
An official website of the United States government
The .gov means it’s official. Federal government websites often end in .gov or .mil. Before sharing sensitive information, make sure you’re on a federal government site.
The site is secure. The https:// ensures that you are connecting to the official website and that any information you provide is encrypted and transmitted securely.
- Publications
- Account settings
Preview improvements coming to the PMC website in October 2024. Learn More or Try it out now .
- Advanced Search
- Journal List
- HHS Author Manuscripts

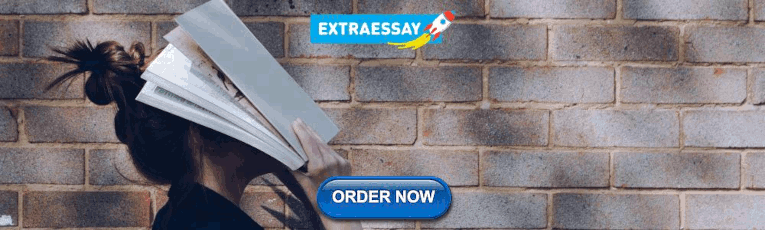
Challenges to Sustainable Safe Drinking Water: A Case Study of Water Quality and Use across Seasons in Rural Communities in Limpopo Province, South Africa
Joshua n. edokpayi.
1 Department of Hydrology and Water Resources, University of Venda, Thohoyandou 0950, South Africa; [email protected]
2 Department of Civil and Environmental Engineering, University of Virginia, Charlottesville, VA 22904, USA; ude.qud@drelhak (D.M.K.); moc.liamg@320hlc (C.L.H.); ude.ainigriv@sm4rfc (C.R.); ude.ainigriv@e9saj (J.A.S.)
Elizabeth T. Rogawski
3 Department of Public Health Sciences, University of Virginia, Charlottesville, VA 22908, USA; ude.ainigriv@m5rte
4 Division of Infectious Diseases & International Health, University of Virginia, Charlottesville, VA 22908, USA; ude.ainigriv.ccm.liamcsh@v8dr
David M. Kahler
5 Center for Environmental Research and Education, Duquesne University, Pittsburgh, PA 15282, USA
Courtney L. Hill
Catherine reynolds.
6 School of Civil and Environmental Engineering, Georgia Institute of Technology, Atlanta, GA 30332, USA
Emanuel Nyathi
7 Department of Animal Science, University of Venda, Thohoyandou 0950, South Africa; [email protected]
James A. Smith
John o. odiyo, amidou samie.
8 Department of Microbiology, University of Venda, Thohoyandou 0950, South Africa; [email protected] (A.S.); [email protected] (P.B.)
Pascal Bessong
Rebecca dillingham.
Author Contributions: Conceived and designed the experiments: J.N.E., E.T.R., D.M.K., C.L.H. Performed the experiments: J.N.E., E.T.R., D.M.K., C.L.H., C.R., E.N. Contributed reagents/materials/analysis tools: P.B., E.N., A.S., R.D., J.A.S., J.O.O. Analyzed the data: J.N.E., E.T.R., D.M.K., C.L.H. Wrote the paper: J.N.E., E.T.R., D.M.K., C.L.H. Participated in the editing of the manuscript: J.N.E., E.T.R., D.M.K., C.L.H., P.B., A.S., R.D., J.A.S., J.O.O., E.N., C.R.
Associated Data
Table S2: Membrane-filtration results for E. Coli and total coliforms of water sources,
Table S3: Anion concentrations (mg/L) of water sources,
Table S4: Major metal concentrations (mg/L) of water sources,
Table S5: Trace metal concentrations μg/L) of water sources.
Consumption of microbial-contaminated water can result in diarrheal illnesses and enteropathy with the heaviest impact upon children below the age of five. We aimed to provide a comprehensive analysis of water quality in a low-resource setting in Limpopo province, South Africa. Surveys were conducted in 405 households in rural communities of Limpopo province to determine their water-use practices, perceptions of water quality, and household water-treatment methods. Drinking water samples were tested from households for microbiological contamination. Water from potential natural sources were tested for physicochemical and microbiological quality in the dry and wet seasons. Most households had their primary water source piped into their yard or used an intermittent public tap. Approximately one third of caregivers perceived that they could get sick from drinking water. All natural water sources tested positive for fecal contamination at some point during each season. The treated municipal supply never tested positive for fecal contamination; however, the treated system does not reach all residents in the valley; furthermore, frequent shutdowns of the treatment systems and intermittent distribution make the treated water unreliable. The increased water quantity in the wet season correlates with increased treated water from municipal taps and a decrease in the average contaminant levels in household water. This research suggests that wet season increases in water quantity result in more treated water in the region and that is reflected in residents’ water-use practices.
1. Introduction
Clean and safe drinking water is vital for human health and can reduce the burden of common illnesses, such as diarrheal disease, especially in young children. Unfortunately, in 2010, it was estimated that 1.8 billion people globally drank water that was not safe [ 1 ]. This scenario is most common in developing countries, and the problem is exacerbated in rural areas [ 1 ]. Significant amounts of time are spent by adults and school children upon water abstraction from various sources [ 2 , 3 ]. It is estimated that, in developing countries, women (64%) and girls (8%) spend billions of hours a year collecting water [ 1 ]. The erratic supply of safe drinking and domestic water often affects good hygiene practices. In most developing countries of the world, inadequate supplies of drinking water can contribute to the underage death of children in the region [ 4 – 10 ].
Storage of collected water from rivers, springs, community stand-pipes, and boreholes is a common practice in communities that lack potable water supplies piped into their homes. Even when water is piped into the home, it is often not available on a continuous basis, and water storage is still necessary. Water is stored in various containers which include jerry cans, buckets, drums, basins and local pots [ 11 – 13 ]. It has been reported that when collection of water from sources of high quality is possible, contamination during transport, handling and storage and poor hygienic practices often results and can cause poor health outcomes [ 11 , 13 – 15 ].
South Africa is a semi-arid country that has limited water resources, and the provision of adequate water-supply systems remains a great challenge. In some of the major cities, access to clean and safe drinking water is comparable to what is found in other developed cities, but this is not the case in some cities, towns and most villages where there is constant erratic supply of potable water, and in some cases, there is no water supply system [ 16 ]. Although access to clean and safe drinking water is stipulated as a constitutional right for all South Africans in the country’s constitution [ 17 , 18 ], sustainable access to a potable water supply by millions of South Africans is lacking.
Residents of communities with inadequate water supply are left with no alternative other than to find local sources of drinking water for themselves. Rural areas are the most affected, and residents resort to the collection of water from wells, ponds, springs, lakes, rivers and rainwater harvesting to meet their domestic water needs [ 19 – 24 ]. Water from such sources is often consumed without any form of treatment [ 12 , 19 , 21 ]. However, these alternative sources of drinking water are often vulnerable to point and non-point sources of pollution and are contaminated frequently by fecal matter [ 5 , 19 , 25 ]. A report by the South African Council for Scientific and Industrial Research clearly showed that almost 2.11 million people in South Africa lack access to any safe water infrastructure. The consumption of water from such unimproved sources without treatment constitutes a major public health risk [ 26 ].
Consumption of contaminated drinking water is a cause of diarrheal disease, a leading cause of child mortality in developing countries with about 700,000 deaths of children under the age of 5 reported in 2011 [ 10 , 27 ]. In South Africa, diarrhea is one of the leading causes of death among young children, and this problem is worst in children infected with HIV (Human Immunodeficiency Virus).
The health risks associated with the consumption of unsafe drinking water are not only related to infectious diseases but also to other environmental components such as fluoride, arsenic, lead, cadmium, nitrates and mercury. Excessive consumption of these substances from contaminated drinking water can lead to cancer, dental and skeletal fluorosis, acute nausea, memory lapses, renal failure, anemia, stunted growth, fetal abnormalities and skin rashes [ 16 , 28 ]. Groundwater contamination with high arsenic concentrations have been reported in Bangladesh, and high fluoride concentrations have been reported in the drinking water from various provinces in South Africa [ 28 – 34 ].
Temporary seasonal variations have been reported to influence the levels of contaminants in various water sources differently. The key environmental drivers across the wet and dry seasons include: volume of water, flow, frequency of rainfall events, storm run-off, evaporation and point sources of pollution [ 35 , 36 ]. An increase in storm-water run-off within a river catchment may increase the level of contaminants due to land-use activities. Increased water volume could lead to a decrease in the concentration of contaminants due to the dilution effect. A low incidence of rainfall and high evaporation can cause a contaminant to concentrate in water. Very few water-quality parameters such as turbidity are expected to be higher in the wet season. Other parameters can vary depending on the key environmental drivers. There is paucity of data on the effect of change across seasons on water-use practices among household in rural areas of developing countries.
The geographic area for this study is located 35 km north of Thohoyandou, in Limpopo Province, South Africa. The area is primarily agricultural, such that water contamination by nitrates is a potential concern. In addition, mining operations in the area may contaminate water sources with heavy metals.
The significance of this study lies in the broad characterization of water-quality parameters that could affect human health, which is not restricted to microbiological analysis. In a rural community, the primary concern of drinking water is the microbiological quality of the water and chemical constituents are often considered not as problematic. This study was designed to evaluate a broad spectrum of water-quality constituents of natural water sources and household drinking water used by residents of rural communities in Limpopo Province. We also aimed to determine how water sources and collection practices change between dry and wet seasons within a one-year sampling period.
2. Materials and Methods
2.1. study design.
A baseline census of 10 villages in the Thulamela Municipality of Limpopo Province was completed to identify all households in which there was at least one healthy child under 3 years of age in the household, the child’s caregiver was at least 16 years of age, and the household did not have a permanent, engineered water-treatment system. 415 households that met these eligibility criteria were enrolled for the purposes of a water-treatment intervention trial. The baseline assessment of water-quality and use practices is reported here. Caregivers of the child under 3 years of age were given a questionnaire concerning demographics, socioeconomic status, water-use practices, sanitation and hygiene practices, and perceptions of water quality and health. In addition, a sample of drinking water was taken from a random selection of 25% of the total enrolled households in the dry (June–August 2016) and wet seasons (January–February 2017). The participant population was sorted by community, as a surrogate for water supply, and one-third from each community was randomly selected by a random number generated within Microsoft Excel (Seattle, WA, USA), which was sampled. The protocol used was approved by the Research Ethics Committee at the University of Venda (SMNS/15/MBY/27/0502) and the Institutional Review Board for Health Sciences Research at the University of Virginia (IRB-HSR #18662). Written informed consent was obtained from all participants and consent documentation was made available in English and Tshivenda. The majority of the baseline surveys were conducted in the dry season (approximately April to October). Six-months later, follow-on surveys were conducted at the height of the wet season (approximately November to March; however, the height of the season in 2016–17 was January to March).
2.2. Regional Description of the Study Area
The communities are located in a valley in the Vhembe District of Limpopo Province, South Africa ( Figure 1 ). The valley surrounds the Mutale River in the Soutpansberg Mountains and is located around 22°47′34′′ S and 30°27′01′′ E, in a tropical environment that exhibits a unimodal dry/wet seasonality ( Figure 2 ). In recent years, the area has received annual precipitation between 400 mm and 1100 mm; more importantly, the timing of the precipitation is highly variable ( Figure 2 ). Specifically, in 2010, the annual precipitation was about 750 mm; however, the majority of the precipitation came in March while, traditionally, the wet season begins earlier, in September or October. The year 2011 had the highest precipitation in the six-year period and had the majority of the rainfall in November. The years 2012 and 2015 began with a typical precipitation pattern; however, the rainfall did not continue as it did in 2013 and 2014. Annual temperature of the area also varies, with the highest temperature always recorded in the wet season ( Figure 3 ). There has been much variability of temperature in past years; however, this is beyond the scope of this study. The abbreviations used in Figure 1 and other figures, including the supplementary data and the type of the various water sources used in this study, are shown in Table 1 .

Map of the study area. The communities are all located within the Mutale River watershed. The rivers are indicated in blue, villages outlined in purple, environmental samples in blue squares, tributaries in green circles (which have intermittent flow), watershed boundary in orange. This heavily agricultural area has cultivated areas along both sides of thee Mutale River for the vast majority of the region; the area is shown with green outlines. There are two identified brick-processing areas shown in brown rectangles. Unfortunately, some sites are so close that the markers overlap (as with CR and IR). The location of the community supplies (CA, CB, and CC) are not shown to protect the privacy of those villages. See supplemental information for Google Earth files.

Precipitation trends in the study area. ( a ) Annual precipitation by hydrologic year. Data quality are presented on a scale of zero to unity where the quantity shown represents the proportion of missing or unreliable data in a year; ( b ) Cumulative precipitation for the last five complete years; ( c ) Average monthly precipitation calculated for years with greater than 90% reliable data (bottom right). All data are presented by the standard Southern hemisphere hydrologic year from July to June numbered with the ending year. Data are from the Nwanedzi Natural Reserve at the Luphephe Dam (17 km from the study area) and fire available through the Republic of South Africa, Department of Water and Sanitation, Hydrologic Services ( http://www.dwa.gov.za/Hydrology/ ).

The mean monthly temperature in the region recorded at Punda Milia. ( a ) Mean monthly temperature based on the means from 1962–1984; ( b ) Mean monthly temperature record. Data are available from the National Oceanic and Aviation Administration (U.S.), National Climatic Data Center, Climate Data Online service ( https://www.ncdc.noaa.gov/cdo-web/ ).
Abbreviations, water sources and type.
Agriculture occupies tine greatest land cover in the valley. Mogt households are engaged in some level of farming. Crops cultivated include maize and vegetables, and tree fruits include mangos and citrus fruits. Livestock is prevalent in the area with chickens, goats, and cattle. Smaller animals typically remain closer to households and larger animals graze throughout the region without boundaries. There are several brick-making facilities in the valley that include excavation, brick-forming and drying.
2.3. Water Sources
Drinking water in the study communities is available from a number of municipal and natural sources. The primary source of drinking water for seven of the villages is treated, municipal water. Two of the villages have community-level boreholes, storage tanks, and distribution tanks. An additional village has a borehole as well; however, residents report that, since its installation, the system has never supplied water.
The water for the treatment facility is drawn from behind a weir in the Mutale River and pumped to a retention basin. The water then undergoes standard treatment that includes pH adjustment, flocculation, settling, filtration, and chlorine disinfection. Water is then pumped to two elevated tanks that supply several adjacent regions, including the study area. Specifically, Branch 1 supplies Tshandama, Pile, Mutodani, Tshapasha and Tshibvumo; Branch 2 supplies an intermediary tank that in turn serves Matshavhawe, Muledane and Thongwe. Households can pay for a metered yard connection for the water used; these yard connections can be connected to household plumbing at the household’s discretion. The treated municipal water service is intermittent. Service in Tshandama and Pile was observed to be constant during the wet season and for only about two to three days per week during the dry season. Service in the remaining communities is two to four days per week during the wet season and about two days per week during the dry season. Furthermore, for the past two years, major repairs in the dry season caused the treated municipal water to cease completely. Households typically stored water for the periods when the treated municipal water was off; however, when the municipal water was unavailable for longer periods or not on the anticipated schedule, households obtained water from natural sources. The community-level boreholes provided water almost constantly but were subject to failure and delays in repairs.
Aside from the municipal sources, many residents of three villages have access to a community installed and operated distribution system that delivers water from the adjacent ephemeral rivers throughout the community (CA, CB, and CC). These systems are constructed with 50 mm to 70 mm (5 to 7 × 10 −2 m) high-density polyethylene pipes. Even these community-level schemes provide water on a schedule and sometimes require repair. Another common source of water for the community is springs. These shallow groundwater sources are common in the valley; however, there are communities that do not have a nearby spring. Some springs have had a pipe placed at the outlet to keep the spring open and facilitate filling containers. Researchers did not observe any constructions around the springs to properly isolate them from further contamination, and they are, therefore, not improved water sources. Pit latrines are common in every household throughout the region. Source (TS) is located near these communities while other springs (OS, LS) are located in agricultural areas. Boreholes provide deep groundwater supplies but require a pump. Such systems provide water as long as there is power for the pump and the well is deep enough to withstand seasonal variations. The two clinics in the study area surveyed each relied on a borehole for their water supply. Some residents also collected water directly from the river. The Mutale River is a perennial river; however, the ephemeral rivers, the Tshiombedi, Madade, Pfaleni, and Tshala Rivers, do not flow in the dry season all the way to the floor of the valley. The Tshala River has a diversion to a lined irrigation canal that always carries water, but there is very little flow that remains in the natural channel.
2.4. Water Sampling
The team of community health workers (CHW) that had previously conducted the MAL-ED (Malnutrition and Enteric Diseases) study in the same region [ 37 ] were recruited to assist with the data collection for this study; specifically, the regional description and water sources. These CHWs have an intimate knowledge of the communities as they are residents and have conducted health research in the area. The CHWs provided information on the location and condition of the various water sources in the study communities.
Water sources were tested during two intensive study periods: one in the dry season (June–August, 2016) and the other in the wet season (January–February, 2017). Water sources for investigation were selected based on identification from resident community health workers. Single samples were taken from all 28 identified drinking water sources in the 10 villages and three days of repeated samples were taken from six sources, which represented a range of sources (e.g., surface, borehole, shallow ground, pond, and municipal treated) in the dry season. Single samples of 17 of the original sources and three days of repeated samples were taken from five sources in the wet season, six months later. Some sources were not resampled because the routes to the sources were flooded, and these sources were likely infrequently used during the wet season due to blocked pathways. The wet and dry season measurements gave two different scenarios for water-use behaviors and allowed the researchers to measure representative water-quality parameters.
2.5. Measurement of Physicochemical Parameters
Physicochemical parameters of source water samples were measured in the field by a YSI Professional Plus meter (YSI Inc., Yellow Springs, OH, USA) for pH, dissolved oxygen and conductivity. The probes and meter was calibrated according to the manufacturer’s instructions. Turbidity was measured in the field with an Orbeco-Hellige portable turbidimeter (Orbeco Hellige, Sarasota, FL, USA) (U.S. Environmental Protection Agency method 180.1) [ 38 ]. The turbidimeter was calibrated according to the manufacturer’s instructions. Measured levels were compared to the South African water-quality standards in the regulations [ 39 ], pursuant to the Water Services Act of 1997.
2.6. Microbiological Water-Quality Analysis
Escherichia coli ( E. coli ) and total coliform bacteria were measured in both source and household water samples by membrane filtration according to U.S. Environmental Protection Agency method 10,029 [ 40 ]. Sample cups of the manifold were immersed in a hot-water bath at 100 °C for 15 min. Reverse osmosis water was flushed through the apparatus to cool the sample cups. Paper filter disks of 47 mm (4.7 × 10 −2 m) diameter and 0.45 μm (4.5 × 10 −7 m) pore size (EMD Millipore, Billerica, MA, USA) were removed from their sterile, individual packages and transferred to the surface of the manifold with forceps with an aseptic technique. Blank tests were run with reverse osmosis dilution water. Two dilutions were tested: full-strength (100 mL sample) and 10 −2 (1 mL sample with 99 mL of sterile dilution water) were passed through the filters; this provides a range of zero to 30,000 CFU/100 mL (colony forming units) for both E. coli and total coliforms. The filter paper was placed in a sterile petri dish with absorbent pad with 2 mL (2 × 10 −6 m 3 ) of selective growth media solution (m-ColiBlue24, EMD Millipore, Billerica, MA, USA). The samples were incubated at 35 °C (308.15 K) for 23–25 h. Colonies were counted on the full-strength sample. If colonies exceeded 300 (the maximum valid count), the dilution count was used. In all tests, the dilution value was expected to be within 10 −2 of the full-strength value and the sample was discarded otherwise.
The distribution of the household bacteria levels was evaluated by the (chi square) χ 2 goodness-of-fit test for various subsets of the data. Subsets of the data were then compared by an unpaired Student’s t-test for statistical significance; specifically, wet versus dry season levels as well as any other subsets that could demonstrate differences within the data.
2.7. Major Metals Analysis
A Thermo ICap 6200 Inductively Coupled Plasma Atomic Emission Spectrometer (ICP-AES, Chemetix Pty Ltd., Johannesburg, South Africa) was used to analyze the major metals in the various samples. The National Institute of Standards and Technology traceable standards (NIST, Gaithersburg, MD, USA) purchased from Inorganic Ventures (INORGANIC VENTURES 300 Technology Drive Christiansburg, Christiansburg, VA, USA) were used to calibrate the instrument for the quantification of selected metals. A NIST-traceable quality control standard from De Bruyn Spectroscopic Solutions, Bryanston, South Africa, were analyzed to verify the accuracy of the calibration before sample analysis, as well as throughout the analysis to monitor drift.
2.8. Trace Metals Analysis
Trace elements were analyzed in source water samples using an Agilent 7900 Quadrupole inductively coupled plasma mass spectrometer (ICP-MS) (Chemetix Pty Ltd., Johannesburg, South Africa). Samples were introduced via a 0.4 mL/min (7 × 10 −9 m 3 s −1 ) micro-mist nebulizer into a Peltier-cooled spray chamber at a temperature of 2 °C (275.15 K), with a carrier gas flow of 1.05 L/min (1.75 × 10 −5 m 3 s −1 ). The elements V, Cr, Mn, Fe, Co, Ni, Cu, Zn, As, Se were analyzed under He-collision mode to remove polyatomic interferences. NIST-traceable standards was used to calibrate the instrument. A NIST-traceable quality control standard of a separate supplier to the main calibration standards was analyzed to verify the accuracy of the calibration before sample analysis.
2.9. Anion Analysis
The anions were analyzed in source-water samples as stated in Edokpayi et al. [ 41 ]. Briefly, an Ion Chromatograph (Metrohm, Johannesburg, South Africa) was used to analyze the concentrations of fluoride, bromide, nitrates, chloride and sulfate. Calibration standards in the range of 1–20 mg/L were prepared from 100 mg/L stock solution containing all the test elements. Prior to analysis, the samples were filtered with a 0.45 μm (4.5 × 10 −7 m) syringe filter. Eluent for the sample run was prepared from sodium bicarbonate and sodium carbonate. A 50 mmol/L sulphuric acid with a flow rate of 0.5 mL/min (8 × 10 −9 m 3 s −1 ) was used as suppressant.
3.1. Socio-Demographic Characteristics of Enrolled Households
We included 405 enrolled households who completed the baseline questionnaire. The majority of caregivers were the mothers (n = 342, 84.4%, median age = 27 years) or grandmothers (n = 51, 12.6%, median age = 50 years) of a young child in the household. Almost all the caregivers had completed at least secondary school education (n = 371, 91.6%). Median monthly income for the entire household was USD$106 (interquartile range (IQR): 71–156). Access to improved sanitation was high. 373 (n = 92.1%) households used an improved pit latrine, and only 19 (n = 4.7%) reported open defecation. However, few households (n = 35,8.6%) reported having a designated place to wash hands near their toilet, and only 29% (n = 119) reported always using soap when washing hands.
Most households had their primary water source ( Table 2 ) piped into their or their neighbor’s yard (dry: n = 226, 62.3%; wet: n = 241, 67.5%) or used a public tap (dry: n = 69, 19.0%; wet: n = 74, 20.7%). A minority (dry: n = 40, 11.0%; wet: n = 19, 5.3%) collected their water directly from rivers, lined canals, or springs. Water was collected by adult women in most households, and it was reported to take a median of 10 min (IQR, both seasons: 5–30) to go to their water source, collect water, and come back in one trip. Three quarters (n = 270, 74.4%) reported that their water source was not continually available in the dry season and two-thirds (n = 234, 65.5%) in the wet season. Almost half (48.9%) reported interruptions in availability that lasted at least 7 days in the dry season and 32.8% in the wet season. Households stored water during interruptions and/or collected water from alternative sources (dry: n = 133, 36.6%; wet: n = 115, 32.2%), which were surface water or shallow groundwater sources (e.g., rivers, lined canals, or springs).
Primary drinking-water sources reported among 363 and 357 households in the study area in the dry and wet seasons, respectively.
Household water was most frequently stored in jerry cans or plastic buckets (n = 363, 89.7%), while 25 households stored water in large drums or plastic tanks (6.2%). Most households reported that their drinking water containers were covered (n = 329, 81.2%), but most used a cup with a handle (n = 281, 69.4%) or their hands (n = 93, 23.0%) for water collection ( Table 3 ). Only 13.3% (n = 54) households reported treating their water, mainly by boiling (n = 22), chlorine (n = 15), or letting the water stand and settle (n = 11).
Mode of water collection from storage containers.
Approximately one-third of caregivers (n = 114, 28.2%) perceived that one can get sick from drinking water (n = 114, 28.2%), and cited diarrhea, schistosomiasis, cholera, fever, vomiting, ear infections, malnutrition, rash, flu and malaria as specific illnesses associated with water. Despite these perceptions, the majority were satisfied with their current water source (n = 297, 73.3%). Those who were unsatisfied cited reasons of insufficient quantity (n = 75), shared water supply (n = 65), uncleanliness (n = 73), cloudiness (n = 47), and bad odor or taste (n = 38).
3.2. Physicochemical and Microbiological Characteristics of the Water Sources
pH and conductivity values ranged between 5.5–7.3 and 24–405 μS/cm in the wet season and 5.8–8.7 and 8–402 μS/cm in the dry season ( Table S1 ). Both pH and conductivity levels were within the recommended limits of the World Health Organization (WHO) for drinking water. The microbiological results and turbidity of the sources tested are presented in Figures Figures4 4 and and5, 5 , and Table S2 , respectively. Microbiological data show contamination with E. coli , a fecal coliform that is potentially pathogenic, and other coliform bacteria.

Membrane filtration results for ( a ) E. coli and ( b ) other coliforms. Data are presented for wet and dry seasons. The four ephemeral rivers (*) have no dry season data because they had no flow; all other sources have the results reported, some of which are zero or near-zero. South African National Standard (SANS 241:1-2015) set the limit of 0 CFU/100 mL for E. coli and 10 CFU/100 mL for total coliforms (CFU/10 −4 m 3 ). Ephemeral rivers that do not flow all the way into the valley are indicated (*) in the dry season.

Turbidity of the water sources in the study area. Two to three measurements were taken during an intensive study period from 13 January 2017 to 4 February 2017 in the wet season and three to four measurements from 5 June 2016 to 15 July 2016 in the dry season. The median measurement of the values is reported here. Ephemeral rivers that do not flow all the way into the valley are indicated (*) in the dry season.
Municipal treated water never showed any detectable colony-forming units (CFU) in a 100 mL sample for E. coli , which is within the Soufh African regulation [ 39 ]. In the wet season, other coliform bacteriaweae detected in the treated wtter (a median valueof 10 CFU/100 mL wac recorded).
Household sample of stored water ( Figure 6 ) show that bacterial contamination levels ranged from no detectable colonies lo the maximum detection level of our protocol of 30,000 CFU/100 mL. There is a trend that total colitorm levels ere lower (during the wet season than the dry season. In the wet season, some communities within the sturdy area had access to constant municipal treated water as monitored by researcher verification of public tap-watcr availebJlity. Othet communities had intermittent access to municipal treated water. Of these honseholds, those that had constant access to treated water at or near their household did have less total coliform in their stored water than those with intermittent services ( Figure 7 ). This neglects the communities that are outside of the municipal treated-water servic e area.

Box-and-whisker plot of total coliform measurements of stored, untreated water in study households in the wet (n = 95) and dry (n = 103) seasons. The box-and-whisker plot indicates the mean (diamond), first, second, and third quartiles (box), and minimum and maximum (whiskers).

Box-and-whisker plot of total coliform measurements of stored water in the wet season in study households in communities that had verified continuous access to municipal treated water versus verified intermittent access.
The total coliform from households in communities with verified continuous treated water had a log-normal distribution (verified by 99%, α = 1 significance level, χ 2 goodness-of-fit test) and were statistically significantly lower (α =1 significance level) than those from households in communities with verified intermittent treated water. Unfortunately, due to the low number of samples from intermittent households, a χ 2 goodness-of-fit test was not meaningful.
3.3. Anion Concentrations
Major anions investigated in the various water sources fell within the recommended guideline values from the WHO [ 42 ]. Fluoride concentrations ranged from below the detection limit (bdl) to 0.82 mg/L in the dry season and to 1.48 mg/L ( Table S3 ) in the wet season. Fluoride levels fell below the threshold limit for fluoride in drinking water from the WHO (1.5 mg/L). Nitrates were also observed within the limit of drinking water, between bdl–17.48 mg/L and bdl–9.72 mg/L in the dry and wet seasons, respectively. Chloride, sulfate and phosphate levels were also present in moderate levels in the various water sources; however, a relatively high concentration of chloride of 462.9 mg/L was determined in the Mutale River in the wet season.
3.4. Trace and Major Elements Composition
Major metals in the various water sources in both seasons complied with the recommended limits of SANS and WHO in drinking water [ 39 , 42 ]. Sodium concentrations in the range of 3.14–41.03 mg/L and 3.02–15.34 mg/L were measured in the wet and the dry seasons, respectively ( Table S4 ). Low values of potassium were measured. Calcium levels ranged between 0.66–33.91 mg/L and 0.53–27.39 mg/L, in the wet and dry seasons, respectively. Low levels of magnesium were also found. Most of the water sources can be classified as soft water owing to the low levels of calcium and magnesium. Aluminium (Al) concentration ranged between 39.18–438 μg/L ( Figure 8 ). Two of the water sources which are community-based water supply systems recorded high levels of Al which exceeded the aesthetic permissible levels of drinking water; others fell within this limit. Similarly, the levels of iron (Fe) varied between 37.30–1354 mg/L and 35.21–1262 mg/L in the wet and the dry seasons, respectively ( Figure 9 ). Some of the sources showed high Fe concentration which exceeded the aesthetic permissible limit of WHO in drinking water [ 42 ]. Two community-based water systems had higher levels of Fe in the wet season as well as the major river in the region (Mutale River) for which high Fe levels were observed in both seasons. One of the clinic boreholes also recorded high levels of Fe above the permissible aesthetic value of (300 mg/L) in both seasons. Temporary seasonal variation was significant only in the levels of Fe and Al. In the wet season, their levels were generally higher than in the dry season. Some other trace metals of concern like Pb, Hg, As, Cd, Cr, Ni, Cu, Mn, Sr were all present at low levels that were below their recommended limits in drinking water for both seasons ( Table S5 ).

Aluminum, measured by an inductively coupled plasma mass spectrometer (ICP-MS), concentration for natural sources in the study area in the wet and dry seasons. The SANS 241 standard is shown (an operational standard is intended for treated water). Sources marked with * are intermittent sources and had no dry-season sample. Other sources have measured concentrations; although they may be too low to plot.

Iron, measured by an ICP-MS, concentration for natural sources in the study area in the wet and dry seasons. The SANS 241 standard is shown. Sources marked with * are intermittent sources and had no dry-season sample. Other sources have measured concentrations; although they may be too low to plot.
4. Discussion
This study provides a comprehensive description of water quality and drinking-water use across seasons in a low-resource community in rural South Africa, including a variety of water sources, ranging from the municipal tap to natural sources and a combination of both when the municipal tap was intermittently available.
Water sources in the study area, aside from the municipal tap, were highly contaminated with E. coli in both the wet and dry seasons; that is, E. coli was above the South African standard (acute health) of 0 CFU/100 mL. It is particularly important to note that E. coli was detected in the boreholes used for water at the local clinics, implying inadequate access to potable water for potentially immunocompromised patients. While the municipal treated water met the E. coli detection limit, the municipal tap did not always fall within the standards of turbidity (≤1 NTU operational and ≤5 NTU aesthetic) and total coliform (≤10 CFU/100 mL) [ 39 ]. These are not direct health risks; however, both measurements can be used to judge the efficacy of the treatment process and suggest that treatment may not have removed other pathogens that were not directly tested, such as protozoan parasites.
While the microbiological contamination of the drinking-water sources was not acceptable, the chemical constituents fell within the South African guidelines [ 39 ]. Calcium, sodium, magnesium and potassium were present in low levels and their concentrations complied with regulatory standards of SANS [ 39 ] and WHO [ 42 ]. Some metals (cadmium, mercury, arsenic and lead) known to be carcinogenic, mutagenic and teratogenic, causing various acute and chronic diseases to humans even at trace levels in drinking water, were investigated and found to be present in very low concentrations that could be of no health risk to the consumers of the various water resources in the region. However, some other metals, such as Al and Fe, were higher in some of the water sources; yet these were still well below the health guidelines for the respective constituent (recommended health levels from SANS and WHO are given as Al < 0.9 mg/L, Fe < 2 mg/L). At these levels, they do not present a health risk but could impart color and significant taste to the water thereby affecting its aesthetic value. Water sources from the community water-supply systems and one of the clinic boreholes recorded higher levels of Al and Fe. The other metals evaluated (copper, zinc, nickel, chromium, Se and Mn) were present in low levels that complied with their recommended limits in drinking water [ 39 , 42 ].
Fluoridation of drinking water is a common practice for oral health in many countries [ 43 ]. The required level of fluoride to reduce incidences of dental caries is in the range of 0.6–0.8 mg/L; however, levels above 1.5 mg/L are associated with dental and skeletal fluorosis [ 43 – 45 ]. The likelihood of fluorosis as a result of high concentration of fluoride is low in these communities, but there could be a high incidence of dental caries since fluoride levels below 0.6 mg/L were measured and some of the water sources did not have fluoride concentrations detectable by the instrument. The National Children’s Health Survey conducted in South Africa showed that 60.3% of children in the age group of 6 years have dental caries. Approximately a third (31.3%) of children aged 4–5 years in Limpopo province have reported cases of dental caries [ 44 , 45 ].
Chloride levels in the water sources do not cause any significant risk to the users except imparting taste to the water for some of the sources that recorded chloride levels above 300 mg/L. Although the study area is characterized by farming activities, the nitrate concentrations measured do not present any health risks. Therefore, the occurrence of methemoglobinemia or blue-baby syndrome as a result of high nitrate levels is unlikely. Other anions were present in moderate levels that would also not constitute any health risks. The levels of all the anions determined in the various sources were lower than the recommended guidelines of WHO [ 42 ].
The microbiological analysis of environmental water sources revealed several trends. Without exception in these samples, bacterial levels in the wet season were higher than in the dry season. This may be caused by greater runoff or infiltration, which carries bacteria from contaminated sources to these water bodies. The upward trend in bacteria in the municipal treated water is not explained by an increase in runoff, but may be due to higher turbidity of the intake for the municipal treated water in the wet season. The treatment facility workers reported to the researchers that they were unable to monitor the quality of the treated water due to instrument failure during the wet season surveillance period.
Water stored in the household showed that the mean total coliform in the wet season was lower than that in the dry season. This trend is opposite to what was observed in the source, or environmental samples. This difference may be explained by the greater availability of treated water in the wet season versus the dry season for approximately 40% of the sampled households ( Figure 7 ). In addition, it is possible that families try to save water during the dry season and do not reject residual water, while the rainy season allows easier washing of the container and for it to be filled with fresh water more regularly.
In the wet season, two communities had consistently treated water available from household connections (usually a tap somewhere in a fence-in yard) or public taps. While the municipal treated water was of lower quality in the wet season than the dry season, the quality was significantly better than most environmental sources.
Another potential explanation is that residents stored their water within their households for a shorter time, which is supported by the use data that showed interruptions in supply were more common and for longer duration in the dry season. The quality of the water stored in households with continuous supply versus intermittent supply also suggests that water availability may play a role in household water quality. This is consistent with research that demonstrates that intermittent water supply introduces contamination into the distribution system in comparison with continuous supply [ 46 ]. Intermittent supply of water may also result in greater quantity and duration of storage at household level, which could increase the likelihood of contamination.
While it has been shown that the quality of water used for drinking in these villages does not meet South African standards, this problem is confounded by evidence from surveys indicating that residents believe they have high-quality water and, therefore, do not use any form of treatment. In the rare case that they do, it is by letting the water stand and settle or by boiling. In addition, even if treated water is collected, there is a risk of recontamination during storage and again when using a cup held by a hand to retrieve water from storage devices, which was common in surveyed homes. In addition, there was little to no detectable residual chlorine in the municipal tap water to prevent recontamination. A previous study performed in an adjacent community showed higher household treatment levels; however, this may have been due to intervention studies in that community (the community in question was excluded from this study because of previous interventions) [ 47 ]. The study also concurred that boiling was the most common method employed.
Given that most of the water from the various sources in this community is contaminated and not treated, there is a high risk of enteric disease in the community. Lack of access to adequate water and sanitation cause exposure to pathogens through water, excreta, toxins, and water-collection and storage pathways, resulting in immense health impacts on communities [ 48 ]. A large burden of death and disability due to lack of access to clean water and sanitation is specifically associated with diarrheal diseases, intestinal helminths, schistosomiasis and trachoma [ 49 ]. While it was found in this study that the study area has a high prevalence of improved sanitation, the likelihood of poor water quality due to intermittent supply and lack of treatment poses a risk of the adverse health effects described. In a previous longitudinal cohort study of children in these villages, most children were exclusively breastfed for only a month or less, and 50% of children had at least one enteropathogen detected in a non-diarrheal stool by three months of age [ 50 ]. Furthermore, the burden of diarrhea was 0.66 episodes per child-year in the first 2 years of life, and stunting prevalence (length-for-age z-score less than −2) in the cohort increased from 12.4% at birth to 35.7% at 24 months [ 50 ]. It is likely that contaminated water contributed to the observed pathogen burden and stunting prevalence in these communities. In summary, microbiological contamination of the drinking water is high in the study area, and risk from other chemical constituents is low. Therefore, engineered solutions should focus more on improving the microbiological quality of the drinking water.
The intermittent supply in municipal tap water, inadequate water quality from alternative sources, and the risk of recontamination during storage suggest a need for a low-cost, point-of-use water-treatment solution to be used at the household level in these communities. Access to clean drinking water will contribute to improving the health of young children who are at highest risk of the morbidity and mortality associated with waterborne diseases. Such an intervention may go beyond the prevention of diarrhea by impacting long-term outcomes such as environmental enteropathy, poor growth and cognitive impairment, which have been associated with long-term exposure to enteropathogens [ 51 ]. This is supported by a recent finding that access to improved water and sanitation was associated with improvements on a receptive vocabulary test at 1, 5 and 8 years of age among Peruvian, Ethiopian, Vietnamese and Indian children [ 52 ]. The implementation of point-of-use water treatment devices would ensure that water is safe to drink before consumption in the homes of these villages, improving child health and development.
5. Conclusions
This study was comprehensive in the assessment of all aspects of water quality and corresponding water-use practices in rural areas of Limpopo Province. The results obtained indicate that microbiological water quality is more likely to have adverse effect on the consumers of natural water without adequate treatment, as E. coli was determined in all the natural water sources. Local needs assessments are critical to understanding local variability in water quality and developing appropriate interventions. Interventions to ensure clean and safe drinking water in rural areas of Limpopo province should, first and foremost, consider microbiological contamination as a priority. Risk-assessment studies of the impact of water quality on human health is, therefore, recommended.
Supplementary Material
Tables s1 through s5.
Table S1: Physical characteristics of water sources. Two to three measurements were taken during an intensive study period from 13 January 2017 to 4 February 2017 in the wet season, and three to four measurements from 5 June 2016 to 15 July 2016 in the dry season. The median measurement of the values is reported here. Sites with missing samples, such as ephemeral rivers that do not flow all the way into the valley in the dry season, are indicated (*). Sites with missing data due to instrument failure are indicated (#). Values that were below the detection limit are indicated (bdl). South African regulation (SANS 241:1-2015) and the World Health Organization Recommended Guidelines for Drinking Water Quality (Fourth Edition) are listed; parameters not listed are indicated (nl),
Acknowledgments:
This project was funded by the Fogarty International Center (FIC) of the National Institutes of Health (NIH) (Award Number D43 TW009359), National Science Foundation (NSF) (Award Number CBET-1438619), the Center for Global Health at the University of Virginia (CGH), and the University of Virginia’s Jefferson Public Fellows (JPC) program. The content is solely the responsibility of the authors and does not represent the official views of the funders. The authors also acknowledge the tireless work of the community field workers who undertook interventions and collected all of the survey data. The authors also acknowledge A. Gaylord, N. Khuliso, S. Mammburu, K. McCain and E. Stinger, who performed much of the water-quality analysis and T. Singh, who supported the laboratory analysis for inorganic materials.
Supplementary Materials: The following are available online at www.mdpi.com/s1 ,
Conflicts of Interest: The authors declare no conflict of interest.
- Open access
- Published: 17 January 2019
Environmental justice and drinking water quality: are there socioeconomic disparities in nitrate levels in U.S. drinking water?
- Laurel A. Schaider ORCID: orcid.org/0000-0003-1988-0012 1 ,
- Lucien Swetschinski 1 ,
- Christopher Campbell 2 &
- Ruthann A. Rudel 1
Environmental Health volume 18 , Article number: 3 ( 2019 ) Cite this article
59k Accesses
98 Citations
190 Altmetric
Metrics details
Low-income and minority communities often face disproportionately high pollutant exposures. The lead crisis in Flint, Michigan, has sparked concern about broader socioeconomic disparities in exposures to drinking water contaminants. Nitrate is commonly found in drinking water, especially in agricultural regions, and epidemiological evidence suggests elevated risk of cancer and birth defects at levels below U.S. EPA’s drinking water standard (10 mg/L NO 3 -N). However, there have been no nationwide assessments of socioeconomic disparities in exposures to nitrate or other contaminants in U.S. drinking water. The goals of this study are to identify determinants of nitrate concentrations in U.S. community water systems (CWSs) and to evaluate disparities related to wealth or race/ethnicity.
We compiled nitrate data from 39,466 U.S. CWSs for 2010–2014. We used EPA’s Safe Drinking Water Information System (SDWIS) to compile CWS characteristics and linked this information with both city- and county-level demographic data gathered from the U.S. Census Bureau. After applying multiple imputation methods to address censored nitrate concentration data, we conducted mixed-effects multivariable regression analyses at national and regional scales.
5.6 million Americans are served by a CWS that had an average nitrate concentration ≥ 5 mg/L NO 3 -N between 2010 and 2014. Extent of agricultural land use and reliance on groundwater sources were significantly associated with nitrate. The percent of Hispanic residents served by each system was significantly associated with nitrate even after accounting for county-level cropland and livestock production, and CWSs in the top quartile of percent Hispanic residents exceeded 5 mg/L nearly three times as often as CWSs serving the lowest quartile. By contrast, the percent of residents living in poverty and percent African American residents were both inversely associated with nitrate.
Conclusions
Epidemiological evidence for health effects associated with drinking water above 5 mg/L NO 3 -N raises concerns about increased risk for the 5.6 million Americans served by public water supplies with average nitrate concentrations above this level. The associations we observed between nitrate concentrations and proportions of Hispanic residents support the need for improved efforts to assist vulnerable communities in addressing contamination and protecting source waters. Future studies can extend our methods to evaluate disparities in exposures to other contaminants and links to health effects.
Peer Review reports
Drinking water quality is regulated in the United States under the Safe Drinking Water Act (SDWA), which establishes national monitoring and reporting requirements and maximum contaminant levels (MCLs) for 88 contaminants. In 2013, 9800 public water systems, serving 26.5 million Americans, had violations of health-based standards [ 1 ]. In 2015, lead contamination in the drinking water supply of Flint, Michigan, caused elevated blood lead levels in children following the use of a new drinking water source, the Flint River, as a cost-saving measure [ 2 ]. Because 60% of Flint’s residents are African American and 40% live below the poverty line, this crisis sparked a nationwide debate about environmental justice—equal treatment and protection from environmental harm regardless of race, ethnicity, or income—and drinking water quality.
Low-income and minority communities often face disproportionate burdens of exposure to contamination sources and environmental pollution, and associations with race and ethnicity persist even after accounting for differences in income [ 3 ]. While few studies have looked for links between drinking water and environmental justice indicators (e.g., poverty, race/ethnicity) [ 4 ], existing studies have found associations between poorer drinking water quality and these indicators [ 4 , 5 , 6 , 7 , 8 ]. Community water systems (CWSs) that serve communities with lower median incomes, lower rates of home ownership, and higher proportions of Hispanic or non-white residents have been associated with higher levels of nitrate and arsenic [ 5 , 6 , 7 ]. Among small rural water systems in Quebec, those serving areas with more material deprivation (based on income, education, and employment) were more likely to have lead levels of health concern and less likely to have advanced water treatment [ 4 ]. Health-based violations of the SDWA were more common in poorer communities with higher proportions of Hispanic or African-American residents; the effects of race and ethnicity were not apparent in more affluent communities [ 8 ]. Environmental justice associations with drinking water have not been consistently observed, and may depend on the spatial scope and individual contaminants studied. For instance, Cory and Rahman [ 9 ] concluded there was limited evidence for environmental justice disparities in exposures to arsenic in water systems in Arizona. The likelihood of studies finding environmental justice associations with the siting of hazardous waste facilities strongly depends on the unit of analysis (e.g., county, census block) and overall scope (e.g., state, national); studies with a small unit of analysis and large scope were most likely to find significant associations [ 10 ]. To date, studies of environmental justice and drinking water contaminants have considered individual states (e.g., Arizona, Oregon) or sections of states (e.g., California’s Central Valley); however a nationwide assessment is lacking.
According to Balazs and Ray’s Drinking Water Disparities Framework [ 11 ], there are a wide range of natural, built, and sociopolitical factors that can cause and perpetuate disparities in water quality, reliability, and infrastructure. Small water supplies, particularly those that serve low-income and minority communities, may have poorer source water quality due to closer proximity to pollution sources. In addition, such supplies may have diminished technical, managerial, and financial (TMF) capacity to properly manage their drinking water, so these systems may lack the resources necessary to comply with testing requirements. Indeed, a nationwide analysis indicated that small CWSs were more likely to have management-related SDWA violations [ 12 ]. When problems are identified, small systems with limited TMF may struggle to address these problems, such as through the installation of new treatment systems or development of better protected sources [ 1 ]. In communities of color, institutional barriers in local planning and zoning practices may lead to lower rates of drinking water and wastewater infrastructure improvement [ 13 ]. These can relate to both internal factors (diminished ability to raise rates for customers) and external factors (ability to apply for loans). These factors are especially apparent in unincorporated areas, which have no tax base and lie outside of the municipal boundaries overseen by county or state entities.
Nitrate is one of the contaminants most frequently found in violation of health-based standards in U.S. drinking water [ 14 ]. Nitrate naturally occurs in aquatic systems at low concentrations (< 1 mg/L NO 3 -N), while concentrations greater than 1 mg/L NO 3 -N are considered elevated above background and indicative of human activity [ 15 ]. Common anthropogenic sources of nitrate include fertilizers used for agricultural production and landscaping, animal manure, wastewater discharges from sewage treatment plants and septic systems, and fossil fuel combustion. Elevated levels of nitrate can signal the presence of other contaminants of concern; a study of over 2000 private wells found that wells with > 1 mg/L NO 3 -N were more likely to have levels of pesticides and volatile organic compounds (VOCs) above one-tenth of an MCL or health-based screening level [ 15 ]. Among public and private wells in sand and gravel aquifers, nitrate concentrations were correlated with pharmaceuticals and other unregulated drinking water contaminants [ 16 , 17 ].
As part of the original implementation of the SDWA in 1974, the U.S. EPA established a nitrate MCL of 10 mg/L NO 3 -N (45 mg/L NO 3 − ) based on case studies of methemoglobinemia in infants who consumed formula mixed with water containing nitrate [ 18 ]. More recent epidemiological studies have found associations between nitrate concentrations in drinking water and bladder cancer [ 19 , 20 ], thyroid cancer [ 21 , 22 ], colon cancer [ 23 , 24 ], kidney cancer [ 25 ], birth defects [ 26 , 27 ], low birth weight [ 28 ], and preterm birth [ 29 , 30 ]. Some of these effects were significant for exposures at or above 5 mg/L, particularly over longer exposure periods [ 21 , 23 , 26 , 27 ]. The International Agency for Research on Cancer classified “ingested nitrate or nitrite under conditions that result in endogenous nitrosation” as a probable human carcinogen (Group 2A) [ 31 ]. Exposure to nitrate in drinking water has also been linked to thyroid dysfunction [ 32 ], although the Agency for Toxic Substances and Disease Registry concluded that there is “limited evidence” for nitrate-induced thyroid dysfunction [ 33 ]. The U.S. EPA’s Integrated Risk Information System (IRIS) is undertaking a broad re-evaluation of the health effects of nitrate and nitrite [ 34 ].
In light of growing epidemiological evidence for nitrate health effects below the MCL and evidence on a local level for socioeconomic disparities in nitrate exposure, our study was designed to evaluate whether nitrate concentrations are elevated in public water supplies that serve communities with higher proportions of low-income and/or minority residents. We hypothesized that CWSs serving communities with higher proportions of Hispanic residents would have higher nitrate levels because 80% of U.S. farmworkers are Hispanic [ 35 ] and because synthetic fertilizers used in agriculture are the largest source of nitrogen inputs in the U.S. [ 36 ]. We also anticipated that the high cost of removing nitrate from contaminated drinking water would lead to socioeconomic disparities in nitrate exposures. This study represents the first investigation of socioeconomic disparities in drinking water contaminants at the national scale and provides new insights into the interplay of system characteristics and demographic parameters.
Water system and demographic data sources
Detailed information about public water systems was gathered from the U.S. EPA’s Safe Drinking Water Information System (SDWIS) [ 37 ]. Our target population were CWSs in each U.S. state that were active at some point between 2010 and 2014. We restricted our analysis to CWSs because these systems serve customers in their homes year-round, whereas non-community systems can serve non-residential settings such as office buildings and campgrounds. We did not include CWSs that purchased their water from another supplier; purchasing water systems are rarely required to test for nitrate and therefore rarely collect nitrate data. In total, we retrieved data for 412,835 systems, of which 42,114 were CWSs active between 2010 and 2014 that did not purchase their water. Relevant characteristics obtained for each system included: activity status, system type (community, non-community, etc.), number of people served, source water type (groundwater or surface water), affiliated wholesaler or purchasing systems, and region served by the system (city, county). CWS system sizes were classified using categories defined by the EPA: very small (≤500 people); small (501–3300); medium (3301–10,000); large (10,001–100,000); and very large (>100,000).
We obtained race, ethnicity, poverty, and home ownership information (2010–2014 five-year estimates) and the proportion of households in urbanized areas (2010 estimates) from the U.S. Census Bureau for each county, census-designated place, and county subdivision in the 50 U.S. states [ 38 , 39 ]. Agricultural data on the amount of livestock (cows, goats, horses, pigs, sheep) per 100 acres and the percent of land area used as cropland were obtained from the U.S. Department of Agriculture’s 2012 Census of Agriculture for each U.S. county [ 40 ]. Demographics and agriculture variables were assumed to remain constant throughout our study period.
Identifying populations served by CWSs
Characterizing the demographics of the communities served by each water system is challenging in part because little information is publicly available on the geographic areas served by each CWS [ 41 ]. Few states provide public access to electronic records documenting the service areas of their public water systems, so we relied on the information included in SDWIS.
SDWIS’s Water System module provides address variables conveying the location of each water system’s “legal entity,” i.e., the mailing address of the administrative personnel associated with the system. Separate variables, city served and county served, describe the areas to which a system directly provides water, and the primacy agency code specifies the agency that has regulatory oversight of the water system (typically a state agency encompassing the cities or counties served). Using SDWIS’s Geographic Area module, which some states primarily use to report the “areas served” parameters, we were able to augment our database’s cities and counties served.
We used the city served and county served fields in SDWIS to determine the areas served by each CWS. SDWIS provided information in the counties served field for > 99% of CWSs ( n = 41,781), but only 48.1% of CWSs reported information in the cities served field ( n = 20,267). By contacting state agencies, we were able to supplement SDWIS data for 1509 CWSs in three states; however, 13 states rarely or never record information in the cities served field (Fig. 1 ). Although administrative address information was available for nearly all water systems, we concluded that such data did not reliably identify the areas served by each CWS. Some system administrator addresses were located hundreds of miles away from the cities served by their affiliated water systems or were located in a different state, and for 40% of the systems with both a city name (pertaining to the administrator’s city) and a city served designated in SDWIS, the two fields shared no overlapping cities. Furthermore, the demographics of the areas associated with the administrative addresses often varied substantially from the demographics of each water system’s cities served (Additional file 1 : Table S1).
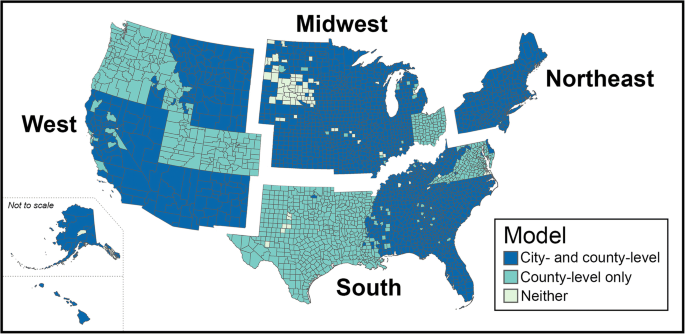
Coverage of the states and regions included in our statistical analyses. Some portions of the country were not included in our analysis either because they did not have a community water system on record or because we were unable to collect nitrate data for that area
Data obtained for wholesale water systems varied widely in whether they included the cities or customers served by downstream purchasing systems. Because purchasing systems were not included in our analysis, it was important that the data for each wholesaler included all of the cities that purchase its water in order to best characterize the population served by each CWS. As described in Additional file 1 , we amended wholesalers’ city served and population served fields to include the area and people served by systems that purchase wholesale water. As a result of these modifications, we expanded data on the cities served for 1245 wholesale systems (174 of which lacked any city served data prior to considering purchased water systems) and adjusted the system size for 220 wholesale systems. Data that we compiled for U.S. CWSs, including service areas and purchaser-seller connections, are provided in Additional files 2 and 3 .
Linking demographic and water system data
Water system characteristics and demographic data were linked by matching the names in the SDWIS city served and county served fields with the geographic names in the Census Bureau demographic data. Because the majority of city served names matched to county subdivisions in the Northeastern U.S. (Pennsylvania and all states to the north and east), county subdivision demographics were prioritized as a match for CWSs in the Northeast. Census-designated places were prioritized in all other U.S. regions, where county subdivision matching was infrequent. Notably, the city served and county served fields are not checked for consistency at a national level, so SDWIS entries are prone to local (rather than official) naming conventions and typographical errors. We used an approximate string matching algorithm based on Jaro-Winkler distance to identify the most similar name in the demographic data for each city served and county served that did not have an identical match. Typographical errors and differences in abbreviation conventions were corrected to improve string matching. All county served names provided by SDWIS were matched to Census Bureau records in this manner. For city served names that did not match or matched multiple Census Bureau records after these corrections, we gathered additional information from online encyclopedias and search engines about individual water systems and geographical locations. In some cases, this additional information allowed us to definitively link city served names from SDWIS with location names in Census Bureau data, while in other cases the names were considered non-matching and were not included in subsequent analyses. In total, we successfully linked demographic data to every CWS that provided a county served (41,781 systems) and 96.8% of CWSs for which we had city served information (21,253 systems).
For CWSs serving multiple cities or counties, we calculated a weighted average for each demographic parameter based on the relative populations of each of the cities or counties served. For instance, if a CWS served five cities, then the proportion of Hispanic residents associated with that CWS was calculated as an average of the proportion of Hispanic residents in each of the five cities, weighted by each city’s population. When demographic information was missing for one or more cities served, as was the case for 262 systems (1.2%), these weighted averages were calculated based on all available demographic data for cities served by that system.
Nitrate data
There is no national database of water contaminant concentration data. Thus, nitrate data were acquired by contacting SDWA-designated primacy agencies in each state through a combination of email and Freedom of Information Act requests and by gathering data from ten state-operated online data portals. In total, we compiled data for over 2.7 million samples analyzed for nitrate and/or nitrate-nitrite (sum of nitrate plus nitrite). Of these, 616,591 samples were collected from our CWSs between 2010 and 2014, covering 40,178 (96.2%) of the systems for which we had county-level demographic data.
Nitrate (NO 3 -N) concentrations were used when available to estimate each CWS’s annual nitrate concentration. However, CWSs in 13 states primarily reported nitrate-nitrite (NO 3 /NO 2 -N) data, and some CWSs reported both. In order to extrapolate NO 3 -N concentrations from NO 3 /NO 2 -N concentrations, we used a univariate linear regression based on all samples for which we could pair by system, sampling date, and sub-facility ( n = 48,925 pairs). This regression ([NO 3 -N] = 0.987*[NO 3 /NO 2 -N], r 2 ≈ 0.93) was used to estimate NO 3 -N concentrations when only NO 3 /NO 2 -N concentrations were provided.
We made additional adjustments to the dataset to address discrepancies in how nitrate data were reported by states. In some cases units (mg/L, μg/L) were either missing or appeared to be misreported (e.g., 0.2 ng/L), complicating the interpretation of sample results, particularly those that substantially diverged from other samples collected from the same system. We developed a protocol to identify outliers that were either misreported or misevaluated based on the average difference between each sample result and other samples from that system. Outliers were confirmed by visual inspection, and we removed 498 of such cases (0.1% of all samples) from the analysis. In addition, 17 states never provided detection limits (DLs) for samples without detectable nitrate. DLs are useful for quantitative analyses of nondetects to constrain the range of possible values for samples below the DL (e.g., < 0.2 mg/L indicates a more limited range of possible concentrations than < 1 mg/L). Among 151,463 nitrate and nitrate-nitrite samples for which DLs were provided, 99.4% of DLs were ≤ 1 mg/L and 17.4% of DLs were exactly 1 mg/L, so 1 mg/L was used as a reasonable upper-bound DL for all nondetects that were missing a DL (12.3% of all samples).
Statistical analyses
We used a mixed-effects linear regression model to evaluate the relationship between nitrate concentrations and demographic, system-characteristic, and land-use data. Model parameters were selected a priori to allow us to explore associations between environmental justice indicators and nitrate concentrations while controlling for possible confounders. Environmental justice indicators included those related to race and ethnicity (percent Hispanic residents, percent non-Hispanic Black residents) and those related to poverty (percent of families with income below the poverty line, percent of home ownership). System characteristics of interest included system size and type of source water (surface water or groundwater). Land use variables (number of livestock per 100 acres, percent of land area used as cropland, percentage of homes in urbanized areas) were included to account for agricultural and wastewater contributions to nitrate source water contamination. State was included as a random effect to capture the influence of different monitoring and reporting practices for drinking water and differences in state regulations of pollutant sources and enforcement. In the baseline model, we used county-level demographics because county served information was provided for nearly all CWSs, offering nearly complete geographical coverage (Fig. 1 ). Collinearity was evaluated by visual inspection of Spearman correlation coefficients (Additional file 1 : Tables S2-S6) and by calculating variance inflation factors (VIFs) for each model. Race, ethnicity, and at least one poverty indicator were retained in all models, and other covariates were retained if they were significantly associated with the outcome ( p < 0.05) or if removing them from the model changed the effect estimates for other covariates by more than 10%.
Nitrate data were right skewed and approximately lognormal. Because nitrate concentrations were below the DL in nearly 30% of water samples, we used a multiple imputation method adapted from Lubin et al. [ 42 ] to address nondetects. Multiple imputation can provide less biased variance estimates relative to a simple substitution method (typically DL/2 or DL/ \( \sqrt{2} \) ) in datasets with more than 10% nondetects [ 42 ]. For each year in which a CWS operated (“system-year”), we calculated a minimum average nitrate concentration, imputing zero for nondetects, and a maximum average nitrate concentration, imputing the DL for nondetects. Together, these values constitute an interval that contains every possible system-year average, and for system-years without nondetect data, these two values are identical. Because we lacked information on the relative contributions of multiple sources to overall water flow within a system, we assumed all sub-facilities and all samples contributed equally to the system-year average. For each system, we then calculated a five-year system average concentration as an interval, with the lower/upper bounds of the interval represented as the average of all of the minima/maxima of system-year intervals, respectively. The ranges of these five-year system average intervals were generally narrow: 67.3% of system averages had a range ≤ 0.2 mg/L, 99.8% had a range ≤ 1 mg/L, and 0.2% (91 systems) had a system average interval range > 1 mg/L.
We used non-parametric bootstrap resampling (1000 bootstrap iterations) and maximum likelihood estimation to estimate means ( \( \overset{\sim }{x} \) ) and standard deviations ( \( \overset{\sim }{\sigma } \) ) of a normal distribution that best fit the natural logarithms of our system average intervals. Natural log-transformed system averages were then randomly imputed in accordance with the proportions of the cumulative distribution function of a normal distribution with mean \( \overset{\sim }{x} \) and standard deviation \( \overset{\sim }{\sigma } \) truncated at the natural logarithms of the system average minimum and maximum. We did not impute values for system averages based entirely on detected concentrations (37.9% of systems) because we could calculate these averages directly. We then evaluated ln-transformed system averages as the outcome variable in a mixed-effects regression model. This procedure (bootstrap resampling, lognormal parameter estimation, imputation, and regression analysis) was repeated five times to determine the consistency of our results. Regression results from each of these five phases were pooled using techniques outlined by Rubin [ 43 ].
To evaluate disparities in drinking water exposures to nitrate levels of health concern, we conducted a separate set of analyses in which we dichotomized the outcome variable. Using the same multiple imputation approach, systems were categorized as “high nitrate” if their estimated system average was ≥5 mg/L, a level associated with adverse health outcomes in some epidemiological studies, and as “low nitrate” otherwise. We used a generalized logistic mixed-effects regression model to evaluate the effects of the same set of predictors, including the state-specific random effect, on the binary nitrate response variable. As before, five sets of regression results were pooled using the Rubin method.
We assessed whether our results depended on geographic region or on the spatial resolution of the demographic data. To evaluate variability across geographic regions, we conducted a similar set of regression analyses stratified into four regions (Midwest, Northeast, South, and West) defined by the U.S. Census Bureau. To evaluate the effect of the spatial scale of the demographic data, we also repeated our analyses using demographic data representing the city or cities served by each CWS. Thirteen states rarely or never report city served values in SDWIS (Fig. 1 ), so a substantial portion (49.4%) of CWSs were not included in this city-level analysis. Since agricultural data were only available at the county level, our analyses using city-level demographics included agricultural variables corresponding to the counties served by each system. Finally, to determine whether differences between the county-level and city-level results were more dependent on the scope (geographic area considered in the analysis) or scale (resolution of the city vs. county demographics), we developed an intermediate third model using county-level demographics for only those CWSs included in our city-level analysis.
All analyses were conducted using R version 3.4.1 [ 44 ].
Of the 42,114 CWSs that did not purchase water from another system and were active between 2010 and 2014, we were able to match 39,466 CWSs to both a complete county-level demographic profile and at least one nitrate measurement from that time period (Additional file 1 : Figure S1). The 39,466 CWSs in our analysis served 233.2 million people, more than 70% of the U.S. population (Table 1 ). Over 90% of the CWSs in our sample served ≤10,000 people (very small, small, and medium size systems); these systems provided water to less than 20% of the population served by public water supplies overall. Nearly 90% of the CWSs in our sample have groundwater sources; however, the majority of the population in our sample area (65%) is served by a CWS with a surface water source.
The majority of estimated annual mean nitrate concentrations were below 1 mg/L for all system sizes, regions, and source water types. However, 1647 CWSs, serving over 5.6 million Americans, had average nitrate concentrations at or above 5 mg/L from 2010 to 2014. The West and Midwest had the highest proportions of high nitrate systems (≥5 mg/L) and the highest 95th percentile nitrate concentrations, while the South had the lowest (Table 1 ). The proportion of high nitrate CWSs was nearly four times higher for systems that rely on groundwater sources compared to CWSs that rely on surface water sources, and the 95th percentile concentration for groundwater systems was more than 2 mg/L higher. Relative to other system sizes, very small systems (≤500 people) had the highest 95th percentile concentration and the highest proportion of high nitrate systems.
Among environmental justice indicators, race and ethnicity differed among regions and across system sizes, while wealth-related factors were less variable. Black and Hispanic residents made up a larger proportion of residents served by very large systems than smaller systems. Systems in the South served the highest proportion of Black residents, and CWSs in the West served the highest proportion of Hispanic residents. The proportion of residents with annual incomes below the U.S. Census poverty threshold did not vary considerably as a function of system size, while rates of home ownership were lowest in counties served by very large systems. Poverty rates were highest in the South and West, and rates of home ownership were lowest in the West.
We observed significant differences in the demographics and land use patterns between high nitrate and low nitrate (mean < 5 mg/L) CWSs (Table 2 ). High nitrate CWSs served nearly twice as many Hispanic residents on average compared to low nitrate CWSs, and CWSs that served the highest proportion of Hispanic residents (top quartile) exceeded 5 mg/L nitrate nearly three times as often as CWSs serving the lowest proportion of Hispanic residents (lowest quartile; Additional file 1 : Figure S2). By contrast, high nitrate CWSs served less than half as many Black residents on average compared to low nitrate CWSs. Rates of poverty and home ownership were marginally, albeit significantly, lower in counties served by systems with higher nitrate concentrations. Counties with the most agricultural and livestock production had higher proportions of high nitrate CWSs than counties with less agricultural and livestock production (Additional file 1 : Figure S2). For instance, 9.1% of CWSs were high nitrate in counties in the top quartile for cropland, while only 1.8% of CWSs were high nitrate in counties in the bottom quartile for cropland.
In our national mixed-effects regression analyses using county-level demographic data, race and ethnicity variables showed similar associations with both system average nitrate concentrations and the likelihood of system averages exceeding 5 mg/L (Table 3 ). A one-unit increase in the percent of Hispanic residents was associated with a 1.8% increase in nitrate concentrations [95% confidence interval (CI): 1.6, 2.0%] and a 1.9% increase in the likelihood of high nitrate concentrations (95% CI: 1.4, 2.4%). By contrast, a one-unit increase in the percent of non-Hispanic Black residents was associated with a 1.3% decrease in nitrate concentrations (95% CI: –1.5, −1.0%) and a 4.3% decrease in the likelihood of high nitrate (95% CI: –5.7, −2.9%). Similar effect estimates were observed in unadjusted models (Additional file 1 : Table S7). Poverty, but not home ownership, was inversely associated with nitrate in the adjusted models, with a one-unit increase in the percent of people with incomes below the poverty line associated with 0.8% lower nitrate concentrations (95% CI: –1.2, −0.3%) and a 2.2% decrease in the likelihood of high nitrate (95% CI: –3.7, −0.7%). In unadjusted models, we observed a similar estimate for the association between poverty and nitrate concentrations but did not observe an association between poverty and likelihood of high nitrate, and in contrast to the adjusted models, home ownership showed significant inverse associations with both outcomes (Additional file 1 : Table S7).
We observed complex relationships between nitrate levels and system size. In the binary model, very small systems had a greater likelihood of high nitrate compared to small and medium systems. By contrast, very small systems were predicted to have lower concentrations than other system sizes when nitrate was evaluated as a continuous variable (compared to very small systems, nitrate concentrations were 9.0% higher in small systems and 51% higher in very large systems; Table 3 ). In unadjusted models, the same observations were noted; very small systems were predicted to have greater likelihood of high nitrate but lower continuous nitrate concentrations than all other system sizes (Additional file 1 : Table S7). For other variables related to water system characteristics and land use, systems relying on groundwater sources had 34% higher nitrate concentrations compared to systems relying on surface water (95% CI: 26, 42%) and were more than four times as likely to have high levels of nitrate (odds ratio = 4.1; 95% CI: 3.0, 5.6). The extent of cropland coverage and livestock production had significant but small associations with nitrate concentrations, while a one-unit increase in the percent of land area used as cropland had a more substantial effect (3.3% increase, 95% CI: 3.0, 3.7%) on the likelihood that a CWS had high nitrate.
The results of our analysis using city-level demographics, based on the subset of CWSs that provided city served information (50.6%, Fig. 1 ), varied in several notable ways from the results of our broader county-level analysis. In the city-level analysis, poverty was not associated with nitrate concentrations (Table 4 ), while home ownership, which was not significant in the national county-level analysis, was associated with lower nitrate. A one unit increase in percent home ownership (roughly equivalent to a one unit decrease in percent renters) was predicted to result in 0.4% lower nitrate (95% CI; −0.6, −0.2%). Similarly, the association between urbanicity and nitrate was significant in the city-level, but not the county-level analysis; a one-unit increase in the percent of households located in urbanized areas was associated with a 0.2% increase in nitrate (95% CI: 0.1, 0.3%).
To evaluate whether differences between the city- and county-level analyses were related to the refined spatial scale of cities or trends specific to the portion of the country that provided city information, we conducted an additional analysis using county-level demographics for only those CWSs that provided city served information (“county-level subset”). Overall, the results of this county-level subset model were similar to the results of the nationwide analysis using county-level data. Although the magnitude of some coefficients in the county-level subset analysis changed relative to the national analysis (for instance, race/ethnicity had approximately half of the effect on nitrate concentrations and poverty had about twice the effect), the statistical significance and directionality of the predictors were comparable (Table 4 ). The results of this comparison suggest that the differences between the county- and city-level analyses are primarily due to different relationships between nitrate levels and demographic predictors at various spatial scales, rather than being an artifact of the part of the country evaluated in the subset analyses.
We included region as a covariate in our nationwide models because of regional differences in nitrate concentrations and demographic characteristics (Table 1 ) and significant differences among some regions in unadjusted models (Additional file 1 : Table S7). In the unadjusted models, the Midwest and West had the highest proportions of high nitrate systems, while the West had higher nitrate concentrations than the Midwest and South. In the adjusted models, the Midwest had significantly lower nitrate concentrations than other regions, while only the West had a higher proportion of high nitrate systems than the Midwest. To investigate potential regional differences in associations among demographics, water system characteristics, land use, and nitrate concentrations, we stratified our nationwide model by region.
In these regionally stratified models using county-level demographics, no single feature had the same impact on system average nitrate concentrations across all four U.S. regions (Table 5 ). The Midwest was the only region in which the percent of cropland was not associated with nitrate and the only region in which percent of Hispanic residents did not have a positive association with nitrate levels. The Midwest also had the strongest effect of urbanicity; a one-unit increase in the percent of urban households was associated with 0.4% lower nitrate (95% CI: –0.6, −0.3%). The percent of non-Hispanic Black residents was only significantly associated with nitrate in the South, where a one-unit increase was associated with a 1.2% reduction in nitrate levels (95% CI: –1.5, −1.0%), nearly the same as for the U.S. as a whole (1.3% reduction). Home ownership had a strong inverse association with nitrate in the West, where a one unit increase in percent home ownership was associated with a 1.4% decline in nitrate concentrations (95% CI: –2.0, −0.7%); in the other three regions, home ownership was not associated with nitrate. The effect of groundwater source water on nitrate concentrations varied substantially across the regions: in the West, systems with groundwater sources had 139% higher levels of nitrate than those with surface water sources (95% CI: 115, 167%), while in the South, systems that relied on groundwater had 17% lower nitrate than systems with surface water sources (95% CI: –24, −8.1%). Nitrate concentrations increased with system size in the Midwest and West, with very large systems in the Midwest having the largest effect (223% increase relative to very small systems, 95% CI: 102, 418%).
This study represents the first nationwide analysis of socioeconomic disparities in exposures to contaminants in public drinking water. We found that 5.6 million Americans relied on a public water supply with an average nitrate concentration ≥ 5 mg/L, one-half of U.S. EPA’s drinking water standard, over the five-year period spanning 2010–2014. Epidemiological studies have suggested that long-term exposure to water with nitrate concentrations above 5 mg/L may be associated with some types of cancer, birth defects, and preterm birth [ 19 , 23 , 27 , 29 ]. We found that the proportion of Hispanic residents was significantly associated with nitrate levels, while the proportion of Black residents was inversely associated with nitrate levels. The associations with poverty and home ownership were mixed; when we used the demographics of the counties served by each water supply, we found that poverty was negatively associated with nitrate, while home ownership, an indicator of wealth and political empowerment, was inversely associated with nitrate when we used city-level demographics. Very small water systems (serving ≤ 500 people) had the highest nitrate levels overall, but after adjusting for demographics and local land use (cropland, livestock production, and urbanicity), very small systems were predicted to have lower nitrate levels than larger systems.
We found that the percent of Hispanic residents was associated with higher nitrate levels in our nationwide analysis and in all U.S. regions except the Midwest. These associations were modest; nationally, a 10% increase in the proportion of Hispanic residents (i.e., increasing from 10 to 20%) served by a CWS was associated with a 19.6% increase in nitrate concentration. Balazs et al. [ 6 ] also saw an association between percent Hispanic residents and nitrate levels in small public water supplies in California’s Central Valley, an agriculturally intensive area. We had hypothesized that proportion of Hispanic residents would be associated with nitrate because many agricultural communities have a high proportion of Hispanic residents. However, our observed association persisted even after we adjusted for agricultural activity by including cropland and livestock production as covariates in our models (Table 3 ) and our nationwide correlation analysis found a negative correlation between the proportion of Hispanic residents and percent cropland (Additional file 1 : Table S2). These results suggest that the association between Hispanic residents and nitrate is not solely explained by proximity to agricultural sources, although adjusting for county-level cropland and livestock production will not eliminate residual confounding of the association between proportion of Hispanic residents and nitrate concentration by agricultural contamination of source waters since our covariates do not account for other factors such as soil type, rates of fertilizer use, and adoption of best management practices to control fertilizer runoff. Nevertheless, while agriculture is the largest source of land-based nitrogen inputs, major sources are also present in urban areas, including wastewater treatment plants, leaking sewer lines, and urban runoff [ 14 ]. Our correlation analysis found a positive correlation between percent Hispanic residents and percent urban households (Additional file 1 : Table S2), suggesting that some of the association between Hispanic residents and nitrate levels may be related to nitrate sources in urban areas.
In addition to proximity to nitrate pollution sources, the observed relationship between proportion of Hispanic residents and nitrate may be indicative of disparities in TMF resources related to source water protection and water treatment. Communities with higher proportions of minority residents, particularly those who are non-native English speakers, may have less political influence and may be disenfranchised from political and budgetary decision-making processes [ 11 ], and therefore may have fewer resources to install new treatment technology or develop new source waters in response to contamination. In our analysis, we are not able to identify the relative importance of proximity to nitrate sources and management-related factors. Nevertheless, our findings are consistent with prior studies in which Hispanic communities were found to have higher drinking water exposures to arsenic, another contaminant regulated under the SDWA. In Oregon, communities served by CWSs in violation of the arsenic MCL had a much higher proportion of Hispanic residents [ 5 ], and in Arizona, the proportion of Hispanic residents served by a public water system was positively associated with the likelihood that that system violated the arsenic MCL [ 9 ]. Since arsenic in groundwater often comes from geogenic rather than anthropogenic sources [ 5 , 7 ], these associations may be indicative of disparities in the ability of communities to afford enhanced drinking water treatment technologies, and taken together, suggest that Hispanic communities may experience elevated exposures to multiple drinking water contaminants.
In contrast to our results for Hispanic residents, we observed that the proportion of Black residents was inversely associated with nitrate on a national level, although this association was only observed in the South in our regional analysis. The inverse association observed nationally may be heavily influenced by the South, which has the lowest proportion of high nitrate systems, the most water systems, and the highest proportion of Black residents of all regions. In the South, the negative relationship between the proportion of Black residents and nitrate levels may be explained in part by biogeochemical factors. Pennino et al. [ 14 ] suggested that the lack of nitrate MCL violations in Louisiana, Mississippi, and Alabama—states that all have > 25% Black residents—may be associated with biological uptake and transformation processes and regional geological factors. The finding of no significant association between the proportion of Black residents and nitrate levels in the West is consistent with findings of Balazs et al. [ 6 ], who observed no significant relationship between non-Hispanic people of color and nitrate levels in California’s Central Valley.
The associations between wealth-related parameters (poverty and home ownership) and nitrate levels differed among U.S. regions and various spatial resolutions of the demographic data. In our national adjusted models using county-level demographics, poverty was inversely associated with nitrate levels while home ownership was not associated with nitrate. By contrast, when we used demographic data corresponding to cities and towns rather than counties, we observed that poverty was not associated with nitrate and that home ownership was inversely associated with nitrate, implying that cities and towns with higher proportions of renters tend to have higher nitrate levels. The effect estimates for poverty and home ownership varied between unadjusted and adjusted models, although our model building approach with a priori variable selection does not indicate which covariates were associated with the most substantial changes in these estimates. As with race/ethnicity, wealth can be expected to relate to levels of contamination in two ways: proximity to pollution sources and ability to treat contaminated source water. While we adjusted our models for agriculture and urbanicity, these variables may not have captured proximity to other important sources of nitrate inputs, such as landfills, industrial facilities, fossil fuel combustion, and home building [ 36 , 45 ]. Such factors may be related to wealth to the extent that they are driven by economic activity. Discrepancies between our city- and county-level analyses could be explained if county-level wealth operated in our model as the best proxy for nitrogen sources not accounted for by other variables, while city-level wealth better represented civic engagement, capacity to raise customer rates, and, by consequence, ability to treat contaminated water.
We hypothesized that smaller water systems would have higher nitrate concentrations. Smaller water systems may have fewer financial and technical resources to address contamination issues when they arise [ 1 ], and the cost of water treatment per household is considerably higher for smaller systems because of a lack of economies of scale [ 9 ]. Indeed, very small systems had higher 95th percentile nitrate concentrations than larger systems and were more likely to exceed 5 mg/L nitrate in unadjusted models. Additionally, after adjusting for demographic and land use parameters, very small systems were more likely to have high nitrate compared to small and medium sized systems. However, in our regression analyses with nitrate as a continuous variable, very small systems had lower nitrate concentrations compared to larger system size categories nationwide (Table 3 ) and in the Midwest, Northeast, and West (Table 5 ). Taken together, these results suggest that larger systems have higher nitrate on average, but that very small systems are more likely than other system sizes to have nitrate concentrations at the high end of the distribution. Previous studies have found inconsistent relationships between system size and contaminant violations. Switzer and Teodoro [ 8 ] identified a negative relationship between the population served by a system and the system’s number of health-based SDWA violations (MCL and treatment technique violations) in a subset of CWSs across the U.S., while Rahman et al. [ 46 ] reported a positive association between MCL violations and the number of people served by water systems in Arizona. In a purely statistical sense, larger water systems may be more likely to detect elevated nitrate levels because they are required to test more frequently and, in the case of groundwater systems, might draw from a greater number of source water wells.
Beyond health-based violations, very small CWSs were reported to have more frequent violations of monitoring and reporting requirements than larger systems [ 47 ]. This observation is consistent with our data: of the 41,781 CWSs we paired with county demographics, very small systems were significantly more likely than larger systems to lack nitrate sample results over the five-year study period (5.2% of very small systems missing nitrate data compared to 1.8% of larger systems; Pearson χ 2 = 301). This difference is unlikely to be due to differences in testing requirements, since CWSs are required to test for nitrate annually or more frequently [ 48 ]. Such difficulties in adequately monitoring drinking water contaminants likely stem from limited financial resources and/or managerial expertise, and may signal concurrent challenges in conforming to SDWA health-based guidelines. In this regard, Balazs and Ray [ 11 ] reported that very small water systems in Fresno County, California, that had failed to monitor for drinking water contaminants under county governance were found to have MCL violations when state officials investigated.
Strengths of our study include the extensive scale and completeness of our dataset for both demographics and water quality data, and our use of information about purchasing water systems to link water quality data with entire areas served by CWSs. A major limitation to our analysis is the potential for exposure misclassification. Because we lacked information about flow volumes from multiple sources within CWSs, we weighted all samples collected for each CWS equally. In some cases, this may have led to overestimates of nitrate concentrations in systems where more contaminated sources are pumped less frequently or only maintained for backup; this overestimation may affect groundwater systems more since we anticipate that they may have more intake points than CWSs with surface water sources. Furthermore, nitrate concentrations may show substantial intra-annual variability, so depending on when nitrate samples were collected within each year, our aggregated metrics may not capture the true average nitrate concentration for each CWS. As with any study of population-level data, we are limited in our ability to draw conclusions about individual exposures and thus limited in our ability to infer causal relationships between the EJ variables of interest and exposure to nitrate in drinking water. Nitrate levels in CWSs may not accurately reflect the exposures among residents in those areas because some residents may rely on bottled water. Use of bottled water may exacerbate disparities in pollutant exposures because lower-income residents have lower ability to pay for bottled water. Another limitation was that we lacked information about cities and towns served for about half of the CWSs in our analysis and so we had to rely on county-level demographics in most analyses, which is a limitation because demographic data for a county may not accurately reflect the demographics of all cities and towns within each county. Our analysis using city-level demographics is limited in its geographic scope, although this did not seem to account for the differences in model results using city- and county-level demographic data. Ideally, we would use census block level information to provide the best resolution of demographic data. However, because geocoded information that specifies the CWS serving each census block is not available, we could not analyze data at the census block level. For small CWSs that serve part of a large city, we used the demographics of the whole city, but the demographics of the population served by the CWS may vary from those of the city overall. Some CWSs were not included in our analysis because we could not match the names of the cities and towns served with locations in the Census Bureau data, although this accounted for a very small proportion of systems. We also could not include unincorporated areas in our city-level analysis because they are not included in Census Bureau data. Finally, we were unable to compile nitrate data for all CWSs. In some cases, this was related to data handling problems; for instance, some records were only available in paper reports, and in other cases, this may reflect a lack of compliance with monitoring requirements, which is more likely for small rural communities who may not be able to afford testing or where there is less enforcement of testing requirements.
Our study did not include the 44 million Americans who rely on a private well for their drinking water, for whom water quality testing is not required under the SDWA. Private wells are shallower than public wells, and shallow wells are more vulnerable to nitrate contamination [ 49 ]. They are also more likely to be located in rural areas and may be in closer proximity to agriculture and livestock production sources. Private well owners are usually not required to test for nitrate or other drinking water contaminants, so their presence may go undetected. Among nearly 4000 private wells tested in rural Wisconsin by a state water quality laboratory, nearly 10% exceeded the nitrate MCL [ 50 ]. There is evidence of environmental justice disparities in communities using private wells or lacking piped-water entirely. A review by VanDerslice [ 41 ] summarized case studies of minority communities reliant on contaminated private wells. For instance, in a low-income Hispanic community of 25,000 in the Yakima Valley in Washington State, more than 10% of private wells exceeded the nitrate MCL [ 51 ]. These case studies are further evidence of impaired water quality in communities reliant on private wells and indicate potential socioeconomic disparities in these communities as well.
This study represents the first nationwide analysis of socioeconomic disparities in exposures to drinking water contaminants, and the framework that we developed in this study can be extended to investigate disparities in exposures to other drinking water contaminants. We found that communities with higher proportions of Hispanic residents tend to be served by community water systems with higher nitrate and greater likelihood of being over 5 mg/L. Our regression analyses indicate that this association is not completely explained by proximity to cropland and livestock production. While > 99% of CWSs do not exceed the nitrate MCL of 10 mg/L, 5.6 million Americans are served by CWSs with nitrate concentrations above 5 mg/L. Nitrate data for private wells, which are even more vulnerable to nitrate contamination, are lacking. Understanding the extent of current exposures, particularly among vulnerable subpopulations, is critical for developing effective strategies to reduce exposures in these communities. Our findings suggest that programs intended to help low-income and small CWSs may not be adequately assisting communities with high proportions of Hispanic residents. Epidemiological evidence for adverse health effects associated with consumption of drinking water above 5 mg/L nitrate raise concerns about increased risk in people exposed at this level and support a re-evaluation of the federal nitrate standard. Even well below the standard, nitrate levels of 1 mg/L or higher are associated with anthropogenic impact; thus nitrate may be an inexpensive indicator to identify drinking water systems that may also contain other contaminants of concern.
Abbreviations
Community water system
Detection limit
United States Environmental Protection Agency
Maximum contaminant level
Massachusetts Water Resources Authority
Nitrate nitrogen
- Safe Drinking Water Act
Safe Drinking Water Information System
Technical, managerial, and financial capacity
U.S. Environmental Protection Agency (US EPA). Providing Safe Drinking Water in America: 2013 National public water systems compliance report. 305-R-15-001. Washington, D.C.: Office of Enforcement and Compliance Assurance. p. 2015.
Hanna-Attisha M, LaChance J, Sadler RC, Schnepp AC. Elevated blood lead levels in children associated with the Flint drinking water crisis: a spatial analysis of risk and public health response. Am J Public Health. 2016;106(2):283–90.
Article Google Scholar
Bullard RD, Johnson GS. Environmental justice: grassroots activism and its impact on public policy decision making. J Soc Issues. 2000;56(3):555–78.
Delpla I, Benmarhnia T, Lebel A, Levallois P, Rodriguez MJ. Investigating social inequalities in exposure to drinking water contaminants in rural areas. Environ Pollut. 2015;207:88–96.
Article CAS Google Scholar
Stone D, Sherman J, Hofeld E. Arsenic in Oregon community water systems: demography matters. Sci Total Environ. 2007;382(1):52–8.
Balazs C, Morello-Frosch R, Hubbard A, Ray I. Social disparities in nitrate-contaminated drinking water in California's San Joaquin Valley. Environ Health Perspect. 2011;119(9):1272–8.
Balazs CL, Morello-Frosch R, Hubbard AE, Ray I. Environmental justice implications of arsenic contamination in California's San Joaquin Valley: a cross-sectional, cluster-design examining exposure and compliance in community drinking water systems. Environ Health. 2012;11:84.
Switzer D, Teodoro MP. The color of drinking water: class, race, ethnicity, and safe drinking water act compliance. J Am Water Works Assoc. 2017;109(9):40–5.
Cory DC, Rahman T. Environmental justice and enforcement of the safe drinking water act: the Arizona arsenic experience. Ecol Econ. 2009;68(6):1825–37.
Baden BM, Noonan DS, Turaga RMR. Scales of justice: is there a geographic bias in environmental equity analysis? J Environ Plan Manag. 2007;50(2):163–85.
Balazs CL, Ray I. The drinking water disparities framework: on the origins and persistence of inequities in exposure. Am J Public Health. 2014;104(4):603–11.
Switzer D, Teodoro MP, Karasik S. The human capital resource challenge: recognizing and overcoming small utility workforce obstacles. J Am Water Works Assoc. 2016;108(8):E416–E24.
Heaney C, Wilson S, Wilson O, Cooper J, Bumpass N, Snipes M. Use of community-owned and -managed research to assess the vulnerability of water and sewer services in marginalized and underserved environmental justice communities. J Environ Health. 2011;74(1):8–17.
Google Scholar
Pennino MJ, Compton JE, Leibowitz SG. Trends in drinking water nitrate violations across the United States. Environ Sci Technol. 2017;51(22):13450–60.
Dubrovsky NM, Burow KR, Clark GM, Gronberg JM, Hamilton PA, Hitt KJ, et al. The Quality of Our Nation’s Water—Nutrients in the Nation’s Streams and Groundwater, 1992–2004. In: Circular 1350. Reston, VA: U.S. Geological Survey; 2010.
Schaider LA, Rudel RA, Ackerman JM, Dunagan SC, Brody JG. Pharmaceuticals, perfluorosurfactants, and other organic wastewater compounds in public drinking water wells in a shallow sand and gravel aquifer. Sci Total Environ. 2014;468:384–93.
Schaider LA, Ackerman JM, Rudel RA. Septic systems as sources of organic wastewater compounds in domestic drinking water wells in a shallow sand and gravel aquifer. Sci Total Environ. 2016;547:470–81.
Maximum Contaminant Levels for Inorganic Contaminants. 40 CFR § 141.62. 2014.
Chiu HF, Tsai SS, Yang CY. Nitrate in drinking water and risk of death from bladder cancer: an ecological case control study in Taiwan. J Toxicol Environ Health A. 2007;70(12):1000–4.
Jones RR, Weyer PJ, DellaValle CT, Inoue-Choi M, Anderson KE, Cantor KP, et al. Nitrate from drinking water and diet and bladder cancer among postmenopausal women in Iowa. Environ Health Perspect. 2016;124(11):1751–8.
Ward MH, Kilfoy BA, Weyer PJ, Anderson KE, Folsom AR, Cerhan JR. Nitrate intake and the risk of thyroid cancer and thyroid disease. Epidemiology. 2010;21(3):389–95.
Kilfoy BA, Zhang YW, Park Y, Holford TR, Schatzkin A, Hollenbeck A, et al. Dietary nitrate and nitrite and the risk of thyroid cancer in the NIH-AARP diet and health study. Int J Cancer. 2011;129(1):160–72.
De Roos AJ, Ward MH, Lynch CF, Cantor KP. Nitrate in public water supplies and the risk of colon and rectum cancers. Epidemiology. 2003;14(6):640–9.
Espejo-Herrera N, Gracia-Lavedan E, Boldo E, Aragones N, Perez-Gomez B, Pollan M, et al. Colorectal cancer risk and nitrate exposure through drinking water and diet. Int J Cancer. 2016;139(2):334–46.
Jones RR, Weyer PJ, DellaValle CT, Robien K, Cantor KP, Krasner S, et al. Ingested nitrate, disinfection by-products, and kidney cancer risk in older women. Epidemiology. 2017;28(5):703–11.
Holtby CE, Guernsey JR, Allen AC, VanLeeuwen JA, Allen VM, Gordon RJ. A population-based case-control study of drinking-water nitrate and congenital anomalies using geographic information systems (GIS) to develop individual-level exposure estimates. Int J Environ Res Public Health. 2014;11(2):1803–23.
Brender JD, Weyer PJ, Romitti PA, Mohanty BP, Shinde MU, Vuong AM, et al. Prenatal nitrate intake from drinking water and selected birth defects in offspring of participants in the National Birth Defects Prevention Study. Environ Health Perspect. 2013;121(9):1083–9.
Migeot V, Albouy-Llaty M, Carles C, Limousi F, Strezlec S, Dupuis A, et al. Drinking-water exposure to a mixture of nitrate and low-dose atrazine metabolites and small-for-gestational age (SGA) babies: a historic cohort study. Environ Res. 2013;122:58–64.
Bukowski J, Somers G, Bryanton J. Agricultural contamination of groundwater as a possible risk factor for growth restriction or prematurity. J Occup Environ Med. 2001;43(4):377–83.
Stayner LT, Almberg K, Jones R, Graber J, Pedersen M, Turyk M. Atrazine and nitrate in drinking water and the risk of preterm delivery and low birth weight in four Midwestern states. Environ Res. 2017;152:294–303.
International Agency for Research on Cancer (IARC). IARC monographs on the evaluation of carcinogenic risks to humans. Volume 94: ingested nitrate and nitrite, and cyanobacterial peptide ToxinsLyon. France: World Health Organization; 2010.
Aschebrook-Kilfoy B, Heltshe SL, Nuckols JR, Sabra MM, Shuldiner AR, Mitchell BD, et al. Modeled nitrate levels in well water supplies and prevalence of abnormal thyroid conditions among the old order Amish in Pennsylvania. Environ Health. 2012;11:6.
Agency for Toxic Substances and Disease Registry (ATSDR). Toxicological Profile for Nitrate and NitriteAtlanta, GA: U.S. Department of Health and Human Services, Public Health Service; 2017.
U.S. Environmental Protection Agency (US EPA). Draft: IRIS Assessment Plan for Nitrate and Nitrite. EPA/635/R-17/331. Washington DC: National Center for Environmental Assessment, Office of Research and Development, U.S. Environmental Protection Agency; 2017.
U.S. Department of Labor. Findings from the National Agricultural Workers Survey (NAWS) 2013-2014: a demographic and employment profile of United States farmworkers. In: Research Report No 12; 2016.
Sobota DJ, Compton JE, Harrison JA. Reactive nitrogen inputs to US lands and waterways: how certain are we about sources and fluxes? Front Ecol Environ. 2013;11(2):82–90.
U.S. Environmental Protection Agency (U.S. EPA). SDWIS application programming Interface model. 2016. https://www.epa.gov/enviro/sdwis-model . Accessed 19 Apr 2017.
U.S. Census Bureau. 2010 Census Summary File 1, Urban and Rural Housing Units (H2). 2011. http://factfinder.census.gov/bkmk/navigation/1.0/en/text_search:H2/d_dataset:DEC_10_SF1/d_people_basic_count:URBAN_RURAL . Accessed 13 Dec 2016.
U.S. Census Bureau. 2010–2014 American Community Survey Data Profiles, 5-year estimates. 2015. https://factfinder.census.gov/bkmk/navigation/1.0/en/d_dataset:ACS_14_5YR/d_product_type:DATA_PROFILE/ . Accessed 13 Dec 2016.
U.S. Department of Agriculture NASS. 2012 Census of agriculture, Agriculture Census Web Maps 2012. https://www.agcensus.usda.gov/Publications/2012/Online_Resources/Ag_Census_Web_Maps/Overview/ . Accessed 12 Dec 2017.
VanDerslice J. Drinking water infrastructure and environmental disparities: evidence and methodological considerations. Am J Public Health. 2011;101(Suppl 1):S109–14.
Lubin JH, Colt JS, Camann D, Davis S, Cerhan JR, Severson RK, et al. Epidemiologic evaluation of measurement data in the presence of detection limits. Environ Health Perspect. 2004;112(17):1691–6.
Rubin DB. Multiple imputation for nonresponse in surveys. New York: Wiley; 1987.
Book Google Scholar
Core R. Team: R: a language and environment for statistical computing. Vienna, Austria: R Foundation for Statistical Computing; 2017.
Wakida FT, Lerner DN. Non-agricultural sources of groundwater nitrate: a review and case study. Water Res. 2005;39(1):3–16.
Rahman T, Kohli M, Megdal S, Aradhyula S, Moxley J. Determinants of environmental noncompliance by public water systems. Contemp Econ Policy. 2010;28(2):264–74.
Wallsten S, Kosec K. The effects of ownership and benchmark competition: an empirical analysis of US water systems. Int J Ind Organ. 2008;26(1):186–205.
Inorganic Chemical Sampling and Analytical Requirements. 40 CFR § 141.23. 2017.
Nolan BT, Hitt KJ. Vulnerability of shallow groundwater and drinking-water wells to nitrate in the United States. Environ Sci Technol. 2006;40(24):7834–40.
Knobeloch L, Gorski P, Christenson M, Anderson H. Private drinking water quality in rural Wisconsin. J Environ Health. 2013;75(7):16–20.
CAS Google Scholar
Washington State Departments of Agriculture Ecology and Health, Yakima County Public Works Department, U.S. Environmental Protection Agency. Lower Yakima Valley Groundwater Quality: Preliminary assessment and recommendations document. In: Ecology publication no. 10-10-009. Olympia, WA; 2010.
Download references
Acknowledgements
We thank state agency officials for providing drinking water data and technical guidance, Julia Udesky for helpful discussion of statistical approaches, and Amanda Hernandez for assistance with data analysis.
This study was funded by the Casey & Family Foundation.
Availability of data and materials
Data used to estimate demographic characteristics can be downloaded using the U.S. Census Bureau’s American Fact Finder ( https://factfinder.census.gov /). For the present study, we compiled information on race/ethnicity (Data Profile 05), poverty (Data Profile 03), home ownership (Table B25003), and urbanicity (Table H2). County-level data on agricultural parameters can be downloaded from the U.S. Department of Agriculture’s 2012 Census of Agriculture website ( https://www.agcensus.usda.gov/Publications/2012/Online_Resources/Ag_Census_Web_Maps/Data_download/index.php ). Datasets gathered from SDWIS describing the characteristics of community water systems in the U.S. (including those features needed to associate wholesalers with the systems that purchase their water) are available for download as Additional files. Nitrate data for U.S. CWSs are available from the corresponding author on reasonable request.
Author information
Authors and affiliations.
Silent Spring Institute, 320 Nevada Street, Suite 302, Newton, MA, 02460, USA
Laurel A. Schaider, Lucien Swetschinski & Ruthann A. Rudel
Environmental Working Group, 1436 U St. NW, Suite 100, Washington, DC, 20009, USA
Christopher Campbell
You can also search for this author in PubMed Google Scholar
Contributions
LAS and LS designed the study, and RAR contributed to the study design. CC and LS gathered and compiled nitrate data from state agencies. LS prepared the dataset and conducted the study’s statistical analysis. LAS and LS interpreted the findings and drafted the manuscript, and all authors contributed to its contents. All authors read and approved the final manuscript.
Corresponding author
Correspondence to Laurel A. Schaider .
Ethics declarations
Ethics approval and consent to participate.
Not applicable
Consent for publication
Competing interests.
The authors declare that they have no competing interests.
Publisher’s Note
Springer Nature remains neutral with regard to jurisdictional claims in published maps and institutional affiliations.
Additional files
Additional file 1:.
Supplemental description of methods and additional tables and figures. Table S1 Number of systems with discrepancies between demographics characterized using SDWIS’s City served and Zip code fields. Table S2 Spearman correlation matrix for county-level predictors, all systems. Table S3 Spearman correlation matrix for county-level predictors, Midwest systems. Table S4 Spearman correlation matrix for county-level predictors, Northeast systems. Table S5 Spearman correlation matrix for county-level predictors, South systems. Table S6 Spearman correlation matrix for county-level predictors, West systems. Table S7 Unadjusted univariate regression model results using nationwide data and county-level demographics. Figure S1 Number of community water systems compiled from SDWIS and subsequently used for regression analyses. Figure S2 Percent of CWSs with mean nitrate ≥5 mg/L by quartile for land use and demographic variables. (PDF 13,659 kb)
Additional file 2:
Data for U.S. community water systems, including service areas and purchaser-seller connections. (PDF 6544 kb)
Additional file 3:
Data glossary for U.S. community water systems. (PDF 1866 kb)
Rights and permissions
Open Access This article is distributed under the terms of the Creative Commons Attribution 4.0 International License ( http://creativecommons.org/licenses/by/4.0/ ), which permits unrestricted use, distribution, and reproduction in any medium, provided you give appropriate credit to the original author(s) and the source, provide a link to the Creative Commons license, and indicate if changes were made. The Creative Commons Public Domain Dedication waiver ( http://creativecommons.org/publicdomain/zero/1.0/ ) applies to the data made available in this article, unless otherwise stated.
Reprints and permissions
About this article
Cite this article.
Schaider, L.A., Swetschinski, L., Campbell, C. et al. Environmental justice and drinking water quality: are there socioeconomic disparities in nitrate levels in U.S. drinking water?. Environ Health 18 , 3 (2019). https://doi.org/10.1186/s12940-018-0442-6
Download citation
Received : 16 July 2018
Accepted : 14 December 2018
Published : 17 January 2019
DOI : https://doi.org/10.1186/s12940-018-0442-6
Share this article
Anyone you share the following link with will be able to read this content:
Sorry, a shareable link is not currently available for this article.
Provided by the Springer Nature SharedIt content-sharing initiative
- Agricultural pollution
- Community water systems
- Disparities
- Drinking water
- Environmental justice
- Water quality
Environmental Health
ISSN: 1476-069X
- General enquiries: [email protected]
- Open access
- Published: 09 September 2022
Water quality index for assessment of drinking groundwater purpose case study: area surrounding Ismailia Canal, Egypt
- Hend Samir Atta ORCID: orcid.org/0000-0001-5529-0664 1 ,
- Maha Abdel-Salam Omar 1 &
- Ahmed Mohamed Tawfik 2
Journal of Engineering and Applied Science volume 69 , Article number: 83 ( 2022 ) Cite this article
6058 Accesses
16 Citations
Metrics details
The dramatic increase of different human activities around and along Ismailia Canal threats the groundwater system. The assessment of groundwater suitability for drinking purpose is needed for groundwater sustainability as a main second source for drinking. The Water Quality Index (WQI) is an approach to identify and assess the drinking groundwater quality suitability.
The analyses are based on Pearson correlation to build the relationship matrix between 20 variables (electrical conductivity (Ec), pH, total dissolved solids (TDS), sodium (Na), potassium (K), calcium (Ca), magnesium (Mg), chloride (Cl), carbonate (CO 3 ), sulphate (SO 4 ), bicarbonate (HCO 3 ), iron (Fe), manganese (Mn), zinc (Zn), copper (Cu), lead (Pb), cobalt (Co), chromium (Cr), cadmium (Cd), and aluminium (Al). Very strong correlation is found at [Ec with Na, SO 4 ] and [Mg with Cl]; strong correlation is found at [TDS with Na, Cl], [Na with Cl, SO 4 ], [K with SO 4 ], [Mg with SO 4 ] and [Cl with SO 4 ], [Fe with Al], [Pb with Al]. The water type is Na–Cl in the southern area due to salinity of the Miocene aquifer and Mg–HCO 3 water type in the northern area due to seepage from Ismailia Canal and excess of irrigation water.
The WQI classification for drinking water quality is assigned with excellent and good groundwater classes between km 10 to km 60, km 80 to km 95 and the adjacent areas around Ismailia Canal. While the rest of WQI classification for drinking water quality is assigned with poor, very poor, undesirable and unfit limits which are assigned between km 67 to km 73 and from km 95 to km 128 along Ismailia Canal.
Introduction
Nowadays, groundwater has become an important source of water in Egypt. Water crises and quality are serious concerns in a lot of countries, particularly in arid and semi-arid regions where water scarcity is widespread, and water quality assessment has received minimal attention [ 3 , 9 ]. So, it is important to assess the quality of water to be used, especially for drinking purposes.
Poor hydrogeological conditions have been encountered causing adverse impacts on threatening the adjacent groundwater aquifer under the Ismailia Canal. The groundwater quality degradation is due to rapid urban development, industrialization, and unwise water use of agricultural water, either groundwater or surface water.
As groundwater quality is affected by several factors, an appropriate study of groundwater aquifers characteristics is an essential step to state a supportable utilization of groundwater resources for future development and requirements [ 11 , 12 ]. It is important that hydrogeochemical information is obtained for the region to help improving the groundwater management practices (sustainability and protection from deterioration) [ 17 ].
Many researchers have paid great attention to groundwater studies. In the current study area, the hydrogeology and physio-hydrochemistry of groundwater in the current study area had been previously discussed by El Fayoumy [ 15 ] and classified the water to NaCl type; Khalil et al. [ 27 ] stated that water had high concentration of Na, Ca, Mg, and K. Geriesh et al. [ 21 ] detected and monitored a waterlogging problem at the Wadi El Tumilate basin, which increased salinity in the area. Singh [ 34 ] studied the problem of salinization on crop yield. Awad et al. [ 7 ] revealed that the groundwater salinity ranges between 303 ppm and 16,638 ppm, increasing northward in the area.
Various statistical concepts were used to understand the water quality parameters [ 24 , 28 , 35 ].
Armanuos et al. [ 4 ] studied the groundwater quality using WQI in the Western Nile Delta, Egypt. They had generated the spatial distribution map of different parameters of water quality. The results of the computed WQI showed that 45.37% and 66.66% of groundwater wells falls into good categories according to WHO and Egypt standards respectively.
Eltarabily et al. [ 19 ] investigate the hydrochemical characteristics of the groundwater at El-Khanka in the eastern Nile Delta to discuss the possibility of groundwater use for agricultural purposes. They used Pearson correlation to deduce the relationship between 13 chemical variables used in their analysis. They concluded that the groundwater is suitable for irrigation use in El-Qalubia Governorate.
The basic goal of WQI is to convert and integrate large numbers of complicated datasets of the physio-hydrochemistry elements with the hydrogeological parameters (which have sensitive effect on the groundwater system) into quantitative and qualitative water quality data, thus contributing to a better understanding and enhancing the evaluation of water quality [ 38 ]. The WQI is calculated by performing a series of computations to convert several values from physicochemical element data into a single value which reflects the water quality level's validity for drinking [ 16 ].
Based on the physicochemical properties of the groundwater, it should be appraised for various uses. One can determine whether groundwater is suitable for use or unsafe based on the maximum allowable concentration, which can be local or international. The type of the material surrounding the groundwater or dissolving from the aquifer matrix is usually reflected in the physicochemical parameters of the groundwater. These metrics are critical in determining groundwater quality and are regarded as a useful tool for determining groundwater chemistry and primary control mechanisms [ 18 ].
The objective of this research is to assess suitability of groundwater quality of the study area around Ismailia Canal for drinking purpose and generating WQI map to help decision-makers and local authorities to use the created WQI map for groundwater in order to avoid the contamination of groundwater and to facilitate in selection safely future development areas around Ismailia Canal.
Description of study area
The study area lies between latitudes 30° 00′ and 31° 00′ North and longitude 31° 00′ and 32° 30′ East. It is bounded by the Nile River in the west, in the east there is the Suez Canal, in the south, there is the Cairo-Ismailia Desert road, and in the north, there are Sharqia and Ismailia Governorates as shown in Fig. 1 . Ismailia Canal passes through the study area. It is considered as the main water resource for the whole Eastern Nile Delta and its fringes. Its intake is driven from the Nile River at Shoubra El Kheima, and its outlet at the Suez Canal. At the intake of the canal, there are large industrial areas, which include the activities of the north Cairo power plant, Amyeria drinking water plant, petroleum companies, Abu Zabaal fertilizer and chemical company, and Egyptian company of Alum. Ismailia Canal has many sources of pollution, which potentially affects and deteriorates the water quality of the canal [ 22 ].
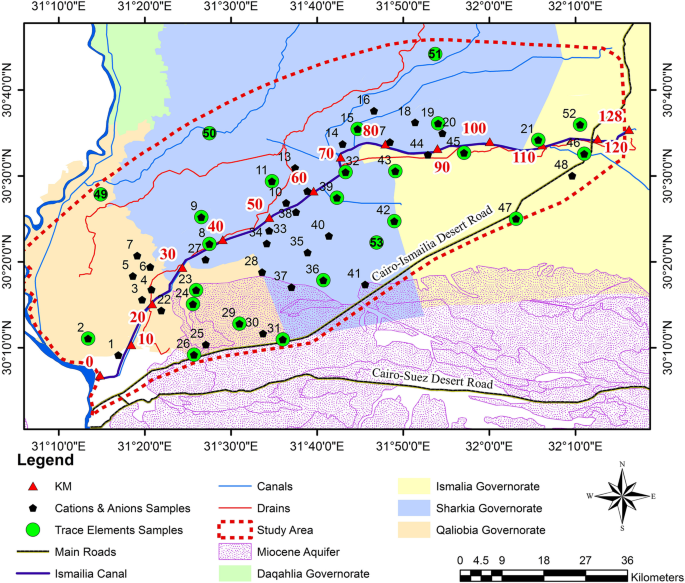
Map of the study area and location of groundwater wells
The topography plays an important role in the direction of groundwater. The ground level in the study area is characterized by a small slope northern Ismailia Canal. It drops gently from around 18 m in the south close to El-Qanater El-Khairia to 2 amsl northward. While southern Ismailia Canal, it is characterized by moderate to high slope. The topography rises from 10 m to more than 200 m in the south direction.
Geology and hydrogeology
The sequence of deposits rocks of wells was investigated through the study of hydrogeological cross-section A-A′ and B-B′ located in Fig. 2 a, b [ 32 ]. Section B-B′ shows that the study area represents two main aquifers that can be distinguished into the Oligocene aquifer (southern portion of the study area) and the Quaternary aquifer (northern portion of the study area). The Oligocene aquifer dominates the area of Cairo-Suez aquifer foothills. The Quaternary occupies the majority of the Eastern Nile Delta. It consists of Pleistocene sand and gravel. It is overlain by Holocene clay. The aquifer is semi-confined (old flood plain) and is phreatic at fringes areas in the southern portion of eastern Nile Delta fringes. The Quaternary aquifer thickness varies from 300 m (northern of the study area) to 0 at the boundary of the Miocene aquifer (south of the study area). The hydraulic conductivity ranges from 60 m/day to 100 m/day [ 8 ]. The transmissivity varies between 10,000 and 20,000 m 2 /day.
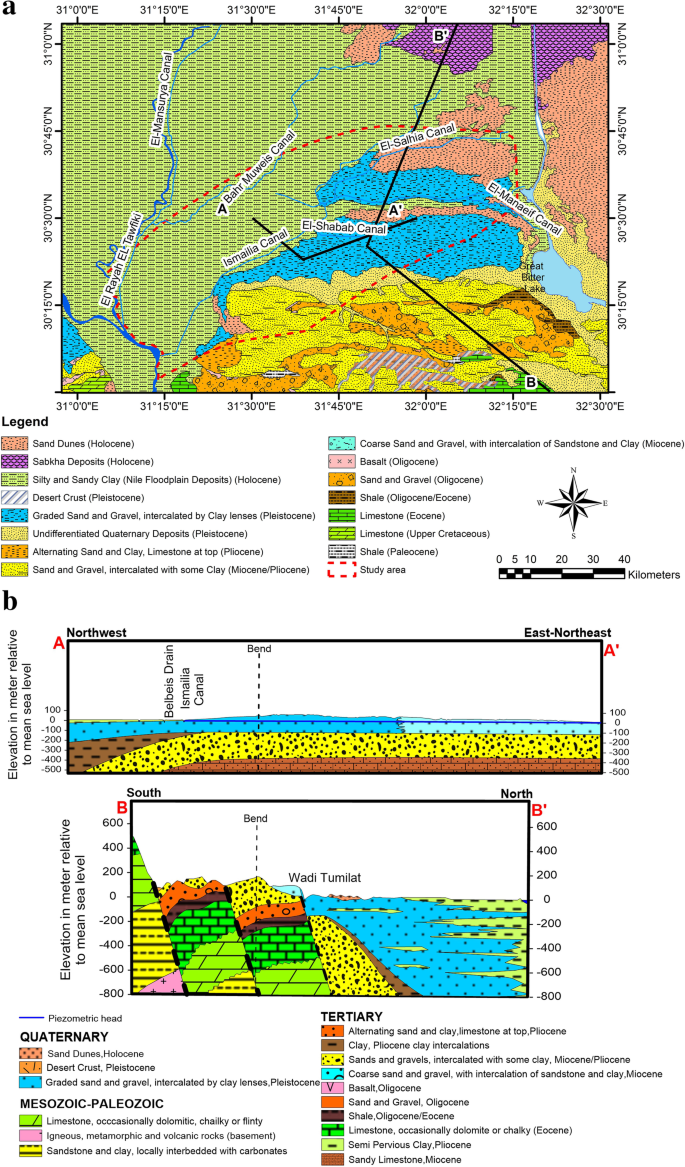
a Geology map of the study area. b Hydrogeological cross-section of the aquifer system (A-A′) and geological cross-section for East of Delta (B-B′)
Groundwater recharge and discharge
The main source of recharge into the aquifer under the study area is the excess drainage surplus (0.5–1.1 mm/day) [ 29 ], in addition to the seepage from irrigation system including Damietta branch and Ismailia Canal.
Groundwater and its movements
In the current research, it was possible to attempt drawing sub-local contour maps for groundwater level with its movement as shown in Fig. 3 . Figure 3 shows the main direction of groundwater flow from south to north. The groundwater levels vary between 5 m and 13 m (above mean sea level). The sensitive areas are affected by (1) the excess drainage surplus from the surface water reclaimed areas which located at low lying areas; (2) the seepage from the Ismailia Canal bed due to the interaction between it and the adjacent groundwater system, and (3) misuse of the irrigation water of the new communities and other issues. Accordingly, a secondary movement was established in a radial direction that is encountered as a source point at the low-lying area (Mullak, Shabab, and Manaief). Groundwater movement acts as a sink at lower groundwater areas (the northern areas of Ismailia Canal located between km 80 to km 90) due to the excessive groundwater extraction. The groundwater level reaches 2 m (AMSL). The groundwater levels range between + 15 m (AMSL) (southern portion of Ismailia Canal and study area near the boundary between the quaternary and Miocene aquifers).
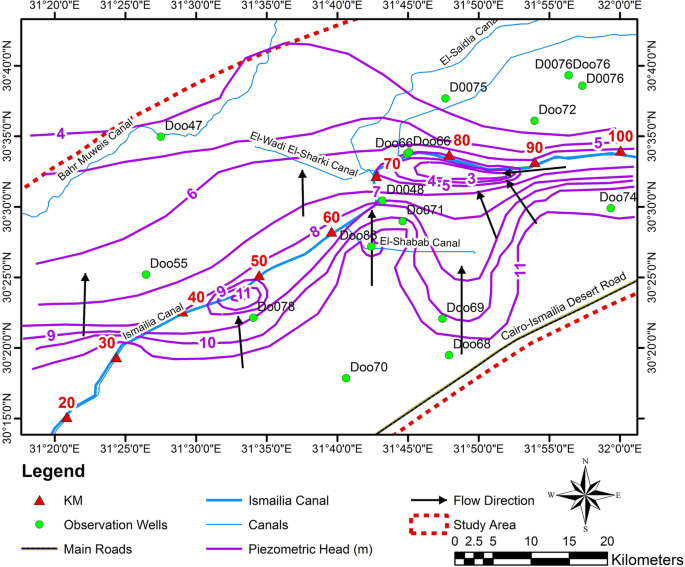
Groundwater flow direction map in the study area (2019)
The assessment of groundwater suitability for drinking purposes is needed and become imperative based on (1) the integration between the effective environmental hydrogeological factors (the selected 9 trace elements Fe, Mn, Zn, Cu, Pb, Co, Cr, Cd, Al) and 11 physio-chemical parameters (major elements of the anions and cations pH, EC, TDS, Na, K, Ca, Mg, Cl, CO 3 , SO 4 , HCO 3 ); (2) evaluation of WQI for drinking water according to WHO [ 36 ] and drinking Egyptian standards limit [ 14 ]; (3) GIS is used as a very helpful tool for mapping the thematic maps to allocate the spatial distribution for some of hydrochemical parameters with reference standards.
The groundwater quality for drinking water suitability is assessed by collecting 53 water samples from an observation well network covering the area of study, as seen in Fig. 1 . The samples were collected after 10 min of pumping and stored in properly washed 2 L of polyethylene bottles in iceboxes until the analyses were finished. The samples for trace elements were acidified with nitric acid to prevent the precipitation of trace elements. They were analyzed by the standard method in the Central Lab of Quality Monitoring according to American Public Health Association [ 2 ].
The water quality index is used as it provides a single number (a grade) that expresses overall water quality at a certain location based on several water quality parameters. It is calculated from different water parameters to evaluate the water quality in the area and its potential for drinking purposes [ 13 , 25 , 31 , 33 ]. Horton [ 23 ] has first used the concept of WQI, which was further developed by many scholars.
The first step of the factor analysis is applying the correlation matrix to measure the degree of the relationship and strength between linearly chemical parameters, using “Pearson correlation matrix” through an excel sheet. The analyses are mainly based on the data from 53 wells for physio-chemical parameters for the major elements and trace elements. Accordingly, it classified the index of correlation into three classes: 95 to 99.9% (very strong correlation); 85 to 94.9% (strong correlation), 70 to 84.9% (moderately), < 70% (weak or negative).
Equation ( 1 ) [ 4 ] is used to calculate WQI for the effective 20 selected parameters of groundwater quality.
In which Q i is the ith quality rating and is given by equation ( 2 ) [ 4 ], W i is the i th relative weight of the parameter i and is given by Eq. ( 3 ) [ 4 ].
Where C i is the i th concentration of water quality parameter and S i is the i th drinking water quality standard according to the guidelines of WHO [ 36 ] and Egypt drinking water standards [ 14 ] in milligram per liter.
Where W i is the relative weight, w i is the weight of i th parameter and n is the number of chemical parameters. The weight of each parameter was assigned ( w i ) according to their relative importance relevant to the water quality as shown in Table 2 , which were figured out from the matrix correlation (Pearson correlation, Table 1 ). Accordingly, it was possible assigning the index for weight ( w i ). Max weight 5 was assigned to very strong effective parameter for EC, K, Na, Mg, and Cl; weight 4 was assigned to a strong effective parameter as TDS, SO 4 ; 3 for a moderate effective parameter as Ca; and weight 2 was assigned to a weak effective parameter like pH, HCO 3, CO 3 , Fe, Cr, Cu, Co, Cd, Pb, Zn, Mn, and Al. Equation ( 2 ) was calculated based on the concertation of the collected samples from representative 53 wells and guidelines of WHO [ 36 ] and Egypt drinking water standards [ 14 ] in milligram per liter. This led to calculation of the relative weight for the weight ( W i ) by equation ( 3 ) of the selected 20 elements (see Table 2 ). Finally, Eq. ( 1 ) is the summation of WQI both the physio-chemical and environmental parameters for each well eventually.
The spatial analysis module GIS software was integrated to generate a map that includes information relating to water quality and its distribution over the study area.
Results and discussion
The basic statistics of groundwater chemistry and permissible limits WHO were presented in Table 3 . It summarized the minimum, maximum, average, med. for all selected 20 parameters and well percentage relevant to the permissible limits for each one; the pH values of groundwater samples ranged from 7.1 to 8.5 with an average value of 7.78 which indicated that the groundwater was alkaline. While TDS ranged from 263 to 5765 mg/l with an average value of 1276 mg/l. Sodium represented the dominant cation in the analyzed groundwater samples as it varied between 31 and 1242 mg/l, with an average value of 270 mg/l. Moreover, sulfate was the most dominant anion which had a broad range (between 12 and 1108 mg/l), with an average value of 184 mg/l. This high sulfate concentration was due to the seepage from excess irrigation water and the dissolution processes of sulfate minerals of soil composition which are rich in the aquifer. Magnesium ranged between 11 and 243 mg/l, with an average value of 43 mg/l. The presence of magnesium normally increased the alkalinity of the soil and groundwater [ 10 , 37 ]. Calcium ranged between 12 and 714 mg/l with a mean value of 119 mg/l. For all the collected groundwater samples, calcium concentration is higher than magnesium. This can be explained by the abundance of carbonate minerals that compose the water-bearing formations as well as ion exchange processes and the precipitation of calcite in the aquifer. Chloride content for groundwater samples varies between 18 and 2662 mg/l with an average value of 423 mg/l. Carbonate was not detected in groundwater, while bicarbonate ranged from 85 to 500 mg/l. Figures 5 , 6 , and 7 were drawn to show the extent of variation between the samples in each well.
Piper diagram [ 30 ] was used to identify the groundwater type in the study area as shown in Fig. 4 . According to the prevailing cations and anions in groundwater samples Na–Cl water type in the southern area due to salinity of the Miocene aquifer, Mg–HCO 3 water type in the northern area due to seepage from Ismailia Canal and excess of irrigation water and there is an interference zone which has a mixed water type between marine water from south and fresh water from north.
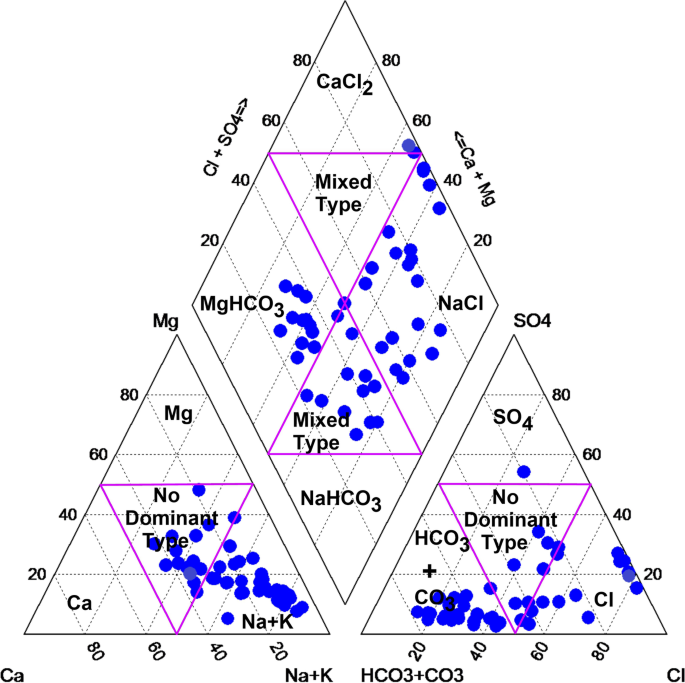
Piper trilinear diagram for the groundwater samples
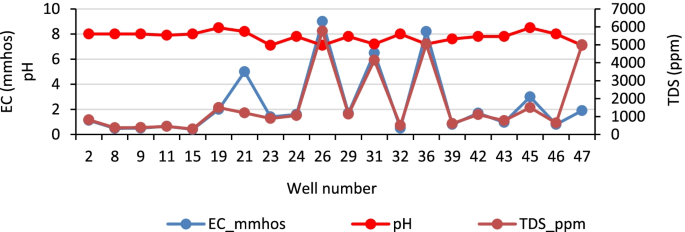
Concentration of selected physio-chemical parameters
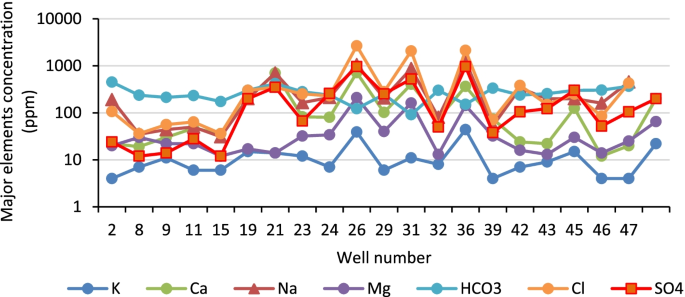
Concentration of major elements
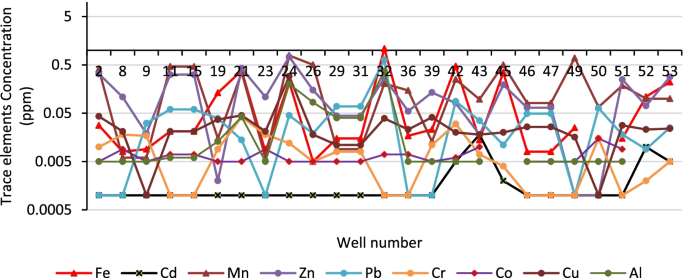
Concentration of trace element
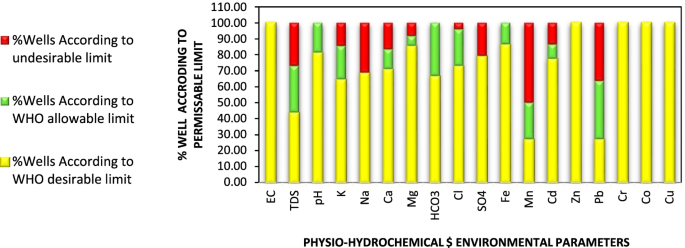
Concentration for 20 elements by percentage of wells (relevant to their limits of WHO for each element)
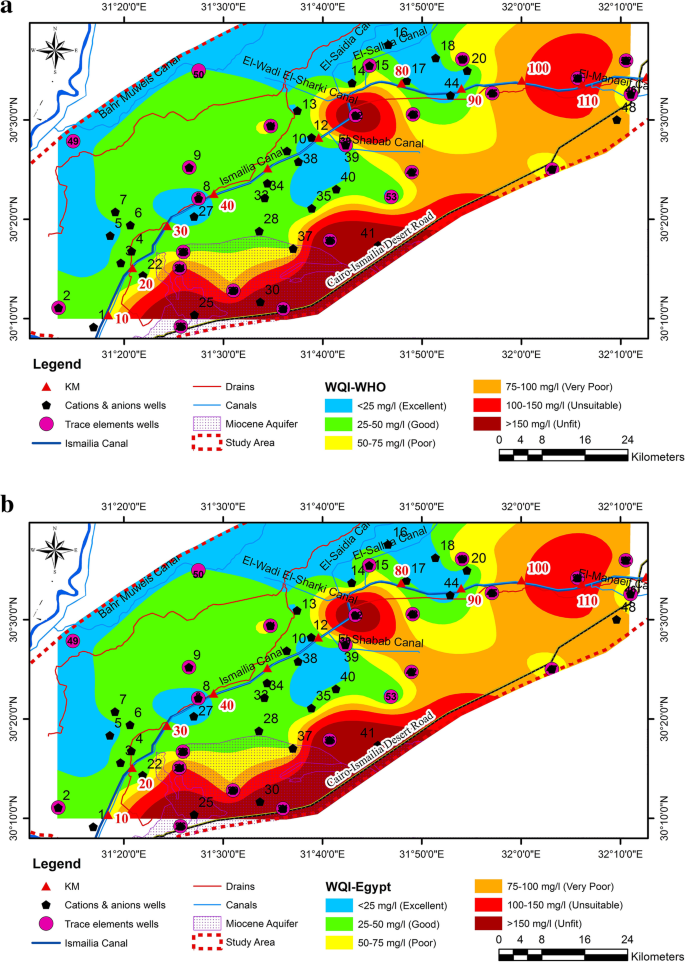
a , b WQI aerial distribution for drinking groundwater suitability for WHO ( a ) and Egyptian standards ( b )
Atta, et al. [ 5 ] revealed that the abundance of Fe, Mn, and Zn in the groundwater is due to geogenic aspects, not pollution sources. Khalil et al. [ 26 ] and Awad et al. [ 6 ] revealed that the source of groundwater in the area is greatly affected by freshwater seepage from canals and excess irrigation water which all agreed with the study.
Table 3 and Fig. 8 showed that 100% of wells for EC were assigned at desirable limits. 43.79% of wells for TDS were assigned at the desirable limit and 27.05% of them at the undesirable limits. While pH, 81.25% were assigned at the desirable limit. The percentage of wells for the aerial distribution of cations concentration assigned at desirable limits ranged between 64.6% for K, 85.45% for Mg, 68.73% for Na, and 70.8% for Ca. While the percentage of wells for the aerial distribution of cations concentration assigned at the undesirable limits ranged between 8.3% for Mg, 31.27% for Na, 14.6% for K, and 16.7% for Ca.
The percentage of wells for the aerial distribution of anions concentration assigned at desirable limits ranged between 72.9% for Cl, 66.7% for HCO 3 , and 79.2% for SO 4 . While the percentage of wells for the aerial distribution of anions concentration assigned at the undesirable limit ranged between 4.2% for Cl, 0% for HCO 3 , and 20.8% for SO 4 as shown in Table 3 and Fig. 8 .
Table 3 and Fig. 8 presented the aerial distribution concentration for 8 sensitive trace elements. The percentage of wells assigned at desirable limits ranged between 100% for (Zn, Cr, and Co), 86% for Fe, 27.3% for Mn, 77.4% for Cd, 27.2% for Pb, and 96% for Al, while the percentage of wells assigned at undesirable limits ranged between 0% for (Fe, Zn, Cr, and Co), 50% for Mn, 13.6% for Cd, 36.4% for Pb, and 4% for Al.
Figure 8 summarizes the results of the concentration for the selected 20 elements (11 physio-hydrochemical characteristics, and 9 sensitive environmental trace elements) by %wells relevant to the limits of WHO for each element.
The water quality index is one of the most important methods to observe groundwater pollution (Alam and Pathak, 2010) [ 1 ] which agreed with the results. It was calculated by using the compared different standard limits of drinking water quality recommended by WHO (2008) and Egyptian Standards (2007). Two values for WQI were calculated and drawn according to these two standards. It was classified into six classes relevant to the drinking groundwater quality classes: excelled water (WQI < 25 mg/l), good water (25–50 mg/l), poor water (50–75 mg/l), very poor water (75–100 mg/l), undesirable water (100–150 mg/l), and unfit water for drinking water (> 150 mg/l) as shown in Fig. 9 a, b. Figure 9 a (WHO classification) indicated that in the most parts of the study area, the good water class was dominant and reached to 35.8%, 28.8% was excellent water; 7.5% were poor water, 11.3% very poor water quality, and 13.3% were unfit water for drinking water. Similarly, for Egyptian Standard classification via WQI, the study area was divided into six classes: Fig. 9 b indicated that 35.8% of groundwater was categorized as excellent water quality, 34% as good water quality, 9.4% as poor water, 5.7% as very poor water, 1.9% as undesirable water and 13.3% as unfit water quality. This assessment was compared to Embaby et al. [ 20 ], who used WQI in the assessment of groundwater quality in El-Salhia Plain East Nile Delta. The study showed that 70% of the analyzed groundwater samples fall in the good class, and the remainder (30%), which were situated in the middle of the plain, was a poor class which mostly agreed with the study.
Conclusions and recommendation
This research studied the groundwater quality assessment for drinking using WQI and concluded that most of observation wells are located within desirable and max. allowable limits.
The groundwater in the study area is alkaline. TDS in groundwater ranged from 263 to 5765 mg/l, with a mean value of 1277 mg/l. Sodium and chloride are the main cation and anion constituents.
The water type is Na–Cl in the southern area due to salinity of the Miocene aquifer, Mg–HCO 3 water type in the northern area due to seepage from Ismailia Canal and excess of irrigation water and there is an interference zone which has a mixed water type between marine water from south and fresh water from north.
The WQI relevant to WHO limits indicated that 23% of wells were located in excellent water quality class that could be used for drinking, irrigation and industrial uses, 38% of wells were located in good water quality class that could be used for domestic, irrigation, and industrial uses, 11% of wells were located in poor water quality class that could be used for irrigation and industrial uses, 8% of wells were located in very poor water quality class that could be used for irrigation, 6% of wells were located in unsuitable water quality class which is restricted for irrigation use and 15% of wells were located in unfit water quality which will require proper treatment before use.
The WQI relevant to Egyptian standard limits indicated that 25% of wells were located in excellent water quality class that could be used for drinking, irrigation, and industrial uses, 43% of wells were located in good water quality class that could be used for domestic, irrigation, and industrial uses, 8% of wells were located in poor water quality class that could be used for irrigation and industrial uses, 6% of wells were located in very poor water quality class that could be used in irrigation, 6% of wells were located in unsuitable water quality class which is restricted for irrigation use and 13% of wells were located in unfit water quality which will require proper treatment before use.
The percentage of wells located at unfit water for drinking were assigned in the Miocene aquifer, and north of Ismailia Canal between km 67 to km 73 and from km 95 to km 128.
It is highly recommended to study the water quality of the Ismailia Canal which may affect the groundwater quality. It is recommended to study the water quality in detail between km 67 to 73 and from km 95 to km 128 as the WQI is unfit in this region and needs more investigations in this region. A full environmental impact assessment should be applied for any future development projects to maximize and sustain the groundwater as a second resource under the area of Ismailia Canal.
Availability of data and materials
The datasets generated and analyzed during the current study are not publicly available because they are part of a PhD thesis and not finished yet but are available from the corresponding author on reasonable request.
Abbreviations
World Health Organization
- Water Quality Index
Electrical conductivity
Total dissolved solids
Bicarbonate
Alam M, Pathak JK (2010) Rapid assessment of water quality index of Ramganga River, Western Uttar Pradesh (India) Using a computer programme. Nat Sci 8(11):1–8
Google Scholar
American Public Health Association (2015) Standard methods for the examination of water sewage and industrial wastes, 23th edn. American Public Health Association, New York
Aragaw TT, Gnanachandrasamy G (2021) Evaluation of groundwater quality for drinking and irrigation purposes using GIS-based water quality index in urban area of Abaya-Chemo sub-basin of Great Rift Valley, Ethiopia. Appl Water Sci 11:148. https://doi.org/10.1007/s13201-021-01482-6
Article Google Scholar
Armanuos A, Negm A, Valeriano OC (2015) Groundwater quality investigation using water quality index and ARCGIS: case study: Western Nile Delta Aquifer, Egypt. Eighteenth International Water Technology Conference, IWTC18, pp 1–10
Atta SA, Afaf AO, Zamzam AH (2003) Hydrogeology and hydrochemistry of the groundwater at Khanka region, Egypt. International Symposium on Future Food Security for Africa, pp 136–155
Awad SR, El Fakharany ZM (2020) Mitigation of waterlogging problem in El-Salhiya area, Egypt. Water Sci J 34(1):1–2. https://doi.org/10.1080/11104929.2019.1709298
Awad SR, Atta SA, El Arabi N (2008) Hydrogeology and quality of groundwater in the Eastern Nile Delta region. Maadi Cultured Association. The fifth International Conference for Water
Awad SR (1999) Environmental studies on groundwater pollution in some localities in Egypt. Ph.D. Thesis. Faculty of Science, Cairo University
Batarseh M, Imreizeeq E, Tilev S, Al Alaween M, Suleiman W, Al Remeithi AM, Al Tamimi MK, Al Alawneh M (2021) Assessment of groundwater quality for irrigation in the arid regions using irrigation water quality index (IWQI) and GIS-Zoning maps: case study from Abu Dhabi Emirate, UAE. Groundwater Sustain Dev 14:100611. https://doi.org/10.1016/j.gsd.2021.100611
Bousser MG, Amarenco P, Chamorro A et al (2011) Terutroban versus aspirin in patients with cerebral ischaemic events (PERFORM): a randomised, double-blind, parallel-group trial. Lancet (London England) 377(9782):2013–2022. https://doi.org/10.1016/S0140-6736(11)60600-4
Carrera-Hernandez JJ, Gaskin SJ (2006) The groundwater-modeling tool for GRASS (GMTG): open source groundwater flow modelling. Comput Geosci 32(3):339–351. https://doi.org/10.1016/j.cageo.2005.06.018
Chenini I, Ben MA (2010) Groundwater recharge study in arid region: an approach using GIS techniques and numerical modeling. Comput Geosci 36(6):801–817. https://doi.org/10.1016/j.cageo.2009.06.014
Chourasia LP (2018) Assessment of ground-water quality using water quality index in and around Korba City, Chhattisgarh, India. Am J Software Eng Appl 7(1):15–21. https://doi.org/10.11648/j.ajsea.20180701.12
Egyptian Higher Committee for Water (2007) Egyptian standards for drinking water and domestic uses. EHCW, Cairo
El Fayoumy IF (1987) Geology of the Quaternary Succession and its Impact on the Groundwater Reservoir in the Nile Delta Region. Submitted to the Bull, Fac. of Sc., Monoufia Univ., Egypt, Egypt
El Osta M, Masoud M, Alqarawy A, Elsayed S, Gad M (2022) Groundwater suitability for drinking and irrigation using water quality indices and multivariate modeling in Makkah Al-Mukarramah Province, Saudi Arabia. Water 14(3):483. https://doi.org/10.3390/w14030483
El Osta M, Masoud M, Ezzeldin H (2020) Assessment of the geochemical evolution of groundwater quality near the El Kharga Oasis, Egypt using NETPATH and water quality indices. Environmental Earth Sciences 81:248. https://doi.org/ https://doi.org/10.1007/s12665-019-8793-z
El Osta M, Niyazi B, Masoud M (2022) Groundwater evolution and vulnerability in semi-arid regions using modeling and GIS tools for sustainable development: case study of Wadi Fatimah, Saudi Arabia. Environ Earth Sci 81:248. https://doi.org/10.1007/s12665-022-10374-0
Eltarabily MG, Negm AM, Yoshimura C, Abdel-Fattah S, Saavedra OC (2018) Quality assessment of southeast Nile delta groundwater for irrigation. Water Resources 45(6):975–991. https://doi.org/10.1134/S0097807818060118
Embaby AA, Beheary MS, Rizk SM (2017) Groundwater quality assessment for drinking and irrigation purposes in El- Salhia Plain East Nile Delta Egypt. Int J Eng Technol Sci 12:51–73 https://www.researchgate.net/publication/330105491_Groundwater_Quality_assessment_For_Drinking_And_Irrigation_Purposes_In_El-Salhia_Plain_East_Nile_Delta_Egypt
Geriesh MH, El-Rayes AE (2001) Municipal contamination of shallow groundwater beneath south Ismailia villages. Fifth international conference on geochemistry. Alexandria University, Egypt, pp 241–253
Geriesh MH, Balke K, El-Bayes A (2008) Problems of drinking water treatment along Ismailia canal province, Egypt. J Zhejiang Univ Sci B 9(3):232–242. https://doi.org/10.1631/jzus.B0710634
Horton RK (1965) An index number system for rating water quality. J Water Pollut Control Fed 37(3):300–306
Isaaks EH, Srivastava RM (1989) An Introduction to Applied Geostatistics. Oxford University Press, New York
Kawo NS, Karuppannan S (2018) Groundwater quality assessment using water quality index and GIS technique in Modjo River Basin, central Ethiopia. J Afr Earth Sci 147:300–311. https://doi.org/10.1016/j.jafrearsci.2018.06.034
Khalil JB, Atta SA (1986) Hydrogeochemistry of groundwater in South of Ismailia canal, Egypt. Egypt J Geol 30(1-2):109–119
Khalil JB, Atta SA, Diab MS (1989) Hydrogeological Studies on the Groundwater Aquifer of the Eastern Part of the Nile Deltaic, Egypt, Water Science, 4th Issue, Egypt. Water Sci:79–90
Kumar D, Ahmed S (2003) Seasonal behaviour of spatial variability of groundwater level in a Granitic Aquifer in Monsoon Climate. Current Sci 84(2):188–196 https://www.jstor.org/stable/24108097
Morsy WS (2009) Environmental management of groundwater resources in the Nile Delta Regio, Ph.D. In: Thesis, Irrigation and Hydraulics Department, Faculty of Engineering, Cairo University, p 102
Piper AM (1944) A graphic representation in the geochemical interpretation of groundwater analyses. Transact Am Geophys Union, USA 25(6):914–928. https://doi.org/10.1029/TR025i006p00914
Rao GS, Nageswararao G (2013) Assessment of ground water quality using water quality index. Arch Environ Sci 7(1):1–5 https://aes.asia.edu.tw/Issues/AES2013/RaoGS2013.pdf
RIGW (1992) Hydrogeological Maps of Egypt, Scale 1: 100,000, Research Institute for Groundwater, National Water Research Center, Egypt.
Prerna S, Meher PK, Kumar A, Gautam P, Misha KP (2014) Changes in water quality index of Ganges river at different locations in Allahabad. Sustain Water Qual Ecol 3:67–76. https://doi.org/10.1016/j.swaqe.2014.10.002
Singh A (2015) Soil salinization and waterlogging: a threat to environment and agricultural sustainability. Ecol Indicat 57(2015):128–130. https://doi.org/10.1016/j.ecolind.2015.04.027
Suk H, Lee K (1999) Characterization of a ground water hydrochemical system through multivariate analysis: clustering into groundwater zones. Ground Water 37(3):358–366. https://doi.org/10.1111/j.1745-6584.1999.tb01112.x
World Health Organization WHO. (2008) Guidelines for drinking water quality. 1st and 2nd Addenda, Geneva, Switzerland, 1(3).
Xu P, Feng W, Qian H, Zhang Q (2019) Hydrogeochemical characterization and irrigation quality assessment of shallow groundwater in the Central-Western Guanzhong Basin, China. Int J Environ Res Public Health 16(9):1492. https://doi.org/10.3390/ijerph16091492
Yogendra K, Puttaiah ET (2008) Determination of water quality index and suitability of an urban waterbody in Shimoga Town, Karnataka. The Proceedings of Taal2007: The 12thWorld Lake Conference, Jaipur, India, pp 342–346
Download references
Acknowledgements
The researchers would like to thank Research Institute for Groundwater that provided us with the necessary data during the study.
No funding has to be declared for this work.
Author information
Authors and affiliations.
Research Institute for Groundwater, El-Kanater El-Khairia, Egypt
Hend Samir Atta & Maha Abdel-Salam Omar
Irrigation and Hydraulics Department, Faculty of Engineering, Cairo University, Cairo, Egypt
Ahmed Mohamed Tawfik
You can also search for this author in PubMed Google Scholar
Contributions
HS: investigation, methodology, writing—original draft. MA: investigation, writing—original draft and reviewing. AM: reviewing and editing. The authors read and approved the final manuscript.
Corresponding author
Correspondence to Hend Samir Atta .
Ethics declarations
Ethics approval and consent to participate.
Not applicable.
Consent for publication
Competing interests.
The authors declare that they have no competing interests.
Additional information
Publisher’s note.
Springer Nature remains neutral with regard to jurisdictional claims in published maps and institutional affiliations.
Rights and permissions
Open Access This article is licensed under a Creative Commons Attribution 4.0 International License, which permits use, sharing, adaptation, distribution and reproduction in any medium or format, as long as you give appropriate credit to the original author(s) and the source, provide a link to the Creative Commons licence, and indicate if changes were made. The images or other third party material in this article are included in the article's Creative Commons licence, unless indicated otherwise in a credit line to the material. If material is not included in the article's Creative Commons licence and your intended use is not permitted by statutory regulation or exceeds the permitted use, you will need to obtain permission directly from the copyright holder. To view a copy of this licence, visit http://creativecommons.org/licenses/by/4.0/ . The Creative Commons Public Domain Dedication waiver ( http://creativecommons.org/publicdomain/zero/1.0/ ) applies to the data made available in this article, unless otherwise stated in a credit line to the data.
Reprints and permissions
About this article
Cite this article.
Atta, H.S., Omar, M.AS. & Tawfik, A.M. Water quality index for assessment of drinking groundwater purpose case study: area surrounding Ismailia Canal, Egypt. J. Eng. Appl. Sci. 69 , 83 (2022). https://doi.org/10.1186/s44147-022-00138-9
Download citation
Received : 19 April 2022
Accepted : 20 July 2022
Published : 09 September 2022
DOI : https://doi.org/10.1186/s44147-022-00138-9
Share this article
Anyone you share the following link with will be able to read this content:
Sorry, a shareable link is not currently available for this article.
Provided by the Springer Nature SharedIt content-sharing initiative
- Groundwater
- Ismailia Canal
- Suitability
Thank you for visiting nature.com. You are using a browser version with limited support for CSS. To obtain the best experience, we recommend you use a more up to date browser (or turn off compatibility mode in Internet Explorer). In the meantime, to ensure continued support, we are displaying the site without styles and JavaScript.
- View all journals
- My Account Login
- Explore content
- About the journal
- Publish with us
- Sign up for alerts
- Open access
- Published: 11 April 2022
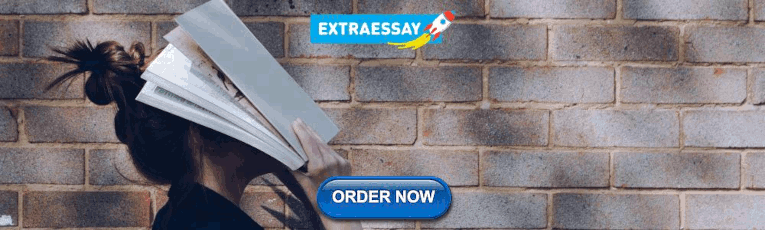
Water quality assessment and evaluation of human health risk of drinking water from source to point of use at Thulamela municipality, Limpopo Province
- N. Luvhimbi 1 ,
- T. G. Tshitangano 1 ,
- J. T. Mabunda 1 ,
- F. C. Olaniyi 1 &
- J. N. Edokpayi 2
Scientific Reports volume 12 , Article number: 6059 ( 2022 ) Cite this article
19k Accesses
19 Citations
1 Altmetric
Metrics details
- Environmental sciences
- Risk factors
Water quality has been linked to health outcomes across the world. This study evaluated the physico-chemical and bacteriological quality of drinking water supplied by the municipality from source to the point of use at Thulamela municipality, Limpopo Province, South Africa; assessed the community practices regarding collection and storage of water and determined the human health risks associated with consumption of the water. Assessment of water quality was carried out on 114 samples. Questionnaires were used to determine the community’s practices of water transportation from source to the point-of-use and storage activities. Many of the households reported constant water supply interruptions and the majority (92.2%) do not treat their water before use. While E. coli and total coliform were not detected in the water samples at source (dam), most of the samples from the street taps and at the point of use (household storage containers) were found to be contaminated with high levels of E. coli and total coliform. The levels of E. coli and total coliform detected during the wet season were higher than the levels detected during the dry season. Trace metals’ levels in the drinking water samples were within permissible range of both the South African National Standards and World Health Organisation. The calculated non-carcinogenic effects using hazard quotient toxicity potential and cumulative hazard index of drinking water through ingestion and dermal pathways were less than unity, implying that consumption of the water could pose no significant non-carcinogenic health risk. Intermittent interruption in municipal water supply and certain water transportation and storage practices by community members increase the risk of water contamination. We recommend a more consistent supply of treated municipal water in Limpopo province and training of residents on hygienic practices of transportation and storage of drinking water from the source to the point of use.
Similar content being viewed by others
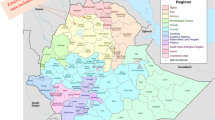
Access to basic drinking water services, safe water storage, and household water treatment practice in rural communities of northwest Ethiopia
Zemichael Gizaw, Mulat Gebrehiwot, … Adane Nigusie
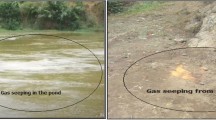
Ecological and health risk assessment of trace metals in water collected from Haripur gas blowout area of Bangladesh
M. Farhad Howladar, Md. Numan Hossain, … Debjani Das
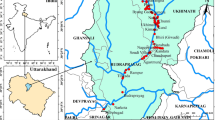
A systematic study on occurrence, risk estimation and health implications of heavy metals in potable water from different sources of Garhwal Himalaya, India
Mukesh Prasad, R. S. Aswal, … R. C. Ramola
Introduction
Water is among the major essential resources for the sustenance of humans, agriculture and industry. Social and economic progress are based and sustained upon this pre-eminent resource 1 . Availability and easy access to safe and quality water is a fundamental human right 2 and availability of clean water and sanitation for all has been listed as one of the goals to be achieved by the year 2030 for sustainable development by the United Nations General Assembly (UNGA) 3 .
The physical, chemical, biological and aesthetic properties of water are the parameters used to describe its quality and determine its capability for a variety of uses including the protection of human health and the aquatic ecosystem. Most of these properties are influenced by constituents that are either dissolved or suspended in water and water quality can be influenced by both natural processes and human activities 4 , 5 . The capacity of a population to safeguard sustainable access to adequate quantities and acceptable quality of water for sustaining livelihoods of human well-being and socioeconomic growth; as well as ensuring protection against pollution and water related disasters; and for conserving ecosystems in a climate of peace and political balance is regarded to as water security 6 .
Although the world’s multitudes have access to water, in numerous places, the available water is seldom safe for human drinking and not obtainable in sufficient quantities to meet basic health needs 7 . The World Health Organization (WHO) estimated that about 1.1 billion people globally drink unsafe water and most diarrheal diseases in the world (88%) is attributed to unsafe water, poor sanitation and unhygienic practices. In addition, the water supply sector is facing enormous challenges due to climate change, global warming and urbanization. Insufficient quantity and poor quality of water have serious impact on sustainable development, especially in developing countries 8 .
The quality of water supplied by the municipality is to be measured against the national standards for drinking water developed by the federal governments and other relevant bodies 9 . These standards considered some attributes to be of primary importance to the quality of drinking water, while others are considered to be of secondary importance. Generally, the guidelines for drinking water quality recommend that faecal indicator bacteria (FIB), especially Escherichia coli ( E. coli ) or thermo tolerant coliform (TTC), should not be found in any 100 mL of drinking water sample 8 .
Despite the availability of these standards and guidelines, numerous WHO and United Nations International Children Emergency Fund (UNICEF) reports have documented faecal contamination of drinking water sources, including enhanced sources of drinking water like the pipe water, especially in low-income countries 10 . Water-related diseases remain the primary cause of a high mortality rate for children under the age of five years worldwide. These problems are specifically seen in rural areas of developing countries. In addition, emerging contaminants and disinfection by-products have been associated with chronic health problems for people in both developed and developing countries 11 . Efforts by governmental and non-governmental organizations to ensure water security and safety in recent years have failed in many areas due to a lack of sustainability of water supply infrastructures 12 .
Water quality, especially regarding the microbiological content, can be compromised during collection, transport, and home storage. Possible sources of drinking water contamination are open field defecation, animal wastes, economic activities (agricultural, industrial and businesses), wastes from residential areas as well as flooding. Any water source, especially is vulnerable to such contamination 13 . Thus, access to a safe source alone does not ensure the quality of water that is consumed, and a good water source alone does not automatically translate to full health benefits in the absence of improved water storage and sanitation 14 . In developing countries, it has been observed that drinking-water frequently becomes re-contaminated following its collection and during storage in homes 15 .
Previous studies in developing countries have identified a progressive contamination of drinking water samples with E. coli and total coliforms from source to the point of use in the households, especially as a result of using dirty containers for collection and storage processes 16 , 17 , 18 . Also, the type of water treatment method employed at household levels, the type of container used to store drinking water, the number of days of water storage, inadequate knowledge and a lack of personal and domestic hygiene have all been linked with levels of water contamination in households 19 , 20 .
In South Africa, many communities have access to treated water supplied by the government. However, the water is more likely to be piped into individual households in the urban than rural areas. In many rural communities, the water is provided through the street taps and residents have to collect from those taps and transport the water to their households. Also, water supply interruptions are frequently experienced in rural communities, hence, the need for long-term water storage. A previous study of water quality in South Africa reported better quality of water at source than the water samples obtained from the household storage containers, showing that water could be contaminated in the process of transporting it from source to the point of use 21 .
This study was conducted in a rural community at Thulamela Municipality, Limpopo province, South Africa, to describe the community’s drinking water handling practices from source to the point of use in the households and evaluate the quality of the water from source (the reservoir), main distribution systems (street taps), yard connections (household taps) and at the point of use (household storage containers). Water quality assessment was done by assessing the microbial contamination and trace metal concentrations, and the possible health risks due to exposure of humans to the harmful pathogens and trace metals in the drinking water were determined.
The study was conducted at Lufule village in Thulamela municipality, Limpopo Province, South Africa. The municipality is situated in the eastern subtropical region of the province. The province is generally hot and humid and it receives much of its rainfall during summer (October–March) 22 . Lufule village is made up of 386 households and a total population of 1, 617 residents 23 . The study area includes Nandoni Dam (main reservoir) which acquires its raw water from Luvuvhu river that flows through Mutoti and Ha-Budeli villages just a few kilometers away from Thohoyandou town. Nandoni dam is where purification process takes place to ensure that the water meets the standards set for drinking water. This dam is the main source of water around the municipality, and it is the one which supplies water to selected areas around the dam, including Lufule village. Water samples for analysis were collected from the dam (D), street taps (ST), household taps (HT) and household storage containers (HSC) (Fig. 1 ).
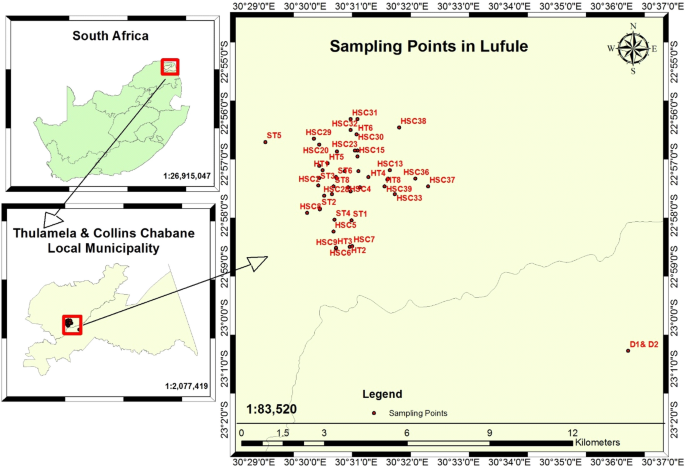
Map of the study area showing water samples’ collection areas.
Research design
This study adopted a quantitative design comprising of field survey and water analysis.
Field survey
The survey was done to identify the selected households and their shared source of drinking water (street taps). The village was divided into 10 quadrants for sampling purposes. From each quadrant, 6 households were randomly selected where questionnaires were distributed and household water samples were also collected for analysis.
Quantitative data collection
A structured interviewer-administered questionnaire was employed for data collection in the selected households. The population of Lufule village residents aged 15–69 years is 1, 026 (Census, 2011). About 10% of the adult population (~ 103) was selected to complete the questionnaires to represent the entire population. However, a total of 120 questionnaires were distributed, to take care of those which might be lacking vital information and therefore would not qualify to be analysed. Adults between the ages of 18 and 69 years were randomly selected to complete the questionnaire which includes questions concerning demographic and socio-economic statuses of the respondents, water use practices, sanitation, hygiene practices as well as perception of water quality and health. The face validity of the instrument was ensured by experts in the Department of Public Health, University of Venda, who reviewed questionnaire and confirmed that the items measure the concepts of interest relevant to the study 24 . Respondents were given time to go through the questionnaire and the researcher was present to clear any misunderstanding that may arise.
Water sampling
Permission to collect water samples from the reservoir tank at the Nandoni water treatment plant and households was obtained from the plant manager and the households’ heads respectively. Two sampling sites were identified at the dam, from where a water sample each was collected during the dry and the wet season. Similarly, 8 sampling sites were identified from the street and household taps, while 60 sampling sites were targeted for the household storage containers. However, only 39 household sites were accessible for sample collection, due to unavailability of the residents at the times of the researcher’s visit. Thus, water samples were collected from a total of 57 sites. Samples were collected from each of the sites during the dry (12th–20th April, 2019) and wet seasons (9th–12th December, 2019) between the hours of 08h00 and 14h30. A total of 114 samples were collected during the sampling period: 4 from the reservoir, 16 from street taps, 16 from household taps and 78 from households’ storage systems. Water samples were collected in 500 mL sterile polyethylene bottles. After collection, the containers were transported to the laboratory on ice in a cooler box. Each of the samples was tested for physico-chemical parameters, microbial parameters and trace metals’ concentration.
Physicochemical parameters’ analysis
Onsite analysis of temperature, pH, Electrical conductivity (EC) and Total Dissolved Solids (TDS) were performed immediately after sampling using a multimeter (model HI “HANNA” instruments), following the standards protocols and methods of American Public Health Association (APHA) 25 . The instrument was calibrated in accordance with the manufacturer’s guideline before taking the measurements. The value of each sample was taken after submerging the probe in the water and held for a couple of minutes to achieve a reliable reading. After measurement of each sample, the probe was rinsed with de-ionized water to avoid cross contamination among different samples.
ICP-OES and ICP-MS analyses of major and trace elements
An inductively coupled plasma optical emission spectrophotometer (ICP-OES) was used to analyse the major metals (Calcium (Ca), Sodium (Na), Potassium (K) and Magnesium (Mg)) in the water samples while inductively coupled plasma mass spectrophotometer (ICP-MS) was used to analyze the trace metals. The instrument was standardized with a multi-element calibration standard IV for ICP for Copper (Cu), Manganese (Mn), Iron (Fe), Chromium (Cr), Cadmium (Cd), Arsenic (As), Nickel (Ni), Zinc (Zn), Lead (Pb) and Cobalt (Co) and analytical precision was checked by frequently analysing the standards as well as blanks. ICP multi Standard solution of 1000 ppm for K, Ca, Mg and Na was prepared with NH 4 OAC for analysis to verify the accuracy of the calibration of the instrument and quantification of selected metals before sample analysis, as well as throughout the analysis to monitor drift.
Microbiological water quality analysis
Analysis of microbial parameters was conducted within 6 h of collection as recommended by APHA 25 . Viable Total coliform and E. coli were quantified in each sample using the IDEXX technique approved by the United States Environmental Protection Agency (USEPA). Colilert media was added to 100 mL sample and mixed until dissolved completely. The solution was poured into an IDEXX Quanti-Tray/2000 and sealed using the Quanti-Tray sealer 26 . The samples were incubated at 35 °C for 24 h. Trays were scanned using a fluorescent UV lamp to count fluorescent wells positive for E. coli concentration and counted with the most probable number (MPN) table provided by the manufacturer 27 .
Health risk assessment
Risk assessment have been estimated for ingestion and dermal pathways. Exposure pathway to water for ingestion and dermal routes are calculated using Eqs. ( 1 ) and ( 2 ) below:
where Exp ing : exposure dose through ingestion of water (mg/kg/day); BW: average body weight (70 kg for adults; 15 kg for children); Exp derm : exposure dose through dermal absorption (mg/kg/day); C water : average concentration of the estimated metals in water (μg/L); IR: ingestion rate in this study (2.0 L/day for adults; 1.0 L/day for children); ED: exposure duration (70 years for adults; and 6 years for children);AT: averaging time (25,550 days for an adult; 2190 days for a child); EF: exposure frequency (365 days/year) SA: exposed skin area (18.000 cm 2 for adults; 6600 cm 2 for children); K p : dermal permeability coefficient in water, (cm/h), 0.001 for Cu, Mn, Fe and Cd, while 0.0006 for Zn; 0.002 for Cr and 0.004 for Pb; ET: exposure time (0.58 h/ day for adults; 1 h/day for children) and CF: unit conversion factor (0.001 L/cm 3 ) 28 .
The hazard quotient (HQ) of non-carcinogenic risk by ingestion pathway can be determined by Eq. ( 3 )
where RfD ing is ingestion toxicity reference dose (mg/kg/day). An HQ under 1 is assumed to be safe and taken as significant non-carcinogenic, but HQ value above 1 may indicate a major potential health concern associated with over-exposure of humans to the contaminants 28 .
The total non-carcinogenic risk is represented by hazard index (HI). HI < 1 means the non-carcinogenic risk is acceptable, while HI > 1 indicates the risk is beyond the acceptable level 29 . The HI of a given pollutant through multiple pathways can be calculated by summing the hazard quotients by Eq. ( 4 ) below.
Carcinogenic risks for ingestion pathway is calculated by Eq. ( 5 ). For the selected metals in the study, carcinogenic risk (CR ing ) can be defined as the probability that an individual will develop cancer during his lifetime due to exposure under specific scenarios 30 .
where CRing is carcinogenic risk via ingestion route and SF ing is the carcinogenic slope factor.
Data analysis
Data obtained from the survey were analysed using Microsoft Excel and presented as descriptive statistics in the form of tables and graphs. The experimental data obtained was compared with the South African National Standards (SANS) 31 and Department of Water Affairs and Forestry (DWAF) 32 guidelines for domestic water use.
Ethics approval and consent to participate
The ethical clearance for this study was granted by the University of Venda Health, Safety and Research Ethics’ Committee (SHS/19/PH/14/1104). Permission to conduct the study was obtained from the Department of Water affairs, Limpopo province, Vhembe district Municipality and the selected households. Respondents were duly informed about the study and informed consent was obtained from all of them. The basic ethical principles of voluntary participation, informed consent, anonymity and confidentiality of respondents were duly complied with during data collection, analysis and reporting.
Consent for publication
Not applicable.
Socio-demographic characteristics of respondents
A total of 120 questionnaires were distributed but only 115 were completed, making a good response rate of 95%. The socio-demographic characteristics of the respondents are presented in Table 1 .
Household water supply
Many households (68.7%) had their primary water source from the municipality piped into their yards, but only 5.2% have the water flowing within their houses. The others have to fetch water at their neighbours’ yards or use the public taps on the streets. When the primary water supply is interrupted (i.e. when there is no water flowing through the pipes within the houses, yards or the public taps due to water rationing activities by the municipality, leakage of water distribution pipes, vandalization of pipes during road maintenance, etc.), the interruption usually lasts between a week or two, during which the respondents resort to other alternative sources. A return trip to the secondary source of water usually takes between 10 and 30 min for more than half of the respondents (53.0%) (Table 2 ).
Water storage and treatment practices at the household
Household water was most frequently stored in plastic buckets (n = 78, 67.8%), but ceramic vessels, metal buckets and other containers are also used for water storage (Fig. 2 ). Most households reported that their drinking water containers were covered (n = 111, 96.5%). More than half (53.9%) of the respondents used cups with handles to collect water from the storage containers whereas 37.4% used cups with no handles. Only 7.8% households reported that they treat their water before use mainly by boiling. Approximately 82.6% of respondent are of the opinion that one cannot get sick from drinking water and only 17.4% knew the risks that come with untreated water, and cited diarrhoea, schistosomiasis, cholera, fever, vomiting, ear infections, malnutrition, rash, flu and malaria as specific illnesses associated with water. Despite these perceptions, the majority (76.5%) were satisfied with their current water source. The few (23.5%) who were not satisfied cited poor quality, uncleanness, cloudiness, bad odour and taste in the water as reasons for their dissatisfaction (Table 3 ).
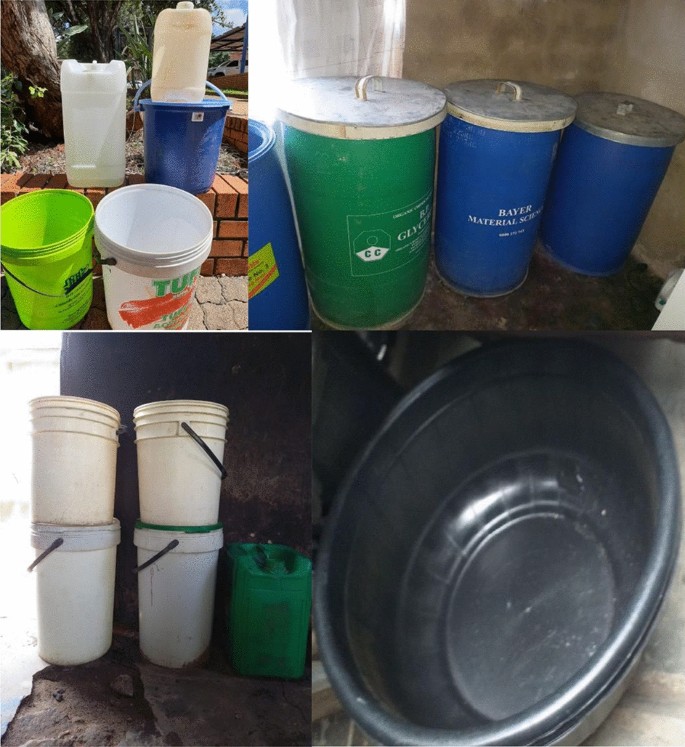
Examples of household water storage containers, some with lids and others without lids (photo from fieldwork).
Sanitation practices at the household level
More than half of the respondents (67%) use pit toilets, whereas only 26.1% use the flush to septic tank system, most of the toilets (93.9%) have a concrete floor. About 76.5% of households do not have designated place to wash their hands, however, all respondents indicated that they always wash their hands with soap or any of its other alternatives before preparing meals and after using the toilet (Table 4 ).
Water samples analysis
The water samples analyses comprise of microbial analysis, physico-chemical analysis and trace metals' parameters.
Microbial analysis
The samples from the reservoir during dry and wet season had 0 MPN/100 mL of total coliform and E. coli and were within the recommended limits of WHO and SANS for drinking water. During the wet season, seven out of the eight water samples collected from the street taps were contaminated with total coliform, while four of the samples taken from the same source were contaminated with total coliform during the dry season. Water samples from street taps 3 and 7 (ST 3 and ST7) were contaminated with total coliform during both seasons, however, the total coliform counts during the wet season were more than the counts during the dry season. None of the samples was contaminated with E. coli during the dry season, however, 2 samples from the street taps (ST3 & ST6) were found to be contaminated with E. coli during the wet season. Samples from household taps showed a similar trend with the street taps—with all samples being contaminated with total coliform during the wet season. Though 7 of the 8 samples taken from the household taps were contaminated with total coliform during the dry season, the samples from the same sources showed a higher level of total coliform in the wet season, with almost all the samples showing contamination at maximum detection levels of more than 2000 MPN/100 mL, except one sample (HT8) which showed a higher level of contamination with total coliform during the dry compared with the wet season. Only one sample (HT4) was found to be contaminated with E. coli during both dry and wet season. This shows that total coliform contamination levels are higher during the wet season than the dry season (Table 5 ).
Water samples from household storage containers (HSC) showed a higher level of total coliform during the wet season than the dry season and more samples were contaminated with E. coli during the wet season also (Table 6 ). A higher level of contamination was recorded for the HSCs compared to the street and household taps.
Physico-chemical analysis
In the reservoir samples, the pH value ranged from 8.37 to 8.45, EC ranged between 183 and 259 µS/cm whereas TDS varied between 118 and 168 mg/L. Similarly, in the street tap samples, pH value ranged from 7.28 and 9.33, EC ranged between 26 and 867 µS/cm whereas TDS varied between 16 and 562 mg/L (Fig. 3 ).
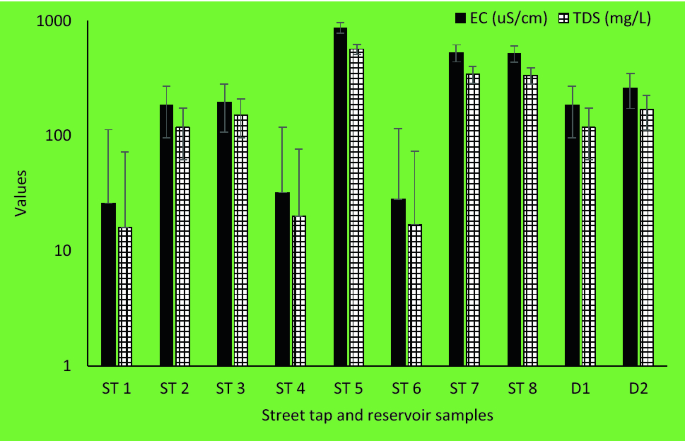
EC and TDS levels for the street taps and reservoir samples.
In the household taps, pH value ranged from 7.70–9.98, EC range between 28–895 µS/cm and TDS varied between 18 and 572 mg/L (Fig. 4 ).
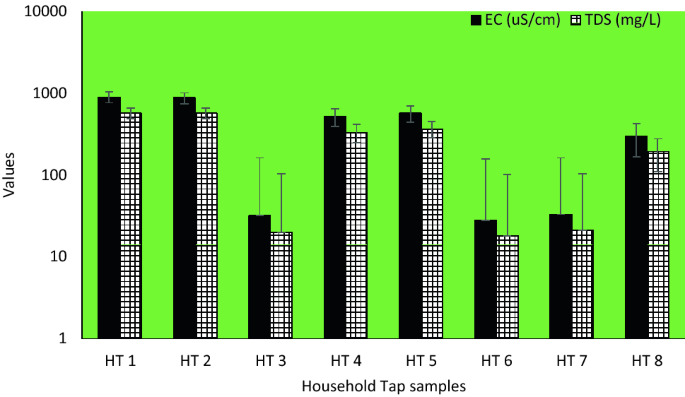
EC and TDS levels for household taps.
In household storage container samples, the pH value ranges from 7.67–9.77, EC ranged between 19–903 µS/cm and TDS values ranged from 12–1148 mg/L (Fig. 5 ).
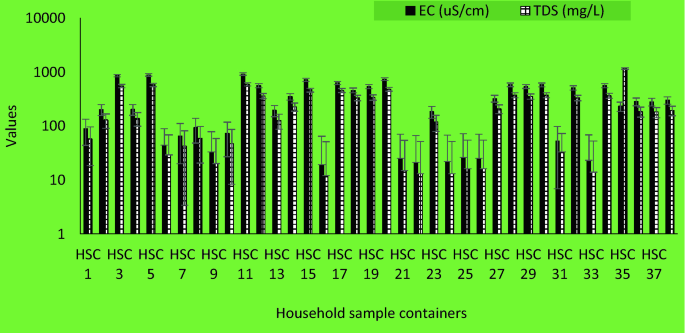
EC and TDS levels for household storage container samples.
Analysis of cations and trace metals in water
To detect the cations’ and trace metals’ concentrations in the water samples, representative samples from each of the sources were selected for analysis. The concentration of Calcium ranged between 2.14 and 31.65 mg/L, Potassium concentration ranged from 0.14 to 1.85 mg/L, Magnesium concentration varied from 1.32 to 16.59 mg/L, Sodium ranged from 0.18 to 12.96 mg/L (Table 7 ).
Trace metals’ analysis
The minimum and maximum concentrations of trace metals (Al, Mn, Fe, Co, Ni, Cu, Zn, As and Pb) present in water samples from selected street taps, household taps and household storage containers are presented in Table 8 .
Hazard quotient (HQ) and carcinogenic risk assessment
Table 9 presents the exposure dosage and hazard quotient (HQ) for ingestion and dermal pathway for metals. The HQ ing and HQ derm for all analyzed trace metals in both children and adults were less than one unit, indicating that there are no potential non-carcinogenic health risks associated with consumption of the water. Table 10 presents the total Hazard Quotient and Health risk index (HI) for trace metals in the water samples, showing that residents of the study area are not susceptible to non-cancer risks due to exposure to trace metals in drinking water. Table 11 presents the cancer risk associated with the levels of Ni, As and Pb in the drinking water samples. The table shows that only the maximum levels of lead had the highest chance of cancer risks for both adults and children.
This study provides information about the quality of drinking water in a selected rural community of Thulamela municipality of Limpopo province, South Africa, taking into consideration the physicochemical, microbiological and trace metals’ parameters of the treated water supplied to the village by the government, through the municipality. Many participants in the study have their primary source of water piped into their yards, while very few have water in their houses. This implies that getting water for household use would involve collecting the water from the yard and then into the storage containers. Those who do not have the taps in their yards have to collect water from the neighbours’ yards or the street taps. This observation is not restricted to the study area, as a similar situation has been observed in other rural communities of Limpopo Province 21 . This need to pass water through multiple containers before the point of use increases the risk of contamination.
Residents of the study area, just like residents of other settlements in Thulamela Municipality 21 , store their drinking water in plastic buckets, ceramic vessels, jerry cans and other containers. Almost all the respondents (96.5%) claim that their water storage vessels are covered and that their drinking water usually stays for less than a week in the storage containers (87.8%). Covering of water storage containers reduces the risk of water contamination from dust or other airborne particles. However, intermittent interruption of municipal water supply lasting for a week or more in the study area and the consequent use of alternative sources of water predispose the residents to various health risks as intermittent interruption in water supply has been linked to higher chances of contamination in the distribution systems, compared with continuous supply; in addition, the alternative sources of water may not be of a good quality as the treated municipal water 33 , 34 , yet, more than half of the respondents in this study (53%) use water directly from source without any form of treatment. This is because many residents in rural communities of Limpopo province believe that the water they drink is of good quality and thus do not need any further treatment 21 . The few who treat their water before drinking mostly use the boiling method. While boiling and other home-based interventions like solar disinfection of water have been reported to improve the quality of drinking water; drinking vessels, like cups, have also been implicated in water re-contamination of treated water at the point of use 16 and most respondents (91.3%) in this study admittedly use cups to collect water from the storage containers. The risk of contamination is even increased when cups without handles are used, where there is a higher chance that the water collector would touch the water in the container with his/her fingers. The Centres for Disease Control and Prevention (CDC) recommends that containers for drinking water should be fitted with a small opening with a cover or a spigot, through which water can be collected while the container remains closed, without dipping any potentially contaminated object into the container 35 . However, it is noteworthy that all the respondents claim to always wash their hands with soap (or its equivalents) and water after using the toilets, a constant practice of hand washing after using the toilet has been associated with a reduced risk of water contamination with E. coli 19 .
Treated water from the dam tested negative for both total coliform and E. coli hence complied with regulatory standards of SANS 31 and WHO 8 . The results could probably be due to the use of chlorine as a disinfectant in the treatment plant. Using disinfectants, pathogenic bacteria from the water can be killed and water made safe for the user. Similar studies have also reported that treated water in urban water treatment plants contains no total coliforms and E. coli 36 . In contrast, treated water sources in rural areas have been reported to have considerable levels of total coliform and E. coli 37 . The reason alluded to this include lack of disinfectant, no residual chlorine in the treated water, high prevalence of open defecation and unhygienic practices in proximity to water sources 38 .
From the water samples collected from the street taps, 62.5% were found to be contaminated with total coliform during the dry season, while the percentage rose to 87.5% during the wet season. The street tap which is about 13 km from the reservoir recorded high levels of total coliform ranging from 1.0 -2000 MPN/100 mL with most of the sites exceeding the WHO guidelines of 10 MPN/100 mL 8 . In both seasons, all the samples tested negative for E. coli , this complies with the WHO guideline of 0 MPN/100 mL. While the water leaving the treatment plant met bacteriological standards, the detection of coliform bacteria in the distribution lines suggest that the water is contaminated in the distribution networks. This could be due to the adherence of bacteria onto biofilms or accidental point source contamination by broken pipes, installation and repair works 39 . Furthermore, the water samples from households’ storage containers were contaminated by total coliform (73% and 85%) and E. coli (10.4% and 13.2%) during the dry and wet season, respectively. Microbiological contamination of household water stored in containers could be due to unhygienic practices occurring between the collection point and the point-of-use 40 , 41 .
Generally, higher levels of contamination were recorded in the wet season than in the dry season. The wet season in Thulamela Municipality is often characterized with increased temperature which could lead to favourable condition for microbial growth. Also, the treatment plant usually makes use of the same amount of chlorine for water purification during both seasons, even though influent water would be of a higher turbidity during the wet season, hence reducing the levels of residual chlorine 42 .
The pH of the analyzed samples from the study area ranged from 7.15 to 9.92. Most of the samples were within the values recommended by SANS (5 to 9.7) and comparable to results from previous similar studies 31 , 43 . Also, the electrical conductivity of all water samples from this study ranged from 28 µS/cm to 903 µS/cm which complied with the recommended value of SANS: < 1700 µS/cm 31 . The presence of dissolved solids such as calcium, chloride, and magnesium in water samples is responsible for its electrical conductivity 44 .
Total dissolved solids are the inorganic salts and small amounts of organic substance, which are present as solution in water 45 . Water has the ability to dissolve a wide range of inorganic and some organic minerals or salts such as potassium, calcium, sodium, bicarbonates, chlorides, magnesium, sulphates, etc. These minerals produced unwanted taste and colour in water 46 . A high TDS value indicates that water is highly mineralised. The recommended TDS value set for drinking water quality is ≤ 1200 mg/L 31 . In this study, the TDS values ranged from 18 mg/L to 572 mg/L. Hence, the TDS of all the household’s storage samples complied with the guidelines and consistent with previous studies 47 .
The analysis of magnesium (1.32 to 16.59 mg/L) and calcium (2.14 to 31.65 mg/L) concentrations showed that they were within the permissible range recommended for drinking water by SANS 31 and WHO 8 . All living organisms depend on magnesium in all types of cells, body tissues and organs for variety of functions while calcium is very important for human cell physiology and bones. Similar studies in Ethiopia and Turkey also showed acceptable levels of these metals in drinking water 46 , 48 . Likewise, the levels of potassium (0.14 to 1.85 mg/L) and sodium (0.18 to 12.96 mg/L) were within the permissible limit of WHO and SANS and may not cause health related problems. Sodium is essential in humans for the regulation of body fluid and electrolytes, and for proper functioning of the nerves and muscles, however, excessive sodium in the body can increase the risk of developing a high blood pressure, cardiovascular diseases and kidney damage 49 , 50 . Potassium is very important for protein synthesis and carbohydrate metabolism, thus, it is very important for normal growth and body building in humans, but, excessive quantity of potassium in the body (hyperkalemia) is characterized with irritability, decreased urine production and cardiac arrest 51 .
Metals like copper (Cu), cobalt (Co) and zinc (Zn) are essential requirements for normal body growth and functions of living organisms, however, in high concentrations, they are considered highly toxic for human and aquatic life 42 . Elevated trace metal(loids) concentrations could deteriorate water quality and pose significant health risks to the public due to their toxicity, persistence, and bio accumulative nature 52 . In this study, the concentrations of Manganese, Cobalt, Nickel and Copper all complied with the recommended concentration by SANS for domestic water use.
Aluminum concentration in the drinking water samples ranged from 1.25—13.46 µg/L. All analysed samples complied with the recommended concentration of ≤ 300 µg/L for domestic water use 31 . The recorded levels of Al in water from this study should not pose any health risk. At a high concentration, aluminium affects the nervous system, and it is linked to several diseases, such as Parkinson’s and Alzheimer’s diseases 53 . Iron (Fe) is an essential element for human health, required for the production of protein haemoglobin, which carries oxygen from our lungs to the other parts of the body. Insufficient or excess levels of iron can have negative effect on body functions 54 . The recommended concentration of iron in drinking water is ≤ 2000 µg/L 31 . In this study, the concentration of iron in the samples ranged from 0.96 to 73.53 µg/L. Similar results were reported by Jamshaid et al. in Khyber Pakhtunkhwa province 55 . A high concentration of Fe in water can give water a metallic taste, even though it is still safe to drink 56 .
The levels of Pb, As and Zn were in the range of 0.02–0.57 µg/L, 0.02–0.17 µg/L, and 2.54–194.96 µg/L, respectively whereas Cr was not detected in the samples collected. The levels recorded complied with the SANS 31 and WHO 8 guidelines for drinking water. Similar results were reported by Mohod and Dhote 57 . Lead is not desirable in drinking water because it is carcinogenic and can cause growth impairment in children 41 . Inorganic arsenic is a confirmed carcinogen and is the most significant chemical contaminant in drinking-water globally 44 . Zinc deficiency can cause loss of appetite, decreased sense of taste and smell, slow wound healing and skin sores 58 . Cr is desirable at low concentration but can be harmful if present in elevated levels.
The hazard quotient (HQ) takes into consideration the oral toxicity reference dose for a trace metal that humans can be exposed to 59 . Health related risk associated with the exposure through ingestion depends on the weight, age and volume of water consumed by an individual. HQ ing and HQ derm for all analyzed trace metals in both children and adults were less than one unit (Table 9 ), indicating that there are no potential non-carcinogenic health risks associated with the consumption of the water from the study area either by children or adults. The calculated average cumulative health risk index (HI) for children and adult was 3.88E-02 and 1.78E-02, respectively. HQ across metals serve as a conservative assessment tool to estimate high-end risk rather than low end-risk in order to protect the public. This served as a screen value to determine whether there is major significant health risk 60 . The results in this study signifies that the population of the investigated area are not susceptible to non-cancer risks due to exposure to trace metals in drinking water. Similar observation has been reported by Bamuwamye et al. after investigating human health risk assessment of trace metals in Kampala (Uganda) drinking water 61 . It should be noted that the hazard index values for children were higher than that of adult, suggesting that children were more susceptible to non-carcinogenic risk from the trace metals.
Drinking water with trace metals such as Pb, As, Cr and Cd could potentially enhance the risk of cancer in human beings 62 , 63 . Long term exposure to low amounts of toxic metals might, consequently, result in many types of cancers. Using As, Ni and Pb carcinogens, the total exposure risks of the residents in Table 11 . For trace metals, an acceptable carcinogenic risk value of less than 1 × 10 −6 is considered as insignificant and the cancer risk can be neglected; while an acceptable carcinogenic risk value of above 1 × 10 –4 is considered as harmful and the cancer risk is worrisome. Amongst the studied trace metals, only the maximum levels of lead for both adults and children had the highest chance of cancer risks (1.93E−03 and 4.46E−03) while Arsenic and Nickel have no chance of cancer risk with values of 3.34E−06; 7.72E−06 and 2.24E−05; 5.18E−05, in both adults and children respectively. The only cancer risk to residents of the studied area could be from the cumulative ingestion of lead in their drinking water. The levels of Pb recorded in this study complied to the SANS guideline value for safe drinking water. While the levels of Pb from the dam and the street pipes were relatively low, higher levels where recorded at household taps and storage containers and this may be due to the kind of storage containers and pipes used in those households. Generally, the water supply is of low Pb levels which should not pose any health risk to the consumers. However, the residents in rural areas should be properly educated on the kind of materials to be used for safe storage of water which should not pose an additional health burden. The likelihood of cancer risk was only associated with the consumption of the highest levels of Pb reported for a life time for adults (set at 70 years) and 6 years for children. Consistent consumption of water from the same source throughout an adult’s lifetime is unlikely as residents in those communities may change their locations at some points, hence reducing the possible risk associated with consistent exposure to the same levels of Pb.
Conclusions
The study shows that as distance increases from the treatment reservoir to distribution points, the cross-contamination rate also increases, therefore, good hygienic practices is required while transporting, storing and using water. Unhygienic handling practices at any point between collection and use contribute to the deterioration of drinking water quality.
The physicochemical, bacteriological quality and trace metals’ concentration of water samples from treated source, street taps and household storage containers were majorly within the permissible range of both WHO and SANS drinking water standards. HQ for both children and adults were less than unity, showing that the drinking water poses less significance health threat to both children and adults. Amongst the studied trace metals, only the maximum level of lead for both adults and children has the highest chance of cancer risks.
We recommend that appropriate measures should be taken to maintain residual free chlorine at the distribution points, supply of municipal treated water should be more consistent in all the rural communities of Thulamela municipality, Limpopo province and residents should be trained on hygienic practices of transportation and storage of drinking water from the source to the point of use.
Data availability
The datasets used and analysed during the current study are available from the first author on reasonable request.
Abbreviations
American Public Health Association
Centres for Disease Control and Prevention
Department of Water Affairs and Forestry
Electrical conductivity
Health risk index
Hazard quotient
Household storage containers
Household taps
Inductively coupled plasma mass spectrophotometer
Inductively coupled plasma optical emission spectrophotometer
Most probable number
South African National Standards
Street taps
Total Dissolved Solids
United Nations General Assembly
United Nations International Children Emergency Fund
United States Environmental Protection Agency
World Health Organization
Taiwo, A.M., Olujimi, O.O., Bamgbose, O. & Arowolo, T.A. Surface water quality monitoring in Nigeria: Situational analysis and future management strategy. In Water Quality Monitoring and Assessment (ed. Voudouris, K) 301–320 (IntechOpen, 2012).
Corcoran, E., et al. Sick water? The central role of wastewater management in sustainable development: A rapid response assessment. United Nations Enviromental Programme UN-HABITAT, GRID-Arendal. https://wedocs.unep.org/20.500.11822/9156 (2010).
United Nations, The 2030 Agenda and the Sustainable Development Goals: An opportunity for Latin America and the Caribbean (LC/G.2681-P/Rev.3), Santiago (2018).
Hubert, E. & Wolkersdorfer, C. Establishing a conversion factor between electrical conductivity and total dissolved solids in South African mine waters. Water S.A. 41 , 490–500 (2015).
Article CAS Google Scholar
Department of Water Affairs (DWA). Groundwater Strategy. Department of Water Affairs: Pretoria, South Africa. 64 (2010).
Lu, Y., Nakicenovic, N., Visbeck, M. & Stevance, A. S. Policy: Five priorities for the UN sustainable development goals. Nature 520 , 432–433 (2015).
Article ADS PubMed Google Scholar
Shaheed, A., Orgil, J., Montgomery, M. A., Jeuland, M. A. & Brown, J. Why, “improved” water sources are not always safe. Bull. World Health Organ. 92 , 283–289 (2014).
Article PubMed PubMed Central Google Scholar
WHO. Guidelines for Drinking Water Quality 4th Edn (World Health Organization, Geneva, Switzerland, 2011). http://apps.who.int/iris/bitstream/10665/44584/1/9789241548151_eng.pdf .
Patil, P. N., Sawant, D. V. & Deshmukh, R. N. Physico-chemical parameters for testing of water—a review. Int. J. Environ. Sci. 3 , 1194–1207 (2012).
CAS Google Scholar
Bain, R. et al. Fecal contamination of drinking-water in low-and middle-income countries: A systematic review and meta-analysis. PLoS Med. 11 , e1001644 (2014).
Younos, T. & Grady, C.A. Potable water, emerging global problems and solutions. In The Handbook of Environmental Chemistry 30 (2014).
Tigabu, A. D., Nicholson, C. F., Collick, A. S. & Steenhuis, T. S. Determinants of household participation in the management of rural water supply systems: A case from Ethiopia. Water Policy. 15 , 985–1000 (2013).
Article Google Scholar
Oljira, G. Investigation of drinking water quality from source to point of distribution: The case of Gimbi Town, in Oromia Regional State of Ethiopia (2015).
Clasen, T., Haller, L., Walker, D., Bartram, J. & Cairncross, S. Cost-effectiveness of water quality interventions for preventing diarrhoeal disease in developing countries. J. Water Health 5 , 599–608 (2007).
Article PubMed Google Scholar
Too, J. K., Sang, W. K., Ng’ang’a, Z. & Ngayo, M. O. Fecal contamination of drinking water in Kericho District, Western Kenya: Role of source and household water handling and hygiene practices. J. Water Health 14 , 662–671 (2016).
Rufener, S., Mausezahl, D., Mosler, H. & Weingartner, R. Quality of drinking-water at source and point-of-consumption—drinking cup as a high potential recontamination risk: A field Study in Bolivia. J. Health Popul. Nutri. 28 , 34–41 (2010).
Google Scholar
Nsubuga, F. N. W., Namutebi, E. N. & Nsubuga-ssenfuma, M. Water resources of Uganda: An assessment and review. Water Resour. Prot. 6 , 1297–1315 (2014).
Rawway, M., Kamel, M. S. & Abdul-raouf, U. M. Microbial and physico-chemical assessment of water quality of the river Nile at Assiut Governorate (Upper Egypt). J. Ecol. Health Environ. 4 , 7–14 (2016).
Agensi, A., Tibyangye, J., Tamale, A., Agwu, E. & Amongi C. Contamination potentials of household water handling and storage practices in Kirundo Subcounty, Kisoro District, Uganda. J. Environ. Public Health. Article ID 7932193, 8 pages (2019).
Mahmud, Z. H. et al. Occurrence of Escherichia coli and faecal coliforms in drinking water at source and household point-of-use in Rohingya camps, Bangladesh. Gut Pathog. 11 , 52. https://doi.org/10.1186/s13099-019-0333-6 (2019).
Edokpayi, J. N. et al. Challenges to sustainable safe drinking water: A case study of water quality and use across seasons in rural communities in Limpopo Province, South Africa. Water 10 , 159 (2018).
Article PubMed PubMed Central CAS Google Scholar
Musyoki, A., Thifhulufhelwi, R. & Murungweni, F. M. The impact of and responses to flooding in Thulamela Municipality, Limpopo Province, South Africa, Jàmbá. J. Disaster Risk Stud. 8 , 1–10 (2016).
Census 2011. Main Place: Lufule. Accessed from Census 2011: Main Place: Lufule (adrianfrith.com) on 30/01/2022.
Bolarinwa, O. A. Principles and methods of validity and reliability testing of questionnaires used in social and health science researches. Niger. Postgrad. Med. J. 22 , 195–201 (2015).
Association, A. P. H. Standard Methods for the Examination of Water and Waste Water 16th edn. (American Public Health Association, Washington, 1992).
Bernardes, C., Bernardes, R., Zimmer, C. & Dorea, C. C. A simple off-grid incubator for microbiological water quality analysis. Water 12 , 240 (2020).
Rich CR, Sellers JM, Taylor HB, IDEXX Laboratories Inc. Chemical reagent test slide. U.S. Patent Application 29/218,589. (2006).
Naveedullah, et al. Concentrations and human health risk assessment of selected heavy metals in surface water of siling reservoir watershed in Zhejiang Province, China. Pol. J. Environ. Stud. 23 , 801–811 (2014).
Wu, J. & Sun, Z. Evaluation of shallow groundwater contamination and associated human health risk in an alluvial plain impacted by agricultural and industrial activities, mid-west China. Expos. Health. 8 , 311–329 (2016).
Wu, L., Zhang, X. & Ju, H. Amperometric glucose sensor based on catalytic reduction of dissolved oxygen at soluble carbon nanofiber. Biosens. Bioelectron. 23 , 479–484 (2007).
Article PubMed CAS Google Scholar
South African National Standard (SANS). 241-1: Drinking Water, Part 1: Microbiological, Physical, Aesthetic and Chemical Determinants. 241-2: 2015 Drinking Water, Part 2: Application of SANS 241-1 (2015).
Department of Water Affairs and Forestry (DWAF). South African Water Quality Guidelines (second edition). Volume 1: Domestic Use, (1996).
Drake, M.J. & Stimpfl, M. Water matters. In Lunar and Planetary Science Conference , Vol. 38, 1179 (2007).
Kumpel, E. & Nelson, K. L. Intermittent water supply: prevalence, practice, and microbial water quality. Environ. Sci. Technol. 50 , 542–553 (2016).
Article ADS CAS PubMed Google Scholar
Centers for Disease Control and Prevention. The safe water system: Safe storage of drinking water. Accessed from CDC Fact Sheet on 30/01/2022 (2012).
Hashmi, I., Farooq, S. & Qaiser, S. Chlorination and water quality monitoring within a public drinking water supply in Rawalpindi Cantt (Westridge and Tench) area, Pakistan. Environ. Monit. Assess. 158 , 393–403 (2009).
Article CAS PubMed Google Scholar
Onyango, A. E., Okoth, M. W., Kunyanga, C. N. & Aliwa, B. O. Microbiological quality and contamination level of water sources in Isiolo country in Kenya. J. Environ. Public Health . 2018 , 2139867 (2018).
Gwimbi, P., George, M. & Ramphalile, M. Bacterial contamination of drinking water sources in rural villages of Mohale Basin, Lesotho: Exposures through neighbourhood sanitation and hygiene practices. Environ. Health Prev. Med. 24 , 33 (2019).
Karikari, A. Y. & Ampofo, J. A. Chlorine treatment effectiveness and physico-chemical and bacteriological characteristics of treated water supplies in distribution networks of Accra-Tema Metropolis, Ghana. Appl. Water Sci. 3 , 535–543 (2013).
Article ADS CAS Google Scholar
Thompson, T., Sobsey, M. & Bartram, J. Providing clean water, keeping water clean: An integrated approach. Int. J. Environ. Health Res. 13 , S89–S94 (2003).
Cronin, A. A., Breslin, N., Gibson, J. & Pedley, S. Monitoring source and domestic water quality in parallel with sanitary risk identification in Northern Mozambique to Prioritise protection interventions. J. Water Health 4 , 333–345 (2006).
Edokpayi, J. N., Enitan, A. M., Mutileni, N. & Odiyo, J. O. Evaluation of water quality and human risk assessment due to heavy metals in groundwater around Muledane area of Vhembe District, Limpopo Province, South Africa. Chem. Cent. J. 12 , 2 (2018).
Article CAS PubMed PubMed Central Google Scholar
Edimeh, P. O., Eneji, I. S., Oketunde, O. F. & Sha’Ato, R. Physico-chemical parameters and some heavy metals content of Rivers Inachalo and Niger in Idah, Kogi State. J. Chem. Soc. Nigeria 36 , 95–101 (2011).
Rahmanian, N., et al . Analysis of physiochemical parameters to evaluate the drinking water quality in the State of Perak, Malaysia. J. Chem. 1–10. Article ID 716125 (2015).
WHO/FAO. Diet, Nutrition, and the Prevention of Chronic Diseases (World Health Organisation, Geneva, 2003).
Meride, Y. & Ayenew, B. Drinking water quality assessment and its effects on resident’s health in Wondo genet campus, Ethiopia. Environ. Syst. Res. 5 , 1 (2016).
Mapoma, H. W. & Xie, X. Basement and alluvial aquifers of Malawi: An overview of groundwater quality and policies. Afr. J. Environ. Sci. Technol. 8 , 190–202 (2014).
Soylak, M., Aydin, F., Saracoglu, S., Elci, L. & Dogan, M. Chemical analysis of drinking water samples from Yozgat. Turkey Pol. J. Environ. Stud. 11 , 151–156 (2002).
Munteanu, C. & Iliuta, A. The role of sodium in the body. Balneo Res. J. 2 , 70–74 (2011).
Strazzullo, P. & Leclercq, C. Sodium. Adv. Nutr. 5 , 188–190 (2014).
Pohl, H. R., Wheeler, J. S. & Murray, H. E. Sodium and potassium in health and disease. Met. Ions Life Sci. 13 , 29–47 (2013).
Muhammad, S., Shah, M. T. & Khan, S. Health risk assessment of heavy metals and their source apportionment in drinking water of Kohistan region, northern Pakistan. Microchem. J. 98 , 334–343 (2011).
Inan-Eroglu, E. & Ayaz, A. Is aluminum exposure a risk factor for neurological disorders?. J. Res. Med. Sci. 23 , 51 (2018).
Milman, N. Prepartum anaemia: Prevention and treatment. Ann. Hematol. 87 , 949–959 (2008).
Jamshaid, M., Khan, A. A., Ahmed, K. & Saleem, M. Heavy metal in drinking water its effect on human health and its treatment techniques—a review. Int. J. Biosci. 12 , 223–240 (2018).
Tagliabue, A., Aumont, O. & Bopp, L. The impact of different external sources of iron on the global carbon cycle. Geophys. Res. Lett. 41 , 920–926 (2014).
Mohod, C. V. & Dhote, J. Review of heavy metals in drinking water and their effect on human health. Int. J. Innov. Res. Technol. Sci. Eng. 2 , 2992–2996 (2013).
Bhowmik, D., Chiranjib, K. P. & Kumar, S. A potential medicinal importance of zinc in human health and chronic. Int. J. Pharm. 1 , 05–11 (2010).
Mahmud, M. A. et al. Low temperature processed ZnO thin film as electron transport layer for efficient perovskite solar cells. Sol. Energy Mater. Sol. Cells. 159 , 251–264 (2017).
Rajan, S. & Ishak, N. S. Estimation of target hazard quotients and potential health risks for metals by consumption of shrimp ( Litopenaeus vannamei ) in Selangor, Malaysia. Sains Malays. 46 , 1825–1830 (2017).
Bamuwamye, M. et al. Human health risk assessment of heavy metals in Kampala (Uganda) drinking water. J. Food Res. 6 , 6–16 (2017).
Saleh, H. N. et al. Carcinogenic and non-carcinogenic risk assessment of heavy metals in groundwater wells in Neyshabur Plain, Iran. Biol. Trace Elem. Res. 190 , 251–261 (2019).
Tani, F. H. & Barrington, S. Zinc and copper uptake by plants under two transpiration rates Part II Buckwheat ( Fagopyrum esculentum L.). Environ. Pollut. 138 , 548–558 (2005).
Download references
Acknowledgements
The authors wish to thank the University of Venda Health, Safety and Research Ethics’ Committee, the Department of Water affairs, Limpopo province and Vhembe district Municipality for granting the permission to conduct this study. We also thank all the respondents from the selected households in Lufule community.
The study was funded by the Research and Publication Committee of the University of Venda (Grant number: SHS/19/PH/14/1104).
Author information
Authors and affiliations.
Department of Public Health, School of Health Sciences, University of Venda, Thohoyandou, 0950, South Africa
N. Luvhimbi, T. G. Tshitangano, J. T. Mabunda & F. C. Olaniyi
Department of Hydrology and Water Resources, School of Environmental Sciences, University of Venda, Thohoyandou, 0950, South Africa
J. N. Edokpayi
You can also search for this author in PubMed Google Scholar
Contributions
L.N. and J.N.E. conceptualized the study, L.N. collected and analysed the data, T.G.T., J.T. M., and J.N.E. supervised the data collection and analysis. F.C.O. drafted the original manuscript, J.N.E. reviewed and edited the original manuscript. All authors approved the final manuscript.
Corresponding author
Correspondence to F. C. Olaniyi .
Ethics declarations
Competing interests.
The authors declare no competing interests.
Additional information
Publisher's note.
Springer Nature remains neutral with regard to jurisdictional claims in published maps and institutional affiliations.
Rights and permissions
Open Access This article is licensed under a Creative Commons Attribution 4.0 International License, which permits use, sharing, adaptation, distribution and reproduction in any medium or format, as long as you give appropriate credit to the original author(s) and the source, provide a link to the Creative Commons licence, and indicate if changes were made. The images or other third party material in this article are included in the article's Creative Commons licence, unless indicated otherwise in a credit line to the material. If material is not included in the article's Creative Commons licence and your intended use is not permitted by statutory regulation or exceeds the permitted use, you will need to obtain permission directly from the copyright holder. To view a copy of this licence, visit http://creativecommons.org/licenses/by/4.0/ .
Reprints and permissions
About this article
Cite this article.
Luvhimbi, N., Tshitangano, T.G., Mabunda, J.T. et al. Water quality assessment and evaluation of human health risk of drinking water from source to point of use at Thulamela municipality, Limpopo Province. Sci Rep 12 , 6059 (2022). https://doi.org/10.1038/s41598-022-10092-4
Download citation
Received : 02 December 2021
Accepted : 30 March 2022
Published : 11 April 2022
DOI : https://doi.org/10.1038/s41598-022-10092-4
Share this article
Anyone you share the following link with will be able to read this content:
Sorry, a shareable link is not currently available for this article.
Provided by the Springer Nature SharedIt content-sharing initiative
This article is cited by
Characterization of natural zeolite and determination of its ion-exchange potential for selected metal ions in water.
- G. M. Wangi
- P. W. Olupot
- R. Kulabako
Environmental Processes (2023)
Relations between personal exposure to elevated concentrations of arsenic in water and soil and blood arsenic levels amongst people living in rural areas in Limpopo, South Africa
- Thandi Kapwata
- Caradee Y. Wright
- Angela Mathee
Environmental Science and Pollution Research (2023)
By submitting a comment you agree to abide by our Terms and Community Guidelines . If you find something abusive or that does not comply with our terms or guidelines please flag it as inappropriate.
Quick links
- Explore articles by subject
- Guide to authors
- Editorial policies
Sign up for the Nature Briefing newsletter — what matters in science, free to your inbox daily.

Change in drinking water quality from catchment to consumers: a case study
- Original Article
- Published: 22 September 2016
- Volume 2 , pages 453–460, ( 2016 )
Cite this article
- G. K. Khadse 1 ,
- P. M. Patni 1 ,
- A. V. Talkhande 1 &
- P. K. Labhasetwar 1
2033 Accesses
3 Citations
Explore all metrics
The study was conducted on the status of water supply at Indore through SDWQ. The performance of the Narmada and Devdharan WTPs was assessed from catchment to consumer for consecutive 7 days during three seasons. No significant change in raw water quality was observed on day-to-day basis. During monsoon, the turbidity of raw water was 690–1530 NTU which was reduced to 0.3–3.7 NTU after treatment. TC and FC were not detected in filtered water. The treated water quality was found within CPHEEO guidelines. At ESR, the residual chlorine was 0.1–0.6 mg/l. During winter, the water from ESR and from consumer ends was free from FC, whereas TC counts at consumer ends were 7–607 CFU/100 ml. During summer, on one occasion, TC and FC counts were found in ESR, whereas at the consumer ends, most of the samples were positive for TC and FC. The variation in bacterial counts amongst the different service reservoirs and consumer ends was due to poor maintenance of ESRs and enroute contamination. The proper maintenance of the distribution network, awareness about the hygienic and sanitary conditions around the public taps and proper storage of water are prerequisites in maintaining safe water supply in the city.
Similar content being viewed by others
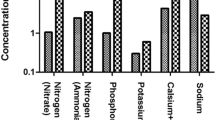
Wastewater Treatment and Reuse: a Review of its Applications and Health Implications
Kavindra Kumar Kesari, Ramendra Soni, … Janne Ruokolainen
Drinking water contamination and treatment techniques
S. Sharma & A. Bhattacharya
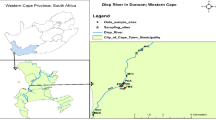
The impact of informal settlement on water quality of Diep River in Dunoon
Babalwa Gqomfa, Thabang Maphanga & Karabo Shale
Avoid common mistakes on your manuscript.
Introduction
Drinking water supply and sanitation are critical water uses for human survival, health and prosperity (WHO 2004 ; Marobhe et al. 2007 ). Almost 900 million people lack access to an improved water supply and 2.6 billion to basic sanitation (WHO and UNICEF 2010 ). The adverse impacts on public health from poor water supply have long been recognized in both developing and developed countries and take the form of disease outbreaks (Esrey et al. 1991 ; Ford 1999 ; Payment and Hunter 2001 ). Water supply access in most developing countries is complex compared to those in developed countries (WHO and UNICEF 2010 ; Howard and Bartram 2005 ) resulting in change in water quality from treatment plant to the distribution system. Initiatives to manage the safety of water do not only support public health, but often promote socioeconomic development and well-being as well (WHO 2013 ). Inspite of significant achievements in water supply and sanitation coverage, many factors render good-quality water unsafe by the time it reaches the consumer. The main problems related to drinking water quality are associated with the conditions of the water supply network (Lehtola et al. 2004 ; Karavoltsosa et al. 2008 ; Farooq et al. 2008 ; Proto et al. 2014 ), during collection and storage (Andrew et al. 2005 ), poor operation management and unsatisfactory sanitary practices; so it becomes obligatory to monitor water quality at each stage of delivery (Khadse et al. 2011a , b , c ). Water quality management and surveillance practices ensure safe water supply to consumers.
It is essential that the service provider and the consumers shoulder combined responsibility to see that needed programs and policies are taken up to achieve objectives and missions of “providing safe and sufficient water to one and all at minimum cost in terms of optimum utilization of available resources, adequate maintenance of existing infrastructures, self sustenance, reduction and loss due to leakage and environmental protections from degradation and pollutions” (WSSP 2004 ). Surveillance of drinking water quality (SDWQ) is the continuous and vigilant public health assessment and overview of the safety and acceptability of drinking water supplies (WHO 1993 ). SDWQ at drinking water treatment plant is necessary to avoid risk from chemical and bacteriological pollutants and to assure consumers that drinking water is safe and can be consumed without any risk (Leeuwen 2000 ). The elements of surveillance include water quality surveillance, study of institutional setup and examination of water for physicochemical and bacteriological parameters. Analysis of these parameters constitutes a risk to human health, others affect the aesthetic quality of the water supplied and others relate to treatment issues (Ratnayaka et al. 2009 ). SDWQ programs identify those interventions that will result in improvements in water supply that will be protective of public health (Lloyd and Bartram 1991 ; Lloyd et al. 1991 , WHO 1997 ; Howard 2002 ) and should identify those communities where water supply improvements will deliver the greatest health gain (Lloyd et al. 1991 ). Public health protection aspects of waterworks should ensure that each component of the system, viz. source, treatment, conveying main, storage and distribution, functions without risk of failures.
The SDWQ also involves inspection of site and sanitary condition of installations. The activities of SDWQ include assessment of design, commissioning and O&M of source, treatment, storage and distribution. The sampling and analysis of water from source to distribution are the main components of SDWQ. The water analysis laboratories of water supply agency, financial and manpower structure are in the purview of SDWQ. The SDWQ is to seek the answer to the question—is the water supply agency in a position to give the specified quality of water to total population within reasonable cost? The SDWQ includes all the activities to seek answer to these questions. It is necessary to ascertain the present status of drinking water quality being supplied in urban areas and to evolve a suitable strategy for future planning for SDWQ. In view of these, in the present paper, the intake water quality of treatment plants, at different treatment stages and at consumer end, was monitored for three different seasons to assess the drinking water quality status of Shimla city which may help in taking appropriate measures for safe drinking water supply to the city.
Indore city is the commercial capital of Madhya Pradesh in India and lies at latitude 22°44′N and longitude 75°50′E. There are two water treatment plants (WTPs) viz. Narmada and Devdharan, which supply treated water to the city. Public Health Engineering Department (PHED) is responsible for O&M of Narmada WTP, and Municipal Corporation is looking after Devdharan WTP and water distribution network of the city. The capacity details of the WTPs are provided in Table 1 . Both the plants follow the conventional method of treatment like addition of coagulant, flash mixing, flocculation, sedimentation, filtration and chlorination. Salient features of WTPs are given in Table 1 and the flow sheets of WTPs are given in Figs. 1 and 2 . The cases of gastroenteritis (296), hepatitis (165), diarrhea (9), jaundice (35) and typhoid (9) were reported during the year 2000–2002 in the city. The water-supplying authority takes the preventive measures in a routine manner. No epidemic due to water-born diseases was reported during the recent past.
Flow diagram of Narmada WTP
Flow diagram of Devdharan WTP
Methodology
Criteria for selecting sampling locations are envisaged with a view to examine water quality starting from raw water at source to consumers end including all the intermediate stages of treatment unit, distribution network and consumer ends (from household tap during water supply) (Tables 2 , 3 ). The city has intermittent water supply for 2–4 h daily. To examine the performance of WTPs, the samples of raw, settled, and filtered water were collected during summer, monsoon and winter seasons for 7 consecutive days from Narmada WTP, whereas Devdharan WTP was assessed during winter only due to non-functioning of WTP during summer and monsoon.
The raw water and treated water samples were collected and analysed for physicochemical parameters and bacteriological quality. The settled water was collected from the overflow of the clariflocculator/settling tank of the WTPs and examined for turbidity and sulphate. The filtered water was collected from the combined channel of the filters after filtration and analysed for turbidity, sulphate, and bacteriological quality. Final treated water after chlorination was collected from the sump of each WTP. To assess the enroute bacteriological contamination in distribution network, water samples were collected from service reservoirs and distribution network for bacteriological analysis. The samples were also collected from consumer end to see the quality of water supplied to the consumer. To monitor the water quality at consumer end, samples from 21 ESR and 21 representative consumer ends were evaluated.
The water quality parameters such as temperature (°C), pH, conductivity (EC) and dissolved oxygen (DO) were measured in the field. For other parameters, samples were preserved by adding appropriate reagents. Samples were filtered and stored in plastic bottles and analysed for major cations (Ca, Mg, Na, K) and major anions (SO 4 , Cl, PO 4 , NO 3 ) as per standard methods (APHA, AWWA, WPCF 1998 , 2005 ). Sodium and potassium were determined by flame photometry. Chloride, total hardness, and total alkalinity were analysed by volumetric methods. Sulphate, nitrate and phosphate were estimated by spectrophotometric technique. Bacteriological tests for total coliform and fecal coliform were undertaken by membrane filter (MF) technique. Knowledge, Attitude and Practice (KAP) survey was conducted which pertains to defined specific and measurable campaign objectives based on the problems identified by the agency. The KAP survey objectives have been achieved separately through a set of questionnaires designed separately for general public, health agencies and managers of water supply agencies. People from different economic and literacy levels comprising various age groups and gender from slum area, civil area, market area were covered during the survey. About 100 people were surveyed for their opinions about drinking water supply in the city.
Results and discussions
Water quality at wtps.
The analytical results for the physicochemical and bacteriological parameters of the water samples collected during three seasons are reported in Tables 2 , 3 and 4 .
Narmada WTP
During winter, it was observed that phase I and phase II units of the WTP were overloaded to the extent of 20 %. The performances of both the units were satisfactory as the raw water quality was fairly good. Alum/PAC addition was discontinued due to low turbidity of raw water. Bacteriological quality of raw water showed the presence of TC in the range of 30–73 CFU/100 ml, while FC was not detected (Table 2 ). TC in the filtered water samples of phase I and phase II units was up to 27 CFU/100 ml and up to 113 CFU/100 ml, respectively, and FC was not detected (Table 2 ).
During summer, the turbidity of raw water was 0.7–1.8 NTU. As the raw water quality was fairly good, Alum/PAC addition was not practised. In raw water, TC and FC were up to 60 CFU/100 ml and up to 8 CFU/100 ml, respectively, (Table 2 ). TC in the filtered water sample of phase I and phase II units was up to 44 and 16 CFU/100 ml, respectively (Table 2 ). FC was not detected in the filtered water.
During monsoon, the river was flooding and the turbidity of raw water was 690–1530 NTU. Primary settling tank brought down the turbidity of raw water to some extent. The applied PAC dose was @20 mg/L and supplemented with 0.1 mg/L dose of catflock. Finished water turbidity was 0.3–3.7 NTU. TC and FC in raw water were in the range of 100–250 and 10–140 CFU/100 ml, respectively (Table 2 ). In filtered water of phase I unit, TC and FC counts were 50–300 CFU/100 ml and up to 80 CFU/100 ml, respectively. In filtered water of phase II unit, TC was up to 20 CFU/100 ml and no FC was observed (Table 2 ). The treated water quality of the plants during all the seasons was found within the desirable limit as per BIS standards (BIS 1991 ).
Devdharan WTP
The raw water turbidity during winter was in the range of 3.0–5.2 NTU, whereas the turbidity of settled and filtered water was in the range of 1.2–3 NTU and 2.4–2.8 NTU, respectively. Settled and filtered water turbidity indicated poor settling of the flocks after alum dosing and malfunctioning of filter beds which may be due to improper back washing. The alum dose was not properly regulated, and the overall maintenance of the plant was not satisfactory. In raw water, TC counts were in the range of 13–310 CFU/100 ml and FC was not detected (Table 2 ). In filtered water, TC was up to 80 CFU/100 ml and FC was not detected (Table 2 ). The finished water quality was within the desirable limit as per BIS standards. During summer and monsoon, the Devdharan WTP was found closed due to non-availability of raw water in Yashwant Sagar Dam.
Water quality of distribution system
After conventional treatment, water from WTP is pumped to elevated service reservoirs (ESR) for distribution. The water quality of 21 ESR (Table 3 ) and 21 consumer ends (Table 4 ) were tested for residual chlorine and samples were analysed for bacteriology. The chlorine solution is added in all the ESR before distribution, and the residual chlorine was found in the range of 0.1–0.6, 0–1 and 0–1.0 mg/l during winter, summer and monsoon, respectively.
During winter, FC was not detected in any water sample from ESR and consumer end, while TC count at consumer ends was 7–607 CFU/100 ml. During summer, on one occasion, TC and FC counts in ESR (T-5) were 80 and 18 CFU/100 ml, respectively. The TC counts present in T-7 and T-20 samples were in the range of 28–48 CFU/100 ml and FC counts were in the range of 4–10 CFU/100. At the consumer ends, out of 21 samples, most of the samples were positive both for TC and FC as 76 and 12 CFU/100 ml, respectively. In monsoon, it was observed that in samples T-6, T-8, T-11 and T-15, TC was up to 196, 104, 580 and 264 CFU/100 ml. At consumer ends, it was observed that TC was up to 700 CFU/100 ml and FC was 48 CFU/100 ml. Presence of TC and FC indicated occasional enroute contamination in the ESR and distribution system.
Perceived state of drinking water pollution
The water quality is good in the opinion of 60 % of consumers. There is a regular water quality control program conducted by the field staff of the water supply agency by carrying out random water sampling of ESR and consumer ends.
Awareness of the SDWQ and information on drinking water standards
Awareness of the SDWQ program amongst general public is 20 %; in water works personnel, it is 35 %, and in health agencies, it is 30 %. Awareness of information on drinking water quality standard was 40 % amongst general public, 80 % amongst waterworks personnel and 90 % amongst health agency staff.
Information on private water supplies
A total of 20 % of the consumer amongst general public informed that they use private water supply through tube wells to meet their additional water demand.
Accountability for the efficient and effective delivery of water supply
10 % of the consumers amongst general public have indicated the satisfaction towards water supply, while 90 % supply efficiency and effective delivery of finished water were reported by 60 % waterworks personnel.
Knowledge of individuals/institution with water supply agency services
A total of 50 % of the consumers amongst general public, especially educated people, were aware of water supply agency services.
Procedure for the complaints against drinking water supply
45 % of the consumers indicated prompt action of waterworks personnel towards the lodged complaint regarding drinking water supply.
Knowledge of sanitary survey
About 80 % of the respondents amongst general public were found aware of cleaning practices and health aspects related to sanitary practices.
Knowledge of preventive measures and health education
65 % of the respondents were aware about the preventive measures to be taken to prevent the water-borne diseases. Yet, there is a need to impart health education to common people, especially illiterates and women.
Status of quality control and surveillance
The raw water sources were well protected from pollution in catchments. Alum bricks are kept in the raw water inlet channel. The dose of chlorine is arbitrary due to absence of dose regulating system, and dosing is done based on experience of the operator. The O&M of Narmada WTP is satisfactory, whereas Devdharan WTP is lacking in O&M. The staff of IMC looks after leakages and maintenance of distribution network. PHED laboratory undertakes the water analysis for the samples sent by the Municipal Corporation who is having well-equipped central water testing laboratory. Laboratory facilities are provided at Narmada WTP, but laboratory at Devdharan WTP did not have adequate equipment/instruments. Raw and treated water quality for pH, alkalinity, hardness, sulphate and residual chlorine are analysed at Narmada WTP and records are maintained properly.
Regular water quality control program is practised by water supply agency by carrying out random water sampling of ESRs and consumer ends. There is no organized leak detection program, and the leakages are repaired on the basis of public complaints and as noticed by water supply personnel. SDWQ program was not in practice. During the study period, 40–50 % water samples for TC and about 20 % water samples for FC were found positive from distribution system; hence, there exists an urgent need to create the infrastructural facility to carry out SDWQ.
The drinking water supply at Indore was assessed at raw water sources, treatment stages, distribution network and consumer ends. From the present study, it is observed that water supplied through the distribution network is of good physicochemical quality; however, 40–50 % water samples for TC and about 20 % water samples for FC were found positive from consumer ends. No significant change in physicochemical parameters of raw water quality was observed on a day-to-day basis. The city had an intermittent water supply. Therefore, during non-supply hours, due to back suction through leakages/damages/faulty jots in the supply lines, some insanitary material might be entering into the pipelines and carried up to the consumer ends, when supply resumes. In the places where the fecal contamination was present at the consumer end, the necessary precautionary measures need to be undertaken to avoid any adverse impact on health. The proper maintenance of the distribution network, awareness about the hygienic and sanitary conditions around the public taps and proper storage of water will definitely help in maintaining water quality within the limits of potable water standards. Thus, the surveillance of water supply and in-time measures to control the contamination along with people’s participation will lead to safety and better health of the community.
Recommendations
Instruments/equipments for flow measurement and head loss indicator need to be repaired and maintained.
Monitor coagulant dosing to avoid carryover of micro-flocs.
Improve backwash to avoid scum formation in settling tank, filter beds.
Ensure requisite quantity of residual chlorine with proper chlorine dose.
Upgradation of water testing laboratories at treatment plant.
O&M aspects of WTPs need due attention.
Andrew FT, Carter RC, Sean FT (2005) Mechanisms leading to post-supply water quality deterioration in rural Honduran communities. Int J Hyg Env Health 208(3):153–161. doi: 10.1016/j.ijheh.2005.01.024
Article Google Scholar
APHA, Awwa, WPCF (1998) Standard methods for examination of water and wastewater, 20th edn. American Public Health Association, Washington, DC
Google Scholar
APHA, Awwa, WPCF (2005) Standard methods for examination of water and wastewater, 21st edn. American Public Health Association, Washington, DC
BIS:10500 (1991) Bureau of Indian Standards (BIS), Guidelines for drinking water quality standards
Esrey SA, Potash JB, Roberts L, Shiff C (1991) Effects of improved water supply and sanitation on ascariasis, diarrhoea, dracunculiasis, hookworm infection, schistosomiasis, and trachoma. Bull World Health Organization 69(5):609–621
Farooq S, Hashmi I, Qazi IA, Qaiser S, Rasheed S (2008) Monitoring of coliforms and chlorine residual in water distribution network of Rawalpindi, Pakistan. Environ Monit Assess 140:339–347. doi: 10.1007/s10661-007-9872-2
Ford TE (1999) Microbiological safety of drinking water: United States and global perspectives. Environ Health Perspect 107(S1):191–206
Howard G (ed) (2002) Water supply surveillance: a reference manual. WEDC, Loughborough
Howard G, Bartram J (2005) Effective water supply surveillance in urban areas of developing countries. J Water Health 3(1):31–43
Karavoltsosa S, Sakellaria A, Mihopoulosb N, Dassenakisa M, Scoullosa MJ (2008) Evaluation of the quality of drinking water in regions of Greece. Desalination 1–3:317–329. doi: 10.1016/j.desal.2007.06.013
Khadse GK, Kalita M, Pimpalkar SN, Labhsetwar PK (2011a) Drinking water quality monitoring and surveillance for safe water supply in Gangtok, India. Environ Monit Assess 178 (1–4): 401–414, 1573–2959. doi: 10.1007/s10661-010-1699-6
Khadse GK, Kalita M, Pimpalkar SN, Labhsetwar PK (2011b) Surveillance of drinking water quality for safe water supply—a case study from Shillong India. Water Resour Manag 25:3321–3342. doi: 10.1007/s1269-011-9858-2
Khadse GK, Kalita M, Labhsetwar PK (2011c) Change in drinking water quality from source to point-of-use and storage: a case study from Guwahati. Environ Monit Assess, India. doi: 10.1007/s10661-011-2344-8
Leeuwen FXRV (2000) Safe drinking water: the toxicologist’s approach. Food Chem Toxicol 38(1):S51–S58
Lehtola MJ, Nissinenb TK, Miettinena IT, Martikainenc PJ, Vartiainen T (2004) Removal of soft deposits from the distribution system improves the drinking water quality. Water Res 38(3):601–610. doi: 10.1016/j.watres.2003.10.054
Lloyd B, Bartram J (1991) Surveillance solutions to microbiological problems in water quality control in developing countries. Wat Sci Technol 24(2):61–75
Lloyd B, Bartram J, Rojas R, Pardon M, Wheeler D, Wedgewood K (1991) Surveillance and improvement of Peruvian drinking water supplies. Robens Institute, DelAgua and ODA, Guildford
Marobhe NJ, Renman G, Jacks G (2007) The study of water supply and traditional water purification knowledge in selected rural villages in Tanzania. Indigenous knowledge systems and sustainable development: relevance for Africa, Emmanuel K. Boon and Luc Hens, Kamla-Raj Enterprises. Tribes and Tribals. vol 1, Chapter 11, 111–120
Payment P, Hunter PR (2001) Endemic and epidemic infectious intestinal disease and its relationship to drinking water. In: Fewtrell L, Bartram J (eds) Water quality: guidelines, standards and health. IWA Publishing, London, pp 61–88
Proto A, Zarrella I, Capacchione C, Motta O (2014) One-year surveillance of the chemical and microbial quality of drinking water shuttled to the Eolian Islands. Water 6:139–149. doi: 10.3390/w6010139
Ratnayaka DD, Brandt MJ, Johnson KM (2009) Chemistry, microbiology and biology of water. Chapter 6, water supply, 6th edn. p 195–266. doi: 10.1016/B978-0-7506-6843-9.00014-7
WHO (1993) Guidelines for drinking-water quality, vol 1. Recommendations, 2nd edn. World Health Organization, Geneva
WHO (1997) Guidelines for drinking-water quality, vol 3. Surveillance and control of community water supplies, 2nd edn. World Health Organization, Geneva
WHO (2004) Guidelines for drinking-water quality, 3rd edn. World Health Organization, Geneva
WHO (2013) Water quality and health strategy 2013–2020. http://www.who.int/watersanitation_health/publications/2013/water_quality_strategy/en/index.html
WHO, UNICEF (2010) Progress on drinking water and sanitation: 2010 update. World Health Organization, Geneva
WSSP (2004) Gangtok-Shillong and South Asia Regional, water supply and sanitation program (WSSP) Citizen’s Charter of Water Security & Public Health Engineering Department, 25 July 2004 EEG301-ID05-004
Download references
Author information
Authors and affiliations.
CSIR–National Environmental Engineering Research Institute, Nagpur, 440020, India
G. K. Khadse, P. M. Patni, A. V. Talkhande & P. K. Labhasetwar
You can also search for this author in PubMed Google Scholar
Corresponding author
Correspondence to G. K. Khadse .
Rights and permissions
Reprints and permissions
About this article
Khadse, G.K., Patni, P.M., Talkhande, A.V. et al. Change in drinking water quality from catchment to consumers: a case study. Sustain. Water Resour. Manag. 2 , 453–460 (2016). https://doi.org/10.1007/s40899-016-0069-0
Download citation
Received : 23 July 2015
Accepted : 07 September 2016
Published : 22 September 2016
Issue Date : December 2016
DOI : https://doi.org/10.1007/s40899-016-0069-0
Share this article
Anyone you share the following link with will be able to read this content:
Sorry, a shareable link is not currently available for this article.
Provided by the Springer Nature SharedIt content-sharing initiative
- Surveillance of drinking water quality (SDWQ)
- Water treatment plant (WTP)
- Total coliforms (TC)
- Fecal coliforms (FC)
- Colony-forming units (CFU)
- Permissible limit
- Find a journal
- Publish with us
- Track your research

Senator Portantino Bill Requiring Robust Study of Microplastics in Drinking Water Passes Senate Committee

For Immediate Release: April 18, 2024
Contact: Lerna Shirinian, (818) 409-0400
Sacramento, CA – SB 1147, authored by Senator Anthony J. Portantino (D – Burbank) that would require the study of the health impacts of microplastics in drinking water, passed the Senate Environmental Quality Committee.
“SB 1147 is an important public health measure that will increase the safety of our drinking water,” stated Senator Portantino. “Microplastics have been identified in rain, drinking water, soil, and air. That’s why more research and action is necessary to understand how to deal with their impact on our health.”
In 2018, there was an average of 325 pieces of microplastics identified in a liter of bottled water. Currently, California’s water bottling facilities do not test for microplastics, nor do they have a method to test for microplastics.
SB 1147 requires all water-bottling plants that produces bottled water for sale must provide an annual report the State Department of Public Health’s Food and Drug branch on the levels of microplastics found in the source water. The bill requires the report to be included with the annual water-bottling plant report and, upon request, be made available to consumers.
SB 1147 also requires the Office of Environmental Health Hazard Assessment (OEHHA) to study the health impacts of microplastics in drinking water, including bottled water, and to evaluate and identify a level of microplastics in those types of water that does not pose a significant risk to health. Additionally, the bill requires OEHHA to adopt a standard methodology, requirements for the testing and reporting of microplastics in drinking water, and to adopt a primary drinking water standard for microplastics.
"Research is increasingly showing that the rapid growth of microplastics presents an existential threat to human health and the environment,” Nick Lapis, Director of Advocacy for Californians Against Waste. “This measure builds on the pioneering work of Senator Portantino's SB 1422 and SB 1263 to go beyond testing and planning to actually adopt protective standards for the water we drink, and it can't come fast enough."
SB 1147 is supported by California Environmental Voters, Californians Against Waste, A Voice For Choice Advocacy, and the California State Coalition of the Climate Reality Project.
Senator Portantino has a history of authoring environmentally friendly water bills. In 2018, he authored SB 1422, the California Safe Drinking Water Act. The bill required the State Water Resources Control Board (SWRCB) to adopt requirements for testing and reporting for four years and to adopt a definition of microplastics in drinking water. The same year, the Governor also signed Senator Portantino’s SB 1263, requiring the California Ocean Protection Council (OPC) to adopt a statewide research strategy and identify early actions to reduce microplastic pollution in California’s marine environment.
SB 230 was also authored by Senator Portantino to improve consumer protection by requiring the State Water Board to gather information to understand the public health risk of contaminants in drinking water. It required the State Water Board to build upon its existing work on Constituents of Emerging Concern (CECs) and improve its knowledge of CECs in drinking water by assessing the state of information. The bill authorized the State Water Board to establish a program to ensure a unified, consistent, and science-based approach for assessing the public health and drinking water consequences of CECs, while identifying which CECs warrant further action.
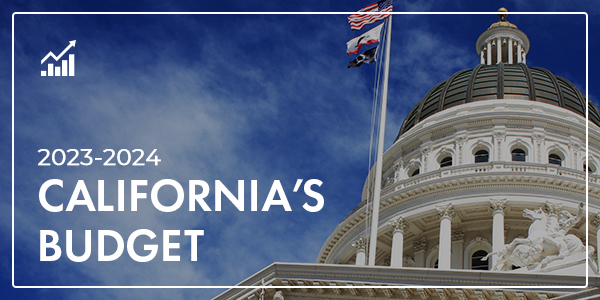
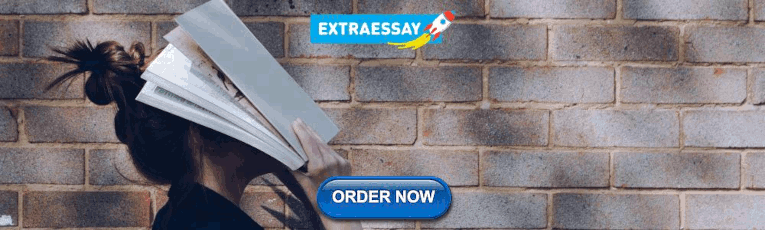
IMAGES
VIDEO
COMMENTS
This study was designed to evaluate a broad spectrum of water-quality constituents of natural water sources and household drinking water used by residents of rural communities in Limpopo Province. We also aimed to determine how water sources and collection practices change between dry and wet seasons within a one-year sampling period.
Using these two measures of poor water quality, we find 2.44% of community water systems, a total of 1165, were Safe Drinking Water Act Serious Violators and 3.37% of Clean Water Act permittees in ...
The investigation reveals that the pH value of surface water was 8.5 which were more than the standard values. The calcium and magnesium content were 398 mg/L and 305.1 mg/L respectively in ground ...
Drinking water quality is regulated in the United States under the Safe Drinking Water Act (SDWA), which establishes national monitoring and reporting requirements and maximum contaminant levels (MCLs) for 88 contaminants. ... Holtby CE, Guernsey JR, Allen AC, VanLeeuwen JA, Allen VM, Gordon RJ. A population-based case-control study of drinking ...
By this study, we compare and contrast the extent to which current state-of-science technology for PWS water quality characterization (Case Study 1) and advanced technologies, HRMS-based wide ...
The WQI classification for drinking water quality is assigned with excellent and good groundwater classes between km 10 to km 60, km 80 to km 95 and the adjacent areas around Ismailia Canal. ... Groundwater quality investigation using water quality index and ARCGIS: case study: Western Nile Delta Aquifer, Egypt. Eighteenth International Water ...
Edokpayi, J. N. et al. Challenges to sustainable safe drinking water: A case study of water quality and use across seasons in rural communities in Limpopo Province, South Africa. Water 10, 159 (2018).
tion levels in water resources. In the present study, modied water quality index (MWQI), integrated water quality index (IWQI), and entropy-weighted water quality index (EWQI) were integrated with statistical analysis for the assessment of drinking water quality in Umunya suburban district, Nigeria. There is no known study that has simultaneously
Drinking water quality assessment is a major issue today, as it is crucial to supply safe drinking water to ensure the well-being of society. Predicting drinking water quality helps strengthen water management and fight water pollution; technologies and practices for drinking water quality assessment are continuously improving; artificial intelligence methods prove their efficiency in this domain.
Consumption of microbial-contaminated water can result in diarrheal illnesses and enteropathy with the heaviest impact upon children below the age of five. We aimed to provide a comprehensive analysis of water quality in a low-resource setting in Limpopo province, South Africa. Surveys were conducted in 405 households in rural communities of Limpopo province to determine their water-use ...
The status of drinking water quality obtained from Wuhan could be generalized to other urbanized areas in China, ... Seasonal and spatial variations of pharmaceuticals and personal care products occurrence and human health risk in drinking water - a case study of China. Sci. Total Environ., 694 (2019), Article 133711, 10.1016/j.scitotenv.2019. ...
The significance of this study lies in the broad characterization of water-quality parameters that could affect human health, which is not restricted to microbiological analysis. In a rural community, the primary concern of drinking water is the microbiological quality of the water and chemical constituents are often considered not as problematic.
community prone to w ater-borne outbreaks. together with detrimental health conditions. This study primarily f ocuses on the. suitability of drinking water quality in. Rajshahi district. The ...
Numerous indicator models have been developed and utilized for the assessment of pollution levels in water resources. In the present study, modified water quality index (MWQI), integrated water quality index (IWQI), and entropy-weighted water quality index (EWQI) were integrated with statistical analysis for the assessment of drinking water quality in Umunya suburban district, Nigeria. There ...
The drinking water supply at Indore was assessed at raw water sources, treatment stages, distribution network and consumer ends. From the present study, it is observed that water supplied through the distribution network is of good physicochemical quality; however, 40-50 % water samples for TC and about 20 % water samples for FC were found positive from consumer ends.
A detailed comparison of the indices also confirmed that the proposed DWQI reduced the eclipsing and sensitivity problems of conventional reputed WQI methodologies and showed the real drinking water quality status with certainty. The methodology developed in the present study clearly improves the Drinking Water Quality Indexing system used to date.
Storage Tank Management to Improve Drinking Water Quality: Case Study. Authors: Vincent Gauthier, Marie-Claude Besner ... Montiel, A., Archenault, M., and Veyrie, A. (1993). "Miniature chlorine micro-sensors for on-line control of drinking water quality in distribution systems." Proc., Water Quality Technol. Conf. Am. Water Works Assoc ...
The aim of the present study is to exhaust the limitations of conventional reputed Water Quality Index (WQI) methodologies through the proposed reliable Drinking Water Quality Indexing (DWQI) system. Slight modifications were carried out in the methodology of the DWQI development which were parameter categorization, development of sub-index with regression statistics and aggregation function ...
Hazard Index (HI): The Hazard Index is a long-established approach that EPA regularly uses to understand health risk from a chemical mixture (i.e., exposure to mul ple chemicals). The HI is made up of a sum of frac ons. Each frac on compares the level of each PFAS measured in the water to the health-based water concentra on.
Understanding drinking water quality is important, given that it is the main factor that determines its suitability for drinking (Wang, 2013; ... Pattern recognition techniques for the evaluation of spatial and temporal variations in water quality. A case study: Suquía River Basin (Cordoba-Argentina) Water. Res., 35 (12) (2001), pp. 2881-2894.
Senator Portantino has a history of authoring environmentally friendly water bills. In 2018, he authored SB 1422, the California Safe Drinking Water Act. The bill required the State Water Resources Control Board (SWRCB) to adopt requirements for testing and reporting for four years and to adopt a definition of microplastics in drinking water.
The sanitary security of drinking water is closely related to human health, but its quality assessment mainly focused on limited types of indicators and relatively restricted time span. The current study was aimed to evaluate the long-term spatial-temporal distribution of municipal drinking water quality and explore the origin of water contamination based on multiple water indicators of 137 ...
Dissolved organic matter (DOM) plays a pivotal role in the biogeochemical cycles of elements and the regulation of forest ecosystem functions. However, studies on the regional and seasonal characteristics of DOM in cold-temperate montane forests are still not comprehensive. In this study, samples of water, soil, and sediment from different sites in the forest drainage basin were collected, and ...