
An official website of the United States government
The .gov means it’s official. Federal government websites often end in .gov or .mil. Before sharing sensitive information, make sure you’re on a federal government site.
The site is secure. The https:// ensures that you are connecting to the official website and that any information you provide is encrypted and transmitted securely.
- Publications
- Account settings
Preview improvements coming to the PMC website in October 2024. Learn More or Try it out now .
- Advanced Search
- Journal List
- Portland Press Opt2Pay

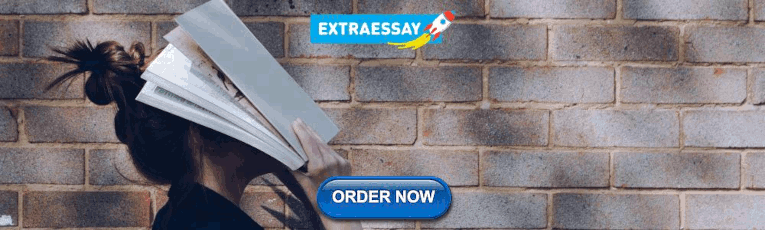
Recent developments in genetic/genomic medicine
Rachel h. horton.
Clinical Ethics and Law, Faculty of Medicine, University of Southampton, Southampton, United Kingdom
Anneke M. Lucassen
Advances in genetic technology are having a major impact in the clinic, and mean that many perceptions of the role and scope of genetic testing are having to change. Genomic testing brings with it a greater opportunity for diagnosis, or predictions of future diagnoses, but also an increased chance of uncertain or unexpected findings, many of which may have impacts for multiple members of a person’s family. In the past, genetic testing was rarely able to provide rapid results, but the increasing speed and availability of genomic testing is changing this, meaning that genomic information is increasingly influencing decisions around patient care in the acute inpatient setting. The landscape of treatment options for genetic conditions is shifting, which has evolving implications for clinical discussions around previously untreatable disorders. Furthermore, the point of access to testing is changing with increasing provision direct to the consumer outside the formal healthcare setting. This review outlines the ways in which genetic medicine is developing in light of technological advances.
Introduction
The past two decades have seen major shifts in our technical ability to sequence genetic information at scale. Historically, genetic testing tended to consist of either highly detailed molecular testing of nominated single genes, or broad genome-wide dosage screening at low resolution, for example karyotyping [ 1 , 2 ]. Genome sequencing was too slow and too expensive to be used in clinical contexts: for example the Human Genome Project, which was 99% complete in 2004, cost three billion dollars and took 13 years to sequence [ 3 ].
More recently, advances in sequencing technology have made it possible to undertake broad genetic testing on an individual patient basis within a clinically useful timeframe, via exome and genome sequencing. Exome tests sequence the entire protein-coding region of the genome, representing less than 2% of the genome but containing approximately 85% of known disease-causing variants [ 4 ]; genome sequencing encompasses the exome but also sequences all the non-protein-coding DNA. Initially implementation of such tests was via clinical research studies such as the Deciphering Developmental Disorders project [ 5 ], but more recently exome sequencing has been utilised as a clinical diagnostic test [ 6 ]. Genome sequencing is also due to transition to being available as a standard NHS test in June 2019, having previously only been available via initiatives such as the 100,000 Genomes Project [ 7 ].
Sequencing technology has improved in depth as well as breadth, and this has been of importance in better understanding cancer. The ability to sequence cancer genomes has led to rapid identification of driver mutations and has helped to work out the complex relationships between different cancer subclones over space and time, demonstrating the enormous heterogeneity of cancers and the difficulty of successfully treating them [ 8 ]. As sequencing techniques have advanced to the level where tiny amounts of tumour or individual cells can be sequenced, it has been possible to identify previously unknown mutational mechanisms, such as chromothripsis 1 [ 9 ] and kataegis 2 [ 10 ].
However, our ability to generate genomic data has substantially outstripped our ability to interpret its significance for an individual, and while improvements in genomic technology are in many cases driving improvements in healthcare, we are also encountering new problems as genomic testing shifts into the clinical setting. The Global Alliance for Genomics and Health (GA4GH) predicts that by 2025, over 60 million people will have had their genome sequenced in a healthcare context [ 11 ], but pathways for managing the output from genome sequencing are still in their infancy. The detailed but unfocused approach of genomic tests gives opportunities to answer questions that go beyond the problems that led to a patient having a test. However, deciding which of the multitude of possible outputs from genomic tests should be considered a ‘result’ at any given time is very challenging, not least because the links between many genetic variants and diseases are often unproven or poorly understood [ 12 ]. Multidisciplinary input and collaboration are increasingly key to interpreting the significance of genomic results. This review discusses the developments in practice that are evolving as a result of increasing use of genomic technologies.
New disease gene discovery and changing concepts of diagnosis
Exome and genome sequencing are powerful diagnostic tools – for example the Deciphering Developmental Disorders project, which recruited patients with severe undiagnosed disorders (who had generally already had any currently available diagnostic genetic testing), achieved a 40% diagnosis rate via trio exome sequencing for the first 1133 family trios in the study [ 13 ]. The search for a diagnosis has often been described as a journey [ 14 ], with parents of children with rare genetic disorders anticipating that a diagnosis may guide treatment, prognosis, acceptance and social support [ 15 ]. However, identification of new rare disease genes may be changing the impact of receiving a diagnosis, and in many cases very little is known about the long-term effects of newly identified genetic conditions.
Historically when making a genetic diagnosis, it has usually been possible to give families some information regarding prognosis, and to provide some parameters as to what to expect for the future, based on previous experience of what has happened for other children affected by the same condition. Now, while in some situations due to strong phenotypic match it is possible to be confident that a child’s rare disease has been caused by pathogenic variants in a recently described rare disease gene, often this provides little information about a child’s future.
We are increasingly in the position of learning about the effects of possible disease-causing variation(s) in a gene through meeting the patients in whom such genetic changes have been discovered. Often these changes will be in a gene newly thought to be linked to developmental disorders and there will be little, if any, published literature to draw on. We then have to speculate whether the genetic change detected is the cause of our patient’s health problems, and whether any additional difficulties that have happened for our patient that have not yet been noted in other patients with changes in the same gene are an extension of the phenotype of the newly described disease gene, or coincidental. In situations like this, we are often unable to give people information about what a new diagnosis might mean for them or their child in the longer term.
This has led to patient support and awareness groups taking on an increasingly important role [ 16 ], as families gather to share their lived experience of newly diagnosed rare genetic conditions, in turn informing clinical services. For example, the charity Unique works with families and professionals to develop specialist information relating to many rare and newly described genetic conditions, and to gather information about their long-term effects, increasing awareness and understanding of what it is like to live with rare genetic conditions. The rapidity with which such information can be gathered is also exemplified by the work of the PURA Syndrome Foundation: in 2014 the first patients with a rare condition called PURA syndrome were described in the medical literature [ 17 ]. Shortly afterwards the PURA Syndrome Foundation was established which has catalysed links between families, clinicians and researchers, greatly improving the speed and quality of research into the condition [ 18 ].
The agnostic approach of exome and genome sequencing is also challenging our previous concepts of existing genetic diagnoses, when apparently pathogenic variants are found in well-described disease genes but the patient’s clinical picture falls outside the boundaries of what we would conventionally expect for a patient affected by that particular genetic condition. For example, loss-of-function variants in SOX2 are known to cause anophthalmia and microphthalmia in addition to other phenotypes such as developmental delay and structural brain anomalies. Eye abnormalities were thought to be a key feature of SOX2 -related disorders, and so SOX2 would only be requested as a genetic test in patients who had absent or small eyes. Recently, via ‘genotype-first’ approaches, loss-of-function SOX2 variants have been found in people with developmental delay but without anophthalmia or microphthalmia, broadening the phenotypic spectrum associated with this gene [ 19 ]. Case Study 1 shows a further example where exome testing has extended previous perceptions of the clinical scope of a genetic condition.
Case Study 1
Redefining our understanding of genetic conditions (fictional case based on eggens et al. [ 20 ]).
An 8-year-old girl was referred to clinical genetics in order to investigate her progressive weakness. She had been floppy as a baby and from the age of 5 years had developed worsening limb weakness with frequent unusual movements, and difficulty in swallowing. Serial brain scans had shown progressive cerebellar atrophy.
Exome testing found that she was homozygous for a variant predicted to disrupt the function of EXOSC3 , a gene associated with pontocerebellar hypoplasia. This diagnosis had never been thought of as she did not have one of the defining characteristics: pontine hypoplasia. Her clinical picture also seemed atypical for this condition – most children with pontocerebellar hypoplasia do not survive infancy.
However, recent research has shown genotype–phenotype correlations in EXOSC3 -mediated pontocerebellar hypoplasia – patients homozygous for p.D132A variants (like this patient had) tend to have a milder clinical course and preservation of the pons. This genetic explanation fitted well in retrospect, but would not have been considered in advance of the exome test.
Key messages
- Many well-recognised genetic conditions may have a wider spectrum of effects than previously thought.
- Patients with genetic conditions identified via genomic tests may not conform to the pattern we expect based on experience of patients with the same condition identified via single gene testing. It can be very difficult to be sure whether this reflects an incorrect diagnosis, or a wider disease spectrum than previously recognised.
In many cases, our understanding of why the same genetic condition may be expressed so differently among different people is at an early stage, and this often makes genetic counselling very challenging, particularly in the prenatal setting. For some genetic conditions, it is becoming possible to provide more personalised risk estimates, based on combining knowledge of a person’s genetic diagnosis, with analysis of other factors that may influence their risk. Personalisation of risk in this way has generally been crude and reliant on clinically obvious characteristics: for example, men with pathogenic BRCA variants have a lower risk of developing breast cancer than women with pathogenic BRCA variants. More recently, genetic testing is being developed to complement ‘key’ genetic test results to provide an increasingly refined personal risk. For example, use of a polygenic risk score using breast cancer and ovarian cancer susceptibility SNPs identified via population GWAS showed large differences in absolute cancer risks between women with pathogenic BRCA variants with higher compared with lower polygenic risk score values [ 21 ]. This has yet to translate into routine clinical practice, but has the potential to help women with pathogenic BRCA variants make more informed decisions about how and when to manage their cancer risk.
The downsides of improved sensitivity: increased uncertainty in what tests mean
The prior probability of any one variant identified via genome sequencing being causative for a patient’s rare disease is extremely low. Attempts to catalogue human genetic variation, for example via the 1000 Genomes Project, show that a typical human genome differs from the reference human genome at 4.1–5 million sites [ 22 ]. Most of these variations will be entirely benign, some may subtly impact on risk of various common diseases, and a very small number will have the potential to cause serious disease either in an individual, or in their children (potentially in combination with variants inherited from their partner).
Genome sequencing identifies the majority of these variants, which then need careful filtering to produce a meaningful output. This has required a significant change in mindset from an era when most variants were identified in the context of carefully chosen single gene sequencing, and so had a much higher prior probability of being causative. There is an increasing shift towards a view that variants should be ‘innocent until proven guilty’ [ 23 ], but there is a lack of consensus regarding how to translate this principle into clinical practice.
There is also considerable discrepancy in how different genetics laboratories interpret the same variants. International guidelines for variant interpretation are helpful but insufficient to remove a great deal of noise when attempting to assign significance to particular findings [ 24 ]. This was illustrated in a recent study comparing variant classification among nine genetic laboratories: although they all used the same guidelines, only 34% of variants were given the same classification by all laboratories, and 22% of variants were classified so differently that different medical interventions would be recommended [ 25 ]. At a lower resolution level, even being sure of the relationship between genes and diseases is often difficult. For example, curation of the 21 genes routinely available on Brugada syndrome gene panels using the ClinGen gene curation scoring matrix found that only one of these genes was definitively linked to Brugada syndrome [ 26 ]. Our improving knowledge of variant interpretation leaves us with a difficult legacy, with many patients having been diagnosed incorrectly with genetic conditions. The effects of this can be far-reaching and difficult to undo, as illustrated by Case Study 2 .
Case Study 2
The legacy of incorrect diagnosis (case reported by ackerman et al. [ 27 ]).
A teenage boy died suddenly and genetic testing was then undertaken for his brother, resulting in the finding of a rare variant in KCNQ1 . On the basis of this test, the living brother was diagnosed with long QT syndrome, and the teenage boy’s sudden death was attributed to long QT syndrome. The living brother had an implantable cardioverter defibrillator inserted, and via cascade genetic testing over 24 relatives were diagnosed as having long QT syndrome, despite having normal QT intervals on ECG.
However, subsequent examination of post-mortem samples found that the boy who died had cardiac features inconsistent with long QT syndrome, did not have the KCNQ1 variant found in the wider family, and instead had a clearly disease-causing de novo variant in DES , a gene linked to cardiomyopathy.
- It is very important to consider whether the clinical picture fits when evaluating variant significance: genetic variants will usually only predict disease well if found in the context of a medical or family history of the relevant disease.
- Incorrect (or inappropriately deterministic) genetic test interpretation can affect the clinical care of a whole family, not just the person being tested.
Although this suggests that we need to be very cautious in making firm genetic diagnoses, it is difficult to know where the threshold should lie for communicating genetic variation of uncertain significance. There is some evidence that people find receiving a variant of uncertain significance surprising and disturbing, and some people misinterpret it as being definitely pathogenic or definitely benign [ 28 ]. However, there is also evidence that many people have a strong desire to receive a broad range of results from genetic testing, including uncertain results, and are uncomfortable with the idea that decisions about non-disclosure might be made without involving them [ 29 ].
The fear is that disclosure of uncertain variants will lead to over-diagnosis and over-management, with variants inappropriately being treated as if pathogenic. Excessive and inappropriate interventions (not to mention anxiety and distress) might then cascade through families, going against one of the fundamental principles of medicine to ‘first do no harm’. However, we also fear missing something or being accused of ‘hiding information’. The result is that we tend to end up in purgatory, documenting uncertain variants on lab reports (though sometimes not) and having lengthy conservations with patients about them (though sometimes not), then tacking on a caveat that ‘maybe this means nothing’. This nominally shifts the responsibility to the next person in the chain but feels unsatisfactory for all concerned.
Uncertainty when to stop looking and what to communicate
Another issue arising from improved sensitivity is the ability to find genetic variants that are unrelated to the clinical problem that a patient presents with, but that may be relevant for their health in other ways. This may be viewed as positive or negative, but working out how to handle this information raises difficult questions. In 2013, the American College of Medical Genetics and Genomics (ACMG) suggested that laboratories should automatically seek and report pathogenic variants in 56 genes associated with ‘medically actionable’ conditions when performing clinical sequencing [ 30 ]. The main rationale was the potential to benefit patients and families by diagnosing disorders where preventative measures and/or treatments were available, with the aim of improving health. However, these recommendations proved controversial. The main debate at the time centred around whether patients should have a right to choose not to know such information [ 31 ]. Subsequent questions about the role of clinicians in offering additional findings, what constitutes a ‘medically actionable’ finding, and what is the predictive value of such findings in the absence of a phenotype or family history of the relevant disorder, are yet to be fully addressed.
Analysis of data from the 1000 Genomes cohort demonstrated that approximately 1% of ‘healthy’ people will have a ‘medically actionable’ finding in one of the 56 genes [ 32 ]. However, what this might mean on an individual basis is often unclear. Most of our knowledge regarding the effects of variation in any given gene has been gathered by observing people who have been identified as having variants in the gene because they were tested as they had a personal history or family history of disease, biasing the sample from which our conclusions are drawn. It is less clear what it might mean to find, for example, an apparently pathogenic variant in a gene linked to cardiomyopathy in a person with no personal or family history of heart problems. This has important implications for ‘cascade screening’, where relatives of a patient affected by a condition with a known genetic cause are offered testing to see whether they have the disease-causing genetic variant that was found in their clinically affected family member (meaning that they may also be at risk of developing the disease). To what extent should testing and subsequent screening be offered in a family based on an incidental finding of a genetic variant thought to be predictive of a particular condition, if there is no clinical evidence that anyone in the family, including the person in whom the genetic variant in question was first identified, is actually affected by it?
Broad genomic testing also has the potential to detect carrier status for recessive and X-linked conditions. From population studies, we know that being a carrier for a genetic condition is very common. For example, a gene panel testing carrier status for 108 recessive disorders in 23453 people found that 24% were carriers for at least one of the 108 disorders, and 5.2% were carriers for multiple disorders [ 33 ]. On a disorder-by-disorder status, being a carrier for a genetic condition is very rare (with notable exceptions such as haemochromatosis and cystic fibrosis), but when considered collectively, it is ‘normal’ to be a carrier for a genetic condition. For most people, being a carrier will have no impact on their life at all. However, if their partner happens to be a carrier for the same condition then the implications could be very profound, as each of their children would have a one in four chance of being affected by the genetic condition. This is particularly relevant for couples who are known to be biologically related [ 34 ], and couples with common ancestry, as they will have a higher chance of both being carriers for the same recessive condition. Carrier screening for various autosomal recessive diseases has been available in some instances for many years, for example screening for carrier status for Tay–Sachs disease for people of Ashkenazi Jewish ancestry has been offered since the 1970s [ 35 , 36 ]. More recently, advances in technology have led to development of expanded carrier screening tests, which check carrier status for multiple diseases simultaneously and are often less targeted towards particular genetic populations [ 37 ].
The increased scope of carrier screening, combined with the recognition that it is very common to be a carrier for one or more recessive genetic conditions, has led to an increasing move to consider carrier results for recessive genetic conditions on a couple basis, where carrier status is only communicated if it would be relevant in the context of a particular relationship (i.e. if both people in a couple are carriers for the same condition) [ 38 ]. This avoids pathologising the status of ‘being a carrier’, recognising that most of us are carriers for some genetic conditions, and conserves resources for genetics services by not flooding the system with large volumes of individual carrier results, most of which will be meaningless in the context of that individual’s life. Objections to this approach are that by not communicating individual carrier results, a person would not know this information for future relationships, and their family could not access cascade screening to see whether they are also carriers. However, these objections could be obviated by widespread adoption of couple carrier testing – a person (or their close relatives) could find out their carrier status if relevant when they next had a couple carrier test in the context of their new relationship. In some ways, this could be seen as comparable with management of infectious disease – lots of healthy people carry MRSA, but very few die of MRSA infection. People are therefore screened at times when they might be especially vulnerable to becoming unwell from MRSA, or when they might pass it on to others at risk, for example when admitted to hospital, rather than being tested at random points when they are generally well.
The expanding remit and availability of genetic technology
‘acute genetics’.
For many years, clinical genetics input has at times influenced acute care, for example in diagnosing trisomies in the neonatal period, or informing the care of babies born with ambiguous genitalia. However in many circumstances, the key contribution of clinical genetics was in providing a post hoc explanation for serious medical problems, rather than in influencing treatment decisions on a real-time basis. This is changing as the availability of exome and genome sequencing increases, as shown by Case Study 3 . A recent study in a neonatal intensive care unit in Texas studied outcomes for 278 infants who were referred for clinical exome sequencing, and found that 36.7% received a genetic diagnosis, and medical management was affected for 52% of infants with diagnoses [ 39 ]. There is increasing evidence that this approach is cost-effective: for example, a prospective study of exome sequencing for infants with suspected monogenic disorders found that standard care achieved an average cost per diagnosis of AU$ 27050, compared with AU$ 5047 for early singleton exome sequencing [ 40 ]. Similarly, ‘real-time’ genetic and genomic testing is making an impact in cancer treatment, where in many cases testing is available to help guide treatment choices by identifying actionable genetic variants in tumours that may respond to specific therapies [ 41 , 42 ].
Case Study 3
Insights from exome testing transforming a clinical course (case from wessex genomic medicine centre [ 43 ]).
A young woman was referred for exome testing having spent months in a coma. From childhood she had experienced sensory problems, and as a young adult she had gone on to develop seizures which deteriorated into status epilepticus, necessitating ventilation on intensive care.
After 3 years during which all other avenues had been explored, analysis of her exome was proposed. An unexpected diagnosis of pyridoxine-dependent epilepsy was found; this had not previously been considered as classically it causes seizures in the first few months of life. She began treatment with pyridoxine (vitamin B 6 ). From that point on she had no further seizures and her clinical situation transformed. Over a 6-month period she was weaned off all of her anti-epileptic drugs, and was able to return to a normal life.
Key message
- Exome or genome tests have the potential to make an enormous difference to clinical care and to people’s lives.
Pharmacogenomics
As well as guiding treatment choice, genetic testing will increasingly influence what doses are prescribed, and whether medications are considered unsuitable in view of a high risk of an adverse reaction. Around the time that the Human Genome Project was completed, there was considerable excitement about the possibility of genetic testing guiding use of medication in the clinic [ 44 , 45 ]. The potential of genotype-driven drug dosing has for the most part yet to be realised, in part because the interaction of the genetic factors involved is sometimes complex, and in part because environmental factors may also have a significant impact on how a person responds to a drug. For example, genotype-driven prescription of warfarin, which has notoriously wide inter-individual variation in dosage requirements, largely remains in the realm of research [ 46 ].
However, for some drugs, pharmacogenomics has already had a significant impact in reducing morbidity and mortality. For example, when the antiretroviral drug abacavir was first introduced, approximately 5% of the people treated developed an idiosyncratic hypersensitivity reaction that could be life-threatening on repeated exposure to the drug [ 47 , 48 ]. Research established that immunologically confirmed hypersensitivity reactions to abacavir only occurred in people with the HLA-B*5701 allele, and a clinical trial went on to show that pre-screening patients to check that they did not have HLA-B*5701 prior to starting the drug led to no confirmed hypersensitivity reactions in the pre-screened arm, while 2.4% of the unscreened patients had reactions [ 49 ]. Patients are now screened for HLA-B*5701 as standard before starting abacavir treatment [ 50 ]. Similar screening is likely to become more widespread as we learn more about genetic risk factors for adverse drug reactions. For example, there are increasing suggestions that the mitochondrial variant m.1555A>G should be checked in patients with cystic fibrosis in order to guide antibiotic treatment choices, in view of the evidence that people with this variant may develop hearing loss when exposed to aminoglycosides [ 51 ].
Evolving options in prenatal genetics
Genetic testing is also being used more extensively in the prenatal setting, in part because of developments in non-invasive prenatal testing and diagnosis, which allow genetic screening or testing of a developing pregnancy by doing a blood test for the mother [ 52 ]. This removes the risk of miscarriage associated with conventional prenatal tests (chorionic villus sampling or amniocentesis). While this is in some ways a stride forward, it raises various ethical issues, as the technical test safety may lead to such testing becoming viewed as routine. This raises the concern that couples will give less careful consideration as to whether they really want to know the results before having such tests, and that women may feel that there is an expectation that they should have testing. The worry is that this could potentially lead to people feeling under pressure to terminate pregnancies in response to genetic test results (including in situations where the clinical implications of the results may be far from clear) [ 53 ].
Widening access to genetic testing within healthcare
The expanding options for genetic testing and the escalating expectation for quick results to drive clinical management mean that testing provision is increasingly being pushed out of highly specialised genetics centres into mainstream medicine. For example, many women with ovarian cancer will now be offered BRCA testing via their oncology team, and only referred to genetics if needed based on the test results [ 54 ]. Genetics appointments now frequently focus on interpretation of tests already done, working out if the test outcome seems to match the clinical problem, and arranging testing and surveillance for family members.
The rise of direct-to-consumer genetic testing
As clinical services have increasingly grown to expect and demand genetic answers for patients with complex health problems, on a broader societal level the hunger for genetic information also seems to be increasing. However this is occurring in the context of a public discourse about personalised/precision medicine and genetics that tend to enthusiastically promote it in a very optimistic light, rarely dwelling on potential concerns and limitations, and therefore potentially sculpting inappropriate expectations from technology that is still being developed [ 55 ].
Direct-to-consumer tests currently sit outside much of the regulation that governs clinical genetic testing, but claim to provide insight into issues as diverse as ancestry, nutrition, athletic ability, and child talent [ 56 ]. Many testing providers also claim to help provide insight on health, though the information provided by many direct-to-consumer companies is far from comprehensive. For example, a recent analysis of 15 direct-to-consumer genetic testing companies advertising to U.K. consumers found that none of them complied with all the U.K. Human Genetics Commission principles for good practice regarding consumer information [ 57 ]. There are also examples that might make us reflect sceptically on the value of these tests – for example a case where a family sent a sample from their dog to a direct-to-consumer testing company designed to provide insights on people’s genetic ‘superpowers’ and received a report which did not mention that the sample was not human but conjectured that the client would be talented at basketball [ 58 ].
‘DIY genetics’ has also risen in popularity, with people asking for raw data from direct-to-consumer companies then processing this themselves via third-party interpretation services, as discussed in Case Study 4 . Approximately 40% of genetic changes in direct-to-consumer test raw data sent for clinical confirmation are false positives [ 59 ], but this is often not appreciated by customers or the doctors they may subsequently visit, leading to anxiety and often inappropriate medical interventions [ 60 ]. However, clearly many people see a value in receiving genetic information and are prepared to pay for this. This marks a shift from genetic testing in order to explain health problems or for people at high risk of developing specific genetic conditions, to testing of healthy people with the rationale of facilitating life planning. This idea has been taken to the extreme with initiatives such as the BabySeq project, exploring the medical, behavioural and economic impacts of integrating genome sequencing into the care of healthy newborns [ 61 ].
Case Study 4
Grime on the crystal ball (fictional case based on moscarello et al. [ 60 ]).
A healthy medical student was given a direct-to-consumer genetic test for Christmas, and explored the raw data from this test using an online interpretation programme, finding a variant in MYBPC3 that was predicted to cause hypertrophic cardiomyopathy. He was understandably worried by this result, taking time off university as he came to terms with it, and giving up running, which he used to really enjoy.
He was seen in a hypertrophic cardiomyopathy clinic and had an expert cardiology assessment including ECG, echocardiogram and review of his family history. He was found to have no clinical evidence of hypertrophic cardiomyopathy, and further genetic testing showed that he did not actually have the disease-causing MYBPC3 variant that the online interpretation programme had identified. However, he continued to feel anxious about his risk of heart problems and decided to give up running permanently.
- Information provided from direct-to-consumer testing may be unreliable, especially where online interpretation programmes are used to further explore the raw data from the test: the level of quality control may be very different from that of accredited genetic laboratories, increasing the likelihood of false positives, false negatives and sample mix-up.
- Many direct-to-consumer genetic tests involve no meaningful pre-test counselling – people are often totally unprepared for the information that might come out of such testing (and are unaware that it might be wrong).
Genetic information as family information
The familial nature of genetic information has always generated discussion as to how to respect the confidentiality of individual patients while ensuring that their close relatives have access to information that may be relevant for their own health and life choices. Clinical guidance in this area has increasingly taken the stance that genetic information should be confidential to families, not individuals (though the personal consequences of having a genetic change for a given individual should be confidential to them alone) [ 62 ].
The consequences of this shift are still being navigated in the clinical setting – research indicates that patients often see genetic information as belonging to their family rather than exclusively to them [ 63 ], but healthcare professionals are often reticent about taking a familial approach to the confidentiality of genetic information in practice, worrying that this stance could disrupt family dynamics or erode patient trust in the health service [ 64 ]. A recent BMJ poll which asked, ‘Are there situations when sharing a patient’s genetic information with relatives without consent is acceptable?’ demonstrated the current split in opinion, with 51% of respondents answering ‘yes’ and 49% ‘no’ [ 65 ]. The personal versus familial nature of genetic information is currently being tested in the courts via the ABC case, which centres around non-disclosure of genetic risk to the daughter of a patient with Huntington’s disease [ 66 ].
Treatment for genetic disorders
One of the most exciting recent developments in genetics and genomics is the prospect of treatment for an increasing number of genetic conditions. However this topic has to be treated with caution as the practical reality for many patients and families is that though promising research is ongoing, meaningful treatment is not possible in many cases. Even in situations where evidence-based treatments have been developed, the expense of many of these therapies risks making them inaccessible.
Many different approaches have been taken to try to treat genetic conditions. Gene therapy, which involves delivering functional genetic code, is one approach but its success has been widely variable, often due to difficulty in developing vectors that can deliver genetic material into affected tissues at sufficiently high levels without being destroyed by the immune system. In certain situations this approach can be highly effective, for example promising results have been achieved in various eye conditions, likely because eyes are small and easily accessible, and have a privileged relationship with the immune system [ 67 ]. In cases aiming to deliver gene therapy to a wider area, such as the lungs or the muscles, treatment attempts have generally proved more challenging [ 68 , 69 ].
Other approaches include use of small molecules to modify various steps in the pathway from gene to functional product. For example, Eteplirsen aims to treat Duchenne muscular dystrophy in certain patients by influencing splicing machinery to skip exon 51 from mature DMD mRNA, restoring a more functional reading frame so that a shortened version of dystrophin can be successfully translated [ 70 ]. Ivacaftor potentiates the action of CFTR channels in some patients with cystic fibrosis (G551D pathogenic variant) [ 71 ]. Enzyme replacement therapy is being trialled to treat children with mucopolysaccharidoses, for example idursulphase infusions in mucopolysaccharidosis type 2 [ 72 ].
While lots of these therapies are very exciting and show demonstrable changes at the molecular level in clinical trials, these cellular changes do not always clearly translate into improvements in clinically relevant outcomes. The therapies are also often hugely expensive, which raises very difficult ethical questions regarding whether limited resources should be spent on such treatments where there is often only limited proof of clinical efficacy.
However the increasing possibility of future treatments for genetic conditions is influencing clinical decisions around the care of very ill children. For example, recently nusinersen has shown promise as a treatment for some children with spinal muscular atrophy, but this may begin to raise new questions about whether interventions such as intubation and tracheostomy should be offered to infants with severe spinal muscular atrophy, where previously these would have been considered medically inappropriate [ 73 ]. This has consequences for the clinical conversations happening when these diagnoses are made. In the past, breaking news of such a diagnosis might flow naturally into discussions around palliation. The possibility of treatment now creates new options to consider, but also new challenges in considering with parents how best to care for their child [ 74 ]. The clinical impact and accessibility of emerging treatments is often very uncertain, but parents may prefer to explore even extremely long-shot treatments over accepting a palliative care pathway route, and may expect or seek crowd funding for experimental treatments for which there is as yet very little, if any, evidence of benefit.
Improving genetic technology has also had a significant impact on fertility services, ranging from pre-implantation genetic diagnosis to mitochondrial donation, offering new options for families affected by genetic conditions [ 75 , 76 ]. Increasing technological capability is set to extend the theoretically possible range of options – for example last year a group in China used the CRISPR/Cas9 system to correct pathogenic variants in the HBB and G6PD genes in human zygotes [ 77 ], though the efficiency and accuracy of the correction procedure was variable. This emerging possibility raises significant ethical issues which need debate. A recent report of the Nuffield Council on Bioethics on genome editing in the context of human reproduction suggested that there may be certain contexts in which this may be ethically acceptable, provided that such interventions were intended to secure the welfare of a person who may be born as a result, and that any such interventions would uphold principles of social justice and solidarity [ 78 ].
Conclusions
Insights from genomic technology have great potential to improve health, but we are currently going through a teething process in learning how to respond to the nebulous information that genomic tests can provide in the clinical setting. In part, this learning process is being driven by patients and families, with patient support groups coming to the fore in an era where we can now make extremely rare diagnoses that link different families across the world, but often have very little information on what this might mean for the future. Our current response to the outcomes from genomic tests is often reactive and ad hoc, partly because we are still learning how to interpret genomic variation and are often unable to gain a consensus on whether genetic variants are clinically significant or not. This situation is exacerbated by the different routes in which genomic information is now accessible – rapid tests to establish diagnosis or plan treatment for patients are now a reality in the real-life clinical setting, but healthy people also have increasing access to commercial tests that claim to provide genetic information to improve health and life planning. This raises particular challenges in the context of a public discourse about genomics that tends to present it as far more predictive and certain than it actually is. Some of the most exciting recent developments in genomic medicine relate to potential future treatments and reproductive options for people and families affected by rare genetic conditions. However hurdles relating to treatment efficacy and optimal timing of treatment, mean that we need to keep these advances in perspective and consider how to research potential treatments responsibly, avoiding creating hype that undermines the ability of families to make a balanced decision whether or not to participate in this research. It is also important to consider financial sustainability, avoiding situations where useful new treatments are developed that remain inaccessible to the patients who need them on account of their cost. To summarise, the introduction of genomic testing is having a big impact on patient care, but raises various issues that need further study and debate in order to help us maximise the potential benefits of genomic medicine while minimising the possible harms.
Acknowledgments
We thank the patient in Case Study 3 for her help with the Case Study box and for sharing her story.
Abbreviations
1 Complex chromosome rearrangements, thought to occur due to single catastrophic events where chromosomes ‘shatter’ and are repaired by error-prone mechanisms.
2 Clusters of localised mutations.
This work was supported by funding from a Wellcome Trust collaborative award [grant number 208053/Z/17/Z (to A.L.)].
Competing interests
The authors declare that there are no competing interests associated with the manuscript.
- Workshops & Institutes
- Curriculum Index
- Research Opportunities
Sign In with Google
Create an Account
Stay informed! Sign up for our newsletter. We will never send you spam or sell your information.
Please verify that you are a teacher
Sign up with Google
Why should I sign up?
Even without an account, you’ll still have free access to most of the award-winning content on Teach.Genetics. Creating an account will give you access to additional content and tools.
Reset Password Email: Reset Password Email
- Exploring Genetics Through Genetic Disorders
Unit Overview
Exploring Genetics Through Genetic Disorders is a three-week, comprehensive genetics curriculum unit that aligns with the Next Generation Science Standards (NGSS). Through paper-based and interactive multimedia lessons, the unit engages students in using models, analyzing skill-level appropriate data from published research, establishing cause and effect relationships, and constructing explanations.
- Complete Genetics curriculum unit
- NGSS-aligned
- In-depth materials for exploring more than 25 real alleles that cause 5 different genetic disorders
- Real data, re-packaged in a way that is easy for students to work with
Suggested Sequence
The unit’s resources were designed to be used in any order, with or without outside lessons. However, we hope you will consider the suggested sequence below. It pulls together the unit’s resources in a way that builds understanding of the mechanisms connecting DNA variations—through effects on proteins, cells, tissues, and organs—to phenotypes.
Step 1: Introduce Traits
GUIDING QUESTION: What shapes the characteristics of all living things?
Step 2: Introduce Genetic Disorders as Traits; Assign Alleles
GUIDING PHENOMENON: Genetic disorders are also traits that vary. Individuals with the same alleles can exhibit different phenotypes.
- Variations in genes lead to variations in traits. Genes code for proteins that shape characteristics at the cellular, tissue, and organism levels.
- Genetic disorders are traits that vary, and they are influenced by the environment. Different alleles have different effects, and even people with the same alleles can have different phenotypes (characteristics).
- When scientists study what happens when a gene is not working correctly, they learn more about the regular function of that gene.
- Distribute one Lab Notebook and one unique Allele Profile to each student (or pair).
- Tell students that for the rest of the unit they will learn about basic concepts, then apply what they learn to their assigned allele. The Lab Notebook will be their guiding framework.
- Have students visit the appropriate genetic disorder page on Learn.Genetics. They should watch the video there, then use their Allele Profiles to fill in the Basic Information section of their Lab Notebooks.
- To help students understand that each allele profile is different, have them compare page 1 of their Allele Profile with a classmate’s.
- You may want to use the Demo Lab Notebook to help students understand what information they should fill in.
- Alpha-1 Antitrypsin Deficiency (pdf)
- Cystic Fibrosis (pdf)
- Hemoglobin Disorders (pdf)
- Hemophilia (pdf)
- Marfan Syndrome (pdf)
- Alpha-1 Antitrypsin Deficiency
- Cystic Fibrosis
- Hemoglobin Disorders
- Marfan Syndrome
- Print the alleles for each disorder on a different color. To save paper, make laminated class sets.
- Hemophilia and hemoglobin disorders are more challenging than the others.
- Within each genetic disorder, Allele #1 is the easiest, and they get more challenging as the numbers go up. You can find more details about each allele in the teacher guide.
- For some disorders, Allele #6 is a bonus. These are meant for students who need an extra challenge, or after they have finished the work for another allele.
- You can hand out the entire Lab Notebook all at once, or in sections as the class progresses through each step.
Step 3: Explore Foundational Content and Apply it to Assigned Alleles
- Complete the activities as a whole group. Follow the links for details and implementation suggestions.
- Their assigned Allele Profile
- The web page for their assigned genetic disorder
- Activities in the corresponding section of the student page on Learn.Genetics (to review as needed)
3a. Mutations and Alleles Foundational Content GUIDING QUESTION: How did the disorder-causing allele arise?
3b. Inheritance Foundational Content GUIDING QUESTION: How is the disorder-causing allele inherited?
3c. Protein Function Foundational Content GUIDING QUESTION: How do genes, and the proteins they code for, cause the disorder?

Parallel section headings on (1) the student page on Learn.Genetics, (2) Allele Profiles, and (3) Lab Notebook keep students oriented.
Step 4: Other Factors
GUIDING QUESTION: How can other genes and environmental factors influence the disorder?
- Through proteins, the information in genes shapes characteristics at the cellular, tissue and organismal levels.
- Environmental factors and variations in other genes interact with disease-causing alleles to produce a range of phenotypes.
- Treatment for a disorder may include any combination of managing environmental factors, medically treating symptoms, or compensating for a missing or altered genetic factor.
- (optional) Revisit and discuss What is an Environmental Factor?
Step 5: Putting it All Together
- Students should already have the information they need in their Lab Notebooks; they just need to find it and fill it in here.

- Their Cause & Effect statements
- Their genetic disorder web page
- Any other relevant section in their lab notebooks
- Each allele is different at the DNA level, because they came about through different mutations. Encourage students to focus on where in their gene the mutation is, and the type of change it causes in the protein.
- Some alleles of the same gene can affect different cell and tissue types, leading to a different set of symptoms at the organism level.
- For all the genetic disorders, some alleles cause more severe symptoms than others.
- There are differences in the data graphs in each Allele Profile. It may be useful to have students compare their data graphs and relate them to the one on the genetic disorder web page.

- A gallery walk of the similarities and differences charts created for each disorder during the Symposium
- A PowerPoint presentation about the disorder at the protein, cellular, tissue, organ, and organism levels, including main differences between the alleles studied
- A "research poster" summarizing the disorder at the protein, cellular, tissue, organ, and organism levels, including main differences between the alleles
- Oral presentations about how their assigned allele causes the disorder

About This Unit
This work was supported by a Science Education Partnership Award (1R25GM021903) from the National Institute of General Medical Sciences of the National Institutes of Health. The content is solely the responsibility of the authors and does not necessarily represent the official views of the National Institutes of Health

Genetic Disorder Research Project: Home
- Evaluating Websites
- Media Literacy
Background Information & Assignment Rubric
A genetic disorder is a disease that is caused by an abnormality in an individual’s DNA. Abnormalities can be as a single-base mutation in just one gene, or they can involve the addition or subtraction of entire chromosomes. In this project, you will research an assigned genetic disease. Then, you will select a role and corresponding format in which to convey your research.
Important Links:
- Genetic Disorder Research Project Assignment Information and Rubric
- HFHS Library Homepage
Genetic Diseases:
Source 1: Database
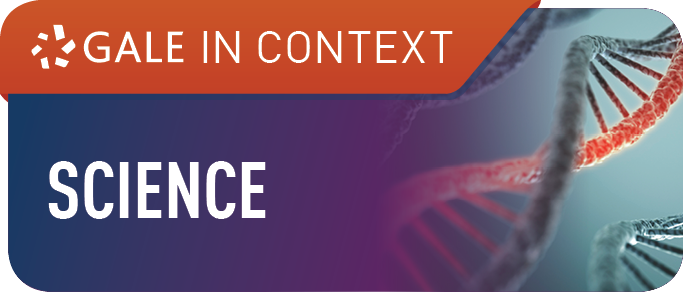
Most articles will be coming from the Gale Encyclopedia of Genetic Disorders . Look for the most recent articles in terms of publication date (2020-2023).
Citing in NoodleTools:

- Click "Cite" button choose APA
- Export to NoodleTools
- Import citation and wait for green confirmation box
- Refresh NoodleTools page
Sources 2 & 3: Websites
Make sure you are using the 7 Layers Of Website Evaluation and the Lateral Reading Methods to evaluate and find two websites that would be good, credible sources.
Citing in NoodleTools:
- Click "New Source" button
- Select Website then Webpage
- Complete the template (check for spelling/grammatical errors)
- Click "Save" button
Creating Your Media Presentation
Depending on your assigned role, you will be creating either an animated slideshow, newsletter, or pamphlet. To create/design these media presentations you will be using the program Canva.
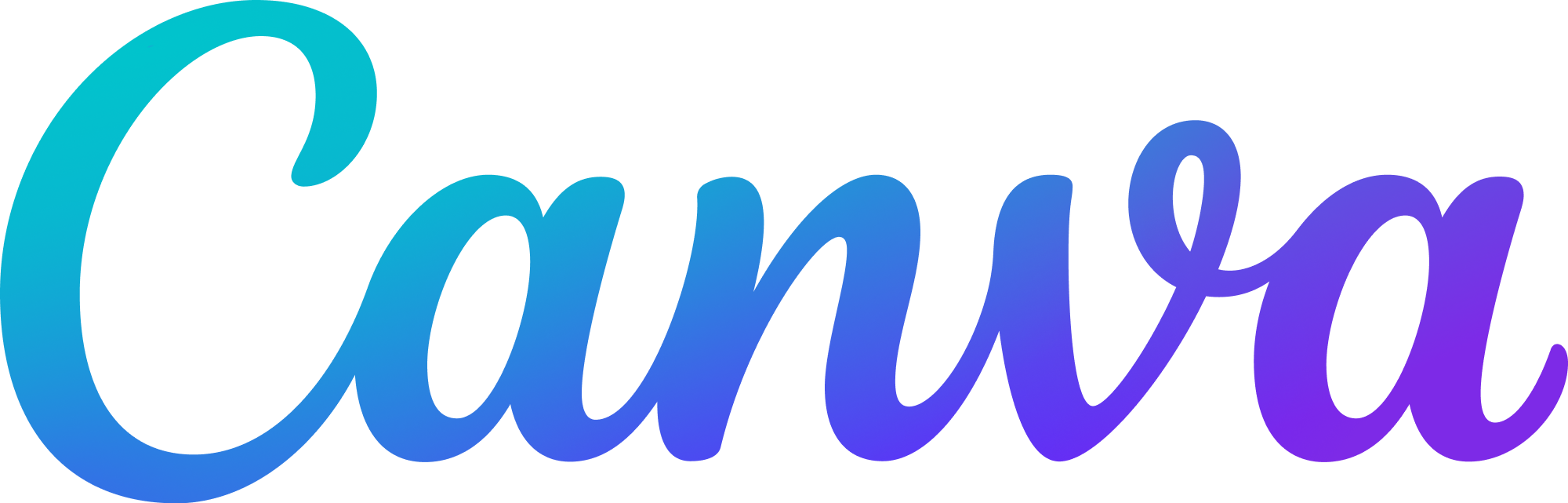
- Next: Evaluating Websites >>
- Last Updated: Feb 23, 2024 10:32 AM
- URL: https://hfhs-hf233.libguides.com/c.php?g=1305555
Please log in to save materials. Log in
- Resource Library
Gallery Walk
- Genetic Disease
- Inheritable Disease
- Research Project
Genetic Disorder Research-Poster Project 2021
Genetic disorder research-poster rubric, genetic disorder slide - tay sachs 2020 (5), human genetics gallery walk, genetic disorder research/powerpoint slide project.
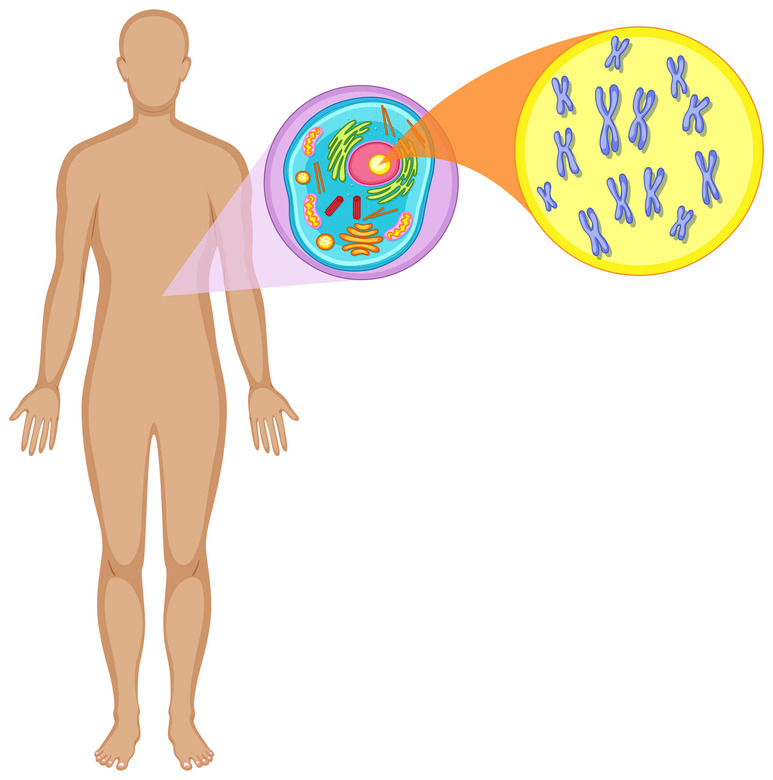
Students will use effective research skills to find and select appropriate information to create a "poster" to inform others about a genetic disorder. They will use their research to create a single PowerPoint slide to be used as a poster or fact sheet that presents information about the genetic disorder they select. The slide will be graded on the information presented, neatness, and legibility. Students will then share their research in a Gallery Walk to learn about the genetic disorders researched by their classmates. As they read/listen to the information presented for each project, they will take notes and provide comments.
Standards : NGSS and Iowa Common Core
HS-LS1.A : Structure and Function - Discplinary Core Ideas
Systems of specialized cells within organisms help them perform the essential functions of life. (HS-LS1-1)
All cells contain genetic information in the form of DNA molecules. Genes are regions in the DNA that contain the instructions that code for the formation of proteins, which carry out most of the work of cells. (HS -LS1-1)
Share Suggestion
Genetic disorder project, lesson plan, grade levels, course, subject.
- Printer Friendly Version
Explain that the information passed from parents to offspring is transmitted by means of genes which are coded in DNA molecules.
Explain the basic process of DNA replication.
Describe the basic processes of transcription and translation.
Explain how crossing over, jumping genes, and deletion and duplication of genes results in genetic variation.
Explain how mutations can alter genetic information and the possible consequences on resultant cells.
Describe how the process of meiosis results in the formation of haploid gametes and analyze the importance of meiosis in sexual reproduction.
\Compare and contrast the function of mitosis and meiosis.
\Illustrate that the sorting and recombining of genes in sexual reproduction results in a great variety of possible gene combinations in offspring.
Describe the basic structure of DNA , including the role of hydrogen bonding.
Explain how the process of DNA replication results in the transmission and conservation of the genetic code.
Describe how transcription and translation result in gene expression.
Differentiate among the end products of replication, transcription, and translation.
Cite evidence to support that the genetic code is universal.
Explain how genetic technologies have impacted the fields of medicine, forensics , and agriculture
Describe how Mendel’s laws of segregation and independent assortment can be observed through patterns of inheritance.
Distinguish among observed inheritance patterns caused by several types of genetic traits (dominant, recessive, codominant, sex-linked, polygenic, incomplete dominance, multiple alleles)
CONSTANCY AND CHANGE
Explain how the processes of replication, transcription, and translation are similar in all organisms. Explain how gene actions, patterns of heredity, and reproduction of cells and organisms account for the continuity of life.
Demonstrate how inherited characteristics can be observed at the molecular, cellular, and organism levels.
Heterozygous
Sex-linked trait
X chromosome
Y chromosome
The primary objective of this project is to allow students to apply their conceptual knowledge of genes and genetic disorders to explore a documented genetic disorder. The students will produce technology enriched projects that will use various media such as digital documents and digital videos to create a final product which will serve to either inform or persuade a predetermined audience.
Lesson Essential Question(s)
Students will utilize five school days (each class period is block schedule for 81 minutes) to complete the activity, in additional to several days provided outside of the class as well. Students will be given a minimum of one day in school to become familiar with their project and explore the library resources available for their use, two days in school to work on their projects within their group and respected roles, and one day in school to generate a cohesive final product. Students will be given approximately two weeks of time outside of the school day to complete the activity and edit their work before it is submitted for grading.
Students will have access to the related technology hardware and software during the school day. Students will also have access to these tools after the scheduled school day and during non-academic times by utilizing the library facilities.
The software available will include but are not limited to Microsoft Word, Microsoft Publisher, Microsoft MovieMaker, and Microsoft Photostory. These are available on all campus computers. Some of these tools are also available at www.windows.com as a free download. If you have any trouble accessing any of the tools you wish to you, please do not hesitate to contact me or ask a computer technician for assistance.
Suggested Instructional Strategies
I will begin the lesson by showing students an exemplary project from one of the previous years. We will discuss the purpose of the lesson and what vehicle the student and their group used to achieve the ultimate goal.
Students will become engaged in their project after exploring the various topics available. Students will look at real case studies and real documented genetic disorders to apply their concepts from the genetics unit.
Students will be provided with the tools necessary to examine the case studies in detail. Students will also have the opportunity to meet with a genetic counselor how they can use as an expert source of information and real life experiences.
Students will be asked to reflect, revisit, revise, and rethink their projects at various points throughout the project. Students will meet as groups and within their respected roles to discuss their topics and evaluate their projects.
Students are asked to evaluate their own experience, the experience within their group, and their experiences within their respected roles.
Students will have the choice of various roles within their respected topics. Students will be able to select a role which is tailored to their unique ability and their level of technology comfort.
Students are given the chance to research their topic and generate a project which is conceptual but also applicable to real life scenarios. Students produce a final product which demonstrates their understanding and application of the course content, as they relate to their given topics.
Instructional Procedures
Students will be asked to work in groups of four to complete this project. Students working within the same group will research the same genetic disorder. Each of the students will assume a role within their respected group. Each group member will be responsible for producing a final product on the same genetic disorder. Students will stay within their groups once the project is started since their research is dependent on the role within each group. Please select your group wisely.
Note – If your school has a literacy coach, please invite them to participate in the RAFTS portion of the project. The students will assume a ROLE, AUDIENCE, FORMAT, and TOPIC, and STRONG VERB for each portion of their project. This practice aligns with Reading Apprenticeship activities.
Introduction
Students will be asked to research a genetic disorder and produce a digital document or video that demonstrates their understanding of the disorder. Students should look at the rubric provided for each of the roles within their group. Each rubric provides a document option (such as a letter) and a technology option (such as a video) for each role. Extra credit is given for students who select the technology related role, but students should assume the additional responsibility for taping and editing their video which may take more time than the traditional document format which is available. Once students are within their groups and their roles are assigned they should research their topic. On the last page of the rubric is a group reference section which listed the number and type of resources which must be found. This will be used as a group to produce a MLA formatted document. One reference section is needed per group, not per student. Since students are working within their group on the same genetic disorder, they should share their resources with their group members.
Formative Assessment
Students will be asked to conference within the group and also within their roles (this will require all students making a video for example to work together or students with the same role within their respected project). Students will also edit and revise their projects using their rubric as a guide within their group. Peer editing is essential in making sure a final product is presentable and meets the requirements on the rubric.
Product Evaluation
Students will submit all projects electronically. Please note the required format on each rubric before submitting your final project. Certain attachments and files will get filtered by the security software within the district so please use only the file formats that are required. Failure to submit your project in the correct file format will result in your project not being graded. Late points may be assessed.
During this activity, students will be monitored to ensure each group is on task and working on their project goal. Students will be asked after each class session they are given time to work on their project to submit a list of questions they may have or help they need for the next work session. This will allow the teacher time to research or explore questions in order to help the students during the next work session.
Related Materials & Resources
Genetic Disorder - Information Sheet.doc Genetic Disorder Project Sign Up Sheet.doc Introduction to Genetic Disorder - RAFTS.ppt
Date Published
Clinical Trials
Genetic disorders.
Displaying 12 studies
The objectives of this study are to perform molecular and/or biochemical testing in individuals with suspected hereditary disorders, and/or their family members, to further elucidate possible mechanisms of disease and/or inheritance, and/or to help yield a final laboratory diagnosis at the judgement of the original ordering healthcare provider.
The primary purpose of this study is to evaluate the effectiveness and safety of ZX008 in subjects with CDD.
The purpose of this study is to survey individuals and families on their experience of receiving a diagnosis of a sex chromosome abnormality and any follow-up services or support they received, and to solicit information on suggested best practices for the return of a diagnosis and subsequent support services in clinical and social care.
The purpose of this study is to identify patients with rare genetic disorders that may potentially benefit from an ASO therapeutic based on the characteristics of their genetic disease and the causative genetic alterations and seek the research and development opportunities to have them developed, to have the safety and toxicity studies performed with the ultimate goal of treating the individual in a future N of 1 clinical trial.
This study will help the investigator determine whether certain genetic mutations, more than others, are a cause of more severe disease in Dent Disease.
The purpose of this study is to investigate whether a new high-resolution analysis of MLCL and/or CL can uncover additional CL subspecies that enhance sensitivity of this ratio or improve the signal-to-noise than previously published studies.
The purpose of this study is to analyze genome-wide methylation patterns in DNA from patients with suspected hereditary disorders but for whom all previous genetic testing has been negative and/or equivocal.
The objectives of this study are to perform genomic testing and trio analysis on specimens from three deceased patients with partial OTC deficiency who died unexpectedly while receiving treatment, along with testing on blood from their 5 parents, to determine whether there were any other genetic factors that may have contributed to sudden, unexpected death of the patient. In addition, medical records analysis and case summary would be carried out for all deceased patients with known partial OTC deficiency.
To consider whether further investigation into the pathogenesis of OTC deficiency is warranted to find previously unidentified risk ...
This laboratory study is looking at genetic mutations and environmental exposure in young patients with retinoblastoma and in their parents and young healthy unrelated volunteers. Gathering information about gene mutations and environmental exposure may help doctors learn more about the causes of retinoblastoma in young patients.
Patients with alpha-1 antitrypsin deficiency are prone to having an imbalance of lung Elastin degradation which render the patient's lung’s susceptible to inflammatory insults. We have postulated that a severe COVID infection would accentuate this inflammatory imbalance and be more likely to lead the patient into a hospitalization event. Our hypothesis would be to determine if this genetic mutation is found more frequently and patients who have been hospitalized with COVID.
The purpose of this study is to form processes to get findings from targeted gene sequencing that can be acted on using two common actionable genetic disorders–familial hyperlipidemia and familial colorectal cancer.
This is an interview study to understand the views of people with the lived experience of 10 different genetic conditions on gene modification therapies, and specifically on prenatal gene editing. Prenatal gene editing is not happening now, but it is possible that prenatal gene editing will be available in the next few years, at least in a research setting, and we want to know your thoughts about the direction this technology is going. We to hope speak with many different stakeholders (patients and their families, clinicians, scientists) with diverse perspectives to understand values and priorities for prenatal gene ...
Mayo Clinic Footer
- Request Appointment
- About Mayo Clinic
- About This Site
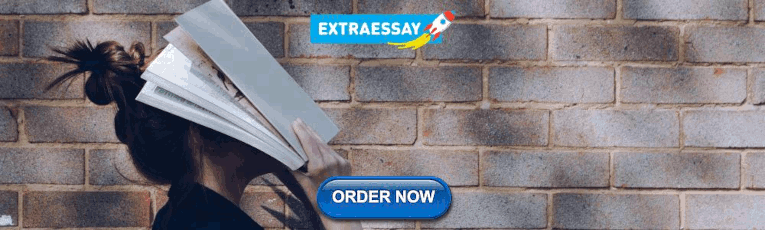
Legal Conditions and Terms
- Terms and Conditions
- Privacy Policy
- Notice of Privacy Practices
- Notice of Nondiscrimination
- Manage Cookies
Advertising
Mayo Clinic is a nonprofit organization and proceeds from Web advertising help support our mission. Mayo Clinic does not endorse any of the third party products and services advertised.
- Advertising and sponsorship policy
- Advertising and sponsorship opportunities
Reprint Permissions
A single copy of these materials may be reprinted for noncommercial personal use only. "Mayo," "Mayo Clinic," "MayoClinic.org," "Mayo Clinic Healthy Living," and the triple-shield Mayo Clinic logo are trademarks of Mayo Foundation for Medical Education and Research.
- Introduction to Genomics
- Educational Resources
- Policy Issues in Genomics
- The Human Genome Project
- Funding Opportunities
- Funded Programs & Projects
- Division and Program Directors
- Scientific Program Analysts
- Contact by Research Area
- News & Events
- Research Areas
- Research investigators
- Research Projects
- Clinical Research
- Data Tools & Resources
- Genomics & Medicine
- Family Health History
- For Patients & Families
- For Health Professionals
- Jobs at NHGRI
- Training at NHGRI
- Funding for Research Training
- Professional Development Programs
- NHGRI Culture
- Social Media
- Broadcast Media
- Image Gallery
- Press Resources
- Organization
- NHGRI Director
- Mission & Vision
- Policies & Guidance
- Institute Advisors
- Strategic Vision
- Leadership Initiatives
- Diversity, Equity, and Inclusion
- Partner with NHGRI
- Staff Search
Genetic Disease Research Branch
The Genetic Disease Research Branch studies the mechanisms by which genetic changes affect the structure and function of gene products leading to human disease.
A major focus of the branch's research lies in understanding how disruptions in signaling pathways and transcription factors contribute to disease. Our investigators use genetics and genomic approaches in both human and mouse systems to identify and better understand pathways involved in human genetic diseases and normal development. Model systems, including genetically altered mice and in vitro cell and organ culture systems, are major components of these investigations. Ongoing efforts include research aimed at understanding genetic contributions to a number of human diseases, particularly those affecting the nervous, immune and musculoskeletal systems. As a major emphasis of their work, our investigators use genetic and genomic tools to understand normal development and differentiation.
Our Research
- Lowe Syndrome Mutation Database
- Pallister-Hall Syndrome
Last updated: March 24, 2022
- Seeking Patient Care?
- John P. Hussman Institute for Human Genomics (HIHG)
- Education and Training
- Division of Genomic Outreach
- Genetics Awareness Project
- What is Genetics?
What is Genetic Research?
Genetic research is the study of human DNA to find out what genes and environmental factors contribute to diseases. If we find out what causes disease, we can better detect disease, better treat disease and hopefully even prevent disease from happening in the first place!
Nearly every disease we know of has a genetic component. Depending on the disease, the genetic contribution may be very large, very small, or, most often, somewhere in between. Researchers are studying nearly every disease to find out how genetic factors may contribute. We inherit our genes from our parents. Genes tell our bodies how to develop and function. There are about 25,000 genes that make the things our bodies need to develop and work. Each gene is made up of DNA. DNA is a very long code that uses only 4 letters. We know that variations in this code can alter the way a gene works. Sometimes the variation is in only one letter. This is referred to as a SNP. Most SNPs don’t cause problems. They either contribute to the normal differences from one person to another, or have no known effect at all. However, a small number of SNPs can alter the way the gene works and contribute to disease. Sometimes the DNA code is altered by pieces that are missing or are extra. The missing or extra pieces may be as small as a one letter or as large as thousands. Depending on what is missing or extra, this may also contribute to health issues.
Research involving families
Diseases such as autism, Alzheimer disease, heart disease, and cancer are common. Approximately 1 out of every 110 children is affected by autism, 1 in 10 adults will develop Alzheimer disease and 1 in 3 adults will develop cancer in their lifetime. Since these are common diseases, we expect to see that sometimes more than one person in a family may have the condition. However, when a family health history is taken, some families have a much higher number of people with a certain complex disease than other families do. For example, imagine a family in which a great-grandmother, grandfather, and mother (three generations) were all affected by Alzheimer disease. We known that closely related members of a family share many of their genes. In fact, we share half our genes with our parents, brothers and sisters, and children! It is likely that there are genes shared by members of that family that are involved in the development of Alzheimer disease. By involving this family in genetic research, scientists can learn which genetic differences are shared by the family members who have Alzheimer disease, but not found in others in the family. These genetic differences narrow down the region where a gene might be. This technique of finding genes using a family is called a family study, or linkage analysis.
Research involving large groups of people
Another way of doing genetic research is to do DNA testing on two large groups of people. One group of people has the disease, and the other does not. Those who do not are sometimes referred to as controls. Most often, the people in this type of study are not related to one another. This technique is called association. Association studies rely on the use of thousands to millions of SNPs (described above) in our DNA. If you could imagine our DNA as huge roadmap, SNPs would be like mile markers on the highway. Scientists know the location of millions of SNPs throughout human DNA. Scientists also know that at the location of these SNPs, there is variation in the DNA code from one person to the next. For example, at one SNP, one person may have a letter T (thymine), while another person has a G (guanine). If a particular DNA SNP is seen more often in those with a disorder, such as autism or Alzheimer disease, it is possible that this SNP is associated with or is near an area of DNA that is associated with the disorder. Association studies are very large projects and require the participation of hundreds to, preferably, thousands of people with a disease and without a disease.
Research involving candidate genes
When trying to find genes that contribute to a disorder, sometimes researchers will look specifically at candidate genes. Candidate genes are those that researchers think might be involved in a disorder either because of what they do, and/or because they are located in an area of the DNA that looks interesting based on family studies or association studies described above. For example, some of the candidate genes that have been looked at for autism are known to be involved in behavior or language.
Diversity in genetic research is crucial!
It is important for all ethnic groups to be represented in genetic research. This is because people of the same ethnic group share many of the same changes and variations in their DNA with each other that they may not share with people of a different ethnic group. If only one ethnic group is involved in genetic research, we learn only about the variations in DNA that are associated with disease in that particular ethnic group.
When genetic research searching for genes involved in breast cancer was just beginning, most women who participated in genetic research studies were Caucasian. That research lead to the discovery of two very important genes (BRCA1 and BRCA2) that are now known to put women who have mutations in these genes at high risk for breast and ovarian cancer. It was found out that many mutations in the BRCA genes can lead to a high risk for cancer. However, only those mutations that were common in the Caucasian population were discovered through the initial research projects. This meant that when a non-Caucasian woman was tested for mutations in the BRCA genes by her doctor, there was a high chance that the results would not be conclusive. After many years of additional research, genetic testing for breast cancer has greatly improved for non-Caucasian women and testing of these genes is now more beneficial for them. By including all ethnic groups in genetic research, all ethnic groups can benefit from the findings of genetic research.
How to get involved in genetic research
At the John P. Hussman Institute for Human Genomics at the University of Miami’s Miller School of Medicine, our research efforts are aimed to ensure that all people, regardless of race or ethnicity, are able to benefit from the research that is done at our institute. Our researchers invite participation from the diverse South Florida community as well as larger national and international communities. Participation in a research study at the HIHG is completely voluntary. While details may vary from study to study, trained members of our team will conduct family history interviews and diagnostic evaluations. In addition, our team members will retrieve a blood or saliva sample. Some research studies ask for one-time participation only, while others request that participants are involved in several appointments. There is no cost or payment for your participation. We maintain the highest standards of confidentiality for all participants and families. Your private information is not given out to other researchers or companies. Your participation will help us to better understand the genetic and environmental causes of diseases and disorders, which may eventually lead to improved treatments and prevention methods, and hopefully a cure. Some research studies offer compensation for participation.
To learn more about the individual research studies ongoing at the HIHG, please visit the HIHG website (www.hihg.org). If you are interested in participating in one of our research projects, please visit the HIHG website or contact us:
Email : [email protected] Telephone : 1-877-686-6444 Fax : 305-243-2396
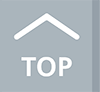

7th Grade Genetic Disorders Project: Home
Project description.
You will be researching an assigned genetic disorder.
- Genetic Disorders Project instruction sheet
- Genetic Disorder Rubric
- Genetic Disorders Creation Guide
Resource Requirements
Information you must include:
- Question 1 o Identify the disorder o What causes it? How can a person inherit the disorder?
- Question 2 o What symptoms, side effects, or harm does it cause to the human body?
- Question 3 o Does this genetic disease or disability mostly affect a certain age group, ethnic group, gender group, etc.? For example: It occurs only in males.
- Question 4 o Are there any treatments, medications, or therapies an individual must go through in order to stay alive?
- Question 5 o What is the life expectancy of someone with this disorder? How long can someone live with the disorder?
- Question 6 o What does a typical day look like for someone with this disorder?
- Works Cited o Works cited page created in Noodle Tools crediting the sites where you found your information.
Find Books in the FWCD Library
Fwcd library catalog, search for books in the library catalog., don't see the book you're looking for ask the librarians if the library can purchase it for the collection. , timeline and due dates.
Tuesday, January 23rd- Project due.

Gale in Context: Middle School provides support for middle school students to complete assignments in literature, science, social studies, history, and more.
- Teens Health
- US National Library of Medicine
- Center for Disease Control
- World Health Organization Under Health Topics search for your topic.
NoodleTools
NoodleTools uses Single Sign-On with your FWCD Google account, so you don't need a separate login and password.
1) Log in to your FWCD email account.
2) Click on the "waffle" (grid) at the top right corner, and keep scrolling until you find the NoodleTools app.
3) Choose your FWCD email account to login.
• NoodleTools Quick Guide for Students
• Tutorials on Using NoodleTools
FWCD Library Database Passwords
- DATABASE PASSWORDS FOR ACCESS FROM HOME (FWCD Google login required)
Login and password information is also available for parents in the Resources section of your FWCD portal account .
- Last Updated: Jan 4, 2024 10:18 AM
- URL: https://fwcd.libguides.com/geneticdisorders
Thank you for visiting nature.com. You are using a browser version with limited support for CSS. To obtain the best experience, we recommend you use a more up to date browser (or turn off compatibility mode in Internet Explorer). In the meantime, to ensure continued support, we are displaying the site without styles and JavaScript.
- View all journals
- Explore content
- About the journal
- Publish with us
- Sign up for alerts
- RESEARCH BRIEFINGS
- 03 April 2024
Five steps to connect genetic risk variants to disease
This is a summary of: Schnitzler, G. R. et al . Convergence of coronary artery disease genes onto endothelial cell programs. Nature 626 , 799–807 (2024) .
Access options
Access Nature and 54 other Nature Portfolio journals
Get Nature+, our best-value online-access subscription
24,99 € / 30 days
cancel any time
Subscribe to this journal
Receive 51 print issues and online access
185,98 € per year
only 3,65 € per issue
Rent or buy this article
Prices vary by article type
Prices may be subject to local taxes which are calculated during checkout
doi: https://doi.org/10.1038/d41586-024-00061-4
Aragam, K. G. et al. Nature Genet. 54 , 1803–1815 (2022).
Article PubMed Google Scholar
Claussnitzer, M. et al. Nature 577 , 179–189 (2020).
Dixit, A. et al. Cell 167 , 1853–1866 (2016).
Adamson, B. et al. Cell 167 , 1867–1882 (2016).
Snellings, D. A. et al. Circ. Res. 129 , 195–215 (2021).
Download references
Reprints and permissions
Related Articles
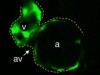
- Cardiovascular biology
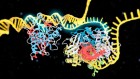
Advanced CRISPR system fixes a deadly mutation in cells
Research Highlight 04 APR 24
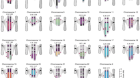
The variation and evolution of complete human centromeres
Article 03 APR 24
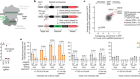
Improving prime editing with an endogenous small RNA-binding protein
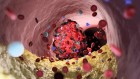
Gut bacteria break down cholesterol — hinting at probiotic treatments
News 02 APR 24
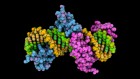
‘Epigenetic’ editing cuts cholesterol in mice
News 28 FEB 24
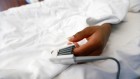
Crackdown on skin-colour bias by fingertip oxygen sensors is coming, hints FDA
News 02 FEB 24
High-Level Talents at the First Affiliated Hospital of Nanchang University
For clinical medicine and basic medicine; basic research of emerging inter-disciplines and medical big data.
Nanchang, Jiangxi, China
The First Affiliated Hospital of Nanchang University
POSTDOCTORAL Fellow -- DEPARTMENT OF Surgery – BIDMC, Harvard Medical School
The Division of Urologic Surgery in the Department of Surgery at Beth Israel Deaconess Medical Center and Harvard Medical School invites applicatio...
Boston, Massachusetts (US)
Director of Research
Applications are invited for the post of Director of Research at Cancer Institute (WIA), Chennai, India.
Chennai, Tamil Nadu (IN)
Cancer Institute (W.I.A)
Postdoctoral Fellow in Human Immunology (wet lab)
Join Atomic Lab in Boston as a postdoc in human immunology for universal flu vaccine project. Expertise in cytometry, cell sorting, scRNAseq.
Boston University Atomic Lab
Research Associate - Neuroscience and Respiratory Physiology
Houston, Texas (US)
Baylor College of Medicine (BCM)
Sign up for the Nature Briefing newsletter — what matters in science, free to your inbox daily.
Quick links
- Explore articles by subject
- Guide to authors
- Editorial policies

‘Race’, anti-racism and biology
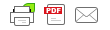
U sing biology to determine the racial ancestry of human remains is racist. Except when it’s done in the name of anti-racism.
We urge all forensic anthropologists to abolish the practice of ancestry estimation.
Biological ancestry, moreover, plays no role in susceptibility to disease. Except when it does.
Despite social scientific perspectives that endorse a nonbiological basis to race, within biomedicine, biological uses of race remain entrenched due to their utility for identifying the causes of the disease.
Artificial intelligence (AI) systems identifying ‘race’ from medical images perpetuate systemic racism. Except when doing so ameliorates racial health disparities.
AI models can predict the demographics of patients, including race, directly from medical images, even though no distinguishing anatomical or physiological features are evident to human clinicians.
Above all, ‘race’ has no basis in biology. Except when it has.
Such contradictory conclusions can be drawn from an increasing number of studies of ‘race’, health and disease. The mixed messaging here is not merely contradictory and confusing; it potentially harms those from racial populations already bearing the brunt of health inequities, while also hindering efforts to close these often deadly divides.
Scientific racism?
To understand why, let’s begin with a standard example of this kind of research — a recent peer-reviewed academic study on apparent racial differences in plague deaths in 14 th -century England. The analysis, based on skeletal remains from three medieval cemeteries in London, found “a significantly higher proportion of people of estimated African affiliation in the plague burials compared to the nonplague burials”. These findings were widely reported in the mainstream media (such as, in the UK, the left-leaning Guardian and the right-leaning Telegraph ), with the BBC reporting the study under the headline, “Black women most likely to die in medieval plague”.
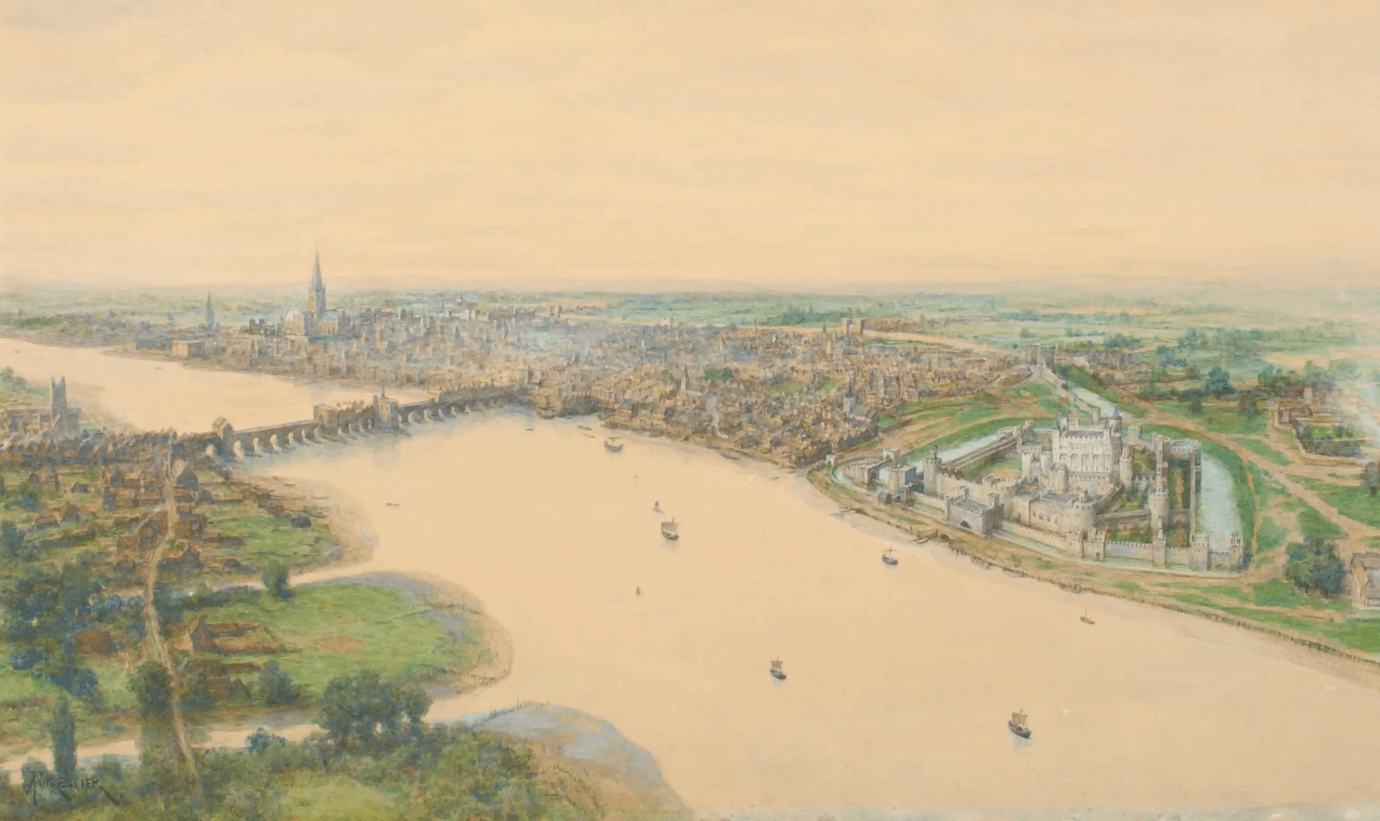
The study’s authors accept that these apparent racial differences in mortality might be an artifice of the small sample sizes (the remains of 145 individuals) used as the basis for the research. Nonetheless, their favored conclusion is that “these findings may reflect premodern structural racism’s devastating effects”. Of most relevance here, however, are two claims repeatedly emphasized throughout the researchers’ paper:
[1] the incorrect and harmful implication that there is a biological basis of race, and … [2] the incorrect inference that there is something inherent to people assigned to a certain racial category that makes them more vulnerable to disease
Racial identity and “decentering”
An obvious initial question, if (as the authors claim) race has no biological basis, is: how was an “estimated African affiliation” of centuries-old skeletal remains ascertained? The study acknowledges that racial affiliations were ultimately based on specific “macromorphoscopic traits” (features of the skull and facial bones) that differ between populations — in this case, “five traits with known heritability: anterior nasal spine, interorbital breadth, nasal aperture width, inferior nasal aperture, and nasal bone contour”.
Given that such traits are themselves the result of divergent biological evolution between geographically distinct ‘racial’ populations, this immediately raises another obvious question: does this not therefore reflect at least some underlying biological basis to race?
The researchers sidestep this issue. Instead, they argue that their analysis “includes data and tools that have been rightly critiqued for their role in perpetuating systemic racism, specifically the use of macromorphoscopic traits”. The authors — “keenly aware of the twinned whiteness of both anthropology and medieval studies” — suggest that to address this suspect historical aspect of their study, “whiteness must be decentered”. They “choose to foreground contexts and complexities, as well as white supremacist genealogies in forensic anthropology and medieval studies, as a form of methodological praxis as process”. In addition, to further “detach” the racial affiliation estimates from the racist “underpinnings of forensic anthropology,” the authors utilize cranial data that is “not attached to definitions of race and ethnicity”.
Although it is unclear what much of this rhetoric means, the authors appear to implicitly adhere to the widely-held ‘skin-deep’ concept of race. This view accepts superficial physical biological differences between racial groups but vehemently rejects the possibility of ‘deeper’ variation, such as cognitive or behavioral differences and in some cases even morphological differences . Such categorization, say many sociologists and cultural anthropologists, is what they call “scientific racism”.
While understandable as a reaction to historical and ongoing (albeit less prevalent) racist attitudes, denying any meaningful biological differences between racial populations can itself exacerbate existing racial disparities. This becomes clear when examining the other claim made above: that it is inherently wrong to assume that “people assigned to a certain racial category” may be more vulnerable to disease.
Disease susceptibility
While emphasizing that “race is a social classification and is not based in biological reality,” the study’s authors also state “that variation by race in susceptibility to and hazard of dying from disease reflects the biological and psychosocial effects of racism”. This seems to suggest a belief that increased vulnerability to a specific disease is the result solely of social behavior and prejudice.
Beyond a doubt, social factors — including racism — play a major role in health disparities between racial groups. But social environment is not solely the cause.
Equally beyond doubt, biological ancestry plays a crucial role in the prevalence and/or virulence of certain diseases in some populations and not others — the West African origins of sickle cell disease (an unfortunate by-product of increased evolved immunity to malaria) is a classic example, as are various hereditary genetic disorders (such Tay-Sachs and Gaucher disease) in Ashkenazi Jewish populations.
Other health disparities may be a combination of environmental factors, such as eating habits, and (biologically-mediated) genetic factors. Black Americans are 20% more likely to get colorectal cancer and die at a much higher rate, in part because, as a group, they consume greater amounts of animal fat than other ‘racial’ groups. Now researchers have identified gene mutations in cancer patients of African ancestry showing they are less likely to respond well to newer treatments.
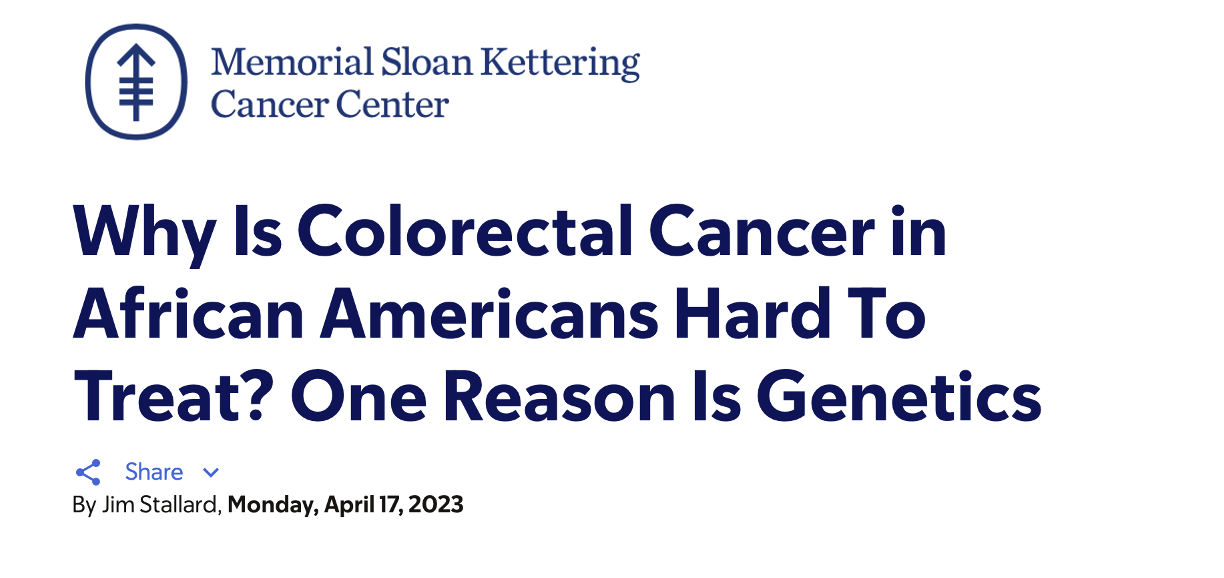
The point is that simply blaming racism for all disparities in disease susceptibility and mortality may blind us to potentially crucial biological or genetic factors.
Exacerbating this issue is the insistence that race has no biological basis beyond, at most, superficial physical traits. This directly contradicts overwhelming evidence of meaningful genetic differences between racial groups (as discussed by GLP director Jon Entine and me with racial differences in the effects of Covid 19 and HIV/Aids). Ignoring this data, (as many scientists did even two years into the pandemic), risks derailing efforts to effectively tackle diseases that disproportionately affect marginalized communities.
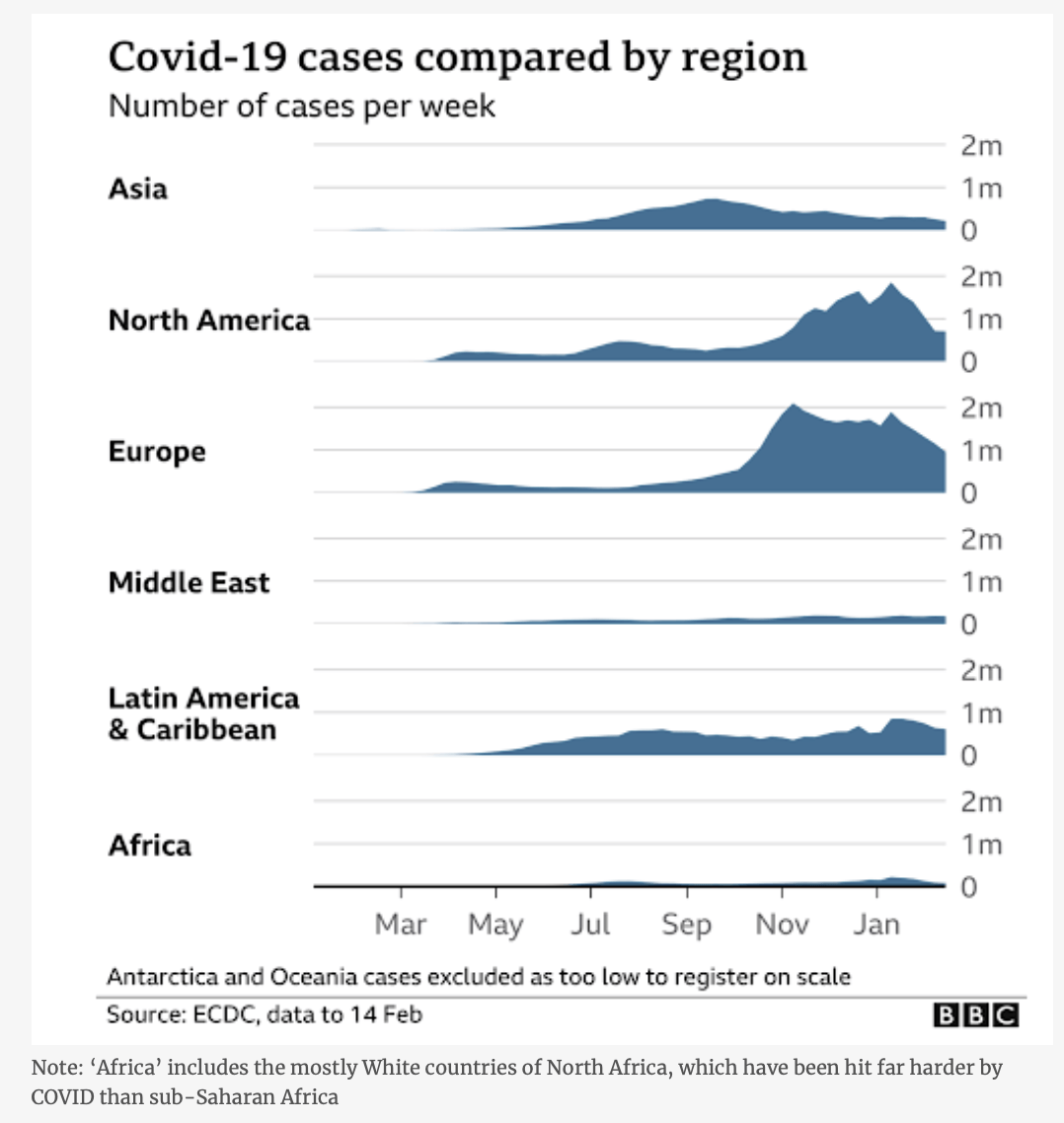
For example, ‘biobanks’ (repositories of human genetic data) are already hugely skewed towards those of European ancestry. Take the UK Biobank, a biomedical database of “genetic, lifestyle and health information and biological samples from half a million UK participants”. While this is an invaluable resource for “the prevention, diagnosis, and treatment of a wide range of serious and life-threatening illnesses,” it only reflects the genetics of the British population as a whole — that is, overwhelmingly northern European. It is much less useful, however, in predicting health outcomes of those from other racial populations, whose genetic traits may differ across thousands of small genetic variations.
As psychologist Jonathan Anomaly points out , the lack of similar biobanks in, say, Africa or South Asia (that is, areas with poor health infrastructure and greater disease prevalence) means many of the most marginalized people in the world will be far less able than Europeans to “mitigate genetic risks through lifestyle changes and early medical interventions”. Further, Anomaly argues, that potential parents from other racial groups attempting in-vitro fertilization will also miss out on the choices increasingly available to prospective European parents.
Research taboos on ‘race’
In explaining this mismatch among different racial groups in the collection and use of useful genetic data, Anomaly points to “taboos surrounding research into genetic differences in socially significant traits” — that is, as highlighted above, the reluctance of many Western academics to accept that biology plays a part in racial variation and in differences in health outcomes.
Anomaly goes on to suggest, “Many social justice advocates say they want to help disadvantaged or poorly performing racial groups. But the taboos they’ve helped create in modern genetics research may end up depriving some ethnic groups of the opportunities that others will have.” (For his thoughtful analysis, Anomaly has been described as a “eugenicist” with “far right connections”.)
The plague study examined above also neatly illustrates another of Anomaly’s claims, that “the scientific establishment in Western liberal democracies has thoroughly absorbed the central dogma holding that race is an illusion, and that racial differences cannot exist”. Evidence of this is, for example, the “ ethical guidance ” by the editors of Nature Human Behaviour that unequivocally states: “Race and ethnicity are sociopolitical constructs. Humans do not have biological races, at least based on modern biological criteria for the identification of geographical races or subspecies.”
How, though, does this square with the “biological criteria’ (i.e., myriad genetic variations) that differentiate racial populations — biological differences that, moreover, have significant impacts on health outcomes?
Edging towards post-modernist nihilism
Fortunately, at least as yet, the scientific establishment has not embraced another feature of activist ‘scholarship’: a relativist approach to ‘truth’ (the idea that factual truth is relative to individuals’ cultural or social background). Consider the authors of the plague study, who claim their work aims to generate “multiple perspectives on truth … enabling a ‘pluralistic approach’ to a myriad of historical truths and methodological discoveries”. While historical events are often open to multiple interpretations, relativism extends and distorts this notion to imply that factual truth (like beauty) lies solely in the eye of the beholder — that ‘facts’ and ‘truth’ are determined by culture entirely. At an extreme, this fosters the insidious belief that science itself lacks objectivity and that so-called scientific ‘facts’ are nothing more than a reflection of Western colonialist biases.
However, by its own logic such relativism is incoherent and self-defeating; any claims made by relativist researchers are no more valid than any alternative ‘perspectives on truth’ that argue the opposite.
Interestingly, the relativist authors of the plague study demonstrate that they do accept some absolute truths — for example, in their claim that “the truth is that race (structural racism) was invented, refined, and rehearsed in medieval England”. The truth, it seems, is relative, except when it suits a specific ideological agenda. Consider the Sokal hoax.
The ‘post-modernist’ takeover of the social sciences began in the 1980s as a precursor to today’s social justice movement. It is marked by a general suspicion of reason and objectivity and an embrace of subjectivism and relativism. It was highlighted by the 1994 “ Sokal hoax ” in which a spoof academic article arguing that reality did not exist was published and lauded in a leading cultural studies journal.
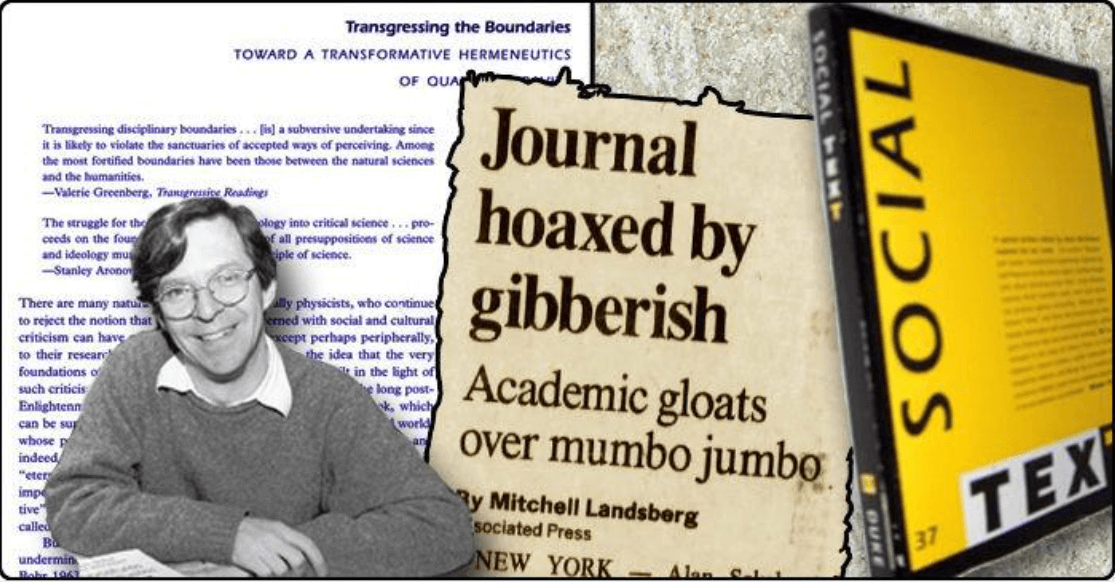
Written deliberately as academic gibberish, his article , “Toward a Transformative Hermeneutics of Quantum Gravity, passed peer review with flying colors, and appeared in one of the notorious post-modernist journals of that era, Social Text. The article’s author, physicist Alan Sokal, later explained he wanted to draw attention to and therefore halt the “wanton abuse of science” by influential sections of the radical left. Unfortunately, in the intervening years, such “ fashionable nonsense ” has become more not less prevalent, and is now creeping deep into the hard science.
‘Race’, discrimination and AI
Before examining other questionable aspects of the plague study example, let’s briefly turn to an actual example of how supposedly ‘progressive’ ideological beliefs about race and racism can have a negative impact on real world scientific practice: recent advances and use of artificial intelligence (AI) technologies in medicine (technologies that, in theory at least, might do away with subjective human bias).
A slew of recent studies indicate that artificial intelligence models “are able to predict a patient’s self-reported race from their medical images” — and, moreover, can do so from images “that contain no indications of race detectable by human experts”.
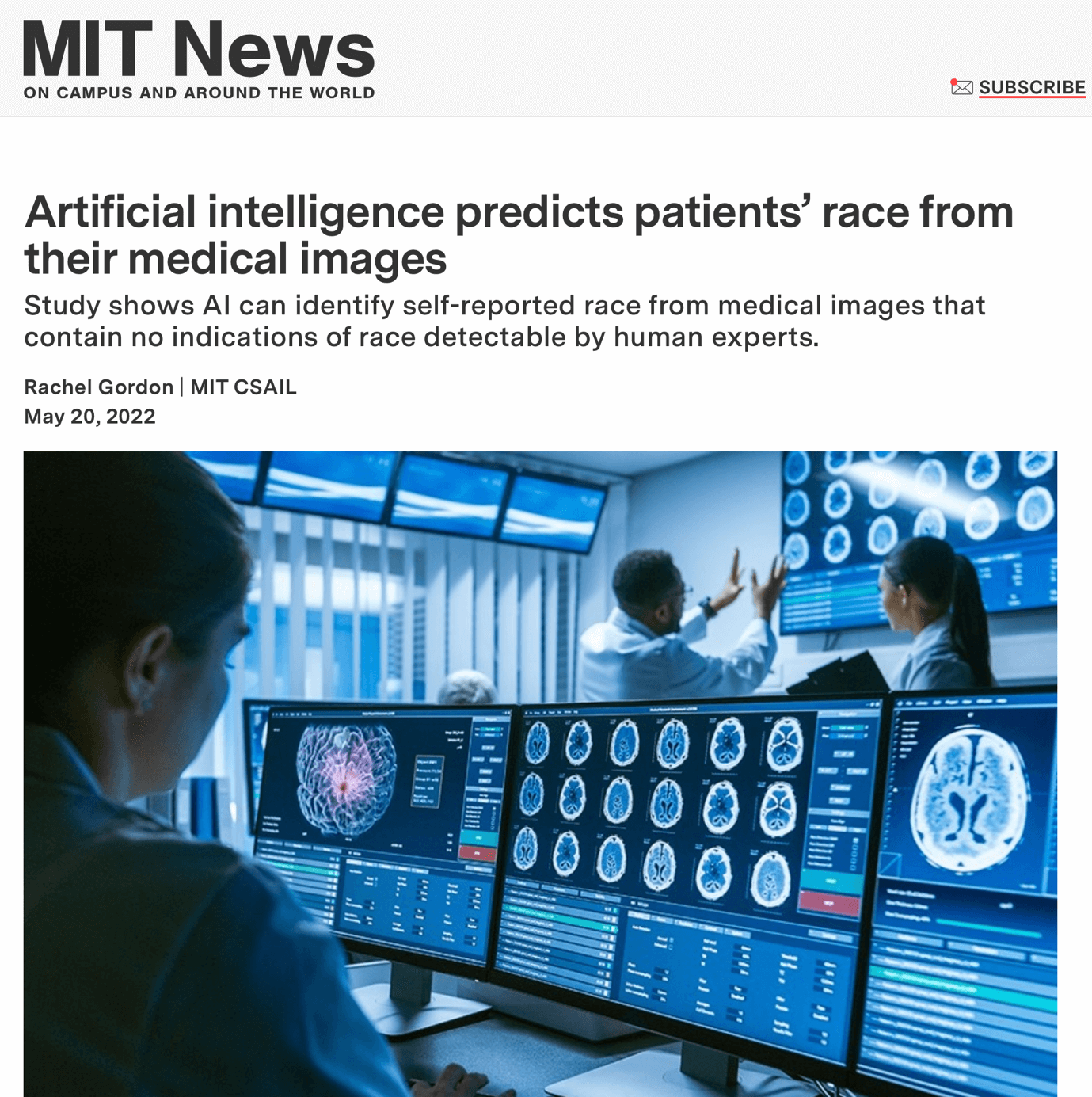
In itself, this seems to undermine the claim that there is no biological basis to race. It’s what we make of this information that determines whether this information is racially harmful or not.
These reports on AI’s uncanny capacity to predict race are also replete with warnings of how this “can perpetuate racial bias in health care” and how it “mirrors unconscious thoughts, racism, and biases … [that] can lead to serious harm”. Likewise, it “raise[s] concerns about the possibility of AI systems to discriminate” and “introduces the potential for AI models to be biased and create racial disparities”. At an extreme, it could even “have catastrophic consequences by propagating deeply rooted societal biases”.
Concerns about how AI might exacerbate racial biases in medicine are not unfounded. Like any tool, artificial intelligence systems are susceptible to misuse and bias — as evidenced, for example, by algorithms that “used health costs as a proxy for health needs” only to falsely conclude “that Black patients are healthier than equally sick white patients, as less money was spent on them”.
But automatically equating this AI’s use or capabilities with societal prejudices (including racism) is misguided. The fact that AI can distinguish race from medical images is inherently neutral; the potential for harm lies not in any predictions themselves, but in how this information is interpreted. Bias and discrimination are not inevitable.
As the erroneous health costs paper also points out, one way to overcome AI’s potential for unintentional bias is through ‘open science’ — for instance, by ensuring relevant AI research and methodology is open for rigorous evaluation by all who use or are impacted by it. Another of the reports above similarly indicates how AI can be a “force for good” by instancing how “algorithms that learn from patients’ pain experiences can find new sources of knee pain in X-rays that disproportionately affect Black patients — and are disproportionately missed by radiologists”.
Again, such knee-jerk rejection of obvious biological aspects of race has the potential to further exacerbate existing unfair disparities. Indeed, fueling suspicions about inherent racism and prejudice within medical science creates a vicious circle by deterring non-white groups from participating in research. Lack of diverse data and over-representation of those with European ancestry — as with the genetic biobanks above — would then, in turn, impede greater appreciation of diseases with higher prevalence in specific non-white ancestry groups, ultimately harming patients who could benefit from targeted treatments.
Given that much of this potentially harmful obsession with racism comes from without the scientific community, medical researchers should perhaps take the following remedy for potential AI prejudice with a large grain of salt: “We need to bring social scientists into the picture.”
Progressive prejudice?
Human behaviorist Ian Leslie is scathing about the social scientists behind the plague victim study and the distorting influence that these kinds of ideas have on the academic discipline of history:
Whole fields of historical study seem to have turned into competitions for who can generate the most eye-catching narrative of identity-based injustice, and if that means making blatantly implausible empirical claims, so be it.
But here this distortion (in addition to the debilitating relativism critiqued above) extends further, into the wider scientific enterprise. As an example: the plague study authors emphasize that “Black Methodologies Matter”. While this may sound suitably progressive, it is a patronizingly racist claim that assumes all black researchers would follow and approve of the same methodologies. While scholars from traditionally underrepresented backgrounds can and do provide valuable insights into previously overlooked biases, framing research along racial or ethnic lines implies there are inherent, monolithic “white” or “black” (or “Asian” or “Native American” or a myriad other) methodologies.
It also segregates science, suggesting that black people are innately suited to studying black subjects, Asians Asian ones, whites white subjects and so on — the kind of thinking that would not have been out of place in apartheid South Africa.
The plague study authors take this one step further by stating their “hope to prioritize the methodologies of Black feminist archaeology”. Ignoring the implication that ‘feminism’ too is monolithic (it isn’t), where does this process stop? What about disabled, say, or transgender feminists from different ethnic groups — do they also have methodologies that must also be “prioritized” in scientific studies?
Ironically, highlighting ever-finer ‘intersections’ of identity simply reflects a standard right-wing trope on the absurdities of identity politics, that of a “disabled, black, lesbian woman” at “the top of a hierarchy of oppression”. The reality is that scientists and researchers are individuals, and the complex interplay of influences reflected in their work is not reducible to simplistic ‘identity’ labels.
Black feminist researchers can certainly bring valid perspectives to science, but the idea of “Black feminist methodologies” is ideological not scientific. Science is universal and cross-cultural, and it does not care about scientists’ identity. Rational, evidence-based scientific inquiry does not discriminate on the basis of who you are. All that matters is whether researchers’ methods and conclusions withstand scrutiny. While racist (or sexist or other prejudiced beliefs) have certainly influenced science — and scientific institutions have indeed followed discriminatory practices in the past — the hallmark of the scientific enterprise is that it is self-correcting.
Eventually, erroneous assumptions are found and weeded out through other scientists’ ongoing rigorous critiques. Thus, the claim that science is inherently ‘white’ or male is ludicrous; indeed, those who trumpet such beliefs perpetuate the prejudiced nonsense that science may be beyond the capabilities of those who are not white or Western or male — or, even more blatantly racist and sexist, that only white males can successfully practice science.
Potential dangers of anti-racist ideology
The study of plague victims exemplifies how anti-racist activism can harm those it purports to support. It also shows how researchers, research efforts and funding can be pushed toward currently fashionable causes rather than towards useful findings.
For instance, fourteenth-century England was a deeply stratified society and it has long been apparent that differences in socio-economic status lead to differences in health outcomes. Health disparities have persisted into the modern era, even as overall health and life spans increase — the rich (of whatever ‘race’) are still healthier and longer-lived than their poorer peers.
Studies of the effects of plague in historical and deeply unequal societies could, therefore, add to our understanding of both the impact and causes of disease on those at different social levels. The more we find out about how and why this occurs, the better able we will be to help those most affected by disease and ill health.
Compare this to the research critiqued above, a study that presupposes (on ideological grounds) that all health disparities are due solely to racism. This automatically precludes other, potentially more significant factors.
A recent example of the limitations of focusing solely on social determinants of health – discussed in greater detail in the Entine/Whittle article cited above – is the contrasting impact of COVID-19 on black people in the US (with disproportionately high mortality) and in Africa (where the impact seemed puzzlingly limited). Ignoring possible genetic factors limits our ability to fully understand the complex interactions between the environment (including racism and marginalization) and biology. This hinders our search for effective solutions.
Similarly, if we refuse to acknowledge any biological differences between racial populations, we will find it difficult to explain, let alone effectively address, the disproportionately high impact of HIV/Aids on black Africans compared to other racial groups. In short, racial identity could be a factor in why some people and not others suffer from disease.
Obsessing over racial categories while trumpeting questionable ideological beliefs does a disservice to science and, more importantly, to those from racial minority groups who currently gain least from advances in scientific and medical practice.
Patrick Whittle has a PhD in philosophy and is a freelance writer with a particular interest in the social and political implications of modern biological science. Follow him at patrickmichaelwhittle.com . Find Patrick on X @WhittlePM
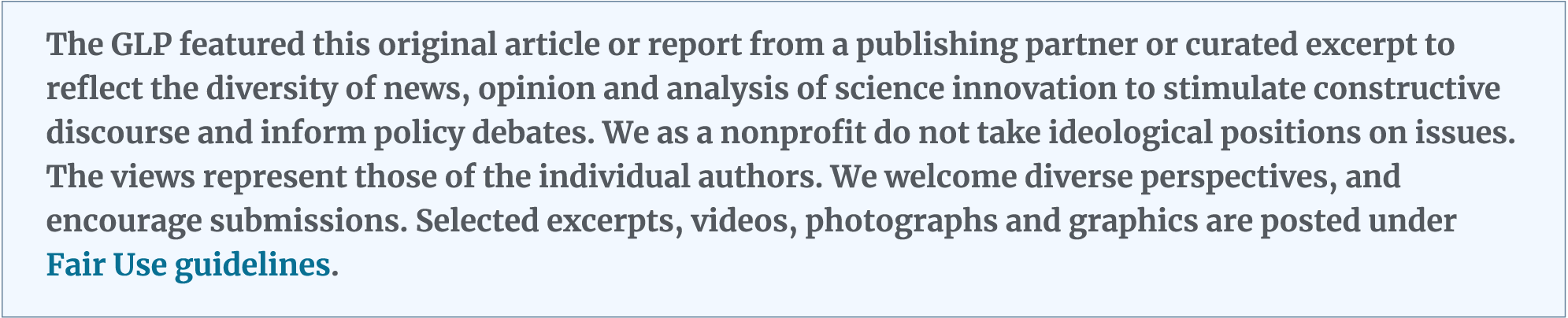
GLP Podcasts & Podcast Videos More...
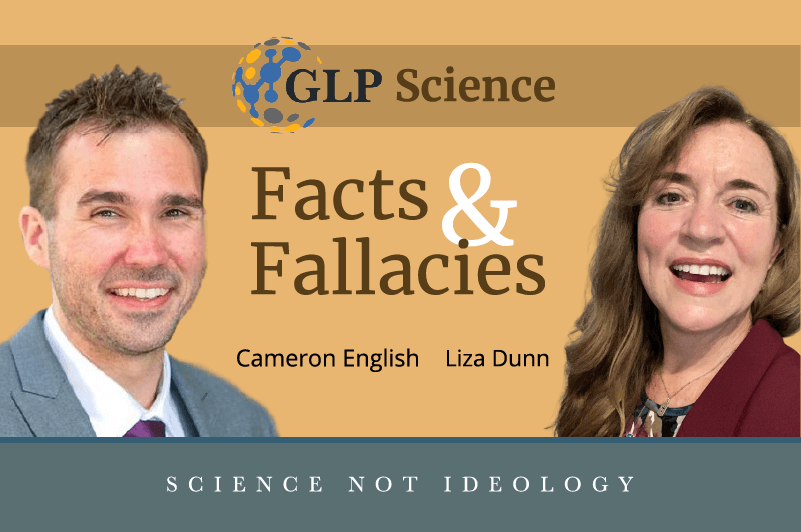
GLP podcast: Dangers of ‘diet weed’; Making insulin in cow’s milk; The conservative case for genetic enhancement
Glp podcast: ge crops have lived up to the hype; growing ‘mini’ organs from stem cells; how do we solve right-wing vaccine hesitancy, videos more....
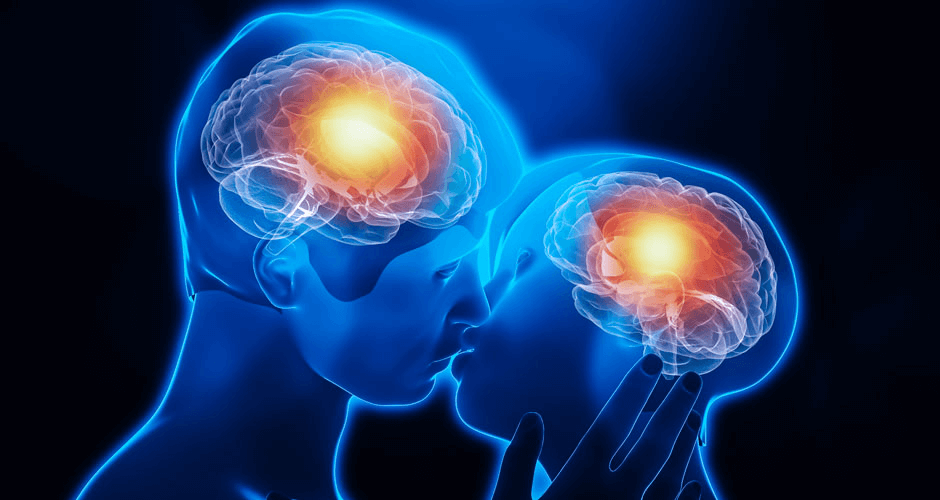
Video: Why does love make us feel so good? Examining its effect on our brains
Bees & pollinators more....
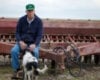
Dissecting claims about Monsanto suing farmers for accidentally planting patented seeds

Analysis: Do neonicotinoid and glyphosate pesticides threaten bees? A reassessment
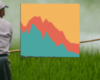
How effective and safe are current-generation pesticides?
Infographics more....

Are pesticide residues on food something to worry about?
Gmo faqs more....
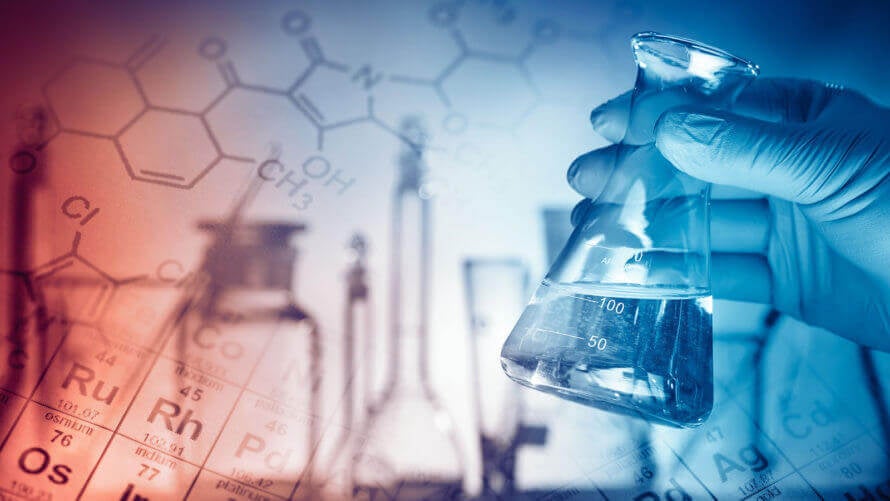
Why is there controversy over GMO foods but not GMO drugs?
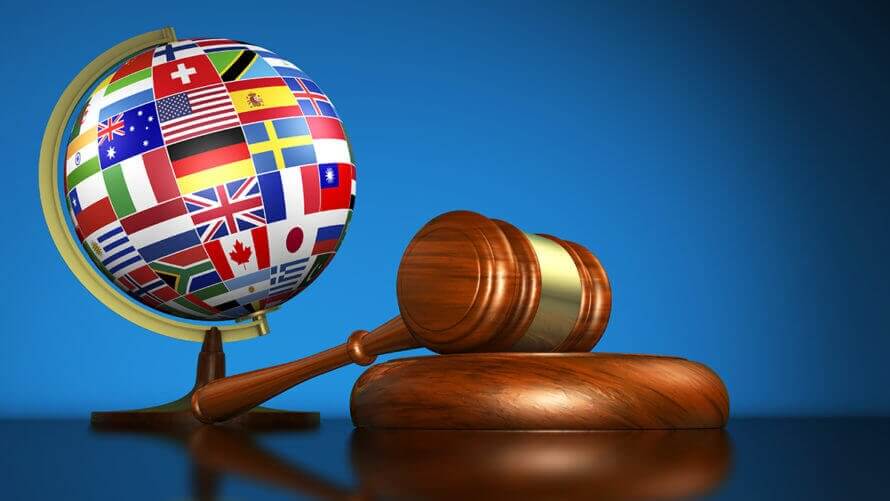
How are GMOs labeled around the world?
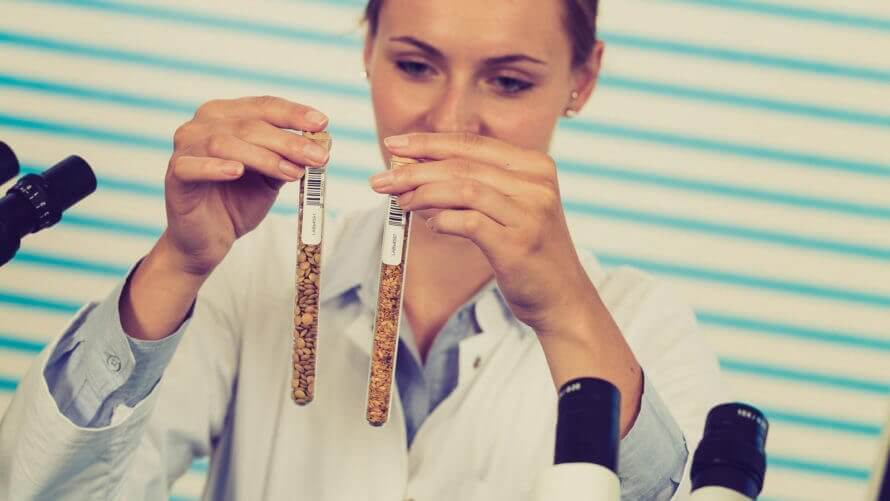
How does genetic engineering differ from conventional breeding?

Alex Jones: Right-wing conspiracy theorist stokes fear of GMOs, pesticides to sell ‘health supplements’

IARC (International Agency for Research on Cancer): Glyphosate cancer determination challenged by world consensus
Most popular.

Newsletter Subscription
- Weekly Newsletter (Wed)
- Daily Digest (Mon, Tue, Thu, Fri)
- Weekly Top Six (Sun)
- Featured Articles Only
- Human Articles Only
- Agriculture Articles Only
- All Types of Content
Get news on human & agricultural genetics and biotechnology delivered to your inbox.
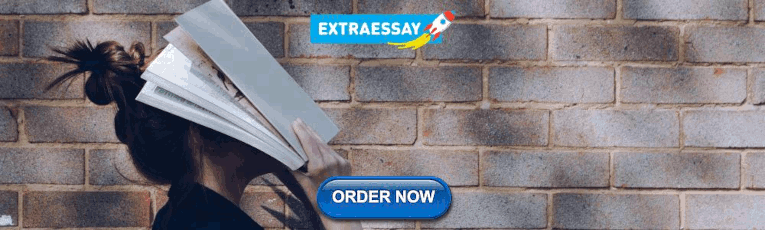
IMAGES
VIDEO
COMMENTS
New disease gene discovery and changing concepts of diagnosis. Exome and genome sequencing are powerful diagnostic tools - for example the Deciphering Developmental Disorders project, which recruited patients with severe undiagnosed disorders (who had generally already had any currently available diagnostic genetic testing), achieved a 40% diagnosis rate via trio exome sequencing for the ...
Unit Overview. Exploring Genetics Through Genetic Disorders is a three-week, comprehensive genetics curriculum unit that aligns with the Next Generation Science Standards (NGSS). Through paper-based and interactive multimedia lessons, the unit engages students in using models, analyzing skill-level appropriate data from published research ...
only treat the symptoms. In this project, you will explore a genetic disorder and present a short Google Slides Presentation to the class about the disorder you researched. You will also turn in two (2) hard copies of a one page personal reflection on the genetic disorder (1 copy each to your science class and Ms. Seymour's LA class. Step 1 ...
A genetic disorder is a disease caused in whole or in part by a change in the DNA sequence away from the normal sequence. Genetic disorders can be caused by a mutation in one gene (monogenic disorder), by mutations in multiple genes (multifactorial inheritance disorder), by a combination of gene mutations and environmental factors, or by damage to chromosomes (changes in the number or ...
I made an example you can use when you are creating the slide. ... Genetic Disorder Research-Poster Project 2021 . File size 36.9 KB. Genetic Disorder Slide - Tay Sachs 2020 (5) File size 644.1 KB. Rubric for Project. A rubric will be used to assess your Genetic Disorder Research and Poster/PowerPoint Slide Project. Use the attached rubric to ...
You will be given a specific genetic disorder found in humans. You will be responsible for giving a short (3-5 minute) presentation that explains each of the following points about your disorder. (*make sure that you can pronounce it!) Record yourself using your cellphone. Make sure your background is free and clear of clutter and noise/music.
Participants in clinical studies help current and future generations. Through these studies, researchers develop new diagnostic tests, more effective treatments, and better ways of managing diseases with genetic components. Participants in studies are actively involved in understanding their disorder and current research.
A genetic disorder is a disease that is caused by an abnormality in an individual's DNA. Abnormalities can be as a single-base mutation in just one gene, or they can involve the addition or subtraction of entire chromosomes. In this project, you will research an assigned genetic disease. Then, you will select a role and corresponding format ...
The Division of Genetic and Genomic Medicine maintains an active basic science and clinical research program. Our laboratory research program focuses on discovering the underlying causes of genetic diseases, understanding the clinical implications of mutations in genes and development of novel approaches for treatment of genetic disorders.
It has previously been estimated that around 42-48% of patients with a severe developmental disorder (DD) have a pathogenic de novo mutation (DNM) in a protein-coding gene 1, 2. However, most of ...
You will use your research to create a single PowerPoint slide to be used as a poster or fact sheet that presents information about the genetic disorder you selected. The slide will be graded on the information presented, neatness, and legibility. We will then share our research in a gallery walk so you can learn about the genetic disorders ...
Genetic disorders are of different types i.e. single-gene disorders, chromosomal disorders, complex disorders. This paper intends to be as an introductory paper for the project "Human genetic ...
The pamphlet must be no larger than 8.5 x 11 unfolded. This brochure should be of professional quality. It must fully inform the reader of all issues pertaining to the genetic disorder. Again, use the questions provided to guide your research. Your brochure will be graded based on accuracy, completeness, and creativity.
Science Fair Project Idea. In a survey conducted from 2007 to 2010, the U.S. Centers for Disease Control and Prevention reported that about 49% of people in the United States had taken at least one prescription drug during the past month, and about 22% of people had taken three or more prescription drugs. People are prescribed drugs all the ...
Other examples of projects focused on community-led and co-designed research with a strong rare disease focus include the work of the National Centre for Indigenous Genomics (https://ncig.anu.edu ...
New approach successfully traces genomic variants back to genetic disorders. Sonja Soo, Ph.D. January 05, 2023 PRESS CONTACT. NIH study shows genotype-first approach uncovers new links to genetic conditions. National Institutes of Health researchers have published an assessment of 13 studies that took a genotype-first approach to patient care.
A genetic disorder is a condition that occurs as a result of a mutation in DNA. ... was a global research project to map the human genome. The project established the sequence of the human genome ...
Students will be asked to work in groups of four to complete this project. Students working within the same group will research the same genetic disorder. Each of the students will assume a role within their respected group. Each group member will be responsible for producing a final product on the same genetic disorder.
The purpose of this study is to form processes to get findings from targeted gene sequencing that can be acted on using two common actionable genetic disorders-familial hyperlipidemia and familial colorectal cancer. This is an interview study to understand the views of people with the lived experience of 10 different genetic conditions on ...
DYRK1A gene linked to heart defects in Down syndrome. A study shows that congenital heart defects in Down syndrome are in part caused by increased dosage of the DYRK1A gene, which lies on ...
A major focus of the branch's research lies in understanding how disruptions in signaling pathways and transcription factors contribute to disease. Our investigators use genetics and genomic approaches in both human and mouse systems to identify and better understand pathways involved in human genetic diseases and normal development. Model ...
Genetic research is the study of human DNA to find out what genes and environmental factors contribute to diseases. If we find out what causes disease, we can better detect disease, better treat disease and hopefully even prevent disease from happening in the first place! Nearly every disease we know of has a genetic component.
Resource Requirements. Information you must include: Question 1 o Identify the disorder o What causes it? How can a person inherit the disorder? Question 2 o What symptoms, side effects, or harm does it cause to the human body? Question 3 o Does this genetic disease or disability mostly affect a certain age group, ethnic group, gender group, etc.?
Our Variant-to-Gene-to-Program (V2G2P) approach consists of five steps. In step one, we choose a cell type relevant to disease. In steps two and three, we use genomic approaches to build maps ...
To understand why, let's begin with a standard example of this kind of research — a recent peer-reviewed academic study on apparent racial differences in plague deaths in 14 th-century England ...