Open Access is an initiative that aims to make scientific research freely available to all. To date our community has made over 100 million downloads. It’s based on principles of collaboration, unobstructed discovery, and, most importantly, scientific progression. As PhD students, we found it difficult to access the research we needed, so we decided to create a new Open Access publisher that levels the playing field for scientists across the world. How? By making research easy to access, and puts the academic needs of the researchers before the business interests of publishers.
We are a community of more than 103,000 authors and editors from 3,291 institutions spanning 160 countries, including Nobel Prize winners and some of the world’s most-cited researchers. Publishing on IntechOpen allows authors to earn citations and find new collaborators, meaning more people see your work not only from your own field of study, but from other related fields too.
Brief introduction to this section that descibes Open Access especially from an IntechOpen perspective
Want to get in touch? Contact our London head office or media team here
Our team is growing all the time, so we’re always on the lookout for smart people who want to help us reshape the world of scientific publishing.
Home > Books > Pipeline Engineering - Design, Failure, and Management
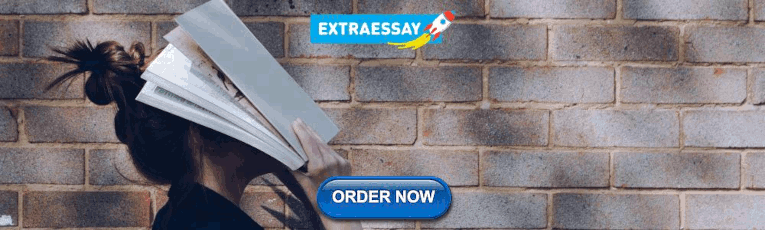
Review on the Stability of the Nanofluids
Submitted: 06 July 2022 Reviewed: 17 August 2022 Published: 27 October 2022
DOI: 10.5772/intechopen.107154
Cite this chapter
There are two ways to cite this chapter:
From the Edited Volume
Pipeline Engineering - Design, Failure, and Management
Edited by Sayeed Rushd and Mohamed Anwar Ismail
To purchase hard copies of this book, please contact the representative in India: CBS Publishers & Distributors Pvt. Ltd. www.cbspd.com | [email protected]
Chapter metrics overview
216 Chapter Downloads
Impact of this chapter
Total Chapter Downloads on intechopen.com

Total Chapter Views on intechopen.com
Both mono and hybrid nanofluids, the engineered colloidal mixture made of the base fluid and nanoparticles, have shown many interesting properties and become a high potential next-generation heat transfer fluid in various engineering applications. The present review focuses on improving the stability of the nanofluids. For this, the present review briefly summarizes the impact of nanofluid preparation on the stability of various nanofluids and described in the following classification; (a) Nanofluid constituent, (b) Nanomaterial synthesis, and (c) Nanofluid synthesis techniques which are well-grouped and thoroughly discussed. Physical mechanisms for heat transfer enhancement using nanofluids are explored as well. Most of the studies reveal that there are significant improvements in the stability of the nanofluids. Hence, there is an excellent opportunity to use stabled nanofluids in various engineering applications. Finally, some useful recommendations are also provided.
- nanomaterial synthesis
Author Information
Sumit kumar singh *.
- Department of Mechanical Engineering, Indian Institute of Technology (B.H.U.), Varanasi, India
*Address all correspondence to: [email protected]
1. Introduction
Nanofluids are engineered by dispersing nanoparticles, having average sizes below 100 nm, in conventional heat transfer fluids. Proper and stable dispersion of even a negligible fraction of particles in nanofluids can offer significant enhancement in the heat transfer properties. Various types of nanoparticles like metals, metal oxides, alloys, allotropes of carbon, ceramics, phase change materials, and metal carbides are being used for preparing nanofluids. In addition to nanofluids, hybrid nanofluids have also gained attention recently due to significant improvement in heat transfer characteristics and stability may be caused by the synergistic effect of hybridization. Heat exchangers that use tubes or pipes often have a circular, rectangular, or elliptical cross-section and are easier to design. Tubular heat exchangers are fairly prevalent in pipeline engineering applications. These heat exchangers might be built to handle fluids under high pressure or to handle pressure differentials between cold and hot fluids. Double-pipe and shell-tube heat exchangers are additional categories that apply to these heat exchangers. Modifying the fluids’ characteristics can also increase the heat exchange rate of a heat exchanger. Due to the fact that stable nanofluids have significantly improved heat transfer characteristics, particularly in terms of thermal conductivity, slip mechanisms, and the nanofin effect, they may be employed in tubular heat exchangers to increase energy efficiency. For preparing mono or hybrid nanofluids, the two-step method is generally used where firstly different nanoparticles or nanocomposites are prepared. Then they are mixed in the base fluid through magnetic or mechanical stirring. After that, the solution is sonicated and then characterized using different techniques to assure the proper (homogeneous) mixing and stability of the hybrid nanofluids. Both mono and hybrid nanofluids are thus prepared to provide improved heat transfer characteristics due to an increase in thermal conductivity, Brownian motion, proper dispersion, agglomeration, solid/liquid interface layering, thermophoresis, the improved thermal network between the solid nanoparticle and fluid molecules, nanofin and nanoporous effects at the heat transfer surface. The reason behind this improvement can be summarized as: (i) More heat transfer surface between nanoparticles and fluid, (ii) Collision between the nanoparticles, (iii) Increment in the thermal conductivity due to the interactive effect of different nanoparticles, and (iv) Proper dispersion of the nanoparticles in the base fluid, creating micro turbulences. Therefore, in hybrid nanofluids, both nanoparticles compromise their properties and provide better thermo-physical, chemical, and rheological properties within the low cost that makes it preferable over nanofluids for different applications. Stability is the main key factor for the performance of nanofluids in various engineering applications. All the thermo-physical properties of nanofluid are dependent on its stability. The unstability of nanofluid can inhibit its performance in several applications such as heat exchangers, chemical industry applications, enhanced oil recovery etc. The unstability of nanofluid is caused due to the propensity of nanoparticles to form a cluster in the fluid. The nanofluids may be broadly categorized into three groups based on the nanoparticle composition, namely: (i) mono-nanofluids (made from one type of nanoparticles), (ii) hybrid nanofluids containing different nanoparticles, and (iii) hybrid nanofluids consisting one solid covered by a layer of another solid (composite nanoparticles).
The current review emphasizes the impact of nanofluid preparation on the stability of various nanofluids and is described in the following classification; (a) Nanofluid constituent, (b) Nanomaterial synthesis, and (c) Nanofluid synthesis techniques.
2. Literature review
The available literature on the preparation, characterization, and stability of mono/hybrid nanofluids are discussed in three sections. In all section, it summarizes the impact of nanofluid preparation on the stability of various nanofluids and described in the following classification; (a) Nanofluid constituent, (b) Nanomaterial synthesis, and (c) Nanofluid synthesis techniques. Exclusive reviews on the heat transfer, pressure drop characteristics, and energy performance of both double-tube and shell-tube heat exchangers using nanofluids are presented in the third and fourth sections.
2.1 Impact of nanofluid preparation
There are two main approaches to synthesize nanofluids: the single-step method and the two-step method. In the one-step method, nanofluid is prepared directly by dispersing nanoparticles in the base fluid without the requirement of numerous steps such as particle drying, storage, etc. Using this method, the stability of nanofluid exhibits most superior compared to the two-step method. But this technique is not beneficial for large scale because of its high production cost. Therefore, the two-step method is the more effective and generally common method of nanofluid preparation. The foremost disadvantage of this process is the control of particle agglomeration tendency. The common application of wide ultrasonication and stirring is the most frequently used method to control agglomeration. Several forces such as Van der Waal attractive force, gravitational force, buoyancy force, and electrostatic repulsive force are acted which lead to destabilization and form sediments. The Van der Waal attractive force and gravitational force work against the stability of any colloidal suspension. Stability is the main key factor for the performance of nanofluids in various engineering applications. All the thermo-physical properties of nanofluid are dependent on its stability. The unstability of nanofluid can inhibit its performance in several applications such as heat exchangers, chemical industry applications, enhanced oil recovery etc. The unstability of nanofluid is caused due to the propensity of nanoparticles to form a cluster in the fluid. For considering a stable nanofluid, agglomeration propensity has to be removed. Some stability evaluation methods are used in literature i.e., sedimentation and centrifugation method, zeta potential measurement, spectral absorbance and transmittance measurement, and dynamic light scattering. Numerous efforts have been made to prepare long-time stable and homogenous nanofluids using various techniques. The current review emphasizes the impact of nanofluid preparation on the stability of various nanofluids and is described in the following classification; (a) Nanofluid constituent, (b) Nanomaterial synthesis, and (c) Nanofluid synthesis techniques.
2.1.1 Nanofluid constituent
2.1.1.1 nanomaterial type.
There are several types of nanofluids: metallic nanofluids (Al, Ag, Cu, Fe, Au), metal oxide nanofluids (Al 2 O 3 , CuO, Fe 3 O 4 , SiO 2 , TiO 2 , ZnO, etc.), and non-metallic nanofluids (SiC, TiC, graphite, diamond, SWCNT/MWCNT, graphene, etc.). Several studies on the impact of the nanofluid constituents on its stability are shown in Table 1 . Xu et al. [ 4 ] prepared hybrid nanofluids with nanoparticles of different masses added with a small amount of SDBS and PEG into DW and observed that 25% Al 2 O 3 + 75% TiO 2 hybrid nanofluid shows good suspension stability. The zeta potential value for the 25% Al 2 O 3 + 75% TiO 2 hybrid nanofluid is found 42.6 mV indicating high stability. Zeta potential means electrostatic repulsion force between nanoparticles and base fluid. High repulsion force indicates high stability of nanofluid, whereby 30mV is generally considered as a benchmark for a stable nanofluid and excellent nanofluid stability may exceed 60 mV. Some studies investigated the impact of functionalizing the nanoparticles surface which reduces aggregation and improves dispersion. Said et al. [ 5 ] studied the stability of Carbon nanofiber (CNF), Functionalized Carbon nanofiber (F-CNF), Reduced graphene oxide (rGO), and F-CNF/rGO nanofluids. The results indicated that hybrid (FCNF/rGO) nanofluid shows higher stability than as compared to CNF, F-CNF, and rGO nanofluids. Also, the sample of CNF almost completely sedimented on 2nd day as shown in the Figure 1 . It is due to the low charge density on the surface of the CNF nanoparticle which leads to the tendency of agglomeration. Said et al. [ 15 ] used acid treatment of CNF to examine the stability. The zeta potential of 0.02 vol. % F-CNF nanofluid was −42.9 and − 41.8 mV after 2 and 90 days which indicates that the stability was improved while the zeta potential of CNF was −16.3 and − 15.5 mV, indicating a relatively unstable dispersion. One way to achieve long-term stability is to adjust the nanofluid pH, away from the isoelectric point (IEP). Thus, IEP differs from one sample to another. These values were prepared in acidic and alkaline ranges using HCl and NaOH solutions and adjusted by pH meter. Kazemi et al. [ 6 ] used two different nanoparticles (GnP, SiO 2 ) with the same base fluid (water) as well as different pH values (3,6,9, and 12) to study the stability of the nanofluids. The results found that SiO 2 /Water nanofluids have good stability at all pH values, especially for samples with pH >3 and GnP/water achieve better stability at higher pH values. Akhgar and Toghraie [ 9 ] examined the stability of water-based MWCNT and TiO 2 nanofluid at different pH (3, 6,9, and 12). The results observed that the nanofluid containing water/TiO 2 with pH = 9 had more stability than the rest of the samples. On the other hand, MWCNT particles are not dispersed in water and are not stable in any pH without any surfactant. Kazemi et al. [ 21 ] compared the stability of three types of nanofluids, G/Water, SiO 2 /Water, and G-SiO 2 /Water and found that SiO 2 /Water nanofluid shows excellent stability at all pH values while G/Water sustainability is poor in lower pH value. Due to better stability in higher pH values, the CMC surfactant can be used to increase pH by creating a negative charge surface for graphene nanoparticles and developing functional groups. Siddiqui et al. [ 8 ] performed a stability study with metal (Cu), oxide (Al 2 O 3 ), and meta-oxide Cu- Al 2 O 3 ) nanofluid containing the same base fluid (DI water). Al 2 O 3 nanofluid exhibits better stability between 0 and 6 h followed by good stability with little particle settling between 6 and 240 h. Cu nanofluid shows poor dispersion stability after 1 hour of preparation. In case of hybrid nanofluid, nanofluid with optimum mixing ratio exhibits relatively better stability. Muthoka et al. [ 14 ] used PCM-DI water as the base fluid and two different nanoparticles (MgO and MWCNT) to examine the stability. They observed that the stability of MgO and 24 wt% base fluid without surfactant showed poor stability after only 24 h while the functionalized MWCNT nanofluid showed no separation after 24 h. Also, it was concluded that the stability of the nanofluid at low temperatures is increased by the use of surfactant. Alawi et al. [ 16 ] synthesized PEG-GnP, PEG-TGr, Al 2 O 3, and SiO 2 water-based nanofluids. They observed the dispersion stabilities of carbon-based nanofluids and metallic oxides nanofluids for 30days, and the results showed the higher dispersibility of the PEG-GnP, PEG-TGr nanofluids in an aqueous media with very low sedimentation. Akbari and Saidi [ 17 ] observed TiO 2 /DW nanofluid shows good stability as compared to GnP/DW nanofluid. Since graphene is an inherently hydrophobic material and the stability of graphene/water nanofluid is not favorable without any surfactants. Boroomandpour et al. [ 22 ] studied the stability of ternary hybrid nanofluids containing MWCNT-TiO 2 -ZnO/DW-EG (80:20) as well as binary and mono nanofluids. They found that all nanofluids have good stability up to 48 h after fabrication and the addition of CTAB surfactant lead to better stability.
Synopsis of the investigations about the impact of the nanofluid constituents on its stability.

Photographs of vials showing the stability of nanofluids for: (a) 1 st day, (b) 2nd day, (c) 30 days, (d) 45 days, (e) 60 days, and (f)180 days [ 5 ].
From the literature reviews on the preparation of nanofluids with different particles, it is found that the stability of water mono/hybrid nanofluid is strongly dependent on the particle shape and size. It is found that the propensity of aggregation is increased with the reduction in particle size and isoelectric point (pH value) decreases with the decrease in particle size. Therefore, the agglomeration process moves toward lesser pH value. The cylindrical-shaped particles sediment faster than spherical and platelet-shaped particles. High aspect ratio nanoparticles are more susceptible to agglomeration.
2.1.1.2 Surfactant type
Addition of different surfactants such as: Anionic (Sodium Dodecyl Sulfate (SDS), Sodium Dodecyl Benzene Sulfonate (SDBS)), Cationic (Cetyltrimethylammonum Bromide (CTAB)), Non-ionic (Span 80, Tween 20) and polymer (Polyvinyl Pyrrolidone (PVP), Poly Vinyl Alcohol (PVA), Gum Arabic (GA)) during nanofluid preparation is an additional way of controlling particle aggregation. A negatively charged suspension may be obtained by using anionic surfactants (SDC, SDS, and SDBS) while a cationic surfactant (CTAB) may contribute a positive charge. The augmentation in stability will achieve by the coating of surfactant on nanoparticles, which leads to a dominating electrostatic repulsion over the van der Waals force and thus prevent nanoparticles from agglomerating. Also, the stability of the nanofluid can be improved by decreasing the sedimentation velocity of the nanoparticles. According to Stokes law, the sedimentation velocity can be reduced by using nanoparticles with smaller diameters. However, when the nanoparticles diameter decreases, the surface energy will be increased which leads increase possibility of agglomeration. The best way to suppress the agglomeration without disturbing the sedimentation velocity is the usage of surfactants. While surfactant addition is an active way to improve the stability of the nanofluids but surfactants may lead to cause some problems. Surfactants may contaminate the heat transfer media. Surfactants may produce foams while heating and cooling are regular processes in heat exchange systems. Additionally, surfactant molecules attributed to the surfaces of nanoparticles might increase the thermal resistance between the nanoparticles and the base fluid, which may hinder the augmentation of the thermal conductivity.
Xian et al. [ 1 ] used three different surfactants, i.e., SDS, CTAB, and SDBS to stabilize the COOH-TiO 2 hybrid nanofluid. They observed that COOH-TiO 2 hybrid nanofluid with CTAB surfactant exhibited the best surfactant to stabilize this hybrid nanofluid. The visual inspection of sedimentation of nanofluids with different surfactants after 40 days is shown in Figure 2 . Almanassra et al. [ 2 ] compared the effect of different types of surfactants on the stability of CNT/water nanofluids. They investigated with three types of surfactants namely, GA, PVP, and SDS and found that the nanofluids with GA as well as PVP surfactants were more stable for more than 6 months. Gum Arabic can be a promising surfactant for stabilizing the CNT in water-based nanofluids. Cacua et al. [ 3 ] found Al 2 O 3 nanofluid with SDBS at 1 CMC and CTAB at 0.5 CMC were the most stable and unstable nanofluids, respectively. Anionic SDBS provides high repulsive forces between nanoparticles. Ouikhalfan et al. [ 7 ] prepared surface-modified TiO 2 nanofluid with two different surfactants (SDS and CTAB). The quick sedimentation was found in non-treated TiO 2 nanofluid after 24 hours of the preparation as shown in Figure 3 . TiO 2 nanofluid with CTAB showed better stability up to several days while the nanofluid with SDS surfactant shows less but overall better dispersion compared to nanofluid with non-treated TiO 2 . Choi et al. [ 10 ] studied the effect of various surfactants as well as the temperature on the stability of water-based MWCNT nanofluid. They prepared nanofluid with four different surfactants, i.e., SDBS, CTAB, SDS, and TX-100 between the temperatures 10°C–80°C. It was observed that for short-term time period (3 h), nanofluids prepared with SDBS, CTAB, and TX-100 show better stability while for long-term time period (1 month), the SDBS and TX-100 nanofluids have the highest suspension stability. On the account of temperature, TX-100, CTAB, and SDS are not suitable surfactants for nanofluids operating from 10 to 85°C. Das et al. [ 11 ] found TiO 2 (Anatase) with SDS and CTAB show excellent stabilization (stable for exceeding 12 h and 24 h) as compared with nanofluid with SDS and acetic acid surfactant. Kuang et al. [ 13 ] prepared nanofluids by dispersing three nanoparticles (i.e., SiOx, Al 2 O 3 , and TiO 2 ) and five different chemical agents i.e., oleic acid (OA), polyacrylic acid (PAA), a cationic, an anionic, and a nonionic surfactant) in base brine solutions. Nanofluids made with the anionic surfactant made the surface slightly more water wet. The results revealed that SiO x nanofluids exhibit stability in all cases while Al 2 O 3 + PAA and Al 2 O 3 + cationic surfactant show the most stability. In case of TiO 2 nanofluid, TiO 2 + PAA show the most stability among all surfactants. Cacua et al. [ 18 ] used UV–vis spectroscopy to examine the stability of Al 2 O 3 with two different surfactants (SDBS and CTAB). The outcome reveals that the nanofluid with SDBS at 1 CMC and that with CTAB at 0.5 CMC achieved the lowest and highest absorbance variation, respectively. Low absorbance variation over time indicates high nanofluid stability. Etedali et al. [ 19 ] investigated the stability of SiO 2 nanofluids with different surfactants, i.e., SLS, CTAB, and Ps 20 through the Zeta potentials test. The results of the Zeta-potential test found that the maximum surface charge for the nanofluids with SLS, CTAB, and Ps20 surfactants were − 87.4, 74.2, and − 97.9, respectively, confirming the stability conditions.

Visual inspection of sedimentation of nanofluids with different surfactants and ultra-sonication time after 40 days. [ 1 ].

Sediment photograph capturing of the nanofluid with (1) nontreated TiO 2 , (2) CTAB-treated TiO 2 nanofluid, and (3) SDS-treated TiO 2 nanofluid [ 7 ].
2.1.1.3 Base fluid type
Gao et al. [ 12 ] prepared GNP nanofluid with three different base fluids namely, EG, DW, and EG/DW and reported that the stability of nanofluid with EG base fluid is better than that of DW-based nanofluid. Figure 1 shows the visual observation of GNP nanofluid with different base fluids. Giwa et al. [ 20 ] used two-step method to prepare Al 2 O 3 -Fe 2 O 3 hybrid nanofluid with two type of base fluid viz., DW and EG/DW. SDS and NaDBS were used as a surfactant. Using UV-visible spectrophotometer, they found DW-based Al 2 O 3 -Fe 2 O 3 were relatively more stable than the EG–DW Al 2 O 3 -Fe 2 O 3 hybrid nanofluid. The absorbance value of the DW-based Al 2 O 3 -Fe 2 O 3 displayed better horizontal straight lines than those of the EG–DW Al 2 O 3 -Fe 2 O 3.
2.2 Nanomaterial synthesis
Ding et al. [ 23 ] prepared the functionalized graphene (ESfG) by adding the graphite powder into the milling jar with steel balls of smaller diameter and the system was filled with SO 3 gas. After removing metallic impurities, the samples were then freeze-dried for 36 hours at −120°C to yield black powder as the final ESfG. The prepared ESfG was stable for several months in water. The sulfonic-acid groups can bond with carbon atoms at the edge of graphite which tends to enhance the stability of ESfG water-based nanofluids. Gul and Firdous [ 24 ] synthesized the graphene oxide nanosheet by the oxidation of graphite using the Hummers method as shown in Figure 4 . In this method the graphite powder was mixed with NaNO 3 , H 2 SO 4, and KMnO 4 and stirred in an ice bath for about 30 min. Finally, the mixture was sonicated and added H 2 O 2 and HCl to quench the reaction and get light yellow graphite oxide. The results found that the highly dispersible nature of GO in water which is fruitful for the preparation of GO nanofluid for multipurpose applications. Li et al. [ 25 ] introduced the β-cyclodextrin(β-CD) onto the surface of MWCNTs by a simple chemical synthesis method. It was found that the introduction of β-CD onto the surface of MWCNTs exhibited better stability of nanofluids. The possibility of aggregation between CD-CNTs is significantly decreased due to the Vander Waals force or steric interrupts between β-CD. Rahimi et al. [ 26 ] treated the hydrophilization of MWCNTs with different concentrated acids. They added the raw MWCNTs into the mixture of H 2 SO 4 and HNO 3 and the mixture was refluxed for 3 hours. The acid-treated MWCNTs were obtained after washing with DI water and dried for 4 hours. Acid-treated MWCNTs suspensions display good stability in water. This is due to the generation of hydroxyl groups on nanotube surfaces. Vozniakovskii et al. [ 27 ] synthesized a hybrid nanomaterial composed of nanodiamonds-multi-walled carbon nanotubes (DND-CNT) using a catalyst chemical vapor deposition (CCVD) method. The results showed that DND-CNT hybrid suspension was stable up to 100 hours while the initial DND began to precipitate after 1 hour. The stability of DND-CNT hybrid particles in water is explained by the opening of a previously closed surface covered with groups with a labile proton, which ensures the stability of the particles of the hybrid material in water.

Synthetic route of graphene oxide by hummers method [ 24 ].
2.3 Nanofluid synthesis technique
Numerous nanofluid stabilization techniques are used for reducing the cluster size of nanoparticles i.e. ultrasonic vibration and ball milling etc. The role of ultrasonication is to break the nanoparticle cluster and create a homogenous mixture. Ultrasonic vibration can be employed in two ways; (a) indirect method (ultrasonic bath), and (b) direct method (probe sonicator). Among these two methods, the probe sonicator offers better results in terms of breaking the particle cluster and lowering the average cluster size. Several studies on the impact of the nanofluid synthesis technique on its stability are shown in Table 2 . Asadi et al. [ 28 ] used two-step method to prepare TiO 2 -CuO hybrid nanofluid. They applied a magnetic stirrer for 1 hour in order to distribute the nanomaterial in the base fluid. Moreover, for breaking the clusters and uniformly distributing the nanoparticles in the base fluid, a probed ultrasonic device was applied for 1 hour. The DLS results ensured the nanoparticles exist in the base fluid, and the phenomenon of agglomeration did not happen. Chen et al. [ 29 ] investigated the impact of sonication time on the stability of the Al 2 O 3 /liquid paraffin nanofluid. They used two-step method with varying the magnetic stirrer time from 10 to 40 minutes and sonication time from 1 to 4 hours. It was found that nanofluids prepared using shorter sonication times show stability for a minimum of 1 month. When increase in sonication time, it breaks the bond between the nano additives and the surfactant which leads to be unstable. Asadi et al. [ 30 ] varied the sonication time from 10 to 80 minutes to measure the stability of MWCNT/water nanofluid. They reported that after the 30th day, the samples subjected to 10, 20, 40, and 60 minutes of ultrasonication showed good stability while the samples subjected to longer time ultrasonication showed the amount of sedimentation leads to having agglomerated particles. Ranjbarzadeh et al. [ 31 ] used magnetic stirrer for 1 hour to mix the SiO 2 nanoparticles in the base fluid and then sonicated for 60 minutes. By visual observation, the result found that no sediments were formed after 6 months. Aberoumand and Jafarimoghaddam [ 32 ] prepared Ag-WO 3 /Transformer oil nanofluid using the first step method. They applied Electrical Explosion Wire (EEW) to prepare the nanofluid. The Zeta potential of applied nanofluids in three different concentrations of 1%, 2%, and 4% was measured. The results indicate the excellent stability of applied hybrid nanofluids. Using the same EEW method, Aberoumand et al. [ 34 ] prepared Ag/water nanofluid and found that with EEW method, the nanofluid maintained their stability for a long time. Dalkılıç et al. [ 33 ] prepared CNT-SiO 2 /DW using two-step methods and the mixture was sonicated for 3 hours. It was found that the sedimentation was not observed up to 48 hours. The raw CNT particles showed poor dispersion stability in the base fluid. SiO 2 particles support and increase the stability of CNTs particles in water. Tests showed that CNT particles exhibit less stability in water without SiO 2 particles and surfactants. Kakavandi and Akbari [ 35 ] used DLS test to examine the distribution of the MWCNT and SiC nanoparticles in the hybrid nanofluids. The results indicated acceptable stability of nanofluids. The hybrid nanofluid was magnetically stirred for 1 hour and then sonicated for 45 minutes. Keyvani et al. [ 36 ] used Ce 2 O/EG nanofluid to examine the stability. The nanofluid was stirred and then exposed to ultrasonic waves for 2 and 7 hours, respectively. The sedimentation of particles was found after 2 weeks. Nanofluid with a higher concentration of particles, nanoparticles led to agglomerate; therefore, the stability of the nanofluid weakened. It was also reported that the stability of the prepared nanofluid with a lower volume fraction of nanoparticles was stable for a longer period of time compared to the nanofluids with a higher volume fraction [ 39 ].
Synopsis of the investigations about the impact of the nanofluid synthesis technique on its stability.
Liu et al. [ 37 ] prepared rGO by the reduction of graphene oxide with L-ascorbic acid as a reductant in an aqueous solution. To prepare rGO, the graphene oxide solution was dispersed in DI water and ultrasonicated for 1 hour. NH 3 -water was then added to control the pH to 10 with sonication for 30 minutes. L-ascorbic acid was added and the mixture was maintained at 95°C for 3 hours for the completion of the reaction. The rGO solution was filtered to obtain rGO on the filter paper. Finally, rGO nanofluids were prepared by sonicating the filtered powder in a certain amount of DI water. The whole process is shown in Figure 5 . The rGO nanofluids exhibited good stability for 10 day without the addition of other dispersants. Ranjbarzadeh et al. [ 38 ] conducted a test to study of pH effects on the stability in acid and alkaline spectrums for GO-SiO 2 /Water hybrid nanofluids. The results observed that the nanofluid, due to the presence of functional groups on the surface of its nanoparticles, shows acceptable stability in all spectrums; however, in the long term, nanofluids with pH > 7 showed better stability. Zeng and Xuan [ 40 ] sonicated the MWCNT-SiO 2 /Ag binary nanofluids for 1 hour and reported that the stability of the binary nanofluid sustained the dispersion stability for 7 days. Gulzar et al. [ 41 ] dispersed hybrid nanopowder (Al 2 O 3 -TiO 2 ) in Therminol-55 oil and the mixture was subjected to high shear stirring at 2500 rpm using a magnetic stirrer for 4 hours. The mixture was then sonicated for 2 hours using a high energy probe sonicator. Oleic acid was used as a surfactant as of better miscibility with Therminol-55 oil. They observed that the value of zeta potential declines with the rise in concentration which may cause agglomeration adequately after a long time. The surfactant which changes the surface charge and increases the repulsive forces between the nanoparticles also contributes to improved stability. Same way, Alarifi et al. [ 42 ] used magnetic stirring for 2 hours and sonicated for 1 hour to prepare a long-term stable MWCNT-TiO 2 /oil nanofluid. The stability of the prepared hybrid nanofluid was observed over 14 days and no sedimentation was found. Akram et al. [ 43 ] checked the stability of CGNP–water nanofluid by zeta potential at different pH values. They prepared this nanofluid after the sonication for 60 minutes and observed that the CGNP nanofluid had high negative values (− 4.42 mV to −49.5 mV) within the pH variations from 1.84 to 10.55. The zeta potential values for the CGNP nanofluids are far from the isoelectric point (i.e., point of zero charges), which indicates that this pH range (2.8–10.55) results in strong electric repulsion forces. Sharafeldin and Grof [ 44 ] did sonication of WO 3 /water nanofluid continuously for about 75 minutes to break the agglomeration between the nanoparticles which leads to well disperse the particles into water. The mean zeta potential value for WO 3 /water nanofluid was −43.12 and a little decrease in the values was observed over the period of 7 days. MWCNT nanofluids suffer from low dispersion and short-time stability which inhibit their practical application. Chen et al. [ 45 ] used a novel method, i.e., a one-pot method by stirred media mill technique. In this method, raw MWCNTs nanoparticles were treated by ball milling to change their morphology, length, and specific surface first. After centrifuging, dry nanoparticles were purified by acid treatment to improve their dispersion in the solution. Thus, the resulting powder was dispersed again in base fluids by ultrasonication and meanwhile, surfactant was added to improve dispersion. The results showed that the milling-treated MWCNT nanofluid exhibited better stability as compared to raw MWCNT and Acid treated MWCNT nanofluid. Ali et al. [ 46 ] investigated the stability of dispersed Al nanoparticles in base fluid (water) prepared by the conventional and the controlled bath temperature two-step methods. The sonication process was taken the same for 4 hours in the range of 10–60°C. The results revealed that the sedimentation behavior of the nanofluids prepared through the controlled bath temperatures of less than 30°C was of dispersed sedimentation type, while those produced by the conventional method and the fixed temperatures of 30°C and higher were of flocculated sedimentation type. Furthermore, increasing the controlled sonication temperature led to an increase in the settling process of the sediments. Also, the rise in nanoparticle concentration was seen to reduce the variation in sedimentation height ratio between the fixed temperature samples. A comparison between the two preparation methods was shown that the 30°C nanofluids had better short- and long-term stability than the conventionally produced suspensions. Mahbubul et al. [ 47 ] varied the sonication time from 1 to 5 hours to study the effect of sonication time on the stability of the 0.5 vol% Al 2 O 3 nanofluids. They observed that with low sonication time or no sonication, the sedimentation rate is higher. It can be concluded that longer ultrasonication reduces the sedimentation of nanoparticles and hence, increases the stability of nanofluids. Mahyari et al. [ 48 ] used probe-type ultrasonicator to achieve the stability of GO-SiC/water-EG hybrid nanofluid. DLS test results with different patterns approved acceptable stability of the nanofluid. Chen et al. [ 49 ] prepared the saline water based magnetic MWCNT nanofluids at different mass concentration from 0 to 0.04 wt% by two-step method. A mechanical stirrer was used at 500 rpm continuously for 30 minutes to mix nanoparticles and water and then the mixture was sonicated thoroughly for 2 hours. Magnetic MWCNTs nanofluids showed high stability in 1000 ppm saline water, and when the solution salinity increased, the original colloidal structure would be destroyed by charge ion. Therefore, the salt-resisting surfactant was added to reinforce the double-layer repulsion and remained the system stable. Okonkwo et al. [ 50 ] prepared the Al 2 O 3 -Fe/Water using two-step methods and measured the stability of nanofluid through the Zeta potential test. Hybrid nanofluid was found significantly more stable at pH values of 12 when compared at any other pH value. Terueal et al. [ 51 ] performed using the liquid phase exfoliation technique starting with bulk MoSe 2 to prepare stable nanofluids. Triton X-100 was used as a surfactant. The suspension underwent sonication in an ultrasound bath for 4 hours with two different frequencies: 80 kHz and 130 kHz. The samples were then centrifuged at 1000 rpm for 10 minutes and again at 4000 rpm for 10 minutes. The results showed that the nanofluid prepared with the frequency of 80 kHz and 130 kHz show the highest extinction coefficient values after 30 days. Higher extinction coefficient values mean the highest amount of nanomaterial in suspension. Li et al. [ 52 ] analyzed the stability of SiO 2 -oleic acid/liquid paraffin nanofluid through the Zeta potential test. The nanofluid was prepared with two-step methods (magnetic stirrer for 30 minute and then sonicated for 1 hour). It was found that the large numbers of SiO 2 nano-sized particles possess maximum value for the total count at values less than −40 mV indicating high stability of SiO2 nano-sized particles in liquid paraffin. Geng et al. [ 53 ] used the DLS test to study the stability of ZnO-MWCNT/Oil nanofluid and found that the nanoparticles are in the nanoscale after the preparation of nano-oil. Li et al. [ 54 ] produced SiO 2 /EG nanofluids by the two-step method with a mass fraction of 0.005–5%. The zeta potential value of the nanofluid was found −56.28 mV and claimed that the nanofluid is stable. Greater the number of particles with a smaller diameter, the higher the probability of stability. Nanofluid cluster formation may lead to the larger diameter of the nanoparticles. As the number of clusters increases, the fluid stability will decrease.

Schematic graph of rGO nanofluids preparation.
3. Conclusion
From the literature, it can be concluded that the stability of suspension of nanoparticles in the base fluid is improved when the nanofluid is synthesized by the one-step method as compared to the two-step method but the preparation of nanofluids by one-step method is difficult and expensive relative than two-step method. The literature also reveals that with low sonication time or no sonication, the sedimentation rate is higher. It can be concluded that longer ultrasonication reduces sedimentation of nanoparticles and hence, increases the stability of nanofluids. There are major tasks, which need to be focused on for selection of mono/hybrid nanofluids and their fabrication process, the stability of hybrid nanofluids. The stabilized nanofluids and their characteristics can increase the heat exchange rate of heat exchangers which are generally used in pipeline engineering. In order to help newcomers and researchers in this field recognize the potential research gap, this review study seeks to provide the latest research and development on stable nanofluids and their applications in pipeline engineering. Due to the lack of understanding of the mechanism of nanofluid at the atomic level, many experimental studies are needed to consider several important issues such as particle migration, agglomeration, and stability.
- 1. Xian HW, Sidik NAC, Saidur R. Impact of different surfactants and ultrasonication time on the stability and thermophysical properties of hybrid nanofluids. International Communications in Heat and Mass Transfer. 2020; 110 :104389
- 2. Almanassra IW, Manasrah AD, Al-Mubaiyedh UA, Al-Ansari T, Malaibari ZO, Atieh MA. An experimental study on stability and thermal conductivity of water/CNTs nanofluids using different surfactants: A comparison study. Journal of Molecular Liquids. 2019; 19 :30397-30396
- 3. Cacua K, Buitrago-Sierra R, Pabon E, Gallego A, Zapata C, Herrera B. Nanofluids stability effect on a thermosyphon thermal performance. International Journal of Thermal Sciences. 2020; 153 :106347
- 4. Xu Q, Liu L, Feng J, Qiao L, Yu C, Shi W, et al. A comparative investigation on the effect of different nanofluids on the thermal performance of two-phase closed thermosyphon. International Journal of Heat and Mass Transfer. 2020; 149 :119189
- 5. Said Z, Abdelkareem MA, Rezk H, Nassef AM, Atwany HZ. Stability, thermophysical and electrical properties of synthesized carbon nanofiber and reduced-graphene oxide-based nanofluids and their hybrid along with fuzzy modeling approach. Powder Technology. 2020; 364 :795-809
- 6. Kazemi I, Sefid M, Afrand M. A novel comparative experimental study on rheological behavior of mono & hybrid nanofluids concerned graphene and silica nano-powders: Characterization, stability and viscosity measurements. Powder Technology. 2020; 366 :216-229
- 7. Ouikhalfan M, Labihi A, Belaqziz M, Chehouani H, Benhamou B, Sarı A, et al. Stability and thermal conductivity enhancement of aqueous nanofluid based on surfactant-modified TiO2. Journal of Dispersion Science and Technology. 2020; 41 :374-382
- 8. Siddiqui F, Tso C, Chan K, Fu S, Chao CY. On trade-off for dispersion stability and thermal transport of Cu-Al2O3 hybrid nanofluid for various mixing ratios. International Journal of Heat and Mass Transfer. 2019; 132 :1200-1216
- 9. Akhgar A, Toghraie D. An experimental study on the stability and thermal conductivity of water-ethylene glycol/TiO2-MWCNTs hybrid nanofluid: Developing a new correlation. Powder Technology. 2018; 338 :806-818
- 10. Choi TJ, Jang SP, Kedzierski M. Effect of surfactants on the stability and solar thermal absorption characteristics of water-based nanofluids with multi-walled carbon nanotubes. International Journal of Heat and Mass Transfer. 2018; 122 :483-490
- 11. Das PK, Mallik AK, Ganguly R, Santra AK. Stability and thermophysical measurements of TiO2 (anatase) nanofluids with different surfactants. Journal of Molecular Liquids. 2018; 254 :98-107
- 12. Gao Y, Wang H, Sasmito AP, Mujumdar AS. Measurement and modeling of thermal conductivity of graphene nanoplatelet water and ethylene glycol base nanofluids. International Journal of Heat and Mass Transfer. 2018; 123 :97-109
- 13. Kuang W, Saraji S, Piri M. A systematic experimental investigation on the synergistic effects of aqueous nanofluids on interfacial properties and their implications for enhanced oil recovery. Fuel. 2018; 220 :849-870
- 14. Muthoka MJ, Xuelai Z, Xioafeng X. Experimental investigation on supercooling, thermal conductivity and stability of nanofluid based composite phase change material. Journal of Energy Storage. 2018; 17 :47-55
- 15. Said Z, Allagui A, Abdelkareem MA, Alawadhi H, Elsaid K. Acid-functionalized carbon nanofibers for high stability, thermoelectrical and electrochemical properties of nanofluids. Journal of Colloid and Interface Science. 2018; 18 :30190-30195
- 16. Alawi OA, Mallah AR, Kazi SN, Sidik NAC, Najafi G. Thermophysical properties and stability of carbon nanostructures and metallic oxides nanofluids. Journal of Thermal Analysis and Calorimetry. 2019; 135 :1545-1562
- 17. Akbari A, Saidi MH. Experimental investigation of nanofluid stability on thermal performance and flow regimes in pulsating heat pipe. Journal of Thermal Analysis and Calorimetry. 2019; 135 :1835-1847
- 18. Cacua K, Ordoñez F, Zapata C, Herrera B, Pabón E, Buitrago-Sierra R. Surfactant concentration and pH effects on the zeta potential values of alumina nanofluids to inspect stability. Colloids and Surfaces, A: Physicochemical and Engineering Aspects. 2019; 583 :123960
- 19. Etedali S, Afrand M, Abdollahi A. Effect of different surfactants on the pool boiling heat transfer of SiO2/deionized water nanofluid on a copper surface. International Journal of Thermal Sciences. 2019; 145 :105977
- 20. Giwa SO, Sharifpur M, Goodarzi M, Alsulami H, Meyer JP. Influence of base fluid, temperature, and concentration on the thermophysical properties of hybrid nanofluids of alumina–ferrofluid: Experimental data, modeling through enhanced ANN, ANFIS, and curve fitting. Journal of Thermal Analysis and Calorimetry. 2020; 6 :4149-4167
- 21. Kazemi I, Sefid M, Afrand M. Improving the thermal conductivity of water by adding mono & hybrid nano-additives containing graphene and silica: A comparative experimental study. International Communications in Heat and Mass Transfer. 2020; 116 :104648
- 22. Boroomandpour A, Toghraie D, Hashemian M. A comprehensive experimental investigation of thermal conductivity of a ternary hybrid nanofluid containing MWCNTs- titania-zinc oxide/water water ethylene (80:20) as well as binary and mono nanofluids. Synthetic Metals. 2020; 268 :116501
- 23. Ding J, Zhao H, Yu H. Graphene nanofluids based on one-step exfoliation and edge-functionalization. Carbon. 2021; 171 :29-35
- 24. Gul T, Firdous K. The experimental study to examine the stable dispersion of the graphene nanoparticles and to look at the GO–H2O nanofluid flow between two rotating disks. Applied Nanoscience. 2018; 8 :1711-1727
- 25. Li X, Zou C, Chen W, Lei X. Experimental investigationof β-cyclodextrinmodified carbon nanotubesnanofluids for solar energy systems: Stability, optical properties and thermal conductivity. Solar Energy Materials & Solar Cells. 2016; 157 :572-579
- 26. Rahimi K, Riahi S, Abbasi M. Effect of host fluid and hydrophilicity of multi-walled carbon nanotubes on stability and CO2 absorption of amine-based and water-based nanofluids. Journal of Environmental Chemical Engineering. 2019; 19 :30703
- 27. Vozniakovskii AA, Kol’tsova TS, Voznyakovskii AP, Kumskov AL, Kidalov SV. Powder hybrid nanomaterial: Detonation nanodiamonds – Carbon nanotubes and its stable reversible water nanofluids. Journal of Colloid and Interface Science. 2020; 565 :305-314
- 28. Asadi A, Alarifi IM, Foong LK. An experimental study on characterization, stability and dynamic viscosity of CuO-TiO2/water hybrid nanofluid. Journal of Molecular Liquids. 2020; 307 :112987
- 29. Chen Z, Shahsavar A, Al-Rashed AA, Afrand M. The impact of sonication and stirring durations on the thermal conductivity of alumina-liquid paraffin nanofluid: An experimental assessment. Powder Technology. 2020; 360 :1134-1142
- 30. Asadi A, Alarifid IM, Ali V, Nguyen HM. An experimental investigation on the effects of ultrasonication time on stability and thermal conductivity of MWCNT-water nanofluid: Finding the optimum ultrasonication time. Ultrasonics Sonochemistry. 2019; 58 :104639
- 31. Ranjbarzadeh R, Kazerouni AM, Bakhtiari R, Asadi A, Afrand M. An experimental study on stability and thermal conductivity of water/silica nanofluid: Eco-friendly production of nanoparticles. Journal of Cleaner Production. 2018; 18 :32938
- 32. Aberoumand S, Jafarimoghaddam A. Tungsten (III) oxide (WO3) – Silver/transformer oil hybrid nanofluid: Preparation, stability, thermal conductivity and dielectric strength. Alexandria Engineering Journal. 2018; 57 :169-174
- 33. Dalkılıç AS, Yalçın G, Küçükyıldırım BO, Öztuna S, Eker AA, Jumpholkul C, et al. Experimental study on the thermal conductivity of water-based CNT-SiO2 hybrid nanofluids. International Communications in Heat and Mass Transfer. 2018; 99 :18-25
- 34. Aberoumand S, Ghamari S, Shabani B. Energy and exergy analysis of a photovoltaic thermal (PV/T) system using nanofluids: An experimental study. Solar Energy. 2018; 165 :167-177
- 35. Kakavandi A, Akbari M. Experimental investigation of thermal conductivity of nanofluids containing of hybrid nanoparticles suspended in binary base fluids and propose a new correlation. International Journal of Heat and Mass Transfer. 2018; 124 :742-751
- 36. Keyvani M, Afrand M, Toghraie D, Reiszadeh M. An experimental study on the thermal conductivity of cerium oxide/ethylene glycol nanofluid: Developing a new correlation. Journal of Molecular Liquids. 2018; 2018 :30794
- 37. Liu X, Wang X, Huang J, Cheng G, He Y. Volumetric solar steam generation enhanced by reduced graphene oxide nanofluid. Applied Energy. 2018; 220 :302-312
- 38. Ranjbarzadeh R, Akhgar A, Musivand S, Afrand M. Effects of graphene oxide’silicon oxide hybrid nanomaterials on rheological behavior of water at various time durations and temperatures: Synthesis, preparation and stability. Powder Technology. 2018; 2018 :30406
- 39. Sharafeldin M, Gróf G. Experimental investigation of flat plate solar collector using CeO2-water nanofluid. Energy Conversion and Management. 2018; 155 :32-41
- 40. Zeng J, Xuan Y. Enhanced solar thermal conversion and thermal conduction of MWCNT-SiO2/Ag binary nanofluids. Applied Energy. 2018; 212 :809-819
- 41. Gulzar O, Qayoum A, Gupta R. Experimental study on stability and rheological behavior of hybrid Al2O3-TiO2 Therminol-55 nanofluids for concentrating solar collectors. Powder Technology. 2019; 352 :436-444
- 42. Alarifi IM, Alkouh AB, Ali V, Nguyen HM, Asadi A. On the rheological properties of MWCNT-TiO2/oil hybrid nanofluid: An experimental investigation on the effects of shear rate, temperature, and solid concentration of nanoparticles. Powder Technology. 2019; 355 :157-162
- 43. Akram N, Sadri R, Kazi SN, Ahmed SM, Zubir MNM, Ridha M, et al. An experimental investigation on the performance of a flat-plate solar collector using eco-friendly treated graphene nanoplatelets–water nanofluids. Journal of Thermal Analysis and Calorimetry. 2019; 138 :609-621
- 44. Sharafeldin M, Grof G. Efficiency of evacuated tube solar collector using WO3/Water nanofluid. Renewable Energy. 2019; 134 :453-460
- 45. Chen W, Zou C, Li X. Application of large-scale prepared MWCNTs nanofluids in solar energy system as volumetric solar absorber. Solar Energy Materials and Solar Cells. 2019; 200 :109931
- 46. Ali N, Teixeira JA, Addali A. Aluminum nanofluids stability: A comparison between the conventional two-step fabrication approach and the controlled sonication bath temperature method. Journal of Nanomaterials. 2019:3930572, https://doi.org/10.1155/2019/3930572
- 47. Mahbubul I, Elcioglu E, Amalina M, Saidur R. Stability, thermophysical properties and performance assessment of alumina–water nanofluid with emphasis on ultrasonication and storage period. Powder Technology. 2019; 345 :668-675
- 48. Mahyari AA, Karimipour A, Afrand M. Effects of dispersed added Graphene Oxide-Silicon Carbide nanoparticles to present a statistical formulation for the mixture thermal properties. Physica A. 2019; 521 :98-112
- 49. Chen W, Zou C, Li X, Liang H. Application of recoverable carbon nanotube nanofluids in solar desalination system: An experimental investigation. Desalination. 2019; 451 :92-101
- 50. Okonkwo EC, Wole-Osho I, Kavaz D, Abid M. Comparison of experimental and theoretical methods of obtaining the thermal properties of alumina/iron mono and hybrid nanofluids. Journal of Molecular Liquids. 2019; 292 :111377
- 51. Teruel M, Aguilar T, Martínez-Merino P, Carrillo-Berdugo I, Gallardo-Bernal JJ, Gómez-Villarejo R, et al. 2D MoSe2-based nanofluids prepared by liquid phase exfoliation for heat transfer applications in concentrating solar power. Solar Energy Materials and Solar Cells. 2019; 200 :109972
- 52. Li Z, Asadi S, Karimipour A, Abdollahi A, Tlili I. Experimental study of temperature and mass fraction effects on thermal conductivity and dynamic viscosity of SiO 2 -oleic acid/liquid paraffin nanofluid. International Communications in Heat and Mass Transfer. 2020; 110 :104436
- 53. Geng Y, Al-Rashed AAAA, Mahmoudi B, Alsagri AS, Shahsavar A, Alebizadehsardari P. Characterization of the nanoparticles, the stability analysis and the evaluation of a new hybrid nano-oil thermal conductivity. Journal of Thermal Analysis and Calorimetry. 2020; 139 :1553-1564
- 54. Li Y, Kalbasi R, Nguyen Q, Afrand M. Effects of sonication duration and nanoparticles concentration on thermal conductivity of silica-ethylene glycol nanofluid under different temperatures: An experimental study. Powder Technology. 2020; 367 :464-473
© 2022 The Author(s). Licensee IntechOpen. This chapter is distributed under the terms of the Creative Commons Attribution 3.0 License , which permits unrestricted use, distribution, and reproduction in any medium, provided the original work is properly cited.
Continue reading from the same book
Pipeline engineering.
Edited by Sayeed Rushd
Published: 01 March 2023
By Satoru Hashimoto
66 downloads
By Mohamed Mohamed Azzam
360 downloads
By Milad Rezvani Rad and Andre McDonald
162 downloads
- Open access
- Published: 20 May 2015
Performance evaluation of nanofluids in solar energy: a review of the recent literature
- Navid Bozorgan 1 &
- Maryam Shafahi 2
Micro and Nano Systems Letters volume 3 , Article number: 5 ( 2015 ) Cite this article
20k Accesses
66 Citations
1 Altmetric
Metrics details
Utilizing nanofluid as an absorber fluid is an effective approach to enhance heat transfer in solar devices. The purpose of this review is to summarize the research done on the nanofluids’ applications in solar thermal engineering systems in recent years. This review article provides comprehensive information for the design of a solar thermal system working at the optimum conditions. This paper identifies the opportunities for future research as well.
Introduction
Energy is an important entity for the economic development of any country. On the other hand, fossil fuels meeting a great portion of the energy demand are scarce and their availability is decreasing continously. Nowadays, solar systems play an important role in the production of energy from renewable sources by converting solar radiation into useful heat or electricity. Considering the environmental protection and great uncertainty over future energy supplies, solar energy is a better alternative energy form in spite of its its slightly higher operation costs. Heat transfer enhancement in solar devices is one of the significant issues in energy saving and compact designs. One of the effictive methods is to replace the working fluid with nanofluids as a novel strategy to improve heat transfer characteristics of the fluid. More recently reserachers have become interested in the use of nanofluids in collectors, water heaters, solar cooling systems, solar cells, solar stills, solar absorption refrigeration systems, and a combination of different solar devices due to higher thermal conductivity of nanofluids and the radiative properties of nanoparticle. How to select suitable nanofluids in solar applications is a key issue. The effectiveness of nanofluids as absorber fluids in a solar device strongly depends on the type of nanoparticles and base fluid, volume fraction of nanoparticles, radiative properties of nanofluids, temperature of the liquid, size and shape of the nanoparticles, pH values, and stability of the nanofluids [ 1 ]. It was found that only a few review papers have discussed the capability of nanofluids to enhance the performance of solar systems [ 2 - 5 ].This paper compiles recent research in this field and identifies many issues that are open or even not commenced to investigate. It is authors’ hope that this review will be useful to determine the effectiveness of nanofluids in solar applications.
Literature review of recent years
Using nanofluids in solar collectors, role of nanoparticles.
Gan et al. [ 6 ] experimently showed that the radiation absorption of Al 2 O 3 nanofluids is less than Aluminuim nanofluids. For nanofluids with Al 2 O 3 particles, the situation is different because of the different optical properties of Al 2 O 3 . The weak radiation absorption of Al 2 O 3 nanoparticles will not result in significant localized convective heat transfer from the particles to the base fluids. The use of Al 2 O 3 /water nanofluid as coolant was simulated for a silicon solar cell using the finite element method by Elmir et al. [ 7 ]. They considered the solar panel as an inclined cavity with a slope of 30°. Application of nanofluids increased the average Nusselt number and rate of cooling. They reported 27% enhancement in the heat transfer rate for 10% alumina nanofluid at Re = 5.
Luo et al. [ 8 ] simulated the performance of a DAC solar collector with nanofluids using a 2D model by solving the radiative transport equations of particulate media and combining conduction and convection heat transfer equations. The nanofluid flows horizontally from right to left in a steady-state solar collector covered with a glass plate. A solar radiation simulator is used to validate their model. They prepared nanofluids by dispersing and oscillating TiO 2 , Al 2 O 3 , Ag, Cu, SiO 2 , graphite nanoparticles, and carbon nanotubes into Texatherm oil. Their results show that the use of nanofluid in solar collector can improve the outlet temperature and efficiency. They also found that the efficiency of most nanofluids are similar and larger than that of oil, except for TiO 2 .
Rahman et al. [ 9 ] performed a numerical study for a triangular shape solar collector with nanofluids by Galerkin weighted residual finite element method for a wide range of Grashof numbers (Gr). The corrugated bottom is kept at a constant high temperature and the side walls of the triangular enclosure are kept at a low temperature as seen in Figure 1 . It is assumed that both fluid phase and nanoparticles are in thermal equilibrium and there is no slip between them. Nanofluid is Newtonian and incompressible, and flow is laminar and unsteady. Constant thermophysical properties are considered for the nanofluid except for the density variation in the buoyancy forces determined by using the Boussinesq approximation. Nevertheless, they have not mentioned the particle’s diameters. The authors concluded that high value of both Gr and solid volume fraction confirms better heat transfer through convection and conduction. Results showed 24.28% improvement for Gr = 10 6 at 10% volume fraction of copper particles. For lower values of Gr number, conduction is the primary mode of heat transfer for any value of solid volume fractions. The results showed that the convective heat transfer performance is better when the solid volume fraction is kept at 0.05 or 0.08. This study also showed that cu-water nanofluid is the best nanofluid for the augmentation of heat transfer.
(a) Schematic of the triangular shape collector (b) 3D view of a solar thermal collector filled with nanofluid [ 9 ].
Faizal et al. [ 10 ] investigated the thermal performance of nanofluid solar collector and its contribution size reduction to estimate the cost saving. Their findings indicated that efficiency of solar collector with nanofluids is calculated by the function of working fluid density, specific heat and mass flow rates. The results confirmed that higher density and lower specific heat of nanofluids offers higher thermal efficiency than water and can reduce the solar collector area about 25.6%, 21.6%, 22.1% and 21.5% for CuO, SiO 2 , TiO 2 and Al 2 O 3 nanofluids as seen in Figure 2 . Hence, it will reduce the weight, energy and cost to manufacture the collector. The average value of 220 MJ embodied energy can be saved for each collector, 2.4 years payback period can be achieved and around 170 kg less CO 2 emissions will be the result of using nanofluid based solar collector compared to a conventional one. Environmental damage cost is also lower with the nanofluid based solar collector.
Percentage of size reduction for solar collector by applying different nanofluids.
Parvin et al. [ 11 ] numerically investigated the effects of the nanoparticle volume fraction (ϕ = 0%, 1%, 3%, 5% and 7%) and the Reynolds number (Re = 200, 400, 600, 800 and 1000) on the temperature distribution, rate of entropy generation, and collector efficiency. The working fluid was incompressible Cu-water nanofluid under a laminar regime. Their findings were as follows: a) Increasing the particles concentration raises the fluid viscosity and decreases the Reynolds number and consequently decreases heat transfer. b) It is important to find the optimum volume fraction of nanoparticle for each application. c) The collector efficiency can be enhanced nearly 2 times by using Ag-water and Cu-water nanofluids with concentration of 3% as seen in Figure 3 d) The entropy generation is enhanced up to ϕ = 3% as seen in Figure 3 . After this level, adding more nanoparticles makes no changes in mean entropy generation.
Collector efficiency (η), mean entropy generation (S) and Bejan number (Be) at various concentrations.
Ladjevardi et al. [ 12 ] numerically studied the effects of using nanofluid on the performance of a solar collector as seen in Figure 4 considering the different diameter and volume fractions of graphite nanoparticles. They observed that in the infrared domain, the water optical characteristics are dominant while in the UV and visible ranges extinction coefficients are dependent on nanoparticle volume fractions. The extinction coefficient is calculated from the absorption and scattering efficiencies in this research. Their numerical results showed that nanofluid collector thermal efficiency increases about 88% compared with the one in pure water collector with the inlet temperature of 313 K. It also can be increased to 227% with the inlet temperature of 333 K.
Schematic of volumetric solar collector.
Filho et al. [ 13 ] studied silver nanoparticles as direct sunlight absorbers for solar thermal applications. Their results showed that the maximum stored thermal energy increases by 52%, 93% and 144% for silver particle concentration of 1.62, 3.25 and 6.5 ppm respectively due to the good photothermal conversion properties of silver nanoparticles. They also observed that the influence of particle concentration on the specific absorption rate (SAR) is only discernable at the initial heating period. It was concluded that reduction in the SAR at higher particle loadings (65 and 650 ppm) might be the result of: (i) The formation of agglomerates and reduction in the intensity of the sunlight into the fluid due to the deposited particles on the surface, (ii) The difference in the absorption efficiency of each particle at different fluid depth, (iii) The heat leak through radiation may become strengthened as the particle concentration exceeds a certain value as seen in Figures 5 , 6 and 7 .
Experimental system: (a) a schematic illustration and (b) a snapshot of the system under direct sunlight on top of a roof.
Comparison of the ratio of stored thermal energy at different concentrations (where b and u refer to thermocouples located at the bottom and upper positions respectively).
Specific absorption rate of silver nanoparticles (where b and u refer to thermocouples located at the bottom and upper positions respectively).
Karami et al. [ 14 ] experimentally showed that aqueous suspension based alkaline functionalized carbon nanotubes (f-CNT), 10 nm in diameter and 5-10 μm in length, has good stability as an absorber fluid in low-temperature Direct Absorption Solar Collector (DASC). The reason is associated with the hydrophilic nature of carboxylate groups. f-CNT considerably reduces the transmittance and enhances the thermal conductivity as seen in Figure 8 . They recommended the use of this kind of nanofluids to absorb the light directly. In this study, f-CNTs was dispersed into the water by an ultrasonic instrument with the volume fractions less than 150 ppm. Higher concentrations produced a black solution which light was not able to pass through it.
Thermal conductivity of f-CNT/water NFs in ambient temperature and 60°C.
Said et al. [ 15 ] found that nanofluids with single wall carbon nanotubes (SWCNTs) in a flat plate solar collector showed the minimum entropy generation compared to the nanofluids prepared by suspending Al 2 O 3 , TiO 2 and SiO 2 nanoparticles in the same base fluid as seen in Figure 9 . They attributed the decrease of the entropy generation to the increase in heat flux on the absorber plate due to the nanoparticles addition. Ultrasonicator and high pressure homogenizer (capacity of up to 2000 bar) is used to disperse the nanoparticles into the water. It was observed the SWCNTs nanofluids could reduce the entropy generation by 4.34% and enhance the heat transfer coefficient by 15.33%. It also had a small penalty in the pumping power by 1.2%.
Change of entropy generation with volume fraction.
Tang et al. [ 16 ] prepared the carbon nanotube/PEG/SiO 2 composites with high thermal conductivity from multiwall carbon nanotubes (MWCNTs), poly (ethylene glycol) (PEG) and inorganic SiO 2 . These composites had higher thermal conductivity than traditional phase-change materials (PCMs) because of the high thermal conductivity of MWCNTs. Their results clearly showed that PEG/ SiO 2 /MWCNT composites can effectively improve the efficiency of solar energy applications.
Saidur et al. [ 17 ] investigated the effects of different parameters on the efficiency of a low-temperature nanofluid-based direct absorption solar collector (DAC) with water and aluminum nanoparticles. One big advantage of using low-temperature systems is that solar collectors can be relatively simple and inexpensive. Additionally, there are a number of working fluids suitable to low-temperature operation. Commonly used base liquids are water, oil, and ethylene glycol. They accounted for the effects of absorption and scattering within the nanofluid to evaluate the intensity distribution within the nanofluid by the radiative transfer equation (RTE). In order to calculate the spectral extinction coefficient of the nanofluid that is sum of scattering coefficient and absorption coefficient, they investigated the optical properties of the based fluid and nanoparticles separately. Their results revealed that Aluminum/water nanofluid with 1% volume fraction improves the solar absorption considerably. They found that the effect of particle size on the optical properties of nanofluid is minimal, but in order to have Rayleigh scattering the size of nanoparticles should be less than 20 nm. They also found that the extinction coefficient is linearly proportionate to volume fraction.
Sokhansefat et al. [ 18 ] numerically investigated the heat transfer enhancement for Al 2 O 3 /synthetic oil nanofluid with concentrations up to 5% in a parabolic trough collector tube at different operational temperatures as seen in Figure 10 . Nanofluid enhanced convective heat transfer coefficient as seen in Figure 11 .
Schematic diagram of the parabolic trough collector and absorber tube.
Mean convective heat transfer coefficient vs.particle concentration at the operational temperatures of 300,400 and 500 K.
Nasrin et al. [ 19 ] performed a numerical study to investigate the influence of Prandtl number on the flow, temperature fields, convective and radiated heat transfer rates, mean bulk temperature of the fluids and average velocity field in a solar with water- Al 2 O 3 nanofluid collector as seen in Figure 12 . The results showed that with increasing Pr from 1.73 to 6.62, the convective heat transfer enhances about 26% and 18% for nanofluid and base fluid respectively whereas the radiation enhances by 8%.
Schematic diagram of the solar collector.
Role of base fluid
Colangelo et al. [ 20 ] experimently showed that the thermal conductivity improvement of the nanofluids with diathermic oil is greater than that with water in high temperature applications such as solar collectores. They observed that the thermal conductivity reduced with increasing the size of nanoparticles.
Hordy et al. [ 21 ] made four different nanofluids by dispersing plasma functionalized multi-walled carbon nanotubes (MWCNTs) in water, ethylene glycol, propylene glycol and Therminol VP-1 heat transfer fluids with the aid of an ultrasonic bath. They examined both the long-term and high-temperature stability of CNT nanofluids for use in direct solar absorption. In this work plasma treatment applied to modify the surface of the MWCNTs to improve their dispersion property within the base fluid. This study reported a quantitative demonstration of the high temperature and long-term stability of ethylene glycol and propylene glycol-based MWCNT nanofluids for solar thermal collectors.
Said et al. [ 22 ] experimentlly investigated the thermal conductivity, viscosity and pressure drop of water, ethylene glycol (EG) and EG + H2O (60:40)-based Al2O3 (13 nm) nanofluids prepared by using ultrasonic dispersion method in the operating temperature range of 25°C to 80°C at low range concentrations of 0.05% to 0.1% for. They observed that deviation of experimental values from estimated values of thermal conductivity of Al 2 O 3 /water nanofluids is considerably high but the experimental values of Al 2 O 3 /EG nanofluids are nearly similar to those of the model calculation as seen in Figure 13 . Their results showd that nanofluids pressure drop at a low concentration flowing in a solar collector is slightly higher than the base fluid.
Thermal conductivity of Al2O3/EG (a) and Al2O3/water (b) nanofluids at different volume fractions and at 25°C.
Liu et al. [ 23 ] experimentally investigated the feasibility of using the graphene (GE)-dispersed nanofluids based on the ionic liquid 1-hexyl-3-methylimidazolium tetrafluoroborate ([HMIM] BF 4 ) in high-temperature heat transfer systems (such as solar collectors). Ionic liquids (ILs) are a group of molten salts with a melting below 100°C as well as a wide liquid temperature range from room temperature to a maximum temperature of 459°C. ILs have excellent thermophysical properties such as good thermal and chemical stability, high density and heat capacity and negligible vapor pressure. In this work, authors showed how to improve the performance of ILs for solar thermal systems. They observed 15.2%-22.9% enhancement in thermal conductivity using 0.06% graphene in the temperature range from 25 to 200°C as seen in Figure 14 . Their results showed that GE is a better nanoadditive for nanofluids than other carbon materials and metal nanoparticles.
Thermal conductivity of [HMIM]BF4 and the GE-dispersed Ionanofluids as a function of temperature.
Variation of specific heat capacity with temperature for the pure and the different nanoparticle concentration of Hitec nanofluid.
The authors attributed this reduction to the self-lubrication characteristic of GE. In addition, the results obtained from the thermogravimetric analysis showed the high thermal stability of GE/BF 4 nanofluids. Their measurements showed that this novel class of nanofluids is very suitable for high temperature applications such as solar collectors.
Ho et al. [ 24 ] found the optimal concentration of alumina nanoparticles in doped molten Hitec (a nitrate salt) by maximizing its specific heat capacity. High-temperature molten salt typically has a high heat capacity and is effective as a working fluid for concentrating solar power (CSP) systems. Their findings are as follows: 1- The addition of less than 2% Al 2 O 3 nanoparticles significantly increases the specific heat of Hitec metal at low temperatures as seen in Figure 15 , 2- For the volume fractions less than or equal to 0.5%, adding Al 2 O 3 nanoparticles has a negative effect on the specific heat in temperature of 335°C, 3- At all temperatures, a concentration of 0.063 wt.% provides the maximum enhancement of specific heat about 19.9%, 4- The scanning electron microscopic (SEM) images show that, even at a relatively low concentration, nanoparticles aggregate as clusters with the size of 0.2 to 0.6 μm in the grain boundaries of Hitec, 5- The findings of this study suggest that the concentration that yields favorable uniform dispersion and optimal pattern of particles or clusters may maximize the specific heat. The simplified model of the solid-fluid interfacial area demonstrates that interfacial area is maximal at a concentration of 0.023 wt.%. As the nanoparticle concentration increases above 0.023 wt. %, the formed clusters become larger and the interfacial area density between the solid clusters and the base fluid decreases which may reduce the increase in specific heat capacity. According to the results obtained from this study, the maximum enhancement of the specific heat capacity occurs at concentration of 0.063 wt.% instead of 0.023 wt.%. Indeed, some agglomeration of nanoparticles forming submicrometer clusters may be the best for the enhancement of specific heat capacity. However, the total interfacial area at concentration of 0.063 wt. % was slightly less than its value at concentration of 0.023 wt. %.
Role of surfactants
Singh et al. [ 25 ] added Cu to commercial solar heat transfer fluids (Therminol 59 (TH59) and Therminol 66 (TH66)) by the combination of temperature and ultrasonic ripening processes. They stated that surfactant selection has an important role in preparing stable nanofluids. Choosing the right surfactant is mainly dependent on the properties of the base fluids and particles. For example, silicon oxide nanoparticles were successfully dispersed in TH66 using benzalkonium chloride (BAC, Acros Organics) as a surfactant but the use of BAC surfactant with Cu nanoparticles did not provide sufficient stability of suspension due to the lack of specific interaction between the nanoparticles and the surfactant molecules. The bi-layer arrangement of surfactant molecules should provide good adhesion to the nanoparticle surface and miscibility with the aromatic solvent. In this work, authors used a combination of oleic acid and BAC and a mixture of octadecyl thiol (ODT) and BAC surfactants to disperse Cu nanoparticles in TH66 and TH59, respectively. They observed that 3D Cu nanoparticle agglomerates do not break by conventional sonication with ultrasound gun without temperature ripening. They showed that a sonication time of about 4 h leads to the effective breakup of Cu agglomerates into individual grains at a 120°C. They also concluded that Cu/TH66 nanofluids appear to be more stable than the Cu/TH59 nanofluids because of the higher dynamic viscosity.
Yousefi et al. [ 26 , 27 ] studied the effect of Al 2 O 3 (15 nm) and MWCNT (10-30 nm) water nanofluid on the efficiency of a flat plate solar collector experimentally. The weight fractions of the nanoparticles were 0.2% and 0.4%, and the experiments were performed with and without Triton X-100 as surfactant. Their findings showed that the surfactant presence in the nanofluid extremely affects solar collector’s efficiency.
Lenert et al. [ 28 ] presented a combined modeling and experimental study to optimize the performance of a cylindrical nano-fluid volumetric receiver. They concluded that the efficiency is more than 35% when nanofluid volumetric receivers are coupled to a power cycle and optimized with respect to the optical thickness and solar exposure time. This study provides an important perspective in the use of nanofluids as volumetric receivers in concentrated solar applications. In this work, 28 nm carbon-coated cobalt (C-Co) nanoparticles dispersed and suspended in Therminol VP-1 after 30 min in a sonication bath without any surfactant.
Role of the pH
Yousefi et al. [ 29 ] investigated the effect of pH of MWCNT-H2O nanofluid on the efficiency of a flat-plate solar collector as seen in Figure 16 . The experiments were carried out using 0.2 wt% MWCNT (10-30 nm) with various pH values (3.5, 6.5 and 9.5) and with Triton X-100 as an additive. They found that increasing or decreasing the pH with respect to the pH of the isoelectric point (IEP) would enhance the positive effect of nanofluids on the efficiency of the solar collector. The collector efficiency enhanced while the differences between the pH of nanofluids and that of isoelectric increased. As the nanofluids become more acidic (lower pH value), more charges are accumulated on the particle surface, leading to lower agglomeration of nanoparticles in the suspension. Consequently, the effective thermal conductivity of the nanofluid increases. In addition, with the increase in pH of the nanofluid, the surface charge of the CNT increases leading to the increase in thermal conductivity and stability of nanofluid.
The efficiency of the flat-plate solar collector with MWCNT nanofluid as base fluid at three pH values as compared with water in0.0333 kg/s mass flow rate.
Using nanofluids in photovoltaic/thermal (PV/T) system
Sardarabadi et al. [ 30 ] performed experiments to study the effects of using SiO2/water nanofluid as a coolant on the thermal and electrical efficiencies of a photovoltaic thermal (PV/T) system. A flat plate solar collector was attached to a PV panel. The tilt angle of the collector was set at a constant value of 32° to maximize the solar collecting area. It was observed that by adding a thermal collector to a PV system, the total exergy for the three cases with pure water, 1% silica/water nanofluid and 3% silica/water nanofluid increased by 19.36%, 22.61% and 24.31%, respectively as seen in Figure 17 . Thermal efficiency of the PV/T collector for the two cases of 1 and 3 wt% of silica/water nanofluid increased 7.6% and 12.8%, respectively.
Exergetic efficiency of the system for the three cases with pure water (a), 1% silica/water nanofluid (b) and 3% silica/water nanofluid (c) during the daily experiment.
Karami et al. [ 31 ] experimentlly investigated the cooling performance of water based Boehmite (AlOOH. xH 2 O) nanofluid in a hybrid photovoltaic (PV) cell. The PV cell is mono-crystalline silicon. Results showed that the nanofluid performed better than water and the average PV surface temperature decreased from 62.29°C to 32.5°C as seen in Figure 18 . They reported that the electrical efficiency falls as the concentration of the nanofluid rises beyond a certain level. The authors attributed this reduction to the high surface activity of nanoparticles and their tendency to agglomeration/clustering at high particle loadings. Table 1 summarizes the results of nanofluids influence on different solar thermal applications
Variation of the average temperatures of the PV surface at various flow rates for water and three different concentrations of nanofluid.
Using nanofluids in solar stills
Kabeel et al. [ 32 ] investigated a small unit for water desalination coupled with nano-fluid-based (Cu/water) solar collector as a heat source as seen in Figure 19 . The system consists of a solar water heater (flat plate solar collector), a mixing tank and a flashing chamber plus a helical heat exchanger and a condenser. The desalination process is based on the evaporation of sea water under a very low pressure (vacuum). The evaporated water is then condensed to obtain fresh water. The simulation results showed that the nanoparticle concentration is an important factor on increasing the fresh water production and decreasing cost. Authors reported that the water cost can be decreased from 16.43 to 11.68 $/m 3 at ϕ = 5% as seen in Figure 20 .
Schematic diagram of single stage flash (SSF) system.
Variations in system productivity and water cost as a function of nano-particle volume fraction.
Kabeel et al. [ 33 ] used Al 2 O 3 nanoparticles with water inside a single basin solar still. Their results showed that using nanofluids improves the solar still water productivity by about 116% and 76% with and without operating the vacuum fan. The authors attributed this increment to the increase of evaporation rate inside the still. Utilizing nanofluid increases the rate of evaporation. In addition, due to this vacuum inside the still the evaporation rate increases further and the productivity increases compared with the still working at atmospheric conditions.
Using nanofluids in solar pond
Al-Nimr et al. [ 34 ] presented a mathematical model to describe the effects of using silver-water nanofluid on the thermal performance of a shallow solar pond (SSP) and showed that the energy stored in the nanofluid pond is about 216% more than the energy stored in the brine pond. The upper layer of the pond is made of mineral oil and the lower layer is made of silver (Ag) water-based nanofluid. Their results showed that for solar radiation of 1000 W/m 2 , the nanofluid pond required a depth less than 25 cm in order to absorb the light, while the brine pond depth must be more than 25 m to absorb the same amount of light. They attributed the increase of stored energy to the increase in thermal conductivity of the base fluid due to the nanoparticles addition that leads to uniform temperature distribution within the layer with reduction in heat losses.
Using nanofluids in the solar collector integrated with open thermosyphon
Liu et al. [ 35 ] experimentally showed that the solar collector integrated with open thermosyphon has a much better collecting performance compared to the collector with concentric tube and its efficiency could be improved by using CuO/water nanofluid as the working fluid as well. Their results showed that the maximum and mean values of the collecting efficiency of the collector with open thermosyphon using nanofluids increased 6.6% and 12.4%, respectively.
Conclusions
Nanofluids have been utilized to improve the efficiency of several solar thermal applications. Theoretical and experimental studies on solar systems proved that the system performance enhances noticeably by using nanofluids. A number of investigations presented the existence of an optimum concentration for nanoparticles in the base fluid. Adding nanoparticles beyond the optimum level no longer enhances the efficiency of the solar system.
Optimal conditions are a function of nanoparticles size and concentration, base fluid, surfactant and pH as discussed throughout this article. Nanofluid utilization in the solar thermal systems is accompanied by important challenges including high cost of production, instability, agglomeration and erosion. This review article is an attempt to elucidate the advantages and disadvantages of nanofluids application in the solar system.
Taylori R, Coulombe S, Otanicar T, Phelan P, Gunawan A, Lv W, Rosengarten G, Prasher R, Tyagi H (2013) Small particles, big impacts: a review of the diverse applications of nanofluids. J Appl Phys 113:011301–011301-19
Article Google Scholar
Mahian O, Kianifar A, Kalogirou SA, Pop I, Wongwises S (2013) A review of the applications of nanofluids in solar energy. Int J Heat Mass Transf 57:582–594
Said Z, Sajid MH, Saidur R, Kamalisarvestani M, Rahim NA (2013) Radiative properties of nanofluids. Int Commun Heat Mass Transfer 46:74–84
Al-Shamani AN, Yazdi MH, Alghoul MA, Abed AM, Ruslan MH, Mat S, Sopian K (2014) Nanofluids for improved efficiency in cooling solar collectors – a review. Renew Sustain Energy Rev 38:348–367
Khullar V, Tyagi H, Phelan PE, Otanicar TP, Singh H, Taylor RA (2013) Solar energy harvesting using nanofluids-based concentrating solar collector. J Nanotechnol Eng Med 3:031003-1-9
Google Scholar
Gan Y, Qiao L (2012) Radiation-enhanced evaporation of ethanol fuel containing suspended metal nanoparticles. Int J Heat Mass Transfer 55:5777–5782
Elmir M, Mehdaoui R, Mojtabi A (2012) Numerical simulation of cooling a solar cell by forced convection in the presence of a nanofluid. Energy Procedia 18:594–603
Luo Z, Wang C, Wei W, Xiao G, Ni M (2014) Performance improvement of a nanofluid solar collector based on direct absorption collection (DAC) concepts. Int J Heat Mass Transfer 75:262–271
Rahman MM, Mojumder S, Saha S, Mekhilef S, Saidur R (2014) Augmentation of natural convection heat transfer in triangular shape solar collector by utilizing water based nanofluids having a corrugated bottom wall. Int Commun Heat Mass Transfer 50:117–127
Faizal M, Saidur R, Mekhilef S, Alim MA (2013) Energy, economic and environmental analysis of metal oxides nanofluid for flat-plate solar collector. Energy Convers Manag 76:162–168
Parvin S, Nasrin R, Alim MA (2014) Heat transfer and entropy generation through nanofluid filled direct absorption solar collector. Int J Heat Mass Transfer 71:386–395
Ladjevardi SM, Asnaghi A, Izadkhast PS, Kashani AH (2013) Applicability of graphite nanofluids in direct solar energy absorption. Sol Energy 94:327–334
Filho EPB, Mendoza OSH, Beicker CLL, Menezes A, Wen D (2014) Experimental investigation of a silver nanoparticle-based direct absorption solar thermal system. Energy Convers Manag 84:261–267
Karami M, AkhavanBahabadi MA, Delfani S, Ghozatloo A (2014) A new application of carbon nanotubes nanofluid as working fluid of low-temperature direct absorption solar collector. Sol Energy Mater Sol Cells 121:114–118
Said Z, Saidur R, Rahim NA, Alim MA (2014) Analyses of exergy efficiency and pumping power for a conventionalflat plate solar collector using SWCNTs based nanofluid. Energy Build 78:1–9
Tang B, Wang Y, Qiu M, Zhang S (2014) A full-band sunlight-driven carbon nanotube/PEG/SiO2 composites for solar energy storage. Sol Energy Mater Sol Cells 123:7–12
Saidur R, Meng TC, Said Z, Hasanuzzaman M, Kamyar A (2012) Evaluation of the effect of nanofluid-based absorbers on direct solar collector. Int J Heat Mass Transfer 55:5899–5907
Sokhansefat T, Kasaeian AB, Kowsary F (2014) Heat transfer enhancement in parabolic trough collector tube using Al2O3/synthetic oilnanofluid. Renew Sustain Energy Rev 33:636–644
Nasrin R, Parvin S, Alim MA (2013) Effect of Prandtl number on free convection in a solar collector filled with nanofluid. Procedia Engineering 56:54–62
Colangelo G, Favale E, de-Risi A, Laforgia D (2012) Results of experimental investigations on the heat conductivity of nanofluids based on diathermic oil for high temperature applications. Appl Energy 97:828–833
Hordy N, Rabilloud D, Meunier JL, Coulombe S (2014) High temperature and long-term stability of carbon nanotube nanofluids for direct absorption solar thermal collectors. Sol Energy 105:82–90
Said Z, Sajid MH, Alim MA, Saidur R, Rahim NA (2013) Experimental investigation of the thermophysical properties of AL2O3-nanofluid and its effect on a flat plate solar collector. Int Commun Heat Mass Transfer 48:99–107
Liu J, Wang F, Zhang L, Fang X, Zhang Z (2014) Thermodynamic properties and thermal stability of ionic liquid-based nanofluids containing graphene as advanced heat transfer fluids for medium-to-high-temperature applications. Renew Energy 63:519–523
Ho MX, Pan C (2014) Optimal concentration of alumina nanoparticles in molten Hitec salt to maximize its specific heat capacity. Int J Heat Mass Transfer 70:174–184
Singh D, Timofeeva EV, Moravek MR, Cingarapu S, Yu W, Fischer T, Mathur S (2014) Use of metallic nanoparticles to improve the thermophysical properties of organic heat transfer fluids used in concentrated solar power. Sol Energy 105:468–478
Yousefi T, Veysi F, Shojaeizadeh E, Zinadini S (2012) An experimental investigation on the effect of Al2O3-H2O nanofluid on the efficiency of flat-plate solar collectors. Renew Energy 39:293–298
Yousefi, F. Veysy, E. Shojaeizadeh, S. Zinadini (2012). An experimental investigation on the effect of MWCNT-H2O nanofluid on the efficiency of flat-plate solar collectors. Experimental Thermal and Fluid Science 207-212
Lenert A, Wang EN (2012) Optimization of nanofluid volumetric receivers for solar thermal energy conversion. Sol Energy 86:253–265
Yousefi T, Shojaeizadeh E, Veysi F, Zinadini S (2012) An experimental investigation on the effect of pH variation of MWCNT–H2O nanofluid on the efficiency of a flat-plate solar collector. Sol Energy 86:771–779
Sardarabadi M, Passandideh-Fard M, Zeinali Heris S (2014) Experimental investigation of the effects of silica/water nanofluid on PV/T (photovoltaic thermal units). Energy 66:264–272
Karami N, Rahimi M (2014) Heat transfer enhancement in a hybrid microchannel-photovoltaic cell using Boehmite nanofluid. Int Commun Heat Mass Transfer 55:45–52
Kabeel AE, El-Said EMS (2014) Applicability of flashing desalination technique for small scale needs using a novel integrated system coupled with nanofluid-based solar collector. Desalination 333:10–22
Kabeel AE, Omara ZM, Essa FA (2014) Enhancement of modified solar still integrated with external condenser using nanofluids: An experimental approach. Energy Convers Manag 78:493–498
Al-Nimr MA, Al-Dafaie AMA (2014) Using nanofluids in enhancing the performance of a novel two-layer solar pond. Energy 68:318–326
Liu ZH, Hu RL, Lu L, Zhao F, Xiao HS (2013) Thermal performance of an open thermosyphon using nanofluid for evacuated tubular high temperature air solar collector. Energy Convers Manag 73:135–143
Download references
Acknowledgements
The authors would like to express their appreciation to the Islamic Azad University of Abadan Branch for providing financial support.
Author information
Authors and affiliations.
Mechanical Engineering Department, Abadan Branch, Islamic Azad University, P.O.B. 666, Abadan, Iran
Navid Bozorgan
Mechanical Engineering Department, California State Polytechnic University, Pomona, California, USA
Maryam Shafahi
You can also search for this author in PubMed Google Scholar
Corresponding author
Correspondence to Navid Bozorgan .
Additional information
Competing interests.
The authors declare that they have no competing interests.
Authors’ contributions
MS conducted the extensive literature review and NB wrote the article. Both authors read and approved the final manuscript.
Rights and permissions
Open Access This article is distributed under the terms of the Creative Commons Attribution 4.0 International License ( https://creativecommons.org/licenses/by/4.0 ), which permits use, duplication, adaptation, distribution, and reproduction in any medium or format, as long as you give appropriate credit to the original author(s) and the source, provide a link to the Creative Commons license, and indicate if changes were made.
Reprints and permissions
About this article
Cite this article.
Bozorgan, N., Shafahi, M. Performance evaluation of nanofluids in solar energy: a review of the recent literature. Micro and Nano Syst Lett 3 , 5 (2015). https://doi.org/10.1186/s40486-015-0014-2
Download citation
Received : 04 September 2014
Accepted : 26 January 2015
Published : 20 May 2015
DOI : https://doi.org/10.1186/s40486-015-0014-2
Share this article
Anyone you share the following link with will be able to read this content:
Sorry, a shareable link is not currently available for this article.
Provided by the Springer Nature SharedIt content-sharing initiative
- Solar energy
- Solar systems
- Heat transfer enhancement
Thank you for visiting nature.com. You are using a browser version with limited support for CSS. To obtain the best experience, we recommend you use a more up to date browser (or turn off compatibility mode in Internet Explorer). In the meantime, to ensure continued support, we are displaying the site without styles and JavaScript.
- View all journals
- My Account Login
- Explore content
- About the journal
- Publish with us
- Sign up for alerts
- Open access
- Published: 30 March 2024
Exploring the surge current degradation of natural ester oil-based nanofluids
- Thomas Tsovilis 1 ,
- George Peppas 2 ,
- Evangelos Staikos 1 ,
- Alexandros Hadjicostas 1 &
- Zacharias Datsios 1
Scientific Reports volume 14 , Article number: 7584 ( 2024 ) Cite this article
580 Accesses
2 Altmetric
Metrics details
- Electrical and electronic engineering
- Energy infrastructure
The surge endurance of natural ester oil-based nanofluids against surge events is investigated experimentally. The focus of this work is the examination, through dielectric spectroscopy measurements, of the alteration of the real and imaginary parts of the complex relative permittivity of iron oxide nanofluids as a result of an accelerated degradation test employing a sequence of repetitive current impulses produced via a 12 kV/6 kA combination wave generator. The target is the exploration of a possible implementation of nanofluids as multipurpose liquids that act, in addition to insulation and coolants, as surge absorption media. Promising experimental results are discussed and compared with those of mineral oil that is widely used as a conventional insulating liquid in power transformers.
Similar content being viewed by others
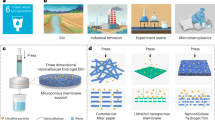
A bio-based nanofibre hydrogel filter for sustainable water purification
Meihui Jiang, Chuyan Jing, … Guihua Yu
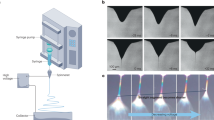
Electrospinning of nanofibres
Dongxiao Ji, Yagai Lin, … Seeram Ramakrishna
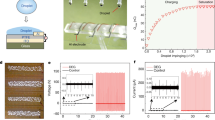
A droplet-based electricity generator with high instantaneous power density
Wanghuai Xu, Huanxi Zheng, … Zuankai Wang
Introduction
Nanofluids show a versatile spectrum of applications since they exhibit an enhancement of physicochemical and electrothermal properties of the base liquid 1 , 2 , 3 , 4 . Actually, the integration of nanoparticles of different shapes, sizes, and chemical compositions results in increased breakdown voltage 5 , 6 , lower partial discharge activity 7 , improved heat transfer 8 , and radiation-proof performance 9 through mechanisms that remain the subject of ongoing investigations. In light of this evidence, natural ester oil-based nanofluids are considered as an environmentally friendly alternative to mineral oils, which are the widely used insulating media and coolants for power transformers 10 .
Currently, the large-scale use of nanofluids in the power industry is impeded by concerns associated with the long-term stability against agglomeration of nanoparticles and consequent sedimentation, as well as the aging due to thermal stress and oxidation 10 . Thus, a series of experimental and theoretical investigations focus on the optimal concentration of nanoparticles in base liquids so as to achieve the advantageous properties of nanofluids that will be maintained for the expected lifetime of the equipment that the liquids will be integrated into 11 .
Although it is well recognized the risk of liquid insulation breakdown due to impinging lightning-related overvoltages 12 , 13 , the energy absorption capability of nanofluids is sparsely reported and the endurance under impulse currents has not been evaluated. This work introduces an experimental setup for an accelerated surge degradation test that involves a sequence of repetitive high-voltage and high-current impulses. This setup differs from conventional multi-stage impulse voltage generators for the determination of the dielectric strength of liquids that produce impulse currents of limited amplitude and charge 14 , 15 which are well below the field experience from lightning electromagnetic pulses; the latter exhibit peak current of several kiloamperes and duration of decades of microseconds 16 . Thus, for the first time with the aid of a combination wave generator and surge-proof test cell, the aging effects of excessive surge current stress are explored by sensing the changes in the complex relative permittivity through dielectric spectroscopy measurements.
Following the global trend for environmentally friendly insulating liquids, that also exhibit low fire hazard and high moisture resistance 17 , this work investigates the surge endurance of natural ester oil-based nanofluids, which are eco-friendly thanks to the biodegradability and nontoxic properties of the vegetable-produced base liquid.
The experimental results of the present study indicate an improved performance of iron oxide nanofluids in terms of surge endurance when compared to natural ester oil base liquid; a discussion is made on the effect of nanoparticles concentration and a comparison is made to surge degradation of mineral oil that is widely used as a conventional insulating liquid in power transformers. This work opens the path for the possible implementation of nanofluids as multipurpose liquid media 18 , 19 with surge absorption capability that may result in various applications in the power industry.
Experimental arrangements
Sample preparation.
The base liquid used for nanofluid preparation is a vegetable-based liquid, Envirotemp FR3 TM . FR3 shows excellent electrothermal properties 20 and constitutes an eco-friendly liquid insulation and coolant that has been used for over 20 years in power transformers. The fluid is non-toxic, as evidenced by acute aquatic and oral toxicity assessments, and exhibits high flash/fire points of ~330/360 °C.
Iron oxide (Fe 2 O 3 ) nanoparticles with a diameter of less than 50 nm, were incorporated into the natural ester oil (FR3). Three concentrations of FR3-based nanofluids, namely 0.005% w/w, 0.05% w/w, and 0.5% w/w, were prepared as illustrated in Fig. 1 . It is noted that the nanofluids exhibit a change in color towards red-brown as the concentration increases; direct visual characterization based on international standards is not applicable. The appropriate quantity of nanoparticles was accurately weighed using a 4-digit scale (Mettler Toledo AB204-S) and added to the natural ester oil by using a 4-hand AtmosBag (Z555525-1EA). Following the integration of nanoparticles into the base liquid, ultrasonication for 4 h was employed with the aid of an ultrasonic cleaner (Branson 1510) to disperse the nanoparticles and homogenize the nanofluids.
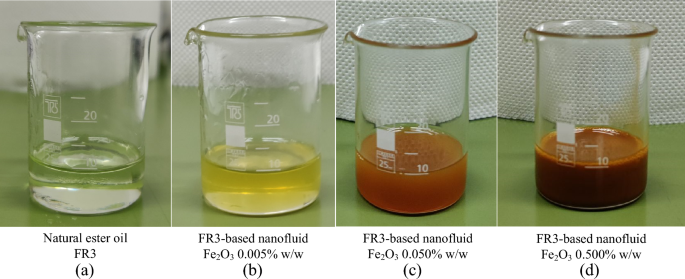
Natural ester oil-based nanofluids of different iron oxide nanoparticle concentrations. ( a ) Natural ester oil FR3, ( b ) FR3-based nanofluid Fe 2 O 3 0.005% w/w, ( c ) FR3-based nanofluid Fe 2 O 3 0.050% w/w, and ( d ) FR3-based nanofluid Fe 2 O 3 0.500% w/w.
Iron oxide nanofluids have been used in the present study in light of the related literature indicating excellent stability and dielectric properties 4 , 21 , 22 ; low-medium nanoparticle concentrations are related to optimal dielectric strength 23 and the upper limit refers to unexplored areas with concentrations that resemble those of metal-oxide additives employed in solid-state semiconductors used in the surge protection industry 24 . Exploring the surge endurance of nanofluids with the used nanoparticle concentrations that differ significantly enables the examination of macroscopic effects on surge degradation and establishes a foundation for subsequent investigations.
Dielectric spectroscopy measurements
The prepared nanofluids were inserted into a fixture suitable for liquids’ characterization (Keysight 16452A) and the real and imaginary parts of the complex relative permittivity were determined using an Impedance Analyzer (Keysight E4990A-120) operating at 0.5 V, as shown in Fig. 2 .
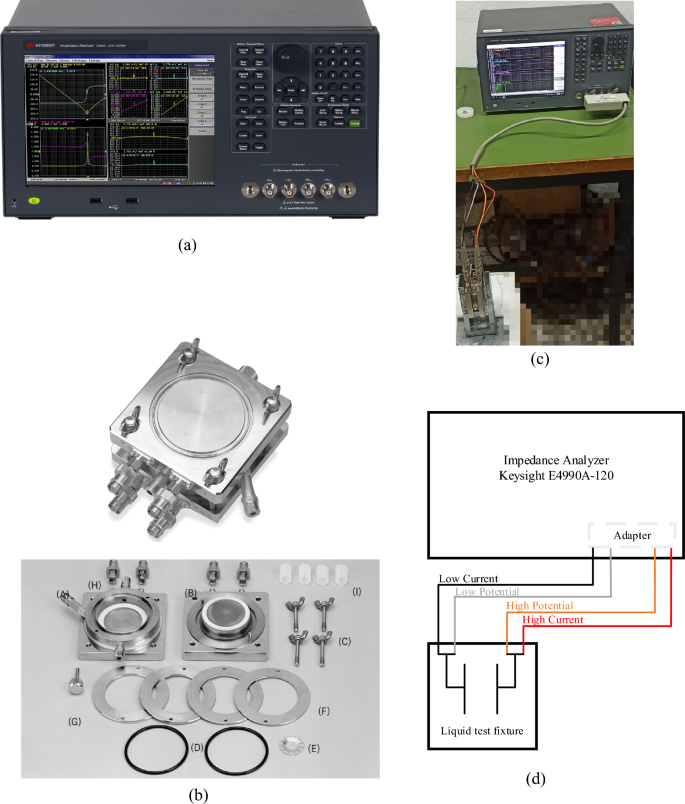
Equipment and experimental setup for dielectric spectroscopy measurements. ( a ) Impedance analyzer, ( b ) liquid test fixture, ( c ) experimental setup, ( d ) schematic diagram.
The dielectric spectroscopy measurements were conducted for a wide frequency range, specifically 50 Hz–5 MHz, covering at the low end (50–400 Hz) the operating frequency of the vast majority of power systems and at the high-frequency domain (10 kHz–5 MHz) the frequencies of transients associated with a wide variety of electromagnetic pulses due to natural (lightning 25 ) and artificial (switching operations 26 , NEMP 27 ) surge events.
The room temperature was maintained constant at 21 °C and the absolute humidity varied between 15–17 g/m 3 during the measurements; we followed the test protocol implemented in a recent study 28 .
Accelerated degradation test
A surge endurance test, simulating accelerated degradation, was conducted to assess the resilience of the natural ester oil-based nanofluids against a sequence of repetitive impulse currents employing the equipment and experimental arrangement depicted in Fig. 3 .
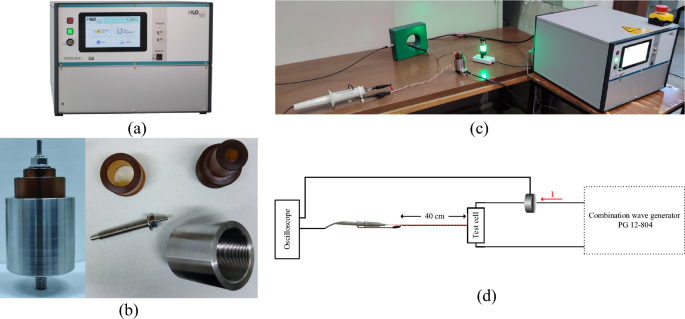
Equipment and experimental setup for accelerated degradation test. ( a ) Combination wave generator, ( b ) custom made test cell, ( c ) experimental setup and ( d ) schematic diagram.
The impulse current was generated with the aid of a 12 kV/6 kA combination wave generator (HILO PG12-804), following UL 1449 29 for low-voltage surge protective devices. At each applied impulse voltage of 12 kV, 1.2/50 μs, a breakdown occurs in the micro gap of the test cell as indicated by the collapse of the voltage, and an impulse current of 6 kA, 8/20 μs is conducted as shown in the oscillogram of Fig. 4 ; it is noted that the impulse current is practically unaffected by the instantaneous breakdown voltage, V b .
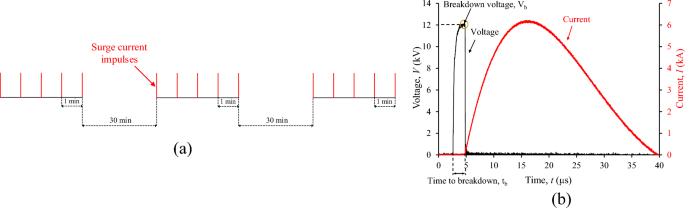
Surge degradation test sequence. ( a ) Sequence of surge current impulses, ( b ) typical impulse voltage and current records.
The accelerated degradation test sequence involves fifteen exponential current impulses to the specially designed metallic test cell that withstands the excessive pressure associated with the shock wave of supersonic propagation of streamer 30 and the post-breakdown discharge current conducted through the integrated liquid medium of 10 ml in volume. Three sets of five surges of current impulses were applied with a time interval of 30 min between them 29 ; successive current impulses within each group were fixed at 1 min.
After the entire sequence of repetitive current impulses, the degraded liquid from the test cell was used to fill the test fixture and enable the dielectric spectroscopy measurements (Fig. 2 ).
Experimental results
The accelerated degradation test results in color darkening that is indicative of the aging of the liquids 31 ; degraded nanofluids are nontransparent with a black color depicted in Fig. 5 . The changes in complex relative permittivity, \({\upvarepsilon }_{r}^{*}\) , which is formulated as the sum of real and imaginary parts as shown in Eq. ( 1 ), were obtained through dielectric spectroscopy measurements, and will be presented in what follows.
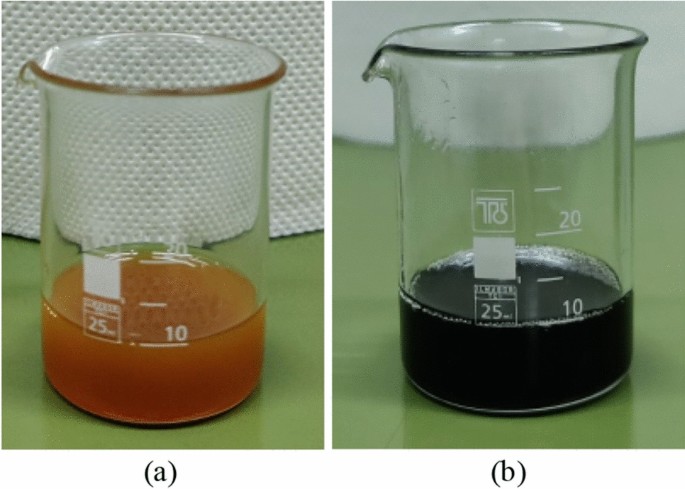
( a ) Untested (virgin) natural ester oil-based nanofluid and ( b ) degraded nanofluid; iron oxide nanoparticle concentration (0.050% w/w).
Real part of relative permittivity
Although the surge degradation of the fluids under study is evident from their color alteration, the change of the real part of relative permittivity, ε r \(^{{\prime}}\) , after the accelerated degradation test is marginal for the base liquid and nanofluids as it can be deduced from Fig. 6 . Considering that the ε r \(^{{\prime}}\) is practically constant for frequencies up to 1 MHz, Fig. 7 illustrates the minimal increase of ε r \(^{{\prime}}\) for the frequency of 25 kHz, which is of high interest in lightning protection technology 25 , revealing a more marked change for the nanofluid with the higher concentration of iron oxide nanoparticles (0.5% w/w).
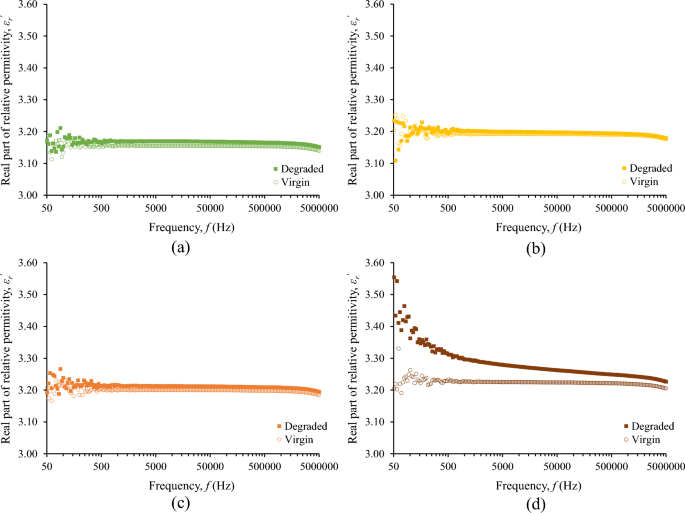
Real part of relative permittivity before and after the accelerated degradation test of natural ester oil-based nanofluids with iron oxides concentration (w/w): ( a ) 0%, ( b ) 0.005%, ( c ) 0.050%, ( d ) 0.500%.
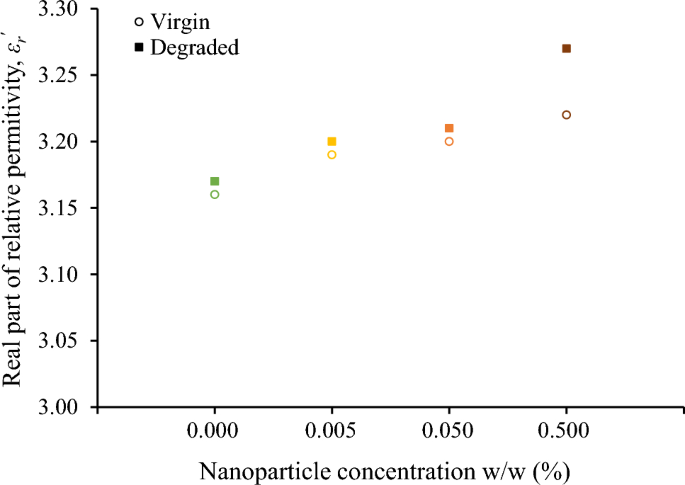
Real part of relative permittivity at 25 kHz for the natural ester oil-based nanofluids under study.
Imaginary part of relative permittivity
In contrast to the minor alterations observed in the real part of relative permittivity, the imaginary part, ε r \(^{{\prime\prime}}\) , an effective parameter reflecting both polarization losses and conduction losses, undergoes substantial changes due to surge degradation, as depicted in Fig. 8 for the frequency of 400 Hz, which is of high interest in aviation industry.
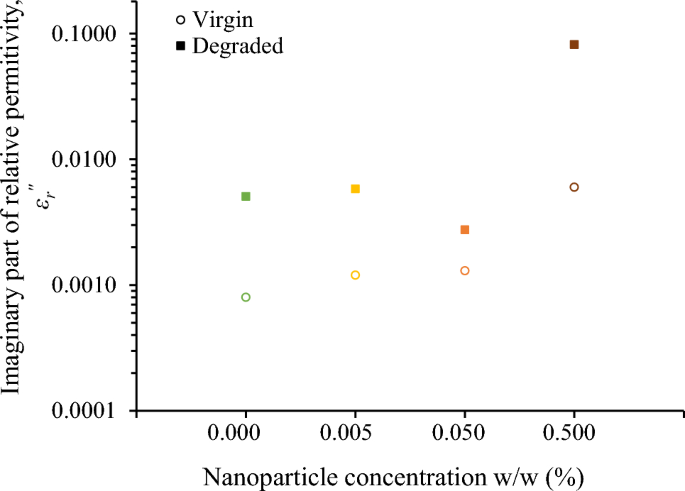
Imaginary part of relative permittivity at 400 Hz for the natural ester oil-based nanofluids under study.
Thus, ε r \(^{{\prime\prime}}\) is a key performance indicator revealing the ageing effects associated with the surge degradation test on the electrical conductivity of the fluids, as described by the following equation:
Figure 9 illustrates the change in electrical conductivity of fluids under study that is more evident at the low-frequency range. This is important since degraded liquids may yield high leakage current in power systems commonly operating at 50/60 Hz. It is noted that the electrical conductivity change is more pronounced for the nanofluid with the higher concentration of iron oxide nanoparticles.
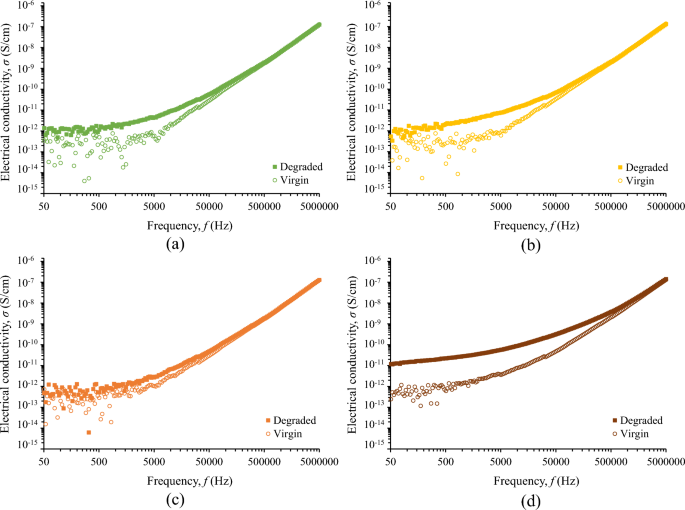
Electrical conductivity before and after the accelerated degradation test of natural ester oil-based nanofluids with iron oxides concentration (w/w): ( a ) 0%, ( b ) 0.005%, ( c ) 0.050%, ( d ) 0.500%.
Discussion on surge endurance of nanofluids and comparison to mineral oil
Fifteen surge current applications (6 kA, 8/20 μs) with a charge transfer of 10 mC/ml each, which is 250 times higher than the charge transfer employed in preliminary experimental investigations of the authors with 2 kA, 1.2/5 μs, that resulted in minor changes in color and impedance of liquid insulation 32 , result in aging of the nanofluids. From Figs. 6 and 9 , it can be deduced that for the diagnosis of aging the change of imaginary part of the complex relative permittivity is more effective than the real part.
Figure 10 reveals that the employed severe surge stress resulted in a significant increase of the electrical conductivity of the fluids under study generally of more than two times at 25 kHz; this change is even more pronounced at lower frequencies where most systems operate, such as 50/60 Hz in power and 400 Hz in aviation industry. This low-frequency performance is crucial, considering that leakage current in operating conditions results in power losses and further electrical degradation of insulating fluids. Nevertheless, the electrical conductivity of degraded liquids exhibits an acceptable conductivity of less than 10 –12 S/cm, for the low-frequency range of 50–400 Hz, as depicted in Fig. 9 with the exception of the nanofluid with the highest iron oxide concentration (0.5% w/w).
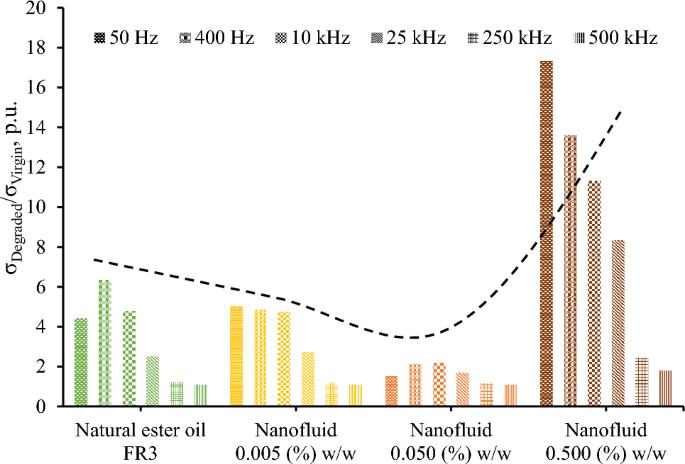
Ratio of electrical conductivity before ( σ Virgin ) and after ( σ Degraded ) the accelerated degradation test of natural ester oil-based nanofluids with different iron oxides concentrations; the dotted line illustrates a qualitative general trend.
It is important to note that the impact of nanoparticle concentration on surge degradation does not exhibit a monotonous trend, with the optimal concentration determined among the investigated levels being 0.05% w/w. A plausible rationale for this observation lies in the hypothesis that medium concentrations of nanoparticles, such as 0.05% w/w, may establish a discharge path for the surge current, thereby mitigating the adverse effects of impulse current stress on the base liquid when compared to natural ester oil and nanofluids with low nanoparticles concentration (0.005% w/w). On the other hand, a further increase in nanoparticle concentration could induce pronounced agglomeration and sedimentation enhanced by surge degradation, leading to a noteworthy increase in electrical conduction through weak links 33 ; this hypothesis is supported by visual observation of sedimentation at surge-degraded FR3 fluid. It is noteworthy that this surge degradation performance necessitates additional investigations (i) into the intricate behaviors of nanoparticles under conditions of elevated current conduction along with the mechanisms associated with aging during surge events and (ii) on the long-term stability of nanofluids against agglomeration of nanoparticles.
Figure 11 illustrates, for comparison purposes, the surge degradation of a mineral oil, Shell Diala S4 ZX-I produced via gas-to-liquid technology, that is commonly employed in the power industry. It is obvious that the surge degradation of mineral oil is 265% higher at 25 kHz than natural ester oil-based nanofluid with medium concentration of nanoparticles (0.05% w/w) which exhibits the highest surge endurance; this is probably associated with the inherent characteristics of the natural ester oil (FR3) and the conductivity of chemical derivatives produced due to the high energy conduction through the different fluids.
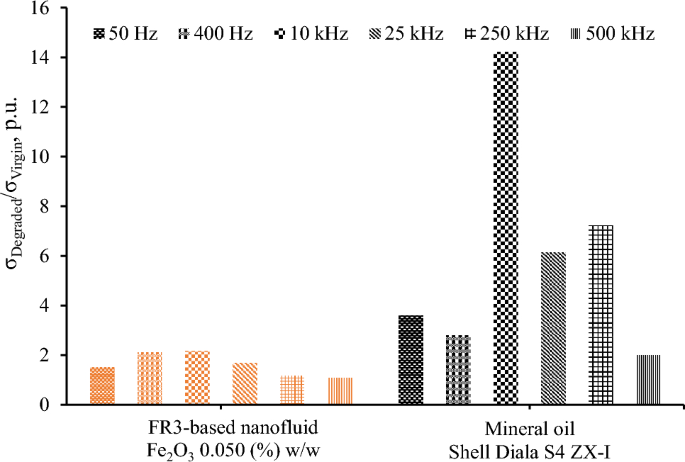
Ratio of electrical conductivity before ( σ Virgin ) and after ( σ Degraded ) the accelerated degradation test of FR3-based nanofluid (0.05% w/w) and mineral oil.
The superior surge endurance demonstrated by the FR3-based nanofluid in comparison to mineral oil, coupled with the satisfactory dielectric performance of natural ester oil-based nanofluids 34 and their fire-resistant properties 17 , establishes a promising framework for the substitution of mineral oils with environmentally friendly liquids facilitated by nanotechnology processes in the forthcoming decades. This prospect, however, necessitates overcoming challenges related to the long-term stability of nanofluids, especially at high nanoparticle concentrations, and addressing aging effects resulting from oxidation and thermal stress 10 , 35 , 36 . Available synthesis methods and nanoparticles’ dispersion techniques 21 , 22 , 37 will make feasible the stability of the surge-proof nanofluids; the shape, size, type, chemical treatment, and moisture content of liquids may be parameters that should be examined by the researchers towards this direction.
Furthermore, the resilience of environmentally friendly nanofluids against surge events opens new horizons for their application as versatile materials, potentially extending to applications such as surge arresters and energy storage systems.
Concluding remarks
The endurance of natural ester oil-based nanofluids against surge events has been experimentally investigated. The evaluation of nanofluid aging has been conducted by examining alterations in the complex relative permittivity resulting from an accelerated degradation test that involves the application of a series of impulse currents of 6 kA, 8/20 μs, with a charge transfer magnitude of 10 mC/ml. Experimental results have shown that:
A non-monotonous impact of nanoparticle concentration on the surge degradation of nanofluids exists. Actually, FR3-based nanofluids containing an iron oxide nanoparticle concentration of 0.05% exhibit superior surge endurance in comparison to base liquid, which is a vegetable oil, as well as to nanofluids with lower nanoparticle concentrations. Notably, a further elevated concentration of nanoparticles dispersed in the natural ester oil leads to a deterioration in the surge endurance of the base liquid. These results warrant further investigation and theoretical elucidation to understand the underlying mechanisms governing the observed phenomena as well as the determination of the optimal concentration.
The encouraging surge endurance exhibited by environmentally friendly nanofluids establishes a promising foundation for the exploration of eco-friendly liquids in a diverse range of applications. This extends beyond their conventional use in power transformers, where mineral oils currently predominate, to encompass potential applications such as surge arresters. The robust performance of green nanofluids under surge conditions positions them as compelling candidates for enhancing the sustainability and resiliency of electrical systems, warranting further consideration and exploration in practical applications.
The base liquid of nanofluids is the Envirotemp FR3 TM with typical dielectric strength shown in Table 1 in standard electrode arrangements. The characteristics of Fe 2 O 3 nanoparticles are provided in Table 2 .
Dielectric spectroscopy measurement procedure
The impedance analyzer, following a typical four-terminal measurement procedure determines the amplitude, | Z |, and phase angle, φ , of the impedance of the samples injected into the liquid test fixture; the latter is connected through an adapter employing a four-cable system (low/high current, low/high potential) as shown in Fig. 2 d.
The liquid test fixture electrode arrangement consists of two parallel circular plates at a distance of 1.3 mm forming a capacitor, which can be represented as a resistance, R , and a capacitance, C , connected in parallel and calculated from measurement results at each frequency f as:
where | Y | and θ , are the amplitude, and phase angle of the admittance that is calculated from the measured | Z | and φ .
To determine the real, ε r ′ , and imaginary, ε r ″ , parts of the complex relative permittivity of each sample two measurements were performed. In the first measurement the fixture is not filled with any liquid and the capacitance of the air gap, C 0 , is obtained from Eq. ( 3b ). In the second measurement the fixture is filled with the liquid sample and the resistance, R L , and capacitance, C L , are determined through Eqs. ( 3a , 3b ). Next ε r ′ , and ε r ″ , are calculated as 38 :
where a is a correction coefficient that is employed to account for the stray capacitance and the edge effects and is calculated for the specific liquid test fixture as 38 :
where \({|\upvarepsilon }_{rm}^{*}|\) , is given by:
Data availability
The datasets used and/or analyzed during the current study are available from the corresponding author on reasonable request.
Cham saard, W., Fawcett, D., Fung, C. C. et al . Synthesis, characterisation and thermo-physical properties of highly stable graphene oxide-based aqueous nanofluids for potential low-temperature direct absorption solar applications. Sci. Rep. 11 , 16549 (2021).
Raza Shah Naqvi, S. M., Farooq, U., Waqas, H. et al . Inspection of thermal jump conditions on nanofluids with nanoparticles and multiple slip effects. Sci. Rep. 12 , 5586 (2022).
Kosinska, A., Balakin, B. V. & Kosinski, P. Exploring the use of nanofluids in pump-free systems for solar thermal applications. Sci. Rep. 13 , 17058 (2023).
Article ADS CAS PubMed PubMed Central Google Scholar
Jiosseu, J. L. et al. Statistical analysis of the impact of FeO 3 and ZnO nanoparticles on the physicochemical and dielectric performance of monoester-based nanofluids. Sci. Rep. 13 , 12328 (2023).
Das, A. K. & Chatterjee, S. Impulse performance of synthetic esters based-nanofluid for power transformer. Mater. Res. 5 (12), 125026 (2018).
Google Scholar
Suhaimi, N. S. et al. Systematical study of multi-walled carbon nanotube nanofluids based disposed transformer oil. Sci. Rep. 10 , 20984 (2020).
Article CAS PubMed PubMed Central Google Scholar
Cianna, A., Sumathi, S. & Jarin, T. Analysis of properties and statistical study on partial discharge inception voltage using normal and Weibull distributions for vegetable oil-based nanofluids. Biomass Conv. Bioref. (2024).
Islam, T. et al. Heat generation/absorption effect on natural convective heat transfer in a wavy triangular cavity filled with nanofluid. Sci. Rep. 13 , 21171 (2023).
Maaza, M. et al. A novel approach for engineering efficient nanofluids by radiolysis. Sci. Rep. 12 , 10767 (2022).
Oparanti, S. O., Fofana, I., Jafari, R., & Zarrougui, R. A state-of-the-art review on green nanofluids for transformer insulation, Journ. Mol. Liq. 396 (2024).
Karatas, M. & Bicen, Y. Nanoparticles for next-generation transformer insulating fluids: A review, Ren. Sust. En. Rev. 167 (2022).
Mikropoulos, P. N., Tsovilis, T. E. & Koutoula, S. G. Lightning performance of distribution transformer feeding GSM base station. IEEE Trans. Power Del. 29 (6), 2570–2579 (2014).
Article Google Scholar
Popov, M., Grcev, L., Hoidalen, H. Kr. et al . Investigation of the overvoltage and fast transient phenomena on transformer terminals by taking into account the grounding effects. IEEE Trans. Ind. Appl. 51 (6), 5218–5227 (2015).
Schon K. High Voltage Measurement Techniques: Fundamentals, Measuring, instruments, and Measuring Methods . 1 st edn, (Springer, 2019).
Shill, D. C., Das, A. K. & Chatterjee, S. Lightning impulse breakdown performance of saturated vs unsaturated vegetable oil and their mixtures with mineral oil. Ind. Crop. Prod. 184 , 115044 (2022).
Article CAS Google Scholar
CIGRE W.G. C4.407. Lightning parameters for engineering applications . Technical Brochure 549 (2013).
CIGRE WG D1.70 TF 3. Dielectric performance of insulating liquids for transformers . Technical Brochure 856 (2021).
Shao, J. J. et al. Self-assembled two-dimensional nanofluidic proton channels with high thermal stability. Nat. Commun. 6 , 7602 (2015).
Article ADS PubMed Google Scholar
Saraswat, M., & Sengwa, R. J. Investigations on the optical, dielectric, electrical, and rheological properties of PEG200/ZnO semiconducting nanofluids for soft matter based technological innovations. Hybr. Adv . 5 (2024).
Emara, M. M. et al. Thermal and dielectric analysis of an ester-based MgO nanofluid. IEEE Trans. Dielectr. Electr. Insul. 30 (5), 2181–2188 (2023).
Mann, S. Self-assembly and transformation of hybrid nano-objects and nanostructures under equilibrium and non-equilibrium conditions. Nat. Mat. 8 , 781–792 (2009).
Sabzi Dizajyekan, B. et al. Preparation of stable colloidal dispersion of surface modified Fe 3 O 4 nanoparticles for magnetic heating applications. Sci. Rep. 14 , 1296 (2024).
Chen, Q., Beroual, A., Sima, W. & Sun, P. AC and lightning impulse breakdown voltage comparative study of mineral oil-based Fe 3 O 4 , Al 2 O 3 , and TiO 2 nanofluids. IEEE Trans. Dielectr. Electr. Insul. 31 (1), 278–287 (2024).
Anas, S. et al. Nanofillers in ZnO based materials: A ‘smart’ technique for developing miniaturized high energy field varistors. J. Mater. Chem. C. 1 , 6455–6462 (2013).
IEC 62305-4. Protection against lightning - Part 4: Electrical and electronic systems within structures (2010).
Das, J. C. Transients in Electrical Systems: Analysis, recognition, and mitigation . 1 st edn, (McGraw Hill, 2010).
Electromagnetic pulse (EMP) protection and resilience guidelines for critical infrastructure and equipment. National Coordinating Center for Communications (NCC), National Cybersecurity and Communications Integration Center Arlington, Virginia, USA, Feb. 2019, Unclassified.
Tsovilis, T. E., Staikos, E. V., Hadjicostas, A. Y. et al . Relative permittivity of natural ester oil-based nanofluids with iron oxide nanoparticles. UPEC 2023 Dublin Ireland, (2023).
UL1449. Surge Protective Devices , (2022).
Lundgaard, L. E., Liu, Q., Lesaint, O., & Madshaven. I. Dielectric performance of transformer liquids—Summary of a CIGRE study. IEEE Electr. Ins. Mag . 39 , 7–16 (2023).
ASTM D1524-15. Visual Examination of Used Electrical Insulating Liquids in the Field (2022).
Tsovilis, T. E. et al. Preliminary results on the surge current withstand capability of natural ester oil. IEEE IAS Annu. Meet. USA (2023).
Book Google Scholar
Küchler, A. High Voltage Engineering . 1 st edn, (Springer Vieweg Berlin, 2018).
Emara, M. M. et al. Thermal and dielectric performance of ester oil-based pentyl-graphene nanofluids. IEEE Trans. Dielectr. Electr. Insul. 29 (2), 510–518 (2022).
CAS Google Scholar
Hassanloo, H., Sadeghzadeh, S. & Ahmadi, R. A new approach to dispersing and stabilizing graphene in aqueous nanofluids of enhanced efficiency of energy-systems. Sci. Rep. 10 , 7707 (2020).
Koutras, K. N. et al. Aging impact on relative permittivity, thermal properties and lightning impulse voltage performance of natural ester oil filled with semiconducting nanoparticles. IEEE Trans. Dielectr. Electr. Insul. 30 (4), 1598–1607 (2023).
Das, S. K., Choi, S. U., Yu, W., & Pradeep, T. Nanofluids: Science and technology . (John Wiley & Sons, Inc. December 2007).
Keysight Technologies. 16452A Liquid Test Fixture: Operation and service manual . 7 th edn, (Keysight Technologies International Japan G.KApr. 2023).
Download references
Acknowledgements
Prof. Thomas Tsovilis and Prof. George Peppas dedicate this work to the memory of Costas Apostolidis, the founder of Raycap, in recognition of his significant contributions to their professional and personal growth. The authors express their sincere gratitude to Mr. Kevin Rapp, Senior Scientist at Cargill, for providing the FR3 natural ester oil. Special thanks are extended to Mrs. Sabine Bowers and Mr. Roberto Fernández Jardón affiliated with Cargill. Prof. Tsovilis would like to thank Mrs. M. Grigoriadou for her valuable administrative support in the research project 11482, funded by H.F.R.I. & SNF.
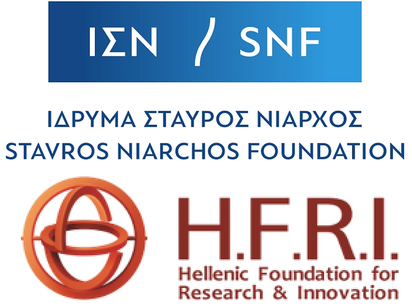
The research project was co-funded by the Stavros Niarchos Foundation (SNF) and the Hellenic Foundation for Research and Innovation (H.F.R.I.) under the 5th Call of “Science and Society” Action—“Always Strive for Excellence—Theodore Papazoglou” (Project Number: 11482).
Author information
Authors and affiliations.
High Voltage Laboratory, School of Electrical and Computer Engineering, Aristotle University of Thessaloniki, 54124, Thessaloniki, Greece
Thomas Tsovilis, Evangelos Staikos, Alexandros Hadjicostas & Zacharias Datsios
School of Electrical and Computer Engineering, Technical University of Crete, 73100, Chania, Greece
George Peppas
You can also search for this author in PubMed Google Scholar
Contributions
T.T.: conceptualization, data analysis and manuscript writing, G.P.: sample preparation, impulse current experiments, data analysis, A.H. and E.S.: conducted experiments, data acquisition, and data analysis, Z.D.: dielectric spectroscopy measurements, data analysis.
Corresponding author
Correspondence to Thomas Tsovilis .
Ethics declarations
Competing interests.
The authors declare no competing interests.
Additional information
Publisher's note.
Springer Nature remains neutral with regard to jurisdictional claims in published maps and institutional affiliations.
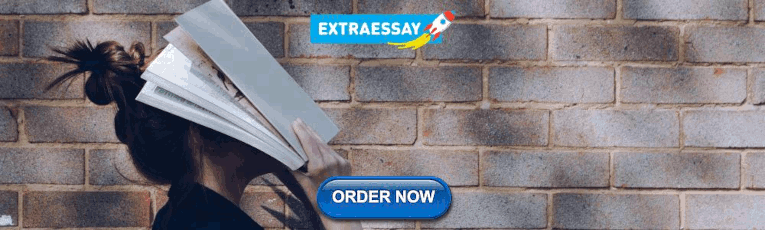
Rights and permissions
Open Access This article is licensed under a Creative Commons Attribution 4.0 International License, which permits use, sharing, adaptation, distribution and reproduction in any medium or format, as long as you give appropriate credit to the original author(s) and the source, provide a link to the Creative Commons licence, and indicate if changes were made. The images or other third party material in this article are included in the article's Creative Commons licence, unless indicated otherwise in a credit line to the material. If material is not included in the article's Creative Commons licence and your intended use is not permitted by statutory regulation or exceeds the permitted use, you will need to obtain permission directly from the copyright holder. To view a copy of this licence, visit http://creativecommons.org/licenses/by/4.0/ .
Reprints and permissions
About this article
Cite this article.
Tsovilis, T., Peppas, G., Staikos, E. et al. Exploring the surge current degradation of natural ester oil-based nanofluids. Sci Rep 14 , 7584 (2024). https://doi.org/10.1038/s41598-024-57575-0
Download citation
Received : 23 January 2024
Accepted : 19 March 2024
Published : 30 March 2024
DOI : https://doi.org/10.1038/s41598-024-57575-0
Share this article
Anyone you share the following link with will be able to read this content:
Sorry, a shareable link is not currently available for this article.
Provided by the Springer Nature SharedIt content-sharing initiative
By submitting a comment you agree to abide by our Terms and Community Guidelines . If you find something abusive or that does not comply with our terms or guidelines please flag it as inappropriate.
Quick links
- Explore articles by subject
- Guide to authors
- Editorial policies
Sign up for the Nature Briefing newsletter — what matters in science, free to your inbox daily.

Help | Advanced Search
Computer Science > Machine Learning
Title: generative ai for architectural design: a literature review.
Abstract: Generative Artificial Intelligence (AI) has pioneered new methodological paradigms in architectural design, significantly expanding the innovative potential and efficiency of the design process. This paper explores the extensive applications of generative AI technologies in architectural design, a trend that has benefited from the rapid development of deep generative models. This article provides a comprehensive review of the basic principles of generative AI and large-scale models and highlights the applications in the generation of 2D images, videos, and 3D models. In addition, by reviewing the latest literature from 2020, this paper scrutinizes the impact of generative AI technologies at different stages of architectural design, from generating initial architectural 3D forms to producing final architectural imagery. The marked trend of research growth indicates an increasing inclination within the architectural design community towards embracing generative AI, thereby catalyzing a shared enthusiasm for research. These research cases and methodologies have not only proven to enhance efficiency and innovation significantly but have also posed challenges to the conventional boundaries of architectural creativity. Finally, we point out new directions for design innovation and articulate fresh trajectories for applying generative AI in the architectural domain. This article provides the first comprehensive literature review about generative AI for architectural design, and we believe this work can facilitate more research work on this significant topic in architecture.
Submission history
Access paper:.
- HTML (experimental)
- Other Formats

References & Citations
- Google Scholar
- Semantic Scholar
BibTeX formatted citation

Bibliographic and Citation Tools
Code, data and media associated with this article, recommenders and search tools.
- Institution
arXivLabs: experimental projects with community collaborators
arXivLabs is a framework that allows collaborators to develop and share new arXiv features directly on our website.
Both individuals and organizations that work with arXivLabs have embraced and accepted our values of openness, community, excellence, and user data privacy. arXiv is committed to these values and only works with partners that adhere to them.
Have an idea for a project that will add value for arXiv's community? Learn more about arXivLabs .
- Share full article
Advertisement
Supported by
Once Upon a Time, the World of Picture Books Came to Life
The tale behind a new museum of children’s literature is equal parts imagination, chutzpah and “The Little Engine That Could.”

By Elisabeth Egan
Photographs and Video by Chase Castor
Elisabeth Egan followed the Rabbit Hole as it was nearing completion. She has written about several of its inhabitants for The Times.
On a crisp Saturday morning that screamed for adventure, a former tin can factory in North Kansas City, Mo., thrummed with the sound of young people climbing, sliding, spinning, jumping, exploring and reading.
Yes, reading.
If you think this is a silent activity, you haven’t spent time in a first grade classroom. And if you think all indoor destinations for young people are sticky, smelly, depressing hellholes, check your assumptions at the unmarked front door.
Welcome to the Rabbit Hole, a brand-new, decade-in-the-making museum of children’s literature founded by the only people with the stamina for such a feat: former bookstore owners. Pete Cowdin and Deb Pettid are long-married artists who share the bullish determination of the Little Red Hen. They’ve transformed the hulking old building into a series of settings lifted straight from the pages of beloved picture books.
Before we get into what the Rabbit Hole is, here’s what it isn’t: a place with touch screens, a ball pit, inscrutable plaques, velvet ropes, a cloying soundtrack or adults in costumes. It doesn’t smell like graham crackers, apple juice or worse (yet). At $16 per person over 2 years old, it also isn’t cheap.
During opening weekend on March 16, the museum was a hive of freckles and gap toothed grins, with visitors ranging in age from newborn to well seasoned. Cries of “Look up here!,” “There’s a path we need to take!” and “There’s Good Dog Carl !” created a pleasant pandemonium. For every child galloping into the 30,000 square foot space, there was an adult hellbent on documenting the moment.
Did you ever have to make a shoe box diorama about your favorite book? If so, you might remember classmates who constructed move-in ready mini kingdoms kitted out with gingham curtains, clothespin people and actual pieces of spaghetti.
Cowdin, Pettid and their team are those students, all grown up.
The main floor of the Rabbit Hole consists of 40 book-themed dioramas blown up to life-size and arranged, Ikea showroom-style, in a space the size of two hockey rinks. The one inspired by John Steptoe’s “ Uptown ” features a pressed tin ceiling, a faux stained-glass window and a jukebox. In the great green room from “ Goodnight Moon ,” you can pick up an old-fashioned phone and hear the illustrator’s son reading the story.

One fictional world blends into the next, allowing characters to rub shoulders in real life just as they do on a shelf. Visitors slid down the pole in “The Fire Cat,” slithered into the gullet of the boa constrictor in “ Where the Sidewalk Ends ” and lounged in a faux bubble bath in “ Harry the Dirty Dog .” There are plenty of familiar faces — Madeline , Strega Nona , Babar — but just as many areas dedicated to worthy titles that don’t feature household names, including “ Crow Boy ,” “ Sam and the Tigers ,” “ Gladiola Garden ” and “ The Zabajaba Jungle .”
Emma Miller, a first-grade teacher, said, “So many of these are books I use in my classroom. It’s immersive and beautiful. I’m overwhelmed.”
As her toddler bolted toward “ Frog and Toad ,” Taylar Brown said, “We love opportunities to explore different sensory things for Mason. He has autism so this is a perfect place for him to find little hiding holes.”
A gaggle of boys reclined on a bean bag in “ Caps for Sale ,” passing around a copy of the book. Identical twins sounded out “ Bread and Jam for Frances ” on the pink rug in the badger’s house. A 3-year-old visiting for the second time listened to her grandfather reading “The Tawny Scrawny Lion.”
Tomy Tran, a father of three from Oklahoma, said, “I’ve been to some of these indoor places and it’s more like a jungle gym. Here, my kids will go into the area, pick up the book and actually start reading it as if they’re in the story.”
All the titles scattered around the museum are available for purchase at the Lucky Rabbit, a bookstore arranged around a cozy amphitheater. Pettid and Cowdin estimate that they’ve sold one book per visitor, with around 650 guests per day following the pink bunny tracks from the parking lot.
Once upon a time, Cowdin and Pettid owned the Reading Reptile, a Kansas City institution known not just for its children’s books but also for its literary installations. When Dav Pilkey came to town, Pettid and Cowdin welcomed him by making a three-and-a-half foot papier-mâché Captain Underpants. Young customers pitched in to build Tooth-Gnasher Superflash or the bread airplane from “In the Night Kitchen.”
One of the store’s devotees was Meg McMath, who continued to visit through college, long after she’d outgrown its offerings (and its chairs). Now 36, McMath traveled from Austin, Texas with her husband and six-month-old son to see the Rabbit Hole. “I’ve cried a few times,” she said.
The Reading Reptile weathered Barnes & Noble superstores and Amazon. Then came “the Harry Potter effect,” Pettid said, “where all of a sudden adults wanted kids to go from picture books to thick chapter books. They skipped from here to there; there was so much they were missing.”
As parents fell under the sway of reading lists for “gifted” kids, story time became yet another proving ground.
“It totally deformed the reading experience,” Cowdin said. Not to mention the scourge of every bookstore: surreptitious photo-snappers who later shopped online.

In 2016, Cowdin and Pettid closed the Reptile to focus on the Rabbit Hole, an idea they’d been percolating for years. They hoped it would be a way to spread the organic bookworm spirit they’d instilled in their five children while dialing up representation for readers who had trouble finding characters who looked like them. The museum would celebrate classics, forgotten gems and quality newcomers. How hard could it be?
Cowdin and Pettid had no experience in the nonprofit world. They knew nothing about fund-raising or construction. They’re ideas people, glass half full types, idealists but also stubborn visionaries. They didn’t want to hand their “dream” — a word they say in quotes — to consultants who knew little about children’s books. Along the way, board members resigned. Their kids grew up. Covid descended. A tree fell on their house and they had to live elsewhere for a year. “I literally have told Pete I quit 20 times,” Pettid said.
“It has not always been pleasant,” Cowdin said. “But it was just like, OK, we’re going to do this and then we’re going to figure out how to do it. And then we just kept figuring it out.”
Little by little, chugging along like “ The Little Engine That Could ,” they raised $15 million and assembled a board who embraced their vision and commitment to Kansas City. They made a wish list of books — “Every ethnicity. Every gender. Every publisher,” Pettid said — and met with rights departments and authors’ estates about acquiring permissions. Most were receptive; some weren’t. (They now have rights to more than 70 titles.)
“A lot of people think a children’s bookstore is very cute,” Pettid said. “They have a small mind for children’s culture. That’s why we had to buy this building.”
For $2 million, they bought the factory from Robert Riccardi, an architect whose family operated a beverage distribution business there for two decades. His firm, Multistudio, worked with Cowdin and Pettid to reimagine the space, which sits on an industrial corner bordered by train tracks, highways and skyline views.
Cowdin and Pettid started experimenting with layouts. Eventually they hired 39 staff members, including 21 full-time artists and fabricators who made everything in the museum from some combination of steel, wood, foam, concrete and papier-mâché.
“My parents are movers and shakers,” Gloria Cowdin said. She’s the middle of the five siblings, named after Frances the badger’s sister — and, yes, that’s her voice reading inside the exhibit. “There’s never been something they’ve wanted to achieve that they haven’t made happen, no matter how crazy.”

During a sneak peek in December, it was hard to imagine how this semi-construction zone would coalesce into a museum. The 22,000 square foot fabrication section was abuzz with drills and saws. A whiteboard showed assembly diagrams and punch lists. (Under “Random jobs,” someone had jotted, “Write Christmas songs.”) The entryway and lower level — known as the grotto and the burrow — were warrens of scaffolding and machinery.
But there were pockets of calm. Kelli Harrod worked on a fresco of trees outside the “ Blueberries for Sal ” kitchen, unfazed by the hubbub. In two years as lead painter, she’d witnessed the Rabbit Hole’s steady growth.
“I remember painting the ‘ Pérez and Martina ’ house before there was insulation,” Harrod said. “I was bundled up in hats, gloves and coats, making sure my hands didn’t shake.”
Leigh Rosser was similarly nonplused while describing his biggest challenge as design fabrication lead. Problem: How to get a dragon and a cloud to fly above a grand staircase in “ My Father’s Dragon .” Solution: “It’s really simple, conceptually” — it didn’t sound simple — “but we’re dealing with weight in the thousands of pounds, mounted up high. We make up things that haven’t been done before, or at least that I’m not aware of.”
Attention to detail extends to floor-bound exhibits. The utensil drawer in “Blueberries for Sal” holds Pete Cowdin’s mother’s egg whisk alongside a jar containing a baby tooth that belonged to Cowdin and Pettid’s oldest daughter, Sally. The tooth is a wink at “ One Morning in Maine ,” an earlier Robert McCloskey book involving a wiggly bicuspid — or was it a molar? If dental records are available, Cowdin and Pettid have consulted them for accuracy.
“With Pete and Deb, it’s about trying to picture what they’re seeing in their minds,” said Brian Selznick , a longtime friend who helped stock the shelves in the Lucky Rabbit. He’s the author of “ The Invention of Hugo Cabret ,” among many other books.
Three months ago, the grotto looked like a desert rock formation studded with pink Chiclets. The burrow, home of Fox Rabbit, the museum’s eponymous mascot, was dark except for sparks blasting from a soldering iron. The floor was covered with tiny metal letters reclaimed from a newly-renovated donor wall at a local museum.
Cowdin and Pettid proudly explained their works-in-progress; these were the parts of the museum that blossomed from seed in their imaginations. But to the naked eye, they had the charm of a bulkhead door leading to a scary basement.
When the museum opened to the public, the grotto and the burrow suddenly made sense. The pink Chiclets are books, more than 3000 of them — molded in silicone, cast in resin — incorporated into the walls, the stairs and the floor. They vary from an inch-and-a-half to three inches thick. As visitors descend into the Rabbit Hole, they can run their fingers over the edges of petrified volumes. They can clamber over rock formations that include layers of books. Or they can curl up and read.
Dennis Butt, another longtime Rabbit Hole employee, molded 92 donated books into the mix, including his own copies of “ The Hobbit ” and “ The Lord of the Rings .” He said, “They’re a little piece of me.”
As for the metal letters, they’re pressed into the walls of a blue-lit tunnel leading up a ramp to the first floor. They spell the first lines of 141 books, including “ Charlotte’s Web ,” “Devil in the Drain” and “ Martha Speaks .” Some were easier to decipher than others, but “Mashed potatoes are to give everybody enough” jumped out. It called to mind another line from “A Hole is to Dig,” Ruth Krauss’s book of first definitions (illustrated by a young Maurice Sendak ): “The world is so you have something to stand on.”
At the Rabbit Hole, books are so you have something to stand on. They’re the bedrock and the foundation; they’re the solid ground.
Cowdin and Pettid have plans to expand into three more floors, adding exhibit space, a print shop, a story lab, a resource library and discovery galleries. An Automat-style cafeteria and George and Martha -themed party and craft room will open soon. A rooftop bar is also in the works.
Of course, museum life isn’t all happily ever after. Certain visitors whined, whinged and wept, especially as they approached the exit. One weary adult said, “Charlie, we did it all.”
Then, “Charlie, it’s time to go.”
And finally, “Fine, Charlie, we’re leaving you here.” Cue hysteria.
But the moral of this story — and the point of the museum, and maybe the point of reading, depending on who you share books with — crystallized in a quiet moment in the great green room. A boy in a Chiefs Super Bowl T-shirt pretended to fall asleep beneath a fleecy blanket. Before closing his eyes, he said, “Goodnight, Grandma. Love you to the moon.”
Elisabeth Egan is a writer and editor at the Times Book Review. She has worked in the world of publishing for 30 years. More about Elisabeth Egan
The Great Read
Here are more fascinating tales you can’t help reading all the way to the end..
Deathbed Visions: Researchers are documenting deathbed visions , a phenomenon that seems to help the dying, as well as those they leave behind.
The Pants Pendulum: Around 2020, the “right” pants began to swing from skinny to wide. But is there even a consensus around trends anymore ?
The Psychic Peril of Mars: NASA is conducting tests on what might be the greatest challenge of a human mission to the red planet: the trauma of isolation .
Saved by a Rescue Dog: He spent 13 years addicted to cocaine. Running a shelter for abused and neglected dogs in New York has kept him sober, but it hasn’t been easy .
An Art Mogul's Fall: After a dramatic rise in business and society, Louise Blouin finds herself unloading a Hamptons dream home in bankruptcy court .

An official website of the United States government
The .gov means it’s official. Federal government websites often end in .gov or .mil. Before sharing sensitive information, make sure you’re on a federal government site.
The site is secure. The https:// ensures that you are connecting to the official website and that any information you provide is encrypted and transmitted securely.
- Publications
- Account settings
Preview improvements coming to the PMC website in October 2024. Learn More or Try it out now .
- Advanced Search
- Journal List
- Nanomaterials (Basel)
- PMC10647477

A Review of Nanofluids as Coolants for Thermal Management Systems in Fuel Cell Vehicles
1 Hubei Key Laboratory of Modern Manufacture Quality Engineering, School of Mechanical Engineering, Hubei University of Technology, Wuhan 430068, China; moc.anis@sxfz_gh
Yadong Deng
2 Hubei Key Laboratory of Advanced Technology for Automotive Components, Wuhan University of Technology, Wuhan 430070, China; moc.anis@gnodaygned
Yiping Wang
3 Hubei Collaborative Innovation Center for Automotive Components Technology, Wuhan University of Technology, Wuhan 430070, China; nc.ude.tuhw@gnipiygnaw
4 Hubei Research Center for New Energy & Intelligent Connected Vehicle, Wuhan University of Technology, Wuhan 430070, China; moc.361@iquhcus
Associated Data
No new data were created or analyzed in this study. Data sharing is not applicable to this article.
With the development of high-power fuel cell vehicles, heat dissipation requirements have become increasingly stringent. Although conventional cooling techniques improve the heat dissipation capacity by increasing the fan rotating speed or radiator dimensions, high energy consumption and limited engine compartment space prevent their implementation. Moreover, the insufficient heat transfer capacity of existing coolants limits the enhancement of heat dissipation performance. Therefore, exploring novel coolants to replace traditional coolants is important. Nanofluids composed of nanoparticles and base liquids are promising alternatives, effectively improving the heat transfer capacity of the base liquid. However, challenges remain that prevent their use in fuel cell vehicles. These include issues regarding the nanofluid stability and cleaning, erosion and abrasion, thermal conductivity, and electrical conductivity. In this review, we summarize the nanofluid applications in oil-fueled, electric, and fuel cell vehicles. Subsequently, we provide a comprehensive literature review of the challenges and future research directions of nanofluids as coolants in fuel cell vehicles. This review demonstrates the potential of nanofluids as an alternative thermal management system that can facilitate transition toward a low-carbon, energy-secure economy. It will serve as a reference for researchers to focus on new areas that could drive the field forward.
1. Introduction
1.1. nanofluid overview.
Nanofluids were first proposed by Professor Choi at Aragon National Laboratory, the United States of America in the early 1990s. They are composed of nanoparticles, metal, or polymer particles of sizes ranging from 1 nm to 100 nm and the base liquid. This is shown in Figure 1 . Nanofluids have a capacity to enhance the heat dissipation performance of the base fluid. They exhibit a variety of unique thermal, optical, electrical, magnetic, and other properties, and they have hence been innovatively utilized in heat transfer engineering and applied in different fields including aerospace, energy, electromechanics, and biomedicine [ 1 ].

Schematic diagram of nanofluid compositions.
The heat transfer enhancement mechanism of a nanofluid involves the addition of nanoparticles to the base fluid, increasing the thermal conductivity of the mixture and thereby improving the heat transfer capacity of the base fluid. In addition, particle-to-particle and wall-to-wall collisions and the mutual motion between the particles and liquid improve the heat transfer characteristics.
However, this is a complicated process in microchannels. Molecular dynamics have been used to analyze the heat transfer mechanism at solid–liquid interfaces. Frank [ 2 , 3 ] investigated the effects of surface irregularities and imperfections on thermal resistance using molecular dynamics (MD). The thermal resistance was reduced by increasing the strength of the solid–liquid interface. Ghorbanian [ 4 ] used nonequilibrium molecular dynamics to analyze the effects of the wall force field and scale. The results proved that wall effects lead to continuum solution deviations. Papanikoulaou [ 5 ] used molecular dynamics to analyze the effects of surface roughness on nanoflows. The results showed that the flow velocity near the wall was reduced with an increasing roughness depth. Liu [ 6 ] explored the transport and structural characteristics of water inside nanotubes using molecular dynamic simulations. The results indicated that thermal conductivity and shear viscosity increased as the pore size decreased. McGaughey [ 7 ] also used molecular dynamics simulations to analyze the heat transport mechanism of silica-based crystals. The simulation results showed that thermal conductivity is related to the scale and temperature of the atomic structure of silica-based crystals. Sun [ 8 ] used the colloidal probe technique to analyze the force profiles of nanoparticles and base fluids. The test results showed that the measured and calculated force curves exhibited an evident slip (10–14 nm).
Nanofluids can be used as coolants in systems with high heat-dissipation requirements. For example, nanofluid applications have been investigated in food processing, solar energy collection, nuclear reactors, and oil recovery. In food processing, nanofluids can be used to increase heat exchanger efficiency and reduce energy consumption. Nanofluids can shorten the thermal processing time and improve the nutritional content of food [ 9 , 10 , 11 , 12 , 13 , 14 ]. In the solar energy collecting field, nanofluids have been proven to increase the collecting efficiency of solar energy collectors, including parabolic trough solar collectors, evacuated tube solar collectors, flat plate solar collectors, and solar cookers [ 15 , 16 , 17 , 18 , 19 , 20 , 21 , 22 ]. This is illustrated in Figure 2 . In the nuclear reactor field, nanofluids as coolants could increase the cooling capacity and maintain safe operation of nuclear reactor cooling systems [ 23 , 24 , 25 , 26 , 27 , 28 , 29 , 30 , 31 , 32 , 33 , 34 , 35 ]. Nanofluids also can be used to improve oil recovery efficiency due to their excellent heat transfer performance [ 36 , 37 , 38 , 39 , 40 , 41 , 42 , 43 , 44 , 45 , 46 , 47 , 48 , 49 , 50 , 51 ]. For example, nanofluid applications in oil-fueled vehicles include their use in cooling, lubrication, air conditioners, and exhaust power generation systems, as shown in Figure 2 .

Nanofluid applications in oil-fueled vehicles.
In 2004, the U.S. Department of Energy used nanofluids in fuel cells, and the heat dissipation capacity of cooling systems could be significantly improved [ 52 ]. Particularly, for fuel cell vehicles, nanofluids have the potential to replace existing coolants and improve the heat transfer capacity of thermal management systems. This paper will explore the challenges and future research directions of nanofluids as coolants in fuel cell vehicles.
1.2. Nanofluid Application in Different Types of Vehicles
1.2.1. nanofluid application in oil-fueled vehicles.
- Nanofluid application in oil-fueled vehicles’ cooling systems
Nanofluids can be used to improve the heat-dissipation capacity of radiators in cooling systems. Many researchers have investigated the mechanism of heat transfer enhancement in radiator tubes using different nanofluids. Table 1 presents a summary of the nanofluid applications in oil-fueled-vehicle cooling systems.
Nanofluid applications in oil-fueled vehicles’ cooling systems [ 53 , 54 , 55 , 56 , 57 , 58 , 59 , 60 , 61 , 62 , 63 , 64 , 65 , 66 , 67 , 68 , 69 , 70 , 71 , 72 , 73 , 74 , 75 , 76 , 77 , 78 , 79 , 80 , 81 , 82 , 83 , 84 , 85 , 86 , 87 , 88 , 89 , 90 , 91 , 92 , 93 , 94 , 95 , 96 , 97 , 98 , 99 ].
According to the literature content in Table 1 above, nanoparticles including Al 2 O 3 , TiO 2 , CuO, SiO 2 , Fe 3 O 4 , Fe 2 O 3 , ZnO, SiC, GnP, CNC, MWCNT, CNT, and graphene have been analyzed in detail. In addition, the thermophysical properties and heat transfer performance of different types of nanofluids have been analyzed in the past. The influencing factors, including concentration, flow rate, temperature, and radiator dimensions, have been compared. Using a nanofluid instead of a conventional coolant has been demonstrated to improve the heat dissipation performance of thermal management systems [ 100 , 101 , 102 , 103 , 104 , 105 , 106 , 107 ].
- Nanofluid applications in oil-fueled vehicles’ air conditioner systems
Sharif [ 108 ] chose a nanofluid as a lubricant to enhance the compressor performance. A SiO 2 /PAG nanofluid was selected as the nano-lubricant. A vehicle air conditioner test rig was used to measure the heat absorption and coefficient of performance (COP). The test results indicated that the maximum increase in the COP was 24% when using the SiO 2 /PAG nanofluid. Redhwan [ 109 ] prepared Al 2 O 3 -SiO 2 /PAG nanofluids and compared the heat transfer performance and tribology enhancement. Zawawi [ 110 ] used an Al 2 O 3 -SiO 2 /PAG nano-lubricant and analyzed its effect on the performance improvement of vehicle air conditioner systems through experimental tests. The test bench is illustrated in Figure 3 . It was composed of a power analyzer, frequency inverter, electric motor, water bath, compressor, data logger, water heater, and condenser.

AAC system experimental test bench [ 110 ].
- Nanofluid applications in oil-fueled vehicles’ lubrication systems
The main reason for the failure of lubricating oils at high temperatures is thermal degradation. Nanofluids can delay the oxidation and burnout of the lubricating oil, which results in a wider temperature range and higher fuel economy. Ali [ 111 ] used a thermal analyzer (TGA/DTG/DSC) to investigate the thermal degradation of lubricating oil. An AVL dynamometer was used to test the engine thermal efficiency with the Al 2 O 3 -TiO 2 /lube nanofluid. Lue [ 112 ] used a two-step method to prepare four different weight fractions of an Al 2 O 3 /lube nanofluid. The densities and viscosities of the nano-lubricant were measured at various temperatures. The test results indicated that the 1.5 wt % Al 2 O 3 /lube nanofluid was the optimal choice for future research. Esfe [ 113 ] proposed a type of MWCNT-ZnO nano-lubricant for light-duty vehicles that can be used to reduce viscosity and effectively improve the engine’s efficiency.
- Nanofluid applications in oil-fueled vehicles’ exhaust power generation systems
Researchers have investigated the possible utilization of waste heat in exhaust pipes because of the large amount of waste heat produced during engine operation. Based on the thermoelectric conversion unit, waste heat can be converted into electricity. For example, Hilmin [ 114 ] prepared a TiO 2 /water nanofluid using a two-step method to increase the efficiency of conversion units in thermoelectric generators (TEGs). The experimental results demonstrated that the TiO 2 /water nanofluid effectively enhanced the conversion efficiency and output power of the TEG. Karana [ 115 ] prepared ZnO/WEG and MgO/WEG nanofluids that were used to improve the efficiency of waste heat recovery for a TEG. The influencing factors included the Reynolds number, total TEG area, nanofluid concentration, and inlet temperature. According to the analysis results, the total TEG area was reduced by 33% with a 1% MgO/WEG nanofluid.
1.2.2. Nanofluid Application in Electric Vehicles
Batteries are the most important power source for electric vehicles. However, maintaining an ordinary operating temperature using a thermal management system is crucial. Nanofluids have been used as a coolant to replace existing coolants, and their performance in improving heat-dissipation capacity has been analyzed. For example, Chen [ 116 ] constructed a battery heating module with a pipe and selected a TiO 2 -CLPHP nanofluid as the coolant. The heating performances of different strategies were investigated. The test results indicated that the 2% TiO 2 /CLPHP nanofluid exhibited the best heat transfer performance. Wu [ 117 ] used a Cu/H 2 O nanofluid with a lattice Boltzmann (LB) model to simulate natural convection in a battery. The problem of a uniformly distributed heat boundary was validated to ensure the accuracy of the model. The results proved that the Cu/H 2 O nanofluid could improve the cooling performance and reduce the temperature difference in a battery thermal management system. Abdelkareem [ 118 ] compared different heat-dissipation capacity-enhancement methods for batteries, including air cooling, liquid cooling, heat pipes, and phase change material and found that the nanofluid effectively enhanced the heat-dissipation capacity. Guo [ 119 ] proposed a type of battery cooling system with a liquid cold plate and analyzed the different factors influencing the heat-dissipation performance. The simulation results showed that the maximum temperature and temperature differences were separately reduced. Adding nanoparticles (Al, Cu, and Ag) to the base fluid could effectively improve the heat transfer performance. Kiani [ 120 ] designed a hybrid cooling system with nanofluid as the coolant. The results indicated that the nanofluid ensured battery safety under stressful conditions. Moreover, many researchers have analyzed the heat-dissipation improvement in battery thermal management systems using nanofluids as coolant [ 121 , 122 , 123 , 124 , 125 , 126 , 127 , 128 , 129 , 130 ].
1.2.3. Nanofluid Application in Fuel Cell Vehicles
In 2004, the U.S. Department of Energy first selected nanofluids as coolants for thermal management systems in fuel cell vehicles. The preparation cost of the nanofluid can be reduced and is close to that of the traditional coolants used in oil-fueled vehicles. Nanofluids can be used as coolants to reduce the radiator dimensions. They can also effectively improve the heat-dissipation capacity of cooling systems [ 52 ]. Many researchers have conducted preliminary studies on the application of nanofluids in fuel cell vehicles. For example, Bargal [ 131 ] analyzed the research progress on nanofluids in a PEMFC and compared different cooling methods for thermal management systems in fuel cell vehicles. Islam [ 132 ] discussed the application of nanofluids as coolants to improve the heat-dissipation capacity of thermal management systems. The results proved that nanofluids can reduce the radiator dimensions and enhance the heat-dissipation capacity. Islam [ 133 ] analyzed a 0.5% ZnO/WEG nanofluid as a coolant to improve the heat-dissipation capacity of thermal management systems. The results showed that the frontal area of the radiator could be reduced by 27% compared to when EG/water was used as a coolant. Islam [ 134 ] investigated the electrical conductivity and heat transfer coefficient of TiO 2 /WEG nanofluid at 0.05–0.5%. The experimental results indicated that the thermal conductivity coefficient increased by 10% with the 0.5% TiO 2 /WEG nanofluid. Zakaria [ 135 ] investigated the heat-dissipation performance of 0.1% and 0.5% Al 2 O 3 /WEG nanofluids in PEMFCs. The experimental results showed that the heat-dissipation power increased by 13.87% with a 0.5% Al 2 O 3 /WEG nanofluid. Zakaria [ 136 ] analyzed the heat transfer performance of 0.1% and 0.5% Al 2 O 3 /WEG nanofluids with different base fluid ratios. The test results indicated that the heat transfer performance of the 0.1% Al 2 O 3 /WEG nanofluid at a 60:40 ratio was better than that at a 50:50 ratio. Zakaria [ 137 ] also analyzed the ratio of thermal conductivities and electrical conductivities of 0.1%, 0.3%, and 0.5% Al 2 O 3 nanofluids. The testing results showed that the thermal conductivity was reduced when the ethylene glycol proportion was increased. They also showed that the electrical conductivity was reduced when the ethylene glycol proportion was increased. In order to assess the significance of Al 2 O 3 nanofluids in an electrically active thermal device, the thermoelectrical conductivity (TEC) ratio was established.
The aforementioned research studies were mainly based on metallic nanoparticles. Boron nitride (BN) is an insulating ceramic with high stability, high thermal conductivity, and good chemical inertness. For example, Ilhan [ 138 ] prepared a nanofluid from boron nitride (BN) and analyzed its heat transfer performance. The test results proved that BN nanofluid has considerable potential for use as a coolant in fuel cell vehicles.
2. Nanofluid Application in Thermal Management Systems of Fuel Cell Vehicles
2.1. thermal management system structure.
The thermal management system of a fuel cell vehicle (FCV) comprises heat dissipation and air conditioning systems. The heat-dissipation system includes heat-generating (fuel cell stack, DC/DC converter, motor, motor controller, and air compressor) and heat-dissipation (radiator, water pump, fan, and expansion tank) components, as shown in Figure 4 .

Thermal management system of a fuel cell vehicle.
A proton-exchange membrane fuel cell (PEMFC) engine comprises an air supply system, control system, cooling system, fuel cell stack, and fuel supply system. Among these, the fuel cell stack is composed of a single PEMFC, which converts chemical into electrical energy. The cooling system of the PEMFC includes a radiator, fan, pump, intercooler, thermostat, and water tank. The gas temperature at the cathode inlet is adjusted using the coolant temperature and flow rate inside the PEMFC. The coolant can be circulated in a cooling loop, and heat is transferred from the interior of the fuel cell. Figure 5 shows a schematic diagram of a PEMFC engine. The systems and components work together to complete the normal operation of the fuel cell engine.

PEMFC engine [ 139 ].
2.2. Cooling Performance Improvement of Thermal Management System
2.2.1. heat-production components.
Many researchers have investigated methods for optimizing the cooling techniques of heat-dissipation components to maintain the ordinary operating temperature of PEMFCs in fuel cell vehicles. As mentioned earlier, the heat-production components of fuel cell vehicles include fuel cells, power batteries, motors, motor controllers, air compressors, and DC/DC converters.
- Cooling techniques for fuel cells
According to the different output powers of fuel cells, their cooling methods can be classified as cathode air, separate airflow, phase change, and liquid cooling. The results are presented in Table 2 . Presently, the output power of fuel cell engines in vehicles is higher than 10 kW. Liquid cooling is the primary cooling technique for fuel cell engines.
Cooling methods for fuel cells [ 140 ].
The heat-dissipation performance can be improved by optimizing the internal flow channel structure and adjusting the fuel cell control strategy. The design optimization of fuel cells includes the use of flow channels, bipolar plates, and polymer film cooling channels. The structure of the flow channel includes serpentine, parallel, wave, and spiral flow channels, as shown in Figure 6 . The efficiency of fuel cells and different cooling fields was analyzed using a numerical method. It was found that the nearest route to the entrance had a worse fluid distribution than the farthest route. Optimization of the flow channel inside the FCs effectively improved the temperature uniformity and heat exchange rate. Thus, this can enhance a fuel cell’s stability, durability, heat-dissipation capacity, and lifetime [ 141 , 142 , 143 ].

Different flow channel structures inside a fuel cell [ 141 ].
In addition, a reasonable control strategy can precisely control the rotational speed of the pump and fan of the cooling system under different operating conditions, ensuring that the fuel cell engines are in the normal operating temperature range to prevent overheating or overcooling. This is helpful in enhancing the operating efficiency of fuel cells [ 144 , 145 ].
- Cooling techniques for batteries
The cooling techniques for batteries include air and liquid cooling. Air cooling can be classified into natural or forced convection. The forced air-cooling technique has the advantages of a simple structure and low manufacturing cost. Moreover, it can increase the heat transfer coefficient on the air side and improve the heat-dissipation capacity relative to natural convection [ 146 ]. Figure 7 shows the schematic of forced air cooling. The battery system is composed of 72 battery cells (270 V and 1400 Wh). The coolant passage between the battery cells is 3 mm, which needs to dissipate a heat flux of 245 W/m 2 .

Schematic diagram of forced air cooling [ 146 ].
Currently, many researchers have investigated the effect of different air-cooling structures and arrangements on cooling performance using theoretical analyses and numerical simulations. For example, the effect of a conical structure on the cooling performance of a battery was investigated. Considering the influencing factors, including the discharge rate, fin arrangement, inlet air temperature, and inlet air flow rate, a prismatic air-cooled module and an aluminum-finned radiator were designed [ 146 , 147 , 148 , 149 , 150 ]. However, the liquid-cooling technique is more effective than the air-cooling technique.
- Cooling techniques for other components
Cooling techniques for the motor and motor controllers include natural, liquid, air, and oil cooling. The cost of air cooling is lower than that of other cooling techniques. However, the heat-dissipation capacity is not as high as that of liquid cooling. The cooling techniques for air compressors include air and liquid cooling. The former cooling method can be improved by optimizing the structure of the air compressor. This cooling method can be improved by enhancing the thermophysical properties of the coolant. Liquid cooling has a better heat-dissipation performance than air cooling. Liquid cooling is also used for DC/DC converters; the cooling can be improved by enhancing the thermophysical properties of the coolant or by improving the structure of the DC/DC converter. Table 3 summarizes the improvement methods for the different heat-production components. Liquid cooling was found to be the best cooling method for all the heat-dissipation components.
Heat-dissipation method and improvement method.
2.2.2. Heat-Dissipation Components
The heat-dissipation components of thermal management systems include radiators, pumps, and fans. For the radiator, heat-dissipation-capacity improvement is mainly achieved by structural and material optimization, where the structure includes fin and flat tube structures [ 151 , 152 , 153 , 154 , 155 , 156 , 157 , 158 , 159 , 160 , 161 , 162 , 163 , 164 , 165 , 166 , 167 ]. Many researchers have investigated the structure and material optimization for radiators. For example, Habibian [ 151 ] investigated the structure of a flat tube and fin of a radiator using numerical analysis. The research results indicated that the louver fin exhibited the best heat-dissipation performance, as shown in Figure 8 . Due to the fact that an automotive vehicle radiator is composed of hundreds of fins and a few tubes, the heat transfer performance and pressure drop can be analyzed in one fin. Vaisi [ 152 ] investigated the air-flow pressure drop and air-side heat transfer characteristics of a louver fin. The analysis results indicated that the heat-dissipation performance of the radiator was affected by its dimensions and Reynolds number.

Structure of louver fin radiator [ 151 ].
Pumps and fans are the main heat-dissipation components of thermal management systems, and they can be improved by optimizing the structure and control strategy. For example, Liu [ 168 ] used a computational fluid dynamics (CFD) method to analyze the heat-dissipation capacity of a seven-blade fan. They considered influential factors, including the blade number, air velocity, and pressure, in the heat-dissipation performance of the fan.
In summary, the radiator, pump, and fan were improved via structural optimization. Structural optimization is more complex and expensive than improving the thermophysical properties of coolants. According to the above literature, there is considerable potential for investigating nanofluids as coolants to improve the heat-dissipation performance of thermal management systems in fuel cell vehicles.
3. Nanofluids as Coolants in Fuel Cell Vehicles
3.1. nanofluid thermophysical properties.
The thermophysical properties of nanofluids, including density, specific heat, and thermal conductivity, have been extensively investigated. The equations for the thermophysical properties are as follows [ 132 ].
Nanofluid density can be calculated as shown in Equation (1):
where ρ n f is the nanofluid density, ρ b f is the base fluid density, ρ p is the nanoparticle density, and φ is the nanofluid volume fraction.
Nanofluid specific heat can be calculated as shown in Equation (2):
where C nf is the nanofluid specific heat, C bf is the base fluid specific heat, C p is the nanoparticle specific heat, and φ is the nanofluid volume fraction.
Nanofluid thermal conductivity was calculated by Hamilton & Crosser as shown in the following Equation (3):
where k nf is the nanofluid thermal conductivity, k bf is the base fluid thermal conductivity, k p is the nanoparticle thermal conductivity, φ is the nanofluid volume fraction, and n is the shape factor.
According to the literature above, ZnO, Al 2 O 3 , TiO 2 , and BN nanoparticles can be added into the base fluid to prepare a new coolant for fuel cell vehicles. The thermophysical parameters of four nanofluids can be seen in Table 4 below. Hence, nanofluids’ thermophysical properties can be calculated by using Equations (1)–(3).
Nanoparticles’ density, specific heat, and thermal conductivity.
The calculation results are presented in Figure 9 below. The nanofluid density increases with an increasing volume fraction. At the same volume fraction, the density of ZnO nanofluid > TiO 2 nanofluid > Al 2 O 3 nanofluid > BN nanofluid. The specific heat of the nanofluid decreases as the volume fraction increases. The specific heat of BN nanofluid > Al 2 O 3 nanofluid > TiO 2 nanofluid > ZnO nanofluid at the same volume fraction. The nanofluid thermal conductivity also increases as the volume fraction increases. At the same volume fraction, the thermal conductivity of BN nanofluid > ZnO nanofluid > Al 2 O 3 nanofluid > TiO 2 nanofluid.

Thermophysical properties of four types of nanofluids.
3.2. Nanofluid Thermal Conductivity Model
Thermal conductivity is one of the most important thermophysical properties used to calculate the heat-dissipation power of a nanofluid. It is affected by different factors, including the nanoparticles’ thermal conductivity, concentration (volume fraction or mass fraction), shape, and size, as well as the base fluid’s thermal conductivity. Several classical thermal conductivity models have been considered. For example, the Maxwell model, which is a thermal conductivity model for solid–liquid and two-phase coolants, was the first proposed. It is a static model for determining the thermal conductivity of monodisperse, low-volume-fraction, and spherical nanoparticles, as shown in Equation (4) [ 169 ]. Subsequently, many researchers proposed thermal conductivity models, including the Braggeman model, Yamada and Ota model, Wasp model, and Hamilton and Crosser model [ 170 ]. The Braggeman model is a type of thermal conductivity model suitable for solid–liquid suspensions with low concentrations. The calculation results obtained using the Braggeman model were close to those of the Maxwell model [ 133 ]. The Hamilton and Crosser model is an extension of the Maxwell model that introduces sphericity to the consideration. The thermal conductivity of solid–liquid suspensions with different nanoparticle shapes were calculated [ 171 ]. The Yamada and Ota model is a modified version of the Hamilton and Crosser model that replaced the (n − 1) factor with the parameter k [ 172 ]. Davis [ 173 ] proposed a thermal conductivity model for nanofluids containing spherical particles. It can be used to calculate the heat transfer rate between solid particles and the thermal conductivity from the nanoparticle to the base fluid.
where K nf is the effective thermal conductivity of the solid–liquid suspension, K p is the thermal conductivity of the nanoparticles, K bf is the thermal conductivity of the base fluid, and φ is the volume fraction of the nanoparticles.
Bruggeman [ 132 ] proposed a thermal conductivity model for a nanofluid in which the particles are distributed randomly. If the volume fraction of the nanofluid is low, the thermal conductivity is close to the experimental results. This model can be seen in Equation (5):
where k p is the thermal conductivity of the nanoparticles, φ is the volume fraction of the nanofluid, and k b is the thermal conductivity of the base fluid.
The Hamilton and Crosser model is extended from the Maxwell model by introducing sphericity, which allows the calculation of the thermal conductivity of solid–liquid suspensions formed by spherical or non-spherical nanoparticles [ 171 ]. This model can be seen in Equation (7):
where n is the shape factor:
where Ψ is the sphericity degree, which is the ratio between a sphere’s surface area and a particle’s surface area at the same volume.
Yamada and Ota modified the Hamilton and Crosser model by replacing the ( n − 1) factor with the parameter k in the equation [ 172 ]. This can be seen in Equation (10):
where k bf is the base fluid thermal conductivity, φ is the nanofluid volume fraction, and k p is the thermal conductivity of the nanoparticles.
When a nanoparticle is cylindrical, the expression is as follows:
where L is the nanoparticle length, and d is the nanoparticle diameter.
Davis proposed a model of thermal conductivity that contains spherical particles. However, Davis’s model is only suitable for calculating the thermal conductivity of spherical particles and a low volume fraction [ 173 ]. It can be seen in Equation (12):
where A p and B p are the constants related to parameters α and p .
However, predicting nanofluid thermal conductivity accurately using the aforementioned thermal conductivity models is difficult, and many researchers have proposed a theory of adsorption layer thermal conductivity, which refers to the liquid layer formed around solid nanoparticles. The thermal conductivity of the adsorption layer is lower than that of the nanoparticles and higher than that of the base fluid. The thermodynamic properties of the nanoparticles can be transferred to the base liquid, which is reflected in the change in the nanofluid’s thermal conductivity [ 174 ]. However, the adsorption layer of a nanofluid is difficult to measure and observe from a microscopic perspective, which is a concept derived from theoretical analyses by researchers. Figure 10 illustrates a schematic of nanoparticles and adsorption layers.

Schematic diagram of nanoparticles and adsorption layers.
The thermal conductivity curve for the adsorption layer includes linear and exponential distribution curves. The model for the linear distribution law of the thermal conductivity within the adsorption layer is shown in Equation (14):
where k l (r) is the thermal conductivity within the adsorption layer, k bf is the base fluid thermal conductivity, r p is the nanoparticle size, t is the adsorption layer thickness, k p is the nanoparticle thermal conductivity, and k l is average thermal conductivity of the nanofluid adsorption layer.
However, there is no unified mathematical equation for calculating the thermal conductivity of nanofluids in fuel cell vehicles. The adsorption layer theory must be used to calculate the nanofluid thermal conductivity more accurately.
3.3. Single and Hybrid Nanofluids
In recent years, two or more nanoparticles have been mixed to prepare multi-mixed nanofluids. Subsequently, the heat-dissipation performances of PEMFCs were investigated. For example, Huang [ 175 ] investigated a hybrid nanofluid of Al 2 O 3 and MWCNTs using experimental analysis. The results showed that heat transfer coefficient of the hybrid nanofluid was slightly higher than that of the Al 2 O 3 nanofluid at the same flow velocity. The hybrid nanofluid had the highest heat transfer coefficient at a given pumping power. Arif [ 176 ] investigated a water-based ternary hybrid nanofluid that included an Al 2 O 3 nanofluid, a CNT nanofluid, and a graphene nanofluid. The analysis results indicated that heat transfer rate of the hybrid nanofluid was increased by 33.67%. Tlili [ 177 ] prepared a hybrid nanofluid that was composed of Ni/Al 2 O 3 nanoparticles and C 2 H 6 O 2 as the base fluid and analyzed the heat transfer performance of the hybrid nanofluid. Devi [ 178 ] used a numerical method to compare the heat transfer rate between a Cu nanofluid and a Cu/Al 2 O 3 nanofluid. The results proved that the heat transfer rate of the Cu/Al 2 O 3 nanofluid was higher than that of the Cu nanofluid under a magnetic field environment. According to Table 1 above, hybrid nanoparticles also include Al 2 O 3 -TiO 2 , TiO 2 -SiO 2 , MWCNT-SiO 2 , Al 2 O 3 -CuO, Fe 3 O 4 -CQD, CuO-CQD, Al 2 O 3 -Ag, Al 2 O 3 -Cu, Al 2 O 3 -SiC, etc. However, current research on hybrid nanofluid applications in PEFMCs only regard the Al 2 O 3 -SiO 2 hybrid nanofluid. For example, Johari [ 179 ] prepared an Al 2 O 3 -SiO 2 hybrid nanofluid with different percentages and analyzed its heat-dissipation capacity using an experimental method. The results proved that the thermal conductivity of the Al 2 O 3 -SiO 2 nanofluid was higher than that of a single nanofluid. Khalid [ 180 ] prepared different percentages of Al 2 O 3 -SiO 2 nanofluids. The experimental results showed that the thermal and electrical conductivity of the Al 2 O 3 -SiO 2 nanofluid decreased as the percentage of Al 2 O 3 increased. Meanwhile, the viscosity of the Al 2 O 3 -SiO 2 nanofluid increased. The test results indicated that the optimal percentage of Al 2 O 3 -SiO 2 nanofluid as a coolant for PEMFC was 10:90.
In addition, many researchers have investigated different types of hybrid nanofluids in electric vehicles [ 181 , 182 , 183 , 184 , 185 ]. It has been proved that hybrid nanofluids have become the future research direction of nanofluid applications in fuel cell vehicles. A summary of the single and hybrid nanofluids is presented in Table 5 below.
Single and hybrid nanofluids in PEFMCs [ 133 , 134 , 135 , 179 , 180 ].
4. The Challenges of Nanofluid Application in Fuel Cell Vehicles
4.1. nanofluid stability and cleaning.
Research on nanofluid applications in fuel cell vehicles has been focused on the heat-dissipation performances of PEFMCs. Although current research has proven that nanofluids can effectively enhance the heat-dissipation capacity of thermal management systems, the stability problem is inevitably considered when nanofluids are applied in fuel cell vehicles. In addition, nanofluid cleaning is a significant challenge, as nanofluid coolants must be replaced at the end-of-life cycle because nanoparticle sediments accumulate in microchannels and corners.
- Nanofluid stability
According to the theory of electrostatic stabilization (Derjaguin Landau Vewey Overbeek), if the van der Waals attraction force is greater than the electrostatic repulsion force, the nanoparticles will collide with each other. This causes the suspended nanoparticles to agglomerate and form clusters. The nanoparticles gradually settle under the influence of gravity. They even block the microchannels and affect the thermal conductivity and viscosity of the nanofluid. From a macroscopic perspective, different influencing factors, such as temperature, concentration, pH, and particle size, lead to destabilization and precipitate formation. Hence, it is necessary to analyze the nanofluid stability from macroscopic and microscopic perspectives.
Nanofluid preparation includes the one-step method and the two-step method. The one-step method involves incorporating nanoparticles directly into the base fluid without intermediate steps, such as nanoparticle drying, storage, and dispersion. This method produces nanofluids with good stability; however, it is only suitable for preparing small quantities of nanofluids because of its high cost. The two-step method refers to the preprocessing of the nanoparticle, which is followed by the mixing of the nanoparticle and the base fluid. This method is suitable for preparing large quantities of nanofluids at a low cost. The stability of this method is not as good as that of the one-step method, and a dispersant is required to maintain good stability [ 186 ]. The preparation equipment is illustrated in Figure 11 below.

Nanofluid preparation equipment.
To solve the nanofluid settlement problem, many researchers have used auxiliary dispersion techniques to improve nanofluid stability. These techniques include physical and chemical dispersions. Physical dispersion involves ball milling, ultrasonic vibration, and magnetic stirring. Ball-milling dispersion refers to the use of planetary ball milling to reduce the average number of clusters. Ultrasonic vibration includes water bath ultrasonic vibration and probe ultrasonic vibration. The latter method is more effective in breaking particle clusters and reducing the average cluster size. A magnetic stirrer can prevent bubble generation during nanofluid preparation by adjusting the stirring speed, which keeps the nanofluid stable for a short time. Chemical dispersion involves the addition of surfactants and pH adjustment. Surfactants can be classified as ionic, nonionic, amphoteric, and complex. The dispersion results are presented in Table 6 .
The research on nanofluid stability [ 187 , 188 , 189 , 190 , 191 , 192 , 193 , 194 ].
According to the summarization in Table 5 , current nanofluid applications in PEMFCs include ZnO nanofluid, Al 2 O 3 nanofluid, TiO 2 nanofluid, and Al 2 O 3 -SiO 2 nanofluid. The stability of these nanofluids have been investigated by some researchers. For example, Choudhary [ 193 ] used zeta measurement and visual inspection methods to analyze an Al 2 O 3 nanofluid’s stability. If the zeta potential was higher, the stability of the Al 2 O 3 nanofluid was better. Witharana [ 195 ] also used zeta measurement, visual inspection, and particle size measurement methods to analyze the stability of a TiO 2 nanofluid. In addition, the effects of sunlight on the stability of the TiO 2 nanofluid were assessed. Sunlight caused the TiO 2 nanofluid’s size to increase up to 45% in three days. The sedimentation velocity is a key parameter to evaluate the stability of a TiO 2 nanofluid. Adil [ 196 ] investigated the effects of a dispersant on the stability of a ZnO nanofluid. Anionic surfactants including SDS, SDBS, and oleic acid were compared. The testing results indicated that using a dispersant, adjusting the pH value, and ultrasonication methods are recommended to increase the stability of a ZnO nanofluid.
Addressing the stability problems of nanofluids is a fundamental requirement for their application in fuel cell vehicles. Although many researchers have elucidated the specific factors affecting nanofluid stability, it is necessary to reveal the hidden laws of these factors. A schematic diagram of nanofluid destabilization and sedimentation is shown in Figure 12 .

Schematic diagram of nanofluid destabilization and sedimentation [ 194 ].
The main factors influencing the stability of nanofluids include the temperature, concentration, pH, and particle size. (1) Temperature: When the temperature changes, the dielectric constant of the base fluid also changes (i.e., a higher dielectric constant indicates a better stability of the nanofluid). (2) Concentration: The average separation distance of the nanoparticle decreases as the concentration increases. The van der Waals attraction also increases. If the Van der Waals attraction is greater than the electrostatic repulsion, the nanoparticles will agglomerate. Hence, a higher nanofluid concentration implies easier settling. (3) The pH affects the charge density on the nanoparticle surface, decreasing the zeta potential. (4) Particle size: This affects the van der Waals attraction and electric double-layer repulsion. When the nanoparticle size is small, the number of atoms on the nanoparticle’s surface is higher. The density increases in the active position. The tendency for aggregation is enhanced, indicating that the nanofluid settles more easily.
- Nanofluid cleaning
According to the literature, the coolant must be replaced every four years in electric vehicles [ 197 ]. Because nanoparticle sedimentation accumulates in the microchannels and corners, the coolant is difficult to clean completely. Generally, the coolant inside a vehicle cooling system must be cleaned in two steps. (1) Coolant appearance inspection: If the coolant undergoes sedimentation and produces an abnormal smell near the end of its useful life, it indicates that the coolant has deteriorated. Therefore, the coolant should be replaced as early as possible. (2) pH value inspection: The pH is affected by additives that can effectively protect the metal and rubber seals in the engine. It is necessary to adjust the pH to maintain the anti-corrosion characteristics of the coolant. Finally, the coolant must be changed under cold conditions. Each branch pipe and joint in the cooling system must be checked for leakages. The engine operation must be maintained and the coolant inside the pipes must be cleaned. Finally, a new coolant is injected into the cooling system [ 198 ].
However, the problem of nanofluid stability is the greatest challenge that prevents its use in fuel cell vehicles. If nanoparticles accumulate in corners, such as in the flat tubes of a radiator, it is difficult to completely clean them. This affects the thermophysical properties of nanofluid coolants.
4.2. Nanofluids in the Cooling Process
4.2.1. nanofluid erosion and abrasion of the microchannel surface.
Nanofluids are composed of nanoparticles (e.g., metal, metal oxide, nonmetal, nonmetal oxide, and nitrides) and base fluids (e.g., water, oil, and ethylene glycol). During circulation in the cooling loop, the nanoparticles impact the surfaces of the microchannels. In the long term, nanoparticles may wear the microchannels, particularly those in the radiator flat tube and the flow channel of PEMFCs, as shown in Figure 13 . The bipolar structure of a PEMFC consists of a membrane electrode, a bipolar plate, and an end plate. The coolant flows through the microchannels of the PEMFC. Heat is generated by the PEMFC and exchanged by the coolant with cold air from the outside. However, during heat exchange, the nanoparticles continuously rub against the surface of the microchannels. In addition, the electrical conductivity of the nanoparticles affects the normal operation of the PEMFC. In the radiator in the cooling system, the nanofluid flows through flat tubes and transfers heat from the inside to the outside. In the flow process, the nanoparticles continuously rub against the surface of the flat tube, which wears the inner surface of the flat tube. The characteristics of the PEMFC and radiators are affected by long-term wear. Hence, it is necessary to reduce the wear effect of the nanofluid as a coolant for PEMFCs and radiators.

Schematic diagram of microchannels in PFMFC and radiator.
Although many researchers have focused on the heat transfer performance of nanofluids, the erosion and abrasion effects of nanofluids on microchannel surfaces have been poorly reported. Eneren [ 199 ] analyzed and compared the abrasion, erosion, and corrosion of metallic surfaces and components caused by nanofluids. Abrasion means that hard particles pass over the surface of a solid metal, causing material loss. Erosion refers to the impact of particles in liquids/gases on a solid surface, causing damage to the solid surface. Corrosion refers to the damage of a solid metallic surface via chemical reactions. These effects are a significant challenge for nanofluid applications in fuel cell vehicles, which require extensive experiments and long-term observations.
4.2.2. Pump Transport Power
The viscosity of the nanofluid increases as the nanofluid concentration increases. This causes the pumping power to exponentially increase. Hence, the nanofluid concentration should not infinitely increase. Karami [ 200 ] investigated the effects of various factors, such as the volume fraction, duct length, Reynolds number, and tube diameter, on pumping power. The study results proved that a high-volume fraction of the nanofluid was not reasonable due to the cost of the pumping power. Ho [ 201 ] analyzed the friction factor, pumping power, average heat transfer coefficient, thermal resistance, and maximum temperature using an Al 2 O 3 /WEG nanofluid as a coolant. The porosity of the nanofluid increased, causing the pumping power to increase. Pandey [ 202 ] tested the heat transfer capacity and frictional losses in a heat exchanger. The experimental results also demonstrated that the pumping power increased as the nanofluid concentration increased. However, some researchers have considered that the pumping power can be ignored because the heat transfer capacity considerably increases when using a nanofluid [ 203 , 204 ]. Therefore, it is challenging to explore the influence of nanofluid viscosity on the pump transport power.
4.2.3. Nanofluid Electrical Conductivity
A nanofluid is a mixture of nanoparticles and a base liquid. However, nanoparticles that include Al 2 O 3 , ZnO, and TiO 2 are metal oxides with electrical conductivity. According to the coolant requirement for PEMFCs, the electrical conductivity should be less than 5 μs/cm. Many researchers have investigated the electrical conductivities of Al 2 O 3 /WEG, ZnO/WEG, and TiO 2 /WEG nanofluids. For example, Khdher [ 205 ] investigated the electrical conductivity of an Al 2 O 3 /bio-glycol nanofluid and measured the electrical conductivity using a measurement apparatus. The test results indicated that adding nanoparticles into the base fluid could increase the electrical conductivity to 53–154 μs/cm at 30–80 °C. Subramaniyan [ 206 ] analyzed the electrical conductivities of three types of nanofluids: TiO 2 /W, TiO 2 /EG, and TiO 2 /PG. The research results showed that the TiO 2 /W nanofluids had higher dielectric constants than EG and PG at the same temperature and frequency, which could be adjusted by changing the volume fraction of the nanofluid. Chilambarasan [ 207 ] selected ZnO/WEG and ZnO/PEG nanofluids as research targets. The electrical conductivity increased when the temperature was increased at all concentrations.
Regarding hybrid nanofluids, Johari [ 180 ] investigated an Al 2 O 3 -SiO 2 /bio-glycol (BG) hybrid nanofluid as a coolant for a PEMFC. Compared to conventional ethylene glycol, green bio-glycol (BG) exhibited a better performance than the base fluid. The thermal conductivity also increased. Consequently, the electrical conductivity decreased. This is shown in Figure 14 . The single SiO 2 nanofluid had the highest electrical conductivity at 268.8 μs/cm, but the single Al 2 O 3 nanofluid’s electrical conductivity was 227.3 μs/cm. The Al 2 O 3 -SiO 2 hybrid nanofluid’s electrical conductivity was lower than that of the single nanofluid. The electrical conductivity of the other mixture ratio of the Al 2 O 3 -SiO 2 hybrid nanofluid was approximate to that of the single Al 2 O 3 nanofluid.

Electrical conductivity of Al 2 O 3 -SiO 2 /BG hybrid nanofluid [ 206 ].
Based on the literature review above, the challenges of nanofluid application in fuel cell vehicles include: (1) nanofluid stability (how to maintain low-term dispersibility of nanoparticles in the base fluid), (2) nanofluid cleaning (how to clean up remaining nanoparticles in microchannels), (3) erosion and abrasion by nanofluids (how to eliminate or reduce erosion and abrasion), (4) nanofluid pump transport power (how to balance a decrease in the heat-dissipation capacity and a decrease in the pumping power), and (5) nanofluid electrical conductivity (how to achieve the requirement of electrical conductivity for coolants in a fuel cell vehicle).
5. Research Directions for Nanofluids in the Future
5.1. nanofluid stability improvement.
Solving nanofluid destabilization and sedimentation problems is a basic requirement for nanofluids’ use in fuel cell vehicles. It is necessary to investigate and analyze the factors influencing nanofluid stability, including the temperature, concentration, pH value, and particle size. However, previous research has only focused on one or two factors, which was difficult to summarize. The nanofluid preparation process includes physical and chemical dispersion techniques, such as ball milling, ultrasonic vibration, magnetic stirring, addition of a surfactant, and adjustment of the pH value. These auxiliary dispersion techniques can effectively improve a nanofluid’s stability. However, it is difficult to summarize the principle of the auxiliary dispersion technique.
5.2. Hybrid Nanofluid Application
For oil-fueled vehicles and electric vehicles, researchers have investigated different types of hybrid nanoparticles, such as GnP-CNC, Al 2 O 3 -TiO 2 , TiO 2 -SiO 2 , MWCNT-SiO 2 , CuO-CNT-graphene, Al 2 O 3 -CNT-graphene, Al 2 O 3 -CuO, Fe 3 O 4 -CQD, CuO-CQD, Al 2 O 3 -Ag, Al 2 O 3 -Cu, Al 2 O 3 -SiC, and Al 2 O 3 -CuO, among others. Current research has verified that hybrid nanofluids have better heat transfer capacities than single nanofluids. However, in fuel cell vehicles, only a few researchers have explored the heat-dissipation performance of an Al 2 O 3 -SiO 2 /WEG nanofluid as a coolant. Hence, it would be of considerable scientific value to study more types of hybrid nanofluids for fuel cell vehicles.
5.3. Reduction in Erosion and Abrasion by Nanofluids
During the circulation of a nanofluid in a cooling system, the nanoparticles impact and wear the surface of the microchannels, which is particularly true for the flat tube and flow channel of PEMFCs. The characteristics of PEMFCs and radiators are affected by long-term wear. However, few researchers have investigated erosion and abrasion of the surfaces of flat radiator tubes and microchannels inside PEMFCs caused by nanofluids. It is important to analyze the impact phenomenon using numerous experiments and observations from macroscopic and microscopic perspectives. Finally, the nanofluid with the lowest impact on erosion and abrasion must be selected for comparison and analysis.
5.4. Thermal Conductivity Model of Nanofluids
The thermal conductivity of a nanofluid is an important thermophysical property. Many researchers have proposed classic thermal conductivity models, such as the Maxwell, Braggeman, Wasp, Hamilton and Crosser, and Yamada and Ota models. There is no unified theoretical model to accurately calculate nanofluid thermal conductivity. A few researchers have proposed a theory on thermal conductivity of the adsorption layer, which refers to the liquid layer formed around solid nanoparticles between the base fluid and the nanoparticles. This reflects the internal heat transfer mechanism of the nanofluid more accurately. Hence, it would be of great scientific value to explore the thermal conductivity of nanofluids using the adsorption layer theory.
5.5. Electrical Conductivity of Nanofluids
According to current research, nanofluid applications in PEMFC include Al 2 O 3 /WEG, ZnO/WEG, and TiO 2 /WEG, which are metal oxides with electrical conductivity. Based on the requirements for coolants for PEMFCs, the electrical conductivity should be less than 5 μs/cm. When nanofluids flow through a PEMFC microchannel, they inevitably contact the inner surface of the BP. If a nanofluid has a high electrical conductivity, it can cause electric leakage inside the PEMFC, affecting the output power of PEMFC. Hence, exploring nanofluids with low electrical conductivities in the future is necessary.
6. Conclusions
With the development of high-power fuel cell stacks, heat-dissipation requirements have become more stringent. Reinforcing the heat transfer performance of the coolant is simpler, has a lower cost, and increases the rotation speed than structural optimization. Nanofluids are a type of heat-dissipation-enhancement coolants that have been applied in different industrial fields. This study summarized the challenges and research directions of nanofluids as coolants in fuel cell vehicles. The main conclusions of this study are as follows.
(1) Nanofluids have great potential as coolants in oil-fueled, pure electric, and fuel cell vehicles. Many researchers have verified this and have highlighted their disadvantages. Nanofluids can be used as coolants, refrigerating fluids, and heat transfer media in oil-fueled vehicle cooling systems, air conditioner systems, and exhaust power generation systems. In pure electric vehicles, nanofluids can be considered as coolants in battery-cooling systems. However, for fuel cell vehicles, the current research has only focused on the heat-dissipation performances of nanofluids in PEMFCs.
(2) The thermal management system of an FCV comprises heat dissipation and air conditioning systems. Heat-dissipation systems can be classified into heat-generation and heat-dissipation systems. Based on the analyses and comparisons performed in numerous studies, the heat transfer capacity of a coolant is better than that of the heat-dissipation system. This further proves that nanofluids have considerable potential in replacing existing coolants.
(3) The thermophysical properties of nanofluids, including the density, specific heat, thermal conductivity, and convective heat transfer coefficient, have been extensively studies. The equations for the thermophysical properties have been analyzed in detail. ZnO/WEG, Al 2 O 3 /WEG, TiO 2 /WEG, and BN/WEG nanofluids were analyzed as coolants in PEMFCs. In this study, four types of nanofluids were selected along with their density, specific heat, thermal conductivity, and convective heat transfer coefficient. Moreover, this study mainly analyzed the thermal conductivity of the nanofluid and the Al 2 O 3 -SiO 2 /WEG nanofluid.
(4) According to a literature review, there are some challenges in using nanofluids as coolants in fuel cell vehicles, including nanofluid stability, cleaning, erosion and abrasion, pump transport power, and electrical conductivity. Among these, nanofluid stability is the biggest obstacle that prevents its use in fuel cell vehicles. Hence, it is necessary to improve the nanofluid preparation methods, which affect the stability of the nanofluid, from macroscopic and microscopic perspectives.
(5) Finally, this study proposed five research directions for using nanofluids as coolants in fuel cell vehicles in the future. They include nanofluid stability improvements, hybrid nanofluid applications, reductions in erosion and abrasion by nanofluids, a nanofluid thermal conductivity model, and nanofluid electrical conductivity.
Nomenclature
Funding statement.
This research was supported by the Scientific Research Foundation for Doctoral Program of Hubei University of Technology (XJ2022001001), the Hubei Key Project of Research and Development Plan (2023BAB088), and the Open-end Fund for Hubei Key Laboratory of Modern Manufacturing Quality Engineering (KFJJ-2023011).
Author Contributions
Conceptualization, F.Z.; methodology, Y.D.; formal analysis, Y.W.; resources, C.S.; writing—original draft preparation, Q.T.; writing—review and editing, Q.T. All authors have read and agreed to the published version of the manuscript.
Data Availability Statement
Conflicts of interest.
The authors declare no conflict of interest.
Disclaimer/Publisher’s Note: The statements, opinions and data contained in all publications are solely those of the individual author(s) and contributor(s) and not of MDPI and/or the editor(s). MDPI and/or the editor(s) disclaim responsibility for any injury to people or property resulting from any ideas, methods, instructions or products referred to in the content.
Application of nanofluids as cutting fluids in machining operations: a brief review
- Review Article
- Published: 03 February 2022
- Volume 13 , pages 4247–4278, ( 2023 )
Cite this article
- Lotfi Ben Said 1 , 2 ,
- Lioua Kolsi ORCID: orcid.org/0000-0003-4368-7458 1 , 3 ,
- Kaouther Ghachem 4 ,
- Mohammed Almeshaal 5 &
- Chemseddine Maatki 5
642 Accesses
9 Citations
Explore all metrics
The evolution of materials of cutting tools and their geometry, is currently leading to a technological development of many other sectors linked to machining. It is, therefore, a progress in the construction of machine tools, the machining of new materials, improvement of cutting fluids (lower toxicity and costs related to the maintenance and treatment of used cutting fluids, chemical formulation). Regarding the cutting fluids, international regulations are moving towards eco-elimination or limiting the use of certain molecules for ecological reasons. In addition, the formulation must be adapted as best as possible to the industrial demand, both in terms of performance and costs. The characteristics of nanofluids meet all these requirements and seem to be a promising solution for major problems encountered when using conventional cutting fluids. The present paper reports a literature review emphasizing essentially the investigations performed during the last 2 years. This literature focuses on the use of nano-lubricant in machining processes such as turning, milling and grinding processes. Analyzing the current review, it has been found that the use of nanofluids especially hybrid ones reduces the tool wear, the surface roughness, cutting forces, and heat generation. However, the optimal choice of nanoparticles and their concentrations that suites more the most severe cutting conditions, needs more attention in future works.
This is a preview of subscription content, log in via an institution to check access.
Access this article
Price includes VAT (Russian Federation)
Instant access to the full article PDF.
Rent this article via DeepDyve
Institutional subscriptions
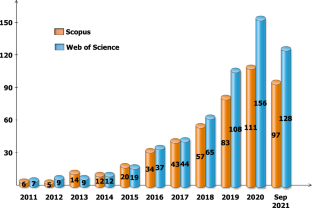
Similar content being viewed by others
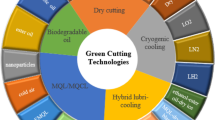
A state-of-the-art review on sustainability evaluation of machining processes involving the effects of advanced cooling technologies
Muhammad Jamil, Ning He, … Navneet Khanna
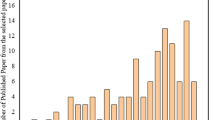
A comprehensive review of studying the influence of laser cutting parameters on surface and kerf quality of metals
Muhammad Alsaadawy, Montasser Dewidar, … Taher A. Shehabeldeen
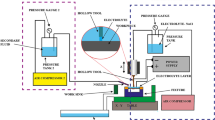
Experimental investigation on the suitability of dual fluid system-assisted ECM (DF-ECM) and the influence of magnet in machining of SS304
Deepak Janardhanan & Hariharan Perianna
Alberts M, Kalaitzidou K, Melkote S (2009) An investigation of graphite nanoplatelets as lubricant in grinding. Int J Mach Tools Manuf 49:966–970
Article Google Scholar
Ali N, Bahman AM, Aljuwayhel NF, Ebrahim SA, Mukherjee S, Alsayegh A (2021) Carbon-based nanofluids and their advances towards heat transfer applications—a review. Nanomaterials 11(6):1628. https://doi.org/10.3390/nano11061628
Article CAS Google Scholar
Ali MAM, Azmi AI, Murad MN, Zain MZM, Khalil ANM, Shuaib NA (2020) Roles of new bio-based nanolubricants towards eco-friendly and improved machinability of Inconel 718 alloys. Tribol Int 144:106106
Anand N, Kumar AS, Paul S (2019) Effect of cutting fluids applied in MQCL mode on machinability of Ti-6Al-4V. J Manuf Process 43:154–163
Anshuman D, Omprakash P, Kumar PS, Sudhansu RD, Bibhuti BB (2019) Performance appraisal of various nanofluids during hard machining of AISI 4340 steel. J Manuf Process 46:248–270
Bakalova T, Svobodová L (2019) Quality assessment of milling technology and the biocidal effects of SiO2 or TiO2 nanoadditives in cooling lubricant emulsions. J Manuf Process 45:509–519
Braga DU, Diniz AE, Miranda GWA, Coppini NL (2003) Minimum lubrication in Al-Si drilling. J Braz Soc Mech Sci Eng 25(1):63–68
Chatha SS, Pal A, Singh T (2016) Performance evaluation of aluminium 6063 drilling under the influence of nanofluid minimum quantity lubrication. J Clean Prod 137:537–545
Che Sidik NA, Samion S, Ghaderian J, Witri Muhammad Yazid MNA (2017) Recent progress on the application of nanofluids in minimum quantity lubrication machining: a review. Int J Heat Mass Transfer 108:79–89
Chetan BC, Behera S, Ghosh PVR (2016) Application of nanofluids during minimum quantity lubrication: a case study in turning process. Tribol Int 101:234–246
Choudhary A, Naskar A, Paul S (2018) An investigation on application of nano-fluids in high speed grinding of sintered alumina. J Manuf Process 35:624–633
Cui X, Li C, Zhang Y, Jia D, Zhao Y, Li R, Cao H (2019) Tribological properties under the grinding wheel and workpiece interface by using graphene nanofluid lubricant. Int J Adv Manuf Technol 104:3943–3958
Dambatta YS, Sayuti M, Sarhan AAD, Hamdi M, Manladan SM, Reddy M (2019) Tribological performance of SiO 2 -based nanofluids in minimum quantity lubrication grinding of Si 3 N 4 ceramic. J Manuf Process 41:135–147
Davim JP, Sreejith PS, Gomes R, Peixoto C (2006) Experimental studies on drilling of aluminium (AA1050) under dry, minimum quantity of lubricant, and floodlubricated conditions. Proc Inst Mech Eng B J Eng Manuf 220(10):1605–1611
De Chiffre L (2002) Surface integrity and part accuracy in reaming and tapping stainless steel with new vegetable based cutting oils. Tribol Int 58(10):865–870
Google Scholar
De Oliveira D, Da Silva RB, Gelamo RV (2019) Influence of multilayer graphene platelet concentration dispersed in semisynthetic oil on the grinding performance of Inconel 718 alloy under various machining conditions. Wear 426–427:1371–1383
Dongzhou J, Changhe L, Yanbin Z, Min Y, Yaogang W, Shuming G, Huajun C (2017) Specific energy and surface roughness of minimum quantity lubrication grinding Ni-based alloy with mixed vegetable oil-based nanofluids. Precis Eng 50:248–262
Elhami S, Razfar MR (2020) Application of nano electrolyte in the electrochemical discharge machining process. Precis Eng 64:34–44
Gajrani KK, Suvin PS, Kailas SV, Sankar MR (2019) Thermal, rheological, wettability and hard machining performance of MoS2 and CaF2 based minimum quantity hybrid nano-green cutting fluids. J Mater Process Technol 266:125–139
Gao T, Zhang X, Li C, Zhang Y, Yang M, Jia D, Ji H, Zhao Y, Li R, Yao P, Zhu L (2020) Surface morphology evaluation of multi-angle 2D ultrasonic vibration integrated with nanofluid minimum quantity lubrication grinding. J Manuf Process 51:44–61
Garg A, Sarma S, Panda BN, Zhang J, Gao L (2016) Study of effect of nanofluid concentration on response characteristics of machining process for cleaner production. J Clean Prod 135:476–489
Ghosh CS, Rao PV (2019) Comparison between sustainable cryogenic techniques and nano-MQL cooling mode in turning of nickel-based alloy. J Clean Prod 231:1036–1049
Guan J, Ding Y, Gao C et al (2021) Experimental study on turning performance of a novel nanofluid prepared by composites of MWCNTs. Int J Adv Manuf Technol 116:2373–2385
Guangxian L, Shuang Y, Nan L, Wencheng P, Cuie W, Songlin D (2019) Quantitative analysis of cooling and lubricating effects of graphene oxide nanofluids in machining titanium alloy Ti6Al4V. J Mater Proces Tech 271:584–598
Gupta MK, Sood PK, Sharma VS (2016) Optimization of machining parameters and cutting fluids during nano-fluid based minimum quantity lubrication turning of titanium alloy by using evolutionary techniques. J Clean Prod 135(1):1276–1288
Hosseini SF, Emami M, Sadeghi MH (2018) An experimental investigation on the effects of minimum quantity nano lubricant application in grinding process of Tungsten carbide. J Manuf Process 35:244–253
Huang WT, Liu WS, Wu DH (2016) Investigations into lubrication in grinding processes using MWCNTs nanofluids with ultrasonic-assisted dispersion. J Clean Prod 137:1553–1559
Huang S, Li X, Yu B, Jiang Z, Huang H (2020) Machining characteristics and mechanism of GO/SiO 2 nanoslurries in fixed abrasive lapping. J Mater Process Tech 277:116444
Joshi KK, Behera RK, Anurag (2018) Effect of minimum quantity lubrication with Al 2 O 3 Nanofluid on Surface Roughness and its prediction using hybrid fuzzy controller in turning operation of Inconel 600. Mater Today: Proc 5:20660–20668
Junankar AA, Yashpal Y, Purohit JK (2021a) Experimental investigation to study the effect of synthesized and characterized monotype and hybrid nanofluids in minimum quantity lubrication assisted turning of bearing steel. Proc Inst Mech Eng J. https://doi.org/10.1177/13506501211038113
Junankar AA, Parate SR, Dethe PK, Dhote NR, Gadkar DG, Gadkar DD, Gajbhiye SA (2021b) Optimization of bearing steel turning parameters under CuO and ZnO nanofluid-MQL using MCDM hybrid approach. Mater Today Proceedings. https://doi.org/10.1016/j.matpr.2021.04.589
Junankar AA, Yashpal JKP et al (2021c) Performance evaluation of Cu nanofluid in bearing steel MQL based turning operation. Mater Today: Proc 44–6:4309–4314
Kakac S, Pramuanjaroenkij A (2009) Review of convective heat transfer enhancement with nanofluids. Int J Heat Mass Transfer 52:3187–3196
Khalilpourazary S, Salehi J (2019) How alumina nanoparticles impact surface characteristics of Al7175 in roller burnishing process. J Manuf Process 39:1–11
Khan AM, Gupta MK, Hegab H, Jamil M, Mia M, He N, Song Q, Liu Z, Pruncu CI (2020a) Energy based cost integrated modelling and sustainability assessment of Al-GnP hybrid nanofluid assisted turning of AISI52100 steel. J Clean Prod 257:120502
Khan AM, Jamil M, Mia M, He N, Zhao W, Gong L (2020b) Sustainability-based performance evaluation of hybrid nanofluid assisted machining. J Clean Prod 257:120541
Kim W-Y, Senguttuvan S, Kim SH, Lee SW, Kim S-M (2021) Numerical study of flow and thermal characteristics in titanium alloy milling with hybrid nanofluid minimum quantity lubrication and cryogenic nitrogen cooling. Int J Heat Mass Transfer 170:121005
Kishawy HA, Hegab H, Deiab I, Eltaggaz A (2019) Sustainability assessment during machining Ti-6Al-4V with nano-additives-based minimum quantity lubrication. J Manuf Mater Process 3:61. https://doi.org/10.3390/jmmp3030061
Kıvak T, Sarıkaya M, Yıldırım ÇV, Şirin Ş (2020) Study on turning performance of PVD TiN coated Al2O3+TiCN ceramic tool under cutting fluid reinforced by nano-sized solid particles. J Manuf Process 56:522–539
Kulkarni HB, Nadakatti MM, Kulkarni SC, Kulkarni RM (2020) Investigations on effect of nanofluid based minimum quantity lubrication technique for surface milling of Al7075-T6 aerospace alloy. Mater Today: Proc 27(1):251–256
CAS Google Scholar
Kumar MS, Krishna VM (2020) An investigation on turning AISI 1018 steel with hybrid biodegradeable nanofluid/MQL incorporated with combinations of CuO-Al 2 O 3 nanoparticles. Mater Today: Proc 24:1577–1584
Kumar A, Ghosh S, Aravindan S (2017) Grinding performance improvement of silicon nitride ceramics by utilizing nanofluids. Ceram Int 43–16:13411–13421
Kumar A, Ghosh S, Aravindan S (2019) Experimental investigations on surface grinding of silicon nitride subjected to mono and hybrid nanofluids. Ceram Int 45(14):17447–17466
Kumar AS, Deb S, Paul S (2020) Tribological characteristics and micro-milling performance of nanoparticle-enhanced water based cutting fluids in minimum quantity lubrication. J Manuf Process 56:766–776
Lee P-H, Kim JW, Lee SW (2018) Experimental characterization on eco-friendly micro-grinding process of titanium alloy using air flow assisted electrospray lubrication with nanofluid. J Clean Prod 201:452–462
Leep HR (1990) Influence of cutting fluids on surface finish of holes drilled into aluminum 390. J Synth Lubr 6(4):325–338
Li B, Li C, Zhang Y, Wang Y, Jia D, Yang M, Zhang N, Wu Q, Han Z, Sun K (2017) Heat transfer performance of MQL grinding with different nanofluids for Ni-based alloys using vegetable oil. J Clean Prod 154:1–11
Li M, Yu T, Zhang R, Yang L, Ma Z, Li B, Wang XZ, Wang W, Zhao J (2020) Experimental evaluation of an eco-friendly grinding process combining minimum quantity lubrication and graphene-enhanced plant-oil-based cutting fluid. J Clean Prod 244:118747
Machado AR, Wallbank J (1997) The effect of extremely low lubricant volumes in machining. Wear 210(1):76–82
Ming L, Tianbiao Y, Lin Y, Hongyu L, Rongchuang Z, Wanshan W (2019) Parameter optimization during minimum quantity lubrication milling of TC4 alloy with graphene-dispersed vegetable-oil-based cutting fluid. J Clean Prod 209:1508–1522
Mohana RG, Dilkush S, Sudhakar I, Anil Babu P (2021) Effect of cutting parameters with dry and MQL nano fluids in turning of EN-36 STEEL. Mater Today Proc 41:1182–1187
Mohd Khalil AN, Azmib AI, Murad MN, Mahboob Ali MA (2018) The effect of cutting parameters on cutting force and tool wear in machining Nickel Titanium Shape Memory Alloy ASTM F2063 under Minimum Quantity Nanolubricant. Procedia CIRP 77:227–230
Mosleh M, Ghaderi M, Shirvani KA, Belk J, Grzina DJ (2017) Performance of cutting nanofluids in tribological testing and conventional drilling. J Manuf Process 25:70–76
Musavi SH, Davoodib B, Niknam SA (2019a) Effects of reinforced nanoparticles with surfactant on surface quality and chip formation morphology in MQL-turning of superalloys. J Manuf Process 40:128–139
Musavi SH, Davoodi B, Niknam SA (2019b) Effects of reinforced nanofluid with nanoparticles on cutting tool wear morphology. J Central South Univ 26(5):1050–1064
Muthusamy Y, Kadirgama K, Rahman MM, Ramasamy D, Sharma KV (2016) Wear analysis when machining AISI 304 with ethylene glycol/TIO 2 nanoparticle-based coolant. Int J Adv Manuf Technol 82:327–340
Najiha MS, Rahman MM, Kadirgama K (2016) Performance of water-based TiO2 nanofluid during the minimum quantity lubrication machining of aluminium alloy, AA6061-T6. J Clean Prod 135:1623–1636
Najman MN, Kasrai M, Bancroft GM (2003) X-ray absorption spectroscopy and atomic force microscopy of films generated from organosulfur extreme-pressure (EP) oil additives. Tribol Lett 14(4):225–235
Nam JS, Lee PH, Lee SW (2011) Experimental characterization of micro-drilling process using nanofluid minimum quantity lubrication. Int J Mach Tools Manuf 51:649–652
Nam J, Kim JW, Kim JS, Lee J, Lee SW (2018) Parametric analysis and optimization of nanofluid minimum quantity lubrication micro-drilling process for titanium alloy (Ti-6Al-4V) using response surface methodology and desirability function. Procedia Manuf 26:403–414
Okokpujie IP, Ohunakin OS, Adelekan DS, Bolu CA, Gill J, Atiba OE, Aghedo OA (2019) Experimental investigation of nano-lubricants effects on temperature distribution of mild steel machining. Procedia Manuf 35:1061–1066
Öndin O, Kıvak T, Sarıkaya M, Yıldırım ÇV (2020) Investigation of the influence of MWCNTs mixed nanofluid on the machinability characteristics of PH 13–8 Mo stainless steel. Tribol Int 148:106323
Padmini R, Vamsi Krishna P, Krishna Mohana Rao G (2016) Effectiveness of vegetable oil based nanofluids as potential cutting fluids in turning AISI 1040 steel. Tribol Int 94:490–501
Padmini R, Krishna PV, Mohana Rao GK (2015) Performance assessment of micro and nano solid lubricant suspensions in vegetable oils during machining. Proc IMechE Part b: J Eng Manuf 229(12):1–9
Padmini R, Krishna PV, Sai M, Satish K (2019) Influence of green nanocutting fluids on machining performance using minimum quantity lubrication technique. Mater Today: Proc 18:1435–1449
Pasam VK, Revuru RS, Gugulothu S (2018) Comparing the performance & viability of nano and microfluids in minimum quantity lubrication for machining AISI1040 steel. Mater Today: Proc 5:8016–8024
Pashmforoush F, Bagherinia RD (2018) Influence of water-based copper nanofluid on wheel loading and surface roughness during grinding of Inconel 738 superalloy. J Clean Prod 178:363–372
Peña-Parás L, Maldonado-Cortésa D, Rodríguez-Villalobos M, Romero-Cantú AG, Montemayor OE, Herreraa M, Trousselle G, González J, Hugler W (2019) Optimization of milling parameters of 1018 steel and nanoparticle additive concentration in cutting fluids for enhancing multi-response characteristics. Wear 426–427:877–886
Peña-Parás L, Maldonado-Cortés D, Villalobos MR, Romero-Cantú AG, Montemayor OE (2020) Enhancing tool life, and reducing power consumption and surface roughness in milling processes by nanolubricants and laser surface texturing. J Clean Prod 253:119836
Peña-Parás L, Rodríguez-Villalobos M, Maldonado-Cortés D, Guajardo M, Rico-Medina CS, Elizondo G, Quintanilla DI (2021) Study of hybrid nanofluids of TiO2 and montmorillonite clay nanoparticles for milling of AISI 4340 steel. Wear 477:203805
Peng R, Tong J, Zhao L, Tang X, Peng X, He X (2021) Molecular dynamics study on the adsorption synergy of MWCNTs/MoS2 nanofluids and its influence of internal-cooling grinding surface integrity. Appl Surface Sci 563:150312
Prashantha Kumar ST, Thirtha Prasada HP, Nagamadhu M, Siddaraju C (2021) Investigate the effect of Al 2 O 3 & CuO nano cutting fluids under MQL technique in turning of DSS-2205. Adv Mater Process Technol. https://doi.org/10.1080/2374068X.2021.1948701
Qu S, Gong Y, Yang Y, Wang W, Liang C, Han B (2020) An investigation of carbon nanofluid minimum quantity lubrication for grinding unidirectional carbon fibre-reinforced ceramic matrix composites. J Clean Prod 249:119353
Rahman SS, Ibne Ashraf MZ, Nurul Amin AKM, Bashar MS, Kabir Ashik MF, Kamruzzaman M (2019) Tuning nanofluids for improved lubrication performance in turning biomedical grade titanium alloy. J Clean Prod 206:180–196
Rao DN, Srikant RR (2006) Influence of emulsifier content on cutting fluid properties. Proc Inst Mech Eng B 220:1803–1806
Rapeti P, Pasam VK, Rao Gurram KM, Revuru RS (2018) Performance evaluation of vegetable oil based nano cutting fluids in machining using Grey Relational Analysis-A step towards sustainable manufacturing. J Clean Prod 172:2862–2875
Reverberi AP, D’Addona DM, Bruzzone AAG, Teti R, Fabiano B (2019) Nanotechnology in machining processes: recent advances. Procedia CIRP 79:3–8
Revuru RS, Pasam VK, Syed I, Paliwal UK (2018) Development of finite element based model for performance evaluation of nano cutting fluids in minimum quantity lubrication. CIRP J Manuf Sci Technol 21:75–85
Sai Geetha CHT, Dash AK, Kavya B, Amrita M (2021) Analysis of hybrid nanofluids in machining AISI 4340 using minimum quantity lubrication. Mater Today: Proc 43:579–586
Salimi-Yasar H, Heris SZ, Shanbedi M, Amiri A, Kameli A (2017a) Experimental investigation of thermal properties of cutting fluid using soluble oil-based TiO2 nanofluid. Powder Technol 310:213–220
Salimi-Yasar H, Heris SZ, Shanbedi M (2017b) Influence of soluble oil-based TiO2 nanofluid on heat transfer performance of cutting fluid. Tribol Int 112:147–154
Sani ASA, Rahim EA, Sharif S, Sasahara H (2019) Machining performance of vegetable oil with phosphonium- and ammonium-based ionic liquids via MQL technique. J Clean Prod 209:947–964
Sarhan AAD, Sayuti M, Hamdi M (2012) Reduction of power and lubricant oil consumption in milling process using a new SiO2 nanolubrication system. Int J Adv Manuf Technol 63:505–512
Satheesh Kumar B, Padmanabhan G, Vamsi Krishna P (2016) Performance assessment of vegetable oil based cutting fluids with extreme pressure additive in machining. J Adv Res Mater Sci 19:1–13
Sen B, Gupta MK, Mia M, Mandal UK, Mondal SP (2020) Wear behaviour of TiAlN coated solid carbide end-mill under alumina enriched minimum quantity palm oil-based lubricating condition. Tribol Int 148:106310
Setti D, Sinha MK, Ghosh S, Rao PV (2015) Performance evaluation of Ti–6Al–4V grinding using chip formation and coefficient of friction under the influence of nanofluids. Int J Mach Tools Manuf 88:237–248
Sharma AK, Tiwar AK, Singha RK, Dixit AR (2016) Tribological investigation of TiO2 nanoparticle based cutting fluid in machining under minimum quantity lubrication (MQL). Mater Today: Proc 3(6):2155–2162
Sharma AK, Tiwari AK, Dixit AR, Singh RK (2017a) Investigation into performance of SiO 2 nanoparticle based cutting fluid in machining process. Mater Today: Proc 4:133–141
Sharma AK, Singh RK, Dixit AR, Tiwari AK (2017b) Novel uses of alumina-MoS2 hybrid nanoparticle enriched cutting fluid in hard turning of AISI 304 steel. J Manuf Process 30:467–482
Sharma AK, Tiwari AK, Dixit AR, Singh RK (2020) Measurement of machining forces and surface roughness in turning of AISI 304 steel using Alumina-MWCNT hybrid nanoparticles enriched cutting fluid. Measurement 150:107078
Shen B, Shih A, Tung S (2008a) Application of nanofluids in minimum quantity lubrication grinding. Tribol Trans 130(51):730–737
Shen B, Malshe AP, Kalita P, Shih AJ (2008b) Performance of novel MoS2 nanoparticles based grinding fluids in minimum quantity lubrication grinding. Trans NAMRI/SME 36:357–364
Shuang Y, John M, Songlin D (2019) Experimental investigation on the performance and mechanism of graphene oxide nanofluids in turning Ti-6Al-4V. J Manuf Process 43:164–174
Shuang Y, Jinjin L, Jiahua Z, Xiangzhi W, John M, Songlin D (2020) Investigation of machining Ti-6Al-4V with graphene oxide nanofluids: tool wear, cutting forces and cutting vibration. J Manuf Process 49:35–49
Singh H, Sharma VS, Singha S, Dogra M (2019) Nanofluids assisted environmental friendly lubricating strategies for the surface grinding of titanium alloy: Ti6Al4V-ELI. J Manuf Process 39:241–249
Singh RK, Sharma AK, Bishwajeet VM, Gaurav K, Nag A, Kumar A, Rai Dixit A, Mandal A, Das AK (2018) Influence of graphene-based nanofluid with minimum quantity lubrication on surface roughness and cutting temperature in turning operation. Mater Today: Proc 5:24578–24586
Singh RK, Sharma AK, Dixit AR, Tiwari AK, Pramanik A, Mandal A (2017) Performance evaluation of alumina-graphene hybrid nano-cutting fluid in hard turning. J Clean Prod 162:830–845
Singh H, Sharma VS, Dogra M (2020) Exploration of graphene assisted vegetables oil based minimum quantity lubrication for surface grinding of TI-6AL-4V-ELI. Tribol Int 144:106113
Sinha MK, Madarkar R, Ghosh S, Venkateswara Rao P (2017) Application of eco- friendly nanofluids during grinding of Inconel 718 through small quantity lubrication. J Clean Prod 141:1359–1375
Sirin E, Kıvak T, Yıldırım ÇV (2021a) Effects of mono/hybrid nanofluid strategies and surfactants on machining performance in the drilling of Hastelloy X. Tribol Int 157:106894
Sirin S, Sarıkaya M, Vakkas Yıldırım Ç, Kıvak T (2021b) Machinability performance of nickel alloy X-750 with SiAlON ceramic cutting tool under dry, MQL and hBN mixed nanofluid-MQL. Tribol Int 153:106673
Şirin Ş, Kıvak T (2019) Performances of different eco-friendly nanofluid lubricants in the milling of Inconel X-750 superalloy. Tribol Int 137:180–192
Sirin S, Kıvak T (2021) Effects of hybrid nanofluids on machining performance in MQL-milling of Inconel X-750 superalloy. J Manuf Process 70:163–176
Surendra DB, Aman K, Sandesh SC, Deepak RU (2021) Investigating a novel Ag/ZnO based hybrid nanofluid for sustainable machining of inconel 718 under nanofluid based minimum quantity lubrication. J Manuf Process 66:313–324
Tiwari A, Agarwal D, Singh A (2021) Computational analysis of machining characteristics of surface using varying concentration of nanofluids (Al 2 O 3 , CuO and TiO 2 ) with MQL. Mater Today: Proc 42:1262–1269
Vamsi Krishna P, Srikant RR, Nageswara Rao D (2010) Experimental investigation on the performance of nanoboric acid suspensions in SAE-40 and coconut oil during turning of AISI 1040 steel. Int J Mach Tools Manuf 50:911–916
Venkatesan K, Devendiran S, Ghazaly NM, Nishanth (2019a) Application of Taguchi-Response surface analysis to optimize the cutting parameters on turning of Inconel X-750 using Nanofluids suspended Al 2 O 3 in coconut oil. Procedia Manuf 30:90–97
Venkatesan K, Mathew AT, Devendiran S, Ghazaly NM, Sanjith S, Raghul R (2019b) Machinability study and multi-response optimization of cutting force, Surface roughness and tool wear on CNC turned Inconel 617 superalloy using Al 2 O 3 Nanofluids in Coconut oil. Procedia Manuf 30:396–403
Venkatesan K, Devendiran S, Ghazaly NM, Rahul R, Mughilan T (2019c) Optimization of cutting parameters on turning of incoloy 800H using Al2O3 nanofluid in coconut oil. Procedia Manuf 30:268–275
Venkatesan K, Devendiran S, Ghazaly NM, Nishanth (2019d) Application of Taguchi-Response surface analysis to optimize the cutting parameters on turning of Inconel X-750 using Nanofluids suspended Al 2 O 3 in coconut oil. Procedia Manufacturing 30:90–97
Venkatesan K, Devendiran S, Sachin D, Swaraj J (2020) Investigation of machinability characteristics and comparative analysis under different machining conditions for sustainable manufacturing. Measurement 154:107425
Virdi RL, Chatha SS, Singh H (2020) Performance evaluation of Inconel 718 under vegetable oils based nanofluids using minimum quantity lubrication grinding. Mater Today: Proc. https://doi.org/10.1016/j.matpr.2020.03.802
Wang Y, Li C, Zhang Y, Yang M, Zhang X, Zhang N, Dai J (2017a) Experimental evaluation on tribological performance of the wheel/workpiece interface in minimum quantity lubrication grinding with different concentrations of Al 2 O 3 nanofluids. J Clean Prod 142–4:3571–3583
Wang Y, Li C, Zhang Y, Li B, Yang M, Zhang X, Guo S, Liu G, Zhai M (2017b) Comparative evaluation of the lubricating properties of vegetable-oil-based nanofluids between frictional test and grinding experiment. J Manuf Process 26:94–104
Yıldırım ÇV (2019) Experimental comparison of the performance of nanofluids, cryogenic and hybrid cooling in turning of Inconel 625. Tribol Int 137:366–378
Yıldırım ÇV, Sarıkaya M, Kıvak T, Şirin Ş (2019) The effect of addition of hBN nanoparticles to nanofluid-MQL on tool wear patterns, tool life, roughness and temperature in turning of Ni-based Inconel 625. Tribol Int 134:443–456
Zhang X, Li C, Zhang Y, Wang Y, Li B, Yang M, Guo S, Liu G, Zhang N (2017) Lubricating property of MQL grinding of Al2O3/SiC mixed nanofluid with different particle sizes and microtopography analysis by cross-correlation. Precis Eng 47:532–545
Zhang J, Li C, Zhang Y, Yang M, Jia D, Liu G, Hou Y, Li R, Zhang N, Wu Q, Cao H (2018) Experimental assessment of an environmentally friendly grinding process using nanofluid minimum quantity lubrication with cryogenic air. J Clean Prod 193:236–248
Zhang Y, Li C, Jia D, Li B, Wang Y, Yang M, Hou Y, Zhang X (2016) Experimental study on the effect of nanoparticle concentration on the lubricating property of nanofluids for MQL grinding of Ni-based Alloy. J Mater Process Technol 232:100–115
Zhang L, Guo X, Zhang K, Wu Y, Huang Q (2020) Enhancing cutting performance of uncoated cemented carbide tools by jointuse of magnetic nanofluids and micro-texture under magnetic field. J Mater Process Tech 284:116764
Zhou C, Guo X, Zhang K, Cheng L, Wu Y (2019a) The coupling effect of micro-groove textures and nanofluids on cutting performance of uncoated cemented carbide tools in milling Ti-6Al-4V. J Mater Process Tech 271:36–45
Zhou R, Fu S, Li H, Yuan D, Tang B, Zhou G (2019b) Experimental study on thermal performance of copper nanofluids in a miniature heat pipe fabricated by wire electrical discharge machining. Appl Thermal Eng 160:113989
Download references
Acknowledgements
This research was funded by the Deanship of Scientific Research at Princess Nourah bint Abdulrahman University through the Fast-track Research Funding Program.
Author information
Authors and affiliations.
Mechanical Engineering Department, College of Engineering, Ha’il University, Ha’il City, Saudi Arabia
Lotfi Ben Said & Lioua Kolsi
Laboratory of Electro-Mechanical Systems (LASEM), National Engineering School of Sfax, University of Sfax, 3038, Sfax, Tunisia
Lotfi Ben Said
Laboratory of Metrology and Energy Systems, National Engineering School, University of Monastir, Monastir, Tunisia
Lioua Kolsi
Department of Industrial Engineering and Systems, College of Engineering, Princess Nourah bint Abdulrahman University, Riyadh, Saudi Arabia
Kaouther Ghachem
Department of Mechanical Engineering, College of Engineering, Imam Mohammad Ibn Saud Islamic University, Riyadh, 11432, Saudi Arabia
Mohammed Almeshaal & Chemseddine Maatki
You can also search for this author in PubMed Google Scholar
Corresponding author
Correspondence to Lioua Kolsi .
Ethics declarations
Conflict of interest.
The authors declare that they have no known competing financial interests or personal relationships that could have appeared to influence the work reported in this paper.
Additional information
Publisher's note.
Springer Nature remains neutral with regard to jurisdictional claims in published maps and institutional affiliations.
Rights and permissions
Reprints and permissions
About this article
Ben Said, L., Kolsi, L., Ghachem, K. et al. Application of nanofluids as cutting fluids in machining operations: a brief review. Appl Nanosci 13 , 4247–4278 (2023). https://doi.org/10.1007/s13204-021-02140-8
Download citation
Received : 03 February 2021
Accepted : 03 October 2021
Published : 03 February 2022
Issue Date : June 2023
DOI : https://doi.org/10.1007/s13204-021-02140-8
Share this article
Anyone you share the following link with will be able to read this content:
Sorry, a shareable link is not currently available for this article.
Provided by the Springer Nature SharedIt content-sharing initiative
- Nano-lubricant
- Nanoparticle
- Find a journal
- Publish with us
- Track your research
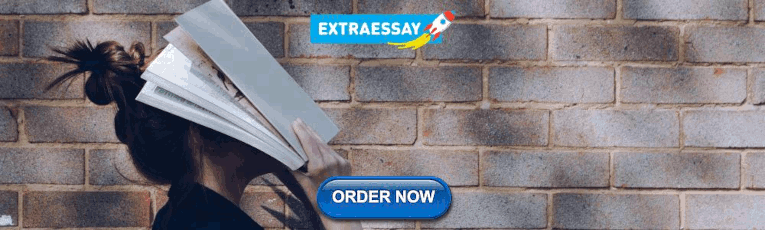
IMAGES
VIDEO
COMMENTS
Literature on experimental specific heats of nanofluids is ve ry limited, In 2009, Namburu [37] reported that several ethylene glycolbased nanofluids exhibit lower specific heat than their respective
Xie et al. [96] studied the relationship between the nanometer layer and the thermal conductivity of nanofluids, and proposed a new prediction model for the thermal conductivity of nanofluids (Eq.(7)) on the basis of considering the interface effect. This model is based on the general solution of the heat conduction equation in spherical coordinates and the new model of the microstructure of ...
Kulkarni DP, Vajjha RS, Das DK, Oliva D (2008) Application of aluminum oxide nanofluids in diesel electric generator as jacket water coolant. Appl Therm Eng 28:1774-1781. Google Scholar Wang XQ, Mujumdar AS (2008) A review on nanofluids—part II: experiments and applications. Brazilian J Chem Eng 25:631-648
The thermal conductivity of NFs appears to be the most researched attribute in the existing literature. NFs are distinct from regular BFs because of their improved thermal conductivity and other novel thermophysical features ... Stability of heat transfer nanofluids - A review. Chem. Bio. Eng. Rev., 5 (5) (2018), pp. 312-333, 10.1002/cben ...
Abstract. Nanofluids are potential heat transfer fluids with enhanced thermophysical properties and heat transfer performance can be applied in many devices for better performances (i.e. energy, heat transfer and other performances). In this paper, a comprehensive literature on the applications and challenges of nanofluids have been compiled ...
In this article, the literature regarding nanofluids is summarized in detail, and the exact solutions for the flow problems of nanofluids are discussed. ... This literature review shows that much work has been done on the exact solutions for the flow problems of nanofluid in different geometries (i.e., flow infinite plate, in a channel and ...
A summary on previously conducted literature review is shown in Table 1. They provide insights on the preparation of nanofluids, enhancements as proclaimed by corresponding researchers, as well as ...
By using nanofluids, the desired thermo-physical properties can be obtained in order to improve the heat transfer property in the system. ... In addition, according to the literature review, minimum entropy generation is an effective way to increase the energy efficiency and improve thermodynamic performance of the thermal system and the use of ...
This work presented a broad literature review concerning nanofluids applied as heat transfer fluids. Based on the present analysis, it is evident that most works are focused on single-phase flows and pool boiling, while fewer studies involving flow boiling can also be found. A variety of physical mechanisms are pointed out by distinct authors ...
It was found that nanofluids fabricated at 5°C lost its stability after 5.55 min but had maintained its form for 7.53 min when applying a 1000 kPa inert pressure to the samples. Moreover, the 15°C samples had achieved a 7.18 min and 6.77 min stability, with and without added pressure, respectively.
Subsequently, we provide a comprehensive literature review of the challenges and future research directions of nanofluids as coolants in fuel cell vehicles. This review demonstrates the potential of nanofluids as an alternative thermal management system that can facilitate transition toward a low-carbon, energy-secure economy.
Both mono and hybrid nanofluids, the engineered colloidal mixture made of the base fluid and nanoparticles, have shown many interesting properties and become a high potential next-generation heat transfer fluid in various engineering applications. The present review focuses on improving the stability of the nanofluids. For this, the present review briefly summarizes the impact of nanofluid ...
The synthesis of nanofluidsfor enhancing thermal properties is essential as they encounter either deposition or aggregation. The application produced various nanofluids like metal oxides, metal carbides, nitrides, and non-metals with or without surfactants [56], [57].The steps involved in synthesising hybrid nanoparticles were shown in Fig. 2.To fabricate solid-state phase materials, high ...
The review is focused on nanosuspensions, as the nanofluids with solid particles. Major applications are focused on nanosuspension, which is the main type of nanofluids. So, concise content about major biomedical applications of nanofluids in drug delivery systems, imaging, and antibacterial activities is presented in this paper.
The reviews include solar collector applications [20, 21], a review of nanofluids in heat exchangers [22, 23], review of nanofluids in heat pipes , radiator cooling , electronic cooling and ... They concluded that from 107 works of literature surveyed, a 15-40% enhancement was recorded with the oxides nanoparticles available back then. Today ...
Utilizing nanofluid as an absorber fluid is an effective approach to enhance heat transfer in solar devices. The purpose of this review is to summarize the research done on the nanofluids' applications in solar thermal engineering systems in recent years. This review article provides comprehensive information for the design of a solar thermal system working at the optimum conditions.
Nanofluids are the colloidal mixtures of the nanoparticles (nano-meter-sized particles) in the base fluids used to improve the heat transfer characteristics suited for the particular application. The nanoparticles used in the nanofluids are mostly made up of metals, oxides, carbides, and carbon nanotubes.
Iron oxide nanofluids have been used in the present study in light of the related literature indicating excellent stability and dielectric properties 4,21,22; low-medium nanoparticle ...
What makes this review paper different from previous reviews is its focus on literature reporting the use of nanofluids in heat exchangers that can be employed in intercoolers. It provides a comprehensive review of the research literature on increasing the efficiency of gas turbines using nanofluids.
However, in the context of the huge amount of literature in the past decade, existing review cannot be used as a technical manual for industrial applications. There are many technical difficulties in establishing a mature production system, which hinder the large-scale application of nanofluids in industrial production.
Fig. 2 shows the rapid growth of nanofluids research in recent years, including US, EU, and China, and more recently, India and South Korea. The potential market for nanofluids for heat transfer applications is estimated to be over 2 billion dollars per year worldwide (CEA, 2007), with prospect of further growth in the next 5-10 years.A critical review of state-of-the-art nanofluids work for ...
This article provides the first comprehensive literature review about generative AI for architectural design, and we believe this work can facilitate more research work on this significant topic in architecture. Comments: 32 pages, 20 figures. Subjects: Machine Learning (cs.LG); Artificial Intelligence (cs.AI) Cite as: arXiv:2404.01335 [cs.LG]
On the museum's roof, three LED rabbits glow pink at night. Chase Castor for The New York Times. Little by little, chugging along like " The Little Engine That Could ," they raised $15 ...
Subsequently, we provide a comprehensive literature review of the challenges and future research directions of nanofluids as coolants in fuel cell vehicles. This review demonstrates the potential of nanofluids as an alternative thermal management system that can facilitate transition toward a low-carbon, energy-secure economy.
To the best of the authors׳ knowledge, no review on hybrid nanofluids has not been done till now. ... [61], [62] of synthesizing hybrid nanofluids and the preparation methods reported in the literature are summarized in Table 1. As shown, mostly the two-step method has been used rather than the one-step method. Here, the preparation methods of ...
This literature focuses on the use of nano-lubricant in machining processes such as turning, milling and grinding processes. Analyzing the current review, it has been found that the use of nanofluids especially hybrid ones reduces the tool wear, the surface roughness, cutting forces, and heat generation.
PDF (0.6 MB) Tools. Share. Abstract: There is a rapidly growing literature on the link between climate change and poverty. This study reviews the existing literature on whether the poor are more exposed to climate shocks and whether they are more adversely affected. About two-thirds of the studies in our analyzed sample find that the poor are ...