
An official website of the United States government
The .gov means it’s official. Federal government websites often end in .gov or .mil. Before sharing sensitive information, make sure you’re on a federal government site.
The site is secure. The https:// ensures that you are connecting to the official website and that any information you provide is encrypted and transmitted securely.
- Publications
- Account settings
Preview improvements coming to the PMC website in October 2024. Learn More or Try it out now .
- Advanced Search
- Journal List
- J Dent (Shiraz)
- v.16(3 Suppl); 2015 Sep
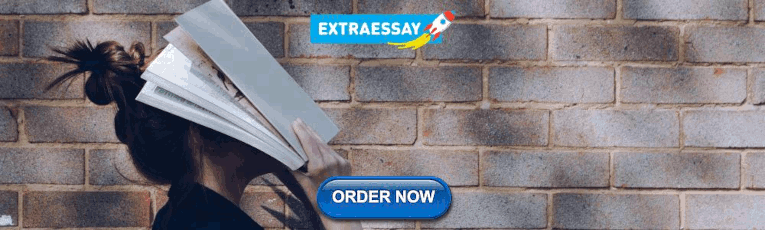
The Effectiveness of Home Water Purification Systems on the Amount of Fluoride in Drinking Water
Behrooz eftekhar.
a Dept. of Endodontic, School of Dentistry, Ahwaz Jondishapoor University of Medical Sciences, Ahwaz, Iran.
Masoume Skini
b Postgraduate Student, Dept. of Endodontic, School of Dentistry, Ahwaz Jondishapoor University of Medical Sciences, Ahwaz, Iran.
Milad Shamohammadi
Jaber ghaffaripour.
c DDS, School of Dentistry, Ahwaz Jondishapoor University of Medical Sciences, Ahwaz, Iran.
Firoozeh Nilchian
d Dental Students Research Center, Dept. of Dental Public Health, School of Dentistry, Isfahan University of Medical Sciences, Isfahan, Iran.
Statement of the Problem
Water purification systems for domestic use have drawn significant attention over the past few years. This can be related to the improvement of public health and concern for water contamination.
The aim of this study was to evaluate whether home water purification systems eliminate the essential materials such as fluoride besides filtrating the heavy ions and other unwanted particles out of water.
Materials and Method
In this experimental study, six most frequently used commercial brands of water purifiers were evaluated and compared. Specimens were collected right before and after setting up the device, and 6 months later. Then, spectrophotometry (the Harrison device) was performed to compare fluoride clearance by each home water cleaner device.
Based on the data collected from all water purification devices in different locations, the amount of fluoride was significantly different before and right after using home water purifier and six months later ( p = 0.001 and p = 0.00, respectively).
The filtration of water significantly decreased its fluoride concentration. The fluoride content of purified water was approximately as much as zero in some cases.
Introduction
Fluoride is a natural element branched from Fluorine. This element can be found in all sorts of water and soil. Out of every kilogram of outer layer of earth, 0.3 gram is fluoride. Mineral waters have more amount of this element compared to other sources.( 1 )
About 60 years ago, Grand Rapids in Michigan State was the first city in which fluoride supplement was synthetically added to tap water. In US, adding fluoride to community water supplies of many cities has improved the oral health of millions of American citizens.( 2 )
Fluoridation of community water supplies is adding a specific amount of fluoride (0.7-1.2 ppm) to water in order to reduce the risk of dental caries. By 2002, almost 170 million Americans were provided with this privilege.( 3 )
Since most of the systemic fluoride is provided through tap water to population, many policies have been established to add fluoride to community water regarding its benefits for teeth and bones.( 4 )
In regions and countries that do not have water-fluoridation technology, there are natural supplements as previously mentioned. For example, Iran has many mineral water supplies that contain considerable amounts of fluoride. Amount of fluoride in natural mineral waters depends on weather conditions; the warmer the weather is, the higher the amount of fluoride can be detected. Mineral waters in southern regions that have warmer weather contain more fluoride. In Iran, the highest amount of fluoride has been found in southeast and northeast areas.
Water purification systems for domestic use have drawn much of attention over the past few years. This can be related to improvement of public health and concerns for water contamination. There are several types of home water purification systems that can be categorized into 3 different groups( 5 ) as filtered systems, systems using UV irradiation, and ion-exchange systems.
The aim of this study was to find out whether domestic water purification systems could eliminate the essential materials such as fluoride besides filtrating the heavy ions and other unwanted particles out of water.
In this study, 6 frequently used commercial brands of water purifiers in Ahwaz were compared. The commercial brands evaluated in the current study were CCK (Ceramic and Ceramic/Carbon Cartridges ; RTX-TS DLM filters, Korea), Soft Water (Ceramic Candles; Alpine TJ Series filters, W9332420, USA), Alkusar (Special media cartridges filters; PRB50-IN, USA), Puricom (Special media cartridges filters; Watts 4.5" x 10" Dual Housing, Korea), Water Safe (Granular Carbon Cartridges filters; LCV (Lead, Cysts, VOC's) (Carbon Block Filter Cartridges, Australia), and Aquafresh (Sediment String-Wound; Poly Spun and Pleated Washable Cartridges filters, K5520, USA). The main drinking water supply for Ahwaz is provided by governmental companies. After making arrangement with certain companies that supported these brands, the devices were setup in 6 different regions of Ahwaz. Samples were collected before and right after setting up the device. To reduce the errors and elevate the accuracy of the module, 5 samples were taken from each device. Another sample was collected from each single device 6 months later. A total of 64 samples were collected including 32 unfiltered (control) and 32 filtered samples of tap water (experimental) from 6 regions in Ahwaz. Fluoride sampling kits (Spands; EW-99574-08Hach ® Test Kits, USA) were used to test the amount of fluoride in sample waters. Samples were all collected in polyethylene sampling containers and were then coded. Spectrophotometry (AvaSpec-ULS2048L- USB2 UARS spectrometer, USA) was performed. In order to measure the characteristics of individual molecules, a mass spectrometer converted them to ions so that they could be moved about and manipulated by external electric and magnetic fields.
Atmospheric pressure was around 760 torr (mm of mercury). The pressure under which ions may be handled is roughly 10 -5 to 10 -8 torr (less than a billionth of an atmosphere). By varying the strength of the magnetic field, ions of different mass can be focused progressively on a detector fixed at the end of a curved tube and also under a high vacuum.
Latin alphabetic words were used to code each commercial device. Numbers were used for samples obtained before and after setting the device.( 6 )
The results were analyzed by using paired sample t-test, with alpha (ɑ) set at 0.05.
The amount of fluoride in water before and after using six brands of water purifier device is summarized in Table 1 .
The amount of fluoride before and after installing water purifier devices
Based on the data gathered from all water purification devices set in different regions, the level of fluoride was significantly different before and after using home water purifier ( p = 0.001). It was found that home water purifiers nearly eliminated fluoride from tap water. Table 2 represents the results of t-test.
Comparison of different study groups with t-test
* p< 0.05 is statistically significant.
Another round of sampling was done 6 months later from the same filters of home water purifier. Details are illustrated in Table 3 and 4.
The amount of fluoride in tap water after 6 months of using a water purification filter
Comparison of the study groups after six mounts with t-test
Fluoride absorption is mostly systemic or local; systemic absorption occurs through eating the element with food, water or fluoride pills, and local absorption by toothpastes and other fluoride-containing hygienic products. In many countries, the highest supply for fluoride absorption is systemic absorption through water consumption.( 6 ) In early 20 th century, the first attempts were made to fluoridate public water supplies, which eventually led to 40% decrease of dental caries in the target population.( 7 )Introduction of water fluoridation in the 1950-1960 and fluoride-containing dental products in the 1970 changed the situation. The main sources of fluoride in established market economies (EME) are drinking water, fluoridated salt, foods and beverages, baby cereals and formulas, fluoride supplements, toothpastes, mouth-rinses, and topical fluorides. Additionally, fluoride in water has a diffusion or halo effect; which means that the drinks and foods manufactured in fluoridated areas are also available to whole population including the residents of non-fluoridated areas.
Although adding fluoride to almost all oral hygienic products has restricted the effect of fluoride water (Halo effect), it is still common to fluoridate the city water supply.( 6 ) In many areas of the world, there is no systematic plan for fluoridation of community water and only the natural sources supply it. Therefore, sometimes the hardness of water and aggregation of different and sometimes poisonous elements drive the population to use bottled water or use home purification devices.
The findings of the present study revealed that all the 6 devices reduced the fluoride in tap water and most of them nearly eliminated it. Different home purification devices have been marketed each of which is claimed to eliminate certain kinds of elements from water.( 9 ) JK Mwabi et al. (2011) used 4 different filters to reduce the hardness and chemical contamination of water in poor villages in Africa, and reported that all of the four filters reduced the fluoride significantly. Bucket filter had the most significant effect and reduced fluoride element 99.9%. These results also indicated that fluoride was the most reduced element of all. Likewise, silver-impregnated porous pot (SIPP) filter reduced 90%-100% of elements.
Clasen et al. ( 5 ) in their study reported that 3 different home purification systems ,the ceramic candle gravity filter, iodine resin gravity filter, and iodine resin faucet filter, reduced bacterial contamination by four logs and decreased ions such as fluoride and arsenic, as well.
Moreover, there are certain methods to reduce the excessive amount of fluoride in the water. One of the best-known methods is absorption technique.( 7 ) Evaluation of 6 different commercial water purifiers has not been done in any other study; therefore, there is no similar study to compare the results exactly. More evaluations are suggested to be performed on home water purification systems, and more strategies should be devised to preserve the essential elements of tap water.
The current study found considerable differences between the amount of fluoride before and after filtration with home purification device; that is filtration significantly decreased the fluoride concentration even as much as 100% in some cases.
Conflict of Interest: None declared
Suggestions or feedback?
MIT News | Massachusetts Institute of Technology
- Machine learning
- Social justice
- Black holes
- Classes and programs
Departments
- Aeronautics and Astronautics
- Brain and Cognitive Sciences
- Architecture
- Political Science
- Mechanical Engineering
Centers, Labs, & Programs
- Abdul Latif Jameel Poverty Action Lab (J-PAL)
- Picower Institute for Learning and Memory
- Lincoln Laboratory
- School of Architecture + Planning
- School of Engineering
- School of Humanities, Arts, and Social Sciences
- Sloan School of Management
- School of Science
- MIT Schwarzman College of Computing
MIT engineers make filters from tree branches to purify drinking water
Press contact :, media download.
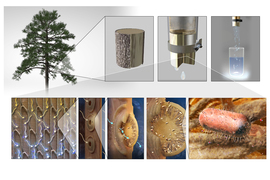
*Terms of Use:
Images for download on the MIT News office website are made available to non-commercial entities, press and the general public under a Creative Commons Attribution Non-Commercial No Derivatives license . You may not alter the images provided, other than to crop them to size. A credit line must be used when reproducing images; if one is not provided below, credit the images to "MIT."
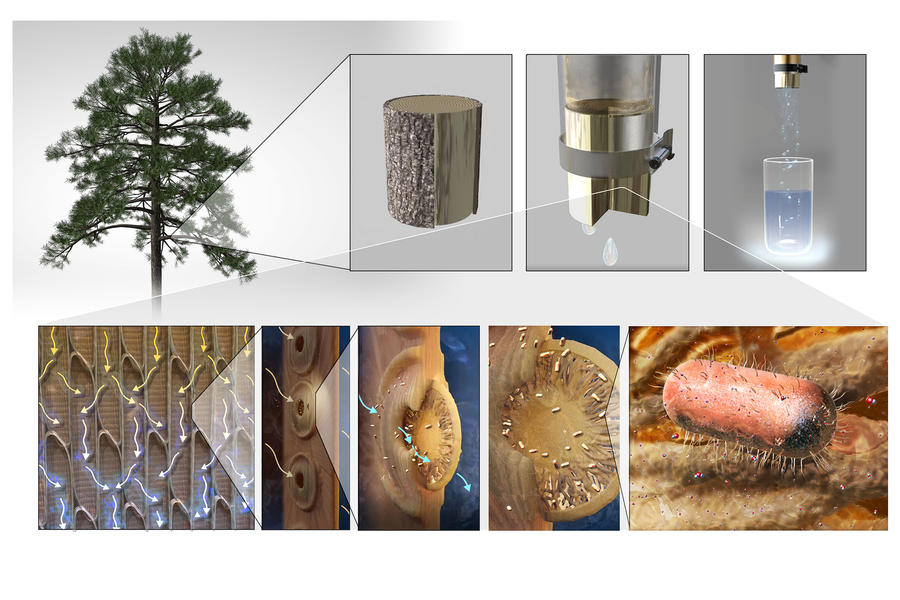
Previous image Next image
The interiors of nonflowering trees such as pine and ginkgo contain sapwood lined with straw-like conduits known as xylem, which draw water up through a tree’s trunk and branches. Xylem conduits are interconnected via thin membranes that act as natural sieves, filtering out bubbles from water and sap.
MIT engineers have been investigating sapwood’s natural filtering ability, and have previously fabricated simple filters from peeled cross-sections of sapwood branches, demonstrating that the low-tech design effectively filters bacteria.
Now, the same team has advanced the technology and shown that it works in real-world situations. They have fabricated new xylem filters that can filter out pathogens such as E. coli and rotavirus in lab tests, and have shown that the filter can remove bacteria from contaminated spring, tap, and groundwater. They also developed simple techniques to extend the filters’ shelf-life, enabling the woody disks to purify water after being stored in a dry form for at least two years.
The researchers took their techniques to India, where they made xylem filters from native trees and tested the filters with local users. Based on their feedback, the team developed a prototype of a simple filtration system, fitted with replaceable xylem filters that purified water at a rate of one liter per hour.
Their results, published today in Nature Communications , show that xylem filters have potential for use in community settings to remove bacteria and viruses from contaminated drinking water.
The researchers are exploring options to make xylem filters available at large scale, particularly in areas where contaminated drinking water is a major cause of disease and death. The team has launched an open-source website , with guidelines for designing and fabricating xylem filters from various tree types. The website is intended to support entrepreneurs, organizations, and leaders to introduce the technology to broader communities, and inspire students to perform their own science experiments with xylem filters.
“Because the raw materials are widely available and the fabrication processes are simple, one could imagine involving communities in procuring, fabricating, and distributing xylem filters,” says Rohit Karnik, professor of mechanical engineering and associate department head for education at MIT. “For places where the only option has been to drink unfiltered water, we expect xylem filters would improve health, and make water drinkable.”
Karnik’s study co-authors are lead author Krithika Ramchander and Luda Wang of MIT’s Department of Mechanical Engineering, and Megha Hegde, Anish Antony, Kendra Leith, and Amy Smith of MIT D-Lab.
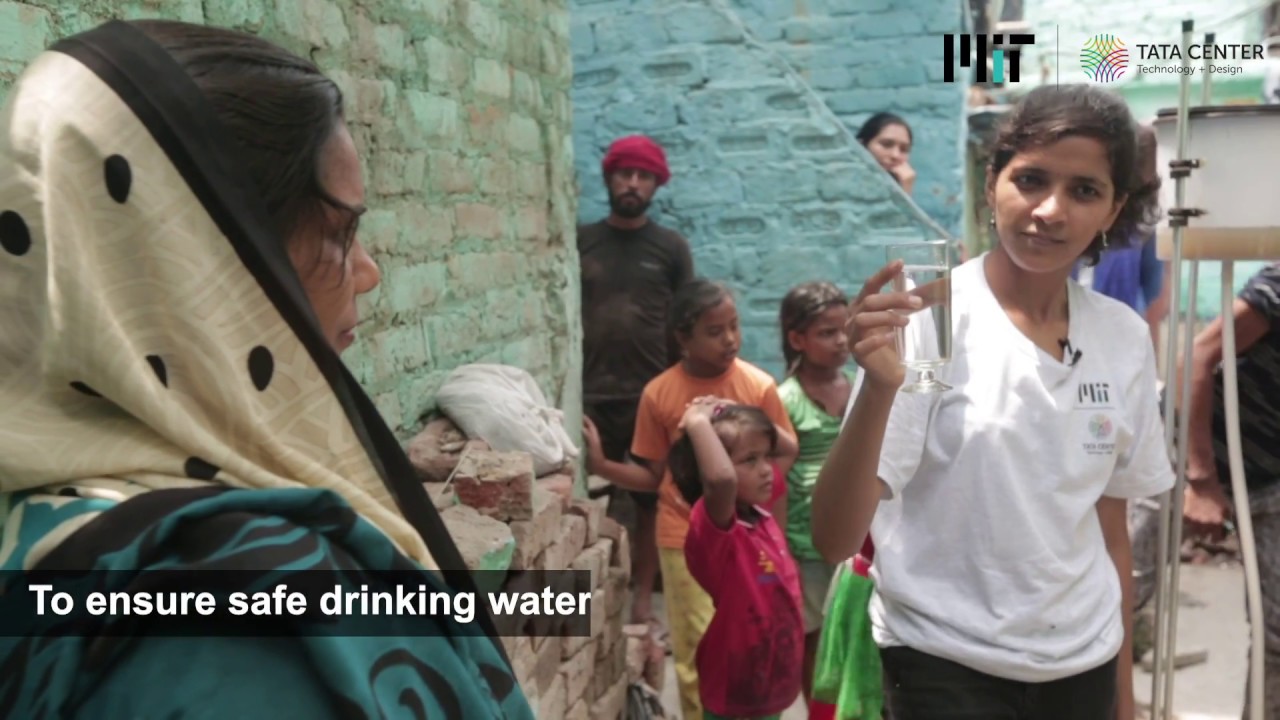
Clearing the way
In their prior studies of xylem, Karnik and his colleagues found that the woody material’s natural filtering ability also came with some natural limitations. As the wood dried, the branches’ sieve-like membranes began to stick to the walls, reducing the filter’s permeance, or ability to allow water to flow through. The filters also appeared to “self-block” over time, building up woody matter that clogged the conduits.
Surprisingly, two simple treatments overcame both limitations. By soaking small cross-sections of sapwood in hot water for an hour, then dipping them in ethanol and letting them dry, Ramchander found that the material retained its permeance, efficiently filtering water without clogging up. Its filtering could also be improved by tailoring a filter’s thickness according to its tree type.
The researchers sliced and treated small cross-sections of white pine from branches around the MIT campus and showed that the resulting filters maintained a permeance comparable to commercial filters, even after being stored for up to two years, significantly extending the filters’ shelf life.
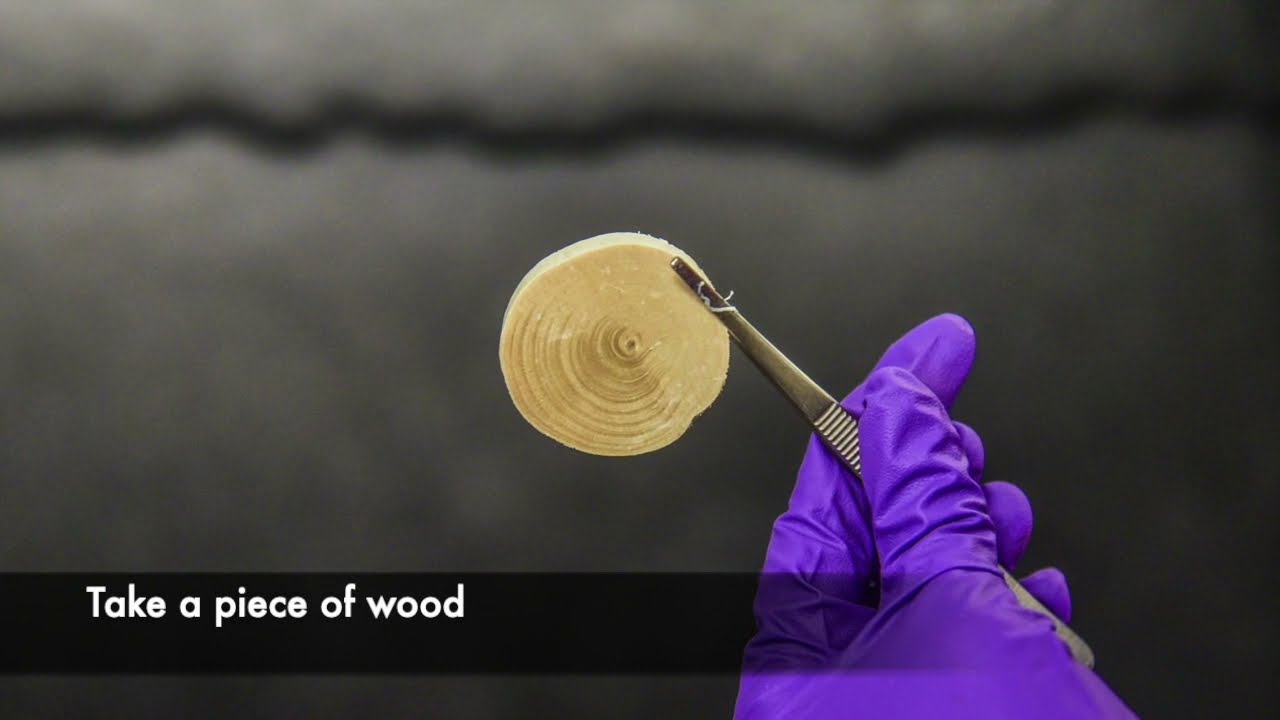
The researchers also tested the filters’ ability to remove contaminants such as E. coli and rotavirus — the most common cause of diarrheal disease. The treated filters removed more than 99 percent of both contaminants, a water treatment level that meets the “ two-star comprehensive protection ” category set by the World Health Organization.
“We think these filters can reasonably address bacterial contaminants,” Ramchander says. “But there are chemical contaminants like arsenic and fluoride where we don’t know the effect yet,” she notes.
Encouraged by their results in the lab, the researchers moved to field-test their designs in India, a country that has experienced the highest mortality rate due to water-borne disease in the world, and where safe and reliable drinking water is inaccessible to more than 160 million people.
Over two years, the engineers, including researchers in the MIT D-Lab, worked in mountain and urban regions, facilitated by local NGOs Himmotthan Society, Shramyog, Peoples Science Institute, and Essmart. They fabricated filters from native pine trees and tested them, along with filters made from ginkgo trees in the U.S., with local drinking water sources. These tests confirmed that the filters effectively removed bacteria found in the local water. The researchers also held interviews, focus groups, and design workshops to understand local communities’ current water practices, and challenges and preferences for water treatment solutions. They also gathered feedback on the design.
“One of the things that scored very high with people was the fact that this filter is a natural material that everyone recognizes,” Hegde says. “We also found that people in low-income households prefer to pay a smaller amount on a daily basis, versus a larger amount less frequently. That was a barrier to using existing filters, because replacement costs were too much.”
With information from more than 1,000 potential users across India, they designed a prototype of a simple filtration system, fitted with a receptacle at the top that users can fill with water. The water flows down a 1-meter-long tube, through a xylem filter, and out through a valve-controlled spout. The xylem filter can be swapped out either daily or weekly, depending on a household’s needs.
The team is exploring ways to produce xylem filters at larger scales, with locally available resources and in a way that would encourage people to practice water purification as part of their daily lives — for instance, by providing replacement filters in affordable, pay-as-you-go packets.
“Xylem filters are made from inexpensive and abundantly available materials, which could be made available at local shops, where people can buy what they need, without requiring an upfront investment as is typical for other water filter cartridges,” Karnik says. “For now, we’ve shown that xylem filters provide performance that’s realistic.”
This research was supported, in part, by the Abdul Latif Jameel Water and Food Systems Lab (J-WAFS) at MIT and the MIT Tata Center for Technology and Design.
Share this news article on:
Press mentions, popular science.
MIT researchers have created a new filter from tree branches that could provide an inexpensive, biodegradable, low-tech option for water purification, writes Shaena Montanari for Popular Science . “We hope that our work empowers such people to further develop and commercialize xylem water filters tailored to local needs to benefit communities around the world,” says Prof. Rohit Karnik.
United Press International (UPI)
UPI reporter Brooks Hays writes that MIT researchers have created a new water filter from tree branches that can remove bacteria. “The filter takes advantage of the natural sieving abilities of xylem -- thin, interconnected membranes found in the sapwood branches of pine, ginkgo and other nonflowering trees,” writes Hays.
Previous item Next item
Related Links
- Rohit Karnik
- Abdul Latif Jameel Water and Food Systems Lab
- Department of Mechanical Engineering
Related Topics
- Abdul Latif Jameel Water and Food Systems Lab (J-WAFS)
- Mechanical engineering
- Public health
- Tata Center
Related Articles
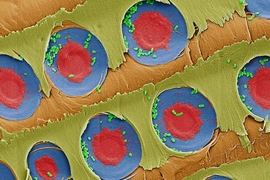
Need a water filter? Peel a tree branch
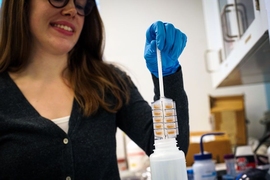
Instrument may enable mail-in testing to detect heavy metals in water
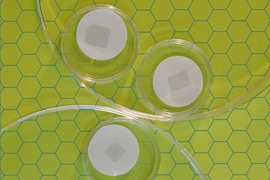
Stamp-sized graphene sheets riddled with holes could be boon for molecular separation
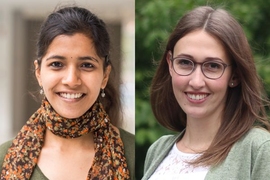
Krithika Ramchander and Andrea Beck awarded J-WAFS fellowships for water solutions
More mit news.
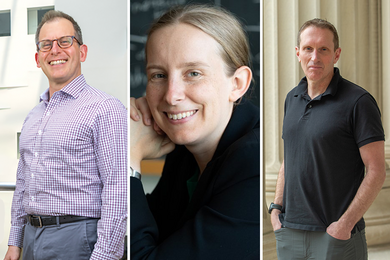
Three from MIT awarded 2024 Guggenheim Fellowships
Read full story →
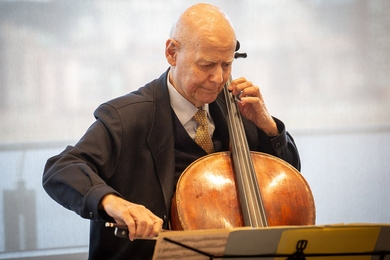
A musical life: Carlos Prieto ’59 in conversation and concert
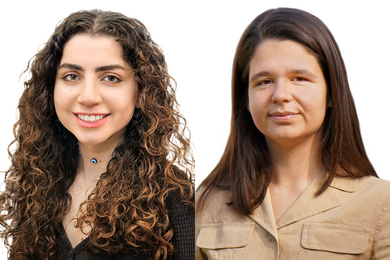
Two from MIT awarded 2024 Paul and Daisy Soros Fellowships for New Americans
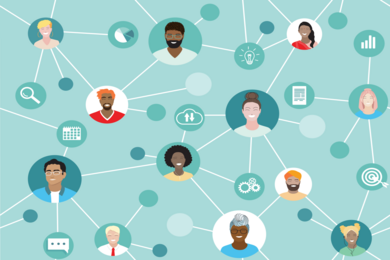
MIT Emerging Talent opens pathways for underserved global learners
The MIT Edgerton Center’s third annual showcase dazzles onlookers
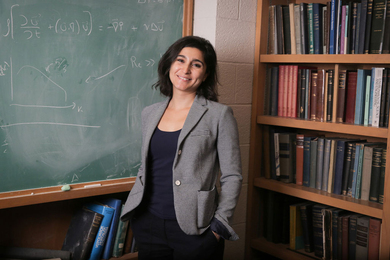
3 Questions: A shared vocabulary for how infectious diseases spread
- More news on MIT News homepage →
Massachusetts Institute of Technology 77 Massachusetts Avenue, Cambridge, MA, USA
- Map (opens in new window)
- Events (opens in new window)
- People (opens in new window)
- Careers (opens in new window)
- Accessibility
- Social Media Hub
- MIT on Facebook
- MIT on YouTube
- MIT on Instagram
Ad-free. Influence-free. Powered by consumers.
The payment for your account couldn't be processed or you've canceled your account with us.
We don’t recognize that sign in. Your username maybe be your email address. Passwords are 6-20 characters with at least one number and letter.
We still don’t recognize that sign in. Retrieve your username. Reset your password.
Forgot your username or password ?
Don’t have an account?
- Account Settings
- My Benefits
- My Products
- Donate Donate
Save products you love, products you own and much more!
Other Membership Benefits:
Suggested Searches
- Become a Member
Car Ratings & Reviews
2024 Top Picks
Car Buying & Pricing
Which Car Brands Make the Best Vehicles?
Car Maintenance & Repair
Car Reliability Guide
Key Topics & News
Listen to the Talking Cars Podcast
Home & Garden
Bed & Bath
Top Picks From CR
Best Mattresses
Lawn & Garden
TOP PICKS FROM CR
Best Lawn Mowers and Tractors
Home Improvement
Home Improvement Essential
Best Wood Stains
Home Safety & Security
HOME SAFETY
Best DIY Home Security Systems
REPAIR OR REPLACE?
What to Do With a Broken Appliance
Small Appliances
Best Small Kitchen Appliances
Laundry & Cleaning
Best Washing Machines
Heating, Cooling & Air
Most Reliable Central Air-Conditioning Systems
Electronics
Home Entertainment
FIND YOUR NEW TV
Home Office
Cheapest Printers for Ink Costs
Smartphones & Wearables
BEST SMARTPHONES
Find the Right Phone for You
Digital Security & Privacy
MEMBER BENEFIT
CR Security Planner
Take Action
- Overview Overview
- Ratings Ratings
- Recommended Recommended
- Buying Guide Buying Guide
- Water Filter Pitchers
- Under-Sink Water Filters
- Reverse Osmosis Water Filters
- Faucet-Mounted Water Filters
- Countertop Water Filters
Water Filter Buying Guide
The water that comes out of your household tap travels through miles of pipes, aquifers, and treatment systems before landing in your glass. One of the easiest ways to boost the quality of that water is by using a water filter, be it under your sink, in your fridge, or on your countertop.
While many people buy water filters simply to improve the taste or appearance of their tap water, filtering your drinking water can also potentially protect you from harmful contaminants. A joint Consumer Reports-Guardian US news organization investigation of the nation’s tap water revealed that 118 of 120 tested locations across the U.S. had detectable amounts of lead, arsenic, or PFAS (per- and polyfluoroalkyl substances linked to significant health problems ) above CR’s recommended maximum levels. Those sampled water systems served more than 19 million people.
An in-home water filter can not only protect against harmful contaminants but also improve the taste of your tap water. Because drinking-water complaints often relate to taste and smell, we test flavor and odor reduction in our current ratings for water filter pitchers, under-sink water filters , faucet-mounted filters , and countertop filters . Our ratings also include reverse osmosis water filters from previous testing.
In addition to refrigerator water filters, the most popular are pitcher-style filters—the kind you fill from the tap and keep in your fridge. They’re easy to use, require no installation, and take up less space than a more permanent option. They’re also relatively economical, with most costing less than $50.
Faucet-mounted water filters attach directly to your tap, while countertop water filters attach to your tap by a hose that diverts water through a small sink-side purifying device equipped with a spout. Both typically filter using granulated activated carbon cartridges that draw away contaminants before water leaves your tap. They work well for apartment dwellers because they don’t require a plumber to install. Faucet-mounted filters often cost less than $50. Countertop filters can cost $80 or much more, depending on the filtration system they use.
Under-sink water filters are more expensive, ranging from less than $100 to $700. They hide discreetly and filter a higher volume of water at a greater flow rate. They may require custom plumbing, or sink or countertop alterations for the water dispenser. But if convenience is important to you, under-sink filters are a good option.
How CR Tests Water Filters
We test a water filter’s flow rate by seeing how long it takes to filter one quart of water. We also give each filter a "clogging" score, based on how much a filter’s flow rate slows down over the stated life of the cartridge.
NSF International and the American National Standards Institute develop filtration standards. If a manufacturer claims that a pitcher meets NSF/ANSI standards for removing specific contaminants, such as chlorine, lead, and PFAS, we verify those claims.
We also check claims of flavor and odor reduction by spiking spring water with commonly found compounds that can make the water smell and taste like a sewage treatment plant, damp soil, metal, or a swimming pool. A trained panel of professional tasters evaluates how successful the filters are at removing those flavors and odors. The best-performing filters work well enough that most people wouldn’t be able to discern a palatable difference between the filtered water and pure spring water.
What's in Your Water?
The Environmental Protection Agency requires community water suppliers to provide a Consumer Confidence Report to customers every July. A CCR states the levels of regulated contaminants detected in the water and how they compare with the EPA’s drinking water standards. If you rent an apartment, contact your building manager or local water company for a copy.
Community water systems providing water to 100,000 people or more must post the reports online . If you use well water, you won’t have a CCR because the EPA doesn’t regulate private wells. In that case, check with the Centers for Disease Control and Prevention for information on well testing and treatment.
The water quality report tells you about the water delivered to your house, but if it was built before lead-free pipes were mandated in 1986, you may want to do your own tests for lead. There’s no safe level of lead exposure, according to the EPA .
Some advocacy groups and consumers feel the same about PFAS (per- and polyfluoroalkyl substances), a group of more than 12,000 synthetic chemicals that persist in the environment and are thought to be detrimental to human health. According to a 2023 study by the U.S. Geological Survey, at least 45 percent of the nation’s tap water is contaminated with PFAS, which are linked to obesity, cancer, learning delays in children, and thyroid disease. In the 2021 CR-Guardian study, almost every test sample had measurable levels of PFAS, and more than 35 percent exceeded the maximum safety threshold specified by CR scientists and other health experts. The Environmental Working Group, an advocacy organization, estimates that more than 3,000 communities in all 50 states and two territories have drinking water contaminated with PFAS .
Tap water crises, state legislation , and public awareness nudged the EPA to propose new federal limits on six different PFAS in drinking water, including PFOA and PFOS, two of the most studied.
Few utilities currently test for PFAS. If yours doesn’t, or if you use well water, you can get your water tested by an EPA-certified lab like SimpleLab or WaterCheck from National Testing Laboratories . But be prepared to shell out a few hundred dollars. In addition to its list of certified labs, the EPA offers a Safe Drinking Water Hotline at 800-426-4791. For more on testing your water, see our interactive guide.
How to Choose a Water Filter
Once you know what’s in your tap water, you can find a filter that best suits your needs.
If tests reveal no harmful contaminants and the only problem with your water is taste and/or odor, check CR’s water filter ratings for filters that score high in our tests for flavor/odor reduction. If contaminants are found, the best way to remove them is with a water filter that’s certified to remove those specific contaminants. That means the filter is independently tested and found to comply with an accepted standard specific to the contaminants and is subject to follow-up testing by the certifying agency to ensure that the product that consumers purchase will perform the same as the product that was tested.
Look at the filter’s packaging for a stamp or seal confirming which contaminants it’s certified to remove. Third-party labs that certify products to NSF/ANSI standards include the National Sanitation Foundation itself, the Water Quality Association ( WQA ), and the International Association of Plumbing & Mechanical Officials (IAPMO).
Our ratings for water filter pitchers and under-sink, faucet-mounted, and countertop water filters identify which are certified for the removal of lead, chlorine, and PFAS. Our ratings of reverse-osmosis water filters indicate which ones passed CR’s tests for the removal of lead and cholorform, an indicator of chlorine.
All filters must be replaced periodically to function effectively. Our ratings allow you to compare the annual cost of replacing filters for each product. For example, replacement filters for the pitchers we test range from $16 to $78. You’ll also see which models have a filter-life indicator, a handy feature that tells you when it’s time to change the filter.
Filtering Your Choices
Water filter pitchers are one of the most widely used types of water filters, but there are other filtration systems available for the home. Factor in your budget, your water consumption, maintenance, and, most importantly, the contaminants found in your water before purchasing a new filter.
Water Filter Pitcher
Water pitchers are great if you’re on a budget, you don’t have the space to install a permanent filter, or you’re renting. But you do need to keep filling them over and over while other types filter constantly. The best water pitcher model we’ve tested does a stellar job of removing chlorine without sacrificing cartridge life or flow rate. Some others are slow, prone to clogging, or have a short filter life. A small pitcher stored in the refrigerator might suffice for one or two people, but a large size—or an installed option that filters continuously—may be more convenient for a family that consumes several gallons of water a day.
Price of tested filters: $17 to $54.
Faucet-Mounted Water Filter
If you’re looking for easy installation, these are a good choice for filtering drinking and cooking water. Simply unscrew the aerator from the threaded tip of your faucet and screw on the filter. Faucet-mounted filters let you switch between filtered and unfiltered water. On the downside, they slow down water flow and don’t fit on all faucets, including those with a pullout sprayer.
Price of tested filters: $15 to $31.
Countertop Water Filter
These filters are also easy to install. Just remove the faucet aerator, then screw the filter hose onto the faucet. This also makes them handy for renters, who might not have a landlord’s okay to do more significant plumbing modifications. Basic models use carbon filtration, while more costly units may use reverse osmosis or ultraviolet light to guard against more serious contaminants. CR’s tests found that countertop filters are less likely to clog than pitcher filters or faucet-mounted filters, but they can clutter a countertop, and they don’t fit all faucets.
Price of tested filters: $80 to $1,179.
Under-Sink Water Filter
Under-sink filters are arguably the most convenient option once you get past the more difficult installation. They don’t clutter the counter (but do take up space under the sink) and they look better than the alternatives. Like countertop filters, these can filter lots of water. But installation may require professional plumbing modifications and drilling a hole through the sink or countertop for the dispenser.
Price of tested filters: $93 to more than $500.
Reverse Osmosis Water Filter
If you’re facing more serious water contamination issues, you may need the more powerful filtration of a reverse osmosis system. Using standard household water pressure, water is forced through a semipermeable membrane, then through filters. Reverse osmosis filters can remove a wide range of contaminants, including dissolved solids. But they can be extremely slow, rob cabinet space, and typically create 3 to 5 gallons of wastewater for every gallon filtered. You must sanitize them with bleach periodically, and the membrane and filters must be replaced, per manufacturer’s recommendation.
Price of tested filters: $155 to $2,200.
Refrigerator Water Filter
Water-and-ice dispensers are common on French door, bottom-freezer, and side-by-side refrigerators. The water line typically runs through a filter inside the refrigerator. Most filters can be easily replaced by simply pushing and twisting. A replacement filter can cost $50 or more, and you’ll need to change it every six months or so. Third-party water filters are widely available at a lower cost but may be less reliable or effective. Depending on the particular filter, fridge filters can reduce many of the same contaminants as a countertop or under-sink filter.
Consumer Reports is not currently testing refrigerator water filters.
Water Filter Brands
The company’s e-Spring countertop filters—which treat water with ultraviolet light technology—are sold through Amway dealers. The brand’s countertop model is priced around $1,000, significantly higher than most of its competitors.
Aqua-Pure is a 3M company that sells a variety of water treatment systems and products, including under-sink, reverse osmosis, whole-house, faucet filters, and replacement filters. It also offers softeners. They’re sold at Amazon, Home Depot, Lowes, and other retailers.
Aquasana’s countertop models use a dual filtration system that includes an ion exchange method in the water filtration process. Its products are sold online, at large retailers like Home Depot and Lowe’s, and at plumbing supply outlets.
The Clorox-owned brand dominates the pitcher category and also offers faucet-mounted models. Brita water filters are sold at national chains such as Home Depot, Lowe’s, Sears, Target, and Walmart, and at hardware and plumbing supply stores, drugstores, and online retailers.
Brondell offers a variety of three-stage countertop, under-counter, and reverse osmosis filtration systems as well as water filter faucets and replacement filters. They’re sold online at Amazon, Wayfair, and on the company’s website.
Crystal Quest offers a selection of water treatment solutions including pitchers, countertop, faucet-mounted, under-sink, reverse osmosis, and whole-house water filter systems.
This company offers a wide range of products. One line of its single-stage filters comes in different sizes, and you can choose a model based on household needs, uses, and size. The Aqua-Cleer line offers a multistage filtration system that uses up to four filters at a time. Culligan filters are sold through many major retailers, such as Home Depot, Walmart, and Ace Hardware, as well as through independent dealers nationwide and online.
Pentair’s Everpure produces whole-house, under-sink, countertop, reverse osmosis, and shower water filters, replacement filters, and water softeners. Its 1-stage, 2-stage, and 3-stage water filters can be found on its website and at plumbing supply stores.
Multipure, the original developer of the solid block carbon filter, makes a variety of residential and commercial filters that it claims remove a broad range of harmful contaminants such as lead, mercury, arsenic, chlorine, asbestos, and PFOA.
This manufacturer offers a wide range of water filter products, such as faucet-mounted filters, filter pitchers, and large-capacity dispensers (the latter of which CR doesn’t currently test). Pur filters are available at national chains such as Home Depot, Lowe’s, Sears, Target, and Walmart, and at hardware and plumbing supply stores, drugstores, and online retailers.
Soma filters are sustainably made from 60 percent plant-based and renewable materials for a lower carbon footprint. The brand says its products filter out chlorine, mercury, zinc, cadmium, copper, and other unwanted tastes and odors through activated coconut shell carbon and charcoal. Soma filters are sold through Crate & Barrel, the Container Store, and directly from the manufacturer.
This brand includes filter pitchers and faucet-mounted filters. They use a multistage filtration process and are sold at most big-box stores, such as Target, Best Buy, and Home Depot.
Sharing is Nice
We respect your privacy . All email addresses you provide will be used just for sending this story.
ZeroWater Extremelife ZFM-400CR
Brita basic saff-100, pelican water systems pdf-450w-p, matterhorn mct-8050cl, home master tmjrf2 jr f2 elite, apex mr 1050, brondell coral uc300 three stage, kirkland signature (costco) water filtration system, soma water filter pitcher, aquasana aq-5200, brita stream rapids ob55, zerowater ready-pour zd-010rp.
See All Ratings
Thank you for visiting nature.com. You are using a browser version with limited support for CSS. To obtain the best experience, we recommend you use a more up to date browser (or turn off compatibility mode in Internet Explorer). In the meantime, to ensure continued support, we are displaying the site without styles and JavaScript.
- View all journals
- Explore content
- About the journal
- Publish with us
- Sign up for alerts
- Research Highlight
- Published: 11 September 2019
CLEAN WATER
Algae-based water filters
- Aimee Guha Roy 1
Nature Sustainability volume 2 , page 788 ( 2019 ) Cite this article
3973 Accesses
1 Altmetric
Metrics details
- Biopolymers
- Developing world
- Water microbiology
- Water resources
ACS Sustain. Chem. Eng . http://doi.org/c9nj (2019)
Access to clean drinking water remains a problem in many developing countries, and affordable point-of-use water treatment is needed to reduce the spread of water-borne diseases. In Bangladesh, piped water is often contaminated and only available for a few hours a day. As such, it must be boiled prior to consumption, but this requires either fuel that may be unavailable to the poorest families or wood that may cause deforestation. One solution is water filtration, but abundant low-cost materials are required.
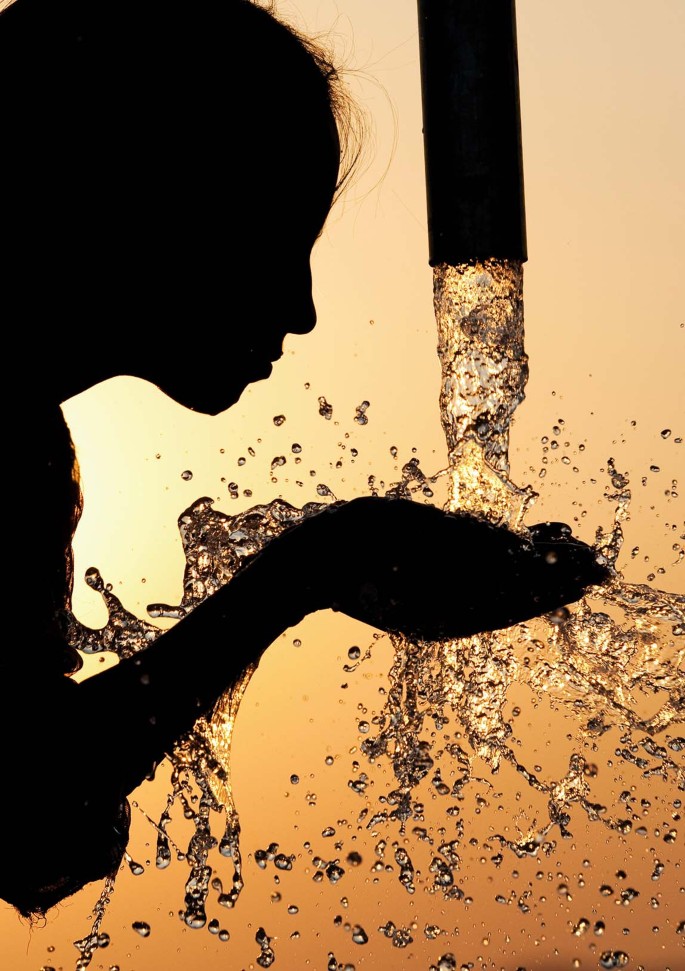
Tim Gainey / Alamy Stock Photo
Olof Gustafsson and colleagues from Uppsala University, Sweden and the University of Dhaka, Bangladesh made filter papers using nanocellulose from a locally available macroalgae called Pithophora . This robust algae thrives in nitrogen- and phosphorus-contaminated water and could be cultivated and harvested in large quantities in Bangladesh. The filter paper’s performance was measured using simulated wastewater containing latex nanobeads and in vitro viruses. The filters also showed complete removal of all infectious pathogens when tested on water samples collected from the Turag River and Dhanmondi Lake in Bangladesh. This study provides a way for locally grown algae to be converted into advanced filters for point-of-use water filtration.
Author information
Authors and affiliations.
Nature Sustainability https://www.nature.com/natsustain
Aimee Guha Roy
You can also search for this author in PubMed Google Scholar
Corresponding author
Correspondence to Aimee Guha Roy .
Rights and permissions
Reprints and permissions
About this article
Cite this article.
Guha Roy, A. Algae-based water filters. Nat Sustain 2 , 788 (2019). https://doi.org/10.1038/s41893-019-0382-3
Download citation
Published : 11 September 2019
Issue Date : September 2019
DOI : https://doi.org/10.1038/s41893-019-0382-3
Share this article
Anyone you share the following link with will be able to read this content:
Sorry, a shareable link is not currently available for this article.
Provided by the Springer Nature SharedIt content-sharing initiative
Quick links
- Explore articles by subject
- Guide to authors
- Editorial policies
Sign up for the Nature Briefing: Anthropocene newsletter — what matters in anthropocene research, free to your inbox weekly.

Microbiology and Drinking Water Filtration
Cite this chapter.
- Gary S. Logsdon 3
Part of the book series: Brock/Springer Series in Contemporary Bioscience ((BROCK/SPRINGER))
882 Accesses
5 Citations
Water filtration research has been undertaken for a variety of reasons. Studies have been performed to develop information for filtration theories and for design of filtration plants to remove suspended matter such as clays, algae, suspended matter in general, and asbestos fibers from water. Filtration studies related to removal of microorganisms have generally been motivated by the need to learn about the removal of pathogens or indicator organisms, or both. Reducing the risk of waterborne disease has been a goal of microbiologically related filtration research for nearly 100 years. This chapter briefly reviews that research and then discusses the results of recent investigations.
This is a preview of subscription content, log in via an institution to check access.
Access this chapter
- Available as PDF
- Read on any device
- Instant download
- Own it forever
- Compact, lightweight edition
- Dispatched in 3 to 5 business days
- Free shipping worldwide - see info
- Durable hardcover edition
Tax calculation will be finalised at checkout
Purchases are for personal use only
Institutional subscriptions
Unable to display preview. Download preview PDF.
Al-Ani, M.Y., Hendricks, D.W., Logsdon, G.S., and Hibler, C.P. 1986. Removing Giardia cysts from low turbidity water by rapid rate filtration. Journal American Water Works Association 78 (5): 66–73.
CAS Google Scholar
Al-Ani, M.Y., McElroy, J.M., Hibler, C.P., and Hendricks. D.W. 1985. Filtration of Giardia Cysts and Other Substances: Volume 3. Rapid Rate Filtration . EPA/600/2-85/027. U.S. Environmental Protection Agency, Cincinnati, Ohio.
Google Scholar
U.S. Army and U.S. Public Health Service. 1944. Report 834. Efficiency of Standard Army Water Purification Equipment and of Diatomite Filters in Removing Cysts of Endamoeba histolytica from Water.
Arozarena, MM . 1979. Removal of Ciardia muris Cysts by Granular Media Filtration. Unpublished Master2019s Thesis, University of Cincinnati, Cincinnati, Ohio.
Baylis, J.R., Gullans, O., and Spector, B.K. 1936. The efficiency of rapid sand filters in removing the cysts of the amoebic dysentery organisms from water. Public Health Reports 51: 1567–1575.
Article CAS Google Scholar
Bellamy, W.D., Silverman, G.P., Hendricks, D.W., and Logsdon, G.S. 1985a. Removing Giardia cysts with slow sand filtration. Journal American Water Works Association 77 (2): 52–60.
Bellamy, W.D., Hendricks, D.W., and Logsdon, G.S. 1985b. Slow sand filtration: influences of selected process variables. Journal American Water Works Association 77 (12): 62–66.
Brazos, B.J., O’Connor, J.T., and Lenau, C.W. 1987. Seasonal effects on total bacterial removals in a rapid sand filtration plant, pp 795–833 in Proceedings of the 1986 Water Quality Technology Conference . American Water Works Association, Denver, Colorado.
Brown, T.S., Malina, J.F., Jr. and Moore, B.D. 1974a. Virus removal by diatomaceous earth filtration-Part 1. Journal American Water Works Association 66 (2): 98–102.
Brown, T.S., Malina, J.F., Jr., and Moore, B.D. 1974b. Virus removal by diatomaecous earth filtration-Part 2. Journal American Water Works Association 66 (12): 735–738.
Bryck, J., Walker, B., and Mills, G. 1987. Giardia removal by slow sand filtration-pilot to full scale, pp. 49–58 in Proceedings AWWA Seminar on Coagulation and Filtration: Pilot to Full Scale , American Water Works Association, Denver, Colorado.
Bucklin, K., and Amirtharajah, A. 1988. The Characteristics of Initial Effluent Quality and Its Implications for the Filter to Waste Procedure . American Water Works Association Research Report. American Water Works Association, Denver, Colorado.
Chang, S.L. and Kabler, P.W. 1958. Removal of coxsackie and bacterial viruses in water by flocculation. Part 1. American Journal of Public Health 48: 51–61.
Cleasby, J.L., Hilmoe, D.J., and Dimitracopoulos, C.J. 1984. Slow sand and direct inline filtration of a surface water. Journal American Water Works Association 76 (12): 44–55.
Cullen, T.R. and Letterman, R.D. 1985. The effect of slow sand filter maintenance on water quality. Journal American Water Works Association 77 (12): 48–55.
DeWalle, F.B., Engeset, J., and Lawrence, W. 1984. Removal of Giardia lamblia Cysts by Drinking Water Treatment Plants. EPA-600/2-84-069. U.S. Environmental Protection Agency, Cincinnati, Ohio.
Edberg, S.C., Allen, M.J., and Smith, D.B. 1988. National field evaluation of a defined substrate method for simultaneous enumeration of total coliforms and Escherichia coli from drinking water: comparison with the standard multiple tube fermentation method. Applied and Environmental Microbiology 54: 1595–1601.
Fayer, R. and Ungar, B.L.P. 1986. Cryptosporidium spp. and Cryptosporidiosis. Microbiological Reviews 50: 458–483.
Fox, K.R., Miltner, R.J., Logsdon, G.S., Dicks, D.L., and Drolet, L.F 1984. Pilot plant studies of slow rate filtration. Journal American Water Works Association 76 (12): 62–68.
Guy, M.D., Mclver, J.D., and Lewis, M.J. 1977. The removal of virus by a pilot treatment plant. Water Research 11: 421–428.
Article Google Scholar
Hazen, A. 1913. The Filtration of Public Water Supplies, 3rd Ed . John Wiley & Sons, New York.
Heer, J.M. 1987. Turbidity Standards, Calibration, and Practice. Water World News March/ April 18–22.
Hendricks, D.W., Seelaus, T. Janonis, B., Mosher, R.R., Gertig, K.R., Williamson-Jones, G.L., Jones, F.E., Alexander, B.D., Saterdal, R., and Blair, J. 1988. Filtration of Giardia Cysts and Other Particles Under Treatment Plant Conditions . American Water Works Association Research Foundation Research Report, American Water Works Association, Denver, Colorado.
Hilmoe, D.J. and Cleasby, J.L. 1986. Comparing constant-rate and declining-rate filtration. Journal American Water Works Association 78 (12): 26–34.
Howell, D.G. 1987. The Evaluation of Montana Surface Water Treatment Plants for Their Ability to Remove Giardia . pp. 437–448. In Proceedings of the 1986 Water Quality Technology Conference , American Water Works Association, Denver, Colorado.
Huisman, L. and Wood, WE. 1974. Slow Sand Filtration . World Health Organization, Geneva, Switzerland.
Johnson, G. 1916. The typhoid toll. Journal American Water Works Association 3:249–326 and 791–868.
Jordan, H.E. 1937. Epidemic amebic dysentery-The Chicago outbreak of 1933. Journal American Water Works Association 29: 133–137.
Lance, J.C., Gerba, C.P., and Melnick, J.L. 1976. Virus movement in soil columns flooded with secondary sewage effluent. Applied and Environmental Microbiology 32: 520–526.
Lange, K.P., Bellamy, W.D., Hendricks, D.W., and Logsdon, G.S. 1986. Diatomaceous earth filtration of Giardia cysts and other substances. Journal American Water Works Association 78 (1): 76–84.
Logsdon, G.S. 1987. Evaluating treatment plants for particulate contaminant removal. Journal American Water Works Association , 79 (9): 82–92.
Logsdon, G.S. and Rice, E.W. 1985. Evaluation of sedimentation and filtration for microorganism removal, pp. 1177–1197 In: Proceedings of the 1985 American Water Works Association Annual Conference , American Water Works Association, Denver, Colorado.
Logsdon, G.S., Symons, J.M., Hoye, R.L. Jr., and Arozarena, M.M. 1981. Alternative filtration methods for removal of cysts and cyst models. Journal American Water Works Association 73: 111–118.
McConnell, L.K. 1984. Evaluation of the Slow Rate Sand Filtration Process for Treatment of Drinking Water Containing Viruses and Bacteria . Unpublished Master of Science Thesis. Utah State University, Logan, Utah.
McCormick, R.F. and King, P.H. 1982. Factors that affect the use of direct filtration in treating surface waters. Journal American Water Works Association 74: 234–242.
Mosher, R.R. and Hendricks. D.W. 1986. Rapid rate filtration of low turbidity water using field-scale pilot filters. Journal American Water Works Association . 78 (12): 42–51.
O’Connor, J.T., Brazos, B.J., Ford, W.C., Plaskett, J.L., and Dusenberg, L.L. 1985. Chemical and Microbiological Evaluations of Drinking Water Systems in Missouri: Summer Conditions, pp. 1199–1225 in: Proceedings of the 1985 American Water Works Association Annual Conference , American Water Works Association, Denver, Colorado.
Ongerth, J. 1988. A study of water treatment practices for the removal of Giardia lamblia cysts, pp. 171–175 in: Proceedings of Conference on Current Research in Drinking Water Treatment, EPA/600/9-88/004, U.S. Environmental Protection Agency, Cincinnati, Ohio.
Poynter, S.F.B. and Slade, J.S. 1977. The removal of viruses by slow sand filtration. Progress in Water Technology 9: 75–88.
Pyper, G.R. 1985. Slow Sand Filter and Package Treatment Plant Evaluation: Operating Costs and Removal of Bacteria, Giardia and Trihalomethanes . EPA-600/2-85-052, U.S. Environmental Protection Agency, Cincinnati, Ohio.
Rao, V.C., Symons, J.M., Ling, A., Wang, P., Metcalf, T.G., Hoff, J.C., and Melnick, J.L. 1988. Removal of hepatitis A virus and rotavirus by drinking water treatment. Journal American Water Worfes Association 80 (2): 59–67.
Robeck, G.G., Clarke, N.A., and Dostal, K.A. 1962. Effectiveness of water treatment processes for virus removal. Journal American Water Works Association 54: 1275–1290.
Sayre, I.M. 1984. Filtration. Journal American Water Works Association 76 (12): 33.
Seelaus, T.J., Hendricks, D.W., and Janonis, B.A. 1986. Design and operation of a slow sand filter. Journal American Water Works Association 78 (12): 35–41.
Slade, J.S. 1978. Enteroviruses in slow sand filtered water. Journal of the Institution of Water Engineers and Scientists 32: 530–536.
Slezak, LA. and Sims, R.C. 1984. The application and effectiveness of slow sand filtration in the United States. Journal American Water Works Association 76 (12): 38–43.
Spector, B.K., Baylis, J.R., and Gullams, O. 1934. Effectiveness of filtration in removing from water, and of chlorine in killing, the causative organism of amoebic dysentery. Public Health Reports 49: 786–800.
Stetler, R.E., Ward, R.L., and Waltrip, S.C. 1984. Enteric virus and indicator bacteria levels in a water treatment system modified to reduce trihalomethane production. Applied and Environmental Microbiology 47: 319–324.
Streeter, H.W. 1927. Studies of the Efficiency of Water Purification Processes. Public Health Bulletin No . 172 , U.S. Public Health Service, Government Printing Office.
Streeter, H.W. 1929. Studies of the Efficiency of Water Purification Processes. Public Health Bulletin 193 . U.S. Public Health Service, Washington, D.C.
Tanner, S.A. 1987. Evaluation of Sand Filters in Northern Idaho . Unpublished Master’s Thesis, University of Washington, Seattle, Washington.
Taylor, E.W 1969–1970. Forty-Fourth Report on the Results of the Bacteriological. Chemical and Biological Examination of the London Waters for the Years 1969–1970 . Metropolitan Water Board, London, England.
Walton, H.W. 1988. Diatomite filtration: how it removes Giardia from water, pp. 113–116 In: Wallis, P.M. and Hammond, B.R. (editors), Advances in Giardia Research , University of Calgary Press, Calgary, Alberta.
Download references
Author information
Authors and affiliations.
Environmental Protection Agency Cincinnati, Ohio, USA
Gary S. Logsdon
You can also search for this author in PubMed Google Scholar
Editor information
Editors and affiliations.
Department of Microbiology, Montana State University, 59717, Bozeman, Montana, USA
Gordon A. McFeters
Rights and permissions
Reprints and permissions
Copyright information
© 1990 Springer-Verlag New York Inc.
About this chapter
Logsdon, G.S. (1990). Microbiology and Drinking Water Filtration. In: McFeters, G.A. (eds) Drinking Water Microbiology. Brock/Springer Series in Contemporary Bioscience. Springer, New York, NY. https://doi.org/10.1007/978-1-4612-4464-6_6
Download citation
DOI : https://doi.org/10.1007/978-1-4612-4464-6_6
Publisher Name : Springer, New York, NY
Print ISBN : 978-1-4612-8786-5
Online ISBN : 978-1-4612-4464-6
eBook Packages : Springer Book Archive
Share this chapter
Anyone you share the following link with will be able to read this content:
Sorry, a shareable link is not currently available for this article.
Provided by the Springer Nature SharedIt content-sharing initiative
- Publish with us
Policies and ethics
- Find a journal
- Track your research
- Share full article

How Much Can a Water Filter Do?
Lead, bacteria and PFAS are among the contaminants cropping up in drinking water.
Credit... Thea Traff for The New York Times
Supported by

By Dana G. Smith
- Published May 30, 2023 Updated July 19, 2023
Over the past few years, water safety crises have cropped up in several cities, including Baltimore , Flint, Mich. , Jackson, Miss. , and Newark, N.J ., where lead or bacteria have leached into tap water, forcing people to rely on bottled water or on boiling their tap water to rid it of pathogens. In New York City, a recent report estimated that one in five people may be drinking lead-contaminated water.
In Wilmington, N.C. , high levels of perfluoroalkyl and polyfluoroalkyl substances, chemicals commonly known as PFAS, were detected in the local watershed. A new study by the U.S. Geological Survey suggests that the problem is widespread, showing that 30 percent of more than 700 tap water samples — from both private wells and public sources — contain at least one PFAS. The chemicals have been linked to a host of health issues, including cancer, liver damage and problems with fertility.
The Environmental Protection Agency proposed new regulations in March that would crack down on drinking water levels of six types of PFAS, substantially lowering the allowable detectable amounts. (Drinking water is not the only source of exposure to PFAS , which show up in food wrappers, cooking pans and waterproof clothing, among other places, but reducing contact wherever possible is advisable.)
These events raise questions about just how safe municipal water supplies in the United States are, and whether additional filtration steps are required even outside of areas experiencing an acute crisis. And if that’s the case, are there home water filters that will help?
Problems with the system
Water sanitation is often listed as one of the greatest health advancements of the 20th century, helping to significantly reduce the death rate from infectious diseases. Water safety standards were enshrined in the Safe Drinking Water Act of 1974, which gives the E.P.A. authority to restrict the amounts of many metals, bacteria, pesticides and other harmful contaminants that can be detected in water. State agencies monitor water treatment plants to ensure they are adhering to the law, and if any violations emerge, they are required to notify consumers within 24 hours. (Owners of private wells are responsible for ensuring that their water is free of contaminants.)

Since the passage of the Safe Drinking Water Act, though, other water-monitoring issues have arisen. For example, most water treatment plants are not set up to remove more modern contaminants, such as PFAS, pharmaceutical drugs and endocrine disrupting chemicals, said Detlef Knappe, a professor of civil, construction and environmental engineering at North Carolina State University, who was one of the first to publish on the Wilmington PFAS problem .
Another concern is whether we are “setting standards at a pace that is reflective of what we know about the science of our water,” said David Cwiertny, a professor of civil and environmental engineering at the University of Iowa. He gave the example of nitrate, an agricultural pollutant that’s present in the water supply in Des Moines . While the local water treatment plant takes steps to remove the contaminant, there are questions about whether the allowable levels could still cause health harms .
Aging infrastructure is also a problem. In several of the recent crises, contamination occurred when lead leached into the water as it traveled through the distribution pipes. National regulations about the amount of lead permitted in pipes have been strengthened over the years, but many old water distribution systems have not been updated and contain unsafe levels.
“Often, things go wrong because of just underinvestment into this type of infrastructure,” Dr. Knappe said. “The rate at which we’re replacing the distribution system pipes in the network is not keeping up with the rate at which the system really needs to be maintained.”
Finally, experts say water treatment plants are not equipped for the extreme weather events that have become more common with climate change. That was part of the problem in Jackson, where flooding caused by heavy rains overpowered one of the city’s treatment plants, resulting in untreated, bacteria-laden water traveling to people’s homes.
The crises in Flint, Baltimore, Jackson and Newark are currently exceptional cases — public water supplies in the U.S. are generally safe, said Thanh Nguyen, a professor of civil and environmental engineering at the University of Illinois, Urbana-Champaign. But “the number of exceptions may increase with time if we don’t” update the infrastructure, she said.
What home filters can do
If there is a known crisis in your area, local officials will provide recommendations for how best to keep yourself safe. If you’re generally concerned about potential contaminants, at-home water filters can help with some issues.
Most filters contain activated carbon to capture contaminants, which can be used in pitchers , refrigerator dispensers, faucet attachments or systems installed under the sink . Activated carbon is good at removing many chemicals and metals but not all (it doesn’t capture nitrate, for example), and it cannot filter out most bacteria.
The American National Standards Institute and NSF — two independent groups that evaluate product performance — have established standards for water filters. Companies aren’t required to make products that meet NSF/ANSI standards, but because “there is no federally regulated requirement,” certification can help to “ensure that the product isn’t a counterfeit or it’s actually effective,” said Kyle Postmus, senior manager of the Global Water Division at NSF.
NSF/ANSI Standard 42 is for aesthetics, such as taste, smell and appearance (people often want to filter out traces of the disinfectant chlorine). Standard 53 focuses on safety, ensuring levels of lead or mercury, as well as some pesticides and industrial chemicals, are below the accepted limit. The certifications are for individual contaminants, and the product should specify all the contaminants it is approved to reduce.
Home filters appear to work decently well for PFAS and can now be NSF/ANSI Standard 53 certified for some of those chemicals, too. In a study published in 2020 , Dr. Knappe and his co-authors found that, on average, pitcher and refrigerator filters that use activated carbon reduced PFAS levels by about 50 percent. More advanced filtration systems that use a process known as reverse osmosis were over 90 percent effective, but they are much more expensive and waste a significant amount of water. A report by the Environmental Working Group found that pitcher filters varied widely in their ability to remove PFAS, with some brands eliminating 100 percent of the chemicals and the worst performer reducing PFAS levels by 22 percent.
Sometimes filters can cause more harm than good. Dr. Nguyen’s research revealed that if water sits in a faucet or under-sink filter for a long period of time, such as overnight, it can actually pick up more contaminants , including lead and bacteria. That’s because the water is essentially bathing in high concentrations of the contaminants that were trapped by the activated carbon. When the faucet is turned on again, the contaminant-infused water comes rushing out. As a result, Dr. Nguyen said that it is important to flush your water filter for at least 10 seconds before drinking from it. Also, be sure to change your filter regularly.
Most of the experts interviewed for this article said that they used an at-home filter, but none said it was essential. Some used filters for taste issues, while others said it was a precautionary measure. “Not everybody needs them, but I can think of a lot of reasons why people might,” Dr. Cwiertny said. “What I would encourage is that people make informed decisions and know why they’re purchasing a device” — for example, for a specific taste concern or for filtering out a known contaminant.
If you’re worried about the quality of your water and want to know whether you should invest in a filter, you can use a home test kit . You can also request a Consumer Confidence Report from the E.P.A. that will detail what’s in the water when it leaves your local treatment plant, although the report does not account for what the water might encounter as it travels through the distribution pipe network.
The experts cautioned that if your region has a known issue with lead or another contaminant, a filter is a bandage on a wound that needs surgery — the larger problem with the pipes or water supply still must be addressed.
An earlier version of this article incorrectly described activated carbon as capturing contaminant particles in drinking water. Activated carbon captures dissolved substances, not particles.
How we handle corrections
Dana G. Smith is a reporter for the Well section, where she has written about everything from psychedelic therapy to exercise trends to Covid-19. More about Dana G. Smith
America’s Vulnerable Water Systems
The Sinking Town: In Arizona’s deeply conservative La Paz County, the most urgent issue facing many voters is not inflation or illegal immigration. It is the water being pumped from under their feet.
Paying the Price: Siemens and other corporations vowed to fix water woes in Mississippi and save cities across the state millions. The deals racked up debt instead , leaving many worse off than before.
A Tax on Groundwater: While American farmers elsewhere can freely pump the water beneath their land, growers in California’s Pajaro Valley pay hefty fees. Experts say the approach is a case study in how to save a vital resource .
A Diet Feeding a Crisis: America’s dietary shift toward far more chicken and cheese in recent decades has taken a major toll on underground water supplies .
First Come, First Served?: As the world warms, California is re-examining claims to its water that are based on a cherished frontier principle and have gone unchallenged for generations.
Jets Powered by Corn: America’s airlines want to replace jet fuel with ethanol to fight global warming. That would require lots of corn, and lots of water .
Advertisement
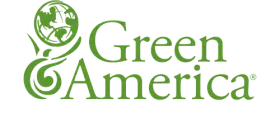
- Take Action
- Other Ways to Give
- Social Justice
Green Living
- For Green Businesses
- Green American Magazine
The Facts About Water Filters

First time here? Check out the most recent and updated version of this article: Bottled Water VS. Tap.
Beverage companies have made a fortune on marketing bottled water on the premise that it’s “pure,” from “pristine, natural sources,” and thereby safer than tap water. Bottled water marketing campaigns have been so successful in making people suspicious of their tap water, that sales skyrocketed 700% between 1997 and 2005. And from 1999 to 2017, per capita bottled water consumption ballooned from 16.2 gallons to 42.1 gallons.
Skyrocketing as well—the environmental degradation, landfill waste, and human rights abuses associated with bottled water. Plus, studies have shown that it’s no safer than tap water. The EPA notes that bottled water, like any water, can be expected to have some contaminants, although that does not make it unsafe.
There’s a much better option for ensuring that the water you and your family drink is as safe as it can be: water filters. Putting a safe water filter in your home is less expensive and far less environmentally damaging than bottled water. And if you choose the right filter, you can minimize or eliminate the contaminants of the highest concern in your area. Here’s what you need to know:
How Safe Is Public Water?
Under the Safe Water Drinking Act, the US Environmental Protection Agency (EPA) is responsible for setting national drinking water standards. The EPA regulates over 80 contaminants—including arsenic, e-coli, cryptosporidia, chlorine, and lead—that may be found in drinking water from public water systems. While the EPA says that 90% of US public water systems meet its standards, you may want to use a water filter to further ensure your water’s safety. A 2015 study by the nonprofit Natural Resources Defense Council (NRDC) found that due to a combination of pollution and deteriorating equipment and pipes, the public water supplies for 18 million Americans have lead violations or other EPA-restricted contaminants (either legal limits or unenforceable suggested limits) and may pose health risks to some residents . So even though it may test fine at its source, public water may still pick up contaminants on the way to your house. Contaminants that snuck into city water supplies studied by the NRDC include rocket fuel, arsenic, lead, fecal waste, and chemical by-products created during water treatment. “Exposure to the contaminants [sometimes found in public and private drinking water] can cause a number of health problems, ranging from nausea and stomach pain to developmental problems and cancer,” notes Physicians for Social Responsibility (PSR) in its booklet, Drinking Water: What Health Care Providers Should Know.
PSR estimates that up to 900,000 people get sick and 900 die in the US per year from contaminated public and private drinking water. Despite the problems with public water, it’s still just as safe as bottled water, despite the billions of dollars beverage companies spend to make you think bottled is better.
Step One: Assess Your Tap Water
There isn’t a one-type-fits-all kind of safe water filter: not every filter type will eliminate every contaminant. You’ll save money and ensure that you’re targeting the contaminants of concern in your area by doing a little research upfront. “Most people purchase the wrong equipment because they skip this very important step, and then they’ve wasted money and resources on a system that isn’t making their water any safer,” says James P. McMahon, owner of Sweetwater, LLC , which provides consulting and products for people wanting to purify their air or water. To start, check your water utility’s “Consumer Confidence Report,” which it must mail to you each year before July 1 by law. The report details where your drinking water comes from, what contaminants have been found in it, and how contaminant levels compare to national standards. You can also call your utility and ask for a copy, or see if it’s online. While your report can tell you what’s going on with the water in your area, only a test of the water coming out of your tap will tell you what you and your family are drinking for sure. If your water comes from a private well, it’s not regulated at all by the EPA, so you should have your water tested annually in late spring (when pesticide runoff will be at its worst), and anytime you notice a change in the color or taste of your water.
Step Two: Find the Best Water Filter
Water filters come in a dizzying variety, from plastic pitcher filters and built-in refrigerator filters, to faucet and under-the-sink filters, to whole-house models that combine a variety of media types and treat all of the water in your house. What type you want depends on your needs. If, after examining your Consumer Confidence Report (or, preferably, your current and several past reports), you find that your water regularly tests better than EPA levels , you may just want a filter that can remove the chemicals your local utility uses to treat the water. These chemicals may or may not show up on your report. Call and ask your utility if it uses chlorine, which can cause neorological and respiratory harm, or chloramine, which can be harmful to circulatory and respiratory systems. Chlorine combines with organic elements during the water treatment process to produce carcinogenic byproducts. The best type of filter to remove chlorine and its byproducts is a combination carbon/KDF adsorption filter (which is a different chemical process than absorption), which range from shower and faucet filters to sink and whole-house filters, like those from Sweetwater. A regular carbon filter won’t remove chloramine, so look for a catalytic carbon filter instead. If you only have one or two contaminants , a smaller unit, such as a countertop or under-the-sink filter, may meet your needs. To find a filter certified to remove the contaminants you’re most concerned about, visit the NSF’s online database . Finally, if you find your water has serious safety issues , consider a multi-stage filter that can tackle a variety of contaminants. Many combine a variety of filter types. Sweetwater sells multi-stage whole-house or sink filters, for example, that combine KDF and carbon adsorption with ultraviolet light, among other steps—and it also sells customized filters.
Step Three: Look at the Labels on Water Filters
Some experts recommend looking for a water filter certified by NSF International, a nonprofit organization that conducts safety testing for the food and water industries. NSF tests and certifies water filters to ensure that they both meet NSF safety standards and are effective at removing contaminants as claimed by the manufacturer. Underwriters Laboratories and the Water Quality Association also offer similar certification, based on NSF standards. NSF has different certifications, so when you read the label, first make sure it says the filter will remove the contaminants you’re most concerned about. A filter certified by NSF to remove chlorine isn’t going to be helpful if you need it to remove nitrates. Then, look for the NSF seal, Underwriters Laboratories’ “UL Water Quality” mark, or the Water Quality Association Gold Seal for added assurance that your filter will actually do what the box claims.
Safe Water for the Future
Filters aren’t perfect—they can be expensive and energy intensive, and the filter cartridges are nearly impossible to recycle. But when you compare throwing away a couple cartridges to the billions of water bottles we toss each year, filters are a preferable option. When it comes to ensuring better water for the future, here are the most important steps: First, we need to stop drinking bottled water. It’s not any safer than tap, and it wastes a mind-boggling number of resources. Then, we need to ask companies to take back and recycle their cartridges. Besides using up resources, filter cartridges trap and hold contaminants. If the cartridges are not disposed of in a sealed landfill, those contaminants could end up right back in the environment. Brita—which sells a popular carbon adsorption pitcher filter, faucet-mounted filters, and cartridges for refrigerator filters does accept recycling shipments of Brita products. If you buy from another manufacturer, research whether their products can be recycled or email them and say you would like them to implement a recycling program.
Finally, US water treatment and distribution systems date back several decades, and they need repairs and upgrades to make water safer for for human and environmental health. While the EPA won’t attach a dollar amount, Dale Kemery, a former EPA spokesman, says more money is needed to make these upgrades. Food and Water Watch is demanding that Congress increase funding to secure our public water system.
That said, public utilities will be using treatment chemicals well into the future, and our systems may never be perfect. Take responsibility for your family’s health by carefully considering whether you need to take additional steps to make your water the healthiest it can be.
FEATURE ARTICLE - JULY/AUGUST 2007 (Updated 2023)
To find more screened green companies offering water filters, search "water purification" in the National Green Pages ™.

Related News
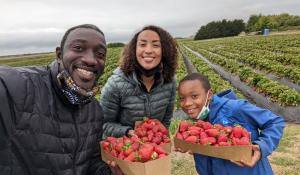
If you own shares in publicly traded companies, you can use your investor power to shape corporate policy for the better.
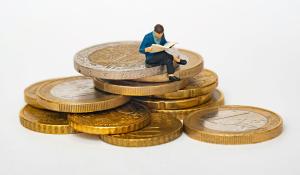
Beyond Lead: Toxins in Toys
Investing in green energy, 10 ways to build community, economic activism is what makes our work powerful. you make our work possible, donate to our matching gift challenge.
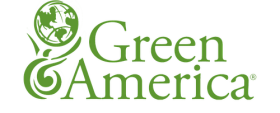
©2024 Green America
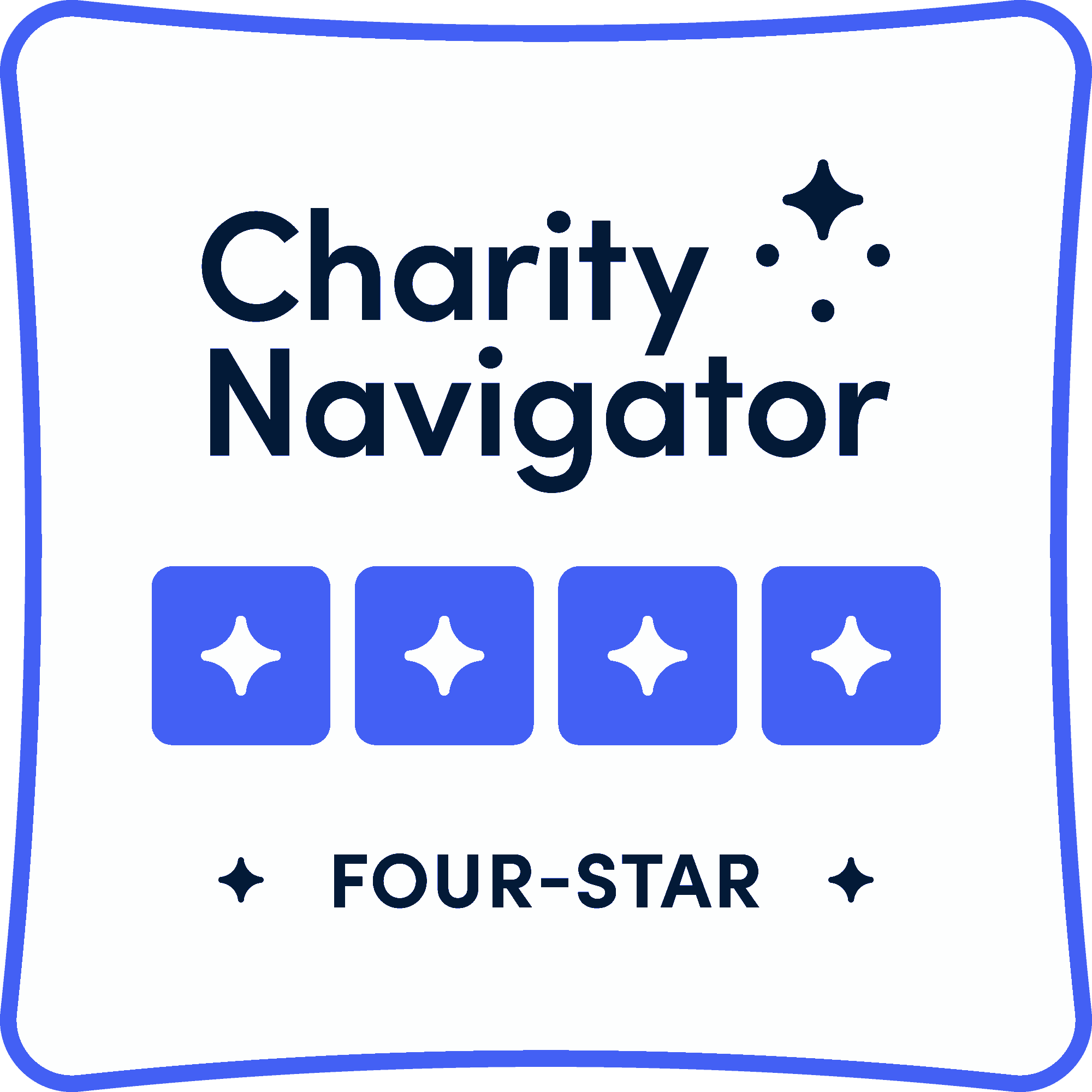
- News & Media
- Get Updates
- Order our Magazine
- Jobs & Internships
- Membership FAQs
- Privacy Policy
- Center for Sustainability Solutions
- Green Business Network
- Green Pages
1612 K Street NW, Suite 1000, Washington DC 20006
Phone: (800) 584-7336 Tax ID/EIN: 52-1660746
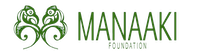
- Terms and Conditions
- Site Credits
Connect With Us
Click through the PLOS taxonomy to find articles in your field.
For more information about PLOS Subject Areas, click here .
Loading metrics
Open Access
Peer-reviewed
Research Article
Research on drinking water purification technologies for household use by reducing total dissolved solids (TDS)
Roles Conceptualization, Data curation, Formal analysis, Investigation, Methodology, Project administration, Resources, Software, Supervision, Validation, Visualization, Writing – original draft, Writing – review & editing
* E-mail: [email protected]
Affiliation Redlands East Valley High School, Redlands, California, United States of America

- Bill B. Wang
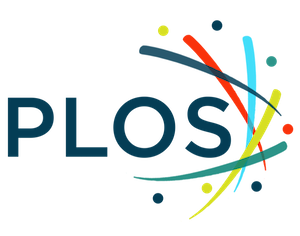
- Published: September 28, 2021
- https://doi.org/10.1371/journal.pone.0257865
- Reader Comments
This study, based in San Bernardino County, Southern California, collected and examined tap water samples within the area to explore the feasibility of adopting non-industrial equipment and methods to reduce water hardness and total dissolved solids(TDS). We investigated how water quality could be improved by utilizing water boiling, activated carbon and sodium bicarbonate additives, as well as electrolysis methods. The results show that heating is effective at lower temperatures rather than long boils, as none of the boiling tests were lower than the original value. Activated carbon is unable to lower TDS, because it is unable to bind to any impurities present in the water. This resulted in an overall TDS increase of 3.5%. However, adding small amounts of sodium bicarbonate(NaHCO 3 ) will further eliminate water hardness by reacting with magnesium ions and improve taste, while increasing the pH. When added to room temperature tap water, there is a continuous increase in TDS of 24.8% at the 30 mg/L mark. The new findings presented in this study showed that electrolysis was the most successful method in eliminating TDS, showing an inverse proportion where an increasing electrical current and duration of electrical lowers more amounts of solids. This method created a maximum decrease in TDS by a maximum of 22.7%, with 3 tests resulting in 15.3–16.6% decreases. Furthermore, when water is heated to a temperature around 50°C (122°F), a decrease in TDS of around 16% was also shown. The reduction of these solids will help lower water hardness and improve the taste of tap water. These results will help direct residents to drink more tap water rather than bottled water with similar taste and health benefits for a cheaper price as well as a reduction on plastic usage.
Citation: Wang BB (2021) Research on drinking water purification technologies for household use by reducing total dissolved solids (TDS). PLoS ONE 16(9): e0257865. https://doi.org/10.1371/journal.pone.0257865
Editor: Mahendra Singh Dhaka, Mohanlal Sukhadia University, INDIA
Received: June 22, 2021; Accepted: September 14, 2021; Published: September 28, 2021
Copyright: © 2021 Bill B. Wang. This is an open access article distributed under the terms of the Creative Commons Attribution License , which permits unrestricted use, distribution, and reproduction in any medium, provided the original author and source are credited.
Data Availability: All relevant data are within the manuscript and its Supporting Information files.
Funding: The author received no specific funding for this work.
Competing interests: The authors have declared that no competing interests exist.
Introduction
The concentration of total dissolved solids(TDS) present in water is one of the most significant factors in giving water taste and also provides important ions such as calcium, magnesium, potassium, and sodium [ 1 – 3 ]. However, water with high TDS measurements usually indicates contamination by human activities, such as soil and agricultural runoff caused by irrigation, unregulated animal grazing and wildlife impacts, environmentally damaging farming methods such as slash and burn agriculture, and the overuse of nitrate-based fertilizer [ 4 , 5 ], etc. Around tourist areas as well as state parks, these factors will slowly add up over time and influence the water sources nearby [ 5 ]. Water that flows through natural springs and waterways with high concentrations of organic salts within minerals and rocks, or groundwater that originates from wells with high salt concentration will also result in higher particle measurements [ 6 ].
Water sources can be contaminated by substances and ions such as nitrate, lead, arsenic, and copper [ 7 , 8 ] and may cause many health problems related to heavy metal consumption and poisoning. Water reservoirs and treatments plants that do not consider water contamination by motor vehicles, as well as locations that struggle to provide the necessary components required for water treatment will be more prone to indirect contamination [ 9 – 11 ]. Many plants are effective in ensuring the quality and reduction of these contaminants, but often leave out the secondary considerations, The United States Environmental Protection Agency(US EPA)’s secondary regulations recommend that TDS should be below 500 mg/L [ 2 ], which is also supported by the World Health Organization(WHO) recommendation of below 600 mg/L and an absolute maximum of less than 1,000 mg/L [ 3 ]. These substances also form calcium or magnesium scales within water boilers, heaters, and pipes, causing excess buildup and drain problems, and nitrate ions may pose a risk to human health by risking the formation of N -nitroso compounds(NOC) and less public knowledge about such substances [ 12 – 15 ]. Nitrates can pose a non-carcinogenic threat to different communities, but continue to slip past water treatment standards [ 15 ]. Furthermore, most people do not tolerate or prefer water with high hardness or chlorine additives [ 16 ], as the taste changes tremendously and becomes unpreferable. Even so, TDS levels are not accounted for in mandatory water regulations, because the essential removal of harmful toxins and heavy metals is what matters the most in water safety. Some companies indicate risks in certain ions and alkali metals, showing how water hardness is mostly disregarded and is not as well treated as commercial water bottling companies [ 17 , 18 ].
In Southern California, water quality is not as well maintained than the northern counties as most treatment plants in violation of a regulation or standard are located in Central-Southern California [ 19 ], with southern counties having the largest number of people affected [ 20 ]. This study is focused on the Redlands area, which has had no state code violations within the last decade [ 21 ]. A previous study has analyzed TDS concentrations throughout the Santa Ana Basin, and found concentrations ranging from 190–600 ppm as treated wastewater and samples obtained from mountain sites, taking into account the urban runoff and untreated groundwater as reasons for elevated levels of TDS but providing no solution in helping reduce TDS [ 22 ]. Also, samples have not been taken directly through home water supplies, where the consumer is most affected. Other water quality studies in this region have been focused on the elimination of perchlorates in soil and groundwater and distribution of nitrates, but such research on chemicals have ceased for the last decade, demonstrated by safe levels of perchlorates and nitrates in water reports [ 23 , 24 ]. In addition to these studies, despite the improving quality of the local water treatment process, people prefer bottled water instead of tap water because of the taste and hardness of tap water [ 25 ]. Although water quality tests are taken and documented regularly, the taste of the water is not a factor to be accounted for in city water supplies, and neither is the residue left behind after boiling water. The residue can build up over time and cause appliance damage or clogs in drainage pipes.
This study will build upon previous analyses of TDS studies and attempt to raise new solutions to help develop a more efficient method in reducing local TDS levels, as well as compare current measurements to previous analyses to determine the magnitude to which local treatment plants have improved and regulated its treatment processes.
Several methods that lower TDS are reviewed: boiling and heating tap water with and without NaHCO₃, absorption by food-grade activated carbon [ 26 , 27 ], and battery-powered electrolysis [ 28 – 30 ]. By obtaining water samples and determining the difference in TDS before and after the listed experiments, we can determine the effectiveness of lowering TDS. The results of this study will provide options for residents and water treatment plants to find ways to maintain the general taste of the tap water, but also preserve the lifespan of accessories and pipelines. By determining a better way to lower TDS and treat water hardness, water standards can be updated to include TDS levels as a mandatory measurement.
Materials and methods
All experiments utilized tap water sourced from Redlands homes. This water is partially supplied from the Mill Creek (Henry Tate) and Santa Ana (Hinckley) Water Sheds/Treatment Plants, as well as local groundwater pumps. Water sampling and sourcing were done at relatively stable temperatures of 26.9°C (80.42°F) through tap water supplies. The average TDS was measured at 159 ppm, which is slightly lower than the reported 175 ppm by the City of Redlands. Permission is obtained by the author from the San Bernardino Municipal Water Department website to permit the testing procedures and the usage of private water treatment devices for the purpose of lowering water hardness and improving taste and odor. The turbidity was reported as 0.03 Nephelometric Turbidity Units (NTU) post-treatment. Residual nitrate measured at 2.3mg/L in groundwater before treatment and 0.2 mg/L after treatment and perchlorate measured at 0.9 μg/L before treatment, barely staying below the standard of 1 μg/L; it was not detected within post-treatment water. Lead content was not detected at all, while copper was detected at 0.15 mg/L.
For each test, all procedures were done indoors under controlled temperatures, and 20 L of fresh water was retrieved before each test. Water samples were taken before each experimental set and measured for TDS and temperature, and all equipment were cleaned thoroughly with purified water before and after each measurement. TDS consists of inorganic salts and organic material present in solution, and consists mostly of calcium, magnesium, sodium, potassium, carbonate, chloride, nitrate, and sulfate ions. These ions can be drawn out by leaving the water to settle, or binding to added ions and purified by directly separating the water and ions. Equipment include a 50 L container, 1 L beakers for water, a graduated cylinder, a stir rod, a measuring spoon, tweezers, a scale, purified water, and a TDS meter. A standard TDS meter is used, operated by measuring the conductivity of the total amount of ionized solids in the water, and is also cleaned in the same manner as aforementioned equipment. The instrument is also calibrated by 3 pH solutions prior to testing.All results were recorded for and then compiled for graphing and analysis.
Heating/Boiling water for various lengths of time
The heating method was selected because heat is able to break down calcium bicarbonate into calcium carbonate ions that are able to settle to the bottom of the sample. Four flasks of 1 L of tap water were each heated to 40°C, 50°C, 60°C, and 80°C (104–176°F) and observed using a laser thermometer. The heated water was then left to cool and measurements were made using a TDS meter at the 5, 10, 20, 30, and 60-minute marks.
For the boiling experiments, five flasks of 1 L of tap water were heated to boil at 100°C (212°F). Each flask, which was labeled corresponding to its boiling duration, was marked with 2, 4, 6, 10, and 20 minutes. Each flask was boiled for its designated time, left to cool under open air, and measurements were made using a TDS meter at the 5, 10, 20, 30, 60, and 120-minute marks. The reason that the boiling experiment was extended to 120 minutes was to allow the water to cool down to room temperature.
Activated carbon as a water purification additive
This test was performed to see if food-grade, powdered activated carbon had any possibility of binding with and settling out residual particles. Activated carbon was measured using a milligram scale and separated into batches of 1, 2, 4, 5, 10, 30, and 50 mg. Each batch of the activated carbon were added to a separate flask of water and stirred for five minutes, and finally left to settle for another five minutes. TDS measurements were recorded after the water settled.
Baking soda as a water purification additive
To lower scale error and increase experimental accuracy, a concentration of 200 mg/L NaHCO₃ solution was made with purified water and pure NaHCO₃. For each part, an initial TDS measurement was taken before each experiment.
In separate flasks of 1 L tap water, each labeled 1, 2, 4, 5, 10, and 30 mg of NaHCO 3 , a batch was added to each flask appropriately and stirred for 5 minutes to ensure that everything dissolved. Measurements were taken after the water was left to settle for another 5 minutes for any TDS to settle.
Next, 6 flasks of 1 L tap water were labeled, with 5 mg (25 mL solution) of NaHCO₃ added to three flasks and 10 mg (50 mL solution) of NaHCO₃ added to the remaining three. One flask from each concentration of NaHCO₃ was boiled for 2 mins., 4 mins., or 6 mins., and then left to cool. A TDS measurement was taken at the 5, 10, 20, 30, 60, and 120-minute marks after removal from heat.
Electrolysis under low voltages
This test was performed because the ionization of the TDS could be manipulated with electricity to isolate an area of water with lower TDS. For this test, two 10cm long graphite pieces were connected via copper wiring to a group of batteries, with each end of the graphite pieces submerged in a beaker of tap water, ~3 cm apart.
Using groups of 1.5 V double-A batteries, 4 beakers with 40mL of tap water were each treated with either 7.5, 9.0, 10.5, and 12.0 V of current. Electrolysis was observed to be present by the bubbling of the water each test, and measurements were taken at the 3, 5, 7, and 10 minute marks.
Results/Discussion
Heating water to various temperatures until the boiling point.
The goal for this test was to use heat to reduce the amount of dissolved oxygen and carbon dioxide within the water, as shown by this chemical equation: Heat: Ca(HCO 3 ) 2 → CaCO 3 ↓ + H 2 O + CO 2 ↑.
This would decompose ions of calcium bicarbonate down into calcium carbonate and water and carbon dioxide byproducts.
Patterns and trends in decreasing temperatures.
The following trend lines are based on a dataset of changes in temperature obtained from the test results and graphed as Fig 1 .
- PPT PowerPoint slide
- PNG larger image
- TIFF original image
https://doi.org/10.1371/journal.pone.0257865.g001
To predict the precise temperature measurements of the tap water at 26.9°C, calculations were made based on Fig 1 . The fitting equations are in the format, y = a.e bx . The values for the fitting coefficients a and b, and correlation coefficient R 2 are listed in Table 1 as column a, b and R 2 . The calculated values and the target temperature are listed in Table 1 .
https://doi.org/10.1371/journal.pone.0257865.t001
Fig 2 was obtained by compiling TDS results with different temperatures and times.
https://doi.org/10.1371/journal.pone.0257865.g002
The fitting equations for Fig 2 are also in the format, y = a.e bx . The fitting coefficients a and b, and correlation coefficient R 2 values are listed in Table 2 . Based on the fitting curves in Fig 2 and the duration to the target temperature in Table 1 , We calculated the TDS at 26.9°C as listed in column calculated TDS in Table 2 based on the values we reported on Fig 2 .
https://doi.org/10.1371/journal.pone.0257865.t002
Based on the heating temperature and the calculated TDS with the same target water temperature, we obtained the following heating temperature vs TDS removal trend line and its corresponding fitting curve in Table 2 .
In Fig 1 , a trend in the rate of cooling is seen, where a higher heating temperature creates a steeper curve. During the first five minutes of cooling, the water cools quicker as the absorbed heat is quickly released into the surrounding environment. By the 10-minute mark, the water begins to cool in a linear rate of change. One detail to note is that the 100°C water cools quicker than the 80°C and eventually cools even faster than the 60°C graph. Table 1 supports this observation as the duration to target temperature begins to decrease from a maximum point of 94.8 mins to 80.95 mins after the 80°C mark.
As shown in Fig 2 , all TDS values decrease as the temperature starts to cool to room temperature, demonstrating a proportional relationship where a lower temperature shows lower TDS. This can partially be explained by the ions settling in the flasks. Visible particles can also be observed during experimentation as small white masses on the bottom, as well as a thin ring that forms where the edge of the water contacts the flask. When the water is heated to 40°C and cooled, a 3.8% decrease in TDS is observed. When 50°C is reached, the TDS drops at its fastest rate from an initial value of 202 ppm to 160 ppm after 60 minutes of settling and cooling. The TDS measurements in these experiements reach a maximum of 204 ppm at the 60°C mark. However, an interesting phenomenon to point out is that the water does not hit a new maximum at 100°C. meaning that TDS reaches a plateau at 60°C. Also, the rate of decrease begins to slow down after 20 minutes, showing that an unknown factor is affecting the rate of decrease. It is also hypothesized that the slight increase in TDS between the 5–20 minute range is caused by a disturbance in the settling of the water, where the temperature starts to decrease at a more gradual and constant rate. The unstable and easy formation of CaCO 3 scaling has also been the subject of a study of antiscaling methods, which also supports the result that temperature is a significant influence for scale formation [ 12 ].
In Table 2 , calculations for TDS and the time it takes for each test to cool were made. Using the data, it is determined that the test with 50°C water decreased the most by 16% from the initial measurement of 159ppm. This means that it is most effective when water is heated between temperatures of 40–60°C when it comes to lowering TDS, with a difference of ~7–16%. When water is heated to temperatures greater than 80°C, the water begins to evaporate, increasing the concentration of the ions, causing the TDS to increase substantially when cooled to room temperature.
Finally, in Fig 3 , a line of best fit of function f(x) = -0.0007x 3 + 0.1641x 2 –10.962x + 369.36 is used with R 2 = 0.9341. Using this function, the local minimum of the graph would be reached at 48.4°C.
https://doi.org/10.1371/journal.pone.0257865.g003
This data shows that heating water at low temperatures (i.e. 40–50°C) may be more beneficial than heating water to higher temperatures. This study segment has not been presented in any section within the United States EPA Report on water management for different residual particles/substances. However, warmer water temperatures are more prone to microorganism growth and algal blooms, requiring more intensive treatment in other areas such as chlorine, ozone, and ultraviolet disinfection.
Using the specific heat capacity equation, we can also determine the amount of energy and voltage needed to heat 1 L of water up to 50°C: Q = mcΔT, where c, the specific heat capacity of water, is 4.186 J/g°C, ΔT, the change in temperature from the experimental maximum to room temperature, is 30°C, and m, the mass of the water, is 1000 g. This means that the amount of energy required will be 125580 J, which is 0.035 kWh or 2.1 kW.
After taking all of the different measurements obtained during TDS testing, and compiling the data onto this plot, Fig 4 is created with a corresponding line of best fit:
https://doi.org/10.1371/journal.pone.0257865.g004
In Fig 4 , it can be observed that the relationship between the temperature of the water and its relative TDS value is a downwards facing parabolic graph. As the temperature increases, the TDS begins to decrease after the steep incline at 50–60°C. The line of best fit is represented by the function f(x) = -0.0142x 2 + 2.258x + 105.84. R 2 = 0.6781. Because the R 2 value is less than expected, factors such as the time spent settling and the reaction rate of the ions should be considered. To determine the specifics within this experiment, deeper research and prolonged studies with more highly accurate analyses must be utilized to solve this problem.
Boiling water for various amounts of time
Trend of boiling duration and rate of cooling..
Using the same methods to create the figures and tables for the previous section, Fig 5 depicts how the duration of time spent boiling water affects how fast the water cools.
https://doi.org/10.1371/journal.pone.0257865.g005
As seen in Fig 5 , within the first 10 minutes of the cooling time, the five different graphs are entwined with each other, with all lines following a similar pattern. However, the graph showing 20 minutes of boiling is much steeper than the other graphs, showing a faster rate of cooling. This data continues to support a previous claim in Fig 2 , as this is most likely represented by a relationship a longer the boil creates a faster cooling curve. This also shows that the first 5 minutes of cooling have the largest deviance compared to any other time frame.
The cooling pattern is hypothesized by possible changes in the orderly structure of the hydrogen bonds in the water molecules, or the decreased heat capacity of water due to the increasing concentration of TDS.
Effect on TDS as boiling duration increases.
In Fig 6 , all lines except for the 20-minute line are clustered in the bottom area of the graph. By excluding the last measurement temporarily due to it being an outlier, we have observed that the difference between the initial and final TDS value of each test decreases.
https://doi.org/10.1371/journal.pone.0257865.g006
Despite following a similar trend of an increase in TDS at the start of the tests and a slow decrease overtime, this experiment had an interesting result, with the final test measuring nearly twice the amount of particles compared to any previous tests at 310 ppm, as shown in Fig 6 . It is confirmed that the long boiling time caused a significant amount of water to evaporate, causing the minerals to be more concentrated, thus resulting in a 300 ppm reading. Fig 6 follows the same trend as Fig 2 , except the TDS reading veers away when the boiling duration reaches 20 minutes. Also, with the long duration of heating, the water has developed an unfavorable taste from intense concentrations of CaCO₃. This also causes a buildup of a thin crust of CaCO₃ and other impurities around the container that is difficult to remove entirely. This finding is in accordance with the introductory statement of hot boiling water causing mineral buildups within pipes and appliances [ 9 ]. A TDS reading of 300ppm is still well below federal secondary standards of TDS, and can still even be compared to bottled water, in which companies may fluctuate and contain 335ppm within their water [ 1 , 2 ].
This experiment continues to stupport that the cooling rate of the water increases as the time spent boiling increases. Based on this test, a prediction can be made in which an increased concentration of dissolved solids lowers the total specific heat capacity of the sample, as the total volume of water decreases. This means that a method can be derived to measure TDS using the heat capacity of a tap water mixture and volume, in addition to current methods of using the electrical conductivity of aqueous ions.
Adding food-grade activated carbon to untreated tap water
Fig 7 presents a line graph with little to no change in TDS, with an initial spike from 157 to 163 ppm. The insoluble carbon remains in the water and shows no benefit.
https://doi.org/10.1371/journal.pone.0257865.g007
The food-grade activated carbon proved no benefit to removing TDS from tap water, and instead added around 5–7 ppm extra, which settled down to around +4 ppm at 120 minutes. The carbon, which is not 100% pure from inorganic compounds and materials present in the carbon, can dissolve into the water, adding to the existing concentration of TDS. Furthermore, household tap water has already been treated in processing facilities using a variety of filters, including carbon, so household charcoal filters are not effective in further reducing dissolved solids [ 18 ].
Adding sodium bicarbonate solution to boiled tap water
As seen in Fig 8 , after adding 1 mg of NaHCO 3 in, the TDS rises to 161 ppm, showing a minuscule increase. When 4 mg was added, the TDS drops down to 158 ppm. Then, when 5 mg was added, a sudden spike to 172 ppm was observed. This means that NaHCO 3 is able to ionize some Ca 2+ and Mg 2+ ions, but also adds Na + back into the water. This also means that adding NaHCO 3 has little to no effect on TDS, with 4mg being the upper limit of effectiveness.
https://doi.org/10.1371/journal.pone.0257865.g008
To examine whether or not the temperature plays a role in the effectiveness in adding NaHCO 3 , a boiling experiment was performed, and the data is graphed in Fig 9 .
https://doi.org/10.1371/journal.pone.0257865.g009
Fig 9 presents the relationship between the amount of common baking soda(NaHCO₃) added, the boiling time involved, and the resulting TDS measurements. After boiling each flask for designated amounts of time, the results showed a downward trend line from a spike but does not reach a TDS value significantly lower than the initial sample. It is apparent that the NaHCO₃ has not lowered the TDS of the boiling water, but instead adds smaller quantities of ions, raising the final value. This additive does not contribute to the lowering of the hardness of the tap water. However, tests boiled with 5 mg/L of baking soda maintained a downward pattern as the water was boiled for an increasing amount of time, compared to the seemingly random graphs of boiling with 10 mg/L.
In some households, however, people often add NaHCO₃ to increase the pH for taste and health benefits. However, as shown in the test results, it is not an effective way of reducing TDS levels in the water [ 10 , 16 ], but instead raises the pH, determined by the concentration added. Even under boiling conditions, the water continues to follow the trend of high growth in TDS, of +25–43 ppm right after boiling and the slow drop in TDS (but maintaining a high concentration) as the particles settle to the bottom.
Utilizing the experimental results, we can summarize that after adding small batches of NaHCO3 and waiting up to 5 minutes will reduce water hardness making it less prone to crystallizing within household appliances such as water brewers. Also, this process raises the pH, which is used more within commercial water companies. However, the cost comes at increasing TDS.
Using electrolysis to treat TDS in tap water
Different voltages were passed through the water to observe the change in TDS overtime, with the data being compiled as Fig 10 .
https://doi.org/10.1371/journal.pone.0257865.g010
The process of electrolysis in this experiment was not to and directly remove the existing TDS, but to separate the water sample into three different areas: the anode, cathode, and an area of clean water between the two nodes [ 19 ]. The anions in the water such as OH - , SO 4 2- , HCO 3 - move to the anode, while the cations such as H + , Ca 2+ 、Mg 2+ 、Na + move to the cathode. The middle area would then be left as an area that is more deprived of such ions, with Fig 10 proving this.
As shown in Fig 10 , electrolysis is effective in lower the TDS within tap water. Despite the lines being extremely tangled and unpredictable, the general trend was a larger decrease with a longer duration of time. At 10 minutes, all lines except 10.5 V are approaching the same value, meaning that the deviation was most likely caused by disturbances to the water during measurement from the low volume of water. With each different voltage test, a decrease of 12.7% for 6.0 V, 14.9% for 9.0 V, 22.7% for 10.5 V. and 19.5% for 12.0 V respectfully were observed. In the treatment of wastewate leachate, a study has shown that with 90 minutes of electrical treatment, 34.58% of TDS content were removed, supporting the effectiveness of electricity and its usage in wastewater treatment [ 29 ].
This experiment concludes that electrolysis is effective in lowering TDS, with the possibility to improve this process by further experimentation, development of a water cleaning system utilizing this cathode-anode setup to process water. This system would be a more specific and limited version of a reverse osmosis system by taking away ions through attraction, rather than a filter.
The Southern Californian tap water supply maintains TDS values below the federal regulations. However, crystalline scale buildup in household appliances is a major issue as it is hard to clean and eliminate. To easily improve the taste and quality of tap water at home as well as eliminating the formation of scales, the following methods were demonstrated as viable:
- By heating water to around 50°C (122°F), TDS and water hardness will decrease the most. Also, the boiling process is effective in killing microorganisms and removing contaminants. This process cannot surpass 10 minutes, as the concentration of the ions in the water is too high, which poses human health risks if consumed. These, along with activated carbon and NaHCO₃ additives, are inefficient methods that have minimal effects for lowering TDS.
- Electrolysis is one of the most effective methods of eliminating TDS. Experiments have proven that increased current and duration of time helps lower TDS. However, this method has yet to be implemented into conventional commercial water filtration systems.
Also, some observations made in these experiments could not be explained, and require further research and experimentation to resolve these problems. The first observation is that TDS and increasing water temperature maintain a parabolic relationship, with a maximum being reached at 80°C, followed by a gradual decrease. The second observation is that when water is boiled for an increased duration of time, the rate of cooling also increases.
This experiment utilized non-professional scientific equipment which are prone to mistakes and less precise. These results may deviate from professionally derived data, and will require further study using more advanced equipment to support these findings.
Ways you can filter out harmful PFAS from drinking water at home
by Kyle Doudrick, The Conversation
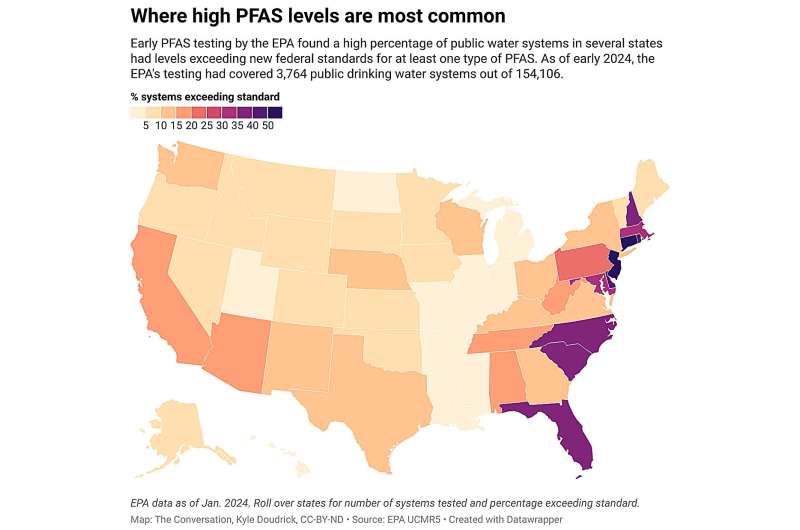
Chemists invented PFAS in the 1930s to make life easier: Nonstick pans, waterproof clothing, grease-resistant food packaging and stain-resistant carpet were all made possible by PFAS. But in recent years, the growing number of health risks found to be connected to these chemicals has become increasingly alarming.
PFAS—perfluoroalkyl and polyfluoroalkyl substances—are now either suspected or known to contribute to thyroid disease, elevated cholesterol, liver damage and cancer , among other health issues .
They can be found in the blood of most Americans and in many drinking water systems , which is why the Environmental Protection Agency in April 2024 finalized the first enforceable federal limits for six types of PFAS in drinking water systems. The limits—between 4 and 10 parts per trillion for PFOS, PFOA, PFHxS, PFNA and GenX—are less than a drop of water in a thousand Olympic-sized swimming pools, which speaks to the chemicals' toxicity. The sixth type, PFBS, is regulated as a mixture using what's known as a hazard index .
Meeting these new limits won't be easy or cheap. And there's another problem: While PFAS can be filtered out of water, these "forever chemicals" are hard to destroy.
My team at the University of Notre Dame works on solving problems involving contaminants in water systems, including PFAS. We explore new technologies to remove PFAS from drinking water and to handle the PFAS waste . Here's a glimpse of the magnitude of the challenge and ways you can reduce PFAS in your own drinking water:
Removing PFAS will cost billions per year
Every five years, the EPA is required to choose 30 unregulated contaminants to monitor in public drinking water systems. Right now, 29 of those 30 contaminants are PFAS. The tests provide a sense of just how widespread PFAS are in water systems and where.
The EPA has taken over 22,500 samples from about 3,800 of the 154,000 public drinking water systems in the U.S. In 22% of those water systems, its testing found at least one of the six newly regulated PFAS, and about 16% of the systems exceeded the new standards. East Coast states had the largest percentage of systems with PFAS levels exceeding the new standards in EPA tests conducted so far.
Under the new EPA rules, public water systems have until 2027 to complete monitoring for PFAS and provide publicly available data. If they find PFAS at concentrations that exceed the new limits, then they must install a treatment system by 2029.
How much that will cost public water systems, and ultimately their customers, is still a big unknown, but it won't be cheap.
The EPA estimated the cost to the nation's public drinking water systems to comply with the news rules at about US$1.5 billion per year. But other estimates suggest the total costs of testing and cleaning up PFAS contamination will be much higher. The American Water Works Association put the cost at over $3.8 billion per year for PFOS and PFOA alone.
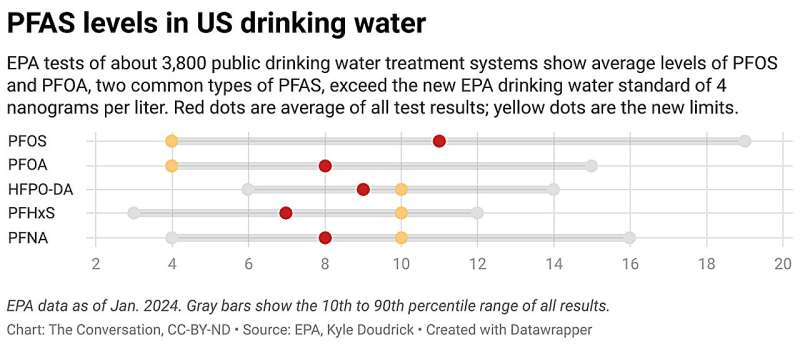
There are more than 5,000 chemicals that are considered PFAS, yet only a few have been studied for their toxicity, and even fewer tested for in drinking water. The United States Geological Survey estimates that nearly half of all tap water is contaminated with PFAS.
Some money for testing and cleanup will come from the federal government . Other funds will come from 3M and DuPont, the leading makers of PFAS. 3M agreed in a settlement to pay between $10.5 billion to $12.5 billion to help reimburse public water systems for some of their PFAS testing and treatment. But public water systems will still bear additional costs, and those costs will be passed on to residents.
Next problem: Disposing of 'forever chemicals'
Another big question is how to dispose of the captured PFAS once they have been filtered out.
Landfills are being considered, but that just pushes the problem to the next generation. PFAS are known as "forever chemicals" for a reason—they are incredibly resilient and don't break down naturally, so they are hard to destroy.
Studies have shown that PFAS can be broken down with energy-intensive technologies. But this comes with steep costs. Incinerators must reach over 1,800 degrees Fahrenheit (1,000 Celsius) to destroy PFAS, and the possibility of creating potentially harmful byproducts is not yet well understood . Other suggested techniques, such as supercritical water oxidation or plasma reactors , have the same drawbacks.
So who is responsible for managing that PFAS waste? Ultimately the responsibility will likely fall on public drinking water systems.
The EPA on April 19, 2024, designated PFOA and PFOS as eligible contaminants for Superfund status , which means companies that are responsible for contaminating sites with those chemicals can be required to pay for cleanup. However, the EPA said it did not intend to go after wastewater treatment plants or public landfills.
Steps to protect your home from PFAS
Your first instinct might be to use bottled water to try to avoid PFAS exposures, but a recent study found that even bottled water can contain these chemicals. And bottled water is regulated by a different federal agency, the Food and Drug Administration, which has no standards for PFAS.
Your best option is to rely on the same technologies that treatment facilities will be using:
Activated carbon is similar to charcoal. Like a sponge, it will capture the PFAS, removing it from the water. This is the same technology in refrigerator filters and in some water pitcher filters, like Brita or PUR. Note that many refrigerator manufacture's filters are not certified for PFAS, so don't assume they will remove PFAS to safe levels.
Ion exchange resin is the same technology found in many home water softeners. Like activated carbon, it captures PFAS from the water, and you can find this technology in many pitcher filter products. If you opt for a whole house treatment system, which a plumber can attach where the water enters the house, ion exchange resin is probably the best choice. But it is expensive.
Reverse osmosis is a membrane technology that only allows water and select compounds to pass through the membrane, while PFAS are blocked. This is commonly installed at the kitchen sink and has been found to be very effective at removing most PFAS in water. It is not practical for whole house treatment, but it is likely to remove a lot of other contaminants as well.
If you have a private well instead of a public drinking water system, that doesn't mean you're safe from PFAS exposure. Wisconsin's Department of Natural Resources estimates that 71% of shallow private wells in that state have some level of PFAS contamination. Using a certified laboratory to test well water for PFAS can run $300-$600 per sample, a cost barrier that will leave many private well owners in the dark.
For all the treatment options, make sure the device you choose is certified for PFAS by a reputable testing agency , and follow the recommended schedule for maintenance and filter replacement. Unfortunately, there is currently no safe way to dispose of the filters, so they go in the trash. No treatment option is perfect, and none is likely to remove all PFAS down to safe levels, but some treatment is better than none.
Explore further
Feedback to editors

Gene linked to epilepsy and autism decoded in new study
9 hours ago

Blood test finds knee osteoarthritis up to eight years before it appears on X-rays

Researchers find pregnancy cytokine levels impact fetal brain development and offspring behavior

Study finds biomarkers for psychiatric symptoms in patients with rare genetic condition 22q
10 hours ago

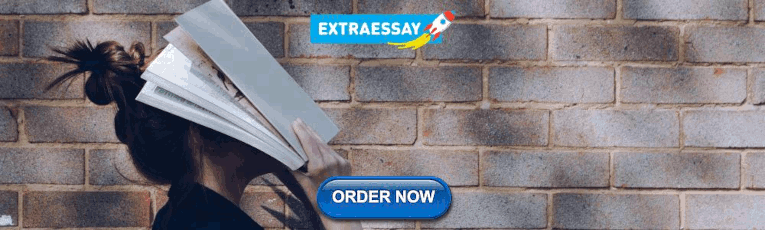
Clinical trial evaluates azithromycin for preventing chronic lung disease in premature babies
11 hours ago

Scientists report that new gene therapy slows down amyotrophic lateral sclerosis disease progression

Using stem cell-derived heart muscle cells to advance heart regenerative therapy

Analysis identifies 50 new genomic regions associated with kidney cancer risk
12 hours ago

Illusion demystifies the way vision works: Experiments imply brightness perception occurs deeper in brain than thought

How buildings influence the microbiome and human health
Related stories.

EPA sets strict limit on PFAS 'forever chemicals' in US drinking water
Apr 10, 2024

Q&A: What the EPA limits on 'forever chemicals' in water mean
Apr 16, 2024

'Forever chemicals' in German drinking water: A hidden threat unveiled
Jan 23, 2024

US says two 'forever chemicals' are hazardous, tells polluters to pay
Apr 19, 2024

US announces tough tap water standards for 'forever chemicals'

'PFAS' no longer sold in US to package greasy food: FDA
Feb 29, 2024
Recommended for you

Homelessness found to be a major issue for many patients in the emergency department

Living at higher altitudes in India linked to increased risk of childhood stunting
Apr 25, 2024

Study reveals racial disparities in COVID-19 testing delays among health care workers

Premature mortality higher among sexual minority women, study finds

Study finds X's (formerly Twitter's) community notes provide accurate, credible answers to vaccine misinformation
Apr 24, 2024
Let us know if there is a problem with our content
Use this form if you have come across a typo, inaccuracy or would like to send an edit request for the content on this page. For general inquiries, please use our contact form . For general feedback, use the public comments section below (please adhere to guidelines ).
Please select the most appropriate category to facilitate processing of your request
Thank you for taking time to provide your feedback to the editors.
Your feedback is important to us. However, we do not guarantee individual replies due to the high volume of messages.
E-mail the story
Your email address is used only to let the recipient know who sent the email. Neither your address nor the recipient's address will be used for any other purpose. The information you enter will appear in your e-mail message and is not retained by Medical Xpress in any form.
Newsletter sign up
Get weekly and/or daily updates delivered to your inbox. You can unsubscribe at any time and we'll never share your details to third parties.
More information Privacy policy
Donate and enjoy an ad-free experience
We keep our content available to everyone. Consider supporting Science X's mission by getting a premium account.
E-mail newsletter
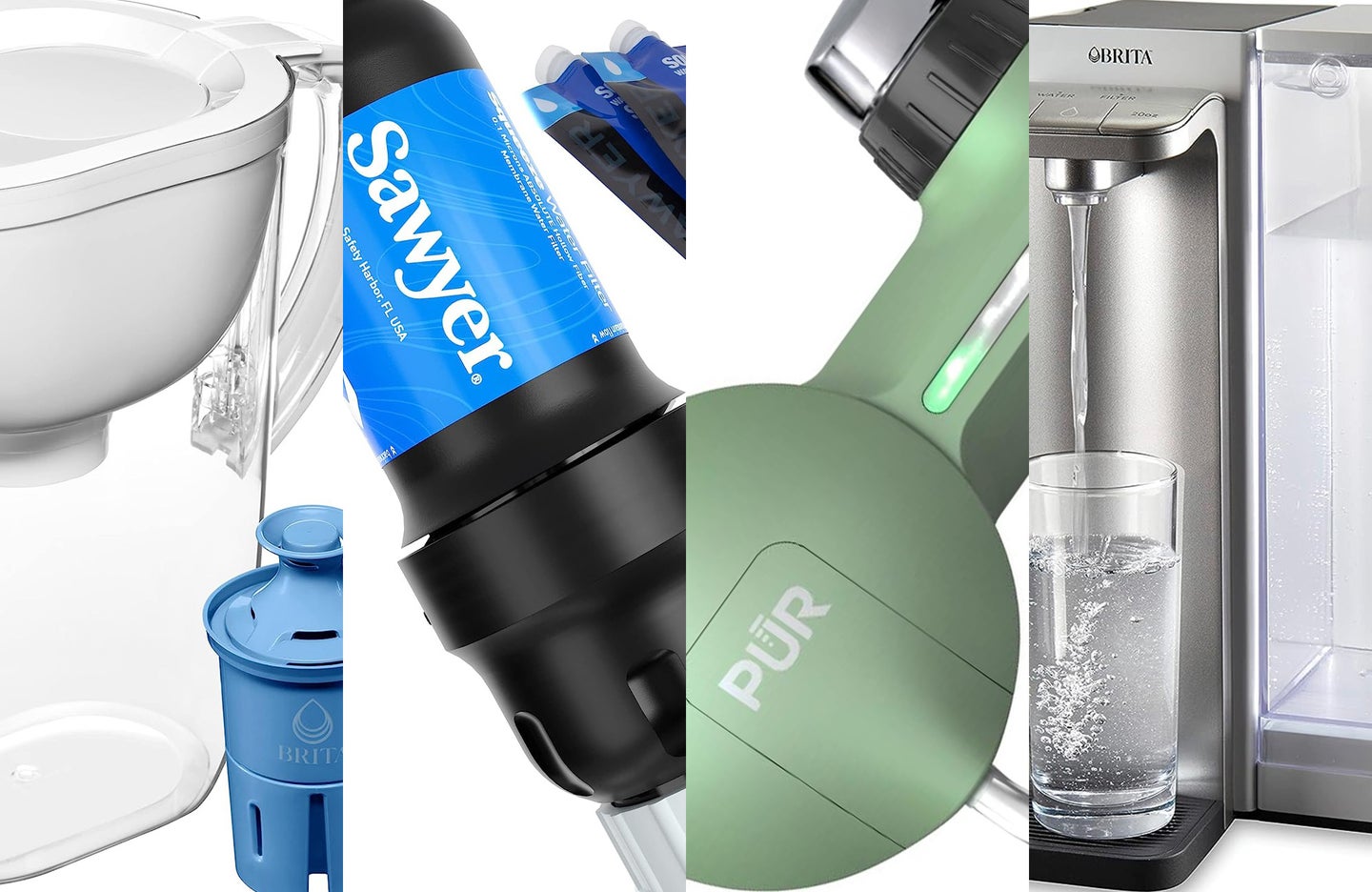
The best water filters of 2024
We filtered through the best options for clean drinking water on the go and at home.
We may earn revenue from the products available on this page and participate in affiliate programs. Learn more ›
Water filters may seem like devices that mostly come up when people talk about travels to the backcountry or other countries, but they can be important tools everywhere. Tap water goes through a fantastic journey from rivers and reservoirs through pipes of many sizes to flow through your faucet. Drinking water in the U.S. from public water systems is among the safest in the world, but many households opt for additional filtration. Filtering often focuses on removing funky tastes, smells, and sediment, though many filtration systems also reduce other potentially harmful chemicals. We dove into specifications and certifications of small portable systems up to countertop water dispensers to surface the best water filters for a glass of fresh-tasting H2O.
- Best overall: Brita Large Water Filter Pitcher
- Best pitcher: Brita Large Stream Filter as You Pour
- Best faucet: PUR PLUS Faucet Mount Water Filtration System
- Best water bottle: LifeStraw Go Series
- Best backpacking: Sawyer Products SP129 Squeeze Water Filtration System
- Best countertop: Brita Hub
- Best budget: PUR PLUS 30-Cup Dispenser
How we chose the best water filters
Water filters come in as many sizes and shapes as you can imagine. However, we winnowed our list of portable and affordable options for households looking to improve their water taste. We kept our renting readers in mind, so homeowners may want to explore permanently installed options like under-sink water filters or whole-house water filters . We’re not a lab, so we opted for products that meet or are certified to standards set by NSF International/American National Standards Institute and the Water Quality Association .
The best water filters: Reviews & Recommendations
The type of water filter that’s best for you depends on how much you want to treat at a time, how long that takes, what you’re treating it for, and how often you’re replacing filters. Are you looking to remove basics like chlorine and sediment? Is a lead a concern? Different filters target a range of contaminants, such as chlorine, particulates, lead, heavy metals, microbes, bacteria, viruses, or PFAS , more commonly known as “forever chemicals.” Some even filter out microplastics , which is a growing concern for many.
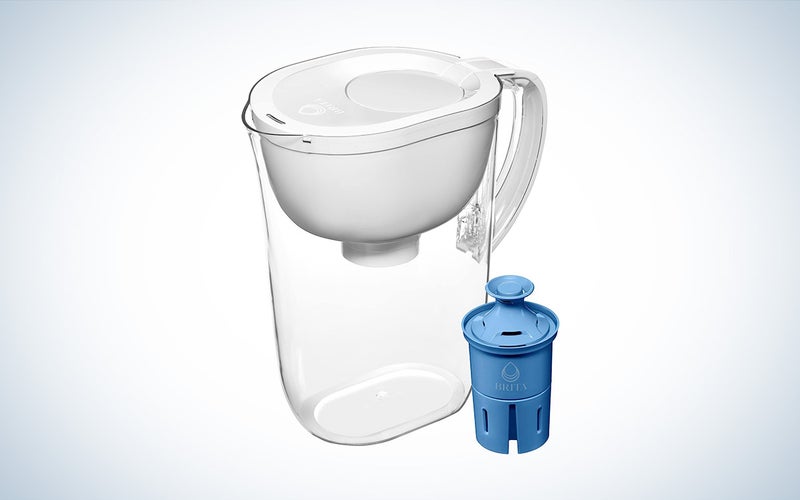
- Dimensions: 10.7 x 5.4 x 10.1 inches
- Weight: 1.3 pounds
- Capacity: 10 cups
- Filter: Brita Elite filters (proprietary active filtering agents)
- Filter lifespan: Replace after 120 gallons or about 6 months
- Inexpensive
- Flip top for easy refilling
- Long-lasting filter
- Electronic filter indicator
- BPA-free container
- Certified NSF/ANSI Standards 42, 53, and 401
- Slow filtering
- Frequent refills for thirsty/large households
Our pick for the best overall water filter is an update on a classic. The Brita Large Water Filter Pitcher is the best for most people because of its low cost (about $35), ease of use, and improved filter. Most of us have likely used a Brita pitcher at one point. This model makes some notable design improvements. Instead of removing the whole lid to refill, simply pop open the flip-top.
The spout features a color-coded system to indicate when to replace a filter. Green is good, yellow indicates replacement is coming up, and red shows it’s time to change. The rest of the form is familiar: The tap water goes into an upper tank, and clean water slowly filters into the bottom reservoir. This model boasts a 10-cup capacity, but households that fill many water bottles daily may find themselves refilling—and waiting—a lot.
The essential improvement in this model is the Brita Elite filter, which catches more contaminants than the standard model and lasts three times longer. You only need to replace the filter once every six months instead of every two, minimizing cost and trash. The filter still results in the crisp, chlorine-free taste Britas are known for and is certified to reduce lead (99 percent), Cadmium, Mercury, Benzene, Asbestos, and more. Many reviewers note that the Elite does have clogging issues, so you may find yourself changing more frequently than the six-month replacement window. Still, the extra protection over Brita’s standard filters seems worth the extra few bucks.
All pitchers have downsides. They don’t purify water, so always start with potable water—and make sure it’s cold or room-temperature, never hot. They’ll need thorough hand washing regularly to keep clean, but most are made of a brittle plastic that chips, clouds, or cracks with age. But they are abundant, readily available, and affordable. Remember to factor in buying and replacing filters as part of the overall expense.
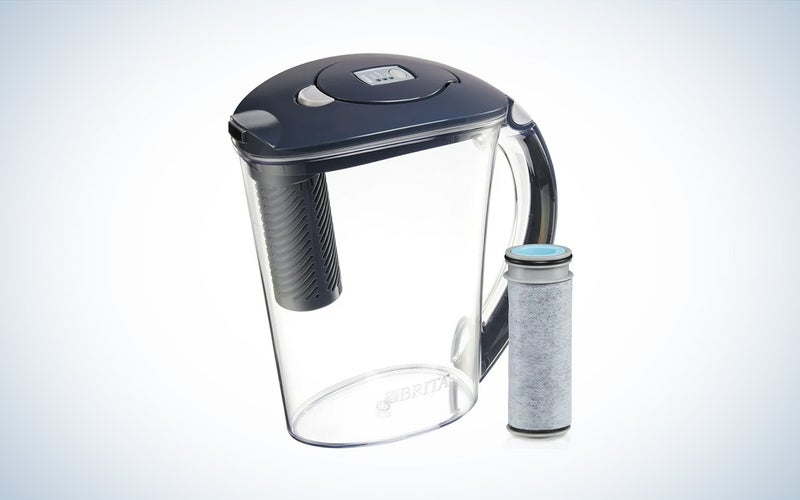
- Dimensions: 10.75 x 5.6 x 10 inches
- Weight: 1.4 pounds
- Filter: Brita Stream filters (activated carbon in a BPA-free housing)
- Filter lifespan: Replace after 40 gallons or about two months
- Low upfront costs
- Filters as you pour
- Certified NSF/ANSI Standards 42 and 53
- Taste/odor-focused filtering
- Frequent filter replacement
Usually, a water filter pitcher has an inner reservoir that you fill, and it slowly drips clean water into the bottom half. You have to wait until gravity does its job before taking a sip. The Brita Large Stream Filter as You Pour bucks that trend. The 10-cup pitcher forces water through an activated carbon filter before pouring out of the spout. Admittedly, waiting a few minutes for filtered water to drip from the reservoir to the rest of the pitcher isn’t the most significant inconvenience—but it is annoying if you’re the person who always seems to grab the pitcher when there’s only half a glass left. (And obviously, we’re often that person.)
This Brita water filter pitcher removes chlorine taste and smell but retains fluoride, minerals, and electrolytes. The filter clicks into a cage that then twists into place on the lid. The lid also houses an electronic indicator to remind you to change filters, which is about every 40 gallons or every two months. Brita Stream replacement filters run about $9 to $10 each, a few bucks more per filter than Brita Standard filters but on par with the longer-lasting Brita Elite filter.

- Dimensions: 6.8 x 2.9 x 5.2 inches
- Capacity: N/A
- Filters: PUR PLUS faucet filters (activated charcoal)
- Filter lifespan: Replace after 100 gallons or 3 months
- Small form factor
- Installs and removes without tools
- Filter change light
- Can switch between filtered/unfiltered water
- May not fit all faucets, especially pull-out or handheld faucets
- Doesn’t filter microbes
Installing a PUR PLUS Faucet Mount Water Filtration System is a low-cost, low-effort filtration system that quickly filters drinking and cooking water. No tools are required: Remove the faucet’s original aerator and washer and replace them with the PUR PLUS Faucet Mount system with the PUR PLUS filter inside. For around $30 to $40, the filtration system helps reduce chlorine taste, odors, and 70 other contaminants, including lead, mercury, and some pesticides. However, the filter does not remove microbes.
The PUR PLUS filter includes activated carbon from coconut shells and a mineral core to replace some natural minerals—like calcium and magnesium—for a fresh taste. The benefit of a faucet filter is that you can pour directly into a pot or a giant gallon water bottle without repeatedly refilling a filtering pitcher or dispenser. And it filters instantly, so you don’t need to wait around. The PUR PLUS Faucet Mount filters last longer than most pitchers, stretching to 100 gallons or every three months. The faucet system comes in several colors, and the horizontal filter positioning looks sleek.
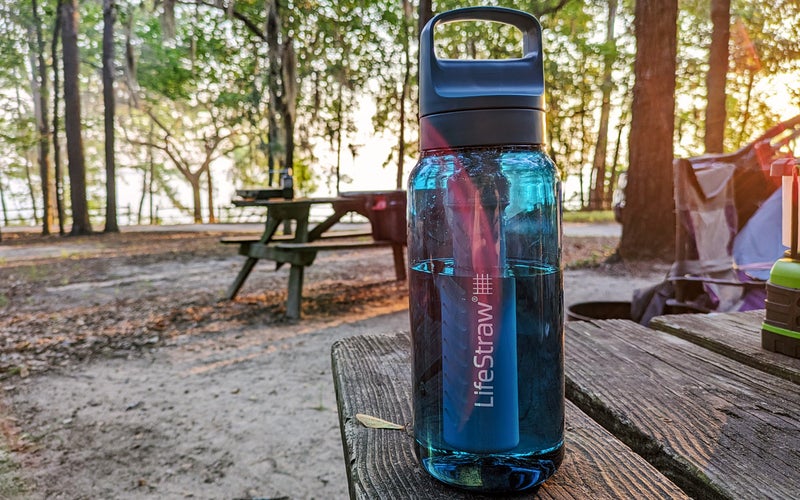
Abby Ferguson
- Dimensions: 3.54 x 3.54 x 10.79 inches
- Weight: 9.9 ounces
- Capacity: 1 liter
- Filter: 2-stage filter system (membrane microfilter and activated carbon)
- Filter lifespan: Membrane microfilter: 1,000 gallons (roughly 5 years), carbon filter: 26 gallons (roughly 2 months)
- Easy to carry
- Available in many colors and 22-ounce capacity
- Cap covers mouthpiece
- Meets NSF/ANSI Standards 42 and P231
- Large size doesn’t fit in cupholders or some backpack pockets
- Two filters will have different replacement schedules
Here at PopSci, we are very much into on-the-go hydration . And carrying a water bottle with a built-in filter can bring peace of mind whether refilling for everyday adventures or traveling to exotic locales. We’ve loved the LifeStraw Go Series for a number of reasons, but largely because of how effective the two-stage filter system is.
The activated charcoal filter helps keep water tasting fresh and smelling, well, not smelling. The membrane microfilter catches little nasties like bacteria, parasites, sand, dirt, and microplastics—all things that could seriously ruin a vacation. It’s important to note that the charcoal filter lasts about two months (26 gallons), while the membrane lasts for five years (or more than 1,000 gallons). There’s no clear way to track both, so you’ll just have to remember.
The plastic version of this water bottle is BPA-free, made of 50 percent recycled plastic, and comes in 1-liter or 22-ounce sizes. The lightweight materials are ideal for hiking or travel. However, if you want to minimize your plastic use or want insulation, the Go Series also comes in a stainless steel version in 24-ounce or 1-liter capacities. The double-walled bottle keeps water cool—a huge part of hitting your daily water goals —but it’s not for hot beverages. All versions include a cover that keeps the mouthpiece clean, which we definitely appreciate when traveling.

- Dimensions: 5.5 x 1.9 x 1.9 inches
- Weight: 2.5 ounces
- Capacity: N/A, but filters up to 32 ounces at a time
- Filter: Hollow fiber filter
- Filter lifespan: Indefinite
- Lightweight
- Includes attachments for different uses
- Lifetime warranty
- Filters down to 0.1-micron absolute filtration
- Reduces bacteria, protozoa, and microplastics
- Can be cleaned and used nearly indefinitely
- Not for chemical contaminants or viruses
- Reviewers warn not to let pouches freeze
- Squeezing and rolling can wear out the pouches
- Occasional backwashing is needed to restore flow rate
PopSci previously identified the Sawyer Products SP129 Squeeze Water Filtration System as a reliable, portable water filter system for backpackers, hikers, and other adventurers. The tiny size makes it easy to throw in your pack without adding excessive weight, which is a must when backpacking.
It’s also very simple to use. To clean water, fill a pouch, attach it to the palm-sized filter, and squeeze the water into your mouth. But that’s just one way the kit works. It also includes a straw and a hydration pack adapter to filter inline. Plus, the filter can attach to most standard water bottles, so you don’t need to fuss with the pouches on trips. Reviewers often suggest avoiding the “squeeze” and letting gravity pull dirty water through the filter to prevent wear and tear.
The system removes most bacteria, protozoa, cysts, and microplastics. In fact, the Sawyer squeeze filter is the only filter on the market that can claim 0.1 absolute microns (every fiber is 0.1 microns in size). For perspective, no harmful bacteria are smaller than that; therefore, none can slip through the filter. As long as you keep the filter clean, this device should last forever. In fact, there are no filters to replace. However, Sawyer recommends periodically backwashing if the flow rate slows, which should restore 98 percent of the flow rate.
And remember, there are right and wrong ways to handle camping water filter storage .

- Dimensions: 12 x 9.5 x 15 inches
- Weight: 8 pounds
- Capacity: 96 ounces
- Filter: Brita Hub filter (carbon block)
- Filter lifespan: 120 gallons or 6 months
- Water reservoir position can change
- No tools for installation
- Frees up fridge space
- Pours 12 or 20 ounces at a time, with one free-flow option
- Filter lasts up to 6 months
- Certified for NSF/ANSI Standards 42, 53, and 401
- Dispenses room-temperature water only
- Requires counter space
- Higher upfront costs
For fast-filtered water and less frequent filter changes, check out the Brita Hub. No installation or plumbing is required for this countertop filter system. Simply plug in the sleek, white Hub, fill the 12-cup water reservoir, and instantly get filtered water free of chlorine tastes or odor. The tradeoff for a filter on the counter is that it will only serve up room-temperature water. But it’s a solid option for people who don’t have the fridge space for clunky pitchers or large dispensers.
The carbon block filters (about $30 each) last up to six months, and an indicator light will give a heads-up when it’s time for a change. The Brita Hub is certified to reduce 70 contaminants, such as lead, some forever chemicals, and select pesticides. The main downside is that a unit will run about $180 (at the time of writing), which is more upfront cost than other options on this list. But if you want a large-capacity option for your counter, the Hub is a great choice.
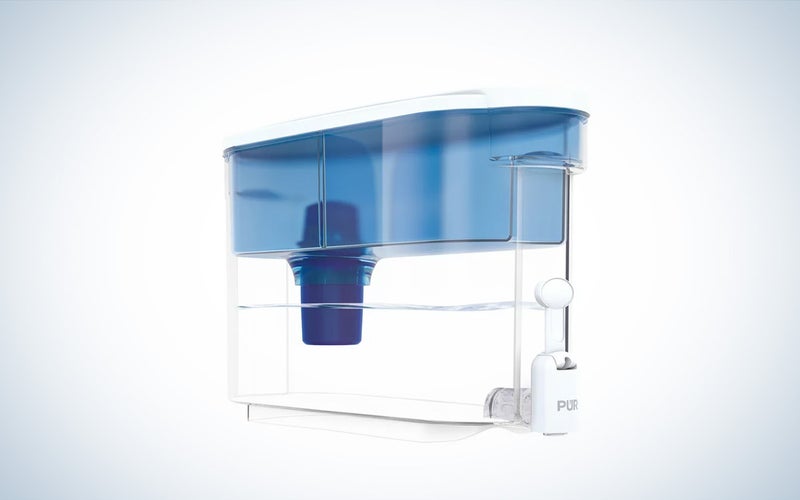
- Dimensions: 11.2 x 6 x 16.1 inches
- Weight: 4.2 pounds
- Capacity: 30 cups
- Filter: PUR PLUS faucet filters (activated charcoal)
- Filter lifespan: Replace after 40 gallons or 2 months
- Filter change indicator
- Slim design
- Large capacity
- Heavy when full
One of the better bargains for water filters is getting a high-capacity dispenser in your fridge. You can snag a PUR PLUS 30-Cup Water Filter Dispenser for the same price as some water filter pitchers. The slim, deep design doesn’t take as much space in a fridge as it feels like it should, though it can be heavy and awkward to put on a shelf when it’s full.
A spigot allows easy access, even if the system is still filtering. The dispenser uses the same activated carbon filters as the PUR PLUS faucet mount system, reducing 70 contaminants, such as lead and microplastics. It’s good for chronically parched or large households, though the filter must be swapped out every two months. Like most water-filtering pitchers, this dispenser isn’t a purifier. It refines already drinkable water and doesn’t remove microbes.
What to consider when buying the best water filters
Choosing water filters depends on where and how much water you plan to drink or use for cooking. That said, you could surround yourself with many options, like a pitcher for home use and a water bottle for running around during the day. While all these options will result in crisp, clean-tasting water, consider if you want your filter to offer additional protection.
We stuck to products with relatively low-cost products, but the ongoing cost of each replacement must be factored into the long-term cost of ownership. There’s no hacking a filter: They must be replaced regularly to remain effective. Most use proprietary filters, so you’ll need to stick with the specific filter made for your product.
Water consumption vs. filter capacity
Compare how much water you drink with how long the filter is supposed to last. Many options above require changes as frequently as two months or 40 gallons. If you’re attempting to drink a gallon per day, that shortens the filter’s lifespan to just over one month. Finding a water filter system that handles a higher volume of water with a longer-lasting filter may be time-saving and cheaper.
Filter type
Most of the filters in this round-up rely on activated carbon, which can absorb chlorine and reduce asbestos, lead, mercury, and volatile organic compounds. Other products use other filtration processes like reverse osmosis, ion exchange, and distillation, which you should consider if installing an under-the-sink or whole-home filter or if you have serious concerns about water quality.
Filtration efficiency
Filter effectiveness varies, so we looked at NSF/ANSI standards. We prefer certified products, which means that NSF International or the Water Quality Association tested and verified the company’s claims. Certification is time-consuming and costly for a company, so we also indicated where outside labs found products that “meet the standards” but aren’t certified.
Here’s what some of the standards mean, but review the manufacturer’s performance data to see the specific contaminants a filter is effective against:
- NSF/ANSI Standard 42: This is a common standard, which indicates a filter can remove chlorine taste and odor or chloramines.
- NSF/ANSI Standard 53: Another common standard that indicates the reduction of some heavy metals like lead, arsenic, and mercury, as well as some pesticides and herbicides.
- NSF/ANSI Standard 401: This indicates the filter removes or reduces up to 15 kinds of “emerging impurities,” such as bisphenol A (BPA), ibuprofen, DEET, microplastics, and some pesticides and herbicides.
- NSF/ANSI Standard P231: This is where purification comes in. This standard means microbiological contaminants like bacteria, viruses, and cysts are reduced or removed.
Q: Do I need a water filter?
Whether you need a water filter should be a straightforward yes or no answer, but it’s not. The U.S. drinking water supply is considered safe, and the Environmental Protection Agency regulates public water systems (this excludes wells). You can check annual water quality reports on EPA’s website , but systems can also experience contamination after leaving a treatment plant or have an acute issue due to recent natural disasters or climate change. Plus, some pollutants aren’t regulated yet, like forever chemicals, which the EPA issued proposed rules for in March . Not sure what may be in your water? You can also look up your zip code on The Environmental Working Group’s database of what’s been measured in tap water or get an at-home water quality test .
Q: What contaminants do water filters remove?
What contaminants water filters remove depends on the individual water filter. Most of the filters in this guide are activated carbon, which can absorb chlorine and reduce asbestos, lead, mercury, and volatile organic compounds. You’ll need to review performance data to see what specific pollutants a filter reduces or removes.
Q: How often should I replace my water filter?
How often you should replace your water filter depends on the filter. Always check the manufacturer’s recommendations, but other factors may reduce the filter’s life. For example, a particularly active or large household may filter 40 gallons of water through a pitcher well before two months.
Final thoughts on the best water filters
Anyone looking for the best water filters has plenty of affordable options to cover a variety of needs, whether lugging around a reliable system in the woods or covering a household’s drinking and cooking needs. It’s hard to go wrong with the filters we recommend from well-known, longtime brands like PUR and Brita. Both brands’ filters are readily available and carry multiple certifications. And don’t rule out getting multiple items—you never know when you’ll be thirsty next.
Why trust us
Popular Science started writing about technology more than 150 years ago. There was no such thing as “gadget writing” when we published our first issue in 1872, but if there was, our mission to demystify the world of innovation for everyday readers means we would have been all over it. Here in the present, PopSci is fully committed to helping readers navigate the increasingly intimidating array of devices on the market right now.
Our writers and editors have combined decades of experience covering and reviewing consumer electronics. We each have our own obsessive specialties—from high-end audio to video games to cameras and beyond—but when we’re reviewing devices outside of our immediate wheelhouses, we do our best to seek out trustworthy voices and opinions to help guide people to the very best recommendations. We know we don’t know everything, but we’re excited to live through the analysis paralysis that internet shopping can spur so readers don’t have to.
Like science, tech, and DIY projects?
Sign up to receive Popular Science's emails and get the highlights.
Are water filtration systems an effective way to get rid of PFAS? Here's what a new study says
N early half of the tap water in the United States is contaminated by PFAS chemicals , or per-and poly-fluoroalkyl substances, a recent report alarmed. The U.S. Environmental Protection Agency has proposed an effort to restrict the group of chemicals, also dubbed "forever chemicals," in food and drinking water to improve public health which could help, but the agency hasn't yet finalized a rule.
In the meantime, Americans can keep themselves safe from the chemicals when grabbing a glass of water from the kitchen sink, in part, by using water filtration systems, one environmental group suggests.
Consumer-available water filtration systems that everyday people can use to rid PFAS from tap water are the center of a new study from the nonprofit activist group Environmental Working Group. Scientists from the group researched the effectiveness of several filtration systems available to consumers across the U.S., and determined that they can serve as a "Band-Aid" to the problem until a longer-term solution comes to form, said Sydney Evans, a senior science analyst at the Environmental Working Group who has led many types of PFAS testing nationwide.
Start the day smarter. Get all the news you need in your inbox each morning.
"The end goal is people wouldn’t have to buy a water filter… but that’s just not the reality," Evans said.
DRINKING WATER IN THE U.S. Nearly half of nation's tap water contains PFAS, a new study finds. Americans living in urban areas are most at risk.
What does the research show?
The group estimated there are "hundreds of brands and models of home water filters" that usually come in six forms: "pitcher or large dispenser, faucet-mounted, faucet-integrated, on-counter, under-sink or whole-house."
Scientists tested 10 different water filtration systems for specific types of PFAS, notably the most common types: perfluorooctanoic acid (PFOA) and perfluorooctane sulfonate (PFOS). The group tested for 25 different types of PFAS using one water sample for each filter, a summary of the research reads.
Most of the water filters the Environmental Working Group researched contain a medium typically made out of granular activated carbon to remove almost all of the PFAS, said Evans.
"To calculate the percentage PFAS reduction achieved by each filter, we contrasted the sum of the total PFAS found in the tap water sample with the PFAS detected in the filtered water," the summary reads.
Evans said she was surprised by how well some of the filters worked adequately removed PFA levels almost as well as through reverse osmosis. And while there's no guarantee they rid of all chemicals, "some kind of reduction is better than nothing," she said.
The systems come with varying price tags. The group is advocating for legislation that restricts and regulates PFA levels from a federal and local level in part so consumers don't have to pay out of pocket to drink safe water.
How effective are water filters?
The Centers for Disease Control and Prevention's website cautions Americans that "no filter eliminates all contaminants" even though water filters can help rid of forever chemicals. The agency says water consumers should not "assume that if the filter removes one contaminant, it also removes others."
"Filtration of contaminants depends highly on the amount of contaminant, size of the contaminant particle, and the charge of the contaminant particle," its website reads.
"Depending on the household’s water needs, pretreatment before filtration may include the addition of coagulants and powdered activated carbon, adjustments in pH or chlorine concentration levels, and other pretreatment processes in order to protect the filter’s membrane surface," it continues.
What are the health risks of drinking water contaminated by PFAS?
Americans who drink water from the faucet contaminated by the chemicals are exposed to various health risks attributed to PFAS, including illnesses like cancer, low birth weight, and thyroid disease.
Location matters when it comes to risk level. A national study from the U.S. Geological Survey released last week shows that people living in urban areas are most at risk of having PFAS in their tap water while those living in some rural regions have a lower chance of consuming those chemicals.
According to a March 2022 survey of 2,246 American adults conducted by Aquasana showed that more than three-fourths of Americans are already filtering their water. The percentage of users grew between three percentage points between 2020 and 2022.
Those surveyed said they were most concerned about lead, chlorine, and chloramines and fearful of bacteria, cysts, and viruses. More than half agreed "they are more concerned about their health because of the pandemic, and 40% said they are "now more concerned about the quality of the unfiltered water in their homes because of COVID-19."
'There is still a lot of work to be done'
Experts are adamant that regulation is the key to keeping people safe from long-term exposure to PFAS.
The Biden administration through the EPA proposed a regulation in March that if finalized, "would regulate PFOA and PFOS as individual contaminants, and will regulate four other PFAS – PFNA, PFHxS, PFBS, and GenX Chemicals – as a mixture," a news release about the proposal reads.
CONCERNED ABOUT YOUR DRINKING WATER Across the US, towns warn of toxic PFAS chemicals in drinking water.
“Communities across this country have suffered far too long from the ever-present threat of PFAS pollution," wrote Michael Regan, an administrator for the EPA, at the time the proposal was announced.
Justin Colacino, an associate professor of environmental health sciences at the University of Michigan, said if the new regulations proposed by the Environmental Protection Agency become reality, it would be "a major public health victory toward reducing exposure to these toxic and persistent chemicals."
He added: "There is still a lot of work to be done, though. With thousands of different PFAS chemicals on the market, we’ll continue to need innovative new strategies to regulate how these chemicals are used in our products to protect the health of people and the environment."
Critics have argued it could cost the nation billions to make that a reality.
What are other ways to stay safe from PFAS?
Aside from the presence of chemicals in tap water, PFAS are commonly used in consumer products like nonstick cookware and linings of fast-food boxes. The Environmental Working Group recommends a few tips Americans can use to stay safe from PFAS in those items, including:
◾ "Skip optional stain-repellent treatment on new carpets and furniture."
◾ "Cut back on fast food and greasy carryout food" because it often comes from wrappers treated with PFAs.
◾ "Be wary of all fabrics labeled stain- or water-repellent."
◾ "Avoid PTFE-based nonstick pans and kitchen utensils."
◾ "Pop popcorn the old-fashioned way – on the stovetop."
Contact Kayla Jimenez at [email protected]. Follow her on Twitter at @kaylajjimenez.
This article originally appeared on USA TODAY: Are water filtration systems an effective way to get rid of PFAS? Here's what a new study says
share this!
April 24, 2024
This article has been reviewed according to Science X's editorial process and policies . Editors have highlighted the following attributes while ensuring the content's credibility:
fact-checked
trusted source
Nanofibers rid water of hazardous dyes: Researchers develop efficient filters based on cellulose waste
by Vienna University of Technology
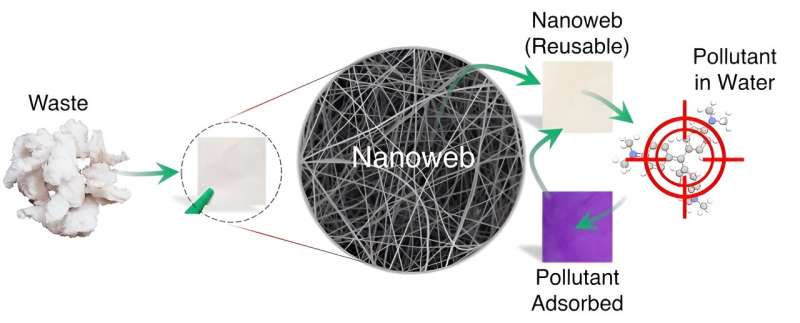
Using waste to purify water may sound counterintuitive. But at TU Wien, this is exactly what has now been achieved. Researchers have developed a special nanostructure to filter a widespread class of harmful dyes from water.
A crucial component is a material that is considered waste: used cellulose , for example in the form of cleaning cloths or paper cups. The cellulose is utilized to coat a fine nano-fabric to create an efficient filter for polluted water . The paper is published in the journal Small Science .
Colored poison in the water
Organic dyes represent the largest group of synthetic dyes, including so-called azo compounds. They are widely used in the textile industry , even in countries where little attention is paid to environmental protection, and the dyes often end up in unfiltered wastewater.
"This is dangerous because such dyes degrade very slowly, they can remain in the water for a long time and pose great danger to humans and nature," says Prof. Günther Rupprechter from the Institute of Materials Chemistry at TU Wien.
There are various materials that can bind such dyes. But that alone is not enough. "If you simply let the polluted water flow over a filter film that can bind dyes, the cleaning effect is low," explains Rupprechter.
"It's much better to create a nanofabric out of lots of tiny fibers and let the water seep through." The water then comes into contact with a much larger surface area, and thus many more organic dye molecules can be bound.
Cellulose waste as a nano-filter
"We are working with semi-crystalline nanocellulose, which can be produced from waste material," says Qaisar Maqbool, first author of the study and postdoc in Rupprechter's research group. "Metal-containing substances are often used for similar purposes. Our material, on the other hand, is completely harmless to the environment, and we can also produce it by upcycling waste paper."
This nano-cellulose is "spun" together with the plastic polyacrylonitrile into nanostructures. However, this requires a lot of technical skill. The team from the TU Wien was successful with a so-called electrospinning process. In this process, the material is sprayed in liquid form , the droplets are electrically charged and sent through an electric field.
"This ensures that the liquid forms extremely fine threads with a diameter of 180 to 200 nanometers during curing," says Rupprechter. These threads form a fine tissue with a high surface area —a so-called "nanoweb." A network of threads can be placed on one square centimeter, with a total surface area of more than 10 cm 2 .
Successful tests
The tests with these cellulose-coated nanostructures were very successful: In three cycles, water contaminated with violet dye was purified, and 95% of the dye was removed. "The dyes remain stored in the nanoweb. You can then either dispose of the entire web or regenerate it, dissolve the stored dyes and reuse the filter fabric," explains Rupprechter.
However, more work needs to be done: evaluating the mechanical properties of the sophisticated nanowebs, conducting biocompatibility tests, assessing sensitivity to more complex pollutants, and achieving scalability to industrial-grade standards.
Now Rupprechter and his research team want to investigate how this dye filter technology can be transferred to other areas of application.
"This technology could also be very interesting for the medical field," Rupprechter believes. "Dialysis, for example, also needs filtering out very specific chemical substances from a liquid." Coated nanofabrics may be useful for such applications.
Provided by Vienna University of Technology
Explore further
Feedback to editors

Optical barcodes expand range of high-resolution sensor
9 hours ago

Ridesourcing platforms thrive on socio-economic inequality, say researchers

Did Vesuvius bury the home of the first Roman emperor?

Florida dolphin found with highly pathogenic avian flu: Report

A new way to study and help prevent landslides

New algorithm cuts through 'noisy' data to better predict tipping points
10 hours ago

Researchers reconstruct landscapes that greeted the first humans in Australia around 65,000 years ago

High-precision blood glucose level prediction achieved by few-molecule reservoir computing
11 hours ago

Enhancing memory technology: Multiferroic nanodots for low-power magnetic storage

Researchers advance detection of gravitational waves to study collisions of neutron stars and black holes
Relevant physicsforums posts, rank copper, brass and bronze by thermal conductivity, inductive heating of a non-conductive reactor's contents.
Apr 18, 2024
System curve and centrifugal pump suitability
Apr 3, 2024
Maximum mass flow in a shell & tube heat exchanger
Air-to-fuel ratio of ethanol.
Mar 27, 2024
Isotopic exchange and SIMS diffusion profile measurement
Mar 20, 2024
More from Materials and Chemical Engineering
Related Stories

Catalysis breakthrough yields self-cleaning wall paint that breaks down air pollutants when exposed to sunlight
Mar 25, 2024

New wood-based technology removes 80% of dye pollutants in wastewater
Mar 23, 2023

New, fully biodegradable cellulose membrane proves effective in oil-water separation
Jun 17, 2022

New method can remove dyes from wastewater
Aug 11, 2022

Gold nanoclusters offer sustainable solution for wastewater polluted by dyes used in many industries
Mar 19, 2024

Cleaning up environmental contaminants with quantum dot technology
Recommended for you.

Diamond dust as a potential alternative to contrast agent gadolinium in magnetic resonance imaging
Apr 25, 2024

Ultrasensitive photonic crystal detects single particles down to 50 nanometers
Apr 24, 2024

Researchers create nanostructures for efficient and sustainable degradation of pollutants

Bioelectronic chip detects vitamins C and D in saliva in under 20 minutes
Let us know if there is a problem with our content.
Use this form if you have come across a typo, inaccuracy or would like to send an edit request for the content on this page. For general inquiries, please use our contact form . For general feedback, use the public comments section below (please adhere to guidelines ).
Please select the most appropriate category to facilitate processing of your request
Thank you for taking time to provide your feedback to the editors.
Your feedback is important to us. However, we do not guarantee individual replies due to the high volume of messages.
E-mail the story
Your email address is used only to let the recipient know who sent the email. Neither your address nor the recipient's address will be used for any other purpose. The information you enter will appear in your e-mail message and is not retained by Phys.org in any form.
Newsletter sign up
Get weekly and/or daily updates delivered to your inbox. You can unsubscribe at any time and we'll never share your details to third parties.
More information Privacy policy
Donate and enjoy an ad-free experience
We keep our content available to everyone. Consider supporting Science X's mission by getting a premium account.
E-mail newsletter
REVIEW article
In-situ optical water quality monitoring sensors—applications, challenges, and future opportunities.
- 1 School of Geography, Earth and Environmental Sciences, University of Birmingham, Birmingham, United Kingdom
- 2 Proteus Instruments Ltd, Bromsgrove, United Kingdom
Water quality issues remain a major cause of global water insecurity, and real-time low-cost monitoring solutions are central to the remediation and management of water pollution. Optical sensors, based on fluorescence, absorbance, scattering and reflectance-based principles, provide effective water quality monitoring (WQM) solutions. However, substantial challenges remain to their wider adoption across scales and environments amid cost and calibration-related concerns. This review discusses the current and future challenges in optical water quality monitoring based on multi-peak fluorescence, full-spectrum absorbance, light-scattering and remotely sensed surface reflectance. We highlight that fluorescence-based sensors can detect relatively low concentrations of aromatic compounds (e.g., proteins and humic acids) and quantify and trace organic pollution (e.g., sewage or industrial effluents). Conversely, absorbance-based sensors (Ultraviolet-Visible-Infra-red, UV-VIS-IR) are suitable for monitoring a wider range of physiochemical variables (e.g., nitrate, dissolved organic carbon and turbidity). Despite being accurate under optimal conditions, measuring fluorescence and absorbance can be demanding in dynamic environments due to ambient temperature and turbidity effects. Scattering-based turbidity sensors provide a detailed understanding of sediment transport and, in conjunction, improve the accuracy of fluorescence and absorbance measurements. Recent advances in micro-sensing components such as mini-spectrometers and light emitting diodes (LEDs), and deep computing provide exciting prospects of in-situ full-spectrum analysis of fluorescence (excitation-emission matrices) and absorbance for improved understanding of interferants to reduce the signal-to-noise ratio, improve detection accuracies of existing pollutants, and enable detection of newer contaminants. We examine the applications combining in-situ spectroscopy and remotely sensed reflectance for scaling Optical WQM in large rivers, lakes and marine bodies to scale from point observations to large water bodies and monitor algal blooms, sediment load, water temperature and oil spills. Lastly, we provide an overview of future applications of optical techniques in detecting emerging contaminants in treated and natural waters. We advocate for greater synergy between industry, academia and public policy for effective pollution control and water management.
1 Introduction
Water pollution is a major challenge to global water security, with >25% of the world’s population unable to access safe drinking water in 2022 ( WHO and UNICEF, 2023 ). After accounting for water quality issues, the fraction increases to 40%, with key hotspots in India, China, the Middle East, the Mediterranean and Mexico ( van Vliet et al., 2021 ; Caretta et al., 2022 ). Real-time and accurate monitoring of critical water pollution variables, including heavy metals, agricultural nutrients [e.g., nitrate and phosphorus, disease-causing bacteria, viruses and emerging contaminants such as microplastics and pesticides, remains a global challenge ( Hannah et al., 2022 )]. The lack of infrastructure, financial and technical resources and regulatory oversight significantly hamper water quality monitoring (WQM) efforts in developing countries. Similarly, despite having financial resources, developed countries are constrained by aging infrastructure, lack of investment, and low compliance and accountability ( Kirschke et al., 2020 ; Hannah et al., 2022 ). Technological improvements can lower some of the financial and infrastructure-related issues and facilitate decision-making by enabling in-situ monitoring of key water quality variables identified under global conventions such as the United Nations’s Sustainable Development Goals (SDGs) 6.3.2, European Union (EU) Water Framework Directive (WFD) and World Health Organization’s (WHO) water quality guidelines ( Warner, 2020 ; European Union, 2021 ; WHO, 2022 ). For example, SDG 6.3.2 details a list of water quality variables, including oxygen, salinity, macronutrients (Nitrogen, Phosphorus) and pH and defines a range of target values that reflect human and ecosystem health perspectives ( Warner, 2020 ). Similarly, in Europe, the WFD focuses on improving the chemical and ecological conditions of both surface and groundwater through monitoring and holistic river basin management ( European Union, 2021 ). However, this monitoring occurs at coarse intervals and often misses critical periods of pollutant transport and transformation (e.g., hot moments; McClain et al., 2003 ). Recent WHO guidelines advocate developing early-warning systems for predicting harmful algal blooms using in-situ monitoring approaches ( WHO, 2022 ).
The technology for in-situ monitoring of physiochemical variables, such as temperature, electrical conductivity, pH, and dissolved oxygen (DO), is well-established ( Banna et al., 2014 ; Silva et al., 2022 ). Recent developments have expanded the potential variables that can be monitored in-situ to include total and dissolved organic carbon (TOC and DOC, respectively), biological and chemical oxygen demand (BOD and COD, respectively), dissolved organic matter (DOM), fecal coliforms, agricultural pollutants (e.g., ammonium, nitrate, and phosphorus), ions (chloride, fluoride) and heavy metals (e.g., iron, arsenic) ( Raich, 2013 ; Banna et al., 2014 ). However, given that human-health-related thresholds are more stringent than those for ecosystem that production and environmental occurrence of emerging contaminants (ECs) continues to increase, there is an urgent requirement for monitoring technology that can detect a wide range of variables accurately and across a broad spectrum of environmental conditions. Alongside sensor technology advances, data transfer (telemetry) and data visualizations support quick and effective stakeholder decision-making ( Gholizadeh et al., 2016 ; Zaidi Farouk et al., 2023 ). Technological advances are also increasingly informing policy-making and riverine ecosystem restoration efforts toward improving the drinking and bathing qualities of rivers, lakes and marine waters ( Johnson et al., 2008 ; Mouchel et al., 2021 ; Tiwari et al., 2021 ).
Recent advances in optical water quality monitoring (OWQM) techniques have improved the understanding of eutrophication and organic pollution in aquatic ecosystems ( Baker, 2002 ; Hudson et al., 2007 ; Thakur and Devi, 2022 ). In-situ optical sensors measuring fluorescence, absorbance, and scattering have become particularly important for aquatic monitoring. Advancements in optical components, i.e., light emitting diode (LED) technology, and miniaturization of sensing elements such as photodiodes, photo-multiplier tubes, charge-coupled device (CCD), and complementary metal-oxide-semiconductor (CMOS) sensors, etc., have made the optical sensors, such as fluorimeter, absorbance and turbidity sensors, smaller in form-factor, lowered their power requirements and improved their field usability ( Mukunda et al., 2022 ; Zainurin et al., 2022 ; Goblirsch et al., 2023 ). Fluorescence spectroscopy relies on the principle of Stokes shift, which is the spectral shift between incident (excitation, ex) light and emitted (em) light (detected orthogonally to incident light) from shorter to longer wavelengths ( Figure 1 ). Full-spectrum applications enable measurement of the fluorescence signature in a three-dimensional excitation-emission matrix (EEM) and use parallel factor analysis (PARAFAC) tools to characterize specific fluorescence peaks associated with specific dissolved organic compounds ( Murphy et al., 2013 ). The key fluorescence peaks include Peak A (ex/em: 260/380–460 nm) and Peak C (ex/em: 350/420–480 nm), both of which have been associated with humic-like material derived from terrestrial environments, Peak B (ex/em: 275/310 nm) and Peak T (ex/em: 275/340 nm), associated with Tyrosine and Tryptophan-like fluorescence (TLF), respectively, and Peak M (ex/em: 310/370–420 nm) associated with humic-like material derived from marine environment. However, EEM applications are still limited primarily to the laboratory, with most in-situ applications focusing on single or dual peak fluorescence to detect dissolved organic compounds (Peak T and Peak C; Khamis et al., 2017 ; Li et al., 2020 ).
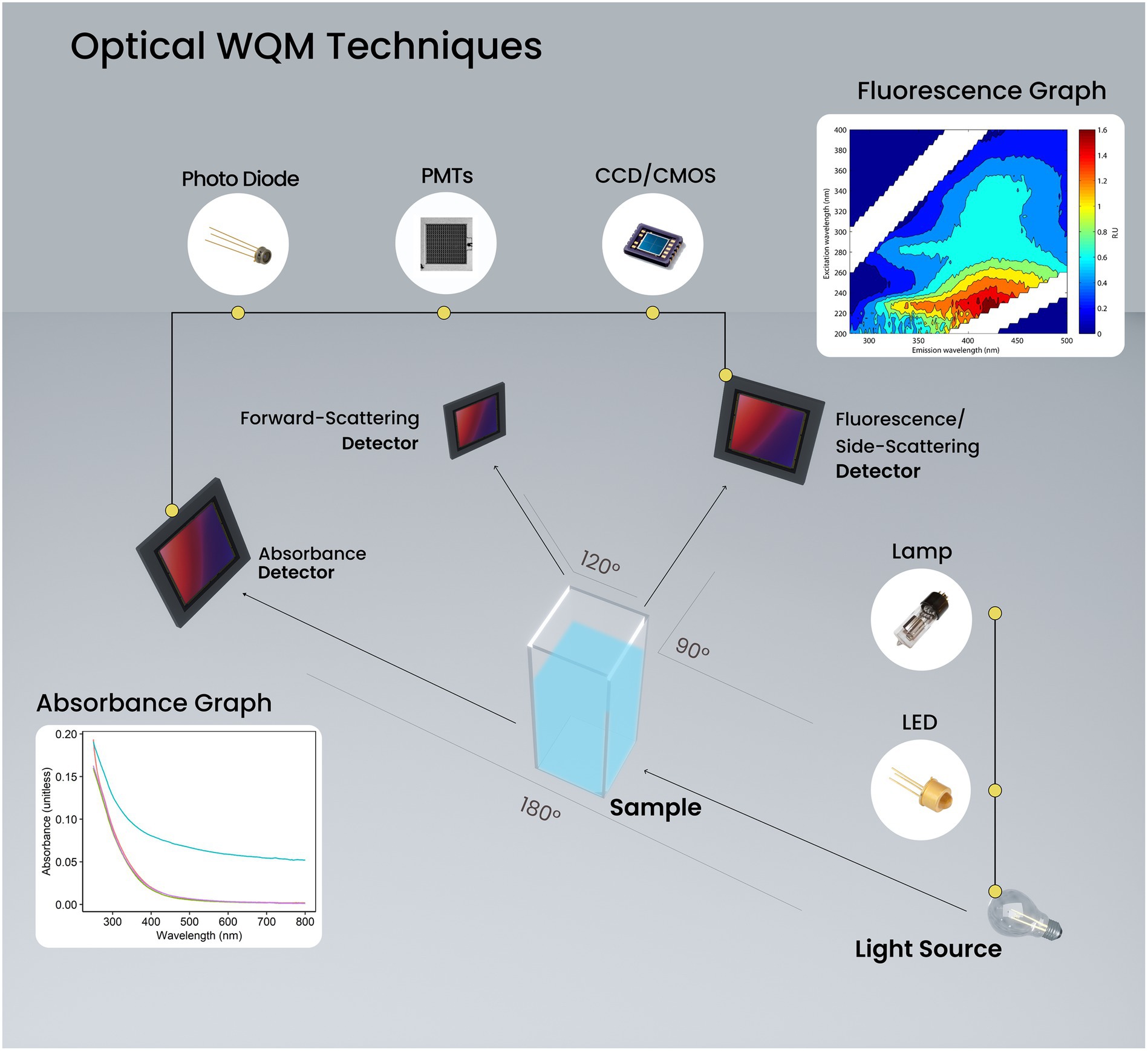
Figure 1 . Schematic diagram showing principles of absorbance, fluorescence and scattering-based probes with optical components and sample results. The optical components include light sources, such as LEDs and lamps, and detectors, such as photodiodes, photo-multiplier tubes (PMTs), and CCD/CMOS sensors. Data visualization is shown as representative graphs of full spectrum absorbance spectra (bottom-left) and fluorescence excitation-emission matrix (EEM, top-right). The sample EEM is adapted from Khamis et al. (2017) .
Absorbance spectroscopy is based on the absorption of incident light at specific wavelengths by the variable of interest, resulting in the loss of transmitted light measured at 180 ° angle. Fluorescence can provide high specificity and sensitivity for a set of organic compounds but can saturate at high concentrations (i.e., the inner-filtering effect) and is prone to interferences due to the sample temperature and turbidity ( Fellman et al., 2010 ; Carstea et al., 2020 ; Kimball et al., 2020 ). Conversely, absorbance can detect a larger pool of organic and inorganic compounds across a wider range of concentrations, albeit with limited specificity ( Dai et al., 2022 ; Carter et al., 2023 ). Nephelometric sensors detect undissolved/suspended solid/sediment particles in water by measuring scattering at different angles to the incident light (0°), including back-scattering (30° ± 15° angle), side-scattering (90° angle) and forward-scattering (120° ± 15° angle) ( Kitchener et al., 2017 ; Droujko et al., 2023 ). These are based on inverse attenuation, i.e., a higher concentration of total suspended solids (TSS) will cause higher scattering and vary considerably with particle size, shape, and wavelength of incident light. The side-scattering-based sensors measure turbidity as a proxy of TSS with good accuracy at low concentrations. In contrast, backward-scattering sensors are accurate at high TSS concentrations, and forward-scattering sensors cover a wider range of concentrations. Together, they provide a detailed understanding of sediment transport in water bodies and are used to improve the accuracy of fluorescence and absorbance measurements ( Khamis et al., 2015 ; Lee et al., 2015 ). However, substantial challenges remain to the large-scale deployment of these technologies, given costs, power requirements and calibration-related concerns. Increasingly, remotely sensed reflectance-based platforms are being used to monitor water quality events, such as algal blooms, in freshwater lakes and marine habitats, but require validation against in-situ ground observations ( Shi et al., 2019 ; Sagan et al., 2020 ; Windle and Silsbe, 2021 ).
Several review papers have summarized recent advances in water quality monitoring ( Raich, 2013 ; Banna et al., 2014 ; Gholizadeh et al., 2016 ; Thakur and Devi, 2022 ; Zainurin et al., 2022 ; Zaidi Farouk et al., 2023 ), and while some have focused explicitly on in-situ optical sensors ( Hudson et al., 2007 ; Carstea et al., 2016 , 2020 ; Gunter et al., 2023 ), there is still a need for deeper understanding of this rapidly evolving technology and its potential for water quality monitoring at different scales. In this review, we provide a concise overview of the current and future challenges in in-situ optical monitoring of freshwater and marine bodies, focusing on fluorescence, full-spectrum absorbance, scattering and reflectance-based techniques. Further, we discuss the available market solutions and data sources using field-deployable optical sensors and their strengths and limitations. Lastly, we provide an overview of future applications of optical techniques in detecting novel/emerging contaminants in water.
2 Status of fluorescence and absorbance spectroscopy for WQM
Early research on the use of fluorescence spectroscopy for WQM used fiber optic sensors for a wide range of applications, including the detection of organic matter (TOC, DOC), oxygen (BOD, DO), biological agents (e.g., bacteria, viruses, algal blooms, etc.) and hydrocarbons ( Scully, 1998 ; Ahmad and Reynolds, 1999 ). The commercial applications of these techniques were either limited to benchtop instruments ( Phillips et al., 1974 ) or in the form of optical fiber-based sensors in the 1980s, which, along with electrochemical techniques such as ion selective electrodes (ISEs), have been widely used for a range of aquatic and industrial monitoring activities for more than half a century ( Frant, 1997 ; Scully, 1998 ; Ahmad and Reynolds, 1999 ). Similarly, in-situ optical turbidity sensors have long been used to measure scattering at different angles to understand the composition of suspended solids in water bodies ( Kitchener et al., 2017 ). In comparison, the application of fluorescence and absorbance spectroscopy for in-situ WQM is a recent trend. The emergence of commercial fluorometers and absorbance probes over the last ~20 years is primarily linked to the development of UV LEDs (<400 nm) and photodetectors ( Johnson and Coletti, 2002 ; Rode et al., 2016 ; Mukunda et al., 2022 ).
Currently, optical water quality monitoring represents a multi-billion US dollar market comprising probes based on various measurement principles, e.g., nephelometry (scattering of light), absorbance and fluorescence. The availability of cost-effective and low-powered LEDs and detectors has driven the expansion of the in-situ OWQM market. Mukunda et al. (2022) provided a comprehensive review of conventional light sources (Arc lamps, Quartz halogen lamps, mercury lamps, etc.) and highlighted cost, bulkiness, short lifespan, drift in output with prolonged flashing and UV phototoxicity as major issues. Conversely, LEDs provide high power, longer lifespan, high signal-to-noise ratio, stable outputs, compact size and low costs, making them ideal for field-deployable OWQM sensors ( Hart and JiJi, 2002 ; Mukunda et al., 2022 ). The early applications of Ultraviolet–Visible-Near Infra-Red (UV–VIS–NIR) LEDs were for biomedical analytical devices and ecophysiological sensing (i.e., plant chlorophyll), which were later adopted for water quality sensors ( Mukunda et al., 2022 ). Early sensors used single or dual-beam LEDs to focus on fluorescence or absorbance measurements for a single variable of interest, e.g., nitrate and TOC, with a second beam often used for turbidity correction. The two major gaps in the wider adoption of LEDs for OWQM are the low efficiency of deep UV (<280 nm) and scattering-induced noise in the signal. Multi-chip LEDs, which position multiple LEDs on a single chip, along with deep learning techniques, provide a better opportunity to understand the sediment composition and develop robust correction factors ( Hart and JiJi, 2002 ; Ighalo et al., 2021 ). However, the cost-effectiveness and power requirements of deep UV LEDs remain a significant bottleneck in developing low-cost OWQM probes.
Similar to LEDs, the use of photodetector technology, such as photodiodes, CCDs, PMTs, and, recently, linear CMOS arrays, for WQM has also evolved significantly ( Spring, 2001 ; Mukunda et al., 2022 ). Photodiodes have a number of desirable properties, namely, low-cost, high specificity, narrow or broad range of wavelengths, low power requirements, and low data processing time. Thus, paired with monochromatic or band-pass filters (which allow transmission of specific wavelengths), low-light photodiodes and PMTs contribute to the affordability and compactness of OQWM probes. Optical detectors can also improve the signal-to-noise ratio by optimizing signal gain and modulating the sensor integration time. However, PMTs and photodiodes have no spatial discrimination, i.e., they convert the intensity of light into an electrical signal and cannot differentiate them according to wavelengths, which limits their applicability to broad spectrum (UV–VIS) sensing ( Spring, 2001 ; Yokota et al., 2021 ). Miniaturizing traditional benchtop spectrometers into mini and micro-spectrometers provides new opportunities for developing field-deployable UV–VIS OWQM probes ( Bouyé et al., 2016 ; Shi et al., 2022 ; Goblirsch et al., 2023 ). The mini/micro-spectrometers use a linear CCD or CMOS imaging sensor in a Czerny-Turner design or micrograting to achieve smaller form factors ( Bouyé et al., 2016 ; Chen et al., 2022 ). However, the significant challenges to adopting mini/micro-spectrometers lie in cost-effectiveness, data analytics and power management.
3 Current in-situ applications of optical WQM
The use of fluorescence and absorbance spectroscopy for in-situ WQM, while relatively new in comparison to electrochemical techniques (ion-selective electrodes have been used for half a century), has become well-established over the past 20 years ( Johnson and Coletti, 2002 ; Rode et al., 2016 ). Fluorescence for monitoring Chlorophyll a and UV absorbance for nitrate measurements are among the most widely cited environmental monitoring applications ( Arndt et al., 2022 ). In this case, the sensors are used to directly measure the variables of interest ( Chlorophyll a and nitrate have specific absorbance and fluorescence spectra). Concurrently, there has been increasing interest in the use of absorbance as a surrogate (or proxy) for other difficult-to-measure variables, including the development of multivariate regression models to quantify DOC/TOC ( Peacock et al., 2014 ; Gaviria Salazar et al., 2023 ), fecal coliforms ( Nowicki et al., 2019 ; Sorensen et al., 2020 ; Dapkus et al., 2023 ), and phosphorus fractions ( Vaughan et al., 2018 ). These approaches have recently utilized emerging data science and machine learning tools to develop more robust proxy models (see Carter et al., 2023 ).
In-situ fluorescence monitoring has been used for both quantitative and qualitative WQM. For example, pollution sources are tracked using fluorescence peaks associated with proteinaceous compounds (e.g., TLF; Baker et al., 2004 ; Lenaker et al., 2023 ) and optical brightening agents ( Finegan and Hasenmueller, 2023 ) as (non-conservative) tracers of wastewater. Fluorescence has also been used to quantify DOC and BOD ( Khamis et al., 2017 , 2021 ) by measuring dual fluorescence peaks (tryptophan and humic-like fluorescence) and turbidity, which is vital for developing reliable proxy models. There has also been growing interest in using fluorescence to quantify microbial contamination in rivers ( Baker et al., 2015 ; Bridgeman et al., 2015 ), groundwater ( Nowicki et al., 2019 ; Sorensen et al., 2020 ; Dapkus et al., 2023 ), and potable water ( Sorensen et al., 2018 ; Gunter et al., 2023 ). However, it is important to note that most studies to date focus on single or dual fluorescence peaks; hence, the associated models tend to be relatively simple compared to those developed for absorbance sensors.
Optical indices (rather than proxy models) have recently emerged as a viable approach for monitoring water quality. There are a large number of fluorescence indices associated with Excitation Emission Matrix spectroscopy ( Fellman et al., 2010 ; Begum et al., 2023 ), which have not yet been implemented for field deployable sensors, although the use of the ratio of peak T:peak C fluorescence has been used as an indicator of wastewater/anthropogenic DOM in an urban river ( Croghan et al., 2021 ). For absorbance, it is possible to calculate a broader range of optical indices using full-spectrum measurements in-situ . For example, the spectral slope, linked to the molecular weight of DOM, has been used to assess carbon quality dynamics across a land cover gradient ( Vaughan et al., 2019 ).
4 Market solutions using fluorescence and absorbance spectroscopy for WQM
In an exhaustive market survey for current probes based on absorbance and fluorescence spectroscopy, we found more than 32 products catering to various environmental, academic, and industrial applications. The survey results are summarized in Supplementary Table S1 . We relied on the datasheets and specifications provided by the retailers to glean the relevant information or lack thereof. The product marketing material (e.g., brochures, webpages, datasheets and manuals) often lacked technological specifications and was thus ignored. However, they provide helpful information on the applications, technology-based demands, supply-side constraints, and insights into their future directions. The surveyed probes were segregated based on the measurement principles: absorbance and luminescence including fluorescence; the light source: LEDs and lamps; the detector: single or arrayed photodiodes; and variables of interest: single or multivariable, design and data post-processing methods.
4.1 Measurement principles
Absorbance-based sensors dominate the market space (72%, N = 31). The single-variable probes (38%) focused on nitrate (NO 3 -N), nitrite (NO 2 -N), DO and DOM. The multivariable probes use specific wavelength LEDs ranging from 200 to 720 nm to observe absorbance, which is converted to equivalent COD, BOD, DOC, TOC, and Total Suspended Solid (TSS) values using calibration algorithms. The absorbance measurements are taken at 180 ° angle from the incident light and are particularly susceptible to changes in turbidity. Thus, most surveyed probes had a turbidity compensation component based on nephelometry principles and temperature compensation. Most probes also offer single or dual-beam (more common) corrections for absorbance using reference blanks.
Luminescence probes (28%) form a smaller proportion of the market and use fluorescence-based sensing for organic matter detection, including TLF, Chromophoric Dissolved Organic Matter (CDOM), Chlorophyll a , etc. and derive other variables such as BOD and even fecal coliforms through statistical extrapolation. Fluorescence measurements are prone to matrix-specific interference, such as inner-filtering effects (IFE) and thermal quenching, creating a non-linear relationship between intensity response and concentrations, especially at high fluorophore concentrations. In ex-situ (laboratory-based) experimental studies, absorbance measurements are used to correct the inner-filtering effects and improve the accuracy of the fluorescence measurements ( Kimball et al., 2020 ). The technique is now widely used in benchtop spectrometers with dual-mode features for absorbance and fluorescence (e.g., Duetta, Horiba Pvt. Ltd.). However, combining absorbance and fluorescence measurements to reduce noise, increase the linear range and measurement repeatability and achieve higher detection accuracies remains challenging for in-situ water quality monitoring probes.
4.2 Light sources and detectors
More than half of the surveyed sensors used LEDs of specific wavelengths, while less than a third preferred lamps, and some provided no information. The availability of affordable deep-UV LEDs (<280 nm, 41%) has led to the development of multiple commercial probes for nitrate, DO and DOM detection. UV–VIS LEDs (315–550 nm, 16%) are frequently used for absorbance and fluorescence measurement of dissolved organic matter (DOM) and algal activities in large water bodies, including marine environments. VIS (~550 nm) and NIR (~850 nm) LEDs are standard for turbidity sensors nowadays. Lamps provide an alternative to multiple LEDs as a single broadband (~190 to ~750 nm) light source, including Xenon flash lamps, Deuterium and Tungsten. In comparison, mercury lamps are used for narrow-band (~254 nm) applications measuring nitrate. They benefit from simpler components and designs and allow simultaneous measurements of multiple variables. However, the tradeoffs with LEDs are in terms of power requirements and component costs, both being considerably higher for lamps. Furthermore, some sensors (24%) use more than one LED in a single-variable probe, in which case the second LED is used for turbidity or absorbance-based corrections.
Approximately two-thirds of the products do not specify the type of detector their probes use. Among the rest, photodiodes are the most common detectors in single or arrayed formations. Like LEDs, advancements in optical technology have made photodiodes affordable and miniaturized them for in-situ deployment. The LED-photodiode specificity allows for precise measurements of specific variables such as nitrate and reduces the scope of interference by other contaminants. However, the specificity limits the potential to account for background interferents, which full spectrum light source and detector combinations can observe and account for in a single measurement ( Goblirsch et al., 2023 ). Current mini-spectrometers in the market are the size of a fingertip (C12880MA and C16767MA, Hamamatsu Pvt. Ltd.) and offer a broad wavelength UV–VIS–NIR range (225–1,000 nm, AFBR-S20M, Broadcom Inc.) in a single body. They use highly-sensitive image sensors such as CCD and CMOS and direct the incident light through optical slits and gratings. Depending on the type of applications, the mini-spectrometers can be tuned to maximum sensitivity at desired blaze angles and are likely to replace the photodiode arrays in future probes aimed at broad-spectrum sensing ( Goblirsch et al., 2023 ). However, only one commercial product (Opus, Trios) is currently mentioned to use a mini-spectrometer (200–360 nm) as the detector, with most probes relying on an array of photodiodes, sensitive to wavelengths of interest as an alternative way of broad-spectrum sensing.
4.3 Sensor components, design, and interface
The design of the optical window of a probe is largely dependent on measurement principles. As explained earlier, the fluorescence and nephelometry measurements are taken at right angles (15–90 ° ) to the incident light, and these probes preferred both incident light and detection inlet at the bottom of the sensor, albeit at a slight angle to each other. The design lends itself to better cleaning and antifouling properties. Conversely, the absorbance probes deploy a notched design, allowing the incident light to be 180 ° to the detector. This design enables path-lengths to be changed as per the application’s requirement and varied between 0.3–50 mm in the surveyed probes. Changeable path lengths are a crucial feature of absorbance probes, which allows them to target specific concentration ranges and resolutions based on the type of applications and environments ( Jensen and Bak, 2002 ; Skouteris et al., 2018 ; Shi et al., 2022 ). For example, probes appealing to drinking water monitoring require higher sensitivity to low concentrations of pollutants and thus need longer path-lengths (10–50 mm), whereas probes serving effluent treatment plants monitor high concentration loads and require shorter path-lengths (0.3–5 mm). However, the notched design is relatively more susceptible to fouling than downward-facing probes and requires active cleaning mechanisms.
Most probes (85%) deployed one or more automatic cleaning methods such as integrated wipers (47%), compressed air (37%), compressed water (9%) and even the use of ultrasonic waves (NiCaVis, Xylem Analytics). Few probes (22%) were solely based on flow-through designs, although many offered them as accessories for drinking water and other process applications. Probes with a flow-through design tend to rely on chemical cleaning or compressed air and have built-in humidity control systems to reduce the noise. Another important approach to minimize the impacts of fouling involves the use of nano-particle coating for optical windows (6%) ( Delgado et al., 2021 ).
The most common method of data interfacing in the probes is via a USB/R232/SI-12 cable, although newer probes come with Bluetooth and WLAN options. Most probes (63%) provided factory calibration of the sensors with optional recommendations of field calibration using standard reagents or comparisons with laboratory-based results. The data analysis and display were primarily server and web-dashboard-based. Despite considerable use in research, fewer probes mention post-processing capabilities or the use of artificial intelligence and machine learning tools, such as Liquid AI ( Ighalo et al., 2021 ; Mustafa et al., 2021 , Supplementary Table S1 ).
5 Challenges and opportunities for WQM using optical spectroscopy
5.1 scaling from in-situ to large water bodies.
Ongoing advances in sensor technology and processing algorithms have led to multiple applications of reflectance spectroscopy for real-time monitoring of water bodies at different scales ( Huang et al., 2018 ). Although cloud obscuration may be problematic for some platforms, the absorption and scattering properties of light are now being routinely monitored by various satellite platforms ( Tyler et al., 2022 ), as well as airborne remote sensing and Unmanned Aerial Vehicles (UAVs) ( Windle and Silsbe, 2021 ). In this context, the Inherent Optical Properties (IOPs) and color of water reflect the composition of optically active constituents in the medium, which include living phytoplankton (i.e., Chlorophyll a ), dissolved organic matter (DOM) and inorganic matter (i.e., dissolved and particulate matter in water), as well as back-scattering by suspended particles. However, the challenges of using and applying remotely sensed optical data lie in resolving the different components of the reflectance signal, i.e., (a) downwelling irradiance, (b) sky radiance, and (c) water-leaving radiance ( Mobley, 2001 ). While these challenges have somewhat constrained the use of remotely sensed optical data for catchment management, the examples summarized below illustrate their increasing potential to study a variety of applied problems, albeit where the real-time outputs are deemed usable after improved quality control and data processing algorithms (e.g., Matthews and Odermatt, 2015 ). For example, with respect to CDOM, which is often the main optical component of inland waters, the algorithms target the blue wavelengths of light, where absorption maxima occur. Still, atmospheric correction algorithms are weakest at this part of the spectrum, while studies of algal blooms focus on red wavelengths, where there is less interference from detrital pigments ( Wynne et al., 2008 ).
The utility of satellite data for WQM can be significantly enhanced by integrating the optical output from remote sensing platforms with in-situ hydrological data, yielding increasing quantities of continuous data. Most sensor platforms are positioned in sun-synchronous, low-Earth orbits ( Tyler et al., 2016 ). Inevitably, there is a tradeoff between spatial, spectral and temporal resolution. Their spatial resolution ranges from coarse (>200 m; AVHRR, MODIS, VIIRS), medium resolution (5–100 m; Landsat, SPOT, Sentinel-2) and high resolution (<5 m; IKONOS, RapidEye, CubeSat). The temporal resolution varies from half a day for some coarse platforms (e.g., MODIS) to between 5 and 26 days for medium-resolution sensors and to potentially daily resolution for platforms such as WorldView and RapidEye ( Huang et al., 2018 ). However, there are ongoing challenges with respect to untangling the multiple (dynamic) relationships between reflectance and absorbance for specific locations and conditions ( Moshtaghi et al., 2021 ).
As the spatial and temporal resolution of optical data has improved, reflectance-based technology has been successfully applied for water quality monitoring in more optically complex environments, such as lake-water bodies ( Kutser et al., 2005 ; Andrzej Urbanski et al., 2016 ; Botha et al., 2020 ), rivers (e.g., Sultana and Dewan, 2021 ), and marine pollution ( Thorhaug et al., 2007 ; Lu et al., 2013 ; Moshtaghi et al., 2021 ). Further work in this area requires paired optical and biogeochemical sampling, as demonstrated by Castagna et al. (2022) in their dataset from nine coastal and inland water bodies in Belgium, which included IOPs (e.g., absorption, scattering, beam attenuation and turbidity) and biogeochemical (e.g., suspended sediment, mineral fraction, particle size distribution, pigment concentration, etc.) properties. Specifically, within boreal lakes, Erlandsson et al. (2012) investigated the UV–VIS absorbance spectra in 983 water bodies in Sweden to report that alkaline lakes generally had lower absorbance and steeper spectral slopes than acidic lakes with a shorter water retention time while providing a comparable (and cost-effective) measure of the quantity and quality of dissolved organic matter (DOM).
Given public health concerns, many studies have investigated the efficacy of using optical sensors to monitor cyanobacteria (algal blooms) in different water bodies. While cyanotoxins cannot be directly quantified, Chlorophyll a can determine algal concentrations ( Stumpf et al., 2016 ), with an absorption peak at 620 nm. Ambrose-Igho et al. (2021) tested algorithms enabling reflectance data from Sentinel-2 for monitoring algal blooms in two reservoirs in Illinois, United States. Coffer et al. (2020) investigated the optical properties of >2,300 lakes of various sizes in the contiguous USA using data from Envisat’s Medium Resolution Imaging Spectrophometer and Sentinel 3 to examine the seasonal growth and decay of algal blooms and using a spectral shape algorithm previously described by Wynne et al. (2008) . The latter suggests that cyanobacteria are present if absorbance at 681 nm is below a baseline (defined by a line drawn between the 665 nm and 709 nm absorbance bands).
This selection of studies illustrates the increasing possibilities of using and applying optical sensors on various platforms to manage water resources at different scales. While problems with data accessibility and interoperability remain (e.g., Agnoli et al., 2023 ), some studies have successfully combined data from other satellite platforms (e.g., Zhao et al., 2020 ), as well as UAVs and in-situ sensors. Shi et al. (2019) highlight the need for automated processing algorithms that can be validated at increasing spatial and temporal resolution. However, the utility of these data is likely to be enhanced when combined with information from other sources, including in-situ monitoring, laboratory analyses and numerical modeling.
5.2 Low-cost sensors—testing and field validation
The recent emergence of low-cost microcontrollers and other electronic components has led to an increase in the development of “Do It Yourself (DIY)” environmental monitoring solutions for varied applications ( Mao et al., 2019 ). A wide range of sensors and associated data loggers have been proposed for monitoring water quantity and quality ( Chan et al., 2021 ), from non-contact water level sensors using ultrasonic ( Pereira et al., 2022 ) or Light Detection and Ranging (Lidar) technology ( Paul et al., 2020 ) to water quality sondes ( Kinar and Brinkmann, 2022 ). However, most developments have been linked to physical or electrochemical sensors, with optical sensors for monitoring water quality largely neglected, except for turbidity ( Kelley et al., 2014 ; Droujko et al., 2023 ).
While there have been some examples of field deployable spectroscopy-based sensors, notably low-cost fluorometers for measuring Chlorophyll a ( Leeuw et al., 2013 ) or organic matter ( Bridgeman et al., 2015 ), examples of absorbance (UV–VIS) based sensors are limited, but note a recent study by Goblirsch et al. (2023) . The lack of UV–VIS probes has been primarily due to technological limitations, such as the challenges associated with LED costs and stability ( Kneissl et al., 2019 ) at the deeper UV wavelengths required for nutrient or organic matter detection ( Etheridge et al., 2014 ). Furthermore, a lack of suitable off-the-shelf detector solutions has impeded the development of lower-cost full-spectrum absorbance sensors ( Ruhala and Zarnetske, 2017 ). Historically, expensive and bulky monochromators have been required for precise measurements, with the recent emergence of lower-cost solutions often lacking the needed range, resolution or measurement repeatability (e.g., AS7262 Visible Spectral Sensor). The few low-cost spectrophotometers/fluorometers reported in the literature leave many critical unanswered questions. Most studies report on short-term field deployments ( Leeuw et al., 2013 ) or laboratory testing ( Power et al., 2023 ). Hence, understanding measurement stability (electronic drift) or fouling rates (devices lack an automated cleaning system) remains limited ( Delgado et al., 2021 ). Further, there has been a lack of multiple devices fabricated to enable cross-comparisons between sensors to quantify inter-sensor measurement uncertainty ( Deutsch et al., 2018 ; Fettweis et al., 2019 ). Also, challenges associated with the calibration of multi-node sensor networks (lower-cost sensors will potentially facilitate more extensive networks of optical sensors) and best practices for using calibration reference standards, mainly when sensors are deployed in remote locations, are poorly defined ( Earp et al., 2011 ).
5.3 Detecting emerging contaminants using spectroscopy
Globally, 27% of the population drinks water contaminated from fecal sources (bio-pollution), of which the majority are in Southeast Asia (34%) and rural areas (41%) ( Bain et al., 2014 ). Changing agricultural practices and urbanization have added or enhanced several emerging contaminants (ECs), such as pesticides, microplastics, pharmaceuticals, etc., creating a cocktail of water pollutants ( Rochman, 2018 ; Sharma et al., 2019 ). Besides their potentially carcinogenic effects, ECs, like microplastics, are also seen as vectors and substratum for pathogenic bacteria, particularly in low-concentration groundwater environments ( Rochman, 2018 ; Ma et al., 2020 ). Thus, detecting ECs in effluents and natural waters is a high priority, although limited to traditional “gold standard” laboratory-based methods using benchtop instruments and techniques ( Manivannan et al., 2022 ). Sgroi et al. (2017) analyzed the EEMs of samples from two river systems in Italy to report significant correlations between known fluorescence indexes and EC groups, e.g., humic-like peaks correlating with sucralose, sulfamethoxazole and carbamazepine, and ibuprofen and caffeine correlating with tyrosine-like (Peak B) fluorescent peaks. Similarly, Wasswa et al. (2019) used the PARAFAC method to analyse fluorescent signals from treated wastewater and natural waters in the USA using benchtop and portable fluorometers and reported significant associations between TLF and ibuprofen (a pharmaceutical drug), diesel and gasoline, and tyrosine-peaks with diesel, gasoline, caffeine, isoxathion (a pesticide) and lopinavir (a pharmaceutical drug). They also recommended using portable fluorometers for in-situ tracking of ECs by proxy associations, especially in waters with high background CDOM and TLF concentrations, such as tertiary wastewater, and during events of high EC contamination, such as spills and leakages. Paradina-Fernández et al. (2023) demonstrated the very-low detection limits achieved by a benchtop fluorometer and PARAFAC modeling while quantifying organic micropollutants (e.g., pharmaceuticals) in surface and wastewater in Sweden. Moshtaghi et al. (2021) used experimental VIS-IR reflectance data to validate the algorithms used to remotely detect marine microplastics. The significant challenges to using fluorescence spectroscopy lie in moderating the fluorescent quenching and reducing inner-filtering effects. Similarly, UV–VIS absorbance measurements could provide additional information for screening ECs in natural waters ( Romão et al., 2017 ).
6 Future of optical WQM sensors
Table 1 summarizes the strengths and challenges of using optical sensors for in-situ water quality monitoring and the potential way forward. Currently, there is a wide range of commercially available sensors for in-situ monitoring of fluorescence and absorbance at high frequency (seconds to minutes resolution) ( Banna et al., 2014 ; Bouyé et al., 2016 ; Mukunda et al., 2022 ). However, these remain expensive and inhibit widespread statutory or regulatory use ( Hannah et al., 2022 ; Mukunda et al., 2022 ). Furthermore, high costs have constrained the development of distributed sensor networks in large river catchments, which could have helped identify sources of pollution, assess propagation and persistence and validate scaling up solutions using remotely sensed datasets. We suggest that recent reductions in component costs, both in terms of light sources (e.g., UV LEDs) and detectors (e.g., mini-spectrometers), previously barriers to the development of lower cost and power sensors, are likely to herald a new wave of affordable optical water quality sensors over the next 5 years ( Goblirsch et al., 2023 ).
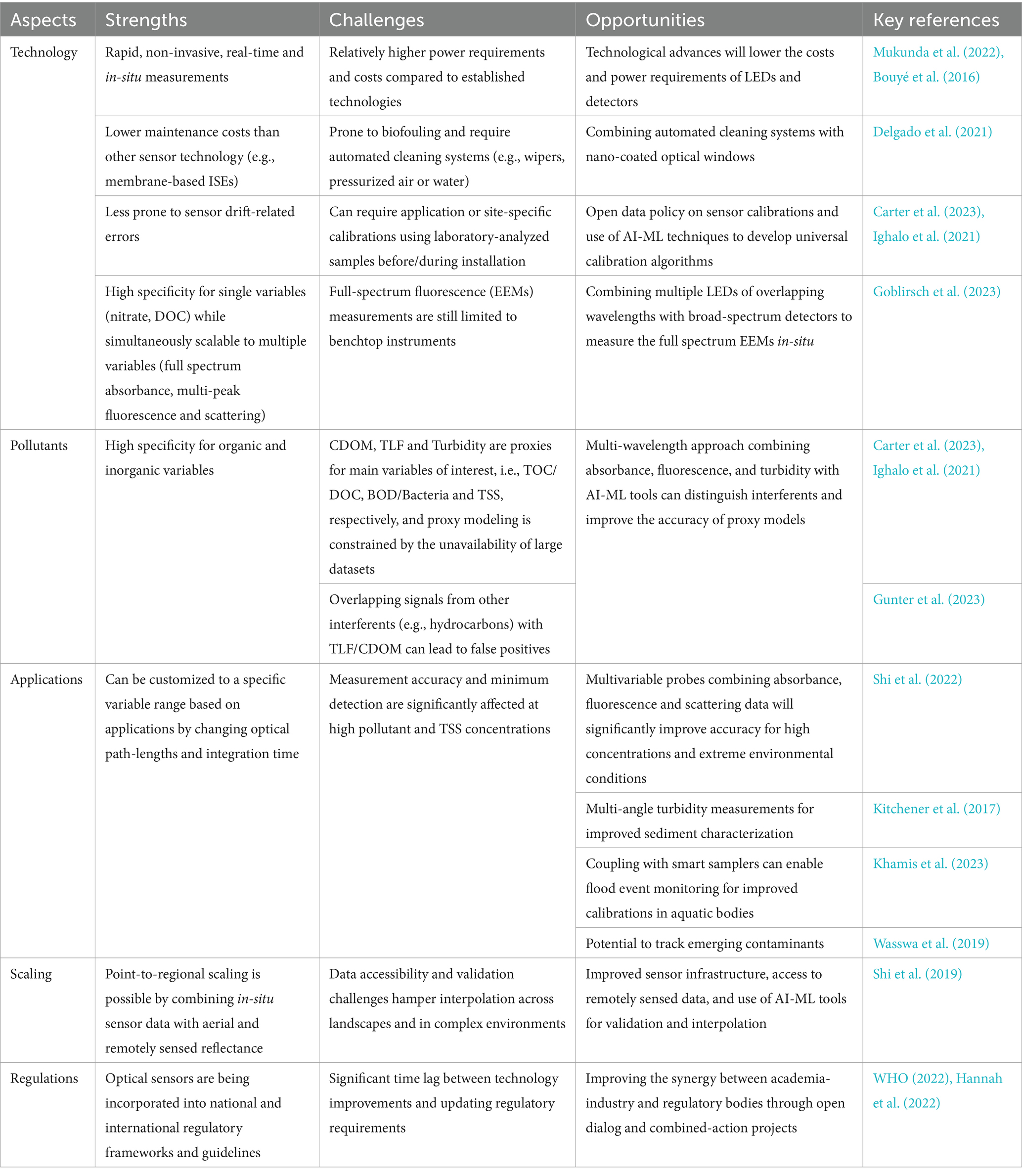
Table 1 . A summary table of the strengths, challenges, and opportunities in OWQM.
Some promising areas for future research involve integrating fluorescence, absorbance, and remote sensing (e.g., reflectance) to increase spatial coverage, particularly in large river networks ( Cao et al., 2022 ; Castagna et al., 2022 ; Fang et al., 2022 ). Future sensor networks (i.e., node locations) should, whenever possible, align with statutory monitoring locations where manual sampling occurs routinely ( Kinar and Brinkmann, 2022 ; Gaviria Salazar et al., 2023 ). This will ensure coupled datasets of laboratory and in-situ measurements, which are of comparable quality and can lead to the development of more rigorous calibration algorithms and proxy models ( Ighalo et al., 2021 ; Paradina-Fernández et al., 2023 ). With the potential for more measurement data from more locations, there is a clear need for the community to embrace emerging artificial intelligence and machine learning (AI-ML) approaches to aid calibration, reduce the signal-to-noise ratio, and capture and correct drift and outliers. It will also facilitate the development of more robust proxy models for hard-to-measure variables such as phosphorus and critical classes of emerging contaminants such as pharmaceuticals and pesticides ( Ighalo et al., 2021 ; Mustafa et al., 2021 ; Carter et al., 2023 ) and microplastics ( Moshtaghi et al., 2021 ). Further, despite remaining unexplored, merging multiple data streams from in-situ WQM, i.e., absorbance, fluorescence, scattering and reflectance, with remotely sensed surface reflectance data can facilitate the development of predictive water quality models and early warning systems for aiding tracking and managing large-scale events, such as, e.g., nutrient enrichment and harmful algal blooms ( Briciu-Burghin et al., 2023 ).
7 Conclusion
In this review, we have highlighted the current state of optical water quality monitoring and identified several challenges and future opportunities. The key concerns are high costs and power requirements, biofouling-induced sensor drift (particularly in dynamic environments with variable turbidity), the need for site-specific calibrations, and the limitation of current commercial WQM probes in performing full-spectrum EEMs in-situ. The rapid growth in UV-LED and full-spectrum detector technologies will likely address these issues. At the same time, the emergence of AI-ML-based data analytics holds the potential to develop universal sensor correction algorithms and proxy models for key variables of interest. Fluorescence spectroscopy may be useful in certain scenarios to detect novel/emerging contaminants in treated and natural waters. We suggest that attention should shift toward multivariable and multi-method (fluorescence, absorbance, scattering and reflectance) optical water quality monitoring, which can enhance existing water management and lead to the development of new applications. Augmenting remotely sensed surface-reflectance datasets with improved on-ground WQM infrastructure would be critical for remote monitoring of large water bodies, including marine and coastal pollution. An emerging application for multi-method monitoring and data fusion is linked to the control and monitoring of stormwater systems and combined sewer overflows. With increasing public awareness and newer environmental laws pushing for stricter monitoring of our water resources, the commercial scope of optical WQM sensors is bound to increase significantly. Thus, we advocate for greater synergy between industry, academia and public policy to ensure technological development supports effective pollution control and water management.
Author contributions
MK: Conceptualization, Formal analysis, Methodology, Visualization, Writing – original draft, Writing – review & editing. KK: Conceptualization, Funding acquisition, Writing – original draft, Writing – review & editing. RS: Funding acquisition, Writing – review & editing. DH: Conceptualization, Funding acquisition, Project administration, Writing – review & editing. CB: Conceptualization, Funding acquisition, Methodology, Writing – original draft, Writing – review & editing.
The author(s) declare that financial support was received for the research, authorship, and/or publication of this article. This research is supported by Innovate UK and represents outcomes from a Knowledge Transfer Partnership (KTP) between The University of Birmingham and Proteus Instruments Limited (KTP no. 13235). KK and CB are also supported by a Natural Environmental Research Council grant, NE/X011178/1.
Acknowledgments
The authors are thankful to Joshua Walmsley-Scotland, Donald Miller and Pillip Tierney at Proteus Instruments Limited for their knowledge-sharing and input. The authors thank Mayank Singh for graphical design support.
Conflict of interest
RS was employed by Proteus Instruments Ltd.
The authors declare that this study received funding from Innovate UK (KTP Grant no. 13235) in partnership with the University of Birmingham and Proteus Instruments Limited. RS is employed by Proteus Instruments Limited; and he was involved in the writing and editing of this article.
The remaining authors declare that the research was conducted in the absence of any commercial or financial relationships that could be construed as a potential conflict of interest.
The author(s) declared that they were an editorial board member of Frontiers, at the time of submission. This had no impact on the peer review process and the final decision.
Publisher’s note
All claims expressed in this article are solely those of the authors and do not necessarily represent those of their affiliated organizations, or those of the publisher, the editors and the reviewers. Any product that may be evaluated in this article, or claim that may be made by its manufacturer, is not guaranteed or endorsed by the publisher.
Supplementary material
The Supplementary material for this article can be found online at: https://www.frontiersin.org/articles/10.3389/frwa.2024.1380133/full#supplementary-material
Agnoli, L., Urquhart, E., Georgantzis, N., Schaeffer, B., Simmons, R., Hoque, B., et al. (2023). Perspectives on user engagement of satellite Earth observation for water quality management. Technol. Forecast. Soc. Change 189:122357. doi: 10.1016/j.techfore.2023.122357
Crossref Full Text | Google Scholar
Ahmad, S. R., and Reynolds, D. M. (1999). Monitoring of water quality using fluorescence technique: Prospect of on-line process control. Water Res. 33, 2069–2074. doi: 10.1016/S0043-1354(98)00435-7
Ambrose-Igho, G., Seyoum, W. M., Perry, W. L., and O'Reilly, C. M. (2021). Spatiotemporal analysis of water quality indicators in small lakes using sentinel-2 satellite data: lake bloomington and evergreen lake, Central Illinois, USA. Environ. Process. 8, 637–660. doi: 10.1007/s40710-021-00519-x
Andrzej Urbanski, J., Wochna, A., Bubak, I., Grzybowski, W., Lukawska-Matuszewska, K., Łącka, M., et al. (2016). Application of Landsat 8 imagery to regional-scale assessment of lake water quality. Int. J. Appl. Earth Obs. Geoinf. 51, 28–36. doi: 10.1016/j.jag.2016.04.004
Arndt, J., Kirchner, J. S., Jewell, K. S., Schluesener, M. P., Wick, A., Ternes, T. A., et al. (2022). Making waves: time for chemical surface water quality monitoring to catch up with its technical potential. Water Res. 213:118168. doi: 10.1016/j.watres.2022.118168
Bain, R., Cronk, R., Hossain, R., Bonjour, S., Onda, K., Wright, J., et al. (2014). Global assessment of exposure to faecal contamination through drinking water based on a systematic review. Trop. Med. Int. Heal. 19, 917–927. doi: 10.1111/tmi.12334
Baker, A. (2002). Fluorescence properties of some farm wastes: Implications for water quality monitoring. Water Res. 36, 189–195. doi: 10.1016/S0043-1354(01)00210-X
Baker, A., Cumberland, S. A., Bradley, C., Buckley, C., and Bridgeman, J. (2015). To what extent can portable fluorescence spectroscopy be used in the real-time assessment of microbial water quality? Sci. Total Environ. 532, 14–19. doi: 10.1016/j.scitotenv.2015.05.114
Baker, A., Ward, D., Lieten, S. H., Periera, R., Simpson, E. C., and Slater, M. (2004). Measurement of protein-like fluorescence in river and waste water using a handheld spectrophotometer. Water Res. 38, 2934–2938. doi: 10.1016/j.watres.2004.04.023
Banna, M. H., Imran, S., Francisque, A., Najjaran, H., Sadiq, R., Rodriguez, M., et al. (2014). Online drinking water quality monitoring: Review on available and emerging technologies. Crit. Rev. Environ. Sci. Technol. 44, 1370–1421. doi: 10.1080/10643389.2013.781936
Begum, M. S., Park, J. H., Yang, L., Shin, K. H., and Hur, J. (2023). Optical and molecular indices of dissolved organic matter for estimating biodegradability and resulting carbon dioxide production in inland waters: A review. Water Res. 228:119362. doi: 10.1016/j.watres.2022.119362
Botha, E. J., Anstee, J. M., Sagar, S., Lehmann, E., and Medeiros, T. A. G. (2020). Classification of Australian waterbodies across a wide range of optical water types. Remote Sens. 12:3018. doi: 10.3390/RS12183018
Bouyé, C., Kolb, H., and d’Humières, B. (2016). Mini and micro spectrometers pave the way to on-field advanced analytics. Photonic Instrum. Eng. III 9754:975408. doi: 10.1117/12.2212384
Briciu-Burghin, C., Power, S., Delgado, A., and Regan, F. (2023). Sensors for Coastal and Ocean Monitoring. Annu. Rev. Anal. Chem. 16, 451–469. doi: 10.1146/annurev-anchem-091922-085746
Bridgeman, J., Baker, A., Brown, D., and Boxall, J. B. (2015). Portable LED fluorescence instrumentation for the rapid assessment of potable water quality. Sci. Total Environ. 524–525, 338–346. doi: 10.1016/j.scitotenv.2015.04.050
Cao, Z., Shen, M., Kutser, T., Liu, M., Qi, T., Ma, J., et al. (2022). What water color parameters could be mapped using MODIS land reflectance products: A global evaluation over coastal and inland waters. Earth Sci. Rev. 232:104154. doi: 10.1016/j.earscirev.2022.104154
Caretta, M. A., Mukherji, A., Arfanuzzaman, M., Betts, R. A., Gelfan, A., Hirabayashi, Y., et al. (2022). “Water” in Climate Change 2022: Impacts, Adaptation and Vulnerability. Contribution of Working Group II to the Sixth Assessment Report of the Intergovernmental Panel on Climate Change . eds. B. R. H.-O. Pörtner, D. C. Roberts, M. Tignor, E. S. Poloczanska, K. Mintenbeck, and A. Alegría, et al. (Cambridge, New York, NY: Cambridge University Press), 551–712.
Google Scholar
Carstea, E. M., Bridgeman, J., Baker, A., and Reynolds, D. M. (2016). Fluorescence spectroscopy for wastewater monitoring: A review. Water Res. 95, 205–219. doi: 10.1016/j.watres.2016.03.021
Carstea, E. M., Popa, C. L., Baker, A., and Bridgeman, J. (2020). In situ fluorescence measurements of dissolved organic matter: A review. Sci. Total Environ. 699:134361. doi: 10.1016/j.scitotenv.2019.134361
Carter, J. B., Huffaker, R., Singh, A., and Bean, E. (2023). HUM: A review of hydrochemical analysis using ultraviolet-visible absorption spectroscopy and machine learning. Sci. Total Environ. 901:165826. doi: 10.1016/j.scitotenv.2023.165826
Castagna, A., Martínez, L. A., Bogorad, M., Daveloose, I., Dasseville, R., Dierssen, H. M., et al. (2022). Optical and biogeochemical properties of diverse Belgian inland and coastal waters. Earth Syst. Sci. Data 14, 2697–2719. doi: 10.5194/essd-14-2697-2022
Chan, K., Schillereff, D. N., Baas, A. C. W., Chadwick, M. A., Main, B., Mulligan, M., et al. (2021). Low-cost electronic sensors for environmental research: Pitfalls and opportunities. Prog. Phys. Geogr. Earth Environ. 45, 305–338. doi: 10.1177/0309133320956567
Chen, S., Jia, Y., Chen, H., Yang, W., Luo, Y., Li, Z., et al. (2022). Dual-wavelength-excitation aerosol fluorescence spectra detection using combined spectrometer with Czerny-Turner design. Spectrochim. Acta A Mol. Biomol. Spectrosc. 277:121260. doi: 10.1016/j.saa.2022.121260
Coffer, M. M., Schaeffer, B. A., Darling, J. A., Urquhart, E. A., and Salls, W. B. (2020). Quantifying national and regional cyanobacterial occurrence in US lakes using satellite remote sensing. Ecol. Indic. 111:105976. doi: 10.1016/j.ecolind.2019.105976
Croghan, D., Khamis, K., Bradley, C., Van Loon, A. F., Sadler, J., and Hannah, D. M. (2021). Combining in-situ fluorometry and distributed rainfall data provides new insights into natural organic matter transport dynamics in an urban river. Sci. Total Environ. 755:142731. doi: 10.1016/j.scitotenv.2020.142731
Dai, Y., Wang, H., Wang, J., Wang, X., Wang, Z., and Ge, X. (2022). Prediction of water quality based on SVR by fluorescence excitation-emission matrix and UV–Vis absorption spectrum. Spectrochim. Acta Part A Mol. Biomol. Spectrosc. 273:121059. doi: 10.1016/j.saa.2022.121059
Dapkus, R. T., Fryar, A. E., Tobin, B. W., Byrne, D. M., Sarker, S. K., Bettel, L., et al. (2023). Utilization of tryptophan-like fluorescence as a proxy for E. coli contamination in a mixed-land-use karst basin. Hydrology 10, 1–12. doi: 10.3390/hydrology10040074
Delgado, A., Briciu-Burghina, C., and Regan, F. (2021). Antifouling strategies for sensors used in water monitoring: Review and future perspectives. Sensors (Switzerland) 21, 1–25. doi: 10.3390/s21020389
Deutsch, E. S., Alameddine, I., and El-Fadel, M. (2018). Monitoring water quality in a hypereutrophic reservoir using Landsat ETM+ and OLI sensors: how transferable are the water quality algorithms? Environ. Monit. Assess. 190:141. doi: 10.1007/s10661-018-6506-9
Droujko, J., Kunz, F., and Molnar, P. (2023). Ötz-T: 3D-printed open-source turbidity sensor with Arduino shield for suspended sediment monitoring. HardwareX 13:e00395. doi: 10.1016/j.ohx.2023.e00395
Earp, A., Hanson, C. E., Ralph, P. J., Brando, V. E., Allen, S., Baird, M., et al. (2011). Review of fluorescent standards for calibration of in situ fluorometers: Recommendations applied in coastal and ocean observing programs. Opt. Express 19:26768. doi: 10.1364/oe.19.026768
Erlandsson, M., Futter, M. N., Kothawala, D. N., and Köhler, S. J. (2012). Variability in spectral absorbance metrics across boreal lake waters. J. Environ. Monit. 14, 2643–2652. doi: 10.1039/c2em30266g
Etheridge, J. R., Birgand, F., Osborne, J. A., Osburn, C. L., Burchell, M. R., and Irving, J. (2014). Using in situ ultraviolet-visual spectroscopy to measure nitrogen, carbon, phosphorus, and suspended solids concentrations at a high frequency in a brackish tidal marsh. Limnol. Oceanogr. Methods 12, 10–22. doi: 10.4319/lom.2014.12.10
European Union (2021). Good-quality water in Europe (EU Water Directive). 13–15. Available at: http://eur-lex.europa.eu/legal-content/EN/LSU/?uri=CELEX:32000L0060 .
Fang, C., Song, K., Paerl, H. W., Jacinthe, P. A., Wen, Z., Liu, G., et al. (2022). Global divergent trends of algal blooms detected by satellite during 1982–2018. Glob. Chang. Biol. 28, 2327–2340. doi: 10.1111/gcb.16077
Fellman, J. B., Hood, E., and Spencer, R. G. M. (2010). Fluorescence spectroscopy opens new windows into dissolved organic matter dynamics in freshwater ecosystems: A review. Limnol. Oceanogr. 55, 2452–2462. doi: 10.4319/lo.2010.55.6.2452
Fettweis, M., Riethmüller, R., Verney, R., Becker, M., Backers, J., Baeye, M., et al. (2019). Uncertainties associated with in situ high-frequency long-term observations of suspended particulate matter concentration using optical and acoustic sensors. Prog. Oceanogr. 178:102162. doi: 10.1016/j.pocean.2019.102162
Finegan, C. R., and Hasenmueller, E. A. (2023). Using in situ measurements of optical brighteners for rapid reconnaissance of wastewater inputs to water resources. Sci. Total Environ. 881:163378. doi: 10.1016/j.scitotenv.2023.163378
Frant, M. S. (1997). Where did ion selective electrodes come from? The story of their development and commercialization. J. Chem. Educ. 74, 159–166. doi: 10.1021/ed074p159
Gaviria Salazar, C., Roebuck, J. A., Myers-Pigg, A. N., and Ziegler, S. (2023). Self-diagnosis of model suitability for continuous measurements of stream-dissolved organic carbon derived from in situ UV–visible spectroscopy. Limnol. Oceanogr. Methods 21, 478–494. doi: 10.1002/lom3.10559
Gholizadeh, M. H., Melesse, A. M., and Reddi, L. (2016). A comprehensive review on water quality parameters estimation using remote sensing techniques. Sensors (Switzerland) 16:1298. doi: 10.3390/s16081298
Goblirsch, T., Mayer, T., Penzel, S., Rudolph, M., and Borsdorf, H. (2023). In situ water quality monitoring using an optical multiparameter sensor probe. Sensors 23:9545. doi: 10.3390/s23239545
Gunter, H., Bradley, C., Hannah, D. M., Manaseki-Holland, S., Stevens, R., and Khamis, K. (2023). Advances in quantifying microbial contamination in potable water: Potential of fluorescence-based sensor technology. Wiley Interdiscip. Rev. Water 10, 1–19. doi: 10.1002/wat2.1622
Hannah, D. M., Abbott, B. W., Khamis, K., Kelleher, C., Lynch, I., Krause, S., et al. (2022). Illuminating the 'invisible water crisis' to address global water pollution challenges. Hydrol. Process. 36, 1–5. doi: 10.1002/hyp.14525
Hart, S. J., and JiJi, R. D. (2002). Light emitting diode excitation emission matrix fluorescence spectroscopy. Analyst 127, 1693–1699. doi: 10.1039/b207660h
Huang, C., Chen, Y., Zhang, S., and Wu, J. (2018). Detecting, extracting, and monitoring surface water from space using optical sensors: a review. Rev. Geophys. 56, 333–360. doi: 10.1029/2018RG000598
Hudson, N., Baker, A., and Reynolds, D. (2007). Fluorescence analysis of dissolved organic matter in natural, waste and polluted waters—a review. River Res. Appl. 23, 631–649. doi: 10.1002/rra
Ighalo, J. O., Adeniyi, A. G., and Marques, G. (2021). Artificial intelligence for surface water quality monitoring and assessment: a systematic literature analysis. Model. Earth Syst. Environ. 7, 669–681. doi: 10.1007/s40808-020-01041-z
Jensen, P. S., and Bak, J. (2002). Near-infrared transmission spectroscopy of aqueous solutions: Influence of optical path-length on signal-to-noise ratio. Appl. Spectrosc. 56, 1600–1606. doi: 10.1366/000370202321115878
Johnson, K. S., and Coletti, L. J. (2002). In situ ultraviolet spectrophotometry for high resolution and long-term monitoring of nitrate, bromide and bisulfide in the ocean. Deep . Res. Part I Oceanogr. Res. Pap. 49, 1291–1305. doi: 10.1016/S0967-0637(02)00020-1
Johnson, E. K., Moran, D., and Vinten, A. J. A. (2008). A framework for valuing the health benefits of improved bathing water quality in the River Irvine catchment. J. Environ. Manag. 87, 633–638. doi: 10.1016/j.jenvman.2007.06.021
Kelley, C. D., Krolick, A., Brunner, L., Burklund, A., Kahn, D., Ball, W. P., et al. (2014). An affordable open-source turbidimeter. Sensors (Switzerland) 14, 7142–7155. doi: 10.3390/s140407142
Khamis, K., Bradley, C., Gunter, H. J., Basevi, G., Stevens, R., and Hannah, D. M. (2021). Calibration of an in-situ fluorescence-based sensor platform for reliable BOD5 measurement in wastewater. Water Sci. Technol. 83, 3075–3091. doi: 10.2166/wst.2021.197
Khamis, K., Bradley, C., Stevens, R., and Hannah, D. M. (2017). Continuous field estimation of dissolved organic carbon concentration and biochemical oxygen demand using dual-wavelength fluorescence, turbidity and temperature. Hydrol. Process. 31, 540–555. doi: 10.1002/hyp.11040
Khamis, K., Ouellet, V., Croghan, D., Hernandez Gonzalez, L. M., Packman, A. I., Hannah, D. M., et al. (2023). The Autobot-WQ: A portable, low-cost autosampler to provide new insight into urban spatio-temporal water quality dynamics. Front. Built Environ. 9:1072757. doi: 10.3389/fbuil.2023.1072757
Khamis, K., Sorensen, J. P. R., Bradley, C., Hannah, D. M., Lapworth, D. J., and Stevens, R. (2015). In situ tryptophan-like fluorometers: Assessing turbidity and temperature effects for freshwater applications. Environ Sci Process Impacts 17, 740–752. doi: 10.1039/c5em00030k
Kimball, J., Chavez, J., Ceresa, L., Kitchner, E., Nurekeyev, Z., Doan, H., et al. (2020). On the origin and correction for inner filter effects in fluorescence Part I: Primary inner filter effect-the proper approach for sample absorbance correction. Methods Appl. Fluoresc. 8:033002. doi: 10.1088/2050-6120/ab947c
Kinar, N. J., and Brinkmann, M. (2022). Development of a sensor and measurement platform for water quality observations: design, sensor integration, 3D printing, and open-source hardware. Environ. Monit. Assess. 194:207. doi: 10.1007/s10661-022-09825-9
Kirschke, S., Avellán, T., Bärlund, I., Bogardi, J. J., Carvalho, L., Chapman, D., et al. (2020). Capacity challenges in water quality monitoring: understanding the role of human development. Environ. Monit. Assess. 192:298. doi: 10.1007/s10661-020-8224-3
Kitchener, B. G. B., Wainwright, J., and Parsons, A. J. (2017). A review of the principles of turbidity measurement. Prog. Phys. Geogr. 41, 620–642. doi: 10.1177/0309133317726540
Kneissl, M., Seong, T. Y., Han, J., and Amano, H. (2019). The emergence and prospects of deep-ultraviolet light-emitting diode technologies. Nat. Photonics 13, 233–244. doi: 10.1038/s41566-019-0359-9
Kutser, T., Pierson, D. C., Kallio, K. Y., Reinart, A., and Sobek, S. (2005). Mapping lake CDOM by satellite remote sensing. Remote Sens. Environ. 94, 535–540. doi: 10.1016/j.rse.2004.11.009
Lee, E. J., Yoo, G. Y., Jeong, Y., Kim, K. U., Park, J. H., and Oh, N. H. (2015). Comparison of UV-VIS and FDOM sensors for in situ monitoring of stream DOC concentrations. Biogeosciences 12, 3109–3118. doi: 10.5194/bg-12-3109-2015
Leeuw, T., Boss, E. S., and Wright, D. L. (2013). In situ measurements of phytoplankton fluorescence using low cost electronics. Sensors (Switzerland) 13, 7872–7883. doi: 10.3390/s130607872
Lenaker, P. L., Corsi, S. R., De Cicco, L. A., Olds, H. T., Dila, D. K., Danz, M. E., et al. (2023). Modeled predictions of human-associated and Faecal-indicator bacteria concentrations and loadings in the Menomonee River, Wisconsin using in-situ optical sensors. PLoS One 18, 1–25. doi: 10.1371/journal.pone.0286851
Li, L., Wang, Y., Zhang, W., Yu, S., Wang, X., and Gao, N. (2020). New advances in fluorescence excitation-emission matrix spectroscopy for the characterization of dissolved organic matter in drinking water treatment: A review. Chem. Eng. J. 381:122676. doi: 10.1016/j.cej.2019.122676
Lu, Y., Li, X., Tian, Q., Zheng, G., Sun, S., Liu, Y., et al. (2013). Progress in marine oil spill optical remote sensing: detected targets, spectral response characteristics, and theories. Mar. Geod. 36, 334–346. doi: 10.1080/01490419.2013.793633
Ma, Y., Wang, L., Wang, T., Chen, Q., and Ji, R. (2020). Microplastics as vectors of chemicals and microorganisms in the environment. In: Particulate Plastics in Terrestrial and Aquatic Environments , 209–230.
Manivannan, B., Nallathambi, G., and Devasena, T. (2022). Alternative methods of monitoring emerging contaminants in water: a review. Environ Sci Process Impacts 24, 2009–2031. doi: 10.1039/d2em00237j
Mao, F., Khamis, K., Krause, S., Clark, J., and Hannah, D. M. (2019). Low-cost environmental sensor networks: recent advances and future directions. Front. Earth Sci. 7, 1–7. doi: 10.3389/feart.2019.00221
Matthews, M. W., and Odermatt, D. (2015). Improved algorithm for routine monitoring of cyanobacteria and eutrophication in inland and near-coastal waters. Remote Sens. Environ. 156, 374–382. doi: 10.1016/j.rse.2014.10.010
McClain, M. E., Boyer, E. W., Dent, C. L., Gergel, S. E., Grimm, N. B., Groffman, P. M., et al. (2003). Biogeochemical hot spots and hot moments at the interface of terrestrial and aquatic ecosystems. Ecosystems 6, 301–312. doi: 10.1007/s10021-003-0161-9
Mobley, C. D. (2001). “Radiative transfer in the ocean” in Encyclopedia of Ocean Sciences , 2321–2330.
Moshtaghi, M., Knaeps, E., Sterckx, S., Garaba, S., and Meire, D. (2021). Spectral reflectance of marine macroplastics in the VNIR and SWIR measured in a controlled environment. Sci. Rep. 11:5436. doi: 10.1038/s41598-021-84867-6
Mouchel, J. M., Lucas, F. S., Moulin, L., Wurtzer, S., Euzen, A., Haghe, J. P., et al. (2021). Bathing activities and microbiological river water quality in the paris area: a long-term perspective. Handb. Environ. Chem. 90, 323–353. doi: 10.1007/698_2019_397
Mukunda, D. C., Joshi, V. K., and Mahato, K. K. (2022). Light emitting diodes (LEDs) in fluorescence-based analytical applications: a review. Appl. Spectrosc. Rev. 57, 1–38. doi: 10.1080/05704928.2020.1835939
Murphy, K. R., Stedmon, C. A., Graeber, D., and Bro, R. (2013). Fluorescence spectroscopy and multi-way techniques, PARAFAC. Anal. Methods 5, 6557–6566. doi: 10.1039/c3ay41160e
Mustafa, H. M., Mustapha, A., Hayder, G., and Salisu, A. (2021). Applications of IoT and artificial intelligence in water quality monitoring and prediction: a review. Proceedings of the 6th International Conference on Computer Technology 2021, 968–975.
Nowicki, S., Lapworth, D. J., Ward, J. S. T., Thomson, P., and Charles, K. (2019). Tryptophan-like fluorescence as a measure of microbial contamination risk in groundwater. Sci. Total Environ. 646, 782–791. doi: 10.1016/j.scitotenv.2018.07.274
Paradina-Fernández, L., Wünsch, U., Bro, R., and Murphy, K. (2023). Direct measurement of organic micropollutants in water and wastewater using fluorescence spectroscopy. ACS EST Water 3, 3905–3915. doi: 10.1021/acsestwater.3c00323
Paul, J. D., Buytaert, W., and Sah, N. (2020). A technical evaluation of lidar-based measurement of river water levels. Water Resour. Res. 56:e2019WR026810. doi: 10.1029/2019WR026810
Peacock, M., Evans, C. D., Fenner, N., Freeman, C., Gough, R., Jones, T. G., et al. (2014). UV-visible absorbance spectroscopy as a proxy for peatland dissolved organic carbon (DOC) quantity and quality: Considerations on wavelength and absorbance degradation. Environ Sci Process Impacts 16, 1445–1461. doi: 10.1039/c4em00108g
Pereira, T. S. R., de Carvalho, T. P., Mendes, T. A., and Formiga, K. T. M. (2022). Evaluation of water level in flowing channels using ultrasonic sensors. Sustain. For. 14, 1–20. doi: 10.3390/su14095512
Phillips, S. L., Mack, D. A., and MacLeod, W. D. (1974). Instrumentation for water quality monitoring. Anal. Chem. 46, 345A–356A. doi: 10.1021/ac60339a715
Power, S. M., Free, L., Delgado, A., Richards, C., Alvarez-Gomez, E., Briciu-Burghina, C., et al. (2023). A novel low-cost plug-and-play multi-spectral LED based fluorometer, with application to chlorophyll detection. Anal. Methods 15, 5474–5482. doi: 10.1039/d3ay00991b
Raich, J. (2013). Review of sensors to monitor water quality. European reference network for critical infrastructure protection (ERNCIP) project. doi: 10.2788/35499
Rochman, C. M. (2018). Microplastics research — from sink to source in freshwater systems. Science 360, 28–29. doi: 10.1126/science.aar7734
Rode, M., Wade, A. J., Cohen, M. J., Hensley, R. T., Bowes, M. J., Kirchner, J. W., et al. (2016). Sensors in the stream: the high-frequency wave of the present. Environ. Sci. Technol. 50, 10297–10307. doi: 10.1021/acs.est.6b02155
Romão, J., Barata, D., Ribeiro, N., Habibovic, P., Fernandes, H., and Mul, G. (2017). High throughput screening of photocatalytic conversion of pharmaceutical contaminants in water. Environ. Pollut. 220, 1199–1207. doi: 10.1016/j.envpol.2016.11.015
Ruhala, S. S., and Zarnetske, J. P. (2017). Using in-situ optical sensors to study dissolved organic carbon dynamics of streams and watersheds: A review. Sci. Total Environ. 575, 713–723. doi: 10.1016/j.scitotenv.2016.09.113
Sagan, V., Peterson, K. T., Maimaitijiang, M., Sidike, P., Sloan, J., Greeling, B. A., et al. (2020). Monitoring inland water quality using remote sensing: potential and limitations of spectral indices, bio-optical simulations, machine learning, and cloud computing. Earth Sci. Rev. 205:103187. doi: 10.1016/j.earscirev.2020.103187
Scully, P. (1998). Optical techniques for water monitoring. Monit. Water Qual. Elsevier Science BV. 15–35. doi: 10.1016/b978-008043340-0/50003-5
Sgroi, M., Roccaro, P., Korshin, G. V., and Vagliasindi, F. G. A. (2017). Monitoring the behavior of emerging contaminants in wastewater-impacted rivers based on the use of fluorescence excitation emission matrixes (EEM). Environ. Sci. Technol. 51, 4306–4316. doi: 10.1021/acs.est.6b05785
Sharma, B. M., Bečanová, J., Scheringer, M., Sharma, A., Bharat, G. K., Whitehead, P. G., et al. (2019). Health and ecological risk assessment of emerging contaminants (pharmaceuticals, personal care products, and artificial sweeteners) in surface and groundwater (drinking water) in the Ganges River Basin, India. Sci. Total Environ. 646, 1459–1467. doi: 10.1016/j.scitotenv.2018.07.235
Shi, Z., Chow, C. W. K., Fabris, R., Liu, J., and Jin, B. (2022). Applications of online UV-vis spectrophotometer for drinking water quality monitoring and process control: a review. Sensors 22, 1–21. doi: 10.3390/s22082987
Shi, K., Zhang, Y., Qin, B., and Zhou, B. (2019). Remote sensing of cyanobacterial blooms in inland waters: present knowledge and future challenges. Sci. Bull. 64, 1540–1556. doi: 10.1016/j.scib.2019.07.002
Silva, G. M. E., Campos, D. F., Brasil, J. A. T., Tremblay, M., Mendiondo, E. M., and Ghiglieno, F. (2022). Advances in technological research for online and in situ water quality monitoring—a review. Sustain. For. 14, 1–28. doi: 10.3390/su14095059
Skouteris, G., Webb, D. P., Shin, K. L. F., and Rahimifard, S. (2018). Assessment of the capability of an optical sensor for in-line real-time wastewater quality analysis in food manufacturing. Water Resour. Ind. 20, 75–81. doi: 10.1016/j.wri.2018.10.002
Sorensen, J. P. R., Baker, A., Cumberland, S. A., Lapworth, D. J., MacDonald, A. M., Pedley, S., et al. (2018). Real-time detection of faecally contaminated drinking water with tryptophan-like fluorescence: defining threshold values. Sci. Total Environ. 622–623, 1250–1257. doi: 10.1016/j.scitotenv.2017.11.162
Sorensen, J. P. R., Diaw, M. T., Pouye, A., Roffo, R., Diongue, D. M. L., Faye, S. C., et al. (2020). In-situ fluorescence spectroscopy indicates total bacterial abundance and dissolved organic carbon. Sci. Total Environ. 738:139419. doi: 10.1016/j.scitotenv.2020.139419
Spring, K. R. (2001). “Detectors for fluorescence microscopy” in Methods in cellular imaging . ed. A. Periasamy, 40–52.
Stumpf, R. P., Davis, T. W., Wynne, T. T., Graham, J. L., Loftin, K. A., Johengen, T. H., et al. (2016). Challenges for mapping cyanotoxin patterns from remote sensing of cyanobacteria. Harmful Algae 54, 160–173. doi: 10.1016/j.hal.2016.01.005
Sultana, M. S., and Dewan, A. (2021). A reflectance-based water quality index and its application to examine degradation of river water quality in a rapidly urbanising megacity. Environ. Adv. 5:100097. doi: 10.1016/j.envadv.2021.100097
Thakur, A., and Devi, P. (2022). A comprehensive review on water quality monitoring devices: materials advances, current status, and future perspective. Crit. Rev. Anal. Chem. 1–26. doi: 10.1080/10408347.2022.2070838
Thorhaug, A., Richardson, A. D., and Berlyn, G. P. (2007). Spectral reflectance of the seagrasses: Thalassia testudinum , Halodule wrightii , Syringodium filiforme and five marine algae. Int. J. Remote Sens. 28, 1487–1501. doi: 10.1080/01431160600954662
Tiwari, A., Oliver, D. M., Bivins, A., Sherchan, S. P., and Pitkänen, T. (2021). Bathing water quality monitoring practices in europe and the United States. Int. J. Environ. Res. Public Health 18:5513. doi: 10.3390/ijerph18115513
Tyler, A., Hunter, P., De Keukelaere, L., Ogashawara, I., and Spyrakos, E. (2022). Remote sensing of inland water quality. Encycl. Inl. Waters Second Ed. 4, 570–584. doi: 10.1016/B978-0-12-819166-8.00213-9
Tyler, A. N., Hunter, P. D., Spyrakos, E., Groom, S., Constantinescu, A. M., and Kitchen, J. (2016). Developments in Earth observation for the assessment and monitoring of inland, transitional, coastal and shelf-sea waters. Sci. Total Environ. 572, 1307–1321. doi: 10.1016/j.scitotenv.2016.01.020
van Vliet, M. T. H., Jones, E. R., Flörke, M., Franssen, W. H. P., Hanasaki, N., Wada, Y., et al. (2021). Global water scarcity including surface water quality and expansions of clean water technologies. Environ. Res. Lett. 16:024020. doi: 10.1088/1748-9326/abbfc3
Vaughan, M. C. H., Bowden, W. B., Shanley, J. B., Vermilyea, A., and Schroth, A. W. (2019). Shining light on the storm: in-stream optics reveal hysteresis of dissolved organic matter character. Biogeochemistry 143, 275–291. doi: 10.1007/s10533-019-00561-w
Vaughan, M. C. H., Bowden, W. B., Shanley, J. B., Vermilyea, A., Wemple, B., and Schroth, A. W. (2018). Using in situ UV-Visible spectrophotometer sensors to quantify riverine phosphorus partitioning and concentration at a high frequency. Limnol. Oceanogr. Methods 16, 840–855. doi: 10.1002/lom3.10287
Warner, S. (2020). SDG Indicator 6.3.2 Technical Guidance Document No. 2: TARGET VALUES. 20200508. Available at: https://communities.unep.org/download/attachments/32407814/CDC_GEMI2_TechDoc2_Targetvalues_20200508.pdf?version=2&modificationDate=1595432590204&api=v2 .
Wasswa, J., Mladenov, N., and Pearce, W. (2019). Assessing the potential of fluorescence spectroscopy to monitor contaminants in source waters and water reuse systems. Environ. Sci. Water Res. Technol. 5, 370–382. doi: 10.1039/c8ew00472b
WHO (2022). Guidelines for drinking-water quality: fourth edition incorporating the first and second addenda. Available at: https://iris.who.int/bitstream/handle/10665/352532/9789240045064-eng.pdf?sequence=1 .
WHO and UNICEF (2023). Progress on Household Drinking Water, Sanitation and Hygiene (Jmp). Available at: http://apps.who.int/bookorders .
Windle, A. E., and Silsbe, G. M. (2021). Evaluation of unoccupied aircraft system (UAS) remote sensing reflectance retrievals for water quality monitoring in coastal waters. Front. Environ. Sci. 9, 1–15. doi: 10.3389/fenvs.2021.674247
Wynne, T. T., Stumpf, R. P., Tomlinson, M. C., Warner, R. A., Tester, P. A., Dyble, J., et al. (2008). Relating spectral shape to cyanobacterial blooms in the Laurentian Great Lakes. Int. J. Remote Sens. 29, 3665–3672. doi: 10.1080/01431160802007640
Yokota, H., Fukasawa, A., Hirano, M., and Ide, T. (2021). Low-light photodetectors for fluorescence microscopy. Appl. Sci. 11:2773. doi: 10.3390/app11062773
Zaidi Farouk, M. I. H., Jamil, Z., and Abdul Latip, M. F. (2023). Towards online surface water quality monitoring technology: A review. Environ. Res. 238:117147. doi: 10.1016/j.envres.2023.117147
Zainurin, S. N., Wan Ismail, W. Z., Mahamud, S. N. I., Ismail, I., Jamaludin, J., Ariffin, K. N. Z., et al. (2022). Advancements in monitoring water quality based on various sensing methods: a systematic review. Int. J. Environ. Res. Public Health 19:14080. doi: 10.3390/ijerph192114080
Zhao, Y., Liu, D., and Wei, X. (2020). Monitoring cyanobacterial harmful algal blooms at high spatiotemporal resolution by fusing Landsat and MODIS imagery. Environ. Adv. 2:100008. doi: 10.1016/j.envadv.2020.100008
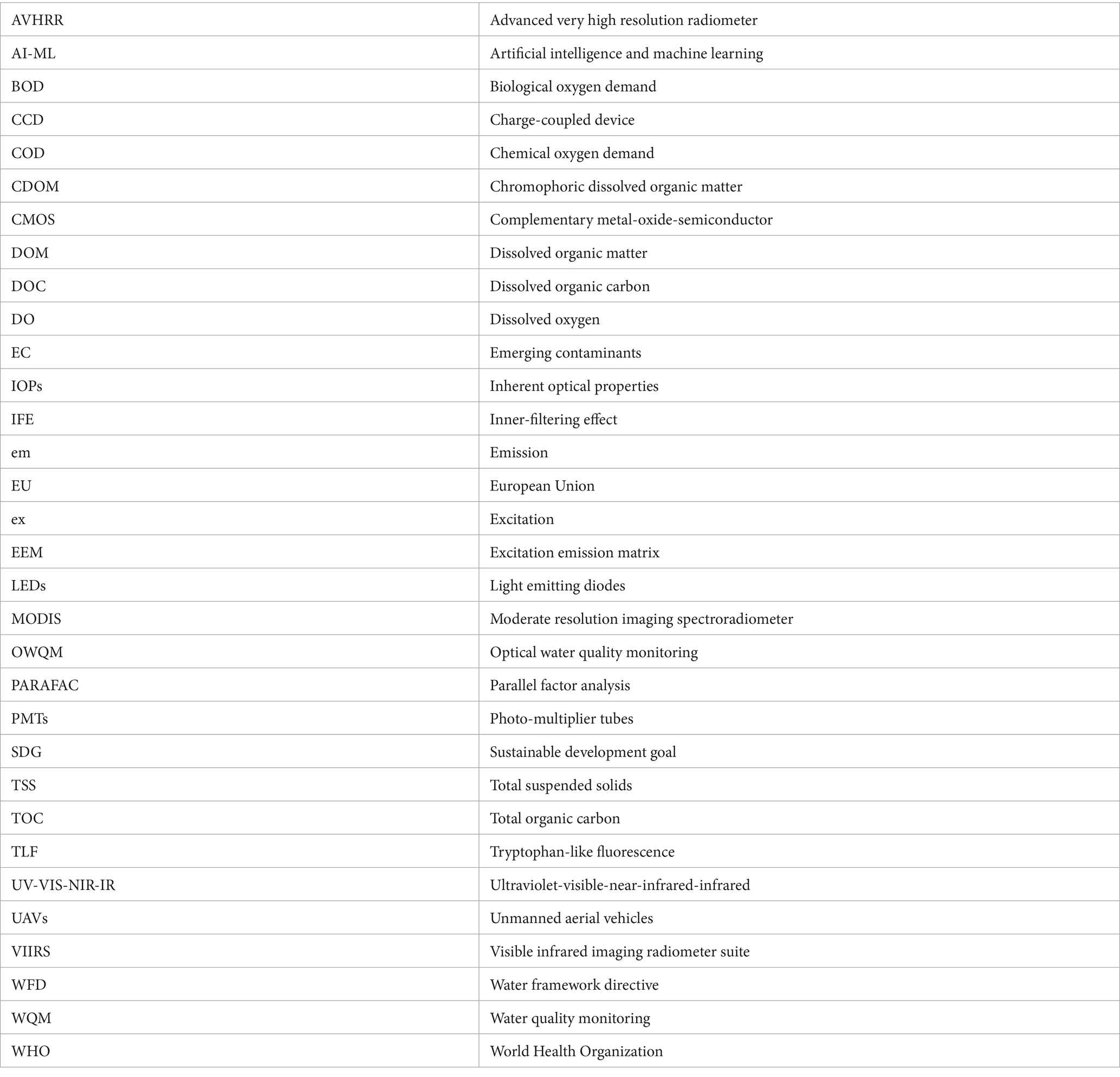
Keywords: optical spectroscopy, fluorescence, absorbance, water quality monitoring, sensors, in-situ
Citation: Kumar M, Khamis K, Stevens R, Hannah DM and Bradley C (2024) In-situ optical water quality monitoring sensors—applications, challenges, and future opportunities. Front. Water . 6:1380133. doi: 10.3389/frwa.2024.1380133
Received: 01 February 2024; Accepted: 04 April 2024; Published: 22 April 2024.
Reviewed by:
Copyright © 2024 Kumar, Khamis, Stevens, Hannah and Bradley. This is an open-access article distributed under the terms of the Creative Commons Attribution License (CC BY) . The use, distribution or reproduction in other forums is permitted, provided the original author(s) and the copyright owner(s) are credited and that the original publication in this journal is cited, in accordance with accepted academic practice. No use, distribution or reproduction is permitted which does not comply with these terms.
*Correspondence: Manish Kumar, [email protected]
† ORCID: Manish Kumar, http://orcid.org/0000-0002-6780-9336 Kieran Khamis, http://orcid.org/0000-0002-5203-3221 Rob Stevens, https://orcid.org/0009-0004-4725-0573 David M. Hannah, http://orcid.org/0000-0003-1714-1240 Chris Bradley, http://orcid.org/0000-0003-4042-867X
Disclaimer: All claims expressed in this article are solely those of the authors and do not necessarily represent those of their affiliated organizations, or those of the publisher, the editors and the reviewers. Any product that may be evaluated in this article or claim that may be made by its manufacturer is not guaranteed or endorsed by the publisher.

Nanofibers rid water of hazardous dyes
Dyes are a major environmental problem. efficient filters have now been developed -- based on cellulose waste.
Dyes, such as those used in the textile industry, are a major environmental problem. At TU Wien, efficient filters have now been developed -- based on cellulose waste. Using waste to purify water may sound counterintuitive. But at TU Wien, this is exactly what has now been achieved: a special nanostructure has been developed to filter a widespread class of harmful dyes from water. A crucial component is a material that is considered waste: used cellulose, for example in the form of cleaning cloths or paper cups. The cellulose is utilized to coat a fine nano-fabric to create an efficient filter for polluted water.
Colored poison in the water
Organic dyes represent the largest group of synthetic dyes, including so-called azo compounds. They are widely used in the textile industry, even in countries where little attention is paid to environmental protection, and the dyes often end up in unfiltered wastewater. "This is dangerous because such dyes degrade very slowly, they can remain in the water for a long time and pose great danger to humans and nature," says Prof. Günther Rupprechter from the Institute of Materials Chemistry at TU Wien.
There are various materials that can bind such dyes. But that alone is not enough. "If you simply let the polluted water flow over a filter film that can bind dyes, the cleaning effect is low," explains Günther Rupprechter. "It's much better to create a nanofabric out of lots of tiny fibers and let the water seep through." The water then comes into contact with a much larger surface area, and thus many more organic dye molecules can be bound.
Cellulose waste as a nano-filter
"We are working with semi-crystalline nanocellulose, which can be produced from waste material," says Qaisar Maqbool, first author of the study and postdoc in Rupprechter's research group. "Metal-containing substances are often used for similar purposes. Our material, on the other hand, is completely harmless to the environment, and we can also produce it by upcycling waste paper."
This nano-cellulose is "spun" together with the plastic polyacrylonitrile into nanostructures. However, this requires a lot of technical skill. The team from the TU Wien was successful with a so-called electrospinning process. In this process, the material is sprayed in liquid form, the droplets are electrically charged and sent through an electric field.
"This ensures that the liquid forms extremely fine threads with a diameter of 180 to 200 nanometers during curing," says Günther Rupprechter. These threads form a fine tissue with a high surface area -- a so-called "nanoweb." A network of threads can be placed on one square centimeter, with a total surface area of more than 10 cm 2 .
Successful tests
The tests with these cellulose-coated nanostructures were very successful: In three cycles, water contaminated with violet dye was purified, and 95% of the dye was removed. "The dyes remain stored in the nanoweb. You can then either dispose of the entire web or regenerate it, dissolve the stored dyes and reuse the filter fabric," explains Günther Rupprechter.
However, more work needs to be done: evaluating the mechanical properties of the sophisticated nanowebs, conducting biocompatibility tests, assessing sensitivity to more complex pollutants, and achieving scalability to industrial-grade standards. Now Rupprechter and his research team want to investigate how this dye filter technology can be transferred to other areas of application. "This technology could also be very interesting for the medical field," Rupprechter believes. "Dialysis, for example, also needs filtering out very specific chemical substances from a liquid." Coated nanofabrics may be useful for such applications.
- Recycling and Waste
- Hazardous Waste
- Environmental Science
- Environmental Issues
- Drought Research
- Sustainability
- Desalination
- Zebra mussel
- Water resources
- Water turbine
Story Source:
Materials provided by Vienna University of Technology . Note: Content may be edited for style and length.
Journal Reference :
- Qaisar Maqbool, Isabella Cavallini, Niusha Lasemi, Simona Sabbatini, Francesca Tittarelli, Günther Rupprechter. Waste‐Valorized Nanowebs for Crystal Violet Removal from Water . Small Science , 2024; 4 (4) DOI: 10.1002/smsc.202300286
Cite This Page :
Explore More
- Advance in Heart Regenerative Therapy
- Bioluminescence in Animals 540 Million Years Ago
- Profound Link Between Diet and Brain Health
- Loneliness Runs Deep Among Parents
- Food in Sight? The Liver Is Ready!
- Acid Reflux Drugs and Risk of Migraine
- Do Cells Have a Hidden Communication System?
- Mice Given Mouse-Rat Brains Can Smell Again
- How Do Birds Flock? New Aerodynamics
- Cancer: Epigenetic Origin Without DNA Mutation
Trending Topics
Strange & offbeat.
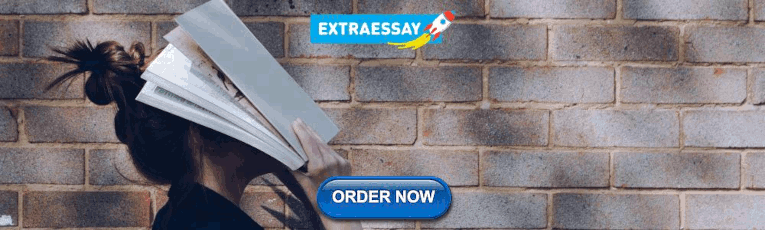
IMAGES
VIDEO
COMMENTS
The filtration of water significantly decreased its fluoride concentration. The fluoride content of purified water was approximately as much as zero in some cases. Keywords: Water Purification, Fluoride, Spectrophotometry. Introduction. Fluoride is a natural element branched from Fluorine. This element can be found in all sorts of water and soil.
The researchers also tested the filters' ability to remove contaminants such as E. coli and rotavirus — the most common cause of diarrheal disease. The treated filters removed more than 99 percent of both contaminants, a water treatment level that meets the "two-star comprehensive protection" category set by the World Health Organization. ...
Nonwoven fabric filters. N. Mao, in Advances in Technical Nonwovens, 2016 10.4.2 Nonwoven water filters. Water filtration is the process of removing or reducing the concentration of particulate matter, including suspended particles, parasites, bacteria, algae, viruses, and fungi, as well as other undesirable chemical and biological contaminants from contaminated water to produce safe and clean ...
In 2019, about 6% of public water utilities in the U.S. had a health-based violation. Due to the high risk of exposure to various contaminants in drinking water, point-of-use (POU) drinking water ...
Faucet-mounted filters often cost less than $50. Countertop filters can cost $80 or much more, depending on the filtration system they use. Under-sink water filters are more expensive, ranging ...
WASHINGTON - New laboratory tests commissioned by the Environmental Working Group found four water filters that reduce the detected " forever chemicals " known as PFAS in sampled drinking water by nearly 100 percent.. The results come as the U.S. Geological Survey announced alarming findings that almost half of the nation's tap water has been contaminated by one or more of the 32 ...
In research published in the journal Nature Communications, a team of Brown University researchers has found a way to orient those gaps, called nanochannels, in a way that makes them more useful for filtering water and other liquids of nanoscale contaminants. "In the last decade, a whole field has sprung up to study these spaces that form ...
Here we evaluated the effectiveness of point-of-use (POU) and point-of-entry (POE) residential drinking water filters in removing a suite of three perfluoroalkyl sulfonic acids, seven perfluoroalkyl carboxylic acids, and six per- and polyfluoroalkyl ether acids in homes in central (n = 61) and southeastern (n = 12) North Carolina. POU systems ...
This robust algae thrives in nitrogen- and phosphorus-contaminated water and could be cultivated and harvested in large quantities in Bangladesh. The filter paper's performance was measured ...
Abstract. Water filtration research has been undertaken for a variety of reasons. Studies have been performed to develop information for filtration theories and for design of filtration plants to remove suspended matter such as clays, algae, suspended matter in general, and asbestos fibers from water. Filtration studies related to removal of ...
Sometimes filters can cause more harm than good. Dr. Nguyen's research revealed that if water sits in a faucet or under-sink filter for a long period of time, such as overnight, it can actually ...
This Special Issue aims to present a summary of important aspects of water filtration, actually scattered in the scientific literature, to a research beginner. Any contribution should be situated in the chain of knowledge on filtration as to help research beginner to identify knowledge gaps. Prof. Dr. Chicgoua Noubactep.
This review paper focuses on the various types of Filtration techniques. available and whic h tec hnique is most suitable for which t ype of region. W e. will study the t ypes of natural ...
Approximately 3 cm3 of sapwood can filter water at the rate of several liters per day, sufficient to meet the clean drinking water needs of one person. ... Swartz C, Wouter Pronk W (2009) Decentralized systems for potable water and the potential of membrane technology. Water Research 43: 245-265. View Article Google Scholar 3. World Health ...
The EPA regulates over 80 contaminants—including arsenic, e-coli, cryptosporidia, chlorine, and lead—that may be found in drinking water from public water systems. While the EPA says that 90% of US public water systems meet its standards, you may want to use a water filter to further ensure your water's safety.
This study, based in San Bernardino County, Southern California, collected and examined tap water samples within the area to explore the feasibility of adopting non-industrial equipment and methods to reduce water hardness and total dissolved solids(TDS). We investigated how water quality could be improved by utilizing water boiling, activated carbon and sodium bicarbonate additives, as well ...
in your drinking water and recommendations for actions you can take. Types of Filters . There are many water filters on the market, but not all filters address PFAS. If you choose to use a filter, be sure to get one that is certified to remove or reduce PFAS in drinking water. As of April 2024, filter certifications focus on removing the ...
LONGEST FILTER LIFE. Travel Berkey Water Filter. KEY STATS: PFAS REDUCTION: 100% | INITIAL COST: $344.00 OVERALL VALUE: FILTER LIFE: 8+ YEARS (6,000 GALLONS) | ONE-YEAR COST: $344.00 This is an expensive filter. But it does offer a few perks for that large upfront cost, including 100 percent elimination of forever chemicals measured in these tests and a useful life of many years.
If EWG's Tap Water Database shows high levels of nitrate in your community, Zero Water may be your best overall choice for a pitcher filter. Pros: This filter removed 100 percent of nitrate. It was also highly effective on most other contaminants, ranking highly in our PFAS filter guide. It has a low initial cost, and the filters are easy to ...
A water filter is a device that removes impurities (such as dirt) from water using a physical barrier, a chemical process, or a biological process. In this environmental engineering science project, you will experiment with a water filter column kit and see how it works to find out how effective it is at filtering color and visible particles ...
This is the same technology in refrigerator filters and in some water pitcher filters, like Brita or PUR. ... Daily science news on research developments and the latest scientific innovations.
Ceramic water filters (CWFs) are promoted in developing nations by World Health Organisation (WHO) to improve water quality. In this study, CWFs were developed from raw materials such as cullet ...
Best overall: Brita Large Water Filter Pitcher. Best pitcher: Brita Large Stream Filter as You Pour. Best faucet: PUR PLUS Faucet Mount Water Filtration System. Best water bottle: LifeStraw Go ...
The group tested for 25 different types of PFAS using one water sample for each filter, a summary of the research reads. Most of the water filters the Environmental Working Group researched ...
Some water pitcher filters work better than the standard filter cartridge. There's a price tag on these systems for sure, but if you want the best-of-the-best, EWG's guide to PFAS water filters ...
Using waste to purify water may sound counterintuitive. But at TU Wien, this is exactly what has now been achieved. Researchers have developed a special nanostructure to filter a widespread class ...
1 Introduction. Water pollution is a major challenge to global water security, with >25% of the world's population unable to access safe drinking water in 2022 (WHO and UNICEF, 2023).After accounting for water quality issues, the fraction increases to 40%, with key hotspots in India, China, the Middle East, the Mediterranean and Mexico (van Vliet et al., 2021; Caretta et al., 2022).
A special nanostructure has been developed to filter a widespread class of harmful dyes from water. A crucial component is a material that is considered waste: used cellulose, for example in the ...