Academia.edu no longer supports Internet Explorer.
To browse Academia.edu and the wider internet faster and more securely, please take a few seconds to upgrade your browser .
Enter the email address you signed up with and we'll email you a reset link.
- We're Hiring!
- Help Center
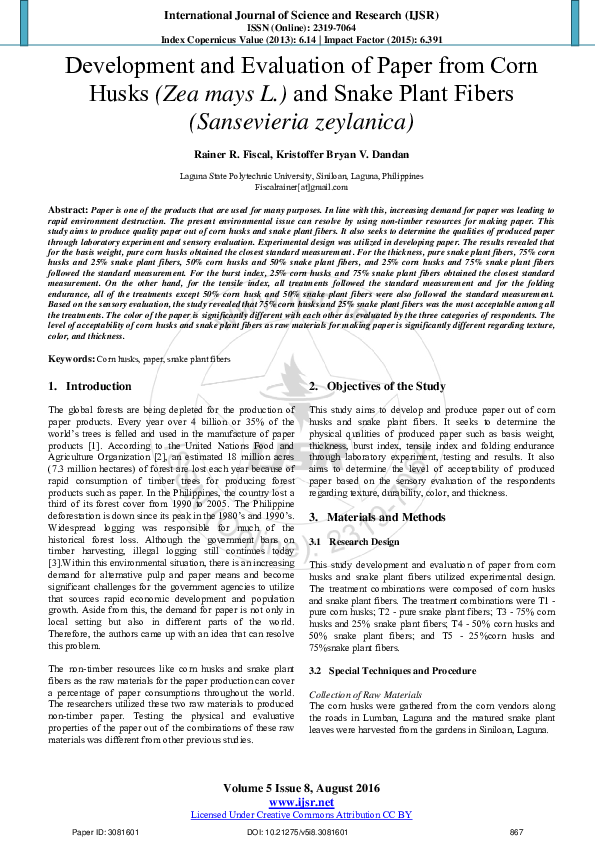
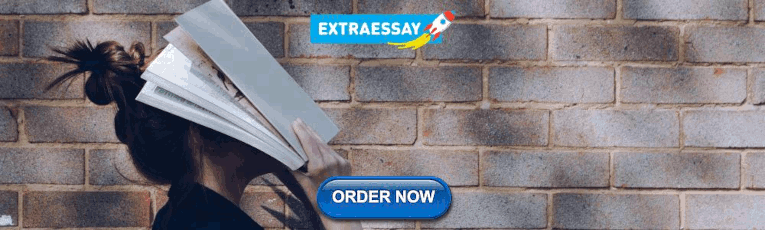
Development and Evaluation of Paper from Corn Husks (Zea mays L.) and Snake Plant Fibers (Sansevieria zeylanica)

Paper is one of the products that are used for many purposes. In line with this, increasing demand for paper was leading to rapid environment destruction. The present environmental issue can resolve by using non-timber resources for making paper. This study aims to produce quality paper out of corn husks and snake plant fibers. It also seeks to determine the qualities of produced paper through laboratory experiment and sensory evaluation. Experimental design was utilized in developing paper. The results revealed that for the basis weight, pure corn husks obtained the closest standard measurement. For the thickness, pure snake plant fibers, 75% corn husks and 25% snake plant fibers, 50% corn husks and 50% snake plant fibers, and 25% corn husks and 75% snake plant fibers followed the standard measurement. For the burst index, 25% corn husks and 75% snake plant fibers obtained the closest standard measurement. On the other hand, for the tensile index, all treatments followed the standa...
Related Papers
Taiwo K A Y O D E Fagbemigun
This study investigates the strength properties of paper samples obtained from pulp blend of corn husk and kenaf bark. Soda pulping of kenaf bark and corn husk was carried out using 18% and 7% alkali respectively. Handmade paper sheets were produced from bleached pulp samples combined in ratios 50% - 50%, 75% - 25% and 25% - 75%. Using a universal testometric machine, strength properties of the papers such as modulus of elasticity, tensile index and tear index were assessed. Results showed that, paper sample from 100% kenaf bark has the highest tear index (20.72 mN.m2/g) and tensile index (7.79 Nm/g), while paper sample from mixture of Kenaf bark and Corn husk (75% - 25%) possess highest modulus of elasticity (42.05 N/mm2). Lowest tensile index (2.77 Nm/g) and modulus of elasticity (12.42 N/mm2) values were observed for paper sample from 100% corn husk. Depending on targeted end use, papers of differing strength properties can be obtained from the combination of kenaf bark and corn husk.
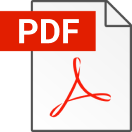
Muhammad Aliuddin Bakar
With paper demand increasing continuously, the deforestation process will be at a higher pace as the main raw material for paper production come from timber tree thus leads to unbalanced ecosystem problems. Using alternative fibre source especially from agriculture waste product for paper production seems like a right approach reducing the deforestation for paper raw material as it is available in abundance and low in cost. Therefore, a study on a physical characteristic of paper made from paddy straw was conducted to see the suitability of paddy straw as a raw material for paper production. Four variety type of paddy straw (MR219, MR220, MR269 and MRQ76) was used as the specimen to compare the physical characteristic of paper produced by different paddy straw variety. For each variety, three samples were treated with various concentrations of NaOH (20%, 25% and 30%) to determine the effect of concentration on paper produced. The material undergoes immersion, cooking, filtering and ...
Journal of Pharmacognosy and Phytochemistry
Dr. Shweta Mariyappanavar
Management of crop residue for industrial application is an upcoming researchable issue for sustainable environment. Locally available eco-friendly renewable resources viz., sugarcane bagasse, sisal fibre and maize husk have been used for development of handmade papers along with cotton rags and waste paper with different blend proportions. Among the papers, bagasse/cotton (60:40) handmade paper possessed significantly greater thickness, more uneven surface, low folding endurance with greater bursting and tears strength. Further, bursting, tear and tensile strength was found to be greater in bagasse/sisal/cotton (40:30:30) handmade paper followed by bagasse/ waste paper/ cotton paper which can be used as packaging materials to disposable paper plates. Effective management and utilization of agriculture crop residue with other cellulosic waste can be aptly suitable for production of eco friendly handmade papers which will provide sustainable enterprise for rural artisans.
PROCEEDINGS OF THE 13TH AUN/SEED-NET REGIONAL CONFERENCE ON MATERIALS (RCM 2020) AND THE 1ST INTERNATIONAL CONFERENCE ON MATERIALS ENGINEERING AND MANUFACTURING (ICMEM 2020)
Marisca Gondokesumo
KRISHNA RAVINDRAN
Wood is a prominent raw material in the production of pulp and paper, but due to the impact of forest depletion on the environment worldwide, research is being focused on non-wood sources as alternative sources of fibre and as a solution to destruction of the environment. The aim of this study therefore, is to investigate the suitability or otherwise of corn husk and plantain stalk which are abundant agro-waste materials in Nigerian environment in pulp and paper production. The pulping of corn husk and plantain stalk was achieved through Chemical Pulping process (Soda pulping) and the pulps obtained were analyzed for their chemical compositions. Also, the scanning electron microscopy (SEM) was used to analyze the surface morphological structure of the pulps produced. The resulting pulps were then used for papermaking and the Mechanical strength of papers obtained was determined. The results of chemical composition analysis for the pulp obtained from corn husk were (3.57%, 41.23%, 10%, 12.04% and 23.00 %) while that of plantain stalk were (11.19%, 57.86%, 10%, 4.60% and 35.96%) for Ash content, Cellulose content, Moisture content, Lignin content and Pulp yield respectively. The result of Mechanical strength analysis of the paper produced are (66.04 g/m 2 , 0.30 mm,5.33 Nm/g,12.83Nm 2 /g,0.13 N/mm 2 ,2.7 mm) from corn husk while that of plantain stalk are (30.99 g/m 2 ,0.08 mm,1.25 Nm/g,5.20Nm 2 /g,2.95 N/mm 2 ,2.20 mm) for Grammage, Thickness, Tensile index, Tear index, Modulus of Elasticity and Elongation at break respectively. The SEM images analysis showed that there is a more condensed and packed arrangement of fibre in corn husk than plantain stalk. The overall results showed that Corn
The threat on forest woods and environmental sustainability has led to an aggressive research into the suitability of several agricultural waste materials for pulp and paper production. Given this, the present study makes an inquest into the paper making potential of cornhusk in Nigeria. Thus, the results of the chemical analysis of the lingo-cellulosic material gives a lignin, cellulose, alcohol-cyclohexane solubility and ash values of about 15%, 44%, 4.57%, and 5.09% respectively. The assessment of fibre morphology of corn husk also confirms that it has short fibres similar to various hardwoods, whose length is <2 mm. Fibre diameter (21.89±5.1 µm), lumen width (6.63±3.5 µm), and cell wall thickness (7.63±2.3 µm) were also determined. Runkel ratio, slenderness ratio, coefficient of flexibility and wall rigidity values were calculated from the fibre dimension. The strength of hand sheet obtained from corn husk was tested and the results show that paper from corn husk has considerable strength. However, it is anticipated that the sheet qualities in terms of mechanical strength and tearing resistance can be improved upon by mixing the pulp of corn husks with pulp of non-wood plants with long fibres.
girja aryal
JPBIO (Jurnal Pendidikan Biologi)
Santhyami Santhyami
High demands for trees as the raw material of paper can disrupt the stability of the environment. It is necessary to find alternatives from other materials which are more environmentally friendly. This study aims to determine the quality of paper made from combining sugarcane bagasse with cassava peels or with corn husks using PVAc or tapioca starch adhesives. A completely randomized 2 x 2 factorial design with five times replication was used. The parameters tested were the tensile and tear resistance of the paper using a micrometer and a universal testing machine. A sensory analysis from panelists was also conducted. The combination of J1P1 produced a paper with the highest tensile strength (11.30 MPa) and the highest tear strength (1.82 MPa). The combination of J2P2 produced a paper with the lowest tensile strength (10.35 MPa) and the lowest tear strength (1.62 MPa). Variance analysis showed that the type of adhesive used showed a significant result on both tensile and tear resist...
Journal of Research in Forestry, Wildlife and Environment
Dr. David Ekhuemelo
Maize husk and stalk were assessed for their fibre characteristics and suitability for pulp and paper production. Fifteen (15) fibres were randomly selected and measured from each representative sample. It was observed that fibre length of maize husk was 1.37mm and that of maize stalk was 1.52mm. The fibre diameter (D) was 30.19 μm for each of the samples. The values of cell wall thickness and lumen width (d) for each sample were 8.82 μm and 13.67 μm respectively. Runkel ratio for maize husk and maize stalks was 1.23 each. This implies that they are feasible for pulp and paper production. The felting rate (slenderness ratio) was 45.93 for maize husk and 50.34 for maize stalk. The elasticity coefficient was 45.30% for maize husk and maize stalk and rigidity coefficients for maize husk and maize stalk was 29.21%. T-test showed that fibre length of maize husk and maize stalk were not significantly different. Keywords: Maize, husk, stalk, pulp, and fibre length.
International Journal of Engineering Research and Technology (IJERT)
IJERT Journal
https://www.ijert.org/investigation-into-the-mechanical-properties-of-bananasugar-cane-fibre-paper-blend https://www.ijert.org/research/investigation-into-the-mechanical-properties-of-bananasugar-cane-fibre-paper-blend-IJERTV3IS110719.pdf This research study was carried out to determine the performance and effect of combining long and short fibre in paper making. Bagasse (short fibre) and banana(long fibre) stem were used for the production of paper in this study. This work took the advantage of waste materials from banana and sugar cane stem which were usually discarded by burning or left to rotten. Hand-sheet method was used for the production, the samples from the products were tested for tear strength, tensile strength, and abrasion resistance. The results show that samples composition from 'A' through F were having considerably good tear resistance value range from 0.20 N/mm 2 to 0.13N/mm 2. For tensile strength samples composition from A through F were having good tensile strength value range from 0.42N/mm 2 to 0.38N/mm2 than the rest. F has the best abrasion resistance property.
RELATED PAPERS
Thu Trang Lê
Kouassi Rufin Kouadio
Biosfer: Jurnal Tadris Biologi
langen puspitawati
Feride Tuğrul
Molecular Brain
Michel Bouvier
Yola Yulanda
Jennifer Wyffels
Isla de Arriarán: revista cultural y …
Juana olga Guerrero
Thin Solid Films
Zoran Djinovic
Paolo Tomassini
Velayudham Ganesan
Jurnal Agrisep
dian retno intan
Journal of Materials Science: Materials in Electronics
mahnaz saraei
Maritza Escobar Villanueva
NIKOLAI NEUSTROEV
Alexis Tabares castillo
JKMP (Jurnal Kebijakan dan Manajemen Publik)
Dr. Uday Dokras
European Political Science Review
European Annals of Allergy and Clinical Immunology
Leonor Carneiro-Leão
Abraham Kebede
João de Pina-Cabral
Fisioterapia e Pesquisa
Valeria Elui
Unri Conference Series: Community Engagement
Yulia Oktavia
Journal of Affective Disorders
Astrid Kamperman
- We're Hiring!
- Help Center
- Find new research papers in:
- Health Sciences
- Earth Sciences
- Cognitive Science
- Mathematics
- Computer Science
- Academia ©2024
Accessibility Links
- Skip to content
- Skip to search IOPscience
- Skip to Journals list
- Accessibility help
- Accessibility Help
Click here to close this panel.

As a society-owned publisher with a legacy of serving scientific communities, we are committed to offering a home to all scientifically valid and rigorously reviewed research. In doing so, we aim to accelerate the dissemination of scientific knowledge and the advancement of scholarly communications to benefit all.
Materials Research Express supports this mission and actively demonstrates our core values of inclusive publishing and trusted science . To find out more about these values and how they can help you publish your next paper with us, visit our journal scope .
Purpose-led Publishing is a coalition of three not-for-profit publishers in the field of physical sciences: AIP Publishing, the American Physical Society and IOP Publishing.
Together, as publishers that will always put purpose above profit, we have defined a set of industry standards that underpin high-quality, ethical scholarly communications.
We are proudly declaring that science is our only shareholder.
Study of raw and chemically treated Sansevieria ehrenbergii fibers for brake pad application
Senthil Kumaran S 1 , Kathiravan Srinivasan 2 , Ponmariappan M 1 , Yashwhanth S 1 , Akshay S 1 and Yuh-Chung Hu 4,3
Published 11 May 2020 • © 2020 The Author(s). Published by IOP Publishing Ltd Materials Research Express , Volume 7 , Number 5 Citation Senthil Kumaran S et al 2020 Mater. Res. Express 7 055102 DOI 10.1088/2053-1591/ab8f48
Article metrics
2161 Total downloads
Share this article
Author e-mails.
Author affiliations
1 Department of Manufacturing Engineering, School of Mechanical Engineering, Vellore Institute of Technology, Vellore - 632 014, Tamil Nadu, India
2 School of Information Technology and Engineering, Vellore Institute of Technology, Vellore - 632 014, Tamil Nadu, India
3 Department of Mechanical and Electromechanical Engineering, National ILan University, No. 1, section 1, Shenlung Rd., ILan City, ILan County, Taiwan
Author notes
4 Author to whom any correspondence should be addressed.
Senthil Kumaran S https://orcid.org/0000-0001-9994-9424
Kathiravan Srinivasan https://orcid.org/0000-0002-9352-0237
Yuh-Chung Hu https://orcid.org/0000-0003-0183-8182
- Received 9 April 2020
- Revised 29 April 2020
- Accepted 1 May 2020
- Published 11 May 2020
Peer review information
Method : Single-anonymous Revisions: 2 Screened for originality? Yes
Buy this article in print
The replacement for synthetic fiber and other materials with natural fiber in the brake pad has become a key research area in many industries. Many production industries are always focused on natural fiber over synthetic fibers because of its advantage of biodegradability, less expensive, availability, and environment friendly. However, natural fibers cannot be used directly in composites because of its poor adhesion behavior between matrix and fiber and observe high water content in the fiber. Further, the excess water content in the inside fiber has been removed by using alkaline, benzoylation, and acetylation treatment. It has been achieved by the same chemical concentration but with different time duration. The Fourier-transform infrared spectroscopy (FT-IR) analysis revealed that the amount of cellulose content after chemical treatment was improved and decrease in other substances like hemicellulose, lignin, and wax content. X-Ray Diffraction (XRD) analysis study confirmed that the rise in the crystalline index (70.41 from 52.7) of alkali-treated SEFs at 30 min. The thermogravimetric analysis study has confirmed that the increase in the degradation temperature of Benzoyl-treated SEFs (387 °C) at 60 min when compared with untreated fiber (320 °C). The Scanning Electron Microscope (SEM) results have confirmed that the elimination of impurities from the fiber surface after chemical treatment. Further, the acetyl- treated fibers have shown that better hydrophobic properties than other treated fibers. In the above tests have been concluded that the chemically treated fibers, as one of the suitable materials for the development of the brake pad application.
Export citation and abstract BibTeX RIS
Original content from this work may be used under the terms of the Creative Commons Attribution 4.0 licence . Any further distribution of this work must maintain attribution to the author(s) and the title of the work, journal citation and DOI.
1. Introduction
Brake pads play a vital role in automotive vehicles. Every vehicle requires brakes to retard or to stop the vehicle. The braking system mainly consists of a drum and pads. When the brakes are applied, the pad comes in contact with the drum, and the friction is generated between brake drum and padding, the vehicle gets retarded. The mechanism of the brake pad is to convert kinetic energy to heat energy through friction. Earlier brake pads were manufactured by using asbestos embedded in a matrix with several other materials. However, due to its carcinogenic nature, the use of asbestos in brake pad has been stopped [ 1 ]. Many researchers studied and developed many materials for brake pad applications, which are free from asbestos-like Semi-metallic, ceramics, glass as a fiber. Usually, brake pads contain various combinations of ingredients of some 5–30 materials, mainly used like abrasives, reinforcement, binders, and friction modifiers for adequate friction and wear performance. Among these, reinforcement plays a vital role as its properties decide the performance of the brake pad. Thus It is essential to choose perfect reinforcement for the brake pad. Reinforcement helps to make the brake pad reliable. Mainly, fibers are used as a reinforcement because it has frictional property, excellent thermal resistance, etc. There are two types of fibers used, natural and synthetic. Synthetic fibers like PVC, potassium Octatitanate, Polyethylene fibers, etc are used in the brake pads and have performed well. Nevertheless, due to health and environmental issues, the usage of these fibers was reduced. The natural fiber was preferred to avoid the usage of synthetic fiber.
Natural fiber plays a vital role as a raw material in many upcoming industries. Due to an increase in environmental issues, Natural fibers have been proved to be better raw materials in many sectors, mainly in the automotive industry. The most common materials used in automotive sectors are synthetic fibers, like carbon and glass [ 2 ]. However, researchers are very much focused on studying the natural fibers as it provided the desired properties like less expensive, lower density, high toughness, harmless, sustainable, soft, and environment friendly features [ 3 ]. Many natural fibers like fibers of Banana, Bamboo, Coconut, Kenaf, etc are used in the day to day applications.
Sansevieria ehrenbergii fibers are obtained from Sansevieria ehrenbergii plant leaves. It is composed of hemicellulose, cellulose, and lignin. The presence of a maximum amount of cellulose content is essential as it helps in efficient reinforcement. Hemicellulose is very hydrophilic and easily gets dissolved in alkali. Lignin is very amorphous and hydrophobic [ 4 ]. The raw fibers cannot be used as the reinforcement in brake pad composite because it does not have the required property like high thermal stability, good strength, etc. To achieve it, we need to eliminate the impurities like hemicellulose, wax, lignin, pectin, etc from the SEF. Thus these fibers are treated with chemicals to enhance their properties. Many researchers have conducted chemical treatment on the fibers. Chemicals like alkaline, acetylation, silane, permanganates acrylation, etc have been done [ 5 ]. In this study, performed three different chemical treatments, mainly Alkaline, Acetylation, and Benzoylation by varying the soaking time for 30 min interval (i.e., 30, 60, 90, 120, 150 min). The effect of various chemical treatments on natural fiber was studied with the help of various testing like TGA, XRD, FTIR, SEM, and water absorption. From this study, it can observe which chemical treatment provides the required result with the duration.
2. Methodology
2.1. materials.
The Sansevieria ehrenbergii plant leaves were collected from the Erode region, Tamil Nadu, South India. This plant is called a Snake grass plant and comes under the Sisal plant family [ 6 ]. Untreated Sansevieria ehrenbergii fibers (SEFs), Sodium hydroxide pellets, concentrated Hydrochloric acid, and deionized water is used in the alkali treatment [ 7 ]. Glacial acetic acid, acetic anhydride, and sulphuric acid are used for Acetylation treatment. Benzoyl chloride and deionized water are used for Benzoylation treatment [ 8 ].
2.2. Fiber extraction
The various process is adopted from the extraction of natural fibers from plants. Retting is a common technique used for fiber extraction. Retting is also called a degumming process. It is an effective method for the separation of fiber from the stem and leaves by removing the outer layer and gum-like material from the surface of the leaves. Different Retting techniques process is available like water retting process, Chemical retting, Spray enzyme retting, and Bio-innovative retting process [ 9 – 11 ]. By using a sharp knife, the Sansevieria ehrenbergii leaves are chopped from Sansevieria ehrenbergii plant. The Sansevieria ehrenbergii leaves were kept inside the water for four days as a part of the soaking process. Then resin-like material and outer layer of the leaf are removed, and single fiber is obtained by manual peeling. The collected fiber is dried under sunlight for 8 h.
2.3. Chemical treatment
2.3.1. alkaline treatment.
For natural fibers to be used as reinforcement in composite, alkaline treatment is commonly used. To increase the surface roughness of the fiber, the alkaline treatment, separates hydrogen bonds from the grid structure. The removal of minor constituents like lignin, hemicellulose, and amorphous cellulose from the outer surface of the fiber to a certain extent [ 7 ]. The hydroxyl group present in natural fiber is ionized to the alkoxide group after the treatment [ 12 ]. This treatment improves fiber matrix interlocking and increase cellulose content in fiber which increase adhesion property, reduce water absorption and thermal stability [ 13 ]
Initially, for this treatment, SE fibers were soaked in 5% (w/v) NaOH solution. The immersion period for fiber in NaOH solution was 30 min, 60 min, 90 min, 120 min, and 150 min. The fiber samples each weighing 6 g are soaked in 200 ml of water containing 10 g of sodium pellets. A total of 5 samples were prepared, and each sample is taken out for 30 min. Then it neutralized by using a few drops of HCl for 3–5 min to prevent a further chemical reaction. These fibers are then oven-dried by placing it in an electric oven and heated at the temperature of 80 °C for 6 h [ 14 ].
2.3.2. Acetylation treatment
The acetylation process was the best method to modify the surface properties of the fiber and also making the fiber hygroscopic. This treatment is most used adequately in textile goods as the rate and extent of swelling is highly reduced [ 15 , 16 ]. The process is applied to the wood cellulose because it improves dimensional accuracies, increases the stability of the cell wall, and also safe environmental degradation [ 17 ]. It is an esterification method in which reaction takes place between an acetyl functional group (CH 3 COO–) and hydroxyl group(–OH). This process can take place with or without the help of a catalyst. During this process, the plasticization of the cellulosic fibers takes place. For the acetylation process, acetic anhydride (CH 3 COOCOCH 3 ) was preferred more than acetic acid because acetic acid does not react adequately with cellulose [ 16 ]. However, before treating with a dioxide, it is treated with acetic acid as anhydride is a poor swelling agent for cellulose. Also, acetic acid increases the rate of the chemical reaction.
The reaction that takes place between the acetic anhydride and the hydroxyl group is the removal of the hydroxyl group. Those are minor constituents like lignin, hemicellulose, and amorphous cellulose [ 18 ]. The reaction does not take place with the crystalline cellulose as it is complicated to remove the strong interchain bonding between them, which is very difficult for any chemicals to react. Also, this reaction results in the generation of by-product acetic acid, which is to be removed before the fiber being along with the lignocellulosic material [ 19 ]. The hygroscopic nature of natural fibers is reduced, which results in dimensional stability. Water absorption mainly depends on factors like fibers hydrophilic nature, temperature, the orientation of the fiber, protection of the surface, exposed surfaces area, loading of the fiber, diffusivity, and amount of void content of the individual components [ 5 ]. This treatment also improves the fiber-matrix adhesion property, which also improves the tensile and thermal properties of the composites [ 20 ]. The chemically treated fibers had developed a very rough surface and also developed the amount of voids present, which provides better mechanical interlocking with the polystyrene matrix [ 21 ]. The chemical reaction is given by
Initially, for this treatment, SE fibers are soaked in 5% (w/v) NaOH solution. Then acetylation treatment was applied for each group of SE fibers in glacial acetic acid concentration solution of 5%. The immersion period for fiber in glacial acetic acid solution was 30 min, 60 min, 90 min, 120 min, and 150 min. The samples each weighing 6 g are to be treated with NaOH and then is neutralized by using HCl concentration [ 22 ]. Then the fibers sample is soaked in 200 ml glacial acetic acid and 2–4 drops of acetic anhydride solution. A total of 5 samples were prepared, and each sample is taken out for 30 min. Then it neutralized by using a few drops of conc. H 2 SO 4 for 3–5 min to prevent further chemical reaction [ 23 ]. These fibers are then oven-dried by placing it in an electric oven and heated at a temperature of 80 °C for 4 h. Figure 1 shows the process of neutralizing the acid-treated fiber. Figure 2 portrays the oven dried fiber.
2.3.3. Benzoylation treatment
Benzoylation treatment is essential and effective in fiber treatment. Usually, Benzoyl chloride was used for the treatment. It contains a benzoyl group, which helps to decrease the hydrophilic property of the fiber. At the beginning of Benzoyl treatment, the fiber is treated with NaOH so that it will trigger the hydroxyl groups, which are present in cellulose and lignin of the fiber [ 24 ]. This treatment improves the adhesion property, reduces water absorption, and increase thermal stability. The following reaction takes place during chemical treatment:-
For this treatment, SE fibers are soaked in 5% (w/v) NaOH solution. Then treated SE fibers are soaked in 5%/v benzoyl chloride solution. The immersion period for fiber in benzoyl chloride solution was 30 min, 60 min, 90 min, 120 min, and 150 min.50 ml of benzoyl chloride is added on 30 grams of SEFs in 1 liter of water for 150 min. At each interval of 30 min, 6 grams of SEFs were taken out and neutralized with deionized water and were cleaned with water and dried in the oven for 4 h at 70 °C [ 25 ]. Figure 3 illustrates the sample that is cleaned after soaking in Benzyl chloride. Figure 4 displays the sample that is dried in an oven.
The Fourier transform infrared analysis of SEFs is done using a Shimadzu IRAffinity-1 spectrometer. In this study, samples are prepared by chopping it approximately for 2–3 mm. The test is conducted in the region from 400 to 4200 cm −1 wavenumber at room temperature of 25 °C and relative humidity of 60%. A total of 16 samples were taken for each example, a resolution between 0.5–16 cm −1 . The chopped 3 mg of dried fiber is mixed with potassium 150 mg of bromide and pelletized. Each sample pellet is kept to record spectra.
X-ray Diffraction analysis is generally used to check the number of cellulosic-crystals present in a mixture. The XRD result was used to determine the percentage of crystallinity and also the crystalline index. The XRD analysis was carried out using a D8 advance machine with the source of Cu-anode ceramic tube and Ni filter as a beta filter. The test was carried out for the finely chopped fiber samples, each weighing 400 mg at room temperature 25 °C and relative humidity of 60%. The machine was operated at the range of 5–90° (2 θ angle range). Materials crystalline index (C.I.) and percentage of crystallinity (%CR) were calculated using the equations ( 1 ) and ( 2 ) respectively, where I22 is the most significant intensity peak of the sample which indicates the crystalline cellulose material peak in the range of 20°–23° and I18 is the smallest peak which means the amorphous crystalline material peak in the range of 16°–19° for the natural fiber [ 26 ].
The %CR only calculates the crystalline area under the curve of the XRD graph, whereas CI is used to calculate the relative amount of crystallinity present in the cellulose [ 27 ].
It is useful to determine the degradation of raw and chemically treated SEFs concerning temperature. The thermograms of SEFs were executed using an SDT Q600 V20.9 Build 20 Jupiter apparatus in a Nitrogen atmosphere to avoid the oxidation, with a heating rate of 20 °C min −1 over a range from 30 °C–800 °C. The experiment can also be done with other noble gases which are not relative.
To evaluate the modification made on the SE fiber surface of untreated and after chemical treatment. SEM is recorded with an instrument which is having an accelerated voltage of 10-kilo Volt, a scale of 20 micrometers, and 500 × magnification for visualizing the surface morphology of SEFs. Fibrous samples were gently secured on an SEM sample holder with carbon tape. A gold layer 5 nm thick was sputter-coated onto the surface of the sample to improve image resolution and to avoid an electrostatic charge. A total of 16 samples were taken.
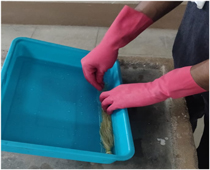
Figure 1. Neutralizing acid-treated fiber.
Download figure:

Figure 2. Oven dried fiber.
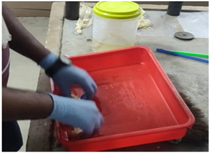
Figure 3. Sample is cleaned after soaking in Benzyl chloride.
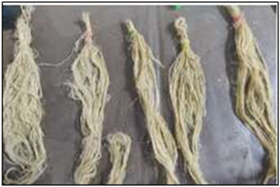
Figure 4. Sample is dried in an oven.
2.8. Water absorption
A water absorption test was performed to check the quantity of water that fiber can absorb under specific conditions. Many factors affect water absorption of fibers like the exposure of fiber in water, temperature, etc. For the test, the fibers were dried in an oven. Each sample of 3 g of fibers was kept in 0.3 l of water at 23 °C for 24 h or until equilibrium. Fibers were removed, squeezed the excess water using the cloth, and weighed. This experiment was carried out only once. The calculation can be done using the given below equation.
3. Results and discussion
FTIR analysis, IR light is sent through each sample material. Some of the infrared light is absorbed in the sample, a wavenumber from 400 to 4200 cm −1 was recorded. It gives information to identify and characterize the functional groups that exist in the sample. The FTIR analysis is done for the untreated and chemically treated SE fibers. FTIR spectrum for Raw, alkaline, Acetyl, and Benzoyl are shown in figures 5 – 7 . Cellulose, lignin, and hemicellulose are the major vibration bands present in both treated and untreated fibers. The broad and deep peak at 3312 cm −1 represents the hydroxyl group with hydrogen bond (refers to cellulose) characteristics of intermolecular bonded hydroxyl (O–H) groups [ 28 ]. The peak intensity of cellulose increases after each chemical treatment. The peak at 2916–2852 cm −1 refers to C–H stretching vibration in the CH 2 group and CH group present in hemicellulose and cellulose components [ 29 ]. The peak of the hemicellulose component noticed only in the raw fiber but perished in chemical-treated fiber spectra. Further, this proves the elimination of hemicellulose content on the SE Fibers surface after chemical treatment. The sharp peak at 1642 cm −1 presences lignin with the carboxylic acid group and C=O vibration [ 30 ].

Figure 5. FTIR peaks for alkaline treated fibers.
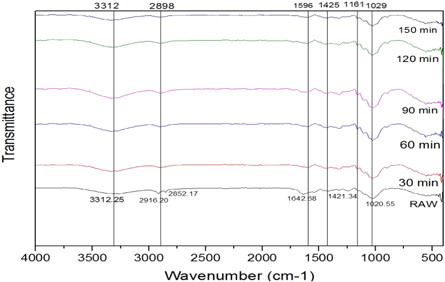
Figure 6. FTIR peaks for acetylation treated fibers.
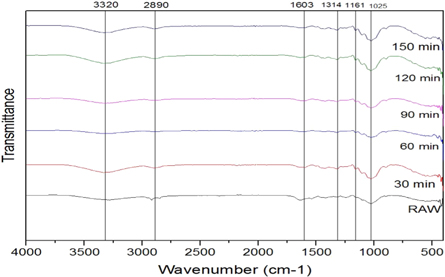
Figure 7. FTIR peaks for Benzoylation treated fibers.
The short peak 1421 cm −1 represents minor constituents like lignin, hemicellulose, and amorphous cellulose with CH 2 bending vibration. The intensities this peak varied for each chemical treatment. In acetyl and benzyl treatment, the intensity of the peaks was much lower than the untreated sample. C–H group bending vibration in the aromatic ring of hemicellulose and lignin in peak 1020 cm −1 [ 31 ]. To increase in cellulose content in SEF proved that the capability of these three chemical treatments, and during the chemical treatment, most of the gangue partials are eliminated. The fibers are changed to hydrophobic nature from hydrophilic after chemical treatment [ 32 ]. Thus chemical treatment improves the adhesion property, reduces water absorption, and increases thermal stability.
The structure of the fibers and its properties are determined mainly by the amount of cellulose and non-cellulosic constituents present in the natural fiber. These also influence the crystallinity of the fiber [ 33 ]. The graphs obtained for the untreated fiber and chemically treated fibers are shown in figures 8 – 11 . Generally, from the figures, we can identify that for untreated and fibers treated with acetic acid, there was the presence of 2 peaks at the 2 θ angle of 18° and 22°, for alkaline and benzyl treated fibers there was the presence of 3 peaks at the range of 13°, 15° and 23°. However, these irregular distributed amorphous peak after chemical treated latter gets merged [ 34 ]. The difference in peak values from the analysis is because of chemical treatment, which causes the generation of disorder in the structure of material [ 35 ].
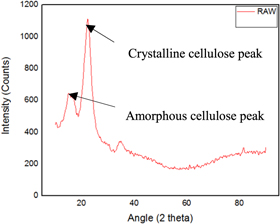
Figure 8. Raw fiber.
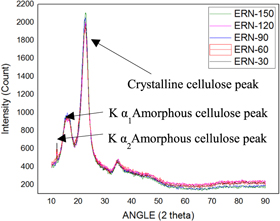
Figure 9. Alkaline Treated.
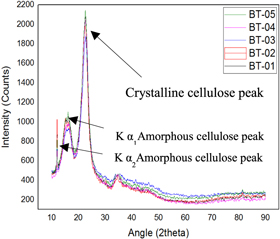
Figure 10. Benzoyl Treated.
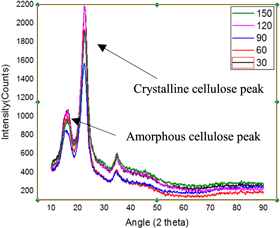
Figure 11. Acetic acid Treated.
Many researchers have already studied the effect of chemical treatment on the lignocellulosic material. Nobuo shiraishi et al [ 36 ] from their observation reported that the chemical reaction takes place with the amorphous region, and the latter proceeds with the rate of diffusion and rate of reaction. Since it cannot diffuse the crystalline region, it reacts with the chain ends, which are on the surface of the crystallites. This results in the opening of some of the bonds of the hydrogen- cellulosic chain and produces amorphous cellulose content. This eventually results in the reduction of crystallinity. Zafeiropoulos et al [ 37 ] observed that chemical treatment increased the crystalline cellulose content of flax fiber because of the removal of impurities and amorphous contents Tserki et al [ 38 ] in their study, observed that even when the flax, hemp, and wood fibers were treated with different chemicals, they still showed the same crystalline index.
Using the equations ( 1 ) and ( 2 ), the CI and crystallinity % are represented in table 1 . From the table 1 , we can identify that for raw fiber the CI and crystallinity % were 0.53 and 63% whereas the chemically treated showed maximum values at a different period, i.e., for alkaline treated fibers the maximum CI and crystallinity % of 0.70 and 68% were identified at 30 min whereas for acetyl treated fibers the maximum CI and crystallinity % of 0.69 and 65% were determined at 90 min whereas for benzyl treated fibers the maximum CI and crystallinity % of 0.67 and 68 were identified at 30 min.
Table 1. Peaks intensities, crystallinity and crystallinity index of raw, NaOH, acetylation and benzoylation treated SEFs.
From the table 1 , we can identify that CI values for all chemically treated fibers, i.e., alkaline, acetyl, and benzyl treated, were nearly the same. This was mainly because when the fibers are treated with different chemicals for the different soaking period, it was found that there was the difference in the concentration in the removal of several impurities, amorphous cellulose, hemicellulose, lignin which were studied in previous observations [ 35 – 37 ]. It was found that alkaline had a maximum crystalline index and the percentage of crystallinity. Also, when compared with the untreated fiber, it showed an increase in values because when the fibers are chemically treated, there was an increment in the amount of crystalline cellulose, which helps in achieving high thermal stability. And also, the removal of non-crystalline materials like lignin, hemicellulose, etc [ 39 ].
Typically, three degradations were observed in various stages during the study of SEF. Firstly, the degradation occurs at 70 °C to 130 °C because of the evaporation of water and other volatile substance present in the SEFs. The other significant degradation (130 °C–275 °C) corresponds to the thermal decomposition of hemicellulose and the degradation of cellulose. The final stage linked to the deterioration of lignin in SEFs. From the table 2 , we can observe that the 1st degradation, i.e., the amount of water evaporation of treated fiber gets decreased when compared to raw fiber. Besides, it can be witnessed from figures 12 – 14 and also from table 2 that the lignin content gets reduced after the chemical treatment. It is also evident that it improved the thermal stability of SEF after chemical treatment as the raw fiber gets degraded at 320 °C. Still, after the chemical treatment, the degradation temperature gets increased up to 330 °C–380 °C. In Alkaline treated fiber, ERN-60(60 min) shows a better result when compared with others. In Benzoyl treated fiber, BT-150(150 min) sample showed a better effect than others as it proves that the treated SEF has more hydrophobicity property compared to the raw fiber and increase in degradation temperature up to 387 °C. In Acetyl treated fiber, EOA-90 (90 min) provides a better result than others in terms of the degradation temperature. Still, water content gets reduced, and it is proved in the water absorption test. It can be observed that the % CR of many samples in XRD is the same; however, the degradation temperature varies, as %CR only indicates a crystalline area in the graph and has no relation with the thermal stability.
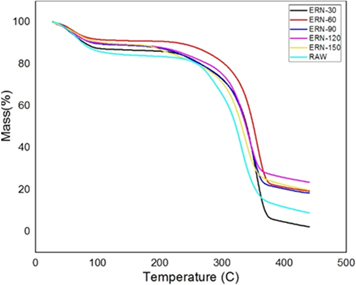
Figure 12. Alkaline treated fiber.
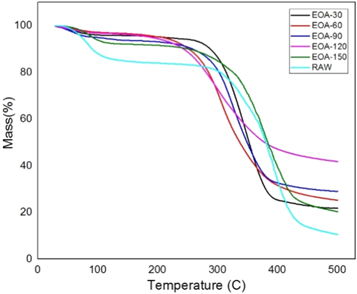
Figure 13. Acetylation treated fiber.
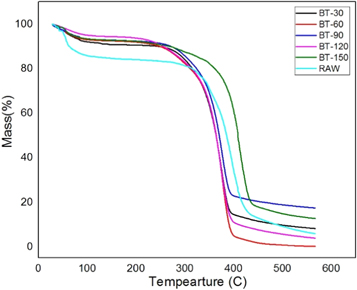
Figure 14. Benzoyl treated fiber.
Table 2. Degradation % and temperature in TGA of raw, NaOH, acetylation and benzoylation treated SEFs.
Thermal stability only depends on CI and crystalline size [ 40 ] . From XRD and FTIR, we witness that there is still a presence of some amorphous content in fibers. Therefore, the major amount of amorphous cellulose region bonds becomes degraded during the thermal treatment, similar to the breaking of C–C bond and other bonds in an amorphous region or some elimination reactions, etc, when compared to those bonds within the crystalline. Besides, it also depends on the packing of the cellulose chain. Further, due to the chemical treatment, the presence of H-bond intensity near the cellulose chains can be seen, and it varies with the different chemical treatments and its soaking period. It makes the cellulose structure more packed, which in turn acts as an obstacle and makes it difficult to break the bond, and thus there is a requirement of high temperature to break it [ 41 , 42 ].
SEM provides the surface morphology of the natural fiber surface. Figure 15 shows the surface image of untreated SEFs. Figures 16 – 18 give the images of SEFs of alkali-treated, Benzyl, and acetylation treated fiber, respectively. The white blanket over the surface of fiber shows the existence of impurities like waxes and other impurities [ 24 ]. By treating SEF with chemical, take out waxes and oil from the outer surface of the fiber and also removal of minor constituents like lignin, hemicellulose, and amorphous cellulose from the outer surface of the fiber to a certain extent and improve roughness on the surface of fibers compared with untreated fiber. Small traces of unwanted materials can be found after alkaline treated fiber, but after Benzyl and acetylation treatment, it removes the unwanted materials from the fiber surface. With an increase in time, the chemical harms the surface of SEF seen on acetyl treated fiber after 60 min. After treatment with benzyl, it is understood that impurities were effectively removed from fiber surface without damaging. The treatment also improves the fiber-matrix adhesion property, which also improves the tensile and thermal properties of the composites [ 20 ]. The chemically treated fibers had developed a very rough surface and also developed amount of voids present which provides good mechanical interlocking with the matrix [ 43 ].
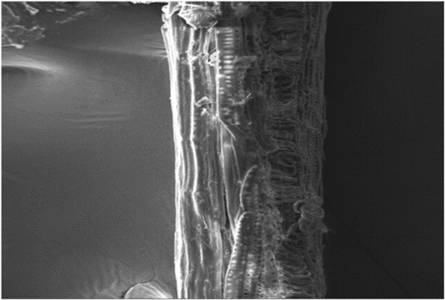
Figure 15. Untreated fiber.
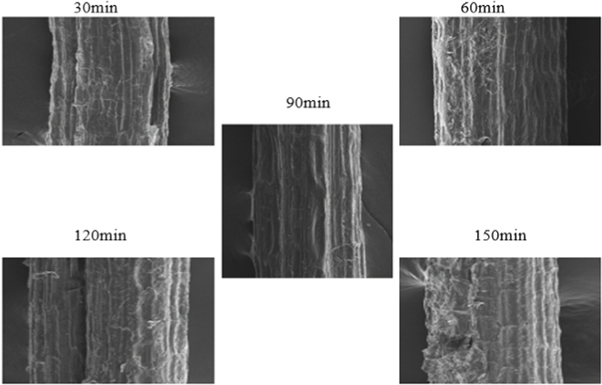
Figure 16. Alkaline treated fiber.
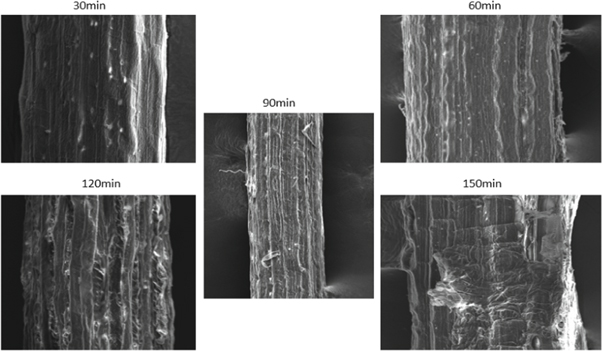
Figure 17. Acetyl treated fiber.
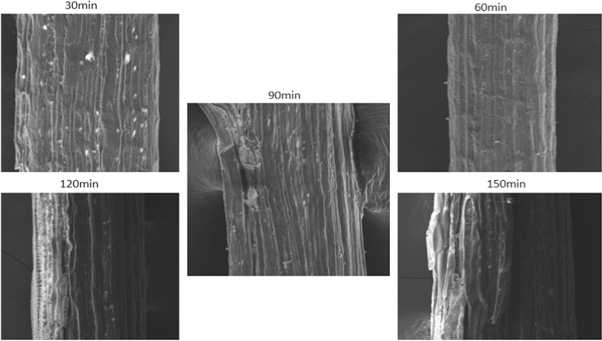
Figure 18. Benzyl treated fiber.
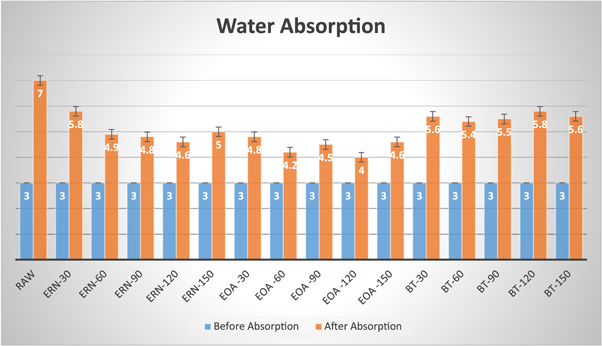
Figure 19. Water absorption graph.
Table 3. Water absorption test of raw, NaOH, acetylation and benzoylation treated SEFs.
3.5. Water absorption
Table 3 shows the water absorption test of raw, NaOH, acetylation and benzoylation treated SEFs. From the table 3 , we can observe that the hydrophobic property of treated fibers is improved (decreased) compared to raw fiber. The reason is due to the removal of hemicellulose with the help of hydrophilic chemicals. In Alkaline treated fiber, ERN-120 (120 min) shows good results of 53.3% absorption compared to others. In Acetylation treated fiber, EOA-120 (120 min) shows 33.3% of absorption, which is better than others. Furthermore, in Benzoyl-treated fiber, BT-60 (60 min) show 80% of absorption, and it is a good result comparing to others. In general, we can say that Acetylation treated fibers exhibit better hydrophobic properties than others. Figure 19 displays the water absorption graph.
4. Conclusion
In this research work, the crystalline property, thermal stability, and surface morphology of SEFs were studied. The FT- IR results showed that chemical treatment removed some contents of impurities and also amorphous cellulose, hemicelluloses, lignin, etc from the SEFs. The thermal stability of the brake pad depends on the crystallinity index of the reinforcement. From the results of the x-ray Diffraction test, it has been found that for alkali-treated fiber, especially when treated for 30 min, showed the crystalline index of 70%. Through TGA, it was determined that the Benzoyl-treated SEFs provided better thermal resistance compared to raw fiber with the thermal stability of 387 °C. From the water absorption test, Acetyl- treated SEFs displayed better hydrophobic property compared to others. The outcome of the results has been confirmed that the chemically treated SEFs more useful for improving the physical, chemical, and thermal properties of the brake pad application. It can be used as a suitable fiber in the composite materials and also the replacement for synthetic fibers in the development brake pad application.

- Search this journal
- Search all journals
Article Sections
- Materials and methods
- Literature cited
Article Figures
Sampled ozone concentrations over time among the plant (chamber) treatments used to evaluate the effectiveness of houseplants in reducing ozone concentrations. When ozone concentrations reached about 200 ppb, the ozone generator was turned off (time = 0) and ozone concentrations among the treatments over the ensuing time were measured (snake = snake plant, pothos = golden pothos, spider = spider plant, control = no plants, 1 ppb = 1 nL·L −1 ).
- View raw image
- Download Powerpoint Slide
Related Content
- Previous Article
- Next Article
Effectiveness of Houseplants in Reducing the Indoor Air Pollutant Ozone
Click on author name to view affiliation information
Three common indoor houseplants, snake plant ( Sansevieria trifasciata ), spider plant ( Chlorophytum comosum ), and golden pothos ( Epipremnum aureum ), were evaluated for their species effectiveness in reducing ozone concentrations in a simulated indoor environment. Continuously stirred tank reactor (CSTR) chambers housed within a greenhouse equipped with a charcoal filtration air supply system were used to simulate an indoor environment in which ozone concentrations could be measured and regulated. Ozone was injected into the chambers and when concentrations reached 200 ± 5 ppb, the ozone-generating system was turned off and ozone concentrations over time (ozone was monitored every 5–6 min in each chamber) were recorded until about <5 ppb were measured in the treatment chamber. On average, ozone depletion time (time from when the ozone generating system was turned off at ≈200 ppb to <5 ppb in the chamber) ranged from 38 to 120 min per evaluation. Ozone depletion rates were higher within chambers that contained plants than within control chambers without plants, but there were no plant species differences.
Indoor air pollution is ranked as one of the world's greatest public health risks ( Wolverton, 1997 ). The United Nations Development Program estimated in 1998 that over 2 million humans die each year due to the persistence of deleterious indoor air ( Brennan and Withgott, 2005 ). It has also been estimated that globally 14 times as many deaths occur from poor indoor air quality compared with ambient air pollution ( Brennan and Withgott, 2005 ).

Because humans in industrialized countries spend about of 80% to 90% of their time indoors ( Orwell et al., 2004 ; Wolverton, 1997 ), negative societal consequences due to polluted indoor air can be great ( Fisk and Rosenfeld, 1997 ). For example, the cost of unhealthy indoor air in Australia has been estimated at $12 billion annually due to losses in productivity, higher medical costs, more absenteeism, and lower earnings ( Wood, 2003 ). In addition, the health burden associated with indoor air pollution does not appear to be equal for developing and developed countries. Australia's Commonwealth Science Council has suggested that 9 of 10 deaths due to indoor air are experienced by the developing world ( Brennan and Withgott, 2005 ).
Ozone (O 3 ), a photochemical oxidant with a redox potential of +2.07 V, is one of the most powerful naturally occurring oxidants ( Maroni et al., 1995 ; Mustafa, 1990 ). It is considered a secondary ambient pollutant and one of the components of tropospheric smog, which can adversely affect human health and property ( Mustafa, 1990 ). Ozone is produced within the troposphere by chemical reactions involving free radicals. It is formed during the reaction of carbon monoxide (CO) and volatile organic compounds (VOCs) in the presence of nitrogen oxides (NO X ), oxygen and sunlight. There is strong evidence to suggest that average ozone concentrations have been rising during the past century as a result of increased input of the precursors of ozone into the atmosphere ( Mustafa, 1990 ). Automobiles are the principal contributors to secondary tropospheric ozone generation ( Maroni et al., 1995 ).
Ozone as an indoor air pollutant can be prevalent in homes and offices due to infiltration of outdoor ambient air indoors ( Weschler, 2000 ). Ozone-emitting equipment such as copy machines, laser printers, ultraviolet lighting, and some electrostatic air purification systems may also contribute to indoor ozone levels ( Maroni et al., 1995 ; Weschler, 2000 ). Ozone generation from appliances such as photocopiers on average yield 5.2 mg·h −1 and laser printers on average produce 1.2 mg·h −1 ; however, concentrations could vary based on equipment maintenance ( Black and Worthan, 1999 ; Weschler, 2000 ). Depending on the air exchange rates between outdoor and indoor environments, indoor air has been reported to contain from 10% to 50% of outdoor values ( Weschler, 2006 ) to five to seven times the contaminant concentrations of ambient urban air ( Brown et al., 1994 ; Orwell et al., 2004 ).
The principal groups at risk to ozone toxicity are organisms in which the primary route of exposure is via inhalation ( Wright and Welbourn, 2002 ). Daily inhalation of indoor ozone for these organisms (such as humans) are estimated to be between 25% and 60% of total ozone intake ( Weschler, 2006 ). Major toxic effects of ozone in humans include alterations in pulmonary function in addition to cellular and biochemical endpoints ( Wright and Welbourn, 2002 ). Exposure to ozone also may result in pulmonary edema, hemorrhage, inflammation, and extensive lesions on the lung tissue, trachea, and upper bronchi ( Mehlman and Borek, 1987 ).
Indoor exposures to ozone are often accompanied by exposure to the products of ozone-initiated oxidative reactions (such as isoprenes, styrenes, terpenes, sesquiterpenes, and unsaturated fatty acids) ( Weschler, 2004 ). The average daily intakes of ozone oxidative products are roughly one-third to twice the average daily intake of ozone alone ( Brown et al., 1994 ). Some of the oxidative products of ozone that are known or suspected to adversely affect human health include formaldehyde, acrolein, hydrogen peroxides, and fine and ultrafine particles ( Weschler, 2004 ). Common indoor sources of reactive chemicals to ozone include occupants themselves, soft woods, linoleums, certain paints, polishes, cleaning products, soiled fabrics, and soiled ventilation filters. Indirect evidence supports connections between human morbidity/mortality and exposures to indoor ozone and its oxidative products ( Weschler, 2006 ).
As indoor air pollution poses concerns for human health, cost-effective and easy-to-implement methods are needed to eliminate or reduce concentrations. Activated charcoal filters reduce air pollutants but installation and maintenance costs can be high ( Wolverton, 1997 ). As an alternative, foliage plants could be used to sequester some types of air pollution, although their effectiveness appears to be plant species specific and air pollutant dependent ( Wolverton, 1986 ).
Wolverton (1984) evaluated common foliage plants in controlled chambers (that simulated indoor environments) for their ability to reduce concentrations of several air pollutants. Depletion rates of known concentrations of air pollutants within the enclosed chambers containing plants were measured. Wolverton concluded that of the taxa selected, common spider plant and golden pothos most effectively reduced various air pollution concentrations (e.g., formaldehyde, nitrogen dioxide, and carbon monoxide) from closed chambers ( Wolverton, 1984 , 1986 ). Because ozone was not one of the indoor air pollutants evaluated in the previous study ( Wolverton, 1984 ) and it is an important indoor air pollutant for which mitigation methods are limited, the objective of the current study was to determine the effectiveness of using common foliage houseplants in reducing concentrations of ozone within a simulated indoor environment.
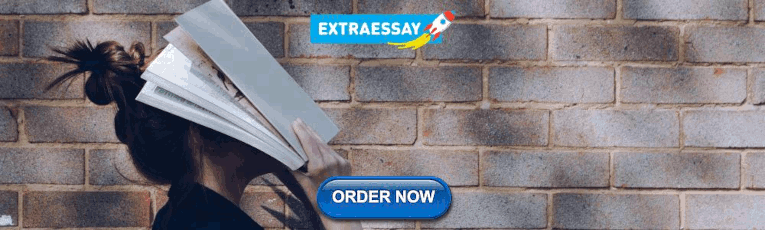
Plant material and growing conditions.
Three plants species, snake plant, spider plant, and golden pothos, were evaluated for their effectiveness in reducing ozone concentrations in a simulated indoor environment. These plant species were chosen because of their popularity as indoor houseplants (primarily due to their low cost, low maintenance, and rich foliage) and their previously reported ability to reduce air pollutants other than ozone ( Wolverton, 1986 , 1997 ).
Plants were grown in greenhouse pots (one plant per pot) containing peatlite commercial growing mix (Sunshine #4; Sun Gro Horticulture, Bellevue, WA) in a greenhouse at The Pennsylvania State University campus in University Park. Watering, fertilization, and pest control were conducted according to recommendations and ensured good healthy plant growth before and during the experiments.
Ozone exposure and monit-oring.
Four continuously stirred tank reactor (CSTR) chambers (2 m 3 volume) covered with clear Teflon® film and situated within a greenhouse equipped with a charcoal filtration air supply system (resulting in ambient levels of ozone within the greenhouse of <5 ppb) were used to simulate an indoor environment in which ozone concentrations were measured and regulated. The CSTR chambers previously described by Heck et al. (1978) had an air flow circulation within the chambers of ≈4.5 m 3 ·min −1 . All of the CSTR chambers were air tight when sealed and were maintained under greenhouse conditions [e.g., photosynthetic photon flux ( PPF ), humidity, and temperature]. Ozone was injected into the chambers via a controllable micrometering system and concentrations were monitored with an ozone analyzer (TECO model 49; Thermo Electron Corp., Hopkinton, MA) and data logger/computer recording system. Impeller fans ensured mixing of the gases within the chambers. Once the ozone concentrations within the chambers reached 200 ± 5 ppb, the ozone-generating system was turned off and the ozone depletion rate (time to <5 ppb) for each chamber was recorded at approximate 5- to 6-min intervals. This depletion rate was used as an indicator that the aerial portion of the plant was assisting in ozone mitigation. Air circulation within the chambers continued during all trials.
Five spider plants, five golden pothos plants, and two snake plants (because of their larger size) per replication were used in this evaluation. During the evaluations, the top part of each pot was enclosed with plastic sheeting in such a manner that only the aerial portion of the plants was exposed to ozone. One chamber that did not contain any plants served as control.
At the completion of the evaluations, leaf surface area and stomatal conductance ( g S ) of the plants were measured. Leaf surface area (cm 2 ) was measured via an area meter (LI-3100; LI-COR, Lincoln, NE) on all the plants within the individual chambers and is reported as leaf area per chamber. G S [water (mol·m −2 ·s −1 )] was measured midleaf on representative midaged leaves (while avoiding large veins or any patterns of variegation) on individual plants via a portable photosynthesis system (LI-6400; LI-COR) equipped with a leaf chamber fluorometer and is reported as g S average per chamber.
Statistical design and analysis.
The experiment was conducted as a completely randomized design with plant species as the main effect replicated six times (trials) over the course of 3 d (27 June 2006, 28 June 2006, and 3 July 2006) under similar environmental conditions ( Table 1 ). Three of the trials were conducted during the late morning ( am ) and three trials were conducted during the early afternoon ( pm ); day and time of day ( am or pm ) were noted for each trial. The plant species evaluated were alternated among specific chambers during the replications over time to exclude any possible chamber effect. The same individual plants within species were used for all trials.
Environmental conditions of temperature and relative humidity in the continuously stirred tank reactor chambers during the six trials conducted in 2006 to test the effectiveness of houseplants in reducing ozone. PPF levels ranged from 150 to 200 μmol·m −2 ·s −1 .

Data were analyzed using Minitab (release 14; Minitab, State College, PA), Excel (Microsoft, Redmond, WA), and SAS (SAS Institute, Cary, NC). Significant results are reported at the level of α = 0.05 (unless otherwise noted). The slope was calculated as the derivative of the depletion curve over time s =[ 0 ( t 0 ) − 0 ( t 1 )]/( t 0 − t 1 ), where o (t) represents the value of ozone at time t. Ozone depletion depends on the uptake of ozone by the plant species, therefore it was not appropriate to use a simple decay formula. The absolute value of slope of the depletion curves (i.e., ozone concentration per time interval), time to 50% reduction of ozone, and time to less than 5 ppb ozone within the chamber (the point at which the trial were ended) were used to account for the ozone depletion rate for each treatment. General Linear Model (GLM) was used to determine the significant factors affecting the depletion curves. Day, time of day ( am or pm ), chamber, and treatments (e.g., species/control) as well as g S and total plant leaf area were all considered in the statistical model.
Analysis of variance of the six trials determined that there was no day or time of day ( am vs. pm ) effect on the ozone depletion rates (as measured as absolute slopes of the curves) among the treatments ( Table 2 ). The negative slopes of the ozone curves from the experimental treatments indicated that ozone was mitigated from the chambers among the four treatments ( Fig. 1 ).
Analysis of variance comparing day, time of day (late morning versus early afternoon), and plant species (snake plant, spider plant, and golden pothos) on absolute slope of the ozone depletion curves. z

Citation: HortTechnology 19, 2; 10.21273/HORTTECH.19.2.286
- Download Figure
There was an effect of treatment on time to <5 ppb (the conclusion of the trial) and absolute slope of the ozone depletion curve ( Table 3 ). Time to <5 ppb among treatments ranged from 38 to 120 min. Any of the plant species treatments evaluated required a shorter time to reach <5 ppb than the control treatment that contained no plants. The absolute slope of the control treatment was the smallest and was significantly different from the golden pothos and snake plant treatments, but similar to the spider plant. There was no statistical difference among treatments in the amount of time for the ozone to reduce to 50% (data not presented).
Influence of selected houseplants on ozone concentrations within chambers over time. Once the ozone concentration within the chambers reached 200 ± 5 ppb, the ozone-generating system was turned off and the ozone concentration for each chamber was recorded at approximate 6-min intervals. The control chamber did not contain any plants.

There were statistical differences in g S ( Table 4 ) among the plant species evaluated. The spider plant had the greatest conductance, while the golden pothos had the least, and snake plant was intermediate.
G S rates and leaf surface area of houseplants evaluated for their effectiveness in reducing ozone concentrations within chambers.

Plant species evaluated in this study (snake plant, spider plant, and golden pothos) were effective in mitigating ozone (compared with a control that did not contain any plants) from the CSTR chambers that were used to simulate a controlled interior environment. These results are consistent to those reported by Wolverton (1984 , 1986) using similar plant species for mitigation of VOCs. Although other studies have suggested differential VOC depletion rates for plant species ( Wolverton, 1984 ), in the current study, we were unable to identify any interspecies differences in depletion rates for ozone.
A plant's ability to reduce concentrations of ozone in its surrounding environment appears to be dependent upon uptake of ozone through the stomata and subsequent detoxification reactions within the intracellular spaces. Therefore, the instantaneous rate at which plant surfaces absorb ozone would depend on the g S and the total leaf surface area of the plant species. Because g S per plant and total leaf area of the plants within the chambers varied among the treatments, it was not possible to determine if g S or total leaf area was the more important plant variable in mitigating ozone. Additional research with plants of varying g S and leaf area as well as alternative nonplant materials that increased the surface area are needed to further understand these variables before more precise plant recommendations can be made.
In previous research, emphasis had been placed on the ability of the indoor plant/substrate microcosm and soil to deplete contaminants more effectively ( Orwell et al., 2004 ; Wolverton and Wolverton, 1993 ). Our study did not consider soil or microorganisms as factors in determining ozone mitigation; prospectively, we plan on exposing soil and microorganisms to ozone to determine their effect of indoor ozone depletion.
In conclusion, common houseplants reduce ozone concentrations in a simulated indoor setting. As a method to reduce airborne contaminants, plant implementation may be cost effective and readily applied throughout the world. In particular, the plant species evaluated in this study were common, inexpensive, and easy to grow and maintain. More information is needed on species effectiveness, the number of plants required per unit area, and other environment interactions (including light, temperature, and humidity) to develop more effective recommendations. Regardless, our results provide relevant evidence of a viable indoor ozone mitigation technique using common indoor plants that could have medical, environmental, and economic implications for developing and developed countries. Because indoor air pollution extensively affects developing countries ( Brennan and Withgott, 2005 ; Smith, 2002 ), using plants as a mitigation method could serve as a cost-effective tool in the developing world where expensive pollution mitigation technology may not be economically feasible.
Black, M.S. & Worthan, A.W. 1999 Emissions from office equipment 454 459 Raw G. , Aizlewood C. & Warren P. Indoor air 99 Vol. 2 Construction Research Communications London
- Search Google Scholar
- Export Citation
Brennan, S. & Withgott, J. 2005 Environment: The science behind the stories Pearson San Francisco
Brown, S.K. , Sim, M.R. , Abramson, M.J. & Gray, C.N. 1994 Concentrations of volatile organic compounds in indoor air: A review Indoor Air 4 123 134
Fisk, W.J. & Rosenfeld, A.H. 1997 Estimates of improved productivity and health from better indoor environments Indoor Air 7 158 172
Heck, W.W. , Philbeck, R.B. & Dunning, J.A. 1978 A continuous stirred tank reactor (CSTR) system for exposing plants to gaseous contaminants: Principles, specifications, construction, and operation U.S. Dept. Agr., Agr. Res. Serv. Publ. No. ARS-S-181
Maroni M. , Seifert B. & Lindvall T. 1995 Indoor air quality: A comprehensive reference book Elsevier Science New York
Mehlman, M.A. & Borek, C. 1987 Toxicity and biochemical mechanisms of ozone Environ. Res. 42 36 53
Mustafa, M.G. 1990 Biochemical basis of ozone toxicity Free Radic. Biol. Med. 9 245 265
Orwell, R.L. , Wood, R.L. , Tarran, J. , Torpy, F. & Burchett, M.D. 2004 Removal of benzene by the indoor plant/substrate microcosm and implications for air quality Water Air Soil Pollut. 157 193 207
Smith, K.R. 2002 Indoor air pollution in developing countries: Recommendations for research Indoor Air 12 198 207
Weschler, C.J. 2000 Ozone in indoor environments: Concentration and chemistry Indoor Air 10 269 288
Weschler, C.J. 2004 New directions: Ozone-initiated reaction products indoors may be more harmful than ozone itself. Atmos Environ. 38 5715 5718
Weschler, C.J. 2006 Ozone's impact on public health: Contributions from indoor exposures to ozone and products of ozone-initiated chemistry. Environ Health Perspect. 114 1489 1496
Wolverton, B.C. 1984 The role of plants and microorganisms in assuring a future supply of clean air and water Natl. Aeronautics Space Admin., Natl. Space Technol. Lab Stennis Space Center, MS
Wolverton, B.C. 1986 Houseplants, indoor air pollutants, and allergic reactions Natl. Aeronautics Space Admin., Natl. Space Technol. Lab Stennis Space Center, MS
Wolverton, B.C. 1997 How to grow fresh air Penguin New York
Wolverton, B.C. & Wolverton, J.D. 1993 Plants and soil micro-organisms: Removal of formaldehyde, xylene and ammonia from the indoor environment J. Mississippi Acad. Sci. 38 2 11 15
Wood, R.A. 2003 Improving the indoor environment for health, well-being and productivity Greening cities: A new urban ecology 30 Apr. 2003 Australian Technology Park Sydney, Australia
Wright, D.A. & Welbourn, P. 2002 Environmental toxicology Cambridge University Press Cambridge, UK
Contributor Notes
We thank James Savage and Jonathan Ferdinand for providing technical assistance.
4 Corresponding author. E-mail: [email protected] .
Headquarters:
1018 Duke Street
Alexandria, VA 22314
Phone : 703.836.4606
Email : [email protected]
© 2018-2024 American Society for Horticultural Science
- [66.249.64.20|185.194.105.172]
- 185.194.105.172
Character limit 500 /500
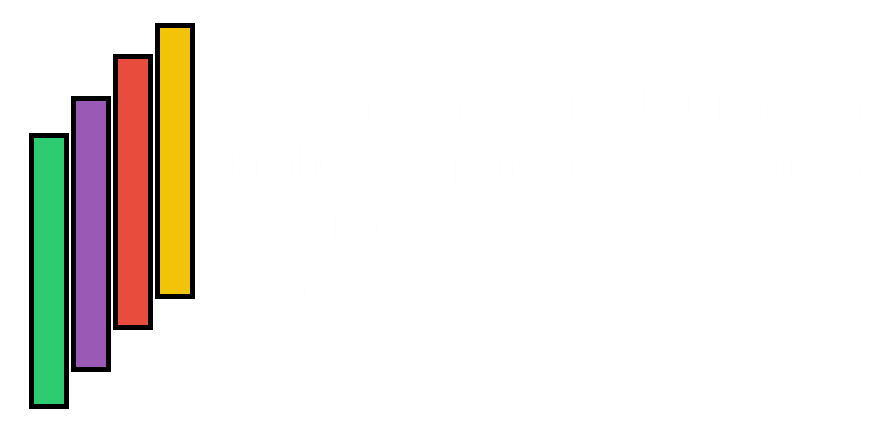
Snake Plant (Sansevieria Trifasciata) Fiber As Alternative Matting In Making Fiberglass
- , Arnie B. Cabral
INTRODUCTION
The growing environmental awareness led to the insight on the use of natural fibers as a sustainable alternative in the composite industry. Continuous research and development in the reinforcement of natural fibers in making composites like fiberglass panels is at its highest trend for engineers and researchers. All of these are for the goal of producing a cost effective with acceptable mechanical properties natural fibers reinforced composites. With the same objective, in this study, the researcher utilized the snake plant (Sansevieria trifasciata) fibers as alternative matting in making fiberglass panels for different industrial application.
The researcher used descriptive and experimental research design in constructing the product, conducting the quality testing, testing the hypothesis and systematically analyzing the data gathered from the ratings of 30 respondents composed of teachers, parents and fiberglass makers from Sta. Cruz and Pagsanjan, Laguna. The statistical tools used were the weighted mean, standard deviation, and ANOVA (F test).
In terms of the Moisture Expansion Test, samples had increased its dimensions with an average percentage of 2.78 %. With regard to the thickness-swelling test of the product, samples increased their dimensions with an average value of 0.83 %. The water Absorption Test of Snake Plant Fiberglass gave the water absorption percentage of the samples an average percentage of 0.22%. The results indicated that the product was water resistant as it can only absorb very little amount of water for a day. However impact test results for snake plant fiberglass suggested that the product could withstand greater forces as the data yields that with 2.75 lbs, 4.96 lbs and 6.61 lbs, recorded descriptions are "no damage”, "slightly deformed”, "deformed" , and "having a crack" . With regard to the buckling point, it was recorded that the product could withstand loads until 250 N before it buckled. It was also found out that there was no significant difference among the ratings made by teachers, parents, and fiberglass makers on the acceptability of Snake Plant Fiberglass in terms of quality, while there was a significant difference on the acceptability of Snake Plant Fiberglass in terms of strength and durability.
DISCUSSIONS
The results suggested that Snake Plant Fiber, which served as alternative matting in making fiberglass, have various uses aside from the focus of this study. The product could be used as construction materials such as decorative tiles, window panes etc. Snake plant fiberglass could also be used as alternative materials in making boats, ships, aeronautic materials and the like which uses fiberglass. Researchers were encouraged to use organic materials other than snake plant fiber as reinforcement on such materials.
Information
- For Readers
- For Authors
- For Librarians
©2017 by Ascendens Asia Pte. Ltd. | NLB Singapore-Registered Publisher.
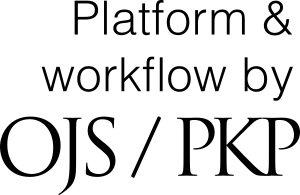

An official website of the United States government
The .gov means it’s official. Federal government websites often end in .gov or .mil. Before sharing sensitive information, make sure you’re on a federal government site.
The site is secure. The https:// ensures that you are connecting to the official website and that any information you provide is encrypted and transmitted securely.
- Publications
- Account settings
Preview improvements coming to the PMC website in October 2024. Learn More or Try it out now .
- Advanced Search
- Journal List
- Gigascience
The rise of genomics in snake venom research: recent advances and future perspectives
Wei-qiao rao.
Department of Biotechnology and Biomedicine, Technical University of Denmark, Søltofts Plads 224, 2800 Kongens Lyngby, Denmark
Department of Mass Spectrometry, Beijing Genomics Institute-Research, 518083, Shenzhen, China
Konstantinos Kalogeropoulos
Morten e allentoft.
Trace and Environmental DNA (TrEnD) Laboratory, School of Molecular and Life Sciences, Curtin University, Kent Street, 6102, Bentley Perth, Australia
Globe Institute, University of Copenhagen, Øster Voldgade 5, 1350, Copenhagen, Denmark
Shyam Gopalakrishnan
Wei-ning zhao, christopher t workman, cecilie knudsen, belén jiménez-mena.
DTU Aqua, Technical University of Denmark, Vejlsøvej 39, 8600, Silkeborg, Denmark
Lorenzo Seneci
Mahsa mousavi-derazmahalleh, timothy p jenkins, esperanza rivera-de-torre, andreas h laustsen, associated data.
Not applicable.
Snake venoms represent a danger to human health, but also a gold mine of bioactive proteins that can be harnessed for drug discovery purposes. The evolution of snakes and their venom has been studied for decades, particularly via traditional morphological and basic genetic methods alongside venom proteomics. However, while the field of genomics has matured rapidly over the past 2 decades, owing to the development of next-generation sequencing technologies, snake genomics remains in its infancy. Here, we provide an overview of the state of the art in snake genomics and discuss its potential implications for studying venom evolution and toxinology. On the basis of current knowledge, gene duplication and positive selection are key mechanisms in the neofunctionalization of snake venom proteins. This makes snake venoms important evolutionary drivers that explain the remarkable venom diversification and adaptive variation observed in these reptiles. Gene duplication and neofunctionalization have also generated a large number of repeat sequences in snake genomes that pose a significant challenge to DNA sequencing, resulting in the need for substantial computational resources and longer sequencing read length for high-quality genome assembly. Fortunately, owing to constantly improving sequencing technologies and computational tools, we are now able to explore the molecular mechanisms of snake venom evolution in unprecedented detail. Such novel insights have the potential to affect the design and development of antivenoms and possibly other drugs, as well as provide new fundamental knowledge on snake biology and evolution.
Snakes (Squamata: Serpentes) represent a monophyletic lineage, comprising ∼3,600 extant species found in all continents, except Antarctica [ 1 , 2 ]. From an evolutionary perspective, these reptiles stand out for their characteristic lack of limbs, elongated body shape, and exclusively carnivorous diet. Even before the advent of genetic approaches, conventional anatomical and morphology-based phylogenetic evidence unambiguously suggested that snakes are nested within lizards, with the Anguimorpha lineage (e.g., monitor lizards, glass lizards, beaded lizards) as their closest relatives [ 3–5 ]. Together with amphisbaenians, snakes and all other lizards thus form the largest branch of terrestrial vertebrates, the squamate reptiles [ 3–5 ]. Snakes have many specialized adaptations compared to other reptile lineages. For example, the evolution of infrared sensing pits in pit vipers (Viperidae: Crotalinae), boas (Boidae), and pythons (Pythonidae), and of a venom apparatus in several snake families (Fig. 1 ), provides these animals with exceptional predatory capabilities despite the loss of limbs and the degradation of visual and auditory perception in many (but not all) species [ 6–8 ]. Moreover, severe jaw modifications and low metabolic rates enable snakes to swallow and digest large prey whole, further consolidating their position as formidable predators [ 9 , 10 ]. Thus, snakes are important model organisms for evolutionary studies and have yielded insights into limb development [ 11–13 ], sex chromosome evolution [ 14 ], and venom evolution [ 15 ].

Schematic diagram of snake evolution based on data from Reptile-database.org [ 16 ]. Snakes (Serpentes) are divided into 3 main infraorders, Scolecophidia, Henophidia, and Alethinophidia, which together encompass ∼24 families (7 shown here). Families comprising venomous species are indicated with a skull and crossbones symbol. Colubridae constitutes the largest family of snakes, encompassing 52% of the ∼3,566 snake species currently described. The total number of currently described venomous snake species is 2,901, predominantly falling within the families Homalopsidae, Lamprophiidae, Colubridae, Elapidae, and Viperidae. Only snake species that have undergone whole-genome sequencing and assembly are listed in this figure.
The development of next-generation sequencing (NGS) technologies in recent decades has allowed researchers to generate large genomic datasets and rendered the assembly and characterization of complete genomes a routine task. Despite the availability of NGS since the early 2000s, the use of these technologies to assemble and study complete snake genomes has been limited, especially when compared to the amount of research that has been conducted in the fields of snake venom proteomics and transcriptomics [ 17 ]. It was not until 2013 that the first snake genomes based on high-throughput sequencing data were published for the Burmese python ( Python bivittatus ), the red-tailed boa ( Boa constrictor constrictor ), and the king cobra ( Ophiophagus hannah ) [ 9 , 18 , 19 ]. Fortunately, snake genome research has eventually gained more attention, with 18 new genomes being released since 2013 and several more currently in progress [ 15 , 20–31 ]. These increased sequencing efforts have already revealed intriguing insights into the regulation and expression of venom-related genes. As an example, a large number of dormant toxin-encoding genes with unknown bioactivity were identified in the Okinawan habu ( Protobothrops flavoviridis ) [ 15 ]. Such discoveries could be of high scientific value and may improve our basic understanding of the interplay between protein function and evolution. Furthermore, because toxins from several animal lineages are known to possess different types of bioactivity, some of them could find utility in a variety of applications, from the development of novel therapeutics [ 32 ] to biopesticides [ 33 ] and molecular research tools [ 34 ]. With only 21 snake genomes publicly available to date, there is great unexplored scientific potential in sequencing and analysing more snake genomes [ 17 , 35 ].
From a broader perspective, having access to a complete or nearly complete assembled genome provides an excellent basis for addressing a wide range of biological research questions. For example, genomic data can be used to predict protein-coding exons [ 36 ] (including exons in genes that recently underwent pseudogenization), non-expressed genes, translated proteins, and microRNA genes [ 37 ]. Genomic data may also allow for the identification of toxin orthologs using comparative studies and homology searches [ 38 ]. Knowledge of homology is crucial for the reliability of functional annotation of genomes and can provide fundamental information on evolution and speciation processes [ 39 , 40 ]. Therefore, complete genomes are crucial to the field of proteomics as well, because the absence of reliable genome-derived protein libraries forces researchers to rely on homologous proteins from other organisms as a benchmark against which to compare newly characterized protein sequences. This results in severely limited accuracy in identifying potentially homologous proteins, which consequently leads to overlooking and/or misrepresenting evolutionary patterns. This is especially relevant considering the likely widespread occurrence of alternative splicing in snake genomes, which gives rise to multiple messenger RNA products that in turn result in various isoforms of a particular toxin [ 41–43 ]. Extensive post-genomic and post-translational modifications are also at play, leading to often remarkable discrepancies between genome, transcriptome, and proteome in terms of expression and sequence identity [ 44–46 ]. Along this line, comparative analysis of whole snake genomes could likely provide invaluable insight on the evolution and structure of the gene regulatory network responsible for the expression of venom genes in these animals (and arguably venomous amniotes in general) [ 47 ].
Several approaches are available to obtain reliable genomic data. Among them is reduced-representation sequencing, in which only a part of the genome is sequenced [ 48 ]. For instance, capture sequencing techniques allow for specific areas of interest (e.g., the exon part of the genome) to be targeted and sequenced at a lower cost compared to whole-genome sequencing (WGS) [ 49 ]. Although less suitable for studying venom genes, restriction site associated–DNA sequencing (RAD-seq) uses restriction enzymes to obtain genome-wide sequencing data, which have recently been used to study population demographic trends that underly venom variation [ 50 ]. Nevertheless, reliable detection of homologous genes across species and/or lineages can be hindered by the acquisition, loss, or pseudogenization of genes [ 40 ]. One way to overcome this challenge is to use WGS, which represents a more comprehensive resource for the detection of homologous genes because it provides the entire genotype of the target organism(s) [ 40 ]. WGS can also provide information on genomic variability of a species, and potentially discover and quantify the extent of selective (e.g., positive/purifying selection and hitchhiking effects) and neutral forces (e.g., genetic drift) driving venom evolution [ 51 ].
This review aims to provide a comprehensive summary of the current knowledge on snake genomics, with a particular focus on the current use and future potential of high-throughput DNA sequencing technologies in the field of snake toxinology. Moreover, we discuss how these technologies can be used to expand our current knowledge on snake venom evolution and toxin diversification.
Current Status of Snake Venom Research
Overview of snake toxin families.
Studies have estimated that between 19,000 and 25,000 toxins are found in venoms from the Elapidae and Viperidae snake families, but only a few thousands have been characterized [ 52 ]. Nonetheless, this body of knowledge has proven sufficient for the systematic classification of snake venom toxins into 63 families, most of which are, however, only found in a small percentage of snake species and/or in negligible amounts within venom mixtures [ 53 ]. The 4 families generally considered to be of highest relevance both from a clinical (human envenoming cases) and an ecological perspective (e.g., prey incapacitation) are the 3-finger toxins (3FTxs), phospholipases A 2 (PLA 2 s), snake venom metalloproteinases (SVMPs), and snake venom serine proteinases (SVSPs). Other widespread snake venom protein families include cysteine-rich secretory proteins (CRISPs), L-amino acid oxidases (LAAOs), and C-type lectin-like proteins (CTLPs) [ 53 ]. An overview of the main snake venom toxin families is provided in Table 1 .
Number of toxin-encoding genes for 22 toxin families in selected venomous and non-venomous reptile species
In venomous snake genomes, the numbers refer to the venom gland genome only. Non-venomous species lack venom glands, and the indicated numbers refer to homologous proteins expressed in other organs.
3FTxs belong to a superfamily of non-enzymatic proteins and are a major component in the venoms of most elapids, while they generally feature less prominently in viperid and colubrid venoms. These toxins have 3 β-stranded loops extending from a central core, contain 4 or 5 conserved disulfide bonds, and cause a wide range of pharmacological effects [ 54–56 ]. A prominent group of 3FTxs, α-neurotoxins, interfere with neuromuscular signal transmission of cholinergic neurons by binding to nicotinic acetylcholine receptors, causing flaccid paralysis [ 53 , 55 ]. Other 3FTxs are toxic to cardiomyocytes and can lead to increased heart rate and ultimately cardiac arrest, while yet others function as calcium channel blockers or platelet aggregation inhibitors [ 54 ].
PLA 2 s are found in the venoms of vipers, elapids, and certain rear-fanged species [ 57–60 ] and exert a wide variety of cytotoxic, myotoxic, cardiotoxic, and neurotoxic effects [ 57 , 58 , 60 ]. Of particular interest is a catalytically inactive, myotoxic category of PLA 2 s stemming from a single substitution of a highly conserved amino acid residue (Asp49 to Lys49/Asn49) [ 57 ]. Both non-catalytic and enzymatic PLA 2 s are able to form heterodimeric complexes with other PLA 2 s or other toxins in certain venoms, whereby their toxicity is greatly potentiated [ 58 ]. Most snake genomes contain multiple PLA 2 genes, which likely originated from repeated gene duplication events [ 60 , 61 ]. These paralogs have diverse pharmacological activities, which were likely acquired through neofunctionalization (i.e., recruitment of a paralog to the venom gland following gene duplication and its subsequent evolution into a toxin-coding gene) [ 62 , 63 ]. Pseudogenization and deletion of PLA 2 genes are also frequent in snakes, making this toxin family one of the most dynamic in terms of evolutionary history [ 28 , 39 , 64 ]. The annotation of more snake genomes, and the likely consequent discovery of more PLA 2 genes, might provide an improved understanding of the evolution and the mechanisms of action of these proteins (including how the phenomenon of toxin synergism has evolved) and potentially assist in the characterization of similar evolutionary processes for other enzymes.
Another major category of enzymes found in snake venoms are SVMPs [ 65 , 66 ]. These proteinases are enzymes that cleave peptide bonds in other proteins, which may result in the degradation or activation of the target [ 66 ]. Zinc-dependent SVMPs are often the major venom component in vipers [ 67 ], and these toxins hydrolyze extracellular matrix components, leading to rupture of capillaries and local and systemic bleeding [ 59 ]. Other clinical manifestations induced by SVMPs include edema, inflammation, myonecrosis, and reduced muscle regeneration [ 67 ]. Additionally, these enzymatic toxins can have anticoagulant, clotting factor–activating, or platelet-aggregating effects [ 68 , 69 ]. SVMPs are divided into 3 distinct classes depending on the domains present in the mature enzymes: P-I (metalloproteinase [M] domain only), P-II (M domain and disintegrin-like domain), and P-III (M domain, disintegrin-like domain, and cysteine-rich domain) [ 65 ]. Elucidation of snake genomes could help shed light on how these enzymes evolved from the ancestral P-III type via loss of domains [ 70–72 ] and postgenomic modifications, acquiring different functions and specificities in the process [ 65 ]. A better understanding of SVMP evolution via snake genomics could also provide insight into the evolutionary process that led to the diversification of SVMPs as a whole from the ancestral A disintegrin and metalloprotease (ADAM) family of metalloproteinases, which play significant roles in all stages of development and survival of higher-order organisms [ 73 ].
Finally, SVSPs are typically present in the venoms of vipers [ 74 ] but can also be found in elapid venoms [ 75 ]. SVSPs contain 2 6-stranded β-barrels and consist of ∼245 amino acid residues. SVSPs also have a unique extended C-terminus that forms a disulfide bridge, which contributes to structural stability [ 76 ]. These toxins can induce blood coagulation through fibrin formation, Factor V activation, prothrombin activation, actin dissolvement, or platelet aggregation; conversely, they can also act as anticoagulants via fibrinolysis, fibrinolytic enzyme activation, or protein C activation [ 59 , 77–79 ]. This toxin family has received increased attention with recent genome studies on P. flavoviridis and B. jararaca , where the evolutionary pathway as well as the molecular regulation of SVSP expression was systematically investigated [ 15 , 29 ].
In summary, snake toxin families are numerous and their pharmacological actions are complex [ 80 ]. Knowledge on the toxicity and structure of different snake toxin families is essential to further our understanding of snake venom evolution, as well as to understand venoms as drug targets for antivenom development. Much knowledge has been gained from venom proteomics and transcriptomics, and new genomics technologies now allow for the investigation of the evolutionary relationships between toxins in different families in unprecedented detail.
State of the art in snake genomics
With the rapid development of high-throughput sequencing technology, large-scale genomic projects have generated rich sequence information data of billions of base pairs and have paved the way for a new era in the field of phylogenetics, whereby the evolutionary history of organisms can be reconstructed from genomic data. The supermatrix method is the most well-known approach for analysing concatenation of multiple gene sequences, and using genomic data sets with improved resolution can potentially mitigate phylogenetic problems previously caused by sampling errors [ 81 ]. However, because only 21 (∼0.6%) of the ∼3,600 existing snake species have undergone WGS so far [ 9 , 15 , 17 , 18 , 20–28 , 30 , 82–85 ], snake genomics will likely develop significantly in the coming years. A complete list of currently available snake genomes is provided in Table 2 .
Whole-genome sequencing studies on snakes, published or in progress
PB stands for PacBio and IL for Illumina.
GC% refers to the percentage of the guanine (G) and cytosine (C) bases in a genome, scaffold N50 is a measure of the assembly quality (see below), DoC is a measure of the depth of coverage (see below), and INSDC ID is the NCBI gene bank accession number of the respective genome.
Available snake genomes differ notably in their assembly and annotation qualities, which makes evaluation of genome quality an important factor in determining the suitability of a genome for addressing a given set of questions. For instance, while estimation of nucleotide composition and genomic repeat content can be achieved from a relatively fragmented genome assembly, high-quality genome assemblies are required for analyses of multi-gene families and regulatory elements [ 88 ]. The reason for this is that the majority of the known venom gene families form tandem-arrayed “gene islands” (significantly enriched in microchromosomes, see, e.g., [ 13 ]), which generally represent a challenge for performing a continuous assembly. To achieve the best quality of assembly of venom genes, the use of long-read technology (e.g., PacBio HiFi or MinIon) is therefore essential (Fig 2 ). Genome assembly quality is assessed using statistics that measure fragmentation of the genome assembly, such as total assembly length, total contig number, contig N50, and scaffold N50. The total length of the assembly represents the total length of all the contigs that are part of the de novo assembled genome. A high total assembly length usually indicates a high-quality genome assembly. The contig N50 expresses the contiguity of the assembled genome. For instance, a contig N50 of 10 kb implies that 50% of the entire genome assembly is contained in contigs that are longer than 10 kb. Thus, a high contig N50 value represents a high-quality assembly without too many gaps. Currently, the contig N50 values of most published snake genomes are <25 kb; exceptions include 7 species with better assembly quality, namely, T. elegans (western terrestrial garter snake; 4,620 kb) [ 89 ], C. tigris (tiger rattlesnake; 2,110 kb) [ 32 ], N. naja (Indian cobra; 304 kb) [ 31 ], H. curtus (Shaw's sea snake; 183 kb) [ 30 ], B. jararaca (Brazilian lancehead; 163.5 kb) [ 29 ], P. textilis (eastern brown snake; 51 kb) [ 84 ], and N. scutatus (tiger snake; 32 kb) [ 83 ].

Schematic representation of the next-generation sequencing pipeline for genomic assembly. (1) Multiple companies have marketed sequencing platforms for genomic and transcriptomic studies, the most commonly used being Illumina (left), PacBio (middle), and Nanopore (right). (2) The 3 platforms differ in read length and accuracy of their generated sequences. Whilst Illumina sequencing generally yields short reads with low error rates, Nanopore sequences are substantially longer (≤2 Mb), yet subject to frequent sequencing errors. Last, PacBio generates sequences with lengths and error rates in between the 2 other platforms. (3) After sequencing, reads are computationally processed and assembled into contigs, which in turn (4) serve as the building blocks for scaffolds.The scaffolds are then aligned and annotated to produce the complete target genome.
Another important parameter is the contig L50, which represents the minimum number of contigs required to cover 50% of the total assembly length. N50 and L50 values can be computed both at the contig and scaffold level. The most complete published snake genomes to date are those of N. naja and C. tigris , which were assembled by combining data obtained from long-read platforms (Pacific Biosciences [PacBio] and Nanopore) and short-read platforms (Illumina), as well as Chicago, Hi-C, and optical mapping in the case of N. naja [ 31 , Fig. 2]. The resulting assemblies have a scaffold N50 reaching a staggering 207.72 Mb ( C. tigris ) and 223.35 Mb ( N. naja ) in length, which is ∼2.5 times greater than the previously assembled human reference genome (87 Mb) [ 31 , 32 , 90 ].
In addition to measures of genome contiguity — such as N50 scores — evaluating the representation of genes in a genome assembly via tools such as BUSCO provides great insight into genome assembly and annotation completeness [ 91 ]. A recent study using 611 published eukaryotic genomes showed that assemblies with high contig and scaffold N50 scores were shown to have high BUSCO values as well. However, the study revealed that assemblies with poor N50 scores may also (albeit rarely) show high BUSCO scores [ 92 ]. One example of this scenario in snakes is the case of the P. flavoviridis genome assembly, where contig N50 was 3.8 kb but percentages of complete and partial coverages for a set of 233 core vertebrate genes were 92.7% and 97.0%, respectively [ 18 ].
Furthermore, much can be learned about the quality of a genome from its reported depth of coverage (DoC). A DoC of 10× implies that each position in the genome has been read on average 10 times from independent sequencing reads. High DoC values imply that each position (i.e., each nucleotide) can be determined with greater confidence. Consequently, the 21 snake genomes published to date or in progress can be categorized into 2 groups: (1) a high DoC group (>50×) comprising B. jararaca (Brazilian lancehead) [ 29 ], C. viridis (prairie rattlesnake) [ 20 , 21 ] , C. horridus (timber rattlesnake) [ 82 ], P. flavoviridis [ 15 ], P. mucrosquamatus (brown-spotted pit viper) [ 24 ], Vipera berus (European adder) [ 25 ], T. baileyi (Tibetan hot-spring snake) [ 23 ], T. sirtalis (common garter snake) [ 85 ], T. elegans (western terrestrial garter snake), P. textilis [ 84 ], C. tigris (tiger rattlesnake) [ 28 ], N. scutatus (tiger snake) [ 93 ], D. acutus (5-paced viper) [ 86 ], B. constrictor (red-tailed boa) [ 19 ] , H. curtus (Shaw's sea snake) [ 30 ], and N. naja [ 27 ]; and (2) a low DoC group (13–40×), which includes S. catenatus (eastern massasauga rattlesnake), C. pyrrhus (southwestern speckled rattlesnake) [ 26 ], P. guttatus (corn snake) [ 22 ], O. hannah [ 18 ], and P. bivittatus [ 9 ]. Unsurprisingly, the earliest published snake genomes are characterized by lower DoCs, whereas the more recently sequenced genomes benefitted from technological advancement and thus generally obtained better coverages. The best example of this is the N. naja genome, which reached a DoC of 250× [ 27 ]—by far the highest DoC reported for a snake genome to date (Table 2 ). This high DoC enabled the discovery of 43 new toxin-encoding genes, some of which are likely to be unique to N. naja [ 27 ].
Genome size (the total amount of DNA contained within 1 copy of a single complete genome [ 94 ]), number of genes, and guanine-cytosine content [ 95 ] vary from species to species and therefore may help elucidate phylogenetic relationships and molecular events (e.g., gene/genome duplication, pseudogenization, gene loss) in the evolution of species. Genome size can vary greatly and is typically correlated with organism size and complexity, as well as with genome repeat content [ 94 ]. The reported genome sizes of snakes range from 1.3 to 1.8 Gb, except for C. pyrrhus (1.1 Gb) and B. jararaca (2.1 Gb) (Table 2 ). This is consistent with previous findings that squamate reptiles and birds generally have smaller genomes than mammals (1–3 Gb for squamates vs 1–2 Gb for birds vs 2–6 Gb for mammals) (Table 3 ) [ 22 ].
Selected genomic features compared across several vertebrate lineages [ 21 ]
Somewhat counterintuitively, genome size is not necessarily correlated with the number of genes in the genome. For example, although the Homo sapiens genome (2.90 Gb) is ∼2 times larger than the T. sirtalis genome (1.42 Gb), the number of genes is similar between the two (20,186 genes for T. sirtalis compared to 21,407 genes for H. sapiens ) [ 87 ]. This implies higher average gene density (genes/Mb) in T. sirtalis than in H. sapiens , which is likely rooted in the larger percentage of repeat elements in the human genome compared to that of T. sirtalis (∼70% and 37.12%, respectively) [ 87 , 96 , 97 ]. Thus, a considerably larger portion of the H. sapiens genome is not composed of protein-coding regions compared to the genome of T. sirtalis , which may compensate for the difference between their genome sizes. Furthermore, even though the average gene length of T. sirtalis (13,384 bp) is significantly smaller than that of H. sapiens (23,247 bp), exon length is comparable between the two (280.12 vs 249.22 bp, respectively) [ 87 ].
Unlike their genome sizes and gene lengths, the genomic GC contents for mammals, birds, and squamates are similar (∼40%) (Table 3 ), and the GC contents of reported snake genomes range from 34.3% to 43.6% (Table 3 ). Interspecies variation in GC content is thought to be caused by selective variation, mutation bias, and biased DNA repair-related recombination [ 95 ]. High GC content might also be an indication of biased sequencing results [ 98 ]. It is advisable to obtain information regarding both genome size and GC content prior to de novo assembly of a genome because these key genomic features can guide the choice of the most appropriate assembly strategy.
Understanding Snake Venom Evolution Through Snake Genomes
Genetic research on snake toxins.
Phylogenetics is the cornerstone of our understanding of evolutionary relationships at all taxonomic levels and provides a historical basis for testing and inferring ecological and evolutionary processes [ 99–102 ]. In the past few decades, snake venom and its evolutionary origins have received considerable attention [ 46 , 103–105 ]. Although there is uncertainty and controversy about the origin of the venom system in squamate reptiles [ 29 , 103 , 106–108 ] a prevalent hypothesis is that the core snake venom system evolved in the common ancestor of snakes and lizards [ 103 ].
Venom is a polygenic trait that has evolved many times in the tree of life, and it serves a role in both prey capture and defence against predators [ 104 , 109 ]. Unlike many polygenic traits [ 110 , 111 ], venom has a relatively direct pathway from transcription of toxin genes to translation into toxin proteins, which are then stored in the venom gland [ 46 , 112 ]. Thus, by combining venom-gland transcriptomics and venom proteomics, we can accurately map the progression from genotype to phenotype in this adaptive trait [ 104 ]. Because transcriptome data will vary depending on the geographical origin of the population, the age and sex of the snake, and time since the last expulsion of venom [ 113 , 114 ] that the snake was subjected to at the time of collection and/or sampling, as well as on the characteristics of the underlying genotype, transcriptomes represent a sample of the spatiotemporally expressed genome and can be used as an entry into genome divergence analysis. Genome divergence analysis takes advantage of whole-genome and/or transcriptome data to reconstruct phylogenies that chart the relationships among snakes, thus representing a precious resource for studies of snake venom evolution.
Structural characteristics of the toxin genes in snake genomes
More than 10,000 species of squamate reptiles have evolved over the past 200 million years, making this clade a major component of the vertebrate lineage [ 115 ]. The number of protein-coding genes is remarkably constant across vertebrates (including snakes), but vertebrate genomes differ considerably in size, structure, and composition [ 21 ]. An important genomic feature in this regard are transposable elements (TEs), which are self-replicating DNA sequences with the ability to insert themselves in new positions in the genome, thereby altering genome structure and gene regulation [ 116 , 117 ]. Having a high abundance of TEs could lead to a high degree of evolvability in structural features of the genome, where pseudogenization and gene duplication may occur more frequently, thus creating opportunities for neofunctionalization. As such, it is perhaps hardly surprising that TEs are consistently involved in the evolution of snake venoms [ 17 , 18 ].
Preliminary research indicates that one of the main differences across snake genomes is the abundance and diversity of TEs, which ranges between 33.0–56.3% in Colubroidea and 28.7–8.7% in non-colubroid snakes [ 20 , 27 , 86 , 87 ]. For comparison, other reptiles, such as members of the order Scincoidea, have a lower variation in their number of TEs (34.3–44.0%) (Table 3 ) [ 21 , 27 , 86 ]. Both abundance and diversity of TEs in snake genomes are exemplified by the genomes of D. acutus and B. jararaca . The former is made up of 13.84% long interspersed elements (LINEs, e.g., CR1, L1, and L2), 7.96% DNA transposons (e.g., hAT and TcMar elements), and 2.59% retrotransposons (e.g., Gypsy and DIRS elements) [ 86 ], whereas the latter comprises 14.6% LINEs with L2/CR1/Rex as the most abundant (8.8% of whole genome). The observed differences in the repeat content cannot be attributed only to varying sequencing technologies, as shown by the comparison of genome assembly qualities between snakes. For instance, while B. constrictor has a higher scaffold N50 (4,505.2 kb) and less total gap length (55,688.38 kb) compared to D. acutus (N50 2,122.2 kb; gap length 82,553.36 kb), the latter shows a higher total TE content (47.47 vs 39.59%) [ 118 ]. The genomes of D. acutus and O. hannah have a fairly low divergence level (<10%) of CR1 and hAT elements from the inferred ancestral consensus sequences, while snakes belonging to more basal-branching clades (e.g., B. constrictor and P. bivittatus ) have >20% divergence level [ 86 ]. Conversely, CR1 and hAT content is >3 times higher in D. acutus and O. hannah than in B. constrictor and P. bivittatus , but the latter 2 species have undergone independent expansion of L2 repeat contents [ 86 ]. Another study that highlights genomic differences in TE content in snakes showed that repeat element abundances in the genomes of D. acutus, T. sirtalis , and O. hannah (all part of the Colubroidea clade) were characterized by a higher CR1-like and DNA transposon content compared to the genome of P. bivittatus [ 87 ]. Overall, repeat elements in the genomes of venomous snakes are generally more active, diverse, and dynamic compared to those of non-venomous species, indicating that different types of TEs may have played multiple important roles in functional regulation of snake genes throughout evolution.
Another TE category that has attracted research attention is microsatellites (short repeated DNA sequences). Microsatellites are so ubiquitous in certain snake species that a snake genome holds the record for containing the highest microsatellite content in any known eukaryote [ 21 ]. Bolstering this claim, a study of 11 viper species found an unprecedented average microsatellite content of 16,214 bp/Mb [ 21 ]. In comparison, the mean microsatellite density of 4 non-venomous snakes was ∼55% of that amount, i.e., 8,953 bp/Mb [ 21 ]. The same study found that the mean genome density of simple sequence repeat (SSR) loci (448–896 loci/Mb) was roughly twice as large in venomous snake microsatellites as in non-venomous snake homologs [ 21 ]. The study further found that the AATAG loci (which tend to be immediately adjacent to CR1-L3 LINEs in colubroid genomes) in venomous colubroids were increased 75-fold compared to other squamate reptiles and 71-fold compared to non-colubroid snakes [ 21 ]. Based on the significant expression of SSRs and LINE-SSR hybrid element content in venomous snakes compared to non-venomous snakes, the study also concluded that SSRs and LINE-SSR hybrid elements may have played key roles in the evolution of snake venoms [ 21 ]. The dynamics and extent of the influence of SSRs and LINE-SSR on venom evolution therefore represent an intriguing venue for further research.
However, microsatellite content alone cannot explain the course of venom evolution. Indeed, another important factor is the chromosomal location of venom genes. What is known about snake chromosomes is largely based on cytogenetic experimental studies, which have revealed that the majority of snakes have 18 chromosomes (8 macrochromosomes and 10 microchromosomes) [ 20 ]. It has been observed that a high proportion of venom genes are located on microchromosomes [ 15 , 21 ], revealing a consistent pattern of homologous chromosomal location for multiple venom gene families arranged in tandem-array gene clusters. For example, 37% of all venom genes in the C. viridis genome and ∼57% (27/47 genes) of all annotated venom-related genes in the P. flavoviridis genome are located on microchromosomes (Fig. 3 ) [ 21 , 22 ]. This is the case for C. tigris as well, with all genes belonging to the major toxin family in the venom of this species (PLA 2 s) located on microchromosome 7 [ 33 ]. Phylogenetic analysis of the 3 most abundant and well-characterized toxin families in C. viridis venom (SVMPs, SVSPs, and PLA 2 s, all located on microchromosomes) revealed that each toxin gene family represents a distinct set of duplicated genes derived from a single ancestral homolog that produced a monophyletic cluster of venomous paraphyletic lineages [ 21 ]. Notably, microchromosomes have higher GC content and faster recombination rates than macrochromosomes [ 21 ], as is evident in the C. viridis genome [ 20 ]. Therefore, it appears that microchromosomes are generally enriched with venom genes, which together with their high recombination rate could explain the huge radiation and rapid evolution of venom-related genes [ 15 ].

Venom-related gene families in the P. flavoviridis genome. (A) Deduced evolutionary history of venom-related gene families through 2 rounds of whole-genome duplication (2R-WGD). An original set of 18 genes (shown in the top box) became 72 (4 copies each). Then, a single copy of each family was likely co-opted to develop toxic functions, resulting in 1 snake venom (SV) copy (shown in a pale red box in the right column) and 3 non-venom (NV) paralogs (shown in the see-through box to the left). (B) Tandem duplications of SVMP genes. (C) Tandem duplications of SVSP genes. (D) Tandem duplications of CTLP genes. Based on Fig. 2 and Fig. S8 from [ 15 ].
Nonetheless, it should be noted that a substantial percentage of toxin-coding genes are found on macrochromosomes as well. This is evident in N. naja , where as many as 16 toxin gene families are located on macrochromosomes [ 32 ]. WGS of other venomous snake species will be essential to investigate how and to what extent chromosomal location of genes influences venom evolution. Interestingly, the chromosome structure of C. viridis is comparable to that of N. naja . In fact, chromosome 4 of N. naja shares syntenic regions with C. viridis chromosomes 3 and 5, and chromosomes 5 and 6 of N. naja are syntenic with chromosome 5 of C. viridis [ 27 ]. This might indicate the occurrence of fusion and fission events, respectively [ 27 ]. The N. naja genome has also been compared to that of O. hannah (another elapid, and thus more closely related to N. naja than C. viridis ), where 139 venom gland toxin genes from the N. naja genome were cross-referenced with genes in the O. hannah genome to find orthologs [ 27 ]. The results showed that 96 of the N. naja genes had counterparts in the O. hannah genome, while 43 did not [ 27 ]. Although some of these 43 genes may be unique to N. naja , others may simply not have been annotated in the O. hannah genome, possibly owing to the high fragmentation of its assembly (which relied on short reads) [ 14 ].
In the future, widespread access to different types of sequencing platforms providing researchers with both short and long reads, complementary tools for genome analysis (Hi-C and CHiCAGO), and higher quality sequence data will likely enable researchers to study snake genomes in greater detail. In turn, this will help elucidate differences and similarities between snake genomes and allow for more fine-grained studies of the structural characteristics of snake venom genes.
Molecular origin and regulation of snake venom genes
Snake venoms and their evolutionary origins have received substantial attention over the past decades, with >15,000 studies published on this topic [ 15 ]. Snake venoms have the dual functions of defense against predators and subduction of prey, with predation typically being the primary function [ 104 ]. This locks snakes and their prey in an evolutionary arms race, where the prey evolves biological strategies that make it resistant to toxins, and snakes are constantly pressured to optimize and adjust the composition of toxins in their venoms [ 104 ]. Indeed, dietary habits have often been indicated as a key driver of adaptive venom evolution in snakes, featuring among the main reasons behind inter- and intraspecific variation in venom composition [ 119 ].
Reports on trophic adaptations of snakes are plentiful. As an example, a study showing that venom variation in the Malayan pit viper ( Calloselasma rhodostoma ) throughout its range is significantly associated with the types of prey locally available [ 120 ]. This is also the case for the Mangrove catsnake ( Boiga dendrophila ), which was found to possess a 3FTx specific for birds and lizards (the bulk of this snake's diet) but scarcely effective on mammals [ 121 ]. However, recent research reported that venom composition in the Mojave rattlesnake ( Crotalus scutulatus ) was associated with environmental factors (e.g., temperature, seasonality) rather than with diet [ 122 ]. This suggests that a more complex scenario of factors could be affecting venom diversity than prey-related drivers alone, as confirmed by the dynamics behind venom variation in the Northern Pacific rattlesnake ( Crotalus oreganus ). In fact, the dichotomy in venom composition observed in this species is consistently influenced not only by coevolution with its prey but also by genetic distance and elevation-based habitat gradients, in a pattern described as “phenotype matching” of venom characteristics to multiple variables in the snake's native ecosystem [ 123 , 124 ]. The genetic basis underlying such complex adaptive processes could likely provide intriguing insight into the influence of natural selection and phylogenetic relatedness on the evolution of a highly dynamic trait such as snake venom. To this end, WGS of snakes will likely be key to conclusively determining the structural and evolutionary features of toxin genes and gene clusters. Analysing such patterns in a comparative framework would then enable researchers to identify similarities and differences in adaptive drivers of venom evolution at all levels of snake taxonomy and phylogeny.
In recent years, venom evolution has been further explored through genome studies on several species of venomous snakes [ 15 , 21 , 27 , 86 ]. One of these studies revealed that the venom gene repertoire of D. acutus has a very different composition from those of O. hannah and the non-venomous A. carolinensis (outgroup) , B. constrictor , and P. bivittatus . These differences are exemplified both by the absence of characteristic venom genes from the D. acutus genome relative to the O. hannah genome and by the increased gene copy number of other venom gene families, including SVMPs, CTLPs, and SVSPs (Table 1 ) [ 86 ]. Expression of most toxin-encoding genes shared by D. acutus and O. hannah (especially older genes derived from the last common ancestor of these species) is limited to venom glands or accessory glands [ 86 ]. Similarly, newer viper-specific toxin genes are expressed in the venom and accessory glands of D. acutus , while equally recent elapid-specific toxin genes are expressed in the venom and accessory glands of O. hannah [ 86 ]. Interestingly, genes closely related to the elapid-specific toxin genes expressed in the venom glands of O. hannah are expressed in the liver of D. acutus , and genes related to viper-specific toxin genes expressed in the venom glands of D. acutus are expressed in pooled organs from O. hannah [ 86 ].
These special expression patterns suggest that these venom genes may originate from metabolic proteins that have undergone subfunctionalization (i.e., paralogs retaining only part of the functional features of the original gene following duplication) or neofunctionalization, as well as that changes in tissue-specific expression have occurred [ 17 , 86 ]. This is in accordance with previous protein-based findings [ 125 , 126 ]. Similarly, analysis of the O. hannah genome demonstrated that the regulatory components of the venomous secretion system may have evolved from the pancreas [ 18 ]. Several mechanisms likely contribute to the enhanced expression of toxin-coding genes in the venom gland. At the chromosome level, methylation and chromatin accessibility were recently shown to play a prominent role in gene regulation in C. tigris . In fact, methylation appears to be significantly more prevalent in non-toxin and unexpressed toxin genes compared to expressed toxin counterparts in the venom gland and pancreas of this species [ 33 ]. Furthermore, chromatin accessibility and methylation levels are positively related with the high expression of toxin genes compared to non-expressed counterparts and non-toxin genes in C. tigris , further supporting a joint role for these 2 factors in toxin gene expression [ 28 ]. Another important factor in regulation and expression of toxin genes is the gene regulatory network associated with them (recently termed “metavenom network”), which comprises ∼3,000 genes that do not code for toxins but actively influence their expression and postgenomic modifications (e.g., protein folding) in the venom gland as housekeeping genes [ 48 ]. Interestingly, this network presents highly conserved elements common to even distantly related lineages such as snakes and venomous mammals; on the other hand, snakes (specifically P. flavoviridis and P. mucrosquamatus ) also displayed several unique regulatory genes that were likely co-opted together with neofunctionalized toxin genes absent in other lineages [ 48 ].
Gene duplication is thought to be one of the main mechanisms behind venom diversification [ 127 ]. The current consensus is that 2 rounds of whole-genome duplication (2R-WGD) occurred during the evolution of vertebrates [ 15 , 128 ]. A study of the P. flavoviridis genome identified 18 families of venom-related genes, including both toxin and non-toxin gene copies. These include metalloproteinases (SVMPs), serine proteases (SVSPs), CTLPs, PLA 2 s, 3FTxs, aminopeptidases (APaseNs), CRISPs, vespryns/SPla and ryanodine receptor domain proteins (Vespryns), 5′-nucleotidases (5Nases), dipeptidyl peptidases (DDPases), hyaluronidases (Hyals), nerve growth factors or neurotrophins (NGF), vascular endothelial growth factors (VEGFs), LAAOs, phosphodiesterases (PDEs), phospholipases B (PLBs), bradykinin-potentiating peptides and C-type natriuretic peptides (BNPs), and glutaminyl peptide cyclotransferases (GPCases). [ 15 ]. The study suggested that 2R-WGD resulted in the creation of 4 paralogs from each of the 18 genes and that during the later evolution of venomous snakes, 1 of these 4 gene copies underwent neo- or subfunctionalization and evolved toxic properties, while the remaining 3 copies did not [ 15 ]. Both the toxin and non-toxin encoding genes subsequently underwent multiplication to different extents (Fig. 4 ) [ 15 ], as is demonstrated by the multiple gene duplication events detected in the SVMP, SVSP, CTLP, PLA 2 , 3FTx, and CRISP gene families in P. flavoviridis and N. naja [ 15 , 27 ]. However, this phenomenon was investigated to the greatest detail in rattlesnakes ( Crotalus spp.), with comparative genomics between species revealing multiple duplication events in neurotoxic PLA 2 genes as well as all SVMP classes. Chromosome mapping of the complete genomes of C. viridis and C. tigris provided further support for the occurrence of this phenomenon, highlighting similar duplication events for both gene families as well as SVSP genes (all of which are arranged in tandem-array single clusters) [ 18 , 26 ].

Syntenic comparison of toxin gene clusters. Comparison showing the 3FTx, CRISP, and SVMP genes in N. naja and C. viridis genomes. Orthologous gene pairs are indicated by the line linked across the corresponding genomic regions. Based on Fig. 4 and Extended Fig. 4 from [ 27 ].
Molecular phylogenetic analysis of P. flavoviridis shows that all toxin genes of a given gene family in this species are homologous to the same toxin gene families found in vipers and elapids, such as P. mucrosquamatus (brown-spotted pit viper) and O. hannah [ 15 ]. The notion that snake toxin genes massively expanded through gene duplication events and underwent sub- and/or neofunctionalization is also supported by other studies [ 18 , 27 , 108 ]. For example, the N. naja genome assembly contributes to our understanding of the origin of multiple unlinked venom gene clusters and provides new and conclusive evidence that each toxin family stems from a unique set of tandem duplicate genes [ 27 ].
While duplication either before or after gene recruitment to the venom gland is an established driving force of venom evolution in snakes, loss of genetic material has been no less pivotal in facilitating diversification of toxin families in certain venomous snake clades. For instance, the interplay between gene duplication and deletion (of entire genes as well as intragenic regions) is remarkable in rattlesnakes ( Crotalus spp.). These pit vipers present signs of multiple independent losses of ancestral genes coding for SVMPs and neurotoxic PLA 2 s—both of which had previously experienced a rampant expansion via repeated duplication episodes—across their phylogenetic tree [ 39 , 71 ]. Intriguingly, different genes underwent deletion among and even within species, such as observed in the western diamondback rattlesnake ( Crotalus atrox ), the Mojave rattlesnake ( C. scutulatus ), and the Southern Pacific rattlesnake ( Crotalus helleri ) [ 39 , 64 , 71 ]. This resulted in great haplotype disparity and differential expression of toxin-encoding genes not only between species but across conspecific individuals as well. WGS of C. tigris further corroborated this pattern, as this species is known for its remarkably simple venom composition largely based on neurotoxic PLA 2 isoforms [ 129 ]. However, the C. tigris genome revealed a deletion of 3 PLA 2 genes on microchromosome 7 and of 10 SVMP genes on microchromosome 1 compared to homologous regions in C. viridis , indicating that even such a simple venom phenotype is the result of extensive genomic modifications over evolutionary time [ 28 ]. This pattern is not limited to rattlesnakes. For instance, the B. jararaca genome also displays a great expansion of SVMP genes via duplication upon recruitment in the venom gland, followed by 2 deletions in the exon 14 region of PII-SVMP genes causing loss of the Cys-rich domain found in PIII-SVMPs [ 29 ]. This observation sheds further light on the genomic processes responsible for evolution and differentiation via domain loss in SVMPs, which has occurred in other viper lineages as well [ 46 ].
Adaptive and neutral evolution in snake venoms
Determining and unraveling the driving factors behind the dynamic evolutionary processes in snake venom gene families has garnered the interest of scientists for decades—a quest that could only benefit from increasing efforts in WGS of venomous snakes. Positive selection appears to be the force behind the evolution of genes involved in predator-prey arms races [ 130 ], and it seems to be pervasive across most toxin-related gene families in snakes. Positive selection leaves a well-defined pattern in the genome, with the accumulation of non-synonymous, amino acid–replacing nucleotide substitutions (denoted by K a ), over synonymous substitutions (K s ) in the gene [ 131 ]. In P. flavoviridis , the K a /K s ratios of the 4 main toxin gene families were consistently > 1 and/or higher than those reported for non-venom genes (SVMPs: 1.047 ± 0.438, SVSPs: 1.253 ± 0.090, CTLPs: 0.871 ± 0.071, PLA 2 s: 1.093 ± 0.062) [ 15 ], suggesting positive selection behind the accelerated evolution of the major toxin gene families in this species. Interestingly, P. flavoviridis also exhibited K a /K s > 1, in the 3FTx and CRISP gene families, which therefore also displayed a tendency towards accelerated evolution despite being present in far fewer copies [ 15 ]. Similarly, a high K a /K s ratio (2.034 ± 0.818) was observed for the 3FTx gene family in the N. naja genome, again pointing towards rapid differentiation and functional diversification for these genes [ 27 ]. Conversely, when K a /K s < 1 it is indicative of either neutral selection (random substitutions that confer neither evolutionary advantages nor disadvantages) or purifying selection (i.e., removal of mutations that usually tend to be deleterious as they appear in conserved areas of the gene). In the P. flavoviridis genome study, all non-dominant toxin gene families had a K a /K s < 1 (mean = 0.512 [SD 0.018]), indicating a more neutral nucleotide substitution and the maintenance of similarity between gene copies [ 15 ]. On the other hand, when examining sequence divergence using venom gland transcriptomes in sidewinder rattlesnakes ( Crotalus cerastes ), data showed evidence of selection being stabilized, which supports that the maintainance of a generalist phenotype is favoured [ 132 ]. It must, however, be noted that despite various methods available for studying selection (see [ 133 ]), relatively few have been applied for the investigation of selection in venom and only in a small number of species [ 15 , 27 , 132 , 134 ]. Therefore, additional studies are required before general conclusions can be drawn.
New -omics tools and methods are rapidly advancing our knowledge of the mechanisms behind venom evolution [ 135 ]. In particular, WGS has introduced advantages to snake venom research, as WGS data can be used to identify structural variants, including inversions (Fig. 3A - -B), B ), insertions (Fig. 3C ), deletions, tandem repeats (Fig. 3A - -C), C ), TEs, and other repeat content [ 21 , 136 ]. An increasing number of studies report venom variation at different levels, such as ontogenetic, within-species, and between-species [ 46 , 131 , 137–140 ]. Once the reference genome of a species is available, population genomics can contribute to the identification of such intra- or interspecific variation. This further enhances the study of venom regulation, helping us to understand the evolution of complex regulatory networks [ 28 ]. Although it is generally acknowledged that positive selection seems to be the main driver behind venom evolution, genomic tools allow zooming in on specific venom-related genes to infer the role of neutral evolutionary processes, i.e., genetic drift or random changes in allele frequencies [ 141 ]. Genetic drift contributes to the accumulation of random neutral variation, which serves as the basis for natural selection to act upon in response to new evolutionary pressures [ 142 ]. Although most research to date has focused on the adaptive processes explaining venom evolution, recent studies have started assessing the role of such neutral forces in shaping venom characteristics. For example, genetic drift was identified as a prominent factor behind sequence divergence in venom genes in P. mucrosquamatus , where dominant toxin-encoding genes displayed relaxed selective constraints for deleterious mutations despite statistically significant rates of positive selection [ 24 ]. Furthermore, it has been shown that variation in expression of the myotoxin, crotamine, in the eastern diamondback rattlesnake ( Crotalus adamanteus ) and the South American rattlesnake ( Crotalus durissus ) is significantly more correlated with differences in number of duplication-derived gene copies between populations than with adaptive divergence in the sequences themselves [ 134 , 143 ].
The strength at which genetic drift acts on the genome is inversely proportional to effective population size ( N e , namely, the number of reproductive individuals that actually produce offspring) [ 142 ]. N e greatly contributes to sequence variation, as the fate of a favourable mutation spreading is controlled by N e and the strength of selection [ 144 , 145 ]. A prime example of this pattern in snake venom evolution is presented by the eastern massasauga rattlesnake ( Sistrurus catenatus ), a threatened species whose range consists of several scattered populations largely isolated from each other. Although the influence of genetic drift on venom evolution in this species is currently weak, it is likely to increase dramatically over time once the impact of drift is augmented due to the low N e found in most populations [ 146 ]. Thus, complete genomes obtained through WGS together with complementary DNA libraries can expand our knowledge of the effects of selection on venom genes, with great potential to either corroborate or challenge the current positive selection–centered view of snake venom evolution.
Conclusions and Perspectives
WGS is a revolutionary advance in genetic research that has only recently been applied to the fields of herpetology and toxinology. Nonetheless, sequencing of complete snake genomes has already shed light on the evolutionary history of toxin-encoding genes as well as their expression patterns in the venom gland. In the future, WGS may be harnessed to obtain a better understanding of the molecular mechanisms involved in snake evolution [ 6 , 103 ], find new bioactive molecules with potential clinical applications, and provide valuable information for antivenom development [ 35 ]. Because only 21 complete snake genomes are currently available, there is ample opportunity for genomic research on the remaining thousands of snake species, including medically relevant venomous representatives. With the increasing power of sequencing technologies, the field of snake genomics is indeed likely to expand significantly in the years to come, with multiple complete genomes already in the process of being sequenced or published. However, this will not come without challenges because the interplay of dietary and environmental factors that has fueled venom diversification via gene duplication, recruitment, and neofunctionalization events makes it difficult to assemble whole venomous snake genomes. Another factor adding to the complexity of de novo genome assembly is the high content of repeat sequences in snake genomes. Some of these challenges might be adequately addressed by using third-generation sequencing technology. As the costs and error rates of this and other approaches decrease, they are certain to be used more widely in snake genome research. In turn, the assembly of more venomous snake genomes will allow us to explore adaptation and venom evolution at all phylogenetic levels, bringing a new perspective to the study of snake genomes and venoms.
Data Availability
Abbreviations.
3FTx: 3-finger toxin; bp: base pairs; BUSCO: Benchmarking Universal Single-Copy Orthologues; CRISP: cysteine-rich secretory protein; CTLP: C-type lectin-like protein; DoC: depth of coverage; Gb: gigabase pairs; kb: kilobase pairs; LAAO: L-amino acid oxidase; LINE: long interspersed element; NCBI: National Center for Biotechnology Information; NGS: next-generation sequencing; PLA 2 : phospholipase A 2 ; SSR: simple sequence repeat; SVMP: snake venom metalloproteinase; SVSP: snake venom serine proteinase; TE: transposable element; WGS: whole-genome sequencing.
Competing Interests
W.R., W.Z., and S.L. are employees at the BGI. The authors declare that they have no other competing interests.
This research was supported by the Beijing Genomics Institute and the Technical University of Denmark. M.E.A. is funded by the Independent Research Fund Denmark (7027–00147B). C.K. is funded by Innovation Fund Denmark (9065–00007B). T.P.J. is funded under Marie Sklodowska-Curie grant agreement No. 713683 (COFUNDfellowsDTU).
Authors’ Contributions
A.H.L. and S.L. conceived the project. A.H.L., W.R., K.K., T.P.J., C.K., C.T.W., W.Z., S.G., L.S., M.M.D., B.J.M., E.R.T., and M.E.A. structured the draft and provided final editing. A.H.L., K.K., T.P.J., and W.R. coordinated and drafted the manuscript and implemented comments provided by all authors. All authors contributed critically to the scientific content. All authors read and approved the final manuscript.
Supplementary Material
Giac024_giga-d-21-00410_original_submission, giac024_giga-d-21-00410_revision_1, giac024_response_to_reviewer_comments_revision_1, giac024_reviewer_1_report_original_submission.
Blair Perry -- 1/18/2022 Reviewed
giac024_Reviewer_2_Report_Original_Submission
Matthew Holding -- 1/18/2022 Reviewed
Contributor Information
Wei-qiao Rao, Department of Biotechnology and Biomedicine, Technical University of Denmark, Søltofts Plads 224, 2800 Kongens Lyngby, Denmark. Department of Mass Spectrometry, Beijing Genomics Institute-Research, 518083, Shenzhen, China.
Konstantinos Kalogeropoulos, Department of Biotechnology and Biomedicine, Technical University of Denmark, Søltofts Plads 224, 2800 Kongens Lyngby, Denmark.
Morten E Allentoft, Trace and Environmental DNA (TrEnD) Laboratory, School of Molecular and Life Sciences, Curtin University, Kent Street, 6102, Bentley Perth, Australia. Globe Institute, University of Copenhagen, Øster Voldgade 5, 1350, Copenhagen, Denmark.
Shyam Gopalakrishnan, Globe Institute, University of Copenhagen, Øster Voldgade 5, 1350, Copenhagen, Denmark.
Wei-ning Zhao, Department of Mass Spectrometry, Beijing Genomics Institute-Research, 518083, Shenzhen, China.
Christopher T Workman, Department of Biotechnology and Biomedicine, Technical University of Denmark, Søltofts Plads 224, 2800 Kongens Lyngby, Denmark.
Cecilie Knudsen, Department of Biotechnology and Biomedicine, Technical University of Denmark, Søltofts Plads 224, 2800 Kongens Lyngby, Denmark.
Belén Jiménez-Mena, DTU Aqua, Technical University of Denmark, Vejlsøvej 39, 8600, Silkeborg, Denmark.
Lorenzo Seneci, Department of Biotechnology and Biomedicine, Technical University of Denmark, Søltofts Plads 224, 2800 Kongens Lyngby, Denmark.
Mahsa Mousavi-Derazmahalleh, Trace and Environmental DNA (TrEnD) Laboratory, School of Molecular and Life Sciences, Curtin University, Kent Street, 6102, Bentley Perth, Australia.
Timothy P Jenkins, Department of Biotechnology and Biomedicine, Technical University of Denmark, Søltofts Plads 224, 2800 Kongens Lyngby, Denmark.
Esperanza Rivera-de-Torre, Department of Biotechnology and Biomedicine, Technical University of Denmark, Søltofts Plads 224, 2800 Kongens Lyngby, Denmark.
Si-qi Liu, Department of Mass Spectrometry, Beijing Genomics Institute-Research, 518083, Shenzhen, China.
Andreas H Laustsen, Department of Biotechnology and Biomedicine, Technical University of Denmark, Søltofts Plads 224, 2800 Kongens Lyngby, Denmark.
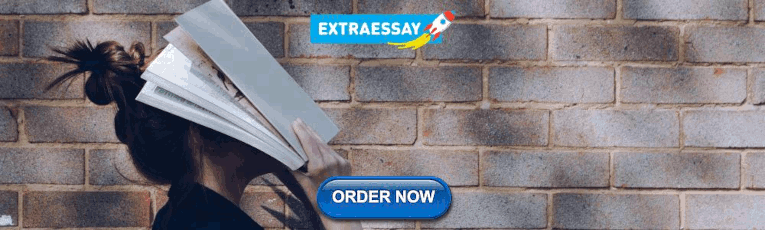
IMAGES
VIDEO
COMMENTS
Sansevieria trifasciata fibre has low costs, a wide availability, a high specific strength, renewability and low density and can. be used as reinforcement in polymer composites. This review. paper ...
The snake plant/epoxy composite was made using a manual hand lay-up process. The epoxy resin and hardener were mixed carefully in an appropriate ratio. Different weight percentage of fibers (10%, 20%, and 30%) were added to the epoxy resin (90%, 80%, and 70%) and hardener mixture to prepare the desired composite.
According to research, Sansevieria trifasciata leaf is frequently used to treat bronchitis, asthma, food poisoning, toxaemia coughs, snake bites and insect bites. The LD50 value was found to be 774.60 mg/kg, and oral dosage of 18,000 mg/kg did not result in animal mortality or any unfavourable behavioural effects.
The produced paper followed the proper standard of 1 mm. Table 2: Sensory evaluation of paper from corn husks and snake plant fibers Treatments Texture Durability Color Thickness 3.67 4.13 4.77 4.40 Treatment 1 A A HA HA 4.03 4.43 2.70 4.30 Treatment 2 A HA FA HA 4.80 4.40 4.23 4.63 Treatment 3 HA HA HA HA 4.30 4.40 4.10 4.60 Treatment 4 HA HA ...
The Sansevieria ehrenbergii plant leaves were collected from the Erode region, Tamil Nadu, South India. This plant is called a Snake grass plant and comes under the Sisal plant family [].Untreated Sansevieria ehrenbergii fibers (SEFs), Sodium hydroxide pellets, concentrated Hydrochloric acid, and deionized water is used in the alkali treatment []. ...
The ethanol and water extracts of Sansevieria trifasciata leaves showed dose-dependent and significant (P < 0.05) increase in pain threshold in tail-immersion test. Moreover, both the extracts (100 - 200 mg/kg) exhibited a dose-dependent inhibition of writhing and also showed a significant (P < 0.001) inhibition of both phases of the formalin ...
INTRODUCTION Paper is used in a variety of application so its demand is increasing. Thus, environmental issues are also increasing. It is said that the consumption of paper has grown 400 percent in the last 40 years and a large number of trees are cut to use in paper industries. Paper pollutes air, land, and water because it contains dyes, inks, and other harmful chemicals.
PDF | On Jan 1, 2017, Julie S Berame and others published Preliminary Phytochemical Screening and Toxicity Test of Leaf and Root Parts of the Snake Plant (Sansevieria trifasciata) | Find, read and ...
Three common indoor houseplants, snake plant (Sansevieria trifasciata), spider plant (Chlorophytum comosum), and golden pothos (Epipremnum aureum), were evaluated for their species effectiveness in reducing ozone concentrations in a simulated indoor environment. Continuously stirred tank reactor (CSTR) chambers housed within a greenhouse equipped with a charcoal filtration air supply system ...
However impact test results for snake plant fiberglass suggested that the product could withstand greater forces as the data yields that with 2.75 lbs, 4.96 lbs and 6.61 lbs, recorded descriptions are "no damage", "slightly deformed", "deformed" , and "having a crack" .
PDF. The study of application of snake plant absorbed carbon dioxide in the offices. The aim of this research was to measure the concentration of carbon dioxide in offices with snake plants. The measuring time is during working time 8.30 am to 4.30 pm, at three offices. The first room was the academic affair at engineering building with volume ...
SNAKE PLANT (Sansevieria trifasciata) FIBER AS A POTENTIAL SOURCE OF PAPER - Free download as Word Doc (.doc / .docx), PDF File (.pdf), Text File (.txt) or read online for free.
The aim of this research is to know the potency of mango seed starch and snake plant fibers as bio-plastic fillers and glycerol concentration as plasticizer on tensile strength and elongation at break, density, and heat resistance. The samples prepared have three treatments and a control group containing…. Expand. View via Publisher. imedpub.com.
SNAKE PLANT RESEARCH.docx - Free download as Word Doc (.doc / .docx), PDF File (.pdf), Text File (.txt) or read online for free. This research proposal aims to determine the potential of using Sansevieria trifasciata (snake plant) fiber as an alternative component for paper production. The study will test 5 treatments that vary the ratio of snake plant fiber to water and starch.
PDF | To know about Snake plant fiber and some of its characteristics | Find, read and cite all the research you need on ResearchGate. Article PDF Available. Literature review. November 2022;
The plant also filters formaldehyde, tri-chloroethylene, xylene, toluene, and benzene from the air. This research work proposes snake plant as a viable option by demonstrating real-time monitoring of CO 2 levels. The comparative study has been shown in section 3.3. The study validates the data by comparing it to research examining human sleep ...
Fortunately, snake genome research has eventually gained more attention, with 18 new genomes being released since 2013 and several more currently in progress [ 15, 20-31 ]. These increased sequencing efforts have already revealed intriguing insights into the regulation and expression of venom-related genes.
SNAKE-PLANT-RESEARCH Final.docx - Free download as Word Doc (.doc / .docx), PDF File (.pdf), Text File (.txt) or read online for free. Scribd is the world's largest social reading and publishing site.