/ijspp.2013-0284 .
Pryor JL, Johnson EC, Roberts WO, et al. Application of evidence-based recommendations for heat acclimation: individual and team sport perspectives. Temperature. 2019;6:37–49. https://doi.org/10.1080/23328940.2018.1516537 .
Racinais S, Alonso JM, Coutts AJ, et al. Consensus recommendations on training and competing in the heat. Br J Sports Med. 2015;49:1164–73. https://doi.org/10.1111/sms.12467 .
Garrett JM, Gunn R, Eston RG, et al. The effects of fatigue on the running profile of elite team sport athletes: a systematic review and meta-analysis. J Sports Med Phys Fitness. 2019;59:1328. https://doi.org/10.23736/s0022-4707.19.09356-3 .
McLaren SJ, Macpherson TW, Coutts AJ, et al. The relationships between internal and external measures of training load and intensity in team sports: a meta-analysis. Sports Med. 2018;48:641–58. https://doi.org/10.1007/s40279-017-0830-z .
Akubat I, Barrett S, Abt G. Integrating the internal and external training loads in soccer. Int J Sports Physiol Perform. 2014;9:457–62. https://doi.org/10.1123/ijspp.2012-0347 .
Atkinson G, Batterham AM. The use of ratios and percentage changes in sports medicine: time for a rethink? Int J Sports Med. 2012;33:505–6. https://doi.org/10.1055/s-0032-1316355 .
Wiseman RM. On the use and misuse of ratios in strategic management research. In: Bergh DD, Ketchen DJ, (editors), Research methodology in strategy and management. Bing: Emerald Group Publishing Limited; 2009: p. 75–110.
Coyne JOC, Gregory Haff G, Coutts AJ, et al. The current state of subjective training load monitoring: a practical perspective and call to action. Sports Med Open. 2018;4:58. https://doi.org/10.1186/s40798-018-0172-x .
Jeffries AC, Wallace L, Coutts AJ, et al. Athlete-reported outcome measures for monitoring training responses: a systematic review of risk of bias and measurement property quality according to the COSMIN guidelines. Int J Sports Physiol Perform. 2020;15:1203–15. https://doi.org/10.1123/ijspp.2020-0386 .
McLaren S, Coutts A, Impellizzeri FM. Perception of effort and subjective monitoring. In: French D, Ronda LT, (editors) NSCA’s essentials of sport science. Champaign, IL, USA: Human Kinetics, Inc.; 2022: p. 231–54.
Borg GA. Psychophysical bases of perceived exertion. Med Sci Sports Exerc. 1982;14:377–81. https://doi.org/10.1249/00005768-198205000-00012 .
Chen MJ, Fan X, Moe ST. Criterion-related validity of the Borg ratings of perceived exertion scale in healthy individuals: a meta-analysis. J Sports Sci. 2002;20:873–99. https://doi.org/10.1080/026404102320761787 .
Lea JWD, O’Driscoll JM, Coleman DA, et al. Validity and reliability of the ‘Isometric Exercise Scale’ (IES) for measuring ratings of perceived exertion during continuous isometric exercise. Sci Rep. 2021;11:5334. https://doi.org/10.1038/s41598-021-84803-8 .
Pageaux B. Perception of effort in exercise science: definition, measurement and perspectives. Eur J Sport Sci. 2016;16:885–94. https://doi.org/10.1080/17461391.2016.1188992 .
Halperin I, Emanuel A. Rating of perceived effort: methodological concerns and future directions. Sports Med. 2020;50:679–87. https://doi.org/10.1007/s40279-019-01229-z .
Sangan HF, Hopker JG, Davison G, et al. The self-paced submaximal run test: associations with the graded exercise test and reliability. Int J Sports Physiol Perform. 2021;16:1865–73. https://doi.org/10.1123/ijspp.2020-0904 .
Lovell R, Halley S, Siegler J, et al. Use of numerically blinded ratings of perceived exertion in soccer: assessing concurrent and construct validity. Int J Sports Physiol Perform. 2020;15:1430–6. https://doi.org/10.1123/ijspp.2019-0740 .
Micklewright D, St Clair Gibson A, Gladwell V, et al. Development and validity of the Rating-of-Fatigue Scale. Sports Med. 2017;47:2375–93. https://doi.org/10.1007/s40279-017-0711-5 .
McLaren SJ, Taylor JM, Macpherson TW, et al. Systematic reductions in differential ratings of perceived exertion across a 2-week repeated-sprint-training intervention that improved soccer players’ high-speed-running abilities. Int J Sports Physiol Perform. 2020;15:1414–21. https://doi.org/10.1123/ijspp.2019-0568 .
Winter EM, Abt G, Brookes FBC, et al. Misuse of “power” and other mechanical terms in sport and exercise science research. J Strength Cond Res. 2016;30:292–300. https://doi.org/10.1519/jsc.0000000000001101 .
Bourdon PC, Cardinale M, Murray A, et al. Monitoring athlete training loads: consensus statement. Int J Sports Physiol Perform. 2017;12:S2–161-S2–170. https://doi.org/10.1123/ijspp.2017-0208 .
Buchheit M, Simpson BM. Player-tracking technology: half-full or half-empty glass? Int J Sports Physiol Perform. 2017;12:S2–35-S2–41. https://doi.org/10.1123/ijspp.2016-0499 .
Nikolaidis PT, Busko K, Afonso J, et al. The effect of maturity on heart rate responses during training and testing in postpubescent female volleyball players. Hum Physiol. 2015;41:636–43. https://doi.org/10.7868/s0131164615060053 .
Younesi S, Rabbani A, Clemente F, et al. Session-to-session variations in external load measures during small-sided games in professional soccer players. Biol Sport. 2021;38:185–93. https://doi.org/10.5114/biolsport.2020.98449 .
Clemente FM, Rabbani A, Kargarfard M, et al. Session-to-session variations of external load measures of youth soccer players in medium-sided games. Int J Environ Res Public Health. 2019;16:3612. https://doi.org/10.3390/ijerph16193612 .
Article PubMed Central Google Scholar
Malone JJ, Lovell R, Varley MC, et al. Unpacking the black box: applications and considerations for using GPS devices in sport. Int J Sports Physiol Perform. 2017;12:S2–18-S2–26. https://doi.org/10.1123/ijspp.2016-0236 .
Verheul J, Nedergaard NJ, Vanrenterghem J, et al. Measuring biomechanical loads in team sports: from lab to field. Sci Med Football. 2020;4:246–52. https://doi.org/10.1080/24733938.2019.1709654 .
Barrett S, Midgley AW, Towlson C, et al. Within-match PlayerLoad™ patterns during a simulated soccer match: potential implications for unit positioning and fatigue management. Int J Sports Physiol Perform. 2016;11:135–40. https://doi.org/10.1123/ijspp.2014-0582 .
Buchheit M, Gray A, Morin J-B. Assessing stride variables and vertical stiffness with GPS-embedded accelerometers: preliminary insights for the monitoring of neuromuscular fatigue on the field. J Sports Sci Med. 2015;14:698–701.
Cormack SJ, Mooney MG, Morgan W, et al. Influence of neuromuscular fatigue on accelerometer load in elite Australian football players. Int J Sports Physiol Perform. 2013;8:373–8. https://doi.org/10.1123/ijspp.8.4.373 .
Hobara H, Inoue K, Gomi K, et al. Continuous change in spring-mass characteristics during a 400m sprint. J Sci Med Sport. 2010;13:256–61. https://doi.org/10.1016/j.jsams.2009.02.002 .
Brughelli M, Cronin J. A review of research on the mechanical stiffness in running and jumping: methodology and implications: mechanical stiffness. Scand J Med Sci Sports. 2008;18:417–26. https://doi.org/10.1111/j.1600-0838.2008.00769.x .
Rowell AE, Aughey RJ, Hopkins WG, et al. Identification of sensitive measures of recovery after external load from football match play. Int J Sports Physiol Perform. 2017;12:969–76. https://doi.org/10.1123/ijspp.2016-0522 .
Silva JR, Ascensão A, Marques F, et al. Neuromuscular function, hormonal and redox status and muscle damage of professional soccer players after a high-level competitive match. Eur J Appl Physiol. 2013;113:2193–201. https://doi.org/10.1007/s00421-013-2633-8 .
Fitzpatrick JF, Akenhead R, Russell M, et al. Sensitivity and reproducibility of a fatigue response in elite youth football players. Sci Med Football. 2019;3:214–20. https://doi.org/10.1080/24733938.2019.1571685 .
Garrett JM, Graham SR, Eston RG, et al. Comparison of a countermovement jump test and submaximal run test to quantify the sensitivity for detecting practically important changes within high-performance Australian Rules football. Int J Sports Physiol Perform. 2020;15:68–72. https://doi.org/10.1123/ijspp.2019-0150 .
Garrett J, Akyildiz Z, Leduc C, et al. Peak running speed can be used to monitor neuromuscular fatigue from a standardized running test in team sport athletes. Res Sports Med. 2021;1–12. https://doi.org/10.1080/15438627.2021.1966012 .
Huygaerts S, Cos F, Cohen DD, et al. Mechanisms of hamstring strain injury: interactions between fatigue, muscle activation and function. Sports (Basel). 2020;8:65. https://doi.org/10.3390/sports8050065 .
Cormack SJ, Newton RU, McGuigan MR, et al. Reliability of measures obtained during single and repeated countermovement jumps. Int J Sports Physiol Perform. 2008;3:131–44. https://doi.org/10.1123/ijspp.3.2.131 .
Barrett S, Midgley A, Reeves M, et al. The within-match patterns of locomotor efficiency during professional soccer match play: implications for injury risk? J Sci Med Sport. 2016;19:810–5. https://doi.org/10.1016/j.jsams.2015.12.514 .
Kelly SJ, Murphy AJ, Watsford ML, et al. Reliability and validity of sports accelerometers during static and dynamic testing. Int J Sports Physiol Perform. 2015;10:106–11. https://doi.org/10.1123/ijspp.2013-0408 .
Eggers TM, Massard TI, Clothier PJ, et al. Measuring vertical stiffness in sport with accelerometers: exercise caution! J Strength Cond Res. 2018;32:1919–22. https://doi.org/10.1519/jsc.0000000000002318 .
Barrett S, Midgley A, Lovell R. PlayerLoad™: reliability, convergent validity, and influence of unit position during treadmill running. Int J Sports Physiol Perform. 2014;9:945–52. https://doi.org/10.1123/ijspp.2013-0418 .
Download references
Acknowledgements
The authors thank the authors who provided additional data for the studies included in our review. We also thank the reviewers for their constructive comments and contributions.
Author information
Authors and affiliations.
School of Health Sciences, Western Sydney University, Sydney, NSW, Australia
Tzlil Shushan & Ric Lovell
Newcastle Falcons Rugby Club, Newcastle upon Tyne, UK
Shaun J. McLaren
Department of Sport and Exercise Sciences, Durham University, Durham, UK
HIIT Science, Revelstoke, BC, Canada
Martin Buchheit
French National Institute of Sport (INSEP), Laboratory of Sport, Expertise and Performance (EA 7370), Paris, France
Kitman Labs, Performance Research Intelligence Initiative, Dublin, Ireland
Institute for Health and Sport, Victoria University, Melbourne, VIC, Australia
Netball Australia, Melbourne, VIC, Australia
Tannath J. Scott
Carnegie Applied Rugby Research (CARR) Centre, Institute for Sport, Physical Activity and Leisure, Carnegie School of Sport, Leeds Beckett University, Leeds, UK
Department of Sport Science Innovation, Playermaker, London, UK
Steve Barrett
You can also search for this author in PubMed Google Scholar
Corresponding author
Correspondence to Tzlil Shushan .
Ethics declarations
Open Access funding enabled and organized by CAUL and its Member Institutions. No sources of funding were used to assist in the preparation of this article.
Conflict of interest
The authors have no conflicts of interest that are directly relevant to the content of this article.
Ethics approval
Not applicable.
Consent to participate
Consent for publication, availability of data and material.
Supplementary materials for this paper are also available on the Open Science Framework at https://osf.io/f9xwj/ .
Code availability
Authors’ contributions.
TS conceived the idea for the article, performed the literature search and data analysis, and wrote the first draft of the manuscript and all subsequent versions. RL and SJM provided significant writing content and revisions to the manuscript. RL, SJM, MB, TJC, and SB contributed substantially to the conceptual direction and content and provided edits and feedback to the manuscript. All authors read and approved the final manuscript. TS coordinated the submission and revision process.
Supplementary Information
Below is the link to the electronic supplementary material.
Supplementary file1 (PDF 104 KB)
Supplementary file2 (pdf 338 kb), supplementary file3 (pdf 273 kb), rights and permissions.
Open Access This article is licensed under a Creative Commons Attribution 4.0 International License, which permits use, sharing, adaptation, distribution and reproduction in any medium or format, as long as you give appropriate credit to the original author(s) and the source, provide a link to the Creative Commons licence, and indicate if changes were made. The images or other third party material in this article are included in the article's Creative Commons licence, unless indicated otherwise in a credit line to the material. If material is not included in the article's Creative Commons licence and your intended use is not permitted by statutory regulation or exceeds the permitted use, you will need to obtain permission directly from the copyright holder. To view a copy of this licence, visit http://creativecommons.org/licenses/by/4.0/ .
Reprints and permissions
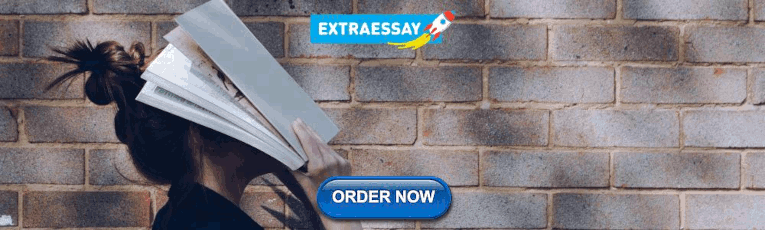
About this article
Shushan, T., McLaren, S.J., Buchheit, M. et al. Submaximal Fitness Tests in Team Sports: A Theoretical Framework for Evaluating Physiological State. Sports Med 52 , 2605–2626 (2022). https://doi.org/10.1007/s40279-022-01712-0
Download citation
Accepted : 22 May 2022
Published : 11 July 2022
Issue Date : November 2022
DOI : https://doi.org/10.1007/s40279-022-01712-0
Share this article
Anyone you share the following link with will be able to read this content:
Sorry, a shareable link is not currently available for this article.
Provided by the Springer Nature SharedIt content-sharing initiative
- Find a journal
- Publish with us
- Track your research

Sports Medicine Research
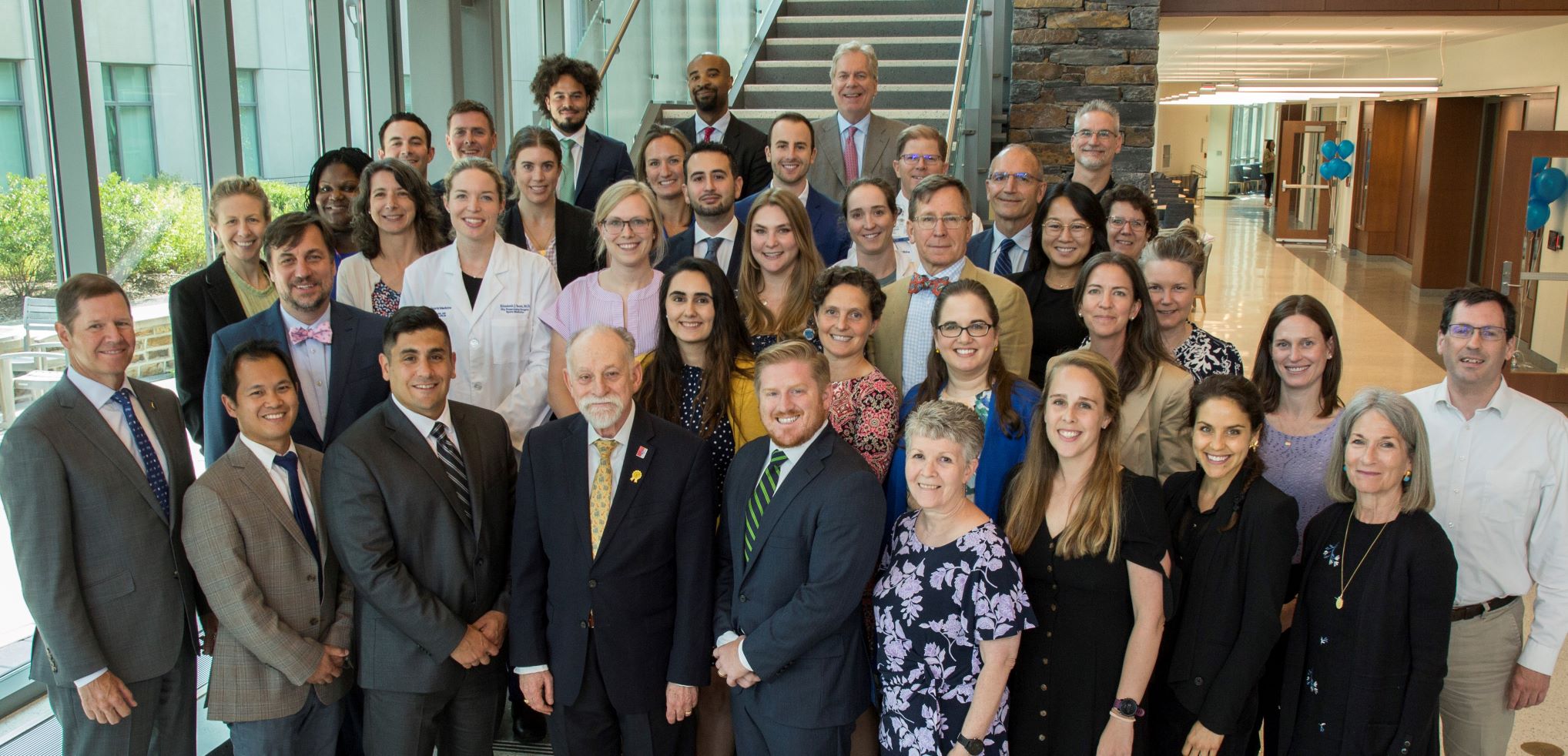
The sports medicine team is dedicated to researching the causes and prevention of injury in order to apply these findings directly to your care. At Duke sports medicine we pride ourselves in fostering and developing the future of research through our fellows and residents who are an integral part of our team. All research conducted within the sports medicine division is IRB approved and regulated.
Our areas of research include knee ( ACL, MPFL, osteochondral treatment, meniscal repair ), shoulder ( anatomic and reverse total shoulder replacement, rotator cuff, instability ), hip ( FAI- , labral injuries ) concussion, orthobiologics ( regenerative medicine ), musculoskeletal ultrasound, return to activity, biomechanics of injury and recovery, and athlete care.
By the Numbers
- Duke Sports Medicine is home to 32 research faculty and staff.
- In the 2022-2023 academic year, Duke Sports Medicine researchers had 87 publications .
- 10 are prospective and actively enrolling.
- Review Article
- Open access
- Published: 01 April 2022
The Training Characteristics of World-Class Distance Runners: An Integration of Scientific Literature and Results-Proven Practice
- Thomas Haugen ORCID: orcid.org/0000-0001-5929-0389 1 ,
- Øyvind Sandbakk 2 , 3 ,
- Stephen Seiler 4 &
- Espen Tønnessen 1
Sports Medicine - Open volume 8 , Article number: 46 ( 2022 ) Cite this article
99k Accesses
30 Citations
175 Altmetric
Metrics details
In this review we integrate the scientific literature and results-proven practice and outline a novel framework for understanding the training and development of elite long-distance performance. Herein, we describe how fundamental training characteristics and well-known training principles are applied. World-leading track runners (i.e., 5000 and 10,000 m) and marathon specialists participate in 9 ± 3 and 6 ± 2 (mean ± SD) annual competitions, respectively. The weekly running distance in the mid-preparation period is in the range 160–220 km for marathoners and 130–190 km for track runners. These differences are mainly explained by more running kilometers on each session for marathon runners. Both groups perform 11–14 sessions per week, and ≥ 80% of the total running volume is performed at low intensity throughout the training year. The training intensity distribution vary across mesocycles and differ between marathon and track runners, but common for both groups is that volume of race-pace running increases as the main competition approaches. The tapering process starts 7–10 days prior to the main competition. While the African runners live and train at high altitude (2000–2500 m above sea level) most of the year, most lowland athletes apply relatively long altitude camps during the preparation period. Overall, this review offers unique insights into the training characteristics of world-class distance runners by integrating scientific literature and results-proven practice, providing a point of departure for future studies related to the training and development in the Olympic long-distance events.
This review bridges the gap between science and results-proven practice regarding how training principles and training methods should be applied for the Olympic long-distance events and identified clear distinctions in training organization between track runners and marathon specialists
The weekly running distance is in the range 160–220 km for marathoners and 130–190 km for track runners, with both groups performing 11–14 sessions per week, and ≥ 80% of the total running volume at low intensity
Training intensity distribution varies across mesocycles and differs between marathon and track runners, but common for both groups is that volume of race-pace running increases as the main competition approaches
Training for long-distance running (LDR) aims to improve the “big three” performance-determining variables: maximum oxygen uptake (VO 2 max; the highest rate at which the body can take up and utilize oxygen during severe exercise), fractional utilization (the ability to sustain a high percentage of VO 2 max when running), and running economy (VO 2 at a given submaximal running velocity). Together, these variables integrate the sustained ability to produce adenosine triphosphate (ATP) aerobically and convert muscular work to power/speed [ 1 , 2 , 3 , 4 , 5 , 6 , 7 , 8 , 9 , 10 , 11 ]. International runners demonstrate different combinations of these determinants, as an “acceptable value” in one variable can be compensated for with extremely high values in the other variables. In addition, a “fourth variable,” neuromuscular power/anaerobic capacity, plays an important role in the decisive end phase of tactical track races [ 12 ]. Further, classic laboratory testing may not capture a “fifth variable,” fatigue resistance associated with specific adaptations that delay muscular deterioration and fatigue and enable maintaining race pace over the final 7–10 km of an elite marathon [ 13 , 14 ]. Different time courses in the development of these performance determinants are very likely. This is exemplified by a case study of former marathon world record holder Paula Radcliffe who improved running economy by ~ 15% between 1991 and 2003, while \(\dot{\text{V}}\) O 2 max remained essentially stable at ~ 70 ml kg −1 min −1 [ 5 ].
Most world-class long-distance runners engage in systematic training for 8–10 years prior to reaching a high international standard [ 15 ]. Different pathways to excellence have been described, as both early and late specialization, and different backgrounds from other sports, can provide a platform for later elite LDR performance [ 15 , 16 , 17 , 18 ]. Several scientific publications during the last two decades have described the training characteristics of world-leading distance runners [ 17 , 18 , 19 , 20 , 21 , 22 , 23 , 24 , 25 , 26 , 27 , 28 , 29 , 30 , 31 ]. However, our understanding of best-practice LDR continues to evolve, and it is fair to say that positive developments in modern long-distance training methods have often been driven by experienced coaches and athletes rather than sports scientists [ 32 ]. Sport scientists have historically found themselves testing hypotheses regarding why elite athletes train as they do rather than driving innovation around the how in the training process. Tightly controlled and adequately powered laboratory studies that span the months-to-years timescales associated with maximizing all the above-mentioned physiological variables impacting LDR performance have been essentially infeasible if not impossible.
Publicly available coaching philosophies and training logs of podium contestants from international athletics championships and world marathon majors constitute a corpus of descriptive training information for the international long-distance community. It is tempting to call this corpus of information made available by international champions a description of training “best practice,” but some of our colleagues in the sports science community would reasonably argue that we can only know that this is results-proven practice, not if it is best practice. Combining and cross-checking data sources from available research evidence and results-proven practice provides a valid point of departure for outlining current training recommendations and for generating new hypotheses to be tested in future research [ 33 , 34 , 35 , 36 ]. This integrative approach also facilitates unique insights into training characteristics that previously have been scarcely investigated, altogether allowing a more holistic picture of “state-of-the-art” LDR training.
The objective of this review is therefore to integrate scientific and results-proven practice literature regarding the training and development of elite LDR performance. Within this context, we will particularly explore areas where the scientific literature offers limited information compared to results-proven training information. Moreover, the distinctions between training characteristics of the most successful marathon runners and track runners (i.e., 5000 and 10,000-m specialists) will be highlighted since they organize their training year differently. Although anchored in the standard Olympic running distances, this review is also relevant for other endurance sports.
Methodological Considerations
The scientific literature supporting this narrative review was obtained from PubMed, using varying combinations of the search terms “endurance,” “long distance,” “marathon,” “training,” “conditioning,” “running,” “elite,” “high performing,” world-class,” “runners, ” and “athletes.” In addition, we searched for non-scientific, publicly available, and English-language training information related to podium contestants from international championships (i.e., Olympic Games [OG], World Championships [WC], and continental championships) and world marathon majors. Most of the training data were obtained from websites (Runner Universe, Sweat Elite, Running Science, LetsRun, and RunnersTribe) dedicated to providing the athletics community an expansive library of information written by top athletes and coaches. Within these websites, all relevant training logs and coaching philosophies were purchased/downloaded and reviewed. Training information from doping-banned athletes or coaches were excluded. Moreover, a Google Search for podium contestants (using athlete name and “training” as search terms) and LDR books was performed. Although we cannot guarantee that relevant data have not been overlooked, the search revealed training logs/information from 59 world-leading athletes and 16 coaches of podium contestants [ 15 , 37 , 38 , 39 , 40 , 41 , 42 , 43 , 44 , 45 , 46 , 47 , 48 , 49 , 50 , 51 , 52 , 53 , 54 , 55 , 56 , 57 , 58 , 59 , 60 , 61 , 62 , 63 , 64 , 65 , 66 , 67 , 68 , 69 , 70 , 71 , 72 , 73 , 74 , 75 , 76 , 77 , 78 , 79 , 80 , 81 , 82 , 83 , 84 , 85 , 86 , 87 , 88 , 89 , 90 , 91 , 92 , 93 , 94 , 95 , 96 , 97 , 98 , 99 , 100 , 101 , 102 , 103 , 104 , 105 , 106 , 107 , 108 , 109 , 110 , 111 , 112 ] (Table 1 ). This information ranged from “typical training week” of various mesocycles to complete annual training logs. Interpretations of longitudinal training logs were weighted more heavily than “short-term” information. Similarly, training information from the 50 s, 60 s, and 70 s was mainly used to provide historical context.
Several limitations to our approach must be acknowledged. Firstly, the inclusion of results-proven training information can be discussed since it is not based on peer-reviewed research. However, elite athletes are systematic in their collection of training “data” and report their training accurately [ 23 , 113 ], justifying the extensive use of training logs as primary or secondary information sources in scientific training characteristics studies within LDR [e.g., 17 – 28 ]. Secondly, an initial review of both the scientific literature and results-proven practice reveals several biases, including a substantial male dominance and focus on a few successful training groups. Additionally, the lack of a common framework (e.g., intensity zones) and terminology can result in misinterpretations. Moreover, the included literature cannot be controlled for possible training prescription–execution differences or changes in training programs over the years. We are also aware that many unsuccessful athletes have applied the same “recipe” as successful runners. Hence, we particularly focus on common key features across varying athlete groups. Finally, the widespread use of doping in international athletics must also be acknowledged [ 114 , 115 ]. The outcomes of this review must therefore be interpreted with these caveats in mind. Sensitive to these limitations, we still contend that integrating scientific evidence and results-proven practice is a strong point of departure for outlining state-of-the-art training recommendations and for generation of new hypotheses to be tested in future research.
Training Periodization and Competition Scheduling
Information about the periodization pattern of LDR training over the course of a year is scarce in the scientific literature. Since Arthur Lydiard introduced his periodization system in the late 1950s [ 46 , 47 , 48 ], leading practitioners typically divide the training year (macrocycle) into distinct, ordered phases (meso- or micro-cycles) with the explicit goal of peaking for major competitions [ 15 , 21 , 26 , 27 , 28 , 39 , 40 , 41 , 42 , 43 , 44 , 45 , 46 , 47 , 48 , 49 , 50 , 51 , 52 , 53 , 54 , 55 , 56 , 57 , 63 , 67 , 73 , 76 , 92 , 94 , 99 , 100 ]. Because track and marathon specialists organize their training year and competition schedule quite differently, we will treat these groups separately in the remainder of this section.
At least three phases are typically organized within a macrocycle for track runners: a preparation period, a competition period, and a transition period. The transition period begins immediately after the conclusion of the outdoor competition season, typically consisting of 1–2 weeks with rest or recreational training/low-intensive running [ 15 , 39 , 40 , 41 , 42 , 43 , 44 , 49 , 53 , 54 , 55 , 63 , 75 , 87 , 94 ], although some athletes may take ~ 4 weeks completely off [ 73 ]. The preparation period is typically broken up into general and specific preparation. In the general preparation period, the focus is high volume to build an aerobic foundation. From the specific preparation period onward, the focus gradually shifts toward higher volume of specific race-pace intensity [ 40 , 41 , 42 , 43 , 44 , 49 , 50 , 51 , 52 , 53 , 54 , 55 , 56 , 72 , 73 , 76 , 92 , 93 , 94 , 112 ]. Such organization of training has also recently been verified as highly effective in the research literature [ 116 ] and bears some resemblance with Matveyev’s traditional periodization model based on the training of successful Soviet athletes during the 1950s and 1960s [ 117 ]. While the Matveyev model suggested a dramatic shift from volume focus to intensity focus as the competition period neared, most track runners maintain a high volume of subthreshold endurance training throughout the preparation and competition period and are careful not to overuse race-pace training or introduce it too early in their annual cycle. This is somewhat in contrast to the research literature, where under-performance caused by overtraining/under-recovery tends to be closely associated with high volumes and/or densities of training rather than reduced volume and increased intensity [ 118 ].
Some track runners apply double periodization (i.e., two peaking phases), consisting of a preparation phase, an indoor or cross-country season, a new preparation phase, and finally an outdoor track competition season (typically lasting 3–4 months, starting in May and ending in September) [ 56 , 57 , 68 ]. However, most world-class track runners apply single periodization; they may participate in cross-country or indoor competitions during their preparation phase but use these competitions as part of their training. A review of the competition schedule for the athletes listed in Table 1 (based on their most successful year in an international championship) revealed that track runners participated in 9 ± 3 (mean ± SD) annual competitions, in which 6 ± 3 where outdoor races prior to OG or WC [ 119 ]. About half of the outdoor races were so-called “under-distances” (1500–3000 m), while the remaining half consisted of 5- or 10,000-m competitions. None of the analyzed track runners competed in “over-distances” (e.g., half-marathon) in the 3–4 preceding months leading up to the OG/WC. The last competition prior to OG/WC was performed 4 ± 2 weeks ahead, and 3 ± 2 additional competitions were performed in the subsequent 2–4 weeks after their most successful championship [ 119 ].
Marathon runners periodize their training year differently. The marathon runners listed in Table 1 participated in 6 ± 2 annual competitions in their most successful year, or ~ 50% fewer races than the track runners. These competitions were distributed across 2 ± 1 marathons (separated by at least 3 months), 1 ± 1 half-marathon(s), and 3 ± 3 races over 5–15 km [ 119 ]. Their last competition prior to OG/WC or a World Marathon Major was performed 10 ± 5 weeks ahead. Marathon runners typically apply double periodization centered around spring and autumn marathons, where the 7–14 days following the marathon competitions are completely training free or very easy [ 15 , 112 ]. The 5–6 preceding months leading up to a marathon are typically divided into general and specific preparation [ 40 , 41 , 42 , 52 , 53 , 54 ]. For track runners, the focus gradually shifts throughout the preparation period from achieving high total running volume to achieving more running volume at or near race pace. Progression is either based on extending the athlete’s accumulated session duration at a goal pace [ 40 , 41 ] or establishing high intensity volume and then slowly increasing pace [ 92 ]. Some marathon runners even apply a reverse linear periodization model, with the highest running volumes registered during the preceding weeks of the tapering phase periods as the competition is approaching [ 112 , 120 ].
The underlying mechanisms for the superiority of specific periodization models in LDR remain unclear, and there is no direct evidence enabling us to compare outcomes across various periodization methodologies [ 121 ]. Although scientific comparisons of different training approaches at a macro-level are challenging to perform, future studies should aim to verify and test the concepts developed by the best practitioners over the last decades.
Training Methods
The specific training methods for LDR consist of varying forms of continuous long runs and interval training (Table 2 ). These training methods bear different labels among practitioners, mainly depending on the intention/goal of the training. For example, “easy runs” are somewhat misguidedly termed “recovery runs” or “regeneration” by some coaches [ 40 , 41 ], assuming that their value is merely to “accelerate recovery” prior to the next hard session. No scientific studies to date support this assumption, but the feeling of recovery might be caused by the low load of such short easy runs, causing very little interference with the ongoing recovery process. However, accumulation of high frequency and volume of low-intensity training (LIT) is considered an important stimulus for inducing peripheral adaptations (e.g., increased mitochondrial biogenesis and capillary density of the skeletal muscle) [ 122 ]. Accumulated volume of low intensity running seems to be a characteristic of those with better running economy [ 123 , 124 ], and continuous running is probably most beneficial in stimulating these adaptations [ 125 ]. High volumes of LIT likely promote better “neural entrainment,” decrease movement variability, and reduce energy cost of movement [ 126 ].
The historical view is that, compared to a high frequency of LIT bouts, high-intensity training (HIT) stimulates central adaptations to a larger degree (e.g., increased stroke volume of the heart) [ 127 , 128 , 129 ]. However, in well-trained athletes that are performing a high total volume of training, further increases in \(\dot{\text{V}}\) O 2 max are not consistently observed after periods of increased HIT [ 130 , 131 , 132 ]. However, there is growing evidence that HIT better stimulates peripheral adaptations in fast-twitch motor units via an adenosine monophosphate (AMP) sensitive signaling pathway [ 133 , 134 ]. In sum, HIT and LIT seem to elicit a complex suite of overlapping and complementary adaptations [ 127 , 135 , 136 , 137 ], justifying the judicious application of varying training intensities for performance development in LDR. Further, it is overly simplistic to dichotomize the LDR training process into “high volume” and “high intensity” phases or training bouts. Whether discussing LIT or HIT, resulting adaptive signaling and stress responses can only be understood when the context of accumulated duration is added. Bill Bowerman, co-founder of Nike and US coach at the 1972 Olympics in Munich where Frank Shorter won the marathon, summarized his training philosophy as follows: 2–3 weekly interval sessions, a weekly long run, and fill the rest with as much LIT as you can handle [ 15 , 38 ]. This simple training description holds true for the training organization of most successful long-distance runners during the last 5 decades (see “ Intensity distribution ” section). However, while the interval sessions are considered “key” sessions for track runners, the training organization for marathoners is most often centered around their weekly “long runs.”
Several successful long-distance runners have supplemented their sport-specific training with alternative locomotion modalities, so-called cross-training, including swimming, biking, cross-country skiing, and workouts on elliptical machines [ 15 , 39 , 57 , 94 ]. Arguments supporting the inclusion of cross-training include injury prevention and avoidance of training monotony [ 138 , 139 ]. Because running is associated with lower total training duration and higher mechanical/ballistic load compared to other locomotion modalities [ 140 ], one could speculate if cross-training should be performed to a larger extent among highly trained long-distance runners to provide the same central and peripheral training stimulus with lower muscular mechanical load. Future long-term studies should aim to investigate the possible aerobic training effects of various types of cross-training.
Less specific training forms such as strength, power and plyometric training in small doses (relative to running training dosage) are commonly applied by world-leading long-distance runners [ 15 , 44 , 56 , 57 , 58 , 60 , 65 , 70 , 93 , 94 , 97 , 104 , 111 ]. Even though these training forms do not duplicate the holistic running movement, they likely target specific neuromuscular qualities that underlie running economy. A review of the results-proven practice shows that such supplementary training is typically implemented as a combination of (1) resistance training using free weights or apparatus (squats, cleans, lunges, step ups, leg press, etc.) without causing noteworthy hypertrophy, (2) circuit training with body mass resistance, (3) core strength/stability (e.g., sit-ups and back exercises), and (4) plyometrics in the form of vertical and/or horizontal multi-jumps on grass, inclines, stairs, hills (e.g., bounding, skipping, squat jumps) or jumping over hurdles [ 15 , 44 , 56 , 57 , 58 , 60 , 65 , 70 , 93 , 94 , 97 , 104 , 111 ]. Overall, this supplementary training is poorly described in terms of resistance loading, sets and repetitions, and caution must therefore be made when drawing conclusions. However, it appears that more strength, power and plyometric training are implemented during early-to-mid preparation (about twice a week) compared to the competition period (typically zero or one weekly session) [ 15 , 44 , 56 , 57 , 58 , 60 , 65 , 70 , 93 , 94 , 97 , 104 , 111 ]. Several studies have shown that strength, power and plyometric training 2–3 times per week can improve running economy in long-distance runners [ 11 , 29 , 141 , 142 , 143 ]. Paula Radcliffe improved her vertical jump performance from 29 to 38 cm between 1996 and 2003, a period where she improved her running economy and marathon performance considerably [ 5 ].
Training Volume
Most world-leading marathon runners train 500–700 h year −1 , while most corresponding track runners are in the range 450–600 h year −1 [ 15 , 40 , 41 , 42 , 43 , 54 , 73 , 76 , 79 , 87 , 94 ]. The relatively broad ranges in training volume are also present in other endurance sports [ 132 , 144 , 145 , 146 , 147 , 148 , 149 , 150 , 151 , 152 , 153 ] and are likely explained by individual differences in mechanical training load tolerance, intensity distribution, risk willingness, training age/career stage, application of cross-training, genetics and perhaps also psychological factors. The present training volume observations are in line with other studies of top-class long- and middle-distance athletes [ 19 , 20 , 21 , 27 , 28 , 34 ], but a larger proportion of middle-distance training is devoted to strength, power, and plyometric training (particularly in 800-m runners) [ 34 ]. Successful endurance athletes in cross-country skiing, biathlon, cycling, triathlon, swimming, and rowing train considerably more (800–1200 h per year) [ 132 , 144 , 145 , 146 , 147 , 148 , 149 , 150 , 151 , 152 , 153 ]. This is likely explained by the fact that LDR is a weight-bearing exercise where rapid plyometric muscle actions put high loads on muscles and tendons during each step. Accordingly, both total training volume and the duration of low-intensity sessions are relatively low for LDR compared to the other endurance sports [ 140 ]. To obtain a relatively high training volume, world-leading athletes seem to compensate by running twice a day most of the week [ 40 , 41 , 56 , 57 , 58 , 59 , 60 , 61 , 62 , 63 , 64 , 65 , 66 , 67 , 68 , 69 , 70 , 71 , 72 , 73 , 74 , 75 , 76 , 79 , 83 , 84 , 85 , 86 , 87 , 88 , 89 , 90 , 91 , 92 , 93 , 94 , 95 , 96 , 97 , 98 , 99 , 100 , 101 , 102 , 103 , 104 , 105 , 106 , 107 , 108 , 109 , 110 , 111 , 112 ].
Many long-distance runners accumulate much of their running kilometers on dirt roads/forest paths instead of paved roads to reduce mechanical loading and maximize training volume. This indicates that the running movement per se is not the main contributor to limited training tolerance, but rather the leg-surface interaction and resulting forces [ 140 ]. Running surface is a specific aspect of training periodization for marathoners. Because major marathons are performed exclusively on hard, paved roads, marathon specialists will build in continuous runs of increasing duration on asphalt or similar hard surfaces as they specifically prepare for these events [ 15 , 41 ].
A discussion of training volume and the constraints created by mechanical interactions between runner and running surface would be incomplete without mentioning running shoes. Recent developments on the footwear front have received massive attention in the LDR community. The “super-shoe” was introduced to road running in 2016 and to track running in 2019, chronologically coincident with a wave of LDR records. These shoes are now subject to strict guidelines and testing [ 154 ]. The footwear features behind these performance improvements include shoe weight, material composition, heel thickness, and bending stiffness, altogether improving running economy (and thereby performance) significantly [ 155 , 156 , 157 , 158 ]. Importantly in the context of LDR training, anecdotal evidence (i.e., our discussions with national-level distance runners) also suggests less muscle soreness and increased training tolerance with the recent shoe technology, altogether facilitating slightly increased running volume. Future studies should investigate how the current rapid development in shoe technology will affect LDR training characteristics.
While most scientific studies tend to only report training volume across macro- and mesocycles [e.g., 17 , 21 , 27 , 28 ], the results-proven practice describes more detailed fluctuations throughout the training year. Because most injuries are attributed to rapid and excessive increases in training load [ 159 , 160 ], elite performers increase the total running volume gradually during the initial 8–12 weeks of the macrocycle. The initial training week is performed with ~ 40–60% of peak weekly running volume, increasing by ~ 5–15 km each week until maximal volume is reached [ 62 , 63 , 90 , 94 , 95 , 100 , 103 ]. This volume progression is mainly achieved by increasing training frequency in the initial phase, then subsequently raised further by lengthening individual training sessions. Variations in training volume progression rate seem to depend on training experience and individual predispositions. The younger the training age, and the longer the transition period, the more careful progression from early to mid-preparation within the macrocycle.
Typical weekly running volume in the mid-preparation period is ~ 160–220 km for marathon runners [ 15 , 85 , 86 , 87 , 88 , 89 , 90 , 91 , 92 , 93 , 94 , 95 , 96 , 97 , 98 , 99 , 100 , 101 , 102 , 103 , 104 , 105 , 106 , 107 , 111 , 112 ] and 130–190 km for track runners [ 56 , 57 , 58 , 59 , 60 , 61 , 62 , 63 , 64 , 65 , 66 , 67 , 68 , 69 , 70 , 71 , 72 , 73 , 74 , 75 , 76 , 112 ], distributed across 11–14 sessions. Peak weekly volume can reach 20–30 km higher values for both groups, but only for short periods (2–3 weeks) of time. These wide ranges must be interpreted in the context of running intensity. Some marathon runners cover “only” 130–150 km wk −1 ; however, a considerably higher proportion of their volume (25–30%) is at or near marathon race pace, compared to others who cover 220–240 km wk −1 , with only 15–20% at or near marathon pace [ 85 , 86 , 87 , 88 , 89 , 90 , 91 , 92 , 93 , 94 , 95 , 96 , 97 , 98 , 99 , 100 , 101 , 102 , 103 , 104 , 105 , 106 , 107 , 112 ]. Training volume in elite LDR increases ≤ 8–10% annually in their late teens and early 20s, before slightly declining and stabilizing in their mid-20s [ 17 , 18 , 49 , 53 , 54 ]. The difference in volume between marathon and track runners is mainly explained by fewer running kilometers per session for track runners, as training frequency is equal for both groups. As shown in Table 2 , some long-run sessions for marathon runners may last up to 60 min longer compared to track runners.
One could argue that the ~ 10% slower running velocity in women [ 161 ] should be compensated for with less covered distance to ensure the same running duration between sexes. A counterargument is that men and women should apply equal distances during practice because they compete in the same disciplines [ 40 , 41 ]. We observed no sex differences in distance covered among the track runners in this study. The analyzed female marathon runners covered ~ 5% (~ 10 km) less distance but trained 30–40 min wk −1 longer than males [ 85 , 86 , 87 , 88 , 89 , 90 , 91 , 92 , 93 , 94 , 95 , 96 , 97 , 98 , 99 , 100 , 101 , 102 , 103 , 104 , 105 , 106 , 107 ]. We can only speculate if the longer training duration is to compensate for the less covered distance.
Overall, total running volume has remained relatively constant among world-leading long-distance runners since the 1950–1960s [ 15 , 46 , 47 , 48 , 78 , 80 , 81 , 82 ]. Some athletes have applied considerably higher volumes (≥ 300 km wk −1 ), seemingly experiencing more challenges related to injury management and fatigue [ 15 ]. Based upon both biomechanical and physiological factors, it is tempting to speculate that lighter athletes tolerate higher running volumes over time compared to their heavier counterparts. Assuming runners spend half the step cycle time on the ground, then the vertical forces exerted upon the ground must be twice the athlete’s body weight. Hence, the higher the body weight, the higher the impact forces during the landing phase. Moreover, slim runners possess superior thermodynamical conditions, as their sweat surface area to heat producing volume ratio increases with decreasing body size [ 162 ].
Intensity Zones
While training volume in endurance sports is straightforward to quantify, training intensity quantification is more complicated. The preponderance of scientific and results-proven practice recommends that intensity scales/zones/domains in LDR should be based on physiological parameters (e.g., heart rate ranges, ventilatory/lactate thresholds), external work rates (running pace or types of training), or perceived exertion [ 17 , 18 , 21 , 22 , 25 , 27 , 28 , 30 , 40 , 41 , 42 , 54 , 112 , 135 , 163 , 164 , 165 ], but no consensus has so far been established. We would argue that this lack of consensus is consistent with an uncomfortable truth; no single intensity parameter performs satisfactorily in isolation as an intensity guide due to (1) intensity–duration interactions and uncoupling of internal and external workload, (2) individual and day-to-day variation, and (3) strain responses that can carry over from preceding workouts and transiently disrupt these relationships [ 13 , 166 , 167 ]. Consequently, combining external load, internal load, and perception regularly during training provides a triangulation of intensity characteristics that is probably complimentary and informative. Whatever intensity parameter that is chosen, describing and comparing training characteristics requires a common intensity scale. To address this, we have developed both a 3- and 7-zone intensity model (Table 3 ). These are mainly anchored around race pace and reflect the practices of world-leading track and marathon runners. In this way, we can analyze their training logs in more detail. Compared to our previously developed intensity scale for 800/1500-m specialists [ 34 ], this version was deemed more representative because (1) lactate production sessions are rarely performed in LDR, (2) long-distance runners present lower blood lactate values within each intensity zone, and (3) long-distance runners exhibit less pronounced velocity declines with increasing training/repetition duration. Admittedly, presenting two “customized” intensity scales when there is overlap among middle- and long-distance performers may be provocative, but we argue that the present scale better reflects the nature of long-distance training. Indeed, standardized intensity zone systems are imperfect tools and have been criticized for several reasons [ 34 , 135 , 168 , 169 ]. However, the potential error sources seem to be outweighed by the improved communication between coach and athlete that a common scale facilitates [ 34 , 135 ]. The intensity scale outlined here (Table 3 ) can be used as a framework for both scientist and practitioners involved in LDR.
Endurance athletes employ varying methods of intensity distribution quantification. These are anchored around blood lactate ranges, running pace references, “time-in-zone” heart rate analysis calibrated against preliminary threshold testing, or the “session goal” approach where each training session is nominally allocated to an intensity zone based on the intensity of the main workout part [ 112 , 135 , 164 , 170 ]. The method of intensity quantification can affect the calculation of the intensity distribution [ 25 , 168 ]. Based on the nature of available results-proven practice [ 15 , 37 , 38 , 39 , 40 , 41 , 42 , 43 , 44 , 45 , 46 , 47 , 48 , 49 , 50 , 51 , 52 , 53 , 54 , 55 , 56 , 57 , 58 , 59 , 60 , 61 , 62 , 63 , 64 , 65 , 66 , 67 , 68 , 69 , 70 , 71 , 72 , 73 , 74 , 75 , 76 , 77 , 78 , 79 , 80 , 81 , 82 , 83 , 84 , 85 , 86 , 87 , 88 , 89 , 90 , 91 , 92 , 93 , 94 , 95 , 96 , 97 , 98 , 99 , 100 , 101 , 102 , 103 , 104 , 105 , 106 , 107 , 108 , 109 , 110 , 111 , 112 ], the time/distance-in-zone approach was applied in this review to assess the intensity distribution for the analyzed running sessions.
Intensity Distribution
The description of training intensity distribution in previous studies of long-distance runners can mainly be categorized into the following three models: (1) The pyramidal model, characterized by a large volume of LIT combined with a small volume of moderate-intensity training (MIT) and an even smaller volume of HIT, (2) the polarized model, where the same large volume of LIT is combined with less MIT and more HIT, and (3) the threshold model, where a relatively larger proportion of training is performed in the threshold intensity range demarcated by lactate/ventilatory thresholds 1 (LT1/VT1) and 2 LT2/VT2 [ 17 , 18 , 21 , 25 , 26 , 28 , 112 , 135 , 163 , 164 , 170 , 171 , 172 ]. Indeed, these intensity distribution definitions have been argued to be vague and inadequate, forming a basis for misinterpretations [ 173 , 174 ]. While previous studies have tended to focus on what model is most optimal for performance based on aggregated data for the entire training year [ 17 , 18 , 21 , 25 , 26 , 28 ], the results-proven practice shows that athletes adjust intensity distribution modestly across meso- and micro-cycles (see later paragraphs in this section). It should also be noted that both MIT- and HIT-training sessions are psychologically and physiologically demanding, requiring increased recovery time between blocks or sessions compared to training at lower intensity. In this context, training at “moderate” intensity is relatively more metabolically demanding in highly trained endurance athletes because they can run at a very high percentage of their v \(\dot{\text{V}}\) O 2 max during MIT-sessions [ 6 , 175 ].
The most consistent training intensity characteristic of elite distance runners is that most of the running distance (≥ 80%) is performed at low intensity throughout the training year (corresponding to zone 1 and 2 in our 7-zone scale) [ 15 , 37 , 38 , 39 , 40 , 41 , 42 , 43 , 44 , 45 , 46 , 47 , 48 , 49 , 50 , 51 , 52 , 53 , 54 , 55 , 56 , 57 , 58 , 59 , 60 , 61 , 62 , 63 , 64 , 65 , 66 , 67 , 68 , 69 , 70 , 71 , 72 , 73 , 74 , 75 , 76 , 77 , 78 , 79 , 80 , 81 , 82 , 83 , 84 , 85 , 86 , 87 , 88 , 89 , 90 , 91 , 92 , 93 , 94 , 95 , 96 , 97 , 98 , 99 , 100 , 101 , 102 , 103 , 104 , 105 , 106 , 107 , 108 , 109 , 110 , 111 , 112 ], in line with previous research [ 15 , 17 , 18 , 19 , 20 , 21 , 22 , 25 , 26 , 27 , 28 , 112 , 135 , 164 , 168 , 169 , 170 , 171 , 172 ]. Most of this training is in turn executed in zone 1, and the duration of the easy runs is very stable throughout the training year. Because zone 2 is closer to marathon pace, a higher proportion of zone 2 is applied by marathon specialists, particularly during the specific preparation period [ 40 , 41 , 85 , 86 , 87 , 88 , 89 , 90 , 91 , 92 , 93 , 94 , 95 , 96 , 97 , 100 ]. Weekly long runs are one of the most important sessions for marathon runners in this period [ 40 , 41 ], typically performed as 30–40 km runs slightly below marathon pace. In contrast, an increasingly higher proportion of LIT is performed in zone 1 for track runners as the competition season approaches [ 41 , 72 , 73 , 74 , 75 , 76 ].
Training in zone 3 (in the 6-zone scale) represents 5–15% of the total running volume in elite long-distance runners [ 15 , 37 , 38 , 39 , 40 , 41 , 42 , 43 , 44 , 45 , 46 , 47 , 48 , 49 , 50 , 51 , 52 , 53 , 54 , 55 , 56 , 57 , 58 , 59 , 60 , 61 , 62 , 63 , 64 , 65 , 66 , 67 , 68 , 69 , 70 , 71 , 72 , 73 , 74 , 75 , 76 , 77 , 78 , 79 , 80 , 81 , 82 , 83 , 84 , 85 , 86 , 87 , 88 , 89 , 90 , 91 , 92 , 93 , 94 , 95 , 96 , 97 , 98 , 99 , 100 , 101 , 102 , 103 , 104 , 105 , 106 , 107 , 108 , 109 , 110 , 111 , 112 ]. However, this proportion can vary across meso- and micro-cycles. There is a trend among marathon runners toward performing a higher proportion of zone-3 training as the major competition approaches [ 40 , 41 , 85 , 86 , 87 , 88 , 89 , 90 , 91 , 92 , 93 , 94 , 95 , 96 , 97 , 100 ]. Track runners seem to follow an opposite organization, as the highest amount of zone-3 training is performed in the early-to-mid preparation period, before decreasing when the competition season is nearing [ 41 , 60 , 72 , 73 , 74 , 75 , 76 ]. According to Casado et al. [ 17 , 18 ], tempo runs (continuous running in zone 2–3 in our model) account for ~ 20% of the total annual running volume in world-class Kenyan long-distance runners, corresponding well with observations of Billat et al. [ 20 ] and data compiled here.
Interval training in zone 4–5 also represents 5–15% of the total running volume, but this proportion is inversely related to zone 3-training. That is, marathon runners perform most training in zones 4–5 in the early-to-mid preparation period before replacing such training with more extensive bouts of zone-3 and upper end of zone-2 training as the major competition approaches [ 40 , 41 , 85 , 86 , 87 , 88 , 89 , 90 , 91 , 92 , 93 , 94 , 95 , 96 , 97 , 100 ]. In contrast, track runners increase the proportion of zone 4–5 training at the expense of zone 3 as the competition season approaches [ 41 , 60 , 72 , 73 , 74 , 75 , 76 ].
During the pre-competition and competition period, most world-class 5000-m runners perform 1–2 weekly interval training sessions in zone 6 or in combination with zone 5 [ 56 , 68 , 72 , 73 , 74 , 75 , 76 ]. These runners may perform 10–20 km weekly in zone 5–6 between May and August, while most marathoners avoid training with such high amounts of lactic/glycolytic energy release [ 40 , 41 , 85 , 86 , 87 , 88 , 89 , 90 , 91 , 92 , 93 , 94 , 95 , 96 , 97 , 100 ].
Distance runners perform sprint training (zone 7 in our model) regularly during the annual cycle, although this accounts for less than 1% of the total running volume [ 15 , 37 , 40 , 42 , 43 , 44 , 49 , 51 , 54 , 55 , 56 , 57 , 58 , 59 , 60 , 66 , 68 , 69 , 70 , 71 , 72 , 73 , 74 , 75 , 76 , 85 , 88 , 90 , 91 , 93 , 94 , 97 , 102 , 103 , 105 , 109 , 110 , 111 ]. Sprint training is considered a supplement rather than the main goal of separate training sessions and is typically performed during the last part of the warm-up or after easy long runs. It is generally assumed that sprint training should be performed without accumulation of fatigue (often indicated by increasing levels of blood lactate). The distances are most commonly in the range 60–120 m, with sufficient recovery between each repetition. Most sprint runs are performed with low to moderate rate of acceleration (i.e., strides, progressive runs, hills sprints, or flying sprints), likely because the energy demands during maximal acceleration greatly exceed those at peak velocity [ 176 ]. However, high amounts of endurance training limit the development of muscular power [ 177 , 178 ], and it is unrealistic to expect significant sprint performance development in elite long-distance runners. Hence, sprint training is mainly performed to minimize the negative impact of aerobic conditioning on maximal sprint speed.
In summary, the annual training intensity distribution is very similar for track runners and marathon specialists, as low intensity volume dominates. However, substantial differences may be present within each mesocycle. Both groups increase the volume of race-pace running as the main competition approaches. Table 4 contrasts case study examples of typical training weeks across the annual cycle for a track runner and marathon specialist.
Tapering in elite sports refers to the marked reduction of total training load prior to important competition(s). This is a short-term balancing act, as tapering strategies are intended to decrease the cumulative effects of fatigue while maintaining fitness [ 179 , 180 ]. Because tapering strategies and outcomes are heavily dependent on the preceding training load, it is often challenging to separate tapering from periodization and training programming in general. According to previous research, a successful taper may enhance competition performance in well-trained endurance athletes by ~ 1–3% [ 179 , 180 , 181 , 182 ]. However, this claim is challenging to verify in elite LDR, as numerous confounding external variables (race tactics/pacing, weather conditions, competitors, etc.) influence performance in many important competitions where runners compete for medals and not for the best possible time [ 183 , 184 , 185 ]. It has also been shown that outstanding performances across a 3-month competition period can be achieved, without tapering for a specific competition, by merely reducing the training substantially in the last 4–5 days prior to each competition [ 73 ].
In cases where major competitions are arranged in warm and/or humid cities, and perhaps also many time zones away from the athletes’ regular location, tapering is integrated with time-, heat-, and humidity-acclimatization processes. For more details related to these topics, we refer readers to previously published reviews [ 186 , 187 , 188 ].
The general scientific guidelines for effective tapering in endurance sports include a 2- to 3-week period with 40–60% reduction in training volume adopting a progressive nonlinear format, while training intensity and frequency are maintained [ 179 , 180 , 181 , 182 ]. However, most long-distance runners do not report a substantial decrease in training volume until the last 7–10 days prior to competition [ 61 , 69 , 74 , 75 , 85 , 86 , 87 , 88 , 89 , 90 , 91 , 92 , 93 , 94 , 95 , 97 ]. Table 5 presents training volume distribution across intensity zones for 10 world-class marathon runners during the countdown to a major competition.
A review of the competition schedule for the athletes listed in Table 1 (based on their most successful year in an international championship) revealed that the last competition was performed 10 ± 5 and 4 ± 2 weeks prior to the season’s main competition for marathon runners and track runners, respectively [ 119 ]. Arrival at the championship destination typically occurs 7–10 days ahead of competition [ 39 , 54 , 57 , 94 ]. The last intensive session (e.g., 10 × 200 m at race pace with optional recoveries) is typically performed 3–5 days ahead of the main championship event [ 40 , 61 , 74 , 75 , 100 ].
Altitude Training
The LDR community became aware of the impact of altitude on endurance performance in the late 1960s and particularly in connection with the 1968 Olympics in Mexico City (2300 m above sea level). Clearly, sufficient altitude acclimatization ahead of endurance competitions at altitudes 1000 m above sea level is required to perform optimally [ 189 , 190 ]. However, many athletes additionally use longer sojourns at altitude to enhance aerobic endurance capacity and thereby performance at sea level, mainly with the goal of increasing red blood cell mass [ 191 ]. Since 1968, > 90% of all OG/WC medals from the 800 m through the marathon have been won by athletes who have lived or systematically trained at altitude [ 9 , 15 , 103 ].
The potential effect of altitude training is influenced by the hypoxic dose, which is a function of the duration of the stay and the altitude [ 192 ]. Most world-class African runners apply the "live high—train high” model, as they live and carry out LIT-, MIT-, and HIT-sessions relatively high (2000–2500 m above sea level) [ 9 ]. Athletes from lowlands typically perform relatively long altitude camps during the preparation period and one camp 2–4 weeks prior to the most important competition, with most emphasis on LIT and MIT-sessions [ 57 , 85 , 100 , 103 , 111 ]. However, the optimal time of return from altitude camps to lowland competition is disputed [ 193 ] and warrants further investigations. The ability to train effectively at altitude may be one feature that distinguishes African runners from their European, American, and Asian competitors [ 9 ]. In all cases, successful use of altitude training by the best long-distance runners is characterized by individualized, well-balanced training load and optimized recovery strategies through adequate sleep, rest and nutritional factors as described in detail elsewhere [e.g., 19 , 194 ].
It has been questioned whether altitude training has positive effects on endurance capacity and sea-level performance beyond the effects achieved with similar training performed at sea level. Here, high-quality scientific evidence is limited, and researchers interpret the current scientific data differently [ 195 , 196 ]. Altitude training research is methodologically demanding due to the difficulty of standardizing the intervention, including control groups, and controlling other psychological and physiological confounders during altitude training. Although research provides limited support for a positive effect of altitude training on sea-level performance in endurance sports, these studies remain scarce and underpowered to detect the small adaptations that may be of importance in elite LDR. This is illustrated through the large individual differences in blood responses documented in connection with altitude training [ 197 ]. Consequently, a nuanced view on altitude training is warranted.
Conclusions
This review integrates the scientific literature and results-proven practice regarding the training and development of world-class LDR performance. Herein, we have outlined a framework for specific characteristics (i.e., training methods, volume, and intensity) and identified the training organization differences between track runners and marathon specialists. Marathon and track runners participate in 6 ± 2 and 9 ± 3 (mean ± SD) annual competitions, respectively. Typical weekly running volume in the mid-preparation period is in the range 160–220 km for marathon runners and 130–190 km for track runners. These differences are mainly explained by fewer running kilometers for each session for track runners, as training frequency (11–14 sessions per week) is equal for both groups. Moreover, ≥ 80% of total running distance is performed at low intensity throughout the training year. In the general preparation period, the focus is to build an aerobic foundation by a large total running volume. From the specific preparation period onward, the volume of race-pace running increases as the main competition approaches. Hence, training intensity distribution models vary across mesocycles and differ between marathon and track runners. While the African runners live and train at high altitude (2000–2500 m above sea level), most lowland athletes apply relatively long altitude camps during the preparation period. The tapering process starts 7–10 days prior to the main competition, typically preceded by a 2–4-week altitude camp. Overall, this review offers novel insights into areas of LDR training that formerly have been scarcely studied in the scientific literature, providing a point of departure for future studies and may serve as a position statement related to the training and development in the Olympic long-distance events.
Availability of Data and Materials
All data and materials support the published claims and comply with field standards.
Code Availability
Not applicable.
Costill DL. The relationship between selected physiological variables and distance running performance. J Sports Med Phys Fitness. 1967;7:61–6.
CAS PubMed Google Scholar
Costill DL. Metabolic responses during distance running. J Appl Physiol. 1970;28:251–5.
Article CAS PubMed Google Scholar
Joyner MJ. Modeling: optimal marathon performance on the basis of physiological factors. J Appl Physiol. 1991;70:683–7.
Joyner M, Coyle EF. Endurance performances: the physiology of champions. J Physiol. 2008;586:35–44.
Jones AM. The physiology of the world record holder for the women’s marathon. Int J Sports Sci Coach. 2006;1:101–16.
Article Google Scholar
Jones AM, Kirby BS, Clark IE, Rice HM, Fulkerson E, Wylie LJ, Wilkerson DP, Vanhatalo A, Wilkins BW. Physiological demands of running at 2-hour marathon race pace. J Appl Physiol. 2021;130:369–79.
Barnes KR, Kilding AE. Running economy: measurement, norms, and determining factors. Sports Med Open. 2015;1:8.
Article PubMed PubMed Central Google Scholar
Larsen HB, Sheel AW. The Kenyan runners. Scand J Med Sci Sports. 2015;25:110–8.
Article PubMed Google Scholar
Wilber RL, Pitsiladis YP. Kenyan and Ethiopian distance runners: What makes them so good? Int J Sports Physiol Perform. 2012;7:92–102.
Joyner MJ, Hunter SK, Lucia A, Jones AM. Physiology and fast marathons. J Appl Physiol. 2020;128:1065–8.
Barnes KR, Kilding AE. Strategies to improve running economy. Sports Med. 2015;45:37–56.
Kirby BS, Winn BJ, Wilkins BW, Jones AM. Interaction of exercise bioenergetics with pacing behavior predicts track distance running performance. J Appl Physiol. 2021;131:1532–42.
Maunder E, Seiler S, Mildenhall MJ, Kilding AE, Plews DJ. The Importance of “durability” in the physiological profiling of endurance athletes. Sports Med. 2021;51:1619–28.
Clark IE, Vanhatalo A, Thompson C, Joseph C, Black MI, Blackwell JR, et al. Dynamics of the power-duration relationship during prolonged endurance exercise and influence of carbohydrate ingestion. J Appl Physiol. 2019;127:726–36.
Sandrock M. Running with the legends: training and racing insights from 21 great runners. Champaign: Human Kinetics; 1996.
Google Scholar
Tjelta LI. Three Norwegian brothers all European 1500 m champions: What is the secret? Int J Sport Sci Coach. 2019;14:694–700.
Casado A, Hanley B, Santos-Concejero J, Ruiz-Pérez LM. World-class long-distance running performances are best predicted by volume of easy runs and deliberate practice of short-interval and tempo runs. J Strength Cond Res. 2019 [Online ahead of print].
Casado A, Hanley B, Ruiz-Pérez LM. Deliberate practice in training differentiates the best Kenyan and Spanish long-distance runners. Eur J Sport Sci. 2020;20:887–95.
Billat VL, Demarle A, Slawinski J, Paiva M, Koralsztein JP. Physical and training characteristics of top-class marathon runners. Med Sci Sports Exerc. 2001;33:2089–97.
Billat V, Lepretre PM, Heugas AM, Laurence MH, Salim D, Koralsztein JP. Training and bioenergetic characteristics in elite male and female Kenyan runners. Med Sci Sports Exerc. 2003;35:297–304.
Tjelta LI. The training of international level distance runners. Int J Sports Sci Coach. 2016;11:122–34.
Stellingwerff T. Case study: nutrition and training periodization in three elite marathon runners. Int J Sport Nutr Exerc Metab. 2012;22:392–400.
Spilsbury KL, Fudge BW, Ingham SA, Faulkner SH, Nimmo MA. Tapering strategies in elite British endurance runners. Eur J Sport Sci. 2015;15:367–73.
Lucia A, Esteve-Lanao J, Oliván J, Gómez-Gallego F, San Juan AF, Santiago C, Pérez M, Chamorro-Viña C, Foster C. Physiological characteristics of the best Eritrean runners-exceptional running economy. Appl Physiol Nutr Metab. 2006;31:530–40.
Kenneally M, Casado A, Gomez-Ezeiza J, Santos-Concejero J. Training intensity distribution analysis by race pace vs. physiological approach in world-class middle- and long-distance runners. Eur J Sport Sci. 2020;20:1–8.
Kenneally M, Casado A, Gomez-Ezeiza J, Santos-Concejero J. Training characteristics of a World Championship 5000-m finalist and multiple continental record holder over the year leading to a World Championship final. Int J Sports Physiol Perform. 2021. [Online ahead of print]
Tjelta LI, Tønnessen E, Enoksen E. Case study of the training of nine times New York marathon winner Grete Waitz. Int J Sports Sci Coach. 2014;9:139–57.
Enoksen E, Tjelta AR, Tjelta LI. Distribution of training volume and intensity of elite male and female track and marathon runners. Int J Sports Sci Coach. 2011;6:273–93.
Balsalobre-Fernández C, Santos-Concejero J, Grivas GV. Effects of strength training on running economy in highly trained runners: a systematic review with meta-analysis of controlled trials. J Strength Cond Res. 2016;30:2361–8.
Billat LV. Interval training for performance: a scientific and empirical practice. Special recommendations for middle- and long-distance running. Part I: aerobic interval training. Sports Med. 2001;31:13–31.
Billat LV. Interval training for performance: a scientific and empirical practice. Special recommendations for middle- and long-distance running. Part II: anaerobic interval training. Sports Med. 2001;31:75–90.
Midgley AW, McNaughton LR, Jones AM. Training to enhance the physiological determinants of long-distance running performance: Can valid recommendations be given to runners and coaches based on current scientific knowledge? Sports Med. 2007;37:857–80.
Haugen T, Seiler S, Sandbakk Ø, Tønnessen E. The training and development of elite sprint performance: an integration of scientific and best practice literature. Sports Med Open. 2019;5:44.
Haugen T, Sandbakk Ø, Enoksen E, Seiler S, Tønnessen E. Crossing the golden training divide: the science and practice of training world-class 800- and 1500-m runners. Sports Med. 2021;51:1835–54.
Haugen T. Key success factors for merging sport science and best practice. Int J Sports Physiol Perform. 2019;15:297.
Haugen T. Best-practice coaches: an untapped resource in sport-science research. Int J Sports Physiol Perform. 2021;16:1215–6.
Nic Bideau. Coaching middle and long distance runners: A commentary. https://beaconhillstriders.co.uk/wp-content/uploads/2015/05/M-L-Distance-Training-Nic-Bideau.pdf . Assessed 1 Sept 2021.
Bowerman B. High performance training for track and field. Champaign: Leisure Press; 1991.
Antonio Cabral. Marathon training—Portuguese style. https://www.runnerprogram.com/product/portuguese-marathon-training-style-antonio-cabral/ . Assessed 1 Sept 2021.
Arcelli E, Canova R. Marathon training: a scientific approach. London: International Athletic Foundation; 1999.
The complete Renato Canova coaching collection. https://www.runnerprogram.com/product/the-complete-renato-canova-coaching-collection/ . Assessed 1 Sept 2021.
Daniels J. Daniel’s running formula. 3rd ed. Champaign: Human Kinetics; 2013.
Davis J. Modern training and physiology for middle and long-distance runners. Philadelphia: Running Writings; 2013.
Brad Hudson training system. https://www.runnerprogram.com/product/brad-hudson-training-system/ . Assessed 1 Sept 2021.
Mihaly Igloi training system. https://www.runnerprogram.com/product/mihaly-igloi-training-system-by-bob-schul/ . Assessed 1 Sept 2021.
Lydiard A, Gilmour G. Running to the top. Oxford: Meyer & Meyer; 1997.
Lydiard A. Running with Lydiard: greatest running coach of all time. 3rd ed. Oxford: Meyer & Meyer Sport; 2017.
Livingstone K. Healthy intelligent training: the proven principles of Arthur Lydiard. Aachen: Meyer & Meyer Fachverlag und Buchhandel GmbH; 2010.
Steve Magness training philosophy. https://www.runnerprogram.com/product/steve-magness-training-philosophy/ . Assessed 1 Sept 2021.
Kim McDonald training system. https://www.runnerprogram.com/product/kim-mcdonald-training-system/ . Assessed 1 Sept 2021.
Terrence Mahon training philosophy. https://www.runnerprogram.com/product/terrence-mahon-training-philosophy/ . Assessed September 1 st 2021.
Gabriele Rosa Marathon training philosophy. https://www.runnerprogram.com/product/gabriele-rosa-training-philosophy/ . Assessed 1 Sept 2021.
Joe Vigil training philosophy. https://www.runnerprogram.com/product/joe-vigil-training-philosophy/ . Assessed September 1 st 2021.
Vigil JI. Road to the top: a systematic approach to training distance runners. 1st ed. Overland Park: Morning Star Communications; 1995.
Chris Wardlaws training system. https://www.runnerprogram.com/product/chris-wardlaws-training-system/ . Assessed 1 Sept 2021.
Said Aouita training program. https://www.runnerprogram.com/product/said-aouita-training-program/ . Assessed 1 Sept 2021.
Dieter Baumann training. https://www.runnerprogram.com/product/dieter-baumann-training-by-isabelle-baumann/ . Assessed 1 Sept 2021.
The making of Joshua Cheptegei and training insights of the Ugandan team. https://www.sweatelite.co/making-joshua-cheptegei-training-insights-ugandan-team-part1/ and https://www.sweatelite.co/making-joshua-cheptegei-training-insights-ugandan-team-part2/ . Assessed September 1 st 2021.
Haile Gebrselassie training. https://runningscience.co.za/elite-athletes-training-log/haile-gebrselassie/ . Assessed 1 Sept 2021.
Bob Kennedy training. https://www.runnerprogram.com/product/bob-kennedy-5000m-training-before-atlanta-og-96/ . Assessed 1 Sept 2021.
Sylvia Kibet training program. https://www.runnerprogram.com/product/sylvia-kibet-training-program-by-renato-canova/ . Assessed 1 Sept 2021.
Florence Kiplagat training program. https://www.runnerprogram.com/product/florence-kiplagat-training-program-renato-canova/ . Assessed 1 Sept 2021.
Susanne Wigene training. https://www.letsrun.com/forum/flat_read.php?thread=948751&page=2 . Assessed 1 Sept 2021.
Sonia O’Sullivan training program. https://www.runnerprogram.com/product/sonia-osullivan-training-program/ . Assessed 1 Sept 2021.
Sifan Hassan training program. https://www.runnerprogram.com/product/sifan-hassan-training-by-honore-hoedt/ . Assessed 1 Sept 2021.
Andy Vernon training. https://runningscience.co.za/elite-athletes-training-log/andy-vernon/ . Assessed 1 Sept 2021.
David Moorcroft training program. https://www.runnerprogram.com/product/david-moorcroft-training-program/ . Assessed 1 Sept 2021.
Bernard Lagat training. https://www.runnerprogram.com/product/james-li-training-bernard-lagat/ . Assessed 1 Sept 2021.
Craig Mottram training program. https://www.runnerprogram.com/product/craig-mottram-training-program/ . Assessed 1 Sept 2021.
Paul Tergat training program. https://www.runnerprogram.com/product/paul-tergat-training-program/ . Assessed 1 Sept 2021.
Caleb Ndiku training program. https://www.runnerprogram.com/product/caleb-ndiku-training-program-by-renato-canova/ . Assessed 1 Sept 2021.
Yobes Ondieki training program. https://www.runnerprogram.com/product/yobes-ondieki-training-program/ . Assessed 1 Sept 2021.
Daniel Komen training program. https://www.runnerprogram.com/product/daniel-komen-training-program/ . Assessed 1 Sept 2021.
Thomas Longosiwa training program. https://www.runnerprogram.com/product/thomas-longosiwa-training-program-by-renato-canova/ . Assessed 1 Sept 2021.
Imane Merga training program. https://www.runnerprogram.com/product/imane-merga-training-program-renato-canova/ . Assessed 1 Sept 2021.
Stephen Cherono training program. https://www.runnerprogram.com/product/stephen-cherono-training-program-renato-canova/ . Assessed 1 Sept 2021.
Kip Keino training. https://runningscience.co.za/elite-athletes-training-log/kipchoge-keino/ . Assessed 1 Sept 2021.
Brendan Foster training program. https://www.runnerprogram.com/product/brendan-foster-training-program/ . Assessed 1 Sept 2021.
Ingrid Kristiansen training. https://www.runnerprogram.com/product/ingrid-kristiansen-training-1986/ . Assessed 1 Sept 2021.
Jim Peters training. https://runningscience.co.za/elite-athletes-training-log/jim-peters/ . Assessed 1 Sept 2021.
Gordon Pirie training. https://www.runnerprogram.com/product/gordon-pirie/ . Assessed 1 Sept 2021.
Ian Stewart training program. https://www.runnerprogram.com/product/ian-stewart-training-program/ . Assessed 1 Sept 2021.
Lasse Viren training. https://www.runnerprogram.com/product/lasse-viren-training-by-rolf-haikkola/ . Assessed 1 Sept 2021.
Grete Waitz training program. https://www.runnerprogram.com/product/grete-waitz-training-program/ . Assessed 1 Sept 2021.
Stefano Baldini training log. https://runningscience.co.za/elite-athletes-training-log/stefano-baldini/ . Assessed 1 Sept 2021.
Gelindo Bordin training log before winning the Olympic Games in Seoul. https://www.runnerprogram.com/product/gelindo-bordin-training-log-before-winning-the-olympic-games-in-seoul/ . Assessed 1 Sept 2021.
Takayuki Inubushi Marathon training. https://www.runnerprogram.com/product/takayuki-inubushi-marathon-training/ . Assessed 1 Sept 2021.
Moses Mosop training log. https://runningscience.co.za/elite-athletes-training-log/moses-mosop/ . Assessed 1 Sept 2021.
Geoffrey Mutai training log. https://www.letsrun.com/forum/flat_read.php?thread=8973493 . Assessed 1 Sept 2021.
Charlie Spedding training log. https://www.runnerprogram.com/product/charlie-spedding-training-log/ . Assessed 1 Sept 2021.
Abel Kirui training. https://www.runnerprogram.com/product/abel-kirui-training-winning-world-championship-gold-renato-canova/ . Assessed 1 Sept 2021.
Eliud Kipchoge training program. https://www.runnerprogram.com/product/eliud-kipchoge-training-programs/ . Assessed 1 Sept 2021.
Mubarak Hassan Shami training. https://www.runnerprogram.com/product/mubarak-hassan-shami-marathon-training/ . Assessed 1 Sept 2021.
Meb Keflezighi training. https://www.runnerprogram.com/product/meb-mortals-run-think-eat-like-champion - marathoner/. Assessed 1 Sept 2021.
Greg Meyer training log. https://runningscience.co.za/elite-athletes-training-log/greg-meyer/ . Assessed 1 Sept 2021.
Steve Jones training program. https://www.runnerprogram.com/product/steve-jones-training-program/ . Assessed 1 Sept 2021.
Kenenisa Bekele’s training. https://www.sweatelite.co/kenenisa-bekeles-training/ . Assessed 1 Sept 2021.
Robert de Castella training program. https://www.runnerprogram.com/product/robert-de-castella-training-program/ . Assessed 1 Sept 2021.
Constantina Dita training program. https://www.runnerprogram.com/product/constantina-dita-training-program/ . Assessed 1 Sept 2021.
Molly Seidel - Strava pro runner profile. https://www.strava.com/pros/bygollymolly . Assessed 1 Sept 2021.
Joyciline Jepkosgei training. https://runningscience.co.za/elite-athletes-training-log/joyciline-jepkosgei/ . Assessed 1 Sept 2021.
Tegla Lourope training program. https://www.runnerprogram.com/product/tegla-loroupe-marathon-training-program/ . Assessed 1 Sept 2021.
Deena Kastor training program. https://www.runnerprogram.com/product/deena-kastor-training-program/ . Assessed 1 Sept 2021.
Lisa Martin training. https://www.runnerstribe.com/expert-advice/training-of-famous-runners/lisa-ondieki-track-and-mileage-to-the-extreme/ . Assessed 1 Sept 2021.
Paula Radcliffe training program. https://www.runnerprogram.com/product/paula-radcliffe-training-program/ . Assessed 1 Sept 2021.
Bill Rodgers training log. https://www.runnerprogram.com/product/bill-rodgers-1977-training-log/ . Assessed 1 Sept 2021.
Toshihiko Seko training log. https://www.runnerprogram.com/product/toshihiko-seko-training-log-1977-1978/ . Assessed 1 Sept 2021.
Brigid Kosgei training. https://www.sweatelite.co/kenyan-elite-training-series-brigid-kosgei-6428-half-marathon-21820-marathon/ . Assessed 1 Sept 2021.
Paul Kosgei training log. https://runningscience.co.za/elite-athletes-training-log/paul-kosgei/ . Assessed 1 Sept 2021.
Rodgers Rop training log. https://www.runnerprogram.com/product/rodgers-rop-training-log/ . Assessed 1 Sept 2021.
Wilson Kipsang—4th fastest marathoner ever—training program. https://www.sweatelite.co/wilson-kipsang-4th-fastest-marathoner-ever-training-program/ . Assessed 1 Sept 2021.
Casado A, Tjelta LI. Training volume and intensity distribution among elite middle- and long-distance runners. In: Blagrove RC, Hayes PR, editors. The science and practice of middle and long distance running. London: Routledge; 2021.
Sylta Ø, Tønnessen E, Seiler S. Do elite endurance athletes report their training accurately? Int J Sports Physiol Perform. 2014;9:85–92.
Faiss R, Saugy J, Zollinger A, Robinson N, Schuetz F, Saugy M, Garnier PY. Prevalence estimate of blood doping in elite track and field athletes during two major international events. Front Physiol. 2020;25(11):160.
Ulrich R, Pope HG Jr, Cléret L, Petróczi A, Nepusz T, Schaffer J, et al. Doping in two elite athletics competitions assessed by randomized-response surveys. Sports Med. 2018;48:211–9.
Filipas L, Bonato M, Gallo G, Codella R. Effects of 16 weeks of pyramidal and polarized training intensity distributions in well-trained endurance runners. Scand J Med Sci Sports. 2022;32:498–511.
Matveyev LP. Periodisierung des sportlichen trainings. 2nd ed. Berlin: Bartels & Wernitz; 1975.
Meeusen R, Duclos M, Foster C, Fry A, Gleeson M, Nieman D, et al. Prevention, diagnosis, and treatment of the overtraining syndrome: joint consensus statement of the European College of Sport Science and the American College of Sports Medicine. Med Sci Sports Exerc. 2013;45:186–205.
World Athletics athlete search. https://www.worldathletics.org/athletes . Assessed 1 Sept 2021.
Kenneally M, Casado A, Santos-Concejero J. The effect of periodization and training intensity distribution on middle-and long-distance running performance: a systematic review. Int J Sports Physiol Perform. 2018;13:1114–21.
Kiely J. Periodization paradigms in the 21st century: evidence-led or tradition-driven? Int J Sports Physiol Perform. 2012;7:242–50.
Bishop D, Botella J, Grantha C. CrossTalk opposing view: exercise training volume is more important than training intensity to promote increases in mitochondrial content. J Physiol. 2019;597:4115–8.
Morgan DW, Bransford DR, Costill DL, Daniels JT, Howley ET, Krahenbuhl GS. Variation in the aerobic demand of running among trained and untrained subjects. Med Sci Sports Exerc. 1995;27:404–9.
Nelson RC, Gregor RJ. Biomechanics of distance running: a longitudinal study. Res Q. 1976;47:417–28.
González-Mohíno F, Santos-Concejero J, Yustres I, González-Ravé JM. The effects of interval and continuous training on the oxygen cost of running in recreational runners: a systematic review and meta-analysis. Sports Med. 2020;50:283–94.
Chapman AR, Vicenzino B, Blanch P, Hodges PW. Is running less skilled in triathletes than runners matched for running training history? Med Sci Sports Exerc. 2008;40:557–65.
Laursen PB. Training for intense exercise performance: High-intensity or high-volume training? Scand J Med Sci Sports. 2010;20:1–10.
Hughes DC, Ellefsen S, Baar K. Adaptations to endurance and strength training. Cold Spring Harb Perspect Med. 2018;8:a029769.
Helgerud J, Høydal K, Wang E, Karlsen T, Berg P, Bjerkaas M, et al. Aerobic high-intensity intervals improve VO2max more than moderate training. Med Sci Sports Exerc. 2007;39:665671.
Losnegaard T, Myklebust H, Spencer M, Hallen J. Seasonal variations in VO 2max , O 2 -cost, O 2 -deficit, and performance in elite cross-country skiers. J Strength Cond Res. 2013;27:1780–90.
Jones AM. A five-year physiological case study of an Olympic runner. Br J Sports Med. 1998;32:39–43.
Article CAS PubMed PubMed Central Google Scholar
Zapico AG, Calderón FJ, Benito PJ, González CB, Parisi A, Pigozzi F, Di Salvo V. Evolution of physiological and haematological parameters with training load in elite male road cyclists: a longitudinal study. J Sports Med Phys Fitness. 2007;47:191–6.
Van der Zaaard S, Brocherie F, Jaspers RT. Under the hood: Skeletal muscle determinants of endurance performance. Front Physiol. 2021;7:19434.
MacInnis MJ, Skelly LE, Gibala MJ. CrossTalk proposal: exercise training intensity is more important than volume to promote increases in human skeletal muscle mitochondrial content. J Physiol. 2019;597:4111–3.
Seiler S. What is best practice for training intensity and duration distribution in endurance athletes? Int J Sports Physiol Perform. 2010;5:276–91.
Talsnes RK, van den Tillaar R, Sandbakk Ø. Effects of increased load of low- versus high-intensity endurance training on performance and physiological adaptations in endurance athletes. Int J Sports Physiol Perf. 2021 [Online ahead of print]
Buchheit M, Laursen PB. High-intensity interval training, solutions to the programming puzzle. Part II: anaerobic energy, neuromuscular load and practical applications. Sports Med. 2013;43:927–54.
Loy SF, Hoffmann JJ, Holland GJ. Benefits and practical use of cross-training in sports. Sports Med. 1995;19:1–8.
Foster C. Monitoring training in athletes with reference to overtraining syndrome. Med Sci Sports Exerc. 1998;30:1164–8.
Sandbakk Ø, Haugen T, Ettema G. The influence of exercise modality on training load management. Int J Sports Physiol Perform. 2021;16:605–8.
Rønnestad BR, Mujika I. Optimizing strength training for running and cycling endurance performance: a review. Scand J Med Sci Sports. 2014;24:603–12.
Blagrove RC, Howatson G, Hayes PR. Effects of strength training on the physiological determinants of middle- and long-distance running performance: a systematic review. Sports Med. 2018;48:1117–49.
Berryman N, Mujika I, Arvisais D, Roubeix M, Binet C, Bosquet L. Strength training for middle- and long-distance performance: a meta-analysis. Int J Sports Physiol Perform. 2018;13:57–63.
Fiskerstrand A, Seiler KS. Training and performance characteristics among Norwegian international rowers 1970–2001. Scand J Med Sci Sports. 2004;14:303–10.
Tønnessen E, Sylta Ø, Haugen T, Hem E, Svendsen I, Seiler S. The road to gold: training and peaking characteristics in the year prior to a gold medal endurance performance. PLoS ONE. 2014;9:e101796.
Solli GS, Tønnessen E, Sandbakk Ø. The training characteristics of the world’s most successful female cross-country skier. Front Physiol. 2017;8:1069.
Sandbakk Ø, Holmberg HC. Physiological capacity and training routines of elite cross-country skiers: approaching the upper limits of human endurance. Int J Sports Physiol Perform. 2017;12:1003–11.
Schumacher OY, Mueller P. The 4000-m team pursuit cycling world record: theoretical and practical aspects. Med Sci Sports Exerc. 2002;34:1029–36.
Gao J. A study on pre-game training characteristics of Chinese elite swimmers. J Beijing Sport Univ. 2008;31:832–4.
Siewierski M. Volume and structure of training loads of top swimmers in direct starting preparation phase for main competition. Pol J Sport Tour. 2010;17:227–32.
Guellich A, Seiler S, Emrich E. Training methods and intensity distribution of young world-class rowers. Int J Sports Physiol Perform. 2009;4:448–60.
Neal CM, Hunter AM, Galloway SD. A 6-month analysis of training intensity distribution and physiological adaptation in Ironman triathletes. J Sports Sci. 2009;29:1515–23.
Mujika I. Olympic preparation of a world-class female triathlete. Int J Sports Physiol Perform. 2014;9:727–31.
World Athletics. Athletic shoe regulations. https://www.worldathletics.org/news/press-releases/new-athletic-shoe-regulations-approved-2022 . Assessed February 28.
Barnes KR, Kilding AE. A randomized crossover study investigating the running economy of highly-trained male and female distance runners in marathon racing shoes versus track spikes. Sports Med. 2019;49:331–42.
Hunter I, McLeod A, Valentine D, Low T, Ward J, Hager R. Running economy, mechanics, and marathon racing shoes. J Sports Sci. 2019;37:2367–73.
Dyer B. A pragmatic approach to resolving technological unfairness: the case of Nike’s Vaporfly and Alphafly running footwear. Sports Med Open. 2020;6:21.
Hébert-Losier K, Finlayson SJ, Driller MW, Dubois B, Esculier JF, Beaven CM. Metabolic and performance responses of male runners wearing 3 types of footwear: Nike Vaporfly 4%, Saucony Endorphin racing flats, and their own shoes. J Sport Health Sci. 2020 [Online ahead of print].
Bertelsen ML, Hulme A, Petersen J, Brund RK, Sørensen H, Finch CF, Parner ET, Nielsen RO. A framework for the etiology of running-related injuries. Scand J Med Sci Sports. 2017;27:1170–80.
Videbaek S, Bueno AM, Nielsen RO, Rasmussen S. Incidence of running-related injuries per 1000 h of running in different types of runners: a systematic review and meta-analysis. Sports Med. 2015;45:1017–26.
Sandbakk Ø, Solli GS, Holmberg HC. Sex differences in world-record performance: the influence of sport discipline and competition duration. Int J Sports Physiol Perform. 2018;13:2–8.
Notley SR, Lamarche DT, Meade RD, Flouris AD, Kenny GP. Revisiting the influence of individual factors on heat exchange during exercise in dry heat using direct calorimetry. Exp Physiol. 2019;104:1038–50.
Seiler KS, Kjerland GØ. Quantifying training intensity distribution in elite endurance athletes: Is there evidence for an “optimal” distribution? Scand J Med Sci Sport. 2006;16:49–56.
Seiler S, Tønnessen E. Intervals, thresholds, and long slow distance: the role of intensity and duration in endurance training. Sportscience. 2009;13:32–53.
Tønnessen E, Svendsen I, Rønnestad B, Hisdal J, Haugen T, Seiler S. The annual training periodization of 8 World Champions in orienteering. Int J Sports Physiol Perform. 2015;10:29–38.
Mann T, Lamberts RP, Lambert MI. Methods of prescribing relative exercise intensity: physiological and practical considerations. Sports Med. 2013;43:613–25.
Le Meur Y, Pichon A, Schaal K, Schmitt L, Louis J, Gueneron J, Vidal PP, Hausswirth C. Evidence of parasympathetic hyperactivity in functionally overreached athletes. Med Sci Sports Exerc. 2013;45:2061–71.
Bellinger P, Arnold B, Minahan C. Quantifying the training-intensity distribution in middle-distance runners: The influence of different methods of training-intensity quantification. Int J Sports Physiol Perform. 2019 [Online ahead of print].
Jamnick NA, Pettitt RW, Granata C, Pyne DB, Bishop DJ. An examination and critique of current methods to determine exercise intensity. Sports Med. 2020;50:1729–56.
Stöggl TL, Sperlich B. The training intensity distribution among well-trained and elite endurance athletes. Front Physiol. 2015;6:295.
Rosenblat MA, Perrotta AS, Vicenzino B. Polarized vs. threshold training intensity distribution on endurance sport performance: a systematic review and meta-analysis of randomized controlled trials. J Strength Cond Res. 2018;33:3491–500.
Kenneally M, Casado A, Santos-Concejero J. The effect of periodization and training intensity distribution on middle- and long-distance running performance: a systematic review. Int J Sports Physiol Perform. 2018;13:1114–21.
Foster C, Casado A, Esteve-Lanao J, Haugen T, Seiler S. Polarized training is optimal for endurance athletes. Med Sci Sports Exerc. 2022 [Online ahead of print].
Burnley M, Bearden SE, Jones AM. Polarized training is not optimal for endurance athletes. Med Sci Sports Exerc. 2022 [Online ahead of print].
Seiler S, Haugen O, Kuffel E. Autonomic recovery after exercise in trained athletes: intensity and duration effects. Med Sci Sports Exerc. 2007;39:1366–73.
di Prampero PE, Fusi S, Sepulcri L, Morin JB, Belli A, Antonutto G. Sprint running: a new energetic approach. J Exp Biol. 2005;208:2809–16.
Wilson JM, Marin PJ, Rhea MR, Wilson SM, Loenneke JP, Anderson JC. Concurrent training: a meta-analysis examining interference of aerobic and resistance exercises. J Strength Cond Res. 2012;26:2293–307.
Nader GA. Concurrent strength and endurance training: from molecules to man. Med Sci Sports Exerc. 2006;38:1965–70.
Mujika I, Padilla S. Scientific bases for precompetition tapering strategies. Med Sci Sports Exerc. 2003;35:1182–7.
Pyne DB, Mujika I, Reilly T. Peaking for optimal performance: Research limitations and future directions. J Sports Sci. 2009;27:195–202.
Mujika I. Intense training: the key to optimal performance before and during the taper. Scand J Med Sci Sports. 2010;20:24–31.
Bosquet L, Montpetit J, Arvisais D, Mujika I. Effects of tapering on performance: a meta-analysis. Med Sci Sports Exerc. 2007;39:1358–65.
Hanley B. Pacing, packing and sex-based differences in Olympic and IAAF World Championship marathons. J Sports Sci. 2016;34:1675–81.
Hanley B, Hettinga FJ. Champions are racers, not pacers: an analysis of qualification patterns of Olympic and IAAF World Championship middle distance runners. J Sports Sci. 2018;36:2614–20.
Casado A, Hanley B, Jiménez-Reyes P, Renfree A. Pacing profiles and tactical behaviors of elite runners. J Sport Health Sci. 2020;S2095–2546(20):30077–86.
Forbes-Robertson S, Dudley E, Vadgama P, Cook C, Drawer S, Kilduff L, et al. Circadian disruption and remedial interventions: effects and interventions for jet lag for athletic peak performance. Sports Med. 2012;42:185–208.
Racinais S, Alonso JM, Coutts AJ, Flouris AD, Girard O, González-Alonso, et al. Consensus recommendations on training and competing in the heat. Br J Sports Med. 2015;49:1164–73.
Racinais S, Casa D, Brocherie F, Ihsan M. Translating science into practice: the perspective of the Doha 2019 IAAF World Championships in the heat. Front Sports Act Living. 2019;1:39.
Burtscher M, Niedermeier M, Burtscher J, Pesta D, Suchy J, Strasser B. Preparation for endurance competitions at altitude: physiological, psychological, dietary and coaching aspects. A narrative review. Front Physiol. 2018;9:1504.
Chapman RF, Laymon AS, Levine BD. Timing of arrival and pre-acclimatization strategies for the endurance athlete competing at moderate to high altitudes. High Alt Med Biol. 2013;14:319–24.
Mujika I, Sharma AP, Stellingwerff T. Contemporary periodization of altitude training for elite endurance athletes: a narrative review. Sports Med. 2019;49:1651–69.
Chapman RF, Karlsen T, Resaland GK, Ge RL, Harber MP, Witkowski S, et al. Defining the “dose” of altitude training: how high to live for optimal sea level performance enhancement. J Appl Physiol. 2014;116:595–603.
Constantini K, Wilhite DP, Chapman RF. A clinician guide to altitude training for optimal endurance exercise performance at sea level. High Alt Med Biol. 2017;18:93–101.
Stellingwerff T, Peeling P, Garvican-Lewis LA, Hall R, Koivisto AE, Heikura IA, Burke LM. Nutrition and altitude: strategies to enhance adaptation, improve performance and maintain health: a narrative review. Sports Med. 2019;49:169–84.
Siebenmann C, Dempsey JA. Hypoxic training is not beneficial in elite athletes. Med Sci Sports Exerc. 2020;52:519–22.
Millet GP, Brocherie F. Hypoxic training is beneficial in elite athletes. Med Sci Sports Exerc. 2020;52:515–8.
Nummela A, Eronen T, Koponen A, Tikkanen H, Peltonen JE. Variability in hemoglobin mass response to altitude training camps. Scand J Med Sci Sports. 2021;31:44–51.
Download references
Acknowledgements
The authors want to thank Renato Canova, Michele Zanini, Sondre Nordstad Moen, and Kristian Ulriksen for their thoughtful and valuable inputs and contributions during a process of “stress testing” our interpretation of elite training practice with top practitioners.
No sources of funding were used to assist in the preparation of this article.
Author information
Authors and affiliations.
School of Health Sciences, Kristiania University College, PB 1190, Sentrum, 0107, Oslo, Norway
Thomas Haugen & Espen Tønnessen
Department of Neuromedicine and Movement Science, Centre for Elite Sports Research, Norwegian University of Science and Technology, 7491, Trondheim, Norway
Øyvind Sandbakk
School of Sport Sciences, UiT The Arctic University of Norway of Health Sciences, Tromsø, Norway
Faculty of Health and Sport Sciences, University of Agder, PB 422, 4604, Kristiansand, Norway
Stephen Seiler
You can also search for this author in PubMed Google Scholar
Contributions
TH, ØS and ET planned the review. TH and ET retrieved the relevant literature. All authors (TH, SS, ØS, EE, and ET) were engaged in drafting and revising the manuscript. All authors read and approved the final version of the manuscript.
Corresponding author
Correspondence to Thomas Haugen .
Ethics declarations
Competing interests.
The authors declare that they have no competing interests.
Additional information
Publisher's note.
Springer Nature remains neutral with regard to jurisdictional claims in published maps and institutional affiliations.
Rights and permissions
Open Access This article is licensed under a Creative Commons Attribution 4.0 International License, which permits use, sharing, adaptation, distribution and reproduction in any medium or format, as long as you give appropriate credit to the original author(s) and the source, provide a link to the Creative Commons licence, and indicate if changes were made. The images or other third party material in this article are included in the article's Creative Commons licence, unless indicated otherwise in a credit line to the material. If material is not included in the article's Creative Commons licence and your intended use is not permitted by statutory regulation or exceeds the permitted use, you will need to obtain permission directly from the copyright holder. To view a copy of this licence, visit http://creativecommons.org/licenses/by/4.0/ .
Reprints and permissions
About this article
Cite this article.
Haugen, T., Sandbakk, Ø., Seiler, S. et al. The Training Characteristics of World-Class Distance Runners: An Integration of Scientific Literature and Results-Proven Practice. Sports Med - Open 8 , 46 (2022). https://doi.org/10.1186/s40798-022-00438-7
Download citation
Received : 18 December 2021
Accepted : 22 March 2022
Published : 01 April 2022
DOI : https://doi.org/10.1186/s40798-022-00438-7
Share this article
Anyone you share the following link with will be able to read this content:
Sorry, a shareable link is not currently available for this article.
Provided by the Springer Nature SharedIt content-sharing initiative
- Training periodization
- Aerobic conditioning
- Olympic athletes
- Training logs
- 123-456-789
- Mon-Fri 8:00 to 2:00
- [email protected]
CEOS Sports Medicine and Research
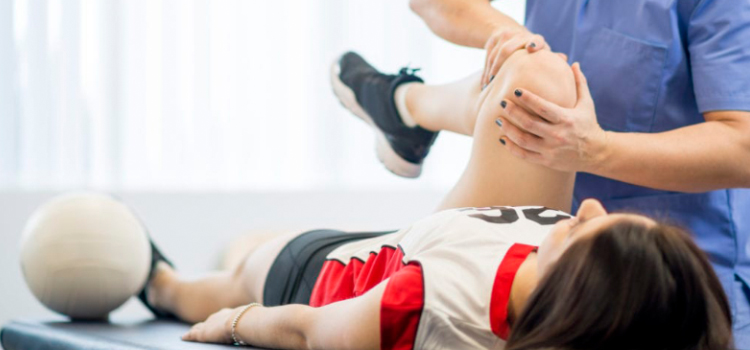
About Journal
CEOS Sports Medicine and Research is an open access, online peer reviewed journal which covers the most active and promising areas of current research in Sports Medicine and Research . This multi-disciplinary journal provides an avenue to less accessible sources to a wide audience of medical researchers and healthcare professionals.
CEOS Sports Medicine and Research invites a well-structured original research, review, case reports, short note and clinical studies from the noted experts to educate the journal readers in all the aspects of Sports Medicine and Research .
CEOS Sports Medicine and Research is an exciting journal that publishes papers of the highest quality and significance in the areas of Sports Medicine and Research .
The scope of the journal includes but not limited to following areas:
- ACL Sprains
- Ankle Sprain
- Athletes’ Physiology
- Bone Fracture
- Doping in Sport
- Exercise Medicine
- Head Injury
- Health Issues in Athletics
- Health Issues in Youth Sports
- Muscle Cramps
- Musculoskeletal Injuries
- Overtraining
- Physical Activity Interventions
- Physical Inactivity
- Runner's Knee
- Shin Splints
- Spinal Cord Injury
- Sports Chiropractic
- Sports Injury
- Sports Nutrition
- Sports Training
- Tennis Elbow
- Ventricular Hypertrophy
- Journal Home
- Editorial Board
- Articles in Process
- Articles in Press
- Current Issue
- Instructions for Authors
- Submit Manuscript
Open Access Journals
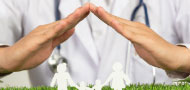
CEOS Family Medicine and Community Health
View Journal Submitmanuscript
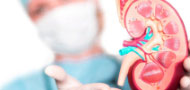
CEOS Urology and Research
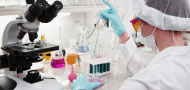
CEOS Journal of Microbiology
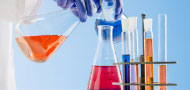
CEOS Chemistry
Student's feedback.
Lorem ipsum dolor sit ametconse ctetur adipisicing elitvolup tatem error sit qui dolorem facilis.
Jonathan Smith
Search
Uw orthopaedic surgery and sports medicine to offer musculoskeletal outcomes research internship.
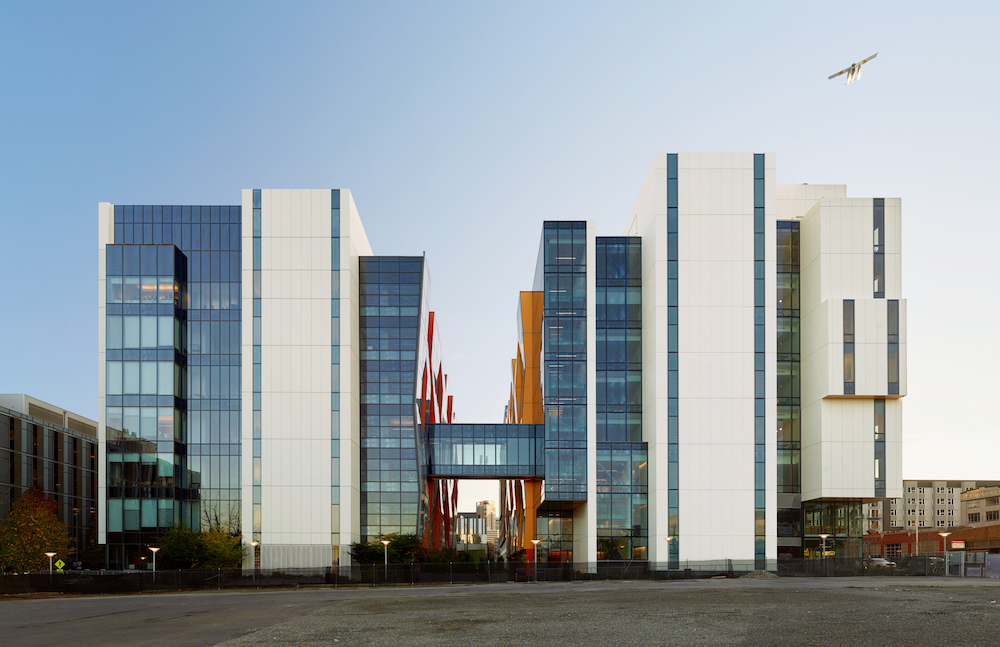
In addition to research co-mentored by departmental and CLEAR affiliated faculty, the internship will include a series of education opportunities whose goal is to enhance the potential for an academic career that incorporates outcomes research. The internship will be supported by a monthly stipend.
Interested applicants (medical students or recent MD graduates) should forward a current CV and a brief (< 300 word) statement summarizing their qualifications and goals for the internship to Calina Garcia ( [email protected] ).
PRIVACY | TERMS OF USE | WEBSITE FEEDBACK
- By Location
- By Specialty
- Foot and Ankle
- Fractures and Trauma
- Hand and Upper Extremity
- Hip and Knee
- Shoulder and Elbow
- Sports Medicine
- UW Medicine Forms
- Orthopaedics Live
- Patient Articles
- Patient Videos
- Related Resources
- Surgical Animations
- Program Overview
- For Applicants
- Meet the Residents
- Orthopaedic E-Books
- Residents Portal and Digital Curriculum
- Fellowships
- Grand Rounds
- Faculty Shadowing
- Medical Student Rotation Evaluation
- Orthopaedic Surgery and Sports Medicine Interest Group
- Preceptorships
- Visiting 4th Year Students
- Continuing Medical Education
- Diversity Visiting Student Program
- Research Labs
- Research Faculty
- Research & AAOS Publications
- Resident Research Intake Form
- Resident Travel Award Requests
- Society Grant Opportunities
- Grants and IRB
- HMC Consent Forms
- Make a Referral
- New User Request Form
- Department eNews
- Arthroplasty Backup Call Schedule
- ASES PJI Database
- EMTRCS Invoice Payment
- Our Mission Statement
- Diversity and Inclusion Resources
- Department Administration
- Developing Orthopaedics
- News and Events
- Orthopaedic Surgery and Sports Medicine Bulletin
- Noteathon Donation

An official website of the United States government
The .gov means it’s official. Federal government websites often end in .gov or .mil. Before sharing sensitive information, make sure you’re on a federal government site.
The site is secure. The https:// ensures that you are connecting to the official website and that any information you provide is encrypted and transmitted securely.
- Publications
- Account settings
Preview improvements coming to the PMC website in October 2024. Learn More or Try it out now .
- Advanced Search
- Journal List
- Front Bioeng Biotechnol
Advances in Regenerative Sports Medicine Research
1 Department of Sports Medicine, Shanghai Jiao Tong University Affiliated Sixth People’s Hospital, Shanghai, China
2 Regenerative Sports Medicine and Translational Youth Science and Technology Innovation Workroom, Shanghai Jiao Tong University School of Medicine, Shanghai, China
3 Regenerative Sports Medicine Lab of the Institute of Microsurgery on Extremities, Shanghai Jiao Tong University Affiliated Sixth People’ Hospital, Shanghai, China
4 National Engineering Research Center for Biomaterials, Sichuan University, Chengdu, China
5 School of Chemistry and Chemical Engineering, Shanghai Engineering Research Center of Pharmaceutical Intelligent Equipment, Shanghai Frontiers Science Research Center for Druggability of Cardiovascular Non-Coding RNA, Institute for Frontier Medical Technology, Shanghai University of Engineering Science, Shanghai, China
Jiangyu Cai
Jinzhong zhao.
Xingge Yu , Shanghai Ninth People’s Hospital, China
Regenerative sports medicine aims to address sports and aging-related conditions in the locomotor system using techniques that induce tissue regeneration. It also involves the treatment of meniscus and ligament injuries in the knee, Achilles’ tendon ruptures, rotator cuff tears, and cartilage and bone defects in various joints, as well as the regeneration of tendon–bone and cartilage–bone interfaces. There has been considerable progress in this field in recent years, resulting in promising steps toward the development of improved treatments as well as the identification of conundrums that require further targeted research. In this review the regeneration techniques currently considered optimal for each area of regenerative sports medicine have been reviewed and the time required for feasible clinical translation has been assessed. This review also provides insights into the direction of future efforts to minimize the gap between basic research and clinical applications.
Introduction
Regenerative medicine utilizes innovative approaches to explore and develop materials that can be used to replace, repair, improve, or reproduce tissues and organs in the human body ( Brody, 2016 ). Sports medicine focuses on aspects of physical health, including the treatment and prevention of exercise-related injuries and aging-related problems that hinder the function of the locomotor system ( Figure 1 ) ( Baby, 2000 ; Foster, 2015 ; Kweon, et al., 2019 ). In the clinical practice of sports medicine, the prevention and treatment of conditions consequently depend on the structural and functional restoration of various related tissues and structures in the locomotor system. When structural integrity cannot be restored through repair, approaches that induce tissue regeneration become necessary, to prevent or delay the use of non-organic structures such as artificial joints. Thus, we explore the field of regenerative sports medicine, which is defined as a science that focuses on the restoration of the structural and functional integrity of the locomotor system, using techniques that induce the regeneration of tissue structures or organs. The approaches currently used in regenerative sports medicine include the utilization of organic and non-organic materials at various structural levels. From a clinical perspective, regenerative sports medicine deals with sports and aging-related conditions in different parts of the locomotor system, such as the menisci, ligaments, tendons, cartilages, and bones.

Sports medicine related injuries.
A meniscus tear is a common knee disorder ( Abrams, et al., 2013 ) that is irreparable in many cases, and consequently, must be addressed via structural restoration with a partial or total meniscectomy to restore its function. Previously, allograft and synthetic menisci have been used with unfavorable or inconsistent clinical results, and thus meniscus regeneration strategies are desirable; however, they require further investigation and development ( Southworth, et al., 2020 ; Li, et al., 2021 ; Veronesi, et al., 2021 ). Knee ligament injuries often result in ligament deficiency and joint instability and necessitate ligament reconstruction ( Pike, et al., 2019 ). To prevent the donor site morbidity that can occur with autografts, the high failure rate with allografts, and the non-graft-bone healing associated with non-receiver transformable synthetic ligaments, receiver transformable regenerative ligaments are considered an alternative choice ( Wang C. et al., 2021 ). Chronic Achilles’ tendon rupture often results in tendon defects that make the direct opposition of the separated tendon ends impossible and graft bridging a necessary choice; consequently, there is demand for regenerative or receiver transformable artificial Achilles’ tendons ( Arshad, et al., 2021 ). Rotator cuff tears are mainly an aging-related condition and quite often irreparable; thus, rotator cuff grafts are required to repair defects and restore the native force chain ( Novi, et al., 2018 ). Though various graft choices are available ( Sunwoo and Murrell, 2020 ), receiver transformable artificial rotator cuff patches are currently considered the ideal option. Sports-related cartilage injuries and osteoarthritis are the main conditions addressed in clinical sports medicine. Clinical approaches such as micro-fractures and autogenous osteochondral graft transplantations are the main strategies used to treat small cartilage defects ( Beck, et al., 2016 ; Guo, et al., 2018 ), while for large cartilage defects, cartilage regeneration is required. Bone defects, fractures, or osteotomies, osteoporosis, and osteonecrosis in the locomotor system, such as glenoid and humeral head bone defects with shoulder dislocations, glenoid bone absorption with severe shoulder osteoarthritis (OA), bone defects with high tibial osteotomy, and tibial plateau depression fractures with knee dislocations, may require bone structure regeneration ( Xie C. et al., 2021 ). In tendon or ligament-to-bone repair, the most important goal is to restore a normal tendon–bone connection with an important fibrocartilage layer ( Tits and Ruffoni, 2021 ). However, after soft tissue-to-bone repair the cartilage layer has been found to reappear inconsistently, which makes tendon–bone interface regeneration a critical issue ( Patel, et al., 2018 ).
In general, regenerative sports medicine has high clinical requirements. In recent years, there has been a large amount of research in this field leading to promising outcomes. In this review, we have assessed the recent progress and assessed the time required for feasible clinical translation of the new techniques and products.
Research Progress in Specific Fields of Regenerative Sports Medicine
Meniscus regeneration, meniscus physiology and the hurdles in regeneration.
The menisci are the semilunar and wedge-shaped fibrocartilaginous tissues between the articular cartilage of the femur and tibia plateau. They have complex 3D structures to absorb shock and distribute its load through collagen fibers which are specifically aligned in a circumferential pattern. The unique zonal phenotypes in the meniscus are histologically and physiologically characterized by two distinct regions: the avascular inner zone (white–white zone), which mainly consists of glycosaminoglycan and type-II collagen with a rounded chondrocyte-like cellular phenotype, and the vascular outer zone (red–red zone), which predominantly contains higher type-I collagen with an elongated ligament-like cellular phenotype. Moreover, these two regions are separated by a middle region (red–white zone), which is a mixture of the inner and outer zones. The limited vascularity of the meniscus indicates a poor healing ability, especially in the avascular white–white zone. Thus, the major hurdles in meniscus regeneration include the inability to replicate its native anisotropic zonal structure and hence, its specialized mechanical function. Moreover, avascularity due to its unique structural properties, and the tibiofemoral articular environment that hinders the healing potency both mechanically and biochemically, have made it challenging for biomedical scientists to create matched engineering constructs for meniscus regeneration.
Surgical Techniques for Meniscus Regeneration
Partial lesions or defects in the meniscus reduce its propensity to heal spontaneously because of mechanical stimuli from the tibiofemoral motions and the avascularity in the white–white zone, leading to degeneration over time. Advances in techniques and tissue engineering strategies have enabled researchers to attempt to repair or regenerate these meniscal defects. Some biological promotion techniques are recommended in clinical scenarios to augment meniscus repair, such as the introduction of bone marrow stem cells using marrow venting techniques, the exogenous addition of fibrin clot, and the stimulation of adjacent healthy meniscus and synovium ( Taylor and Rodeo, 2013 ; Dean, et al., 2017 ; Kwon, et al., 2019 ). Notably, concurrent anterior cruciate ligament (ACL) reconstruction that used bone tunnel to release the cells and growth factors from the bone marrow, has been proven to enhance meniscal repair ( Chahla, et al., 2017 ; Dean, et al., 2017 ; Westermann, et al., 2017 ; Tagliero, et al., 2018 ; DePhillipo, et al., 2019 ). Moreover, partial meniscus replacements offer promising approaches to treat patients with segmental meniscus defects. Both collagen meniscus implants (CMI) from the USA and polyurethane polymeric implants (Actifit) from Europe have been shown to improve clinical outcomes and substantially relieve pain in patients with meniscus defects in both medium- and long-term follow-ups ( van Tienen, et al., 2009 ; Bulgheroni, et al., 2015 ).
Tissue Engineering Strategies for Meniscus Regeneration
Current surgical techniques have failed to promote meniscus regeneration, while many natural or synthetic materials, such as decellularized extracellular matrices, alginate, hyaluronan, polylactides, polyglycolides, and silk have been successfully utilized as scaffolds for meniscus engineering ( Makris, et al., 2011 ). Among these scaffolds, decellularized extracellular matrices derived from the white–white and red–red regions of the meniscus have been shown to promote the differentiation of MSCs toward fibroblastic and fibrochondrocyte phenotypes ( Shimomura, et al., 2017 ). Other types include injectable hydrogels that can be used to address structural defects due to their ability to form structural adaptations ( Athanasiou, et al., 2013 ; Liu, et al., 2017 ). While scaffolds are beneficial due to their ability to incorporate growth factors and their initial mechanical stability, they also indicate recapitulation of the mechanical and biochemical architecture of the native meniscus, with matched stiffness and ingredient gradients ( Higashioka, et al., 2014 ; Steele, et al., 2014 ; Zhu, et al., 2018 ; Zitnay, et al., 2018 ). Notably, when engineering menisci for regeneration, although an obvious choice for cell source might be fibrochondrocytes, regeneration effects are best when fibrochondrocytes are cocultured with other cell subsets ( Hadidi, et al., 2016 ; Koh, et al., 2017 ; Son and Levenston, 2017 ; Sasaki, et al., 2018 ; Xie, et al., 2018 ). Zhang C. H. et al. (2018) used a pre-mechanically stimulated poly (ε-caprolactone) (PCL) scaffold, cocultured with rabbit bone marrow stem cells, for meniscus replacement and found that the pretreated scaffold was a better choice for inducing tissue regeneration ( Figure 2 ).

Orchestrated biomechanical, structural, and biochemical stimuli for engineering anisotropic meniscus. (A) Schematic diagrams for reconstruction of functional anisotropic meniscus; (B) Gross view and low-magnification immunofluorescence (IF) images of native or regenerated menisci at 24 weeks after in vivo implantation in rabbit knees. Green, COL-1; red, COL-2. Copyright 2019 American Association for the Advancement of Science.
Current Clinical Studies and the Challenges in Clinically Translating Meniscus Regeneration
Currently, a few scaffolds for meniscus engineering are undergoing clinical trials with a focus on cell-based therapies. Cell Bandage, a collagen sponge embedded with autologous bone marrow-derived MSCs, was applied in clinical practice to close the torn edges and defects of the meniscus, and is assumed to potentially promote healing. In another clinical trial in humans, a chondrogenic composed of allogeneic bone marrow-derived MSCs was administered to the knee, and reported to effectively decrease visual analog scale pain scores. While meniscus repair products for clinical applications are currently lacking, preliminary outcomes suggest that cell-based therapies are a positive and promising road ahead; however, they have also identified challenges that must be overcome. In the clinical translation of engineered meniscus products, an insufficient source of autologous cells is the primary issue. The use of non-articular cells, however, seems to be a potential strategy to alleviate the scarcity of cells for autologous meniscus therapies ( Makris, et al., 2011 ; Utomo, et al., 2016 ). Additionally, the high-quality autologous neo-tissues required to consistently regenerate the meniscus are difficult to obtain, as demonstrated by the large biological variability observed between donors ( Martin, et al., 2017 ; Vapniarsky, et al., 2018 ; Kwon, et al., 2019 ). Therefore, well-characterized allogeneic tissues and cell banks should be established to enable suitable neo-tissues to be provided stably and avoid disease transmission, and this is likely to solve the intractable problem of biological variability. Furthermore, mechanical biomimicry when engineering the meniscus should be achieved, as the native meniscus allows for frictionless tibiofemoral joint movement and load distribution, which may be related to positive long-term healing outcomes ( Elder and Athanasiou, 2009 ; MacBarb, et al., 2013 ; Huwe, et al., 2018 ). If the meniscus can be successfully generated after overcoming the aforementioned challenges, then the avascular white–white zone of the meniscus leads to difficulties in both implant protection and its integration into existing native tissues ( Arvayo, et al., 2018 ; Vapniarsky, et al., 2018 ). For surgeons and biomechanical researchers, developing appropriate techniques and protocols to enhance the vascular supply to implants should be a priority ( Vapniarsky, et al., 2018 ; Kwon, et al., 2019 ). In addition to vascularity, the engineering meniscus must also adjust to the inflammatory microenvironment, especially in an injured or diseased joint with its complex biochemical conditions. Therefore, modifications by decellularization and antigen removal when engineering the meniscus are required to minimize the immunoreaction of xenogeneic or allogeneic menisci to ensure implant survival and integration. Li et al . (2021) fabricated silk/graphene oxide-based meniscus scaffolds, which consisted of tannic acid and Sr 2+ . The scaffold exerts anti-inflammatory and reactive oxygen species elimination effects, which protect against cartilage degeneration and delay OA development after meniscus injury.
The promising progression which will ultimately lead to the application of tissue-engineered therapies for meniscus regeneration in clinical practice, is evident in current clinical trials. In the near future, tissue engineering strategies may rapidly emerge for the development of meniscus regeneration products, which could potentially provide long-term solutions for patients.
Cruciate Ligament Regeneration
Common strategies for cruciate ligament regeneration.
Knee crucial ligament injuries are common in sports medicine, and often occur during adolescence and young adulthood ( Petersonand Krabak, 2014 ). Ligament reconstruction is the main solution to prevent subsequent cartilage and meniscus damage, thus improving quality of life ( Mastrokalos, et al., 2005 ; Petersonand Krabak, 2014 ). Clinically, autografts and allografts are the two most common graft types used for surgical ligament reconstruction ( Cai J. Y. et al., 2021 ). However, donor site morbidity remains an inevitable problem associated with their use ( Yilgor, et al., 2012 ), and allografts carry additional risks of disease transmission, infection, rejection, low availability and quality, and high failure rates ( Jackson, et al., 1993 ). Xenografts [porcine bone-patellar tendon–bone (BTB)] were harvested by Galili et al. and treated with recombinant alpha-galactosidase and glutaraldehyde for ACL reconstruction ( Galili and Stone, 2021 ). The authors have completed preclinical trials with monkeys and progressed to clinical trials, and they have reported no significant differences in the functional performances of the porcine BTB group and cadaveric allograft group at the 24 months follow up, if the missing/contaminated cases were excluded ( Stone, et al., 2007 ; Van Der Merwe, et al., 2020 ). The potential advantages of the xenografts are that they could help address the quality concerns and availability problems that occur with allografts. However, like allografts, xenografts also have the disadvantages including the possibility for disease transmission, infection, and rejection. Moreover, the process of utilizing an animal originated graft with human tissue, namely graft “humanization,” is difficult and will require further investigation ( Van Der Merwe, et al., 2020 ). To address this, artificial ligaments have been developed in recent years. To date, those ligaments that are clinically available, have been made of non-degradable materials or non-receiver transformable materials, such as polyethylene terephthalate (PET) and polyethylene, which are characterized by their hydrophobic properties and inferior biocompatibility and lead to poor graft-host bone healing after implantation ( Figure 3 ) ( Ai, et al., 2017 ; Cai J. et al., 2021 ). The development of receiver transformable artificial ligaments is another scope and direction for future ligament research.

Electrodeposition of calcium phosphate onto polyethylene terephthalate artificial ligament enhances graft-bone integration after anterior cruciate ligament reconstruction. (A) Electrodeposition of calcium phosphate onto polyethylene terephthalate artificial ligament; (B) The viability and SEM morphology of MC3T3-E1 in the PET, PET/BM-CaP and PET/ED-CaP groups; (C) Micro-CT analysis of the PET, PET/BM-CaP and PET/ED-CaP groups at 12 weeks after surgery; (D) Masson and toluidine blue staining results of pathological sections in the PET, PET/BM-CaP and PET/ED-CaP groups at 6 and 12 weeks after surgery. Copyright 2021 Elsevier.
Artificial Materials for Cruciate Ligament Regeneration
Teuschl et al. ( Teuschl, et al., 2016 ) fabricated novel degradable silk fiber-based artificial ligaments and used biological materials, biodegradable polymers, and composite materials in ligament fabrication for ACL reconstruction in a sheep model. The silk ligaments could induce new tissue ingrowth and stimulate ACL regeneration in vivo . However, the balance between the degradation rate of the materials and the regeneration and remodeling rate of the tissues was not controllable. Furthermore, it is unknown whether the regenerated tissues could maintain the function of the knee at a level similar to that of the native ACL, as functional recovery and a return to sports cannot be fully evaluated in quadruped animal models.
The combined use of receiver transformable and non-transformable materials is an additional option. Mengsteab et al. ( Mengsteab, et al., 2020 ) incorporated PET fibers into the poly (L-lactic) acid (PLLA) bioengineered ACL matrix to fabricate a PET/PLLA hybrid ligament. The hybrid ligament demonstrated great peak loads and promoted the regeneration of ACL in a rabbit model.
Future Perspectives
Despite these encouraging results, further work is required to optimize the properties of newly developed grafts for crucial ligament reconstruction of the knee. We believe that decellularized scaffolds with ready-made collagen and degradable artificial ligaments are the two most promising graft types for ligament reconstruction in future clinical practice. However, prior to application, issues regarding ligament development must be addressed, as it is vital that the host-graph response be regulated and controlled. Moreover, there is a need to explore the regenerative competent microenvironment, which is the articular cavity that can induce tissue ingrowth into the graft. For clinical use, the functional assessment of the knee is more important than the regeneration and healing assessment, as the regenerated or remodeled ligament should be able to mimic the function of the native ligament.
Achilles Tendon Regeneration
Surgical techniques for achilles tendon repair.
End-to-end repair of the chronic Achilles tendon is appropriate when the gap is 2 cm or less, while the V-Y technique, turndown flaps, autograft tendon transfer, and reconstructions using allograft, xenogeneic, or synthetic biomaterials are required for larger defects with or without the preservation of the paratenon ( Kraeutler, et al., 2017 ; Maffulli, et al., 2018 ; Muller, et al., 2018 ; Chen and Hunt, 2019 ). Regardless of the multiple surgical management strategies, the ideal treatment for tendon injury is to promote Achilles tendon regeneration after gap formation ( Sun, et al., 2018 ).
Strategies for Achilles Tendon Repair
The literature on Achilles tendon regeneration is limited mostly to laboratory studies using porcine small intestinal submucosa ( Badylak, et al., 1995 ), acellular tendon matrix ( Gungormus, et al., 2015 ; Zhang C. H. et al., 2018 ), and collagen ( Sun, et al., 2018 ) or collagen gel ( Shen, et al., 2010 ) as scaffolds. Moreover, exogenous cell transplantations such as for tenocytes ( Gungormus, et al., 2015 ) and human amniotic epithelial cells ( Barboni, et al., 2018 ) have been applied but restricted by the cell source, immune rejection, ethics, and injured microenvironment ( Figure 4 ) ( Harris, et al., 2004 ; Sun, et al., 2018 ). Chemokines like SDF-1α and recombinant SDF-1α containing a collagen-binding domain (CBD) have also been reported to promote endogenous tendon regeneration by inducing extracellular matrix production and avoiding the above drawbacks of exogenous cell transplantation in clinical applications ( Shen, et al., 2010 ; Sun, et al., 2018 ). The local application of combined ascorbic acid and T 3 also showed the potential benefits for accelerated tendon healing ( Oliva, et al., 2019 ). Platelet-rich fibrin (PRF), with the delivery of cytokine and growth factor, induced more organized collagen fibers in vivo and promoted tenocyte viability and tenogenic phenotypic differentiation in vitro ( Wong, et al., 2020 ). Similarly, platelet-rich plasma (PRP) is widely used and has been proven to be effective for tendon healing in vivo ( Chiou, et al., 2015 ). However, Zhang et al. ( Zhang C. H. et al., 2018 ) found that the combined PRP was no better at repair-augmenting effects than the scaffolds alone for Achilles tendon regeneration.

Therapeutic potential of hAECs for early Achilles tendon defect repair through regeneration. (A) Circular defects of 5 mm created in the Achilles tendons. One defect was filled with fibrin glue, whereas the contralateral with 10 × 106 PKH26-stained cells suspended in fibrin glue (bottom); (B) Representative haematoxylin–eosin-, Herovici and immunofluorescent staining of CTR (control) and human amniotic epithelial cell (hAEC)-treated tendons. (C) Key functions associated with genes found to be up-regulated in hAECs and the top-scored network; (D) Key functions associated with genes found to be down-regulated in hAECs and the top-scored network. Copyright 2017 Wiley.
Promising laboratory findings reported in the literature suggest that there will be important implications for the practical application and clinical translation of tendon regeneration ( Cai C. et al., 2021 ; Cai et al., 2022 ; Wang et al., 2022 ). However, few clinical studies have been reported to date, and most are case reports and small case series ( Chen and Hunt, 2019 ) with a few translational animal models (dog, horse, etc .) ( Sun, et al., 2018 ; Badylak, et al., 1995 ; Gungormus, et al., 2015 ; Zhang C. H. et al., 2018 ; Shen, et al., 2010 ). Additionally, therapeutic perspectives are not achievable until the critical challenges relating to the scaffold, cell, or chemokine use (source, induction condition, genomic compatibility, dose, etc .) are solved. The combination of scaffold implanted with cell or chemokines, however, is encouraging for future studies and promising for human clinics.
Rotator Cuff Regeneration
Treatment of rotator cuff repairs is an ongoing challenge.
Rotator cuff defects are the main issues that occur in shoulder repair, and the rate of surgical failure is reportedly up to 94%, especially for large and massive tears after simple repairs ( McElvany, et al., 2015 ; Lewington, et al., 2017 ; Saveh-Shemshaki, et al., 2019 ). Various scaffolds have been used to replace the native tissue structures in rotator cuff repairs. Most scaffolds consist chiefly of extracellular matrix and chemical polymer, which provides a bridge for connecting tendon and bone tissues, and adsorbs the fibroblast secreted collagen matrix ( Guevara, et al., 2020 ). These scaffolds have been utilized in rotator cuff tendon tissue engineering for several decades ( Steinhaus, et al., 2016 ; Zhao, et al., 2017 ). They usually combine bioactive substances to promote rotator cuff regeneration, such as stem cells and growth factors. There are currently three types of rotator cuff tendon scaffold used: xeno-patches, allo-matrices, and synthetic films ( Steinhaus, et al., 2016 ; Coons and Alan, 2006 ; Wang D. et al., 2021 ). However, imperfect tissue regeneration is an ongoing problem.
Xeno-Patches for Rotator Cuff Repair
Xeno-patches extracted from extracellular matrices are effective bioactive scaffolds for tendon engineering and can be applied in surgical implantations to rotator cuff defects ( McGovern, et al., 2018 ). The acellular xenografts derived from the porcine dermis and small intestine were used in a large animal model for infraspinatus repair to evaluate the effects of tendon regeneration. Nicholson et al. ( Nicholson, et al., 2007 ) found that intestinal and porcine dermis patches were almost replaced by tendon-like tissues at 24-week, but a foreign body reaction was observed in the conjunction site of the tendon and xenograft. Ultimately, the cause of failure was the same for the dermal and intestinal groups. The potential immune reaction and associated chronic foreign body responses were the main concerns. This reaction may result from the residual DNA in the Xeno-tissue, even if processed by decellularization. Another problem is the hyper acute rejection caused by α-Gal. The α-Gal epitope exists in non-primate mammals ( Naso, et al., 2011 ; Platts-Mills, et al., 2021 ), and therefore, the epitope antibody is produced in humans, which specifically binds to xeno-tissue.
Allo-Matrices for Rotator Cuff Repair
Allo-matrices originated from decellularized cadaveric human tissues and were found to have the capability to bridge tendon tissue defects, with a low risk of tissue-scaffold rejection ( Fini, et al., 2012 ). A study by Adams et al. ( Adams, et al., 2006 ) explored the histological and biomechanical processes of allo-matrices, by utilizing allo-dermal matrices to bridge tendon and bone in an animal model for infraspinatus repair. The fibroblast infiltration and new collagen deposition were surrounded by dermal matrices 6 weeks after implantation, and at 24 weeks, a more mature tendon-like tissue was formed in the allo-dermal group. The biomechanical properties of the regenerated tissues were promising. However, there were only small-scale clinical trials conducted to evaluate their performance ( Zhao, et al., 2017 ). Even though no serious allo-matrix related complications were observed, and clinical outcomes appeared to be good, some potential problems still existed. Similar to the xeno-patches, there were concerns about the residual DNA. The residual DNA may cause immune inflammatory reactions and increase the proliferation of the scaring tissue ( Lewington, et al., 2017 ). There is also evidence that the mechanical properties of the allogenic matrices are decreased when compared with that of the auto-tendons. To better induce tendon–bone interface regeneration, Chen et al. (2019) added recombinant SDF-1α to the decellularized bone–fibrocartilage–tendon composite and injected synovium-derived mesenchymal stem cells (SMSCs) into the repair site. They found that the fabricated scaffold was better at recruiting the SMSCs and resulted in well regeneration of the tendon–bone interface 8 weeks after surgery. However, the option proposed in this study is too complicated for clinical application. Neither the addition of recombinant SDF-1α nor the injection of stem cells has been approved by the administration ( Figure 5 ).

Functional decellularized fibrocartilaginous matrix graft for rotator cuff enthesis regeneration: A novel technique to avoid in-vitro loading of cells. (A) Developing a cell-free graft with chemotaxis to recruit postoperative injected cells; (B) Macroscopic observation, histological analysis, and synchrotron radiation-Fourier transform infrared spectroscopy analysis of the book-type nature fibrocartilage tissues and C-SDF-1α/BDFM, sections stained with hematoxylin and eosin (H&E), DAPI, toluidine blue (TB), and picrosirius red (PR); (C , D) Histological analyses of regenerated fibrocartilage during RC healing. Copyright 2020 Elsevier.
Synthetic Polymers for Rotator Cuff Repair
Owing to the ongoing focus on immune reactions for both the xeno-patches and allo-matrices, synthetic polymers that are of great interest for tendon regeneration engineering have been identified ( Qiu, et al., 2021 ; Zhao, et al., 2021 ). Degradable polymers, including PLLA, poly-dioxanone (PDO), and poly (lactic-co-glycolic) acid (PLGA) have been used as supports to create multifunctional scaffolds. These synthetic films consisting of regularly arranged fibers exhibit stronger mechanical characteristics than the scaffolds derived from bio-tissues ( Gachon and Mesquida, 2021 ). The controllable arrangement plays a role in cell migration, with fibroblasts well aligned with the axis of the polymer fibers when compared to the un-aligned films in a random orientation. The polymer film had fewer immune responses when compared with that of the xeno-and allo-patches, indicating that it could be a potential scaffold to bridge the tendon gap. Yokoya et al. ( Yokoya, et al., 2012 ) used the PLGA sheet to repair the full-thickness defect of the rotator cuff in a rabbit model and observed that a greater proportion of type-I collagen was generated in the PLGA sheet with mesenchymal stem cells. The repaired site also had a better ultimate strength when compared with that of the controls without mesenchymal stem cells. Studies that have explored synthetic films have achieved encouraging results, but the products of the polymer film degradation were identified as a concern ( Silva, et al., 2020 ). High levels of chemical composite have a toxic effect on fibroblast proliferation and inhibit collagen deposition. These toxic effects vary with different polymers, and therefore, more research is required to ensure that the degradation product levels are safe.
These bio-scaffolds are assumed to offer ideal structural binding sites for tissue integration. Synthetic films have the advantage of mechanical properties and low rates of immune reaction. Several scaffolds have emerged in recent years; however, they have not yet been used in routine clinical surgery ( Zurina, et al., 2020 ). The ideal scaffold should be able to meet the mechanical strength of the cuff tendon and provide bioactive binding sites that promote fibroblast-mediated healing and tendon regeneration.
Cartilage and Osteochondral Regeneration
Simple cartilage regeneration.
The common types of joint bone defects include partial cartilage injury, full-thickness cartilage injury, and osteochondral defect ( Chow, et al., 2004 ; Shkhyan, et al., 2018 ; Kato, et al., 2019 ). The repair and regeneration of damaged cartilage tissue is one of the most challenging problems in the field of tissue engineering and regenerative medicine.
Until now, promoting cartilage regeneration has been one of the greatest difficulties in the field of regenerative medicine, due to the limited self-healing ability of cartilage tissues ( Wang, et al., 2020 ; Zhang, et al., 2020 ). Natural and synthetic substances are the two main biomaterials used to restore cartilage ( Chae, et al., 2021 ; Qiao, et al., 2021 ; Schuurmans, et al., 2021 ). The aim is mainly to improve cell adhesion and promote the growth and dynamic migration of regenerative tissues. Scaffolds are not only regarded as physical substrates, but in the biological environment, scaffolds are related to each other through clear chemical exchanges and physical stimulation through cells and adjacent tissues. Therefore, scaffolds are mainly used to support cell culture, infiltration, proliferation, and differentiation caused by signal factors and mechanical stimulation ( Gardner, et al., 2016 ; Lynch, et al., 2016 ). Scaffolds are dissimilated into categories such as nanomaterials, biomimetic materials, biological enhancers, and hydrogels, and they can be used to bind chondrocytes or can be placed at cartilage defect sites. Hua et al. (2021) fabricated multifunctional hybrid optical crosslinking (HPC) hydrogels by photopolymerization and photopolymerization of imine crosslinking. Loaded with chondrocytes, the scaffold was used for cartilage defect repairing through arthroscopy in a pig model. Six months after implantation, an ideal layer of cartilage was regenerated. However, the development of an optimal scaffold that can induce cartilage regeneration is ongoing. The other critical issue in isolated cartilage regeneration is how to increase the adherence of the regenerated cartilage to the bone underneath.
Scaffolds for Osteochondral Regeneration
A method to repair osteochondral defects, the terminal stage of a cartilage defects, is urgently required ( Eldridge, et al., 2020 ; Hall, et al., 2021 ; Kim, et al., 2021 ). At present, serious defects can only be treated by arthroplasty ( Pirosa, et al., 2021 ; Xie J. et al., 2021 ). However, the commonly used artificial joints based on non-degradable materials, such as metals and ceramics have some disadvantages, including their high cost, limited biocompatibility, foreign body rejection, and long-term loosening ( Labek, et al., 2011 ; Valdes, et al., 2012 ; Sakellariou, et al., 2016 ). Achieve bone–cartilage composite tissue regeneration and permanent joint physiological function reconstruction remains a challenging problem. Using biomimetic scaffolds to induce in situ osteochondral regeneration is expected to be an important option for future joint function reconstruction ( Figure 6 ) ( Radhakrishnan, et al., 2018 ; Chen et al., 2019 ; Xu, et al., 2019 ; Zhu, et al., 2019 ). The tissue engineering of osteochondral integrated scaffolds imitates a normal osteochondral structure, as well as the natural osteochondral components in composition, to achieve a double bionics structure and components, which will eventually enable the effective repair and regeneration of osteochondral defects. However, due to the complex anatomical structure and component content of normal bone and cartilage, as well as the dynamic changes in time and space that occur in the regeneration area, the repair and regeneration of the osteochondral defect area is not just a simple “filling” of new tissue. It requires subchondral bone regeneration to support hyaline cartilage, and hyaline cartilage is closely combined with bone to generate cartilage–bone interface integration and thus the concurrent regeneration of both cartilage and bone.

3D printing of a lithium-calcium-silicate crystal bioscaffold with dual bioactivities for osteochondral interface reconstruction. (A) Schematic illustration of application of Li2Ca4Si4O13 scaffolds for osteochondral reconstruction; (B) SEM images of 3D-printed Li 2 Ca 4 Si 4 O 13 scaffolds after fabrication and (C) after soaking in the simulated body fluids for 14 days; Macro-photographs showed the defects in the control group and the other two experimental groups ( D 1 : blank control without scaffolds, E 1 : pure β-TCP scaffolds, F 1 : Li 2 Ca 4 Si 4 O 13 scaffolds) at 12 weeks of post-surgery; (D 2 – F 2 ) showed 2D projection images of the three experimental groups at week 12; (D 3 – F 3 ) showed the transverse view of 3D reconstruction images of the three experimental groups at week 12; (D 4 – F 4 ) showed the sagittal view of 3D reconstruction images of the three experimental groups at week 12. Copyright 2019 Elsevier.
To achieve a breakthrough in osteochondral regeneration, we must focus on preparing integrated bionic scaffolds that accurately simulate the microenvironment of osteochondral regeneration, and solve the following key scientific problems: 1 ) accurate simulation of 3D shapes, multi gradient structures, regional specific matrix components, and the microenvironment factors of joints to prepare a bionic osteochondral scaffold ( Choe, et al., 2021 ; Lee, et al., 2021 ; Liu, et al., 2021 ); 2 ) achievement of osteochondral tissue regeneration and biological joint construction in vitro ; and 3 ) realization of the industrialization of integrated bionic stents and clinical transformations of the biological joints. The accumulation of separate tissue regeneration research for the cartilage and bone and the application of emerging cutting-edge technologies in recent years has facilitated breakthroughs regarding these technical problems.
At present, osteochondral scaffolds are commonly used in experimental research and clinical applications including natural biomaterial scaffolds, synthetic scaffolds, bioceramic scaffolds, bioactive glass, extracellular matrix scaffolds, and composite scaffolds.
The biological and mechanical properties of various tissue-engineered osteochondral scaffolds are different due to their different components and structures. For example, although natural biological scaffolds have the advantage of good biocompatibility, high cell affinity and degradability, which are conducive to adhesion and proliferation following the infiltration and recruitment of cells, they also have disadvantages, such as poor mechanical properties, rapid degradation rates, and limited sources. Synthetic scaffolds and bioceramics have good mechanical properties, strong plasticity, controllable degradation, and unrestricted wide sources. Their corresponding disadvantages are poor biocompatibility, low cell affinity, lack of hydrophilicity of some scaffolds, and their degradation products may have certain toxicities. With the development of tissue engineering, to overcome the shortcomings of single materials, two or more materials have been combined according to the principles of complementary characteristics and advantages, to design an ideal scaffold that can meet the needs of osteochondral tissue engineering.
Composite scaffolds combine the advantages of individual scaffolds, such as a controllable degradation rate, good cell compatibility, good hydrophilicity, and appropriate biomechanical strength. Su et al. (2021) prepared cartilage layers via collagen II and chitosan with a pore diameter of approximately 100 μm and a bone layer via the PLGA with a pore diameter of 500 μm. The chondrocytes labeled by nano magnetic particles were planted on this biphasic scaffold to observe their growth, proliferation, and distribution on the scaffold, to further investigate the effects of this method on the repair and regeneration of bone and cartilage. The experimental results showed that the combination of a scaffold structure and cells labeled by novel technology has good application prospects for repairing regenerated osteochondral defects.
Biomedical Material Fabrication in Osteochondral Regeneration
Rapid progress in biomedical material fabrication has been made in the field of osteochondral regeneration, including 3D bioprinting, electrospinning, aerogels, hydrogels, and drug loaded microspheres ( Deng, et al., 2021 ; Gao, et al., 2021 ; Jiang, et al., 2021 ). However, there are still some challenges, such as the accuracy and stability of 3D biological printing technologies and the flexibility and function of the products. A potential clinical application of bioprinting is to develop “ in vivo bioprinting” technology, which can accurately “print” cell materials on the damaged parts with the help of handheld print heads, to directly repair cartilage defects of different shapes and thicknesses. This technique has great potential for the development of individualized treatment plans and will help to eliminate the need for a secondary surgery.
In addition to 3D printing technologies, tissue-engineered hydrogel scaffolds have also been utilized in cartilage repair. However, it is difficult for hydrogels to meet the advantages of high porosity, good mechanical properties, toxicity, biocompatibility, and a controllable degradation cycle. Most of the hydrogels can only satisfy one or two advantages. A composite hydrogel that can synchronize the degradation rate with the regeneration rate of cartilage tissue could be promising as a repairing material for the treatment of cartilage defects.
The applicational prospects for tissue engineering electrospinning scaffolds is optimistic, with high porosity and bionic extracellular matrix structures, but there are still many problems that must be addressed, such as: 1 ) electrospinning scaffolds seriously affecting the adhesion, proliferation, and differentiation of seed cells on materials; 2 ) solving the contradictions between the mechanical strength and degradation rates of materials; and 3 ) unclear the teratogenicity and tumorigenicity of materials in the human body.
While the tissue engineering of osteochondral integrated scaffolds can solve some of the existing problems in traditional treatments, there are also shortcomings. If there is no in-depth study on the mechanisms for the repair and regeneration of osteochondral integrated scaffolds, it is impossible to clarify the repair and regeneration mechanisms for the defect area from a microscopic cellular and molecular level. Moreover, the osteochondral integrated scaffold has double bionics for its structure and composition, which cannot be compared with the normal osteochondral structure at both biological and mechanical levels; further, special materials similar to the natural osteochondral structure-cannot be found. In addition, the calcified layer and tidal line play important roles in the structure of bone and cartilage, but the integrated bionic scaffold is still difficult to completely biomimic these unique structures. Therefore, at present, the problem of the calcification of the cartilage layer and easy separation between the two layers of biphasic and multiphasic scaffolds has not yet been solved. Nevertheless, use of new preparation technologies and methods, such as 3D printing and electrospinning technologies, discovery or synthesis of new scaffold materials, and cooperation between medicine, industry, materials, biological structures, biomechanics, and integrated bionic scaffolds can finally solve the clinical scientific problem of osteochondral defects.
Bone Regeneration
Bone defects, fractures or osteotomies, osteoporosis, and osteonecrosis in the locomotor system may require bone-structure restoration to achieve regeneration ( McFarland, et al., 2016 ; Khira and Salama, 2017 ; Choi and Rhee, 2017 ; Zhang Y. et al., 2018 ). Some examples of such cases are glenoid and humeral head bone defects occurring in shoulder dislocations, glenoid bone absorption in cases of severe shoulder osteoarthritis (OA), bone defects occurring in high tibial osteotomy, and tibial plateau depression fractures in cases of knee dislocation. Bone regeneration is defined as the process wherein bone-grafting materials are replaced by newly formed bone ( Wei et al., 2022 ). Till now, autologous grafts have been suggested as the gold standard for bone regeneration. However, the accompanying donor-site complications and the limited availability of autografts hamper their extensive use in clinical applications. Meanwhile, allogeneic bone grafts are challenged by vascularization issues and disease transmission risks ( Giannoudis, et al., 2005 ; Laurencin, et al., 2006 ). Thus, there is an urgent need for the development of biomaterials in sports medicine. There are different strategies used to treat bone defects, such as 1 ) simple artificial bone material, 2 ) artificial bone material with bioactive factors, and 3 ) artificial bone material with stem cells.
The ideal scaffold would have an appropriate hierarchical architecture that would permit normal metabolic activity as well as the migration, proliferation, and differentiation of cells together with angiogenesis and bone ingrowth. As an example, a highly porous scaffold would have a greater surface area that would promote improved osteogenic effects by allowing greater mass exchange and adsorption of growth factors ( Wu, et al., 2014 ; Tang, et al., 2016 ). The pore size of the scaffold is also critical for good bone regeneration, as the presence of smaller pores leads to hypoxic conditions that promote pre-osteogenic osteochondral formation while larger interconnected pores promote directional osteogenesis ( Karageorgiou and kaplan, 2005 ; Zhang, et al., 2013 ). Porous surfaces also stimulate interactions and linkages between the implant and the bone, and the pore size is critical for bone integration. For example, it was found that while 300-μm pores produced the most lamellar bone, the process of osseointegration was longer than with 200-μm pores ( Xu, et al., 2011 ).
In this review, we have focused on research over the past 5 years into the optimization of the architectural, chemical, and surface features of bone graft substitutes (BGS) for the promotion of bone regeneration and osteointegration. Three-dimensional printing permits the creation of BGS tailored for the individual patient by optimization of both mechanical and structural characteristics. The optimizing structure permits specific correspondence between the BGS and the patient’s body, leading to more rapid postoperative recovery ( Tan, et al., 2017 ). Although titanium is most commonly used for 3D printing, materials such as bioceramics and polymers such as polyetheretherketon (PEEK), which allow custom design, are only being investigated at the pre-clinical stage at the moment ( Mustafa, et al., 2011 ; Li, et al., 2017a ; Yang, et al., 2017 ). The issues that are being addressed in the use of these novel ceramic materials include optimal mechanical characteristics, architectural design, and chemical properties to enhance both porosity and degradability. Optimal surface characteristics are vital for osteogenic cell adhesion to the BGS, leading to the promotion of new bone growth ( Colquhoun and Tanner, 2015 ; Fernandez-Yague, et al., 2015 ; Babaie and Bhaduri, 2018 ). These properties can be manipulated by coatings that promote bone regeneration.
As bone repair is a complex process that is dependent on various growth factors, we discuss the application of active biomolecules for promoting bone repair ( Krishnan, et al., 2006 ; Carragee, et al., 2011 ; Polak, et al., 2011 ; Kim, et al., 2012 ; Santo, et al., 2013 ; Mumith, et al., 2017 ). These applications can be divided into three approaches are three approaches: 1 ) the application of recombinant growth factors, individually or as mixtures, together with a natural or calcium phosphate matrix, such as BMP-2 (Infuse bone graft), BMP-7 (OP-1 putty), and rhPGDF-BB (Augment bone graft ® ); 2 ) the use of ECM-derived peptides targeting cellular receptors, such as B2A (B2A2-K-NS) and P-15; 3 ) the use of small molecules targeting pathways that influence none mass, including parathyroid hormone (PTH), Nel-like molecule-1 (NELL-1), and LIM mineralization protein-1 (LMP-1). These molecules may affect bone mass directly or indirectly by inhibiting negative modulators of bone mass and thus promoting increased bone mass.
The third approach used stem cells as part of a cell-based construct. This requires the presence of progenitor cells allowing the formation of new tissue through interaction with host cells, stimulatory factors, and support providing cells with 3D cues for new tissue formation. The progenitor cells used include bone marrow stromal cells (BMSCs), adipose-derived mesenchymal cells (ASCs) and periosteum-derived stem cells (PDSCs) ( Ingber, et al., 2006 ; Bolander, et al., 2016 ; Bolander, et al., 2017 ). However, the necessity of pre-incubating the biomaterial with the cells complicates the engineering process considerably and also reduces the viability of the cells, leading to high production costs. In addition, the scaffolds may not have sufficient ability to promote vascularization in vivo and may thus be unable to maintain cell and tissue viability ( Lenas, et al., 2009 ). Because of these issues, this approach has not proved popular in clinical practice.
Bone tissue engineering approaches were devised to address the shortcomings of bone grafts and alloplasts and to promote the repair of bone defects and fractures. Both biological derivatives and synthetic materials have been used for scaffold fabrication, singly or in combination. The developments in the field include the use of scaffolds together with gene therapy and stimulatory factors ( Farberg, et al., 2012 ; Pountos, et al., 2016 ; El Bialy, et al., 2017 ; Yan, et al., 2019 ), while recent advances in the 3D printing of scaffolds open new directions for effective bone regeneration ( Ding, et al., 2013 ; Gong, et al., 2015 ; Lee, et al., 2017 ; Li, et al., 2018 ) ( Figure 7 ). Nevertheless, many challenges remain. Recent reports have emphasized the importance of local microenvironments for the success of these scaffolds. In addition, more understanding of the precise functions of the active biomolecular constituents, such as their influence on inflammation or bone precursor cells, is required. The precise control and delivery of these molecules in the correct doses are necessary to prevent undesirable side effects. There is intensive research in the form of pre-clinical studies to understand the underlying mechanisms of these therapies and their effective applications. Translation to clinical practice also requires many regulatory steps and costs.

Vascularized 3D printed scaffolds for promoting bone regeneration. (A) Schematic diagram of bridging deferoxamine (DFO) on the surface of 3D printed polycaprolactone (PCL) scaffold and its biological function for bone regeneration in bone defect model; (B) Micro-CT analysis of the effect of scaffolds on bone repair in vivo ; (C) Representative images of hematoxylin-eosin (HE) staining of the decalcificated bones slice, showing the new formed tissue including the fibrous tissue (F), newly mineralized bone tissue (NB) and the structure of scaffolds (S). Copyright 2019 Elsevier.
Tendon–Bone Interface Regeneration
Poor tendon–bone interface healing after tendon/ligament reconstruction.
One of the main problems in the functional reconstruction of tendons and ligaments is the poor healing of the tendon–bone interface ( Yang, et al., 2017 ; Xing, et al., 2020 , Zhu, C., et al., 2021). Natural tendon–bone interfaces can be categorized into four portions: tendon, uncalcified fibrocartilage, calcified fibrocartilage, and bone ( Weimin, et al., 2013 ; Liu, et al., 2019 ). The transition of the structures effectively prevents the structural damage caused by a sudden tension, by gradually distributing tension across the interface ( Lu and Thomopoulos, 2013 ). From a mechanical perspective, this structure perfectly connects ligament and bone tissue with an elasticity modulus of 200 MPa and 20 GPa, respectively, which also increases the strength of the insertion to avoid the avulsion of the tendon. However, after injury, even with proper surgical treatments, scar tissue takes the place of the transitional structure in the tendon–bone interface, which greatly decreases its mechanical properties (Zhu, J., et al., 2021). Hence, inducing the regeneration of natural tendon–bone interfaces is a major issue when treating tendon–bone interface injuries.
Scaffolds Fabricated for Tendon–Bone Interface Regeneration
A decellularized extracellular matrix is a common option for tendon–bone interface regeneration, as it possesses ideal biocompatibility and a natural microstructure. Through a combination of physical, chemical, and enzymatic treatments, Su et al . (2019) successfully fabricated decellularized triphasic hierarchical bone–fibrocartilage–tendon composites, with the preservation of natural microstructures and mineralization. After 8 weeks implantation occurred into the tibia bone tunnel, and the fabricated decellularized bone–fibrocartilage–tendon composite induced a notably larger amount of bone regeneration in the bone tunnel when compared with that of simple decellularized tendon tissue. However, it is still unclear whether the decellularized bone–fibrocartilage–tendon composite can induce cartilage regeneration between the tendon–bone interface, which is the main issue in tendon–bone interface regeneration. Additionally, the fabrication procedure of decellularized bone–fibrocartilage–tendon composite is relatively complicated. It is quite difficult to achieve a balance between the preservation of the natural microstructure and the elimination of remnant cell debris and consequently, there is no current gold standard method for the field.
Decellularized small intestinal submucosa (SIS) has been generated for use as a commercial medical implant as numerous studies have indicated that many bioactive factors can be preserved, even after the decellularization procedure ( Meng, et al., 2021 ; Singh, et al., 2021 ). However, unlike the decellularized bone–fibrocartilage–tendon composite, decellularized SIS scaffolds lack the required microstructures between the tendon–bone interface. Even though decellularized SIS has been successfully used in many other tissue repair processes, recent clinical studies jointly suggested that the use of decellularized SIS did not ( Su, et al., 2021 )result in better clinical results when used for rotator cuff repair surgery ( Iannotti, et al., 2006 ; Bryant, et al., 2016 ). The retear rate and American Shoulder and Elbow Surgeons shoulder score were not improved, which indicated that the tendon–bone interface regeneration was not properly induced by a decellularized SIS scaffold ( Sclamberg, et al., 2004 ).
When compared with a natural extracellular matrix, artificial polymer scaffolds have advantages, including good quality control reliability and microstructure adjustability. Nevertheless, unlike a natural extracellular matrix, the artificial polymer scaffold lacks biocompatibility, biodegradability, and inducibility; highlighting the need to select appropriate materials. PLGA and PCL are common material options that have been deemed safe by the Food and Drug Administration ( Li, et al., 2015 ). By electrospinning, the fabricated scaffold is equipped with the similar microstructure when compared with that of a natural extracellular matrix ( Hua, et al., 2021 ). However, the microstructures of the tendon–bone interfaces are very different from the extracellular matrices of other tissues ( Rossetti, et al., 2017 ). The orientation of fiber in the extracellular matrix of the tendon–bone interface changes from aligned-to-random, suggesting that the fabricated scaffold should also mimic the transitional microstructure differences ( Deymier-Black, et al., 2015 ). Xie C. et al. (2021) fabricated electrospun scaffolds with aligned-to-random microstructures and morphologies of the tendon fibroblasts were cultured on the scaffold, and were organized and haphazardly oriented, respectively. In addition to the differences in the microstructures, there is also a gradient density for calcium in the tendon–bone interface. As PLGA and PCL are bioinert materials, which cannot induce bone regeneration, bioactive factors were commonly added to the scaffolds. In a previous study, we produced a PCL scaffold combined with gradient calcium phosphate silicate (CPS) content and found that the multilayer gradient composite effectively increased tendon–bone healing at the tear site, where better tissue cellularity and gradient mineralized cartilage formation were observed ( Su, et al., 2021 ). Wang D. et al. (2021) crosslinked nanofibrous scaffolds to fabricate a scaffold, which simulates the microstructure of the natural tendon-to-bone interface, and 3 months after implantation in rabbit, it fully regenerated the unique 3D structure of the tendon-to-bone interface ( Figure 8 ). However, the aforementioned studies were also too complicated for application in industrial production. Controlling the fiber orientation transition and gradient density of calcium is difficult using the current industry techniques.

Crimped nanofiber scaffold mimicking tendon-to-bone interface for fatty-infiltrated massive rotator cuff repair. (A) Schemata of fabrication of the crimped nanofibrous scaffold for massive rotator cuff tear repairing; (B) Representative micro-computed tomography (micro-CT) images of the proximal humerus and quantitative analysis. Copyright 2022 Elsevier.
Expectations in Tendon–Bone Interface Regeneration
To date, no medical implant for tendon–bone healing has been developed. Considering the difficulties in balancing the biocompatibility, biodegradability, inducibility, microstructure, and gradient density of the calcium, the time frame for a relevant implant is not short; however, it will be improved with time. Previous studies have either presented procedures that are too sophisticated or added bioactive materials not approved by the Food and Drug Administration. Scaffolds that can effectively induce tendon–bone interface healing, are easy to manufacture, and are highly reliable, are urgently required.
Materials for Sports Medicine Injury
Autografts are recommended as the “gold standard” for most common sports medicine injuries, including ACL tears and bone defects ( Cho, 1975 ; Clancy, et al., 1982 ; Howe, et al., 1991 ). Although autografts contribute to an increased healing rate, both structurally and functionally, donor site injury and limited tissue sources restrict their applications ( Piva, et al., 2009 ). Allografts can not only reduce surgery time but also reduce damage and complication at the donor site. However, a long-term follow-up study indicated that, when compared with autografts, allografts could prolong the post-surgery recovery time, increase the incidence of infection, and the risk of spreading infectious diseases ( Dahlstedt, et al., 1989 ; Good, et al., 1989 ; Greenberg, et al., 2010 ).
Natural biological materials are one of the major material sources of tissue engineering. SIS is a natural, acellular, degenerable, extracellular collagen matrix material which is mainly composed of helically interweaving type-I and -III collagen ( Badylak, et al., 1989 ; Badylak, et al., 1995 ). Studies confirmed that using SI as an ACL supplementary repair could contribute to neuro-vascularization and cell growth ( Nguyen, et al., 2015 ). However, Badylak et al. (1989) found that there was a reduced tensile resistance in the mucous membrane of the small intestine after 3 months in the experiment for goat ACL reconstruction, which could not meet the prerequisite in clinical biomechanics. Silk is a natural material and highly recommended for its desirable biocompatibility and mechanical strength ( Li, et al., 2014 ; Teuschl, et al., 2016 ). However, the uncontrollable speed of silk degeneration, limited cellular affinity, and unstable mechanical strength immediately after surgery all imposed restrictions on its application ( Soong and Kenyon, 1984 ).
Non-degradable polyester materials, such as PET, are another option as they are equipped with high mechanical strength. Ligament advanced reinforcement system (LARS) is a representative product of non-degradable artificial ligaments and it was advantageous to the patients who needed to be back to the field with a high-level performance. Considering its disadvantages, such as poor hydrophilicity, inertness, and no osteogenic active ingredient, the LARS ligament would lead to the formation of a scar at the ligament-bone interface and loss of long-term effectiveness ( Li, et al., 2012 ; Tiefenboeck, et al., 2015 ). Some studies have focused on surface coatings to improve bioinert material defects. Studies have shown that coating the surface of LARS ligaments with fibroin or hydroxyapatite can effectively promote the degradation of LARS ligaments and the tendon–bone healing of inert material in the bone tunnel ( Ai, et al., 2017 ; Cai, et al., 2018 ; Wang, et al., 2018 ). However, there are still problems with the current technique, such as nonuniform surface coating on the ligament and binding force, which greatly limits the clinical applications of the products.
Single polymer/macromolecular based scaffolds (such as polylactic acid and poly (caprolactone)) have been recently developed and are being explored for potential clinical use (Correia Pinto, et al., 2017; Erisken, et al., 2008 ; Zhang, et al., 2005 ). Such scaffolds can be equipped with relatively good mechanical properties and structural machinability which can be adjusted for different requirements. Research has indicated that they can gradually degrade in 600 days and maintain a certain degree of strength, which provides stable conditions for tissue regeneration ( Blaker, et al., 2011 ). Most of the absorbable polymer materials have a low hydrophilicity and cellular affinity. Furthermore, polylactic acid can produce acid metabolites during the degeneration process and thus cannot form real tissue inductivity ( Lu, et al., 2005 ; Cardwell, et al., 2014 ). For successful tissue engineering, the single polymer/macromolecular based scaffolds should meet several criteria ( Zhao, et al., 2014a ; Zhao, et al., 2014b ; Zhao, et al., 2015 ; Li, et al., 2017b ). First, good biocompatibility and mechanical properties should be confirmed in vitro to lay the foundation for further in vivo implantation. During degeneration, the initial, middle-stage, and final grafts should cooperate with induced regenerative tissues to support sufficient strength. Finally, the toxicity of the degeneration product should be strictly limited.
There is a high demand for tissue regeneration techniques in the field of sports medicine, which make regenerative sports medicine crucial for the treatment of injuries and diseases in the locomotor system. There are several conundrums, however, that are limiting the application of these techniques ( Tables 1 , ,2). 2 ). The first issue is how to restore like for like, i.e., how to recreate tissues that mimic the native tissues in both structure and function. The second issue is how to fabricate a structure that can overcome the defects of the native structure to ensure that its function is improved and the potential for reinjury is reduced.
Common sports medicine injuries and their promising treatment methods.
Promising treatments’ advantage and disadvantage.
For meniscus regeneration, tissue engineering strategies could potentially generate a meniscus like cellular or acellular structure with or without growth factors. Progress has already been made in restoring its native anisotropy and zonal organization. Further research should be conducted to understand how to restore its hooping effect from the collagen structure and the connection between the anterior and posterior horns and the tibia, as well as how to ensure its healing to the peripheral capsule ligament and how to obtain sustained vitalization of the neonatal structure to reduce the risk of reinjury.
For cruciate ligament, rotator cuff, and Achilles’ tendon regeneration, the goal is to obtain a viable type-I collagen dominated fibrous structure that can heal to the bone and soft tissue it connects. For the collagen regeneration-inducing technique that utilizes degradable synthetic material as the main construct, there is still a long way to go before it will be able to induce the regeneration of sufficient volumes of collagen. The collagen transformation-inducing technique is more practical with readily available collagen. However, vitalizing the structure in the intraarticular environment, obtaining a mature ligament or tendon structure with sufficient final strength, and achieving satisfactory ligament or tendon – bone healing requires further investigation.
Osteochondral regeneration induced with a biomimetic scaffold is a promising strategy to solve the problem of cartilage regeneration and cartilage–bone adhesion. Progress has been achieved in the development of a scaffold simulate native osteochondral construct with regard to its microstructure, components, and bioactive stimulator. Breakthroughs are still required, however, to obtain a scaffold that can induce bone, cartilage, and the bone–cartilage interface with desired biological and mechanical features throughout the regeneration induction process, and to obtain semi-finished or finished osteochondral products that can be directly used for implantation.
In bone regeneration, obtaining an appropriate scaffold and bioactive molecules does not seem to be a problem. However, precisely controlling the release of the bioactive molecules to avoid undesirable side effects requires further investigation.
For tendon–bone interface regeneration, the goal is to obtain a layer of fibrocartilage between the tendon and the bone. However, neither simple structure materials nor complicated scaffolds can be used clinically to achieve this goal.
The increasing incidence of sports medicine injury is posing clinical challenges to surgeons worldwide. Approaches in the field of regenerative sports medicine will present promising options for the structural and functional restoration of the locomotor system. Unlike tissue regeneration in other systems of the body, the to-be-regenerated structure in the locomotor system should be capable of bearing various types of forces during regeneration without exhibiting obvious deformation and should restore the native connection of the regenerated structure to the different surrounding tissues. The future of sports medicine injury repairing scaffolds relies on using optimal components for the structural materials, bionic 3D structures, which simulate natural structures, and long-lasting viability of the regenerated structure with bioactivity.
Author Contributions
LW: Reviewing articles, Writing and original draft, Format conducting.JJ: Reviewing articles, Writing and original draft. HL: Reviewing articles, Writing and original draft. TZ: Reviewing articles, Writing and original draft. JCa: Reviewing articles, Writing and original draft. WS: Reviewing articles, Writing and original draft. JCh: Reviewing articles, Writing and original draft. JX: Reviewing articles, Writing and original draft. YL: Reviewing articles, Writing and original draft. JW: Reviewing articles, Writing and original draft. KZ: Conceptualization, Supervision, Writing - review and editing, Project administration. JZ: Funding acquisition, Conceptualization, Supervision, Writing - review and editing, Project administration.
This work was supported by National Natural Science Foundation of China (Grant No. 81902186, 81671920, 31972923, 81871753, 81772341), National Key Research and Development Program of China (Grant No. 2018YFC1106200, 2018YFC1106201, 2018YFC1106202), and Technology Support Project of Science and Technology Commission of Shanghai Municipality of China (Grant No. 19441901700, 19441901701, 19441901702, 18441902800).
Conflict of Interest
The authors declare that the research was conducted in the absence of any commercial or financial relationships that could be construed as a potential conflict of interest.
The handling editor, KL, and Reviewer, XY, declared a shared parent affiliation with the authors LW, JJ, JC, WS, JCh, JX, YL, JW, JZ, at the time of review.
Publisher’s Note
All claims expressed in this article are solely those of the authors and do not necessarily represent those of their affiliated organizations, or those of the publisher, the editors and the reviewers. Any product that may be evaluated in this article, or claim that may be made by its manufacturer, is not guaranteed or endorsed by the publisher.
- Abrams G. D., Frank R. M., Gupta A. K., Harris J. D., McCormick F. M., Cole B. J. (2013). Trends in Meniscus Repair and Meniscectomy in the United States, 2005-2011 . Am. J. Sports Med. 41 ( 10 ), 2333–2339. 10.1177/0363546513495641 [ PubMed ] [ CrossRef ] [ Google Scholar ]
- Adams J. E., Zobitz M. E., Reach J. S., An K. N., Steinmann S. P. (2006). Rotator Cuff Repair Using an Acellular Dermal Matrix Graft: an In Vivo Study in a Canine Model . Arthroscopy 22 ( 7 ). 10.1016/j.arthro.2006.03.016 [ PubMed ] [ CrossRef ] [ Google Scholar ]
- Ai C., Sheng D., Chen J., Cai J., Wang S., Jiang J., et al. (2017). Surface Modification of Vascular Endothelial Growth Factor-Loaded Silk Fibroin to Improve Biological Performance of Ultra-high-molecular-weight Polyethylene via Promoting Angiogenesis . Int. J. Nanomedicine 12 . 10.2147/IJN.S148845 [ PMC free article ] [ PubMed ] [ CrossRef ] [ Google Scholar ]
- Arshad Z., Lau E. J. S., Leow S. H., Bhatia M. (2021). Management of Chronic Achilles Ruptures: a Scoping Review . Int. Orthop . 10.1007/s00264-021-05102-5 [ PMC free article ] [ PubMed ] [ CrossRef ] [ Google Scholar ]
- Arvayo A. L., Wong I. J., Dragoo J. L., Levenston M. E. (2018). Enhancing Integration of Articular Cartilage Grafts via Photochemical Bonding . J. Orthop. Res. 36 ( 9 ). 10.1002/jor.23898 [ PubMed ] [ CrossRef ] [ Google Scholar ]
- Athanasiou K. A., Eswaramoorthy R., Hadidi P., Hu J. C. (2013). Self-organization and the Self-Assembling Process in Tissue Engineering . Annu. Rev. Biomed. Eng. 15 . 10.1146/annurev-bioeng-071812-152423 [ PMC free article ] [ PubMed ] [ CrossRef ] [ Google Scholar ]
- Babaie E., Bhaduri S. B. (2018). Fabrication Aspects of Porous Biomaterials in Orthopedic Applications: A Review . ACS Biomater. Sci. Eng. 4 ( 1 ). 10.1021/acsbiomaterials.7b00615 [ PubMed ] [ CrossRef ] [ Google Scholar ]
- Baby B. (2000). What Is Sports Medicine? Medical Students Don't Know . Br. J. Sports Med. 34 ( 1 ), 73. 10.1136/bjsm.34.1.73-a [ PMC free article ] [ PubMed ] [ CrossRef ] [ Google Scholar ]
- Badylak S. F., Lantz G. C., Coffey A., Geddes L. A. (1989). Small Intestinal Submucosa as a Large Diameter Vascular Graft in the Dog . J. Surg. Res. 47 ( 1 ). 10.1016/0022-4804(89)90050-4 [ PubMed ] [ CrossRef ] [ Google Scholar ]
- Badylak S. F., Tullius R., Kokini K., Shelbourne K. D., Klootwyk T., Voytik S. L., et al. (1995). The Use of Xenogeneic Small Intestinal Submucosa as a Biomaterial for Achilles Tendon Repair in a Dog Model . J. Biomed. Mater Res. 29 ( 8 ). 10.1002/jbm.820290809 [ PubMed ] [ CrossRef ] [ Google Scholar ]
- Barboni B., Russo V., Gatta V., Bernabò N., Berardinelli P., Mauro A., et al. (2018). Therapeutic Potential of hAECs for Early Achilles Tendon Defect Repair through Regeneration . J. Tissue Eng. Regen. Med. 12 ( 3 ). 10.1002/term.2584 [ PubMed ] [ CrossRef ] [ Google Scholar ]
- Beck A., Murphy D. J., Carey-Smith R., Wood D. J., Zheng M. H. (2016). Treatment of Articular Cartilage Defects with Microfracture and Autologous Matrix-Induced Chondrogenesis Leads to Extensive Subchondral Bone Cyst Formation in a Sheep Model . Am. J. Sports Med. 44 ( 10 ), 2629–2643. 10.1177/0363546516652619 [ PubMed ] [ CrossRef ] [ Google Scholar ]
- Blaker J. J., Nazhat S. N., Maquet V., Boccaccini A. R. (2011). Long-term In Vitro Degradation of PDLLA/bioglass Bone Scaffolds in Acellular Simulated Body Fluid . Acta Biomater. 7 ( 2 ). 10.1016/j.actbio.2010.09.013 [ PubMed ] [ CrossRef ] [ Google Scholar ]
- Bolander J., Ji W., Geris L., Bloemen V., Chai Y. C., Schrooten J., et al. (2016). The Combined Mechanism of Bone Morphogenetic Protein- and Calcium Phosphate-Induced Skeletal Tissue Formation by Human Periosteum Derived Cells . Eur. Cell Mater 31 . 10.22203/ecm.v031a02 [ PubMed ] [ CrossRef ] [ Google Scholar ]
- Bolander J., Ji W., Leijten J., Teixeira L. M., Bloemen V., Lambrechts D., et al. (2017). Healing of a Large Long-Bone Defect through Serum-free In Vitro Priming of Human Periosteum-Derived Cells . Stem Cell Rep. 8 ( 3 ). 10.1016/j.stemcr.2017.01.005 [ PMC free article ] [ PubMed ] [ CrossRef ] [ Google Scholar ]
- Brody H. (2016). Regenerative Medicine . Nature 540 ( 7632 ). 10.1038/540s49a [ PubMed ] [ CrossRef ] [ Google Scholar ]
- Bryant D., Holtby R., Willits K., Litchfield R., Drosdowech D., Spouge A., et al. (2016). A Randomized Clinical Trial to Compare the Effectiveness of Rotator Cuff Repair with or without Augmentation Using Porcine Small Intestine Submucosa for Patients with Moderate to Large Rotator Cuff Tears: a Pilot Study . J. Shoulder Elb. Surg. 25 ( 10 ). 10.1016/j.jse.2016.06.006 [ PubMed ] [ CrossRef ] [ Google Scholar ]
- Bulgheroni E., Grassi A., Bulgheroni P., Marcheggiani Muccioli G. M., Zaffagnini S., Marcacci M. (2015). Long-term Outcomes of Medial CMI Implant versus Partial Medial Meniscectomy in Patients with Concomitant ACL Reconstruction . Knee Surg. Sports Traumatol. Arthrosc. 23 ( 11 ). 10.1007/s00167-014-3136-9 [ PubMed ] [ CrossRef ] [ Google Scholar ]
- Cai C., Wang W., Liang J., Li Y., Lu M., Cui W., et al. (2021). MMP-2 Responsive Unidirectional Hydrogel-Electrospun Patch Loading TGF-β1 siRNA Polyplexes for Peritendinous Anti-Adhesion . Adv. Funct. Mater. 31 ( 6 ). 10.1002/adfm.202008364 [ CrossRef ] [ Google Scholar ]
- Cai C., Zhang X., Li Y., Liu X., Wang S., Lu M., et al. (2022). Self-Healing Hydrogel Embodied with Macrophage-Regulation and Responsive-Gene-Silencing Properties for Synergistic Prevention of Peritendinous Adhesion . Adv. Mater. 34 ( 5 ). 10.1002/adma.202106564 [ PubMed ] [ CrossRef ] [ Google Scholar ]
- Cai J., Wang J., Ye K., Li D., Ai C., Sheng D., et al. (2018). Dual-layer Aligned-Random Nanofibrous Scaffolds for Improving Gradient Microstructure of Tendon-To-Bone Healing in a Rabbit Extra-articular Model . Int. J. Nanomedicine 13 . 10.2147/IJN.S165633 [ PMC free article ] [ PubMed ] [ CrossRef ] [ Google Scholar ]
- Cai J. Y., Xu J. J., Kang Y. H., Li Y. F., Wang L. R., Yan X. Y., et al. (2021). Acceleration of Ligamentization and Osseointegration Processes after Anterior Cruciate Ligament Reconstruction with Autologous Tissue-Engineered Polyethylene Terephthalate Graft . Ann. Transl. Med. 9 ( 9 ). 10.21037/atm-20-8048 [ PMC free article ] [ PubMed ] [ CrossRef ] [ Google Scholar ]
- Cai J., Zhang Q., Chen J., Jiang J., Mo X., He C., et al. (2021). Electrodeposition of Calcium Phosphate onto Polyethylene Terephthalate Artificial Ligament Enhances Graft-Bone Integration after Anterior Cruciate Ligament Reconstruction . Bioact. Mater 6 ( 3 ). 10.1016/j.bioactmat.2020.08.037 [ PMC free article ] [ PubMed ] [ CrossRef ] [ Google Scholar ]
- Cardwell R. D., Dahlgren L. A., Goldstein A. S. (2014). Electrospun Fibre Diameter, Not Alignment, Affects Mesenchymal Stem Cell Differentiation into the Tendon/ligament Lineage . J. Tissue Eng. Regen. Med. 8 ( 12 ). 10.1002/term.1589 [ PubMed ] [ CrossRef ] [ Google Scholar ]
- Carragee E. J., Hurwitz E. L., Weiner B. K. (2011). A Critical Review of Recombinant Human Bone Morphogenetic Protein-2 Trials in Spinal Surgery: Emerging Safety Concerns and Lessons Learned . Spine J. 11 ( 6 ). 10.1016/j.spinee.2011.04.023 [ PubMed ] [ CrossRef ] [ Google Scholar ]
- Chae S., Lee S. S., Choi Y. J., Hong D. H., Gao G., Wang J. H., et al. (2021). 3D Cell-Printing of Biocompatible and Functional Meniscus Constructs Using Meniscus-Derived Bioink . Amsterdam: Biomaterials, 267. [ PubMed ] [ Google Scholar ]
- Chahla J., Dean C. S., Matheny L. M., Mitchell J. J., Cinque M. E., LaPrade R. F. (2017). Outcomes of Inside-Out Meniscal Repair in the Setting of Multiligament Reconstruction in the Knee . Am. J. Sports Med. 45 ( 9 ). 10.1177/0363546517698944 [ PubMed ] [ CrossRef ] [ Google Scholar ]
- Chen C., Hunt K. J. (2019). Open Reconstructive Strategies for Chronic Achilles Tendon Ruptures . Foot Ankle Clin. 24 ( 3 ). 10.1016/j.fcl.2019.04.002 [ PubMed ] [ CrossRef ] [ Google Scholar ]
- Chen L., Deng C., Li J., Yao Q., Chang J., Wang L., et al. (2019). 3D Printing of a Lithium-Calcium-Silicate Crystal Bioscaffold with Dual Bioactivities for Osteochondral Interface Reconstruction . Amsterdam: Biomaterials. [ PubMed ] [ Google Scholar ]
- Chiou G. J., Crowe C., McGoldrick R., Hui K., Pham H., Chang J. (2015). Optimization of an Injectable Tendon Hydrogel: the Effects of Platelet-Rich Plasma and Adipose-Derived Stem Cells on Tendon Healing In Vivo . Tissue Eng. Part A 21 ( 9-10 ). 10.1089/ten.TEA.2014.0490 [ PubMed ] [ CrossRef ] [ Google Scholar ]
- Cho K. O. (1975). Reconstruction of the Anterior Cruciate Ligament by Semitendinosus Tenodesis . J. Bone & Jt. Surg. Am. Volume) 57 ( 5 ). 10.2106/00004623-197557050-00003 [ PubMed ] [ CrossRef ] [ Google Scholar ]
- Choe R., Devoy E., Jabari E., Packer J. D., Fisher J. P. (2021). Biomechanical Aspects of Osteochondral Regeneration: Implications and Strategies for 3D Bioprinting . New York: Tissue Eng Part B Rev. [ PMC free article ] [ PubMed ] [ Google Scholar ]
- Choi Y. Y., Rhee S. J. (2017). Medial Opening Wedge High Tibial Osteotomy in Patients with Posttraumatic Medial Tibial Plateau Bone Defect: A Case Report . Int. J. Surg. Case Rep. 41 . 10.1016/j.ijscr.2017.10.020 [ PMC free article ] [ PubMed ] [ CrossRef ] [ Google Scholar ]
- Chow J. C., Hantes M. E., Houle J. B., Zalavras C. G. (2004). Arthroscopic Autogenous Osteochondral Transplantation for Treating Knee Cartilage Defects: a 2- to 5-year Follow-Up Study . Arthroscopy 20 ( 7 ). 10.1016/j.arthro.2004.06.005 [ PubMed ] [ CrossRef ] [ Google Scholar ]
- Clancy W. G., Jr., Nelson D. A., Reider B., Narechania R. G. (1982). Anterior Cruciate Ligament Reconstruction Using One-Third of the Patellar Ligament, Augmented by Extra-articular Tendon Transfers . J. Bone & Jt. Surg. Am. Volume) 64 ( 3 ). 10.2106/00004623-198264030-00004 [ PubMed ] [ CrossRef ] [ Google Scholar ]
- Colquhoun R., Tanner K. E. (2015). Mechanical Behaviour of Degradable Phosphate Glass Fibres and Composites-A Review . Biomed. Mater 11 ( 1 ). 10.1088/1748-6041/11/1/014105 [ PubMed ] [ CrossRef ] [ Google Scholar ]
- Coons D. A., Alan Barber F. (2006). Tendon Graft Substitutes-Rotator Cuff Patches . Sports Med. Arthrosc. Rev. 14 ( 3 ). 10.1097/00132585-200609000-00011 [ PubMed ] [ CrossRef ] [ Google Scholar ]
- Correia Pinto V., Costa-Almeida R., Rodrigues I., Guardao L., Soares R., Miranda Guedes R. (2017). Exploring the In Vitro and In Vivo Compatibility of PLA, PLA/GNP and PLA/CNT-COOH Biodegradable Nanocomposites: Prospects for Tendon and Ligament Applications . J. Biomed. Mater Res. A 105 ( 8 ). 10.1002/jbm.a.36075 [ PubMed ] [ CrossRef ] [ Google Scholar ]
- Dahlstedt L. J., Netz P., Dalen N. (1989). Poor Results of Bovine Xenograft for Knee Cruciate Ligament Repair . Acta Orthop. Scand. 60 ( 1 ). 10.3109/17453678909150079 [ PubMed ] [ CrossRef ] [ Google Scholar ]
- Dean C. S., Chahla J., Matheny L. M., Mitchell J. J., LaPrade R. F. (2017). Outcomes after Biologically Augmented Isolated Meniscal Repair with Marrow Venting Are Comparable with Those after Meniscal Repair with Concomitant Anterior Cruciate Ligament Reconstruction . Am. J. Sports Med. 45 ( 6 ). 10.1177/0363546516686968 [ PubMed ] [ CrossRef ] [ Google Scholar ]
- Deng C., Yang J., He H., Ma Z., Wang W., Zhang Y., et al. (2021). 3D Bio-Printed Biphasic Scaffolds with Dual Modification of Silk Fibroin for the Integrated Repair of Osteochondral Defects . Biomater. Sci. 9 ( 14 ). 10.1039/d1bm00535a [ PubMed ] [ CrossRef ] [ Google Scholar ]
- DePhillipo N. N., Dekker T. J., Aman Z. S., Bernholt D., Grantham W. J., LaPrade R. F. (2019). Incidence and Healing Rates of Meniscal Tears in Patients Undergoing Repair during the First Stage of 2-Stage Revision Anterior Cruciate Ligament Reconstruction . Am. J. Sports Med. 47 ( 14 ). 10.1177/0363546519878421 [ PubMed ] [ CrossRef ] [ Google Scholar ]
- Deymier-Black A. C., Pasteris J. D., Genin G. M., Thomopoulos S. (2015). Allometry of the Tendon Enthesis: Mechanisms of Load Transfer between Tendon and Bone . J. Biomech. Eng. 137 ( 11 ). 10.1115/1.4031571 [ PMC free article ] [ PubMed ] [ CrossRef ] [ Google Scholar ]
- Ding C., Qiao Z., Jiang W., Li H., Wei J., Zhou G., et al. (2013). Regeneration of a Goat Femoral Head Using a Tissue-specific, Biphasic Scaffold Fabricated with CAD/CAM Technology . Biomaterials 34 ( 28 ). 10.1016/j.biomaterials.2013.05.038 [ PubMed ] [ CrossRef ] [ Google Scholar ]
- El Bialy I., Jiskoot W., Reza Nejadnik M. (2017). Formulation, Delivery and Stability of Bone Morphogenetic Proteins for Effective Bone Regeneration . Pharm. Res. 34 ( 6 ). 10.1007/s11095-017-2147-x [ PMC free article ] [ PubMed ] [ CrossRef ] [ Google Scholar ]
- Elder B. D., Athanasiou K. A. (2009). Systematic Assessment of Growth Factor Treatment on Biochemical and Biomechanical Properties of Engineered Articular Cartilage Constructs . Osteoarthr. Cartil. 17 ( 1 ). 10.1016/j.joca.2008.05.006 [ PMC free article ] [ PubMed ] [ CrossRef ] [ Google Scholar ]
- Eldridge S. E., Barawi A., Wang H., Roelofs A. J., Kaneva M., Guan Z., et al. (2020). Agrin Induces Long-Term Osteochondral Regeneration by Supporting Repair Morphogenesis . Sci. Transl. Med. 12 ( 559 ). 10.1126/scitranslmed.aax9086 [ PubMed ] [ CrossRef ] [ Google Scholar ]
- Erisken C., Kalyon D. M., Wang H. (2008). Functionally Graded Electrospun Polycaprolactone and Beta-Tricalcium Phosphate Nanocomposites for Tissue Engineering Applications . Biomaterials 29 ( 30 ). 10.1016/j.biomaterials.2008.06.022 [ PubMed ] [ CrossRef ] [ Google Scholar ]
- Farberg A. S., Jing X. L., Monson L. A., Donneys A., Tchanque-Fossuo C. N., Deshpande S. S., et al. (2012). Deferoxamine Reverses Radiation Induced Hypovascularity during Bone Regeneration and Repair in the Murine Mandible . Bone 50 ( 5 ). 10.1016/j.bone.2012.01.019 [ PMC free article ] [ PubMed ] [ CrossRef ] [ Google Scholar ]
- Fernandez-Yague M. A., Abbah S. A., McNamara L., Zeugolis D. I., Pandit A., Biggs M. J. (2015). Biomimetic Approaches in Bone Tissue Engineering: Integrating Biological and Physicomechanical Strategies . Adv. Drug Deliv. Rev. 84 . 10.1016/j.addr.2014.09.005 [ PubMed ] [ CrossRef ] [ Google Scholar ]
- Fini M., Bondioli E., Castagna A., Torricelli P., Giavaresi G., Rotini R., et al. (2012). Decellularized Human Dermis to Treat Massive Rotator Cuff Tears: In Vitro Evaluations . Connect. Tissue Res. 53 ( 4 ). 10.3109/03008207.2011.649929 [ PubMed ] [ CrossRef ] [ Google Scholar ]
- Foster T. E. (2015). Redefining the Value of Sports Medicine . Am. J. Sports Med. 43 ( 7 ), 1573–1574. 10.1177/0363546515590803 [ PubMed ] [ CrossRef ] [ Google Scholar ]
- Gachon E., Mesquida P. (2021). Mechanical Strain Alters the Surface Charge of Collagen Fibrils . ACS Nano . 10.1021/acsnano.1c00682 [ PubMed ] [ CrossRef ] [ Google Scholar ]
- Galili U., Stone K. R. (2021). In Situ "Humanization" of Porcine Bioprostheses: Demonstration of Tendon Bioprostheses Conversion into Human ACL and Possible Implications for Heart Valve Bioprostheses . Bioeng. (Basel) 8 ( 1 ). 10.3390/bioengineering8010010 [ PMC free article ] [ PubMed ] [ CrossRef ] [ Google Scholar ]
- Gao J., Ding X., Yu X., Chen X., Zhang X., Cui S., et al. (2021). Cell-Free Bilayered Porous Scaffolds for Osteochondral Regeneration Fabricated by Continuous 3D-Printing Using Nascent Physical Hydrogel as Ink . Adv. Healthc. Mater 10 ( 3 ). 10.1002/adhm.202001404 [ PubMed ] [ CrossRef ] [ Google Scholar ]
- Gardner O. F., Fahy N., Alini M., Stoddart M. J. (2016). Differences in Human Mesenchymal Stem Cell Secretomes during Chondrogenic Induction . Eur. Cell Mater 31 . 10.22203/ecm.v031a15 [ PubMed ] [ CrossRef ] [ Google Scholar ]
- Giannoudis P. V., Dinopoulos H., Tsiridis E. (2005). Bone Substitutes: an Update . Injury 36 ( Suppl. 3 ). 10.1016/j.injury.2005.07.029 [ PubMed ] [ CrossRef ] [ Google Scholar ]
- Gong T., Xie J., Liao J., Zhang T., Lin S., Lin Y. (2015). Nanomaterials and Bone Regeneration . Bone Res. 3 . 10.1038/boneres.2015.29 [ PMC free article ] [ PubMed ] [ CrossRef ] [ Google Scholar ]
- Good L., Odensten M., Pettersson L., Gillquist J. (1989). Failure of a Bovine Xenograft for Reconstruction of the Anterior Cruciate Ligament . Acta Orthop. Scand. 60 ( 1 ). 10.3109/17453678909150080 [ PubMed ] [ CrossRef ] [ Google Scholar ]
- Greenberg D. D., Robertson M., Vallurupalli S., White R. A., Allen W. C. (2010). Allograft Compared with Autograft Infection Rates in Primary Anterior Cruciate Ligament Reconstruction . J. Bone & Jt. Surg. Am. Volume) 92 ( 14 ). 10.2106/JBJS.I.00456 [ PubMed ] [ CrossRef ] [ Google Scholar ]
- Guevara J. A., Entezari V., Ho J. C., Derwin K. A., Iannotti J. P., Ricchetti E. T. (2020). An Update on Surgical Management of the Repairable Large-To-Massive Rotator Cuff Tear . J. Bone Jt. Surg. Am. 102 ( 19 ). 10.2106/JBJS.20.00177 [ PubMed ] [ CrossRef ] [ Google Scholar ]
- Gungormus C., Kolankaya D., Aydin E. (2015). Histopathological and Biomechanical Evaluation of Tenocyte Seeded Allografts on Rat Achilles Tendon Regeneration . Amsterdam: Biomaterials, 51. [ PubMed ] [ Google Scholar ]
- Guo T., Noshin M., Baker H. B., Taskoy E., Meredith S. J., Tang Q., et al. (2018). 3D Printed Biofunctionalized Scaffolds for Microfracture Repair of Cartilage Defects . Amsterdam: Biomaterials, 185. [ PMC free article ] [ PubMed ] [ Google Scholar ]
- Hadidi P., Paschos N. K., Huang B. J., Aryaei A., Hu J. C., Athanasiou K. A. (2016). Tendon and Ligament as Novel Cell Sources for Engineering the Knee Meniscus . Osteoarthr. Cartil. 24 ( 12 ). 10.1016/j.joca.2016.07.006 [ PMC free article ] [ PubMed ] [ CrossRef ] [ Google Scholar ]
- Hall G. N., Tam W. L., Andrikopoulos K. S., Casas-Fraile L., Voyiatzis G. A., Geris L., et al. (2021). Patterned, Organoid-Based Cartilaginous Implants Exhibit Zone Specific Functionality Forming Osteochondral-like Tissues in Vivo . Amsterdam: Biomaterials, 273. [ PubMed ] [ Google Scholar ]
- Harris M. T., Butler D. L., Boivin G. P., Florer J. B., Schantz E. J., Wenstrup R. J. (2004). Mesenchymal Stem Cells Used for Rabbit Tendon Repair Can Form Ectopic Bone and Express Alkaline Phosphatase Activity in Constructs . J. Orthop. Res. 22 ( 5 ). 10.1016/j.orthres.2004.02.012 [ PubMed ] [ CrossRef ] [ Google Scholar ]
- Higashioka M. M., Chen J. A., Hu J. C., Athanasiou K. A. (2014). Building an Anisotropic Meniscus with Zonal Variations . Tissue Eng. Part A 20 ( 1-2 ). 10.1089/ten.TEA.2013.0098 [ PMC free article ] [ PubMed ] [ CrossRef ] [ Google Scholar ]
- Howe J. G., Johnson R. J., Kaplan M. J., Fleming B., Jarvinen M. (1991). Anterior Cruciate Ligament Reconstruction Using Quadriceps Patellar Tendon Graft. Part I. Long-Term Followup . Am. J. Sports Med. 19 ( 5 ). 10.1177/036354659101900505 [ PubMed ] [ CrossRef ] [ Google Scholar ]
- Hua Y., Xia H., Jia L., Zhao J., Zhao D., Yan X., et al. (2021). Ultrafast, Tough, and Adhesive Hydrogel Based on Hybrid Photocrosslinking for Articular Cartilage Repair in Water-Filled Arthroscopy . Sci. Adv. 7 ( 35 ). 10.1126/sciadv.abg0628 [ PMC free article ] [ PubMed ] [ CrossRef ] [ Google Scholar ]
- Huwe L. W., Brown W. E., Hu J. C., Athanasiou K. A. (2018). Characterization of Costal Cartilage and its Suitability as a Cell Source for Articular Cartilage Tissue Engineering . J. Tissue Eng. Regen. Med. 12 ( 5 ). 10.1002/term.2630 [ PMC free article ] [ PubMed ] [ CrossRef ] [ Google Scholar ]
- Iannotti J. P., Codsi M. J., Kwon Y. W., Derwin K., Ciccone J., Brems J. J. (2006). Porcine Small Intestine Submucosa Augmentation of Surgical Repair of Chronic Two-Tendon Rotator Cuff Tears. A Randomized, Controlled Trial . J. Bone Jt. Surg. Am. 88 ( 6 ). 10.2106/JBJS.E.00524 [ PubMed ] [ CrossRef ] [ Google Scholar ]
- Ingber D. E., Mow V. C., Butler D., Niklason L., Huard J., Mao J., et al. (2006). Tissue Engineering and Developmental Biology: Going Biomimetic . Tissue Eng. 12 ( 12 ). 10.1089/ten.2006.12.3265 [ PubMed ] [ CrossRef ] [ Google Scholar ]
- Jackson D. W., Grood E. S., Goldstein J. D., Rosen M. A., Kurzweil P. R., Cummings J. F., et al. (1993). A Comparison of Patellar Tendon Autograft and Allograft Used for Anterior Cruciate Ligament Reconstruction in the Goat Model . Am. J. Sports Med. 21 ( 2 ). 10.1177/036354659302100203 [ PubMed ] [ CrossRef ] [ Google Scholar ]
- Jiang G., Li S., Yu K., He B., Hong J., Xu T., et al. (2021). A 3D-Printed PRP-GelMA Hydrogel Promotes Osteochondral Regeneration through M2 Macrophage Polarization in a Rabbit Model . Acta Biomater. 128 . 10.1016/j.actbio.2021.04.010 [ PubMed ] [ CrossRef ] [ Google Scholar ]
- Karageorgiou V., Kaplan D. (2005). Porosity of 3D Biomaterial Scaffolds and Osteogenesis . Biomaterials 26 ( 27 ). 10.1016/j.biomaterials.2005.02.002 [ PubMed ] [ CrossRef ] [ Google Scholar ]
- Kato Y., Chavez J., Yamada S., Hattori S., Takazawa S., Ohuchi H. (2019). A Large Knee Osteochondral Lesion Treated Using a Combination of Osteochondral Autograft Transfer and Second-Generation Autologous Chondrocyte Implantation: A Case Report . Regen. Ther. 10 . 10.1016/j.reth.2018.10.002 [ PMC free article ] [ PubMed ] [ CrossRef ] [ Google Scholar ]
- Khira Y. M., Salama A. M. (2017). Treatment of Locked Posterior Shoulder Dislocation with Bone Defect . Orthopedics 40 ( 3 ). 10.3928/01477447-20170308-07 [ PubMed ] [ CrossRef ] [ Google Scholar ]
- Kim H. S., Mandakhbayar N., Kim H. W., Leong K. W., Yoo H. S. (2021). Protein-reactive Nanofibrils Decorated with Cartilage-Derived Decellularized Extracellular Matrix for Osteochondral Defects . Amsterdam: Biomaterials, 269. [ PubMed ] [ Google Scholar ]
- Kim J. W., Jung I. H., Lee K. I., Jung U. W., Kim C. S., Choi S. H., et al. (2012). Volumetric Bone Regenerative Efficacy of Biphasic Calcium Phosphate-Collagen Composite Block Loaded with rhBMP-2 in Vertical Bone Augmentation Model of a Rabbit Calvarium . J. Biomed. Mater Res. A 100 ( 12 ). 10.1002/jbm.a.34278 [ PubMed ] [ CrossRef ] [ Google Scholar ]
- Koh R. H., Jin Y., Kang B. J., Hwang N. S. (2017). Chondrogenically Primed Tonsil-Derived Mesenchymal Stem Cells Encapsulated in Riboflavin-Induced Photocrosslinking Collagen-Hyaluronic Acid Hydrogel for Meniscus Tissue Repairs . Acta Biomater. 53 . 10.1016/j.actbio.2017.01.081 [ PubMed ] [ CrossRef ] [ Google Scholar ]
- Kraeutler M. J., Purcell J. M., Hunt K. J. (2017). Chronic Achilles Tendon Ruptures . Foot Ankle Int. 38 ( 8 ). 10.1177/1071100717709570 [ PubMed ] [ CrossRef ] [ Google Scholar ]
- Krishnan V., Bryant H. U., Macdougald O. A. (2006). Regulation of Bone Mass by Wnt Signaling . J. Clin. Investig. 116 ( 5 ). 10.1172/JCI28551 [ PMC free article ] [ PubMed ] [ CrossRef ] [ Google Scholar ]
- Kweon C. Y., Hagen M. S., Gee A. O. (2019). What's New in Sports Medicine . J. Bone Jt. Surg. Am. 101 ( 8 ), 669–674. 10.2106/JBJS.19.00007 [ PubMed ] [ CrossRef ] [ Google Scholar ]
- Kwon H., Brown W. E., Lee C. A., Wang D., Paschos N., Hu J. C., et al. (2019). Surgical and Tissue Engineering Strategies for Articular Cartilage and Meniscus Repair . Nat. Rev. Rheumatol. 15 ( 9 ). 10.1038/s41584-019-0255-1 [ PMC free article ] [ PubMed ] [ CrossRef ] [ Google Scholar ]
- Labek G., Thaler M., Janda W., Agreiter M., Stockl B. (2011). Revision Rates after Total Joint Replacement: Cumulative Results from Worldwide Joint Register Datasets . J. Bone Jt. Surg. Br. 93 ( 3 ). 10.1302/0301-620X.93B3.25467 [ PubMed ] [ CrossRef ] [ Google Scholar ]
- Laurencin C., Khan Y., El-Amin S. F. (2006). Bone Graft Substitutes . Expert Rev. Med. Devices 3 ( 1 ). 10.1586/17434440.3.1.49 [ PubMed ] [ CrossRef ] [ Google Scholar ]
- Lee S. H., Moon J. H., Jeong C. M., Bae E. B., Park C. E., Jeon G. R., et al. (20172017). The Mechanical Properties and Biometrical Effect of 3D Preformed Titanium Membrane for Guided Bone Regeneration on Alveolar Bone Defect . Biomed. Res. Int. 10.1155/2017/7102123 [ PMC free article ] [ PubMed ] [ CrossRef ] [ Google Scholar ]
- Lee S. J., Nam Y., Rim Y. A., Lee K., Ju J. H., Kim D. S. (2021). Perichondrium-inspired Permeable Nanofibrous Tube Well Promoting Differentiation of hiPSC-Derived Pellet toward Hyaline-like Cartilage Pellet . Biofabrication . 10.1088/1758-5090/ac1e76 [ PubMed ] [ CrossRef ] [ Google Scholar ]
- Lenas P., Moos M., Luyten F. P. (2009). Developmental Engineering: a New Paradigm for the Design and Manufacturing of Cell-Based Products. Part I: from Three-Dimensional Cell Growth to Biomimetics of In Vivo Development . Tissue Eng. Part B Rev. 15 ( 4 ). 10.1089/ten.TEB.2008.0575 [ PubMed ] [ CrossRef ] [ Google Scholar ]
- Lewington M. R., Ferguson D. P., Smith T. D., Burks R., Coady C., Wong I. H. (2017). Graft Utilization in the Bridging Reconstruction of Irreparable Rotator Cuff Tears: A Systematic Review . Am. J. Sports Med. 45 ( 13 ). 10.1177/0363546517694355 [ PubMed ] [ CrossRef ] [ Google Scholar ]
- Li H., Li P., Yang Z., Gao C., Fu L., Liao Z., et al. (2021). Meniscal Regenerative Scaffolds Based on Biopolymers and Polymers: Recent Status and Applications . Front. Cell Dev. Biol. 9 . 10.3389/fcell.2021.661802 [ PMC free article ] [ PubMed ] [ CrossRef ] [ Google Scholar ]
- Li H., Yao Z., Jiang J., Hua Y., Chen J., Li Y., et al. (2012). Biologic Failure of a Ligament Advanced Reinforcement System Artificial Ligament in Anterior Cruciate Ligament Reconstruction: a Report of Serious Knee Synovitis . Arthroscopy 28 ( 4 ). 10.1016/j.arthro.2011.12.008 [ PubMed ] [ CrossRef ] [ Google Scholar ]
- Li T., Peng M., Yang Z., Zhou X., Deng Y., Jiang C., et al. (2018). 3D-printed IFN-Gamma-Loading Calcium Silicate-Beta-Tricalcium Phosphate Scaffold Sequentially Activates M1 and M2 Polarization of Macrophages to Promote Vascularization of Tissue Engineering Bone . Acta Biomater. 71 . 10.1016/j.actbio.2018.03.012 [ PubMed ] [ CrossRef ] [ Google Scholar ]
- Li X., Cheng R., Sun Z., Su W., Pan G., Zhao S., et al. (2017b). Flexible Bipolar Nanofibrous Membranes for Improving Gradient Microstructure in Tendon-To-Bone Healing . Acta Biomater. 61 . 10.1016/j.actbio.2017.07.044 [ PubMed ] [ CrossRef ] [ Google Scholar ]
- Li X., He J., Bian W., Li Z., Zhang W., Li D., et al. (2014). A Novel Silk-Based Artificial Ligament and Tricalcium Phosphate/polyether Ether Ketone Anchor for Anterior Cruciate Ligament Reconstruction - Safety and Efficacy in a Porcine Model . Acta Biomater. 10 ( 8 ). 10.1016/j.actbio.2014.05.015 [ PubMed ] [ CrossRef ] [ Google Scholar ]
- Li X., Wang Y., Zhao Y., Liu J., Xiao S., Mao K. (2017a). Multilevel 3D Printing Implant for Reconstructing Cervical Spine with Metastatic Papillary Thyroid Carcinoma . Spine (Phila Pa 1976) ( 22 ), 42. 10.1097/BRS.0000000000002229 [ PubMed ] [ CrossRef ] [ Google Scholar ]
- Li Y. H., Wang Z. D., Wang W., Ding C. W., Zhang H. X., Li J. M. (2015). The Biocompatibility of Calcium Phosphate Cements Containing Alendronate-Loaded PLGA Microparticles In Vitro . Exp. Biol. Med. (Maywood) 240 ( 11 ). 10.1177/1535370215579142 [ PMC free article ] [ PubMed ] [ CrossRef ] [ Google Scholar ]
- Liu M., Zeng X., Ma C., Yi H., Ali Z., Mou X., et al. (2017). Injectable Hydrogels for Cartilage and Bone Tissue Engineering . Bone Res. 5 . 10.1038/boneres.2017.14 [ PMC free article ] [ PubMed ] [ CrossRef ] [ Google Scholar ]
- Liu Q., Yu Y., Reisdorf R. L., Qi J., Lu C. K., Berglund L. J., et al. (2019). Engineered Tendon-Fibrocartilage-Bone Composite and Bone Marrow-Derived Mesenchymal Stem Cell Sheet Augmentation Promotes Rotator Cuff Healing in a Non-weight-bearing Canine Model . Amsterdam: Biomaterials. [ PubMed ] [ Google Scholar ]
- Liu S., Li P., Liu X., Wang P., Xue W., Ren Y., et al. (2021). Bioinspired Mineral-Polymeric Hybrid Hyaluronic Acid/poly (Gamma-glutamic Acid) Hydrogels as Tunable Scaffolds for Stem Cells Differentiation . Carbohydr. Polym. 264 . 10.1016/j.carbpol.2021.118048 [ PubMed ] [ CrossRef ] [ Google Scholar ]
- Lu H. H., Cooper J. A., Jr., Manuel S., Freeman J. W., Attawia M. A., Ko F. K., et al. (2005). Anterior Cruciate Ligament Regeneration Using Braided Biodegradable Scaffolds: In Vitro Optimization Studies . Biomaterials 26 ( 23 ). 10.1016/j.biomaterials.2004.11.050 [ PubMed ] [ CrossRef ] [ Google Scholar ]
- Lu H. H., Thomopoulos S. (2013). Functional Attachment of Soft Tissues to Bone: Development, Healing, and Tissue Engineering . Annu. Rev. Biomed. Eng. 15 . 10.1146/annurev-bioeng-071910-124656 [ PMC free article ] [ PubMed ] [ CrossRef ] [ Google Scholar ]
- Lynch M. E., Chiou A. E., Lee M. J., Marcott S. C., Polamraju P. V., Lee Y., et al. (2016). Three-Dimensional Mechanical Loading Modulates the Osteogenic Response of Mesenchymal Stem Cells to Tumor-Derived Soluble Signals . Tissue Eng. Part A 22 ( 15-16 ). 10.1089/ten.TEA.2016.0153 [ PMC free article ] [ PubMed ] [ CrossRef ] [ Google Scholar ]
- MacBarb R. F., Chen A. L., Hu J. C., Athanasiou K. A. (2013). Engineering Functional Anisotropy in Fibrocartilage Neotissues . Biomaterials 34 ( 38 ). 10.1016/j.biomaterials.2013.09.026 [ PMC free article ] [ PubMed ] [ CrossRef ] [ Google Scholar ]
- Maffulli N., Oliva F., Maffulli G. D., Buono A. D., Gougoulias N. (2018). Surgical Management of Chronic Achilles Tendon Ruptures Using Less Invasive Techniques . Foot Ankle Surg. 24 ( 2 ). 10.1016/j.fas.2017.02.002 [ PubMed ] [ CrossRef ] [ Google Scholar ]
- Makris E. A., Hadidi P., Athanasiou K. A. (2011). The Knee Meniscus: Structure-Function, Pathophysiology, Current Repair Techniques, and Prospects for Regeneration . Biomaterials 32 ( 30 ). 10.1016/j.biomaterials.2011.06.037 [ PMC free article ] [ PubMed ] [ CrossRef ] [ Google Scholar ]
- Martin F., Lehmann M., Sack U., Anderer U. (2017). Featured Article: In Vitro Development of Personalized Cartilage Microtissues Uncovers an Individualized Differentiation Capacity of Human Chondrocytes . Exp. Biol. Med. (Maywood) 242 ( 18 ). 10.1177/1535370217728498 [ PMC free article ] [ PubMed ] [ CrossRef ] [ Google Scholar ]
- Mastrokalos D. S., Springer J., Siebold R., Paessler H. H. (2005). Donor Site Morbidity and Return to the Preinjury Activity Level after Anterior Cruciate Ligament Reconstruction Using Ipsilateral and Contralateral Patellar Tendon Autograft: a Retrospective, Nonrandomized Study . Am. J. Sports Med. 33 ( 1 ). 10.1177/0363546504265926 [ PubMed ] [ CrossRef ] [ Google Scholar ]
- McElvany M. D., McGoldrick E., Gee A. O., Neradilek M. B., Matsen F. A. (2015). Rotator Cuff Repair: Published Evidence on Factors Associated with Repair Integrity and Clinical Outcome . Am. J. Sports Med. 43 ( 2 ). 10.1177/0363546514529644 [ PubMed ] [ CrossRef ] [ Google Scholar ]
- McFarland E. G., Huri G., Hyun Y. S., Petersen S. A., Srikumaran U. (2016). Reverse Total Shoulder Arthroplasty without Bone-Grafting for Severe Glenoid Bone Loss in Patients with Osteoarthritis and Intact Rotator Cuff . J. Bone Jt. Surg. Am. 98 ( 21 ). 10.2106/JBJS.15.01181 [ PubMed ] [ CrossRef ] [ Google Scholar ]
- McGovern J. A., Griffin M., Hutmacher D. W. (2018). Animal Models for Bone Tissue Engineering and Modelling Disease . Dis. Model. Mech. 11 ( 4 ). 10.1242/dmm.033084 [ PMC free article ] [ PubMed ] [ CrossRef ] [ Google Scholar ]
- Meng C., Su W., Liu M., Yao S., Ding Q., Yu K., et al. (2021). Controlled Delivery of Bone Morphogenic Protein-2-Related Peptide from Mineralised Extracellular Matrix-Based Scaffold Induces Bone Regeneration . Mater Sci. Eng. C Mater Biol. Appl. 126 . 10.1016/j.msec.2021.112182 [ PubMed ] [ CrossRef ] [ Google Scholar ]
- Mengsteab P. Y., Otsuka T., McClinton A., Shemshaki N. S., Shah S., Kan H. M., et al. (2020). Mechanically Superior Matrices Promote Osteointegration and Regeneration of Anterior Cruciate Ligament Tissue in Rabbits . Proc. Natl. Acad. Sci. U. S. A. 117 ( 46 ). 10.1073/pnas.2012347117 [ PMC free article ] [ PubMed ] [ CrossRef ] [ Google Scholar ]
- Muller S. A., Evans C. H., Heisterbach P. E., Majewski M. (2018). The Role of the Paratenon in Achilles Tendon Healing: A Study in Rats . Am. J. Sports Med. 46 ( 5 ). 10.1177/0363546518756093 [ PubMed ] [ CrossRef ] [ Google Scholar ]
- Mumith A., Coathup M., Chimutengwende-Gordon M., Aston W., Briggs T., Blunn G. (2017). Augmenting the Osseointegration of Endoprostheses Using Laser-Sintered Porous Collars: an In Vivo Study . Bone Jt. J. 99-B ( 2 ). 10.1302/0301-620X.99B2.BJJ-2016-0584.R1 [ PubMed ] [ CrossRef ] [ Google Scholar ]
- Mustafa S. F., Evans P. L., Bocca A., Patton D. W., Sugar A. W., Baxter P. W. (2011). Customized Titanium Reconstruction of Post-traumatic Orbital Wall Defects: a Review of 22 Cases . Int. J. Oral Maxillofac. Surg. 40 ( 12 ). 10.1016/j.ijom.2011.04.020 [ PubMed ] [ CrossRef ] [ Google Scholar ]
- Naso F., Gandaglia A., Iop L., Spina M., Gerosa G. (2011). First Quantitative Assay of Alpha-Gal in Soft Tissues: Presence and Distribution of the Epitope before and after Cell Removal from Xenogeneic Heart Valves . Acta Biomater. 7 ( 4 ). 10.1016/j.actbio.2010.11.030 [ PubMed ] [ CrossRef ] [ Google Scholar ]
- Nguyen D. T., Dellbrugge S., Tak P. P., Woo S. L., Blankevoort L., van Dijk N. C. (2015). Histological Characteristics of Ligament Healing after Bio-Enhanced Repair of the Transected Goat ACL . J. Exp. Orthop. 2 ( 1 ). 10.1186/s40634-015-0021-5 [ PMC free article ] [ PubMed ] [ CrossRef ] [ Google Scholar ]
- Nicholson G. P., Breur G. J., Van Sickle D., Yao J. Q., Kim J., Blanchard C. R. (2007). Evaluation of a Cross-Linked Acellular Porcine Dermal Patch for Rotator Cuff Repair Augmentation in an Ovine Model . J. Shoulder Elb. Surg. 16 ( 5 Suppl. l ). 10.1016/j.jse.2007.03.010 [ PubMed ] [ CrossRef ] [ Google Scholar ]
- Novi M., Kumar A., Paladini P., Porcellini G., Merolla G. (2018). Irreparable Rotator Cuff Tears: Challenges and Solutions . Orthop. Res. Rev. 10 , 93–103. 10.2147/ORR.S151259 [ PMC free article ] [ PubMed ] [ CrossRef ] [ Google Scholar ]
- Oliva F., Maffulli N., Gissi C., Veronesi F., Calciano L., Fini M., et al. (2019). Combined Ascorbic Acid and T3 Produce Better Healing Compared to Bone Marrow Mesenchymal Stem Cells in an Achilles Tendon Injury Rat Model: a Proof of Concept Study . J. Orthop. Surg. Res. 14 ( 1 ). 10.1186/s13018-019-1098-9 [ PMC free article ] [ PubMed ] [ CrossRef ] [ Google Scholar ]
- Patel S., Caldwell J. M., Doty S. B., Levine W. N., Rodeo S., Soslowsky L. J. (2018) Integrating Soft and Hard Tissues via Interface Tissue Engineering . J. Orthop. Res. , 36 ( 4 ). 10.1002/jor.23810 [ PMC free article ] [ PubMed ] [ CrossRef ] [ Google Scholar ]
- Peterson J. R., Krabak B. J. (2014). Anterior Cruciate Ligament Injury: Mechanisms of Injury and Strategies for Injury Prevention . Phys. Med. Rehabil. Clin. N. Am. 25 ( 4 ). 10.1016/j.pmr.2014.06.010 [ PubMed ] [ CrossRef ] [ Google Scholar ]
- Pike A. N., Patzkowski J. C., Bottoni C. R. (2019). Meniscal and Chondral Pathology Associated with Anterior Cruciate Ligament Injuries . J. Am. Acad. Orthop. Surg. 27 ( 3 ), 75–84. 10.5435/JAAOS-D-17-00670 [ PubMed ] [ CrossRef ] [ Google Scholar ]
- Pirosa A., Gottardi R., Alexander P. G., Puppi D., Chiellini F., Tuan R. S. (2021). An in Vitro Chondro-Osteo-Vascular Triphasic Model of the Osteochondral Complex . Amsterdam: Biomaterials, 272. [ PubMed ] [ Google Scholar ]
- Piva S. R., Childs J. D., Klucinec B. M., Irrgang J. J., Almeida G. J., Fitzgerald G. K. (2009). Patella Fracture during Rehabilitation after Bone-Patellar Tendon-Bone Anterior Cruciate Ligament Reconstruction: 2 Case Reports . J. Orthop. Sports Phys. Ther. 39 ( 4 ). 10.2519/jospt.2009.2864 [ PubMed ] [ CrossRef ] [ Google Scholar ]
- Platts-Mills T. A., Hilger C., Jappe U., Hage M., Gadermaier G., Spillner E., et al. (2021). Carbohydrate Epitopes Currently Recognized as Targets for IgE Antibodies . Allergy. [ PMC free article ] [ PubMed ] [ Google Scholar ]
- Polak S. J., Levengood S. K., Wheeler M. B., Maki A. J., Clark S. G., Johnson A. J. (2011). Analysis of the Roles of Microporosity and BMP-2 on Multiple Measures of Bone Regeneration and Healing in Calcium Phosphate Scaffolds . Acta Biomater. 7 ( 4 ). 10.1016/j.actbio.2010.12.030 [ PubMed ] [ CrossRef ] [ Google Scholar ]
- Pountos I., Panteli M., Lampropoulos A., Jones E., Calori G. M., Giannoudis P. V. (2016). The Role of Peptides in Bone Healing and Regeneration: a Systematic Review . BMC Med. 14 . 10.1186/s12916-016-0646-y [ PMC free article ] [ PubMed ] [ CrossRef ] [ Google Scholar ]
- Qiao Z., Lian M., Han Y., Sun B., Zhang X., Jiang W., et al. (2021). Bioinspired Stratified Electrowritten Fiber-Reinforced Hydrogel Constructs with Layer-specific Induction Capacity for Functional Osteochondral Regeneration . Amsterdam: Biomaterials, 266. [ PubMed ] [ Google Scholar ]
- Qiu J., Ahn J., Qin D., Thomopoulos S., Xia Y. (2021). Biomimetic Scaffolds with a Mineral Gradient and Funnel-Shaped Channels for Spatially Controllable Osteogenesis . Adv. Healthc. Mater . [ PubMed ] [ Google Scholar ]
- Radhakrishnan J., Manigandan A., Chinnaswamy P., Subramanian A., Sethuraman S. (2018). Gradient Nano-Engineered in Situ Forming Composite Hydrogel for Osteochondral Regeneration . Amsterdam: Biomaterials, 162. [ PubMed ] [ Google Scholar ]
- Rossetti L., Kuntz L. A., Kunold E., Schock J., Muller K. W., Grabmayr H., et al. (2017). The Microstructure and Micromechanics of the Tendon-Bone Insertion . Nat. Mater 16 ( 6 ). 10.1038/nmat4863 [ PubMed ] [ CrossRef ] [ Google Scholar ]
- Sakellariou V. I., Poultsides L. A., Ma Y., Bae J., Liu S., Sculco T. P. (2016). Risk Assessment for Chronic Pain and Patient Satisfaction after Total Knee Arthroplasty . Orthopedics 39 ( 1 ). 10.3928/01477447-20151228-06 [ PubMed ] [ CrossRef ] [ Google Scholar ]
- Santo V. E., Gomes M. E., Mano J. F., Reis R. L. (2013). Controlled Release Strategies for Bone, Cartilage, and Osteochondral Engineering--Part I: Recapitulation of Native Tissue Healing and Variables for the Design of Delivery Systems . Tissue Eng. Part B Rev. 19 ( 4 ). 10.1089/ten.TEB.2012.0138 [ PMC free article ] [ PubMed ] [ CrossRef ] [ Google Scholar ]
- Sasaki H., Rothrauff B. B., Alexander P. G., Lin H., Gottardi R., Fu F. H., et al. (2018). In Vitro Repair of Meniscal Radial Tear with Hydrogels Seeded with Adipose Stem Cells and TGF-Beta3 . Am. J. Sports Med. 46 ( 10 ). 10.1177/0363546518782973 [ PubMed ] [ CrossRef ] [ Google Scholar ]
- Saveh-Shemshaki N., Laurencin S. N. L., Laurencin C. T. (2019). Nanofiber-based Matrices for Rotator Cuff Regenerative Engineering . Acta Biomater. 94 . 10.1016/j.actbio.2019.05.041 [ PubMed ] [ CrossRef ] [ Google Scholar ]
- Schuurmans C. C. L., Mihajlovic M., Hiemstra C., Ito K., Hennink W. E., Vermonden T. (2021). Hyaluronic Acid and Chondroitin Sulfate (Meth)acrylate-based Hydrogels for Tissue Engineering: Synthesis, Characteristics and Pre-clinical Evaluation . Amsterdam: Biomaterials, 268. [ PubMed ] [ Google Scholar ]
- Sclamberg S. G., Tibone J. E., Itamura J. M., Kasraeian S. (2004). Six-month Magnetic Resonance Imaging Follow-Up of Large and Massive Rotator Cuff Repairs Reinforced with Porcine Small Intestinal Submucosa . J. Shoulder Elb. Surg. 13 ( 5 ). 10.1016/j.jse.2004.03.005 [ PubMed ] [ CrossRef ] [ Google Scholar ]
- Shen W., Chen X., Chen J., Yin Z., Heng B. C., Chen W., et al. (2010). The Effect of Incorporation of Exogenous Stromal Cell-Derived Factor-1 Alpha within a Knitted Silk-Collagen Sponge Scaffold on Tendon Regeneration . Biomaterials 31 ( 28 ). 10.1016/j.biomaterials.2010.05.040 [ PubMed ] [ CrossRef ] [ Google Scholar ]
- Shimomura K., Rothrauff B. B., Tuan R. S. (2017). Region-Specific Effect of the Decellularized Meniscus Extracellular Matrix on Mesenchymal Stem Cell-Based Meniscus Tissue Engineering . Am. J. Sports Med. 45 ( 3 ). 10.1177/0363546516674184 [ PubMed ] [ CrossRef ] [ Google Scholar ]
- Shkhyan R., Van Handel B., Bogdanov J., Lee S., Yu Y., Scheinberg M., et al. (2018). Drug-induced Modulation of Gp130 Signalling Prevents Articular Cartilage Degeneration and Promotes Repair . Ann. Rheum. Dis. 77 ( 5 ). 10.1136/annrheumdis-2017-212037 [ PMC free article ] [ PubMed ] [ CrossRef ] [ Google Scholar ]
- Silva M., Ferreira F. N., Alves N. M., Paiva M. C. (2020). Biodegradable Polymer Nanocomposites for Ligament/tendon Tissue Engineering . J. Nanobiotechnology 18 ( 1 ). 10.1186/s12951-019-0556-1 [ PMC free article ] [ PubMed ] [ CrossRef ] [ Google Scholar ]
- Singh H., Purohit S. D., Bhaskar R., Yadav I., Bhushan S., Gupta M. K., et al. (2021). Biomatrix from Goat-Waste in Sponge/gel/powder Form for Tissue Engineering and Synergistic Effect of Nanoceria . Biomed. Mater 16 ( 2 ). 10.1088/1748-605x/abdb74 [ PubMed ] [ CrossRef ] [ Google Scholar ]
- Son M. S., Levenston M. E. (2017). Quantitative Tracking of Passage and 3D Culture Effects on Chondrocyte and Fibrochondrocyte Gene Expression . J. Tissue Eng. Regen. Med. 11 ( 4 ). 10.1002/term.2022 [ PubMed ] [ CrossRef ] [ Google Scholar ]
- Soong H. K., Kenyon K. R. (1984). Adverse Reactions to Virgin Silk Sutures in Cataract Surgery . Ophthalmology 91 ( 5 ). 10.1016/s0161-6420(84)34273-7 [ PubMed ] [ CrossRef ] [ Google Scholar ]
- Southworth T. M., Naveen N. B., Tauro T. M., Chahla J., Cole B. J. (2020). Meniscal Allograft Transplants . Clin. Sports Med. 39 ( 1 ), 93–123. 10.1016/j.csm.2019.08.013 [ PubMed ] [ CrossRef ] [ Google Scholar ]
- Steele J. A., McCullen S. D., Callanan A., Autefage H., Accardi M. A., Dini D., et al. (2014). Combinatorial Scaffold Morphologies for Zonal Articular Cartilage Engineering . Acta Biomater. 10 ( 5 ). 10.1016/j.actbio.2013.12.030 [ PMC free article ] [ PubMed ] [ CrossRef ] [ Google Scholar ]
- Steinhaus M. E., Makhni E. C., Cole B. J., Romeo A. A., Verma N. N. (2016). Outcomes after Patch Use in Rotator Cuff Repair . Arthroscopy 32 ( 8 ). 10.1016/j.arthro.2016.02.009 [ PubMed ] [ CrossRef ] [ Google Scholar ]
- Stone K. R., Walgenbach A. W., Turek T. J., Somers D. L., Wicomb W., Galili U. (2007). Anterior Cruciate Ligament Reconstruction with a Porcine Xenograft: a Serologic, Histologic, and Biomechanical Study in Primates . Arthroscopy 23 ( 4 ). 10.1016/j.arthro.2006.12.024 [ PubMed ] [ CrossRef ] [ Google Scholar ]
- Su W., Guo J., Xu J., Huang K., Chen J., Jiang J., et al. (2021). Gradient Composite Film with Calcium Phosphate Silicate for Improved Tendon-To-Bone Intergration . Chem. Eng. J. 404 . 10.1016/j.cej.2020.126473 [ CrossRef ] [ Google Scholar ]
- Sun J., Mou C., Shi Q., Chen B., Hou X., Zhang W., et al. (2018). Controlled Release of Collagen-Binding SDF-1alpha from the Collagen Scaffold Promoted Tendon Regeneration in a Rat Achilles Tendon Defect Model . Amsterdam: Biomaterials, 162. [ PubMed ] [ Google Scholar ]
- Sunwoo J. Y., Murrell G. A. C. (2020). Interposition Graft Repair of Irreparable Rotator Cuff Tears: A Review of Biomechanics and Clinical Outcomes . J. Am. Acad. Orthop. Surg. 28 ( 19 ), e829. 10.5435/JAAOS-D-19-00500 [ PubMed ] [ CrossRef ] [ Google Scholar ]
- Tagliero A. J., Desai V. S., Kennedy N. I., Camp C. L., Stuart M. J., Levy B. A., et al. (2018). Seventeen-Year Follow-Up after Meniscal Repair with Concomitant Anterior Cruciate Ligament Reconstruction in a Pediatric and Adolescent Population . Am. J. Sports Med. 46 ( 14 ). 10.1177/0363546518803934 [ PubMed ] [ CrossRef ] [ Google Scholar ]
- Tan X. P., Tan Y. J., Chow C. S. L., Tor S. B., Yeong W. Y. (2017). Metallic Powder-Bed Based 3D Printing of Cellular Scaffolds for Orthopaedic Implants: A State-Of-The-Art Review on Manufacturing, Topological Design, Mechanical Properties and Biocompatibility . Mater Sci. Eng. C Mater Biol. Appl. 76 . 10.1016/j.msec.2017.02.094 [ PubMed ] [ CrossRef ] [ Google Scholar ]
- Tang W., Lin D., Yu Y., Niu H., Guo H., Yuan Y., et al. (2016). Bioinspired Trimodal Macro/micro/nano-Porous Scaffolds Loading rhBMP-2 for Complete Regeneration of Critical Size Bone Defect . Acta Biomater. 32 . 10.1016/j.actbio.2015.12.006 [ PubMed ] [ CrossRef ] [ Google Scholar ]
- Taylor S. A., Rodeo S. A. (2013). Augmentation Techniques for Isolated Meniscal Tears . Curr. Rev. Musculoskelet. Med. 6 ( 2 ). 10.1007/s12178-013-9165-z [ PMC free article ] [ PubMed ] [ CrossRef ] [ Google Scholar ]
- Teuschl A., Heimel P., Nurnberger S., van Griensven M., Redl H., Nau T. (2016). A Novel Silk Fiber-Based Scaffold for Regeneration of the Anterior Cruciate Ligament: Histological Results from a Study in Sheep . Am. J. Sports Med. 44 ( 6 ). 10.1177/0363546516631954 [ PubMed ] [ CrossRef ] [ Google Scholar ]
- Tiefenboeck T. M., Thurmaier E., Tiefenboeck M. M., Ostermann R. C., Joestl J., Winnisch M., et al. (2015). Clinical and Functional Outcome after Anterior Cruciate Ligament Reconstruction Using the LARS System at a Minimum Follow-Up of 10 Years . Knee 22 ( 6 ). 10.1016/j.knee.2015.06.003 [ PubMed ] [ CrossRef ] [ Google Scholar ]
- Tits A., Ruffoni D. (2021). Joining Soft Tissues to Bone: Insights from Modeling and Simulations . Bone Rep. 14 , 100742. 10.1016/j.bonr.2020.100742 [ PMC free article ] [ PubMed ] [ CrossRef ] [ Google Scholar ]
- Utomo L., van Osch G. J., Bayon Y., Verhaar J. A., Bastiaansen-Jenniskens Y. M. (2016). Guiding Synovial Inflammation by Macrophage Phenotype Modulation: an In Vitro Study towards a Therapy for Osteoarthritis . Osteoarthr. Cartil. 24 ( 9 ). 10.1016/j.joca.2016.04.013 [ PubMed ] [ CrossRef ] [ Google Scholar ]
- Valdes A. M., Doherty S. A., Zhang W., Muir K. R., Maciewicz R. A., Doherty M. (2012). Inverse Relationship between Preoperative Radiographic Severity and Postoperative Pain in Patients with Osteoarthritis Who Have Undergone Total Joint Arthroplasty . Semin. Arthritis Rheum. 41 ( 4 ). 10.1016/j.semarthrit.2011.07.002 [ PubMed ] [ CrossRef ] [ Google Scholar ]
- Van Der Merwe W., Lind M., Fauno P., Van Egmond K., Zaffagnini S., Marcacci M., et al. (2020). Xenograft for Anterior Cruciate Ligament Reconstruction Was Associated with High Graft Processing Infection . J. Exp. Orthop. 7 ( 1 ). 10.1186/s40634-020-00292-0 [ PMC free article ] [ PubMed ] [ CrossRef ] [ Google Scholar ]
- van Tienen T. G., Hannink G., Buma P. (2009). Meniscus Replacement Using Synthetic Materials . Clin. Sports Med. 28 ( 1 ). 10.1016/j.csm.2008.08.003 [ PubMed ] [ CrossRef ] [ Google Scholar ]
- Vapniarsky N., Huwe L. W., Arzi B., Houghton M. K., Wong M. E., Wilson J. W., et al. (2018). Tissue Engineering toward Temporomandibular Joint Disc Regeneration . Sci. Transl. Med. 10 ( 446 ). 10.1126/scitranslmed.aaq1802 [ PMC free article ] [ PubMed ] [ CrossRef ] [ Google Scholar ]
- Veronesi F., Di Matteo B., Vitale N. D., Filardo G., Visani A., Kon E., et al. (2021). Biosynthetic Scaffolds for Partial Meniscal Loss: A Systematic Review from Animal Models to Clinical Practice . Bioact. Mater 6 ( 11 ). 10.1016/j.bioactmat.2021.03.033 [ PMC free article ] [ PubMed ] [ CrossRef ] [ Google Scholar ]
- Wang C., Hu Y., Zhang S., Ruan D., Huang Z., He P., et al. (2021). Application of Stem Cell Therapy for ACL Graft Regeneration . Stem Cells Int. 10.1155/2021/6641818 [ PMC free article ] [ PubMed ] [ CrossRef ] [ Google Scholar ]
- Wang D., Zhang X., Huang S., Liu Y., Fu B. S., Mak K. K., et al. (2021). Engineering Multi-Tissue Units for Regenerative Medicine: Bone-Tendon-Muscle Units of the Rotator Cuff . Amsterdam: Biomaterials, 272. [ PubMed ] [ Google Scholar ]
- Wang S., Ge Y., Ai C., Jiang J., Cai J., Sheng D., et al. (2018). Enhance the Biocompatibility and Osseointegration of Polyethylene Terephthalate Ligament by Plasma Spraying with Hydroxyapatite In Vitro and In Vivo . Int. J. Nanomedicine 13 . 10.2147/IJN.S162466 [ PMC free article ] [ PubMed ] [ CrossRef ] [ Google Scholar ]
- Wang S., Lu M., Wang W., Yu S., Yu R., Cai C., et al. (2022). Macrophage Polarization Modulated by NF-kappaB in Polylactide Membranes-Treated Peritendinous Adhesion . Small 18 ( 3 ). 10.1002/smll.202104112 [ PubMed ] [ CrossRef ] [ Google Scholar ]
- Wang Z., Han L., Sun T., Ma J., Sun S., Ma L., et al. (2020). Extracellular Matrix Derived from Allogenic Decellularized Bone Marrow Mesenchymal Stem Cell Sheets for the Reconstruction of Osteochondral Defects in Rabbits . Acta Biomater. 118 . 10.1016/j.actbio.2020.10.022 [ PubMed ] [ CrossRef ] [ Google Scholar ]
- Wei H., Cui J., Lin K., Xie J., Wang X. (2022). Recent Advances in Smart Stimuli-Responsive Biomaterials for Bone Therapeutics and Regeneration . Bone Res. 10 ( 1 ). 10.1038/s41413-021-00180-y [ PMC free article ] [ PubMed ] [ CrossRef ] [ Google Scholar ]
- Weimin P., Dan L., Yiyong W., Yunyu H., Li Z. (2013). Tendon-to-bone Healing Using an Injectable Calcium Phosphate Cement Combined with Bone Xenograft/BMP Composite . Biomaterials 34 ( 38 ). 10.1016/j.biomaterials.2013.09.018 [ PubMed ] [ CrossRef ] [ Google Scholar ]
- Westermann R. W., Duchman K. R., Amendola A., Glass N., Wolf B. R. (2017). All-Inside versus Inside-Out Meniscal Repair with Concurrent Anterior Cruciate Ligament Reconstruction: A Meta-Regression Analysis . Am. J. Sports Med. 45 ( 3 ). 10.1177/0363546516642220 [ PubMed ] [ CrossRef ] [ Google Scholar ]
- Wong C. C., Huang Y. M., Chen C. H., Lin F. H., Yeh Y. Y., Bai M. Y. (2020). Cytokine and Growth Factor Delivery from Implanted Platelet-Rich Fibrin Enhances Rabbit Achilles Tendon Healing . Int. J. Mol. Sci. 21 ( 9 ). 10.3390/ijms21093221 [ PMC free article ] [ PubMed ] [ CrossRef ] [ Google Scholar ]
- Wu Z., Tang T., Guo H., Tang S., Niu Y., Zhang J., et al. (2014). In Vitro degradability, Bioactivity and Cell Responses to Mesoporous Magnesium Silicate for the Induction of Bone Regeneration . Colloids Surf. B Biointerfaces 120 . 10.1016/j.colsurfb.2014.04.010 [ PubMed ] [ CrossRef ] [ Google Scholar ]
- Xie C., Ye J., Liang R., Yao X., Wu X., Koh Y., et al. (2021). Advanced Strategies of Biomimetic Tissue-Engineered Grafts for Bone Regeneration . Adv. Healthc. Mater 10 ( 14 ). 10.1002/adhm.202100408 [ PubMed ] [ CrossRef ] [ Google Scholar ]
- Xie J., Wang Y., Lu L., Liu L., Yu X., Pei F. (2021). Cellular Senescence in Knee Osteoarthritis: Molecular Mechanisms and Therapeutic Implications . Ageing Res. Rev. 70 . 10.1016/j.arr.2021.101413 [ PubMed ] [ CrossRef ] [ Google Scholar ]
- Xie X., Zhu J., Hu X., Dai L., Fu X., Zhang J., et al. (2018). A Co-culture System of Rat Synovial Stem Cells and Meniscus Cells Promotes Cell Proliferation and Differentiation as Compared to Mono-Culture . Sci. Rep. 8 ( 1 ). 10.1038/s41598-018-25709-w [ PMC free article ] [ PubMed ] [ CrossRef ] [ Google Scholar ]
- Xing S. G., Zhou Y. L., Yang Q. Q., Ju F., Zhang L., Tang J. B. (2020). Effects of Nanoparticle-Mediated Growth Factor Gene Transfer to the Injured Microenvironment on the Tendon-To-Bone Healing Strength . Biomater. Sci. 8 ( 23 ). 10.1039/d0bm01222j [ PubMed ] [ CrossRef ] [ Google Scholar ]
- Xu C., Su P., Chen X., Meng Y., Yu W., Xiang A. P., et al. (2011). Biocompatibility and Osteogenesis of Biomimetic Bioglass-Collagen-Phosphatidylserine Composite Scaffolds for Bone Tissue Engineering . Biomaterials 32 ( 4 ). 10.1016/j.biomaterials.2010.09.068 [ PubMed ] [ CrossRef ] [ Google Scholar ]
- Xu J., Feng Q., Lin S., Yuan W., Li R., Li J., et al. (2019). Injectable Stem Cell-Laden Supramolecular Hydrogels Enhance in Situ Osteochondral Regeneration via the Sustained Co-delivery of Hydrophilic and Hydrophobic Chondrogenic Molecules . Amsterdam: Biomaterials, 210. [ PubMed ] [ Google Scholar ]
- Yan Y., Chen H., Zhang H., Guo C., Yang K., Chen K., et al. (2019). Vascularized 3D Printed Scaffolds for Promoting Bone Regeneration . Amsterdam: Biomaterials, 190–191. [ PubMed ] [ Google Scholar ]
- Yang F., Chen C., Zhou Q., Gong Y., Li R., Li C., et al. (2017). Laser Beam Melting 3D Printing of Ti6Al4V Based Porous Structured Dental Implants: Fabrication, Biocompatibility Analysis and Photoelastic Study . Sci. Rep. 7 . 10.1038/srep45360 [ PMC free article ] [ PubMed ] [ CrossRef ] [ Google Scholar ]
- Yilgor C., Yilgor Huri P., Huri G. (20122012). Tissue Engineering Strategies in Ligament Regeneration . Stem Cells Int. 10.1155/2012/374676 [ PMC free article ] [ PubMed ] [ CrossRef ] [ Google Scholar ]
- Yokoya S., Mochizuki Y., Natsu K., Omae H., Nagata Y., Ochi M. (2012). Rotator Cuff Regeneration Using a Bioabsorbable Material with Bone Marrow-Derived Mesenchymal Stem Cells in a Rabbit Model . Am. J. Sports Med. 40 ( 6 ). 10.1177/0363546512442343 [ PubMed ] [ CrossRef ] [ Google Scholar ]
- Zhang C. H., Jiang Y. L., Ning L. J., Li Q., Fu W. L., Zhang Y. J., et al. (2018). Evaluation of Decellularized Bovine Tendon Sheets for Achilles Tendon Defect Reconstruction in a Rabbit Model . Am. J. Sports Med. 46 ( 11 ). 10.1177/0363546518787515 [ PubMed ] [ CrossRef ] [ Google Scholar ]
- Zhang J., Zhou H., Yang K., Yuan Y., Liu C. (2013). RhBMP-2-loaded Calcium Silicate/calcium Phosphate Cement Scaffold with Hierarchically Porous Structure for Enhanced Bone Tissue Regeneration . Biomaterials 34 ( 37 ). 10.1016/j.biomaterials.2013.08.059 [ PubMed ] [ CrossRef ] [ Google Scholar ]
- Zhang M., Shi J., Xie M., Wen J., Niibe K., Zhang X., et al. (2020). Recapitulation of Cartilage/bone Formation Using iPSCs via Biomimetic 3D Rotary Culture Approach for Developmental Engineering . Amsterdam: Biomaterials, 260. [ PubMed ] [ Google Scholar ]
- Zhang Y., Song L., Li X., Fang J. (2018). Flexion-valgus Unicondylar Tibial Plateau Depression Fracture Pattern: Classification and Treatment . Injury 49 ( 4 ). 10.1016/j.injury.2018.03.009 [ PubMed ] [ CrossRef ] [ Google Scholar ]
- Zhang Y. Z., Venugopal J., Huang Z. M., Lim C. T., Ramakrishna S. (2005). Characterization of the Surface Biocompatibility of the Electrospun PCL-Collagen Nanofibers Using Fibroblasts . Biomacromolecules 6 ( 5 ). 10.1021/bm050314k [ PubMed ] [ CrossRef ] [ Google Scholar ]
- Zhao S., Peng L., Xie G., Li D., Zhao J., Ning C. (2014a). Effect of the Interposition of Calcium Phosphate Materials on Tendon-Bone Healing during Repair of Chronic Rotator Cuff Tear . Am. J. Sports Med. 42 ( 8 ). 10.1177/0363546514532781 [ PubMed ] [ CrossRef ] [ Google Scholar ]
- Zhao S., Su W., Shah V., Hobson D., Yildirimer L., Yeung K. W. K., et al. (2017). Biomaterials Based Strategies for Rotator Cuff Repair . Colloids Surf. B Biointerfaces 157 . 10.1016/j.colsurfb.2017.06.004 [ PubMed ] [ CrossRef ] [ Google Scholar ]
- Zhao S., Xie X., Pan G., Shen P., Zhao J., Cui W. (2015). Healing Improvement after Rotator Cuff Repair Using Gelatin-Grafted poly(L-Lactide) Electrospun Fibrous Membranes . J. Surg. Res. 193 ( 1 ). 10.1016/j.jss.2014.08.019 [ PubMed ] [ CrossRef ] [ Google Scholar ]
- Zhao S., Zhao J., Dong S., Huangfu X., Li B., Yang H., et al. (2014b). Biological Augmentation of Rotator Cuff Repair Using bFGF-Loaded Electrospun Poly(lactide-Co-Glycolide) Fibrous Membranes . Int. J. Nanomedicine 9 . 10.2147/IJN.S59536 [ PMC free article ] [ PubMed ] [ CrossRef ] [ Google Scholar ]
- Zhao X., Chen X., Yuk H., Lin S., Liu X., Parada G. (2021). Soft Materials by Design: Unconventional Polymer Networks Give Extreme Properties . Chem. Rev. 121 ( 8 ). 10.1021/acs.chemrev.0c01088 [ PMC free article ] [ PubMed ] [ CrossRef ] [ Google Scholar ]
- Zhu C., Qiu J., Thomopoulos S., Xia Y. (2021). Augmenting Tendon-To-Bone Repair with Functionally Graded Scaffolds . Adv. Healthc. Mater 10 ( 9 ). 10.1002/adhm.202002269 [ PMC free article ] [ PubMed ] [ CrossRef ] [ Google Scholar ]
- Zhu D., Tong X., Trinh P., Yang F. (2018). Mimicking Cartilage Tissue Zonal Organization by Engineering Tissue-Scale Gradient Hydrogels as 3D Cell Niche . Tissue Eng. Part A 24 ( 1-2 ). 10.1089/ten.TEA.2016.0453 [ PMC free article ] [ PubMed ] [ CrossRef ] [ Google Scholar ]
- Zhu J., Shao J., Chen Y., Zhao G., Li L., Fu Q., et al. (20212021). Fibrin Glue-Kartogenin Complex Promotes the Regeneration of the Tendon-Bone Interface in Rotator Cuff Injury . Stem Cells Int. 10.1155/2021/6640424 [ PMC free article ] [ PubMed ] [ CrossRef ] [ Google Scholar ]
- Zhu Y., Kong L., Farhadi F., Xia W., Chang J., He Y., et al. (2019). An Injectable Continuous Stratified Structurally and Functionally Biomimetic Construct for Enhancing Osteochondral Regeneration . Amsterdam: Biomaterials. [ PubMed ] [ Google Scholar ]
- Zitnay J. L., Reese S. P., Tran G., Farhang N., Bowles R. D., Weiss J. A. (2018). Fabrication of Dense Anisotropic Collagen Scaffolds Using Biaxial Compression . Acta Biomater. 65 . 10.1016/j.actbio.2017.11.017 [ PMC free article ] [ PubMed ] [ CrossRef ] [ Google Scholar ]
- Zurina I. M., Presniakova V. S., Butnaru D. V., Svistunov A. A., Timashev P. S., Rochev Y. A. (2020). Tissue Engineering Using a Combined Cell Sheet Technology and Scaffolding Approach . Acta Biomater. 113 . 10.1016/j.actbio.2020.06.016 [ PubMed ] [ CrossRef ] [ Google Scholar ]
Study of trans athletes concludes blanket sports bans are a mistake
The lead researcher of a landmark IOC-funded study looking at strength, power and aerobic capacity of trans athletes tells Outsports that sports federations should consider its findings carefully.
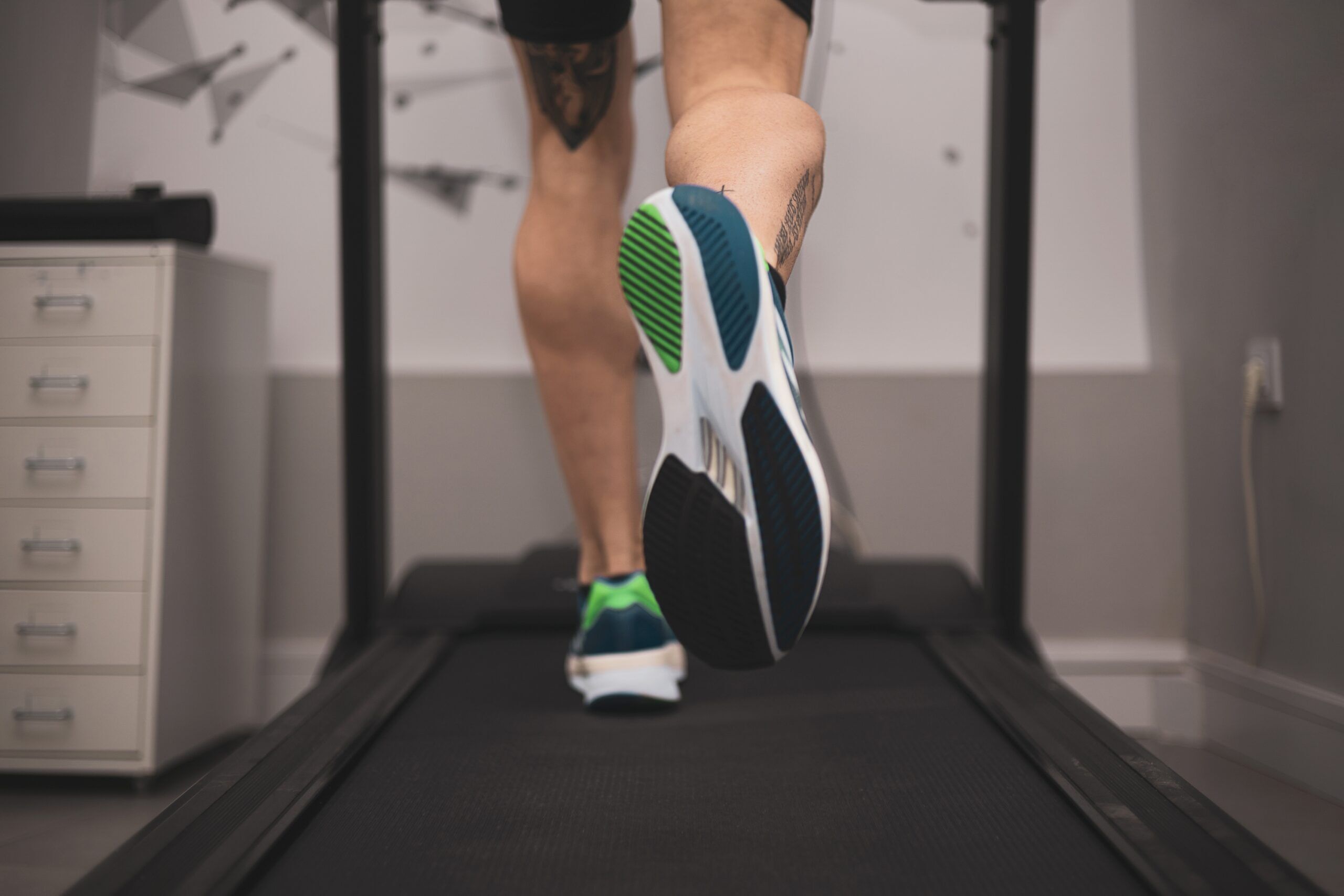
Results from a first-of-its-kind study comparing the strength, power and aerobic capacity of transgender athletes with cisgender athletes have been published in a major medical journal , with the authors cautioning sports bodies not to pass blanket bans without doing proper research.
Researchers invited 23 trans women and 12 trans men to undergo a series of performance tests in laboratory conditions, while also putting 21 cis women and 19 cis men through the same tests.
In certain cardiovascular tests, the trans women performed worse than the cis women, and were found to have less lower-body strength, according to the study published Wednesday in the British Journal of Sports Medicine.
Related NAIA accused of bowing to ‘political pressure’ over ban on trans women student-athletes The small colleges governing body has decided to exclude transgender women student-athletes, with Chris Mosier among those critical of the ban. By Karleigh Webb | April 9, 2024 6 truths and myths about Lia Thomas, trans athletes and women’s swimming There is lots of information, and falsehoods, shared about Penn swimmer Lia Thomas. By Cyd Zeigler | January 6, 2022
Get off the sidelines and into the game Our weekly playbook is packed with everything from locker room chatter to pressing LGBTQ sports issues. Weekender * Weekly Newsletter * Promotions and Partner Emails * Sign Up
Lead researcher Professor Yannis Pitsiladis told Outsports “the main takeaway message is the requirement of international federations (and their “experts”) to treat trans women very differently to cis men.”
Pitsiladis is on the Medical and Scientific Commission of the International Olympic Committee, which helped to fund the research via a grant.
He added: “It follows that research conducted comparing biological men to biological women is almost irrelevant in this debate and evidence from such comparisons should not be used to inform policy as is the case by many “armchair professors” advocating the default ban position.
“Our study also stresses the need for studies to be conducted in trained individuals / athletes. The use of non-trained individuals / non-athletes (cis and trans) is also of limited to no use.”
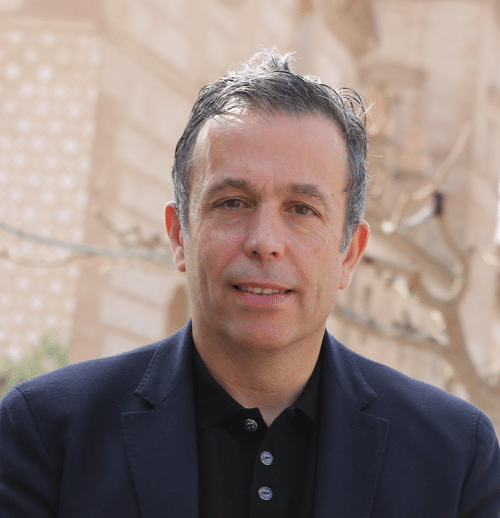
All the athletes involved in the tests were required to participate in competitive sports or take part in physical training at least three times a week. The average age was 34 years old.
Meanwhile, all 35 trans athletes had to have completed more than one year of gender affirmation hormone therapy (GAHT).
Of the full cohort, 36% were active in endurance sports, 26% in team sports and 38% in power sports, with none of those involved competing at national or international level.
The study was designed by another of the lead researchers, Blair Hamilton of the University of Brighton, with support from colleagues at Heriot-Watt University in Edinburgh, the University of Rome and Hong Kong Baptist University. Pitsiladis is based at HKBU.
🚨NEW #OriginalResearch Strength, power and aerobic capacity of transgender athletes: a cross-sectional study 👉 A comparison of laboratory measures of transgender athletes to their cisgender counterparts 👉 What can we learn from this? #OpenAccess 👉 https://t.co/h2u05XymoZ pic.twitter.com/LfkcEkL9iM — British Journal of Sports Medicine (BJSM) (@BJSM_BMJ) April 11, 2024
The tests assessed body composition, lung function, cardiopulmonary exercise testing, strength and lower body power.
Among the results was a determination that the trans women athletes had decreased lung function compared to the cis women athletes.
In addition, the bone density of the trans women athletes was found to be equivalent to that of the cis women. Bone density is linked to muscle strength.
The researchers say their findings “reveal notable disparities in fat mass, fat-free mass, laboratory sports performance measures and hand-grip strength measures between cisgender male and transgender female athletes.
“These differences underscore the inadequacy of using cisgender male athletes as proxies for transgender women athletes.”
Pointing to guidance issued by the IOC in November 2021 , which moved away from a previous policy that required competing athletes who are trans to undergo “medically unnecessary” procedures or treatment, they added: “Based on these limited findings, we recommend that transgender women athletes be evaluated as their own demographic group, in accordance with the principles outlined in Article 6.1b of the IOC Framework on Fairness, Inclusion and Non-Discrimination based on Gender Identity and Sex Variations.”
The authors also concluded: “This research shows the potential complexity of transgender athlete physiology and its effects on the laboratory measures of physical performance.”
Noting the shortcomings of their cross-sectional, lab-based study, which had a limited sample size, they added: “A long-term longitudinal study is needed to confirm whether these findings are directly related to GAHT.”
Sports governing bodies will be invited to study the data, with the researchers also emphasizing the need for sports-specific studies to inform policy making.
Prof. Pitsiladis was a guest speaker Tuesday at SportAccord in Birmingham, U.K., a major conference for international sports federations. He spoke on a roundtable panel titled “Examining the implementation and development of rules for transgender athletes in International Federations.”
Academic + medical experts, sports leaders, and lawyers are now discussing the complex topic of rules for #transgender athletes in International Federations at LawAccord. #SportAccord #PowerOfSport #sportslaw pic.twitter.com/jougYcTm2F — SportAccord World Sport & Business Summit (@sportaccord) April 9, 2024
Asked by Outsports what might happen next after this week’s publication, Prof. Pitsiladis said he hoped it would help to convince the IOC to contribute funding that would allow for further research that takes a multicentre approach, involving many international federations.
He noted how smaller sports federations with less resources or expertise feel “somewhat lost” on the topic of trans inclusion and need more assistance. “Their only real option is to follow the big federations and hope for the best,” he said.
Pitsiladis believes the newly-published study can have an impact on inclusion, but is cautious over how others will interpret the results.
“It’s my expectation that it may become a little easier for some federations developing their policies to reject the default position adopted by some of the large federations to ban trans women athletes in the absence of scientific data to support such a position.
“But I suspect most will follow the positions of the large federations to ban. Also, it’s unlikely that those large federations will change their position as they are now too invested and they don’t really look at the science or evidence.
“Their wish is mainly to appease their membership and the decisions being taken are mainly justified by politics and dictates, rather than science.”
More Like This
Joanna harper: world rugby’s proposed transban is based on ‘false assumptions’, caster semenya appeals discriminatory rule: ‘the iaaf will not drug me’, mandatory hormone therapy for caster semenya is an affront to all women.
Score weekly updates Your go-to newsletter for LGBTQ sports scoops and major plays. Weekender * Weekly Newsletter * Promotions and Partner Emails * Sign Up
More in science
Trans athlete, author and researcher Joanna Harper suspects decision-makers at World Rugby “had their minds made up before they called the meeting.”
South African Caster Semenya appealed IAAF rules that effectively ban her from the event where twice she won Olympic gold.
South African Olympic champion Caster Semenya has few options other than appeal again, quit or undergo testosterone suppression
How to avoid choking under pressure
From Scientific American comes research into avoiding choking under pressure, be it in sports, on the job or anywhere else in your life. Some concepts seem counterintuitive, but have been borne out somewhat by studies. We choke under pressure because such conditions thwart the normal brain processing of tasks that are so well learned they […]
Latest Articles on Outsports
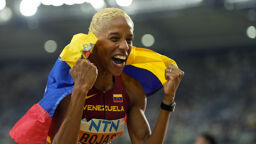
Yulimar Rojas, the out defending Olympic champion, will miss the Paris Summer Olympics
Yulimar Rojas, the defending Olympic champ and world record holder, will miss Paris after a devasting injury.
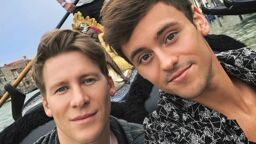
Tom Daley discovers his mom initially thought he was setting Lance Black up with her
On a new podcast interview, Daley shares a cute new detail about why his mom assumed he was talking up his boyfriend before she learned he was gay.
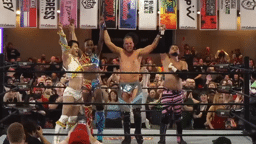
The top 30 LGBTQ matches of WrestleMania Week 2024
Pro wrestling's biggest week got a huge boost in LGBTQ presence all-around, with dozens of out wrestlers highlighted.
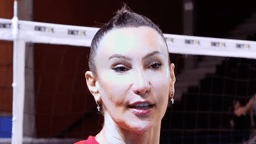
Tifanny Abreu faces do-or-die playoff match Friday
Trans volleyball star Tiffany Abreu looks to end a Superliga semifinal jinx in Brazil and get revenge on a rival.
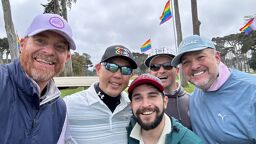
Gay golf pro says his sport’s culture continues to be ‘straight,’ ahead of The Masters
Greg Fitzgerald is a golf pro in the San Francisco Bay area who has hosted a Major. He's also gay and sees the sport as not particularly inclusive.
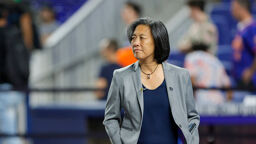
Talkin’ Gaysball: Karma reels in the Marlins after their treatment of female GM Kim Ng
Maybe Miami should've thought twice before undercutting MLB's first female GM. Plus, Magic Mike pants and Thirst Trap of the Week.
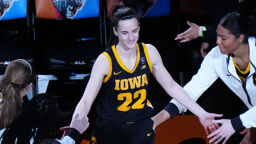
Caitlin Clark and the WNBA going to the next level would be the best revenge on OutKick
After OutKick tried to turn the NCAA Championship into an anti-trans moment, Clark and the WNBA have a chance to turn them into an object of ridicule.
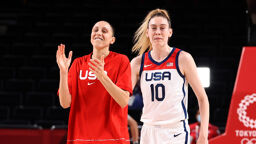
Ahead of the WNBA Draft, 2 players have thrown a little shade at Caitlin Clark. Why?
The WNBA Draft for 2024 is upon us. As Caitlin Clark will be the first pick, Diana Taurasi and Breanna Stewart rained on the parade a bit.
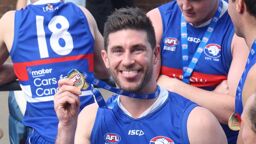
Pro athlete gets ban for using anti-gay slur during match – this out gay athlete calls it ‘significant’ and ‘major step’
Jeremy Finlayson will sit three games after using an anti-gay slur. Michael O’Donnell, who plays in a lower league, applauds the decision.
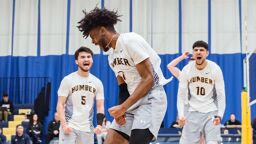
The makeup MVP – why this gay college athlete is ‘tough as nails’
Marcus Morgan helped power Humber College's volleyball team to a fourth consecutive provincial title in Canada, a year after experiencing homophobia on court.
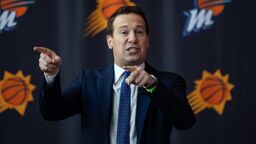
Did Phoenix Suns and Mercury owner Mat Ishbia use a profane gay slur? Or is it a deep fake?
Phoenix Suns and Mercury owner Mat Ishbia is being questioned about a recording some say features him using a gay slur.
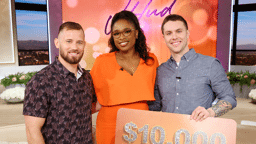
Jennifer Hudson shocked a gay diver when she helped him meet his hero on national TV
Ryan Dafforn and Ford McLiney met through Outsports, with each of them sharing their coming-out stories as gay athletes. JHud finally brought them together
Privacy Overview
- Partnerships
Diagnostic and Support Services
- Imaging Services
- Laboratories
- Remote Patient Monitoring
- Private Duty Home Care
- Primary Care
- Family Medicine
- Internal Medicine
Specialty Care
- Bleeding and Clotting Disorders
- Endocrinology
- Gastroenterology
- Heart and Vascular
- Vascular Imaging and Surgery
- Hematology-Oncology
- Infectious Disease
- Neurology and Ophthalmology
- Comprehensive Headache and Facial Pain Center
- Muscular Dystrophy Clinic
- Myasthenia Gravis Clinic
- Neuro-Endovascular Surgery | Interventional Neurology
- Peripheral Neuropathy Clinic
- Pediatric Specialty
- Adolescent & Young Adult Oncology
- Pediatric Hematology & Oncology
- Pediatric Infectious Disease
- Occupational and Environmental Medicine
- Osteopathic Manipulative Medicine
- Physical Medicine and Rehabilitation
- Physical & Occupational Therapy
- Subspecialty Care | Adult & Pediatric
- Allergy and Immunology
- Comprehensive Bronchopulmonary Dysplasia Center
- Cystic Fibrosis Center
- Pediatric Pulmonology
- Pulmonary Function Laboratory
- Sickle Cell Lifespan
- Sports Medicine
- Breast Oncology
- Colorectal Surgery
- Endocrine Surgery
- Foot and Ankle Surgery
- Hand Surgery
- Orthopedic Surgery
- Plastic and Reconstructive Surgery
- Spine Surgery
- Surgical Oncology
- Financial Services
- Pay My Bill
- Financial Assistance
- Health and Community Resources
- Language Assistance Services
- Medical Records
- MyMSUHealth
- Non-discrimination Notice
- Patient Rights
- Symptom Checker
- Affiliate Clinics
- Referring Guidelines
- Services and Treatments
- Affiliates Clinics
- Patient & Visitor Information
- COVID-19 Testing at MSU
- Telehealth Services at MSU Health Care
- For Health Professionals
- For MSUHC Providers & Staff
MSU researchers find more action needed to prevent arthritis
April 11, 2024 - MSUToday
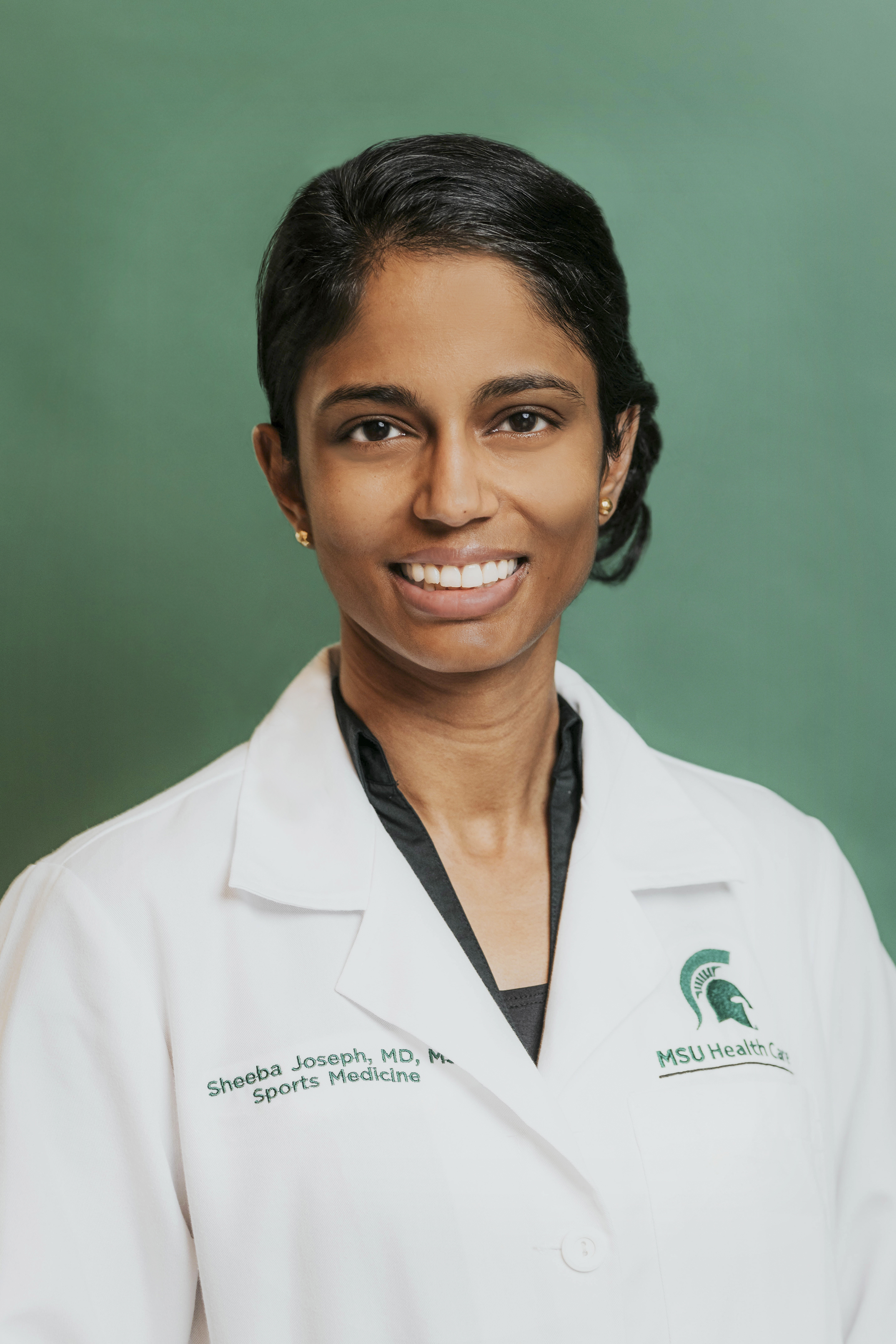
Originally published April 8, 2024 on MSUToday
The prevalence of early knee osteoarthritis (OA) symptoms faced by patients after anterior cruciate ligament (ACL) reconstruction is staggering — but not much is being done to address it according to new research published by scholars from Michigan State University’s Department of Kinesiology .
The study – published by the Journal of Athletic Training in January of 2024 – shows these symptoms persist throughout the first year following surgery and need to be addressed with early intervention.
The co-authors of the study with MSU affiliations include Ashley Triplett , assistant professor in the College of Education; Sheeba Joseph , associate professor, Colleges of Human and Osteopathic Medicine; Francesca Genoese , doctoral student in the Department of Kinesiology; Michael Shingles and Andrew Schorfhaar of Sparrow Hospital, alums of the College of Osteopathic Medicine.
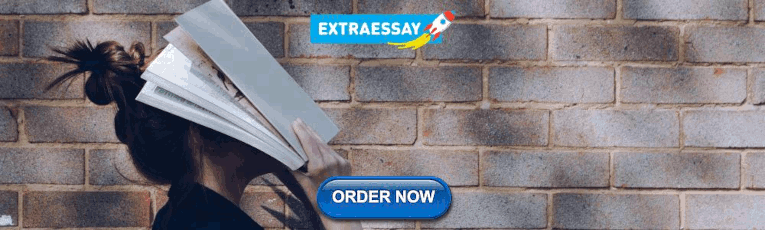
IMAGES
VIDEO
COMMENTS
Research in Sports Medicine is a broad journal that aims to bridge the gap between all professionals in the fields of sports medicine. The journal serves an international audience and is of interest to professionals worldwide. The journal covers major aspects of sports medicine and sports science - prevention, management, and rehabilitation of sports, exercise and physical activity related ...
Research in Sports Medicine is a broad journal that aims to bridge the gap between all professionals in the fields of sports medicine. The journal serves an international audience and is of interest to professionals worldwide. The journal covers major aspects of sports medicine and sports science - prevention, management, and rehabilitation of sports, exercise and physical activity related ...
The American Journal of Sports Medicine, founded in 1972, is the official publication of the American Orthopaedic Society for Sports Medicine.It contains original articles addressed to orthopaedic surgeons specializing in sports medicine, and to team physicians, athletic trainers, and physical therapists focusing on the causes and effects of injury or disease resulting from or affected by ...
Sports Medicine Research investigates all aspects of sports injury evaluation, treatment and prevention to provide optimal care to those involved in sports- or fitness-related activities. Recent work has addressed neuromuscular interventions to prevent anterior cruciate ligament (ACL) injuries, the use of subsymptom exercise to return athletes ...
As such, optimising performance, improving the best players' availability, and decreasing the risk of injury have become the main thrusts of sports science and sports medicine when tied to high-performance teams. 5. Sports science research can help lead to evidence-based approaches that will allow athletes and active individuals to exercise ...
Sports medicine of athletes research, including blood, biomedical imaging informatics, and activity monitor has been a research hotspots in recent years. Through scientometric analysis of the past 20 years, we know the blood, biomedical imaging informatics, and activity monitor is the focus of future research. The USA, Australia, and England ...
The issues of replication and scientific transparency have been raised in exercise and sports science research. A potential means to address the replication crisis and enhance research reliability is to improv... Ting-Yu Lin, Ting-Yu Chueh and Tsung-Min Hung. Sports Medicine - Open 2023 9 :114.
The American College of Sports Medicine (ACSM) promotes and integrates scientific research, education, and practical applications of sports medicine and exercise science to maintain and enhance physical performance, fitness, health, and quality of life.
Sports Medicine also welcomes the submission of high-quality original research in the above fields. As a hybrid journal, Sports Medicine does not charge authors to publish using the traditional subscription-based publishing route, but does offer the option to publish accepted articles open access if authors so wish or if their funders require ...
British Journal of Sports Medicine (BJSM) is a Plan S compliant Transformative Journal. British Journal of Sports Medicine (BJSM) is a multimedia portal for authoritative original research, systematic reviews, consensus statements and debate in sport and exercise medicine (SEM). We define sport and exercise medicine broadly. BJSM's web, print, video and audio material serves the ...
Among the fastest-growing areas of research within sports medicine, orthopaedic surgery, and medicine in general is biologics. In sports medicine, biologic research over the past year has focused primarily on PRP and cellular therapies. Notably, the heterogeneity of biologic preparations, in particular PRP, make evaluating the literature difficult.
Medicine & Science in Sports & Exercise (MSSE), ACSM's flagship monthly peer-reviewed journal, is the leading multidisciplinary original research journal for members. Each issue features original investigations, clinical studies and comprehensive reviews on current topics in sports medicine and exercise science.
Sports Medicine is a relatively new topic in medicine and includes a variety of medical and paramedical fields. Although sports medicine is mistakenly thought to be mainly for sports professionals/athletes, it actually encompasses the entire population, including the active and non-active healthy populations, as well as the sick [].Sports medicine also engages amateur sportsmen and strives to ...
by Jeffrey B. Driban | Nov 6, 2023. The Biological Basis of Sex Differences in Athletic Performance: Consensus Statement for the American College of Sports Medicine. Posts and discussions regarding the latest research relevant to clinicians and students interested in sports medicine. Sports Med Res.
The American Sports Medicine Institute (ASMI) is a national and international leader in sports medicine research related to clinical and surgical outcomes, biomechanics, and rehabilitation. The foci of ongoing studies at ASMI includes both clinical and biomechanical research, and our team includes researchers with expertise in motion capture ...
Aims and scope. Sports Medicine - Open focuses on original research and definitive reviews in the field of sport and exercise medicine. The Journal includes medical and scientific research relating to: Sporting performance enhancement including nutrition, equipment and training. Medical syndromes associated with sport and exercise.
Team-sports staff often administer non-exhaustive exercise assessments with a view to evaluating physiological state, to inform decision making on athlete management (e.g., future training or recovery). Submaximal fitness tests have become prominent in team-sports settings for observing responses to a standardized physical stimulus, likely because of their time-efficient nature, relative ease ...
At Duke sports medicine we pride ourselves in fostering and developing the future of research through our fellows and residents who are an integral part of our team. All research conducted within the sports medicine division is IRB approved and regulated. Our areas of research include knee ( ACL, MPFL, osteochondral treatment, meniscal repair ...
In this review we integrate the scientific literature and results-proven practice and outline a novel framework for understanding the training and development of elite long-distance performance. Herein, we describe how fundamental training characteristics and well-known training principles are applied. World-leading track runners (i.e., 5000 and 10,000 m) and marathon specialists participate ...
Additional sports supplementation research efforts have focused on the impact of genetic polymorphisms, primarily CYP1A2 and ADORA2A , which are polymorphisms of the Cytochrome P450 system ... This review aims to guide sports medicine providers, dieticians, coaches, and athletic trainers to adhere to evidence-based research and begin the ...
Protecting health is a team sport — yet the public health and clinical care systems meant to advance this goal have been siloed for too long.
A total of 1414 patients underwent randomization (701 to the TAVI group and 713 to the SAVR group). The mean (±SD) age of the patients was 74±4 years; 57% were men, and the median Society of ...
Sports psychiatry is a young field of medicine and psychiatry that focuses on mental health among athletes, and sports and exercise within psychiatry and mental disorders. However, the development of sports psychiatry and its fields of activity vary from region to region and are not uniform yet.
About Journal. CEOS Sports Medicine and Research is an open access, online peer reviewed journal which covers the most active and promising areas of current research in Sports Medicine and Research. This multi-disciplinary journal provides an avenue to less accessible sources to a wide audience of medical researchers and healthcare professionals.
The University of Washington Department of Orthopaedic Surgery and Sports Medicine, in partnership with the CLEAR outcomes research center at the University of Washington, are pleased to offer a 1 yr internship focused on musculoskeletal outcomes research.. In addition to research co-mentored by departmental and CLEAR affiliated faculty, the internship will include a series of education ...
Introduction. Regenerative medicine utilizes innovative approaches to explore and develop materials that can be used to replace, repair, improve, or reproduce tissues and organs in the human body (Brody, 2016).Sports medicine focuses on aspects of physical health, including the treatment and prevention of exercise-related injuries and aging-related problems that hinder the function of the ...
A total of 75 transgender and cisgender athletes were invited to take part in the research, which is the first study of trans athlete laboratory sports performance. | Yiistocking / Shutterstock
New research from Michigan State University's Department of Kinesiology sheds light on the prevalence of early knee osteoarthritis (OA) symptoms in patients following anterior cruciate ligament (ACL) reconstruction surgery. Findings indicate that symptoms persist throughout the first year post-surgery, emphasizing the need for early intervention to prevent long-term decline and function.