Have a language expert improve your writing
Run a free plagiarism check in 10 minutes, generate accurate citations for free.
- Knowledge Base
- Academic writing
- How to write a lab report
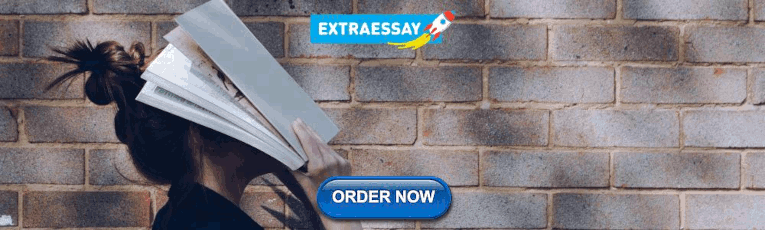
How To Write A Lab Report | Step-by-Step Guide & Examples
Published on May 20, 2021 by Pritha Bhandari . Revised on July 23, 2023.
A lab report conveys the aim, methods, results, and conclusions of a scientific experiment. The main purpose of a lab report is to demonstrate your understanding of the scientific method by performing and evaluating a hands-on lab experiment. This type of assignment is usually shorter than a research paper .
Lab reports are commonly used in science, technology, engineering, and mathematics (STEM) fields. This article focuses on how to structure and write a lab report.
Instantly correct all language mistakes in your text
Upload your document to correct all your mistakes in minutes
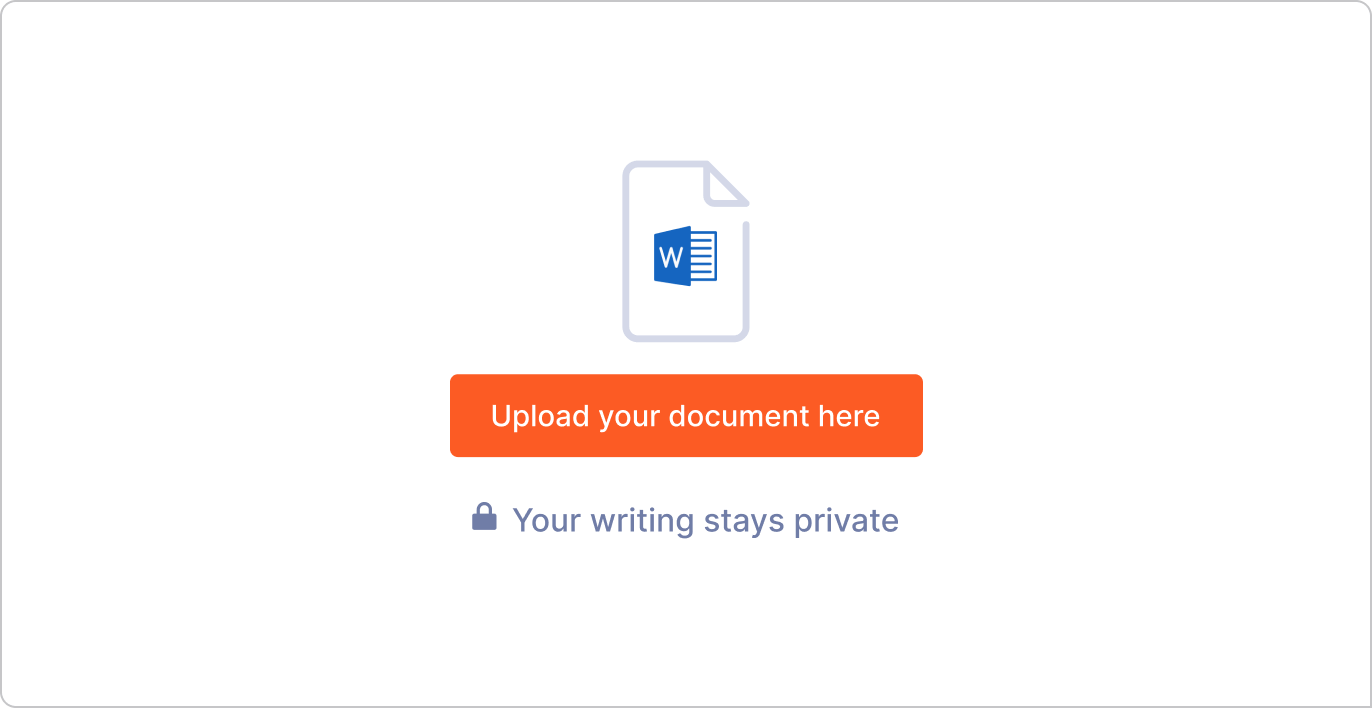
Table of contents
Structuring a lab report, introduction, other interesting articles, frequently asked questions about lab reports.
The sections of a lab report can vary between scientific fields and course requirements, but they usually contain the purpose, methods, and findings of a lab experiment .
Each section of a lab report has its own purpose.
- Title: expresses the topic of your study
- Abstract : summarizes your research aims, methods, results, and conclusions
- Introduction: establishes the context needed to understand the topic
- Method: describes the materials and procedures used in the experiment
- Results: reports all descriptive and inferential statistical analyses
- Discussion: interprets and evaluates results and identifies limitations
- Conclusion: sums up the main findings of your experiment
- References: list of all sources cited using a specific style (e.g. APA )
- Appendices : contains lengthy materials, procedures, tables or figures
Although most lab reports contain these sections, some sections can be omitted or combined with others. For example, some lab reports contain a brief section on research aims instead of an introduction, and a separate conclusion is not always required.
If you’re not sure, it’s best to check your lab report requirements with your instructor.
Check for common mistakes
Use the best grammar checker available to check for common mistakes in your text.
Fix mistakes for free
Your title provides the first impression of your lab report – effective titles communicate the topic and/or the findings of your study in specific terms.
Create a title that directly conveys the main focus or purpose of your study. It doesn’t need to be creative or thought-provoking, but it should be informative.
- The effects of varying nitrogen levels on tomato plant height.
- Testing the universality of the McGurk effect.
- Comparing the viscosity of common liquids found in kitchens.
An abstract condenses a lab report into a brief overview of about 150–300 words. It should provide readers with a compact version of the research aims, the methods and materials used, the main results, and the final conclusion.
Think of it as a way of giving readers a preview of your full lab report. Write the abstract last, in the past tense, after you’ve drafted all the other sections of your report, so you’ll be able to succinctly summarize each section.
To write a lab report abstract, use these guiding questions:
- What is the wider context of your study?
- What research question were you trying to answer?
- How did you perform the experiment?
- What did your results show?
- How did you interpret your results?
- What is the importance of your findings?
Nitrogen is a necessary nutrient for high quality plants. Tomatoes, one of the most consumed fruits worldwide, rely on nitrogen for healthy leaves and stems to grow fruit. This experiment tested whether nitrogen levels affected tomato plant height in a controlled setting. It was expected that higher levels of nitrogen fertilizer would yield taller tomato plants.
Levels of nitrogen fertilizer were varied between three groups of tomato plants. The control group did not receive any nitrogen fertilizer, while one experimental group received low levels of nitrogen fertilizer, and a second experimental group received high levels of nitrogen fertilizer. All plants were grown from seeds, and heights were measured 50 days into the experiment.
The effects of nitrogen levels on plant height were tested between groups using an ANOVA. The plants with the highest level of nitrogen fertilizer were the tallest, while the plants with low levels of nitrogen exceeded the control group plants in height. In line with expectations and previous findings, the effects of nitrogen levels on plant height were statistically significant. This study strengthens the importance of nitrogen for tomato plants.
Your lab report introduction should set the scene for your experiment. One way to write your introduction is with a funnel (an inverted triangle) structure:
- Start with the broad, general research topic
- Narrow your topic down your specific study focus
- End with a clear research question
Begin by providing background information on your research topic and explaining why it’s important in a broad real-world or theoretical context. Describe relevant previous research on your topic and note how your study may confirm it or expand it, or fill a gap in the research field.
This lab experiment builds on previous research from Haque, Paul, and Sarker (2011), who demonstrated that tomato plant yield increased at higher levels of nitrogen. However, the present research focuses on plant height as a growth indicator and uses a lab-controlled setting instead.
Next, go into detail on the theoretical basis for your study and describe any directly relevant laws or equations that you’ll be using. State your main research aims and expectations by outlining your hypotheses .
Based on the importance of nitrogen for tomato plants, the primary hypothesis was that the plants with the high levels of nitrogen would grow the tallest. The secondary hypothesis was that plants with low levels of nitrogen would grow taller than plants with no nitrogen.
Your introduction doesn’t need to be long, but you may need to organize it into a few paragraphs or with subheadings such as “Research Context” or “Research Aims.”
Prevent plagiarism. Run a free check.
A lab report Method section details the steps you took to gather and analyze data. Give enough detail so that others can follow or evaluate your procedures. Write this section in the past tense. If you need to include any long lists of procedural steps or materials, place them in the Appendices section but refer to them in the text here.
You should describe your experimental design, your subjects, materials, and specific procedures used for data collection and analysis.
Experimental design
Briefly note whether your experiment is a within-subjects or between-subjects design, and describe how your sample units were assigned to conditions if relevant.
A between-subjects design with three groups of tomato plants was used. The control group did not receive any nitrogen fertilizer. The first experimental group received a low level of nitrogen fertilizer, while the second experimental group received a high level of nitrogen fertilizer.
Describe human subjects in terms of demographic characteristics, and animal or plant subjects in terms of genetic background. Note the total number of subjects as well as the number of subjects per condition or per group. You should also state how you recruited subjects for your study.
List the equipment or materials you used to gather data and state the model names for any specialized equipment.
List of materials
35 Tomato seeds
15 plant pots (15 cm tall)
Light lamps (50,000 lux)
Nitrogen fertilizer
Measuring tape
Describe your experimental settings and conditions in detail. You can provide labelled diagrams or images of the exact set-up necessary for experimental equipment. State how extraneous variables were controlled through restriction or by fixing them at a certain level (e.g., keeping the lab at room temperature).
Light levels were fixed throughout the experiment, and the plants were exposed to 12 hours of light a day. Temperature was restricted to between 23 and 25℃. The pH and carbon levels of the soil were also held constant throughout the experiment as these variables could influence plant height. The plants were grown in rooms free of insects or other pests, and they were spaced out adequately.
Your experimental procedure should describe the exact steps you took to gather data in chronological order. You’ll need to provide enough information so that someone else can replicate your procedure, but you should also be concise. Place detailed information in the appendices where appropriate.
In a lab experiment, you’ll often closely follow a lab manual to gather data. Some instructors will allow you to simply reference the manual and state whether you changed any steps based on practical considerations. Other instructors may want you to rewrite the lab manual procedures as complete sentences in coherent paragraphs, while noting any changes to the steps that you applied in practice.
If you’re performing extensive data analysis, be sure to state your planned analysis methods as well. This includes the types of tests you’ll perform and any programs or software you’ll use for calculations (if relevant).
First, tomato seeds were sown in wooden flats containing soil about 2 cm below the surface. Each seed was kept 3-5 cm apart. The flats were covered to keep the soil moist until germination. The seedlings were removed and transplanted to pots 8 days later, with a maximum of 2 plants to a pot. Each pot was watered once a day to keep the soil moist.
The nitrogen fertilizer treatment was applied to the plant pots 12 days after transplantation. The control group received no treatment, while the first experimental group received a low concentration, and the second experimental group received a high concentration. There were 5 pots in each group, and each plant pot was labelled to indicate the group the plants belonged to.
50 days after the start of the experiment, plant height was measured for all plants. A measuring tape was used to record the length of the plant from ground level to the top of the tallest leaf.
In your results section, you should report the results of any statistical analysis procedures that you undertook. You should clearly state how the results of statistical tests support or refute your initial hypotheses.
The main results to report include:
- any descriptive statistics
- statistical test results
- the significance of the test results
- estimates of standard error or confidence intervals
The mean heights of the plants in the control group, low nitrogen group, and high nitrogen groups were 20.3, 25.1, and 29.6 cm respectively. A one-way ANOVA was applied to calculate the effect of nitrogen fertilizer level on plant height. The results demonstrated statistically significant ( p = .03) height differences between groups.
Next, post-hoc tests were performed to assess the primary and secondary hypotheses. In support of the primary hypothesis, the high nitrogen group plants were significantly taller than the low nitrogen group and the control group plants. Similarly, the results supported the secondary hypothesis: the low nitrogen plants were taller than the control group plants.
These results can be reported in the text or in tables and figures. Use text for highlighting a few key results, but present large sets of numbers in tables, or show relationships between variables with graphs.
You should also include sample calculations in the Results section for complex experiments. For each sample calculation, provide a brief description of what it does and use clear symbols. Present your raw data in the Appendices section and refer to it to highlight any outliers or trends.
The Discussion section will help demonstrate your understanding of the experimental process and your critical thinking skills.
In this section, you can:
- Interpret your results
- Compare your findings with your expectations
- Identify any sources of experimental error
- Explain any unexpected results
- Suggest possible improvements for further studies
Interpreting your results involves clarifying how your results help you answer your main research question. Report whether your results support your hypotheses.
- Did you measure what you sought out to measure?
- Were your analysis procedures appropriate for this type of data?
Compare your findings with other research and explain any key differences in findings.
- Are your results in line with those from previous studies or your classmates’ results? Why or why not?
An effective Discussion section will also highlight the strengths and limitations of a study.
- Did you have high internal validity or reliability?
- How did you establish these aspects of your study?
When describing limitations, use specific examples. For example, if random error contributed substantially to the measurements in your study, state the particular sources of error (e.g., imprecise apparatus) and explain ways to improve them.
The results support the hypothesis that nitrogen levels affect plant height, with increasing levels producing taller plants. These statistically significant results are taken together with previous research to support the importance of nitrogen as a nutrient for tomato plant growth.
However, unlike previous studies, this study focused on plant height as an indicator of plant growth in the present experiment. Importantly, plant height may not always reflect plant health or fruit yield, so measuring other indicators would have strengthened the study findings.
Another limitation of the study is the plant height measurement technique, as the measuring tape was not suitable for plants with extreme curvature. Future studies may focus on measuring plant height in different ways.
The main strengths of this study were the controls for extraneous variables, such as pH and carbon levels of the soil. All other factors that could affect plant height were tightly controlled to isolate the effects of nitrogen levels, resulting in high internal validity for this study.
Your conclusion should be the final section of your lab report. Here, you’ll summarize the findings of your experiment, with a brief overview of the strengths and limitations, and implications of your study for further research.
Some lab reports may omit a Conclusion section because it overlaps with the Discussion section, but you should check with your instructor before doing so.
If you want to know more about AI for academic writing, AI tools, or fallacies make sure to check out some of our other articles with explanations and examples or go directly to our tools!
- Ad hominem fallacy
- Post hoc fallacy
- Appeal to authority fallacy
- False cause fallacy
- Sunk cost fallacy
- Deep learning
- Generative AI
- Machine learning
- Reinforcement learning
- Supervised vs. unsupervised learning
(AI) Tools
- Grammar Checker
- Paraphrasing Tool
- Text Summarizer
- AI Detector
- Plagiarism Checker
- Citation Generator
A lab report conveys the aim, methods, results, and conclusions of a scientific experiment . Lab reports are commonly assigned in science, technology, engineering, and mathematics (STEM) fields.
The purpose of a lab report is to demonstrate your understanding of the scientific method with a hands-on lab experiment. Course instructors will often provide you with an experimental design and procedure. Your task is to write up how you actually performed the experiment and evaluate the outcome.
In contrast, a research paper requires you to independently develop an original argument. It involves more in-depth research and interpretation of sources and data.
A lab report is usually shorter than a research paper.
The sections of a lab report can vary between scientific fields and course requirements, but it usually contains the following:
- Abstract: summarizes your research aims, methods, results, and conclusions
- References: list of all sources cited using a specific style (e.g. APA)
- Appendices: contains lengthy materials, procedures, tables or figures
The results chapter or section simply and objectively reports what you found, without speculating on why you found these results. The discussion interprets the meaning of the results, puts them in context, and explains why they matter.
In qualitative research , results and discussion are sometimes combined. But in quantitative research , it’s considered important to separate the objective results from your interpretation of them.
Cite this Scribbr article
If you want to cite this source, you can copy and paste the citation or click the “Cite this Scribbr article” button to automatically add the citation to our free Citation Generator.
Bhandari, P. (2023, July 23). How To Write A Lab Report | Step-by-Step Guide & Examples. Scribbr. Retrieved April 1, 2024, from https://www.scribbr.com/academic-writing/lab-report/
Is this article helpful?
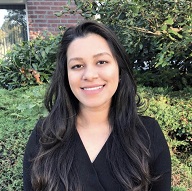
Pritha Bhandari
Other students also liked, guide to experimental design | overview, steps, & examples, how to write an apa methods section, how to write an apa results section, "i thought ai proofreading was useless but..".
I've been using Scribbr for years now and I know it's a service that won't disappoint. It does a good job spotting mistakes”
Tips on How to Write a Lab Report: A Full Guide

What Is a Lab Report
Let's start with a burning question: what is lab report? A lab report is an overview of your scientific experiment. It describes what you did (the course of the experiment), how you did it (what equipment and materials you used), and what outcome your experiment led to.
If you take any science classes involving a lab experiment – or full-fledged laboratory courses, you'll have to do your share of lab report writing.
Unlike the format of case study writing , lab reports have to follow a different structure. They, along with other lab report guidelines, are likely defined by your instructor. Your lab notebook may also contain the requirements.
But if it's not your case, here's what to include in a lab report:
- title page;
- introduction;
- equipment and materials list;
- conclusion;
- appendices.
If this structure looks intimidating now, don't worry: we'll break down every component below.
Format for Lab Reports
Different instructors require different formats for lab reports. So, look through the requirements you've received and see if a science lab report format is specified.
If no format is specified, see if your school, college, or university has specific formatting guidelines or a lab report template to follow.
If that's also not the case, then you can choose the most common formatting style for research papers and lab reports alike: the APA (American Psychology Association) format. Other options include the MLA (Modern Language Association) and Chicago styles.
APA Lab Report Style
Let's break down the main particularities of using the APA style for lab reports. When it comes to the lab report outline, this style dictates that you should include the following:
- a title page;
- an abstract;
- sources (as a References page).
How to format references under the APA format deserves a separate blog post. But here's a short example:
Smith, J. (2021). A lab report introduction guide. Cambridge Press.
To cite this source in the text, style it like this: (Smith, 2021)
As for the text formatting, here are the key APA guidelines to keep in mind:
- page margins: 1" (on all sides);
- indent: 0.5";
- page number: in the upper right corner;
- spacing: double;
- font: Times New Roman 12 pt.
How Long Should a Lab Report Be?
The appropriate report length depends heavily on the kind of experiment conducted – and on the requirements set by your instructor. That said, most lab reports are five to ten pages long, in our experience. That includes all the raw data, appendices, and graphs.
Need a lab report example? You'll find three below!
What's the Difference Between Lab Reports & Research Papers?
While lab report format and structure are similar to that of a research paper, they differ. But unfortunately, in our work as a college essay writing service , we see them confused often enough.
The key differences between lab reports and research papers are:
- Lab reports require you to conduct a hands-on experiment, while research papers are focused on the interpretation of existing data;
- A lab report's purpose is to show that you understand the scientific methods central to the experimental procedure – that's why the lab report template is different, too;
- A lab experiment doesn't require you to have an original hypothesis or argument;
- Research papers are usually longer than lab reports.
How to Do a Lab Report: Outline
Like with any other papers, from SWOT analysis to case studies, writing lab reports is easier when you have a clear college lab report outline in front of you. Luckily for you, the lab report structure is the same in most cases.
So, here's how to do a lab report – follow this outline (unless your instructor's requirements contradict it!):
- Title page: your name, course, instructor, and the report title;
- Abstract: a short description of the key findings and their significance;
- Introduction: the purpose of the lab experiment and its background information;
- Methods and materials: what you used during the experiment (e.g., a lab manual, certain reagents, etc.);
- Procedure: the detailed description of the lab experiment;
- Results: the outcome of your experiment and its interpretation;
- Conclusion: what your findings may mean for the field;
- References: the list of your sources;
- Appendices: raw data, calculations, graphs, etc.
Feeling Stuck With Your Lab Report?
Let our qualified writers handle it for you and relieve you of this burden!
Guide on How to Write a Lab Report
If the outline above is overwhelming at first, don't worry! As a paper and essay writing service , we've had our share of experience in writing lab reports. Today, we'd like to share this experience with you in this lab report guide.
So, below you'll find everything you need to know on how to write a good lab report, along with handy lab report guidelines!
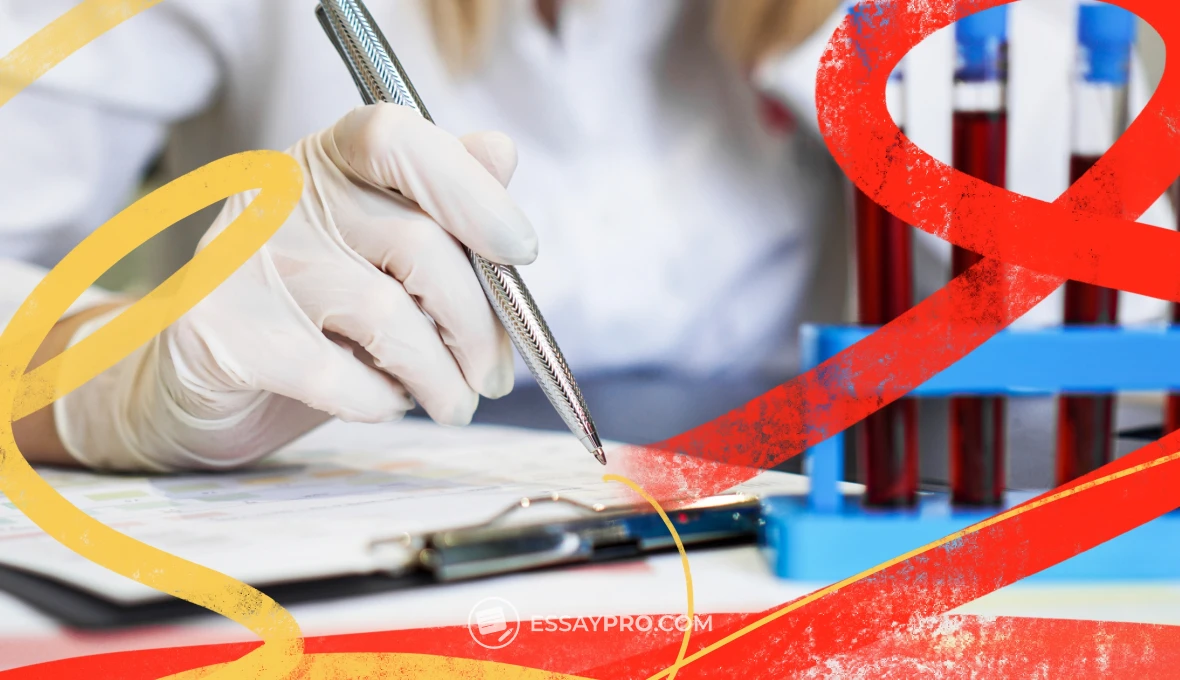
Lab Report Title Page
The lab report title page should include your name, student code, and any lab partners you may have had. It should also contain the date of the experiment and the title of your report.
The title length should be less than ten words. You'll also need to include the name of the academic supervisor in your lab report title page if you have one.
This paragraph describes your experiment, its main point, and its findings in a nutshell. Here are several guidelines on how to write an abstract for a lab report:
- Keep it under 200 words;
- Start with the purpose of your experiment;
- Describe the experimental procedure;
- State the results;
- Include 2-3 keywords (optional).
Lab Report Introduction
The first paragraph is where you explain your hypothesis and the purpose of your experiment. You can also add any previous research on the matter and any background information worth including. Here's a short lab report introduction example with a hypothesis:
This experiment examined the correlation between the levels of CO2 and the rate of photosynthesis in Chlorella algae. The latter was quantified by measuring the levels of RuBisCO.
Equipment (Methods and Materials)
Next in the lab report structure is the equipment section (also known as methods and materials). This is where you mention your lab manual, methods used during the experimental procedure, and the materials list.
In this part of the report, ensure to include all the details of the experimental procedure. It should provide readers with everything they need to know to replicate your study.
Procedure (with Graphs & Figures)
This part is, perhaps, the easiest (unlike how to write a hypothesis for a lab report). You should simply document the course of the lab experiment step-by-step, in chronological order.
This is usually a significant part of the report, taking up most of it. So make sure to provide detailed information on your hands-on experience!
Results Section
This is the overview of your experiment's findings (also known as the discussion section). Here's how to write a results section for a lab report:
- Discuss the outcome of the experiment;
- Explain how it pertains to your hypothesis (whether it proves or disproves it);
- Keep it brief and concise.
Note . You might notice that describing future work or further studies is absent from the tips on how to write the discussion section of a lab report. That's because it's a part of the conclusion, not the discussion.
This is where you sum up the results of your experiment and draw any major conclusions. You may also suggest future laboratory experiments or further research.
Here's how to write a conclusion for a lab report in three steps:
- Explain the results of your experiment;
- Determine their significance – and any limitations to the experimental design;
- Suggest future studies (if applicable).
The conclusion part of lab reports is typically short. So, don't worry if you can't write a lengthy one – you don't have to!
This is the part of your lab report outline where you list all of the sources you relied on in your lab experiments. It should include your lab manual, along with any relevant recommended reading from your course. You may also include any extra sources you used.
Remember to format your references list according to the formatting style you have to follow. Apart from every entry's formatting, you'll also have to present your references in alphabetical order based on the author's last name (for APA lab reports).
Finally, any lab report format includes appendices – your figures and graphs, in other words. This is where you add your raw data in tables, complete calculations, charts, etc.
Keep in mind: just like with sources, you need to cite each of the appendices in the main body of the report. Remember to format the appendix and its citation according to the chosen formatting style.
Lab Report Examples
As a paper and dissertation writing service , we know that sometimes it's better to see a great example of how to write a lab report once than to read dozens of tips. So, we've asked our lab report writing service to prepare a lab report template for three disciplines: chemistry, biology, and science.
Look at these samples if you keep wondering how to do a lab report! But keep in mind: you won't be able to use them as-is. So instead, use them as examples for your writing.
Note . References to lab manuals are made up – you should refer to the one you use in the experiment!
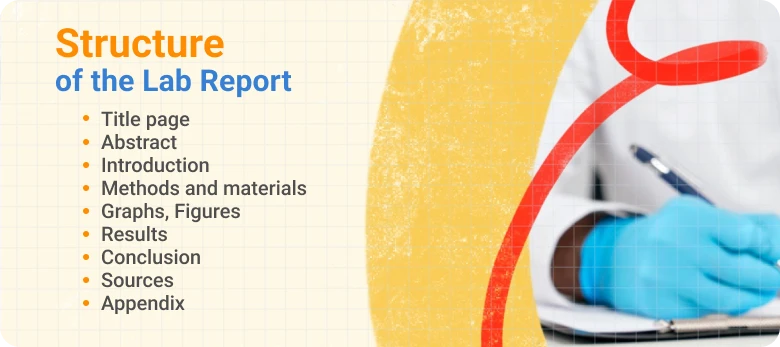
How to Write a Formal Lab Report for Chemistry?
The same lab report guidelines listed above apply to chemistry lab reports. Here's a short example that includes a lab report introduction, equipment, procedure, results, and references for an electrolysis reaction.
How to Write a Lab Report for Biology?
Next up in your lab report guide, it's a biology lab report! Like in any other lab report, its main point is to describe your experiment and explain its findings. Below you can find an example of one biology lab report that seeks to explain how to extract DNA from sliced fruit and make it visible to the naked eye.
How to Write a Science Lab Report?
Finally, let's look at a general science lab report. In this case, the science lab report format is the same as for other disciplines: start with the introduction and hypothesis, describe the equipment and procedure, and explain the outcome.
Here's a science lab report example on testing the density of different juices.
7 More Tips on How to Write a Lab Report
Need some more guidance on writing lab reports? Then, we've got you covered! Here are seven more tips on writing an excellent report:
- Carefully examine your lab manual before starting the experiment;
- Take detailed notes throughout the process;
- Be conscious of any limitations of your experimental design – and mention them in conclusion;
- Stick to the lab report structure defined by your instructor;
- Be transparent about any experimental error that may occur;
- Search for examples if you feel stuck with writing lab reports;
- Triple-check your lab report before submitting it: look for formatting issues, sources forgotten, and grammar and syntax mistakes.
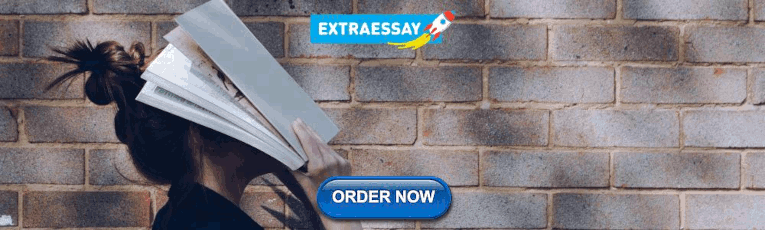
Need Qualified Help With an Assignment?
You're seconds away from it! Hire a professional writer now – and stop worrying about a deadline coming up!
Related Articles
.webp)
Purdue Online Writing Lab Purdue OWL® College of Liberal Arts
Welcome to the Purdue Online Writing Lab
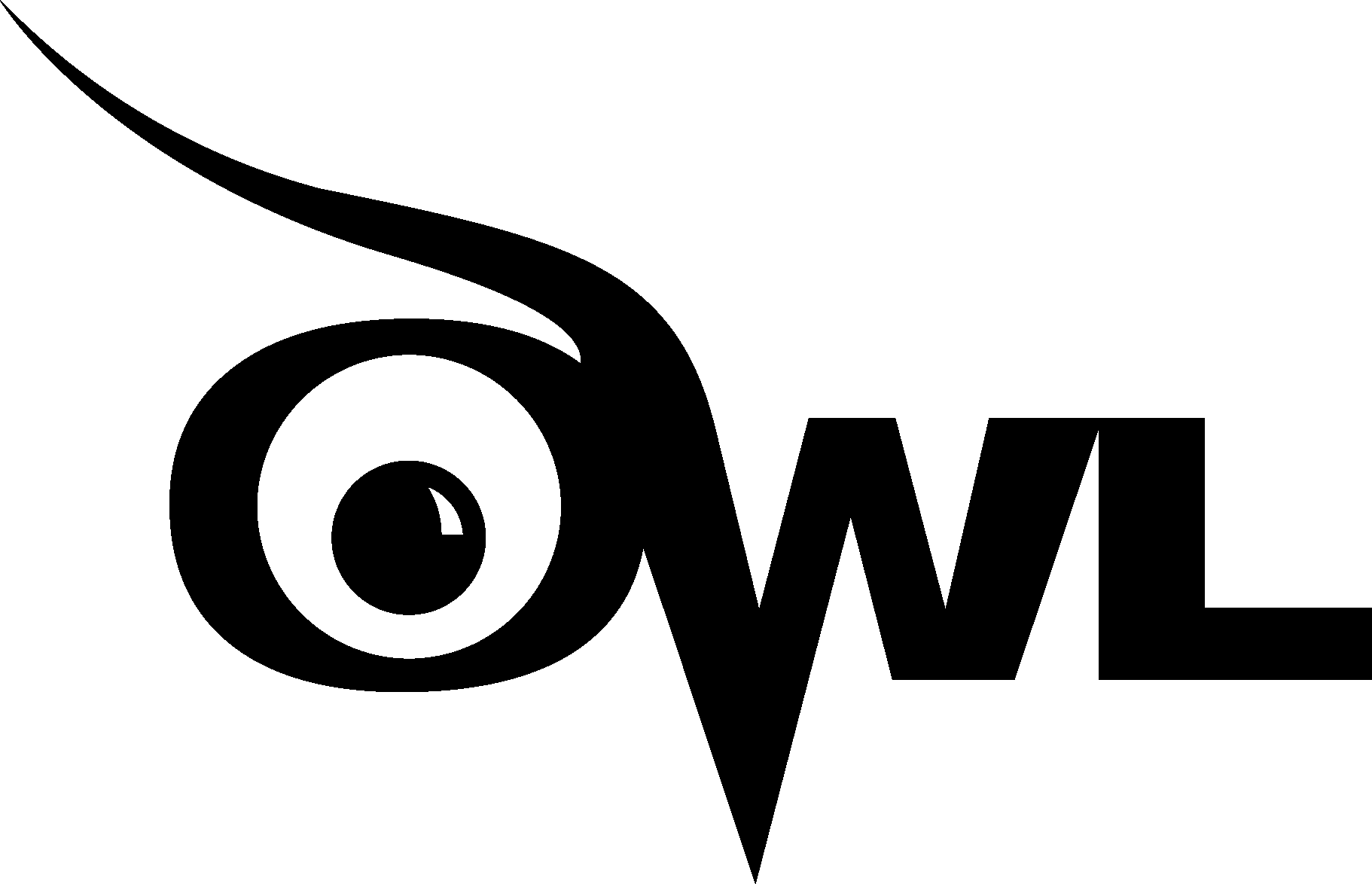
Welcome to the Purdue OWL
This page is brought to you by the OWL at Purdue University. When printing this page, you must include the entire legal notice.
Copyright ©1995-2018 by The Writing Lab & The OWL at Purdue and Purdue University. All rights reserved. This material may not be published, reproduced, broadcast, rewritten, or redistributed without permission. Use of this site constitutes acceptance of our terms and conditions of fair use.
The Online Writing Lab at Purdue University houses writing resources and instructional material, and we provide these as a free service of the Writing Lab at Purdue. Students, members of the community, and users worldwide will find information to assist with many writing projects. Teachers and trainers may use this material for in-class and out-of-class instruction.
The Purdue On-Campus Writing Lab and Purdue Online Writing Lab assist clients in their development as writers—no matter what their skill level—with on-campus consultations, online participation, and community engagement. The Purdue Writing Lab serves the Purdue, West Lafayette, campus and coordinates with local literacy initiatives. The Purdue OWL offers global support through online reference materials and services.
A Message From the Assistant Director of Content Development
The Purdue OWL® is committed to supporting students, instructors, and writers by offering a wide range of resources that are developed and revised with them in mind. To do this, the OWL team is always exploring possibilties for a better design, allowing accessibility and user experience to guide our process. As the OWL undergoes some changes, we welcome your feedback and suggestions by email at any time.
Please don't hesitate to contact us via our contact page if you have any questions or comments.
All the best,
Social Media
Facebook twitter.

America's Lab Report: Investigations in High School Science (2006)
Chapter: 1 introduction, history, and definition of laboratories, 1 introduction, history, and definition of laboratories.
Science laboratories have been part of high school education for two centuries, yet a clear articulation of their role in student learning of science remains elusive. This report evaluates the evidence about the role of laboratories in helping students attain science learning goals and discusses factors that currently limit science learning in high school laboratories. In this chap-
ter, the committee presents its charge, reviews the history of science laboratories in U.S. high schools, defines laboratories, and outlines the organization of the report.
CHARGE TO THE COMMITTEE
In the National Science Foundation (NSF) Authorization Act of 2002 (P.L. 107-368, authorizing funding for fiscal years 2003-2007), Congress called on NSF to launch a secondary school systemic initiative. The initiative was to “promote scientific and technological literacy” and to “meet the mathematics and science needs of students at risk of not achieving State student academic achievement standards.” Congress directed NSF to provide grants for such activities as “laboratory improvement and provision of instrumentation as part of a comprehensive program to enhance the quality of mathematics, science, engineering, and technology instruction” (P.L. 107-368, Section 8-E). In response, NSF turned to the National Research Council (NRC) of the National Academies. NSF requested that the NRC
nominate a committee to review the status of and future directions for the role of high school science laboratories in promoting the teaching and learning of science for all students. This committee will guide the conduct of a study and author a consensus report that will provide guidance on the question of the role and purpose of high school science laboratories with an emphasis on future directions…. Among the questions that may guide these activities are:
What is the current state of science laboratories and what do we know about how they are used in high schools?
What examples or alternatives are there to traditional approaches to labs and what is the evidence base as to their effectiveness?
If labs in high school never existed (i.e., if they were to be planned and designed de novo), what would that experience look like now, given modern advances in the natural and learning sciences?
In what ways can the integration of technologies into the curriculum augment and extend a new vision of high school science labs? What is known about high school science labs based on principles of design?
How do the structures and policies of high schools (course scheduling, curricular design, textbook adoption, and resource deployment) influence the organization of science labs? What kinds of changes might be needed in the infrastructure of high schools to enhance the effectiveness of science labs?
What are the costs (e.g., financial, personnel, space, scheduling) associated with different models of high school science labs? How might a new vision of laboratory experiences for high school students influence those costs?
In what way does the growing interdisciplinary nature of the work of scientists help to shape discussions of laboratories as contexts in high school for science learning?
How do high school lab experiences align with both middle school and postsecondary education? How is the role of teaching labs changing in the nation’s colleges and universities? Would a redesign of high school science labs enhance or limit articulation between high school and college-level science education?
The NRC convened the Committee on High School Science Laboratories: Role and Vision to address this charge.
SCOPE OF THE STUDY
The committee carried out its charge through an iterative process of gathering information, deliberating on it, identifying gaps and questions, gathering further information to fill these gaps, and holding further discussions. In the search for relevant information, the committee held three public fact-finding meetings, reviewed published reports and unpublished research, searched the Internet, and commissioned experts to prepare and present papers. At a fourth, private meeting, the committee intensely analyzed and discussed its findings and conclusions over the course of three days. Although the committee considered information from a variety of sources, its final report gives most weight to research published in peer-reviewed journals and books.
At an early stage in its deliberations, the committee chose to focus primarily on “the role of high school laboratories in promoting the teaching and learning of science for all students.” The committee soon became frustrated by the limited research evidence on the role of laboratories in learning. To address one of many problems in the research evidence—a lack of agreement about what constitutes a laboratory and about the purposes of laboratory education—the committee commissioned a paper to analyze the alternative definitions and goals of laboratories.
The committee developed a concept map outlining the main themes of the study (see Figure 1-1 ) and organized the three fact-finding meetings to gather information on each of these themes. For example, reflecting the committee’s focus on student learning (“how students learn science” on the concept map), all three fact-finding meetings included researchers who had developed innovative approaches to high school science laboratories. We also commissioned two experts to present papers reviewing available research on the role of laboratories in students’ learning of science.
At the fact-finding meetings, some researchers presented evidence of student learning following exposure to sequences of instruction that included laboratory experiences; others provided data on how various technologies
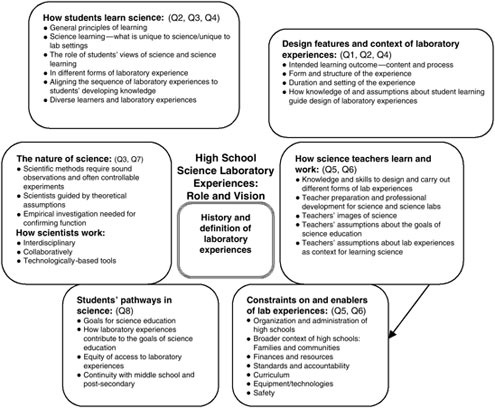
FIGURE 1-1 High school science laboratory experiences: Role and vision. Concept map with references to guiding questions in committee charge.
contribute to student learning in the laboratory. Responding to the congressional mandate to meet the mathematics and science needs of students at risk of not achieving state student academic achievement standards, the third fact-finding meeting included researchers who have studied laboratory teaching and learning among diverse students. Taken together, all of these activities enabled the committee to address questions 2, 3, and 4 of the charge.
The committee took several steps to ensure that the study reflected the current realities of science laboratories in U.S high schools, addressing the themes of “how science teachers learn and work” and “constraints and enablers of laboratory experiences” on the concept map. At the first fact-finding meeting, representatives of associations of scientists and science teachers described their efforts to help science teachers learn to lead effective labora-
tory activities. They noted constraints on laboratory learning, including poorly designed, overcrowded laboratory classrooms and inadequate preparation of science teachers. This first meeting also included a presentation about laboratory scheduling, supplies, and equipment drawn from a national survey of science teachers conducted in 2000. At the second fact-finding meeting, an architect spoke about the design of laboratory facilities, and a sociologist described how the organization of work and authority in schools may enable or constrain innovative approaches to laboratory teaching. Two meetings included panel discussions about laboratory teaching among groups of science teachers and school administrators. Through these presentations, review of additional literature, and internal discussions, the committee was able to respond to questions 1, 5, and 6 of the charge. The agendas for each fact-finding meeting, including the guiding questions that were sent to each presenter, appear in Appendix A .
The committee recognized that the question in its charge about the increasingly interdisciplinary nature of science (question 7) is important to the future of science and to high school science laboratories. In presentations and commissioned papers, several experts offered suggestions for how laboratory activities could be designed to more accurately reflect the work of scientists and to improve students’ understanding of the way scientists work today. Based on our analysis of this information, the committee partially addresses this question from the perspective of how scientists conduct their work (in this chapter). The committee also identifies design principles for laboratory activities that may increase students’ understanding of the nature of science (in Chapter 3 ). However, in order to maintain our focus on the key question of student learning in laboratories, the committee did not fully address question 7.
Another important question in the committee’s charge (question 8) addresses the alignment of laboratory learning in middle school, high school, and undergraduate science education. Within the short time frame of this study, the committee focused on identifying, assembling, and analyzing the limited research available on high school science laboratories and did not attempt to do the same analysis for middle school and undergraduate science laboratories. However, this report does discuss several studies of student laboratory learning in middle school (see Chapter 3 ) and describes undergraduate science laboratories briefly in its analysis of the preparation of high school science teachers (see in Chapter 5 ). The committee thinks questions about the alignment of laboratory learning merit more sustained attention than was possible in this study.
During the course of our deliberations, other important questions emerged. For example, it is apparent that the scientific community is engaged in an array of efforts to strengthen teaching and learning in high school science laboratories, but little information is available on the extent
of these efforts and on their effectiveness at enhancing student learning. As a result, we address the role of the scientific community in high school laboratories only briefly in Chapters 1 and 5 . Another issue that arose over the course of this study is laboratory safety. We became convinced that laboratory safety is critical, but we did not fully analyze safety issues, which lay outside our charge. Finally, although engaging students in design or engineering laboratory activities appears to hold promising connections with science laboratory activities, the committee did not explore this possibility. Although all of these issues and questions are important, taking time and energy to address them would have deterred us from a central focus on the role of high school laboratories in promoting the teaching and learning of science for all students.
One important step in defining the scope of the study was to review the history of laboratories. Examining the history of laboratory education helped to illuminate persistent tensions, provided insight into approaches to be avoided in the future, and allowed the committee to more clearly frame key questions for the future.
HISTORY OF LABORATORY EDUCATION
The history of laboratories in U.S. high schools has been affected by changing views of the nature of science and by society’s changing goals for science education. Between 1850 and the present, educators, scientists, and the public have, at different times, placed more or less emphasis on three sometimes-competing goals for school science education: (1) a theoretical emphasis, stressing the structure of scientific disciplines, the benefits of basic scientific research, and the importance of preparing young people for higher education in science; (2) an applied or practical emphasis, stressing high school students’ ability to understand and apply the science and workings of everyday things; and (3) a liberal or contextual emphasis, stressing the historical development and cultural implications of science (Matthews, 1994). These changing goals have affected the nature and extent of laboratory education.
By the mid-19th century, British writers and philosophers had articulated a view of science as an inductive process (Mill, 1843; Whewell, 1840, 1858). They believed that scientists engaged in painstaking observation of nature to identify and accumulate facts, and only very cautiously did they draw conclusions from these facts to propose new theories. British and American scientists portrayed the newest scientific discoveries—such as the laws of thermodynamics and Darwin’s theory of evolution—to an increas-
ingly interested public as certain knowledge derived through well-established inductive methods. However, scientists and teachers made few efforts to teach students about these methods. High school and undergraduate science courses, like those in history and other subjects, were taught through lectures and textbooks, followed by rote memorization and recitation (Rudolph, 2005). Lecturers emphasized student knowledge of the facts, and science laboratories were not yet accepted as part of higher education. For example, when Benjamin Silliman set up the first chemistry laboratory at Yale in 1847, he paid rent to the college for use of the building and equipped it at his own expense (Whitman, 1898, p. 201). Few students were allowed into these laboratories, which were reserved for scientists’ research, although some apparatus from the laboratory was occasionally brought into the lecture room for demonstrations.
During the 1880s, the situation changed rapidly. Influenced by the example of chemist Justus von Liebig in Germany, leading American universities embraced the German model. In this model, laboratories played a central role as the setting for faculty research and for advanced scientific study by students. Johns Hopkins University established itself as a research institution with student laboratories. Other leading colleges and universities followed suit, and high schools—which were just being established as educational institutions—soon began to create student science laboratories as well.
The primary goal of these early high school laboratories was to prepare students for higher science education in college and university laboratories. The National Education Association produced an influential report noting the “absolute necessity of laboratory work” in the high school science curriculum (National Education Association, 1894) in order to prepare students for undergraduate science studies. As demand for secondary school teachers trained in laboratory methods grew, colleges and universities began offering summer laboratory courses for teachers. In 1895, a zoology professor at Brown University described “large and increasing attendance at our summer schools,” which focused on the dissection of cats and other animals (Bump, 1895, p. 260).
In these early years, American educators emphasized the theoretical, disciplinary goals of science education in order to prepare graduates for further science education. Because of this emphasis, high schools quickly embraced a detailed list of 40 physics experiments published by Harvard instructor Edwin Hall (Harvard University, 1889). The list outlined the experiments, procedures, and equipment necessary to successfully complete all 40 experiments as a condition of admission to study physics at Harvard. Scientific supply companies began selling complete sets of the required equipment to schools and successful completion of the exercises was soon required for admission to study physics at other colleges and universities (Rudolph, 2005).
At that time, most educators and scientists believed that participating in laboratory experiments would help students learn methods of accurate observation and inductive reasoning. However, the focus on prescribing specific experiments and procedures, illustrated by the embrace of the Harvard list, limited the effectiveness of early laboratory education. In the rush to specify laboratory experiments, procedures, and equipment, little attention had been paid to how students might learn from these experiences. Students were expected to simply absorb the methods of inductive reasoning by carrying out experiments according to prescribed procedures (Rudolph, 2005).
Between 1890 and 1910, as U.S. high schools expanded rapidly to absorb a huge influx of new students, a backlash began to develop against the prevailing approach to laboratory education. In a 1901 lecture at the New England Association of College and Secondary Schools, G. Stanley Hall, one of the first American psychologists, criticized high school physics education based on the Harvard list, saying that “boys of this age … want more dynamic physics” (Hall, 1901). Building on Hall’s critique, University of Chicago physicist Charles Mann and other members of the Central Association for Science and Mathematics Teaching launched a complete overhaul of high school physics teaching. Mann and others attacked the “dry bones” of the Harvard experiments, calling for a high school physics curriculum with more personal and social relevance to students. One described lab work as “at best a very artificial means of supplying experiences upon which to build physical concepts” (Woodhull, 1909). Other educators argued that science teaching could be improved by providing more historical perspective, and high schools began reducing the number of laboratory exercises.
By 1910, a clear tension had emerged between those emphasizing laboratory experiments and reformers favoring an emphasis on interesting, practical science content in high school science. However, the focus on content also led to problems, as students became overwhelmed with “interesting” facts. New York’s experience illustrates this tension. In 1890, the New York State Regents exam included questions asking students to design experiments (Champagne and Shiland, 2004). In 1905, the state introduced a new syllabus of physics topics. The content to be covered was so extensive that, over the course of a year, an average of half an hour could be devoted to each topic, virtually eliminating the possibility of including laboratory activities (Matthews, 1994). An outcry to return to more experimentation in science courses resulted, and in 1910 New York State instituted a requirement for 30 science laboratory sessions taking double periods in the syllabus for Regents science courses (courses preparing students for the New York State Regents examinations) (Champagne and Shiland, 2004).
In an influential speech to the American Association for the Advancement of Science (AAAS) in 1909, philosopher and educator John Dewey proposed a solution to the tension between advocates for more laboratory
experimentation and advocates for science education emphasizing practical content. While criticizing science teaching focused strictly on covering large amounts of known content, Dewey also pointed to the flaws in rigid laboratory exercises: “A student may acquire laboratory methods as so much isolated and final stuff, just as he may so acquire material from a textbook…. Many a student had acquired dexterity and skill in laboratory methods without it ever occurring to him that they have anything to do with constructing beliefs that are alone worthy of the title of knowledge” (Dewey, 1910b). Dewey believed that people should leave school with some understanding of the kinds of evidence required to substantiate scientific beliefs. However, he never explicitly described his view of the process by which scientists develop and substantiate such evidence.
In 1910, Dewey wrote a short textbook aimed at helping teachers deal with students as individuals despite rapidly growing enrollments. He analyzed what he called “a complete act of thought,” including five steps: (1) a felt difficulty, (2) its location and definition, (3) suggestion of possible solution, (4) development by reasoning of the bearing of the suggestion, and (5) further observation and experiment leading to its acceptance or rejection (Dewey, 1910a, pp. 68-78). Educators quickly misinterpreted these five steps as a description of the scientific method that could be applied to practical problems. In 1918, William Kilpatrick of Teachers College published a seminal article on the “project method,” which used Dewey’s five steps to address problems of everyday life. The article was eventually reprinted 60,000 times as reformers embraced the idea of engaging students with practical problems, while at the same time teaching them about what were seen as the methods of science (Rudolph, 2005).
During the 1920s, reform-minded teachers struggled to use the project method. Faced with ever-larger classes and state requirements for coverage of science content, they began to look for lists of specific projects that students could undertake, the procedures they could use, and the expected results. Soon, standardized lists of projects were published, and students who had previously been freed from rigid laboratory procedures were now engaged in rigid, specified projects, leading one writer to observe, “the project is little more than a new cloak for the inductive method” (Downing, 1919, p. 571).
Despite these unresolved tensions, laboratory education had become firmly established, and growing numbers of future high school teachers were instructed in teaching laboratory activities. For example, a 1925 textbook for preservice science teachers included a chapter titled “Place of Laboratory Work in the Teaching of Science” followed by three additional chapters on how to teach laboratory science (Brownell and Wade, 1925). Over the following decades, high school science education (including laboratory education) increasingly emphasized practical goals and the benefits of science in everyday life. During World War II, as scientists focused on federally funded
research programs aimed at defense and public health needs, high school science education also emphasized applications of scientific knowledge (Rudolph, 2002).
Changing Goals of Science Education
Following World War II, the flood of “baby boomers” strained the physical and financial resources of public schools. Requests for increased taxes and bond issues led to increasing questions about public schooling. Some academics and policy makers began to criticize the “life adjustment” high school curriculum, which had been designed to meet adolescents’ social, personal, and vocational needs. Instead, they called for a renewed emphasis on the academic disciplines. At the same time, the nation was shaken by the Soviet Union’s explosion of an atomic bomb and the communist takeover of China. By the early 1950s, some federal policy makers began to view a more rigorous, academic high school science curriculum as critical to respond to the Soviet threat.
In 1956, physicist Jerrold Zacharias received a small grant from NSF to establish the Physical Science Study Committee (PSSC) in order to develop a curriculum focusing on physics as a scientific discipline. When the Union of Soviet Socialist Republics launched the space satellite Sputnik the following year, those who had argued that U.S. science education was not rigorous enough appeared vindicated, and a new era of science education began.
Although most historians believe that the overriding goal of the post-Sputnik science education reforms was to create a new generation of U.S. scientists and engineers capable of defending the nation from the Soviet Union, the actual goals were more complex and varied (Rudolph, 2002). Clearly, Congress, the president, and NSF were focused on the goal of preparing more scientists and engineers, as reflected in NSF director Alan Waterman’s 1957 statement (National Science Foundation, 1957, pp. xv-xvi):
Our schools and colleges are badly in need of modern science laboratories and laboratory, demonstration, and research equipment. Most important of all, we need more trained scientists and engineers in many special fields, and especially very many more competent, fully trained teachers of science, notably in our secondary schools. Undoubtedly, by a determined campaign, we can accomplish these ends in our traditional way, but how soon? The process is usually a lengthy one, and there is no time to be lost. Therefore, the pressing question is how quickly can our people act to accomplish these things?
The scientists, however, had another agenda. Over the course of World War II, their research had become increasingly dependent on federal fund-
ing and influenced by federal needs. In physics, for example, federally funded efforts to develop nuclear weapons led research to focus increasingly at the atomic level. In order to maintain public funding while reducing unwanted public pressure on research directions, the scientists sought to use curriculum redesign as a way to build the public’s faith in the expertise of professional scientists (Rudolph, 2002). They wanted to emphasize the humanistic aspects of science, portraying science as an essential element in a broad liberal education. Some scientists sought to reach not only the select group who might become future scientists but also a slightly larger group of elite, mostly white male students who would be future leaders in government and business. They hoped to help these students appreciate the empirical grounding of scientific knowledge and to value and appreciate the role of science in society (Rudolph, 2002).
Changing Views of the Nature of Science
While this shift in the goals of science education was taking place, historians and philosophers were proposing new views of science. In 1958, British chemist Michael Polanyi questioned the ideal of scientific detachment and objectivity, arguing that scientific discovery relies on the personal participation and the creative, original thoughts of scientists (Polanyi, 1958). In the United States, geneticist and science educator Joseph Schwab suggested that scientific methods were specific to each discipline and that all scientific “inquiry” (his term for scientific research) was guided by the current theories and concepts within the discipline (Schwab, 1964). Publication of The Structure of Scientific Revolutions (Kuhn, 1962) a few years later fueled the debate about whether science was truly rational, and whether theory or observation was more important to the scientific enterprise. Over time, this debate subsided, as historians and philosophers of science came to focus on the process of scientific discovery. Increasingly, they recognized that this process involves deductive reasoning (developing inferences from known scientific principles and theories) as well as inductive reasoning (proceeding from particular observations to reach more general theories or conclusions).
Development of New Science Curricula
Although these changing views of the nature of science later led to changes in science education, they had little influence in the immediate aftermath of Sputnik. With NSF support, scientists led a flurry of curriculum development over the next three decades (Matthews, 1994). In addition to the physics text developed by the PSSC, the Biological Sciences Curriculum Study (BSCS) created biology curricula, the Chemical Education Materials group created chemistry materials, and groups of physicists created Intro-
ductory Physical Science and Project Physics. By 1975, NSF supported 28 science curriculum reform projects.
By 1977 over 60 percent of school districts had adopted at least one of the new curricula (Rudolph, 2002). The PSSC program employed high school teachers to train their peers in how to use the curriculum, reaching over half of all high school physics teachers by the late 1960s. However, due to implementation problems that we discuss further below, most schools soon shifted to other texts, and the federal goal of attracting a larger proportion of students to undergraduate science was not achieved (Linn, 1997).
Dissemination of the NSF-funded curriculum development efforts was limited by several weaknesses. Some curriculum developers tried to “teacher proof” their curricula, providing detailed texts, teacher guides, and filmstrips designed to ensure that students faithfully carried out the experiments as intended (Matthews, 1994). Physics teacher and curriculum developer Arnold Arons attributed the limited implementation of most of the NSF-funded curricula to lack of logistical support for science teachers and inadequate teacher training, since “curricular materials, however skilful and imaginative, cannot ‘teach themselves’” (Arons, 1983, p. 117). Case studies showed that schools were slow to change in response to the new curricula and highlighted the central role of the teacher in carrying them out (Stake and Easley, 1978). In his analysis of Project Physics, Welch concluded that the new curriculum accounted for only 5 percent of the variance in student achievement, while other factors, such as teacher effectiveness, student ability, and time on task, played a larger role (Welch, 1979).
Despite their limited diffusion, the new curricula pioneered important new approaches to science education, including elevating the role of laboratory activities in order to help students understand the nature of modern scientific research (Rudolph, 2002). For example, in the PSSC curriculum, Massachusetts Institute of Technology physicist Jerrold Zacharias coordinated laboratory activities with the textbook in order to deepen students’ understanding of the links between theory and experiments. As part of that curriculum, students experimented with a ripple tank, generating wave patterns in water in order to gain understanding of wave models of light. A new definition of the scientific laboratory informed these efforts. The PSSC text explained that a “laboratory” was a way of thinking about scientific investigations—an intellectual process rather than a building with specialized equipment (Rudolph, 2002, p. 131).
The new approach to using laboratory experiences was also apparent in the Science Curriculum Improvement Study. The study group drew on the developmental psychology of Jean Piaget to integrate laboratory experiences with other forms of instruction in a “learning cycle” (Atkin and Karplus, 1962). The learning cycle included (1) exploration of a concept, often through a laboratory experiment; (2) conceptual invention, in which the student or
TABLE 1-1 New Approaches Included in Post-Sputnik Science Curricula
teacher (or both) derived the concept from the experimental data, usually during a classroom discussion; and (3) concept application in which the student applied the concept (Karplus and Their, 1967). Evaluations of the instructional materials, which were targeted to elementary school students, revealed that they were more successful than traditional forms of science instruction at enhancing students’ understanding of science concepts, their understanding of the processes of science, and their positive attitudes toward science (Abraham, 1998). Subsequently, the learning cycle approach was applied to development of science curricula for high school and undergraduate students. Research into these more recent curricula confirms that “merely providing students with hands-on laboratory experiences is not by itself enough” (Abraham, 1998, p. 520) to motivate and help them understand science concepts and the nature of science.
In sum, the new approach of integrating laboratory experiences represented a marked change from earlier science education. In contrast to earlier curricula, which included laboratory experiences as secondary applications of concepts previously addressed by the teacher, the new curricula integrated laboratory activities into class routines in order to emphasize the nature and processes of science (Shymansky, Kyle, and Alport, 1983; see Table 1-1 ). Large meta-analyses of evaluations of the post-Sputnik curricula (Shymansky et al., 1983; Shymansky, Hedges, and Woodworth, 1990) found they were more effective than the traditional curriculum in boosting students’ science achievement and interest in science. As we discuss in Chapter 3 , current designs of science curricula that integrate laboratory experiences
into ongoing classroom instruction have proven effective in enhancing students’ science achievement and interest in science.
Discovery Learning and Inquiry
One offshoot of the curriculum development efforts in the 1960s and 1970s was the development of an approach to science learning termed “discovery learning.” In 1959, Harvard cognitive psychologist Jerome Bruner began to develop his ideas about discovery learning as director of an NRC committee convened to evaluate the new NSF-funded curricula. In a book drawing in part on that experience, Bruner suggested that young students are active problem solvers, ready and motivated to learn science by their natural interest in the material world (Bruner, 1960). He argued that children should not be taught isolated science facts, but rather should be helped to discover the structures, or underlying concepts and theories, of science. Bruner’s emphasis on helping students to understand the theoretical structures of the scientific disciplines became confounded with the idea of engaging students with the physical structures of natural phenomena in the laboratory (Matthews, 1994). Developers of NSF-funded curricula embraced this interpretation of Bruner’s ideas, as it leant support to their emphasis on laboratory activities.
On the basis of his observation that scientific knowledge was changing rapidly through large-scale research and development during this postwar period, Joseph Schwab advocated the closely related idea of an “inquiry approach” to science education (Rudolph, 2003). In a seminal article, Schwab argued against teaching science facts, which he termed a “rhetoric of conclusions” (Schwab, 1962, p. 25). Instead, he proposed that teachers engage students with materials that would motivate them to learn about natural phenomena through inquiry while also learning about some of the strengths and weaknesses of the processes of scientific inquiry. He developed a framework to describe the inquiry approach in a biology laboratory. At the highest level of inquiry, the student simply confronts the “raw phenomenon” (Schwab, 1962, p. 55) with no guidance. At the other end of the spectrum, biology students would experience low levels of inquiry, or none at all, if the laboratory manual provides the problem to be investigated, the methods to address the problem, and the solutions. When Herron applied Schwab’s framework to analyze the laboratory manuals included in the PSSC and the BSCS curricula, he found that most of the manuals provided extensive guidance to students and thus did not follow the inquiry approach (Herron, 1971).
The NRC defines inquiry somewhat differently in the National Science Education Standards . Rather than using “inquiry” as an indicator of the amount of guidance provided to students, the NRC described inquiry as
encompassing both “the diverse ways in which scientists study the natural world” (National Research Council, 1996, p. 23) and also students’ activities that support the learning of science concepts and the processes of science. In the NRC definition, student inquiry may include reading about known scientific theories and ideas, posing questions, planning investigations, making observations, using tools to gather and analyze data, proposing explanations, reviewing known theories and concepts in light of empirical data, and communicating the results. The Standards caution that emphasizing inquiry does not mean relying on a single approach to science teaching, suggesting that teachers use a variety of strategies, including reading, laboratory activities, and other approaches to help students learn science (National Research Council, 1996).
Diversity in Schools
During the 1950s, as some scientists developed new science curricula for teaching a small group of mostly white male students, other Americans were much more concerned about the weak quality of racially segregated schools for black children. In 1954, the Supreme Court ruled unanimously that the Topeka, Kansas Board of Education was in violation of the U.S. Constitution because it provided black students with “separate but equal” education. Schools in both the North and the South changed dramatically as formerly all-white schools were integrated. Following the example of the civil rights movement, in the 1970s and the 1980s the women’s liberation movement sought improved education and employment opportunities for girls and women, including opportunities in science. In response, some educators began to seek ways to improve science education for all students, regardless of their race or gender.
1975 to Present
By 1975, the United States had put a man on the moon, concerns about the “space race” had subsided, and substantial NSF funding for science education reform ended. These changes, together with increased concern for equity in science education, heralded a shift in society’s goals for science education. Science educators became less focused on the goal of disciplinary knowledge for science specialists and began to place greater emphasis on a liberal, humanistic view of science education.
Many of the tensions evident in the first 100 years of U.S. high school laboratories have continued over the past 30 years. Scientists, educators, and policy makers continue to disagree about the nature of science, the goals of science education, and the role of the curriculum and the teacher in student
learning. Within this larger dialogue, debate about the value of laboratory activities continues.
Changing Goals for Science Education
National reports issued during the 1980s and 1990s illustrate new views of the nature of science and increased emphasis on liberal goals for science education. In Science for All Americans , the AAAS advocated the achievement of scientific literacy by all U.S. high school students, in order to increase their awareness and understanding of science and the natural world and to develop their ability to think scientifically (American Association for the Advancement of Science, 1989). This seminal report described science as tentative (striving toward objectivity within the constraints of human fallibility) and as a social enterprise, while also discussing the durability of scientific theories, the importance of logical reasoning, and the lack of a single scientific method. In the ongoing debate about the coverage of science content, the AAAS took the position that “curricula must be changed to reduce the sheer amount of material covered” (American Association for the Advancement of Science, 1989, p. 5). Four years later, the AAAS published Benchmarks for Science Literacy , which identified expected competencies at each school grade level in each of the earlier report’s 10 areas of scientific literacy (American Association for the Advancement of Science, 1993).
The NRC’s National Science Education Standards (National Research Council, 1996) built on the AAAS reports, opening with the statement: “This nation has established as a goal that all students should achieve scientific literacy” (p. ix). The NRC proposed national science standards for high school students designed to help all students develop (1) abilities necessary to do scientific inquiry and (2) understandings about scientific inquiry (National Research Council, 1996, p. 173).
In the standards, the NRC suggested a new approach to laboratories that went beyond simply engaging students in experiments. The NRC explicitly recognized that laboratory investigations should be learning experiences, stating that high school students must “actively participate in scientific investigations, and … use the cognitive and manipulative skills associated with the formulation of scientific explanations” (National Research Council, 1996, p. 173).
According to the standards, regardless of the scientific investigation performed, students must use evidence, apply logic, and construct an argument for their proposed explanations. These standards emphasize the importance of creating scientific arguments and explanations for observations made in the laboratory.
While most educators, scientists, and policy makers now agree that scientific literacy for all students is the primary goal of high school science
education, the secondary goals of preparing the future scientific and technical workforce and including science as an essential part of a broad liberal education remain important. In 2004, the NSF National Science Board released a report describing a “troubling decline” in the number of U.S. citizens training to become scientists and engineers at a time when many current scientists and engineers are soon to retire. NSF called for improvements in science education to reverse these trends, which “threaten the economic welfare and security of our country” (National Science Foundation, 2004, p. 1). Another recent study found that secure, well-paying jobs that do not require postsecondary education nonetheless require abilities that may be developed in science laboratories. These include the ability to use inductive and deductive reasoning to arrive at valid conclusions; distinguish among facts and opinions; identify false premises in an argument; and use mathematics to solve problems (Achieve, 2004).
Achieving the goal of scientific literacy for all students, as well as motivating some students to study further in science, may require diverse approaches for the increasingly diverse body of science students, as we discuss in Chapter 2 .
Changing Role of Teachers and Curriculum
Over the past 20 years, science educators have increasingly recognized the complementary roles of curriculum and teachers in helping students learn science. Both evaluations of NSF-funded curricula from the 1960s and more recent research on science learning have highlighted the important role of the teacher in helping students learn through laboratory activities. Cognitive psychologists and science educators have found that the teacher’s expectations, interventions, and actions can help students develop understanding of scientific concepts and ideas (Driver, 1995; Penner, Lehrer, and Schauble, 1998; Roth and Roychoudhury, 1993). In response to this growing awareness, some school districts and institutions of higher education have made efforts to improve laboratory education for current teachers as well as to improve the undergraduate education of future teachers (National Research Council, 2001).
In the early 1980s, NSF began again to fund the development of laboratory-centered high school science curricula. Today, several publishers offer comprehensive packages developed with NSF support, including textbooks, teacher guides, and laboratory materials (and, in some cases, videos and web sites). In 2001, one earth science curriculum, five physical science curricula, five life science curricula, and six integrated science curricula were available for sale, while several others in various science disciplines were still under development (Biological Sciences Curriculum Study, 2001). In contrast to the curriculum development approach of the 1960s, teachers have played an important role in developing and field-testing these newer
curricula and in designing the teacher professional development courses that accompany most of them. However, as in the 1960s and 1970s, only a few of these NSF-funded curricula have been widely adopted. Private publishers have also developed a multitude of new textbooks, laboratory manuals, and laboratory equipment kits in response to the national education standards and the growing national concern about scientific literacy. Nevertheless, most schools today use science curricula that have not been developed, field-tested, or refined on the basis of specific education research (see Chapter 2 ).
CURRENT DEBATES
Clearly, the United States needs high school graduates with scientific literacy—both to meet the economy’s need for skilled workers and future scientists and to develop the scientific habits of mind that can help citizens in their everyday lives. Science is also important as part of a liberal high school education that conveys an important aspect of modern culture. However, the value of laboratory experiences in meeting these national goals has not been clearly established.
Researchers agree neither on the desired learning outcomes of laboratory experiences nor on whether those outcomes are attained. For example, on the basis of a 1978 review of over 80 studies, Bates concluded that there was no conclusive answer to the question, “What does the laboratory accomplish that could not be accomplished as well by less expensive and less time-consuming alternatives?” (Bates, 1978, p. 75). Some experts have suggested that the only contribution of laboratories lies in helping students develop skills in manipulating equipment and acquiring a feel for phenomena but that laboratories cannot help students understand science concepts (Woolnough, 1983; Klopfer, 1990). Others, however, argue that laboratory experiences have the potential to help students understand complex science concepts, but the potential has not been realized (Tobin, 1990; Gunstone and Champagne, 1990).
These debates in the research are reflected in practice. On one hand, most states and school districts continue to invest in laboratory facilities and equipment, many undergraduate institutions require completion of laboratory courses to qualify for admission, and some states require completion of science laboratory courses as a condition of high school graduation. On the other hand, in early 2004, the California Department of Education considered draft criteria for the evaluation of science instructional materials that reflected skepticism about the value of laboratory experiences or other hands-on learning activities. The proposed criteria would have required materials to demonstrate that the state science standards could be comprehensively covered with hands-on activities composing no more than 20 to 25 percent
of instructional time (Linn, 2004). However, in response to opposition, the criteria were changed to require that the instructional materials would comprehensively cover the California science standards with “hands-on activities composing at least 20 to 25 percent of the science instructional program” (California Department of Education, 2004, p. 4, italics added).
The growing variety in laboratory experiences—which may be designed to achieve a variety of different learning outcomes—poses a challenge to resolving these debates. In a recent review of the literature, Hofstein and Lunetta (2004, p. 46) call attention to this variety:
The assumption that laboratory experiences help students understand materials, phenomena, concepts, models and relationships, almost independent of the nature of the laboratory experience, continues to be widespread in spite of sparse data from carefully designed and conducted studies.
As a first step toward understanding the nature of the laboratory experience, the committee developed a definition and a typology of high school science laboratory experiences.
DEFINITION OF LABORATORY EXPERIENCES
Rapid developments in science, technology, and cognitive research have made the traditional definition of science laboratories—as rooms in which students use special equipment to carry out well-defined procedures—obsolete. The committee gathered information on a wide variety of approaches to laboratory education, arriving at the term “laboratory experiences” to describe teaching and learning that may take place in a laboratory room or in other settings:
Laboratory experiences provide opportunities for students to interact directly with the material world (or with data drawn from the material world), using the tools, data collection techniques, models, and theories of science.
This definition includes the following student activities:
Physical manipulation of the real-world substances or systems under investigation. This may include such activities as chemistry experiments, plant or animal dissections in biology, and investigation of rocks or minerals for identification in earth science.
Interaction with simulations. Physical models have been used throughout the history of science teaching (Lunetta, 1998). Today, students can work
with computerized models, or simulations, representing aspects of natural phenomena that cannot be observed directly, because they are very large, very small, very slow, very fast, or very complex. Using simulations, students may model the interaction of molecules in chemistry or manipulate models of cells, animal or plant systems, wave motion, weather patterns, or geological formations.
Interaction with data drawn from the real world. Students may interact with real-world data that are obtained and represented in a variety of forms. For example, they may study photographs to examine characteristics of the moon or other heavenly bodies or analyze emission and absorption spectra in the light from stars. Data may be incorporated in films, DVDs, computer programs, or other formats.
Access to large databases. In many fields of science, researchers have arranged for empirical data to be normalized and aggregated—for example, genome databases, astronomy image collections, databases of climatic events over long time periods, biological field observations. With the help of the Internet, some students sitting in science class can now access these authentic and timely scientific data. Students can manipulate and analyze these data drawn from the real world in new forms of laboratory experiences (Bell, 2005).
Remote access to scientific instruments and observations. A few classrooms around the nation experience laboratory activities enabled by Internet links to remote instruments. Some students and teachers study insects by accessing and controlling an environmental scanning electron microscope (Thakkar et al., 2000), while others control automated telescopes (Gould, 2004).
Although we include all of these types of direct and indirect interaction with the material world in this definition, it does not include student manipulation or analysis of data created by a teacher to replace or substitute for direct interaction with the material world. For example, if a physics teacher presented students with a constructed data set on the weight and required pulling force for boxes pulled across desks with different surfaces, asking the students to analyze these data, the students’ problem-solving activity would not constitute a laboratory experience according to the committee’s definition.
Previous Definitions of Laboratories
In developing its definition, the committee reviewed previous definitions of student laboratories. Hegarty-Hazel (1990, p. 4) defined laboratory work as:
a form of practical work taking place in a purposely assigned environment where students engage in planned learning experiences … [and] interact
with materials to observe and understand phenomena (Some forms of practical work such as field trips are thus excluded).
Lunetta defined laboratories as “experiences in school settings in which students interact with materials to observe and understand the natural world” (Lunetta, 1998, p. 249). However, these definitions include only students’ direct interactions with natural phenomena, whereas we include both such direct interactions and also student interactions with data drawn from the material world. In addition, these earlier definitions confine laboratory experiences to schools or other “purposely assigned environments,” but our definition encompasses student observation and manipulation of natural phenomena in a variety of settings, including science museums and science centers, school gardens, local streams, or nearby geological formations. The committee’s definition also includes students who work as interns in research laboratories, after school or during the summer months. All of these experiences, as well as those that take place in traditional school science laboratories, are included in our definition of laboratory experiences.
Variety in Laboratory Experiences
Both the preceding review of the history of laboratories and the committee’s review of the evidence of student learning in laboratories reveal the limitations of engaging students in replicating the work of scientists. It has become increasingly clear that it is not realistic to expect students to arrive at accepted scientific concepts and ideas by simply experiencing some aspects of scientific research (Millar, 2004). While recognizing these limitations, the committee thinks that laboratory experiences should at least partially reflect the range of activities involved in real scientific research. Providing students with opportunities to participate in a range of scientific activities represents a step toward achieving the learning goals of laboratories identified in Chapter 3 . 1
Historians and philosophers of science now recognize that the well-ordered scientific method taught in many high school classes does not exist. Scientists’ empirical research in the laboratory or the field is one part of a larger process that may include reading and attending conferences to stay abreast of current developments in the discipline and to present work in progress. As Schwab recognized (1964), the “structure” of current theories and concepts in a discipline acts as a guide to further empirical research. The work of scientists may include formulating research questions, generat-
ing alternative hypotheses, designing and conducting investigations, and building and revising models to explain the results of their investigations. The process of evaluating and revising models may generate new questions and new investigations (see Table 1-2 ). Recent studies of science indicate that scientists’ interactions with their peers, particularly their response to questions from other scientists, as well as their use of analogies in formulating hypotheses and solving problems, and their responses to unexplained results, all influence their success in making discoveries (Dunbar, 2000). Some scientists concentrate their efforts on developing theory, reading, or conducting thought experiments, while others specialize in direct interactions with the material world (Bell, 2005).
Student laboratory experiences that reflect these aspects of the work of scientists would include learning about the most current concepts and theories through reading, lectures, or discussions; formulating questions; designing and carrying out investigations; creating and revising explanatory models; and presenting their evolving ideas and scientific arguments to others for discussion and evaluation (see Table 1-3 ).
Currently, however, most high schools provide a narrow range of laboratory activities, engaging students primarily in using tools to make observations and gather data, often in order to verify established scientific knowledge. Students rarely have opportunities to formulate research questions or to build and revise explanatory models (see Chapter 4 ).
ORGANIZATION OF THE REPORT
The ability of high school science laboratories to help improve all citizens’ understanding and appreciation of science and prepare the next generation of scientists and engineers is affected by the context in which laboratory experiences take place. Laboratory experiences do not take place in isolation, but are part of the larger fabric of students’ experiences during their high school years. Following this introduction, Chapter 2 describes recent trends in U.S. science education and policies influencing science education, including laboratory experiences. In Chapter 3 we turn to a review of available evidence on student learning in laboratories and identify principles for design of effective laboratory learning environments. Chapter 4 describes current laboratory experiences in U.S. high schools, and Chapter 5 discusses teacher and school readiness for laboratory experiences. In Chapter 6 , we describe the current state of laboratory facilities, equipment, and safety. Finally, in Chapter 7 , we present our conclusions and an agenda designed to help laboratory experiences fulfill their potential role in the high school science curriculum.
TABLE 1-2 A Typology of Scientists’ Activities
TABLE 1-3 A Typology of School Laboratory Experiences
Since the late 19th century, high school students in the United States have carried out laboratory investigations as part of their science classes. Since that time, changes in science, education, and American society have influenced the role of laboratory experiences in the high school science curriculum. At the turn of the 20th century, high school science laboratory experiences were designed primarily to prepare a select group of young people for further scientific study at research universities. During the period between World War I and World War II, many high schools emphasized the more practical aspects of science, engaging students in laboratory projects related to daily life. In the 1950s and 1960s, science curricula were redesigned to integrate laboratory experiences into classroom instruction, with the goal of increasing public appreciation of science.
Policy makers, scientists, and educators agree that high school graduates today, more than ever, need a basic understanding of science and technology to function effectively in an increasingly complex, technological society. They seek to help students understand the nature of science and to develop both the inductive and deductive reasoning skills that scientists apply in their work. However, researchers and educators do not agree on how to define high school science laboratories or on their purposes, hampering the accumulation of evidence that might guide improvements in laboratory education. Gaps in the research and in capturing the knowledge of expert science teachers make it difficult to reach precise conclusions on the best approaches to laboratory teaching and learning.
In order to provide a focus for the study, the committee defines laboratory experiences as follows: laboratory experiences provide opportunities for students to interact directly with the material world (or with data drawn from the material world), using the tools, data collection techniques, models, and theories of science. This definition includes a variety of types of laboratory experiences, reflecting the range of activities that scientists engage in. The following chapters discuss the educational context; laboratory experiences and student learning; current laboratory experiences, teacher and school readiness, facilities, equipment, and safety; and laboratory experiences for the 21st century.
Abraham, M.R. (1998). The learning cycle approach as a strategy for instruction in science. In B.J. Fraser and K.G. Tobin (Eds.), International handbook of science education . London, England: Kluwer Academic.
Achieve. (2004). Ready or not: Creating a high school diploma that counts . (The American Diploma Project.) Washington, DC: Author.
American Association for the Advancement of Science. (1989). Science for all Americans . Washington, DC: Author.
American Association for the Advancement of Science. (1993). Benchmarks for science literacy . Washington, DC: Author.
Arons, A. (1983). Achieving wider scientific literacy. Daedalus , 112 (2), 91-122.
Atkin, J.M., and Karplus, R. (1962). Discovery or invention? The Science Teacher , 29 , 45-51.
Bates, G.R. (1978). The role of the laboratory in science teaching. School Science and Mathematics , 83 , 165-169.
Bell, P. (2005). The school science laboratory: Considerations of learning, technology , and scientific practice . Paper prepared for the Committee on High School Science Laboratories: Role and Vision. Available at: http://www7.nationalacademies.org/bose/July_12-13_2004_High_School_Labs_Meeting_Agenda.html .
Biological Sciences Curriculum Study. (2001). Profiles in science: A guide to NSF - funded high school instructional materials . Colorado Springs, CO: Author. Available at: http://www.bscs.org/page.asp?id=Professional_Development|Resources|Profiles_In_Science [accessed Sept. 2004].
Brownell, H., and Wade, F.B. (1925). The teaching of science and the science teacher . New York: Century Company.
Bruner, J.S. (1960). The process of education . New York: Vintage.
Bump, H.C. (1895). Laboratory teaching of large classes—Zoology. [Letter to the editor]. Science, New Series , 1 (10), 260.
California Department of Education. (2004). Criteria for evaluating instructional materials in science, kindergarten through grade eight . Available at: http://www.cde.ca.gov/ci/sc/cf/documents/scicriteria04.pdf [accessed Nov. 2004].
Champagne, A., and Shiland, T. (2004). Large-scale assessment and the high school science laboratory . Presentation to the Committee on High School Science Laboratories: Role and Vision, June 3-4, National Research Council, Washington, DC. Available at: http://www7.nationalacademies.org/bose/June_3-4_2004_High_School_Labs_Meeting_Agenda.html [accessed Jan. 2004].
Davies, K. (2001). Cracking the genome: Inside the race to unlock human DNA . New York: Free Press.
Dewey, J. (1910a). How we think . Boston, MA: D.C. Heath.
Dewey, J. (1910b). Science as subject-matter and method. Science, New Series , 31 , 122, 125.
Downing, E.R. (1919). The scientific method and the problems of school science. School and Society , 10 , 571.
Driver, R. (1995). Constructivist approaches to science teaching. In L.P. Steffe and J. Gale (Eds.), Constructivism in education (pp. 385-400). Hillsdale, NJ: Lawrence Erlbaum.
Driver, R., Leach, J., Millar, R., and Scott, P. (1996). Young people’s images of science . Buckingham, U.K.: Open University Press.
Dunbar, K. (1993). Concept discovery in a scientific domain. Cognitive Science , 17 , 397-434.
Dunbar, K. (2000). How scientists think in the real world: Implications for science education. Journal of Applied Developmental Psychology 21 (1), 49-58.
Duschl, R. (2004). The HS lab experience: Reconsidering the role of evidence, explanation, and the language of science . Paper prepared for the Committee on High School Science Laboratories: Role and Vision, July 12-13, National Research Council, Washington, DC. Available at: http://www7.nationalacademies.org/bose/July_12-13_2004_High_School_Labs_Meeting_Agenda.html [accessed Nov. 2004].
Gould, R. (2004). About micro observatory . Cambridge, MA: Harvard University. Available at: http://mo-www.harvard.edu/MicroObservatory/ [accessed Sept. 2004].
Gugliotta, G. (2004). Space sugar a clue to life’s origins. The Washington Post , September 27.
Gunstone, R.F., and Champagne, A.B. (1990). Promoting conceptual change in the laboratory. In E. Hegarty-Hazel (Ed.), The student laboratory and the science curriculum (pp. 159-182). London, England: Routledge.
Hall, G.S. (1901). How far is the present high-school and early college training adapted to the nature and needs of adolescents? School Review , 9 , 652.
Harvard University. (1889). Descriptive list of elementary physical experiments intended for use in preparing students for Harvard College . Cambridge, MA: Author.
Hegarty-Hazel, E. (1990). Overview. In E. Hegarty-Hazel (Ed.), The student laboratory and the science curriculum . London, England: Routledge.
Herron, M.D. (1971). The nature of scientific enquiry. School Review, 79, 171-212.
Hilosky, A., Sutman, F., and Schmuckler, J. (1998). Is laboratory-based instruction in beginning college-level chemistry worth the effort and expense? Journal of Chemical Education , 75 (1), 103.
Hofstein, A., and Lunetta, V.N. (2004). The laboratory in science education: Foundations for the twenty-first century. Science Education , 88 , 28-54.
Hollis, J.M., Jewell, P.R., Lovas, F.J., and Remijan, J. (2004, September 20). Green Bank telescope observations of interstellar glycolaldehyde: Low-temperature sugar. Astrophysical Journal , 613 , L45-L48.
Hull, D. (1988). Science as a process . Chicago: University of Chicago Press.
Karplus, R., and Their, H.D. (1967). A new look at elementary school science . Chicago: Rand McNally.
Klopfer, L.E. (1990). Learning scientific enquiry in the student laboratory. In E. Hegarty-Hazel (Ed.), The student laboratory and the science curriculum (pp. 95-118). London, England: Routledge.
Kuhn, T.S. (1962). The structure of scientific revolutions . Chicago: University of Chicago Press.
Linn, M. (2004). High school science laboratories: How can technology contribute? Presentation to the Committee on High School Science Laboratories: Role and Vision. June 3-4, National Research Council, Washington, DC. Available at: http://www7.nationalacademies.org/bose/June_3-4_2004_High_School_Labs_Meeting_Agenda.html [accessed April 2005].
Linn, M.C. (1997). The role of the laboratory in science learning. Elementary School Journal , 97 (4), 401-417.
Longino, H. (1990). Science as social knowledge . Princeton, NJ: Princeton University Press.
Longino, H. (1994). The fate of knowledge in social theories of science. In F.F. Schmitt (Ed.), Socializing epistemology: The social dimensions of knowledge (pp. 135-158). Lanham, MD: Rowman and Littlefield.
Lunetta, V. (1998). The school science laboratory: Historical perspectives and contexts for contemporary teaching . In B.J. Fraser and K.G. Tobin (Eds.), International handbook of science education (pp. 249-262). London, England: Kluwer Academic.
Matthews, M.R. (1994). Science teaching: The role of history and philosophy of science . New York: Routledge.
Merton, R.K., and Barber, E. (2004). The travels and adventures of serendipity . Princeton, NJ: Princeton University Press.
Mill, J.S. (1963). System of logic, ratiocinative and inductive. In J.M. Robson (Ed.), Collected works of John Stuart Mill . Toronto: University of Toronto Press (Original work published 1843).
Millar, R. (2004). The role of practical work in the teaching and learning of science . Paper prepared for the Committee on High School Science Laboratories: Role and Vision, June 3-4, National Research Council, Washington, DC. Available at: http://www7.nationalacademies.org/bose/June3-4_2004_High_School_Labs_Meeting_Agenda.html [accessed April 2005].
National Education Association. (1894). Report of the Committee of Ten on secondary school studies . New York: American Book Company.
National Research Council. (1996). National science education standards . National Committee on Science Education Standards and Assessment, Center for Science, Mathematics, and Engineering Education. Washington, DC: National Academy Press.
National Research Council. (2001). Educating teachers of science, mathematics, and technology: New practices for the new millennium . Committee on Science and Mathematics Teacher Preparation, Center for Education. Washington, DC: National Academy Press.
National Science Foundation. (1957). Annual report . Available at: http://www.nsf.gov/pubs/1957/annualreports/start.htm [accessed Nov. 2004].
National Science Foundation. (2004). An emerging and critical problem of the science and engineering workforce . Washington, DC: Author. Available at: http://www.nsf.gov/sbe/srs/nsb0407/start.htm [accessed Sept. 2003].
Penner, D.E., Lehrer, R., and Schauble, L. (1998). From physical models to biomechanics: A design based modeling approach. Journal of the Learning Sciences , 7 , 429-449.
Polanyi, M. (1958). Personal knowledge: Towards a post-critical philosophy . London, England: Routledge.
Roth, W.M., and Roychoudhury, A. (1993). The development of science process skills in authentic contexts. Journal of Research in Science Teaching , 30 , 127-152.
Rudolph, J.L. (2002). Scientists in the classroom: The cold war reconstruction of American science education . New York: Palgrave.
Rudolph, J.L. (2003). Portraying epistemology: School science in historical context. Science Education , 87 , 64-79.
Rudolph, J.L. (2005). Epistemology for the masses: The origins of the “scientific method” in American schools. History of Education Quarterly , 45(2), 341-376.
Schwab, J.J. (1962). The teaching of science as enquiry. In P.F. Brandwein (Ed.), The teaching of science (pp. 1-103). Cambridge, MA: Harvard University Press.
Schwab, J.J. (1964). The structure of the natural sciences. In G.W. Ford and L. Pugno (Eds.), The structure of knowledge and the curriculum (pp. 31-49). Chicago: Rand McNally.
Shymansky, J.A., Hedges, L.B., and Woodworth, G. (1990). A re-assessment of the effects of inquiry-based science curriculum of the sixties on student achievement. Journal of Research in Science Teaching , 20 , 387-404.
Shymansky, J.A., Kyle, W.C., and Alport, J.M. (1983). The effects of new science curricula on student performance. Journal of Research in Science Teaching , 20 , 387-404.
Stake, R.E., and Easley, J.A. (1978). Case studies in science education . Center for Instructional Research and Curriculum Evaluation and Committee on Culture and Cognition. Urbana: University of Illinois at Urbana-Champagne.
Thakkar, U., Carragher, B., Carroll, L., Conway, C., Grosser, B., Kisseberth, N., Potter, C.S., Robinson, S., Sinn-Hanlon, J., Stone, D., and Weber, D. (2000). Formative evaluation of Bugscope: A sustainable world wide laboratory for K-12 . Paper prepared for the annual meeting of the American Educational Research Association, Special Interest Group on Advanced Technologies for Learning, April 24-28, New Orleans, LA. Available at: http://bugscope.beckman.uiuc.edu/publications/index.htm#papers [accessed May 2005].
Tobin, K. 1990. Research on science laboratory activities: In pursuit of better questions and answers to improve learning. School Science and Mathematics , 90 (5), 403-418.
Welch, W.W. (1979). Twenty years of science education development: A look back. Review of Research in Education , 7 , 282-306.
Whewell, W. (1840). Philosophy of the inductive sciences, founded upon their history . London, England: John W. Parker.
Whewell, W. (1858). The history of the inductive sciences, from the earliest to the present time (3rd edition). New York: Appleton.
White, B.Y., and Frederiksen, J.R. (1998). Inquiry, modeling, and metacognition: Making science accessible to all students. Cognition and Instruction , 16 (1), 3-118.
Whitman, F.P. (1898). The beginnings of laboratory teaching in America. Science , New Series , 8 (190), 201-206.
Woodhull, J.F. (1909). How the public will solve the problems of science teaching. School Science and Mathematics , 9 , 276.
Woolnough, B.E. (1983). Exercises, investigations and experiences. Physics Education , 18 , 60-63.
Laboratory experiences as a part of most U.S. high school science curricula have been taken for granted for decades, but they have rarely been carefully examined. What do they contribute to science learning? What can they contribute to science learning? What is the current status of labs in our nation�s high schools as a context for learning science? This book looks at a range of questions about how laboratory experiences fit into U.S. high schools:
- What is effective laboratory teaching?
- What does research tell us about learning in high school science labs?
- How should student learning in laboratory experiences be assessed?
- Do all student have access to laboratory experiences?
- What changes need to be made to improve laboratory experiences for high school students?
- How can school organization contribute to effective laboratory teaching?
With increased attention to the U.S. education system and student outcomes, no part of the high school curriculum should escape scrutiny. This timely book investigates factors that influence a high school laboratory experience, looking closely at what currently takes place and what the goals of those experiences are and should be. Science educators, school administrators, policy makers, and parents will all benefit from a better understanding of the need for laboratory experiences to be an integral part of the science curriculum—and how that can be accomplished.
Welcome to OpenBook!
You're looking at OpenBook, NAP.edu's online reading room since 1999. Based on feedback from you, our users, we've made some improvements that make it easier than ever to read thousands of publications on our website.
Do you want to take a quick tour of the OpenBook's features?
Show this book's table of contents , where you can jump to any chapter by name.
...or use these buttons to go back to the previous chapter or skip to the next one.
Jump up to the previous page or down to the next one. Also, you can type in a page number and press Enter to go directly to that page in the book.
Switch between the Original Pages , where you can read the report as it appeared in print, and Text Pages for the web version, where you can highlight and search the text.
To search the entire text of this book, type in your search term here and press Enter .
Share a link to this book page on your preferred social network or via email.
View our suggested citation for this chapter.
Ready to take your reading offline? Click here to buy this book in print or download it as a free PDF, if available.
Get Email Updates
Do you enjoy reading reports from the Academies online for free ? Sign up for email notifications and we'll let you know about new publications in your areas of interest when they're released.
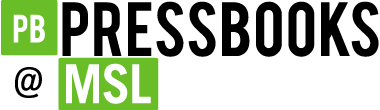
Chapter 3: Literacies across the disciplines
3.3.4 The impact of student attitudes on laboratory reports (research essay)
Sydney Arnold
English 102, November 2020
In a majority of science laboratory classes students will be required to write a lab report. I have taken four chemistry labs and three biology labs in college and I was required to write lab reports every week for these labs. At first, I found writing these reports to be a struggle as I have never written one before college. Many other students I know struggled in the beginning and some still do. Over time I found it much easier to write lab reports. It is a necessary skill that is learned over time and with practice, but many students struggle with it.
Generally, a lab report is written in a format that is similar to a science journal article. The lab report is split into different sections which includes the title, introduction, materials and methods, results, discussion, and conclusion. The title emphasizes the focus of the experiment. From my experiences the title has the same name as the lab that was done. The introduction presents the general objectives of the experiment and provides important background information about the specific science topic the experiment is on. The materials and methods section provides what materials were used in the experiment and the steps that were taken to complete the experiment. The results section summarizes the data that was collected using graphs, charts, math, or descriptions of the physical/chemical changes that occurred. The discussion section discusses the results that were found and compares them to what the true results should be. This section also explains what could have went wrong in the experiment that made the results not perfect and what could be done next time to improve results. Lastly, the conclusion summarizes the main objectives of the experiment, important results, and what could be done to improve results in the future.
When people think of doing a science laboratory class, they generally do not think about the writing that is involved in them. It is believed that learning to write a lab report is one of the most important things in a science laboratory class. The main reasons learning to write lab report is important is because it helps students understand the concepts that were done in the experiment and it prepares them for future careers that will require them to write scientific journals. Literacy is especially important when it comes to a lab report. The writer needs to be able to effectively write about the specific topic, their research findings, and the conclusions drawn from the experiment. To do this, the writer needs to understand what they are writing about and be able to write for an audience specifically in the science field. It is important to be able to do this because scientific journals are the primary means of communicating important results to other scientists.
A large percentage of students have negative attitudes towards writing lab reports. The article “How Attitudes Affect Grades” states “An attitude is some state of mind about an object, fact, or situation.”. Lab reports cause many students to have stress, anxiety, and general negative attitudes which is shown to lead to lower grades. I have personally heard more students say they hate writing lab reports than students saying they like them or do not mind them. I have also heard students say they think lab reports are a waste of time. My own attitudes about writing lab reports were negative at first because I did not understand how to write one and they were intimidating. This led me to procrastinate when it came to writing lab reports and it caused me to have stress which just made me not want to do it even more. This made me rush my lab reports and not do my best work which means I did not get the best grade I could have gotten. Negative attitudes affect what we expect of ourselves which affects our actions. This leads to limited performance, lower motivation, and inhibits learning. It is said that attitude is equally as important as ability for success. The article “Effects of Students Attitude on Their Performance” states “Success is 80% attitude and 20% aptitude.”.
The article “The Relationships between University Students’ Chemistry Laboratory Anxiety, Attitudes, and Self-Efficacy Beliefs” explains a study that was done on negative feelings and how they affected the performance of student in a chemistry laboratory. This article states “Affective dimensions of learning such as anxiety, attitudes, and self-efficacy are perceived as important predictors of student performance in laboratory situations.” (p1-2). Chemistry laboratory anxiety is caused by many things which include previous bad experiences in science classes, exposure to anxious science teachers who taught in elementary and secondary schools, lack of role models, gender and racial stereotyping, and the stereotyping of scientists in the media. This study found that “attitude can account for nearly 30% of the variance in achievement.” (p.3) and found students with negative attitudes obtained lower examination marks. Self-efficacy is a person’s beliefs about their ability to successfully perform a given task. This determines if a person will do the given task, the amount of effort towards the task, and how much persistence they have when faced with obstacles. Students with high self-efficacy choose more challenging tasks, use more effort, and do not easily give up. Students with low self-efficacy avoid challenging takes, use minimal effort, and have a higher chance of giving up. Many of my articles have stated that there needs to be change on how writing lab reports are taught so students have better attitudes toward them and receive better grades.
There have been multiple studies done on different approaches to writing a laboratory report to increase positive attitudes in students and increase their grades. I will be going over six different studies that focused on attitudes and grades of college students. The first study is “Developing Technical Writing Skills in the Physical Chemistry Laboratory: A Progressive Approach Employing Peer Review” by Derek Gragson and John Hagen. The authors developed an approach that uses peer review and revision components for the lab reports. The three principles the authors believe are essential to improving technical writing skill include: “less is sometimes more, initial guidance on writing and expectations that is gradually reduced leads to autonomy, and experience with the review and revision processes is essential to developing writing skills.” (62). Students were given a Calibrated Peer Review (CPR) tool and Integrated Writing Guide (IWG) for the class. The IWG allowed students to become critical readers of their own work and helped them become better at peer reviewing (64). The CPR and writing cycle mimic the process of how journal articles are written as a real-world chemist (64). For the first experiment students were required to write and abstract and materials section on their own which was later peer reviewed (62). Then students grouped up and wrote a formal report for the experiment (62). The authors have seen significant improvements in the quality of the lab reports. This believe to be due to the mixture of the IWG, CPR, and writing cycle (65).
The second study is “Creative Report Writing in Undergraduate Organic Chemistry Laboratory Inspires Nonmajors” by Maged Henary, Eric Owens, and Joseph Tawney. The authors believe that a more creative approach to writing a lab report is beneficial for new organic chemistry students as it promotes appreciation and understanding of presented material (90). This method “encourages students to be creative and serves as a gentle introduction into writing laboratory reports and helps alleviate the start to the unfamiliar task of scientific writing.” (91). For this approach students are still required to correctly annotate and describe their compounds, but they can do so by telling a story rather than doing so in a traditional way. For example, a student could write a story about living in a fairytale land but the story must still contain the concepts learned from the experiment and the compounds from the experiment. It has been shown students using this approach demonstrated a higher degree of understanding and makes them excited for the course (92). The students were given a survey in which they had to answer questions on a scale of 1-5 on whether they disagreed or agreed with a statement (92). This survey showed that students excitement and understanding of the material increased, they came out of the class more prepared and knowledgeable, and half the students even said they were more likely to consider a career in chemistry (92).
The third study is “Writing Activities Embedded in Bioscience Laboratory Courses to Change Students’ Attitudes and Enhance their Scientific Writing” by Susan Lee, Kyra Woods, and Kathryn Tonissen. The authors suggest using an approach that utilizes in-course writing activities (195). The authors suggest that a challenge in teaching a science course is finding ways to effectively engage students with scientific communication and writing (193). A suggested barrier to this is students tend to like doing experiments but do not enjoy writing (193). The writing activities used in the laboratory were made to engage students, make connections to their future careers, promote collaborative learning, and teach them how to provide a basic model for scientific writing (196). Completing each activity allowed the students to construct their laboratory report while performing their experiment (196). The students were also able to get advice from a tutor or course coordinator during this time (196). Each writing assignment came with a checklist that focused on the requirements for a scientific paper and collaborative learning was encouraged by peer review (196). The first activity aimed to make sure students read the laboratory manual, completed background research, and understood the point of the project (197). The second activity focused on how to present figures in the results section of the laboratory report (197). The final activity involved the discussion section of the laboratory report (197). A survey was given to students and the results showed there was a high increase in confidence of students ability to write a scientific report, an increase in confidence for finding journal articles using databases, and students found the activities useful and had positive learning outcomes (197-198).
The fourth study is “Inquiry-Based Writing in the Laboratory Course” by Cary Moskovitz and David Kellogg. The authors believe that inquiry-based writing is beneficial to students. The authors believe that for inquiry-based writing to be successful, there needs to be three modifications to the inquiry lab (919). These modifications include using forms of writing in the laboratory report that are similar to writing used by scientists, writing tasks need to be aligned with what is going on in the laboratory so students have meaningful things to say, and lastly students need to write for a real audience (919). This first step in inquiry-based writing is to assign writing activities that use the form scientists use (919). The second step is aligning student writing with lab activities (920). This step has students present and discuss results (920). The last step is to provide students with a real audience for their work (920). This step shows students the constraints faced by real scientists (920). The authors also suggest that instructors need to shift from graders to scientists, so students are required to make scientific arguments rather than just reproduce scientific arguments (920). This approach has shown that students are more likely to find tasks meaningful and engaging (920).
The fifth study is “Using Journal Articles to Teach Writing Skills for Laboratory Reports in General Chemistry” by Luanne Tilstra. Tilstra uses an approach that teaches students how to properly construct a report and helps them understand the chemical concepts (762). This specific approach is used for students taking their first college chemistry course (762). In the first week of the quarter students are asked to find an article from the Journal of the American Chemical Society and create a properly formatted citation (762). This allows students to practice what is considered a low-level writing skill (762). As the quarter goes on, the writing tasks get harder. For the second report of the quarter, the students begin a higher level of writing such as classification and organization of data (763). Students are also required to write a critique on someone else’s work. The third report asks students to write a discussion section (763). The fourth report focuses on making good figures and using the high-level writing skill of critical analysis (763). This approach has shown that students do a better job at describing their observations, it lowered complaining, and it increased their writing confidence (764).
The last study is “Stepwise Approach to Writing Journal-Style Lab Reports in the Organic Chemistry Course Sequence” by Jay Wackerly. Wackerly believes using a stepwise approach would be beneficial to students as it helps build rhetorical skills in scientific and technical writing (76). This approach is based on the framework of stepwise writing, collaborative writing, journal-style reports, and imitation (76). The stepwise approach has students basically write their report in steps from the lowest skill to the highest skill throughout the semester (76). For each lab, it is required to write a post laboratory assignment (78). The students first experience report writing one-third into the semester (78). Students are asked to supply aspects of the procedure and data gathered during the experiment which is known as the “results” (78). For the second report, students wrote the results and discussion which increases the writing level from low to medium (78). The final reports are full reports which is a high level of writing (78). Increasing the writing complexity overtime allowed students to learn the expectations of journal-style writing and gave them a smoother transition into organic chemistry from a general chemistry lab (79). Most students wrote their reports with their lab partner which is an important skill to have for many careers (79). Students also were asked to peer review other work, which is also an essential skill to many careers (79). Students wrote their reports in the style of The Journal of Organic Chemistry format (79). This allowed students to be “introduced to technical writing in a manner that “real scientists” use to communicate information.” (80). This approach showed improvement in students’ writings (78). Students felt that this approach improved their writing skills and made them feel like better scientists (81).
There have also been other methods than changing the way lab reports are taught that have been studied and can help improve students’ attitudes and thus improve their grades. The article “The relationships between University Students’ Chemistry Laboratory Anxiety, Attitudes, and Self-Efficacy Beliefs” by Izzet Kurbanoglu and Ahmet Akim states “Studetnts’ chemistry attitudes are important factors highly associated with chemistry success and motivation.” (p.6). Students who have positive attitudes towards chemistry have a higher chance of sustaining their efforts and want to be involved in learning tasks. The article also states, “students’ self-efficacy beliefs play an integral role in their academic motivation, learning, and achievement.” (p.6). It has been shown that students who believe they can by successful academically ten to have a greater interest in academic work, set higher goals, use greater effort, and are more resilient to difficulties.
The article “How Attitudes Affect Grades” by Dennis Congos claims it is possible to change an attitude (p.2). The article specifically states “For success in acquiring attitudes that promote your success you must be willing to admit and face the truth about yourself and admit and face the truth about what you are willing to change.” (p.2). From personal experience, I also believe this to be true. You first must admit that something is wrong and commit to a change to see improvement. This article has provided a model that has exercises/questions to help someone see how they can replace their negative attitudes that could be limiting their success. There are eight exercises/questions in the model. The first on asks, “Identify a negative or limiting attitude you have about something related to college and write it down.” (p.2). This question can be about literally anything involving college whether it be stress, social situations, or even pressure from family. To make any change you must identify what is causing the negative attitudes. The second question asks, “Declare to yourself that you intend to change.” (p.2) This step requires you to write a statement that states your intention to change an attitude. It then requires you to re-word the limiting attitude in a positive way. This is important to do because generally you tend to do what you tell yourself to do which influences attitudes. The third question asks, “List 3 people who you believe currently have the positive attitude you recorded in question 2.” (p.2). This step is important because we tend to be like those who we surround ourselves with and who we admire.
Question four asks, “List 3 different behaviors or actions you can do tat could lead to other to believe that you have new attitude.” (p.2). This step is important because practicing a certain attitude can help you become better at it and thus improve the attitude overall. Question five asks, “Describe 3 situations in which you commonly find yourself where you could practice the 3 behaviors or actions listed above.” (p.2). More practice means the attitude will become a habitual habit. Question six asks, “List 3 people you could talk to about changing and becoming more the person you want to be.” (p.3). Telling someone your intentions generally makes you follow what you said you will do. Question seven asks, “List 3 times during the day when you intend to visualize what you will be like and how you will feel once you acquire this new attitude.” (p.3). Visualizing yourself doing something tends to encourage you to do that thing. The last question asks, “Write down at least one way to reward yourself for acting in a way that demonstrates your new attitude.” (p.3). Rewards tend to encourage you to continue to repeat whatever it is you are rewarding yourself for. Using this model could improve negative attitudes which could increase grades.
The article “Utility Value Intervention in a College Biology Lab: The Impact on Motivation” by Kevin Curry Jr., Dan Spencer, Ondra Pesout, and Kimberly Pigford compared the outcomes of students who had utility value intervention in the biology lab and those who did not. These authors believe that “requiring students to generate their own utility value toward a task, followed by written reflection, increases students’ maintained and situational interest for biology laboratory reports.” (p.232). The authors believe that science graduates often have poor scientific writing and critical thinking skills due to students being unmotivated (p.233). The interventions used in this study were used to motivate students to engage with the course content rather than motivate students to engage in the writing task itself (p.234). Utility value represents and person’s view on the usefulness of scientific writing or future goals (p.234). It has been shown that high levels of utility value are positively related to academic outcomes (p.235). High levels of utility value have also shown positive results in math, social science, writing, and educational psychology (p.235).
Before further explaining this study, it is important to understand a couple of terms. Firstly, anything that is “directly-communicated” refers to information that was supplied to students with value related information (p.235). Lastly, anything that is “self-generated” refers to the values that students provided themselves (p.235). Throughout the semester participants were given 5 five-minute interventions that had a theme involving the utility value of scientific writing (p.239). Self-reported measures were completed through an online survey (p.240). There were four groups included in this study. The first group was the directly-communicated group. Students received external information about the utility value of lab reports and did not have a follow-up exercise (p.240). The self-generated group had students create their own thoughts of the utility value of lab reports using self-reflective brainstorming sessions (p.240). No videos were used in this group. The hybrid group was a combination of direct communication and self-generation of utility value of lab reports (p.240). Lastly, the control group were not exposed to the utility value of lab reports during the interventions (p.240).
Results have shown that the self-generated and hybrid group showed a higher utility value than the control group by the end of the semester (p.242). The self-generated group also reported a significantly higher situation interest compared to the directly-communicated group (p246.). Compared to the control group, the self-generated group showed the greatest promise for increasing motivation toward laboratory reports (p.246). These results suggest that using brainstorming sessions and written reflections show the greatest promise for increasing the value of scientific writing in a college laboratory (p.248).
Even though many of the studies I explained have shown promising results, there is still more research that is needed to continue to either prove or refute these results. The article “Using Journal Articles to Teach Writing Skills for Laboratory Reports in General Chemistry” explained that future studies could show that students who create data tables learn more about how the data fits together to solve a problem (p.764). The article “Creative Report Writing in Undergraduate Organic Chemistry Laboratory Inspires Nonmajors” mentions having students in lower-level chemistry classes use this technique to see if the results are similar (p.95). The article “The Relationships between University Students’ Chemistry Laboratory Anxiety, Attitudes, and self-efficacy” mentions that future studies should involve more student populations and use more than just correlational statistics (p.8).
The article “Utility Value Interventions in a College Biology Lab: The Impact on Motivation” brough up multiple options for future studies which include exploring the casual effect of utility value, should be conducted in an authentic classroom context, account for individuals’ confidence in tasks and the degree of personal connections a task and their life, consider students’ perceptions of autonomy when designing directly-communicated and hybrid interventions, explore changes in value across shorter periods of time, expand the study design to include an additional condition that involves brainstorming followed by a control task, integrate similar types of interventions within other aspects of the course such as class lectures, value-enhancing activities that take place only in the classroom environment, utilize qualitative analysis to compare essay between the self-generated and directly-communicated group, and examine the impact of utility interventions on college students’ scientific writing and their academic performance in the science field (p.235-236, 247-248). Many of the other articles have stated similar studies need to be done but with a larger participant size.
Many articles have stated there needs to be a change in how laboratory reports are taught so students will have more positive attitudes which increases their performance and grades. It has been shown that student attitudes can make a big impact on grades. Students with negative attitudes tend to have lower grades and performance while those with positive attitudes have higher grades and performance. So far, there have been multiple studies on the different types of teaching and also other methods that can be used to improve student attitudes through self-reflection. Although these studies show very promising results, there still needs to be further research to have more evidence of these positive effects.
Congos, Dennis. How Attitudes Affect Grades – SARC Online • UCF . 2011, academicsuccess.ucf.edu/sarconline/wp-content/uploads/sites/32/2017/07/How_Attitudes_Affect_Grades11.pdf.
Curry, Kevin W., et al. “Utility Value Interventions in a College Biology Lab: The Impact on Motivation.” Journal of Research in Science Teaching , vol. 57, no. 2, 29 July 2019, pp. 232–252., doi:10.1002/tea.21592.
Kurbanoglu, N. Izzet, and Ahmet Akim. “The Relationships between University Students’ Chemistry Laboratory Anxiety, Attitudes, and Self-Efficacy Beliefs.” Australian Journal of Teacher Education , vol. 35, no. 8, 2010, pp. 1–9., doi:10.14221/ajte.2010v35n8.4.
Murray, Dr., and Anna Rockowitz. “Writing Across the Curriculum: Writing Lab Reports.” Hunter College , www.hunter.cuny.edu/rwc/repository/files/WAC/Writing-Lab-Reports.pdf.
Student. “Effects of Students Attitude on Their Performance.” UKEssays.com , Nov. 2018, www.ukessays.com/essays/psychology/effects-of-students-attitude-on-their-performance-psychology-essay.php.
Gragson, Derek E., and John P. Hagen. “Developing Technical Writing Skills in the Physical Chemistry Laboratory: A Progressive Approach Employing Peer Review.” Journal of Chemical Education , vol. 87, no. 1, 2010, pp. 62–65., doi:10.1021/ed800015t.
Henary, Maged, et al. “Creative Report Writing in Undergraduate Organic Chemistry Laboratory Inspires Nonmajors.” Journal of Chemical Education , vol. 92, no. 1, 5 Nov. 2014, pp. 90–95., doi:10.1021/ed5002619.
Lee, Susan E., et al. “Writing Activities Embedded In Bioscience Laboratory Courses To Change Students’ Attitudes And Enhance Their Scientific Writing.” EURASIA Journal of Mathematics, Science and Technology Education , vol. 7, no. 3, 21 Mar. 2011, pp. 193–202., doi:10.12973/ejmste/75191.
Moskovitz, C., and D. Kellogg. “Inquiry-Based Writing in the Laboratory Course.” Science , vol. 332, no. 6032, 19 May 2011, pp. 919–920., doi:10.1126/science.1200353.
Tilstra, Luanne. “Using Journal Articles to Teach Writing Skills for Laboratory Reports in General Chemistry.” Journal of Chemical Education , vol. 78, no. 6, June 2001, pp. 762–764., doi:10.1021/ed078p762.
Wackerly, Jay Wm. “Stepwise Approach To Writing Journal-Style Lab Reports in the Organic Chemistry Course Sequence.” Journal of Chemical Education , vol. 95, no. 1, 20 Nov. 2017, pp. 76–83., doi:10.1021/acs.jchemed.6b00630.
Understanding Literacy in Our Lives by Sydney Arnold is licensed under a Creative Commons Attribution-NonCommercial-NoDerivatives 4.0 International License , except where otherwise noted.
Share This Book
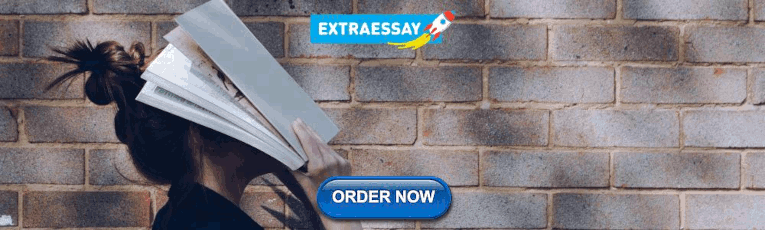
IMAGES
VIDEO
COMMENTS
Introduction. Your lab report introduction should set the scene for your experiment. One way to write your introduction is with a funnel (an inverted triangle) structure: Start with the broad, general research topic. Narrow your topic down your specific study focus. End with a clear research question.
The science learning goals of laboratory experiences include enhancing mastery of science subject matter, developing scientific reasoning abilities, increasing understanding of the complexity and ambiguity of empirical work, developing practical skills, increasing understanding of the nature of science, cultivating interest in science and science learning, and improving teamwork abilities.
When it comes to the lab report outline, this style dictates that you should include the following: a title page; an abstract; sources (as a References page). How to format references under the APA format deserves a separate blog post. But here's a short example: Smith, J. (2021). A lab report introduction guide. Cambridge Press.
Mission. The Purdue On-Campus Writing Lab and Purdue Online Writing Lab assist clients in their development as writers—no matter what their skill level—with on-campus consultations, online participation, and community engagement. The Purdue Writing Lab serves the Purdue, West Lafayette, campus and coordinates with local literacy initiatives.
Lab Safety Essay: If you’re an aspiring chemist or lab technician, then this essay is for you! There are many dangers that surround the lab, and you must be aware of them if you want to prevent injury or even death. This essay explores the safest and most effective methods of keeping yourself and your coworkers safe while in a lab.
The scientific lab report, or research paper, is the cornerstone of professional discussion in the sciences. Professional journals and college departments use it as a basic template to report findings. It is in this format that findings and new experiments are submitted for peer review. Professionalism is a required aspect of this type of writing.
Some laboratory experiences may engage students in formulating and assessing the importance of alternative questions. Using laboratory tools and procedures. Some laboratory experiences may be designed primarily to develop students’ skills in making measurements and safely and correctly handling materials and equipment (Lunetta, 1998).
Clean the samples thoroughly using ethanol to remove any impurities or oils. Weigh each sample accurately using a digital scale and record the initial weight. Prepare a 3% NaCl solution by dissolving 30 g of NaCl in 1000 mL of deionized water. Pour 250 mL of the 3% NaCl solution into each beaker.
Tips on Writing Laboratory Report Essay. Students who take science degrees will have to take a few lab courses where they are asked to do an experiment and write a research report to demonstrate their understanding. In the research report, students must explain about the experiment and describe whether their findings agree to the hypothesis.
3.2.2 Culinary literacy and its impact on society (synthesis essay) 3.3.1 Writing in a chemistry lab (argument from experience) 3.3.2 Different approaches to writing in a science lab (synthesis) 3.3.3 The impact of student attitudes on laboratory reports (prospectus) 3.3.4 The impact of student attitudes on laboratory reports (research essay)