- 0 Shopping Cart
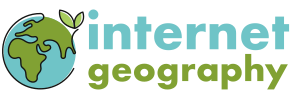
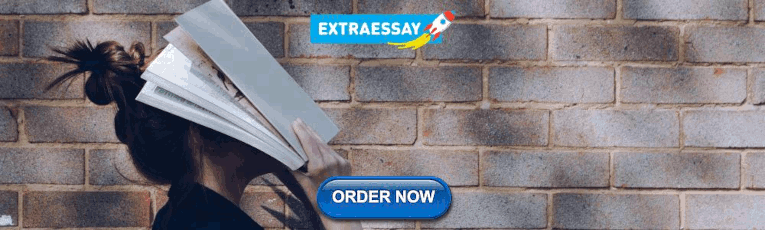
Geography Case Studies
All of our geography case studies in one place
Coastal Erosion
Use the images below to find out more about each case study.
The Holderness Coast
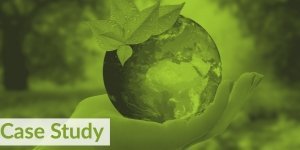
The Dorset Coast
Happisburgh
Coastal Management
Sandscaping at Bacton, Norfolk
Coastal Realignment Donna Nook
Coastal Realignment Medmerry
Coastal Deposition
Spurn Point
Blakeney Point Spit
Earthquakes
Amatrice Earthquake Case Study
Chile Earthquake 2010
Christchurch Earthquake
Haiti Earthquake
Japan Earthquake 2011
L’Aquila Earthquake
Lombok Indonesia Earthquake 2018
Nepal Earthquake 2015
Sulawesi, Indonesia Earthquake and Tsunami 2018
New Zealand 2016
Malaysia Causes of Deforestation
Malaysia Impacts of Deforestation
Alaska Case Study
Epping Forest Case Study
Sahara Desert Case Study
Svalbard Case Study
Thar Desert Case Study
Western Desert Case Study
Energy Resources
Chambamontera Micro-hydro Scheme
Extreme Weather in the UK
Beast from the East Case Study
Storm Ciera Case Study
Food Resources
Almería, Spain: a large-scale agricultural development
The Indus Basin Irrigation System: a large-scale agricultural development
Sustainable food supplies in a LIC – Bangladesh
Sustainable food supplies in a LIC – Makueni, Kenya
Landforms on the River Tees
Landforms on the River Severn
Indus River Basin (CIE)
River Flooding
Jubilee River Flood Management Scheme
Banbury Flood Management Scheme
Boscastle Floods
Kerala Flood 2018
Wainfleet Floods 2019
The Somerset Levels Flood Case Study
UK Floods Case Study November 2019
River Management
The Three Gorges Dam
Mekong River
The Changing Economic World
How can the growth of tourism reduce the development gap? Jamaica Case Study
How can the growth of tourism reduce the development gap? Tunisia Case Study
India Case Study of Development
Nigeria – A NEE
Torr Quarry
Nissan Sunderland
The London Sustainable Industries Park (London SIP)
Tropical Storms
Beast from the East
Hurricane Andrew
Cyclone Eline
Cyclone Idai Case Study
Typhoon Haiyan 2013
Hurricane Irma 2017
Typhoon Jebi 2018
Hurricane Florence 2018
Typhoon Mangkhut 2018
Urban Issues
Birmingham – Edexcel B
Urban Growth in Brazil – Rio de Janeiro
Urban Growth in India – Mumbai
Urban Growth in Nigeria – Lagos
London – A Case Study of a UK City
Inner City Redevelopment – London Docklands
Sustainable Urban Living – Freiburg
Sustainable Urban Living – East Village
Sustainable Urban Transport Bristol Case Study
Bristol – A major UK city
Volcanic Eruptions
Eyjafjallajokull – 2010
Mount Merapi – 2010
Mount Pinatubo – 1991
Sakurajima Case Study
Nyiragongo Case Study
Water Resources
Hitosa, Ethiopia – A local water supply scheme in an LIC
The South-North Water Transfer Project, China
Wakal River Basin Project
Lesotho Large-Scale Water Transfer Scheme
Share this:
- Click to share on Twitter (Opens in new window)
- Click to share on Facebook (Opens in new window)
- Click to share on Pinterest (Opens in new window)
- Click to email a link to a friend (Opens in new window)
- Click to share on WhatsApp (Opens in new window)
- Click to print (Opens in new window)
Please Support Internet Geography
If you've found the resources on this site useful please consider making a secure donation via PayPal to support the development of the site. The site is self-funded and your support is really appreciated.
Search Internet Geography
Top posts and pages.

Latest Blog Entries

Pin It on Pinterest
- Click to share
- Print Friendly
- Find Flashcards
- Why It Works
- Tutors & resellers
- Content partnerships
- Teachers & professors
- Employee training
Brainscape's Knowledge Genome TM
Entrance exams, professional certifications.
- Foreign Languages
- Medical & Nursing
Humanities & Social Studies
Mathematics, health & fitness, business & finance, technology & engineering, food & beverage, random knowledge, see full index.
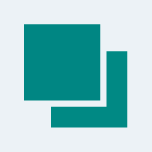
- User generated content
a-level geography case study nile delta-low energy environment
This class was created by Brainscape user esme appleby. Visit their profile to learn more about the creator.
Decks in this class (5)
More about a-level geography case study nile delta-low energy environment.
- Class purpose General learning
Learn faster with Brainscape on your web, iPhone, or Android device. Study esme appleby's A-level Geography case study Nile Delta-low energy environment flashcards for their Redmaids class now!
How studying works.
Brainscape's adaptive web mobile flashcards system will drill you on your weaknesses, using a pattern guaranteed to help you learn more in less time.
Add your own flashcards.
Either request "Edit" access from the author, or make a copy of the class to edit as your own. And you can always create a totally new class of your own too!
What's Brainscape anyway?
Brainscape is a digital flashcards platform where you can find, create, share, and study any subject on the planet.
We use an adaptive study algorithm that is proven to help you learn faster and remember longer....
Looking for something else?
U.s. geography.
- 226 flashcards
- 20,415 learners
World Geography
- 1,050 flashcards
- 265,857 learners
A Level OCR Geography Paper 2 Case St...
- 60 flashcards
Energy - Eduqas A Level Geography
- 218 flashcards
- 13 learners
- Corporate Training
- Teachers & Schools
- Android App
- Help Center
- Law Education
- All Subjects A-Z
- All Certified Classes
- Earn Money!

An official website of the United States government
The .gov means it’s official. Federal government websites often end in .gov or .mil. Before sharing sensitive information, make sure you’re on a federal government site.
The site is secure. The https:// ensures that you are connecting to the official website and that any information you provide is encrypted and transmitted securely.
- Publications
- Account settings
Preview improvements coming to the PMC website in October 2024. Learn More or Try it out now .
- Advanced Search
- Journal List
Investigation of the physical-geographical characteristics of river delta with geophysical and satellite data. The case study of Pineios River, Greece
Georgia s. mitsika.
a National and Kapodistrian University of Athens, Department of Geology and Geoenvironment, Division of Geophysics-Geothermy, Panepistiomioupoli Zografou, Greece
John D. Alexopoulos
Emmanuel vassilakis.
b National and Kapodistrian University of Athens, Department of Geology and Geoenvironment, Division of Geography-Climatology, Panepistiomioupoli Zografou, Greece
Spyridon Dilalos
Serafim e. poulos, associated data.
- Data will be made available on request.
This paper presents the methodology of an applied geophysical and remote sensing research at river deltas for their subsurface and surface structure and its application in the deltaic plain of Pineios River (Thessaly, Greece). The scope is to primarily calculate the thickness of deltaic post alpine deposits, with the contribution of Transient Electromagnetic Method (TEM), but also identify the surficial characteristics (biotic/ abiotic) of the area with the appropriate combination of spectral bands and spectral indices. Regarding the remote sensing approach, some geomorphological features were outlined. This was managed from six false color composites of the area, produced by combining spectral bands and additional five false color composites by combining several spectral indices.
- • The results of the TEM method along with their statistical analysis provided important information regarding the spatial distribution and thickness of the lithological units along the deltaic plain.
- • The combination of spectral bands 8, 7, 6 seems to adumbrate the hydrographic network of the area and even detects possible changes in the inflow.
- • The combination of spectral indices MSAVI2, WV-BI, WV-WI was helpful enough to geomorphological mapping of the deltaic plain.
Graphical abstarct

Specifications table
Method details
Introduction
More than half a billion people live on or close to deltas, often in large cities [1] , [2] . There are numerous advantages for societal development established by the existence of Deltas, such as a smooth topography, available fresh resources, good transportation via waterways and the nearby coast, productive soils for agriculture, and a valuable biodiversity and recreational value through, such as beaches, wetland ecosystems and coastal forests [3] . Potential threats such as sea level rise and subsidence come from both environmental change but also human intervention [2] . Taking into account that sea level rise, sand depletion, groundwater pumping and human pressure on delta land are expected to increase, conventional approaches to control delta landscapes may become unsustainable [4] . The determination of the subsurface geological structure is crucial for modeling the paleo-relief where the river delta has been created on, but also allows the delineation of the river's flow paths [5] .
Remote sensing techniques in combination with field data and Geographic Information Systems (GIS) have been used in various agricultural applications or research [6] , [7] , [8] , [9] . With the accessibility of global remote sensing databases, the physical attributes of deltaic systems are becoming increasingly better quantified [10] , [11] , [12] , [13] , [14] . Geophysical surveys have been conducted in many deltaic regions and coastal zones around the world [15] , [16] , [17] , [18] , [19] , [20] , [21] , [22] .
Regarding the presented case study of Pineios river, the authors have already discussed the results and the interpretation from the application of several geophysical studies, including the Vertical Electrical Soundings (VES), the Transient Electromagnetic Soundings (TEM) and gravity measurements [23] , [24] , [25] , [26] , [27] .
Pineios River is the largest Greek river with its drainage basin within the Greek borders. It is also the only river in Greece with reduced flow control (in only less than 10% of its catchment) [28] . Its delta has been recognized as an environmentally sensitive coastal zone protected by the NATURA 2000 network (RAMSAR convention). It is characterized by great fresh surface water availability supporting a valuable biodiversity of fauna and flora [29] . It includes an estuary with riparian woodland, riparian forests marshes, freshwater lakes, coastal zones and sand dunes. The delta of Pineios River is characterized by the expanding touristic exploitation of the coastline and the extensive agricultural cultivation [30] , [31] .
The relief of the deltaic plain is flat and incorporates meanders, radial riverbeds and isolated parts of old riverbeds, which function mainly during flood events, revealing the continuous geomorphological evolution of the plain over the years [32] . In Fig. 1 , the coverage of the largest part of the deltaic plain by alluvial sediments is obvious, while sand dunes and coastal sediments exist along coastal areas. The northern (Olympus Mt.) and western (Pyrgetos alluvial fan) boundaries of the deltaic plain comprise Neogene deposits (conglomerates and marls) and Pleistocene formations (screes, debris cones and fluvial terraces). The southern margin (Ossa Mt.) is surrounded by metamorphic rocks, such as dolomites, crystalline limestones, marbles, shales, gneisses, blue schists and ophiolites [33] . Based on the authors of [34] the Neogene formations have undergone severe tectonic deformation leading to several block rotations with values of 10–30 ο , towards south. The NW dipping normal fault zone located south of Omolio settlement strikes WSW-ENE and appears to play a substantial role in the rotation of the Neogene formations.

Geological map of the study area, modified by [33] , along with the location of the Transient Electromagnetic soundings and boreholes of [35] .
From a hydrogeological aspect, groundwater occurs in the alluvial sediments of the deltaic plain of Pineios River, which based on [25] , is not identified throughout the entire deltaic basin, but only at its central and northern parts. Below the alluvial sediments, a clay formation occurs, as revealed from wells logs, along with a deeper confined aquifer consisting mainly by conglomerates. Therefore, two major aquifer systems are defined: the phreatic and the confined deep aquifer.
Methodology
Our investigation along the deltaic plain of Pineios River is comprised of two parts, the subsurface investigation with geophysical methods and the surficial one, with the contribution of satellite image processing. The coordinate system used in all data is the Greek Geodetic Reference System 1987 (GGRS87/Greek Grid).
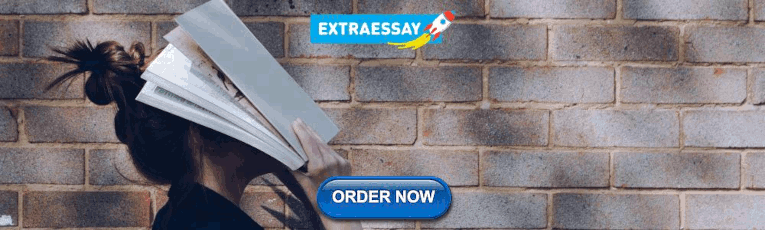
Geophysical survey for subsurface investigation
A geophysical survey has been conducted along the deltaic area of Pineios River for the delineation of the subsurface regime and hydrogeological characteristics. In this paper, we will deal with the results of the Transient Electromagnetic Method (TEM) that has been carried out in the area along with other geophysical methods in previous studies, such as Vertical Electrical Soundings (VES) and gravity measurements. The main objective of this geophysical survey was to calculate the thickness of the deltaic deposits followed by the deposition of the transgressive sediments.
The Transient Electromagnetic Method (TEM) has been used for hydrogeological applications [36] , [37] , [38] and for the determination of the subsurface geological structure as well [39] , [40] , [41] . Over the last decades the TEM method has become increasingly popular for environmental and groundwater investigation [42] , [43] . TEM is a method highly sensitive to low-resistivity layers, successfully applied in the past by other researchers, especially for mapping coastal aquifers and seawater intrusion [44] , [45] .
A total of 127 TEM soundings ( Fig.1 ) were acquired across the entire deltaic valley. The TEM measurements were acquired with ABEM's WalkTEM instrument. The field configuration for data acquisition was comprised of a 40 m x 40 m square transmitter loop with two in-loop antennas, the RC-200 (10 m x 10 m) and the RC-5 (0.5 m x 0.5 m) each with different receiver areas. In Fig. 2 the described configuration array deployed in the field is presented.

The set-up of in-loop field configuration (left) and a sounding location during data acquisition.
The processing of the TEM soundings and their inversion were carried out with ViewTEM software leading to their corresponding multilayer resistivity models. Each multi-layer resistivity model was transformed into a few-layer resistivity model. An example of this procedure is presented in Fig. 3 , where the ‘Resistivity– Elevation’ chart is illustrated, presenting the same TEM sounding in both ways; as a multi-layer resistivity model and as a four-layer geophysical model. The layers’ boundaries and resistivity values are given in Table 1 .

The resistivities calculated for the same TEM sounding as a multi-layer model (blue color) and as a four-layer model (red color).
Geophysical layers extracted from the four-layer resistivity model of the above TEM sounding.
The geological interpretation of the geophysical data was achieved calibrated with available drilling data ( Fig. 1 ) from [27] . Beyond that, the interpretation of the TEM soundings, was also cross-checked based on the published results of the geoelectrical method carried out by the authors [23] , [24] , [25] , [26] but also with the recently published results by [28] regarding the investigation of the deeper geological and tectonic structure of the deltaic plain by gravity measurements.
The surface lithology in the deltaic plain that is structured by alluvial deposits or sand dunes (at the coastal area) ( Fig. 1 ) have been investigated with resistivity values ranging between 17 and 45 Ohm.m and 66–120 Ohm.m respectively. The underlaying lithological layer, observed throughout the study area, corresponds to an impermeable clay formation, with resistivity of 1–7 Ohm.m. These two lithological units comprise the deltaic deposits. The third lithological unit, investigated by almost all TEM soundings (114/127 soundings), is consisted mainly of sands and gravels with small amount of clay and represents the transgressive deposits of the last interglacial period and is characterized by resistivity values of 10–30 Ohm.m. In many TEM soundings, an additional layer was also investigated, corresponding either to the Neogene formations that appear west and northwest of the deltaic plain, or the roof of the alpine basement (Blue schists or Crystalline Limestones).
Satellite image processing for surficial investigation
A very high resolution WordlView-2 image acquired on 27/07/2013 has been processed. The 8-band multispectral imagery was at 2 m resolution and the panchromatic imagery was at 50 cm resolution. The high spatial resolution enables the discrimination of fine details and the high spectral resolution provides detailed information on such diverse areas. The processing of WorldView-2 satellite images was performed with the ERDAS Imagine 2014 software, as well as the calculation of ten spectral indices ( Table 2 ).
The Spectral Indices calculated for the study area.
The objective of the surface investigation was to identify the appropriate combination of spectral bands / spectral indices to highlight various biotic / abiotic characteristics of the delta. As a result, six false color composites of the area were produced combining spectral bands and additional five false color composites by combining several spectral indices. The spectral band and spectral indices combinations used in this study along with their benefits are given in Tables 3 and and4 4 respectively.
The benefits of the six false color composites of the area that were produced combining spectral bands.
The benefits of the five false color composites of the area that were produced combining spectral indices.
In Fig. 4 we can clearly distinguish the different land uses in this area of the delta where Pineios River exits Tempi valley. The combination used is spectral bands 8, 7, 6. The roads, railway, settlements, bare soils and rocky areas appear in blue and grayish shades, while yellow, orange and red colors represent different types of vegetation and crops. Particularly, we can note the contrast presented between the riparian vegetation and the surrounding area, an element that delineates the hydrographic network. The river appears dark blue and black.

False color composite by the combination of spectral bands 8, 7, 6. This combination highlights the drainage network due to the riparian vegetation (yellow) while built-up areas and bare soils appear blue.
The combination of spectral indices MSAVI2, WV-BI, and WV-WI is shown in Fig. 5 . This is a very detailed imagery of the deltaic plain north of Stomio settlement. Vegetation appears in red color while built-up areas and roads in blue. The beach along with the sand dunes and the beach ridges with orientation NE-SW, are very well defined.

False color composite by the combination of spectral indices MSAVI2, WV-BI, and WV-WI. The beach is clearly distinguished from other bare soils. The beach ridges and sand dunes appear very detailed.
Results and discussion
Our main interest of the applied methodology and this paper is the deltaic deposits. Taking into consideration the elevation of each TEM station and its model, the absolute heights of the top and the base of the alluvial deposits were calculated individually, as well as the point thickness of the deposits. The same procedure was also followed for the clay formation.
The map in Fig. 6 shows the spatial distribution of the thickness for the alluvial deposits along the delta plain. The formation has an average thickness of 11.1 m, the mean value was calculated 9.5 and the standard deviation equal to 6.28. The minimum value is 1.3 m and the maximum value is 28 m. Based on the standard deviation and the mean value calculated for the alluvial deposits, we categorized their spatial distribution along the deltaic area in three zones/classes (yellow, orange and red). These 3 classes are the following: i) ≤ 4.86 m ii) 4.86 - 17.4 m iii) ≥ 17.4 m. The first (yellow areas) and third (red area) classes include values of thickness beyond a standard deviation from the mean value. The highest values of thickness (greater than 15 m) of the alluvial deposits are located in the areas west of Kouloura settlement and southwest of Palaiopyrgos settlement (red zone). Respectively, the smallest values of thickness (less than 5 m) can be observed north and southeast (yellow zone). The alluvial deposits covering the rest of the deltaic area have been identified with thickness ranging between 4.86 and 17.4 m (orange zone).

Map presenting the distribution of the thickness of the alluvial deposits in the investigation area. The classes’ limits were calculated based on the standard deviation and mean values of the formation.
In addition, a statistical analysis of these data was performed for the calculation of important characteristics, but also a short and clear presentation that will enhance the spatial presentation of the results. The Natural Neighbor spatial interpolation method was used to construct the raster data.
The Histogram Graph of Fig. 7 refers to the values calculated for the thickness of the Clay Formation throughout the study area. We observe that the formation has an average thickness of 31.8 m, the median was calculated 31 and the standard deviation equal to 9. The minimum value is 13 m and the maximum 52 m. Regarding the spatial distribution of the thickness of Clay Formation in the deltaic plain ( Fig. 8 ), the highest values are observed throughout the central and eastern part of Pineios River deltaic plain. The formation exceeds 45 m of thickness in the area south and east of Kouloura settlement.

The results of the statistical analysis performed for the Clay Formation.

Map presenting the distribution of the thickness of the clay formations in the investigation area. The classes’ limits were calculated based on the standard deviation and mean values of the formation.
Based on the values of the standard deviation and the mean value for Clay Formation, TEM soundings were again classified in the following 3 classes of thickness: i) ≤ 22.8 m, ii) 22.8 - 40.8 m iii) ≥ 40.8 m. The map in Fig. 8 shows the result of this classification spatially distributed along the deltaic plain. The first (yellow areas) and third class (red areas) include values beyond a standard deviation from the mean value. Small values are limited mainly in the southern part of the deltaic plain, parallel to the slopes of Ossa Mt. but also in the western part of the deltaic plain, in the area north of Omolio settlement. A small appearance of values of the first class is found in the north, in Paliomana settlement. Values of the third class occupy the area of the present estuary and inland the settlement of Kouloura.
The ratio ‘Thickness of Clay Formation / Thickness of Alluvium Deposits’ was also calculated in order to compare the distribution of thickness of the two formations appearing in the study area. The minimum value of the ratio is 0.74 and the maximum 14.2. The average value of the ratio is 4.04, the median 3.12 and the standard deviation 2.71. The map in Fig. 9 presents the spatial distribution of the ‘Thickness of Clay Formation / Thickness of Alluvial Deposits’ ratio along the deltaic plain. We observe that the ratio gets its maximum values in the areas of the present and past estuary (north of Stomio settlement) as well as further north in the area of Paliomana settlement. We can also notice one sounding location with high ratio value north of Omolio settlement.

Distribution of the values of the ratio ‘Thickness of Clay Formation / Thickness of Alluvial Deposits’.
Conclusions
In general, the applied methodology provided valuable data for the subsurface structure of a deltaic plain along with important information regarding its surficial characteristics. The statistical analysis of the geophysical interpretation can provide even better understanding of the river delta structure and can contribute to the understanding of the physical-geographical characteristics of a river delta area.
Regarding the results and the interpretation of the geophysical survey, new information regarding the subsurface structure has been obtained. The average thickness of the Alluvial deposits’ formation has been estimated to 11 m and is hosting the shallow unconfined aquifer. These deposits were formed during the growth of Pineios River Delta that began 4–5.000 years BP. Regarding the Clay formation, which is an impermeable formation, its average thickness has been calculated equal to 32 m. Basically, it represents the pro-deltaic fine-grained deposits of the growing delta during the Upper Holocene. Finally, the transgressive deposits which are consisted mainly of sands and gravels with small amount of clay, host an aquifer, artesian in places, and overlays the Neogene formations which can be considered as the paleosurface during the last glacial period (sea level was 120–130 m below present sea level).
The statistical analysis of the thickness of the lithological units of the deltaic area and their ratio thickness, also revealed some important results. More specifically, the greater values of thickness of the Alluvial deposits are limited to the central part of the deltaic plain and can be associated with the frequent flooding phenomena that are being observed in this area. On the other hand, the smallest thickness of this formation is located i) in the area north in the deltaic plain, where many meanders and isolated parts of old riverbeds are present, ii) in the area of the present estuary and iii) in the old estuary area, north of the Stomio settlement. Regarding the clay formation, small thickness values mainly appear i) in the southern part of the deltaic plain near Ossa Mt.’s slopes, ii) in the western part in the deltaic plain north of the settlement of Omolio and iii) in the north in Paliomana settlement. On the other hand, the high values of clay thickness appear i) in the eastern part of the Pineios Delta, ii) at the present estuary area and iii) inland, around Kouloura settlement. This seems to be consistent with the interpretation of the marine acoustic survey conducted in the area [52] , which has estimated the thickness of the deltaic deposits in the central part of the Pineios Delta up to-35 m.
The Clay Formation Thickness/Alluvial deposits Thickness ratio gets its maximum values in the areas where the fluvial processes dominate through the mainstream or the past tributaries. These locations are: i) the area of the present estuary, ii) the old estuaries (Stomio settlement) and iii) further north in the area with the appearance of the large number of meanders. Finally, one TEM sounding, remote from the areas just indicated, that appears with high ratio value (north of Omolio settlement) coincides with the location where Pineios River changes abruptly two times its direction of flow .
Regarding the surface investigation of the area, we came up with some conclusions, based on the results of the combinations of the spectral bands and indices that we applied. More specifically, we observed that the combination of spectral bands 8, 7, 6 can be used to clearly distinguish the land uses with the high degree of contrast between vegetation, water, and built-up features. The vegetation is colored yellow and orange while the bare soils, rocky areas and built-up features appear blue and gray, something that greatly outlines the hydrographic network of the area as a result of the intense difference of the riparian vegetation. This can be very useful in monitoring the whole basin area and detecting possible changes in the inflow, like stream piracy that can change the routing of water and sediment and may have an effect on landscape evolution. Moreover, the combination of spectral indices MSAVI2, WV-BI, WV-WI can be very helpful to geomorphological mapping of the deltaic plain, given the fact that many features such as the beach ridges, sand dunes and the part of the sandy beach appear very detailed with this combination of spectral indices.
CRediT authorship contribution statement
Georgia S. Mitsika: Methodology, Validation, Formal analysis, Investigation, Data curation, Writing – original draft, Writing – review & editing, Visualization. John D. Alexopoulos: Conceptualization, Methodology, Validation, Formal analysis, Investigation, Resources, Data curation, Writing – review & editing, Supervision, Project administration. Emmanuel Vassilakis: Conceptualization, Methodology, Resources, Data curation, Writing – review & editing, Supervision. Spyridon Dilalos: Conceptualization, Methodology, Validation, Formal analysis, Investigation, Data curation, Writing – original draft, Writing – review & editing. Serafim E. Poulos: Conceptualization, Methodology, Resources, Writing – review & editing, Supervision, Project administration, Funding acquisition.
Declaration of Competing Interest
The authors declare that they have no known competing financial interests or personal relationships that could have appeared to influence the work reported in this paper.
Acknowledgments
A significant part of this work was financially supported by the project AGROCLIMA (11SYN_3_1913) that is funded by the Action “Cooperation 2011–2015″ of the Operational Program "Competitiveness and Entrepreneurship” co-funded by the European Regional Development Fund (ERDF) and the General Secretariat for Research and Technology (Hellenic Ministry of Education) and project DAPHNE (MIS: 375908), which was co-funded by Greece and the European Union through the O. P. “Education and lifelong learning, 2007–2013″ of the Ministry of Education and Religious Affairs. The authors would also like to thank Mr. Mavroulis S., Ms. Kaplanidi H., Ms. Kalaboki E., Mr Farangitakis P., Mr. Bazionis G. and Mr. Andreadis O. for their valuable contribution to the field survey.
Related research article: Dilalos S., Alexopoulos J.D., Vassilakis Em., Poulos S.E. (2022). Investigation of the structural control of a deltaic valley with geophysical methods. The case study of Pineios river delta (Thessaly, Greece), Journal of Applied Geophysics, 202, 104652, https://doi.org/10.1016/j.jappgeo.2022.104652
Data Availability

- Action (WP)
- Case studies
- Local Authorities

Select your language
The Rhone river delta - France
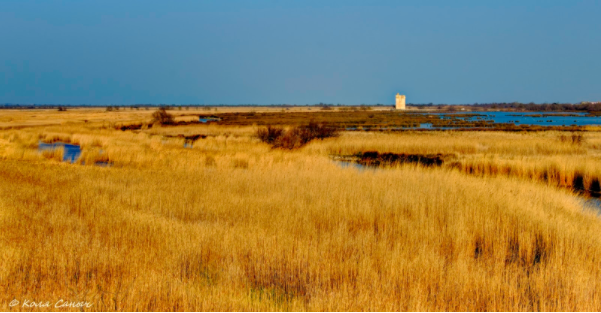
With an area of over 930 km 2 , the Rhone river mouth (southern France) is the largest river delta of the western Mediterranean . This zone, known as Camargue, is a natural park characterized by a vast plain facing the Mediterranean Sea, where outflow the two main arms of the Rhone river named the Grand Rhône (in the eastern side) and the Petit Rhône (in the western side). A large brackish lagoon is separated from the sea by sandbars and encircled by reed-covered marshes, surrounded by large cultivated areas. The well-known historical city of Arles, famous for the roman age buildings, is the main settlement of this region. Along the coast are the small maritime villages of Saintes-Maries-de-la-Mer, Le Grau-du-Roi, Aigues-Mortes and Port-Saint-Louis-du-Rhône, among the others, which are highly exposed to erosion and sea storms.
Observed phenomena associated with Sea Level Rise (SLR)
The formation of the Rhone river delta developed during the last 7000 years in response to sea-level rise, after the last glacial maximum of 20.000 years ago. The geomorphological features of this area are associated with the evolution of the delta and land subsidence . The latter is associated with the compaction of soft sediments transported by the river. This area is often subjected to floods because it is placed at very low elevation above the sea level and land is poorly sloping toward the sea, thus prone to river overflows in case of heavy rains . In addition, it is very sensible to Sea Level Rise because the most part of this area is lying at less than 1 m above the present mean sea level or even at the mean or slightly below the sea-level.
Effects and open questions
During the last decades were built breakwaters and barriers to protect the coast against frequent storms that caused flooding and coastal retreat. Due to marine erosion and land subsidence, these structures require frequent maintenance and restoration works, especially after extreme events. For its features, this zone is highly prone to be flooded by sea level rise by 2100 in particular with land subsidence, which is amplifying the effects of beach retreat and erosion.
Thank you for visiting nature.com. You are using a browser version with limited support for CSS. To obtain the best experience, we recommend you use a more up to date browser (or turn off compatibility mode in Internet Explorer). In the meantime, to ensure continued support, we are displaying the site without styles and JavaScript.
- View all journals
- My Account Login
- Explore content
- About the journal
- Publish with us
- Sign up for alerts
- Open access
- Published: 28 March 2024
New water accounting reveals why the Colorado River no longer reaches the sea
- Brian D. Richter ORCID: orcid.org/0000-0001-7216-1397 1 , 2 ,
- Gambhir Lamsal ORCID: orcid.org/0000-0002-2593-8949 3 ,
- Landon Marston ORCID: orcid.org/0000-0001-9116-1691 3 ,
- Sameer Dhakal ORCID: orcid.org/0000-0003-4941-1559 3 ,
- Laljeet Singh Sangha ORCID: orcid.org/0000-0002-0986-1785 4 ,
- Richard R. Rushforth 4 ,
- Dongyang Wei ORCID: orcid.org/0000-0003-0384-4340 5 ,
- Benjamin L. Ruddell 4 ,
- Kyle Frankel Davis ORCID: orcid.org/0000-0003-4504-1407 5 , 6 ,
- Astrid Hernandez-Cruz ORCID: orcid.org/0000-0003-0776-5105 7 ,
- Samuel Sandoval-Solis 8 &
- John C. Schmidt 9
Communications Earth & Environment volume 5 , Article number: 134 ( 2024 ) Cite this article
8461 Accesses
728 Altmetric
Metrics details
- Water resources
Persistent overuse of water supplies from the Colorado River during recent decades has substantially depleted large storage reservoirs and triggered mandatory cutbacks in water use. The river holds critical importance to more than 40 million people and more than two million hectares of cropland. Therefore, a full accounting of where the river’s water goes en route to its delta is necessary. Detailed knowledge of how and where the river’s water is used can aid design of strategies and plans for bringing water use into balance with available supplies. Here we apply authoritative primary data sources and modeled crop and riparian/wetland evapotranspiration estimates to compile a water budget based on average consumptive water use during 2000–2019. Overall water consumption includes both direct human uses in the municipal, commercial, industrial, and agricultural sectors, as well as indirect water losses to reservoir evaporation and water consumed through riparian/wetland evapotranspiration. Irrigated agriculture is responsible for 74% of direct human uses and 52% of overall water consumption. Water consumed for agriculture amounts to three times all other direct uses combined. Cattle feed crops including alfalfa and other grass hays account for 46% of all direct water consumption.
Similar content being viewed by others
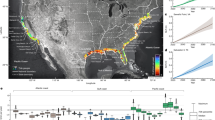
Disappearing cities on US coasts
Leonard O. Ohenhen, Manoochehr Shirzaei, … Robert J. Nicholls
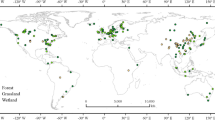
Meta-analysis shows the impacts of ecological restoration on greenhouse gas emissions
Tiehu He, Weixin Ding, … Quanfa Zhang
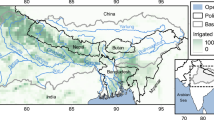
Irrigation-driven groundwater depletion in the Ganges-Brahmaputra basin decreases the streamflow in the Bay of Bengal
Fadji Z. Maina, Augusto Getirana, … Ravi Appana
Introduction
Barely a trickle of water is left of the iconic Colorado River of the American Southwest as it approaches its outlet in the Gulf of California in Mexico after watering many cities and farms along its 2330-kilometer course. There were a few years in the 1980s in which enormous snowfall in the Rocky Mountains produced a deluge of spring snowmelt runoff capable of escaping full capture for human uses, but for most of the past 60 years the river’s water has been fully consumed before reaching its delta 1 , 2 . In fact, the river was overconsumed (i.e., total annual water consumption exceeding runoff supplies) in 16 of 21 years during 2000–2020 3 , requiring large withdrawals of water stored in Lake Mead and Lake Powell to accommodate the deficits. An average annual overdraft of 10% during this period 2 caused these reservoirs– the two largest in the US – to drop to three-quarters empty by the end of 2022 4 , triggering urgent policy decisions on where to cut consumption.
Despite the river’s importance to more than 40 million people and more than two million hectares (>5 million acres) of cropland—producing most of the vegetable produce for American and Canadian plates in wintertime and also feeding many additional people worldwide via exports—a full sectoral and crop-specific accounting of where all that water goes en route to its delta has never been attempted, until now. Detailed knowledge of how and where the river’s water is used can aid design of strategies and plans for bringing water use into balance with available supplies.
There are interesting historical reasons to explain why this full water budget accounting has not been accomplished previously, beginning a full century ago when the apportionment of rights to use the river’s water within the United States was inscribed into the Colorado River Compact of 1922 5 . That Compact was ambiguous and confusing in its allocation of water inflowing to the Colorado River from the Gila River basin in New Mexico and Arizona 6 , even though it accounts for 24% of the drainage area of the Colorado River Basin (Fig. 1 ). Because of intense disagreements over the rights to the Gila and other tributaries entering the Colorado River downstream of the Grand Canyon, the Compact negotiators decided to leave the allocation of those waters rights to a later time so that the Compact could proceed 6 . Arizona’s formal rights to the Gila and other Arizona tributaries were finally affirmed in a US Supreme Court decision in 1963 that also specified the volumes of Colorado River water allocated to California, Arizona, and Nevada 7 . Because the rights to the Gila’s waters lie outside of the Compact allocations, the Gila has not been included in formal accounting of the Colorado River Basin water budget to date 8 . Additionally, the Compact did not specify how much water Mexico—at the river’s downstream end—should receive. Mexico’s share of the river was not formalized until 22 years later, in the 1944 international treaty on “Utilization of the Waters of the Colorado and Tijuana Rivers and of the Rio Grande” (1944 Water Treaty) 9 . As a result of these political circumstances, full accounting for direct water consumption at the sectoral level—in which water use is accounted according to categories such as municipal, industrial, commercial, or agricultural uses—has not previously been compiled for the Gila River basin’s water, and sectoral accounting for Mexico was not published until 2023 10 .
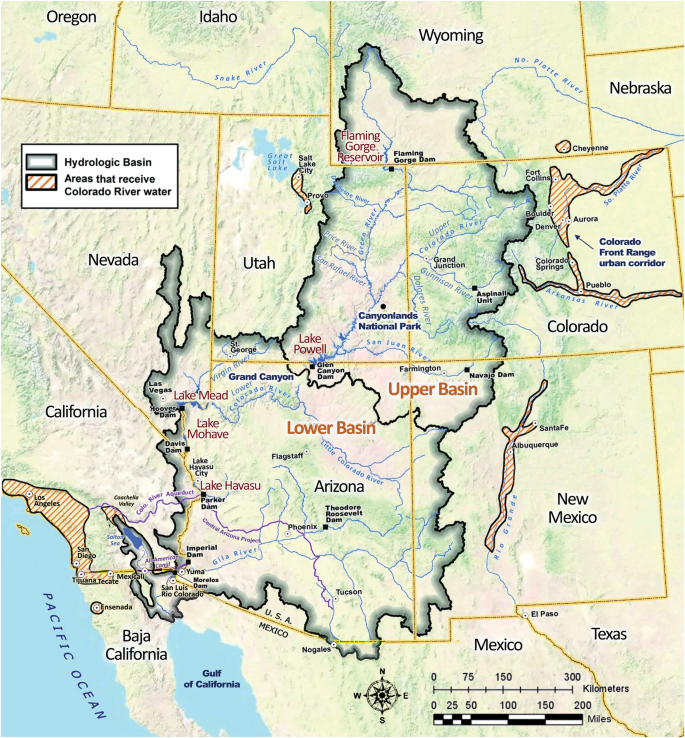
The physical boundary of the Colorado River Basin is outlined in black. Hatched areas outside of the basin boundary receive Colorado River water via inter-basin transfers (also known as ‘exports’). The Gila River basin is situated in the far southern portion of the CRB in Arizona, New Mexico, and Mexico. Map courtesy of Center for Colorado River Studies, Utah State University.
The US Bureau of Reclamation (“Reclamation”)—which owns and operates massive water infrastructure in the Colorado River Basin—has served as the primary accountant of Colorado River water. In 2012, the agency produced a “Colorado River Basin Water Supply and Demand Study” 8 that accounted for both the sectoral uses of water within the basin’s physical boundaries within the US as well as river water exported outside of the basin (Fig. 1 ). But Reclamation did not attempt to account for water generated from the Gila River basin because of that sub-basin’s exclusion from the Colorado River Compact, and it did not attempt to explain how water crossing the border into Mexico is used. The agency estimated riparian vegetation evapotranspiration for the lower Colorado River but not the remainder of the extensive river system. Richter et al. 11 published a water budget for the Colorado River that included sectoral and crop-specific water consumption but it too did not include water used in Mexico, nor reservoir evaporation or riparian evapotranspiration, and it did not account for water exported outside of the Colorado River Basin’s physical boundary as illustrated in Fig. 1 . Given that nearly one-fifth (19%) of the river’s water is exported from the basin or used in Mexico, and that the Gila is a major tributary to the Colorado, this incomplete accounting has led to inaccuracies and misinterpretations of “where the Colorado River’s water goes” and has created uncertainty in discussions based on the numbers. This paper provides fuller accounting of the fate of all river water during 2000–2019, including averaged annual consumption in each of the sub-basins including exports, consumption in major sectors of the economy, consumption in the production of specific types of crops, and water consumed by reservoir evaporation and riparian/wetland evapotranspiration.
Rising awareness of water overuse and prolonged drought has driven intensifying dialog among the seven US states sharing the basin’s waters as well as between the United States, Mexico, and 30 tribal nations within the US. Since 2000, six legal agreements affecting the US states and two international agreements with Mexico have had the effect of reducing water use from the Colorado River 7 :
In 2001, the US Secretary of the Interior issued a set of “Interim Surplus Guidelines” to reduce California’s water use by 14% to bring the state within its allocation as determined in the 1963 US Supreme Court case mentioned previously. A subsequent “Quantification Settlement Agreement” executed in 2003 spelled out details about how California was going to achieve the targeted reduction.
In 2007, the US Secretary of the Interior adopted a set of “Colorado River Interim Guidelines for Lower Basin Shortages and the Coordinated Operations for Lake Powell and Lake Mead” that reduced water deliveries to Arizona and Nevada when Lake Mead drops to specified levels, with increasing cutbacks as levels decline.
In 2012, the US and Mexican federal governments signed an addendum to the 1944 Water Treaty known as Minute 319 that reduced deliveries to Mexico as Lake Mead elevations fall.
In 2017, the US and Mexican federal governments established a “Binational Water Scarcity Contingency Plan” as part of Minute 323 that provides for deeper cuts in deliveries to Mexico under specified low reservoir elevations in Lake Mead.i
In 2019, the three Lower Basin states and the US Secretary of the Interior agreed to commitments under the “Lower Basin Drought Contingency Plan” that further reduced water deliveries beyond the levels set in 2007 and added specifications for deeper cuts as Lake Mead drops to levels lower than anticipated in the 2007 Guidelines.
In 2023, the states of California, Arizona and Nevada committed to further reductions in water use through the year 2026 12 .
With each of the above agreements, overall water consumption has been reduced but many scientists assert that these reductions still fall substantially short of balancing consumptive use with 21st century water supplies 2 , 13 . With all of these agreements—excepting the Interim Surplus Guidelines of 2001—set to expire in 2026, management of the Colorado River’s binational water supply is now at a crucial point, emphasizing the need for comprehensive water budget accounting.
Our tabulation of the Colorado River’s full water consumption budget (Table 1 ) provides accounting for all direct human uses of water as either agricultural or MCI (municipal, commercial, industrial), as well as indirect losses of water to reservoir evaporation and evapotranspiration from riparian or wetland vegetation including in the Salton Sea and in a wetland in Mexico (Cienega de Santa Clara) that receives agricultural return flows from irrigated areas in Arizona. We explicitly note that all estimates represent consumptive use , resulting from the subtraction of return flows from total water withdrawals. Table 2 provides a summary based only on direct human uses and does not include indirect consumption of water. We have provided Tables 1 and 2 in English units in our Supplementary Information as Tables SI-1 and SI-2 . We have lumped municipal, commercial, and industrial (MCI) uses together because these sub-categories of consumption are not consistently differentiated within official water delivery data for cities utilizing Colorado River water. More detail on urban water use by cities dependent on the river is available in Richter 14 , among other studies.
We differentiated water consumption geographically using the ‘accounting units’ mapped in Fig. 2 , which are based on the Colorado River Basin map as revised by Schmidt 15 ; importantly, these accounting units align spatially with Reclamation’s accounting systems for the Upper Basin and Lower Basin as described in our Methods, thereby enabling readers accustomed to Reclamation’s water-use reports to easily comprehend our accounting. We have also accounted for all water consumed within the Colorado River Basin boundaries as well as water exported via inter-basin transfers. Water exported outside of the basin includes 47 individual inter-basin transfer systems (i.e., canals, pipelines, pumps) that in aggregate export ~12% of the river’s water. We note that the Imperial Irrigation District of southern California is often counted as a recipient of exported water, but we have followed the rationale of Schmidt 15 by including it as an interior part of the Lower Basin even though it receives its Colorado River water via the All American Canal (Fig. 2 ).
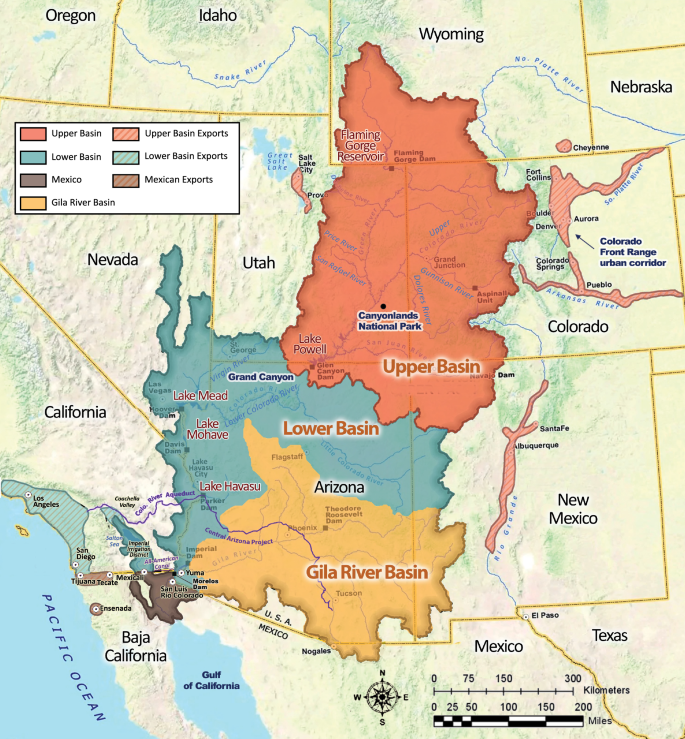
The water budget estimates presented in Tables 1 and 2 are summarized for each of the seven “accounting units” displayed here.
These results confirm previous findings that irrigated agriculture is the dominant consumer of Colorado River water. Irrigated agriculture accounts for 52% of overall consumption (Table 1 ; Figs. 3 and 4 ) and 74% of direct human consumption (Table 2 ) of water from the Colorado River Basin. As highlighted in Richter et al. 11 , cattle-feed crops (alfalfa and other hay) are the dominant water-consuming crops dependent upon irrigation water from the basin (Tables 1 and 2 ; Figs. 3 and 4 ). Those crops account for 32% of all water consumed from the basin, 46% of all direct water consumption, and 62% of all agricultural water consumed (Table 1 ; Fig. 3 ). The percentage of water consumed by irrigated crops is greatest in Mexico, where they account for 86% of all direct human uses (Table 2 ) and 80% of total water consumed (Table 1 ). Cattle-feed crops consume 90% of all water used by irrigated agriculture within the Upper Basin, where the consumed volume associated with these cattle-feed crops amounts to more than three times what is consumed for municipal, commercial, or industrial uses combined.
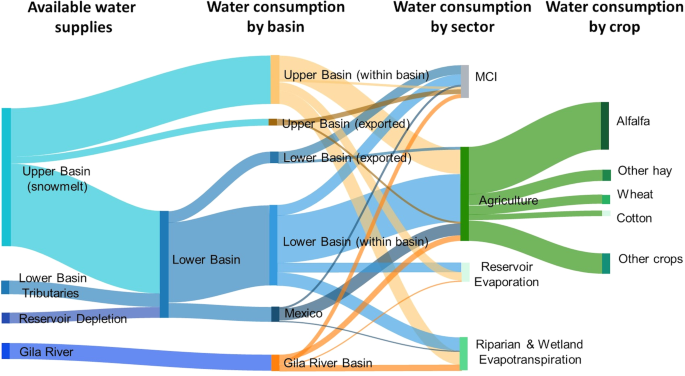
All estimates based on 2000–2019 averages. Both agriculture and MCI (municipal, commercial, and industrial) uses are herein referred to as “direct human uses.” “Indirect uses” include both reservoir evaporation as well as evapotranspiration by riparian/wetland vegetation.
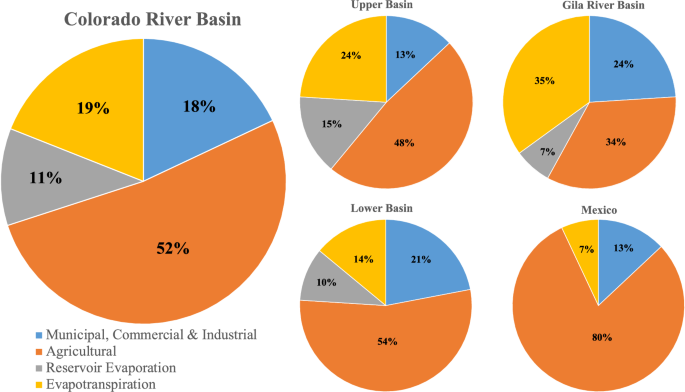
Water consumed by each sector in the Colorado River Basin and sub-basins (including exports), based on 2000–2019 averages.
Another important finding is that a substantial volume of water (19%) is consumed in supporting the natural environment through riparian and wetland vegetation evapotranspiration along river courses. This analysis—made possible because of recent mapping of riparian vegetation in the Colorado River Basin 16 —is an important addition to the water budget of the Colorado River Basin, given that the only previous accounting for riparian vegetation consumption has limited to the mainstem of the Colorado River below Hoover Dam and does not include vegetation upstream of Hoover Dam nor vegetation along tributary rivers 17 . Given that many of these habitats and associated species have been lost or became imperiled due to river flow depletion 18 —including the river’s vast delta ecosystem in Mexico—an ecologically sustainable approach to water management would need to allow more water to remain in the river system to support riparian and aquatic ecosystems. Additionally, 11% of all water consumed in the Colorado River Basin is lost through evaporation from reservoirs.
It is also important to note a fairly high degree of inter-annual variability in each sector of water use; for example, the range of values portrayed for the four water budget sectors shown in Fig. 5 equates to 24–47% of their 20-year averages. Also notable is a decrease in water consumed in the Lower Basin between the years 2000 and 2019 for both the MCI (−38%) and agricultural sectors (−15%), which can in part be attributed to the policy agreements summarized previously that have mandated water-use reductions.
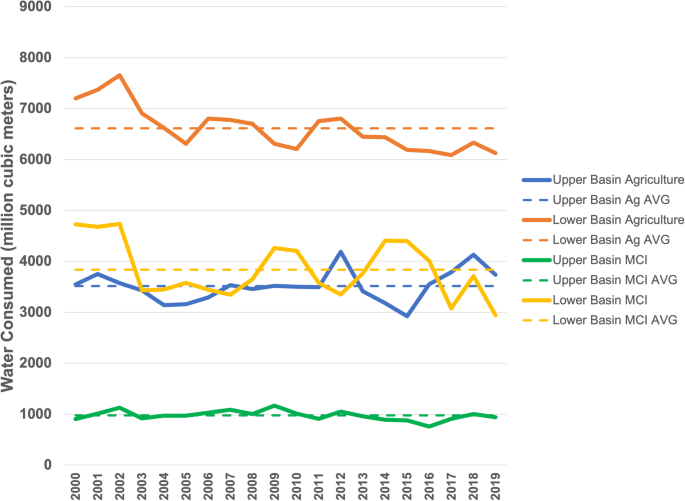
Inter-annual variability of water consumption within the Lower and Upper Basins, including water exported from these basins. The average (AVG) values shown are used in the water budgets detailed in Tables 1 and 2 .
The water accounting in Richter et al. 11 received a great deal of media attention including a front-page story in the New York Times 19 . These stories focused primarily on our conclusion that more than half (53%) of water consumed in the Colorado River Basin was attributable to cattle-feed crops (alfalfa and other hays) supporting beef and dairy production. However, that tabulation of the river’s water budget had notable shortcomings, as discussed previously. In this more complete accounting that includes Colorado River water exported outside of the basin’s physical boundary as well as indirect water consumption, we find that irrigated agriculture consumes half (52%) of all Colorado River Basin water, and the portion of direct consumption going to cattle-feed crops dropped from 53% as reported in Richter et al. 11 to 46% in this revised analysis.
These differences are explained by the fact that we now account for all exported water and also include indirect losses of water to reservoir evaporation and riparian/wetland evapotranspiration in our revised accounting, as well as improvements in our estimation of crop-water consumption. However, the punch line of our 2020 paper does not change fundamentally. Irrigated agriculture is the dominant consumer of water from the Colorado River, and 62% of agricultural water consumption goes to alfalfa and grass hay production.
Richter et al. 20 found that alfalfa and grass hay were the largest water consumers in 57% of all sub-basins across the western US, and their production is increasing in many western regions. Alfalfa is favored for its ability to tolerate variable climate conditions, especially its ability to persist under greatly reduced irrigation during droughts and its ability to recover production quickly after full irrigation is resumed, acting as a “shock absorber” for agricultural production under unpredictable drought conditions. The plant is also valued for fixing nitrogen in soils, reducing fertilizer costs. Perhaps most importantly, labor costs are comparatively low because alfalfa is mechanically harvested. Alfalfa is increasing in demand and price as a feed crop in the growing dairy industry of the region 21 . Any efforts to reduce water consumed by alfalfa—either through shifting to alternative lower-water crops or through compensated fallowing 20 —will need to compete with these attributes.
This new accounting provides a more comprehensive and complete understanding of how the Colorado River Basin’s water is consumed. During our study period of 2000–2019, an estimated average of 23.7 billion cubic meters (19.3 million acre-feet) of water was consumed each year before reaching its now-dry delta in Mexico. Schmidt et al. 2 have estimated that a reduction in consumptive use in the Upper and Lower Basins of 3–4 billion cubic meters (2.4–3.2 million acre-feet) per year—equivalent to 22–29% of direct use in those basins—will be necessary to stabilize reservoir levels, and an additional reduction of 1–3 billion cubic meters (~811,000–2.4 million acre-feet) per year will likely be needed by 2050 as climate warming continues to reduce runoff in the Colorado River Basin.
We hope that this new accounting will add clarity and a useful informational foundation to the public dialog and political negotiations over Colorado River Basin water allocations and cutbacks that are presently underway 2 . Because a persistent drought and intensifying aridification in the region has placed both people and river ecosystems in danger of water shortages in recent decades, knowledge of where the water goes will be essential in the design of policies for bringing the basin into a sustainable water supply-demand balance.
The data sources and analytical approaches used in this study are summarized below. Unless otherwise noted, all data were assembled for each year from 2000–2019 and then averaged. We acknowledge some inconsistency in the manner in which water consumption is measured or estimated across the various data sources and sectors used in this study, as discussed below, and each of these different approaches entail some degree of inaccuracy or uncertainty. We also note that technical measurement or estimation approaches change over time, and new approaches can yield differing results. For instance, the Upper Colorado River Commission is exploring new approaches for estimating crop evapotranspiration in the Upper Basin 22 . When new estimates become available we will update our water budget accordingly.
MCI and agricultural water consumption
The primary source of data on aggregate MCI (municipal, commercial, and industrial) and agricultural water consumption from the Upper and Lower Basins was the US Bureau of Reclamation. Water consumed from the Upper Basin is published in Reclamation’s five-year reports entitled “Colorado River—Upper Basin Consumptive Uses and Losses.” 23 These annual data have been compiled into a single spreadsheet used for this study 24 . Because measurements of agricultural diversions and return flows in the Upper Basin are not sufficiently complete to allow direct calculation of consumptive use, theoretical and indirect methods are used as described in the Consumptive Uses and Losses reports 25 . Reclamation performs these estimates for Colorado, Wyoming, and Utah, but the State of New Mexico provides its own estimates that are collaboratively reviewed with Reclamation staff. The consumptive use of water in thermoelectric power generation in the Upper Basin is provided to Reclamation by the power companies managing each generation facility. Reclamation derives estimates of consumptive use for municipal and industrial purposes from the US Geological Survey’s reporting series (published every 5 years) titled “Estimated Use of Water in the United States” at an 8-digit watershed scale 26 .
Use of shallow alluvial groundwater is included in the water accounting compiled by Reclamation but use of deeper groundwater sources—such as in Mexico and the Gila River Basin—is explicitly excluded in their accounting, and in ours. Reclamation staff involved with water accounting for the Upper and Lower Basins assume that groundwater use counted in their data reports is sourced from aquifers that are hydraulically connected to rivers and streams in the CRB (James Prairie, US Bureau of Reclamation, personal communication, 2023); because of this high connectivity, much of the groundwater being consumed is likely being sourced from river capture as discussed in Jasechko et al. 27 and Wiele et al. 28 and is soon recharged during higher river flows.
Water consumed from the Lower Basin (excluding water supplied by the Gila River Basin) is published in Reclamation’s annual reports entitled “Colorado River Accounting and Water Use Report: Arizona, California, and Nevada.” 3 These consumptive use data are based on measured deliveries and return flows for each individual water user. These data are either measured by Reclamation or provided to the agency by individual water users, tribes, states, and federal agencies 29 . When not explicitly stated in Reclamation reports, attribution of water volumes to MCI or agricultural uses was based on information obtained from each water user’s website, information provided directly by the water user, or information on export water use provided in Siddik et al. 30 . Water use by entities using less than 1.23 million cubic meters (1000 acre-feet) per year on average was allocated to MCI and agricultural uses according to the overall MCI-agricultural percentages calculated within each sub-basin indicated in Tables 1 and 2 for users of greater than 1.23 million cubic meters/year.
Disaggregation of water consumption by sector was particularly important and challenging for the Central Arizona Project given that this canal accounts for 21% of all direct water consumption in the Lower Basin. Reclamation accounts for the volumes of annual diversions into the Central Arizona Project canal but the structure serves 1071 water delivery subcontracts. We classified every unique Central Arizona Project subcontract delivery between 2000–2019 by its final water use to derive an estimated split between agricultural and MCI uses. Central Arizona Project subcontract delivery data were obtained from the current and archived versions of the project’s website summaries in addition to being directly obtained from the agency through a public information request. Subcontract deliveries were classified based on the final end use, including long-term and temporary leases of project water. This accounting also includes the storage of water in groundwater basins for later MCI or agricultural use. Additionally, water allocated to Native American agricultural uses that was subsequently leased to cities was classified as an MCI use.
Data for the Gila River basin was obtained from two sources. The Arizona Department of Water Resources has published data for surface water use in five “Active Management Areas” (AMAs) located in the Gila River basin: Prescott AMA, Phoenix AMA, Pinal AMA, Tucson AMA, and Santa Cruz AMA 31 . The water-use data for these AMAs is compiled from annual reports submitted by each water user (contractor) and then reviewed by the Arizona Department of Water Resources. The AMA water-use data are categorized by purpose of use, facilitating our separation into MCI and agricultural uses. These data are additionally categorized by water source; only surface water sourced from the Gila River hydrologic system was counted (deep groundwater use was not). The AMA data were supplemented with data for the upper Gila River basin provided by the University of Arizona 32 . We have assumed that all water supplied by the Gila River Basin is fully consumed, as the river is almost always completely dry in its lower reaches (less than 1% flows out of the basin into the Colorado River, on average 33 ).
Data for Mexico were obtained from Hernandez-Cruz et al. 10 based on estimates for 2008–2015. Agricultural demands were estimated from annual reports of irrigated area and water use published by the Ministry of Agriculture and the evapotranspiration estimates of the principal crops published by the National Institute for Forestry, Animal Husbandry, and Agricultural Research of Mexico 10 . The average annual volume of Colorado River water consumption in Mexico estimated by these researchers is within 1% of the cross-border delivery volume estimated by the Bureau of Reclamation for 2000–2019 in its Colorado River Accounting and Water Use Reports 3 .
Exported water consumption
Annual average inter-basin transfer volumes for each of 46 canals and pipelines exporting water outside of the Upper Basin were obtained from Reclamation’s Consumptive Uses and Losses spreadsheet 34 . Data for the Colorado River Aqueduct in the Lower Basin were obtained from Siddik et al. 30 Data for exported water in Mexico was available from Hernandez-Cruz et al. 10 . We assigned any seepage or evaporation losses from inter-basin transfers to their proportional end uses. All uses of exported water are considered to be consumptive uses with respect to the Colorado River, because none of the water exported out of the basin is returned to the Colorado River Basin.
We relied on data from Siddik et al. (2023) to identify whether the water exported out of the Colorado River Basin was for only MCI or agricultural use. When more than one water use purpose was identified, as well as for all major inter-basin transfers, we used government and inter-basin transfer project websites or information obtained directly from the project operator or water manager to determine the volume of water transferred and the end uses. Major recipients of exported water include the Coachella Valley Water District (California); Metropolitan Water District of Southern California (particularly for San Diego County, California); Northern Colorado Water Conservancy District; City of Denver (Colorado); the Central Utah Project; City of Albuquerque (New Mexico); and the Middle Rio Grande Conservancy District (New Mexico). We did not pursue sectoral water-use information for 17 of the 46 Upper Basin inter-basin transfers due to their relatively low volumes of water transferred by each system (<247,000 cubic meters or 2000 acre-feet), and instead assigned the average MCI or agricultural percentage (72% MCI, 28% agricultural) from all other inter-basin transfers in the Upper Basin. The export volume of these 17 inter-basin transfers sums to 9.76 million cubic meters (7910 acre-feet) per year, equivalent to 1% of the total volume exported from the Upper Basin.
Reservoir evaporation
Evaporation estimates for the Upper Basin and Lower Basin are based upon Reclamation’s HydroData repository 35 . Reclamation’s evaporation estimates are based on the standardized Penman-Monteith equation as described in the “Lower Colorado River Annual Summaries of Evapotranspiration and Evaporation” reports 17 . The Penman-Monteith estimates are based on pan evaporation measurements. Evaporation estimates for the Salt River Project reservoirs in the Gila River basin were provided by the Salt River Project in Arizona (Charlie Ester, personal communication, 2023).
Another consideration with reservoirs is the volume of water that seeps into the banks or sediments surrounding the reservoir when reservoir levels are high, but then drains back into the reservoir as water levels decline 36 . This has the effect of either exacerbating reservoir losses (consumptive use) or offsetting evaporation when bank seepage flows back into a reservoir. The flow of water into and out of reservoir banks is non-trivial; during 1999–2008, an estimated 247 million cubic meters (200,000 acre-feet) of water drained from the canyon walls surrounding Lake Powell into the reservoir each year, providing additional water supply 36 . However, the annual rate of alternating gains or losses has not been sufficiently measured at any of the basin’s reservoirs and therefore is not included in Tables 1 and 2 .
Riparian and wetland vegetation evapotranspiration
We exported the total annual evapotranspiration depth at a 30 meter resolution from OpenET 37 using Google Earth Engine from 2016 to 2019 to align with OpenET’s data availability starting in 2016. Total annual precipitation depths, sourced from gridMET 38 , were resampled to align with the evapotranspiration raster resolution. Subsequently, a conservative estimate of the annual water depth utilized by riparian vegetation from the river was derived by subtracting the annual precipitation raster from the evapotranspiration raster for each year. Positive differentials, indicative of river-derived evapotranspiration, were then multiplied by the riparian vegetation area as identified in the CO-RIP 16 dataset to estimate the total annual volumetric water consumption by riparian vegetation across the Upper, Lower, and Gila River Basins. The annual volumetric water consumption calculated over four years were finally averaged to get riparian vegetation evapotranspiration in the three basins. Because the entire flow of the Colorado River is diverted into the Canal Alimentador Central near the international border, very little riparian evapotranspiration occurs along the river south of the international border in the Mexico basin.
In addition to water consumed by riparian evapotranspiration within the Lower Basin, the Salton Sea receives agricultural drain water from both the Imperial Irrigation District and the Coachella Valley Irrigation District, stormwater drainage from the Coachella Valley, and inflows from the New and Alamo Rivers 39 . Combined inflows to the Sea during 2015–2019 were added to our estimates of riparian/wetland evapotranspiration in the Lower Basin.
Similarly, Mexico receives drainage water from the Wellton–Mohawk bypass drain originating in southern Arizona that empties into the Cienega de Santa Clara (a wetland); this drainage water is included as riparian/wetland evapotranspiration in the Mexico basin.
Crop-specific water consumption
The volumes of total agricultural consumption reported for each sub-basin in Tables 1 and 2 were obtained from the same data sources described above for MCI consumption and exported water. The portion (%) of those agricultural consumption volumes going to each individual crop was then allocated according to percentage estimates of each crop’s water consumption in each accounting unit using methods described in Richter et al. 20 and detailed here.
Monthly crop water requirements during 1981–2019 for 13 individual crops, representing 68.8% of total irrigated area in the US in 2019, were estimated using the AquaCrop-OS model (Table SI- 3 ) 40 . For 17 additional crops representing about 25.4% of the total irrigated area, we used a simple crop growth model following Marston et al. 41 as crop parameters needed to run AquaCrop-OS were not available. A list of the crops included in this study is shown in Table SI- 3 . The crop water requirements used in Richter et al. 11 were based on a simplistic crop growth model, often using seasonal crop coefficients whereas we use AquaCrop-OS 40 , a robust crop growth model, to produce more realistic crop growth and crop water estimates for major crops. AquaCrop-OS is an open-source version of the AquaCrop model 42 , a crop growth model capable of simulating herbaceous crops. Additionally, we leverage detailed local data unique to the US, including planting dates and subcounty irrigated crop areas, to produce estimates at a finer spatial resolution than the previous study. We obtained crop-specific planting dates from USDA 43 progress data at the state level. For crops that did not have USDA crop progress data, we used data from FAO 44 and CUP+ model 45 for planting dates. We used climate data (precipitation, minimum and maximum air temperature, reference ET) from gridMET 38 , soil texture data from ISRIC 46 database and crop parameters from AquaCrop-OS to run the model. The modeled crop water requirement was partitioned into blue and green components following the framework from Hoekestra et al. 47 , assuming that blue and green water consumed on a given day is proportional to the amount of green and blue water soil moisture available on that day. When applying a simple crop growth model, daily gridded (2.5 arc minutes) crop-specific evapotranspiration (ETc) was computed by taking the product of reference evapotranspiration (ETo) and crop coefficient (Kc), where ETo was obtained from gridMET. Crop coefficients were calculated using planting dates and crop coefficient curves from FAO and CUP+ model. Kc was set to zero outside of the growing season. We partitioned the daily ETc into blue and green components by following the methods from ref. 41 It is assumed that the crop water demands are met by irrigation whenever it exceeds effective precipitation (the latter calculated using the USDA Soil Conservation Service method (USDA, 1968 48 ). We obtained county level harvested area from USDA 43 and disaggregated to sub-county level using Cropland Data Layer (CDL) 49 and Landsat-based National Irrigation Dataset (LANID) 50 . The CDL is an annual raster layer that provides crop-specific land cover data, while the LANID provides irrigation status information. The CDL and LANID raster were multiplied and aggregated to 2.5 arc minutes to match the AquaCrop-OS output. We produced a gridded crop area map by using this resulting product as weights to disaggregate county level area. CDL is unavailable before 2008. Therefore, we used land use data from ref. 51 in combination with average CDL map and county level harvested area to produce gridded crop harvested area. We computed volumetric water consumption by multiplying the crop water requirement depth by the corresponding crop harvested area.
Data availability
All data compiled and analyzed in this study are publicly available as cited and linked in our Methods section. Our compilation of these data is also available from Hydroshare at: http://www.hydroshare.org/resource/2098ae29ae704d9aacfd08e030690392 .
Code availability
All model code and software used in this study have been accessed from sources cited in our Methods section. We used AquaCrop-OS (v5.0a), an open source version of AquaCrop crop growth model, to run crop simulations. This model is publicly available at http://www.aquacropos.com/ . For estimating riparian evapotranspiration, we used ArcGIS Pro 3.1.3 on the Google Earth Engine. Riparian vegetation distribution maps were sourced from Dryad at https://doi.org/10.5061/dryad.3g55sv8 .
Stromberg, J. C., Andersen, D. C. & Scott, M. L. Riparian floodplain wetlands of the arid and semiarid southwest In Wetland Habitats of North America: Ecology and Conservation Concern s , Chapter 24, pp. 343–356. (University of California Press, 2012). https://www.ucpress.edu/book/9780520271647/wetland-habitats-of-north-america .
Schmidt, J. C., Yackulic, C. B. & Kuhn, E. The Colorado River water crisis: Its origin and the future. WIREs Water https://doi.org/10.1002/wat2.1672 (2023).
Article Google Scholar
Colorado River Accounting and Water Use Report: Arizona, California, and Nevada. Interior Region 8: Lower Colorado Basin (US Bureau of Reclamation, 2023). Annual reports available under “Water Accounting Reports” at https://www.usbr.gov/lc/region/g4000/wtracct.html .
Water Operations: Historic Data (US Bureau of Reclamation, 2023). https://www.usbr.gov/rsvrWater/HistoricalApp.html .
Colorado River Compact , 1922 . US Bureau of Reclamation. https://www.usbr.gov/lc/region/pao/pdfiles/crcompct.pdf .
Kuhn, E. & Fleck, J. Science Be Dammed:How Ignoring Inconvenient Science Drained the Colorado River (The University of Arizona Press, 2019) https://uapress.arizona.edu/book/science-be-dammed .
Castle, A. & Fleck, J. The Risk of Curtailment under the Colorado River Compact ( https://doi.org/10.2139/ssrn.3483654 (2019).
US Bureau of Reclamation. Colorado River Basin Water Supply and Demand Study: Technical Report C – Water Demand Assessment https://www.usbr.gov/lc/region/programs/crbstudy/finalreport/Technical%20Report%20C%20-%20Water%20Demand%20Assessment/TR-C-Water_Demand_Assessmemt_FINAL.pdf (2012).
Utilization of the Waters of the Colorado and Tijuana Rivers and of the Rio Grande . International Treaty between the United States and Mexico, February 3, 1944. (International Boundary and Waters Commission, 1944). https://www.ibwc.gov/wp-content/uploads/2022/11/1944Treaty.pdf .
Hernández-Cruz, A. et al. Assessing water management strategies under water scarcity in the Mexican portion of the Colorado River Basin. J. Water Resour. Plan. Manag. 149 , 04023042 (2023).
Richter, B. D. et al. Water scarcity and fish imperilment driven by beef production. Nat. Sustain. 3 , 319–328 (2020).
Biden-Harris Administration announces historic Consensus System Conservation Proposal to protect the Colorado River Basin . US Department of the Interior, May 22, 2023. https://www.doi.gov/pressreleases/biden-harris-administration-announces-historic-consensus-system-conservation-proposal .
Wheeler, K. G. et al. What will it take to stabilize the Colorado River? Science 377 , 373–375 (2022).
Article ADS CAS PubMed Google Scholar
Richter, B. D. Decoupling urban water use from population growth in the Colorado River Basin. J. Water Plan. Manag. 149 , 2 (2023).
Google Scholar
Schmidt, J. C. Maps Matter: A few suggested changes to the Colorado River basin base map . Center for Colorado River Studies. (Utah State University, 2022).
Woodward, B. D. et al. Co-Rip: A riparian vegetation and corridor extent dataset for Colorado river basin streams and rivers. ISPRS Int. J. Geo Inform. 7 , 397 (2018).
Article ADS Google Scholar
Lower Colorado River Annual Summaries of Evapotranspiration and Evaporation . (US Bureau of Reclamation, Lower Colorado Region, 2023). https://www.usbr.gov/lc/region/g4000/wtracct.html .
Richter, B. D., Powell, E. M., Lystash, T. & Faggert, M. Protection and restoration of freshwater ecosystems. Chapter 5 in Miller, Kathleen A., Alan F. Hamlet, Douglas S. Kenney, and Kelly T. Redmond (Eds.) Water Policy and Planning in a Variable and Changing Climate . (CRC Press - Taylor & Francis Group, 2016).
Shao, Elena. “The Colorado River is shrinking. See what’s using all the water.” New York Times , May 22, 2023. https://www.nytimes.com/interactive/2023/05/22/climate/colorado-river-water.html .
Richter, B. D., et al. Alleviating water scarcity by optimizing crop mixes. Nat. Water . https://doi.org/10.1038/s44221-023-00155-9 .
Njuki, E. U.S. dairy productivity increased faster in large farms and across southwestern states . U.S. Economic Research Service, US Department of Agriculture, March 22, 2022. https://www.ers.usda.gov/amber-waves/2022/march/u-s-dairy-productivity-increased-faster-in-large-farms-and-across-southwestern-states/ .
Mefford, B. & Prairie J., eds. Assessing Agricultural Consumptive Use in the Upper Colorado River Basin - Phase III Report U.S. Bureau of Reclamation and the Upper Colorado River Commission. http://www.ucrcommission.com/reports-studies/ (2022).
Upper Basin Consumptive Uses and Losses (Bureau of Reclamation). Annual reports available at https://www.usbr.gov/uc/envdocs/plans.html .
Bureau of Reclamation. “Consumptive Uses and Losses spreadsheet 1971–2020” Colorado River Basin Natural Flow and Salt Data, Supporting data for consumptive uses and losses computation. https://www.usbr.gov/lc/region/g4000/NaturalFlow/documentation.html .
Upper Colorado River Basin Consumptive Uses and Losses Report 2016–2020 . US Department of Interior: Bureau of Reclamation. Five year reports available under “Colorado River-Consumptive Uses and Losses Reports” at https://www.usbr.gov/uc/envdocs/plans.html .
Estimated Use of Water in the United States . US Department of Interior: US Geological Survey. Reports available every five years at https://www.usgs.gov/mission-areas/water-resources/science/water-use-united-states .
Jasechko, S. et al. Widespread potential loss of streamflow into underlying aquifers across the USA. Nature 591 , 391–395 (2021).
Wiele, S. M., Leake, S. A., Owen-Joyce, S. J. & and McGuire, E. H. Update of the Accounting Surface Along the Lower Colorado River US Department of the Interior: US Geological Survey Scientific Investigations Report 2008–5113 (2008).
Bruce, B. W., et al. Comparison of U.S. Geological Survey and Bureau of Reclamation water-use reporting in the Colorado River Basin U.S. Geological Survey Scientific Investigations Report 2018–5021 . https://doi.org/10.3133/sir20185021 (2018).
Siddik, M. A. B., Dickson, K. E., Rising, J., Ruddell, B. L. & Marston, L. T. Interbasin water transfers in the United States and Canada. Sci. Data 10 , 27 (2023). Data spreadsheet provided by M.A.B. Siddik.
Article PubMed PubMed Central Google Scholar
Active Management Areas : AMA Annual Supply and Demand Dashboard (Arizona Department of Water Resources, 2023). https://azwater.gov/ama/ama-data .
Lacroix, K. M. et al. Wet water and paper water in the Upper Gila River Watershed https://extension.arizona.edu/sites/extension.arizona.edu/files/pubs/az1708-2016_0.pdf The University of Arizona Cooperative Extension, AZ1708. Data spreadsheet provided by A. Hullinger (2016).
Surface-Water Annual Statistics for the Nation: Gila River at Dome, Arizona . US Geological Survey. Available at https://waterdata.usgs.gov/nwis/annual/?referred_module=sw&site_no=09520500&por_09520500_5810=19975,00060,5810,1905,2024&year_type=C&format=html_table&date_format=YYYY-MM-DD&rdb_compression=file&submitted_form=parameter_selection_list .
Consumptive Uses and Losses spreadsheet 1971–2020 . Bureau of Reclamation, Colorado River Basin Natural Flow and Salt Data, Supporting data for consumptive uses and losses computation. https://www.usbr.gov/lc/region/g4000/NaturalFlow/documentation.html .
HydroData: Reservoir Data . US Bureau of Reclamation. https://www.usbr.gov/uc/water/ .
Myers, T. Loss rates from Lake Powell and their impact on management of the Colorado River. J. Am. Water Resour. Assoc. 49 , 1213–1224 (2013).
Melton, F. S. et al. OpenET: filling a critical data gap in water management for the western United States. J. Am. Water Resour. Assoc. 58 , 971–994 (2022).
Abatzoglou, J. T. Development of gridded surface meteorological data for ecological applications and modelling. Int. J. Climatol. 33 , 121–131 (2013).
Salton Sea Management Program: Long-Range Plan Public Draft (2022). California Natural Resources Agency. https://saltonsea.ca.gov/wp-content/uploads/2022/12/Salton-Sea-Long-Range-Plan-Public-Draft-Dec-2022.pdf .
Foster, T. et al. AquaCrop-OS: an open source version of FAO’s crop water productivity model. Agricul. Water Manag. 181 , 18–22 (2017).
Marston, L. T., et al. Reducing water scarcity by improving water productivity in the United States. Environ. Res. Lett. 15 https://doi.org/10.1088/1748-9326/ab9d39 (2020).
Steduto, P., Hsiao, T. C., Fereres, E. & Raes, D. Crop yield response to water (2012). 1028. Rome: Food and Agriculture Organization of the United Nations.
USDA, National Agricultural Statistics Service. “Quick Stats.” http://quickstats.nass.usda.gov .
Allen, R. G., Pereira, L. S., Raes, D. & Smith, M. FAO Irrigation and drainage paper No. 56 56, (e156. Food and Agriculture Organization of the United Nations, Rome, 1998).
Orange, M. N., Scott Matyac, J. & Snyder, R. L. Consumptive use program (CUP) model. IV Int. Symp. Irrig. Horticult. Crops 664 , 461–468 (2003).
Hengl, T. et al. SoilGrids250m: Global gridded soil information based on machine learning. PLoS One 12 , e0169748 (2017).
Hoekstra, A. Y. Green-blue water accounting in a soil water balance. Adv. Water Resour. 129 , 112–117 (2019).
USDA (US Department of Agriculture). A Method for Estimating Volume and Rate of Runoff in Small Watersheds . SCS-TP-149. Washington DC: Soil Conservation Service (1968).
Johnson, D. M., & Mueller, R. 2010. “Cropland Data Layer.” https://nassgeodata.gmu.edu/CropScape/ .
Xie, Y., Gibbs, H. K. & Lark, T. J. Landsat-based Irrigation Dataset (LANID): 30m resolution maps of irrigation distribution, frequency, and change for the US, 1997–2017. Earth Syst. Sci. Data 13 , 5689–5710 (2021).
Sohl, T. et al. Modeled historical land use and land cover for the conterminous United States. J. Land Use Sci. 11 , 476–499 (2016).
Download references
Acknowledgements
This paper is dedicated to our colleague Jack Schmidt in recognition of his retirement and enormous contributions to the science and management of the Colorado River. The authors thank James Prairie of the US Bureau of Reclamation, Luke Shawcross of the Northern Colorado Water Conservancy District, Charlie Ester of the Salt River Project, and Brian Woodward of the University of California Cooperative Extension for their assistance in accessing data used in this study. The authors also thank Rhett Larson at the Sandra Day O’Connor School of Law at Arizona State University for their review of Arizona water budget data, and the Central Arizona Project for providing delivery data by each subcontract. G.L., L.M., and K.F.D. acknowledge support by the United States Department of Agriculture National Institute of Food and Agriculture grant 2022-67019-37180. L.T.M. acknowledges the support the National Science Foundation grant CBET-2144169 and the Foundation for Food and Agriculture Research Grant No. FF-NIA19-0000000084. R.R.R. acknowledges the support the National Science Foundation grant CBET-2115169.
Author information
Authors and affiliations.
World Wildlife Fund, 1250 24th St NW, Washington, DC, 20037, USA
Brian D. Richter
Sustainable Waters, Crozet, Virginia, 22932, USA
The Charles E.Via, Jr, Department of Civil and Environmental Engineering, Virginia Tech, Blacksburg, VA, 24061, USA
Gambhir Lamsal, Landon Marston & Sameer Dhakal
Northern Arizona University, Flagstaff, AZ, 86011, USA
Laljeet Singh Sangha, Richard R. Rushforth & Benjamin L. Ruddell
Department of Geography and Spatial Sciences, University of Delaware, Newark, DE, 19716, USA
Dongyang Wei & Kyle Frankel Davis
Department of Plant and Soil Sciences, University of Delaware, Newark, DE, 19716, USA
Kyle Frankel Davis
Instituto de Investigaciones Oceanologicas, Universidad Autonoma de Baja California, Ensenada, Baja California, México
Astrid Hernandez-Cruz
Department of Land, Air and Water Resources, Univeristy of California at Davis, Davis, CA, 95616, USA
Samuel Sandoval-Solis
Center for Colorado River Studies, Utah State University, Logan, UT, 84322, USA
John C. Schmidt
You can also search for this author in PubMed Google Scholar
Contributions
B.D.R. designed the study, compiled and analyzed data, wrote the manuscript and supervised co-author contributions. G.L. compiled all crop data, estimated crop evapotranspiration, and prepared figures. S.D. compiled all riparian vegetation data and estimated riparian evapotranspiration. L.S.S. and R.R.R. accessed, compiled, and analyzed data from the Central Arizona Project. D.W. compiled data and prepared figures. A.H.-C. and S.S.-S. compiled and analyzed data for Mexico. J.C.S. compiled and analyzed reservoir evaporation data and edited the manuscript. L.M., B.L.R., and K.F.D. supervised data compilation and analysis and edited the manuscript.
Corresponding author
Correspondence to Brian D. Richter .
Ethics declarations
Competing interests.
The authors declare no competing interests.
Peer review
Peer review information.
Communications Earth & Environment thanks James Booker and Becky Bolinger for their contribution to the peer review of this work. Primary Handling Editors: Aliénor Lavergne and Carolina Ortiz Guerrero. A peer review file is available.
Additional information
Publisher’s note Springer Nature remains neutral with regard to jurisdictional claims in published maps and institutional affiliations.
Supplementary information
Peer review file, supplementary information, rights and permissions.
Open Access This article is licensed under a Creative Commons Attribution 4.0 International License, which permits use, sharing, adaptation, distribution and reproduction in any medium or format, as long as you give appropriate credit to the original author(s) and the source, provide a link to the Creative Commons licence, and indicate if changes were made. The images or other third party material in this article are included in the article’s Creative Commons licence, unless indicated otherwise in a credit line to the material. If material is not included in the article’s Creative Commons licence and your intended use is not permitted by statutory regulation or exceeds the permitted use, you will need to obtain permission directly from the copyright holder. To view a copy of this licence, visit http://creativecommons.org/licenses/by/4.0/ .
Reprints and permissions
About this article
Cite this article.
Richter, B.D., Lamsal, G., Marston, L. et al. New water accounting reveals why the Colorado River no longer reaches the sea. Commun Earth Environ 5 , 134 (2024). https://doi.org/10.1038/s43247-024-01291-0
Download citation
Received : 03 October 2023
Accepted : 27 February 2024
Published : 28 March 2024
DOI : https://doi.org/10.1038/s43247-024-01291-0
Share this article
Anyone you share the following link with will be able to read this content:
Sorry, a shareable link is not currently available for this article.
Provided by the Springer Nature SharedIt content-sharing initiative
By submitting a comment you agree to abide by our Terms and Community Guidelines . If you find something abusive or that does not comply with our terms or guidelines please flag it as inappropriate.
Quick links
- Explore articles by subject
- Guide to authors
- Editorial policies
Sign up for the Nature Briefing newsletter — what matters in science, free to your inbox daily.

- International
- Schools directory
- Resources Jobs Schools directory News Search
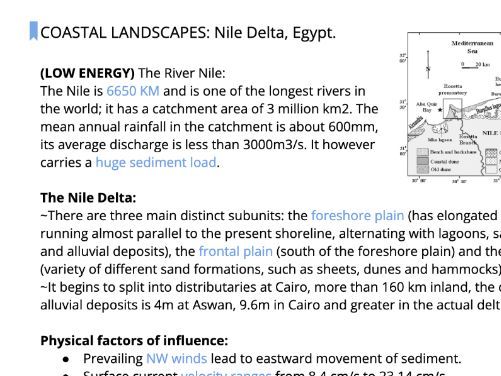
OCR A Level Geography Case Studies
Subject: Geography
Age range: 16+
Resource type: Assessment and revision

Last updated
11 August 2023
- Share through email
- Share through twitter
- Share through linkedin
- Share through facebook
- Share through pinterest
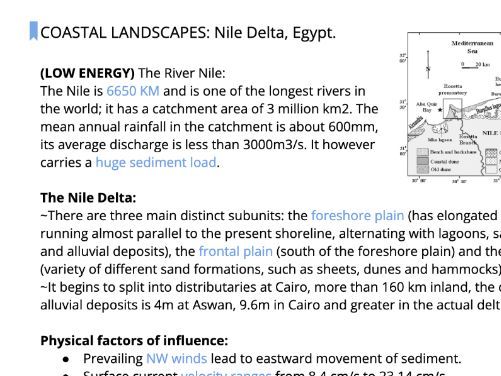
All of the case studies from OCR A level geography.
COASTAL LANDSCAPES Nile Delta, Egypt (extra) Rhone Delta, France Flambrough Head, Yorkshire Sandbanks, Dorset Mangawhai-Pakiri, New Zealand
EARTH’S LIFE SUPPORT SYSTEMS The Amazon Rainforest The Alaskan Arctic Tundra
CHANGING SPACES, MAKING PLACES. Lympstone, Devon Toxteth, Liverpool Jembatan Besi, Jakarta, Indonesia Northwood, Irvine, Southern California Birmingham Metropolitan Region Barcelona, Spain
GLOBAL MIGRATION Brazil, an EDC The USA, A HIC Laos (Lao PDR)
HUMAN RIGHTS Human rights in India Global governance in Afghanistan The DRC, an LIDC
HAZARDOUS EARTH Living with volcanoes - Japan/ Indonesia Living with earthquakes - Japan Living with earthquakes - Nepal Living with earthquakes - Haiti (extra) Living with volcanoes - Italy/ Indonesia Living with earthquakes - Haiti Living with earthquakes - Japan
DISEASE DILEMMAS Air pollution and cancer in India Malaria in Ethiopia Cancer in the UK Cholera epidemic - Haiti Rosy Periwinkle/ GlaxoSmithKline
Tes paid licence How can I reuse this?
Your rating is required to reflect your happiness.
It's good to leave some feedback.
Something went wrong, please try again later.
This resource hasn't been reviewed yet
To ensure quality for our reviews, only customers who have purchased this resource can review it
Report this resource to let us know if it violates our terms and conditions. Our customer service team will review your report and will be in touch.
Not quite what you were looking for? Search by keyword to find the right resource:
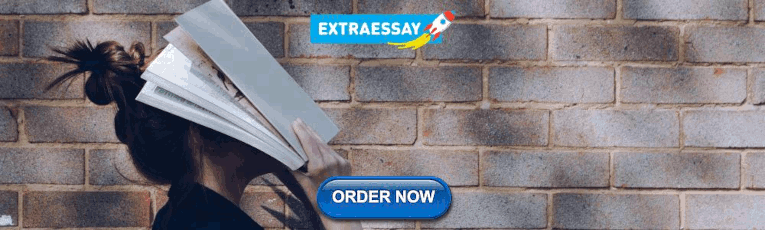
IMAGES
VIDEO
COMMENTS
Give some information about the river Nile -. 6650km long - one of the longest rivers in the world. catchment area of over 3 million km2. mean annual rainfall in the catchment is 600mm - average discharge is less than 3000m3/s - one of the lowest of the worlds great rivers. Despite low discharge it carries a huge sediment load.
Coasts Case Study: Nile Delta in a Snap! Unlock the full A-level Geography course at https://bit.ly/34Ei9Mb created by Isabella, Geography expert at SnapRevi...
The delta is very gently sloping. The coastal beaches are narrow with low dunes behind them. In the nearshore area there are longshore bars - brackish water removes sand and deposits it. Between the bars are longshore troughs. Behind the bars and dunes are lagoons. CASE STUDY: Low Energy Coastline - The Rhone Delta, France. What is the location ...
Share this: Geography Case Studies - A wide selection of geography case studies to support you with GCSE Geography revision, homework and research.
1) Region was an alluvial plain with seasonally active braided river channels. 2) Sea level begins to rise and alluvial plain deposits were reworked. 3) The delta changed from a river-dominated system to a wave-dominated cuspate delta. 4) Increased coastal erosion and marine flooding of the northern delta plain. (Aswan Dam).
Three case studies, Lake Chad, the Aral Sea region, and the Nile Delta, representing various geographic and socio-political settings, were selected to perform the multitemporal analysis.
Resource type: Worksheet/Activity. File previews. pdf, 213.93 KB. pptx, 1.58 MB. A case study of the coastal recession at the Nile Delta for Edexcel A Level Geography. Introduction to the location of the Delta. Importance of the Delta for the region. Risks facing the Delta. Human actions and Physical factors affecting the Delta.
OCR A level Geography The Nile Delta, Case study of a low energy coastline. Subject: Geography. Age range: 16+. Resource type: Unit of work. File previews. pdf, 1.47 MB. A* Powerpoint resource on the Nile Delta- an example of a low energy coastline. The powerpoint gives an in depth guide to the Nile Delta following all the specification points ...
5.8C - Conflicts. The potential for conflicts to occur between users within a country, and internationally over local and trans-boundary water sources. (Nile or Mekong) (P: Role of different players) When the demand for water overtakes the available supply and there are key stakeholders desperate for that water, there is potential for conflict ...
EUROSION Case Study 2 1. GENERAL DESCRIPTION OF THE AREA The Rhone delta is located on the north-western coast of the Mediterranean, at about latitude 43°30 N. The deltaic plain covers an area of 1,740 km² and is situated downstream of an extensive catchment area (95,500 km²). 1.1 Physical process level 1.1.1 Classification
The Niger Delta region falls within the tropical rain forest zone of Nigeria. The region spanning over 20,000 square kilometers hosts about 25% of the Nigerian population. About 2,370 square kilometers ... This case study is divided into three units and is designed to take three teaching classes of two hours each. The first class will be ...
A. The quality of life should improve with better health. - Clean water to drink. Study Case Study Pearl River Delta flashcards from Nanou Sabourian's class online, or in Brainscape's iPhone or Android app. Learn faster with spaced repetition.
the nile delta case study. Background: Click the card to flip 👆. -The Nile delta has many low energy coastal features: -it is 6650 km in length. -Over the 3,000 years, deposition has exceeded marine erosion rates. -the river carries a huge sediment load. 30% clay, 40% silt and 30% fine sand. Click the card to flip 👆. 1 / 9.
CIE ALevel Case Study The Niger Delta: Environmental Impact. Course. A Level Geography. Institution. A Level Geography. A detailed case study on the impact of unsustainable energy practices in the Niger Delta and how these practices have considerably impacted the environment in the Niger Delta. Preview 1 out of 2 pages.
Study esme appleby's A-level Geography case study Nile Delta-low energy environment flashcards for their Redmaids class now! How studying works. Brainscape's adaptive web mobile flashcards system will drill you on your weaknesses, using a pattern guaranteed to help you learn more in less time.
An investigation of the impact of the climate change on river deltas: case study: the delta of r. Pineios (Thessaly, Greece) - concept and preliminary results on surface water environmental state. In: 13th International Conference on Environmental Science and Technology .
OCR A Level Geography - Coastal landscapes case studies - The Nile Delta. 8 terms. Harry-James-Preview. Physical Geography: 2.b. Low energy coastline case study. 26 terms. ashleyxtan. Preview. 1.2 Case Study: The Nile Delta & Saltburn to Flamborough Head. Teacher 65 terms. Mr-Longden. Preview. marine midterm vocab. 151 terms. Emma_Jasperson08 ...
With an area of over 930 km2, the Rhone river mouth (southern France) is the largest river delta of the western Mediterranean.This zone, known as Camargue, is a natural park characterized by a vast plain facing the Mediterranean Sea, where outflow the two main arms of the Rhone river named the Grand Rhône (in the eastern side) and the Petit Rhône (in the western side).
Age range: 16+. Resource type: Other. File previews. docx, 15.11 KB. A* level geography notes. Tes paid licence How can I reuse this? This resource hasn't been reviewed yet. To ensure quality for our reviews, only customers who have purchased this resource can review it. Report this resource to let us know if it violates our terms and conditions.
Example Case Study: Pearl River Delta, China. Located in South-East China, the Pearl River is China's third longest river ... At a time when the study of geography has never been more important, Bridgette is passionate about creating content which supports students in achieving their potential in geography and builds their confidence.
During our study period of 2000-2019, an estimated average of 23.7 billion cubic meters (19.3 million acre-feet) of water was consumed each year before reaching its now-dry delta in Mexico.
All of the case studies from OCR A level geography. Including… COASTAL LANDSCAPES Nile Delta, Egypt (extra) Rhone Delta, France Flambrough Head, Yorkshire Sandbanks, Dorset Mangawhai-Pakiri, New Zealand. EARTH'S LIFE SUPPORT SYSTEMS The Amazon Rainforest The Alaskan Arctic Tundra. CHANGING SPACES, MAKING PLACES. Lympstone, Devon Toxteth ...
Nearly 50 million km2 of global land experiences seasonal transitions from predominantly frozen to thawed conditions, significantly impacting various ecosystems and hydrologic processes. In this study, we assessed the capability to retrieve surface freeze-thaw (FT) conditions using Sentinel-1 synthetic aperture radar (SAR) data time series at two agro-forested study sites, St-Marthe and St ...
3). Concentration of mercury and aluminium increases as go up the food chain: fish = 1 unit, bird eats 20 fish = 20 units, fox eats 5 birds = 100 units. Tree-dwelling birds and animals suffer due to loss of habitat. 4). Increased acidity in lakes/rivers kills fish and plants. Impacts on buildings. 1).
Study with Quizlet and memorize flashcards containing terms like The Niger delta covers _____, making up about _____ of Nigeria's land area. It contains of ____ of Africa's remaining mangroves., A report published in _____ estimated that over _____ of oil had been spilled in the delta over the last 50 years., The report, compiled by WFF, says the delta is in: and more.